Type safe retained render world (#15756)
# Objective
In the Render World, there are a number of collections that are derived
from Main World entities and are used to drive rendering. The most
notable are:
- `VisibleEntities`, which is generated in the `check_visibility` system
and contains visible entities for a view.
- `ExtractedInstances`, which maps entity ids to asset ids.
In the old model, these collections were trivially kept in sync -- any
extracted phase item could look itself up because the render entity id
was guaranteed to always match the corresponding main world id.
After #15320, this became much more complicated, and was leading to a
number of subtle bugs in the Render World. The main rendering systems,
i.e. `queue_material_meshes` and `queue_material2d_meshes`, follow a
similar pattern:
```rust
for visible_entity in visible_entities.iter::<With<Mesh2d>>() {
let Some(mesh_instance) = render_mesh_instances.get_mut(visible_entity) else {
continue;
};
// Look some more stuff up and specialize the pipeline...
let bin_key = Opaque2dBinKey {
pipeline: pipeline_id,
draw_function: draw_opaque_2d,
asset_id: mesh_instance.mesh_asset_id.into(),
material_bind_group_id: material_2d.get_bind_group_id().0,
};
opaque_phase.add(
bin_key,
*visible_entity,
BinnedRenderPhaseType::mesh(mesh_instance.automatic_batching),
);
}
```
In this case, `visible_entities` and `render_mesh_instances` are both
collections that are created and keyed by Main World entity ids, and so
this lookup happens to work by coincidence. However, there is a major
unintentional bug here: namely, because `visible_entities` is a
collection of Main World ids, the phase item being queued is created
with a Main World id rather than its correct Render World id.
This happens to not break mesh rendering because the render commands
used for drawing meshes do not access the `ItemQuery` parameter, but
demonstrates the confusion that is now possible: our UI phase items are
correctly being queued with Render World ids while our meshes aren't.
Additionally, this makes it very easy and error prone to use the wrong
entity id to look up things like assets. For example, if instead we
ignored visibility checks and queued our meshes via a query, we'd have
to be extra careful to use `&MainEntity` instead of the natural
`Entity`.
## Solution
Make all collections that are derived from Main World data use
`MainEntity` as their key, to ensure type safety and avoid accidentally
looking up data with the wrong entity id:
```rust
pub type MainEntityHashMap<V> = hashbrown::HashMap<MainEntity, V, EntityHash>;
```
Additionally, we make all `PhaseItem` be able to provide both their Main
and Render World ids, to allow render phase implementors maximum
flexibility as to what id should be used to look up data.
You can think of this like tracking at the type level whether something
in the Render World should use it's "primary key", i.e. entity id, or
needs to use a foreign key, i.e. `MainEntity`.
## Testing
##### TODO:
This will require extensive testing to make sure things didn't break!
Additionally, some extraction logic has become more complicated and
needs to be checked for regressions.
## Migration Guide
With the advent of the retained render world, collections that contain
references to `Entity` that are extracted into the render world have
been changed to contain `MainEntity` in order to prevent errors where a
render world entity id is used to look up an item by accident. Custom
rendering code may need to be changed to query for `&MainEntity` in
order to look up the correct item from such a collection. Additionally,
users who implement their own extraction logic for collections of main
world entity should strongly consider extracting into a different
collection that uses `MainEntity` as a key.
Additionally, render phases now require specifying both the `Entity` and
`MainEntity` for a given `PhaseItem`. Custom render phases should ensure
`MainEntity` is available when queuing a phase item.
2024-10-10 18:47:04 +00:00
|
|
|
use self::{irradiance_volume::IrradianceVolume, prelude::EnvironmentMapLight};
|
2024-03-25 19:08:27 +00:00
|
|
|
#[cfg(feature = "meshlet")]
|
|
|
|
use crate::meshlet::{
|
|
|
|
prepare_material_meshlet_meshes_main_opaque_pass, queue_material_meshlet_meshes,
|
Meshlet software raster + start of cleanup (#14623)
# Objective
- Faster meshlet rasterization path for small triangles
- Avoid having to allocate and write out a triangle buffer
- Refactor gpu_scene.rs
## Solution
- Replace the 32bit visbuffer texture with a 64bit visbuffer buffer,
where the left 32 bits encode depth, and the right 32 bits encode the
existing cluster + triangle IDs. Can't use 64bit textures, wgpu/naga
doesn't support atomic ops on textures yet.
- Instead of writing out a buffer of packed cluster + triangle IDs (per
triangle) to raster, the culling pass now writes out a buffer of just
cluster IDs (per cluster, so less memory allocated, cheaper to write
out).
- Clusters for software raster are allocated from the left side
- Clusters for hardware raster are allocated in the same buffer, from
the right side
- The buffer size is fixed at MeshletPlugin build time, and should be
set to a reasonable value for your scene (no warning on overflow, and no
good way to determine what value you need outside of renderdoc - I plan
to fix this in a future PR adding a meshlet stats overlay)
- Currently I don't have a heuristic for software vs hardware raster
selection for each cluster. The existing code is just a placeholder. I
need to profile on a release scene and come up with a heuristic,
probably in a future PR.
- The culling shader is getting pretty hard to follow at this point, but
I don't want to spend time improving it as the entire shader/pass is
getting rewritten/replaced in the near future.
- Software raster is a compute workgroup per-cluster. Each workgroup
loads and transforms the <=64 vertices of the cluster, and then
rasterizes the <=64 triangles of the cluster.
- Two variants are implemented: Scanline for clusters with any larger
triangles (still smaller than hardware is good at), and brute-force for
very very tiny triangles
- Once the shader determines that a pixel should be filled in, it does
an atomicMax() on the visbuffer to store the results, copying how Nanite
works
- On devices with a low max workgroups per dispatch limit, an extra
compute pass is inserted before software raster to convert from a 1d to
2d dispatch (I don't think 3d would ever be necessary).
- I haven't implemented the top-left rule or subpixel precision yet, I'm
leaving that for a future PR since I get usable results without it for
now
- Resources used:
https://kristoffer-dyrkorn.github.io/triangle-rasterizer and chapters
6-8 of
https://fgiesen.wordpress.com/2013/02/17/optimizing-sw-occlusion-culling-index
- Hardware raster now spawns 64*3 vertex invocations per meshlet,
instead of the actual meshlet vertex count. Extra invocations just
early-exit.
- While this is slower than the existing system, hardware draws should
be rare now that software raster is usable, and it saves a ton of memory
using the unified cluster ID buffer. This would be fixed if wgpu had
support for mesh shaders.
- Instead of writing to a color+depth attachment, the hardware raster
pass also does the same atomic visbuffer writes that software raster
uses.
- We have to bind a dummy render target anyways, as wgpu doesn't
currently support render passes without any attachments
- Material IDs are no longer written out during the main rasterization
passes.
- If we had async compute queues, we could overlap the software and
hardware raster passes.
- New material and depth resolve passes run at the end of the visbuffer
node, and write out view depth and material ID depth textures
### Misc changes
- Fixed cluster culling importing, but never actually using the previous
view uniforms when doing occlusion culling
- Fixed incorrectly adding the LOD error twice when building the meshlet
mesh
- Splitup gpu_scene module into meshlet_mesh_manager, instance_manager,
and resource_manager
- resource_manager is still too complex and inefficient (extract and
prepare are way too expensive). I plan on improving this in a future PR,
but for now ResourceManager is mostly a 1:1 port of the leftover
MeshletGpuScene bits.
- Material draw passes have been renamed to the more accurate material
shade pass, as well as some other misc renaming (in the future, these
will be compute shaders even, and not actual draw calls)
---
## Migration Guide
- TBD (ask me at the end of the release for meshlet changes as a whole)
---------
Co-authored-by: vero <email@atlasdostal.com>
2024-08-26 17:54:34 +00:00
|
|
|
InstanceManager,
|
2024-03-25 19:08:27 +00:00
|
|
|
};
|
Implement irradiance volumes. (#10268)
# Objective
Bevy could benefit from *irradiance volumes*, also known as *voxel
global illumination* or simply as light probes (though this term is not
preferred, as multiple techniques can be called light probes).
Irradiance volumes are a form of baked global illumination; they work by
sampling the light at the centers of each voxel within a cuboid. At
runtime, the voxels surrounding the fragment center are sampled and
interpolated to produce indirect diffuse illumination.
## Solution
This is divided into two sections. The first is copied and pasted from
the irradiance volume module documentation and describes the technique.
The second part consists of notes on the implementation.
### Overview
An *irradiance volume* is a cuboid voxel region consisting of
regularly-spaced precomputed samples of diffuse indirect light. They're
ideal if you have a dynamic object such as a character that can move
about
static non-moving geometry such as a level in a game, and you want that
dynamic object to be affected by the light bouncing off that static
geometry.
To use irradiance volumes, you need to precompute, or *bake*, the
indirect
light in your scene. Bevy doesn't currently come with a way to do this.
Fortunately, [Blender] provides a [baking tool] as part of the Eevee
renderer, and its irradiance volumes are compatible with those used by
Bevy.
The [`bevy-baked-gi`] project provides a tool, `export-blender-gi`, that
can
extract the baked irradiance volumes from the Blender `.blend` file and
package them up into a `.ktx2` texture for use by the engine. See the
documentation in the `bevy-baked-gi` project for more details as to this
workflow.
Like all light probes in Bevy, irradiance volumes are 1×1×1 cubes that
can
be arbitrarily scaled, rotated, and positioned in a scene with the
[`bevy_transform::components::Transform`] component. The 3D voxel grid
will
be stretched to fill the interior of the cube, and the illumination from
the
irradiance volume will apply to all fragments within that bounding
region.
Bevy's irradiance volumes are based on Valve's [*ambient cubes*] as used
in
*Half-Life 2* ([Mitchell 2006], slide 27). These encode a single color
of
light from the six 3D cardinal directions and blend the sides together
according to the surface normal.
The primary reason for choosing ambient cubes is to match Blender, so
that
its Eevee renderer can be used for baking. However, they also have some
advantages over the common second-order spherical harmonics approach:
ambient cubes don't suffer from ringing artifacts, they are smaller (6
colors for ambient cubes as opposed to 9 for spherical harmonics), and
evaluation is faster. A smaller basis allows for a denser grid of voxels
with the same storage requirements.
If you wish to use a tool other than `export-blender-gi` to produce the
irradiance volumes, you'll need to pack the irradiance volumes in the
following format. The irradiance volume of resolution *(Rx, Ry, Rz)* is
expected to be a 3D texture of dimensions *(Rx, 2Ry, 3Rz)*. The
unnormalized
texture coordinate *(s, t, p)* of the voxel at coordinate *(x, y, z)*
with
side *S* ∈ *{-X, +X, -Y, +Y, -Z, +Z}* is as follows:
```text
s = x
t = y + ⎰ 0 if S ∈ {-X, -Y, -Z}
⎱ Ry if S ∈ {+X, +Y, +Z}
⎧ 0 if S ∈ {-X, +X}
p = z + ⎨ Rz if S ∈ {-Y, +Y}
⎩ 2Rz if S ∈ {-Z, +Z}
```
Visually, in a left-handed coordinate system with Y up, viewed from the
right, the 3D texture looks like a stacked series of voxel grids, one
for
each cube side, in this order:
| **+X** | **+Y** | **+Z** |
| ------ | ------ | ------ |
| **-X** | **-Y** | **-Z** |
A terminology note: Other engines may refer to irradiance volumes as
*voxel
global illumination*, *VXGI*, or simply as *light probes*. Sometimes
*light
probe* refers to what Bevy calls a reflection probe. In Bevy, *light
probe*
is a generic term that encompasses all cuboid bounding regions that
capture
indirect illumination, whether based on voxels or not.
Note that, if binding arrays aren't supported (e.g. on WebGPU or WebGL
2),
then only the closest irradiance volume to the view will be taken into
account during rendering.
[*ambient cubes*]:
https://advances.realtimerendering.com/s2006/Mitchell-ShadingInValvesSourceEngine.pdf
[Mitchell 2006]:
https://advances.realtimerendering.com/s2006/Mitchell-ShadingInValvesSourceEngine.pdf
[Blender]: http://blender.org/
[baking tool]:
https://docs.blender.org/manual/en/latest/render/eevee/render_settings/indirect_lighting.html
[`bevy-baked-gi`]: https://github.com/pcwalton/bevy-baked-gi
### Implementation notes
This patch generalizes light probes so as to reuse as much code as
possible between irradiance volumes and the existing reflection probes.
This approach was chosen because both techniques share numerous
similarities:
1. Both irradiance volumes and reflection probes are cuboid bounding
regions.
2. Both are responsible for providing baked indirect light.
3. Both techniques involve presenting a variable number of textures to
the shader from which indirect light is sampled. (In the current
implementation, this uses binding arrays.)
4. Both irradiance volumes and reflection probes require gathering and
sorting probes by distance on CPU.
5. Both techniques require the GPU to search through a list of bounding
regions.
6. Both will eventually want to have falloff so that we can smoothly
blend as objects enter and exit the probes' influence ranges. (This is
not implemented yet to keep this patch relatively small and reviewable.)
To do this, we generalize most of the methods in the reflection probes
patch #11366 to be generic over a trait, `LightProbeComponent`. This
trait is implemented by both `EnvironmentMapLight` (for reflection
probes) and `IrradianceVolume` (for irradiance volumes). Using a trait
will allow us to add more types of light probes in the future. In
particular, I highly suspect we will want real-time reflection planes
for mirrors in the future, which can be easily slotted into this
framework.
## Changelog
> This section is optional. If this was a trivial fix, or has no
externally-visible impact, you can delete this section.
### Added
* A new `IrradianceVolume` asset type is available for baked voxelized
light probes. You can bake the global illumination using Blender or
another tool of your choice and use it in Bevy to apply indirect
illumination to dynamic objects.
2024-02-06 23:23:20 +00:00
|
|
|
use crate::*;
|
2024-04-09 13:26:34 +00:00
|
|
|
use bevy_asset::{Asset, AssetId, AssetServer};
|
2022-10-26 20:13:59 +00:00
|
|
|
use bevy_core_pipeline::{
|
`StandardMaterial` Light Transmission (#8015)
# Objective
<img width="1920" alt="Screenshot 2023-04-26 at 01 07 34"
src="https://user-images.githubusercontent.com/418473/234467578-0f34187b-5863-4ea1-88e9-7a6bb8ce8da3.png">
This PR adds both diffuse and specular light transmission capabilities
to the `StandardMaterial`, with support for screen space refractions.
This enables realistically representing a wide range of real-world
materials, such as:
- Glass; (Including frosted glass)
- Transparent and translucent plastics;
- Various liquids and gels;
- Gemstones;
- Marble;
- Wax;
- Paper;
- Leaves;
- Porcelain.
Unlike existing support for transparency, light transmission does not
rely on fixed function alpha blending, and therefore works with both
`AlphaMode::Opaque` and `AlphaMode::Mask` materials.
## Solution
- Introduces a number of transmission related fields in the
`StandardMaterial`;
- For specular transmission:
- Adds logic to take a view main texture snapshot after the opaque
phase; (in order to perform screen space refractions)
- Introduces a new `Transmissive3d` phase to the renderer, to which all
meshes with `transmission > 0.0` materials are sent.
- Calculates a light exit point (of the approximate mesh volume) using
`ior` and `thickness` properties
- Samples the snapshot texture with an adaptive number of taps across a
`roughness`-controlled radius enabling “blurry” refractions
- For diffuse transmission:
- Approximates transmitted diffuse light by using a second, flipped +
displaced, diffuse-only Lambertian lobe for each light source.
## To Do
- [x] Figure out where `fresnel_mix()` is taking place, if at all, and
where `dielectric_specular` is being calculated, if at all, and update
them to use the `ior` value (Not a blocker, just a nice-to-have for more
correct BSDF)
- To the _best of my knowledge, this is now taking place, after
964340cdd. The fresnel mix is actually "split" into two parts in our
implementation, one `(1 - fresnel(...))` in the transmission, and
`fresnel()` in the light implementations. A surface with more
reflectance now will produce slightly dimmer transmission towards the
grazing angle, as more of the light gets reflected.
- [x] Add `transmission_texture`
- [x] Add `diffuse_transmission_texture`
- [x] Add `thickness_texture`
- [x] Add `attenuation_distance` and `attenuation_color`
- [x] Connect values to glTF loader
- [x] `transmission` and `transmission_texture`
- [x] `thickness` and `thickness_texture`
- [x] `ior`
- [ ] `diffuse_transmission` and `diffuse_transmission_texture` (needs
upstream support in `gltf` crate, not a blocker)
- [x] Add support for multiple screen space refraction “steps”
- [x] Conditionally create no transmission snapshot texture at all if
`steps == 0`
- [x] Conditionally enable/disable screen space refraction transmission
snapshots
- [x] Read from depth pre-pass to prevent refracting pixels in front of
the light exit point
- [x] Use `interleaved_gradient_noise()` function for sampling blur in a
way that benefits from TAA
- [x] Drill down a TAA `#define`, tweak some aspects of the effect
conditionally based on it
- [x] Remove const array that's crashing under HLSL (unless a new `naga`
release with https://github.com/gfx-rs/naga/pull/2496 comes out before
we merge this)
- [ ] Look into alternatives to the `switch` hack for dynamically
indexing the const array (might not be needed, compilers seem to be
decent at expanding it)
- [ ] Add pipeline keys for gating transmission (do we really want/need
this?)
- [x] Tweak some material field/function names?
## A Note on Texture Packing
_This was originally added as a comment to the
`specular_transmission_texture`, `thickness_texture` and
`diffuse_transmission_texture` documentation, I removed it since it was
more confusing than helpful, and will likely be made redundant/will need
to be updated once we have a better infrastructure for preprocessing
assets_
Due to how channels are mapped, you can more efficiently use a single
shared texture image
for configuring the following:
- R - `specular_transmission_texture`
- G - `thickness_texture`
- B - _unused_
- A - `diffuse_transmission_texture`
The `KHR_materials_diffuse_transmission` glTF extension also defines a
`diffuseTransmissionColorTexture`,
that _we don't currently support_. One might choose to pack the
intensity and color textures together,
using RGB for the color and A for the intensity, in which case this
packing advice doesn't really apply.
---
## Changelog
- Added a new `Transmissive3d` render phase for rendering specular
transmissive materials with screen space refractions
- Added rendering support for transmitted environment map light on the
`StandardMaterial` as a fallback for screen space refractions
- Added `diffuse_transmission`, `specular_transmission`, `thickness`,
`ior`, `attenuation_distance` and `attenuation_color` to the
`StandardMaterial`
- Added `diffuse_transmission_texture`, `specular_transmission_texture`,
`thickness_texture` to the `StandardMaterial`, gated behind a new
`pbr_transmission_textures` cargo feature (off by default, for maximum
hardware compatibility)
- Added `Camera3d::screen_space_specular_transmission_steps` for
controlling the number of “layers of transparency” rendered for
transmissive objects
- Added a `TransmittedShadowReceiver` component for enabling shadows in
(diffusely) transmitted light. (disabled by default, as it requires
carefully setting up the `thickness` to avoid self-shadow artifacts)
- Added support for the `KHR_materials_transmission`,
`KHR_materials_ior` and `KHR_materials_volume` glTF extensions
- Renamed items related to temporal jitter for greater consistency
## Migration Guide
- `SsaoPipelineKey::temporal_noise` has been renamed to
`SsaoPipelineKey::temporal_jitter`
- The `TAA` shader def (controlled by the presence of the
`TemporalAntiAliasSettings` component in the camera) has been replaced
with the `TEMPORAL_JITTER` shader def (controlled by the presence of the
`TemporalJitter` component in the camera)
- `MeshPipelineKey::TAA` has been replaced by
`MeshPipelineKey::TEMPORAL_JITTER`
- The `TEMPORAL_NOISE` shader def has been consolidated with
`TEMPORAL_JITTER`
2023-10-31 20:59:02 +00:00
|
|
|
core_3d::{
|
Improve performance by binning together opaque items instead of sorting them. (#12453)
Today, we sort all entities added to all phases, even the phases that
don't strictly need sorting, such as the opaque and shadow phases. This
results in a performance loss because our `PhaseItem`s are rather large
in memory, so sorting is slow. Additionally, determining the boundaries
of batches is an O(n) process.
This commit makes Bevy instead applicable place phase items into *bins*
keyed by *bin keys*, which have the invariant that everything in the
same bin is potentially batchable. This makes determining batch
boundaries O(1), because everything in the same bin can be batched.
Instead of sorting each entity, we now sort only the bin keys. This
drops the sorting time to near-zero on workloads with few bins like
`many_cubes --no-frustum-culling`. Memory usage is improved too, with
batch boundaries and dynamic indices now implicit instead of explicit.
The improved memory usage results in a significant win even on
unbatchable workloads like `many_cubes --no-frustum-culling
--vary-material-data-per-instance`, presumably due to cache effects.
Not all phases can be binned; some, such as transparent and transmissive
phases, must still be sorted. To handle this, this commit splits
`PhaseItem` into `BinnedPhaseItem` and `SortedPhaseItem`. Most of the
logic that today deals with `PhaseItem`s has been moved to
`SortedPhaseItem`. `BinnedPhaseItem` has the new logic.
Frame time results (in ms/frame) are as follows:
| Benchmark | `binning` | `main` | Speedup |
| ------------------------ | --------- | ------- | ------- |
| `many_cubes -nfc -vpi` | 232.179 | 312.123 | 34.43% |
| `many_cubes -nfc` | 25.874 | 30.117 | 16.40% |
| `many_foxes` | 3.276 | 3.515 | 7.30% |
(`-nfc` is short for `--no-frustum-culling`; `-vpi` is short for
`--vary-per-instance`.)
---
## Changelog
### Changed
* Render phases have been split into binned and sorted phases. Binned
phases, such as the common opaque phase, achieve improved CPU
performance by avoiding the sorting step.
## Migration Guide
- `PhaseItem` has been split into `BinnedPhaseItem` and
`SortedPhaseItem`. If your code has custom `PhaseItem`s, you will need
to migrate them to one of these two types. `SortedPhaseItem` requires
the fewest code changes, but you may want to pick `BinnedPhaseItem` if
your phase doesn't require sorting, as that enables higher performance.
## Tracy graphs
`many-cubes --no-frustum-culling`, `main` branch:
<img width="1064" alt="Screenshot 2024-03-12 180037"
src="https://github.com/bevyengine/bevy/assets/157897/e1180ce8-8e89-46d2-85e3-f59f72109a55">
`many-cubes --no-frustum-culling`, this branch:
<img width="1064" alt="Screenshot 2024-03-12 180011"
src="https://github.com/bevyengine/bevy/assets/157897/0899f036-6075-44c5-a972-44d95895f46c">
You can see that `batch_and_prepare_binned_render_phase` is a much
smaller fraction of the time. Zooming in on that function, with yellow
being this branch and red being `main`, we see:
<img width="1064" alt="Screenshot 2024-03-12 175832"
src="https://github.com/bevyengine/bevy/assets/157897/0dfc8d3f-49f4-496e-8825-a66e64d356d0">
The binning happens in `queue_material_meshes`. Again with yellow being
this branch and red being `main`:
<img width="1064" alt="Screenshot 2024-03-12 175755"
src="https://github.com/bevyengine/bevy/assets/157897/b9b20dc1-11c8-400c-a6cc-1c2e09c1bb96">
We can see that there is a small regression in `queue_material_meshes`
performance, but it's not nearly enough to outweigh the large gains in
`batch_and_prepare_binned_render_phase`.
---------
Co-authored-by: James Liu <contact@jamessliu.com>
2024-03-30 02:55:02 +00:00
|
|
|
AlphaMask3d, Camera3d, Opaque3d, Opaque3dBinKey, ScreenSpaceTransmissionQuality,
|
|
|
|
Transmissive3d, Transparent3d,
|
|
|
|
},
|
2024-10-07 23:50:28 +00:00
|
|
|
oit::OrderIndependentTransparencySettings,
|
Improve performance by binning together opaque items instead of sorting them. (#12453)
Today, we sort all entities added to all phases, even the phases that
don't strictly need sorting, such as the opaque and shadow phases. This
results in a performance loss because our `PhaseItem`s are rather large
in memory, so sorting is slow. Additionally, determining the boundaries
of batches is an O(n) process.
This commit makes Bevy instead applicable place phase items into *bins*
keyed by *bin keys*, which have the invariant that everything in the
same bin is potentially batchable. This makes determining batch
boundaries O(1), because everything in the same bin can be batched.
Instead of sorting each entity, we now sort only the bin keys. This
drops the sorting time to near-zero on workloads with few bins like
`many_cubes --no-frustum-culling`. Memory usage is improved too, with
batch boundaries and dynamic indices now implicit instead of explicit.
The improved memory usage results in a significant win even on
unbatchable workloads like `many_cubes --no-frustum-culling
--vary-material-data-per-instance`, presumably due to cache effects.
Not all phases can be binned; some, such as transparent and transmissive
phases, must still be sorted. To handle this, this commit splits
`PhaseItem` into `BinnedPhaseItem` and `SortedPhaseItem`. Most of the
logic that today deals with `PhaseItem`s has been moved to
`SortedPhaseItem`. `BinnedPhaseItem` has the new logic.
Frame time results (in ms/frame) are as follows:
| Benchmark | `binning` | `main` | Speedup |
| ------------------------ | --------- | ------- | ------- |
| `many_cubes -nfc -vpi` | 232.179 | 312.123 | 34.43% |
| `many_cubes -nfc` | 25.874 | 30.117 | 16.40% |
| `many_foxes` | 3.276 | 3.515 | 7.30% |
(`-nfc` is short for `--no-frustum-culling`; `-vpi` is short for
`--vary-per-instance`.)
---
## Changelog
### Changed
* Render phases have been split into binned and sorted phases. Binned
phases, such as the common opaque phase, achieve improved CPU
performance by avoiding the sorting step.
## Migration Guide
- `PhaseItem` has been split into `BinnedPhaseItem` and
`SortedPhaseItem`. If your code has custom `PhaseItem`s, you will need
to migrate them to one of these two types. `SortedPhaseItem` requires
the fewest code changes, but you may want to pick `BinnedPhaseItem` if
your phase doesn't require sorting, as that enables higher performance.
## Tracy graphs
`many-cubes --no-frustum-culling`, `main` branch:
<img width="1064" alt="Screenshot 2024-03-12 180037"
src="https://github.com/bevyengine/bevy/assets/157897/e1180ce8-8e89-46d2-85e3-f59f72109a55">
`many-cubes --no-frustum-culling`, this branch:
<img width="1064" alt="Screenshot 2024-03-12 180011"
src="https://github.com/bevyengine/bevy/assets/157897/0899f036-6075-44c5-a972-44d95895f46c">
You can see that `batch_and_prepare_binned_render_phase` is a much
smaller fraction of the time. Zooming in on that function, with yellow
being this branch and red being `main`, we see:
<img width="1064" alt="Screenshot 2024-03-12 175832"
src="https://github.com/bevyengine/bevy/assets/157897/0dfc8d3f-49f4-496e-8825-a66e64d356d0">
The binning happens in `queue_material_meshes`. Again with yellow being
this branch and red being `main`:
<img width="1064" alt="Screenshot 2024-03-12 175755"
src="https://github.com/bevyengine/bevy/assets/157897/b9b20dc1-11c8-400c-a6cc-1c2e09c1bb96">
We can see that there is a small regression in `queue_material_meshes`
performance, but it's not nearly enough to outweigh the large gains in
`batch_and_prepare_binned_render_phase`.
---------
Co-authored-by: James Liu <contact@jamessliu.com>
2024-03-30 02:55:02 +00:00
|
|
|
prepass::{
|
|
|
|
DeferredPrepass, DepthPrepass, MotionVectorPrepass, NormalPrepass, OpaqueNoLightmap3dBinKey,
|
`StandardMaterial` Light Transmission (#8015)
# Objective
<img width="1920" alt="Screenshot 2023-04-26 at 01 07 34"
src="https://user-images.githubusercontent.com/418473/234467578-0f34187b-5863-4ea1-88e9-7a6bb8ce8da3.png">
This PR adds both diffuse and specular light transmission capabilities
to the `StandardMaterial`, with support for screen space refractions.
This enables realistically representing a wide range of real-world
materials, such as:
- Glass; (Including frosted glass)
- Transparent and translucent plastics;
- Various liquids and gels;
- Gemstones;
- Marble;
- Wax;
- Paper;
- Leaves;
- Porcelain.
Unlike existing support for transparency, light transmission does not
rely on fixed function alpha blending, and therefore works with both
`AlphaMode::Opaque` and `AlphaMode::Mask` materials.
## Solution
- Introduces a number of transmission related fields in the
`StandardMaterial`;
- For specular transmission:
- Adds logic to take a view main texture snapshot after the opaque
phase; (in order to perform screen space refractions)
- Introduces a new `Transmissive3d` phase to the renderer, to which all
meshes with `transmission > 0.0` materials are sent.
- Calculates a light exit point (of the approximate mesh volume) using
`ior` and `thickness` properties
- Samples the snapshot texture with an adaptive number of taps across a
`roughness`-controlled radius enabling “blurry” refractions
- For diffuse transmission:
- Approximates transmitted diffuse light by using a second, flipped +
displaced, diffuse-only Lambertian lobe for each light source.
## To Do
- [x] Figure out where `fresnel_mix()` is taking place, if at all, and
where `dielectric_specular` is being calculated, if at all, and update
them to use the `ior` value (Not a blocker, just a nice-to-have for more
correct BSDF)
- To the _best of my knowledge, this is now taking place, after
964340cdd. The fresnel mix is actually "split" into two parts in our
implementation, one `(1 - fresnel(...))` in the transmission, and
`fresnel()` in the light implementations. A surface with more
reflectance now will produce slightly dimmer transmission towards the
grazing angle, as more of the light gets reflected.
- [x] Add `transmission_texture`
- [x] Add `diffuse_transmission_texture`
- [x] Add `thickness_texture`
- [x] Add `attenuation_distance` and `attenuation_color`
- [x] Connect values to glTF loader
- [x] `transmission` and `transmission_texture`
- [x] `thickness` and `thickness_texture`
- [x] `ior`
- [ ] `diffuse_transmission` and `diffuse_transmission_texture` (needs
upstream support in `gltf` crate, not a blocker)
- [x] Add support for multiple screen space refraction “steps”
- [x] Conditionally create no transmission snapshot texture at all if
`steps == 0`
- [x] Conditionally enable/disable screen space refraction transmission
snapshots
- [x] Read from depth pre-pass to prevent refracting pixels in front of
the light exit point
- [x] Use `interleaved_gradient_noise()` function for sampling blur in a
way that benefits from TAA
- [x] Drill down a TAA `#define`, tweak some aspects of the effect
conditionally based on it
- [x] Remove const array that's crashing under HLSL (unless a new `naga`
release with https://github.com/gfx-rs/naga/pull/2496 comes out before
we merge this)
- [ ] Look into alternatives to the `switch` hack for dynamically
indexing the const array (might not be needed, compilers seem to be
decent at expanding it)
- [ ] Add pipeline keys for gating transmission (do we really want/need
this?)
- [x] Tweak some material field/function names?
## A Note on Texture Packing
_This was originally added as a comment to the
`specular_transmission_texture`, `thickness_texture` and
`diffuse_transmission_texture` documentation, I removed it since it was
more confusing than helpful, and will likely be made redundant/will need
to be updated once we have a better infrastructure for preprocessing
assets_
Due to how channels are mapped, you can more efficiently use a single
shared texture image
for configuring the following:
- R - `specular_transmission_texture`
- G - `thickness_texture`
- B - _unused_
- A - `diffuse_transmission_texture`
The `KHR_materials_diffuse_transmission` glTF extension also defines a
`diffuseTransmissionColorTexture`,
that _we don't currently support_. One might choose to pack the
intensity and color textures together,
using RGB for the color and A for the intensity, in which case this
packing advice doesn't really apply.
---
## Changelog
- Added a new `Transmissive3d` render phase for rendering specular
transmissive materials with screen space refractions
- Added rendering support for transmitted environment map light on the
`StandardMaterial` as a fallback for screen space refractions
- Added `diffuse_transmission`, `specular_transmission`, `thickness`,
`ior`, `attenuation_distance` and `attenuation_color` to the
`StandardMaterial`
- Added `diffuse_transmission_texture`, `specular_transmission_texture`,
`thickness_texture` to the `StandardMaterial`, gated behind a new
`pbr_transmission_textures` cargo feature (off by default, for maximum
hardware compatibility)
- Added `Camera3d::screen_space_specular_transmission_steps` for
controlling the number of “layers of transparency” rendered for
transmissive objects
- Added a `TransmittedShadowReceiver` component for enabling shadows in
(diffusely) transmitted light. (disabled by default, as it requires
carefully setting up the `thickness` to avoid self-shadow artifacts)
- Added support for the `KHR_materials_transmission`,
`KHR_materials_ior` and `KHR_materials_volume` glTF extensions
- Renamed items related to temporal jitter for greater consistency
## Migration Guide
- `SsaoPipelineKey::temporal_noise` has been renamed to
`SsaoPipelineKey::temporal_jitter`
- The `TAA` shader def (controlled by the presence of the
`TemporalAntiAliasSettings` component in the camera) has been replaced
with the `TEMPORAL_JITTER` shader def (controlled by the presence of the
`TemporalJitter` component in the camera)
- `MeshPipelineKey::TAA` has been replaced by
`MeshPipelineKey::TEMPORAL_JITTER`
- The `TEMPORAL_NOISE` shader def has been consolidated with
`TEMPORAL_JITTER`
2023-10-31 20:59:02 +00:00
|
|
|
},
|
2023-02-19 20:38:13 +00:00
|
|
|
tonemapping::{DebandDither, Tonemapping},
|
2022-10-26 20:13:59 +00:00
|
|
|
};
|
Make `Resource` trait opt-in, requiring `#[derive(Resource)]` V2 (#5577)
*This PR description is an edited copy of #5007, written by @alice-i-cecile.*
# Objective
Follow-up to https://github.com/bevyengine/bevy/pull/2254. The `Resource` trait currently has a blanket implementation for all types that meet its bounds.
While ergonomic, this results in several drawbacks:
* it is possible to make confusing, silent mistakes such as inserting a function pointer (Foo) rather than a value (Foo::Bar) as a resource
* it is challenging to discover if a type is intended to be used as a resource
* we cannot later add customization options (see the [RFC](https://github.com/bevyengine/rfcs/blob/main/rfcs/27-derive-component.md) for the equivalent choice for Component).
* dependencies can use the same Rust type as a resource in invisibly conflicting ways
* raw Rust types used as resources cannot preserve privacy appropriately, as anyone able to access that type can read and write to internal values
* we cannot capture a definitive list of possible resources to display to users in an editor
## Notes to reviewers
* Review this commit-by-commit; there's effectively no back-tracking and there's a lot of churn in some of these commits.
*ira: My commits are not as well organized :')*
* I've relaxed the bound on Local to Send + Sync + 'static: I don't think these concerns apply there, so this can keep things simple. Storing e.g. a u32 in a Local is fine, because there's a variable name attached explaining what it does.
* I think this is a bad place for the Resource trait to live, but I've left it in place to make reviewing easier. IMO that's best tackled with https://github.com/bevyengine/bevy/issues/4981.
## Changelog
`Resource` is no longer automatically implemented for all matching types. Instead, use the new `#[derive(Resource)]` macro.
## Migration Guide
Add `#[derive(Resource)]` to all types you are using as a resource.
If you are using a third party type as a resource, wrap it in a tuple struct to bypass orphan rules. Consider deriving `Deref` and `DerefMut` to improve ergonomics.
`ClearColor` no longer implements `Component`. Using `ClearColor` as a component in 0.8 did nothing.
Use the `ClearColorConfig` in the `Camera3d` and `Camera2d` components instead.
Co-authored-by: Alice <alice.i.cecile@gmail.com>
Co-authored-by: Alice Cecile <alice.i.cecile@gmail.com>
Co-authored-by: devil-ira <justthecooldude@gmail.com>
Co-authored-by: Carter Anderson <mcanders1@gmail.com>
2022-08-08 21:36:35 +00:00
|
|
|
use bevy_derive::{Deref, DerefMut};
|
2021-12-25 21:45:43 +00:00
|
|
|
use bevy_ecs::{
|
Migrate engine to Schedule v3 (#7267)
Huge thanks to @maniwani, @devil-ira, @hymm, @cart, @superdump and @jakobhellermann for the help with this PR.
# Objective
- Followup #6587.
- Minimal integration for the Stageless Scheduling RFC: https://github.com/bevyengine/rfcs/pull/45
## Solution
- [x] Remove old scheduling module
- [x] Migrate new methods to no longer use extension methods
- [x] Fix compiler errors
- [x] Fix benchmarks
- [x] Fix examples
- [x] Fix docs
- [x] Fix tests
## Changelog
### Added
- a large number of methods on `App` to work with schedules ergonomically
- the `CoreSchedule` enum
- `App::add_extract_system` via the `RenderingAppExtension` trait extension method
- the private `prepare_view_uniforms` system now has a public system set for scheduling purposes, called `ViewSet::PrepareUniforms`
### Removed
- stages, and all code that mentions stages
- states have been dramatically simplified, and no longer use a stack
- `RunCriteriaLabel`
- `AsSystemLabel` trait
- `on_hierarchy_reports_enabled` run criteria (now just uses an ad hoc resource checking run condition)
- systems in `RenderSet/Stage::Extract` no longer warn when they do not read data from the main world
- `RunCriteriaLabel`
- `transform_propagate_system_set`: this was a nonstandard pattern that didn't actually provide enough control. The systems are already `pub`: the docs have been updated to ensure that the third-party usage is clear.
### Changed
- `System::default_labels` is now `System::default_system_sets`.
- `App::add_default_labels` is now `App::add_default_sets`
- `CoreStage` and `StartupStage` enums are now `CoreSet` and `StartupSet`
- `App::add_system_set` was renamed to `App::add_systems`
- The `StartupSchedule` label is now defined as part of the `CoreSchedules` enum
- `.label(SystemLabel)` is now referred to as `.in_set(SystemSet)`
- `SystemLabel` trait was replaced by `SystemSet`
- `SystemTypeIdLabel<T>` was replaced by `SystemSetType<T>`
- The `ReportHierarchyIssue` resource now has a public constructor (`new`), and implements `PartialEq`
- Fixed time steps now use a schedule (`CoreSchedule::FixedTimeStep`) rather than a run criteria.
- Adding rendering extraction systems now panics rather than silently failing if no subapp with the `RenderApp` label is found.
- the `calculate_bounds` system, with the `CalculateBounds` label, is now in `CoreSet::Update`, rather than in `CoreSet::PostUpdate` before commands are applied.
- `SceneSpawnerSystem` now runs under `CoreSet::Update`, rather than `CoreStage::PreUpdate.at_end()`.
- `bevy_pbr::add_clusters` is no longer an exclusive system
- the top level `bevy_ecs::schedule` module was replaced with `bevy_ecs::scheduling`
- `tick_global_task_pools_on_main_thread` is no longer run as an exclusive system. Instead, it has been replaced by `tick_global_task_pools`, which uses a `NonSend` resource to force running on the main thread.
## Migration Guide
- Calls to `.label(MyLabel)` should be replaced with `.in_set(MySet)`
- Stages have been removed. Replace these with system sets, and then add command flushes using the `apply_system_buffers` exclusive system where needed.
- The `CoreStage`, `StartupStage, `RenderStage` and `AssetStage` enums have been replaced with `CoreSet`, `StartupSet, `RenderSet` and `AssetSet`. The same scheduling guarantees have been preserved.
- Systems are no longer added to `CoreSet::Update` by default. Add systems manually if this behavior is needed, although you should consider adding your game logic systems to `CoreSchedule::FixedTimestep` instead for more reliable framerate-independent behavior.
- Similarly, startup systems are no longer part of `StartupSet::Startup` by default. In most cases, this won't matter to you.
- For example, `add_system_to_stage(CoreStage::PostUpdate, my_system)` should be replaced with
- `add_system(my_system.in_set(CoreSet::PostUpdate)`
- When testing systems or otherwise running them in a headless fashion, simply construct and run a schedule using `Schedule::new()` and `World::run_schedule` rather than constructing stages
- Run criteria have been renamed to run conditions. These can now be combined with each other and with states.
- Looping run criteria and state stacks have been removed. Use an exclusive system that runs a schedule if you need this level of control over system control flow.
- For app-level control flow over which schedules get run when (such as for rollback networking), create your own schedule and insert it under the `CoreSchedule::Outer` label.
- Fixed timesteps are now evaluated in a schedule, rather than controlled via run criteria. The `run_fixed_timestep` system runs this schedule between `CoreSet::First` and `CoreSet::PreUpdate` by default.
- Command flush points introduced by `AssetStage` have been removed. If you were relying on these, add them back manually.
- Adding extract systems is now typically done directly on the main app. Make sure the `RenderingAppExtension` trait is in scope, then call `app.add_extract_system(my_system)`.
- the `calculate_bounds` system, with the `CalculateBounds` label, is now in `CoreSet::Update`, rather than in `CoreSet::PostUpdate` before commands are applied. You may need to order your movement systems to occur before this system in order to avoid system order ambiguities in culling behavior.
- the `RenderLabel` `AppLabel` was renamed to `RenderApp` for clarity
- `App::add_state` now takes 0 arguments: the starting state is set based on the `Default` impl.
- Instead of creating `SystemSet` containers for systems that run in stages, simply use `.on_enter::<State::Variant>()` or its `on_exit` or `on_update` siblings.
- `SystemLabel` derives should be replaced with `SystemSet`. You will also need to add the `Debug`, `PartialEq`, `Eq`, and `Hash` traits to satisfy the new trait bounds.
- `with_run_criteria` has been renamed to `run_if`. Run criteria have been renamed to run conditions for clarity, and should now simply return a bool.
- States have been dramatically simplified: there is no longer a "state stack". To queue a transition to the next state, call `NextState::set`
## TODO
- [x] remove dead methods on App and World
- [x] add `App::add_system_to_schedule` and `App::add_systems_to_schedule`
- [x] avoid adding the default system set at inappropriate times
- [x] remove any accidental cycles in the default plugins schedule
- [x] migrate benchmarks
- [x] expose explicit labels for the built-in command flush points
- [x] migrate engine code
- [x] remove all mentions of stages from the docs
- [x] verify docs for States
- [x] fix uses of exclusive systems that use .end / .at_start / .before_commands
- [x] migrate RenderStage and AssetStage
- [x] migrate examples
- [x] ensure that transform propagation is exported in a sufficiently public way (the systems are already pub)
- [x] ensure that on_enter schedules are run at least once before the main app
- [x] re-enable opt-in to execution order ambiguities
- [x] revert change to `update_bounds` to ensure it runs in `PostUpdate`
- [x] test all examples
- [x] unbreak directional lights
- [x] unbreak shadows (see 3d_scene, 3d_shape, lighting, transparaency_3d examples)
- [x] game menu example shows loading screen and menu simultaneously
- [x] display settings menu is a blank screen
- [x] `without_winit` example panics
- [x] ensure all tests pass
- [x] SubApp doc test fails
- [x] runs_spawn_local tasks fails
- [x] [Fix panic_when_hierachy_cycle test hanging](https://github.com/alice-i-cecile/bevy/pull/120)
## Points of Difficulty and Controversy
**Reviewers, please give feedback on these and look closely**
1. Default sets, from the RFC, have been removed. These added a tremendous amount of implicit complexity and result in hard to debug scheduling errors. They're going to be tackled in the form of "base sets" by @cart in a followup.
2. The outer schedule controls which schedule is run when `App::update` is called.
3. I implemented `Label for `Box<dyn Label>` for our label types. This enables us to store schedule labels in concrete form, and then later run them. I ran into the same set of problems when working with one-shot systems. We've previously investigated this pattern in depth, and it does not appear to lead to extra indirection with nested boxes.
4. `SubApp::update` simply runs the default schedule once. This sucks, but this whole API is incomplete and this was the minimal changeset.
5. `time_system` and `tick_global_task_pools_on_main_thread` no longer use exclusive systems to attempt to force scheduling order
6. Implemetnation strategy for fixed timesteps
7. `AssetStage` was migrated to `AssetSet` without reintroducing command flush points. These did not appear to be used, and it's nice to remove these bottlenecks.
8. Migration of `bevy_render/lib.rs` and pipelined rendering. The logic here is unusually tricky, as we have complex scheduling requirements.
## Future Work (ideally before 0.10)
- Rename schedule_v3 module to schedule or scheduling
- Add a derive macro to states, and likely a `EnumIter` trait of some form
- Figure out what exactly to do with the "systems added should basically work by default" problem
- Improve ergonomics for working with fixed timesteps and states
- Polish FixedTime API to match Time
- Rebase and merge #7415
- Resolve all internal ambiguities (blocked on better tools, especially #7442)
- Add "base sets" to replace the removed default sets.
2023-02-06 02:04:50 +00:00
|
|
|
prelude::*,
|
Use EntityHashMap<Entity, T> for render world entity storage for better performance (#9903)
# Objective
- Improve rendering performance, particularly by avoiding the large
system commands costs of using the ECS in the way that the render world
does.
## Solution
- Define `EntityHasher` that calculates a hash from the
`Entity.to_bits()` by `i | (i.wrapping_mul(0x517cc1b727220a95) << 32)`.
`0x517cc1b727220a95` is something like `u64::MAX / N` for N that gives a
value close to π and that works well for hashing. Thanks for @SkiFire13
for the suggestion and to @nicopap for alternative suggestions and
discussion. This approach comes from `rustc-hash` (a.k.a. `FxHasher`)
with some tweaks for the case of hashing an `Entity`. `FxHasher` and
`SeaHasher` were also tested but were significantly slower.
- Define `EntityHashMap` type that uses the `EntityHashser`
- Use `EntityHashMap<Entity, T>` for render world entity storage,
including:
- `RenderMaterialInstances` - contains the `AssetId<M>` of the material
associated with the entity. Also for 2D.
- `RenderMeshInstances` - contains mesh transforms, flags and properties
about mesh entities. Also for 2D.
- `SkinIndices` and `MorphIndices` - contains the skin and morph index
for an entity, respectively
- `ExtractedSprites`
- `ExtractedUiNodes`
## Benchmarks
All benchmarks have been conducted on an M1 Max connected to AC power.
The tests are run for 1500 frames. The 1000th frame is captured for
comparison to check for visual regressions. There were none.
### 2D Meshes
`bevymark --benchmark --waves 160 --per-wave 1000 --mode mesh2d`
#### `--ordered-z`
This test spawns the 2D meshes with z incrementing back to front, which
is the ideal arrangement allocation order as it matches the sorted
render order which means lookups have a high cache hit rate.
<img width="1112" alt="Screenshot 2023-09-27 at 07 50 45"
src="https://github.com/bevyengine/bevy/assets/302146/e140bc98-7091-4a3b-8ae1-ab75d16d2ccb">
-39.1% median frame time.
#### Random
This test spawns the 2D meshes with random z. This not only makes the
batching and transparent 2D pass lookups get a lot of cache misses, it
also currently means that the meshes are almost certain to not be
batchable.
<img width="1108" alt="Screenshot 2023-09-27 at 07 51 28"
src="https://github.com/bevyengine/bevy/assets/302146/29c2e813-645a-43ce-982a-55df4bf7d8c4">
-7.2% median frame time.
### 3D Meshes
`many_cubes --benchmark`
<img width="1112" alt="Screenshot 2023-09-27 at 07 51 57"
src="https://github.com/bevyengine/bevy/assets/302146/1a729673-3254-4e2a-9072-55e27c69f0fc">
-7.7% median frame time.
### Sprites
**NOTE: On `main` sprites are using `SparseSet<Entity, T>`!**
`bevymark --benchmark --waves 160 --per-wave 1000 --mode sprite`
#### `--ordered-z`
This test spawns the sprites with z incrementing back to front, which is
the ideal arrangement allocation order as it matches the sorted render
order which means lookups have a high cache hit rate.
<img width="1116" alt="Screenshot 2023-09-27 at 07 52 31"
src="https://github.com/bevyengine/bevy/assets/302146/bc8eab90-e375-4d31-b5cd-f55f6f59ab67">
+13.0% median frame time.
#### Random
This test spawns the sprites with random z. This makes the batching and
transparent 2D pass lookups get a lot of cache misses.
<img width="1109" alt="Screenshot 2023-09-27 at 07 53 01"
src="https://github.com/bevyengine/bevy/assets/302146/22073f5d-99a7-49b0-9584-d3ac3eac3033">
+0.6% median frame time.
### UI
**NOTE: On `main` UI is using `SparseSet<Entity, T>`!**
`many_buttons`
<img width="1111" alt="Screenshot 2023-09-27 at 07 53 26"
src="https://github.com/bevyengine/bevy/assets/302146/66afd56d-cbe4-49e7-8b64-2f28f6043d85">
+15.1% median frame time.
## Alternatives
- Cart originally suggested trying out `SparseSet<Entity, T>` and indeed
that is slightly faster under ideal conditions. However,
`PassHashMap<Entity, T>` has better worst case performance when data is
randomly distributed, rather than in sorted render order, and does not
have the worst case memory usage that `SparseSet`'s dense `Vec<usize>`
that maps from the `Entity` index to sparse index into `Vec<T>`. This
dense `Vec` has to be as large as the largest Entity index used with the
`SparseSet`.
- I also tested `PassHashMap<u32, T>`, intending to use `Entity.index()`
as the key, but this proved to sometimes be slower and mostly no
different.
- The only outstanding approach that has not been implemented and tested
is to _not_ clear the render world of its entities each frame. That has
its own problems, though they could perhaps be solved.
- Performance-wise, if the entities and their component data were not
cleared, then they would incur table moves on spawn, and should not
thereafter, rather just their component data would be overwritten.
Ideally we would have a neat way of either updating data in-place via
`&mut T` queries, or inserting components if not present. This would
likely be quite cumbersome to have to remember to do everywhere, but
perhaps it only needs to be done in the more performance-sensitive
systems.
- The main problem to solve however is that we want to both maintain a
mapping between main world entities and render world entities, be able
to run the render app and world in parallel with the main app and world
for pipelined rendering, and at the same time be able to spawn entities
in the render world in such a way that those Entity ids do not collide
with those spawned in the main world. This is potentially quite
solvable, but could well be a lot of ECS work to do it in a way that
makes sense.
---
## Changelog
- Changed: Component data for entities to be drawn are no longer stored
on entities in the render world. Instead, data is stored in a
`EntityHashMap<Entity, T>` in various resources. This brings significant
performance benefits due to the way the render app clears entities every
frame. Resources of most interest are `RenderMeshInstances` and
`RenderMaterialInstances`, and their 2D counterparts.
## Migration Guide
Previously the render app extracted mesh entities and their component
data from the main world and stored them as entities and components in
the render world. Now they are extracted into essentially
`EntityHashMap<Entity, T>` where `T` are structs containing an
appropriate group of data. This means that while extract set systems
will continue to run extract queries against the main world they will
store their data in hash maps. Also, systems in later sets will either
need to look up entities in the available resources such as
`RenderMeshInstances`, or maintain their own `EntityHashMap<Entity, T>`
for their own data.
Before:
```rust
fn queue_custom(
material_meshes: Query<(Entity, &MeshTransforms, &Handle<Mesh>), With<InstanceMaterialData>>,
) {
...
for (entity, mesh_transforms, mesh_handle) in &material_meshes {
...
}
}
```
After:
```rust
fn queue_custom(
render_mesh_instances: Res<RenderMeshInstances>,
instance_entities: Query<Entity, With<InstanceMaterialData>>,
) {
...
for entity in &instance_entities {
let Some(mesh_instance) = render_mesh_instances.get(&entity) else { continue; };
// The mesh handle in `AssetId<Mesh>` form, and the `MeshTransforms` can now
// be found in `mesh_instance` which is a `RenderMeshInstance`
...
}
}
```
---------
Co-authored-by: robtfm <50659922+robtfm@users.noreply.github.com>
2023-09-27 08:28:28 +00:00
|
|
|
system::{lifetimeless::SRes, SystemParamItem},
|
2021-12-25 21:45:43 +00:00
|
|
|
};
|
2024-09-27 00:59:59 +00:00
|
|
|
use bevy_reflect::std_traits::ReflectDefault;
|
|
|
|
use bevy_reflect::Reflect;
|
Type safe retained render world (#15756)
# Objective
In the Render World, there are a number of collections that are derived
from Main World entities and are used to drive rendering. The most
notable are:
- `VisibleEntities`, which is generated in the `check_visibility` system
and contains visible entities for a view.
- `ExtractedInstances`, which maps entity ids to asset ids.
In the old model, these collections were trivially kept in sync -- any
extracted phase item could look itself up because the render entity id
was guaranteed to always match the corresponding main world id.
After #15320, this became much more complicated, and was leading to a
number of subtle bugs in the Render World. The main rendering systems,
i.e. `queue_material_meshes` and `queue_material2d_meshes`, follow a
similar pattern:
```rust
for visible_entity in visible_entities.iter::<With<Mesh2d>>() {
let Some(mesh_instance) = render_mesh_instances.get_mut(visible_entity) else {
continue;
};
// Look some more stuff up and specialize the pipeline...
let bin_key = Opaque2dBinKey {
pipeline: pipeline_id,
draw_function: draw_opaque_2d,
asset_id: mesh_instance.mesh_asset_id.into(),
material_bind_group_id: material_2d.get_bind_group_id().0,
};
opaque_phase.add(
bin_key,
*visible_entity,
BinnedRenderPhaseType::mesh(mesh_instance.automatic_batching),
);
}
```
In this case, `visible_entities` and `render_mesh_instances` are both
collections that are created and keyed by Main World entity ids, and so
this lookup happens to work by coincidence. However, there is a major
unintentional bug here: namely, because `visible_entities` is a
collection of Main World ids, the phase item being queued is created
with a Main World id rather than its correct Render World id.
This happens to not break mesh rendering because the render commands
used for drawing meshes do not access the `ItemQuery` parameter, but
demonstrates the confusion that is now possible: our UI phase items are
correctly being queued with Render World ids while our meshes aren't.
Additionally, this makes it very easy and error prone to use the wrong
entity id to look up things like assets. For example, if instead we
ignored visibility checks and queued our meshes via a query, we'd have
to be extra careful to use `&MainEntity` instead of the natural
`Entity`.
## Solution
Make all collections that are derived from Main World data use
`MainEntity` as their key, to ensure type safety and avoid accidentally
looking up data with the wrong entity id:
```rust
pub type MainEntityHashMap<V> = hashbrown::HashMap<MainEntity, V, EntityHash>;
```
Additionally, we make all `PhaseItem` be able to provide both their Main
and Render World ids, to allow render phase implementors maximum
flexibility as to what id should be used to look up data.
You can think of this like tracking at the type level whether something
in the Render World should use it's "primary key", i.e. entity id, or
needs to use a foreign key, i.e. `MainEntity`.
## Testing
##### TODO:
This will require extensive testing to make sure things didn't break!
Additionally, some extraction logic has become more complicated and
needs to be checked for regressions.
## Migration Guide
With the advent of the retained render world, collections that contain
references to `Entity` that are extracted into the render world have
been changed to contain `MainEntity` in order to prevent errors where a
render world entity id is used to look up an item by accident. Custom
rendering code may need to be changed to query for `&MainEntity` in
order to look up the correct item from such a collection. Additionally,
users who implement their own extraction logic for collections of main
world entity should strongly consider extracting into a different
collection that uses `MainEntity` as a key.
Additionally, render phases now require specifying both the `Entity` and
`MainEntity` for a given `PhaseItem`. Custom render phases should ensure
`MainEntity` is available when queuing a phase item.
2024-10-10 18:47:04 +00:00
|
|
|
use bevy_render::sync_world::MainEntityHashMap;
|
|
|
|
use bevy_render::view::RenderVisibleEntities;
|
2021-12-14 03:58:23 +00:00
|
|
|
use bevy_render::{
|
`StandardMaterial` Light Transmission (#8015)
# Objective
<img width="1920" alt="Screenshot 2023-04-26 at 01 07 34"
src="https://user-images.githubusercontent.com/418473/234467578-0f34187b-5863-4ea1-88e9-7a6bb8ce8da3.png">
This PR adds both diffuse and specular light transmission capabilities
to the `StandardMaterial`, with support for screen space refractions.
This enables realistically representing a wide range of real-world
materials, such as:
- Glass; (Including frosted glass)
- Transparent and translucent plastics;
- Various liquids and gels;
- Gemstones;
- Marble;
- Wax;
- Paper;
- Leaves;
- Porcelain.
Unlike existing support for transparency, light transmission does not
rely on fixed function alpha blending, and therefore works with both
`AlphaMode::Opaque` and `AlphaMode::Mask` materials.
## Solution
- Introduces a number of transmission related fields in the
`StandardMaterial`;
- For specular transmission:
- Adds logic to take a view main texture snapshot after the opaque
phase; (in order to perform screen space refractions)
- Introduces a new `Transmissive3d` phase to the renderer, to which all
meshes with `transmission > 0.0` materials are sent.
- Calculates a light exit point (of the approximate mesh volume) using
`ior` and `thickness` properties
- Samples the snapshot texture with an adaptive number of taps across a
`roughness`-controlled radius enabling “blurry” refractions
- For diffuse transmission:
- Approximates transmitted diffuse light by using a second, flipped +
displaced, diffuse-only Lambertian lobe for each light source.
## To Do
- [x] Figure out where `fresnel_mix()` is taking place, if at all, and
where `dielectric_specular` is being calculated, if at all, and update
them to use the `ior` value (Not a blocker, just a nice-to-have for more
correct BSDF)
- To the _best of my knowledge, this is now taking place, after
964340cdd. The fresnel mix is actually "split" into two parts in our
implementation, one `(1 - fresnel(...))` in the transmission, and
`fresnel()` in the light implementations. A surface with more
reflectance now will produce slightly dimmer transmission towards the
grazing angle, as more of the light gets reflected.
- [x] Add `transmission_texture`
- [x] Add `diffuse_transmission_texture`
- [x] Add `thickness_texture`
- [x] Add `attenuation_distance` and `attenuation_color`
- [x] Connect values to glTF loader
- [x] `transmission` and `transmission_texture`
- [x] `thickness` and `thickness_texture`
- [x] `ior`
- [ ] `diffuse_transmission` and `diffuse_transmission_texture` (needs
upstream support in `gltf` crate, not a blocker)
- [x] Add support for multiple screen space refraction “steps”
- [x] Conditionally create no transmission snapshot texture at all if
`steps == 0`
- [x] Conditionally enable/disable screen space refraction transmission
snapshots
- [x] Read from depth pre-pass to prevent refracting pixels in front of
the light exit point
- [x] Use `interleaved_gradient_noise()` function for sampling blur in a
way that benefits from TAA
- [x] Drill down a TAA `#define`, tweak some aspects of the effect
conditionally based on it
- [x] Remove const array that's crashing under HLSL (unless a new `naga`
release with https://github.com/gfx-rs/naga/pull/2496 comes out before
we merge this)
- [ ] Look into alternatives to the `switch` hack for dynamically
indexing the const array (might not be needed, compilers seem to be
decent at expanding it)
- [ ] Add pipeline keys for gating transmission (do we really want/need
this?)
- [x] Tweak some material field/function names?
## A Note on Texture Packing
_This was originally added as a comment to the
`specular_transmission_texture`, `thickness_texture` and
`diffuse_transmission_texture` documentation, I removed it since it was
more confusing than helpful, and will likely be made redundant/will need
to be updated once we have a better infrastructure for preprocessing
assets_
Due to how channels are mapped, you can more efficiently use a single
shared texture image
for configuring the following:
- R - `specular_transmission_texture`
- G - `thickness_texture`
- B - _unused_
- A - `diffuse_transmission_texture`
The `KHR_materials_diffuse_transmission` glTF extension also defines a
`diffuseTransmissionColorTexture`,
that _we don't currently support_. One might choose to pack the
intensity and color textures together,
using RGB for the color and A for the intensity, in which case this
packing advice doesn't really apply.
---
## Changelog
- Added a new `Transmissive3d` render phase for rendering specular
transmissive materials with screen space refractions
- Added rendering support for transmitted environment map light on the
`StandardMaterial` as a fallback for screen space refractions
- Added `diffuse_transmission`, `specular_transmission`, `thickness`,
`ior`, `attenuation_distance` and `attenuation_color` to the
`StandardMaterial`
- Added `diffuse_transmission_texture`, `specular_transmission_texture`,
`thickness_texture` to the `StandardMaterial`, gated behind a new
`pbr_transmission_textures` cargo feature (off by default, for maximum
hardware compatibility)
- Added `Camera3d::screen_space_specular_transmission_steps` for
controlling the number of “layers of transparency” rendered for
transmissive objects
- Added a `TransmittedShadowReceiver` component for enabling shadows in
(diffusely) transmitted light. (disabled by default, as it requires
carefully setting up the `thickness` to avoid self-shadow artifacts)
- Added support for the `KHR_materials_transmission`,
`KHR_materials_ior` and `KHR_materials_volume` glTF extensions
- Renamed items related to temporal jitter for greater consistency
## Migration Guide
- `SsaoPipelineKey::temporal_noise` has been renamed to
`SsaoPipelineKey::temporal_jitter`
- The `TAA` shader def (controlled by the presence of the
`TemporalAntiAliasSettings` component in the camera) has been replaced
with the `TEMPORAL_JITTER` shader def (controlled by the presence of the
`TemporalJitter` component in the camera)
- `MeshPipelineKey::TAA` has been replaced by
`MeshPipelineKey::TEMPORAL_JITTER`
- The `TEMPORAL_NOISE` shader def has been consolidated with
`TEMPORAL_JITTER`
2023-10-31 20:59:02 +00:00
|
|
|
camera::TemporalJitter,
|
2023-10-12 22:10:38 +00:00
|
|
|
extract_resource::ExtractResource,
|
Migrate meshes and materials to required components (#15524)
# Objective
A big step in the migration to required components: meshes and
materials!
## Solution
As per the [selected
proposal](https://hackmd.io/@bevy/required_components/%2Fj9-PnF-2QKK0on1KQ29UWQ):
- Deprecate `MaterialMesh2dBundle`, `MaterialMeshBundle`, and
`PbrBundle`.
- Add `Mesh2d` and `Mesh3d` components, which wrap a `Handle<Mesh>`.
- Add `MeshMaterial2d<M: Material2d>` and `MeshMaterial3d<M: Material>`,
which wrap a `Handle<M>`.
- Meshes *without* a mesh material should be rendered with a default
material. The existence of a material is determined by
`HasMaterial2d`/`HasMaterial3d`, which is required by
`MeshMaterial2d`/`MeshMaterial3d`. This gets around problems with the
generics.
Previously:
```rust
commands.spawn(MaterialMesh2dBundle {
mesh: meshes.add(Circle::new(100.0)).into(),
material: materials.add(Color::srgb(7.5, 0.0, 7.5)),
transform: Transform::from_translation(Vec3::new(-200., 0., 0.)),
..default()
});
```
Now:
```rust
commands.spawn((
Mesh2d(meshes.add(Circle::new(100.0))),
MeshMaterial2d(materials.add(Color::srgb(7.5, 0.0, 7.5))),
Transform::from_translation(Vec3::new(-200., 0., 0.)),
));
```
If the mesh material is missing, previously nothing was rendered. Now,
it renders a white default `ColorMaterial` in 2D and a
`StandardMaterial` in 3D (this can be overridden). Below, only every
other entity has a material:


Why white? This is still open for discussion, but I think white makes
sense for a *default* material, while *invalid* asset handles pointing
to nothing should have something like a pink material to indicate that
something is broken (I don't handle that in this PR yet). This is kind
of a mix of Godot and Unity: Godot just renders a white material for
non-existent materials, while Unity renders nothing when no materials
exist, but renders pink for invalid materials. I can also change the
default material to pink if that is preferable though.
## Testing
I ran some 2D and 3D examples to test if anything changed visually. I
have not tested all examples or features yet however. If anyone wants to
test more extensively, it would be appreciated!
## Implementation Notes
- The relationship between `bevy_render` and `bevy_pbr` is weird here.
`bevy_render` needs `Mesh3d` for its own systems, but `bevy_pbr` has all
of the material logic, and `bevy_render` doesn't depend on it. I feel
like the two crates should be refactored in some way, but I think that's
out of scope for this PR.
- I didn't migrate meshlets to required components yet. That can
probably be done in a follow-up, as this is already a huge PR.
- It is becoming increasingly clear to me that we really, *really* want
to disallow raw asset handles as components. They caused me a *ton* of
headache here already, and it took me a long time to find every place
that queried for them or inserted them directly on entities, since there
were no compiler errors for it. If we don't remove the `Component`
derive, I expect raw asset handles to be a *huge* footgun for users as
we transition to wrapper components, especially as handles as components
have been the norm so far. I personally consider this to be a blocker
for 0.15: we need to migrate to wrapper components for asset handles
everywhere, and remove the `Component` derive. Also see
https://github.com/bevyengine/bevy/issues/14124.
---
## Migration Guide
Asset handles for meshes and mesh materials must now be wrapped in the
`Mesh2d` and `MeshMaterial2d` or `Mesh3d` and `MeshMaterial3d`
components for 2D and 3D respectively. Raw handles as components no
longer render meshes.
Additionally, `MaterialMesh2dBundle`, `MaterialMeshBundle`, and
`PbrBundle` have been deprecated. Instead, use the mesh and material
components directly.
Previously:
```rust
commands.spawn(MaterialMesh2dBundle {
mesh: meshes.add(Circle::new(100.0)).into(),
material: materials.add(Color::srgb(7.5, 0.0, 7.5)),
transform: Transform::from_translation(Vec3::new(-200., 0., 0.)),
..default()
});
```
Now:
```rust
commands.spawn((
Mesh2d(meshes.add(Circle::new(100.0))),
MeshMaterial2d(materials.add(Color::srgb(7.5, 0.0, 7.5))),
Transform::from_translation(Vec3::new(-200., 0., 0.)),
));
```
If the mesh material is missing, a white default material is now used.
Previously, nothing was rendered if the material was missing.
The `WithMesh2d` and `WithMesh3d` query filter type aliases have also
been removed. Simply use `With<Mesh2d>` or `With<Mesh3d>`.
---------
Co-authored-by: Tim Blackbird <justthecooldude@gmail.com>
Co-authored-by: Carter Anderson <mcanders1@gmail.com>
2024-10-01 21:33:17 +00:00
|
|
|
mesh::{Mesh3d, MeshVertexBufferLayoutRef, RenderMesh},
|
2024-04-09 13:26:34 +00:00
|
|
|
render_asset::{PrepareAssetError, RenderAsset, RenderAssetPlugin, RenderAssets},
|
2023-10-25 08:40:55 +00:00
|
|
|
render_phase::*,
|
|
|
|
render_resource::*,
|
2021-12-14 03:58:23 +00:00
|
|
|
renderer::RenderDevice,
|
Type safe retained render world (#15756)
# Objective
In the Render World, there are a number of collections that are derived
from Main World entities and are used to drive rendering. The most
notable are:
- `VisibleEntities`, which is generated in the `check_visibility` system
and contains visible entities for a view.
- `ExtractedInstances`, which maps entity ids to asset ids.
In the old model, these collections were trivially kept in sync -- any
extracted phase item could look itself up because the render entity id
was guaranteed to always match the corresponding main world id.
After #15320, this became much more complicated, and was leading to a
number of subtle bugs in the Render World. The main rendering systems,
i.e. `queue_material_meshes` and `queue_material2d_meshes`, follow a
similar pattern:
```rust
for visible_entity in visible_entities.iter::<With<Mesh2d>>() {
let Some(mesh_instance) = render_mesh_instances.get_mut(visible_entity) else {
continue;
};
// Look some more stuff up and specialize the pipeline...
let bin_key = Opaque2dBinKey {
pipeline: pipeline_id,
draw_function: draw_opaque_2d,
asset_id: mesh_instance.mesh_asset_id.into(),
material_bind_group_id: material_2d.get_bind_group_id().0,
};
opaque_phase.add(
bin_key,
*visible_entity,
BinnedRenderPhaseType::mesh(mesh_instance.automatic_batching),
);
}
```
In this case, `visible_entities` and `render_mesh_instances` are both
collections that are created and keyed by Main World entity ids, and so
this lookup happens to work by coincidence. However, there is a major
unintentional bug here: namely, because `visible_entities` is a
collection of Main World ids, the phase item being queued is created
with a Main World id rather than its correct Render World id.
This happens to not break mesh rendering because the render commands
used for drawing meshes do not access the `ItemQuery` parameter, but
demonstrates the confusion that is now possible: our UI phase items are
correctly being queued with Render World ids while our meshes aren't.
Additionally, this makes it very easy and error prone to use the wrong
entity id to look up things like assets. For example, if instead we
ignored visibility checks and queued our meshes via a query, we'd have
to be extra careful to use `&MainEntity` instead of the natural
`Entity`.
## Solution
Make all collections that are derived from Main World data use
`MainEntity` as their key, to ensure type safety and avoid accidentally
looking up data with the wrong entity id:
```rust
pub type MainEntityHashMap<V> = hashbrown::HashMap<MainEntity, V, EntityHash>;
```
Additionally, we make all `PhaseItem` be able to provide both their Main
and Render World ids, to allow render phase implementors maximum
flexibility as to what id should be used to look up data.
You can think of this like tracking at the type level whether something
in the Render World should use it's "primary key", i.e. entity id, or
needs to use a foreign key, i.e. `MainEntity`.
## Testing
##### TODO:
This will require extensive testing to make sure things didn't break!
Additionally, some extraction logic has become more complicated and
needs to be checked for regressions.
## Migration Guide
With the advent of the retained render world, collections that contain
references to `Entity` that are extracted into the render world have
been changed to contain `MainEntity` in order to prevent errors where a
render world entity id is used to look up an item by accident. Custom
rendering code may need to be changed to query for `&MainEntity` in
order to look up the correct item from such a collection. Additionally,
users who implement their own extraction logic for collections of main
world entity should strongly consider extracting into a different
collection that uses `MainEntity` as a key.
Additionally, render phases now require specifying both the `Entity` and
`MainEntity` for a given `PhaseItem`. Custom render phases should ensure
`MainEntity` is available when queuing a phase item.
2024-10-10 18:47:04 +00:00
|
|
|
view::{ExtractedView, Msaa, RenderVisibilityRanges, ViewVisibility},
|
Migrate meshes and materials to required components (#15524)
# Objective
A big step in the migration to required components: meshes and
materials!
## Solution
As per the [selected
proposal](https://hackmd.io/@bevy/required_components/%2Fj9-PnF-2QKK0on1KQ29UWQ):
- Deprecate `MaterialMesh2dBundle`, `MaterialMeshBundle`, and
`PbrBundle`.
- Add `Mesh2d` and `Mesh3d` components, which wrap a `Handle<Mesh>`.
- Add `MeshMaterial2d<M: Material2d>` and `MeshMaterial3d<M: Material>`,
which wrap a `Handle<M>`.
- Meshes *without* a mesh material should be rendered with a default
material. The existence of a material is determined by
`HasMaterial2d`/`HasMaterial3d`, which is required by
`MeshMaterial2d`/`MeshMaterial3d`. This gets around problems with the
generics.
Previously:
```rust
commands.spawn(MaterialMesh2dBundle {
mesh: meshes.add(Circle::new(100.0)).into(),
material: materials.add(Color::srgb(7.5, 0.0, 7.5)),
transform: Transform::from_translation(Vec3::new(-200., 0., 0.)),
..default()
});
```
Now:
```rust
commands.spawn((
Mesh2d(meshes.add(Circle::new(100.0))),
MeshMaterial2d(materials.add(Color::srgb(7.5, 0.0, 7.5))),
Transform::from_translation(Vec3::new(-200., 0., 0.)),
));
```
If the mesh material is missing, previously nothing was rendered. Now,
it renders a white default `ColorMaterial` in 2D and a
`StandardMaterial` in 3D (this can be overridden). Below, only every
other entity has a material:


Why white? This is still open for discussion, but I think white makes
sense for a *default* material, while *invalid* asset handles pointing
to nothing should have something like a pink material to indicate that
something is broken (I don't handle that in this PR yet). This is kind
of a mix of Godot and Unity: Godot just renders a white material for
non-existent materials, while Unity renders nothing when no materials
exist, but renders pink for invalid materials. I can also change the
default material to pink if that is preferable though.
## Testing
I ran some 2D and 3D examples to test if anything changed visually. I
have not tested all examples or features yet however. If anyone wants to
test more extensively, it would be appreciated!
## Implementation Notes
- The relationship between `bevy_render` and `bevy_pbr` is weird here.
`bevy_render` needs `Mesh3d` for its own systems, but `bevy_pbr` has all
of the material logic, and `bevy_render` doesn't depend on it. I feel
like the two crates should be refactored in some way, but I think that's
out of scope for this PR.
- I didn't migrate meshlets to required components yet. That can
probably be done in a follow-up, as this is already a huge PR.
- It is becoming increasingly clear to me that we really, *really* want
to disallow raw asset handles as components. They caused me a *ton* of
headache here already, and it took me a long time to find every place
that queried for them or inserted them directly on entities, since there
were no compiler errors for it. If we don't remove the `Component`
derive, I expect raw asset handles to be a *huge* footgun for users as
we transition to wrapper components, especially as handles as components
have been the norm so far. I personally consider this to be a blocker
for 0.15: we need to migrate to wrapper components for asset handles
everywhere, and remove the `Component` derive. Also see
https://github.com/bevyengine/bevy/issues/14124.
---
## Migration Guide
Asset handles for meshes and mesh materials must now be wrapped in the
`Mesh2d` and `MeshMaterial2d` or `Mesh3d` and `MeshMaterial3d`
components for 2D and 3D respectively. Raw handles as components no
longer render meshes.
Additionally, `MaterialMesh2dBundle`, `MaterialMeshBundle`, and
`PbrBundle` have been deprecated. Instead, use the mesh and material
components directly.
Previously:
```rust
commands.spawn(MaterialMesh2dBundle {
mesh: meshes.add(Circle::new(100.0)).into(),
material: materials.add(Color::srgb(7.5, 0.0, 7.5)),
transform: Transform::from_translation(Vec3::new(-200., 0., 0.)),
..default()
});
```
Now:
```rust
commands.spawn((
Mesh2d(meshes.add(Circle::new(100.0))),
MeshMaterial2d(materials.add(Color::srgb(7.5, 0.0, 7.5))),
Transform::from_translation(Vec3::new(-200., 0., 0.)),
));
```
If the mesh material is missing, a white default material is now used.
Previously, nothing was rendered if the material was missing.
The `WithMesh2d` and `WithMesh3d` query filter type aliases have also
been removed. Simply use `With<Mesh2d>` or `With<Mesh3d>`.
---------
Co-authored-by: Tim Blackbird <justthecooldude@gmail.com>
Co-authored-by: Carter Anderson <mcanders1@gmail.com>
2024-10-01 21:33:17 +00:00
|
|
|
Extract,
|
2021-12-14 03:58:23 +00:00
|
|
|
};
|
2024-04-09 13:26:34 +00:00
|
|
|
use bevy_utils::tracing::error;
|
2024-09-27 00:59:59 +00:00
|
|
|
use core::{
|
2024-09-24 11:42:59 +00:00
|
|
|
hash::Hash,
|
|
|
|
marker::PhantomData,
|
|
|
|
num::NonZero,
|
|
|
|
sync::atomic::{AtomicU32, Ordering},
|
|
|
|
};
|
2020-02-11 17:31:49 +00:00
|
|
|
|
Migrate meshes and materials to required components (#15524)
# Objective
A big step in the migration to required components: meshes and
materials!
## Solution
As per the [selected
proposal](https://hackmd.io/@bevy/required_components/%2Fj9-PnF-2QKK0on1KQ29UWQ):
- Deprecate `MaterialMesh2dBundle`, `MaterialMeshBundle`, and
`PbrBundle`.
- Add `Mesh2d` and `Mesh3d` components, which wrap a `Handle<Mesh>`.
- Add `MeshMaterial2d<M: Material2d>` and `MeshMaterial3d<M: Material>`,
which wrap a `Handle<M>`.
- Meshes *without* a mesh material should be rendered with a default
material. The existence of a material is determined by
`HasMaterial2d`/`HasMaterial3d`, which is required by
`MeshMaterial2d`/`MeshMaterial3d`. This gets around problems with the
generics.
Previously:
```rust
commands.spawn(MaterialMesh2dBundle {
mesh: meshes.add(Circle::new(100.0)).into(),
material: materials.add(Color::srgb(7.5, 0.0, 7.5)),
transform: Transform::from_translation(Vec3::new(-200., 0., 0.)),
..default()
});
```
Now:
```rust
commands.spawn((
Mesh2d(meshes.add(Circle::new(100.0))),
MeshMaterial2d(materials.add(Color::srgb(7.5, 0.0, 7.5))),
Transform::from_translation(Vec3::new(-200., 0., 0.)),
));
```
If the mesh material is missing, previously nothing was rendered. Now,
it renders a white default `ColorMaterial` in 2D and a
`StandardMaterial` in 3D (this can be overridden). Below, only every
other entity has a material:


Why white? This is still open for discussion, but I think white makes
sense for a *default* material, while *invalid* asset handles pointing
to nothing should have something like a pink material to indicate that
something is broken (I don't handle that in this PR yet). This is kind
of a mix of Godot and Unity: Godot just renders a white material for
non-existent materials, while Unity renders nothing when no materials
exist, but renders pink for invalid materials. I can also change the
default material to pink if that is preferable though.
## Testing
I ran some 2D and 3D examples to test if anything changed visually. I
have not tested all examples or features yet however. If anyone wants to
test more extensively, it would be appreciated!
## Implementation Notes
- The relationship between `bevy_render` and `bevy_pbr` is weird here.
`bevy_render` needs `Mesh3d` for its own systems, but `bevy_pbr` has all
of the material logic, and `bevy_render` doesn't depend on it. I feel
like the two crates should be refactored in some way, but I think that's
out of scope for this PR.
- I didn't migrate meshlets to required components yet. That can
probably be done in a follow-up, as this is already a huge PR.
- It is becoming increasingly clear to me that we really, *really* want
to disallow raw asset handles as components. They caused me a *ton* of
headache here already, and it took me a long time to find every place
that queried for them or inserted them directly on entities, since there
were no compiler errors for it. If we don't remove the `Component`
derive, I expect raw asset handles to be a *huge* footgun for users as
we transition to wrapper components, especially as handles as components
have been the norm so far. I personally consider this to be a blocker
for 0.15: we need to migrate to wrapper components for asset handles
everywhere, and remove the `Component` derive. Also see
https://github.com/bevyengine/bevy/issues/14124.
---
## Migration Guide
Asset handles for meshes and mesh materials must now be wrapped in the
`Mesh2d` and `MeshMaterial2d` or `Mesh3d` and `MeshMaterial3d`
components for 2D and 3D respectively. Raw handles as components no
longer render meshes.
Additionally, `MaterialMesh2dBundle`, `MaterialMeshBundle`, and
`PbrBundle` have been deprecated. Instead, use the mesh and material
components directly.
Previously:
```rust
commands.spawn(MaterialMesh2dBundle {
mesh: meshes.add(Circle::new(100.0)).into(),
material: materials.add(Color::srgb(7.5, 0.0, 7.5)),
transform: Transform::from_translation(Vec3::new(-200., 0., 0.)),
..default()
});
```
Now:
```rust
commands.spawn((
Mesh2d(meshes.add(Circle::new(100.0))),
MeshMaterial2d(materials.add(Color::srgb(7.5, 0.0, 7.5))),
Transform::from_translation(Vec3::new(-200., 0., 0.)),
));
```
If the mesh material is missing, a white default material is now used.
Previously, nothing was rendered if the material was missing.
The `WithMesh2d` and `WithMesh3d` query filter type aliases have also
been removed. Simply use `With<Mesh2d>` or `With<Mesh3d>`.
---------
Co-authored-by: Tim Blackbird <justthecooldude@gmail.com>
Co-authored-by: Carter Anderson <mcanders1@gmail.com>
2024-10-01 21:33:17 +00:00
|
|
|
/// Materials are used alongside [`MaterialPlugin`], [`Mesh3d`], and [`MeshMaterial3d`]
|
2021-12-25 21:45:43 +00:00
|
|
|
/// to spawn entities that are rendered with a specific [`Material`] type. They serve as an easy to use high level
|
Migrate meshes and materials to required components (#15524)
# Objective
A big step in the migration to required components: meshes and
materials!
## Solution
As per the [selected
proposal](https://hackmd.io/@bevy/required_components/%2Fj9-PnF-2QKK0on1KQ29UWQ):
- Deprecate `MaterialMesh2dBundle`, `MaterialMeshBundle`, and
`PbrBundle`.
- Add `Mesh2d` and `Mesh3d` components, which wrap a `Handle<Mesh>`.
- Add `MeshMaterial2d<M: Material2d>` and `MeshMaterial3d<M: Material>`,
which wrap a `Handle<M>`.
- Meshes *without* a mesh material should be rendered with a default
material. The existence of a material is determined by
`HasMaterial2d`/`HasMaterial3d`, which is required by
`MeshMaterial2d`/`MeshMaterial3d`. This gets around problems with the
generics.
Previously:
```rust
commands.spawn(MaterialMesh2dBundle {
mesh: meshes.add(Circle::new(100.0)).into(),
material: materials.add(Color::srgb(7.5, 0.0, 7.5)),
transform: Transform::from_translation(Vec3::new(-200., 0., 0.)),
..default()
});
```
Now:
```rust
commands.spawn((
Mesh2d(meshes.add(Circle::new(100.0))),
MeshMaterial2d(materials.add(Color::srgb(7.5, 0.0, 7.5))),
Transform::from_translation(Vec3::new(-200., 0., 0.)),
));
```
If the mesh material is missing, previously nothing was rendered. Now,
it renders a white default `ColorMaterial` in 2D and a
`StandardMaterial` in 3D (this can be overridden). Below, only every
other entity has a material:


Why white? This is still open for discussion, but I think white makes
sense for a *default* material, while *invalid* asset handles pointing
to nothing should have something like a pink material to indicate that
something is broken (I don't handle that in this PR yet). This is kind
of a mix of Godot and Unity: Godot just renders a white material for
non-existent materials, while Unity renders nothing when no materials
exist, but renders pink for invalid materials. I can also change the
default material to pink if that is preferable though.
## Testing
I ran some 2D and 3D examples to test if anything changed visually. I
have not tested all examples or features yet however. If anyone wants to
test more extensively, it would be appreciated!
## Implementation Notes
- The relationship between `bevy_render` and `bevy_pbr` is weird here.
`bevy_render` needs `Mesh3d` for its own systems, but `bevy_pbr` has all
of the material logic, and `bevy_render` doesn't depend on it. I feel
like the two crates should be refactored in some way, but I think that's
out of scope for this PR.
- I didn't migrate meshlets to required components yet. That can
probably be done in a follow-up, as this is already a huge PR.
- It is becoming increasingly clear to me that we really, *really* want
to disallow raw asset handles as components. They caused me a *ton* of
headache here already, and it took me a long time to find every place
that queried for them or inserted them directly on entities, since there
were no compiler errors for it. If we don't remove the `Component`
derive, I expect raw asset handles to be a *huge* footgun for users as
we transition to wrapper components, especially as handles as components
have been the norm so far. I personally consider this to be a blocker
for 0.15: we need to migrate to wrapper components for asset handles
everywhere, and remove the `Component` derive. Also see
https://github.com/bevyengine/bevy/issues/14124.
---
## Migration Guide
Asset handles for meshes and mesh materials must now be wrapped in the
`Mesh2d` and `MeshMaterial2d` or `Mesh3d` and `MeshMaterial3d`
components for 2D and 3D respectively. Raw handles as components no
longer render meshes.
Additionally, `MaterialMesh2dBundle`, `MaterialMeshBundle`, and
`PbrBundle` have been deprecated. Instead, use the mesh and material
components directly.
Previously:
```rust
commands.spawn(MaterialMesh2dBundle {
mesh: meshes.add(Circle::new(100.0)).into(),
material: materials.add(Color::srgb(7.5, 0.0, 7.5)),
transform: Transform::from_translation(Vec3::new(-200., 0., 0.)),
..default()
});
```
Now:
```rust
commands.spawn((
Mesh2d(meshes.add(Circle::new(100.0))),
MeshMaterial2d(materials.add(Color::srgb(7.5, 0.0, 7.5))),
Transform::from_translation(Vec3::new(-200., 0., 0.)),
));
```
If the mesh material is missing, a white default material is now used.
Previously, nothing was rendered if the material was missing.
The `WithMesh2d` and `WithMesh3d` query filter type aliases have also
been removed. Simply use `With<Mesh2d>` or `With<Mesh3d>`.
---------
Co-authored-by: Tim Blackbird <justthecooldude@gmail.com>
Co-authored-by: Carter Anderson <mcanders1@gmail.com>
2024-10-01 21:33:17 +00:00
|
|
|
/// way to render [`Mesh3d`] entities with custom shader logic.
|
Better Materials: AsBindGroup trait and derive, simpler Material trait (#5053)
# Objective
This PR reworks Bevy's Material system, making the user experience of defining Materials _much_ nicer. Bevy's previous material system leaves a lot to be desired:
* Materials require manually implementing the `RenderAsset` trait, which involves manually generating the bind group, handling gpu buffer data transfer, looking up image textures, etc. Even the simplest single-texture material involves writing ~80 unnecessary lines of code. This was never the long term plan.
* There are two material traits, which is confusing, hard to document, and often redundant: `Material` and `SpecializedMaterial`. `Material` implicitly implements `SpecializedMaterial`, and `SpecializedMaterial` is used in most high level apis to support both use cases. Most users shouldn't need to think about specialization at all (I consider it a "power-user tool"), so the fact that `SpecializedMaterial` is front-and-center in our apis is a miss.
* Implementing either material trait involves a lot of "type soup". The "prepared asset" parameter is particularly heinous: `&<Self as RenderAsset>::PreparedAsset`. Defining vertex and fragment shaders is also more verbose than it needs to be.
## Solution
Say hello to the new `Material` system:
```rust
#[derive(AsBindGroup, TypeUuid, Debug, Clone)]
#[uuid = "f690fdae-d598-45ab-8225-97e2a3f056e0"]
pub struct CoolMaterial {
#[uniform(0)]
color: Color,
#[texture(1)]
#[sampler(2)]
color_texture: Handle<Image>,
}
impl Material for CoolMaterial {
fn fragment_shader() -> ShaderRef {
"cool_material.wgsl".into()
}
}
```
Thats it! This same material would have required [~80 lines of complicated "type heavy" code](https://github.com/bevyengine/bevy/blob/v0.7.0/examples/shader/shader_material.rs) in the old Material system. Now it is just 14 lines of simple, readable code.
This is thanks to a new consolidated `Material` trait and the new `AsBindGroup` trait / derive.
### The new `Material` trait
The old "split" `Material` and `SpecializedMaterial` traits have been removed in favor of a new consolidated `Material` trait. All of the functions on the trait are optional.
The difficulty of implementing `Material` has been reduced by simplifying dataflow and removing type complexity:
```rust
// Old
impl Material for CustomMaterial {
fn fragment_shader(asset_server: &AssetServer) -> Option<Handle<Shader>> {
Some(asset_server.load("custom_material.wgsl"))
}
fn alpha_mode(render_asset: &<Self as RenderAsset>::PreparedAsset) -> AlphaMode {
render_asset.alpha_mode
}
}
// New
impl Material for CustomMaterial {
fn fragment_shader() -> ShaderRef {
"custom_material.wgsl".into()
}
fn alpha_mode(&self) -> AlphaMode {
self.alpha_mode
}
}
```
Specialization is still supported, but it is hidden by default under the `specialize()` function (more on this later).
### The `AsBindGroup` trait / derive
The `Material` trait now requires the `AsBindGroup` derive. This can be implemented manually relatively easily, but deriving it will almost always be preferable.
Field attributes like `uniform` and `texture` are used to define which fields should be bindings,
what their binding type is, and what index they should be bound at:
```rust
#[derive(AsBindGroup)]
struct CoolMaterial {
#[uniform(0)]
color: Color,
#[texture(1)]
#[sampler(2)]
color_texture: Handle<Image>,
}
```
In WGSL shaders, the binding looks like this:
```wgsl
struct CoolMaterial {
color: vec4<f32>;
};
[[group(1), binding(0)]]
var<uniform> material: CoolMaterial;
[[group(1), binding(1)]]
var color_texture: texture_2d<f32>;
[[group(1), binding(2)]]
var color_sampler: sampler;
```
Note that the "group" index is determined by the usage context. It is not defined in `AsBindGroup`. Bevy material bind groups are bound to group 1.
The following field-level attributes are supported:
* `uniform(BINDING_INDEX)`
* The field will be converted to a shader-compatible type using the `ShaderType` trait, written to a `Buffer`, and bound as a uniform. It can also be derived for custom structs.
* `texture(BINDING_INDEX)`
* This field's `Handle<Image>` will be used to look up the matching `Texture` gpu resource, which will be bound as a texture in shaders. The field will be assumed to implement `Into<Option<Handle<Image>>>`. In practice, most fields should be a `Handle<Image>` or `Option<Handle<Image>>`. If the value of an `Option<Handle<Image>>` is `None`, the new `FallbackImage` resource will be used instead. This attribute can be used in conjunction with a `sampler` binding attribute (with a different binding index).
* `sampler(BINDING_INDEX)`
* Behaves exactly like the `texture` attribute, but sets the Image's sampler binding instead of the texture.
Note that fields without field-level binding attributes will be ignored.
```rust
#[derive(AsBindGroup)]
struct CoolMaterial {
#[uniform(0)]
color: Color,
this_field_is_ignored: String,
}
```
As mentioned above, `Option<Handle<Image>>` is also supported:
```rust
#[derive(AsBindGroup)]
struct CoolMaterial {
#[uniform(0)]
color: Color,
#[texture(1)]
#[sampler(2)]
color_texture: Option<Handle<Image>>,
}
```
This is useful if you want a texture to be optional. When the value is `None`, the `FallbackImage` will be used for the binding instead, which defaults to "pure white".
Field uniforms with the same binding index will be combined into a single binding:
```rust
#[derive(AsBindGroup)]
struct CoolMaterial {
#[uniform(0)]
color: Color,
#[uniform(0)]
roughness: f32,
}
```
In WGSL shaders, the binding would look like this:
```wgsl
struct CoolMaterial {
color: vec4<f32>;
roughness: f32;
};
[[group(1), binding(0)]]
var<uniform> material: CoolMaterial;
```
Some less common scenarios will require "struct-level" attributes. These are the currently supported struct-level attributes:
* `uniform(BINDING_INDEX, ConvertedShaderType)`
* Similar to the field-level `uniform` attribute, but instead the entire `AsBindGroup` value is converted to `ConvertedShaderType`, which must implement `ShaderType`. This is useful if more complicated conversion logic is required.
* `bind_group_data(DataType)`
* The `AsBindGroup` type will be converted to some `DataType` using `Into<DataType>` and stored as `AsBindGroup::Data` as part of the `AsBindGroup::as_bind_group` call. This is useful if data needs to be stored alongside the generated bind group, such as a unique identifier for a material's bind group. The most common use case for this attribute is "shader pipeline specialization".
The previous `CoolMaterial` example illustrating "combining multiple field-level uniform attributes with the same binding index" can
also be equivalently represented with a single struct-level uniform attribute:
```rust
#[derive(AsBindGroup)]
#[uniform(0, CoolMaterialUniform)]
struct CoolMaterial {
color: Color,
roughness: f32,
}
#[derive(ShaderType)]
struct CoolMaterialUniform {
color: Color,
roughness: f32,
}
impl From<&CoolMaterial> for CoolMaterialUniform {
fn from(material: &CoolMaterial) -> CoolMaterialUniform {
CoolMaterialUniform {
color: material.color,
roughness: material.roughness,
}
}
}
```
### Material Specialization
Material shader specialization is now _much_ simpler:
```rust
#[derive(AsBindGroup, TypeUuid, Debug, Clone)]
#[uuid = "f690fdae-d598-45ab-8225-97e2a3f056e0"]
#[bind_group_data(CoolMaterialKey)]
struct CoolMaterial {
#[uniform(0)]
color: Color,
is_red: bool,
}
#[derive(Copy, Clone, Hash, Eq, PartialEq)]
struct CoolMaterialKey {
is_red: bool,
}
impl From<&CoolMaterial> for CoolMaterialKey {
fn from(material: &CoolMaterial) -> CoolMaterialKey {
CoolMaterialKey {
is_red: material.is_red,
}
}
}
impl Material for CoolMaterial {
fn fragment_shader() -> ShaderRef {
"cool_material.wgsl".into()
}
fn specialize(
pipeline: &MaterialPipeline<Self>,
descriptor: &mut RenderPipelineDescriptor,
layout: &MeshVertexBufferLayout,
key: MaterialPipelineKey<Self>,
) -> Result<(), SpecializedMeshPipelineError> {
if key.bind_group_data.is_red {
let fragment = descriptor.fragment.as_mut().unwrap();
fragment.shader_defs.push("IS_RED".to_string());
}
Ok(())
}
}
```
Setting `bind_group_data` is not required for specialization (it defaults to `()`). Scenarios like "custom vertex attributes" also benefit from this system:
```rust
impl Material for CustomMaterial {
fn vertex_shader() -> ShaderRef {
"custom_material.wgsl".into()
}
fn fragment_shader() -> ShaderRef {
"custom_material.wgsl".into()
}
fn specialize(
pipeline: &MaterialPipeline<Self>,
descriptor: &mut RenderPipelineDescriptor,
layout: &MeshVertexBufferLayout,
key: MaterialPipelineKey<Self>,
) -> Result<(), SpecializedMeshPipelineError> {
let vertex_layout = layout.get_layout(&[
Mesh::ATTRIBUTE_POSITION.at_shader_location(0),
ATTRIBUTE_BLEND_COLOR.at_shader_location(1),
])?;
descriptor.vertex.buffers = vec![vertex_layout];
Ok(())
}
}
```
### Ported `StandardMaterial` to the new `Material` system
Bevy's built-in PBR material uses the new Material system (including the AsBindGroup derive):
```rust
#[derive(AsBindGroup, Debug, Clone, TypeUuid)]
#[uuid = "7494888b-c082-457b-aacf-517228cc0c22"]
#[bind_group_data(StandardMaterialKey)]
#[uniform(0, StandardMaterialUniform)]
pub struct StandardMaterial {
pub base_color: Color,
#[texture(1)]
#[sampler(2)]
pub base_color_texture: Option<Handle<Image>>,
/* other fields omitted for brevity */
```
### Ported Bevy examples to the new `Material` system
The overall complexity of Bevy's "custom shader examples" has gone down significantly. Take a look at the diffs if you want a dopamine spike.
Please note that while this PR has a net increase in "lines of code", most of those extra lines come from added documentation. There is a significant reduction
in the overall complexity of the code (even accounting for the new derive logic).
---
## Changelog
### Added
* `AsBindGroup` trait and derive, which make it much easier to transfer data to the gpu and generate bind groups for a given type.
### Changed
* The old `Material` and `SpecializedMaterial` traits have been replaced by a consolidated (much simpler) `Material` trait. Materials no longer implement `RenderAsset`.
* `StandardMaterial` was ported to the new material system. There are no user-facing api changes to the `StandardMaterial` struct api, but it now implements `AsBindGroup` and `Material` instead of `RenderAsset` and `SpecializedMaterial`.
## Migration Guide
The Material system has been reworked to be much simpler. We've removed a lot of boilerplate with the new `AsBindGroup` derive and the `Material` trait is simpler as well!
### Bevy 0.7 (old)
```rust
#[derive(Debug, Clone, TypeUuid)]
#[uuid = "f690fdae-d598-45ab-8225-97e2a3f056e0"]
pub struct CustomMaterial {
color: Color,
color_texture: Handle<Image>,
}
#[derive(Clone)]
pub struct GpuCustomMaterial {
_buffer: Buffer,
bind_group: BindGroup,
}
impl RenderAsset for CustomMaterial {
type ExtractedAsset = CustomMaterial;
type PreparedAsset = GpuCustomMaterial;
type Param = (SRes<RenderDevice>, SRes<MaterialPipeline<Self>>);
fn extract_asset(&self) -> Self::ExtractedAsset {
self.clone()
}
fn prepare_asset(
extracted_asset: Self::ExtractedAsset,
(render_device, material_pipeline): &mut SystemParamItem<Self::Param>,
) -> Result<Self::PreparedAsset, PrepareAssetError<Self::ExtractedAsset>> {
let color = Vec4::from_slice(&extracted_asset.color.as_linear_rgba_f32());
let byte_buffer = [0u8; Vec4::SIZE.get() as usize];
let mut buffer = encase::UniformBuffer::new(byte_buffer);
buffer.write(&color).unwrap();
let buffer = render_device.create_buffer_with_data(&BufferInitDescriptor {
contents: buffer.as_ref(),
label: None,
usage: BufferUsages::UNIFORM | BufferUsages::COPY_DST,
});
let (texture_view, texture_sampler) = if let Some(result) = material_pipeline
.mesh_pipeline
.get_image_texture(gpu_images, &Some(extracted_asset.color_texture.clone()))
{
result
} else {
return Err(PrepareAssetError::RetryNextUpdate(extracted_asset));
};
let bind_group = render_device.create_bind_group(&BindGroupDescriptor {
entries: &[
BindGroupEntry {
binding: 0,
resource: buffer.as_entire_binding(),
},
BindGroupEntry {
binding: 0,
resource: BindingResource::TextureView(texture_view),
},
BindGroupEntry {
binding: 1,
resource: BindingResource::Sampler(texture_sampler),
},
],
label: None,
layout: &material_pipeline.material_layout,
});
Ok(GpuCustomMaterial {
_buffer: buffer,
bind_group,
})
}
}
impl Material for CustomMaterial {
fn fragment_shader(asset_server: &AssetServer) -> Option<Handle<Shader>> {
Some(asset_server.load("custom_material.wgsl"))
}
fn bind_group(render_asset: &<Self as RenderAsset>::PreparedAsset) -> &BindGroup {
&render_asset.bind_group
}
fn bind_group_layout(render_device: &RenderDevice) -> BindGroupLayout {
render_device.create_bind_group_layout(&BindGroupLayoutDescriptor {
entries: &[
BindGroupLayoutEntry {
binding: 0,
visibility: ShaderStages::FRAGMENT,
ty: BindingType::Buffer {
ty: BufferBindingType::Uniform,
has_dynamic_offset: false,
min_binding_size: Some(Vec4::min_size()),
},
count: None,
},
BindGroupLayoutEntry {
binding: 1,
visibility: ShaderStages::FRAGMENT,
ty: BindingType::Texture {
multisampled: false,
sample_type: TextureSampleType::Float { filterable: true },
view_dimension: TextureViewDimension::D2Array,
},
count: None,
},
BindGroupLayoutEntry {
binding: 2,
visibility: ShaderStages::FRAGMENT,
ty: BindingType::Sampler(SamplerBindingType::Filtering),
count: None,
},
],
label: None,
})
}
}
```
### Bevy 0.8 (new)
```rust
impl Material for CustomMaterial {
fn fragment_shader() -> ShaderRef {
"custom_material.wgsl".into()
}
}
#[derive(AsBindGroup, TypeUuid, Debug, Clone)]
#[uuid = "f690fdae-d598-45ab-8225-97e2a3f056e0"]
pub struct CustomMaterial {
#[uniform(0)]
color: Color,
#[texture(1)]
#[sampler(2)]
color_texture: Handle<Image>,
}
```
## Future Work
* Add support for more binding types (cubemaps, buffers, etc). This PR intentionally includes a bare minimum number of binding types to keep "reviewability" in check.
* Consider optionally eliding binding indices using binding names. `AsBindGroup` could pass in (optional?) reflection info as a "hint".
* This would make it possible for the derive to do this:
```rust
#[derive(AsBindGroup)]
pub struct CustomMaterial {
#[uniform]
color: Color,
#[texture]
#[sampler]
color_texture: Option<Handle<Image>>,
alpha_mode: AlphaMode,
}
```
* Or this
```rust
#[derive(AsBindGroup)]
pub struct CustomMaterial {
#[binding]
color: Color,
#[binding]
color_texture: Option<Handle<Image>>,
alpha_mode: AlphaMode,
}
```
* Or even this (if we flip to "include bindings by default")
```rust
#[derive(AsBindGroup)]
pub struct CustomMaterial {
color: Color,
color_texture: Option<Handle<Image>>,
#[binding(ignore)]
alpha_mode: AlphaMode,
}
```
* If we add the option to define custom draw functions for materials (which could be done in a type-erased way), I think that would be enough to support extra non-material bindings. Worth considering!
2022-06-30 23:48:46 +00:00
|
|
|
///
|
|
|
|
/// Materials must implement [`AsBindGroup`] to define how data will be transferred to the GPU and bound in shaders.
|
|
|
|
/// [`AsBindGroup`] can be derived, which makes generating bindings straightforward. See the [`AsBindGroup`] docs for details.
|
|
|
|
///
|
|
|
|
/// # Example
|
|
|
|
///
|
Migrate meshes and materials to required components (#15524)
# Objective
A big step in the migration to required components: meshes and
materials!
## Solution
As per the [selected
proposal](https://hackmd.io/@bevy/required_components/%2Fj9-PnF-2QKK0on1KQ29UWQ):
- Deprecate `MaterialMesh2dBundle`, `MaterialMeshBundle`, and
`PbrBundle`.
- Add `Mesh2d` and `Mesh3d` components, which wrap a `Handle<Mesh>`.
- Add `MeshMaterial2d<M: Material2d>` and `MeshMaterial3d<M: Material>`,
which wrap a `Handle<M>`.
- Meshes *without* a mesh material should be rendered with a default
material. The existence of a material is determined by
`HasMaterial2d`/`HasMaterial3d`, which is required by
`MeshMaterial2d`/`MeshMaterial3d`. This gets around problems with the
generics.
Previously:
```rust
commands.spawn(MaterialMesh2dBundle {
mesh: meshes.add(Circle::new(100.0)).into(),
material: materials.add(Color::srgb(7.5, 0.0, 7.5)),
transform: Transform::from_translation(Vec3::new(-200., 0., 0.)),
..default()
});
```
Now:
```rust
commands.spawn((
Mesh2d(meshes.add(Circle::new(100.0))),
MeshMaterial2d(materials.add(Color::srgb(7.5, 0.0, 7.5))),
Transform::from_translation(Vec3::new(-200., 0., 0.)),
));
```
If the mesh material is missing, previously nothing was rendered. Now,
it renders a white default `ColorMaterial` in 2D and a
`StandardMaterial` in 3D (this can be overridden). Below, only every
other entity has a material:


Why white? This is still open for discussion, but I think white makes
sense for a *default* material, while *invalid* asset handles pointing
to nothing should have something like a pink material to indicate that
something is broken (I don't handle that in this PR yet). This is kind
of a mix of Godot and Unity: Godot just renders a white material for
non-existent materials, while Unity renders nothing when no materials
exist, but renders pink for invalid materials. I can also change the
default material to pink if that is preferable though.
## Testing
I ran some 2D and 3D examples to test if anything changed visually. I
have not tested all examples or features yet however. If anyone wants to
test more extensively, it would be appreciated!
## Implementation Notes
- The relationship between `bevy_render` and `bevy_pbr` is weird here.
`bevy_render` needs `Mesh3d` for its own systems, but `bevy_pbr` has all
of the material logic, and `bevy_render` doesn't depend on it. I feel
like the two crates should be refactored in some way, but I think that's
out of scope for this PR.
- I didn't migrate meshlets to required components yet. That can
probably be done in a follow-up, as this is already a huge PR.
- It is becoming increasingly clear to me that we really, *really* want
to disallow raw asset handles as components. They caused me a *ton* of
headache here already, and it took me a long time to find every place
that queried for them or inserted them directly on entities, since there
were no compiler errors for it. If we don't remove the `Component`
derive, I expect raw asset handles to be a *huge* footgun for users as
we transition to wrapper components, especially as handles as components
have been the norm so far. I personally consider this to be a blocker
for 0.15: we need to migrate to wrapper components for asset handles
everywhere, and remove the `Component` derive. Also see
https://github.com/bevyengine/bevy/issues/14124.
---
## Migration Guide
Asset handles for meshes and mesh materials must now be wrapped in the
`Mesh2d` and `MeshMaterial2d` or `Mesh3d` and `MeshMaterial3d`
components for 2D and 3D respectively. Raw handles as components no
longer render meshes.
Additionally, `MaterialMesh2dBundle`, `MaterialMeshBundle`, and
`PbrBundle` have been deprecated. Instead, use the mesh and material
components directly.
Previously:
```rust
commands.spawn(MaterialMesh2dBundle {
mesh: meshes.add(Circle::new(100.0)).into(),
material: materials.add(Color::srgb(7.5, 0.0, 7.5)),
transform: Transform::from_translation(Vec3::new(-200., 0., 0.)),
..default()
});
```
Now:
```rust
commands.spawn((
Mesh2d(meshes.add(Circle::new(100.0))),
MeshMaterial2d(materials.add(Color::srgb(7.5, 0.0, 7.5))),
Transform::from_translation(Vec3::new(-200., 0., 0.)),
));
```
If the mesh material is missing, a white default material is now used.
Previously, nothing was rendered if the material was missing.
The `WithMesh2d` and `WithMesh3d` query filter type aliases have also
been removed. Simply use `With<Mesh2d>` or `With<Mesh3d>`.
---------
Co-authored-by: Tim Blackbird <justthecooldude@gmail.com>
Co-authored-by: Carter Anderson <mcanders1@gmail.com>
2024-10-01 21:33:17 +00:00
|
|
|
/// Here is a simple [`Material`] implementation. The [`AsBindGroup`] derive has many features. To see what else is available,
|
Better Materials: AsBindGroup trait and derive, simpler Material trait (#5053)
# Objective
This PR reworks Bevy's Material system, making the user experience of defining Materials _much_ nicer. Bevy's previous material system leaves a lot to be desired:
* Materials require manually implementing the `RenderAsset` trait, which involves manually generating the bind group, handling gpu buffer data transfer, looking up image textures, etc. Even the simplest single-texture material involves writing ~80 unnecessary lines of code. This was never the long term plan.
* There are two material traits, which is confusing, hard to document, and often redundant: `Material` and `SpecializedMaterial`. `Material` implicitly implements `SpecializedMaterial`, and `SpecializedMaterial` is used in most high level apis to support both use cases. Most users shouldn't need to think about specialization at all (I consider it a "power-user tool"), so the fact that `SpecializedMaterial` is front-and-center in our apis is a miss.
* Implementing either material trait involves a lot of "type soup". The "prepared asset" parameter is particularly heinous: `&<Self as RenderAsset>::PreparedAsset`. Defining vertex and fragment shaders is also more verbose than it needs to be.
## Solution
Say hello to the new `Material` system:
```rust
#[derive(AsBindGroup, TypeUuid, Debug, Clone)]
#[uuid = "f690fdae-d598-45ab-8225-97e2a3f056e0"]
pub struct CoolMaterial {
#[uniform(0)]
color: Color,
#[texture(1)]
#[sampler(2)]
color_texture: Handle<Image>,
}
impl Material for CoolMaterial {
fn fragment_shader() -> ShaderRef {
"cool_material.wgsl".into()
}
}
```
Thats it! This same material would have required [~80 lines of complicated "type heavy" code](https://github.com/bevyengine/bevy/blob/v0.7.0/examples/shader/shader_material.rs) in the old Material system. Now it is just 14 lines of simple, readable code.
This is thanks to a new consolidated `Material` trait and the new `AsBindGroup` trait / derive.
### The new `Material` trait
The old "split" `Material` and `SpecializedMaterial` traits have been removed in favor of a new consolidated `Material` trait. All of the functions on the trait are optional.
The difficulty of implementing `Material` has been reduced by simplifying dataflow and removing type complexity:
```rust
// Old
impl Material for CustomMaterial {
fn fragment_shader(asset_server: &AssetServer) -> Option<Handle<Shader>> {
Some(asset_server.load("custom_material.wgsl"))
}
fn alpha_mode(render_asset: &<Self as RenderAsset>::PreparedAsset) -> AlphaMode {
render_asset.alpha_mode
}
}
// New
impl Material for CustomMaterial {
fn fragment_shader() -> ShaderRef {
"custom_material.wgsl".into()
}
fn alpha_mode(&self) -> AlphaMode {
self.alpha_mode
}
}
```
Specialization is still supported, but it is hidden by default under the `specialize()` function (more on this later).
### The `AsBindGroup` trait / derive
The `Material` trait now requires the `AsBindGroup` derive. This can be implemented manually relatively easily, but deriving it will almost always be preferable.
Field attributes like `uniform` and `texture` are used to define which fields should be bindings,
what their binding type is, and what index they should be bound at:
```rust
#[derive(AsBindGroup)]
struct CoolMaterial {
#[uniform(0)]
color: Color,
#[texture(1)]
#[sampler(2)]
color_texture: Handle<Image>,
}
```
In WGSL shaders, the binding looks like this:
```wgsl
struct CoolMaterial {
color: vec4<f32>;
};
[[group(1), binding(0)]]
var<uniform> material: CoolMaterial;
[[group(1), binding(1)]]
var color_texture: texture_2d<f32>;
[[group(1), binding(2)]]
var color_sampler: sampler;
```
Note that the "group" index is determined by the usage context. It is not defined in `AsBindGroup`. Bevy material bind groups are bound to group 1.
The following field-level attributes are supported:
* `uniform(BINDING_INDEX)`
* The field will be converted to a shader-compatible type using the `ShaderType` trait, written to a `Buffer`, and bound as a uniform. It can also be derived for custom structs.
* `texture(BINDING_INDEX)`
* This field's `Handle<Image>` will be used to look up the matching `Texture` gpu resource, which will be bound as a texture in shaders. The field will be assumed to implement `Into<Option<Handle<Image>>>`. In practice, most fields should be a `Handle<Image>` or `Option<Handle<Image>>`. If the value of an `Option<Handle<Image>>` is `None`, the new `FallbackImage` resource will be used instead. This attribute can be used in conjunction with a `sampler` binding attribute (with a different binding index).
* `sampler(BINDING_INDEX)`
* Behaves exactly like the `texture` attribute, but sets the Image's sampler binding instead of the texture.
Note that fields without field-level binding attributes will be ignored.
```rust
#[derive(AsBindGroup)]
struct CoolMaterial {
#[uniform(0)]
color: Color,
this_field_is_ignored: String,
}
```
As mentioned above, `Option<Handle<Image>>` is also supported:
```rust
#[derive(AsBindGroup)]
struct CoolMaterial {
#[uniform(0)]
color: Color,
#[texture(1)]
#[sampler(2)]
color_texture: Option<Handle<Image>>,
}
```
This is useful if you want a texture to be optional. When the value is `None`, the `FallbackImage` will be used for the binding instead, which defaults to "pure white".
Field uniforms with the same binding index will be combined into a single binding:
```rust
#[derive(AsBindGroup)]
struct CoolMaterial {
#[uniform(0)]
color: Color,
#[uniform(0)]
roughness: f32,
}
```
In WGSL shaders, the binding would look like this:
```wgsl
struct CoolMaterial {
color: vec4<f32>;
roughness: f32;
};
[[group(1), binding(0)]]
var<uniform> material: CoolMaterial;
```
Some less common scenarios will require "struct-level" attributes. These are the currently supported struct-level attributes:
* `uniform(BINDING_INDEX, ConvertedShaderType)`
* Similar to the field-level `uniform` attribute, but instead the entire `AsBindGroup` value is converted to `ConvertedShaderType`, which must implement `ShaderType`. This is useful if more complicated conversion logic is required.
* `bind_group_data(DataType)`
* The `AsBindGroup` type will be converted to some `DataType` using `Into<DataType>` and stored as `AsBindGroup::Data` as part of the `AsBindGroup::as_bind_group` call. This is useful if data needs to be stored alongside the generated bind group, such as a unique identifier for a material's bind group. The most common use case for this attribute is "shader pipeline specialization".
The previous `CoolMaterial` example illustrating "combining multiple field-level uniform attributes with the same binding index" can
also be equivalently represented with a single struct-level uniform attribute:
```rust
#[derive(AsBindGroup)]
#[uniform(0, CoolMaterialUniform)]
struct CoolMaterial {
color: Color,
roughness: f32,
}
#[derive(ShaderType)]
struct CoolMaterialUniform {
color: Color,
roughness: f32,
}
impl From<&CoolMaterial> for CoolMaterialUniform {
fn from(material: &CoolMaterial) -> CoolMaterialUniform {
CoolMaterialUniform {
color: material.color,
roughness: material.roughness,
}
}
}
```
### Material Specialization
Material shader specialization is now _much_ simpler:
```rust
#[derive(AsBindGroup, TypeUuid, Debug, Clone)]
#[uuid = "f690fdae-d598-45ab-8225-97e2a3f056e0"]
#[bind_group_data(CoolMaterialKey)]
struct CoolMaterial {
#[uniform(0)]
color: Color,
is_red: bool,
}
#[derive(Copy, Clone, Hash, Eq, PartialEq)]
struct CoolMaterialKey {
is_red: bool,
}
impl From<&CoolMaterial> for CoolMaterialKey {
fn from(material: &CoolMaterial) -> CoolMaterialKey {
CoolMaterialKey {
is_red: material.is_red,
}
}
}
impl Material for CoolMaterial {
fn fragment_shader() -> ShaderRef {
"cool_material.wgsl".into()
}
fn specialize(
pipeline: &MaterialPipeline<Self>,
descriptor: &mut RenderPipelineDescriptor,
layout: &MeshVertexBufferLayout,
key: MaterialPipelineKey<Self>,
) -> Result<(), SpecializedMeshPipelineError> {
if key.bind_group_data.is_red {
let fragment = descriptor.fragment.as_mut().unwrap();
fragment.shader_defs.push("IS_RED".to_string());
}
Ok(())
}
}
```
Setting `bind_group_data` is not required for specialization (it defaults to `()`). Scenarios like "custom vertex attributes" also benefit from this system:
```rust
impl Material for CustomMaterial {
fn vertex_shader() -> ShaderRef {
"custom_material.wgsl".into()
}
fn fragment_shader() -> ShaderRef {
"custom_material.wgsl".into()
}
fn specialize(
pipeline: &MaterialPipeline<Self>,
descriptor: &mut RenderPipelineDescriptor,
layout: &MeshVertexBufferLayout,
key: MaterialPipelineKey<Self>,
) -> Result<(), SpecializedMeshPipelineError> {
let vertex_layout = layout.get_layout(&[
Mesh::ATTRIBUTE_POSITION.at_shader_location(0),
ATTRIBUTE_BLEND_COLOR.at_shader_location(1),
])?;
descriptor.vertex.buffers = vec![vertex_layout];
Ok(())
}
}
```
### Ported `StandardMaterial` to the new `Material` system
Bevy's built-in PBR material uses the new Material system (including the AsBindGroup derive):
```rust
#[derive(AsBindGroup, Debug, Clone, TypeUuid)]
#[uuid = "7494888b-c082-457b-aacf-517228cc0c22"]
#[bind_group_data(StandardMaterialKey)]
#[uniform(0, StandardMaterialUniform)]
pub struct StandardMaterial {
pub base_color: Color,
#[texture(1)]
#[sampler(2)]
pub base_color_texture: Option<Handle<Image>>,
/* other fields omitted for brevity */
```
### Ported Bevy examples to the new `Material` system
The overall complexity of Bevy's "custom shader examples" has gone down significantly. Take a look at the diffs if you want a dopamine spike.
Please note that while this PR has a net increase in "lines of code", most of those extra lines come from added documentation. There is a significant reduction
in the overall complexity of the code (even accounting for the new derive logic).
---
## Changelog
### Added
* `AsBindGroup` trait and derive, which make it much easier to transfer data to the gpu and generate bind groups for a given type.
### Changed
* The old `Material` and `SpecializedMaterial` traits have been replaced by a consolidated (much simpler) `Material` trait. Materials no longer implement `RenderAsset`.
* `StandardMaterial` was ported to the new material system. There are no user-facing api changes to the `StandardMaterial` struct api, but it now implements `AsBindGroup` and `Material` instead of `RenderAsset` and `SpecializedMaterial`.
## Migration Guide
The Material system has been reworked to be much simpler. We've removed a lot of boilerplate with the new `AsBindGroup` derive and the `Material` trait is simpler as well!
### Bevy 0.7 (old)
```rust
#[derive(Debug, Clone, TypeUuid)]
#[uuid = "f690fdae-d598-45ab-8225-97e2a3f056e0"]
pub struct CustomMaterial {
color: Color,
color_texture: Handle<Image>,
}
#[derive(Clone)]
pub struct GpuCustomMaterial {
_buffer: Buffer,
bind_group: BindGroup,
}
impl RenderAsset for CustomMaterial {
type ExtractedAsset = CustomMaterial;
type PreparedAsset = GpuCustomMaterial;
type Param = (SRes<RenderDevice>, SRes<MaterialPipeline<Self>>);
fn extract_asset(&self) -> Self::ExtractedAsset {
self.clone()
}
fn prepare_asset(
extracted_asset: Self::ExtractedAsset,
(render_device, material_pipeline): &mut SystemParamItem<Self::Param>,
) -> Result<Self::PreparedAsset, PrepareAssetError<Self::ExtractedAsset>> {
let color = Vec4::from_slice(&extracted_asset.color.as_linear_rgba_f32());
let byte_buffer = [0u8; Vec4::SIZE.get() as usize];
let mut buffer = encase::UniformBuffer::new(byte_buffer);
buffer.write(&color).unwrap();
let buffer = render_device.create_buffer_with_data(&BufferInitDescriptor {
contents: buffer.as_ref(),
label: None,
usage: BufferUsages::UNIFORM | BufferUsages::COPY_DST,
});
let (texture_view, texture_sampler) = if let Some(result) = material_pipeline
.mesh_pipeline
.get_image_texture(gpu_images, &Some(extracted_asset.color_texture.clone()))
{
result
} else {
return Err(PrepareAssetError::RetryNextUpdate(extracted_asset));
};
let bind_group = render_device.create_bind_group(&BindGroupDescriptor {
entries: &[
BindGroupEntry {
binding: 0,
resource: buffer.as_entire_binding(),
},
BindGroupEntry {
binding: 0,
resource: BindingResource::TextureView(texture_view),
},
BindGroupEntry {
binding: 1,
resource: BindingResource::Sampler(texture_sampler),
},
],
label: None,
layout: &material_pipeline.material_layout,
});
Ok(GpuCustomMaterial {
_buffer: buffer,
bind_group,
})
}
}
impl Material for CustomMaterial {
fn fragment_shader(asset_server: &AssetServer) -> Option<Handle<Shader>> {
Some(asset_server.load("custom_material.wgsl"))
}
fn bind_group(render_asset: &<Self as RenderAsset>::PreparedAsset) -> &BindGroup {
&render_asset.bind_group
}
fn bind_group_layout(render_device: &RenderDevice) -> BindGroupLayout {
render_device.create_bind_group_layout(&BindGroupLayoutDescriptor {
entries: &[
BindGroupLayoutEntry {
binding: 0,
visibility: ShaderStages::FRAGMENT,
ty: BindingType::Buffer {
ty: BufferBindingType::Uniform,
has_dynamic_offset: false,
min_binding_size: Some(Vec4::min_size()),
},
count: None,
},
BindGroupLayoutEntry {
binding: 1,
visibility: ShaderStages::FRAGMENT,
ty: BindingType::Texture {
multisampled: false,
sample_type: TextureSampleType::Float { filterable: true },
view_dimension: TextureViewDimension::D2Array,
},
count: None,
},
BindGroupLayoutEntry {
binding: 2,
visibility: ShaderStages::FRAGMENT,
ty: BindingType::Sampler(SamplerBindingType::Filtering),
count: None,
},
],
label: None,
})
}
}
```
### Bevy 0.8 (new)
```rust
impl Material for CustomMaterial {
fn fragment_shader() -> ShaderRef {
"custom_material.wgsl".into()
}
}
#[derive(AsBindGroup, TypeUuid, Debug, Clone)]
#[uuid = "f690fdae-d598-45ab-8225-97e2a3f056e0"]
pub struct CustomMaterial {
#[uniform(0)]
color: Color,
#[texture(1)]
#[sampler(2)]
color_texture: Handle<Image>,
}
```
## Future Work
* Add support for more binding types (cubemaps, buffers, etc). This PR intentionally includes a bare minimum number of binding types to keep "reviewability" in check.
* Consider optionally eliding binding indices using binding names. `AsBindGroup` could pass in (optional?) reflection info as a "hint".
* This would make it possible for the derive to do this:
```rust
#[derive(AsBindGroup)]
pub struct CustomMaterial {
#[uniform]
color: Color,
#[texture]
#[sampler]
color_texture: Option<Handle<Image>>,
alpha_mode: AlphaMode,
}
```
* Or this
```rust
#[derive(AsBindGroup)]
pub struct CustomMaterial {
#[binding]
color: Color,
#[binding]
color_texture: Option<Handle<Image>>,
alpha_mode: AlphaMode,
}
```
* Or even this (if we flip to "include bindings by default")
```rust
#[derive(AsBindGroup)]
pub struct CustomMaterial {
color: Color,
color_texture: Option<Handle<Image>>,
#[binding(ignore)]
alpha_mode: AlphaMode,
}
```
* If we add the option to define custom draw functions for materials (which could be done in a type-erased way), I think that would be enough to support extra non-material bindings. Worth considering!
2022-06-30 23:48:46 +00:00
|
|
|
/// check out the [`AsBindGroup`] documentation.
|
Migrate meshes and materials to required components (#15524)
# Objective
A big step in the migration to required components: meshes and
materials!
## Solution
As per the [selected
proposal](https://hackmd.io/@bevy/required_components/%2Fj9-PnF-2QKK0on1KQ29UWQ):
- Deprecate `MaterialMesh2dBundle`, `MaterialMeshBundle`, and
`PbrBundle`.
- Add `Mesh2d` and `Mesh3d` components, which wrap a `Handle<Mesh>`.
- Add `MeshMaterial2d<M: Material2d>` and `MeshMaterial3d<M: Material>`,
which wrap a `Handle<M>`.
- Meshes *without* a mesh material should be rendered with a default
material. The existence of a material is determined by
`HasMaterial2d`/`HasMaterial3d`, which is required by
`MeshMaterial2d`/`MeshMaterial3d`. This gets around problems with the
generics.
Previously:
```rust
commands.spawn(MaterialMesh2dBundle {
mesh: meshes.add(Circle::new(100.0)).into(),
material: materials.add(Color::srgb(7.5, 0.0, 7.5)),
transform: Transform::from_translation(Vec3::new(-200., 0., 0.)),
..default()
});
```
Now:
```rust
commands.spawn((
Mesh2d(meshes.add(Circle::new(100.0))),
MeshMaterial2d(materials.add(Color::srgb(7.5, 0.0, 7.5))),
Transform::from_translation(Vec3::new(-200., 0., 0.)),
));
```
If the mesh material is missing, previously nothing was rendered. Now,
it renders a white default `ColorMaterial` in 2D and a
`StandardMaterial` in 3D (this can be overridden). Below, only every
other entity has a material:


Why white? This is still open for discussion, but I think white makes
sense for a *default* material, while *invalid* asset handles pointing
to nothing should have something like a pink material to indicate that
something is broken (I don't handle that in this PR yet). This is kind
of a mix of Godot and Unity: Godot just renders a white material for
non-existent materials, while Unity renders nothing when no materials
exist, but renders pink for invalid materials. I can also change the
default material to pink if that is preferable though.
## Testing
I ran some 2D and 3D examples to test if anything changed visually. I
have not tested all examples or features yet however. If anyone wants to
test more extensively, it would be appreciated!
## Implementation Notes
- The relationship between `bevy_render` and `bevy_pbr` is weird here.
`bevy_render` needs `Mesh3d` for its own systems, but `bevy_pbr` has all
of the material logic, and `bevy_render` doesn't depend on it. I feel
like the two crates should be refactored in some way, but I think that's
out of scope for this PR.
- I didn't migrate meshlets to required components yet. That can
probably be done in a follow-up, as this is already a huge PR.
- It is becoming increasingly clear to me that we really, *really* want
to disallow raw asset handles as components. They caused me a *ton* of
headache here already, and it took me a long time to find every place
that queried for them or inserted them directly on entities, since there
were no compiler errors for it. If we don't remove the `Component`
derive, I expect raw asset handles to be a *huge* footgun for users as
we transition to wrapper components, especially as handles as components
have been the norm so far. I personally consider this to be a blocker
for 0.15: we need to migrate to wrapper components for asset handles
everywhere, and remove the `Component` derive. Also see
https://github.com/bevyengine/bevy/issues/14124.
---
## Migration Guide
Asset handles for meshes and mesh materials must now be wrapped in the
`Mesh2d` and `MeshMaterial2d` or `Mesh3d` and `MeshMaterial3d`
components for 2D and 3D respectively. Raw handles as components no
longer render meshes.
Additionally, `MaterialMesh2dBundle`, `MaterialMeshBundle`, and
`PbrBundle` have been deprecated. Instead, use the mesh and material
components directly.
Previously:
```rust
commands.spawn(MaterialMesh2dBundle {
mesh: meshes.add(Circle::new(100.0)).into(),
material: materials.add(Color::srgb(7.5, 0.0, 7.5)),
transform: Transform::from_translation(Vec3::new(-200., 0., 0.)),
..default()
});
```
Now:
```rust
commands.spawn((
Mesh2d(meshes.add(Circle::new(100.0))),
MeshMaterial2d(materials.add(Color::srgb(7.5, 0.0, 7.5))),
Transform::from_translation(Vec3::new(-200., 0., 0.)),
));
```
If the mesh material is missing, a white default material is now used.
Previously, nothing was rendered if the material was missing.
The `WithMesh2d` and `WithMesh3d` query filter type aliases have also
been removed. Simply use `With<Mesh2d>` or `With<Mesh3d>`.
---------
Co-authored-by: Tim Blackbird <justthecooldude@gmail.com>
Co-authored-by: Carter Anderson <mcanders1@gmail.com>
2024-10-01 21:33:17 +00:00
|
|
|
///
|
Better Materials: AsBindGroup trait and derive, simpler Material trait (#5053)
# Objective
This PR reworks Bevy's Material system, making the user experience of defining Materials _much_ nicer. Bevy's previous material system leaves a lot to be desired:
* Materials require manually implementing the `RenderAsset` trait, which involves manually generating the bind group, handling gpu buffer data transfer, looking up image textures, etc. Even the simplest single-texture material involves writing ~80 unnecessary lines of code. This was never the long term plan.
* There are two material traits, which is confusing, hard to document, and often redundant: `Material` and `SpecializedMaterial`. `Material` implicitly implements `SpecializedMaterial`, and `SpecializedMaterial` is used in most high level apis to support both use cases. Most users shouldn't need to think about specialization at all (I consider it a "power-user tool"), so the fact that `SpecializedMaterial` is front-and-center in our apis is a miss.
* Implementing either material trait involves a lot of "type soup". The "prepared asset" parameter is particularly heinous: `&<Self as RenderAsset>::PreparedAsset`. Defining vertex and fragment shaders is also more verbose than it needs to be.
## Solution
Say hello to the new `Material` system:
```rust
#[derive(AsBindGroup, TypeUuid, Debug, Clone)]
#[uuid = "f690fdae-d598-45ab-8225-97e2a3f056e0"]
pub struct CoolMaterial {
#[uniform(0)]
color: Color,
#[texture(1)]
#[sampler(2)]
color_texture: Handle<Image>,
}
impl Material for CoolMaterial {
fn fragment_shader() -> ShaderRef {
"cool_material.wgsl".into()
}
}
```
Thats it! This same material would have required [~80 lines of complicated "type heavy" code](https://github.com/bevyengine/bevy/blob/v0.7.0/examples/shader/shader_material.rs) in the old Material system. Now it is just 14 lines of simple, readable code.
This is thanks to a new consolidated `Material` trait and the new `AsBindGroup` trait / derive.
### The new `Material` trait
The old "split" `Material` and `SpecializedMaterial` traits have been removed in favor of a new consolidated `Material` trait. All of the functions on the trait are optional.
The difficulty of implementing `Material` has been reduced by simplifying dataflow and removing type complexity:
```rust
// Old
impl Material for CustomMaterial {
fn fragment_shader(asset_server: &AssetServer) -> Option<Handle<Shader>> {
Some(asset_server.load("custom_material.wgsl"))
}
fn alpha_mode(render_asset: &<Self as RenderAsset>::PreparedAsset) -> AlphaMode {
render_asset.alpha_mode
}
}
// New
impl Material for CustomMaterial {
fn fragment_shader() -> ShaderRef {
"custom_material.wgsl".into()
}
fn alpha_mode(&self) -> AlphaMode {
self.alpha_mode
}
}
```
Specialization is still supported, but it is hidden by default under the `specialize()` function (more on this later).
### The `AsBindGroup` trait / derive
The `Material` trait now requires the `AsBindGroup` derive. This can be implemented manually relatively easily, but deriving it will almost always be preferable.
Field attributes like `uniform` and `texture` are used to define which fields should be bindings,
what their binding type is, and what index they should be bound at:
```rust
#[derive(AsBindGroup)]
struct CoolMaterial {
#[uniform(0)]
color: Color,
#[texture(1)]
#[sampler(2)]
color_texture: Handle<Image>,
}
```
In WGSL shaders, the binding looks like this:
```wgsl
struct CoolMaterial {
color: vec4<f32>;
};
[[group(1), binding(0)]]
var<uniform> material: CoolMaterial;
[[group(1), binding(1)]]
var color_texture: texture_2d<f32>;
[[group(1), binding(2)]]
var color_sampler: sampler;
```
Note that the "group" index is determined by the usage context. It is not defined in `AsBindGroup`. Bevy material bind groups are bound to group 1.
The following field-level attributes are supported:
* `uniform(BINDING_INDEX)`
* The field will be converted to a shader-compatible type using the `ShaderType` trait, written to a `Buffer`, and bound as a uniform. It can also be derived for custom structs.
* `texture(BINDING_INDEX)`
* This field's `Handle<Image>` will be used to look up the matching `Texture` gpu resource, which will be bound as a texture in shaders. The field will be assumed to implement `Into<Option<Handle<Image>>>`. In practice, most fields should be a `Handle<Image>` or `Option<Handle<Image>>`. If the value of an `Option<Handle<Image>>` is `None`, the new `FallbackImage` resource will be used instead. This attribute can be used in conjunction with a `sampler` binding attribute (with a different binding index).
* `sampler(BINDING_INDEX)`
* Behaves exactly like the `texture` attribute, but sets the Image's sampler binding instead of the texture.
Note that fields without field-level binding attributes will be ignored.
```rust
#[derive(AsBindGroup)]
struct CoolMaterial {
#[uniform(0)]
color: Color,
this_field_is_ignored: String,
}
```
As mentioned above, `Option<Handle<Image>>` is also supported:
```rust
#[derive(AsBindGroup)]
struct CoolMaterial {
#[uniform(0)]
color: Color,
#[texture(1)]
#[sampler(2)]
color_texture: Option<Handle<Image>>,
}
```
This is useful if you want a texture to be optional. When the value is `None`, the `FallbackImage` will be used for the binding instead, which defaults to "pure white".
Field uniforms with the same binding index will be combined into a single binding:
```rust
#[derive(AsBindGroup)]
struct CoolMaterial {
#[uniform(0)]
color: Color,
#[uniform(0)]
roughness: f32,
}
```
In WGSL shaders, the binding would look like this:
```wgsl
struct CoolMaterial {
color: vec4<f32>;
roughness: f32;
};
[[group(1), binding(0)]]
var<uniform> material: CoolMaterial;
```
Some less common scenarios will require "struct-level" attributes. These are the currently supported struct-level attributes:
* `uniform(BINDING_INDEX, ConvertedShaderType)`
* Similar to the field-level `uniform` attribute, but instead the entire `AsBindGroup` value is converted to `ConvertedShaderType`, which must implement `ShaderType`. This is useful if more complicated conversion logic is required.
* `bind_group_data(DataType)`
* The `AsBindGroup` type will be converted to some `DataType` using `Into<DataType>` and stored as `AsBindGroup::Data` as part of the `AsBindGroup::as_bind_group` call. This is useful if data needs to be stored alongside the generated bind group, such as a unique identifier for a material's bind group. The most common use case for this attribute is "shader pipeline specialization".
The previous `CoolMaterial` example illustrating "combining multiple field-level uniform attributes with the same binding index" can
also be equivalently represented with a single struct-level uniform attribute:
```rust
#[derive(AsBindGroup)]
#[uniform(0, CoolMaterialUniform)]
struct CoolMaterial {
color: Color,
roughness: f32,
}
#[derive(ShaderType)]
struct CoolMaterialUniform {
color: Color,
roughness: f32,
}
impl From<&CoolMaterial> for CoolMaterialUniform {
fn from(material: &CoolMaterial) -> CoolMaterialUniform {
CoolMaterialUniform {
color: material.color,
roughness: material.roughness,
}
}
}
```
### Material Specialization
Material shader specialization is now _much_ simpler:
```rust
#[derive(AsBindGroup, TypeUuid, Debug, Clone)]
#[uuid = "f690fdae-d598-45ab-8225-97e2a3f056e0"]
#[bind_group_data(CoolMaterialKey)]
struct CoolMaterial {
#[uniform(0)]
color: Color,
is_red: bool,
}
#[derive(Copy, Clone, Hash, Eq, PartialEq)]
struct CoolMaterialKey {
is_red: bool,
}
impl From<&CoolMaterial> for CoolMaterialKey {
fn from(material: &CoolMaterial) -> CoolMaterialKey {
CoolMaterialKey {
is_red: material.is_red,
}
}
}
impl Material for CoolMaterial {
fn fragment_shader() -> ShaderRef {
"cool_material.wgsl".into()
}
fn specialize(
pipeline: &MaterialPipeline<Self>,
descriptor: &mut RenderPipelineDescriptor,
layout: &MeshVertexBufferLayout,
key: MaterialPipelineKey<Self>,
) -> Result<(), SpecializedMeshPipelineError> {
if key.bind_group_data.is_red {
let fragment = descriptor.fragment.as_mut().unwrap();
fragment.shader_defs.push("IS_RED".to_string());
}
Ok(())
}
}
```
Setting `bind_group_data` is not required for specialization (it defaults to `()`). Scenarios like "custom vertex attributes" also benefit from this system:
```rust
impl Material for CustomMaterial {
fn vertex_shader() -> ShaderRef {
"custom_material.wgsl".into()
}
fn fragment_shader() -> ShaderRef {
"custom_material.wgsl".into()
}
fn specialize(
pipeline: &MaterialPipeline<Self>,
descriptor: &mut RenderPipelineDescriptor,
layout: &MeshVertexBufferLayout,
key: MaterialPipelineKey<Self>,
) -> Result<(), SpecializedMeshPipelineError> {
let vertex_layout = layout.get_layout(&[
Mesh::ATTRIBUTE_POSITION.at_shader_location(0),
ATTRIBUTE_BLEND_COLOR.at_shader_location(1),
])?;
descriptor.vertex.buffers = vec![vertex_layout];
Ok(())
}
}
```
### Ported `StandardMaterial` to the new `Material` system
Bevy's built-in PBR material uses the new Material system (including the AsBindGroup derive):
```rust
#[derive(AsBindGroup, Debug, Clone, TypeUuid)]
#[uuid = "7494888b-c082-457b-aacf-517228cc0c22"]
#[bind_group_data(StandardMaterialKey)]
#[uniform(0, StandardMaterialUniform)]
pub struct StandardMaterial {
pub base_color: Color,
#[texture(1)]
#[sampler(2)]
pub base_color_texture: Option<Handle<Image>>,
/* other fields omitted for brevity */
```
### Ported Bevy examples to the new `Material` system
The overall complexity of Bevy's "custom shader examples" has gone down significantly. Take a look at the diffs if you want a dopamine spike.
Please note that while this PR has a net increase in "lines of code", most of those extra lines come from added documentation. There is a significant reduction
in the overall complexity of the code (even accounting for the new derive logic).
---
## Changelog
### Added
* `AsBindGroup` trait and derive, which make it much easier to transfer data to the gpu and generate bind groups for a given type.
### Changed
* The old `Material` and `SpecializedMaterial` traits have been replaced by a consolidated (much simpler) `Material` trait. Materials no longer implement `RenderAsset`.
* `StandardMaterial` was ported to the new material system. There are no user-facing api changes to the `StandardMaterial` struct api, but it now implements `AsBindGroup` and `Material` instead of `RenderAsset` and `SpecializedMaterial`.
## Migration Guide
The Material system has been reworked to be much simpler. We've removed a lot of boilerplate with the new `AsBindGroup` derive and the `Material` trait is simpler as well!
### Bevy 0.7 (old)
```rust
#[derive(Debug, Clone, TypeUuid)]
#[uuid = "f690fdae-d598-45ab-8225-97e2a3f056e0"]
pub struct CustomMaterial {
color: Color,
color_texture: Handle<Image>,
}
#[derive(Clone)]
pub struct GpuCustomMaterial {
_buffer: Buffer,
bind_group: BindGroup,
}
impl RenderAsset for CustomMaterial {
type ExtractedAsset = CustomMaterial;
type PreparedAsset = GpuCustomMaterial;
type Param = (SRes<RenderDevice>, SRes<MaterialPipeline<Self>>);
fn extract_asset(&self) -> Self::ExtractedAsset {
self.clone()
}
fn prepare_asset(
extracted_asset: Self::ExtractedAsset,
(render_device, material_pipeline): &mut SystemParamItem<Self::Param>,
) -> Result<Self::PreparedAsset, PrepareAssetError<Self::ExtractedAsset>> {
let color = Vec4::from_slice(&extracted_asset.color.as_linear_rgba_f32());
let byte_buffer = [0u8; Vec4::SIZE.get() as usize];
let mut buffer = encase::UniformBuffer::new(byte_buffer);
buffer.write(&color).unwrap();
let buffer = render_device.create_buffer_with_data(&BufferInitDescriptor {
contents: buffer.as_ref(),
label: None,
usage: BufferUsages::UNIFORM | BufferUsages::COPY_DST,
});
let (texture_view, texture_sampler) = if let Some(result) = material_pipeline
.mesh_pipeline
.get_image_texture(gpu_images, &Some(extracted_asset.color_texture.clone()))
{
result
} else {
return Err(PrepareAssetError::RetryNextUpdate(extracted_asset));
};
let bind_group = render_device.create_bind_group(&BindGroupDescriptor {
entries: &[
BindGroupEntry {
binding: 0,
resource: buffer.as_entire_binding(),
},
BindGroupEntry {
binding: 0,
resource: BindingResource::TextureView(texture_view),
},
BindGroupEntry {
binding: 1,
resource: BindingResource::Sampler(texture_sampler),
},
],
label: None,
layout: &material_pipeline.material_layout,
});
Ok(GpuCustomMaterial {
_buffer: buffer,
bind_group,
})
}
}
impl Material for CustomMaterial {
fn fragment_shader(asset_server: &AssetServer) -> Option<Handle<Shader>> {
Some(asset_server.load("custom_material.wgsl"))
}
fn bind_group(render_asset: &<Self as RenderAsset>::PreparedAsset) -> &BindGroup {
&render_asset.bind_group
}
fn bind_group_layout(render_device: &RenderDevice) -> BindGroupLayout {
render_device.create_bind_group_layout(&BindGroupLayoutDescriptor {
entries: &[
BindGroupLayoutEntry {
binding: 0,
visibility: ShaderStages::FRAGMENT,
ty: BindingType::Buffer {
ty: BufferBindingType::Uniform,
has_dynamic_offset: false,
min_binding_size: Some(Vec4::min_size()),
},
count: None,
},
BindGroupLayoutEntry {
binding: 1,
visibility: ShaderStages::FRAGMENT,
ty: BindingType::Texture {
multisampled: false,
sample_type: TextureSampleType::Float { filterable: true },
view_dimension: TextureViewDimension::D2Array,
},
count: None,
},
BindGroupLayoutEntry {
binding: 2,
visibility: ShaderStages::FRAGMENT,
ty: BindingType::Sampler(SamplerBindingType::Filtering),
count: None,
},
],
label: None,
})
}
}
```
### Bevy 0.8 (new)
```rust
impl Material for CustomMaterial {
fn fragment_shader() -> ShaderRef {
"custom_material.wgsl".into()
}
}
#[derive(AsBindGroup, TypeUuid, Debug, Clone)]
#[uuid = "f690fdae-d598-45ab-8225-97e2a3f056e0"]
pub struct CustomMaterial {
#[uniform(0)]
color: Color,
#[texture(1)]
#[sampler(2)]
color_texture: Handle<Image>,
}
```
## Future Work
* Add support for more binding types (cubemaps, buffers, etc). This PR intentionally includes a bare minimum number of binding types to keep "reviewability" in check.
* Consider optionally eliding binding indices using binding names. `AsBindGroup` could pass in (optional?) reflection info as a "hint".
* This would make it possible for the derive to do this:
```rust
#[derive(AsBindGroup)]
pub struct CustomMaterial {
#[uniform]
color: Color,
#[texture]
#[sampler]
color_texture: Option<Handle<Image>>,
alpha_mode: AlphaMode,
}
```
* Or this
```rust
#[derive(AsBindGroup)]
pub struct CustomMaterial {
#[binding]
color: Color,
#[binding]
color_texture: Option<Handle<Image>>,
alpha_mode: AlphaMode,
}
```
* Or even this (if we flip to "include bindings by default")
```rust
#[derive(AsBindGroup)]
pub struct CustomMaterial {
color: Color,
color_texture: Option<Handle<Image>>,
#[binding(ignore)]
alpha_mode: AlphaMode,
}
```
* If we add the option to define custom draw functions for materials (which could be done in a type-erased way), I think that would be enough to support extra non-material bindings. Worth considering!
2022-06-30 23:48:46 +00:00
|
|
|
/// ```
|
Migrate meshes and materials to required components (#15524)
# Objective
A big step in the migration to required components: meshes and
materials!
## Solution
As per the [selected
proposal](https://hackmd.io/@bevy/required_components/%2Fj9-PnF-2QKK0on1KQ29UWQ):
- Deprecate `MaterialMesh2dBundle`, `MaterialMeshBundle`, and
`PbrBundle`.
- Add `Mesh2d` and `Mesh3d` components, which wrap a `Handle<Mesh>`.
- Add `MeshMaterial2d<M: Material2d>` and `MeshMaterial3d<M: Material>`,
which wrap a `Handle<M>`.
- Meshes *without* a mesh material should be rendered with a default
material. The existence of a material is determined by
`HasMaterial2d`/`HasMaterial3d`, which is required by
`MeshMaterial2d`/`MeshMaterial3d`. This gets around problems with the
generics.
Previously:
```rust
commands.spawn(MaterialMesh2dBundle {
mesh: meshes.add(Circle::new(100.0)).into(),
material: materials.add(Color::srgb(7.5, 0.0, 7.5)),
transform: Transform::from_translation(Vec3::new(-200., 0., 0.)),
..default()
});
```
Now:
```rust
commands.spawn((
Mesh2d(meshes.add(Circle::new(100.0))),
MeshMaterial2d(materials.add(Color::srgb(7.5, 0.0, 7.5))),
Transform::from_translation(Vec3::new(-200., 0., 0.)),
));
```
If the mesh material is missing, previously nothing was rendered. Now,
it renders a white default `ColorMaterial` in 2D and a
`StandardMaterial` in 3D (this can be overridden). Below, only every
other entity has a material:


Why white? This is still open for discussion, but I think white makes
sense for a *default* material, while *invalid* asset handles pointing
to nothing should have something like a pink material to indicate that
something is broken (I don't handle that in this PR yet). This is kind
of a mix of Godot and Unity: Godot just renders a white material for
non-existent materials, while Unity renders nothing when no materials
exist, but renders pink for invalid materials. I can also change the
default material to pink if that is preferable though.
## Testing
I ran some 2D and 3D examples to test if anything changed visually. I
have not tested all examples or features yet however. If anyone wants to
test more extensively, it would be appreciated!
## Implementation Notes
- The relationship between `bevy_render` and `bevy_pbr` is weird here.
`bevy_render` needs `Mesh3d` for its own systems, but `bevy_pbr` has all
of the material logic, and `bevy_render` doesn't depend on it. I feel
like the two crates should be refactored in some way, but I think that's
out of scope for this PR.
- I didn't migrate meshlets to required components yet. That can
probably be done in a follow-up, as this is already a huge PR.
- It is becoming increasingly clear to me that we really, *really* want
to disallow raw asset handles as components. They caused me a *ton* of
headache here already, and it took me a long time to find every place
that queried for them or inserted them directly on entities, since there
were no compiler errors for it. If we don't remove the `Component`
derive, I expect raw asset handles to be a *huge* footgun for users as
we transition to wrapper components, especially as handles as components
have been the norm so far. I personally consider this to be a blocker
for 0.15: we need to migrate to wrapper components for asset handles
everywhere, and remove the `Component` derive. Also see
https://github.com/bevyengine/bevy/issues/14124.
---
## Migration Guide
Asset handles for meshes and mesh materials must now be wrapped in the
`Mesh2d` and `MeshMaterial2d` or `Mesh3d` and `MeshMaterial3d`
components for 2D and 3D respectively. Raw handles as components no
longer render meshes.
Additionally, `MaterialMesh2dBundle`, `MaterialMeshBundle`, and
`PbrBundle` have been deprecated. Instead, use the mesh and material
components directly.
Previously:
```rust
commands.spawn(MaterialMesh2dBundle {
mesh: meshes.add(Circle::new(100.0)).into(),
material: materials.add(Color::srgb(7.5, 0.0, 7.5)),
transform: Transform::from_translation(Vec3::new(-200., 0., 0.)),
..default()
});
```
Now:
```rust
commands.spawn((
Mesh2d(meshes.add(Circle::new(100.0))),
MeshMaterial2d(materials.add(Color::srgb(7.5, 0.0, 7.5))),
Transform::from_translation(Vec3::new(-200., 0., 0.)),
));
```
If the mesh material is missing, a white default material is now used.
Previously, nothing was rendered if the material was missing.
The `WithMesh2d` and `WithMesh3d` query filter type aliases have also
been removed. Simply use `With<Mesh2d>` or `With<Mesh3d>`.
---------
Co-authored-by: Tim Blackbird <justthecooldude@gmail.com>
Co-authored-by: Carter Anderson <mcanders1@gmail.com>
2024-10-01 21:33:17 +00:00
|
|
|
/// # use bevy_pbr::{Material, MeshMaterial3d};
|
Better Materials: AsBindGroup trait and derive, simpler Material trait (#5053)
# Objective
This PR reworks Bevy's Material system, making the user experience of defining Materials _much_ nicer. Bevy's previous material system leaves a lot to be desired:
* Materials require manually implementing the `RenderAsset` trait, which involves manually generating the bind group, handling gpu buffer data transfer, looking up image textures, etc. Even the simplest single-texture material involves writing ~80 unnecessary lines of code. This was never the long term plan.
* There are two material traits, which is confusing, hard to document, and often redundant: `Material` and `SpecializedMaterial`. `Material` implicitly implements `SpecializedMaterial`, and `SpecializedMaterial` is used in most high level apis to support both use cases. Most users shouldn't need to think about specialization at all (I consider it a "power-user tool"), so the fact that `SpecializedMaterial` is front-and-center in our apis is a miss.
* Implementing either material trait involves a lot of "type soup". The "prepared asset" parameter is particularly heinous: `&<Self as RenderAsset>::PreparedAsset`. Defining vertex and fragment shaders is also more verbose than it needs to be.
## Solution
Say hello to the new `Material` system:
```rust
#[derive(AsBindGroup, TypeUuid, Debug, Clone)]
#[uuid = "f690fdae-d598-45ab-8225-97e2a3f056e0"]
pub struct CoolMaterial {
#[uniform(0)]
color: Color,
#[texture(1)]
#[sampler(2)]
color_texture: Handle<Image>,
}
impl Material for CoolMaterial {
fn fragment_shader() -> ShaderRef {
"cool_material.wgsl".into()
}
}
```
Thats it! This same material would have required [~80 lines of complicated "type heavy" code](https://github.com/bevyengine/bevy/blob/v0.7.0/examples/shader/shader_material.rs) in the old Material system. Now it is just 14 lines of simple, readable code.
This is thanks to a new consolidated `Material` trait and the new `AsBindGroup` trait / derive.
### The new `Material` trait
The old "split" `Material` and `SpecializedMaterial` traits have been removed in favor of a new consolidated `Material` trait. All of the functions on the trait are optional.
The difficulty of implementing `Material` has been reduced by simplifying dataflow and removing type complexity:
```rust
// Old
impl Material for CustomMaterial {
fn fragment_shader(asset_server: &AssetServer) -> Option<Handle<Shader>> {
Some(asset_server.load("custom_material.wgsl"))
}
fn alpha_mode(render_asset: &<Self as RenderAsset>::PreparedAsset) -> AlphaMode {
render_asset.alpha_mode
}
}
// New
impl Material for CustomMaterial {
fn fragment_shader() -> ShaderRef {
"custom_material.wgsl".into()
}
fn alpha_mode(&self) -> AlphaMode {
self.alpha_mode
}
}
```
Specialization is still supported, but it is hidden by default under the `specialize()` function (more on this later).
### The `AsBindGroup` trait / derive
The `Material` trait now requires the `AsBindGroup` derive. This can be implemented manually relatively easily, but deriving it will almost always be preferable.
Field attributes like `uniform` and `texture` are used to define which fields should be bindings,
what their binding type is, and what index they should be bound at:
```rust
#[derive(AsBindGroup)]
struct CoolMaterial {
#[uniform(0)]
color: Color,
#[texture(1)]
#[sampler(2)]
color_texture: Handle<Image>,
}
```
In WGSL shaders, the binding looks like this:
```wgsl
struct CoolMaterial {
color: vec4<f32>;
};
[[group(1), binding(0)]]
var<uniform> material: CoolMaterial;
[[group(1), binding(1)]]
var color_texture: texture_2d<f32>;
[[group(1), binding(2)]]
var color_sampler: sampler;
```
Note that the "group" index is determined by the usage context. It is not defined in `AsBindGroup`. Bevy material bind groups are bound to group 1.
The following field-level attributes are supported:
* `uniform(BINDING_INDEX)`
* The field will be converted to a shader-compatible type using the `ShaderType` trait, written to a `Buffer`, and bound as a uniform. It can also be derived for custom structs.
* `texture(BINDING_INDEX)`
* This field's `Handle<Image>` will be used to look up the matching `Texture` gpu resource, which will be bound as a texture in shaders. The field will be assumed to implement `Into<Option<Handle<Image>>>`. In practice, most fields should be a `Handle<Image>` or `Option<Handle<Image>>`. If the value of an `Option<Handle<Image>>` is `None`, the new `FallbackImage` resource will be used instead. This attribute can be used in conjunction with a `sampler` binding attribute (with a different binding index).
* `sampler(BINDING_INDEX)`
* Behaves exactly like the `texture` attribute, but sets the Image's sampler binding instead of the texture.
Note that fields without field-level binding attributes will be ignored.
```rust
#[derive(AsBindGroup)]
struct CoolMaterial {
#[uniform(0)]
color: Color,
this_field_is_ignored: String,
}
```
As mentioned above, `Option<Handle<Image>>` is also supported:
```rust
#[derive(AsBindGroup)]
struct CoolMaterial {
#[uniform(0)]
color: Color,
#[texture(1)]
#[sampler(2)]
color_texture: Option<Handle<Image>>,
}
```
This is useful if you want a texture to be optional. When the value is `None`, the `FallbackImage` will be used for the binding instead, which defaults to "pure white".
Field uniforms with the same binding index will be combined into a single binding:
```rust
#[derive(AsBindGroup)]
struct CoolMaterial {
#[uniform(0)]
color: Color,
#[uniform(0)]
roughness: f32,
}
```
In WGSL shaders, the binding would look like this:
```wgsl
struct CoolMaterial {
color: vec4<f32>;
roughness: f32;
};
[[group(1), binding(0)]]
var<uniform> material: CoolMaterial;
```
Some less common scenarios will require "struct-level" attributes. These are the currently supported struct-level attributes:
* `uniform(BINDING_INDEX, ConvertedShaderType)`
* Similar to the field-level `uniform` attribute, but instead the entire `AsBindGroup` value is converted to `ConvertedShaderType`, which must implement `ShaderType`. This is useful if more complicated conversion logic is required.
* `bind_group_data(DataType)`
* The `AsBindGroup` type will be converted to some `DataType` using `Into<DataType>` and stored as `AsBindGroup::Data` as part of the `AsBindGroup::as_bind_group` call. This is useful if data needs to be stored alongside the generated bind group, such as a unique identifier for a material's bind group. The most common use case for this attribute is "shader pipeline specialization".
The previous `CoolMaterial` example illustrating "combining multiple field-level uniform attributes with the same binding index" can
also be equivalently represented with a single struct-level uniform attribute:
```rust
#[derive(AsBindGroup)]
#[uniform(0, CoolMaterialUniform)]
struct CoolMaterial {
color: Color,
roughness: f32,
}
#[derive(ShaderType)]
struct CoolMaterialUniform {
color: Color,
roughness: f32,
}
impl From<&CoolMaterial> for CoolMaterialUniform {
fn from(material: &CoolMaterial) -> CoolMaterialUniform {
CoolMaterialUniform {
color: material.color,
roughness: material.roughness,
}
}
}
```
### Material Specialization
Material shader specialization is now _much_ simpler:
```rust
#[derive(AsBindGroup, TypeUuid, Debug, Clone)]
#[uuid = "f690fdae-d598-45ab-8225-97e2a3f056e0"]
#[bind_group_data(CoolMaterialKey)]
struct CoolMaterial {
#[uniform(0)]
color: Color,
is_red: bool,
}
#[derive(Copy, Clone, Hash, Eq, PartialEq)]
struct CoolMaterialKey {
is_red: bool,
}
impl From<&CoolMaterial> for CoolMaterialKey {
fn from(material: &CoolMaterial) -> CoolMaterialKey {
CoolMaterialKey {
is_red: material.is_red,
}
}
}
impl Material for CoolMaterial {
fn fragment_shader() -> ShaderRef {
"cool_material.wgsl".into()
}
fn specialize(
pipeline: &MaterialPipeline<Self>,
descriptor: &mut RenderPipelineDescriptor,
layout: &MeshVertexBufferLayout,
key: MaterialPipelineKey<Self>,
) -> Result<(), SpecializedMeshPipelineError> {
if key.bind_group_data.is_red {
let fragment = descriptor.fragment.as_mut().unwrap();
fragment.shader_defs.push("IS_RED".to_string());
}
Ok(())
}
}
```
Setting `bind_group_data` is not required for specialization (it defaults to `()`). Scenarios like "custom vertex attributes" also benefit from this system:
```rust
impl Material for CustomMaterial {
fn vertex_shader() -> ShaderRef {
"custom_material.wgsl".into()
}
fn fragment_shader() -> ShaderRef {
"custom_material.wgsl".into()
}
fn specialize(
pipeline: &MaterialPipeline<Self>,
descriptor: &mut RenderPipelineDescriptor,
layout: &MeshVertexBufferLayout,
key: MaterialPipelineKey<Self>,
) -> Result<(), SpecializedMeshPipelineError> {
let vertex_layout = layout.get_layout(&[
Mesh::ATTRIBUTE_POSITION.at_shader_location(0),
ATTRIBUTE_BLEND_COLOR.at_shader_location(1),
])?;
descriptor.vertex.buffers = vec![vertex_layout];
Ok(())
}
}
```
### Ported `StandardMaterial` to the new `Material` system
Bevy's built-in PBR material uses the new Material system (including the AsBindGroup derive):
```rust
#[derive(AsBindGroup, Debug, Clone, TypeUuid)]
#[uuid = "7494888b-c082-457b-aacf-517228cc0c22"]
#[bind_group_data(StandardMaterialKey)]
#[uniform(0, StandardMaterialUniform)]
pub struct StandardMaterial {
pub base_color: Color,
#[texture(1)]
#[sampler(2)]
pub base_color_texture: Option<Handle<Image>>,
/* other fields omitted for brevity */
```
### Ported Bevy examples to the new `Material` system
The overall complexity of Bevy's "custom shader examples" has gone down significantly. Take a look at the diffs if you want a dopamine spike.
Please note that while this PR has a net increase in "lines of code", most of those extra lines come from added documentation. There is a significant reduction
in the overall complexity of the code (even accounting for the new derive logic).
---
## Changelog
### Added
* `AsBindGroup` trait and derive, which make it much easier to transfer data to the gpu and generate bind groups for a given type.
### Changed
* The old `Material` and `SpecializedMaterial` traits have been replaced by a consolidated (much simpler) `Material` trait. Materials no longer implement `RenderAsset`.
* `StandardMaterial` was ported to the new material system. There are no user-facing api changes to the `StandardMaterial` struct api, but it now implements `AsBindGroup` and `Material` instead of `RenderAsset` and `SpecializedMaterial`.
## Migration Guide
The Material system has been reworked to be much simpler. We've removed a lot of boilerplate with the new `AsBindGroup` derive and the `Material` trait is simpler as well!
### Bevy 0.7 (old)
```rust
#[derive(Debug, Clone, TypeUuid)]
#[uuid = "f690fdae-d598-45ab-8225-97e2a3f056e0"]
pub struct CustomMaterial {
color: Color,
color_texture: Handle<Image>,
}
#[derive(Clone)]
pub struct GpuCustomMaterial {
_buffer: Buffer,
bind_group: BindGroup,
}
impl RenderAsset for CustomMaterial {
type ExtractedAsset = CustomMaterial;
type PreparedAsset = GpuCustomMaterial;
type Param = (SRes<RenderDevice>, SRes<MaterialPipeline<Self>>);
fn extract_asset(&self) -> Self::ExtractedAsset {
self.clone()
}
fn prepare_asset(
extracted_asset: Self::ExtractedAsset,
(render_device, material_pipeline): &mut SystemParamItem<Self::Param>,
) -> Result<Self::PreparedAsset, PrepareAssetError<Self::ExtractedAsset>> {
let color = Vec4::from_slice(&extracted_asset.color.as_linear_rgba_f32());
let byte_buffer = [0u8; Vec4::SIZE.get() as usize];
let mut buffer = encase::UniformBuffer::new(byte_buffer);
buffer.write(&color).unwrap();
let buffer = render_device.create_buffer_with_data(&BufferInitDescriptor {
contents: buffer.as_ref(),
label: None,
usage: BufferUsages::UNIFORM | BufferUsages::COPY_DST,
});
let (texture_view, texture_sampler) = if let Some(result) = material_pipeline
.mesh_pipeline
.get_image_texture(gpu_images, &Some(extracted_asset.color_texture.clone()))
{
result
} else {
return Err(PrepareAssetError::RetryNextUpdate(extracted_asset));
};
let bind_group = render_device.create_bind_group(&BindGroupDescriptor {
entries: &[
BindGroupEntry {
binding: 0,
resource: buffer.as_entire_binding(),
},
BindGroupEntry {
binding: 0,
resource: BindingResource::TextureView(texture_view),
},
BindGroupEntry {
binding: 1,
resource: BindingResource::Sampler(texture_sampler),
},
],
label: None,
layout: &material_pipeline.material_layout,
});
Ok(GpuCustomMaterial {
_buffer: buffer,
bind_group,
})
}
}
impl Material for CustomMaterial {
fn fragment_shader(asset_server: &AssetServer) -> Option<Handle<Shader>> {
Some(asset_server.load("custom_material.wgsl"))
}
fn bind_group(render_asset: &<Self as RenderAsset>::PreparedAsset) -> &BindGroup {
&render_asset.bind_group
}
fn bind_group_layout(render_device: &RenderDevice) -> BindGroupLayout {
render_device.create_bind_group_layout(&BindGroupLayoutDescriptor {
entries: &[
BindGroupLayoutEntry {
binding: 0,
visibility: ShaderStages::FRAGMENT,
ty: BindingType::Buffer {
ty: BufferBindingType::Uniform,
has_dynamic_offset: false,
min_binding_size: Some(Vec4::min_size()),
},
count: None,
},
BindGroupLayoutEntry {
binding: 1,
visibility: ShaderStages::FRAGMENT,
ty: BindingType::Texture {
multisampled: false,
sample_type: TextureSampleType::Float { filterable: true },
view_dimension: TextureViewDimension::D2Array,
},
count: None,
},
BindGroupLayoutEntry {
binding: 2,
visibility: ShaderStages::FRAGMENT,
ty: BindingType::Sampler(SamplerBindingType::Filtering),
count: None,
},
],
label: None,
})
}
}
```
### Bevy 0.8 (new)
```rust
impl Material for CustomMaterial {
fn fragment_shader() -> ShaderRef {
"custom_material.wgsl".into()
}
}
#[derive(AsBindGroup, TypeUuid, Debug, Clone)]
#[uuid = "f690fdae-d598-45ab-8225-97e2a3f056e0"]
pub struct CustomMaterial {
#[uniform(0)]
color: Color,
#[texture(1)]
#[sampler(2)]
color_texture: Handle<Image>,
}
```
## Future Work
* Add support for more binding types (cubemaps, buffers, etc). This PR intentionally includes a bare minimum number of binding types to keep "reviewability" in check.
* Consider optionally eliding binding indices using binding names. `AsBindGroup` could pass in (optional?) reflection info as a "hint".
* This would make it possible for the derive to do this:
```rust
#[derive(AsBindGroup)]
pub struct CustomMaterial {
#[uniform]
color: Color,
#[texture]
#[sampler]
color_texture: Option<Handle<Image>>,
alpha_mode: AlphaMode,
}
```
* Or this
```rust
#[derive(AsBindGroup)]
pub struct CustomMaterial {
#[binding]
color: Color,
#[binding]
color_texture: Option<Handle<Image>>,
alpha_mode: AlphaMode,
}
```
* Or even this (if we flip to "include bindings by default")
```rust
#[derive(AsBindGroup)]
pub struct CustomMaterial {
color: Color,
color_texture: Option<Handle<Image>>,
#[binding(ignore)]
alpha_mode: AlphaMode,
}
```
* If we add the option to define custom draw functions for materials (which could be done in a type-erased way), I think that would be enough to support extra non-material bindings. Worth considering!
2022-06-30 23:48:46 +00:00
|
|
|
/// # use bevy_ecs::prelude::*;
|
2024-11-10 06:54:38 +00:00
|
|
|
/// # use bevy_image::Image;
|
2024-01-25 16:16:58 +00:00
|
|
|
/// # use bevy_reflect::TypePath;
|
2024-11-10 06:54:38 +00:00
|
|
|
/// # use bevy_render::{mesh::{Mesh, Mesh3d}, render_resource::{AsBindGroup, ShaderRef}};
|
Migrate from `LegacyColor` to `bevy_color::Color` (#12163)
# Objective
- As part of the migration process we need to a) see the end effect of
the migration on user ergonomics b) check for serious perf regressions
c) actually migrate the code
- To accomplish this, I'm going to attempt to migrate all of the
remaining user-facing usages of `LegacyColor` in one PR, being careful
to keep a clean commit history.
- Fixes #12056.
## Solution
I've chosen to use the polymorphic `Color` type as our standard
user-facing API.
- [x] Migrate `bevy_gizmos`.
- [x] Take `impl Into<Color>` in all `bevy_gizmos` APIs
- [x] Migrate sprites
- [x] Migrate UI
- [x] Migrate `ColorMaterial`
- [x] Migrate `MaterialMesh2D`
- [x] Migrate fog
- [x] Migrate lights
- [x] Migrate StandardMaterial
- [x] Migrate wireframes
- [x] Migrate clear color
- [x] Migrate text
- [x] Migrate gltf loader
- [x] Register color types for reflection
- [x] Remove `LegacyColor`
- [x] Make sure CI passes
Incidental improvements to ease migration:
- added `Color::srgba_u8`, `Color::srgba_from_array` and friends
- added `set_alpha`, `is_fully_transparent` and `is_fully_opaque` to the
`Alpha` trait
- add and immediately deprecate (lol) `Color::rgb` and friends in favor
of more explicit and consistent `Color::srgb`
- standardized on white and black for most example text colors
- added vector field traits to `LinearRgba`: ~~`Add`, `Sub`,
`AddAssign`, `SubAssign`,~~ `Mul<f32>` and `Div<f32>`. Multiplications
and divisions do not scale alpha. `Add` and `Sub` have been cut from
this PR.
- added `LinearRgba` and `Srgba` `RED/GREEN/BLUE`
- added `LinearRgba_to_f32_array` and `LinearRgba::to_u32`
## Migration Guide
Bevy's color types have changed! Wherever you used a
`bevy::render::Color`, a `bevy::color::Color` is used instead.
These are quite similar! Both are enums storing a color in a specific
color space (or to be more precise, using a specific color model).
However, each of the different color models now has its own type.
TODO...
- `Color::rgba`, `Color::rgb`, `Color::rbga_u8`, `Color::rgb_u8`,
`Color::rgb_from_array` are now `Color::srgba`, `Color::srgb`,
`Color::srgba_u8`, `Color::srgb_u8` and `Color::srgb_from_array`.
- `Color::set_a` and `Color::a` is now `Color::set_alpha` and
`Color::alpha`. These are part of the `Alpha` trait in `bevy_color`.
- `Color::is_fully_transparent` is now part of the `Alpha` trait in
`bevy_color`
- `Color::r`, `Color::set_r`, `Color::with_r` and the equivalents for
`g`, `b` `h`, `s` and `l` have been removed due to causing silent
relatively expensive conversions. Convert your `Color` into the desired
color space, perform your operations there, and then convert it back
into a polymorphic `Color` enum.
- `Color::hex` is now `Srgba::hex`. Call `.into` or construct a
`Color::Srgba` variant manually to convert it.
- `WireframeMaterial`, `ExtractedUiNode`, `ExtractedDirectionalLight`,
`ExtractedPointLight`, `ExtractedSpotLight` and `ExtractedSprite` now
store a `LinearRgba`, rather than a polymorphic `Color`
- `Color::rgb_linear` and `Color::rgba_linear` are now
`Color::linear_rgb` and `Color::linear_rgba`
- The various CSS color constants are no longer stored directly on
`Color`. Instead, they're defined in the `Srgba` color space, and
accessed via `bevy::color::palettes::css`. Call `.into()` on them to
convert them into a `Color` for quick debugging use, and consider using
the much prettier `tailwind` palette for prototyping.
- The `LIME_GREEN` color has been renamed to `LIMEGREEN` to comply with
the standard naming.
- Vector field arithmetic operations on `Color` (add, subtract, multiply
and divide by a f32) have been removed. Instead, convert your colors
into `LinearRgba` space, and perform your operations explicitly there.
This is particularly relevant when working with emissive or HDR colors,
whose color channel values are routinely outside of the ordinary 0 to 1
range.
- `Color::as_linear_rgba_f32` has been removed. Call
`LinearRgba::to_f32_array` instead, converting if needed.
- `Color::as_linear_rgba_u32` has been removed. Call
`LinearRgba::to_u32` instead, converting if needed.
- Several other color conversion methods to transform LCH or HSL colors
into float arrays or `Vec` types have been removed. Please reimplement
these externally or open a PR to re-add them if you found them
particularly useful.
- Various methods on `Color` such as `rgb` or `hsl` to convert the color
into a specific color space have been removed. Convert into
`LinearRgba`, then to the color space of your choice.
- Various implicitly-converting color value methods on `Color` such as
`r`, `g`, `b` or `h` have been removed. Please convert it into the color
space of your choice, then check these properties.
- `Color` no longer implements `AsBindGroup`. Store a `LinearRgba`
internally instead to avoid conversion costs.
---------
Co-authored-by: Alice Cecile <alice.i.cecil@gmail.com>
Co-authored-by: Afonso Lage <lage.afonso@gmail.com>
Co-authored-by: Rob Parrett <robparrett@gmail.com>
Co-authored-by: Zachary Harrold <zac@harrold.com.au>
2024-02-29 19:35:12 +00:00
|
|
|
/// # use bevy_color::LinearRgba;
|
|
|
|
/// # use bevy_color::palettes::basic::RED;
|
Bevy Asset V2 (#8624)
# Bevy Asset V2 Proposal
## Why Does Bevy Need A New Asset System?
Asset pipelines are a central part of the gamedev process. Bevy's
current asset system is missing a number of features that make it
non-viable for many classes of gamedev. After plenty of discussions and
[a long community feedback
period](https://github.com/bevyengine/bevy/discussions/3972), we've
identified a number missing features:
* **Asset Preprocessing**: it should be possible to "preprocess" /
"compile" / "crunch" assets at "development time" rather than when the
game starts up. This enables offloading expensive work from deployed
apps, faster asset loading, less runtime memory usage, etc.
* **Per-Asset Loader Settings**: Individual assets cannot define their
own loaders that override the defaults. Additionally, they cannot
provide per-asset settings to their loaders. This is a huge limitation,
as many asset types don't provide all information necessary for Bevy
_inside_ the asset. For example, a raw PNG image says nothing about how
it should be sampled (ex: linear vs nearest).
* **Asset `.meta` files**: assets should have configuration files stored
adjacent to the asset in question, which allows the user to configure
asset-type-specific settings. These settings should be accessible during
the pre-processing phase. Modifying a `.meta` file should trigger a
re-processing / re-load of the asset. It should be possible to configure
asset loaders from the meta file.
* **Processed Asset Hot Reloading**: Changes to processed assets (or
their dependencies) should result in re-processing them and re-loading
the results in live Bevy Apps.
* **Asset Dependency Tracking**: The current bevy_asset has no good way
to wait for asset dependencies to load. It punts this as an exercise for
consumers of the loader apis, which is unreasonable and error prone.
There should be easy, ergonomic ways to wait for assets to load and
block some logic on an asset's entire dependency tree loading.
* **Runtime Asset Loading**: it should be (optionally) possible to load
arbitrary assets dynamically at runtime. This necessitates being able to
deploy and run the asset server alongside Bevy Apps on _all platforms_.
For example, we should be able to invoke the shader compiler at runtime,
stream scenes from sources like the internet, etc. To keep deployed
binaries (and startup times) small, the runtime asset server
configuration should be configurable with different settings compared to
the "pre processor asset server".
* **Multiple Backends**: It should be possible to load assets from
arbitrary sources (filesystems, the internet, remote asset serves, etc).
* **Asset Packing**: It should be possible to deploy assets in
compressed "packs", which makes it easier and more efficient to
distribute assets with Bevy Apps.
* **Asset Handoff**: It should be possible to hold a "live" asset
handle, which correlates to runtime data, without actually holding the
asset in memory. Ex: it must be possible to hold a reference to a GPU
mesh generated from a "mesh asset" without keeping the mesh data in CPU
memory
* **Per-Platform Processed Assets**: Different platforms and app
distributions have different capabilities and requirements. Some
platforms need lower asset resolutions or different asset formats to
operate within the hardware constraints of the platform. It should be
possible to define per-platform asset processing profiles. And it should
be possible to deploy only the assets required for a given platform.
These features have architectural implications that are significant
enough to require a full rewrite. The current Bevy Asset implementation
got us this far, but it can take us no farther. This PR defines a brand
new asset system that implements most of these features, while laying
the foundations for the remaining features to be built.
## Bevy Asset V2
Here is a quick overview of the features introduced in this PR.
* **Asset Preprocessing**: Preprocess assets at development time into
more efficient (and configurable) representations
* **Dependency Aware**: Dependencies required to process an asset are
tracked. If an asset's processed dependency changes, it will be
reprocessed
* **Hot Reprocessing/Reloading**: detect changes to asset source files,
reprocess them if they have changed, and then hot-reload them in Bevy
Apps.
* **Only Process Changes**: Assets are only re-processed when their
source file (or meta file) has changed. This uses hashing and timestamps
to avoid processing assets that haven't changed.
* **Transactional and Reliable**: Uses write-ahead logging (a technique
commonly used by databases) to recover from crashes / forced-exits.
Whenever possible it avoids full-reprocessing / only uncompleted
transactions will be reprocessed. When the processor is running in
parallel with a Bevy App, processor asset writes block Bevy App asset
reads. Reading metadata + asset bytes is guaranteed to be transactional
/ correctly paired.
* **Portable / Run anywhere / Database-free**: The processor does not
rely on an in-memory database (although it uses some database techniques
for reliability). This is important because pretty much all in-memory
databases have unsupported platforms or build complications.
* **Configure Processor Defaults Per File Type**: You can say "use this
processor for all files of this type".
* **Custom Processors**: The `Processor` trait is flexible and
unopinionated. It can be implemented by downstream plugins.
* **LoadAndSave Processors**: Most asset processing scenarios can be
expressed as "run AssetLoader A, save the results using AssetSaver X,
and then load the result using AssetLoader B". For example, load this
png image using `PngImageLoader`, which produces an `Image` asset and
then save it using `CompressedImageSaver` (which also produces an
`Image` asset, but in a compressed format), which takes an `Image` asset
as input. This means if you have an `AssetLoader` for an asset, you are
already half way there! It also means that you can share AssetSavers
across multiple loaders. Because `CompressedImageSaver` accepts Bevy's
generic Image asset as input, it means you can also use it with some
future `JpegImageLoader`.
* **Loader and Saver Settings**: Asset Loaders and Savers can now define
their own settings types, which are passed in as input when an asset is
loaded / saved. Each asset can define its own settings.
* **Asset `.meta` files**: configure asset loaders, their settings,
enable/disable processing, and configure processor settings
* **Runtime Asset Dependency Tracking** Runtime asset dependencies (ex:
if an asset contains a `Handle<Image>`) are tracked by the asset server.
An event is emitted when an asset and all of its dependencies have been
loaded
* **Unprocessed Asset Loading**: Assets do not require preprocessing.
They can be loaded directly. A processed asset is just a "normal" asset
with some extra metadata. Asset Loaders don't need to know or care about
whether or not an asset was processed.
* **Async Asset IO**: Asset readers/writers use async non-blocking
interfaces. Note that because Rust doesn't yet support async traits,
there is a bit of manual Boxing / Future boilerplate. This will
hopefully be removed in the near future when Rust gets async traits.
* **Pluggable Asset Readers and Writers**: Arbitrary asset source
readers/writers are supported, both by the processor and the asset
server.
* **Better Asset Handles**
* **Single Arc Tree**: Asset Handles now use a single arc tree that
represents the lifetime of the asset. This makes their implementation
simpler, more efficient, and allows us to cheaply attach metadata to
handles. Ex: the AssetPath of a handle is now directly accessible on the
handle itself!
* **Const Typed Handles**: typed handles can be constructed in a const
context. No more weird "const untyped converted to typed at runtime"
patterns!
* **Handles and Ids are Smaller / Faster To Hash / Compare**: Typed
`Handle<T>` is now much smaller in memory and `AssetId<T>` is even
smaller.
* **Weak Handle Usage Reduction**: In general Handles are now considered
to be "strong". Bevy features that previously used "weak `Handle<T>`"
have been ported to `AssetId<T>`, which makes it statically clear that
the features do not hold strong handles (while retaining strong type
information). Currently Handle::Weak still exists, but it is very
possible that we can remove that entirely.
* **Efficient / Dense Asset Ids**: Assets now have efficient dense
runtime asset ids, which means we can avoid expensive hash lookups.
Assets are stored in Vecs instead of HashMaps. There are now typed and
untyped ids, which means we no longer need to store dynamic type
information in the ID for typed handles. "AssetPathId" (which was a
nightmare from a performance and correctness standpoint) has been
entirely removed in favor of dense ids (which are retrieved for a path
on load)
* **Direct Asset Loading, with Dependency Tracking**: Assets that are
defined at runtime can still have their dependencies tracked by the
Asset Server (ex: if you create a material at runtime, you can still
wait for its textures to load). This is accomplished via the (currently
optional) "asset dependency visitor" trait. This system can also be used
to define a set of assets to load, then wait for those assets to load.
* **Async folder loading**: Folder loading also uses this system and
immediately returns a handle to the LoadedFolder asset, which means
folder loading no longer blocks on directory traversals.
* **Improved Loader Interface**: Loaders now have a specific "top level
asset type", which makes returning the top-level asset simpler and
statically typed.
* **Basic Image Settings and Processing**: Image assets can now be
processed into the gpu-friendly Basic Universal format. The ImageLoader
now has a setting to define what format the image should be loaded as.
Note that this is just a minimal MVP ... plenty of additional work to do
here. To demo this, enable the `basis-universal` feature and turn on
asset processing.
* **Simpler Audio Play / AudioSink API**: Asset handle providers are
cloneable, which means the Audio resource can mint its own handles. This
means you can now do `let sink_handle = audio.play(music)` instead of
`let sink_handle = audio_sinks.get_handle(audio.play(music))`. Note that
this might still be replaced by
https://github.com/bevyengine/bevy/pull/8424.
**Removed Handle Casting From Engine Features**: Ex: FontAtlases no
longer use casting between handle types
## Using The New Asset System
### Normal Unprocessed Asset Loading
By default the `AssetPlugin` does not use processing. It behaves pretty
much the same way as the old system.
If you are defining a custom asset, first derive `Asset`:
```rust
#[derive(Asset)]
struct Thing {
value: String,
}
```
Initialize the asset:
```rust
app.init_asset:<Thing>()
```
Implement a new `AssetLoader` for it:
```rust
#[derive(Default)]
struct ThingLoader;
#[derive(Serialize, Deserialize, Default)]
pub struct ThingSettings {
some_setting: bool,
}
impl AssetLoader for ThingLoader {
type Asset = Thing;
type Settings = ThingSettings;
fn load<'a>(
&'a self,
reader: &'a mut Reader,
settings: &'a ThingSettings,
load_context: &'a mut LoadContext,
) -> BoxedFuture<'a, Result<Thing, anyhow::Error>> {
Box::pin(async move {
let mut bytes = Vec::new();
reader.read_to_end(&mut bytes).await?;
// convert bytes to value somehow
Ok(Thing {
value
})
})
}
fn extensions(&self) -> &[&str] {
&["thing"]
}
}
```
Note that this interface will get much cleaner once Rust gets support
for async traits. `Reader` is an async futures_io::AsyncRead. You can
stream bytes as they come in or read them all into a `Vec<u8>`,
depending on the context. You can use `let handle =
load_context.load(path)` to kick off a dependency load, retrieve a
handle, and register the dependency for the asset.
Then just register the loader in your Bevy app:
```rust
app.init_asset_loader::<ThingLoader>()
```
Now just add your `Thing` asset files into the `assets` folder and load
them like this:
```rust
fn system(asset_server: Res<AssetServer>) {
let handle = Handle<Thing> = asset_server.load("cool.thing");
}
```
You can check load states directly via the asset server:
```rust
if asset_server.load_state(&handle) == LoadState::Loaded { }
```
You can also listen for events:
```rust
fn system(mut events: EventReader<AssetEvent<Thing>>, handle: Res<SomeThingHandle>) {
for event in events.iter() {
if event.is_loaded_with_dependencies(&handle) {
}
}
}
```
Note the new `AssetEvent::LoadedWithDependencies`, which only fires when
the asset is loaded _and_ all dependencies (and their dependencies) have
loaded.
Unlike the old asset system, for a given asset path all `Handle<T>`
values point to the same underlying Arc. This means Handles can cheaply
hold more asset information, such as the AssetPath:
```rust
// prints the AssetPath of the handle
info!("{:?}", handle.path())
```
### Processed Assets
Asset processing can be enabled via the `AssetPlugin`. When developing
Bevy Apps with processed assets, do this:
```rust
app.add_plugins(DefaultPlugins.set(AssetPlugin::processed_dev()))
```
This runs the `AssetProcessor` in the background with hot-reloading. It
reads assets from the `assets` folder, processes them, and writes them
to the `.imported_assets` folder. Asset loads in the Bevy App will wait
for a processed version of the asset to become available. If an asset in
the `assets` folder changes, it will be reprocessed and hot-reloaded in
the Bevy App.
When deploying processed Bevy apps, do this:
```rust
app.add_plugins(DefaultPlugins.set(AssetPlugin::processed()))
```
This does not run the `AssetProcessor` in the background. It behaves
like `AssetPlugin::unprocessed()`, but reads assets from
`.imported_assets`.
When the `AssetProcessor` is running, it will populate sibling `.meta`
files for assets in the `assets` folder. Meta files for assets that do
not have a processor configured look like this:
```rust
(
meta_format_version: "1.0",
asset: Load(
loader: "bevy_render::texture::image_loader::ImageLoader",
settings: (
format: FromExtension,
),
),
)
```
This is metadata for an image asset. For example, if you have
`assets/my_sprite.png`, this could be the metadata stored at
`assets/my_sprite.png.meta`. Meta files are totally optional. If no
metadata exists, the default settings will be used.
In short, this file says "load this asset with the ImageLoader and use
the file extension to determine the image type". This type of meta file
is supported in all AssetPlugin modes. If in `Unprocessed` mode, the
asset (with the meta settings) will be loaded directly. If in
`ProcessedDev` mode, the asset file will be copied directly to the
`.imported_assets` folder. The meta will also be copied directly to the
`.imported_assets` folder, but with one addition:
```rust
(
meta_format_version: "1.0",
processed_info: Some((
hash: 12415480888597742505,
full_hash: 14344495437905856884,
process_dependencies: [],
)),
asset: Load(
loader: "bevy_render::texture::image_loader::ImageLoader",
settings: (
format: FromExtension,
),
),
)
```
`processed_info` contains `hash` (a direct hash of the asset and meta
bytes), `full_hash` (a hash of `hash` and the hashes of all
`process_dependencies`), and `process_dependencies` (the `path` and
`full_hash` of every process_dependency). A "process dependency" is an
asset dependency that is _directly_ used when processing the asset.
Images do not have process dependencies, so this is empty.
When the processor is enabled, you can use the `Process` metadata
config:
```rust
(
meta_format_version: "1.0",
asset: Process(
processor: "bevy_asset::processor::process::LoadAndSave<bevy_render::texture::image_loader::ImageLoader, bevy_render::texture::compressed_image_saver::CompressedImageSaver>",
settings: (
loader_settings: (
format: FromExtension,
),
saver_settings: (
generate_mipmaps: true,
),
),
),
)
```
This configures the asset to use the `LoadAndSave` processor, which runs
an AssetLoader and feeds the result into an AssetSaver (which saves the
given Asset and defines a loader to load it with). (for terseness
LoadAndSave will likely get a shorter/friendlier type name when [Stable
Type Paths](#7184) lands). `LoadAndSave` is likely to be the most common
processor type, but arbitrary processors are supported.
`CompressedImageSaver` saves an `Image` in the Basis Universal format
and configures the ImageLoader to load it as basis universal. The
`AssetProcessor` will read this meta, run it through the LoadAndSave
processor, and write the basis-universal version of the image to
`.imported_assets`. The final metadata will look like this:
```rust
(
meta_format_version: "1.0",
processed_info: Some((
hash: 905599590923828066,
full_hash: 9948823010183819117,
process_dependencies: [],
)),
asset: Load(
loader: "bevy_render::texture::image_loader::ImageLoader",
settings: (
format: Format(Basis),
),
),
)
```
To try basis-universal processing out in Bevy examples, (for example
`sprite.rs`), change `add_plugins(DefaultPlugins)` to
`add_plugins(DefaultPlugins.set(AssetPlugin::processed_dev()))` and run
with the `basis-universal` feature enabled: `cargo run
--features=basis-universal --example sprite`.
To create a custom processor, there are two main paths:
1. Use the `LoadAndSave` processor with an existing `AssetLoader`.
Implement the `AssetSaver` trait, register the processor using
`asset_processor.register_processor::<LoadAndSave<ImageLoader,
CompressedImageSaver>>(image_saver.into())`.
2. Implement the `Process` trait directly and register it using:
`asset_processor.register_processor(thing_processor)`.
You can configure default processors for file extensions like this:
```rust
asset_processor.set_default_processor::<ThingProcessor>("thing")
```
There is one more metadata type to be aware of:
```rust
(
meta_format_version: "1.0",
asset: Ignore,
)
```
This will ignore the asset during processing / prevent it from being
written to `.imported_assets`.
The AssetProcessor stores a transaction log at `.imported_assets/log`
and uses it to gracefully recover from unexpected stops. This means you
can force-quit the processor (and Bevy Apps running the processor in
parallel) at arbitrary times!
`.imported_assets` is "local state". It should _not_ be checked into
source control. It should also be considered "read only". In practice,
you _can_ modify processed assets and processed metadata if you really
need to test something. But those modifications will not be represented
in the hashes of the assets, so the processed state will be "out of
sync" with the source assets. The processor _will not_ fix this for you.
Either revert the change after you have tested it, or delete the
processed files so they can be re-populated.
## Open Questions
There are a number of open questions to be discussed. We should decide
if they need to be addressed in this PR and if so, how we will address
them:
### Implied Dependencies vs Dependency Enumeration
There are currently two ways to populate asset dependencies:
* **Implied via AssetLoaders**: if an AssetLoader loads an asset (and
retrieves a handle), a dependency is added to the list.
* **Explicit via the optional Asset::visit_dependencies**: if
`server.load_asset(my_asset)` is called, it will call
`my_asset.visit_dependencies`, which will grab dependencies that have
been manually defined for the asset via the Asset trait impl (which can
be derived).
This means that defining explicit dependencies is optional for "loaded
assets". And the list of dependencies is always accurate because loaders
can only produce Handles if they register dependencies. If an asset was
loaded with an AssetLoader, it only uses the implied dependencies. If an
asset was created at runtime and added with
`asset_server.load_asset(MyAsset)`, it will use
`Asset::visit_dependencies`.
However this can create a behavior mismatch between loaded assets and
equivalent "created at runtime" assets if `Assets::visit_dependencies`
doesn't exactly match the dependencies produced by the AssetLoader. This
behavior mismatch can be resolved by completely removing "implied loader
dependencies" and requiring `Asset::visit_dependencies` to supply
dependency data. But this creates two problems:
* It makes defining loaded assets harder and more error prone: Devs must
remember to manually annotate asset dependencies with `#[dependency]`
when deriving `Asset`. For more complicated assets (such as scenes), the
derive likely wouldn't be sufficient and a manual `visit_dependencies`
impl would be required.
* Removes the ability to immediately kick off dependency loads: When
AssetLoaders retrieve a Handle, they also immediately kick off an asset
load for the handle, which means it can start loading in parallel
_before_ the asset finishes loading. For large assets, this could be
significant. (although this could be mitigated for processed assets if
we store dependencies in the processed meta file and load them ahead of
time)
### Eager ProcessorDev Asset Loading
I made a controversial call in the interest of fast startup times ("time
to first pixel") for the "processor dev mode configuration". When
initializing the AssetProcessor, current processed versions of unchanged
assets are yielded immediately, even if their dependencies haven't been
checked yet for reprocessing. This means that
non-current-state-of-filesystem-but-previously-valid assets might be
returned to the App first, then hot-reloaded if/when their dependencies
change and the asset is reprocessed.
Is this behavior desirable? There is largely one alternative: do not
yield an asset from the processor to the app until all of its
dependencies have been checked for changes. In some common cases (load
dependency has not changed since last run) this will increase startup
time. The main question is "by how much" and is that slower startup time
worth it in the interest of only yielding assets that are true to the
current state of the filesystem. Should this be configurable? I'm
starting to think we should only yield an asset after its (historical)
dependencies have been checked for changes + processed as necessary, but
I'm curious what you all think.
### Paths Are Currently The Only Canonical ID / Do We Want Asset UUIDs?
In this implementation AssetPaths are the only canonical asset
identifier (just like the previous Bevy Asset system and Godot). Moving
assets will result in re-scans (and currently reprocessing, although
reprocessing can easily be avoided with some changes). Asset
renames/moves will break code and assets that rely on specific paths,
unless those paths are fixed up.
Do we want / need "stable asset uuids"? Introducing them is very
possible:
1. Generate a UUID and include it in .meta files
2. Support UUID in AssetPath
3. Generate "asset indices" which are loaded on startup and map UUIDs to
paths.
4 (maybe). Consider only supporting UUIDs for processed assets so we can
generate quick-to-load indices instead of scanning meta files.
The main "pro" is that assets referencing UUIDs don't need to be
migrated when a path changes. The main "con" is that UUIDs cannot be
"lazily resolved" like paths. They need a full view of all assets to
answer the question "does this UUID exist". Which means UUIDs require
the AssetProcessor to fully finish startup scans before saying an asset
doesnt exist. And they essentially require asset pre-processing to use
in apps, because scanning all asset metadata files at runtime to resolve
a UUID is not viable for medium-to-large apps. It really requires a
pre-generated UUID index, which must be loaded before querying for
assets.
I personally think this should be investigated in a separate PR. Paths
aren't going anywhere ... _everyone_ uses filesystems (and
filesystem-like apis) to manage their asset source files. I consider
them permanent canonical asset information. Additionally, they behave
well for both processed and unprocessed asset modes. Given that Bevy is
supporting both, this feels like the right canonical ID to start with.
UUIDS (and maybe even other indexed-identifier types) can be added later
as necessary.
### Folder / File Naming Conventions
All asset processing config currently lives in the `.imported_assets`
folder. The processor transaction log is in `.imported_assets/log`.
Processed assets are added to `.imported_assets/Default`, which will
make migrating to processed asset profiles (ex: a
`.imported_assets/Mobile` profile) a non-breaking change. It also allows
us to create top-level files like `.imported_assets/log` without it
being interpreted as an asset. Meta files currently have a `.meta`
suffix. Do we like these names and conventions?
### Should the `AssetPlugin::processed_dev` configuration enable
`watch_for_changes` automatically?
Currently it does (which I think makes sense), but it does make it the
only configuration that enables watch_for_changes by default.
### Discuss on_loaded High Level Interface:
This PR includes a very rough "proof of concept" `on_loaded` system
adapter that uses the `LoadedWithDependencies` event in combination with
`asset_server.load_asset` dependency tracking to support this pattern
```rust
fn main() {
App::new()
.init_asset::<MyAssets>()
.add_systems(Update, on_loaded(create_array_texture))
.run();
}
#[derive(Asset, Clone)]
struct MyAssets {
#[dependency]
picture_of_my_cat: Handle<Image>,
#[dependency]
picture_of_my_other_cat: Handle<Image>,
}
impl FromWorld for ArrayTexture {
fn from_world(world: &mut World) -> Self {
picture_of_my_cat: server.load("meow.png"),
picture_of_my_other_cat: server.load("meeeeeeeow.png"),
}
}
fn spawn_cat(In(my_assets): In<MyAssets>, mut commands: Commands) {
commands.spawn(SpriteBundle {
texture: my_assets.picture_of_my_cat.clone(),
..default()
});
commands.spawn(SpriteBundle {
texture: my_assets.picture_of_my_other_cat.clone(),
..default()
});
}
```
The implementation is _very_ rough. And it is currently unsafe because
`bevy_ecs` doesn't expose some internals to do this safely from inside
`bevy_asset`. There are plenty of unanswered questions like:
* "do we add a Loadable" derive? (effectively automate the FromWorld
implementation above)
* Should `MyAssets` even be an Asset? (largely implemented this way
because it elegantly builds on `server.load_asset(MyAsset { .. })`
dependency tracking).
We should think hard about what our ideal API looks like (and if this is
a pattern we want to support). Not necessarily something we need to
solve in this PR. The current `on_loaded` impl should probably be
removed from this PR before merging.
## Clarifying Questions
### What about Assets as Entities?
This Bevy Asset V2 proposal implementation initially stored Assets as
ECS Entities. Instead of `AssetId<T>` + the `Assets<T>` resource it used
`Entity` as the asset id and Asset values were just ECS components.
There are plenty of compelling reasons to do this:
1. Easier to inline assets in Bevy Scenes (as they are "just" normal
entities + components)
2. More flexible queries: use the power of the ECS to filter assets (ex:
`Query<Mesh, With<Tree>>`).
3. Extensible. Users can add arbitrary component data to assets.
4. Things like "component visualization tools" work out of the box to
visualize asset data.
However Assets as Entities has a ton of caveats right now:
* We need to be able to allocate entity ids without a direct World
reference (aka rework id allocator in Entities ... i worked around this
in my prototypes by just pre allocating big chunks of entities)
* We want asset change events in addition to ECS change tracking ... how
do we populate them when mutations can come from anywhere? Do we use
Changed queries? This would require iterating over the change data for
all assets every frame. Is this acceptable or should we implement a new
"event based" component change detection option?
* Reconciling manually created assets with asset-system managed assets
has some nuance (ex: are they "loaded" / do they also have that
component metadata?)
* "how do we handle "static" / default entity handles" (ties in to the
Entity Indices discussion:
https://github.com/bevyengine/bevy/discussions/8319). This is necessary
for things like "built in" assets and default handles in things like
SpriteBundle.
* Storing asset information as a component makes it easy to "invalidate"
asset state by removing the component (or forcing modifications).
Ideally we have ways to lock this down (some combination of Rust type
privacy and ECS validation)
In practice, how we store and identify assets is a reasonably
superficial change (porting off of Assets as Entities and implementing
dedicated storage + ids took less than a day). So once we sort out the
remaining challenges the flip should be straightforward. Additionally, I
do still have "Assets as Entities" in my commit history, so we can reuse
that work. I personally think "assets as entities" is a good endgame,
but it also doesn't provide _significant_ value at the moment and it
certainly isn't ready yet with the current state of things.
### Why not Distill?
[Distill](https://github.com/amethyst/distill) is a high quality fully
featured asset system built in Rust. It is very natural to ask "why not
just use Distill?".
It is also worth calling out that for awhile, [we planned on adopting
Distill / I signed off on
it](https://github.com/bevyengine/bevy/issues/708).
However I think Bevy has a number of constraints that make Distill
adoption suboptimal:
* **Architectural Simplicity:**
* Distill's processor requires an in-memory database (lmdb) and RPC
networked API (using Cap'n Proto). Each of these introduces API
complexity that increases maintenance burden and "code grokability".
Ignoring tests, documentation, and examples, Distill has 24,237 lines of
Rust code (including generated code for RPC + database interactions). If
you ignore generated code, it has 11,499 lines.
* Bevy builds the AssetProcessor and AssetServer using pluggable
AssetReader/AssetWriter Rust traits with simple io interfaces. They do
not necessitate databases or RPC interfaces (although Readers/Writers
could use them if that is desired). Bevy Asset V2 (at the time of
writing this PR) is 5,384 lines of Rust code (ignoring tests,
documentation, and examples). Grain of salt: Distill does have more
features currently (ex: Asset Packing, GUIDS, remote-out-of-process
asset processor). I do plan to implement these features in Bevy Asset V2
and I personally highly doubt they will meaningfully close the 6115
lines-of-code gap.
* This complexity gap (which while illustrated by lines of code, is much
bigger than just that) is noteworthy to me. Bevy should be hackable and
there are pillars of Distill that are very hard to understand and
extend. This is a matter of opinion (and Bevy Asset V2 also has
complicated areas), but I think Bevy Asset V2 is much more approachable
for the average developer.
* Necessary disclaimer: counting lines of code is an extremely rough
complexity metric. Read the code and form your own opinions.
* **Optional Asset Processing:** Not all Bevy Apps (or Bevy App
developers) need / want asset preprocessing. Processing increases the
complexity of the development environment by introducing things like
meta files, imported asset storage, running processors in the
background, waiting for processing to finish, etc. Distill _requires_
preprocessing to work. With Bevy Asset V2 processing is fully opt-in.
The AssetServer isn't directly aware of asset processors at all.
AssetLoaders only care about converting bytes to runtime Assets ... they
don't know or care if the bytes were pre-processed or not. Processing is
"elegantly" (forgive my self-congratulatory phrasing) layered on top and
builds on the existing Asset system primitives.
* **Direct Filesystem Access to Processed Asset State:** Distill stores
processed assets in a database. This makes debugging / inspecting the
processed outputs harder (either requires special tooling to query the
database or they need to be "deployed" to be inspected). Bevy Asset V2,
on the other hand, stores processed assets in the filesystem (by default
... this is configurable). This makes interacting with the processed
state more natural. Note that both Godot and Unity's new asset system
store processed assets in the filesystem.
* **Portability**: Because Distill's processor uses lmdb and RPC
networking, it cannot be run on certain platforms (ex: lmdb is a
non-rust dependency that cannot run on the web, some platforms don't
support running network servers). Bevy should be able to process assets
everywhere (ex: run the Bevy Editor on the web, compile + process
shaders on mobile, etc). Distill does partially mitigate this problem by
supporting "streaming" assets via the RPC protocol, but this is not a
full solve from my perspective. And Bevy Asset V2 can (in theory) also
stream assets (without requiring RPC, although this isn't implemented
yet)
Note that I _do_ still think Distill would be a solid asset system for
Bevy. But I think the approach in this PR is a better solve for Bevy's
specific "asset system requirements".
### Doesn't async-fs just shim requests to "sync" `std::fs`? What is the
point?
"True async file io" has limited / spotty platform support. async-fs
(and the rust async ecosystem generally ... ex Tokio) currently use
async wrappers over std::fs that offload blocking requests to separate
threads. This may feel unsatisfying, but it _does_ still provide value
because it prevents our task pools from blocking on file system
operations (which would prevent progress when there are many tasks to
do, but all threads in a pool are currently blocking on file system
ops).
Additionally, using async APIs for our AssetReaders and AssetWriters
also provides value because we can later add support for "true async
file io" for platforms that support it. _And_ we can implement other
"true async io" asset backends (such as networked asset io).
## Draft TODO
- [x] Fill in missing filesystem event APIs: file removed event (which
is expressed as dangling RenameFrom events in some cases), file/folder
renamed event
- [x] Assets without loaders are not moved to the processed folder. This
breaks things like referenced `.bin` files for GLTFs. This should be
configurable per-non-asset-type.
- [x] Initial implementation of Reflect and FromReflect for Handle. The
"deserialization" parity bar is low here as this only worked with static
UUIDs in the old impl ... this is a non-trivial problem. Either we add a
Handle::AssetPath variant that gets "upgraded" to a strong handle on
scene load or we use a separate AssetRef type for Bevy scenes (which is
converted to a runtime Handle on load). This deserves its own discussion
in a different pr.
- [x] Populate read_asset_bytes hash when run by the processor (a bit of
a special case .. when run by the processor the processed meta will
contain the hash so we don't need to compute it on the spot, but we
don't want/need to read the meta when run by the main AssetServer)
- [x] Delay hot reloading: currently filesystem events are handled
immediately, which creates timing issues in some cases. For example hot
reloading images can sometimes break because the image isn't finished
writing. We should add a delay, likely similar to the [implementation in
this PR](https://github.com/bevyengine/bevy/pull/8503).
- [x] Port old platform-specific AssetIo implementations to the new
AssetReader interface (currently missing Android and web)
- [x] Resolve on_loaded unsafety (either by removing the API entirely or
removing the unsafe)
- [x] Runtime loader setting overrides
- [x] Remove remaining unwraps that should be error-handled. There are
number of TODOs here
- [x] Pretty AssetPath Display impl
- [x] Document more APIs
- [x] Resolve spurious "reloading because it has changed" events (to
repro run load_gltf with `processed_dev()`)
- [x] load_dependency hot reloading currently only works for processed
assets. If processing is disabled, load_dependency changes are not hot
reloaded.
- [x] Replace AssetInfo dependency load/fail counters with
`loading_dependencies: HashSet<UntypedAssetId>` to prevent reloads from
(potentially) breaking counters. Storing this will also enable
"dependency reloaded" events (see [Next Steps](#next-steps))
- [x] Re-add filesystem watcher cargo feature gate (currently it is not
optional)
- [ ] Migration Guide
- [ ] Changelog
## Followup TODO
- [ ] Replace "eager unchanged processed asset loading" behavior with
"don't returned unchanged processed asset until dependencies have been
checked".
- [ ] Add true `Ignore` AssetAction that does not copy the asset to the
imported_assets folder.
- [ ] Finish "live asset unloading" (ex: free up CPU asset memory after
uploading an image to the GPU), rethink RenderAssets, and port renderer
features. The `Assets` collection uses `Option<T>` for asset storage to
support its removal. (1) the Option might not actually be necessary ...
might be able to just remove from the collection entirely (2) need to
finalize removal apis
- [ ] Try replacing the "channel based" asset id recycling with
something a bit more efficient (ex: we might be able to use raw atomic
ints with some cleverness)
- [ ] Consider adding UUIDs to processed assets (scoped just to helping
identify moved assets ... not exposed to load queries ... see [Next
Steps](#next-steps))
- [ ] Store "last modified" source asset and meta timestamps in
processed meta files to enable skipping expensive hashing when the file
wasn't changed
- [ ] Fix "slow loop" handle drop fix
- [ ] Migrate to TypeName
- [x] Handle "loader preregistration". See #9429
## Next Steps
* **Configurable per-type defaults for AssetMeta**: It should be
possible to add configuration like "all png image meta should default to
using nearest sampling" (currently this hard-coded per-loader/processor
Settings::default() impls). Also see the "Folder Meta" bullet point.
* **Avoid Reprocessing on Asset Renames / Moves**: See the "canonical
asset ids" discussion in [Open Questions](#open-questions) and the
relevant bullet point in [Draft TODO](#draft-todo). Even without
canonical ids, folder renames could avoid reprocessing in some cases.
* **Multiple Asset Sources**: Expand AssetPath to support "asset source
names" and support multiple AssetReaders in the asset server (ex:
`webserver://some_path/image.png` backed by an Http webserver
AssetReader). The "default" asset reader would use normal
`some_path/image.png` paths. Ideally this works in combination with
multiple AssetWatchers for hot-reloading
* **Stable Type Names**: this pr removes the TypeUuid requirement from
assets in favor of `std::any::type_name`. This makes defining assets
easier (no need to generate a new uuid / use weird proc macro syntax).
It also makes reading meta files easier (because things have "friendly
names"). We also use type names for components in scene files. If they
are good enough for components, they are good enough for assets. And
consistency across Bevy pillars is desirable. However,
`std::any::type_name` is not guaranteed to be stable (although in
practice it is). We've developed a [stable type
path](https://github.com/bevyengine/bevy/pull/7184) to resolve this,
which should be adopted when it is ready.
* **Command Line Interface**: It should be possible to run the asset
processor in a separate process from the command line. This will also
require building a network-server-backed AssetReader to communicate
between the app and the processor. We've been planning to build a "bevy
cli" for awhile. This seems like a good excuse to build it.
* **Asset Packing**: This is largely an additive feature, so it made
sense to me to punt this until we've laid the foundations in this PR.
* **Per-Platform Processed Assets**: It should be possible to generate
assets for multiple platforms by supporting multiple "processor
profiles" per asset (ex: compress with format X on PC and Y on iOS). I
think there should probably be arbitrary "profiles" (which can be
separate from actual platforms), which are then assigned to a given
platform when generating the final asset distribution for that platform.
Ex: maybe devs want a "Mobile" profile that is shared between iOS and
Android. Or a "LowEnd" profile shared between web and mobile.
* **Versioning and Migrations**: Assets, Loaders, Savers, and Processors
need to have versions to determine if their schema is valid. If an asset
/ loader version is incompatible with the current version expected at
runtime, the processor should be able to migrate them. I think we should
try using Bevy Reflect for this, as it would allow us to load the old
version as a dynamic Reflect type without actually having the old Rust
type. It would also allow us to define "patches" to migrate between
versions (Bevy Reflect devs are currently working on patching). The
`.meta` file already has its own format version. Migrating that to new
versions should also be possible.
* **Real Copy-on-write AssetPaths**: Rust's actual Cow (clone-on-write
type) currently used by AssetPath can still result in String clones that
aren't actually necessary (cloning an Owned Cow clones the contents).
Bevy's asset system requires cloning AssetPaths in a number of places,
which result in actual clones of the internal Strings. This is not
efficient. AssetPath internals should be reworked to exhibit truer
cow-like-behavior that reduces String clones to the absolute minimum.
* **Consider processor-less processing**: In theory the AssetServer
could run processors "inline" even if the background AssetProcessor is
disabled. If we decide this is actually desirable, we could add this.
But I don't think its a priority in the short or medium term.
* **Pre-emptive dependency loading**: We could encode dependencies in
processed meta files, which could then be used by the Asset Server to
kick of dependency loads as early as possible (prior to starting the
actual asset load). Is this desirable? How much time would this save in
practice?
* **Optimize Processor With UntypedAssetIds**: The processor exclusively
uses AssetPath to identify assets currently. It might be possible to
swap these out for UntypedAssetIds in some places, which are smaller /
cheaper to hash and compare.
* **One to Many Asset Processing**: An asset source file that produces
many assets currently must be processed into a single "processed" asset
source. If labeled assets can be written separately they can each have
their own configured savers _and_ they could be loaded more granularly.
Definitely worth exploring!
* **Automatically Track "Runtime-only" Asset Dependencies**: Right now,
tracking "created at runtime" asset dependencies requires adding them
via `asset_server.load_asset(StandardMaterial::default())`. I think with
some cleverness we could also do this for
`materials.add(StandardMaterial::default())`, making tracking work
"everywhere". There are challenges here relating to change detection /
ensuring the server is made aware of dependency changes. This could be
expensive in some cases.
* **"Dependency Changed" events**: Some assets have runtime artifacts
that need to be re-generated when one of their dependencies change (ex:
regenerate a material's bind group when a Texture needs to change). We
are generating the dependency graph so we can definitely produce these
events. Buuuuut generating these events will have a cost / they could be
high frequency for some assets, so we might want this to be opt-in for
specific cases.
* **Investigate Storing More Information In Handles**: Handles can now
store arbitrary information, which makes it cheaper and easier to
access. How much should we move into them? Canonical asset load states
(via atomics)? (`handle.is_loaded()` would be very cool). Should we
store the entire asset and remove the `Assets<T>` collection?
(`Arc<RwLock<Option<Image>>>`?)
* **Support processing and loading files without extensions**: This is a
pretty arbitrary restriction and could be supported with very minimal
changes.
* **Folder Meta**: It would be nice if we could define per folder
processor configuration defaults (likely in a `.meta` or `.folder_meta`
file). Things like "default to linear filtering for all Images in this
folder".
* **Replace async_broadcast with event-listener?** This might be
approximately drop-in for some uses and it feels more light weight
* **Support Running the AssetProcessor on the Web**: Most of the hard
work is done here, but there are some easy straggling TODOs (make the
transaction log an interface instead of a direct file writer so we can
write a web storage backend, implement an AssetReader/AssetWriter that
reads/writes to something like LocalStorage).
* **Consider identifying and preventing circular dependencies**: This is
especially important for "processor dependencies", as processing will
silently never finish in these cases.
* **Built-in/Inlined Asset Hot Reloading**: This PR regresses
"built-in/inlined" asset hot reloading (previously provided by the
DebugAssetServer). I'm intentionally punting this because I think it can
be cleanly implemented with "multiple asset sources" by registering a
"debug asset source" (ex: `debug://bevy_pbr/src/render/pbr.wgsl` asset
paths) in combination with an AssetWatcher for that asset source and
support for "manually loading pats with asset bytes instead of
AssetReaders". The old DebugAssetServer was quite nasty and I'd love to
avoid that hackery going forward.
* **Investigate ways to remove double-parsing meta files**: Parsing meta
files currently involves parsing once with "minimal" versions of the
meta file to extract the type name of the loader/processor config, then
parsing again to parse the "full" meta. This is suboptimal. We should be
able to define custom deserializers that (1) assume the loader/processor
type name comes first (2) dynamically looks up the loader/processor
registrations to deserialize settings in-line (similar to components in
the bevy scene format). Another alternative: deserialize as dynamic
Reflect objects and then convert.
* **More runtime loading configuration**: Support using the Handle type
as a hint to select an asset loader (instead of relying on AssetPath
extensions)
* **More high level Processor trait implementations**: For example, it
might be worth adding support for arbitrary chains of "asset transforms"
that modify an in-memory asset representation between loading and
saving. (ex: load a Mesh, run a `subdivide_mesh` transform, followed by
a `flip_normals` transform, then save the mesh to an efficient
compressed format).
* **Bevy Scene Handle Deserialization**: (see the relevant [Draft TODO
item](#draft-todo) for context)
* **Explore High Level Load Interfaces**: See [this
discussion](#discuss-on_loaded-high-level-interface) for one prototype.
* **Asset Streaming**: It would be great if we could stream Assets (ex:
stream a long video file piece by piece)
* **ID Exchanging**: In this PR Asset Handles/AssetIds are bigger than
they need to be because they have a Uuid enum variant. If we implement
an "id exchanging" system that trades Uuids for "efficient runtime ids",
we can cut down on the size of AssetIds, making them more efficient.
This has some open design questions, such as how to spawn entities with
"default" handle values (as these wouldn't have access to the exchange
api in the current system).
* **Asset Path Fixup Tooling**: Assets that inline asset paths inside
them will break when an asset moves. The asset system provides the
functionality to detect when paths break. We should build a framework
that enables formats to define "path migrations". This is especially
important for scene files. For editor-generated files, we should also
consider using UUIDs (see other bullet point) to avoid the need to
migrate in these cases.
---------
Co-authored-by: BeastLe9enD <beastle9end@outlook.de>
Co-authored-by: Mike <mike.hsu@gmail.com>
Co-authored-by: Nicola Papale <nicopap@users.noreply.github.com>
2023-09-07 02:07:27 +00:00
|
|
|
/// # use bevy_asset::{Handle, AssetServer, Assets, Asset};
|
Migrate meshes and materials to required components (#15524)
# Objective
A big step in the migration to required components: meshes and
materials!
## Solution
As per the [selected
proposal](https://hackmd.io/@bevy/required_components/%2Fj9-PnF-2QKK0on1KQ29UWQ):
- Deprecate `MaterialMesh2dBundle`, `MaterialMeshBundle`, and
`PbrBundle`.
- Add `Mesh2d` and `Mesh3d` components, which wrap a `Handle<Mesh>`.
- Add `MeshMaterial2d<M: Material2d>` and `MeshMaterial3d<M: Material>`,
which wrap a `Handle<M>`.
- Meshes *without* a mesh material should be rendered with a default
material. The existence of a material is determined by
`HasMaterial2d`/`HasMaterial3d`, which is required by
`MeshMaterial2d`/`MeshMaterial3d`. This gets around problems with the
generics.
Previously:
```rust
commands.spawn(MaterialMesh2dBundle {
mesh: meshes.add(Circle::new(100.0)).into(),
material: materials.add(Color::srgb(7.5, 0.0, 7.5)),
transform: Transform::from_translation(Vec3::new(-200., 0., 0.)),
..default()
});
```
Now:
```rust
commands.spawn((
Mesh2d(meshes.add(Circle::new(100.0))),
MeshMaterial2d(materials.add(Color::srgb(7.5, 0.0, 7.5))),
Transform::from_translation(Vec3::new(-200., 0., 0.)),
));
```
If the mesh material is missing, previously nothing was rendered. Now,
it renders a white default `ColorMaterial` in 2D and a
`StandardMaterial` in 3D (this can be overridden). Below, only every
other entity has a material:


Why white? This is still open for discussion, but I think white makes
sense for a *default* material, while *invalid* asset handles pointing
to nothing should have something like a pink material to indicate that
something is broken (I don't handle that in this PR yet). This is kind
of a mix of Godot and Unity: Godot just renders a white material for
non-existent materials, while Unity renders nothing when no materials
exist, but renders pink for invalid materials. I can also change the
default material to pink if that is preferable though.
## Testing
I ran some 2D and 3D examples to test if anything changed visually. I
have not tested all examples or features yet however. If anyone wants to
test more extensively, it would be appreciated!
## Implementation Notes
- The relationship between `bevy_render` and `bevy_pbr` is weird here.
`bevy_render` needs `Mesh3d` for its own systems, but `bevy_pbr` has all
of the material logic, and `bevy_render` doesn't depend on it. I feel
like the two crates should be refactored in some way, but I think that's
out of scope for this PR.
- I didn't migrate meshlets to required components yet. That can
probably be done in a follow-up, as this is already a huge PR.
- It is becoming increasingly clear to me that we really, *really* want
to disallow raw asset handles as components. They caused me a *ton* of
headache here already, and it took me a long time to find every place
that queried for them or inserted them directly on entities, since there
were no compiler errors for it. If we don't remove the `Component`
derive, I expect raw asset handles to be a *huge* footgun for users as
we transition to wrapper components, especially as handles as components
have been the norm so far. I personally consider this to be a blocker
for 0.15: we need to migrate to wrapper components for asset handles
everywhere, and remove the `Component` derive. Also see
https://github.com/bevyengine/bevy/issues/14124.
---
## Migration Guide
Asset handles for meshes and mesh materials must now be wrapped in the
`Mesh2d` and `MeshMaterial2d` or `Mesh3d` and `MeshMaterial3d`
components for 2D and 3D respectively. Raw handles as components no
longer render meshes.
Additionally, `MaterialMesh2dBundle`, `MaterialMeshBundle`, and
`PbrBundle` have been deprecated. Instead, use the mesh and material
components directly.
Previously:
```rust
commands.spawn(MaterialMesh2dBundle {
mesh: meshes.add(Circle::new(100.0)).into(),
material: materials.add(Color::srgb(7.5, 0.0, 7.5)),
transform: Transform::from_translation(Vec3::new(-200., 0., 0.)),
..default()
});
```
Now:
```rust
commands.spawn((
Mesh2d(meshes.add(Circle::new(100.0))),
MeshMaterial2d(materials.add(Color::srgb(7.5, 0.0, 7.5))),
Transform::from_translation(Vec3::new(-200., 0., 0.)),
));
```
If the mesh material is missing, a white default material is now used.
Previously, nothing was rendered if the material was missing.
The `WithMesh2d` and `WithMesh3d` query filter type aliases have also
been removed. Simply use `With<Mesh2d>` or `With<Mesh3d>`.
---------
Co-authored-by: Tim Blackbird <justthecooldude@gmail.com>
Co-authored-by: Carter Anderson <mcanders1@gmail.com>
2024-10-01 21:33:17 +00:00
|
|
|
/// # use bevy_math::primitives::Capsule3d;
|
|
|
|
/// #
|
Bevy Asset V2 (#8624)
# Bevy Asset V2 Proposal
## Why Does Bevy Need A New Asset System?
Asset pipelines are a central part of the gamedev process. Bevy's
current asset system is missing a number of features that make it
non-viable for many classes of gamedev. After plenty of discussions and
[a long community feedback
period](https://github.com/bevyengine/bevy/discussions/3972), we've
identified a number missing features:
* **Asset Preprocessing**: it should be possible to "preprocess" /
"compile" / "crunch" assets at "development time" rather than when the
game starts up. This enables offloading expensive work from deployed
apps, faster asset loading, less runtime memory usage, etc.
* **Per-Asset Loader Settings**: Individual assets cannot define their
own loaders that override the defaults. Additionally, they cannot
provide per-asset settings to their loaders. This is a huge limitation,
as many asset types don't provide all information necessary for Bevy
_inside_ the asset. For example, a raw PNG image says nothing about how
it should be sampled (ex: linear vs nearest).
* **Asset `.meta` files**: assets should have configuration files stored
adjacent to the asset in question, which allows the user to configure
asset-type-specific settings. These settings should be accessible during
the pre-processing phase. Modifying a `.meta` file should trigger a
re-processing / re-load of the asset. It should be possible to configure
asset loaders from the meta file.
* **Processed Asset Hot Reloading**: Changes to processed assets (or
their dependencies) should result in re-processing them and re-loading
the results in live Bevy Apps.
* **Asset Dependency Tracking**: The current bevy_asset has no good way
to wait for asset dependencies to load. It punts this as an exercise for
consumers of the loader apis, which is unreasonable and error prone.
There should be easy, ergonomic ways to wait for assets to load and
block some logic on an asset's entire dependency tree loading.
* **Runtime Asset Loading**: it should be (optionally) possible to load
arbitrary assets dynamically at runtime. This necessitates being able to
deploy and run the asset server alongside Bevy Apps on _all platforms_.
For example, we should be able to invoke the shader compiler at runtime,
stream scenes from sources like the internet, etc. To keep deployed
binaries (and startup times) small, the runtime asset server
configuration should be configurable with different settings compared to
the "pre processor asset server".
* **Multiple Backends**: It should be possible to load assets from
arbitrary sources (filesystems, the internet, remote asset serves, etc).
* **Asset Packing**: It should be possible to deploy assets in
compressed "packs", which makes it easier and more efficient to
distribute assets with Bevy Apps.
* **Asset Handoff**: It should be possible to hold a "live" asset
handle, which correlates to runtime data, without actually holding the
asset in memory. Ex: it must be possible to hold a reference to a GPU
mesh generated from a "mesh asset" without keeping the mesh data in CPU
memory
* **Per-Platform Processed Assets**: Different platforms and app
distributions have different capabilities and requirements. Some
platforms need lower asset resolutions or different asset formats to
operate within the hardware constraints of the platform. It should be
possible to define per-platform asset processing profiles. And it should
be possible to deploy only the assets required for a given platform.
These features have architectural implications that are significant
enough to require a full rewrite. The current Bevy Asset implementation
got us this far, but it can take us no farther. This PR defines a brand
new asset system that implements most of these features, while laying
the foundations for the remaining features to be built.
## Bevy Asset V2
Here is a quick overview of the features introduced in this PR.
* **Asset Preprocessing**: Preprocess assets at development time into
more efficient (and configurable) representations
* **Dependency Aware**: Dependencies required to process an asset are
tracked. If an asset's processed dependency changes, it will be
reprocessed
* **Hot Reprocessing/Reloading**: detect changes to asset source files,
reprocess them if they have changed, and then hot-reload them in Bevy
Apps.
* **Only Process Changes**: Assets are only re-processed when their
source file (or meta file) has changed. This uses hashing and timestamps
to avoid processing assets that haven't changed.
* **Transactional and Reliable**: Uses write-ahead logging (a technique
commonly used by databases) to recover from crashes / forced-exits.
Whenever possible it avoids full-reprocessing / only uncompleted
transactions will be reprocessed. When the processor is running in
parallel with a Bevy App, processor asset writes block Bevy App asset
reads. Reading metadata + asset bytes is guaranteed to be transactional
/ correctly paired.
* **Portable / Run anywhere / Database-free**: The processor does not
rely on an in-memory database (although it uses some database techniques
for reliability). This is important because pretty much all in-memory
databases have unsupported platforms or build complications.
* **Configure Processor Defaults Per File Type**: You can say "use this
processor for all files of this type".
* **Custom Processors**: The `Processor` trait is flexible and
unopinionated. It can be implemented by downstream plugins.
* **LoadAndSave Processors**: Most asset processing scenarios can be
expressed as "run AssetLoader A, save the results using AssetSaver X,
and then load the result using AssetLoader B". For example, load this
png image using `PngImageLoader`, which produces an `Image` asset and
then save it using `CompressedImageSaver` (which also produces an
`Image` asset, but in a compressed format), which takes an `Image` asset
as input. This means if you have an `AssetLoader` for an asset, you are
already half way there! It also means that you can share AssetSavers
across multiple loaders. Because `CompressedImageSaver` accepts Bevy's
generic Image asset as input, it means you can also use it with some
future `JpegImageLoader`.
* **Loader and Saver Settings**: Asset Loaders and Savers can now define
their own settings types, which are passed in as input when an asset is
loaded / saved. Each asset can define its own settings.
* **Asset `.meta` files**: configure asset loaders, their settings,
enable/disable processing, and configure processor settings
* **Runtime Asset Dependency Tracking** Runtime asset dependencies (ex:
if an asset contains a `Handle<Image>`) are tracked by the asset server.
An event is emitted when an asset and all of its dependencies have been
loaded
* **Unprocessed Asset Loading**: Assets do not require preprocessing.
They can be loaded directly. A processed asset is just a "normal" asset
with some extra metadata. Asset Loaders don't need to know or care about
whether or not an asset was processed.
* **Async Asset IO**: Asset readers/writers use async non-blocking
interfaces. Note that because Rust doesn't yet support async traits,
there is a bit of manual Boxing / Future boilerplate. This will
hopefully be removed in the near future when Rust gets async traits.
* **Pluggable Asset Readers and Writers**: Arbitrary asset source
readers/writers are supported, both by the processor and the asset
server.
* **Better Asset Handles**
* **Single Arc Tree**: Asset Handles now use a single arc tree that
represents the lifetime of the asset. This makes their implementation
simpler, more efficient, and allows us to cheaply attach metadata to
handles. Ex: the AssetPath of a handle is now directly accessible on the
handle itself!
* **Const Typed Handles**: typed handles can be constructed in a const
context. No more weird "const untyped converted to typed at runtime"
patterns!
* **Handles and Ids are Smaller / Faster To Hash / Compare**: Typed
`Handle<T>` is now much smaller in memory and `AssetId<T>` is even
smaller.
* **Weak Handle Usage Reduction**: In general Handles are now considered
to be "strong". Bevy features that previously used "weak `Handle<T>`"
have been ported to `AssetId<T>`, which makes it statically clear that
the features do not hold strong handles (while retaining strong type
information). Currently Handle::Weak still exists, but it is very
possible that we can remove that entirely.
* **Efficient / Dense Asset Ids**: Assets now have efficient dense
runtime asset ids, which means we can avoid expensive hash lookups.
Assets are stored in Vecs instead of HashMaps. There are now typed and
untyped ids, which means we no longer need to store dynamic type
information in the ID for typed handles. "AssetPathId" (which was a
nightmare from a performance and correctness standpoint) has been
entirely removed in favor of dense ids (which are retrieved for a path
on load)
* **Direct Asset Loading, with Dependency Tracking**: Assets that are
defined at runtime can still have their dependencies tracked by the
Asset Server (ex: if you create a material at runtime, you can still
wait for its textures to load). This is accomplished via the (currently
optional) "asset dependency visitor" trait. This system can also be used
to define a set of assets to load, then wait for those assets to load.
* **Async folder loading**: Folder loading also uses this system and
immediately returns a handle to the LoadedFolder asset, which means
folder loading no longer blocks on directory traversals.
* **Improved Loader Interface**: Loaders now have a specific "top level
asset type", which makes returning the top-level asset simpler and
statically typed.
* **Basic Image Settings and Processing**: Image assets can now be
processed into the gpu-friendly Basic Universal format. The ImageLoader
now has a setting to define what format the image should be loaded as.
Note that this is just a minimal MVP ... plenty of additional work to do
here. To demo this, enable the `basis-universal` feature and turn on
asset processing.
* **Simpler Audio Play / AudioSink API**: Asset handle providers are
cloneable, which means the Audio resource can mint its own handles. This
means you can now do `let sink_handle = audio.play(music)` instead of
`let sink_handle = audio_sinks.get_handle(audio.play(music))`. Note that
this might still be replaced by
https://github.com/bevyengine/bevy/pull/8424.
**Removed Handle Casting From Engine Features**: Ex: FontAtlases no
longer use casting between handle types
## Using The New Asset System
### Normal Unprocessed Asset Loading
By default the `AssetPlugin` does not use processing. It behaves pretty
much the same way as the old system.
If you are defining a custom asset, first derive `Asset`:
```rust
#[derive(Asset)]
struct Thing {
value: String,
}
```
Initialize the asset:
```rust
app.init_asset:<Thing>()
```
Implement a new `AssetLoader` for it:
```rust
#[derive(Default)]
struct ThingLoader;
#[derive(Serialize, Deserialize, Default)]
pub struct ThingSettings {
some_setting: bool,
}
impl AssetLoader for ThingLoader {
type Asset = Thing;
type Settings = ThingSettings;
fn load<'a>(
&'a self,
reader: &'a mut Reader,
settings: &'a ThingSettings,
load_context: &'a mut LoadContext,
) -> BoxedFuture<'a, Result<Thing, anyhow::Error>> {
Box::pin(async move {
let mut bytes = Vec::new();
reader.read_to_end(&mut bytes).await?;
// convert bytes to value somehow
Ok(Thing {
value
})
})
}
fn extensions(&self) -> &[&str] {
&["thing"]
}
}
```
Note that this interface will get much cleaner once Rust gets support
for async traits. `Reader` is an async futures_io::AsyncRead. You can
stream bytes as they come in or read them all into a `Vec<u8>`,
depending on the context. You can use `let handle =
load_context.load(path)` to kick off a dependency load, retrieve a
handle, and register the dependency for the asset.
Then just register the loader in your Bevy app:
```rust
app.init_asset_loader::<ThingLoader>()
```
Now just add your `Thing` asset files into the `assets` folder and load
them like this:
```rust
fn system(asset_server: Res<AssetServer>) {
let handle = Handle<Thing> = asset_server.load("cool.thing");
}
```
You can check load states directly via the asset server:
```rust
if asset_server.load_state(&handle) == LoadState::Loaded { }
```
You can also listen for events:
```rust
fn system(mut events: EventReader<AssetEvent<Thing>>, handle: Res<SomeThingHandle>) {
for event in events.iter() {
if event.is_loaded_with_dependencies(&handle) {
}
}
}
```
Note the new `AssetEvent::LoadedWithDependencies`, which only fires when
the asset is loaded _and_ all dependencies (and their dependencies) have
loaded.
Unlike the old asset system, for a given asset path all `Handle<T>`
values point to the same underlying Arc. This means Handles can cheaply
hold more asset information, such as the AssetPath:
```rust
// prints the AssetPath of the handle
info!("{:?}", handle.path())
```
### Processed Assets
Asset processing can be enabled via the `AssetPlugin`. When developing
Bevy Apps with processed assets, do this:
```rust
app.add_plugins(DefaultPlugins.set(AssetPlugin::processed_dev()))
```
This runs the `AssetProcessor` in the background with hot-reloading. It
reads assets from the `assets` folder, processes them, and writes them
to the `.imported_assets` folder. Asset loads in the Bevy App will wait
for a processed version of the asset to become available. If an asset in
the `assets` folder changes, it will be reprocessed and hot-reloaded in
the Bevy App.
When deploying processed Bevy apps, do this:
```rust
app.add_plugins(DefaultPlugins.set(AssetPlugin::processed()))
```
This does not run the `AssetProcessor` in the background. It behaves
like `AssetPlugin::unprocessed()`, but reads assets from
`.imported_assets`.
When the `AssetProcessor` is running, it will populate sibling `.meta`
files for assets in the `assets` folder. Meta files for assets that do
not have a processor configured look like this:
```rust
(
meta_format_version: "1.0",
asset: Load(
loader: "bevy_render::texture::image_loader::ImageLoader",
settings: (
format: FromExtension,
),
),
)
```
This is metadata for an image asset. For example, if you have
`assets/my_sprite.png`, this could be the metadata stored at
`assets/my_sprite.png.meta`. Meta files are totally optional. If no
metadata exists, the default settings will be used.
In short, this file says "load this asset with the ImageLoader and use
the file extension to determine the image type". This type of meta file
is supported in all AssetPlugin modes. If in `Unprocessed` mode, the
asset (with the meta settings) will be loaded directly. If in
`ProcessedDev` mode, the asset file will be copied directly to the
`.imported_assets` folder. The meta will also be copied directly to the
`.imported_assets` folder, but with one addition:
```rust
(
meta_format_version: "1.0",
processed_info: Some((
hash: 12415480888597742505,
full_hash: 14344495437905856884,
process_dependencies: [],
)),
asset: Load(
loader: "bevy_render::texture::image_loader::ImageLoader",
settings: (
format: FromExtension,
),
),
)
```
`processed_info` contains `hash` (a direct hash of the asset and meta
bytes), `full_hash` (a hash of `hash` and the hashes of all
`process_dependencies`), and `process_dependencies` (the `path` and
`full_hash` of every process_dependency). A "process dependency" is an
asset dependency that is _directly_ used when processing the asset.
Images do not have process dependencies, so this is empty.
When the processor is enabled, you can use the `Process` metadata
config:
```rust
(
meta_format_version: "1.0",
asset: Process(
processor: "bevy_asset::processor::process::LoadAndSave<bevy_render::texture::image_loader::ImageLoader, bevy_render::texture::compressed_image_saver::CompressedImageSaver>",
settings: (
loader_settings: (
format: FromExtension,
),
saver_settings: (
generate_mipmaps: true,
),
),
),
)
```
This configures the asset to use the `LoadAndSave` processor, which runs
an AssetLoader and feeds the result into an AssetSaver (which saves the
given Asset and defines a loader to load it with). (for terseness
LoadAndSave will likely get a shorter/friendlier type name when [Stable
Type Paths](#7184) lands). `LoadAndSave` is likely to be the most common
processor type, but arbitrary processors are supported.
`CompressedImageSaver` saves an `Image` in the Basis Universal format
and configures the ImageLoader to load it as basis universal. The
`AssetProcessor` will read this meta, run it through the LoadAndSave
processor, and write the basis-universal version of the image to
`.imported_assets`. The final metadata will look like this:
```rust
(
meta_format_version: "1.0",
processed_info: Some((
hash: 905599590923828066,
full_hash: 9948823010183819117,
process_dependencies: [],
)),
asset: Load(
loader: "bevy_render::texture::image_loader::ImageLoader",
settings: (
format: Format(Basis),
),
),
)
```
To try basis-universal processing out in Bevy examples, (for example
`sprite.rs`), change `add_plugins(DefaultPlugins)` to
`add_plugins(DefaultPlugins.set(AssetPlugin::processed_dev()))` and run
with the `basis-universal` feature enabled: `cargo run
--features=basis-universal --example sprite`.
To create a custom processor, there are two main paths:
1. Use the `LoadAndSave` processor with an existing `AssetLoader`.
Implement the `AssetSaver` trait, register the processor using
`asset_processor.register_processor::<LoadAndSave<ImageLoader,
CompressedImageSaver>>(image_saver.into())`.
2. Implement the `Process` trait directly and register it using:
`asset_processor.register_processor(thing_processor)`.
You can configure default processors for file extensions like this:
```rust
asset_processor.set_default_processor::<ThingProcessor>("thing")
```
There is one more metadata type to be aware of:
```rust
(
meta_format_version: "1.0",
asset: Ignore,
)
```
This will ignore the asset during processing / prevent it from being
written to `.imported_assets`.
The AssetProcessor stores a transaction log at `.imported_assets/log`
and uses it to gracefully recover from unexpected stops. This means you
can force-quit the processor (and Bevy Apps running the processor in
parallel) at arbitrary times!
`.imported_assets` is "local state". It should _not_ be checked into
source control. It should also be considered "read only". In practice,
you _can_ modify processed assets and processed metadata if you really
need to test something. But those modifications will not be represented
in the hashes of the assets, so the processed state will be "out of
sync" with the source assets. The processor _will not_ fix this for you.
Either revert the change after you have tested it, or delete the
processed files so they can be re-populated.
## Open Questions
There are a number of open questions to be discussed. We should decide
if they need to be addressed in this PR and if so, how we will address
them:
### Implied Dependencies vs Dependency Enumeration
There are currently two ways to populate asset dependencies:
* **Implied via AssetLoaders**: if an AssetLoader loads an asset (and
retrieves a handle), a dependency is added to the list.
* **Explicit via the optional Asset::visit_dependencies**: if
`server.load_asset(my_asset)` is called, it will call
`my_asset.visit_dependencies`, which will grab dependencies that have
been manually defined for the asset via the Asset trait impl (which can
be derived).
This means that defining explicit dependencies is optional for "loaded
assets". And the list of dependencies is always accurate because loaders
can only produce Handles if they register dependencies. If an asset was
loaded with an AssetLoader, it only uses the implied dependencies. If an
asset was created at runtime and added with
`asset_server.load_asset(MyAsset)`, it will use
`Asset::visit_dependencies`.
However this can create a behavior mismatch between loaded assets and
equivalent "created at runtime" assets if `Assets::visit_dependencies`
doesn't exactly match the dependencies produced by the AssetLoader. This
behavior mismatch can be resolved by completely removing "implied loader
dependencies" and requiring `Asset::visit_dependencies` to supply
dependency data. But this creates two problems:
* It makes defining loaded assets harder and more error prone: Devs must
remember to manually annotate asset dependencies with `#[dependency]`
when deriving `Asset`. For more complicated assets (such as scenes), the
derive likely wouldn't be sufficient and a manual `visit_dependencies`
impl would be required.
* Removes the ability to immediately kick off dependency loads: When
AssetLoaders retrieve a Handle, they also immediately kick off an asset
load for the handle, which means it can start loading in parallel
_before_ the asset finishes loading. For large assets, this could be
significant. (although this could be mitigated for processed assets if
we store dependencies in the processed meta file and load them ahead of
time)
### Eager ProcessorDev Asset Loading
I made a controversial call in the interest of fast startup times ("time
to first pixel") for the "processor dev mode configuration". When
initializing the AssetProcessor, current processed versions of unchanged
assets are yielded immediately, even if their dependencies haven't been
checked yet for reprocessing. This means that
non-current-state-of-filesystem-but-previously-valid assets might be
returned to the App first, then hot-reloaded if/when their dependencies
change and the asset is reprocessed.
Is this behavior desirable? There is largely one alternative: do not
yield an asset from the processor to the app until all of its
dependencies have been checked for changes. In some common cases (load
dependency has not changed since last run) this will increase startup
time. The main question is "by how much" and is that slower startup time
worth it in the interest of only yielding assets that are true to the
current state of the filesystem. Should this be configurable? I'm
starting to think we should only yield an asset after its (historical)
dependencies have been checked for changes + processed as necessary, but
I'm curious what you all think.
### Paths Are Currently The Only Canonical ID / Do We Want Asset UUIDs?
In this implementation AssetPaths are the only canonical asset
identifier (just like the previous Bevy Asset system and Godot). Moving
assets will result in re-scans (and currently reprocessing, although
reprocessing can easily be avoided with some changes). Asset
renames/moves will break code and assets that rely on specific paths,
unless those paths are fixed up.
Do we want / need "stable asset uuids"? Introducing them is very
possible:
1. Generate a UUID and include it in .meta files
2. Support UUID in AssetPath
3. Generate "asset indices" which are loaded on startup and map UUIDs to
paths.
4 (maybe). Consider only supporting UUIDs for processed assets so we can
generate quick-to-load indices instead of scanning meta files.
The main "pro" is that assets referencing UUIDs don't need to be
migrated when a path changes. The main "con" is that UUIDs cannot be
"lazily resolved" like paths. They need a full view of all assets to
answer the question "does this UUID exist". Which means UUIDs require
the AssetProcessor to fully finish startup scans before saying an asset
doesnt exist. And they essentially require asset pre-processing to use
in apps, because scanning all asset metadata files at runtime to resolve
a UUID is not viable for medium-to-large apps. It really requires a
pre-generated UUID index, which must be loaded before querying for
assets.
I personally think this should be investigated in a separate PR. Paths
aren't going anywhere ... _everyone_ uses filesystems (and
filesystem-like apis) to manage their asset source files. I consider
them permanent canonical asset information. Additionally, they behave
well for both processed and unprocessed asset modes. Given that Bevy is
supporting both, this feels like the right canonical ID to start with.
UUIDS (and maybe even other indexed-identifier types) can be added later
as necessary.
### Folder / File Naming Conventions
All asset processing config currently lives in the `.imported_assets`
folder. The processor transaction log is in `.imported_assets/log`.
Processed assets are added to `.imported_assets/Default`, which will
make migrating to processed asset profiles (ex: a
`.imported_assets/Mobile` profile) a non-breaking change. It also allows
us to create top-level files like `.imported_assets/log` without it
being interpreted as an asset. Meta files currently have a `.meta`
suffix. Do we like these names and conventions?
### Should the `AssetPlugin::processed_dev` configuration enable
`watch_for_changes` automatically?
Currently it does (which I think makes sense), but it does make it the
only configuration that enables watch_for_changes by default.
### Discuss on_loaded High Level Interface:
This PR includes a very rough "proof of concept" `on_loaded` system
adapter that uses the `LoadedWithDependencies` event in combination with
`asset_server.load_asset` dependency tracking to support this pattern
```rust
fn main() {
App::new()
.init_asset::<MyAssets>()
.add_systems(Update, on_loaded(create_array_texture))
.run();
}
#[derive(Asset, Clone)]
struct MyAssets {
#[dependency]
picture_of_my_cat: Handle<Image>,
#[dependency]
picture_of_my_other_cat: Handle<Image>,
}
impl FromWorld for ArrayTexture {
fn from_world(world: &mut World) -> Self {
picture_of_my_cat: server.load("meow.png"),
picture_of_my_other_cat: server.load("meeeeeeeow.png"),
}
}
fn spawn_cat(In(my_assets): In<MyAssets>, mut commands: Commands) {
commands.spawn(SpriteBundle {
texture: my_assets.picture_of_my_cat.clone(),
..default()
});
commands.spawn(SpriteBundle {
texture: my_assets.picture_of_my_other_cat.clone(),
..default()
});
}
```
The implementation is _very_ rough. And it is currently unsafe because
`bevy_ecs` doesn't expose some internals to do this safely from inside
`bevy_asset`. There are plenty of unanswered questions like:
* "do we add a Loadable" derive? (effectively automate the FromWorld
implementation above)
* Should `MyAssets` even be an Asset? (largely implemented this way
because it elegantly builds on `server.load_asset(MyAsset { .. })`
dependency tracking).
We should think hard about what our ideal API looks like (and if this is
a pattern we want to support). Not necessarily something we need to
solve in this PR. The current `on_loaded` impl should probably be
removed from this PR before merging.
## Clarifying Questions
### What about Assets as Entities?
This Bevy Asset V2 proposal implementation initially stored Assets as
ECS Entities. Instead of `AssetId<T>` + the `Assets<T>` resource it used
`Entity` as the asset id and Asset values were just ECS components.
There are plenty of compelling reasons to do this:
1. Easier to inline assets in Bevy Scenes (as they are "just" normal
entities + components)
2. More flexible queries: use the power of the ECS to filter assets (ex:
`Query<Mesh, With<Tree>>`).
3. Extensible. Users can add arbitrary component data to assets.
4. Things like "component visualization tools" work out of the box to
visualize asset data.
However Assets as Entities has a ton of caveats right now:
* We need to be able to allocate entity ids without a direct World
reference (aka rework id allocator in Entities ... i worked around this
in my prototypes by just pre allocating big chunks of entities)
* We want asset change events in addition to ECS change tracking ... how
do we populate them when mutations can come from anywhere? Do we use
Changed queries? This would require iterating over the change data for
all assets every frame. Is this acceptable or should we implement a new
"event based" component change detection option?
* Reconciling manually created assets with asset-system managed assets
has some nuance (ex: are they "loaded" / do they also have that
component metadata?)
* "how do we handle "static" / default entity handles" (ties in to the
Entity Indices discussion:
https://github.com/bevyengine/bevy/discussions/8319). This is necessary
for things like "built in" assets and default handles in things like
SpriteBundle.
* Storing asset information as a component makes it easy to "invalidate"
asset state by removing the component (or forcing modifications).
Ideally we have ways to lock this down (some combination of Rust type
privacy and ECS validation)
In practice, how we store and identify assets is a reasonably
superficial change (porting off of Assets as Entities and implementing
dedicated storage + ids took less than a day). So once we sort out the
remaining challenges the flip should be straightforward. Additionally, I
do still have "Assets as Entities" in my commit history, so we can reuse
that work. I personally think "assets as entities" is a good endgame,
but it also doesn't provide _significant_ value at the moment and it
certainly isn't ready yet with the current state of things.
### Why not Distill?
[Distill](https://github.com/amethyst/distill) is a high quality fully
featured asset system built in Rust. It is very natural to ask "why not
just use Distill?".
It is also worth calling out that for awhile, [we planned on adopting
Distill / I signed off on
it](https://github.com/bevyengine/bevy/issues/708).
However I think Bevy has a number of constraints that make Distill
adoption suboptimal:
* **Architectural Simplicity:**
* Distill's processor requires an in-memory database (lmdb) and RPC
networked API (using Cap'n Proto). Each of these introduces API
complexity that increases maintenance burden and "code grokability".
Ignoring tests, documentation, and examples, Distill has 24,237 lines of
Rust code (including generated code for RPC + database interactions). If
you ignore generated code, it has 11,499 lines.
* Bevy builds the AssetProcessor and AssetServer using pluggable
AssetReader/AssetWriter Rust traits with simple io interfaces. They do
not necessitate databases or RPC interfaces (although Readers/Writers
could use them if that is desired). Bevy Asset V2 (at the time of
writing this PR) is 5,384 lines of Rust code (ignoring tests,
documentation, and examples). Grain of salt: Distill does have more
features currently (ex: Asset Packing, GUIDS, remote-out-of-process
asset processor). I do plan to implement these features in Bevy Asset V2
and I personally highly doubt they will meaningfully close the 6115
lines-of-code gap.
* This complexity gap (which while illustrated by lines of code, is much
bigger than just that) is noteworthy to me. Bevy should be hackable and
there are pillars of Distill that are very hard to understand and
extend. This is a matter of opinion (and Bevy Asset V2 also has
complicated areas), but I think Bevy Asset V2 is much more approachable
for the average developer.
* Necessary disclaimer: counting lines of code is an extremely rough
complexity metric. Read the code and form your own opinions.
* **Optional Asset Processing:** Not all Bevy Apps (or Bevy App
developers) need / want asset preprocessing. Processing increases the
complexity of the development environment by introducing things like
meta files, imported asset storage, running processors in the
background, waiting for processing to finish, etc. Distill _requires_
preprocessing to work. With Bevy Asset V2 processing is fully opt-in.
The AssetServer isn't directly aware of asset processors at all.
AssetLoaders only care about converting bytes to runtime Assets ... they
don't know or care if the bytes were pre-processed or not. Processing is
"elegantly" (forgive my self-congratulatory phrasing) layered on top and
builds on the existing Asset system primitives.
* **Direct Filesystem Access to Processed Asset State:** Distill stores
processed assets in a database. This makes debugging / inspecting the
processed outputs harder (either requires special tooling to query the
database or they need to be "deployed" to be inspected). Bevy Asset V2,
on the other hand, stores processed assets in the filesystem (by default
... this is configurable). This makes interacting with the processed
state more natural. Note that both Godot and Unity's new asset system
store processed assets in the filesystem.
* **Portability**: Because Distill's processor uses lmdb and RPC
networking, it cannot be run on certain platforms (ex: lmdb is a
non-rust dependency that cannot run on the web, some platforms don't
support running network servers). Bevy should be able to process assets
everywhere (ex: run the Bevy Editor on the web, compile + process
shaders on mobile, etc). Distill does partially mitigate this problem by
supporting "streaming" assets via the RPC protocol, but this is not a
full solve from my perspective. And Bevy Asset V2 can (in theory) also
stream assets (without requiring RPC, although this isn't implemented
yet)
Note that I _do_ still think Distill would be a solid asset system for
Bevy. But I think the approach in this PR is a better solve for Bevy's
specific "asset system requirements".
### Doesn't async-fs just shim requests to "sync" `std::fs`? What is the
point?
"True async file io" has limited / spotty platform support. async-fs
(and the rust async ecosystem generally ... ex Tokio) currently use
async wrappers over std::fs that offload blocking requests to separate
threads. This may feel unsatisfying, but it _does_ still provide value
because it prevents our task pools from blocking on file system
operations (which would prevent progress when there are many tasks to
do, but all threads in a pool are currently blocking on file system
ops).
Additionally, using async APIs for our AssetReaders and AssetWriters
also provides value because we can later add support for "true async
file io" for platforms that support it. _And_ we can implement other
"true async io" asset backends (such as networked asset io).
## Draft TODO
- [x] Fill in missing filesystem event APIs: file removed event (which
is expressed as dangling RenameFrom events in some cases), file/folder
renamed event
- [x] Assets without loaders are not moved to the processed folder. This
breaks things like referenced `.bin` files for GLTFs. This should be
configurable per-non-asset-type.
- [x] Initial implementation of Reflect and FromReflect for Handle. The
"deserialization" parity bar is low here as this only worked with static
UUIDs in the old impl ... this is a non-trivial problem. Either we add a
Handle::AssetPath variant that gets "upgraded" to a strong handle on
scene load or we use a separate AssetRef type for Bevy scenes (which is
converted to a runtime Handle on load). This deserves its own discussion
in a different pr.
- [x] Populate read_asset_bytes hash when run by the processor (a bit of
a special case .. when run by the processor the processed meta will
contain the hash so we don't need to compute it on the spot, but we
don't want/need to read the meta when run by the main AssetServer)
- [x] Delay hot reloading: currently filesystem events are handled
immediately, which creates timing issues in some cases. For example hot
reloading images can sometimes break because the image isn't finished
writing. We should add a delay, likely similar to the [implementation in
this PR](https://github.com/bevyengine/bevy/pull/8503).
- [x] Port old platform-specific AssetIo implementations to the new
AssetReader interface (currently missing Android and web)
- [x] Resolve on_loaded unsafety (either by removing the API entirely or
removing the unsafe)
- [x] Runtime loader setting overrides
- [x] Remove remaining unwraps that should be error-handled. There are
number of TODOs here
- [x] Pretty AssetPath Display impl
- [x] Document more APIs
- [x] Resolve spurious "reloading because it has changed" events (to
repro run load_gltf with `processed_dev()`)
- [x] load_dependency hot reloading currently only works for processed
assets. If processing is disabled, load_dependency changes are not hot
reloaded.
- [x] Replace AssetInfo dependency load/fail counters with
`loading_dependencies: HashSet<UntypedAssetId>` to prevent reloads from
(potentially) breaking counters. Storing this will also enable
"dependency reloaded" events (see [Next Steps](#next-steps))
- [x] Re-add filesystem watcher cargo feature gate (currently it is not
optional)
- [ ] Migration Guide
- [ ] Changelog
## Followup TODO
- [ ] Replace "eager unchanged processed asset loading" behavior with
"don't returned unchanged processed asset until dependencies have been
checked".
- [ ] Add true `Ignore` AssetAction that does not copy the asset to the
imported_assets folder.
- [ ] Finish "live asset unloading" (ex: free up CPU asset memory after
uploading an image to the GPU), rethink RenderAssets, and port renderer
features. The `Assets` collection uses `Option<T>` for asset storage to
support its removal. (1) the Option might not actually be necessary ...
might be able to just remove from the collection entirely (2) need to
finalize removal apis
- [ ] Try replacing the "channel based" asset id recycling with
something a bit more efficient (ex: we might be able to use raw atomic
ints with some cleverness)
- [ ] Consider adding UUIDs to processed assets (scoped just to helping
identify moved assets ... not exposed to load queries ... see [Next
Steps](#next-steps))
- [ ] Store "last modified" source asset and meta timestamps in
processed meta files to enable skipping expensive hashing when the file
wasn't changed
- [ ] Fix "slow loop" handle drop fix
- [ ] Migrate to TypeName
- [x] Handle "loader preregistration". See #9429
## Next Steps
* **Configurable per-type defaults for AssetMeta**: It should be
possible to add configuration like "all png image meta should default to
using nearest sampling" (currently this hard-coded per-loader/processor
Settings::default() impls). Also see the "Folder Meta" bullet point.
* **Avoid Reprocessing on Asset Renames / Moves**: See the "canonical
asset ids" discussion in [Open Questions](#open-questions) and the
relevant bullet point in [Draft TODO](#draft-todo). Even without
canonical ids, folder renames could avoid reprocessing in some cases.
* **Multiple Asset Sources**: Expand AssetPath to support "asset source
names" and support multiple AssetReaders in the asset server (ex:
`webserver://some_path/image.png` backed by an Http webserver
AssetReader). The "default" asset reader would use normal
`some_path/image.png` paths. Ideally this works in combination with
multiple AssetWatchers for hot-reloading
* **Stable Type Names**: this pr removes the TypeUuid requirement from
assets in favor of `std::any::type_name`. This makes defining assets
easier (no need to generate a new uuid / use weird proc macro syntax).
It also makes reading meta files easier (because things have "friendly
names"). We also use type names for components in scene files. If they
are good enough for components, they are good enough for assets. And
consistency across Bevy pillars is desirable. However,
`std::any::type_name` is not guaranteed to be stable (although in
practice it is). We've developed a [stable type
path](https://github.com/bevyengine/bevy/pull/7184) to resolve this,
which should be adopted when it is ready.
* **Command Line Interface**: It should be possible to run the asset
processor in a separate process from the command line. This will also
require building a network-server-backed AssetReader to communicate
between the app and the processor. We've been planning to build a "bevy
cli" for awhile. This seems like a good excuse to build it.
* **Asset Packing**: This is largely an additive feature, so it made
sense to me to punt this until we've laid the foundations in this PR.
* **Per-Platform Processed Assets**: It should be possible to generate
assets for multiple platforms by supporting multiple "processor
profiles" per asset (ex: compress with format X on PC and Y on iOS). I
think there should probably be arbitrary "profiles" (which can be
separate from actual platforms), which are then assigned to a given
platform when generating the final asset distribution for that platform.
Ex: maybe devs want a "Mobile" profile that is shared between iOS and
Android. Or a "LowEnd" profile shared between web and mobile.
* **Versioning and Migrations**: Assets, Loaders, Savers, and Processors
need to have versions to determine if their schema is valid. If an asset
/ loader version is incompatible with the current version expected at
runtime, the processor should be able to migrate them. I think we should
try using Bevy Reflect for this, as it would allow us to load the old
version as a dynamic Reflect type without actually having the old Rust
type. It would also allow us to define "patches" to migrate between
versions (Bevy Reflect devs are currently working on patching). The
`.meta` file already has its own format version. Migrating that to new
versions should also be possible.
* **Real Copy-on-write AssetPaths**: Rust's actual Cow (clone-on-write
type) currently used by AssetPath can still result in String clones that
aren't actually necessary (cloning an Owned Cow clones the contents).
Bevy's asset system requires cloning AssetPaths in a number of places,
which result in actual clones of the internal Strings. This is not
efficient. AssetPath internals should be reworked to exhibit truer
cow-like-behavior that reduces String clones to the absolute minimum.
* **Consider processor-less processing**: In theory the AssetServer
could run processors "inline" even if the background AssetProcessor is
disabled. If we decide this is actually desirable, we could add this.
But I don't think its a priority in the short or medium term.
* **Pre-emptive dependency loading**: We could encode dependencies in
processed meta files, which could then be used by the Asset Server to
kick of dependency loads as early as possible (prior to starting the
actual asset load). Is this desirable? How much time would this save in
practice?
* **Optimize Processor With UntypedAssetIds**: The processor exclusively
uses AssetPath to identify assets currently. It might be possible to
swap these out for UntypedAssetIds in some places, which are smaller /
cheaper to hash and compare.
* **One to Many Asset Processing**: An asset source file that produces
many assets currently must be processed into a single "processed" asset
source. If labeled assets can be written separately they can each have
their own configured savers _and_ they could be loaded more granularly.
Definitely worth exploring!
* **Automatically Track "Runtime-only" Asset Dependencies**: Right now,
tracking "created at runtime" asset dependencies requires adding them
via `asset_server.load_asset(StandardMaterial::default())`. I think with
some cleverness we could also do this for
`materials.add(StandardMaterial::default())`, making tracking work
"everywhere". There are challenges here relating to change detection /
ensuring the server is made aware of dependency changes. This could be
expensive in some cases.
* **"Dependency Changed" events**: Some assets have runtime artifacts
that need to be re-generated when one of their dependencies change (ex:
regenerate a material's bind group when a Texture needs to change). We
are generating the dependency graph so we can definitely produce these
events. Buuuuut generating these events will have a cost / they could be
high frequency for some assets, so we might want this to be opt-in for
specific cases.
* **Investigate Storing More Information In Handles**: Handles can now
store arbitrary information, which makes it cheaper and easier to
access. How much should we move into them? Canonical asset load states
(via atomics)? (`handle.is_loaded()` would be very cool). Should we
store the entire asset and remove the `Assets<T>` collection?
(`Arc<RwLock<Option<Image>>>`?)
* **Support processing and loading files without extensions**: This is a
pretty arbitrary restriction and could be supported with very minimal
changes.
* **Folder Meta**: It would be nice if we could define per folder
processor configuration defaults (likely in a `.meta` or `.folder_meta`
file). Things like "default to linear filtering for all Images in this
folder".
* **Replace async_broadcast with event-listener?** This might be
approximately drop-in for some uses and it feels more light weight
* **Support Running the AssetProcessor on the Web**: Most of the hard
work is done here, but there are some easy straggling TODOs (make the
transaction log an interface instead of a direct file writer so we can
write a web storage backend, implement an AssetReader/AssetWriter that
reads/writes to something like LocalStorage).
* **Consider identifying and preventing circular dependencies**: This is
especially important for "processor dependencies", as processing will
silently never finish in these cases.
* **Built-in/Inlined Asset Hot Reloading**: This PR regresses
"built-in/inlined" asset hot reloading (previously provided by the
DebugAssetServer). I'm intentionally punting this because I think it can
be cleanly implemented with "multiple asset sources" by registering a
"debug asset source" (ex: `debug://bevy_pbr/src/render/pbr.wgsl` asset
paths) in combination with an AssetWatcher for that asset source and
support for "manually loading pats with asset bytes instead of
AssetReaders". The old DebugAssetServer was quite nasty and I'd love to
avoid that hackery going forward.
* **Investigate ways to remove double-parsing meta files**: Parsing meta
files currently involves parsing once with "minimal" versions of the
meta file to extract the type name of the loader/processor config, then
parsing again to parse the "full" meta. This is suboptimal. We should be
able to define custom deserializers that (1) assume the loader/processor
type name comes first (2) dynamically looks up the loader/processor
registrations to deserialize settings in-line (similar to components in
the bevy scene format). Another alternative: deserialize as dynamic
Reflect objects and then convert.
* **More runtime loading configuration**: Support using the Handle type
as a hint to select an asset loader (instead of relying on AssetPath
extensions)
* **More high level Processor trait implementations**: For example, it
might be worth adding support for arbitrary chains of "asset transforms"
that modify an in-memory asset representation between loading and
saving. (ex: load a Mesh, run a `subdivide_mesh` transform, followed by
a `flip_normals` transform, then save the mesh to an efficient
compressed format).
* **Bevy Scene Handle Deserialization**: (see the relevant [Draft TODO
item](#draft-todo) for context)
* **Explore High Level Load Interfaces**: See [this
discussion](#discuss-on_loaded-high-level-interface) for one prototype.
* **Asset Streaming**: It would be great if we could stream Assets (ex:
stream a long video file piece by piece)
* **ID Exchanging**: In this PR Asset Handles/AssetIds are bigger than
they need to be because they have a Uuid enum variant. If we implement
an "id exchanging" system that trades Uuids for "efficient runtime ids",
we can cut down on the size of AssetIds, making them more efficient.
This has some open design questions, such as how to spawn entities with
"default" handle values (as these wouldn't have access to the exchange
api in the current system).
* **Asset Path Fixup Tooling**: Assets that inline asset paths inside
them will break when an asset moves. The asset system provides the
functionality to detect when paths break. We should build a framework
that enables formats to define "path migrations". This is especially
important for scene files. For editor-generated files, we should also
consider using UUIDs (see other bullet point) to avoid the need to
migrate in these cases.
---------
Co-authored-by: BeastLe9enD <beastle9end@outlook.de>
Co-authored-by: Mike <mike.hsu@gmail.com>
Co-authored-by: Nicola Papale <nicopap@users.noreply.github.com>
2023-09-07 02:07:27 +00:00
|
|
|
/// #[derive(AsBindGroup, Debug, Clone, Asset, TypePath)]
|
Better Materials: AsBindGroup trait and derive, simpler Material trait (#5053)
# Objective
This PR reworks Bevy's Material system, making the user experience of defining Materials _much_ nicer. Bevy's previous material system leaves a lot to be desired:
* Materials require manually implementing the `RenderAsset` trait, which involves manually generating the bind group, handling gpu buffer data transfer, looking up image textures, etc. Even the simplest single-texture material involves writing ~80 unnecessary lines of code. This was never the long term plan.
* There are two material traits, which is confusing, hard to document, and often redundant: `Material` and `SpecializedMaterial`. `Material` implicitly implements `SpecializedMaterial`, and `SpecializedMaterial` is used in most high level apis to support both use cases. Most users shouldn't need to think about specialization at all (I consider it a "power-user tool"), so the fact that `SpecializedMaterial` is front-and-center in our apis is a miss.
* Implementing either material trait involves a lot of "type soup". The "prepared asset" parameter is particularly heinous: `&<Self as RenderAsset>::PreparedAsset`. Defining vertex and fragment shaders is also more verbose than it needs to be.
## Solution
Say hello to the new `Material` system:
```rust
#[derive(AsBindGroup, TypeUuid, Debug, Clone)]
#[uuid = "f690fdae-d598-45ab-8225-97e2a3f056e0"]
pub struct CoolMaterial {
#[uniform(0)]
color: Color,
#[texture(1)]
#[sampler(2)]
color_texture: Handle<Image>,
}
impl Material for CoolMaterial {
fn fragment_shader() -> ShaderRef {
"cool_material.wgsl".into()
}
}
```
Thats it! This same material would have required [~80 lines of complicated "type heavy" code](https://github.com/bevyengine/bevy/blob/v0.7.0/examples/shader/shader_material.rs) in the old Material system. Now it is just 14 lines of simple, readable code.
This is thanks to a new consolidated `Material` trait and the new `AsBindGroup` trait / derive.
### The new `Material` trait
The old "split" `Material` and `SpecializedMaterial` traits have been removed in favor of a new consolidated `Material` trait. All of the functions on the trait are optional.
The difficulty of implementing `Material` has been reduced by simplifying dataflow and removing type complexity:
```rust
// Old
impl Material for CustomMaterial {
fn fragment_shader(asset_server: &AssetServer) -> Option<Handle<Shader>> {
Some(asset_server.load("custom_material.wgsl"))
}
fn alpha_mode(render_asset: &<Self as RenderAsset>::PreparedAsset) -> AlphaMode {
render_asset.alpha_mode
}
}
// New
impl Material for CustomMaterial {
fn fragment_shader() -> ShaderRef {
"custom_material.wgsl".into()
}
fn alpha_mode(&self) -> AlphaMode {
self.alpha_mode
}
}
```
Specialization is still supported, but it is hidden by default under the `specialize()` function (more on this later).
### The `AsBindGroup` trait / derive
The `Material` trait now requires the `AsBindGroup` derive. This can be implemented manually relatively easily, but deriving it will almost always be preferable.
Field attributes like `uniform` and `texture` are used to define which fields should be bindings,
what their binding type is, and what index they should be bound at:
```rust
#[derive(AsBindGroup)]
struct CoolMaterial {
#[uniform(0)]
color: Color,
#[texture(1)]
#[sampler(2)]
color_texture: Handle<Image>,
}
```
In WGSL shaders, the binding looks like this:
```wgsl
struct CoolMaterial {
color: vec4<f32>;
};
[[group(1), binding(0)]]
var<uniform> material: CoolMaterial;
[[group(1), binding(1)]]
var color_texture: texture_2d<f32>;
[[group(1), binding(2)]]
var color_sampler: sampler;
```
Note that the "group" index is determined by the usage context. It is not defined in `AsBindGroup`. Bevy material bind groups are bound to group 1.
The following field-level attributes are supported:
* `uniform(BINDING_INDEX)`
* The field will be converted to a shader-compatible type using the `ShaderType` trait, written to a `Buffer`, and bound as a uniform. It can also be derived for custom structs.
* `texture(BINDING_INDEX)`
* This field's `Handle<Image>` will be used to look up the matching `Texture` gpu resource, which will be bound as a texture in shaders. The field will be assumed to implement `Into<Option<Handle<Image>>>`. In practice, most fields should be a `Handle<Image>` or `Option<Handle<Image>>`. If the value of an `Option<Handle<Image>>` is `None`, the new `FallbackImage` resource will be used instead. This attribute can be used in conjunction with a `sampler` binding attribute (with a different binding index).
* `sampler(BINDING_INDEX)`
* Behaves exactly like the `texture` attribute, but sets the Image's sampler binding instead of the texture.
Note that fields without field-level binding attributes will be ignored.
```rust
#[derive(AsBindGroup)]
struct CoolMaterial {
#[uniform(0)]
color: Color,
this_field_is_ignored: String,
}
```
As mentioned above, `Option<Handle<Image>>` is also supported:
```rust
#[derive(AsBindGroup)]
struct CoolMaterial {
#[uniform(0)]
color: Color,
#[texture(1)]
#[sampler(2)]
color_texture: Option<Handle<Image>>,
}
```
This is useful if you want a texture to be optional. When the value is `None`, the `FallbackImage` will be used for the binding instead, which defaults to "pure white".
Field uniforms with the same binding index will be combined into a single binding:
```rust
#[derive(AsBindGroup)]
struct CoolMaterial {
#[uniform(0)]
color: Color,
#[uniform(0)]
roughness: f32,
}
```
In WGSL shaders, the binding would look like this:
```wgsl
struct CoolMaterial {
color: vec4<f32>;
roughness: f32;
};
[[group(1), binding(0)]]
var<uniform> material: CoolMaterial;
```
Some less common scenarios will require "struct-level" attributes. These are the currently supported struct-level attributes:
* `uniform(BINDING_INDEX, ConvertedShaderType)`
* Similar to the field-level `uniform` attribute, but instead the entire `AsBindGroup` value is converted to `ConvertedShaderType`, which must implement `ShaderType`. This is useful if more complicated conversion logic is required.
* `bind_group_data(DataType)`
* The `AsBindGroup` type will be converted to some `DataType` using `Into<DataType>` and stored as `AsBindGroup::Data` as part of the `AsBindGroup::as_bind_group` call. This is useful if data needs to be stored alongside the generated bind group, such as a unique identifier for a material's bind group. The most common use case for this attribute is "shader pipeline specialization".
The previous `CoolMaterial` example illustrating "combining multiple field-level uniform attributes with the same binding index" can
also be equivalently represented with a single struct-level uniform attribute:
```rust
#[derive(AsBindGroup)]
#[uniform(0, CoolMaterialUniform)]
struct CoolMaterial {
color: Color,
roughness: f32,
}
#[derive(ShaderType)]
struct CoolMaterialUniform {
color: Color,
roughness: f32,
}
impl From<&CoolMaterial> for CoolMaterialUniform {
fn from(material: &CoolMaterial) -> CoolMaterialUniform {
CoolMaterialUniform {
color: material.color,
roughness: material.roughness,
}
}
}
```
### Material Specialization
Material shader specialization is now _much_ simpler:
```rust
#[derive(AsBindGroup, TypeUuid, Debug, Clone)]
#[uuid = "f690fdae-d598-45ab-8225-97e2a3f056e0"]
#[bind_group_data(CoolMaterialKey)]
struct CoolMaterial {
#[uniform(0)]
color: Color,
is_red: bool,
}
#[derive(Copy, Clone, Hash, Eq, PartialEq)]
struct CoolMaterialKey {
is_red: bool,
}
impl From<&CoolMaterial> for CoolMaterialKey {
fn from(material: &CoolMaterial) -> CoolMaterialKey {
CoolMaterialKey {
is_red: material.is_red,
}
}
}
impl Material for CoolMaterial {
fn fragment_shader() -> ShaderRef {
"cool_material.wgsl".into()
}
fn specialize(
pipeline: &MaterialPipeline<Self>,
descriptor: &mut RenderPipelineDescriptor,
layout: &MeshVertexBufferLayout,
key: MaterialPipelineKey<Self>,
) -> Result<(), SpecializedMeshPipelineError> {
if key.bind_group_data.is_red {
let fragment = descriptor.fragment.as_mut().unwrap();
fragment.shader_defs.push("IS_RED".to_string());
}
Ok(())
}
}
```
Setting `bind_group_data` is not required for specialization (it defaults to `()`). Scenarios like "custom vertex attributes" also benefit from this system:
```rust
impl Material for CustomMaterial {
fn vertex_shader() -> ShaderRef {
"custom_material.wgsl".into()
}
fn fragment_shader() -> ShaderRef {
"custom_material.wgsl".into()
}
fn specialize(
pipeline: &MaterialPipeline<Self>,
descriptor: &mut RenderPipelineDescriptor,
layout: &MeshVertexBufferLayout,
key: MaterialPipelineKey<Self>,
) -> Result<(), SpecializedMeshPipelineError> {
let vertex_layout = layout.get_layout(&[
Mesh::ATTRIBUTE_POSITION.at_shader_location(0),
ATTRIBUTE_BLEND_COLOR.at_shader_location(1),
])?;
descriptor.vertex.buffers = vec![vertex_layout];
Ok(())
}
}
```
### Ported `StandardMaterial` to the new `Material` system
Bevy's built-in PBR material uses the new Material system (including the AsBindGroup derive):
```rust
#[derive(AsBindGroup, Debug, Clone, TypeUuid)]
#[uuid = "7494888b-c082-457b-aacf-517228cc0c22"]
#[bind_group_data(StandardMaterialKey)]
#[uniform(0, StandardMaterialUniform)]
pub struct StandardMaterial {
pub base_color: Color,
#[texture(1)]
#[sampler(2)]
pub base_color_texture: Option<Handle<Image>>,
/* other fields omitted for brevity */
```
### Ported Bevy examples to the new `Material` system
The overall complexity of Bevy's "custom shader examples" has gone down significantly. Take a look at the diffs if you want a dopamine spike.
Please note that while this PR has a net increase in "lines of code", most of those extra lines come from added documentation. There is a significant reduction
in the overall complexity of the code (even accounting for the new derive logic).
---
## Changelog
### Added
* `AsBindGroup` trait and derive, which make it much easier to transfer data to the gpu and generate bind groups for a given type.
### Changed
* The old `Material` and `SpecializedMaterial` traits have been replaced by a consolidated (much simpler) `Material` trait. Materials no longer implement `RenderAsset`.
* `StandardMaterial` was ported to the new material system. There are no user-facing api changes to the `StandardMaterial` struct api, but it now implements `AsBindGroup` and `Material` instead of `RenderAsset` and `SpecializedMaterial`.
## Migration Guide
The Material system has been reworked to be much simpler. We've removed a lot of boilerplate with the new `AsBindGroup` derive and the `Material` trait is simpler as well!
### Bevy 0.7 (old)
```rust
#[derive(Debug, Clone, TypeUuid)]
#[uuid = "f690fdae-d598-45ab-8225-97e2a3f056e0"]
pub struct CustomMaterial {
color: Color,
color_texture: Handle<Image>,
}
#[derive(Clone)]
pub struct GpuCustomMaterial {
_buffer: Buffer,
bind_group: BindGroup,
}
impl RenderAsset for CustomMaterial {
type ExtractedAsset = CustomMaterial;
type PreparedAsset = GpuCustomMaterial;
type Param = (SRes<RenderDevice>, SRes<MaterialPipeline<Self>>);
fn extract_asset(&self) -> Self::ExtractedAsset {
self.clone()
}
fn prepare_asset(
extracted_asset: Self::ExtractedAsset,
(render_device, material_pipeline): &mut SystemParamItem<Self::Param>,
) -> Result<Self::PreparedAsset, PrepareAssetError<Self::ExtractedAsset>> {
let color = Vec4::from_slice(&extracted_asset.color.as_linear_rgba_f32());
let byte_buffer = [0u8; Vec4::SIZE.get() as usize];
let mut buffer = encase::UniformBuffer::new(byte_buffer);
buffer.write(&color).unwrap();
let buffer = render_device.create_buffer_with_data(&BufferInitDescriptor {
contents: buffer.as_ref(),
label: None,
usage: BufferUsages::UNIFORM | BufferUsages::COPY_DST,
});
let (texture_view, texture_sampler) = if let Some(result) = material_pipeline
.mesh_pipeline
.get_image_texture(gpu_images, &Some(extracted_asset.color_texture.clone()))
{
result
} else {
return Err(PrepareAssetError::RetryNextUpdate(extracted_asset));
};
let bind_group = render_device.create_bind_group(&BindGroupDescriptor {
entries: &[
BindGroupEntry {
binding: 0,
resource: buffer.as_entire_binding(),
},
BindGroupEntry {
binding: 0,
resource: BindingResource::TextureView(texture_view),
},
BindGroupEntry {
binding: 1,
resource: BindingResource::Sampler(texture_sampler),
},
],
label: None,
layout: &material_pipeline.material_layout,
});
Ok(GpuCustomMaterial {
_buffer: buffer,
bind_group,
})
}
}
impl Material for CustomMaterial {
fn fragment_shader(asset_server: &AssetServer) -> Option<Handle<Shader>> {
Some(asset_server.load("custom_material.wgsl"))
}
fn bind_group(render_asset: &<Self as RenderAsset>::PreparedAsset) -> &BindGroup {
&render_asset.bind_group
}
fn bind_group_layout(render_device: &RenderDevice) -> BindGroupLayout {
render_device.create_bind_group_layout(&BindGroupLayoutDescriptor {
entries: &[
BindGroupLayoutEntry {
binding: 0,
visibility: ShaderStages::FRAGMENT,
ty: BindingType::Buffer {
ty: BufferBindingType::Uniform,
has_dynamic_offset: false,
min_binding_size: Some(Vec4::min_size()),
},
count: None,
},
BindGroupLayoutEntry {
binding: 1,
visibility: ShaderStages::FRAGMENT,
ty: BindingType::Texture {
multisampled: false,
sample_type: TextureSampleType::Float { filterable: true },
view_dimension: TextureViewDimension::D2Array,
},
count: None,
},
BindGroupLayoutEntry {
binding: 2,
visibility: ShaderStages::FRAGMENT,
ty: BindingType::Sampler(SamplerBindingType::Filtering),
count: None,
},
],
label: None,
})
}
}
```
### Bevy 0.8 (new)
```rust
impl Material for CustomMaterial {
fn fragment_shader() -> ShaderRef {
"custom_material.wgsl".into()
}
}
#[derive(AsBindGroup, TypeUuid, Debug, Clone)]
#[uuid = "f690fdae-d598-45ab-8225-97e2a3f056e0"]
pub struct CustomMaterial {
#[uniform(0)]
color: Color,
#[texture(1)]
#[sampler(2)]
color_texture: Handle<Image>,
}
```
## Future Work
* Add support for more binding types (cubemaps, buffers, etc). This PR intentionally includes a bare minimum number of binding types to keep "reviewability" in check.
* Consider optionally eliding binding indices using binding names. `AsBindGroup` could pass in (optional?) reflection info as a "hint".
* This would make it possible for the derive to do this:
```rust
#[derive(AsBindGroup)]
pub struct CustomMaterial {
#[uniform]
color: Color,
#[texture]
#[sampler]
color_texture: Option<Handle<Image>>,
alpha_mode: AlphaMode,
}
```
* Or this
```rust
#[derive(AsBindGroup)]
pub struct CustomMaterial {
#[binding]
color: Color,
#[binding]
color_texture: Option<Handle<Image>>,
alpha_mode: AlphaMode,
}
```
* Or even this (if we flip to "include bindings by default")
```rust
#[derive(AsBindGroup)]
pub struct CustomMaterial {
color: Color,
color_texture: Option<Handle<Image>>,
#[binding(ignore)]
alpha_mode: AlphaMode,
}
```
* If we add the option to define custom draw functions for materials (which could be done in a type-erased way), I think that would be enough to support extra non-material bindings. Worth considering!
2022-06-30 23:48:46 +00:00
|
|
|
/// pub struct CustomMaterial {
|
|
|
|
/// // Uniform bindings must implement `ShaderType`, which will be used to convert the value to
|
|
|
|
/// // its shader-compatible equivalent. Most core math types already implement `ShaderType`.
|
|
|
|
/// #[uniform(0)]
|
Migrate from `LegacyColor` to `bevy_color::Color` (#12163)
# Objective
- As part of the migration process we need to a) see the end effect of
the migration on user ergonomics b) check for serious perf regressions
c) actually migrate the code
- To accomplish this, I'm going to attempt to migrate all of the
remaining user-facing usages of `LegacyColor` in one PR, being careful
to keep a clean commit history.
- Fixes #12056.
## Solution
I've chosen to use the polymorphic `Color` type as our standard
user-facing API.
- [x] Migrate `bevy_gizmos`.
- [x] Take `impl Into<Color>` in all `bevy_gizmos` APIs
- [x] Migrate sprites
- [x] Migrate UI
- [x] Migrate `ColorMaterial`
- [x] Migrate `MaterialMesh2D`
- [x] Migrate fog
- [x] Migrate lights
- [x] Migrate StandardMaterial
- [x] Migrate wireframes
- [x] Migrate clear color
- [x] Migrate text
- [x] Migrate gltf loader
- [x] Register color types for reflection
- [x] Remove `LegacyColor`
- [x] Make sure CI passes
Incidental improvements to ease migration:
- added `Color::srgba_u8`, `Color::srgba_from_array` and friends
- added `set_alpha`, `is_fully_transparent` and `is_fully_opaque` to the
`Alpha` trait
- add and immediately deprecate (lol) `Color::rgb` and friends in favor
of more explicit and consistent `Color::srgb`
- standardized on white and black for most example text colors
- added vector field traits to `LinearRgba`: ~~`Add`, `Sub`,
`AddAssign`, `SubAssign`,~~ `Mul<f32>` and `Div<f32>`. Multiplications
and divisions do not scale alpha. `Add` and `Sub` have been cut from
this PR.
- added `LinearRgba` and `Srgba` `RED/GREEN/BLUE`
- added `LinearRgba_to_f32_array` and `LinearRgba::to_u32`
## Migration Guide
Bevy's color types have changed! Wherever you used a
`bevy::render::Color`, a `bevy::color::Color` is used instead.
These are quite similar! Both are enums storing a color in a specific
color space (or to be more precise, using a specific color model).
However, each of the different color models now has its own type.
TODO...
- `Color::rgba`, `Color::rgb`, `Color::rbga_u8`, `Color::rgb_u8`,
`Color::rgb_from_array` are now `Color::srgba`, `Color::srgb`,
`Color::srgba_u8`, `Color::srgb_u8` and `Color::srgb_from_array`.
- `Color::set_a` and `Color::a` is now `Color::set_alpha` and
`Color::alpha`. These are part of the `Alpha` trait in `bevy_color`.
- `Color::is_fully_transparent` is now part of the `Alpha` trait in
`bevy_color`
- `Color::r`, `Color::set_r`, `Color::with_r` and the equivalents for
`g`, `b` `h`, `s` and `l` have been removed due to causing silent
relatively expensive conversions. Convert your `Color` into the desired
color space, perform your operations there, and then convert it back
into a polymorphic `Color` enum.
- `Color::hex` is now `Srgba::hex`. Call `.into` or construct a
`Color::Srgba` variant manually to convert it.
- `WireframeMaterial`, `ExtractedUiNode`, `ExtractedDirectionalLight`,
`ExtractedPointLight`, `ExtractedSpotLight` and `ExtractedSprite` now
store a `LinearRgba`, rather than a polymorphic `Color`
- `Color::rgb_linear` and `Color::rgba_linear` are now
`Color::linear_rgb` and `Color::linear_rgba`
- The various CSS color constants are no longer stored directly on
`Color`. Instead, they're defined in the `Srgba` color space, and
accessed via `bevy::color::palettes::css`. Call `.into()` on them to
convert them into a `Color` for quick debugging use, and consider using
the much prettier `tailwind` palette for prototyping.
- The `LIME_GREEN` color has been renamed to `LIMEGREEN` to comply with
the standard naming.
- Vector field arithmetic operations on `Color` (add, subtract, multiply
and divide by a f32) have been removed. Instead, convert your colors
into `LinearRgba` space, and perform your operations explicitly there.
This is particularly relevant when working with emissive or HDR colors,
whose color channel values are routinely outside of the ordinary 0 to 1
range.
- `Color::as_linear_rgba_f32` has been removed. Call
`LinearRgba::to_f32_array` instead, converting if needed.
- `Color::as_linear_rgba_u32` has been removed. Call
`LinearRgba::to_u32` instead, converting if needed.
- Several other color conversion methods to transform LCH or HSL colors
into float arrays or `Vec` types have been removed. Please reimplement
these externally or open a PR to re-add them if you found them
particularly useful.
- Various methods on `Color` such as `rgb` or `hsl` to convert the color
into a specific color space have been removed. Convert into
`LinearRgba`, then to the color space of your choice.
- Various implicitly-converting color value methods on `Color` such as
`r`, `g`, `b` or `h` have been removed. Please convert it into the color
space of your choice, then check these properties.
- `Color` no longer implements `AsBindGroup`. Store a `LinearRgba`
internally instead to avoid conversion costs.
---------
Co-authored-by: Alice Cecile <alice.i.cecil@gmail.com>
Co-authored-by: Afonso Lage <lage.afonso@gmail.com>
Co-authored-by: Rob Parrett <robparrett@gmail.com>
Co-authored-by: Zachary Harrold <zac@harrold.com.au>
2024-02-29 19:35:12 +00:00
|
|
|
/// color: LinearRgba,
|
Better Materials: AsBindGroup trait and derive, simpler Material trait (#5053)
# Objective
This PR reworks Bevy's Material system, making the user experience of defining Materials _much_ nicer. Bevy's previous material system leaves a lot to be desired:
* Materials require manually implementing the `RenderAsset` trait, which involves manually generating the bind group, handling gpu buffer data transfer, looking up image textures, etc. Even the simplest single-texture material involves writing ~80 unnecessary lines of code. This was never the long term plan.
* There are two material traits, which is confusing, hard to document, and often redundant: `Material` and `SpecializedMaterial`. `Material` implicitly implements `SpecializedMaterial`, and `SpecializedMaterial` is used in most high level apis to support both use cases. Most users shouldn't need to think about specialization at all (I consider it a "power-user tool"), so the fact that `SpecializedMaterial` is front-and-center in our apis is a miss.
* Implementing either material trait involves a lot of "type soup". The "prepared asset" parameter is particularly heinous: `&<Self as RenderAsset>::PreparedAsset`. Defining vertex and fragment shaders is also more verbose than it needs to be.
## Solution
Say hello to the new `Material` system:
```rust
#[derive(AsBindGroup, TypeUuid, Debug, Clone)]
#[uuid = "f690fdae-d598-45ab-8225-97e2a3f056e0"]
pub struct CoolMaterial {
#[uniform(0)]
color: Color,
#[texture(1)]
#[sampler(2)]
color_texture: Handle<Image>,
}
impl Material for CoolMaterial {
fn fragment_shader() -> ShaderRef {
"cool_material.wgsl".into()
}
}
```
Thats it! This same material would have required [~80 lines of complicated "type heavy" code](https://github.com/bevyengine/bevy/blob/v0.7.0/examples/shader/shader_material.rs) in the old Material system. Now it is just 14 lines of simple, readable code.
This is thanks to a new consolidated `Material` trait and the new `AsBindGroup` trait / derive.
### The new `Material` trait
The old "split" `Material` and `SpecializedMaterial` traits have been removed in favor of a new consolidated `Material` trait. All of the functions on the trait are optional.
The difficulty of implementing `Material` has been reduced by simplifying dataflow and removing type complexity:
```rust
// Old
impl Material for CustomMaterial {
fn fragment_shader(asset_server: &AssetServer) -> Option<Handle<Shader>> {
Some(asset_server.load("custom_material.wgsl"))
}
fn alpha_mode(render_asset: &<Self as RenderAsset>::PreparedAsset) -> AlphaMode {
render_asset.alpha_mode
}
}
// New
impl Material for CustomMaterial {
fn fragment_shader() -> ShaderRef {
"custom_material.wgsl".into()
}
fn alpha_mode(&self) -> AlphaMode {
self.alpha_mode
}
}
```
Specialization is still supported, but it is hidden by default under the `specialize()` function (more on this later).
### The `AsBindGroup` trait / derive
The `Material` trait now requires the `AsBindGroup` derive. This can be implemented manually relatively easily, but deriving it will almost always be preferable.
Field attributes like `uniform` and `texture` are used to define which fields should be bindings,
what their binding type is, and what index they should be bound at:
```rust
#[derive(AsBindGroup)]
struct CoolMaterial {
#[uniform(0)]
color: Color,
#[texture(1)]
#[sampler(2)]
color_texture: Handle<Image>,
}
```
In WGSL shaders, the binding looks like this:
```wgsl
struct CoolMaterial {
color: vec4<f32>;
};
[[group(1), binding(0)]]
var<uniform> material: CoolMaterial;
[[group(1), binding(1)]]
var color_texture: texture_2d<f32>;
[[group(1), binding(2)]]
var color_sampler: sampler;
```
Note that the "group" index is determined by the usage context. It is not defined in `AsBindGroup`. Bevy material bind groups are bound to group 1.
The following field-level attributes are supported:
* `uniform(BINDING_INDEX)`
* The field will be converted to a shader-compatible type using the `ShaderType` trait, written to a `Buffer`, and bound as a uniform. It can also be derived for custom structs.
* `texture(BINDING_INDEX)`
* This field's `Handle<Image>` will be used to look up the matching `Texture` gpu resource, which will be bound as a texture in shaders. The field will be assumed to implement `Into<Option<Handle<Image>>>`. In practice, most fields should be a `Handle<Image>` or `Option<Handle<Image>>`. If the value of an `Option<Handle<Image>>` is `None`, the new `FallbackImage` resource will be used instead. This attribute can be used in conjunction with a `sampler` binding attribute (with a different binding index).
* `sampler(BINDING_INDEX)`
* Behaves exactly like the `texture` attribute, but sets the Image's sampler binding instead of the texture.
Note that fields without field-level binding attributes will be ignored.
```rust
#[derive(AsBindGroup)]
struct CoolMaterial {
#[uniform(0)]
color: Color,
this_field_is_ignored: String,
}
```
As mentioned above, `Option<Handle<Image>>` is also supported:
```rust
#[derive(AsBindGroup)]
struct CoolMaterial {
#[uniform(0)]
color: Color,
#[texture(1)]
#[sampler(2)]
color_texture: Option<Handle<Image>>,
}
```
This is useful if you want a texture to be optional. When the value is `None`, the `FallbackImage` will be used for the binding instead, which defaults to "pure white".
Field uniforms with the same binding index will be combined into a single binding:
```rust
#[derive(AsBindGroup)]
struct CoolMaterial {
#[uniform(0)]
color: Color,
#[uniform(0)]
roughness: f32,
}
```
In WGSL shaders, the binding would look like this:
```wgsl
struct CoolMaterial {
color: vec4<f32>;
roughness: f32;
};
[[group(1), binding(0)]]
var<uniform> material: CoolMaterial;
```
Some less common scenarios will require "struct-level" attributes. These are the currently supported struct-level attributes:
* `uniform(BINDING_INDEX, ConvertedShaderType)`
* Similar to the field-level `uniform` attribute, but instead the entire `AsBindGroup` value is converted to `ConvertedShaderType`, which must implement `ShaderType`. This is useful if more complicated conversion logic is required.
* `bind_group_data(DataType)`
* The `AsBindGroup` type will be converted to some `DataType` using `Into<DataType>` and stored as `AsBindGroup::Data` as part of the `AsBindGroup::as_bind_group` call. This is useful if data needs to be stored alongside the generated bind group, such as a unique identifier for a material's bind group. The most common use case for this attribute is "shader pipeline specialization".
The previous `CoolMaterial` example illustrating "combining multiple field-level uniform attributes with the same binding index" can
also be equivalently represented with a single struct-level uniform attribute:
```rust
#[derive(AsBindGroup)]
#[uniform(0, CoolMaterialUniform)]
struct CoolMaterial {
color: Color,
roughness: f32,
}
#[derive(ShaderType)]
struct CoolMaterialUniform {
color: Color,
roughness: f32,
}
impl From<&CoolMaterial> for CoolMaterialUniform {
fn from(material: &CoolMaterial) -> CoolMaterialUniform {
CoolMaterialUniform {
color: material.color,
roughness: material.roughness,
}
}
}
```
### Material Specialization
Material shader specialization is now _much_ simpler:
```rust
#[derive(AsBindGroup, TypeUuid, Debug, Clone)]
#[uuid = "f690fdae-d598-45ab-8225-97e2a3f056e0"]
#[bind_group_data(CoolMaterialKey)]
struct CoolMaterial {
#[uniform(0)]
color: Color,
is_red: bool,
}
#[derive(Copy, Clone, Hash, Eq, PartialEq)]
struct CoolMaterialKey {
is_red: bool,
}
impl From<&CoolMaterial> for CoolMaterialKey {
fn from(material: &CoolMaterial) -> CoolMaterialKey {
CoolMaterialKey {
is_red: material.is_red,
}
}
}
impl Material for CoolMaterial {
fn fragment_shader() -> ShaderRef {
"cool_material.wgsl".into()
}
fn specialize(
pipeline: &MaterialPipeline<Self>,
descriptor: &mut RenderPipelineDescriptor,
layout: &MeshVertexBufferLayout,
key: MaterialPipelineKey<Self>,
) -> Result<(), SpecializedMeshPipelineError> {
if key.bind_group_data.is_red {
let fragment = descriptor.fragment.as_mut().unwrap();
fragment.shader_defs.push("IS_RED".to_string());
}
Ok(())
}
}
```
Setting `bind_group_data` is not required for specialization (it defaults to `()`). Scenarios like "custom vertex attributes" also benefit from this system:
```rust
impl Material for CustomMaterial {
fn vertex_shader() -> ShaderRef {
"custom_material.wgsl".into()
}
fn fragment_shader() -> ShaderRef {
"custom_material.wgsl".into()
}
fn specialize(
pipeline: &MaterialPipeline<Self>,
descriptor: &mut RenderPipelineDescriptor,
layout: &MeshVertexBufferLayout,
key: MaterialPipelineKey<Self>,
) -> Result<(), SpecializedMeshPipelineError> {
let vertex_layout = layout.get_layout(&[
Mesh::ATTRIBUTE_POSITION.at_shader_location(0),
ATTRIBUTE_BLEND_COLOR.at_shader_location(1),
])?;
descriptor.vertex.buffers = vec![vertex_layout];
Ok(())
}
}
```
### Ported `StandardMaterial` to the new `Material` system
Bevy's built-in PBR material uses the new Material system (including the AsBindGroup derive):
```rust
#[derive(AsBindGroup, Debug, Clone, TypeUuid)]
#[uuid = "7494888b-c082-457b-aacf-517228cc0c22"]
#[bind_group_data(StandardMaterialKey)]
#[uniform(0, StandardMaterialUniform)]
pub struct StandardMaterial {
pub base_color: Color,
#[texture(1)]
#[sampler(2)]
pub base_color_texture: Option<Handle<Image>>,
/* other fields omitted for brevity */
```
### Ported Bevy examples to the new `Material` system
The overall complexity of Bevy's "custom shader examples" has gone down significantly. Take a look at the diffs if you want a dopamine spike.
Please note that while this PR has a net increase in "lines of code", most of those extra lines come from added documentation. There is a significant reduction
in the overall complexity of the code (even accounting for the new derive logic).
---
## Changelog
### Added
* `AsBindGroup` trait and derive, which make it much easier to transfer data to the gpu and generate bind groups for a given type.
### Changed
* The old `Material` and `SpecializedMaterial` traits have been replaced by a consolidated (much simpler) `Material` trait. Materials no longer implement `RenderAsset`.
* `StandardMaterial` was ported to the new material system. There are no user-facing api changes to the `StandardMaterial` struct api, but it now implements `AsBindGroup` and `Material` instead of `RenderAsset` and `SpecializedMaterial`.
## Migration Guide
The Material system has been reworked to be much simpler. We've removed a lot of boilerplate with the new `AsBindGroup` derive and the `Material` trait is simpler as well!
### Bevy 0.7 (old)
```rust
#[derive(Debug, Clone, TypeUuid)]
#[uuid = "f690fdae-d598-45ab-8225-97e2a3f056e0"]
pub struct CustomMaterial {
color: Color,
color_texture: Handle<Image>,
}
#[derive(Clone)]
pub struct GpuCustomMaterial {
_buffer: Buffer,
bind_group: BindGroup,
}
impl RenderAsset for CustomMaterial {
type ExtractedAsset = CustomMaterial;
type PreparedAsset = GpuCustomMaterial;
type Param = (SRes<RenderDevice>, SRes<MaterialPipeline<Self>>);
fn extract_asset(&self) -> Self::ExtractedAsset {
self.clone()
}
fn prepare_asset(
extracted_asset: Self::ExtractedAsset,
(render_device, material_pipeline): &mut SystemParamItem<Self::Param>,
) -> Result<Self::PreparedAsset, PrepareAssetError<Self::ExtractedAsset>> {
let color = Vec4::from_slice(&extracted_asset.color.as_linear_rgba_f32());
let byte_buffer = [0u8; Vec4::SIZE.get() as usize];
let mut buffer = encase::UniformBuffer::new(byte_buffer);
buffer.write(&color).unwrap();
let buffer = render_device.create_buffer_with_data(&BufferInitDescriptor {
contents: buffer.as_ref(),
label: None,
usage: BufferUsages::UNIFORM | BufferUsages::COPY_DST,
});
let (texture_view, texture_sampler) = if let Some(result) = material_pipeline
.mesh_pipeline
.get_image_texture(gpu_images, &Some(extracted_asset.color_texture.clone()))
{
result
} else {
return Err(PrepareAssetError::RetryNextUpdate(extracted_asset));
};
let bind_group = render_device.create_bind_group(&BindGroupDescriptor {
entries: &[
BindGroupEntry {
binding: 0,
resource: buffer.as_entire_binding(),
},
BindGroupEntry {
binding: 0,
resource: BindingResource::TextureView(texture_view),
},
BindGroupEntry {
binding: 1,
resource: BindingResource::Sampler(texture_sampler),
},
],
label: None,
layout: &material_pipeline.material_layout,
});
Ok(GpuCustomMaterial {
_buffer: buffer,
bind_group,
})
}
}
impl Material for CustomMaterial {
fn fragment_shader(asset_server: &AssetServer) -> Option<Handle<Shader>> {
Some(asset_server.load("custom_material.wgsl"))
}
fn bind_group(render_asset: &<Self as RenderAsset>::PreparedAsset) -> &BindGroup {
&render_asset.bind_group
}
fn bind_group_layout(render_device: &RenderDevice) -> BindGroupLayout {
render_device.create_bind_group_layout(&BindGroupLayoutDescriptor {
entries: &[
BindGroupLayoutEntry {
binding: 0,
visibility: ShaderStages::FRAGMENT,
ty: BindingType::Buffer {
ty: BufferBindingType::Uniform,
has_dynamic_offset: false,
min_binding_size: Some(Vec4::min_size()),
},
count: None,
},
BindGroupLayoutEntry {
binding: 1,
visibility: ShaderStages::FRAGMENT,
ty: BindingType::Texture {
multisampled: false,
sample_type: TextureSampleType::Float { filterable: true },
view_dimension: TextureViewDimension::D2Array,
},
count: None,
},
BindGroupLayoutEntry {
binding: 2,
visibility: ShaderStages::FRAGMENT,
ty: BindingType::Sampler(SamplerBindingType::Filtering),
count: None,
},
],
label: None,
})
}
}
```
### Bevy 0.8 (new)
```rust
impl Material for CustomMaterial {
fn fragment_shader() -> ShaderRef {
"custom_material.wgsl".into()
}
}
#[derive(AsBindGroup, TypeUuid, Debug, Clone)]
#[uuid = "f690fdae-d598-45ab-8225-97e2a3f056e0"]
pub struct CustomMaterial {
#[uniform(0)]
color: Color,
#[texture(1)]
#[sampler(2)]
color_texture: Handle<Image>,
}
```
## Future Work
* Add support for more binding types (cubemaps, buffers, etc). This PR intentionally includes a bare minimum number of binding types to keep "reviewability" in check.
* Consider optionally eliding binding indices using binding names. `AsBindGroup` could pass in (optional?) reflection info as a "hint".
* This would make it possible for the derive to do this:
```rust
#[derive(AsBindGroup)]
pub struct CustomMaterial {
#[uniform]
color: Color,
#[texture]
#[sampler]
color_texture: Option<Handle<Image>>,
alpha_mode: AlphaMode,
}
```
* Or this
```rust
#[derive(AsBindGroup)]
pub struct CustomMaterial {
#[binding]
color: Color,
#[binding]
color_texture: Option<Handle<Image>>,
alpha_mode: AlphaMode,
}
```
* Or even this (if we flip to "include bindings by default")
```rust
#[derive(AsBindGroup)]
pub struct CustomMaterial {
color: Color,
color_texture: Option<Handle<Image>>,
#[binding(ignore)]
alpha_mode: AlphaMode,
}
```
* If we add the option to define custom draw functions for materials (which could be done in a type-erased way), I think that would be enough to support extra non-material bindings. Worth considering!
2022-06-30 23:48:46 +00:00
|
|
|
/// // Images can be bound as textures in shaders. If the Image's sampler is also needed, just
|
|
|
|
/// // add the sampler attribute with a different binding index.
|
|
|
|
/// #[texture(1)]
|
|
|
|
/// #[sampler(2)]
|
|
|
|
/// color_texture: Handle<Image>,
|
|
|
|
/// }
|
|
|
|
///
|
|
|
|
/// // All functions on `Material` have default impls. You only need to implement the
|
|
|
|
/// // functions that are relevant for your material.
|
|
|
|
/// impl Material for CustomMaterial {
|
|
|
|
/// fn fragment_shader() -> ShaderRef {
|
|
|
|
/// "shaders/custom_material.wgsl".into()
|
|
|
|
/// }
|
|
|
|
/// }
|
|
|
|
///
|
Migrate meshes and materials to required components (#15524)
# Objective
A big step in the migration to required components: meshes and
materials!
## Solution
As per the [selected
proposal](https://hackmd.io/@bevy/required_components/%2Fj9-PnF-2QKK0on1KQ29UWQ):
- Deprecate `MaterialMesh2dBundle`, `MaterialMeshBundle`, and
`PbrBundle`.
- Add `Mesh2d` and `Mesh3d` components, which wrap a `Handle<Mesh>`.
- Add `MeshMaterial2d<M: Material2d>` and `MeshMaterial3d<M: Material>`,
which wrap a `Handle<M>`.
- Meshes *without* a mesh material should be rendered with a default
material. The existence of a material is determined by
`HasMaterial2d`/`HasMaterial3d`, which is required by
`MeshMaterial2d`/`MeshMaterial3d`. This gets around problems with the
generics.
Previously:
```rust
commands.spawn(MaterialMesh2dBundle {
mesh: meshes.add(Circle::new(100.0)).into(),
material: materials.add(Color::srgb(7.5, 0.0, 7.5)),
transform: Transform::from_translation(Vec3::new(-200., 0., 0.)),
..default()
});
```
Now:
```rust
commands.spawn((
Mesh2d(meshes.add(Circle::new(100.0))),
MeshMaterial2d(materials.add(Color::srgb(7.5, 0.0, 7.5))),
Transform::from_translation(Vec3::new(-200., 0., 0.)),
));
```
If the mesh material is missing, previously nothing was rendered. Now,
it renders a white default `ColorMaterial` in 2D and a
`StandardMaterial` in 3D (this can be overridden). Below, only every
other entity has a material:


Why white? This is still open for discussion, but I think white makes
sense for a *default* material, while *invalid* asset handles pointing
to nothing should have something like a pink material to indicate that
something is broken (I don't handle that in this PR yet). This is kind
of a mix of Godot and Unity: Godot just renders a white material for
non-existent materials, while Unity renders nothing when no materials
exist, but renders pink for invalid materials. I can also change the
default material to pink if that is preferable though.
## Testing
I ran some 2D and 3D examples to test if anything changed visually. I
have not tested all examples or features yet however. If anyone wants to
test more extensively, it would be appreciated!
## Implementation Notes
- The relationship between `bevy_render` and `bevy_pbr` is weird here.
`bevy_render` needs `Mesh3d` for its own systems, but `bevy_pbr` has all
of the material logic, and `bevy_render` doesn't depend on it. I feel
like the two crates should be refactored in some way, but I think that's
out of scope for this PR.
- I didn't migrate meshlets to required components yet. That can
probably be done in a follow-up, as this is already a huge PR.
- It is becoming increasingly clear to me that we really, *really* want
to disallow raw asset handles as components. They caused me a *ton* of
headache here already, and it took me a long time to find every place
that queried for them or inserted them directly on entities, since there
were no compiler errors for it. If we don't remove the `Component`
derive, I expect raw asset handles to be a *huge* footgun for users as
we transition to wrapper components, especially as handles as components
have been the norm so far. I personally consider this to be a blocker
for 0.15: we need to migrate to wrapper components for asset handles
everywhere, and remove the `Component` derive. Also see
https://github.com/bevyengine/bevy/issues/14124.
---
## Migration Guide
Asset handles for meshes and mesh materials must now be wrapped in the
`Mesh2d` and `MeshMaterial2d` or `Mesh3d` and `MeshMaterial3d`
components for 2D and 3D respectively. Raw handles as components no
longer render meshes.
Additionally, `MaterialMesh2dBundle`, `MaterialMeshBundle`, and
`PbrBundle` have been deprecated. Instead, use the mesh and material
components directly.
Previously:
```rust
commands.spawn(MaterialMesh2dBundle {
mesh: meshes.add(Circle::new(100.0)).into(),
material: materials.add(Color::srgb(7.5, 0.0, 7.5)),
transform: Transform::from_translation(Vec3::new(-200., 0., 0.)),
..default()
});
```
Now:
```rust
commands.spawn((
Mesh2d(meshes.add(Circle::new(100.0))),
MeshMaterial2d(materials.add(Color::srgb(7.5, 0.0, 7.5))),
Transform::from_translation(Vec3::new(-200., 0., 0.)),
));
```
If the mesh material is missing, a white default material is now used.
Previously, nothing was rendered if the material was missing.
The `WithMesh2d` and `WithMesh3d` query filter type aliases have also
been removed. Simply use `With<Mesh2d>` or `With<Mesh3d>`.
---------
Co-authored-by: Tim Blackbird <justthecooldude@gmail.com>
Co-authored-by: Carter Anderson <mcanders1@gmail.com>
2024-10-01 21:33:17 +00:00
|
|
|
/// // Spawn an entity with a mesh using `CustomMaterial`.
|
|
|
|
/// fn setup(
|
|
|
|
/// mut commands: Commands,
|
|
|
|
/// mut meshes: ResMut<Assets<Mesh>>,
|
|
|
|
/// mut materials: ResMut<Assets<CustomMaterial>>,
|
|
|
|
/// asset_server: Res<AssetServer>
|
|
|
|
/// ) {
|
|
|
|
/// commands.spawn((
|
|
|
|
/// Mesh3d(meshes.add(Capsule3d::default())),
|
|
|
|
/// MeshMaterial3d(materials.add(CustomMaterial {
|
Migrate from `LegacyColor` to `bevy_color::Color` (#12163)
# Objective
- As part of the migration process we need to a) see the end effect of
the migration on user ergonomics b) check for serious perf regressions
c) actually migrate the code
- To accomplish this, I'm going to attempt to migrate all of the
remaining user-facing usages of `LegacyColor` in one PR, being careful
to keep a clean commit history.
- Fixes #12056.
## Solution
I've chosen to use the polymorphic `Color` type as our standard
user-facing API.
- [x] Migrate `bevy_gizmos`.
- [x] Take `impl Into<Color>` in all `bevy_gizmos` APIs
- [x] Migrate sprites
- [x] Migrate UI
- [x] Migrate `ColorMaterial`
- [x] Migrate `MaterialMesh2D`
- [x] Migrate fog
- [x] Migrate lights
- [x] Migrate StandardMaterial
- [x] Migrate wireframes
- [x] Migrate clear color
- [x] Migrate text
- [x] Migrate gltf loader
- [x] Register color types for reflection
- [x] Remove `LegacyColor`
- [x] Make sure CI passes
Incidental improvements to ease migration:
- added `Color::srgba_u8`, `Color::srgba_from_array` and friends
- added `set_alpha`, `is_fully_transparent` and `is_fully_opaque` to the
`Alpha` trait
- add and immediately deprecate (lol) `Color::rgb` and friends in favor
of more explicit and consistent `Color::srgb`
- standardized on white and black for most example text colors
- added vector field traits to `LinearRgba`: ~~`Add`, `Sub`,
`AddAssign`, `SubAssign`,~~ `Mul<f32>` and `Div<f32>`. Multiplications
and divisions do not scale alpha. `Add` and `Sub` have been cut from
this PR.
- added `LinearRgba` and `Srgba` `RED/GREEN/BLUE`
- added `LinearRgba_to_f32_array` and `LinearRgba::to_u32`
## Migration Guide
Bevy's color types have changed! Wherever you used a
`bevy::render::Color`, a `bevy::color::Color` is used instead.
These are quite similar! Both are enums storing a color in a specific
color space (or to be more precise, using a specific color model).
However, each of the different color models now has its own type.
TODO...
- `Color::rgba`, `Color::rgb`, `Color::rbga_u8`, `Color::rgb_u8`,
`Color::rgb_from_array` are now `Color::srgba`, `Color::srgb`,
`Color::srgba_u8`, `Color::srgb_u8` and `Color::srgb_from_array`.
- `Color::set_a` and `Color::a` is now `Color::set_alpha` and
`Color::alpha`. These are part of the `Alpha` trait in `bevy_color`.
- `Color::is_fully_transparent` is now part of the `Alpha` trait in
`bevy_color`
- `Color::r`, `Color::set_r`, `Color::with_r` and the equivalents for
`g`, `b` `h`, `s` and `l` have been removed due to causing silent
relatively expensive conversions. Convert your `Color` into the desired
color space, perform your operations there, and then convert it back
into a polymorphic `Color` enum.
- `Color::hex` is now `Srgba::hex`. Call `.into` or construct a
`Color::Srgba` variant manually to convert it.
- `WireframeMaterial`, `ExtractedUiNode`, `ExtractedDirectionalLight`,
`ExtractedPointLight`, `ExtractedSpotLight` and `ExtractedSprite` now
store a `LinearRgba`, rather than a polymorphic `Color`
- `Color::rgb_linear` and `Color::rgba_linear` are now
`Color::linear_rgb` and `Color::linear_rgba`
- The various CSS color constants are no longer stored directly on
`Color`. Instead, they're defined in the `Srgba` color space, and
accessed via `bevy::color::palettes::css`. Call `.into()` on them to
convert them into a `Color` for quick debugging use, and consider using
the much prettier `tailwind` palette for prototyping.
- The `LIME_GREEN` color has been renamed to `LIMEGREEN` to comply with
the standard naming.
- Vector field arithmetic operations on `Color` (add, subtract, multiply
and divide by a f32) have been removed. Instead, convert your colors
into `LinearRgba` space, and perform your operations explicitly there.
This is particularly relevant when working with emissive or HDR colors,
whose color channel values are routinely outside of the ordinary 0 to 1
range.
- `Color::as_linear_rgba_f32` has been removed. Call
`LinearRgba::to_f32_array` instead, converting if needed.
- `Color::as_linear_rgba_u32` has been removed. Call
`LinearRgba::to_u32` instead, converting if needed.
- Several other color conversion methods to transform LCH or HSL colors
into float arrays or `Vec` types have been removed. Please reimplement
these externally or open a PR to re-add them if you found them
particularly useful.
- Various methods on `Color` such as `rgb` or `hsl` to convert the color
into a specific color space have been removed. Convert into
`LinearRgba`, then to the color space of your choice.
- Various implicitly-converting color value methods on `Color` such as
`r`, `g`, `b` or `h` have been removed. Please convert it into the color
space of your choice, then check these properties.
- `Color` no longer implements `AsBindGroup`. Store a `LinearRgba`
internally instead to avoid conversion costs.
---------
Co-authored-by: Alice Cecile <alice.i.cecil@gmail.com>
Co-authored-by: Afonso Lage <lage.afonso@gmail.com>
Co-authored-by: Rob Parrett <robparrett@gmail.com>
Co-authored-by: Zachary Harrold <zac@harrold.com.au>
2024-02-29 19:35:12 +00:00
|
|
|
/// color: RED.into(),
|
Better Materials: AsBindGroup trait and derive, simpler Material trait (#5053)
# Objective
This PR reworks Bevy's Material system, making the user experience of defining Materials _much_ nicer. Bevy's previous material system leaves a lot to be desired:
* Materials require manually implementing the `RenderAsset` trait, which involves manually generating the bind group, handling gpu buffer data transfer, looking up image textures, etc. Even the simplest single-texture material involves writing ~80 unnecessary lines of code. This was never the long term plan.
* There are two material traits, which is confusing, hard to document, and often redundant: `Material` and `SpecializedMaterial`. `Material` implicitly implements `SpecializedMaterial`, and `SpecializedMaterial` is used in most high level apis to support both use cases. Most users shouldn't need to think about specialization at all (I consider it a "power-user tool"), so the fact that `SpecializedMaterial` is front-and-center in our apis is a miss.
* Implementing either material trait involves a lot of "type soup". The "prepared asset" parameter is particularly heinous: `&<Self as RenderAsset>::PreparedAsset`. Defining vertex and fragment shaders is also more verbose than it needs to be.
## Solution
Say hello to the new `Material` system:
```rust
#[derive(AsBindGroup, TypeUuid, Debug, Clone)]
#[uuid = "f690fdae-d598-45ab-8225-97e2a3f056e0"]
pub struct CoolMaterial {
#[uniform(0)]
color: Color,
#[texture(1)]
#[sampler(2)]
color_texture: Handle<Image>,
}
impl Material for CoolMaterial {
fn fragment_shader() -> ShaderRef {
"cool_material.wgsl".into()
}
}
```
Thats it! This same material would have required [~80 lines of complicated "type heavy" code](https://github.com/bevyengine/bevy/blob/v0.7.0/examples/shader/shader_material.rs) in the old Material system. Now it is just 14 lines of simple, readable code.
This is thanks to a new consolidated `Material` trait and the new `AsBindGroup` trait / derive.
### The new `Material` trait
The old "split" `Material` and `SpecializedMaterial` traits have been removed in favor of a new consolidated `Material` trait. All of the functions on the trait are optional.
The difficulty of implementing `Material` has been reduced by simplifying dataflow and removing type complexity:
```rust
// Old
impl Material for CustomMaterial {
fn fragment_shader(asset_server: &AssetServer) -> Option<Handle<Shader>> {
Some(asset_server.load("custom_material.wgsl"))
}
fn alpha_mode(render_asset: &<Self as RenderAsset>::PreparedAsset) -> AlphaMode {
render_asset.alpha_mode
}
}
// New
impl Material for CustomMaterial {
fn fragment_shader() -> ShaderRef {
"custom_material.wgsl".into()
}
fn alpha_mode(&self) -> AlphaMode {
self.alpha_mode
}
}
```
Specialization is still supported, but it is hidden by default under the `specialize()` function (more on this later).
### The `AsBindGroup` trait / derive
The `Material` trait now requires the `AsBindGroup` derive. This can be implemented manually relatively easily, but deriving it will almost always be preferable.
Field attributes like `uniform` and `texture` are used to define which fields should be bindings,
what their binding type is, and what index they should be bound at:
```rust
#[derive(AsBindGroup)]
struct CoolMaterial {
#[uniform(0)]
color: Color,
#[texture(1)]
#[sampler(2)]
color_texture: Handle<Image>,
}
```
In WGSL shaders, the binding looks like this:
```wgsl
struct CoolMaterial {
color: vec4<f32>;
};
[[group(1), binding(0)]]
var<uniform> material: CoolMaterial;
[[group(1), binding(1)]]
var color_texture: texture_2d<f32>;
[[group(1), binding(2)]]
var color_sampler: sampler;
```
Note that the "group" index is determined by the usage context. It is not defined in `AsBindGroup`. Bevy material bind groups are bound to group 1.
The following field-level attributes are supported:
* `uniform(BINDING_INDEX)`
* The field will be converted to a shader-compatible type using the `ShaderType` trait, written to a `Buffer`, and bound as a uniform. It can also be derived for custom structs.
* `texture(BINDING_INDEX)`
* This field's `Handle<Image>` will be used to look up the matching `Texture` gpu resource, which will be bound as a texture in shaders. The field will be assumed to implement `Into<Option<Handle<Image>>>`. In practice, most fields should be a `Handle<Image>` or `Option<Handle<Image>>`. If the value of an `Option<Handle<Image>>` is `None`, the new `FallbackImage` resource will be used instead. This attribute can be used in conjunction with a `sampler` binding attribute (with a different binding index).
* `sampler(BINDING_INDEX)`
* Behaves exactly like the `texture` attribute, but sets the Image's sampler binding instead of the texture.
Note that fields without field-level binding attributes will be ignored.
```rust
#[derive(AsBindGroup)]
struct CoolMaterial {
#[uniform(0)]
color: Color,
this_field_is_ignored: String,
}
```
As mentioned above, `Option<Handle<Image>>` is also supported:
```rust
#[derive(AsBindGroup)]
struct CoolMaterial {
#[uniform(0)]
color: Color,
#[texture(1)]
#[sampler(2)]
color_texture: Option<Handle<Image>>,
}
```
This is useful if you want a texture to be optional. When the value is `None`, the `FallbackImage` will be used for the binding instead, which defaults to "pure white".
Field uniforms with the same binding index will be combined into a single binding:
```rust
#[derive(AsBindGroup)]
struct CoolMaterial {
#[uniform(0)]
color: Color,
#[uniform(0)]
roughness: f32,
}
```
In WGSL shaders, the binding would look like this:
```wgsl
struct CoolMaterial {
color: vec4<f32>;
roughness: f32;
};
[[group(1), binding(0)]]
var<uniform> material: CoolMaterial;
```
Some less common scenarios will require "struct-level" attributes. These are the currently supported struct-level attributes:
* `uniform(BINDING_INDEX, ConvertedShaderType)`
* Similar to the field-level `uniform` attribute, but instead the entire `AsBindGroup` value is converted to `ConvertedShaderType`, which must implement `ShaderType`. This is useful if more complicated conversion logic is required.
* `bind_group_data(DataType)`
* The `AsBindGroup` type will be converted to some `DataType` using `Into<DataType>` and stored as `AsBindGroup::Data` as part of the `AsBindGroup::as_bind_group` call. This is useful if data needs to be stored alongside the generated bind group, such as a unique identifier for a material's bind group. The most common use case for this attribute is "shader pipeline specialization".
The previous `CoolMaterial` example illustrating "combining multiple field-level uniform attributes with the same binding index" can
also be equivalently represented with a single struct-level uniform attribute:
```rust
#[derive(AsBindGroup)]
#[uniform(0, CoolMaterialUniform)]
struct CoolMaterial {
color: Color,
roughness: f32,
}
#[derive(ShaderType)]
struct CoolMaterialUniform {
color: Color,
roughness: f32,
}
impl From<&CoolMaterial> for CoolMaterialUniform {
fn from(material: &CoolMaterial) -> CoolMaterialUniform {
CoolMaterialUniform {
color: material.color,
roughness: material.roughness,
}
}
}
```
### Material Specialization
Material shader specialization is now _much_ simpler:
```rust
#[derive(AsBindGroup, TypeUuid, Debug, Clone)]
#[uuid = "f690fdae-d598-45ab-8225-97e2a3f056e0"]
#[bind_group_data(CoolMaterialKey)]
struct CoolMaterial {
#[uniform(0)]
color: Color,
is_red: bool,
}
#[derive(Copy, Clone, Hash, Eq, PartialEq)]
struct CoolMaterialKey {
is_red: bool,
}
impl From<&CoolMaterial> for CoolMaterialKey {
fn from(material: &CoolMaterial) -> CoolMaterialKey {
CoolMaterialKey {
is_red: material.is_red,
}
}
}
impl Material for CoolMaterial {
fn fragment_shader() -> ShaderRef {
"cool_material.wgsl".into()
}
fn specialize(
pipeline: &MaterialPipeline<Self>,
descriptor: &mut RenderPipelineDescriptor,
layout: &MeshVertexBufferLayout,
key: MaterialPipelineKey<Self>,
) -> Result<(), SpecializedMeshPipelineError> {
if key.bind_group_data.is_red {
let fragment = descriptor.fragment.as_mut().unwrap();
fragment.shader_defs.push("IS_RED".to_string());
}
Ok(())
}
}
```
Setting `bind_group_data` is not required for specialization (it defaults to `()`). Scenarios like "custom vertex attributes" also benefit from this system:
```rust
impl Material for CustomMaterial {
fn vertex_shader() -> ShaderRef {
"custom_material.wgsl".into()
}
fn fragment_shader() -> ShaderRef {
"custom_material.wgsl".into()
}
fn specialize(
pipeline: &MaterialPipeline<Self>,
descriptor: &mut RenderPipelineDescriptor,
layout: &MeshVertexBufferLayout,
key: MaterialPipelineKey<Self>,
) -> Result<(), SpecializedMeshPipelineError> {
let vertex_layout = layout.get_layout(&[
Mesh::ATTRIBUTE_POSITION.at_shader_location(0),
ATTRIBUTE_BLEND_COLOR.at_shader_location(1),
])?;
descriptor.vertex.buffers = vec![vertex_layout];
Ok(())
}
}
```
### Ported `StandardMaterial` to the new `Material` system
Bevy's built-in PBR material uses the new Material system (including the AsBindGroup derive):
```rust
#[derive(AsBindGroup, Debug, Clone, TypeUuid)]
#[uuid = "7494888b-c082-457b-aacf-517228cc0c22"]
#[bind_group_data(StandardMaterialKey)]
#[uniform(0, StandardMaterialUniform)]
pub struct StandardMaterial {
pub base_color: Color,
#[texture(1)]
#[sampler(2)]
pub base_color_texture: Option<Handle<Image>>,
/* other fields omitted for brevity */
```
### Ported Bevy examples to the new `Material` system
The overall complexity of Bevy's "custom shader examples" has gone down significantly. Take a look at the diffs if you want a dopamine spike.
Please note that while this PR has a net increase in "lines of code", most of those extra lines come from added documentation. There is a significant reduction
in the overall complexity of the code (even accounting for the new derive logic).
---
## Changelog
### Added
* `AsBindGroup` trait and derive, which make it much easier to transfer data to the gpu and generate bind groups for a given type.
### Changed
* The old `Material` and `SpecializedMaterial` traits have been replaced by a consolidated (much simpler) `Material` trait. Materials no longer implement `RenderAsset`.
* `StandardMaterial` was ported to the new material system. There are no user-facing api changes to the `StandardMaterial` struct api, but it now implements `AsBindGroup` and `Material` instead of `RenderAsset` and `SpecializedMaterial`.
## Migration Guide
The Material system has been reworked to be much simpler. We've removed a lot of boilerplate with the new `AsBindGroup` derive and the `Material` trait is simpler as well!
### Bevy 0.7 (old)
```rust
#[derive(Debug, Clone, TypeUuid)]
#[uuid = "f690fdae-d598-45ab-8225-97e2a3f056e0"]
pub struct CustomMaterial {
color: Color,
color_texture: Handle<Image>,
}
#[derive(Clone)]
pub struct GpuCustomMaterial {
_buffer: Buffer,
bind_group: BindGroup,
}
impl RenderAsset for CustomMaterial {
type ExtractedAsset = CustomMaterial;
type PreparedAsset = GpuCustomMaterial;
type Param = (SRes<RenderDevice>, SRes<MaterialPipeline<Self>>);
fn extract_asset(&self) -> Self::ExtractedAsset {
self.clone()
}
fn prepare_asset(
extracted_asset: Self::ExtractedAsset,
(render_device, material_pipeline): &mut SystemParamItem<Self::Param>,
) -> Result<Self::PreparedAsset, PrepareAssetError<Self::ExtractedAsset>> {
let color = Vec4::from_slice(&extracted_asset.color.as_linear_rgba_f32());
let byte_buffer = [0u8; Vec4::SIZE.get() as usize];
let mut buffer = encase::UniformBuffer::new(byte_buffer);
buffer.write(&color).unwrap();
let buffer = render_device.create_buffer_with_data(&BufferInitDescriptor {
contents: buffer.as_ref(),
label: None,
usage: BufferUsages::UNIFORM | BufferUsages::COPY_DST,
});
let (texture_view, texture_sampler) = if let Some(result) = material_pipeline
.mesh_pipeline
.get_image_texture(gpu_images, &Some(extracted_asset.color_texture.clone()))
{
result
} else {
return Err(PrepareAssetError::RetryNextUpdate(extracted_asset));
};
let bind_group = render_device.create_bind_group(&BindGroupDescriptor {
entries: &[
BindGroupEntry {
binding: 0,
resource: buffer.as_entire_binding(),
},
BindGroupEntry {
binding: 0,
resource: BindingResource::TextureView(texture_view),
},
BindGroupEntry {
binding: 1,
resource: BindingResource::Sampler(texture_sampler),
},
],
label: None,
layout: &material_pipeline.material_layout,
});
Ok(GpuCustomMaterial {
_buffer: buffer,
bind_group,
})
}
}
impl Material for CustomMaterial {
fn fragment_shader(asset_server: &AssetServer) -> Option<Handle<Shader>> {
Some(asset_server.load("custom_material.wgsl"))
}
fn bind_group(render_asset: &<Self as RenderAsset>::PreparedAsset) -> &BindGroup {
&render_asset.bind_group
}
fn bind_group_layout(render_device: &RenderDevice) -> BindGroupLayout {
render_device.create_bind_group_layout(&BindGroupLayoutDescriptor {
entries: &[
BindGroupLayoutEntry {
binding: 0,
visibility: ShaderStages::FRAGMENT,
ty: BindingType::Buffer {
ty: BufferBindingType::Uniform,
has_dynamic_offset: false,
min_binding_size: Some(Vec4::min_size()),
},
count: None,
},
BindGroupLayoutEntry {
binding: 1,
visibility: ShaderStages::FRAGMENT,
ty: BindingType::Texture {
multisampled: false,
sample_type: TextureSampleType::Float { filterable: true },
view_dimension: TextureViewDimension::D2Array,
},
count: None,
},
BindGroupLayoutEntry {
binding: 2,
visibility: ShaderStages::FRAGMENT,
ty: BindingType::Sampler(SamplerBindingType::Filtering),
count: None,
},
],
label: None,
})
}
}
```
### Bevy 0.8 (new)
```rust
impl Material for CustomMaterial {
fn fragment_shader() -> ShaderRef {
"custom_material.wgsl".into()
}
}
#[derive(AsBindGroup, TypeUuid, Debug, Clone)]
#[uuid = "f690fdae-d598-45ab-8225-97e2a3f056e0"]
pub struct CustomMaterial {
#[uniform(0)]
color: Color,
#[texture(1)]
#[sampler(2)]
color_texture: Handle<Image>,
}
```
## Future Work
* Add support for more binding types (cubemaps, buffers, etc). This PR intentionally includes a bare minimum number of binding types to keep "reviewability" in check.
* Consider optionally eliding binding indices using binding names. `AsBindGroup` could pass in (optional?) reflection info as a "hint".
* This would make it possible for the derive to do this:
```rust
#[derive(AsBindGroup)]
pub struct CustomMaterial {
#[uniform]
color: Color,
#[texture]
#[sampler]
color_texture: Option<Handle<Image>>,
alpha_mode: AlphaMode,
}
```
* Or this
```rust
#[derive(AsBindGroup)]
pub struct CustomMaterial {
#[binding]
color: Color,
#[binding]
color_texture: Option<Handle<Image>>,
alpha_mode: AlphaMode,
}
```
* Or even this (if we flip to "include bindings by default")
```rust
#[derive(AsBindGroup)]
pub struct CustomMaterial {
color: Color,
color_texture: Option<Handle<Image>>,
#[binding(ignore)]
alpha_mode: AlphaMode,
}
```
* If we add the option to define custom draw functions for materials (which could be done in a type-erased way), I think that would be enough to support extra non-material bindings. Worth considering!
2022-06-30 23:48:46 +00:00
|
|
|
/// color_texture: asset_server.load("some_image.png"),
|
Migrate meshes and materials to required components (#15524)
# Objective
A big step in the migration to required components: meshes and
materials!
## Solution
As per the [selected
proposal](https://hackmd.io/@bevy/required_components/%2Fj9-PnF-2QKK0on1KQ29UWQ):
- Deprecate `MaterialMesh2dBundle`, `MaterialMeshBundle`, and
`PbrBundle`.
- Add `Mesh2d` and `Mesh3d` components, which wrap a `Handle<Mesh>`.
- Add `MeshMaterial2d<M: Material2d>` and `MeshMaterial3d<M: Material>`,
which wrap a `Handle<M>`.
- Meshes *without* a mesh material should be rendered with a default
material. The existence of a material is determined by
`HasMaterial2d`/`HasMaterial3d`, which is required by
`MeshMaterial2d`/`MeshMaterial3d`. This gets around problems with the
generics.
Previously:
```rust
commands.spawn(MaterialMesh2dBundle {
mesh: meshes.add(Circle::new(100.0)).into(),
material: materials.add(Color::srgb(7.5, 0.0, 7.5)),
transform: Transform::from_translation(Vec3::new(-200., 0., 0.)),
..default()
});
```
Now:
```rust
commands.spawn((
Mesh2d(meshes.add(Circle::new(100.0))),
MeshMaterial2d(materials.add(Color::srgb(7.5, 0.0, 7.5))),
Transform::from_translation(Vec3::new(-200., 0., 0.)),
));
```
If the mesh material is missing, previously nothing was rendered. Now,
it renders a white default `ColorMaterial` in 2D and a
`StandardMaterial` in 3D (this can be overridden). Below, only every
other entity has a material:


Why white? This is still open for discussion, but I think white makes
sense for a *default* material, while *invalid* asset handles pointing
to nothing should have something like a pink material to indicate that
something is broken (I don't handle that in this PR yet). This is kind
of a mix of Godot and Unity: Godot just renders a white material for
non-existent materials, while Unity renders nothing when no materials
exist, but renders pink for invalid materials. I can also change the
default material to pink if that is preferable though.
## Testing
I ran some 2D and 3D examples to test if anything changed visually. I
have not tested all examples or features yet however. If anyone wants to
test more extensively, it would be appreciated!
## Implementation Notes
- The relationship between `bevy_render` and `bevy_pbr` is weird here.
`bevy_render` needs `Mesh3d` for its own systems, but `bevy_pbr` has all
of the material logic, and `bevy_render` doesn't depend on it. I feel
like the two crates should be refactored in some way, but I think that's
out of scope for this PR.
- I didn't migrate meshlets to required components yet. That can
probably be done in a follow-up, as this is already a huge PR.
- It is becoming increasingly clear to me that we really, *really* want
to disallow raw asset handles as components. They caused me a *ton* of
headache here already, and it took me a long time to find every place
that queried for them or inserted them directly on entities, since there
were no compiler errors for it. If we don't remove the `Component`
derive, I expect raw asset handles to be a *huge* footgun for users as
we transition to wrapper components, especially as handles as components
have been the norm so far. I personally consider this to be a blocker
for 0.15: we need to migrate to wrapper components for asset handles
everywhere, and remove the `Component` derive. Also see
https://github.com/bevyengine/bevy/issues/14124.
---
## Migration Guide
Asset handles for meshes and mesh materials must now be wrapped in the
`Mesh2d` and `MeshMaterial2d` or `Mesh3d` and `MeshMaterial3d`
components for 2D and 3D respectively. Raw handles as components no
longer render meshes.
Additionally, `MaterialMesh2dBundle`, `MaterialMeshBundle`, and
`PbrBundle` have been deprecated. Instead, use the mesh and material
components directly.
Previously:
```rust
commands.spawn(MaterialMesh2dBundle {
mesh: meshes.add(Circle::new(100.0)).into(),
material: materials.add(Color::srgb(7.5, 0.0, 7.5)),
transform: Transform::from_translation(Vec3::new(-200., 0., 0.)),
..default()
});
```
Now:
```rust
commands.spawn((
Mesh2d(meshes.add(Circle::new(100.0))),
MeshMaterial2d(materials.add(Color::srgb(7.5, 0.0, 7.5))),
Transform::from_translation(Vec3::new(-200., 0., 0.)),
));
```
If the mesh material is missing, a white default material is now used.
Previously, nothing was rendered if the material was missing.
The `WithMesh2d` and `WithMesh3d` query filter type aliases have also
been removed. Simply use `With<Mesh2d>` or `With<Mesh3d>`.
---------
Co-authored-by: Tim Blackbird <justthecooldude@gmail.com>
Co-authored-by: Carter Anderson <mcanders1@gmail.com>
2024-10-01 21:33:17 +00:00
|
|
|
/// })),
|
|
|
|
/// ));
|
Better Materials: AsBindGroup trait and derive, simpler Material trait (#5053)
# Objective
This PR reworks Bevy's Material system, making the user experience of defining Materials _much_ nicer. Bevy's previous material system leaves a lot to be desired:
* Materials require manually implementing the `RenderAsset` trait, which involves manually generating the bind group, handling gpu buffer data transfer, looking up image textures, etc. Even the simplest single-texture material involves writing ~80 unnecessary lines of code. This was never the long term plan.
* There are two material traits, which is confusing, hard to document, and often redundant: `Material` and `SpecializedMaterial`. `Material` implicitly implements `SpecializedMaterial`, and `SpecializedMaterial` is used in most high level apis to support both use cases. Most users shouldn't need to think about specialization at all (I consider it a "power-user tool"), so the fact that `SpecializedMaterial` is front-and-center in our apis is a miss.
* Implementing either material trait involves a lot of "type soup". The "prepared asset" parameter is particularly heinous: `&<Self as RenderAsset>::PreparedAsset`. Defining vertex and fragment shaders is also more verbose than it needs to be.
## Solution
Say hello to the new `Material` system:
```rust
#[derive(AsBindGroup, TypeUuid, Debug, Clone)]
#[uuid = "f690fdae-d598-45ab-8225-97e2a3f056e0"]
pub struct CoolMaterial {
#[uniform(0)]
color: Color,
#[texture(1)]
#[sampler(2)]
color_texture: Handle<Image>,
}
impl Material for CoolMaterial {
fn fragment_shader() -> ShaderRef {
"cool_material.wgsl".into()
}
}
```
Thats it! This same material would have required [~80 lines of complicated "type heavy" code](https://github.com/bevyengine/bevy/blob/v0.7.0/examples/shader/shader_material.rs) in the old Material system. Now it is just 14 lines of simple, readable code.
This is thanks to a new consolidated `Material` trait and the new `AsBindGroup` trait / derive.
### The new `Material` trait
The old "split" `Material` and `SpecializedMaterial` traits have been removed in favor of a new consolidated `Material` trait. All of the functions on the trait are optional.
The difficulty of implementing `Material` has been reduced by simplifying dataflow and removing type complexity:
```rust
// Old
impl Material for CustomMaterial {
fn fragment_shader(asset_server: &AssetServer) -> Option<Handle<Shader>> {
Some(asset_server.load("custom_material.wgsl"))
}
fn alpha_mode(render_asset: &<Self as RenderAsset>::PreparedAsset) -> AlphaMode {
render_asset.alpha_mode
}
}
// New
impl Material for CustomMaterial {
fn fragment_shader() -> ShaderRef {
"custom_material.wgsl".into()
}
fn alpha_mode(&self) -> AlphaMode {
self.alpha_mode
}
}
```
Specialization is still supported, but it is hidden by default under the `specialize()` function (more on this later).
### The `AsBindGroup` trait / derive
The `Material` trait now requires the `AsBindGroup` derive. This can be implemented manually relatively easily, but deriving it will almost always be preferable.
Field attributes like `uniform` and `texture` are used to define which fields should be bindings,
what their binding type is, and what index they should be bound at:
```rust
#[derive(AsBindGroup)]
struct CoolMaterial {
#[uniform(0)]
color: Color,
#[texture(1)]
#[sampler(2)]
color_texture: Handle<Image>,
}
```
In WGSL shaders, the binding looks like this:
```wgsl
struct CoolMaterial {
color: vec4<f32>;
};
[[group(1), binding(0)]]
var<uniform> material: CoolMaterial;
[[group(1), binding(1)]]
var color_texture: texture_2d<f32>;
[[group(1), binding(2)]]
var color_sampler: sampler;
```
Note that the "group" index is determined by the usage context. It is not defined in `AsBindGroup`. Bevy material bind groups are bound to group 1.
The following field-level attributes are supported:
* `uniform(BINDING_INDEX)`
* The field will be converted to a shader-compatible type using the `ShaderType` trait, written to a `Buffer`, and bound as a uniform. It can also be derived for custom structs.
* `texture(BINDING_INDEX)`
* This field's `Handle<Image>` will be used to look up the matching `Texture` gpu resource, which will be bound as a texture in shaders. The field will be assumed to implement `Into<Option<Handle<Image>>>`. In practice, most fields should be a `Handle<Image>` or `Option<Handle<Image>>`. If the value of an `Option<Handle<Image>>` is `None`, the new `FallbackImage` resource will be used instead. This attribute can be used in conjunction with a `sampler` binding attribute (with a different binding index).
* `sampler(BINDING_INDEX)`
* Behaves exactly like the `texture` attribute, but sets the Image's sampler binding instead of the texture.
Note that fields without field-level binding attributes will be ignored.
```rust
#[derive(AsBindGroup)]
struct CoolMaterial {
#[uniform(0)]
color: Color,
this_field_is_ignored: String,
}
```
As mentioned above, `Option<Handle<Image>>` is also supported:
```rust
#[derive(AsBindGroup)]
struct CoolMaterial {
#[uniform(0)]
color: Color,
#[texture(1)]
#[sampler(2)]
color_texture: Option<Handle<Image>>,
}
```
This is useful if you want a texture to be optional. When the value is `None`, the `FallbackImage` will be used for the binding instead, which defaults to "pure white".
Field uniforms with the same binding index will be combined into a single binding:
```rust
#[derive(AsBindGroup)]
struct CoolMaterial {
#[uniform(0)]
color: Color,
#[uniform(0)]
roughness: f32,
}
```
In WGSL shaders, the binding would look like this:
```wgsl
struct CoolMaterial {
color: vec4<f32>;
roughness: f32;
};
[[group(1), binding(0)]]
var<uniform> material: CoolMaterial;
```
Some less common scenarios will require "struct-level" attributes. These are the currently supported struct-level attributes:
* `uniform(BINDING_INDEX, ConvertedShaderType)`
* Similar to the field-level `uniform` attribute, but instead the entire `AsBindGroup` value is converted to `ConvertedShaderType`, which must implement `ShaderType`. This is useful if more complicated conversion logic is required.
* `bind_group_data(DataType)`
* The `AsBindGroup` type will be converted to some `DataType` using `Into<DataType>` and stored as `AsBindGroup::Data` as part of the `AsBindGroup::as_bind_group` call. This is useful if data needs to be stored alongside the generated bind group, such as a unique identifier for a material's bind group. The most common use case for this attribute is "shader pipeline specialization".
The previous `CoolMaterial` example illustrating "combining multiple field-level uniform attributes with the same binding index" can
also be equivalently represented with a single struct-level uniform attribute:
```rust
#[derive(AsBindGroup)]
#[uniform(0, CoolMaterialUniform)]
struct CoolMaterial {
color: Color,
roughness: f32,
}
#[derive(ShaderType)]
struct CoolMaterialUniform {
color: Color,
roughness: f32,
}
impl From<&CoolMaterial> for CoolMaterialUniform {
fn from(material: &CoolMaterial) -> CoolMaterialUniform {
CoolMaterialUniform {
color: material.color,
roughness: material.roughness,
}
}
}
```
### Material Specialization
Material shader specialization is now _much_ simpler:
```rust
#[derive(AsBindGroup, TypeUuid, Debug, Clone)]
#[uuid = "f690fdae-d598-45ab-8225-97e2a3f056e0"]
#[bind_group_data(CoolMaterialKey)]
struct CoolMaterial {
#[uniform(0)]
color: Color,
is_red: bool,
}
#[derive(Copy, Clone, Hash, Eq, PartialEq)]
struct CoolMaterialKey {
is_red: bool,
}
impl From<&CoolMaterial> for CoolMaterialKey {
fn from(material: &CoolMaterial) -> CoolMaterialKey {
CoolMaterialKey {
is_red: material.is_red,
}
}
}
impl Material for CoolMaterial {
fn fragment_shader() -> ShaderRef {
"cool_material.wgsl".into()
}
fn specialize(
pipeline: &MaterialPipeline<Self>,
descriptor: &mut RenderPipelineDescriptor,
layout: &MeshVertexBufferLayout,
key: MaterialPipelineKey<Self>,
) -> Result<(), SpecializedMeshPipelineError> {
if key.bind_group_data.is_red {
let fragment = descriptor.fragment.as_mut().unwrap();
fragment.shader_defs.push("IS_RED".to_string());
}
Ok(())
}
}
```
Setting `bind_group_data` is not required for specialization (it defaults to `()`). Scenarios like "custom vertex attributes" also benefit from this system:
```rust
impl Material for CustomMaterial {
fn vertex_shader() -> ShaderRef {
"custom_material.wgsl".into()
}
fn fragment_shader() -> ShaderRef {
"custom_material.wgsl".into()
}
fn specialize(
pipeline: &MaterialPipeline<Self>,
descriptor: &mut RenderPipelineDescriptor,
layout: &MeshVertexBufferLayout,
key: MaterialPipelineKey<Self>,
) -> Result<(), SpecializedMeshPipelineError> {
let vertex_layout = layout.get_layout(&[
Mesh::ATTRIBUTE_POSITION.at_shader_location(0),
ATTRIBUTE_BLEND_COLOR.at_shader_location(1),
])?;
descriptor.vertex.buffers = vec![vertex_layout];
Ok(())
}
}
```
### Ported `StandardMaterial` to the new `Material` system
Bevy's built-in PBR material uses the new Material system (including the AsBindGroup derive):
```rust
#[derive(AsBindGroup, Debug, Clone, TypeUuid)]
#[uuid = "7494888b-c082-457b-aacf-517228cc0c22"]
#[bind_group_data(StandardMaterialKey)]
#[uniform(0, StandardMaterialUniform)]
pub struct StandardMaterial {
pub base_color: Color,
#[texture(1)]
#[sampler(2)]
pub base_color_texture: Option<Handle<Image>>,
/* other fields omitted for brevity */
```
### Ported Bevy examples to the new `Material` system
The overall complexity of Bevy's "custom shader examples" has gone down significantly. Take a look at the diffs if you want a dopamine spike.
Please note that while this PR has a net increase in "lines of code", most of those extra lines come from added documentation. There is a significant reduction
in the overall complexity of the code (even accounting for the new derive logic).
---
## Changelog
### Added
* `AsBindGroup` trait and derive, which make it much easier to transfer data to the gpu and generate bind groups for a given type.
### Changed
* The old `Material` and `SpecializedMaterial` traits have been replaced by a consolidated (much simpler) `Material` trait. Materials no longer implement `RenderAsset`.
* `StandardMaterial` was ported to the new material system. There are no user-facing api changes to the `StandardMaterial` struct api, but it now implements `AsBindGroup` and `Material` instead of `RenderAsset` and `SpecializedMaterial`.
## Migration Guide
The Material system has been reworked to be much simpler. We've removed a lot of boilerplate with the new `AsBindGroup` derive and the `Material` trait is simpler as well!
### Bevy 0.7 (old)
```rust
#[derive(Debug, Clone, TypeUuid)]
#[uuid = "f690fdae-d598-45ab-8225-97e2a3f056e0"]
pub struct CustomMaterial {
color: Color,
color_texture: Handle<Image>,
}
#[derive(Clone)]
pub struct GpuCustomMaterial {
_buffer: Buffer,
bind_group: BindGroup,
}
impl RenderAsset for CustomMaterial {
type ExtractedAsset = CustomMaterial;
type PreparedAsset = GpuCustomMaterial;
type Param = (SRes<RenderDevice>, SRes<MaterialPipeline<Self>>);
fn extract_asset(&self) -> Self::ExtractedAsset {
self.clone()
}
fn prepare_asset(
extracted_asset: Self::ExtractedAsset,
(render_device, material_pipeline): &mut SystemParamItem<Self::Param>,
) -> Result<Self::PreparedAsset, PrepareAssetError<Self::ExtractedAsset>> {
let color = Vec4::from_slice(&extracted_asset.color.as_linear_rgba_f32());
let byte_buffer = [0u8; Vec4::SIZE.get() as usize];
let mut buffer = encase::UniformBuffer::new(byte_buffer);
buffer.write(&color).unwrap();
let buffer = render_device.create_buffer_with_data(&BufferInitDescriptor {
contents: buffer.as_ref(),
label: None,
usage: BufferUsages::UNIFORM | BufferUsages::COPY_DST,
});
let (texture_view, texture_sampler) = if let Some(result) = material_pipeline
.mesh_pipeline
.get_image_texture(gpu_images, &Some(extracted_asset.color_texture.clone()))
{
result
} else {
return Err(PrepareAssetError::RetryNextUpdate(extracted_asset));
};
let bind_group = render_device.create_bind_group(&BindGroupDescriptor {
entries: &[
BindGroupEntry {
binding: 0,
resource: buffer.as_entire_binding(),
},
BindGroupEntry {
binding: 0,
resource: BindingResource::TextureView(texture_view),
},
BindGroupEntry {
binding: 1,
resource: BindingResource::Sampler(texture_sampler),
},
],
label: None,
layout: &material_pipeline.material_layout,
});
Ok(GpuCustomMaterial {
_buffer: buffer,
bind_group,
})
}
}
impl Material for CustomMaterial {
fn fragment_shader(asset_server: &AssetServer) -> Option<Handle<Shader>> {
Some(asset_server.load("custom_material.wgsl"))
}
fn bind_group(render_asset: &<Self as RenderAsset>::PreparedAsset) -> &BindGroup {
&render_asset.bind_group
}
fn bind_group_layout(render_device: &RenderDevice) -> BindGroupLayout {
render_device.create_bind_group_layout(&BindGroupLayoutDescriptor {
entries: &[
BindGroupLayoutEntry {
binding: 0,
visibility: ShaderStages::FRAGMENT,
ty: BindingType::Buffer {
ty: BufferBindingType::Uniform,
has_dynamic_offset: false,
min_binding_size: Some(Vec4::min_size()),
},
count: None,
},
BindGroupLayoutEntry {
binding: 1,
visibility: ShaderStages::FRAGMENT,
ty: BindingType::Texture {
multisampled: false,
sample_type: TextureSampleType::Float { filterable: true },
view_dimension: TextureViewDimension::D2Array,
},
count: None,
},
BindGroupLayoutEntry {
binding: 2,
visibility: ShaderStages::FRAGMENT,
ty: BindingType::Sampler(SamplerBindingType::Filtering),
count: None,
},
],
label: None,
})
}
}
```
### Bevy 0.8 (new)
```rust
impl Material for CustomMaterial {
fn fragment_shader() -> ShaderRef {
"custom_material.wgsl".into()
}
}
#[derive(AsBindGroup, TypeUuid, Debug, Clone)]
#[uuid = "f690fdae-d598-45ab-8225-97e2a3f056e0"]
pub struct CustomMaterial {
#[uniform(0)]
color: Color,
#[texture(1)]
#[sampler(2)]
color_texture: Handle<Image>,
}
```
## Future Work
* Add support for more binding types (cubemaps, buffers, etc). This PR intentionally includes a bare minimum number of binding types to keep "reviewability" in check.
* Consider optionally eliding binding indices using binding names. `AsBindGroup` could pass in (optional?) reflection info as a "hint".
* This would make it possible for the derive to do this:
```rust
#[derive(AsBindGroup)]
pub struct CustomMaterial {
#[uniform]
color: Color,
#[texture]
#[sampler]
color_texture: Option<Handle<Image>>,
alpha_mode: AlphaMode,
}
```
* Or this
```rust
#[derive(AsBindGroup)]
pub struct CustomMaterial {
#[binding]
color: Color,
#[binding]
color_texture: Option<Handle<Image>>,
alpha_mode: AlphaMode,
}
```
* Or even this (if we flip to "include bindings by default")
```rust
#[derive(AsBindGroup)]
pub struct CustomMaterial {
color: Color,
color_texture: Option<Handle<Image>>,
#[binding(ignore)]
alpha_mode: AlphaMode,
}
```
* If we add the option to define custom draw functions for materials (which could be done in a type-erased way), I think that would be enough to support extra non-material bindings. Worth considering!
2022-06-30 23:48:46 +00:00
|
|
|
/// }
|
|
|
|
/// ```
|
Migrate meshes and materials to required components (#15524)
# Objective
A big step in the migration to required components: meshes and
materials!
## Solution
As per the [selected
proposal](https://hackmd.io/@bevy/required_components/%2Fj9-PnF-2QKK0on1KQ29UWQ):
- Deprecate `MaterialMesh2dBundle`, `MaterialMeshBundle`, and
`PbrBundle`.
- Add `Mesh2d` and `Mesh3d` components, which wrap a `Handle<Mesh>`.
- Add `MeshMaterial2d<M: Material2d>` and `MeshMaterial3d<M: Material>`,
which wrap a `Handle<M>`.
- Meshes *without* a mesh material should be rendered with a default
material. The existence of a material is determined by
`HasMaterial2d`/`HasMaterial3d`, which is required by
`MeshMaterial2d`/`MeshMaterial3d`. This gets around problems with the
generics.
Previously:
```rust
commands.spawn(MaterialMesh2dBundle {
mesh: meshes.add(Circle::new(100.0)).into(),
material: materials.add(Color::srgb(7.5, 0.0, 7.5)),
transform: Transform::from_translation(Vec3::new(-200., 0., 0.)),
..default()
});
```
Now:
```rust
commands.spawn((
Mesh2d(meshes.add(Circle::new(100.0))),
MeshMaterial2d(materials.add(Color::srgb(7.5, 0.0, 7.5))),
Transform::from_translation(Vec3::new(-200., 0., 0.)),
));
```
If the mesh material is missing, previously nothing was rendered. Now,
it renders a white default `ColorMaterial` in 2D and a
`StandardMaterial` in 3D (this can be overridden). Below, only every
other entity has a material:


Why white? This is still open for discussion, but I think white makes
sense for a *default* material, while *invalid* asset handles pointing
to nothing should have something like a pink material to indicate that
something is broken (I don't handle that in this PR yet). This is kind
of a mix of Godot and Unity: Godot just renders a white material for
non-existent materials, while Unity renders nothing when no materials
exist, but renders pink for invalid materials. I can also change the
default material to pink if that is preferable though.
## Testing
I ran some 2D and 3D examples to test if anything changed visually. I
have not tested all examples or features yet however. If anyone wants to
test more extensively, it would be appreciated!
## Implementation Notes
- The relationship between `bevy_render` and `bevy_pbr` is weird here.
`bevy_render` needs `Mesh3d` for its own systems, but `bevy_pbr` has all
of the material logic, and `bevy_render` doesn't depend on it. I feel
like the two crates should be refactored in some way, but I think that's
out of scope for this PR.
- I didn't migrate meshlets to required components yet. That can
probably be done in a follow-up, as this is already a huge PR.
- It is becoming increasingly clear to me that we really, *really* want
to disallow raw asset handles as components. They caused me a *ton* of
headache here already, and it took me a long time to find every place
that queried for them or inserted them directly on entities, since there
were no compiler errors for it. If we don't remove the `Component`
derive, I expect raw asset handles to be a *huge* footgun for users as
we transition to wrapper components, especially as handles as components
have been the norm so far. I personally consider this to be a blocker
for 0.15: we need to migrate to wrapper components for asset handles
everywhere, and remove the `Component` derive. Also see
https://github.com/bevyengine/bevy/issues/14124.
---
## Migration Guide
Asset handles for meshes and mesh materials must now be wrapped in the
`Mesh2d` and `MeshMaterial2d` or `Mesh3d` and `MeshMaterial3d`
components for 2D and 3D respectively. Raw handles as components no
longer render meshes.
Additionally, `MaterialMesh2dBundle`, `MaterialMeshBundle`, and
`PbrBundle` have been deprecated. Instead, use the mesh and material
components directly.
Previously:
```rust
commands.spawn(MaterialMesh2dBundle {
mesh: meshes.add(Circle::new(100.0)).into(),
material: materials.add(Color::srgb(7.5, 0.0, 7.5)),
transform: Transform::from_translation(Vec3::new(-200., 0., 0.)),
..default()
});
```
Now:
```rust
commands.spawn((
Mesh2d(meshes.add(Circle::new(100.0))),
MeshMaterial2d(materials.add(Color::srgb(7.5, 0.0, 7.5))),
Transform::from_translation(Vec3::new(-200., 0., 0.)),
));
```
If the mesh material is missing, a white default material is now used.
Previously, nothing was rendered if the material was missing.
The `WithMesh2d` and `WithMesh3d` query filter type aliases have also
been removed. Simply use `With<Mesh2d>` or `With<Mesh3d>`.
---------
Co-authored-by: Tim Blackbird <justthecooldude@gmail.com>
Co-authored-by: Carter Anderson <mcanders1@gmail.com>
2024-10-01 21:33:17 +00:00
|
|
|
///
|
Better Materials: AsBindGroup trait and derive, simpler Material trait (#5053)
# Objective
This PR reworks Bevy's Material system, making the user experience of defining Materials _much_ nicer. Bevy's previous material system leaves a lot to be desired:
* Materials require manually implementing the `RenderAsset` trait, which involves manually generating the bind group, handling gpu buffer data transfer, looking up image textures, etc. Even the simplest single-texture material involves writing ~80 unnecessary lines of code. This was never the long term plan.
* There are two material traits, which is confusing, hard to document, and often redundant: `Material` and `SpecializedMaterial`. `Material` implicitly implements `SpecializedMaterial`, and `SpecializedMaterial` is used in most high level apis to support both use cases. Most users shouldn't need to think about specialization at all (I consider it a "power-user tool"), so the fact that `SpecializedMaterial` is front-and-center in our apis is a miss.
* Implementing either material trait involves a lot of "type soup". The "prepared asset" parameter is particularly heinous: `&<Self as RenderAsset>::PreparedAsset`. Defining vertex and fragment shaders is also more verbose than it needs to be.
## Solution
Say hello to the new `Material` system:
```rust
#[derive(AsBindGroup, TypeUuid, Debug, Clone)]
#[uuid = "f690fdae-d598-45ab-8225-97e2a3f056e0"]
pub struct CoolMaterial {
#[uniform(0)]
color: Color,
#[texture(1)]
#[sampler(2)]
color_texture: Handle<Image>,
}
impl Material for CoolMaterial {
fn fragment_shader() -> ShaderRef {
"cool_material.wgsl".into()
}
}
```
Thats it! This same material would have required [~80 lines of complicated "type heavy" code](https://github.com/bevyengine/bevy/blob/v0.7.0/examples/shader/shader_material.rs) in the old Material system. Now it is just 14 lines of simple, readable code.
This is thanks to a new consolidated `Material` trait and the new `AsBindGroup` trait / derive.
### The new `Material` trait
The old "split" `Material` and `SpecializedMaterial` traits have been removed in favor of a new consolidated `Material` trait. All of the functions on the trait are optional.
The difficulty of implementing `Material` has been reduced by simplifying dataflow and removing type complexity:
```rust
// Old
impl Material for CustomMaterial {
fn fragment_shader(asset_server: &AssetServer) -> Option<Handle<Shader>> {
Some(asset_server.load("custom_material.wgsl"))
}
fn alpha_mode(render_asset: &<Self as RenderAsset>::PreparedAsset) -> AlphaMode {
render_asset.alpha_mode
}
}
// New
impl Material for CustomMaterial {
fn fragment_shader() -> ShaderRef {
"custom_material.wgsl".into()
}
fn alpha_mode(&self) -> AlphaMode {
self.alpha_mode
}
}
```
Specialization is still supported, but it is hidden by default under the `specialize()` function (more on this later).
### The `AsBindGroup` trait / derive
The `Material` trait now requires the `AsBindGroup` derive. This can be implemented manually relatively easily, but deriving it will almost always be preferable.
Field attributes like `uniform` and `texture` are used to define which fields should be bindings,
what their binding type is, and what index they should be bound at:
```rust
#[derive(AsBindGroup)]
struct CoolMaterial {
#[uniform(0)]
color: Color,
#[texture(1)]
#[sampler(2)]
color_texture: Handle<Image>,
}
```
In WGSL shaders, the binding looks like this:
```wgsl
struct CoolMaterial {
color: vec4<f32>;
};
[[group(1), binding(0)]]
var<uniform> material: CoolMaterial;
[[group(1), binding(1)]]
var color_texture: texture_2d<f32>;
[[group(1), binding(2)]]
var color_sampler: sampler;
```
Note that the "group" index is determined by the usage context. It is not defined in `AsBindGroup`. Bevy material bind groups are bound to group 1.
The following field-level attributes are supported:
* `uniform(BINDING_INDEX)`
* The field will be converted to a shader-compatible type using the `ShaderType` trait, written to a `Buffer`, and bound as a uniform. It can also be derived for custom structs.
* `texture(BINDING_INDEX)`
* This field's `Handle<Image>` will be used to look up the matching `Texture` gpu resource, which will be bound as a texture in shaders. The field will be assumed to implement `Into<Option<Handle<Image>>>`. In practice, most fields should be a `Handle<Image>` or `Option<Handle<Image>>`. If the value of an `Option<Handle<Image>>` is `None`, the new `FallbackImage` resource will be used instead. This attribute can be used in conjunction with a `sampler` binding attribute (with a different binding index).
* `sampler(BINDING_INDEX)`
* Behaves exactly like the `texture` attribute, but sets the Image's sampler binding instead of the texture.
Note that fields without field-level binding attributes will be ignored.
```rust
#[derive(AsBindGroup)]
struct CoolMaterial {
#[uniform(0)]
color: Color,
this_field_is_ignored: String,
}
```
As mentioned above, `Option<Handle<Image>>` is also supported:
```rust
#[derive(AsBindGroup)]
struct CoolMaterial {
#[uniform(0)]
color: Color,
#[texture(1)]
#[sampler(2)]
color_texture: Option<Handle<Image>>,
}
```
This is useful if you want a texture to be optional. When the value is `None`, the `FallbackImage` will be used for the binding instead, which defaults to "pure white".
Field uniforms with the same binding index will be combined into a single binding:
```rust
#[derive(AsBindGroup)]
struct CoolMaterial {
#[uniform(0)]
color: Color,
#[uniform(0)]
roughness: f32,
}
```
In WGSL shaders, the binding would look like this:
```wgsl
struct CoolMaterial {
color: vec4<f32>;
roughness: f32;
};
[[group(1), binding(0)]]
var<uniform> material: CoolMaterial;
```
Some less common scenarios will require "struct-level" attributes. These are the currently supported struct-level attributes:
* `uniform(BINDING_INDEX, ConvertedShaderType)`
* Similar to the field-level `uniform` attribute, but instead the entire `AsBindGroup` value is converted to `ConvertedShaderType`, which must implement `ShaderType`. This is useful if more complicated conversion logic is required.
* `bind_group_data(DataType)`
* The `AsBindGroup` type will be converted to some `DataType` using `Into<DataType>` and stored as `AsBindGroup::Data` as part of the `AsBindGroup::as_bind_group` call. This is useful if data needs to be stored alongside the generated bind group, such as a unique identifier for a material's bind group. The most common use case for this attribute is "shader pipeline specialization".
The previous `CoolMaterial` example illustrating "combining multiple field-level uniform attributes with the same binding index" can
also be equivalently represented with a single struct-level uniform attribute:
```rust
#[derive(AsBindGroup)]
#[uniform(0, CoolMaterialUniform)]
struct CoolMaterial {
color: Color,
roughness: f32,
}
#[derive(ShaderType)]
struct CoolMaterialUniform {
color: Color,
roughness: f32,
}
impl From<&CoolMaterial> for CoolMaterialUniform {
fn from(material: &CoolMaterial) -> CoolMaterialUniform {
CoolMaterialUniform {
color: material.color,
roughness: material.roughness,
}
}
}
```
### Material Specialization
Material shader specialization is now _much_ simpler:
```rust
#[derive(AsBindGroup, TypeUuid, Debug, Clone)]
#[uuid = "f690fdae-d598-45ab-8225-97e2a3f056e0"]
#[bind_group_data(CoolMaterialKey)]
struct CoolMaterial {
#[uniform(0)]
color: Color,
is_red: bool,
}
#[derive(Copy, Clone, Hash, Eq, PartialEq)]
struct CoolMaterialKey {
is_red: bool,
}
impl From<&CoolMaterial> for CoolMaterialKey {
fn from(material: &CoolMaterial) -> CoolMaterialKey {
CoolMaterialKey {
is_red: material.is_red,
}
}
}
impl Material for CoolMaterial {
fn fragment_shader() -> ShaderRef {
"cool_material.wgsl".into()
}
fn specialize(
pipeline: &MaterialPipeline<Self>,
descriptor: &mut RenderPipelineDescriptor,
layout: &MeshVertexBufferLayout,
key: MaterialPipelineKey<Self>,
) -> Result<(), SpecializedMeshPipelineError> {
if key.bind_group_data.is_red {
let fragment = descriptor.fragment.as_mut().unwrap();
fragment.shader_defs.push("IS_RED".to_string());
}
Ok(())
}
}
```
Setting `bind_group_data` is not required for specialization (it defaults to `()`). Scenarios like "custom vertex attributes" also benefit from this system:
```rust
impl Material for CustomMaterial {
fn vertex_shader() -> ShaderRef {
"custom_material.wgsl".into()
}
fn fragment_shader() -> ShaderRef {
"custom_material.wgsl".into()
}
fn specialize(
pipeline: &MaterialPipeline<Self>,
descriptor: &mut RenderPipelineDescriptor,
layout: &MeshVertexBufferLayout,
key: MaterialPipelineKey<Self>,
) -> Result<(), SpecializedMeshPipelineError> {
let vertex_layout = layout.get_layout(&[
Mesh::ATTRIBUTE_POSITION.at_shader_location(0),
ATTRIBUTE_BLEND_COLOR.at_shader_location(1),
])?;
descriptor.vertex.buffers = vec![vertex_layout];
Ok(())
}
}
```
### Ported `StandardMaterial` to the new `Material` system
Bevy's built-in PBR material uses the new Material system (including the AsBindGroup derive):
```rust
#[derive(AsBindGroup, Debug, Clone, TypeUuid)]
#[uuid = "7494888b-c082-457b-aacf-517228cc0c22"]
#[bind_group_data(StandardMaterialKey)]
#[uniform(0, StandardMaterialUniform)]
pub struct StandardMaterial {
pub base_color: Color,
#[texture(1)]
#[sampler(2)]
pub base_color_texture: Option<Handle<Image>>,
/* other fields omitted for brevity */
```
### Ported Bevy examples to the new `Material` system
The overall complexity of Bevy's "custom shader examples" has gone down significantly. Take a look at the diffs if you want a dopamine spike.
Please note that while this PR has a net increase in "lines of code", most of those extra lines come from added documentation. There is a significant reduction
in the overall complexity of the code (even accounting for the new derive logic).
---
## Changelog
### Added
* `AsBindGroup` trait and derive, which make it much easier to transfer data to the gpu and generate bind groups for a given type.
### Changed
* The old `Material` and `SpecializedMaterial` traits have been replaced by a consolidated (much simpler) `Material` trait. Materials no longer implement `RenderAsset`.
* `StandardMaterial` was ported to the new material system. There are no user-facing api changes to the `StandardMaterial` struct api, but it now implements `AsBindGroup` and `Material` instead of `RenderAsset` and `SpecializedMaterial`.
## Migration Guide
The Material system has been reworked to be much simpler. We've removed a lot of boilerplate with the new `AsBindGroup` derive and the `Material` trait is simpler as well!
### Bevy 0.7 (old)
```rust
#[derive(Debug, Clone, TypeUuid)]
#[uuid = "f690fdae-d598-45ab-8225-97e2a3f056e0"]
pub struct CustomMaterial {
color: Color,
color_texture: Handle<Image>,
}
#[derive(Clone)]
pub struct GpuCustomMaterial {
_buffer: Buffer,
bind_group: BindGroup,
}
impl RenderAsset for CustomMaterial {
type ExtractedAsset = CustomMaterial;
type PreparedAsset = GpuCustomMaterial;
type Param = (SRes<RenderDevice>, SRes<MaterialPipeline<Self>>);
fn extract_asset(&self) -> Self::ExtractedAsset {
self.clone()
}
fn prepare_asset(
extracted_asset: Self::ExtractedAsset,
(render_device, material_pipeline): &mut SystemParamItem<Self::Param>,
) -> Result<Self::PreparedAsset, PrepareAssetError<Self::ExtractedAsset>> {
let color = Vec4::from_slice(&extracted_asset.color.as_linear_rgba_f32());
let byte_buffer = [0u8; Vec4::SIZE.get() as usize];
let mut buffer = encase::UniformBuffer::new(byte_buffer);
buffer.write(&color).unwrap();
let buffer = render_device.create_buffer_with_data(&BufferInitDescriptor {
contents: buffer.as_ref(),
label: None,
usage: BufferUsages::UNIFORM | BufferUsages::COPY_DST,
});
let (texture_view, texture_sampler) = if let Some(result) = material_pipeline
.mesh_pipeline
.get_image_texture(gpu_images, &Some(extracted_asset.color_texture.clone()))
{
result
} else {
return Err(PrepareAssetError::RetryNextUpdate(extracted_asset));
};
let bind_group = render_device.create_bind_group(&BindGroupDescriptor {
entries: &[
BindGroupEntry {
binding: 0,
resource: buffer.as_entire_binding(),
},
BindGroupEntry {
binding: 0,
resource: BindingResource::TextureView(texture_view),
},
BindGroupEntry {
binding: 1,
resource: BindingResource::Sampler(texture_sampler),
},
],
label: None,
layout: &material_pipeline.material_layout,
});
Ok(GpuCustomMaterial {
_buffer: buffer,
bind_group,
})
}
}
impl Material for CustomMaterial {
fn fragment_shader(asset_server: &AssetServer) -> Option<Handle<Shader>> {
Some(asset_server.load("custom_material.wgsl"))
}
fn bind_group(render_asset: &<Self as RenderAsset>::PreparedAsset) -> &BindGroup {
&render_asset.bind_group
}
fn bind_group_layout(render_device: &RenderDevice) -> BindGroupLayout {
render_device.create_bind_group_layout(&BindGroupLayoutDescriptor {
entries: &[
BindGroupLayoutEntry {
binding: 0,
visibility: ShaderStages::FRAGMENT,
ty: BindingType::Buffer {
ty: BufferBindingType::Uniform,
has_dynamic_offset: false,
min_binding_size: Some(Vec4::min_size()),
},
count: None,
},
BindGroupLayoutEntry {
binding: 1,
visibility: ShaderStages::FRAGMENT,
ty: BindingType::Texture {
multisampled: false,
sample_type: TextureSampleType::Float { filterable: true },
view_dimension: TextureViewDimension::D2Array,
},
count: None,
},
BindGroupLayoutEntry {
binding: 2,
visibility: ShaderStages::FRAGMENT,
ty: BindingType::Sampler(SamplerBindingType::Filtering),
count: None,
},
],
label: None,
})
}
}
```
### Bevy 0.8 (new)
```rust
impl Material for CustomMaterial {
fn fragment_shader() -> ShaderRef {
"custom_material.wgsl".into()
}
}
#[derive(AsBindGroup, TypeUuid, Debug, Clone)]
#[uuid = "f690fdae-d598-45ab-8225-97e2a3f056e0"]
pub struct CustomMaterial {
#[uniform(0)]
color: Color,
#[texture(1)]
#[sampler(2)]
color_texture: Handle<Image>,
}
```
## Future Work
* Add support for more binding types (cubemaps, buffers, etc). This PR intentionally includes a bare minimum number of binding types to keep "reviewability" in check.
* Consider optionally eliding binding indices using binding names. `AsBindGroup` could pass in (optional?) reflection info as a "hint".
* This would make it possible for the derive to do this:
```rust
#[derive(AsBindGroup)]
pub struct CustomMaterial {
#[uniform]
color: Color,
#[texture]
#[sampler]
color_texture: Option<Handle<Image>>,
alpha_mode: AlphaMode,
}
```
* Or this
```rust
#[derive(AsBindGroup)]
pub struct CustomMaterial {
#[binding]
color: Color,
#[binding]
color_texture: Option<Handle<Image>>,
alpha_mode: AlphaMode,
}
```
* Or even this (if we flip to "include bindings by default")
```rust
#[derive(AsBindGroup)]
pub struct CustomMaterial {
color: Color,
color_texture: Option<Handle<Image>>,
#[binding(ignore)]
alpha_mode: AlphaMode,
}
```
* If we add the option to define custom draw functions for materials (which could be done in a type-erased way), I think that would be enough to support extra non-material bindings. Worth considering!
2022-06-30 23:48:46 +00:00
|
|
|
/// In WGSL shaders, the material's binding would look like this:
|
|
|
|
///
|
|
|
|
/// ```wgsl
|
2023-11-28 22:26:22 +00:00
|
|
|
/// @group(2) @binding(0) var<uniform> color: vec4<f32>;
|
|
|
|
/// @group(2) @binding(1) var color_texture: texture_2d<f32>;
|
|
|
|
/// @group(2) @binding(2) var color_sampler: sampler;
|
Better Materials: AsBindGroup trait and derive, simpler Material trait (#5053)
# Objective
This PR reworks Bevy's Material system, making the user experience of defining Materials _much_ nicer. Bevy's previous material system leaves a lot to be desired:
* Materials require manually implementing the `RenderAsset` trait, which involves manually generating the bind group, handling gpu buffer data transfer, looking up image textures, etc. Even the simplest single-texture material involves writing ~80 unnecessary lines of code. This was never the long term plan.
* There are two material traits, which is confusing, hard to document, and often redundant: `Material` and `SpecializedMaterial`. `Material` implicitly implements `SpecializedMaterial`, and `SpecializedMaterial` is used in most high level apis to support both use cases. Most users shouldn't need to think about specialization at all (I consider it a "power-user tool"), so the fact that `SpecializedMaterial` is front-and-center in our apis is a miss.
* Implementing either material trait involves a lot of "type soup". The "prepared asset" parameter is particularly heinous: `&<Self as RenderAsset>::PreparedAsset`. Defining vertex and fragment shaders is also more verbose than it needs to be.
## Solution
Say hello to the new `Material` system:
```rust
#[derive(AsBindGroup, TypeUuid, Debug, Clone)]
#[uuid = "f690fdae-d598-45ab-8225-97e2a3f056e0"]
pub struct CoolMaterial {
#[uniform(0)]
color: Color,
#[texture(1)]
#[sampler(2)]
color_texture: Handle<Image>,
}
impl Material for CoolMaterial {
fn fragment_shader() -> ShaderRef {
"cool_material.wgsl".into()
}
}
```
Thats it! This same material would have required [~80 lines of complicated "type heavy" code](https://github.com/bevyengine/bevy/blob/v0.7.0/examples/shader/shader_material.rs) in the old Material system. Now it is just 14 lines of simple, readable code.
This is thanks to a new consolidated `Material` trait and the new `AsBindGroup` trait / derive.
### The new `Material` trait
The old "split" `Material` and `SpecializedMaterial` traits have been removed in favor of a new consolidated `Material` trait. All of the functions on the trait are optional.
The difficulty of implementing `Material` has been reduced by simplifying dataflow and removing type complexity:
```rust
// Old
impl Material for CustomMaterial {
fn fragment_shader(asset_server: &AssetServer) -> Option<Handle<Shader>> {
Some(asset_server.load("custom_material.wgsl"))
}
fn alpha_mode(render_asset: &<Self as RenderAsset>::PreparedAsset) -> AlphaMode {
render_asset.alpha_mode
}
}
// New
impl Material for CustomMaterial {
fn fragment_shader() -> ShaderRef {
"custom_material.wgsl".into()
}
fn alpha_mode(&self) -> AlphaMode {
self.alpha_mode
}
}
```
Specialization is still supported, but it is hidden by default under the `specialize()` function (more on this later).
### The `AsBindGroup` trait / derive
The `Material` trait now requires the `AsBindGroup` derive. This can be implemented manually relatively easily, but deriving it will almost always be preferable.
Field attributes like `uniform` and `texture` are used to define which fields should be bindings,
what their binding type is, and what index they should be bound at:
```rust
#[derive(AsBindGroup)]
struct CoolMaterial {
#[uniform(0)]
color: Color,
#[texture(1)]
#[sampler(2)]
color_texture: Handle<Image>,
}
```
In WGSL shaders, the binding looks like this:
```wgsl
struct CoolMaterial {
color: vec4<f32>;
};
[[group(1), binding(0)]]
var<uniform> material: CoolMaterial;
[[group(1), binding(1)]]
var color_texture: texture_2d<f32>;
[[group(1), binding(2)]]
var color_sampler: sampler;
```
Note that the "group" index is determined by the usage context. It is not defined in `AsBindGroup`. Bevy material bind groups are bound to group 1.
The following field-level attributes are supported:
* `uniform(BINDING_INDEX)`
* The field will be converted to a shader-compatible type using the `ShaderType` trait, written to a `Buffer`, and bound as a uniform. It can also be derived for custom structs.
* `texture(BINDING_INDEX)`
* This field's `Handle<Image>` will be used to look up the matching `Texture` gpu resource, which will be bound as a texture in shaders. The field will be assumed to implement `Into<Option<Handle<Image>>>`. In practice, most fields should be a `Handle<Image>` or `Option<Handle<Image>>`. If the value of an `Option<Handle<Image>>` is `None`, the new `FallbackImage` resource will be used instead. This attribute can be used in conjunction with a `sampler` binding attribute (with a different binding index).
* `sampler(BINDING_INDEX)`
* Behaves exactly like the `texture` attribute, but sets the Image's sampler binding instead of the texture.
Note that fields without field-level binding attributes will be ignored.
```rust
#[derive(AsBindGroup)]
struct CoolMaterial {
#[uniform(0)]
color: Color,
this_field_is_ignored: String,
}
```
As mentioned above, `Option<Handle<Image>>` is also supported:
```rust
#[derive(AsBindGroup)]
struct CoolMaterial {
#[uniform(0)]
color: Color,
#[texture(1)]
#[sampler(2)]
color_texture: Option<Handle<Image>>,
}
```
This is useful if you want a texture to be optional. When the value is `None`, the `FallbackImage` will be used for the binding instead, which defaults to "pure white".
Field uniforms with the same binding index will be combined into a single binding:
```rust
#[derive(AsBindGroup)]
struct CoolMaterial {
#[uniform(0)]
color: Color,
#[uniform(0)]
roughness: f32,
}
```
In WGSL shaders, the binding would look like this:
```wgsl
struct CoolMaterial {
color: vec4<f32>;
roughness: f32;
};
[[group(1), binding(0)]]
var<uniform> material: CoolMaterial;
```
Some less common scenarios will require "struct-level" attributes. These are the currently supported struct-level attributes:
* `uniform(BINDING_INDEX, ConvertedShaderType)`
* Similar to the field-level `uniform` attribute, but instead the entire `AsBindGroup` value is converted to `ConvertedShaderType`, which must implement `ShaderType`. This is useful if more complicated conversion logic is required.
* `bind_group_data(DataType)`
* The `AsBindGroup` type will be converted to some `DataType` using `Into<DataType>` and stored as `AsBindGroup::Data` as part of the `AsBindGroup::as_bind_group` call. This is useful if data needs to be stored alongside the generated bind group, such as a unique identifier for a material's bind group. The most common use case for this attribute is "shader pipeline specialization".
The previous `CoolMaterial` example illustrating "combining multiple field-level uniform attributes with the same binding index" can
also be equivalently represented with a single struct-level uniform attribute:
```rust
#[derive(AsBindGroup)]
#[uniform(0, CoolMaterialUniform)]
struct CoolMaterial {
color: Color,
roughness: f32,
}
#[derive(ShaderType)]
struct CoolMaterialUniform {
color: Color,
roughness: f32,
}
impl From<&CoolMaterial> for CoolMaterialUniform {
fn from(material: &CoolMaterial) -> CoolMaterialUniform {
CoolMaterialUniform {
color: material.color,
roughness: material.roughness,
}
}
}
```
### Material Specialization
Material shader specialization is now _much_ simpler:
```rust
#[derive(AsBindGroup, TypeUuid, Debug, Clone)]
#[uuid = "f690fdae-d598-45ab-8225-97e2a3f056e0"]
#[bind_group_data(CoolMaterialKey)]
struct CoolMaterial {
#[uniform(0)]
color: Color,
is_red: bool,
}
#[derive(Copy, Clone, Hash, Eq, PartialEq)]
struct CoolMaterialKey {
is_red: bool,
}
impl From<&CoolMaterial> for CoolMaterialKey {
fn from(material: &CoolMaterial) -> CoolMaterialKey {
CoolMaterialKey {
is_red: material.is_red,
}
}
}
impl Material for CoolMaterial {
fn fragment_shader() -> ShaderRef {
"cool_material.wgsl".into()
}
fn specialize(
pipeline: &MaterialPipeline<Self>,
descriptor: &mut RenderPipelineDescriptor,
layout: &MeshVertexBufferLayout,
key: MaterialPipelineKey<Self>,
) -> Result<(), SpecializedMeshPipelineError> {
if key.bind_group_data.is_red {
let fragment = descriptor.fragment.as_mut().unwrap();
fragment.shader_defs.push("IS_RED".to_string());
}
Ok(())
}
}
```
Setting `bind_group_data` is not required for specialization (it defaults to `()`). Scenarios like "custom vertex attributes" also benefit from this system:
```rust
impl Material for CustomMaterial {
fn vertex_shader() -> ShaderRef {
"custom_material.wgsl".into()
}
fn fragment_shader() -> ShaderRef {
"custom_material.wgsl".into()
}
fn specialize(
pipeline: &MaterialPipeline<Self>,
descriptor: &mut RenderPipelineDescriptor,
layout: &MeshVertexBufferLayout,
key: MaterialPipelineKey<Self>,
) -> Result<(), SpecializedMeshPipelineError> {
let vertex_layout = layout.get_layout(&[
Mesh::ATTRIBUTE_POSITION.at_shader_location(0),
ATTRIBUTE_BLEND_COLOR.at_shader_location(1),
])?;
descriptor.vertex.buffers = vec![vertex_layout];
Ok(())
}
}
```
### Ported `StandardMaterial` to the new `Material` system
Bevy's built-in PBR material uses the new Material system (including the AsBindGroup derive):
```rust
#[derive(AsBindGroup, Debug, Clone, TypeUuid)]
#[uuid = "7494888b-c082-457b-aacf-517228cc0c22"]
#[bind_group_data(StandardMaterialKey)]
#[uniform(0, StandardMaterialUniform)]
pub struct StandardMaterial {
pub base_color: Color,
#[texture(1)]
#[sampler(2)]
pub base_color_texture: Option<Handle<Image>>,
/* other fields omitted for brevity */
```
### Ported Bevy examples to the new `Material` system
The overall complexity of Bevy's "custom shader examples" has gone down significantly. Take a look at the diffs if you want a dopamine spike.
Please note that while this PR has a net increase in "lines of code", most of those extra lines come from added documentation. There is a significant reduction
in the overall complexity of the code (even accounting for the new derive logic).
---
## Changelog
### Added
* `AsBindGroup` trait and derive, which make it much easier to transfer data to the gpu and generate bind groups for a given type.
### Changed
* The old `Material` and `SpecializedMaterial` traits have been replaced by a consolidated (much simpler) `Material` trait. Materials no longer implement `RenderAsset`.
* `StandardMaterial` was ported to the new material system. There are no user-facing api changes to the `StandardMaterial` struct api, but it now implements `AsBindGroup` and `Material` instead of `RenderAsset` and `SpecializedMaterial`.
## Migration Guide
The Material system has been reworked to be much simpler. We've removed a lot of boilerplate with the new `AsBindGroup` derive and the `Material` trait is simpler as well!
### Bevy 0.7 (old)
```rust
#[derive(Debug, Clone, TypeUuid)]
#[uuid = "f690fdae-d598-45ab-8225-97e2a3f056e0"]
pub struct CustomMaterial {
color: Color,
color_texture: Handle<Image>,
}
#[derive(Clone)]
pub struct GpuCustomMaterial {
_buffer: Buffer,
bind_group: BindGroup,
}
impl RenderAsset for CustomMaterial {
type ExtractedAsset = CustomMaterial;
type PreparedAsset = GpuCustomMaterial;
type Param = (SRes<RenderDevice>, SRes<MaterialPipeline<Self>>);
fn extract_asset(&self) -> Self::ExtractedAsset {
self.clone()
}
fn prepare_asset(
extracted_asset: Self::ExtractedAsset,
(render_device, material_pipeline): &mut SystemParamItem<Self::Param>,
) -> Result<Self::PreparedAsset, PrepareAssetError<Self::ExtractedAsset>> {
let color = Vec4::from_slice(&extracted_asset.color.as_linear_rgba_f32());
let byte_buffer = [0u8; Vec4::SIZE.get() as usize];
let mut buffer = encase::UniformBuffer::new(byte_buffer);
buffer.write(&color).unwrap();
let buffer = render_device.create_buffer_with_data(&BufferInitDescriptor {
contents: buffer.as_ref(),
label: None,
usage: BufferUsages::UNIFORM | BufferUsages::COPY_DST,
});
let (texture_view, texture_sampler) = if let Some(result) = material_pipeline
.mesh_pipeline
.get_image_texture(gpu_images, &Some(extracted_asset.color_texture.clone()))
{
result
} else {
return Err(PrepareAssetError::RetryNextUpdate(extracted_asset));
};
let bind_group = render_device.create_bind_group(&BindGroupDescriptor {
entries: &[
BindGroupEntry {
binding: 0,
resource: buffer.as_entire_binding(),
},
BindGroupEntry {
binding: 0,
resource: BindingResource::TextureView(texture_view),
},
BindGroupEntry {
binding: 1,
resource: BindingResource::Sampler(texture_sampler),
},
],
label: None,
layout: &material_pipeline.material_layout,
});
Ok(GpuCustomMaterial {
_buffer: buffer,
bind_group,
})
}
}
impl Material for CustomMaterial {
fn fragment_shader(asset_server: &AssetServer) -> Option<Handle<Shader>> {
Some(asset_server.load("custom_material.wgsl"))
}
fn bind_group(render_asset: &<Self as RenderAsset>::PreparedAsset) -> &BindGroup {
&render_asset.bind_group
}
fn bind_group_layout(render_device: &RenderDevice) -> BindGroupLayout {
render_device.create_bind_group_layout(&BindGroupLayoutDescriptor {
entries: &[
BindGroupLayoutEntry {
binding: 0,
visibility: ShaderStages::FRAGMENT,
ty: BindingType::Buffer {
ty: BufferBindingType::Uniform,
has_dynamic_offset: false,
min_binding_size: Some(Vec4::min_size()),
},
count: None,
},
BindGroupLayoutEntry {
binding: 1,
visibility: ShaderStages::FRAGMENT,
ty: BindingType::Texture {
multisampled: false,
sample_type: TextureSampleType::Float { filterable: true },
view_dimension: TextureViewDimension::D2Array,
},
count: None,
},
BindGroupLayoutEntry {
binding: 2,
visibility: ShaderStages::FRAGMENT,
ty: BindingType::Sampler(SamplerBindingType::Filtering),
count: None,
},
],
label: None,
})
}
}
```
### Bevy 0.8 (new)
```rust
impl Material for CustomMaterial {
fn fragment_shader() -> ShaderRef {
"custom_material.wgsl".into()
}
}
#[derive(AsBindGroup, TypeUuid, Debug, Clone)]
#[uuid = "f690fdae-d598-45ab-8225-97e2a3f056e0"]
pub struct CustomMaterial {
#[uniform(0)]
color: Color,
#[texture(1)]
#[sampler(2)]
color_texture: Handle<Image>,
}
```
## Future Work
* Add support for more binding types (cubemaps, buffers, etc). This PR intentionally includes a bare minimum number of binding types to keep "reviewability" in check.
* Consider optionally eliding binding indices using binding names. `AsBindGroup` could pass in (optional?) reflection info as a "hint".
* This would make it possible for the derive to do this:
```rust
#[derive(AsBindGroup)]
pub struct CustomMaterial {
#[uniform]
color: Color,
#[texture]
#[sampler]
color_texture: Option<Handle<Image>>,
alpha_mode: AlphaMode,
}
```
* Or this
```rust
#[derive(AsBindGroup)]
pub struct CustomMaterial {
#[binding]
color: Color,
#[binding]
color_texture: Option<Handle<Image>>,
alpha_mode: AlphaMode,
}
```
* Or even this (if we flip to "include bindings by default")
```rust
#[derive(AsBindGroup)]
pub struct CustomMaterial {
color: Color,
color_texture: Option<Handle<Image>>,
#[binding(ignore)]
alpha_mode: AlphaMode,
}
```
* If we add the option to define custom draw functions for materials (which could be done in a type-erased way), I think that would be enough to support extra non-material bindings. Worth considering!
2022-06-30 23:48:46 +00:00
|
|
|
/// ```
|
Bevy Asset V2 (#8624)
# Bevy Asset V2 Proposal
## Why Does Bevy Need A New Asset System?
Asset pipelines are a central part of the gamedev process. Bevy's
current asset system is missing a number of features that make it
non-viable for many classes of gamedev. After plenty of discussions and
[a long community feedback
period](https://github.com/bevyengine/bevy/discussions/3972), we've
identified a number missing features:
* **Asset Preprocessing**: it should be possible to "preprocess" /
"compile" / "crunch" assets at "development time" rather than when the
game starts up. This enables offloading expensive work from deployed
apps, faster asset loading, less runtime memory usage, etc.
* **Per-Asset Loader Settings**: Individual assets cannot define their
own loaders that override the defaults. Additionally, they cannot
provide per-asset settings to their loaders. This is a huge limitation,
as many asset types don't provide all information necessary for Bevy
_inside_ the asset. For example, a raw PNG image says nothing about how
it should be sampled (ex: linear vs nearest).
* **Asset `.meta` files**: assets should have configuration files stored
adjacent to the asset in question, which allows the user to configure
asset-type-specific settings. These settings should be accessible during
the pre-processing phase. Modifying a `.meta` file should trigger a
re-processing / re-load of the asset. It should be possible to configure
asset loaders from the meta file.
* **Processed Asset Hot Reloading**: Changes to processed assets (or
their dependencies) should result in re-processing them and re-loading
the results in live Bevy Apps.
* **Asset Dependency Tracking**: The current bevy_asset has no good way
to wait for asset dependencies to load. It punts this as an exercise for
consumers of the loader apis, which is unreasonable and error prone.
There should be easy, ergonomic ways to wait for assets to load and
block some logic on an asset's entire dependency tree loading.
* **Runtime Asset Loading**: it should be (optionally) possible to load
arbitrary assets dynamically at runtime. This necessitates being able to
deploy and run the asset server alongside Bevy Apps on _all platforms_.
For example, we should be able to invoke the shader compiler at runtime,
stream scenes from sources like the internet, etc. To keep deployed
binaries (and startup times) small, the runtime asset server
configuration should be configurable with different settings compared to
the "pre processor asset server".
* **Multiple Backends**: It should be possible to load assets from
arbitrary sources (filesystems, the internet, remote asset serves, etc).
* **Asset Packing**: It should be possible to deploy assets in
compressed "packs", which makes it easier and more efficient to
distribute assets with Bevy Apps.
* **Asset Handoff**: It should be possible to hold a "live" asset
handle, which correlates to runtime data, without actually holding the
asset in memory. Ex: it must be possible to hold a reference to a GPU
mesh generated from a "mesh asset" without keeping the mesh data in CPU
memory
* **Per-Platform Processed Assets**: Different platforms and app
distributions have different capabilities and requirements. Some
platforms need lower asset resolutions or different asset formats to
operate within the hardware constraints of the platform. It should be
possible to define per-platform asset processing profiles. And it should
be possible to deploy only the assets required for a given platform.
These features have architectural implications that are significant
enough to require a full rewrite. The current Bevy Asset implementation
got us this far, but it can take us no farther. This PR defines a brand
new asset system that implements most of these features, while laying
the foundations for the remaining features to be built.
## Bevy Asset V2
Here is a quick overview of the features introduced in this PR.
* **Asset Preprocessing**: Preprocess assets at development time into
more efficient (and configurable) representations
* **Dependency Aware**: Dependencies required to process an asset are
tracked. If an asset's processed dependency changes, it will be
reprocessed
* **Hot Reprocessing/Reloading**: detect changes to asset source files,
reprocess them if they have changed, and then hot-reload them in Bevy
Apps.
* **Only Process Changes**: Assets are only re-processed when their
source file (or meta file) has changed. This uses hashing and timestamps
to avoid processing assets that haven't changed.
* **Transactional and Reliable**: Uses write-ahead logging (a technique
commonly used by databases) to recover from crashes / forced-exits.
Whenever possible it avoids full-reprocessing / only uncompleted
transactions will be reprocessed. When the processor is running in
parallel with a Bevy App, processor asset writes block Bevy App asset
reads. Reading metadata + asset bytes is guaranteed to be transactional
/ correctly paired.
* **Portable / Run anywhere / Database-free**: The processor does not
rely on an in-memory database (although it uses some database techniques
for reliability). This is important because pretty much all in-memory
databases have unsupported platforms or build complications.
* **Configure Processor Defaults Per File Type**: You can say "use this
processor for all files of this type".
* **Custom Processors**: The `Processor` trait is flexible and
unopinionated. It can be implemented by downstream plugins.
* **LoadAndSave Processors**: Most asset processing scenarios can be
expressed as "run AssetLoader A, save the results using AssetSaver X,
and then load the result using AssetLoader B". For example, load this
png image using `PngImageLoader`, which produces an `Image` asset and
then save it using `CompressedImageSaver` (which also produces an
`Image` asset, but in a compressed format), which takes an `Image` asset
as input. This means if you have an `AssetLoader` for an asset, you are
already half way there! It also means that you can share AssetSavers
across multiple loaders. Because `CompressedImageSaver` accepts Bevy's
generic Image asset as input, it means you can also use it with some
future `JpegImageLoader`.
* **Loader and Saver Settings**: Asset Loaders and Savers can now define
their own settings types, which are passed in as input when an asset is
loaded / saved. Each asset can define its own settings.
* **Asset `.meta` files**: configure asset loaders, their settings,
enable/disable processing, and configure processor settings
* **Runtime Asset Dependency Tracking** Runtime asset dependencies (ex:
if an asset contains a `Handle<Image>`) are tracked by the asset server.
An event is emitted when an asset and all of its dependencies have been
loaded
* **Unprocessed Asset Loading**: Assets do not require preprocessing.
They can be loaded directly. A processed asset is just a "normal" asset
with some extra metadata. Asset Loaders don't need to know or care about
whether or not an asset was processed.
* **Async Asset IO**: Asset readers/writers use async non-blocking
interfaces. Note that because Rust doesn't yet support async traits,
there is a bit of manual Boxing / Future boilerplate. This will
hopefully be removed in the near future when Rust gets async traits.
* **Pluggable Asset Readers and Writers**: Arbitrary asset source
readers/writers are supported, both by the processor and the asset
server.
* **Better Asset Handles**
* **Single Arc Tree**: Asset Handles now use a single arc tree that
represents the lifetime of the asset. This makes their implementation
simpler, more efficient, and allows us to cheaply attach metadata to
handles. Ex: the AssetPath of a handle is now directly accessible on the
handle itself!
* **Const Typed Handles**: typed handles can be constructed in a const
context. No more weird "const untyped converted to typed at runtime"
patterns!
* **Handles and Ids are Smaller / Faster To Hash / Compare**: Typed
`Handle<T>` is now much smaller in memory and `AssetId<T>` is even
smaller.
* **Weak Handle Usage Reduction**: In general Handles are now considered
to be "strong". Bevy features that previously used "weak `Handle<T>`"
have been ported to `AssetId<T>`, which makes it statically clear that
the features do not hold strong handles (while retaining strong type
information). Currently Handle::Weak still exists, but it is very
possible that we can remove that entirely.
* **Efficient / Dense Asset Ids**: Assets now have efficient dense
runtime asset ids, which means we can avoid expensive hash lookups.
Assets are stored in Vecs instead of HashMaps. There are now typed and
untyped ids, which means we no longer need to store dynamic type
information in the ID for typed handles. "AssetPathId" (which was a
nightmare from a performance and correctness standpoint) has been
entirely removed in favor of dense ids (which are retrieved for a path
on load)
* **Direct Asset Loading, with Dependency Tracking**: Assets that are
defined at runtime can still have their dependencies tracked by the
Asset Server (ex: if you create a material at runtime, you can still
wait for its textures to load). This is accomplished via the (currently
optional) "asset dependency visitor" trait. This system can also be used
to define a set of assets to load, then wait for those assets to load.
* **Async folder loading**: Folder loading also uses this system and
immediately returns a handle to the LoadedFolder asset, which means
folder loading no longer blocks on directory traversals.
* **Improved Loader Interface**: Loaders now have a specific "top level
asset type", which makes returning the top-level asset simpler and
statically typed.
* **Basic Image Settings and Processing**: Image assets can now be
processed into the gpu-friendly Basic Universal format. The ImageLoader
now has a setting to define what format the image should be loaded as.
Note that this is just a minimal MVP ... plenty of additional work to do
here. To demo this, enable the `basis-universal` feature and turn on
asset processing.
* **Simpler Audio Play / AudioSink API**: Asset handle providers are
cloneable, which means the Audio resource can mint its own handles. This
means you can now do `let sink_handle = audio.play(music)` instead of
`let sink_handle = audio_sinks.get_handle(audio.play(music))`. Note that
this might still be replaced by
https://github.com/bevyengine/bevy/pull/8424.
**Removed Handle Casting From Engine Features**: Ex: FontAtlases no
longer use casting between handle types
## Using The New Asset System
### Normal Unprocessed Asset Loading
By default the `AssetPlugin` does not use processing. It behaves pretty
much the same way as the old system.
If you are defining a custom asset, first derive `Asset`:
```rust
#[derive(Asset)]
struct Thing {
value: String,
}
```
Initialize the asset:
```rust
app.init_asset:<Thing>()
```
Implement a new `AssetLoader` for it:
```rust
#[derive(Default)]
struct ThingLoader;
#[derive(Serialize, Deserialize, Default)]
pub struct ThingSettings {
some_setting: bool,
}
impl AssetLoader for ThingLoader {
type Asset = Thing;
type Settings = ThingSettings;
fn load<'a>(
&'a self,
reader: &'a mut Reader,
settings: &'a ThingSettings,
load_context: &'a mut LoadContext,
) -> BoxedFuture<'a, Result<Thing, anyhow::Error>> {
Box::pin(async move {
let mut bytes = Vec::new();
reader.read_to_end(&mut bytes).await?;
// convert bytes to value somehow
Ok(Thing {
value
})
})
}
fn extensions(&self) -> &[&str] {
&["thing"]
}
}
```
Note that this interface will get much cleaner once Rust gets support
for async traits. `Reader` is an async futures_io::AsyncRead. You can
stream bytes as they come in or read them all into a `Vec<u8>`,
depending on the context. You can use `let handle =
load_context.load(path)` to kick off a dependency load, retrieve a
handle, and register the dependency for the asset.
Then just register the loader in your Bevy app:
```rust
app.init_asset_loader::<ThingLoader>()
```
Now just add your `Thing` asset files into the `assets` folder and load
them like this:
```rust
fn system(asset_server: Res<AssetServer>) {
let handle = Handle<Thing> = asset_server.load("cool.thing");
}
```
You can check load states directly via the asset server:
```rust
if asset_server.load_state(&handle) == LoadState::Loaded { }
```
You can also listen for events:
```rust
fn system(mut events: EventReader<AssetEvent<Thing>>, handle: Res<SomeThingHandle>) {
for event in events.iter() {
if event.is_loaded_with_dependencies(&handle) {
}
}
}
```
Note the new `AssetEvent::LoadedWithDependencies`, which only fires when
the asset is loaded _and_ all dependencies (and their dependencies) have
loaded.
Unlike the old asset system, for a given asset path all `Handle<T>`
values point to the same underlying Arc. This means Handles can cheaply
hold more asset information, such as the AssetPath:
```rust
// prints the AssetPath of the handle
info!("{:?}", handle.path())
```
### Processed Assets
Asset processing can be enabled via the `AssetPlugin`. When developing
Bevy Apps with processed assets, do this:
```rust
app.add_plugins(DefaultPlugins.set(AssetPlugin::processed_dev()))
```
This runs the `AssetProcessor` in the background with hot-reloading. It
reads assets from the `assets` folder, processes them, and writes them
to the `.imported_assets` folder. Asset loads in the Bevy App will wait
for a processed version of the asset to become available. If an asset in
the `assets` folder changes, it will be reprocessed and hot-reloaded in
the Bevy App.
When deploying processed Bevy apps, do this:
```rust
app.add_plugins(DefaultPlugins.set(AssetPlugin::processed()))
```
This does not run the `AssetProcessor` in the background. It behaves
like `AssetPlugin::unprocessed()`, but reads assets from
`.imported_assets`.
When the `AssetProcessor` is running, it will populate sibling `.meta`
files for assets in the `assets` folder. Meta files for assets that do
not have a processor configured look like this:
```rust
(
meta_format_version: "1.0",
asset: Load(
loader: "bevy_render::texture::image_loader::ImageLoader",
settings: (
format: FromExtension,
),
),
)
```
This is metadata for an image asset. For example, if you have
`assets/my_sprite.png`, this could be the metadata stored at
`assets/my_sprite.png.meta`. Meta files are totally optional. If no
metadata exists, the default settings will be used.
In short, this file says "load this asset with the ImageLoader and use
the file extension to determine the image type". This type of meta file
is supported in all AssetPlugin modes. If in `Unprocessed` mode, the
asset (with the meta settings) will be loaded directly. If in
`ProcessedDev` mode, the asset file will be copied directly to the
`.imported_assets` folder. The meta will also be copied directly to the
`.imported_assets` folder, but with one addition:
```rust
(
meta_format_version: "1.0",
processed_info: Some((
hash: 12415480888597742505,
full_hash: 14344495437905856884,
process_dependencies: [],
)),
asset: Load(
loader: "bevy_render::texture::image_loader::ImageLoader",
settings: (
format: FromExtension,
),
),
)
```
`processed_info` contains `hash` (a direct hash of the asset and meta
bytes), `full_hash` (a hash of `hash` and the hashes of all
`process_dependencies`), and `process_dependencies` (the `path` and
`full_hash` of every process_dependency). A "process dependency" is an
asset dependency that is _directly_ used when processing the asset.
Images do not have process dependencies, so this is empty.
When the processor is enabled, you can use the `Process` metadata
config:
```rust
(
meta_format_version: "1.0",
asset: Process(
processor: "bevy_asset::processor::process::LoadAndSave<bevy_render::texture::image_loader::ImageLoader, bevy_render::texture::compressed_image_saver::CompressedImageSaver>",
settings: (
loader_settings: (
format: FromExtension,
),
saver_settings: (
generate_mipmaps: true,
),
),
),
)
```
This configures the asset to use the `LoadAndSave` processor, which runs
an AssetLoader and feeds the result into an AssetSaver (which saves the
given Asset and defines a loader to load it with). (for terseness
LoadAndSave will likely get a shorter/friendlier type name when [Stable
Type Paths](#7184) lands). `LoadAndSave` is likely to be the most common
processor type, but arbitrary processors are supported.
`CompressedImageSaver` saves an `Image` in the Basis Universal format
and configures the ImageLoader to load it as basis universal. The
`AssetProcessor` will read this meta, run it through the LoadAndSave
processor, and write the basis-universal version of the image to
`.imported_assets`. The final metadata will look like this:
```rust
(
meta_format_version: "1.0",
processed_info: Some((
hash: 905599590923828066,
full_hash: 9948823010183819117,
process_dependencies: [],
)),
asset: Load(
loader: "bevy_render::texture::image_loader::ImageLoader",
settings: (
format: Format(Basis),
),
),
)
```
To try basis-universal processing out in Bevy examples, (for example
`sprite.rs`), change `add_plugins(DefaultPlugins)` to
`add_plugins(DefaultPlugins.set(AssetPlugin::processed_dev()))` and run
with the `basis-universal` feature enabled: `cargo run
--features=basis-universal --example sprite`.
To create a custom processor, there are two main paths:
1. Use the `LoadAndSave` processor with an existing `AssetLoader`.
Implement the `AssetSaver` trait, register the processor using
`asset_processor.register_processor::<LoadAndSave<ImageLoader,
CompressedImageSaver>>(image_saver.into())`.
2. Implement the `Process` trait directly and register it using:
`asset_processor.register_processor(thing_processor)`.
You can configure default processors for file extensions like this:
```rust
asset_processor.set_default_processor::<ThingProcessor>("thing")
```
There is one more metadata type to be aware of:
```rust
(
meta_format_version: "1.0",
asset: Ignore,
)
```
This will ignore the asset during processing / prevent it from being
written to `.imported_assets`.
The AssetProcessor stores a transaction log at `.imported_assets/log`
and uses it to gracefully recover from unexpected stops. This means you
can force-quit the processor (and Bevy Apps running the processor in
parallel) at arbitrary times!
`.imported_assets` is "local state". It should _not_ be checked into
source control. It should also be considered "read only". In practice,
you _can_ modify processed assets and processed metadata if you really
need to test something. But those modifications will not be represented
in the hashes of the assets, so the processed state will be "out of
sync" with the source assets. The processor _will not_ fix this for you.
Either revert the change after you have tested it, or delete the
processed files so they can be re-populated.
## Open Questions
There are a number of open questions to be discussed. We should decide
if they need to be addressed in this PR and if so, how we will address
them:
### Implied Dependencies vs Dependency Enumeration
There are currently two ways to populate asset dependencies:
* **Implied via AssetLoaders**: if an AssetLoader loads an asset (and
retrieves a handle), a dependency is added to the list.
* **Explicit via the optional Asset::visit_dependencies**: if
`server.load_asset(my_asset)` is called, it will call
`my_asset.visit_dependencies`, which will grab dependencies that have
been manually defined for the asset via the Asset trait impl (which can
be derived).
This means that defining explicit dependencies is optional for "loaded
assets". And the list of dependencies is always accurate because loaders
can only produce Handles if they register dependencies. If an asset was
loaded with an AssetLoader, it only uses the implied dependencies. If an
asset was created at runtime and added with
`asset_server.load_asset(MyAsset)`, it will use
`Asset::visit_dependencies`.
However this can create a behavior mismatch between loaded assets and
equivalent "created at runtime" assets if `Assets::visit_dependencies`
doesn't exactly match the dependencies produced by the AssetLoader. This
behavior mismatch can be resolved by completely removing "implied loader
dependencies" and requiring `Asset::visit_dependencies` to supply
dependency data. But this creates two problems:
* It makes defining loaded assets harder and more error prone: Devs must
remember to manually annotate asset dependencies with `#[dependency]`
when deriving `Asset`. For more complicated assets (such as scenes), the
derive likely wouldn't be sufficient and a manual `visit_dependencies`
impl would be required.
* Removes the ability to immediately kick off dependency loads: When
AssetLoaders retrieve a Handle, they also immediately kick off an asset
load for the handle, which means it can start loading in parallel
_before_ the asset finishes loading. For large assets, this could be
significant. (although this could be mitigated for processed assets if
we store dependencies in the processed meta file and load them ahead of
time)
### Eager ProcessorDev Asset Loading
I made a controversial call in the interest of fast startup times ("time
to first pixel") for the "processor dev mode configuration". When
initializing the AssetProcessor, current processed versions of unchanged
assets are yielded immediately, even if their dependencies haven't been
checked yet for reprocessing. This means that
non-current-state-of-filesystem-but-previously-valid assets might be
returned to the App first, then hot-reloaded if/when their dependencies
change and the asset is reprocessed.
Is this behavior desirable? There is largely one alternative: do not
yield an asset from the processor to the app until all of its
dependencies have been checked for changes. In some common cases (load
dependency has not changed since last run) this will increase startup
time. The main question is "by how much" and is that slower startup time
worth it in the interest of only yielding assets that are true to the
current state of the filesystem. Should this be configurable? I'm
starting to think we should only yield an asset after its (historical)
dependencies have been checked for changes + processed as necessary, but
I'm curious what you all think.
### Paths Are Currently The Only Canonical ID / Do We Want Asset UUIDs?
In this implementation AssetPaths are the only canonical asset
identifier (just like the previous Bevy Asset system and Godot). Moving
assets will result in re-scans (and currently reprocessing, although
reprocessing can easily be avoided with some changes). Asset
renames/moves will break code and assets that rely on specific paths,
unless those paths are fixed up.
Do we want / need "stable asset uuids"? Introducing them is very
possible:
1. Generate a UUID and include it in .meta files
2. Support UUID in AssetPath
3. Generate "asset indices" which are loaded on startup and map UUIDs to
paths.
4 (maybe). Consider only supporting UUIDs for processed assets so we can
generate quick-to-load indices instead of scanning meta files.
The main "pro" is that assets referencing UUIDs don't need to be
migrated when a path changes. The main "con" is that UUIDs cannot be
"lazily resolved" like paths. They need a full view of all assets to
answer the question "does this UUID exist". Which means UUIDs require
the AssetProcessor to fully finish startup scans before saying an asset
doesnt exist. And they essentially require asset pre-processing to use
in apps, because scanning all asset metadata files at runtime to resolve
a UUID is not viable for medium-to-large apps. It really requires a
pre-generated UUID index, which must be loaded before querying for
assets.
I personally think this should be investigated in a separate PR. Paths
aren't going anywhere ... _everyone_ uses filesystems (and
filesystem-like apis) to manage their asset source files. I consider
them permanent canonical asset information. Additionally, they behave
well for both processed and unprocessed asset modes. Given that Bevy is
supporting both, this feels like the right canonical ID to start with.
UUIDS (and maybe even other indexed-identifier types) can be added later
as necessary.
### Folder / File Naming Conventions
All asset processing config currently lives in the `.imported_assets`
folder. The processor transaction log is in `.imported_assets/log`.
Processed assets are added to `.imported_assets/Default`, which will
make migrating to processed asset profiles (ex: a
`.imported_assets/Mobile` profile) a non-breaking change. It also allows
us to create top-level files like `.imported_assets/log` without it
being interpreted as an asset. Meta files currently have a `.meta`
suffix. Do we like these names and conventions?
### Should the `AssetPlugin::processed_dev` configuration enable
`watch_for_changes` automatically?
Currently it does (which I think makes sense), but it does make it the
only configuration that enables watch_for_changes by default.
### Discuss on_loaded High Level Interface:
This PR includes a very rough "proof of concept" `on_loaded` system
adapter that uses the `LoadedWithDependencies` event in combination with
`asset_server.load_asset` dependency tracking to support this pattern
```rust
fn main() {
App::new()
.init_asset::<MyAssets>()
.add_systems(Update, on_loaded(create_array_texture))
.run();
}
#[derive(Asset, Clone)]
struct MyAssets {
#[dependency]
picture_of_my_cat: Handle<Image>,
#[dependency]
picture_of_my_other_cat: Handle<Image>,
}
impl FromWorld for ArrayTexture {
fn from_world(world: &mut World) -> Self {
picture_of_my_cat: server.load("meow.png"),
picture_of_my_other_cat: server.load("meeeeeeeow.png"),
}
}
fn spawn_cat(In(my_assets): In<MyAssets>, mut commands: Commands) {
commands.spawn(SpriteBundle {
texture: my_assets.picture_of_my_cat.clone(),
..default()
});
commands.spawn(SpriteBundle {
texture: my_assets.picture_of_my_other_cat.clone(),
..default()
});
}
```
The implementation is _very_ rough. And it is currently unsafe because
`bevy_ecs` doesn't expose some internals to do this safely from inside
`bevy_asset`. There are plenty of unanswered questions like:
* "do we add a Loadable" derive? (effectively automate the FromWorld
implementation above)
* Should `MyAssets` even be an Asset? (largely implemented this way
because it elegantly builds on `server.load_asset(MyAsset { .. })`
dependency tracking).
We should think hard about what our ideal API looks like (and if this is
a pattern we want to support). Not necessarily something we need to
solve in this PR. The current `on_loaded` impl should probably be
removed from this PR before merging.
## Clarifying Questions
### What about Assets as Entities?
This Bevy Asset V2 proposal implementation initially stored Assets as
ECS Entities. Instead of `AssetId<T>` + the `Assets<T>` resource it used
`Entity` as the asset id and Asset values were just ECS components.
There are plenty of compelling reasons to do this:
1. Easier to inline assets in Bevy Scenes (as they are "just" normal
entities + components)
2. More flexible queries: use the power of the ECS to filter assets (ex:
`Query<Mesh, With<Tree>>`).
3. Extensible. Users can add arbitrary component data to assets.
4. Things like "component visualization tools" work out of the box to
visualize asset data.
However Assets as Entities has a ton of caveats right now:
* We need to be able to allocate entity ids without a direct World
reference (aka rework id allocator in Entities ... i worked around this
in my prototypes by just pre allocating big chunks of entities)
* We want asset change events in addition to ECS change tracking ... how
do we populate them when mutations can come from anywhere? Do we use
Changed queries? This would require iterating over the change data for
all assets every frame. Is this acceptable or should we implement a new
"event based" component change detection option?
* Reconciling manually created assets with asset-system managed assets
has some nuance (ex: are they "loaded" / do they also have that
component metadata?)
* "how do we handle "static" / default entity handles" (ties in to the
Entity Indices discussion:
https://github.com/bevyengine/bevy/discussions/8319). This is necessary
for things like "built in" assets and default handles in things like
SpriteBundle.
* Storing asset information as a component makes it easy to "invalidate"
asset state by removing the component (or forcing modifications).
Ideally we have ways to lock this down (some combination of Rust type
privacy and ECS validation)
In practice, how we store and identify assets is a reasonably
superficial change (porting off of Assets as Entities and implementing
dedicated storage + ids took less than a day). So once we sort out the
remaining challenges the flip should be straightforward. Additionally, I
do still have "Assets as Entities" in my commit history, so we can reuse
that work. I personally think "assets as entities" is a good endgame,
but it also doesn't provide _significant_ value at the moment and it
certainly isn't ready yet with the current state of things.
### Why not Distill?
[Distill](https://github.com/amethyst/distill) is a high quality fully
featured asset system built in Rust. It is very natural to ask "why not
just use Distill?".
It is also worth calling out that for awhile, [we planned on adopting
Distill / I signed off on
it](https://github.com/bevyengine/bevy/issues/708).
However I think Bevy has a number of constraints that make Distill
adoption suboptimal:
* **Architectural Simplicity:**
* Distill's processor requires an in-memory database (lmdb) and RPC
networked API (using Cap'n Proto). Each of these introduces API
complexity that increases maintenance burden and "code grokability".
Ignoring tests, documentation, and examples, Distill has 24,237 lines of
Rust code (including generated code for RPC + database interactions). If
you ignore generated code, it has 11,499 lines.
* Bevy builds the AssetProcessor and AssetServer using pluggable
AssetReader/AssetWriter Rust traits with simple io interfaces. They do
not necessitate databases or RPC interfaces (although Readers/Writers
could use them if that is desired). Bevy Asset V2 (at the time of
writing this PR) is 5,384 lines of Rust code (ignoring tests,
documentation, and examples). Grain of salt: Distill does have more
features currently (ex: Asset Packing, GUIDS, remote-out-of-process
asset processor). I do plan to implement these features in Bevy Asset V2
and I personally highly doubt they will meaningfully close the 6115
lines-of-code gap.
* This complexity gap (which while illustrated by lines of code, is much
bigger than just that) is noteworthy to me. Bevy should be hackable and
there are pillars of Distill that are very hard to understand and
extend. This is a matter of opinion (and Bevy Asset V2 also has
complicated areas), but I think Bevy Asset V2 is much more approachable
for the average developer.
* Necessary disclaimer: counting lines of code is an extremely rough
complexity metric. Read the code and form your own opinions.
* **Optional Asset Processing:** Not all Bevy Apps (or Bevy App
developers) need / want asset preprocessing. Processing increases the
complexity of the development environment by introducing things like
meta files, imported asset storage, running processors in the
background, waiting for processing to finish, etc. Distill _requires_
preprocessing to work. With Bevy Asset V2 processing is fully opt-in.
The AssetServer isn't directly aware of asset processors at all.
AssetLoaders only care about converting bytes to runtime Assets ... they
don't know or care if the bytes were pre-processed or not. Processing is
"elegantly" (forgive my self-congratulatory phrasing) layered on top and
builds on the existing Asset system primitives.
* **Direct Filesystem Access to Processed Asset State:** Distill stores
processed assets in a database. This makes debugging / inspecting the
processed outputs harder (either requires special tooling to query the
database or they need to be "deployed" to be inspected). Bevy Asset V2,
on the other hand, stores processed assets in the filesystem (by default
... this is configurable). This makes interacting with the processed
state more natural. Note that both Godot and Unity's new asset system
store processed assets in the filesystem.
* **Portability**: Because Distill's processor uses lmdb and RPC
networking, it cannot be run on certain platforms (ex: lmdb is a
non-rust dependency that cannot run on the web, some platforms don't
support running network servers). Bevy should be able to process assets
everywhere (ex: run the Bevy Editor on the web, compile + process
shaders on mobile, etc). Distill does partially mitigate this problem by
supporting "streaming" assets via the RPC protocol, but this is not a
full solve from my perspective. And Bevy Asset V2 can (in theory) also
stream assets (without requiring RPC, although this isn't implemented
yet)
Note that I _do_ still think Distill would be a solid asset system for
Bevy. But I think the approach in this PR is a better solve for Bevy's
specific "asset system requirements".
### Doesn't async-fs just shim requests to "sync" `std::fs`? What is the
point?
"True async file io" has limited / spotty platform support. async-fs
(and the rust async ecosystem generally ... ex Tokio) currently use
async wrappers over std::fs that offload blocking requests to separate
threads. This may feel unsatisfying, but it _does_ still provide value
because it prevents our task pools from blocking on file system
operations (which would prevent progress when there are many tasks to
do, but all threads in a pool are currently blocking on file system
ops).
Additionally, using async APIs for our AssetReaders and AssetWriters
also provides value because we can later add support for "true async
file io" for platforms that support it. _And_ we can implement other
"true async io" asset backends (such as networked asset io).
## Draft TODO
- [x] Fill in missing filesystem event APIs: file removed event (which
is expressed as dangling RenameFrom events in some cases), file/folder
renamed event
- [x] Assets without loaders are not moved to the processed folder. This
breaks things like referenced `.bin` files for GLTFs. This should be
configurable per-non-asset-type.
- [x] Initial implementation of Reflect and FromReflect for Handle. The
"deserialization" parity bar is low here as this only worked with static
UUIDs in the old impl ... this is a non-trivial problem. Either we add a
Handle::AssetPath variant that gets "upgraded" to a strong handle on
scene load or we use a separate AssetRef type for Bevy scenes (which is
converted to a runtime Handle on load). This deserves its own discussion
in a different pr.
- [x] Populate read_asset_bytes hash when run by the processor (a bit of
a special case .. when run by the processor the processed meta will
contain the hash so we don't need to compute it on the spot, but we
don't want/need to read the meta when run by the main AssetServer)
- [x] Delay hot reloading: currently filesystem events are handled
immediately, which creates timing issues in some cases. For example hot
reloading images can sometimes break because the image isn't finished
writing. We should add a delay, likely similar to the [implementation in
this PR](https://github.com/bevyengine/bevy/pull/8503).
- [x] Port old platform-specific AssetIo implementations to the new
AssetReader interface (currently missing Android and web)
- [x] Resolve on_loaded unsafety (either by removing the API entirely or
removing the unsafe)
- [x] Runtime loader setting overrides
- [x] Remove remaining unwraps that should be error-handled. There are
number of TODOs here
- [x] Pretty AssetPath Display impl
- [x] Document more APIs
- [x] Resolve spurious "reloading because it has changed" events (to
repro run load_gltf with `processed_dev()`)
- [x] load_dependency hot reloading currently only works for processed
assets. If processing is disabled, load_dependency changes are not hot
reloaded.
- [x] Replace AssetInfo dependency load/fail counters with
`loading_dependencies: HashSet<UntypedAssetId>` to prevent reloads from
(potentially) breaking counters. Storing this will also enable
"dependency reloaded" events (see [Next Steps](#next-steps))
- [x] Re-add filesystem watcher cargo feature gate (currently it is not
optional)
- [ ] Migration Guide
- [ ] Changelog
## Followup TODO
- [ ] Replace "eager unchanged processed asset loading" behavior with
"don't returned unchanged processed asset until dependencies have been
checked".
- [ ] Add true `Ignore` AssetAction that does not copy the asset to the
imported_assets folder.
- [ ] Finish "live asset unloading" (ex: free up CPU asset memory after
uploading an image to the GPU), rethink RenderAssets, and port renderer
features. The `Assets` collection uses `Option<T>` for asset storage to
support its removal. (1) the Option might not actually be necessary ...
might be able to just remove from the collection entirely (2) need to
finalize removal apis
- [ ] Try replacing the "channel based" asset id recycling with
something a bit more efficient (ex: we might be able to use raw atomic
ints with some cleverness)
- [ ] Consider adding UUIDs to processed assets (scoped just to helping
identify moved assets ... not exposed to load queries ... see [Next
Steps](#next-steps))
- [ ] Store "last modified" source asset and meta timestamps in
processed meta files to enable skipping expensive hashing when the file
wasn't changed
- [ ] Fix "slow loop" handle drop fix
- [ ] Migrate to TypeName
- [x] Handle "loader preregistration". See #9429
## Next Steps
* **Configurable per-type defaults for AssetMeta**: It should be
possible to add configuration like "all png image meta should default to
using nearest sampling" (currently this hard-coded per-loader/processor
Settings::default() impls). Also see the "Folder Meta" bullet point.
* **Avoid Reprocessing on Asset Renames / Moves**: See the "canonical
asset ids" discussion in [Open Questions](#open-questions) and the
relevant bullet point in [Draft TODO](#draft-todo). Even without
canonical ids, folder renames could avoid reprocessing in some cases.
* **Multiple Asset Sources**: Expand AssetPath to support "asset source
names" and support multiple AssetReaders in the asset server (ex:
`webserver://some_path/image.png` backed by an Http webserver
AssetReader). The "default" asset reader would use normal
`some_path/image.png` paths. Ideally this works in combination with
multiple AssetWatchers for hot-reloading
* **Stable Type Names**: this pr removes the TypeUuid requirement from
assets in favor of `std::any::type_name`. This makes defining assets
easier (no need to generate a new uuid / use weird proc macro syntax).
It also makes reading meta files easier (because things have "friendly
names"). We also use type names for components in scene files. If they
are good enough for components, they are good enough for assets. And
consistency across Bevy pillars is desirable. However,
`std::any::type_name` is not guaranteed to be stable (although in
practice it is). We've developed a [stable type
path](https://github.com/bevyengine/bevy/pull/7184) to resolve this,
which should be adopted when it is ready.
* **Command Line Interface**: It should be possible to run the asset
processor in a separate process from the command line. This will also
require building a network-server-backed AssetReader to communicate
between the app and the processor. We've been planning to build a "bevy
cli" for awhile. This seems like a good excuse to build it.
* **Asset Packing**: This is largely an additive feature, so it made
sense to me to punt this until we've laid the foundations in this PR.
* **Per-Platform Processed Assets**: It should be possible to generate
assets for multiple platforms by supporting multiple "processor
profiles" per asset (ex: compress with format X on PC and Y on iOS). I
think there should probably be arbitrary "profiles" (which can be
separate from actual platforms), which are then assigned to a given
platform when generating the final asset distribution for that platform.
Ex: maybe devs want a "Mobile" profile that is shared between iOS and
Android. Or a "LowEnd" profile shared between web and mobile.
* **Versioning and Migrations**: Assets, Loaders, Savers, and Processors
need to have versions to determine if their schema is valid. If an asset
/ loader version is incompatible with the current version expected at
runtime, the processor should be able to migrate them. I think we should
try using Bevy Reflect for this, as it would allow us to load the old
version as a dynamic Reflect type without actually having the old Rust
type. It would also allow us to define "patches" to migrate between
versions (Bevy Reflect devs are currently working on patching). The
`.meta` file already has its own format version. Migrating that to new
versions should also be possible.
* **Real Copy-on-write AssetPaths**: Rust's actual Cow (clone-on-write
type) currently used by AssetPath can still result in String clones that
aren't actually necessary (cloning an Owned Cow clones the contents).
Bevy's asset system requires cloning AssetPaths in a number of places,
which result in actual clones of the internal Strings. This is not
efficient. AssetPath internals should be reworked to exhibit truer
cow-like-behavior that reduces String clones to the absolute minimum.
* **Consider processor-less processing**: In theory the AssetServer
could run processors "inline" even if the background AssetProcessor is
disabled. If we decide this is actually desirable, we could add this.
But I don't think its a priority in the short or medium term.
* **Pre-emptive dependency loading**: We could encode dependencies in
processed meta files, which could then be used by the Asset Server to
kick of dependency loads as early as possible (prior to starting the
actual asset load). Is this desirable? How much time would this save in
practice?
* **Optimize Processor With UntypedAssetIds**: The processor exclusively
uses AssetPath to identify assets currently. It might be possible to
swap these out for UntypedAssetIds in some places, which are smaller /
cheaper to hash and compare.
* **One to Many Asset Processing**: An asset source file that produces
many assets currently must be processed into a single "processed" asset
source. If labeled assets can be written separately they can each have
their own configured savers _and_ they could be loaded more granularly.
Definitely worth exploring!
* **Automatically Track "Runtime-only" Asset Dependencies**: Right now,
tracking "created at runtime" asset dependencies requires adding them
via `asset_server.load_asset(StandardMaterial::default())`. I think with
some cleverness we could also do this for
`materials.add(StandardMaterial::default())`, making tracking work
"everywhere". There are challenges here relating to change detection /
ensuring the server is made aware of dependency changes. This could be
expensive in some cases.
* **"Dependency Changed" events**: Some assets have runtime artifacts
that need to be re-generated when one of their dependencies change (ex:
regenerate a material's bind group when a Texture needs to change). We
are generating the dependency graph so we can definitely produce these
events. Buuuuut generating these events will have a cost / they could be
high frequency for some assets, so we might want this to be opt-in for
specific cases.
* **Investigate Storing More Information In Handles**: Handles can now
store arbitrary information, which makes it cheaper and easier to
access. How much should we move into them? Canonical asset load states
(via atomics)? (`handle.is_loaded()` would be very cool). Should we
store the entire asset and remove the `Assets<T>` collection?
(`Arc<RwLock<Option<Image>>>`?)
* **Support processing and loading files without extensions**: This is a
pretty arbitrary restriction and could be supported with very minimal
changes.
* **Folder Meta**: It would be nice if we could define per folder
processor configuration defaults (likely in a `.meta` or `.folder_meta`
file). Things like "default to linear filtering for all Images in this
folder".
* **Replace async_broadcast with event-listener?** This might be
approximately drop-in for some uses and it feels more light weight
* **Support Running the AssetProcessor on the Web**: Most of the hard
work is done here, but there are some easy straggling TODOs (make the
transaction log an interface instead of a direct file writer so we can
write a web storage backend, implement an AssetReader/AssetWriter that
reads/writes to something like LocalStorage).
* **Consider identifying and preventing circular dependencies**: This is
especially important for "processor dependencies", as processing will
silently never finish in these cases.
* **Built-in/Inlined Asset Hot Reloading**: This PR regresses
"built-in/inlined" asset hot reloading (previously provided by the
DebugAssetServer). I'm intentionally punting this because I think it can
be cleanly implemented with "multiple asset sources" by registering a
"debug asset source" (ex: `debug://bevy_pbr/src/render/pbr.wgsl` asset
paths) in combination with an AssetWatcher for that asset source and
support for "manually loading pats with asset bytes instead of
AssetReaders". The old DebugAssetServer was quite nasty and I'd love to
avoid that hackery going forward.
* **Investigate ways to remove double-parsing meta files**: Parsing meta
files currently involves parsing once with "minimal" versions of the
meta file to extract the type name of the loader/processor config, then
parsing again to parse the "full" meta. This is suboptimal. We should be
able to define custom deserializers that (1) assume the loader/processor
type name comes first (2) dynamically looks up the loader/processor
registrations to deserialize settings in-line (similar to components in
the bevy scene format). Another alternative: deserialize as dynamic
Reflect objects and then convert.
* **More runtime loading configuration**: Support using the Handle type
as a hint to select an asset loader (instead of relying on AssetPath
extensions)
* **More high level Processor trait implementations**: For example, it
might be worth adding support for arbitrary chains of "asset transforms"
that modify an in-memory asset representation between loading and
saving. (ex: load a Mesh, run a `subdivide_mesh` transform, followed by
a `flip_normals` transform, then save the mesh to an efficient
compressed format).
* **Bevy Scene Handle Deserialization**: (see the relevant [Draft TODO
item](#draft-todo) for context)
* **Explore High Level Load Interfaces**: See [this
discussion](#discuss-on_loaded-high-level-interface) for one prototype.
* **Asset Streaming**: It would be great if we could stream Assets (ex:
stream a long video file piece by piece)
* **ID Exchanging**: In this PR Asset Handles/AssetIds are bigger than
they need to be because they have a Uuid enum variant. If we implement
an "id exchanging" system that trades Uuids for "efficient runtime ids",
we can cut down on the size of AssetIds, making them more efficient.
This has some open design questions, such as how to spawn entities with
"default" handle values (as these wouldn't have access to the exchange
api in the current system).
* **Asset Path Fixup Tooling**: Assets that inline asset paths inside
them will break when an asset moves. The asset system provides the
functionality to detect when paths break. We should build a framework
that enables formats to define "path migrations". This is especially
important for scene files. For editor-generated files, we should also
consider using UUIDs (see other bullet point) to avoid the need to
migrate in these cases.
---------
Co-authored-by: BeastLe9enD <beastle9end@outlook.de>
Co-authored-by: Mike <mike.hsu@gmail.com>
Co-authored-by: Nicola Papale <nicopap@users.noreply.github.com>
2023-09-07 02:07:27 +00:00
|
|
|
pub trait Material: Asset + AsBindGroup + Clone + Sized {
|
Better Materials: AsBindGroup trait and derive, simpler Material trait (#5053)
# Objective
This PR reworks Bevy's Material system, making the user experience of defining Materials _much_ nicer. Bevy's previous material system leaves a lot to be desired:
* Materials require manually implementing the `RenderAsset` trait, which involves manually generating the bind group, handling gpu buffer data transfer, looking up image textures, etc. Even the simplest single-texture material involves writing ~80 unnecessary lines of code. This was never the long term plan.
* There are two material traits, which is confusing, hard to document, and often redundant: `Material` and `SpecializedMaterial`. `Material` implicitly implements `SpecializedMaterial`, and `SpecializedMaterial` is used in most high level apis to support both use cases. Most users shouldn't need to think about specialization at all (I consider it a "power-user tool"), so the fact that `SpecializedMaterial` is front-and-center in our apis is a miss.
* Implementing either material trait involves a lot of "type soup". The "prepared asset" parameter is particularly heinous: `&<Self as RenderAsset>::PreparedAsset`. Defining vertex and fragment shaders is also more verbose than it needs to be.
## Solution
Say hello to the new `Material` system:
```rust
#[derive(AsBindGroup, TypeUuid, Debug, Clone)]
#[uuid = "f690fdae-d598-45ab-8225-97e2a3f056e0"]
pub struct CoolMaterial {
#[uniform(0)]
color: Color,
#[texture(1)]
#[sampler(2)]
color_texture: Handle<Image>,
}
impl Material for CoolMaterial {
fn fragment_shader() -> ShaderRef {
"cool_material.wgsl".into()
}
}
```
Thats it! This same material would have required [~80 lines of complicated "type heavy" code](https://github.com/bevyengine/bevy/blob/v0.7.0/examples/shader/shader_material.rs) in the old Material system. Now it is just 14 lines of simple, readable code.
This is thanks to a new consolidated `Material` trait and the new `AsBindGroup` trait / derive.
### The new `Material` trait
The old "split" `Material` and `SpecializedMaterial` traits have been removed in favor of a new consolidated `Material` trait. All of the functions on the trait are optional.
The difficulty of implementing `Material` has been reduced by simplifying dataflow and removing type complexity:
```rust
// Old
impl Material for CustomMaterial {
fn fragment_shader(asset_server: &AssetServer) -> Option<Handle<Shader>> {
Some(asset_server.load("custom_material.wgsl"))
}
fn alpha_mode(render_asset: &<Self as RenderAsset>::PreparedAsset) -> AlphaMode {
render_asset.alpha_mode
}
}
// New
impl Material for CustomMaterial {
fn fragment_shader() -> ShaderRef {
"custom_material.wgsl".into()
}
fn alpha_mode(&self) -> AlphaMode {
self.alpha_mode
}
}
```
Specialization is still supported, but it is hidden by default under the `specialize()` function (more on this later).
### The `AsBindGroup` trait / derive
The `Material` trait now requires the `AsBindGroup` derive. This can be implemented manually relatively easily, but deriving it will almost always be preferable.
Field attributes like `uniform` and `texture` are used to define which fields should be bindings,
what their binding type is, and what index they should be bound at:
```rust
#[derive(AsBindGroup)]
struct CoolMaterial {
#[uniform(0)]
color: Color,
#[texture(1)]
#[sampler(2)]
color_texture: Handle<Image>,
}
```
In WGSL shaders, the binding looks like this:
```wgsl
struct CoolMaterial {
color: vec4<f32>;
};
[[group(1), binding(0)]]
var<uniform> material: CoolMaterial;
[[group(1), binding(1)]]
var color_texture: texture_2d<f32>;
[[group(1), binding(2)]]
var color_sampler: sampler;
```
Note that the "group" index is determined by the usage context. It is not defined in `AsBindGroup`. Bevy material bind groups are bound to group 1.
The following field-level attributes are supported:
* `uniform(BINDING_INDEX)`
* The field will be converted to a shader-compatible type using the `ShaderType` trait, written to a `Buffer`, and bound as a uniform. It can also be derived for custom structs.
* `texture(BINDING_INDEX)`
* This field's `Handle<Image>` will be used to look up the matching `Texture` gpu resource, which will be bound as a texture in shaders. The field will be assumed to implement `Into<Option<Handle<Image>>>`. In practice, most fields should be a `Handle<Image>` or `Option<Handle<Image>>`. If the value of an `Option<Handle<Image>>` is `None`, the new `FallbackImage` resource will be used instead. This attribute can be used in conjunction with a `sampler` binding attribute (with a different binding index).
* `sampler(BINDING_INDEX)`
* Behaves exactly like the `texture` attribute, but sets the Image's sampler binding instead of the texture.
Note that fields without field-level binding attributes will be ignored.
```rust
#[derive(AsBindGroup)]
struct CoolMaterial {
#[uniform(0)]
color: Color,
this_field_is_ignored: String,
}
```
As mentioned above, `Option<Handle<Image>>` is also supported:
```rust
#[derive(AsBindGroup)]
struct CoolMaterial {
#[uniform(0)]
color: Color,
#[texture(1)]
#[sampler(2)]
color_texture: Option<Handle<Image>>,
}
```
This is useful if you want a texture to be optional. When the value is `None`, the `FallbackImage` will be used for the binding instead, which defaults to "pure white".
Field uniforms with the same binding index will be combined into a single binding:
```rust
#[derive(AsBindGroup)]
struct CoolMaterial {
#[uniform(0)]
color: Color,
#[uniform(0)]
roughness: f32,
}
```
In WGSL shaders, the binding would look like this:
```wgsl
struct CoolMaterial {
color: vec4<f32>;
roughness: f32;
};
[[group(1), binding(0)]]
var<uniform> material: CoolMaterial;
```
Some less common scenarios will require "struct-level" attributes. These are the currently supported struct-level attributes:
* `uniform(BINDING_INDEX, ConvertedShaderType)`
* Similar to the field-level `uniform` attribute, but instead the entire `AsBindGroup` value is converted to `ConvertedShaderType`, which must implement `ShaderType`. This is useful if more complicated conversion logic is required.
* `bind_group_data(DataType)`
* The `AsBindGroup` type will be converted to some `DataType` using `Into<DataType>` and stored as `AsBindGroup::Data` as part of the `AsBindGroup::as_bind_group` call. This is useful if data needs to be stored alongside the generated bind group, such as a unique identifier for a material's bind group. The most common use case for this attribute is "shader pipeline specialization".
The previous `CoolMaterial` example illustrating "combining multiple field-level uniform attributes with the same binding index" can
also be equivalently represented with a single struct-level uniform attribute:
```rust
#[derive(AsBindGroup)]
#[uniform(0, CoolMaterialUniform)]
struct CoolMaterial {
color: Color,
roughness: f32,
}
#[derive(ShaderType)]
struct CoolMaterialUniform {
color: Color,
roughness: f32,
}
impl From<&CoolMaterial> for CoolMaterialUniform {
fn from(material: &CoolMaterial) -> CoolMaterialUniform {
CoolMaterialUniform {
color: material.color,
roughness: material.roughness,
}
}
}
```
### Material Specialization
Material shader specialization is now _much_ simpler:
```rust
#[derive(AsBindGroup, TypeUuid, Debug, Clone)]
#[uuid = "f690fdae-d598-45ab-8225-97e2a3f056e0"]
#[bind_group_data(CoolMaterialKey)]
struct CoolMaterial {
#[uniform(0)]
color: Color,
is_red: bool,
}
#[derive(Copy, Clone, Hash, Eq, PartialEq)]
struct CoolMaterialKey {
is_red: bool,
}
impl From<&CoolMaterial> for CoolMaterialKey {
fn from(material: &CoolMaterial) -> CoolMaterialKey {
CoolMaterialKey {
is_red: material.is_red,
}
}
}
impl Material for CoolMaterial {
fn fragment_shader() -> ShaderRef {
"cool_material.wgsl".into()
}
fn specialize(
pipeline: &MaterialPipeline<Self>,
descriptor: &mut RenderPipelineDescriptor,
layout: &MeshVertexBufferLayout,
key: MaterialPipelineKey<Self>,
) -> Result<(), SpecializedMeshPipelineError> {
if key.bind_group_data.is_red {
let fragment = descriptor.fragment.as_mut().unwrap();
fragment.shader_defs.push("IS_RED".to_string());
}
Ok(())
}
}
```
Setting `bind_group_data` is not required for specialization (it defaults to `()`). Scenarios like "custom vertex attributes" also benefit from this system:
```rust
impl Material for CustomMaterial {
fn vertex_shader() -> ShaderRef {
"custom_material.wgsl".into()
}
fn fragment_shader() -> ShaderRef {
"custom_material.wgsl".into()
}
fn specialize(
pipeline: &MaterialPipeline<Self>,
descriptor: &mut RenderPipelineDescriptor,
layout: &MeshVertexBufferLayout,
key: MaterialPipelineKey<Self>,
) -> Result<(), SpecializedMeshPipelineError> {
let vertex_layout = layout.get_layout(&[
Mesh::ATTRIBUTE_POSITION.at_shader_location(0),
ATTRIBUTE_BLEND_COLOR.at_shader_location(1),
])?;
descriptor.vertex.buffers = vec![vertex_layout];
Ok(())
}
}
```
### Ported `StandardMaterial` to the new `Material` system
Bevy's built-in PBR material uses the new Material system (including the AsBindGroup derive):
```rust
#[derive(AsBindGroup, Debug, Clone, TypeUuid)]
#[uuid = "7494888b-c082-457b-aacf-517228cc0c22"]
#[bind_group_data(StandardMaterialKey)]
#[uniform(0, StandardMaterialUniform)]
pub struct StandardMaterial {
pub base_color: Color,
#[texture(1)]
#[sampler(2)]
pub base_color_texture: Option<Handle<Image>>,
/* other fields omitted for brevity */
```
### Ported Bevy examples to the new `Material` system
The overall complexity of Bevy's "custom shader examples" has gone down significantly. Take a look at the diffs if you want a dopamine spike.
Please note that while this PR has a net increase in "lines of code", most of those extra lines come from added documentation. There is a significant reduction
in the overall complexity of the code (even accounting for the new derive logic).
---
## Changelog
### Added
* `AsBindGroup` trait and derive, which make it much easier to transfer data to the gpu and generate bind groups for a given type.
### Changed
* The old `Material` and `SpecializedMaterial` traits have been replaced by a consolidated (much simpler) `Material` trait. Materials no longer implement `RenderAsset`.
* `StandardMaterial` was ported to the new material system. There are no user-facing api changes to the `StandardMaterial` struct api, but it now implements `AsBindGroup` and `Material` instead of `RenderAsset` and `SpecializedMaterial`.
## Migration Guide
The Material system has been reworked to be much simpler. We've removed a lot of boilerplate with the new `AsBindGroup` derive and the `Material` trait is simpler as well!
### Bevy 0.7 (old)
```rust
#[derive(Debug, Clone, TypeUuid)]
#[uuid = "f690fdae-d598-45ab-8225-97e2a3f056e0"]
pub struct CustomMaterial {
color: Color,
color_texture: Handle<Image>,
}
#[derive(Clone)]
pub struct GpuCustomMaterial {
_buffer: Buffer,
bind_group: BindGroup,
}
impl RenderAsset for CustomMaterial {
type ExtractedAsset = CustomMaterial;
type PreparedAsset = GpuCustomMaterial;
type Param = (SRes<RenderDevice>, SRes<MaterialPipeline<Self>>);
fn extract_asset(&self) -> Self::ExtractedAsset {
self.clone()
}
fn prepare_asset(
extracted_asset: Self::ExtractedAsset,
(render_device, material_pipeline): &mut SystemParamItem<Self::Param>,
) -> Result<Self::PreparedAsset, PrepareAssetError<Self::ExtractedAsset>> {
let color = Vec4::from_slice(&extracted_asset.color.as_linear_rgba_f32());
let byte_buffer = [0u8; Vec4::SIZE.get() as usize];
let mut buffer = encase::UniformBuffer::new(byte_buffer);
buffer.write(&color).unwrap();
let buffer = render_device.create_buffer_with_data(&BufferInitDescriptor {
contents: buffer.as_ref(),
label: None,
usage: BufferUsages::UNIFORM | BufferUsages::COPY_DST,
});
let (texture_view, texture_sampler) = if let Some(result) = material_pipeline
.mesh_pipeline
.get_image_texture(gpu_images, &Some(extracted_asset.color_texture.clone()))
{
result
} else {
return Err(PrepareAssetError::RetryNextUpdate(extracted_asset));
};
let bind_group = render_device.create_bind_group(&BindGroupDescriptor {
entries: &[
BindGroupEntry {
binding: 0,
resource: buffer.as_entire_binding(),
},
BindGroupEntry {
binding: 0,
resource: BindingResource::TextureView(texture_view),
},
BindGroupEntry {
binding: 1,
resource: BindingResource::Sampler(texture_sampler),
},
],
label: None,
layout: &material_pipeline.material_layout,
});
Ok(GpuCustomMaterial {
_buffer: buffer,
bind_group,
})
}
}
impl Material for CustomMaterial {
fn fragment_shader(asset_server: &AssetServer) -> Option<Handle<Shader>> {
Some(asset_server.load("custom_material.wgsl"))
}
fn bind_group(render_asset: &<Self as RenderAsset>::PreparedAsset) -> &BindGroup {
&render_asset.bind_group
}
fn bind_group_layout(render_device: &RenderDevice) -> BindGroupLayout {
render_device.create_bind_group_layout(&BindGroupLayoutDescriptor {
entries: &[
BindGroupLayoutEntry {
binding: 0,
visibility: ShaderStages::FRAGMENT,
ty: BindingType::Buffer {
ty: BufferBindingType::Uniform,
has_dynamic_offset: false,
min_binding_size: Some(Vec4::min_size()),
},
count: None,
},
BindGroupLayoutEntry {
binding: 1,
visibility: ShaderStages::FRAGMENT,
ty: BindingType::Texture {
multisampled: false,
sample_type: TextureSampleType::Float { filterable: true },
view_dimension: TextureViewDimension::D2Array,
},
count: None,
},
BindGroupLayoutEntry {
binding: 2,
visibility: ShaderStages::FRAGMENT,
ty: BindingType::Sampler(SamplerBindingType::Filtering),
count: None,
},
],
label: None,
})
}
}
```
### Bevy 0.8 (new)
```rust
impl Material for CustomMaterial {
fn fragment_shader() -> ShaderRef {
"custom_material.wgsl".into()
}
}
#[derive(AsBindGroup, TypeUuid, Debug, Clone)]
#[uuid = "f690fdae-d598-45ab-8225-97e2a3f056e0"]
pub struct CustomMaterial {
#[uniform(0)]
color: Color,
#[texture(1)]
#[sampler(2)]
color_texture: Handle<Image>,
}
```
## Future Work
* Add support for more binding types (cubemaps, buffers, etc). This PR intentionally includes a bare minimum number of binding types to keep "reviewability" in check.
* Consider optionally eliding binding indices using binding names. `AsBindGroup` could pass in (optional?) reflection info as a "hint".
* This would make it possible for the derive to do this:
```rust
#[derive(AsBindGroup)]
pub struct CustomMaterial {
#[uniform]
color: Color,
#[texture]
#[sampler]
color_texture: Option<Handle<Image>>,
alpha_mode: AlphaMode,
}
```
* Or this
```rust
#[derive(AsBindGroup)]
pub struct CustomMaterial {
#[binding]
color: Color,
#[binding]
color_texture: Option<Handle<Image>>,
alpha_mode: AlphaMode,
}
```
* Or even this (if we flip to "include bindings by default")
```rust
#[derive(AsBindGroup)]
pub struct CustomMaterial {
color: Color,
color_texture: Option<Handle<Image>>,
#[binding(ignore)]
alpha_mode: AlphaMode,
}
```
* If we add the option to define custom draw functions for materials (which could be done in a type-erased way), I think that would be enough to support extra non-material bindings. Worth considering!
2022-06-30 23:48:46 +00:00
|
|
|
/// Returns this material's vertex shader. If [`ShaderRef::Default`] is returned, the default mesh vertex shader
|
|
|
|
/// will be used.
|
|
|
|
fn vertex_shader() -> ShaderRef {
|
|
|
|
ShaderRef::Default
|
2020-02-11 17:31:49 +00:00
|
|
|
}
|
2020-08-01 00:10:29 +00:00
|
|
|
|
Better Materials: AsBindGroup trait and derive, simpler Material trait (#5053)
# Objective
This PR reworks Bevy's Material system, making the user experience of defining Materials _much_ nicer. Bevy's previous material system leaves a lot to be desired:
* Materials require manually implementing the `RenderAsset` trait, which involves manually generating the bind group, handling gpu buffer data transfer, looking up image textures, etc. Even the simplest single-texture material involves writing ~80 unnecessary lines of code. This was never the long term plan.
* There are two material traits, which is confusing, hard to document, and often redundant: `Material` and `SpecializedMaterial`. `Material` implicitly implements `SpecializedMaterial`, and `SpecializedMaterial` is used in most high level apis to support both use cases. Most users shouldn't need to think about specialization at all (I consider it a "power-user tool"), so the fact that `SpecializedMaterial` is front-and-center in our apis is a miss.
* Implementing either material trait involves a lot of "type soup". The "prepared asset" parameter is particularly heinous: `&<Self as RenderAsset>::PreparedAsset`. Defining vertex and fragment shaders is also more verbose than it needs to be.
## Solution
Say hello to the new `Material` system:
```rust
#[derive(AsBindGroup, TypeUuid, Debug, Clone)]
#[uuid = "f690fdae-d598-45ab-8225-97e2a3f056e0"]
pub struct CoolMaterial {
#[uniform(0)]
color: Color,
#[texture(1)]
#[sampler(2)]
color_texture: Handle<Image>,
}
impl Material for CoolMaterial {
fn fragment_shader() -> ShaderRef {
"cool_material.wgsl".into()
}
}
```
Thats it! This same material would have required [~80 lines of complicated "type heavy" code](https://github.com/bevyengine/bevy/blob/v0.7.0/examples/shader/shader_material.rs) in the old Material system. Now it is just 14 lines of simple, readable code.
This is thanks to a new consolidated `Material` trait and the new `AsBindGroup` trait / derive.
### The new `Material` trait
The old "split" `Material` and `SpecializedMaterial` traits have been removed in favor of a new consolidated `Material` trait. All of the functions on the trait are optional.
The difficulty of implementing `Material` has been reduced by simplifying dataflow and removing type complexity:
```rust
// Old
impl Material for CustomMaterial {
fn fragment_shader(asset_server: &AssetServer) -> Option<Handle<Shader>> {
Some(asset_server.load("custom_material.wgsl"))
}
fn alpha_mode(render_asset: &<Self as RenderAsset>::PreparedAsset) -> AlphaMode {
render_asset.alpha_mode
}
}
// New
impl Material for CustomMaterial {
fn fragment_shader() -> ShaderRef {
"custom_material.wgsl".into()
}
fn alpha_mode(&self) -> AlphaMode {
self.alpha_mode
}
}
```
Specialization is still supported, but it is hidden by default under the `specialize()` function (more on this later).
### The `AsBindGroup` trait / derive
The `Material` trait now requires the `AsBindGroup` derive. This can be implemented manually relatively easily, but deriving it will almost always be preferable.
Field attributes like `uniform` and `texture` are used to define which fields should be bindings,
what their binding type is, and what index they should be bound at:
```rust
#[derive(AsBindGroup)]
struct CoolMaterial {
#[uniform(0)]
color: Color,
#[texture(1)]
#[sampler(2)]
color_texture: Handle<Image>,
}
```
In WGSL shaders, the binding looks like this:
```wgsl
struct CoolMaterial {
color: vec4<f32>;
};
[[group(1), binding(0)]]
var<uniform> material: CoolMaterial;
[[group(1), binding(1)]]
var color_texture: texture_2d<f32>;
[[group(1), binding(2)]]
var color_sampler: sampler;
```
Note that the "group" index is determined by the usage context. It is not defined in `AsBindGroup`. Bevy material bind groups are bound to group 1.
The following field-level attributes are supported:
* `uniform(BINDING_INDEX)`
* The field will be converted to a shader-compatible type using the `ShaderType` trait, written to a `Buffer`, and bound as a uniform. It can also be derived for custom structs.
* `texture(BINDING_INDEX)`
* This field's `Handle<Image>` will be used to look up the matching `Texture` gpu resource, which will be bound as a texture in shaders. The field will be assumed to implement `Into<Option<Handle<Image>>>`. In practice, most fields should be a `Handle<Image>` or `Option<Handle<Image>>`. If the value of an `Option<Handle<Image>>` is `None`, the new `FallbackImage` resource will be used instead. This attribute can be used in conjunction with a `sampler` binding attribute (with a different binding index).
* `sampler(BINDING_INDEX)`
* Behaves exactly like the `texture` attribute, but sets the Image's sampler binding instead of the texture.
Note that fields without field-level binding attributes will be ignored.
```rust
#[derive(AsBindGroup)]
struct CoolMaterial {
#[uniform(0)]
color: Color,
this_field_is_ignored: String,
}
```
As mentioned above, `Option<Handle<Image>>` is also supported:
```rust
#[derive(AsBindGroup)]
struct CoolMaterial {
#[uniform(0)]
color: Color,
#[texture(1)]
#[sampler(2)]
color_texture: Option<Handle<Image>>,
}
```
This is useful if you want a texture to be optional. When the value is `None`, the `FallbackImage` will be used for the binding instead, which defaults to "pure white".
Field uniforms with the same binding index will be combined into a single binding:
```rust
#[derive(AsBindGroup)]
struct CoolMaterial {
#[uniform(0)]
color: Color,
#[uniform(0)]
roughness: f32,
}
```
In WGSL shaders, the binding would look like this:
```wgsl
struct CoolMaterial {
color: vec4<f32>;
roughness: f32;
};
[[group(1), binding(0)]]
var<uniform> material: CoolMaterial;
```
Some less common scenarios will require "struct-level" attributes. These are the currently supported struct-level attributes:
* `uniform(BINDING_INDEX, ConvertedShaderType)`
* Similar to the field-level `uniform` attribute, but instead the entire `AsBindGroup` value is converted to `ConvertedShaderType`, which must implement `ShaderType`. This is useful if more complicated conversion logic is required.
* `bind_group_data(DataType)`
* The `AsBindGroup` type will be converted to some `DataType` using `Into<DataType>` and stored as `AsBindGroup::Data` as part of the `AsBindGroup::as_bind_group` call. This is useful if data needs to be stored alongside the generated bind group, such as a unique identifier for a material's bind group. The most common use case for this attribute is "shader pipeline specialization".
The previous `CoolMaterial` example illustrating "combining multiple field-level uniform attributes with the same binding index" can
also be equivalently represented with a single struct-level uniform attribute:
```rust
#[derive(AsBindGroup)]
#[uniform(0, CoolMaterialUniform)]
struct CoolMaterial {
color: Color,
roughness: f32,
}
#[derive(ShaderType)]
struct CoolMaterialUniform {
color: Color,
roughness: f32,
}
impl From<&CoolMaterial> for CoolMaterialUniform {
fn from(material: &CoolMaterial) -> CoolMaterialUniform {
CoolMaterialUniform {
color: material.color,
roughness: material.roughness,
}
}
}
```
### Material Specialization
Material shader specialization is now _much_ simpler:
```rust
#[derive(AsBindGroup, TypeUuid, Debug, Clone)]
#[uuid = "f690fdae-d598-45ab-8225-97e2a3f056e0"]
#[bind_group_data(CoolMaterialKey)]
struct CoolMaterial {
#[uniform(0)]
color: Color,
is_red: bool,
}
#[derive(Copy, Clone, Hash, Eq, PartialEq)]
struct CoolMaterialKey {
is_red: bool,
}
impl From<&CoolMaterial> for CoolMaterialKey {
fn from(material: &CoolMaterial) -> CoolMaterialKey {
CoolMaterialKey {
is_red: material.is_red,
}
}
}
impl Material for CoolMaterial {
fn fragment_shader() -> ShaderRef {
"cool_material.wgsl".into()
}
fn specialize(
pipeline: &MaterialPipeline<Self>,
descriptor: &mut RenderPipelineDescriptor,
layout: &MeshVertexBufferLayout,
key: MaterialPipelineKey<Self>,
) -> Result<(), SpecializedMeshPipelineError> {
if key.bind_group_data.is_red {
let fragment = descriptor.fragment.as_mut().unwrap();
fragment.shader_defs.push("IS_RED".to_string());
}
Ok(())
}
}
```
Setting `bind_group_data` is not required for specialization (it defaults to `()`). Scenarios like "custom vertex attributes" also benefit from this system:
```rust
impl Material for CustomMaterial {
fn vertex_shader() -> ShaderRef {
"custom_material.wgsl".into()
}
fn fragment_shader() -> ShaderRef {
"custom_material.wgsl".into()
}
fn specialize(
pipeline: &MaterialPipeline<Self>,
descriptor: &mut RenderPipelineDescriptor,
layout: &MeshVertexBufferLayout,
key: MaterialPipelineKey<Self>,
) -> Result<(), SpecializedMeshPipelineError> {
let vertex_layout = layout.get_layout(&[
Mesh::ATTRIBUTE_POSITION.at_shader_location(0),
ATTRIBUTE_BLEND_COLOR.at_shader_location(1),
])?;
descriptor.vertex.buffers = vec![vertex_layout];
Ok(())
}
}
```
### Ported `StandardMaterial` to the new `Material` system
Bevy's built-in PBR material uses the new Material system (including the AsBindGroup derive):
```rust
#[derive(AsBindGroup, Debug, Clone, TypeUuid)]
#[uuid = "7494888b-c082-457b-aacf-517228cc0c22"]
#[bind_group_data(StandardMaterialKey)]
#[uniform(0, StandardMaterialUniform)]
pub struct StandardMaterial {
pub base_color: Color,
#[texture(1)]
#[sampler(2)]
pub base_color_texture: Option<Handle<Image>>,
/* other fields omitted for brevity */
```
### Ported Bevy examples to the new `Material` system
The overall complexity of Bevy's "custom shader examples" has gone down significantly. Take a look at the diffs if you want a dopamine spike.
Please note that while this PR has a net increase in "lines of code", most of those extra lines come from added documentation. There is a significant reduction
in the overall complexity of the code (even accounting for the new derive logic).
---
## Changelog
### Added
* `AsBindGroup` trait and derive, which make it much easier to transfer data to the gpu and generate bind groups for a given type.
### Changed
* The old `Material` and `SpecializedMaterial` traits have been replaced by a consolidated (much simpler) `Material` trait. Materials no longer implement `RenderAsset`.
* `StandardMaterial` was ported to the new material system. There are no user-facing api changes to the `StandardMaterial` struct api, but it now implements `AsBindGroup` and `Material` instead of `RenderAsset` and `SpecializedMaterial`.
## Migration Guide
The Material system has been reworked to be much simpler. We've removed a lot of boilerplate with the new `AsBindGroup` derive and the `Material` trait is simpler as well!
### Bevy 0.7 (old)
```rust
#[derive(Debug, Clone, TypeUuid)]
#[uuid = "f690fdae-d598-45ab-8225-97e2a3f056e0"]
pub struct CustomMaterial {
color: Color,
color_texture: Handle<Image>,
}
#[derive(Clone)]
pub struct GpuCustomMaterial {
_buffer: Buffer,
bind_group: BindGroup,
}
impl RenderAsset for CustomMaterial {
type ExtractedAsset = CustomMaterial;
type PreparedAsset = GpuCustomMaterial;
type Param = (SRes<RenderDevice>, SRes<MaterialPipeline<Self>>);
fn extract_asset(&self) -> Self::ExtractedAsset {
self.clone()
}
fn prepare_asset(
extracted_asset: Self::ExtractedAsset,
(render_device, material_pipeline): &mut SystemParamItem<Self::Param>,
) -> Result<Self::PreparedAsset, PrepareAssetError<Self::ExtractedAsset>> {
let color = Vec4::from_slice(&extracted_asset.color.as_linear_rgba_f32());
let byte_buffer = [0u8; Vec4::SIZE.get() as usize];
let mut buffer = encase::UniformBuffer::new(byte_buffer);
buffer.write(&color).unwrap();
let buffer = render_device.create_buffer_with_data(&BufferInitDescriptor {
contents: buffer.as_ref(),
label: None,
usage: BufferUsages::UNIFORM | BufferUsages::COPY_DST,
});
let (texture_view, texture_sampler) = if let Some(result) = material_pipeline
.mesh_pipeline
.get_image_texture(gpu_images, &Some(extracted_asset.color_texture.clone()))
{
result
} else {
return Err(PrepareAssetError::RetryNextUpdate(extracted_asset));
};
let bind_group = render_device.create_bind_group(&BindGroupDescriptor {
entries: &[
BindGroupEntry {
binding: 0,
resource: buffer.as_entire_binding(),
},
BindGroupEntry {
binding: 0,
resource: BindingResource::TextureView(texture_view),
},
BindGroupEntry {
binding: 1,
resource: BindingResource::Sampler(texture_sampler),
},
],
label: None,
layout: &material_pipeline.material_layout,
});
Ok(GpuCustomMaterial {
_buffer: buffer,
bind_group,
})
}
}
impl Material for CustomMaterial {
fn fragment_shader(asset_server: &AssetServer) -> Option<Handle<Shader>> {
Some(asset_server.load("custom_material.wgsl"))
}
fn bind_group(render_asset: &<Self as RenderAsset>::PreparedAsset) -> &BindGroup {
&render_asset.bind_group
}
fn bind_group_layout(render_device: &RenderDevice) -> BindGroupLayout {
render_device.create_bind_group_layout(&BindGroupLayoutDescriptor {
entries: &[
BindGroupLayoutEntry {
binding: 0,
visibility: ShaderStages::FRAGMENT,
ty: BindingType::Buffer {
ty: BufferBindingType::Uniform,
has_dynamic_offset: false,
min_binding_size: Some(Vec4::min_size()),
},
count: None,
},
BindGroupLayoutEntry {
binding: 1,
visibility: ShaderStages::FRAGMENT,
ty: BindingType::Texture {
multisampled: false,
sample_type: TextureSampleType::Float { filterable: true },
view_dimension: TextureViewDimension::D2Array,
},
count: None,
},
BindGroupLayoutEntry {
binding: 2,
visibility: ShaderStages::FRAGMENT,
ty: BindingType::Sampler(SamplerBindingType::Filtering),
count: None,
},
],
label: None,
})
}
}
```
### Bevy 0.8 (new)
```rust
impl Material for CustomMaterial {
fn fragment_shader() -> ShaderRef {
"custom_material.wgsl".into()
}
}
#[derive(AsBindGroup, TypeUuid, Debug, Clone)]
#[uuid = "f690fdae-d598-45ab-8225-97e2a3f056e0"]
pub struct CustomMaterial {
#[uniform(0)]
color: Color,
#[texture(1)]
#[sampler(2)]
color_texture: Handle<Image>,
}
```
## Future Work
* Add support for more binding types (cubemaps, buffers, etc). This PR intentionally includes a bare minimum number of binding types to keep "reviewability" in check.
* Consider optionally eliding binding indices using binding names. `AsBindGroup` could pass in (optional?) reflection info as a "hint".
* This would make it possible for the derive to do this:
```rust
#[derive(AsBindGroup)]
pub struct CustomMaterial {
#[uniform]
color: Color,
#[texture]
#[sampler]
color_texture: Option<Handle<Image>>,
alpha_mode: AlphaMode,
}
```
* Or this
```rust
#[derive(AsBindGroup)]
pub struct CustomMaterial {
#[binding]
color: Color,
#[binding]
color_texture: Option<Handle<Image>>,
alpha_mode: AlphaMode,
}
```
* Or even this (if we flip to "include bindings by default")
```rust
#[derive(AsBindGroup)]
pub struct CustomMaterial {
color: Color,
color_texture: Option<Handle<Image>>,
#[binding(ignore)]
alpha_mode: AlphaMode,
}
```
* If we add the option to define custom draw functions for materials (which could be done in a type-erased way), I think that would be enough to support extra non-material bindings. Worth considering!
2022-06-30 23:48:46 +00:00
|
|
|
/// Returns this material's fragment shader. If [`ShaderRef::Default`] is returned, the default mesh fragment shader
|
|
|
|
/// will be used.
|
2021-12-25 21:45:43 +00:00
|
|
|
#[allow(unused_variables)]
|
Better Materials: AsBindGroup trait and derive, simpler Material trait (#5053)
# Objective
This PR reworks Bevy's Material system, making the user experience of defining Materials _much_ nicer. Bevy's previous material system leaves a lot to be desired:
* Materials require manually implementing the `RenderAsset` trait, which involves manually generating the bind group, handling gpu buffer data transfer, looking up image textures, etc. Even the simplest single-texture material involves writing ~80 unnecessary lines of code. This was never the long term plan.
* There are two material traits, which is confusing, hard to document, and often redundant: `Material` and `SpecializedMaterial`. `Material` implicitly implements `SpecializedMaterial`, and `SpecializedMaterial` is used in most high level apis to support both use cases. Most users shouldn't need to think about specialization at all (I consider it a "power-user tool"), so the fact that `SpecializedMaterial` is front-and-center in our apis is a miss.
* Implementing either material trait involves a lot of "type soup". The "prepared asset" parameter is particularly heinous: `&<Self as RenderAsset>::PreparedAsset`. Defining vertex and fragment shaders is also more verbose than it needs to be.
## Solution
Say hello to the new `Material` system:
```rust
#[derive(AsBindGroup, TypeUuid, Debug, Clone)]
#[uuid = "f690fdae-d598-45ab-8225-97e2a3f056e0"]
pub struct CoolMaterial {
#[uniform(0)]
color: Color,
#[texture(1)]
#[sampler(2)]
color_texture: Handle<Image>,
}
impl Material for CoolMaterial {
fn fragment_shader() -> ShaderRef {
"cool_material.wgsl".into()
}
}
```
Thats it! This same material would have required [~80 lines of complicated "type heavy" code](https://github.com/bevyengine/bevy/blob/v0.7.0/examples/shader/shader_material.rs) in the old Material system. Now it is just 14 lines of simple, readable code.
This is thanks to a new consolidated `Material` trait and the new `AsBindGroup` trait / derive.
### The new `Material` trait
The old "split" `Material` and `SpecializedMaterial` traits have been removed in favor of a new consolidated `Material` trait. All of the functions on the trait are optional.
The difficulty of implementing `Material` has been reduced by simplifying dataflow and removing type complexity:
```rust
// Old
impl Material for CustomMaterial {
fn fragment_shader(asset_server: &AssetServer) -> Option<Handle<Shader>> {
Some(asset_server.load("custom_material.wgsl"))
}
fn alpha_mode(render_asset: &<Self as RenderAsset>::PreparedAsset) -> AlphaMode {
render_asset.alpha_mode
}
}
// New
impl Material for CustomMaterial {
fn fragment_shader() -> ShaderRef {
"custom_material.wgsl".into()
}
fn alpha_mode(&self) -> AlphaMode {
self.alpha_mode
}
}
```
Specialization is still supported, but it is hidden by default under the `specialize()` function (more on this later).
### The `AsBindGroup` trait / derive
The `Material` trait now requires the `AsBindGroup` derive. This can be implemented manually relatively easily, but deriving it will almost always be preferable.
Field attributes like `uniform` and `texture` are used to define which fields should be bindings,
what their binding type is, and what index they should be bound at:
```rust
#[derive(AsBindGroup)]
struct CoolMaterial {
#[uniform(0)]
color: Color,
#[texture(1)]
#[sampler(2)]
color_texture: Handle<Image>,
}
```
In WGSL shaders, the binding looks like this:
```wgsl
struct CoolMaterial {
color: vec4<f32>;
};
[[group(1), binding(0)]]
var<uniform> material: CoolMaterial;
[[group(1), binding(1)]]
var color_texture: texture_2d<f32>;
[[group(1), binding(2)]]
var color_sampler: sampler;
```
Note that the "group" index is determined by the usage context. It is not defined in `AsBindGroup`. Bevy material bind groups are bound to group 1.
The following field-level attributes are supported:
* `uniform(BINDING_INDEX)`
* The field will be converted to a shader-compatible type using the `ShaderType` trait, written to a `Buffer`, and bound as a uniform. It can also be derived for custom structs.
* `texture(BINDING_INDEX)`
* This field's `Handle<Image>` will be used to look up the matching `Texture` gpu resource, which will be bound as a texture in shaders. The field will be assumed to implement `Into<Option<Handle<Image>>>`. In practice, most fields should be a `Handle<Image>` or `Option<Handle<Image>>`. If the value of an `Option<Handle<Image>>` is `None`, the new `FallbackImage` resource will be used instead. This attribute can be used in conjunction with a `sampler` binding attribute (with a different binding index).
* `sampler(BINDING_INDEX)`
* Behaves exactly like the `texture` attribute, but sets the Image's sampler binding instead of the texture.
Note that fields without field-level binding attributes will be ignored.
```rust
#[derive(AsBindGroup)]
struct CoolMaterial {
#[uniform(0)]
color: Color,
this_field_is_ignored: String,
}
```
As mentioned above, `Option<Handle<Image>>` is also supported:
```rust
#[derive(AsBindGroup)]
struct CoolMaterial {
#[uniform(0)]
color: Color,
#[texture(1)]
#[sampler(2)]
color_texture: Option<Handle<Image>>,
}
```
This is useful if you want a texture to be optional. When the value is `None`, the `FallbackImage` will be used for the binding instead, which defaults to "pure white".
Field uniforms with the same binding index will be combined into a single binding:
```rust
#[derive(AsBindGroup)]
struct CoolMaterial {
#[uniform(0)]
color: Color,
#[uniform(0)]
roughness: f32,
}
```
In WGSL shaders, the binding would look like this:
```wgsl
struct CoolMaterial {
color: vec4<f32>;
roughness: f32;
};
[[group(1), binding(0)]]
var<uniform> material: CoolMaterial;
```
Some less common scenarios will require "struct-level" attributes. These are the currently supported struct-level attributes:
* `uniform(BINDING_INDEX, ConvertedShaderType)`
* Similar to the field-level `uniform` attribute, but instead the entire `AsBindGroup` value is converted to `ConvertedShaderType`, which must implement `ShaderType`. This is useful if more complicated conversion logic is required.
* `bind_group_data(DataType)`
* The `AsBindGroup` type will be converted to some `DataType` using `Into<DataType>` and stored as `AsBindGroup::Data` as part of the `AsBindGroup::as_bind_group` call. This is useful if data needs to be stored alongside the generated bind group, such as a unique identifier for a material's bind group. The most common use case for this attribute is "shader pipeline specialization".
The previous `CoolMaterial` example illustrating "combining multiple field-level uniform attributes with the same binding index" can
also be equivalently represented with a single struct-level uniform attribute:
```rust
#[derive(AsBindGroup)]
#[uniform(0, CoolMaterialUniform)]
struct CoolMaterial {
color: Color,
roughness: f32,
}
#[derive(ShaderType)]
struct CoolMaterialUniform {
color: Color,
roughness: f32,
}
impl From<&CoolMaterial> for CoolMaterialUniform {
fn from(material: &CoolMaterial) -> CoolMaterialUniform {
CoolMaterialUniform {
color: material.color,
roughness: material.roughness,
}
}
}
```
### Material Specialization
Material shader specialization is now _much_ simpler:
```rust
#[derive(AsBindGroup, TypeUuid, Debug, Clone)]
#[uuid = "f690fdae-d598-45ab-8225-97e2a3f056e0"]
#[bind_group_data(CoolMaterialKey)]
struct CoolMaterial {
#[uniform(0)]
color: Color,
is_red: bool,
}
#[derive(Copy, Clone, Hash, Eq, PartialEq)]
struct CoolMaterialKey {
is_red: bool,
}
impl From<&CoolMaterial> for CoolMaterialKey {
fn from(material: &CoolMaterial) -> CoolMaterialKey {
CoolMaterialKey {
is_red: material.is_red,
}
}
}
impl Material for CoolMaterial {
fn fragment_shader() -> ShaderRef {
"cool_material.wgsl".into()
}
fn specialize(
pipeline: &MaterialPipeline<Self>,
descriptor: &mut RenderPipelineDescriptor,
layout: &MeshVertexBufferLayout,
key: MaterialPipelineKey<Self>,
) -> Result<(), SpecializedMeshPipelineError> {
if key.bind_group_data.is_red {
let fragment = descriptor.fragment.as_mut().unwrap();
fragment.shader_defs.push("IS_RED".to_string());
}
Ok(())
}
}
```
Setting `bind_group_data` is not required for specialization (it defaults to `()`). Scenarios like "custom vertex attributes" also benefit from this system:
```rust
impl Material for CustomMaterial {
fn vertex_shader() -> ShaderRef {
"custom_material.wgsl".into()
}
fn fragment_shader() -> ShaderRef {
"custom_material.wgsl".into()
}
fn specialize(
pipeline: &MaterialPipeline<Self>,
descriptor: &mut RenderPipelineDescriptor,
layout: &MeshVertexBufferLayout,
key: MaterialPipelineKey<Self>,
) -> Result<(), SpecializedMeshPipelineError> {
let vertex_layout = layout.get_layout(&[
Mesh::ATTRIBUTE_POSITION.at_shader_location(0),
ATTRIBUTE_BLEND_COLOR.at_shader_location(1),
])?;
descriptor.vertex.buffers = vec![vertex_layout];
Ok(())
}
}
```
### Ported `StandardMaterial` to the new `Material` system
Bevy's built-in PBR material uses the new Material system (including the AsBindGroup derive):
```rust
#[derive(AsBindGroup, Debug, Clone, TypeUuid)]
#[uuid = "7494888b-c082-457b-aacf-517228cc0c22"]
#[bind_group_data(StandardMaterialKey)]
#[uniform(0, StandardMaterialUniform)]
pub struct StandardMaterial {
pub base_color: Color,
#[texture(1)]
#[sampler(2)]
pub base_color_texture: Option<Handle<Image>>,
/* other fields omitted for brevity */
```
### Ported Bevy examples to the new `Material` system
The overall complexity of Bevy's "custom shader examples" has gone down significantly. Take a look at the diffs if you want a dopamine spike.
Please note that while this PR has a net increase in "lines of code", most of those extra lines come from added documentation. There is a significant reduction
in the overall complexity of the code (even accounting for the new derive logic).
---
## Changelog
### Added
* `AsBindGroup` trait and derive, which make it much easier to transfer data to the gpu and generate bind groups for a given type.
### Changed
* The old `Material` and `SpecializedMaterial` traits have been replaced by a consolidated (much simpler) `Material` trait. Materials no longer implement `RenderAsset`.
* `StandardMaterial` was ported to the new material system. There are no user-facing api changes to the `StandardMaterial` struct api, but it now implements `AsBindGroup` and `Material` instead of `RenderAsset` and `SpecializedMaterial`.
## Migration Guide
The Material system has been reworked to be much simpler. We've removed a lot of boilerplate with the new `AsBindGroup` derive and the `Material` trait is simpler as well!
### Bevy 0.7 (old)
```rust
#[derive(Debug, Clone, TypeUuid)]
#[uuid = "f690fdae-d598-45ab-8225-97e2a3f056e0"]
pub struct CustomMaterial {
color: Color,
color_texture: Handle<Image>,
}
#[derive(Clone)]
pub struct GpuCustomMaterial {
_buffer: Buffer,
bind_group: BindGroup,
}
impl RenderAsset for CustomMaterial {
type ExtractedAsset = CustomMaterial;
type PreparedAsset = GpuCustomMaterial;
type Param = (SRes<RenderDevice>, SRes<MaterialPipeline<Self>>);
fn extract_asset(&self) -> Self::ExtractedAsset {
self.clone()
}
fn prepare_asset(
extracted_asset: Self::ExtractedAsset,
(render_device, material_pipeline): &mut SystemParamItem<Self::Param>,
) -> Result<Self::PreparedAsset, PrepareAssetError<Self::ExtractedAsset>> {
let color = Vec4::from_slice(&extracted_asset.color.as_linear_rgba_f32());
let byte_buffer = [0u8; Vec4::SIZE.get() as usize];
let mut buffer = encase::UniformBuffer::new(byte_buffer);
buffer.write(&color).unwrap();
let buffer = render_device.create_buffer_with_data(&BufferInitDescriptor {
contents: buffer.as_ref(),
label: None,
usage: BufferUsages::UNIFORM | BufferUsages::COPY_DST,
});
let (texture_view, texture_sampler) = if let Some(result) = material_pipeline
.mesh_pipeline
.get_image_texture(gpu_images, &Some(extracted_asset.color_texture.clone()))
{
result
} else {
return Err(PrepareAssetError::RetryNextUpdate(extracted_asset));
};
let bind_group = render_device.create_bind_group(&BindGroupDescriptor {
entries: &[
BindGroupEntry {
binding: 0,
resource: buffer.as_entire_binding(),
},
BindGroupEntry {
binding: 0,
resource: BindingResource::TextureView(texture_view),
},
BindGroupEntry {
binding: 1,
resource: BindingResource::Sampler(texture_sampler),
},
],
label: None,
layout: &material_pipeline.material_layout,
});
Ok(GpuCustomMaterial {
_buffer: buffer,
bind_group,
})
}
}
impl Material for CustomMaterial {
fn fragment_shader(asset_server: &AssetServer) -> Option<Handle<Shader>> {
Some(asset_server.load("custom_material.wgsl"))
}
fn bind_group(render_asset: &<Self as RenderAsset>::PreparedAsset) -> &BindGroup {
&render_asset.bind_group
}
fn bind_group_layout(render_device: &RenderDevice) -> BindGroupLayout {
render_device.create_bind_group_layout(&BindGroupLayoutDescriptor {
entries: &[
BindGroupLayoutEntry {
binding: 0,
visibility: ShaderStages::FRAGMENT,
ty: BindingType::Buffer {
ty: BufferBindingType::Uniform,
has_dynamic_offset: false,
min_binding_size: Some(Vec4::min_size()),
},
count: None,
},
BindGroupLayoutEntry {
binding: 1,
visibility: ShaderStages::FRAGMENT,
ty: BindingType::Texture {
multisampled: false,
sample_type: TextureSampleType::Float { filterable: true },
view_dimension: TextureViewDimension::D2Array,
},
count: None,
},
BindGroupLayoutEntry {
binding: 2,
visibility: ShaderStages::FRAGMENT,
ty: BindingType::Sampler(SamplerBindingType::Filtering),
count: None,
},
],
label: None,
})
}
}
```
### Bevy 0.8 (new)
```rust
impl Material for CustomMaterial {
fn fragment_shader() -> ShaderRef {
"custom_material.wgsl".into()
}
}
#[derive(AsBindGroup, TypeUuid, Debug, Clone)]
#[uuid = "f690fdae-d598-45ab-8225-97e2a3f056e0"]
pub struct CustomMaterial {
#[uniform(0)]
color: Color,
#[texture(1)]
#[sampler(2)]
color_texture: Handle<Image>,
}
```
## Future Work
* Add support for more binding types (cubemaps, buffers, etc). This PR intentionally includes a bare minimum number of binding types to keep "reviewability" in check.
* Consider optionally eliding binding indices using binding names. `AsBindGroup` could pass in (optional?) reflection info as a "hint".
* This would make it possible for the derive to do this:
```rust
#[derive(AsBindGroup)]
pub struct CustomMaterial {
#[uniform]
color: Color,
#[texture]
#[sampler]
color_texture: Option<Handle<Image>>,
alpha_mode: AlphaMode,
}
```
* Or this
```rust
#[derive(AsBindGroup)]
pub struct CustomMaterial {
#[binding]
color: Color,
#[binding]
color_texture: Option<Handle<Image>>,
alpha_mode: AlphaMode,
}
```
* Or even this (if we flip to "include bindings by default")
```rust
#[derive(AsBindGroup)]
pub struct CustomMaterial {
color: Color,
color_texture: Option<Handle<Image>>,
#[binding(ignore)]
alpha_mode: AlphaMode,
}
```
* If we add the option to define custom draw functions for materials (which could be done in a type-erased way), I think that would be enough to support extra non-material bindings. Worth considering!
2022-06-30 23:48:46 +00:00
|
|
|
fn fragment_shader() -> ShaderRef {
|
|
|
|
ShaderRef::Default
|
2021-12-25 21:45:43 +00:00
|
|
|
}
|
|
|
|
|
|
|
|
/// Returns this material's [`AlphaMode`]. Defaults to [`AlphaMode::Opaque`].
|
|
|
|
#[inline]
|
Better Materials: AsBindGroup trait and derive, simpler Material trait (#5053)
# Objective
This PR reworks Bevy's Material system, making the user experience of defining Materials _much_ nicer. Bevy's previous material system leaves a lot to be desired:
* Materials require manually implementing the `RenderAsset` trait, which involves manually generating the bind group, handling gpu buffer data transfer, looking up image textures, etc. Even the simplest single-texture material involves writing ~80 unnecessary lines of code. This was never the long term plan.
* There are two material traits, which is confusing, hard to document, and often redundant: `Material` and `SpecializedMaterial`. `Material` implicitly implements `SpecializedMaterial`, and `SpecializedMaterial` is used in most high level apis to support both use cases. Most users shouldn't need to think about specialization at all (I consider it a "power-user tool"), so the fact that `SpecializedMaterial` is front-and-center in our apis is a miss.
* Implementing either material trait involves a lot of "type soup". The "prepared asset" parameter is particularly heinous: `&<Self as RenderAsset>::PreparedAsset`. Defining vertex and fragment shaders is also more verbose than it needs to be.
## Solution
Say hello to the new `Material` system:
```rust
#[derive(AsBindGroup, TypeUuid, Debug, Clone)]
#[uuid = "f690fdae-d598-45ab-8225-97e2a3f056e0"]
pub struct CoolMaterial {
#[uniform(0)]
color: Color,
#[texture(1)]
#[sampler(2)]
color_texture: Handle<Image>,
}
impl Material for CoolMaterial {
fn fragment_shader() -> ShaderRef {
"cool_material.wgsl".into()
}
}
```
Thats it! This same material would have required [~80 lines of complicated "type heavy" code](https://github.com/bevyengine/bevy/blob/v0.7.0/examples/shader/shader_material.rs) in the old Material system. Now it is just 14 lines of simple, readable code.
This is thanks to a new consolidated `Material` trait and the new `AsBindGroup` trait / derive.
### The new `Material` trait
The old "split" `Material` and `SpecializedMaterial` traits have been removed in favor of a new consolidated `Material` trait. All of the functions on the trait are optional.
The difficulty of implementing `Material` has been reduced by simplifying dataflow and removing type complexity:
```rust
// Old
impl Material for CustomMaterial {
fn fragment_shader(asset_server: &AssetServer) -> Option<Handle<Shader>> {
Some(asset_server.load("custom_material.wgsl"))
}
fn alpha_mode(render_asset: &<Self as RenderAsset>::PreparedAsset) -> AlphaMode {
render_asset.alpha_mode
}
}
// New
impl Material for CustomMaterial {
fn fragment_shader() -> ShaderRef {
"custom_material.wgsl".into()
}
fn alpha_mode(&self) -> AlphaMode {
self.alpha_mode
}
}
```
Specialization is still supported, but it is hidden by default under the `specialize()` function (more on this later).
### The `AsBindGroup` trait / derive
The `Material` trait now requires the `AsBindGroup` derive. This can be implemented manually relatively easily, but deriving it will almost always be preferable.
Field attributes like `uniform` and `texture` are used to define which fields should be bindings,
what their binding type is, and what index they should be bound at:
```rust
#[derive(AsBindGroup)]
struct CoolMaterial {
#[uniform(0)]
color: Color,
#[texture(1)]
#[sampler(2)]
color_texture: Handle<Image>,
}
```
In WGSL shaders, the binding looks like this:
```wgsl
struct CoolMaterial {
color: vec4<f32>;
};
[[group(1), binding(0)]]
var<uniform> material: CoolMaterial;
[[group(1), binding(1)]]
var color_texture: texture_2d<f32>;
[[group(1), binding(2)]]
var color_sampler: sampler;
```
Note that the "group" index is determined by the usage context. It is not defined in `AsBindGroup`. Bevy material bind groups are bound to group 1.
The following field-level attributes are supported:
* `uniform(BINDING_INDEX)`
* The field will be converted to a shader-compatible type using the `ShaderType` trait, written to a `Buffer`, and bound as a uniform. It can also be derived for custom structs.
* `texture(BINDING_INDEX)`
* This field's `Handle<Image>` will be used to look up the matching `Texture` gpu resource, which will be bound as a texture in shaders. The field will be assumed to implement `Into<Option<Handle<Image>>>`. In practice, most fields should be a `Handle<Image>` or `Option<Handle<Image>>`. If the value of an `Option<Handle<Image>>` is `None`, the new `FallbackImage` resource will be used instead. This attribute can be used in conjunction with a `sampler` binding attribute (with a different binding index).
* `sampler(BINDING_INDEX)`
* Behaves exactly like the `texture` attribute, but sets the Image's sampler binding instead of the texture.
Note that fields without field-level binding attributes will be ignored.
```rust
#[derive(AsBindGroup)]
struct CoolMaterial {
#[uniform(0)]
color: Color,
this_field_is_ignored: String,
}
```
As mentioned above, `Option<Handle<Image>>` is also supported:
```rust
#[derive(AsBindGroup)]
struct CoolMaterial {
#[uniform(0)]
color: Color,
#[texture(1)]
#[sampler(2)]
color_texture: Option<Handle<Image>>,
}
```
This is useful if you want a texture to be optional. When the value is `None`, the `FallbackImage` will be used for the binding instead, which defaults to "pure white".
Field uniforms with the same binding index will be combined into a single binding:
```rust
#[derive(AsBindGroup)]
struct CoolMaterial {
#[uniform(0)]
color: Color,
#[uniform(0)]
roughness: f32,
}
```
In WGSL shaders, the binding would look like this:
```wgsl
struct CoolMaterial {
color: vec4<f32>;
roughness: f32;
};
[[group(1), binding(0)]]
var<uniform> material: CoolMaterial;
```
Some less common scenarios will require "struct-level" attributes. These are the currently supported struct-level attributes:
* `uniform(BINDING_INDEX, ConvertedShaderType)`
* Similar to the field-level `uniform` attribute, but instead the entire `AsBindGroup` value is converted to `ConvertedShaderType`, which must implement `ShaderType`. This is useful if more complicated conversion logic is required.
* `bind_group_data(DataType)`
* The `AsBindGroup` type will be converted to some `DataType` using `Into<DataType>` and stored as `AsBindGroup::Data` as part of the `AsBindGroup::as_bind_group` call. This is useful if data needs to be stored alongside the generated bind group, such as a unique identifier for a material's bind group. The most common use case for this attribute is "shader pipeline specialization".
The previous `CoolMaterial` example illustrating "combining multiple field-level uniform attributes with the same binding index" can
also be equivalently represented with a single struct-level uniform attribute:
```rust
#[derive(AsBindGroup)]
#[uniform(0, CoolMaterialUniform)]
struct CoolMaterial {
color: Color,
roughness: f32,
}
#[derive(ShaderType)]
struct CoolMaterialUniform {
color: Color,
roughness: f32,
}
impl From<&CoolMaterial> for CoolMaterialUniform {
fn from(material: &CoolMaterial) -> CoolMaterialUniform {
CoolMaterialUniform {
color: material.color,
roughness: material.roughness,
}
}
}
```
### Material Specialization
Material shader specialization is now _much_ simpler:
```rust
#[derive(AsBindGroup, TypeUuid, Debug, Clone)]
#[uuid = "f690fdae-d598-45ab-8225-97e2a3f056e0"]
#[bind_group_data(CoolMaterialKey)]
struct CoolMaterial {
#[uniform(0)]
color: Color,
is_red: bool,
}
#[derive(Copy, Clone, Hash, Eq, PartialEq)]
struct CoolMaterialKey {
is_red: bool,
}
impl From<&CoolMaterial> for CoolMaterialKey {
fn from(material: &CoolMaterial) -> CoolMaterialKey {
CoolMaterialKey {
is_red: material.is_red,
}
}
}
impl Material for CoolMaterial {
fn fragment_shader() -> ShaderRef {
"cool_material.wgsl".into()
}
fn specialize(
pipeline: &MaterialPipeline<Self>,
descriptor: &mut RenderPipelineDescriptor,
layout: &MeshVertexBufferLayout,
key: MaterialPipelineKey<Self>,
) -> Result<(), SpecializedMeshPipelineError> {
if key.bind_group_data.is_red {
let fragment = descriptor.fragment.as_mut().unwrap();
fragment.shader_defs.push("IS_RED".to_string());
}
Ok(())
}
}
```
Setting `bind_group_data` is not required for specialization (it defaults to `()`). Scenarios like "custom vertex attributes" also benefit from this system:
```rust
impl Material for CustomMaterial {
fn vertex_shader() -> ShaderRef {
"custom_material.wgsl".into()
}
fn fragment_shader() -> ShaderRef {
"custom_material.wgsl".into()
}
fn specialize(
pipeline: &MaterialPipeline<Self>,
descriptor: &mut RenderPipelineDescriptor,
layout: &MeshVertexBufferLayout,
key: MaterialPipelineKey<Self>,
) -> Result<(), SpecializedMeshPipelineError> {
let vertex_layout = layout.get_layout(&[
Mesh::ATTRIBUTE_POSITION.at_shader_location(0),
ATTRIBUTE_BLEND_COLOR.at_shader_location(1),
])?;
descriptor.vertex.buffers = vec![vertex_layout];
Ok(())
}
}
```
### Ported `StandardMaterial` to the new `Material` system
Bevy's built-in PBR material uses the new Material system (including the AsBindGroup derive):
```rust
#[derive(AsBindGroup, Debug, Clone, TypeUuid)]
#[uuid = "7494888b-c082-457b-aacf-517228cc0c22"]
#[bind_group_data(StandardMaterialKey)]
#[uniform(0, StandardMaterialUniform)]
pub struct StandardMaterial {
pub base_color: Color,
#[texture(1)]
#[sampler(2)]
pub base_color_texture: Option<Handle<Image>>,
/* other fields omitted for brevity */
```
### Ported Bevy examples to the new `Material` system
The overall complexity of Bevy's "custom shader examples" has gone down significantly. Take a look at the diffs if you want a dopamine spike.
Please note that while this PR has a net increase in "lines of code", most of those extra lines come from added documentation. There is a significant reduction
in the overall complexity of the code (even accounting for the new derive logic).
---
## Changelog
### Added
* `AsBindGroup` trait and derive, which make it much easier to transfer data to the gpu and generate bind groups for a given type.
### Changed
* The old `Material` and `SpecializedMaterial` traits have been replaced by a consolidated (much simpler) `Material` trait. Materials no longer implement `RenderAsset`.
* `StandardMaterial` was ported to the new material system. There are no user-facing api changes to the `StandardMaterial` struct api, but it now implements `AsBindGroup` and `Material` instead of `RenderAsset` and `SpecializedMaterial`.
## Migration Guide
The Material system has been reworked to be much simpler. We've removed a lot of boilerplate with the new `AsBindGroup` derive and the `Material` trait is simpler as well!
### Bevy 0.7 (old)
```rust
#[derive(Debug, Clone, TypeUuid)]
#[uuid = "f690fdae-d598-45ab-8225-97e2a3f056e0"]
pub struct CustomMaterial {
color: Color,
color_texture: Handle<Image>,
}
#[derive(Clone)]
pub struct GpuCustomMaterial {
_buffer: Buffer,
bind_group: BindGroup,
}
impl RenderAsset for CustomMaterial {
type ExtractedAsset = CustomMaterial;
type PreparedAsset = GpuCustomMaterial;
type Param = (SRes<RenderDevice>, SRes<MaterialPipeline<Self>>);
fn extract_asset(&self) -> Self::ExtractedAsset {
self.clone()
}
fn prepare_asset(
extracted_asset: Self::ExtractedAsset,
(render_device, material_pipeline): &mut SystemParamItem<Self::Param>,
) -> Result<Self::PreparedAsset, PrepareAssetError<Self::ExtractedAsset>> {
let color = Vec4::from_slice(&extracted_asset.color.as_linear_rgba_f32());
let byte_buffer = [0u8; Vec4::SIZE.get() as usize];
let mut buffer = encase::UniformBuffer::new(byte_buffer);
buffer.write(&color).unwrap();
let buffer = render_device.create_buffer_with_data(&BufferInitDescriptor {
contents: buffer.as_ref(),
label: None,
usage: BufferUsages::UNIFORM | BufferUsages::COPY_DST,
});
let (texture_view, texture_sampler) = if let Some(result) = material_pipeline
.mesh_pipeline
.get_image_texture(gpu_images, &Some(extracted_asset.color_texture.clone()))
{
result
} else {
return Err(PrepareAssetError::RetryNextUpdate(extracted_asset));
};
let bind_group = render_device.create_bind_group(&BindGroupDescriptor {
entries: &[
BindGroupEntry {
binding: 0,
resource: buffer.as_entire_binding(),
},
BindGroupEntry {
binding: 0,
resource: BindingResource::TextureView(texture_view),
},
BindGroupEntry {
binding: 1,
resource: BindingResource::Sampler(texture_sampler),
},
],
label: None,
layout: &material_pipeline.material_layout,
});
Ok(GpuCustomMaterial {
_buffer: buffer,
bind_group,
})
}
}
impl Material for CustomMaterial {
fn fragment_shader(asset_server: &AssetServer) -> Option<Handle<Shader>> {
Some(asset_server.load("custom_material.wgsl"))
}
fn bind_group(render_asset: &<Self as RenderAsset>::PreparedAsset) -> &BindGroup {
&render_asset.bind_group
}
fn bind_group_layout(render_device: &RenderDevice) -> BindGroupLayout {
render_device.create_bind_group_layout(&BindGroupLayoutDescriptor {
entries: &[
BindGroupLayoutEntry {
binding: 0,
visibility: ShaderStages::FRAGMENT,
ty: BindingType::Buffer {
ty: BufferBindingType::Uniform,
has_dynamic_offset: false,
min_binding_size: Some(Vec4::min_size()),
},
count: None,
},
BindGroupLayoutEntry {
binding: 1,
visibility: ShaderStages::FRAGMENT,
ty: BindingType::Texture {
multisampled: false,
sample_type: TextureSampleType::Float { filterable: true },
view_dimension: TextureViewDimension::D2Array,
},
count: None,
},
BindGroupLayoutEntry {
binding: 2,
visibility: ShaderStages::FRAGMENT,
ty: BindingType::Sampler(SamplerBindingType::Filtering),
count: None,
},
],
label: None,
})
}
}
```
### Bevy 0.8 (new)
```rust
impl Material for CustomMaterial {
fn fragment_shader() -> ShaderRef {
"custom_material.wgsl".into()
}
}
#[derive(AsBindGroup, TypeUuid, Debug, Clone)]
#[uuid = "f690fdae-d598-45ab-8225-97e2a3f056e0"]
pub struct CustomMaterial {
#[uniform(0)]
color: Color,
#[texture(1)]
#[sampler(2)]
color_texture: Handle<Image>,
}
```
## Future Work
* Add support for more binding types (cubemaps, buffers, etc). This PR intentionally includes a bare minimum number of binding types to keep "reviewability" in check.
* Consider optionally eliding binding indices using binding names. `AsBindGroup` could pass in (optional?) reflection info as a "hint".
* This would make it possible for the derive to do this:
```rust
#[derive(AsBindGroup)]
pub struct CustomMaterial {
#[uniform]
color: Color,
#[texture]
#[sampler]
color_texture: Option<Handle<Image>>,
alpha_mode: AlphaMode,
}
```
* Or this
```rust
#[derive(AsBindGroup)]
pub struct CustomMaterial {
#[binding]
color: Color,
#[binding]
color_texture: Option<Handle<Image>>,
alpha_mode: AlphaMode,
}
```
* Or even this (if we flip to "include bindings by default")
```rust
#[derive(AsBindGroup)]
pub struct CustomMaterial {
color: Color,
color_texture: Option<Handle<Image>>,
#[binding(ignore)]
alpha_mode: AlphaMode,
}
```
* If we add the option to define custom draw functions for materials (which could be done in a type-erased way), I think that would be enough to support extra non-material bindings. Worth considering!
2022-06-30 23:48:46 +00:00
|
|
|
fn alpha_mode(&self) -> AlphaMode {
|
|
|
|
AlphaMode::Opaque
|
2020-08-01 00:10:29 +00:00
|
|
|
}
|
Mesh vertex buffer layouts (#3959)
This PR makes a number of changes to how meshes and vertex attributes are handled, which the goal of enabling easy and flexible custom vertex attributes:
* Reworks the `Mesh` type to use the newly added `VertexAttribute` internally
* `VertexAttribute` defines the name, a unique `VertexAttributeId`, and a `VertexFormat`
* `VertexAttributeId` is used to produce consistent sort orders for vertex buffer generation, replacing the more expensive and often surprising "name based sorting"
* Meshes can be used to generate a `MeshVertexBufferLayout`, which defines the layout of the gpu buffer produced by the mesh. `MeshVertexBufferLayouts` can then be used to generate actual `VertexBufferLayouts` according to the requirements of a specific pipeline. This decoupling of "mesh layout" vs "pipeline vertex buffer layout" is what enables custom attributes. We don't need to standardize _mesh layouts_ or contort meshes to meet the needs of a specific pipeline. As long as the mesh has what the pipeline needs, it will work transparently.
* Mesh-based pipelines now specialize on `&MeshVertexBufferLayout` via the new `SpecializedMeshPipeline` trait (which behaves like `SpecializedPipeline`, but adds `&MeshVertexBufferLayout`). The integrity of the pipeline cache is maintained because the `MeshVertexBufferLayout` is treated as part of the key (which is fully abstracted from implementers of the trait ... no need to add any additional info to the specialization key).
* Hashing `MeshVertexBufferLayout` is too expensive to do for every entity, every frame. To make this scalable, I added a generalized "pre-hashing" solution to `bevy_utils`: `Hashed<T>` keys and `PreHashMap<K, V>` (which uses `Hashed<T>` internally) . Why didn't I just do the quick and dirty in-place "pre-compute hash and use that u64 as a key in a hashmap" that we've done in the past? Because its wrong! Hashes by themselves aren't enough because two different values can produce the same hash. Re-hashing a hash is even worse! I decided to build a generalized solution because this pattern has come up in the past and we've chosen to do the wrong thing. Now we can do the right thing! This did unfortunately require pulling in `hashbrown` and using that in `bevy_utils`, because avoiding re-hashes requires the `raw_entry_mut` api, which isn't stabilized yet (and may never be ... `entry_ref` has favor now, but also isn't available yet). If std's HashMap ever provides the tools we need, we can move back to that. Note that adding `hashbrown` doesn't increase our dependency count because it was already in our tree. I will probably break these changes out into their own PR.
* Specializing on `MeshVertexBufferLayout` has one non-obvious behavior: it can produce identical pipelines for two different MeshVertexBufferLayouts. To optimize the number of active pipelines / reduce re-binds while drawing, I de-duplicate pipelines post-specialization using the final `VertexBufferLayout` as the key. For example, consider a pipeline that needs the layout `(position, normal)` and is specialized using two meshes: `(position, normal, uv)` and `(position, normal, other_vec2)`. If both of these meshes result in `(position, normal)` specializations, we can use the same pipeline! Now we do. Cool!
To briefly illustrate, this is what the relevant section of `MeshPipeline`'s specialization code looks like now:
```rust
impl SpecializedMeshPipeline for MeshPipeline {
type Key = MeshPipelineKey;
fn specialize(
&self,
key: Self::Key,
layout: &MeshVertexBufferLayout,
) -> RenderPipelineDescriptor {
let mut vertex_attributes = vec![
Mesh::ATTRIBUTE_POSITION.at_shader_location(0),
Mesh::ATTRIBUTE_NORMAL.at_shader_location(1),
Mesh::ATTRIBUTE_UV_0.at_shader_location(2),
];
let mut shader_defs = Vec::new();
if layout.contains(Mesh::ATTRIBUTE_TANGENT) {
shader_defs.push(String::from("VERTEX_TANGENTS"));
vertex_attributes.push(Mesh::ATTRIBUTE_TANGENT.at_shader_location(3));
}
let vertex_buffer_layout = layout
.get_layout(&vertex_attributes)
.expect("Mesh is missing a vertex attribute");
```
Notice that this is _much_ simpler than it was before. And now any mesh with any layout can be used with this pipeline, provided it has vertex postions, normals, and uvs. We even got to remove `HAS_TANGENTS` from MeshPipelineKey and `has_tangents` from `GpuMesh`, because that information is redundant with `MeshVertexBufferLayout`.
This is still a draft because I still need to:
* Add more docs
* Experiment with adding error handling to mesh pipeline specialization (which would print errors at runtime when a mesh is missing a vertex attribute required by a pipeline). If it doesn't tank perf, we'll keep it.
* Consider breaking out the PreHash / hashbrown changes into a separate PR.
* Add an example illustrating this change
* Verify that the "mesh-specialized pipeline de-duplication code" works properly
Please dont yell at me for not doing these things yet :) Just trying to get this in peoples' hands asap.
Alternative to #3120
Fixes #3030
Co-authored-by: Carter Anderson <mcanders1@gmail.com>
2022-02-23 23:21:13 +00:00
|
|
|
|
2023-10-12 22:10:38 +00:00
|
|
|
/// Returns if this material should be rendered by the deferred or forward renderer.
|
|
|
|
/// for `AlphaMode::Opaque` or `AlphaMode::Mask` materials.
|
|
|
|
/// If `OpaqueRendererMethod::Auto`, it will default to what is selected in the `DefaultOpaqueRendererMethod` resource.
|
2022-05-31 02:02:49 +00:00
|
|
|
#[inline]
|
2023-10-12 22:10:38 +00:00
|
|
|
fn opaque_render_method(&self) -> OpaqueRendererMethod {
|
|
|
|
OpaqueRendererMethod::Forward
|
|
|
|
}
|
|
|
|
|
|
|
|
#[inline]
|
|
|
|
/// Add a bias to the view depth of the mesh which can be used to force a specific render order.
|
2023-03-04 12:29:09 +00:00
|
|
|
/// for meshes with similar depth, to avoid z-fighting.
|
|
|
|
/// The bias is in depth-texture units so large values may be needed to overcome small depth differences.
|
Better Materials: AsBindGroup trait and derive, simpler Material trait (#5053)
# Objective
This PR reworks Bevy's Material system, making the user experience of defining Materials _much_ nicer. Bevy's previous material system leaves a lot to be desired:
* Materials require manually implementing the `RenderAsset` trait, which involves manually generating the bind group, handling gpu buffer data transfer, looking up image textures, etc. Even the simplest single-texture material involves writing ~80 unnecessary lines of code. This was never the long term plan.
* There are two material traits, which is confusing, hard to document, and often redundant: `Material` and `SpecializedMaterial`. `Material` implicitly implements `SpecializedMaterial`, and `SpecializedMaterial` is used in most high level apis to support both use cases. Most users shouldn't need to think about specialization at all (I consider it a "power-user tool"), so the fact that `SpecializedMaterial` is front-and-center in our apis is a miss.
* Implementing either material trait involves a lot of "type soup". The "prepared asset" parameter is particularly heinous: `&<Self as RenderAsset>::PreparedAsset`. Defining vertex and fragment shaders is also more verbose than it needs to be.
## Solution
Say hello to the new `Material` system:
```rust
#[derive(AsBindGroup, TypeUuid, Debug, Clone)]
#[uuid = "f690fdae-d598-45ab-8225-97e2a3f056e0"]
pub struct CoolMaterial {
#[uniform(0)]
color: Color,
#[texture(1)]
#[sampler(2)]
color_texture: Handle<Image>,
}
impl Material for CoolMaterial {
fn fragment_shader() -> ShaderRef {
"cool_material.wgsl".into()
}
}
```
Thats it! This same material would have required [~80 lines of complicated "type heavy" code](https://github.com/bevyengine/bevy/blob/v0.7.0/examples/shader/shader_material.rs) in the old Material system. Now it is just 14 lines of simple, readable code.
This is thanks to a new consolidated `Material` trait and the new `AsBindGroup` trait / derive.
### The new `Material` trait
The old "split" `Material` and `SpecializedMaterial` traits have been removed in favor of a new consolidated `Material` trait. All of the functions on the trait are optional.
The difficulty of implementing `Material` has been reduced by simplifying dataflow and removing type complexity:
```rust
// Old
impl Material for CustomMaterial {
fn fragment_shader(asset_server: &AssetServer) -> Option<Handle<Shader>> {
Some(asset_server.load("custom_material.wgsl"))
}
fn alpha_mode(render_asset: &<Self as RenderAsset>::PreparedAsset) -> AlphaMode {
render_asset.alpha_mode
}
}
// New
impl Material for CustomMaterial {
fn fragment_shader() -> ShaderRef {
"custom_material.wgsl".into()
}
fn alpha_mode(&self) -> AlphaMode {
self.alpha_mode
}
}
```
Specialization is still supported, but it is hidden by default under the `specialize()` function (more on this later).
### The `AsBindGroup` trait / derive
The `Material` trait now requires the `AsBindGroup` derive. This can be implemented manually relatively easily, but deriving it will almost always be preferable.
Field attributes like `uniform` and `texture` are used to define which fields should be bindings,
what their binding type is, and what index they should be bound at:
```rust
#[derive(AsBindGroup)]
struct CoolMaterial {
#[uniform(0)]
color: Color,
#[texture(1)]
#[sampler(2)]
color_texture: Handle<Image>,
}
```
In WGSL shaders, the binding looks like this:
```wgsl
struct CoolMaterial {
color: vec4<f32>;
};
[[group(1), binding(0)]]
var<uniform> material: CoolMaterial;
[[group(1), binding(1)]]
var color_texture: texture_2d<f32>;
[[group(1), binding(2)]]
var color_sampler: sampler;
```
Note that the "group" index is determined by the usage context. It is not defined in `AsBindGroup`. Bevy material bind groups are bound to group 1.
The following field-level attributes are supported:
* `uniform(BINDING_INDEX)`
* The field will be converted to a shader-compatible type using the `ShaderType` trait, written to a `Buffer`, and bound as a uniform. It can also be derived for custom structs.
* `texture(BINDING_INDEX)`
* This field's `Handle<Image>` will be used to look up the matching `Texture` gpu resource, which will be bound as a texture in shaders. The field will be assumed to implement `Into<Option<Handle<Image>>>`. In practice, most fields should be a `Handle<Image>` or `Option<Handle<Image>>`. If the value of an `Option<Handle<Image>>` is `None`, the new `FallbackImage` resource will be used instead. This attribute can be used in conjunction with a `sampler` binding attribute (with a different binding index).
* `sampler(BINDING_INDEX)`
* Behaves exactly like the `texture` attribute, but sets the Image's sampler binding instead of the texture.
Note that fields without field-level binding attributes will be ignored.
```rust
#[derive(AsBindGroup)]
struct CoolMaterial {
#[uniform(0)]
color: Color,
this_field_is_ignored: String,
}
```
As mentioned above, `Option<Handle<Image>>` is also supported:
```rust
#[derive(AsBindGroup)]
struct CoolMaterial {
#[uniform(0)]
color: Color,
#[texture(1)]
#[sampler(2)]
color_texture: Option<Handle<Image>>,
}
```
This is useful if you want a texture to be optional. When the value is `None`, the `FallbackImage` will be used for the binding instead, which defaults to "pure white".
Field uniforms with the same binding index will be combined into a single binding:
```rust
#[derive(AsBindGroup)]
struct CoolMaterial {
#[uniform(0)]
color: Color,
#[uniform(0)]
roughness: f32,
}
```
In WGSL shaders, the binding would look like this:
```wgsl
struct CoolMaterial {
color: vec4<f32>;
roughness: f32;
};
[[group(1), binding(0)]]
var<uniform> material: CoolMaterial;
```
Some less common scenarios will require "struct-level" attributes. These are the currently supported struct-level attributes:
* `uniform(BINDING_INDEX, ConvertedShaderType)`
* Similar to the field-level `uniform` attribute, but instead the entire `AsBindGroup` value is converted to `ConvertedShaderType`, which must implement `ShaderType`. This is useful if more complicated conversion logic is required.
* `bind_group_data(DataType)`
* The `AsBindGroup` type will be converted to some `DataType` using `Into<DataType>` and stored as `AsBindGroup::Data` as part of the `AsBindGroup::as_bind_group` call. This is useful if data needs to be stored alongside the generated bind group, such as a unique identifier for a material's bind group. The most common use case for this attribute is "shader pipeline specialization".
The previous `CoolMaterial` example illustrating "combining multiple field-level uniform attributes with the same binding index" can
also be equivalently represented with a single struct-level uniform attribute:
```rust
#[derive(AsBindGroup)]
#[uniform(0, CoolMaterialUniform)]
struct CoolMaterial {
color: Color,
roughness: f32,
}
#[derive(ShaderType)]
struct CoolMaterialUniform {
color: Color,
roughness: f32,
}
impl From<&CoolMaterial> for CoolMaterialUniform {
fn from(material: &CoolMaterial) -> CoolMaterialUniform {
CoolMaterialUniform {
color: material.color,
roughness: material.roughness,
}
}
}
```
### Material Specialization
Material shader specialization is now _much_ simpler:
```rust
#[derive(AsBindGroup, TypeUuid, Debug, Clone)]
#[uuid = "f690fdae-d598-45ab-8225-97e2a3f056e0"]
#[bind_group_data(CoolMaterialKey)]
struct CoolMaterial {
#[uniform(0)]
color: Color,
is_red: bool,
}
#[derive(Copy, Clone, Hash, Eq, PartialEq)]
struct CoolMaterialKey {
is_red: bool,
}
impl From<&CoolMaterial> for CoolMaterialKey {
fn from(material: &CoolMaterial) -> CoolMaterialKey {
CoolMaterialKey {
is_red: material.is_red,
}
}
}
impl Material for CoolMaterial {
fn fragment_shader() -> ShaderRef {
"cool_material.wgsl".into()
}
fn specialize(
pipeline: &MaterialPipeline<Self>,
descriptor: &mut RenderPipelineDescriptor,
layout: &MeshVertexBufferLayout,
key: MaterialPipelineKey<Self>,
) -> Result<(), SpecializedMeshPipelineError> {
if key.bind_group_data.is_red {
let fragment = descriptor.fragment.as_mut().unwrap();
fragment.shader_defs.push("IS_RED".to_string());
}
Ok(())
}
}
```
Setting `bind_group_data` is not required for specialization (it defaults to `()`). Scenarios like "custom vertex attributes" also benefit from this system:
```rust
impl Material for CustomMaterial {
fn vertex_shader() -> ShaderRef {
"custom_material.wgsl".into()
}
fn fragment_shader() -> ShaderRef {
"custom_material.wgsl".into()
}
fn specialize(
pipeline: &MaterialPipeline<Self>,
descriptor: &mut RenderPipelineDescriptor,
layout: &MeshVertexBufferLayout,
key: MaterialPipelineKey<Self>,
) -> Result<(), SpecializedMeshPipelineError> {
let vertex_layout = layout.get_layout(&[
Mesh::ATTRIBUTE_POSITION.at_shader_location(0),
ATTRIBUTE_BLEND_COLOR.at_shader_location(1),
])?;
descriptor.vertex.buffers = vec![vertex_layout];
Ok(())
}
}
```
### Ported `StandardMaterial` to the new `Material` system
Bevy's built-in PBR material uses the new Material system (including the AsBindGroup derive):
```rust
#[derive(AsBindGroup, Debug, Clone, TypeUuid)]
#[uuid = "7494888b-c082-457b-aacf-517228cc0c22"]
#[bind_group_data(StandardMaterialKey)]
#[uniform(0, StandardMaterialUniform)]
pub struct StandardMaterial {
pub base_color: Color,
#[texture(1)]
#[sampler(2)]
pub base_color_texture: Option<Handle<Image>>,
/* other fields omitted for brevity */
```
### Ported Bevy examples to the new `Material` system
The overall complexity of Bevy's "custom shader examples" has gone down significantly. Take a look at the diffs if you want a dopamine spike.
Please note that while this PR has a net increase in "lines of code", most of those extra lines come from added documentation. There is a significant reduction
in the overall complexity of the code (even accounting for the new derive logic).
---
## Changelog
### Added
* `AsBindGroup` trait and derive, which make it much easier to transfer data to the gpu and generate bind groups for a given type.
### Changed
* The old `Material` and `SpecializedMaterial` traits have been replaced by a consolidated (much simpler) `Material` trait. Materials no longer implement `RenderAsset`.
* `StandardMaterial` was ported to the new material system. There are no user-facing api changes to the `StandardMaterial` struct api, but it now implements `AsBindGroup` and `Material` instead of `RenderAsset` and `SpecializedMaterial`.
## Migration Guide
The Material system has been reworked to be much simpler. We've removed a lot of boilerplate with the new `AsBindGroup` derive and the `Material` trait is simpler as well!
### Bevy 0.7 (old)
```rust
#[derive(Debug, Clone, TypeUuid)]
#[uuid = "f690fdae-d598-45ab-8225-97e2a3f056e0"]
pub struct CustomMaterial {
color: Color,
color_texture: Handle<Image>,
}
#[derive(Clone)]
pub struct GpuCustomMaterial {
_buffer: Buffer,
bind_group: BindGroup,
}
impl RenderAsset for CustomMaterial {
type ExtractedAsset = CustomMaterial;
type PreparedAsset = GpuCustomMaterial;
type Param = (SRes<RenderDevice>, SRes<MaterialPipeline<Self>>);
fn extract_asset(&self) -> Self::ExtractedAsset {
self.clone()
}
fn prepare_asset(
extracted_asset: Self::ExtractedAsset,
(render_device, material_pipeline): &mut SystemParamItem<Self::Param>,
) -> Result<Self::PreparedAsset, PrepareAssetError<Self::ExtractedAsset>> {
let color = Vec4::from_slice(&extracted_asset.color.as_linear_rgba_f32());
let byte_buffer = [0u8; Vec4::SIZE.get() as usize];
let mut buffer = encase::UniformBuffer::new(byte_buffer);
buffer.write(&color).unwrap();
let buffer = render_device.create_buffer_with_data(&BufferInitDescriptor {
contents: buffer.as_ref(),
label: None,
usage: BufferUsages::UNIFORM | BufferUsages::COPY_DST,
});
let (texture_view, texture_sampler) = if let Some(result) = material_pipeline
.mesh_pipeline
.get_image_texture(gpu_images, &Some(extracted_asset.color_texture.clone()))
{
result
} else {
return Err(PrepareAssetError::RetryNextUpdate(extracted_asset));
};
let bind_group = render_device.create_bind_group(&BindGroupDescriptor {
entries: &[
BindGroupEntry {
binding: 0,
resource: buffer.as_entire_binding(),
},
BindGroupEntry {
binding: 0,
resource: BindingResource::TextureView(texture_view),
},
BindGroupEntry {
binding: 1,
resource: BindingResource::Sampler(texture_sampler),
},
],
label: None,
layout: &material_pipeline.material_layout,
});
Ok(GpuCustomMaterial {
_buffer: buffer,
bind_group,
})
}
}
impl Material for CustomMaterial {
fn fragment_shader(asset_server: &AssetServer) -> Option<Handle<Shader>> {
Some(asset_server.load("custom_material.wgsl"))
}
fn bind_group(render_asset: &<Self as RenderAsset>::PreparedAsset) -> &BindGroup {
&render_asset.bind_group
}
fn bind_group_layout(render_device: &RenderDevice) -> BindGroupLayout {
render_device.create_bind_group_layout(&BindGroupLayoutDescriptor {
entries: &[
BindGroupLayoutEntry {
binding: 0,
visibility: ShaderStages::FRAGMENT,
ty: BindingType::Buffer {
ty: BufferBindingType::Uniform,
has_dynamic_offset: false,
min_binding_size: Some(Vec4::min_size()),
},
count: None,
},
BindGroupLayoutEntry {
binding: 1,
visibility: ShaderStages::FRAGMENT,
ty: BindingType::Texture {
multisampled: false,
sample_type: TextureSampleType::Float { filterable: true },
view_dimension: TextureViewDimension::D2Array,
},
count: None,
},
BindGroupLayoutEntry {
binding: 2,
visibility: ShaderStages::FRAGMENT,
ty: BindingType::Sampler(SamplerBindingType::Filtering),
count: None,
},
],
label: None,
})
}
}
```
### Bevy 0.8 (new)
```rust
impl Material for CustomMaterial {
fn fragment_shader() -> ShaderRef {
"custom_material.wgsl".into()
}
}
#[derive(AsBindGroup, TypeUuid, Debug, Clone)]
#[uuid = "f690fdae-d598-45ab-8225-97e2a3f056e0"]
pub struct CustomMaterial {
#[uniform(0)]
color: Color,
#[texture(1)]
#[sampler(2)]
color_texture: Handle<Image>,
}
```
## Future Work
* Add support for more binding types (cubemaps, buffers, etc). This PR intentionally includes a bare minimum number of binding types to keep "reviewability" in check.
* Consider optionally eliding binding indices using binding names. `AsBindGroup` could pass in (optional?) reflection info as a "hint".
* This would make it possible for the derive to do this:
```rust
#[derive(AsBindGroup)]
pub struct CustomMaterial {
#[uniform]
color: Color,
#[texture]
#[sampler]
color_texture: Option<Handle<Image>>,
alpha_mode: AlphaMode,
}
```
* Or this
```rust
#[derive(AsBindGroup)]
pub struct CustomMaterial {
#[binding]
color: Color,
#[binding]
color_texture: Option<Handle<Image>>,
alpha_mode: AlphaMode,
}
```
* Or even this (if we flip to "include bindings by default")
```rust
#[derive(AsBindGroup)]
pub struct CustomMaterial {
color: Color,
color_texture: Option<Handle<Image>>,
#[binding(ignore)]
alpha_mode: AlphaMode,
}
```
* If we add the option to define custom draw functions for materials (which could be done in a type-erased way), I think that would be enough to support extra non-material bindings. Worth considering!
2022-06-30 23:48:46 +00:00
|
|
|
fn depth_bias(&self) -> f32 {
|
2022-05-31 02:02:49 +00:00
|
|
|
0.0
|
|
|
|
}
|
|
|
|
|
`StandardMaterial` Light Transmission (#8015)
# Objective
<img width="1920" alt="Screenshot 2023-04-26 at 01 07 34"
src="https://user-images.githubusercontent.com/418473/234467578-0f34187b-5863-4ea1-88e9-7a6bb8ce8da3.png">
This PR adds both diffuse and specular light transmission capabilities
to the `StandardMaterial`, with support for screen space refractions.
This enables realistically representing a wide range of real-world
materials, such as:
- Glass; (Including frosted glass)
- Transparent and translucent plastics;
- Various liquids and gels;
- Gemstones;
- Marble;
- Wax;
- Paper;
- Leaves;
- Porcelain.
Unlike existing support for transparency, light transmission does not
rely on fixed function alpha blending, and therefore works with both
`AlphaMode::Opaque` and `AlphaMode::Mask` materials.
## Solution
- Introduces a number of transmission related fields in the
`StandardMaterial`;
- For specular transmission:
- Adds logic to take a view main texture snapshot after the opaque
phase; (in order to perform screen space refractions)
- Introduces a new `Transmissive3d` phase to the renderer, to which all
meshes with `transmission > 0.0` materials are sent.
- Calculates a light exit point (of the approximate mesh volume) using
`ior` and `thickness` properties
- Samples the snapshot texture with an adaptive number of taps across a
`roughness`-controlled radius enabling “blurry” refractions
- For diffuse transmission:
- Approximates transmitted diffuse light by using a second, flipped +
displaced, diffuse-only Lambertian lobe for each light source.
## To Do
- [x] Figure out where `fresnel_mix()` is taking place, if at all, and
where `dielectric_specular` is being calculated, if at all, and update
them to use the `ior` value (Not a blocker, just a nice-to-have for more
correct BSDF)
- To the _best of my knowledge, this is now taking place, after
964340cdd. The fresnel mix is actually "split" into two parts in our
implementation, one `(1 - fresnel(...))` in the transmission, and
`fresnel()` in the light implementations. A surface with more
reflectance now will produce slightly dimmer transmission towards the
grazing angle, as more of the light gets reflected.
- [x] Add `transmission_texture`
- [x] Add `diffuse_transmission_texture`
- [x] Add `thickness_texture`
- [x] Add `attenuation_distance` and `attenuation_color`
- [x] Connect values to glTF loader
- [x] `transmission` and `transmission_texture`
- [x] `thickness` and `thickness_texture`
- [x] `ior`
- [ ] `diffuse_transmission` and `diffuse_transmission_texture` (needs
upstream support in `gltf` crate, not a blocker)
- [x] Add support for multiple screen space refraction “steps”
- [x] Conditionally create no transmission snapshot texture at all if
`steps == 0`
- [x] Conditionally enable/disable screen space refraction transmission
snapshots
- [x] Read from depth pre-pass to prevent refracting pixels in front of
the light exit point
- [x] Use `interleaved_gradient_noise()` function for sampling blur in a
way that benefits from TAA
- [x] Drill down a TAA `#define`, tweak some aspects of the effect
conditionally based on it
- [x] Remove const array that's crashing under HLSL (unless a new `naga`
release with https://github.com/gfx-rs/naga/pull/2496 comes out before
we merge this)
- [ ] Look into alternatives to the `switch` hack for dynamically
indexing the const array (might not be needed, compilers seem to be
decent at expanding it)
- [ ] Add pipeline keys for gating transmission (do we really want/need
this?)
- [x] Tweak some material field/function names?
## A Note on Texture Packing
_This was originally added as a comment to the
`specular_transmission_texture`, `thickness_texture` and
`diffuse_transmission_texture` documentation, I removed it since it was
more confusing than helpful, and will likely be made redundant/will need
to be updated once we have a better infrastructure for preprocessing
assets_
Due to how channels are mapped, you can more efficiently use a single
shared texture image
for configuring the following:
- R - `specular_transmission_texture`
- G - `thickness_texture`
- B - _unused_
- A - `diffuse_transmission_texture`
The `KHR_materials_diffuse_transmission` glTF extension also defines a
`diffuseTransmissionColorTexture`,
that _we don't currently support_. One might choose to pack the
intensity and color textures together,
using RGB for the color and A for the intensity, in which case this
packing advice doesn't really apply.
---
## Changelog
- Added a new `Transmissive3d` render phase for rendering specular
transmissive materials with screen space refractions
- Added rendering support for transmitted environment map light on the
`StandardMaterial` as a fallback for screen space refractions
- Added `diffuse_transmission`, `specular_transmission`, `thickness`,
`ior`, `attenuation_distance` and `attenuation_color` to the
`StandardMaterial`
- Added `diffuse_transmission_texture`, `specular_transmission_texture`,
`thickness_texture` to the `StandardMaterial`, gated behind a new
`pbr_transmission_textures` cargo feature (off by default, for maximum
hardware compatibility)
- Added `Camera3d::screen_space_specular_transmission_steps` for
controlling the number of “layers of transparency” rendered for
transmissive objects
- Added a `TransmittedShadowReceiver` component for enabling shadows in
(diffusely) transmitted light. (disabled by default, as it requires
carefully setting up the `thickness` to avoid self-shadow artifacts)
- Added support for the `KHR_materials_transmission`,
`KHR_materials_ior` and `KHR_materials_volume` glTF extensions
- Renamed items related to temporal jitter for greater consistency
## Migration Guide
- `SsaoPipelineKey::temporal_noise` has been renamed to
`SsaoPipelineKey::temporal_jitter`
- The `TAA` shader def (controlled by the presence of the
`TemporalAntiAliasSettings` component in the camera) has been replaced
with the `TEMPORAL_JITTER` shader def (controlled by the presence of the
`TemporalJitter` component in the camera)
- `MeshPipelineKey::TAA` has been replaced by
`MeshPipelineKey::TEMPORAL_JITTER`
- The `TEMPORAL_NOISE` shader def has been consolidated with
`TEMPORAL_JITTER`
2023-10-31 20:59:02 +00:00
|
|
|
#[inline]
|
|
|
|
/// Returns whether the material would like to read from [`ViewTransmissionTexture`](bevy_core_pipeline::core_3d::ViewTransmissionTexture).
|
|
|
|
///
|
|
|
|
/// This allows taking color output from the [`Opaque3d`] pass as an input, (for screen-space transmission) but requires
|
|
|
|
/// rendering to take place in a separate [`Transmissive3d`] pass.
|
|
|
|
fn reads_view_transmission_texture(&self) -> bool {
|
|
|
|
false
|
|
|
|
}
|
|
|
|
|
2023-01-19 22:11:13 +00:00
|
|
|
/// Returns this material's prepass vertex shader. If [`ShaderRef::Default`] is returned, the default prepass vertex shader
|
|
|
|
/// will be used.
|
2023-10-16 13:39:17 +00:00
|
|
|
///
|
|
|
|
/// This is used for the various [prepasses](bevy_core_pipeline::prepass) as well as for generating the depth maps
|
|
|
|
/// required for shadow mapping.
|
2023-01-19 22:11:13 +00:00
|
|
|
fn prepass_vertex_shader() -> ShaderRef {
|
|
|
|
ShaderRef::Default
|
|
|
|
}
|
|
|
|
|
|
|
|
/// Returns this material's prepass fragment shader. If [`ShaderRef::Default`] is returned, the default prepass fragment shader
|
|
|
|
/// will be used.
|
2023-10-16 13:39:17 +00:00
|
|
|
///
|
|
|
|
/// This is used for the various [prepasses](bevy_core_pipeline::prepass) as well as for generating the depth maps
|
|
|
|
/// required for shadow mapping.
|
2023-01-19 22:11:13 +00:00
|
|
|
#[allow(unused_variables)]
|
|
|
|
fn prepass_fragment_shader() -> ShaderRef {
|
|
|
|
ShaderRef::Default
|
|
|
|
}
|
|
|
|
|
2023-10-12 22:10:38 +00:00
|
|
|
/// Returns this material's deferred vertex shader. If [`ShaderRef::Default`] is returned, the default deferred vertex shader
|
|
|
|
/// will be used.
|
|
|
|
fn deferred_vertex_shader() -> ShaderRef {
|
|
|
|
ShaderRef::Default
|
|
|
|
}
|
|
|
|
|
|
|
|
/// Returns this material's deferred fragment shader. If [`ShaderRef::Default`] is returned, the default deferred fragment shader
|
|
|
|
/// will be used.
|
|
|
|
#[allow(unused_variables)]
|
|
|
|
fn deferred_fragment_shader() -> ShaderRef {
|
|
|
|
ShaderRef::Default
|
|
|
|
}
|
|
|
|
|
2024-03-25 19:08:27 +00:00
|
|
|
/// Returns this material's [`crate::meshlet::MeshletMesh`] fragment shader. If [`ShaderRef::Default`] is returned,
|
|
|
|
/// the default meshlet mesh fragment shader will be used.
|
|
|
|
///
|
|
|
|
/// This is part of an experimental feature, and is unnecessary to implement unless you are using `MeshletMesh`'s.
|
2024-09-29 18:39:25 +00:00
|
|
|
///
|
|
|
|
/// See [`crate::meshlet::MeshletMesh`] for limitations.
|
2024-03-25 19:08:27 +00:00
|
|
|
#[allow(unused_variables)]
|
|
|
|
#[cfg(feature = "meshlet")]
|
|
|
|
fn meshlet_mesh_fragment_shader() -> ShaderRef {
|
|
|
|
ShaderRef::Default
|
|
|
|
}
|
|
|
|
|
|
|
|
/// Returns this material's [`crate::meshlet::MeshletMesh`] prepass fragment shader. If [`ShaderRef::Default`] is returned,
|
|
|
|
/// the default meshlet mesh prepass fragment shader will be used.
|
|
|
|
///
|
|
|
|
/// This is part of an experimental feature, and is unnecessary to implement unless you are using `MeshletMesh`'s.
|
2024-09-29 18:39:25 +00:00
|
|
|
///
|
|
|
|
/// See [`crate::meshlet::MeshletMesh`] for limitations.
|
2024-03-25 19:08:27 +00:00
|
|
|
#[allow(unused_variables)]
|
|
|
|
#[cfg(feature = "meshlet")]
|
|
|
|
fn meshlet_mesh_prepass_fragment_shader() -> ShaderRef {
|
|
|
|
ShaderRef::Default
|
|
|
|
}
|
|
|
|
|
|
|
|
/// Returns this material's [`crate::meshlet::MeshletMesh`] deferred fragment shader. If [`ShaderRef::Default`] is returned,
|
|
|
|
/// the default meshlet mesh deferred fragment shader will be used.
|
|
|
|
///
|
|
|
|
/// This is part of an experimental feature, and is unnecessary to implement unless you are using `MeshletMesh`'s.
|
2024-09-29 18:39:25 +00:00
|
|
|
///
|
|
|
|
/// See [`crate::meshlet::MeshletMesh`] for limitations.
|
2024-03-25 19:08:27 +00:00
|
|
|
#[allow(unused_variables)]
|
|
|
|
#[cfg(feature = "meshlet")]
|
|
|
|
fn meshlet_mesh_deferred_fragment_shader() -> ShaderRef {
|
|
|
|
ShaderRef::Default
|
|
|
|
}
|
|
|
|
|
Better Materials: AsBindGroup trait and derive, simpler Material trait (#5053)
# Objective
This PR reworks Bevy's Material system, making the user experience of defining Materials _much_ nicer. Bevy's previous material system leaves a lot to be desired:
* Materials require manually implementing the `RenderAsset` trait, which involves manually generating the bind group, handling gpu buffer data transfer, looking up image textures, etc. Even the simplest single-texture material involves writing ~80 unnecessary lines of code. This was never the long term plan.
* There are two material traits, which is confusing, hard to document, and often redundant: `Material` and `SpecializedMaterial`. `Material` implicitly implements `SpecializedMaterial`, and `SpecializedMaterial` is used in most high level apis to support both use cases. Most users shouldn't need to think about specialization at all (I consider it a "power-user tool"), so the fact that `SpecializedMaterial` is front-and-center in our apis is a miss.
* Implementing either material trait involves a lot of "type soup". The "prepared asset" parameter is particularly heinous: `&<Self as RenderAsset>::PreparedAsset`. Defining vertex and fragment shaders is also more verbose than it needs to be.
## Solution
Say hello to the new `Material` system:
```rust
#[derive(AsBindGroup, TypeUuid, Debug, Clone)]
#[uuid = "f690fdae-d598-45ab-8225-97e2a3f056e0"]
pub struct CoolMaterial {
#[uniform(0)]
color: Color,
#[texture(1)]
#[sampler(2)]
color_texture: Handle<Image>,
}
impl Material for CoolMaterial {
fn fragment_shader() -> ShaderRef {
"cool_material.wgsl".into()
}
}
```
Thats it! This same material would have required [~80 lines of complicated "type heavy" code](https://github.com/bevyengine/bevy/blob/v0.7.0/examples/shader/shader_material.rs) in the old Material system. Now it is just 14 lines of simple, readable code.
This is thanks to a new consolidated `Material` trait and the new `AsBindGroup` trait / derive.
### The new `Material` trait
The old "split" `Material` and `SpecializedMaterial` traits have been removed in favor of a new consolidated `Material` trait. All of the functions on the trait are optional.
The difficulty of implementing `Material` has been reduced by simplifying dataflow and removing type complexity:
```rust
// Old
impl Material for CustomMaterial {
fn fragment_shader(asset_server: &AssetServer) -> Option<Handle<Shader>> {
Some(asset_server.load("custom_material.wgsl"))
}
fn alpha_mode(render_asset: &<Self as RenderAsset>::PreparedAsset) -> AlphaMode {
render_asset.alpha_mode
}
}
// New
impl Material for CustomMaterial {
fn fragment_shader() -> ShaderRef {
"custom_material.wgsl".into()
}
fn alpha_mode(&self) -> AlphaMode {
self.alpha_mode
}
}
```
Specialization is still supported, but it is hidden by default under the `specialize()` function (more on this later).
### The `AsBindGroup` trait / derive
The `Material` trait now requires the `AsBindGroup` derive. This can be implemented manually relatively easily, but deriving it will almost always be preferable.
Field attributes like `uniform` and `texture` are used to define which fields should be bindings,
what their binding type is, and what index they should be bound at:
```rust
#[derive(AsBindGroup)]
struct CoolMaterial {
#[uniform(0)]
color: Color,
#[texture(1)]
#[sampler(2)]
color_texture: Handle<Image>,
}
```
In WGSL shaders, the binding looks like this:
```wgsl
struct CoolMaterial {
color: vec4<f32>;
};
[[group(1), binding(0)]]
var<uniform> material: CoolMaterial;
[[group(1), binding(1)]]
var color_texture: texture_2d<f32>;
[[group(1), binding(2)]]
var color_sampler: sampler;
```
Note that the "group" index is determined by the usage context. It is not defined in `AsBindGroup`. Bevy material bind groups are bound to group 1.
The following field-level attributes are supported:
* `uniform(BINDING_INDEX)`
* The field will be converted to a shader-compatible type using the `ShaderType` trait, written to a `Buffer`, and bound as a uniform. It can also be derived for custom structs.
* `texture(BINDING_INDEX)`
* This field's `Handle<Image>` will be used to look up the matching `Texture` gpu resource, which will be bound as a texture in shaders. The field will be assumed to implement `Into<Option<Handle<Image>>>`. In practice, most fields should be a `Handle<Image>` or `Option<Handle<Image>>`. If the value of an `Option<Handle<Image>>` is `None`, the new `FallbackImage` resource will be used instead. This attribute can be used in conjunction with a `sampler` binding attribute (with a different binding index).
* `sampler(BINDING_INDEX)`
* Behaves exactly like the `texture` attribute, but sets the Image's sampler binding instead of the texture.
Note that fields without field-level binding attributes will be ignored.
```rust
#[derive(AsBindGroup)]
struct CoolMaterial {
#[uniform(0)]
color: Color,
this_field_is_ignored: String,
}
```
As mentioned above, `Option<Handle<Image>>` is also supported:
```rust
#[derive(AsBindGroup)]
struct CoolMaterial {
#[uniform(0)]
color: Color,
#[texture(1)]
#[sampler(2)]
color_texture: Option<Handle<Image>>,
}
```
This is useful if you want a texture to be optional. When the value is `None`, the `FallbackImage` will be used for the binding instead, which defaults to "pure white".
Field uniforms with the same binding index will be combined into a single binding:
```rust
#[derive(AsBindGroup)]
struct CoolMaterial {
#[uniform(0)]
color: Color,
#[uniform(0)]
roughness: f32,
}
```
In WGSL shaders, the binding would look like this:
```wgsl
struct CoolMaterial {
color: vec4<f32>;
roughness: f32;
};
[[group(1), binding(0)]]
var<uniform> material: CoolMaterial;
```
Some less common scenarios will require "struct-level" attributes. These are the currently supported struct-level attributes:
* `uniform(BINDING_INDEX, ConvertedShaderType)`
* Similar to the field-level `uniform` attribute, but instead the entire `AsBindGroup` value is converted to `ConvertedShaderType`, which must implement `ShaderType`. This is useful if more complicated conversion logic is required.
* `bind_group_data(DataType)`
* The `AsBindGroup` type will be converted to some `DataType` using `Into<DataType>` and stored as `AsBindGroup::Data` as part of the `AsBindGroup::as_bind_group` call. This is useful if data needs to be stored alongside the generated bind group, such as a unique identifier for a material's bind group. The most common use case for this attribute is "shader pipeline specialization".
The previous `CoolMaterial` example illustrating "combining multiple field-level uniform attributes with the same binding index" can
also be equivalently represented with a single struct-level uniform attribute:
```rust
#[derive(AsBindGroup)]
#[uniform(0, CoolMaterialUniform)]
struct CoolMaterial {
color: Color,
roughness: f32,
}
#[derive(ShaderType)]
struct CoolMaterialUniform {
color: Color,
roughness: f32,
}
impl From<&CoolMaterial> for CoolMaterialUniform {
fn from(material: &CoolMaterial) -> CoolMaterialUniform {
CoolMaterialUniform {
color: material.color,
roughness: material.roughness,
}
}
}
```
### Material Specialization
Material shader specialization is now _much_ simpler:
```rust
#[derive(AsBindGroup, TypeUuid, Debug, Clone)]
#[uuid = "f690fdae-d598-45ab-8225-97e2a3f056e0"]
#[bind_group_data(CoolMaterialKey)]
struct CoolMaterial {
#[uniform(0)]
color: Color,
is_red: bool,
}
#[derive(Copy, Clone, Hash, Eq, PartialEq)]
struct CoolMaterialKey {
is_red: bool,
}
impl From<&CoolMaterial> for CoolMaterialKey {
fn from(material: &CoolMaterial) -> CoolMaterialKey {
CoolMaterialKey {
is_red: material.is_red,
}
}
}
impl Material for CoolMaterial {
fn fragment_shader() -> ShaderRef {
"cool_material.wgsl".into()
}
fn specialize(
pipeline: &MaterialPipeline<Self>,
descriptor: &mut RenderPipelineDescriptor,
layout: &MeshVertexBufferLayout,
key: MaterialPipelineKey<Self>,
) -> Result<(), SpecializedMeshPipelineError> {
if key.bind_group_data.is_red {
let fragment = descriptor.fragment.as_mut().unwrap();
fragment.shader_defs.push("IS_RED".to_string());
}
Ok(())
}
}
```
Setting `bind_group_data` is not required for specialization (it defaults to `()`). Scenarios like "custom vertex attributes" also benefit from this system:
```rust
impl Material for CustomMaterial {
fn vertex_shader() -> ShaderRef {
"custom_material.wgsl".into()
}
fn fragment_shader() -> ShaderRef {
"custom_material.wgsl".into()
}
fn specialize(
pipeline: &MaterialPipeline<Self>,
descriptor: &mut RenderPipelineDescriptor,
layout: &MeshVertexBufferLayout,
key: MaterialPipelineKey<Self>,
) -> Result<(), SpecializedMeshPipelineError> {
let vertex_layout = layout.get_layout(&[
Mesh::ATTRIBUTE_POSITION.at_shader_location(0),
ATTRIBUTE_BLEND_COLOR.at_shader_location(1),
])?;
descriptor.vertex.buffers = vec![vertex_layout];
Ok(())
}
}
```
### Ported `StandardMaterial` to the new `Material` system
Bevy's built-in PBR material uses the new Material system (including the AsBindGroup derive):
```rust
#[derive(AsBindGroup, Debug, Clone, TypeUuid)]
#[uuid = "7494888b-c082-457b-aacf-517228cc0c22"]
#[bind_group_data(StandardMaterialKey)]
#[uniform(0, StandardMaterialUniform)]
pub struct StandardMaterial {
pub base_color: Color,
#[texture(1)]
#[sampler(2)]
pub base_color_texture: Option<Handle<Image>>,
/* other fields omitted for brevity */
```
### Ported Bevy examples to the new `Material` system
The overall complexity of Bevy's "custom shader examples" has gone down significantly. Take a look at the diffs if you want a dopamine spike.
Please note that while this PR has a net increase in "lines of code", most of those extra lines come from added documentation. There is a significant reduction
in the overall complexity of the code (even accounting for the new derive logic).
---
## Changelog
### Added
* `AsBindGroup` trait and derive, which make it much easier to transfer data to the gpu and generate bind groups for a given type.
### Changed
* The old `Material` and `SpecializedMaterial` traits have been replaced by a consolidated (much simpler) `Material` trait. Materials no longer implement `RenderAsset`.
* `StandardMaterial` was ported to the new material system. There are no user-facing api changes to the `StandardMaterial` struct api, but it now implements `AsBindGroup` and `Material` instead of `RenderAsset` and `SpecializedMaterial`.
## Migration Guide
The Material system has been reworked to be much simpler. We've removed a lot of boilerplate with the new `AsBindGroup` derive and the `Material` trait is simpler as well!
### Bevy 0.7 (old)
```rust
#[derive(Debug, Clone, TypeUuid)]
#[uuid = "f690fdae-d598-45ab-8225-97e2a3f056e0"]
pub struct CustomMaterial {
color: Color,
color_texture: Handle<Image>,
}
#[derive(Clone)]
pub struct GpuCustomMaterial {
_buffer: Buffer,
bind_group: BindGroup,
}
impl RenderAsset for CustomMaterial {
type ExtractedAsset = CustomMaterial;
type PreparedAsset = GpuCustomMaterial;
type Param = (SRes<RenderDevice>, SRes<MaterialPipeline<Self>>);
fn extract_asset(&self) -> Self::ExtractedAsset {
self.clone()
}
fn prepare_asset(
extracted_asset: Self::ExtractedAsset,
(render_device, material_pipeline): &mut SystemParamItem<Self::Param>,
) -> Result<Self::PreparedAsset, PrepareAssetError<Self::ExtractedAsset>> {
let color = Vec4::from_slice(&extracted_asset.color.as_linear_rgba_f32());
let byte_buffer = [0u8; Vec4::SIZE.get() as usize];
let mut buffer = encase::UniformBuffer::new(byte_buffer);
buffer.write(&color).unwrap();
let buffer = render_device.create_buffer_with_data(&BufferInitDescriptor {
contents: buffer.as_ref(),
label: None,
usage: BufferUsages::UNIFORM | BufferUsages::COPY_DST,
});
let (texture_view, texture_sampler) = if let Some(result) = material_pipeline
.mesh_pipeline
.get_image_texture(gpu_images, &Some(extracted_asset.color_texture.clone()))
{
result
} else {
return Err(PrepareAssetError::RetryNextUpdate(extracted_asset));
};
let bind_group = render_device.create_bind_group(&BindGroupDescriptor {
entries: &[
BindGroupEntry {
binding: 0,
resource: buffer.as_entire_binding(),
},
BindGroupEntry {
binding: 0,
resource: BindingResource::TextureView(texture_view),
},
BindGroupEntry {
binding: 1,
resource: BindingResource::Sampler(texture_sampler),
},
],
label: None,
layout: &material_pipeline.material_layout,
});
Ok(GpuCustomMaterial {
_buffer: buffer,
bind_group,
})
}
}
impl Material for CustomMaterial {
fn fragment_shader(asset_server: &AssetServer) -> Option<Handle<Shader>> {
Some(asset_server.load("custom_material.wgsl"))
}
fn bind_group(render_asset: &<Self as RenderAsset>::PreparedAsset) -> &BindGroup {
&render_asset.bind_group
}
fn bind_group_layout(render_device: &RenderDevice) -> BindGroupLayout {
render_device.create_bind_group_layout(&BindGroupLayoutDescriptor {
entries: &[
BindGroupLayoutEntry {
binding: 0,
visibility: ShaderStages::FRAGMENT,
ty: BindingType::Buffer {
ty: BufferBindingType::Uniform,
has_dynamic_offset: false,
min_binding_size: Some(Vec4::min_size()),
},
count: None,
},
BindGroupLayoutEntry {
binding: 1,
visibility: ShaderStages::FRAGMENT,
ty: BindingType::Texture {
multisampled: false,
sample_type: TextureSampleType::Float { filterable: true },
view_dimension: TextureViewDimension::D2Array,
},
count: None,
},
BindGroupLayoutEntry {
binding: 2,
visibility: ShaderStages::FRAGMENT,
ty: BindingType::Sampler(SamplerBindingType::Filtering),
count: None,
},
],
label: None,
})
}
}
```
### Bevy 0.8 (new)
```rust
impl Material for CustomMaterial {
fn fragment_shader() -> ShaderRef {
"custom_material.wgsl".into()
}
}
#[derive(AsBindGroup, TypeUuid, Debug, Clone)]
#[uuid = "f690fdae-d598-45ab-8225-97e2a3f056e0"]
pub struct CustomMaterial {
#[uniform(0)]
color: Color,
#[texture(1)]
#[sampler(2)]
color_texture: Handle<Image>,
}
```
## Future Work
* Add support for more binding types (cubemaps, buffers, etc). This PR intentionally includes a bare minimum number of binding types to keep "reviewability" in check.
* Consider optionally eliding binding indices using binding names. `AsBindGroup` could pass in (optional?) reflection info as a "hint".
* This would make it possible for the derive to do this:
```rust
#[derive(AsBindGroup)]
pub struct CustomMaterial {
#[uniform]
color: Color,
#[texture]
#[sampler]
color_texture: Option<Handle<Image>>,
alpha_mode: AlphaMode,
}
```
* Or this
```rust
#[derive(AsBindGroup)]
pub struct CustomMaterial {
#[binding]
color: Color,
#[binding]
color_texture: Option<Handle<Image>>,
alpha_mode: AlphaMode,
}
```
* Or even this (if we flip to "include bindings by default")
```rust
#[derive(AsBindGroup)]
pub struct CustomMaterial {
color: Color,
color_texture: Option<Handle<Image>>,
#[binding(ignore)]
alpha_mode: AlphaMode,
}
```
* If we add the option to define custom draw functions for materials (which could be done in a type-erased way), I think that would be enough to support extra non-material bindings. Worth considering!
2022-06-30 23:48:46 +00:00
|
|
|
/// Customizes the default [`RenderPipelineDescriptor`] for a specific entity using the entity's
|
2024-03-01 20:56:21 +00:00
|
|
|
/// [`MaterialPipelineKey`] and [`MeshVertexBufferLayoutRef`] as input.
|
Mesh vertex buffer layouts (#3959)
This PR makes a number of changes to how meshes and vertex attributes are handled, which the goal of enabling easy and flexible custom vertex attributes:
* Reworks the `Mesh` type to use the newly added `VertexAttribute` internally
* `VertexAttribute` defines the name, a unique `VertexAttributeId`, and a `VertexFormat`
* `VertexAttributeId` is used to produce consistent sort orders for vertex buffer generation, replacing the more expensive and often surprising "name based sorting"
* Meshes can be used to generate a `MeshVertexBufferLayout`, which defines the layout of the gpu buffer produced by the mesh. `MeshVertexBufferLayouts` can then be used to generate actual `VertexBufferLayouts` according to the requirements of a specific pipeline. This decoupling of "mesh layout" vs "pipeline vertex buffer layout" is what enables custom attributes. We don't need to standardize _mesh layouts_ or contort meshes to meet the needs of a specific pipeline. As long as the mesh has what the pipeline needs, it will work transparently.
* Mesh-based pipelines now specialize on `&MeshVertexBufferLayout` via the new `SpecializedMeshPipeline` trait (which behaves like `SpecializedPipeline`, but adds `&MeshVertexBufferLayout`). The integrity of the pipeline cache is maintained because the `MeshVertexBufferLayout` is treated as part of the key (which is fully abstracted from implementers of the trait ... no need to add any additional info to the specialization key).
* Hashing `MeshVertexBufferLayout` is too expensive to do for every entity, every frame. To make this scalable, I added a generalized "pre-hashing" solution to `bevy_utils`: `Hashed<T>` keys and `PreHashMap<K, V>` (which uses `Hashed<T>` internally) . Why didn't I just do the quick and dirty in-place "pre-compute hash and use that u64 as a key in a hashmap" that we've done in the past? Because its wrong! Hashes by themselves aren't enough because two different values can produce the same hash. Re-hashing a hash is even worse! I decided to build a generalized solution because this pattern has come up in the past and we've chosen to do the wrong thing. Now we can do the right thing! This did unfortunately require pulling in `hashbrown` and using that in `bevy_utils`, because avoiding re-hashes requires the `raw_entry_mut` api, which isn't stabilized yet (and may never be ... `entry_ref` has favor now, but also isn't available yet). If std's HashMap ever provides the tools we need, we can move back to that. Note that adding `hashbrown` doesn't increase our dependency count because it was already in our tree. I will probably break these changes out into their own PR.
* Specializing on `MeshVertexBufferLayout` has one non-obvious behavior: it can produce identical pipelines for two different MeshVertexBufferLayouts. To optimize the number of active pipelines / reduce re-binds while drawing, I de-duplicate pipelines post-specialization using the final `VertexBufferLayout` as the key. For example, consider a pipeline that needs the layout `(position, normal)` and is specialized using two meshes: `(position, normal, uv)` and `(position, normal, other_vec2)`. If both of these meshes result in `(position, normal)` specializations, we can use the same pipeline! Now we do. Cool!
To briefly illustrate, this is what the relevant section of `MeshPipeline`'s specialization code looks like now:
```rust
impl SpecializedMeshPipeline for MeshPipeline {
type Key = MeshPipelineKey;
fn specialize(
&self,
key: Self::Key,
layout: &MeshVertexBufferLayout,
) -> RenderPipelineDescriptor {
let mut vertex_attributes = vec![
Mesh::ATTRIBUTE_POSITION.at_shader_location(0),
Mesh::ATTRIBUTE_NORMAL.at_shader_location(1),
Mesh::ATTRIBUTE_UV_0.at_shader_location(2),
];
let mut shader_defs = Vec::new();
if layout.contains(Mesh::ATTRIBUTE_TANGENT) {
shader_defs.push(String::from("VERTEX_TANGENTS"));
vertex_attributes.push(Mesh::ATTRIBUTE_TANGENT.at_shader_location(3));
}
let vertex_buffer_layout = layout
.get_layout(&vertex_attributes)
.expect("Mesh is missing a vertex attribute");
```
Notice that this is _much_ simpler than it was before. And now any mesh with any layout can be used with this pipeline, provided it has vertex postions, normals, and uvs. We even got to remove `HAS_TANGENTS` from MeshPipelineKey and `has_tangents` from `GpuMesh`, because that information is redundant with `MeshVertexBufferLayout`.
This is still a draft because I still need to:
* Add more docs
* Experiment with adding error handling to mesh pipeline specialization (which would print errors at runtime when a mesh is missing a vertex attribute required by a pipeline). If it doesn't tank perf, we'll keep it.
* Consider breaking out the PreHash / hashbrown changes into a separate PR.
* Add an example illustrating this change
* Verify that the "mesh-specialized pipeline de-duplication code" works properly
Please dont yell at me for not doing these things yet :) Just trying to get this in peoples' hands asap.
Alternative to #3120
Fixes #3030
Co-authored-by: Carter Anderson <mcanders1@gmail.com>
2022-02-23 23:21:13 +00:00
|
|
|
#[allow(unused_variables)]
|
|
|
|
#[inline]
|
|
|
|
fn specialize(
|
2022-04-07 16:16:35 +00:00
|
|
|
pipeline: &MaterialPipeline<Self>,
|
Mesh vertex buffer layouts (#3959)
This PR makes a number of changes to how meshes and vertex attributes are handled, which the goal of enabling easy and flexible custom vertex attributes:
* Reworks the `Mesh` type to use the newly added `VertexAttribute` internally
* `VertexAttribute` defines the name, a unique `VertexAttributeId`, and a `VertexFormat`
* `VertexAttributeId` is used to produce consistent sort orders for vertex buffer generation, replacing the more expensive and often surprising "name based sorting"
* Meshes can be used to generate a `MeshVertexBufferLayout`, which defines the layout of the gpu buffer produced by the mesh. `MeshVertexBufferLayouts` can then be used to generate actual `VertexBufferLayouts` according to the requirements of a specific pipeline. This decoupling of "mesh layout" vs "pipeline vertex buffer layout" is what enables custom attributes. We don't need to standardize _mesh layouts_ or contort meshes to meet the needs of a specific pipeline. As long as the mesh has what the pipeline needs, it will work transparently.
* Mesh-based pipelines now specialize on `&MeshVertexBufferLayout` via the new `SpecializedMeshPipeline` trait (which behaves like `SpecializedPipeline`, but adds `&MeshVertexBufferLayout`). The integrity of the pipeline cache is maintained because the `MeshVertexBufferLayout` is treated as part of the key (which is fully abstracted from implementers of the trait ... no need to add any additional info to the specialization key).
* Hashing `MeshVertexBufferLayout` is too expensive to do for every entity, every frame. To make this scalable, I added a generalized "pre-hashing" solution to `bevy_utils`: `Hashed<T>` keys and `PreHashMap<K, V>` (which uses `Hashed<T>` internally) . Why didn't I just do the quick and dirty in-place "pre-compute hash and use that u64 as a key in a hashmap" that we've done in the past? Because its wrong! Hashes by themselves aren't enough because two different values can produce the same hash. Re-hashing a hash is even worse! I decided to build a generalized solution because this pattern has come up in the past and we've chosen to do the wrong thing. Now we can do the right thing! This did unfortunately require pulling in `hashbrown` and using that in `bevy_utils`, because avoiding re-hashes requires the `raw_entry_mut` api, which isn't stabilized yet (and may never be ... `entry_ref` has favor now, but also isn't available yet). If std's HashMap ever provides the tools we need, we can move back to that. Note that adding `hashbrown` doesn't increase our dependency count because it was already in our tree. I will probably break these changes out into their own PR.
* Specializing on `MeshVertexBufferLayout` has one non-obvious behavior: it can produce identical pipelines for two different MeshVertexBufferLayouts. To optimize the number of active pipelines / reduce re-binds while drawing, I de-duplicate pipelines post-specialization using the final `VertexBufferLayout` as the key. For example, consider a pipeline that needs the layout `(position, normal)` and is specialized using two meshes: `(position, normal, uv)` and `(position, normal, other_vec2)`. If both of these meshes result in `(position, normal)` specializations, we can use the same pipeline! Now we do. Cool!
To briefly illustrate, this is what the relevant section of `MeshPipeline`'s specialization code looks like now:
```rust
impl SpecializedMeshPipeline for MeshPipeline {
type Key = MeshPipelineKey;
fn specialize(
&self,
key: Self::Key,
layout: &MeshVertexBufferLayout,
) -> RenderPipelineDescriptor {
let mut vertex_attributes = vec![
Mesh::ATTRIBUTE_POSITION.at_shader_location(0),
Mesh::ATTRIBUTE_NORMAL.at_shader_location(1),
Mesh::ATTRIBUTE_UV_0.at_shader_location(2),
];
let mut shader_defs = Vec::new();
if layout.contains(Mesh::ATTRIBUTE_TANGENT) {
shader_defs.push(String::from("VERTEX_TANGENTS"));
vertex_attributes.push(Mesh::ATTRIBUTE_TANGENT.at_shader_location(3));
}
let vertex_buffer_layout = layout
.get_layout(&vertex_attributes)
.expect("Mesh is missing a vertex attribute");
```
Notice that this is _much_ simpler than it was before. And now any mesh with any layout can be used with this pipeline, provided it has vertex postions, normals, and uvs. We even got to remove `HAS_TANGENTS` from MeshPipelineKey and `has_tangents` from `GpuMesh`, because that information is redundant with `MeshVertexBufferLayout`.
This is still a draft because I still need to:
* Add more docs
* Experiment with adding error handling to mesh pipeline specialization (which would print errors at runtime when a mesh is missing a vertex attribute required by a pipeline). If it doesn't tank perf, we'll keep it.
* Consider breaking out the PreHash / hashbrown changes into a separate PR.
* Add an example illustrating this change
* Verify that the "mesh-specialized pipeline de-duplication code" works properly
Please dont yell at me for not doing these things yet :) Just trying to get this in peoples' hands asap.
Alternative to #3120
Fixes #3030
Co-authored-by: Carter Anderson <mcanders1@gmail.com>
2022-02-23 23:21:13 +00:00
|
|
|
descriptor: &mut RenderPipelineDescriptor,
|
2024-03-01 20:56:21 +00:00
|
|
|
layout: &MeshVertexBufferLayoutRef,
|
Better Materials: AsBindGroup trait and derive, simpler Material trait (#5053)
# Objective
This PR reworks Bevy's Material system, making the user experience of defining Materials _much_ nicer. Bevy's previous material system leaves a lot to be desired:
* Materials require manually implementing the `RenderAsset` trait, which involves manually generating the bind group, handling gpu buffer data transfer, looking up image textures, etc. Even the simplest single-texture material involves writing ~80 unnecessary lines of code. This was never the long term plan.
* There are two material traits, which is confusing, hard to document, and often redundant: `Material` and `SpecializedMaterial`. `Material` implicitly implements `SpecializedMaterial`, and `SpecializedMaterial` is used in most high level apis to support both use cases. Most users shouldn't need to think about specialization at all (I consider it a "power-user tool"), so the fact that `SpecializedMaterial` is front-and-center in our apis is a miss.
* Implementing either material trait involves a lot of "type soup". The "prepared asset" parameter is particularly heinous: `&<Self as RenderAsset>::PreparedAsset`. Defining vertex and fragment shaders is also more verbose than it needs to be.
## Solution
Say hello to the new `Material` system:
```rust
#[derive(AsBindGroup, TypeUuid, Debug, Clone)]
#[uuid = "f690fdae-d598-45ab-8225-97e2a3f056e0"]
pub struct CoolMaterial {
#[uniform(0)]
color: Color,
#[texture(1)]
#[sampler(2)]
color_texture: Handle<Image>,
}
impl Material for CoolMaterial {
fn fragment_shader() -> ShaderRef {
"cool_material.wgsl".into()
}
}
```
Thats it! This same material would have required [~80 lines of complicated "type heavy" code](https://github.com/bevyengine/bevy/blob/v0.7.0/examples/shader/shader_material.rs) in the old Material system. Now it is just 14 lines of simple, readable code.
This is thanks to a new consolidated `Material` trait and the new `AsBindGroup` trait / derive.
### The new `Material` trait
The old "split" `Material` and `SpecializedMaterial` traits have been removed in favor of a new consolidated `Material` trait. All of the functions on the trait are optional.
The difficulty of implementing `Material` has been reduced by simplifying dataflow and removing type complexity:
```rust
// Old
impl Material for CustomMaterial {
fn fragment_shader(asset_server: &AssetServer) -> Option<Handle<Shader>> {
Some(asset_server.load("custom_material.wgsl"))
}
fn alpha_mode(render_asset: &<Self as RenderAsset>::PreparedAsset) -> AlphaMode {
render_asset.alpha_mode
}
}
// New
impl Material for CustomMaterial {
fn fragment_shader() -> ShaderRef {
"custom_material.wgsl".into()
}
fn alpha_mode(&self) -> AlphaMode {
self.alpha_mode
}
}
```
Specialization is still supported, but it is hidden by default under the `specialize()` function (more on this later).
### The `AsBindGroup` trait / derive
The `Material` trait now requires the `AsBindGroup` derive. This can be implemented manually relatively easily, but deriving it will almost always be preferable.
Field attributes like `uniform` and `texture` are used to define which fields should be bindings,
what their binding type is, and what index they should be bound at:
```rust
#[derive(AsBindGroup)]
struct CoolMaterial {
#[uniform(0)]
color: Color,
#[texture(1)]
#[sampler(2)]
color_texture: Handle<Image>,
}
```
In WGSL shaders, the binding looks like this:
```wgsl
struct CoolMaterial {
color: vec4<f32>;
};
[[group(1), binding(0)]]
var<uniform> material: CoolMaterial;
[[group(1), binding(1)]]
var color_texture: texture_2d<f32>;
[[group(1), binding(2)]]
var color_sampler: sampler;
```
Note that the "group" index is determined by the usage context. It is not defined in `AsBindGroup`. Bevy material bind groups are bound to group 1.
The following field-level attributes are supported:
* `uniform(BINDING_INDEX)`
* The field will be converted to a shader-compatible type using the `ShaderType` trait, written to a `Buffer`, and bound as a uniform. It can also be derived for custom structs.
* `texture(BINDING_INDEX)`
* This field's `Handle<Image>` will be used to look up the matching `Texture` gpu resource, which will be bound as a texture in shaders. The field will be assumed to implement `Into<Option<Handle<Image>>>`. In practice, most fields should be a `Handle<Image>` or `Option<Handle<Image>>`. If the value of an `Option<Handle<Image>>` is `None`, the new `FallbackImage` resource will be used instead. This attribute can be used in conjunction with a `sampler` binding attribute (with a different binding index).
* `sampler(BINDING_INDEX)`
* Behaves exactly like the `texture` attribute, but sets the Image's sampler binding instead of the texture.
Note that fields without field-level binding attributes will be ignored.
```rust
#[derive(AsBindGroup)]
struct CoolMaterial {
#[uniform(0)]
color: Color,
this_field_is_ignored: String,
}
```
As mentioned above, `Option<Handle<Image>>` is also supported:
```rust
#[derive(AsBindGroup)]
struct CoolMaterial {
#[uniform(0)]
color: Color,
#[texture(1)]
#[sampler(2)]
color_texture: Option<Handle<Image>>,
}
```
This is useful if you want a texture to be optional. When the value is `None`, the `FallbackImage` will be used for the binding instead, which defaults to "pure white".
Field uniforms with the same binding index will be combined into a single binding:
```rust
#[derive(AsBindGroup)]
struct CoolMaterial {
#[uniform(0)]
color: Color,
#[uniform(0)]
roughness: f32,
}
```
In WGSL shaders, the binding would look like this:
```wgsl
struct CoolMaterial {
color: vec4<f32>;
roughness: f32;
};
[[group(1), binding(0)]]
var<uniform> material: CoolMaterial;
```
Some less common scenarios will require "struct-level" attributes. These are the currently supported struct-level attributes:
* `uniform(BINDING_INDEX, ConvertedShaderType)`
* Similar to the field-level `uniform` attribute, but instead the entire `AsBindGroup` value is converted to `ConvertedShaderType`, which must implement `ShaderType`. This is useful if more complicated conversion logic is required.
* `bind_group_data(DataType)`
* The `AsBindGroup` type will be converted to some `DataType` using `Into<DataType>` and stored as `AsBindGroup::Data` as part of the `AsBindGroup::as_bind_group` call. This is useful if data needs to be stored alongside the generated bind group, such as a unique identifier for a material's bind group. The most common use case for this attribute is "shader pipeline specialization".
The previous `CoolMaterial` example illustrating "combining multiple field-level uniform attributes with the same binding index" can
also be equivalently represented with a single struct-level uniform attribute:
```rust
#[derive(AsBindGroup)]
#[uniform(0, CoolMaterialUniform)]
struct CoolMaterial {
color: Color,
roughness: f32,
}
#[derive(ShaderType)]
struct CoolMaterialUniform {
color: Color,
roughness: f32,
}
impl From<&CoolMaterial> for CoolMaterialUniform {
fn from(material: &CoolMaterial) -> CoolMaterialUniform {
CoolMaterialUniform {
color: material.color,
roughness: material.roughness,
}
}
}
```
### Material Specialization
Material shader specialization is now _much_ simpler:
```rust
#[derive(AsBindGroup, TypeUuid, Debug, Clone)]
#[uuid = "f690fdae-d598-45ab-8225-97e2a3f056e0"]
#[bind_group_data(CoolMaterialKey)]
struct CoolMaterial {
#[uniform(0)]
color: Color,
is_red: bool,
}
#[derive(Copy, Clone, Hash, Eq, PartialEq)]
struct CoolMaterialKey {
is_red: bool,
}
impl From<&CoolMaterial> for CoolMaterialKey {
fn from(material: &CoolMaterial) -> CoolMaterialKey {
CoolMaterialKey {
is_red: material.is_red,
}
}
}
impl Material for CoolMaterial {
fn fragment_shader() -> ShaderRef {
"cool_material.wgsl".into()
}
fn specialize(
pipeline: &MaterialPipeline<Self>,
descriptor: &mut RenderPipelineDescriptor,
layout: &MeshVertexBufferLayout,
key: MaterialPipelineKey<Self>,
) -> Result<(), SpecializedMeshPipelineError> {
if key.bind_group_data.is_red {
let fragment = descriptor.fragment.as_mut().unwrap();
fragment.shader_defs.push("IS_RED".to_string());
}
Ok(())
}
}
```
Setting `bind_group_data` is not required for specialization (it defaults to `()`). Scenarios like "custom vertex attributes" also benefit from this system:
```rust
impl Material for CustomMaterial {
fn vertex_shader() -> ShaderRef {
"custom_material.wgsl".into()
}
fn fragment_shader() -> ShaderRef {
"custom_material.wgsl".into()
}
fn specialize(
pipeline: &MaterialPipeline<Self>,
descriptor: &mut RenderPipelineDescriptor,
layout: &MeshVertexBufferLayout,
key: MaterialPipelineKey<Self>,
) -> Result<(), SpecializedMeshPipelineError> {
let vertex_layout = layout.get_layout(&[
Mesh::ATTRIBUTE_POSITION.at_shader_location(0),
ATTRIBUTE_BLEND_COLOR.at_shader_location(1),
])?;
descriptor.vertex.buffers = vec![vertex_layout];
Ok(())
}
}
```
### Ported `StandardMaterial` to the new `Material` system
Bevy's built-in PBR material uses the new Material system (including the AsBindGroup derive):
```rust
#[derive(AsBindGroup, Debug, Clone, TypeUuid)]
#[uuid = "7494888b-c082-457b-aacf-517228cc0c22"]
#[bind_group_data(StandardMaterialKey)]
#[uniform(0, StandardMaterialUniform)]
pub struct StandardMaterial {
pub base_color: Color,
#[texture(1)]
#[sampler(2)]
pub base_color_texture: Option<Handle<Image>>,
/* other fields omitted for brevity */
```
### Ported Bevy examples to the new `Material` system
The overall complexity of Bevy's "custom shader examples" has gone down significantly. Take a look at the diffs if you want a dopamine spike.
Please note that while this PR has a net increase in "lines of code", most of those extra lines come from added documentation. There is a significant reduction
in the overall complexity of the code (even accounting for the new derive logic).
---
## Changelog
### Added
* `AsBindGroup` trait and derive, which make it much easier to transfer data to the gpu and generate bind groups for a given type.
### Changed
* The old `Material` and `SpecializedMaterial` traits have been replaced by a consolidated (much simpler) `Material` trait. Materials no longer implement `RenderAsset`.
* `StandardMaterial` was ported to the new material system. There are no user-facing api changes to the `StandardMaterial` struct api, but it now implements `AsBindGroup` and `Material` instead of `RenderAsset` and `SpecializedMaterial`.
## Migration Guide
The Material system has been reworked to be much simpler. We've removed a lot of boilerplate with the new `AsBindGroup` derive and the `Material` trait is simpler as well!
### Bevy 0.7 (old)
```rust
#[derive(Debug, Clone, TypeUuid)]
#[uuid = "f690fdae-d598-45ab-8225-97e2a3f056e0"]
pub struct CustomMaterial {
color: Color,
color_texture: Handle<Image>,
}
#[derive(Clone)]
pub struct GpuCustomMaterial {
_buffer: Buffer,
bind_group: BindGroup,
}
impl RenderAsset for CustomMaterial {
type ExtractedAsset = CustomMaterial;
type PreparedAsset = GpuCustomMaterial;
type Param = (SRes<RenderDevice>, SRes<MaterialPipeline<Self>>);
fn extract_asset(&self) -> Self::ExtractedAsset {
self.clone()
}
fn prepare_asset(
extracted_asset: Self::ExtractedAsset,
(render_device, material_pipeline): &mut SystemParamItem<Self::Param>,
) -> Result<Self::PreparedAsset, PrepareAssetError<Self::ExtractedAsset>> {
let color = Vec4::from_slice(&extracted_asset.color.as_linear_rgba_f32());
let byte_buffer = [0u8; Vec4::SIZE.get() as usize];
let mut buffer = encase::UniformBuffer::new(byte_buffer);
buffer.write(&color).unwrap();
let buffer = render_device.create_buffer_with_data(&BufferInitDescriptor {
contents: buffer.as_ref(),
label: None,
usage: BufferUsages::UNIFORM | BufferUsages::COPY_DST,
});
let (texture_view, texture_sampler) = if let Some(result) = material_pipeline
.mesh_pipeline
.get_image_texture(gpu_images, &Some(extracted_asset.color_texture.clone()))
{
result
} else {
return Err(PrepareAssetError::RetryNextUpdate(extracted_asset));
};
let bind_group = render_device.create_bind_group(&BindGroupDescriptor {
entries: &[
BindGroupEntry {
binding: 0,
resource: buffer.as_entire_binding(),
},
BindGroupEntry {
binding: 0,
resource: BindingResource::TextureView(texture_view),
},
BindGroupEntry {
binding: 1,
resource: BindingResource::Sampler(texture_sampler),
},
],
label: None,
layout: &material_pipeline.material_layout,
});
Ok(GpuCustomMaterial {
_buffer: buffer,
bind_group,
})
}
}
impl Material for CustomMaterial {
fn fragment_shader(asset_server: &AssetServer) -> Option<Handle<Shader>> {
Some(asset_server.load("custom_material.wgsl"))
}
fn bind_group(render_asset: &<Self as RenderAsset>::PreparedAsset) -> &BindGroup {
&render_asset.bind_group
}
fn bind_group_layout(render_device: &RenderDevice) -> BindGroupLayout {
render_device.create_bind_group_layout(&BindGroupLayoutDescriptor {
entries: &[
BindGroupLayoutEntry {
binding: 0,
visibility: ShaderStages::FRAGMENT,
ty: BindingType::Buffer {
ty: BufferBindingType::Uniform,
has_dynamic_offset: false,
min_binding_size: Some(Vec4::min_size()),
},
count: None,
},
BindGroupLayoutEntry {
binding: 1,
visibility: ShaderStages::FRAGMENT,
ty: BindingType::Texture {
multisampled: false,
sample_type: TextureSampleType::Float { filterable: true },
view_dimension: TextureViewDimension::D2Array,
},
count: None,
},
BindGroupLayoutEntry {
binding: 2,
visibility: ShaderStages::FRAGMENT,
ty: BindingType::Sampler(SamplerBindingType::Filtering),
count: None,
},
],
label: None,
})
}
}
```
### Bevy 0.8 (new)
```rust
impl Material for CustomMaterial {
fn fragment_shader() -> ShaderRef {
"custom_material.wgsl".into()
}
}
#[derive(AsBindGroup, TypeUuid, Debug, Clone)]
#[uuid = "f690fdae-d598-45ab-8225-97e2a3f056e0"]
pub struct CustomMaterial {
#[uniform(0)]
color: Color,
#[texture(1)]
#[sampler(2)]
color_texture: Handle<Image>,
}
```
## Future Work
* Add support for more binding types (cubemaps, buffers, etc). This PR intentionally includes a bare minimum number of binding types to keep "reviewability" in check.
* Consider optionally eliding binding indices using binding names. `AsBindGroup` could pass in (optional?) reflection info as a "hint".
* This would make it possible for the derive to do this:
```rust
#[derive(AsBindGroup)]
pub struct CustomMaterial {
#[uniform]
color: Color,
#[texture]
#[sampler]
color_texture: Option<Handle<Image>>,
alpha_mode: AlphaMode,
}
```
* Or this
```rust
#[derive(AsBindGroup)]
pub struct CustomMaterial {
#[binding]
color: Color,
#[binding]
color_texture: Option<Handle<Image>>,
alpha_mode: AlphaMode,
}
```
* Or even this (if we flip to "include bindings by default")
```rust
#[derive(AsBindGroup)]
pub struct CustomMaterial {
color: Color,
color_texture: Option<Handle<Image>>,
#[binding(ignore)]
alpha_mode: AlphaMode,
}
```
* If we add the option to define custom draw functions for materials (which could be done in a type-erased way), I think that would be enough to support extra non-material bindings. Worth considering!
2022-06-30 23:48:46 +00:00
|
|
|
key: MaterialPipelineKey<Self>,
|
Mesh vertex buffer layouts (#3959)
This PR makes a number of changes to how meshes and vertex attributes are handled, which the goal of enabling easy and flexible custom vertex attributes:
* Reworks the `Mesh` type to use the newly added `VertexAttribute` internally
* `VertexAttribute` defines the name, a unique `VertexAttributeId`, and a `VertexFormat`
* `VertexAttributeId` is used to produce consistent sort orders for vertex buffer generation, replacing the more expensive and often surprising "name based sorting"
* Meshes can be used to generate a `MeshVertexBufferLayout`, which defines the layout of the gpu buffer produced by the mesh. `MeshVertexBufferLayouts` can then be used to generate actual `VertexBufferLayouts` according to the requirements of a specific pipeline. This decoupling of "mesh layout" vs "pipeline vertex buffer layout" is what enables custom attributes. We don't need to standardize _mesh layouts_ or contort meshes to meet the needs of a specific pipeline. As long as the mesh has what the pipeline needs, it will work transparently.
* Mesh-based pipelines now specialize on `&MeshVertexBufferLayout` via the new `SpecializedMeshPipeline` trait (which behaves like `SpecializedPipeline`, but adds `&MeshVertexBufferLayout`). The integrity of the pipeline cache is maintained because the `MeshVertexBufferLayout` is treated as part of the key (which is fully abstracted from implementers of the trait ... no need to add any additional info to the specialization key).
* Hashing `MeshVertexBufferLayout` is too expensive to do for every entity, every frame. To make this scalable, I added a generalized "pre-hashing" solution to `bevy_utils`: `Hashed<T>` keys and `PreHashMap<K, V>` (which uses `Hashed<T>` internally) . Why didn't I just do the quick and dirty in-place "pre-compute hash and use that u64 as a key in a hashmap" that we've done in the past? Because its wrong! Hashes by themselves aren't enough because two different values can produce the same hash. Re-hashing a hash is even worse! I decided to build a generalized solution because this pattern has come up in the past and we've chosen to do the wrong thing. Now we can do the right thing! This did unfortunately require pulling in `hashbrown` and using that in `bevy_utils`, because avoiding re-hashes requires the `raw_entry_mut` api, which isn't stabilized yet (and may never be ... `entry_ref` has favor now, but also isn't available yet). If std's HashMap ever provides the tools we need, we can move back to that. Note that adding `hashbrown` doesn't increase our dependency count because it was already in our tree. I will probably break these changes out into their own PR.
* Specializing on `MeshVertexBufferLayout` has one non-obvious behavior: it can produce identical pipelines for two different MeshVertexBufferLayouts. To optimize the number of active pipelines / reduce re-binds while drawing, I de-duplicate pipelines post-specialization using the final `VertexBufferLayout` as the key. For example, consider a pipeline that needs the layout `(position, normal)` and is specialized using two meshes: `(position, normal, uv)` and `(position, normal, other_vec2)`. If both of these meshes result in `(position, normal)` specializations, we can use the same pipeline! Now we do. Cool!
To briefly illustrate, this is what the relevant section of `MeshPipeline`'s specialization code looks like now:
```rust
impl SpecializedMeshPipeline for MeshPipeline {
type Key = MeshPipelineKey;
fn specialize(
&self,
key: Self::Key,
layout: &MeshVertexBufferLayout,
) -> RenderPipelineDescriptor {
let mut vertex_attributes = vec![
Mesh::ATTRIBUTE_POSITION.at_shader_location(0),
Mesh::ATTRIBUTE_NORMAL.at_shader_location(1),
Mesh::ATTRIBUTE_UV_0.at_shader_location(2),
];
let mut shader_defs = Vec::new();
if layout.contains(Mesh::ATTRIBUTE_TANGENT) {
shader_defs.push(String::from("VERTEX_TANGENTS"));
vertex_attributes.push(Mesh::ATTRIBUTE_TANGENT.at_shader_location(3));
}
let vertex_buffer_layout = layout
.get_layout(&vertex_attributes)
.expect("Mesh is missing a vertex attribute");
```
Notice that this is _much_ simpler than it was before. And now any mesh with any layout can be used with this pipeline, provided it has vertex postions, normals, and uvs. We even got to remove `HAS_TANGENTS` from MeshPipelineKey and `has_tangents` from `GpuMesh`, because that information is redundant with `MeshVertexBufferLayout`.
This is still a draft because I still need to:
* Add more docs
* Experiment with adding error handling to mesh pipeline specialization (which would print errors at runtime when a mesh is missing a vertex attribute required by a pipeline). If it doesn't tank perf, we'll keep it.
* Consider breaking out the PreHash / hashbrown changes into a separate PR.
* Add an example illustrating this change
* Verify that the "mesh-specialized pipeline de-duplication code" works properly
Please dont yell at me for not doing these things yet :) Just trying to get this in peoples' hands asap.
Alternative to #3120
Fixes #3030
Co-authored-by: Carter Anderson <mcanders1@gmail.com>
2022-02-23 23:21:13 +00:00
|
|
|
) -> Result<(), SpecializedMeshPipelineError> {
|
|
|
|
Ok(())
|
|
|
|
}
|
2020-08-01 00:10:29 +00:00
|
|
|
}
|
|
|
|
|
Better Materials: AsBindGroup trait and derive, simpler Material trait (#5053)
# Objective
This PR reworks Bevy's Material system, making the user experience of defining Materials _much_ nicer. Bevy's previous material system leaves a lot to be desired:
* Materials require manually implementing the `RenderAsset` trait, which involves manually generating the bind group, handling gpu buffer data transfer, looking up image textures, etc. Even the simplest single-texture material involves writing ~80 unnecessary lines of code. This was never the long term plan.
* There are two material traits, which is confusing, hard to document, and often redundant: `Material` and `SpecializedMaterial`. `Material` implicitly implements `SpecializedMaterial`, and `SpecializedMaterial` is used in most high level apis to support both use cases. Most users shouldn't need to think about specialization at all (I consider it a "power-user tool"), so the fact that `SpecializedMaterial` is front-and-center in our apis is a miss.
* Implementing either material trait involves a lot of "type soup". The "prepared asset" parameter is particularly heinous: `&<Self as RenderAsset>::PreparedAsset`. Defining vertex and fragment shaders is also more verbose than it needs to be.
## Solution
Say hello to the new `Material` system:
```rust
#[derive(AsBindGroup, TypeUuid, Debug, Clone)]
#[uuid = "f690fdae-d598-45ab-8225-97e2a3f056e0"]
pub struct CoolMaterial {
#[uniform(0)]
color: Color,
#[texture(1)]
#[sampler(2)]
color_texture: Handle<Image>,
}
impl Material for CoolMaterial {
fn fragment_shader() -> ShaderRef {
"cool_material.wgsl".into()
}
}
```
Thats it! This same material would have required [~80 lines of complicated "type heavy" code](https://github.com/bevyengine/bevy/blob/v0.7.0/examples/shader/shader_material.rs) in the old Material system. Now it is just 14 lines of simple, readable code.
This is thanks to a new consolidated `Material` trait and the new `AsBindGroup` trait / derive.
### The new `Material` trait
The old "split" `Material` and `SpecializedMaterial` traits have been removed in favor of a new consolidated `Material` trait. All of the functions on the trait are optional.
The difficulty of implementing `Material` has been reduced by simplifying dataflow and removing type complexity:
```rust
// Old
impl Material for CustomMaterial {
fn fragment_shader(asset_server: &AssetServer) -> Option<Handle<Shader>> {
Some(asset_server.load("custom_material.wgsl"))
}
fn alpha_mode(render_asset: &<Self as RenderAsset>::PreparedAsset) -> AlphaMode {
render_asset.alpha_mode
}
}
// New
impl Material for CustomMaterial {
fn fragment_shader() -> ShaderRef {
"custom_material.wgsl".into()
}
fn alpha_mode(&self) -> AlphaMode {
self.alpha_mode
}
}
```
Specialization is still supported, but it is hidden by default under the `specialize()` function (more on this later).
### The `AsBindGroup` trait / derive
The `Material` trait now requires the `AsBindGroup` derive. This can be implemented manually relatively easily, but deriving it will almost always be preferable.
Field attributes like `uniform` and `texture` are used to define which fields should be bindings,
what their binding type is, and what index they should be bound at:
```rust
#[derive(AsBindGroup)]
struct CoolMaterial {
#[uniform(0)]
color: Color,
#[texture(1)]
#[sampler(2)]
color_texture: Handle<Image>,
}
```
In WGSL shaders, the binding looks like this:
```wgsl
struct CoolMaterial {
color: vec4<f32>;
};
[[group(1), binding(0)]]
var<uniform> material: CoolMaterial;
[[group(1), binding(1)]]
var color_texture: texture_2d<f32>;
[[group(1), binding(2)]]
var color_sampler: sampler;
```
Note that the "group" index is determined by the usage context. It is not defined in `AsBindGroup`. Bevy material bind groups are bound to group 1.
The following field-level attributes are supported:
* `uniform(BINDING_INDEX)`
* The field will be converted to a shader-compatible type using the `ShaderType` trait, written to a `Buffer`, and bound as a uniform. It can also be derived for custom structs.
* `texture(BINDING_INDEX)`
* This field's `Handle<Image>` will be used to look up the matching `Texture` gpu resource, which will be bound as a texture in shaders. The field will be assumed to implement `Into<Option<Handle<Image>>>`. In practice, most fields should be a `Handle<Image>` or `Option<Handle<Image>>`. If the value of an `Option<Handle<Image>>` is `None`, the new `FallbackImage` resource will be used instead. This attribute can be used in conjunction with a `sampler` binding attribute (with a different binding index).
* `sampler(BINDING_INDEX)`
* Behaves exactly like the `texture` attribute, but sets the Image's sampler binding instead of the texture.
Note that fields without field-level binding attributes will be ignored.
```rust
#[derive(AsBindGroup)]
struct CoolMaterial {
#[uniform(0)]
color: Color,
this_field_is_ignored: String,
}
```
As mentioned above, `Option<Handle<Image>>` is also supported:
```rust
#[derive(AsBindGroup)]
struct CoolMaterial {
#[uniform(0)]
color: Color,
#[texture(1)]
#[sampler(2)]
color_texture: Option<Handle<Image>>,
}
```
This is useful if you want a texture to be optional. When the value is `None`, the `FallbackImage` will be used for the binding instead, which defaults to "pure white".
Field uniforms with the same binding index will be combined into a single binding:
```rust
#[derive(AsBindGroup)]
struct CoolMaterial {
#[uniform(0)]
color: Color,
#[uniform(0)]
roughness: f32,
}
```
In WGSL shaders, the binding would look like this:
```wgsl
struct CoolMaterial {
color: vec4<f32>;
roughness: f32;
};
[[group(1), binding(0)]]
var<uniform> material: CoolMaterial;
```
Some less common scenarios will require "struct-level" attributes. These are the currently supported struct-level attributes:
* `uniform(BINDING_INDEX, ConvertedShaderType)`
* Similar to the field-level `uniform` attribute, but instead the entire `AsBindGroup` value is converted to `ConvertedShaderType`, which must implement `ShaderType`. This is useful if more complicated conversion logic is required.
* `bind_group_data(DataType)`
* The `AsBindGroup` type will be converted to some `DataType` using `Into<DataType>` and stored as `AsBindGroup::Data` as part of the `AsBindGroup::as_bind_group` call. This is useful if data needs to be stored alongside the generated bind group, such as a unique identifier for a material's bind group. The most common use case for this attribute is "shader pipeline specialization".
The previous `CoolMaterial` example illustrating "combining multiple field-level uniform attributes with the same binding index" can
also be equivalently represented with a single struct-level uniform attribute:
```rust
#[derive(AsBindGroup)]
#[uniform(0, CoolMaterialUniform)]
struct CoolMaterial {
color: Color,
roughness: f32,
}
#[derive(ShaderType)]
struct CoolMaterialUniform {
color: Color,
roughness: f32,
}
impl From<&CoolMaterial> for CoolMaterialUniform {
fn from(material: &CoolMaterial) -> CoolMaterialUniform {
CoolMaterialUniform {
color: material.color,
roughness: material.roughness,
}
}
}
```
### Material Specialization
Material shader specialization is now _much_ simpler:
```rust
#[derive(AsBindGroup, TypeUuid, Debug, Clone)]
#[uuid = "f690fdae-d598-45ab-8225-97e2a3f056e0"]
#[bind_group_data(CoolMaterialKey)]
struct CoolMaterial {
#[uniform(0)]
color: Color,
is_red: bool,
}
#[derive(Copy, Clone, Hash, Eq, PartialEq)]
struct CoolMaterialKey {
is_red: bool,
}
impl From<&CoolMaterial> for CoolMaterialKey {
fn from(material: &CoolMaterial) -> CoolMaterialKey {
CoolMaterialKey {
is_red: material.is_red,
}
}
}
impl Material for CoolMaterial {
fn fragment_shader() -> ShaderRef {
"cool_material.wgsl".into()
}
fn specialize(
pipeline: &MaterialPipeline<Self>,
descriptor: &mut RenderPipelineDescriptor,
layout: &MeshVertexBufferLayout,
key: MaterialPipelineKey<Self>,
) -> Result<(), SpecializedMeshPipelineError> {
if key.bind_group_data.is_red {
let fragment = descriptor.fragment.as_mut().unwrap();
fragment.shader_defs.push("IS_RED".to_string());
}
Ok(())
}
}
```
Setting `bind_group_data` is not required for specialization (it defaults to `()`). Scenarios like "custom vertex attributes" also benefit from this system:
```rust
impl Material for CustomMaterial {
fn vertex_shader() -> ShaderRef {
"custom_material.wgsl".into()
}
fn fragment_shader() -> ShaderRef {
"custom_material.wgsl".into()
}
fn specialize(
pipeline: &MaterialPipeline<Self>,
descriptor: &mut RenderPipelineDescriptor,
layout: &MeshVertexBufferLayout,
key: MaterialPipelineKey<Self>,
) -> Result<(), SpecializedMeshPipelineError> {
let vertex_layout = layout.get_layout(&[
Mesh::ATTRIBUTE_POSITION.at_shader_location(0),
ATTRIBUTE_BLEND_COLOR.at_shader_location(1),
])?;
descriptor.vertex.buffers = vec![vertex_layout];
Ok(())
}
}
```
### Ported `StandardMaterial` to the new `Material` system
Bevy's built-in PBR material uses the new Material system (including the AsBindGroup derive):
```rust
#[derive(AsBindGroup, Debug, Clone, TypeUuid)]
#[uuid = "7494888b-c082-457b-aacf-517228cc0c22"]
#[bind_group_data(StandardMaterialKey)]
#[uniform(0, StandardMaterialUniform)]
pub struct StandardMaterial {
pub base_color: Color,
#[texture(1)]
#[sampler(2)]
pub base_color_texture: Option<Handle<Image>>,
/* other fields omitted for brevity */
```
### Ported Bevy examples to the new `Material` system
The overall complexity of Bevy's "custom shader examples" has gone down significantly. Take a look at the diffs if you want a dopamine spike.
Please note that while this PR has a net increase in "lines of code", most of those extra lines come from added documentation. There is a significant reduction
in the overall complexity of the code (even accounting for the new derive logic).
---
## Changelog
### Added
* `AsBindGroup` trait and derive, which make it much easier to transfer data to the gpu and generate bind groups for a given type.
### Changed
* The old `Material` and `SpecializedMaterial` traits have been replaced by a consolidated (much simpler) `Material` trait. Materials no longer implement `RenderAsset`.
* `StandardMaterial` was ported to the new material system. There are no user-facing api changes to the `StandardMaterial` struct api, but it now implements `AsBindGroup` and `Material` instead of `RenderAsset` and `SpecializedMaterial`.
## Migration Guide
The Material system has been reworked to be much simpler. We've removed a lot of boilerplate with the new `AsBindGroup` derive and the `Material` trait is simpler as well!
### Bevy 0.7 (old)
```rust
#[derive(Debug, Clone, TypeUuid)]
#[uuid = "f690fdae-d598-45ab-8225-97e2a3f056e0"]
pub struct CustomMaterial {
color: Color,
color_texture: Handle<Image>,
}
#[derive(Clone)]
pub struct GpuCustomMaterial {
_buffer: Buffer,
bind_group: BindGroup,
}
impl RenderAsset for CustomMaterial {
type ExtractedAsset = CustomMaterial;
type PreparedAsset = GpuCustomMaterial;
type Param = (SRes<RenderDevice>, SRes<MaterialPipeline<Self>>);
fn extract_asset(&self) -> Self::ExtractedAsset {
self.clone()
}
fn prepare_asset(
extracted_asset: Self::ExtractedAsset,
(render_device, material_pipeline): &mut SystemParamItem<Self::Param>,
) -> Result<Self::PreparedAsset, PrepareAssetError<Self::ExtractedAsset>> {
let color = Vec4::from_slice(&extracted_asset.color.as_linear_rgba_f32());
let byte_buffer = [0u8; Vec4::SIZE.get() as usize];
let mut buffer = encase::UniformBuffer::new(byte_buffer);
buffer.write(&color).unwrap();
let buffer = render_device.create_buffer_with_data(&BufferInitDescriptor {
contents: buffer.as_ref(),
label: None,
usage: BufferUsages::UNIFORM | BufferUsages::COPY_DST,
});
let (texture_view, texture_sampler) = if let Some(result) = material_pipeline
.mesh_pipeline
.get_image_texture(gpu_images, &Some(extracted_asset.color_texture.clone()))
{
result
} else {
return Err(PrepareAssetError::RetryNextUpdate(extracted_asset));
};
let bind_group = render_device.create_bind_group(&BindGroupDescriptor {
entries: &[
BindGroupEntry {
binding: 0,
resource: buffer.as_entire_binding(),
},
BindGroupEntry {
binding: 0,
resource: BindingResource::TextureView(texture_view),
},
BindGroupEntry {
binding: 1,
resource: BindingResource::Sampler(texture_sampler),
},
],
label: None,
layout: &material_pipeline.material_layout,
});
Ok(GpuCustomMaterial {
_buffer: buffer,
bind_group,
})
}
}
impl Material for CustomMaterial {
fn fragment_shader(asset_server: &AssetServer) -> Option<Handle<Shader>> {
Some(asset_server.load("custom_material.wgsl"))
}
fn bind_group(render_asset: &<Self as RenderAsset>::PreparedAsset) -> &BindGroup {
&render_asset.bind_group
}
fn bind_group_layout(render_device: &RenderDevice) -> BindGroupLayout {
render_device.create_bind_group_layout(&BindGroupLayoutDescriptor {
entries: &[
BindGroupLayoutEntry {
binding: 0,
visibility: ShaderStages::FRAGMENT,
ty: BindingType::Buffer {
ty: BufferBindingType::Uniform,
has_dynamic_offset: false,
min_binding_size: Some(Vec4::min_size()),
},
count: None,
},
BindGroupLayoutEntry {
binding: 1,
visibility: ShaderStages::FRAGMENT,
ty: BindingType::Texture {
multisampled: false,
sample_type: TextureSampleType::Float { filterable: true },
view_dimension: TextureViewDimension::D2Array,
},
count: None,
},
BindGroupLayoutEntry {
binding: 2,
visibility: ShaderStages::FRAGMENT,
ty: BindingType::Sampler(SamplerBindingType::Filtering),
count: None,
},
],
label: None,
})
}
}
```
### Bevy 0.8 (new)
```rust
impl Material for CustomMaterial {
fn fragment_shader() -> ShaderRef {
"custom_material.wgsl".into()
}
}
#[derive(AsBindGroup, TypeUuid, Debug, Clone)]
#[uuid = "f690fdae-d598-45ab-8225-97e2a3f056e0"]
pub struct CustomMaterial {
#[uniform(0)]
color: Color,
#[texture(1)]
#[sampler(2)]
color_texture: Handle<Image>,
}
```
## Future Work
* Add support for more binding types (cubemaps, buffers, etc). This PR intentionally includes a bare minimum number of binding types to keep "reviewability" in check.
* Consider optionally eliding binding indices using binding names. `AsBindGroup` could pass in (optional?) reflection info as a "hint".
* This would make it possible for the derive to do this:
```rust
#[derive(AsBindGroup)]
pub struct CustomMaterial {
#[uniform]
color: Color,
#[texture]
#[sampler]
color_texture: Option<Handle<Image>>,
alpha_mode: AlphaMode,
}
```
* Or this
```rust
#[derive(AsBindGroup)]
pub struct CustomMaterial {
#[binding]
color: Color,
#[binding]
color_texture: Option<Handle<Image>>,
alpha_mode: AlphaMode,
}
```
* Or even this (if we flip to "include bindings by default")
```rust
#[derive(AsBindGroup)]
pub struct CustomMaterial {
color: Color,
color_texture: Option<Handle<Image>>,
#[binding(ignore)]
alpha_mode: AlphaMode,
}
```
* If we add the option to define custom draw functions for materials (which could be done in a type-erased way), I think that would be enough to support extra non-material bindings. Worth considering!
2022-06-30 23:48:46 +00:00
|
|
|
/// Adds the necessary ECS resources and render logic to enable rendering entities using the given [`Material`]
|
|
|
|
/// asset type.
|
2023-01-19 22:11:13 +00:00
|
|
|
pub struct MaterialPlugin<M: Material> {
|
|
|
|
/// Controls if the prepass is enabled for the Material.
|
|
|
|
/// For more information about what a prepass is, see the [`bevy_core_pipeline::prepass`] docs.
|
|
|
|
///
|
|
|
|
/// When it is enabled, it will automatically add the [`PrepassPlugin`]
|
|
|
|
/// required to make the prepass work on this Material.
|
|
|
|
pub prepass_enabled: bool,
|
2024-03-18 17:54:41 +00:00
|
|
|
/// Controls if shadows are enabled for the Material.
|
|
|
|
pub shadows_enabled: bool,
|
2023-01-19 22:11:13 +00:00
|
|
|
pub _marker: PhantomData<M>,
|
|
|
|
}
|
2021-12-14 03:58:23 +00:00
|
|
|
|
Better Materials: AsBindGroup trait and derive, simpler Material trait (#5053)
# Objective
This PR reworks Bevy's Material system, making the user experience of defining Materials _much_ nicer. Bevy's previous material system leaves a lot to be desired:
* Materials require manually implementing the `RenderAsset` trait, which involves manually generating the bind group, handling gpu buffer data transfer, looking up image textures, etc. Even the simplest single-texture material involves writing ~80 unnecessary lines of code. This was never the long term plan.
* There are two material traits, which is confusing, hard to document, and often redundant: `Material` and `SpecializedMaterial`. `Material` implicitly implements `SpecializedMaterial`, and `SpecializedMaterial` is used in most high level apis to support both use cases. Most users shouldn't need to think about specialization at all (I consider it a "power-user tool"), so the fact that `SpecializedMaterial` is front-and-center in our apis is a miss.
* Implementing either material trait involves a lot of "type soup". The "prepared asset" parameter is particularly heinous: `&<Self as RenderAsset>::PreparedAsset`. Defining vertex and fragment shaders is also more verbose than it needs to be.
## Solution
Say hello to the new `Material` system:
```rust
#[derive(AsBindGroup, TypeUuid, Debug, Clone)]
#[uuid = "f690fdae-d598-45ab-8225-97e2a3f056e0"]
pub struct CoolMaterial {
#[uniform(0)]
color: Color,
#[texture(1)]
#[sampler(2)]
color_texture: Handle<Image>,
}
impl Material for CoolMaterial {
fn fragment_shader() -> ShaderRef {
"cool_material.wgsl".into()
}
}
```
Thats it! This same material would have required [~80 lines of complicated "type heavy" code](https://github.com/bevyengine/bevy/blob/v0.7.0/examples/shader/shader_material.rs) in the old Material system. Now it is just 14 lines of simple, readable code.
This is thanks to a new consolidated `Material` trait and the new `AsBindGroup` trait / derive.
### The new `Material` trait
The old "split" `Material` and `SpecializedMaterial` traits have been removed in favor of a new consolidated `Material` trait. All of the functions on the trait are optional.
The difficulty of implementing `Material` has been reduced by simplifying dataflow and removing type complexity:
```rust
// Old
impl Material for CustomMaterial {
fn fragment_shader(asset_server: &AssetServer) -> Option<Handle<Shader>> {
Some(asset_server.load("custom_material.wgsl"))
}
fn alpha_mode(render_asset: &<Self as RenderAsset>::PreparedAsset) -> AlphaMode {
render_asset.alpha_mode
}
}
// New
impl Material for CustomMaterial {
fn fragment_shader() -> ShaderRef {
"custom_material.wgsl".into()
}
fn alpha_mode(&self) -> AlphaMode {
self.alpha_mode
}
}
```
Specialization is still supported, but it is hidden by default under the `specialize()` function (more on this later).
### The `AsBindGroup` trait / derive
The `Material` trait now requires the `AsBindGroup` derive. This can be implemented manually relatively easily, but deriving it will almost always be preferable.
Field attributes like `uniform` and `texture` are used to define which fields should be bindings,
what their binding type is, and what index they should be bound at:
```rust
#[derive(AsBindGroup)]
struct CoolMaterial {
#[uniform(0)]
color: Color,
#[texture(1)]
#[sampler(2)]
color_texture: Handle<Image>,
}
```
In WGSL shaders, the binding looks like this:
```wgsl
struct CoolMaterial {
color: vec4<f32>;
};
[[group(1), binding(0)]]
var<uniform> material: CoolMaterial;
[[group(1), binding(1)]]
var color_texture: texture_2d<f32>;
[[group(1), binding(2)]]
var color_sampler: sampler;
```
Note that the "group" index is determined by the usage context. It is not defined in `AsBindGroup`. Bevy material bind groups are bound to group 1.
The following field-level attributes are supported:
* `uniform(BINDING_INDEX)`
* The field will be converted to a shader-compatible type using the `ShaderType` trait, written to a `Buffer`, and bound as a uniform. It can also be derived for custom structs.
* `texture(BINDING_INDEX)`
* This field's `Handle<Image>` will be used to look up the matching `Texture` gpu resource, which will be bound as a texture in shaders. The field will be assumed to implement `Into<Option<Handle<Image>>>`. In practice, most fields should be a `Handle<Image>` or `Option<Handle<Image>>`. If the value of an `Option<Handle<Image>>` is `None`, the new `FallbackImage` resource will be used instead. This attribute can be used in conjunction with a `sampler` binding attribute (with a different binding index).
* `sampler(BINDING_INDEX)`
* Behaves exactly like the `texture` attribute, but sets the Image's sampler binding instead of the texture.
Note that fields without field-level binding attributes will be ignored.
```rust
#[derive(AsBindGroup)]
struct CoolMaterial {
#[uniform(0)]
color: Color,
this_field_is_ignored: String,
}
```
As mentioned above, `Option<Handle<Image>>` is also supported:
```rust
#[derive(AsBindGroup)]
struct CoolMaterial {
#[uniform(0)]
color: Color,
#[texture(1)]
#[sampler(2)]
color_texture: Option<Handle<Image>>,
}
```
This is useful if you want a texture to be optional. When the value is `None`, the `FallbackImage` will be used for the binding instead, which defaults to "pure white".
Field uniforms with the same binding index will be combined into a single binding:
```rust
#[derive(AsBindGroup)]
struct CoolMaterial {
#[uniform(0)]
color: Color,
#[uniform(0)]
roughness: f32,
}
```
In WGSL shaders, the binding would look like this:
```wgsl
struct CoolMaterial {
color: vec4<f32>;
roughness: f32;
};
[[group(1), binding(0)]]
var<uniform> material: CoolMaterial;
```
Some less common scenarios will require "struct-level" attributes. These are the currently supported struct-level attributes:
* `uniform(BINDING_INDEX, ConvertedShaderType)`
* Similar to the field-level `uniform` attribute, but instead the entire `AsBindGroup` value is converted to `ConvertedShaderType`, which must implement `ShaderType`. This is useful if more complicated conversion logic is required.
* `bind_group_data(DataType)`
* The `AsBindGroup` type will be converted to some `DataType` using `Into<DataType>` and stored as `AsBindGroup::Data` as part of the `AsBindGroup::as_bind_group` call. This is useful if data needs to be stored alongside the generated bind group, such as a unique identifier for a material's bind group. The most common use case for this attribute is "shader pipeline specialization".
The previous `CoolMaterial` example illustrating "combining multiple field-level uniform attributes with the same binding index" can
also be equivalently represented with a single struct-level uniform attribute:
```rust
#[derive(AsBindGroup)]
#[uniform(0, CoolMaterialUniform)]
struct CoolMaterial {
color: Color,
roughness: f32,
}
#[derive(ShaderType)]
struct CoolMaterialUniform {
color: Color,
roughness: f32,
}
impl From<&CoolMaterial> for CoolMaterialUniform {
fn from(material: &CoolMaterial) -> CoolMaterialUniform {
CoolMaterialUniform {
color: material.color,
roughness: material.roughness,
}
}
}
```
### Material Specialization
Material shader specialization is now _much_ simpler:
```rust
#[derive(AsBindGroup, TypeUuid, Debug, Clone)]
#[uuid = "f690fdae-d598-45ab-8225-97e2a3f056e0"]
#[bind_group_data(CoolMaterialKey)]
struct CoolMaterial {
#[uniform(0)]
color: Color,
is_red: bool,
}
#[derive(Copy, Clone, Hash, Eq, PartialEq)]
struct CoolMaterialKey {
is_red: bool,
}
impl From<&CoolMaterial> for CoolMaterialKey {
fn from(material: &CoolMaterial) -> CoolMaterialKey {
CoolMaterialKey {
is_red: material.is_red,
}
}
}
impl Material for CoolMaterial {
fn fragment_shader() -> ShaderRef {
"cool_material.wgsl".into()
}
fn specialize(
pipeline: &MaterialPipeline<Self>,
descriptor: &mut RenderPipelineDescriptor,
layout: &MeshVertexBufferLayout,
key: MaterialPipelineKey<Self>,
) -> Result<(), SpecializedMeshPipelineError> {
if key.bind_group_data.is_red {
let fragment = descriptor.fragment.as_mut().unwrap();
fragment.shader_defs.push("IS_RED".to_string());
}
Ok(())
}
}
```
Setting `bind_group_data` is not required for specialization (it defaults to `()`). Scenarios like "custom vertex attributes" also benefit from this system:
```rust
impl Material for CustomMaterial {
fn vertex_shader() -> ShaderRef {
"custom_material.wgsl".into()
}
fn fragment_shader() -> ShaderRef {
"custom_material.wgsl".into()
}
fn specialize(
pipeline: &MaterialPipeline<Self>,
descriptor: &mut RenderPipelineDescriptor,
layout: &MeshVertexBufferLayout,
key: MaterialPipelineKey<Self>,
) -> Result<(), SpecializedMeshPipelineError> {
let vertex_layout = layout.get_layout(&[
Mesh::ATTRIBUTE_POSITION.at_shader_location(0),
ATTRIBUTE_BLEND_COLOR.at_shader_location(1),
])?;
descriptor.vertex.buffers = vec![vertex_layout];
Ok(())
}
}
```
### Ported `StandardMaterial` to the new `Material` system
Bevy's built-in PBR material uses the new Material system (including the AsBindGroup derive):
```rust
#[derive(AsBindGroup, Debug, Clone, TypeUuid)]
#[uuid = "7494888b-c082-457b-aacf-517228cc0c22"]
#[bind_group_data(StandardMaterialKey)]
#[uniform(0, StandardMaterialUniform)]
pub struct StandardMaterial {
pub base_color: Color,
#[texture(1)]
#[sampler(2)]
pub base_color_texture: Option<Handle<Image>>,
/* other fields omitted for brevity */
```
### Ported Bevy examples to the new `Material` system
The overall complexity of Bevy's "custom shader examples" has gone down significantly. Take a look at the diffs if you want a dopamine spike.
Please note that while this PR has a net increase in "lines of code", most of those extra lines come from added documentation. There is a significant reduction
in the overall complexity of the code (even accounting for the new derive logic).
---
## Changelog
### Added
* `AsBindGroup` trait and derive, which make it much easier to transfer data to the gpu and generate bind groups for a given type.
### Changed
* The old `Material` and `SpecializedMaterial` traits have been replaced by a consolidated (much simpler) `Material` trait. Materials no longer implement `RenderAsset`.
* `StandardMaterial` was ported to the new material system. There are no user-facing api changes to the `StandardMaterial` struct api, but it now implements `AsBindGroup` and `Material` instead of `RenderAsset` and `SpecializedMaterial`.
## Migration Guide
The Material system has been reworked to be much simpler. We've removed a lot of boilerplate with the new `AsBindGroup` derive and the `Material` trait is simpler as well!
### Bevy 0.7 (old)
```rust
#[derive(Debug, Clone, TypeUuid)]
#[uuid = "f690fdae-d598-45ab-8225-97e2a3f056e0"]
pub struct CustomMaterial {
color: Color,
color_texture: Handle<Image>,
}
#[derive(Clone)]
pub struct GpuCustomMaterial {
_buffer: Buffer,
bind_group: BindGroup,
}
impl RenderAsset for CustomMaterial {
type ExtractedAsset = CustomMaterial;
type PreparedAsset = GpuCustomMaterial;
type Param = (SRes<RenderDevice>, SRes<MaterialPipeline<Self>>);
fn extract_asset(&self) -> Self::ExtractedAsset {
self.clone()
}
fn prepare_asset(
extracted_asset: Self::ExtractedAsset,
(render_device, material_pipeline): &mut SystemParamItem<Self::Param>,
) -> Result<Self::PreparedAsset, PrepareAssetError<Self::ExtractedAsset>> {
let color = Vec4::from_slice(&extracted_asset.color.as_linear_rgba_f32());
let byte_buffer = [0u8; Vec4::SIZE.get() as usize];
let mut buffer = encase::UniformBuffer::new(byte_buffer);
buffer.write(&color).unwrap();
let buffer = render_device.create_buffer_with_data(&BufferInitDescriptor {
contents: buffer.as_ref(),
label: None,
usage: BufferUsages::UNIFORM | BufferUsages::COPY_DST,
});
let (texture_view, texture_sampler) = if let Some(result) = material_pipeline
.mesh_pipeline
.get_image_texture(gpu_images, &Some(extracted_asset.color_texture.clone()))
{
result
} else {
return Err(PrepareAssetError::RetryNextUpdate(extracted_asset));
};
let bind_group = render_device.create_bind_group(&BindGroupDescriptor {
entries: &[
BindGroupEntry {
binding: 0,
resource: buffer.as_entire_binding(),
},
BindGroupEntry {
binding: 0,
resource: BindingResource::TextureView(texture_view),
},
BindGroupEntry {
binding: 1,
resource: BindingResource::Sampler(texture_sampler),
},
],
label: None,
layout: &material_pipeline.material_layout,
});
Ok(GpuCustomMaterial {
_buffer: buffer,
bind_group,
})
}
}
impl Material for CustomMaterial {
fn fragment_shader(asset_server: &AssetServer) -> Option<Handle<Shader>> {
Some(asset_server.load("custom_material.wgsl"))
}
fn bind_group(render_asset: &<Self as RenderAsset>::PreparedAsset) -> &BindGroup {
&render_asset.bind_group
}
fn bind_group_layout(render_device: &RenderDevice) -> BindGroupLayout {
render_device.create_bind_group_layout(&BindGroupLayoutDescriptor {
entries: &[
BindGroupLayoutEntry {
binding: 0,
visibility: ShaderStages::FRAGMENT,
ty: BindingType::Buffer {
ty: BufferBindingType::Uniform,
has_dynamic_offset: false,
min_binding_size: Some(Vec4::min_size()),
},
count: None,
},
BindGroupLayoutEntry {
binding: 1,
visibility: ShaderStages::FRAGMENT,
ty: BindingType::Texture {
multisampled: false,
sample_type: TextureSampleType::Float { filterable: true },
view_dimension: TextureViewDimension::D2Array,
},
count: None,
},
BindGroupLayoutEntry {
binding: 2,
visibility: ShaderStages::FRAGMENT,
ty: BindingType::Sampler(SamplerBindingType::Filtering),
count: None,
},
],
label: None,
})
}
}
```
### Bevy 0.8 (new)
```rust
impl Material for CustomMaterial {
fn fragment_shader() -> ShaderRef {
"custom_material.wgsl".into()
}
}
#[derive(AsBindGroup, TypeUuid, Debug, Clone)]
#[uuid = "f690fdae-d598-45ab-8225-97e2a3f056e0"]
pub struct CustomMaterial {
#[uniform(0)]
color: Color,
#[texture(1)]
#[sampler(2)]
color_texture: Handle<Image>,
}
```
## Future Work
* Add support for more binding types (cubemaps, buffers, etc). This PR intentionally includes a bare minimum number of binding types to keep "reviewability" in check.
* Consider optionally eliding binding indices using binding names. `AsBindGroup` could pass in (optional?) reflection info as a "hint".
* This would make it possible for the derive to do this:
```rust
#[derive(AsBindGroup)]
pub struct CustomMaterial {
#[uniform]
color: Color,
#[texture]
#[sampler]
color_texture: Option<Handle<Image>>,
alpha_mode: AlphaMode,
}
```
* Or this
```rust
#[derive(AsBindGroup)]
pub struct CustomMaterial {
#[binding]
color: Color,
#[binding]
color_texture: Option<Handle<Image>>,
alpha_mode: AlphaMode,
}
```
* Or even this (if we flip to "include bindings by default")
```rust
#[derive(AsBindGroup)]
pub struct CustomMaterial {
color: Color,
color_texture: Option<Handle<Image>>,
#[binding(ignore)]
alpha_mode: AlphaMode,
}
```
* If we add the option to define custom draw functions for materials (which could be done in a type-erased way), I think that would be enough to support extra non-material bindings. Worth considering!
2022-06-30 23:48:46 +00:00
|
|
|
impl<M: Material> Default for MaterialPlugin<M> {
|
2021-12-25 21:45:43 +00:00
|
|
|
fn default() -> Self {
|
2023-01-19 22:11:13 +00:00
|
|
|
Self {
|
|
|
|
prepass_enabled: true,
|
2024-03-18 17:54:41 +00:00
|
|
|
shadows_enabled: true,
|
2023-01-19 22:11:13 +00:00
|
|
|
_marker: Default::default(),
|
|
|
|
}
|
2021-12-25 21:45:43 +00:00
|
|
|
}
|
|
|
|
}
|
|
|
|
|
Better Materials: AsBindGroup trait and derive, simpler Material trait (#5053)
# Objective
This PR reworks Bevy's Material system, making the user experience of defining Materials _much_ nicer. Bevy's previous material system leaves a lot to be desired:
* Materials require manually implementing the `RenderAsset` trait, which involves manually generating the bind group, handling gpu buffer data transfer, looking up image textures, etc. Even the simplest single-texture material involves writing ~80 unnecessary lines of code. This was never the long term plan.
* There are two material traits, which is confusing, hard to document, and often redundant: `Material` and `SpecializedMaterial`. `Material` implicitly implements `SpecializedMaterial`, and `SpecializedMaterial` is used in most high level apis to support both use cases. Most users shouldn't need to think about specialization at all (I consider it a "power-user tool"), so the fact that `SpecializedMaterial` is front-and-center in our apis is a miss.
* Implementing either material trait involves a lot of "type soup". The "prepared asset" parameter is particularly heinous: `&<Self as RenderAsset>::PreparedAsset`. Defining vertex and fragment shaders is also more verbose than it needs to be.
## Solution
Say hello to the new `Material` system:
```rust
#[derive(AsBindGroup, TypeUuid, Debug, Clone)]
#[uuid = "f690fdae-d598-45ab-8225-97e2a3f056e0"]
pub struct CoolMaterial {
#[uniform(0)]
color: Color,
#[texture(1)]
#[sampler(2)]
color_texture: Handle<Image>,
}
impl Material for CoolMaterial {
fn fragment_shader() -> ShaderRef {
"cool_material.wgsl".into()
}
}
```
Thats it! This same material would have required [~80 lines of complicated "type heavy" code](https://github.com/bevyengine/bevy/blob/v0.7.0/examples/shader/shader_material.rs) in the old Material system. Now it is just 14 lines of simple, readable code.
This is thanks to a new consolidated `Material` trait and the new `AsBindGroup` trait / derive.
### The new `Material` trait
The old "split" `Material` and `SpecializedMaterial` traits have been removed in favor of a new consolidated `Material` trait. All of the functions on the trait are optional.
The difficulty of implementing `Material` has been reduced by simplifying dataflow and removing type complexity:
```rust
// Old
impl Material for CustomMaterial {
fn fragment_shader(asset_server: &AssetServer) -> Option<Handle<Shader>> {
Some(asset_server.load("custom_material.wgsl"))
}
fn alpha_mode(render_asset: &<Self as RenderAsset>::PreparedAsset) -> AlphaMode {
render_asset.alpha_mode
}
}
// New
impl Material for CustomMaterial {
fn fragment_shader() -> ShaderRef {
"custom_material.wgsl".into()
}
fn alpha_mode(&self) -> AlphaMode {
self.alpha_mode
}
}
```
Specialization is still supported, but it is hidden by default under the `specialize()` function (more on this later).
### The `AsBindGroup` trait / derive
The `Material` trait now requires the `AsBindGroup` derive. This can be implemented manually relatively easily, but deriving it will almost always be preferable.
Field attributes like `uniform` and `texture` are used to define which fields should be bindings,
what their binding type is, and what index they should be bound at:
```rust
#[derive(AsBindGroup)]
struct CoolMaterial {
#[uniform(0)]
color: Color,
#[texture(1)]
#[sampler(2)]
color_texture: Handle<Image>,
}
```
In WGSL shaders, the binding looks like this:
```wgsl
struct CoolMaterial {
color: vec4<f32>;
};
[[group(1), binding(0)]]
var<uniform> material: CoolMaterial;
[[group(1), binding(1)]]
var color_texture: texture_2d<f32>;
[[group(1), binding(2)]]
var color_sampler: sampler;
```
Note that the "group" index is determined by the usage context. It is not defined in `AsBindGroup`. Bevy material bind groups are bound to group 1.
The following field-level attributes are supported:
* `uniform(BINDING_INDEX)`
* The field will be converted to a shader-compatible type using the `ShaderType` trait, written to a `Buffer`, and bound as a uniform. It can also be derived for custom structs.
* `texture(BINDING_INDEX)`
* This field's `Handle<Image>` will be used to look up the matching `Texture` gpu resource, which will be bound as a texture in shaders. The field will be assumed to implement `Into<Option<Handle<Image>>>`. In practice, most fields should be a `Handle<Image>` or `Option<Handle<Image>>`. If the value of an `Option<Handle<Image>>` is `None`, the new `FallbackImage` resource will be used instead. This attribute can be used in conjunction with a `sampler` binding attribute (with a different binding index).
* `sampler(BINDING_INDEX)`
* Behaves exactly like the `texture` attribute, but sets the Image's sampler binding instead of the texture.
Note that fields without field-level binding attributes will be ignored.
```rust
#[derive(AsBindGroup)]
struct CoolMaterial {
#[uniform(0)]
color: Color,
this_field_is_ignored: String,
}
```
As mentioned above, `Option<Handle<Image>>` is also supported:
```rust
#[derive(AsBindGroup)]
struct CoolMaterial {
#[uniform(0)]
color: Color,
#[texture(1)]
#[sampler(2)]
color_texture: Option<Handle<Image>>,
}
```
This is useful if you want a texture to be optional. When the value is `None`, the `FallbackImage` will be used for the binding instead, which defaults to "pure white".
Field uniforms with the same binding index will be combined into a single binding:
```rust
#[derive(AsBindGroup)]
struct CoolMaterial {
#[uniform(0)]
color: Color,
#[uniform(0)]
roughness: f32,
}
```
In WGSL shaders, the binding would look like this:
```wgsl
struct CoolMaterial {
color: vec4<f32>;
roughness: f32;
};
[[group(1), binding(0)]]
var<uniform> material: CoolMaterial;
```
Some less common scenarios will require "struct-level" attributes. These are the currently supported struct-level attributes:
* `uniform(BINDING_INDEX, ConvertedShaderType)`
* Similar to the field-level `uniform` attribute, but instead the entire `AsBindGroup` value is converted to `ConvertedShaderType`, which must implement `ShaderType`. This is useful if more complicated conversion logic is required.
* `bind_group_data(DataType)`
* The `AsBindGroup` type will be converted to some `DataType` using `Into<DataType>` and stored as `AsBindGroup::Data` as part of the `AsBindGroup::as_bind_group` call. This is useful if data needs to be stored alongside the generated bind group, such as a unique identifier for a material's bind group. The most common use case for this attribute is "shader pipeline specialization".
The previous `CoolMaterial` example illustrating "combining multiple field-level uniform attributes with the same binding index" can
also be equivalently represented with a single struct-level uniform attribute:
```rust
#[derive(AsBindGroup)]
#[uniform(0, CoolMaterialUniform)]
struct CoolMaterial {
color: Color,
roughness: f32,
}
#[derive(ShaderType)]
struct CoolMaterialUniform {
color: Color,
roughness: f32,
}
impl From<&CoolMaterial> for CoolMaterialUniform {
fn from(material: &CoolMaterial) -> CoolMaterialUniform {
CoolMaterialUniform {
color: material.color,
roughness: material.roughness,
}
}
}
```
### Material Specialization
Material shader specialization is now _much_ simpler:
```rust
#[derive(AsBindGroup, TypeUuid, Debug, Clone)]
#[uuid = "f690fdae-d598-45ab-8225-97e2a3f056e0"]
#[bind_group_data(CoolMaterialKey)]
struct CoolMaterial {
#[uniform(0)]
color: Color,
is_red: bool,
}
#[derive(Copy, Clone, Hash, Eq, PartialEq)]
struct CoolMaterialKey {
is_red: bool,
}
impl From<&CoolMaterial> for CoolMaterialKey {
fn from(material: &CoolMaterial) -> CoolMaterialKey {
CoolMaterialKey {
is_red: material.is_red,
}
}
}
impl Material for CoolMaterial {
fn fragment_shader() -> ShaderRef {
"cool_material.wgsl".into()
}
fn specialize(
pipeline: &MaterialPipeline<Self>,
descriptor: &mut RenderPipelineDescriptor,
layout: &MeshVertexBufferLayout,
key: MaterialPipelineKey<Self>,
) -> Result<(), SpecializedMeshPipelineError> {
if key.bind_group_data.is_red {
let fragment = descriptor.fragment.as_mut().unwrap();
fragment.shader_defs.push("IS_RED".to_string());
}
Ok(())
}
}
```
Setting `bind_group_data` is not required for specialization (it defaults to `()`). Scenarios like "custom vertex attributes" also benefit from this system:
```rust
impl Material for CustomMaterial {
fn vertex_shader() -> ShaderRef {
"custom_material.wgsl".into()
}
fn fragment_shader() -> ShaderRef {
"custom_material.wgsl".into()
}
fn specialize(
pipeline: &MaterialPipeline<Self>,
descriptor: &mut RenderPipelineDescriptor,
layout: &MeshVertexBufferLayout,
key: MaterialPipelineKey<Self>,
) -> Result<(), SpecializedMeshPipelineError> {
let vertex_layout = layout.get_layout(&[
Mesh::ATTRIBUTE_POSITION.at_shader_location(0),
ATTRIBUTE_BLEND_COLOR.at_shader_location(1),
])?;
descriptor.vertex.buffers = vec![vertex_layout];
Ok(())
}
}
```
### Ported `StandardMaterial` to the new `Material` system
Bevy's built-in PBR material uses the new Material system (including the AsBindGroup derive):
```rust
#[derive(AsBindGroup, Debug, Clone, TypeUuid)]
#[uuid = "7494888b-c082-457b-aacf-517228cc0c22"]
#[bind_group_data(StandardMaterialKey)]
#[uniform(0, StandardMaterialUniform)]
pub struct StandardMaterial {
pub base_color: Color,
#[texture(1)]
#[sampler(2)]
pub base_color_texture: Option<Handle<Image>>,
/* other fields omitted for brevity */
```
### Ported Bevy examples to the new `Material` system
The overall complexity of Bevy's "custom shader examples" has gone down significantly. Take a look at the diffs if you want a dopamine spike.
Please note that while this PR has a net increase in "lines of code", most of those extra lines come from added documentation. There is a significant reduction
in the overall complexity of the code (even accounting for the new derive logic).
---
## Changelog
### Added
* `AsBindGroup` trait and derive, which make it much easier to transfer data to the gpu and generate bind groups for a given type.
### Changed
* The old `Material` and `SpecializedMaterial` traits have been replaced by a consolidated (much simpler) `Material` trait. Materials no longer implement `RenderAsset`.
* `StandardMaterial` was ported to the new material system. There are no user-facing api changes to the `StandardMaterial` struct api, but it now implements `AsBindGroup` and `Material` instead of `RenderAsset` and `SpecializedMaterial`.
## Migration Guide
The Material system has been reworked to be much simpler. We've removed a lot of boilerplate with the new `AsBindGroup` derive and the `Material` trait is simpler as well!
### Bevy 0.7 (old)
```rust
#[derive(Debug, Clone, TypeUuid)]
#[uuid = "f690fdae-d598-45ab-8225-97e2a3f056e0"]
pub struct CustomMaterial {
color: Color,
color_texture: Handle<Image>,
}
#[derive(Clone)]
pub struct GpuCustomMaterial {
_buffer: Buffer,
bind_group: BindGroup,
}
impl RenderAsset for CustomMaterial {
type ExtractedAsset = CustomMaterial;
type PreparedAsset = GpuCustomMaterial;
type Param = (SRes<RenderDevice>, SRes<MaterialPipeline<Self>>);
fn extract_asset(&self) -> Self::ExtractedAsset {
self.clone()
}
fn prepare_asset(
extracted_asset: Self::ExtractedAsset,
(render_device, material_pipeline): &mut SystemParamItem<Self::Param>,
) -> Result<Self::PreparedAsset, PrepareAssetError<Self::ExtractedAsset>> {
let color = Vec4::from_slice(&extracted_asset.color.as_linear_rgba_f32());
let byte_buffer = [0u8; Vec4::SIZE.get() as usize];
let mut buffer = encase::UniformBuffer::new(byte_buffer);
buffer.write(&color).unwrap();
let buffer = render_device.create_buffer_with_data(&BufferInitDescriptor {
contents: buffer.as_ref(),
label: None,
usage: BufferUsages::UNIFORM | BufferUsages::COPY_DST,
});
let (texture_view, texture_sampler) = if let Some(result) = material_pipeline
.mesh_pipeline
.get_image_texture(gpu_images, &Some(extracted_asset.color_texture.clone()))
{
result
} else {
return Err(PrepareAssetError::RetryNextUpdate(extracted_asset));
};
let bind_group = render_device.create_bind_group(&BindGroupDescriptor {
entries: &[
BindGroupEntry {
binding: 0,
resource: buffer.as_entire_binding(),
},
BindGroupEntry {
binding: 0,
resource: BindingResource::TextureView(texture_view),
},
BindGroupEntry {
binding: 1,
resource: BindingResource::Sampler(texture_sampler),
},
],
label: None,
layout: &material_pipeline.material_layout,
});
Ok(GpuCustomMaterial {
_buffer: buffer,
bind_group,
})
}
}
impl Material for CustomMaterial {
fn fragment_shader(asset_server: &AssetServer) -> Option<Handle<Shader>> {
Some(asset_server.load("custom_material.wgsl"))
}
fn bind_group(render_asset: &<Self as RenderAsset>::PreparedAsset) -> &BindGroup {
&render_asset.bind_group
}
fn bind_group_layout(render_device: &RenderDevice) -> BindGroupLayout {
render_device.create_bind_group_layout(&BindGroupLayoutDescriptor {
entries: &[
BindGroupLayoutEntry {
binding: 0,
visibility: ShaderStages::FRAGMENT,
ty: BindingType::Buffer {
ty: BufferBindingType::Uniform,
has_dynamic_offset: false,
min_binding_size: Some(Vec4::min_size()),
},
count: None,
},
BindGroupLayoutEntry {
binding: 1,
visibility: ShaderStages::FRAGMENT,
ty: BindingType::Texture {
multisampled: false,
sample_type: TextureSampleType::Float { filterable: true },
view_dimension: TextureViewDimension::D2Array,
},
count: None,
},
BindGroupLayoutEntry {
binding: 2,
visibility: ShaderStages::FRAGMENT,
ty: BindingType::Sampler(SamplerBindingType::Filtering),
count: None,
},
],
label: None,
})
}
}
```
### Bevy 0.8 (new)
```rust
impl Material for CustomMaterial {
fn fragment_shader() -> ShaderRef {
"custom_material.wgsl".into()
}
}
#[derive(AsBindGroup, TypeUuid, Debug, Clone)]
#[uuid = "f690fdae-d598-45ab-8225-97e2a3f056e0"]
pub struct CustomMaterial {
#[uniform(0)]
color: Color,
#[texture(1)]
#[sampler(2)]
color_texture: Handle<Image>,
}
```
## Future Work
* Add support for more binding types (cubemaps, buffers, etc). This PR intentionally includes a bare minimum number of binding types to keep "reviewability" in check.
* Consider optionally eliding binding indices using binding names. `AsBindGroup` could pass in (optional?) reflection info as a "hint".
* This would make it possible for the derive to do this:
```rust
#[derive(AsBindGroup)]
pub struct CustomMaterial {
#[uniform]
color: Color,
#[texture]
#[sampler]
color_texture: Option<Handle<Image>>,
alpha_mode: AlphaMode,
}
```
* Or this
```rust
#[derive(AsBindGroup)]
pub struct CustomMaterial {
#[binding]
color: Color,
#[binding]
color_texture: Option<Handle<Image>>,
alpha_mode: AlphaMode,
}
```
* Or even this (if we flip to "include bindings by default")
```rust
#[derive(AsBindGroup)]
pub struct CustomMaterial {
color: Color,
color_texture: Option<Handle<Image>>,
#[binding(ignore)]
alpha_mode: AlphaMode,
}
```
* If we add the option to define custom draw functions for materials (which could be done in a type-erased way), I think that would be enough to support extra non-material bindings. Worth considering!
2022-06-30 23:48:46 +00:00
|
|
|
impl<M: Material> Plugin for MaterialPlugin<M>
|
|
|
|
where
|
|
|
|
M::Data: PartialEq + Eq + Hash + Clone,
|
|
|
|
{
|
2021-12-14 03:58:23 +00:00
|
|
|
fn build(&self, app: &mut App) {
|
Migrate meshes and materials to required components (#15524)
# Objective
A big step in the migration to required components: meshes and
materials!
## Solution
As per the [selected
proposal](https://hackmd.io/@bevy/required_components/%2Fj9-PnF-2QKK0on1KQ29UWQ):
- Deprecate `MaterialMesh2dBundle`, `MaterialMeshBundle`, and
`PbrBundle`.
- Add `Mesh2d` and `Mesh3d` components, which wrap a `Handle<Mesh>`.
- Add `MeshMaterial2d<M: Material2d>` and `MeshMaterial3d<M: Material>`,
which wrap a `Handle<M>`.
- Meshes *without* a mesh material should be rendered with a default
material. The existence of a material is determined by
`HasMaterial2d`/`HasMaterial3d`, which is required by
`MeshMaterial2d`/`MeshMaterial3d`. This gets around problems with the
generics.
Previously:
```rust
commands.spawn(MaterialMesh2dBundle {
mesh: meshes.add(Circle::new(100.0)).into(),
material: materials.add(Color::srgb(7.5, 0.0, 7.5)),
transform: Transform::from_translation(Vec3::new(-200., 0., 0.)),
..default()
});
```
Now:
```rust
commands.spawn((
Mesh2d(meshes.add(Circle::new(100.0))),
MeshMaterial2d(materials.add(Color::srgb(7.5, 0.0, 7.5))),
Transform::from_translation(Vec3::new(-200., 0., 0.)),
));
```
If the mesh material is missing, previously nothing was rendered. Now,
it renders a white default `ColorMaterial` in 2D and a
`StandardMaterial` in 3D (this can be overridden). Below, only every
other entity has a material:


Why white? This is still open for discussion, but I think white makes
sense for a *default* material, while *invalid* asset handles pointing
to nothing should have something like a pink material to indicate that
something is broken (I don't handle that in this PR yet). This is kind
of a mix of Godot and Unity: Godot just renders a white material for
non-existent materials, while Unity renders nothing when no materials
exist, but renders pink for invalid materials. I can also change the
default material to pink if that is preferable though.
## Testing
I ran some 2D and 3D examples to test if anything changed visually. I
have not tested all examples or features yet however. If anyone wants to
test more extensively, it would be appreciated!
## Implementation Notes
- The relationship between `bevy_render` and `bevy_pbr` is weird here.
`bevy_render` needs `Mesh3d` for its own systems, but `bevy_pbr` has all
of the material logic, and `bevy_render` doesn't depend on it. I feel
like the two crates should be refactored in some way, but I think that's
out of scope for this PR.
- I didn't migrate meshlets to required components yet. That can
probably be done in a follow-up, as this is already a huge PR.
- It is becoming increasingly clear to me that we really, *really* want
to disallow raw asset handles as components. They caused me a *ton* of
headache here already, and it took me a long time to find every place
that queried for them or inserted them directly on entities, since there
were no compiler errors for it. If we don't remove the `Component`
derive, I expect raw asset handles to be a *huge* footgun for users as
we transition to wrapper components, especially as handles as components
have been the norm so far. I personally consider this to be a blocker
for 0.15: we need to migrate to wrapper components for asset handles
everywhere, and remove the `Component` derive. Also see
https://github.com/bevyengine/bevy/issues/14124.
---
## Migration Guide
Asset handles for meshes and mesh materials must now be wrapped in the
`Mesh2d` and `MeshMaterial2d` or `Mesh3d` and `MeshMaterial3d`
components for 2D and 3D respectively. Raw handles as components no
longer render meshes.
Additionally, `MaterialMesh2dBundle`, `MaterialMeshBundle`, and
`PbrBundle` have been deprecated. Instead, use the mesh and material
components directly.
Previously:
```rust
commands.spawn(MaterialMesh2dBundle {
mesh: meshes.add(Circle::new(100.0)).into(),
material: materials.add(Color::srgb(7.5, 0.0, 7.5)),
transform: Transform::from_translation(Vec3::new(-200., 0., 0.)),
..default()
});
```
Now:
```rust
commands.spawn((
Mesh2d(meshes.add(Circle::new(100.0))),
MeshMaterial2d(materials.add(Color::srgb(7.5, 0.0, 7.5))),
Transform::from_translation(Vec3::new(-200., 0., 0.)),
));
```
If the mesh material is missing, a white default material is now used.
Previously, nothing was rendered if the material was missing.
The `WithMesh2d` and `WithMesh3d` query filter type aliases have also
been removed. Simply use `With<Mesh2d>` or `With<Mesh3d>`.
---------
Co-authored-by: Tim Blackbird <justthecooldude@gmail.com>
Co-authored-by: Carter Anderson <mcanders1@gmail.com>
2024-10-01 21:33:17 +00:00
|
|
|
app.init_asset::<M>()
|
|
|
|
.register_type::<MeshMaterial3d<M>>()
|
|
|
|
.add_plugins(RenderAssetPlugin::<PreparedMaterial<M>>::default());
|
2023-01-19 22:11:13 +00:00
|
|
|
|
2024-03-31 03:16:10 +00:00
|
|
|
if let Some(render_app) = app.get_sub_app_mut(RenderApp) {
|
2021-12-25 21:45:43 +00:00
|
|
|
render_app
|
2023-03-02 08:21:21 +00:00
|
|
|
.init_resource::<DrawFunctions<Shadow>>()
|
2024-10-09 17:12:27 +00:00
|
|
|
.init_resource::<RenderMaterialInstances<M>>()
|
2023-03-02 08:21:21 +00:00
|
|
|
.add_render_command::<Shadow, DrawPrepass<M>>()
|
`StandardMaterial` Light Transmission (#8015)
# Objective
<img width="1920" alt="Screenshot 2023-04-26 at 01 07 34"
src="https://user-images.githubusercontent.com/418473/234467578-0f34187b-5863-4ea1-88e9-7a6bb8ce8da3.png">
This PR adds both diffuse and specular light transmission capabilities
to the `StandardMaterial`, with support for screen space refractions.
This enables realistically representing a wide range of real-world
materials, such as:
- Glass; (Including frosted glass)
- Transparent and translucent plastics;
- Various liquids and gels;
- Gemstones;
- Marble;
- Wax;
- Paper;
- Leaves;
- Porcelain.
Unlike existing support for transparency, light transmission does not
rely on fixed function alpha blending, and therefore works with both
`AlphaMode::Opaque` and `AlphaMode::Mask` materials.
## Solution
- Introduces a number of transmission related fields in the
`StandardMaterial`;
- For specular transmission:
- Adds logic to take a view main texture snapshot after the opaque
phase; (in order to perform screen space refractions)
- Introduces a new `Transmissive3d` phase to the renderer, to which all
meshes with `transmission > 0.0` materials are sent.
- Calculates a light exit point (of the approximate mesh volume) using
`ior` and `thickness` properties
- Samples the snapshot texture with an adaptive number of taps across a
`roughness`-controlled radius enabling “blurry” refractions
- For diffuse transmission:
- Approximates transmitted diffuse light by using a second, flipped +
displaced, diffuse-only Lambertian lobe for each light source.
## To Do
- [x] Figure out where `fresnel_mix()` is taking place, if at all, and
where `dielectric_specular` is being calculated, if at all, and update
them to use the `ior` value (Not a blocker, just a nice-to-have for more
correct BSDF)
- To the _best of my knowledge, this is now taking place, after
964340cdd. The fresnel mix is actually "split" into two parts in our
implementation, one `(1 - fresnel(...))` in the transmission, and
`fresnel()` in the light implementations. A surface with more
reflectance now will produce slightly dimmer transmission towards the
grazing angle, as more of the light gets reflected.
- [x] Add `transmission_texture`
- [x] Add `diffuse_transmission_texture`
- [x] Add `thickness_texture`
- [x] Add `attenuation_distance` and `attenuation_color`
- [x] Connect values to glTF loader
- [x] `transmission` and `transmission_texture`
- [x] `thickness` and `thickness_texture`
- [x] `ior`
- [ ] `diffuse_transmission` and `diffuse_transmission_texture` (needs
upstream support in `gltf` crate, not a blocker)
- [x] Add support for multiple screen space refraction “steps”
- [x] Conditionally create no transmission snapshot texture at all if
`steps == 0`
- [x] Conditionally enable/disable screen space refraction transmission
snapshots
- [x] Read from depth pre-pass to prevent refracting pixels in front of
the light exit point
- [x] Use `interleaved_gradient_noise()` function for sampling blur in a
way that benefits from TAA
- [x] Drill down a TAA `#define`, tweak some aspects of the effect
conditionally based on it
- [x] Remove const array that's crashing under HLSL (unless a new `naga`
release with https://github.com/gfx-rs/naga/pull/2496 comes out before
we merge this)
- [ ] Look into alternatives to the `switch` hack for dynamically
indexing the const array (might not be needed, compilers seem to be
decent at expanding it)
- [ ] Add pipeline keys for gating transmission (do we really want/need
this?)
- [x] Tweak some material field/function names?
## A Note on Texture Packing
_This was originally added as a comment to the
`specular_transmission_texture`, `thickness_texture` and
`diffuse_transmission_texture` documentation, I removed it since it was
more confusing than helpful, and will likely be made redundant/will need
to be updated once we have a better infrastructure for preprocessing
assets_
Due to how channels are mapped, you can more efficiently use a single
shared texture image
for configuring the following:
- R - `specular_transmission_texture`
- G - `thickness_texture`
- B - _unused_
- A - `diffuse_transmission_texture`
The `KHR_materials_diffuse_transmission` glTF extension also defines a
`diffuseTransmissionColorTexture`,
that _we don't currently support_. One might choose to pack the
intensity and color textures together,
using RGB for the color and A for the intensity, in which case this
packing advice doesn't really apply.
---
## Changelog
- Added a new `Transmissive3d` render phase for rendering specular
transmissive materials with screen space refractions
- Added rendering support for transmitted environment map light on the
`StandardMaterial` as a fallback for screen space refractions
- Added `diffuse_transmission`, `specular_transmission`, `thickness`,
`ior`, `attenuation_distance` and `attenuation_color` to the
`StandardMaterial`
- Added `diffuse_transmission_texture`, `specular_transmission_texture`,
`thickness_texture` to the `StandardMaterial`, gated behind a new
`pbr_transmission_textures` cargo feature (off by default, for maximum
hardware compatibility)
- Added `Camera3d::screen_space_specular_transmission_steps` for
controlling the number of “layers of transparency” rendered for
transmissive objects
- Added a `TransmittedShadowReceiver` component for enabling shadows in
(diffusely) transmitted light. (disabled by default, as it requires
carefully setting up the `thickness` to avoid self-shadow artifacts)
- Added support for the `KHR_materials_transmission`,
`KHR_materials_ior` and `KHR_materials_volume` glTF extensions
- Renamed items related to temporal jitter for greater consistency
## Migration Guide
- `SsaoPipelineKey::temporal_noise` has been renamed to
`SsaoPipelineKey::temporal_jitter`
- The `TAA` shader def (controlled by the presence of the
`TemporalAntiAliasSettings` component in the camera) has been replaced
with the `TEMPORAL_JITTER` shader def (controlled by the presence of the
`TemporalJitter` component in the camera)
- `MeshPipelineKey::TAA` has been replaced by
`MeshPipelineKey::TEMPORAL_JITTER`
- The `TEMPORAL_NOISE` shader def has been consolidated with
`TEMPORAL_JITTER`
2023-10-31 20:59:02 +00:00
|
|
|
.add_render_command::<Transmissive3d, DrawMaterial<M>>()
|
2021-12-25 21:45:43 +00:00
|
|
|
.add_render_command::<Transparent3d, DrawMaterial<M>>()
|
|
|
|
.add_render_command::<Opaque3d, DrawMaterial<M>>()
|
|
|
|
.add_render_command::<AlphaMask3d, DrawMaterial<M>>()
|
Mesh vertex buffer layouts (#3959)
This PR makes a number of changes to how meshes and vertex attributes are handled, which the goal of enabling easy and flexible custom vertex attributes:
* Reworks the `Mesh` type to use the newly added `VertexAttribute` internally
* `VertexAttribute` defines the name, a unique `VertexAttributeId`, and a `VertexFormat`
* `VertexAttributeId` is used to produce consistent sort orders for vertex buffer generation, replacing the more expensive and often surprising "name based sorting"
* Meshes can be used to generate a `MeshVertexBufferLayout`, which defines the layout of the gpu buffer produced by the mesh. `MeshVertexBufferLayouts` can then be used to generate actual `VertexBufferLayouts` according to the requirements of a specific pipeline. This decoupling of "mesh layout" vs "pipeline vertex buffer layout" is what enables custom attributes. We don't need to standardize _mesh layouts_ or contort meshes to meet the needs of a specific pipeline. As long as the mesh has what the pipeline needs, it will work transparently.
* Mesh-based pipelines now specialize on `&MeshVertexBufferLayout` via the new `SpecializedMeshPipeline` trait (which behaves like `SpecializedPipeline`, but adds `&MeshVertexBufferLayout`). The integrity of the pipeline cache is maintained because the `MeshVertexBufferLayout` is treated as part of the key (which is fully abstracted from implementers of the trait ... no need to add any additional info to the specialization key).
* Hashing `MeshVertexBufferLayout` is too expensive to do for every entity, every frame. To make this scalable, I added a generalized "pre-hashing" solution to `bevy_utils`: `Hashed<T>` keys and `PreHashMap<K, V>` (which uses `Hashed<T>` internally) . Why didn't I just do the quick and dirty in-place "pre-compute hash and use that u64 as a key in a hashmap" that we've done in the past? Because its wrong! Hashes by themselves aren't enough because two different values can produce the same hash. Re-hashing a hash is even worse! I decided to build a generalized solution because this pattern has come up in the past and we've chosen to do the wrong thing. Now we can do the right thing! This did unfortunately require pulling in `hashbrown` and using that in `bevy_utils`, because avoiding re-hashes requires the `raw_entry_mut` api, which isn't stabilized yet (and may never be ... `entry_ref` has favor now, but also isn't available yet). If std's HashMap ever provides the tools we need, we can move back to that. Note that adding `hashbrown` doesn't increase our dependency count because it was already in our tree. I will probably break these changes out into their own PR.
* Specializing on `MeshVertexBufferLayout` has one non-obvious behavior: it can produce identical pipelines for two different MeshVertexBufferLayouts. To optimize the number of active pipelines / reduce re-binds while drawing, I de-duplicate pipelines post-specialization using the final `VertexBufferLayout` as the key. For example, consider a pipeline that needs the layout `(position, normal)` and is specialized using two meshes: `(position, normal, uv)` and `(position, normal, other_vec2)`. If both of these meshes result in `(position, normal)` specializations, we can use the same pipeline! Now we do. Cool!
To briefly illustrate, this is what the relevant section of `MeshPipeline`'s specialization code looks like now:
```rust
impl SpecializedMeshPipeline for MeshPipeline {
type Key = MeshPipelineKey;
fn specialize(
&self,
key: Self::Key,
layout: &MeshVertexBufferLayout,
) -> RenderPipelineDescriptor {
let mut vertex_attributes = vec![
Mesh::ATTRIBUTE_POSITION.at_shader_location(0),
Mesh::ATTRIBUTE_NORMAL.at_shader_location(1),
Mesh::ATTRIBUTE_UV_0.at_shader_location(2),
];
let mut shader_defs = Vec::new();
if layout.contains(Mesh::ATTRIBUTE_TANGENT) {
shader_defs.push(String::from("VERTEX_TANGENTS"));
vertex_attributes.push(Mesh::ATTRIBUTE_TANGENT.at_shader_location(3));
}
let vertex_buffer_layout = layout
.get_layout(&vertex_attributes)
.expect("Mesh is missing a vertex attribute");
```
Notice that this is _much_ simpler than it was before. And now any mesh with any layout can be used with this pipeline, provided it has vertex postions, normals, and uvs. We even got to remove `HAS_TANGENTS` from MeshPipelineKey and `has_tangents` from `GpuMesh`, because that information is redundant with `MeshVertexBufferLayout`.
This is still a draft because I still need to:
* Add more docs
* Experiment with adding error handling to mesh pipeline specialization (which would print errors at runtime when a mesh is missing a vertex attribute required by a pipeline). If it doesn't tank perf, we'll keep it.
* Consider breaking out the PreHash / hashbrown changes into a separate PR.
* Add an example illustrating this change
* Verify that the "mesh-specialized pipeline de-duplication code" works properly
Please dont yell at me for not doing these things yet :) Just trying to get this in peoples' hands asap.
Alternative to #3120
Fixes #3030
Co-authored-by: Carter Anderson <mcanders1@gmail.com>
2022-02-23 23:21:13 +00:00
|
|
|
.init_resource::<SpecializedMeshPipelines<MaterialPipeline<M>>>()
|
Revert default mesh materials (#15930)
# Objective
Closes #15799.
Many rendering people and maintainers are in favor of reverting default
mesh materials added in #15524, especially as the migration to required
component is already large and heavily breaking.
## Solution
Revert default mesh materials, and adjust docs accordingly.
- Remove `extract_default_materials`
- Remove `clear_material_instances`, and move the logic back into
`extract_mesh_materials`
- Remove `HasMaterial2d` and `HasMaterial3d`
- Change default material handles back to pink instead of white
- 2D uses `Color::srgb(1.0, 0.0, 1.0)`, while 3D uses `Color::srgb(1.0,
0.0, 0.5)`. Not sure if this is intended.
There is now no indication at all about missing materials for `Mesh2d`
and `Mesh3d`. Having a mesh without a material renders nothing.
## Testing
I ran `2d_shapes`, `mesh2d_manual`, and `3d_shapes`, with and without
mesh material components.
2024-10-15 19:47:40 +00:00
|
|
|
.add_systems(ExtractSchedule, extract_mesh_materials::<M>)
|
2023-03-18 01:45:34 +00:00
|
|
|
.add_systems(
|
|
|
|
Render,
|
2024-04-09 13:26:34 +00:00
|
|
|
queue_material_meshes::<M>
|
|
|
|
.in_set(RenderSet::QueueMeshes)
|
|
|
|
.after(prepare_assets::<PreparedMaterial<M>>),
|
2023-03-18 01:45:34 +00:00
|
|
|
);
|
2024-03-18 17:54:41 +00:00
|
|
|
|
|
|
|
if self.shadows_enabled {
|
|
|
|
render_app.add_systems(
|
|
|
|
Render,
|
2024-04-09 13:26:34 +00:00
|
|
|
queue_shadows::<M>
|
2024-03-18 17:54:41 +00:00
|
|
|
.in_set(RenderSet::QueueMeshes)
|
2024-04-09 13:26:34 +00:00
|
|
|
.after(prepare_assets::<PreparedMaterial<M>>),
|
2024-03-18 17:54:41 +00:00
|
|
|
);
|
|
|
|
}
|
2024-03-25 19:08:27 +00:00
|
|
|
|
|
|
|
#[cfg(feature = "meshlet")]
|
|
|
|
render_app.add_systems(
|
|
|
|
Render,
|
Fix MeshletMesh material system ordering (#14016)
# Objective
- Fixes #13811 (probably, I lost my test code...)
## Solution
- Turns out that Queue and PrepareAssets are _not_ ordered. We should
probably either rethink our system sets (again), or improve the
documentation here. For reference, I've included the current ordering
below.
- The `prepare_meshlet_meshes_X` systems need to run after
`prepare_assets::<PreparedMaterial<M>>`, and have also been moved to
QueueMeshes.
```rust
schedule.configure_sets(
(
ExtractCommands,
ManageViews,
Queue,
PhaseSort,
Prepare,
Render,
Cleanup,
)
.chain(),
);
schedule.configure_sets((ExtractCommands, PrepareAssets, Prepare).chain());
schedule.configure_sets(QueueMeshes.in_set(Queue).after(prepare_assets::<GpuMesh>));
schedule.configure_sets(
(PrepareResources, PrepareResourcesFlush, PrepareBindGroups)
.chain()
.in_set(Prepare),
);
```
## Testing
- Ambiguity checker to make sure I don't have ambiguous system ordering
2024-06-25 18:17:52 +00:00
|
|
|
queue_material_meshlet_meshes::<M>
|
|
|
|
.in_set(RenderSet::QueueMeshes)
|
Meshlet software raster + start of cleanup (#14623)
# Objective
- Faster meshlet rasterization path for small triangles
- Avoid having to allocate and write out a triangle buffer
- Refactor gpu_scene.rs
## Solution
- Replace the 32bit visbuffer texture with a 64bit visbuffer buffer,
where the left 32 bits encode depth, and the right 32 bits encode the
existing cluster + triangle IDs. Can't use 64bit textures, wgpu/naga
doesn't support atomic ops on textures yet.
- Instead of writing out a buffer of packed cluster + triangle IDs (per
triangle) to raster, the culling pass now writes out a buffer of just
cluster IDs (per cluster, so less memory allocated, cheaper to write
out).
- Clusters for software raster are allocated from the left side
- Clusters for hardware raster are allocated in the same buffer, from
the right side
- The buffer size is fixed at MeshletPlugin build time, and should be
set to a reasonable value for your scene (no warning on overflow, and no
good way to determine what value you need outside of renderdoc - I plan
to fix this in a future PR adding a meshlet stats overlay)
- Currently I don't have a heuristic for software vs hardware raster
selection for each cluster. The existing code is just a placeholder. I
need to profile on a release scene and come up with a heuristic,
probably in a future PR.
- The culling shader is getting pretty hard to follow at this point, but
I don't want to spend time improving it as the entire shader/pass is
getting rewritten/replaced in the near future.
- Software raster is a compute workgroup per-cluster. Each workgroup
loads and transforms the <=64 vertices of the cluster, and then
rasterizes the <=64 triangles of the cluster.
- Two variants are implemented: Scanline for clusters with any larger
triangles (still smaller than hardware is good at), and brute-force for
very very tiny triangles
- Once the shader determines that a pixel should be filled in, it does
an atomicMax() on the visbuffer to store the results, copying how Nanite
works
- On devices with a low max workgroups per dispatch limit, an extra
compute pass is inserted before software raster to convert from a 1d to
2d dispatch (I don't think 3d would ever be necessary).
- I haven't implemented the top-left rule or subpixel precision yet, I'm
leaving that for a future PR since I get usable results without it for
now
- Resources used:
https://kristoffer-dyrkorn.github.io/triangle-rasterizer and chapters
6-8 of
https://fgiesen.wordpress.com/2013/02/17/optimizing-sw-occlusion-culling-index
- Hardware raster now spawns 64*3 vertex invocations per meshlet,
instead of the actual meshlet vertex count. Extra invocations just
early-exit.
- While this is slower than the existing system, hardware draws should
be rare now that software raster is usable, and it saves a ton of memory
using the unified cluster ID buffer. This would be fixed if wgpu had
support for mesh shaders.
- Instead of writing to a color+depth attachment, the hardware raster
pass also does the same atomic visbuffer writes that software raster
uses.
- We have to bind a dummy render target anyways, as wgpu doesn't
currently support render passes without any attachments
- Material IDs are no longer written out during the main rasterization
passes.
- If we had async compute queues, we could overlap the software and
hardware raster passes.
- New material and depth resolve passes run at the end of the visbuffer
node, and write out view depth and material ID depth textures
### Misc changes
- Fixed cluster culling importing, but never actually using the previous
view uniforms when doing occlusion culling
- Fixed incorrectly adding the LOD error twice when building the meshlet
mesh
- Splitup gpu_scene module into meshlet_mesh_manager, instance_manager,
and resource_manager
- resource_manager is still too complex and inefficient (extract and
prepare are way too expensive). I plan on improving this in a future PR,
but for now ResourceManager is mostly a 1:1 port of the leftover
MeshletGpuScene bits.
- Material draw passes have been renamed to the more accurate material
shade pass, as well as some other misc renaming (in the future, these
will be compute shaders even, and not actual draw calls)
---
## Migration Guide
- TBD (ask me at the end of the release for meshlet changes as a whole)
---------
Co-authored-by: vero <email@atlasdostal.com>
2024-08-26 17:54:34 +00:00
|
|
|
.run_if(resource_exists::<InstanceManager>),
|
Fix MeshletMesh material system ordering (#14016)
# Objective
- Fixes #13811 (probably, I lost my test code...)
## Solution
- Turns out that Queue and PrepareAssets are _not_ ordered. We should
probably either rethink our system sets (again), or improve the
documentation here. For reference, I've included the current ordering
below.
- The `prepare_meshlet_meshes_X` systems need to run after
`prepare_assets::<PreparedMaterial<M>>`, and have also been moved to
QueueMeshes.
```rust
schedule.configure_sets(
(
ExtractCommands,
ManageViews,
Queue,
PhaseSort,
Prepare,
Render,
Cleanup,
)
.chain(),
);
schedule.configure_sets((ExtractCommands, PrepareAssets, Prepare).chain());
schedule.configure_sets(QueueMeshes.in_set(Queue).after(prepare_assets::<GpuMesh>));
schedule.configure_sets(
(PrepareResources, PrepareResourcesFlush, PrepareBindGroups)
.chain()
.in_set(Prepare),
);
```
## Testing
- Ambiguity checker to make sure I don't have ambiguous system ordering
2024-06-25 18:17:52 +00:00
|
|
|
);
|
|
|
|
|
|
|
|
#[cfg(feature = "meshlet")]
|
|
|
|
render_app.add_systems(
|
|
|
|
Render,
|
|
|
|
prepare_material_meshlet_meshes_main_opaque_pass::<M>
|
|
|
|
.in_set(RenderSet::QueueMeshes)
|
|
|
|
.after(prepare_assets::<PreparedMaterial<M>>)
|
|
|
|
.before(queue_material_meshlet_meshes::<M>)
|
Meshlet software raster + start of cleanup (#14623)
# Objective
- Faster meshlet rasterization path for small triangles
- Avoid having to allocate and write out a triangle buffer
- Refactor gpu_scene.rs
## Solution
- Replace the 32bit visbuffer texture with a 64bit visbuffer buffer,
where the left 32 bits encode depth, and the right 32 bits encode the
existing cluster + triangle IDs. Can't use 64bit textures, wgpu/naga
doesn't support atomic ops on textures yet.
- Instead of writing out a buffer of packed cluster + triangle IDs (per
triangle) to raster, the culling pass now writes out a buffer of just
cluster IDs (per cluster, so less memory allocated, cheaper to write
out).
- Clusters for software raster are allocated from the left side
- Clusters for hardware raster are allocated in the same buffer, from
the right side
- The buffer size is fixed at MeshletPlugin build time, and should be
set to a reasonable value for your scene (no warning on overflow, and no
good way to determine what value you need outside of renderdoc - I plan
to fix this in a future PR adding a meshlet stats overlay)
- Currently I don't have a heuristic for software vs hardware raster
selection for each cluster. The existing code is just a placeholder. I
need to profile on a release scene and come up with a heuristic,
probably in a future PR.
- The culling shader is getting pretty hard to follow at this point, but
I don't want to spend time improving it as the entire shader/pass is
getting rewritten/replaced in the near future.
- Software raster is a compute workgroup per-cluster. Each workgroup
loads and transforms the <=64 vertices of the cluster, and then
rasterizes the <=64 triangles of the cluster.
- Two variants are implemented: Scanline for clusters with any larger
triangles (still smaller than hardware is good at), and brute-force for
very very tiny triangles
- Once the shader determines that a pixel should be filled in, it does
an atomicMax() on the visbuffer to store the results, copying how Nanite
works
- On devices with a low max workgroups per dispatch limit, an extra
compute pass is inserted before software raster to convert from a 1d to
2d dispatch (I don't think 3d would ever be necessary).
- I haven't implemented the top-left rule or subpixel precision yet, I'm
leaving that for a future PR since I get usable results without it for
now
- Resources used:
https://kristoffer-dyrkorn.github.io/triangle-rasterizer and chapters
6-8 of
https://fgiesen.wordpress.com/2013/02/17/optimizing-sw-occlusion-culling-index
- Hardware raster now spawns 64*3 vertex invocations per meshlet,
instead of the actual meshlet vertex count. Extra invocations just
early-exit.
- While this is slower than the existing system, hardware draws should
be rare now that software raster is usable, and it saves a ton of memory
using the unified cluster ID buffer. This would be fixed if wgpu had
support for mesh shaders.
- Instead of writing to a color+depth attachment, the hardware raster
pass also does the same atomic visbuffer writes that software raster
uses.
- We have to bind a dummy render target anyways, as wgpu doesn't
currently support render passes without any attachments
- Material IDs are no longer written out during the main rasterization
passes.
- If we had async compute queues, we could overlap the software and
hardware raster passes.
- New material and depth resolve passes run at the end of the visbuffer
node, and write out view depth and material ID depth textures
### Misc changes
- Fixed cluster culling importing, but never actually using the previous
view uniforms when doing occlusion culling
- Fixed incorrectly adding the LOD error twice when building the meshlet
mesh
- Splitup gpu_scene module into meshlet_mesh_manager, instance_manager,
and resource_manager
- resource_manager is still too complex and inefficient (extract and
prepare are way too expensive). I plan on improving this in a future PR,
but for now ResourceManager is mostly a 1:1 port of the leftover
MeshletGpuScene bits.
- Material draw passes have been renamed to the more accurate material
shade pass, as well as some other misc renaming (in the future, these
will be compute shaders even, and not actual draw calls)
---
## Migration Guide
- TBD (ask me at the end of the release for meshlet changes as a whole)
---------
Co-authored-by: vero <email@atlasdostal.com>
2024-08-26 17:54:34 +00:00
|
|
|
.run_if(resource_exists::<InstanceManager>),
|
2024-03-25 19:08:27 +00:00
|
|
|
);
|
2021-12-25 21:45:43 +00:00
|
|
|
}
|
2023-01-19 22:11:13 +00:00
|
|
|
|
2024-03-18 17:54:41 +00:00
|
|
|
if self.shadows_enabled || self.prepass_enabled {
|
|
|
|
// PrepassPipelinePlugin is required for shadow mapping and the optional PrepassPlugin
|
|
|
|
app.add_plugins(PrepassPipelinePlugin::<M>::default());
|
|
|
|
}
|
2023-03-03 15:08:54 +00:00
|
|
|
|
2023-01-19 22:11:13 +00:00
|
|
|
if self.prepass_enabled {
|
2023-06-21 20:51:03 +00:00
|
|
|
app.add_plugins(PrepassPlugin::<M>::default());
|
2023-01-19 22:11:13 +00:00
|
|
|
}
|
2021-12-14 03:58:23 +00:00
|
|
|
}
|
Webgpu support (#8336)
# Objective
- Support WebGPU
- alternative to #5027 that doesn't need any async / await
- fixes #8315
- Surprise fix #7318
## Solution
### For async renderer initialisation
- Update the plugin lifecycle:
- app builds the plugin
- calls `plugin.build`
- registers the plugin
- app starts the event loop
- event loop waits for `ready` of all registered plugins in the same
order
- returns `true` by default
- then call all `finish` then all `cleanup` in the same order as
registered
- then execute the schedule
In the case of the renderer, to avoid anything async:
- building the renderer plugin creates a detached task that will send
back the initialised renderer through a mutex in a resource
- `ready` will wait for the renderer to be present in the resource
- `finish` will take that renderer and place it in the expected
resources by other plugins
- other plugins (that expect the renderer to be available) `finish` are
called and they are able to set up their pipelines
- `cleanup` is called, only custom one is still for pipeline rendering
### For WebGPU support
- update the `build-wasm-example` script to support passing `--api
webgpu` that will build the example with WebGPU support
- feature for webgl2 was always enabled when building for wasm. it's now
in the default feature list and enabled on all platforms, so check for
this feature must also check that the target_arch is `wasm32`
---
## Migration Guide
- `Plugin::setup` has been renamed `Plugin::cleanup`
- `Plugin::finish` has been added, and plugins adding pipelines should
do it in this function instead of `Plugin::build`
```rust
// Before
impl Plugin for MyPlugin {
fn build(&self, app: &mut App) {
app.insert_resource::<MyResource>
.add_systems(Update, my_system);
let render_app = match app.get_sub_app_mut(RenderApp) {
Ok(render_app) => render_app,
Err(_) => return,
};
render_app
.init_resource::<RenderResourceNeedingDevice>()
.init_resource::<OtherRenderResource>();
}
}
// After
impl Plugin for MyPlugin {
fn build(&self, app: &mut App) {
app.insert_resource::<MyResource>
.add_systems(Update, my_system);
let render_app = match app.get_sub_app_mut(RenderApp) {
Ok(render_app) => render_app,
Err(_) => return,
};
render_app
.init_resource::<OtherRenderResource>();
}
fn finish(&self, app: &mut App) {
let render_app = match app.get_sub_app_mut(RenderApp) {
Ok(render_app) => render_app,
Err(_) => return,
};
render_app
.init_resource::<RenderResourceNeedingDevice>();
}
}
```
2023-05-04 22:07:57 +00:00
|
|
|
|
|
|
|
fn finish(&self, app: &mut App) {
|
2024-03-31 03:16:10 +00:00
|
|
|
if let Some(render_app) = app.get_sub_app_mut(RenderApp) {
|
Webgpu support (#8336)
# Objective
- Support WebGPU
- alternative to #5027 that doesn't need any async / await
- fixes #8315
- Surprise fix #7318
## Solution
### For async renderer initialisation
- Update the plugin lifecycle:
- app builds the plugin
- calls `plugin.build`
- registers the plugin
- app starts the event loop
- event loop waits for `ready` of all registered plugins in the same
order
- returns `true` by default
- then call all `finish` then all `cleanup` in the same order as
registered
- then execute the schedule
In the case of the renderer, to avoid anything async:
- building the renderer plugin creates a detached task that will send
back the initialised renderer through a mutex in a resource
- `ready` will wait for the renderer to be present in the resource
- `finish` will take that renderer and place it in the expected
resources by other plugins
- other plugins (that expect the renderer to be available) `finish` are
called and they are able to set up their pipelines
- `cleanup` is called, only custom one is still for pipeline rendering
### For WebGPU support
- update the `build-wasm-example` script to support passing `--api
webgpu` that will build the example with WebGPU support
- feature for webgl2 was always enabled when building for wasm. it's now
in the default feature list and enabled on all platforms, so check for
this feature must also check that the target_arch is `wasm32`
---
## Migration Guide
- `Plugin::setup` has been renamed `Plugin::cleanup`
- `Plugin::finish` has been added, and plugins adding pipelines should
do it in this function instead of `Plugin::build`
```rust
// Before
impl Plugin for MyPlugin {
fn build(&self, app: &mut App) {
app.insert_resource::<MyResource>
.add_systems(Update, my_system);
let render_app = match app.get_sub_app_mut(RenderApp) {
Ok(render_app) => render_app,
Err(_) => return,
};
render_app
.init_resource::<RenderResourceNeedingDevice>()
.init_resource::<OtherRenderResource>();
}
}
// After
impl Plugin for MyPlugin {
fn build(&self, app: &mut App) {
app.insert_resource::<MyResource>
.add_systems(Update, my_system);
let render_app = match app.get_sub_app_mut(RenderApp) {
Ok(render_app) => render_app,
Err(_) => return,
};
render_app
.init_resource::<OtherRenderResource>();
}
fn finish(&self, app: &mut App) {
let render_app = match app.get_sub_app_mut(RenderApp) {
Ok(render_app) => render_app,
Err(_) => return,
};
render_app
.init_resource::<RenderResourceNeedingDevice>();
}
}
```
2023-05-04 22:07:57 +00:00
|
|
|
render_app.init_resource::<MaterialPipeline<M>>();
|
|
|
|
}
|
|
|
|
}
|
2021-12-14 03:58:23 +00:00
|
|
|
}
|
|
|
|
|
Better Materials: AsBindGroup trait and derive, simpler Material trait (#5053)
# Objective
This PR reworks Bevy's Material system, making the user experience of defining Materials _much_ nicer. Bevy's previous material system leaves a lot to be desired:
* Materials require manually implementing the `RenderAsset` trait, which involves manually generating the bind group, handling gpu buffer data transfer, looking up image textures, etc. Even the simplest single-texture material involves writing ~80 unnecessary lines of code. This was never the long term plan.
* There are two material traits, which is confusing, hard to document, and often redundant: `Material` and `SpecializedMaterial`. `Material` implicitly implements `SpecializedMaterial`, and `SpecializedMaterial` is used in most high level apis to support both use cases. Most users shouldn't need to think about specialization at all (I consider it a "power-user tool"), so the fact that `SpecializedMaterial` is front-and-center in our apis is a miss.
* Implementing either material trait involves a lot of "type soup". The "prepared asset" parameter is particularly heinous: `&<Self as RenderAsset>::PreparedAsset`. Defining vertex and fragment shaders is also more verbose than it needs to be.
## Solution
Say hello to the new `Material` system:
```rust
#[derive(AsBindGroup, TypeUuid, Debug, Clone)]
#[uuid = "f690fdae-d598-45ab-8225-97e2a3f056e0"]
pub struct CoolMaterial {
#[uniform(0)]
color: Color,
#[texture(1)]
#[sampler(2)]
color_texture: Handle<Image>,
}
impl Material for CoolMaterial {
fn fragment_shader() -> ShaderRef {
"cool_material.wgsl".into()
}
}
```
Thats it! This same material would have required [~80 lines of complicated "type heavy" code](https://github.com/bevyengine/bevy/blob/v0.7.0/examples/shader/shader_material.rs) in the old Material system. Now it is just 14 lines of simple, readable code.
This is thanks to a new consolidated `Material` trait and the new `AsBindGroup` trait / derive.
### The new `Material` trait
The old "split" `Material` and `SpecializedMaterial` traits have been removed in favor of a new consolidated `Material` trait. All of the functions on the trait are optional.
The difficulty of implementing `Material` has been reduced by simplifying dataflow and removing type complexity:
```rust
// Old
impl Material for CustomMaterial {
fn fragment_shader(asset_server: &AssetServer) -> Option<Handle<Shader>> {
Some(asset_server.load("custom_material.wgsl"))
}
fn alpha_mode(render_asset: &<Self as RenderAsset>::PreparedAsset) -> AlphaMode {
render_asset.alpha_mode
}
}
// New
impl Material for CustomMaterial {
fn fragment_shader() -> ShaderRef {
"custom_material.wgsl".into()
}
fn alpha_mode(&self) -> AlphaMode {
self.alpha_mode
}
}
```
Specialization is still supported, but it is hidden by default under the `specialize()` function (more on this later).
### The `AsBindGroup` trait / derive
The `Material` trait now requires the `AsBindGroup` derive. This can be implemented manually relatively easily, but deriving it will almost always be preferable.
Field attributes like `uniform` and `texture` are used to define which fields should be bindings,
what their binding type is, and what index they should be bound at:
```rust
#[derive(AsBindGroup)]
struct CoolMaterial {
#[uniform(0)]
color: Color,
#[texture(1)]
#[sampler(2)]
color_texture: Handle<Image>,
}
```
In WGSL shaders, the binding looks like this:
```wgsl
struct CoolMaterial {
color: vec4<f32>;
};
[[group(1), binding(0)]]
var<uniform> material: CoolMaterial;
[[group(1), binding(1)]]
var color_texture: texture_2d<f32>;
[[group(1), binding(2)]]
var color_sampler: sampler;
```
Note that the "group" index is determined by the usage context. It is not defined in `AsBindGroup`. Bevy material bind groups are bound to group 1.
The following field-level attributes are supported:
* `uniform(BINDING_INDEX)`
* The field will be converted to a shader-compatible type using the `ShaderType` trait, written to a `Buffer`, and bound as a uniform. It can also be derived for custom structs.
* `texture(BINDING_INDEX)`
* This field's `Handle<Image>` will be used to look up the matching `Texture` gpu resource, which will be bound as a texture in shaders. The field will be assumed to implement `Into<Option<Handle<Image>>>`. In practice, most fields should be a `Handle<Image>` or `Option<Handle<Image>>`. If the value of an `Option<Handle<Image>>` is `None`, the new `FallbackImage` resource will be used instead. This attribute can be used in conjunction with a `sampler` binding attribute (with a different binding index).
* `sampler(BINDING_INDEX)`
* Behaves exactly like the `texture` attribute, but sets the Image's sampler binding instead of the texture.
Note that fields without field-level binding attributes will be ignored.
```rust
#[derive(AsBindGroup)]
struct CoolMaterial {
#[uniform(0)]
color: Color,
this_field_is_ignored: String,
}
```
As mentioned above, `Option<Handle<Image>>` is also supported:
```rust
#[derive(AsBindGroup)]
struct CoolMaterial {
#[uniform(0)]
color: Color,
#[texture(1)]
#[sampler(2)]
color_texture: Option<Handle<Image>>,
}
```
This is useful if you want a texture to be optional. When the value is `None`, the `FallbackImage` will be used for the binding instead, which defaults to "pure white".
Field uniforms with the same binding index will be combined into a single binding:
```rust
#[derive(AsBindGroup)]
struct CoolMaterial {
#[uniform(0)]
color: Color,
#[uniform(0)]
roughness: f32,
}
```
In WGSL shaders, the binding would look like this:
```wgsl
struct CoolMaterial {
color: vec4<f32>;
roughness: f32;
};
[[group(1), binding(0)]]
var<uniform> material: CoolMaterial;
```
Some less common scenarios will require "struct-level" attributes. These are the currently supported struct-level attributes:
* `uniform(BINDING_INDEX, ConvertedShaderType)`
* Similar to the field-level `uniform` attribute, but instead the entire `AsBindGroup` value is converted to `ConvertedShaderType`, which must implement `ShaderType`. This is useful if more complicated conversion logic is required.
* `bind_group_data(DataType)`
* The `AsBindGroup` type will be converted to some `DataType` using `Into<DataType>` and stored as `AsBindGroup::Data` as part of the `AsBindGroup::as_bind_group` call. This is useful if data needs to be stored alongside the generated bind group, such as a unique identifier for a material's bind group. The most common use case for this attribute is "shader pipeline specialization".
The previous `CoolMaterial` example illustrating "combining multiple field-level uniform attributes with the same binding index" can
also be equivalently represented with a single struct-level uniform attribute:
```rust
#[derive(AsBindGroup)]
#[uniform(0, CoolMaterialUniform)]
struct CoolMaterial {
color: Color,
roughness: f32,
}
#[derive(ShaderType)]
struct CoolMaterialUniform {
color: Color,
roughness: f32,
}
impl From<&CoolMaterial> for CoolMaterialUniform {
fn from(material: &CoolMaterial) -> CoolMaterialUniform {
CoolMaterialUniform {
color: material.color,
roughness: material.roughness,
}
}
}
```
### Material Specialization
Material shader specialization is now _much_ simpler:
```rust
#[derive(AsBindGroup, TypeUuid, Debug, Clone)]
#[uuid = "f690fdae-d598-45ab-8225-97e2a3f056e0"]
#[bind_group_data(CoolMaterialKey)]
struct CoolMaterial {
#[uniform(0)]
color: Color,
is_red: bool,
}
#[derive(Copy, Clone, Hash, Eq, PartialEq)]
struct CoolMaterialKey {
is_red: bool,
}
impl From<&CoolMaterial> for CoolMaterialKey {
fn from(material: &CoolMaterial) -> CoolMaterialKey {
CoolMaterialKey {
is_red: material.is_red,
}
}
}
impl Material for CoolMaterial {
fn fragment_shader() -> ShaderRef {
"cool_material.wgsl".into()
}
fn specialize(
pipeline: &MaterialPipeline<Self>,
descriptor: &mut RenderPipelineDescriptor,
layout: &MeshVertexBufferLayout,
key: MaterialPipelineKey<Self>,
) -> Result<(), SpecializedMeshPipelineError> {
if key.bind_group_data.is_red {
let fragment = descriptor.fragment.as_mut().unwrap();
fragment.shader_defs.push("IS_RED".to_string());
}
Ok(())
}
}
```
Setting `bind_group_data` is not required for specialization (it defaults to `()`). Scenarios like "custom vertex attributes" also benefit from this system:
```rust
impl Material for CustomMaterial {
fn vertex_shader() -> ShaderRef {
"custom_material.wgsl".into()
}
fn fragment_shader() -> ShaderRef {
"custom_material.wgsl".into()
}
fn specialize(
pipeline: &MaterialPipeline<Self>,
descriptor: &mut RenderPipelineDescriptor,
layout: &MeshVertexBufferLayout,
key: MaterialPipelineKey<Self>,
) -> Result<(), SpecializedMeshPipelineError> {
let vertex_layout = layout.get_layout(&[
Mesh::ATTRIBUTE_POSITION.at_shader_location(0),
ATTRIBUTE_BLEND_COLOR.at_shader_location(1),
])?;
descriptor.vertex.buffers = vec![vertex_layout];
Ok(())
}
}
```
### Ported `StandardMaterial` to the new `Material` system
Bevy's built-in PBR material uses the new Material system (including the AsBindGroup derive):
```rust
#[derive(AsBindGroup, Debug, Clone, TypeUuid)]
#[uuid = "7494888b-c082-457b-aacf-517228cc0c22"]
#[bind_group_data(StandardMaterialKey)]
#[uniform(0, StandardMaterialUniform)]
pub struct StandardMaterial {
pub base_color: Color,
#[texture(1)]
#[sampler(2)]
pub base_color_texture: Option<Handle<Image>>,
/* other fields omitted for brevity */
```
### Ported Bevy examples to the new `Material` system
The overall complexity of Bevy's "custom shader examples" has gone down significantly. Take a look at the diffs if you want a dopamine spike.
Please note that while this PR has a net increase in "lines of code", most of those extra lines come from added documentation. There is a significant reduction
in the overall complexity of the code (even accounting for the new derive logic).
---
## Changelog
### Added
* `AsBindGroup` trait and derive, which make it much easier to transfer data to the gpu and generate bind groups for a given type.
### Changed
* The old `Material` and `SpecializedMaterial` traits have been replaced by a consolidated (much simpler) `Material` trait. Materials no longer implement `RenderAsset`.
* `StandardMaterial` was ported to the new material system. There are no user-facing api changes to the `StandardMaterial` struct api, but it now implements `AsBindGroup` and `Material` instead of `RenderAsset` and `SpecializedMaterial`.
## Migration Guide
The Material system has been reworked to be much simpler. We've removed a lot of boilerplate with the new `AsBindGroup` derive and the `Material` trait is simpler as well!
### Bevy 0.7 (old)
```rust
#[derive(Debug, Clone, TypeUuid)]
#[uuid = "f690fdae-d598-45ab-8225-97e2a3f056e0"]
pub struct CustomMaterial {
color: Color,
color_texture: Handle<Image>,
}
#[derive(Clone)]
pub struct GpuCustomMaterial {
_buffer: Buffer,
bind_group: BindGroup,
}
impl RenderAsset for CustomMaterial {
type ExtractedAsset = CustomMaterial;
type PreparedAsset = GpuCustomMaterial;
type Param = (SRes<RenderDevice>, SRes<MaterialPipeline<Self>>);
fn extract_asset(&self) -> Self::ExtractedAsset {
self.clone()
}
fn prepare_asset(
extracted_asset: Self::ExtractedAsset,
(render_device, material_pipeline): &mut SystemParamItem<Self::Param>,
) -> Result<Self::PreparedAsset, PrepareAssetError<Self::ExtractedAsset>> {
let color = Vec4::from_slice(&extracted_asset.color.as_linear_rgba_f32());
let byte_buffer = [0u8; Vec4::SIZE.get() as usize];
let mut buffer = encase::UniformBuffer::new(byte_buffer);
buffer.write(&color).unwrap();
let buffer = render_device.create_buffer_with_data(&BufferInitDescriptor {
contents: buffer.as_ref(),
label: None,
usage: BufferUsages::UNIFORM | BufferUsages::COPY_DST,
});
let (texture_view, texture_sampler) = if let Some(result) = material_pipeline
.mesh_pipeline
.get_image_texture(gpu_images, &Some(extracted_asset.color_texture.clone()))
{
result
} else {
return Err(PrepareAssetError::RetryNextUpdate(extracted_asset));
};
let bind_group = render_device.create_bind_group(&BindGroupDescriptor {
entries: &[
BindGroupEntry {
binding: 0,
resource: buffer.as_entire_binding(),
},
BindGroupEntry {
binding: 0,
resource: BindingResource::TextureView(texture_view),
},
BindGroupEntry {
binding: 1,
resource: BindingResource::Sampler(texture_sampler),
},
],
label: None,
layout: &material_pipeline.material_layout,
});
Ok(GpuCustomMaterial {
_buffer: buffer,
bind_group,
})
}
}
impl Material for CustomMaterial {
fn fragment_shader(asset_server: &AssetServer) -> Option<Handle<Shader>> {
Some(asset_server.load("custom_material.wgsl"))
}
fn bind_group(render_asset: &<Self as RenderAsset>::PreparedAsset) -> &BindGroup {
&render_asset.bind_group
}
fn bind_group_layout(render_device: &RenderDevice) -> BindGroupLayout {
render_device.create_bind_group_layout(&BindGroupLayoutDescriptor {
entries: &[
BindGroupLayoutEntry {
binding: 0,
visibility: ShaderStages::FRAGMENT,
ty: BindingType::Buffer {
ty: BufferBindingType::Uniform,
has_dynamic_offset: false,
min_binding_size: Some(Vec4::min_size()),
},
count: None,
},
BindGroupLayoutEntry {
binding: 1,
visibility: ShaderStages::FRAGMENT,
ty: BindingType::Texture {
multisampled: false,
sample_type: TextureSampleType::Float { filterable: true },
view_dimension: TextureViewDimension::D2Array,
},
count: None,
},
BindGroupLayoutEntry {
binding: 2,
visibility: ShaderStages::FRAGMENT,
ty: BindingType::Sampler(SamplerBindingType::Filtering),
count: None,
},
],
label: None,
})
}
}
```
### Bevy 0.8 (new)
```rust
impl Material for CustomMaterial {
fn fragment_shader() -> ShaderRef {
"custom_material.wgsl".into()
}
}
#[derive(AsBindGroup, TypeUuid, Debug, Clone)]
#[uuid = "f690fdae-d598-45ab-8225-97e2a3f056e0"]
pub struct CustomMaterial {
#[uniform(0)]
color: Color,
#[texture(1)]
#[sampler(2)]
color_texture: Handle<Image>,
}
```
## Future Work
* Add support for more binding types (cubemaps, buffers, etc). This PR intentionally includes a bare minimum number of binding types to keep "reviewability" in check.
* Consider optionally eliding binding indices using binding names. `AsBindGroup` could pass in (optional?) reflection info as a "hint".
* This would make it possible for the derive to do this:
```rust
#[derive(AsBindGroup)]
pub struct CustomMaterial {
#[uniform]
color: Color,
#[texture]
#[sampler]
color_texture: Option<Handle<Image>>,
alpha_mode: AlphaMode,
}
```
* Or this
```rust
#[derive(AsBindGroup)]
pub struct CustomMaterial {
#[binding]
color: Color,
#[binding]
color_texture: Option<Handle<Image>>,
alpha_mode: AlphaMode,
}
```
* Or even this (if we flip to "include bindings by default")
```rust
#[derive(AsBindGroup)]
pub struct CustomMaterial {
color: Color,
color_texture: Option<Handle<Image>>,
#[binding(ignore)]
alpha_mode: AlphaMode,
}
```
* If we add the option to define custom draw functions for materials (which could be done in a type-erased way), I think that would be enough to support extra non-material bindings. Worth considering!
2022-06-30 23:48:46 +00:00
|
|
|
/// A key uniquely identifying a specialized [`MaterialPipeline`].
|
|
|
|
pub struct MaterialPipelineKey<M: Material> {
|
2022-04-18 10:11:14 +00:00
|
|
|
pub mesh_key: MeshPipelineKey,
|
Better Materials: AsBindGroup trait and derive, simpler Material trait (#5053)
# Objective
This PR reworks Bevy's Material system, making the user experience of defining Materials _much_ nicer. Bevy's previous material system leaves a lot to be desired:
* Materials require manually implementing the `RenderAsset` trait, which involves manually generating the bind group, handling gpu buffer data transfer, looking up image textures, etc. Even the simplest single-texture material involves writing ~80 unnecessary lines of code. This was never the long term plan.
* There are two material traits, which is confusing, hard to document, and often redundant: `Material` and `SpecializedMaterial`. `Material` implicitly implements `SpecializedMaterial`, and `SpecializedMaterial` is used in most high level apis to support both use cases. Most users shouldn't need to think about specialization at all (I consider it a "power-user tool"), so the fact that `SpecializedMaterial` is front-and-center in our apis is a miss.
* Implementing either material trait involves a lot of "type soup". The "prepared asset" parameter is particularly heinous: `&<Self as RenderAsset>::PreparedAsset`. Defining vertex and fragment shaders is also more verbose than it needs to be.
## Solution
Say hello to the new `Material` system:
```rust
#[derive(AsBindGroup, TypeUuid, Debug, Clone)]
#[uuid = "f690fdae-d598-45ab-8225-97e2a3f056e0"]
pub struct CoolMaterial {
#[uniform(0)]
color: Color,
#[texture(1)]
#[sampler(2)]
color_texture: Handle<Image>,
}
impl Material for CoolMaterial {
fn fragment_shader() -> ShaderRef {
"cool_material.wgsl".into()
}
}
```
Thats it! This same material would have required [~80 lines of complicated "type heavy" code](https://github.com/bevyengine/bevy/blob/v0.7.0/examples/shader/shader_material.rs) in the old Material system. Now it is just 14 lines of simple, readable code.
This is thanks to a new consolidated `Material` trait and the new `AsBindGroup` trait / derive.
### The new `Material` trait
The old "split" `Material` and `SpecializedMaterial` traits have been removed in favor of a new consolidated `Material` trait. All of the functions on the trait are optional.
The difficulty of implementing `Material` has been reduced by simplifying dataflow and removing type complexity:
```rust
// Old
impl Material for CustomMaterial {
fn fragment_shader(asset_server: &AssetServer) -> Option<Handle<Shader>> {
Some(asset_server.load("custom_material.wgsl"))
}
fn alpha_mode(render_asset: &<Self as RenderAsset>::PreparedAsset) -> AlphaMode {
render_asset.alpha_mode
}
}
// New
impl Material for CustomMaterial {
fn fragment_shader() -> ShaderRef {
"custom_material.wgsl".into()
}
fn alpha_mode(&self) -> AlphaMode {
self.alpha_mode
}
}
```
Specialization is still supported, but it is hidden by default under the `specialize()` function (more on this later).
### The `AsBindGroup` trait / derive
The `Material` trait now requires the `AsBindGroup` derive. This can be implemented manually relatively easily, but deriving it will almost always be preferable.
Field attributes like `uniform` and `texture` are used to define which fields should be bindings,
what their binding type is, and what index they should be bound at:
```rust
#[derive(AsBindGroup)]
struct CoolMaterial {
#[uniform(0)]
color: Color,
#[texture(1)]
#[sampler(2)]
color_texture: Handle<Image>,
}
```
In WGSL shaders, the binding looks like this:
```wgsl
struct CoolMaterial {
color: vec4<f32>;
};
[[group(1), binding(0)]]
var<uniform> material: CoolMaterial;
[[group(1), binding(1)]]
var color_texture: texture_2d<f32>;
[[group(1), binding(2)]]
var color_sampler: sampler;
```
Note that the "group" index is determined by the usage context. It is not defined in `AsBindGroup`. Bevy material bind groups are bound to group 1.
The following field-level attributes are supported:
* `uniform(BINDING_INDEX)`
* The field will be converted to a shader-compatible type using the `ShaderType` trait, written to a `Buffer`, and bound as a uniform. It can also be derived for custom structs.
* `texture(BINDING_INDEX)`
* This field's `Handle<Image>` will be used to look up the matching `Texture` gpu resource, which will be bound as a texture in shaders. The field will be assumed to implement `Into<Option<Handle<Image>>>`. In practice, most fields should be a `Handle<Image>` or `Option<Handle<Image>>`. If the value of an `Option<Handle<Image>>` is `None`, the new `FallbackImage` resource will be used instead. This attribute can be used in conjunction with a `sampler` binding attribute (with a different binding index).
* `sampler(BINDING_INDEX)`
* Behaves exactly like the `texture` attribute, but sets the Image's sampler binding instead of the texture.
Note that fields without field-level binding attributes will be ignored.
```rust
#[derive(AsBindGroup)]
struct CoolMaterial {
#[uniform(0)]
color: Color,
this_field_is_ignored: String,
}
```
As mentioned above, `Option<Handle<Image>>` is also supported:
```rust
#[derive(AsBindGroup)]
struct CoolMaterial {
#[uniform(0)]
color: Color,
#[texture(1)]
#[sampler(2)]
color_texture: Option<Handle<Image>>,
}
```
This is useful if you want a texture to be optional. When the value is `None`, the `FallbackImage` will be used for the binding instead, which defaults to "pure white".
Field uniforms with the same binding index will be combined into a single binding:
```rust
#[derive(AsBindGroup)]
struct CoolMaterial {
#[uniform(0)]
color: Color,
#[uniform(0)]
roughness: f32,
}
```
In WGSL shaders, the binding would look like this:
```wgsl
struct CoolMaterial {
color: vec4<f32>;
roughness: f32;
};
[[group(1), binding(0)]]
var<uniform> material: CoolMaterial;
```
Some less common scenarios will require "struct-level" attributes. These are the currently supported struct-level attributes:
* `uniform(BINDING_INDEX, ConvertedShaderType)`
* Similar to the field-level `uniform` attribute, but instead the entire `AsBindGroup` value is converted to `ConvertedShaderType`, which must implement `ShaderType`. This is useful if more complicated conversion logic is required.
* `bind_group_data(DataType)`
* The `AsBindGroup` type will be converted to some `DataType` using `Into<DataType>` and stored as `AsBindGroup::Data` as part of the `AsBindGroup::as_bind_group` call. This is useful if data needs to be stored alongside the generated bind group, such as a unique identifier for a material's bind group. The most common use case for this attribute is "shader pipeline specialization".
The previous `CoolMaterial` example illustrating "combining multiple field-level uniform attributes with the same binding index" can
also be equivalently represented with a single struct-level uniform attribute:
```rust
#[derive(AsBindGroup)]
#[uniform(0, CoolMaterialUniform)]
struct CoolMaterial {
color: Color,
roughness: f32,
}
#[derive(ShaderType)]
struct CoolMaterialUniform {
color: Color,
roughness: f32,
}
impl From<&CoolMaterial> for CoolMaterialUniform {
fn from(material: &CoolMaterial) -> CoolMaterialUniform {
CoolMaterialUniform {
color: material.color,
roughness: material.roughness,
}
}
}
```
### Material Specialization
Material shader specialization is now _much_ simpler:
```rust
#[derive(AsBindGroup, TypeUuid, Debug, Clone)]
#[uuid = "f690fdae-d598-45ab-8225-97e2a3f056e0"]
#[bind_group_data(CoolMaterialKey)]
struct CoolMaterial {
#[uniform(0)]
color: Color,
is_red: bool,
}
#[derive(Copy, Clone, Hash, Eq, PartialEq)]
struct CoolMaterialKey {
is_red: bool,
}
impl From<&CoolMaterial> for CoolMaterialKey {
fn from(material: &CoolMaterial) -> CoolMaterialKey {
CoolMaterialKey {
is_red: material.is_red,
}
}
}
impl Material for CoolMaterial {
fn fragment_shader() -> ShaderRef {
"cool_material.wgsl".into()
}
fn specialize(
pipeline: &MaterialPipeline<Self>,
descriptor: &mut RenderPipelineDescriptor,
layout: &MeshVertexBufferLayout,
key: MaterialPipelineKey<Self>,
) -> Result<(), SpecializedMeshPipelineError> {
if key.bind_group_data.is_red {
let fragment = descriptor.fragment.as_mut().unwrap();
fragment.shader_defs.push("IS_RED".to_string());
}
Ok(())
}
}
```
Setting `bind_group_data` is not required for specialization (it defaults to `()`). Scenarios like "custom vertex attributes" also benefit from this system:
```rust
impl Material for CustomMaterial {
fn vertex_shader() -> ShaderRef {
"custom_material.wgsl".into()
}
fn fragment_shader() -> ShaderRef {
"custom_material.wgsl".into()
}
fn specialize(
pipeline: &MaterialPipeline<Self>,
descriptor: &mut RenderPipelineDescriptor,
layout: &MeshVertexBufferLayout,
key: MaterialPipelineKey<Self>,
) -> Result<(), SpecializedMeshPipelineError> {
let vertex_layout = layout.get_layout(&[
Mesh::ATTRIBUTE_POSITION.at_shader_location(0),
ATTRIBUTE_BLEND_COLOR.at_shader_location(1),
])?;
descriptor.vertex.buffers = vec![vertex_layout];
Ok(())
}
}
```
### Ported `StandardMaterial` to the new `Material` system
Bevy's built-in PBR material uses the new Material system (including the AsBindGroup derive):
```rust
#[derive(AsBindGroup, Debug, Clone, TypeUuid)]
#[uuid = "7494888b-c082-457b-aacf-517228cc0c22"]
#[bind_group_data(StandardMaterialKey)]
#[uniform(0, StandardMaterialUniform)]
pub struct StandardMaterial {
pub base_color: Color,
#[texture(1)]
#[sampler(2)]
pub base_color_texture: Option<Handle<Image>>,
/* other fields omitted for brevity */
```
### Ported Bevy examples to the new `Material` system
The overall complexity of Bevy's "custom shader examples" has gone down significantly. Take a look at the diffs if you want a dopamine spike.
Please note that while this PR has a net increase in "lines of code", most of those extra lines come from added documentation. There is a significant reduction
in the overall complexity of the code (even accounting for the new derive logic).
---
## Changelog
### Added
* `AsBindGroup` trait and derive, which make it much easier to transfer data to the gpu and generate bind groups for a given type.
### Changed
* The old `Material` and `SpecializedMaterial` traits have been replaced by a consolidated (much simpler) `Material` trait. Materials no longer implement `RenderAsset`.
* `StandardMaterial` was ported to the new material system. There are no user-facing api changes to the `StandardMaterial` struct api, but it now implements `AsBindGroup` and `Material` instead of `RenderAsset` and `SpecializedMaterial`.
## Migration Guide
The Material system has been reworked to be much simpler. We've removed a lot of boilerplate with the new `AsBindGroup` derive and the `Material` trait is simpler as well!
### Bevy 0.7 (old)
```rust
#[derive(Debug, Clone, TypeUuid)]
#[uuid = "f690fdae-d598-45ab-8225-97e2a3f056e0"]
pub struct CustomMaterial {
color: Color,
color_texture: Handle<Image>,
}
#[derive(Clone)]
pub struct GpuCustomMaterial {
_buffer: Buffer,
bind_group: BindGroup,
}
impl RenderAsset for CustomMaterial {
type ExtractedAsset = CustomMaterial;
type PreparedAsset = GpuCustomMaterial;
type Param = (SRes<RenderDevice>, SRes<MaterialPipeline<Self>>);
fn extract_asset(&self) -> Self::ExtractedAsset {
self.clone()
}
fn prepare_asset(
extracted_asset: Self::ExtractedAsset,
(render_device, material_pipeline): &mut SystemParamItem<Self::Param>,
) -> Result<Self::PreparedAsset, PrepareAssetError<Self::ExtractedAsset>> {
let color = Vec4::from_slice(&extracted_asset.color.as_linear_rgba_f32());
let byte_buffer = [0u8; Vec4::SIZE.get() as usize];
let mut buffer = encase::UniformBuffer::new(byte_buffer);
buffer.write(&color).unwrap();
let buffer = render_device.create_buffer_with_data(&BufferInitDescriptor {
contents: buffer.as_ref(),
label: None,
usage: BufferUsages::UNIFORM | BufferUsages::COPY_DST,
});
let (texture_view, texture_sampler) = if let Some(result) = material_pipeline
.mesh_pipeline
.get_image_texture(gpu_images, &Some(extracted_asset.color_texture.clone()))
{
result
} else {
return Err(PrepareAssetError::RetryNextUpdate(extracted_asset));
};
let bind_group = render_device.create_bind_group(&BindGroupDescriptor {
entries: &[
BindGroupEntry {
binding: 0,
resource: buffer.as_entire_binding(),
},
BindGroupEntry {
binding: 0,
resource: BindingResource::TextureView(texture_view),
},
BindGroupEntry {
binding: 1,
resource: BindingResource::Sampler(texture_sampler),
},
],
label: None,
layout: &material_pipeline.material_layout,
});
Ok(GpuCustomMaterial {
_buffer: buffer,
bind_group,
})
}
}
impl Material for CustomMaterial {
fn fragment_shader(asset_server: &AssetServer) -> Option<Handle<Shader>> {
Some(asset_server.load("custom_material.wgsl"))
}
fn bind_group(render_asset: &<Self as RenderAsset>::PreparedAsset) -> &BindGroup {
&render_asset.bind_group
}
fn bind_group_layout(render_device: &RenderDevice) -> BindGroupLayout {
render_device.create_bind_group_layout(&BindGroupLayoutDescriptor {
entries: &[
BindGroupLayoutEntry {
binding: 0,
visibility: ShaderStages::FRAGMENT,
ty: BindingType::Buffer {
ty: BufferBindingType::Uniform,
has_dynamic_offset: false,
min_binding_size: Some(Vec4::min_size()),
},
count: None,
},
BindGroupLayoutEntry {
binding: 1,
visibility: ShaderStages::FRAGMENT,
ty: BindingType::Texture {
multisampled: false,
sample_type: TextureSampleType::Float { filterable: true },
view_dimension: TextureViewDimension::D2Array,
},
count: None,
},
BindGroupLayoutEntry {
binding: 2,
visibility: ShaderStages::FRAGMENT,
ty: BindingType::Sampler(SamplerBindingType::Filtering),
count: None,
},
],
label: None,
})
}
}
```
### Bevy 0.8 (new)
```rust
impl Material for CustomMaterial {
fn fragment_shader() -> ShaderRef {
"custom_material.wgsl".into()
}
}
#[derive(AsBindGroup, TypeUuid, Debug, Clone)]
#[uuid = "f690fdae-d598-45ab-8225-97e2a3f056e0"]
pub struct CustomMaterial {
#[uniform(0)]
color: Color,
#[texture(1)]
#[sampler(2)]
color_texture: Handle<Image>,
}
```
## Future Work
* Add support for more binding types (cubemaps, buffers, etc). This PR intentionally includes a bare minimum number of binding types to keep "reviewability" in check.
* Consider optionally eliding binding indices using binding names. `AsBindGroup` could pass in (optional?) reflection info as a "hint".
* This would make it possible for the derive to do this:
```rust
#[derive(AsBindGroup)]
pub struct CustomMaterial {
#[uniform]
color: Color,
#[texture]
#[sampler]
color_texture: Option<Handle<Image>>,
alpha_mode: AlphaMode,
}
```
* Or this
```rust
#[derive(AsBindGroup)]
pub struct CustomMaterial {
#[binding]
color: Color,
#[binding]
color_texture: Option<Handle<Image>>,
alpha_mode: AlphaMode,
}
```
* Or even this (if we flip to "include bindings by default")
```rust
#[derive(AsBindGroup)]
pub struct CustomMaterial {
color: Color,
color_texture: Option<Handle<Image>>,
#[binding(ignore)]
alpha_mode: AlphaMode,
}
```
* If we add the option to define custom draw functions for materials (which could be done in a type-erased way), I think that would be enough to support extra non-material bindings. Worth considering!
2022-06-30 23:48:46 +00:00
|
|
|
pub bind_group_data: M::Data,
|
|
|
|
}
|
|
|
|
|
|
|
|
impl<M: Material> Eq for MaterialPipelineKey<M> where M::Data: PartialEq {}
|
|
|
|
|
|
|
|
impl<M: Material> PartialEq for MaterialPipelineKey<M>
|
|
|
|
where
|
|
|
|
M::Data: PartialEq,
|
|
|
|
{
|
|
|
|
fn eq(&self, other: &Self) -> bool {
|
|
|
|
self.mesh_key == other.mesh_key && self.bind_group_data == other.bind_group_data
|
|
|
|
}
|
|
|
|
}
|
|
|
|
|
|
|
|
impl<M: Material> Clone for MaterialPipelineKey<M>
|
|
|
|
where
|
|
|
|
M::Data: Clone,
|
|
|
|
{
|
|
|
|
fn clone(&self) -> Self {
|
|
|
|
Self {
|
|
|
|
mesh_key: self.mesh_key,
|
|
|
|
bind_group_data: self.bind_group_data.clone(),
|
|
|
|
}
|
|
|
|
}
|
|
|
|
}
|
|
|
|
|
|
|
|
impl<M: Material> Hash for MaterialPipelineKey<M>
|
|
|
|
where
|
|
|
|
M::Data: Hash,
|
|
|
|
{
|
2024-09-27 00:59:59 +00:00
|
|
|
fn hash<H: core::hash::Hasher>(&self, state: &mut H) {
|
Better Materials: AsBindGroup trait and derive, simpler Material trait (#5053)
# Objective
This PR reworks Bevy's Material system, making the user experience of defining Materials _much_ nicer. Bevy's previous material system leaves a lot to be desired:
* Materials require manually implementing the `RenderAsset` trait, which involves manually generating the bind group, handling gpu buffer data transfer, looking up image textures, etc. Even the simplest single-texture material involves writing ~80 unnecessary lines of code. This was never the long term plan.
* There are two material traits, which is confusing, hard to document, and often redundant: `Material` and `SpecializedMaterial`. `Material` implicitly implements `SpecializedMaterial`, and `SpecializedMaterial` is used in most high level apis to support both use cases. Most users shouldn't need to think about specialization at all (I consider it a "power-user tool"), so the fact that `SpecializedMaterial` is front-and-center in our apis is a miss.
* Implementing either material trait involves a lot of "type soup". The "prepared asset" parameter is particularly heinous: `&<Self as RenderAsset>::PreparedAsset`. Defining vertex and fragment shaders is also more verbose than it needs to be.
## Solution
Say hello to the new `Material` system:
```rust
#[derive(AsBindGroup, TypeUuid, Debug, Clone)]
#[uuid = "f690fdae-d598-45ab-8225-97e2a3f056e0"]
pub struct CoolMaterial {
#[uniform(0)]
color: Color,
#[texture(1)]
#[sampler(2)]
color_texture: Handle<Image>,
}
impl Material for CoolMaterial {
fn fragment_shader() -> ShaderRef {
"cool_material.wgsl".into()
}
}
```
Thats it! This same material would have required [~80 lines of complicated "type heavy" code](https://github.com/bevyengine/bevy/blob/v0.7.0/examples/shader/shader_material.rs) in the old Material system. Now it is just 14 lines of simple, readable code.
This is thanks to a new consolidated `Material` trait and the new `AsBindGroup` trait / derive.
### The new `Material` trait
The old "split" `Material` and `SpecializedMaterial` traits have been removed in favor of a new consolidated `Material` trait. All of the functions on the trait are optional.
The difficulty of implementing `Material` has been reduced by simplifying dataflow and removing type complexity:
```rust
// Old
impl Material for CustomMaterial {
fn fragment_shader(asset_server: &AssetServer) -> Option<Handle<Shader>> {
Some(asset_server.load("custom_material.wgsl"))
}
fn alpha_mode(render_asset: &<Self as RenderAsset>::PreparedAsset) -> AlphaMode {
render_asset.alpha_mode
}
}
// New
impl Material for CustomMaterial {
fn fragment_shader() -> ShaderRef {
"custom_material.wgsl".into()
}
fn alpha_mode(&self) -> AlphaMode {
self.alpha_mode
}
}
```
Specialization is still supported, but it is hidden by default under the `specialize()` function (more on this later).
### The `AsBindGroup` trait / derive
The `Material` trait now requires the `AsBindGroup` derive. This can be implemented manually relatively easily, but deriving it will almost always be preferable.
Field attributes like `uniform` and `texture` are used to define which fields should be bindings,
what their binding type is, and what index they should be bound at:
```rust
#[derive(AsBindGroup)]
struct CoolMaterial {
#[uniform(0)]
color: Color,
#[texture(1)]
#[sampler(2)]
color_texture: Handle<Image>,
}
```
In WGSL shaders, the binding looks like this:
```wgsl
struct CoolMaterial {
color: vec4<f32>;
};
[[group(1), binding(0)]]
var<uniform> material: CoolMaterial;
[[group(1), binding(1)]]
var color_texture: texture_2d<f32>;
[[group(1), binding(2)]]
var color_sampler: sampler;
```
Note that the "group" index is determined by the usage context. It is not defined in `AsBindGroup`. Bevy material bind groups are bound to group 1.
The following field-level attributes are supported:
* `uniform(BINDING_INDEX)`
* The field will be converted to a shader-compatible type using the `ShaderType` trait, written to a `Buffer`, and bound as a uniform. It can also be derived for custom structs.
* `texture(BINDING_INDEX)`
* This field's `Handle<Image>` will be used to look up the matching `Texture` gpu resource, which will be bound as a texture in shaders. The field will be assumed to implement `Into<Option<Handle<Image>>>`. In practice, most fields should be a `Handle<Image>` or `Option<Handle<Image>>`. If the value of an `Option<Handle<Image>>` is `None`, the new `FallbackImage` resource will be used instead. This attribute can be used in conjunction with a `sampler` binding attribute (with a different binding index).
* `sampler(BINDING_INDEX)`
* Behaves exactly like the `texture` attribute, but sets the Image's sampler binding instead of the texture.
Note that fields without field-level binding attributes will be ignored.
```rust
#[derive(AsBindGroup)]
struct CoolMaterial {
#[uniform(0)]
color: Color,
this_field_is_ignored: String,
}
```
As mentioned above, `Option<Handle<Image>>` is also supported:
```rust
#[derive(AsBindGroup)]
struct CoolMaterial {
#[uniform(0)]
color: Color,
#[texture(1)]
#[sampler(2)]
color_texture: Option<Handle<Image>>,
}
```
This is useful if you want a texture to be optional. When the value is `None`, the `FallbackImage` will be used for the binding instead, which defaults to "pure white".
Field uniforms with the same binding index will be combined into a single binding:
```rust
#[derive(AsBindGroup)]
struct CoolMaterial {
#[uniform(0)]
color: Color,
#[uniform(0)]
roughness: f32,
}
```
In WGSL shaders, the binding would look like this:
```wgsl
struct CoolMaterial {
color: vec4<f32>;
roughness: f32;
};
[[group(1), binding(0)]]
var<uniform> material: CoolMaterial;
```
Some less common scenarios will require "struct-level" attributes. These are the currently supported struct-level attributes:
* `uniform(BINDING_INDEX, ConvertedShaderType)`
* Similar to the field-level `uniform` attribute, but instead the entire `AsBindGroup` value is converted to `ConvertedShaderType`, which must implement `ShaderType`. This is useful if more complicated conversion logic is required.
* `bind_group_data(DataType)`
* The `AsBindGroup` type will be converted to some `DataType` using `Into<DataType>` and stored as `AsBindGroup::Data` as part of the `AsBindGroup::as_bind_group` call. This is useful if data needs to be stored alongside the generated bind group, such as a unique identifier for a material's bind group. The most common use case for this attribute is "shader pipeline specialization".
The previous `CoolMaterial` example illustrating "combining multiple field-level uniform attributes with the same binding index" can
also be equivalently represented with a single struct-level uniform attribute:
```rust
#[derive(AsBindGroup)]
#[uniform(0, CoolMaterialUniform)]
struct CoolMaterial {
color: Color,
roughness: f32,
}
#[derive(ShaderType)]
struct CoolMaterialUniform {
color: Color,
roughness: f32,
}
impl From<&CoolMaterial> for CoolMaterialUniform {
fn from(material: &CoolMaterial) -> CoolMaterialUniform {
CoolMaterialUniform {
color: material.color,
roughness: material.roughness,
}
}
}
```
### Material Specialization
Material shader specialization is now _much_ simpler:
```rust
#[derive(AsBindGroup, TypeUuid, Debug, Clone)]
#[uuid = "f690fdae-d598-45ab-8225-97e2a3f056e0"]
#[bind_group_data(CoolMaterialKey)]
struct CoolMaterial {
#[uniform(0)]
color: Color,
is_red: bool,
}
#[derive(Copy, Clone, Hash, Eq, PartialEq)]
struct CoolMaterialKey {
is_red: bool,
}
impl From<&CoolMaterial> for CoolMaterialKey {
fn from(material: &CoolMaterial) -> CoolMaterialKey {
CoolMaterialKey {
is_red: material.is_red,
}
}
}
impl Material for CoolMaterial {
fn fragment_shader() -> ShaderRef {
"cool_material.wgsl".into()
}
fn specialize(
pipeline: &MaterialPipeline<Self>,
descriptor: &mut RenderPipelineDescriptor,
layout: &MeshVertexBufferLayout,
key: MaterialPipelineKey<Self>,
) -> Result<(), SpecializedMeshPipelineError> {
if key.bind_group_data.is_red {
let fragment = descriptor.fragment.as_mut().unwrap();
fragment.shader_defs.push("IS_RED".to_string());
}
Ok(())
}
}
```
Setting `bind_group_data` is not required for specialization (it defaults to `()`). Scenarios like "custom vertex attributes" also benefit from this system:
```rust
impl Material for CustomMaterial {
fn vertex_shader() -> ShaderRef {
"custom_material.wgsl".into()
}
fn fragment_shader() -> ShaderRef {
"custom_material.wgsl".into()
}
fn specialize(
pipeline: &MaterialPipeline<Self>,
descriptor: &mut RenderPipelineDescriptor,
layout: &MeshVertexBufferLayout,
key: MaterialPipelineKey<Self>,
) -> Result<(), SpecializedMeshPipelineError> {
let vertex_layout = layout.get_layout(&[
Mesh::ATTRIBUTE_POSITION.at_shader_location(0),
ATTRIBUTE_BLEND_COLOR.at_shader_location(1),
])?;
descriptor.vertex.buffers = vec![vertex_layout];
Ok(())
}
}
```
### Ported `StandardMaterial` to the new `Material` system
Bevy's built-in PBR material uses the new Material system (including the AsBindGroup derive):
```rust
#[derive(AsBindGroup, Debug, Clone, TypeUuid)]
#[uuid = "7494888b-c082-457b-aacf-517228cc0c22"]
#[bind_group_data(StandardMaterialKey)]
#[uniform(0, StandardMaterialUniform)]
pub struct StandardMaterial {
pub base_color: Color,
#[texture(1)]
#[sampler(2)]
pub base_color_texture: Option<Handle<Image>>,
/* other fields omitted for brevity */
```
### Ported Bevy examples to the new `Material` system
The overall complexity of Bevy's "custom shader examples" has gone down significantly. Take a look at the diffs if you want a dopamine spike.
Please note that while this PR has a net increase in "lines of code", most of those extra lines come from added documentation. There is a significant reduction
in the overall complexity of the code (even accounting for the new derive logic).
---
## Changelog
### Added
* `AsBindGroup` trait and derive, which make it much easier to transfer data to the gpu and generate bind groups for a given type.
### Changed
* The old `Material` and `SpecializedMaterial` traits have been replaced by a consolidated (much simpler) `Material` trait. Materials no longer implement `RenderAsset`.
* `StandardMaterial` was ported to the new material system. There are no user-facing api changes to the `StandardMaterial` struct api, but it now implements `AsBindGroup` and `Material` instead of `RenderAsset` and `SpecializedMaterial`.
## Migration Guide
The Material system has been reworked to be much simpler. We've removed a lot of boilerplate with the new `AsBindGroup` derive and the `Material` trait is simpler as well!
### Bevy 0.7 (old)
```rust
#[derive(Debug, Clone, TypeUuid)]
#[uuid = "f690fdae-d598-45ab-8225-97e2a3f056e0"]
pub struct CustomMaterial {
color: Color,
color_texture: Handle<Image>,
}
#[derive(Clone)]
pub struct GpuCustomMaterial {
_buffer: Buffer,
bind_group: BindGroup,
}
impl RenderAsset for CustomMaterial {
type ExtractedAsset = CustomMaterial;
type PreparedAsset = GpuCustomMaterial;
type Param = (SRes<RenderDevice>, SRes<MaterialPipeline<Self>>);
fn extract_asset(&self) -> Self::ExtractedAsset {
self.clone()
}
fn prepare_asset(
extracted_asset: Self::ExtractedAsset,
(render_device, material_pipeline): &mut SystemParamItem<Self::Param>,
) -> Result<Self::PreparedAsset, PrepareAssetError<Self::ExtractedAsset>> {
let color = Vec4::from_slice(&extracted_asset.color.as_linear_rgba_f32());
let byte_buffer = [0u8; Vec4::SIZE.get() as usize];
let mut buffer = encase::UniformBuffer::new(byte_buffer);
buffer.write(&color).unwrap();
let buffer = render_device.create_buffer_with_data(&BufferInitDescriptor {
contents: buffer.as_ref(),
label: None,
usage: BufferUsages::UNIFORM | BufferUsages::COPY_DST,
});
let (texture_view, texture_sampler) = if let Some(result) = material_pipeline
.mesh_pipeline
.get_image_texture(gpu_images, &Some(extracted_asset.color_texture.clone()))
{
result
} else {
return Err(PrepareAssetError::RetryNextUpdate(extracted_asset));
};
let bind_group = render_device.create_bind_group(&BindGroupDescriptor {
entries: &[
BindGroupEntry {
binding: 0,
resource: buffer.as_entire_binding(),
},
BindGroupEntry {
binding: 0,
resource: BindingResource::TextureView(texture_view),
},
BindGroupEntry {
binding: 1,
resource: BindingResource::Sampler(texture_sampler),
},
],
label: None,
layout: &material_pipeline.material_layout,
});
Ok(GpuCustomMaterial {
_buffer: buffer,
bind_group,
})
}
}
impl Material for CustomMaterial {
fn fragment_shader(asset_server: &AssetServer) -> Option<Handle<Shader>> {
Some(asset_server.load("custom_material.wgsl"))
}
fn bind_group(render_asset: &<Self as RenderAsset>::PreparedAsset) -> &BindGroup {
&render_asset.bind_group
}
fn bind_group_layout(render_device: &RenderDevice) -> BindGroupLayout {
render_device.create_bind_group_layout(&BindGroupLayoutDescriptor {
entries: &[
BindGroupLayoutEntry {
binding: 0,
visibility: ShaderStages::FRAGMENT,
ty: BindingType::Buffer {
ty: BufferBindingType::Uniform,
has_dynamic_offset: false,
min_binding_size: Some(Vec4::min_size()),
},
count: None,
},
BindGroupLayoutEntry {
binding: 1,
visibility: ShaderStages::FRAGMENT,
ty: BindingType::Texture {
multisampled: false,
sample_type: TextureSampleType::Float { filterable: true },
view_dimension: TextureViewDimension::D2Array,
},
count: None,
},
BindGroupLayoutEntry {
binding: 2,
visibility: ShaderStages::FRAGMENT,
ty: BindingType::Sampler(SamplerBindingType::Filtering),
count: None,
},
],
label: None,
})
}
}
```
### Bevy 0.8 (new)
```rust
impl Material for CustomMaterial {
fn fragment_shader() -> ShaderRef {
"custom_material.wgsl".into()
}
}
#[derive(AsBindGroup, TypeUuid, Debug, Clone)]
#[uuid = "f690fdae-d598-45ab-8225-97e2a3f056e0"]
pub struct CustomMaterial {
#[uniform(0)]
color: Color,
#[texture(1)]
#[sampler(2)]
color_texture: Handle<Image>,
}
```
## Future Work
* Add support for more binding types (cubemaps, buffers, etc). This PR intentionally includes a bare minimum number of binding types to keep "reviewability" in check.
* Consider optionally eliding binding indices using binding names. `AsBindGroup` could pass in (optional?) reflection info as a "hint".
* This would make it possible for the derive to do this:
```rust
#[derive(AsBindGroup)]
pub struct CustomMaterial {
#[uniform]
color: Color,
#[texture]
#[sampler]
color_texture: Option<Handle<Image>>,
alpha_mode: AlphaMode,
}
```
* Or this
```rust
#[derive(AsBindGroup)]
pub struct CustomMaterial {
#[binding]
color: Color,
#[binding]
color_texture: Option<Handle<Image>>,
alpha_mode: AlphaMode,
}
```
* Or even this (if we flip to "include bindings by default")
```rust
#[derive(AsBindGroup)]
pub struct CustomMaterial {
color: Color,
color_texture: Option<Handle<Image>>,
#[binding(ignore)]
alpha_mode: AlphaMode,
}
```
* If we add the option to define custom draw functions for materials (which could be done in a type-erased way), I think that would be enough to support extra non-material bindings. Worth considering!
2022-06-30 23:48:46 +00:00
|
|
|
self.mesh_key.hash(state);
|
|
|
|
self.bind_group_data.hash(state);
|
|
|
|
}
|
Mesh vertex buffer layouts (#3959)
This PR makes a number of changes to how meshes and vertex attributes are handled, which the goal of enabling easy and flexible custom vertex attributes:
* Reworks the `Mesh` type to use the newly added `VertexAttribute` internally
* `VertexAttribute` defines the name, a unique `VertexAttributeId`, and a `VertexFormat`
* `VertexAttributeId` is used to produce consistent sort orders for vertex buffer generation, replacing the more expensive and often surprising "name based sorting"
* Meshes can be used to generate a `MeshVertexBufferLayout`, which defines the layout of the gpu buffer produced by the mesh. `MeshVertexBufferLayouts` can then be used to generate actual `VertexBufferLayouts` according to the requirements of a specific pipeline. This decoupling of "mesh layout" vs "pipeline vertex buffer layout" is what enables custom attributes. We don't need to standardize _mesh layouts_ or contort meshes to meet the needs of a specific pipeline. As long as the mesh has what the pipeline needs, it will work transparently.
* Mesh-based pipelines now specialize on `&MeshVertexBufferLayout` via the new `SpecializedMeshPipeline` trait (which behaves like `SpecializedPipeline`, but adds `&MeshVertexBufferLayout`). The integrity of the pipeline cache is maintained because the `MeshVertexBufferLayout` is treated as part of the key (which is fully abstracted from implementers of the trait ... no need to add any additional info to the specialization key).
* Hashing `MeshVertexBufferLayout` is too expensive to do for every entity, every frame. To make this scalable, I added a generalized "pre-hashing" solution to `bevy_utils`: `Hashed<T>` keys and `PreHashMap<K, V>` (which uses `Hashed<T>` internally) . Why didn't I just do the quick and dirty in-place "pre-compute hash and use that u64 as a key in a hashmap" that we've done in the past? Because its wrong! Hashes by themselves aren't enough because two different values can produce the same hash. Re-hashing a hash is even worse! I decided to build a generalized solution because this pattern has come up in the past and we've chosen to do the wrong thing. Now we can do the right thing! This did unfortunately require pulling in `hashbrown` and using that in `bevy_utils`, because avoiding re-hashes requires the `raw_entry_mut` api, which isn't stabilized yet (and may never be ... `entry_ref` has favor now, but also isn't available yet). If std's HashMap ever provides the tools we need, we can move back to that. Note that adding `hashbrown` doesn't increase our dependency count because it was already in our tree. I will probably break these changes out into their own PR.
* Specializing on `MeshVertexBufferLayout` has one non-obvious behavior: it can produce identical pipelines for two different MeshVertexBufferLayouts. To optimize the number of active pipelines / reduce re-binds while drawing, I de-duplicate pipelines post-specialization using the final `VertexBufferLayout` as the key. For example, consider a pipeline that needs the layout `(position, normal)` and is specialized using two meshes: `(position, normal, uv)` and `(position, normal, other_vec2)`. If both of these meshes result in `(position, normal)` specializations, we can use the same pipeline! Now we do. Cool!
To briefly illustrate, this is what the relevant section of `MeshPipeline`'s specialization code looks like now:
```rust
impl SpecializedMeshPipeline for MeshPipeline {
type Key = MeshPipelineKey;
fn specialize(
&self,
key: Self::Key,
layout: &MeshVertexBufferLayout,
) -> RenderPipelineDescriptor {
let mut vertex_attributes = vec![
Mesh::ATTRIBUTE_POSITION.at_shader_location(0),
Mesh::ATTRIBUTE_NORMAL.at_shader_location(1),
Mesh::ATTRIBUTE_UV_0.at_shader_location(2),
];
let mut shader_defs = Vec::new();
if layout.contains(Mesh::ATTRIBUTE_TANGENT) {
shader_defs.push(String::from("VERTEX_TANGENTS"));
vertex_attributes.push(Mesh::ATTRIBUTE_TANGENT.at_shader_location(3));
}
let vertex_buffer_layout = layout
.get_layout(&vertex_attributes)
.expect("Mesh is missing a vertex attribute");
```
Notice that this is _much_ simpler than it was before. And now any mesh with any layout can be used with this pipeline, provided it has vertex postions, normals, and uvs. We even got to remove `HAS_TANGENTS` from MeshPipelineKey and `has_tangents` from `GpuMesh`, because that information is redundant with `MeshVertexBufferLayout`.
This is still a draft because I still need to:
* Add more docs
* Experiment with adding error handling to mesh pipeline specialization (which would print errors at runtime when a mesh is missing a vertex attribute required by a pipeline). If it doesn't tank perf, we'll keep it.
* Consider breaking out the PreHash / hashbrown changes into a separate PR.
* Add an example illustrating this change
* Verify that the "mesh-specialized pipeline de-duplication code" works properly
Please dont yell at me for not doing these things yet :) Just trying to get this in peoples' hands asap.
Alternative to #3120
Fixes #3030
Co-authored-by: Carter Anderson <mcanders1@gmail.com>
2022-02-23 23:21:13 +00:00
|
|
|
}
|
|
|
|
|
Better Materials: AsBindGroup trait and derive, simpler Material trait (#5053)
# Objective
This PR reworks Bevy's Material system, making the user experience of defining Materials _much_ nicer. Bevy's previous material system leaves a lot to be desired:
* Materials require manually implementing the `RenderAsset` trait, which involves manually generating the bind group, handling gpu buffer data transfer, looking up image textures, etc. Even the simplest single-texture material involves writing ~80 unnecessary lines of code. This was never the long term plan.
* There are two material traits, which is confusing, hard to document, and often redundant: `Material` and `SpecializedMaterial`. `Material` implicitly implements `SpecializedMaterial`, and `SpecializedMaterial` is used in most high level apis to support both use cases. Most users shouldn't need to think about specialization at all (I consider it a "power-user tool"), so the fact that `SpecializedMaterial` is front-and-center in our apis is a miss.
* Implementing either material trait involves a lot of "type soup". The "prepared asset" parameter is particularly heinous: `&<Self as RenderAsset>::PreparedAsset`. Defining vertex and fragment shaders is also more verbose than it needs to be.
## Solution
Say hello to the new `Material` system:
```rust
#[derive(AsBindGroup, TypeUuid, Debug, Clone)]
#[uuid = "f690fdae-d598-45ab-8225-97e2a3f056e0"]
pub struct CoolMaterial {
#[uniform(0)]
color: Color,
#[texture(1)]
#[sampler(2)]
color_texture: Handle<Image>,
}
impl Material for CoolMaterial {
fn fragment_shader() -> ShaderRef {
"cool_material.wgsl".into()
}
}
```
Thats it! This same material would have required [~80 lines of complicated "type heavy" code](https://github.com/bevyengine/bevy/blob/v0.7.0/examples/shader/shader_material.rs) in the old Material system. Now it is just 14 lines of simple, readable code.
This is thanks to a new consolidated `Material` trait and the new `AsBindGroup` trait / derive.
### The new `Material` trait
The old "split" `Material` and `SpecializedMaterial` traits have been removed in favor of a new consolidated `Material` trait. All of the functions on the trait are optional.
The difficulty of implementing `Material` has been reduced by simplifying dataflow and removing type complexity:
```rust
// Old
impl Material for CustomMaterial {
fn fragment_shader(asset_server: &AssetServer) -> Option<Handle<Shader>> {
Some(asset_server.load("custom_material.wgsl"))
}
fn alpha_mode(render_asset: &<Self as RenderAsset>::PreparedAsset) -> AlphaMode {
render_asset.alpha_mode
}
}
// New
impl Material for CustomMaterial {
fn fragment_shader() -> ShaderRef {
"custom_material.wgsl".into()
}
fn alpha_mode(&self) -> AlphaMode {
self.alpha_mode
}
}
```
Specialization is still supported, but it is hidden by default under the `specialize()` function (more on this later).
### The `AsBindGroup` trait / derive
The `Material` trait now requires the `AsBindGroup` derive. This can be implemented manually relatively easily, but deriving it will almost always be preferable.
Field attributes like `uniform` and `texture` are used to define which fields should be bindings,
what their binding type is, and what index they should be bound at:
```rust
#[derive(AsBindGroup)]
struct CoolMaterial {
#[uniform(0)]
color: Color,
#[texture(1)]
#[sampler(2)]
color_texture: Handle<Image>,
}
```
In WGSL shaders, the binding looks like this:
```wgsl
struct CoolMaterial {
color: vec4<f32>;
};
[[group(1), binding(0)]]
var<uniform> material: CoolMaterial;
[[group(1), binding(1)]]
var color_texture: texture_2d<f32>;
[[group(1), binding(2)]]
var color_sampler: sampler;
```
Note that the "group" index is determined by the usage context. It is not defined in `AsBindGroup`. Bevy material bind groups are bound to group 1.
The following field-level attributes are supported:
* `uniform(BINDING_INDEX)`
* The field will be converted to a shader-compatible type using the `ShaderType` trait, written to a `Buffer`, and bound as a uniform. It can also be derived for custom structs.
* `texture(BINDING_INDEX)`
* This field's `Handle<Image>` will be used to look up the matching `Texture` gpu resource, which will be bound as a texture in shaders. The field will be assumed to implement `Into<Option<Handle<Image>>>`. In practice, most fields should be a `Handle<Image>` or `Option<Handle<Image>>`. If the value of an `Option<Handle<Image>>` is `None`, the new `FallbackImage` resource will be used instead. This attribute can be used in conjunction with a `sampler` binding attribute (with a different binding index).
* `sampler(BINDING_INDEX)`
* Behaves exactly like the `texture` attribute, but sets the Image's sampler binding instead of the texture.
Note that fields without field-level binding attributes will be ignored.
```rust
#[derive(AsBindGroup)]
struct CoolMaterial {
#[uniform(0)]
color: Color,
this_field_is_ignored: String,
}
```
As mentioned above, `Option<Handle<Image>>` is also supported:
```rust
#[derive(AsBindGroup)]
struct CoolMaterial {
#[uniform(0)]
color: Color,
#[texture(1)]
#[sampler(2)]
color_texture: Option<Handle<Image>>,
}
```
This is useful if you want a texture to be optional. When the value is `None`, the `FallbackImage` will be used for the binding instead, which defaults to "pure white".
Field uniforms with the same binding index will be combined into a single binding:
```rust
#[derive(AsBindGroup)]
struct CoolMaterial {
#[uniform(0)]
color: Color,
#[uniform(0)]
roughness: f32,
}
```
In WGSL shaders, the binding would look like this:
```wgsl
struct CoolMaterial {
color: vec4<f32>;
roughness: f32;
};
[[group(1), binding(0)]]
var<uniform> material: CoolMaterial;
```
Some less common scenarios will require "struct-level" attributes. These are the currently supported struct-level attributes:
* `uniform(BINDING_INDEX, ConvertedShaderType)`
* Similar to the field-level `uniform` attribute, but instead the entire `AsBindGroup` value is converted to `ConvertedShaderType`, which must implement `ShaderType`. This is useful if more complicated conversion logic is required.
* `bind_group_data(DataType)`
* The `AsBindGroup` type will be converted to some `DataType` using `Into<DataType>` and stored as `AsBindGroup::Data` as part of the `AsBindGroup::as_bind_group` call. This is useful if data needs to be stored alongside the generated bind group, such as a unique identifier for a material's bind group. The most common use case for this attribute is "shader pipeline specialization".
The previous `CoolMaterial` example illustrating "combining multiple field-level uniform attributes with the same binding index" can
also be equivalently represented with a single struct-level uniform attribute:
```rust
#[derive(AsBindGroup)]
#[uniform(0, CoolMaterialUniform)]
struct CoolMaterial {
color: Color,
roughness: f32,
}
#[derive(ShaderType)]
struct CoolMaterialUniform {
color: Color,
roughness: f32,
}
impl From<&CoolMaterial> for CoolMaterialUniform {
fn from(material: &CoolMaterial) -> CoolMaterialUniform {
CoolMaterialUniform {
color: material.color,
roughness: material.roughness,
}
}
}
```
### Material Specialization
Material shader specialization is now _much_ simpler:
```rust
#[derive(AsBindGroup, TypeUuid, Debug, Clone)]
#[uuid = "f690fdae-d598-45ab-8225-97e2a3f056e0"]
#[bind_group_data(CoolMaterialKey)]
struct CoolMaterial {
#[uniform(0)]
color: Color,
is_red: bool,
}
#[derive(Copy, Clone, Hash, Eq, PartialEq)]
struct CoolMaterialKey {
is_red: bool,
}
impl From<&CoolMaterial> for CoolMaterialKey {
fn from(material: &CoolMaterial) -> CoolMaterialKey {
CoolMaterialKey {
is_red: material.is_red,
}
}
}
impl Material for CoolMaterial {
fn fragment_shader() -> ShaderRef {
"cool_material.wgsl".into()
}
fn specialize(
pipeline: &MaterialPipeline<Self>,
descriptor: &mut RenderPipelineDescriptor,
layout: &MeshVertexBufferLayout,
key: MaterialPipelineKey<Self>,
) -> Result<(), SpecializedMeshPipelineError> {
if key.bind_group_data.is_red {
let fragment = descriptor.fragment.as_mut().unwrap();
fragment.shader_defs.push("IS_RED".to_string());
}
Ok(())
}
}
```
Setting `bind_group_data` is not required for specialization (it defaults to `()`). Scenarios like "custom vertex attributes" also benefit from this system:
```rust
impl Material for CustomMaterial {
fn vertex_shader() -> ShaderRef {
"custom_material.wgsl".into()
}
fn fragment_shader() -> ShaderRef {
"custom_material.wgsl".into()
}
fn specialize(
pipeline: &MaterialPipeline<Self>,
descriptor: &mut RenderPipelineDescriptor,
layout: &MeshVertexBufferLayout,
key: MaterialPipelineKey<Self>,
) -> Result<(), SpecializedMeshPipelineError> {
let vertex_layout = layout.get_layout(&[
Mesh::ATTRIBUTE_POSITION.at_shader_location(0),
ATTRIBUTE_BLEND_COLOR.at_shader_location(1),
])?;
descriptor.vertex.buffers = vec![vertex_layout];
Ok(())
}
}
```
### Ported `StandardMaterial` to the new `Material` system
Bevy's built-in PBR material uses the new Material system (including the AsBindGroup derive):
```rust
#[derive(AsBindGroup, Debug, Clone, TypeUuid)]
#[uuid = "7494888b-c082-457b-aacf-517228cc0c22"]
#[bind_group_data(StandardMaterialKey)]
#[uniform(0, StandardMaterialUniform)]
pub struct StandardMaterial {
pub base_color: Color,
#[texture(1)]
#[sampler(2)]
pub base_color_texture: Option<Handle<Image>>,
/* other fields omitted for brevity */
```
### Ported Bevy examples to the new `Material` system
The overall complexity of Bevy's "custom shader examples" has gone down significantly. Take a look at the diffs if you want a dopamine spike.
Please note that while this PR has a net increase in "lines of code", most of those extra lines come from added documentation. There is a significant reduction
in the overall complexity of the code (even accounting for the new derive logic).
---
## Changelog
### Added
* `AsBindGroup` trait and derive, which make it much easier to transfer data to the gpu and generate bind groups for a given type.
### Changed
* The old `Material` and `SpecializedMaterial` traits have been replaced by a consolidated (much simpler) `Material` trait. Materials no longer implement `RenderAsset`.
* `StandardMaterial` was ported to the new material system. There are no user-facing api changes to the `StandardMaterial` struct api, but it now implements `AsBindGroup` and `Material` instead of `RenderAsset` and `SpecializedMaterial`.
## Migration Guide
The Material system has been reworked to be much simpler. We've removed a lot of boilerplate with the new `AsBindGroup` derive and the `Material` trait is simpler as well!
### Bevy 0.7 (old)
```rust
#[derive(Debug, Clone, TypeUuid)]
#[uuid = "f690fdae-d598-45ab-8225-97e2a3f056e0"]
pub struct CustomMaterial {
color: Color,
color_texture: Handle<Image>,
}
#[derive(Clone)]
pub struct GpuCustomMaterial {
_buffer: Buffer,
bind_group: BindGroup,
}
impl RenderAsset for CustomMaterial {
type ExtractedAsset = CustomMaterial;
type PreparedAsset = GpuCustomMaterial;
type Param = (SRes<RenderDevice>, SRes<MaterialPipeline<Self>>);
fn extract_asset(&self) -> Self::ExtractedAsset {
self.clone()
}
fn prepare_asset(
extracted_asset: Self::ExtractedAsset,
(render_device, material_pipeline): &mut SystemParamItem<Self::Param>,
) -> Result<Self::PreparedAsset, PrepareAssetError<Self::ExtractedAsset>> {
let color = Vec4::from_slice(&extracted_asset.color.as_linear_rgba_f32());
let byte_buffer = [0u8; Vec4::SIZE.get() as usize];
let mut buffer = encase::UniformBuffer::new(byte_buffer);
buffer.write(&color).unwrap();
let buffer = render_device.create_buffer_with_data(&BufferInitDescriptor {
contents: buffer.as_ref(),
label: None,
usage: BufferUsages::UNIFORM | BufferUsages::COPY_DST,
});
let (texture_view, texture_sampler) = if let Some(result) = material_pipeline
.mesh_pipeline
.get_image_texture(gpu_images, &Some(extracted_asset.color_texture.clone()))
{
result
} else {
return Err(PrepareAssetError::RetryNextUpdate(extracted_asset));
};
let bind_group = render_device.create_bind_group(&BindGroupDescriptor {
entries: &[
BindGroupEntry {
binding: 0,
resource: buffer.as_entire_binding(),
},
BindGroupEntry {
binding: 0,
resource: BindingResource::TextureView(texture_view),
},
BindGroupEntry {
binding: 1,
resource: BindingResource::Sampler(texture_sampler),
},
],
label: None,
layout: &material_pipeline.material_layout,
});
Ok(GpuCustomMaterial {
_buffer: buffer,
bind_group,
})
}
}
impl Material for CustomMaterial {
fn fragment_shader(asset_server: &AssetServer) -> Option<Handle<Shader>> {
Some(asset_server.load("custom_material.wgsl"))
}
fn bind_group(render_asset: &<Self as RenderAsset>::PreparedAsset) -> &BindGroup {
&render_asset.bind_group
}
fn bind_group_layout(render_device: &RenderDevice) -> BindGroupLayout {
render_device.create_bind_group_layout(&BindGroupLayoutDescriptor {
entries: &[
BindGroupLayoutEntry {
binding: 0,
visibility: ShaderStages::FRAGMENT,
ty: BindingType::Buffer {
ty: BufferBindingType::Uniform,
has_dynamic_offset: false,
min_binding_size: Some(Vec4::min_size()),
},
count: None,
},
BindGroupLayoutEntry {
binding: 1,
visibility: ShaderStages::FRAGMENT,
ty: BindingType::Texture {
multisampled: false,
sample_type: TextureSampleType::Float { filterable: true },
view_dimension: TextureViewDimension::D2Array,
},
count: None,
},
BindGroupLayoutEntry {
binding: 2,
visibility: ShaderStages::FRAGMENT,
ty: BindingType::Sampler(SamplerBindingType::Filtering),
count: None,
},
],
label: None,
})
}
}
```
### Bevy 0.8 (new)
```rust
impl Material for CustomMaterial {
fn fragment_shader() -> ShaderRef {
"custom_material.wgsl".into()
}
}
#[derive(AsBindGroup, TypeUuid, Debug, Clone)]
#[uuid = "f690fdae-d598-45ab-8225-97e2a3f056e0"]
pub struct CustomMaterial {
#[uniform(0)]
color: Color,
#[texture(1)]
#[sampler(2)]
color_texture: Handle<Image>,
}
```
## Future Work
* Add support for more binding types (cubemaps, buffers, etc). This PR intentionally includes a bare minimum number of binding types to keep "reviewability" in check.
* Consider optionally eliding binding indices using binding names. `AsBindGroup` could pass in (optional?) reflection info as a "hint".
* This would make it possible for the derive to do this:
```rust
#[derive(AsBindGroup)]
pub struct CustomMaterial {
#[uniform]
color: Color,
#[texture]
#[sampler]
color_texture: Option<Handle<Image>>,
alpha_mode: AlphaMode,
}
```
* Or this
```rust
#[derive(AsBindGroup)]
pub struct CustomMaterial {
#[binding]
color: Color,
#[binding]
color_texture: Option<Handle<Image>>,
alpha_mode: AlphaMode,
}
```
* Or even this (if we flip to "include bindings by default")
```rust
#[derive(AsBindGroup)]
pub struct CustomMaterial {
color: Color,
color_texture: Option<Handle<Image>>,
#[binding(ignore)]
alpha_mode: AlphaMode,
}
```
* If we add the option to define custom draw functions for materials (which could be done in a type-erased way), I think that would be enough to support extra non-material bindings. Worth considering!
2022-06-30 23:48:46 +00:00
|
|
|
/// Render pipeline data for a given [`Material`].
|
Make `Resource` trait opt-in, requiring `#[derive(Resource)]` V2 (#5577)
*This PR description is an edited copy of #5007, written by @alice-i-cecile.*
# Objective
Follow-up to https://github.com/bevyengine/bevy/pull/2254. The `Resource` trait currently has a blanket implementation for all types that meet its bounds.
While ergonomic, this results in several drawbacks:
* it is possible to make confusing, silent mistakes such as inserting a function pointer (Foo) rather than a value (Foo::Bar) as a resource
* it is challenging to discover if a type is intended to be used as a resource
* we cannot later add customization options (see the [RFC](https://github.com/bevyengine/rfcs/blob/main/rfcs/27-derive-component.md) for the equivalent choice for Component).
* dependencies can use the same Rust type as a resource in invisibly conflicting ways
* raw Rust types used as resources cannot preserve privacy appropriately, as anyone able to access that type can read and write to internal values
* we cannot capture a definitive list of possible resources to display to users in an editor
## Notes to reviewers
* Review this commit-by-commit; there's effectively no back-tracking and there's a lot of churn in some of these commits.
*ira: My commits are not as well organized :')*
* I've relaxed the bound on Local to Send + Sync + 'static: I don't think these concerns apply there, so this can keep things simple. Storing e.g. a u32 in a Local is fine, because there's a variable name attached explaining what it does.
* I think this is a bad place for the Resource trait to live, but I've left it in place to make reviewing easier. IMO that's best tackled with https://github.com/bevyengine/bevy/issues/4981.
## Changelog
`Resource` is no longer automatically implemented for all matching types. Instead, use the new `#[derive(Resource)]` macro.
## Migration Guide
Add `#[derive(Resource)]` to all types you are using as a resource.
If you are using a third party type as a resource, wrap it in a tuple struct to bypass orphan rules. Consider deriving `Deref` and `DerefMut` to improve ergonomics.
`ClearColor` no longer implements `Component`. Using `ClearColor` as a component in 0.8 did nothing.
Use the `ClearColorConfig` in the `Camera3d` and `Camera2d` components instead.
Co-authored-by: Alice <alice.i.cecile@gmail.com>
Co-authored-by: Alice Cecile <alice.i.cecile@gmail.com>
Co-authored-by: devil-ira <justthecooldude@gmail.com>
Co-authored-by: Carter Anderson <mcanders1@gmail.com>
2022-08-08 21:36:35 +00:00
|
|
|
#[derive(Resource)]
|
Better Materials: AsBindGroup trait and derive, simpler Material trait (#5053)
# Objective
This PR reworks Bevy's Material system, making the user experience of defining Materials _much_ nicer. Bevy's previous material system leaves a lot to be desired:
* Materials require manually implementing the `RenderAsset` trait, which involves manually generating the bind group, handling gpu buffer data transfer, looking up image textures, etc. Even the simplest single-texture material involves writing ~80 unnecessary lines of code. This was never the long term plan.
* There are two material traits, which is confusing, hard to document, and often redundant: `Material` and `SpecializedMaterial`. `Material` implicitly implements `SpecializedMaterial`, and `SpecializedMaterial` is used in most high level apis to support both use cases. Most users shouldn't need to think about specialization at all (I consider it a "power-user tool"), so the fact that `SpecializedMaterial` is front-and-center in our apis is a miss.
* Implementing either material trait involves a lot of "type soup". The "prepared asset" parameter is particularly heinous: `&<Self as RenderAsset>::PreparedAsset`. Defining vertex and fragment shaders is also more verbose than it needs to be.
## Solution
Say hello to the new `Material` system:
```rust
#[derive(AsBindGroup, TypeUuid, Debug, Clone)]
#[uuid = "f690fdae-d598-45ab-8225-97e2a3f056e0"]
pub struct CoolMaterial {
#[uniform(0)]
color: Color,
#[texture(1)]
#[sampler(2)]
color_texture: Handle<Image>,
}
impl Material for CoolMaterial {
fn fragment_shader() -> ShaderRef {
"cool_material.wgsl".into()
}
}
```
Thats it! This same material would have required [~80 lines of complicated "type heavy" code](https://github.com/bevyengine/bevy/blob/v0.7.0/examples/shader/shader_material.rs) in the old Material system. Now it is just 14 lines of simple, readable code.
This is thanks to a new consolidated `Material` trait and the new `AsBindGroup` trait / derive.
### The new `Material` trait
The old "split" `Material` and `SpecializedMaterial` traits have been removed in favor of a new consolidated `Material` trait. All of the functions on the trait are optional.
The difficulty of implementing `Material` has been reduced by simplifying dataflow and removing type complexity:
```rust
// Old
impl Material for CustomMaterial {
fn fragment_shader(asset_server: &AssetServer) -> Option<Handle<Shader>> {
Some(asset_server.load("custom_material.wgsl"))
}
fn alpha_mode(render_asset: &<Self as RenderAsset>::PreparedAsset) -> AlphaMode {
render_asset.alpha_mode
}
}
// New
impl Material for CustomMaterial {
fn fragment_shader() -> ShaderRef {
"custom_material.wgsl".into()
}
fn alpha_mode(&self) -> AlphaMode {
self.alpha_mode
}
}
```
Specialization is still supported, but it is hidden by default under the `specialize()` function (more on this later).
### The `AsBindGroup` trait / derive
The `Material` trait now requires the `AsBindGroup` derive. This can be implemented manually relatively easily, but deriving it will almost always be preferable.
Field attributes like `uniform` and `texture` are used to define which fields should be bindings,
what their binding type is, and what index they should be bound at:
```rust
#[derive(AsBindGroup)]
struct CoolMaterial {
#[uniform(0)]
color: Color,
#[texture(1)]
#[sampler(2)]
color_texture: Handle<Image>,
}
```
In WGSL shaders, the binding looks like this:
```wgsl
struct CoolMaterial {
color: vec4<f32>;
};
[[group(1), binding(0)]]
var<uniform> material: CoolMaterial;
[[group(1), binding(1)]]
var color_texture: texture_2d<f32>;
[[group(1), binding(2)]]
var color_sampler: sampler;
```
Note that the "group" index is determined by the usage context. It is not defined in `AsBindGroup`. Bevy material bind groups are bound to group 1.
The following field-level attributes are supported:
* `uniform(BINDING_INDEX)`
* The field will be converted to a shader-compatible type using the `ShaderType` trait, written to a `Buffer`, and bound as a uniform. It can also be derived for custom structs.
* `texture(BINDING_INDEX)`
* This field's `Handle<Image>` will be used to look up the matching `Texture` gpu resource, which will be bound as a texture in shaders. The field will be assumed to implement `Into<Option<Handle<Image>>>`. In practice, most fields should be a `Handle<Image>` or `Option<Handle<Image>>`. If the value of an `Option<Handle<Image>>` is `None`, the new `FallbackImage` resource will be used instead. This attribute can be used in conjunction with a `sampler` binding attribute (with a different binding index).
* `sampler(BINDING_INDEX)`
* Behaves exactly like the `texture` attribute, but sets the Image's sampler binding instead of the texture.
Note that fields without field-level binding attributes will be ignored.
```rust
#[derive(AsBindGroup)]
struct CoolMaterial {
#[uniform(0)]
color: Color,
this_field_is_ignored: String,
}
```
As mentioned above, `Option<Handle<Image>>` is also supported:
```rust
#[derive(AsBindGroup)]
struct CoolMaterial {
#[uniform(0)]
color: Color,
#[texture(1)]
#[sampler(2)]
color_texture: Option<Handle<Image>>,
}
```
This is useful if you want a texture to be optional. When the value is `None`, the `FallbackImage` will be used for the binding instead, which defaults to "pure white".
Field uniforms with the same binding index will be combined into a single binding:
```rust
#[derive(AsBindGroup)]
struct CoolMaterial {
#[uniform(0)]
color: Color,
#[uniform(0)]
roughness: f32,
}
```
In WGSL shaders, the binding would look like this:
```wgsl
struct CoolMaterial {
color: vec4<f32>;
roughness: f32;
};
[[group(1), binding(0)]]
var<uniform> material: CoolMaterial;
```
Some less common scenarios will require "struct-level" attributes. These are the currently supported struct-level attributes:
* `uniform(BINDING_INDEX, ConvertedShaderType)`
* Similar to the field-level `uniform` attribute, but instead the entire `AsBindGroup` value is converted to `ConvertedShaderType`, which must implement `ShaderType`. This is useful if more complicated conversion logic is required.
* `bind_group_data(DataType)`
* The `AsBindGroup` type will be converted to some `DataType` using `Into<DataType>` and stored as `AsBindGroup::Data` as part of the `AsBindGroup::as_bind_group` call. This is useful if data needs to be stored alongside the generated bind group, such as a unique identifier for a material's bind group. The most common use case for this attribute is "shader pipeline specialization".
The previous `CoolMaterial` example illustrating "combining multiple field-level uniform attributes with the same binding index" can
also be equivalently represented with a single struct-level uniform attribute:
```rust
#[derive(AsBindGroup)]
#[uniform(0, CoolMaterialUniform)]
struct CoolMaterial {
color: Color,
roughness: f32,
}
#[derive(ShaderType)]
struct CoolMaterialUniform {
color: Color,
roughness: f32,
}
impl From<&CoolMaterial> for CoolMaterialUniform {
fn from(material: &CoolMaterial) -> CoolMaterialUniform {
CoolMaterialUniform {
color: material.color,
roughness: material.roughness,
}
}
}
```
### Material Specialization
Material shader specialization is now _much_ simpler:
```rust
#[derive(AsBindGroup, TypeUuid, Debug, Clone)]
#[uuid = "f690fdae-d598-45ab-8225-97e2a3f056e0"]
#[bind_group_data(CoolMaterialKey)]
struct CoolMaterial {
#[uniform(0)]
color: Color,
is_red: bool,
}
#[derive(Copy, Clone, Hash, Eq, PartialEq)]
struct CoolMaterialKey {
is_red: bool,
}
impl From<&CoolMaterial> for CoolMaterialKey {
fn from(material: &CoolMaterial) -> CoolMaterialKey {
CoolMaterialKey {
is_red: material.is_red,
}
}
}
impl Material for CoolMaterial {
fn fragment_shader() -> ShaderRef {
"cool_material.wgsl".into()
}
fn specialize(
pipeline: &MaterialPipeline<Self>,
descriptor: &mut RenderPipelineDescriptor,
layout: &MeshVertexBufferLayout,
key: MaterialPipelineKey<Self>,
) -> Result<(), SpecializedMeshPipelineError> {
if key.bind_group_data.is_red {
let fragment = descriptor.fragment.as_mut().unwrap();
fragment.shader_defs.push("IS_RED".to_string());
}
Ok(())
}
}
```
Setting `bind_group_data` is not required for specialization (it defaults to `()`). Scenarios like "custom vertex attributes" also benefit from this system:
```rust
impl Material for CustomMaterial {
fn vertex_shader() -> ShaderRef {
"custom_material.wgsl".into()
}
fn fragment_shader() -> ShaderRef {
"custom_material.wgsl".into()
}
fn specialize(
pipeline: &MaterialPipeline<Self>,
descriptor: &mut RenderPipelineDescriptor,
layout: &MeshVertexBufferLayout,
key: MaterialPipelineKey<Self>,
) -> Result<(), SpecializedMeshPipelineError> {
let vertex_layout = layout.get_layout(&[
Mesh::ATTRIBUTE_POSITION.at_shader_location(0),
ATTRIBUTE_BLEND_COLOR.at_shader_location(1),
])?;
descriptor.vertex.buffers = vec![vertex_layout];
Ok(())
}
}
```
### Ported `StandardMaterial` to the new `Material` system
Bevy's built-in PBR material uses the new Material system (including the AsBindGroup derive):
```rust
#[derive(AsBindGroup, Debug, Clone, TypeUuid)]
#[uuid = "7494888b-c082-457b-aacf-517228cc0c22"]
#[bind_group_data(StandardMaterialKey)]
#[uniform(0, StandardMaterialUniform)]
pub struct StandardMaterial {
pub base_color: Color,
#[texture(1)]
#[sampler(2)]
pub base_color_texture: Option<Handle<Image>>,
/* other fields omitted for brevity */
```
### Ported Bevy examples to the new `Material` system
The overall complexity of Bevy's "custom shader examples" has gone down significantly. Take a look at the diffs if you want a dopamine spike.
Please note that while this PR has a net increase in "lines of code", most of those extra lines come from added documentation. There is a significant reduction
in the overall complexity of the code (even accounting for the new derive logic).
---
## Changelog
### Added
* `AsBindGroup` trait and derive, which make it much easier to transfer data to the gpu and generate bind groups for a given type.
### Changed
* The old `Material` and `SpecializedMaterial` traits have been replaced by a consolidated (much simpler) `Material` trait. Materials no longer implement `RenderAsset`.
* `StandardMaterial` was ported to the new material system. There are no user-facing api changes to the `StandardMaterial` struct api, but it now implements `AsBindGroup` and `Material` instead of `RenderAsset` and `SpecializedMaterial`.
## Migration Guide
The Material system has been reworked to be much simpler. We've removed a lot of boilerplate with the new `AsBindGroup` derive and the `Material` trait is simpler as well!
### Bevy 0.7 (old)
```rust
#[derive(Debug, Clone, TypeUuid)]
#[uuid = "f690fdae-d598-45ab-8225-97e2a3f056e0"]
pub struct CustomMaterial {
color: Color,
color_texture: Handle<Image>,
}
#[derive(Clone)]
pub struct GpuCustomMaterial {
_buffer: Buffer,
bind_group: BindGroup,
}
impl RenderAsset for CustomMaterial {
type ExtractedAsset = CustomMaterial;
type PreparedAsset = GpuCustomMaterial;
type Param = (SRes<RenderDevice>, SRes<MaterialPipeline<Self>>);
fn extract_asset(&self) -> Self::ExtractedAsset {
self.clone()
}
fn prepare_asset(
extracted_asset: Self::ExtractedAsset,
(render_device, material_pipeline): &mut SystemParamItem<Self::Param>,
) -> Result<Self::PreparedAsset, PrepareAssetError<Self::ExtractedAsset>> {
let color = Vec4::from_slice(&extracted_asset.color.as_linear_rgba_f32());
let byte_buffer = [0u8; Vec4::SIZE.get() as usize];
let mut buffer = encase::UniformBuffer::new(byte_buffer);
buffer.write(&color).unwrap();
let buffer = render_device.create_buffer_with_data(&BufferInitDescriptor {
contents: buffer.as_ref(),
label: None,
usage: BufferUsages::UNIFORM | BufferUsages::COPY_DST,
});
let (texture_view, texture_sampler) = if let Some(result) = material_pipeline
.mesh_pipeline
.get_image_texture(gpu_images, &Some(extracted_asset.color_texture.clone()))
{
result
} else {
return Err(PrepareAssetError::RetryNextUpdate(extracted_asset));
};
let bind_group = render_device.create_bind_group(&BindGroupDescriptor {
entries: &[
BindGroupEntry {
binding: 0,
resource: buffer.as_entire_binding(),
},
BindGroupEntry {
binding: 0,
resource: BindingResource::TextureView(texture_view),
},
BindGroupEntry {
binding: 1,
resource: BindingResource::Sampler(texture_sampler),
},
],
label: None,
layout: &material_pipeline.material_layout,
});
Ok(GpuCustomMaterial {
_buffer: buffer,
bind_group,
})
}
}
impl Material for CustomMaterial {
fn fragment_shader(asset_server: &AssetServer) -> Option<Handle<Shader>> {
Some(asset_server.load("custom_material.wgsl"))
}
fn bind_group(render_asset: &<Self as RenderAsset>::PreparedAsset) -> &BindGroup {
&render_asset.bind_group
}
fn bind_group_layout(render_device: &RenderDevice) -> BindGroupLayout {
render_device.create_bind_group_layout(&BindGroupLayoutDescriptor {
entries: &[
BindGroupLayoutEntry {
binding: 0,
visibility: ShaderStages::FRAGMENT,
ty: BindingType::Buffer {
ty: BufferBindingType::Uniform,
has_dynamic_offset: false,
min_binding_size: Some(Vec4::min_size()),
},
count: None,
},
BindGroupLayoutEntry {
binding: 1,
visibility: ShaderStages::FRAGMENT,
ty: BindingType::Texture {
multisampled: false,
sample_type: TextureSampleType::Float { filterable: true },
view_dimension: TextureViewDimension::D2Array,
},
count: None,
},
BindGroupLayoutEntry {
binding: 2,
visibility: ShaderStages::FRAGMENT,
ty: BindingType::Sampler(SamplerBindingType::Filtering),
count: None,
},
],
label: None,
})
}
}
```
### Bevy 0.8 (new)
```rust
impl Material for CustomMaterial {
fn fragment_shader() -> ShaderRef {
"custom_material.wgsl".into()
}
}
#[derive(AsBindGroup, TypeUuid, Debug, Clone)]
#[uuid = "f690fdae-d598-45ab-8225-97e2a3f056e0"]
pub struct CustomMaterial {
#[uniform(0)]
color: Color,
#[texture(1)]
#[sampler(2)]
color_texture: Handle<Image>,
}
```
## Future Work
* Add support for more binding types (cubemaps, buffers, etc). This PR intentionally includes a bare minimum number of binding types to keep "reviewability" in check.
* Consider optionally eliding binding indices using binding names. `AsBindGroup` could pass in (optional?) reflection info as a "hint".
* This would make it possible for the derive to do this:
```rust
#[derive(AsBindGroup)]
pub struct CustomMaterial {
#[uniform]
color: Color,
#[texture]
#[sampler]
color_texture: Option<Handle<Image>>,
alpha_mode: AlphaMode,
}
```
* Or this
```rust
#[derive(AsBindGroup)]
pub struct CustomMaterial {
#[binding]
color: Color,
#[binding]
color_texture: Option<Handle<Image>>,
alpha_mode: AlphaMode,
}
```
* Or even this (if we flip to "include bindings by default")
```rust
#[derive(AsBindGroup)]
pub struct CustomMaterial {
color: Color,
color_texture: Option<Handle<Image>>,
#[binding(ignore)]
alpha_mode: AlphaMode,
}
```
* If we add the option to define custom draw functions for materials (which could be done in a type-erased way), I think that would be enough to support extra non-material bindings. Worth considering!
2022-06-30 23:48:46 +00:00
|
|
|
pub struct MaterialPipeline<M: Material> {
|
2021-12-25 21:45:43 +00:00
|
|
|
pub mesh_pipeline: MeshPipeline,
|
|
|
|
pub material_layout: BindGroupLayout,
|
|
|
|
pub vertex_shader: Option<Handle<Shader>>,
|
|
|
|
pub fragment_shader: Option<Handle<Shader>>,
|
2023-10-17 21:28:08 +00:00
|
|
|
pub marker: PhantomData<M>,
|
2021-12-14 03:58:23 +00:00
|
|
|
}
|
|
|
|
|
2023-01-14 18:33:38 +00:00
|
|
|
impl<M: Material> Clone for MaterialPipeline<M> {
|
|
|
|
fn clone(&self) -> Self {
|
|
|
|
Self {
|
|
|
|
mesh_pipeline: self.mesh_pipeline.clone(),
|
|
|
|
material_layout: self.material_layout.clone(),
|
|
|
|
vertex_shader: self.vertex_shader.clone(),
|
|
|
|
fragment_shader: self.fragment_shader.clone(),
|
|
|
|
marker: PhantomData,
|
|
|
|
}
|
|
|
|
}
|
|
|
|
}
|
|
|
|
|
Better Materials: AsBindGroup trait and derive, simpler Material trait (#5053)
# Objective
This PR reworks Bevy's Material system, making the user experience of defining Materials _much_ nicer. Bevy's previous material system leaves a lot to be desired:
* Materials require manually implementing the `RenderAsset` trait, which involves manually generating the bind group, handling gpu buffer data transfer, looking up image textures, etc. Even the simplest single-texture material involves writing ~80 unnecessary lines of code. This was never the long term plan.
* There are two material traits, which is confusing, hard to document, and often redundant: `Material` and `SpecializedMaterial`. `Material` implicitly implements `SpecializedMaterial`, and `SpecializedMaterial` is used in most high level apis to support both use cases. Most users shouldn't need to think about specialization at all (I consider it a "power-user tool"), so the fact that `SpecializedMaterial` is front-and-center in our apis is a miss.
* Implementing either material trait involves a lot of "type soup". The "prepared asset" parameter is particularly heinous: `&<Self as RenderAsset>::PreparedAsset`. Defining vertex and fragment shaders is also more verbose than it needs to be.
## Solution
Say hello to the new `Material` system:
```rust
#[derive(AsBindGroup, TypeUuid, Debug, Clone)]
#[uuid = "f690fdae-d598-45ab-8225-97e2a3f056e0"]
pub struct CoolMaterial {
#[uniform(0)]
color: Color,
#[texture(1)]
#[sampler(2)]
color_texture: Handle<Image>,
}
impl Material for CoolMaterial {
fn fragment_shader() -> ShaderRef {
"cool_material.wgsl".into()
}
}
```
Thats it! This same material would have required [~80 lines of complicated "type heavy" code](https://github.com/bevyengine/bevy/blob/v0.7.0/examples/shader/shader_material.rs) in the old Material system. Now it is just 14 lines of simple, readable code.
This is thanks to a new consolidated `Material` trait and the new `AsBindGroup` trait / derive.
### The new `Material` trait
The old "split" `Material` and `SpecializedMaterial` traits have been removed in favor of a new consolidated `Material` trait. All of the functions on the trait are optional.
The difficulty of implementing `Material` has been reduced by simplifying dataflow and removing type complexity:
```rust
// Old
impl Material for CustomMaterial {
fn fragment_shader(asset_server: &AssetServer) -> Option<Handle<Shader>> {
Some(asset_server.load("custom_material.wgsl"))
}
fn alpha_mode(render_asset: &<Self as RenderAsset>::PreparedAsset) -> AlphaMode {
render_asset.alpha_mode
}
}
// New
impl Material for CustomMaterial {
fn fragment_shader() -> ShaderRef {
"custom_material.wgsl".into()
}
fn alpha_mode(&self) -> AlphaMode {
self.alpha_mode
}
}
```
Specialization is still supported, but it is hidden by default under the `specialize()` function (more on this later).
### The `AsBindGroup` trait / derive
The `Material` trait now requires the `AsBindGroup` derive. This can be implemented manually relatively easily, but deriving it will almost always be preferable.
Field attributes like `uniform` and `texture` are used to define which fields should be bindings,
what their binding type is, and what index they should be bound at:
```rust
#[derive(AsBindGroup)]
struct CoolMaterial {
#[uniform(0)]
color: Color,
#[texture(1)]
#[sampler(2)]
color_texture: Handle<Image>,
}
```
In WGSL shaders, the binding looks like this:
```wgsl
struct CoolMaterial {
color: vec4<f32>;
};
[[group(1), binding(0)]]
var<uniform> material: CoolMaterial;
[[group(1), binding(1)]]
var color_texture: texture_2d<f32>;
[[group(1), binding(2)]]
var color_sampler: sampler;
```
Note that the "group" index is determined by the usage context. It is not defined in `AsBindGroup`. Bevy material bind groups are bound to group 1.
The following field-level attributes are supported:
* `uniform(BINDING_INDEX)`
* The field will be converted to a shader-compatible type using the `ShaderType` trait, written to a `Buffer`, and bound as a uniform. It can also be derived for custom structs.
* `texture(BINDING_INDEX)`
* This field's `Handle<Image>` will be used to look up the matching `Texture` gpu resource, which will be bound as a texture in shaders. The field will be assumed to implement `Into<Option<Handle<Image>>>`. In practice, most fields should be a `Handle<Image>` or `Option<Handle<Image>>`. If the value of an `Option<Handle<Image>>` is `None`, the new `FallbackImage` resource will be used instead. This attribute can be used in conjunction with a `sampler` binding attribute (with a different binding index).
* `sampler(BINDING_INDEX)`
* Behaves exactly like the `texture` attribute, but sets the Image's sampler binding instead of the texture.
Note that fields without field-level binding attributes will be ignored.
```rust
#[derive(AsBindGroup)]
struct CoolMaterial {
#[uniform(0)]
color: Color,
this_field_is_ignored: String,
}
```
As mentioned above, `Option<Handle<Image>>` is also supported:
```rust
#[derive(AsBindGroup)]
struct CoolMaterial {
#[uniform(0)]
color: Color,
#[texture(1)]
#[sampler(2)]
color_texture: Option<Handle<Image>>,
}
```
This is useful if you want a texture to be optional. When the value is `None`, the `FallbackImage` will be used for the binding instead, which defaults to "pure white".
Field uniforms with the same binding index will be combined into a single binding:
```rust
#[derive(AsBindGroup)]
struct CoolMaterial {
#[uniform(0)]
color: Color,
#[uniform(0)]
roughness: f32,
}
```
In WGSL shaders, the binding would look like this:
```wgsl
struct CoolMaterial {
color: vec4<f32>;
roughness: f32;
};
[[group(1), binding(0)]]
var<uniform> material: CoolMaterial;
```
Some less common scenarios will require "struct-level" attributes. These are the currently supported struct-level attributes:
* `uniform(BINDING_INDEX, ConvertedShaderType)`
* Similar to the field-level `uniform` attribute, but instead the entire `AsBindGroup` value is converted to `ConvertedShaderType`, which must implement `ShaderType`. This is useful if more complicated conversion logic is required.
* `bind_group_data(DataType)`
* The `AsBindGroup` type will be converted to some `DataType` using `Into<DataType>` and stored as `AsBindGroup::Data` as part of the `AsBindGroup::as_bind_group` call. This is useful if data needs to be stored alongside the generated bind group, such as a unique identifier for a material's bind group. The most common use case for this attribute is "shader pipeline specialization".
The previous `CoolMaterial` example illustrating "combining multiple field-level uniform attributes with the same binding index" can
also be equivalently represented with a single struct-level uniform attribute:
```rust
#[derive(AsBindGroup)]
#[uniform(0, CoolMaterialUniform)]
struct CoolMaterial {
color: Color,
roughness: f32,
}
#[derive(ShaderType)]
struct CoolMaterialUniform {
color: Color,
roughness: f32,
}
impl From<&CoolMaterial> for CoolMaterialUniform {
fn from(material: &CoolMaterial) -> CoolMaterialUniform {
CoolMaterialUniform {
color: material.color,
roughness: material.roughness,
}
}
}
```
### Material Specialization
Material shader specialization is now _much_ simpler:
```rust
#[derive(AsBindGroup, TypeUuid, Debug, Clone)]
#[uuid = "f690fdae-d598-45ab-8225-97e2a3f056e0"]
#[bind_group_data(CoolMaterialKey)]
struct CoolMaterial {
#[uniform(0)]
color: Color,
is_red: bool,
}
#[derive(Copy, Clone, Hash, Eq, PartialEq)]
struct CoolMaterialKey {
is_red: bool,
}
impl From<&CoolMaterial> for CoolMaterialKey {
fn from(material: &CoolMaterial) -> CoolMaterialKey {
CoolMaterialKey {
is_red: material.is_red,
}
}
}
impl Material for CoolMaterial {
fn fragment_shader() -> ShaderRef {
"cool_material.wgsl".into()
}
fn specialize(
pipeline: &MaterialPipeline<Self>,
descriptor: &mut RenderPipelineDescriptor,
layout: &MeshVertexBufferLayout,
key: MaterialPipelineKey<Self>,
) -> Result<(), SpecializedMeshPipelineError> {
if key.bind_group_data.is_red {
let fragment = descriptor.fragment.as_mut().unwrap();
fragment.shader_defs.push("IS_RED".to_string());
}
Ok(())
}
}
```
Setting `bind_group_data` is not required for specialization (it defaults to `()`). Scenarios like "custom vertex attributes" also benefit from this system:
```rust
impl Material for CustomMaterial {
fn vertex_shader() -> ShaderRef {
"custom_material.wgsl".into()
}
fn fragment_shader() -> ShaderRef {
"custom_material.wgsl".into()
}
fn specialize(
pipeline: &MaterialPipeline<Self>,
descriptor: &mut RenderPipelineDescriptor,
layout: &MeshVertexBufferLayout,
key: MaterialPipelineKey<Self>,
) -> Result<(), SpecializedMeshPipelineError> {
let vertex_layout = layout.get_layout(&[
Mesh::ATTRIBUTE_POSITION.at_shader_location(0),
ATTRIBUTE_BLEND_COLOR.at_shader_location(1),
])?;
descriptor.vertex.buffers = vec![vertex_layout];
Ok(())
}
}
```
### Ported `StandardMaterial` to the new `Material` system
Bevy's built-in PBR material uses the new Material system (including the AsBindGroup derive):
```rust
#[derive(AsBindGroup, Debug, Clone, TypeUuid)]
#[uuid = "7494888b-c082-457b-aacf-517228cc0c22"]
#[bind_group_data(StandardMaterialKey)]
#[uniform(0, StandardMaterialUniform)]
pub struct StandardMaterial {
pub base_color: Color,
#[texture(1)]
#[sampler(2)]
pub base_color_texture: Option<Handle<Image>>,
/* other fields omitted for brevity */
```
### Ported Bevy examples to the new `Material` system
The overall complexity of Bevy's "custom shader examples" has gone down significantly. Take a look at the diffs if you want a dopamine spike.
Please note that while this PR has a net increase in "lines of code", most of those extra lines come from added documentation. There is a significant reduction
in the overall complexity of the code (even accounting for the new derive logic).
---
## Changelog
### Added
* `AsBindGroup` trait and derive, which make it much easier to transfer data to the gpu and generate bind groups for a given type.
### Changed
* The old `Material` and `SpecializedMaterial` traits have been replaced by a consolidated (much simpler) `Material` trait. Materials no longer implement `RenderAsset`.
* `StandardMaterial` was ported to the new material system. There are no user-facing api changes to the `StandardMaterial` struct api, but it now implements `AsBindGroup` and `Material` instead of `RenderAsset` and `SpecializedMaterial`.
## Migration Guide
The Material system has been reworked to be much simpler. We've removed a lot of boilerplate with the new `AsBindGroup` derive and the `Material` trait is simpler as well!
### Bevy 0.7 (old)
```rust
#[derive(Debug, Clone, TypeUuid)]
#[uuid = "f690fdae-d598-45ab-8225-97e2a3f056e0"]
pub struct CustomMaterial {
color: Color,
color_texture: Handle<Image>,
}
#[derive(Clone)]
pub struct GpuCustomMaterial {
_buffer: Buffer,
bind_group: BindGroup,
}
impl RenderAsset for CustomMaterial {
type ExtractedAsset = CustomMaterial;
type PreparedAsset = GpuCustomMaterial;
type Param = (SRes<RenderDevice>, SRes<MaterialPipeline<Self>>);
fn extract_asset(&self) -> Self::ExtractedAsset {
self.clone()
}
fn prepare_asset(
extracted_asset: Self::ExtractedAsset,
(render_device, material_pipeline): &mut SystemParamItem<Self::Param>,
) -> Result<Self::PreparedAsset, PrepareAssetError<Self::ExtractedAsset>> {
let color = Vec4::from_slice(&extracted_asset.color.as_linear_rgba_f32());
let byte_buffer = [0u8; Vec4::SIZE.get() as usize];
let mut buffer = encase::UniformBuffer::new(byte_buffer);
buffer.write(&color).unwrap();
let buffer = render_device.create_buffer_with_data(&BufferInitDescriptor {
contents: buffer.as_ref(),
label: None,
usage: BufferUsages::UNIFORM | BufferUsages::COPY_DST,
});
let (texture_view, texture_sampler) = if let Some(result) = material_pipeline
.mesh_pipeline
.get_image_texture(gpu_images, &Some(extracted_asset.color_texture.clone()))
{
result
} else {
return Err(PrepareAssetError::RetryNextUpdate(extracted_asset));
};
let bind_group = render_device.create_bind_group(&BindGroupDescriptor {
entries: &[
BindGroupEntry {
binding: 0,
resource: buffer.as_entire_binding(),
},
BindGroupEntry {
binding: 0,
resource: BindingResource::TextureView(texture_view),
},
BindGroupEntry {
binding: 1,
resource: BindingResource::Sampler(texture_sampler),
},
],
label: None,
layout: &material_pipeline.material_layout,
});
Ok(GpuCustomMaterial {
_buffer: buffer,
bind_group,
})
}
}
impl Material for CustomMaterial {
fn fragment_shader(asset_server: &AssetServer) -> Option<Handle<Shader>> {
Some(asset_server.load("custom_material.wgsl"))
}
fn bind_group(render_asset: &<Self as RenderAsset>::PreparedAsset) -> &BindGroup {
&render_asset.bind_group
}
fn bind_group_layout(render_device: &RenderDevice) -> BindGroupLayout {
render_device.create_bind_group_layout(&BindGroupLayoutDescriptor {
entries: &[
BindGroupLayoutEntry {
binding: 0,
visibility: ShaderStages::FRAGMENT,
ty: BindingType::Buffer {
ty: BufferBindingType::Uniform,
has_dynamic_offset: false,
min_binding_size: Some(Vec4::min_size()),
},
count: None,
},
BindGroupLayoutEntry {
binding: 1,
visibility: ShaderStages::FRAGMENT,
ty: BindingType::Texture {
multisampled: false,
sample_type: TextureSampleType::Float { filterable: true },
view_dimension: TextureViewDimension::D2Array,
},
count: None,
},
BindGroupLayoutEntry {
binding: 2,
visibility: ShaderStages::FRAGMENT,
ty: BindingType::Sampler(SamplerBindingType::Filtering),
count: None,
},
],
label: None,
})
}
}
```
### Bevy 0.8 (new)
```rust
impl Material for CustomMaterial {
fn fragment_shader() -> ShaderRef {
"custom_material.wgsl".into()
}
}
#[derive(AsBindGroup, TypeUuid, Debug, Clone)]
#[uuid = "f690fdae-d598-45ab-8225-97e2a3f056e0"]
pub struct CustomMaterial {
#[uniform(0)]
color: Color,
#[texture(1)]
#[sampler(2)]
color_texture: Handle<Image>,
}
```
## Future Work
* Add support for more binding types (cubemaps, buffers, etc). This PR intentionally includes a bare minimum number of binding types to keep "reviewability" in check.
* Consider optionally eliding binding indices using binding names. `AsBindGroup` could pass in (optional?) reflection info as a "hint".
* This would make it possible for the derive to do this:
```rust
#[derive(AsBindGroup)]
pub struct CustomMaterial {
#[uniform]
color: Color,
#[texture]
#[sampler]
color_texture: Option<Handle<Image>>,
alpha_mode: AlphaMode,
}
```
* Or this
```rust
#[derive(AsBindGroup)]
pub struct CustomMaterial {
#[binding]
color: Color,
#[binding]
color_texture: Option<Handle<Image>>,
alpha_mode: AlphaMode,
}
```
* Or even this (if we flip to "include bindings by default")
```rust
#[derive(AsBindGroup)]
pub struct CustomMaterial {
color: Color,
color_texture: Option<Handle<Image>>,
#[binding(ignore)]
alpha_mode: AlphaMode,
}
```
* If we add the option to define custom draw functions for materials (which could be done in a type-erased way), I think that would be enough to support extra non-material bindings. Worth considering!
2022-06-30 23:48:46 +00:00
|
|
|
impl<M: Material> SpecializedMeshPipeline for MaterialPipeline<M>
|
|
|
|
where
|
|
|
|
M::Data: PartialEq + Eq + Hash + Clone,
|
|
|
|
{
|
|
|
|
type Key = MaterialPipelineKey<M>;
|
2021-12-14 03:58:23 +00:00
|
|
|
|
Mesh vertex buffer layouts (#3959)
This PR makes a number of changes to how meshes and vertex attributes are handled, which the goal of enabling easy and flexible custom vertex attributes:
* Reworks the `Mesh` type to use the newly added `VertexAttribute` internally
* `VertexAttribute` defines the name, a unique `VertexAttributeId`, and a `VertexFormat`
* `VertexAttributeId` is used to produce consistent sort orders for vertex buffer generation, replacing the more expensive and often surprising "name based sorting"
* Meshes can be used to generate a `MeshVertexBufferLayout`, which defines the layout of the gpu buffer produced by the mesh. `MeshVertexBufferLayouts` can then be used to generate actual `VertexBufferLayouts` according to the requirements of a specific pipeline. This decoupling of "mesh layout" vs "pipeline vertex buffer layout" is what enables custom attributes. We don't need to standardize _mesh layouts_ or contort meshes to meet the needs of a specific pipeline. As long as the mesh has what the pipeline needs, it will work transparently.
* Mesh-based pipelines now specialize on `&MeshVertexBufferLayout` via the new `SpecializedMeshPipeline` trait (which behaves like `SpecializedPipeline`, but adds `&MeshVertexBufferLayout`). The integrity of the pipeline cache is maintained because the `MeshVertexBufferLayout` is treated as part of the key (which is fully abstracted from implementers of the trait ... no need to add any additional info to the specialization key).
* Hashing `MeshVertexBufferLayout` is too expensive to do for every entity, every frame. To make this scalable, I added a generalized "pre-hashing" solution to `bevy_utils`: `Hashed<T>` keys and `PreHashMap<K, V>` (which uses `Hashed<T>` internally) . Why didn't I just do the quick and dirty in-place "pre-compute hash and use that u64 as a key in a hashmap" that we've done in the past? Because its wrong! Hashes by themselves aren't enough because two different values can produce the same hash. Re-hashing a hash is even worse! I decided to build a generalized solution because this pattern has come up in the past and we've chosen to do the wrong thing. Now we can do the right thing! This did unfortunately require pulling in `hashbrown` and using that in `bevy_utils`, because avoiding re-hashes requires the `raw_entry_mut` api, which isn't stabilized yet (and may never be ... `entry_ref` has favor now, but also isn't available yet). If std's HashMap ever provides the tools we need, we can move back to that. Note that adding `hashbrown` doesn't increase our dependency count because it was already in our tree. I will probably break these changes out into their own PR.
* Specializing on `MeshVertexBufferLayout` has one non-obvious behavior: it can produce identical pipelines for two different MeshVertexBufferLayouts. To optimize the number of active pipelines / reduce re-binds while drawing, I de-duplicate pipelines post-specialization using the final `VertexBufferLayout` as the key. For example, consider a pipeline that needs the layout `(position, normal)` and is specialized using two meshes: `(position, normal, uv)` and `(position, normal, other_vec2)`. If both of these meshes result in `(position, normal)` specializations, we can use the same pipeline! Now we do. Cool!
To briefly illustrate, this is what the relevant section of `MeshPipeline`'s specialization code looks like now:
```rust
impl SpecializedMeshPipeline for MeshPipeline {
type Key = MeshPipelineKey;
fn specialize(
&self,
key: Self::Key,
layout: &MeshVertexBufferLayout,
) -> RenderPipelineDescriptor {
let mut vertex_attributes = vec![
Mesh::ATTRIBUTE_POSITION.at_shader_location(0),
Mesh::ATTRIBUTE_NORMAL.at_shader_location(1),
Mesh::ATTRIBUTE_UV_0.at_shader_location(2),
];
let mut shader_defs = Vec::new();
if layout.contains(Mesh::ATTRIBUTE_TANGENT) {
shader_defs.push(String::from("VERTEX_TANGENTS"));
vertex_attributes.push(Mesh::ATTRIBUTE_TANGENT.at_shader_location(3));
}
let vertex_buffer_layout = layout
.get_layout(&vertex_attributes)
.expect("Mesh is missing a vertex attribute");
```
Notice that this is _much_ simpler than it was before. And now any mesh with any layout can be used with this pipeline, provided it has vertex postions, normals, and uvs. We even got to remove `HAS_TANGENTS` from MeshPipelineKey and `has_tangents` from `GpuMesh`, because that information is redundant with `MeshVertexBufferLayout`.
This is still a draft because I still need to:
* Add more docs
* Experiment with adding error handling to mesh pipeline specialization (which would print errors at runtime when a mesh is missing a vertex attribute required by a pipeline). If it doesn't tank perf, we'll keep it.
* Consider breaking out the PreHash / hashbrown changes into a separate PR.
* Add an example illustrating this change
* Verify that the "mesh-specialized pipeline de-duplication code" works properly
Please dont yell at me for not doing these things yet :) Just trying to get this in peoples' hands asap.
Alternative to #3120
Fixes #3030
Co-authored-by: Carter Anderson <mcanders1@gmail.com>
2022-02-23 23:21:13 +00:00
|
|
|
fn specialize(
|
|
|
|
&self,
|
|
|
|
key: Self::Key,
|
2024-03-01 20:56:21 +00:00
|
|
|
layout: &MeshVertexBufferLayoutRef,
|
Mesh vertex buffer layouts (#3959)
This PR makes a number of changes to how meshes and vertex attributes are handled, which the goal of enabling easy and flexible custom vertex attributes:
* Reworks the `Mesh` type to use the newly added `VertexAttribute` internally
* `VertexAttribute` defines the name, a unique `VertexAttributeId`, and a `VertexFormat`
* `VertexAttributeId` is used to produce consistent sort orders for vertex buffer generation, replacing the more expensive and often surprising "name based sorting"
* Meshes can be used to generate a `MeshVertexBufferLayout`, which defines the layout of the gpu buffer produced by the mesh. `MeshVertexBufferLayouts` can then be used to generate actual `VertexBufferLayouts` according to the requirements of a specific pipeline. This decoupling of "mesh layout" vs "pipeline vertex buffer layout" is what enables custom attributes. We don't need to standardize _mesh layouts_ or contort meshes to meet the needs of a specific pipeline. As long as the mesh has what the pipeline needs, it will work transparently.
* Mesh-based pipelines now specialize on `&MeshVertexBufferLayout` via the new `SpecializedMeshPipeline` trait (which behaves like `SpecializedPipeline`, but adds `&MeshVertexBufferLayout`). The integrity of the pipeline cache is maintained because the `MeshVertexBufferLayout` is treated as part of the key (which is fully abstracted from implementers of the trait ... no need to add any additional info to the specialization key).
* Hashing `MeshVertexBufferLayout` is too expensive to do for every entity, every frame. To make this scalable, I added a generalized "pre-hashing" solution to `bevy_utils`: `Hashed<T>` keys and `PreHashMap<K, V>` (which uses `Hashed<T>` internally) . Why didn't I just do the quick and dirty in-place "pre-compute hash and use that u64 as a key in a hashmap" that we've done in the past? Because its wrong! Hashes by themselves aren't enough because two different values can produce the same hash. Re-hashing a hash is even worse! I decided to build a generalized solution because this pattern has come up in the past and we've chosen to do the wrong thing. Now we can do the right thing! This did unfortunately require pulling in `hashbrown` and using that in `bevy_utils`, because avoiding re-hashes requires the `raw_entry_mut` api, which isn't stabilized yet (and may never be ... `entry_ref` has favor now, but also isn't available yet). If std's HashMap ever provides the tools we need, we can move back to that. Note that adding `hashbrown` doesn't increase our dependency count because it was already in our tree. I will probably break these changes out into their own PR.
* Specializing on `MeshVertexBufferLayout` has one non-obvious behavior: it can produce identical pipelines for two different MeshVertexBufferLayouts. To optimize the number of active pipelines / reduce re-binds while drawing, I de-duplicate pipelines post-specialization using the final `VertexBufferLayout` as the key. For example, consider a pipeline that needs the layout `(position, normal)` and is specialized using two meshes: `(position, normal, uv)` and `(position, normal, other_vec2)`. If both of these meshes result in `(position, normal)` specializations, we can use the same pipeline! Now we do. Cool!
To briefly illustrate, this is what the relevant section of `MeshPipeline`'s specialization code looks like now:
```rust
impl SpecializedMeshPipeline for MeshPipeline {
type Key = MeshPipelineKey;
fn specialize(
&self,
key: Self::Key,
layout: &MeshVertexBufferLayout,
) -> RenderPipelineDescriptor {
let mut vertex_attributes = vec![
Mesh::ATTRIBUTE_POSITION.at_shader_location(0),
Mesh::ATTRIBUTE_NORMAL.at_shader_location(1),
Mesh::ATTRIBUTE_UV_0.at_shader_location(2),
];
let mut shader_defs = Vec::new();
if layout.contains(Mesh::ATTRIBUTE_TANGENT) {
shader_defs.push(String::from("VERTEX_TANGENTS"));
vertex_attributes.push(Mesh::ATTRIBUTE_TANGENT.at_shader_location(3));
}
let vertex_buffer_layout = layout
.get_layout(&vertex_attributes)
.expect("Mesh is missing a vertex attribute");
```
Notice that this is _much_ simpler than it was before. And now any mesh with any layout can be used with this pipeline, provided it has vertex postions, normals, and uvs. We even got to remove `HAS_TANGENTS` from MeshPipelineKey and `has_tangents` from `GpuMesh`, because that information is redundant with `MeshVertexBufferLayout`.
This is still a draft because I still need to:
* Add more docs
* Experiment with adding error handling to mesh pipeline specialization (which would print errors at runtime when a mesh is missing a vertex attribute required by a pipeline). If it doesn't tank perf, we'll keep it.
* Consider breaking out the PreHash / hashbrown changes into a separate PR.
* Add an example illustrating this change
* Verify that the "mesh-specialized pipeline de-duplication code" works properly
Please dont yell at me for not doing these things yet :) Just trying to get this in peoples' hands asap.
Alternative to #3120
Fixes #3030
Co-authored-by: Carter Anderson <mcanders1@gmail.com>
2022-02-23 23:21:13 +00:00
|
|
|
) -> Result<RenderPipelineDescriptor, SpecializedMeshPipelineError> {
|
|
|
|
let mut descriptor = self.mesh_pipeline.specialize(key.mesh_key, layout)?;
|
2021-12-25 21:45:43 +00:00
|
|
|
if let Some(vertex_shader) = &self.vertex_shader {
|
|
|
|
descriptor.vertex.shader = vertex_shader.clone();
|
2021-12-14 03:58:23 +00:00
|
|
|
}
|
2021-12-25 21:45:43 +00:00
|
|
|
|
|
|
|
if let Some(fragment_shader) = &self.fragment_shader {
|
|
|
|
descriptor.fragment.as_mut().unwrap().shader = fragment_shader.clone();
|
2021-12-14 03:58:23 +00:00
|
|
|
}
|
2022-03-29 18:31:13 +00:00
|
|
|
|
2023-11-28 22:26:22 +00:00
|
|
|
descriptor.layout.insert(2, self.material_layout.clone());
|
2021-12-25 21:45:43 +00:00
|
|
|
|
Better Materials: AsBindGroup trait and derive, simpler Material trait (#5053)
# Objective
This PR reworks Bevy's Material system, making the user experience of defining Materials _much_ nicer. Bevy's previous material system leaves a lot to be desired:
* Materials require manually implementing the `RenderAsset` trait, which involves manually generating the bind group, handling gpu buffer data transfer, looking up image textures, etc. Even the simplest single-texture material involves writing ~80 unnecessary lines of code. This was never the long term plan.
* There are two material traits, which is confusing, hard to document, and often redundant: `Material` and `SpecializedMaterial`. `Material` implicitly implements `SpecializedMaterial`, and `SpecializedMaterial` is used in most high level apis to support both use cases. Most users shouldn't need to think about specialization at all (I consider it a "power-user tool"), so the fact that `SpecializedMaterial` is front-and-center in our apis is a miss.
* Implementing either material trait involves a lot of "type soup". The "prepared asset" parameter is particularly heinous: `&<Self as RenderAsset>::PreparedAsset`. Defining vertex and fragment shaders is also more verbose than it needs to be.
## Solution
Say hello to the new `Material` system:
```rust
#[derive(AsBindGroup, TypeUuid, Debug, Clone)]
#[uuid = "f690fdae-d598-45ab-8225-97e2a3f056e0"]
pub struct CoolMaterial {
#[uniform(0)]
color: Color,
#[texture(1)]
#[sampler(2)]
color_texture: Handle<Image>,
}
impl Material for CoolMaterial {
fn fragment_shader() -> ShaderRef {
"cool_material.wgsl".into()
}
}
```
Thats it! This same material would have required [~80 lines of complicated "type heavy" code](https://github.com/bevyengine/bevy/blob/v0.7.0/examples/shader/shader_material.rs) in the old Material system. Now it is just 14 lines of simple, readable code.
This is thanks to a new consolidated `Material` trait and the new `AsBindGroup` trait / derive.
### The new `Material` trait
The old "split" `Material` and `SpecializedMaterial` traits have been removed in favor of a new consolidated `Material` trait. All of the functions on the trait are optional.
The difficulty of implementing `Material` has been reduced by simplifying dataflow and removing type complexity:
```rust
// Old
impl Material for CustomMaterial {
fn fragment_shader(asset_server: &AssetServer) -> Option<Handle<Shader>> {
Some(asset_server.load("custom_material.wgsl"))
}
fn alpha_mode(render_asset: &<Self as RenderAsset>::PreparedAsset) -> AlphaMode {
render_asset.alpha_mode
}
}
// New
impl Material for CustomMaterial {
fn fragment_shader() -> ShaderRef {
"custom_material.wgsl".into()
}
fn alpha_mode(&self) -> AlphaMode {
self.alpha_mode
}
}
```
Specialization is still supported, but it is hidden by default under the `specialize()` function (more on this later).
### The `AsBindGroup` trait / derive
The `Material` trait now requires the `AsBindGroup` derive. This can be implemented manually relatively easily, but deriving it will almost always be preferable.
Field attributes like `uniform` and `texture` are used to define which fields should be bindings,
what their binding type is, and what index they should be bound at:
```rust
#[derive(AsBindGroup)]
struct CoolMaterial {
#[uniform(0)]
color: Color,
#[texture(1)]
#[sampler(2)]
color_texture: Handle<Image>,
}
```
In WGSL shaders, the binding looks like this:
```wgsl
struct CoolMaterial {
color: vec4<f32>;
};
[[group(1), binding(0)]]
var<uniform> material: CoolMaterial;
[[group(1), binding(1)]]
var color_texture: texture_2d<f32>;
[[group(1), binding(2)]]
var color_sampler: sampler;
```
Note that the "group" index is determined by the usage context. It is not defined in `AsBindGroup`. Bevy material bind groups are bound to group 1.
The following field-level attributes are supported:
* `uniform(BINDING_INDEX)`
* The field will be converted to a shader-compatible type using the `ShaderType` trait, written to a `Buffer`, and bound as a uniform. It can also be derived for custom structs.
* `texture(BINDING_INDEX)`
* This field's `Handle<Image>` will be used to look up the matching `Texture` gpu resource, which will be bound as a texture in shaders. The field will be assumed to implement `Into<Option<Handle<Image>>>`. In practice, most fields should be a `Handle<Image>` or `Option<Handle<Image>>`. If the value of an `Option<Handle<Image>>` is `None`, the new `FallbackImage` resource will be used instead. This attribute can be used in conjunction with a `sampler` binding attribute (with a different binding index).
* `sampler(BINDING_INDEX)`
* Behaves exactly like the `texture` attribute, but sets the Image's sampler binding instead of the texture.
Note that fields without field-level binding attributes will be ignored.
```rust
#[derive(AsBindGroup)]
struct CoolMaterial {
#[uniform(0)]
color: Color,
this_field_is_ignored: String,
}
```
As mentioned above, `Option<Handle<Image>>` is also supported:
```rust
#[derive(AsBindGroup)]
struct CoolMaterial {
#[uniform(0)]
color: Color,
#[texture(1)]
#[sampler(2)]
color_texture: Option<Handle<Image>>,
}
```
This is useful if you want a texture to be optional. When the value is `None`, the `FallbackImage` will be used for the binding instead, which defaults to "pure white".
Field uniforms with the same binding index will be combined into a single binding:
```rust
#[derive(AsBindGroup)]
struct CoolMaterial {
#[uniform(0)]
color: Color,
#[uniform(0)]
roughness: f32,
}
```
In WGSL shaders, the binding would look like this:
```wgsl
struct CoolMaterial {
color: vec4<f32>;
roughness: f32;
};
[[group(1), binding(0)]]
var<uniform> material: CoolMaterial;
```
Some less common scenarios will require "struct-level" attributes. These are the currently supported struct-level attributes:
* `uniform(BINDING_INDEX, ConvertedShaderType)`
* Similar to the field-level `uniform` attribute, but instead the entire `AsBindGroup` value is converted to `ConvertedShaderType`, which must implement `ShaderType`. This is useful if more complicated conversion logic is required.
* `bind_group_data(DataType)`
* The `AsBindGroup` type will be converted to some `DataType` using `Into<DataType>` and stored as `AsBindGroup::Data` as part of the `AsBindGroup::as_bind_group` call. This is useful if data needs to be stored alongside the generated bind group, such as a unique identifier for a material's bind group. The most common use case for this attribute is "shader pipeline specialization".
The previous `CoolMaterial` example illustrating "combining multiple field-level uniform attributes with the same binding index" can
also be equivalently represented with a single struct-level uniform attribute:
```rust
#[derive(AsBindGroup)]
#[uniform(0, CoolMaterialUniform)]
struct CoolMaterial {
color: Color,
roughness: f32,
}
#[derive(ShaderType)]
struct CoolMaterialUniform {
color: Color,
roughness: f32,
}
impl From<&CoolMaterial> for CoolMaterialUniform {
fn from(material: &CoolMaterial) -> CoolMaterialUniform {
CoolMaterialUniform {
color: material.color,
roughness: material.roughness,
}
}
}
```
### Material Specialization
Material shader specialization is now _much_ simpler:
```rust
#[derive(AsBindGroup, TypeUuid, Debug, Clone)]
#[uuid = "f690fdae-d598-45ab-8225-97e2a3f056e0"]
#[bind_group_data(CoolMaterialKey)]
struct CoolMaterial {
#[uniform(0)]
color: Color,
is_red: bool,
}
#[derive(Copy, Clone, Hash, Eq, PartialEq)]
struct CoolMaterialKey {
is_red: bool,
}
impl From<&CoolMaterial> for CoolMaterialKey {
fn from(material: &CoolMaterial) -> CoolMaterialKey {
CoolMaterialKey {
is_red: material.is_red,
}
}
}
impl Material for CoolMaterial {
fn fragment_shader() -> ShaderRef {
"cool_material.wgsl".into()
}
fn specialize(
pipeline: &MaterialPipeline<Self>,
descriptor: &mut RenderPipelineDescriptor,
layout: &MeshVertexBufferLayout,
key: MaterialPipelineKey<Self>,
) -> Result<(), SpecializedMeshPipelineError> {
if key.bind_group_data.is_red {
let fragment = descriptor.fragment.as_mut().unwrap();
fragment.shader_defs.push("IS_RED".to_string());
}
Ok(())
}
}
```
Setting `bind_group_data` is not required for specialization (it defaults to `()`). Scenarios like "custom vertex attributes" also benefit from this system:
```rust
impl Material for CustomMaterial {
fn vertex_shader() -> ShaderRef {
"custom_material.wgsl".into()
}
fn fragment_shader() -> ShaderRef {
"custom_material.wgsl".into()
}
fn specialize(
pipeline: &MaterialPipeline<Self>,
descriptor: &mut RenderPipelineDescriptor,
layout: &MeshVertexBufferLayout,
key: MaterialPipelineKey<Self>,
) -> Result<(), SpecializedMeshPipelineError> {
let vertex_layout = layout.get_layout(&[
Mesh::ATTRIBUTE_POSITION.at_shader_location(0),
ATTRIBUTE_BLEND_COLOR.at_shader_location(1),
])?;
descriptor.vertex.buffers = vec![vertex_layout];
Ok(())
}
}
```
### Ported `StandardMaterial` to the new `Material` system
Bevy's built-in PBR material uses the new Material system (including the AsBindGroup derive):
```rust
#[derive(AsBindGroup, Debug, Clone, TypeUuid)]
#[uuid = "7494888b-c082-457b-aacf-517228cc0c22"]
#[bind_group_data(StandardMaterialKey)]
#[uniform(0, StandardMaterialUniform)]
pub struct StandardMaterial {
pub base_color: Color,
#[texture(1)]
#[sampler(2)]
pub base_color_texture: Option<Handle<Image>>,
/* other fields omitted for brevity */
```
### Ported Bevy examples to the new `Material` system
The overall complexity of Bevy's "custom shader examples" has gone down significantly. Take a look at the diffs if you want a dopamine spike.
Please note that while this PR has a net increase in "lines of code", most of those extra lines come from added documentation. There is a significant reduction
in the overall complexity of the code (even accounting for the new derive logic).
---
## Changelog
### Added
* `AsBindGroup` trait and derive, which make it much easier to transfer data to the gpu and generate bind groups for a given type.
### Changed
* The old `Material` and `SpecializedMaterial` traits have been replaced by a consolidated (much simpler) `Material` trait. Materials no longer implement `RenderAsset`.
* `StandardMaterial` was ported to the new material system. There are no user-facing api changes to the `StandardMaterial` struct api, but it now implements `AsBindGroup` and `Material` instead of `RenderAsset` and `SpecializedMaterial`.
## Migration Guide
The Material system has been reworked to be much simpler. We've removed a lot of boilerplate with the new `AsBindGroup` derive and the `Material` trait is simpler as well!
### Bevy 0.7 (old)
```rust
#[derive(Debug, Clone, TypeUuid)]
#[uuid = "f690fdae-d598-45ab-8225-97e2a3f056e0"]
pub struct CustomMaterial {
color: Color,
color_texture: Handle<Image>,
}
#[derive(Clone)]
pub struct GpuCustomMaterial {
_buffer: Buffer,
bind_group: BindGroup,
}
impl RenderAsset for CustomMaterial {
type ExtractedAsset = CustomMaterial;
type PreparedAsset = GpuCustomMaterial;
type Param = (SRes<RenderDevice>, SRes<MaterialPipeline<Self>>);
fn extract_asset(&self) -> Self::ExtractedAsset {
self.clone()
}
fn prepare_asset(
extracted_asset: Self::ExtractedAsset,
(render_device, material_pipeline): &mut SystemParamItem<Self::Param>,
) -> Result<Self::PreparedAsset, PrepareAssetError<Self::ExtractedAsset>> {
let color = Vec4::from_slice(&extracted_asset.color.as_linear_rgba_f32());
let byte_buffer = [0u8; Vec4::SIZE.get() as usize];
let mut buffer = encase::UniformBuffer::new(byte_buffer);
buffer.write(&color).unwrap();
let buffer = render_device.create_buffer_with_data(&BufferInitDescriptor {
contents: buffer.as_ref(),
label: None,
usage: BufferUsages::UNIFORM | BufferUsages::COPY_DST,
});
let (texture_view, texture_sampler) = if let Some(result) = material_pipeline
.mesh_pipeline
.get_image_texture(gpu_images, &Some(extracted_asset.color_texture.clone()))
{
result
} else {
return Err(PrepareAssetError::RetryNextUpdate(extracted_asset));
};
let bind_group = render_device.create_bind_group(&BindGroupDescriptor {
entries: &[
BindGroupEntry {
binding: 0,
resource: buffer.as_entire_binding(),
},
BindGroupEntry {
binding: 0,
resource: BindingResource::TextureView(texture_view),
},
BindGroupEntry {
binding: 1,
resource: BindingResource::Sampler(texture_sampler),
},
],
label: None,
layout: &material_pipeline.material_layout,
});
Ok(GpuCustomMaterial {
_buffer: buffer,
bind_group,
})
}
}
impl Material for CustomMaterial {
fn fragment_shader(asset_server: &AssetServer) -> Option<Handle<Shader>> {
Some(asset_server.load("custom_material.wgsl"))
}
fn bind_group(render_asset: &<Self as RenderAsset>::PreparedAsset) -> &BindGroup {
&render_asset.bind_group
}
fn bind_group_layout(render_device: &RenderDevice) -> BindGroupLayout {
render_device.create_bind_group_layout(&BindGroupLayoutDescriptor {
entries: &[
BindGroupLayoutEntry {
binding: 0,
visibility: ShaderStages::FRAGMENT,
ty: BindingType::Buffer {
ty: BufferBindingType::Uniform,
has_dynamic_offset: false,
min_binding_size: Some(Vec4::min_size()),
},
count: None,
},
BindGroupLayoutEntry {
binding: 1,
visibility: ShaderStages::FRAGMENT,
ty: BindingType::Texture {
multisampled: false,
sample_type: TextureSampleType::Float { filterable: true },
view_dimension: TextureViewDimension::D2Array,
},
count: None,
},
BindGroupLayoutEntry {
binding: 2,
visibility: ShaderStages::FRAGMENT,
ty: BindingType::Sampler(SamplerBindingType::Filtering),
count: None,
},
],
label: None,
})
}
}
```
### Bevy 0.8 (new)
```rust
impl Material for CustomMaterial {
fn fragment_shader() -> ShaderRef {
"custom_material.wgsl".into()
}
}
#[derive(AsBindGroup, TypeUuid, Debug, Clone)]
#[uuid = "f690fdae-d598-45ab-8225-97e2a3f056e0"]
pub struct CustomMaterial {
#[uniform(0)]
color: Color,
#[texture(1)]
#[sampler(2)]
color_texture: Handle<Image>,
}
```
## Future Work
* Add support for more binding types (cubemaps, buffers, etc). This PR intentionally includes a bare minimum number of binding types to keep "reviewability" in check.
* Consider optionally eliding binding indices using binding names. `AsBindGroup` could pass in (optional?) reflection info as a "hint".
* This would make it possible for the derive to do this:
```rust
#[derive(AsBindGroup)]
pub struct CustomMaterial {
#[uniform]
color: Color,
#[texture]
#[sampler]
color_texture: Option<Handle<Image>>,
alpha_mode: AlphaMode,
}
```
* Or this
```rust
#[derive(AsBindGroup)]
pub struct CustomMaterial {
#[binding]
color: Color,
#[binding]
color_texture: Option<Handle<Image>>,
alpha_mode: AlphaMode,
}
```
* Or even this (if we flip to "include bindings by default")
```rust
#[derive(AsBindGroup)]
pub struct CustomMaterial {
color: Color,
color_texture: Option<Handle<Image>>,
#[binding(ignore)]
alpha_mode: AlphaMode,
}
```
* If we add the option to define custom draw functions for materials (which could be done in a type-erased way), I think that would be enough to support extra non-material bindings. Worth considering!
2022-06-30 23:48:46 +00:00
|
|
|
M::specialize(self, &mut descriptor, layout, key)?;
|
Mesh vertex buffer layouts (#3959)
This PR makes a number of changes to how meshes and vertex attributes are handled, which the goal of enabling easy and flexible custom vertex attributes:
* Reworks the `Mesh` type to use the newly added `VertexAttribute` internally
* `VertexAttribute` defines the name, a unique `VertexAttributeId`, and a `VertexFormat`
* `VertexAttributeId` is used to produce consistent sort orders for vertex buffer generation, replacing the more expensive and often surprising "name based sorting"
* Meshes can be used to generate a `MeshVertexBufferLayout`, which defines the layout of the gpu buffer produced by the mesh. `MeshVertexBufferLayouts` can then be used to generate actual `VertexBufferLayouts` according to the requirements of a specific pipeline. This decoupling of "mesh layout" vs "pipeline vertex buffer layout" is what enables custom attributes. We don't need to standardize _mesh layouts_ or contort meshes to meet the needs of a specific pipeline. As long as the mesh has what the pipeline needs, it will work transparently.
* Mesh-based pipelines now specialize on `&MeshVertexBufferLayout` via the new `SpecializedMeshPipeline` trait (which behaves like `SpecializedPipeline`, but adds `&MeshVertexBufferLayout`). The integrity of the pipeline cache is maintained because the `MeshVertexBufferLayout` is treated as part of the key (which is fully abstracted from implementers of the trait ... no need to add any additional info to the specialization key).
* Hashing `MeshVertexBufferLayout` is too expensive to do for every entity, every frame. To make this scalable, I added a generalized "pre-hashing" solution to `bevy_utils`: `Hashed<T>` keys and `PreHashMap<K, V>` (which uses `Hashed<T>` internally) . Why didn't I just do the quick and dirty in-place "pre-compute hash and use that u64 as a key in a hashmap" that we've done in the past? Because its wrong! Hashes by themselves aren't enough because two different values can produce the same hash. Re-hashing a hash is even worse! I decided to build a generalized solution because this pattern has come up in the past and we've chosen to do the wrong thing. Now we can do the right thing! This did unfortunately require pulling in `hashbrown` and using that in `bevy_utils`, because avoiding re-hashes requires the `raw_entry_mut` api, which isn't stabilized yet (and may never be ... `entry_ref` has favor now, but also isn't available yet). If std's HashMap ever provides the tools we need, we can move back to that. Note that adding `hashbrown` doesn't increase our dependency count because it was already in our tree. I will probably break these changes out into their own PR.
* Specializing on `MeshVertexBufferLayout` has one non-obvious behavior: it can produce identical pipelines for two different MeshVertexBufferLayouts. To optimize the number of active pipelines / reduce re-binds while drawing, I de-duplicate pipelines post-specialization using the final `VertexBufferLayout` as the key. For example, consider a pipeline that needs the layout `(position, normal)` and is specialized using two meshes: `(position, normal, uv)` and `(position, normal, other_vec2)`. If both of these meshes result in `(position, normal)` specializations, we can use the same pipeline! Now we do. Cool!
To briefly illustrate, this is what the relevant section of `MeshPipeline`'s specialization code looks like now:
```rust
impl SpecializedMeshPipeline for MeshPipeline {
type Key = MeshPipelineKey;
fn specialize(
&self,
key: Self::Key,
layout: &MeshVertexBufferLayout,
) -> RenderPipelineDescriptor {
let mut vertex_attributes = vec![
Mesh::ATTRIBUTE_POSITION.at_shader_location(0),
Mesh::ATTRIBUTE_NORMAL.at_shader_location(1),
Mesh::ATTRIBUTE_UV_0.at_shader_location(2),
];
let mut shader_defs = Vec::new();
if layout.contains(Mesh::ATTRIBUTE_TANGENT) {
shader_defs.push(String::from("VERTEX_TANGENTS"));
vertex_attributes.push(Mesh::ATTRIBUTE_TANGENT.at_shader_location(3));
}
let vertex_buffer_layout = layout
.get_layout(&vertex_attributes)
.expect("Mesh is missing a vertex attribute");
```
Notice that this is _much_ simpler than it was before. And now any mesh with any layout can be used with this pipeline, provided it has vertex postions, normals, and uvs. We even got to remove `HAS_TANGENTS` from MeshPipelineKey and `has_tangents` from `GpuMesh`, because that information is redundant with `MeshVertexBufferLayout`.
This is still a draft because I still need to:
* Add more docs
* Experiment with adding error handling to mesh pipeline specialization (which would print errors at runtime when a mesh is missing a vertex attribute required by a pipeline). If it doesn't tank perf, we'll keep it.
* Consider breaking out the PreHash / hashbrown changes into a separate PR.
* Add an example illustrating this change
* Verify that the "mesh-specialized pipeline de-duplication code" works properly
Please dont yell at me for not doing these things yet :) Just trying to get this in peoples' hands asap.
Alternative to #3120
Fixes #3030
Co-authored-by: Carter Anderson <mcanders1@gmail.com>
2022-02-23 23:21:13 +00:00
|
|
|
Ok(descriptor)
|
2021-12-25 21:45:43 +00:00
|
|
|
}
|
|
|
|
}
|
|
|
|
|
Better Materials: AsBindGroup trait and derive, simpler Material trait (#5053)
# Objective
This PR reworks Bevy's Material system, making the user experience of defining Materials _much_ nicer. Bevy's previous material system leaves a lot to be desired:
* Materials require manually implementing the `RenderAsset` trait, which involves manually generating the bind group, handling gpu buffer data transfer, looking up image textures, etc. Even the simplest single-texture material involves writing ~80 unnecessary lines of code. This was never the long term plan.
* There are two material traits, which is confusing, hard to document, and often redundant: `Material` and `SpecializedMaterial`. `Material` implicitly implements `SpecializedMaterial`, and `SpecializedMaterial` is used in most high level apis to support both use cases. Most users shouldn't need to think about specialization at all (I consider it a "power-user tool"), so the fact that `SpecializedMaterial` is front-and-center in our apis is a miss.
* Implementing either material trait involves a lot of "type soup". The "prepared asset" parameter is particularly heinous: `&<Self as RenderAsset>::PreparedAsset`. Defining vertex and fragment shaders is also more verbose than it needs to be.
## Solution
Say hello to the new `Material` system:
```rust
#[derive(AsBindGroup, TypeUuid, Debug, Clone)]
#[uuid = "f690fdae-d598-45ab-8225-97e2a3f056e0"]
pub struct CoolMaterial {
#[uniform(0)]
color: Color,
#[texture(1)]
#[sampler(2)]
color_texture: Handle<Image>,
}
impl Material for CoolMaterial {
fn fragment_shader() -> ShaderRef {
"cool_material.wgsl".into()
}
}
```
Thats it! This same material would have required [~80 lines of complicated "type heavy" code](https://github.com/bevyengine/bevy/blob/v0.7.0/examples/shader/shader_material.rs) in the old Material system. Now it is just 14 lines of simple, readable code.
This is thanks to a new consolidated `Material` trait and the new `AsBindGroup` trait / derive.
### The new `Material` trait
The old "split" `Material` and `SpecializedMaterial` traits have been removed in favor of a new consolidated `Material` trait. All of the functions on the trait are optional.
The difficulty of implementing `Material` has been reduced by simplifying dataflow and removing type complexity:
```rust
// Old
impl Material for CustomMaterial {
fn fragment_shader(asset_server: &AssetServer) -> Option<Handle<Shader>> {
Some(asset_server.load("custom_material.wgsl"))
}
fn alpha_mode(render_asset: &<Self as RenderAsset>::PreparedAsset) -> AlphaMode {
render_asset.alpha_mode
}
}
// New
impl Material for CustomMaterial {
fn fragment_shader() -> ShaderRef {
"custom_material.wgsl".into()
}
fn alpha_mode(&self) -> AlphaMode {
self.alpha_mode
}
}
```
Specialization is still supported, but it is hidden by default under the `specialize()` function (more on this later).
### The `AsBindGroup` trait / derive
The `Material` trait now requires the `AsBindGroup` derive. This can be implemented manually relatively easily, but deriving it will almost always be preferable.
Field attributes like `uniform` and `texture` are used to define which fields should be bindings,
what their binding type is, and what index they should be bound at:
```rust
#[derive(AsBindGroup)]
struct CoolMaterial {
#[uniform(0)]
color: Color,
#[texture(1)]
#[sampler(2)]
color_texture: Handle<Image>,
}
```
In WGSL shaders, the binding looks like this:
```wgsl
struct CoolMaterial {
color: vec4<f32>;
};
[[group(1), binding(0)]]
var<uniform> material: CoolMaterial;
[[group(1), binding(1)]]
var color_texture: texture_2d<f32>;
[[group(1), binding(2)]]
var color_sampler: sampler;
```
Note that the "group" index is determined by the usage context. It is not defined in `AsBindGroup`. Bevy material bind groups are bound to group 1.
The following field-level attributes are supported:
* `uniform(BINDING_INDEX)`
* The field will be converted to a shader-compatible type using the `ShaderType` trait, written to a `Buffer`, and bound as a uniform. It can also be derived for custom structs.
* `texture(BINDING_INDEX)`
* This field's `Handle<Image>` will be used to look up the matching `Texture` gpu resource, which will be bound as a texture in shaders. The field will be assumed to implement `Into<Option<Handle<Image>>>`. In practice, most fields should be a `Handle<Image>` or `Option<Handle<Image>>`. If the value of an `Option<Handle<Image>>` is `None`, the new `FallbackImage` resource will be used instead. This attribute can be used in conjunction with a `sampler` binding attribute (with a different binding index).
* `sampler(BINDING_INDEX)`
* Behaves exactly like the `texture` attribute, but sets the Image's sampler binding instead of the texture.
Note that fields without field-level binding attributes will be ignored.
```rust
#[derive(AsBindGroup)]
struct CoolMaterial {
#[uniform(0)]
color: Color,
this_field_is_ignored: String,
}
```
As mentioned above, `Option<Handle<Image>>` is also supported:
```rust
#[derive(AsBindGroup)]
struct CoolMaterial {
#[uniform(0)]
color: Color,
#[texture(1)]
#[sampler(2)]
color_texture: Option<Handle<Image>>,
}
```
This is useful if you want a texture to be optional. When the value is `None`, the `FallbackImage` will be used for the binding instead, which defaults to "pure white".
Field uniforms with the same binding index will be combined into a single binding:
```rust
#[derive(AsBindGroup)]
struct CoolMaterial {
#[uniform(0)]
color: Color,
#[uniform(0)]
roughness: f32,
}
```
In WGSL shaders, the binding would look like this:
```wgsl
struct CoolMaterial {
color: vec4<f32>;
roughness: f32;
};
[[group(1), binding(0)]]
var<uniform> material: CoolMaterial;
```
Some less common scenarios will require "struct-level" attributes. These are the currently supported struct-level attributes:
* `uniform(BINDING_INDEX, ConvertedShaderType)`
* Similar to the field-level `uniform` attribute, but instead the entire `AsBindGroup` value is converted to `ConvertedShaderType`, which must implement `ShaderType`. This is useful if more complicated conversion logic is required.
* `bind_group_data(DataType)`
* The `AsBindGroup` type will be converted to some `DataType` using `Into<DataType>` and stored as `AsBindGroup::Data` as part of the `AsBindGroup::as_bind_group` call. This is useful if data needs to be stored alongside the generated bind group, such as a unique identifier for a material's bind group. The most common use case for this attribute is "shader pipeline specialization".
The previous `CoolMaterial` example illustrating "combining multiple field-level uniform attributes with the same binding index" can
also be equivalently represented with a single struct-level uniform attribute:
```rust
#[derive(AsBindGroup)]
#[uniform(0, CoolMaterialUniform)]
struct CoolMaterial {
color: Color,
roughness: f32,
}
#[derive(ShaderType)]
struct CoolMaterialUniform {
color: Color,
roughness: f32,
}
impl From<&CoolMaterial> for CoolMaterialUniform {
fn from(material: &CoolMaterial) -> CoolMaterialUniform {
CoolMaterialUniform {
color: material.color,
roughness: material.roughness,
}
}
}
```
### Material Specialization
Material shader specialization is now _much_ simpler:
```rust
#[derive(AsBindGroup, TypeUuid, Debug, Clone)]
#[uuid = "f690fdae-d598-45ab-8225-97e2a3f056e0"]
#[bind_group_data(CoolMaterialKey)]
struct CoolMaterial {
#[uniform(0)]
color: Color,
is_red: bool,
}
#[derive(Copy, Clone, Hash, Eq, PartialEq)]
struct CoolMaterialKey {
is_red: bool,
}
impl From<&CoolMaterial> for CoolMaterialKey {
fn from(material: &CoolMaterial) -> CoolMaterialKey {
CoolMaterialKey {
is_red: material.is_red,
}
}
}
impl Material for CoolMaterial {
fn fragment_shader() -> ShaderRef {
"cool_material.wgsl".into()
}
fn specialize(
pipeline: &MaterialPipeline<Self>,
descriptor: &mut RenderPipelineDescriptor,
layout: &MeshVertexBufferLayout,
key: MaterialPipelineKey<Self>,
) -> Result<(), SpecializedMeshPipelineError> {
if key.bind_group_data.is_red {
let fragment = descriptor.fragment.as_mut().unwrap();
fragment.shader_defs.push("IS_RED".to_string());
}
Ok(())
}
}
```
Setting `bind_group_data` is not required for specialization (it defaults to `()`). Scenarios like "custom vertex attributes" also benefit from this system:
```rust
impl Material for CustomMaterial {
fn vertex_shader() -> ShaderRef {
"custom_material.wgsl".into()
}
fn fragment_shader() -> ShaderRef {
"custom_material.wgsl".into()
}
fn specialize(
pipeline: &MaterialPipeline<Self>,
descriptor: &mut RenderPipelineDescriptor,
layout: &MeshVertexBufferLayout,
key: MaterialPipelineKey<Self>,
) -> Result<(), SpecializedMeshPipelineError> {
let vertex_layout = layout.get_layout(&[
Mesh::ATTRIBUTE_POSITION.at_shader_location(0),
ATTRIBUTE_BLEND_COLOR.at_shader_location(1),
])?;
descriptor.vertex.buffers = vec![vertex_layout];
Ok(())
}
}
```
### Ported `StandardMaterial` to the new `Material` system
Bevy's built-in PBR material uses the new Material system (including the AsBindGroup derive):
```rust
#[derive(AsBindGroup, Debug, Clone, TypeUuid)]
#[uuid = "7494888b-c082-457b-aacf-517228cc0c22"]
#[bind_group_data(StandardMaterialKey)]
#[uniform(0, StandardMaterialUniform)]
pub struct StandardMaterial {
pub base_color: Color,
#[texture(1)]
#[sampler(2)]
pub base_color_texture: Option<Handle<Image>>,
/* other fields omitted for brevity */
```
### Ported Bevy examples to the new `Material` system
The overall complexity of Bevy's "custom shader examples" has gone down significantly. Take a look at the diffs if you want a dopamine spike.
Please note that while this PR has a net increase in "lines of code", most of those extra lines come from added documentation. There is a significant reduction
in the overall complexity of the code (even accounting for the new derive logic).
---
## Changelog
### Added
* `AsBindGroup` trait and derive, which make it much easier to transfer data to the gpu and generate bind groups for a given type.
### Changed
* The old `Material` and `SpecializedMaterial` traits have been replaced by a consolidated (much simpler) `Material` trait. Materials no longer implement `RenderAsset`.
* `StandardMaterial` was ported to the new material system. There are no user-facing api changes to the `StandardMaterial` struct api, but it now implements `AsBindGroup` and `Material` instead of `RenderAsset` and `SpecializedMaterial`.
## Migration Guide
The Material system has been reworked to be much simpler. We've removed a lot of boilerplate with the new `AsBindGroup` derive and the `Material` trait is simpler as well!
### Bevy 0.7 (old)
```rust
#[derive(Debug, Clone, TypeUuid)]
#[uuid = "f690fdae-d598-45ab-8225-97e2a3f056e0"]
pub struct CustomMaterial {
color: Color,
color_texture: Handle<Image>,
}
#[derive(Clone)]
pub struct GpuCustomMaterial {
_buffer: Buffer,
bind_group: BindGroup,
}
impl RenderAsset for CustomMaterial {
type ExtractedAsset = CustomMaterial;
type PreparedAsset = GpuCustomMaterial;
type Param = (SRes<RenderDevice>, SRes<MaterialPipeline<Self>>);
fn extract_asset(&self) -> Self::ExtractedAsset {
self.clone()
}
fn prepare_asset(
extracted_asset: Self::ExtractedAsset,
(render_device, material_pipeline): &mut SystemParamItem<Self::Param>,
) -> Result<Self::PreparedAsset, PrepareAssetError<Self::ExtractedAsset>> {
let color = Vec4::from_slice(&extracted_asset.color.as_linear_rgba_f32());
let byte_buffer = [0u8; Vec4::SIZE.get() as usize];
let mut buffer = encase::UniformBuffer::new(byte_buffer);
buffer.write(&color).unwrap();
let buffer = render_device.create_buffer_with_data(&BufferInitDescriptor {
contents: buffer.as_ref(),
label: None,
usage: BufferUsages::UNIFORM | BufferUsages::COPY_DST,
});
let (texture_view, texture_sampler) = if let Some(result) = material_pipeline
.mesh_pipeline
.get_image_texture(gpu_images, &Some(extracted_asset.color_texture.clone()))
{
result
} else {
return Err(PrepareAssetError::RetryNextUpdate(extracted_asset));
};
let bind_group = render_device.create_bind_group(&BindGroupDescriptor {
entries: &[
BindGroupEntry {
binding: 0,
resource: buffer.as_entire_binding(),
},
BindGroupEntry {
binding: 0,
resource: BindingResource::TextureView(texture_view),
},
BindGroupEntry {
binding: 1,
resource: BindingResource::Sampler(texture_sampler),
},
],
label: None,
layout: &material_pipeline.material_layout,
});
Ok(GpuCustomMaterial {
_buffer: buffer,
bind_group,
})
}
}
impl Material for CustomMaterial {
fn fragment_shader(asset_server: &AssetServer) -> Option<Handle<Shader>> {
Some(asset_server.load("custom_material.wgsl"))
}
fn bind_group(render_asset: &<Self as RenderAsset>::PreparedAsset) -> &BindGroup {
&render_asset.bind_group
}
fn bind_group_layout(render_device: &RenderDevice) -> BindGroupLayout {
render_device.create_bind_group_layout(&BindGroupLayoutDescriptor {
entries: &[
BindGroupLayoutEntry {
binding: 0,
visibility: ShaderStages::FRAGMENT,
ty: BindingType::Buffer {
ty: BufferBindingType::Uniform,
has_dynamic_offset: false,
min_binding_size: Some(Vec4::min_size()),
},
count: None,
},
BindGroupLayoutEntry {
binding: 1,
visibility: ShaderStages::FRAGMENT,
ty: BindingType::Texture {
multisampled: false,
sample_type: TextureSampleType::Float { filterable: true },
view_dimension: TextureViewDimension::D2Array,
},
count: None,
},
BindGroupLayoutEntry {
binding: 2,
visibility: ShaderStages::FRAGMENT,
ty: BindingType::Sampler(SamplerBindingType::Filtering),
count: None,
},
],
label: None,
})
}
}
```
### Bevy 0.8 (new)
```rust
impl Material for CustomMaterial {
fn fragment_shader() -> ShaderRef {
"custom_material.wgsl".into()
}
}
#[derive(AsBindGroup, TypeUuid, Debug, Clone)]
#[uuid = "f690fdae-d598-45ab-8225-97e2a3f056e0"]
pub struct CustomMaterial {
#[uniform(0)]
color: Color,
#[texture(1)]
#[sampler(2)]
color_texture: Handle<Image>,
}
```
## Future Work
* Add support for more binding types (cubemaps, buffers, etc). This PR intentionally includes a bare minimum number of binding types to keep "reviewability" in check.
* Consider optionally eliding binding indices using binding names. `AsBindGroup` could pass in (optional?) reflection info as a "hint".
* This would make it possible for the derive to do this:
```rust
#[derive(AsBindGroup)]
pub struct CustomMaterial {
#[uniform]
color: Color,
#[texture]
#[sampler]
color_texture: Option<Handle<Image>>,
alpha_mode: AlphaMode,
}
```
* Or this
```rust
#[derive(AsBindGroup)]
pub struct CustomMaterial {
#[binding]
color: Color,
#[binding]
color_texture: Option<Handle<Image>>,
alpha_mode: AlphaMode,
}
```
* Or even this (if we flip to "include bindings by default")
```rust
#[derive(AsBindGroup)]
pub struct CustomMaterial {
color: Color,
color_texture: Option<Handle<Image>>,
#[binding(ignore)]
alpha_mode: AlphaMode,
}
```
* If we add the option to define custom draw functions for materials (which could be done in a type-erased way), I think that would be enough to support extra non-material bindings. Worth considering!
2022-06-30 23:48:46 +00:00
|
|
|
impl<M: Material> FromWorld for MaterialPipeline<M> {
|
2021-12-25 21:45:43 +00:00
|
|
|
fn from_world(world: &mut World) -> Self {
|
2022-02-27 22:37:18 +00:00
|
|
|
let asset_server = world.resource::<AssetServer>();
|
|
|
|
let render_device = world.resource::<RenderDevice>();
|
2021-12-25 21:45:43 +00:00
|
|
|
|
|
|
|
MaterialPipeline {
|
2022-02-27 22:37:18 +00:00
|
|
|
mesh_pipeline: world.resource::<MeshPipeline>().clone(),
|
Better Materials: AsBindGroup trait and derive, simpler Material trait (#5053)
# Objective
This PR reworks Bevy's Material system, making the user experience of defining Materials _much_ nicer. Bevy's previous material system leaves a lot to be desired:
* Materials require manually implementing the `RenderAsset` trait, which involves manually generating the bind group, handling gpu buffer data transfer, looking up image textures, etc. Even the simplest single-texture material involves writing ~80 unnecessary lines of code. This was never the long term plan.
* There are two material traits, which is confusing, hard to document, and often redundant: `Material` and `SpecializedMaterial`. `Material` implicitly implements `SpecializedMaterial`, and `SpecializedMaterial` is used in most high level apis to support both use cases. Most users shouldn't need to think about specialization at all (I consider it a "power-user tool"), so the fact that `SpecializedMaterial` is front-and-center in our apis is a miss.
* Implementing either material trait involves a lot of "type soup". The "prepared asset" parameter is particularly heinous: `&<Self as RenderAsset>::PreparedAsset`. Defining vertex and fragment shaders is also more verbose than it needs to be.
## Solution
Say hello to the new `Material` system:
```rust
#[derive(AsBindGroup, TypeUuid, Debug, Clone)]
#[uuid = "f690fdae-d598-45ab-8225-97e2a3f056e0"]
pub struct CoolMaterial {
#[uniform(0)]
color: Color,
#[texture(1)]
#[sampler(2)]
color_texture: Handle<Image>,
}
impl Material for CoolMaterial {
fn fragment_shader() -> ShaderRef {
"cool_material.wgsl".into()
}
}
```
Thats it! This same material would have required [~80 lines of complicated "type heavy" code](https://github.com/bevyengine/bevy/blob/v0.7.0/examples/shader/shader_material.rs) in the old Material system. Now it is just 14 lines of simple, readable code.
This is thanks to a new consolidated `Material` trait and the new `AsBindGroup` trait / derive.
### The new `Material` trait
The old "split" `Material` and `SpecializedMaterial` traits have been removed in favor of a new consolidated `Material` trait. All of the functions on the trait are optional.
The difficulty of implementing `Material` has been reduced by simplifying dataflow and removing type complexity:
```rust
// Old
impl Material for CustomMaterial {
fn fragment_shader(asset_server: &AssetServer) -> Option<Handle<Shader>> {
Some(asset_server.load("custom_material.wgsl"))
}
fn alpha_mode(render_asset: &<Self as RenderAsset>::PreparedAsset) -> AlphaMode {
render_asset.alpha_mode
}
}
// New
impl Material for CustomMaterial {
fn fragment_shader() -> ShaderRef {
"custom_material.wgsl".into()
}
fn alpha_mode(&self) -> AlphaMode {
self.alpha_mode
}
}
```
Specialization is still supported, but it is hidden by default under the `specialize()` function (more on this later).
### The `AsBindGroup` trait / derive
The `Material` trait now requires the `AsBindGroup` derive. This can be implemented manually relatively easily, but deriving it will almost always be preferable.
Field attributes like `uniform` and `texture` are used to define which fields should be bindings,
what their binding type is, and what index they should be bound at:
```rust
#[derive(AsBindGroup)]
struct CoolMaterial {
#[uniform(0)]
color: Color,
#[texture(1)]
#[sampler(2)]
color_texture: Handle<Image>,
}
```
In WGSL shaders, the binding looks like this:
```wgsl
struct CoolMaterial {
color: vec4<f32>;
};
[[group(1), binding(0)]]
var<uniform> material: CoolMaterial;
[[group(1), binding(1)]]
var color_texture: texture_2d<f32>;
[[group(1), binding(2)]]
var color_sampler: sampler;
```
Note that the "group" index is determined by the usage context. It is not defined in `AsBindGroup`. Bevy material bind groups are bound to group 1.
The following field-level attributes are supported:
* `uniform(BINDING_INDEX)`
* The field will be converted to a shader-compatible type using the `ShaderType` trait, written to a `Buffer`, and bound as a uniform. It can also be derived for custom structs.
* `texture(BINDING_INDEX)`
* This field's `Handle<Image>` will be used to look up the matching `Texture` gpu resource, which will be bound as a texture in shaders. The field will be assumed to implement `Into<Option<Handle<Image>>>`. In practice, most fields should be a `Handle<Image>` or `Option<Handle<Image>>`. If the value of an `Option<Handle<Image>>` is `None`, the new `FallbackImage` resource will be used instead. This attribute can be used in conjunction with a `sampler` binding attribute (with a different binding index).
* `sampler(BINDING_INDEX)`
* Behaves exactly like the `texture` attribute, but sets the Image's sampler binding instead of the texture.
Note that fields without field-level binding attributes will be ignored.
```rust
#[derive(AsBindGroup)]
struct CoolMaterial {
#[uniform(0)]
color: Color,
this_field_is_ignored: String,
}
```
As mentioned above, `Option<Handle<Image>>` is also supported:
```rust
#[derive(AsBindGroup)]
struct CoolMaterial {
#[uniform(0)]
color: Color,
#[texture(1)]
#[sampler(2)]
color_texture: Option<Handle<Image>>,
}
```
This is useful if you want a texture to be optional. When the value is `None`, the `FallbackImage` will be used for the binding instead, which defaults to "pure white".
Field uniforms with the same binding index will be combined into a single binding:
```rust
#[derive(AsBindGroup)]
struct CoolMaterial {
#[uniform(0)]
color: Color,
#[uniform(0)]
roughness: f32,
}
```
In WGSL shaders, the binding would look like this:
```wgsl
struct CoolMaterial {
color: vec4<f32>;
roughness: f32;
};
[[group(1), binding(0)]]
var<uniform> material: CoolMaterial;
```
Some less common scenarios will require "struct-level" attributes. These are the currently supported struct-level attributes:
* `uniform(BINDING_INDEX, ConvertedShaderType)`
* Similar to the field-level `uniform` attribute, but instead the entire `AsBindGroup` value is converted to `ConvertedShaderType`, which must implement `ShaderType`. This is useful if more complicated conversion logic is required.
* `bind_group_data(DataType)`
* The `AsBindGroup` type will be converted to some `DataType` using `Into<DataType>` and stored as `AsBindGroup::Data` as part of the `AsBindGroup::as_bind_group` call. This is useful if data needs to be stored alongside the generated bind group, such as a unique identifier for a material's bind group. The most common use case for this attribute is "shader pipeline specialization".
The previous `CoolMaterial` example illustrating "combining multiple field-level uniform attributes with the same binding index" can
also be equivalently represented with a single struct-level uniform attribute:
```rust
#[derive(AsBindGroup)]
#[uniform(0, CoolMaterialUniform)]
struct CoolMaterial {
color: Color,
roughness: f32,
}
#[derive(ShaderType)]
struct CoolMaterialUniform {
color: Color,
roughness: f32,
}
impl From<&CoolMaterial> for CoolMaterialUniform {
fn from(material: &CoolMaterial) -> CoolMaterialUniform {
CoolMaterialUniform {
color: material.color,
roughness: material.roughness,
}
}
}
```
### Material Specialization
Material shader specialization is now _much_ simpler:
```rust
#[derive(AsBindGroup, TypeUuid, Debug, Clone)]
#[uuid = "f690fdae-d598-45ab-8225-97e2a3f056e0"]
#[bind_group_data(CoolMaterialKey)]
struct CoolMaterial {
#[uniform(0)]
color: Color,
is_red: bool,
}
#[derive(Copy, Clone, Hash, Eq, PartialEq)]
struct CoolMaterialKey {
is_red: bool,
}
impl From<&CoolMaterial> for CoolMaterialKey {
fn from(material: &CoolMaterial) -> CoolMaterialKey {
CoolMaterialKey {
is_red: material.is_red,
}
}
}
impl Material for CoolMaterial {
fn fragment_shader() -> ShaderRef {
"cool_material.wgsl".into()
}
fn specialize(
pipeline: &MaterialPipeline<Self>,
descriptor: &mut RenderPipelineDescriptor,
layout: &MeshVertexBufferLayout,
key: MaterialPipelineKey<Self>,
) -> Result<(), SpecializedMeshPipelineError> {
if key.bind_group_data.is_red {
let fragment = descriptor.fragment.as_mut().unwrap();
fragment.shader_defs.push("IS_RED".to_string());
}
Ok(())
}
}
```
Setting `bind_group_data` is not required for specialization (it defaults to `()`). Scenarios like "custom vertex attributes" also benefit from this system:
```rust
impl Material for CustomMaterial {
fn vertex_shader() -> ShaderRef {
"custom_material.wgsl".into()
}
fn fragment_shader() -> ShaderRef {
"custom_material.wgsl".into()
}
fn specialize(
pipeline: &MaterialPipeline<Self>,
descriptor: &mut RenderPipelineDescriptor,
layout: &MeshVertexBufferLayout,
key: MaterialPipelineKey<Self>,
) -> Result<(), SpecializedMeshPipelineError> {
let vertex_layout = layout.get_layout(&[
Mesh::ATTRIBUTE_POSITION.at_shader_location(0),
ATTRIBUTE_BLEND_COLOR.at_shader_location(1),
])?;
descriptor.vertex.buffers = vec![vertex_layout];
Ok(())
}
}
```
### Ported `StandardMaterial` to the new `Material` system
Bevy's built-in PBR material uses the new Material system (including the AsBindGroup derive):
```rust
#[derive(AsBindGroup, Debug, Clone, TypeUuid)]
#[uuid = "7494888b-c082-457b-aacf-517228cc0c22"]
#[bind_group_data(StandardMaterialKey)]
#[uniform(0, StandardMaterialUniform)]
pub struct StandardMaterial {
pub base_color: Color,
#[texture(1)]
#[sampler(2)]
pub base_color_texture: Option<Handle<Image>>,
/* other fields omitted for brevity */
```
### Ported Bevy examples to the new `Material` system
The overall complexity of Bevy's "custom shader examples" has gone down significantly. Take a look at the diffs if you want a dopamine spike.
Please note that while this PR has a net increase in "lines of code", most of those extra lines come from added documentation. There is a significant reduction
in the overall complexity of the code (even accounting for the new derive logic).
---
## Changelog
### Added
* `AsBindGroup` trait and derive, which make it much easier to transfer data to the gpu and generate bind groups for a given type.
### Changed
* The old `Material` and `SpecializedMaterial` traits have been replaced by a consolidated (much simpler) `Material` trait. Materials no longer implement `RenderAsset`.
* `StandardMaterial` was ported to the new material system. There are no user-facing api changes to the `StandardMaterial` struct api, but it now implements `AsBindGroup` and `Material` instead of `RenderAsset` and `SpecializedMaterial`.
## Migration Guide
The Material system has been reworked to be much simpler. We've removed a lot of boilerplate with the new `AsBindGroup` derive and the `Material` trait is simpler as well!
### Bevy 0.7 (old)
```rust
#[derive(Debug, Clone, TypeUuid)]
#[uuid = "f690fdae-d598-45ab-8225-97e2a3f056e0"]
pub struct CustomMaterial {
color: Color,
color_texture: Handle<Image>,
}
#[derive(Clone)]
pub struct GpuCustomMaterial {
_buffer: Buffer,
bind_group: BindGroup,
}
impl RenderAsset for CustomMaterial {
type ExtractedAsset = CustomMaterial;
type PreparedAsset = GpuCustomMaterial;
type Param = (SRes<RenderDevice>, SRes<MaterialPipeline<Self>>);
fn extract_asset(&self) -> Self::ExtractedAsset {
self.clone()
}
fn prepare_asset(
extracted_asset: Self::ExtractedAsset,
(render_device, material_pipeline): &mut SystemParamItem<Self::Param>,
) -> Result<Self::PreparedAsset, PrepareAssetError<Self::ExtractedAsset>> {
let color = Vec4::from_slice(&extracted_asset.color.as_linear_rgba_f32());
let byte_buffer = [0u8; Vec4::SIZE.get() as usize];
let mut buffer = encase::UniformBuffer::new(byte_buffer);
buffer.write(&color).unwrap();
let buffer = render_device.create_buffer_with_data(&BufferInitDescriptor {
contents: buffer.as_ref(),
label: None,
usage: BufferUsages::UNIFORM | BufferUsages::COPY_DST,
});
let (texture_view, texture_sampler) = if let Some(result) = material_pipeline
.mesh_pipeline
.get_image_texture(gpu_images, &Some(extracted_asset.color_texture.clone()))
{
result
} else {
return Err(PrepareAssetError::RetryNextUpdate(extracted_asset));
};
let bind_group = render_device.create_bind_group(&BindGroupDescriptor {
entries: &[
BindGroupEntry {
binding: 0,
resource: buffer.as_entire_binding(),
},
BindGroupEntry {
binding: 0,
resource: BindingResource::TextureView(texture_view),
},
BindGroupEntry {
binding: 1,
resource: BindingResource::Sampler(texture_sampler),
},
],
label: None,
layout: &material_pipeline.material_layout,
});
Ok(GpuCustomMaterial {
_buffer: buffer,
bind_group,
})
}
}
impl Material for CustomMaterial {
fn fragment_shader(asset_server: &AssetServer) -> Option<Handle<Shader>> {
Some(asset_server.load("custom_material.wgsl"))
}
fn bind_group(render_asset: &<Self as RenderAsset>::PreparedAsset) -> &BindGroup {
&render_asset.bind_group
}
fn bind_group_layout(render_device: &RenderDevice) -> BindGroupLayout {
render_device.create_bind_group_layout(&BindGroupLayoutDescriptor {
entries: &[
BindGroupLayoutEntry {
binding: 0,
visibility: ShaderStages::FRAGMENT,
ty: BindingType::Buffer {
ty: BufferBindingType::Uniform,
has_dynamic_offset: false,
min_binding_size: Some(Vec4::min_size()),
},
count: None,
},
BindGroupLayoutEntry {
binding: 1,
visibility: ShaderStages::FRAGMENT,
ty: BindingType::Texture {
multisampled: false,
sample_type: TextureSampleType::Float { filterable: true },
view_dimension: TextureViewDimension::D2Array,
},
count: None,
},
BindGroupLayoutEntry {
binding: 2,
visibility: ShaderStages::FRAGMENT,
ty: BindingType::Sampler(SamplerBindingType::Filtering),
count: None,
},
],
label: None,
})
}
}
```
### Bevy 0.8 (new)
```rust
impl Material for CustomMaterial {
fn fragment_shader() -> ShaderRef {
"custom_material.wgsl".into()
}
}
#[derive(AsBindGroup, TypeUuid, Debug, Clone)]
#[uuid = "f690fdae-d598-45ab-8225-97e2a3f056e0"]
pub struct CustomMaterial {
#[uniform(0)]
color: Color,
#[texture(1)]
#[sampler(2)]
color_texture: Handle<Image>,
}
```
## Future Work
* Add support for more binding types (cubemaps, buffers, etc). This PR intentionally includes a bare minimum number of binding types to keep "reviewability" in check.
* Consider optionally eliding binding indices using binding names. `AsBindGroup` could pass in (optional?) reflection info as a "hint".
* This would make it possible for the derive to do this:
```rust
#[derive(AsBindGroup)]
pub struct CustomMaterial {
#[uniform]
color: Color,
#[texture]
#[sampler]
color_texture: Option<Handle<Image>>,
alpha_mode: AlphaMode,
}
```
* Or this
```rust
#[derive(AsBindGroup)]
pub struct CustomMaterial {
#[binding]
color: Color,
#[binding]
color_texture: Option<Handle<Image>>,
alpha_mode: AlphaMode,
}
```
* Or even this (if we flip to "include bindings by default")
```rust
#[derive(AsBindGroup)]
pub struct CustomMaterial {
color: Color,
color_texture: Option<Handle<Image>>,
#[binding(ignore)]
alpha_mode: AlphaMode,
}
```
* If we add the option to define custom draw functions for materials (which could be done in a type-erased way), I think that would be enough to support extra non-material bindings. Worth considering!
2022-06-30 23:48:46 +00:00
|
|
|
material_layout: M::bind_group_layout(render_device),
|
|
|
|
vertex_shader: match M::vertex_shader() {
|
|
|
|
ShaderRef::Default => None,
|
|
|
|
ShaderRef::Handle(handle) => Some(handle),
|
|
|
|
ShaderRef::Path(path) => Some(asset_server.load(path)),
|
|
|
|
},
|
|
|
|
fragment_shader: match M::fragment_shader() {
|
|
|
|
ShaderRef::Default => None,
|
|
|
|
ShaderRef::Handle(handle) => Some(handle),
|
|
|
|
ShaderRef::Path(path) => Some(asset_server.load(path)),
|
|
|
|
},
|
2021-12-25 21:45:43 +00:00
|
|
|
marker: PhantomData,
|
2021-12-14 03:58:23 +00:00
|
|
|
}
|
2021-12-25 21:45:43 +00:00
|
|
|
}
|
|
|
|
}
|
|
|
|
|
|
|
|
type DrawMaterial<M> = (
|
|
|
|
SetItemPipeline,
|
|
|
|
SetMeshViewBindGroup<0>,
|
2023-11-28 22:26:22 +00:00
|
|
|
SetMeshBindGroup<1>,
|
|
|
|
SetMaterialBindGroup<M, 2>,
|
2021-12-25 21:45:43 +00:00
|
|
|
DrawMesh,
|
|
|
|
);
|
|
|
|
|
Better Materials: AsBindGroup trait and derive, simpler Material trait (#5053)
# Objective
This PR reworks Bevy's Material system, making the user experience of defining Materials _much_ nicer. Bevy's previous material system leaves a lot to be desired:
* Materials require manually implementing the `RenderAsset` trait, which involves manually generating the bind group, handling gpu buffer data transfer, looking up image textures, etc. Even the simplest single-texture material involves writing ~80 unnecessary lines of code. This was never the long term plan.
* There are two material traits, which is confusing, hard to document, and often redundant: `Material` and `SpecializedMaterial`. `Material` implicitly implements `SpecializedMaterial`, and `SpecializedMaterial` is used in most high level apis to support both use cases. Most users shouldn't need to think about specialization at all (I consider it a "power-user tool"), so the fact that `SpecializedMaterial` is front-and-center in our apis is a miss.
* Implementing either material trait involves a lot of "type soup". The "prepared asset" parameter is particularly heinous: `&<Self as RenderAsset>::PreparedAsset`. Defining vertex and fragment shaders is also more verbose than it needs to be.
## Solution
Say hello to the new `Material` system:
```rust
#[derive(AsBindGroup, TypeUuid, Debug, Clone)]
#[uuid = "f690fdae-d598-45ab-8225-97e2a3f056e0"]
pub struct CoolMaterial {
#[uniform(0)]
color: Color,
#[texture(1)]
#[sampler(2)]
color_texture: Handle<Image>,
}
impl Material for CoolMaterial {
fn fragment_shader() -> ShaderRef {
"cool_material.wgsl".into()
}
}
```
Thats it! This same material would have required [~80 lines of complicated "type heavy" code](https://github.com/bevyengine/bevy/blob/v0.7.0/examples/shader/shader_material.rs) in the old Material system. Now it is just 14 lines of simple, readable code.
This is thanks to a new consolidated `Material` trait and the new `AsBindGroup` trait / derive.
### The new `Material` trait
The old "split" `Material` and `SpecializedMaterial` traits have been removed in favor of a new consolidated `Material` trait. All of the functions on the trait are optional.
The difficulty of implementing `Material` has been reduced by simplifying dataflow and removing type complexity:
```rust
// Old
impl Material for CustomMaterial {
fn fragment_shader(asset_server: &AssetServer) -> Option<Handle<Shader>> {
Some(asset_server.load("custom_material.wgsl"))
}
fn alpha_mode(render_asset: &<Self as RenderAsset>::PreparedAsset) -> AlphaMode {
render_asset.alpha_mode
}
}
// New
impl Material for CustomMaterial {
fn fragment_shader() -> ShaderRef {
"custom_material.wgsl".into()
}
fn alpha_mode(&self) -> AlphaMode {
self.alpha_mode
}
}
```
Specialization is still supported, but it is hidden by default under the `specialize()` function (more on this later).
### The `AsBindGroup` trait / derive
The `Material` trait now requires the `AsBindGroup` derive. This can be implemented manually relatively easily, but deriving it will almost always be preferable.
Field attributes like `uniform` and `texture` are used to define which fields should be bindings,
what their binding type is, and what index they should be bound at:
```rust
#[derive(AsBindGroup)]
struct CoolMaterial {
#[uniform(0)]
color: Color,
#[texture(1)]
#[sampler(2)]
color_texture: Handle<Image>,
}
```
In WGSL shaders, the binding looks like this:
```wgsl
struct CoolMaterial {
color: vec4<f32>;
};
[[group(1), binding(0)]]
var<uniform> material: CoolMaterial;
[[group(1), binding(1)]]
var color_texture: texture_2d<f32>;
[[group(1), binding(2)]]
var color_sampler: sampler;
```
Note that the "group" index is determined by the usage context. It is not defined in `AsBindGroup`. Bevy material bind groups are bound to group 1.
The following field-level attributes are supported:
* `uniform(BINDING_INDEX)`
* The field will be converted to a shader-compatible type using the `ShaderType` trait, written to a `Buffer`, and bound as a uniform. It can also be derived for custom structs.
* `texture(BINDING_INDEX)`
* This field's `Handle<Image>` will be used to look up the matching `Texture` gpu resource, which will be bound as a texture in shaders. The field will be assumed to implement `Into<Option<Handle<Image>>>`. In practice, most fields should be a `Handle<Image>` or `Option<Handle<Image>>`. If the value of an `Option<Handle<Image>>` is `None`, the new `FallbackImage` resource will be used instead. This attribute can be used in conjunction with a `sampler` binding attribute (with a different binding index).
* `sampler(BINDING_INDEX)`
* Behaves exactly like the `texture` attribute, but sets the Image's sampler binding instead of the texture.
Note that fields without field-level binding attributes will be ignored.
```rust
#[derive(AsBindGroup)]
struct CoolMaterial {
#[uniform(0)]
color: Color,
this_field_is_ignored: String,
}
```
As mentioned above, `Option<Handle<Image>>` is also supported:
```rust
#[derive(AsBindGroup)]
struct CoolMaterial {
#[uniform(0)]
color: Color,
#[texture(1)]
#[sampler(2)]
color_texture: Option<Handle<Image>>,
}
```
This is useful if you want a texture to be optional. When the value is `None`, the `FallbackImage` will be used for the binding instead, which defaults to "pure white".
Field uniforms with the same binding index will be combined into a single binding:
```rust
#[derive(AsBindGroup)]
struct CoolMaterial {
#[uniform(0)]
color: Color,
#[uniform(0)]
roughness: f32,
}
```
In WGSL shaders, the binding would look like this:
```wgsl
struct CoolMaterial {
color: vec4<f32>;
roughness: f32;
};
[[group(1), binding(0)]]
var<uniform> material: CoolMaterial;
```
Some less common scenarios will require "struct-level" attributes. These are the currently supported struct-level attributes:
* `uniform(BINDING_INDEX, ConvertedShaderType)`
* Similar to the field-level `uniform` attribute, but instead the entire `AsBindGroup` value is converted to `ConvertedShaderType`, which must implement `ShaderType`. This is useful if more complicated conversion logic is required.
* `bind_group_data(DataType)`
* The `AsBindGroup` type will be converted to some `DataType` using `Into<DataType>` and stored as `AsBindGroup::Data` as part of the `AsBindGroup::as_bind_group` call. This is useful if data needs to be stored alongside the generated bind group, such as a unique identifier for a material's bind group. The most common use case for this attribute is "shader pipeline specialization".
The previous `CoolMaterial` example illustrating "combining multiple field-level uniform attributes with the same binding index" can
also be equivalently represented with a single struct-level uniform attribute:
```rust
#[derive(AsBindGroup)]
#[uniform(0, CoolMaterialUniform)]
struct CoolMaterial {
color: Color,
roughness: f32,
}
#[derive(ShaderType)]
struct CoolMaterialUniform {
color: Color,
roughness: f32,
}
impl From<&CoolMaterial> for CoolMaterialUniform {
fn from(material: &CoolMaterial) -> CoolMaterialUniform {
CoolMaterialUniform {
color: material.color,
roughness: material.roughness,
}
}
}
```
### Material Specialization
Material shader specialization is now _much_ simpler:
```rust
#[derive(AsBindGroup, TypeUuid, Debug, Clone)]
#[uuid = "f690fdae-d598-45ab-8225-97e2a3f056e0"]
#[bind_group_data(CoolMaterialKey)]
struct CoolMaterial {
#[uniform(0)]
color: Color,
is_red: bool,
}
#[derive(Copy, Clone, Hash, Eq, PartialEq)]
struct CoolMaterialKey {
is_red: bool,
}
impl From<&CoolMaterial> for CoolMaterialKey {
fn from(material: &CoolMaterial) -> CoolMaterialKey {
CoolMaterialKey {
is_red: material.is_red,
}
}
}
impl Material for CoolMaterial {
fn fragment_shader() -> ShaderRef {
"cool_material.wgsl".into()
}
fn specialize(
pipeline: &MaterialPipeline<Self>,
descriptor: &mut RenderPipelineDescriptor,
layout: &MeshVertexBufferLayout,
key: MaterialPipelineKey<Self>,
) -> Result<(), SpecializedMeshPipelineError> {
if key.bind_group_data.is_red {
let fragment = descriptor.fragment.as_mut().unwrap();
fragment.shader_defs.push("IS_RED".to_string());
}
Ok(())
}
}
```
Setting `bind_group_data` is not required for specialization (it defaults to `()`). Scenarios like "custom vertex attributes" also benefit from this system:
```rust
impl Material for CustomMaterial {
fn vertex_shader() -> ShaderRef {
"custom_material.wgsl".into()
}
fn fragment_shader() -> ShaderRef {
"custom_material.wgsl".into()
}
fn specialize(
pipeline: &MaterialPipeline<Self>,
descriptor: &mut RenderPipelineDescriptor,
layout: &MeshVertexBufferLayout,
key: MaterialPipelineKey<Self>,
) -> Result<(), SpecializedMeshPipelineError> {
let vertex_layout = layout.get_layout(&[
Mesh::ATTRIBUTE_POSITION.at_shader_location(0),
ATTRIBUTE_BLEND_COLOR.at_shader_location(1),
])?;
descriptor.vertex.buffers = vec![vertex_layout];
Ok(())
}
}
```
### Ported `StandardMaterial` to the new `Material` system
Bevy's built-in PBR material uses the new Material system (including the AsBindGroup derive):
```rust
#[derive(AsBindGroup, Debug, Clone, TypeUuid)]
#[uuid = "7494888b-c082-457b-aacf-517228cc0c22"]
#[bind_group_data(StandardMaterialKey)]
#[uniform(0, StandardMaterialUniform)]
pub struct StandardMaterial {
pub base_color: Color,
#[texture(1)]
#[sampler(2)]
pub base_color_texture: Option<Handle<Image>>,
/* other fields omitted for brevity */
```
### Ported Bevy examples to the new `Material` system
The overall complexity of Bevy's "custom shader examples" has gone down significantly. Take a look at the diffs if you want a dopamine spike.
Please note that while this PR has a net increase in "lines of code", most of those extra lines come from added documentation. There is a significant reduction
in the overall complexity of the code (even accounting for the new derive logic).
---
## Changelog
### Added
* `AsBindGroup` trait and derive, which make it much easier to transfer data to the gpu and generate bind groups for a given type.
### Changed
* The old `Material` and `SpecializedMaterial` traits have been replaced by a consolidated (much simpler) `Material` trait. Materials no longer implement `RenderAsset`.
* `StandardMaterial` was ported to the new material system. There are no user-facing api changes to the `StandardMaterial` struct api, but it now implements `AsBindGroup` and `Material` instead of `RenderAsset` and `SpecializedMaterial`.
## Migration Guide
The Material system has been reworked to be much simpler. We've removed a lot of boilerplate with the new `AsBindGroup` derive and the `Material` trait is simpler as well!
### Bevy 0.7 (old)
```rust
#[derive(Debug, Clone, TypeUuid)]
#[uuid = "f690fdae-d598-45ab-8225-97e2a3f056e0"]
pub struct CustomMaterial {
color: Color,
color_texture: Handle<Image>,
}
#[derive(Clone)]
pub struct GpuCustomMaterial {
_buffer: Buffer,
bind_group: BindGroup,
}
impl RenderAsset for CustomMaterial {
type ExtractedAsset = CustomMaterial;
type PreparedAsset = GpuCustomMaterial;
type Param = (SRes<RenderDevice>, SRes<MaterialPipeline<Self>>);
fn extract_asset(&self) -> Self::ExtractedAsset {
self.clone()
}
fn prepare_asset(
extracted_asset: Self::ExtractedAsset,
(render_device, material_pipeline): &mut SystemParamItem<Self::Param>,
) -> Result<Self::PreparedAsset, PrepareAssetError<Self::ExtractedAsset>> {
let color = Vec4::from_slice(&extracted_asset.color.as_linear_rgba_f32());
let byte_buffer = [0u8; Vec4::SIZE.get() as usize];
let mut buffer = encase::UniformBuffer::new(byte_buffer);
buffer.write(&color).unwrap();
let buffer = render_device.create_buffer_with_data(&BufferInitDescriptor {
contents: buffer.as_ref(),
label: None,
usage: BufferUsages::UNIFORM | BufferUsages::COPY_DST,
});
let (texture_view, texture_sampler) = if let Some(result) = material_pipeline
.mesh_pipeline
.get_image_texture(gpu_images, &Some(extracted_asset.color_texture.clone()))
{
result
} else {
return Err(PrepareAssetError::RetryNextUpdate(extracted_asset));
};
let bind_group = render_device.create_bind_group(&BindGroupDescriptor {
entries: &[
BindGroupEntry {
binding: 0,
resource: buffer.as_entire_binding(),
},
BindGroupEntry {
binding: 0,
resource: BindingResource::TextureView(texture_view),
},
BindGroupEntry {
binding: 1,
resource: BindingResource::Sampler(texture_sampler),
},
],
label: None,
layout: &material_pipeline.material_layout,
});
Ok(GpuCustomMaterial {
_buffer: buffer,
bind_group,
})
}
}
impl Material for CustomMaterial {
fn fragment_shader(asset_server: &AssetServer) -> Option<Handle<Shader>> {
Some(asset_server.load("custom_material.wgsl"))
}
fn bind_group(render_asset: &<Self as RenderAsset>::PreparedAsset) -> &BindGroup {
&render_asset.bind_group
}
fn bind_group_layout(render_device: &RenderDevice) -> BindGroupLayout {
render_device.create_bind_group_layout(&BindGroupLayoutDescriptor {
entries: &[
BindGroupLayoutEntry {
binding: 0,
visibility: ShaderStages::FRAGMENT,
ty: BindingType::Buffer {
ty: BufferBindingType::Uniform,
has_dynamic_offset: false,
min_binding_size: Some(Vec4::min_size()),
},
count: None,
},
BindGroupLayoutEntry {
binding: 1,
visibility: ShaderStages::FRAGMENT,
ty: BindingType::Texture {
multisampled: false,
sample_type: TextureSampleType::Float { filterable: true },
view_dimension: TextureViewDimension::D2Array,
},
count: None,
},
BindGroupLayoutEntry {
binding: 2,
visibility: ShaderStages::FRAGMENT,
ty: BindingType::Sampler(SamplerBindingType::Filtering),
count: None,
},
],
label: None,
})
}
}
```
### Bevy 0.8 (new)
```rust
impl Material for CustomMaterial {
fn fragment_shader() -> ShaderRef {
"custom_material.wgsl".into()
}
}
#[derive(AsBindGroup, TypeUuid, Debug, Clone)]
#[uuid = "f690fdae-d598-45ab-8225-97e2a3f056e0"]
pub struct CustomMaterial {
#[uniform(0)]
color: Color,
#[texture(1)]
#[sampler(2)]
color_texture: Handle<Image>,
}
```
## Future Work
* Add support for more binding types (cubemaps, buffers, etc). This PR intentionally includes a bare minimum number of binding types to keep "reviewability" in check.
* Consider optionally eliding binding indices using binding names. `AsBindGroup` could pass in (optional?) reflection info as a "hint".
* This would make it possible for the derive to do this:
```rust
#[derive(AsBindGroup)]
pub struct CustomMaterial {
#[uniform]
color: Color,
#[texture]
#[sampler]
color_texture: Option<Handle<Image>>,
alpha_mode: AlphaMode,
}
```
* Or this
```rust
#[derive(AsBindGroup)]
pub struct CustomMaterial {
#[binding]
color: Color,
#[binding]
color_texture: Option<Handle<Image>>,
alpha_mode: AlphaMode,
}
```
* Or even this (if we flip to "include bindings by default")
```rust
#[derive(AsBindGroup)]
pub struct CustomMaterial {
color: Color,
color_texture: Option<Handle<Image>>,
#[binding(ignore)]
alpha_mode: AlphaMode,
}
```
* If we add the option to define custom draw functions for materials (which could be done in a type-erased way), I think that would be enough to support extra non-material bindings. Worth considering!
2022-06-30 23:48:46 +00:00
|
|
|
/// Sets the bind group for a given [`Material`] at the configured `I` index.
|
|
|
|
pub struct SetMaterialBindGroup<M: Material, const I: usize>(PhantomData<M>);
|
2023-01-04 01:13:30 +00:00
|
|
|
impl<P: PhaseItem, M: Material, const I: usize> RenderCommand<P> for SetMaterialBindGroup<M, I> {
|
2024-04-09 13:26:34 +00:00
|
|
|
type Param = (
|
|
|
|
SRes<RenderAssets<PreparedMaterial<M>>>,
|
|
|
|
SRes<RenderMaterialInstances<M>>,
|
|
|
|
);
|
2024-01-22 15:01:55 +00:00
|
|
|
type ViewQuery = ();
|
|
|
|
type ItemQuery = ();
|
2023-01-04 01:13:30 +00:00
|
|
|
|
|
|
|
#[inline]
|
2021-12-25 21:45:43 +00:00
|
|
|
fn render<'w>(
|
Use EntityHashMap<Entity, T> for render world entity storage for better performance (#9903)
# Objective
- Improve rendering performance, particularly by avoiding the large
system commands costs of using the ECS in the way that the render world
does.
## Solution
- Define `EntityHasher` that calculates a hash from the
`Entity.to_bits()` by `i | (i.wrapping_mul(0x517cc1b727220a95) << 32)`.
`0x517cc1b727220a95` is something like `u64::MAX / N` for N that gives a
value close to π and that works well for hashing. Thanks for @SkiFire13
for the suggestion and to @nicopap for alternative suggestions and
discussion. This approach comes from `rustc-hash` (a.k.a. `FxHasher`)
with some tweaks for the case of hashing an `Entity`. `FxHasher` and
`SeaHasher` were also tested but were significantly slower.
- Define `EntityHashMap` type that uses the `EntityHashser`
- Use `EntityHashMap<Entity, T>` for render world entity storage,
including:
- `RenderMaterialInstances` - contains the `AssetId<M>` of the material
associated with the entity. Also for 2D.
- `RenderMeshInstances` - contains mesh transforms, flags and properties
about mesh entities. Also for 2D.
- `SkinIndices` and `MorphIndices` - contains the skin and morph index
for an entity, respectively
- `ExtractedSprites`
- `ExtractedUiNodes`
## Benchmarks
All benchmarks have been conducted on an M1 Max connected to AC power.
The tests are run for 1500 frames. The 1000th frame is captured for
comparison to check for visual regressions. There were none.
### 2D Meshes
`bevymark --benchmark --waves 160 --per-wave 1000 --mode mesh2d`
#### `--ordered-z`
This test spawns the 2D meshes with z incrementing back to front, which
is the ideal arrangement allocation order as it matches the sorted
render order which means lookups have a high cache hit rate.
<img width="1112" alt="Screenshot 2023-09-27 at 07 50 45"
src="https://github.com/bevyengine/bevy/assets/302146/e140bc98-7091-4a3b-8ae1-ab75d16d2ccb">
-39.1% median frame time.
#### Random
This test spawns the 2D meshes with random z. This not only makes the
batching and transparent 2D pass lookups get a lot of cache misses, it
also currently means that the meshes are almost certain to not be
batchable.
<img width="1108" alt="Screenshot 2023-09-27 at 07 51 28"
src="https://github.com/bevyengine/bevy/assets/302146/29c2e813-645a-43ce-982a-55df4bf7d8c4">
-7.2% median frame time.
### 3D Meshes
`many_cubes --benchmark`
<img width="1112" alt="Screenshot 2023-09-27 at 07 51 57"
src="https://github.com/bevyengine/bevy/assets/302146/1a729673-3254-4e2a-9072-55e27c69f0fc">
-7.7% median frame time.
### Sprites
**NOTE: On `main` sprites are using `SparseSet<Entity, T>`!**
`bevymark --benchmark --waves 160 --per-wave 1000 --mode sprite`
#### `--ordered-z`
This test spawns the sprites with z incrementing back to front, which is
the ideal arrangement allocation order as it matches the sorted render
order which means lookups have a high cache hit rate.
<img width="1116" alt="Screenshot 2023-09-27 at 07 52 31"
src="https://github.com/bevyengine/bevy/assets/302146/bc8eab90-e375-4d31-b5cd-f55f6f59ab67">
+13.0% median frame time.
#### Random
This test spawns the sprites with random z. This makes the batching and
transparent 2D pass lookups get a lot of cache misses.
<img width="1109" alt="Screenshot 2023-09-27 at 07 53 01"
src="https://github.com/bevyengine/bevy/assets/302146/22073f5d-99a7-49b0-9584-d3ac3eac3033">
+0.6% median frame time.
### UI
**NOTE: On `main` UI is using `SparseSet<Entity, T>`!**
`many_buttons`
<img width="1111" alt="Screenshot 2023-09-27 at 07 53 26"
src="https://github.com/bevyengine/bevy/assets/302146/66afd56d-cbe4-49e7-8b64-2f28f6043d85">
+15.1% median frame time.
## Alternatives
- Cart originally suggested trying out `SparseSet<Entity, T>` and indeed
that is slightly faster under ideal conditions. However,
`PassHashMap<Entity, T>` has better worst case performance when data is
randomly distributed, rather than in sorted render order, and does not
have the worst case memory usage that `SparseSet`'s dense `Vec<usize>`
that maps from the `Entity` index to sparse index into `Vec<T>`. This
dense `Vec` has to be as large as the largest Entity index used with the
`SparseSet`.
- I also tested `PassHashMap<u32, T>`, intending to use `Entity.index()`
as the key, but this proved to sometimes be slower and mostly no
different.
- The only outstanding approach that has not been implemented and tested
is to _not_ clear the render world of its entities each frame. That has
its own problems, though they could perhaps be solved.
- Performance-wise, if the entities and their component data were not
cleared, then they would incur table moves on spawn, and should not
thereafter, rather just their component data would be overwritten.
Ideally we would have a neat way of either updating data in-place via
`&mut T` queries, or inserting components if not present. This would
likely be quite cumbersome to have to remember to do everywhere, but
perhaps it only needs to be done in the more performance-sensitive
systems.
- The main problem to solve however is that we want to both maintain a
mapping between main world entities and render world entities, be able
to run the render app and world in parallel with the main app and world
for pipelined rendering, and at the same time be able to spawn entities
in the render world in such a way that those Entity ids do not collide
with those spawned in the main world. This is potentially quite
solvable, but could well be a lot of ECS work to do it in a way that
makes sense.
---
## Changelog
- Changed: Component data for entities to be drawn are no longer stored
on entities in the render world. Instead, data is stored in a
`EntityHashMap<Entity, T>` in various resources. This brings significant
performance benefits due to the way the render app clears entities every
frame. Resources of most interest are `RenderMeshInstances` and
`RenderMaterialInstances`, and their 2D counterparts.
## Migration Guide
Previously the render app extracted mesh entities and their component
data from the main world and stored them as entities and components in
the render world. Now they are extracted into essentially
`EntityHashMap<Entity, T>` where `T` are structs containing an
appropriate group of data. This means that while extract set systems
will continue to run extract queries against the main world they will
store their data in hash maps. Also, systems in later sets will either
need to look up entities in the available resources such as
`RenderMeshInstances`, or maintain their own `EntityHashMap<Entity, T>`
for their own data.
Before:
```rust
fn queue_custom(
material_meshes: Query<(Entity, &MeshTransforms, &Handle<Mesh>), With<InstanceMaterialData>>,
) {
...
for (entity, mesh_transforms, mesh_handle) in &material_meshes {
...
}
}
```
After:
```rust
fn queue_custom(
render_mesh_instances: Res<RenderMeshInstances>,
instance_entities: Query<Entity, With<InstanceMaterialData>>,
) {
...
for entity in &instance_entities {
let Some(mesh_instance) = render_mesh_instances.get(&entity) else { continue; };
// The mesh handle in `AssetId<Mesh>` form, and the `MeshTransforms` can now
// be found in `mesh_instance` which is a `RenderMeshInstance`
...
}
}
```
---------
Co-authored-by: robtfm <50659922+robtfm@users.noreply.github.com>
2023-09-27 08:28:28 +00:00
|
|
|
item: &P,
|
2023-01-04 01:13:30 +00:00
|
|
|
_view: (),
|
2024-02-10 10:46:10 +00:00
|
|
|
_item_query: Option<()>,
|
Use EntityHashMap<Entity, T> for render world entity storage for better performance (#9903)
# Objective
- Improve rendering performance, particularly by avoiding the large
system commands costs of using the ECS in the way that the render world
does.
## Solution
- Define `EntityHasher` that calculates a hash from the
`Entity.to_bits()` by `i | (i.wrapping_mul(0x517cc1b727220a95) << 32)`.
`0x517cc1b727220a95` is something like `u64::MAX / N` for N that gives a
value close to π and that works well for hashing. Thanks for @SkiFire13
for the suggestion and to @nicopap for alternative suggestions and
discussion. This approach comes from `rustc-hash` (a.k.a. `FxHasher`)
with some tweaks for the case of hashing an `Entity`. `FxHasher` and
`SeaHasher` were also tested but were significantly slower.
- Define `EntityHashMap` type that uses the `EntityHashser`
- Use `EntityHashMap<Entity, T>` for render world entity storage,
including:
- `RenderMaterialInstances` - contains the `AssetId<M>` of the material
associated with the entity. Also for 2D.
- `RenderMeshInstances` - contains mesh transforms, flags and properties
about mesh entities. Also for 2D.
- `SkinIndices` and `MorphIndices` - contains the skin and morph index
for an entity, respectively
- `ExtractedSprites`
- `ExtractedUiNodes`
## Benchmarks
All benchmarks have been conducted on an M1 Max connected to AC power.
The tests are run for 1500 frames. The 1000th frame is captured for
comparison to check for visual regressions. There were none.
### 2D Meshes
`bevymark --benchmark --waves 160 --per-wave 1000 --mode mesh2d`
#### `--ordered-z`
This test spawns the 2D meshes with z incrementing back to front, which
is the ideal arrangement allocation order as it matches the sorted
render order which means lookups have a high cache hit rate.
<img width="1112" alt="Screenshot 2023-09-27 at 07 50 45"
src="https://github.com/bevyengine/bevy/assets/302146/e140bc98-7091-4a3b-8ae1-ab75d16d2ccb">
-39.1% median frame time.
#### Random
This test spawns the 2D meshes with random z. This not only makes the
batching and transparent 2D pass lookups get a lot of cache misses, it
also currently means that the meshes are almost certain to not be
batchable.
<img width="1108" alt="Screenshot 2023-09-27 at 07 51 28"
src="https://github.com/bevyengine/bevy/assets/302146/29c2e813-645a-43ce-982a-55df4bf7d8c4">
-7.2% median frame time.
### 3D Meshes
`many_cubes --benchmark`
<img width="1112" alt="Screenshot 2023-09-27 at 07 51 57"
src="https://github.com/bevyengine/bevy/assets/302146/1a729673-3254-4e2a-9072-55e27c69f0fc">
-7.7% median frame time.
### Sprites
**NOTE: On `main` sprites are using `SparseSet<Entity, T>`!**
`bevymark --benchmark --waves 160 --per-wave 1000 --mode sprite`
#### `--ordered-z`
This test spawns the sprites with z incrementing back to front, which is
the ideal arrangement allocation order as it matches the sorted render
order which means lookups have a high cache hit rate.
<img width="1116" alt="Screenshot 2023-09-27 at 07 52 31"
src="https://github.com/bevyengine/bevy/assets/302146/bc8eab90-e375-4d31-b5cd-f55f6f59ab67">
+13.0% median frame time.
#### Random
This test spawns the sprites with random z. This makes the batching and
transparent 2D pass lookups get a lot of cache misses.
<img width="1109" alt="Screenshot 2023-09-27 at 07 53 01"
src="https://github.com/bevyengine/bevy/assets/302146/22073f5d-99a7-49b0-9584-d3ac3eac3033">
+0.6% median frame time.
### UI
**NOTE: On `main` UI is using `SparseSet<Entity, T>`!**
`many_buttons`
<img width="1111" alt="Screenshot 2023-09-27 at 07 53 26"
src="https://github.com/bevyengine/bevy/assets/302146/66afd56d-cbe4-49e7-8b64-2f28f6043d85">
+15.1% median frame time.
## Alternatives
- Cart originally suggested trying out `SparseSet<Entity, T>` and indeed
that is slightly faster under ideal conditions. However,
`PassHashMap<Entity, T>` has better worst case performance when data is
randomly distributed, rather than in sorted render order, and does not
have the worst case memory usage that `SparseSet`'s dense `Vec<usize>`
that maps from the `Entity` index to sparse index into `Vec<T>`. This
dense `Vec` has to be as large as the largest Entity index used with the
`SparseSet`.
- I also tested `PassHashMap<u32, T>`, intending to use `Entity.index()`
as the key, but this proved to sometimes be slower and mostly no
different.
- The only outstanding approach that has not been implemented and tested
is to _not_ clear the render world of its entities each frame. That has
its own problems, though they could perhaps be solved.
- Performance-wise, if the entities and their component data were not
cleared, then they would incur table moves on spawn, and should not
thereafter, rather just their component data would be overwritten.
Ideally we would have a neat way of either updating data in-place via
`&mut T` queries, or inserting components if not present. This would
likely be quite cumbersome to have to remember to do everywhere, but
perhaps it only needs to be done in the more performance-sensitive
systems.
- The main problem to solve however is that we want to both maintain a
mapping between main world entities and render world entities, be able
to run the render app and world in parallel with the main app and world
for pipelined rendering, and at the same time be able to spawn entities
in the render world in such a way that those Entity ids do not collide
with those spawned in the main world. This is potentially quite
solvable, but could well be a lot of ECS work to do it in a way that
makes sense.
---
## Changelog
- Changed: Component data for entities to be drawn are no longer stored
on entities in the render world. Instead, data is stored in a
`EntityHashMap<Entity, T>` in various resources. This brings significant
performance benefits due to the way the render app clears entities every
frame. Resources of most interest are `RenderMeshInstances` and
`RenderMaterialInstances`, and their 2D counterparts.
## Migration Guide
Previously the render app extracted mesh entities and their component
data from the main world and stored them as entities and components in
the render world. Now they are extracted into essentially
`EntityHashMap<Entity, T>` where `T` are structs containing an
appropriate group of data. This means that while extract set systems
will continue to run extract queries against the main world they will
store their data in hash maps. Also, systems in later sets will either
need to look up entities in the available resources such as
`RenderMeshInstances`, or maintain their own `EntityHashMap<Entity, T>`
for their own data.
Before:
```rust
fn queue_custom(
material_meshes: Query<(Entity, &MeshTransforms, &Handle<Mesh>), With<InstanceMaterialData>>,
) {
...
for (entity, mesh_transforms, mesh_handle) in &material_meshes {
...
}
}
```
After:
```rust
fn queue_custom(
render_mesh_instances: Res<RenderMeshInstances>,
instance_entities: Query<Entity, With<InstanceMaterialData>>,
) {
...
for entity in &instance_entities {
let Some(mesh_instance) = render_mesh_instances.get(&entity) else { continue; };
// The mesh handle in `AssetId<Mesh>` form, and the `MeshTransforms` can now
// be found in `mesh_instance` which is a `RenderMeshInstance`
...
}
}
```
---------
Co-authored-by: robtfm <50659922+robtfm@users.noreply.github.com>
2023-09-27 08:28:28 +00:00
|
|
|
(materials, material_instances): SystemParamItem<'w, '_, Self::Param>,
|
2021-12-25 21:45:43 +00:00
|
|
|
pass: &mut TrackedRenderPass<'w>,
|
|
|
|
) -> RenderCommandResult {
|
Use EntityHashMap<Entity, T> for render world entity storage for better performance (#9903)
# Objective
- Improve rendering performance, particularly by avoiding the large
system commands costs of using the ECS in the way that the render world
does.
## Solution
- Define `EntityHasher` that calculates a hash from the
`Entity.to_bits()` by `i | (i.wrapping_mul(0x517cc1b727220a95) << 32)`.
`0x517cc1b727220a95` is something like `u64::MAX / N` for N that gives a
value close to π and that works well for hashing. Thanks for @SkiFire13
for the suggestion and to @nicopap for alternative suggestions and
discussion. This approach comes from `rustc-hash` (a.k.a. `FxHasher`)
with some tweaks for the case of hashing an `Entity`. `FxHasher` and
`SeaHasher` were also tested but were significantly slower.
- Define `EntityHashMap` type that uses the `EntityHashser`
- Use `EntityHashMap<Entity, T>` for render world entity storage,
including:
- `RenderMaterialInstances` - contains the `AssetId<M>` of the material
associated with the entity. Also for 2D.
- `RenderMeshInstances` - contains mesh transforms, flags and properties
about mesh entities. Also for 2D.
- `SkinIndices` and `MorphIndices` - contains the skin and morph index
for an entity, respectively
- `ExtractedSprites`
- `ExtractedUiNodes`
## Benchmarks
All benchmarks have been conducted on an M1 Max connected to AC power.
The tests are run for 1500 frames. The 1000th frame is captured for
comparison to check for visual regressions. There were none.
### 2D Meshes
`bevymark --benchmark --waves 160 --per-wave 1000 --mode mesh2d`
#### `--ordered-z`
This test spawns the 2D meshes with z incrementing back to front, which
is the ideal arrangement allocation order as it matches the sorted
render order which means lookups have a high cache hit rate.
<img width="1112" alt="Screenshot 2023-09-27 at 07 50 45"
src="https://github.com/bevyengine/bevy/assets/302146/e140bc98-7091-4a3b-8ae1-ab75d16d2ccb">
-39.1% median frame time.
#### Random
This test spawns the 2D meshes with random z. This not only makes the
batching and transparent 2D pass lookups get a lot of cache misses, it
also currently means that the meshes are almost certain to not be
batchable.
<img width="1108" alt="Screenshot 2023-09-27 at 07 51 28"
src="https://github.com/bevyengine/bevy/assets/302146/29c2e813-645a-43ce-982a-55df4bf7d8c4">
-7.2% median frame time.
### 3D Meshes
`many_cubes --benchmark`
<img width="1112" alt="Screenshot 2023-09-27 at 07 51 57"
src="https://github.com/bevyengine/bevy/assets/302146/1a729673-3254-4e2a-9072-55e27c69f0fc">
-7.7% median frame time.
### Sprites
**NOTE: On `main` sprites are using `SparseSet<Entity, T>`!**
`bevymark --benchmark --waves 160 --per-wave 1000 --mode sprite`
#### `--ordered-z`
This test spawns the sprites with z incrementing back to front, which is
the ideal arrangement allocation order as it matches the sorted render
order which means lookups have a high cache hit rate.
<img width="1116" alt="Screenshot 2023-09-27 at 07 52 31"
src="https://github.com/bevyengine/bevy/assets/302146/bc8eab90-e375-4d31-b5cd-f55f6f59ab67">
+13.0% median frame time.
#### Random
This test spawns the sprites with random z. This makes the batching and
transparent 2D pass lookups get a lot of cache misses.
<img width="1109" alt="Screenshot 2023-09-27 at 07 53 01"
src="https://github.com/bevyengine/bevy/assets/302146/22073f5d-99a7-49b0-9584-d3ac3eac3033">
+0.6% median frame time.
### UI
**NOTE: On `main` UI is using `SparseSet<Entity, T>`!**
`many_buttons`
<img width="1111" alt="Screenshot 2023-09-27 at 07 53 26"
src="https://github.com/bevyengine/bevy/assets/302146/66afd56d-cbe4-49e7-8b64-2f28f6043d85">
+15.1% median frame time.
## Alternatives
- Cart originally suggested trying out `SparseSet<Entity, T>` and indeed
that is slightly faster under ideal conditions. However,
`PassHashMap<Entity, T>` has better worst case performance when data is
randomly distributed, rather than in sorted render order, and does not
have the worst case memory usage that `SparseSet`'s dense `Vec<usize>`
that maps from the `Entity` index to sparse index into `Vec<T>`. This
dense `Vec` has to be as large as the largest Entity index used with the
`SparseSet`.
- I also tested `PassHashMap<u32, T>`, intending to use `Entity.index()`
as the key, but this proved to sometimes be slower and mostly no
different.
- The only outstanding approach that has not been implemented and tested
is to _not_ clear the render world of its entities each frame. That has
its own problems, though they could perhaps be solved.
- Performance-wise, if the entities and their component data were not
cleared, then they would incur table moves on spawn, and should not
thereafter, rather just their component data would be overwritten.
Ideally we would have a neat way of either updating data in-place via
`&mut T` queries, or inserting components if not present. This would
likely be quite cumbersome to have to remember to do everywhere, but
perhaps it only needs to be done in the more performance-sensitive
systems.
- The main problem to solve however is that we want to both maintain a
mapping between main world entities and render world entities, be able
to run the render app and world in parallel with the main app and world
for pipelined rendering, and at the same time be able to spawn entities
in the render world in such a way that those Entity ids do not collide
with those spawned in the main world. This is potentially quite
solvable, but could well be a lot of ECS work to do it in a way that
makes sense.
---
## Changelog
- Changed: Component data for entities to be drawn are no longer stored
on entities in the render world. Instead, data is stored in a
`EntityHashMap<Entity, T>` in various resources. This brings significant
performance benefits due to the way the render app clears entities every
frame. Resources of most interest are `RenderMeshInstances` and
`RenderMaterialInstances`, and their 2D counterparts.
## Migration Guide
Previously the render app extracted mesh entities and their component
data from the main world and stored them as entities and components in
the render world. Now they are extracted into essentially
`EntityHashMap<Entity, T>` where `T` are structs containing an
appropriate group of data. This means that while extract set systems
will continue to run extract queries against the main world they will
store their data in hash maps. Also, systems in later sets will either
need to look up entities in the available resources such as
`RenderMeshInstances`, or maintain their own `EntityHashMap<Entity, T>`
for their own data.
Before:
```rust
fn queue_custom(
material_meshes: Query<(Entity, &MeshTransforms, &Handle<Mesh>), With<InstanceMaterialData>>,
) {
...
for (entity, mesh_transforms, mesh_handle) in &material_meshes {
...
}
}
```
After:
```rust
fn queue_custom(
render_mesh_instances: Res<RenderMeshInstances>,
instance_entities: Query<Entity, With<InstanceMaterialData>>,
) {
...
for entity in &instance_entities {
let Some(mesh_instance) = render_mesh_instances.get(&entity) else { continue; };
// The mesh handle in `AssetId<Mesh>` form, and the `MeshTransforms` can now
// be found in `mesh_instance` which is a `RenderMeshInstance`
...
}
}
```
---------
Co-authored-by: robtfm <50659922+robtfm@users.noreply.github.com>
2023-09-27 08:28:28 +00:00
|
|
|
let materials = materials.into_inner();
|
|
|
|
let material_instances = material_instances.into_inner();
|
|
|
|
|
Type safe retained render world (#15756)
# Objective
In the Render World, there are a number of collections that are derived
from Main World entities and are used to drive rendering. The most
notable are:
- `VisibleEntities`, which is generated in the `check_visibility` system
and contains visible entities for a view.
- `ExtractedInstances`, which maps entity ids to asset ids.
In the old model, these collections were trivially kept in sync -- any
extracted phase item could look itself up because the render entity id
was guaranteed to always match the corresponding main world id.
After #15320, this became much more complicated, and was leading to a
number of subtle bugs in the Render World. The main rendering systems,
i.e. `queue_material_meshes` and `queue_material2d_meshes`, follow a
similar pattern:
```rust
for visible_entity in visible_entities.iter::<With<Mesh2d>>() {
let Some(mesh_instance) = render_mesh_instances.get_mut(visible_entity) else {
continue;
};
// Look some more stuff up and specialize the pipeline...
let bin_key = Opaque2dBinKey {
pipeline: pipeline_id,
draw_function: draw_opaque_2d,
asset_id: mesh_instance.mesh_asset_id.into(),
material_bind_group_id: material_2d.get_bind_group_id().0,
};
opaque_phase.add(
bin_key,
*visible_entity,
BinnedRenderPhaseType::mesh(mesh_instance.automatic_batching),
);
}
```
In this case, `visible_entities` and `render_mesh_instances` are both
collections that are created and keyed by Main World entity ids, and so
this lookup happens to work by coincidence. However, there is a major
unintentional bug here: namely, because `visible_entities` is a
collection of Main World ids, the phase item being queued is created
with a Main World id rather than its correct Render World id.
This happens to not break mesh rendering because the render commands
used for drawing meshes do not access the `ItemQuery` parameter, but
demonstrates the confusion that is now possible: our UI phase items are
correctly being queued with Render World ids while our meshes aren't.
Additionally, this makes it very easy and error prone to use the wrong
entity id to look up things like assets. For example, if instead we
ignored visibility checks and queued our meshes via a query, we'd have
to be extra careful to use `&MainEntity` instead of the natural
`Entity`.
## Solution
Make all collections that are derived from Main World data use
`MainEntity` as their key, to ensure type safety and avoid accidentally
looking up data with the wrong entity id:
```rust
pub type MainEntityHashMap<V> = hashbrown::HashMap<MainEntity, V, EntityHash>;
```
Additionally, we make all `PhaseItem` be able to provide both their Main
and Render World ids, to allow render phase implementors maximum
flexibility as to what id should be used to look up data.
You can think of this like tracking at the type level whether something
in the Render World should use it's "primary key", i.e. entity id, or
needs to use a foreign key, i.e. `MainEntity`.
## Testing
##### TODO:
This will require extensive testing to make sure things didn't break!
Additionally, some extraction logic has become more complicated and
needs to be checked for regressions.
## Migration Guide
With the advent of the retained render world, collections that contain
references to `Entity` that are extracted into the render world have
been changed to contain `MainEntity` in order to prevent errors where a
render world entity id is used to look up an item by accident. Custom
rendering code may need to be changed to query for `&MainEntity` in
order to look up the correct item from such a collection. Additionally,
users who implement their own extraction logic for collections of main
world entity should strongly consider extracting into a different
collection that uses `MainEntity` as a key.
Additionally, render phases now require specifying both the `Entity` and
`MainEntity` for a given `PhaseItem`. Custom render phases should ensure
`MainEntity` is available when queuing a phase item.
2024-10-10 18:47:04 +00:00
|
|
|
let Some(material_asset_id) = material_instances.get(&item.main_entity()) else {
|
2024-07-22 19:22:30 +00:00
|
|
|
return RenderCommandResult::Skip;
|
Use EntityHashMap<Entity, T> for render world entity storage for better performance (#9903)
# Objective
- Improve rendering performance, particularly by avoiding the large
system commands costs of using the ECS in the way that the render world
does.
## Solution
- Define `EntityHasher` that calculates a hash from the
`Entity.to_bits()` by `i | (i.wrapping_mul(0x517cc1b727220a95) << 32)`.
`0x517cc1b727220a95` is something like `u64::MAX / N` for N that gives a
value close to π and that works well for hashing. Thanks for @SkiFire13
for the suggestion and to @nicopap for alternative suggestions and
discussion. This approach comes from `rustc-hash` (a.k.a. `FxHasher`)
with some tweaks for the case of hashing an `Entity`. `FxHasher` and
`SeaHasher` were also tested but were significantly slower.
- Define `EntityHashMap` type that uses the `EntityHashser`
- Use `EntityHashMap<Entity, T>` for render world entity storage,
including:
- `RenderMaterialInstances` - contains the `AssetId<M>` of the material
associated with the entity. Also for 2D.
- `RenderMeshInstances` - contains mesh transforms, flags and properties
about mesh entities. Also for 2D.
- `SkinIndices` and `MorphIndices` - contains the skin and morph index
for an entity, respectively
- `ExtractedSprites`
- `ExtractedUiNodes`
## Benchmarks
All benchmarks have been conducted on an M1 Max connected to AC power.
The tests are run for 1500 frames. The 1000th frame is captured for
comparison to check for visual regressions. There were none.
### 2D Meshes
`bevymark --benchmark --waves 160 --per-wave 1000 --mode mesh2d`
#### `--ordered-z`
This test spawns the 2D meshes with z incrementing back to front, which
is the ideal arrangement allocation order as it matches the sorted
render order which means lookups have a high cache hit rate.
<img width="1112" alt="Screenshot 2023-09-27 at 07 50 45"
src="https://github.com/bevyengine/bevy/assets/302146/e140bc98-7091-4a3b-8ae1-ab75d16d2ccb">
-39.1% median frame time.
#### Random
This test spawns the 2D meshes with random z. This not only makes the
batching and transparent 2D pass lookups get a lot of cache misses, it
also currently means that the meshes are almost certain to not be
batchable.
<img width="1108" alt="Screenshot 2023-09-27 at 07 51 28"
src="https://github.com/bevyengine/bevy/assets/302146/29c2e813-645a-43ce-982a-55df4bf7d8c4">
-7.2% median frame time.
### 3D Meshes
`many_cubes --benchmark`
<img width="1112" alt="Screenshot 2023-09-27 at 07 51 57"
src="https://github.com/bevyengine/bevy/assets/302146/1a729673-3254-4e2a-9072-55e27c69f0fc">
-7.7% median frame time.
### Sprites
**NOTE: On `main` sprites are using `SparseSet<Entity, T>`!**
`bevymark --benchmark --waves 160 --per-wave 1000 --mode sprite`
#### `--ordered-z`
This test spawns the sprites with z incrementing back to front, which is
the ideal arrangement allocation order as it matches the sorted render
order which means lookups have a high cache hit rate.
<img width="1116" alt="Screenshot 2023-09-27 at 07 52 31"
src="https://github.com/bevyengine/bevy/assets/302146/bc8eab90-e375-4d31-b5cd-f55f6f59ab67">
+13.0% median frame time.
#### Random
This test spawns the sprites with random z. This makes the batching and
transparent 2D pass lookups get a lot of cache misses.
<img width="1109" alt="Screenshot 2023-09-27 at 07 53 01"
src="https://github.com/bevyengine/bevy/assets/302146/22073f5d-99a7-49b0-9584-d3ac3eac3033">
+0.6% median frame time.
### UI
**NOTE: On `main` UI is using `SparseSet<Entity, T>`!**
`many_buttons`
<img width="1111" alt="Screenshot 2023-09-27 at 07 53 26"
src="https://github.com/bevyengine/bevy/assets/302146/66afd56d-cbe4-49e7-8b64-2f28f6043d85">
+15.1% median frame time.
## Alternatives
- Cart originally suggested trying out `SparseSet<Entity, T>` and indeed
that is slightly faster under ideal conditions. However,
`PassHashMap<Entity, T>` has better worst case performance when data is
randomly distributed, rather than in sorted render order, and does not
have the worst case memory usage that `SparseSet`'s dense `Vec<usize>`
that maps from the `Entity` index to sparse index into `Vec<T>`. This
dense `Vec` has to be as large as the largest Entity index used with the
`SparseSet`.
- I also tested `PassHashMap<u32, T>`, intending to use `Entity.index()`
as the key, but this proved to sometimes be slower and mostly no
different.
- The only outstanding approach that has not been implemented and tested
is to _not_ clear the render world of its entities each frame. That has
its own problems, though they could perhaps be solved.
- Performance-wise, if the entities and their component data were not
cleared, then they would incur table moves on spawn, and should not
thereafter, rather just their component data would be overwritten.
Ideally we would have a neat way of either updating data in-place via
`&mut T` queries, or inserting components if not present. This would
likely be quite cumbersome to have to remember to do everywhere, but
perhaps it only needs to be done in the more performance-sensitive
systems.
- The main problem to solve however is that we want to both maintain a
mapping between main world entities and render world entities, be able
to run the render app and world in parallel with the main app and world
for pipelined rendering, and at the same time be able to spawn entities
in the render world in such a way that those Entity ids do not collide
with those spawned in the main world. This is potentially quite
solvable, but could well be a lot of ECS work to do it in a way that
makes sense.
---
## Changelog
- Changed: Component data for entities to be drawn are no longer stored
on entities in the render world. Instead, data is stored in a
`EntityHashMap<Entity, T>` in various resources. This brings significant
performance benefits due to the way the render app clears entities every
frame. Resources of most interest are `RenderMeshInstances` and
`RenderMaterialInstances`, and their 2D counterparts.
## Migration Guide
Previously the render app extracted mesh entities and their component
data from the main world and stored them as entities and components in
the render world. Now they are extracted into essentially
`EntityHashMap<Entity, T>` where `T` are structs containing an
appropriate group of data. This means that while extract set systems
will continue to run extract queries against the main world they will
store their data in hash maps. Also, systems in later sets will either
need to look up entities in the available resources such as
`RenderMeshInstances`, or maintain their own `EntityHashMap<Entity, T>`
for their own data.
Before:
```rust
fn queue_custom(
material_meshes: Query<(Entity, &MeshTransforms, &Handle<Mesh>), With<InstanceMaterialData>>,
) {
...
for (entity, mesh_transforms, mesh_handle) in &material_meshes {
...
}
}
```
After:
```rust
fn queue_custom(
render_mesh_instances: Res<RenderMeshInstances>,
instance_entities: Query<Entity, With<InstanceMaterialData>>,
) {
...
for entity in &instance_entities {
let Some(mesh_instance) = render_mesh_instances.get(&entity) else { continue; };
// The mesh handle in `AssetId<Mesh>` form, and the `MeshTransforms` can now
// be found in `mesh_instance` which is a `RenderMeshInstance`
...
}
}
```
---------
Co-authored-by: robtfm <50659922+robtfm@users.noreply.github.com>
2023-09-27 08:28:28 +00:00
|
|
|
};
|
2024-04-09 13:26:34 +00:00
|
|
|
let Some(material) = materials.get(*material_asset_id) else {
|
2024-07-22 19:22:30 +00:00
|
|
|
return RenderCommandResult::Skip;
|
Use EntityHashMap<Entity, T> for render world entity storage for better performance (#9903)
# Objective
- Improve rendering performance, particularly by avoiding the large
system commands costs of using the ECS in the way that the render world
does.
## Solution
- Define `EntityHasher` that calculates a hash from the
`Entity.to_bits()` by `i | (i.wrapping_mul(0x517cc1b727220a95) << 32)`.
`0x517cc1b727220a95` is something like `u64::MAX / N` for N that gives a
value close to π and that works well for hashing. Thanks for @SkiFire13
for the suggestion and to @nicopap for alternative suggestions and
discussion. This approach comes from `rustc-hash` (a.k.a. `FxHasher`)
with some tweaks for the case of hashing an `Entity`. `FxHasher` and
`SeaHasher` were also tested but were significantly slower.
- Define `EntityHashMap` type that uses the `EntityHashser`
- Use `EntityHashMap<Entity, T>` for render world entity storage,
including:
- `RenderMaterialInstances` - contains the `AssetId<M>` of the material
associated with the entity. Also for 2D.
- `RenderMeshInstances` - contains mesh transforms, flags and properties
about mesh entities. Also for 2D.
- `SkinIndices` and `MorphIndices` - contains the skin and morph index
for an entity, respectively
- `ExtractedSprites`
- `ExtractedUiNodes`
## Benchmarks
All benchmarks have been conducted on an M1 Max connected to AC power.
The tests are run for 1500 frames. The 1000th frame is captured for
comparison to check for visual regressions. There were none.
### 2D Meshes
`bevymark --benchmark --waves 160 --per-wave 1000 --mode mesh2d`
#### `--ordered-z`
This test spawns the 2D meshes with z incrementing back to front, which
is the ideal arrangement allocation order as it matches the sorted
render order which means lookups have a high cache hit rate.
<img width="1112" alt="Screenshot 2023-09-27 at 07 50 45"
src="https://github.com/bevyengine/bevy/assets/302146/e140bc98-7091-4a3b-8ae1-ab75d16d2ccb">
-39.1% median frame time.
#### Random
This test spawns the 2D meshes with random z. This not only makes the
batching and transparent 2D pass lookups get a lot of cache misses, it
also currently means that the meshes are almost certain to not be
batchable.
<img width="1108" alt="Screenshot 2023-09-27 at 07 51 28"
src="https://github.com/bevyengine/bevy/assets/302146/29c2e813-645a-43ce-982a-55df4bf7d8c4">
-7.2% median frame time.
### 3D Meshes
`many_cubes --benchmark`
<img width="1112" alt="Screenshot 2023-09-27 at 07 51 57"
src="https://github.com/bevyengine/bevy/assets/302146/1a729673-3254-4e2a-9072-55e27c69f0fc">
-7.7% median frame time.
### Sprites
**NOTE: On `main` sprites are using `SparseSet<Entity, T>`!**
`bevymark --benchmark --waves 160 --per-wave 1000 --mode sprite`
#### `--ordered-z`
This test spawns the sprites with z incrementing back to front, which is
the ideal arrangement allocation order as it matches the sorted render
order which means lookups have a high cache hit rate.
<img width="1116" alt="Screenshot 2023-09-27 at 07 52 31"
src="https://github.com/bevyengine/bevy/assets/302146/bc8eab90-e375-4d31-b5cd-f55f6f59ab67">
+13.0% median frame time.
#### Random
This test spawns the sprites with random z. This makes the batching and
transparent 2D pass lookups get a lot of cache misses.
<img width="1109" alt="Screenshot 2023-09-27 at 07 53 01"
src="https://github.com/bevyengine/bevy/assets/302146/22073f5d-99a7-49b0-9584-d3ac3eac3033">
+0.6% median frame time.
### UI
**NOTE: On `main` UI is using `SparseSet<Entity, T>`!**
`many_buttons`
<img width="1111" alt="Screenshot 2023-09-27 at 07 53 26"
src="https://github.com/bevyengine/bevy/assets/302146/66afd56d-cbe4-49e7-8b64-2f28f6043d85">
+15.1% median frame time.
## Alternatives
- Cart originally suggested trying out `SparseSet<Entity, T>` and indeed
that is slightly faster under ideal conditions. However,
`PassHashMap<Entity, T>` has better worst case performance when data is
randomly distributed, rather than in sorted render order, and does not
have the worst case memory usage that `SparseSet`'s dense `Vec<usize>`
that maps from the `Entity` index to sparse index into `Vec<T>`. This
dense `Vec` has to be as large as the largest Entity index used with the
`SparseSet`.
- I also tested `PassHashMap<u32, T>`, intending to use `Entity.index()`
as the key, but this proved to sometimes be slower and mostly no
different.
- The only outstanding approach that has not been implemented and tested
is to _not_ clear the render world of its entities each frame. That has
its own problems, though they could perhaps be solved.
- Performance-wise, if the entities and their component data were not
cleared, then they would incur table moves on spawn, and should not
thereafter, rather just their component data would be overwritten.
Ideally we would have a neat way of either updating data in-place via
`&mut T` queries, or inserting components if not present. This would
likely be quite cumbersome to have to remember to do everywhere, but
perhaps it only needs to be done in the more performance-sensitive
systems.
- The main problem to solve however is that we want to both maintain a
mapping between main world entities and render world entities, be able
to run the render app and world in parallel with the main app and world
for pipelined rendering, and at the same time be able to spawn entities
in the render world in such a way that those Entity ids do not collide
with those spawned in the main world. This is potentially quite
solvable, but could well be a lot of ECS work to do it in a way that
makes sense.
---
## Changelog
- Changed: Component data for entities to be drawn are no longer stored
on entities in the render world. Instead, data is stored in a
`EntityHashMap<Entity, T>` in various resources. This brings significant
performance benefits due to the way the render app clears entities every
frame. Resources of most interest are `RenderMeshInstances` and
`RenderMaterialInstances`, and their 2D counterparts.
## Migration Guide
Previously the render app extracted mesh entities and their component
data from the main world and stored them as entities and components in
the render world. Now they are extracted into essentially
`EntityHashMap<Entity, T>` where `T` are structs containing an
appropriate group of data. This means that while extract set systems
will continue to run extract queries against the main world they will
store their data in hash maps. Also, systems in later sets will either
need to look up entities in the available resources such as
`RenderMeshInstances`, or maintain their own `EntityHashMap<Entity, T>`
for their own data.
Before:
```rust
fn queue_custom(
material_meshes: Query<(Entity, &MeshTransforms, &Handle<Mesh>), With<InstanceMaterialData>>,
) {
...
for (entity, mesh_transforms, mesh_handle) in &material_meshes {
...
}
}
```
After:
```rust
fn queue_custom(
render_mesh_instances: Res<RenderMeshInstances>,
instance_entities: Query<Entity, With<InstanceMaterialData>>,
) {
...
for entity in &instance_entities {
let Some(mesh_instance) = render_mesh_instances.get(&entity) else { continue; };
// The mesh handle in `AssetId<Mesh>` form, and the `MeshTransforms` can now
// be found in `mesh_instance` which is a `RenderMeshInstance`
...
}
}
```
---------
Co-authored-by: robtfm <50659922+robtfm@users.noreply.github.com>
2023-09-27 08:28:28 +00:00
|
|
|
};
|
Better Materials: AsBindGroup trait and derive, simpler Material trait (#5053)
# Objective
This PR reworks Bevy's Material system, making the user experience of defining Materials _much_ nicer. Bevy's previous material system leaves a lot to be desired:
* Materials require manually implementing the `RenderAsset` trait, which involves manually generating the bind group, handling gpu buffer data transfer, looking up image textures, etc. Even the simplest single-texture material involves writing ~80 unnecessary lines of code. This was never the long term plan.
* There are two material traits, which is confusing, hard to document, and often redundant: `Material` and `SpecializedMaterial`. `Material` implicitly implements `SpecializedMaterial`, and `SpecializedMaterial` is used in most high level apis to support both use cases. Most users shouldn't need to think about specialization at all (I consider it a "power-user tool"), so the fact that `SpecializedMaterial` is front-and-center in our apis is a miss.
* Implementing either material trait involves a lot of "type soup". The "prepared asset" parameter is particularly heinous: `&<Self as RenderAsset>::PreparedAsset`. Defining vertex and fragment shaders is also more verbose than it needs to be.
## Solution
Say hello to the new `Material` system:
```rust
#[derive(AsBindGroup, TypeUuid, Debug, Clone)]
#[uuid = "f690fdae-d598-45ab-8225-97e2a3f056e0"]
pub struct CoolMaterial {
#[uniform(0)]
color: Color,
#[texture(1)]
#[sampler(2)]
color_texture: Handle<Image>,
}
impl Material for CoolMaterial {
fn fragment_shader() -> ShaderRef {
"cool_material.wgsl".into()
}
}
```
Thats it! This same material would have required [~80 lines of complicated "type heavy" code](https://github.com/bevyengine/bevy/blob/v0.7.0/examples/shader/shader_material.rs) in the old Material system. Now it is just 14 lines of simple, readable code.
This is thanks to a new consolidated `Material` trait and the new `AsBindGroup` trait / derive.
### The new `Material` trait
The old "split" `Material` and `SpecializedMaterial` traits have been removed in favor of a new consolidated `Material` trait. All of the functions on the trait are optional.
The difficulty of implementing `Material` has been reduced by simplifying dataflow and removing type complexity:
```rust
// Old
impl Material for CustomMaterial {
fn fragment_shader(asset_server: &AssetServer) -> Option<Handle<Shader>> {
Some(asset_server.load("custom_material.wgsl"))
}
fn alpha_mode(render_asset: &<Self as RenderAsset>::PreparedAsset) -> AlphaMode {
render_asset.alpha_mode
}
}
// New
impl Material for CustomMaterial {
fn fragment_shader() -> ShaderRef {
"custom_material.wgsl".into()
}
fn alpha_mode(&self) -> AlphaMode {
self.alpha_mode
}
}
```
Specialization is still supported, but it is hidden by default under the `specialize()` function (more on this later).
### The `AsBindGroup` trait / derive
The `Material` trait now requires the `AsBindGroup` derive. This can be implemented manually relatively easily, but deriving it will almost always be preferable.
Field attributes like `uniform` and `texture` are used to define which fields should be bindings,
what their binding type is, and what index they should be bound at:
```rust
#[derive(AsBindGroup)]
struct CoolMaterial {
#[uniform(0)]
color: Color,
#[texture(1)]
#[sampler(2)]
color_texture: Handle<Image>,
}
```
In WGSL shaders, the binding looks like this:
```wgsl
struct CoolMaterial {
color: vec4<f32>;
};
[[group(1), binding(0)]]
var<uniform> material: CoolMaterial;
[[group(1), binding(1)]]
var color_texture: texture_2d<f32>;
[[group(1), binding(2)]]
var color_sampler: sampler;
```
Note that the "group" index is determined by the usage context. It is not defined in `AsBindGroup`. Bevy material bind groups are bound to group 1.
The following field-level attributes are supported:
* `uniform(BINDING_INDEX)`
* The field will be converted to a shader-compatible type using the `ShaderType` trait, written to a `Buffer`, and bound as a uniform. It can also be derived for custom structs.
* `texture(BINDING_INDEX)`
* This field's `Handle<Image>` will be used to look up the matching `Texture` gpu resource, which will be bound as a texture in shaders. The field will be assumed to implement `Into<Option<Handle<Image>>>`. In practice, most fields should be a `Handle<Image>` or `Option<Handle<Image>>`. If the value of an `Option<Handle<Image>>` is `None`, the new `FallbackImage` resource will be used instead. This attribute can be used in conjunction with a `sampler` binding attribute (with a different binding index).
* `sampler(BINDING_INDEX)`
* Behaves exactly like the `texture` attribute, but sets the Image's sampler binding instead of the texture.
Note that fields without field-level binding attributes will be ignored.
```rust
#[derive(AsBindGroup)]
struct CoolMaterial {
#[uniform(0)]
color: Color,
this_field_is_ignored: String,
}
```
As mentioned above, `Option<Handle<Image>>` is also supported:
```rust
#[derive(AsBindGroup)]
struct CoolMaterial {
#[uniform(0)]
color: Color,
#[texture(1)]
#[sampler(2)]
color_texture: Option<Handle<Image>>,
}
```
This is useful if you want a texture to be optional. When the value is `None`, the `FallbackImage` will be used for the binding instead, which defaults to "pure white".
Field uniforms with the same binding index will be combined into a single binding:
```rust
#[derive(AsBindGroup)]
struct CoolMaterial {
#[uniform(0)]
color: Color,
#[uniform(0)]
roughness: f32,
}
```
In WGSL shaders, the binding would look like this:
```wgsl
struct CoolMaterial {
color: vec4<f32>;
roughness: f32;
};
[[group(1), binding(0)]]
var<uniform> material: CoolMaterial;
```
Some less common scenarios will require "struct-level" attributes. These are the currently supported struct-level attributes:
* `uniform(BINDING_INDEX, ConvertedShaderType)`
* Similar to the field-level `uniform` attribute, but instead the entire `AsBindGroup` value is converted to `ConvertedShaderType`, which must implement `ShaderType`. This is useful if more complicated conversion logic is required.
* `bind_group_data(DataType)`
* The `AsBindGroup` type will be converted to some `DataType` using `Into<DataType>` and stored as `AsBindGroup::Data` as part of the `AsBindGroup::as_bind_group` call. This is useful if data needs to be stored alongside the generated bind group, such as a unique identifier for a material's bind group. The most common use case for this attribute is "shader pipeline specialization".
The previous `CoolMaterial` example illustrating "combining multiple field-level uniform attributes with the same binding index" can
also be equivalently represented with a single struct-level uniform attribute:
```rust
#[derive(AsBindGroup)]
#[uniform(0, CoolMaterialUniform)]
struct CoolMaterial {
color: Color,
roughness: f32,
}
#[derive(ShaderType)]
struct CoolMaterialUniform {
color: Color,
roughness: f32,
}
impl From<&CoolMaterial> for CoolMaterialUniform {
fn from(material: &CoolMaterial) -> CoolMaterialUniform {
CoolMaterialUniform {
color: material.color,
roughness: material.roughness,
}
}
}
```
### Material Specialization
Material shader specialization is now _much_ simpler:
```rust
#[derive(AsBindGroup, TypeUuid, Debug, Clone)]
#[uuid = "f690fdae-d598-45ab-8225-97e2a3f056e0"]
#[bind_group_data(CoolMaterialKey)]
struct CoolMaterial {
#[uniform(0)]
color: Color,
is_red: bool,
}
#[derive(Copy, Clone, Hash, Eq, PartialEq)]
struct CoolMaterialKey {
is_red: bool,
}
impl From<&CoolMaterial> for CoolMaterialKey {
fn from(material: &CoolMaterial) -> CoolMaterialKey {
CoolMaterialKey {
is_red: material.is_red,
}
}
}
impl Material for CoolMaterial {
fn fragment_shader() -> ShaderRef {
"cool_material.wgsl".into()
}
fn specialize(
pipeline: &MaterialPipeline<Self>,
descriptor: &mut RenderPipelineDescriptor,
layout: &MeshVertexBufferLayout,
key: MaterialPipelineKey<Self>,
) -> Result<(), SpecializedMeshPipelineError> {
if key.bind_group_data.is_red {
let fragment = descriptor.fragment.as_mut().unwrap();
fragment.shader_defs.push("IS_RED".to_string());
}
Ok(())
}
}
```
Setting `bind_group_data` is not required for specialization (it defaults to `()`). Scenarios like "custom vertex attributes" also benefit from this system:
```rust
impl Material for CustomMaterial {
fn vertex_shader() -> ShaderRef {
"custom_material.wgsl".into()
}
fn fragment_shader() -> ShaderRef {
"custom_material.wgsl".into()
}
fn specialize(
pipeline: &MaterialPipeline<Self>,
descriptor: &mut RenderPipelineDescriptor,
layout: &MeshVertexBufferLayout,
key: MaterialPipelineKey<Self>,
) -> Result<(), SpecializedMeshPipelineError> {
let vertex_layout = layout.get_layout(&[
Mesh::ATTRIBUTE_POSITION.at_shader_location(0),
ATTRIBUTE_BLEND_COLOR.at_shader_location(1),
])?;
descriptor.vertex.buffers = vec![vertex_layout];
Ok(())
}
}
```
### Ported `StandardMaterial` to the new `Material` system
Bevy's built-in PBR material uses the new Material system (including the AsBindGroup derive):
```rust
#[derive(AsBindGroup, Debug, Clone, TypeUuid)]
#[uuid = "7494888b-c082-457b-aacf-517228cc0c22"]
#[bind_group_data(StandardMaterialKey)]
#[uniform(0, StandardMaterialUniform)]
pub struct StandardMaterial {
pub base_color: Color,
#[texture(1)]
#[sampler(2)]
pub base_color_texture: Option<Handle<Image>>,
/* other fields omitted for brevity */
```
### Ported Bevy examples to the new `Material` system
The overall complexity of Bevy's "custom shader examples" has gone down significantly. Take a look at the diffs if you want a dopamine spike.
Please note that while this PR has a net increase in "lines of code", most of those extra lines come from added documentation. There is a significant reduction
in the overall complexity of the code (even accounting for the new derive logic).
---
## Changelog
### Added
* `AsBindGroup` trait and derive, which make it much easier to transfer data to the gpu and generate bind groups for a given type.
### Changed
* The old `Material` and `SpecializedMaterial` traits have been replaced by a consolidated (much simpler) `Material` trait. Materials no longer implement `RenderAsset`.
* `StandardMaterial` was ported to the new material system. There are no user-facing api changes to the `StandardMaterial` struct api, but it now implements `AsBindGroup` and `Material` instead of `RenderAsset` and `SpecializedMaterial`.
## Migration Guide
The Material system has been reworked to be much simpler. We've removed a lot of boilerplate with the new `AsBindGroup` derive and the `Material` trait is simpler as well!
### Bevy 0.7 (old)
```rust
#[derive(Debug, Clone, TypeUuid)]
#[uuid = "f690fdae-d598-45ab-8225-97e2a3f056e0"]
pub struct CustomMaterial {
color: Color,
color_texture: Handle<Image>,
}
#[derive(Clone)]
pub struct GpuCustomMaterial {
_buffer: Buffer,
bind_group: BindGroup,
}
impl RenderAsset for CustomMaterial {
type ExtractedAsset = CustomMaterial;
type PreparedAsset = GpuCustomMaterial;
type Param = (SRes<RenderDevice>, SRes<MaterialPipeline<Self>>);
fn extract_asset(&self) -> Self::ExtractedAsset {
self.clone()
}
fn prepare_asset(
extracted_asset: Self::ExtractedAsset,
(render_device, material_pipeline): &mut SystemParamItem<Self::Param>,
) -> Result<Self::PreparedAsset, PrepareAssetError<Self::ExtractedAsset>> {
let color = Vec4::from_slice(&extracted_asset.color.as_linear_rgba_f32());
let byte_buffer = [0u8; Vec4::SIZE.get() as usize];
let mut buffer = encase::UniformBuffer::new(byte_buffer);
buffer.write(&color).unwrap();
let buffer = render_device.create_buffer_with_data(&BufferInitDescriptor {
contents: buffer.as_ref(),
label: None,
usage: BufferUsages::UNIFORM | BufferUsages::COPY_DST,
});
let (texture_view, texture_sampler) = if let Some(result) = material_pipeline
.mesh_pipeline
.get_image_texture(gpu_images, &Some(extracted_asset.color_texture.clone()))
{
result
} else {
return Err(PrepareAssetError::RetryNextUpdate(extracted_asset));
};
let bind_group = render_device.create_bind_group(&BindGroupDescriptor {
entries: &[
BindGroupEntry {
binding: 0,
resource: buffer.as_entire_binding(),
},
BindGroupEntry {
binding: 0,
resource: BindingResource::TextureView(texture_view),
},
BindGroupEntry {
binding: 1,
resource: BindingResource::Sampler(texture_sampler),
},
],
label: None,
layout: &material_pipeline.material_layout,
});
Ok(GpuCustomMaterial {
_buffer: buffer,
bind_group,
})
}
}
impl Material for CustomMaterial {
fn fragment_shader(asset_server: &AssetServer) -> Option<Handle<Shader>> {
Some(asset_server.load("custom_material.wgsl"))
}
fn bind_group(render_asset: &<Self as RenderAsset>::PreparedAsset) -> &BindGroup {
&render_asset.bind_group
}
fn bind_group_layout(render_device: &RenderDevice) -> BindGroupLayout {
render_device.create_bind_group_layout(&BindGroupLayoutDescriptor {
entries: &[
BindGroupLayoutEntry {
binding: 0,
visibility: ShaderStages::FRAGMENT,
ty: BindingType::Buffer {
ty: BufferBindingType::Uniform,
has_dynamic_offset: false,
min_binding_size: Some(Vec4::min_size()),
},
count: None,
},
BindGroupLayoutEntry {
binding: 1,
visibility: ShaderStages::FRAGMENT,
ty: BindingType::Texture {
multisampled: false,
sample_type: TextureSampleType::Float { filterable: true },
view_dimension: TextureViewDimension::D2Array,
},
count: None,
},
BindGroupLayoutEntry {
binding: 2,
visibility: ShaderStages::FRAGMENT,
ty: BindingType::Sampler(SamplerBindingType::Filtering),
count: None,
},
],
label: None,
})
}
}
```
### Bevy 0.8 (new)
```rust
impl Material for CustomMaterial {
fn fragment_shader() -> ShaderRef {
"custom_material.wgsl".into()
}
}
#[derive(AsBindGroup, TypeUuid, Debug, Clone)]
#[uuid = "f690fdae-d598-45ab-8225-97e2a3f056e0"]
pub struct CustomMaterial {
#[uniform(0)]
color: Color,
#[texture(1)]
#[sampler(2)]
color_texture: Handle<Image>,
}
```
## Future Work
* Add support for more binding types (cubemaps, buffers, etc). This PR intentionally includes a bare minimum number of binding types to keep "reviewability" in check.
* Consider optionally eliding binding indices using binding names. `AsBindGroup` could pass in (optional?) reflection info as a "hint".
* This would make it possible for the derive to do this:
```rust
#[derive(AsBindGroup)]
pub struct CustomMaterial {
#[uniform]
color: Color,
#[texture]
#[sampler]
color_texture: Option<Handle<Image>>,
alpha_mode: AlphaMode,
}
```
* Or this
```rust
#[derive(AsBindGroup)]
pub struct CustomMaterial {
#[binding]
color: Color,
#[binding]
color_texture: Option<Handle<Image>>,
alpha_mode: AlphaMode,
}
```
* Or even this (if we flip to "include bindings by default")
```rust
#[derive(AsBindGroup)]
pub struct CustomMaterial {
color: Color,
color_texture: Option<Handle<Image>>,
#[binding(ignore)]
alpha_mode: AlphaMode,
}
```
* If we add the option to define custom draw functions for materials (which could be done in a type-erased way), I think that would be enough to support extra non-material bindings. Worth considering!
2022-06-30 23:48:46 +00:00
|
|
|
pass.set_bind_group(I, &material.bind_group, &[]);
|
2021-12-25 21:45:43 +00:00
|
|
|
RenderCommandResult::Success
|
|
|
|
}
|
|
|
|
}
|
|
|
|
|
2024-10-09 17:12:27 +00:00
|
|
|
/// Stores all extracted instances of a [`Material`] in the render world.
|
|
|
|
#[derive(Resource, Deref, DerefMut)]
|
Type safe retained render world (#15756)
# Objective
In the Render World, there are a number of collections that are derived
from Main World entities and are used to drive rendering. The most
notable are:
- `VisibleEntities`, which is generated in the `check_visibility` system
and contains visible entities for a view.
- `ExtractedInstances`, which maps entity ids to asset ids.
In the old model, these collections were trivially kept in sync -- any
extracted phase item could look itself up because the render entity id
was guaranteed to always match the corresponding main world id.
After #15320, this became much more complicated, and was leading to a
number of subtle bugs in the Render World. The main rendering systems,
i.e. `queue_material_meshes` and `queue_material2d_meshes`, follow a
similar pattern:
```rust
for visible_entity in visible_entities.iter::<With<Mesh2d>>() {
let Some(mesh_instance) = render_mesh_instances.get_mut(visible_entity) else {
continue;
};
// Look some more stuff up and specialize the pipeline...
let bin_key = Opaque2dBinKey {
pipeline: pipeline_id,
draw_function: draw_opaque_2d,
asset_id: mesh_instance.mesh_asset_id.into(),
material_bind_group_id: material_2d.get_bind_group_id().0,
};
opaque_phase.add(
bin_key,
*visible_entity,
BinnedRenderPhaseType::mesh(mesh_instance.automatic_batching),
);
}
```
In this case, `visible_entities` and `render_mesh_instances` are both
collections that are created and keyed by Main World entity ids, and so
this lookup happens to work by coincidence. However, there is a major
unintentional bug here: namely, because `visible_entities` is a
collection of Main World ids, the phase item being queued is created
with a Main World id rather than its correct Render World id.
This happens to not break mesh rendering because the render commands
used for drawing meshes do not access the `ItemQuery` parameter, but
demonstrates the confusion that is now possible: our UI phase items are
correctly being queued with Render World ids while our meshes aren't.
Additionally, this makes it very easy and error prone to use the wrong
entity id to look up things like assets. For example, if instead we
ignored visibility checks and queued our meshes via a query, we'd have
to be extra careful to use `&MainEntity` instead of the natural
`Entity`.
## Solution
Make all collections that are derived from Main World data use
`MainEntity` as their key, to ensure type safety and avoid accidentally
looking up data with the wrong entity id:
```rust
pub type MainEntityHashMap<V> = hashbrown::HashMap<MainEntity, V, EntityHash>;
```
Additionally, we make all `PhaseItem` be able to provide both their Main
and Render World ids, to allow render phase implementors maximum
flexibility as to what id should be used to look up data.
You can think of this like tracking at the type level whether something
in the Render World should use it's "primary key", i.e. entity id, or
needs to use a foreign key, i.e. `MainEntity`.
## Testing
##### TODO:
This will require extensive testing to make sure things didn't break!
Additionally, some extraction logic has become more complicated and
needs to be checked for regressions.
## Migration Guide
With the advent of the retained render world, collections that contain
references to `Entity` that are extracted into the render world have
been changed to contain `MainEntity` in order to prevent errors where a
render world entity id is used to look up an item by accident. Custom
rendering code may need to be changed to query for `&MainEntity` in
order to look up the correct item from such a collection. Additionally,
users who implement their own extraction logic for collections of main
world entity should strongly consider extracting into a different
collection that uses `MainEntity` as a key.
Additionally, render phases now require specifying both the `Entity` and
`MainEntity` for a given `PhaseItem`. Custom render phases should ensure
`MainEntity` is available when queuing a phase item.
2024-10-10 18:47:04 +00:00
|
|
|
pub struct RenderMaterialInstances<M: Material>(pub MainEntityHashMap<AssetId<M>>);
|
2024-10-09 17:12:27 +00:00
|
|
|
|
|
|
|
impl<M: Material> Default for RenderMaterialInstances<M> {
|
|
|
|
fn default() -> Self {
|
|
|
|
Self(Default::default())
|
|
|
|
}
|
|
|
|
}
|
Use EntityHashMap<Entity, T> for render world entity storage for better performance (#9903)
# Objective
- Improve rendering performance, particularly by avoiding the large
system commands costs of using the ECS in the way that the render world
does.
## Solution
- Define `EntityHasher` that calculates a hash from the
`Entity.to_bits()` by `i | (i.wrapping_mul(0x517cc1b727220a95) << 32)`.
`0x517cc1b727220a95` is something like `u64::MAX / N` for N that gives a
value close to π and that works well for hashing. Thanks for @SkiFire13
for the suggestion and to @nicopap for alternative suggestions and
discussion. This approach comes from `rustc-hash` (a.k.a. `FxHasher`)
with some tweaks for the case of hashing an `Entity`. `FxHasher` and
`SeaHasher` were also tested but were significantly slower.
- Define `EntityHashMap` type that uses the `EntityHashser`
- Use `EntityHashMap<Entity, T>` for render world entity storage,
including:
- `RenderMaterialInstances` - contains the `AssetId<M>` of the material
associated with the entity. Also for 2D.
- `RenderMeshInstances` - contains mesh transforms, flags and properties
about mesh entities. Also for 2D.
- `SkinIndices` and `MorphIndices` - contains the skin and morph index
for an entity, respectively
- `ExtractedSprites`
- `ExtractedUiNodes`
## Benchmarks
All benchmarks have been conducted on an M1 Max connected to AC power.
The tests are run for 1500 frames. The 1000th frame is captured for
comparison to check for visual regressions. There were none.
### 2D Meshes
`bevymark --benchmark --waves 160 --per-wave 1000 --mode mesh2d`
#### `--ordered-z`
This test spawns the 2D meshes with z incrementing back to front, which
is the ideal arrangement allocation order as it matches the sorted
render order which means lookups have a high cache hit rate.
<img width="1112" alt="Screenshot 2023-09-27 at 07 50 45"
src="https://github.com/bevyengine/bevy/assets/302146/e140bc98-7091-4a3b-8ae1-ab75d16d2ccb">
-39.1% median frame time.
#### Random
This test spawns the 2D meshes with random z. This not only makes the
batching and transparent 2D pass lookups get a lot of cache misses, it
also currently means that the meshes are almost certain to not be
batchable.
<img width="1108" alt="Screenshot 2023-09-27 at 07 51 28"
src="https://github.com/bevyengine/bevy/assets/302146/29c2e813-645a-43ce-982a-55df4bf7d8c4">
-7.2% median frame time.
### 3D Meshes
`many_cubes --benchmark`
<img width="1112" alt="Screenshot 2023-09-27 at 07 51 57"
src="https://github.com/bevyengine/bevy/assets/302146/1a729673-3254-4e2a-9072-55e27c69f0fc">
-7.7% median frame time.
### Sprites
**NOTE: On `main` sprites are using `SparseSet<Entity, T>`!**
`bevymark --benchmark --waves 160 --per-wave 1000 --mode sprite`
#### `--ordered-z`
This test spawns the sprites with z incrementing back to front, which is
the ideal arrangement allocation order as it matches the sorted render
order which means lookups have a high cache hit rate.
<img width="1116" alt="Screenshot 2023-09-27 at 07 52 31"
src="https://github.com/bevyengine/bevy/assets/302146/bc8eab90-e375-4d31-b5cd-f55f6f59ab67">
+13.0% median frame time.
#### Random
This test spawns the sprites with random z. This makes the batching and
transparent 2D pass lookups get a lot of cache misses.
<img width="1109" alt="Screenshot 2023-09-27 at 07 53 01"
src="https://github.com/bevyengine/bevy/assets/302146/22073f5d-99a7-49b0-9584-d3ac3eac3033">
+0.6% median frame time.
### UI
**NOTE: On `main` UI is using `SparseSet<Entity, T>`!**
`many_buttons`
<img width="1111" alt="Screenshot 2023-09-27 at 07 53 26"
src="https://github.com/bevyengine/bevy/assets/302146/66afd56d-cbe4-49e7-8b64-2f28f6043d85">
+15.1% median frame time.
## Alternatives
- Cart originally suggested trying out `SparseSet<Entity, T>` and indeed
that is slightly faster under ideal conditions. However,
`PassHashMap<Entity, T>` has better worst case performance when data is
randomly distributed, rather than in sorted render order, and does not
have the worst case memory usage that `SparseSet`'s dense `Vec<usize>`
that maps from the `Entity` index to sparse index into `Vec<T>`. This
dense `Vec` has to be as large as the largest Entity index used with the
`SparseSet`.
- I also tested `PassHashMap<u32, T>`, intending to use `Entity.index()`
as the key, but this proved to sometimes be slower and mostly no
different.
- The only outstanding approach that has not been implemented and tested
is to _not_ clear the render world of its entities each frame. That has
its own problems, though they could perhaps be solved.
- Performance-wise, if the entities and their component data were not
cleared, then they would incur table moves on spawn, and should not
thereafter, rather just their component data would be overwritten.
Ideally we would have a neat way of either updating data in-place via
`&mut T` queries, or inserting components if not present. This would
likely be quite cumbersome to have to remember to do everywhere, but
perhaps it only needs to be done in the more performance-sensitive
systems.
- The main problem to solve however is that we want to both maintain a
mapping between main world entities and render world entities, be able
to run the render app and world in parallel with the main app and world
for pipelined rendering, and at the same time be able to spawn entities
in the render world in such a way that those Entity ids do not collide
with those spawned in the main world. This is potentially quite
solvable, but could well be a lot of ECS work to do it in a way that
makes sense.
---
## Changelog
- Changed: Component data for entities to be drawn are no longer stored
on entities in the render world. Instead, data is stored in a
`EntityHashMap<Entity, T>` in various resources. This brings significant
performance benefits due to the way the render app clears entities every
frame. Resources of most interest are `RenderMeshInstances` and
`RenderMaterialInstances`, and their 2D counterparts.
## Migration Guide
Previously the render app extracted mesh entities and their component
data from the main world and stored them as entities and components in
the render world. Now they are extracted into essentially
`EntityHashMap<Entity, T>` where `T` are structs containing an
appropriate group of data. This means that while extract set systems
will continue to run extract queries against the main world they will
store their data in hash maps. Also, systems in later sets will either
need to look up entities in the available resources such as
`RenderMeshInstances`, or maintain their own `EntityHashMap<Entity, T>`
for their own data.
Before:
```rust
fn queue_custom(
material_meshes: Query<(Entity, &MeshTransforms, &Handle<Mesh>), With<InstanceMaterialData>>,
) {
...
for (entity, mesh_transforms, mesh_handle) in &material_meshes {
...
}
}
```
After:
```rust
fn queue_custom(
render_mesh_instances: Res<RenderMeshInstances>,
instance_entities: Query<Entity, With<InstanceMaterialData>>,
) {
...
for entity in &instance_entities {
let Some(mesh_instance) = render_mesh_instances.get(&entity) else { continue; };
// The mesh handle in `AssetId<Mesh>` form, and the `MeshTransforms` can now
// be found in `mesh_instance` which is a `RenderMeshInstance`
...
}
}
```
---------
Co-authored-by: robtfm <50659922+robtfm@users.noreply.github.com>
2023-09-27 08:28:28 +00:00
|
|
|
|
2024-04-15 20:37:52 +00:00
|
|
|
pub const fn alpha_mode_pipeline_key(alpha_mode: AlphaMode, msaa: &Msaa) -> MeshPipelineKey {
|
2023-09-20 20:18:55 +00:00
|
|
|
match alpha_mode {
|
|
|
|
// Premultiplied and Add share the same pipeline key
|
|
|
|
// They're made distinct in the PBR shader, via `premultiply_alpha()`
|
|
|
|
AlphaMode::Premultiplied | AlphaMode::Add => MeshPipelineKey::BLEND_PREMULTIPLIED_ALPHA,
|
|
|
|
AlphaMode::Blend => MeshPipelineKey::BLEND_ALPHA,
|
|
|
|
AlphaMode::Multiply => MeshPipelineKey::BLEND_MULTIPLY,
|
|
|
|
AlphaMode::Mask(_) => MeshPipelineKey::MAY_DISCARD,
|
2024-04-15 20:37:52 +00:00
|
|
|
AlphaMode::AlphaToCoverage => match *msaa {
|
|
|
|
Msaa::Off => MeshPipelineKey::MAY_DISCARD,
|
|
|
|
_ => MeshPipelineKey::BLEND_ALPHA_TO_COVERAGE,
|
|
|
|
},
|
2023-09-20 20:18:55 +00:00
|
|
|
_ => MeshPipelineKey::NONE,
|
|
|
|
}
|
|
|
|
}
|
|
|
|
|
2023-12-30 23:27:48 +00:00
|
|
|
pub const fn tonemapping_pipeline_key(tonemapping: Tonemapping) -> MeshPipelineKey {
|
2023-09-20 20:18:55 +00:00
|
|
|
match tonemapping {
|
|
|
|
Tonemapping::None => MeshPipelineKey::TONEMAP_METHOD_NONE,
|
|
|
|
Tonemapping::Reinhard => MeshPipelineKey::TONEMAP_METHOD_REINHARD,
|
|
|
|
Tonemapping::ReinhardLuminance => MeshPipelineKey::TONEMAP_METHOD_REINHARD_LUMINANCE,
|
|
|
|
Tonemapping::AcesFitted => MeshPipelineKey::TONEMAP_METHOD_ACES_FITTED,
|
|
|
|
Tonemapping::AgX => MeshPipelineKey::TONEMAP_METHOD_AGX,
|
|
|
|
Tonemapping::SomewhatBoringDisplayTransform => {
|
|
|
|
MeshPipelineKey::TONEMAP_METHOD_SOMEWHAT_BORING_DISPLAY_TRANSFORM
|
|
|
|
}
|
|
|
|
Tonemapping::TonyMcMapface => MeshPipelineKey::TONEMAP_METHOD_TONY_MC_MAPFACE,
|
|
|
|
Tonemapping::BlenderFilmic => MeshPipelineKey::TONEMAP_METHOD_BLENDER_FILMIC,
|
|
|
|
}
|
|
|
|
}
|
|
|
|
|
2023-12-30 23:27:48 +00:00
|
|
|
pub const fn screen_space_specular_transmission_pipeline_key(
|
`StandardMaterial` Light Transmission (#8015)
# Objective
<img width="1920" alt="Screenshot 2023-04-26 at 01 07 34"
src="https://user-images.githubusercontent.com/418473/234467578-0f34187b-5863-4ea1-88e9-7a6bb8ce8da3.png">
This PR adds both diffuse and specular light transmission capabilities
to the `StandardMaterial`, with support for screen space refractions.
This enables realistically representing a wide range of real-world
materials, such as:
- Glass; (Including frosted glass)
- Transparent and translucent plastics;
- Various liquids and gels;
- Gemstones;
- Marble;
- Wax;
- Paper;
- Leaves;
- Porcelain.
Unlike existing support for transparency, light transmission does not
rely on fixed function alpha blending, and therefore works with both
`AlphaMode::Opaque` and `AlphaMode::Mask` materials.
## Solution
- Introduces a number of transmission related fields in the
`StandardMaterial`;
- For specular transmission:
- Adds logic to take a view main texture snapshot after the opaque
phase; (in order to perform screen space refractions)
- Introduces a new `Transmissive3d` phase to the renderer, to which all
meshes with `transmission > 0.0` materials are sent.
- Calculates a light exit point (of the approximate mesh volume) using
`ior` and `thickness` properties
- Samples the snapshot texture with an adaptive number of taps across a
`roughness`-controlled radius enabling “blurry” refractions
- For diffuse transmission:
- Approximates transmitted diffuse light by using a second, flipped +
displaced, diffuse-only Lambertian lobe for each light source.
## To Do
- [x] Figure out where `fresnel_mix()` is taking place, if at all, and
where `dielectric_specular` is being calculated, if at all, and update
them to use the `ior` value (Not a blocker, just a nice-to-have for more
correct BSDF)
- To the _best of my knowledge, this is now taking place, after
964340cdd. The fresnel mix is actually "split" into two parts in our
implementation, one `(1 - fresnel(...))` in the transmission, and
`fresnel()` in the light implementations. A surface with more
reflectance now will produce slightly dimmer transmission towards the
grazing angle, as more of the light gets reflected.
- [x] Add `transmission_texture`
- [x] Add `diffuse_transmission_texture`
- [x] Add `thickness_texture`
- [x] Add `attenuation_distance` and `attenuation_color`
- [x] Connect values to glTF loader
- [x] `transmission` and `transmission_texture`
- [x] `thickness` and `thickness_texture`
- [x] `ior`
- [ ] `diffuse_transmission` and `diffuse_transmission_texture` (needs
upstream support in `gltf` crate, not a blocker)
- [x] Add support for multiple screen space refraction “steps”
- [x] Conditionally create no transmission snapshot texture at all if
`steps == 0`
- [x] Conditionally enable/disable screen space refraction transmission
snapshots
- [x] Read from depth pre-pass to prevent refracting pixels in front of
the light exit point
- [x] Use `interleaved_gradient_noise()` function for sampling blur in a
way that benefits from TAA
- [x] Drill down a TAA `#define`, tweak some aspects of the effect
conditionally based on it
- [x] Remove const array that's crashing under HLSL (unless a new `naga`
release with https://github.com/gfx-rs/naga/pull/2496 comes out before
we merge this)
- [ ] Look into alternatives to the `switch` hack for dynamically
indexing the const array (might not be needed, compilers seem to be
decent at expanding it)
- [ ] Add pipeline keys for gating transmission (do we really want/need
this?)
- [x] Tweak some material field/function names?
## A Note on Texture Packing
_This was originally added as a comment to the
`specular_transmission_texture`, `thickness_texture` and
`diffuse_transmission_texture` documentation, I removed it since it was
more confusing than helpful, and will likely be made redundant/will need
to be updated once we have a better infrastructure for preprocessing
assets_
Due to how channels are mapped, you can more efficiently use a single
shared texture image
for configuring the following:
- R - `specular_transmission_texture`
- G - `thickness_texture`
- B - _unused_
- A - `diffuse_transmission_texture`
The `KHR_materials_diffuse_transmission` glTF extension also defines a
`diffuseTransmissionColorTexture`,
that _we don't currently support_. One might choose to pack the
intensity and color textures together,
using RGB for the color and A for the intensity, in which case this
packing advice doesn't really apply.
---
## Changelog
- Added a new `Transmissive3d` render phase for rendering specular
transmissive materials with screen space refractions
- Added rendering support for transmitted environment map light on the
`StandardMaterial` as a fallback for screen space refractions
- Added `diffuse_transmission`, `specular_transmission`, `thickness`,
`ior`, `attenuation_distance` and `attenuation_color` to the
`StandardMaterial`
- Added `diffuse_transmission_texture`, `specular_transmission_texture`,
`thickness_texture` to the `StandardMaterial`, gated behind a new
`pbr_transmission_textures` cargo feature (off by default, for maximum
hardware compatibility)
- Added `Camera3d::screen_space_specular_transmission_steps` for
controlling the number of “layers of transparency” rendered for
transmissive objects
- Added a `TransmittedShadowReceiver` component for enabling shadows in
(diffusely) transmitted light. (disabled by default, as it requires
carefully setting up the `thickness` to avoid self-shadow artifacts)
- Added support for the `KHR_materials_transmission`,
`KHR_materials_ior` and `KHR_materials_volume` glTF extensions
- Renamed items related to temporal jitter for greater consistency
## Migration Guide
- `SsaoPipelineKey::temporal_noise` has been renamed to
`SsaoPipelineKey::temporal_jitter`
- The `TAA` shader def (controlled by the presence of the
`TemporalAntiAliasSettings` component in the camera) has been replaced
with the `TEMPORAL_JITTER` shader def (controlled by the presence of the
`TemporalJitter` component in the camera)
- `MeshPipelineKey::TAA` has been replaced by
`MeshPipelineKey::TEMPORAL_JITTER`
- The `TEMPORAL_NOISE` shader def has been consolidated with
`TEMPORAL_JITTER`
2023-10-31 20:59:02 +00:00
|
|
|
screen_space_transmissive_blur_quality: ScreenSpaceTransmissionQuality,
|
|
|
|
) -> MeshPipelineKey {
|
|
|
|
match screen_space_transmissive_blur_quality {
|
|
|
|
ScreenSpaceTransmissionQuality::Low => {
|
|
|
|
MeshPipelineKey::SCREEN_SPACE_SPECULAR_TRANSMISSION_LOW
|
|
|
|
}
|
|
|
|
ScreenSpaceTransmissionQuality::Medium => {
|
|
|
|
MeshPipelineKey::SCREEN_SPACE_SPECULAR_TRANSMISSION_MEDIUM
|
|
|
|
}
|
|
|
|
ScreenSpaceTransmissionQuality::High => {
|
|
|
|
MeshPipelineKey::SCREEN_SPACE_SPECULAR_TRANSMISSION_HIGH
|
|
|
|
}
|
|
|
|
ScreenSpaceTransmissionQuality::Ultra => {
|
|
|
|
MeshPipelineKey::SCREEN_SPACE_SPECULAR_TRANSMISSION_ULTRA
|
|
|
|
}
|
|
|
|
}
|
|
|
|
}
|
|
|
|
|
Migrate meshes and materials to required components (#15524)
# Objective
A big step in the migration to required components: meshes and
materials!
## Solution
As per the [selected
proposal](https://hackmd.io/@bevy/required_components/%2Fj9-PnF-2QKK0on1KQ29UWQ):
- Deprecate `MaterialMesh2dBundle`, `MaterialMeshBundle`, and
`PbrBundle`.
- Add `Mesh2d` and `Mesh3d` components, which wrap a `Handle<Mesh>`.
- Add `MeshMaterial2d<M: Material2d>` and `MeshMaterial3d<M: Material>`,
which wrap a `Handle<M>`.
- Meshes *without* a mesh material should be rendered with a default
material. The existence of a material is determined by
`HasMaterial2d`/`HasMaterial3d`, which is required by
`MeshMaterial2d`/`MeshMaterial3d`. This gets around problems with the
generics.
Previously:
```rust
commands.spawn(MaterialMesh2dBundle {
mesh: meshes.add(Circle::new(100.0)).into(),
material: materials.add(Color::srgb(7.5, 0.0, 7.5)),
transform: Transform::from_translation(Vec3::new(-200., 0., 0.)),
..default()
});
```
Now:
```rust
commands.spawn((
Mesh2d(meshes.add(Circle::new(100.0))),
MeshMaterial2d(materials.add(Color::srgb(7.5, 0.0, 7.5))),
Transform::from_translation(Vec3::new(-200., 0., 0.)),
));
```
If the mesh material is missing, previously nothing was rendered. Now,
it renders a white default `ColorMaterial` in 2D and a
`StandardMaterial` in 3D (this can be overridden). Below, only every
other entity has a material:


Why white? This is still open for discussion, but I think white makes
sense for a *default* material, while *invalid* asset handles pointing
to nothing should have something like a pink material to indicate that
something is broken (I don't handle that in this PR yet). This is kind
of a mix of Godot and Unity: Godot just renders a white material for
non-existent materials, while Unity renders nothing when no materials
exist, but renders pink for invalid materials. I can also change the
default material to pink if that is preferable though.
## Testing
I ran some 2D and 3D examples to test if anything changed visually. I
have not tested all examples or features yet however. If anyone wants to
test more extensively, it would be appreciated!
## Implementation Notes
- The relationship between `bevy_render` and `bevy_pbr` is weird here.
`bevy_render` needs `Mesh3d` for its own systems, but `bevy_pbr` has all
of the material logic, and `bevy_render` doesn't depend on it. I feel
like the two crates should be refactored in some way, but I think that's
out of scope for this PR.
- I didn't migrate meshlets to required components yet. That can
probably be done in a follow-up, as this is already a huge PR.
- It is becoming increasingly clear to me that we really, *really* want
to disallow raw asset handles as components. They caused me a *ton* of
headache here already, and it took me a long time to find every place
that queried for them or inserted them directly on entities, since there
were no compiler errors for it. If we don't remove the `Component`
derive, I expect raw asset handles to be a *huge* footgun for users as
we transition to wrapper components, especially as handles as components
have been the norm so far. I personally consider this to be a blocker
for 0.15: we need to migrate to wrapper components for asset handles
everywhere, and remove the `Component` derive. Also see
https://github.com/bevyengine/bevy/issues/14124.
---
## Migration Guide
Asset handles for meshes and mesh materials must now be wrapped in the
`Mesh2d` and `MeshMaterial2d` or `Mesh3d` and `MeshMaterial3d`
components for 2D and 3D respectively. Raw handles as components no
longer render meshes.
Additionally, `MaterialMesh2dBundle`, `MaterialMeshBundle`, and
`PbrBundle` have been deprecated. Instead, use the mesh and material
components directly.
Previously:
```rust
commands.spawn(MaterialMesh2dBundle {
mesh: meshes.add(Circle::new(100.0)).into(),
material: materials.add(Color::srgb(7.5, 0.0, 7.5)),
transform: Transform::from_translation(Vec3::new(-200., 0., 0.)),
..default()
});
```
Now:
```rust
commands.spawn((
Mesh2d(meshes.add(Circle::new(100.0))),
MeshMaterial2d(materials.add(Color::srgb(7.5, 0.0, 7.5))),
Transform::from_translation(Vec3::new(-200., 0., 0.)),
));
```
If the mesh material is missing, a white default material is now used.
Previously, nothing was rendered if the material was missing.
The `WithMesh2d` and `WithMesh3d` query filter type aliases have also
been removed. Simply use `With<Mesh2d>` or `With<Mesh3d>`.
---------
Co-authored-by: Tim Blackbird <justthecooldude@gmail.com>
Co-authored-by: Carter Anderson <mcanders1@gmail.com>
2024-10-01 21:33:17 +00:00
|
|
|
fn extract_mesh_materials<M: Material>(
|
|
|
|
mut material_instances: ResMut<RenderMaterialInstances<M>>,
|
2024-10-09 15:39:10 +00:00
|
|
|
query: Extract<Query<(Entity, &ViewVisibility, &MeshMaterial3d<M>)>>,
|
Migrate meshes and materials to required components (#15524)
# Objective
A big step in the migration to required components: meshes and
materials!
## Solution
As per the [selected
proposal](https://hackmd.io/@bevy/required_components/%2Fj9-PnF-2QKK0on1KQ29UWQ):
- Deprecate `MaterialMesh2dBundle`, `MaterialMeshBundle`, and
`PbrBundle`.
- Add `Mesh2d` and `Mesh3d` components, which wrap a `Handle<Mesh>`.
- Add `MeshMaterial2d<M: Material2d>` and `MeshMaterial3d<M: Material>`,
which wrap a `Handle<M>`.
- Meshes *without* a mesh material should be rendered with a default
material. The existence of a material is determined by
`HasMaterial2d`/`HasMaterial3d`, which is required by
`MeshMaterial2d`/`MeshMaterial3d`. This gets around problems with the
generics.
Previously:
```rust
commands.spawn(MaterialMesh2dBundle {
mesh: meshes.add(Circle::new(100.0)).into(),
material: materials.add(Color::srgb(7.5, 0.0, 7.5)),
transform: Transform::from_translation(Vec3::new(-200., 0., 0.)),
..default()
});
```
Now:
```rust
commands.spawn((
Mesh2d(meshes.add(Circle::new(100.0))),
MeshMaterial2d(materials.add(Color::srgb(7.5, 0.0, 7.5))),
Transform::from_translation(Vec3::new(-200., 0., 0.)),
));
```
If the mesh material is missing, previously nothing was rendered. Now,
it renders a white default `ColorMaterial` in 2D and a
`StandardMaterial` in 3D (this can be overridden). Below, only every
other entity has a material:


Why white? This is still open for discussion, but I think white makes
sense for a *default* material, while *invalid* asset handles pointing
to nothing should have something like a pink material to indicate that
something is broken (I don't handle that in this PR yet). This is kind
of a mix of Godot and Unity: Godot just renders a white material for
non-existent materials, while Unity renders nothing when no materials
exist, but renders pink for invalid materials. I can also change the
default material to pink if that is preferable though.
## Testing
I ran some 2D and 3D examples to test if anything changed visually. I
have not tested all examples or features yet however. If anyone wants to
test more extensively, it would be appreciated!
## Implementation Notes
- The relationship between `bevy_render` and `bevy_pbr` is weird here.
`bevy_render` needs `Mesh3d` for its own systems, but `bevy_pbr` has all
of the material logic, and `bevy_render` doesn't depend on it. I feel
like the two crates should be refactored in some way, but I think that's
out of scope for this PR.
- I didn't migrate meshlets to required components yet. That can
probably be done in a follow-up, as this is already a huge PR.
- It is becoming increasingly clear to me that we really, *really* want
to disallow raw asset handles as components. They caused me a *ton* of
headache here already, and it took me a long time to find every place
that queried for them or inserted them directly on entities, since there
were no compiler errors for it. If we don't remove the `Component`
derive, I expect raw asset handles to be a *huge* footgun for users as
we transition to wrapper components, especially as handles as components
have been the norm so far. I personally consider this to be a blocker
for 0.15: we need to migrate to wrapper components for asset handles
everywhere, and remove the `Component` derive. Also see
https://github.com/bevyengine/bevy/issues/14124.
---
## Migration Guide
Asset handles for meshes and mesh materials must now be wrapped in the
`Mesh2d` and `MeshMaterial2d` or `Mesh3d` and `MeshMaterial3d`
components for 2D and 3D respectively. Raw handles as components no
longer render meshes.
Additionally, `MaterialMesh2dBundle`, `MaterialMeshBundle`, and
`PbrBundle` have been deprecated. Instead, use the mesh and material
components directly.
Previously:
```rust
commands.spawn(MaterialMesh2dBundle {
mesh: meshes.add(Circle::new(100.0)).into(),
material: materials.add(Color::srgb(7.5, 0.0, 7.5)),
transform: Transform::from_translation(Vec3::new(-200., 0., 0.)),
..default()
});
```
Now:
```rust
commands.spawn((
Mesh2d(meshes.add(Circle::new(100.0))),
MeshMaterial2d(materials.add(Color::srgb(7.5, 0.0, 7.5))),
Transform::from_translation(Vec3::new(-200., 0., 0.)),
));
```
If the mesh material is missing, a white default material is now used.
Previously, nothing was rendered if the material was missing.
The `WithMesh2d` and `WithMesh3d` query filter type aliases have also
been removed. Simply use `With<Mesh2d>` or `With<Mesh3d>`.
---------
Co-authored-by: Tim Blackbird <justthecooldude@gmail.com>
Co-authored-by: Carter Anderson <mcanders1@gmail.com>
2024-10-01 21:33:17 +00:00
|
|
|
) {
|
Revert default mesh materials (#15930)
# Objective
Closes #15799.
Many rendering people and maintainers are in favor of reverting default
mesh materials added in #15524, especially as the migration to required
component is already large and heavily breaking.
## Solution
Revert default mesh materials, and adjust docs accordingly.
- Remove `extract_default_materials`
- Remove `clear_material_instances`, and move the logic back into
`extract_mesh_materials`
- Remove `HasMaterial2d` and `HasMaterial3d`
- Change default material handles back to pink instead of white
- 2D uses `Color::srgb(1.0, 0.0, 1.0)`, while 3D uses `Color::srgb(1.0,
0.0, 0.5)`. Not sure if this is intended.
There is now no indication at all about missing materials for `Mesh2d`
and `Mesh3d`. Having a mesh without a material renders nothing.
## Testing
I ran `2d_shapes`, `mesh2d_manual`, and `3d_shapes`, with and without
mesh material components.
2024-10-15 19:47:40 +00:00
|
|
|
material_instances.clear();
|
|
|
|
|
Migrate meshes and materials to required components (#15524)
# Objective
A big step in the migration to required components: meshes and
materials!
## Solution
As per the [selected
proposal](https://hackmd.io/@bevy/required_components/%2Fj9-PnF-2QKK0on1KQ29UWQ):
- Deprecate `MaterialMesh2dBundle`, `MaterialMeshBundle`, and
`PbrBundle`.
- Add `Mesh2d` and `Mesh3d` components, which wrap a `Handle<Mesh>`.
- Add `MeshMaterial2d<M: Material2d>` and `MeshMaterial3d<M: Material>`,
which wrap a `Handle<M>`.
- Meshes *without* a mesh material should be rendered with a default
material. The existence of a material is determined by
`HasMaterial2d`/`HasMaterial3d`, which is required by
`MeshMaterial2d`/`MeshMaterial3d`. This gets around problems with the
generics.
Previously:
```rust
commands.spawn(MaterialMesh2dBundle {
mesh: meshes.add(Circle::new(100.0)).into(),
material: materials.add(Color::srgb(7.5, 0.0, 7.5)),
transform: Transform::from_translation(Vec3::new(-200., 0., 0.)),
..default()
});
```
Now:
```rust
commands.spawn((
Mesh2d(meshes.add(Circle::new(100.0))),
MeshMaterial2d(materials.add(Color::srgb(7.5, 0.0, 7.5))),
Transform::from_translation(Vec3::new(-200., 0., 0.)),
));
```
If the mesh material is missing, previously nothing was rendered. Now,
it renders a white default `ColorMaterial` in 2D and a
`StandardMaterial` in 3D (this can be overridden). Below, only every
other entity has a material:


Why white? This is still open for discussion, but I think white makes
sense for a *default* material, while *invalid* asset handles pointing
to nothing should have something like a pink material to indicate that
something is broken (I don't handle that in this PR yet). This is kind
of a mix of Godot and Unity: Godot just renders a white material for
non-existent materials, while Unity renders nothing when no materials
exist, but renders pink for invalid materials. I can also change the
default material to pink if that is preferable though.
## Testing
I ran some 2D and 3D examples to test if anything changed visually. I
have not tested all examples or features yet however. If anyone wants to
test more extensively, it would be appreciated!
## Implementation Notes
- The relationship between `bevy_render` and `bevy_pbr` is weird here.
`bevy_render` needs `Mesh3d` for its own systems, but `bevy_pbr` has all
of the material logic, and `bevy_render` doesn't depend on it. I feel
like the two crates should be refactored in some way, but I think that's
out of scope for this PR.
- I didn't migrate meshlets to required components yet. That can
probably be done in a follow-up, as this is already a huge PR.
- It is becoming increasingly clear to me that we really, *really* want
to disallow raw asset handles as components. They caused me a *ton* of
headache here already, and it took me a long time to find every place
that queried for them or inserted them directly on entities, since there
were no compiler errors for it. If we don't remove the `Component`
derive, I expect raw asset handles to be a *huge* footgun for users as
we transition to wrapper components, especially as handles as components
have been the norm so far. I personally consider this to be a blocker
for 0.15: we need to migrate to wrapper components for asset handles
everywhere, and remove the `Component` derive. Also see
https://github.com/bevyengine/bevy/issues/14124.
---
## Migration Guide
Asset handles for meshes and mesh materials must now be wrapped in the
`Mesh2d` and `MeshMaterial2d` or `Mesh3d` and `MeshMaterial3d`
components for 2D and 3D respectively. Raw handles as components no
longer render meshes.
Additionally, `MaterialMesh2dBundle`, `MaterialMeshBundle`, and
`PbrBundle` have been deprecated. Instead, use the mesh and material
components directly.
Previously:
```rust
commands.spawn(MaterialMesh2dBundle {
mesh: meshes.add(Circle::new(100.0)).into(),
material: materials.add(Color::srgb(7.5, 0.0, 7.5)),
transform: Transform::from_translation(Vec3::new(-200., 0., 0.)),
..default()
});
```
Now:
```rust
commands.spawn((
Mesh2d(meshes.add(Circle::new(100.0))),
MeshMaterial2d(materials.add(Color::srgb(7.5, 0.0, 7.5))),
Transform::from_translation(Vec3::new(-200., 0., 0.)),
));
```
If the mesh material is missing, a white default material is now used.
Previously, nothing was rendered if the material was missing.
The `WithMesh2d` and `WithMesh3d` query filter type aliases have also
been removed. Simply use `With<Mesh2d>` or `With<Mesh3d>`.
---------
Co-authored-by: Tim Blackbird <justthecooldude@gmail.com>
Co-authored-by: Carter Anderson <mcanders1@gmail.com>
2024-10-01 21:33:17 +00:00
|
|
|
for (entity, view_visibility, material) in &query {
|
|
|
|
if view_visibility.get() {
|
Type safe retained render world (#15756)
# Objective
In the Render World, there are a number of collections that are derived
from Main World entities and are used to drive rendering. The most
notable are:
- `VisibleEntities`, which is generated in the `check_visibility` system
and contains visible entities for a view.
- `ExtractedInstances`, which maps entity ids to asset ids.
In the old model, these collections were trivially kept in sync -- any
extracted phase item could look itself up because the render entity id
was guaranteed to always match the corresponding main world id.
After #15320, this became much more complicated, and was leading to a
number of subtle bugs in the Render World. The main rendering systems,
i.e. `queue_material_meshes` and `queue_material2d_meshes`, follow a
similar pattern:
```rust
for visible_entity in visible_entities.iter::<With<Mesh2d>>() {
let Some(mesh_instance) = render_mesh_instances.get_mut(visible_entity) else {
continue;
};
// Look some more stuff up and specialize the pipeline...
let bin_key = Opaque2dBinKey {
pipeline: pipeline_id,
draw_function: draw_opaque_2d,
asset_id: mesh_instance.mesh_asset_id.into(),
material_bind_group_id: material_2d.get_bind_group_id().0,
};
opaque_phase.add(
bin_key,
*visible_entity,
BinnedRenderPhaseType::mesh(mesh_instance.automatic_batching),
);
}
```
In this case, `visible_entities` and `render_mesh_instances` are both
collections that are created and keyed by Main World entity ids, and so
this lookup happens to work by coincidence. However, there is a major
unintentional bug here: namely, because `visible_entities` is a
collection of Main World ids, the phase item being queued is created
with a Main World id rather than its correct Render World id.
This happens to not break mesh rendering because the render commands
used for drawing meshes do not access the `ItemQuery` parameter, but
demonstrates the confusion that is now possible: our UI phase items are
correctly being queued with Render World ids while our meshes aren't.
Additionally, this makes it very easy and error prone to use the wrong
entity id to look up things like assets. For example, if instead we
ignored visibility checks and queued our meshes via a query, we'd have
to be extra careful to use `&MainEntity` instead of the natural
`Entity`.
## Solution
Make all collections that are derived from Main World data use
`MainEntity` as their key, to ensure type safety and avoid accidentally
looking up data with the wrong entity id:
```rust
pub type MainEntityHashMap<V> = hashbrown::HashMap<MainEntity, V, EntityHash>;
```
Additionally, we make all `PhaseItem` be able to provide both their Main
and Render World ids, to allow render phase implementors maximum
flexibility as to what id should be used to look up data.
You can think of this like tracking at the type level whether something
in the Render World should use it's "primary key", i.e. entity id, or
needs to use a foreign key, i.e. `MainEntity`.
## Testing
##### TODO:
This will require extensive testing to make sure things didn't break!
Additionally, some extraction logic has become more complicated and
needs to be checked for regressions.
## Migration Guide
With the advent of the retained render world, collections that contain
references to `Entity` that are extracted into the render world have
been changed to contain `MainEntity` in order to prevent errors where a
render world entity id is used to look up an item by accident. Custom
rendering code may need to be changed to query for `&MainEntity` in
order to look up the correct item from such a collection. Additionally,
users who implement their own extraction logic for collections of main
world entity should strongly consider extracting into a different
collection that uses `MainEntity` as a key.
Additionally, render phases now require specifying both the `Entity` and
`MainEntity` for a given `PhaseItem`. Custom render phases should ensure
`MainEntity` is available when queuing a phase item.
2024-10-10 18:47:04 +00:00
|
|
|
material_instances.insert(entity.into(), material.id());
|
Migrate meshes and materials to required components (#15524)
# Objective
A big step in the migration to required components: meshes and
materials!
## Solution
As per the [selected
proposal](https://hackmd.io/@bevy/required_components/%2Fj9-PnF-2QKK0on1KQ29UWQ):
- Deprecate `MaterialMesh2dBundle`, `MaterialMeshBundle`, and
`PbrBundle`.
- Add `Mesh2d` and `Mesh3d` components, which wrap a `Handle<Mesh>`.
- Add `MeshMaterial2d<M: Material2d>` and `MeshMaterial3d<M: Material>`,
which wrap a `Handle<M>`.
- Meshes *without* a mesh material should be rendered with a default
material. The existence of a material is determined by
`HasMaterial2d`/`HasMaterial3d`, which is required by
`MeshMaterial2d`/`MeshMaterial3d`. This gets around problems with the
generics.
Previously:
```rust
commands.spawn(MaterialMesh2dBundle {
mesh: meshes.add(Circle::new(100.0)).into(),
material: materials.add(Color::srgb(7.5, 0.0, 7.5)),
transform: Transform::from_translation(Vec3::new(-200., 0., 0.)),
..default()
});
```
Now:
```rust
commands.spawn((
Mesh2d(meshes.add(Circle::new(100.0))),
MeshMaterial2d(materials.add(Color::srgb(7.5, 0.0, 7.5))),
Transform::from_translation(Vec3::new(-200., 0., 0.)),
));
```
If the mesh material is missing, previously nothing was rendered. Now,
it renders a white default `ColorMaterial` in 2D and a
`StandardMaterial` in 3D (this can be overridden). Below, only every
other entity has a material:


Why white? This is still open for discussion, but I think white makes
sense for a *default* material, while *invalid* asset handles pointing
to nothing should have something like a pink material to indicate that
something is broken (I don't handle that in this PR yet). This is kind
of a mix of Godot and Unity: Godot just renders a white material for
non-existent materials, while Unity renders nothing when no materials
exist, but renders pink for invalid materials. I can also change the
default material to pink if that is preferable though.
## Testing
I ran some 2D and 3D examples to test if anything changed visually. I
have not tested all examples or features yet however. If anyone wants to
test more extensively, it would be appreciated!
## Implementation Notes
- The relationship between `bevy_render` and `bevy_pbr` is weird here.
`bevy_render` needs `Mesh3d` for its own systems, but `bevy_pbr` has all
of the material logic, and `bevy_render` doesn't depend on it. I feel
like the two crates should be refactored in some way, but I think that's
out of scope for this PR.
- I didn't migrate meshlets to required components yet. That can
probably be done in a follow-up, as this is already a huge PR.
- It is becoming increasingly clear to me that we really, *really* want
to disallow raw asset handles as components. They caused me a *ton* of
headache here already, and it took me a long time to find every place
that queried for them or inserted them directly on entities, since there
were no compiler errors for it. If we don't remove the `Component`
derive, I expect raw asset handles to be a *huge* footgun for users as
we transition to wrapper components, especially as handles as components
have been the norm so far. I personally consider this to be a blocker
for 0.15: we need to migrate to wrapper components for asset handles
everywhere, and remove the `Component` derive. Also see
https://github.com/bevyengine/bevy/issues/14124.
---
## Migration Guide
Asset handles for meshes and mesh materials must now be wrapped in the
`Mesh2d` and `MeshMaterial2d` or `Mesh3d` and `MeshMaterial3d`
components for 2D and 3D respectively. Raw handles as components no
longer render meshes.
Additionally, `MaterialMesh2dBundle`, `MaterialMeshBundle`, and
`PbrBundle` have been deprecated. Instead, use the mesh and material
components directly.
Previously:
```rust
commands.spawn(MaterialMesh2dBundle {
mesh: meshes.add(Circle::new(100.0)).into(),
material: materials.add(Color::srgb(7.5, 0.0, 7.5)),
transform: Transform::from_translation(Vec3::new(-200., 0., 0.)),
..default()
});
```
Now:
```rust
commands.spawn((
Mesh2d(meshes.add(Circle::new(100.0))),
MeshMaterial2d(materials.add(Color::srgb(7.5, 0.0, 7.5))),
Transform::from_translation(Vec3::new(-200., 0., 0.)),
));
```
If the mesh material is missing, a white default material is now used.
Previously, nothing was rendered if the material was missing.
The `WithMesh2d` and `WithMesh3d` query filter type aliases have also
been removed. Simply use `With<Mesh2d>` or `With<Mesh3d>`.
---------
Co-authored-by: Tim Blackbird <justthecooldude@gmail.com>
Co-authored-by: Carter Anderson <mcanders1@gmail.com>
2024-10-01 21:33:17 +00:00
|
|
|
}
|
|
|
|
}
|
|
|
|
}
|
|
|
|
|
Generate `MeshUniform`s on the GPU via compute shader where available. (#12773)
Currently, `MeshUniform`s are rather large: 160 bytes. They're also
somewhat expensive to compute, because they involve taking the inverse
of a 3x4 matrix. Finally, if a mesh is present in multiple views, that
mesh will have a separate `MeshUniform` for each and every view, which
is wasteful.
This commit fixes these issues by introducing the concept of a *mesh
input uniform* and adding a *mesh uniform building* compute shader pass.
The `MeshInputUniform` is simply the minimum amount of data needed for
the GPU to compute the full `MeshUniform`. Most of this data is just the
transform and is therefore only 64 bytes. `MeshInputUniform`s are
computed during the *extraction* phase, much like skins are today, in
order to avoid needlessly copying transforms around on CPU. (In fact,
the render app has been changed to only store the translation of each
mesh; it no longer cares about any other part of the transform, which is
stored only on the GPU and the main world.) Before rendering, the
`build_mesh_uniforms` pass runs to expand the `MeshInputUniform`s to the
full `MeshUniform`.
The mesh uniform building pass does the following, all on GPU:
1. Copy the appropriate fields of the `MeshInputUniform` to the
`MeshUniform` slot. If a single mesh is present in multiple views, this
effectively duplicates it into each view.
2. Compute the inverse transpose of the model transform, used for
transforming normals.
3. If applicable, copy the mesh's transform from the previous frame for
TAA. To support this, we double-buffer the `MeshInputUniform`s over two
frames and swap the buffers each frame. The `MeshInputUniform`s for the
current frame contain the index of that mesh's `MeshInputUniform` for
the previous frame.
This commit produces wins in virtually every CPU part of the pipeline:
`extract_meshes`, `queue_material_meshes`,
`batch_and_prepare_render_phase`, and especially
`write_batched_instance_buffer` are all faster. Shrinking the amount of
CPU data that has to be shuffled around speeds up the entire rendering
process.
| Benchmark | This branch | `main` | Speedup |
|------------------------|-------------|---------|---------|
| `many_cubes -nfc` | 17.259 | 24.529 | 42.12% |
| `many_cubes -nfc -vpi` | 302.116 | 312.123 | 3.31% |
| `many_foxes` | 3.227 | 3.515 | 8.92% |
Because mesh uniform building requires compute shader, and WebGL 2 has
no compute shader, the existing CPU mesh uniform building code has been
left as-is. Many types now have both CPU mesh uniform building and GPU
mesh uniform building modes. Developers can opt into the old CPU mesh
uniform building by setting the `use_gpu_uniform_builder` option on
`PbrPlugin` to `false`.
Below are graphs of the CPU portions of `many-cubes
--no-frustum-culling`. Yellow is this branch, red is `main`.
`extract_meshes`:

It's notable that we get a small win even though we're now writing to a
GPU buffer.
`queue_material_meshes`:

There's a bit of a regression here; not sure what's causing it. In any
case it's very outweighed by the other gains.
`batch_and_prepare_render_phase`:

There's a huge win here, enough to make batching basically drop off the
profile.
`write_batched_instance_buffer`:

There's a massive improvement here, as expected. Note that a lot of it
simply comes from the fact that `MeshInputUniform` is `Pod`. (This isn't
a maintainability problem in my view because `MeshInputUniform` is so
simple: just 16 tightly-packed words.)
## Changelog
### Added
* Per-mesh instance data is now generated on GPU with a compute shader
instead of CPU, resulting in rendering performance improvements on
platforms where compute shaders are supported.
## Migration guide
* Custom render phases now need multiple systems beyond just
`batch_and_prepare_render_phase`. Code that was previously creating
custom render phases should now add a `BinnedRenderPhasePlugin` or
`SortedRenderPhasePlugin` as appropriate instead of directly adding
`batch_and_prepare_render_phase`.
2024-04-10 05:33:32 +00:00
|
|
|
/// For each view, iterates over all the meshes visible from that view and adds
|
|
|
|
/// them to [`BinnedRenderPhase`]s or [`SortedRenderPhase`]s as appropriate.
|
2021-12-25 21:45:43 +00:00
|
|
|
#[allow(clippy::too_many_arguments)]
|
Better Materials: AsBindGroup trait and derive, simpler Material trait (#5053)
# Objective
This PR reworks Bevy's Material system, making the user experience of defining Materials _much_ nicer. Bevy's previous material system leaves a lot to be desired:
* Materials require manually implementing the `RenderAsset` trait, which involves manually generating the bind group, handling gpu buffer data transfer, looking up image textures, etc. Even the simplest single-texture material involves writing ~80 unnecessary lines of code. This was never the long term plan.
* There are two material traits, which is confusing, hard to document, and often redundant: `Material` and `SpecializedMaterial`. `Material` implicitly implements `SpecializedMaterial`, and `SpecializedMaterial` is used in most high level apis to support both use cases. Most users shouldn't need to think about specialization at all (I consider it a "power-user tool"), so the fact that `SpecializedMaterial` is front-and-center in our apis is a miss.
* Implementing either material trait involves a lot of "type soup". The "prepared asset" parameter is particularly heinous: `&<Self as RenderAsset>::PreparedAsset`. Defining vertex and fragment shaders is also more verbose than it needs to be.
## Solution
Say hello to the new `Material` system:
```rust
#[derive(AsBindGroup, TypeUuid, Debug, Clone)]
#[uuid = "f690fdae-d598-45ab-8225-97e2a3f056e0"]
pub struct CoolMaterial {
#[uniform(0)]
color: Color,
#[texture(1)]
#[sampler(2)]
color_texture: Handle<Image>,
}
impl Material for CoolMaterial {
fn fragment_shader() -> ShaderRef {
"cool_material.wgsl".into()
}
}
```
Thats it! This same material would have required [~80 lines of complicated "type heavy" code](https://github.com/bevyengine/bevy/blob/v0.7.0/examples/shader/shader_material.rs) in the old Material system. Now it is just 14 lines of simple, readable code.
This is thanks to a new consolidated `Material` trait and the new `AsBindGroup` trait / derive.
### The new `Material` trait
The old "split" `Material` and `SpecializedMaterial` traits have been removed in favor of a new consolidated `Material` trait. All of the functions on the trait are optional.
The difficulty of implementing `Material` has been reduced by simplifying dataflow and removing type complexity:
```rust
// Old
impl Material for CustomMaterial {
fn fragment_shader(asset_server: &AssetServer) -> Option<Handle<Shader>> {
Some(asset_server.load("custom_material.wgsl"))
}
fn alpha_mode(render_asset: &<Self as RenderAsset>::PreparedAsset) -> AlphaMode {
render_asset.alpha_mode
}
}
// New
impl Material for CustomMaterial {
fn fragment_shader() -> ShaderRef {
"custom_material.wgsl".into()
}
fn alpha_mode(&self) -> AlphaMode {
self.alpha_mode
}
}
```
Specialization is still supported, but it is hidden by default under the `specialize()` function (more on this later).
### The `AsBindGroup` trait / derive
The `Material` trait now requires the `AsBindGroup` derive. This can be implemented manually relatively easily, but deriving it will almost always be preferable.
Field attributes like `uniform` and `texture` are used to define which fields should be bindings,
what their binding type is, and what index they should be bound at:
```rust
#[derive(AsBindGroup)]
struct CoolMaterial {
#[uniform(0)]
color: Color,
#[texture(1)]
#[sampler(2)]
color_texture: Handle<Image>,
}
```
In WGSL shaders, the binding looks like this:
```wgsl
struct CoolMaterial {
color: vec4<f32>;
};
[[group(1), binding(0)]]
var<uniform> material: CoolMaterial;
[[group(1), binding(1)]]
var color_texture: texture_2d<f32>;
[[group(1), binding(2)]]
var color_sampler: sampler;
```
Note that the "group" index is determined by the usage context. It is not defined in `AsBindGroup`. Bevy material bind groups are bound to group 1.
The following field-level attributes are supported:
* `uniform(BINDING_INDEX)`
* The field will be converted to a shader-compatible type using the `ShaderType` trait, written to a `Buffer`, and bound as a uniform. It can also be derived for custom structs.
* `texture(BINDING_INDEX)`
* This field's `Handle<Image>` will be used to look up the matching `Texture` gpu resource, which will be bound as a texture in shaders. The field will be assumed to implement `Into<Option<Handle<Image>>>`. In practice, most fields should be a `Handle<Image>` or `Option<Handle<Image>>`. If the value of an `Option<Handle<Image>>` is `None`, the new `FallbackImage` resource will be used instead. This attribute can be used in conjunction with a `sampler` binding attribute (with a different binding index).
* `sampler(BINDING_INDEX)`
* Behaves exactly like the `texture` attribute, but sets the Image's sampler binding instead of the texture.
Note that fields without field-level binding attributes will be ignored.
```rust
#[derive(AsBindGroup)]
struct CoolMaterial {
#[uniform(0)]
color: Color,
this_field_is_ignored: String,
}
```
As mentioned above, `Option<Handle<Image>>` is also supported:
```rust
#[derive(AsBindGroup)]
struct CoolMaterial {
#[uniform(0)]
color: Color,
#[texture(1)]
#[sampler(2)]
color_texture: Option<Handle<Image>>,
}
```
This is useful if you want a texture to be optional. When the value is `None`, the `FallbackImage` will be used for the binding instead, which defaults to "pure white".
Field uniforms with the same binding index will be combined into a single binding:
```rust
#[derive(AsBindGroup)]
struct CoolMaterial {
#[uniform(0)]
color: Color,
#[uniform(0)]
roughness: f32,
}
```
In WGSL shaders, the binding would look like this:
```wgsl
struct CoolMaterial {
color: vec4<f32>;
roughness: f32;
};
[[group(1), binding(0)]]
var<uniform> material: CoolMaterial;
```
Some less common scenarios will require "struct-level" attributes. These are the currently supported struct-level attributes:
* `uniform(BINDING_INDEX, ConvertedShaderType)`
* Similar to the field-level `uniform` attribute, but instead the entire `AsBindGroup` value is converted to `ConvertedShaderType`, which must implement `ShaderType`. This is useful if more complicated conversion logic is required.
* `bind_group_data(DataType)`
* The `AsBindGroup` type will be converted to some `DataType` using `Into<DataType>` and stored as `AsBindGroup::Data` as part of the `AsBindGroup::as_bind_group` call. This is useful if data needs to be stored alongside the generated bind group, such as a unique identifier for a material's bind group. The most common use case for this attribute is "shader pipeline specialization".
The previous `CoolMaterial` example illustrating "combining multiple field-level uniform attributes with the same binding index" can
also be equivalently represented with a single struct-level uniform attribute:
```rust
#[derive(AsBindGroup)]
#[uniform(0, CoolMaterialUniform)]
struct CoolMaterial {
color: Color,
roughness: f32,
}
#[derive(ShaderType)]
struct CoolMaterialUniform {
color: Color,
roughness: f32,
}
impl From<&CoolMaterial> for CoolMaterialUniform {
fn from(material: &CoolMaterial) -> CoolMaterialUniform {
CoolMaterialUniform {
color: material.color,
roughness: material.roughness,
}
}
}
```
### Material Specialization
Material shader specialization is now _much_ simpler:
```rust
#[derive(AsBindGroup, TypeUuid, Debug, Clone)]
#[uuid = "f690fdae-d598-45ab-8225-97e2a3f056e0"]
#[bind_group_data(CoolMaterialKey)]
struct CoolMaterial {
#[uniform(0)]
color: Color,
is_red: bool,
}
#[derive(Copy, Clone, Hash, Eq, PartialEq)]
struct CoolMaterialKey {
is_red: bool,
}
impl From<&CoolMaterial> for CoolMaterialKey {
fn from(material: &CoolMaterial) -> CoolMaterialKey {
CoolMaterialKey {
is_red: material.is_red,
}
}
}
impl Material for CoolMaterial {
fn fragment_shader() -> ShaderRef {
"cool_material.wgsl".into()
}
fn specialize(
pipeline: &MaterialPipeline<Self>,
descriptor: &mut RenderPipelineDescriptor,
layout: &MeshVertexBufferLayout,
key: MaterialPipelineKey<Self>,
) -> Result<(), SpecializedMeshPipelineError> {
if key.bind_group_data.is_red {
let fragment = descriptor.fragment.as_mut().unwrap();
fragment.shader_defs.push("IS_RED".to_string());
}
Ok(())
}
}
```
Setting `bind_group_data` is not required for specialization (it defaults to `()`). Scenarios like "custom vertex attributes" also benefit from this system:
```rust
impl Material for CustomMaterial {
fn vertex_shader() -> ShaderRef {
"custom_material.wgsl".into()
}
fn fragment_shader() -> ShaderRef {
"custom_material.wgsl".into()
}
fn specialize(
pipeline: &MaterialPipeline<Self>,
descriptor: &mut RenderPipelineDescriptor,
layout: &MeshVertexBufferLayout,
key: MaterialPipelineKey<Self>,
) -> Result<(), SpecializedMeshPipelineError> {
let vertex_layout = layout.get_layout(&[
Mesh::ATTRIBUTE_POSITION.at_shader_location(0),
ATTRIBUTE_BLEND_COLOR.at_shader_location(1),
])?;
descriptor.vertex.buffers = vec![vertex_layout];
Ok(())
}
}
```
### Ported `StandardMaterial` to the new `Material` system
Bevy's built-in PBR material uses the new Material system (including the AsBindGroup derive):
```rust
#[derive(AsBindGroup, Debug, Clone, TypeUuid)]
#[uuid = "7494888b-c082-457b-aacf-517228cc0c22"]
#[bind_group_data(StandardMaterialKey)]
#[uniform(0, StandardMaterialUniform)]
pub struct StandardMaterial {
pub base_color: Color,
#[texture(1)]
#[sampler(2)]
pub base_color_texture: Option<Handle<Image>>,
/* other fields omitted for brevity */
```
### Ported Bevy examples to the new `Material` system
The overall complexity of Bevy's "custom shader examples" has gone down significantly. Take a look at the diffs if you want a dopamine spike.
Please note that while this PR has a net increase in "lines of code", most of those extra lines come from added documentation. There is a significant reduction
in the overall complexity of the code (even accounting for the new derive logic).
---
## Changelog
### Added
* `AsBindGroup` trait and derive, which make it much easier to transfer data to the gpu and generate bind groups for a given type.
### Changed
* The old `Material` and `SpecializedMaterial` traits have been replaced by a consolidated (much simpler) `Material` trait. Materials no longer implement `RenderAsset`.
* `StandardMaterial` was ported to the new material system. There are no user-facing api changes to the `StandardMaterial` struct api, but it now implements `AsBindGroup` and `Material` instead of `RenderAsset` and `SpecializedMaterial`.
## Migration Guide
The Material system has been reworked to be much simpler. We've removed a lot of boilerplate with the new `AsBindGroup` derive and the `Material` trait is simpler as well!
### Bevy 0.7 (old)
```rust
#[derive(Debug, Clone, TypeUuid)]
#[uuid = "f690fdae-d598-45ab-8225-97e2a3f056e0"]
pub struct CustomMaterial {
color: Color,
color_texture: Handle<Image>,
}
#[derive(Clone)]
pub struct GpuCustomMaterial {
_buffer: Buffer,
bind_group: BindGroup,
}
impl RenderAsset for CustomMaterial {
type ExtractedAsset = CustomMaterial;
type PreparedAsset = GpuCustomMaterial;
type Param = (SRes<RenderDevice>, SRes<MaterialPipeline<Self>>);
fn extract_asset(&self) -> Self::ExtractedAsset {
self.clone()
}
fn prepare_asset(
extracted_asset: Self::ExtractedAsset,
(render_device, material_pipeline): &mut SystemParamItem<Self::Param>,
) -> Result<Self::PreparedAsset, PrepareAssetError<Self::ExtractedAsset>> {
let color = Vec4::from_slice(&extracted_asset.color.as_linear_rgba_f32());
let byte_buffer = [0u8; Vec4::SIZE.get() as usize];
let mut buffer = encase::UniformBuffer::new(byte_buffer);
buffer.write(&color).unwrap();
let buffer = render_device.create_buffer_with_data(&BufferInitDescriptor {
contents: buffer.as_ref(),
label: None,
usage: BufferUsages::UNIFORM | BufferUsages::COPY_DST,
});
let (texture_view, texture_sampler) = if let Some(result) = material_pipeline
.mesh_pipeline
.get_image_texture(gpu_images, &Some(extracted_asset.color_texture.clone()))
{
result
} else {
return Err(PrepareAssetError::RetryNextUpdate(extracted_asset));
};
let bind_group = render_device.create_bind_group(&BindGroupDescriptor {
entries: &[
BindGroupEntry {
binding: 0,
resource: buffer.as_entire_binding(),
},
BindGroupEntry {
binding: 0,
resource: BindingResource::TextureView(texture_view),
},
BindGroupEntry {
binding: 1,
resource: BindingResource::Sampler(texture_sampler),
},
],
label: None,
layout: &material_pipeline.material_layout,
});
Ok(GpuCustomMaterial {
_buffer: buffer,
bind_group,
})
}
}
impl Material for CustomMaterial {
fn fragment_shader(asset_server: &AssetServer) -> Option<Handle<Shader>> {
Some(asset_server.load("custom_material.wgsl"))
}
fn bind_group(render_asset: &<Self as RenderAsset>::PreparedAsset) -> &BindGroup {
&render_asset.bind_group
}
fn bind_group_layout(render_device: &RenderDevice) -> BindGroupLayout {
render_device.create_bind_group_layout(&BindGroupLayoutDescriptor {
entries: &[
BindGroupLayoutEntry {
binding: 0,
visibility: ShaderStages::FRAGMENT,
ty: BindingType::Buffer {
ty: BufferBindingType::Uniform,
has_dynamic_offset: false,
min_binding_size: Some(Vec4::min_size()),
},
count: None,
},
BindGroupLayoutEntry {
binding: 1,
visibility: ShaderStages::FRAGMENT,
ty: BindingType::Texture {
multisampled: false,
sample_type: TextureSampleType::Float { filterable: true },
view_dimension: TextureViewDimension::D2Array,
},
count: None,
},
BindGroupLayoutEntry {
binding: 2,
visibility: ShaderStages::FRAGMENT,
ty: BindingType::Sampler(SamplerBindingType::Filtering),
count: None,
},
],
label: None,
})
}
}
```
### Bevy 0.8 (new)
```rust
impl Material for CustomMaterial {
fn fragment_shader() -> ShaderRef {
"custom_material.wgsl".into()
}
}
#[derive(AsBindGroup, TypeUuid, Debug, Clone)]
#[uuid = "f690fdae-d598-45ab-8225-97e2a3f056e0"]
pub struct CustomMaterial {
#[uniform(0)]
color: Color,
#[texture(1)]
#[sampler(2)]
color_texture: Handle<Image>,
}
```
## Future Work
* Add support for more binding types (cubemaps, buffers, etc). This PR intentionally includes a bare minimum number of binding types to keep "reviewability" in check.
* Consider optionally eliding binding indices using binding names. `AsBindGroup` could pass in (optional?) reflection info as a "hint".
* This would make it possible for the derive to do this:
```rust
#[derive(AsBindGroup)]
pub struct CustomMaterial {
#[uniform]
color: Color,
#[texture]
#[sampler]
color_texture: Option<Handle<Image>>,
alpha_mode: AlphaMode,
}
```
* Or this
```rust
#[derive(AsBindGroup)]
pub struct CustomMaterial {
#[binding]
color: Color,
#[binding]
color_texture: Option<Handle<Image>>,
alpha_mode: AlphaMode,
}
```
* Or even this (if we flip to "include bindings by default")
```rust
#[derive(AsBindGroup)]
pub struct CustomMaterial {
color: Color,
color_texture: Option<Handle<Image>>,
#[binding(ignore)]
alpha_mode: AlphaMode,
}
```
* If we add the option to define custom draw functions for materials (which could be done in a type-erased way), I think that would be enough to support extra non-material bindings. Worth considering!
2022-06-30 23:48:46 +00:00
|
|
|
pub fn queue_material_meshes<M: Material>(
|
2024-05-21 18:23:04 +00:00
|
|
|
(
|
|
|
|
opaque_draw_functions,
|
|
|
|
alpha_mask_draw_functions,
|
|
|
|
transmissive_draw_functions,
|
|
|
|
transparent_draw_functions,
|
|
|
|
): (
|
|
|
|
Res<DrawFunctions<Opaque3d>>,
|
|
|
|
Res<DrawFunctions<AlphaMask3d>>,
|
|
|
|
Res<DrawFunctions<Transmissive3d>>,
|
|
|
|
Res<DrawFunctions<Transparent3d>>,
|
|
|
|
),
|
2021-12-25 21:45:43 +00:00
|
|
|
material_pipeline: Res<MaterialPipeline<M>>,
|
Mesh vertex buffer layouts (#3959)
This PR makes a number of changes to how meshes and vertex attributes are handled, which the goal of enabling easy and flexible custom vertex attributes:
* Reworks the `Mesh` type to use the newly added `VertexAttribute` internally
* `VertexAttribute` defines the name, a unique `VertexAttributeId`, and a `VertexFormat`
* `VertexAttributeId` is used to produce consistent sort orders for vertex buffer generation, replacing the more expensive and often surprising "name based sorting"
* Meshes can be used to generate a `MeshVertexBufferLayout`, which defines the layout of the gpu buffer produced by the mesh. `MeshVertexBufferLayouts` can then be used to generate actual `VertexBufferLayouts` according to the requirements of a specific pipeline. This decoupling of "mesh layout" vs "pipeline vertex buffer layout" is what enables custom attributes. We don't need to standardize _mesh layouts_ or contort meshes to meet the needs of a specific pipeline. As long as the mesh has what the pipeline needs, it will work transparently.
* Mesh-based pipelines now specialize on `&MeshVertexBufferLayout` via the new `SpecializedMeshPipeline` trait (which behaves like `SpecializedPipeline`, but adds `&MeshVertexBufferLayout`). The integrity of the pipeline cache is maintained because the `MeshVertexBufferLayout` is treated as part of the key (which is fully abstracted from implementers of the trait ... no need to add any additional info to the specialization key).
* Hashing `MeshVertexBufferLayout` is too expensive to do for every entity, every frame. To make this scalable, I added a generalized "pre-hashing" solution to `bevy_utils`: `Hashed<T>` keys and `PreHashMap<K, V>` (which uses `Hashed<T>` internally) . Why didn't I just do the quick and dirty in-place "pre-compute hash and use that u64 as a key in a hashmap" that we've done in the past? Because its wrong! Hashes by themselves aren't enough because two different values can produce the same hash. Re-hashing a hash is even worse! I decided to build a generalized solution because this pattern has come up in the past and we've chosen to do the wrong thing. Now we can do the right thing! This did unfortunately require pulling in `hashbrown` and using that in `bevy_utils`, because avoiding re-hashes requires the `raw_entry_mut` api, which isn't stabilized yet (and may never be ... `entry_ref` has favor now, but also isn't available yet). If std's HashMap ever provides the tools we need, we can move back to that. Note that adding `hashbrown` doesn't increase our dependency count because it was already in our tree. I will probably break these changes out into their own PR.
* Specializing on `MeshVertexBufferLayout` has one non-obvious behavior: it can produce identical pipelines for two different MeshVertexBufferLayouts. To optimize the number of active pipelines / reduce re-binds while drawing, I de-duplicate pipelines post-specialization using the final `VertexBufferLayout` as the key. For example, consider a pipeline that needs the layout `(position, normal)` and is specialized using two meshes: `(position, normal, uv)` and `(position, normal, other_vec2)`. If both of these meshes result in `(position, normal)` specializations, we can use the same pipeline! Now we do. Cool!
To briefly illustrate, this is what the relevant section of `MeshPipeline`'s specialization code looks like now:
```rust
impl SpecializedMeshPipeline for MeshPipeline {
type Key = MeshPipelineKey;
fn specialize(
&self,
key: Self::Key,
layout: &MeshVertexBufferLayout,
) -> RenderPipelineDescriptor {
let mut vertex_attributes = vec![
Mesh::ATTRIBUTE_POSITION.at_shader_location(0),
Mesh::ATTRIBUTE_NORMAL.at_shader_location(1),
Mesh::ATTRIBUTE_UV_0.at_shader_location(2),
];
let mut shader_defs = Vec::new();
if layout.contains(Mesh::ATTRIBUTE_TANGENT) {
shader_defs.push(String::from("VERTEX_TANGENTS"));
vertex_attributes.push(Mesh::ATTRIBUTE_TANGENT.at_shader_location(3));
}
let vertex_buffer_layout = layout
.get_layout(&vertex_attributes)
.expect("Mesh is missing a vertex attribute");
```
Notice that this is _much_ simpler than it was before. And now any mesh with any layout can be used with this pipeline, provided it has vertex postions, normals, and uvs. We even got to remove `HAS_TANGENTS` from MeshPipelineKey and `has_tangents` from `GpuMesh`, because that information is redundant with `MeshVertexBufferLayout`.
This is still a draft because I still need to:
* Add more docs
* Experiment with adding error handling to mesh pipeline specialization (which would print errors at runtime when a mesh is missing a vertex attribute required by a pipeline). If it doesn't tank perf, we'll keep it.
* Consider breaking out the PreHash / hashbrown changes into a separate PR.
* Add an example illustrating this change
* Verify that the "mesh-specialized pipeline de-duplication code" works properly
Please dont yell at me for not doing these things yet :) Just trying to get this in peoples' hands asap.
Alternative to #3120
Fixes #3030
Co-authored-by: Carter Anderson <mcanders1@gmail.com>
2022-02-23 23:21:13 +00:00
|
|
|
mut pipelines: ResMut<SpecializedMeshPipelines<MaterialPipeline<M>>>,
|
2023-01-16 15:41:14 +00:00
|
|
|
pipeline_cache: Res<PipelineCache>,
|
Pack multiple vertex and index arrays together into growable buffers. (#14257)
This commit uses the [`offset-allocator`] crate to combine vertex and
index arrays from different meshes into single buffers. Since the
primary source of `wgpu` overhead is from validation and synchronization
when switching buffers, this significantly improves Bevy's rendering
performance on many scenes.
This patch is a more flexible version of #13218, which also used slabs.
Unlike #13218, which used slabs of a fixed size, this commit implements
slabs that start small and can grow. In addition to reducing memory
usage, supporting slab growth reduces the number of vertex and index
buffer switches that need to happen during rendering, leading to
improved performance. To prevent pathological fragmentation behavior,
slabs are capped to a maximum size, and mesh arrays that are too large
get their own dedicated slabs.
As an additional improvement over #13218, this commit allows the
application to customize all allocator heuristics. The
`MeshAllocatorSettings` resource contains values that adjust the minimum
and maximum slab sizes, the cutoff point at which meshes get their own
dedicated slabs, and the rate at which slabs grow. Hopefully-sensible
defaults have been chosen for each value.
Unfortunately, WebGL 2 doesn't support the *base vertex* feature, which
is necessary to pack vertex arrays from different meshes into the same
buffer. `wgpu` represents this restriction as the downlevel flag
`BASE_VERTEX`. This patch detects that bit and ensures that all vertex
buffers get dedicated slabs on that platform. Even on WebGL 2, though,
we can combine all *index* arrays into single buffers to reduce buffer
changes, and we do so.
The following measurements are on Bistro:
Overall frame time improves from 8.74 ms to 5.53 ms (1.58x speedup):

Render system time improves from 6.57 ms to 3.54 ms (1.86x speedup):

Opaque pass time improves from 4.64 ms to 2.33 ms (1.99x speedup):

## Migration Guide
### Changed
* Vertex and index buffers for meshes may now be packed alongside other
buffers, for performance.
* `GpuMesh` has been renamed to `RenderMesh`, to reflect the fact that
it no longer directly stores handles to GPU objects.
* Because meshes no longer have their own vertex and index buffers, the
responsibility for the buffers has moved from `GpuMesh` (now called
`RenderMesh`) to the `MeshAllocator` resource. To access the vertex data
for a mesh, use `MeshAllocator::mesh_vertex_slice`. To access the index
data for a mesh, use `MeshAllocator::mesh_index_slice`.
[`offset-allocator`]: https://github.com/pcwalton/offset-allocator
2024-07-16 20:33:15 +00:00
|
|
|
render_meshes: Res<RenderAssets<RenderMesh>>,
|
2024-04-09 13:26:34 +00:00
|
|
|
render_materials: Res<RenderAssets<PreparedMaterial<M>>>,
|
2024-02-20 00:12:41 +00:00
|
|
|
render_mesh_instances: Res<RenderMeshInstances>,
|
Use EntityHashMap<Entity, T> for render world entity storage for better performance (#9903)
# Objective
- Improve rendering performance, particularly by avoiding the large
system commands costs of using the ECS in the way that the render world
does.
## Solution
- Define `EntityHasher` that calculates a hash from the
`Entity.to_bits()` by `i | (i.wrapping_mul(0x517cc1b727220a95) << 32)`.
`0x517cc1b727220a95` is something like `u64::MAX / N` for N that gives a
value close to π and that works well for hashing. Thanks for @SkiFire13
for the suggestion and to @nicopap for alternative suggestions and
discussion. This approach comes from `rustc-hash` (a.k.a. `FxHasher`)
with some tweaks for the case of hashing an `Entity`. `FxHasher` and
`SeaHasher` were also tested but were significantly slower.
- Define `EntityHashMap` type that uses the `EntityHashser`
- Use `EntityHashMap<Entity, T>` for render world entity storage,
including:
- `RenderMaterialInstances` - contains the `AssetId<M>` of the material
associated with the entity. Also for 2D.
- `RenderMeshInstances` - contains mesh transforms, flags and properties
about mesh entities. Also for 2D.
- `SkinIndices` and `MorphIndices` - contains the skin and morph index
for an entity, respectively
- `ExtractedSprites`
- `ExtractedUiNodes`
## Benchmarks
All benchmarks have been conducted on an M1 Max connected to AC power.
The tests are run for 1500 frames. The 1000th frame is captured for
comparison to check for visual regressions. There were none.
### 2D Meshes
`bevymark --benchmark --waves 160 --per-wave 1000 --mode mesh2d`
#### `--ordered-z`
This test spawns the 2D meshes with z incrementing back to front, which
is the ideal arrangement allocation order as it matches the sorted
render order which means lookups have a high cache hit rate.
<img width="1112" alt="Screenshot 2023-09-27 at 07 50 45"
src="https://github.com/bevyengine/bevy/assets/302146/e140bc98-7091-4a3b-8ae1-ab75d16d2ccb">
-39.1% median frame time.
#### Random
This test spawns the 2D meshes with random z. This not only makes the
batching and transparent 2D pass lookups get a lot of cache misses, it
also currently means that the meshes are almost certain to not be
batchable.
<img width="1108" alt="Screenshot 2023-09-27 at 07 51 28"
src="https://github.com/bevyengine/bevy/assets/302146/29c2e813-645a-43ce-982a-55df4bf7d8c4">
-7.2% median frame time.
### 3D Meshes
`many_cubes --benchmark`
<img width="1112" alt="Screenshot 2023-09-27 at 07 51 57"
src="https://github.com/bevyengine/bevy/assets/302146/1a729673-3254-4e2a-9072-55e27c69f0fc">
-7.7% median frame time.
### Sprites
**NOTE: On `main` sprites are using `SparseSet<Entity, T>`!**
`bevymark --benchmark --waves 160 --per-wave 1000 --mode sprite`
#### `--ordered-z`
This test spawns the sprites with z incrementing back to front, which is
the ideal arrangement allocation order as it matches the sorted render
order which means lookups have a high cache hit rate.
<img width="1116" alt="Screenshot 2023-09-27 at 07 52 31"
src="https://github.com/bevyengine/bevy/assets/302146/bc8eab90-e375-4d31-b5cd-f55f6f59ab67">
+13.0% median frame time.
#### Random
This test spawns the sprites with random z. This makes the batching and
transparent 2D pass lookups get a lot of cache misses.
<img width="1109" alt="Screenshot 2023-09-27 at 07 53 01"
src="https://github.com/bevyengine/bevy/assets/302146/22073f5d-99a7-49b0-9584-d3ac3eac3033">
+0.6% median frame time.
### UI
**NOTE: On `main` UI is using `SparseSet<Entity, T>`!**
`many_buttons`
<img width="1111" alt="Screenshot 2023-09-27 at 07 53 26"
src="https://github.com/bevyengine/bevy/assets/302146/66afd56d-cbe4-49e7-8b64-2f28f6043d85">
+15.1% median frame time.
## Alternatives
- Cart originally suggested trying out `SparseSet<Entity, T>` and indeed
that is slightly faster under ideal conditions. However,
`PassHashMap<Entity, T>` has better worst case performance when data is
randomly distributed, rather than in sorted render order, and does not
have the worst case memory usage that `SparseSet`'s dense `Vec<usize>`
that maps from the `Entity` index to sparse index into `Vec<T>`. This
dense `Vec` has to be as large as the largest Entity index used with the
`SparseSet`.
- I also tested `PassHashMap<u32, T>`, intending to use `Entity.index()`
as the key, but this proved to sometimes be slower and mostly no
different.
- The only outstanding approach that has not been implemented and tested
is to _not_ clear the render world of its entities each frame. That has
its own problems, though they could perhaps be solved.
- Performance-wise, if the entities and their component data were not
cleared, then they would incur table moves on spawn, and should not
thereafter, rather just their component data would be overwritten.
Ideally we would have a neat way of either updating data in-place via
`&mut T` queries, or inserting components if not present. This would
likely be quite cumbersome to have to remember to do everywhere, but
perhaps it only needs to be done in the more performance-sensitive
systems.
- The main problem to solve however is that we want to both maintain a
mapping between main world entities and render world entities, be able
to run the render app and world in parallel with the main app and world
for pipelined rendering, and at the same time be able to spawn entities
in the render world in such a way that those Entity ids do not collide
with those spawned in the main world. This is potentially quite
solvable, but could well be a lot of ECS work to do it in a way that
makes sense.
---
## Changelog
- Changed: Component data for entities to be drawn are no longer stored
on entities in the render world. Instead, data is stored in a
`EntityHashMap<Entity, T>` in various resources. This brings significant
performance benefits due to the way the render app clears entities every
frame. Resources of most interest are `RenderMeshInstances` and
`RenderMaterialInstances`, and their 2D counterparts.
## Migration Guide
Previously the render app extracted mesh entities and their component
data from the main world and stored them as entities and components in
the render world. Now they are extracted into essentially
`EntityHashMap<Entity, T>` where `T` are structs containing an
appropriate group of data. This means that while extract set systems
will continue to run extract queries against the main world they will
store their data in hash maps. Also, systems in later sets will either
need to look up entities in the available resources such as
`RenderMeshInstances`, or maintain their own `EntityHashMap<Entity, T>`
for their own data.
Before:
```rust
fn queue_custom(
material_meshes: Query<(Entity, &MeshTransforms, &Handle<Mesh>), With<InstanceMaterialData>>,
) {
...
for (entity, mesh_transforms, mesh_handle) in &material_meshes {
...
}
}
```
After:
```rust
fn queue_custom(
render_mesh_instances: Res<RenderMeshInstances>,
instance_entities: Query<Entity, With<InstanceMaterialData>>,
) {
...
for entity in &instance_entities {
let Some(mesh_instance) = render_mesh_instances.get(&entity) else { continue; };
// The mesh handle in `AssetId<Mesh>` form, and the `MeshTransforms` can now
// be found in `mesh_instance` which is a `RenderMeshInstance`
...
}
}
```
---------
Co-authored-by: robtfm <50659922+robtfm@users.noreply.github.com>
2023-09-27 08:28:28 +00:00
|
|
|
render_material_instances: Res<RenderMaterialInstances<M>>,
|
Implement lightmaps. (#10231)

# Objective
Lightmaps, textures that store baked global illumination, have been a
mainstay of real-time graphics for decades. Bevy currently has no
support for them, so this pull request implements them.
## Solution
The new `Lightmap` component can be attached to any entity that contains
a `Handle<Mesh>` and a `StandardMaterial`. When present, it will be
applied in the PBR shader. Because multiple lightmaps are frequently
packed into atlases, each lightmap may have its own UV boundaries within
its texture. An `exposure` field is also provided, to control the
brightness of the lightmap.
Note that this PR doesn't provide any way to bake the lightmaps. That
can be done with [The Lightmapper] or another solution, such as Unity's
Bakery.
---
## Changelog
### Added
* A new component, `Lightmap`, is available, for baked global
illumination. If your mesh has a second UV channel (UV1), and you attach
this component to the entity with that mesh, Bevy will apply the texture
referenced in the lightmap.
[The Lightmapper]: https://github.com/Naxela/The_Lightmapper
---------
Co-authored-by: Carter Anderson <mcanders1@gmail.com>
2024-01-02 20:38:47 +00:00
|
|
|
render_lightmaps: Res<RenderLightmaps>,
|
Implement visibility ranges, also known as hierarchical levels of detail (HLODs). (#12916)
Implement visibility ranges, also known as hierarchical levels of detail
(HLODs).
This commit introduces a new component, `VisibilityRange`, which allows
developers to specify camera distances in which meshes are to be shown
and hidden. Hiding meshes happens early in the rendering pipeline, so
this feature can be used for level of detail optimization. Additionally,
this feature is properly evaluated per-view, so different views can show
different levels of detail.
This feature differs from proper mesh LODs, which can be implemented
later. Engines generally implement true mesh LODs later in the pipeline;
they're typically more efficient than HLODs with GPU-driven rendering.
However, mesh LODs are more limited than HLODs, because they require the
lower levels of detail to be meshes with the same vertex layout and
shader (and perhaps the same material) as the original mesh. Games often
want to use objects other than meshes to replace distant models, such as
*octahedral imposters* or *billboard imposters*.
The reason why the feature is called *hierarchical level of detail* is
that HLODs can replace multiple meshes with a single mesh when the
camera is far away. This can be useful for reducing drawcall count. Note
that `VisibilityRange` doesn't automatically propagate down to children;
it must be placed on every mesh.
Crossfading between different levels of detail is supported, using the
standard 4x4 ordered dithering pattern from [1]. The shader code to
compute the dithering patterns should be well-optimized. The dithering
code is only active when visibility ranges are in use for the mesh in
question, so that we don't lose early Z.
Cascaded shadow maps show the HLOD level of the view they're associated
with. Point light and spot light shadow maps, which have no CSMs,
display all HLOD levels that are visible in any view. To support this
efficiently and avoid doing visibility checks multiple times, we
precalculate all visible HLOD levels for each entity with a
`VisibilityRange` during the `check_visibility_range` system.
A new example, `visibility_range`, has been added to the tree, as well
as a new low-poly version of the flight helmet model to go with it. It
demonstrates use of the visibility range feature to provide levels of
detail.
[1]: https://en.wikipedia.org/wiki/Ordered_dithering#Threshold_map
[^1]: Unreal doesn't have a feature that exactly corresponds to
visibility ranges, but Unreal's HLOD system serves roughly the same
purpose.
## Changelog
### Added
* A new `VisibilityRange` component is available to conditionally enable
entity visibility at camera distances, with optional crossfade support.
This can be used to implement different levels of detail (LODs).
## Screenshots
High-poly model:

Low-poly model up close:

Crossfading between the two:

---------
Co-authored-by: Carter Anderson <mcanders1@gmail.com>
2024-05-03 00:11:35 +00:00
|
|
|
render_visibility_ranges: Res<RenderVisibilityRanges>,
|
2024-05-21 18:23:04 +00:00
|
|
|
mut opaque_render_phases: ResMut<ViewBinnedRenderPhases<Opaque3d>>,
|
|
|
|
mut alpha_mask_render_phases: ResMut<ViewBinnedRenderPhases<AlphaMask3d>>,
|
|
|
|
mut transmissive_render_phases: ResMut<ViewSortedRenderPhases<Transmissive3d>>,
|
|
|
|
mut transparent_render_phases: ResMut<ViewSortedRenderPhases<Transparent3d>>,
|
2024-08-28 11:38:38 +00:00
|
|
|
views: Query<(
|
2024-05-21 18:23:04 +00:00
|
|
|
Entity,
|
2021-12-25 21:45:43 +00:00
|
|
|
&ExtractedView,
|
Type safe retained render world (#15756)
# Objective
In the Render World, there are a number of collections that are derived
from Main World entities and are used to drive rendering. The most
notable are:
- `VisibleEntities`, which is generated in the `check_visibility` system
and contains visible entities for a view.
- `ExtractedInstances`, which maps entity ids to asset ids.
In the old model, these collections were trivially kept in sync -- any
extracted phase item could look itself up because the render entity id
was guaranteed to always match the corresponding main world id.
After #15320, this became much more complicated, and was leading to a
number of subtle bugs in the Render World. The main rendering systems,
i.e. `queue_material_meshes` and `queue_material2d_meshes`, follow a
similar pattern:
```rust
for visible_entity in visible_entities.iter::<With<Mesh2d>>() {
let Some(mesh_instance) = render_mesh_instances.get_mut(visible_entity) else {
continue;
};
// Look some more stuff up and specialize the pipeline...
let bin_key = Opaque2dBinKey {
pipeline: pipeline_id,
draw_function: draw_opaque_2d,
asset_id: mesh_instance.mesh_asset_id.into(),
material_bind_group_id: material_2d.get_bind_group_id().0,
};
opaque_phase.add(
bin_key,
*visible_entity,
BinnedRenderPhaseType::mesh(mesh_instance.automatic_batching),
);
}
```
In this case, `visible_entities` and `render_mesh_instances` are both
collections that are created and keyed by Main World entity ids, and so
this lookup happens to work by coincidence. However, there is a major
unintentional bug here: namely, because `visible_entities` is a
collection of Main World ids, the phase item being queued is created
with a Main World id rather than its correct Render World id.
This happens to not break mesh rendering because the render commands
used for drawing meshes do not access the `ItemQuery` parameter, but
demonstrates the confusion that is now possible: our UI phase items are
correctly being queued with Render World ids while our meshes aren't.
Additionally, this makes it very easy and error prone to use the wrong
entity id to look up things like assets. For example, if instead we
ignored visibility checks and queued our meshes via a query, we'd have
to be extra careful to use `&MainEntity` instead of the natural
`Entity`.
## Solution
Make all collections that are derived from Main World data use
`MainEntity` as their key, to ensure type safety and avoid accidentally
looking up data with the wrong entity id:
```rust
pub type MainEntityHashMap<V> = hashbrown::HashMap<MainEntity, V, EntityHash>;
```
Additionally, we make all `PhaseItem` be able to provide both their Main
and Render World ids, to allow render phase implementors maximum
flexibility as to what id should be used to look up data.
You can think of this like tracking at the type level whether something
in the Render World should use it's "primary key", i.e. entity id, or
needs to use a foreign key, i.e. `MainEntity`.
## Testing
##### TODO:
This will require extensive testing to make sure things didn't break!
Additionally, some extraction logic has become more complicated and
needs to be checked for regressions.
## Migration Guide
With the advent of the retained render world, collections that contain
references to `Entity` that are extracted into the render world have
been changed to contain `MainEntity` in order to prevent errors where a
render world entity id is used to look up an item by accident. Custom
rendering code may need to be changed to query for `&MainEntity` in
order to look up the correct item from such a collection. Additionally,
users who implement their own extraction logic for collections of main
world entity should strongly consider extracting into a different
collection that uses `MainEntity` as a key.
Additionally, render phases now require specifying both the `Entity` and
`MainEntity` for a given `PhaseItem`. Custom render phases should ensure
`MainEntity` is available when queuing a phase item.
2024-10-10 18:47:04 +00:00
|
|
|
&RenderVisibleEntities,
|
2024-07-22 18:28:23 +00:00
|
|
|
&Msaa,
|
2022-10-26 20:13:59 +00:00
|
|
|
Option<&Tonemapping>,
|
2023-02-19 20:38:13 +00:00
|
|
|
Option<&DebandDither>,
|
2023-10-07 17:13:29 +00:00
|
|
|
Option<&ShadowFilteringMethod>,
|
Rename rendering components for improved consistency and clarity (#15035)
# Objective
The names of numerous rendering components in Bevy are inconsistent and
a bit confusing. Relevant names include:
- `AutoExposureSettings`
- `AutoExposureSettingsUniform`
- `BloomSettings`
- `BloomUniform` (no `Settings`)
- `BloomPrefilterSettings`
- `ChromaticAberration` (no `Settings`)
- `ContrastAdaptiveSharpeningSettings`
- `DepthOfFieldSettings`
- `DepthOfFieldUniform` (no `Settings`)
- `FogSettings`
- `SmaaSettings`, `Fxaa`, `TemporalAntiAliasSettings` (really
inconsistent??)
- `ScreenSpaceAmbientOcclusionSettings`
- `ScreenSpaceReflectionsSettings`
- `VolumetricFogSettings`
Firstly, there's a lot of inconsistency between `Foo`/`FooSettings` and
`FooUniform`/`FooSettingsUniform` and whether names are abbreviated or
not.
Secondly, the `Settings` post-fix seems unnecessary and a bit confusing
semantically, since it makes it seem like the component is mostly just
auxiliary configuration instead of the core *thing* that actually
enables the feature. This will be an even bigger problem once bundles
like `TemporalAntiAliasBundle` are deprecated in favor of required
components, as users will expect a component named `TemporalAntiAlias`
(or similar), not `TemporalAntiAliasSettings`.
## Solution
Drop the `Settings` post-fix from the component names, and change some
names to be more consistent.
- `AutoExposure`
- `AutoExposureUniform`
- `Bloom`
- `BloomUniform`
- `BloomPrefilter`
- `ChromaticAberration`
- `ContrastAdaptiveSharpening`
- `DepthOfField`
- `DepthOfFieldUniform`
- `DistanceFog`
- `Smaa`, `Fxaa`, `TemporalAntiAliasing` (note: we might want to change
to `Taa`, see "Discussion")
- `ScreenSpaceAmbientOcclusion`
- `ScreenSpaceReflections`
- `VolumetricFog`
I kept the old names as deprecated type aliases to make migration a bit
less painful for users. We should remove them after the next release.
(And let me know if I should just... not add them at all)
I also added some very basic docs for a few types where they were
missing, like on `Fxaa` and `DepthOfField`.
## Discussion
- `TemporalAntiAliasing` is still inconsistent with `Smaa` and `Fxaa`.
Consensus [on
Discord](https://discord.com/channels/691052431525675048/743663924229963868/1280601167209955431)
seemed to be that renaming to `Taa` would probably be fine, but I think
it's a bit more controversial, and it would've required renaming a lot
of related types like `TemporalAntiAliasNode`,
`TemporalAntiAliasBundle`, and `TemporalAntiAliasPlugin`, so I think
it's better to leave to a follow-up.
- I think `Fog` should probably have a more specific name like
`DistanceFog` considering it seems to be distinct from `VolumetricFog`.
~~This should probably be done in a follow-up though, so I just removed
the `Settings` post-fix for now.~~ (done)
---
## Migration Guide
Many rendering components have been renamed for improved consistency and
clarity.
- `AutoExposureSettings` → `AutoExposure`
- `BloomSettings` → `Bloom`
- `BloomPrefilterSettings` → `BloomPrefilter`
- `ContrastAdaptiveSharpeningSettings` → `ContrastAdaptiveSharpening`
- `DepthOfFieldSettings` → `DepthOfField`
- `FogSettings` → `DistanceFog`
- `SmaaSettings` → `Smaa`
- `TemporalAntiAliasSettings` → `TemporalAntiAliasing`
- `ScreenSpaceAmbientOcclusionSettings` → `ScreenSpaceAmbientOcclusion`
- `ScreenSpaceReflectionsSettings` → `ScreenSpaceReflections`
- `VolumetricFogSettings` → `VolumetricFog`
---------
Co-authored-by: Carter Anderson <mcanders1@gmail.com>
2024-09-10 01:11:46 +00:00
|
|
|
Has<ScreenSpaceAmbientOcclusion>,
|
`*_PREPASS` Shader Def Cleanup (#10136)
# Objective
- This PR aims to make the various `*_PREPASS` shader defs we have
(`NORMAL_PREPASS`, `DEPTH_PREPASS`, `MOTION_VECTORS_PREPASS` AND
`DEFERRED_PREPASS`) easier to use and understand:
- So that their meaning is now consistent across all contexts; (“prepass
X is enabled for the current view”)
- So that they're also consistently set across all contexts.
- It also aims to enable us to (with a follow up PR) to conditionally
gate the `BindGroupEntry` and `BindGroupLayoutEntry` items associated
with these prepasses, saving us up to 4 texture slots in WebGL
(currently globally limited to 16 per shader, regardless of bind groups)
## Solution
- We now consistently set these from `PrepassPipeline`, the
`MeshPipeline` and the `DeferredLightingPipeline`, we also set their
`MeshPipelineKey`s;
- We introduce `PREPASS_PIPELINE`, `MESH_PIPELINE` and
`DEFERRED_LIGHTING_PIPELINE` that can be used to detect where the code
is running, without overloading the meanings of the prepass shader defs;
- We also gate the WGSL functions in `bevy_pbr::prepass_utils` with
`#ifdef`s for their respective shader defs, so that shader code can
provide a fallback whenever they're not available.
- This allows us to conditionally include the bindings for these prepass
textures (My next PR, which will hopefully unblock #8015)
- @robtfm mentioned [these were being used to prevent accessing the same
binding as read/write in the
prepass](https://discord.com/channels/691052431525675048/743663924229963868/1163270458393759814),
however even after reversing the `#ifndef`s I had no issues running the
code, so perhaps the compiler is already smart enough even without tree
shaking to know they're not being used, thanks to `#ifdef
PREPASS_PIPELINE`?
## Comparison
### Before
| Shader Def | `PrepassPipeline` | `MeshPipeline` |
`DeferredLightingPipeline` |
| ------------------------ | ----------------- | -------------- |
-------------------------- |
| `NORMAL_PREPASS` | Yes | No | No |
| `DEPTH_PREPASS` | Yes | No | No |
| `MOTION_VECTORS_PREPASS` | Yes | No | No |
| `DEFERRED_PREPASS` | Yes | No | No |
| View Key | `PrepassPipeline` | `MeshPipeline` |
`DeferredLightingPipeline` |
| ------------------------ | ----------------- | -------------- |
-------------------------- |
| `NORMAL_PREPASS` | Yes | Yes | No |
| `DEPTH_PREPASS` | Yes | No | No |
| `MOTION_VECTORS_PREPASS` | Yes | No | No |
| `DEFERRED_PREPASS` | Yes | Yes\* | No |
\* Accidentally was being set twice, once with only
`deferred_prepass.is_some()` as a condition,
and once with `deferred_p repass.is_some() && !forward` as a condition.
### After
| Shader Def | `PrepassPipeline` | `MeshPipeline` |
`DeferredLightingPipeline` |
| ---------------------------- | ----------------- | --------------- |
-------------------------- |
| `NORMAL_PREPASS` | Yes | Yes | Yes |
| `DEPTH_PREPASS` | Yes | Yes | Yes |
| `MOTION_VECTORS_PREPASS` | Yes | Yes | Yes |
| `DEFERRED_PREPASS` | Yes | Yes | Unconditionally |
| `PREPASS_PIPELINE` | Unconditionally | No | No |
| `MESH_PIPELINE` | No | Unconditionally | No |
| `DEFERRED_LIGHTING_PIPELINE` | No | No | Unconditionally |
| View Key | `PrepassPipeline` | `MeshPipeline` |
`DeferredLightingPipeline` |
| ------------------------ | ----------------- | -------------- |
-------------------------- |
| `NORMAL_PREPASS` | Yes | Yes | Yes |
| `DEPTH_PREPASS` | Yes | Yes | Yes |
| `MOTION_VECTORS_PREPASS` | Yes | Yes | Yes |
| `DEFERRED_PREPASS` | Yes | Yes | Unconditionally |
---
## Changelog
- Cleaned up WGSL `*_PREPASS` shader defs so they're now consistently
used everywhere;
- Introduced `PREPASS_PIPELINE`, `MESH_PIPELINE` and
`DEFERRED_LIGHTING_PIPELINE` WGSL shader defs for conditionally
compiling logic based the current pipeline;
- WGSL functions from `bevy_pbr::prepass_utils` are now guarded with
`#ifdef` based on the currently enabled prepasses;
## Migration Guide
- When using functions from `bevy_pbr::prepass_utils`
(`prepass_depth()`, `prepass_normal()`, `prepass_motion_vector()`) in
contexts where these prepasses might be disabled, you should now wrap
your calls with the appropriate `#ifdef` guards, (`#ifdef
DEPTH_PREPASS`, `#ifdef NORMAL_PREPASS`, `#ifdef MOTION_VECTOR_PREPASS`)
providing fallback logic where applicable.
---------
Co-authored-by: Carter Anderson <mcanders1@gmail.com>
Co-authored-by: IceSentry <IceSentry@users.noreply.github.com>
2023-10-17 00:16:21 +00:00
|
|
|
(
|
|
|
|
Has<NormalPrepass>,
|
|
|
|
Has<DepthPrepass>,
|
|
|
|
Has<MotionVectorPrepass>,
|
|
|
|
Has<DeferredPrepass>,
|
|
|
|
),
|
`StandardMaterial` Light Transmission (#8015)
# Objective
<img width="1920" alt="Screenshot 2023-04-26 at 01 07 34"
src="https://user-images.githubusercontent.com/418473/234467578-0f34187b-5863-4ea1-88e9-7a6bb8ce8da3.png">
This PR adds both diffuse and specular light transmission capabilities
to the `StandardMaterial`, with support for screen space refractions.
This enables realistically representing a wide range of real-world
materials, such as:
- Glass; (Including frosted glass)
- Transparent and translucent plastics;
- Various liquids and gels;
- Gemstones;
- Marble;
- Wax;
- Paper;
- Leaves;
- Porcelain.
Unlike existing support for transparency, light transmission does not
rely on fixed function alpha blending, and therefore works with both
`AlphaMode::Opaque` and `AlphaMode::Mask` materials.
## Solution
- Introduces a number of transmission related fields in the
`StandardMaterial`;
- For specular transmission:
- Adds logic to take a view main texture snapshot after the opaque
phase; (in order to perform screen space refractions)
- Introduces a new `Transmissive3d` phase to the renderer, to which all
meshes with `transmission > 0.0` materials are sent.
- Calculates a light exit point (of the approximate mesh volume) using
`ior` and `thickness` properties
- Samples the snapshot texture with an adaptive number of taps across a
`roughness`-controlled radius enabling “blurry” refractions
- For diffuse transmission:
- Approximates transmitted diffuse light by using a second, flipped +
displaced, diffuse-only Lambertian lobe for each light source.
## To Do
- [x] Figure out where `fresnel_mix()` is taking place, if at all, and
where `dielectric_specular` is being calculated, if at all, and update
them to use the `ior` value (Not a blocker, just a nice-to-have for more
correct BSDF)
- To the _best of my knowledge, this is now taking place, after
964340cdd. The fresnel mix is actually "split" into two parts in our
implementation, one `(1 - fresnel(...))` in the transmission, and
`fresnel()` in the light implementations. A surface with more
reflectance now will produce slightly dimmer transmission towards the
grazing angle, as more of the light gets reflected.
- [x] Add `transmission_texture`
- [x] Add `diffuse_transmission_texture`
- [x] Add `thickness_texture`
- [x] Add `attenuation_distance` and `attenuation_color`
- [x] Connect values to glTF loader
- [x] `transmission` and `transmission_texture`
- [x] `thickness` and `thickness_texture`
- [x] `ior`
- [ ] `diffuse_transmission` and `diffuse_transmission_texture` (needs
upstream support in `gltf` crate, not a blocker)
- [x] Add support for multiple screen space refraction “steps”
- [x] Conditionally create no transmission snapshot texture at all if
`steps == 0`
- [x] Conditionally enable/disable screen space refraction transmission
snapshots
- [x] Read from depth pre-pass to prevent refracting pixels in front of
the light exit point
- [x] Use `interleaved_gradient_noise()` function for sampling blur in a
way that benefits from TAA
- [x] Drill down a TAA `#define`, tweak some aspects of the effect
conditionally based on it
- [x] Remove const array that's crashing under HLSL (unless a new `naga`
release with https://github.com/gfx-rs/naga/pull/2496 comes out before
we merge this)
- [ ] Look into alternatives to the `switch` hack for dynamically
indexing the const array (might not be needed, compilers seem to be
decent at expanding it)
- [ ] Add pipeline keys for gating transmission (do we really want/need
this?)
- [x] Tweak some material field/function names?
## A Note on Texture Packing
_This was originally added as a comment to the
`specular_transmission_texture`, `thickness_texture` and
`diffuse_transmission_texture` documentation, I removed it since it was
more confusing than helpful, and will likely be made redundant/will need
to be updated once we have a better infrastructure for preprocessing
assets_
Due to how channels are mapped, you can more efficiently use a single
shared texture image
for configuring the following:
- R - `specular_transmission_texture`
- G - `thickness_texture`
- B - _unused_
- A - `diffuse_transmission_texture`
The `KHR_materials_diffuse_transmission` glTF extension also defines a
`diffuseTransmissionColorTexture`,
that _we don't currently support_. One might choose to pack the
intensity and color textures together,
using RGB for the color and A for the intensity, in which case this
packing advice doesn't really apply.
---
## Changelog
- Added a new `Transmissive3d` render phase for rendering specular
transmissive materials with screen space refractions
- Added rendering support for transmitted environment map light on the
`StandardMaterial` as a fallback for screen space refractions
- Added `diffuse_transmission`, `specular_transmission`, `thickness`,
`ior`, `attenuation_distance` and `attenuation_color` to the
`StandardMaterial`
- Added `diffuse_transmission_texture`, `specular_transmission_texture`,
`thickness_texture` to the `StandardMaterial`, gated behind a new
`pbr_transmission_textures` cargo feature (off by default, for maximum
hardware compatibility)
- Added `Camera3d::screen_space_specular_transmission_steps` for
controlling the number of “layers of transparency” rendered for
transmissive objects
- Added a `TransmittedShadowReceiver` component for enabling shadows in
(diffusely) transmitted light. (disabled by default, as it requires
carefully setting up the `thickness` to avoid self-shadow artifacts)
- Added support for the `KHR_materials_transmission`,
`KHR_materials_ior` and `KHR_materials_volume` glTF extensions
- Renamed items related to temporal jitter for greater consistency
## Migration Guide
- `SsaoPipelineKey::temporal_noise` has been renamed to
`SsaoPipelineKey::temporal_jitter`
- The `TAA` shader def (controlled by the presence of the
`TemporalAntiAliasSettings` component in the camera) has been replaced
with the `TEMPORAL_JITTER` shader def (controlled by the presence of the
`TemporalJitter` component in the camera)
- `MeshPipelineKey::TAA` has been replaced by
`MeshPipelineKey::TEMPORAL_JITTER`
- The `TEMPORAL_NOISE` shader def has been consolidated with
`TEMPORAL_JITTER`
2023-10-31 20:59:02 +00:00
|
|
|
Option<&Camera3d>,
|
2023-12-30 23:27:48 +00:00
|
|
|
Has<TemporalJitter>,
|
2023-10-24 21:26:19 +00:00
|
|
|
Option<&Projection>,
|
Implement irradiance volumes. (#10268)
# Objective
Bevy could benefit from *irradiance volumes*, also known as *voxel
global illumination* or simply as light probes (though this term is not
preferred, as multiple techniques can be called light probes).
Irradiance volumes are a form of baked global illumination; they work by
sampling the light at the centers of each voxel within a cuboid. At
runtime, the voxels surrounding the fragment center are sampled and
interpolated to produce indirect diffuse illumination.
## Solution
This is divided into two sections. The first is copied and pasted from
the irradiance volume module documentation and describes the technique.
The second part consists of notes on the implementation.
### Overview
An *irradiance volume* is a cuboid voxel region consisting of
regularly-spaced precomputed samples of diffuse indirect light. They're
ideal if you have a dynamic object such as a character that can move
about
static non-moving geometry such as a level in a game, and you want that
dynamic object to be affected by the light bouncing off that static
geometry.
To use irradiance volumes, you need to precompute, or *bake*, the
indirect
light in your scene. Bevy doesn't currently come with a way to do this.
Fortunately, [Blender] provides a [baking tool] as part of the Eevee
renderer, and its irradiance volumes are compatible with those used by
Bevy.
The [`bevy-baked-gi`] project provides a tool, `export-blender-gi`, that
can
extract the baked irradiance volumes from the Blender `.blend` file and
package them up into a `.ktx2` texture for use by the engine. See the
documentation in the `bevy-baked-gi` project for more details as to this
workflow.
Like all light probes in Bevy, irradiance volumes are 1×1×1 cubes that
can
be arbitrarily scaled, rotated, and positioned in a scene with the
[`bevy_transform::components::Transform`] component. The 3D voxel grid
will
be stretched to fill the interior of the cube, and the illumination from
the
irradiance volume will apply to all fragments within that bounding
region.
Bevy's irradiance volumes are based on Valve's [*ambient cubes*] as used
in
*Half-Life 2* ([Mitchell 2006], slide 27). These encode a single color
of
light from the six 3D cardinal directions and blend the sides together
according to the surface normal.
The primary reason for choosing ambient cubes is to match Blender, so
that
its Eevee renderer can be used for baking. However, they also have some
advantages over the common second-order spherical harmonics approach:
ambient cubes don't suffer from ringing artifacts, they are smaller (6
colors for ambient cubes as opposed to 9 for spherical harmonics), and
evaluation is faster. A smaller basis allows for a denser grid of voxels
with the same storage requirements.
If you wish to use a tool other than `export-blender-gi` to produce the
irradiance volumes, you'll need to pack the irradiance volumes in the
following format. The irradiance volume of resolution *(Rx, Ry, Rz)* is
expected to be a 3D texture of dimensions *(Rx, 2Ry, 3Rz)*. The
unnormalized
texture coordinate *(s, t, p)* of the voxel at coordinate *(x, y, z)*
with
side *S* ∈ *{-X, +X, -Y, +Y, -Z, +Z}* is as follows:
```text
s = x
t = y + ⎰ 0 if S ∈ {-X, -Y, -Z}
⎱ Ry if S ∈ {+X, +Y, +Z}
⎧ 0 if S ∈ {-X, +X}
p = z + ⎨ Rz if S ∈ {-Y, +Y}
⎩ 2Rz if S ∈ {-Z, +Z}
```
Visually, in a left-handed coordinate system with Y up, viewed from the
right, the 3D texture looks like a stacked series of voxel grids, one
for
each cube side, in this order:
| **+X** | **+Y** | **+Z** |
| ------ | ------ | ------ |
| **-X** | **-Y** | **-Z** |
A terminology note: Other engines may refer to irradiance volumes as
*voxel
global illumination*, *VXGI*, or simply as *light probes*. Sometimes
*light
probe* refers to what Bevy calls a reflection probe. In Bevy, *light
probe*
is a generic term that encompasses all cuboid bounding regions that
capture
indirect illumination, whether based on voxels or not.
Note that, if binding arrays aren't supported (e.g. on WebGPU or WebGL
2),
then only the closest irradiance volume to the view will be taken into
account during rendering.
[*ambient cubes*]:
https://advances.realtimerendering.com/s2006/Mitchell-ShadingInValvesSourceEngine.pdf
[Mitchell 2006]:
https://advances.realtimerendering.com/s2006/Mitchell-ShadingInValvesSourceEngine.pdf
[Blender]: http://blender.org/
[baking tool]:
https://docs.blender.org/manual/en/latest/render/eevee/render_settings/indirect_lighting.html
[`bevy-baked-gi`]: https://github.com/pcwalton/bevy-baked-gi
### Implementation notes
This patch generalizes light probes so as to reuse as much code as
possible between irradiance volumes and the existing reflection probes.
This approach was chosen because both techniques share numerous
similarities:
1. Both irradiance volumes and reflection probes are cuboid bounding
regions.
2. Both are responsible for providing baked indirect light.
3. Both techniques involve presenting a variable number of textures to
the shader from which indirect light is sampled. (In the current
implementation, this uses binding arrays.)
4. Both irradiance volumes and reflection probes require gathering and
sorting probes by distance on CPU.
5. Both techniques require the GPU to search through a list of bounding
regions.
6. Both will eventually want to have falloff so that we can smoothly
blend as objects enter and exit the probes' influence ranges. (This is
not implemented yet to keep this patch relatively small and reviewable.)
To do this, we generalize most of the methods in the reflection probes
patch #11366 to be generic over a trait, `LightProbeComponent`. This
trait is implemented by both `EnvironmentMapLight` (for reflection
probes) and `IrradianceVolume` (for irradiance volumes). Using a trait
will allow us to add more types of light probes in the future. In
particular, I highly suspect we will want real-time reflection planes
for mirrors in the future, which can be easily slotted into this
framework.
## Changelog
> This section is optional. If this was a trivial fix, or has no
externally-visible impact, you can delete this section.
### Added
* A new `IrradianceVolume` asset type is available for baked voxelized
light probes. You can bake the global illumination using Blender or
another tool of your choice and use it in Bevy to apply indirect
illumination to dynamic objects.
2024-02-06 23:23:20 +00:00
|
|
|
(
|
|
|
|
Has<RenderViewLightProbes<EnvironmentMapLight>>,
|
|
|
|
Has<RenderViewLightProbes<IrradianceVolume>>,
|
|
|
|
),
|
2024-10-07 23:50:28 +00:00
|
|
|
Has<OrderIndependentTransparencySettings>,
|
2021-12-25 21:45:43 +00:00
|
|
|
)>,
|
Better Materials: AsBindGroup trait and derive, simpler Material trait (#5053)
# Objective
This PR reworks Bevy's Material system, making the user experience of defining Materials _much_ nicer. Bevy's previous material system leaves a lot to be desired:
* Materials require manually implementing the `RenderAsset` trait, which involves manually generating the bind group, handling gpu buffer data transfer, looking up image textures, etc. Even the simplest single-texture material involves writing ~80 unnecessary lines of code. This was never the long term plan.
* There are two material traits, which is confusing, hard to document, and often redundant: `Material` and `SpecializedMaterial`. `Material` implicitly implements `SpecializedMaterial`, and `SpecializedMaterial` is used in most high level apis to support both use cases. Most users shouldn't need to think about specialization at all (I consider it a "power-user tool"), so the fact that `SpecializedMaterial` is front-and-center in our apis is a miss.
* Implementing either material trait involves a lot of "type soup". The "prepared asset" parameter is particularly heinous: `&<Self as RenderAsset>::PreparedAsset`. Defining vertex and fragment shaders is also more verbose than it needs to be.
## Solution
Say hello to the new `Material` system:
```rust
#[derive(AsBindGroup, TypeUuid, Debug, Clone)]
#[uuid = "f690fdae-d598-45ab-8225-97e2a3f056e0"]
pub struct CoolMaterial {
#[uniform(0)]
color: Color,
#[texture(1)]
#[sampler(2)]
color_texture: Handle<Image>,
}
impl Material for CoolMaterial {
fn fragment_shader() -> ShaderRef {
"cool_material.wgsl".into()
}
}
```
Thats it! This same material would have required [~80 lines of complicated "type heavy" code](https://github.com/bevyengine/bevy/blob/v0.7.0/examples/shader/shader_material.rs) in the old Material system. Now it is just 14 lines of simple, readable code.
This is thanks to a new consolidated `Material` trait and the new `AsBindGroup` trait / derive.
### The new `Material` trait
The old "split" `Material` and `SpecializedMaterial` traits have been removed in favor of a new consolidated `Material` trait. All of the functions on the trait are optional.
The difficulty of implementing `Material` has been reduced by simplifying dataflow and removing type complexity:
```rust
// Old
impl Material for CustomMaterial {
fn fragment_shader(asset_server: &AssetServer) -> Option<Handle<Shader>> {
Some(asset_server.load("custom_material.wgsl"))
}
fn alpha_mode(render_asset: &<Self as RenderAsset>::PreparedAsset) -> AlphaMode {
render_asset.alpha_mode
}
}
// New
impl Material for CustomMaterial {
fn fragment_shader() -> ShaderRef {
"custom_material.wgsl".into()
}
fn alpha_mode(&self) -> AlphaMode {
self.alpha_mode
}
}
```
Specialization is still supported, but it is hidden by default under the `specialize()` function (more on this later).
### The `AsBindGroup` trait / derive
The `Material` trait now requires the `AsBindGroup` derive. This can be implemented manually relatively easily, but deriving it will almost always be preferable.
Field attributes like `uniform` and `texture` are used to define which fields should be bindings,
what their binding type is, and what index they should be bound at:
```rust
#[derive(AsBindGroup)]
struct CoolMaterial {
#[uniform(0)]
color: Color,
#[texture(1)]
#[sampler(2)]
color_texture: Handle<Image>,
}
```
In WGSL shaders, the binding looks like this:
```wgsl
struct CoolMaterial {
color: vec4<f32>;
};
[[group(1), binding(0)]]
var<uniform> material: CoolMaterial;
[[group(1), binding(1)]]
var color_texture: texture_2d<f32>;
[[group(1), binding(2)]]
var color_sampler: sampler;
```
Note that the "group" index is determined by the usage context. It is not defined in `AsBindGroup`. Bevy material bind groups are bound to group 1.
The following field-level attributes are supported:
* `uniform(BINDING_INDEX)`
* The field will be converted to a shader-compatible type using the `ShaderType` trait, written to a `Buffer`, and bound as a uniform. It can also be derived for custom structs.
* `texture(BINDING_INDEX)`
* This field's `Handle<Image>` will be used to look up the matching `Texture` gpu resource, which will be bound as a texture in shaders. The field will be assumed to implement `Into<Option<Handle<Image>>>`. In practice, most fields should be a `Handle<Image>` or `Option<Handle<Image>>`. If the value of an `Option<Handle<Image>>` is `None`, the new `FallbackImage` resource will be used instead. This attribute can be used in conjunction with a `sampler` binding attribute (with a different binding index).
* `sampler(BINDING_INDEX)`
* Behaves exactly like the `texture` attribute, but sets the Image's sampler binding instead of the texture.
Note that fields without field-level binding attributes will be ignored.
```rust
#[derive(AsBindGroup)]
struct CoolMaterial {
#[uniform(0)]
color: Color,
this_field_is_ignored: String,
}
```
As mentioned above, `Option<Handle<Image>>` is also supported:
```rust
#[derive(AsBindGroup)]
struct CoolMaterial {
#[uniform(0)]
color: Color,
#[texture(1)]
#[sampler(2)]
color_texture: Option<Handle<Image>>,
}
```
This is useful if you want a texture to be optional. When the value is `None`, the `FallbackImage` will be used for the binding instead, which defaults to "pure white".
Field uniforms with the same binding index will be combined into a single binding:
```rust
#[derive(AsBindGroup)]
struct CoolMaterial {
#[uniform(0)]
color: Color,
#[uniform(0)]
roughness: f32,
}
```
In WGSL shaders, the binding would look like this:
```wgsl
struct CoolMaterial {
color: vec4<f32>;
roughness: f32;
};
[[group(1), binding(0)]]
var<uniform> material: CoolMaterial;
```
Some less common scenarios will require "struct-level" attributes. These are the currently supported struct-level attributes:
* `uniform(BINDING_INDEX, ConvertedShaderType)`
* Similar to the field-level `uniform` attribute, but instead the entire `AsBindGroup` value is converted to `ConvertedShaderType`, which must implement `ShaderType`. This is useful if more complicated conversion logic is required.
* `bind_group_data(DataType)`
* The `AsBindGroup` type will be converted to some `DataType` using `Into<DataType>` and stored as `AsBindGroup::Data` as part of the `AsBindGroup::as_bind_group` call. This is useful if data needs to be stored alongside the generated bind group, such as a unique identifier for a material's bind group. The most common use case for this attribute is "shader pipeline specialization".
The previous `CoolMaterial` example illustrating "combining multiple field-level uniform attributes with the same binding index" can
also be equivalently represented with a single struct-level uniform attribute:
```rust
#[derive(AsBindGroup)]
#[uniform(0, CoolMaterialUniform)]
struct CoolMaterial {
color: Color,
roughness: f32,
}
#[derive(ShaderType)]
struct CoolMaterialUniform {
color: Color,
roughness: f32,
}
impl From<&CoolMaterial> for CoolMaterialUniform {
fn from(material: &CoolMaterial) -> CoolMaterialUniform {
CoolMaterialUniform {
color: material.color,
roughness: material.roughness,
}
}
}
```
### Material Specialization
Material shader specialization is now _much_ simpler:
```rust
#[derive(AsBindGroup, TypeUuid, Debug, Clone)]
#[uuid = "f690fdae-d598-45ab-8225-97e2a3f056e0"]
#[bind_group_data(CoolMaterialKey)]
struct CoolMaterial {
#[uniform(0)]
color: Color,
is_red: bool,
}
#[derive(Copy, Clone, Hash, Eq, PartialEq)]
struct CoolMaterialKey {
is_red: bool,
}
impl From<&CoolMaterial> for CoolMaterialKey {
fn from(material: &CoolMaterial) -> CoolMaterialKey {
CoolMaterialKey {
is_red: material.is_red,
}
}
}
impl Material for CoolMaterial {
fn fragment_shader() -> ShaderRef {
"cool_material.wgsl".into()
}
fn specialize(
pipeline: &MaterialPipeline<Self>,
descriptor: &mut RenderPipelineDescriptor,
layout: &MeshVertexBufferLayout,
key: MaterialPipelineKey<Self>,
) -> Result<(), SpecializedMeshPipelineError> {
if key.bind_group_data.is_red {
let fragment = descriptor.fragment.as_mut().unwrap();
fragment.shader_defs.push("IS_RED".to_string());
}
Ok(())
}
}
```
Setting `bind_group_data` is not required for specialization (it defaults to `()`). Scenarios like "custom vertex attributes" also benefit from this system:
```rust
impl Material for CustomMaterial {
fn vertex_shader() -> ShaderRef {
"custom_material.wgsl".into()
}
fn fragment_shader() -> ShaderRef {
"custom_material.wgsl".into()
}
fn specialize(
pipeline: &MaterialPipeline<Self>,
descriptor: &mut RenderPipelineDescriptor,
layout: &MeshVertexBufferLayout,
key: MaterialPipelineKey<Self>,
) -> Result<(), SpecializedMeshPipelineError> {
let vertex_layout = layout.get_layout(&[
Mesh::ATTRIBUTE_POSITION.at_shader_location(0),
ATTRIBUTE_BLEND_COLOR.at_shader_location(1),
])?;
descriptor.vertex.buffers = vec![vertex_layout];
Ok(())
}
}
```
### Ported `StandardMaterial` to the new `Material` system
Bevy's built-in PBR material uses the new Material system (including the AsBindGroup derive):
```rust
#[derive(AsBindGroup, Debug, Clone, TypeUuid)]
#[uuid = "7494888b-c082-457b-aacf-517228cc0c22"]
#[bind_group_data(StandardMaterialKey)]
#[uniform(0, StandardMaterialUniform)]
pub struct StandardMaterial {
pub base_color: Color,
#[texture(1)]
#[sampler(2)]
pub base_color_texture: Option<Handle<Image>>,
/* other fields omitted for brevity */
```
### Ported Bevy examples to the new `Material` system
The overall complexity of Bevy's "custom shader examples" has gone down significantly. Take a look at the diffs if you want a dopamine spike.
Please note that while this PR has a net increase in "lines of code", most of those extra lines come from added documentation. There is a significant reduction
in the overall complexity of the code (even accounting for the new derive logic).
---
## Changelog
### Added
* `AsBindGroup` trait and derive, which make it much easier to transfer data to the gpu and generate bind groups for a given type.
### Changed
* The old `Material` and `SpecializedMaterial` traits have been replaced by a consolidated (much simpler) `Material` trait. Materials no longer implement `RenderAsset`.
* `StandardMaterial` was ported to the new material system. There are no user-facing api changes to the `StandardMaterial` struct api, but it now implements `AsBindGroup` and `Material` instead of `RenderAsset` and `SpecializedMaterial`.
## Migration Guide
The Material system has been reworked to be much simpler. We've removed a lot of boilerplate with the new `AsBindGroup` derive and the `Material` trait is simpler as well!
### Bevy 0.7 (old)
```rust
#[derive(Debug, Clone, TypeUuid)]
#[uuid = "f690fdae-d598-45ab-8225-97e2a3f056e0"]
pub struct CustomMaterial {
color: Color,
color_texture: Handle<Image>,
}
#[derive(Clone)]
pub struct GpuCustomMaterial {
_buffer: Buffer,
bind_group: BindGroup,
}
impl RenderAsset for CustomMaterial {
type ExtractedAsset = CustomMaterial;
type PreparedAsset = GpuCustomMaterial;
type Param = (SRes<RenderDevice>, SRes<MaterialPipeline<Self>>);
fn extract_asset(&self) -> Self::ExtractedAsset {
self.clone()
}
fn prepare_asset(
extracted_asset: Self::ExtractedAsset,
(render_device, material_pipeline): &mut SystemParamItem<Self::Param>,
) -> Result<Self::PreparedAsset, PrepareAssetError<Self::ExtractedAsset>> {
let color = Vec4::from_slice(&extracted_asset.color.as_linear_rgba_f32());
let byte_buffer = [0u8; Vec4::SIZE.get() as usize];
let mut buffer = encase::UniformBuffer::new(byte_buffer);
buffer.write(&color).unwrap();
let buffer = render_device.create_buffer_with_data(&BufferInitDescriptor {
contents: buffer.as_ref(),
label: None,
usage: BufferUsages::UNIFORM | BufferUsages::COPY_DST,
});
let (texture_view, texture_sampler) = if let Some(result) = material_pipeline
.mesh_pipeline
.get_image_texture(gpu_images, &Some(extracted_asset.color_texture.clone()))
{
result
} else {
return Err(PrepareAssetError::RetryNextUpdate(extracted_asset));
};
let bind_group = render_device.create_bind_group(&BindGroupDescriptor {
entries: &[
BindGroupEntry {
binding: 0,
resource: buffer.as_entire_binding(),
},
BindGroupEntry {
binding: 0,
resource: BindingResource::TextureView(texture_view),
},
BindGroupEntry {
binding: 1,
resource: BindingResource::Sampler(texture_sampler),
},
],
label: None,
layout: &material_pipeline.material_layout,
});
Ok(GpuCustomMaterial {
_buffer: buffer,
bind_group,
})
}
}
impl Material for CustomMaterial {
fn fragment_shader(asset_server: &AssetServer) -> Option<Handle<Shader>> {
Some(asset_server.load("custom_material.wgsl"))
}
fn bind_group(render_asset: &<Self as RenderAsset>::PreparedAsset) -> &BindGroup {
&render_asset.bind_group
}
fn bind_group_layout(render_device: &RenderDevice) -> BindGroupLayout {
render_device.create_bind_group_layout(&BindGroupLayoutDescriptor {
entries: &[
BindGroupLayoutEntry {
binding: 0,
visibility: ShaderStages::FRAGMENT,
ty: BindingType::Buffer {
ty: BufferBindingType::Uniform,
has_dynamic_offset: false,
min_binding_size: Some(Vec4::min_size()),
},
count: None,
},
BindGroupLayoutEntry {
binding: 1,
visibility: ShaderStages::FRAGMENT,
ty: BindingType::Texture {
multisampled: false,
sample_type: TextureSampleType::Float { filterable: true },
view_dimension: TextureViewDimension::D2Array,
},
count: None,
},
BindGroupLayoutEntry {
binding: 2,
visibility: ShaderStages::FRAGMENT,
ty: BindingType::Sampler(SamplerBindingType::Filtering),
count: None,
},
],
label: None,
})
}
}
```
### Bevy 0.8 (new)
```rust
impl Material for CustomMaterial {
fn fragment_shader() -> ShaderRef {
"custom_material.wgsl".into()
}
}
#[derive(AsBindGroup, TypeUuid, Debug, Clone)]
#[uuid = "f690fdae-d598-45ab-8225-97e2a3f056e0"]
pub struct CustomMaterial {
#[uniform(0)]
color: Color,
#[texture(1)]
#[sampler(2)]
color_texture: Handle<Image>,
}
```
## Future Work
* Add support for more binding types (cubemaps, buffers, etc). This PR intentionally includes a bare minimum number of binding types to keep "reviewability" in check.
* Consider optionally eliding binding indices using binding names. `AsBindGroup` could pass in (optional?) reflection info as a "hint".
* This would make it possible for the derive to do this:
```rust
#[derive(AsBindGroup)]
pub struct CustomMaterial {
#[uniform]
color: Color,
#[texture]
#[sampler]
color_texture: Option<Handle<Image>>,
alpha_mode: AlphaMode,
}
```
* Or this
```rust
#[derive(AsBindGroup)]
pub struct CustomMaterial {
#[binding]
color: Color,
#[binding]
color_texture: Option<Handle<Image>>,
alpha_mode: AlphaMode,
}
```
* Or even this (if we flip to "include bindings by default")
```rust
#[derive(AsBindGroup)]
pub struct CustomMaterial {
color: Color,
color_texture: Option<Handle<Image>>,
#[binding(ignore)]
alpha_mode: AlphaMode,
}
```
* If we add the option to define custom draw functions for materials (which could be done in a type-erased way), I think that would be enough to support extra non-material bindings. Worth considering!
2022-06-30 23:48:46 +00:00
|
|
|
) where
|
|
|
|
M::Data: PartialEq + Eq + Hash + Clone,
|
|
|
|
{
|
2022-10-26 20:13:59 +00:00
|
|
|
for (
|
2024-05-21 18:23:04 +00:00
|
|
|
view_entity,
|
2022-10-26 20:13:59 +00:00
|
|
|
view,
|
|
|
|
visible_entities,
|
2024-07-22 18:28:23 +00:00
|
|
|
msaa,
|
2022-10-26 20:13:59 +00:00
|
|
|
tonemapping,
|
2023-02-19 20:38:13 +00:00
|
|
|
dither,
|
2023-10-07 17:13:29 +00:00
|
|
|
shadow_filter_method,
|
Screen Space Ambient Occlusion (SSAO) MVP (#7402)

# Objective
- Add Screen space ambient occlusion (SSAO). SSAO approximates
small-scale, local occlusion of _indirect_ diffuse light between
objects. SSAO does not apply to direct lighting, such as point or
directional lights.
- This darkens creases, e.g. on staircases, and gives nice contact
shadows where objects meet, giving entities a more "grounded" feel.
- Closes https://github.com/bevyengine/bevy/issues/3632.
## Solution
- Implement the GTAO algorithm.
-
https://www.activision.com/cdn/research/Practical_Real_Time_Strategies_for_Accurate_Indirect_Occlusion_NEW%20VERSION_COLOR.pdf
-
https://blog.selfshadow.com/publications/s2016-shading-course/activision/s2016_pbs_activision_occlusion.pdf
- Source code heavily based on [Intel's
XeGTAO](https://github.com/GameTechDev/XeGTAO/blob/0d177ce06bfa642f64d8af4de1197ad1bcb862d4/Source/Rendering/Shaders/XeGTAO.hlsli).
- Add an SSAO bevy example.
## Algorithm Overview
* Run a depth and normal prepass
* Create downscaled mips of the depth texture (preprocess_depths pass)
* GTAO pass - for each pixel, take several random samples from the
depth+normal buffers, reconstruct world position, raytrace in screen
space to estimate occlusion. Rather then doing completely random samples
on a hemisphere, you choose random _slices_ of the hemisphere, and then
can analytically compute the full occlusion of that slice. Also compute
edges based on depth differences here.
* Spatial denoise pass - bilateral blur, using edge detection to not
blur over edges. This is the final SSAO result.
* Main pass - if SSAO exists, sample the SSAO texture, and set occlusion
to be the minimum of ssao/material occlusion. This then feeds into the
rest of the PBR shader as normal.
---
## Future Improvements
- Maybe remove the low quality preset for now (too noisy)
- WebGPU fallback (see below)
- Faster depth->world position (see reverted code)
- Bent normals
- Try interleaved gradient noise or spatiotemporal blue noise
- Replace the spatial denoiser with a combined spatial+temporal denoiser
- Render at half resolution and use a bilateral upsample
- Better multibounce approximation
(https://drive.google.com/file/d/1SyagcEVplIm2KkRD3WQYSO9O0Iyi1hfy/view)
## Far-Future Performance Improvements
- F16 math (missing naga-wgsl support
https://github.com/gfx-rs/naga/issues/1884)
- Faster coordinate space conversion for normals
- Faster depth mipchain creation
(https://github.com/GPUOpen-Effects/FidelityFX-SPD) (wgpu/naga does not
currently support subgroup ops)
- Deinterleaved SSAO for better cache efficiency
(https://developer.nvidia.com/sites/default/files/akamai/gameworks/samples/DeinterleavedTexturing.pdf)
## Other Interesting Papers
- Visibility bitmask
(https://link.springer.com/article/10.1007/s00371-022-02703-y,
https://cdrinmatane.github.io/posts/cgspotlight-slides/)
- Screen space diffuse lighting
(https://github.com/Patapom/GodComplex/blob/master/Tests/TestHBIL/2018%20Mayaux%20-%20Horizon-Based%20Indirect%20Lighting%20(HBIL).pdf)
## Platform Support
* SSAO currently does not work on DirectX12 due to issues with wgpu and
naga:
* https://github.com/gfx-rs/wgpu/pull/3798
* https://github.com/gfx-rs/naga/pull/2353
* SSAO currently does not work on WebGPU because r16float is not a valid
storage texture format
https://gpuweb.github.io/gpuweb/wgsl/#storage-texel-formats. We can fix
this with a fallback to r32float.
---
## Changelog
- Added ScreenSpaceAmbientOcclusionSettings,
ScreenSpaceAmbientOcclusionQualityLevel, and
ScreenSpaceAmbientOcclusionBundle
---------
Co-authored-by: IceSentry <c.giguere42@gmail.com>
Co-authored-by: IceSentry <IceSentry@users.noreply.github.com>
Co-authored-by: Daniel Chia <danstryder@gmail.com>
Co-authored-by: Elabajaba <Elabajaba@users.noreply.github.com>
Co-authored-by: Robert Swain <robert.swain@gmail.com>
Co-authored-by: robtfm <50659922+robtfm@users.noreply.github.com>
Co-authored-by: Brandon Dyer <brandondyer64@gmail.com>
Co-authored-by: Edgar Geier <geieredgar@gmail.com>
Co-authored-by: Nicola Papale <nicopap@users.noreply.github.com>
Co-authored-by: Carter Anderson <mcanders1@gmail.com>
2023-06-18 21:05:55 +00:00
|
|
|
ssao,
|
`*_PREPASS` Shader Def Cleanup (#10136)
# Objective
- This PR aims to make the various `*_PREPASS` shader defs we have
(`NORMAL_PREPASS`, `DEPTH_PREPASS`, `MOTION_VECTORS_PREPASS` AND
`DEFERRED_PREPASS`) easier to use and understand:
- So that their meaning is now consistent across all contexts; (“prepass
X is enabled for the current view”)
- So that they're also consistently set across all contexts.
- It also aims to enable us to (with a follow up PR) to conditionally
gate the `BindGroupEntry` and `BindGroupLayoutEntry` items associated
with these prepasses, saving us up to 4 texture slots in WebGL
(currently globally limited to 16 per shader, regardless of bind groups)
## Solution
- We now consistently set these from `PrepassPipeline`, the
`MeshPipeline` and the `DeferredLightingPipeline`, we also set their
`MeshPipelineKey`s;
- We introduce `PREPASS_PIPELINE`, `MESH_PIPELINE` and
`DEFERRED_LIGHTING_PIPELINE` that can be used to detect where the code
is running, without overloading the meanings of the prepass shader defs;
- We also gate the WGSL functions in `bevy_pbr::prepass_utils` with
`#ifdef`s for their respective shader defs, so that shader code can
provide a fallback whenever they're not available.
- This allows us to conditionally include the bindings for these prepass
textures (My next PR, which will hopefully unblock #8015)
- @robtfm mentioned [these were being used to prevent accessing the same
binding as read/write in the
prepass](https://discord.com/channels/691052431525675048/743663924229963868/1163270458393759814),
however even after reversing the `#ifndef`s I had no issues running the
code, so perhaps the compiler is already smart enough even without tree
shaking to know they're not being used, thanks to `#ifdef
PREPASS_PIPELINE`?
## Comparison
### Before
| Shader Def | `PrepassPipeline` | `MeshPipeline` |
`DeferredLightingPipeline` |
| ------------------------ | ----------------- | -------------- |
-------------------------- |
| `NORMAL_PREPASS` | Yes | No | No |
| `DEPTH_PREPASS` | Yes | No | No |
| `MOTION_VECTORS_PREPASS` | Yes | No | No |
| `DEFERRED_PREPASS` | Yes | No | No |
| View Key | `PrepassPipeline` | `MeshPipeline` |
`DeferredLightingPipeline` |
| ------------------------ | ----------------- | -------------- |
-------------------------- |
| `NORMAL_PREPASS` | Yes | Yes | No |
| `DEPTH_PREPASS` | Yes | No | No |
| `MOTION_VECTORS_PREPASS` | Yes | No | No |
| `DEFERRED_PREPASS` | Yes | Yes\* | No |
\* Accidentally was being set twice, once with only
`deferred_prepass.is_some()` as a condition,
and once with `deferred_p repass.is_some() && !forward` as a condition.
### After
| Shader Def | `PrepassPipeline` | `MeshPipeline` |
`DeferredLightingPipeline` |
| ---------------------------- | ----------------- | --------------- |
-------------------------- |
| `NORMAL_PREPASS` | Yes | Yes | Yes |
| `DEPTH_PREPASS` | Yes | Yes | Yes |
| `MOTION_VECTORS_PREPASS` | Yes | Yes | Yes |
| `DEFERRED_PREPASS` | Yes | Yes | Unconditionally |
| `PREPASS_PIPELINE` | Unconditionally | No | No |
| `MESH_PIPELINE` | No | Unconditionally | No |
| `DEFERRED_LIGHTING_PIPELINE` | No | No | Unconditionally |
| View Key | `PrepassPipeline` | `MeshPipeline` |
`DeferredLightingPipeline` |
| ------------------------ | ----------------- | -------------- |
-------------------------- |
| `NORMAL_PREPASS` | Yes | Yes | Yes |
| `DEPTH_PREPASS` | Yes | Yes | Yes |
| `MOTION_VECTORS_PREPASS` | Yes | Yes | Yes |
| `DEFERRED_PREPASS` | Yes | Yes | Unconditionally |
---
## Changelog
- Cleaned up WGSL `*_PREPASS` shader defs so they're now consistently
used everywhere;
- Introduced `PREPASS_PIPELINE`, `MESH_PIPELINE` and
`DEFERRED_LIGHTING_PIPELINE` WGSL shader defs for conditionally
compiling logic based the current pipeline;
- WGSL functions from `bevy_pbr::prepass_utils` are now guarded with
`#ifdef` based on the currently enabled prepasses;
## Migration Guide
- When using functions from `bevy_pbr::prepass_utils`
(`prepass_depth()`, `prepass_normal()`, `prepass_motion_vector()`) in
contexts where these prepasses might be disabled, you should now wrap
your calls with the appropriate `#ifdef` guards, (`#ifdef
DEPTH_PREPASS`, `#ifdef NORMAL_PREPASS`, `#ifdef MOTION_VECTOR_PREPASS`)
providing fallback logic where applicable.
---------
Co-authored-by: Carter Anderson <mcanders1@gmail.com>
Co-authored-by: IceSentry <IceSentry@users.noreply.github.com>
2023-10-17 00:16:21 +00:00
|
|
|
(normal_prepass, depth_prepass, motion_vector_prepass, deferred_prepass),
|
`StandardMaterial` Light Transmission (#8015)
# Objective
<img width="1920" alt="Screenshot 2023-04-26 at 01 07 34"
src="https://user-images.githubusercontent.com/418473/234467578-0f34187b-5863-4ea1-88e9-7a6bb8ce8da3.png">
This PR adds both diffuse and specular light transmission capabilities
to the `StandardMaterial`, with support for screen space refractions.
This enables realistically representing a wide range of real-world
materials, such as:
- Glass; (Including frosted glass)
- Transparent and translucent plastics;
- Various liquids and gels;
- Gemstones;
- Marble;
- Wax;
- Paper;
- Leaves;
- Porcelain.
Unlike existing support for transparency, light transmission does not
rely on fixed function alpha blending, and therefore works with both
`AlphaMode::Opaque` and `AlphaMode::Mask` materials.
## Solution
- Introduces a number of transmission related fields in the
`StandardMaterial`;
- For specular transmission:
- Adds logic to take a view main texture snapshot after the opaque
phase; (in order to perform screen space refractions)
- Introduces a new `Transmissive3d` phase to the renderer, to which all
meshes with `transmission > 0.0` materials are sent.
- Calculates a light exit point (of the approximate mesh volume) using
`ior` and `thickness` properties
- Samples the snapshot texture with an adaptive number of taps across a
`roughness`-controlled radius enabling “blurry” refractions
- For diffuse transmission:
- Approximates transmitted diffuse light by using a second, flipped +
displaced, diffuse-only Lambertian lobe for each light source.
## To Do
- [x] Figure out where `fresnel_mix()` is taking place, if at all, and
where `dielectric_specular` is being calculated, if at all, and update
them to use the `ior` value (Not a blocker, just a nice-to-have for more
correct BSDF)
- To the _best of my knowledge, this is now taking place, after
964340cdd. The fresnel mix is actually "split" into two parts in our
implementation, one `(1 - fresnel(...))` in the transmission, and
`fresnel()` in the light implementations. A surface with more
reflectance now will produce slightly dimmer transmission towards the
grazing angle, as more of the light gets reflected.
- [x] Add `transmission_texture`
- [x] Add `diffuse_transmission_texture`
- [x] Add `thickness_texture`
- [x] Add `attenuation_distance` and `attenuation_color`
- [x] Connect values to glTF loader
- [x] `transmission` and `transmission_texture`
- [x] `thickness` and `thickness_texture`
- [x] `ior`
- [ ] `diffuse_transmission` and `diffuse_transmission_texture` (needs
upstream support in `gltf` crate, not a blocker)
- [x] Add support for multiple screen space refraction “steps”
- [x] Conditionally create no transmission snapshot texture at all if
`steps == 0`
- [x] Conditionally enable/disable screen space refraction transmission
snapshots
- [x] Read from depth pre-pass to prevent refracting pixels in front of
the light exit point
- [x] Use `interleaved_gradient_noise()` function for sampling blur in a
way that benefits from TAA
- [x] Drill down a TAA `#define`, tweak some aspects of the effect
conditionally based on it
- [x] Remove const array that's crashing under HLSL (unless a new `naga`
release with https://github.com/gfx-rs/naga/pull/2496 comes out before
we merge this)
- [ ] Look into alternatives to the `switch` hack for dynamically
indexing the const array (might not be needed, compilers seem to be
decent at expanding it)
- [ ] Add pipeline keys for gating transmission (do we really want/need
this?)
- [x] Tweak some material field/function names?
## A Note on Texture Packing
_This was originally added as a comment to the
`specular_transmission_texture`, `thickness_texture` and
`diffuse_transmission_texture` documentation, I removed it since it was
more confusing than helpful, and will likely be made redundant/will need
to be updated once we have a better infrastructure for preprocessing
assets_
Due to how channels are mapped, you can more efficiently use a single
shared texture image
for configuring the following:
- R - `specular_transmission_texture`
- G - `thickness_texture`
- B - _unused_
- A - `diffuse_transmission_texture`
The `KHR_materials_diffuse_transmission` glTF extension also defines a
`diffuseTransmissionColorTexture`,
that _we don't currently support_. One might choose to pack the
intensity and color textures together,
using RGB for the color and A for the intensity, in which case this
packing advice doesn't really apply.
---
## Changelog
- Added a new `Transmissive3d` render phase for rendering specular
transmissive materials with screen space refractions
- Added rendering support for transmitted environment map light on the
`StandardMaterial` as a fallback for screen space refractions
- Added `diffuse_transmission`, `specular_transmission`, `thickness`,
`ior`, `attenuation_distance` and `attenuation_color` to the
`StandardMaterial`
- Added `diffuse_transmission_texture`, `specular_transmission_texture`,
`thickness_texture` to the `StandardMaterial`, gated behind a new
`pbr_transmission_textures` cargo feature (off by default, for maximum
hardware compatibility)
- Added `Camera3d::screen_space_specular_transmission_steps` for
controlling the number of “layers of transparency” rendered for
transmissive objects
- Added a `TransmittedShadowReceiver` component for enabling shadows in
(diffusely) transmitted light. (disabled by default, as it requires
carefully setting up the `thickness` to avoid self-shadow artifacts)
- Added support for the `KHR_materials_transmission`,
`KHR_materials_ior` and `KHR_materials_volume` glTF extensions
- Renamed items related to temporal jitter for greater consistency
## Migration Guide
- `SsaoPipelineKey::temporal_noise` has been renamed to
`SsaoPipelineKey::temporal_jitter`
- The `TAA` shader def (controlled by the presence of the
`TemporalAntiAliasSettings` component in the camera) has been replaced
with the `TEMPORAL_JITTER` shader def (controlled by the presence of the
`TemporalJitter` component in the camera)
- `MeshPipelineKey::TAA` has been replaced by
`MeshPipelineKey::TEMPORAL_JITTER`
- The `TEMPORAL_NOISE` shader def has been consolidated with
`TEMPORAL_JITTER`
2023-10-31 20:59:02 +00:00
|
|
|
camera_3d,
|
|
|
|
temporal_jitter,
|
2023-10-24 21:26:19 +00:00
|
|
|
projection,
|
Implement irradiance volumes. (#10268)
# Objective
Bevy could benefit from *irradiance volumes*, also known as *voxel
global illumination* or simply as light probes (though this term is not
preferred, as multiple techniques can be called light probes).
Irradiance volumes are a form of baked global illumination; they work by
sampling the light at the centers of each voxel within a cuboid. At
runtime, the voxels surrounding the fragment center are sampled and
interpolated to produce indirect diffuse illumination.
## Solution
This is divided into two sections. The first is copied and pasted from
the irradiance volume module documentation and describes the technique.
The second part consists of notes on the implementation.
### Overview
An *irradiance volume* is a cuboid voxel region consisting of
regularly-spaced precomputed samples of diffuse indirect light. They're
ideal if you have a dynamic object such as a character that can move
about
static non-moving geometry such as a level in a game, and you want that
dynamic object to be affected by the light bouncing off that static
geometry.
To use irradiance volumes, you need to precompute, or *bake*, the
indirect
light in your scene. Bevy doesn't currently come with a way to do this.
Fortunately, [Blender] provides a [baking tool] as part of the Eevee
renderer, and its irradiance volumes are compatible with those used by
Bevy.
The [`bevy-baked-gi`] project provides a tool, `export-blender-gi`, that
can
extract the baked irradiance volumes from the Blender `.blend` file and
package them up into a `.ktx2` texture for use by the engine. See the
documentation in the `bevy-baked-gi` project for more details as to this
workflow.
Like all light probes in Bevy, irradiance volumes are 1×1×1 cubes that
can
be arbitrarily scaled, rotated, and positioned in a scene with the
[`bevy_transform::components::Transform`] component. The 3D voxel grid
will
be stretched to fill the interior of the cube, and the illumination from
the
irradiance volume will apply to all fragments within that bounding
region.
Bevy's irradiance volumes are based on Valve's [*ambient cubes*] as used
in
*Half-Life 2* ([Mitchell 2006], slide 27). These encode a single color
of
light from the six 3D cardinal directions and blend the sides together
according to the surface normal.
The primary reason for choosing ambient cubes is to match Blender, so
that
its Eevee renderer can be used for baking. However, they also have some
advantages over the common second-order spherical harmonics approach:
ambient cubes don't suffer from ringing artifacts, they are smaller (6
colors for ambient cubes as opposed to 9 for spherical harmonics), and
evaluation is faster. A smaller basis allows for a denser grid of voxels
with the same storage requirements.
If you wish to use a tool other than `export-blender-gi` to produce the
irradiance volumes, you'll need to pack the irradiance volumes in the
following format. The irradiance volume of resolution *(Rx, Ry, Rz)* is
expected to be a 3D texture of dimensions *(Rx, 2Ry, 3Rz)*. The
unnormalized
texture coordinate *(s, t, p)* of the voxel at coordinate *(x, y, z)*
with
side *S* ∈ *{-X, +X, -Y, +Y, -Z, +Z}* is as follows:
```text
s = x
t = y + ⎰ 0 if S ∈ {-X, -Y, -Z}
⎱ Ry if S ∈ {+X, +Y, +Z}
⎧ 0 if S ∈ {-X, +X}
p = z + ⎨ Rz if S ∈ {-Y, +Y}
⎩ 2Rz if S ∈ {-Z, +Z}
```
Visually, in a left-handed coordinate system with Y up, viewed from the
right, the 3D texture looks like a stacked series of voxel grids, one
for
each cube side, in this order:
| **+X** | **+Y** | **+Z** |
| ------ | ------ | ------ |
| **-X** | **-Y** | **-Z** |
A terminology note: Other engines may refer to irradiance volumes as
*voxel
global illumination*, *VXGI*, or simply as *light probes*. Sometimes
*light
probe* refers to what Bevy calls a reflection probe. In Bevy, *light
probe*
is a generic term that encompasses all cuboid bounding regions that
capture
indirect illumination, whether based on voxels or not.
Note that, if binding arrays aren't supported (e.g. on WebGPU or WebGL
2),
then only the closest irradiance volume to the view will be taken into
account during rendering.
[*ambient cubes*]:
https://advances.realtimerendering.com/s2006/Mitchell-ShadingInValvesSourceEngine.pdf
[Mitchell 2006]:
https://advances.realtimerendering.com/s2006/Mitchell-ShadingInValvesSourceEngine.pdf
[Blender]: http://blender.org/
[baking tool]:
https://docs.blender.org/manual/en/latest/render/eevee/render_settings/indirect_lighting.html
[`bevy-baked-gi`]: https://github.com/pcwalton/bevy-baked-gi
### Implementation notes
This patch generalizes light probes so as to reuse as much code as
possible between irradiance volumes and the existing reflection probes.
This approach was chosen because both techniques share numerous
similarities:
1. Both irradiance volumes and reflection probes are cuboid bounding
regions.
2. Both are responsible for providing baked indirect light.
3. Both techniques involve presenting a variable number of textures to
the shader from which indirect light is sampled. (In the current
implementation, this uses binding arrays.)
4. Both irradiance volumes and reflection probes require gathering and
sorting probes by distance on CPU.
5. Both techniques require the GPU to search through a list of bounding
regions.
6. Both will eventually want to have falloff so that we can smoothly
blend as objects enter and exit the probes' influence ranges. (This is
not implemented yet to keep this patch relatively small and reviewable.)
To do this, we generalize most of the methods in the reflection probes
patch #11366 to be generic over a trait, `LightProbeComponent`. This
trait is implemented by both `EnvironmentMapLight` (for reflection
probes) and `IrradianceVolume` (for irradiance volumes). Using a trait
will allow us to add more types of light probes in the future. In
particular, I highly suspect we will want real-time reflection planes
for mirrors in the future, which can be easily slotted into this
framework.
## Changelog
> This section is optional. If this was a trivial fix, or has no
externally-visible impact, you can delete this section.
### Added
* A new `IrradianceVolume` asset type is available for baked voxelized
light probes. You can bake the global illumination using Blender or
another tool of your choice and use it in Bevy to apply indirect
illumination to dynamic objects.
2024-02-06 23:23:20 +00:00
|
|
|
(has_environment_maps, has_irradiance_volumes),
|
2024-10-07 23:50:28 +00:00
|
|
|
has_oit,
|
2024-08-28 11:38:38 +00:00
|
|
|
) in &views
|
2021-12-25 21:45:43 +00:00
|
|
|
{
|
2024-05-21 18:23:04 +00:00
|
|
|
let (
|
|
|
|
Some(opaque_phase),
|
|
|
|
Some(alpha_mask_phase),
|
|
|
|
Some(transmissive_phase),
|
|
|
|
Some(transparent_phase),
|
|
|
|
) = (
|
|
|
|
opaque_render_phases.get_mut(&view_entity),
|
|
|
|
alpha_mask_render_phases.get_mut(&view_entity),
|
|
|
|
transmissive_render_phases.get_mut(&view_entity),
|
|
|
|
transparent_render_phases.get_mut(&view_entity),
|
|
|
|
)
|
|
|
|
else {
|
|
|
|
continue;
|
|
|
|
};
|
|
|
|
|
2022-11-28 13:54:13 +00:00
|
|
|
let draw_opaque_pbr = opaque_draw_functions.read().id::<DrawMaterial<M>>();
|
|
|
|
let draw_alpha_mask_pbr = alpha_mask_draw_functions.read().id::<DrawMaterial<M>>();
|
`StandardMaterial` Light Transmission (#8015)
# Objective
<img width="1920" alt="Screenshot 2023-04-26 at 01 07 34"
src="https://user-images.githubusercontent.com/418473/234467578-0f34187b-5863-4ea1-88e9-7a6bb8ce8da3.png">
This PR adds both diffuse and specular light transmission capabilities
to the `StandardMaterial`, with support for screen space refractions.
This enables realistically representing a wide range of real-world
materials, such as:
- Glass; (Including frosted glass)
- Transparent and translucent plastics;
- Various liquids and gels;
- Gemstones;
- Marble;
- Wax;
- Paper;
- Leaves;
- Porcelain.
Unlike existing support for transparency, light transmission does not
rely on fixed function alpha blending, and therefore works with both
`AlphaMode::Opaque` and `AlphaMode::Mask` materials.
## Solution
- Introduces a number of transmission related fields in the
`StandardMaterial`;
- For specular transmission:
- Adds logic to take a view main texture snapshot after the opaque
phase; (in order to perform screen space refractions)
- Introduces a new `Transmissive3d` phase to the renderer, to which all
meshes with `transmission > 0.0` materials are sent.
- Calculates a light exit point (of the approximate mesh volume) using
`ior` and `thickness` properties
- Samples the snapshot texture with an adaptive number of taps across a
`roughness`-controlled radius enabling “blurry” refractions
- For diffuse transmission:
- Approximates transmitted diffuse light by using a second, flipped +
displaced, diffuse-only Lambertian lobe for each light source.
## To Do
- [x] Figure out where `fresnel_mix()` is taking place, if at all, and
where `dielectric_specular` is being calculated, if at all, and update
them to use the `ior` value (Not a blocker, just a nice-to-have for more
correct BSDF)
- To the _best of my knowledge, this is now taking place, after
964340cdd. The fresnel mix is actually "split" into two parts in our
implementation, one `(1 - fresnel(...))` in the transmission, and
`fresnel()` in the light implementations. A surface with more
reflectance now will produce slightly dimmer transmission towards the
grazing angle, as more of the light gets reflected.
- [x] Add `transmission_texture`
- [x] Add `diffuse_transmission_texture`
- [x] Add `thickness_texture`
- [x] Add `attenuation_distance` and `attenuation_color`
- [x] Connect values to glTF loader
- [x] `transmission` and `transmission_texture`
- [x] `thickness` and `thickness_texture`
- [x] `ior`
- [ ] `diffuse_transmission` and `diffuse_transmission_texture` (needs
upstream support in `gltf` crate, not a blocker)
- [x] Add support for multiple screen space refraction “steps”
- [x] Conditionally create no transmission snapshot texture at all if
`steps == 0`
- [x] Conditionally enable/disable screen space refraction transmission
snapshots
- [x] Read from depth pre-pass to prevent refracting pixels in front of
the light exit point
- [x] Use `interleaved_gradient_noise()` function for sampling blur in a
way that benefits from TAA
- [x] Drill down a TAA `#define`, tweak some aspects of the effect
conditionally based on it
- [x] Remove const array that's crashing under HLSL (unless a new `naga`
release with https://github.com/gfx-rs/naga/pull/2496 comes out before
we merge this)
- [ ] Look into alternatives to the `switch` hack for dynamically
indexing the const array (might not be needed, compilers seem to be
decent at expanding it)
- [ ] Add pipeline keys for gating transmission (do we really want/need
this?)
- [x] Tweak some material field/function names?
## A Note on Texture Packing
_This was originally added as a comment to the
`specular_transmission_texture`, `thickness_texture` and
`diffuse_transmission_texture` documentation, I removed it since it was
more confusing than helpful, and will likely be made redundant/will need
to be updated once we have a better infrastructure for preprocessing
assets_
Due to how channels are mapped, you can more efficiently use a single
shared texture image
for configuring the following:
- R - `specular_transmission_texture`
- G - `thickness_texture`
- B - _unused_
- A - `diffuse_transmission_texture`
The `KHR_materials_diffuse_transmission` glTF extension also defines a
`diffuseTransmissionColorTexture`,
that _we don't currently support_. One might choose to pack the
intensity and color textures together,
using RGB for the color and A for the intensity, in which case this
packing advice doesn't really apply.
---
## Changelog
- Added a new `Transmissive3d` render phase for rendering specular
transmissive materials with screen space refractions
- Added rendering support for transmitted environment map light on the
`StandardMaterial` as a fallback for screen space refractions
- Added `diffuse_transmission`, `specular_transmission`, `thickness`,
`ior`, `attenuation_distance` and `attenuation_color` to the
`StandardMaterial`
- Added `diffuse_transmission_texture`, `specular_transmission_texture`,
`thickness_texture` to the `StandardMaterial`, gated behind a new
`pbr_transmission_textures` cargo feature (off by default, for maximum
hardware compatibility)
- Added `Camera3d::screen_space_specular_transmission_steps` for
controlling the number of “layers of transparency” rendered for
transmissive objects
- Added a `TransmittedShadowReceiver` component for enabling shadows in
(diffusely) transmitted light. (disabled by default, as it requires
carefully setting up the `thickness` to avoid self-shadow artifacts)
- Added support for the `KHR_materials_transmission`,
`KHR_materials_ior` and `KHR_materials_volume` glTF extensions
- Renamed items related to temporal jitter for greater consistency
## Migration Guide
- `SsaoPipelineKey::temporal_noise` has been renamed to
`SsaoPipelineKey::temporal_jitter`
- The `TAA` shader def (controlled by the presence of the
`TemporalAntiAliasSettings` component in the camera) has been replaced
with the `TEMPORAL_JITTER` shader def (controlled by the presence of the
`TemporalJitter` component in the camera)
- `MeshPipelineKey::TAA` has been replaced by
`MeshPipelineKey::TEMPORAL_JITTER`
- The `TEMPORAL_NOISE` shader def has been consolidated with
`TEMPORAL_JITTER`
2023-10-31 20:59:02 +00:00
|
|
|
let draw_transmissive_pbr = transmissive_draw_functions.read().id::<DrawMaterial<M>>();
|
2022-11-28 13:54:13 +00:00
|
|
|
let draw_transparent_pbr = transparent_draw_functions.read().id::<DrawMaterial<M>>();
|
2021-12-25 21:45:43 +00:00
|
|
|
|
2023-01-20 14:25:21 +00:00
|
|
|
let mut view_key = MeshPipelineKey::from_msaa_samples(msaa.samples())
|
|
|
|
| MeshPipelineKey::from_hdr(view.hdr);
|
2022-10-26 20:13:59 +00:00
|
|
|
|
`*_PREPASS` Shader Def Cleanup (#10136)
# Objective
- This PR aims to make the various `*_PREPASS` shader defs we have
(`NORMAL_PREPASS`, `DEPTH_PREPASS`, `MOTION_VECTORS_PREPASS` AND
`DEFERRED_PREPASS`) easier to use and understand:
- So that their meaning is now consistent across all contexts; (“prepass
X is enabled for the current view”)
- So that they're also consistently set across all contexts.
- It also aims to enable us to (with a follow up PR) to conditionally
gate the `BindGroupEntry` and `BindGroupLayoutEntry` items associated
with these prepasses, saving us up to 4 texture slots in WebGL
(currently globally limited to 16 per shader, regardless of bind groups)
## Solution
- We now consistently set these from `PrepassPipeline`, the
`MeshPipeline` and the `DeferredLightingPipeline`, we also set their
`MeshPipelineKey`s;
- We introduce `PREPASS_PIPELINE`, `MESH_PIPELINE` and
`DEFERRED_LIGHTING_PIPELINE` that can be used to detect where the code
is running, without overloading the meanings of the prepass shader defs;
- We also gate the WGSL functions in `bevy_pbr::prepass_utils` with
`#ifdef`s for their respective shader defs, so that shader code can
provide a fallback whenever they're not available.
- This allows us to conditionally include the bindings for these prepass
textures (My next PR, which will hopefully unblock #8015)
- @robtfm mentioned [these were being used to prevent accessing the same
binding as read/write in the
prepass](https://discord.com/channels/691052431525675048/743663924229963868/1163270458393759814),
however even after reversing the `#ifndef`s I had no issues running the
code, so perhaps the compiler is already smart enough even without tree
shaking to know they're not being used, thanks to `#ifdef
PREPASS_PIPELINE`?
## Comparison
### Before
| Shader Def | `PrepassPipeline` | `MeshPipeline` |
`DeferredLightingPipeline` |
| ------------------------ | ----------------- | -------------- |
-------------------------- |
| `NORMAL_PREPASS` | Yes | No | No |
| `DEPTH_PREPASS` | Yes | No | No |
| `MOTION_VECTORS_PREPASS` | Yes | No | No |
| `DEFERRED_PREPASS` | Yes | No | No |
| View Key | `PrepassPipeline` | `MeshPipeline` |
`DeferredLightingPipeline` |
| ------------------------ | ----------------- | -------------- |
-------------------------- |
| `NORMAL_PREPASS` | Yes | Yes | No |
| `DEPTH_PREPASS` | Yes | No | No |
| `MOTION_VECTORS_PREPASS` | Yes | No | No |
| `DEFERRED_PREPASS` | Yes | Yes\* | No |
\* Accidentally was being set twice, once with only
`deferred_prepass.is_some()` as a condition,
and once with `deferred_p repass.is_some() && !forward` as a condition.
### After
| Shader Def | `PrepassPipeline` | `MeshPipeline` |
`DeferredLightingPipeline` |
| ---------------------------- | ----------------- | --------------- |
-------------------------- |
| `NORMAL_PREPASS` | Yes | Yes | Yes |
| `DEPTH_PREPASS` | Yes | Yes | Yes |
| `MOTION_VECTORS_PREPASS` | Yes | Yes | Yes |
| `DEFERRED_PREPASS` | Yes | Yes | Unconditionally |
| `PREPASS_PIPELINE` | Unconditionally | No | No |
| `MESH_PIPELINE` | No | Unconditionally | No |
| `DEFERRED_LIGHTING_PIPELINE` | No | No | Unconditionally |
| View Key | `PrepassPipeline` | `MeshPipeline` |
`DeferredLightingPipeline` |
| ------------------------ | ----------------- | -------------- |
-------------------------- |
| `NORMAL_PREPASS` | Yes | Yes | Yes |
| `DEPTH_PREPASS` | Yes | Yes | Yes |
| `MOTION_VECTORS_PREPASS` | Yes | Yes | Yes |
| `DEFERRED_PREPASS` | Yes | Yes | Unconditionally |
---
## Changelog
- Cleaned up WGSL `*_PREPASS` shader defs so they're now consistently
used everywhere;
- Introduced `PREPASS_PIPELINE`, `MESH_PIPELINE` and
`DEFERRED_LIGHTING_PIPELINE` WGSL shader defs for conditionally
compiling logic based the current pipeline;
- WGSL functions from `bevy_pbr::prepass_utils` are now guarded with
`#ifdef` based on the currently enabled prepasses;
## Migration Guide
- When using functions from `bevy_pbr::prepass_utils`
(`prepass_depth()`, `prepass_normal()`, `prepass_motion_vector()`) in
contexts where these prepasses might be disabled, you should now wrap
your calls with the appropriate `#ifdef` guards, (`#ifdef
DEPTH_PREPASS`, `#ifdef NORMAL_PREPASS`, `#ifdef MOTION_VECTOR_PREPASS`)
providing fallback logic where applicable.
---------
Co-authored-by: Carter Anderson <mcanders1@gmail.com>
Co-authored-by: IceSentry <IceSentry@users.noreply.github.com>
2023-10-17 00:16:21 +00:00
|
|
|
if normal_prepass {
|
2023-03-29 18:04:40 +00:00
|
|
|
view_key |= MeshPipelineKey::NORMAL_PREPASS;
|
|
|
|
}
|
2023-10-12 22:10:38 +00:00
|
|
|
|
`*_PREPASS` Shader Def Cleanup (#10136)
# Objective
- This PR aims to make the various `*_PREPASS` shader defs we have
(`NORMAL_PREPASS`, `DEPTH_PREPASS`, `MOTION_VECTORS_PREPASS` AND
`DEFERRED_PREPASS`) easier to use and understand:
- So that their meaning is now consistent across all contexts; (“prepass
X is enabled for the current view”)
- So that they're also consistently set across all contexts.
- It also aims to enable us to (with a follow up PR) to conditionally
gate the `BindGroupEntry` and `BindGroupLayoutEntry` items associated
with these prepasses, saving us up to 4 texture slots in WebGL
(currently globally limited to 16 per shader, regardless of bind groups)
## Solution
- We now consistently set these from `PrepassPipeline`, the
`MeshPipeline` and the `DeferredLightingPipeline`, we also set their
`MeshPipelineKey`s;
- We introduce `PREPASS_PIPELINE`, `MESH_PIPELINE` and
`DEFERRED_LIGHTING_PIPELINE` that can be used to detect where the code
is running, without overloading the meanings of the prepass shader defs;
- We also gate the WGSL functions in `bevy_pbr::prepass_utils` with
`#ifdef`s for their respective shader defs, so that shader code can
provide a fallback whenever they're not available.
- This allows us to conditionally include the bindings for these prepass
textures (My next PR, which will hopefully unblock #8015)
- @robtfm mentioned [these were being used to prevent accessing the same
binding as read/write in the
prepass](https://discord.com/channels/691052431525675048/743663924229963868/1163270458393759814),
however even after reversing the `#ifndef`s I had no issues running the
code, so perhaps the compiler is already smart enough even without tree
shaking to know they're not being used, thanks to `#ifdef
PREPASS_PIPELINE`?
## Comparison
### Before
| Shader Def | `PrepassPipeline` | `MeshPipeline` |
`DeferredLightingPipeline` |
| ------------------------ | ----------------- | -------------- |
-------------------------- |
| `NORMAL_PREPASS` | Yes | No | No |
| `DEPTH_PREPASS` | Yes | No | No |
| `MOTION_VECTORS_PREPASS` | Yes | No | No |
| `DEFERRED_PREPASS` | Yes | No | No |
| View Key | `PrepassPipeline` | `MeshPipeline` |
`DeferredLightingPipeline` |
| ------------------------ | ----------------- | -------------- |
-------------------------- |
| `NORMAL_PREPASS` | Yes | Yes | No |
| `DEPTH_PREPASS` | Yes | No | No |
| `MOTION_VECTORS_PREPASS` | Yes | No | No |
| `DEFERRED_PREPASS` | Yes | Yes\* | No |
\* Accidentally was being set twice, once with only
`deferred_prepass.is_some()` as a condition,
and once with `deferred_p repass.is_some() && !forward` as a condition.
### After
| Shader Def | `PrepassPipeline` | `MeshPipeline` |
`DeferredLightingPipeline` |
| ---------------------------- | ----------------- | --------------- |
-------------------------- |
| `NORMAL_PREPASS` | Yes | Yes | Yes |
| `DEPTH_PREPASS` | Yes | Yes | Yes |
| `MOTION_VECTORS_PREPASS` | Yes | Yes | Yes |
| `DEFERRED_PREPASS` | Yes | Yes | Unconditionally |
| `PREPASS_PIPELINE` | Unconditionally | No | No |
| `MESH_PIPELINE` | No | Unconditionally | No |
| `DEFERRED_LIGHTING_PIPELINE` | No | No | Unconditionally |
| View Key | `PrepassPipeline` | `MeshPipeline` |
`DeferredLightingPipeline` |
| ------------------------ | ----------------- | -------------- |
-------------------------- |
| `NORMAL_PREPASS` | Yes | Yes | Yes |
| `DEPTH_PREPASS` | Yes | Yes | Yes |
| `MOTION_VECTORS_PREPASS` | Yes | Yes | Yes |
| `DEFERRED_PREPASS` | Yes | Yes | Unconditionally |
---
## Changelog
- Cleaned up WGSL `*_PREPASS` shader defs so they're now consistently
used everywhere;
- Introduced `PREPASS_PIPELINE`, `MESH_PIPELINE` and
`DEFERRED_LIGHTING_PIPELINE` WGSL shader defs for conditionally
compiling logic based the current pipeline;
- WGSL functions from `bevy_pbr::prepass_utils` are now guarded with
`#ifdef` based on the currently enabled prepasses;
## Migration Guide
- When using functions from `bevy_pbr::prepass_utils`
(`prepass_depth()`, `prepass_normal()`, `prepass_motion_vector()`) in
contexts where these prepasses might be disabled, you should now wrap
your calls with the appropriate `#ifdef` guards, (`#ifdef
DEPTH_PREPASS`, `#ifdef NORMAL_PREPASS`, `#ifdef MOTION_VECTOR_PREPASS`)
providing fallback logic where applicable.
---------
Co-authored-by: Carter Anderson <mcanders1@gmail.com>
Co-authored-by: IceSentry <IceSentry@users.noreply.github.com>
2023-10-17 00:16:21 +00:00
|
|
|
if depth_prepass {
|
|
|
|
view_key |= MeshPipelineKey::DEPTH_PREPASS;
|
|
|
|
}
|
|
|
|
|
|
|
|
if motion_vector_prepass {
|
|
|
|
view_key |= MeshPipelineKey::MOTION_VECTOR_PREPASS;
|
|
|
|
}
|
|
|
|
|
|
|
|
if deferred_prepass {
|
2023-10-12 22:10:38 +00:00
|
|
|
view_key |= MeshPipelineKey::DEFERRED_PREPASS;
|
|
|
|
}
|
|
|
|
|
2023-12-30 23:27:48 +00:00
|
|
|
if temporal_jitter {
|
`StandardMaterial` Light Transmission (#8015)
# Objective
<img width="1920" alt="Screenshot 2023-04-26 at 01 07 34"
src="https://user-images.githubusercontent.com/418473/234467578-0f34187b-5863-4ea1-88e9-7a6bb8ce8da3.png">
This PR adds both diffuse and specular light transmission capabilities
to the `StandardMaterial`, with support for screen space refractions.
This enables realistically representing a wide range of real-world
materials, such as:
- Glass; (Including frosted glass)
- Transparent and translucent plastics;
- Various liquids and gels;
- Gemstones;
- Marble;
- Wax;
- Paper;
- Leaves;
- Porcelain.
Unlike existing support for transparency, light transmission does not
rely on fixed function alpha blending, and therefore works with both
`AlphaMode::Opaque` and `AlphaMode::Mask` materials.
## Solution
- Introduces a number of transmission related fields in the
`StandardMaterial`;
- For specular transmission:
- Adds logic to take a view main texture snapshot after the opaque
phase; (in order to perform screen space refractions)
- Introduces a new `Transmissive3d` phase to the renderer, to which all
meshes with `transmission > 0.0` materials are sent.
- Calculates a light exit point (of the approximate mesh volume) using
`ior` and `thickness` properties
- Samples the snapshot texture with an adaptive number of taps across a
`roughness`-controlled radius enabling “blurry” refractions
- For diffuse transmission:
- Approximates transmitted diffuse light by using a second, flipped +
displaced, diffuse-only Lambertian lobe for each light source.
## To Do
- [x] Figure out where `fresnel_mix()` is taking place, if at all, and
where `dielectric_specular` is being calculated, if at all, and update
them to use the `ior` value (Not a blocker, just a nice-to-have for more
correct BSDF)
- To the _best of my knowledge, this is now taking place, after
964340cdd. The fresnel mix is actually "split" into two parts in our
implementation, one `(1 - fresnel(...))` in the transmission, and
`fresnel()` in the light implementations. A surface with more
reflectance now will produce slightly dimmer transmission towards the
grazing angle, as more of the light gets reflected.
- [x] Add `transmission_texture`
- [x] Add `diffuse_transmission_texture`
- [x] Add `thickness_texture`
- [x] Add `attenuation_distance` and `attenuation_color`
- [x] Connect values to glTF loader
- [x] `transmission` and `transmission_texture`
- [x] `thickness` and `thickness_texture`
- [x] `ior`
- [ ] `diffuse_transmission` and `diffuse_transmission_texture` (needs
upstream support in `gltf` crate, not a blocker)
- [x] Add support for multiple screen space refraction “steps”
- [x] Conditionally create no transmission snapshot texture at all if
`steps == 0`
- [x] Conditionally enable/disable screen space refraction transmission
snapshots
- [x] Read from depth pre-pass to prevent refracting pixels in front of
the light exit point
- [x] Use `interleaved_gradient_noise()` function for sampling blur in a
way that benefits from TAA
- [x] Drill down a TAA `#define`, tweak some aspects of the effect
conditionally based on it
- [x] Remove const array that's crashing under HLSL (unless a new `naga`
release with https://github.com/gfx-rs/naga/pull/2496 comes out before
we merge this)
- [ ] Look into alternatives to the `switch` hack for dynamically
indexing the const array (might not be needed, compilers seem to be
decent at expanding it)
- [ ] Add pipeline keys for gating transmission (do we really want/need
this?)
- [x] Tweak some material field/function names?
## A Note on Texture Packing
_This was originally added as a comment to the
`specular_transmission_texture`, `thickness_texture` and
`diffuse_transmission_texture` documentation, I removed it since it was
more confusing than helpful, and will likely be made redundant/will need
to be updated once we have a better infrastructure for preprocessing
assets_
Due to how channels are mapped, you can more efficiently use a single
shared texture image
for configuring the following:
- R - `specular_transmission_texture`
- G - `thickness_texture`
- B - _unused_
- A - `diffuse_transmission_texture`
The `KHR_materials_diffuse_transmission` glTF extension also defines a
`diffuseTransmissionColorTexture`,
that _we don't currently support_. One might choose to pack the
intensity and color textures together,
using RGB for the color and A for the intensity, in which case this
packing advice doesn't really apply.
---
## Changelog
- Added a new `Transmissive3d` render phase for rendering specular
transmissive materials with screen space refractions
- Added rendering support for transmitted environment map light on the
`StandardMaterial` as a fallback for screen space refractions
- Added `diffuse_transmission`, `specular_transmission`, `thickness`,
`ior`, `attenuation_distance` and `attenuation_color` to the
`StandardMaterial`
- Added `diffuse_transmission_texture`, `specular_transmission_texture`,
`thickness_texture` to the `StandardMaterial`, gated behind a new
`pbr_transmission_textures` cargo feature (off by default, for maximum
hardware compatibility)
- Added `Camera3d::screen_space_specular_transmission_steps` for
controlling the number of “layers of transparency” rendered for
transmissive objects
- Added a `TransmittedShadowReceiver` component for enabling shadows in
(diffusely) transmitted light. (disabled by default, as it requires
carefully setting up the `thickness` to avoid self-shadow artifacts)
- Added support for the `KHR_materials_transmission`,
`KHR_materials_ior` and `KHR_materials_volume` glTF extensions
- Renamed items related to temporal jitter for greater consistency
## Migration Guide
- `SsaoPipelineKey::temporal_noise` has been renamed to
`SsaoPipelineKey::temporal_jitter`
- The `TAA` shader def (controlled by the presence of the
`TemporalAntiAliasSettings` component in the camera) has been replaced
with the `TEMPORAL_JITTER` shader def (controlled by the presence of the
`TemporalJitter` component in the camera)
- `MeshPipelineKey::TAA` has been replaced by
`MeshPipelineKey::TEMPORAL_JITTER`
- The `TEMPORAL_NOISE` shader def has been consolidated with
`TEMPORAL_JITTER`
2023-10-31 20:59:02 +00:00
|
|
|
view_key |= MeshPipelineKey::TEMPORAL_JITTER;
|
Apply codebase changes in preparation for `StandardMaterial` transmission (#8704)
# Objective
- Make #8015 easier to review;
## Solution
- This commit contains changes not directly related to transmission
required by #8015, in easier-to-review, one-change-per-commit form.
---
## Changelog
### Fixed
- Clear motion vector prepass using `0.0` instead of `1.0`, to avoid TAA
artifacts on transparent objects against the background;
### Added
- The `E` mathematical constant is now available for use in shaders,
exposed under `bevy_pbr::utils`;
- A new `TAA` shader def is now available, for conditionally enabling
shader logic via `#ifdef` when TAA is enabled; (e.g. for jittering
texture samples)
- A new `FallbackImageZero` resource is introduced, for when a fallback
image filled with zeroes is required;
- A new `RenderPhase<I>::render_range()` method is introduced, for
render phases that need to render their items in multiple parceled out
“steps”;
### Changed
- The `MainTargetTextures` struct now holds both `Texture` and
`TextureViews` for the main textures;
- The fog shader functions under `bevy_pbr::fog` now take the a `Fog`
structure as their first argument, instead of relying on the global
`fog` uniform;
- The main textures can now be used as copy sources;
## Migration Guide
- `ViewTarget::main_texture()` and `ViewTarget::main_texture_other()`
now return `&Texture` instead of `&TextureView`. If you were relying on
these methods, replace your usage with
`ViewTarget::main_texture_view()`and
`ViewTarget::main_texture_other_view()`, respectively;
- `ViewTarget::sampled_main_texture()` now returns `Option<&Texture>`
instead of a `Option<&TextureView>`. If you were relying on this method,
replace your usage with `ViewTarget::sampled_main_texture_view()`;
- The `apply_fog()`, `linear_fog()`, `exponential_fog()`,
`exponential_squared_fog()` and `atmospheric_fog()` functions now take a
configurable `Fog` struct. If you were relying on them, update your
usage by adding the global `fog` uniform as their first argument;
2023-05-30 14:21:53 +00:00
|
|
|
}
|
`StandardMaterial` Light Transmission (#8015)
# Objective
<img width="1920" alt="Screenshot 2023-04-26 at 01 07 34"
src="https://user-images.githubusercontent.com/418473/234467578-0f34187b-5863-4ea1-88e9-7a6bb8ce8da3.png">
This PR adds both diffuse and specular light transmission capabilities
to the `StandardMaterial`, with support for screen space refractions.
This enables realistically representing a wide range of real-world
materials, such as:
- Glass; (Including frosted glass)
- Transparent and translucent plastics;
- Various liquids and gels;
- Gemstones;
- Marble;
- Wax;
- Paper;
- Leaves;
- Porcelain.
Unlike existing support for transparency, light transmission does not
rely on fixed function alpha blending, and therefore works with both
`AlphaMode::Opaque` and `AlphaMode::Mask` materials.
## Solution
- Introduces a number of transmission related fields in the
`StandardMaterial`;
- For specular transmission:
- Adds logic to take a view main texture snapshot after the opaque
phase; (in order to perform screen space refractions)
- Introduces a new `Transmissive3d` phase to the renderer, to which all
meshes with `transmission > 0.0` materials are sent.
- Calculates a light exit point (of the approximate mesh volume) using
`ior` and `thickness` properties
- Samples the snapshot texture with an adaptive number of taps across a
`roughness`-controlled radius enabling “blurry” refractions
- For diffuse transmission:
- Approximates transmitted diffuse light by using a second, flipped +
displaced, diffuse-only Lambertian lobe for each light source.
## To Do
- [x] Figure out where `fresnel_mix()` is taking place, if at all, and
where `dielectric_specular` is being calculated, if at all, and update
them to use the `ior` value (Not a blocker, just a nice-to-have for more
correct BSDF)
- To the _best of my knowledge, this is now taking place, after
964340cdd. The fresnel mix is actually "split" into two parts in our
implementation, one `(1 - fresnel(...))` in the transmission, and
`fresnel()` in the light implementations. A surface with more
reflectance now will produce slightly dimmer transmission towards the
grazing angle, as more of the light gets reflected.
- [x] Add `transmission_texture`
- [x] Add `diffuse_transmission_texture`
- [x] Add `thickness_texture`
- [x] Add `attenuation_distance` and `attenuation_color`
- [x] Connect values to glTF loader
- [x] `transmission` and `transmission_texture`
- [x] `thickness` and `thickness_texture`
- [x] `ior`
- [ ] `diffuse_transmission` and `diffuse_transmission_texture` (needs
upstream support in `gltf` crate, not a blocker)
- [x] Add support for multiple screen space refraction “steps”
- [x] Conditionally create no transmission snapshot texture at all if
`steps == 0`
- [x] Conditionally enable/disable screen space refraction transmission
snapshots
- [x] Read from depth pre-pass to prevent refracting pixels in front of
the light exit point
- [x] Use `interleaved_gradient_noise()` function for sampling blur in a
way that benefits from TAA
- [x] Drill down a TAA `#define`, tweak some aspects of the effect
conditionally based on it
- [x] Remove const array that's crashing under HLSL (unless a new `naga`
release with https://github.com/gfx-rs/naga/pull/2496 comes out before
we merge this)
- [ ] Look into alternatives to the `switch` hack for dynamically
indexing the const array (might not be needed, compilers seem to be
decent at expanding it)
- [ ] Add pipeline keys for gating transmission (do we really want/need
this?)
- [x] Tweak some material field/function names?
## A Note on Texture Packing
_This was originally added as a comment to the
`specular_transmission_texture`, `thickness_texture` and
`diffuse_transmission_texture` documentation, I removed it since it was
more confusing than helpful, and will likely be made redundant/will need
to be updated once we have a better infrastructure for preprocessing
assets_
Due to how channels are mapped, you can more efficiently use a single
shared texture image
for configuring the following:
- R - `specular_transmission_texture`
- G - `thickness_texture`
- B - _unused_
- A - `diffuse_transmission_texture`
The `KHR_materials_diffuse_transmission` glTF extension also defines a
`diffuseTransmissionColorTexture`,
that _we don't currently support_. One might choose to pack the
intensity and color textures together,
using RGB for the color and A for the intensity, in which case this
packing advice doesn't really apply.
---
## Changelog
- Added a new `Transmissive3d` render phase for rendering specular
transmissive materials with screen space refractions
- Added rendering support for transmitted environment map light on the
`StandardMaterial` as a fallback for screen space refractions
- Added `diffuse_transmission`, `specular_transmission`, `thickness`,
`ior`, `attenuation_distance` and `attenuation_color` to the
`StandardMaterial`
- Added `diffuse_transmission_texture`, `specular_transmission_texture`,
`thickness_texture` to the `StandardMaterial`, gated behind a new
`pbr_transmission_textures` cargo feature (off by default, for maximum
hardware compatibility)
- Added `Camera3d::screen_space_specular_transmission_steps` for
controlling the number of “layers of transparency” rendered for
transmissive objects
- Added a `TransmittedShadowReceiver` component for enabling shadows in
(diffusely) transmitted light. (disabled by default, as it requires
carefully setting up the `thickness` to avoid self-shadow artifacts)
- Added support for the `KHR_materials_transmission`,
`KHR_materials_ior` and `KHR_materials_volume` glTF extensions
- Renamed items related to temporal jitter for greater consistency
## Migration Guide
- `SsaoPipelineKey::temporal_noise` has been renamed to
`SsaoPipelineKey::temporal_jitter`
- The `TAA` shader def (controlled by the presence of the
`TemporalAntiAliasSettings` component in the camera) has been replaced
with the `TEMPORAL_JITTER` shader def (controlled by the presence of the
`TemporalJitter` component in the camera)
- `MeshPipelineKey::TAA` has been replaced by
`MeshPipelineKey::TEMPORAL_JITTER`
- The `TEMPORAL_NOISE` shader def has been consolidated with
`TEMPORAL_JITTER`
2023-10-31 20:59:02 +00:00
|
|
|
|
Implement minimal reflection probes (fixed macOS, iOS, and Android). (#11366)
This pull request re-submits #10057, which was backed out for breaking
macOS, iOS, and Android. I've tested this version on macOS and Android
and on the iOS simulator.
# Objective
This pull request implements *reflection probes*, which generalize
environment maps to allow for multiple environment maps in the same
scene, each of which has an axis-aligned bounding box. This is a
standard feature of physically-based renderers and was inspired by [the
corresponding feature in Blender's Eevee renderer].
## Solution
This is a minimal implementation of reflection probes that allows
artists to define cuboid bounding regions associated with environment
maps. For every view, on every frame, a system builds up a list of the
nearest 4 reflection probes that are within the view's frustum and
supplies that list to the shader. The PBR fragment shader searches
through the list, finds the first containing reflection probe, and uses
it for indirect lighting, falling back to the view's environment map if
none is found. Both forward and deferred renderers are fully supported.
A reflection probe is an entity with a pair of components, *LightProbe*
and *EnvironmentMapLight* (as well as the standard *SpatialBundle*, to
position it in the world). The *LightProbe* component (along with the
*Transform*) defines the bounding region, while the
*EnvironmentMapLight* component specifies the associated diffuse and
specular cubemaps.
A frequent question is "why two components instead of just one?" The
advantages of this setup are:
1. It's readily extensible to other types of light probes, in particular
*irradiance volumes* (also known as ambient cubes or voxel global
illumination), which use the same approach of bounding cuboids. With a
single component that applies to both reflection probes and irradiance
volumes, we can share the logic that implements falloff and blending
between multiple light probes between both of those features.
2. It reduces duplication between the existing *EnvironmentMapLight* and
these new reflection probes. Systems can treat environment maps attached
to cameras the same way they treat environment maps applied to
reflection probes if they wish.
Internally, we gather up all environment maps in the scene and place
them in a cubemap array. At present, this means that all environment
maps must have the same size, mipmap count, and texture format. A
warning is emitted if this restriction is violated. We could potentially
relax this in the future as part of the automatic mipmap generation
work, which could easily do texture format conversion as part of its
preprocessing.
An easy way to generate reflection probe cubemaps is to bake them in
Blender and use the `export-blender-gi` tool that's part of the
[`bevy-baked-gi`] project. This tool takes a `.blend` file containing
baked cubemaps as input and exports cubemap images, pre-filtered with an
embedded fork of the [glTF IBL Sampler], alongside a corresponding
`.scn.ron` file that the scene spawner can use to recreate the
reflection probes.
Note that this is intentionally a minimal implementation, to aid
reviewability. Known issues are:
* Reflection probes are basically unsupported on WebGL 2, because WebGL
2 has no cubemap arrays. (Strictly speaking, you can have precisely one
reflection probe in the scene if you have no other cubemaps anywhere,
but this isn't very useful.)
* Reflection probes have no falloff, so reflections will abruptly change
when objects move from one bounding region to another.
* As mentioned before, all cubemaps in the world of a given type
(diffuse or specular) must have the same size, format, and mipmap count.
Future work includes:
* Blending between multiple reflection probes.
* A falloff/fade-out region so that reflected objects disappear
gradually instead of vanishing all at once.
* Irradiance volumes for voxel-based global illumination. This should
reuse much of the reflection probe logic, as they're both GI techniques
based on cuboid bounding regions.
* Support for WebGL 2, by breaking batches when reflection probes are
used.
These issues notwithstanding, I think it's best to land this with
roughly the current set of functionality, because this patch is useful
as is and adding everything above would make the pull request
significantly larger and harder to review.
---
## Changelog
### Added
* A new *LightProbe* component is available that specifies a bounding
region that an *EnvironmentMapLight* applies to. The combination of a
*LightProbe* and an *EnvironmentMapLight* offers *reflection probe*
functionality similar to that available in other engines.
[the corresponding feature in Blender's Eevee renderer]:
https://docs.blender.org/manual/en/latest/render/eevee/light_probes/reflection_cubemaps.html
[`bevy-baked-gi`]: https://github.com/pcwalton/bevy-baked-gi
[glTF IBL Sampler]: https://github.com/KhronosGroup/glTF-IBL-Sampler
2024-01-19 07:33:52 +00:00
|
|
|
if has_environment_maps {
|
EnvironmentMapLight, BRDF Improvements (#7051)
(Before)
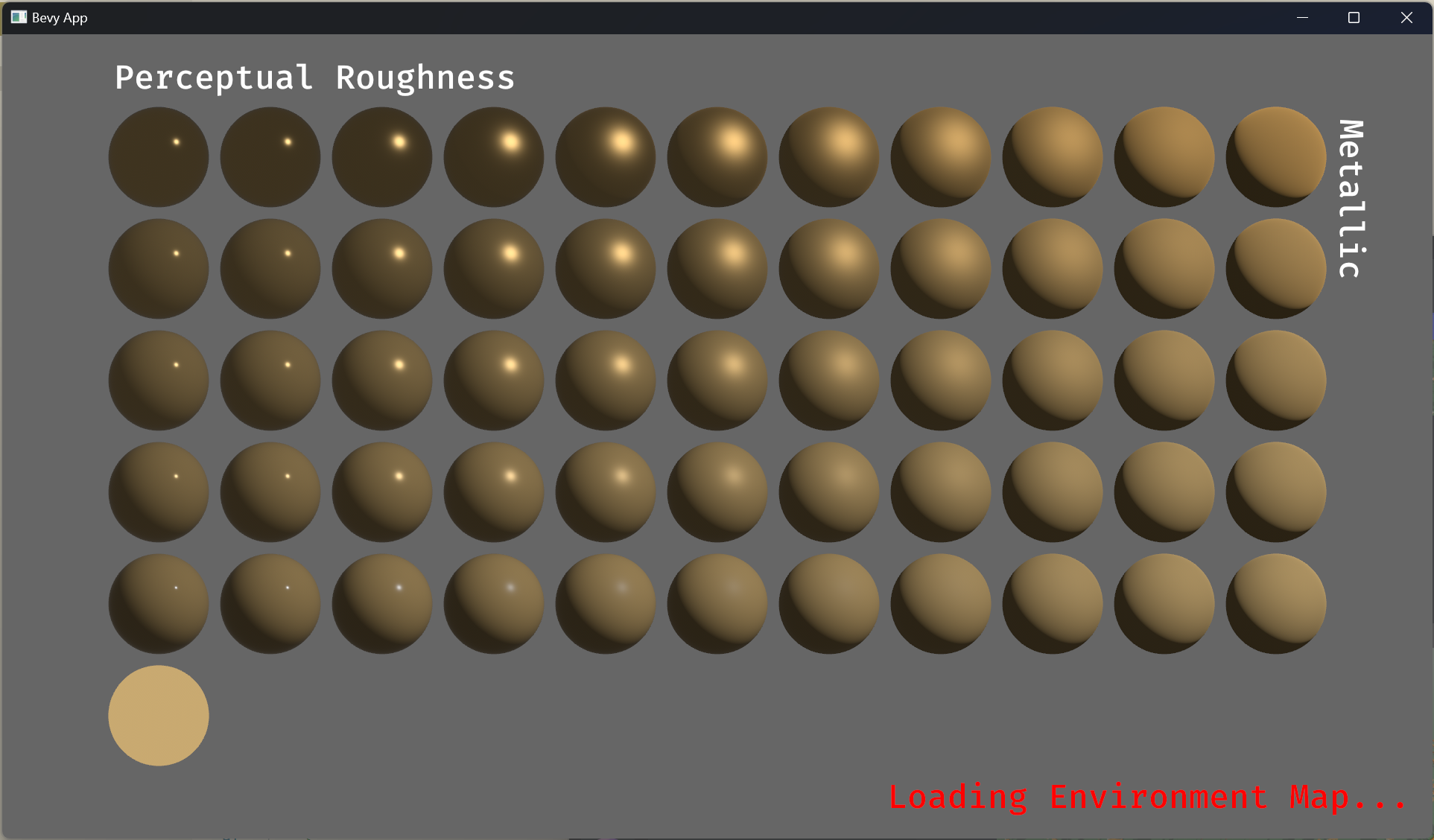
(After)
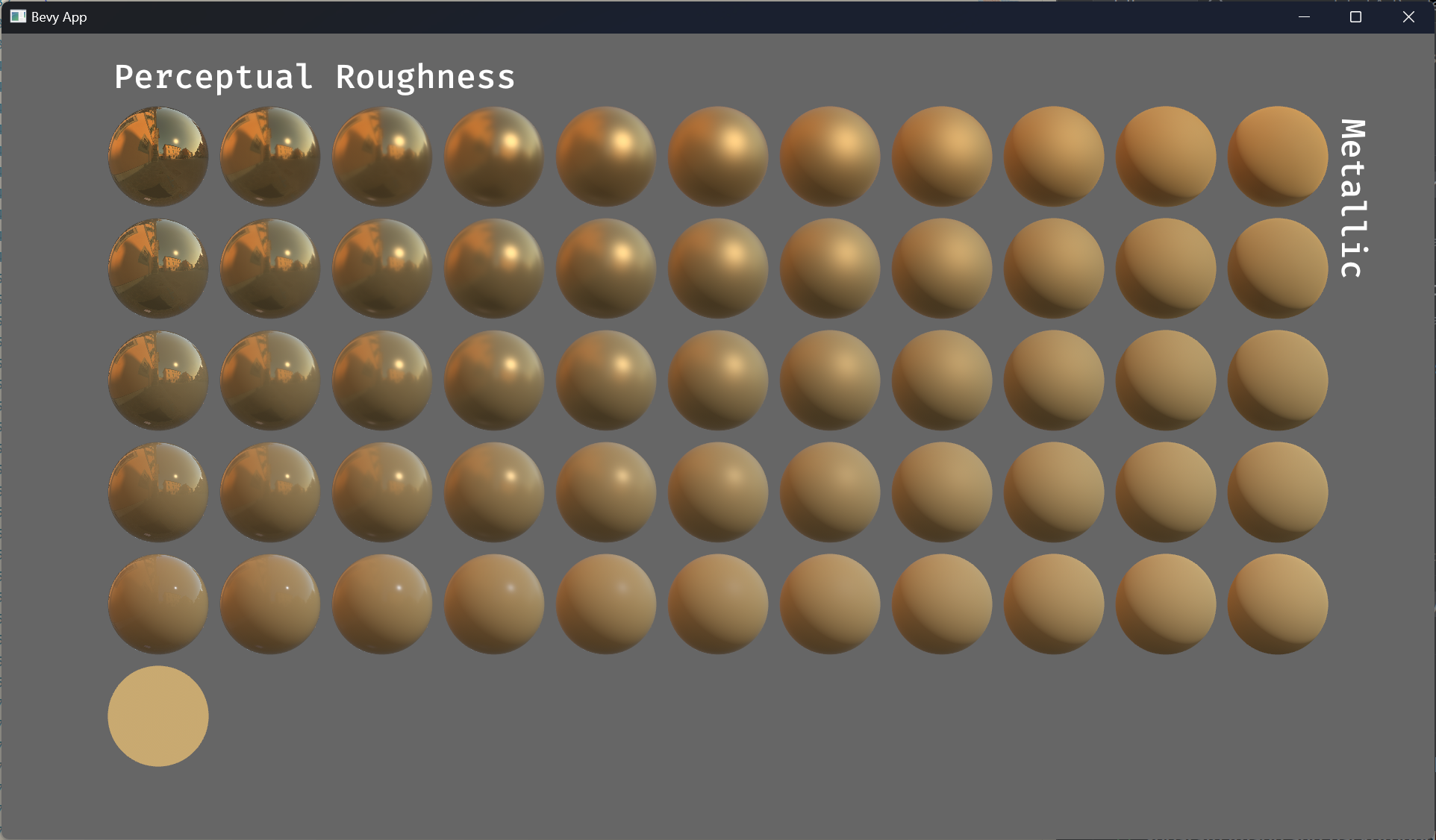
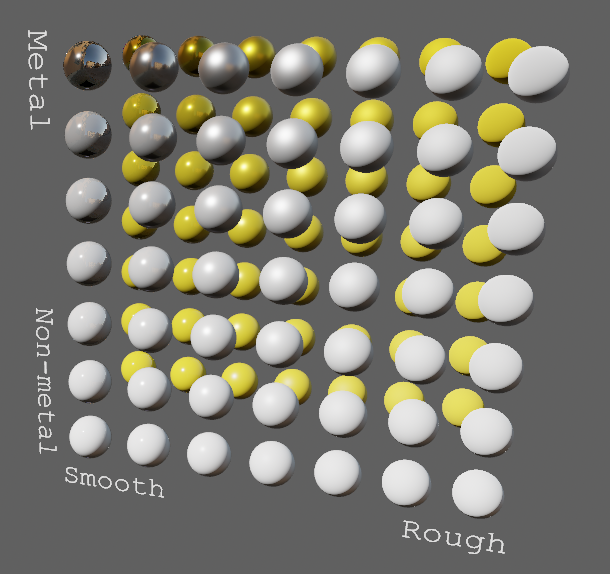
# Objective
- Improve lighting; especially reflections.
- Closes https://github.com/bevyengine/bevy/issues/4581.
## Solution
- Implement environment maps, providing better ambient light.
- Add microfacet multibounce approximation for specular highlights from Filament.
- Occlusion is no longer incorrectly applied to direct lighting. It now only applies to diffuse indirect light. Unsure if it's also supposed to apply to specular indirect light - the glTF specification just says "indirect light". In the case of ambient occlusion, for instance, that's usually only calculated as diffuse though. For now, I'm choosing to apply this just to indirect diffuse light, and not specular.
- Modified the PBR example to use an environment map, and have labels.
- Added `FallbackImageCubemap`.
## Implementation
- IBL technique references can be found in environment_map.wgsl.
- It's more accurate to use a LUT for the scale/bias. Filament has a good reference on generating this LUT. For now, I just used an analytic approximation.
- For now, environment maps must first be prefiltered outside of bevy using a 3rd party tool. See the `EnvironmentMap` documentation.
- Eventually, we should have our own prefiltering code, so that we can have dynamically changing environment maps, as well as let users drop in an HDR image and use asset preprocessing to create the needed textures using only bevy.
---
## Changelog
- Added an `EnvironmentMapLight` camera component that adds additional ambient light to a scene.
- StandardMaterials will now appear brighter and more saturated at high roughness, due to internal material changes. This is more physically correct.
- Fixed StandardMaterial occlusion being incorrectly applied to direct lighting.
- Added `FallbackImageCubemap`.
Co-authored-by: IceSentry <c.giguere42@gmail.com>
Co-authored-by: James Liu <contact@jamessliu.com>
Co-authored-by: Rob Parrett <robparrett@gmail.com>
2023-02-09 16:46:32 +00:00
|
|
|
view_key |= MeshPipelineKey::ENVIRONMENT_MAP;
|
|
|
|
}
|
2023-10-07 17:13:29 +00:00
|
|
|
|
Implement irradiance volumes. (#10268)
# Objective
Bevy could benefit from *irradiance volumes*, also known as *voxel
global illumination* or simply as light probes (though this term is not
preferred, as multiple techniques can be called light probes).
Irradiance volumes are a form of baked global illumination; they work by
sampling the light at the centers of each voxel within a cuboid. At
runtime, the voxels surrounding the fragment center are sampled and
interpolated to produce indirect diffuse illumination.
## Solution
This is divided into two sections. The first is copied and pasted from
the irradiance volume module documentation and describes the technique.
The second part consists of notes on the implementation.
### Overview
An *irradiance volume* is a cuboid voxel region consisting of
regularly-spaced precomputed samples of diffuse indirect light. They're
ideal if you have a dynamic object such as a character that can move
about
static non-moving geometry such as a level in a game, and you want that
dynamic object to be affected by the light bouncing off that static
geometry.
To use irradiance volumes, you need to precompute, or *bake*, the
indirect
light in your scene. Bevy doesn't currently come with a way to do this.
Fortunately, [Blender] provides a [baking tool] as part of the Eevee
renderer, and its irradiance volumes are compatible with those used by
Bevy.
The [`bevy-baked-gi`] project provides a tool, `export-blender-gi`, that
can
extract the baked irradiance volumes from the Blender `.blend` file and
package them up into a `.ktx2` texture for use by the engine. See the
documentation in the `bevy-baked-gi` project for more details as to this
workflow.
Like all light probes in Bevy, irradiance volumes are 1×1×1 cubes that
can
be arbitrarily scaled, rotated, and positioned in a scene with the
[`bevy_transform::components::Transform`] component. The 3D voxel grid
will
be stretched to fill the interior of the cube, and the illumination from
the
irradiance volume will apply to all fragments within that bounding
region.
Bevy's irradiance volumes are based on Valve's [*ambient cubes*] as used
in
*Half-Life 2* ([Mitchell 2006], slide 27). These encode a single color
of
light from the six 3D cardinal directions and blend the sides together
according to the surface normal.
The primary reason for choosing ambient cubes is to match Blender, so
that
its Eevee renderer can be used for baking. However, they also have some
advantages over the common second-order spherical harmonics approach:
ambient cubes don't suffer from ringing artifacts, they are smaller (6
colors for ambient cubes as opposed to 9 for spherical harmonics), and
evaluation is faster. A smaller basis allows for a denser grid of voxels
with the same storage requirements.
If you wish to use a tool other than `export-blender-gi` to produce the
irradiance volumes, you'll need to pack the irradiance volumes in the
following format. The irradiance volume of resolution *(Rx, Ry, Rz)* is
expected to be a 3D texture of dimensions *(Rx, 2Ry, 3Rz)*. The
unnormalized
texture coordinate *(s, t, p)* of the voxel at coordinate *(x, y, z)*
with
side *S* ∈ *{-X, +X, -Y, +Y, -Z, +Z}* is as follows:
```text
s = x
t = y + ⎰ 0 if S ∈ {-X, -Y, -Z}
⎱ Ry if S ∈ {+X, +Y, +Z}
⎧ 0 if S ∈ {-X, +X}
p = z + ⎨ Rz if S ∈ {-Y, +Y}
⎩ 2Rz if S ∈ {-Z, +Z}
```
Visually, in a left-handed coordinate system with Y up, viewed from the
right, the 3D texture looks like a stacked series of voxel grids, one
for
each cube side, in this order:
| **+X** | **+Y** | **+Z** |
| ------ | ------ | ------ |
| **-X** | **-Y** | **-Z** |
A terminology note: Other engines may refer to irradiance volumes as
*voxel
global illumination*, *VXGI*, or simply as *light probes*. Sometimes
*light
probe* refers to what Bevy calls a reflection probe. In Bevy, *light
probe*
is a generic term that encompasses all cuboid bounding regions that
capture
indirect illumination, whether based on voxels or not.
Note that, if binding arrays aren't supported (e.g. on WebGPU or WebGL
2),
then only the closest irradiance volume to the view will be taken into
account during rendering.
[*ambient cubes*]:
https://advances.realtimerendering.com/s2006/Mitchell-ShadingInValvesSourceEngine.pdf
[Mitchell 2006]:
https://advances.realtimerendering.com/s2006/Mitchell-ShadingInValvesSourceEngine.pdf
[Blender]: http://blender.org/
[baking tool]:
https://docs.blender.org/manual/en/latest/render/eevee/render_settings/indirect_lighting.html
[`bevy-baked-gi`]: https://github.com/pcwalton/bevy-baked-gi
### Implementation notes
This patch generalizes light probes so as to reuse as much code as
possible between irradiance volumes and the existing reflection probes.
This approach was chosen because both techniques share numerous
similarities:
1. Both irradiance volumes and reflection probes are cuboid bounding
regions.
2. Both are responsible for providing baked indirect light.
3. Both techniques involve presenting a variable number of textures to
the shader from which indirect light is sampled. (In the current
implementation, this uses binding arrays.)
4. Both irradiance volumes and reflection probes require gathering and
sorting probes by distance on CPU.
5. Both techniques require the GPU to search through a list of bounding
regions.
6. Both will eventually want to have falloff so that we can smoothly
blend as objects enter and exit the probes' influence ranges. (This is
not implemented yet to keep this patch relatively small and reviewable.)
To do this, we generalize most of the methods in the reflection probes
patch #11366 to be generic over a trait, `LightProbeComponent`. This
trait is implemented by both `EnvironmentMapLight` (for reflection
probes) and `IrradianceVolume` (for irradiance volumes). Using a trait
will allow us to add more types of light probes in the future. In
particular, I highly suspect we will want real-time reflection planes
for mirrors in the future, which can be easily slotted into this
framework.
## Changelog
> This section is optional. If this was a trivial fix, or has no
externally-visible impact, you can delete this section.
### Added
* A new `IrradianceVolume` asset type is available for baked voxelized
light probes. You can bake the global illumination using Blender or
another tool of your choice and use it in Bevy to apply indirect
illumination to dynamic objects.
2024-02-06 23:23:20 +00:00
|
|
|
if has_irradiance_volumes {
|
|
|
|
view_key |= MeshPipelineKey::IRRADIANCE_VOLUME;
|
|
|
|
}
|
|
|
|
|
2024-10-07 23:50:28 +00:00
|
|
|
if has_oit {
|
|
|
|
view_key |= MeshPipelineKey::OIT_ENABLED;
|
|
|
|
}
|
|
|
|
|
2023-10-24 21:26:19 +00:00
|
|
|
if let Some(projection) = projection {
|
|
|
|
view_key |= match projection {
|
|
|
|
Projection::Perspective(_) => MeshPipelineKey::VIEW_PROJECTION_PERSPECTIVE,
|
|
|
|
Projection::Orthographic(_) => MeshPipelineKey::VIEW_PROJECTION_ORTHOGRAPHIC,
|
|
|
|
};
|
|
|
|
}
|
|
|
|
|
2023-10-07 17:13:29 +00:00
|
|
|
match shadow_filter_method.unwrap_or(&ShadowFilteringMethod::default()) {
|
|
|
|
ShadowFilteringMethod::Hardware2x2 => {
|
|
|
|
view_key |= MeshPipelineKey::SHADOW_FILTER_METHOD_HARDWARE_2X2;
|
|
|
|
}
|
Implement percentage-closer filtering (PCF) for point lights. (#12910)
I ported the two existing PCF techniques to the cubemap domain as best I
could. Generally, the technique is to create a 2D orthonormal basis
using Gram-Schmidt normalization, then apply the technique over that
basis. The results look fine, though the shadow bias often needs
adjusting.
For comparison, Unity uses a 4-tap pattern for PCF on point lights of
(1, 1, 1), (-1, -1, 1), (-1, 1, -1), (1, -1, -1). I tried this but
didn't like the look, so I went with the design above, which ports the
2D techniques to the 3D domain. There's surprisingly little material on
point light PCF.
I've gone through every example using point lights and verified that the
shadow maps look fine, adjusting biases as necessary.
Fixes #3628.
---
## Changelog
### Added
* Shadows from point lights now support percentage-closer filtering
(PCF), and as a result look less aliased.
### Changed
* `ShadowFilteringMethod::Castano13` and
`ShadowFilteringMethod::Jimenez14` have been renamed to
`ShadowFilteringMethod::Gaussian` and `ShadowFilteringMethod::Temporal`
respectively.
## Migration Guide
* `ShadowFilteringMethod::Castano13` and
`ShadowFilteringMethod::Jimenez14` have been renamed to
`ShadowFilteringMethod::Gaussian` and `ShadowFilteringMethod::Temporal`
respectively.
2024-04-10 20:16:08 +00:00
|
|
|
ShadowFilteringMethod::Gaussian => {
|
|
|
|
view_key |= MeshPipelineKey::SHADOW_FILTER_METHOD_GAUSSIAN;
|
2023-10-07 17:13:29 +00:00
|
|
|
}
|
Implement percentage-closer filtering (PCF) for point lights. (#12910)
I ported the two existing PCF techniques to the cubemap domain as best I
could. Generally, the technique is to create a 2D orthonormal basis
using Gram-Schmidt normalization, then apply the technique over that
basis. The results look fine, though the shadow bias often needs
adjusting.
For comparison, Unity uses a 4-tap pattern for PCF on point lights of
(1, 1, 1), (-1, -1, 1), (-1, 1, -1), (1, -1, -1). I tried this but
didn't like the look, so I went with the design above, which ports the
2D techniques to the 3D domain. There's surprisingly little material on
point light PCF.
I've gone through every example using point lights and verified that the
shadow maps look fine, adjusting biases as necessary.
Fixes #3628.
---
## Changelog
### Added
* Shadows from point lights now support percentage-closer filtering
(PCF), and as a result look less aliased.
### Changed
* `ShadowFilteringMethod::Castano13` and
`ShadowFilteringMethod::Jimenez14` have been renamed to
`ShadowFilteringMethod::Gaussian` and `ShadowFilteringMethod::Temporal`
respectively.
## Migration Guide
* `ShadowFilteringMethod::Castano13` and
`ShadowFilteringMethod::Jimenez14` have been renamed to
`ShadowFilteringMethod::Gaussian` and `ShadowFilteringMethod::Temporal`
respectively.
2024-04-10 20:16:08 +00:00
|
|
|
ShadowFilteringMethod::Temporal => {
|
|
|
|
view_key |= MeshPipelineKey::SHADOW_FILTER_METHOD_TEMPORAL;
|
2023-10-07 17:13:29 +00:00
|
|
|
}
|
|
|
|
}
|
|
|
|
|
2023-02-19 20:38:13 +00:00
|
|
|
if !view.hdr {
|
|
|
|
if let Some(tonemapping) = tonemapping {
|
2022-10-26 20:13:59 +00:00
|
|
|
view_key |= MeshPipelineKey::TONEMAP_IN_SHADER;
|
2023-09-20 20:18:55 +00:00
|
|
|
view_key |= tonemapping_pipeline_key(*tonemapping);
|
2023-02-19 20:38:13 +00:00
|
|
|
}
|
|
|
|
if let Some(DebandDither::Enabled) = dither {
|
|
|
|
view_key |= MeshPipelineKey::DEBAND_DITHER;
|
2022-10-26 20:13:59 +00:00
|
|
|
}
|
|
|
|
}
|
2023-12-30 23:27:48 +00:00
|
|
|
if ssao {
|
Screen Space Ambient Occlusion (SSAO) MVP (#7402)

# Objective
- Add Screen space ambient occlusion (SSAO). SSAO approximates
small-scale, local occlusion of _indirect_ diffuse light between
objects. SSAO does not apply to direct lighting, such as point or
directional lights.
- This darkens creases, e.g. on staircases, and gives nice contact
shadows where objects meet, giving entities a more "grounded" feel.
- Closes https://github.com/bevyengine/bevy/issues/3632.
## Solution
- Implement the GTAO algorithm.
-
https://www.activision.com/cdn/research/Practical_Real_Time_Strategies_for_Accurate_Indirect_Occlusion_NEW%20VERSION_COLOR.pdf
-
https://blog.selfshadow.com/publications/s2016-shading-course/activision/s2016_pbs_activision_occlusion.pdf
- Source code heavily based on [Intel's
XeGTAO](https://github.com/GameTechDev/XeGTAO/blob/0d177ce06bfa642f64d8af4de1197ad1bcb862d4/Source/Rendering/Shaders/XeGTAO.hlsli).
- Add an SSAO bevy example.
## Algorithm Overview
* Run a depth and normal prepass
* Create downscaled mips of the depth texture (preprocess_depths pass)
* GTAO pass - for each pixel, take several random samples from the
depth+normal buffers, reconstruct world position, raytrace in screen
space to estimate occlusion. Rather then doing completely random samples
on a hemisphere, you choose random _slices_ of the hemisphere, and then
can analytically compute the full occlusion of that slice. Also compute
edges based on depth differences here.
* Spatial denoise pass - bilateral blur, using edge detection to not
blur over edges. This is the final SSAO result.
* Main pass - if SSAO exists, sample the SSAO texture, and set occlusion
to be the minimum of ssao/material occlusion. This then feeds into the
rest of the PBR shader as normal.
---
## Future Improvements
- Maybe remove the low quality preset for now (too noisy)
- WebGPU fallback (see below)
- Faster depth->world position (see reverted code)
- Bent normals
- Try interleaved gradient noise or spatiotemporal blue noise
- Replace the spatial denoiser with a combined spatial+temporal denoiser
- Render at half resolution and use a bilateral upsample
- Better multibounce approximation
(https://drive.google.com/file/d/1SyagcEVplIm2KkRD3WQYSO9O0Iyi1hfy/view)
## Far-Future Performance Improvements
- F16 math (missing naga-wgsl support
https://github.com/gfx-rs/naga/issues/1884)
- Faster coordinate space conversion for normals
- Faster depth mipchain creation
(https://github.com/GPUOpen-Effects/FidelityFX-SPD) (wgpu/naga does not
currently support subgroup ops)
- Deinterleaved SSAO for better cache efficiency
(https://developer.nvidia.com/sites/default/files/akamai/gameworks/samples/DeinterleavedTexturing.pdf)
## Other Interesting Papers
- Visibility bitmask
(https://link.springer.com/article/10.1007/s00371-022-02703-y,
https://cdrinmatane.github.io/posts/cgspotlight-slides/)
- Screen space diffuse lighting
(https://github.com/Patapom/GodComplex/blob/master/Tests/TestHBIL/2018%20Mayaux%20-%20Horizon-Based%20Indirect%20Lighting%20(HBIL).pdf)
## Platform Support
* SSAO currently does not work on DirectX12 due to issues with wgpu and
naga:
* https://github.com/gfx-rs/wgpu/pull/3798
* https://github.com/gfx-rs/naga/pull/2353
* SSAO currently does not work on WebGPU because r16float is not a valid
storage texture format
https://gpuweb.github.io/gpuweb/wgsl/#storage-texel-formats. We can fix
this with a fallback to r32float.
---
## Changelog
- Added ScreenSpaceAmbientOcclusionSettings,
ScreenSpaceAmbientOcclusionQualityLevel, and
ScreenSpaceAmbientOcclusionBundle
---------
Co-authored-by: IceSentry <c.giguere42@gmail.com>
Co-authored-by: IceSentry <IceSentry@users.noreply.github.com>
Co-authored-by: Daniel Chia <danstryder@gmail.com>
Co-authored-by: Elabajaba <Elabajaba@users.noreply.github.com>
Co-authored-by: Robert Swain <robert.swain@gmail.com>
Co-authored-by: robtfm <50659922+robtfm@users.noreply.github.com>
Co-authored-by: Brandon Dyer <brandondyer64@gmail.com>
Co-authored-by: Edgar Geier <geieredgar@gmail.com>
Co-authored-by: Nicola Papale <nicopap@users.noreply.github.com>
Co-authored-by: Carter Anderson <mcanders1@gmail.com>
2023-06-18 21:05:55 +00:00
|
|
|
view_key |= MeshPipelineKey::SCREEN_SPACE_AMBIENT_OCCLUSION;
|
|
|
|
}
|
`StandardMaterial` Light Transmission (#8015)
# Objective
<img width="1920" alt="Screenshot 2023-04-26 at 01 07 34"
src="https://user-images.githubusercontent.com/418473/234467578-0f34187b-5863-4ea1-88e9-7a6bb8ce8da3.png">
This PR adds both diffuse and specular light transmission capabilities
to the `StandardMaterial`, with support for screen space refractions.
This enables realistically representing a wide range of real-world
materials, such as:
- Glass; (Including frosted glass)
- Transparent and translucent plastics;
- Various liquids and gels;
- Gemstones;
- Marble;
- Wax;
- Paper;
- Leaves;
- Porcelain.
Unlike existing support for transparency, light transmission does not
rely on fixed function alpha blending, and therefore works with both
`AlphaMode::Opaque` and `AlphaMode::Mask` materials.
## Solution
- Introduces a number of transmission related fields in the
`StandardMaterial`;
- For specular transmission:
- Adds logic to take a view main texture snapshot after the opaque
phase; (in order to perform screen space refractions)
- Introduces a new `Transmissive3d` phase to the renderer, to which all
meshes with `transmission > 0.0` materials are sent.
- Calculates a light exit point (of the approximate mesh volume) using
`ior` and `thickness` properties
- Samples the snapshot texture with an adaptive number of taps across a
`roughness`-controlled radius enabling “blurry” refractions
- For diffuse transmission:
- Approximates transmitted diffuse light by using a second, flipped +
displaced, diffuse-only Lambertian lobe for each light source.
## To Do
- [x] Figure out where `fresnel_mix()` is taking place, if at all, and
where `dielectric_specular` is being calculated, if at all, and update
them to use the `ior` value (Not a blocker, just a nice-to-have for more
correct BSDF)
- To the _best of my knowledge, this is now taking place, after
964340cdd. The fresnel mix is actually "split" into two parts in our
implementation, one `(1 - fresnel(...))` in the transmission, and
`fresnel()` in the light implementations. A surface with more
reflectance now will produce slightly dimmer transmission towards the
grazing angle, as more of the light gets reflected.
- [x] Add `transmission_texture`
- [x] Add `diffuse_transmission_texture`
- [x] Add `thickness_texture`
- [x] Add `attenuation_distance` and `attenuation_color`
- [x] Connect values to glTF loader
- [x] `transmission` and `transmission_texture`
- [x] `thickness` and `thickness_texture`
- [x] `ior`
- [ ] `diffuse_transmission` and `diffuse_transmission_texture` (needs
upstream support in `gltf` crate, not a blocker)
- [x] Add support for multiple screen space refraction “steps”
- [x] Conditionally create no transmission snapshot texture at all if
`steps == 0`
- [x] Conditionally enable/disable screen space refraction transmission
snapshots
- [x] Read from depth pre-pass to prevent refracting pixels in front of
the light exit point
- [x] Use `interleaved_gradient_noise()` function for sampling blur in a
way that benefits from TAA
- [x] Drill down a TAA `#define`, tweak some aspects of the effect
conditionally based on it
- [x] Remove const array that's crashing under HLSL (unless a new `naga`
release with https://github.com/gfx-rs/naga/pull/2496 comes out before
we merge this)
- [ ] Look into alternatives to the `switch` hack for dynamically
indexing the const array (might not be needed, compilers seem to be
decent at expanding it)
- [ ] Add pipeline keys for gating transmission (do we really want/need
this?)
- [x] Tweak some material field/function names?
## A Note on Texture Packing
_This was originally added as a comment to the
`specular_transmission_texture`, `thickness_texture` and
`diffuse_transmission_texture` documentation, I removed it since it was
more confusing than helpful, and will likely be made redundant/will need
to be updated once we have a better infrastructure for preprocessing
assets_
Due to how channels are mapped, you can more efficiently use a single
shared texture image
for configuring the following:
- R - `specular_transmission_texture`
- G - `thickness_texture`
- B - _unused_
- A - `diffuse_transmission_texture`
The `KHR_materials_diffuse_transmission` glTF extension also defines a
`diffuseTransmissionColorTexture`,
that _we don't currently support_. One might choose to pack the
intensity and color textures together,
using RGB for the color and A for the intensity, in which case this
packing advice doesn't really apply.
---
## Changelog
- Added a new `Transmissive3d` render phase for rendering specular
transmissive materials with screen space refractions
- Added rendering support for transmitted environment map light on the
`StandardMaterial` as a fallback for screen space refractions
- Added `diffuse_transmission`, `specular_transmission`, `thickness`,
`ior`, `attenuation_distance` and `attenuation_color` to the
`StandardMaterial`
- Added `diffuse_transmission_texture`, `specular_transmission_texture`,
`thickness_texture` to the `StandardMaterial`, gated behind a new
`pbr_transmission_textures` cargo feature (off by default, for maximum
hardware compatibility)
- Added `Camera3d::screen_space_specular_transmission_steps` for
controlling the number of “layers of transparency” rendered for
transmissive objects
- Added a `TransmittedShadowReceiver` component for enabling shadows in
(diffusely) transmitted light. (disabled by default, as it requires
carefully setting up the `thickness` to avoid self-shadow artifacts)
- Added support for the `KHR_materials_transmission`,
`KHR_materials_ior` and `KHR_materials_volume` glTF extensions
- Renamed items related to temporal jitter for greater consistency
## Migration Guide
- `SsaoPipelineKey::temporal_noise` has been renamed to
`SsaoPipelineKey::temporal_jitter`
- The `TAA` shader def (controlled by the presence of the
`TemporalAntiAliasSettings` component in the camera) has been replaced
with the `TEMPORAL_JITTER` shader def (controlled by the presence of the
`TemporalJitter` component in the camera)
- `MeshPipelineKey::TAA` has been replaced by
`MeshPipelineKey::TEMPORAL_JITTER`
- The `TEMPORAL_NOISE` shader def has been consolidated with
`TEMPORAL_JITTER`
2023-10-31 20:59:02 +00:00
|
|
|
if let Some(camera_3d) = camera_3d {
|
|
|
|
view_key |= screen_space_specular_transmission_pipeline_key(
|
|
|
|
camera_3d.screen_space_specular_transmission_quality,
|
|
|
|
);
|
|
|
|
}
|
2024-03-01 20:56:21 +00:00
|
|
|
|
EnvironmentMapLight, BRDF Improvements (#7051)
(Before)
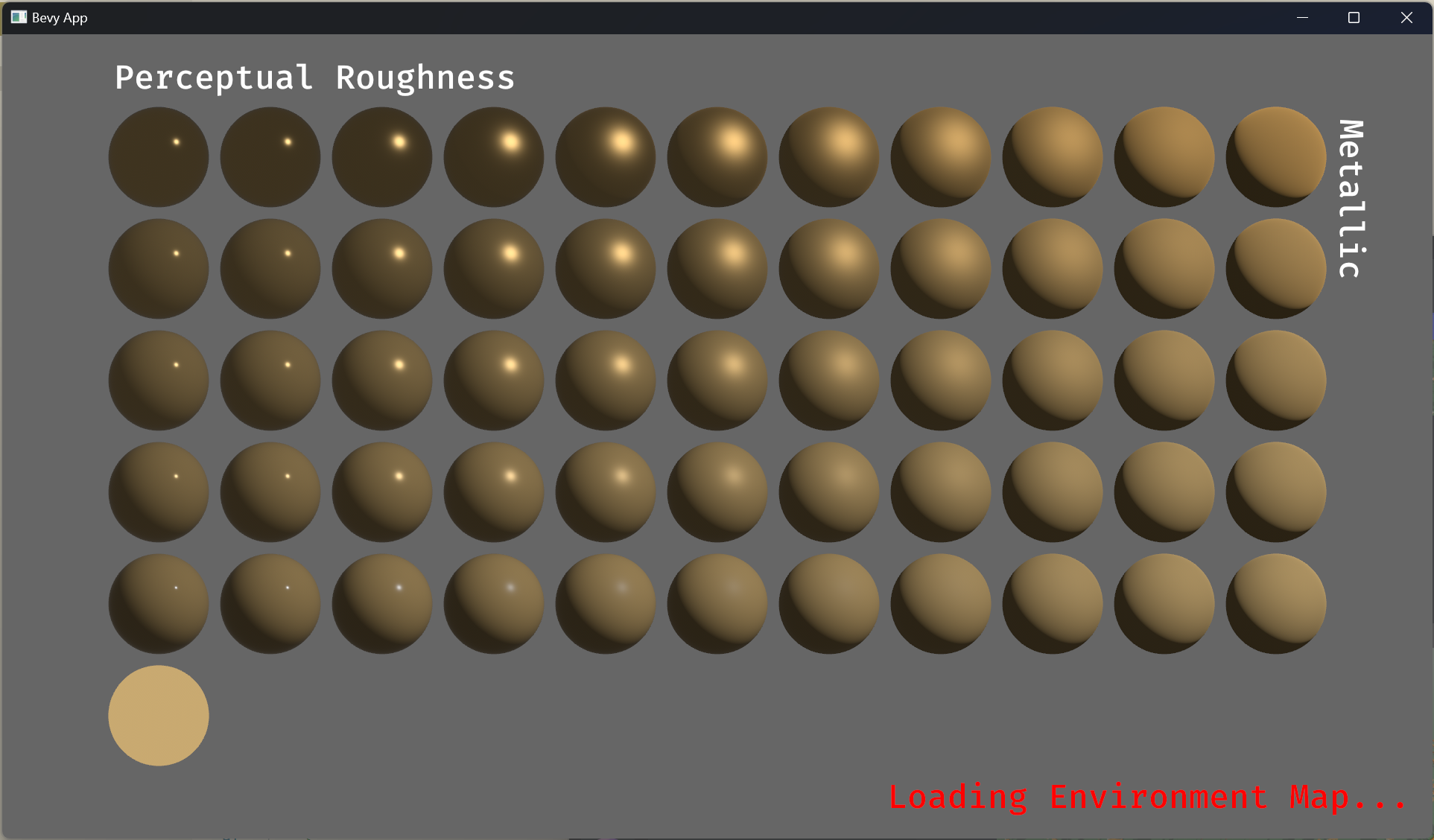
(After)
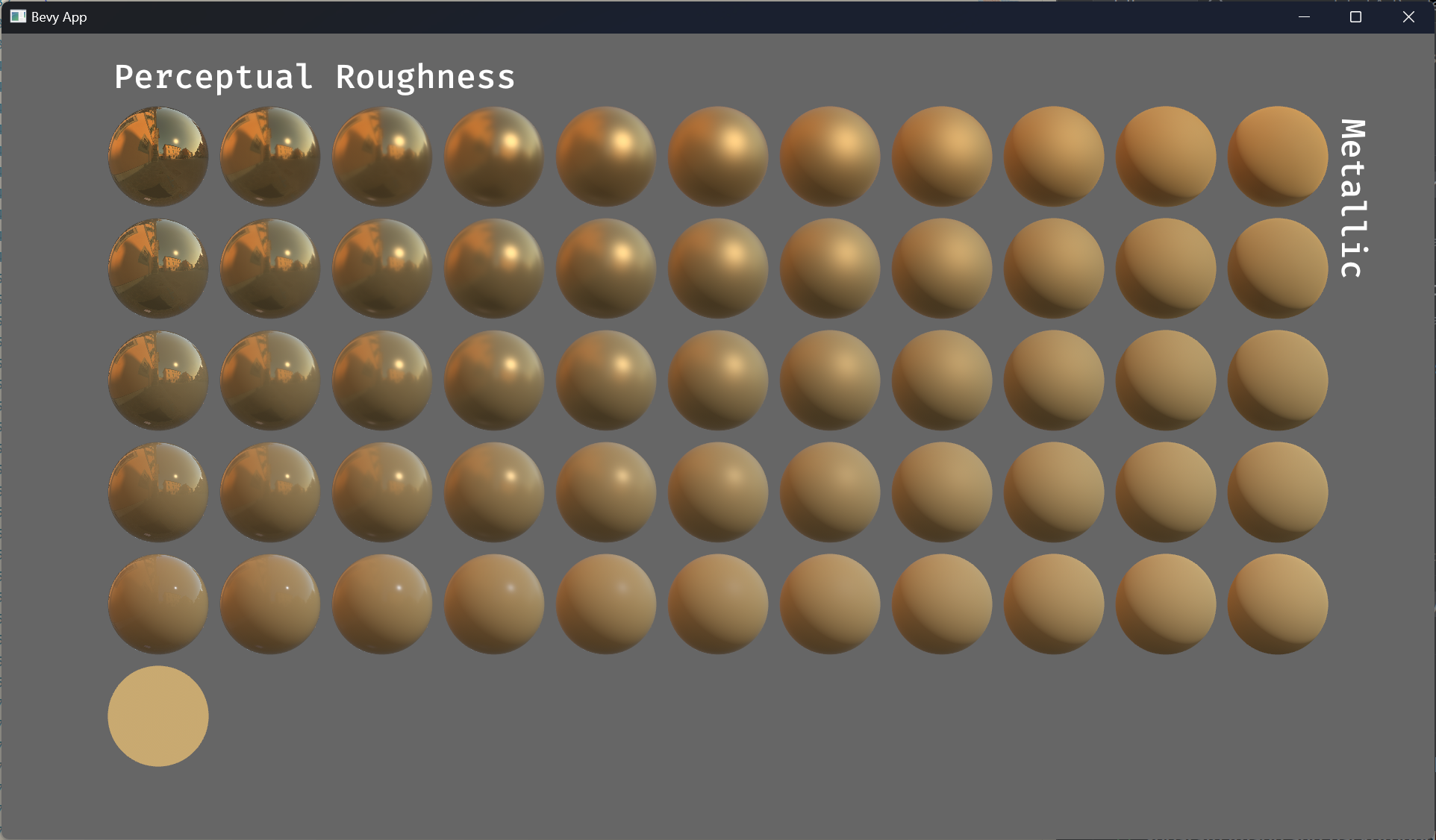
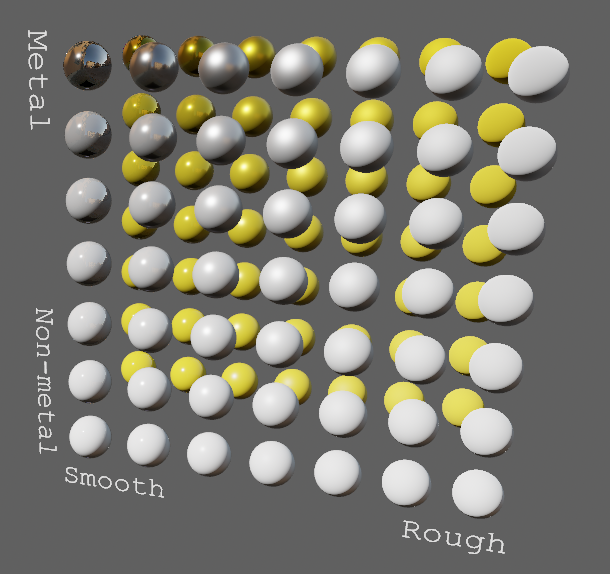
# Objective
- Improve lighting; especially reflections.
- Closes https://github.com/bevyengine/bevy/issues/4581.
## Solution
- Implement environment maps, providing better ambient light.
- Add microfacet multibounce approximation for specular highlights from Filament.
- Occlusion is no longer incorrectly applied to direct lighting. It now only applies to diffuse indirect light. Unsure if it's also supposed to apply to specular indirect light - the glTF specification just says "indirect light". In the case of ambient occlusion, for instance, that's usually only calculated as diffuse though. For now, I'm choosing to apply this just to indirect diffuse light, and not specular.
- Modified the PBR example to use an environment map, and have labels.
- Added `FallbackImageCubemap`.
## Implementation
- IBL technique references can be found in environment_map.wgsl.
- It's more accurate to use a LUT for the scale/bias. Filament has a good reference on generating this LUT. For now, I just used an analytic approximation.
- For now, environment maps must first be prefiltered outside of bevy using a 3rd party tool. See the `EnvironmentMap` documentation.
- Eventually, we should have our own prefiltering code, so that we can have dynamically changing environment maps, as well as let users drop in an HDR image and use asset preprocessing to create the needed textures using only bevy.
---
## Changelog
- Added an `EnvironmentMapLight` camera component that adds additional ambient light to a scene.
- StandardMaterials will now appear brighter and more saturated at high roughness, due to internal material changes. This is more physically correct.
- Fixed StandardMaterial occlusion being incorrectly applied to direct lighting.
- Added `FallbackImageCubemap`.
Co-authored-by: IceSentry <c.giguere42@gmail.com>
Co-authored-by: James Liu <contact@jamessliu.com>
Co-authored-by: Rob Parrett <robparrett@gmail.com>
2023-02-09 16:46:32 +00:00
|
|
|
let rangefinder = view.rangefinder3d();
|
Type safe retained render world (#15756)
# Objective
In the Render World, there are a number of collections that are derived
from Main World entities and are used to drive rendering. The most
notable are:
- `VisibleEntities`, which is generated in the `check_visibility` system
and contains visible entities for a view.
- `ExtractedInstances`, which maps entity ids to asset ids.
In the old model, these collections were trivially kept in sync -- any
extracted phase item could look itself up because the render entity id
was guaranteed to always match the corresponding main world id.
After #15320, this became much more complicated, and was leading to a
number of subtle bugs in the Render World. The main rendering systems,
i.e. `queue_material_meshes` and `queue_material2d_meshes`, follow a
similar pattern:
```rust
for visible_entity in visible_entities.iter::<With<Mesh2d>>() {
let Some(mesh_instance) = render_mesh_instances.get_mut(visible_entity) else {
continue;
};
// Look some more stuff up and specialize the pipeline...
let bin_key = Opaque2dBinKey {
pipeline: pipeline_id,
draw_function: draw_opaque_2d,
asset_id: mesh_instance.mesh_asset_id.into(),
material_bind_group_id: material_2d.get_bind_group_id().0,
};
opaque_phase.add(
bin_key,
*visible_entity,
BinnedRenderPhaseType::mesh(mesh_instance.automatic_batching),
);
}
```
In this case, `visible_entities` and `render_mesh_instances` are both
collections that are created and keyed by Main World entity ids, and so
this lookup happens to work by coincidence. However, there is a major
unintentional bug here: namely, because `visible_entities` is a
collection of Main World ids, the phase item being queued is created
with a Main World id rather than its correct Render World id.
This happens to not break mesh rendering because the render commands
used for drawing meshes do not access the `ItemQuery` parameter, but
demonstrates the confusion that is now possible: our UI phase items are
correctly being queued with Render World ids while our meshes aren't.
Additionally, this makes it very easy and error prone to use the wrong
entity id to look up things like assets. For example, if instead we
ignored visibility checks and queued our meshes via a query, we'd have
to be extra careful to use `&MainEntity` instead of the natural
`Entity`.
## Solution
Make all collections that are derived from Main World data use
`MainEntity` as their key, to ensure type safety and avoid accidentally
looking up data with the wrong entity id:
```rust
pub type MainEntityHashMap<V> = hashbrown::HashMap<MainEntity, V, EntityHash>;
```
Additionally, we make all `PhaseItem` be able to provide both their Main
and Render World ids, to allow render phase implementors maximum
flexibility as to what id should be used to look up data.
You can think of this like tracking at the type level whether something
in the Render World should use it's "primary key", i.e. entity id, or
needs to use a foreign key, i.e. `MainEntity`.
## Testing
##### TODO:
This will require extensive testing to make sure things didn't break!
Additionally, some extraction logic has become more complicated and
needs to be checked for regressions.
## Migration Guide
With the advent of the retained render world, collections that contain
references to `Entity` that are extracted into the render world have
been changed to contain `MainEntity` in order to prevent errors where a
render world entity id is used to look up an item by accident. Custom
rendering code may need to be changed to query for `&MainEntity` in
order to look up the correct item from such a collection. Additionally,
users who implement their own extraction logic for collections of main
world entity should strongly consider extracting into a different
collection that uses `MainEntity` as a key.
Additionally, render phases now require specifying both the `Entity` and
`MainEntity` for a given `PhaseItem`. Custom render phases should ensure
`MainEntity` is available when queuing a phase item.
2024-10-10 18:47:04 +00:00
|
|
|
for (render_entity, visible_entity) in visible_entities.iter::<With<Mesh3d>>() {
|
Use EntityHashMap<Entity, T> for render world entity storage for better performance (#9903)
# Objective
- Improve rendering performance, particularly by avoiding the large
system commands costs of using the ECS in the way that the render world
does.
## Solution
- Define `EntityHasher` that calculates a hash from the
`Entity.to_bits()` by `i | (i.wrapping_mul(0x517cc1b727220a95) << 32)`.
`0x517cc1b727220a95` is something like `u64::MAX / N` for N that gives a
value close to π and that works well for hashing. Thanks for @SkiFire13
for the suggestion and to @nicopap for alternative suggestions and
discussion. This approach comes from `rustc-hash` (a.k.a. `FxHasher`)
with some tweaks for the case of hashing an `Entity`. `FxHasher` and
`SeaHasher` were also tested but were significantly slower.
- Define `EntityHashMap` type that uses the `EntityHashser`
- Use `EntityHashMap<Entity, T>` for render world entity storage,
including:
- `RenderMaterialInstances` - contains the `AssetId<M>` of the material
associated with the entity. Also for 2D.
- `RenderMeshInstances` - contains mesh transforms, flags and properties
about mesh entities. Also for 2D.
- `SkinIndices` and `MorphIndices` - contains the skin and morph index
for an entity, respectively
- `ExtractedSprites`
- `ExtractedUiNodes`
## Benchmarks
All benchmarks have been conducted on an M1 Max connected to AC power.
The tests are run for 1500 frames. The 1000th frame is captured for
comparison to check for visual regressions. There were none.
### 2D Meshes
`bevymark --benchmark --waves 160 --per-wave 1000 --mode mesh2d`
#### `--ordered-z`
This test spawns the 2D meshes with z incrementing back to front, which
is the ideal arrangement allocation order as it matches the sorted
render order which means lookups have a high cache hit rate.
<img width="1112" alt="Screenshot 2023-09-27 at 07 50 45"
src="https://github.com/bevyengine/bevy/assets/302146/e140bc98-7091-4a3b-8ae1-ab75d16d2ccb">
-39.1% median frame time.
#### Random
This test spawns the 2D meshes with random z. This not only makes the
batching and transparent 2D pass lookups get a lot of cache misses, it
also currently means that the meshes are almost certain to not be
batchable.
<img width="1108" alt="Screenshot 2023-09-27 at 07 51 28"
src="https://github.com/bevyengine/bevy/assets/302146/29c2e813-645a-43ce-982a-55df4bf7d8c4">
-7.2% median frame time.
### 3D Meshes
`many_cubes --benchmark`
<img width="1112" alt="Screenshot 2023-09-27 at 07 51 57"
src="https://github.com/bevyengine/bevy/assets/302146/1a729673-3254-4e2a-9072-55e27c69f0fc">
-7.7% median frame time.
### Sprites
**NOTE: On `main` sprites are using `SparseSet<Entity, T>`!**
`bevymark --benchmark --waves 160 --per-wave 1000 --mode sprite`
#### `--ordered-z`
This test spawns the sprites with z incrementing back to front, which is
the ideal arrangement allocation order as it matches the sorted render
order which means lookups have a high cache hit rate.
<img width="1116" alt="Screenshot 2023-09-27 at 07 52 31"
src="https://github.com/bevyengine/bevy/assets/302146/bc8eab90-e375-4d31-b5cd-f55f6f59ab67">
+13.0% median frame time.
#### Random
This test spawns the sprites with random z. This makes the batching and
transparent 2D pass lookups get a lot of cache misses.
<img width="1109" alt="Screenshot 2023-09-27 at 07 53 01"
src="https://github.com/bevyengine/bevy/assets/302146/22073f5d-99a7-49b0-9584-d3ac3eac3033">
+0.6% median frame time.
### UI
**NOTE: On `main` UI is using `SparseSet<Entity, T>`!**
`many_buttons`
<img width="1111" alt="Screenshot 2023-09-27 at 07 53 26"
src="https://github.com/bevyengine/bevy/assets/302146/66afd56d-cbe4-49e7-8b64-2f28f6043d85">
+15.1% median frame time.
## Alternatives
- Cart originally suggested trying out `SparseSet<Entity, T>` and indeed
that is slightly faster under ideal conditions. However,
`PassHashMap<Entity, T>` has better worst case performance when data is
randomly distributed, rather than in sorted render order, and does not
have the worst case memory usage that `SparseSet`'s dense `Vec<usize>`
that maps from the `Entity` index to sparse index into `Vec<T>`. This
dense `Vec` has to be as large as the largest Entity index used with the
`SparseSet`.
- I also tested `PassHashMap<u32, T>`, intending to use `Entity.index()`
as the key, but this proved to sometimes be slower and mostly no
different.
- The only outstanding approach that has not been implemented and tested
is to _not_ clear the render world of its entities each frame. That has
its own problems, though they could perhaps be solved.
- Performance-wise, if the entities and their component data were not
cleared, then they would incur table moves on spawn, and should not
thereafter, rather just their component data would be overwritten.
Ideally we would have a neat way of either updating data in-place via
`&mut T` queries, or inserting components if not present. This would
likely be quite cumbersome to have to remember to do everywhere, but
perhaps it only needs to be done in the more performance-sensitive
systems.
- The main problem to solve however is that we want to both maintain a
mapping between main world entities and render world entities, be able
to run the render app and world in parallel with the main app and world
for pipelined rendering, and at the same time be able to spawn entities
in the render world in such a way that those Entity ids do not collide
with those spawned in the main world. This is potentially quite
solvable, but could well be a lot of ECS work to do it in a way that
makes sense.
---
## Changelog
- Changed: Component data for entities to be drawn are no longer stored
on entities in the render world. Instead, data is stored in a
`EntityHashMap<Entity, T>` in various resources. This brings significant
performance benefits due to the way the render app clears entities every
frame. Resources of most interest are `RenderMeshInstances` and
`RenderMaterialInstances`, and their 2D counterparts.
## Migration Guide
Previously the render app extracted mesh entities and their component
data from the main world and stored them as entities and components in
the render world. Now they are extracted into essentially
`EntityHashMap<Entity, T>` where `T` are structs containing an
appropriate group of data. This means that while extract set systems
will continue to run extract queries against the main world they will
store their data in hash maps. Also, systems in later sets will either
need to look up entities in the available resources such as
`RenderMeshInstances`, or maintain their own `EntityHashMap<Entity, T>`
for their own data.
Before:
```rust
fn queue_custom(
material_meshes: Query<(Entity, &MeshTransforms, &Handle<Mesh>), With<InstanceMaterialData>>,
) {
...
for (entity, mesh_transforms, mesh_handle) in &material_meshes {
...
}
}
```
After:
```rust
fn queue_custom(
render_mesh_instances: Res<RenderMeshInstances>,
instance_entities: Query<Entity, With<InstanceMaterialData>>,
) {
...
for entity in &instance_entities {
let Some(mesh_instance) = render_mesh_instances.get(&entity) else { continue; };
// The mesh handle in `AssetId<Mesh>` form, and the `MeshTransforms` can now
// be found in `mesh_instance` which is a `RenderMeshInstance`
...
}
}
```
---------
Co-authored-by: robtfm <50659922+robtfm@users.noreply.github.com>
2023-09-27 08:28:28 +00:00
|
|
|
let Some(material_asset_id) = render_material_instances.get(visible_entity) else {
|
|
|
|
continue;
|
|
|
|
};
|
Generate `MeshUniform`s on the GPU via compute shader where available. (#12773)
Currently, `MeshUniform`s are rather large: 160 bytes. They're also
somewhat expensive to compute, because they involve taking the inverse
of a 3x4 matrix. Finally, if a mesh is present in multiple views, that
mesh will have a separate `MeshUniform` for each and every view, which
is wasteful.
This commit fixes these issues by introducing the concept of a *mesh
input uniform* and adding a *mesh uniform building* compute shader pass.
The `MeshInputUniform` is simply the minimum amount of data needed for
the GPU to compute the full `MeshUniform`. Most of this data is just the
transform and is therefore only 64 bytes. `MeshInputUniform`s are
computed during the *extraction* phase, much like skins are today, in
order to avoid needlessly copying transforms around on CPU. (In fact,
the render app has been changed to only store the translation of each
mesh; it no longer cares about any other part of the transform, which is
stored only on the GPU and the main world.) Before rendering, the
`build_mesh_uniforms` pass runs to expand the `MeshInputUniform`s to the
full `MeshUniform`.
The mesh uniform building pass does the following, all on GPU:
1. Copy the appropriate fields of the `MeshInputUniform` to the
`MeshUniform` slot. If a single mesh is present in multiple views, this
effectively duplicates it into each view.
2. Compute the inverse transpose of the model transform, used for
transforming normals.
3. If applicable, copy the mesh's transform from the previous frame for
TAA. To support this, we double-buffer the `MeshInputUniform`s over two
frames and swap the buffers each frame. The `MeshInputUniform`s for the
current frame contain the index of that mesh's `MeshInputUniform` for
the previous frame.
This commit produces wins in virtually every CPU part of the pipeline:
`extract_meshes`, `queue_material_meshes`,
`batch_and_prepare_render_phase`, and especially
`write_batched_instance_buffer` are all faster. Shrinking the amount of
CPU data that has to be shuffled around speeds up the entire rendering
process.
| Benchmark | This branch | `main` | Speedup |
|------------------------|-------------|---------|---------|
| `many_cubes -nfc` | 17.259 | 24.529 | 42.12% |
| `many_cubes -nfc -vpi` | 302.116 | 312.123 | 3.31% |
| `many_foxes` | 3.227 | 3.515 | 8.92% |
Because mesh uniform building requires compute shader, and WebGL 2 has
no compute shader, the existing CPU mesh uniform building code has been
left as-is. Many types now have both CPU mesh uniform building and GPU
mesh uniform building modes. Developers can opt into the old CPU mesh
uniform building by setting the `use_gpu_uniform_builder` option on
`PbrPlugin` to `false`.
Below are graphs of the CPU portions of `many-cubes
--no-frustum-culling`. Yellow is this branch, red is `main`.
`extract_meshes`:

It's notable that we get a small win even though we're now writing to a
GPU buffer.
`queue_material_meshes`:

There's a bit of a regression here; not sure what's causing it. In any
case it's very outweighed by the other gains.
`batch_and_prepare_render_phase`:

There's a huge win here, enough to make batching basically drop off the
profile.
`write_batched_instance_buffer`:

There's a massive improvement here, as expected. Note that a lot of it
simply comes from the fact that `MeshInputUniform` is `Pod`. (This isn't
a maintainability problem in my view because `MeshInputUniform` is so
simple: just 16 tightly-packed words.)
## Changelog
### Added
* Per-mesh instance data is now generated on GPU with a compute shader
instead of CPU, resulting in rendering performance improvements on
platforms where compute shaders are supported.
## Migration guide
* Custom render phases now need multiple systems beyond just
`batch_and_prepare_render_phase`. Code that was previously creating
custom render phases should now add a `BinnedRenderPhasePlugin` or
`SortedRenderPhasePlugin` as appropriate instead of directly adding
`batch_and_prepare_render_phase`.
2024-04-10 05:33:32 +00:00
|
|
|
let Some(mesh_instance) = render_mesh_instances.render_mesh_queue_data(*visible_entity)
|
|
|
|
else {
|
2023-09-20 20:18:55 +00:00
|
|
|
continue;
|
|
|
|
};
|
Use EntityHashMap<Entity, T> for render world entity storage for better performance (#9903)
# Objective
- Improve rendering performance, particularly by avoiding the large
system commands costs of using the ECS in the way that the render world
does.
## Solution
- Define `EntityHasher` that calculates a hash from the
`Entity.to_bits()` by `i | (i.wrapping_mul(0x517cc1b727220a95) << 32)`.
`0x517cc1b727220a95` is something like `u64::MAX / N` for N that gives a
value close to π and that works well for hashing. Thanks for @SkiFire13
for the suggestion and to @nicopap for alternative suggestions and
discussion. This approach comes from `rustc-hash` (a.k.a. `FxHasher`)
with some tweaks for the case of hashing an `Entity`. `FxHasher` and
`SeaHasher` were also tested but were significantly slower.
- Define `EntityHashMap` type that uses the `EntityHashser`
- Use `EntityHashMap<Entity, T>` for render world entity storage,
including:
- `RenderMaterialInstances` - contains the `AssetId<M>` of the material
associated with the entity. Also for 2D.
- `RenderMeshInstances` - contains mesh transforms, flags and properties
about mesh entities. Also for 2D.
- `SkinIndices` and `MorphIndices` - contains the skin and morph index
for an entity, respectively
- `ExtractedSprites`
- `ExtractedUiNodes`
## Benchmarks
All benchmarks have been conducted on an M1 Max connected to AC power.
The tests are run for 1500 frames. The 1000th frame is captured for
comparison to check for visual regressions. There were none.
### 2D Meshes
`bevymark --benchmark --waves 160 --per-wave 1000 --mode mesh2d`
#### `--ordered-z`
This test spawns the 2D meshes with z incrementing back to front, which
is the ideal arrangement allocation order as it matches the sorted
render order which means lookups have a high cache hit rate.
<img width="1112" alt="Screenshot 2023-09-27 at 07 50 45"
src="https://github.com/bevyengine/bevy/assets/302146/e140bc98-7091-4a3b-8ae1-ab75d16d2ccb">
-39.1% median frame time.
#### Random
This test spawns the 2D meshes with random z. This not only makes the
batching and transparent 2D pass lookups get a lot of cache misses, it
also currently means that the meshes are almost certain to not be
batchable.
<img width="1108" alt="Screenshot 2023-09-27 at 07 51 28"
src="https://github.com/bevyengine/bevy/assets/302146/29c2e813-645a-43ce-982a-55df4bf7d8c4">
-7.2% median frame time.
### 3D Meshes
`many_cubes --benchmark`
<img width="1112" alt="Screenshot 2023-09-27 at 07 51 57"
src="https://github.com/bevyengine/bevy/assets/302146/1a729673-3254-4e2a-9072-55e27c69f0fc">
-7.7% median frame time.
### Sprites
**NOTE: On `main` sprites are using `SparseSet<Entity, T>`!**
`bevymark --benchmark --waves 160 --per-wave 1000 --mode sprite`
#### `--ordered-z`
This test spawns the sprites with z incrementing back to front, which is
the ideal arrangement allocation order as it matches the sorted render
order which means lookups have a high cache hit rate.
<img width="1116" alt="Screenshot 2023-09-27 at 07 52 31"
src="https://github.com/bevyengine/bevy/assets/302146/bc8eab90-e375-4d31-b5cd-f55f6f59ab67">
+13.0% median frame time.
#### Random
This test spawns the sprites with random z. This makes the batching and
transparent 2D pass lookups get a lot of cache misses.
<img width="1109" alt="Screenshot 2023-09-27 at 07 53 01"
src="https://github.com/bevyengine/bevy/assets/302146/22073f5d-99a7-49b0-9584-d3ac3eac3033">
+0.6% median frame time.
### UI
**NOTE: On `main` UI is using `SparseSet<Entity, T>`!**
`many_buttons`
<img width="1111" alt="Screenshot 2023-09-27 at 07 53 26"
src="https://github.com/bevyengine/bevy/assets/302146/66afd56d-cbe4-49e7-8b64-2f28f6043d85">
+15.1% median frame time.
## Alternatives
- Cart originally suggested trying out `SparseSet<Entity, T>` and indeed
that is slightly faster under ideal conditions. However,
`PassHashMap<Entity, T>` has better worst case performance when data is
randomly distributed, rather than in sorted render order, and does not
have the worst case memory usage that `SparseSet`'s dense `Vec<usize>`
that maps from the `Entity` index to sparse index into `Vec<T>`. This
dense `Vec` has to be as large as the largest Entity index used with the
`SparseSet`.
- I also tested `PassHashMap<u32, T>`, intending to use `Entity.index()`
as the key, but this proved to sometimes be slower and mostly no
different.
- The only outstanding approach that has not been implemented and tested
is to _not_ clear the render world of its entities each frame. That has
its own problems, though they could perhaps be solved.
- Performance-wise, if the entities and their component data were not
cleared, then they would incur table moves on spawn, and should not
thereafter, rather just their component data would be overwritten.
Ideally we would have a neat way of either updating data in-place via
`&mut T` queries, or inserting components if not present. This would
likely be quite cumbersome to have to remember to do everywhere, but
perhaps it only needs to be done in the more performance-sensitive
systems.
- The main problem to solve however is that we want to both maintain a
mapping between main world entities and render world entities, be able
to run the render app and world in parallel with the main app and world
for pipelined rendering, and at the same time be able to spawn entities
in the render world in such a way that those Entity ids do not collide
with those spawned in the main world. This is potentially quite
solvable, but could well be a lot of ECS work to do it in a way that
makes sense.
---
## Changelog
- Changed: Component data for entities to be drawn are no longer stored
on entities in the render world. Instead, data is stored in a
`EntityHashMap<Entity, T>` in various resources. This brings significant
performance benefits due to the way the render app clears entities every
frame. Resources of most interest are `RenderMeshInstances` and
`RenderMaterialInstances`, and their 2D counterparts.
## Migration Guide
Previously the render app extracted mesh entities and their component
data from the main world and stored them as entities and components in
the render world. Now they are extracted into essentially
`EntityHashMap<Entity, T>` where `T` are structs containing an
appropriate group of data. This means that while extract set systems
will continue to run extract queries against the main world they will
store their data in hash maps. Also, systems in later sets will either
need to look up entities in the available resources such as
`RenderMeshInstances`, or maintain their own `EntityHashMap<Entity, T>`
for their own data.
Before:
```rust
fn queue_custom(
material_meshes: Query<(Entity, &MeshTransforms, &Handle<Mesh>), With<InstanceMaterialData>>,
) {
...
for (entity, mesh_transforms, mesh_handle) in &material_meshes {
...
}
}
```
After:
```rust
fn queue_custom(
render_mesh_instances: Res<RenderMeshInstances>,
instance_entities: Query<Entity, With<InstanceMaterialData>>,
) {
...
for entity in &instance_entities {
let Some(mesh_instance) = render_mesh_instances.get(&entity) else { continue; };
// The mesh handle in `AssetId<Mesh>` form, and the `MeshTransforms` can now
// be found in `mesh_instance` which is a `RenderMeshInstance`
...
}
}
```
---------
Co-authored-by: robtfm <50659922+robtfm@users.noreply.github.com>
2023-09-27 08:28:28 +00:00
|
|
|
let Some(mesh) = render_meshes.get(mesh_instance.mesh_asset_id) else {
|
2023-09-20 20:18:55 +00:00
|
|
|
continue;
|
|
|
|
};
|
2024-04-09 13:26:34 +00:00
|
|
|
let Some(material) = render_materials.get(*material_asset_id) else {
|
2023-09-20 20:18:55 +00:00
|
|
|
continue;
|
|
|
|
};
|
2023-10-12 22:10:38 +00:00
|
|
|
|
2024-07-22 18:28:23 +00:00
|
|
|
let mut mesh_pipeline_key_bits = material.properties.mesh_pipeline_key_bits;
|
|
|
|
mesh_pipeline_key_bits.insert(alpha_mode_pipeline_key(
|
|
|
|
material.properties.alpha_mode,
|
|
|
|
msaa,
|
|
|
|
));
|
Micro-optimize `queue_material_meshes`, primarily to remove bit manipulation. (#12791)
This commit makes the following optimizations:
## `MeshPipelineKey`/`BaseMeshPipelineKey` split
`MeshPipelineKey` has been split into `BaseMeshPipelineKey`, which lives
in `bevy_render` and `MeshPipelineKey`, which lives in `bevy_pbr`.
Conceptually, `BaseMeshPipelineKey` is a superclass of
`MeshPipelineKey`. For `BaseMeshPipelineKey`, the bits start at the
highest (most significant) bit and grow downward toward the lowest bit;
for `MeshPipelineKey`, the bits start at the lowest bit and grow upward
toward the highest bit. This prevents them from colliding.
The goal of this is to avoid having to reassemble bits of the pipeline
key for every mesh every frame. Instead, we can just use a bitwise or
operation to combine the pieces that make up a `MeshPipelineKey`.
## `specialize_slow`
Previously, all of `specialize()` was marked as `#[inline]`. This
bloated `queue_material_meshes` unnecessarily, as a large chunk of it
ended up being a slow path that was rarely hit. This commit refactors
the function to move the slow path to `specialize_slow()`.
Together, these two changes shave about 5% off `queue_material_meshes`:

## Migration Guide
- The `primitive_topology` field on `GpuMesh` is now an accessor method:
`GpuMesh::primitive_topology()`.
- For performance reasons, `MeshPipelineKey` has been split into
`BaseMeshPipelineKey`, which lives in `bevy_render`, and
`MeshPipelineKey`, which lives in `bevy_pbr`. These two should be
combined with bitwise-or to produce the final `MeshPipelineKey`.
2024-04-01 21:58:53 +00:00
|
|
|
let mut mesh_key = view_key
|
|
|
|
| MeshPipelineKey::from_bits_retain(mesh.key_bits.bits())
|
2024-07-22 18:28:23 +00:00
|
|
|
| mesh_pipeline_key_bits;
|
2023-09-20 20:18:55 +00:00
|
|
|
|
Improve performance by binning together opaque items instead of sorting them. (#12453)
Today, we sort all entities added to all phases, even the phases that
don't strictly need sorting, such as the opaque and shadow phases. This
results in a performance loss because our `PhaseItem`s are rather large
in memory, so sorting is slow. Additionally, determining the boundaries
of batches is an O(n) process.
This commit makes Bevy instead applicable place phase items into *bins*
keyed by *bin keys*, which have the invariant that everything in the
same bin is potentially batchable. This makes determining batch
boundaries O(1), because everything in the same bin can be batched.
Instead of sorting each entity, we now sort only the bin keys. This
drops the sorting time to near-zero on workloads with few bins like
`many_cubes --no-frustum-culling`. Memory usage is improved too, with
batch boundaries and dynamic indices now implicit instead of explicit.
The improved memory usage results in a significant win even on
unbatchable workloads like `many_cubes --no-frustum-culling
--vary-material-data-per-instance`, presumably due to cache effects.
Not all phases can be binned; some, such as transparent and transmissive
phases, must still be sorted. To handle this, this commit splits
`PhaseItem` into `BinnedPhaseItem` and `SortedPhaseItem`. Most of the
logic that today deals with `PhaseItem`s has been moved to
`SortedPhaseItem`. `BinnedPhaseItem` has the new logic.
Frame time results (in ms/frame) are as follows:
| Benchmark | `binning` | `main` | Speedup |
| ------------------------ | --------- | ------- | ------- |
| `many_cubes -nfc -vpi` | 232.179 | 312.123 | 34.43% |
| `many_cubes -nfc` | 25.874 | 30.117 | 16.40% |
| `many_foxes` | 3.276 | 3.515 | 7.30% |
(`-nfc` is short for `--no-frustum-culling`; `-vpi` is short for
`--vary-per-instance`.)
---
## Changelog
### Changed
* Render phases have been split into binned and sorted phases. Binned
phases, such as the common opaque phase, achieve improved CPU
performance by avoiding the sorting step.
## Migration Guide
- `PhaseItem` has been split into `BinnedPhaseItem` and
`SortedPhaseItem`. If your code has custom `PhaseItem`s, you will need
to migrate them to one of these two types. `SortedPhaseItem` requires
the fewest code changes, but you may want to pick `BinnedPhaseItem` if
your phase doesn't require sorting, as that enables higher performance.
## Tracy graphs
`many-cubes --no-frustum-culling`, `main` branch:
<img width="1064" alt="Screenshot 2024-03-12 180037"
src="https://github.com/bevyengine/bevy/assets/157897/e1180ce8-8e89-46d2-85e3-f59f72109a55">
`many-cubes --no-frustum-culling`, this branch:
<img width="1064" alt="Screenshot 2024-03-12 180011"
src="https://github.com/bevyengine/bevy/assets/157897/0899f036-6075-44c5-a972-44d95895f46c">
You can see that `batch_and_prepare_binned_render_phase` is a much
smaller fraction of the time. Zooming in on that function, with yellow
being this branch and red being `main`, we see:
<img width="1064" alt="Screenshot 2024-03-12 175832"
src="https://github.com/bevyengine/bevy/assets/157897/0dfc8d3f-49f4-496e-8825-a66e64d356d0">
The binning happens in `queue_material_meshes`. Again with yellow being
this branch and red being `main`:
<img width="1064" alt="Screenshot 2024-03-12 175755"
src="https://github.com/bevyengine/bevy/assets/157897/b9b20dc1-11c8-400c-a6cc-1c2e09c1bb96">
We can see that there is a small regression in `queue_material_meshes`
performance, but it's not nearly enough to outweigh the large gains in
`batch_and_prepare_binned_render_phase`.
---------
Co-authored-by: James Liu <contact@jamessliu.com>
2024-03-30 02:55:02 +00:00
|
|
|
let lightmap_image = render_lightmaps
|
Implement lightmaps. (#10231)

# Objective
Lightmaps, textures that store baked global illumination, have been a
mainstay of real-time graphics for decades. Bevy currently has no
support for them, so this pull request implements them.
## Solution
The new `Lightmap` component can be attached to any entity that contains
a `Handle<Mesh>` and a `StandardMaterial`. When present, it will be
applied in the PBR shader. Because multiple lightmaps are frequently
packed into atlases, each lightmap may have its own UV boundaries within
its texture. An `exposure` field is also provided, to control the
brightness of the lightmap.
Note that this PR doesn't provide any way to bake the lightmaps. That
can be done with [The Lightmapper] or another solution, such as Unity's
Bakery.
---
## Changelog
### Added
* A new component, `Lightmap`, is available, for baked global
illumination. If your mesh has a second UV channel (UV1), and you attach
this component to the entity with that mesh, Bevy will apply the texture
referenced in the lightmap.
[The Lightmapper]: https://github.com/Naxela/The_Lightmapper
---------
Co-authored-by: Carter Anderson <mcanders1@gmail.com>
2024-01-02 20:38:47 +00:00
|
|
|
.render_lightmaps
|
Improve performance by binning together opaque items instead of sorting them. (#12453)
Today, we sort all entities added to all phases, even the phases that
don't strictly need sorting, such as the opaque and shadow phases. This
results in a performance loss because our `PhaseItem`s are rather large
in memory, so sorting is slow. Additionally, determining the boundaries
of batches is an O(n) process.
This commit makes Bevy instead applicable place phase items into *bins*
keyed by *bin keys*, which have the invariant that everything in the
same bin is potentially batchable. This makes determining batch
boundaries O(1), because everything in the same bin can be batched.
Instead of sorting each entity, we now sort only the bin keys. This
drops the sorting time to near-zero on workloads with few bins like
`many_cubes --no-frustum-culling`. Memory usage is improved too, with
batch boundaries and dynamic indices now implicit instead of explicit.
The improved memory usage results in a significant win even on
unbatchable workloads like `many_cubes --no-frustum-culling
--vary-material-data-per-instance`, presumably due to cache effects.
Not all phases can be binned; some, such as transparent and transmissive
phases, must still be sorted. To handle this, this commit splits
`PhaseItem` into `BinnedPhaseItem` and `SortedPhaseItem`. Most of the
logic that today deals with `PhaseItem`s has been moved to
`SortedPhaseItem`. `BinnedPhaseItem` has the new logic.
Frame time results (in ms/frame) are as follows:
| Benchmark | `binning` | `main` | Speedup |
| ------------------------ | --------- | ------- | ------- |
| `many_cubes -nfc -vpi` | 232.179 | 312.123 | 34.43% |
| `many_cubes -nfc` | 25.874 | 30.117 | 16.40% |
| `many_foxes` | 3.276 | 3.515 | 7.30% |
(`-nfc` is short for `--no-frustum-culling`; `-vpi` is short for
`--vary-per-instance`.)
---
## Changelog
### Changed
* Render phases have been split into binned and sorted phases. Binned
phases, such as the common opaque phase, achieve improved CPU
performance by avoiding the sorting step.
## Migration Guide
- `PhaseItem` has been split into `BinnedPhaseItem` and
`SortedPhaseItem`. If your code has custom `PhaseItem`s, you will need
to migrate them to one of these two types. `SortedPhaseItem` requires
the fewest code changes, but you may want to pick `BinnedPhaseItem` if
your phase doesn't require sorting, as that enables higher performance.
## Tracy graphs
`many-cubes --no-frustum-culling`, `main` branch:
<img width="1064" alt="Screenshot 2024-03-12 180037"
src="https://github.com/bevyengine/bevy/assets/157897/e1180ce8-8e89-46d2-85e3-f59f72109a55">
`many-cubes --no-frustum-culling`, this branch:
<img width="1064" alt="Screenshot 2024-03-12 180011"
src="https://github.com/bevyengine/bevy/assets/157897/0899f036-6075-44c5-a972-44d95895f46c">
You can see that `batch_and_prepare_binned_render_phase` is a much
smaller fraction of the time. Zooming in on that function, with yellow
being this branch and red being `main`, we see:
<img width="1064" alt="Screenshot 2024-03-12 175832"
src="https://github.com/bevyengine/bevy/assets/157897/0dfc8d3f-49f4-496e-8825-a66e64d356d0">
The binning happens in `queue_material_meshes`. Again with yellow being
this branch and red being `main`:
<img width="1064" alt="Screenshot 2024-03-12 175755"
src="https://github.com/bevyengine/bevy/assets/157897/b9b20dc1-11c8-400c-a6cc-1c2e09c1bb96">
We can see that there is a small regression in `queue_material_meshes`
performance, but it's not nearly enough to outweigh the large gains in
`batch_and_prepare_binned_render_phase`.
---------
Co-authored-by: James Liu <contact@jamessliu.com>
2024-03-30 02:55:02 +00:00
|
|
|
.get(visible_entity)
|
|
|
|
.map(|lightmap| lightmap.image);
|
|
|
|
if lightmap_image.is_some() {
|
Implement lightmaps. (#10231)

# Objective
Lightmaps, textures that store baked global illumination, have been a
mainstay of real-time graphics for decades. Bevy currently has no
support for them, so this pull request implements them.
## Solution
The new `Lightmap` component can be attached to any entity that contains
a `Handle<Mesh>` and a `StandardMaterial`. When present, it will be
applied in the PBR shader. Because multiple lightmaps are frequently
packed into atlases, each lightmap may have its own UV boundaries within
its texture. An `exposure` field is also provided, to control the
brightness of the lightmap.
Note that this PR doesn't provide any way to bake the lightmaps. That
can be done with [The Lightmapper] or another solution, such as Unity's
Bakery.
---
## Changelog
### Added
* A new component, `Lightmap`, is available, for baked global
illumination. If your mesh has a second UV channel (UV1), and you attach
this component to the entity with that mesh, Bevy will apply the texture
referenced in the lightmap.
[The Lightmapper]: https://github.com/Naxela/The_Lightmapper
---------
Co-authored-by: Carter Anderson <mcanders1@gmail.com>
2024-01-02 20:38:47 +00:00
|
|
|
mesh_key |= MeshPipelineKey::LIGHTMAPPED;
|
|
|
|
}
|
|
|
|
|
Implement visibility ranges, also known as hierarchical levels of detail (HLODs). (#12916)
Implement visibility ranges, also known as hierarchical levels of detail
(HLODs).
This commit introduces a new component, `VisibilityRange`, which allows
developers to specify camera distances in which meshes are to be shown
and hidden. Hiding meshes happens early in the rendering pipeline, so
this feature can be used for level of detail optimization. Additionally,
this feature is properly evaluated per-view, so different views can show
different levels of detail.
This feature differs from proper mesh LODs, which can be implemented
later. Engines generally implement true mesh LODs later in the pipeline;
they're typically more efficient than HLODs with GPU-driven rendering.
However, mesh LODs are more limited than HLODs, because they require the
lower levels of detail to be meshes with the same vertex layout and
shader (and perhaps the same material) as the original mesh. Games often
want to use objects other than meshes to replace distant models, such as
*octahedral imposters* or *billboard imposters*.
The reason why the feature is called *hierarchical level of detail* is
that HLODs can replace multiple meshes with a single mesh when the
camera is far away. This can be useful for reducing drawcall count. Note
that `VisibilityRange` doesn't automatically propagate down to children;
it must be placed on every mesh.
Crossfading between different levels of detail is supported, using the
standard 4x4 ordered dithering pattern from [1]. The shader code to
compute the dithering patterns should be well-optimized. The dithering
code is only active when visibility ranges are in use for the mesh in
question, so that we don't lose early Z.
Cascaded shadow maps show the HLOD level of the view they're associated
with. Point light and spot light shadow maps, which have no CSMs,
display all HLOD levels that are visible in any view. To support this
efficiently and avoid doing visibility checks multiple times, we
precalculate all visible HLOD levels for each entity with a
`VisibilityRange` during the `check_visibility_range` system.
A new example, `visibility_range`, has been added to the tree, as well
as a new low-poly version of the flight helmet model to go with it. It
demonstrates use of the visibility range feature to provide levels of
detail.
[1]: https://en.wikipedia.org/wiki/Ordered_dithering#Threshold_map
[^1]: Unreal doesn't have a feature that exactly corresponds to
visibility ranges, but Unreal's HLOD system serves roughly the same
purpose.
## Changelog
### Added
* A new `VisibilityRange` component is available to conditionally enable
entity visibility at camera distances, with optional crossfade support.
This can be used to implement different levels of detail (LODs).
## Screenshots
High-poly model:

Low-poly model up close:

Crossfading between the two:

---------
Co-authored-by: Carter Anderson <mcanders1@gmail.com>
2024-05-03 00:11:35 +00:00
|
|
|
if render_visibility_ranges.entity_has_crossfading_visibility_ranges(*visible_entity) {
|
|
|
|
mesh_key |= MeshPipelineKey::VISIBILITY_RANGE_DITHER;
|
|
|
|
}
|
|
|
|
|
Implement motion vectors and TAA for skinned meshes and meshes with morph targets. (#13572)
This is a revamped equivalent to #9902, though it shares none of the
code. It handles all special cases that I've tested correctly.
The overall technique consists of double-buffering the joint matrix and
morph weights buffers, as most of the previous attempts to solve this
problem did. The process is generally straightforward. Note that, to
avoid regressing the ability of mesh extraction, skin extraction, and
morph target extraction to run in parallel, I had to add a new system to
rendering, `set_mesh_motion_vector_flags`. The comment there explains
the details; it generally runs very quickly.
I've tested this with modified versions of the `animated_fox`,
`morph_targets`, and `many_foxes` examples that add TAA, and the patch
works. To avoid bloating those examples, I didn't add switches for TAA
to them.
Addresses points (1) and (2) of #8423.
## Changelog
### Fixed
* Motion vectors, and therefore TAA, are now supported for meshes with
skins and/or morph targets.
2024-05-31 17:02:28 +00:00
|
|
|
if motion_vector_prepass {
|
|
|
|
// If the previous frame have skins or morph targets, note that.
|
|
|
|
if mesh_instance
|
|
|
|
.flags
|
|
|
|
.contains(RenderMeshInstanceFlags::HAS_PREVIOUS_SKIN)
|
|
|
|
{
|
|
|
|
mesh_key |= MeshPipelineKey::HAS_PREVIOUS_SKIN;
|
|
|
|
}
|
|
|
|
if mesh_instance
|
|
|
|
.flags
|
|
|
|
.contains(RenderMeshInstanceFlags::HAS_PREVIOUS_MORPH)
|
|
|
|
{
|
|
|
|
mesh_key |= MeshPipelineKey::HAS_PREVIOUS_MORPH;
|
|
|
|
}
|
|
|
|
}
|
|
|
|
|
2023-09-20 20:18:55 +00:00
|
|
|
let pipeline_id = pipelines.specialize(
|
|
|
|
&pipeline_cache,
|
|
|
|
&material_pipeline,
|
|
|
|
MaterialPipelineKey {
|
|
|
|
mesh_key,
|
|
|
|
bind_group_data: material.key.clone(),
|
|
|
|
},
|
|
|
|
&mesh.layout,
|
|
|
|
);
|
|
|
|
let pipeline_id = match pipeline_id {
|
|
|
|
Ok(id) => id,
|
|
|
|
Err(err) => {
|
|
|
|
error!("{}", err);
|
|
|
|
continue;
|
|
|
|
}
|
|
|
|
};
|
|
|
|
|
2024-02-20 00:12:41 +00:00
|
|
|
mesh_instance
|
|
|
|
.material_bind_group_id
|
|
|
|
.set(material.get_bind_group_id());
|
Automatic batching/instancing of draw commands (#9685)
# Objective
- Implement the foundations of automatic batching/instancing of draw
commands as the next step from #89
- NOTE: More performance improvements will come when more data is
managed and bound in ways that do not require rebinding such as mesh,
material, and texture data.
## Solution
- The core idea for batching of draw commands is to check whether any of
the information that has to be passed when encoding a draw command
changes between two things that are being drawn according to the sorted
render phase order. These should be things like the pipeline, bind
groups and their dynamic offsets, index/vertex buffers, and so on.
- The following assumptions have been made:
- Only entities with prepared assets (pipelines, materials, meshes) are
queued to phases
- View bindings are constant across a phase for a given draw function as
phases are per-view
- `batch_and_prepare_render_phase` is the only system that performs this
batching and has sole responsibility for preparing the per-object data.
As such the mesh binding and dynamic offsets are assumed to only vary as
a result of the `batch_and_prepare_render_phase` system, e.g. due to
having to split data across separate uniform bindings within the same
buffer due to the maximum uniform buffer binding size.
- Implement `GpuArrayBuffer` for `Mesh2dUniform` to store Mesh2dUniform
in arrays in GPU buffers rather than each one being at a dynamic offset
in a uniform buffer. This is the same optimisation that was made for 3D
not long ago.
- Change batch size for a range in `PhaseItem`, adding API for getting
or mutating the range. This is more flexible than a size as the length
of the range can be used in place of the size, but the start and end can
be otherwise whatever is needed.
- Add an optional mesh bind group dynamic offset to `PhaseItem`. This
avoids having to do a massive table move just to insert
`GpuArrayBufferIndex` components.
## Benchmarks
All tests have been run on an M1 Max on AC power. `bevymark` and
`many_cubes` were modified to use 1920x1080 with a scale factor of 1. I
run a script that runs a separate Tracy capture process, and then runs
the bevy example with `--features bevy_ci_testing,trace_tracy` and
`CI_TESTING_CONFIG=../benchmark.ron` with the contents of
`../benchmark.ron`:
```rust
(
exit_after: Some(1500)
)
```
...in order to run each test for 1500 frames.
The recent changes to `many_cubes` and `bevymark` added reproducible
random number generation so that with the same settings, the same rng
will occur. They also added benchmark modes that use a fixed delta time
for animations. Combined this means that the same frames should be
rendered both on main and on the branch.
The graphs compare main (yellow) to this PR (red).
### 3D Mesh `many_cubes --benchmark`
<img width="1411" alt="Screenshot 2023-09-03 at 23 42 10"
src="https://github.com/bevyengine/bevy/assets/302146/2088716a-c918-486c-8129-090b26fd2bc4">
The mesh and material are the same for all instances. This is basically
the best case for the initial batching implementation as it results in 1
draw for the ~11.7k visible meshes. It gives a ~30% reduction in median
frame time.
The 1000th frame is identical using the flip tool:

```
Mean: 0.000000
Weighted median: 0.000000
1st weighted quartile: 0.000000
3rd weighted quartile: 0.000000
Min: 0.000000
Max: 0.000000
Evaluation time: 0.4615 seconds
```
### 3D Mesh `many_cubes --benchmark --material-texture-count 10`
<img width="1404" alt="Screenshot 2023-09-03 at 23 45 18"
src="https://github.com/bevyengine/bevy/assets/302146/5ee9c447-5bd2-45c6-9706-ac5ff8916daf">
This run uses 10 different materials by varying their textures. The
materials are randomly selected, and there is no sorting by material
bind group for opaque 3D so any batching is 'random'. The PR produces a
~5% reduction in median frame time. If we were to sort the opaque phase
by the material bind group, then this should be a lot faster. This
produces about 10.5k draws for the 11.7k visible entities. This makes
sense as randomly selecting from 10 materials gives a chance that two
adjacent entities randomly select the same material and can be batched.
The 1000th frame is identical in flip:
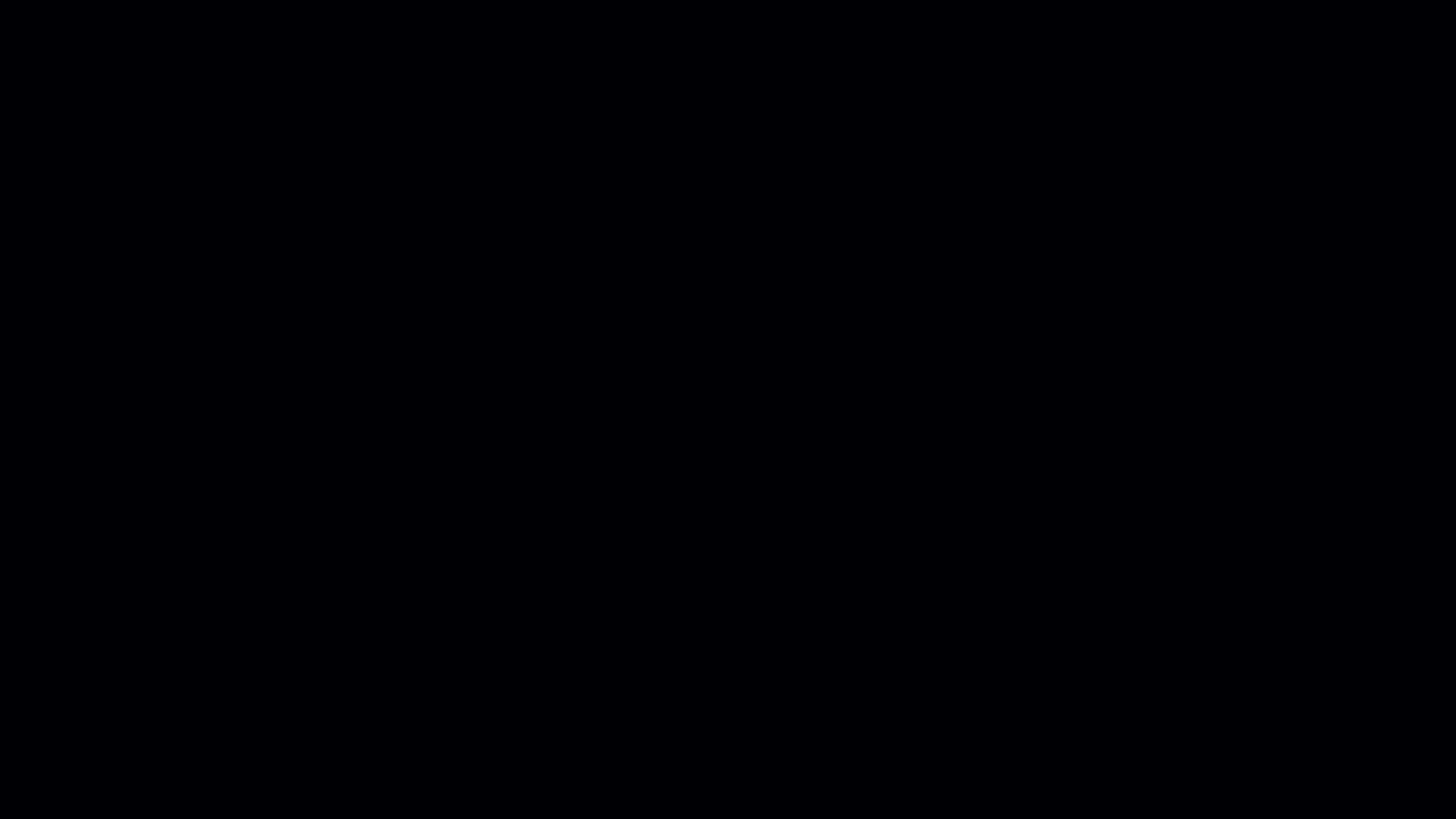
```
Mean: 0.000000
Weighted median: 0.000000
1st weighted quartile: 0.000000
3rd weighted quartile: 0.000000
Min: 0.000000
Max: 0.000000
Evaluation time: 0.4537 seconds
```
### 3D Mesh `many_cubes --benchmark --vary-per-instance`
<img width="1394" alt="Screenshot 2023-09-03 at 23 48 44"
src="https://github.com/bevyengine/bevy/assets/302146/f02a816b-a444-4c18-a96a-63b5436f3b7f">
This run varies the material data per instance by randomly-generating
its colour. This is the worst case for batching and that it performs
about the same as `main` is a good thing as it demonstrates that the
batching has minimal overhead when dealing with ~11k visible mesh
entities.
The 1000th frame is identical according to flip:
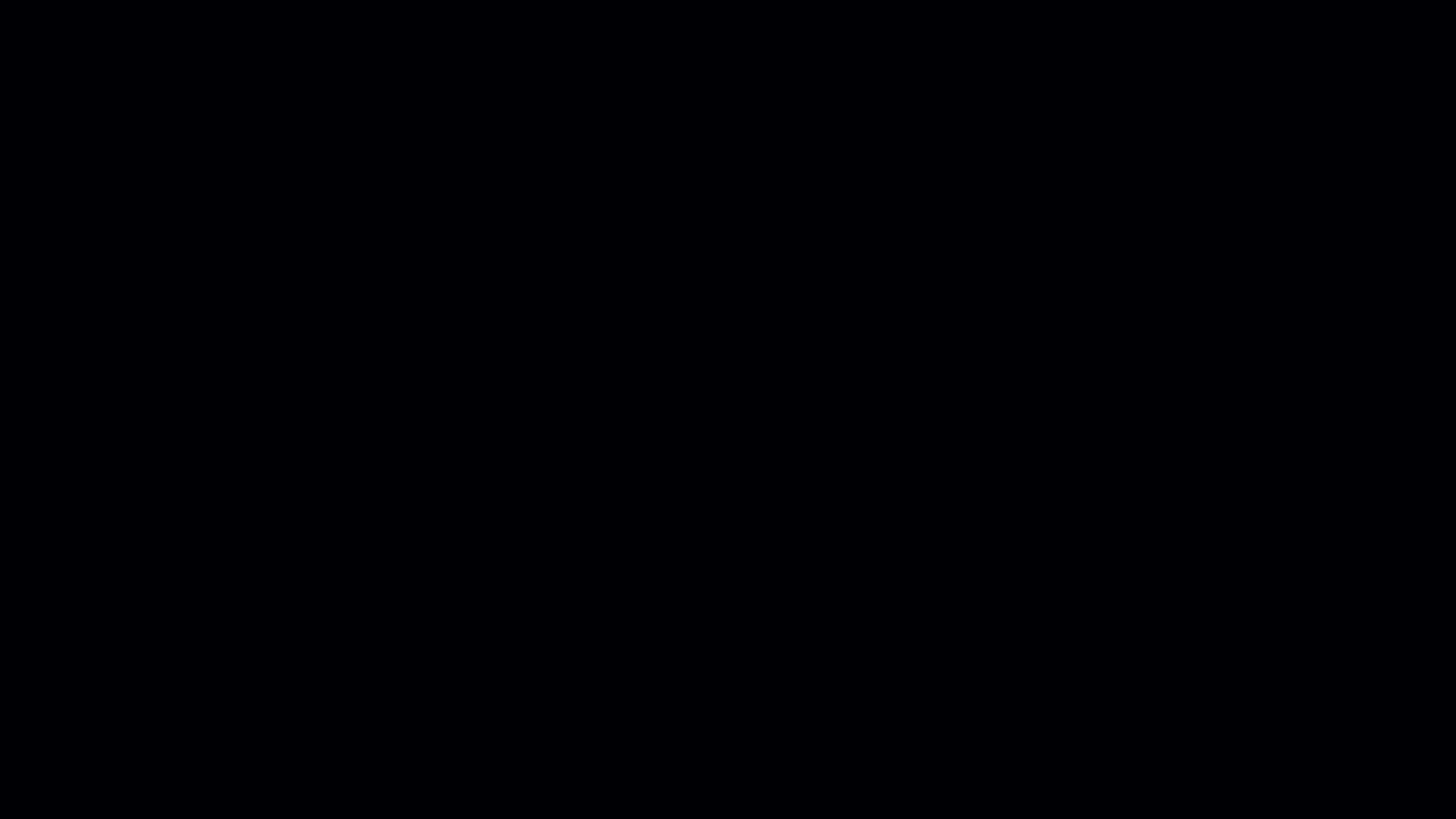
```
Mean: 0.000000
Weighted median: 0.000000
1st weighted quartile: 0.000000
3rd weighted quartile: 0.000000
Min: 0.000000
Max: 0.000000
Evaluation time: 0.4568 seconds
```
### 2D Mesh `bevymark --benchmark --waves 160 --per-wave 1000 --mode
mesh2d`
<img width="1412" alt="Screenshot 2023-09-03 at 23 59 56"
src="https://github.com/bevyengine/bevy/assets/302146/cb02ae07-237b-4646-ae9f-fda4dafcbad4">
This spawns 160 waves of 1000 quad meshes that are shaded with
ColorMaterial. Each wave has a different material so 160 waves currently
should result in 160 batches. This results in a 50% reduction in median
frame time.
Capturing a screenshot of the 1000th frame main vs PR gives:

```
Mean: 0.001222
Weighted median: 0.750432
1st weighted quartile: 0.453494
3rd weighted quartile: 0.969758
Min: 0.000000
Max: 0.990296
Evaluation time: 0.4255 seconds
```
So they seem to produce the same results. I also double-checked the
number of draws. `main` does 160000 draws, and the PR does 160, as
expected.
### 2D Mesh `bevymark --benchmark --waves 160 --per-wave 1000 --mode
mesh2d --material-texture-count 10`
<img width="1392" alt="Screenshot 2023-09-04 at 00 09 22"
src="https://github.com/bevyengine/bevy/assets/302146/4358da2e-ce32-4134-82df-3ab74c40849c">
This generates 10 textures and generates materials for each of those and
then selects one material per wave. The median frame time is reduced by
50%. Similar to the plain run above, this produces 160 draws on the PR
and 160000 on `main` and the 1000th frame is identical (ignoring the fps
counter text overlay).

```
Mean: 0.002877
Weighted median: 0.964980
1st weighted quartile: 0.668871
3rd weighted quartile: 0.982749
Min: 0.000000
Max: 0.992377
Evaluation time: 0.4301 seconds
```
### 2D Mesh `bevymark --benchmark --waves 160 --per-wave 1000 --mode
mesh2d --vary-per-instance`
<img width="1396" alt="Screenshot 2023-09-04 at 00 13 53"
src="https://github.com/bevyengine/bevy/assets/302146/b2198b18-3439-47ad-919a-cdabe190facb">
This creates unique materials per instance by randomly-generating the
material's colour. This is the worst case for 2D batching. Somehow, this
PR manages a 7% reduction in median frame time. Both main and this PR
issue 160000 draws.
The 1000th frame is the same:

```
Mean: 0.001214
Weighted median: 0.937499
1st weighted quartile: 0.635467
3rd weighted quartile: 0.979085
Min: 0.000000
Max: 0.988971
Evaluation time: 0.4462 seconds
```
### 2D Sprite `bevymark --benchmark --waves 160 --per-wave 1000 --mode
sprite`
<img width="1396" alt="Screenshot 2023-09-04 at 12 21 12"
src="https://github.com/bevyengine/bevy/assets/302146/8b31e915-d6be-4cac-abf5-c6a4da9c3d43">
This just spawns 160 waves of 1000 sprites. There should be and is no
notable difference between main and the PR.
### 2D Sprite `bevymark --benchmark --waves 160 --per-wave 1000 --mode
sprite --material-texture-count 10`
<img width="1389" alt="Screenshot 2023-09-04 at 12 36 08"
src="https://github.com/bevyengine/bevy/assets/302146/45fe8d6d-c901-4062-a349-3693dd044413">
This spawns the sprites selecting a texture at random per instance from
the 10 generated textures. This has no significant change vs main and
shouldn't.
### 2D Sprite `bevymark --benchmark --waves 160 --per-wave 1000 --mode
sprite --vary-per-instance`
<img width="1401" alt="Screenshot 2023-09-04 at 12 29 52"
src="https://github.com/bevyengine/bevy/assets/302146/762c5c60-352e-471f-8dbe-bbf10e24ebd6">
This sets the sprite colour as being unique per instance. This can still
all be drawn using one batch. There should be no difference but the PR
produces median frame times that are 4% higher. Investigation showed no
clear sources of cost, rather a mix of give and take that should not
happen. It seems like noise in the results.
### Summary
| Benchmark | % change in median frame time |
| ------------- | ------------- |
| many_cubes | 🟩 -30% |
| many_cubes 10 materials | 🟩 -5% |
| many_cubes unique materials | 🟩 ~0% |
| bevymark mesh2d | 🟩 -50% |
| bevymark mesh2d 10 materials | 🟩 -50% |
| bevymark mesh2d unique materials | 🟩 -7% |
| bevymark sprite | 🟥 2% |
| bevymark sprite 10 materials | 🟥 0.6% |
| bevymark sprite unique materials | 🟥 4.1% |
---
## Changelog
- Added: 2D and 3D mesh entities that share the same mesh and material
(same textures, same data) are now batched into the same draw command
for better performance.
---------
Co-authored-by: robtfm <50659922+robtfm@users.noreply.github.com>
Co-authored-by: Nicola Papale <nico@nicopap.ch>
2023-09-21 22:12:34 +00:00
|
|
|
|
2024-04-15 20:37:52 +00:00
|
|
|
match mesh_key
|
|
|
|
.intersection(MeshPipelineKey::BLEND_RESERVED_BITS | MeshPipelineKey::MAY_DISCARD)
|
|
|
|
{
|
|
|
|
MeshPipelineKey::BLEND_OPAQUE | MeshPipelineKey::BLEND_ALPHA_TO_COVERAGE => {
|
`StandardMaterial` Light Transmission (#8015)
# Objective
<img width="1920" alt="Screenshot 2023-04-26 at 01 07 34"
src="https://user-images.githubusercontent.com/418473/234467578-0f34187b-5863-4ea1-88e9-7a6bb8ce8da3.png">
This PR adds both diffuse and specular light transmission capabilities
to the `StandardMaterial`, with support for screen space refractions.
This enables realistically representing a wide range of real-world
materials, such as:
- Glass; (Including frosted glass)
- Transparent and translucent plastics;
- Various liquids and gels;
- Gemstones;
- Marble;
- Wax;
- Paper;
- Leaves;
- Porcelain.
Unlike existing support for transparency, light transmission does not
rely on fixed function alpha blending, and therefore works with both
`AlphaMode::Opaque` and `AlphaMode::Mask` materials.
## Solution
- Introduces a number of transmission related fields in the
`StandardMaterial`;
- For specular transmission:
- Adds logic to take a view main texture snapshot after the opaque
phase; (in order to perform screen space refractions)
- Introduces a new `Transmissive3d` phase to the renderer, to which all
meshes with `transmission > 0.0` materials are sent.
- Calculates a light exit point (of the approximate mesh volume) using
`ior` and `thickness` properties
- Samples the snapshot texture with an adaptive number of taps across a
`roughness`-controlled radius enabling “blurry” refractions
- For diffuse transmission:
- Approximates transmitted diffuse light by using a second, flipped +
displaced, diffuse-only Lambertian lobe for each light source.
## To Do
- [x] Figure out where `fresnel_mix()` is taking place, if at all, and
where `dielectric_specular` is being calculated, if at all, and update
them to use the `ior` value (Not a blocker, just a nice-to-have for more
correct BSDF)
- To the _best of my knowledge, this is now taking place, after
964340cdd. The fresnel mix is actually "split" into two parts in our
implementation, one `(1 - fresnel(...))` in the transmission, and
`fresnel()` in the light implementations. A surface with more
reflectance now will produce slightly dimmer transmission towards the
grazing angle, as more of the light gets reflected.
- [x] Add `transmission_texture`
- [x] Add `diffuse_transmission_texture`
- [x] Add `thickness_texture`
- [x] Add `attenuation_distance` and `attenuation_color`
- [x] Connect values to glTF loader
- [x] `transmission` and `transmission_texture`
- [x] `thickness` and `thickness_texture`
- [x] `ior`
- [ ] `diffuse_transmission` and `diffuse_transmission_texture` (needs
upstream support in `gltf` crate, not a blocker)
- [x] Add support for multiple screen space refraction “steps”
- [x] Conditionally create no transmission snapshot texture at all if
`steps == 0`
- [x] Conditionally enable/disable screen space refraction transmission
snapshots
- [x] Read from depth pre-pass to prevent refracting pixels in front of
the light exit point
- [x] Use `interleaved_gradient_noise()` function for sampling blur in a
way that benefits from TAA
- [x] Drill down a TAA `#define`, tweak some aspects of the effect
conditionally based on it
- [x] Remove const array that's crashing under HLSL (unless a new `naga`
release with https://github.com/gfx-rs/naga/pull/2496 comes out before
we merge this)
- [ ] Look into alternatives to the `switch` hack for dynamically
indexing the const array (might not be needed, compilers seem to be
decent at expanding it)
- [ ] Add pipeline keys for gating transmission (do we really want/need
this?)
- [x] Tweak some material field/function names?
## A Note on Texture Packing
_This was originally added as a comment to the
`specular_transmission_texture`, `thickness_texture` and
`diffuse_transmission_texture` documentation, I removed it since it was
more confusing than helpful, and will likely be made redundant/will need
to be updated once we have a better infrastructure for preprocessing
assets_
Due to how channels are mapped, you can more efficiently use a single
shared texture image
for configuring the following:
- R - `specular_transmission_texture`
- G - `thickness_texture`
- B - _unused_
- A - `diffuse_transmission_texture`
The `KHR_materials_diffuse_transmission` glTF extension also defines a
`diffuseTransmissionColorTexture`,
that _we don't currently support_. One might choose to pack the
intensity and color textures together,
using RGB for the color and A for the intensity, in which case this
packing advice doesn't really apply.
---
## Changelog
- Added a new `Transmissive3d` render phase for rendering specular
transmissive materials with screen space refractions
- Added rendering support for transmitted environment map light on the
`StandardMaterial` as a fallback for screen space refractions
- Added `diffuse_transmission`, `specular_transmission`, `thickness`,
`ior`, `attenuation_distance` and `attenuation_color` to the
`StandardMaterial`
- Added `diffuse_transmission_texture`, `specular_transmission_texture`,
`thickness_texture` to the `StandardMaterial`, gated behind a new
`pbr_transmission_textures` cargo feature (off by default, for maximum
hardware compatibility)
- Added `Camera3d::screen_space_specular_transmission_steps` for
controlling the number of “layers of transparency” rendered for
transmissive objects
- Added a `TransmittedShadowReceiver` component for enabling shadows in
(diffusely) transmitted light. (disabled by default, as it requires
carefully setting up the `thickness` to avoid self-shadow artifacts)
- Added support for the `KHR_materials_transmission`,
`KHR_materials_ior` and `KHR_materials_volume` glTF extensions
- Renamed items related to temporal jitter for greater consistency
## Migration Guide
- `SsaoPipelineKey::temporal_noise` has been renamed to
`SsaoPipelineKey::temporal_jitter`
- The `TAA` shader def (controlled by the presence of the
`TemporalAntiAliasSettings` component in the camera) has been replaced
with the `TEMPORAL_JITTER` shader def (controlled by the presence of the
`TemporalJitter` component in the camera)
- `MeshPipelineKey::TAA` has been replaced by
`MeshPipelineKey::TEMPORAL_JITTER`
- The `TEMPORAL_NOISE` shader def has been consolidated with
`TEMPORAL_JITTER`
2023-10-31 20:59:02 +00:00
|
|
|
if material.properties.reads_view_transmission_texture {
|
Generate `MeshUniform`s on the GPU via compute shader where available. (#12773)
Currently, `MeshUniform`s are rather large: 160 bytes. They're also
somewhat expensive to compute, because they involve taking the inverse
of a 3x4 matrix. Finally, if a mesh is present in multiple views, that
mesh will have a separate `MeshUniform` for each and every view, which
is wasteful.
This commit fixes these issues by introducing the concept of a *mesh
input uniform* and adding a *mesh uniform building* compute shader pass.
The `MeshInputUniform` is simply the minimum amount of data needed for
the GPU to compute the full `MeshUniform`. Most of this data is just the
transform and is therefore only 64 bytes. `MeshInputUniform`s are
computed during the *extraction* phase, much like skins are today, in
order to avoid needlessly copying transforms around on CPU. (In fact,
the render app has been changed to only store the translation of each
mesh; it no longer cares about any other part of the transform, which is
stored only on the GPU and the main world.) Before rendering, the
`build_mesh_uniforms` pass runs to expand the `MeshInputUniform`s to the
full `MeshUniform`.
The mesh uniform building pass does the following, all on GPU:
1. Copy the appropriate fields of the `MeshInputUniform` to the
`MeshUniform` slot. If a single mesh is present in multiple views, this
effectively duplicates it into each view.
2. Compute the inverse transpose of the model transform, used for
transforming normals.
3. If applicable, copy the mesh's transform from the previous frame for
TAA. To support this, we double-buffer the `MeshInputUniform`s over two
frames and swap the buffers each frame. The `MeshInputUniform`s for the
current frame contain the index of that mesh's `MeshInputUniform` for
the previous frame.
This commit produces wins in virtually every CPU part of the pipeline:
`extract_meshes`, `queue_material_meshes`,
`batch_and_prepare_render_phase`, and especially
`write_batched_instance_buffer` are all faster. Shrinking the amount of
CPU data that has to be shuffled around speeds up the entire rendering
process.
| Benchmark | This branch | `main` | Speedup |
|------------------------|-------------|---------|---------|
| `many_cubes -nfc` | 17.259 | 24.529 | 42.12% |
| `many_cubes -nfc -vpi` | 302.116 | 312.123 | 3.31% |
| `many_foxes` | 3.227 | 3.515 | 8.92% |
Because mesh uniform building requires compute shader, and WebGL 2 has
no compute shader, the existing CPU mesh uniform building code has been
left as-is. Many types now have both CPU mesh uniform building and GPU
mesh uniform building modes. Developers can opt into the old CPU mesh
uniform building by setting the `use_gpu_uniform_builder` option on
`PbrPlugin` to `false`.
Below are graphs of the CPU portions of `many-cubes
--no-frustum-culling`. Yellow is this branch, red is `main`.
`extract_meshes`:

It's notable that we get a small win even though we're now writing to a
GPU buffer.
`queue_material_meshes`:

There's a bit of a regression here; not sure what's causing it. In any
case it's very outweighed by the other gains.
`batch_and_prepare_render_phase`:

There's a huge win here, enough to make batching basically drop off the
profile.
`write_batched_instance_buffer`:

There's a massive improvement here, as expected. Note that a lot of it
simply comes from the fact that `MeshInputUniform` is `Pod`. (This isn't
a maintainability problem in my view because `MeshInputUniform` is so
simple: just 16 tightly-packed words.)
## Changelog
### Added
* Per-mesh instance data is now generated on GPU with a compute shader
instead of CPU, resulting in rendering performance improvements on
platforms where compute shaders are supported.
## Migration guide
* Custom render phases now need multiple systems beyond just
`batch_and_prepare_render_phase`. Code that was previously creating
custom render phases should now add a `BinnedRenderPhasePlugin` or
`SortedRenderPhasePlugin` as appropriate instead of directly adding
`batch_and_prepare_render_phase`.
2024-04-10 05:33:32 +00:00
|
|
|
let distance = rangefinder.distance_translation(&mesh_instance.translation)
|
2024-02-05 22:12:22 +00:00
|
|
|
+ material.properties.depth_bias;
|
`StandardMaterial` Light Transmission (#8015)
# Objective
<img width="1920" alt="Screenshot 2023-04-26 at 01 07 34"
src="https://user-images.githubusercontent.com/418473/234467578-0f34187b-5863-4ea1-88e9-7a6bb8ce8da3.png">
This PR adds both diffuse and specular light transmission capabilities
to the `StandardMaterial`, with support for screen space refractions.
This enables realistically representing a wide range of real-world
materials, such as:
- Glass; (Including frosted glass)
- Transparent and translucent plastics;
- Various liquids and gels;
- Gemstones;
- Marble;
- Wax;
- Paper;
- Leaves;
- Porcelain.
Unlike existing support for transparency, light transmission does not
rely on fixed function alpha blending, and therefore works with both
`AlphaMode::Opaque` and `AlphaMode::Mask` materials.
## Solution
- Introduces a number of transmission related fields in the
`StandardMaterial`;
- For specular transmission:
- Adds logic to take a view main texture snapshot after the opaque
phase; (in order to perform screen space refractions)
- Introduces a new `Transmissive3d` phase to the renderer, to which all
meshes with `transmission > 0.0` materials are sent.
- Calculates a light exit point (of the approximate mesh volume) using
`ior` and `thickness` properties
- Samples the snapshot texture with an adaptive number of taps across a
`roughness`-controlled radius enabling “blurry” refractions
- For diffuse transmission:
- Approximates transmitted diffuse light by using a second, flipped +
displaced, diffuse-only Lambertian lobe for each light source.
## To Do
- [x] Figure out where `fresnel_mix()` is taking place, if at all, and
where `dielectric_specular` is being calculated, if at all, and update
them to use the `ior` value (Not a blocker, just a nice-to-have for more
correct BSDF)
- To the _best of my knowledge, this is now taking place, after
964340cdd. The fresnel mix is actually "split" into two parts in our
implementation, one `(1 - fresnel(...))` in the transmission, and
`fresnel()` in the light implementations. A surface with more
reflectance now will produce slightly dimmer transmission towards the
grazing angle, as more of the light gets reflected.
- [x] Add `transmission_texture`
- [x] Add `diffuse_transmission_texture`
- [x] Add `thickness_texture`
- [x] Add `attenuation_distance` and `attenuation_color`
- [x] Connect values to glTF loader
- [x] `transmission` and `transmission_texture`
- [x] `thickness` and `thickness_texture`
- [x] `ior`
- [ ] `diffuse_transmission` and `diffuse_transmission_texture` (needs
upstream support in `gltf` crate, not a blocker)
- [x] Add support for multiple screen space refraction “steps”
- [x] Conditionally create no transmission snapshot texture at all if
`steps == 0`
- [x] Conditionally enable/disable screen space refraction transmission
snapshots
- [x] Read from depth pre-pass to prevent refracting pixels in front of
the light exit point
- [x] Use `interleaved_gradient_noise()` function for sampling blur in a
way that benefits from TAA
- [x] Drill down a TAA `#define`, tweak some aspects of the effect
conditionally based on it
- [x] Remove const array that's crashing under HLSL (unless a new `naga`
release with https://github.com/gfx-rs/naga/pull/2496 comes out before
we merge this)
- [ ] Look into alternatives to the `switch` hack for dynamically
indexing the const array (might not be needed, compilers seem to be
decent at expanding it)
- [ ] Add pipeline keys for gating transmission (do we really want/need
this?)
- [x] Tweak some material field/function names?
## A Note on Texture Packing
_This was originally added as a comment to the
`specular_transmission_texture`, `thickness_texture` and
`diffuse_transmission_texture` documentation, I removed it since it was
more confusing than helpful, and will likely be made redundant/will need
to be updated once we have a better infrastructure for preprocessing
assets_
Due to how channels are mapped, you can more efficiently use a single
shared texture image
for configuring the following:
- R - `specular_transmission_texture`
- G - `thickness_texture`
- B - _unused_
- A - `diffuse_transmission_texture`
The `KHR_materials_diffuse_transmission` glTF extension also defines a
`diffuseTransmissionColorTexture`,
that _we don't currently support_. One might choose to pack the
intensity and color textures together,
using RGB for the color and A for the intensity, in which case this
packing advice doesn't really apply.
---
## Changelog
- Added a new `Transmissive3d` render phase for rendering specular
transmissive materials with screen space refractions
- Added rendering support for transmitted environment map light on the
`StandardMaterial` as a fallback for screen space refractions
- Added `diffuse_transmission`, `specular_transmission`, `thickness`,
`ior`, `attenuation_distance` and `attenuation_color` to the
`StandardMaterial`
- Added `diffuse_transmission_texture`, `specular_transmission_texture`,
`thickness_texture` to the `StandardMaterial`, gated behind a new
`pbr_transmission_textures` cargo feature (off by default, for maximum
hardware compatibility)
- Added `Camera3d::screen_space_specular_transmission_steps` for
controlling the number of “layers of transparency” rendered for
transmissive objects
- Added a `TransmittedShadowReceiver` component for enabling shadows in
(diffusely) transmitted light. (disabled by default, as it requires
carefully setting up the `thickness` to avoid self-shadow artifacts)
- Added support for the `KHR_materials_transmission`,
`KHR_materials_ior` and `KHR_materials_volume` glTF extensions
- Renamed items related to temporal jitter for greater consistency
## Migration Guide
- `SsaoPipelineKey::temporal_noise` has been renamed to
`SsaoPipelineKey::temporal_jitter`
- The `TAA` shader def (controlled by the presence of the
`TemporalAntiAliasSettings` component in the camera) has been replaced
with the `TEMPORAL_JITTER` shader def (controlled by the presence of the
`TemporalJitter` component in the camera)
- `MeshPipelineKey::TAA` has been replaced by
`MeshPipelineKey::TEMPORAL_JITTER`
- The `TEMPORAL_NOISE` shader def has been consolidated with
`TEMPORAL_JITTER`
2023-10-31 20:59:02 +00:00
|
|
|
transmissive_phase.add(Transmissive3d {
|
Type safe retained render world (#15756)
# Objective
In the Render World, there are a number of collections that are derived
from Main World entities and are used to drive rendering. The most
notable are:
- `VisibleEntities`, which is generated in the `check_visibility` system
and contains visible entities for a view.
- `ExtractedInstances`, which maps entity ids to asset ids.
In the old model, these collections were trivially kept in sync -- any
extracted phase item could look itself up because the render entity id
was guaranteed to always match the corresponding main world id.
After #15320, this became much more complicated, and was leading to a
number of subtle bugs in the Render World. The main rendering systems,
i.e. `queue_material_meshes` and `queue_material2d_meshes`, follow a
similar pattern:
```rust
for visible_entity in visible_entities.iter::<With<Mesh2d>>() {
let Some(mesh_instance) = render_mesh_instances.get_mut(visible_entity) else {
continue;
};
// Look some more stuff up and specialize the pipeline...
let bin_key = Opaque2dBinKey {
pipeline: pipeline_id,
draw_function: draw_opaque_2d,
asset_id: mesh_instance.mesh_asset_id.into(),
material_bind_group_id: material_2d.get_bind_group_id().0,
};
opaque_phase.add(
bin_key,
*visible_entity,
BinnedRenderPhaseType::mesh(mesh_instance.automatic_batching),
);
}
```
In this case, `visible_entities` and `render_mesh_instances` are both
collections that are created and keyed by Main World entity ids, and so
this lookup happens to work by coincidence. However, there is a major
unintentional bug here: namely, because `visible_entities` is a
collection of Main World ids, the phase item being queued is created
with a Main World id rather than its correct Render World id.
This happens to not break mesh rendering because the render commands
used for drawing meshes do not access the `ItemQuery` parameter, but
demonstrates the confusion that is now possible: our UI phase items are
correctly being queued with Render World ids while our meshes aren't.
Additionally, this makes it very easy and error prone to use the wrong
entity id to look up things like assets. For example, if instead we
ignored visibility checks and queued our meshes via a query, we'd have
to be extra careful to use `&MainEntity` instead of the natural
`Entity`.
## Solution
Make all collections that are derived from Main World data use
`MainEntity` as their key, to ensure type safety and avoid accidentally
looking up data with the wrong entity id:
```rust
pub type MainEntityHashMap<V> = hashbrown::HashMap<MainEntity, V, EntityHash>;
```
Additionally, we make all `PhaseItem` be able to provide both their Main
and Render World ids, to allow render phase implementors maximum
flexibility as to what id should be used to look up data.
You can think of this like tracking at the type level whether something
in the Render World should use it's "primary key", i.e. entity id, or
needs to use a foreign key, i.e. `MainEntity`.
## Testing
##### TODO:
This will require extensive testing to make sure things didn't break!
Additionally, some extraction logic has become more complicated and
needs to be checked for regressions.
## Migration Guide
With the advent of the retained render world, collections that contain
references to `Entity` that are extracted into the render world have
been changed to contain `MainEntity` in order to prevent errors where a
render world entity id is used to look up an item by accident. Custom
rendering code may need to be changed to query for `&MainEntity` in
order to look up the correct item from such a collection. Additionally,
users who implement their own extraction logic for collections of main
world entity should strongly consider extracting into a different
collection that uses `MainEntity` as a key.
Additionally, render phases now require specifying both the `Entity` and
`MainEntity` for a given `PhaseItem`. Custom render phases should ensure
`MainEntity` is available when queuing a phase item.
2024-10-10 18:47:04 +00:00
|
|
|
entity: (*render_entity, *visible_entity),
|
`StandardMaterial` Light Transmission (#8015)
# Objective
<img width="1920" alt="Screenshot 2023-04-26 at 01 07 34"
src="https://user-images.githubusercontent.com/418473/234467578-0f34187b-5863-4ea1-88e9-7a6bb8ce8da3.png">
This PR adds both diffuse and specular light transmission capabilities
to the `StandardMaterial`, with support for screen space refractions.
This enables realistically representing a wide range of real-world
materials, such as:
- Glass; (Including frosted glass)
- Transparent and translucent plastics;
- Various liquids and gels;
- Gemstones;
- Marble;
- Wax;
- Paper;
- Leaves;
- Porcelain.
Unlike existing support for transparency, light transmission does not
rely on fixed function alpha blending, and therefore works with both
`AlphaMode::Opaque` and `AlphaMode::Mask` materials.
## Solution
- Introduces a number of transmission related fields in the
`StandardMaterial`;
- For specular transmission:
- Adds logic to take a view main texture snapshot after the opaque
phase; (in order to perform screen space refractions)
- Introduces a new `Transmissive3d` phase to the renderer, to which all
meshes with `transmission > 0.0` materials are sent.
- Calculates a light exit point (of the approximate mesh volume) using
`ior` and `thickness` properties
- Samples the snapshot texture with an adaptive number of taps across a
`roughness`-controlled radius enabling “blurry” refractions
- For diffuse transmission:
- Approximates transmitted diffuse light by using a second, flipped +
displaced, diffuse-only Lambertian lobe for each light source.
## To Do
- [x] Figure out where `fresnel_mix()` is taking place, if at all, and
where `dielectric_specular` is being calculated, if at all, and update
them to use the `ior` value (Not a blocker, just a nice-to-have for more
correct BSDF)
- To the _best of my knowledge, this is now taking place, after
964340cdd. The fresnel mix is actually "split" into two parts in our
implementation, one `(1 - fresnel(...))` in the transmission, and
`fresnel()` in the light implementations. A surface with more
reflectance now will produce slightly dimmer transmission towards the
grazing angle, as more of the light gets reflected.
- [x] Add `transmission_texture`
- [x] Add `diffuse_transmission_texture`
- [x] Add `thickness_texture`
- [x] Add `attenuation_distance` and `attenuation_color`
- [x] Connect values to glTF loader
- [x] `transmission` and `transmission_texture`
- [x] `thickness` and `thickness_texture`
- [x] `ior`
- [ ] `diffuse_transmission` and `diffuse_transmission_texture` (needs
upstream support in `gltf` crate, not a blocker)
- [x] Add support for multiple screen space refraction “steps”
- [x] Conditionally create no transmission snapshot texture at all if
`steps == 0`
- [x] Conditionally enable/disable screen space refraction transmission
snapshots
- [x] Read from depth pre-pass to prevent refracting pixels in front of
the light exit point
- [x] Use `interleaved_gradient_noise()` function for sampling blur in a
way that benefits from TAA
- [x] Drill down a TAA `#define`, tweak some aspects of the effect
conditionally based on it
- [x] Remove const array that's crashing under HLSL (unless a new `naga`
release with https://github.com/gfx-rs/naga/pull/2496 comes out before
we merge this)
- [ ] Look into alternatives to the `switch` hack for dynamically
indexing the const array (might not be needed, compilers seem to be
decent at expanding it)
- [ ] Add pipeline keys for gating transmission (do we really want/need
this?)
- [x] Tweak some material field/function names?
## A Note on Texture Packing
_This was originally added as a comment to the
`specular_transmission_texture`, `thickness_texture` and
`diffuse_transmission_texture` documentation, I removed it since it was
more confusing than helpful, and will likely be made redundant/will need
to be updated once we have a better infrastructure for preprocessing
assets_
Due to how channels are mapped, you can more efficiently use a single
shared texture image
for configuring the following:
- R - `specular_transmission_texture`
- G - `thickness_texture`
- B - _unused_
- A - `diffuse_transmission_texture`
The `KHR_materials_diffuse_transmission` glTF extension also defines a
`diffuseTransmissionColorTexture`,
that _we don't currently support_. One might choose to pack the
intensity and color textures together,
using RGB for the color and A for the intensity, in which case this
packing advice doesn't really apply.
---
## Changelog
- Added a new `Transmissive3d` render phase for rendering specular
transmissive materials with screen space refractions
- Added rendering support for transmitted environment map light on the
`StandardMaterial` as a fallback for screen space refractions
- Added `diffuse_transmission`, `specular_transmission`, `thickness`,
`ior`, `attenuation_distance` and `attenuation_color` to the
`StandardMaterial`
- Added `diffuse_transmission_texture`, `specular_transmission_texture`,
`thickness_texture` to the `StandardMaterial`, gated behind a new
`pbr_transmission_textures` cargo feature (off by default, for maximum
hardware compatibility)
- Added `Camera3d::screen_space_specular_transmission_steps` for
controlling the number of “layers of transparency” rendered for
transmissive objects
- Added a `TransmittedShadowReceiver` component for enabling shadows in
(diffusely) transmitted light. (disabled by default, as it requires
carefully setting up the `thickness` to avoid self-shadow artifacts)
- Added support for the `KHR_materials_transmission`,
`KHR_materials_ior` and `KHR_materials_volume` glTF extensions
- Renamed items related to temporal jitter for greater consistency
## Migration Guide
- `SsaoPipelineKey::temporal_noise` has been renamed to
`SsaoPipelineKey::temporal_jitter`
- The `TAA` shader def (controlled by the presence of the
`TemporalAntiAliasSettings` component in the camera) has been replaced
with the `TEMPORAL_JITTER` shader def (controlled by the presence of the
`TemporalJitter` component in the camera)
- `MeshPipelineKey::TAA` has been replaced by
`MeshPipelineKey::TEMPORAL_JITTER`
- The `TEMPORAL_NOISE` shader def has been consolidated with
`TEMPORAL_JITTER`
2023-10-31 20:59:02 +00:00
|
|
|
draw_function: draw_transmissive_pbr,
|
|
|
|
pipeline: pipeline_id,
|
|
|
|
distance,
|
|
|
|
batch_range: 0..1,
|
Implement GPU frustum culling. (#12889)
This commit implements opt-in GPU frustum culling, built on top of the
infrastructure in https://github.com/bevyengine/bevy/pull/12773. To
enable it on a camera, add the `GpuCulling` component to it. To
additionally disable CPU frustum culling, add the `NoCpuCulling`
component. Note that adding `GpuCulling` without `NoCpuCulling`
*currently* does nothing useful. The reason why `GpuCulling` doesn't
automatically imply `NoCpuCulling` is that I intend to follow this patch
up with GPU two-phase occlusion culling, and CPU frustum culling plus
GPU occlusion culling seems like a very commonly-desired mode.
Adding the `GpuCulling` component to a view puts that view into
*indirect mode*. This mode makes all drawcalls indirect, relying on the
mesh preprocessing shader to allocate instances dynamically. In indirect
mode, the `PreprocessWorkItem` `output_index` points not to a
`MeshUniform` instance slot but instead to a set of `wgpu`
`IndirectParameters`, from which it allocates an instance slot
dynamically if frustum culling succeeds. Batch building has been updated
to allocate and track indirect parameter slots, and the AABBs are now
supplied to the GPU as `MeshCullingData`.
A small amount of code relating to the frustum culling has been borrowed
from meshlets and moved into `maths.wgsl`. Note that standard Bevy
frustum culling uses AABBs, while meshlets use bounding spheres; this
means that not as much code can be shared as one might think.
This patch doesn't provide any way to perform GPU culling on shadow
maps, to avoid making this patch bigger than it already is. That can be
a followup.
## Changelog
### Added
* Frustum culling can now optionally be done on the GPU. To enable it,
add the `GpuCulling` component to a camera.
* To disable CPU frustum culling, add `NoCpuCulling` to a camera. Note
that `GpuCulling` doesn't automatically imply `NoCpuCulling`.
2024-04-28 12:50:00 +00:00
|
|
|
extra_index: PhaseItemExtraIndex::NONE,
|
`StandardMaterial` Light Transmission (#8015)
# Objective
<img width="1920" alt="Screenshot 2023-04-26 at 01 07 34"
src="https://user-images.githubusercontent.com/418473/234467578-0f34187b-5863-4ea1-88e9-7a6bb8ce8da3.png">
This PR adds both diffuse and specular light transmission capabilities
to the `StandardMaterial`, with support for screen space refractions.
This enables realistically representing a wide range of real-world
materials, such as:
- Glass; (Including frosted glass)
- Transparent and translucent plastics;
- Various liquids and gels;
- Gemstones;
- Marble;
- Wax;
- Paper;
- Leaves;
- Porcelain.
Unlike existing support for transparency, light transmission does not
rely on fixed function alpha blending, and therefore works with both
`AlphaMode::Opaque` and `AlphaMode::Mask` materials.
## Solution
- Introduces a number of transmission related fields in the
`StandardMaterial`;
- For specular transmission:
- Adds logic to take a view main texture snapshot after the opaque
phase; (in order to perform screen space refractions)
- Introduces a new `Transmissive3d` phase to the renderer, to which all
meshes with `transmission > 0.0` materials are sent.
- Calculates a light exit point (of the approximate mesh volume) using
`ior` and `thickness` properties
- Samples the snapshot texture with an adaptive number of taps across a
`roughness`-controlled radius enabling “blurry” refractions
- For diffuse transmission:
- Approximates transmitted diffuse light by using a second, flipped +
displaced, diffuse-only Lambertian lobe for each light source.
## To Do
- [x] Figure out where `fresnel_mix()` is taking place, if at all, and
where `dielectric_specular` is being calculated, if at all, and update
them to use the `ior` value (Not a blocker, just a nice-to-have for more
correct BSDF)
- To the _best of my knowledge, this is now taking place, after
964340cdd. The fresnel mix is actually "split" into two parts in our
implementation, one `(1 - fresnel(...))` in the transmission, and
`fresnel()` in the light implementations. A surface with more
reflectance now will produce slightly dimmer transmission towards the
grazing angle, as more of the light gets reflected.
- [x] Add `transmission_texture`
- [x] Add `diffuse_transmission_texture`
- [x] Add `thickness_texture`
- [x] Add `attenuation_distance` and `attenuation_color`
- [x] Connect values to glTF loader
- [x] `transmission` and `transmission_texture`
- [x] `thickness` and `thickness_texture`
- [x] `ior`
- [ ] `diffuse_transmission` and `diffuse_transmission_texture` (needs
upstream support in `gltf` crate, not a blocker)
- [x] Add support for multiple screen space refraction “steps”
- [x] Conditionally create no transmission snapshot texture at all if
`steps == 0`
- [x] Conditionally enable/disable screen space refraction transmission
snapshots
- [x] Read from depth pre-pass to prevent refracting pixels in front of
the light exit point
- [x] Use `interleaved_gradient_noise()` function for sampling blur in a
way that benefits from TAA
- [x] Drill down a TAA `#define`, tweak some aspects of the effect
conditionally based on it
- [x] Remove const array that's crashing under HLSL (unless a new `naga`
release with https://github.com/gfx-rs/naga/pull/2496 comes out before
we merge this)
- [ ] Look into alternatives to the `switch` hack for dynamically
indexing the const array (might not be needed, compilers seem to be
decent at expanding it)
- [ ] Add pipeline keys for gating transmission (do we really want/need
this?)
- [x] Tweak some material field/function names?
## A Note on Texture Packing
_This was originally added as a comment to the
`specular_transmission_texture`, `thickness_texture` and
`diffuse_transmission_texture` documentation, I removed it since it was
more confusing than helpful, and will likely be made redundant/will need
to be updated once we have a better infrastructure for preprocessing
assets_
Due to how channels are mapped, you can more efficiently use a single
shared texture image
for configuring the following:
- R - `specular_transmission_texture`
- G - `thickness_texture`
- B - _unused_
- A - `diffuse_transmission_texture`
The `KHR_materials_diffuse_transmission` glTF extension also defines a
`diffuseTransmissionColorTexture`,
that _we don't currently support_. One might choose to pack the
intensity and color textures together,
using RGB for the color and A for the intensity, in which case this
packing advice doesn't really apply.
---
## Changelog
- Added a new `Transmissive3d` render phase for rendering specular
transmissive materials with screen space refractions
- Added rendering support for transmitted environment map light on the
`StandardMaterial` as a fallback for screen space refractions
- Added `diffuse_transmission`, `specular_transmission`, `thickness`,
`ior`, `attenuation_distance` and `attenuation_color` to the
`StandardMaterial`
- Added `diffuse_transmission_texture`, `specular_transmission_texture`,
`thickness_texture` to the `StandardMaterial`, gated behind a new
`pbr_transmission_textures` cargo feature (off by default, for maximum
hardware compatibility)
- Added `Camera3d::screen_space_specular_transmission_steps` for
controlling the number of “layers of transparency” rendered for
transmissive objects
- Added a `TransmittedShadowReceiver` component for enabling shadows in
(diffusely) transmitted light. (disabled by default, as it requires
carefully setting up the `thickness` to avoid self-shadow artifacts)
- Added support for the `KHR_materials_transmission`,
`KHR_materials_ior` and `KHR_materials_volume` glTF extensions
- Renamed items related to temporal jitter for greater consistency
## Migration Guide
- `SsaoPipelineKey::temporal_noise` has been renamed to
`SsaoPipelineKey::temporal_jitter`
- The `TAA` shader def (controlled by the presence of the
`TemporalAntiAliasSettings` component in the camera) has been replaced
with the `TEMPORAL_JITTER` shader def (controlled by the presence of the
`TemporalJitter` component in the camera)
- `MeshPipelineKey::TAA` has been replaced by
`MeshPipelineKey::TEMPORAL_JITTER`
- The `TEMPORAL_NOISE` shader def has been consolidated with
`TEMPORAL_JITTER`
2023-10-31 20:59:02 +00:00
|
|
|
});
|
Micro-optimize `queue_material_meshes`, primarily to remove bit manipulation. (#12791)
This commit makes the following optimizations:
## `MeshPipelineKey`/`BaseMeshPipelineKey` split
`MeshPipelineKey` has been split into `BaseMeshPipelineKey`, which lives
in `bevy_render` and `MeshPipelineKey`, which lives in `bevy_pbr`.
Conceptually, `BaseMeshPipelineKey` is a superclass of
`MeshPipelineKey`. For `BaseMeshPipelineKey`, the bits start at the
highest (most significant) bit and grow downward toward the lowest bit;
for `MeshPipelineKey`, the bits start at the lowest bit and grow upward
toward the highest bit. This prevents them from colliding.
The goal of this is to avoid having to reassemble bits of the pipeline
key for every mesh every frame. Instead, we can just use a bitwise or
operation to combine the pieces that make up a `MeshPipelineKey`.
## `specialize_slow`
Previously, all of `specialize()` was marked as `#[inline]`. This
bloated `queue_material_meshes` unnecessarily, as a large chunk of it
ended up being a slow path that was rarely hit. This commit refactors
the function to move the slow path to `specialize_slow()`.
Together, these two changes shave about 5% off `queue_material_meshes`:

## Migration Guide
- The `primitive_topology` field on `GpuMesh` is now an accessor method:
`GpuMesh::primitive_topology()`.
- For performance reasons, `MeshPipelineKey` has been split into
`BaseMeshPipelineKey`, which lives in `bevy_render`, and
`MeshPipelineKey`, which lives in `bevy_pbr`. These two should be
combined with bitwise-or to produce the final `MeshPipelineKey`.
2024-04-01 21:58:53 +00:00
|
|
|
} else if material.properties.render_method == OpaqueRendererMethod::Forward {
|
Improve performance by binning together opaque items instead of sorting them. (#12453)
Today, we sort all entities added to all phases, even the phases that
don't strictly need sorting, such as the opaque and shadow phases. This
results in a performance loss because our `PhaseItem`s are rather large
in memory, so sorting is slow. Additionally, determining the boundaries
of batches is an O(n) process.
This commit makes Bevy instead applicable place phase items into *bins*
keyed by *bin keys*, which have the invariant that everything in the
same bin is potentially batchable. This makes determining batch
boundaries O(1), because everything in the same bin can be batched.
Instead of sorting each entity, we now sort only the bin keys. This
drops the sorting time to near-zero on workloads with few bins like
`many_cubes --no-frustum-culling`. Memory usage is improved too, with
batch boundaries and dynamic indices now implicit instead of explicit.
The improved memory usage results in a significant win even on
unbatchable workloads like `many_cubes --no-frustum-culling
--vary-material-data-per-instance`, presumably due to cache effects.
Not all phases can be binned; some, such as transparent and transmissive
phases, must still be sorted. To handle this, this commit splits
`PhaseItem` into `BinnedPhaseItem` and `SortedPhaseItem`. Most of the
logic that today deals with `PhaseItem`s has been moved to
`SortedPhaseItem`. `BinnedPhaseItem` has the new logic.
Frame time results (in ms/frame) are as follows:
| Benchmark | `binning` | `main` | Speedup |
| ------------------------ | --------- | ------- | ------- |
| `many_cubes -nfc -vpi` | 232.179 | 312.123 | 34.43% |
| `many_cubes -nfc` | 25.874 | 30.117 | 16.40% |
| `many_foxes` | 3.276 | 3.515 | 7.30% |
(`-nfc` is short for `--no-frustum-culling`; `-vpi` is short for
`--vary-per-instance`.)
---
## Changelog
### Changed
* Render phases have been split into binned and sorted phases. Binned
phases, such as the common opaque phase, achieve improved CPU
performance by avoiding the sorting step.
## Migration Guide
- `PhaseItem` has been split into `BinnedPhaseItem` and
`SortedPhaseItem`. If your code has custom `PhaseItem`s, you will need
to migrate them to one of these two types. `SortedPhaseItem` requires
the fewest code changes, but you may want to pick `BinnedPhaseItem` if
your phase doesn't require sorting, as that enables higher performance.
## Tracy graphs
`many-cubes --no-frustum-culling`, `main` branch:
<img width="1064" alt="Screenshot 2024-03-12 180037"
src="https://github.com/bevyengine/bevy/assets/157897/e1180ce8-8e89-46d2-85e3-f59f72109a55">
`many-cubes --no-frustum-culling`, this branch:
<img width="1064" alt="Screenshot 2024-03-12 180011"
src="https://github.com/bevyengine/bevy/assets/157897/0899f036-6075-44c5-a972-44d95895f46c">
You can see that `batch_and_prepare_binned_render_phase` is a much
smaller fraction of the time. Zooming in on that function, with yellow
being this branch and red being `main`, we see:
<img width="1064" alt="Screenshot 2024-03-12 175832"
src="https://github.com/bevyengine/bevy/assets/157897/0dfc8d3f-49f4-496e-8825-a66e64d356d0">
The binning happens in `queue_material_meshes`. Again with yellow being
this branch and red being `main`:
<img width="1064" alt="Screenshot 2024-03-12 175755"
src="https://github.com/bevyengine/bevy/assets/157897/b9b20dc1-11c8-400c-a6cc-1c2e09c1bb96">
We can see that there is a small regression in `queue_material_meshes`
performance, but it's not nearly enough to outweigh the large gains in
`batch_and_prepare_binned_render_phase`.
---------
Co-authored-by: James Liu <contact@jamessliu.com>
2024-03-30 02:55:02 +00:00
|
|
|
let bin_key = Opaque3dBinKey {
|
2023-10-12 22:10:38 +00:00
|
|
|
draw_function: draw_opaque_pbr,
|
|
|
|
pipeline: pipeline_id,
|
Allow phase items not associated with meshes to be binned. (#14029)
As reported in #14004, many third-party plugins, such as Hanabi, enqueue
entities that don't have meshes into render phases. However, the
introduction of indirect mode added a dependency on mesh-specific data,
breaking this workflow. This is because GPU preprocessing requires that
the render phases manage indirect draw parameters, which don't apply to
objects that aren't meshes. The existing code skips over binned entities
that don't have indirect draw parameters, which causes the rendering to
be skipped for such objects.
To support this workflow, this commit adds a new field,
`non_mesh_items`, to `BinnedRenderPhase`. This field contains a simple
list of (bin key, entity) pairs. After drawing batchable and unbatchable
objects, the non-mesh items are drawn one after another. Bevy itself
doesn't enqueue any items into this list; it exists solely for the
application and/or plugins to use.
Additionally, this commit switches the asset ID in the standard bin keys
to be an untyped asset ID rather than that of a mesh. This allows more
flexibility, allowing bins to be keyed off any type of asset.
This patch adds a new example, `custom_phase_item`, which simultaneously
serves to demonstrate how to use this new feature and to act as a
regression test so this doesn't break again.
Fixes #14004.
## Changelog
### Added
* `BinnedRenderPhase` now contains a `non_mesh_items` field for plugins
to add custom items to.
2024-06-27 16:13:03 +00:00
|
|
|
asset_id: mesh_instance.mesh_asset_id.into(),
|
Improve performance by binning together opaque items instead of sorting them. (#12453)
Today, we sort all entities added to all phases, even the phases that
don't strictly need sorting, such as the opaque and shadow phases. This
results in a performance loss because our `PhaseItem`s are rather large
in memory, so sorting is slow. Additionally, determining the boundaries
of batches is an O(n) process.
This commit makes Bevy instead applicable place phase items into *bins*
keyed by *bin keys*, which have the invariant that everything in the
same bin is potentially batchable. This makes determining batch
boundaries O(1), because everything in the same bin can be batched.
Instead of sorting each entity, we now sort only the bin keys. This
drops the sorting time to near-zero on workloads with few bins like
`many_cubes --no-frustum-culling`. Memory usage is improved too, with
batch boundaries and dynamic indices now implicit instead of explicit.
The improved memory usage results in a significant win even on
unbatchable workloads like `many_cubes --no-frustum-culling
--vary-material-data-per-instance`, presumably due to cache effects.
Not all phases can be binned; some, such as transparent and transmissive
phases, must still be sorted. To handle this, this commit splits
`PhaseItem` into `BinnedPhaseItem` and `SortedPhaseItem`. Most of the
logic that today deals with `PhaseItem`s has been moved to
`SortedPhaseItem`. `BinnedPhaseItem` has the new logic.
Frame time results (in ms/frame) are as follows:
| Benchmark | `binning` | `main` | Speedup |
| ------------------------ | --------- | ------- | ------- |
| `many_cubes -nfc -vpi` | 232.179 | 312.123 | 34.43% |
| `many_cubes -nfc` | 25.874 | 30.117 | 16.40% |
| `many_foxes` | 3.276 | 3.515 | 7.30% |
(`-nfc` is short for `--no-frustum-culling`; `-vpi` is short for
`--vary-per-instance`.)
---
## Changelog
### Changed
* Render phases have been split into binned and sorted phases. Binned
phases, such as the common opaque phase, achieve improved CPU
performance by avoiding the sorting step.
## Migration Guide
- `PhaseItem` has been split into `BinnedPhaseItem` and
`SortedPhaseItem`. If your code has custom `PhaseItem`s, you will need
to migrate them to one of these two types. `SortedPhaseItem` requires
the fewest code changes, but you may want to pick `BinnedPhaseItem` if
your phase doesn't require sorting, as that enables higher performance.
## Tracy graphs
`many-cubes --no-frustum-culling`, `main` branch:
<img width="1064" alt="Screenshot 2024-03-12 180037"
src="https://github.com/bevyengine/bevy/assets/157897/e1180ce8-8e89-46d2-85e3-f59f72109a55">
`many-cubes --no-frustum-culling`, this branch:
<img width="1064" alt="Screenshot 2024-03-12 180011"
src="https://github.com/bevyengine/bevy/assets/157897/0899f036-6075-44c5-a972-44d95895f46c">
You can see that `batch_and_prepare_binned_render_phase` is a much
smaller fraction of the time. Zooming in on that function, with yellow
being this branch and red being `main`, we see:
<img width="1064" alt="Screenshot 2024-03-12 175832"
src="https://github.com/bevyengine/bevy/assets/157897/0dfc8d3f-49f4-496e-8825-a66e64d356d0">
The binning happens in `queue_material_meshes`. Again with yellow being
this branch and red being `main`:
<img width="1064" alt="Screenshot 2024-03-12 175755"
src="https://github.com/bevyengine/bevy/assets/157897/b9b20dc1-11c8-400c-a6cc-1c2e09c1bb96">
We can see that there is a small regression in `queue_material_meshes`
performance, but it's not nearly enough to outweigh the large gains in
`batch_and_prepare_binned_render_phase`.
---------
Co-authored-by: James Liu <contact@jamessliu.com>
2024-03-30 02:55:02 +00:00
|
|
|
material_bind_group_id: material.get_bind_group_id().0,
|
|
|
|
lightmap_image,
|
|
|
|
};
|
Allow phase items not associated with meshes to be binned. (#14029)
As reported in #14004, many third-party plugins, such as Hanabi, enqueue
entities that don't have meshes into render phases. However, the
introduction of indirect mode added a dependency on mesh-specific data,
breaking this workflow. This is because GPU preprocessing requires that
the render phases manage indirect draw parameters, which don't apply to
objects that aren't meshes. The existing code skips over binned entities
that don't have indirect draw parameters, which causes the rendering to
be skipped for such objects.
To support this workflow, this commit adds a new field,
`non_mesh_items`, to `BinnedRenderPhase`. This field contains a simple
list of (bin key, entity) pairs. After drawing batchable and unbatchable
objects, the non-mesh items are drawn one after another. Bevy itself
doesn't enqueue any items into this list; it exists solely for the
application and/or plugins to use.
Additionally, this commit switches the asset ID in the standard bin keys
to be an untyped asset ID rather than that of a mesh. This allows more
flexibility, allowing bins to be keyed off any type of asset.
This patch adds a new example, `custom_phase_item`, which simultaneously
serves to demonstrate how to use this new feature and to act as a
regression test so this doesn't break again.
Fixes #14004.
## Changelog
### Added
* `BinnedRenderPhase` now contains a `non_mesh_items` field for plugins
to add custom items to.
2024-06-27 16:13:03 +00:00
|
|
|
opaque_phase.add(
|
|
|
|
bin_key,
|
Type safe retained render world (#15756)
# Objective
In the Render World, there are a number of collections that are derived
from Main World entities and are used to drive rendering. The most
notable are:
- `VisibleEntities`, which is generated in the `check_visibility` system
and contains visible entities for a view.
- `ExtractedInstances`, which maps entity ids to asset ids.
In the old model, these collections were trivially kept in sync -- any
extracted phase item could look itself up because the render entity id
was guaranteed to always match the corresponding main world id.
After #15320, this became much more complicated, and was leading to a
number of subtle bugs in the Render World. The main rendering systems,
i.e. `queue_material_meshes` and `queue_material2d_meshes`, follow a
similar pattern:
```rust
for visible_entity in visible_entities.iter::<With<Mesh2d>>() {
let Some(mesh_instance) = render_mesh_instances.get_mut(visible_entity) else {
continue;
};
// Look some more stuff up and specialize the pipeline...
let bin_key = Opaque2dBinKey {
pipeline: pipeline_id,
draw_function: draw_opaque_2d,
asset_id: mesh_instance.mesh_asset_id.into(),
material_bind_group_id: material_2d.get_bind_group_id().0,
};
opaque_phase.add(
bin_key,
*visible_entity,
BinnedRenderPhaseType::mesh(mesh_instance.automatic_batching),
);
}
```
In this case, `visible_entities` and `render_mesh_instances` are both
collections that are created and keyed by Main World entity ids, and so
this lookup happens to work by coincidence. However, there is a major
unintentional bug here: namely, because `visible_entities` is a
collection of Main World ids, the phase item being queued is created
with a Main World id rather than its correct Render World id.
This happens to not break mesh rendering because the render commands
used for drawing meshes do not access the `ItemQuery` parameter, but
demonstrates the confusion that is now possible: our UI phase items are
correctly being queued with Render World ids while our meshes aren't.
Additionally, this makes it very easy and error prone to use the wrong
entity id to look up things like assets. For example, if instead we
ignored visibility checks and queued our meshes via a query, we'd have
to be extra careful to use `&MainEntity` instead of the natural
`Entity`.
## Solution
Make all collections that are derived from Main World data use
`MainEntity` as their key, to ensure type safety and avoid accidentally
looking up data with the wrong entity id:
```rust
pub type MainEntityHashMap<V> = hashbrown::HashMap<MainEntity, V, EntityHash>;
```
Additionally, we make all `PhaseItem` be able to provide both their Main
and Render World ids, to allow render phase implementors maximum
flexibility as to what id should be used to look up data.
You can think of this like tracking at the type level whether something
in the Render World should use it's "primary key", i.e. entity id, or
needs to use a foreign key, i.e. `MainEntity`.
## Testing
##### TODO:
This will require extensive testing to make sure things didn't break!
Additionally, some extraction logic has become more complicated and
needs to be checked for regressions.
## Migration Guide
With the advent of the retained render world, collections that contain
references to `Entity` that are extracted into the render world have
been changed to contain `MainEntity` in order to prevent errors where a
render world entity id is used to look up an item by accident. Custom
rendering code may need to be changed to query for `&MainEntity` in
order to look up the correct item from such a collection. Additionally,
users who implement their own extraction logic for collections of main
world entity should strongly consider extracting into a different
collection that uses `MainEntity` as a key.
Additionally, render phases now require specifying both the `Entity` and
`MainEntity` for a given `PhaseItem`. Custom render phases should ensure
`MainEntity` is available when queuing a phase item.
2024-10-10 18:47:04 +00:00
|
|
|
(*render_entity, *visible_entity),
|
Allow phase items not associated with meshes to be binned. (#14029)
As reported in #14004, many third-party plugins, such as Hanabi, enqueue
entities that don't have meshes into render phases. However, the
introduction of indirect mode added a dependency on mesh-specific data,
breaking this workflow. This is because GPU preprocessing requires that
the render phases manage indirect draw parameters, which don't apply to
objects that aren't meshes. The existing code skips over binned entities
that don't have indirect draw parameters, which causes the rendering to
be skipped for such objects.
To support this workflow, this commit adds a new field,
`non_mesh_items`, to `BinnedRenderPhase`. This field contains a simple
list of (bin key, entity) pairs. After drawing batchable and unbatchable
objects, the non-mesh items are drawn one after another. Bevy itself
doesn't enqueue any items into this list; it exists solely for the
application and/or plugins to use.
Additionally, this commit switches the asset ID in the standard bin keys
to be an untyped asset ID rather than that of a mesh. This allows more
flexibility, allowing bins to be keyed off any type of asset.
This patch adds a new example, `custom_phase_item`, which simultaneously
serves to demonstrate how to use this new feature and to act as a
regression test so this doesn't break again.
Fixes #14004.
## Changelog
### Added
* `BinnedRenderPhase` now contains a `non_mesh_items` field for plugins
to add custom items to.
2024-06-27 16:13:03 +00:00
|
|
|
BinnedRenderPhaseType::mesh(mesh_instance.should_batch()),
|
|
|
|
);
|
2023-10-12 22:10:38 +00:00
|
|
|
}
|
2023-09-20 20:18:55 +00:00
|
|
|
}
|
2024-04-15 20:37:52 +00:00
|
|
|
// Alpha mask
|
|
|
|
MeshPipelineKey::MAY_DISCARD => {
|
`StandardMaterial` Light Transmission (#8015)
# Objective
<img width="1920" alt="Screenshot 2023-04-26 at 01 07 34"
src="https://user-images.githubusercontent.com/418473/234467578-0f34187b-5863-4ea1-88e9-7a6bb8ce8da3.png">
This PR adds both diffuse and specular light transmission capabilities
to the `StandardMaterial`, with support for screen space refractions.
This enables realistically representing a wide range of real-world
materials, such as:
- Glass; (Including frosted glass)
- Transparent and translucent plastics;
- Various liquids and gels;
- Gemstones;
- Marble;
- Wax;
- Paper;
- Leaves;
- Porcelain.
Unlike existing support for transparency, light transmission does not
rely on fixed function alpha blending, and therefore works with both
`AlphaMode::Opaque` and `AlphaMode::Mask` materials.
## Solution
- Introduces a number of transmission related fields in the
`StandardMaterial`;
- For specular transmission:
- Adds logic to take a view main texture snapshot after the opaque
phase; (in order to perform screen space refractions)
- Introduces a new `Transmissive3d` phase to the renderer, to which all
meshes with `transmission > 0.0` materials are sent.
- Calculates a light exit point (of the approximate mesh volume) using
`ior` and `thickness` properties
- Samples the snapshot texture with an adaptive number of taps across a
`roughness`-controlled radius enabling “blurry” refractions
- For diffuse transmission:
- Approximates transmitted diffuse light by using a second, flipped +
displaced, diffuse-only Lambertian lobe for each light source.
## To Do
- [x] Figure out where `fresnel_mix()` is taking place, if at all, and
where `dielectric_specular` is being calculated, if at all, and update
them to use the `ior` value (Not a blocker, just a nice-to-have for more
correct BSDF)
- To the _best of my knowledge, this is now taking place, after
964340cdd. The fresnel mix is actually "split" into two parts in our
implementation, one `(1 - fresnel(...))` in the transmission, and
`fresnel()` in the light implementations. A surface with more
reflectance now will produce slightly dimmer transmission towards the
grazing angle, as more of the light gets reflected.
- [x] Add `transmission_texture`
- [x] Add `diffuse_transmission_texture`
- [x] Add `thickness_texture`
- [x] Add `attenuation_distance` and `attenuation_color`
- [x] Connect values to glTF loader
- [x] `transmission` and `transmission_texture`
- [x] `thickness` and `thickness_texture`
- [x] `ior`
- [ ] `diffuse_transmission` and `diffuse_transmission_texture` (needs
upstream support in `gltf` crate, not a blocker)
- [x] Add support for multiple screen space refraction “steps”
- [x] Conditionally create no transmission snapshot texture at all if
`steps == 0`
- [x] Conditionally enable/disable screen space refraction transmission
snapshots
- [x] Read from depth pre-pass to prevent refracting pixels in front of
the light exit point
- [x] Use `interleaved_gradient_noise()` function for sampling blur in a
way that benefits from TAA
- [x] Drill down a TAA `#define`, tweak some aspects of the effect
conditionally based on it
- [x] Remove const array that's crashing under HLSL (unless a new `naga`
release with https://github.com/gfx-rs/naga/pull/2496 comes out before
we merge this)
- [ ] Look into alternatives to the `switch` hack for dynamically
indexing the const array (might not be needed, compilers seem to be
decent at expanding it)
- [ ] Add pipeline keys for gating transmission (do we really want/need
this?)
- [x] Tweak some material field/function names?
## A Note on Texture Packing
_This was originally added as a comment to the
`specular_transmission_texture`, `thickness_texture` and
`diffuse_transmission_texture` documentation, I removed it since it was
more confusing than helpful, and will likely be made redundant/will need
to be updated once we have a better infrastructure for preprocessing
assets_
Due to how channels are mapped, you can more efficiently use a single
shared texture image
for configuring the following:
- R - `specular_transmission_texture`
- G - `thickness_texture`
- B - _unused_
- A - `diffuse_transmission_texture`
The `KHR_materials_diffuse_transmission` glTF extension also defines a
`diffuseTransmissionColorTexture`,
that _we don't currently support_. One might choose to pack the
intensity and color textures together,
using RGB for the color and A for the intensity, in which case this
packing advice doesn't really apply.
---
## Changelog
- Added a new `Transmissive3d` render phase for rendering specular
transmissive materials with screen space refractions
- Added rendering support for transmitted environment map light on the
`StandardMaterial` as a fallback for screen space refractions
- Added `diffuse_transmission`, `specular_transmission`, `thickness`,
`ior`, `attenuation_distance` and `attenuation_color` to the
`StandardMaterial`
- Added `diffuse_transmission_texture`, `specular_transmission_texture`,
`thickness_texture` to the `StandardMaterial`, gated behind a new
`pbr_transmission_textures` cargo feature (off by default, for maximum
hardware compatibility)
- Added `Camera3d::screen_space_specular_transmission_steps` for
controlling the number of “layers of transparency” rendered for
transmissive objects
- Added a `TransmittedShadowReceiver` component for enabling shadows in
(diffusely) transmitted light. (disabled by default, as it requires
carefully setting up the `thickness` to avoid self-shadow artifacts)
- Added support for the `KHR_materials_transmission`,
`KHR_materials_ior` and `KHR_materials_volume` glTF extensions
- Renamed items related to temporal jitter for greater consistency
## Migration Guide
- `SsaoPipelineKey::temporal_noise` has been renamed to
`SsaoPipelineKey::temporal_jitter`
- The `TAA` shader def (controlled by the presence of the
`TemporalAntiAliasSettings` component in the camera) has been replaced
with the `TEMPORAL_JITTER` shader def (controlled by the presence of the
`TemporalJitter` component in the camera)
- `MeshPipelineKey::TAA` has been replaced by
`MeshPipelineKey::TEMPORAL_JITTER`
- The `TEMPORAL_NOISE` shader def has been consolidated with
`TEMPORAL_JITTER`
2023-10-31 20:59:02 +00:00
|
|
|
if material.properties.reads_view_transmission_texture {
|
Generate `MeshUniform`s on the GPU via compute shader where available. (#12773)
Currently, `MeshUniform`s are rather large: 160 bytes. They're also
somewhat expensive to compute, because they involve taking the inverse
of a 3x4 matrix. Finally, if a mesh is present in multiple views, that
mesh will have a separate `MeshUniform` for each and every view, which
is wasteful.
This commit fixes these issues by introducing the concept of a *mesh
input uniform* and adding a *mesh uniform building* compute shader pass.
The `MeshInputUniform` is simply the minimum amount of data needed for
the GPU to compute the full `MeshUniform`. Most of this data is just the
transform and is therefore only 64 bytes. `MeshInputUniform`s are
computed during the *extraction* phase, much like skins are today, in
order to avoid needlessly copying transforms around on CPU. (In fact,
the render app has been changed to only store the translation of each
mesh; it no longer cares about any other part of the transform, which is
stored only on the GPU and the main world.) Before rendering, the
`build_mesh_uniforms` pass runs to expand the `MeshInputUniform`s to the
full `MeshUniform`.
The mesh uniform building pass does the following, all on GPU:
1. Copy the appropriate fields of the `MeshInputUniform` to the
`MeshUniform` slot. If a single mesh is present in multiple views, this
effectively duplicates it into each view.
2. Compute the inverse transpose of the model transform, used for
transforming normals.
3. If applicable, copy the mesh's transform from the previous frame for
TAA. To support this, we double-buffer the `MeshInputUniform`s over two
frames and swap the buffers each frame. The `MeshInputUniform`s for the
current frame contain the index of that mesh's `MeshInputUniform` for
the previous frame.
This commit produces wins in virtually every CPU part of the pipeline:
`extract_meshes`, `queue_material_meshes`,
`batch_and_prepare_render_phase`, and especially
`write_batched_instance_buffer` are all faster. Shrinking the amount of
CPU data that has to be shuffled around speeds up the entire rendering
process.
| Benchmark | This branch | `main` | Speedup |
|------------------------|-------------|---------|---------|
| `many_cubes -nfc` | 17.259 | 24.529 | 42.12% |
| `many_cubes -nfc -vpi` | 302.116 | 312.123 | 3.31% |
| `many_foxes` | 3.227 | 3.515 | 8.92% |
Because mesh uniform building requires compute shader, and WebGL 2 has
no compute shader, the existing CPU mesh uniform building code has been
left as-is. Many types now have both CPU mesh uniform building and GPU
mesh uniform building modes. Developers can opt into the old CPU mesh
uniform building by setting the `use_gpu_uniform_builder` option on
`PbrPlugin` to `false`.
Below are graphs of the CPU portions of `many-cubes
--no-frustum-culling`. Yellow is this branch, red is `main`.
`extract_meshes`:

It's notable that we get a small win even though we're now writing to a
GPU buffer.
`queue_material_meshes`:

There's a bit of a regression here; not sure what's causing it. In any
case it's very outweighed by the other gains.
`batch_and_prepare_render_phase`:

There's a huge win here, enough to make batching basically drop off the
profile.
`write_batched_instance_buffer`:

There's a massive improvement here, as expected. Note that a lot of it
simply comes from the fact that `MeshInputUniform` is `Pod`. (This isn't
a maintainability problem in my view because `MeshInputUniform` is so
simple: just 16 tightly-packed words.)
## Changelog
### Added
* Per-mesh instance data is now generated on GPU with a compute shader
instead of CPU, resulting in rendering performance improvements on
platforms where compute shaders are supported.
## Migration guide
* Custom render phases now need multiple systems beyond just
`batch_and_prepare_render_phase`. Code that was previously creating
custom render phases should now add a `BinnedRenderPhasePlugin` or
`SortedRenderPhasePlugin` as appropriate instead of directly adding
`batch_and_prepare_render_phase`.
2024-04-10 05:33:32 +00:00
|
|
|
let distance = rangefinder.distance_translation(&mesh_instance.translation)
|
2024-02-26 11:14:59 +00:00
|
|
|
+ material.properties.depth_bias;
|
`StandardMaterial` Light Transmission (#8015)
# Objective
<img width="1920" alt="Screenshot 2023-04-26 at 01 07 34"
src="https://user-images.githubusercontent.com/418473/234467578-0f34187b-5863-4ea1-88e9-7a6bb8ce8da3.png">
This PR adds both diffuse and specular light transmission capabilities
to the `StandardMaterial`, with support for screen space refractions.
This enables realistically representing a wide range of real-world
materials, such as:
- Glass; (Including frosted glass)
- Transparent and translucent plastics;
- Various liquids and gels;
- Gemstones;
- Marble;
- Wax;
- Paper;
- Leaves;
- Porcelain.
Unlike existing support for transparency, light transmission does not
rely on fixed function alpha blending, and therefore works with both
`AlphaMode::Opaque` and `AlphaMode::Mask` materials.
## Solution
- Introduces a number of transmission related fields in the
`StandardMaterial`;
- For specular transmission:
- Adds logic to take a view main texture snapshot after the opaque
phase; (in order to perform screen space refractions)
- Introduces a new `Transmissive3d` phase to the renderer, to which all
meshes with `transmission > 0.0` materials are sent.
- Calculates a light exit point (of the approximate mesh volume) using
`ior` and `thickness` properties
- Samples the snapshot texture with an adaptive number of taps across a
`roughness`-controlled radius enabling “blurry” refractions
- For diffuse transmission:
- Approximates transmitted diffuse light by using a second, flipped +
displaced, diffuse-only Lambertian lobe for each light source.
## To Do
- [x] Figure out where `fresnel_mix()` is taking place, if at all, and
where `dielectric_specular` is being calculated, if at all, and update
them to use the `ior` value (Not a blocker, just a nice-to-have for more
correct BSDF)
- To the _best of my knowledge, this is now taking place, after
964340cdd. The fresnel mix is actually "split" into two parts in our
implementation, one `(1 - fresnel(...))` in the transmission, and
`fresnel()` in the light implementations. A surface with more
reflectance now will produce slightly dimmer transmission towards the
grazing angle, as more of the light gets reflected.
- [x] Add `transmission_texture`
- [x] Add `diffuse_transmission_texture`
- [x] Add `thickness_texture`
- [x] Add `attenuation_distance` and `attenuation_color`
- [x] Connect values to glTF loader
- [x] `transmission` and `transmission_texture`
- [x] `thickness` and `thickness_texture`
- [x] `ior`
- [ ] `diffuse_transmission` and `diffuse_transmission_texture` (needs
upstream support in `gltf` crate, not a blocker)
- [x] Add support for multiple screen space refraction “steps”
- [x] Conditionally create no transmission snapshot texture at all if
`steps == 0`
- [x] Conditionally enable/disable screen space refraction transmission
snapshots
- [x] Read from depth pre-pass to prevent refracting pixels in front of
the light exit point
- [x] Use `interleaved_gradient_noise()` function for sampling blur in a
way that benefits from TAA
- [x] Drill down a TAA `#define`, tweak some aspects of the effect
conditionally based on it
- [x] Remove const array that's crashing under HLSL (unless a new `naga`
release with https://github.com/gfx-rs/naga/pull/2496 comes out before
we merge this)
- [ ] Look into alternatives to the `switch` hack for dynamically
indexing the const array (might not be needed, compilers seem to be
decent at expanding it)
- [ ] Add pipeline keys for gating transmission (do we really want/need
this?)
- [x] Tweak some material field/function names?
## A Note on Texture Packing
_This was originally added as a comment to the
`specular_transmission_texture`, `thickness_texture` and
`diffuse_transmission_texture` documentation, I removed it since it was
more confusing than helpful, and will likely be made redundant/will need
to be updated once we have a better infrastructure for preprocessing
assets_
Due to how channels are mapped, you can more efficiently use a single
shared texture image
for configuring the following:
- R - `specular_transmission_texture`
- G - `thickness_texture`
- B - _unused_
- A - `diffuse_transmission_texture`
The `KHR_materials_diffuse_transmission` glTF extension also defines a
`diffuseTransmissionColorTexture`,
that _we don't currently support_. One might choose to pack the
intensity and color textures together,
using RGB for the color and A for the intensity, in which case this
packing advice doesn't really apply.
---
## Changelog
- Added a new `Transmissive3d` render phase for rendering specular
transmissive materials with screen space refractions
- Added rendering support for transmitted environment map light on the
`StandardMaterial` as a fallback for screen space refractions
- Added `diffuse_transmission`, `specular_transmission`, `thickness`,
`ior`, `attenuation_distance` and `attenuation_color` to the
`StandardMaterial`
- Added `diffuse_transmission_texture`, `specular_transmission_texture`,
`thickness_texture` to the `StandardMaterial`, gated behind a new
`pbr_transmission_textures` cargo feature (off by default, for maximum
hardware compatibility)
- Added `Camera3d::screen_space_specular_transmission_steps` for
controlling the number of “layers of transparency” rendered for
transmissive objects
- Added a `TransmittedShadowReceiver` component for enabling shadows in
(diffusely) transmitted light. (disabled by default, as it requires
carefully setting up the `thickness` to avoid self-shadow artifacts)
- Added support for the `KHR_materials_transmission`,
`KHR_materials_ior` and `KHR_materials_volume` glTF extensions
- Renamed items related to temporal jitter for greater consistency
## Migration Guide
- `SsaoPipelineKey::temporal_noise` has been renamed to
`SsaoPipelineKey::temporal_jitter`
- The `TAA` shader def (controlled by the presence of the
`TemporalAntiAliasSettings` component in the camera) has been replaced
with the `TEMPORAL_JITTER` shader def (controlled by the presence of the
`TemporalJitter` component in the camera)
- `MeshPipelineKey::TAA` has been replaced by
`MeshPipelineKey::TEMPORAL_JITTER`
- The `TEMPORAL_NOISE` shader def has been consolidated with
`TEMPORAL_JITTER`
2023-10-31 20:59:02 +00:00
|
|
|
transmissive_phase.add(Transmissive3d {
|
Type safe retained render world (#15756)
# Objective
In the Render World, there are a number of collections that are derived
from Main World entities and are used to drive rendering. The most
notable are:
- `VisibleEntities`, which is generated in the `check_visibility` system
and contains visible entities for a view.
- `ExtractedInstances`, which maps entity ids to asset ids.
In the old model, these collections were trivially kept in sync -- any
extracted phase item could look itself up because the render entity id
was guaranteed to always match the corresponding main world id.
After #15320, this became much more complicated, and was leading to a
number of subtle bugs in the Render World. The main rendering systems,
i.e. `queue_material_meshes` and `queue_material2d_meshes`, follow a
similar pattern:
```rust
for visible_entity in visible_entities.iter::<With<Mesh2d>>() {
let Some(mesh_instance) = render_mesh_instances.get_mut(visible_entity) else {
continue;
};
// Look some more stuff up and specialize the pipeline...
let bin_key = Opaque2dBinKey {
pipeline: pipeline_id,
draw_function: draw_opaque_2d,
asset_id: mesh_instance.mesh_asset_id.into(),
material_bind_group_id: material_2d.get_bind_group_id().0,
};
opaque_phase.add(
bin_key,
*visible_entity,
BinnedRenderPhaseType::mesh(mesh_instance.automatic_batching),
);
}
```
In this case, `visible_entities` and `render_mesh_instances` are both
collections that are created and keyed by Main World entity ids, and so
this lookup happens to work by coincidence. However, there is a major
unintentional bug here: namely, because `visible_entities` is a
collection of Main World ids, the phase item being queued is created
with a Main World id rather than its correct Render World id.
This happens to not break mesh rendering because the render commands
used for drawing meshes do not access the `ItemQuery` parameter, but
demonstrates the confusion that is now possible: our UI phase items are
correctly being queued with Render World ids while our meshes aren't.
Additionally, this makes it very easy and error prone to use the wrong
entity id to look up things like assets. For example, if instead we
ignored visibility checks and queued our meshes via a query, we'd have
to be extra careful to use `&MainEntity` instead of the natural
`Entity`.
## Solution
Make all collections that are derived from Main World data use
`MainEntity` as their key, to ensure type safety and avoid accidentally
looking up data with the wrong entity id:
```rust
pub type MainEntityHashMap<V> = hashbrown::HashMap<MainEntity, V, EntityHash>;
```
Additionally, we make all `PhaseItem` be able to provide both their Main
and Render World ids, to allow render phase implementors maximum
flexibility as to what id should be used to look up data.
You can think of this like tracking at the type level whether something
in the Render World should use it's "primary key", i.e. entity id, or
needs to use a foreign key, i.e. `MainEntity`.
## Testing
##### TODO:
This will require extensive testing to make sure things didn't break!
Additionally, some extraction logic has become more complicated and
needs to be checked for regressions.
## Migration Guide
With the advent of the retained render world, collections that contain
references to `Entity` that are extracted into the render world have
been changed to contain `MainEntity` in order to prevent errors where a
render world entity id is used to look up an item by accident. Custom
rendering code may need to be changed to query for `&MainEntity` in
order to look up the correct item from such a collection. Additionally,
users who implement their own extraction logic for collections of main
world entity should strongly consider extracting into a different
collection that uses `MainEntity` as a key.
Additionally, render phases now require specifying both the `Entity` and
`MainEntity` for a given `PhaseItem`. Custom render phases should ensure
`MainEntity` is available when queuing a phase item.
2024-10-10 18:47:04 +00:00
|
|
|
entity: (*render_entity, *visible_entity),
|
`StandardMaterial` Light Transmission (#8015)
# Objective
<img width="1920" alt="Screenshot 2023-04-26 at 01 07 34"
src="https://user-images.githubusercontent.com/418473/234467578-0f34187b-5863-4ea1-88e9-7a6bb8ce8da3.png">
This PR adds both diffuse and specular light transmission capabilities
to the `StandardMaterial`, with support for screen space refractions.
This enables realistically representing a wide range of real-world
materials, such as:
- Glass; (Including frosted glass)
- Transparent and translucent plastics;
- Various liquids and gels;
- Gemstones;
- Marble;
- Wax;
- Paper;
- Leaves;
- Porcelain.
Unlike existing support for transparency, light transmission does not
rely on fixed function alpha blending, and therefore works with both
`AlphaMode::Opaque` and `AlphaMode::Mask` materials.
## Solution
- Introduces a number of transmission related fields in the
`StandardMaterial`;
- For specular transmission:
- Adds logic to take a view main texture snapshot after the opaque
phase; (in order to perform screen space refractions)
- Introduces a new `Transmissive3d` phase to the renderer, to which all
meshes with `transmission > 0.0` materials are sent.
- Calculates a light exit point (of the approximate mesh volume) using
`ior` and `thickness` properties
- Samples the snapshot texture with an adaptive number of taps across a
`roughness`-controlled radius enabling “blurry” refractions
- For diffuse transmission:
- Approximates transmitted diffuse light by using a second, flipped +
displaced, diffuse-only Lambertian lobe for each light source.
## To Do
- [x] Figure out where `fresnel_mix()` is taking place, if at all, and
where `dielectric_specular` is being calculated, if at all, and update
them to use the `ior` value (Not a blocker, just a nice-to-have for more
correct BSDF)
- To the _best of my knowledge, this is now taking place, after
964340cdd. The fresnel mix is actually "split" into two parts in our
implementation, one `(1 - fresnel(...))` in the transmission, and
`fresnel()` in the light implementations. A surface with more
reflectance now will produce slightly dimmer transmission towards the
grazing angle, as more of the light gets reflected.
- [x] Add `transmission_texture`
- [x] Add `diffuse_transmission_texture`
- [x] Add `thickness_texture`
- [x] Add `attenuation_distance` and `attenuation_color`
- [x] Connect values to glTF loader
- [x] `transmission` and `transmission_texture`
- [x] `thickness` and `thickness_texture`
- [x] `ior`
- [ ] `diffuse_transmission` and `diffuse_transmission_texture` (needs
upstream support in `gltf` crate, not a blocker)
- [x] Add support for multiple screen space refraction “steps”
- [x] Conditionally create no transmission snapshot texture at all if
`steps == 0`
- [x] Conditionally enable/disable screen space refraction transmission
snapshots
- [x] Read from depth pre-pass to prevent refracting pixels in front of
the light exit point
- [x] Use `interleaved_gradient_noise()` function for sampling blur in a
way that benefits from TAA
- [x] Drill down a TAA `#define`, tweak some aspects of the effect
conditionally based on it
- [x] Remove const array that's crashing under HLSL (unless a new `naga`
release with https://github.com/gfx-rs/naga/pull/2496 comes out before
we merge this)
- [ ] Look into alternatives to the `switch` hack for dynamically
indexing the const array (might not be needed, compilers seem to be
decent at expanding it)
- [ ] Add pipeline keys for gating transmission (do we really want/need
this?)
- [x] Tweak some material field/function names?
## A Note on Texture Packing
_This was originally added as a comment to the
`specular_transmission_texture`, `thickness_texture` and
`diffuse_transmission_texture` documentation, I removed it since it was
more confusing than helpful, and will likely be made redundant/will need
to be updated once we have a better infrastructure for preprocessing
assets_
Due to how channels are mapped, you can more efficiently use a single
shared texture image
for configuring the following:
- R - `specular_transmission_texture`
- G - `thickness_texture`
- B - _unused_
- A - `diffuse_transmission_texture`
The `KHR_materials_diffuse_transmission` glTF extension also defines a
`diffuseTransmissionColorTexture`,
that _we don't currently support_. One might choose to pack the
intensity and color textures together,
using RGB for the color and A for the intensity, in which case this
packing advice doesn't really apply.
---
## Changelog
- Added a new `Transmissive3d` render phase for rendering specular
transmissive materials with screen space refractions
- Added rendering support for transmitted environment map light on the
`StandardMaterial` as a fallback for screen space refractions
- Added `diffuse_transmission`, `specular_transmission`, `thickness`,
`ior`, `attenuation_distance` and `attenuation_color` to the
`StandardMaterial`
- Added `diffuse_transmission_texture`, `specular_transmission_texture`,
`thickness_texture` to the `StandardMaterial`, gated behind a new
`pbr_transmission_textures` cargo feature (off by default, for maximum
hardware compatibility)
- Added `Camera3d::screen_space_specular_transmission_steps` for
controlling the number of “layers of transparency” rendered for
transmissive objects
- Added a `TransmittedShadowReceiver` component for enabling shadows in
(diffusely) transmitted light. (disabled by default, as it requires
carefully setting up the `thickness` to avoid self-shadow artifacts)
- Added support for the `KHR_materials_transmission`,
`KHR_materials_ior` and `KHR_materials_volume` glTF extensions
- Renamed items related to temporal jitter for greater consistency
## Migration Guide
- `SsaoPipelineKey::temporal_noise` has been renamed to
`SsaoPipelineKey::temporal_jitter`
- The `TAA` shader def (controlled by the presence of the
`TemporalAntiAliasSettings` component in the camera) has been replaced
with the `TEMPORAL_JITTER` shader def (controlled by the presence of the
`TemporalJitter` component in the camera)
- `MeshPipelineKey::TAA` has been replaced by
`MeshPipelineKey::TEMPORAL_JITTER`
- The `TEMPORAL_NOISE` shader def has been consolidated with
`TEMPORAL_JITTER`
2023-10-31 20:59:02 +00:00
|
|
|
draw_function: draw_transmissive_pbr,
|
|
|
|
pipeline: pipeline_id,
|
|
|
|
distance,
|
|
|
|
batch_range: 0..1,
|
Implement GPU frustum culling. (#12889)
This commit implements opt-in GPU frustum culling, built on top of the
infrastructure in https://github.com/bevyengine/bevy/pull/12773. To
enable it on a camera, add the `GpuCulling` component to it. To
additionally disable CPU frustum culling, add the `NoCpuCulling`
component. Note that adding `GpuCulling` without `NoCpuCulling`
*currently* does nothing useful. The reason why `GpuCulling` doesn't
automatically imply `NoCpuCulling` is that I intend to follow this patch
up with GPU two-phase occlusion culling, and CPU frustum culling plus
GPU occlusion culling seems like a very commonly-desired mode.
Adding the `GpuCulling` component to a view puts that view into
*indirect mode*. This mode makes all drawcalls indirect, relying on the
mesh preprocessing shader to allocate instances dynamically. In indirect
mode, the `PreprocessWorkItem` `output_index` points not to a
`MeshUniform` instance slot but instead to a set of `wgpu`
`IndirectParameters`, from which it allocates an instance slot
dynamically if frustum culling succeeds. Batch building has been updated
to allocate and track indirect parameter slots, and the AABBs are now
supplied to the GPU as `MeshCullingData`.
A small amount of code relating to the frustum culling has been borrowed
from meshlets and moved into `maths.wgsl`. Note that standard Bevy
frustum culling uses AABBs, while meshlets use bounding spheres; this
means that not as much code can be shared as one might think.
This patch doesn't provide any way to perform GPU culling on shadow
maps, to avoid making this patch bigger than it already is. That can be
a followup.
## Changelog
### Added
* Frustum culling can now optionally be done on the GPU. To enable it,
add the `GpuCulling` component to a camera.
* To disable CPU frustum culling, add `NoCpuCulling` to a camera. Note
that `GpuCulling` doesn't automatically imply `NoCpuCulling`.
2024-04-28 12:50:00 +00:00
|
|
|
extra_index: PhaseItemExtraIndex::NONE,
|
`StandardMaterial` Light Transmission (#8015)
# Objective
<img width="1920" alt="Screenshot 2023-04-26 at 01 07 34"
src="https://user-images.githubusercontent.com/418473/234467578-0f34187b-5863-4ea1-88e9-7a6bb8ce8da3.png">
This PR adds both diffuse and specular light transmission capabilities
to the `StandardMaterial`, with support for screen space refractions.
This enables realistically representing a wide range of real-world
materials, such as:
- Glass; (Including frosted glass)
- Transparent and translucent plastics;
- Various liquids and gels;
- Gemstones;
- Marble;
- Wax;
- Paper;
- Leaves;
- Porcelain.
Unlike existing support for transparency, light transmission does not
rely on fixed function alpha blending, and therefore works with both
`AlphaMode::Opaque` and `AlphaMode::Mask` materials.
## Solution
- Introduces a number of transmission related fields in the
`StandardMaterial`;
- For specular transmission:
- Adds logic to take a view main texture snapshot after the opaque
phase; (in order to perform screen space refractions)
- Introduces a new `Transmissive3d` phase to the renderer, to which all
meshes with `transmission > 0.0` materials are sent.
- Calculates a light exit point (of the approximate mesh volume) using
`ior` and `thickness` properties
- Samples the snapshot texture with an adaptive number of taps across a
`roughness`-controlled radius enabling “blurry” refractions
- For diffuse transmission:
- Approximates transmitted diffuse light by using a second, flipped +
displaced, diffuse-only Lambertian lobe for each light source.
## To Do
- [x] Figure out where `fresnel_mix()` is taking place, if at all, and
where `dielectric_specular` is being calculated, if at all, and update
them to use the `ior` value (Not a blocker, just a nice-to-have for more
correct BSDF)
- To the _best of my knowledge, this is now taking place, after
964340cdd. The fresnel mix is actually "split" into two parts in our
implementation, one `(1 - fresnel(...))` in the transmission, and
`fresnel()` in the light implementations. A surface with more
reflectance now will produce slightly dimmer transmission towards the
grazing angle, as more of the light gets reflected.
- [x] Add `transmission_texture`
- [x] Add `diffuse_transmission_texture`
- [x] Add `thickness_texture`
- [x] Add `attenuation_distance` and `attenuation_color`
- [x] Connect values to glTF loader
- [x] `transmission` and `transmission_texture`
- [x] `thickness` and `thickness_texture`
- [x] `ior`
- [ ] `diffuse_transmission` and `diffuse_transmission_texture` (needs
upstream support in `gltf` crate, not a blocker)
- [x] Add support for multiple screen space refraction “steps”
- [x] Conditionally create no transmission snapshot texture at all if
`steps == 0`
- [x] Conditionally enable/disable screen space refraction transmission
snapshots
- [x] Read from depth pre-pass to prevent refracting pixels in front of
the light exit point
- [x] Use `interleaved_gradient_noise()` function for sampling blur in a
way that benefits from TAA
- [x] Drill down a TAA `#define`, tweak some aspects of the effect
conditionally based on it
- [x] Remove const array that's crashing under HLSL (unless a new `naga`
release with https://github.com/gfx-rs/naga/pull/2496 comes out before
we merge this)
- [ ] Look into alternatives to the `switch` hack for dynamically
indexing the const array (might not be needed, compilers seem to be
decent at expanding it)
- [ ] Add pipeline keys for gating transmission (do we really want/need
this?)
- [x] Tweak some material field/function names?
## A Note on Texture Packing
_This was originally added as a comment to the
`specular_transmission_texture`, `thickness_texture` and
`diffuse_transmission_texture` documentation, I removed it since it was
more confusing than helpful, and will likely be made redundant/will need
to be updated once we have a better infrastructure for preprocessing
assets_
Due to how channels are mapped, you can more efficiently use a single
shared texture image
for configuring the following:
- R - `specular_transmission_texture`
- G - `thickness_texture`
- B - _unused_
- A - `diffuse_transmission_texture`
The `KHR_materials_diffuse_transmission` glTF extension also defines a
`diffuseTransmissionColorTexture`,
that _we don't currently support_. One might choose to pack the
intensity and color textures together,
using RGB for the color and A for the intensity, in which case this
packing advice doesn't really apply.
---
## Changelog
- Added a new `Transmissive3d` render phase for rendering specular
transmissive materials with screen space refractions
- Added rendering support for transmitted environment map light on the
`StandardMaterial` as a fallback for screen space refractions
- Added `diffuse_transmission`, `specular_transmission`, `thickness`,
`ior`, `attenuation_distance` and `attenuation_color` to the
`StandardMaterial`
- Added `diffuse_transmission_texture`, `specular_transmission_texture`,
`thickness_texture` to the `StandardMaterial`, gated behind a new
`pbr_transmission_textures` cargo feature (off by default, for maximum
hardware compatibility)
- Added `Camera3d::screen_space_specular_transmission_steps` for
controlling the number of “layers of transparency” rendered for
transmissive objects
- Added a `TransmittedShadowReceiver` component for enabling shadows in
(diffusely) transmitted light. (disabled by default, as it requires
carefully setting up the `thickness` to avoid self-shadow artifacts)
- Added support for the `KHR_materials_transmission`,
`KHR_materials_ior` and `KHR_materials_volume` glTF extensions
- Renamed items related to temporal jitter for greater consistency
## Migration Guide
- `SsaoPipelineKey::temporal_noise` has been renamed to
`SsaoPipelineKey::temporal_jitter`
- The `TAA` shader def (controlled by the presence of the
`TemporalAntiAliasSettings` component in the camera) has been replaced
with the `TEMPORAL_JITTER` shader def (controlled by the presence of the
`TemporalJitter` component in the camera)
- `MeshPipelineKey::TAA` has been replaced by
`MeshPipelineKey::TEMPORAL_JITTER`
- The `TEMPORAL_NOISE` shader def has been consolidated with
`TEMPORAL_JITTER`
2023-10-31 20:59:02 +00:00
|
|
|
});
|
Micro-optimize `queue_material_meshes`, primarily to remove bit manipulation. (#12791)
This commit makes the following optimizations:
## `MeshPipelineKey`/`BaseMeshPipelineKey` split
`MeshPipelineKey` has been split into `BaseMeshPipelineKey`, which lives
in `bevy_render` and `MeshPipelineKey`, which lives in `bevy_pbr`.
Conceptually, `BaseMeshPipelineKey` is a superclass of
`MeshPipelineKey`. For `BaseMeshPipelineKey`, the bits start at the
highest (most significant) bit and grow downward toward the lowest bit;
for `MeshPipelineKey`, the bits start at the lowest bit and grow upward
toward the highest bit. This prevents them from colliding.
The goal of this is to avoid having to reassemble bits of the pipeline
key for every mesh every frame. Instead, we can just use a bitwise or
operation to combine the pieces that make up a `MeshPipelineKey`.
## `specialize_slow`
Previously, all of `specialize()` was marked as `#[inline]`. This
bloated `queue_material_meshes` unnecessarily, as a large chunk of it
ended up being a slow path that was rarely hit. This commit refactors
the function to move the slow path to `specialize_slow()`.
Together, these two changes shave about 5% off `queue_material_meshes`:

## Migration Guide
- The `primitive_topology` field on `GpuMesh` is now an accessor method:
`GpuMesh::primitive_topology()`.
- For performance reasons, `MeshPipelineKey` has been split into
`BaseMeshPipelineKey`, which lives in `bevy_render`, and
`MeshPipelineKey`, which lives in `bevy_pbr`. These two should be
combined with bitwise-or to produce the final `MeshPipelineKey`.
2024-04-01 21:58:53 +00:00
|
|
|
} else if material.properties.render_method == OpaqueRendererMethod::Forward {
|
Improve performance by binning together opaque items instead of sorting them. (#12453)
Today, we sort all entities added to all phases, even the phases that
don't strictly need sorting, such as the opaque and shadow phases. This
results in a performance loss because our `PhaseItem`s are rather large
in memory, so sorting is slow. Additionally, determining the boundaries
of batches is an O(n) process.
This commit makes Bevy instead applicable place phase items into *bins*
keyed by *bin keys*, which have the invariant that everything in the
same bin is potentially batchable. This makes determining batch
boundaries O(1), because everything in the same bin can be batched.
Instead of sorting each entity, we now sort only the bin keys. This
drops the sorting time to near-zero on workloads with few bins like
`many_cubes --no-frustum-culling`. Memory usage is improved too, with
batch boundaries and dynamic indices now implicit instead of explicit.
The improved memory usage results in a significant win even on
unbatchable workloads like `many_cubes --no-frustum-culling
--vary-material-data-per-instance`, presumably due to cache effects.
Not all phases can be binned; some, such as transparent and transmissive
phases, must still be sorted. To handle this, this commit splits
`PhaseItem` into `BinnedPhaseItem` and `SortedPhaseItem`. Most of the
logic that today deals with `PhaseItem`s has been moved to
`SortedPhaseItem`. `BinnedPhaseItem` has the new logic.
Frame time results (in ms/frame) are as follows:
| Benchmark | `binning` | `main` | Speedup |
| ------------------------ | --------- | ------- | ------- |
| `many_cubes -nfc -vpi` | 232.179 | 312.123 | 34.43% |
| `many_cubes -nfc` | 25.874 | 30.117 | 16.40% |
| `many_foxes` | 3.276 | 3.515 | 7.30% |
(`-nfc` is short for `--no-frustum-culling`; `-vpi` is short for
`--vary-per-instance`.)
---
## Changelog
### Changed
* Render phases have been split into binned and sorted phases. Binned
phases, such as the common opaque phase, achieve improved CPU
performance by avoiding the sorting step.
## Migration Guide
- `PhaseItem` has been split into `BinnedPhaseItem` and
`SortedPhaseItem`. If your code has custom `PhaseItem`s, you will need
to migrate them to one of these two types. `SortedPhaseItem` requires
the fewest code changes, but you may want to pick `BinnedPhaseItem` if
your phase doesn't require sorting, as that enables higher performance.
## Tracy graphs
`many-cubes --no-frustum-culling`, `main` branch:
<img width="1064" alt="Screenshot 2024-03-12 180037"
src="https://github.com/bevyengine/bevy/assets/157897/e1180ce8-8e89-46d2-85e3-f59f72109a55">
`many-cubes --no-frustum-culling`, this branch:
<img width="1064" alt="Screenshot 2024-03-12 180011"
src="https://github.com/bevyengine/bevy/assets/157897/0899f036-6075-44c5-a972-44d95895f46c">
You can see that `batch_and_prepare_binned_render_phase` is a much
smaller fraction of the time. Zooming in on that function, with yellow
being this branch and red being `main`, we see:
<img width="1064" alt="Screenshot 2024-03-12 175832"
src="https://github.com/bevyengine/bevy/assets/157897/0dfc8d3f-49f4-496e-8825-a66e64d356d0">
The binning happens in `queue_material_meshes`. Again with yellow being
this branch and red being `main`:
<img width="1064" alt="Screenshot 2024-03-12 175755"
src="https://github.com/bevyengine/bevy/assets/157897/b9b20dc1-11c8-400c-a6cc-1c2e09c1bb96">
We can see that there is a small regression in `queue_material_meshes`
performance, but it's not nearly enough to outweigh the large gains in
`batch_and_prepare_binned_render_phase`.
---------
Co-authored-by: James Liu <contact@jamessliu.com>
2024-03-30 02:55:02 +00:00
|
|
|
let bin_key = OpaqueNoLightmap3dBinKey {
|
2023-10-12 22:10:38 +00:00
|
|
|
draw_function: draw_alpha_mask_pbr,
|
|
|
|
pipeline: pipeline_id,
|
Allow phase items not associated with meshes to be binned. (#14029)
As reported in #14004, many third-party plugins, such as Hanabi, enqueue
entities that don't have meshes into render phases. However, the
introduction of indirect mode added a dependency on mesh-specific data,
breaking this workflow. This is because GPU preprocessing requires that
the render phases manage indirect draw parameters, which don't apply to
objects that aren't meshes. The existing code skips over binned entities
that don't have indirect draw parameters, which causes the rendering to
be skipped for such objects.
To support this workflow, this commit adds a new field,
`non_mesh_items`, to `BinnedRenderPhase`. This field contains a simple
list of (bin key, entity) pairs. After drawing batchable and unbatchable
objects, the non-mesh items are drawn one after another. Bevy itself
doesn't enqueue any items into this list; it exists solely for the
application and/or plugins to use.
Additionally, this commit switches the asset ID in the standard bin keys
to be an untyped asset ID rather than that of a mesh. This allows more
flexibility, allowing bins to be keyed off any type of asset.
This patch adds a new example, `custom_phase_item`, which simultaneously
serves to demonstrate how to use this new feature and to act as a
regression test so this doesn't break again.
Fixes #14004.
## Changelog
### Added
* `BinnedRenderPhase` now contains a `non_mesh_items` field for plugins
to add custom items to.
2024-06-27 16:13:03 +00:00
|
|
|
asset_id: mesh_instance.mesh_asset_id.into(),
|
Improve performance by binning together opaque items instead of sorting them. (#12453)
Today, we sort all entities added to all phases, even the phases that
don't strictly need sorting, such as the opaque and shadow phases. This
results in a performance loss because our `PhaseItem`s are rather large
in memory, so sorting is slow. Additionally, determining the boundaries
of batches is an O(n) process.
This commit makes Bevy instead applicable place phase items into *bins*
keyed by *bin keys*, which have the invariant that everything in the
same bin is potentially batchable. This makes determining batch
boundaries O(1), because everything in the same bin can be batched.
Instead of sorting each entity, we now sort only the bin keys. This
drops the sorting time to near-zero on workloads with few bins like
`many_cubes --no-frustum-culling`. Memory usage is improved too, with
batch boundaries and dynamic indices now implicit instead of explicit.
The improved memory usage results in a significant win even on
unbatchable workloads like `many_cubes --no-frustum-culling
--vary-material-data-per-instance`, presumably due to cache effects.
Not all phases can be binned; some, such as transparent and transmissive
phases, must still be sorted. To handle this, this commit splits
`PhaseItem` into `BinnedPhaseItem` and `SortedPhaseItem`. Most of the
logic that today deals with `PhaseItem`s has been moved to
`SortedPhaseItem`. `BinnedPhaseItem` has the new logic.
Frame time results (in ms/frame) are as follows:
| Benchmark | `binning` | `main` | Speedup |
| ------------------------ | --------- | ------- | ------- |
| `many_cubes -nfc -vpi` | 232.179 | 312.123 | 34.43% |
| `many_cubes -nfc` | 25.874 | 30.117 | 16.40% |
| `many_foxes` | 3.276 | 3.515 | 7.30% |
(`-nfc` is short for `--no-frustum-culling`; `-vpi` is short for
`--vary-per-instance`.)
---
## Changelog
### Changed
* Render phases have been split into binned and sorted phases. Binned
phases, such as the common opaque phase, achieve improved CPU
performance by avoiding the sorting step.
## Migration Guide
- `PhaseItem` has been split into `BinnedPhaseItem` and
`SortedPhaseItem`. If your code has custom `PhaseItem`s, you will need
to migrate them to one of these two types. `SortedPhaseItem` requires
the fewest code changes, but you may want to pick `BinnedPhaseItem` if
your phase doesn't require sorting, as that enables higher performance.
## Tracy graphs
`many-cubes --no-frustum-culling`, `main` branch:
<img width="1064" alt="Screenshot 2024-03-12 180037"
src="https://github.com/bevyengine/bevy/assets/157897/e1180ce8-8e89-46d2-85e3-f59f72109a55">
`many-cubes --no-frustum-culling`, this branch:
<img width="1064" alt="Screenshot 2024-03-12 180011"
src="https://github.com/bevyengine/bevy/assets/157897/0899f036-6075-44c5-a972-44d95895f46c">
You can see that `batch_and_prepare_binned_render_phase` is a much
smaller fraction of the time. Zooming in on that function, with yellow
being this branch and red being `main`, we see:
<img width="1064" alt="Screenshot 2024-03-12 175832"
src="https://github.com/bevyengine/bevy/assets/157897/0dfc8d3f-49f4-496e-8825-a66e64d356d0">
The binning happens in `queue_material_meshes`. Again with yellow being
this branch and red being `main`:
<img width="1064" alt="Screenshot 2024-03-12 175755"
src="https://github.com/bevyengine/bevy/assets/157897/b9b20dc1-11c8-400c-a6cc-1c2e09c1bb96">
We can see that there is a small regression in `queue_material_meshes`
performance, but it's not nearly enough to outweigh the large gains in
`batch_and_prepare_binned_render_phase`.
---------
Co-authored-by: James Liu <contact@jamessliu.com>
2024-03-30 02:55:02 +00:00
|
|
|
material_bind_group_id: material.get_bind_group_id().0,
|
|
|
|
};
|
|
|
|
alpha_mask_phase.add(
|
|
|
|
bin_key,
|
Type safe retained render world (#15756)
# Objective
In the Render World, there are a number of collections that are derived
from Main World entities and are used to drive rendering. The most
notable are:
- `VisibleEntities`, which is generated in the `check_visibility` system
and contains visible entities for a view.
- `ExtractedInstances`, which maps entity ids to asset ids.
In the old model, these collections were trivially kept in sync -- any
extracted phase item could look itself up because the render entity id
was guaranteed to always match the corresponding main world id.
After #15320, this became much more complicated, and was leading to a
number of subtle bugs in the Render World. The main rendering systems,
i.e. `queue_material_meshes` and `queue_material2d_meshes`, follow a
similar pattern:
```rust
for visible_entity in visible_entities.iter::<With<Mesh2d>>() {
let Some(mesh_instance) = render_mesh_instances.get_mut(visible_entity) else {
continue;
};
// Look some more stuff up and specialize the pipeline...
let bin_key = Opaque2dBinKey {
pipeline: pipeline_id,
draw_function: draw_opaque_2d,
asset_id: mesh_instance.mesh_asset_id.into(),
material_bind_group_id: material_2d.get_bind_group_id().0,
};
opaque_phase.add(
bin_key,
*visible_entity,
BinnedRenderPhaseType::mesh(mesh_instance.automatic_batching),
);
}
```
In this case, `visible_entities` and `render_mesh_instances` are both
collections that are created and keyed by Main World entity ids, and so
this lookup happens to work by coincidence. However, there is a major
unintentional bug here: namely, because `visible_entities` is a
collection of Main World ids, the phase item being queued is created
with a Main World id rather than its correct Render World id.
This happens to not break mesh rendering because the render commands
used for drawing meshes do not access the `ItemQuery` parameter, but
demonstrates the confusion that is now possible: our UI phase items are
correctly being queued with Render World ids while our meshes aren't.
Additionally, this makes it very easy and error prone to use the wrong
entity id to look up things like assets. For example, if instead we
ignored visibility checks and queued our meshes via a query, we'd have
to be extra careful to use `&MainEntity` instead of the natural
`Entity`.
## Solution
Make all collections that are derived from Main World data use
`MainEntity` as their key, to ensure type safety and avoid accidentally
looking up data with the wrong entity id:
```rust
pub type MainEntityHashMap<V> = hashbrown::HashMap<MainEntity, V, EntityHash>;
```
Additionally, we make all `PhaseItem` be able to provide both their Main
and Render World ids, to allow render phase implementors maximum
flexibility as to what id should be used to look up data.
You can think of this like tracking at the type level whether something
in the Render World should use it's "primary key", i.e. entity id, or
needs to use a foreign key, i.e. `MainEntity`.
## Testing
##### TODO:
This will require extensive testing to make sure things didn't break!
Additionally, some extraction logic has become more complicated and
needs to be checked for regressions.
## Migration Guide
With the advent of the retained render world, collections that contain
references to `Entity` that are extracted into the render world have
been changed to contain `MainEntity` in order to prevent errors where a
render world entity id is used to look up an item by accident. Custom
rendering code may need to be changed to query for `&MainEntity` in
order to look up the correct item from such a collection. Additionally,
users who implement their own extraction logic for collections of main
world entity should strongly consider extracting into a different
collection that uses `MainEntity` as a key.
Additionally, render phases now require specifying both the `Entity` and
`MainEntity` for a given `PhaseItem`. Custom render phases should ensure
`MainEntity` is available when queuing a phase item.
2024-10-10 18:47:04 +00:00
|
|
|
(*render_entity, *visible_entity),
|
Allow phase items not associated with meshes to be binned. (#14029)
As reported in #14004, many third-party plugins, such as Hanabi, enqueue
entities that don't have meshes into render phases. However, the
introduction of indirect mode added a dependency on mesh-specific data,
breaking this workflow. This is because GPU preprocessing requires that
the render phases manage indirect draw parameters, which don't apply to
objects that aren't meshes. The existing code skips over binned entities
that don't have indirect draw parameters, which causes the rendering to
be skipped for such objects.
To support this workflow, this commit adds a new field,
`non_mesh_items`, to `BinnedRenderPhase`. This field contains a simple
list of (bin key, entity) pairs. After drawing batchable and unbatchable
objects, the non-mesh items are drawn one after another. Bevy itself
doesn't enqueue any items into this list; it exists solely for the
application and/or plugins to use.
Additionally, this commit switches the asset ID in the standard bin keys
to be an untyped asset ID rather than that of a mesh. This allows more
flexibility, allowing bins to be keyed off any type of asset.
This patch adds a new example, `custom_phase_item`, which simultaneously
serves to demonstrate how to use this new feature and to act as a
regression test so this doesn't break again.
Fixes #14004.
## Changelog
### Added
* `BinnedRenderPhase` now contains a `non_mesh_items` field for plugins
to add custom items to.
2024-06-27 16:13:03 +00:00
|
|
|
BinnedRenderPhaseType::mesh(mesh_instance.should_batch()),
|
Improve performance by binning together opaque items instead of sorting them. (#12453)
Today, we sort all entities added to all phases, even the phases that
don't strictly need sorting, such as the opaque and shadow phases. This
results in a performance loss because our `PhaseItem`s are rather large
in memory, so sorting is slow. Additionally, determining the boundaries
of batches is an O(n) process.
This commit makes Bevy instead applicable place phase items into *bins*
keyed by *bin keys*, which have the invariant that everything in the
same bin is potentially batchable. This makes determining batch
boundaries O(1), because everything in the same bin can be batched.
Instead of sorting each entity, we now sort only the bin keys. This
drops the sorting time to near-zero on workloads with few bins like
`many_cubes --no-frustum-culling`. Memory usage is improved too, with
batch boundaries and dynamic indices now implicit instead of explicit.
The improved memory usage results in a significant win even on
unbatchable workloads like `many_cubes --no-frustum-culling
--vary-material-data-per-instance`, presumably due to cache effects.
Not all phases can be binned; some, such as transparent and transmissive
phases, must still be sorted. To handle this, this commit splits
`PhaseItem` into `BinnedPhaseItem` and `SortedPhaseItem`. Most of the
logic that today deals with `PhaseItem`s has been moved to
`SortedPhaseItem`. `BinnedPhaseItem` has the new logic.
Frame time results (in ms/frame) are as follows:
| Benchmark | `binning` | `main` | Speedup |
| ------------------------ | --------- | ------- | ------- |
| `many_cubes -nfc -vpi` | 232.179 | 312.123 | 34.43% |
| `many_cubes -nfc` | 25.874 | 30.117 | 16.40% |
| `many_foxes` | 3.276 | 3.515 | 7.30% |
(`-nfc` is short for `--no-frustum-culling`; `-vpi` is short for
`--vary-per-instance`.)
---
## Changelog
### Changed
* Render phases have been split into binned and sorted phases. Binned
phases, such as the common opaque phase, achieve improved CPU
performance by avoiding the sorting step.
## Migration Guide
- `PhaseItem` has been split into `BinnedPhaseItem` and
`SortedPhaseItem`. If your code has custom `PhaseItem`s, you will need
to migrate them to one of these two types. `SortedPhaseItem` requires
the fewest code changes, but you may want to pick `BinnedPhaseItem` if
your phase doesn't require sorting, as that enables higher performance.
## Tracy graphs
`many-cubes --no-frustum-culling`, `main` branch:
<img width="1064" alt="Screenshot 2024-03-12 180037"
src="https://github.com/bevyengine/bevy/assets/157897/e1180ce8-8e89-46d2-85e3-f59f72109a55">
`many-cubes --no-frustum-culling`, this branch:
<img width="1064" alt="Screenshot 2024-03-12 180011"
src="https://github.com/bevyengine/bevy/assets/157897/0899f036-6075-44c5-a972-44d95895f46c">
You can see that `batch_and_prepare_binned_render_phase` is a much
smaller fraction of the time. Zooming in on that function, with yellow
being this branch and red being `main`, we see:
<img width="1064" alt="Screenshot 2024-03-12 175832"
src="https://github.com/bevyengine/bevy/assets/157897/0dfc8d3f-49f4-496e-8825-a66e64d356d0">
The binning happens in `queue_material_meshes`. Again with yellow being
this branch and red being `main`:
<img width="1064" alt="Screenshot 2024-03-12 175755"
src="https://github.com/bevyengine/bevy/assets/157897/b9b20dc1-11c8-400c-a6cc-1c2e09c1bb96">
We can see that there is a small regression in `queue_material_meshes`
performance, but it's not nearly enough to outweigh the large gains in
`batch_and_prepare_binned_render_phase`.
---------
Co-authored-by: James Liu <contact@jamessliu.com>
2024-03-30 02:55:02 +00:00
|
|
|
);
|
2023-10-12 22:10:38 +00:00
|
|
|
}
|
2023-09-20 20:18:55 +00:00
|
|
|
}
|
2024-04-15 20:37:52 +00:00
|
|
|
_ => {
|
Generate `MeshUniform`s on the GPU via compute shader where available. (#12773)
Currently, `MeshUniform`s are rather large: 160 bytes. They're also
somewhat expensive to compute, because they involve taking the inverse
of a 3x4 matrix. Finally, if a mesh is present in multiple views, that
mesh will have a separate `MeshUniform` for each and every view, which
is wasteful.
This commit fixes these issues by introducing the concept of a *mesh
input uniform* and adding a *mesh uniform building* compute shader pass.
The `MeshInputUniform` is simply the minimum amount of data needed for
the GPU to compute the full `MeshUniform`. Most of this data is just the
transform and is therefore only 64 bytes. `MeshInputUniform`s are
computed during the *extraction* phase, much like skins are today, in
order to avoid needlessly copying transforms around on CPU. (In fact,
the render app has been changed to only store the translation of each
mesh; it no longer cares about any other part of the transform, which is
stored only on the GPU and the main world.) Before rendering, the
`build_mesh_uniforms` pass runs to expand the `MeshInputUniform`s to the
full `MeshUniform`.
The mesh uniform building pass does the following, all on GPU:
1. Copy the appropriate fields of the `MeshInputUniform` to the
`MeshUniform` slot. If a single mesh is present in multiple views, this
effectively duplicates it into each view.
2. Compute the inverse transpose of the model transform, used for
transforming normals.
3. If applicable, copy the mesh's transform from the previous frame for
TAA. To support this, we double-buffer the `MeshInputUniform`s over two
frames and swap the buffers each frame. The `MeshInputUniform`s for the
current frame contain the index of that mesh's `MeshInputUniform` for
the previous frame.
This commit produces wins in virtually every CPU part of the pipeline:
`extract_meshes`, `queue_material_meshes`,
`batch_and_prepare_render_phase`, and especially
`write_batched_instance_buffer` are all faster. Shrinking the amount of
CPU data that has to be shuffled around speeds up the entire rendering
process.
| Benchmark | This branch | `main` | Speedup |
|------------------------|-------------|---------|---------|
| `many_cubes -nfc` | 17.259 | 24.529 | 42.12% |
| `many_cubes -nfc -vpi` | 302.116 | 312.123 | 3.31% |
| `many_foxes` | 3.227 | 3.515 | 8.92% |
Because mesh uniform building requires compute shader, and WebGL 2 has
no compute shader, the existing CPU mesh uniform building code has been
left as-is. Many types now have both CPU mesh uniform building and GPU
mesh uniform building modes. Developers can opt into the old CPU mesh
uniform building by setting the `use_gpu_uniform_builder` option on
`PbrPlugin` to `false`.
Below are graphs of the CPU portions of `many-cubes
--no-frustum-culling`. Yellow is this branch, red is `main`.
`extract_meshes`:

It's notable that we get a small win even though we're now writing to a
GPU buffer.
`queue_material_meshes`:

There's a bit of a regression here; not sure what's causing it. In any
case it's very outweighed by the other gains.
`batch_and_prepare_render_phase`:

There's a huge win here, enough to make batching basically drop off the
profile.
`write_batched_instance_buffer`:

There's a massive improvement here, as expected. Note that a lot of it
simply comes from the fact that `MeshInputUniform` is `Pod`. (This isn't
a maintainability problem in my view because `MeshInputUniform` is so
simple: just 16 tightly-packed words.)
## Changelog
### Added
* Per-mesh instance data is now generated on GPU with a compute shader
instead of CPU, resulting in rendering performance improvements on
platforms where compute shaders are supported.
## Migration guide
* Custom render phases now need multiple systems beyond just
`batch_and_prepare_render_phase`. Code that was previously creating
custom render phases should now add a `BinnedRenderPhasePlugin` or
`SortedRenderPhasePlugin` as appropriate instead of directly adding
`batch_and_prepare_render_phase`.
2024-04-10 05:33:32 +00:00
|
|
|
let distance = rangefinder.distance_translation(&mesh_instance.translation)
|
2024-02-05 22:12:22 +00:00
|
|
|
+ material.properties.depth_bias;
|
2023-09-20 20:18:55 +00:00
|
|
|
transparent_phase.add(Transparent3d {
|
Type safe retained render world (#15756)
# Objective
In the Render World, there are a number of collections that are derived
from Main World entities and are used to drive rendering. The most
notable are:
- `VisibleEntities`, which is generated in the `check_visibility` system
and contains visible entities for a view.
- `ExtractedInstances`, which maps entity ids to asset ids.
In the old model, these collections were trivially kept in sync -- any
extracted phase item could look itself up because the render entity id
was guaranteed to always match the corresponding main world id.
After #15320, this became much more complicated, and was leading to a
number of subtle bugs in the Render World. The main rendering systems,
i.e. `queue_material_meshes` and `queue_material2d_meshes`, follow a
similar pattern:
```rust
for visible_entity in visible_entities.iter::<With<Mesh2d>>() {
let Some(mesh_instance) = render_mesh_instances.get_mut(visible_entity) else {
continue;
};
// Look some more stuff up and specialize the pipeline...
let bin_key = Opaque2dBinKey {
pipeline: pipeline_id,
draw_function: draw_opaque_2d,
asset_id: mesh_instance.mesh_asset_id.into(),
material_bind_group_id: material_2d.get_bind_group_id().0,
};
opaque_phase.add(
bin_key,
*visible_entity,
BinnedRenderPhaseType::mesh(mesh_instance.automatic_batching),
);
}
```
In this case, `visible_entities` and `render_mesh_instances` are both
collections that are created and keyed by Main World entity ids, and so
this lookup happens to work by coincidence. However, there is a major
unintentional bug here: namely, because `visible_entities` is a
collection of Main World ids, the phase item being queued is created
with a Main World id rather than its correct Render World id.
This happens to not break mesh rendering because the render commands
used for drawing meshes do not access the `ItemQuery` parameter, but
demonstrates the confusion that is now possible: our UI phase items are
correctly being queued with Render World ids while our meshes aren't.
Additionally, this makes it very easy and error prone to use the wrong
entity id to look up things like assets. For example, if instead we
ignored visibility checks and queued our meshes via a query, we'd have
to be extra careful to use `&MainEntity` instead of the natural
`Entity`.
## Solution
Make all collections that are derived from Main World data use
`MainEntity` as their key, to ensure type safety and avoid accidentally
looking up data with the wrong entity id:
```rust
pub type MainEntityHashMap<V> = hashbrown::HashMap<MainEntity, V, EntityHash>;
```
Additionally, we make all `PhaseItem` be able to provide both their Main
and Render World ids, to allow render phase implementors maximum
flexibility as to what id should be used to look up data.
You can think of this like tracking at the type level whether something
in the Render World should use it's "primary key", i.e. entity id, or
needs to use a foreign key, i.e. `MainEntity`.
## Testing
##### TODO:
This will require extensive testing to make sure things didn't break!
Additionally, some extraction logic has become more complicated and
needs to be checked for regressions.
## Migration Guide
With the advent of the retained render world, collections that contain
references to `Entity` that are extracted into the render world have
been changed to contain `MainEntity` in order to prevent errors where a
render world entity id is used to look up an item by accident. Custom
rendering code may need to be changed to query for `&MainEntity` in
order to look up the correct item from such a collection. Additionally,
users who implement their own extraction logic for collections of main
world entity should strongly consider extracting into a different
collection that uses `MainEntity` as a key.
Additionally, render phases now require specifying both the `Entity` and
`MainEntity` for a given `PhaseItem`. Custom render phases should ensure
`MainEntity` is available when queuing a phase item.
2024-10-10 18:47:04 +00:00
|
|
|
entity: (*render_entity, *visible_entity),
|
2023-09-20 20:18:55 +00:00
|
|
|
draw_function: draw_transparent_pbr,
|
|
|
|
pipeline: pipeline_id,
|
|
|
|
distance,
|
Automatic batching/instancing of draw commands (#9685)
# Objective
- Implement the foundations of automatic batching/instancing of draw
commands as the next step from #89
- NOTE: More performance improvements will come when more data is
managed and bound in ways that do not require rebinding such as mesh,
material, and texture data.
## Solution
- The core idea for batching of draw commands is to check whether any of
the information that has to be passed when encoding a draw command
changes between two things that are being drawn according to the sorted
render phase order. These should be things like the pipeline, bind
groups and their dynamic offsets, index/vertex buffers, and so on.
- The following assumptions have been made:
- Only entities with prepared assets (pipelines, materials, meshes) are
queued to phases
- View bindings are constant across a phase for a given draw function as
phases are per-view
- `batch_and_prepare_render_phase` is the only system that performs this
batching and has sole responsibility for preparing the per-object data.
As such the mesh binding and dynamic offsets are assumed to only vary as
a result of the `batch_and_prepare_render_phase` system, e.g. due to
having to split data across separate uniform bindings within the same
buffer due to the maximum uniform buffer binding size.
- Implement `GpuArrayBuffer` for `Mesh2dUniform` to store Mesh2dUniform
in arrays in GPU buffers rather than each one being at a dynamic offset
in a uniform buffer. This is the same optimisation that was made for 3D
not long ago.
- Change batch size for a range in `PhaseItem`, adding API for getting
or mutating the range. This is more flexible than a size as the length
of the range can be used in place of the size, but the start and end can
be otherwise whatever is needed.
- Add an optional mesh bind group dynamic offset to `PhaseItem`. This
avoids having to do a massive table move just to insert
`GpuArrayBufferIndex` components.
## Benchmarks
All tests have been run on an M1 Max on AC power. `bevymark` and
`many_cubes` were modified to use 1920x1080 with a scale factor of 1. I
run a script that runs a separate Tracy capture process, and then runs
the bevy example with `--features bevy_ci_testing,trace_tracy` and
`CI_TESTING_CONFIG=../benchmark.ron` with the contents of
`../benchmark.ron`:
```rust
(
exit_after: Some(1500)
)
```
...in order to run each test for 1500 frames.
The recent changes to `many_cubes` and `bevymark` added reproducible
random number generation so that with the same settings, the same rng
will occur. They also added benchmark modes that use a fixed delta time
for animations. Combined this means that the same frames should be
rendered both on main and on the branch.
The graphs compare main (yellow) to this PR (red).
### 3D Mesh `many_cubes --benchmark`
<img width="1411" alt="Screenshot 2023-09-03 at 23 42 10"
src="https://github.com/bevyengine/bevy/assets/302146/2088716a-c918-486c-8129-090b26fd2bc4">
The mesh and material are the same for all instances. This is basically
the best case for the initial batching implementation as it results in 1
draw for the ~11.7k visible meshes. It gives a ~30% reduction in median
frame time.
The 1000th frame is identical using the flip tool:

```
Mean: 0.000000
Weighted median: 0.000000
1st weighted quartile: 0.000000
3rd weighted quartile: 0.000000
Min: 0.000000
Max: 0.000000
Evaluation time: 0.4615 seconds
```
### 3D Mesh `many_cubes --benchmark --material-texture-count 10`
<img width="1404" alt="Screenshot 2023-09-03 at 23 45 18"
src="https://github.com/bevyengine/bevy/assets/302146/5ee9c447-5bd2-45c6-9706-ac5ff8916daf">
This run uses 10 different materials by varying their textures. The
materials are randomly selected, and there is no sorting by material
bind group for opaque 3D so any batching is 'random'. The PR produces a
~5% reduction in median frame time. If we were to sort the opaque phase
by the material bind group, then this should be a lot faster. This
produces about 10.5k draws for the 11.7k visible entities. This makes
sense as randomly selecting from 10 materials gives a chance that two
adjacent entities randomly select the same material and can be batched.
The 1000th frame is identical in flip:
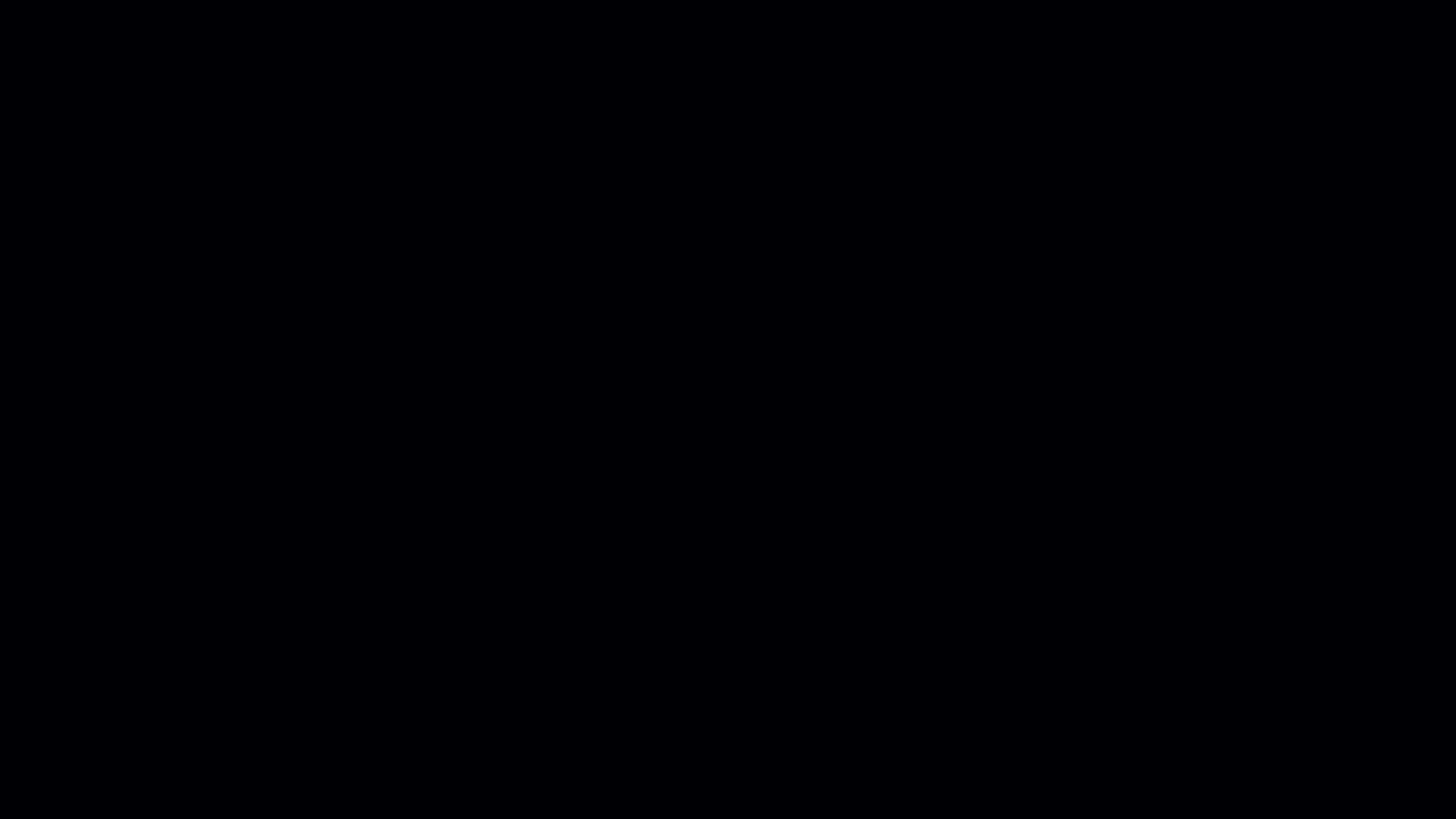
```
Mean: 0.000000
Weighted median: 0.000000
1st weighted quartile: 0.000000
3rd weighted quartile: 0.000000
Min: 0.000000
Max: 0.000000
Evaluation time: 0.4537 seconds
```
### 3D Mesh `many_cubes --benchmark --vary-per-instance`
<img width="1394" alt="Screenshot 2023-09-03 at 23 48 44"
src="https://github.com/bevyengine/bevy/assets/302146/f02a816b-a444-4c18-a96a-63b5436f3b7f">
This run varies the material data per instance by randomly-generating
its colour. This is the worst case for batching and that it performs
about the same as `main` is a good thing as it demonstrates that the
batching has minimal overhead when dealing with ~11k visible mesh
entities.
The 1000th frame is identical according to flip:
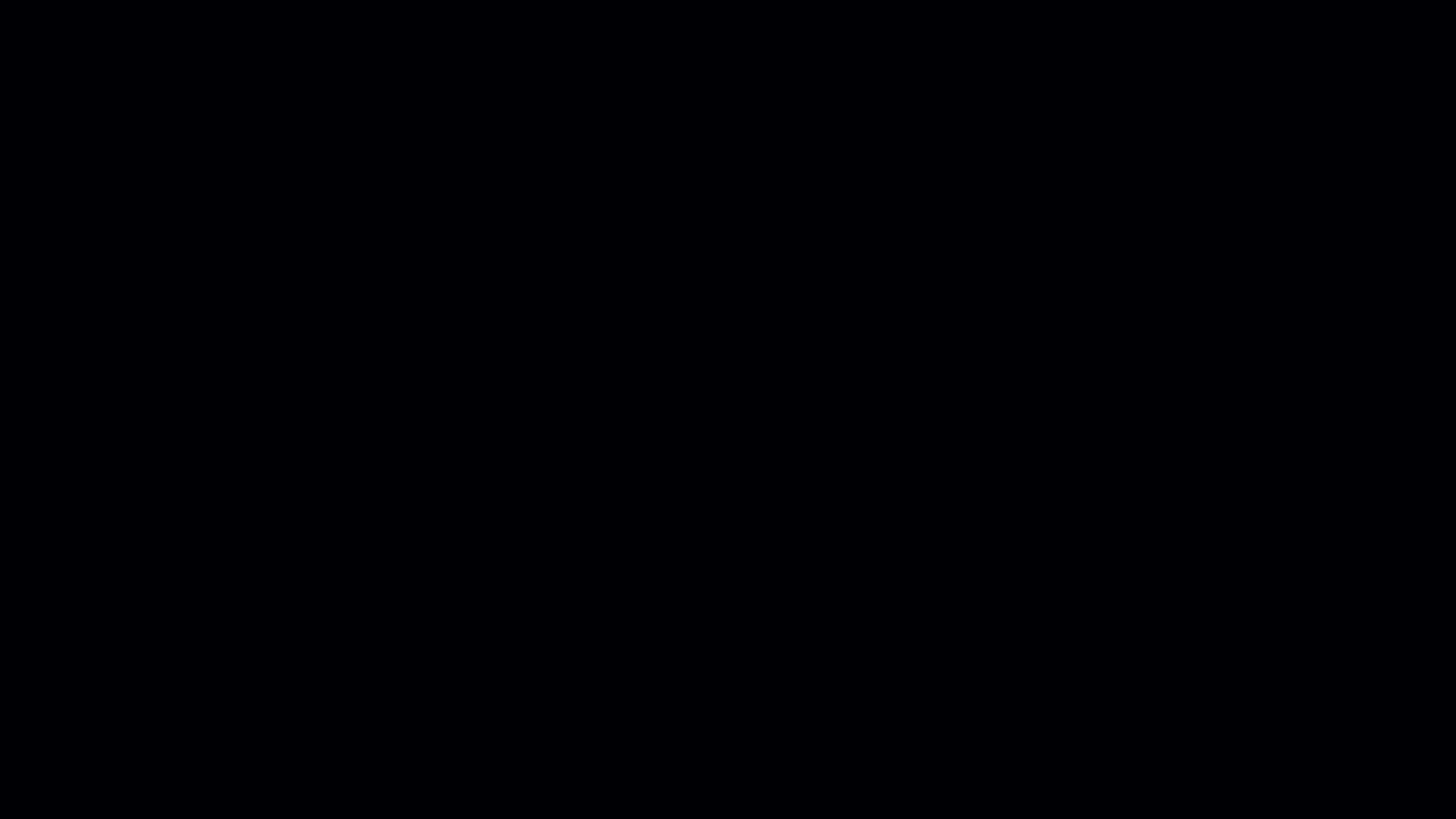
```
Mean: 0.000000
Weighted median: 0.000000
1st weighted quartile: 0.000000
3rd weighted quartile: 0.000000
Min: 0.000000
Max: 0.000000
Evaluation time: 0.4568 seconds
```
### 2D Mesh `bevymark --benchmark --waves 160 --per-wave 1000 --mode
mesh2d`
<img width="1412" alt="Screenshot 2023-09-03 at 23 59 56"
src="https://github.com/bevyengine/bevy/assets/302146/cb02ae07-237b-4646-ae9f-fda4dafcbad4">
This spawns 160 waves of 1000 quad meshes that are shaded with
ColorMaterial. Each wave has a different material so 160 waves currently
should result in 160 batches. This results in a 50% reduction in median
frame time.
Capturing a screenshot of the 1000th frame main vs PR gives:

```
Mean: 0.001222
Weighted median: 0.750432
1st weighted quartile: 0.453494
3rd weighted quartile: 0.969758
Min: 0.000000
Max: 0.990296
Evaluation time: 0.4255 seconds
```
So they seem to produce the same results. I also double-checked the
number of draws. `main` does 160000 draws, and the PR does 160, as
expected.
### 2D Mesh `bevymark --benchmark --waves 160 --per-wave 1000 --mode
mesh2d --material-texture-count 10`
<img width="1392" alt="Screenshot 2023-09-04 at 00 09 22"
src="https://github.com/bevyengine/bevy/assets/302146/4358da2e-ce32-4134-82df-3ab74c40849c">
This generates 10 textures and generates materials for each of those and
then selects one material per wave. The median frame time is reduced by
50%. Similar to the plain run above, this produces 160 draws on the PR
and 160000 on `main` and the 1000th frame is identical (ignoring the fps
counter text overlay).

```
Mean: 0.002877
Weighted median: 0.964980
1st weighted quartile: 0.668871
3rd weighted quartile: 0.982749
Min: 0.000000
Max: 0.992377
Evaluation time: 0.4301 seconds
```
### 2D Mesh `bevymark --benchmark --waves 160 --per-wave 1000 --mode
mesh2d --vary-per-instance`
<img width="1396" alt="Screenshot 2023-09-04 at 00 13 53"
src="https://github.com/bevyengine/bevy/assets/302146/b2198b18-3439-47ad-919a-cdabe190facb">
This creates unique materials per instance by randomly-generating the
material's colour. This is the worst case for 2D batching. Somehow, this
PR manages a 7% reduction in median frame time. Both main and this PR
issue 160000 draws.
The 1000th frame is the same:

```
Mean: 0.001214
Weighted median: 0.937499
1st weighted quartile: 0.635467
3rd weighted quartile: 0.979085
Min: 0.000000
Max: 0.988971
Evaluation time: 0.4462 seconds
```
### 2D Sprite `bevymark --benchmark --waves 160 --per-wave 1000 --mode
sprite`
<img width="1396" alt="Screenshot 2023-09-04 at 12 21 12"
src="https://github.com/bevyengine/bevy/assets/302146/8b31e915-d6be-4cac-abf5-c6a4da9c3d43">
This just spawns 160 waves of 1000 sprites. There should be and is no
notable difference between main and the PR.
### 2D Sprite `bevymark --benchmark --waves 160 --per-wave 1000 --mode
sprite --material-texture-count 10`
<img width="1389" alt="Screenshot 2023-09-04 at 12 36 08"
src="https://github.com/bevyengine/bevy/assets/302146/45fe8d6d-c901-4062-a349-3693dd044413">
This spawns the sprites selecting a texture at random per instance from
the 10 generated textures. This has no significant change vs main and
shouldn't.
### 2D Sprite `bevymark --benchmark --waves 160 --per-wave 1000 --mode
sprite --vary-per-instance`
<img width="1401" alt="Screenshot 2023-09-04 at 12 29 52"
src="https://github.com/bevyengine/bevy/assets/302146/762c5c60-352e-471f-8dbe-bbf10e24ebd6">
This sets the sprite colour as being unique per instance. This can still
all be drawn using one batch. There should be no difference but the PR
produces median frame times that are 4% higher. Investigation showed no
clear sources of cost, rather a mix of give and take that should not
happen. It seems like noise in the results.
### Summary
| Benchmark | % change in median frame time |
| ------------- | ------------- |
| many_cubes | 🟩 -30% |
| many_cubes 10 materials | 🟩 -5% |
| many_cubes unique materials | 🟩 ~0% |
| bevymark mesh2d | 🟩 -50% |
| bevymark mesh2d 10 materials | 🟩 -50% |
| bevymark mesh2d unique materials | 🟩 -7% |
| bevymark sprite | 🟥 2% |
| bevymark sprite 10 materials | 🟥 0.6% |
| bevymark sprite unique materials | 🟥 4.1% |
---
## Changelog
- Added: 2D and 3D mesh entities that share the same mesh and material
(same textures, same data) are now batched into the same draw command
for better performance.
---------
Co-authored-by: robtfm <50659922+robtfm@users.noreply.github.com>
Co-authored-by: Nicola Papale <nico@nicopap.ch>
2023-09-21 22:12:34 +00:00
|
|
|
batch_range: 0..1,
|
Implement GPU frustum culling. (#12889)
This commit implements opt-in GPU frustum culling, built on top of the
infrastructure in https://github.com/bevyengine/bevy/pull/12773. To
enable it on a camera, add the `GpuCulling` component to it. To
additionally disable CPU frustum culling, add the `NoCpuCulling`
component. Note that adding `GpuCulling` without `NoCpuCulling`
*currently* does nothing useful. The reason why `GpuCulling` doesn't
automatically imply `NoCpuCulling` is that I intend to follow this patch
up with GPU two-phase occlusion culling, and CPU frustum culling plus
GPU occlusion culling seems like a very commonly-desired mode.
Adding the `GpuCulling` component to a view puts that view into
*indirect mode*. This mode makes all drawcalls indirect, relying on the
mesh preprocessing shader to allocate instances dynamically. In indirect
mode, the `PreprocessWorkItem` `output_index` points not to a
`MeshUniform` instance slot but instead to a set of `wgpu`
`IndirectParameters`, from which it allocates an instance slot
dynamically if frustum culling succeeds. Batch building has been updated
to allocate and track indirect parameter slots, and the AABBs are now
supplied to the GPU as `MeshCullingData`.
A small amount of code relating to the frustum culling has been borrowed
from meshlets and moved into `maths.wgsl`. Note that standard Bevy
frustum culling uses AABBs, while meshlets use bounding spheres; this
means that not as much code can be shared as one might think.
This patch doesn't provide any way to perform GPU culling on shadow
maps, to avoid making this patch bigger than it already is. That can be
a followup.
## Changelog
### Added
* Frustum culling can now optionally be done on the GPU. To enable it,
add the `GpuCulling` component to a camera.
* To disable CPU frustum culling, add `NoCpuCulling` to a camera. Note
that `GpuCulling` doesn't automatically imply `NoCpuCulling`.
2024-04-28 12:50:00 +00:00
|
|
|
extra_index: PhaseItemExtraIndex::NONE,
|
2023-09-20 20:18:55 +00:00
|
|
|
});
|
2021-12-25 21:45:43 +00:00
|
|
|
}
|
2021-12-14 03:58:23 +00:00
|
|
|
}
|
2021-12-25 21:45:43 +00:00
|
|
|
}
|
2021-12-14 03:58:23 +00:00
|
|
|
}
|
|
|
|
}
|
Better Materials: AsBindGroup trait and derive, simpler Material trait (#5053)
# Objective
This PR reworks Bevy's Material system, making the user experience of defining Materials _much_ nicer. Bevy's previous material system leaves a lot to be desired:
* Materials require manually implementing the `RenderAsset` trait, which involves manually generating the bind group, handling gpu buffer data transfer, looking up image textures, etc. Even the simplest single-texture material involves writing ~80 unnecessary lines of code. This was never the long term plan.
* There are two material traits, which is confusing, hard to document, and often redundant: `Material` and `SpecializedMaterial`. `Material` implicitly implements `SpecializedMaterial`, and `SpecializedMaterial` is used in most high level apis to support both use cases. Most users shouldn't need to think about specialization at all (I consider it a "power-user tool"), so the fact that `SpecializedMaterial` is front-and-center in our apis is a miss.
* Implementing either material trait involves a lot of "type soup". The "prepared asset" parameter is particularly heinous: `&<Self as RenderAsset>::PreparedAsset`. Defining vertex and fragment shaders is also more verbose than it needs to be.
## Solution
Say hello to the new `Material` system:
```rust
#[derive(AsBindGroup, TypeUuid, Debug, Clone)]
#[uuid = "f690fdae-d598-45ab-8225-97e2a3f056e0"]
pub struct CoolMaterial {
#[uniform(0)]
color: Color,
#[texture(1)]
#[sampler(2)]
color_texture: Handle<Image>,
}
impl Material for CoolMaterial {
fn fragment_shader() -> ShaderRef {
"cool_material.wgsl".into()
}
}
```
Thats it! This same material would have required [~80 lines of complicated "type heavy" code](https://github.com/bevyengine/bevy/blob/v0.7.0/examples/shader/shader_material.rs) in the old Material system. Now it is just 14 lines of simple, readable code.
This is thanks to a new consolidated `Material` trait and the new `AsBindGroup` trait / derive.
### The new `Material` trait
The old "split" `Material` and `SpecializedMaterial` traits have been removed in favor of a new consolidated `Material` trait. All of the functions on the trait are optional.
The difficulty of implementing `Material` has been reduced by simplifying dataflow and removing type complexity:
```rust
// Old
impl Material for CustomMaterial {
fn fragment_shader(asset_server: &AssetServer) -> Option<Handle<Shader>> {
Some(asset_server.load("custom_material.wgsl"))
}
fn alpha_mode(render_asset: &<Self as RenderAsset>::PreparedAsset) -> AlphaMode {
render_asset.alpha_mode
}
}
// New
impl Material for CustomMaterial {
fn fragment_shader() -> ShaderRef {
"custom_material.wgsl".into()
}
fn alpha_mode(&self) -> AlphaMode {
self.alpha_mode
}
}
```
Specialization is still supported, but it is hidden by default under the `specialize()` function (more on this later).
### The `AsBindGroup` trait / derive
The `Material` trait now requires the `AsBindGroup` derive. This can be implemented manually relatively easily, but deriving it will almost always be preferable.
Field attributes like `uniform` and `texture` are used to define which fields should be bindings,
what their binding type is, and what index they should be bound at:
```rust
#[derive(AsBindGroup)]
struct CoolMaterial {
#[uniform(0)]
color: Color,
#[texture(1)]
#[sampler(2)]
color_texture: Handle<Image>,
}
```
In WGSL shaders, the binding looks like this:
```wgsl
struct CoolMaterial {
color: vec4<f32>;
};
[[group(1), binding(0)]]
var<uniform> material: CoolMaterial;
[[group(1), binding(1)]]
var color_texture: texture_2d<f32>;
[[group(1), binding(2)]]
var color_sampler: sampler;
```
Note that the "group" index is determined by the usage context. It is not defined in `AsBindGroup`. Bevy material bind groups are bound to group 1.
The following field-level attributes are supported:
* `uniform(BINDING_INDEX)`
* The field will be converted to a shader-compatible type using the `ShaderType` trait, written to a `Buffer`, and bound as a uniform. It can also be derived for custom structs.
* `texture(BINDING_INDEX)`
* This field's `Handle<Image>` will be used to look up the matching `Texture` gpu resource, which will be bound as a texture in shaders. The field will be assumed to implement `Into<Option<Handle<Image>>>`. In practice, most fields should be a `Handle<Image>` or `Option<Handle<Image>>`. If the value of an `Option<Handle<Image>>` is `None`, the new `FallbackImage` resource will be used instead. This attribute can be used in conjunction with a `sampler` binding attribute (with a different binding index).
* `sampler(BINDING_INDEX)`
* Behaves exactly like the `texture` attribute, but sets the Image's sampler binding instead of the texture.
Note that fields without field-level binding attributes will be ignored.
```rust
#[derive(AsBindGroup)]
struct CoolMaterial {
#[uniform(0)]
color: Color,
this_field_is_ignored: String,
}
```
As mentioned above, `Option<Handle<Image>>` is also supported:
```rust
#[derive(AsBindGroup)]
struct CoolMaterial {
#[uniform(0)]
color: Color,
#[texture(1)]
#[sampler(2)]
color_texture: Option<Handle<Image>>,
}
```
This is useful if you want a texture to be optional. When the value is `None`, the `FallbackImage` will be used for the binding instead, which defaults to "pure white".
Field uniforms with the same binding index will be combined into a single binding:
```rust
#[derive(AsBindGroup)]
struct CoolMaterial {
#[uniform(0)]
color: Color,
#[uniform(0)]
roughness: f32,
}
```
In WGSL shaders, the binding would look like this:
```wgsl
struct CoolMaterial {
color: vec4<f32>;
roughness: f32;
};
[[group(1), binding(0)]]
var<uniform> material: CoolMaterial;
```
Some less common scenarios will require "struct-level" attributes. These are the currently supported struct-level attributes:
* `uniform(BINDING_INDEX, ConvertedShaderType)`
* Similar to the field-level `uniform` attribute, but instead the entire `AsBindGroup` value is converted to `ConvertedShaderType`, which must implement `ShaderType`. This is useful if more complicated conversion logic is required.
* `bind_group_data(DataType)`
* The `AsBindGroup` type will be converted to some `DataType` using `Into<DataType>` and stored as `AsBindGroup::Data` as part of the `AsBindGroup::as_bind_group` call. This is useful if data needs to be stored alongside the generated bind group, such as a unique identifier for a material's bind group. The most common use case for this attribute is "shader pipeline specialization".
The previous `CoolMaterial` example illustrating "combining multiple field-level uniform attributes with the same binding index" can
also be equivalently represented with a single struct-level uniform attribute:
```rust
#[derive(AsBindGroup)]
#[uniform(0, CoolMaterialUniform)]
struct CoolMaterial {
color: Color,
roughness: f32,
}
#[derive(ShaderType)]
struct CoolMaterialUniform {
color: Color,
roughness: f32,
}
impl From<&CoolMaterial> for CoolMaterialUniform {
fn from(material: &CoolMaterial) -> CoolMaterialUniform {
CoolMaterialUniform {
color: material.color,
roughness: material.roughness,
}
}
}
```
### Material Specialization
Material shader specialization is now _much_ simpler:
```rust
#[derive(AsBindGroup, TypeUuid, Debug, Clone)]
#[uuid = "f690fdae-d598-45ab-8225-97e2a3f056e0"]
#[bind_group_data(CoolMaterialKey)]
struct CoolMaterial {
#[uniform(0)]
color: Color,
is_red: bool,
}
#[derive(Copy, Clone, Hash, Eq, PartialEq)]
struct CoolMaterialKey {
is_red: bool,
}
impl From<&CoolMaterial> for CoolMaterialKey {
fn from(material: &CoolMaterial) -> CoolMaterialKey {
CoolMaterialKey {
is_red: material.is_red,
}
}
}
impl Material for CoolMaterial {
fn fragment_shader() -> ShaderRef {
"cool_material.wgsl".into()
}
fn specialize(
pipeline: &MaterialPipeline<Self>,
descriptor: &mut RenderPipelineDescriptor,
layout: &MeshVertexBufferLayout,
key: MaterialPipelineKey<Self>,
) -> Result<(), SpecializedMeshPipelineError> {
if key.bind_group_data.is_red {
let fragment = descriptor.fragment.as_mut().unwrap();
fragment.shader_defs.push("IS_RED".to_string());
}
Ok(())
}
}
```
Setting `bind_group_data` is not required for specialization (it defaults to `()`). Scenarios like "custom vertex attributes" also benefit from this system:
```rust
impl Material for CustomMaterial {
fn vertex_shader() -> ShaderRef {
"custom_material.wgsl".into()
}
fn fragment_shader() -> ShaderRef {
"custom_material.wgsl".into()
}
fn specialize(
pipeline: &MaterialPipeline<Self>,
descriptor: &mut RenderPipelineDescriptor,
layout: &MeshVertexBufferLayout,
key: MaterialPipelineKey<Self>,
) -> Result<(), SpecializedMeshPipelineError> {
let vertex_layout = layout.get_layout(&[
Mesh::ATTRIBUTE_POSITION.at_shader_location(0),
ATTRIBUTE_BLEND_COLOR.at_shader_location(1),
])?;
descriptor.vertex.buffers = vec![vertex_layout];
Ok(())
}
}
```
### Ported `StandardMaterial` to the new `Material` system
Bevy's built-in PBR material uses the new Material system (including the AsBindGroup derive):
```rust
#[derive(AsBindGroup, Debug, Clone, TypeUuid)]
#[uuid = "7494888b-c082-457b-aacf-517228cc0c22"]
#[bind_group_data(StandardMaterialKey)]
#[uniform(0, StandardMaterialUniform)]
pub struct StandardMaterial {
pub base_color: Color,
#[texture(1)]
#[sampler(2)]
pub base_color_texture: Option<Handle<Image>>,
/* other fields omitted for brevity */
```
### Ported Bevy examples to the new `Material` system
The overall complexity of Bevy's "custom shader examples" has gone down significantly. Take a look at the diffs if you want a dopamine spike.
Please note that while this PR has a net increase in "lines of code", most of those extra lines come from added documentation. There is a significant reduction
in the overall complexity of the code (even accounting for the new derive logic).
---
## Changelog
### Added
* `AsBindGroup` trait and derive, which make it much easier to transfer data to the gpu and generate bind groups for a given type.
### Changed
* The old `Material` and `SpecializedMaterial` traits have been replaced by a consolidated (much simpler) `Material` trait. Materials no longer implement `RenderAsset`.
* `StandardMaterial` was ported to the new material system. There are no user-facing api changes to the `StandardMaterial` struct api, but it now implements `AsBindGroup` and `Material` instead of `RenderAsset` and `SpecializedMaterial`.
## Migration Guide
The Material system has been reworked to be much simpler. We've removed a lot of boilerplate with the new `AsBindGroup` derive and the `Material` trait is simpler as well!
### Bevy 0.7 (old)
```rust
#[derive(Debug, Clone, TypeUuid)]
#[uuid = "f690fdae-d598-45ab-8225-97e2a3f056e0"]
pub struct CustomMaterial {
color: Color,
color_texture: Handle<Image>,
}
#[derive(Clone)]
pub struct GpuCustomMaterial {
_buffer: Buffer,
bind_group: BindGroup,
}
impl RenderAsset for CustomMaterial {
type ExtractedAsset = CustomMaterial;
type PreparedAsset = GpuCustomMaterial;
type Param = (SRes<RenderDevice>, SRes<MaterialPipeline<Self>>);
fn extract_asset(&self) -> Self::ExtractedAsset {
self.clone()
}
fn prepare_asset(
extracted_asset: Self::ExtractedAsset,
(render_device, material_pipeline): &mut SystemParamItem<Self::Param>,
) -> Result<Self::PreparedAsset, PrepareAssetError<Self::ExtractedAsset>> {
let color = Vec4::from_slice(&extracted_asset.color.as_linear_rgba_f32());
let byte_buffer = [0u8; Vec4::SIZE.get() as usize];
let mut buffer = encase::UniformBuffer::new(byte_buffer);
buffer.write(&color).unwrap();
let buffer = render_device.create_buffer_with_data(&BufferInitDescriptor {
contents: buffer.as_ref(),
label: None,
usage: BufferUsages::UNIFORM | BufferUsages::COPY_DST,
});
let (texture_view, texture_sampler) = if let Some(result) = material_pipeline
.mesh_pipeline
.get_image_texture(gpu_images, &Some(extracted_asset.color_texture.clone()))
{
result
} else {
return Err(PrepareAssetError::RetryNextUpdate(extracted_asset));
};
let bind_group = render_device.create_bind_group(&BindGroupDescriptor {
entries: &[
BindGroupEntry {
binding: 0,
resource: buffer.as_entire_binding(),
},
BindGroupEntry {
binding: 0,
resource: BindingResource::TextureView(texture_view),
},
BindGroupEntry {
binding: 1,
resource: BindingResource::Sampler(texture_sampler),
},
],
label: None,
layout: &material_pipeline.material_layout,
});
Ok(GpuCustomMaterial {
_buffer: buffer,
bind_group,
})
}
}
impl Material for CustomMaterial {
fn fragment_shader(asset_server: &AssetServer) -> Option<Handle<Shader>> {
Some(asset_server.load("custom_material.wgsl"))
}
fn bind_group(render_asset: &<Self as RenderAsset>::PreparedAsset) -> &BindGroup {
&render_asset.bind_group
}
fn bind_group_layout(render_device: &RenderDevice) -> BindGroupLayout {
render_device.create_bind_group_layout(&BindGroupLayoutDescriptor {
entries: &[
BindGroupLayoutEntry {
binding: 0,
visibility: ShaderStages::FRAGMENT,
ty: BindingType::Buffer {
ty: BufferBindingType::Uniform,
has_dynamic_offset: false,
min_binding_size: Some(Vec4::min_size()),
},
count: None,
},
BindGroupLayoutEntry {
binding: 1,
visibility: ShaderStages::FRAGMENT,
ty: BindingType::Texture {
multisampled: false,
sample_type: TextureSampleType::Float { filterable: true },
view_dimension: TextureViewDimension::D2Array,
},
count: None,
},
BindGroupLayoutEntry {
binding: 2,
visibility: ShaderStages::FRAGMENT,
ty: BindingType::Sampler(SamplerBindingType::Filtering),
count: None,
},
],
label: None,
})
}
}
```
### Bevy 0.8 (new)
```rust
impl Material for CustomMaterial {
fn fragment_shader() -> ShaderRef {
"custom_material.wgsl".into()
}
}
#[derive(AsBindGroup, TypeUuid, Debug, Clone)]
#[uuid = "f690fdae-d598-45ab-8225-97e2a3f056e0"]
pub struct CustomMaterial {
#[uniform(0)]
color: Color,
#[texture(1)]
#[sampler(2)]
color_texture: Handle<Image>,
}
```
## Future Work
* Add support for more binding types (cubemaps, buffers, etc). This PR intentionally includes a bare minimum number of binding types to keep "reviewability" in check.
* Consider optionally eliding binding indices using binding names. `AsBindGroup` could pass in (optional?) reflection info as a "hint".
* This would make it possible for the derive to do this:
```rust
#[derive(AsBindGroup)]
pub struct CustomMaterial {
#[uniform]
color: Color,
#[texture]
#[sampler]
color_texture: Option<Handle<Image>>,
alpha_mode: AlphaMode,
}
```
* Or this
```rust
#[derive(AsBindGroup)]
pub struct CustomMaterial {
#[binding]
color: Color,
#[binding]
color_texture: Option<Handle<Image>>,
alpha_mode: AlphaMode,
}
```
* Or even this (if we flip to "include bindings by default")
```rust
#[derive(AsBindGroup)]
pub struct CustomMaterial {
color: Color,
color_texture: Option<Handle<Image>>,
#[binding(ignore)]
alpha_mode: AlphaMode,
}
```
* If we add the option to define custom draw functions for materials (which could be done in a type-erased way), I think that would be enough to support extra non-material bindings. Worth considering!
2022-06-30 23:48:46 +00:00
|
|
|
|
2023-10-12 22:10:38 +00:00
|
|
|
/// Default render method used for opaque materials.
|
|
|
|
#[derive(Default, Resource, Clone, Debug, ExtractResource, Reflect)]
|
2024-09-15 16:07:30 +00:00
|
|
|
#[reflect(Resource, Default, Debug)]
|
2023-10-12 22:10:38 +00:00
|
|
|
pub struct DefaultOpaqueRendererMethod(OpaqueRendererMethod);
|
|
|
|
|
|
|
|
impl DefaultOpaqueRendererMethod {
|
|
|
|
pub fn forward() -> Self {
|
|
|
|
DefaultOpaqueRendererMethod(OpaqueRendererMethod::Forward)
|
|
|
|
}
|
|
|
|
|
|
|
|
pub fn deferred() -> Self {
|
|
|
|
DefaultOpaqueRendererMethod(OpaqueRendererMethod::Deferred)
|
|
|
|
}
|
|
|
|
|
|
|
|
pub fn set_to_forward(&mut self) {
|
|
|
|
self.0 = OpaqueRendererMethod::Forward;
|
|
|
|
}
|
|
|
|
|
|
|
|
pub fn set_to_deferred(&mut self) {
|
|
|
|
self.0 = OpaqueRendererMethod::Deferred;
|
|
|
|
}
|
|
|
|
}
|
|
|
|
|
|
|
|
/// Render method used for opaque materials.
|
|
|
|
///
|
|
|
|
/// The forward rendering main pass draws each mesh entity and shades it according to its
|
|
|
|
/// corresponding material and the lights that affect it. Some render features like Screen Space
|
|
|
|
/// Ambient Occlusion require running depth and normal prepasses, that are 'deferred'-like
|
|
|
|
/// prepasses over all mesh entities to populate depth and normal textures. This means that when
|
|
|
|
/// using render features that require running prepasses, multiple passes over all visible geometry
|
|
|
|
/// are required. This can be slow if there is a lot of geometry that cannot be batched into few
|
|
|
|
/// draws.
|
|
|
|
///
|
|
|
|
/// Deferred rendering runs a prepass to gather not only geometric information like depth and
|
|
|
|
/// normals, but also all the material properties like base color, emissive color, reflectance,
|
|
|
|
/// metalness, etc, and writes them into a deferred 'g-buffer' texture. The deferred main pass is
|
|
|
|
/// then a fullscreen pass that reads data from these textures and executes shading. This allows
|
|
|
|
/// for one pass over geometry, but is at the cost of not being able to use MSAA, and has heavier
|
|
|
|
/// bandwidth usage which can be unsuitable for low end mobile or other bandwidth-constrained devices.
|
|
|
|
///
|
|
|
|
/// If a material indicates `OpaqueRendererMethod::Auto`, `DefaultOpaqueRendererMethod` will be used.
|
Micro-optimize `queue_material_meshes`, primarily to remove bit manipulation. (#12791)
This commit makes the following optimizations:
## `MeshPipelineKey`/`BaseMeshPipelineKey` split
`MeshPipelineKey` has been split into `BaseMeshPipelineKey`, which lives
in `bevy_render` and `MeshPipelineKey`, which lives in `bevy_pbr`.
Conceptually, `BaseMeshPipelineKey` is a superclass of
`MeshPipelineKey`. For `BaseMeshPipelineKey`, the bits start at the
highest (most significant) bit and grow downward toward the lowest bit;
for `MeshPipelineKey`, the bits start at the lowest bit and grow upward
toward the highest bit. This prevents them from colliding.
The goal of this is to avoid having to reassemble bits of the pipeline
key for every mesh every frame. Instead, we can just use a bitwise or
operation to combine the pieces that make up a `MeshPipelineKey`.
## `specialize_slow`
Previously, all of `specialize()` was marked as `#[inline]`. This
bloated `queue_material_meshes` unnecessarily, as a large chunk of it
ended up being a slow path that was rarely hit. This commit refactors
the function to move the slow path to `specialize_slow()`.
Together, these two changes shave about 5% off `queue_material_meshes`:

## Migration Guide
- The `primitive_topology` field on `GpuMesh` is now an accessor method:
`GpuMesh::primitive_topology()`.
- For performance reasons, `MeshPipelineKey` has been split into
`BaseMeshPipelineKey`, which lives in `bevy_render`, and
`MeshPipelineKey`, which lives in `bevy_pbr`. These two should be
combined with bitwise-or to produce the final `MeshPipelineKey`.
2024-04-01 21:58:53 +00:00
|
|
|
#[derive(Default, Clone, Copy, Debug, PartialEq, Reflect)]
|
2023-10-12 22:10:38 +00:00
|
|
|
pub enum OpaqueRendererMethod {
|
|
|
|
#[default]
|
|
|
|
Forward,
|
|
|
|
Deferred,
|
|
|
|
Auto,
|
|
|
|
}
|
|
|
|
|
Better Materials: AsBindGroup trait and derive, simpler Material trait (#5053)
# Objective
This PR reworks Bevy's Material system, making the user experience of defining Materials _much_ nicer. Bevy's previous material system leaves a lot to be desired:
* Materials require manually implementing the `RenderAsset` trait, which involves manually generating the bind group, handling gpu buffer data transfer, looking up image textures, etc. Even the simplest single-texture material involves writing ~80 unnecessary lines of code. This was never the long term plan.
* There are two material traits, which is confusing, hard to document, and often redundant: `Material` and `SpecializedMaterial`. `Material` implicitly implements `SpecializedMaterial`, and `SpecializedMaterial` is used in most high level apis to support both use cases. Most users shouldn't need to think about specialization at all (I consider it a "power-user tool"), so the fact that `SpecializedMaterial` is front-and-center in our apis is a miss.
* Implementing either material trait involves a lot of "type soup". The "prepared asset" parameter is particularly heinous: `&<Self as RenderAsset>::PreparedAsset`. Defining vertex and fragment shaders is also more verbose than it needs to be.
## Solution
Say hello to the new `Material` system:
```rust
#[derive(AsBindGroup, TypeUuid, Debug, Clone)]
#[uuid = "f690fdae-d598-45ab-8225-97e2a3f056e0"]
pub struct CoolMaterial {
#[uniform(0)]
color: Color,
#[texture(1)]
#[sampler(2)]
color_texture: Handle<Image>,
}
impl Material for CoolMaterial {
fn fragment_shader() -> ShaderRef {
"cool_material.wgsl".into()
}
}
```
Thats it! This same material would have required [~80 lines of complicated "type heavy" code](https://github.com/bevyengine/bevy/blob/v0.7.0/examples/shader/shader_material.rs) in the old Material system. Now it is just 14 lines of simple, readable code.
This is thanks to a new consolidated `Material` trait and the new `AsBindGroup` trait / derive.
### The new `Material` trait
The old "split" `Material` and `SpecializedMaterial` traits have been removed in favor of a new consolidated `Material` trait. All of the functions on the trait are optional.
The difficulty of implementing `Material` has been reduced by simplifying dataflow and removing type complexity:
```rust
// Old
impl Material for CustomMaterial {
fn fragment_shader(asset_server: &AssetServer) -> Option<Handle<Shader>> {
Some(asset_server.load("custom_material.wgsl"))
}
fn alpha_mode(render_asset: &<Self as RenderAsset>::PreparedAsset) -> AlphaMode {
render_asset.alpha_mode
}
}
// New
impl Material for CustomMaterial {
fn fragment_shader() -> ShaderRef {
"custom_material.wgsl".into()
}
fn alpha_mode(&self) -> AlphaMode {
self.alpha_mode
}
}
```
Specialization is still supported, but it is hidden by default under the `specialize()` function (more on this later).
### The `AsBindGroup` trait / derive
The `Material` trait now requires the `AsBindGroup` derive. This can be implemented manually relatively easily, but deriving it will almost always be preferable.
Field attributes like `uniform` and `texture` are used to define which fields should be bindings,
what their binding type is, and what index they should be bound at:
```rust
#[derive(AsBindGroup)]
struct CoolMaterial {
#[uniform(0)]
color: Color,
#[texture(1)]
#[sampler(2)]
color_texture: Handle<Image>,
}
```
In WGSL shaders, the binding looks like this:
```wgsl
struct CoolMaterial {
color: vec4<f32>;
};
[[group(1), binding(0)]]
var<uniform> material: CoolMaterial;
[[group(1), binding(1)]]
var color_texture: texture_2d<f32>;
[[group(1), binding(2)]]
var color_sampler: sampler;
```
Note that the "group" index is determined by the usage context. It is not defined in `AsBindGroup`. Bevy material bind groups are bound to group 1.
The following field-level attributes are supported:
* `uniform(BINDING_INDEX)`
* The field will be converted to a shader-compatible type using the `ShaderType` trait, written to a `Buffer`, and bound as a uniform. It can also be derived for custom structs.
* `texture(BINDING_INDEX)`
* This field's `Handle<Image>` will be used to look up the matching `Texture` gpu resource, which will be bound as a texture in shaders. The field will be assumed to implement `Into<Option<Handle<Image>>>`. In practice, most fields should be a `Handle<Image>` or `Option<Handle<Image>>`. If the value of an `Option<Handle<Image>>` is `None`, the new `FallbackImage` resource will be used instead. This attribute can be used in conjunction with a `sampler` binding attribute (with a different binding index).
* `sampler(BINDING_INDEX)`
* Behaves exactly like the `texture` attribute, but sets the Image's sampler binding instead of the texture.
Note that fields without field-level binding attributes will be ignored.
```rust
#[derive(AsBindGroup)]
struct CoolMaterial {
#[uniform(0)]
color: Color,
this_field_is_ignored: String,
}
```
As mentioned above, `Option<Handle<Image>>` is also supported:
```rust
#[derive(AsBindGroup)]
struct CoolMaterial {
#[uniform(0)]
color: Color,
#[texture(1)]
#[sampler(2)]
color_texture: Option<Handle<Image>>,
}
```
This is useful if you want a texture to be optional. When the value is `None`, the `FallbackImage` will be used for the binding instead, which defaults to "pure white".
Field uniforms with the same binding index will be combined into a single binding:
```rust
#[derive(AsBindGroup)]
struct CoolMaterial {
#[uniform(0)]
color: Color,
#[uniform(0)]
roughness: f32,
}
```
In WGSL shaders, the binding would look like this:
```wgsl
struct CoolMaterial {
color: vec4<f32>;
roughness: f32;
};
[[group(1), binding(0)]]
var<uniform> material: CoolMaterial;
```
Some less common scenarios will require "struct-level" attributes. These are the currently supported struct-level attributes:
* `uniform(BINDING_INDEX, ConvertedShaderType)`
* Similar to the field-level `uniform` attribute, but instead the entire `AsBindGroup` value is converted to `ConvertedShaderType`, which must implement `ShaderType`. This is useful if more complicated conversion logic is required.
* `bind_group_data(DataType)`
* The `AsBindGroup` type will be converted to some `DataType` using `Into<DataType>` and stored as `AsBindGroup::Data` as part of the `AsBindGroup::as_bind_group` call. This is useful if data needs to be stored alongside the generated bind group, such as a unique identifier for a material's bind group. The most common use case for this attribute is "shader pipeline specialization".
The previous `CoolMaterial` example illustrating "combining multiple field-level uniform attributes with the same binding index" can
also be equivalently represented with a single struct-level uniform attribute:
```rust
#[derive(AsBindGroup)]
#[uniform(0, CoolMaterialUniform)]
struct CoolMaterial {
color: Color,
roughness: f32,
}
#[derive(ShaderType)]
struct CoolMaterialUniform {
color: Color,
roughness: f32,
}
impl From<&CoolMaterial> for CoolMaterialUniform {
fn from(material: &CoolMaterial) -> CoolMaterialUniform {
CoolMaterialUniform {
color: material.color,
roughness: material.roughness,
}
}
}
```
### Material Specialization
Material shader specialization is now _much_ simpler:
```rust
#[derive(AsBindGroup, TypeUuid, Debug, Clone)]
#[uuid = "f690fdae-d598-45ab-8225-97e2a3f056e0"]
#[bind_group_data(CoolMaterialKey)]
struct CoolMaterial {
#[uniform(0)]
color: Color,
is_red: bool,
}
#[derive(Copy, Clone, Hash, Eq, PartialEq)]
struct CoolMaterialKey {
is_red: bool,
}
impl From<&CoolMaterial> for CoolMaterialKey {
fn from(material: &CoolMaterial) -> CoolMaterialKey {
CoolMaterialKey {
is_red: material.is_red,
}
}
}
impl Material for CoolMaterial {
fn fragment_shader() -> ShaderRef {
"cool_material.wgsl".into()
}
fn specialize(
pipeline: &MaterialPipeline<Self>,
descriptor: &mut RenderPipelineDescriptor,
layout: &MeshVertexBufferLayout,
key: MaterialPipelineKey<Self>,
) -> Result<(), SpecializedMeshPipelineError> {
if key.bind_group_data.is_red {
let fragment = descriptor.fragment.as_mut().unwrap();
fragment.shader_defs.push("IS_RED".to_string());
}
Ok(())
}
}
```
Setting `bind_group_data` is not required for specialization (it defaults to `()`). Scenarios like "custom vertex attributes" also benefit from this system:
```rust
impl Material for CustomMaterial {
fn vertex_shader() -> ShaderRef {
"custom_material.wgsl".into()
}
fn fragment_shader() -> ShaderRef {
"custom_material.wgsl".into()
}
fn specialize(
pipeline: &MaterialPipeline<Self>,
descriptor: &mut RenderPipelineDescriptor,
layout: &MeshVertexBufferLayout,
key: MaterialPipelineKey<Self>,
) -> Result<(), SpecializedMeshPipelineError> {
let vertex_layout = layout.get_layout(&[
Mesh::ATTRIBUTE_POSITION.at_shader_location(0),
ATTRIBUTE_BLEND_COLOR.at_shader_location(1),
])?;
descriptor.vertex.buffers = vec![vertex_layout];
Ok(())
}
}
```
### Ported `StandardMaterial` to the new `Material` system
Bevy's built-in PBR material uses the new Material system (including the AsBindGroup derive):
```rust
#[derive(AsBindGroup, Debug, Clone, TypeUuid)]
#[uuid = "7494888b-c082-457b-aacf-517228cc0c22"]
#[bind_group_data(StandardMaterialKey)]
#[uniform(0, StandardMaterialUniform)]
pub struct StandardMaterial {
pub base_color: Color,
#[texture(1)]
#[sampler(2)]
pub base_color_texture: Option<Handle<Image>>,
/* other fields omitted for brevity */
```
### Ported Bevy examples to the new `Material` system
The overall complexity of Bevy's "custom shader examples" has gone down significantly. Take a look at the diffs if you want a dopamine spike.
Please note that while this PR has a net increase in "lines of code", most of those extra lines come from added documentation. There is a significant reduction
in the overall complexity of the code (even accounting for the new derive logic).
---
## Changelog
### Added
* `AsBindGroup` trait and derive, which make it much easier to transfer data to the gpu and generate bind groups for a given type.
### Changed
* The old `Material` and `SpecializedMaterial` traits have been replaced by a consolidated (much simpler) `Material` trait. Materials no longer implement `RenderAsset`.
* `StandardMaterial` was ported to the new material system. There are no user-facing api changes to the `StandardMaterial` struct api, but it now implements `AsBindGroup` and `Material` instead of `RenderAsset` and `SpecializedMaterial`.
## Migration Guide
The Material system has been reworked to be much simpler. We've removed a lot of boilerplate with the new `AsBindGroup` derive and the `Material` trait is simpler as well!
### Bevy 0.7 (old)
```rust
#[derive(Debug, Clone, TypeUuid)]
#[uuid = "f690fdae-d598-45ab-8225-97e2a3f056e0"]
pub struct CustomMaterial {
color: Color,
color_texture: Handle<Image>,
}
#[derive(Clone)]
pub struct GpuCustomMaterial {
_buffer: Buffer,
bind_group: BindGroup,
}
impl RenderAsset for CustomMaterial {
type ExtractedAsset = CustomMaterial;
type PreparedAsset = GpuCustomMaterial;
type Param = (SRes<RenderDevice>, SRes<MaterialPipeline<Self>>);
fn extract_asset(&self) -> Self::ExtractedAsset {
self.clone()
}
fn prepare_asset(
extracted_asset: Self::ExtractedAsset,
(render_device, material_pipeline): &mut SystemParamItem<Self::Param>,
) -> Result<Self::PreparedAsset, PrepareAssetError<Self::ExtractedAsset>> {
let color = Vec4::from_slice(&extracted_asset.color.as_linear_rgba_f32());
let byte_buffer = [0u8; Vec4::SIZE.get() as usize];
let mut buffer = encase::UniformBuffer::new(byte_buffer);
buffer.write(&color).unwrap();
let buffer = render_device.create_buffer_with_data(&BufferInitDescriptor {
contents: buffer.as_ref(),
label: None,
usage: BufferUsages::UNIFORM | BufferUsages::COPY_DST,
});
let (texture_view, texture_sampler) = if let Some(result) = material_pipeline
.mesh_pipeline
.get_image_texture(gpu_images, &Some(extracted_asset.color_texture.clone()))
{
result
} else {
return Err(PrepareAssetError::RetryNextUpdate(extracted_asset));
};
let bind_group = render_device.create_bind_group(&BindGroupDescriptor {
entries: &[
BindGroupEntry {
binding: 0,
resource: buffer.as_entire_binding(),
},
BindGroupEntry {
binding: 0,
resource: BindingResource::TextureView(texture_view),
},
BindGroupEntry {
binding: 1,
resource: BindingResource::Sampler(texture_sampler),
},
],
label: None,
layout: &material_pipeline.material_layout,
});
Ok(GpuCustomMaterial {
_buffer: buffer,
bind_group,
})
}
}
impl Material for CustomMaterial {
fn fragment_shader(asset_server: &AssetServer) -> Option<Handle<Shader>> {
Some(asset_server.load("custom_material.wgsl"))
}
fn bind_group(render_asset: &<Self as RenderAsset>::PreparedAsset) -> &BindGroup {
&render_asset.bind_group
}
fn bind_group_layout(render_device: &RenderDevice) -> BindGroupLayout {
render_device.create_bind_group_layout(&BindGroupLayoutDescriptor {
entries: &[
BindGroupLayoutEntry {
binding: 0,
visibility: ShaderStages::FRAGMENT,
ty: BindingType::Buffer {
ty: BufferBindingType::Uniform,
has_dynamic_offset: false,
min_binding_size: Some(Vec4::min_size()),
},
count: None,
},
BindGroupLayoutEntry {
binding: 1,
visibility: ShaderStages::FRAGMENT,
ty: BindingType::Texture {
multisampled: false,
sample_type: TextureSampleType::Float { filterable: true },
view_dimension: TextureViewDimension::D2Array,
},
count: None,
},
BindGroupLayoutEntry {
binding: 2,
visibility: ShaderStages::FRAGMENT,
ty: BindingType::Sampler(SamplerBindingType::Filtering),
count: None,
},
],
label: None,
})
}
}
```
### Bevy 0.8 (new)
```rust
impl Material for CustomMaterial {
fn fragment_shader() -> ShaderRef {
"custom_material.wgsl".into()
}
}
#[derive(AsBindGroup, TypeUuid, Debug, Clone)]
#[uuid = "f690fdae-d598-45ab-8225-97e2a3f056e0"]
pub struct CustomMaterial {
#[uniform(0)]
color: Color,
#[texture(1)]
#[sampler(2)]
color_texture: Handle<Image>,
}
```
## Future Work
* Add support for more binding types (cubemaps, buffers, etc). This PR intentionally includes a bare minimum number of binding types to keep "reviewability" in check.
* Consider optionally eliding binding indices using binding names. `AsBindGroup` could pass in (optional?) reflection info as a "hint".
* This would make it possible for the derive to do this:
```rust
#[derive(AsBindGroup)]
pub struct CustomMaterial {
#[uniform]
color: Color,
#[texture]
#[sampler]
color_texture: Option<Handle<Image>>,
alpha_mode: AlphaMode,
}
```
* Or this
```rust
#[derive(AsBindGroup)]
pub struct CustomMaterial {
#[binding]
color: Color,
#[binding]
color_texture: Option<Handle<Image>>,
alpha_mode: AlphaMode,
}
```
* Or even this (if we flip to "include bindings by default")
```rust
#[derive(AsBindGroup)]
pub struct CustomMaterial {
color: Color,
color_texture: Option<Handle<Image>>,
#[binding(ignore)]
alpha_mode: AlphaMode,
}
```
* If we add the option to define custom draw functions for materials (which could be done in a type-erased way), I think that would be enough to support extra non-material bindings. Worth considering!
2022-06-30 23:48:46 +00:00
|
|
|
/// Common [`Material`] properties, calculated for a specific material instance.
|
|
|
|
pub struct MaterialProperties {
|
2023-10-12 22:10:38 +00:00
|
|
|
/// Is this material should be rendered by the deferred renderer when.
|
2024-04-16 02:46:46 +00:00
|
|
|
/// [`AlphaMode::Opaque`] or [`AlphaMode::Mask`]
|
2023-10-12 22:10:38 +00:00
|
|
|
pub render_method: OpaqueRendererMethod,
|
Better Materials: AsBindGroup trait and derive, simpler Material trait (#5053)
# Objective
This PR reworks Bevy's Material system, making the user experience of defining Materials _much_ nicer. Bevy's previous material system leaves a lot to be desired:
* Materials require manually implementing the `RenderAsset` trait, which involves manually generating the bind group, handling gpu buffer data transfer, looking up image textures, etc. Even the simplest single-texture material involves writing ~80 unnecessary lines of code. This was never the long term plan.
* There are two material traits, which is confusing, hard to document, and often redundant: `Material` and `SpecializedMaterial`. `Material` implicitly implements `SpecializedMaterial`, and `SpecializedMaterial` is used in most high level apis to support both use cases. Most users shouldn't need to think about specialization at all (I consider it a "power-user tool"), so the fact that `SpecializedMaterial` is front-and-center in our apis is a miss.
* Implementing either material trait involves a lot of "type soup". The "prepared asset" parameter is particularly heinous: `&<Self as RenderAsset>::PreparedAsset`. Defining vertex and fragment shaders is also more verbose than it needs to be.
## Solution
Say hello to the new `Material` system:
```rust
#[derive(AsBindGroup, TypeUuid, Debug, Clone)]
#[uuid = "f690fdae-d598-45ab-8225-97e2a3f056e0"]
pub struct CoolMaterial {
#[uniform(0)]
color: Color,
#[texture(1)]
#[sampler(2)]
color_texture: Handle<Image>,
}
impl Material for CoolMaterial {
fn fragment_shader() -> ShaderRef {
"cool_material.wgsl".into()
}
}
```
Thats it! This same material would have required [~80 lines of complicated "type heavy" code](https://github.com/bevyengine/bevy/blob/v0.7.0/examples/shader/shader_material.rs) in the old Material system. Now it is just 14 lines of simple, readable code.
This is thanks to a new consolidated `Material` trait and the new `AsBindGroup` trait / derive.
### The new `Material` trait
The old "split" `Material` and `SpecializedMaterial` traits have been removed in favor of a new consolidated `Material` trait. All of the functions on the trait are optional.
The difficulty of implementing `Material` has been reduced by simplifying dataflow and removing type complexity:
```rust
// Old
impl Material for CustomMaterial {
fn fragment_shader(asset_server: &AssetServer) -> Option<Handle<Shader>> {
Some(asset_server.load("custom_material.wgsl"))
}
fn alpha_mode(render_asset: &<Self as RenderAsset>::PreparedAsset) -> AlphaMode {
render_asset.alpha_mode
}
}
// New
impl Material for CustomMaterial {
fn fragment_shader() -> ShaderRef {
"custom_material.wgsl".into()
}
fn alpha_mode(&self) -> AlphaMode {
self.alpha_mode
}
}
```
Specialization is still supported, but it is hidden by default under the `specialize()` function (more on this later).
### The `AsBindGroup` trait / derive
The `Material` trait now requires the `AsBindGroup` derive. This can be implemented manually relatively easily, but deriving it will almost always be preferable.
Field attributes like `uniform` and `texture` are used to define which fields should be bindings,
what their binding type is, and what index they should be bound at:
```rust
#[derive(AsBindGroup)]
struct CoolMaterial {
#[uniform(0)]
color: Color,
#[texture(1)]
#[sampler(2)]
color_texture: Handle<Image>,
}
```
In WGSL shaders, the binding looks like this:
```wgsl
struct CoolMaterial {
color: vec4<f32>;
};
[[group(1), binding(0)]]
var<uniform> material: CoolMaterial;
[[group(1), binding(1)]]
var color_texture: texture_2d<f32>;
[[group(1), binding(2)]]
var color_sampler: sampler;
```
Note that the "group" index is determined by the usage context. It is not defined in `AsBindGroup`. Bevy material bind groups are bound to group 1.
The following field-level attributes are supported:
* `uniform(BINDING_INDEX)`
* The field will be converted to a shader-compatible type using the `ShaderType` trait, written to a `Buffer`, and bound as a uniform. It can also be derived for custom structs.
* `texture(BINDING_INDEX)`
* This field's `Handle<Image>` will be used to look up the matching `Texture` gpu resource, which will be bound as a texture in shaders. The field will be assumed to implement `Into<Option<Handle<Image>>>`. In practice, most fields should be a `Handle<Image>` or `Option<Handle<Image>>`. If the value of an `Option<Handle<Image>>` is `None`, the new `FallbackImage` resource will be used instead. This attribute can be used in conjunction with a `sampler` binding attribute (with a different binding index).
* `sampler(BINDING_INDEX)`
* Behaves exactly like the `texture` attribute, but sets the Image's sampler binding instead of the texture.
Note that fields without field-level binding attributes will be ignored.
```rust
#[derive(AsBindGroup)]
struct CoolMaterial {
#[uniform(0)]
color: Color,
this_field_is_ignored: String,
}
```
As mentioned above, `Option<Handle<Image>>` is also supported:
```rust
#[derive(AsBindGroup)]
struct CoolMaterial {
#[uniform(0)]
color: Color,
#[texture(1)]
#[sampler(2)]
color_texture: Option<Handle<Image>>,
}
```
This is useful if you want a texture to be optional. When the value is `None`, the `FallbackImage` will be used for the binding instead, which defaults to "pure white".
Field uniforms with the same binding index will be combined into a single binding:
```rust
#[derive(AsBindGroup)]
struct CoolMaterial {
#[uniform(0)]
color: Color,
#[uniform(0)]
roughness: f32,
}
```
In WGSL shaders, the binding would look like this:
```wgsl
struct CoolMaterial {
color: vec4<f32>;
roughness: f32;
};
[[group(1), binding(0)]]
var<uniform> material: CoolMaterial;
```
Some less common scenarios will require "struct-level" attributes. These are the currently supported struct-level attributes:
* `uniform(BINDING_INDEX, ConvertedShaderType)`
* Similar to the field-level `uniform` attribute, but instead the entire `AsBindGroup` value is converted to `ConvertedShaderType`, which must implement `ShaderType`. This is useful if more complicated conversion logic is required.
* `bind_group_data(DataType)`
* The `AsBindGroup` type will be converted to some `DataType` using `Into<DataType>` and stored as `AsBindGroup::Data` as part of the `AsBindGroup::as_bind_group` call. This is useful if data needs to be stored alongside the generated bind group, such as a unique identifier for a material's bind group. The most common use case for this attribute is "shader pipeline specialization".
The previous `CoolMaterial` example illustrating "combining multiple field-level uniform attributes with the same binding index" can
also be equivalently represented with a single struct-level uniform attribute:
```rust
#[derive(AsBindGroup)]
#[uniform(0, CoolMaterialUniform)]
struct CoolMaterial {
color: Color,
roughness: f32,
}
#[derive(ShaderType)]
struct CoolMaterialUniform {
color: Color,
roughness: f32,
}
impl From<&CoolMaterial> for CoolMaterialUniform {
fn from(material: &CoolMaterial) -> CoolMaterialUniform {
CoolMaterialUniform {
color: material.color,
roughness: material.roughness,
}
}
}
```
### Material Specialization
Material shader specialization is now _much_ simpler:
```rust
#[derive(AsBindGroup, TypeUuid, Debug, Clone)]
#[uuid = "f690fdae-d598-45ab-8225-97e2a3f056e0"]
#[bind_group_data(CoolMaterialKey)]
struct CoolMaterial {
#[uniform(0)]
color: Color,
is_red: bool,
}
#[derive(Copy, Clone, Hash, Eq, PartialEq)]
struct CoolMaterialKey {
is_red: bool,
}
impl From<&CoolMaterial> for CoolMaterialKey {
fn from(material: &CoolMaterial) -> CoolMaterialKey {
CoolMaterialKey {
is_red: material.is_red,
}
}
}
impl Material for CoolMaterial {
fn fragment_shader() -> ShaderRef {
"cool_material.wgsl".into()
}
fn specialize(
pipeline: &MaterialPipeline<Self>,
descriptor: &mut RenderPipelineDescriptor,
layout: &MeshVertexBufferLayout,
key: MaterialPipelineKey<Self>,
) -> Result<(), SpecializedMeshPipelineError> {
if key.bind_group_data.is_red {
let fragment = descriptor.fragment.as_mut().unwrap();
fragment.shader_defs.push("IS_RED".to_string());
}
Ok(())
}
}
```
Setting `bind_group_data` is not required for specialization (it defaults to `()`). Scenarios like "custom vertex attributes" also benefit from this system:
```rust
impl Material for CustomMaterial {
fn vertex_shader() -> ShaderRef {
"custom_material.wgsl".into()
}
fn fragment_shader() -> ShaderRef {
"custom_material.wgsl".into()
}
fn specialize(
pipeline: &MaterialPipeline<Self>,
descriptor: &mut RenderPipelineDescriptor,
layout: &MeshVertexBufferLayout,
key: MaterialPipelineKey<Self>,
) -> Result<(), SpecializedMeshPipelineError> {
let vertex_layout = layout.get_layout(&[
Mesh::ATTRIBUTE_POSITION.at_shader_location(0),
ATTRIBUTE_BLEND_COLOR.at_shader_location(1),
])?;
descriptor.vertex.buffers = vec![vertex_layout];
Ok(())
}
}
```
### Ported `StandardMaterial` to the new `Material` system
Bevy's built-in PBR material uses the new Material system (including the AsBindGroup derive):
```rust
#[derive(AsBindGroup, Debug, Clone, TypeUuid)]
#[uuid = "7494888b-c082-457b-aacf-517228cc0c22"]
#[bind_group_data(StandardMaterialKey)]
#[uniform(0, StandardMaterialUniform)]
pub struct StandardMaterial {
pub base_color: Color,
#[texture(1)]
#[sampler(2)]
pub base_color_texture: Option<Handle<Image>>,
/* other fields omitted for brevity */
```
### Ported Bevy examples to the new `Material` system
The overall complexity of Bevy's "custom shader examples" has gone down significantly. Take a look at the diffs if you want a dopamine spike.
Please note that while this PR has a net increase in "lines of code", most of those extra lines come from added documentation. There is a significant reduction
in the overall complexity of the code (even accounting for the new derive logic).
---
## Changelog
### Added
* `AsBindGroup` trait and derive, which make it much easier to transfer data to the gpu and generate bind groups for a given type.
### Changed
* The old `Material` and `SpecializedMaterial` traits have been replaced by a consolidated (much simpler) `Material` trait. Materials no longer implement `RenderAsset`.
* `StandardMaterial` was ported to the new material system. There are no user-facing api changes to the `StandardMaterial` struct api, but it now implements `AsBindGroup` and `Material` instead of `RenderAsset` and `SpecializedMaterial`.
## Migration Guide
The Material system has been reworked to be much simpler. We've removed a lot of boilerplate with the new `AsBindGroup` derive and the `Material` trait is simpler as well!
### Bevy 0.7 (old)
```rust
#[derive(Debug, Clone, TypeUuid)]
#[uuid = "f690fdae-d598-45ab-8225-97e2a3f056e0"]
pub struct CustomMaterial {
color: Color,
color_texture: Handle<Image>,
}
#[derive(Clone)]
pub struct GpuCustomMaterial {
_buffer: Buffer,
bind_group: BindGroup,
}
impl RenderAsset for CustomMaterial {
type ExtractedAsset = CustomMaterial;
type PreparedAsset = GpuCustomMaterial;
type Param = (SRes<RenderDevice>, SRes<MaterialPipeline<Self>>);
fn extract_asset(&self) -> Self::ExtractedAsset {
self.clone()
}
fn prepare_asset(
extracted_asset: Self::ExtractedAsset,
(render_device, material_pipeline): &mut SystemParamItem<Self::Param>,
) -> Result<Self::PreparedAsset, PrepareAssetError<Self::ExtractedAsset>> {
let color = Vec4::from_slice(&extracted_asset.color.as_linear_rgba_f32());
let byte_buffer = [0u8; Vec4::SIZE.get() as usize];
let mut buffer = encase::UniformBuffer::new(byte_buffer);
buffer.write(&color).unwrap();
let buffer = render_device.create_buffer_with_data(&BufferInitDescriptor {
contents: buffer.as_ref(),
label: None,
usage: BufferUsages::UNIFORM | BufferUsages::COPY_DST,
});
let (texture_view, texture_sampler) = if let Some(result) = material_pipeline
.mesh_pipeline
.get_image_texture(gpu_images, &Some(extracted_asset.color_texture.clone()))
{
result
} else {
return Err(PrepareAssetError::RetryNextUpdate(extracted_asset));
};
let bind_group = render_device.create_bind_group(&BindGroupDescriptor {
entries: &[
BindGroupEntry {
binding: 0,
resource: buffer.as_entire_binding(),
},
BindGroupEntry {
binding: 0,
resource: BindingResource::TextureView(texture_view),
},
BindGroupEntry {
binding: 1,
resource: BindingResource::Sampler(texture_sampler),
},
],
label: None,
layout: &material_pipeline.material_layout,
});
Ok(GpuCustomMaterial {
_buffer: buffer,
bind_group,
})
}
}
impl Material for CustomMaterial {
fn fragment_shader(asset_server: &AssetServer) -> Option<Handle<Shader>> {
Some(asset_server.load("custom_material.wgsl"))
}
fn bind_group(render_asset: &<Self as RenderAsset>::PreparedAsset) -> &BindGroup {
&render_asset.bind_group
}
fn bind_group_layout(render_device: &RenderDevice) -> BindGroupLayout {
render_device.create_bind_group_layout(&BindGroupLayoutDescriptor {
entries: &[
BindGroupLayoutEntry {
binding: 0,
visibility: ShaderStages::FRAGMENT,
ty: BindingType::Buffer {
ty: BufferBindingType::Uniform,
has_dynamic_offset: false,
min_binding_size: Some(Vec4::min_size()),
},
count: None,
},
BindGroupLayoutEntry {
binding: 1,
visibility: ShaderStages::FRAGMENT,
ty: BindingType::Texture {
multisampled: false,
sample_type: TextureSampleType::Float { filterable: true },
view_dimension: TextureViewDimension::D2Array,
},
count: None,
},
BindGroupLayoutEntry {
binding: 2,
visibility: ShaderStages::FRAGMENT,
ty: BindingType::Sampler(SamplerBindingType::Filtering),
count: None,
},
],
label: None,
})
}
}
```
### Bevy 0.8 (new)
```rust
impl Material for CustomMaterial {
fn fragment_shader() -> ShaderRef {
"custom_material.wgsl".into()
}
}
#[derive(AsBindGroup, TypeUuid, Debug, Clone)]
#[uuid = "f690fdae-d598-45ab-8225-97e2a3f056e0"]
pub struct CustomMaterial {
#[uniform(0)]
color: Color,
#[texture(1)]
#[sampler(2)]
color_texture: Handle<Image>,
}
```
## Future Work
* Add support for more binding types (cubemaps, buffers, etc). This PR intentionally includes a bare minimum number of binding types to keep "reviewability" in check.
* Consider optionally eliding binding indices using binding names. `AsBindGroup` could pass in (optional?) reflection info as a "hint".
* This would make it possible for the derive to do this:
```rust
#[derive(AsBindGroup)]
pub struct CustomMaterial {
#[uniform]
color: Color,
#[texture]
#[sampler]
color_texture: Option<Handle<Image>>,
alpha_mode: AlphaMode,
}
```
* Or this
```rust
#[derive(AsBindGroup)]
pub struct CustomMaterial {
#[binding]
color: Color,
#[binding]
color_texture: Option<Handle<Image>>,
alpha_mode: AlphaMode,
}
```
* Or even this (if we flip to "include bindings by default")
```rust
#[derive(AsBindGroup)]
pub struct CustomMaterial {
color: Color,
color_texture: Option<Handle<Image>>,
#[binding(ignore)]
alpha_mode: AlphaMode,
}
```
* If we add the option to define custom draw functions for materials (which could be done in a type-erased way), I think that would be enough to support extra non-material bindings. Worth considering!
2022-06-30 23:48:46 +00:00
|
|
|
/// The [`AlphaMode`] of this material.
|
|
|
|
pub alpha_mode: AlphaMode,
|
Micro-optimize `queue_material_meshes`, primarily to remove bit manipulation. (#12791)
This commit makes the following optimizations:
## `MeshPipelineKey`/`BaseMeshPipelineKey` split
`MeshPipelineKey` has been split into `BaseMeshPipelineKey`, which lives
in `bevy_render` and `MeshPipelineKey`, which lives in `bevy_pbr`.
Conceptually, `BaseMeshPipelineKey` is a superclass of
`MeshPipelineKey`. For `BaseMeshPipelineKey`, the bits start at the
highest (most significant) bit and grow downward toward the lowest bit;
for `MeshPipelineKey`, the bits start at the lowest bit and grow upward
toward the highest bit. This prevents them from colliding.
The goal of this is to avoid having to reassemble bits of the pipeline
key for every mesh every frame. Instead, we can just use a bitwise or
operation to combine the pieces that make up a `MeshPipelineKey`.
## `specialize_slow`
Previously, all of `specialize()` was marked as `#[inline]`. This
bloated `queue_material_meshes` unnecessarily, as a large chunk of it
ended up being a slow path that was rarely hit. This commit refactors
the function to move the slow path to `specialize_slow()`.
Together, these two changes shave about 5% off `queue_material_meshes`:

## Migration Guide
- The `primitive_topology` field on `GpuMesh` is now an accessor method:
`GpuMesh::primitive_topology()`.
- For performance reasons, `MeshPipelineKey` has been split into
`BaseMeshPipelineKey`, which lives in `bevy_render`, and
`MeshPipelineKey`, which lives in `bevy_pbr`. These two should be
combined with bitwise-or to produce the final `MeshPipelineKey`.
2024-04-01 21:58:53 +00:00
|
|
|
/// The bits in the [`MeshPipelineKey`] for this material.
|
|
|
|
///
|
|
|
|
/// These are precalculated so that we can just "or" them together in
|
|
|
|
/// [`queue_material_meshes`].
|
|
|
|
pub mesh_pipeline_key_bits: MeshPipelineKey,
|
Better Materials: AsBindGroup trait and derive, simpler Material trait (#5053)
# Objective
This PR reworks Bevy's Material system, making the user experience of defining Materials _much_ nicer. Bevy's previous material system leaves a lot to be desired:
* Materials require manually implementing the `RenderAsset` trait, which involves manually generating the bind group, handling gpu buffer data transfer, looking up image textures, etc. Even the simplest single-texture material involves writing ~80 unnecessary lines of code. This was never the long term plan.
* There are two material traits, which is confusing, hard to document, and often redundant: `Material` and `SpecializedMaterial`. `Material` implicitly implements `SpecializedMaterial`, and `SpecializedMaterial` is used in most high level apis to support both use cases. Most users shouldn't need to think about specialization at all (I consider it a "power-user tool"), so the fact that `SpecializedMaterial` is front-and-center in our apis is a miss.
* Implementing either material trait involves a lot of "type soup". The "prepared asset" parameter is particularly heinous: `&<Self as RenderAsset>::PreparedAsset`. Defining vertex and fragment shaders is also more verbose than it needs to be.
## Solution
Say hello to the new `Material` system:
```rust
#[derive(AsBindGroup, TypeUuid, Debug, Clone)]
#[uuid = "f690fdae-d598-45ab-8225-97e2a3f056e0"]
pub struct CoolMaterial {
#[uniform(0)]
color: Color,
#[texture(1)]
#[sampler(2)]
color_texture: Handle<Image>,
}
impl Material for CoolMaterial {
fn fragment_shader() -> ShaderRef {
"cool_material.wgsl".into()
}
}
```
Thats it! This same material would have required [~80 lines of complicated "type heavy" code](https://github.com/bevyengine/bevy/blob/v0.7.0/examples/shader/shader_material.rs) in the old Material system. Now it is just 14 lines of simple, readable code.
This is thanks to a new consolidated `Material` trait and the new `AsBindGroup` trait / derive.
### The new `Material` trait
The old "split" `Material` and `SpecializedMaterial` traits have been removed in favor of a new consolidated `Material` trait. All of the functions on the trait are optional.
The difficulty of implementing `Material` has been reduced by simplifying dataflow and removing type complexity:
```rust
// Old
impl Material for CustomMaterial {
fn fragment_shader(asset_server: &AssetServer) -> Option<Handle<Shader>> {
Some(asset_server.load("custom_material.wgsl"))
}
fn alpha_mode(render_asset: &<Self as RenderAsset>::PreparedAsset) -> AlphaMode {
render_asset.alpha_mode
}
}
// New
impl Material for CustomMaterial {
fn fragment_shader() -> ShaderRef {
"custom_material.wgsl".into()
}
fn alpha_mode(&self) -> AlphaMode {
self.alpha_mode
}
}
```
Specialization is still supported, but it is hidden by default under the `specialize()` function (more on this later).
### The `AsBindGroup` trait / derive
The `Material` trait now requires the `AsBindGroup` derive. This can be implemented manually relatively easily, but deriving it will almost always be preferable.
Field attributes like `uniform` and `texture` are used to define which fields should be bindings,
what their binding type is, and what index they should be bound at:
```rust
#[derive(AsBindGroup)]
struct CoolMaterial {
#[uniform(0)]
color: Color,
#[texture(1)]
#[sampler(2)]
color_texture: Handle<Image>,
}
```
In WGSL shaders, the binding looks like this:
```wgsl
struct CoolMaterial {
color: vec4<f32>;
};
[[group(1), binding(0)]]
var<uniform> material: CoolMaterial;
[[group(1), binding(1)]]
var color_texture: texture_2d<f32>;
[[group(1), binding(2)]]
var color_sampler: sampler;
```
Note that the "group" index is determined by the usage context. It is not defined in `AsBindGroup`. Bevy material bind groups are bound to group 1.
The following field-level attributes are supported:
* `uniform(BINDING_INDEX)`
* The field will be converted to a shader-compatible type using the `ShaderType` trait, written to a `Buffer`, and bound as a uniform. It can also be derived for custom structs.
* `texture(BINDING_INDEX)`
* This field's `Handle<Image>` will be used to look up the matching `Texture` gpu resource, which will be bound as a texture in shaders. The field will be assumed to implement `Into<Option<Handle<Image>>>`. In practice, most fields should be a `Handle<Image>` or `Option<Handle<Image>>`. If the value of an `Option<Handle<Image>>` is `None`, the new `FallbackImage` resource will be used instead. This attribute can be used in conjunction with a `sampler` binding attribute (with a different binding index).
* `sampler(BINDING_INDEX)`
* Behaves exactly like the `texture` attribute, but sets the Image's sampler binding instead of the texture.
Note that fields without field-level binding attributes will be ignored.
```rust
#[derive(AsBindGroup)]
struct CoolMaterial {
#[uniform(0)]
color: Color,
this_field_is_ignored: String,
}
```
As mentioned above, `Option<Handle<Image>>` is also supported:
```rust
#[derive(AsBindGroup)]
struct CoolMaterial {
#[uniform(0)]
color: Color,
#[texture(1)]
#[sampler(2)]
color_texture: Option<Handle<Image>>,
}
```
This is useful if you want a texture to be optional. When the value is `None`, the `FallbackImage` will be used for the binding instead, which defaults to "pure white".
Field uniforms with the same binding index will be combined into a single binding:
```rust
#[derive(AsBindGroup)]
struct CoolMaterial {
#[uniform(0)]
color: Color,
#[uniform(0)]
roughness: f32,
}
```
In WGSL shaders, the binding would look like this:
```wgsl
struct CoolMaterial {
color: vec4<f32>;
roughness: f32;
};
[[group(1), binding(0)]]
var<uniform> material: CoolMaterial;
```
Some less common scenarios will require "struct-level" attributes. These are the currently supported struct-level attributes:
* `uniform(BINDING_INDEX, ConvertedShaderType)`
* Similar to the field-level `uniform` attribute, but instead the entire `AsBindGroup` value is converted to `ConvertedShaderType`, which must implement `ShaderType`. This is useful if more complicated conversion logic is required.
* `bind_group_data(DataType)`
* The `AsBindGroup` type will be converted to some `DataType` using `Into<DataType>` and stored as `AsBindGroup::Data` as part of the `AsBindGroup::as_bind_group` call. This is useful if data needs to be stored alongside the generated bind group, such as a unique identifier for a material's bind group. The most common use case for this attribute is "shader pipeline specialization".
The previous `CoolMaterial` example illustrating "combining multiple field-level uniform attributes with the same binding index" can
also be equivalently represented with a single struct-level uniform attribute:
```rust
#[derive(AsBindGroup)]
#[uniform(0, CoolMaterialUniform)]
struct CoolMaterial {
color: Color,
roughness: f32,
}
#[derive(ShaderType)]
struct CoolMaterialUniform {
color: Color,
roughness: f32,
}
impl From<&CoolMaterial> for CoolMaterialUniform {
fn from(material: &CoolMaterial) -> CoolMaterialUniform {
CoolMaterialUniform {
color: material.color,
roughness: material.roughness,
}
}
}
```
### Material Specialization
Material shader specialization is now _much_ simpler:
```rust
#[derive(AsBindGroup, TypeUuid, Debug, Clone)]
#[uuid = "f690fdae-d598-45ab-8225-97e2a3f056e0"]
#[bind_group_data(CoolMaterialKey)]
struct CoolMaterial {
#[uniform(0)]
color: Color,
is_red: bool,
}
#[derive(Copy, Clone, Hash, Eq, PartialEq)]
struct CoolMaterialKey {
is_red: bool,
}
impl From<&CoolMaterial> for CoolMaterialKey {
fn from(material: &CoolMaterial) -> CoolMaterialKey {
CoolMaterialKey {
is_red: material.is_red,
}
}
}
impl Material for CoolMaterial {
fn fragment_shader() -> ShaderRef {
"cool_material.wgsl".into()
}
fn specialize(
pipeline: &MaterialPipeline<Self>,
descriptor: &mut RenderPipelineDescriptor,
layout: &MeshVertexBufferLayout,
key: MaterialPipelineKey<Self>,
) -> Result<(), SpecializedMeshPipelineError> {
if key.bind_group_data.is_red {
let fragment = descriptor.fragment.as_mut().unwrap();
fragment.shader_defs.push("IS_RED".to_string());
}
Ok(())
}
}
```
Setting `bind_group_data` is not required for specialization (it defaults to `()`). Scenarios like "custom vertex attributes" also benefit from this system:
```rust
impl Material for CustomMaterial {
fn vertex_shader() -> ShaderRef {
"custom_material.wgsl".into()
}
fn fragment_shader() -> ShaderRef {
"custom_material.wgsl".into()
}
fn specialize(
pipeline: &MaterialPipeline<Self>,
descriptor: &mut RenderPipelineDescriptor,
layout: &MeshVertexBufferLayout,
key: MaterialPipelineKey<Self>,
) -> Result<(), SpecializedMeshPipelineError> {
let vertex_layout = layout.get_layout(&[
Mesh::ATTRIBUTE_POSITION.at_shader_location(0),
ATTRIBUTE_BLEND_COLOR.at_shader_location(1),
])?;
descriptor.vertex.buffers = vec![vertex_layout];
Ok(())
}
}
```
### Ported `StandardMaterial` to the new `Material` system
Bevy's built-in PBR material uses the new Material system (including the AsBindGroup derive):
```rust
#[derive(AsBindGroup, Debug, Clone, TypeUuid)]
#[uuid = "7494888b-c082-457b-aacf-517228cc0c22"]
#[bind_group_data(StandardMaterialKey)]
#[uniform(0, StandardMaterialUniform)]
pub struct StandardMaterial {
pub base_color: Color,
#[texture(1)]
#[sampler(2)]
pub base_color_texture: Option<Handle<Image>>,
/* other fields omitted for brevity */
```
### Ported Bevy examples to the new `Material` system
The overall complexity of Bevy's "custom shader examples" has gone down significantly. Take a look at the diffs if you want a dopamine spike.
Please note that while this PR has a net increase in "lines of code", most of those extra lines come from added documentation. There is a significant reduction
in the overall complexity of the code (even accounting for the new derive logic).
---
## Changelog
### Added
* `AsBindGroup` trait and derive, which make it much easier to transfer data to the gpu and generate bind groups for a given type.
### Changed
* The old `Material` and `SpecializedMaterial` traits have been replaced by a consolidated (much simpler) `Material` trait. Materials no longer implement `RenderAsset`.
* `StandardMaterial` was ported to the new material system. There are no user-facing api changes to the `StandardMaterial` struct api, but it now implements `AsBindGroup` and `Material` instead of `RenderAsset` and `SpecializedMaterial`.
## Migration Guide
The Material system has been reworked to be much simpler. We've removed a lot of boilerplate with the new `AsBindGroup` derive and the `Material` trait is simpler as well!
### Bevy 0.7 (old)
```rust
#[derive(Debug, Clone, TypeUuid)]
#[uuid = "f690fdae-d598-45ab-8225-97e2a3f056e0"]
pub struct CustomMaterial {
color: Color,
color_texture: Handle<Image>,
}
#[derive(Clone)]
pub struct GpuCustomMaterial {
_buffer: Buffer,
bind_group: BindGroup,
}
impl RenderAsset for CustomMaterial {
type ExtractedAsset = CustomMaterial;
type PreparedAsset = GpuCustomMaterial;
type Param = (SRes<RenderDevice>, SRes<MaterialPipeline<Self>>);
fn extract_asset(&self) -> Self::ExtractedAsset {
self.clone()
}
fn prepare_asset(
extracted_asset: Self::ExtractedAsset,
(render_device, material_pipeline): &mut SystemParamItem<Self::Param>,
) -> Result<Self::PreparedAsset, PrepareAssetError<Self::ExtractedAsset>> {
let color = Vec4::from_slice(&extracted_asset.color.as_linear_rgba_f32());
let byte_buffer = [0u8; Vec4::SIZE.get() as usize];
let mut buffer = encase::UniformBuffer::new(byte_buffer);
buffer.write(&color).unwrap();
let buffer = render_device.create_buffer_with_data(&BufferInitDescriptor {
contents: buffer.as_ref(),
label: None,
usage: BufferUsages::UNIFORM | BufferUsages::COPY_DST,
});
let (texture_view, texture_sampler) = if let Some(result) = material_pipeline
.mesh_pipeline
.get_image_texture(gpu_images, &Some(extracted_asset.color_texture.clone()))
{
result
} else {
return Err(PrepareAssetError::RetryNextUpdate(extracted_asset));
};
let bind_group = render_device.create_bind_group(&BindGroupDescriptor {
entries: &[
BindGroupEntry {
binding: 0,
resource: buffer.as_entire_binding(),
},
BindGroupEntry {
binding: 0,
resource: BindingResource::TextureView(texture_view),
},
BindGroupEntry {
binding: 1,
resource: BindingResource::Sampler(texture_sampler),
},
],
label: None,
layout: &material_pipeline.material_layout,
});
Ok(GpuCustomMaterial {
_buffer: buffer,
bind_group,
})
}
}
impl Material for CustomMaterial {
fn fragment_shader(asset_server: &AssetServer) -> Option<Handle<Shader>> {
Some(asset_server.load("custom_material.wgsl"))
}
fn bind_group(render_asset: &<Self as RenderAsset>::PreparedAsset) -> &BindGroup {
&render_asset.bind_group
}
fn bind_group_layout(render_device: &RenderDevice) -> BindGroupLayout {
render_device.create_bind_group_layout(&BindGroupLayoutDescriptor {
entries: &[
BindGroupLayoutEntry {
binding: 0,
visibility: ShaderStages::FRAGMENT,
ty: BindingType::Buffer {
ty: BufferBindingType::Uniform,
has_dynamic_offset: false,
min_binding_size: Some(Vec4::min_size()),
},
count: None,
},
BindGroupLayoutEntry {
binding: 1,
visibility: ShaderStages::FRAGMENT,
ty: BindingType::Texture {
multisampled: false,
sample_type: TextureSampleType::Float { filterable: true },
view_dimension: TextureViewDimension::D2Array,
},
count: None,
},
BindGroupLayoutEntry {
binding: 2,
visibility: ShaderStages::FRAGMENT,
ty: BindingType::Sampler(SamplerBindingType::Filtering),
count: None,
},
],
label: None,
})
}
}
```
### Bevy 0.8 (new)
```rust
impl Material for CustomMaterial {
fn fragment_shader() -> ShaderRef {
"custom_material.wgsl".into()
}
}
#[derive(AsBindGroup, TypeUuid, Debug, Clone)]
#[uuid = "f690fdae-d598-45ab-8225-97e2a3f056e0"]
pub struct CustomMaterial {
#[uniform(0)]
color: Color,
#[texture(1)]
#[sampler(2)]
color_texture: Handle<Image>,
}
```
## Future Work
* Add support for more binding types (cubemaps, buffers, etc). This PR intentionally includes a bare minimum number of binding types to keep "reviewability" in check.
* Consider optionally eliding binding indices using binding names. `AsBindGroup` could pass in (optional?) reflection info as a "hint".
* This would make it possible for the derive to do this:
```rust
#[derive(AsBindGroup)]
pub struct CustomMaterial {
#[uniform]
color: Color,
#[texture]
#[sampler]
color_texture: Option<Handle<Image>>,
alpha_mode: AlphaMode,
}
```
* Or this
```rust
#[derive(AsBindGroup)]
pub struct CustomMaterial {
#[binding]
color: Color,
#[binding]
color_texture: Option<Handle<Image>>,
alpha_mode: AlphaMode,
}
```
* Or even this (if we flip to "include bindings by default")
```rust
#[derive(AsBindGroup)]
pub struct CustomMaterial {
color: Color,
color_texture: Option<Handle<Image>>,
#[binding(ignore)]
alpha_mode: AlphaMode,
}
```
* If we add the option to define custom draw functions for materials (which could be done in a type-erased way), I think that would be enough to support extra non-material bindings. Worth considering!
2022-06-30 23:48:46 +00:00
|
|
|
/// Add a bias to the view depth of the mesh which can be used to force a specific render order
|
|
|
|
/// for meshes with equal depth, to avoid z-fighting.
|
2023-03-04 12:29:09 +00:00
|
|
|
/// The bias is in depth-texture units so large values may be needed to overcome small depth differences.
|
Better Materials: AsBindGroup trait and derive, simpler Material trait (#5053)
# Objective
This PR reworks Bevy's Material system, making the user experience of defining Materials _much_ nicer. Bevy's previous material system leaves a lot to be desired:
* Materials require manually implementing the `RenderAsset` trait, which involves manually generating the bind group, handling gpu buffer data transfer, looking up image textures, etc. Even the simplest single-texture material involves writing ~80 unnecessary lines of code. This was never the long term plan.
* There are two material traits, which is confusing, hard to document, and often redundant: `Material` and `SpecializedMaterial`. `Material` implicitly implements `SpecializedMaterial`, and `SpecializedMaterial` is used in most high level apis to support both use cases. Most users shouldn't need to think about specialization at all (I consider it a "power-user tool"), so the fact that `SpecializedMaterial` is front-and-center in our apis is a miss.
* Implementing either material trait involves a lot of "type soup". The "prepared asset" parameter is particularly heinous: `&<Self as RenderAsset>::PreparedAsset`. Defining vertex and fragment shaders is also more verbose than it needs to be.
## Solution
Say hello to the new `Material` system:
```rust
#[derive(AsBindGroup, TypeUuid, Debug, Clone)]
#[uuid = "f690fdae-d598-45ab-8225-97e2a3f056e0"]
pub struct CoolMaterial {
#[uniform(0)]
color: Color,
#[texture(1)]
#[sampler(2)]
color_texture: Handle<Image>,
}
impl Material for CoolMaterial {
fn fragment_shader() -> ShaderRef {
"cool_material.wgsl".into()
}
}
```
Thats it! This same material would have required [~80 lines of complicated "type heavy" code](https://github.com/bevyengine/bevy/blob/v0.7.0/examples/shader/shader_material.rs) in the old Material system. Now it is just 14 lines of simple, readable code.
This is thanks to a new consolidated `Material` trait and the new `AsBindGroup` trait / derive.
### The new `Material` trait
The old "split" `Material` and `SpecializedMaterial` traits have been removed in favor of a new consolidated `Material` trait. All of the functions on the trait are optional.
The difficulty of implementing `Material` has been reduced by simplifying dataflow and removing type complexity:
```rust
// Old
impl Material for CustomMaterial {
fn fragment_shader(asset_server: &AssetServer) -> Option<Handle<Shader>> {
Some(asset_server.load("custom_material.wgsl"))
}
fn alpha_mode(render_asset: &<Self as RenderAsset>::PreparedAsset) -> AlphaMode {
render_asset.alpha_mode
}
}
// New
impl Material for CustomMaterial {
fn fragment_shader() -> ShaderRef {
"custom_material.wgsl".into()
}
fn alpha_mode(&self) -> AlphaMode {
self.alpha_mode
}
}
```
Specialization is still supported, but it is hidden by default under the `specialize()` function (more on this later).
### The `AsBindGroup` trait / derive
The `Material` trait now requires the `AsBindGroup` derive. This can be implemented manually relatively easily, but deriving it will almost always be preferable.
Field attributes like `uniform` and `texture` are used to define which fields should be bindings,
what their binding type is, and what index they should be bound at:
```rust
#[derive(AsBindGroup)]
struct CoolMaterial {
#[uniform(0)]
color: Color,
#[texture(1)]
#[sampler(2)]
color_texture: Handle<Image>,
}
```
In WGSL shaders, the binding looks like this:
```wgsl
struct CoolMaterial {
color: vec4<f32>;
};
[[group(1), binding(0)]]
var<uniform> material: CoolMaterial;
[[group(1), binding(1)]]
var color_texture: texture_2d<f32>;
[[group(1), binding(2)]]
var color_sampler: sampler;
```
Note that the "group" index is determined by the usage context. It is not defined in `AsBindGroup`. Bevy material bind groups are bound to group 1.
The following field-level attributes are supported:
* `uniform(BINDING_INDEX)`
* The field will be converted to a shader-compatible type using the `ShaderType` trait, written to a `Buffer`, and bound as a uniform. It can also be derived for custom structs.
* `texture(BINDING_INDEX)`
* This field's `Handle<Image>` will be used to look up the matching `Texture` gpu resource, which will be bound as a texture in shaders. The field will be assumed to implement `Into<Option<Handle<Image>>>`. In practice, most fields should be a `Handle<Image>` or `Option<Handle<Image>>`. If the value of an `Option<Handle<Image>>` is `None`, the new `FallbackImage` resource will be used instead. This attribute can be used in conjunction with a `sampler` binding attribute (with a different binding index).
* `sampler(BINDING_INDEX)`
* Behaves exactly like the `texture` attribute, but sets the Image's sampler binding instead of the texture.
Note that fields without field-level binding attributes will be ignored.
```rust
#[derive(AsBindGroup)]
struct CoolMaterial {
#[uniform(0)]
color: Color,
this_field_is_ignored: String,
}
```
As mentioned above, `Option<Handle<Image>>` is also supported:
```rust
#[derive(AsBindGroup)]
struct CoolMaterial {
#[uniform(0)]
color: Color,
#[texture(1)]
#[sampler(2)]
color_texture: Option<Handle<Image>>,
}
```
This is useful if you want a texture to be optional. When the value is `None`, the `FallbackImage` will be used for the binding instead, which defaults to "pure white".
Field uniforms with the same binding index will be combined into a single binding:
```rust
#[derive(AsBindGroup)]
struct CoolMaterial {
#[uniform(0)]
color: Color,
#[uniform(0)]
roughness: f32,
}
```
In WGSL shaders, the binding would look like this:
```wgsl
struct CoolMaterial {
color: vec4<f32>;
roughness: f32;
};
[[group(1), binding(0)]]
var<uniform> material: CoolMaterial;
```
Some less common scenarios will require "struct-level" attributes. These are the currently supported struct-level attributes:
* `uniform(BINDING_INDEX, ConvertedShaderType)`
* Similar to the field-level `uniform` attribute, but instead the entire `AsBindGroup` value is converted to `ConvertedShaderType`, which must implement `ShaderType`. This is useful if more complicated conversion logic is required.
* `bind_group_data(DataType)`
* The `AsBindGroup` type will be converted to some `DataType` using `Into<DataType>` and stored as `AsBindGroup::Data` as part of the `AsBindGroup::as_bind_group` call. This is useful if data needs to be stored alongside the generated bind group, such as a unique identifier for a material's bind group. The most common use case for this attribute is "shader pipeline specialization".
The previous `CoolMaterial` example illustrating "combining multiple field-level uniform attributes with the same binding index" can
also be equivalently represented with a single struct-level uniform attribute:
```rust
#[derive(AsBindGroup)]
#[uniform(0, CoolMaterialUniform)]
struct CoolMaterial {
color: Color,
roughness: f32,
}
#[derive(ShaderType)]
struct CoolMaterialUniform {
color: Color,
roughness: f32,
}
impl From<&CoolMaterial> for CoolMaterialUniform {
fn from(material: &CoolMaterial) -> CoolMaterialUniform {
CoolMaterialUniform {
color: material.color,
roughness: material.roughness,
}
}
}
```
### Material Specialization
Material shader specialization is now _much_ simpler:
```rust
#[derive(AsBindGroup, TypeUuid, Debug, Clone)]
#[uuid = "f690fdae-d598-45ab-8225-97e2a3f056e0"]
#[bind_group_data(CoolMaterialKey)]
struct CoolMaterial {
#[uniform(0)]
color: Color,
is_red: bool,
}
#[derive(Copy, Clone, Hash, Eq, PartialEq)]
struct CoolMaterialKey {
is_red: bool,
}
impl From<&CoolMaterial> for CoolMaterialKey {
fn from(material: &CoolMaterial) -> CoolMaterialKey {
CoolMaterialKey {
is_red: material.is_red,
}
}
}
impl Material for CoolMaterial {
fn fragment_shader() -> ShaderRef {
"cool_material.wgsl".into()
}
fn specialize(
pipeline: &MaterialPipeline<Self>,
descriptor: &mut RenderPipelineDescriptor,
layout: &MeshVertexBufferLayout,
key: MaterialPipelineKey<Self>,
) -> Result<(), SpecializedMeshPipelineError> {
if key.bind_group_data.is_red {
let fragment = descriptor.fragment.as_mut().unwrap();
fragment.shader_defs.push("IS_RED".to_string());
}
Ok(())
}
}
```
Setting `bind_group_data` is not required for specialization (it defaults to `()`). Scenarios like "custom vertex attributes" also benefit from this system:
```rust
impl Material for CustomMaterial {
fn vertex_shader() -> ShaderRef {
"custom_material.wgsl".into()
}
fn fragment_shader() -> ShaderRef {
"custom_material.wgsl".into()
}
fn specialize(
pipeline: &MaterialPipeline<Self>,
descriptor: &mut RenderPipelineDescriptor,
layout: &MeshVertexBufferLayout,
key: MaterialPipelineKey<Self>,
) -> Result<(), SpecializedMeshPipelineError> {
let vertex_layout = layout.get_layout(&[
Mesh::ATTRIBUTE_POSITION.at_shader_location(0),
ATTRIBUTE_BLEND_COLOR.at_shader_location(1),
])?;
descriptor.vertex.buffers = vec![vertex_layout];
Ok(())
}
}
```
### Ported `StandardMaterial` to the new `Material` system
Bevy's built-in PBR material uses the new Material system (including the AsBindGroup derive):
```rust
#[derive(AsBindGroup, Debug, Clone, TypeUuid)]
#[uuid = "7494888b-c082-457b-aacf-517228cc0c22"]
#[bind_group_data(StandardMaterialKey)]
#[uniform(0, StandardMaterialUniform)]
pub struct StandardMaterial {
pub base_color: Color,
#[texture(1)]
#[sampler(2)]
pub base_color_texture: Option<Handle<Image>>,
/* other fields omitted for brevity */
```
### Ported Bevy examples to the new `Material` system
The overall complexity of Bevy's "custom shader examples" has gone down significantly. Take a look at the diffs if you want a dopamine spike.
Please note that while this PR has a net increase in "lines of code", most of those extra lines come from added documentation. There is a significant reduction
in the overall complexity of the code (even accounting for the new derive logic).
---
## Changelog
### Added
* `AsBindGroup` trait and derive, which make it much easier to transfer data to the gpu and generate bind groups for a given type.
### Changed
* The old `Material` and `SpecializedMaterial` traits have been replaced by a consolidated (much simpler) `Material` trait. Materials no longer implement `RenderAsset`.
* `StandardMaterial` was ported to the new material system. There are no user-facing api changes to the `StandardMaterial` struct api, but it now implements `AsBindGroup` and `Material` instead of `RenderAsset` and `SpecializedMaterial`.
## Migration Guide
The Material system has been reworked to be much simpler. We've removed a lot of boilerplate with the new `AsBindGroup` derive and the `Material` trait is simpler as well!
### Bevy 0.7 (old)
```rust
#[derive(Debug, Clone, TypeUuid)]
#[uuid = "f690fdae-d598-45ab-8225-97e2a3f056e0"]
pub struct CustomMaterial {
color: Color,
color_texture: Handle<Image>,
}
#[derive(Clone)]
pub struct GpuCustomMaterial {
_buffer: Buffer,
bind_group: BindGroup,
}
impl RenderAsset for CustomMaterial {
type ExtractedAsset = CustomMaterial;
type PreparedAsset = GpuCustomMaterial;
type Param = (SRes<RenderDevice>, SRes<MaterialPipeline<Self>>);
fn extract_asset(&self) -> Self::ExtractedAsset {
self.clone()
}
fn prepare_asset(
extracted_asset: Self::ExtractedAsset,
(render_device, material_pipeline): &mut SystemParamItem<Self::Param>,
) -> Result<Self::PreparedAsset, PrepareAssetError<Self::ExtractedAsset>> {
let color = Vec4::from_slice(&extracted_asset.color.as_linear_rgba_f32());
let byte_buffer = [0u8; Vec4::SIZE.get() as usize];
let mut buffer = encase::UniformBuffer::new(byte_buffer);
buffer.write(&color).unwrap();
let buffer = render_device.create_buffer_with_data(&BufferInitDescriptor {
contents: buffer.as_ref(),
label: None,
usage: BufferUsages::UNIFORM | BufferUsages::COPY_DST,
});
let (texture_view, texture_sampler) = if let Some(result) = material_pipeline
.mesh_pipeline
.get_image_texture(gpu_images, &Some(extracted_asset.color_texture.clone()))
{
result
} else {
return Err(PrepareAssetError::RetryNextUpdate(extracted_asset));
};
let bind_group = render_device.create_bind_group(&BindGroupDescriptor {
entries: &[
BindGroupEntry {
binding: 0,
resource: buffer.as_entire_binding(),
},
BindGroupEntry {
binding: 0,
resource: BindingResource::TextureView(texture_view),
},
BindGroupEntry {
binding: 1,
resource: BindingResource::Sampler(texture_sampler),
},
],
label: None,
layout: &material_pipeline.material_layout,
});
Ok(GpuCustomMaterial {
_buffer: buffer,
bind_group,
})
}
}
impl Material for CustomMaterial {
fn fragment_shader(asset_server: &AssetServer) -> Option<Handle<Shader>> {
Some(asset_server.load("custom_material.wgsl"))
}
fn bind_group(render_asset: &<Self as RenderAsset>::PreparedAsset) -> &BindGroup {
&render_asset.bind_group
}
fn bind_group_layout(render_device: &RenderDevice) -> BindGroupLayout {
render_device.create_bind_group_layout(&BindGroupLayoutDescriptor {
entries: &[
BindGroupLayoutEntry {
binding: 0,
visibility: ShaderStages::FRAGMENT,
ty: BindingType::Buffer {
ty: BufferBindingType::Uniform,
has_dynamic_offset: false,
min_binding_size: Some(Vec4::min_size()),
},
count: None,
},
BindGroupLayoutEntry {
binding: 1,
visibility: ShaderStages::FRAGMENT,
ty: BindingType::Texture {
multisampled: false,
sample_type: TextureSampleType::Float { filterable: true },
view_dimension: TextureViewDimension::D2Array,
},
count: None,
},
BindGroupLayoutEntry {
binding: 2,
visibility: ShaderStages::FRAGMENT,
ty: BindingType::Sampler(SamplerBindingType::Filtering),
count: None,
},
],
label: None,
})
}
}
```
### Bevy 0.8 (new)
```rust
impl Material for CustomMaterial {
fn fragment_shader() -> ShaderRef {
"custom_material.wgsl".into()
}
}
#[derive(AsBindGroup, TypeUuid, Debug, Clone)]
#[uuid = "f690fdae-d598-45ab-8225-97e2a3f056e0"]
pub struct CustomMaterial {
#[uniform(0)]
color: Color,
#[texture(1)]
#[sampler(2)]
color_texture: Handle<Image>,
}
```
## Future Work
* Add support for more binding types (cubemaps, buffers, etc). This PR intentionally includes a bare minimum number of binding types to keep "reviewability" in check.
* Consider optionally eliding binding indices using binding names. `AsBindGroup` could pass in (optional?) reflection info as a "hint".
* This would make it possible for the derive to do this:
```rust
#[derive(AsBindGroup)]
pub struct CustomMaterial {
#[uniform]
color: Color,
#[texture]
#[sampler]
color_texture: Option<Handle<Image>>,
alpha_mode: AlphaMode,
}
```
* Or this
```rust
#[derive(AsBindGroup)]
pub struct CustomMaterial {
#[binding]
color: Color,
#[binding]
color_texture: Option<Handle<Image>>,
alpha_mode: AlphaMode,
}
```
* Or even this (if we flip to "include bindings by default")
```rust
#[derive(AsBindGroup)]
pub struct CustomMaterial {
color: Color,
color_texture: Option<Handle<Image>>,
#[binding(ignore)]
alpha_mode: AlphaMode,
}
```
* If we add the option to define custom draw functions for materials (which could be done in a type-erased way), I think that would be enough to support extra non-material bindings. Worth considering!
2022-06-30 23:48:46 +00:00
|
|
|
pub depth_bias: f32,
|
`StandardMaterial` Light Transmission (#8015)
# Objective
<img width="1920" alt="Screenshot 2023-04-26 at 01 07 34"
src="https://user-images.githubusercontent.com/418473/234467578-0f34187b-5863-4ea1-88e9-7a6bb8ce8da3.png">
This PR adds both diffuse and specular light transmission capabilities
to the `StandardMaterial`, with support for screen space refractions.
This enables realistically representing a wide range of real-world
materials, such as:
- Glass; (Including frosted glass)
- Transparent and translucent plastics;
- Various liquids and gels;
- Gemstones;
- Marble;
- Wax;
- Paper;
- Leaves;
- Porcelain.
Unlike existing support for transparency, light transmission does not
rely on fixed function alpha blending, and therefore works with both
`AlphaMode::Opaque` and `AlphaMode::Mask` materials.
## Solution
- Introduces a number of transmission related fields in the
`StandardMaterial`;
- For specular transmission:
- Adds logic to take a view main texture snapshot after the opaque
phase; (in order to perform screen space refractions)
- Introduces a new `Transmissive3d` phase to the renderer, to which all
meshes with `transmission > 0.0` materials are sent.
- Calculates a light exit point (of the approximate mesh volume) using
`ior` and `thickness` properties
- Samples the snapshot texture with an adaptive number of taps across a
`roughness`-controlled radius enabling “blurry” refractions
- For diffuse transmission:
- Approximates transmitted diffuse light by using a second, flipped +
displaced, diffuse-only Lambertian lobe for each light source.
## To Do
- [x] Figure out where `fresnel_mix()` is taking place, if at all, and
where `dielectric_specular` is being calculated, if at all, and update
them to use the `ior` value (Not a blocker, just a nice-to-have for more
correct BSDF)
- To the _best of my knowledge, this is now taking place, after
964340cdd. The fresnel mix is actually "split" into two parts in our
implementation, one `(1 - fresnel(...))` in the transmission, and
`fresnel()` in the light implementations. A surface with more
reflectance now will produce slightly dimmer transmission towards the
grazing angle, as more of the light gets reflected.
- [x] Add `transmission_texture`
- [x] Add `diffuse_transmission_texture`
- [x] Add `thickness_texture`
- [x] Add `attenuation_distance` and `attenuation_color`
- [x] Connect values to glTF loader
- [x] `transmission` and `transmission_texture`
- [x] `thickness` and `thickness_texture`
- [x] `ior`
- [ ] `diffuse_transmission` and `diffuse_transmission_texture` (needs
upstream support in `gltf` crate, not a blocker)
- [x] Add support for multiple screen space refraction “steps”
- [x] Conditionally create no transmission snapshot texture at all if
`steps == 0`
- [x] Conditionally enable/disable screen space refraction transmission
snapshots
- [x] Read from depth pre-pass to prevent refracting pixels in front of
the light exit point
- [x] Use `interleaved_gradient_noise()` function for sampling blur in a
way that benefits from TAA
- [x] Drill down a TAA `#define`, tweak some aspects of the effect
conditionally based on it
- [x] Remove const array that's crashing under HLSL (unless a new `naga`
release with https://github.com/gfx-rs/naga/pull/2496 comes out before
we merge this)
- [ ] Look into alternatives to the `switch` hack for dynamically
indexing the const array (might not be needed, compilers seem to be
decent at expanding it)
- [ ] Add pipeline keys for gating transmission (do we really want/need
this?)
- [x] Tweak some material field/function names?
## A Note on Texture Packing
_This was originally added as a comment to the
`specular_transmission_texture`, `thickness_texture` and
`diffuse_transmission_texture` documentation, I removed it since it was
more confusing than helpful, and will likely be made redundant/will need
to be updated once we have a better infrastructure for preprocessing
assets_
Due to how channels are mapped, you can more efficiently use a single
shared texture image
for configuring the following:
- R - `specular_transmission_texture`
- G - `thickness_texture`
- B - _unused_
- A - `diffuse_transmission_texture`
The `KHR_materials_diffuse_transmission` glTF extension also defines a
`diffuseTransmissionColorTexture`,
that _we don't currently support_. One might choose to pack the
intensity and color textures together,
using RGB for the color and A for the intensity, in which case this
packing advice doesn't really apply.
---
## Changelog
- Added a new `Transmissive3d` render phase for rendering specular
transmissive materials with screen space refractions
- Added rendering support for transmitted environment map light on the
`StandardMaterial` as a fallback for screen space refractions
- Added `diffuse_transmission`, `specular_transmission`, `thickness`,
`ior`, `attenuation_distance` and `attenuation_color` to the
`StandardMaterial`
- Added `diffuse_transmission_texture`, `specular_transmission_texture`,
`thickness_texture` to the `StandardMaterial`, gated behind a new
`pbr_transmission_textures` cargo feature (off by default, for maximum
hardware compatibility)
- Added `Camera3d::screen_space_specular_transmission_steps` for
controlling the number of “layers of transparency” rendered for
transmissive objects
- Added a `TransmittedShadowReceiver` component for enabling shadows in
(diffusely) transmitted light. (disabled by default, as it requires
carefully setting up the `thickness` to avoid self-shadow artifacts)
- Added support for the `KHR_materials_transmission`,
`KHR_materials_ior` and `KHR_materials_volume` glTF extensions
- Renamed items related to temporal jitter for greater consistency
## Migration Guide
- `SsaoPipelineKey::temporal_noise` has been renamed to
`SsaoPipelineKey::temporal_jitter`
- The `TAA` shader def (controlled by the presence of the
`TemporalAntiAliasSettings` component in the camera) has been replaced
with the `TEMPORAL_JITTER` shader def (controlled by the presence of the
`TemporalJitter` component in the camera)
- `MeshPipelineKey::TAA` has been replaced by
`MeshPipelineKey::TEMPORAL_JITTER`
- The `TEMPORAL_NOISE` shader def has been consolidated with
`TEMPORAL_JITTER`
2023-10-31 20:59:02 +00:00
|
|
|
/// Whether the material would like to read from [`ViewTransmissionTexture`](bevy_core_pipeline::core_3d::ViewTransmissionTexture).
|
|
|
|
///
|
|
|
|
/// This allows taking color output from the [`Opaque3d`] pass as an input, (for screen-space transmission) but requires
|
|
|
|
/// rendering to take place in a separate [`Transmissive3d`] pass.
|
|
|
|
pub reads_view_transmission_texture: bool,
|
Better Materials: AsBindGroup trait and derive, simpler Material trait (#5053)
# Objective
This PR reworks Bevy's Material system, making the user experience of defining Materials _much_ nicer. Bevy's previous material system leaves a lot to be desired:
* Materials require manually implementing the `RenderAsset` trait, which involves manually generating the bind group, handling gpu buffer data transfer, looking up image textures, etc. Even the simplest single-texture material involves writing ~80 unnecessary lines of code. This was never the long term plan.
* There are two material traits, which is confusing, hard to document, and often redundant: `Material` and `SpecializedMaterial`. `Material` implicitly implements `SpecializedMaterial`, and `SpecializedMaterial` is used in most high level apis to support both use cases. Most users shouldn't need to think about specialization at all (I consider it a "power-user tool"), so the fact that `SpecializedMaterial` is front-and-center in our apis is a miss.
* Implementing either material trait involves a lot of "type soup". The "prepared asset" parameter is particularly heinous: `&<Self as RenderAsset>::PreparedAsset`. Defining vertex and fragment shaders is also more verbose than it needs to be.
## Solution
Say hello to the new `Material` system:
```rust
#[derive(AsBindGroup, TypeUuid, Debug, Clone)]
#[uuid = "f690fdae-d598-45ab-8225-97e2a3f056e0"]
pub struct CoolMaterial {
#[uniform(0)]
color: Color,
#[texture(1)]
#[sampler(2)]
color_texture: Handle<Image>,
}
impl Material for CoolMaterial {
fn fragment_shader() -> ShaderRef {
"cool_material.wgsl".into()
}
}
```
Thats it! This same material would have required [~80 lines of complicated "type heavy" code](https://github.com/bevyengine/bevy/blob/v0.7.0/examples/shader/shader_material.rs) in the old Material system. Now it is just 14 lines of simple, readable code.
This is thanks to a new consolidated `Material` trait and the new `AsBindGroup` trait / derive.
### The new `Material` trait
The old "split" `Material` and `SpecializedMaterial` traits have been removed in favor of a new consolidated `Material` trait. All of the functions on the trait are optional.
The difficulty of implementing `Material` has been reduced by simplifying dataflow and removing type complexity:
```rust
// Old
impl Material for CustomMaterial {
fn fragment_shader(asset_server: &AssetServer) -> Option<Handle<Shader>> {
Some(asset_server.load("custom_material.wgsl"))
}
fn alpha_mode(render_asset: &<Self as RenderAsset>::PreparedAsset) -> AlphaMode {
render_asset.alpha_mode
}
}
// New
impl Material for CustomMaterial {
fn fragment_shader() -> ShaderRef {
"custom_material.wgsl".into()
}
fn alpha_mode(&self) -> AlphaMode {
self.alpha_mode
}
}
```
Specialization is still supported, but it is hidden by default under the `specialize()` function (more on this later).
### The `AsBindGroup` trait / derive
The `Material` trait now requires the `AsBindGroup` derive. This can be implemented manually relatively easily, but deriving it will almost always be preferable.
Field attributes like `uniform` and `texture` are used to define which fields should be bindings,
what their binding type is, and what index they should be bound at:
```rust
#[derive(AsBindGroup)]
struct CoolMaterial {
#[uniform(0)]
color: Color,
#[texture(1)]
#[sampler(2)]
color_texture: Handle<Image>,
}
```
In WGSL shaders, the binding looks like this:
```wgsl
struct CoolMaterial {
color: vec4<f32>;
};
[[group(1), binding(0)]]
var<uniform> material: CoolMaterial;
[[group(1), binding(1)]]
var color_texture: texture_2d<f32>;
[[group(1), binding(2)]]
var color_sampler: sampler;
```
Note that the "group" index is determined by the usage context. It is not defined in `AsBindGroup`. Bevy material bind groups are bound to group 1.
The following field-level attributes are supported:
* `uniform(BINDING_INDEX)`
* The field will be converted to a shader-compatible type using the `ShaderType` trait, written to a `Buffer`, and bound as a uniform. It can also be derived for custom structs.
* `texture(BINDING_INDEX)`
* This field's `Handle<Image>` will be used to look up the matching `Texture` gpu resource, which will be bound as a texture in shaders. The field will be assumed to implement `Into<Option<Handle<Image>>>`. In practice, most fields should be a `Handle<Image>` or `Option<Handle<Image>>`. If the value of an `Option<Handle<Image>>` is `None`, the new `FallbackImage` resource will be used instead. This attribute can be used in conjunction with a `sampler` binding attribute (with a different binding index).
* `sampler(BINDING_INDEX)`
* Behaves exactly like the `texture` attribute, but sets the Image's sampler binding instead of the texture.
Note that fields without field-level binding attributes will be ignored.
```rust
#[derive(AsBindGroup)]
struct CoolMaterial {
#[uniform(0)]
color: Color,
this_field_is_ignored: String,
}
```
As mentioned above, `Option<Handle<Image>>` is also supported:
```rust
#[derive(AsBindGroup)]
struct CoolMaterial {
#[uniform(0)]
color: Color,
#[texture(1)]
#[sampler(2)]
color_texture: Option<Handle<Image>>,
}
```
This is useful if you want a texture to be optional. When the value is `None`, the `FallbackImage` will be used for the binding instead, which defaults to "pure white".
Field uniforms with the same binding index will be combined into a single binding:
```rust
#[derive(AsBindGroup)]
struct CoolMaterial {
#[uniform(0)]
color: Color,
#[uniform(0)]
roughness: f32,
}
```
In WGSL shaders, the binding would look like this:
```wgsl
struct CoolMaterial {
color: vec4<f32>;
roughness: f32;
};
[[group(1), binding(0)]]
var<uniform> material: CoolMaterial;
```
Some less common scenarios will require "struct-level" attributes. These are the currently supported struct-level attributes:
* `uniform(BINDING_INDEX, ConvertedShaderType)`
* Similar to the field-level `uniform` attribute, but instead the entire `AsBindGroup` value is converted to `ConvertedShaderType`, which must implement `ShaderType`. This is useful if more complicated conversion logic is required.
* `bind_group_data(DataType)`
* The `AsBindGroup` type will be converted to some `DataType` using `Into<DataType>` and stored as `AsBindGroup::Data` as part of the `AsBindGroup::as_bind_group` call. This is useful if data needs to be stored alongside the generated bind group, such as a unique identifier for a material's bind group. The most common use case for this attribute is "shader pipeline specialization".
The previous `CoolMaterial` example illustrating "combining multiple field-level uniform attributes with the same binding index" can
also be equivalently represented with a single struct-level uniform attribute:
```rust
#[derive(AsBindGroup)]
#[uniform(0, CoolMaterialUniform)]
struct CoolMaterial {
color: Color,
roughness: f32,
}
#[derive(ShaderType)]
struct CoolMaterialUniform {
color: Color,
roughness: f32,
}
impl From<&CoolMaterial> for CoolMaterialUniform {
fn from(material: &CoolMaterial) -> CoolMaterialUniform {
CoolMaterialUniform {
color: material.color,
roughness: material.roughness,
}
}
}
```
### Material Specialization
Material shader specialization is now _much_ simpler:
```rust
#[derive(AsBindGroup, TypeUuid, Debug, Clone)]
#[uuid = "f690fdae-d598-45ab-8225-97e2a3f056e0"]
#[bind_group_data(CoolMaterialKey)]
struct CoolMaterial {
#[uniform(0)]
color: Color,
is_red: bool,
}
#[derive(Copy, Clone, Hash, Eq, PartialEq)]
struct CoolMaterialKey {
is_red: bool,
}
impl From<&CoolMaterial> for CoolMaterialKey {
fn from(material: &CoolMaterial) -> CoolMaterialKey {
CoolMaterialKey {
is_red: material.is_red,
}
}
}
impl Material for CoolMaterial {
fn fragment_shader() -> ShaderRef {
"cool_material.wgsl".into()
}
fn specialize(
pipeline: &MaterialPipeline<Self>,
descriptor: &mut RenderPipelineDescriptor,
layout: &MeshVertexBufferLayout,
key: MaterialPipelineKey<Self>,
) -> Result<(), SpecializedMeshPipelineError> {
if key.bind_group_data.is_red {
let fragment = descriptor.fragment.as_mut().unwrap();
fragment.shader_defs.push("IS_RED".to_string());
}
Ok(())
}
}
```
Setting `bind_group_data` is not required for specialization (it defaults to `()`). Scenarios like "custom vertex attributes" also benefit from this system:
```rust
impl Material for CustomMaterial {
fn vertex_shader() -> ShaderRef {
"custom_material.wgsl".into()
}
fn fragment_shader() -> ShaderRef {
"custom_material.wgsl".into()
}
fn specialize(
pipeline: &MaterialPipeline<Self>,
descriptor: &mut RenderPipelineDescriptor,
layout: &MeshVertexBufferLayout,
key: MaterialPipelineKey<Self>,
) -> Result<(), SpecializedMeshPipelineError> {
let vertex_layout = layout.get_layout(&[
Mesh::ATTRIBUTE_POSITION.at_shader_location(0),
ATTRIBUTE_BLEND_COLOR.at_shader_location(1),
])?;
descriptor.vertex.buffers = vec![vertex_layout];
Ok(())
}
}
```
### Ported `StandardMaterial` to the new `Material` system
Bevy's built-in PBR material uses the new Material system (including the AsBindGroup derive):
```rust
#[derive(AsBindGroup, Debug, Clone, TypeUuid)]
#[uuid = "7494888b-c082-457b-aacf-517228cc0c22"]
#[bind_group_data(StandardMaterialKey)]
#[uniform(0, StandardMaterialUniform)]
pub struct StandardMaterial {
pub base_color: Color,
#[texture(1)]
#[sampler(2)]
pub base_color_texture: Option<Handle<Image>>,
/* other fields omitted for brevity */
```
### Ported Bevy examples to the new `Material` system
The overall complexity of Bevy's "custom shader examples" has gone down significantly. Take a look at the diffs if you want a dopamine spike.
Please note that while this PR has a net increase in "lines of code", most of those extra lines come from added documentation. There is a significant reduction
in the overall complexity of the code (even accounting for the new derive logic).
---
## Changelog
### Added
* `AsBindGroup` trait and derive, which make it much easier to transfer data to the gpu and generate bind groups for a given type.
### Changed
* The old `Material` and `SpecializedMaterial` traits have been replaced by a consolidated (much simpler) `Material` trait. Materials no longer implement `RenderAsset`.
* `StandardMaterial` was ported to the new material system. There are no user-facing api changes to the `StandardMaterial` struct api, but it now implements `AsBindGroup` and `Material` instead of `RenderAsset` and `SpecializedMaterial`.
## Migration Guide
The Material system has been reworked to be much simpler. We've removed a lot of boilerplate with the new `AsBindGroup` derive and the `Material` trait is simpler as well!
### Bevy 0.7 (old)
```rust
#[derive(Debug, Clone, TypeUuid)]
#[uuid = "f690fdae-d598-45ab-8225-97e2a3f056e0"]
pub struct CustomMaterial {
color: Color,
color_texture: Handle<Image>,
}
#[derive(Clone)]
pub struct GpuCustomMaterial {
_buffer: Buffer,
bind_group: BindGroup,
}
impl RenderAsset for CustomMaterial {
type ExtractedAsset = CustomMaterial;
type PreparedAsset = GpuCustomMaterial;
type Param = (SRes<RenderDevice>, SRes<MaterialPipeline<Self>>);
fn extract_asset(&self) -> Self::ExtractedAsset {
self.clone()
}
fn prepare_asset(
extracted_asset: Self::ExtractedAsset,
(render_device, material_pipeline): &mut SystemParamItem<Self::Param>,
) -> Result<Self::PreparedAsset, PrepareAssetError<Self::ExtractedAsset>> {
let color = Vec4::from_slice(&extracted_asset.color.as_linear_rgba_f32());
let byte_buffer = [0u8; Vec4::SIZE.get() as usize];
let mut buffer = encase::UniformBuffer::new(byte_buffer);
buffer.write(&color).unwrap();
let buffer = render_device.create_buffer_with_data(&BufferInitDescriptor {
contents: buffer.as_ref(),
label: None,
usage: BufferUsages::UNIFORM | BufferUsages::COPY_DST,
});
let (texture_view, texture_sampler) = if let Some(result) = material_pipeline
.mesh_pipeline
.get_image_texture(gpu_images, &Some(extracted_asset.color_texture.clone()))
{
result
} else {
return Err(PrepareAssetError::RetryNextUpdate(extracted_asset));
};
let bind_group = render_device.create_bind_group(&BindGroupDescriptor {
entries: &[
BindGroupEntry {
binding: 0,
resource: buffer.as_entire_binding(),
},
BindGroupEntry {
binding: 0,
resource: BindingResource::TextureView(texture_view),
},
BindGroupEntry {
binding: 1,
resource: BindingResource::Sampler(texture_sampler),
},
],
label: None,
layout: &material_pipeline.material_layout,
});
Ok(GpuCustomMaterial {
_buffer: buffer,
bind_group,
})
}
}
impl Material for CustomMaterial {
fn fragment_shader(asset_server: &AssetServer) -> Option<Handle<Shader>> {
Some(asset_server.load("custom_material.wgsl"))
}
fn bind_group(render_asset: &<Self as RenderAsset>::PreparedAsset) -> &BindGroup {
&render_asset.bind_group
}
fn bind_group_layout(render_device: &RenderDevice) -> BindGroupLayout {
render_device.create_bind_group_layout(&BindGroupLayoutDescriptor {
entries: &[
BindGroupLayoutEntry {
binding: 0,
visibility: ShaderStages::FRAGMENT,
ty: BindingType::Buffer {
ty: BufferBindingType::Uniform,
has_dynamic_offset: false,
min_binding_size: Some(Vec4::min_size()),
},
count: None,
},
BindGroupLayoutEntry {
binding: 1,
visibility: ShaderStages::FRAGMENT,
ty: BindingType::Texture {
multisampled: false,
sample_type: TextureSampleType::Float { filterable: true },
view_dimension: TextureViewDimension::D2Array,
},
count: None,
},
BindGroupLayoutEntry {
binding: 2,
visibility: ShaderStages::FRAGMENT,
ty: BindingType::Sampler(SamplerBindingType::Filtering),
count: None,
},
],
label: None,
})
}
}
```
### Bevy 0.8 (new)
```rust
impl Material for CustomMaterial {
fn fragment_shader() -> ShaderRef {
"custom_material.wgsl".into()
}
}
#[derive(AsBindGroup, TypeUuid, Debug, Clone)]
#[uuid = "f690fdae-d598-45ab-8225-97e2a3f056e0"]
pub struct CustomMaterial {
#[uniform(0)]
color: Color,
#[texture(1)]
#[sampler(2)]
color_texture: Handle<Image>,
}
```
## Future Work
* Add support for more binding types (cubemaps, buffers, etc). This PR intentionally includes a bare minimum number of binding types to keep "reviewability" in check.
* Consider optionally eliding binding indices using binding names. `AsBindGroup` could pass in (optional?) reflection info as a "hint".
* This would make it possible for the derive to do this:
```rust
#[derive(AsBindGroup)]
pub struct CustomMaterial {
#[uniform]
color: Color,
#[texture]
#[sampler]
color_texture: Option<Handle<Image>>,
alpha_mode: AlphaMode,
}
```
* Or this
```rust
#[derive(AsBindGroup)]
pub struct CustomMaterial {
#[binding]
color: Color,
#[binding]
color_texture: Option<Handle<Image>>,
alpha_mode: AlphaMode,
}
```
* Or even this (if we flip to "include bindings by default")
```rust
#[derive(AsBindGroup)]
pub struct CustomMaterial {
color: Color,
color_texture: Option<Handle<Image>>,
#[binding(ignore)]
alpha_mode: AlphaMode,
}
```
* If we add the option to define custom draw functions for materials (which could be done in a type-erased way), I think that would be enough to support extra non-material bindings. Worth considering!
2022-06-30 23:48:46 +00:00
|
|
|
}
|
|
|
|
|
|
|
|
/// Data prepared for a [`Material`] instance.
|
|
|
|
pub struct PreparedMaterial<T: Material> {
|
2023-10-17 21:28:08 +00:00
|
|
|
pub bindings: Vec<(u32, OwnedBindingResource)>,
|
Better Materials: AsBindGroup trait and derive, simpler Material trait (#5053)
# Objective
This PR reworks Bevy's Material system, making the user experience of defining Materials _much_ nicer. Bevy's previous material system leaves a lot to be desired:
* Materials require manually implementing the `RenderAsset` trait, which involves manually generating the bind group, handling gpu buffer data transfer, looking up image textures, etc. Even the simplest single-texture material involves writing ~80 unnecessary lines of code. This was never the long term plan.
* There are two material traits, which is confusing, hard to document, and often redundant: `Material` and `SpecializedMaterial`. `Material` implicitly implements `SpecializedMaterial`, and `SpecializedMaterial` is used in most high level apis to support both use cases. Most users shouldn't need to think about specialization at all (I consider it a "power-user tool"), so the fact that `SpecializedMaterial` is front-and-center in our apis is a miss.
* Implementing either material trait involves a lot of "type soup". The "prepared asset" parameter is particularly heinous: `&<Self as RenderAsset>::PreparedAsset`. Defining vertex and fragment shaders is also more verbose than it needs to be.
## Solution
Say hello to the new `Material` system:
```rust
#[derive(AsBindGroup, TypeUuid, Debug, Clone)]
#[uuid = "f690fdae-d598-45ab-8225-97e2a3f056e0"]
pub struct CoolMaterial {
#[uniform(0)]
color: Color,
#[texture(1)]
#[sampler(2)]
color_texture: Handle<Image>,
}
impl Material for CoolMaterial {
fn fragment_shader() -> ShaderRef {
"cool_material.wgsl".into()
}
}
```
Thats it! This same material would have required [~80 lines of complicated "type heavy" code](https://github.com/bevyengine/bevy/blob/v0.7.0/examples/shader/shader_material.rs) in the old Material system. Now it is just 14 lines of simple, readable code.
This is thanks to a new consolidated `Material` trait and the new `AsBindGroup` trait / derive.
### The new `Material` trait
The old "split" `Material` and `SpecializedMaterial` traits have been removed in favor of a new consolidated `Material` trait. All of the functions on the trait are optional.
The difficulty of implementing `Material` has been reduced by simplifying dataflow and removing type complexity:
```rust
// Old
impl Material for CustomMaterial {
fn fragment_shader(asset_server: &AssetServer) -> Option<Handle<Shader>> {
Some(asset_server.load("custom_material.wgsl"))
}
fn alpha_mode(render_asset: &<Self as RenderAsset>::PreparedAsset) -> AlphaMode {
render_asset.alpha_mode
}
}
// New
impl Material for CustomMaterial {
fn fragment_shader() -> ShaderRef {
"custom_material.wgsl".into()
}
fn alpha_mode(&self) -> AlphaMode {
self.alpha_mode
}
}
```
Specialization is still supported, but it is hidden by default under the `specialize()` function (more on this later).
### The `AsBindGroup` trait / derive
The `Material` trait now requires the `AsBindGroup` derive. This can be implemented manually relatively easily, but deriving it will almost always be preferable.
Field attributes like `uniform` and `texture` are used to define which fields should be bindings,
what their binding type is, and what index they should be bound at:
```rust
#[derive(AsBindGroup)]
struct CoolMaterial {
#[uniform(0)]
color: Color,
#[texture(1)]
#[sampler(2)]
color_texture: Handle<Image>,
}
```
In WGSL shaders, the binding looks like this:
```wgsl
struct CoolMaterial {
color: vec4<f32>;
};
[[group(1), binding(0)]]
var<uniform> material: CoolMaterial;
[[group(1), binding(1)]]
var color_texture: texture_2d<f32>;
[[group(1), binding(2)]]
var color_sampler: sampler;
```
Note that the "group" index is determined by the usage context. It is not defined in `AsBindGroup`. Bevy material bind groups are bound to group 1.
The following field-level attributes are supported:
* `uniform(BINDING_INDEX)`
* The field will be converted to a shader-compatible type using the `ShaderType` trait, written to a `Buffer`, and bound as a uniform. It can also be derived for custom structs.
* `texture(BINDING_INDEX)`
* This field's `Handle<Image>` will be used to look up the matching `Texture` gpu resource, which will be bound as a texture in shaders. The field will be assumed to implement `Into<Option<Handle<Image>>>`. In practice, most fields should be a `Handle<Image>` or `Option<Handle<Image>>`. If the value of an `Option<Handle<Image>>` is `None`, the new `FallbackImage` resource will be used instead. This attribute can be used in conjunction with a `sampler` binding attribute (with a different binding index).
* `sampler(BINDING_INDEX)`
* Behaves exactly like the `texture` attribute, but sets the Image's sampler binding instead of the texture.
Note that fields without field-level binding attributes will be ignored.
```rust
#[derive(AsBindGroup)]
struct CoolMaterial {
#[uniform(0)]
color: Color,
this_field_is_ignored: String,
}
```
As mentioned above, `Option<Handle<Image>>` is also supported:
```rust
#[derive(AsBindGroup)]
struct CoolMaterial {
#[uniform(0)]
color: Color,
#[texture(1)]
#[sampler(2)]
color_texture: Option<Handle<Image>>,
}
```
This is useful if you want a texture to be optional. When the value is `None`, the `FallbackImage` will be used for the binding instead, which defaults to "pure white".
Field uniforms with the same binding index will be combined into a single binding:
```rust
#[derive(AsBindGroup)]
struct CoolMaterial {
#[uniform(0)]
color: Color,
#[uniform(0)]
roughness: f32,
}
```
In WGSL shaders, the binding would look like this:
```wgsl
struct CoolMaterial {
color: vec4<f32>;
roughness: f32;
};
[[group(1), binding(0)]]
var<uniform> material: CoolMaterial;
```
Some less common scenarios will require "struct-level" attributes. These are the currently supported struct-level attributes:
* `uniform(BINDING_INDEX, ConvertedShaderType)`
* Similar to the field-level `uniform` attribute, but instead the entire `AsBindGroup` value is converted to `ConvertedShaderType`, which must implement `ShaderType`. This is useful if more complicated conversion logic is required.
* `bind_group_data(DataType)`
* The `AsBindGroup` type will be converted to some `DataType` using `Into<DataType>` and stored as `AsBindGroup::Data` as part of the `AsBindGroup::as_bind_group` call. This is useful if data needs to be stored alongside the generated bind group, such as a unique identifier for a material's bind group. The most common use case for this attribute is "shader pipeline specialization".
The previous `CoolMaterial` example illustrating "combining multiple field-level uniform attributes with the same binding index" can
also be equivalently represented with a single struct-level uniform attribute:
```rust
#[derive(AsBindGroup)]
#[uniform(0, CoolMaterialUniform)]
struct CoolMaterial {
color: Color,
roughness: f32,
}
#[derive(ShaderType)]
struct CoolMaterialUniform {
color: Color,
roughness: f32,
}
impl From<&CoolMaterial> for CoolMaterialUniform {
fn from(material: &CoolMaterial) -> CoolMaterialUniform {
CoolMaterialUniform {
color: material.color,
roughness: material.roughness,
}
}
}
```
### Material Specialization
Material shader specialization is now _much_ simpler:
```rust
#[derive(AsBindGroup, TypeUuid, Debug, Clone)]
#[uuid = "f690fdae-d598-45ab-8225-97e2a3f056e0"]
#[bind_group_data(CoolMaterialKey)]
struct CoolMaterial {
#[uniform(0)]
color: Color,
is_red: bool,
}
#[derive(Copy, Clone, Hash, Eq, PartialEq)]
struct CoolMaterialKey {
is_red: bool,
}
impl From<&CoolMaterial> for CoolMaterialKey {
fn from(material: &CoolMaterial) -> CoolMaterialKey {
CoolMaterialKey {
is_red: material.is_red,
}
}
}
impl Material for CoolMaterial {
fn fragment_shader() -> ShaderRef {
"cool_material.wgsl".into()
}
fn specialize(
pipeline: &MaterialPipeline<Self>,
descriptor: &mut RenderPipelineDescriptor,
layout: &MeshVertexBufferLayout,
key: MaterialPipelineKey<Self>,
) -> Result<(), SpecializedMeshPipelineError> {
if key.bind_group_data.is_red {
let fragment = descriptor.fragment.as_mut().unwrap();
fragment.shader_defs.push("IS_RED".to_string());
}
Ok(())
}
}
```
Setting `bind_group_data` is not required for specialization (it defaults to `()`). Scenarios like "custom vertex attributes" also benefit from this system:
```rust
impl Material for CustomMaterial {
fn vertex_shader() -> ShaderRef {
"custom_material.wgsl".into()
}
fn fragment_shader() -> ShaderRef {
"custom_material.wgsl".into()
}
fn specialize(
pipeline: &MaterialPipeline<Self>,
descriptor: &mut RenderPipelineDescriptor,
layout: &MeshVertexBufferLayout,
key: MaterialPipelineKey<Self>,
) -> Result<(), SpecializedMeshPipelineError> {
let vertex_layout = layout.get_layout(&[
Mesh::ATTRIBUTE_POSITION.at_shader_location(0),
ATTRIBUTE_BLEND_COLOR.at_shader_location(1),
])?;
descriptor.vertex.buffers = vec![vertex_layout];
Ok(())
}
}
```
### Ported `StandardMaterial` to the new `Material` system
Bevy's built-in PBR material uses the new Material system (including the AsBindGroup derive):
```rust
#[derive(AsBindGroup, Debug, Clone, TypeUuid)]
#[uuid = "7494888b-c082-457b-aacf-517228cc0c22"]
#[bind_group_data(StandardMaterialKey)]
#[uniform(0, StandardMaterialUniform)]
pub struct StandardMaterial {
pub base_color: Color,
#[texture(1)]
#[sampler(2)]
pub base_color_texture: Option<Handle<Image>>,
/* other fields omitted for brevity */
```
### Ported Bevy examples to the new `Material` system
The overall complexity of Bevy's "custom shader examples" has gone down significantly. Take a look at the diffs if you want a dopamine spike.
Please note that while this PR has a net increase in "lines of code", most of those extra lines come from added documentation. There is a significant reduction
in the overall complexity of the code (even accounting for the new derive logic).
---
## Changelog
### Added
* `AsBindGroup` trait and derive, which make it much easier to transfer data to the gpu and generate bind groups for a given type.
### Changed
* The old `Material` and `SpecializedMaterial` traits have been replaced by a consolidated (much simpler) `Material` trait. Materials no longer implement `RenderAsset`.
* `StandardMaterial` was ported to the new material system. There are no user-facing api changes to the `StandardMaterial` struct api, but it now implements `AsBindGroup` and `Material` instead of `RenderAsset` and `SpecializedMaterial`.
## Migration Guide
The Material system has been reworked to be much simpler. We've removed a lot of boilerplate with the new `AsBindGroup` derive and the `Material` trait is simpler as well!
### Bevy 0.7 (old)
```rust
#[derive(Debug, Clone, TypeUuid)]
#[uuid = "f690fdae-d598-45ab-8225-97e2a3f056e0"]
pub struct CustomMaterial {
color: Color,
color_texture: Handle<Image>,
}
#[derive(Clone)]
pub struct GpuCustomMaterial {
_buffer: Buffer,
bind_group: BindGroup,
}
impl RenderAsset for CustomMaterial {
type ExtractedAsset = CustomMaterial;
type PreparedAsset = GpuCustomMaterial;
type Param = (SRes<RenderDevice>, SRes<MaterialPipeline<Self>>);
fn extract_asset(&self) -> Self::ExtractedAsset {
self.clone()
}
fn prepare_asset(
extracted_asset: Self::ExtractedAsset,
(render_device, material_pipeline): &mut SystemParamItem<Self::Param>,
) -> Result<Self::PreparedAsset, PrepareAssetError<Self::ExtractedAsset>> {
let color = Vec4::from_slice(&extracted_asset.color.as_linear_rgba_f32());
let byte_buffer = [0u8; Vec4::SIZE.get() as usize];
let mut buffer = encase::UniformBuffer::new(byte_buffer);
buffer.write(&color).unwrap();
let buffer = render_device.create_buffer_with_data(&BufferInitDescriptor {
contents: buffer.as_ref(),
label: None,
usage: BufferUsages::UNIFORM | BufferUsages::COPY_DST,
});
let (texture_view, texture_sampler) = if let Some(result) = material_pipeline
.mesh_pipeline
.get_image_texture(gpu_images, &Some(extracted_asset.color_texture.clone()))
{
result
} else {
return Err(PrepareAssetError::RetryNextUpdate(extracted_asset));
};
let bind_group = render_device.create_bind_group(&BindGroupDescriptor {
entries: &[
BindGroupEntry {
binding: 0,
resource: buffer.as_entire_binding(),
},
BindGroupEntry {
binding: 0,
resource: BindingResource::TextureView(texture_view),
},
BindGroupEntry {
binding: 1,
resource: BindingResource::Sampler(texture_sampler),
},
],
label: None,
layout: &material_pipeline.material_layout,
});
Ok(GpuCustomMaterial {
_buffer: buffer,
bind_group,
})
}
}
impl Material for CustomMaterial {
fn fragment_shader(asset_server: &AssetServer) -> Option<Handle<Shader>> {
Some(asset_server.load("custom_material.wgsl"))
}
fn bind_group(render_asset: &<Self as RenderAsset>::PreparedAsset) -> &BindGroup {
&render_asset.bind_group
}
fn bind_group_layout(render_device: &RenderDevice) -> BindGroupLayout {
render_device.create_bind_group_layout(&BindGroupLayoutDescriptor {
entries: &[
BindGroupLayoutEntry {
binding: 0,
visibility: ShaderStages::FRAGMENT,
ty: BindingType::Buffer {
ty: BufferBindingType::Uniform,
has_dynamic_offset: false,
min_binding_size: Some(Vec4::min_size()),
},
count: None,
},
BindGroupLayoutEntry {
binding: 1,
visibility: ShaderStages::FRAGMENT,
ty: BindingType::Texture {
multisampled: false,
sample_type: TextureSampleType::Float { filterable: true },
view_dimension: TextureViewDimension::D2Array,
},
count: None,
},
BindGroupLayoutEntry {
binding: 2,
visibility: ShaderStages::FRAGMENT,
ty: BindingType::Sampler(SamplerBindingType::Filtering),
count: None,
},
],
label: None,
})
}
}
```
### Bevy 0.8 (new)
```rust
impl Material for CustomMaterial {
fn fragment_shader() -> ShaderRef {
"custom_material.wgsl".into()
}
}
#[derive(AsBindGroup, TypeUuid, Debug, Clone)]
#[uuid = "f690fdae-d598-45ab-8225-97e2a3f056e0"]
pub struct CustomMaterial {
#[uniform(0)]
color: Color,
#[texture(1)]
#[sampler(2)]
color_texture: Handle<Image>,
}
```
## Future Work
* Add support for more binding types (cubemaps, buffers, etc). This PR intentionally includes a bare minimum number of binding types to keep "reviewability" in check.
* Consider optionally eliding binding indices using binding names. `AsBindGroup` could pass in (optional?) reflection info as a "hint".
* This would make it possible for the derive to do this:
```rust
#[derive(AsBindGroup)]
pub struct CustomMaterial {
#[uniform]
color: Color,
#[texture]
#[sampler]
color_texture: Option<Handle<Image>>,
alpha_mode: AlphaMode,
}
```
* Or this
```rust
#[derive(AsBindGroup)]
pub struct CustomMaterial {
#[binding]
color: Color,
#[binding]
color_texture: Option<Handle<Image>>,
alpha_mode: AlphaMode,
}
```
* Or even this (if we flip to "include bindings by default")
```rust
#[derive(AsBindGroup)]
pub struct CustomMaterial {
color: Color,
color_texture: Option<Handle<Image>>,
#[binding(ignore)]
alpha_mode: AlphaMode,
}
```
* If we add the option to define custom draw functions for materials (which could be done in a type-erased way), I think that would be enough to support extra non-material bindings. Worth considering!
2022-06-30 23:48:46 +00:00
|
|
|
pub bind_group: BindGroup,
|
|
|
|
pub key: T::Data,
|
|
|
|
pub properties: MaterialProperties,
|
|
|
|
}
|
|
|
|
|
2024-04-09 13:26:34 +00:00
|
|
|
impl<M: Material> RenderAsset for PreparedMaterial<M> {
|
|
|
|
type SourceAsset = M;
|
|
|
|
|
|
|
|
type Param = (
|
|
|
|
SRes<RenderDevice>,
|
|
|
|
SRes<MaterialPipeline<M>>,
|
|
|
|
SRes<DefaultOpaqueRendererMethod>,
|
2024-08-25 20:16:34 +00:00
|
|
|
M::Param,
|
2024-04-09 13:26:34 +00:00
|
|
|
);
|
|
|
|
|
|
|
|
fn prepare_asset(
|
|
|
|
material: Self::SourceAsset,
|
2024-08-25 20:16:34 +00:00
|
|
|
(render_device, pipeline, default_opaque_render_method, ref mut material_param): &mut SystemParamItem<Self::Param>,
|
2024-04-09 13:26:34 +00:00
|
|
|
) -> Result<Self, PrepareAssetError<Self::SourceAsset>> {
|
2024-08-25 20:16:34 +00:00
|
|
|
match material.as_bind_group(&pipeline.material_layout, render_device, material_param) {
|
2024-04-09 13:26:34 +00:00
|
|
|
Ok(prepared) => {
|
|
|
|
let method = match material.opaque_render_method() {
|
|
|
|
OpaqueRendererMethod::Forward => OpaqueRendererMethod::Forward,
|
|
|
|
OpaqueRendererMethod::Deferred => OpaqueRendererMethod::Deferred,
|
|
|
|
OpaqueRendererMethod::Auto => default_opaque_render_method.0,
|
|
|
|
};
|
|
|
|
let mut mesh_pipeline_key_bits = MeshPipelineKey::empty();
|
|
|
|
mesh_pipeline_key_bits.set(
|
|
|
|
MeshPipelineKey::READS_VIEW_TRANSMISSION_TEXTURE,
|
|
|
|
material.reads_view_transmission_texture(),
|
|
|
|
);
|
|
|
|
|
|
|
|
Ok(PreparedMaterial {
|
|
|
|
bindings: prepared.bindings,
|
|
|
|
bind_group: prepared.bind_group,
|
|
|
|
key: prepared.data,
|
|
|
|
properties: MaterialProperties {
|
|
|
|
alpha_mode: material.alpha_mode(),
|
|
|
|
depth_bias: material.depth_bias(),
|
|
|
|
reads_view_transmission_texture: mesh_pipeline_key_bits
|
|
|
|
.contains(MeshPipelineKey::READS_VIEW_TRANSMISSION_TEXTURE),
|
|
|
|
render_method: method,
|
|
|
|
mesh_pipeline_key_bits,
|
|
|
|
},
|
|
|
|
})
|
|
|
|
}
|
|
|
|
Err(AsBindGroupError::RetryNextUpdate) => {
|
|
|
|
Err(PrepareAssetError::RetryNextUpdate(material))
|
|
|
|
}
|
2024-08-21 01:41:31 +00:00
|
|
|
Err(other) => Err(PrepareAssetError::AsBindGroupError(other)),
|
2024-04-09 13:26:34 +00:00
|
|
|
}
|
|
|
|
}
|
|
|
|
}
|
|
|
|
|
Automatic batching/instancing of draw commands (#9685)
# Objective
- Implement the foundations of automatic batching/instancing of draw
commands as the next step from #89
- NOTE: More performance improvements will come when more data is
managed and bound in ways that do not require rebinding such as mesh,
material, and texture data.
## Solution
- The core idea for batching of draw commands is to check whether any of
the information that has to be passed when encoding a draw command
changes between two things that are being drawn according to the sorted
render phase order. These should be things like the pipeline, bind
groups and their dynamic offsets, index/vertex buffers, and so on.
- The following assumptions have been made:
- Only entities with prepared assets (pipelines, materials, meshes) are
queued to phases
- View bindings are constant across a phase for a given draw function as
phases are per-view
- `batch_and_prepare_render_phase` is the only system that performs this
batching and has sole responsibility for preparing the per-object data.
As such the mesh binding and dynamic offsets are assumed to only vary as
a result of the `batch_and_prepare_render_phase` system, e.g. due to
having to split data across separate uniform bindings within the same
buffer due to the maximum uniform buffer binding size.
- Implement `GpuArrayBuffer` for `Mesh2dUniform` to store Mesh2dUniform
in arrays in GPU buffers rather than each one being at a dynamic offset
in a uniform buffer. This is the same optimisation that was made for 3D
not long ago.
- Change batch size for a range in `PhaseItem`, adding API for getting
or mutating the range. This is more flexible than a size as the length
of the range can be used in place of the size, but the start and end can
be otherwise whatever is needed.
- Add an optional mesh bind group dynamic offset to `PhaseItem`. This
avoids having to do a massive table move just to insert
`GpuArrayBufferIndex` components.
## Benchmarks
All tests have been run on an M1 Max on AC power. `bevymark` and
`many_cubes` were modified to use 1920x1080 with a scale factor of 1. I
run a script that runs a separate Tracy capture process, and then runs
the bevy example with `--features bevy_ci_testing,trace_tracy` and
`CI_TESTING_CONFIG=../benchmark.ron` with the contents of
`../benchmark.ron`:
```rust
(
exit_after: Some(1500)
)
```
...in order to run each test for 1500 frames.
The recent changes to `many_cubes` and `bevymark` added reproducible
random number generation so that with the same settings, the same rng
will occur. They also added benchmark modes that use a fixed delta time
for animations. Combined this means that the same frames should be
rendered both on main and on the branch.
The graphs compare main (yellow) to this PR (red).
### 3D Mesh `many_cubes --benchmark`
<img width="1411" alt="Screenshot 2023-09-03 at 23 42 10"
src="https://github.com/bevyengine/bevy/assets/302146/2088716a-c918-486c-8129-090b26fd2bc4">
The mesh and material are the same for all instances. This is basically
the best case for the initial batching implementation as it results in 1
draw for the ~11.7k visible meshes. It gives a ~30% reduction in median
frame time.
The 1000th frame is identical using the flip tool:

```
Mean: 0.000000
Weighted median: 0.000000
1st weighted quartile: 0.000000
3rd weighted quartile: 0.000000
Min: 0.000000
Max: 0.000000
Evaluation time: 0.4615 seconds
```
### 3D Mesh `many_cubes --benchmark --material-texture-count 10`
<img width="1404" alt="Screenshot 2023-09-03 at 23 45 18"
src="https://github.com/bevyengine/bevy/assets/302146/5ee9c447-5bd2-45c6-9706-ac5ff8916daf">
This run uses 10 different materials by varying their textures. The
materials are randomly selected, and there is no sorting by material
bind group for opaque 3D so any batching is 'random'. The PR produces a
~5% reduction in median frame time. If we were to sort the opaque phase
by the material bind group, then this should be a lot faster. This
produces about 10.5k draws for the 11.7k visible entities. This makes
sense as randomly selecting from 10 materials gives a chance that two
adjacent entities randomly select the same material and can be batched.
The 1000th frame is identical in flip:
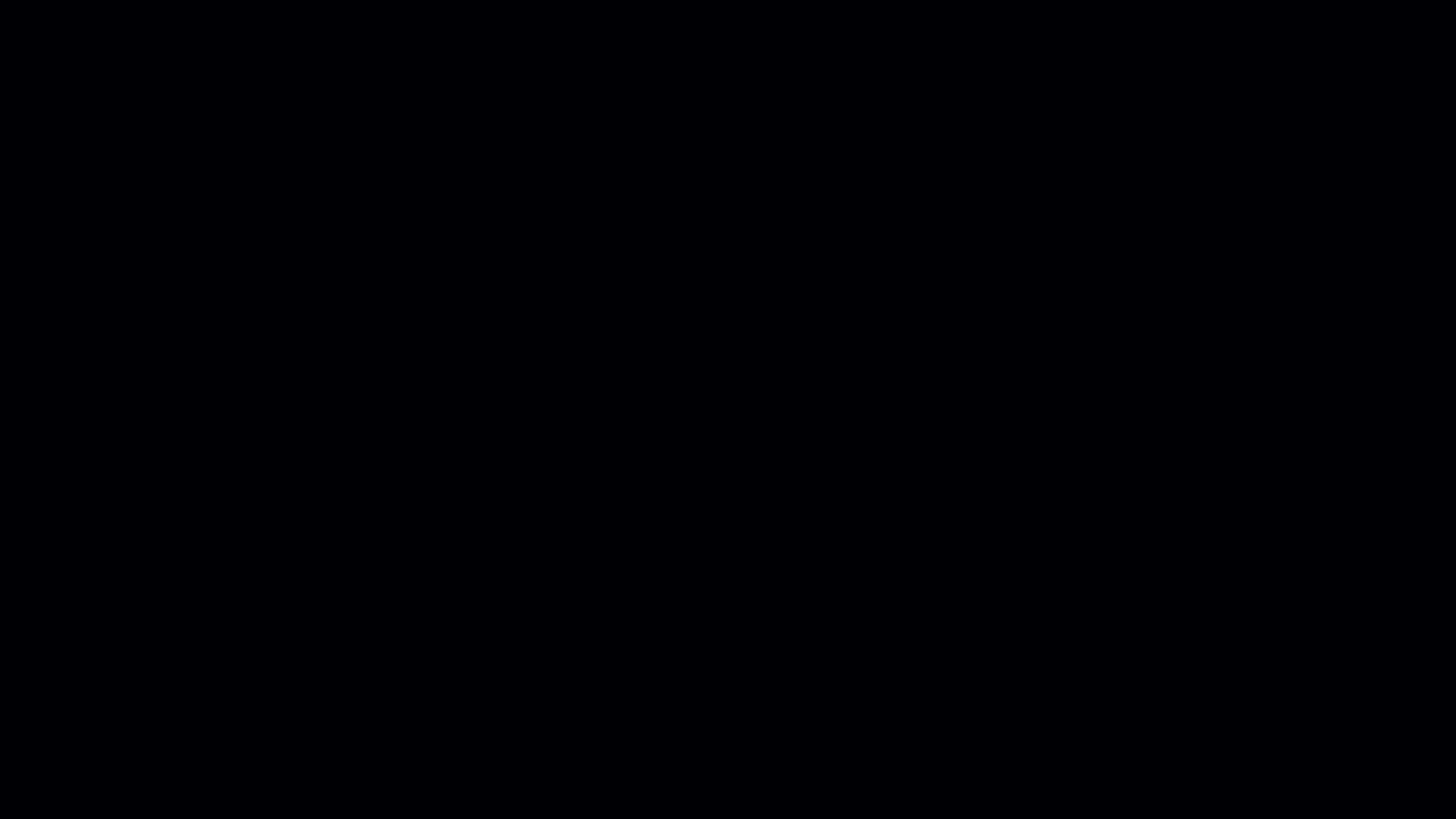
```
Mean: 0.000000
Weighted median: 0.000000
1st weighted quartile: 0.000000
3rd weighted quartile: 0.000000
Min: 0.000000
Max: 0.000000
Evaluation time: 0.4537 seconds
```
### 3D Mesh `many_cubes --benchmark --vary-per-instance`
<img width="1394" alt="Screenshot 2023-09-03 at 23 48 44"
src="https://github.com/bevyengine/bevy/assets/302146/f02a816b-a444-4c18-a96a-63b5436f3b7f">
This run varies the material data per instance by randomly-generating
its colour. This is the worst case for batching and that it performs
about the same as `main` is a good thing as it demonstrates that the
batching has minimal overhead when dealing with ~11k visible mesh
entities.
The 1000th frame is identical according to flip:
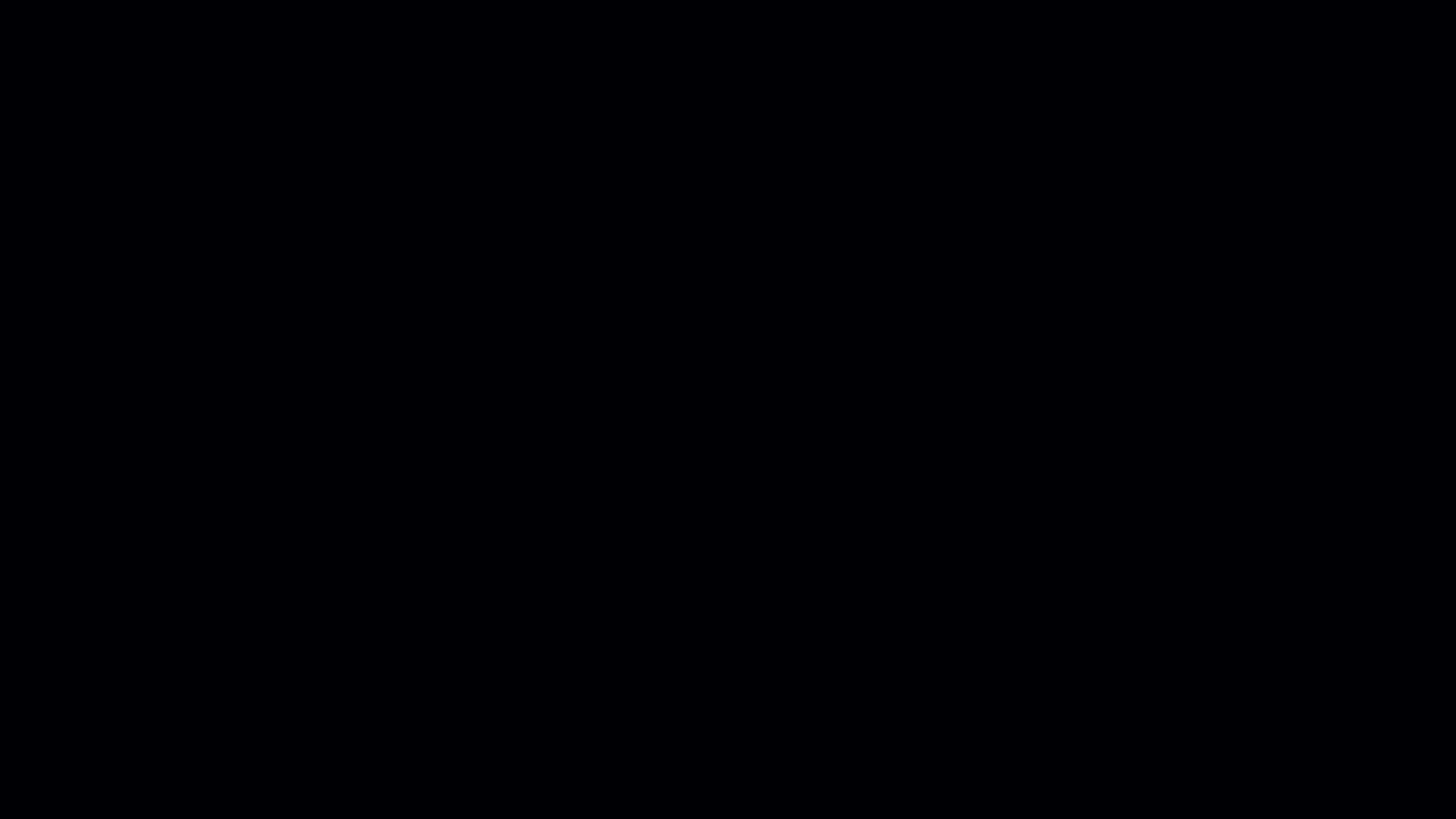
```
Mean: 0.000000
Weighted median: 0.000000
1st weighted quartile: 0.000000
3rd weighted quartile: 0.000000
Min: 0.000000
Max: 0.000000
Evaluation time: 0.4568 seconds
```
### 2D Mesh `bevymark --benchmark --waves 160 --per-wave 1000 --mode
mesh2d`
<img width="1412" alt="Screenshot 2023-09-03 at 23 59 56"
src="https://github.com/bevyengine/bevy/assets/302146/cb02ae07-237b-4646-ae9f-fda4dafcbad4">
This spawns 160 waves of 1000 quad meshes that are shaded with
ColorMaterial. Each wave has a different material so 160 waves currently
should result in 160 batches. This results in a 50% reduction in median
frame time.
Capturing a screenshot of the 1000th frame main vs PR gives:

```
Mean: 0.001222
Weighted median: 0.750432
1st weighted quartile: 0.453494
3rd weighted quartile: 0.969758
Min: 0.000000
Max: 0.990296
Evaluation time: 0.4255 seconds
```
So they seem to produce the same results. I also double-checked the
number of draws. `main` does 160000 draws, and the PR does 160, as
expected.
### 2D Mesh `bevymark --benchmark --waves 160 --per-wave 1000 --mode
mesh2d --material-texture-count 10`
<img width="1392" alt="Screenshot 2023-09-04 at 00 09 22"
src="https://github.com/bevyengine/bevy/assets/302146/4358da2e-ce32-4134-82df-3ab74c40849c">
This generates 10 textures and generates materials for each of those and
then selects one material per wave. The median frame time is reduced by
50%. Similar to the plain run above, this produces 160 draws on the PR
and 160000 on `main` and the 1000th frame is identical (ignoring the fps
counter text overlay).

```
Mean: 0.002877
Weighted median: 0.964980
1st weighted quartile: 0.668871
3rd weighted quartile: 0.982749
Min: 0.000000
Max: 0.992377
Evaluation time: 0.4301 seconds
```
### 2D Mesh `bevymark --benchmark --waves 160 --per-wave 1000 --mode
mesh2d --vary-per-instance`
<img width="1396" alt="Screenshot 2023-09-04 at 00 13 53"
src="https://github.com/bevyengine/bevy/assets/302146/b2198b18-3439-47ad-919a-cdabe190facb">
This creates unique materials per instance by randomly-generating the
material's colour. This is the worst case for 2D batching. Somehow, this
PR manages a 7% reduction in median frame time. Both main and this PR
issue 160000 draws.
The 1000th frame is the same:

```
Mean: 0.001214
Weighted median: 0.937499
1st weighted quartile: 0.635467
3rd weighted quartile: 0.979085
Min: 0.000000
Max: 0.988971
Evaluation time: 0.4462 seconds
```
### 2D Sprite `bevymark --benchmark --waves 160 --per-wave 1000 --mode
sprite`
<img width="1396" alt="Screenshot 2023-09-04 at 12 21 12"
src="https://github.com/bevyengine/bevy/assets/302146/8b31e915-d6be-4cac-abf5-c6a4da9c3d43">
This just spawns 160 waves of 1000 sprites. There should be and is no
notable difference between main and the PR.
### 2D Sprite `bevymark --benchmark --waves 160 --per-wave 1000 --mode
sprite --material-texture-count 10`
<img width="1389" alt="Screenshot 2023-09-04 at 12 36 08"
src="https://github.com/bevyengine/bevy/assets/302146/45fe8d6d-c901-4062-a349-3693dd044413">
This spawns the sprites selecting a texture at random per instance from
the 10 generated textures. This has no significant change vs main and
shouldn't.
### 2D Sprite `bevymark --benchmark --waves 160 --per-wave 1000 --mode
sprite --vary-per-instance`
<img width="1401" alt="Screenshot 2023-09-04 at 12 29 52"
src="https://github.com/bevyengine/bevy/assets/302146/762c5c60-352e-471f-8dbe-bbf10e24ebd6">
This sets the sprite colour as being unique per instance. This can still
all be drawn using one batch. There should be no difference but the PR
produces median frame times that are 4% higher. Investigation showed no
clear sources of cost, rather a mix of give and take that should not
happen. It seems like noise in the results.
### Summary
| Benchmark | % change in median frame time |
| ------------- | ------------- |
| many_cubes | 🟩 -30% |
| many_cubes 10 materials | 🟩 -5% |
| many_cubes unique materials | 🟩 ~0% |
| bevymark mesh2d | 🟩 -50% |
| bevymark mesh2d 10 materials | 🟩 -50% |
| bevymark mesh2d unique materials | 🟩 -7% |
| bevymark sprite | 🟥 2% |
| bevymark sprite 10 materials | 🟥 0.6% |
| bevymark sprite unique materials | 🟥 4.1% |
---
## Changelog
- Added: 2D and 3D mesh entities that share the same mesh and material
(same textures, same data) are now batched into the same draw command
for better performance.
---------
Co-authored-by: robtfm <50659922+robtfm@users.noreply.github.com>
Co-authored-by: Nicola Papale <nico@nicopap.ch>
2023-09-21 22:12:34 +00:00
|
|
|
#[derive(Component, Clone, Copy, Default, PartialEq, Eq, Deref, DerefMut)]
|
2024-02-23 06:13:37 +00:00
|
|
|
pub struct MaterialBindGroupId(pub Option<BindGroupId>);
|
|
|
|
|
|
|
|
impl MaterialBindGroupId {
|
|
|
|
pub fn new(id: BindGroupId) -> Self {
|
|
|
|
Self(Some(id))
|
|
|
|
}
|
|
|
|
}
|
|
|
|
|
|
|
|
impl From<BindGroup> for MaterialBindGroupId {
|
|
|
|
fn from(value: BindGroup) -> Self {
|
|
|
|
Self::new(value.id())
|
|
|
|
}
|
|
|
|
}
|
Automatic batching/instancing of draw commands (#9685)
# Objective
- Implement the foundations of automatic batching/instancing of draw
commands as the next step from #89
- NOTE: More performance improvements will come when more data is
managed and bound in ways that do not require rebinding such as mesh,
material, and texture data.
## Solution
- The core idea for batching of draw commands is to check whether any of
the information that has to be passed when encoding a draw command
changes between two things that are being drawn according to the sorted
render phase order. These should be things like the pipeline, bind
groups and their dynamic offsets, index/vertex buffers, and so on.
- The following assumptions have been made:
- Only entities with prepared assets (pipelines, materials, meshes) are
queued to phases
- View bindings are constant across a phase for a given draw function as
phases are per-view
- `batch_and_prepare_render_phase` is the only system that performs this
batching and has sole responsibility for preparing the per-object data.
As such the mesh binding and dynamic offsets are assumed to only vary as
a result of the `batch_and_prepare_render_phase` system, e.g. due to
having to split data across separate uniform bindings within the same
buffer due to the maximum uniform buffer binding size.
- Implement `GpuArrayBuffer` for `Mesh2dUniform` to store Mesh2dUniform
in arrays in GPU buffers rather than each one being at a dynamic offset
in a uniform buffer. This is the same optimisation that was made for 3D
not long ago.
- Change batch size for a range in `PhaseItem`, adding API for getting
or mutating the range. This is more flexible than a size as the length
of the range can be used in place of the size, but the start and end can
be otherwise whatever is needed.
- Add an optional mesh bind group dynamic offset to `PhaseItem`. This
avoids having to do a massive table move just to insert
`GpuArrayBufferIndex` components.
## Benchmarks
All tests have been run on an M1 Max on AC power. `bevymark` and
`many_cubes` were modified to use 1920x1080 with a scale factor of 1. I
run a script that runs a separate Tracy capture process, and then runs
the bevy example with `--features bevy_ci_testing,trace_tracy` and
`CI_TESTING_CONFIG=../benchmark.ron` with the contents of
`../benchmark.ron`:
```rust
(
exit_after: Some(1500)
)
```
...in order to run each test for 1500 frames.
The recent changes to `many_cubes` and `bevymark` added reproducible
random number generation so that with the same settings, the same rng
will occur. They also added benchmark modes that use a fixed delta time
for animations. Combined this means that the same frames should be
rendered both on main and on the branch.
The graphs compare main (yellow) to this PR (red).
### 3D Mesh `many_cubes --benchmark`
<img width="1411" alt="Screenshot 2023-09-03 at 23 42 10"
src="https://github.com/bevyengine/bevy/assets/302146/2088716a-c918-486c-8129-090b26fd2bc4">
The mesh and material are the same for all instances. This is basically
the best case for the initial batching implementation as it results in 1
draw for the ~11.7k visible meshes. It gives a ~30% reduction in median
frame time.
The 1000th frame is identical using the flip tool:

```
Mean: 0.000000
Weighted median: 0.000000
1st weighted quartile: 0.000000
3rd weighted quartile: 0.000000
Min: 0.000000
Max: 0.000000
Evaluation time: 0.4615 seconds
```
### 3D Mesh `many_cubes --benchmark --material-texture-count 10`
<img width="1404" alt="Screenshot 2023-09-03 at 23 45 18"
src="https://github.com/bevyengine/bevy/assets/302146/5ee9c447-5bd2-45c6-9706-ac5ff8916daf">
This run uses 10 different materials by varying their textures. The
materials are randomly selected, and there is no sorting by material
bind group for opaque 3D so any batching is 'random'. The PR produces a
~5% reduction in median frame time. If we were to sort the opaque phase
by the material bind group, then this should be a lot faster. This
produces about 10.5k draws for the 11.7k visible entities. This makes
sense as randomly selecting from 10 materials gives a chance that two
adjacent entities randomly select the same material and can be batched.
The 1000th frame is identical in flip:
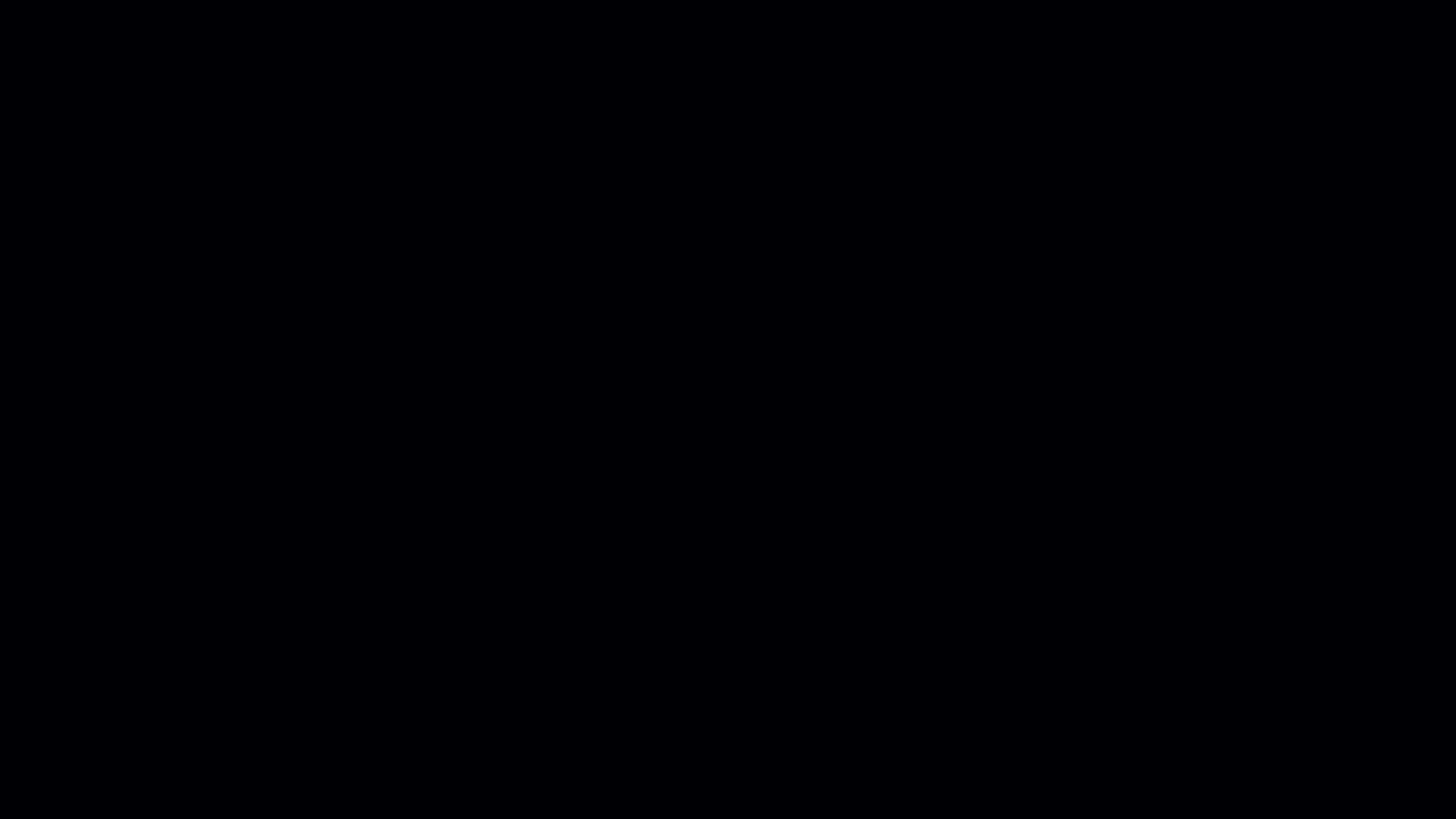
```
Mean: 0.000000
Weighted median: 0.000000
1st weighted quartile: 0.000000
3rd weighted quartile: 0.000000
Min: 0.000000
Max: 0.000000
Evaluation time: 0.4537 seconds
```
### 3D Mesh `many_cubes --benchmark --vary-per-instance`
<img width="1394" alt="Screenshot 2023-09-03 at 23 48 44"
src="https://github.com/bevyengine/bevy/assets/302146/f02a816b-a444-4c18-a96a-63b5436f3b7f">
This run varies the material data per instance by randomly-generating
its colour. This is the worst case for batching and that it performs
about the same as `main` is a good thing as it demonstrates that the
batching has minimal overhead when dealing with ~11k visible mesh
entities.
The 1000th frame is identical according to flip:
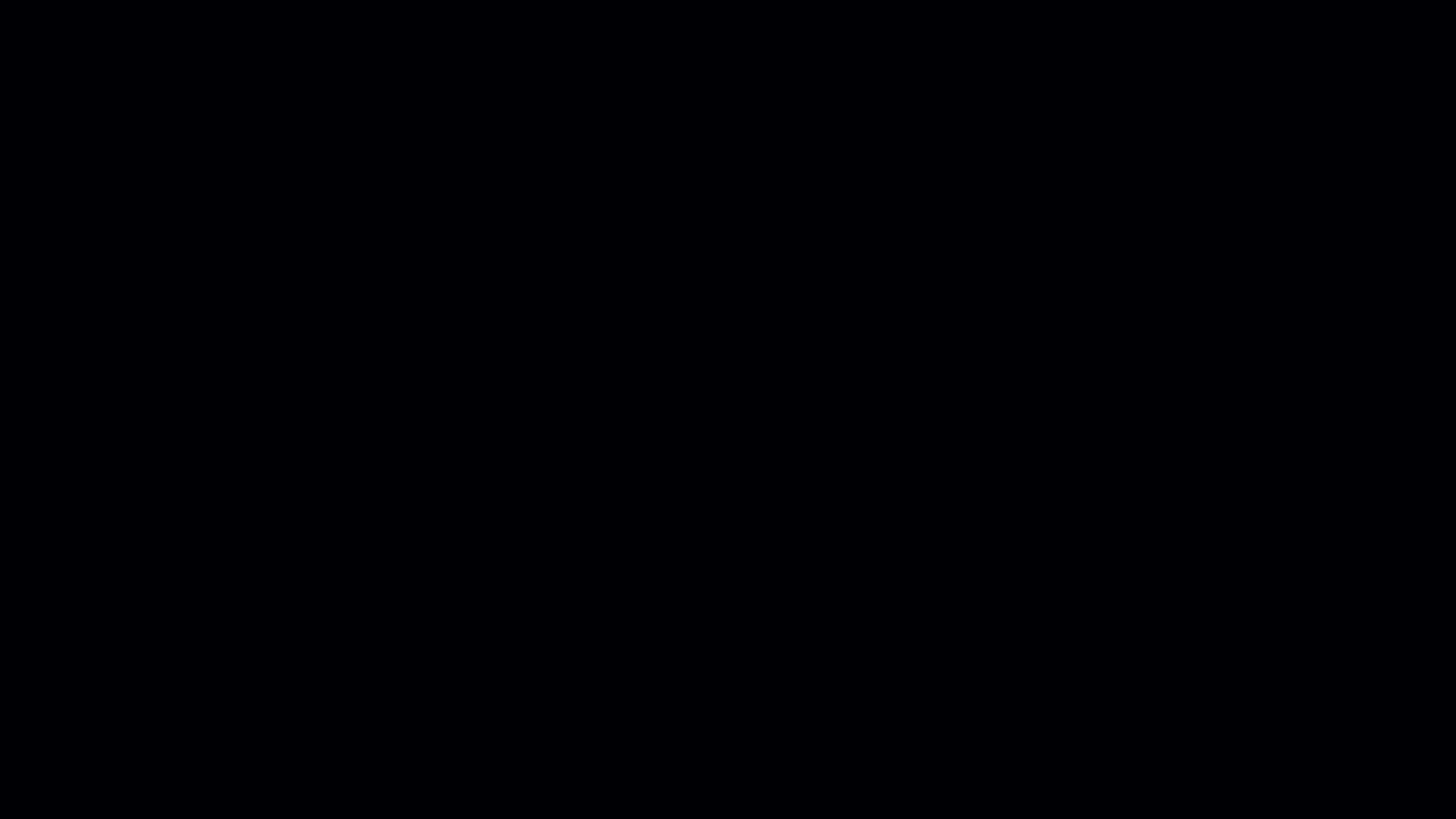
```
Mean: 0.000000
Weighted median: 0.000000
1st weighted quartile: 0.000000
3rd weighted quartile: 0.000000
Min: 0.000000
Max: 0.000000
Evaluation time: 0.4568 seconds
```
### 2D Mesh `bevymark --benchmark --waves 160 --per-wave 1000 --mode
mesh2d`
<img width="1412" alt="Screenshot 2023-09-03 at 23 59 56"
src="https://github.com/bevyengine/bevy/assets/302146/cb02ae07-237b-4646-ae9f-fda4dafcbad4">
This spawns 160 waves of 1000 quad meshes that are shaded with
ColorMaterial. Each wave has a different material so 160 waves currently
should result in 160 batches. This results in a 50% reduction in median
frame time.
Capturing a screenshot of the 1000th frame main vs PR gives:

```
Mean: 0.001222
Weighted median: 0.750432
1st weighted quartile: 0.453494
3rd weighted quartile: 0.969758
Min: 0.000000
Max: 0.990296
Evaluation time: 0.4255 seconds
```
So they seem to produce the same results. I also double-checked the
number of draws. `main` does 160000 draws, and the PR does 160, as
expected.
### 2D Mesh `bevymark --benchmark --waves 160 --per-wave 1000 --mode
mesh2d --material-texture-count 10`
<img width="1392" alt="Screenshot 2023-09-04 at 00 09 22"
src="https://github.com/bevyengine/bevy/assets/302146/4358da2e-ce32-4134-82df-3ab74c40849c">
This generates 10 textures and generates materials for each of those and
then selects one material per wave. The median frame time is reduced by
50%. Similar to the plain run above, this produces 160 draws on the PR
and 160000 on `main` and the 1000th frame is identical (ignoring the fps
counter text overlay).

```
Mean: 0.002877
Weighted median: 0.964980
1st weighted quartile: 0.668871
3rd weighted quartile: 0.982749
Min: 0.000000
Max: 0.992377
Evaluation time: 0.4301 seconds
```
### 2D Mesh `bevymark --benchmark --waves 160 --per-wave 1000 --mode
mesh2d --vary-per-instance`
<img width="1396" alt="Screenshot 2023-09-04 at 00 13 53"
src="https://github.com/bevyengine/bevy/assets/302146/b2198b18-3439-47ad-919a-cdabe190facb">
This creates unique materials per instance by randomly-generating the
material's colour. This is the worst case for 2D batching. Somehow, this
PR manages a 7% reduction in median frame time. Both main and this PR
issue 160000 draws.
The 1000th frame is the same:

```
Mean: 0.001214
Weighted median: 0.937499
1st weighted quartile: 0.635467
3rd weighted quartile: 0.979085
Min: 0.000000
Max: 0.988971
Evaluation time: 0.4462 seconds
```
### 2D Sprite `bevymark --benchmark --waves 160 --per-wave 1000 --mode
sprite`
<img width="1396" alt="Screenshot 2023-09-04 at 12 21 12"
src="https://github.com/bevyengine/bevy/assets/302146/8b31e915-d6be-4cac-abf5-c6a4da9c3d43">
This just spawns 160 waves of 1000 sprites. There should be and is no
notable difference between main and the PR.
### 2D Sprite `bevymark --benchmark --waves 160 --per-wave 1000 --mode
sprite --material-texture-count 10`
<img width="1389" alt="Screenshot 2023-09-04 at 12 36 08"
src="https://github.com/bevyengine/bevy/assets/302146/45fe8d6d-c901-4062-a349-3693dd044413">
This spawns the sprites selecting a texture at random per instance from
the 10 generated textures. This has no significant change vs main and
shouldn't.
### 2D Sprite `bevymark --benchmark --waves 160 --per-wave 1000 --mode
sprite --vary-per-instance`
<img width="1401" alt="Screenshot 2023-09-04 at 12 29 52"
src="https://github.com/bevyengine/bevy/assets/302146/762c5c60-352e-471f-8dbe-bbf10e24ebd6">
This sets the sprite colour as being unique per instance. This can still
all be drawn using one batch. There should be no difference but the PR
produces median frame times that are 4% higher. Investigation showed no
clear sources of cost, rather a mix of give and take that should not
happen. It seems like noise in the results.
### Summary
| Benchmark | % change in median frame time |
| ------------- | ------------- |
| many_cubes | 🟩 -30% |
| many_cubes 10 materials | 🟩 -5% |
| many_cubes unique materials | 🟩 ~0% |
| bevymark mesh2d | 🟩 -50% |
| bevymark mesh2d 10 materials | 🟩 -50% |
| bevymark mesh2d unique materials | 🟩 -7% |
| bevymark sprite | 🟥 2% |
| bevymark sprite 10 materials | 🟥 0.6% |
| bevymark sprite unique materials | 🟥 4.1% |
---
## Changelog
- Added: 2D and 3D mesh entities that share the same mesh and material
(same textures, same data) are now batched into the same draw command
for better performance.
---------
Co-authored-by: robtfm <50659922+robtfm@users.noreply.github.com>
Co-authored-by: Nicola Papale <nico@nicopap.ch>
2023-09-21 22:12:34 +00:00
|
|
|
|
2024-02-20 00:12:41 +00:00
|
|
|
/// An atomic version of [`MaterialBindGroupId`] that can be read from and written to
|
|
|
|
/// safely from multiple threads.
|
|
|
|
#[derive(Default)]
|
|
|
|
pub struct AtomicMaterialBindGroupId(AtomicU32);
|
|
|
|
|
|
|
|
impl AtomicMaterialBindGroupId {
|
2024-02-22 18:55:22 +00:00
|
|
|
/// Stores a value atomically. Uses [`Ordering::Relaxed`] so there is zero guarantee of ordering
|
2024-02-20 00:12:41 +00:00
|
|
|
/// relative to other operations.
|
|
|
|
///
|
|
|
|
/// See also: [`AtomicU32::store`].
|
|
|
|
pub fn set(&self, id: MaterialBindGroupId) {
|
|
|
|
let id = if let Some(id) = id.0 {
|
2024-08-30 02:37:47 +00:00
|
|
|
NonZero::<u32>::from(id).get()
|
2024-02-20 00:12:41 +00:00
|
|
|
} else {
|
|
|
|
0
|
|
|
|
};
|
|
|
|
self.0.store(id, Ordering::Relaxed);
|
|
|
|
}
|
|
|
|
|
2024-02-22 18:55:22 +00:00
|
|
|
/// Loads a value atomically. Uses [`Ordering::Relaxed`] so there is zero guarantee of ordering
|
2024-02-20 00:12:41 +00:00
|
|
|
/// relative to other operations.
|
|
|
|
///
|
|
|
|
/// See also: [`AtomicU32::load`].
|
|
|
|
pub fn get(&self) -> MaterialBindGroupId {
|
2024-08-30 02:37:47 +00:00
|
|
|
MaterialBindGroupId(
|
|
|
|
NonZero::<u32>::new(self.0.load(Ordering::Relaxed)).map(BindGroupId::from),
|
|
|
|
)
|
2024-02-20 00:12:41 +00:00
|
|
|
}
|
|
|
|
}
|
|
|
|
|
Automatic batching/instancing of draw commands (#9685)
# Objective
- Implement the foundations of automatic batching/instancing of draw
commands as the next step from #89
- NOTE: More performance improvements will come when more data is
managed and bound in ways that do not require rebinding such as mesh,
material, and texture data.
## Solution
- The core idea for batching of draw commands is to check whether any of
the information that has to be passed when encoding a draw command
changes between two things that are being drawn according to the sorted
render phase order. These should be things like the pipeline, bind
groups and their dynamic offsets, index/vertex buffers, and so on.
- The following assumptions have been made:
- Only entities with prepared assets (pipelines, materials, meshes) are
queued to phases
- View bindings are constant across a phase for a given draw function as
phases are per-view
- `batch_and_prepare_render_phase` is the only system that performs this
batching and has sole responsibility for preparing the per-object data.
As such the mesh binding and dynamic offsets are assumed to only vary as
a result of the `batch_and_prepare_render_phase` system, e.g. due to
having to split data across separate uniform bindings within the same
buffer due to the maximum uniform buffer binding size.
- Implement `GpuArrayBuffer` for `Mesh2dUniform` to store Mesh2dUniform
in arrays in GPU buffers rather than each one being at a dynamic offset
in a uniform buffer. This is the same optimisation that was made for 3D
not long ago.
- Change batch size for a range in `PhaseItem`, adding API for getting
or mutating the range. This is more flexible than a size as the length
of the range can be used in place of the size, but the start and end can
be otherwise whatever is needed.
- Add an optional mesh bind group dynamic offset to `PhaseItem`. This
avoids having to do a massive table move just to insert
`GpuArrayBufferIndex` components.
## Benchmarks
All tests have been run on an M1 Max on AC power. `bevymark` and
`many_cubes` were modified to use 1920x1080 with a scale factor of 1. I
run a script that runs a separate Tracy capture process, and then runs
the bevy example with `--features bevy_ci_testing,trace_tracy` and
`CI_TESTING_CONFIG=../benchmark.ron` with the contents of
`../benchmark.ron`:
```rust
(
exit_after: Some(1500)
)
```
...in order to run each test for 1500 frames.
The recent changes to `many_cubes` and `bevymark` added reproducible
random number generation so that with the same settings, the same rng
will occur. They also added benchmark modes that use a fixed delta time
for animations. Combined this means that the same frames should be
rendered both on main and on the branch.
The graphs compare main (yellow) to this PR (red).
### 3D Mesh `many_cubes --benchmark`
<img width="1411" alt="Screenshot 2023-09-03 at 23 42 10"
src="https://github.com/bevyengine/bevy/assets/302146/2088716a-c918-486c-8129-090b26fd2bc4">
The mesh and material are the same for all instances. This is basically
the best case for the initial batching implementation as it results in 1
draw for the ~11.7k visible meshes. It gives a ~30% reduction in median
frame time.
The 1000th frame is identical using the flip tool:

```
Mean: 0.000000
Weighted median: 0.000000
1st weighted quartile: 0.000000
3rd weighted quartile: 0.000000
Min: 0.000000
Max: 0.000000
Evaluation time: 0.4615 seconds
```
### 3D Mesh `many_cubes --benchmark --material-texture-count 10`
<img width="1404" alt="Screenshot 2023-09-03 at 23 45 18"
src="https://github.com/bevyengine/bevy/assets/302146/5ee9c447-5bd2-45c6-9706-ac5ff8916daf">
This run uses 10 different materials by varying their textures. The
materials are randomly selected, and there is no sorting by material
bind group for opaque 3D so any batching is 'random'. The PR produces a
~5% reduction in median frame time. If we were to sort the opaque phase
by the material bind group, then this should be a lot faster. This
produces about 10.5k draws for the 11.7k visible entities. This makes
sense as randomly selecting from 10 materials gives a chance that two
adjacent entities randomly select the same material and can be batched.
The 1000th frame is identical in flip:
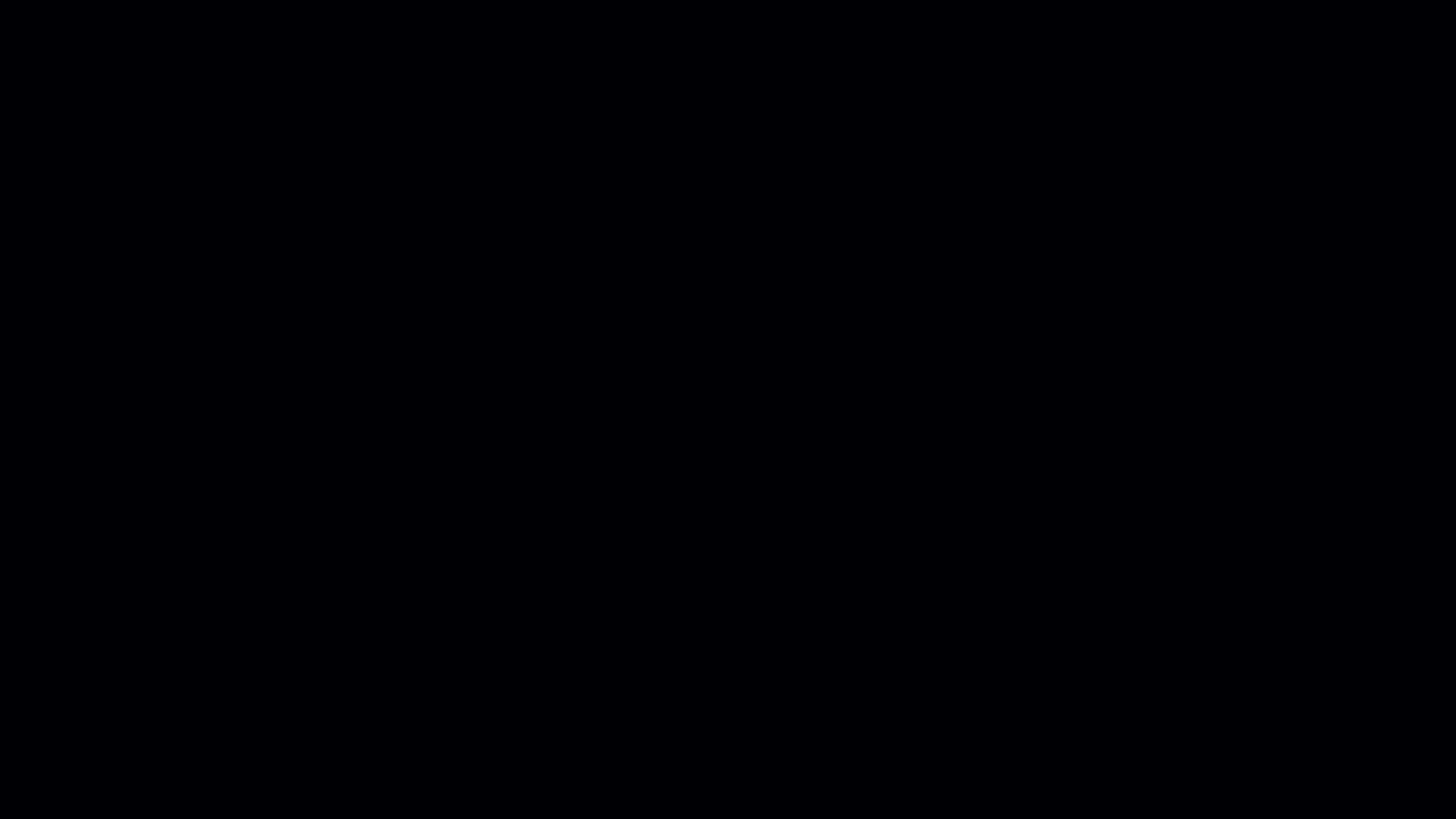
```
Mean: 0.000000
Weighted median: 0.000000
1st weighted quartile: 0.000000
3rd weighted quartile: 0.000000
Min: 0.000000
Max: 0.000000
Evaluation time: 0.4537 seconds
```
### 3D Mesh `many_cubes --benchmark --vary-per-instance`
<img width="1394" alt="Screenshot 2023-09-03 at 23 48 44"
src="https://github.com/bevyengine/bevy/assets/302146/f02a816b-a444-4c18-a96a-63b5436f3b7f">
This run varies the material data per instance by randomly-generating
its colour. This is the worst case for batching and that it performs
about the same as `main` is a good thing as it demonstrates that the
batching has minimal overhead when dealing with ~11k visible mesh
entities.
The 1000th frame is identical according to flip:
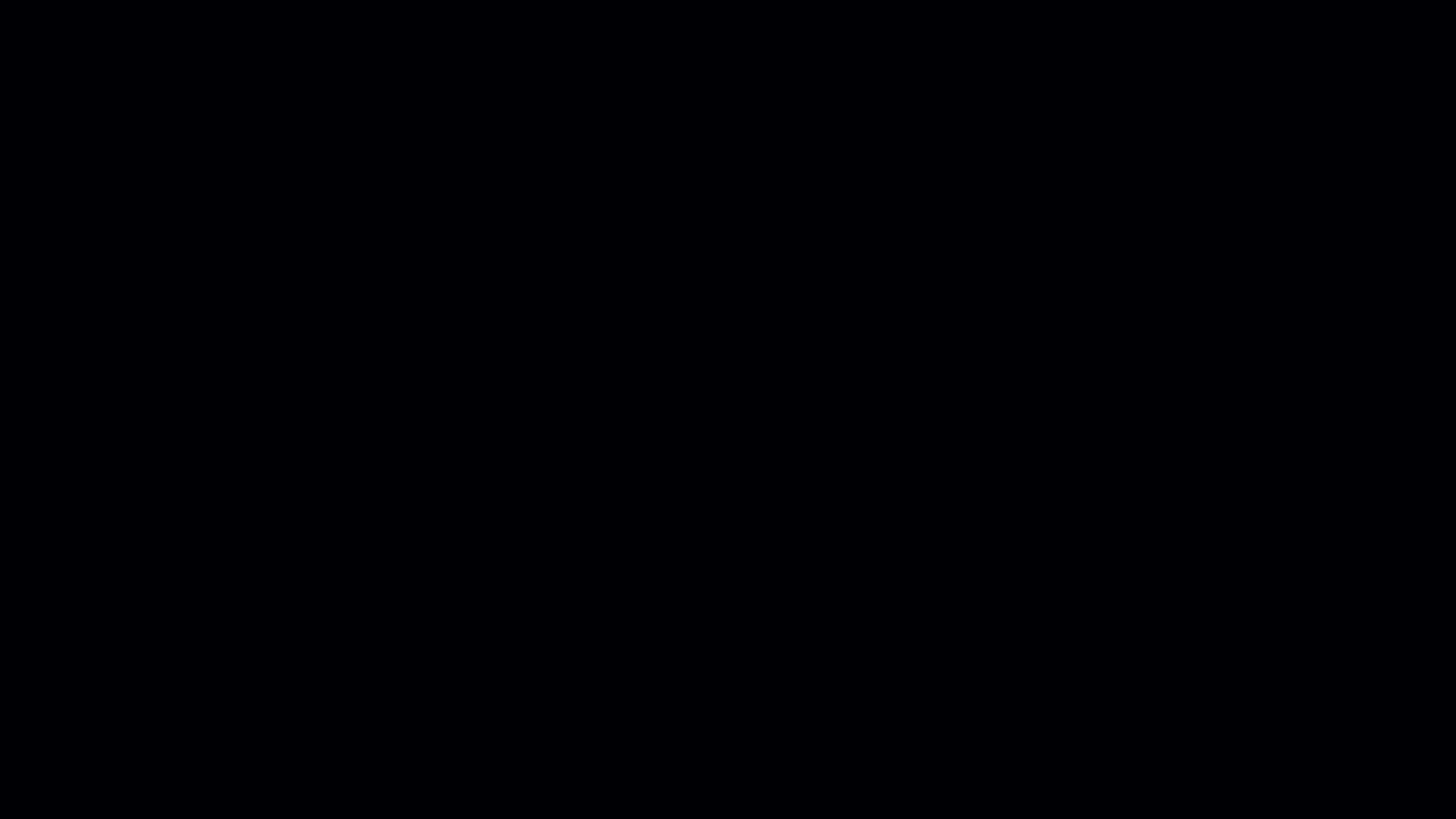
```
Mean: 0.000000
Weighted median: 0.000000
1st weighted quartile: 0.000000
3rd weighted quartile: 0.000000
Min: 0.000000
Max: 0.000000
Evaluation time: 0.4568 seconds
```
### 2D Mesh `bevymark --benchmark --waves 160 --per-wave 1000 --mode
mesh2d`
<img width="1412" alt="Screenshot 2023-09-03 at 23 59 56"
src="https://github.com/bevyengine/bevy/assets/302146/cb02ae07-237b-4646-ae9f-fda4dafcbad4">
This spawns 160 waves of 1000 quad meshes that are shaded with
ColorMaterial. Each wave has a different material so 160 waves currently
should result in 160 batches. This results in a 50% reduction in median
frame time.
Capturing a screenshot of the 1000th frame main vs PR gives:

```
Mean: 0.001222
Weighted median: 0.750432
1st weighted quartile: 0.453494
3rd weighted quartile: 0.969758
Min: 0.000000
Max: 0.990296
Evaluation time: 0.4255 seconds
```
So they seem to produce the same results. I also double-checked the
number of draws. `main` does 160000 draws, and the PR does 160, as
expected.
### 2D Mesh `bevymark --benchmark --waves 160 --per-wave 1000 --mode
mesh2d --material-texture-count 10`
<img width="1392" alt="Screenshot 2023-09-04 at 00 09 22"
src="https://github.com/bevyengine/bevy/assets/302146/4358da2e-ce32-4134-82df-3ab74c40849c">
This generates 10 textures and generates materials for each of those and
then selects one material per wave. The median frame time is reduced by
50%. Similar to the plain run above, this produces 160 draws on the PR
and 160000 on `main` and the 1000th frame is identical (ignoring the fps
counter text overlay).

```
Mean: 0.002877
Weighted median: 0.964980
1st weighted quartile: 0.668871
3rd weighted quartile: 0.982749
Min: 0.000000
Max: 0.992377
Evaluation time: 0.4301 seconds
```
### 2D Mesh `bevymark --benchmark --waves 160 --per-wave 1000 --mode
mesh2d --vary-per-instance`
<img width="1396" alt="Screenshot 2023-09-04 at 00 13 53"
src="https://github.com/bevyengine/bevy/assets/302146/b2198b18-3439-47ad-919a-cdabe190facb">
This creates unique materials per instance by randomly-generating the
material's colour. This is the worst case for 2D batching. Somehow, this
PR manages a 7% reduction in median frame time. Both main and this PR
issue 160000 draws.
The 1000th frame is the same:

```
Mean: 0.001214
Weighted median: 0.937499
1st weighted quartile: 0.635467
3rd weighted quartile: 0.979085
Min: 0.000000
Max: 0.988971
Evaluation time: 0.4462 seconds
```
### 2D Sprite `bevymark --benchmark --waves 160 --per-wave 1000 --mode
sprite`
<img width="1396" alt="Screenshot 2023-09-04 at 12 21 12"
src="https://github.com/bevyengine/bevy/assets/302146/8b31e915-d6be-4cac-abf5-c6a4da9c3d43">
This just spawns 160 waves of 1000 sprites. There should be and is no
notable difference between main and the PR.
### 2D Sprite `bevymark --benchmark --waves 160 --per-wave 1000 --mode
sprite --material-texture-count 10`
<img width="1389" alt="Screenshot 2023-09-04 at 12 36 08"
src="https://github.com/bevyengine/bevy/assets/302146/45fe8d6d-c901-4062-a349-3693dd044413">
This spawns the sprites selecting a texture at random per instance from
the 10 generated textures. This has no significant change vs main and
shouldn't.
### 2D Sprite `bevymark --benchmark --waves 160 --per-wave 1000 --mode
sprite --vary-per-instance`
<img width="1401" alt="Screenshot 2023-09-04 at 12 29 52"
src="https://github.com/bevyengine/bevy/assets/302146/762c5c60-352e-471f-8dbe-bbf10e24ebd6">
This sets the sprite colour as being unique per instance. This can still
all be drawn using one batch. There should be no difference but the PR
produces median frame times that are 4% higher. Investigation showed no
clear sources of cost, rather a mix of give and take that should not
happen. It seems like noise in the results.
### Summary
| Benchmark | % change in median frame time |
| ------------- | ------------- |
| many_cubes | 🟩 -30% |
| many_cubes 10 materials | 🟩 -5% |
| many_cubes unique materials | 🟩 ~0% |
| bevymark mesh2d | 🟩 -50% |
| bevymark mesh2d 10 materials | 🟩 -50% |
| bevymark mesh2d unique materials | 🟩 -7% |
| bevymark sprite | 🟥 2% |
| bevymark sprite 10 materials | 🟥 0.6% |
| bevymark sprite unique materials | 🟥 4.1% |
---
## Changelog
- Added: 2D and 3D mesh entities that share the same mesh and material
(same textures, same data) are now batched into the same draw command
for better performance.
---------
Co-authored-by: robtfm <50659922+robtfm@users.noreply.github.com>
Co-authored-by: Nicola Papale <nico@nicopap.ch>
2023-09-21 22:12:34 +00:00
|
|
|
impl<T: Material> PreparedMaterial<T> {
|
|
|
|
pub fn get_bind_group_id(&self) -> MaterialBindGroupId {
|
|
|
|
MaterialBindGroupId(Some(self.bind_group.id()))
|
|
|
|
}
|
|
|
|
}
|