2023-01-11 15:41:54 +00:00
pub use crate ::change_detection ::{ NonSendMut , Res , ResMut } ;
2020-11-08 20:34:05 +00:00
use crate ::{
Bevy ECS V2 (#1525)
# Bevy ECS V2
This is a rewrite of Bevy ECS (basically everything but the new executor/schedule, which are already awesome). The overall goal was to improve the performance and versatility of Bevy ECS. Here is a quick bulleted list of changes before we dive into the details:
* Complete World rewrite
* Multiple component storage types:
* Tables: fast cache friendly iteration, slower add/removes (previously called Archetypes)
* Sparse Sets: fast add/remove, slower iteration
* Stateful Queries (caches query results for faster iteration. fragmented iteration is _fast_ now)
* Stateful System Params (caches expensive operations. inspired by @DJMcNab's work in #1364)
* Configurable System Params (users can set configuration when they construct their systems. once again inspired by @DJMcNab's work)
* Archetypes are now "just metadata", component storage is separate
* Archetype Graph (for faster archetype changes)
* Component Metadata
* Configure component storage type
* Retrieve information about component size/type/name/layout/send-ness/etc
* Components are uniquely identified by a densely packed ComponentId
* TypeIds are now totally optional (which should make implementing scripting easier)
* Super fast "for_each" query iterators
* Merged Resources into World. Resources are now just a special type of component
* EntityRef/EntityMut builder apis (more efficient and more ergonomic)
* Fast bitset-backed `Access<T>` replaces old hashmap-based approach everywhere
* Query conflicts are determined by component access instead of archetype component access (to avoid random failures at runtime)
* With/Without are still taken into account for conflicts, so this should still be comfy to use
* Much simpler `IntoSystem` impl
* Significantly reduced the amount of hashing throughout the ecs in favor of Sparse Sets (indexed by densely packed ArchetypeId, ComponentId, BundleId, and TableId)
* Safety Improvements
* Entity reservation uses a normal world reference instead of unsafe transmute
* QuerySets no longer transmute lifetimes
* Made traits "unsafe" where relevant
* More thorough safety docs
* WorldCell
* Exposes safe mutable access to multiple resources at a time in a World
* Replaced "catch all" `System::update_archetypes(world: &World)` with `System::new_archetype(archetype: &Archetype)`
* Simpler Bundle implementation
* Replaced slow "remove_bundle_one_by_one" used as fallback for Commands::remove_bundle with fast "remove_bundle_intersection"
* Removed `Mut<T>` query impl. it is better to only support one way: `&mut T`
* Removed with() from `Flags<T>` in favor of `Option<Flags<T>>`, which allows querying for flags to be "filtered" by default
* Components now have is_send property (currently only resources support non-send)
* More granular module organization
* New `RemovedComponents<T>` SystemParam that replaces `query.removed::<T>()`
* `world.resource_scope()` for mutable access to resources and world at the same time
* WorldQuery and QueryFilter traits unified. FilterFetch trait added to enable "short circuit" filtering. Auto impled for cases that don't need it
* Significantly slimmed down SystemState in favor of individual SystemParam state
* System Commands changed from `commands: &mut Commands` back to `mut commands: Commands` (to allow Commands to have a World reference)
Fixes #1320
## `World` Rewrite
This is a from-scratch rewrite of `World` that fills the niche that `hecs` used to. Yes, this means Bevy ECS is no longer a "fork" of hecs. We're going out our own!
(the only shared code between the projects is the entity id allocator, which is already basically ideal)
A huge shout out to @SanderMertens (author of [flecs](https://github.com/SanderMertens/flecs)) for sharing some great ideas with me (specifically hybrid ecs storage and archetype graphs). He also helped advise on a number of implementation details.
## Component Storage (The Problem)
Two ECS storage paradigms have gained a lot of traction over the years:
* **Archetypal ECS**:
* Stores components in "tables" with static schemas. Each "column" stores components of a given type. Each "row" is an entity.
* Each "archetype" has its own table. Adding/removing an entity's component changes the archetype.
* Enables super-fast Query iteration due to its cache-friendly data layout
* Comes at the cost of more expensive add/remove operations for an Entity's components, because all components need to be copied to the new archetype's "table"
* **Sparse Set ECS**:
* Stores components of the same type in densely packed arrays, which are sparsely indexed by densely packed unsigned integers (Entity ids)
* Query iteration is slower than Archetypal ECS because each entity's component could be at any position in the sparse set. This "random access" pattern isn't cache friendly. Additionally, there is an extra layer of indirection because you must first map the entity id to an index in the component array.
* Adding/removing components is a cheap, constant time operation
Bevy ECS V1, hecs, legion, flec, and Unity DOTS are all "archetypal ecs-es". I personally think "archetypal" storage is a good default for game engines. An entity's archetype doesn't need to change frequently in general, and it creates "fast by default" query iteration (which is a much more common operation). It is also "self optimizing". Users don't need to think about optimizing component layouts for iteration performance. It "just works" without any extra boilerplate.
Shipyard and EnTT are "sparse set ecs-es". They employ "packing" as a way to work around the "suboptimal by default" iteration performance for specific sets of components. This helps, but I didn't think this was a good choice for a general purpose engine like Bevy because:
1. "packs" conflict with each other. If bevy decides to internally pack the Transform and GlobalTransform components, users are then blocked if they want to pack some custom component with Transform.
2. users need to take manual action to optimize
Developers selecting an ECS framework are stuck with a hard choice. Select an "archetypal" framework with "fast iteration everywhere" but without the ability to cheaply add/remove components, or select a "sparse set" framework to cheaply add/remove components but with slower iteration performance.
## Hybrid Component Storage (The Solution)
In Bevy ECS V2, we get to have our cake and eat it too. It now has _both_ of the component storage types above (and more can be added later if needed):
* **Tables** (aka "archetypal" storage)
* The default storage. If you don't configure anything, this is what you get
* Fast iteration by default
* Slower add/remove operations
* **Sparse Sets**
* Opt-in
* Slower iteration
* Faster add/remove operations
These storage types complement each other perfectly. By default Query iteration is fast. If developers know that they want to add/remove a component at high frequencies, they can set the storage to "sparse set":
```rust
world.register_component(
ComponentDescriptor::new::<MyComponent>(StorageType::SparseSet)
).unwrap();
```
## Archetypes
Archetypes are now "just metadata" ... they no longer store components directly. They do store:
* The `ComponentId`s of each of the Archetype's components (and that component's storage type)
* Archetypes are uniquely defined by their component layouts
* For example: entities with "table" components `[A, B, C]` _and_ "sparse set" components `[D, E]` will always be in the same archetype.
* The `TableId` associated with the archetype
* For now each archetype has exactly one table (which can have no components),
* There is a 1->Many relationship from Tables->Archetypes. A given table could have any number of archetype components stored in it:
* Ex: an entity with "table storage" components `[A, B, C]` and "sparse set" components `[D, E]` will share the same `[A, B, C]` table as an entity with `[A, B, C]` table component and `[F]` sparse set components.
* This 1->Many relationship is how we preserve fast "cache friendly" iteration performance when possible (more on this later)
* A list of entities that are in the archetype and the row id of the table they are in
* ArchetypeComponentIds
* unique densely packed identifiers for (ArchetypeId, ComponentId) pairs
* used by the schedule executor for cheap system access control
* "Archetype Graph Edges" (see the next section)
## The "Archetype Graph"
Archetype changes in Bevy (and a number of other archetypal ecs-es) have historically been expensive to compute. First, you need to allocate a new vector of the entity's current component ids, add or remove components based on the operation performed, sort it (to ensure it is order-independent), then hash it to find the archetype (if it exists). And thats all before we get to the _already_ expensive full copy of all components to the new table storage.
The solution is to build a "graph" of archetypes to cache these results. @SanderMertens first exposed me to the idea (and he got it from @gjroelofs, who came up with it). They propose adding directed edges between archetypes for add/remove component operations. If `ComponentId`s are densely packed, you can use sparse sets to cheaply jump between archetypes.
Bevy takes this one step further by using add/remove `Bundle` edges instead of `Component` edges. Bevy encourages the use of `Bundles` to group add/remove operations. This is largely for "clearer game logic" reasons, but it also helps cut down on the number of archetype changes required. `Bundles` now also have densely-packed `BundleId`s. This allows us to use a _single_ edge for each bundle operation (rather than needing to traverse N edges ... one for each component). Single component operations are also bundles, so this is strictly an improvement over a "component only" graph.
As a result, an operation that used to be _heavy_ (both for allocations and compute) is now two dirt-cheap array lookups and zero allocations.
## Stateful Queries
World queries are now stateful. This allows us to:
1. Cache archetype (and table) matches
* This resolves another issue with (naive) archetypal ECS: query performance getting worse as the number of archetypes goes up (and fragmentation occurs).
2. Cache Fetch and Filter state
* The expensive parts of fetch/filter operations (such as hashing the TypeId to find the ComponentId) now only happen once when the Query is first constructed
3. Incrementally build up state
* When new archetypes are added, we only process the new archetypes (no need to rebuild state for old archetypes)
As a result, the direct `World` query api now looks like this:
```rust
let mut query = world.query::<(&A, &mut B)>();
for (a, mut b) in query.iter_mut(&mut world) {
}
```
Requiring `World` to generate stateful queries (rather than letting the `QueryState` type be constructed separately) allows us to ensure that _all_ queries are properly initialized (and the relevant world state, such as ComponentIds). This enables QueryState to remove branches from its operations that check for initialization status (and also enables query.iter() to take an immutable world reference because it doesn't need to initialize anything in world).
However in systems, this is a non-breaking change. State management is done internally by the relevant SystemParam.
## Stateful SystemParams
Like Queries, `SystemParams` now also cache state. For example, `Query` system params store the "stateful query" state mentioned above. Commands store their internal `CommandQueue`. This means you can now safely use as many separate `Commands` parameters in your system as you want. `Local<T>` system params store their `T` value in their state (instead of in Resources).
SystemParam state also enabled a significant slim-down of SystemState. It is much nicer to look at now.
Per-SystemParam state naturally insulates us from an "aliased mut" class of errors we have hit in the past (ex: using multiple `Commands` system params).
(credit goes to @DJMcNab for the initial idea and draft pr here #1364)
## Configurable SystemParams
@DJMcNab also had the great idea to make SystemParams configurable. This allows users to provide some initial configuration / values for system parameters (when possible). Most SystemParams have no config (the config type is `()`), but the `Local<T>` param now supports user-provided parameters:
```rust
fn foo(value: Local<usize>) {
}
app.add_system(foo.system().config(|c| c.0 = Some(10)));
```
## Uber Fast "for_each" Query Iterators
Developers now have the choice to use a fast "for_each" iterator, which yields ~1.5-3x iteration speed improvements for "fragmented iteration", and minor ~1.2x iteration speed improvements for unfragmented iteration.
```rust
fn system(query: Query<(&A, &mut B)>) {
// you now have the option to do this for a speed boost
query.for_each_mut(|(a, mut b)| {
});
// however normal iterators are still available
for (a, mut b) in query.iter_mut() {
}
}
```
I think in most cases we should continue to encourage "normal" iterators as they are more flexible and more "rust idiomatic". But when that extra "oomf" is needed, it makes sense to use `for_each`.
We should also consider using `for_each` for internal bevy systems to give our users a nice speed boost (but that should be a separate pr).
## Component Metadata
`World` now has a `Components` collection, which is accessible via `world.components()`. This stores mappings from `ComponentId` to `ComponentInfo`, as well as `TypeId` to `ComponentId` mappings (where relevant). `ComponentInfo` stores information about the component, such as ComponentId, TypeId, memory layout, send-ness (currently limited to resources), and storage type.
## Significantly Cheaper `Access<T>`
We used to use `TypeAccess<TypeId>` to manage read/write component/archetype-component access. This was expensive because TypeIds must be hashed and compared individually. The parallel executor got around this by "condensing" type ids into bitset-backed access types. This worked, but it had to be re-generated from the `TypeAccess<TypeId>`sources every time archetypes changed.
This pr removes TypeAccess in favor of faster bitset access everywhere. We can do this thanks to the move to densely packed `ComponentId`s and `ArchetypeComponentId`s.
## Merged Resources into World
Resources had a lot of redundant functionality with Components. They stored typed data, they had access control, they had unique ids, they were queryable via SystemParams, etc. In fact the _only_ major difference between them was that they were unique (and didn't correlate to an entity).
Separate resources also had the downside of requiring a separate set of access controls, which meant the parallel executor needed to compare more bitsets per system and manage more state.
I initially got the "separate resources" idea from `legion`. I think that design was motivated by the fact that it made the direct world query/resource lifetime interactions more manageable. It certainly made our lives easier when using Resources alongside hecs/bevy_ecs. However we already have a construct for safely and ergonomically managing in-world lifetimes: systems (which use `Access<T>` internally).
This pr merges Resources into World:
```rust
world.insert_resource(1);
world.insert_resource(2.0);
let a = world.get_resource::<i32>().unwrap();
let mut b = world.get_resource_mut::<f64>().unwrap();
*b = 3.0;
```
Resources are now just a special kind of component. They have their own ComponentIds (and their own resource TypeId->ComponentId scope, so they don't conflict wit components of the same type). They are stored in a special "resource archetype", which stores components inside the archetype using a new `unique_components` sparse set (note that this sparse set could later be used to implement Tags). This allows us to keep the code size small by reusing existing datastructures (namely Column, Archetype, ComponentFlags, and ComponentInfo). This allows us the executor to use a single `Access<ArchetypeComponentId>` per system. It should also make scripting language integration easier.
_But_ this merge did create problems for people directly interacting with `World`. What if you need mutable access to multiple resources at the same time? `world.get_resource_mut()` borrows World mutably!
## WorldCell
WorldCell applies the `Access<ArchetypeComponentId>` concept to direct world access:
```rust
let world_cell = world.cell();
let a = world_cell.get_resource_mut::<i32>().unwrap();
let b = world_cell.get_resource_mut::<f64>().unwrap();
```
This adds cheap runtime checks (a sparse set lookup of `ArchetypeComponentId` and a counter) to ensure that world accesses do not conflict with each other. Each operation returns a `WorldBorrow<'w, T>` or `WorldBorrowMut<'w, T>` wrapper type, which will release the relevant ArchetypeComponentId resources when dropped.
World caches the access sparse set (and only one cell can exist at a time), so `world.cell()` is a cheap operation.
WorldCell does _not_ use atomic operations. It is non-send, does a mutable borrow of world to prevent other accesses, and uses a simple `Rc<RefCell<ArchetypeComponentAccess>>` wrapper in each WorldBorrow pointer.
The api is currently limited to resource access, but it can and should be extended to queries / entity component access.
## Resource Scopes
WorldCell does not yet support component queries, and even when it does there are sometimes legitimate reasons to want a mutable world ref _and_ a mutable resource ref (ex: bevy_render and bevy_scene both need this). In these cases we could always drop down to the unsafe `world.get_resource_unchecked_mut()`, but that is not ideal!
Instead developers can use a "resource scope"
```rust
world.resource_scope(|world: &mut World, a: &mut A| {
})
```
This temporarily removes the `A` resource from `World`, provides mutable pointers to both, and re-adds A to World when finished. Thanks to the move to ComponentIds/sparse sets, this is a cheap operation.
If multiple resources are required, scopes can be nested. We could also consider adding a "resource tuple" to the api if this pattern becomes common and the boilerplate gets nasty.
## Query Conflicts Use ComponentId Instead of ArchetypeComponentId
For safety reasons, systems cannot contain queries that conflict with each other without wrapping them in a QuerySet. On bevy `main`, we use ArchetypeComponentIds to determine conflicts. This is nice because it can take into account filters:
```rust
// these queries will never conflict due to their filters
fn filter_system(a: Query<&mut A, With<B>>, b: Query<&mut B, Without<B>>) {
}
```
But it also has a significant downside:
```rust
// these queries will not conflict _until_ an entity with A, B, and C is spawned
fn maybe_conflicts_system(a: Query<(&mut A, &C)>, b: Query<(&mut A, &B)>) {
}
```
The system above will panic at runtime if an entity with A, B, and C is spawned. This makes it hard to trust that your game logic will run without crashing.
In this pr, I switched to using `ComponentId` instead. This _is_ more constraining. `maybe_conflicts_system` will now always fail, but it will do it consistently at startup. Naively, it would also _disallow_ `filter_system`, which would be a significant downgrade in usability. Bevy has a number of internal systems that rely on disjoint queries and I expect it to be a common pattern in userspace.
To resolve this, I added a new `FilteredAccess<T>` type, which wraps `Access<T>` and adds with/without filters. If two `FilteredAccess` have with/without values that prove they are disjoint, they will no longer conflict.
## EntityRef / EntityMut
World entity operations on `main` require that the user passes in an `entity` id to each operation:
```rust
let entity = world.spawn((A, )); // create a new entity with A
world.get::<A>(entity);
world.insert(entity, (B, C));
world.insert_one(entity, D);
```
This means that each operation needs to look up the entity location / verify its validity. The initial spawn operation also requires a Bundle as input. This can be awkward when no components are required (or one component is required).
These operations have been replaced by `EntityRef` and `EntityMut`, which are "builder-style" wrappers around world that provide read and read/write operations on a single, pre-validated entity:
```rust
// spawn now takes no inputs and returns an EntityMut
let entity = world.spawn()
.insert(A) // insert a single component into the entity
.insert_bundle((B, C)) // insert a bundle of components into the entity
.id() // id returns the Entity id
// Returns EntityMut (or panics if the entity does not exist)
world.entity_mut(entity)
.insert(D)
.insert_bundle(SomeBundle::default());
{
// returns EntityRef (or panics if the entity does not exist)
let d = world.entity(entity)
.get::<D>() // gets the D component
.unwrap();
// world.get still exists for ergonomics
let d = world.get::<D>(entity).unwrap();
}
// These variants return Options if you want to check existence instead of panicing
world.get_entity_mut(entity)
.unwrap()
.insert(E);
if let Some(entity_ref) = world.get_entity(entity) {
let d = entity_ref.get::<D>().unwrap();
}
```
This _does not_ affect the current Commands api or terminology. I think that should be a separate conversation as that is a much larger breaking change.
## Safety Improvements
* Entity reservation in Commands uses a normal world borrow instead of an unsafe transmute
* QuerySets no longer transmutes lifetimes
* Made traits "unsafe" when implementing a trait incorrectly could cause unsafety
* More thorough safety docs
## RemovedComponents SystemParam
The old approach to querying removed components: `query.removed:<T>()` was confusing because it had no connection to the query itself. I replaced it with the following, which is both clearer and allows us to cache the ComponentId mapping in the SystemParamState:
```rust
fn system(removed: RemovedComponents<T>) {
for entity in removed.iter() {
}
}
```
## Simpler Bundle implementation
Bundles are no longer responsible for sorting (or deduping) TypeInfo. They are just a simple ordered list of component types / data. This makes the implementation smaller and opens the door to an easy "nested bundle" implementation in the future (which i might even add in this pr). Duplicate detection is now done once per bundle type by World the first time a bundle is used.
## Unified WorldQuery and QueryFilter types
(don't worry they are still separate type _parameters_ in Queries .. this is a non-breaking change)
WorldQuery and QueryFilter were already basically identical apis. With the addition of `FetchState` and more storage-specific fetch methods, the overlap was even clearer (and the redundancy more painful).
QueryFilters are now just `F: WorldQuery where F::Fetch: FilterFetch`. FilterFetch requires `Fetch<Item = bool>` and adds new "short circuit" variants of fetch methods. This enables a filter tuple like `(With<A>, Without<B>, Changed<C>)` to stop evaluating the filter after the first mismatch is encountered. FilterFetch is automatically implemented for `Fetch` implementations that return bool.
This forces fetch implementations that return things like `(bool, bool, bool)` (such as the filter above) to manually implement FilterFetch and decide whether or not to short-circuit.
## More Granular Modules
World no longer globs all of the internal modules together. It now exports `core`, `system`, and `schedule` separately. I'm also considering exporting `core` submodules directly as that is still pretty "glob-ey" and unorganized (feedback welcome here).
## Remaining Draft Work (to be done in this pr)
* ~~panic on conflicting WorldQuery fetches (&A, &mut A)~~
* ~~bevy `main` and hecs both currently allow this, but we should protect against it if possible~~
* ~~batch_iter / par_iter (currently stubbed out)~~
* ~~ChangedRes~~
* ~~I skipped this while we sort out #1313. This pr should be adapted to account for whatever we land on there~~.
* ~~The `Archetypes` and `Tables` collections use hashes of sorted lists of component ids to uniquely identify each archetype/table. This hash is then used as the key in a HashMap to look up the relevant ArchetypeId or TableId. (which doesn't handle hash collisions properly)~~
* ~~It is currently unsafe to generate a Query from "World A", then use it on "World B" (despite the api claiming it is safe). We should probably close this gap. This could be done by adding a randomly generated WorldId to each world, then storing that id in each Query. They could then be compared to each other on each `query.do_thing(&world)` operation. This _does_ add an extra branch to each query operation, so I'm open to other suggestions if people have them.~~
* ~~Nested Bundles (if i find time)~~
## Potential Future Work
* Expand WorldCell to support queries.
* Consider not allocating in the empty archetype on `world.spawn()`
* ex: return something like EntityMutUninit, which turns into EntityMut after an `insert` or `insert_bundle` op
* this actually regressed performance last time i tried it, but in theory it should be faster
* Optimize SparseSet::insert (see `PERF` comment on insert)
* Replace SparseArray `Option<T>` with T::MAX to cut down on branching
* would enable cheaper get_unchecked() operations
* upstream fixedbitset optimizations
* fixedbitset could be allocation free for small block counts (store blocks in a SmallVec)
* fixedbitset could have a const constructor
* Consider implementing Tags (archetype-specific by-value data that affects archetype identity)
* ex: ArchetypeA could have `[A, B, C]` table components and `[D(1)]` "tag" component. ArchetypeB could have `[A, B, C]` table components and a `[D(2)]` tag component. The archetypes are different, despite both having D tags because the value inside D is different.
* this could potentially build on top of the `archetype.unique_components` added in this pr for resource storage.
* Consider reverting `all_tuples` proc macro in favor of the old `macro_rules` implementation
* all_tuples is more flexible and produces cleaner documentation (the macro_rules version produces weird type parameter orders due to parser constraints)
* but unfortunately all_tuples also appears to make Rust Analyzer sad/slow when working inside of `bevy_ecs` (does not affect user code)
* Consider "resource queries" and/or "mixed resource and entity component queries" as an alternative to WorldCell
* this is basically just "systems" so maybe it's not worth it
* Add more world ops
* `world.clear()`
* `world.reserve<T: Bundle>(count: usize)`
* Try using the old archetype allocation strategy (allocate new memory on resize and copy everything over). I expect this to improve batch insertion performance at the cost of unbatched performance. But thats just a guess. I'm not an allocation perf pro :)
* Adapt Commands apis for consistency with new World apis
## Benchmarks
key:
* `bevy_old`: bevy `main` branch
* `bevy`: this branch
* `_foreach`: uses an optimized for_each iterator
* ` _sparse`: uses sparse set storage (if unspecified assume table storage)
* `_system`: runs inside a system (if unspecified assume test happens via direct world ops)
### Simple Insert (from ecs_bench_suite)
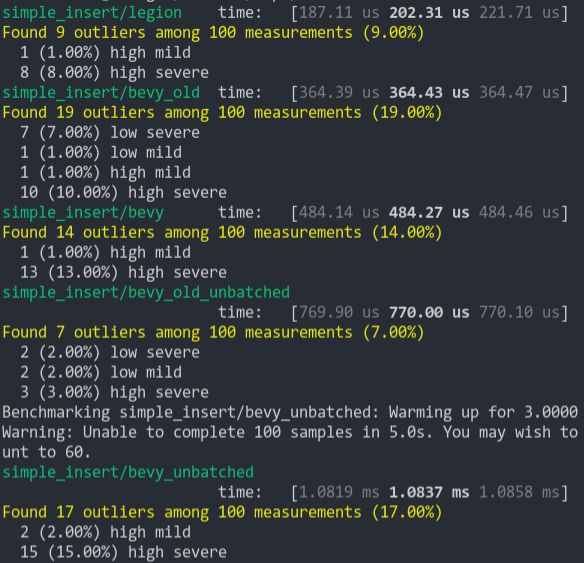
### Simpler Iter (from ecs_bench_suite)
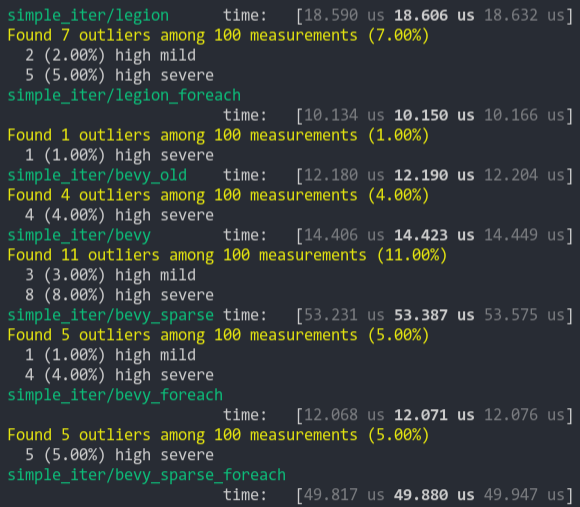
### Fragment Iter (from ecs_bench_suite)
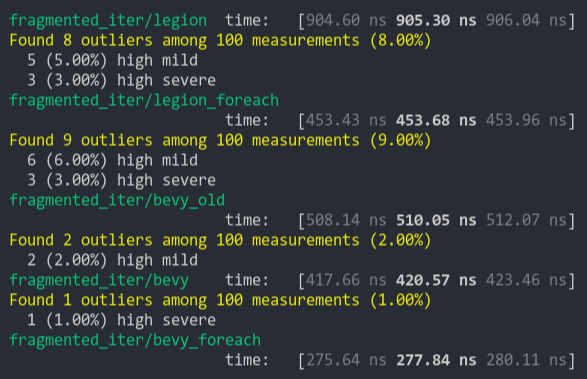
### Sparse Fragmented Iter
Iterate a query that matches 5 entities from a single matching archetype, but there are 100 unmatching archetypes
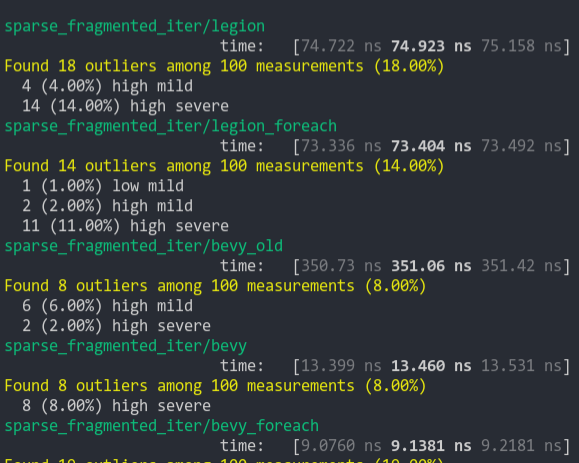
### Schedule (from ecs_bench_suite)
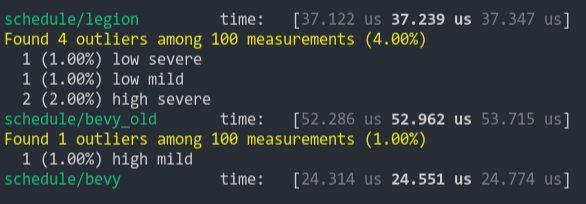
### Add Remove Component (from ecs_bench_suite)
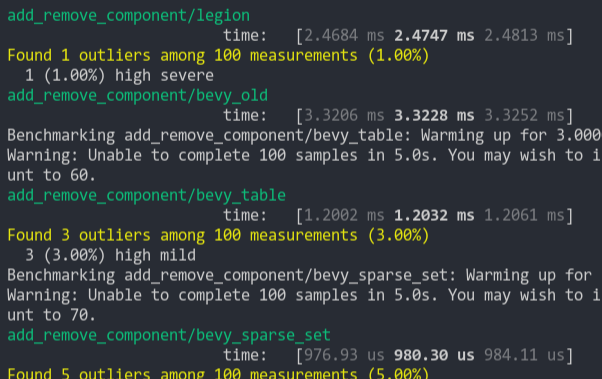
### Add Remove Component Big
Same as the test above, but each entity has 5 "large" matrix components and 1 "large" matrix component is added and removed
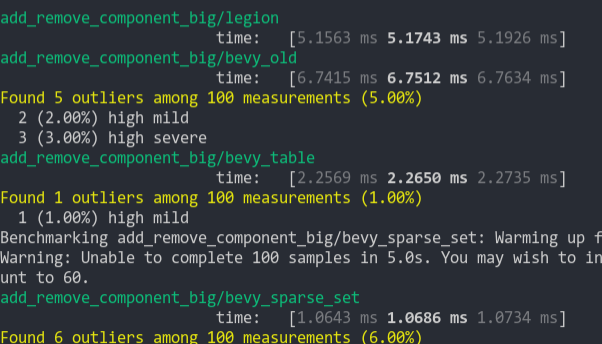
### Get Component
Looks up a single component value a large number of times
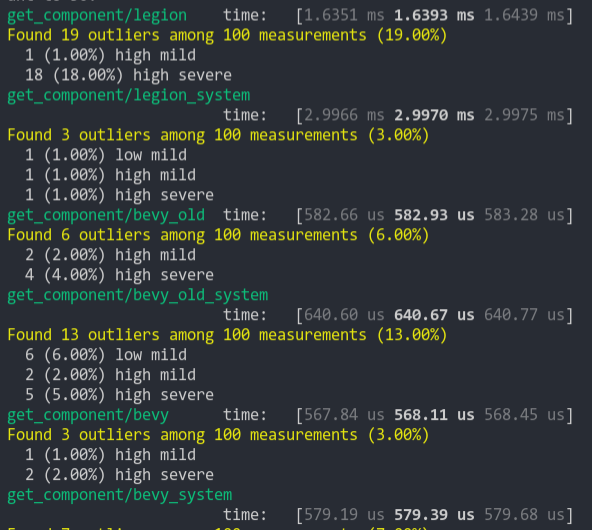
2021-03-05 07:54:35 +00:00
archetype ::{ Archetype , Archetypes } ,
bundle ::Bundles ,
2023-01-11 15:41:54 +00:00
change_detection ::{ Ticks , TicksMut } ,
2023-02-04 20:53:37 +00:00
component ::{ ComponentId , ComponentTicks , Components } ,
entity ::Entities ,
Add new SystemState and rename old SystemState to SystemMeta (#2283)
This enables `SystemParams` to be used outside of function systems. Anything can create and store `SystemState`, which enables efficient "param state cached" access to `SystemParams`.
It adds a `ReadOnlySystemParamFetch` trait, which enables safe `SystemState::get` calls without unique world access.
I renamed the old `SystemState` to `SystemMeta` to enable us to mirror the `QueryState` naming convention (but I'm happy to discuss alternative names if people have other ideas). I initially pitched this as `ParamState`, but given that it needs to include full system metadata, that doesn't feel like a particularly accurate name.
```rust
#[derive(Eq, PartialEq, Debug)]
struct A(usize);
#[derive(Eq, PartialEq, Debug)]
struct B(usize);
let mut world = World::default();
world.insert_resource(A(42));
world.spawn().insert(B(7));
// we get nice lifetime elision when declaring the type on the left hand side
let mut system_state: SystemState<(Res<A>, Query<&B>)> = SystemState::new(&mut world);
let (a, query) = system_state.get(&world);
assert_eq!(*a, A(42), "returned resource matches initial value");
assert_eq!(
*query.single().unwrap(),
B(7),
"returned component matches initial value"
);
// mutable system params require unique world access
let mut system_state: SystemState<(ResMut<A>, Query<&mut B>)> = SystemState::new(&mut world);
let (a, query) = system_state.get_mut(&mut world);
// static lifetimes are required when declaring inside of structs
struct SomeContainer {
state: SystemState<(Res<'static, A>, Res<'static, B>)>
}
// this can be shortened using type aliases, which will be useful for complex param tuples
type MyParams<'a> = (Res<'a, A>, Res<'a, B>);
struct SomeContainer {
state: SystemState<MyParams<'static>>
}
// It is the user's responsibility to call SystemState::apply(world) for parameters that queue up work
let mut system_state: SystemState<(Commands, Query<&B>)> = SystemState::new(&mut world);
{
let (mut commands, query) = system_state.get(&world);
commands.insert_resource(3.14);
}
system_state.apply(&mut world);
```
## Future Work
* Actually use SystemState inside FunctionSystem. This would be trivial, but it requires FunctionSystem to wrap SystemState in Option in its current form (which complicates system metadata lookup). I'd prefer to hold off until we adopt something like the later designs linked in #1364, which enable us to contruct Systems using a World reference (and also remove the need for `.system`).
* Consider a "scoped" approach to automatically call SystemState::apply when systems params are no longer being used (either a container type with a Drop impl, or a function that takes a closure for user logic operating on params).
2021-06-02 19:57:38 +00:00
query ::{
Replace `ReadOnlyFetch` with `ReadOnlyWorldQuery` (#4626)
# Objective
- Fix a type inference regression introduced by #3001
- Make read only bounds on world queries more user friendly
ptrification required you to write `Q::Fetch: ReadOnlyFetch` as `for<'w> QueryFetch<'w, Q>: ReadOnlyFetch` which has the same type inference problem as `for<'w> QueryFetch<'w, Q>: FilterFetch<'w>` had, i.e. the following code would error:
```rust
#[derive(Component)]
struct Foo;
fn bar(a: Query<(&Foo, Without<Foo>)>) {
foo(a);
}
fn foo<Q: WorldQuery>(a: Query<Q, ()>)
where
for<'w> QueryFetch<'w, Q>: ReadOnlyFetch,
{
}
```
`for<..>` bounds are also rather user unfriendly..
## Solution
Remove the `ReadOnlyFetch` trait in favour of a `ReadOnlyWorldQuery` trait, and remove `WorldQueryGats::ReadOnlyFetch` in favor of `WorldQuery::ReadOnly` allowing the previous code snippet to be written as:
```rust
#[derive(Component)]
struct Foo;
fn bar(a: Query<(&Foo, Without<Foo>)>) {
foo(a);
}
fn foo<Q: ReadOnlyWorldQuery>(a: Query<Q, ()>) {}
```
This avoids the `for<...>` bound which makes the code simpler and also fixes the type inference issue.
The reason for moving the two functions out of `FetchState` and into `WorldQuery` is to allow the world query `&mut T` to share a `State` with the `&T` world query so that it can have `type ReadOnly = &T`. Presumably it would be possible to instead have a `ReadOnlyRefMut<T>` world query and then do `type ReadOnly = ReadOnlyRefMut<T>` much like how (before this PR) we had a `ReadOnlyWriteFetch<T>`. A side benefit of the current solution in this PR is that it will likely make it easier in the future to support an API such as `Query<&mut T> -> Query<&T>`. The primary benefit IMO is just that `ReadOnlyRefMut<T>` and its associated fetch would have to reimplement all of the logic that the `&T` world query impl does but this solution avoids that :)
---
## Changelog/Migration Guide
The trait `ReadOnlyFetch` has been replaced with `ReadOnlyWorldQuery` along with the `WorldQueryGats::ReadOnlyFetch` assoc type which has been replaced with `<WorldQuery::ReadOnly as WorldQueryGats>::Fetch`
- Any where clauses such as `QueryFetch<Q>: ReadOnlyFetch` should be replaced with `Q: ReadOnlyWorldQuery`.
- Any custom world query impls should implement `ReadOnlyWorldQuery` insead of `ReadOnlyFetch`
Functions `update_component_access` and `update_archetype_component_access` have been moved from the `FetchState` trait to `WorldQuery`
- Any callers should now call `Q::update_component_access(state` instead of `state.update_component_access` (and `update_archetype_component_access` respectively)
- Any custom world query impls should move the functions from the `FetchState` impl to `WorldQuery` impl
`WorldQuery` has been made an `unsafe trait`, `FetchState` has been made a safe `trait`. (I think this is how it should have always been, but regardless this is _definitely_ necessary now that the two functions have been moved to `WorldQuery`)
- If you have a custom `FetchState` impl make it a normal `impl` instead of `unsafe impl`
- If you have a custom `WorldQuery` impl make it an `unsafe impl`, if your code was sound before it is going to still be sound
2022-06-13 23:35:54 +00:00
Access , FilteredAccess , FilteredAccessSet , QueryState , ReadOnlyWorldQuery , WorldQuery ,
Add new SystemState and rename old SystemState to SystemMeta (#2283)
This enables `SystemParams` to be used outside of function systems. Anything can create and store `SystemState`, which enables efficient "param state cached" access to `SystemParams`.
It adds a `ReadOnlySystemParamFetch` trait, which enables safe `SystemState::get` calls without unique world access.
I renamed the old `SystemState` to `SystemMeta` to enable us to mirror the `QueryState` naming convention (but I'm happy to discuss alternative names if people have other ideas). I initially pitched this as `ParamState`, but given that it needs to include full system metadata, that doesn't feel like a particularly accurate name.
```rust
#[derive(Eq, PartialEq, Debug)]
struct A(usize);
#[derive(Eq, PartialEq, Debug)]
struct B(usize);
let mut world = World::default();
world.insert_resource(A(42));
world.spawn().insert(B(7));
// we get nice lifetime elision when declaring the type on the left hand side
let mut system_state: SystemState<(Res<A>, Query<&B>)> = SystemState::new(&mut world);
let (a, query) = system_state.get(&world);
assert_eq!(*a, A(42), "returned resource matches initial value");
assert_eq!(
*query.single().unwrap(),
B(7),
"returned component matches initial value"
);
// mutable system params require unique world access
let mut system_state: SystemState<(ResMut<A>, Query<&mut B>)> = SystemState::new(&mut world);
let (a, query) = system_state.get_mut(&mut world);
// static lifetimes are required when declaring inside of structs
struct SomeContainer {
state: SystemState<(Res<'static, A>, Res<'static, B>)>
}
// this can be shortened using type aliases, which will be useful for complex param tuples
type MyParams<'a> = (Res<'a, A>, Res<'a, B>);
struct SomeContainer {
state: SystemState<MyParams<'static>>
}
// It is the user's responsibility to call SystemState::apply(world) for parameters that queue up work
let mut system_state: SystemState<(Commands, Query<&B>)> = SystemState::new(&mut world);
{
let (mut commands, query) = system_state.get(&world);
commands.insert_resource(3.14);
}
system_state.apply(&mut world);
```
## Future Work
* Actually use SystemState inside FunctionSystem. This would be trivial, but it requires FunctionSystem to wrap SystemState in Option in its current form (which complicates system metadata lookup). I'd prefer to hold off until we adopt something like the later designs linked in #1364, which enable us to contruct Systems using a World reference (and also remove the need for `.system`).
* Consider a "scoped" approach to automatically call SystemState::apply when systems params are no longer being used (either a container type with a Drop impl, or a function that takes a closure for user logic operating on params).
2021-06-02 19:57:38 +00:00
} ,
Add a `SystemParam` primitive for deferred mutations; allow `#[derive]`ing more types of SystemParam (#6817)
# Objective
One pattern to increase parallelism is deferred mutation: instead of directly mutating the world (and preventing other systems from running at the same time), you queue up operations to be applied to the world at the end of the stage. The most common example of this pattern uses the `Commands` SystemParam.
In order to avoid the overhead associated with commands, some power users may want to add their own deferred mutation behavior. To do this, you must implement the unsafe trait `SystemParam`, which interfaces with engine internals in a way that we'd like users to be able to avoid.
## Solution
Add the `Deferred<T>` primitive `SystemParam`, which encapsulates the deferred mutation pattern.
This can be combined with other types of `SystemParam` to safely and ergonomically create powerful custom types.
Essentially, this is just a variant of `Local<T>` which can run code at the end of the stage.
This type is used in the engine to derive `Commands` and `ParallelCommands`, which removes a bunch of unsafe boilerplate.
### Example
```rust
use bevy_ecs::system::{Deferred, SystemBuffer};
/// Sends events with a delay, but may run in parallel with other event writers.
#[derive(SystemParam)]
pub struct BufferedEventWriter<'s, E: Event> {
queue: Deferred<'s, EventQueue<E>>,
}
struct EventQueue<E>(Vec<E>);
impl<'s, E: Event> BufferedEventWriter<'s, E> {
/// Queues up an event to be sent at the end of this stage.
pub fn send(&mut self, event: E) {
self.queue.0.push(event);
}
}
// The `SystemBuffer` trait controls how [`Deferred`] gets applied at the end of the stage.
impl<E: Event> SystemBuffer for EventQueue<E> {
fn apply(&mut self, world: &mut World) {
let mut events = world.resource_mut::<Events<E>>();
for e in self.0.drain(..) {
events.send(e);
}
}
}
```
---
## Changelog
+ Added the `SystemParam` type `Deferred<T>`, which can be used to defer `World` mutations. Powered by the new trait `SystemBuffer`.
2023-02-06 21:57:57 +00:00
system ::{ Query , SystemMeta } ,
Bevy ECS V2 (#1525)
# Bevy ECS V2
This is a rewrite of Bevy ECS (basically everything but the new executor/schedule, which are already awesome). The overall goal was to improve the performance and versatility of Bevy ECS. Here is a quick bulleted list of changes before we dive into the details:
* Complete World rewrite
* Multiple component storage types:
* Tables: fast cache friendly iteration, slower add/removes (previously called Archetypes)
* Sparse Sets: fast add/remove, slower iteration
* Stateful Queries (caches query results for faster iteration. fragmented iteration is _fast_ now)
* Stateful System Params (caches expensive operations. inspired by @DJMcNab's work in #1364)
* Configurable System Params (users can set configuration when they construct their systems. once again inspired by @DJMcNab's work)
* Archetypes are now "just metadata", component storage is separate
* Archetype Graph (for faster archetype changes)
* Component Metadata
* Configure component storage type
* Retrieve information about component size/type/name/layout/send-ness/etc
* Components are uniquely identified by a densely packed ComponentId
* TypeIds are now totally optional (which should make implementing scripting easier)
* Super fast "for_each" query iterators
* Merged Resources into World. Resources are now just a special type of component
* EntityRef/EntityMut builder apis (more efficient and more ergonomic)
* Fast bitset-backed `Access<T>` replaces old hashmap-based approach everywhere
* Query conflicts are determined by component access instead of archetype component access (to avoid random failures at runtime)
* With/Without are still taken into account for conflicts, so this should still be comfy to use
* Much simpler `IntoSystem` impl
* Significantly reduced the amount of hashing throughout the ecs in favor of Sparse Sets (indexed by densely packed ArchetypeId, ComponentId, BundleId, and TableId)
* Safety Improvements
* Entity reservation uses a normal world reference instead of unsafe transmute
* QuerySets no longer transmute lifetimes
* Made traits "unsafe" where relevant
* More thorough safety docs
* WorldCell
* Exposes safe mutable access to multiple resources at a time in a World
* Replaced "catch all" `System::update_archetypes(world: &World)` with `System::new_archetype(archetype: &Archetype)`
* Simpler Bundle implementation
* Replaced slow "remove_bundle_one_by_one" used as fallback for Commands::remove_bundle with fast "remove_bundle_intersection"
* Removed `Mut<T>` query impl. it is better to only support one way: `&mut T`
* Removed with() from `Flags<T>` in favor of `Option<Flags<T>>`, which allows querying for flags to be "filtered" by default
* Components now have is_send property (currently only resources support non-send)
* More granular module organization
* New `RemovedComponents<T>` SystemParam that replaces `query.removed::<T>()`
* `world.resource_scope()` for mutable access to resources and world at the same time
* WorldQuery and QueryFilter traits unified. FilterFetch trait added to enable "short circuit" filtering. Auto impled for cases that don't need it
* Significantly slimmed down SystemState in favor of individual SystemParam state
* System Commands changed from `commands: &mut Commands` back to `mut commands: Commands` (to allow Commands to have a World reference)
Fixes #1320
## `World` Rewrite
This is a from-scratch rewrite of `World` that fills the niche that `hecs` used to. Yes, this means Bevy ECS is no longer a "fork" of hecs. We're going out our own!
(the only shared code between the projects is the entity id allocator, which is already basically ideal)
A huge shout out to @SanderMertens (author of [flecs](https://github.com/SanderMertens/flecs)) for sharing some great ideas with me (specifically hybrid ecs storage and archetype graphs). He also helped advise on a number of implementation details.
## Component Storage (The Problem)
Two ECS storage paradigms have gained a lot of traction over the years:
* **Archetypal ECS**:
* Stores components in "tables" with static schemas. Each "column" stores components of a given type. Each "row" is an entity.
* Each "archetype" has its own table. Adding/removing an entity's component changes the archetype.
* Enables super-fast Query iteration due to its cache-friendly data layout
* Comes at the cost of more expensive add/remove operations for an Entity's components, because all components need to be copied to the new archetype's "table"
* **Sparse Set ECS**:
* Stores components of the same type in densely packed arrays, which are sparsely indexed by densely packed unsigned integers (Entity ids)
* Query iteration is slower than Archetypal ECS because each entity's component could be at any position in the sparse set. This "random access" pattern isn't cache friendly. Additionally, there is an extra layer of indirection because you must first map the entity id to an index in the component array.
* Adding/removing components is a cheap, constant time operation
Bevy ECS V1, hecs, legion, flec, and Unity DOTS are all "archetypal ecs-es". I personally think "archetypal" storage is a good default for game engines. An entity's archetype doesn't need to change frequently in general, and it creates "fast by default" query iteration (which is a much more common operation). It is also "self optimizing". Users don't need to think about optimizing component layouts for iteration performance. It "just works" without any extra boilerplate.
Shipyard and EnTT are "sparse set ecs-es". They employ "packing" as a way to work around the "suboptimal by default" iteration performance for specific sets of components. This helps, but I didn't think this was a good choice for a general purpose engine like Bevy because:
1. "packs" conflict with each other. If bevy decides to internally pack the Transform and GlobalTransform components, users are then blocked if they want to pack some custom component with Transform.
2. users need to take manual action to optimize
Developers selecting an ECS framework are stuck with a hard choice. Select an "archetypal" framework with "fast iteration everywhere" but without the ability to cheaply add/remove components, or select a "sparse set" framework to cheaply add/remove components but with slower iteration performance.
## Hybrid Component Storage (The Solution)
In Bevy ECS V2, we get to have our cake and eat it too. It now has _both_ of the component storage types above (and more can be added later if needed):
* **Tables** (aka "archetypal" storage)
* The default storage. If you don't configure anything, this is what you get
* Fast iteration by default
* Slower add/remove operations
* **Sparse Sets**
* Opt-in
* Slower iteration
* Faster add/remove operations
These storage types complement each other perfectly. By default Query iteration is fast. If developers know that they want to add/remove a component at high frequencies, they can set the storage to "sparse set":
```rust
world.register_component(
ComponentDescriptor::new::<MyComponent>(StorageType::SparseSet)
).unwrap();
```
## Archetypes
Archetypes are now "just metadata" ... they no longer store components directly. They do store:
* The `ComponentId`s of each of the Archetype's components (and that component's storage type)
* Archetypes are uniquely defined by their component layouts
* For example: entities with "table" components `[A, B, C]` _and_ "sparse set" components `[D, E]` will always be in the same archetype.
* The `TableId` associated with the archetype
* For now each archetype has exactly one table (which can have no components),
* There is a 1->Many relationship from Tables->Archetypes. A given table could have any number of archetype components stored in it:
* Ex: an entity with "table storage" components `[A, B, C]` and "sparse set" components `[D, E]` will share the same `[A, B, C]` table as an entity with `[A, B, C]` table component and `[F]` sparse set components.
* This 1->Many relationship is how we preserve fast "cache friendly" iteration performance when possible (more on this later)
* A list of entities that are in the archetype and the row id of the table they are in
* ArchetypeComponentIds
* unique densely packed identifiers for (ArchetypeId, ComponentId) pairs
* used by the schedule executor for cheap system access control
* "Archetype Graph Edges" (see the next section)
## The "Archetype Graph"
Archetype changes in Bevy (and a number of other archetypal ecs-es) have historically been expensive to compute. First, you need to allocate a new vector of the entity's current component ids, add or remove components based on the operation performed, sort it (to ensure it is order-independent), then hash it to find the archetype (if it exists). And thats all before we get to the _already_ expensive full copy of all components to the new table storage.
The solution is to build a "graph" of archetypes to cache these results. @SanderMertens first exposed me to the idea (and he got it from @gjroelofs, who came up with it). They propose adding directed edges between archetypes for add/remove component operations. If `ComponentId`s are densely packed, you can use sparse sets to cheaply jump between archetypes.
Bevy takes this one step further by using add/remove `Bundle` edges instead of `Component` edges. Bevy encourages the use of `Bundles` to group add/remove operations. This is largely for "clearer game logic" reasons, but it also helps cut down on the number of archetype changes required. `Bundles` now also have densely-packed `BundleId`s. This allows us to use a _single_ edge for each bundle operation (rather than needing to traverse N edges ... one for each component). Single component operations are also bundles, so this is strictly an improvement over a "component only" graph.
As a result, an operation that used to be _heavy_ (both for allocations and compute) is now two dirt-cheap array lookups and zero allocations.
## Stateful Queries
World queries are now stateful. This allows us to:
1. Cache archetype (and table) matches
* This resolves another issue with (naive) archetypal ECS: query performance getting worse as the number of archetypes goes up (and fragmentation occurs).
2. Cache Fetch and Filter state
* The expensive parts of fetch/filter operations (such as hashing the TypeId to find the ComponentId) now only happen once when the Query is first constructed
3. Incrementally build up state
* When new archetypes are added, we only process the new archetypes (no need to rebuild state for old archetypes)
As a result, the direct `World` query api now looks like this:
```rust
let mut query = world.query::<(&A, &mut B)>();
for (a, mut b) in query.iter_mut(&mut world) {
}
```
Requiring `World` to generate stateful queries (rather than letting the `QueryState` type be constructed separately) allows us to ensure that _all_ queries are properly initialized (and the relevant world state, such as ComponentIds). This enables QueryState to remove branches from its operations that check for initialization status (and also enables query.iter() to take an immutable world reference because it doesn't need to initialize anything in world).
However in systems, this is a non-breaking change. State management is done internally by the relevant SystemParam.
## Stateful SystemParams
Like Queries, `SystemParams` now also cache state. For example, `Query` system params store the "stateful query" state mentioned above. Commands store their internal `CommandQueue`. This means you can now safely use as many separate `Commands` parameters in your system as you want. `Local<T>` system params store their `T` value in their state (instead of in Resources).
SystemParam state also enabled a significant slim-down of SystemState. It is much nicer to look at now.
Per-SystemParam state naturally insulates us from an "aliased mut" class of errors we have hit in the past (ex: using multiple `Commands` system params).
(credit goes to @DJMcNab for the initial idea and draft pr here #1364)
## Configurable SystemParams
@DJMcNab also had the great idea to make SystemParams configurable. This allows users to provide some initial configuration / values for system parameters (when possible). Most SystemParams have no config (the config type is `()`), but the `Local<T>` param now supports user-provided parameters:
```rust
fn foo(value: Local<usize>) {
}
app.add_system(foo.system().config(|c| c.0 = Some(10)));
```
## Uber Fast "for_each" Query Iterators
Developers now have the choice to use a fast "for_each" iterator, which yields ~1.5-3x iteration speed improvements for "fragmented iteration", and minor ~1.2x iteration speed improvements for unfragmented iteration.
```rust
fn system(query: Query<(&A, &mut B)>) {
// you now have the option to do this for a speed boost
query.for_each_mut(|(a, mut b)| {
});
// however normal iterators are still available
for (a, mut b) in query.iter_mut() {
}
}
```
I think in most cases we should continue to encourage "normal" iterators as they are more flexible and more "rust idiomatic". But when that extra "oomf" is needed, it makes sense to use `for_each`.
We should also consider using `for_each` for internal bevy systems to give our users a nice speed boost (but that should be a separate pr).
## Component Metadata
`World` now has a `Components` collection, which is accessible via `world.components()`. This stores mappings from `ComponentId` to `ComponentInfo`, as well as `TypeId` to `ComponentId` mappings (where relevant). `ComponentInfo` stores information about the component, such as ComponentId, TypeId, memory layout, send-ness (currently limited to resources), and storage type.
## Significantly Cheaper `Access<T>`
We used to use `TypeAccess<TypeId>` to manage read/write component/archetype-component access. This was expensive because TypeIds must be hashed and compared individually. The parallel executor got around this by "condensing" type ids into bitset-backed access types. This worked, but it had to be re-generated from the `TypeAccess<TypeId>`sources every time archetypes changed.
This pr removes TypeAccess in favor of faster bitset access everywhere. We can do this thanks to the move to densely packed `ComponentId`s and `ArchetypeComponentId`s.
## Merged Resources into World
Resources had a lot of redundant functionality with Components. They stored typed data, they had access control, they had unique ids, they were queryable via SystemParams, etc. In fact the _only_ major difference between them was that they were unique (and didn't correlate to an entity).
Separate resources also had the downside of requiring a separate set of access controls, which meant the parallel executor needed to compare more bitsets per system and manage more state.
I initially got the "separate resources" idea from `legion`. I think that design was motivated by the fact that it made the direct world query/resource lifetime interactions more manageable. It certainly made our lives easier when using Resources alongside hecs/bevy_ecs. However we already have a construct for safely and ergonomically managing in-world lifetimes: systems (which use `Access<T>` internally).
This pr merges Resources into World:
```rust
world.insert_resource(1);
world.insert_resource(2.0);
let a = world.get_resource::<i32>().unwrap();
let mut b = world.get_resource_mut::<f64>().unwrap();
*b = 3.0;
```
Resources are now just a special kind of component. They have their own ComponentIds (and their own resource TypeId->ComponentId scope, so they don't conflict wit components of the same type). They are stored in a special "resource archetype", which stores components inside the archetype using a new `unique_components` sparse set (note that this sparse set could later be used to implement Tags). This allows us to keep the code size small by reusing existing datastructures (namely Column, Archetype, ComponentFlags, and ComponentInfo). This allows us the executor to use a single `Access<ArchetypeComponentId>` per system. It should also make scripting language integration easier.
_But_ this merge did create problems for people directly interacting with `World`. What if you need mutable access to multiple resources at the same time? `world.get_resource_mut()` borrows World mutably!
## WorldCell
WorldCell applies the `Access<ArchetypeComponentId>` concept to direct world access:
```rust
let world_cell = world.cell();
let a = world_cell.get_resource_mut::<i32>().unwrap();
let b = world_cell.get_resource_mut::<f64>().unwrap();
```
This adds cheap runtime checks (a sparse set lookup of `ArchetypeComponentId` and a counter) to ensure that world accesses do not conflict with each other. Each operation returns a `WorldBorrow<'w, T>` or `WorldBorrowMut<'w, T>` wrapper type, which will release the relevant ArchetypeComponentId resources when dropped.
World caches the access sparse set (and only one cell can exist at a time), so `world.cell()` is a cheap operation.
WorldCell does _not_ use atomic operations. It is non-send, does a mutable borrow of world to prevent other accesses, and uses a simple `Rc<RefCell<ArchetypeComponentAccess>>` wrapper in each WorldBorrow pointer.
The api is currently limited to resource access, but it can and should be extended to queries / entity component access.
## Resource Scopes
WorldCell does not yet support component queries, and even when it does there are sometimes legitimate reasons to want a mutable world ref _and_ a mutable resource ref (ex: bevy_render and bevy_scene both need this). In these cases we could always drop down to the unsafe `world.get_resource_unchecked_mut()`, but that is not ideal!
Instead developers can use a "resource scope"
```rust
world.resource_scope(|world: &mut World, a: &mut A| {
})
```
This temporarily removes the `A` resource from `World`, provides mutable pointers to both, and re-adds A to World when finished. Thanks to the move to ComponentIds/sparse sets, this is a cheap operation.
If multiple resources are required, scopes can be nested. We could also consider adding a "resource tuple" to the api if this pattern becomes common and the boilerplate gets nasty.
## Query Conflicts Use ComponentId Instead of ArchetypeComponentId
For safety reasons, systems cannot contain queries that conflict with each other without wrapping them in a QuerySet. On bevy `main`, we use ArchetypeComponentIds to determine conflicts. This is nice because it can take into account filters:
```rust
// these queries will never conflict due to their filters
fn filter_system(a: Query<&mut A, With<B>>, b: Query<&mut B, Without<B>>) {
}
```
But it also has a significant downside:
```rust
// these queries will not conflict _until_ an entity with A, B, and C is spawned
fn maybe_conflicts_system(a: Query<(&mut A, &C)>, b: Query<(&mut A, &B)>) {
}
```
The system above will panic at runtime if an entity with A, B, and C is spawned. This makes it hard to trust that your game logic will run without crashing.
In this pr, I switched to using `ComponentId` instead. This _is_ more constraining. `maybe_conflicts_system` will now always fail, but it will do it consistently at startup. Naively, it would also _disallow_ `filter_system`, which would be a significant downgrade in usability. Bevy has a number of internal systems that rely on disjoint queries and I expect it to be a common pattern in userspace.
To resolve this, I added a new `FilteredAccess<T>` type, which wraps `Access<T>` and adds with/without filters. If two `FilteredAccess` have with/without values that prove they are disjoint, they will no longer conflict.
## EntityRef / EntityMut
World entity operations on `main` require that the user passes in an `entity` id to each operation:
```rust
let entity = world.spawn((A, )); // create a new entity with A
world.get::<A>(entity);
world.insert(entity, (B, C));
world.insert_one(entity, D);
```
This means that each operation needs to look up the entity location / verify its validity. The initial spawn operation also requires a Bundle as input. This can be awkward when no components are required (or one component is required).
These operations have been replaced by `EntityRef` and `EntityMut`, which are "builder-style" wrappers around world that provide read and read/write operations on a single, pre-validated entity:
```rust
// spawn now takes no inputs and returns an EntityMut
let entity = world.spawn()
.insert(A) // insert a single component into the entity
.insert_bundle((B, C)) // insert a bundle of components into the entity
.id() // id returns the Entity id
// Returns EntityMut (or panics if the entity does not exist)
world.entity_mut(entity)
.insert(D)
.insert_bundle(SomeBundle::default());
{
// returns EntityRef (or panics if the entity does not exist)
let d = world.entity(entity)
.get::<D>() // gets the D component
.unwrap();
// world.get still exists for ergonomics
let d = world.get::<D>(entity).unwrap();
}
// These variants return Options if you want to check existence instead of panicing
world.get_entity_mut(entity)
.unwrap()
.insert(E);
if let Some(entity_ref) = world.get_entity(entity) {
let d = entity_ref.get::<D>().unwrap();
}
```
This _does not_ affect the current Commands api or terminology. I think that should be a separate conversation as that is a much larger breaking change.
## Safety Improvements
* Entity reservation in Commands uses a normal world borrow instead of an unsafe transmute
* QuerySets no longer transmutes lifetimes
* Made traits "unsafe" when implementing a trait incorrectly could cause unsafety
* More thorough safety docs
## RemovedComponents SystemParam
The old approach to querying removed components: `query.removed:<T>()` was confusing because it had no connection to the query itself. I replaced it with the following, which is both clearer and allows us to cache the ComponentId mapping in the SystemParamState:
```rust
fn system(removed: RemovedComponents<T>) {
for entity in removed.iter() {
}
}
```
## Simpler Bundle implementation
Bundles are no longer responsible for sorting (or deduping) TypeInfo. They are just a simple ordered list of component types / data. This makes the implementation smaller and opens the door to an easy "nested bundle" implementation in the future (which i might even add in this pr). Duplicate detection is now done once per bundle type by World the first time a bundle is used.
## Unified WorldQuery and QueryFilter types
(don't worry they are still separate type _parameters_ in Queries .. this is a non-breaking change)
WorldQuery and QueryFilter were already basically identical apis. With the addition of `FetchState` and more storage-specific fetch methods, the overlap was even clearer (and the redundancy more painful).
QueryFilters are now just `F: WorldQuery where F::Fetch: FilterFetch`. FilterFetch requires `Fetch<Item = bool>` and adds new "short circuit" variants of fetch methods. This enables a filter tuple like `(With<A>, Without<B>, Changed<C>)` to stop evaluating the filter after the first mismatch is encountered. FilterFetch is automatically implemented for `Fetch` implementations that return bool.
This forces fetch implementations that return things like `(bool, bool, bool)` (such as the filter above) to manually implement FilterFetch and decide whether or not to short-circuit.
## More Granular Modules
World no longer globs all of the internal modules together. It now exports `core`, `system`, and `schedule` separately. I'm also considering exporting `core` submodules directly as that is still pretty "glob-ey" and unorganized (feedback welcome here).
## Remaining Draft Work (to be done in this pr)
* ~~panic on conflicting WorldQuery fetches (&A, &mut A)~~
* ~~bevy `main` and hecs both currently allow this, but we should protect against it if possible~~
* ~~batch_iter / par_iter (currently stubbed out)~~
* ~~ChangedRes~~
* ~~I skipped this while we sort out #1313. This pr should be adapted to account for whatever we land on there~~.
* ~~The `Archetypes` and `Tables` collections use hashes of sorted lists of component ids to uniquely identify each archetype/table. This hash is then used as the key in a HashMap to look up the relevant ArchetypeId or TableId. (which doesn't handle hash collisions properly)~~
* ~~It is currently unsafe to generate a Query from "World A", then use it on "World B" (despite the api claiming it is safe). We should probably close this gap. This could be done by adding a randomly generated WorldId to each world, then storing that id in each Query. They could then be compared to each other on each `query.do_thing(&world)` operation. This _does_ add an extra branch to each query operation, so I'm open to other suggestions if people have them.~~
* ~~Nested Bundles (if i find time)~~
## Potential Future Work
* Expand WorldCell to support queries.
* Consider not allocating in the empty archetype on `world.spawn()`
* ex: return something like EntityMutUninit, which turns into EntityMut after an `insert` or `insert_bundle` op
* this actually regressed performance last time i tried it, but in theory it should be faster
* Optimize SparseSet::insert (see `PERF` comment on insert)
* Replace SparseArray `Option<T>` with T::MAX to cut down on branching
* would enable cheaper get_unchecked() operations
* upstream fixedbitset optimizations
* fixedbitset could be allocation free for small block counts (store blocks in a SmallVec)
* fixedbitset could have a const constructor
* Consider implementing Tags (archetype-specific by-value data that affects archetype identity)
* ex: ArchetypeA could have `[A, B, C]` table components and `[D(1)]` "tag" component. ArchetypeB could have `[A, B, C]` table components and a `[D(2)]` tag component. The archetypes are different, despite both having D tags because the value inside D is different.
* this could potentially build on top of the `archetype.unique_components` added in this pr for resource storage.
* Consider reverting `all_tuples` proc macro in favor of the old `macro_rules` implementation
* all_tuples is more flexible and produces cleaner documentation (the macro_rules version produces weird type parameter orders due to parser constraints)
* but unfortunately all_tuples also appears to make Rust Analyzer sad/slow when working inside of `bevy_ecs` (does not affect user code)
* Consider "resource queries" and/or "mixed resource and entity component queries" as an alternative to WorldCell
* this is basically just "systems" so maybe it's not worth it
* Add more world ops
* `world.clear()`
* `world.reserve<T: Bundle>(count: usize)`
* Try using the old archetype allocation strategy (allocate new memory on resize and copy everything over). I expect this to improve batch insertion performance at the cost of unbatched performance. But thats just a guess. I'm not an allocation perf pro :)
* Adapt Commands apis for consistency with new World apis
## Benchmarks
key:
* `bevy_old`: bevy `main` branch
* `bevy`: this branch
* `_foreach`: uses an optimized for_each iterator
* ` _sparse`: uses sparse set storage (if unspecified assume table storage)
* `_system`: runs inside a system (if unspecified assume test happens via direct world ops)
### Simple Insert (from ecs_bench_suite)
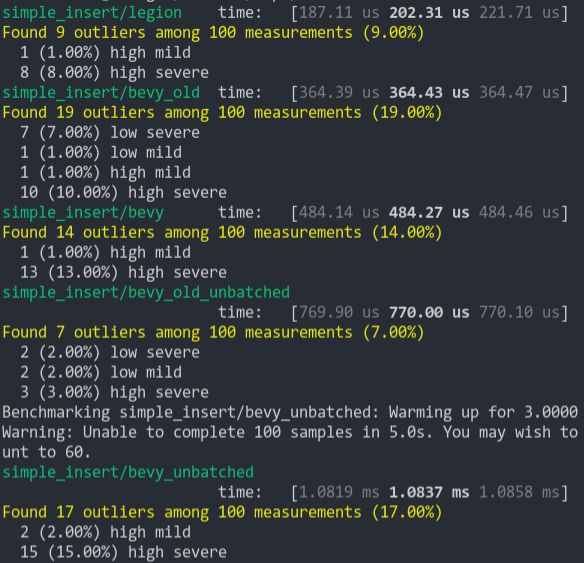
### Simpler Iter (from ecs_bench_suite)
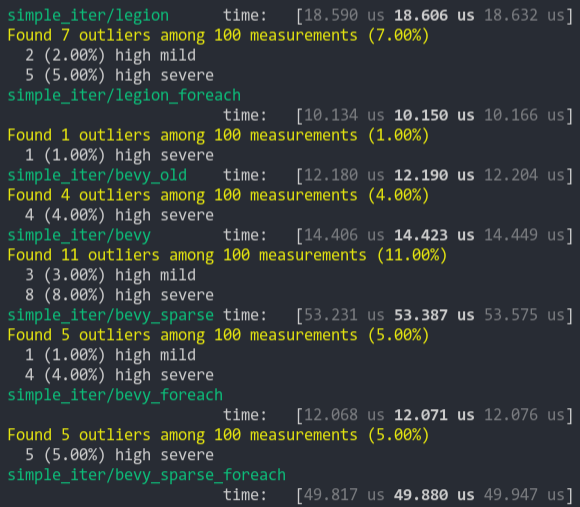
### Fragment Iter (from ecs_bench_suite)
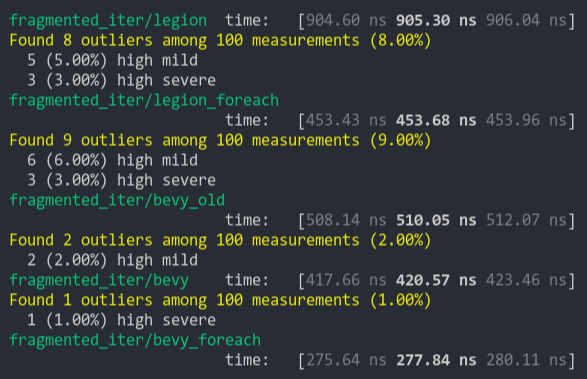
### Sparse Fragmented Iter
Iterate a query that matches 5 entities from a single matching archetype, but there are 100 unmatching archetypes
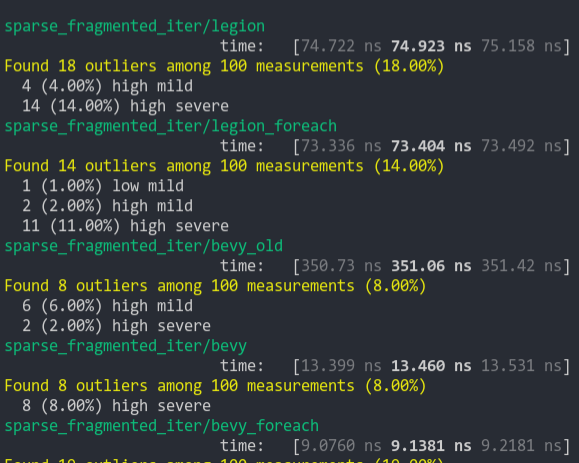
### Schedule (from ecs_bench_suite)
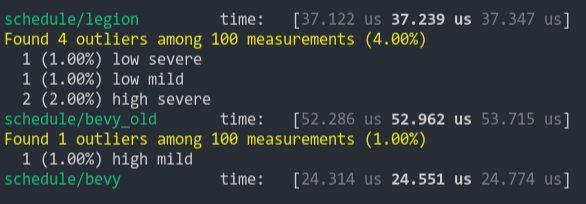
### Add Remove Component (from ecs_bench_suite)
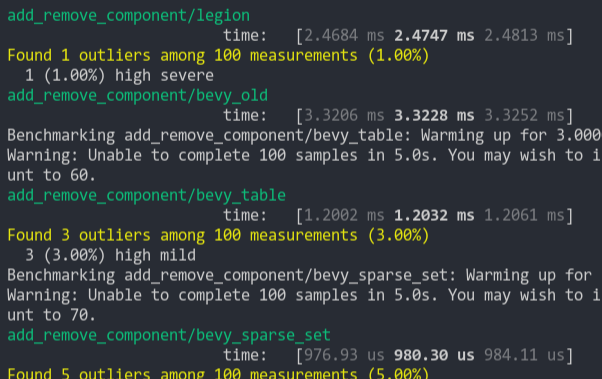
### Add Remove Component Big
Same as the test above, but each entity has 5 "large" matrix components and 1 "large" matrix component is added and removed
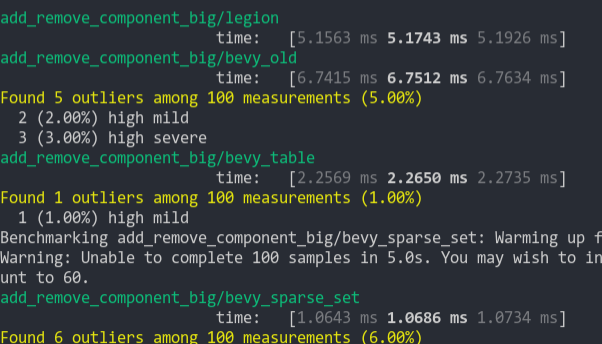
### Get Component
Looks up a single component value a large number of times
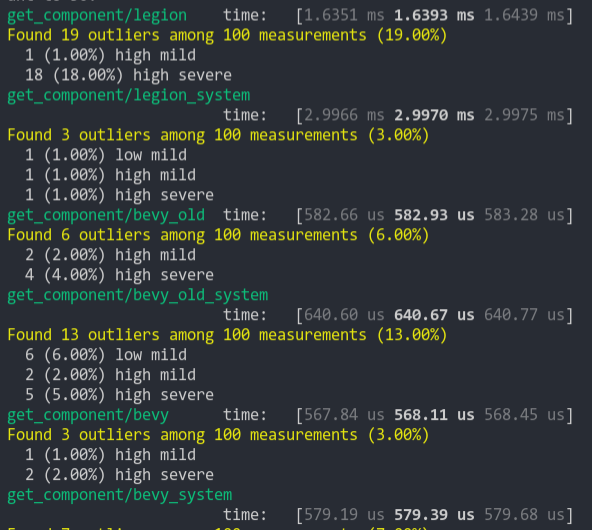
2021-03-05 07:54:35 +00:00
world ::{ FromWorld , World } ,
} ;
2023-02-16 17:09:44 +00:00
use bevy_ecs_macros ::impl_param_set ;
Make `Resource` trait opt-in, requiring `#[derive(Resource)]` V2 (#5577)
*This PR description is an edited copy of #5007, written by @alice-i-cecile.*
# Objective
Follow-up to https://github.com/bevyengine/bevy/pull/2254. The `Resource` trait currently has a blanket implementation for all types that meet its bounds.
While ergonomic, this results in several drawbacks:
* it is possible to make confusing, silent mistakes such as inserting a function pointer (Foo) rather than a value (Foo::Bar) as a resource
* it is challenging to discover if a type is intended to be used as a resource
* we cannot later add customization options (see the [RFC](https://github.com/bevyengine/rfcs/blob/main/rfcs/27-derive-component.md) for the equivalent choice for Component).
* dependencies can use the same Rust type as a resource in invisibly conflicting ways
* raw Rust types used as resources cannot preserve privacy appropriately, as anyone able to access that type can read and write to internal values
* we cannot capture a definitive list of possible resources to display to users in an editor
## Notes to reviewers
* Review this commit-by-commit; there's effectively no back-tracking and there's a lot of churn in some of these commits.
*ira: My commits are not as well organized :')*
* I've relaxed the bound on Local to Send + Sync + 'static: I don't think these concerns apply there, so this can keep things simple. Storing e.g. a u32 in a Local is fine, because there's a variable name attached explaining what it does.
* I think this is a bad place for the Resource trait to live, but I've left it in place to make reviewing easier. IMO that's best tackled with https://github.com/bevyengine/bevy/issues/4981.
## Changelog
`Resource` is no longer automatically implemented for all matching types. Instead, use the new `#[derive(Resource)]` macro.
## Migration Guide
Add `#[derive(Resource)]` to all types you are using as a resource.
If you are using a third party type as a resource, wrap it in a tuple struct to bypass orphan rules. Consider deriving `Deref` and `DerefMut` to improve ergonomics.
`ClearColor` no longer implements `Component`. Using `ClearColor` as a component in 0.8 did nothing.
Use the `ClearColorConfig` in the `Camera3d` and `Camera2d` components instead.
Co-authored-by: Alice <alice.i.cecile@gmail.com>
Co-authored-by: Alice Cecile <alice.i.cecile@gmail.com>
Co-authored-by: devil-ira <justthecooldude@gmail.com>
Co-authored-by: Carter Anderson <mcanders1@gmail.com>
2022-08-08 21:36:35 +00:00
pub use bevy_ecs_macros ::Resource ;
Bevy ECS V2 (#1525)
# Bevy ECS V2
This is a rewrite of Bevy ECS (basically everything but the new executor/schedule, which are already awesome). The overall goal was to improve the performance and versatility of Bevy ECS. Here is a quick bulleted list of changes before we dive into the details:
* Complete World rewrite
* Multiple component storage types:
* Tables: fast cache friendly iteration, slower add/removes (previously called Archetypes)
* Sparse Sets: fast add/remove, slower iteration
* Stateful Queries (caches query results for faster iteration. fragmented iteration is _fast_ now)
* Stateful System Params (caches expensive operations. inspired by @DJMcNab's work in #1364)
* Configurable System Params (users can set configuration when they construct their systems. once again inspired by @DJMcNab's work)
* Archetypes are now "just metadata", component storage is separate
* Archetype Graph (for faster archetype changes)
* Component Metadata
* Configure component storage type
* Retrieve information about component size/type/name/layout/send-ness/etc
* Components are uniquely identified by a densely packed ComponentId
* TypeIds are now totally optional (which should make implementing scripting easier)
* Super fast "for_each" query iterators
* Merged Resources into World. Resources are now just a special type of component
* EntityRef/EntityMut builder apis (more efficient and more ergonomic)
* Fast bitset-backed `Access<T>` replaces old hashmap-based approach everywhere
* Query conflicts are determined by component access instead of archetype component access (to avoid random failures at runtime)
* With/Without are still taken into account for conflicts, so this should still be comfy to use
* Much simpler `IntoSystem` impl
* Significantly reduced the amount of hashing throughout the ecs in favor of Sparse Sets (indexed by densely packed ArchetypeId, ComponentId, BundleId, and TableId)
* Safety Improvements
* Entity reservation uses a normal world reference instead of unsafe transmute
* QuerySets no longer transmute lifetimes
* Made traits "unsafe" where relevant
* More thorough safety docs
* WorldCell
* Exposes safe mutable access to multiple resources at a time in a World
* Replaced "catch all" `System::update_archetypes(world: &World)` with `System::new_archetype(archetype: &Archetype)`
* Simpler Bundle implementation
* Replaced slow "remove_bundle_one_by_one" used as fallback for Commands::remove_bundle with fast "remove_bundle_intersection"
* Removed `Mut<T>` query impl. it is better to only support one way: `&mut T`
* Removed with() from `Flags<T>` in favor of `Option<Flags<T>>`, which allows querying for flags to be "filtered" by default
* Components now have is_send property (currently only resources support non-send)
* More granular module organization
* New `RemovedComponents<T>` SystemParam that replaces `query.removed::<T>()`
* `world.resource_scope()` for mutable access to resources and world at the same time
* WorldQuery and QueryFilter traits unified. FilterFetch trait added to enable "short circuit" filtering. Auto impled for cases that don't need it
* Significantly slimmed down SystemState in favor of individual SystemParam state
* System Commands changed from `commands: &mut Commands` back to `mut commands: Commands` (to allow Commands to have a World reference)
Fixes #1320
## `World` Rewrite
This is a from-scratch rewrite of `World` that fills the niche that `hecs` used to. Yes, this means Bevy ECS is no longer a "fork" of hecs. We're going out our own!
(the only shared code between the projects is the entity id allocator, which is already basically ideal)
A huge shout out to @SanderMertens (author of [flecs](https://github.com/SanderMertens/flecs)) for sharing some great ideas with me (specifically hybrid ecs storage and archetype graphs). He also helped advise on a number of implementation details.
## Component Storage (The Problem)
Two ECS storage paradigms have gained a lot of traction over the years:
* **Archetypal ECS**:
* Stores components in "tables" with static schemas. Each "column" stores components of a given type. Each "row" is an entity.
* Each "archetype" has its own table. Adding/removing an entity's component changes the archetype.
* Enables super-fast Query iteration due to its cache-friendly data layout
* Comes at the cost of more expensive add/remove operations for an Entity's components, because all components need to be copied to the new archetype's "table"
* **Sparse Set ECS**:
* Stores components of the same type in densely packed arrays, which are sparsely indexed by densely packed unsigned integers (Entity ids)
* Query iteration is slower than Archetypal ECS because each entity's component could be at any position in the sparse set. This "random access" pattern isn't cache friendly. Additionally, there is an extra layer of indirection because you must first map the entity id to an index in the component array.
* Adding/removing components is a cheap, constant time operation
Bevy ECS V1, hecs, legion, flec, and Unity DOTS are all "archetypal ecs-es". I personally think "archetypal" storage is a good default for game engines. An entity's archetype doesn't need to change frequently in general, and it creates "fast by default" query iteration (which is a much more common operation). It is also "self optimizing". Users don't need to think about optimizing component layouts for iteration performance. It "just works" without any extra boilerplate.
Shipyard and EnTT are "sparse set ecs-es". They employ "packing" as a way to work around the "suboptimal by default" iteration performance for specific sets of components. This helps, but I didn't think this was a good choice for a general purpose engine like Bevy because:
1. "packs" conflict with each other. If bevy decides to internally pack the Transform and GlobalTransform components, users are then blocked if they want to pack some custom component with Transform.
2. users need to take manual action to optimize
Developers selecting an ECS framework are stuck with a hard choice. Select an "archetypal" framework with "fast iteration everywhere" but without the ability to cheaply add/remove components, or select a "sparse set" framework to cheaply add/remove components but with slower iteration performance.
## Hybrid Component Storage (The Solution)
In Bevy ECS V2, we get to have our cake and eat it too. It now has _both_ of the component storage types above (and more can be added later if needed):
* **Tables** (aka "archetypal" storage)
* The default storage. If you don't configure anything, this is what you get
* Fast iteration by default
* Slower add/remove operations
* **Sparse Sets**
* Opt-in
* Slower iteration
* Faster add/remove operations
These storage types complement each other perfectly. By default Query iteration is fast. If developers know that they want to add/remove a component at high frequencies, they can set the storage to "sparse set":
```rust
world.register_component(
ComponentDescriptor::new::<MyComponent>(StorageType::SparseSet)
).unwrap();
```
## Archetypes
Archetypes are now "just metadata" ... they no longer store components directly. They do store:
* The `ComponentId`s of each of the Archetype's components (and that component's storage type)
* Archetypes are uniquely defined by their component layouts
* For example: entities with "table" components `[A, B, C]` _and_ "sparse set" components `[D, E]` will always be in the same archetype.
* The `TableId` associated with the archetype
* For now each archetype has exactly one table (which can have no components),
* There is a 1->Many relationship from Tables->Archetypes. A given table could have any number of archetype components stored in it:
* Ex: an entity with "table storage" components `[A, B, C]` and "sparse set" components `[D, E]` will share the same `[A, B, C]` table as an entity with `[A, B, C]` table component and `[F]` sparse set components.
* This 1->Many relationship is how we preserve fast "cache friendly" iteration performance when possible (more on this later)
* A list of entities that are in the archetype and the row id of the table they are in
* ArchetypeComponentIds
* unique densely packed identifiers for (ArchetypeId, ComponentId) pairs
* used by the schedule executor for cheap system access control
* "Archetype Graph Edges" (see the next section)
## The "Archetype Graph"
Archetype changes in Bevy (and a number of other archetypal ecs-es) have historically been expensive to compute. First, you need to allocate a new vector of the entity's current component ids, add or remove components based on the operation performed, sort it (to ensure it is order-independent), then hash it to find the archetype (if it exists). And thats all before we get to the _already_ expensive full copy of all components to the new table storage.
The solution is to build a "graph" of archetypes to cache these results. @SanderMertens first exposed me to the idea (and he got it from @gjroelofs, who came up with it). They propose adding directed edges between archetypes for add/remove component operations. If `ComponentId`s are densely packed, you can use sparse sets to cheaply jump between archetypes.
Bevy takes this one step further by using add/remove `Bundle` edges instead of `Component` edges. Bevy encourages the use of `Bundles` to group add/remove operations. This is largely for "clearer game logic" reasons, but it also helps cut down on the number of archetype changes required. `Bundles` now also have densely-packed `BundleId`s. This allows us to use a _single_ edge for each bundle operation (rather than needing to traverse N edges ... one for each component). Single component operations are also bundles, so this is strictly an improvement over a "component only" graph.
As a result, an operation that used to be _heavy_ (both for allocations and compute) is now two dirt-cheap array lookups and zero allocations.
## Stateful Queries
World queries are now stateful. This allows us to:
1. Cache archetype (and table) matches
* This resolves another issue with (naive) archetypal ECS: query performance getting worse as the number of archetypes goes up (and fragmentation occurs).
2. Cache Fetch and Filter state
* The expensive parts of fetch/filter operations (such as hashing the TypeId to find the ComponentId) now only happen once when the Query is first constructed
3. Incrementally build up state
* When new archetypes are added, we only process the new archetypes (no need to rebuild state for old archetypes)
As a result, the direct `World` query api now looks like this:
```rust
let mut query = world.query::<(&A, &mut B)>();
for (a, mut b) in query.iter_mut(&mut world) {
}
```
Requiring `World` to generate stateful queries (rather than letting the `QueryState` type be constructed separately) allows us to ensure that _all_ queries are properly initialized (and the relevant world state, such as ComponentIds). This enables QueryState to remove branches from its operations that check for initialization status (and also enables query.iter() to take an immutable world reference because it doesn't need to initialize anything in world).
However in systems, this is a non-breaking change. State management is done internally by the relevant SystemParam.
## Stateful SystemParams
Like Queries, `SystemParams` now also cache state. For example, `Query` system params store the "stateful query" state mentioned above. Commands store their internal `CommandQueue`. This means you can now safely use as many separate `Commands` parameters in your system as you want. `Local<T>` system params store their `T` value in their state (instead of in Resources).
SystemParam state also enabled a significant slim-down of SystemState. It is much nicer to look at now.
Per-SystemParam state naturally insulates us from an "aliased mut" class of errors we have hit in the past (ex: using multiple `Commands` system params).
(credit goes to @DJMcNab for the initial idea and draft pr here #1364)
## Configurable SystemParams
@DJMcNab also had the great idea to make SystemParams configurable. This allows users to provide some initial configuration / values for system parameters (when possible). Most SystemParams have no config (the config type is `()`), but the `Local<T>` param now supports user-provided parameters:
```rust
fn foo(value: Local<usize>) {
}
app.add_system(foo.system().config(|c| c.0 = Some(10)));
```
## Uber Fast "for_each" Query Iterators
Developers now have the choice to use a fast "for_each" iterator, which yields ~1.5-3x iteration speed improvements for "fragmented iteration", and minor ~1.2x iteration speed improvements for unfragmented iteration.
```rust
fn system(query: Query<(&A, &mut B)>) {
// you now have the option to do this for a speed boost
query.for_each_mut(|(a, mut b)| {
});
// however normal iterators are still available
for (a, mut b) in query.iter_mut() {
}
}
```
I think in most cases we should continue to encourage "normal" iterators as they are more flexible and more "rust idiomatic". But when that extra "oomf" is needed, it makes sense to use `for_each`.
We should also consider using `for_each` for internal bevy systems to give our users a nice speed boost (but that should be a separate pr).
## Component Metadata
`World` now has a `Components` collection, which is accessible via `world.components()`. This stores mappings from `ComponentId` to `ComponentInfo`, as well as `TypeId` to `ComponentId` mappings (where relevant). `ComponentInfo` stores information about the component, such as ComponentId, TypeId, memory layout, send-ness (currently limited to resources), and storage type.
## Significantly Cheaper `Access<T>`
We used to use `TypeAccess<TypeId>` to manage read/write component/archetype-component access. This was expensive because TypeIds must be hashed and compared individually. The parallel executor got around this by "condensing" type ids into bitset-backed access types. This worked, but it had to be re-generated from the `TypeAccess<TypeId>`sources every time archetypes changed.
This pr removes TypeAccess in favor of faster bitset access everywhere. We can do this thanks to the move to densely packed `ComponentId`s and `ArchetypeComponentId`s.
## Merged Resources into World
Resources had a lot of redundant functionality with Components. They stored typed data, they had access control, they had unique ids, they were queryable via SystemParams, etc. In fact the _only_ major difference between them was that they were unique (and didn't correlate to an entity).
Separate resources also had the downside of requiring a separate set of access controls, which meant the parallel executor needed to compare more bitsets per system and manage more state.
I initially got the "separate resources" idea from `legion`. I think that design was motivated by the fact that it made the direct world query/resource lifetime interactions more manageable. It certainly made our lives easier when using Resources alongside hecs/bevy_ecs. However we already have a construct for safely and ergonomically managing in-world lifetimes: systems (which use `Access<T>` internally).
This pr merges Resources into World:
```rust
world.insert_resource(1);
world.insert_resource(2.0);
let a = world.get_resource::<i32>().unwrap();
let mut b = world.get_resource_mut::<f64>().unwrap();
*b = 3.0;
```
Resources are now just a special kind of component. They have their own ComponentIds (and their own resource TypeId->ComponentId scope, so they don't conflict wit components of the same type). They are stored in a special "resource archetype", which stores components inside the archetype using a new `unique_components` sparse set (note that this sparse set could later be used to implement Tags). This allows us to keep the code size small by reusing existing datastructures (namely Column, Archetype, ComponentFlags, and ComponentInfo). This allows us the executor to use a single `Access<ArchetypeComponentId>` per system. It should also make scripting language integration easier.
_But_ this merge did create problems for people directly interacting with `World`. What if you need mutable access to multiple resources at the same time? `world.get_resource_mut()` borrows World mutably!
## WorldCell
WorldCell applies the `Access<ArchetypeComponentId>` concept to direct world access:
```rust
let world_cell = world.cell();
let a = world_cell.get_resource_mut::<i32>().unwrap();
let b = world_cell.get_resource_mut::<f64>().unwrap();
```
This adds cheap runtime checks (a sparse set lookup of `ArchetypeComponentId` and a counter) to ensure that world accesses do not conflict with each other. Each operation returns a `WorldBorrow<'w, T>` or `WorldBorrowMut<'w, T>` wrapper type, which will release the relevant ArchetypeComponentId resources when dropped.
World caches the access sparse set (and only one cell can exist at a time), so `world.cell()` is a cheap operation.
WorldCell does _not_ use atomic operations. It is non-send, does a mutable borrow of world to prevent other accesses, and uses a simple `Rc<RefCell<ArchetypeComponentAccess>>` wrapper in each WorldBorrow pointer.
The api is currently limited to resource access, but it can and should be extended to queries / entity component access.
## Resource Scopes
WorldCell does not yet support component queries, and even when it does there are sometimes legitimate reasons to want a mutable world ref _and_ a mutable resource ref (ex: bevy_render and bevy_scene both need this). In these cases we could always drop down to the unsafe `world.get_resource_unchecked_mut()`, but that is not ideal!
Instead developers can use a "resource scope"
```rust
world.resource_scope(|world: &mut World, a: &mut A| {
})
```
This temporarily removes the `A` resource from `World`, provides mutable pointers to both, and re-adds A to World when finished. Thanks to the move to ComponentIds/sparse sets, this is a cheap operation.
If multiple resources are required, scopes can be nested. We could also consider adding a "resource tuple" to the api if this pattern becomes common and the boilerplate gets nasty.
## Query Conflicts Use ComponentId Instead of ArchetypeComponentId
For safety reasons, systems cannot contain queries that conflict with each other without wrapping them in a QuerySet. On bevy `main`, we use ArchetypeComponentIds to determine conflicts. This is nice because it can take into account filters:
```rust
// these queries will never conflict due to their filters
fn filter_system(a: Query<&mut A, With<B>>, b: Query<&mut B, Without<B>>) {
}
```
But it also has a significant downside:
```rust
// these queries will not conflict _until_ an entity with A, B, and C is spawned
fn maybe_conflicts_system(a: Query<(&mut A, &C)>, b: Query<(&mut A, &B)>) {
}
```
The system above will panic at runtime if an entity with A, B, and C is spawned. This makes it hard to trust that your game logic will run without crashing.
In this pr, I switched to using `ComponentId` instead. This _is_ more constraining. `maybe_conflicts_system` will now always fail, but it will do it consistently at startup. Naively, it would also _disallow_ `filter_system`, which would be a significant downgrade in usability. Bevy has a number of internal systems that rely on disjoint queries and I expect it to be a common pattern in userspace.
To resolve this, I added a new `FilteredAccess<T>` type, which wraps `Access<T>` and adds with/without filters. If two `FilteredAccess` have with/without values that prove they are disjoint, they will no longer conflict.
## EntityRef / EntityMut
World entity operations on `main` require that the user passes in an `entity` id to each operation:
```rust
let entity = world.spawn((A, )); // create a new entity with A
world.get::<A>(entity);
world.insert(entity, (B, C));
world.insert_one(entity, D);
```
This means that each operation needs to look up the entity location / verify its validity. The initial spawn operation also requires a Bundle as input. This can be awkward when no components are required (or one component is required).
These operations have been replaced by `EntityRef` and `EntityMut`, which are "builder-style" wrappers around world that provide read and read/write operations on a single, pre-validated entity:
```rust
// spawn now takes no inputs and returns an EntityMut
let entity = world.spawn()
.insert(A) // insert a single component into the entity
.insert_bundle((B, C)) // insert a bundle of components into the entity
.id() // id returns the Entity id
// Returns EntityMut (or panics if the entity does not exist)
world.entity_mut(entity)
.insert(D)
.insert_bundle(SomeBundle::default());
{
// returns EntityRef (or panics if the entity does not exist)
let d = world.entity(entity)
.get::<D>() // gets the D component
.unwrap();
// world.get still exists for ergonomics
let d = world.get::<D>(entity).unwrap();
}
// These variants return Options if you want to check existence instead of panicing
world.get_entity_mut(entity)
.unwrap()
.insert(E);
if let Some(entity_ref) = world.get_entity(entity) {
let d = entity_ref.get::<D>().unwrap();
}
```
This _does not_ affect the current Commands api or terminology. I think that should be a separate conversation as that is a much larger breaking change.
## Safety Improvements
* Entity reservation in Commands uses a normal world borrow instead of an unsafe transmute
* QuerySets no longer transmutes lifetimes
* Made traits "unsafe" when implementing a trait incorrectly could cause unsafety
* More thorough safety docs
## RemovedComponents SystemParam
The old approach to querying removed components: `query.removed:<T>()` was confusing because it had no connection to the query itself. I replaced it with the following, which is both clearer and allows us to cache the ComponentId mapping in the SystemParamState:
```rust
fn system(removed: RemovedComponents<T>) {
for entity in removed.iter() {
}
}
```
## Simpler Bundle implementation
Bundles are no longer responsible for sorting (or deduping) TypeInfo. They are just a simple ordered list of component types / data. This makes the implementation smaller and opens the door to an easy "nested bundle" implementation in the future (which i might even add in this pr). Duplicate detection is now done once per bundle type by World the first time a bundle is used.
## Unified WorldQuery and QueryFilter types
(don't worry they are still separate type _parameters_ in Queries .. this is a non-breaking change)
WorldQuery and QueryFilter were already basically identical apis. With the addition of `FetchState` and more storage-specific fetch methods, the overlap was even clearer (and the redundancy more painful).
QueryFilters are now just `F: WorldQuery where F::Fetch: FilterFetch`. FilterFetch requires `Fetch<Item = bool>` and adds new "short circuit" variants of fetch methods. This enables a filter tuple like `(With<A>, Without<B>, Changed<C>)` to stop evaluating the filter after the first mismatch is encountered. FilterFetch is automatically implemented for `Fetch` implementations that return bool.
This forces fetch implementations that return things like `(bool, bool, bool)` (such as the filter above) to manually implement FilterFetch and decide whether or not to short-circuit.
## More Granular Modules
World no longer globs all of the internal modules together. It now exports `core`, `system`, and `schedule` separately. I'm also considering exporting `core` submodules directly as that is still pretty "glob-ey" and unorganized (feedback welcome here).
## Remaining Draft Work (to be done in this pr)
* ~~panic on conflicting WorldQuery fetches (&A, &mut A)~~
* ~~bevy `main` and hecs both currently allow this, but we should protect against it if possible~~
* ~~batch_iter / par_iter (currently stubbed out)~~
* ~~ChangedRes~~
* ~~I skipped this while we sort out #1313. This pr should be adapted to account for whatever we land on there~~.
* ~~The `Archetypes` and `Tables` collections use hashes of sorted lists of component ids to uniquely identify each archetype/table. This hash is then used as the key in a HashMap to look up the relevant ArchetypeId or TableId. (which doesn't handle hash collisions properly)~~
* ~~It is currently unsafe to generate a Query from "World A", then use it on "World B" (despite the api claiming it is safe). We should probably close this gap. This could be done by adding a randomly generated WorldId to each world, then storing that id in each Query. They could then be compared to each other on each `query.do_thing(&world)` operation. This _does_ add an extra branch to each query operation, so I'm open to other suggestions if people have them.~~
* ~~Nested Bundles (if i find time)~~
## Potential Future Work
* Expand WorldCell to support queries.
* Consider not allocating in the empty archetype on `world.spawn()`
* ex: return something like EntityMutUninit, which turns into EntityMut after an `insert` or `insert_bundle` op
* this actually regressed performance last time i tried it, but in theory it should be faster
* Optimize SparseSet::insert (see `PERF` comment on insert)
* Replace SparseArray `Option<T>` with T::MAX to cut down on branching
* would enable cheaper get_unchecked() operations
* upstream fixedbitset optimizations
* fixedbitset could be allocation free for small block counts (store blocks in a SmallVec)
* fixedbitset could have a const constructor
* Consider implementing Tags (archetype-specific by-value data that affects archetype identity)
* ex: ArchetypeA could have `[A, B, C]` table components and `[D(1)]` "tag" component. ArchetypeB could have `[A, B, C]` table components and a `[D(2)]` tag component. The archetypes are different, despite both having D tags because the value inside D is different.
* this could potentially build on top of the `archetype.unique_components` added in this pr for resource storage.
* Consider reverting `all_tuples` proc macro in favor of the old `macro_rules` implementation
* all_tuples is more flexible and produces cleaner documentation (the macro_rules version produces weird type parameter orders due to parser constraints)
* but unfortunately all_tuples also appears to make Rust Analyzer sad/slow when working inside of `bevy_ecs` (does not affect user code)
* Consider "resource queries" and/or "mixed resource and entity component queries" as an alternative to WorldCell
* this is basically just "systems" so maybe it's not worth it
* Add more world ops
* `world.clear()`
* `world.reserve<T: Bundle>(count: usize)`
* Try using the old archetype allocation strategy (allocate new memory on resize and copy everything over). I expect this to improve batch insertion performance at the cost of unbatched performance. But thats just a guess. I'm not an allocation perf pro :)
* Adapt Commands apis for consistency with new World apis
## Benchmarks
key:
* `bevy_old`: bevy `main` branch
* `bevy`: this branch
* `_foreach`: uses an optimized for_each iterator
* ` _sparse`: uses sparse set storage (if unspecified assume table storage)
* `_system`: runs inside a system (if unspecified assume test happens via direct world ops)
### Simple Insert (from ecs_bench_suite)
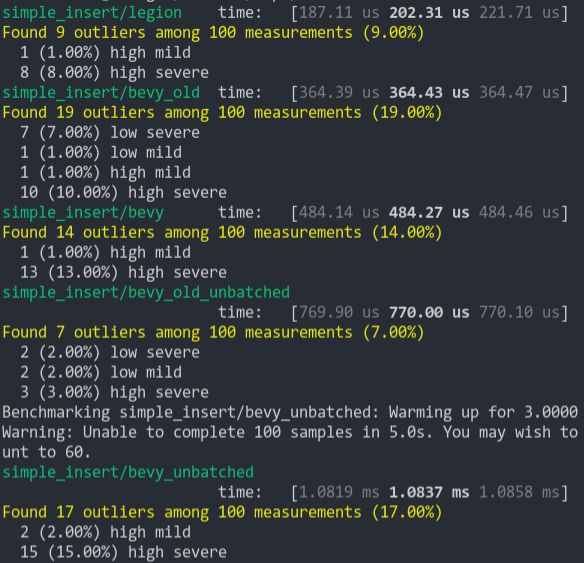
### Simpler Iter (from ecs_bench_suite)
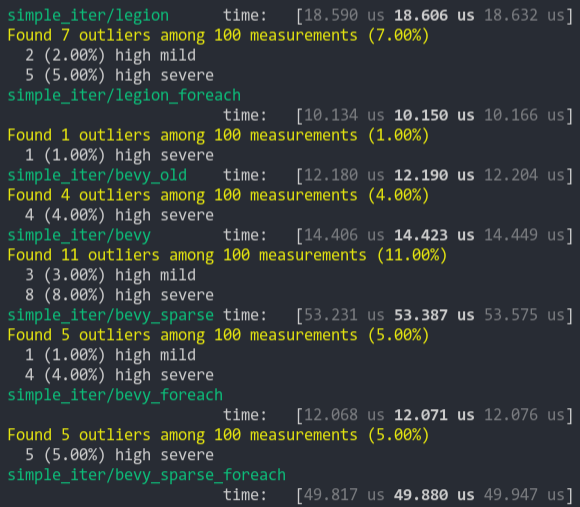
### Fragment Iter (from ecs_bench_suite)
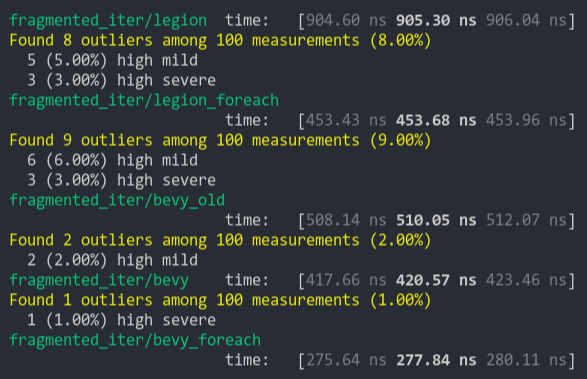
### Sparse Fragmented Iter
Iterate a query that matches 5 entities from a single matching archetype, but there are 100 unmatching archetypes
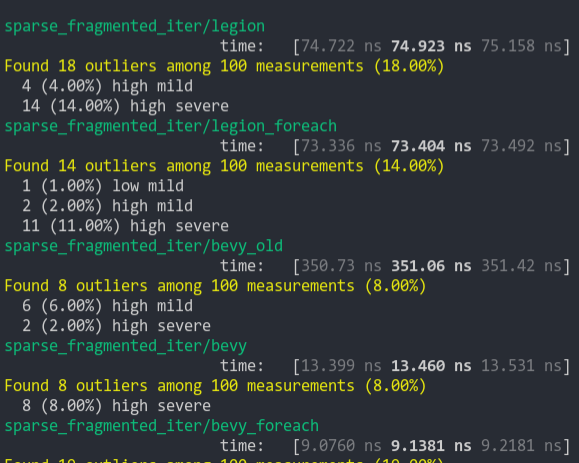
### Schedule (from ecs_bench_suite)
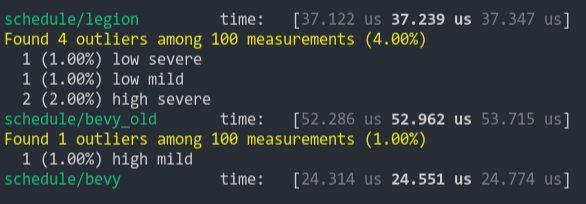
### Add Remove Component (from ecs_bench_suite)
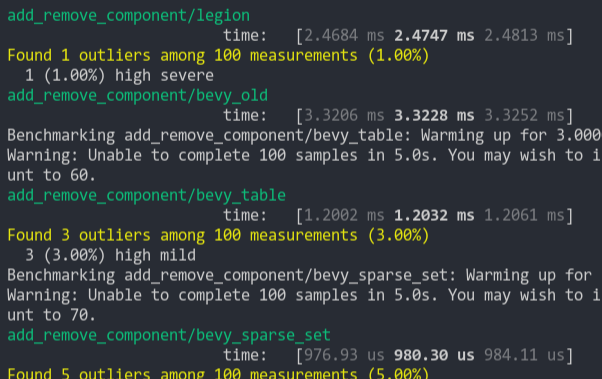
### Add Remove Component Big
Same as the test above, but each entity has 5 "large" matrix components and 1 "large" matrix component is added and removed
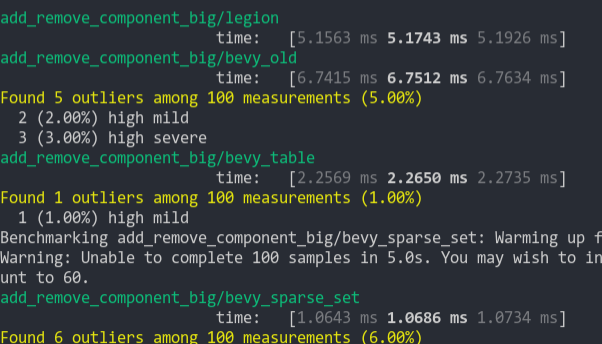
### Get Component
Looks up a single component value a large number of times
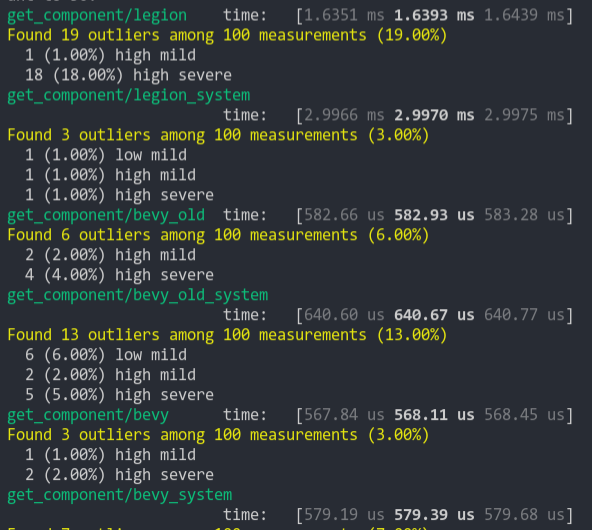
2021-03-05 07:54:35 +00:00
pub use bevy_ecs_macros ::SystemParam ;
2022-05-04 19:16:10 +00:00
use bevy_ptr ::UnsafeCellDeref ;
2023-02-16 17:09:44 +00:00
use bevy_utils ::{ all_tuples , synccell ::SyncCell } ;
Bevy ECS V2 (#1525)
# Bevy ECS V2
This is a rewrite of Bevy ECS (basically everything but the new executor/schedule, which are already awesome). The overall goal was to improve the performance and versatility of Bevy ECS. Here is a quick bulleted list of changes before we dive into the details:
* Complete World rewrite
* Multiple component storage types:
* Tables: fast cache friendly iteration, slower add/removes (previously called Archetypes)
* Sparse Sets: fast add/remove, slower iteration
* Stateful Queries (caches query results for faster iteration. fragmented iteration is _fast_ now)
* Stateful System Params (caches expensive operations. inspired by @DJMcNab's work in #1364)
* Configurable System Params (users can set configuration when they construct their systems. once again inspired by @DJMcNab's work)
* Archetypes are now "just metadata", component storage is separate
* Archetype Graph (for faster archetype changes)
* Component Metadata
* Configure component storage type
* Retrieve information about component size/type/name/layout/send-ness/etc
* Components are uniquely identified by a densely packed ComponentId
* TypeIds are now totally optional (which should make implementing scripting easier)
* Super fast "for_each" query iterators
* Merged Resources into World. Resources are now just a special type of component
* EntityRef/EntityMut builder apis (more efficient and more ergonomic)
* Fast bitset-backed `Access<T>` replaces old hashmap-based approach everywhere
* Query conflicts are determined by component access instead of archetype component access (to avoid random failures at runtime)
* With/Without are still taken into account for conflicts, so this should still be comfy to use
* Much simpler `IntoSystem` impl
* Significantly reduced the amount of hashing throughout the ecs in favor of Sparse Sets (indexed by densely packed ArchetypeId, ComponentId, BundleId, and TableId)
* Safety Improvements
* Entity reservation uses a normal world reference instead of unsafe transmute
* QuerySets no longer transmute lifetimes
* Made traits "unsafe" where relevant
* More thorough safety docs
* WorldCell
* Exposes safe mutable access to multiple resources at a time in a World
* Replaced "catch all" `System::update_archetypes(world: &World)` with `System::new_archetype(archetype: &Archetype)`
* Simpler Bundle implementation
* Replaced slow "remove_bundle_one_by_one" used as fallback for Commands::remove_bundle with fast "remove_bundle_intersection"
* Removed `Mut<T>` query impl. it is better to only support one way: `&mut T`
* Removed with() from `Flags<T>` in favor of `Option<Flags<T>>`, which allows querying for flags to be "filtered" by default
* Components now have is_send property (currently only resources support non-send)
* More granular module organization
* New `RemovedComponents<T>` SystemParam that replaces `query.removed::<T>()`
* `world.resource_scope()` for mutable access to resources and world at the same time
* WorldQuery and QueryFilter traits unified. FilterFetch trait added to enable "short circuit" filtering. Auto impled for cases that don't need it
* Significantly slimmed down SystemState in favor of individual SystemParam state
* System Commands changed from `commands: &mut Commands` back to `mut commands: Commands` (to allow Commands to have a World reference)
Fixes #1320
## `World` Rewrite
This is a from-scratch rewrite of `World` that fills the niche that `hecs` used to. Yes, this means Bevy ECS is no longer a "fork" of hecs. We're going out our own!
(the only shared code between the projects is the entity id allocator, which is already basically ideal)
A huge shout out to @SanderMertens (author of [flecs](https://github.com/SanderMertens/flecs)) for sharing some great ideas with me (specifically hybrid ecs storage and archetype graphs). He also helped advise on a number of implementation details.
## Component Storage (The Problem)
Two ECS storage paradigms have gained a lot of traction over the years:
* **Archetypal ECS**:
* Stores components in "tables" with static schemas. Each "column" stores components of a given type. Each "row" is an entity.
* Each "archetype" has its own table. Adding/removing an entity's component changes the archetype.
* Enables super-fast Query iteration due to its cache-friendly data layout
* Comes at the cost of more expensive add/remove operations for an Entity's components, because all components need to be copied to the new archetype's "table"
* **Sparse Set ECS**:
* Stores components of the same type in densely packed arrays, which are sparsely indexed by densely packed unsigned integers (Entity ids)
* Query iteration is slower than Archetypal ECS because each entity's component could be at any position in the sparse set. This "random access" pattern isn't cache friendly. Additionally, there is an extra layer of indirection because you must first map the entity id to an index in the component array.
* Adding/removing components is a cheap, constant time operation
Bevy ECS V1, hecs, legion, flec, and Unity DOTS are all "archetypal ecs-es". I personally think "archetypal" storage is a good default for game engines. An entity's archetype doesn't need to change frequently in general, and it creates "fast by default" query iteration (which is a much more common operation). It is also "self optimizing". Users don't need to think about optimizing component layouts for iteration performance. It "just works" without any extra boilerplate.
Shipyard and EnTT are "sparse set ecs-es". They employ "packing" as a way to work around the "suboptimal by default" iteration performance for specific sets of components. This helps, but I didn't think this was a good choice for a general purpose engine like Bevy because:
1. "packs" conflict with each other. If bevy decides to internally pack the Transform and GlobalTransform components, users are then blocked if they want to pack some custom component with Transform.
2. users need to take manual action to optimize
Developers selecting an ECS framework are stuck with a hard choice. Select an "archetypal" framework with "fast iteration everywhere" but without the ability to cheaply add/remove components, or select a "sparse set" framework to cheaply add/remove components but with slower iteration performance.
## Hybrid Component Storage (The Solution)
In Bevy ECS V2, we get to have our cake and eat it too. It now has _both_ of the component storage types above (and more can be added later if needed):
* **Tables** (aka "archetypal" storage)
* The default storage. If you don't configure anything, this is what you get
* Fast iteration by default
* Slower add/remove operations
* **Sparse Sets**
* Opt-in
* Slower iteration
* Faster add/remove operations
These storage types complement each other perfectly. By default Query iteration is fast. If developers know that they want to add/remove a component at high frequencies, they can set the storage to "sparse set":
```rust
world.register_component(
ComponentDescriptor::new::<MyComponent>(StorageType::SparseSet)
).unwrap();
```
## Archetypes
Archetypes are now "just metadata" ... they no longer store components directly. They do store:
* The `ComponentId`s of each of the Archetype's components (and that component's storage type)
* Archetypes are uniquely defined by their component layouts
* For example: entities with "table" components `[A, B, C]` _and_ "sparse set" components `[D, E]` will always be in the same archetype.
* The `TableId` associated with the archetype
* For now each archetype has exactly one table (which can have no components),
* There is a 1->Many relationship from Tables->Archetypes. A given table could have any number of archetype components stored in it:
* Ex: an entity with "table storage" components `[A, B, C]` and "sparse set" components `[D, E]` will share the same `[A, B, C]` table as an entity with `[A, B, C]` table component and `[F]` sparse set components.
* This 1->Many relationship is how we preserve fast "cache friendly" iteration performance when possible (more on this later)
* A list of entities that are in the archetype and the row id of the table they are in
* ArchetypeComponentIds
* unique densely packed identifiers for (ArchetypeId, ComponentId) pairs
* used by the schedule executor for cheap system access control
* "Archetype Graph Edges" (see the next section)
## The "Archetype Graph"
Archetype changes in Bevy (and a number of other archetypal ecs-es) have historically been expensive to compute. First, you need to allocate a new vector of the entity's current component ids, add or remove components based on the operation performed, sort it (to ensure it is order-independent), then hash it to find the archetype (if it exists). And thats all before we get to the _already_ expensive full copy of all components to the new table storage.
The solution is to build a "graph" of archetypes to cache these results. @SanderMertens first exposed me to the idea (and he got it from @gjroelofs, who came up with it). They propose adding directed edges between archetypes for add/remove component operations. If `ComponentId`s are densely packed, you can use sparse sets to cheaply jump between archetypes.
Bevy takes this one step further by using add/remove `Bundle` edges instead of `Component` edges. Bevy encourages the use of `Bundles` to group add/remove operations. This is largely for "clearer game logic" reasons, but it also helps cut down on the number of archetype changes required. `Bundles` now also have densely-packed `BundleId`s. This allows us to use a _single_ edge for each bundle operation (rather than needing to traverse N edges ... one for each component). Single component operations are also bundles, so this is strictly an improvement over a "component only" graph.
As a result, an operation that used to be _heavy_ (both for allocations and compute) is now two dirt-cheap array lookups and zero allocations.
## Stateful Queries
World queries are now stateful. This allows us to:
1. Cache archetype (and table) matches
* This resolves another issue with (naive) archetypal ECS: query performance getting worse as the number of archetypes goes up (and fragmentation occurs).
2. Cache Fetch and Filter state
* The expensive parts of fetch/filter operations (such as hashing the TypeId to find the ComponentId) now only happen once when the Query is first constructed
3. Incrementally build up state
* When new archetypes are added, we only process the new archetypes (no need to rebuild state for old archetypes)
As a result, the direct `World` query api now looks like this:
```rust
let mut query = world.query::<(&A, &mut B)>();
for (a, mut b) in query.iter_mut(&mut world) {
}
```
Requiring `World` to generate stateful queries (rather than letting the `QueryState` type be constructed separately) allows us to ensure that _all_ queries are properly initialized (and the relevant world state, such as ComponentIds). This enables QueryState to remove branches from its operations that check for initialization status (and also enables query.iter() to take an immutable world reference because it doesn't need to initialize anything in world).
However in systems, this is a non-breaking change. State management is done internally by the relevant SystemParam.
## Stateful SystemParams
Like Queries, `SystemParams` now also cache state. For example, `Query` system params store the "stateful query" state mentioned above. Commands store their internal `CommandQueue`. This means you can now safely use as many separate `Commands` parameters in your system as you want. `Local<T>` system params store their `T` value in their state (instead of in Resources).
SystemParam state also enabled a significant slim-down of SystemState. It is much nicer to look at now.
Per-SystemParam state naturally insulates us from an "aliased mut" class of errors we have hit in the past (ex: using multiple `Commands` system params).
(credit goes to @DJMcNab for the initial idea and draft pr here #1364)
## Configurable SystemParams
@DJMcNab also had the great idea to make SystemParams configurable. This allows users to provide some initial configuration / values for system parameters (when possible). Most SystemParams have no config (the config type is `()`), but the `Local<T>` param now supports user-provided parameters:
```rust
fn foo(value: Local<usize>) {
}
app.add_system(foo.system().config(|c| c.0 = Some(10)));
```
## Uber Fast "for_each" Query Iterators
Developers now have the choice to use a fast "for_each" iterator, which yields ~1.5-3x iteration speed improvements for "fragmented iteration", and minor ~1.2x iteration speed improvements for unfragmented iteration.
```rust
fn system(query: Query<(&A, &mut B)>) {
// you now have the option to do this for a speed boost
query.for_each_mut(|(a, mut b)| {
});
// however normal iterators are still available
for (a, mut b) in query.iter_mut() {
}
}
```
I think in most cases we should continue to encourage "normal" iterators as they are more flexible and more "rust idiomatic". But when that extra "oomf" is needed, it makes sense to use `for_each`.
We should also consider using `for_each` for internal bevy systems to give our users a nice speed boost (but that should be a separate pr).
## Component Metadata
`World` now has a `Components` collection, which is accessible via `world.components()`. This stores mappings from `ComponentId` to `ComponentInfo`, as well as `TypeId` to `ComponentId` mappings (where relevant). `ComponentInfo` stores information about the component, such as ComponentId, TypeId, memory layout, send-ness (currently limited to resources), and storage type.
## Significantly Cheaper `Access<T>`
We used to use `TypeAccess<TypeId>` to manage read/write component/archetype-component access. This was expensive because TypeIds must be hashed and compared individually. The parallel executor got around this by "condensing" type ids into bitset-backed access types. This worked, but it had to be re-generated from the `TypeAccess<TypeId>`sources every time archetypes changed.
This pr removes TypeAccess in favor of faster bitset access everywhere. We can do this thanks to the move to densely packed `ComponentId`s and `ArchetypeComponentId`s.
## Merged Resources into World
Resources had a lot of redundant functionality with Components. They stored typed data, they had access control, they had unique ids, they were queryable via SystemParams, etc. In fact the _only_ major difference between them was that they were unique (and didn't correlate to an entity).
Separate resources also had the downside of requiring a separate set of access controls, which meant the parallel executor needed to compare more bitsets per system and manage more state.
I initially got the "separate resources" idea from `legion`. I think that design was motivated by the fact that it made the direct world query/resource lifetime interactions more manageable. It certainly made our lives easier when using Resources alongside hecs/bevy_ecs. However we already have a construct for safely and ergonomically managing in-world lifetimes: systems (which use `Access<T>` internally).
This pr merges Resources into World:
```rust
world.insert_resource(1);
world.insert_resource(2.0);
let a = world.get_resource::<i32>().unwrap();
let mut b = world.get_resource_mut::<f64>().unwrap();
*b = 3.0;
```
Resources are now just a special kind of component. They have their own ComponentIds (and their own resource TypeId->ComponentId scope, so they don't conflict wit components of the same type). They are stored in a special "resource archetype", which stores components inside the archetype using a new `unique_components` sparse set (note that this sparse set could later be used to implement Tags). This allows us to keep the code size small by reusing existing datastructures (namely Column, Archetype, ComponentFlags, and ComponentInfo). This allows us the executor to use a single `Access<ArchetypeComponentId>` per system. It should also make scripting language integration easier.
_But_ this merge did create problems for people directly interacting with `World`. What if you need mutable access to multiple resources at the same time? `world.get_resource_mut()` borrows World mutably!
## WorldCell
WorldCell applies the `Access<ArchetypeComponentId>` concept to direct world access:
```rust
let world_cell = world.cell();
let a = world_cell.get_resource_mut::<i32>().unwrap();
let b = world_cell.get_resource_mut::<f64>().unwrap();
```
This adds cheap runtime checks (a sparse set lookup of `ArchetypeComponentId` and a counter) to ensure that world accesses do not conflict with each other. Each operation returns a `WorldBorrow<'w, T>` or `WorldBorrowMut<'w, T>` wrapper type, which will release the relevant ArchetypeComponentId resources when dropped.
World caches the access sparse set (and only one cell can exist at a time), so `world.cell()` is a cheap operation.
WorldCell does _not_ use atomic operations. It is non-send, does a mutable borrow of world to prevent other accesses, and uses a simple `Rc<RefCell<ArchetypeComponentAccess>>` wrapper in each WorldBorrow pointer.
The api is currently limited to resource access, but it can and should be extended to queries / entity component access.
## Resource Scopes
WorldCell does not yet support component queries, and even when it does there are sometimes legitimate reasons to want a mutable world ref _and_ a mutable resource ref (ex: bevy_render and bevy_scene both need this). In these cases we could always drop down to the unsafe `world.get_resource_unchecked_mut()`, but that is not ideal!
Instead developers can use a "resource scope"
```rust
world.resource_scope(|world: &mut World, a: &mut A| {
})
```
This temporarily removes the `A` resource from `World`, provides mutable pointers to both, and re-adds A to World when finished. Thanks to the move to ComponentIds/sparse sets, this is a cheap operation.
If multiple resources are required, scopes can be nested. We could also consider adding a "resource tuple" to the api if this pattern becomes common and the boilerplate gets nasty.
## Query Conflicts Use ComponentId Instead of ArchetypeComponentId
For safety reasons, systems cannot contain queries that conflict with each other without wrapping them in a QuerySet. On bevy `main`, we use ArchetypeComponentIds to determine conflicts. This is nice because it can take into account filters:
```rust
// these queries will never conflict due to their filters
fn filter_system(a: Query<&mut A, With<B>>, b: Query<&mut B, Without<B>>) {
}
```
But it also has a significant downside:
```rust
// these queries will not conflict _until_ an entity with A, B, and C is spawned
fn maybe_conflicts_system(a: Query<(&mut A, &C)>, b: Query<(&mut A, &B)>) {
}
```
The system above will panic at runtime if an entity with A, B, and C is spawned. This makes it hard to trust that your game logic will run without crashing.
In this pr, I switched to using `ComponentId` instead. This _is_ more constraining. `maybe_conflicts_system` will now always fail, but it will do it consistently at startup. Naively, it would also _disallow_ `filter_system`, which would be a significant downgrade in usability. Bevy has a number of internal systems that rely on disjoint queries and I expect it to be a common pattern in userspace.
To resolve this, I added a new `FilteredAccess<T>` type, which wraps `Access<T>` and adds with/without filters. If two `FilteredAccess` have with/without values that prove they are disjoint, they will no longer conflict.
## EntityRef / EntityMut
World entity operations on `main` require that the user passes in an `entity` id to each operation:
```rust
let entity = world.spawn((A, )); // create a new entity with A
world.get::<A>(entity);
world.insert(entity, (B, C));
world.insert_one(entity, D);
```
This means that each operation needs to look up the entity location / verify its validity. The initial spawn operation also requires a Bundle as input. This can be awkward when no components are required (or one component is required).
These operations have been replaced by `EntityRef` and `EntityMut`, which are "builder-style" wrappers around world that provide read and read/write operations on a single, pre-validated entity:
```rust
// spawn now takes no inputs and returns an EntityMut
let entity = world.spawn()
.insert(A) // insert a single component into the entity
.insert_bundle((B, C)) // insert a bundle of components into the entity
.id() // id returns the Entity id
// Returns EntityMut (or panics if the entity does not exist)
world.entity_mut(entity)
.insert(D)
.insert_bundle(SomeBundle::default());
{
// returns EntityRef (or panics if the entity does not exist)
let d = world.entity(entity)
.get::<D>() // gets the D component
.unwrap();
// world.get still exists for ergonomics
let d = world.get::<D>(entity).unwrap();
}
// These variants return Options if you want to check existence instead of panicing
world.get_entity_mut(entity)
.unwrap()
.insert(E);
if let Some(entity_ref) = world.get_entity(entity) {
let d = entity_ref.get::<D>().unwrap();
}
```
This _does not_ affect the current Commands api or terminology. I think that should be a separate conversation as that is a much larger breaking change.
## Safety Improvements
* Entity reservation in Commands uses a normal world borrow instead of an unsafe transmute
* QuerySets no longer transmutes lifetimes
* Made traits "unsafe" when implementing a trait incorrectly could cause unsafety
* More thorough safety docs
## RemovedComponents SystemParam
The old approach to querying removed components: `query.removed:<T>()` was confusing because it had no connection to the query itself. I replaced it with the following, which is both clearer and allows us to cache the ComponentId mapping in the SystemParamState:
```rust
fn system(removed: RemovedComponents<T>) {
for entity in removed.iter() {
}
}
```
## Simpler Bundle implementation
Bundles are no longer responsible for sorting (or deduping) TypeInfo. They are just a simple ordered list of component types / data. This makes the implementation smaller and opens the door to an easy "nested bundle" implementation in the future (which i might even add in this pr). Duplicate detection is now done once per bundle type by World the first time a bundle is used.
## Unified WorldQuery and QueryFilter types
(don't worry they are still separate type _parameters_ in Queries .. this is a non-breaking change)
WorldQuery and QueryFilter were already basically identical apis. With the addition of `FetchState` and more storage-specific fetch methods, the overlap was even clearer (and the redundancy more painful).
QueryFilters are now just `F: WorldQuery where F::Fetch: FilterFetch`. FilterFetch requires `Fetch<Item = bool>` and adds new "short circuit" variants of fetch methods. This enables a filter tuple like `(With<A>, Without<B>, Changed<C>)` to stop evaluating the filter after the first mismatch is encountered. FilterFetch is automatically implemented for `Fetch` implementations that return bool.
This forces fetch implementations that return things like `(bool, bool, bool)` (such as the filter above) to manually implement FilterFetch and decide whether or not to short-circuit.
## More Granular Modules
World no longer globs all of the internal modules together. It now exports `core`, `system`, and `schedule` separately. I'm also considering exporting `core` submodules directly as that is still pretty "glob-ey" and unorganized (feedback welcome here).
## Remaining Draft Work (to be done in this pr)
* ~~panic on conflicting WorldQuery fetches (&A, &mut A)~~
* ~~bevy `main` and hecs both currently allow this, but we should protect against it if possible~~
* ~~batch_iter / par_iter (currently stubbed out)~~
* ~~ChangedRes~~
* ~~I skipped this while we sort out #1313. This pr should be adapted to account for whatever we land on there~~.
* ~~The `Archetypes` and `Tables` collections use hashes of sorted lists of component ids to uniquely identify each archetype/table. This hash is then used as the key in a HashMap to look up the relevant ArchetypeId or TableId. (which doesn't handle hash collisions properly)~~
* ~~It is currently unsafe to generate a Query from "World A", then use it on "World B" (despite the api claiming it is safe). We should probably close this gap. This could be done by adding a randomly generated WorldId to each world, then storing that id in each Query. They could then be compared to each other on each `query.do_thing(&world)` operation. This _does_ add an extra branch to each query operation, so I'm open to other suggestions if people have them.~~
* ~~Nested Bundles (if i find time)~~
## Potential Future Work
* Expand WorldCell to support queries.
* Consider not allocating in the empty archetype on `world.spawn()`
* ex: return something like EntityMutUninit, which turns into EntityMut after an `insert` or `insert_bundle` op
* this actually regressed performance last time i tried it, but in theory it should be faster
* Optimize SparseSet::insert (see `PERF` comment on insert)
* Replace SparseArray `Option<T>` with T::MAX to cut down on branching
* would enable cheaper get_unchecked() operations
* upstream fixedbitset optimizations
* fixedbitset could be allocation free for small block counts (store blocks in a SmallVec)
* fixedbitset could have a const constructor
* Consider implementing Tags (archetype-specific by-value data that affects archetype identity)
* ex: ArchetypeA could have `[A, B, C]` table components and `[D(1)]` "tag" component. ArchetypeB could have `[A, B, C]` table components and a `[D(2)]` tag component. The archetypes are different, despite both having D tags because the value inside D is different.
* this could potentially build on top of the `archetype.unique_components` added in this pr for resource storage.
* Consider reverting `all_tuples` proc macro in favor of the old `macro_rules` implementation
* all_tuples is more flexible and produces cleaner documentation (the macro_rules version produces weird type parameter orders due to parser constraints)
* but unfortunately all_tuples also appears to make Rust Analyzer sad/slow when working inside of `bevy_ecs` (does not affect user code)
* Consider "resource queries" and/or "mixed resource and entity component queries" as an alternative to WorldCell
* this is basically just "systems" so maybe it's not worth it
* Add more world ops
* `world.clear()`
* `world.reserve<T: Bundle>(count: usize)`
* Try using the old archetype allocation strategy (allocate new memory on resize and copy everything over). I expect this to improve batch insertion performance at the cost of unbatched performance. But thats just a guess. I'm not an allocation perf pro :)
* Adapt Commands apis for consistency with new World apis
## Benchmarks
key:
* `bevy_old`: bevy `main` branch
* `bevy`: this branch
* `_foreach`: uses an optimized for_each iterator
* ` _sparse`: uses sparse set storage (if unspecified assume table storage)
* `_system`: runs inside a system (if unspecified assume test happens via direct world ops)
### Simple Insert (from ecs_bench_suite)
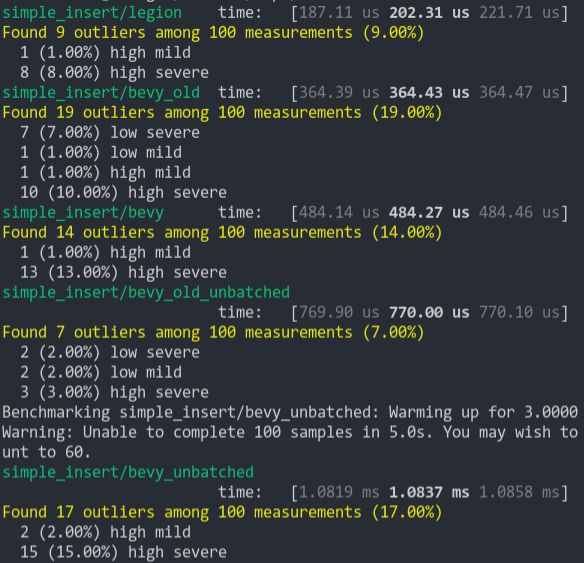
### Simpler Iter (from ecs_bench_suite)
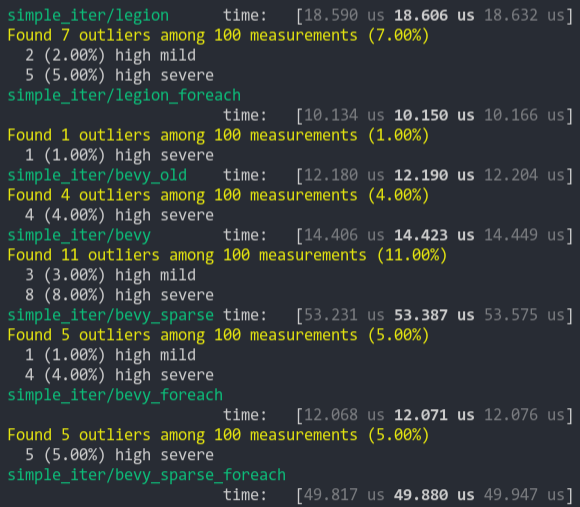
### Fragment Iter (from ecs_bench_suite)
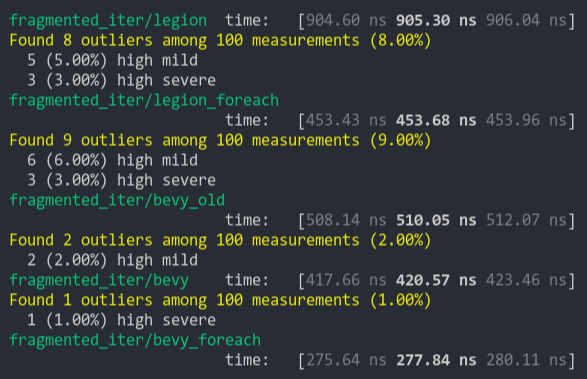
### Sparse Fragmented Iter
Iterate a query that matches 5 entities from a single matching archetype, but there are 100 unmatching archetypes
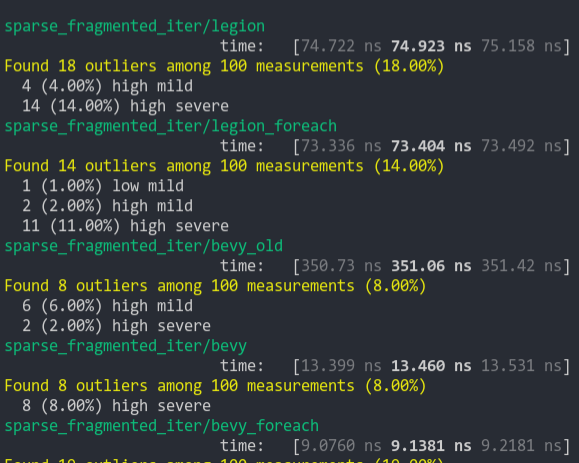
### Schedule (from ecs_bench_suite)
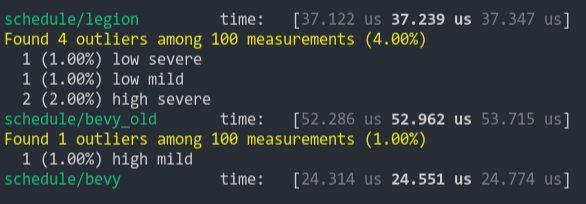
### Add Remove Component (from ecs_bench_suite)
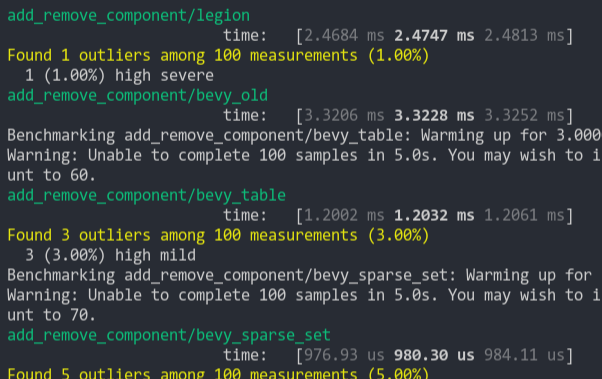
### Add Remove Component Big
Same as the test above, but each entity has 5 "large" matrix components and 1 "large" matrix component is added and removed
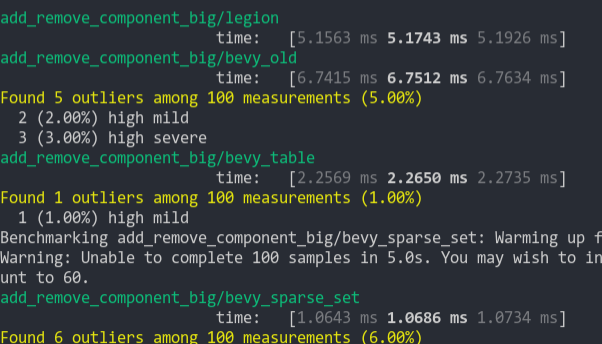
### Get Component
Looks up a single component value a large number of times
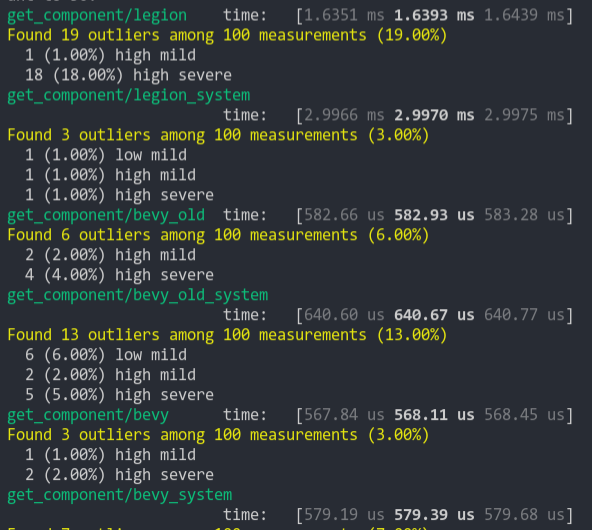
2021-03-05 07:54:35 +00:00
use std ::{
2022-08-18 18:31:12 +00:00
borrow ::Cow ,
2021-05-01 02:32:32 +00:00
fmt ::Debug ,
Bevy ECS V2 (#1525)
# Bevy ECS V2
This is a rewrite of Bevy ECS (basically everything but the new executor/schedule, which are already awesome). The overall goal was to improve the performance and versatility of Bevy ECS. Here is a quick bulleted list of changes before we dive into the details:
* Complete World rewrite
* Multiple component storage types:
* Tables: fast cache friendly iteration, slower add/removes (previously called Archetypes)
* Sparse Sets: fast add/remove, slower iteration
* Stateful Queries (caches query results for faster iteration. fragmented iteration is _fast_ now)
* Stateful System Params (caches expensive operations. inspired by @DJMcNab's work in #1364)
* Configurable System Params (users can set configuration when they construct their systems. once again inspired by @DJMcNab's work)
* Archetypes are now "just metadata", component storage is separate
* Archetype Graph (for faster archetype changes)
* Component Metadata
* Configure component storage type
* Retrieve information about component size/type/name/layout/send-ness/etc
* Components are uniquely identified by a densely packed ComponentId
* TypeIds are now totally optional (which should make implementing scripting easier)
* Super fast "for_each" query iterators
* Merged Resources into World. Resources are now just a special type of component
* EntityRef/EntityMut builder apis (more efficient and more ergonomic)
* Fast bitset-backed `Access<T>` replaces old hashmap-based approach everywhere
* Query conflicts are determined by component access instead of archetype component access (to avoid random failures at runtime)
* With/Without are still taken into account for conflicts, so this should still be comfy to use
* Much simpler `IntoSystem` impl
* Significantly reduced the amount of hashing throughout the ecs in favor of Sparse Sets (indexed by densely packed ArchetypeId, ComponentId, BundleId, and TableId)
* Safety Improvements
* Entity reservation uses a normal world reference instead of unsafe transmute
* QuerySets no longer transmute lifetimes
* Made traits "unsafe" where relevant
* More thorough safety docs
* WorldCell
* Exposes safe mutable access to multiple resources at a time in a World
* Replaced "catch all" `System::update_archetypes(world: &World)` with `System::new_archetype(archetype: &Archetype)`
* Simpler Bundle implementation
* Replaced slow "remove_bundle_one_by_one" used as fallback for Commands::remove_bundle with fast "remove_bundle_intersection"
* Removed `Mut<T>` query impl. it is better to only support one way: `&mut T`
* Removed with() from `Flags<T>` in favor of `Option<Flags<T>>`, which allows querying for flags to be "filtered" by default
* Components now have is_send property (currently only resources support non-send)
* More granular module organization
* New `RemovedComponents<T>` SystemParam that replaces `query.removed::<T>()`
* `world.resource_scope()` for mutable access to resources and world at the same time
* WorldQuery and QueryFilter traits unified. FilterFetch trait added to enable "short circuit" filtering. Auto impled for cases that don't need it
* Significantly slimmed down SystemState in favor of individual SystemParam state
* System Commands changed from `commands: &mut Commands` back to `mut commands: Commands` (to allow Commands to have a World reference)
Fixes #1320
## `World` Rewrite
This is a from-scratch rewrite of `World` that fills the niche that `hecs` used to. Yes, this means Bevy ECS is no longer a "fork" of hecs. We're going out our own!
(the only shared code between the projects is the entity id allocator, which is already basically ideal)
A huge shout out to @SanderMertens (author of [flecs](https://github.com/SanderMertens/flecs)) for sharing some great ideas with me (specifically hybrid ecs storage and archetype graphs). He also helped advise on a number of implementation details.
## Component Storage (The Problem)
Two ECS storage paradigms have gained a lot of traction over the years:
* **Archetypal ECS**:
* Stores components in "tables" with static schemas. Each "column" stores components of a given type. Each "row" is an entity.
* Each "archetype" has its own table. Adding/removing an entity's component changes the archetype.
* Enables super-fast Query iteration due to its cache-friendly data layout
* Comes at the cost of more expensive add/remove operations for an Entity's components, because all components need to be copied to the new archetype's "table"
* **Sparse Set ECS**:
* Stores components of the same type in densely packed arrays, which are sparsely indexed by densely packed unsigned integers (Entity ids)
* Query iteration is slower than Archetypal ECS because each entity's component could be at any position in the sparse set. This "random access" pattern isn't cache friendly. Additionally, there is an extra layer of indirection because you must first map the entity id to an index in the component array.
* Adding/removing components is a cheap, constant time operation
Bevy ECS V1, hecs, legion, flec, and Unity DOTS are all "archetypal ecs-es". I personally think "archetypal" storage is a good default for game engines. An entity's archetype doesn't need to change frequently in general, and it creates "fast by default" query iteration (which is a much more common operation). It is also "self optimizing". Users don't need to think about optimizing component layouts for iteration performance. It "just works" without any extra boilerplate.
Shipyard and EnTT are "sparse set ecs-es". They employ "packing" as a way to work around the "suboptimal by default" iteration performance for specific sets of components. This helps, but I didn't think this was a good choice for a general purpose engine like Bevy because:
1. "packs" conflict with each other. If bevy decides to internally pack the Transform and GlobalTransform components, users are then blocked if they want to pack some custom component with Transform.
2. users need to take manual action to optimize
Developers selecting an ECS framework are stuck with a hard choice. Select an "archetypal" framework with "fast iteration everywhere" but without the ability to cheaply add/remove components, or select a "sparse set" framework to cheaply add/remove components but with slower iteration performance.
## Hybrid Component Storage (The Solution)
In Bevy ECS V2, we get to have our cake and eat it too. It now has _both_ of the component storage types above (and more can be added later if needed):
* **Tables** (aka "archetypal" storage)
* The default storage. If you don't configure anything, this is what you get
* Fast iteration by default
* Slower add/remove operations
* **Sparse Sets**
* Opt-in
* Slower iteration
* Faster add/remove operations
These storage types complement each other perfectly. By default Query iteration is fast. If developers know that they want to add/remove a component at high frequencies, they can set the storage to "sparse set":
```rust
world.register_component(
ComponentDescriptor::new::<MyComponent>(StorageType::SparseSet)
).unwrap();
```
## Archetypes
Archetypes are now "just metadata" ... they no longer store components directly. They do store:
* The `ComponentId`s of each of the Archetype's components (and that component's storage type)
* Archetypes are uniquely defined by their component layouts
* For example: entities with "table" components `[A, B, C]` _and_ "sparse set" components `[D, E]` will always be in the same archetype.
* The `TableId` associated with the archetype
* For now each archetype has exactly one table (which can have no components),
* There is a 1->Many relationship from Tables->Archetypes. A given table could have any number of archetype components stored in it:
* Ex: an entity with "table storage" components `[A, B, C]` and "sparse set" components `[D, E]` will share the same `[A, B, C]` table as an entity with `[A, B, C]` table component and `[F]` sparse set components.
* This 1->Many relationship is how we preserve fast "cache friendly" iteration performance when possible (more on this later)
* A list of entities that are in the archetype and the row id of the table they are in
* ArchetypeComponentIds
* unique densely packed identifiers for (ArchetypeId, ComponentId) pairs
* used by the schedule executor for cheap system access control
* "Archetype Graph Edges" (see the next section)
## The "Archetype Graph"
Archetype changes in Bevy (and a number of other archetypal ecs-es) have historically been expensive to compute. First, you need to allocate a new vector of the entity's current component ids, add or remove components based on the operation performed, sort it (to ensure it is order-independent), then hash it to find the archetype (if it exists). And thats all before we get to the _already_ expensive full copy of all components to the new table storage.
The solution is to build a "graph" of archetypes to cache these results. @SanderMertens first exposed me to the idea (and he got it from @gjroelofs, who came up with it). They propose adding directed edges between archetypes for add/remove component operations. If `ComponentId`s are densely packed, you can use sparse sets to cheaply jump between archetypes.
Bevy takes this one step further by using add/remove `Bundle` edges instead of `Component` edges. Bevy encourages the use of `Bundles` to group add/remove operations. This is largely for "clearer game logic" reasons, but it also helps cut down on the number of archetype changes required. `Bundles` now also have densely-packed `BundleId`s. This allows us to use a _single_ edge for each bundle operation (rather than needing to traverse N edges ... one for each component). Single component operations are also bundles, so this is strictly an improvement over a "component only" graph.
As a result, an operation that used to be _heavy_ (both for allocations and compute) is now two dirt-cheap array lookups and zero allocations.
## Stateful Queries
World queries are now stateful. This allows us to:
1. Cache archetype (and table) matches
* This resolves another issue with (naive) archetypal ECS: query performance getting worse as the number of archetypes goes up (and fragmentation occurs).
2. Cache Fetch and Filter state
* The expensive parts of fetch/filter operations (such as hashing the TypeId to find the ComponentId) now only happen once when the Query is first constructed
3. Incrementally build up state
* When new archetypes are added, we only process the new archetypes (no need to rebuild state for old archetypes)
As a result, the direct `World` query api now looks like this:
```rust
let mut query = world.query::<(&A, &mut B)>();
for (a, mut b) in query.iter_mut(&mut world) {
}
```
Requiring `World` to generate stateful queries (rather than letting the `QueryState` type be constructed separately) allows us to ensure that _all_ queries are properly initialized (and the relevant world state, such as ComponentIds). This enables QueryState to remove branches from its operations that check for initialization status (and also enables query.iter() to take an immutable world reference because it doesn't need to initialize anything in world).
However in systems, this is a non-breaking change. State management is done internally by the relevant SystemParam.
## Stateful SystemParams
Like Queries, `SystemParams` now also cache state. For example, `Query` system params store the "stateful query" state mentioned above. Commands store their internal `CommandQueue`. This means you can now safely use as many separate `Commands` parameters in your system as you want. `Local<T>` system params store their `T` value in their state (instead of in Resources).
SystemParam state also enabled a significant slim-down of SystemState. It is much nicer to look at now.
Per-SystemParam state naturally insulates us from an "aliased mut" class of errors we have hit in the past (ex: using multiple `Commands` system params).
(credit goes to @DJMcNab for the initial idea and draft pr here #1364)
## Configurable SystemParams
@DJMcNab also had the great idea to make SystemParams configurable. This allows users to provide some initial configuration / values for system parameters (when possible). Most SystemParams have no config (the config type is `()`), but the `Local<T>` param now supports user-provided parameters:
```rust
fn foo(value: Local<usize>) {
}
app.add_system(foo.system().config(|c| c.0 = Some(10)));
```
## Uber Fast "for_each" Query Iterators
Developers now have the choice to use a fast "for_each" iterator, which yields ~1.5-3x iteration speed improvements for "fragmented iteration", and minor ~1.2x iteration speed improvements for unfragmented iteration.
```rust
fn system(query: Query<(&A, &mut B)>) {
// you now have the option to do this for a speed boost
query.for_each_mut(|(a, mut b)| {
});
// however normal iterators are still available
for (a, mut b) in query.iter_mut() {
}
}
```
I think in most cases we should continue to encourage "normal" iterators as they are more flexible and more "rust idiomatic". But when that extra "oomf" is needed, it makes sense to use `for_each`.
We should also consider using `for_each` for internal bevy systems to give our users a nice speed boost (but that should be a separate pr).
## Component Metadata
`World` now has a `Components` collection, which is accessible via `world.components()`. This stores mappings from `ComponentId` to `ComponentInfo`, as well as `TypeId` to `ComponentId` mappings (where relevant). `ComponentInfo` stores information about the component, such as ComponentId, TypeId, memory layout, send-ness (currently limited to resources), and storage type.
## Significantly Cheaper `Access<T>`
We used to use `TypeAccess<TypeId>` to manage read/write component/archetype-component access. This was expensive because TypeIds must be hashed and compared individually. The parallel executor got around this by "condensing" type ids into bitset-backed access types. This worked, but it had to be re-generated from the `TypeAccess<TypeId>`sources every time archetypes changed.
This pr removes TypeAccess in favor of faster bitset access everywhere. We can do this thanks to the move to densely packed `ComponentId`s and `ArchetypeComponentId`s.
## Merged Resources into World
Resources had a lot of redundant functionality with Components. They stored typed data, they had access control, they had unique ids, they were queryable via SystemParams, etc. In fact the _only_ major difference between them was that they were unique (and didn't correlate to an entity).
Separate resources also had the downside of requiring a separate set of access controls, which meant the parallel executor needed to compare more bitsets per system and manage more state.
I initially got the "separate resources" idea from `legion`. I think that design was motivated by the fact that it made the direct world query/resource lifetime interactions more manageable. It certainly made our lives easier when using Resources alongside hecs/bevy_ecs. However we already have a construct for safely and ergonomically managing in-world lifetimes: systems (which use `Access<T>` internally).
This pr merges Resources into World:
```rust
world.insert_resource(1);
world.insert_resource(2.0);
let a = world.get_resource::<i32>().unwrap();
let mut b = world.get_resource_mut::<f64>().unwrap();
*b = 3.0;
```
Resources are now just a special kind of component. They have their own ComponentIds (and their own resource TypeId->ComponentId scope, so they don't conflict wit components of the same type). They are stored in a special "resource archetype", which stores components inside the archetype using a new `unique_components` sparse set (note that this sparse set could later be used to implement Tags). This allows us to keep the code size small by reusing existing datastructures (namely Column, Archetype, ComponentFlags, and ComponentInfo). This allows us the executor to use a single `Access<ArchetypeComponentId>` per system. It should also make scripting language integration easier.
_But_ this merge did create problems for people directly interacting with `World`. What if you need mutable access to multiple resources at the same time? `world.get_resource_mut()` borrows World mutably!
## WorldCell
WorldCell applies the `Access<ArchetypeComponentId>` concept to direct world access:
```rust
let world_cell = world.cell();
let a = world_cell.get_resource_mut::<i32>().unwrap();
let b = world_cell.get_resource_mut::<f64>().unwrap();
```
This adds cheap runtime checks (a sparse set lookup of `ArchetypeComponentId` and a counter) to ensure that world accesses do not conflict with each other. Each operation returns a `WorldBorrow<'w, T>` or `WorldBorrowMut<'w, T>` wrapper type, which will release the relevant ArchetypeComponentId resources when dropped.
World caches the access sparse set (and only one cell can exist at a time), so `world.cell()` is a cheap operation.
WorldCell does _not_ use atomic operations. It is non-send, does a mutable borrow of world to prevent other accesses, and uses a simple `Rc<RefCell<ArchetypeComponentAccess>>` wrapper in each WorldBorrow pointer.
The api is currently limited to resource access, but it can and should be extended to queries / entity component access.
## Resource Scopes
WorldCell does not yet support component queries, and even when it does there are sometimes legitimate reasons to want a mutable world ref _and_ a mutable resource ref (ex: bevy_render and bevy_scene both need this). In these cases we could always drop down to the unsafe `world.get_resource_unchecked_mut()`, but that is not ideal!
Instead developers can use a "resource scope"
```rust
world.resource_scope(|world: &mut World, a: &mut A| {
})
```
This temporarily removes the `A` resource from `World`, provides mutable pointers to both, and re-adds A to World when finished. Thanks to the move to ComponentIds/sparse sets, this is a cheap operation.
If multiple resources are required, scopes can be nested. We could also consider adding a "resource tuple" to the api if this pattern becomes common and the boilerplate gets nasty.
## Query Conflicts Use ComponentId Instead of ArchetypeComponentId
For safety reasons, systems cannot contain queries that conflict with each other without wrapping them in a QuerySet. On bevy `main`, we use ArchetypeComponentIds to determine conflicts. This is nice because it can take into account filters:
```rust
// these queries will never conflict due to their filters
fn filter_system(a: Query<&mut A, With<B>>, b: Query<&mut B, Without<B>>) {
}
```
But it also has a significant downside:
```rust
// these queries will not conflict _until_ an entity with A, B, and C is spawned
fn maybe_conflicts_system(a: Query<(&mut A, &C)>, b: Query<(&mut A, &B)>) {
}
```
The system above will panic at runtime if an entity with A, B, and C is spawned. This makes it hard to trust that your game logic will run without crashing.
In this pr, I switched to using `ComponentId` instead. This _is_ more constraining. `maybe_conflicts_system` will now always fail, but it will do it consistently at startup. Naively, it would also _disallow_ `filter_system`, which would be a significant downgrade in usability. Bevy has a number of internal systems that rely on disjoint queries and I expect it to be a common pattern in userspace.
To resolve this, I added a new `FilteredAccess<T>` type, which wraps `Access<T>` and adds with/without filters. If two `FilteredAccess` have with/without values that prove they are disjoint, they will no longer conflict.
## EntityRef / EntityMut
World entity operations on `main` require that the user passes in an `entity` id to each operation:
```rust
let entity = world.spawn((A, )); // create a new entity with A
world.get::<A>(entity);
world.insert(entity, (B, C));
world.insert_one(entity, D);
```
This means that each operation needs to look up the entity location / verify its validity. The initial spawn operation also requires a Bundle as input. This can be awkward when no components are required (or one component is required).
These operations have been replaced by `EntityRef` and `EntityMut`, which are "builder-style" wrappers around world that provide read and read/write operations on a single, pre-validated entity:
```rust
// spawn now takes no inputs and returns an EntityMut
let entity = world.spawn()
.insert(A) // insert a single component into the entity
.insert_bundle((B, C)) // insert a bundle of components into the entity
.id() // id returns the Entity id
// Returns EntityMut (or panics if the entity does not exist)
world.entity_mut(entity)
.insert(D)
.insert_bundle(SomeBundle::default());
{
// returns EntityRef (or panics if the entity does not exist)
let d = world.entity(entity)
.get::<D>() // gets the D component
.unwrap();
// world.get still exists for ergonomics
let d = world.get::<D>(entity).unwrap();
}
// These variants return Options if you want to check existence instead of panicing
world.get_entity_mut(entity)
.unwrap()
.insert(E);
if let Some(entity_ref) = world.get_entity(entity) {
let d = entity_ref.get::<D>().unwrap();
}
```
This _does not_ affect the current Commands api or terminology. I think that should be a separate conversation as that is a much larger breaking change.
## Safety Improvements
* Entity reservation in Commands uses a normal world borrow instead of an unsafe transmute
* QuerySets no longer transmutes lifetimes
* Made traits "unsafe" when implementing a trait incorrectly could cause unsafety
* More thorough safety docs
## RemovedComponents SystemParam
The old approach to querying removed components: `query.removed:<T>()` was confusing because it had no connection to the query itself. I replaced it with the following, which is both clearer and allows us to cache the ComponentId mapping in the SystemParamState:
```rust
fn system(removed: RemovedComponents<T>) {
for entity in removed.iter() {
}
}
```
## Simpler Bundle implementation
Bundles are no longer responsible for sorting (or deduping) TypeInfo. They are just a simple ordered list of component types / data. This makes the implementation smaller and opens the door to an easy "nested bundle" implementation in the future (which i might even add in this pr). Duplicate detection is now done once per bundle type by World the first time a bundle is used.
## Unified WorldQuery and QueryFilter types
(don't worry they are still separate type _parameters_ in Queries .. this is a non-breaking change)
WorldQuery and QueryFilter were already basically identical apis. With the addition of `FetchState` and more storage-specific fetch methods, the overlap was even clearer (and the redundancy more painful).
QueryFilters are now just `F: WorldQuery where F::Fetch: FilterFetch`. FilterFetch requires `Fetch<Item = bool>` and adds new "short circuit" variants of fetch methods. This enables a filter tuple like `(With<A>, Without<B>, Changed<C>)` to stop evaluating the filter after the first mismatch is encountered. FilterFetch is automatically implemented for `Fetch` implementations that return bool.
This forces fetch implementations that return things like `(bool, bool, bool)` (such as the filter above) to manually implement FilterFetch and decide whether or not to short-circuit.
## More Granular Modules
World no longer globs all of the internal modules together. It now exports `core`, `system`, and `schedule` separately. I'm also considering exporting `core` submodules directly as that is still pretty "glob-ey" and unorganized (feedback welcome here).
## Remaining Draft Work (to be done in this pr)
* ~~panic on conflicting WorldQuery fetches (&A, &mut A)~~
* ~~bevy `main` and hecs both currently allow this, but we should protect against it if possible~~
* ~~batch_iter / par_iter (currently stubbed out)~~
* ~~ChangedRes~~
* ~~I skipped this while we sort out #1313. This pr should be adapted to account for whatever we land on there~~.
* ~~The `Archetypes` and `Tables` collections use hashes of sorted lists of component ids to uniquely identify each archetype/table. This hash is then used as the key in a HashMap to look up the relevant ArchetypeId or TableId. (which doesn't handle hash collisions properly)~~
* ~~It is currently unsafe to generate a Query from "World A", then use it on "World B" (despite the api claiming it is safe). We should probably close this gap. This could be done by adding a randomly generated WorldId to each world, then storing that id in each Query. They could then be compared to each other on each `query.do_thing(&world)` operation. This _does_ add an extra branch to each query operation, so I'm open to other suggestions if people have them.~~
* ~~Nested Bundles (if i find time)~~
## Potential Future Work
* Expand WorldCell to support queries.
* Consider not allocating in the empty archetype on `world.spawn()`
* ex: return something like EntityMutUninit, which turns into EntityMut after an `insert` or `insert_bundle` op
* this actually regressed performance last time i tried it, but in theory it should be faster
* Optimize SparseSet::insert (see `PERF` comment on insert)
* Replace SparseArray `Option<T>` with T::MAX to cut down on branching
* would enable cheaper get_unchecked() operations
* upstream fixedbitset optimizations
* fixedbitset could be allocation free for small block counts (store blocks in a SmallVec)
* fixedbitset could have a const constructor
* Consider implementing Tags (archetype-specific by-value data that affects archetype identity)
* ex: ArchetypeA could have `[A, B, C]` table components and `[D(1)]` "tag" component. ArchetypeB could have `[A, B, C]` table components and a `[D(2)]` tag component. The archetypes are different, despite both having D tags because the value inside D is different.
* this could potentially build on top of the `archetype.unique_components` added in this pr for resource storage.
* Consider reverting `all_tuples` proc macro in favor of the old `macro_rules` implementation
* all_tuples is more flexible and produces cleaner documentation (the macro_rules version produces weird type parameter orders due to parser constraints)
* but unfortunately all_tuples also appears to make Rust Analyzer sad/slow when working inside of `bevy_ecs` (does not affect user code)
* Consider "resource queries" and/or "mixed resource and entity component queries" as an alternative to WorldCell
* this is basically just "systems" so maybe it's not worth it
* Add more world ops
* `world.clear()`
* `world.reserve<T: Bundle>(count: usize)`
* Try using the old archetype allocation strategy (allocate new memory on resize and copy everything over). I expect this to improve batch insertion performance at the cost of unbatched performance. But thats just a guess. I'm not an allocation perf pro :)
* Adapt Commands apis for consistency with new World apis
## Benchmarks
key:
* `bevy_old`: bevy `main` branch
* `bevy`: this branch
* `_foreach`: uses an optimized for_each iterator
* ` _sparse`: uses sparse set storage (if unspecified assume table storage)
* `_system`: runs inside a system (if unspecified assume test happens via direct world ops)
### Simple Insert (from ecs_bench_suite)
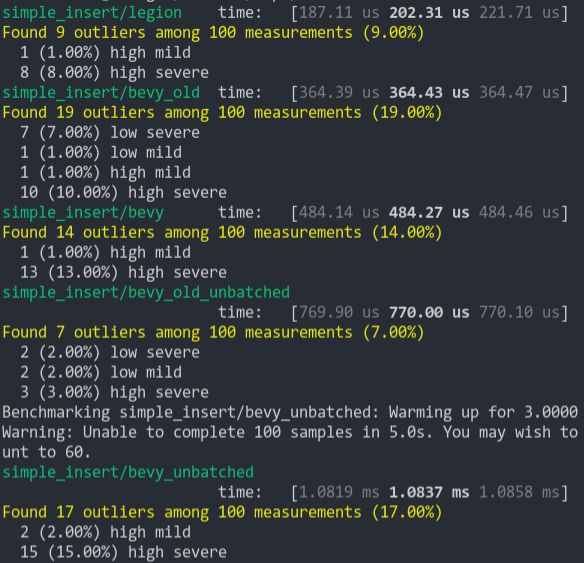
### Simpler Iter (from ecs_bench_suite)
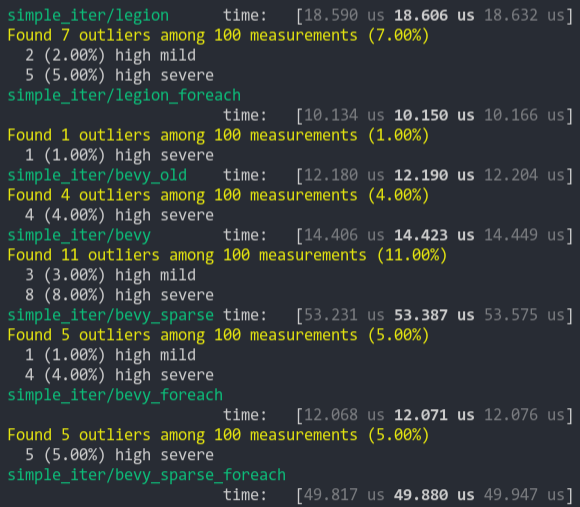
### Fragment Iter (from ecs_bench_suite)
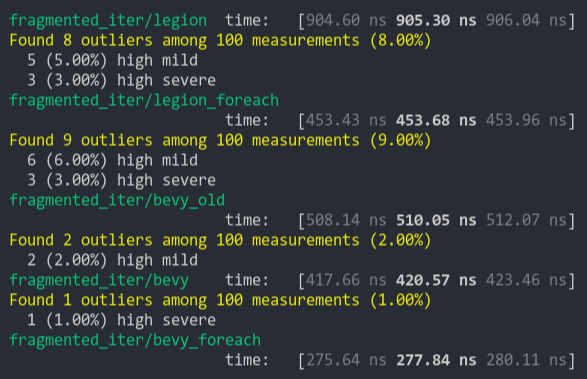
### Sparse Fragmented Iter
Iterate a query that matches 5 entities from a single matching archetype, but there are 100 unmatching archetypes
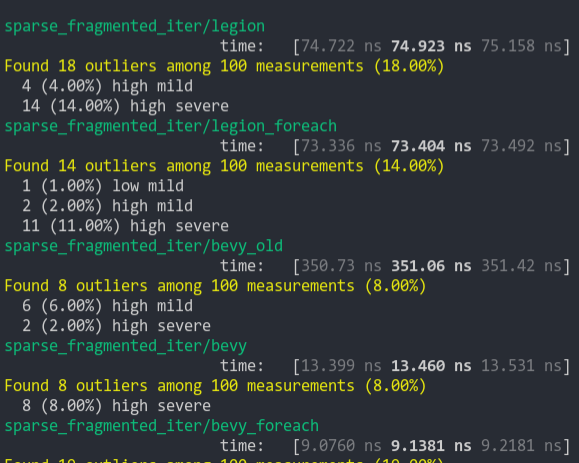
### Schedule (from ecs_bench_suite)
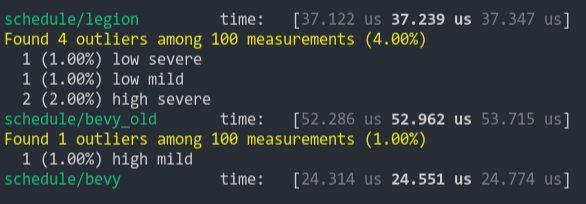
### Add Remove Component (from ecs_bench_suite)
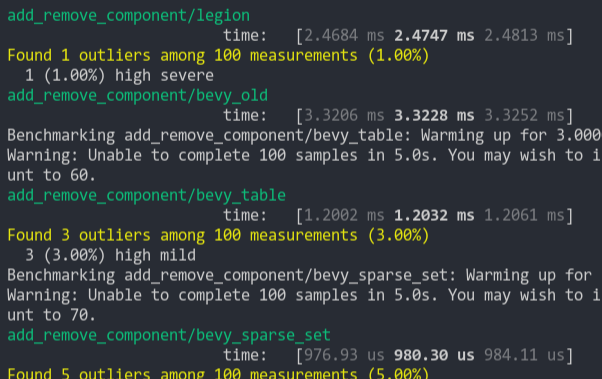
### Add Remove Component Big
Same as the test above, but each entity has 5 "large" matrix components and 1 "large" matrix component is added and removed
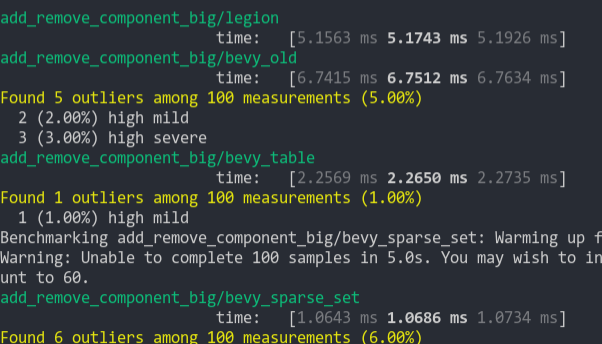
### Get Component
Looks up a single component value a large number of times
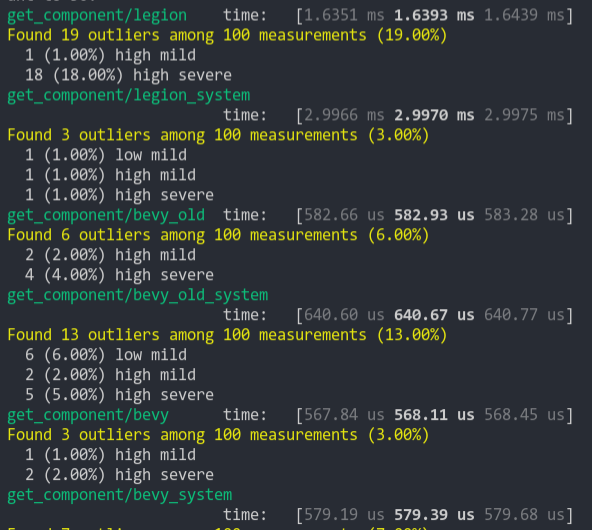
2021-03-05 07:54:35 +00:00
ops ::{ Deref , DerefMut } ,
2020-11-08 20:34:05 +00:00
} ;
2021-03-03 03:11:11 +00:00
2021-04-15 20:36:16 +00:00
/// A parameter that can be used in a [`System`](super::System).
2021-03-03 03:11:11 +00:00
///
/// # Derive
2021-04-15 20:36:16 +00:00
///
2022-05-17 22:24:50 +00:00
/// This trait can be derived with the [`derive@super::SystemParam`] macro.
/// This macro only works if each field on the derived struct implements [`SystemParam`].
/// Note: There are additional requirements on the field types.
/// See the *Generic `SystemParam`s* section for details and workarounds of the probable
/// cause if this derive causes an error to be emitted.
///
Make the `SystemParam` derive macro more flexible (#6694)
# Objective
Currently, the `SystemParam` derive forces you to declare the lifetime parameters `<'w, 's>`, even if you don't use them.
If you don't follow this structure, the error message is quite nasty.
### Example (before):
```rust
#[derive(SystemParam)]
pub struct EventWriter<'w, 's, E: Event> {
events: ResMut<'w, Events<E>>,
// The derive forces us to declare the `'s` lifetime even though we don't use it,
// so we have to add this `PhantomData` to please rustc.
#[system_param(ignore)]
_marker: PhantomData<&'s ()>,
}
```
## Solution
* Allow the user to omit either lifetime.
* Emit a descriptive error if any lifetimes used are invalid.
### Example (after):
```rust
#[derive(SystemParam)]
pub struct EventWriter<'w, E: Event> {
events: ResMut<'w, Events<E>>,
}
```
---
## Changelog
* The `SystemParam` derive is now more flexible, allowing you to omit unused lifetime parameters.
2022-12-05 20:15:03 +00:00
/// Derived `SystemParam` structs may have two lifetimes: `'w` for data stored in the [`World`],
/// and `'s` for data stored in the parameter's state.
2022-05-17 22:24:50 +00:00
///
/// ## Attributes
///
/// `#[system_param(ignore)]`:
/// Can be added to any field in the struct. Fields decorated with this attribute
2022-08-09 16:53:27 +00:00
/// will be created with the default value upon realisation.
Make the `SystemParam` derive macro more flexible (#6694)
# Objective
Currently, the `SystemParam` derive forces you to declare the lifetime parameters `<'w, 's>`, even if you don't use them.
If you don't follow this structure, the error message is quite nasty.
### Example (before):
```rust
#[derive(SystemParam)]
pub struct EventWriter<'w, 's, E: Event> {
events: ResMut<'w, Events<E>>,
// The derive forces us to declare the `'s` lifetime even though we don't use it,
// so we have to add this `PhantomData` to please rustc.
#[system_param(ignore)]
_marker: PhantomData<&'s ()>,
}
```
## Solution
* Allow the user to omit either lifetime.
* Emit a descriptive error if any lifetimes used are invalid.
### Example (after):
```rust
#[derive(SystemParam)]
pub struct EventWriter<'w, E: Event> {
events: ResMut<'w, Events<E>>,
}
```
---
## Changelog
* The `SystemParam` derive is now more flexible, allowing you to omit unused lifetime parameters.
2022-12-05 20:15:03 +00:00
/// This is most useful for `PhantomData` fields, such as markers for generic types.
2022-05-17 22:24:50 +00:00
///
/// # Example
2021-03-03 03:11:11 +00:00
///
/// ```
/// # use bevy_ecs::prelude::*;
Make `Resource` trait opt-in, requiring `#[derive(Resource)]` V2 (#5577)
*This PR description is an edited copy of #5007, written by @alice-i-cecile.*
# Objective
Follow-up to https://github.com/bevyengine/bevy/pull/2254. The `Resource` trait currently has a blanket implementation for all types that meet its bounds.
While ergonomic, this results in several drawbacks:
* it is possible to make confusing, silent mistakes such as inserting a function pointer (Foo) rather than a value (Foo::Bar) as a resource
* it is challenging to discover if a type is intended to be used as a resource
* we cannot later add customization options (see the [RFC](https://github.com/bevyengine/rfcs/blob/main/rfcs/27-derive-component.md) for the equivalent choice for Component).
* dependencies can use the same Rust type as a resource in invisibly conflicting ways
* raw Rust types used as resources cannot preserve privacy appropriately, as anyone able to access that type can read and write to internal values
* we cannot capture a definitive list of possible resources to display to users in an editor
## Notes to reviewers
* Review this commit-by-commit; there's effectively no back-tracking and there's a lot of churn in some of these commits.
*ira: My commits are not as well organized :')*
* I've relaxed the bound on Local to Send + Sync + 'static: I don't think these concerns apply there, so this can keep things simple. Storing e.g. a u32 in a Local is fine, because there's a variable name attached explaining what it does.
* I think this is a bad place for the Resource trait to live, but I've left it in place to make reviewing easier. IMO that's best tackled with https://github.com/bevyengine/bevy/issues/4981.
## Changelog
`Resource` is no longer automatically implemented for all matching types. Instead, use the new `#[derive(Resource)]` macro.
## Migration Guide
Add `#[derive(Resource)]` to all types you are using as a resource.
If you are using a third party type as a resource, wrap it in a tuple struct to bypass orphan rules. Consider deriving `Deref` and `DerefMut` to improve ergonomics.
`ClearColor` no longer implements `Component`. Using `ClearColor` as a component in 0.8 did nothing.
Use the `ClearColorConfig` in the `Camera3d` and `Camera2d` components instead.
Co-authored-by: Alice <alice.i.cecile@gmail.com>
Co-authored-by: Alice Cecile <alice.i.cecile@gmail.com>
Co-authored-by: devil-ira <justthecooldude@gmail.com>
Co-authored-by: Carter Anderson <mcanders1@gmail.com>
2022-08-08 21:36:35 +00:00
/// # #[derive(Resource)]
/// # struct SomeResource;
System Param Lifetime Split (#2605)
# Objective
Enable using exact World lifetimes during read-only access . This is motivated by the new renderer's need to allow read-only world-only queries to outlive the query itself (but still be constrained by the world lifetime).
For example:
https://github.com/bevyengine/bevy/blob/115b170d1f11a91146bb6d6e9684dceb8b21f786/pipelined/bevy_pbr2/src/render/mod.rs#L774
## Solution
Split out SystemParam state and world lifetimes and pipe those lifetimes up to read-only Query ops (and add into_inner for Res). According to every safety test I've run so far (except one), this is safe (see the temporary safety test commit). Note that changing the mutable variants to the new lifetimes would allow aliased mutable pointers (try doing that to see how it affects the temporary safety tests).
The new state lifetime on SystemParam does make `#[derive(SystemParam)]` more cumbersome (the current impl requires PhantomData if you don't use both lifetimes). We can make this better by detecting whether or not a lifetime is used in the derive and adjusting accordingly, but that should probably be done in its own pr.
## Why is this a draft?
The new lifetimes break QuerySet safety in one very specific case (see the query_set system in system_safety_test). We need to solve this before we can use the lifetimes given.
This is due to the fact that QuerySet is just a wrapper over Query, which now relies on world lifetimes instead of `&self` lifetimes to prevent aliasing (but in systems, each Query has its own implied lifetime, not a centralized world lifetime). I believe the fix is to rewrite QuerySet to have its own World lifetime (and own the internal reference). This will complicate the impl a bit, but I think it is doable. I'm curious if anyone else has better ideas.
Personally, I think these new lifetimes need to happen. We've gotta have a way to directly tie read-only World queries to the World lifetime. The new renderer is the first place this has come up, but I doubt it will be the last. Worst case scenario we can come up with a second `WorldLifetimeQuery<Q, F = ()>` parameter to enable these read-only scenarios, but I'd rather not add another type to the type zoo.
2021-08-15 20:51:53 +00:00
/// use std::marker::PhantomData;
Bevy ECS V2 (#1525)
# Bevy ECS V2
This is a rewrite of Bevy ECS (basically everything but the new executor/schedule, which are already awesome). The overall goal was to improve the performance and versatility of Bevy ECS. Here is a quick bulleted list of changes before we dive into the details:
* Complete World rewrite
* Multiple component storage types:
* Tables: fast cache friendly iteration, slower add/removes (previously called Archetypes)
* Sparse Sets: fast add/remove, slower iteration
* Stateful Queries (caches query results for faster iteration. fragmented iteration is _fast_ now)
* Stateful System Params (caches expensive operations. inspired by @DJMcNab's work in #1364)
* Configurable System Params (users can set configuration when they construct their systems. once again inspired by @DJMcNab's work)
* Archetypes are now "just metadata", component storage is separate
* Archetype Graph (for faster archetype changes)
* Component Metadata
* Configure component storage type
* Retrieve information about component size/type/name/layout/send-ness/etc
* Components are uniquely identified by a densely packed ComponentId
* TypeIds are now totally optional (which should make implementing scripting easier)
* Super fast "for_each" query iterators
* Merged Resources into World. Resources are now just a special type of component
* EntityRef/EntityMut builder apis (more efficient and more ergonomic)
* Fast bitset-backed `Access<T>` replaces old hashmap-based approach everywhere
* Query conflicts are determined by component access instead of archetype component access (to avoid random failures at runtime)
* With/Without are still taken into account for conflicts, so this should still be comfy to use
* Much simpler `IntoSystem` impl
* Significantly reduced the amount of hashing throughout the ecs in favor of Sparse Sets (indexed by densely packed ArchetypeId, ComponentId, BundleId, and TableId)
* Safety Improvements
* Entity reservation uses a normal world reference instead of unsafe transmute
* QuerySets no longer transmute lifetimes
* Made traits "unsafe" where relevant
* More thorough safety docs
* WorldCell
* Exposes safe mutable access to multiple resources at a time in a World
* Replaced "catch all" `System::update_archetypes(world: &World)` with `System::new_archetype(archetype: &Archetype)`
* Simpler Bundle implementation
* Replaced slow "remove_bundle_one_by_one" used as fallback for Commands::remove_bundle with fast "remove_bundle_intersection"
* Removed `Mut<T>` query impl. it is better to only support one way: `&mut T`
* Removed with() from `Flags<T>` in favor of `Option<Flags<T>>`, which allows querying for flags to be "filtered" by default
* Components now have is_send property (currently only resources support non-send)
* More granular module organization
* New `RemovedComponents<T>` SystemParam that replaces `query.removed::<T>()`
* `world.resource_scope()` for mutable access to resources and world at the same time
* WorldQuery and QueryFilter traits unified. FilterFetch trait added to enable "short circuit" filtering. Auto impled for cases that don't need it
* Significantly slimmed down SystemState in favor of individual SystemParam state
* System Commands changed from `commands: &mut Commands` back to `mut commands: Commands` (to allow Commands to have a World reference)
Fixes #1320
## `World` Rewrite
This is a from-scratch rewrite of `World` that fills the niche that `hecs` used to. Yes, this means Bevy ECS is no longer a "fork" of hecs. We're going out our own!
(the only shared code between the projects is the entity id allocator, which is already basically ideal)
A huge shout out to @SanderMertens (author of [flecs](https://github.com/SanderMertens/flecs)) for sharing some great ideas with me (specifically hybrid ecs storage and archetype graphs). He also helped advise on a number of implementation details.
## Component Storage (The Problem)
Two ECS storage paradigms have gained a lot of traction over the years:
* **Archetypal ECS**:
* Stores components in "tables" with static schemas. Each "column" stores components of a given type. Each "row" is an entity.
* Each "archetype" has its own table. Adding/removing an entity's component changes the archetype.
* Enables super-fast Query iteration due to its cache-friendly data layout
* Comes at the cost of more expensive add/remove operations for an Entity's components, because all components need to be copied to the new archetype's "table"
* **Sparse Set ECS**:
* Stores components of the same type in densely packed arrays, which are sparsely indexed by densely packed unsigned integers (Entity ids)
* Query iteration is slower than Archetypal ECS because each entity's component could be at any position in the sparse set. This "random access" pattern isn't cache friendly. Additionally, there is an extra layer of indirection because you must first map the entity id to an index in the component array.
* Adding/removing components is a cheap, constant time operation
Bevy ECS V1, hecs, legion, flec, and Unity DOTS are all "archetypal ecs-es". I personally think "archetypal" storage is a good default for game engines. An entity's archetype doesn't need to change frequently in general, and it creates "fast by default" query iteration (which is a much more common operation). It is also "self optimizing". Users don't need to think about optimizing component layouts for iteration performance. It "just works" without any extra boilerplate.
Shipyard and EnTT are "sparse set ecs-es". They employ "packing" as a way to work around the "suboptimal by default" iteration performance for specific sets of components. This helps, but I didn't think this was a good choice for a general purpose engine like Bevy because:
1. "packs" conflict with each other. If bevy decides to internally pack the Transform and GlobalTransform components, users are then blocked if they want to pack some custom component with Transform.
2. users need to take manual action to optimize
Developers selecting an ECS framework are stuck with a hard choice. Select an "archetypal" framework with "fast iteration everywhere" but without the ability to cheaply add/remove components, or select a "sparse set" framework to cheaply add/remove components but with slower iteration performance.
## Hybrid Component Storage (The Solution)
In Bevy ECS V2, we get to have our cake and eat it too. It now has _both_ of the component storage types above (and more can be added later if needed):
* **Tables** (aka "archetypal" storage)
* The default storage. If you don't configure anything, this is what you get
* Fast iteration by default
* Slower add/remove operations
* **Sparse Sets**
* Opt-in
* Slower iteration
* Faster add/remove operations
These storage types complement each other perfectly. By default Query iteration is fast. If developers know that they want to add/remove a component at high frequencies, they can set the storage to "sparse set":
```rust
world.register_component(
ComponentDescriptor::new::<MyComponent>(StorageType::SparseSet)
).unwrap();
```
## Archetypes
Archetypes are now "just metadata" ... they no longer store components directly. They do store:
* The `ComponentId`s of each of the Archetype's components (and that component's storage type)
* Archetypes are uniquely defined by their component layouts
* For example: entities with "table" components `[A, B, C]` _and_ "sparse set" components `[D, E]` will always be in the same archetype.
* The `TableId` associated with the archetype
* For now each archetype has exactly one table (which can have no components),
* There is a 1->Many relationship from Tables->Archetypes. A given table could have any number of archetype components stored in it:
* Ex: an entity with "table storage" components `[A, B, C]` and "sparse set" components `[D, E]` will share the same `[A, B, C]` table as an entity with `[A, B, C]` table component and `[F]` sparse set components.
* This 1->Many relationship is how we preserve fast "cache friendly" iteration performance when possible (more on this later)
* A list of entities that are in the archetype and the row id of the table they are in
* ArchetypeComponentIds
* unique densely packed identifiers for (ArchetypeId, ComponentId) pairs
* used by the schedule executor for cheap system access control
* "Archetype Graph Edges" (see the next section)
## The "Archetype Graph"
Archetype changes in Bevy (and a number of other archetypal ecs-es) have historically been expensive to compute. First, you need to allocate a new vector of the entity's current component ids, add or remove components based on the operation performed, sort it (to ensure it is order-independent), then hash it to find the archetype (if it exists). And thats all before we get to the _already_ expensive full copy of all components to the new table storage.
The solution is to build a "graph" of archetypes to cache these results. @SanderMertens first exposed me to the idea (and he got it from @gjroelofs, who came up with it). They propose adding directed edges between archetypes for add/remove component operations. If `ComponentId`s are densely packed, you can use sparse sets to cheaply jump between archetypes.
Bevy takes this one step further by using add/remove `Bundle` edges instead of `Component` edges. Bevy encourages the use of `Bundles` to group add/remove operations. This is largely for "clearer game logic" reasons, but it also helps cut down on the number of archetype changes required. `Bundles` now also have densely-packed `BundleId`s. This allows us to use a _single_ edge for each bundle operation (rather than needing to traverse N edges ... one for each component). Single component operations are also bundles, so this is strictly an improvement over a "component only" graph.
As a result, an operation that used to be _heavy_ (both for allocations and compute) is now two dirt-cheap array lookups and zero allocations.
## Stateful Queries
World queries are now stateful. This allows us to:
1. Cache archetype (and table) matches
* This resolves another issue with (naive) archetypal ECS: query performance getting worse as the number of archetypes goes up (and fragmentation occurs).
2. Cache Fetch and Filter state
* The expensive parts of fetch/filter operations (such as hashing the TypeId to find the ComponentId) now only happen once when the Query is first constructed
3. Incrementally build up state
* When new archetypes are added, we only process the new archetypes (no need to rebuild state for old archetypes)
As a result, the direct `World` query api now looks like this:
```rust
let mut query = world.query::<(&A, &mut B)>();
for (a, mut b) in query.iter_mut(&mut world) {
}
```
Requiring `World` to generate stateful queries (rather than letting the `QueryState` type be constructed separately) allows us to ensure that _all_ queries are properly initialized (and the relevant world state, such as ComponentIds). This enables QueryState to remove branches from its operations that check for initialization status (and also enables query.iter() to take an immutable world reference because it doesn't need to initialize anything in world).
However in systems, this is a non-breaking change. State management is done internally by the relevant SystemParam.
## Stateful SystemParams
Like Queries, `SystemParams` now also cache state. For example, `Query` system params store the "stateful query" state mentioned above. Commands store their internal `CommandQueue`. This means you can now safely use as many separate `Commands` parameters in your system as you want. `Local<T>` system params store their `T` value in their state (instead of in Resources).
SystemParam state also enabled a significant slim-down of SystemState. It is much nicer to look at now.
Per-SystemParam state naturally insulates us from an "aliased mut" class of errors we have hit in the past (ex: using multiple `Commands` system params).
(credit goes to @DJMcNab for the initial idea and draft pr here #1364)
## Configurable SystemParams
@DJMcNab also had the great idea to make SystemParams configurable. This allows users to provide some initial configuration / values for system parameters (when possible). Most SystemParams have no config (the config type is `()`), but the `Local<T>` param now supports user-provided parameters:
```rust
fn foo(value: Local<usize>) {
}
app.add_system(foo.system().config(|c| c.0 = Some(10)));
```
## Uber Fast "for_each" Query Iterators
Developers now have the choice to use a fast "for_each" iterator, which yields ~1.5-3x iteration speed improvements for "fragmented iteration", and minor ~1.2x iteration speed improvements for unfragmented iteration.
```rust
fn system(query: Query<(&A, &mut B)>) {
// you now have the option to do this for a speed boost
query.for_each_mut(|(a, mut b)| {
});
// however normal iterators are still available
for (a, mut b) in query.iter_mut() {
}
}
```
I think in most cases we should continue to encourage "normal" iterators as they are more flexible and more "rust idiomatic". But when that extra "oomf" is needed, it makes sense to use `for_each`.
We should also consider using `for_each` for internal bevy systems to give our users a nice speed boost (but that should be a separate pr).
## Component Metadata
`World` now has a `Components` collection, which is accessible via `world.components()`. This stores mappings from `ComponentId` to `ComponentInfo`, as well as `TypeId` to `ComponentId` mappings (where relevant). `ComponentInfo` stores information about the component, such as ComponentId, TypeId, memory layout, send-ness (currently limited to resources), and storage type.
## Significantly Cheaper `Access<T>`
We used to use `TypeAccess<TypeId>` to manage read/write component/archetype-component access. This was expensive because TypeIds must be hashed and compared individually. The parallel executor got around this by "condensing" type ids into bitset-backed access types. This worked, but it had to be re-generated from the `TypeAccess<TypeId>`sources every time archetypes changed.
This pr removes TypeAccess in favor of faster bitset access everywhere. We can do this thanks to the move to densely packed `ComponentId`s and `ArchetypeComponentId`s.
## Merged Resources into World
Resources had a lot of redundant functionality with Components. They stored typed data, they had access control, they had unique ids, they were queryable via SystemParams, etc. In fact the _only_ major difference between them was that they were unique (and didn't correlate to an entity).
Separate resources also had the downside of requiring a separate set of access controls, which meant the parallel executor needed to compare more bitsets per system and manage more state.
I initially got the "separate resources" idea from `legion`. I think that design was motivated by the fact that it made the direct world query/resource lifetime interactions more manageable. It certainly made our lives easier when using Resources alongside hecs/bevy_ecs. However we already have a construct for safely and ergonomically managing in-world lifetimes: systems (which use `Access<T>` internally).
This pr merges Resources into World:
```rust
world.insert_resource(1);
world.insert_resource(2.0);
let a = world.get_resource::<i32>().unwrap();
let mut b = world.get_resource_mut::<f64>().unwrap();
*b = 3.0;
```
Resources are now just a special kind of component. They have their own ComponentIds (and their own resource TypeId->ComponentId scope, so they don't conflict wit components of the same type). They are stored in a special "resource archetype", which stores components inside the archetype using a new `unique_components` sparse set (note that this sparse set could later be used to implement Tags). This allows us to keep the code size small by reusing existing datastructures (namely Column, Archetype, ComponentFlags, and ComponentInfo). This allows us the executor to use a single `Access<ArchetypeComponentId>` per system. It should also make scripting language integration easier.
_But_ this merge did create problems for people directly interacting with `World`. What if you need mutable access to multiple resources at the same time? `world.get_resource_mut()` borrows World mutably!
## WorldCell
WorldCell applies the `Access<ArchetypeComponentId>` concept to direct world access:
```rust
let world_cell = world.cell();
let a = world_cell.get_resource_mut::<i32>().unwrap();
let b = world_cell.get_resource_mut::<f64>().unwrap();
```
This adds cheap runtime checks (a sparse set lookup of `ArchetypeComponentId` and a counter) to ensure that world accesses do not conflict with each other. Each operation returns a `WorldBorrow<'w, T>` or `WorldBorrowMut<'w, T>` wrapper type, which will release the relevant ArchetypeComponentId resources when dropped.
World caches the access sparse set (and only one cell can exist at a time), so `world.cell()` is a cheap operation.
WorldCell does _not_ use atomic operations. It is non-send, does a mutable borrow of world to prevent other accesses, and uses a simple `Rc<RefCell<ArchetypeComponentAccess>>` wrapper in each WorldBorrow pointer.
The api is currently limited to resource access, but it can and should be extended to queries / entity component access.
## Resource Scopes
WorldCell does not yet support component queries, and even when it does there are sometimes legitimate reasons to want a mutable world ref _and_ a mutable resource ref (ex: bevy_render and bevy_scene both need this). In these cases we could always drop down to the unsafe `world.get_resource_unchecked_mut()`, but that is not ideal!
Instead developers can use a "resource scope"
```rust
world.resource_scope(|world: &mut World, a: &mut A| {
})
```
This temporarily removes the `A` resource from `World`, provides mutable pointers to both, and re-adds A to World when finished. Thanks to the move to ComponentIds/sparse sets, this is a cheap operation.
If multiple resources are required, scopes can be nested. We could also consider adding a "resource tuple" to the api if this pattern becomes common and the boilerplate gets nasty.
## Query Conflicts Use ComponentId Instead of ArchetypeComponentId
For safety reasons, systems cannot contain queries that conflict with each other without wrapping them in a QuerySet. On bevy `main`, we use ArchetypeComponentIds to determine conflicts. This is nice because it can take into account filters:
```rust
// these queries will never conflict due to their filters
fn filter_system(a: Query<&mut A, With<B>>, b: Query<&mut B, Without<B>>) {
}
```
But it also has a significant downside:
```rust
// these queries will not conflict _until_ an entity with A, B, and C is spawned
fn maybe_conflicts_system(a: Query<(&mut A, &C)>, b: Query<(&mut A, &B)>) {
}
```
The system above will panic at runtime if an entity with A, B, and C is spawned. This makes it hard to trust that your game logic will run without crashing.
In this pr, I switched to using `ComponentId` instead. This _is_ more constraining. `maybe_conflicts_system` will now always fail, but it will do it consistently at startup. Naively, it would also _disallow_ `filter_system`, which would be a significant downgrade in usability. Bevy has a number of internal systems that rely on disjoint queries and I expect it to be a common pattern in userspace.
To resolve this, I added a new `FilteredAccess<T>` type, which wraps `Access<T>` and adds with/without filters. If two `FilteredAccess` have with/without values that prove they are disjoint, they will no longer conflict.
## EntityRef / EntityMut
World entity operations on `main` require that the user passes in an `entity` id to each operation:
```rust
let entity = world.spawn((A, )); // create a new entity with A
world.get::<A>(entity);
world.insert(entity, (B, C));
world.insert_one(entity, D);
```
This means that each operation needs to look up the entity location / verify its validity. The initial spawn operation also requires a Bundle as input. This can be awkward when no components are required (or one component is required).
These operations have been replaced by `EntityRef` and `EntityMut`, which are "builder-style" wrappers around world that provide read and read/write operations on a single, pre-validated entity:
```rust
// spawn now takes no inputs and returns an EntityMut
let entity = world.spawn()
.insert(A) // insert a single component into the entity
.insert_bundle((B, C)) // insert a bundle of components into the entity
.id() // id returns the Entity id
// Returns EntityMut (or panics if the entity does not exist)
world.entity_mut(entity)
.insert(D)
.insert_bundle(SomeBundle::default());
{
// returns EntityRef (or panics if the entity does not exist)
let d = world.entity(entity)
.get::<D>() // gets the D component
.unwrap();
// world.get still exists for ergonomics
let d = world.get::<D>(entity).unwrap();
}
// These variants return Options if you want to check existence instead of panicing
world.get_entity_mut(entity)
.unwrap()
.insert(E);
if let Some(entity_ref) = world.get_entity(entity) {
let d = entity_ref.get::<D>().unwrap();
}
```
This _does not_ affect the current Commands api or terminology. I think that should be a separate conversation as that is a much larger breaking change.
## Safety Improvements
* Entity reservation in Commands uses a normal world borrow instead of an unsafe transmute
* QuerySets no longer transmutes lifetimes
* Made traits "unsafe" when implementing a trait incorrectly could cause unsafety
* More thorough safety docs
## RemovedComponents SystemParam
The old approach to querying removed components: `query.removed:<T>()` was confusing because it had no connection to the query itself. I replaced it with the following, which is both clearer and allows us to cache the ComponentId mapping in the SystemParamState:
```rust
fn system(removed: RemovedComponents<T>) {
for entity in removed.iter() {
}
}
```
## Simpler Bundle implementation
Bundles are no longer responsible for sorting (or deduping) TypeInfo. They are just a simple ordered list of component types / data. This makes the implementation smaller and opens the door to an easy "nested bundle" implementation in the future (which i might even add in this pr). Duplicate detection is now done once per bundle type by World the first time a bundle is used.
## Unified WorldQuery and QueryFilter types
(don't worry they are still separate type _parameters_ in Queries .. this is a non-breaking change)
WorldQuery and QueryFilter were already basically identical apis. With the addition of `FetchState` and more storage-specific fetch methods, the overlap was even clearer (and the redundancy more painful).
QueryFilters are now just `F: WorldQuery where F::Fetch: FilterFetch`. FilterFetch requires `Fetch<Item = bool>` and adds new "short circuit" variants of fetch methods. This enables a filter tuple like `(With<A>, Without<B>, Changed<C>)` to stop evaluating the filter after the first mismatch is encountered. FilterFetch is automatically implemented for `Fetch` implementations that return bool.
This forces fetch implementations that return things like `(bool, bool, bool)` (such as the filter above) to manually implement FilterFetch and decide whether or not to short-circuit.
## More Granular Modules
World no longer globs all of the internal modules together. It now exports `core`, `system`, and `schedule` separately. I'm also considering exporting `core` submodules directly as that is still pretty "glob-ey" and unorganized (feedback welcome here).
## Remaining Draft Work (to be done in this pr)
* ~~panic on conflicting WorldQuery fetches (&A, &mut A)~~
* ~~bevy `main` and hecs both currently allow this, but we should protect against it if possible~~
* ~~batch_iter / par_iter (currently stubbed out)~~
* ~~ChangedRes~~
* ~~I skipped this while we sort out #1313. This pr should be adapted to account for whatever we land on there~~.
* ~~The `Archetypes` and `Tables` collections use hashes of sorted lists of component ids to uniquely identify each archetype/table. This hash is then used as the key in a HashMap to look up the relevant ArchetypeId or TableId. (which doesn't handle hash collisions properly)~~
* ~~It is currently unsafe to generate a Query from "World A", then use it on "World B" (despite the api claiming it is safe). We should probably close this gap. This could be done by adding a randomly generated WorldId to each world, then storing that id in each Query. They could then be compared to each other on each `query.do_thing(&world)` operation. This _does_ add an extra branch to each query operation, so I'm open to other suggestions if people have them.~~
* ~~Nested Bundles (if i find time)~~
## Potential Future Work
* Expand WorldCell to support queries.
* Consider not allocating in the empty archetype on `world.spawn()`
* ex: return something like EntityMutUninit, which turns into EntityMut after an `insert` or `insert_bundle` op
* this actually regressed performance last time i tried it, but in theory it should be faster
* Optimize SparseSet::insert (see `PERF` comment on insert)
* Replace SparseArray `Option<T>` with T::MAX to cut down on branching
* would enable cheaper get_unchecked() operations
* upstream fixedbitset optimizations
* fixedbitset could be allocation free for small block counts (store blocks in a SmallVec)
* fixedbitset could have a const constructor
* Consider implementing Tags (archetype-specific by-value data that affects archetype identity)
* ex: ArchetypeA could have `[A, B, C]` table components and `[D(1)]` "tag" component. ArchetypeB could have `[A, B, C]` table components and a `[D(2)]` tag component. The archetypes are different, despite both having D tags because the value inside D is different.
* this could potentially build on top of the `archetype.unique_components` added in this pr for resource storage.
* Consider reverting `all_tuples` proc macro in favor of the old `macro_rules` implementation
* all_tuples is more flexible and produces cleaner documentation (the macro_rules version produces weird type parameter orders due to parser constraints)
* but unfortunately all_tuples also appears to make Rust Analyzer sad/slow when working inside of `bevy_ecs` (does not affect user code)
* Consider "resource queries" and/or "mixed resource and entity component queries" as an alternative to WorldCell
* this is basically just "systems" so maybe it's not worth it
* Add more world ops
* `world.clear()`
* `world.reserve<T: Bundle>(count: usize)`
* Try using the old archetype allocation strategy (allocate new memory on resize and copy everything over). I expect this to improve batch insertion performance at the cost of unbatched performance. But thats just a guess. I'm not an allocation perf pro :)
* Adapt Commands apis for consistency with new World apis
## Benchmarks
key:
* `bevy_old`: bevy `main` branch
* `bevy`: this branch
* `_foreach`: uses an optimized for_each iterator
* ` _sparse`: uses sparse set storage (if unspecified assume table storage)
* `_system`: runs inside a system (if unspecified assume test happens via direct world ops)
### Simple Insert (from ecs_bench_suite)
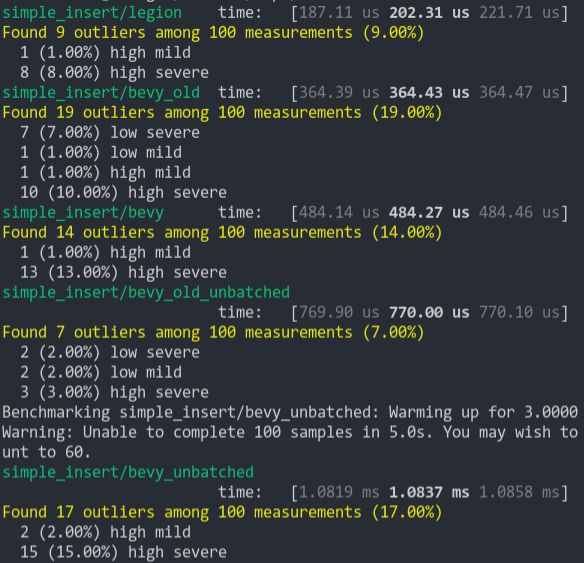
### Simpler Iter (from ecs_bench_suite)
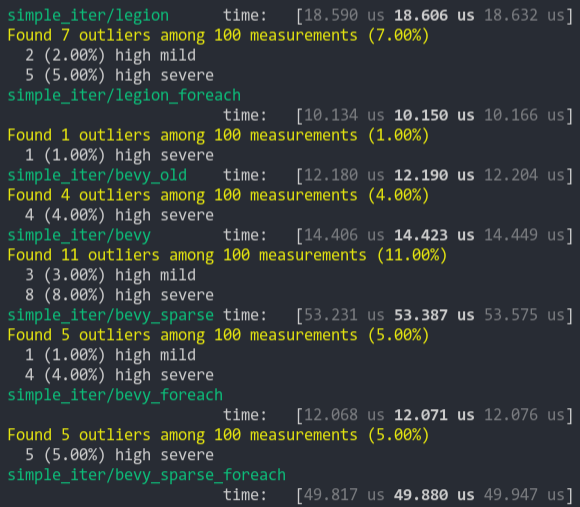
### Fragment Iter (from ecs_bench_suite)
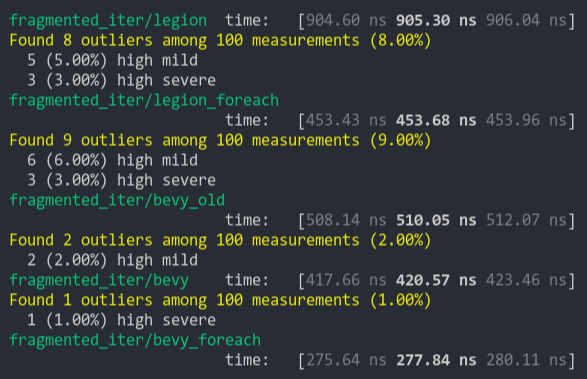
### Sparse Fragmented Iter
Iterate a query that matches 5 entities from a single matching archetype, but there are 100 unmatching archetypes
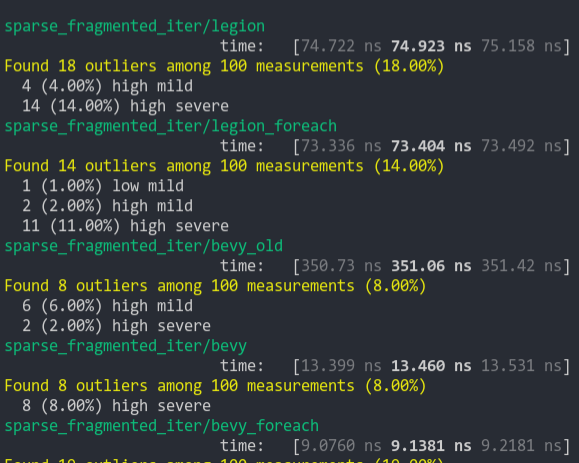
### Schedule (from ecs_bench_suite)
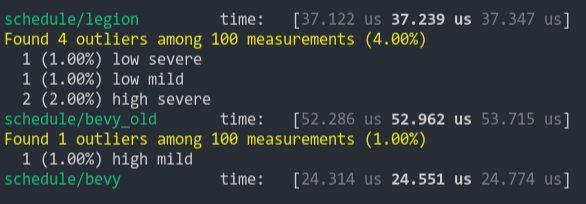
### Add Remove Component (from ecs_bench_suite)
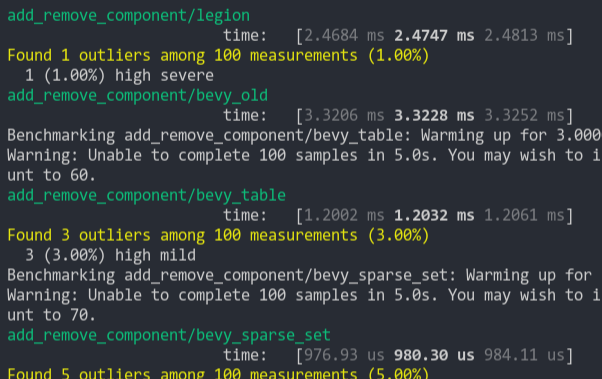
### Add Remove Component Big
Same as the test above, but each entity has 5 "large" matrix components and 1 "large" matrix component is added and removed
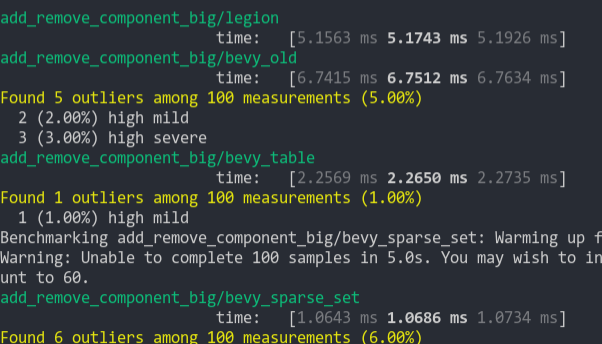
### Get Component
Looks up a single component value a large number of times
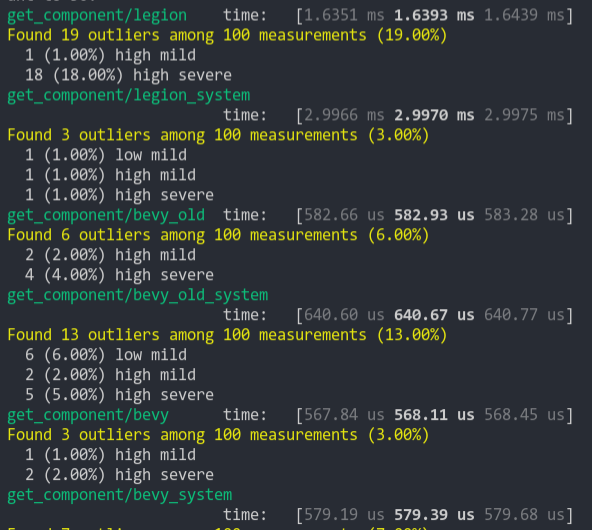
2021-03-05 07:54:35 +00:00
/// use bevy_ecs::system::SystemParam;
2021-03-03 03:11:11 +00:00
///
/// #[derive(SystemParam)]
Make the `SystemParam` derive macro more flexible (#6694)
# Objective
Currently, the `SystemParam` derive forces you to declare the lifetime parameters `<'w, 's>`, even if you don't use them.
If you don't follow this structure, the error message is quite nasty.
### Example (before):
```rust
#[derive(SystemParam)]
pub struct EventWriter<'w, 's, E: Event> {
events: ResMut<'w, Events<E>>,
// The derive forces us to declare the `'s` lifetime even though we don't use it,
// so we have to add this `PhantomData` to please rustc.
#[system_param(ignore)]
_marker: PhantomData<&'s ()>,
}
```
## Solution
* Allow the user to omit either lifetime.
* Emit a descriptive error if any lifetimes used are invalid.
### Example (after):
```rust
#[derive(SystemParam)]
pub struct EventWriter<'w, E: Event> {
events: ResMut<'w, Events<E>>,
}
```
---
## Changelog
* The `SystemParam` derive is now more flexible, allowing you to omit unused lifetime parameters.
2022-12-05 20:15:03 +00:00
/// struct MyParam<'w, Marker: 'static> {
Make `Resource` trait opt-in, requiring `#[derive(Resource)]` V2 (#5577)
*This PR description is an edited copy of #5007, written by @alice-i-cecile.*
# Objective
Follow-up to https://github.com/bevyengine/bevy/pull/2254. The `Resource` trait currently has a blanket implementation for all types that meet its bounds.
While ergonomic, this results in several drawbacks:
* it is possible to make confusing, silent mistakes such as inserting a function pointer (Foo) rather than a value (Foo::Bar) as a resource
* it is challenging to discover if a type is intended to be used as a resource
* we cannot later add customization options (see the [RFC](https://github.com/bevyengine/rfcs/blob/main/rfcs/27-derive-component.md) for the equivalent choice for Component).
* dependencies can use the same Rust type as a resource in invisibly conflicting ways
* raw Rust types used as resources cannot preserve privacy appropriately, as anyone able to access that type can read and write to internal values
* we cannot capture a definitive list of possible resources to display to users in an editor
## Notes to reviewers
* Review this commit-by-commit; there's effectively no back-tracking and there's a lot of churn in some of these commits.
*ira: My commits are not as well organized :')*
* I've relaxed the bound on Local to Send + Sync + 'static: I don't think these concerns apply there, so this can keep things simple. Storing e.g. a u32 in a Local is fine, because there's a variable name attached explaining what it does.
* I think this is a bad place for the Resource trait to live, but I've left it in place to make reviewing easier. IMO that's best tackled with https://github.com/bevyengine/bevy/issues/4981.
## Changelog
`Resource` is no longer automatically implemented for all matching types. Instead, use the new `#[derive(Resource)]` macro.
## Migration Guide
Add `#[derive(Resource)]` to all types you are using as a resource.
If you are using a third party type as a resource, wrap it in a tuple struct to bypass orphan rules. Consider deriving `Deref` and `DerefMut` to improve ergonomics.
`ClearColor` no longer implements `Component`. Using `ClearColor` as a component in 0.8 did nothing.
Use the `ClearColorConfig` in the `Camera3d` and `Camera2d` components instead.
Co-authored-by: Alice <alice.i.cecile@gmail.com>
Co-authored-by: Alice Cecile <alice.i.cecile@gmail.com>
Co-authored-by: devil-ira <justthecooldude@gmail.com>
Co-authored-by: Carter Anderson <mcanders1@gmail.com>
2022-08-08 21:36:35 +00:00
/// foo: Res<'w, SomeResource>,
System Param Lifetime Split (#2605)
# Objective
Enable using exact World lifetimes during read-only access . This is motivated by the new renderer's need to allow read-only world-only queries to outlive the query itself (but still be constrained by the world lifetime).
For example:
https://github.com/bevyengine/bevy/blob/115b170d1f11a91146bb6d6e9684dceb8b21f786/pipelined/bevy_pbr2/src/render/mod.rs#L774
## Solution
Split out SystemParam state and world lifetimes and pipe those lifetimes up to read-only Query ops (and add into_inner for Res). According to every safety test I've run so far (except one), this is safe (see the temporary safety test commit). Note that changing the mutable variants to the new lifetimes would allow aliased mutable pointers (try doing that to see how it affects the temporary safety tests).
The new state lifetime on SystemParam does make `#[derive(SystemParam)]` more cumbersome (the current impl requires PhantomData if you don't use both lifetimes). We can make this better by detecting whether or not a lifetime is used in the derive and adjusting accordingly, but that should probably be done in its own pr.
## Why is this a draft?
The new lifetimes break QuerySet safety in one very specific case (see the query_set system in system_safety_test). We need to solve this before we can use the lifetimes given.
This is due to the fact that QuerySet is just a wrapper over Query, which now relies on world lifetimes instead of `&self` lifetimes to prevent aliasing (but in systems, each Query has its own implied lifetime, not a centralized world lifetime). I believe the fix is to rewrite QuerySet to have its own World lifetime (and own the internal reference). This will complicate the impl a bit, but I think it is doable. I'm curious if anyone else has better ideas.
Personally, I think these new lifetimes need to happen. We've gotta have a way to directly tie read-only World queries to the World lifetime. The new renderer is the first place this has come up, but I doubt it will be the last. Worst case scenario we can come up with a second `WorldLifetimeQuery<Q, F = ()>` parameter to enable these read-only scenarios, but I'd rather not add another type to the type zoo.
2021-08-15 20:51:53 +00:00
/// #[system_param(ignore)]
Make the `SystemParam` derive macro more flexible (#6694)
# Objective
Currently, the `SystemParam` derive forces you to declare the lifetime parameters `<'w, 's>`, even if you don't use them.
If you don't follow this structure, the error message is quite nasty.
### Example (before):
```rust
#[derive(SystemParam)]
pub struct EventWriter<'w, 's, E: Event> {
events: ResMut<'w, Events<E>>,
// The derive forces us to declare the `'s` lifetime even though we don't use it,
// so we have to add this `PhantomData` to please rustc.
#[system_param(ignore)]
_marker: PhantomData<&'s ()>,
}
```
## Solution
* Allow the user to omit either lifetime.
* Emit a descriptive error if any lifetimes used are invalid.
### Example (after):
```rust
#[derive(SystemParam)]
pub struct EventWriter<'w, E: Event> {
events: ResMut<'w, Events<E>>,
}
```
---
## Changelog
* The `SystemParam` derive is now more flexible, allowing you to omit unused lifetime parameters.
2022-12-05 20:15:03 +00:00
/// marker: PhantomData<Marker>,
2021-03-03 03:11:11 +00:00
/// }
///
Make the `SystemParam` derive macro more flexible (#6694)
# Objective
Currently, the `SystemParam` derive forces you to declare the lifetime parameters `<'w, 's>`, even if you don't use them.
If you don't follow this structure, the error message is quite nasty.
### Example (before):
```rust
#[derive(SystemParam)]
pub struct EventWriter<'w, 's, E: Event> {
events: ResMut<'w, Events<E>>,
// The derive forces us to declare the `'s` lifetime even though we don't use it,
// so we have to add this `PhantomData` to please rustc.
#[system_param(ignore)]
_marker: PhantomData<&'s ()>,
}
```
## Solution
* Allow the user to omit either lifetime.
* Emit a descriptive error if any lifetimes used are invalid.
### Example (after):
```rust
#[derive(SystemParam)]
pub struct EventWriter<'w, E: Event> {
events: ResMut<'w, Events<E>>,
}
```
---
## Changelog
* The `SystemParam` derive is now more flexible, allowing you to omit unused lifetime parameters.
2022-12-05 20:15:03 +00:00
/// fn my_system<T: 'static>(param: MyParam<T>) {
2021-03-03 03:11:11 +00:00
/// // Access the resource through `param.foo`
/// }
2021-04-16 19:13:08 +00:00
///
Make the `SystemParam` derive macro more flexible (#6694)
# Objective
Currently, the `SystemParam` derive forces you to declare the lifetime parameters `<'w, 's>`, even if you don't use them.
If you don't follow this structure, the error message is quite nasty.
### Example (before):
```rust
#[derive(SystemParam)]
pub struct EventWriter<'w, 's, E: Event> {
events: ResMut<'w, Events<E>>,
// The derive forces us to declare the `'s` lifetime even though we don't use it,
// so we have to add this `PhantomData` to please rustc.
#[system_param(ignore)]
_marker: PhantomData<&'s ()>,
}
```
## Solution
* Allow the user to omit either lifetime.
* Emit a descriptive error if any lifetimes used are invalid.
### Example (after):
```rust
#[derive(SystemParam)]
pub struct EventWriter<'w, E: Event> {
events: ResMut<'w, Events<E>>,
}
```
---
## Changelog
* The `SystemParam` derive is now more flexible, allowing you to omit unused lifetime parameters.
2022-12-05 20:15:03 +00:00
/// # bevy_ecs::system::assert_is_system(my_system::<()>);
2021-03-03 03:11:11 +00:00
/// ```
2022-05-17 22:24:50 +00:00
///
/// # Generic `SystemParam`s
///
/// When using the derive macro, you may see an error in the form of:
///
/// ```text
/// expected ... [ParamType]
Remove the `SystemParamState` trait and remove types like `ResState` (#6919)
Spiritual successor to #5205.
Actual successor to #6865.
# Objective
Currently, system params are defined using three traits: `SystemParam`, `ReadOnlySystemParam`, `SystemParamState`. The behavior for each param is specified by the `SystemParamState` trait, while `SystemParam` simply defers to the state.
Splitting the traits in this way makes it easier to implement within macros, but it increases the cognitive load. Worst of all, this approach requires each `MySystemParam` to have a public `MySystemParamState` type associated with it.
## Solution
* Merge the trait `SystemParamState` into `SystemParam`.
* Remove all trivial `SystemParam` state types.
* `OptionNonSendMutState<T>`: you will not be missed.
---
- [x] Fix/resolve the remaining test failure.
## Changelog
* Removed the trait `SystemParamState`, merging its functionality into `SystemParam`.
## Migration Guide
**Note**: this should replace the migration guide for #6865.
This is relative to Bevy 0.9, not main.
The traits `SystemParamState` and `SystemParamFetch` have been removed, and their functionality has been transferred to `SystemParam`.
```rust
// Before (0.9)
impl SystemParam for MyParam<'_, '_> {
type State = MyParamState;
}
unsafe impl SystemParamState for MyParamState {
fn init(world: &mut World, system_meta: &mut SystemMeta) -> Self { ... }
}
unsafe impl<'w, 's> SystemParamFetch<'w, 's> for MyParamState {
type Item = MyParam<'w, 's>;
fn get_param(&mut self, ...) -> Self::Item;
}
unsafe impl ReadOnlySystemParamFetch for MyParamState { }
// After (0.10)
unsafe impl SystemParam for MyParam<'_, '_> {
type State = MyParamState;
type Item<'w, 's> = MyParam<'w, 's>;
fn init_state(world: &mut World, system_meta: &mut SystemMeta) -> Self::State { ... }
fn get_param<'w, 's>(state: &mut Self::State, ...) -> Self::Item<'w, 's>;
}
unsafe impl ReadOnlySystemParam for MyParam<'_, '_> { }
```
The trait `ReadOnlySystemParamFetch` has been replaced with `ReadOnlySystemParam`.
```rust
// Before
unsafe impl ReadOnlySystemParamFetch for MyParamState {}
// After
unsafe impl ReadOnlySystemParam for MyParam<'_, '_> {}
```
2023-01-07 23:20:32 +00:00
/// found associated type `<[ParamType] as SystemParam>::Item<'_, '_>`
2022-05-17 22:24:50 +00:00
/// ```
/// where `[ParamType]` is the type of one of your fields.
/// To solve this error, you can wrap the field of type `[ParamType]` with [`StaticSystemParam`]
/// (i.e. `StaticSystemParam<[ParamType]>`).
///
/// ## Details
///
/// The derive macro requires that the [`SystemParam`] implementation of
Remove the `SystemParamState` trait and remove types like `ResState` (#6919)
Spiritual successor to #5205.
Actual successor to #6865.
# Objective
Currently, system params are defined using three traits: `SystemParam`, `ReadOnlySystemParam`, `SystemParamState`. The behavior for each param is specified by the `SystemParamState` trait, while `SystemParam` simply defers to the state.
Splitting the traits in this way makes it easier to implement within macros, but it increases the cognitive load. Worst of all, this approach requires each `MySystemParam` to have a public `MySystemParamState` type associated with it.
## Solution
* Merge the trait `SystemParamState` into `SystemParam`.
* Remove all trivial `SystemParam` state types.
* `OptionNonSendMutState<T>`: you will not be missed.
---
- [x] Fix/resolve the remaining test failure.
## Changelog
* Removed the trait `SystemParamState`, merging its functionality into `SystemParam`.
## Migration Guide
**Note**: this should replace the migration guide for #6865.
This is relative to Bevy 0.9, not main.
The traits `SystemParamState` and `SystemParamFetch` have been removed, and their functionality has been transferred to `SystemParam`.
```rust
// Before (0.9)
impl SystemParam for MyParam<'_, '_> {
type State = MyParamState;
}
unsafe impl SystemParamState for MyParamState {
fn init(world: &mut World, system_meta: &mut SystemMeta) -> Self { ... }
}
unsafe impl<'w, 's> SystemParamFetch<'w, 's> for MyParamState {
type Item = MyParam<'w, 's>;
fn get_param(&mut self, ...) -> Self::Item;
}
unsafe impl ReadOnlySystemParamFetch for MyParamState { }
// After (0.10)
unsafe impl SystemParam for MyParam<'_, '_> {
type State = MyParamState;
type Item<'w, 's> = MyParam<'w, 's>;
fn init_state(world: &mut World, system_meta: &mut SystemMeta) -> Self::State { ... }
fn get_param<'w, 's>(state: &mut Self::State, ...) -> Self::Item<'w, 's>;
}
unsafe impl ReadOnlySystemParam for MyParam<'_, '_> { }
```
The trait `ReadOnlySystemParamFetch` has been replaced with `ReadOnlySystemParam`.
```rust
// Before
unsafe impl ReadOnlySystemParamFetch for MyParamState {}
// After
unsafe impl ReadOnlySystemParam for MyParam<'_, '_> {}
```
2023-01-07 23:20:32 +00:00
/// each field `F`'s [`Item`](`SystemParam::Item`)'s is itself `F`
2022-05-17 22:24:50 +00:00
/// (ignoring lifetimes for simplicity).
/// This assumption is due to type inference reasons, so that the derived [`SystemParam`] can be
/// used as an argument to a function system.
/// If the compiler cannot validate this property for `[ParamType]`, it will error in the form shown above.
///
/// This will most commonly occur when working with `SystemParam`s generically, as the requirement
/// has not been proven to the compiler.
2022-12-11 18:34:13 +00:00
///
/// # `!Sync` Resources
/// A `!Sync` type cannot implement `Resource`. However, it is possible to wrap a `Send` but not `Sync`
/// type in [`SyncCell`] or the currently unstable [`Exclusive`] to make it `Sync`. This forces only
/// having mutable access (`&mut T` only, never `&T`), but makes it safe to reference across multiple
/// threads.
///
/// This will fail to compile since `RefCell` is `!Sync`.
/// ```compile_fail
/// # use std::cell::RefCell;
/// # use bevy_ecs::system::Resource;
///
/// #[derive(Resource)]
/// struct NotSync {
/// counter: RefCell<usize>,
/// }
/// ```
///
/// This will compile since the `RefCell` is wrapped with `SyncCell`.
/// ```
/// # use std::cell::RefCell;
/// # use bevy_ecs::system::Resource;
/// use bevy_utils::synccell::SyncCell;
///
/// #[derive(Resource)]
/// struct ActuallySync {
/// counter: SyncCell<RefCell<usize>>,
/// }
/// ```
///
/// [`SyncCell`]: bevy_utils::synccell::SyncCell
/// [`Exclusive`]: https://doc.rust-lang.org/nightly/std/sync/struct.Exclusive.html
2021-04-15 20:36:16 +00:00
///
Bevy ECS V2 (#1525)
# Bevy ECS V2
This is a rewrite of Bevy ECS (basically everything but the new executor/schedule, which are already awesome). The overall goal was to improve the performance and versatility of Bevy ECS. Here is a quick bulleted list of changes before we dive into the details:
* Complete World rewrite
* Multiple component storage types:
* Tables: fast cache friendly iteration, slower add/removes (previously called Archetypes)
* Sparse Sets: fast add/remove, slower iteration
* Stateful Queries (caches query results for faster iteration. fragmented iteration is _fast_ now)
* Stateful System Params (caches expensive operations. inspired by @DJMcNab's work in #1364)
* Configurable System Params (users can set configuration when they construct their systems. once again inspired by @DJMcNab's work)
* Archetypes are now "just metadata", component storage is separate
* Archetype Graph (for faster archetype changes)
* Component Metadata
* Configure component storage type
* Retrieve information about component size/type/name/layout/send-ness/etc
* Components are uniquely identified by a densely packed ComponentId
* TypeIds are now totally optional (which should make implementing scripting easier)
* Super fast "for_each" query iterators
* Merged Resources into World. Resources are now just a special type of component
* EntityRef/EntityMut builder apis (more efficient and more ergonomic)
* Fast bitset-backed `Access<T>` replaces old hashmap-based approach everywhere
* Query conflicts are determined by component access instead of archetype component access (to avoid random failures at runtime)
* With/Without are still taken into account for conflicts, so this should still be comfy to use
* Much simpler `IntoSystem` impl
* Significantly reduced the amount of hashing throughout the ecs in favor of Sparse Sets (indexed by densely packed ArchetypeId, ComponentId, BundleId, and TableId)
* Safety Improvements
* Entity reservation uses a normal world reference instead of unsafe transmute
* QuerySets no longer transmute lifetimes
* Made traits "unsafe" where relevant
* More thorough safety docs
* WorldCell
* Exposes safe mutable access to multiple resources at a time in a World
* Replaced "catch all" `System::update_archetypes(world: &World)` with `System::new_archetype(archetype: &Archetype)`
* Simpler Bundle implementation
* Replaced slow "remove_bundle_one_by_one" used as fallback for Commands::remove_bundle with fast "remove_bundle_intersection"
* Removed `Mut<T>` query impl. it is better to only support one way: `&mut T`
* Removed with() from `Flags<T>` in favor of `Option<Flags<T>>`, which allows querying for flags to be "filtered" by default
* Components now have is_send property (currently only resources support non-send)
* More granular module organization
* New `RemovedComponents<T>` SystemParam that replaces `query.removed::<T>()`
* `world.resource_scope()` for mutable access to resources and world at the same time
* WorldQuery and QueryFilter traits unified. FilterFetch trait added to enable "short circuit" filtering. Auto impled for cases that don't need it
* Significantly slimmed down SystemState in favor of individual SystemParam state
* System Commands changed from `commands: &mut Commands` back to `mut commands: Commands` (to allow Commands to have a World reference)
Fixes #1320
## `World` Rewrite
This is a from-scratch rewrite of `World` that fills the niche that `hecs` used to. Yes, this means Bevy ECS is no longer a "fork" of hecs. We're going out our own!
(the only shared code between the projects is the entity id allocator, which is already basically ideal)
A huge shout out to @SanderMertens (author of [flecs](https://github.com/SanderMertens/flecs)) for sharing some great ideas with me (specifically hybrid ecs storage and archetype graphs). He also helped advise on a number of implementation details.
## Component Storage (The Problem)
Two ECS storage paradigms have gained a lot of traction over the years:
* **Archetypal ECS**:
* Stores components in "tables" with static schemas. Each "column" stores components of a given type. Each "row" is an entity.
* Each "archetype" has its own table. Adding/removing an entity's component changes the archetype.
* Enables super-fast Query iteration due to its cache-friendly data layout
* Comes at the cost of more expensive add/remove operations for an Entity's components, because all components need to be copied to the new archetype's "table"
* **Sparse Set ECS**:
* Stores components of the same type in densely packed arrays, which are sparsely indexed by densely packed unsigned integers (Entity ids)
* Query iteration is slower than Archetypal ECS because each entity's component could be at any position in the sparse set. This "random access" pattern isn't cache friendly. Additionally, there is an extra layer of indirection because you must first map the entity id to an index in the component array.
* Adding/removing components is a cheap, constant time operation
Bevy ECS V1, hecs, legion, flec, and Unity DOTS are all "archetypal ecs-es". I personally think "archetypal" storage is a good default for game engines. An entity's archetype doesn't need to change frequently in general, and it creates "fast by default" query iteration (which is a much more common operation). It is also "self optimizing". Users don't need to think about optimizing component layouts for iteration performance. It "just works" without any extra boilerplate.
Shipyard and EnTT are "sparse set ecs-es". They employ "packing" as a way to work around the "suboptimal by default" iteration performance for specific sets of components. This helps, but I didn't think this was a good choice for a general purpose engine like Bevy because:
1. "packs" conflict with each other. If bevy decides to internally pack the Transform and GlobalTransform components, users are then blocked if they want to pack some custom component with Transform.
2. users need to take manual action to optimize
Developers selecting an ECS framework are stuck with a hard choice. Select an "archetypal" framework with "fast iteration everywhere" but without the ability to cheaply add/remove components, or select a "sparse set" framework to cheaply add/remove components but with slower iteration performance.
## Hybrid Component Storage (The Solution)
In Bevy ECS V2, we get to have our cake and eat it too. It now has _both_ of the component storage types above (and more can be added later if needed):
* **Tables** (aka "archetypal" storage)
* The default storage. If you don't configure anything, this is what you get
* Fast iteration by default
* Slower add/remove operations
* **Sparse Sets**
* Opt-in
* Slower iteration
* Faster add/remove operations
These storage types complement each other perfectly. By default Query iteration is fast. If developers know that they want to add/remove a component at high frequencies, they can set the storage to "sparse set":
```rust
world.register_component(
ComponentDescriptor::new::<MyComponent>(StorageType::SparseSet)
).unwrap();
```
## Archetypes
Archetypes are now "just metadata" ... they no longer store components directly. They do store:
* The `ComponentId`s of each of the Archetype's components (and that component's storage type)
* Archetypes are uniquely defined by their component layouts
* For example: entities with "table" components `[A, B, C]` _and_ "sparse set" components `[D, E]` will always be in the same archetype.
* The `TableId` associated with the archetype
* For now each archetype has exactly one table (which can have no components),
* There is a 1->Many relationship from Tables->Archetypes. A given table could have any number of archetype components stored in it:
* Ex: an entity with "table storage" components `[A, B, C]` and "sparse set" components `[D, E]` will share the same `[A, B, C]` table as an entity with `[A, B, C]` table component and `[F]` sparse set components.
* This 1->Many relationship is how we preserve fast "cache friendly" iteration performance when possible (more on this later)
* A list of entities that are in the archetype and the row id of the table they are in
* ArchetypeComponentIds
* unique densely packed identifiers for (ArchetypeId, ComponentId) pairs
* used by the schedule executor for cheap system access control
* "Archetype Graph Edges" (see the next section)
## The "Archetype Graph"
Archetype changes in Bevy (and a number of other archetypal ecs-es) have historically been expensive to compute. First, you need to allocate a new vector of the entity's current component ids, add or remove components based on the operation performed, sort it (to ensure it is order-independent), then hash it to find the archetype (if it exists). And thats all before we get to the _already_ expensive full copy of all components to the new table storage.
The solution is to build a "graph" of archetypes to cache these results. @SanderMertens first exposed me to the idea (and he got it from @gjroelofs, who came up with it). They propose adding directed edges between archetypes for add/remove component operations. If `ComponentId`s are densely packed, you can use sparse sets to cheaply jump between archetypes.
Bevy takes this one step further by using add/remove `Bundle` edges instead of `Component` edges. Bevy encourages the use of `Bundles` to group add/remove operations. This is largely for "clearer game logic" reasons, but it also helps cut down on the number of archetype changes required. `Bundles` now also have densely-packed `BundleId`s. This allows us to use a _single_ edge for each bundle operation (rather than needing to traverse N edges ... one for each component). Single component operations are also bundles, so this is strictly an improvement over a "component only" graph.
As a result, an operation that used to be _heavy_ (both for allocations and compute) is now two dirt-cheap array lookups and zero allocations.
## Stateful Queries
World queries are now stateful. This allows us to:
1. Cache archetype (and table) matches
* This resolves another issue with (naive) archetypal ECS: query performance getting worse as the number of archetypes goes up (and fragmentation occurs).
2. Cache Fetch and Filter state
* The expensive parts of fetch/filter operations (such as hashing the TypeId to find the ComponentId) now only happen once when the Query is first constructed
3. Incrementally build up state
* When new archetypes are added, we only process the new archetypes (no need to rebuild state for old archetypes)
As a result, the direct `World` query api now looks like this:
```rust
let mut query = world.query::<(&A, &mut B)>();
for (a, mut b) in query.iter_mut(&mut world) {
}
```
Requiring `World` to generate stateful queries (rather than letting the `QueryState` type be constructed separately) allows us to ensure that _all_ queries are properly initialized (and the relevant world state, such as ComponentIds). This enables QueryState to remove branches from its operations that check for initialization status (and also enables query.iter() to take an immutable world reference because it doesn't need to initialize anything in world).
However in systems, this is a non-breaking change. State management is done internally by the relevant SystemParam.
## Stateful SystemParams
Like Queries, `SystemParams` now also cache state. For example, `Query` system params store the "stateful query" state mentioned above. Commands store their internal `CommandQueue`. This means you can now safely use as many separate `Commands` parameters in your system as you want. `Local<T>` system params store their `T` value in their state (instead of in Resources).
SystemParam state also enabled a significant slim-down of SystemState. It is much nicer to look at now.
Per-SystemParam state naturally insulates us from an "aliased mut" class of errors we have hit in the past (ex: using multiple `Commands` system params).
(credit goes to @DJMcNab for the initial idea and draft pr here #1364)
## Configurable SystemParams
@DJMcNab also had the great idea to make SystemParams configurable. This allows users to provide some initial configuration / values for system parameters (when possible). Most SystemParams have no config (the config type is `()`), but the `Local<T>` param now supports user-provided parameters:
```rust
fn foo(value: Local<usize>) {
}
app.add_system(foo.system().config(|c| c.0 = Some(10)));
```
## Uber Fast "for_each" Query Iterators
Developers now have the choice to use a fast "for_each" iterator, which yields ~1.5-3x iteration speed improvements for "fragmented iteration", and minor ~1.2x iteration speed improvements for unfragmented iteration.
```rust
fn system(query: Query<(&A, &mut B)>) {
// you now have the option to do this for a speed boost
query.for_each_mut(|(a, mut b)| {
});
// however normal iterators are still available
for (a, mut b) in query.iter_mut() {
}
}
```
I think in most cases we should continue to encourage "normal" iterators as they are more flexible and more "rust idiomatic". But when that extra "oomf" is needed, it makes sense to use `for_each`.
We should also consider using `for_each` for internal bevy systems to give our users a nice speed boost (but that should be a separate pr).
## Component Metadata
`World` now has a `Components` collection, which is accessible via `world.components()`. This stores mappings from `ComponentId` to `ComponentInfo`, as well as `TypeId` to `ComponentId` mappings (where relevant). `ComponentInfo` stores information about the component, such as ComponentId, TypeId, memory layout, send-ness (currently limited to resources), and storage type.
## Significantly Cheaper `Access<T>`
We used to use `TypeAccess<TypeId>` to manage read/write component/archetype-component access. This was expensive because TypeIds must be hashed and compared individually. The parallel executor got around this by "condensing" type ids into bitset-backed access types. This worked, but it had to be re-generated from the `TypeAccess<TypeId>`sources every time archetypes changed.
This pr removes TypeAccess in favor of faster bitset access everywhere. We can do this thanks to the move to densely packed `ComponentId`s and `ArchetypeComponentId`s.
## Merged Resources into World
Resources had a lot of redundant functionality with Components. They stored typed data, they had access control, they had unique ids, they were queryable via SystemParams, etc. In fact the _only_ major difference between them was that they were unique (and didn't correlate to an entity).
Separate resources also had the downside of requiring a separate set of access controls, which meant the parallel executor needed to compare more bitsets per system and manage more state.
I initially got the "separate resources" idea from `legion`. I think that design was motivated by the fact that it made the direct world query/resource lifetime interactions more manageable. It certainly made our lives easier when using Resources alongside hecs/bevy_ecs. However we already have a construct for safely and ergonomically managing in-world lifetimes: systems (which use `Access<T>` internally).
This pr merges Resources into World:
```rust
world.insert_resource(1);
world.insert_resource(2.0);
let a = world.get_resource::<i32>().unwrap();
let mut b = world.get_resource_mut::<f64>().unwrap();
*b = 3.0;
```
Resources are now just a special kind of component. They have their own ComponentIds (and their own resource TypeId->ComponentId scope, so they don't conflict wit components of the same type). They are stored in a special "resource archetype", which stores components inside the archetype using a new `unique_components` sparse set (note that this sparse set could later be used to implement Tags). This allows us to keep the code size small by reusing existing datastructures (namely Column, Archetype, ComponentFlags, and ComponentInfo). This allows us the executor to use a single `Access<ArchetypeComponentId>` per system. It should also make scripting language integration easier.
_But_ this merge did create problems for people directly interacting with `World`. What if you need mutable access to multiple resources at the same time? `world.get_resource_mut()` borrows World mutably!
## WorldCell
WorldCell applies the `Access<ArchetypeComponentId>` concept to direct world access:
```rust
let world_cell = world.cell();
let a = world_cell.get_resource_mut::<i32>().unwrap();
let b = world_cell.get_resource_mut::<f64>().unwrap();
```
This adds cheap runtime checks (a sparse set lookup of `ArchetypeComponentId` and a counter) to ensure that world accesses do not conflict with each other. Each operation returns a `WorldBorrow<'w, T>` or `WorldBorrowMut<'w, T>` wrapper type, which will release the relevant ArchetypeComponentId resources when dropped.
World caches the access sparse set (and only one cell can exist at a time), so `world.cell()` is a cheap operation.
WorldCell does _not_ use atomic operations. It is non-send, does a mutable borrow of world to prevent other accesses, and uses a simple `Rc<RefCell<ArchetypeComponentAccess>>` wrapper in each WorldBorrow pointer.
The api is currently limited to resource access, but it can and should be extended to queries / entity component access.
## Resource Scopes
WorldCell does not yet support component queries, and even when it does there are sometimes legitimate reasons to want a mutable world ref _and_ a mutable resource ref (ex: bevy_render and bevy_scene both need this). In these cases we could always drop down to the unsafe `world.get_resource_unchecked_mut()`, but that is not ideal!
Instead developers can use a "resource scope"
```rust
world.resource_scope(|world: &mut World, a: &mut A| {
})
```
This temporarily removes the `A` resource from `World`, provides mutable pointers to both, and re-adds A to World when finished. Thanks to the move to ComponentIds/sparse sets, this is a cheap operation.
If multiple resources are required, scopes can be nested. We could also consider adding a "resource tuple" to the api if this pattern becomes common and the boilerplate gets nasty.
## Query Conflicts Use ComponentId Instead of ArchetypeComponentId
For safety reasons, systems cannot contain queries that conflict with each other without wrapping them in a QuerySet. On bevy `main`, we use ArchetypeComponentIds to determine conflicts. This is nice because it can take into account filters:
```rust
// these queries will never conflict due to their filters
fn filter_system(a: Query<&mut A, With<B>>, b: Query<&mut B, Without<B>>) {
}
```
But it also has a significant downside:
```rust
// these queries will not conflict _until_ an entity with A, B, and C is spawned
fn maybe_conflicts_system(a: Query<(&mut A, &C)>, b: Query<(&mut A, &B)>) {
}
```
The system above will panic at runtime if an entity with A, B, and C is spawned. This makes it hard to trust that your game logic will run without crashing.
In this pr, I switched to using `ComponentId` instead. This _is_ more constraining. `maybe_conflicts_system` will now always fail, but it will do it consistently at startup. Naively, it would also _disallow_ `filter_system`, which would be a significant downgrade in usability. Bevy has a number of internal systems that rely on disjoint queries and I expect it to be a common pattern in userspace.
To resolve this, I added a new `FilteredAccess<T>` type, which wraps `Access<T>` and adds with/without filters. If two `FilteredAccess` have with/without values that prove they are disjoint, they will no longer conflict.
## EntityRef / EntityMut
World entity operations on `main` require that the user passes in an `entity` id to each operation:
```rust
let entity = world.spawn((A, )); // create a new entity with A
world.get::<A>(entity);
world.insert(entity, (B, C));
world.insert_one(entity, D);
```
This means that each operation needs to look up the entity location / verify its validity. The initial spawn operation also requires a Bundle as input. This can be awkward when no components are required (or one component is required).
These operations have been replaced by `EntityRef` and `EntityMut`, which are "builder-style" wrappers around world that provide read and read/write operations on a single, pre-validated entity:
```rust
// spawn now takes no inputs and returns an EntityMut
let entity = world.spawn()
.insert(A) // insert a single component into the entity
.insert_bundle((B, C)) // insert a bundle of components into the entity
.id() // id returns the Entity id
// Returns EntityMut (or panics if the entity does not exist)
world.entity_mut(entity)
.insert(D)
.insert_bundle(SomeBundle::default());
{
// returns EntityRef (or panics if the entity does not exist)
let d = world.entity(entity)
.get::<D>() // gets the D component
.unwrap();
// world.get still exists for ergonomics
let d = world.get::<D>(entity).unwrap();
}
// These variants return Options if you want to check existence instead of panicing
world.get_entity_mut(entity)
.unwrap()
.insert(E);
if let Some(entity_ref) = world.get_entity(entity) {
let d = entity_ref.get::<D>().unwrap();
}
```
This _does not_ affect the current Commands api or terminology. I think that should be a separate conversation as that is a much larger breaking change.
## Safety Improvements
* Entity reservation in Commands uses a normal world borrow instead of an unsafe transmute
* QuerySets no longer transmutes lifetimes
* Made traits "unsafe" when implementing a trait incorrectly could cause unsafety
* More thorough safety docs
## RemovedComponents SystemParam
The old approach to querying removed components: `query.removed:<T>()` was confusing because it had no connection to the query itself. I replaced it with the following, which is both clearer and allows us to cache the ComponentId mapping in the SystemParamState:
```rust
fn system(removed: RemovedComponents<T>) {
for entity in removed.iter() {
}
}
```
## Simpler Bundle implementation
Bundles are no longer responsible for sorting (or deduping) TypeInfo. They are just a simple ordered list of component types / data. This makes the implementation smaller and opens the door to an easy "nested bundle" implementation in the future (which i might even add in this pr). Duplicate detection is now done once per bundle type by World the first time a bundle is used.
## Unified WorldQuery and QueryFilter types
(don't worry they are still separate type _parameters_ in Queries .. this is a non-breaking change)
WorldQuery and QueryFilter were already basically identical apis. With the addition of `FetchState` and more storage-specific fetch methods, the overlap was even clearer (and the redundancy more painful).
QueryFilters are now just `F: WorldQuery where F::Fetch: FilterFetch`. FilterFetch requires `Fetch<Item = bool>` and adds new "short circuit" variants of fetch methods. This enables a filter tuple like `(With<A>, Without<B>, Changed<C>)` to stop evaluating the filter after the first mismatch is encountered. FilterFetch is automatically implemented for `Fetch` implementations that return bool.
This forces fetch implementations that return things like `(bool, bool, bool)` (such as the filter above) to manually implement FilterFetch and decide whether or not to short-circuit.
## More Granular Modules
World no longer globs all of the internal modules together. It now exports `core`, `system`, and `schedule` separately. I'm also considering exporting `core` submodules directly as that is still pretty "glob-ey" and unorganized (feedback welcome here).
## Remaining Draft Work (to be done in this pr)
* ~~panic on conflicting WorldQuery fetches (&A, &mut A)~~
* ~~bevy `main` and hecs both currently allow this, but we should protect against it if possible~~
* ~~batch_iter / par_iter (currently stubbed out)~~
* ~~ChangedRes~~
* ~~I skipped this while we sort out #1313. This pr should be adapted to account for whatever we land on there~~.
* ~~The `Archetypes` and `Tables` collections use hashes of sorted lists of component ids to uniquely identify each archetype/table. This hash is then used as the key in a HashMap to look up the relevant ArchetypeId or TableId. (which doesn't handle hash collisions properly)~~
* ~~It is currently unsafe to generate a Query from "World A", then use it on "World B" (despite the api claiming it is safe). We should probably close this gap. This could be done by adding a randomly generated WorldId to each world, then storing that id in each Query. They could then be compared to each other on each `query.do_thing(&world)` operation. This _does_ add an extra branch to each query operation, so I'm open to other suggestions if people have them.~~
* ~~Nested Bundles (if i find time)~~
## Potential Future Work
* Expand WorldCell to support queries.
* Consider not allocating in the empty archetype on `world.spawn()`
* ex: return something like EntityMutUninit, which turns into EntityMut after an `insert` or `insert_bundle` op
* this actually regressed performance last time i tried it, but in theory it should be faster
* Optimize SparseSet::insert (see `PERF` comment on insert)
* Replace SparseArray `Option<T>` with T::MAX to cut down on branching
* would enable cheaper get_unchecked() operations
* upstream fixedbitset optimizations
* fixedbitset could be allocation free for small block counts (store blocks in a SmallVec)
* fixedbitset could have a const constructor
* Consider implementing Tags (archetype-specific by-value data that affects archetype identity)
* ex: ArchetypeA could have `[A, B, C]` table components and `[D(1)]` "tag" component. ArchetypeB could have `[A, B, C]` table components and a `[D(2)]` tag component. The archetypes are different, despite both having D tags because the value inside D is different.
* this could potentially build on top of the `archetype.unique_components` added in this pr for resource storage.
* Consider reverting `all_tuples` proc macro in favor of the old `macro_rules` implementation
* all_tuples is more flexible and produces cleaner documentation (the macro_rules version produces weird type parameter orders due to parser constraints)
* but unfortunately all_tuples also appears to make Rust Analyzer sad/slow when working inside of `bevy_ecs` (does not affect user code)
* Consider "resource queries" and/or "mixed resource and entity component queries" as an alternative to WorldCell
* this is basically just "systems" so maybe it's not worth it
* Add more world ops
* `world.clear()`
* `world.reserve<T: Bundle>(count: usize)`
* Try using the old archetype allocation strategy (allocate new memory on resize and copy everything over). I expect this to improve batch insertion performance at the cost of unbatched performance. But thats just a guess. I'm not an allocation perf pro :)
* Adapt Commands apis for consistency with new World apis
## Benchmarks
key:
* `bevy_old`: bevy `main` branch
* `bevy`: this branch
* `_foreach`: uses an optimized for_each iterator
* ` _sparse`: uses sparse set storage (if unspecified assume table storage)
* `_system`: runs inside a system (if unspecified assume test happens via direct world ops)
### Simple Insert (from ecs_bench_suite)
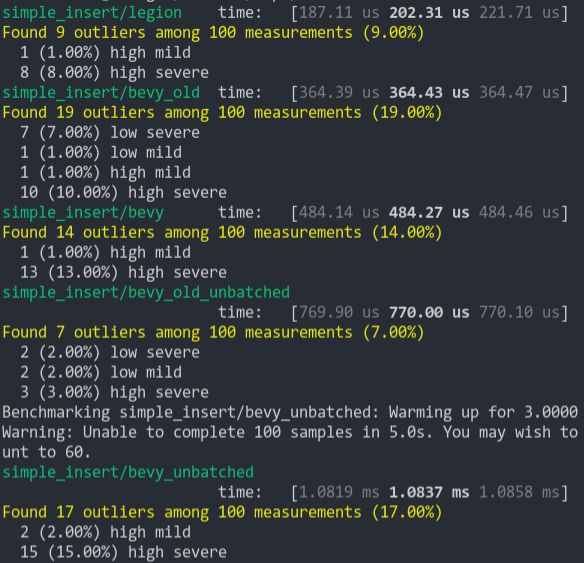
### Simpler Iter (from ecs_bench_suite)
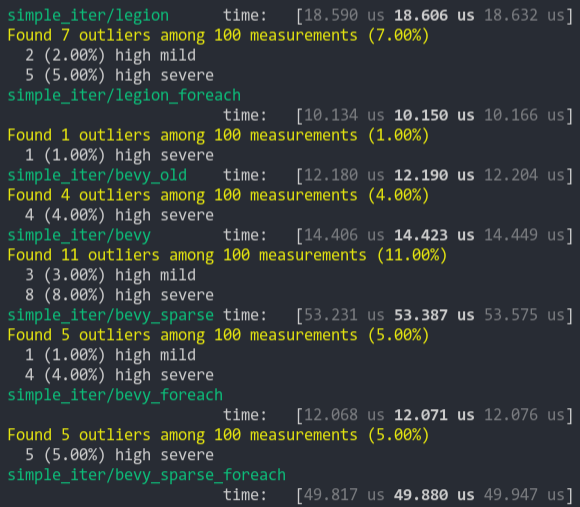
### Fragment Iter (from ecs_bench_suite)
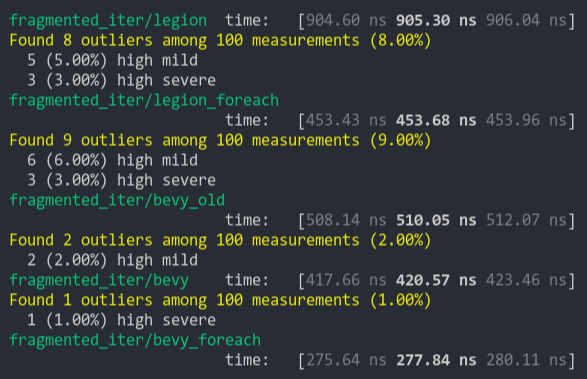
### Sparse Fragmented Iter
Iterate a query that matches 5 entities from a single matching archetype, but there are 100 unmatching archetypes
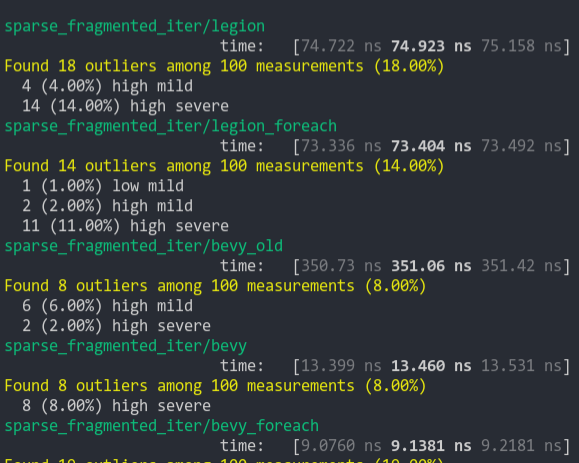
### Schedule (from ecs_bench_suite)
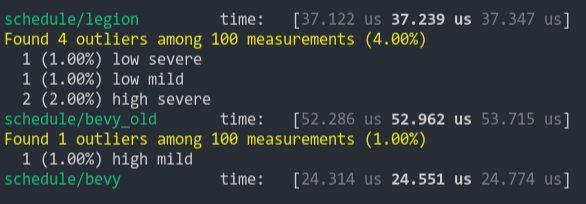
### Add Remove Component (from ecs_bench_suite)
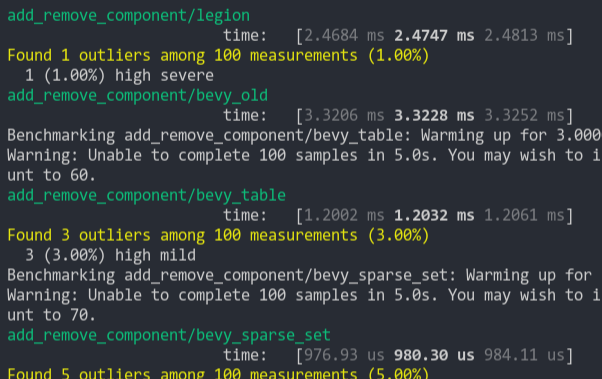
### Add Remove Component Big
Same as the test above, but each entity has 5 "large" matrix components and 1 "large" matrix component is added and removed
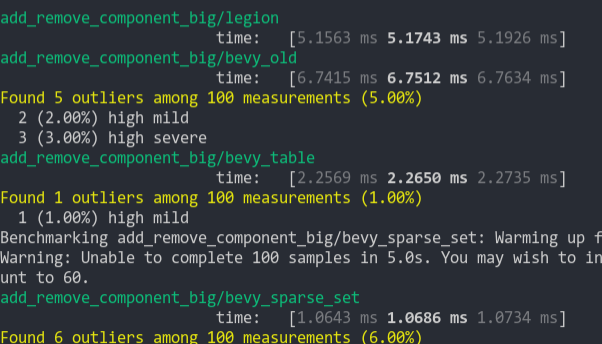
### Get Component
Looks up a single component value a large number of times
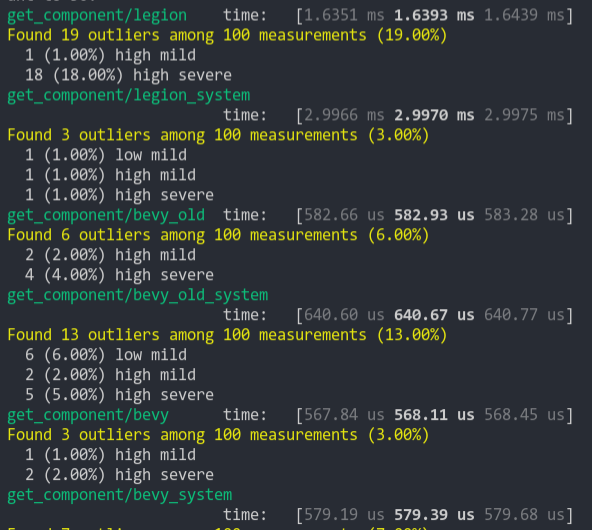
2021-03-05 07:54:35 +00:00
/// # Safety
2021-04-15 20:36:16 +00:00
///
2023-01-20 13:39:23 +00:00
/// The implementor must ensure the following is true.
/// - [`SystemParam::init_state`] correctly registers all [`World`] accesses used
/// by [`SystemParam::get_param`] with the provided [`system_meta`](SystemMeta).
/// - None of the world accesses may conflict with any prior accesses registered
/// on `system_meta`.
Remove the `SystemParamState` trait and remove types like `ResState` (#6919)
Spiritual successor to #5205.
Actual successor to #6865.
# Objective
Currently, system params are defined using three traits: `SystemParam`, `ReadOnlySystemParam`, `SystemParamState`. The behavior for each param is specified by the `SystemParamState` trait, while `SystemParam` simply defers to the state.
Splitting the traits in this way makes it easier to implement within macros, but it increases the cognitive load. Worst of all, this approach requires each `MySystemParam` to have a public `MySystemParamState` type associated with it.
## Solution
* Merge the trait `SystemParamState` into `SystemParam`.
* Remove all trivial `SystemParam` state types.
* `OptionNonSendMutState<T>`: you will not be missed.
---
- [x] Fix/resolve the remaining test failure.
## Changelog
* Removed the trait `SystemParamState`, merging its functionality into `SystemParam`.
## Migration Guide
**Note**: this should replace the migration guide for #6865.
This is relative to Bevy 0.9, not main.
The traits `SystemParamState` and `SystemParamFetch` have been removed, and their functionality has been transferred to `SystemParam`.
```rust
// Before (0.9)
impl SystemParam for MyParam<'_, '_> {
type State = MyParamState;
}
unsafe impl SystemParamState for MyParamState {
fn init(world: &mut World, system_meta: &mut SystemMeta) -> Self { ... }
}
unsafe impl<'w, 's> SystemParamFetch<'w, 's> for MyParamState {
type Item = MyParam<'w, 's>;
fn get_param(&mut self, ...) -> Self::Item;
}
unsafe impl ReadOnlySystemParamFetch for MyParamState { }
// After (0.10)
unsafe impl SystemParam for MyParam<'_, '_> {
type State = MyParamState;
type Item<'w, 's> = MyParam<'w, 's>;
fn init_state(world: &mut World, system_meta: &mut SystemMeta) -> Self::State { ... }
fn get_param<'w, 's>(state: &mut Self::State, ...) -> Self::Item<'w, 's>;
}
unsafe impl ReadOnlySystemParam for MyParam<'_, '_> { }
```
The trait `ReadOnlySystemParamFetch` has been replaced with `ReadOnlySystemParam`.
```rust
// Before
unsafe impl ReadOnlySystemParamFetch for MyParamState {}
// After
unsafe impl ReadOnlySystemParam for MyParam<'_, '_> {}
```
2023-01-07 23:20:32 +00:00
pub unsafe trait SystemParam : Sized {
/// Used to store data which persists across invocations of a system.
type State : Send + Sync + 'static ;
/// The item type returned when constructing this system param.
/// The value of this associated type should be `Self`, instantiated with new lifetimes.
///
/// You could think of `SystemParam::Item<'w, 's>` as being an *operation* that changes the lifetimes bound to `Self`.
type Item < ' world , ' state > : SystemParam < State = Self ::State > ;
/// Registers any [`World`] access used by this [`SystemParam`]
/// and creates a new instance of this param's [`State`](Self::State).
fn init_state ( world : & mut World , system_meta : & mut SystemMeta ) -> Self ::State ;
/// For the specified [`Archetype`], registers the components accessed by this [`SystemParam`] (if applicable).
Bevy ECS V2 (#1525)
# Bevy ECS V2
This is a rewrite of Bevy ECS (basically everything but the new executor/schedule, which are already awesome). The overall goal was to improve the performance and versatility of Bevy ECS. Here is a quick bulleted list of changes before we dive into the details:
* Complete World rewrite
* Multiple component storage types:
* Tables: fast cache friendly iteration, slower add/removes (previously called Archetypes)
* Sparse Sets: fast add/remove, slower iteration
* Stateful Queries (caches query results for faster iteration. fragmented iteration is _fast_ now)
* Stateful System Params (caches expensive operations. inspired by @DJMcNab's work in #1364)
* Configurable System Params (users can set configuration when they construct their systems. once again inspired by @DJMcNab's work)
* Archetypes are now "just metadata", component storage is separate
* Archetype Graph (for faster archetype changes)
* Component Metadata
* Configure component storage type
* Retrieve information about component size/type/name/layout/send-ness/etc
* Components are uniquely identified by a densely packed ComponentId
* TypeIds are now totally optional (which should make implementing scripting easier)
* Super fast "for_each" query iterators
* Merged Resources into World. Resources are now just a special type of component
* EntityRef/EntityMut builder apis (more efficient and more ergonomic)
* Fast bitset-backed `Access<T>` replaces old hashmap-based approach everywhere
* Query conflicts are determined by component access instead of archetype component access (to avoid random failures at runtime)
* With/Without are still taken into account for conflicts, so this should still be comfy to use
* Much simpler `IntoSystem` impl
* Significantly reduced the amount of hashing throughout the ecs in favor of Sparse Sets (indexed by densely packed ArchetypeId, ComponentId, BundleId, and TableId)
* Safety Improvements
* Entity reservation uses a normal world reference instead of unsafe transmute
* QuerySets no longer transmute lifetimes
* Made traits "unsafe" where relevant
* More thorough safety docs
* WorldCell
* Exposes safe mutable access to multiple resources at a time in a World
* Replaced "catch all" `System::update_archetypes(world: &World)` with `System::new_archetype(archetype: &Archetype)`
* Simpler Bundle implementation
* Replaced slow "remove_bundle_one_by_one" used as fallback for Commands::remove_bundle with fast "remove_bundle_intersection"
* Removed `Mut<T>` query impl. it is better to only support one way: `&mut T`
* Removed with() from `Flags<T>` in favor of `Option<Flags<T>>`, which allows querying for flags to be "filtered" by default
* Components now have is_send property (currently only resources support non-send)
* More granular module organization
* New `RemovedComponents<T>` SystemParam that replaces `query.removed::<T>()`
* `world.resource_scope()` for mutable access to resources and world at the same time
* WorldQuery and QueryFilter traits unified. FilterFetch trait added to enable "short circuit" filtering. Auto impled for cases that don't need it
* Significantly slimmed down SystemState in favor of individual SystemParam state
* System Commands changed from `commands: &mut Commands` back to `mut commands: Commands` (to allow Commands to have a World reference)
Fixes #1320
## `World` Rewrite
This is a from-scratch rewrite of `World` that fills the niche that `hecs` used to. Yes, this means Bevy ECS is no longer a "fork" of hecs. We're going out our own!
(the only shared code between the projects is the entity id allocator, which is already basically ideal)
A huge shout out to @SanderMertens (author of [flecs](https://github.com/SanderMertens/flecs)) for sharing some great ideas with me (specifically hybrid ecs storage and archetype graphs). He also helped advise on a number of implementation details.
## Component Storage (The Problem)
Two ECS storage paradigms have gained a lot of traction over the years:
* **Archetypal ECS**:
* Stores components in "tables" with static schemas. Each "column" stores components of a given type. Each "row" is an entity.
* Each "archetype" has its own table. Adding/removing an entity's component changes the archetype.
* Enables super-fast Query iteration due to its cache-friendly data layout
* Comes at the cost of more expensive add/remove operations for an Entity's components, because all components need to be copied to the new archetype's "table"
* **Sparse Set ECS**:
* Stores components of the same type in densely packed arrays, which are sparsely indexed by densely packed unsigned integers (Entity ids)
* Query iteration is slower than Archetypal ECS because each entity's component could be at any position in the sparse set. This "random access" pattern isn't cache friendly. Additionally, there is an extra layer of indirection because you must first map the entity id to an index in the component array.
* Adding/removing components is a cheap, constant time operation
Bevy ECS V1, hecs, legion, flec, and Unity DOTS are all "archetypal ecs-es". I personally think "archetypal" storage is a good default for game engines. An entity's archetype doesn't need to change frequently in general, and it creates "fast by default" query iteration (which is a much more common operation). It is also "self optimizing". Users don't need to think about optimizing component layouts for iteration performance. It "just works" without any extra boilerplate.
Shipyard and EnTT are "sparse set ecs-es". They employ "packing" as a way to work around the "suboptimal by default" iteration performance for specific sets of components. This helps, but I didn't think this was a good choice for a general purpose engine like Bevy because:
1. "packs" conflict with each other. If bevy decides to internally pack the Transform and GlobalTransform components, users are then blocked if they want to pack some custom component with Transform.
2. users need to take manual action to optimize
Developers selecting an ECS framework are stuck with a hard choice. Select an "archetypal" framework with "fast iteration everywhere" but without the ability to cheaply add/remove components, or select a "sparse set" framework to cheaply add/remove components but with slower iteration performance.
## Hybrid Component Storage (The Solution)
In Bevy ECS V2, we get to have our cake and eat it too. It now has _both_ of the component storage types above (and more can be added later if needed):
* **Tables** (aka "archetypal" storage)
* The default storage. If you don't configure anything, this is what you get
* Fast iteration by default
* Slower add/remove operations
* **Sparse Sets**
* Opt-in
* Slower iteration
* Faster add/remove operations
These storage types complement each other perfectly. By default Query iteration is fast. If developers know that they want to add/remove a component at high frequencies, they can set the storage to "sparse set":
```rust
world.register_component(
ComponentDescriptor::new::<MyComponent>(StorageType::SparseSet)
).unwrap();
```
## Archetypes
Archetypes are now "just metadata" ... they no longer store components directly. They do store:
* The `ComponentId`s of each of the Archetype's components (and that component's storage type)
* Archetypes are uniquely defined by their component layouts
* For example: entities with "table" components `[A, B, C]` _and_ "sparse set" components `[D, E]` will always be in the same archetype.
* The `TableId` associated with the archetype
* For now each archetype has exactly one table (which can have no components),
* There is a 1->Many relationship from Tables->Archetypes. A given table could have any number of archetype components stored in it:
* Ex: an entity with "table storage" components `[A, B, C]` and "sparse set" components `[D, E]` will share the same `[A, B, C]` table as an entity with `[A, B, C]` table component and `[F]` sparse set components.
* This 1->Many relationship is how we preserve fast "cache friendly" iteration performance when possible (more on this later)
* A list of entities that are in the archetype and the row id of the table they are in
* ArchetypeComponentIds
* unique densely packed identifiers for (ArchetypeId, ComponentId) pairs
* used by the schedule executor for cheap system access control
* "Archetype Graph Edges" (see the next section)
## The "Archetype Graph"
Archetype changes in Bevy (and a number of other archetypal ecs-es) have historically been expensive to compute. First, you need to allocate a new vector of the entity's current component ids, add or remove components based on the operation performed, sort it (to ensure it is order-independent), then hash it to find the archetype (if it exists). And thats all before we get to the _already_ expensive full copy of all components to the new table storage.
The solution is to build a "graph" of archetypes to cache these results. @SanderMertens first exposed me to the idea (and he got it from @gjroelofs, who came up with it). They propose adding directed edges between archetypes for add/remove component operations. If `ComponentId`s are densely packed, you can use sparse sets to cheaply jump between archetypes.
Bevy takes this one step further by using add/remove `Bundle` edges instead of `Component` edges. Bevy encourages the use of `Bundles` to group add/remove operations. This is largely for "clearer game logic" reasons, but it also helps cut down on the number of archetype changes required. `Bundles` now also have densely-packed `BundleId`s. This allows us to use a _single_ edge for each bundle operation (rather than needing to traverse N edges ... one for each component). Single component operations are also bundles, so this is strictly an improvement over a "component only" graph.
As a result, an operation that used to be _heavy_ (both for allocations and compute) is now two dirt-cheap array lookups and zero allocations.
## Stateful Queries
World queries are now stateful. This allows us to:
1. Cache archetype (and table) matches
* This resolves another issue with (naive) archetypal ECS: query performance getting worse as the number of archetypes goes up (and fragmentation occurs).
2. Cache Fetch and Filter state
* The expensive parts of fetch/filter operations (such as hashing the TypeId to find the ComponentId) now only happen once when the Query is first constructed
3. Incrementally build up state
* When new archetypes are added, we only process the new archetypes (no need to rebuild state for old archetypes)
As a result, the direct `World` query api now looks like this:
```rust
let mut query = world.query::<(&A, &mut B)>();
for (a, mut b) in query.iter_mut(&mut world) {
}
```
Requiring `World` to generate stateful queries (rather than letting the `QueryState` type be constructed separately) allows us to ensure that _all_ queries are properly initialized (and the relevant world state, such as ComponentIds). This enables QueryState to remove branches from its operations that check for initialization status (and also enables query.iter() to take an immutable world reference because it doesn't need to initialize anything in world).
However in systems, this is a non-breaking change. State management is done internally by the relevant SystemParam.
## Stateful SystemParams
Like Queries, `SystemParams` now also cache state. For example, `Query` system params store the "stateful query" state mentioned above. Commands store their internal `CommandQueue`. This means you can now safely use as many separate `Commands` parameters in your system as you want. `Local<T>` system params store their `T` value in their state (instead of in Resources).
SystemParam state also enabled a significant slim-down of SystemState. It is much nicer to look at now.
Per-SystemParam state naturally insulates us from an "aliased mut" class of errors we have hit in the past (ex: using multiple `Commands` system params).
(credit goes to @DJMcNab for the initial idea and draft pr here #1364)
## Configurable SystemParams
@DJMcNab also had the great idea to make SystemParams configurable. This allows users to provide some initial configuration / values for system parameters (when possible). Most SystemParams have no config (the config type is `()`), but the `Local<T>` param now supports user-provided parameters:
```rust
fn foo(value: Local<usize>) {
}
app.add_system(foo.system().config(|c| c.0 = Some(10)));
```
## Uber Fast "for_each" Query Iterators
Developers now have the choice to use a fast "for_each" iterator, which yields ~1.5-3x iteration speed improvements for "fragmented iteration", and minor ~1.2x iteration speed improvements for unfragmented iteration.
```rust
fn system(query: Query<(&A, &mut B)>) {
// you now have the option to do this for a speed boost
query.for_each_mut(|(a, mut b)| {
});
// however normal iterators are still available
for (a, mut b) in query.iter_mut() {
}
}
```
I think in most cases we should continue to encourage "normal" iterators as they are more flexible and more "rust idiomatic". But when that extra "oomf" is needed, it makes sense to use `for_each`.
We should also consider using `for_each` for internal bevy systems to give our users a nice speed boost (but that should be a separate pr).
## Component Metadata
`World` now has a `Components` collection, which is accessible via `world.components()`. This stores mappings from `ComponentId` to `ComponentInfo`, as well as `TypeId` to `ComponentId` mappings (where relevant). `ComponentInfo` stores information about the component, such as ComponentId, TypeId, memory layout, send-ness (currently limited to resources), and storage type.
## Significantly Cheaper `Access<T>`
We used to use `TypeAccess<TypeId>` to manage read/write component/archetype-component access. This was expensive because TypeIds must be hashed and compared individually. The parallel executor got around this by "condensing" type ids into bitset-backed access types. This worked, but it had to be re-generated from the `TypeAccess<TypeId>`sources every time archetypes changed.
This pr removes TypeAccess in favor of faster bitset access everywhere. We can do this thanks to the move to densely packed `ComponentId`s and `ArchetypeComponentId`s.
## Merged Resources into World
Resources had a lot of redundant functionality with Components. They stored typed data, they had access control, they had unique ids, they were queryable via SystemParams, etc. In fact the _only_ major difference between them was that they were unique (and didn't correlate to an entity).
Separate resources also had the downside of requiring a separate set of access controls, which meant the parallel executor needed to compare more bitsets per system and manage more state.
I initially got the "separate resources" idea from `legion`. I think that design was motivated by the fact that it made the direct world query/resource lifetime interactions more manageable. It certainly made our lives easier when using Resources alongside hecs/bevy_ecs. However we already have a construct for safely and ergonomically managing in-world lifetimes: systems (which use `Access<T>` internally).
This pr merges Resources into World:
```rust
world.insert_resource(1);
world.insert_resource(2.0);
let a = world.get_resource::<i32>().unwrap();
let mut b = world.get_resource_mut::<f64>().unwrap();
*b = 3.0;
```
Resources are now just a special kind of component. They have their own ComponentIds (and their own resource TypeId->ComponentId scope, so they don't conflict wit components of the same type). They are stored in a special "resource archetype", which stores components inside the archetype using a new `unique_components` sparse set (note that this sparse set could later be used to implement Tags). This allows us to keep the code size small by reusing existing datastructures (namely Column, Archetype, ComponentFlags, and ComponentInfo). This allows us the executor to use a single `Access<ArchetypeComponentId>` per system. It should also make scripting language integration easier.
_But_ this merge did create problems for people directly interacting with `World`. What if you need mutable access to multiple resources at the same time? `world.get_resource_mut()` borrows World mutably!
## WorldCell
WorldCell applies the `Access<ArchetypeComponentId>` concept to direct world access:
```rust
let world_cell = world.cell();
let a = world_cell.get_resource_mut::<i32>().unwrap();
let b = world_cell.get_resource_mut::<f64>().unwrap();
```
This adds cheap runtime checks (a sparse set lookup of `ArchetypeComponentId` and a counter) to ensure that world accesses do not conflict with each other. Each operation returns a `WorldBorrow<'w, T>` or `WorldBorrowMut<'w, T>` wrapper type, which will release the relevant ArchetypeComponentId resources when dropped.
World caches the access sparse set (and only one cell can exist at a time), so `world.cell()` is a cheap operation.
WorldCell does _not_ use atomic operations. It is non-send, does a mutable borrow of world to prevent other accesses, and uses a simple `Rc<RefCell<ArchetypeComponentAccess>>` wrapper in each WorldBorrow pointer.
The api is currently limited to resource access, but it can and should be extended to queries / entity component access.
## Resource Scopes
WorldCell does not yet support component queries, and even when it does there are sometimes legitimate reasons to want a mutable world ref _and_ a mutable resource ref (ex: bevy_render and bevy_scene both need this). In these cases we could always drop down to the unsafe `world.get_resource_unchecked_mut()`, but that is not ideal!
Instead developers can use a "resource scope"
```rust
world.resource_scope(|world: &mut World, a: &mut A| {
})
```
This temporarily removes the `A` resource from `World`, provides mutable pointers to both, and re-adds A to World when finished. Thanks to the move to ComponentIds/sparse sets, this is a cheap operation.
If multiple resources are required, scopes can be nested. We could also consider adding a "resource tuple" to the api if this pattern becomes common and the boilerplate gets nasty.
## Query Conflicts Use ComponentId Instead of ArchetypeComponentId
For safety reasons, systems cannot contain queries that conflict with each other without wrapping them in a QuerySet. On bevy `main`, we use ArchetypeComponentIds to determine conflicts. This is nice because it can take into account filters:
```rust
// these queries will never conflict due to their filters
fn filter_system(a: Query<&mut A, With<B>>, b: Query<&mut B, Without<B>>) {
}
```
But it also has a significant downside:
```rust
// these queries will not conflict _until_ an entity with A, B, and C is spawned
fn maybe_conflicts_system(a: Query<(&mut A, &C)>, b: Query<(&mut A, &B)>) {
}
```
The system above will panic at runtime if an entity with A, B, and C is spawned. This makes it hard to trust that your game logic will run without crashing.
In this pr, I switched to using `ComponentId` instead. This _is_ more constraining. `maybe_conflicts_system` will now always fail, but it will do it consistently at startup. Naively, it would also _disallow_ `filter_system`, which would be a significant downgrade in usability. Bevy has a number of internal systems that rely on disjoint queries and I expect it to be a common pattern in userspace.
To resolve this, I added a new `FilteredAccess<T>` type, which wraps `Access<T>` and adds with/without filters. If two `FilteredAccess` have with/without values that prove they are disjoint, they will no longer conflict.
## EntityRef / EntityMut
World entity operations on `main` require that the user passes in an `entity` id to each operation:
```rust
let entity = world.spawn((A, )); // create a new entity with A
world.get::<A>(entity);
world.insert(entity, (B, C));
world.insert_one(entity, D);
```
This means that each operation needs to look up the entity location / verify its validity. The initial spawn operation also requires a Bundle as input. This can be awkward when no components are required (or one component is required).
These operations have been replaced by `EntityRef` and `EntityMut`, which are "builder-style" wrappers around world that provide read and read/write operations on a single, pre-validated entity:
```rust
// spawn now takes no inputs and returns an EntityMut
let entity = world.spawn()
.insert(A) // insert a single component into the entity
.insert_bundle((B, C)) // insert a bundle of components into the entity
.id() // id returns the Entity id
// Returns EntityMut (or panics if the entity does not exist)
world.entity_mut(entity)
.insert(D)
.insert_bundle(SomeBundle::default());
{
// returns EntityRef (or panics if the entity does not exist)
let d = world.entity(entity)
.get::<D>() // gets the D component
.unwrap();
// world.get still exists for ergonomics
let d = world.get::<D>(entity).unwrap();
}
// These variants return Options if you want to check existence instead of panicing
world.get_entity_mut(entity)
.unwrap()
.insert(E);
if let Some(entity_ref) = world.get_entity(entity) {
let d = entity_ref.get::<D>().unwrap();
}
```
This _does not_ affect the current Commands api or terminology. I think that should be a separate conversation as that is a much larger breaking change.
## Safety Improvements
* Entity reservation in Commands uses a normal world borrow instead of an unsafe transmute
* QuerySets no longer transmutes lifetimes
* Made traits "unsafe" when implementing a trait incorrectly could cause unsafety
* More thorough safety docs
## RemovedComponents SystemParam
The old approach to querying removed components: `query.removed:<T>()` was confusing because it had no connection to the query itself. I replaced it with the following, which is both clearer and allows us to cache the ComponentId mapping in the SystemParamState:
```rust
fn system(removed: RemovedComponents<T>) {
for entity in removed.iter() {
}
}
```
## Simpler Bundle implementation
Bundles are no longer responsible for sorting (or deduping) TypeInfo. They are just a simple ordered list of component types / data. This makes the implementation smaller and opens the door to an easy "nested bundle" implementation in the future (which i might even add in this pr). Duplicate detection is now done once per bundle type by World the first time a bundle is used.
## Unified WorldQuery and QueryFilter types
(don't worry they are still separate type _parameters_ in Queries .. this is a non-breaking change)
WorldQuery and QueryFilter were already basically identical apis. With the addition of `FetchState` and more storage-specific fetch methods, the overlap was even clearer (and the redundancy more painful).
QueryFilters are now just `F: WorldQuery where F::Fetch: FilterFetch`. FilterFetch requires `Fetch<Item = bool>` and adds new "short circuit" variants of fetch methods. This enables a filter tuple like `(With<A>, Without<B>, Changed<C>)` to stop evaluating the filter after the first mismatch is encountered. FilterFetch is automatically implemented for `Fetch` implementations that return bool.
This forces fetch implementations that return things like `(bool, bool, bool)` (such as the filter above) to manually implement FilterFetch and decide whether or not to short-circuit.
## More Granular Modules
World no longer globs all of the internal modules together. It now exports `core`, `system`, and `schedule` separately. I'm also considering exporting `core` submodules directly as that is still pretty "glob-ey" and unorganized (feedback welcome here).
## Remaining Draft Work (to be done in this pr)
* ~~panic on conflicting WorldQuery fetches (&A, &mut A)~~
* ~~bevy `main` and hecs both currently allow this, but we should protect against it if possible~~
* ~~batch_iter / par_iter (currently stubbed out)~~
* ~~ChangedRes~~
* ~~I skipped this while we sort out #1313. This pr should be adapted to account for whatever we land on there~~.
* ~~The `Archetypes` and `Tables` collections use hashes of sorted lists of component ids to uniquely identify each archetype/table. This hash is then used as the key in a HashMap to look up the relevant ArchetypeId or TableId. (which doesn't handle hash collisions properly)~~
* ~~It is currently unsafe to generate a Query from "World A", then use it on "World B" (despite the api claiming it is safe). We should probably close this gap. This could be done by adding a randomly generated WorldId to each world, then storing that id in each Query. They could then be compared to each other on each `query.do_thing(&world)` operation. This _does_ add an extra branch to each query operation, so I'm open to other suggestions if people have them.~~
* ~~Nested Bundles (if i find time)~~
## Potential Future Work
* Expand WorldCell to support queries.
* Consider not allocating in the empty archetype on `world.spawn()`
* ex: return something like EntityMutUninit, which turns into EntityMut after an `insert` or `insert_bundle` op
* this actually regressed performance last time i tried it, but in theory it should be faster
* Optimize SparseSet::insert (see `PERF` comment on insert)
* Replace SparseArray `Option<T>` with T::MAX to cut down on branching
* would enable cheaper get_unchecked() operations
* upstream fixedbitset optimizations
* fixedbitset could be allocation free for small block counts (store blocks in a SmallVec)
* fixedbitset could have a const constructor
* Consider implementing Tags (archetype-specific by-value data that affects archetype identity)
* ex: ArchetypeA could have `[A, B, C]` table components and `[D(1)]` "tag" component. ArchetypeB could have `[A, B, C]` table components and a `[D(2)]` tag component. The archetypes are different, despite both having D tags because the value inside D is different.
* this could potentially build on top of the `archetype.unique_components` added in this pr for resource storage.
* Consider reverting `all_tuples` proc macro in favor of the old `macro_rules` implementation
* all_tuples is more flexible and produces cleaner documentation (the macro_rules version produces weird type parameter orders due to parser constraints)
* but unfortunately all_tuples also appears to make Rust Analyzer sad/slow when working inside of `bevy_ecs` (does not affect user code)
* Consider "resource queries" and/or "mixed resource and entity component queries" as an alternative to WorldCell
* this is basically just "systems" so maybe it's not worth it
* Add more world ops
* `world.clear()`
* `world.reserve<T: Bundle>(count: usize)`
* Try using the old archetype allocation strategy (allocate new memory on resize and copy everything over). I expect this to improve batch insertion performance at the cost of unbatched performance. But thats just a guess. I'm not an allocation perf pro :)
* Adapt Commands apis for consistency with new World apis
## Benchmarks
key:
* `bevy_old`: bevy `main` branch
* `bevy`: this branch
* `_foreach`: uses an optimized for_each iterator
* ` _sparse`: uses sparse set storage (if unspecified assume table storage)
* `_system`: runs inside a system (if unspecified assume test happens via direct world ops)
### Simple Insert (from ecs_bench_suite)
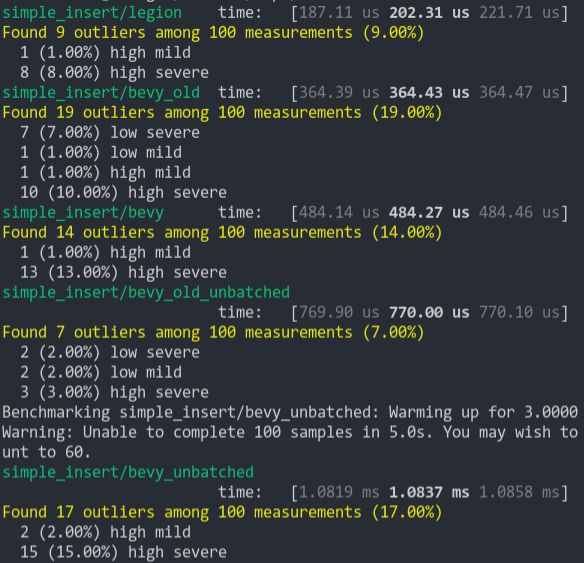
### Simpler Iter (from ecs_bench_suite)
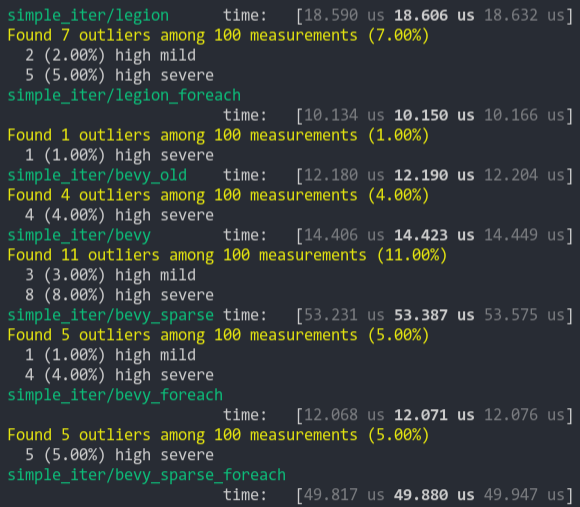
### Fragment Iter (from ecs_bench_suite)
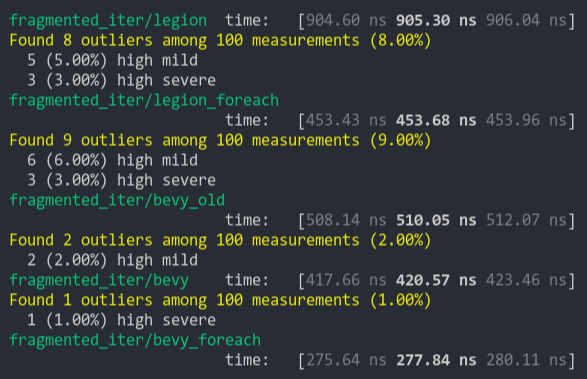
### Sparse Fragmented Iter
Iterate a query that matches 5 entities from a single matching archetype, but there are 100 unmatching archetypes
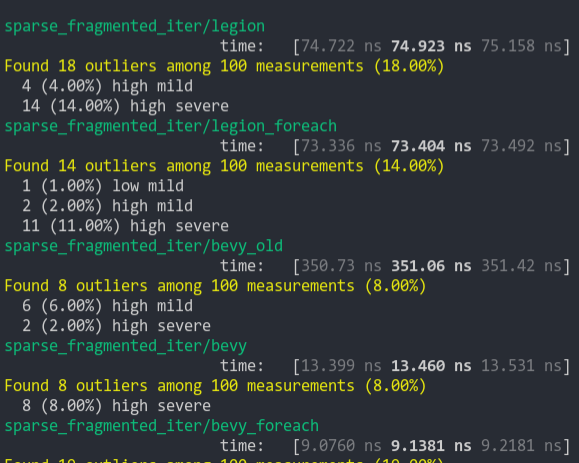
### Schedule (from ecs_bench_suite)
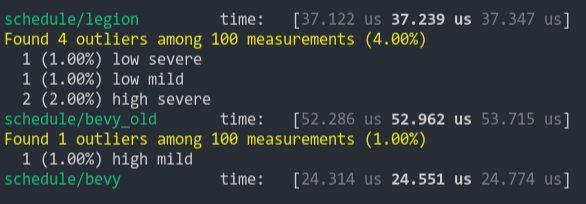
### Add Remove Component (from ecs_bench_suite)
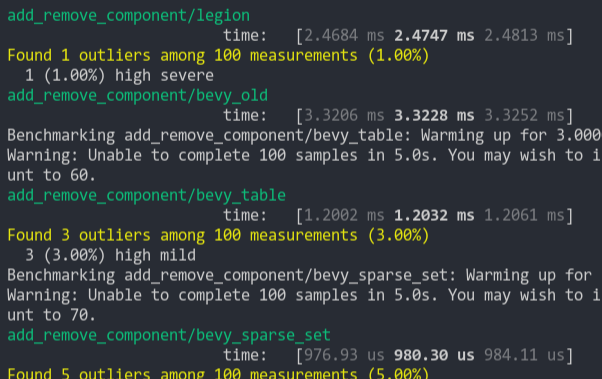
### Add Remove Component Big
Same as the test above, but each entity has 5 "large" matrix components and 1 "large" matrix component is added and removed
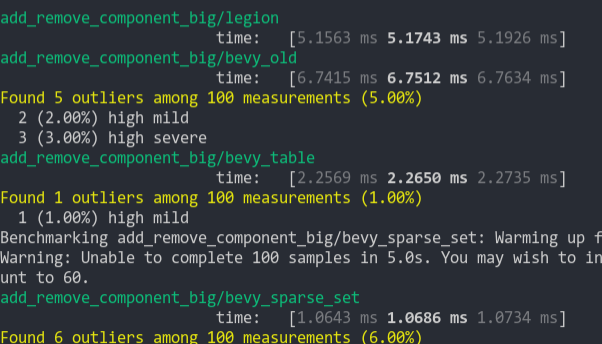
### Get Component
Looks up a single component value a large number of times
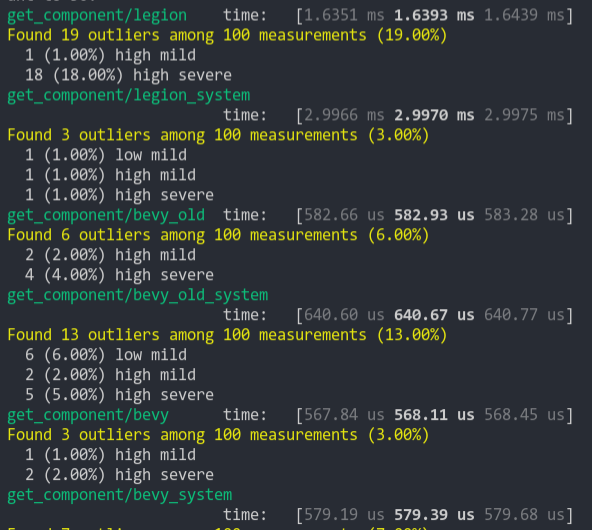
2021-03-05 07:54:35 +00:00
#[ inline ]
Remove the `SystemParamState` trait and remove types like `ResState` (#6919)
Spiritual successor to #5205.
Actual successor to #6865.
# Objective
Currently, system params are defined using three traits: `SystemParam`, `ReadOnlySystemParam`, `SystemParamState`. The behavior for each param is specified by the `SystemParamState` trait, while `SystemParam` simply defers to the state.
Splitting the traits in this way makes it easier to implement within macros, but it increases the cognitive load. Worst of all, this approach requires each `MySystemParam` to have a public `MySystemParamState` type associated with it.
## Solution
* Merge the trait `SystemParamState` into `SystemParam`.
* Remove all trivial `SystemParam` state types.
* `OptionNonSendMutState<T>`: you will not be missed.
---
- [x] Fix/resolve the remaining test failure.
## Changelog
* Removed the trait `SystemParamState`, merging its functionality into `SystemParam`.
## Migration Guide
**Note**: this should replace the migration guide for #6865.
This is relative to Bevy 0.9, not main.
The traits `SystemParamState` and `SystemParamFetch` have been removed, and their functionality has been transferred to `SystemParam`.
```rust
// Before (0.9)
impl SystemParam for MyParam<'_, '_> {
type State = MyParamState;
}
unsafe impl SystemParamState for MyParamState {
fn init(world: &mut World, system_meta: &mut SystemMeta) -> Self { ... }
}
unsafe impl<'w, 's> SystemParamFetch<'w, 's> for MyParamState {
type Item = MyParam<'w, 's>;
fn get_param(&mut self, ...) -> Self::Item;
}
unsafe impl ReadOnlySystemParamFetch for MyParamState { }
// After (0.10)
unsafe impl SystemParam for MyParam<'_, '_> {
type State = MyParamState;
type Item<'w, 's> = MyParam<'w, 's>;
fn init_state(world: &mut World, system_meta: &mut SystemMeta) -> Self::State { ... }
fn get_param<'w, 's>(state: &mut Self::State, ...) -> Self::Item<'w, 's>;
}
unsafe impl ReadOnlySystemParam for MyParam<'_, '_> { }
```
The trait `ReadOnlySystemParamFetch` has been replaced with `ReadOnlySystemParam`.
```rust
// Before
unsafe impl ReadOnlySystemParamFetch for MyParamState {}
// After
unsafe impl ReadOnlySystemParam for MyParam<'_, '_> {}
```
2023-01-07 23:20:32 +00:00
fn new_archetype (
_state : & mut Self ::State ,
_archetype : & Archetype ,
_system_meta : & mut SystemMeta ,
) {
}
/// Applies any deferred mutations stored in this [`SystemParam`]'s state.
Migrate engine to Schedule v3 (#7267)
Huge thanks to @maniwani, @devil-ira, @hymm, @cart, @superdump and @jakobhellermann for the help with this PR.
# Objective
- Followup #6587.
- Minimal integration for the Stageless Scheduling RFC: https://github.com/bevyengine/rfcs/pull/45
## Solution
- [x] Remove old scheduling module
- [x] Migrate new methods to no longer use extension methods
- [x] Fix compiler errors
- [x] Fix benchmarks
- [x] Fix examples
- [x] Fix docs
- [x] Fix tests
## Changelog
### Added
- a large number of methods on `App` to work with schedules ergonomically
- the `CoreSchedule` enum
- `App::add_extract_system` via the `RenderingAppExtension` trait extension method
- the private `prepare_view_uniforms` system now has a public system set for scheduling purposes, called `ViewSet::PrepareUniforms`
### Removed
- stages, and all code that mentions stages
- states have been dramatically simplified, and no longer use a stack
- `RunCriteriaLabel`
- `AsSystemLabel` trait
- `on_hierarchy_reports_enabled` run criteria (now just uses an ad hoc resource checking run condition)
- systems in `RenderSet/Stage::Extract` no longer warn when they do not read data from the main world
- `RunCriteriaLabel`
- `transform_propagate_system_set`: this was a nonstandard pattern that didn't actually provide enough control. The systems are already `pub`: the docs have been updated to ensure that the third-party usage is clear.
### Changed
- `System::default_labels` is now `System::default_system_sets`.
- `App::add_default_labels` is now `App::add_default_sets`
- `CoreStage` and `StartupStage` enums are now `CoreSet` and `StartupSet`
- `App::add_system_set` was renamed to `App::add_systems`
- The `StartupSchedule` label is now defined as part of the `CoreSchedules` enum
- `.label(SystemLabel)` is now referred to as `.in_set(SystemSet)`
- `SystemLabel` trait was replaced by `SystemSet`
- `SystemTypeIdLabel<T>` was replaced by `SystemSetType<T>`
- The `ReportHierarchyIssue` resource now has a public constructor (`new`), and implements `PartialEq`
- Fixed time steps now use a schedule (`CoreSchedule::FixedTimeStep`) rather than a run criteria.
- Adding rendering extraction systems now panics rather than silently failing if no subapp with the `RenderApp` label is found.
- the `calculate_bounds` system, with the `CalculateBounds` label, is now in `CoreSet::Update`, rather than in `CoreSet::PostUpdate` before commands are applied.
- `SceneSpawnerSystem` now runs under `CoreSet::Update`, rather than `CoreStage::PreUpdate.at_end()`.
- `bevy_pbr::add_clusters` is no longer an exclusive system
- the top level `bevy_ecs::schedule` module was replaced with `bevy_ecs::scheduling`
- `tick_global_task_pools_on_main_thread` is no longer run as an exclusive system. Instead, it has been replaced by `tick_global_task_pools`, which uses a `NonSend` resource to force running on the main thread.
## Migration Guide
- Calls to `.label(MyLabel)` should be replaced with `.in_set(MySet)`
- Stages have been removed. Replace these with system sets, and then add command flushes using the `apply_system_buffers` exclusive system where needed.
- The `CoreStage`, `StartupStage, `RenderStage` and `AssetStage` enums have been replaced with `CoreSet`, `StartupSet, `RenderSet` and `AssetSet`. The same scheduling guarantees have been preserved.
- Systems are no longer added to `CoreSet::Update` by default. Add systems manually if this behavior is needed, although you should consider adding your game logic systems to `CoreSchedule::FixedTimestep` instead for more reliable framerate-independent behavior.
- Similarly, startup systems are no longer part of `StartupSet::Startup` by default. In most cases, this won't matter to you.
- For example, `add_system_to_stage(CoreStage::PostUpdate, my_system)` should be replaced with
- `add_system(my_system.in_set(CoreSet::PostUpdate)`
- When testing systems or otherwise running them in a headless fashion, simply construct and run a schedule using `Schedule::new()` and `World::run_schedule` rather than constructing stages
- Run criteria have been renamed to run conditions. These can now be combined with each other and with states.
- Looping run criteria and state stacks have been removed. Use an exclusive system that runs a schedule if you need this level of control over system control flow.
- For app-level control flow over which schedules get run when (such as for rollback networking), create your own schedule and insert it under the `CoreSchedule::Outer` label.
- Fixed timesteps are now evaluated in a schedule, rather than controlled via run criteria. The `run_fixed_timestep` system runs this schedule between `CoreSet::First` and `CoreSet::PreUpdate` by default.
- Command flush points introduced by `AssetStage` have been removed. If you were relying on these, add them back manually.
- Adding extract systems is now typically done directly on the main app. Make sure the `RenderingAppExtension` trait is in scope, then call `app.add_extract_system(my_system)`.
- the `calculate_bounds` system, with the `CalculateBounds` label, is now in `CoreSet::Update`, rather than in `CoreSet::PostUpdate` before commands are applied. You may need to order your movement systems to occur before this system in order to avoid system order ambiguities in culling behavior.
- the `RenderLabel` `AppLabel` was renamed to `RenderApp` for clarity
- `App::add_state` now takes 0 arguments: the starting state is set based on the `Default` impl.
- Instead of creating `SystemSet` containers for systems that run in stages, simply use `.on_enter::<State::Variant>()` or its `on_exit` or `on_update` siblings.
- `SystemLabel` derives should be replaced with `SystemSet`. You will also need to add the `Debug`, `PartialEq`, `Eq`, and `Hash` traits to satisfy the new trait bounds.
- `with_run_criteria` has been renamed to `run_if`. Run criteria have been renamed to run conditions for clarity, and should now simply return a bool.
- States have been dramatically simplified: there is no longer a "state stack". To queue a transition to the next state, call `NextState::set`
## TODO
- [x] remove dead methods on App and World
- [x] add `App::add_system_to_schedule` and `App::add_systems_to_schedule`
- [x] avoid adding the default system set at inappropriate times
- [x] remove any accidental cycles in the default plugins schedule
- [x] migrate benchmarks
- [x] expose explicit labels for the built-in command flush points
- [x] migrate engine code
- [x] remove all mentions of stages from the docs
- [x] verify docs for States
- [x] fix uses of exclusive systems that use .end / .at_start / .before_commands
- [x] migrate RenderStage and AssetStage
- [x] migrate examples
- [x] ensure that transform propagation is exported in a sufficiently public way (the systems are already pub)
- [x] ensure that on_enter schedules are run at least once before the main app
- [x] re-enable opt-in to execution order ambiguities
- [x] revert change to `update_bounds` to ensure it runs in `PostUpdate`
- [x] test all examples
- [x] unbreak directional lights
- [x] unbreak shadows (see 3d_scene, 3d_shape, lighting, transparaency_3d examples)
- [x] game menu example shows loading screen and menu simultaneously
- [x] display settings menu is a blank screen
- [x] `without_winit` example panics
- [x] ensure all tests pass
- [x] SubApp doc test fails
- [x] runs_spawn_local tasks fails
- [x] [Fix panic_when_hierachy_cycle test hanging](https://github.com/alice-i-cecile/bevy/pull/120)
## Points of Difficulty and Controversy
**Reviewers, please give feedback on these and look closely**
1. Default sets, from the RFC, have been removed. These added a tremendous amount of implicit complexity and result in hard to debug scheduling errors. They're going to be tackled in the form of "base sets" by @cart in a followup.
2. The outer schedule controls which schedule is run when `App::update` is called.
3. I implemented `Label for `Box<dyn Label>` for our label types. This enables us to store schedule labels in concrete form, and then later run them. I ran into the same set of problems when working with one-shot systems. We've previously investigated this pattern in depth, and it does not appear to lead to extra indirection with nested boxes.
4. `SubApp::update` simply runs the default schedule once. This sucks, but this whole API is incomplete and this was the minimal changeset.
5. `time_system` and `tick_global_task_pools_on_main_thread` no longer use exclusive systems to attempt to force scheduling order
6. Implemetnation strategy for fixed timesteps
7. `AssetStage` was migrated to `AssetSet` without reintroducing command flush points. These did not appear to be used, and it's nice to remove these bottlenecks.
8. Migration of `bevy_render/lib.rs` and pipelined rendering. The logic here is unusually tricky, as we have complex scheduling requirements.
## Future Work (ideally before 0.10)
- Rename schedule_v3 module to schedule or scheduling
- Add a derive macro to states, and likely a `EnumIter` trait of some form
- Figure out what exactly to do with the "systems added should basically work by default" problem
- Improve ergonomics for working with fixed timesteps and states
- Polish FixedTime API to match Time
- Rebase and merge #7415
- Resolve all internal ambiguities (blocked on better tools, especially #7442)
- Add "base sets" to replace the removed default sets.
2023-02-06 02:04:50 +00:00
/// This is used to apply [`Commands`] during [`apply_system_buffers`](crate::prelude::apply_system_buffers).
Add a `SystemParam` primitive for deferred mutations; allow `#[derive]`ing more types of SystemParam (#6817)
# Objective
One pattern to increase parallelism is deferred mutation: instead of directly mutating the world (and preventing other systems from running at the same time), you queue up operations to be applied to the world at the end of the stage. The most common example of this pattern uses the `Commands` SystemParam.
In order to avoid the overhead associated with commands, some power users may want to add their own deferred mutation behavior. To do this, you must implement the unsafe trait `SystemParam`, which interfaces with engine internals in a way that we'd like users to be able to avoid.
## Solution
Add the `Deferred<T>` primitive `SystemParam`, which encapsulates the deferred mutation pattern.
This can be combined with other types of `SystemParam` to safely and ergonomically create powerful custom types.
Essentially, this is just a variant of `Local<T>` which can run code at the end of the stage.
This type is used in the engine to derive `Commands` and `ParallelCommands`, which removes a bunch of unsafe boilerplate.
### Example
```rust
use bevy_ecs::system::{Deferred, SystemBuffer};
/// Sends events with a delay, but may run in parallel with other event writers.
#[derive(SystemParam)]
pub struct BufferedEventWriter<'s, E: Event> {
queue: Deferred<'s, EventQueue<E>>,
}
struct EventQueue<E>(Vec<E>);
impl<'s, E: Event> BufferedEventWriter<'s, E> {
/// Queues up an event to be sent at the end of this stage.
pub fn send(&mut self, event: E) {
self.queue.0.push(event);
}
}
// The `SystemBuffer` trait controls how [`Deferred`] gets applied at the end of the stage.
impl<E: Event> SystemBuffer for EventQueue<E> {
fn apply(&mut self, world: &mut World) {
let mut events = world.resource_mut::<Events<E>>();
for e in self.0.drain(..) {
events.send(e);
}
}
}
```
---
## Changelog
+ Added the `SystemParam` type `Deferred<T>`, which can be used to defer `World` mutations. Powered by the new trait `SystemBuffer`.
2023-02-06 21:57:57 +00:00
///
/// [`Commands`]: crate::prelude::Commands
Bevy ECS V2 (#1525)
# Bevy ECS V2
This is a rewrite of Bevy ECS (basically everything but the new executor/schedule, which are already awesome). The overall goal was to improve the performance and versatility of Bevy ECS. Here is a quick bulleted list of changes before we dive into the details:
* Complete World rewrite
* Multiple component storage types:
* Tables: fast cache friendly iteration, slower add/removes (previously called Archetypes)
* Sparse Sets: fast add/remove, slower iteration
* Stateful Queries (caches query results for faster iteration. fragmented iteration is _fast_ now)
* Stateful System Params (caches expensive operations. inspired by @DJMcNab's work in #1364)
* Configurable System Params (users can set configuration when they construct their systems. once again inspired by @DJMcNab's work)
* Archetypes are now "just metadata", component storage is separate
* Archetype Graph (for faster archetype changes)
* Component Metadata
* Configure component storage type
* Retrieve information about component size/type/name/layout/send-ness/etc
* Components are uniquely identified by a densely packed ComponentId
* TypeIds are now totally optional (which should make implementing scripting easier)
* Super fast "for_each" query iterators
* Merged Resources into World. Resources are now just a special type of component
* EntityRef/EntityMut builder apis (more efficient and more ergonomic)
* Fast bitset-backed `Access<T>` replaces old hashmap-based approach everywhere
* Query conflicts are determined by component access instead of archetype component access (to avoid random failures at runtime)
* With/Without are still taken into account for conflicts, so this should still be comfy to use
* Much simpler `IntoSystem` impl
* Significantly reduced the amount of hashing throughout the ecs in favor of Sparse Sets (indexed by densely packed ArchetypeId, ComponentId, BundleId, and TableId)
* Safety Improvements
* Entity reservation uses a normal world reference instead of unsafe transmute
* QuerySets no longer transmute lifetimes
* Made traits "unsafe" where relevant
* More thorough safety docs
* WorldCell
* Exposes safe mutable access to multiple resources at a time in a World
* Replaced "catch all" `System::update_archetypes(world: &World)` with `System::new_archetype(archetype: &Archetype)`
* Simpler Bundle implementation
* Replaced slow "remove_bundle_one_by_one" used as fallback for Commands::remove_bundle with fast "remove_bundle_intersection"
* Removed `Mut<T>` query impl. it is better to only support one way: `&mut T`
* Removed with() from `Flags<T>` in favor of `Option<Flags<T>>`, which allows querying for flags to be "filtered" by default
* Components now have is_send property (currently only resources support non-send)
* More granular module organization
* New `RemovedComponents<T>` SystemParam that replaces `query.removed::<T>()`
* `world.resource_scope()` for mutable access to resources and world at the same time
* WorldQuery and QueryFilter traits unified. FilterFetch trait added to enable "short circuit" filtering. Auto impled for cases that don't need it
* Significantly slimmed down SystemState in favor of individual SystemParam state
* System Commands changed from `commands: &mut Commands` back to `mut commands: Commands` (to allow Commands to have a World reference)
Fixes #1320
## `World` Rewrite
This is a from-scratch rewrite of `World` that fills the niche that `hecs` used to. Yes, this means Bevy ECS is no longer a "fork" of hecs. We're going out our own!
(the only shared code between the projects is the entity id allocator, which is already basically ideal)
A huge shout out to @SanderMertens (author of [flecs](https://github.com/SanderMertens/flecs)) for sharing some great ideas with me (specifically hybrid ecs storage and archetype graphs). He also helped advise on a number of implementation details.
## Component Storage (The Problem)
Two ECS storage paradigms have gained a lot of traction over the years:
* **Archetypal ECS**:
* Stores components in "tables" with static schemas. Each "column" stores components of a given type. Each "row" is an entity.
* Each "archetype" has its own table. Adding/removing an entity's component changes the archetype.
* Enables super-fast Query iteration due to its cache-friendly data layout
* Comes at the cost of more expensive add/remove operations for an Entity's components, because all components need to be copied to the new archetype's "table"
* **Sparse Set ECS**:
* Stores components of the same type in densely packed arrays, which are sparsely indexed by densely packed unsigned integers (Entity ids)
* Query iteration is slower than Archetypal ECS because each entity's component could be at any position in the sparse set. This "random access" pattern isn't cache friendly. Additionally, there is an extra layer of indirection because you must first map the entity id to an index in the component array.
* Adding/removing components is a cheap, constant time operation
Bevy ECS V1, hecs, legion, flec, and Unity DOTS are all "archetypal ecs-es". I personally think "archetypal" storage is a good default for game engines. An entity's archetype doesn't need to change frequently in general, and it creates "fast by default" query iteration (which is a much more common operation). It is also "self optimizing". Users don't need to think about optimizing component layouts for iteration performance. It "just works" without any extra boilerplate.
Shipyard and EnTT are "sparse set ecs-es". They employ "packing" as a way to work around the "suboptimal by default" iteration performance for specific sets of components. This helps, but I didn't think this was a good choice for a general purpose engine like Bevy because:
1. "packs" conflict with each other. If bevy decides to internally pack the Transform and GlobalTransform components, users are then blocked if they want to pack some custom component with Transform.
2. users need to take manual action to optimize
Developers selecting an ECS framework are stuck with a hard choice. Select an "archetypal" framework with "fast iteration everywhere" but without the ability to cheaply add/remove components, or select a "sparse set" framework to cheaply add/remove components but with slower iteration performance.
## Hybrid Component Storage (The Solution)
In Bevy ECS V2, we get to have our cake and eat it too. It now has _both_ of the component storage types above (and more can be added later if needed):
* **Tables** (aka "archetypal" storage)
* The default storage. If you don't configure anything, this is what you get
* Fast iteration by default
* Slower add/remove operations
* **Sparse Sets**
* Opt-in
* Slower iteration
* Faster add/remove operations
These storage types complement each other perfectly. By default Query iteration is fast. If developers know that they want to add/remove a component at high frequencies, they can set the storage to "sparse set":
```rust
world.register_component(
ComponentDescriptor::new::<MyComponent>(StorageType::SparseSet)
).unwrap();
```
## Archetypes
Archetypes are now "just metadata" ... they no longer store components directly. They do store:
* The `ComponentId`s of each of the Archetype's components (and that component's storage type)
* Archetypes are uniquely defined by their component layouts
* For example: entities with "table" components `[A, B, C]` _and_ "sparse set" components `[D, E]` will always be in the same archetype.
* The `TableId` associated with the archetype
* For now each archetype has exactly one table (which can have no components),
* There is a 1->Many relationship from Tables->Archetypes. A given table could have any number of archetype components stored in it:
* Ex: an entity with "table storage" components `[A, B, C]` and "sparse set" components `[D, E]` will share the same `[A, B, C]` table as an entity with `[A, B, C]` table component and `[F]` sparse set components.
* This 1->Many relationship is how we preserve fast "cache friendly" iteration performance when possible (more on this later)
* A list of entities that are in the archetype and the row id of the table they are in
* ArchetypeComponentIds
* unique densely packed identifiers for (ArchetypeId, ComponentId) pairs
* used by the schedule executor for cheap system access control
* "Archetype Graph Edges" (see the next section)
## The "Archetype Graph"
Archetype changes in Bevy (and a number of other archetypal ecs-es) have historically been expensive to compute. First, you need to allocate a new vector of the entity's current component ids, add or remove components based on the operation performed, sort it (to ensure it is order-independent), then hash it to find the archetype (if it exists). And thats all before we get to the _already_ expensive full copy of all components to the new table storage.
The solution is to build a "graph" of archetypes to cache these results. @SanderMertens first exposed me to the idea (and he got it from @gjroelofs, who came up with it). They propose adding directed edges between archetypes for add/remove component operations. If `ComponentId`s are densely packed, you can use sparse sets to cheaply jump between archetypes.
Bevy takes this one step further by using add/remove `Bundle` edges instead of `Component` edges. Bevy encourages the use of `Bundles` to group add/remove operations. This is largely for "clearer game logic" reasons, but it also helps cut down on the number of archetype changes required. `Bundles` now also have densely-packed `BundleId`s. This allows us to use a _single_ edge for each bundle operation (rather than needing to traverse N edges ... one for each component). Single component operations are also bundles, so this is strictly an improvement over a "component only" graph.
As a result, an operation that used to be _heavy_ (both for allocations and compute) is now two dirt-cheap array lookups and zero allocations.
## Stateful Queries
World queries are now stateful. This allows us to:
1. Cache archetype (and table) matches
* This resolves another issue with (naive) archetypal ECS: query performance getting worse as the number of archetypes goes up (and fragmentation occurs).
2. Cache Fetch and Filter state
* The expensive parts of fetch/filter operations (such as hashing the TypeId to find the ComponentId) now only happen once when the Query is first constructed
3. Incrementally build up state
* When new archetypes are added, we only process the new archetypes (no need to rebuild state for old archetypes)
As a result, the direct `World` query api now looks like this:
```rust
let mut query = world.query::<(&A, &mut B)>();
for (a, mut b) in query.iter_mut(&mut world) {
}
```
Requiring `World` to generate stateful queries (rather than letting the `QueryState` type be constructed separately) allows us to ensure that _all_ queries are properly initialized (and the relevant world state, such as ComponentIds). This enables QueryState to remove branches from its operations that check for initialization status (and also enables query.iter() to take an immutable world reference because it doesn't need to initialize anything in world).
However in systems, this is a non-breaking change. State management is done internally by the relevant SystemParam.
## Stateful SystemParams
Like Queries, `SystemParams` now also cache state. For example, `Query` system params store the "stateful query" state mentioned above. Commands store their internal `CommandQueue`. This means you can now safely use as many separate `Commands` parameters in your system as you want. `Local<T>` system params store their `T` value in their state (instead of in Resources).
SystemParam state also enabled a significant slim-down of SystemState. It is much nicer to look at now.
Per-SystemParam state naturally insulates us from an "aliased mut" class of errors we have hit in the past (ex: using multiple `Commands` system params).
(credit goes to @DJMcNab for the initial idea and draft pr here #1364)
## Configurable SystemParams
@DJMcNab also had the great idea to make SystemParams configurable. This allows users to provide some initial configuration / values for system parameters (when possible). Most SystemParams have no config (the config type is `()`), but the `Local<T>` param now supports user-provided parameters:
```rust
fn foo(value: Local<usize>) {
}
app.add_system(foo.system().config(|c| c.0 = Some(10)));
```
## Uber Fast "for_each" Query Iterators
Developers now have the choice to use a fast "for_each" iterator, which yields ~1.5-3x iteration speed improvements for "fragmented iteration", and minor ~1.2x iteration speed improvements for unfragmented iteration.
```rust
fn system(query: Query<(&A, &mut B)>) {
// you now have the option to do this for a speed boost
query.for_each_mut(|(a, mut b)| {
});
// however normal iterators are still available
for (a, mut b) in query.iter_mut() {
}
}
```
I think in most cases we should continue to encourage "normal" iterators as they are more flexible and more "rust idiomatic". But when that extra "oomf" is needed, it makes sense to use `for_each`.
We should also consider using `for_each` for internal bevy systems to give our users a nice speed boost (but that should be a separate pr).
## Component Metadata
`World` now has a `Components` collection, which is accessible via `world.components()`. This stores mappings from `ComponentId` to `ComponentInfo`, as well as `TypeId` to `ComponentId` mappings (where relevant). `ComponentInfo` stores information about the component, such as ComponentId, TypeId, memory layout, send-ness (currently limited to resources), and storage type.
## Significantly Cheaper `Access<T>`
We used to use `TypeAccess<TypeId>` to manage read/write component/archetype-component access. This was expensive because TypeIds must be hashed and compared individually. The parallel executor got around this by "condensing" type ids into bitset-backed access types. This worked, but it had to be re-generated from the `TypeAccess<TypeId>`sources every time archetypes changed.
This pr removes TypeAccess in favor of faster bitset access everywhere. We can do this thanks to the move to densely packed `ComponentId`s and `ArchetypeComponentId`s.
## Merged Resources into World
Resources had a lot of redundant functionality with Components. They stored typed data, they had access control, they had unique ids, they were queryable via SystemParams, etc. In fact the _only_ major difference between them was that they were unique (and didn't correlate to an entity).
Separate resources also had the downside of requiring a separate set of access controls, which meant the parallel executor needed to compare more bitsets per system and manage more state.
I initially got the "separate resources" idea from `legion`. I think that design was motivated by the fact that it made the direct world query/resource lifetime interactions more manageable. It certainly made our lives easier when using Resources alongside hecs/bevy_ecs. However we already have a construct for safely and ergonomically managing in-world lifetimes: systems (which use `Access<T>` internally).
This pr merges Resources into World:
```rust
world.insert_resource(1);
world.insert_resource(2.0);
let a = world.get_resource::<i32>().unwrap();
let mut b = world.get_resource_mut::<f64>().unwrap();
*b = 3.0;
```
Resources are now just a special kind of component. They have their own ComponentIds (and their own resource TypeId->ComponentId scope, so they don't conflict wit components of the same type). They are stored in a special "resource archetype", which stores components inside the archetype using a new `unique_components` sparse set (note that this sparse set could later be used to implement Tags). This allows us to keep the code size small by reusing existing datastructures (namely Column, Archetype, ComponentFlags, and ComponentInfo). This allows us the executor to use a single `Access<ArchetypeComponentId>` per system. It should also make scripting language integration easier.
_But_ this merge did create problems for people directly interacting with `World`. What if you need mutable access to multiple resources at the same time? `world.get_resource_mut()` borrows World mutably!
## WorldCell
WorldCell applies the `Access<ArchetypeComponentId>` concept to direct world access:
```rust
let world_cell = world.cell();
let a = world_cell.get_resource_mut::<i32>().unwrap();
let b = world_cell.get_resource_mut::<f64>().unwrap();
```
This adds cheap runtime checks (a sparse set lookup of `ArchetypeComponentId` and a counter) to ensure that world accesses do not conflict with each other. Each operation returns a `WorldBorrow<'w, T>` or `WorldBorrowMut<'w, T>` wrapper type, which will release the relevant ArchetypeComponentId resources when dropped.
World caches the access sparse set (and only one cell can exist at a time), so `world.cell()` is a cheap operation.
WorldCell does _not_ use atomic operations. It is non-send, does a mutable borrow of world to prevent other accesses, and uses a simple `Rc<RefCell<ArchetypeComponentAccess>>` wrapper in each WorldBorrow pointer.
The api is currently limited to resource access, but it can and should be extended to queries / entity component access.
## Resource Scopes
WorldCell does not yet support component queries, and even when it does there are sometimes legitimate reasons to want a mutable world ref _and_ a mutable resource ref (ex: bevy_render and bevy_scene both need this). In these cases we could always drop down to the unsafe `world.get_resource_unchecked_mut()`, but that is not ideal!
Instead developers can use a "resource scope"
```rust
world.resource_scope(|world: &mut World, a: &mut A| {
})
```
This temporarily removes the `A` resource from `World`, provides mutable pointers to both, and re-adds A to World when finished. Thanks to the move to ComponentIds/sparse sets, this is a cheap operation.
If multiple resources are required, scopes can be nested. We could also consider adding a "resource tuple" to the api if this pattern becomes common and the boilerplate gets nasty.
## Query Conflicts Use ComponentId Instead of ArchetypeComponentId
For safety reasons, systems cannot contain queries that conflict with each other without wrapping them in a QuerySet. On bevy `main`, we use ArchetypeComponentIds to determine conflicts. This is nice because it can take into account filters:
```rust
// these queries will never conflict due to their filters
fn filter_system(a: Query<&mut A, With<B>>, b: Query<&mut B, Without<B>>) {
}
```
But it also has a significant downside:
```rust
// these queries will not conflict _until_ an entity with A, B, and C is spawned
fn maybe_conflicts_system(a: Query<(&mut A, &C)>, b: Query<(&mut A, &B)>) {
}
```
The system above will panic at runtime if an entity with A, B, and C is spawned. This makes it hard to trust that your game logic will run without crashing.
In this pr, I switched to using `ComponentId` instead. This _is_ more constraining. `maybe_conflicts_system` will now always fail, but it will do it consistently at startup. Naively, it would also _disallow_ `filter_system`, which would be a significant downgrade in usability. Bevy has a number of internal systems that rely on disjoint queries and I expect it to be a common pattern in userspace.
To resolve this, I added a new `FilteredAccess<T>` type, which wraps `Access<T>` and adds with/without filters. If two `FilteredAccess` have with/without values that prove they are disjoint, they will no longer conflict.
## EntityRef / EntityMut
World entity operations on `main` require that the user passes in an `entity` id to each operation:
```rust
let entity = world.spawn((A, )); // create a new entity with A
world.get::<A>(entity);
world.insert(entity, (B, C));
world.insert_one(entity, D);
```
This means that each operation needs to look up the entity location / verify its validity. The initial spawn operation also requires a Bundle as input. This can be awkward when no components are required (or one component is required).
These operations have been replaced by `EntityRef` and `EntityMut`, which are "builder-style" wrappers around world that provide read and read/write operations on a single, pre-validated entity:
```rust
// spawn now takes no inputs and returns an EntityMut
let entity = world.spawn()
.insert(A) // insert a single component into the entity
.insert_bundle((B, C)) // insert a bundle of components into the entity
.id() // id returns the Entity id
// Returns EntityMut (or panics if the entity does not exist)
world.entity_mut(entity)
.insert(D)
.insert_bundle(SomeBundle::default());
{
// returns EntityRef (or panics if the entity does not exist)
let d = world.entity(entity)
.get::<D>() // gets the D component
.unwrap();
// world.get still exists for ergonomics
let d = world.get::<D>(entity).unwrap();
}
// These variants return Options if you want to check existence instead of panicing
world.get_entity_mut(entity)
.unwrap()
.insert(E);
if let Some(entity_ref) = world.get_entity(entity) {
let d = entity_ref.get::<D>().unwrap();
}
```
This _does not_ affect the current Commands api or terminology. I think that should be a separate conversation as that is a much larger breaking change.
## Safety Improvements
* Entity reservation in Commands uses a normal world borrow instead of an unsafe transmute
* QuerySets no longer transmutes lifetimes
* Made traits "unsafe" when implementing a trait incorrectly could cause unsafety
* More thorough safety docs
## RemovedComponents SystemParam
The old approach to querying removed components: `query.removed:<T>()` was confusing because it had no connection to the query itself. I replaced it with the following, which is both clearer and allows us to cache the ComponentId mapping in the SystemParamState:
```rust
fn system(removed: RemovedComponents<T>) {
for entity in removed.iter() {
}
}
```
## Simpler Bundle implementation
Bundles are no longer responsible for sorting (or deduping) TypeInfo. They are just a simple ordered list of component types / data. This makes the implementation smaller and opens the door to an easy "nested bundle" implementation in the future (which i might even add in this pr). Duplicate detection is now done once per bundle type by World the first time a bundle is used.
## Unified WorldQuery and QueryFilter types
(don't worry they are still separate type _parameters_ in Queries .. this is a non-breaking change)
WorldQuery and QueryFilter were already basically identical apis. With the addition of `FetchState` and more storage-specific fetch methods, the overlap was even clearer (and the redundancy more painful).
QueryFilters are now just `F: WorldQuery where F::Fetch: FilterFetch`. FilterFetch requires `Fetch<Item = bool>` and adds new "short circuit" variants of fetch methods. This enables a filter tuple like `(With<A>, Without<B>, Changed<C>)` to stop evaluating the filter after the first mismatch is encountered. FilterFetch is automatically implemented for `Fetch` implementations that return bool.
This forces fetch implementations that return things like `(bool, bool, bool)` (such as the filter above) to manually implement FilterFetch and decide whether or not to short-circuit.
## More Granular Modules
World no longer globs all of the internal modules together. It now exports `core`, `system`, and `schedule` separately. I'm also considering exporting `core` submodules directly as that is still pretty "glob-ey" and unorganized (feedback welcome here).
## Remaining Draft Work (to be done in this pr)
* ~~panic on conflicting WorldQuery fetches (&A, &mut A)~~
* ~~bevy `main` and hecs both currently allow this, but we should protect against it if possible~~
* ~~batch_iter / par_iter (currently stubbed out)~~
* ~~ChangedRes~~
* ~~I skipped this while we sort out #1313. This pr should be adapted to account for whatever we land on there~~.
* ~~The `Archetypes` and `Tables` collections use hashes of sorted lists of component ids to uniquely identify each archetype/table. This hash is then used as the key in a HashMap to look up the relevant ArchetypeId or TableId. (which doesn't handle hash collisions properly)~~
* ~~It is currently unsafe to generate a Query from "World A", then use it on "World B" (despite the api claiming it is safe). We should probably close this gap. This could be done by adding a randomly generated WorldId to each world, then storing that id in each Query. They could then be compared to each other on each `query.do_thing(&world)` operation. This _does_ add an extra branch to each query operation, so I'm open to other suggestions if people have them.~~
* ~~Nested Bundles (if i find time)~~
## Potential Future Work
* Expand WorldCell to support queries.
* Consider not allocating in the empty archetype on `world.spawn()`
* ex: return something like EntityMutUninit, which turns into EntityMut after an `insert` or `insert_bundle` op
* this actually regressed performance last time i tried it, but in theory it should be faster
* Optimize SparseSet::insert (see `PERF` comment on insert)
* Replace SparseArray `Option<T>` with T::MAX to cut down on branching
* would enable cheaper get_unchecked() operations
* upstream fixedbitset optimizations
* fixedbitset could be allocation free for small block counts (store blocks in a SmallVec)
* fixedbitset could have a const constructor
* Consider implementing Tags (archetype-specific by-value data that affects archetype identity)
* ex: ArchetypeA could have `[A, B, C]` table components and `[D(1)]` "tag" component. ArchetypeB could have `[A, B, C]` table components and a `[D(2)]` tag component. The archetypes are different, despite both having D tags because the value inside D is different.
* this could potentially build on top of the `archetype.unique_components` added in this pr for resource storage.
* Consider reverting `all_tuples` proc macro in favor of the old `macro_rules` implementation
* all_tuples is more flexible and produces cleaner documentation (the macro_rules version produces weird type parameter orders due to parser constraints)
* but unfortunately all_tuples also appears to make Rust Analyzer sad/slow when working inside of `bevy_ecs` (does not affect user code)
* Consider "resource queries" and/or "mixed resource and entity component queries" as an alternative to WorldCell
* this is basically just "systems" so maybe it's not worth it
* Add more world ops
* `world.clear()`
* `world.reserve<T: Bundle>(count: usize)`
* Try using the old archetype allocation strategy (allocate new memory on resize and copy everything over). I expect this to improve batch insertion performance at the cost of unbatched performance. But thats just a guess. I'm not an allocation perf pro :)
* Adapt Commands apis for consistency with new World apis
## Benchmarks
key:
* `bevy_old`: bevy `main` branch
* `bevy`: this branch
* `_foreach`: uses an optimized for_each iterator
* ` _sparse`: uses sparse set storage (if unspecified assume table storage)
* `_system`: runs inside a system (if unspecified assume test happens via direct world ops)
### Simple Insert (from ecs_bench_suite)
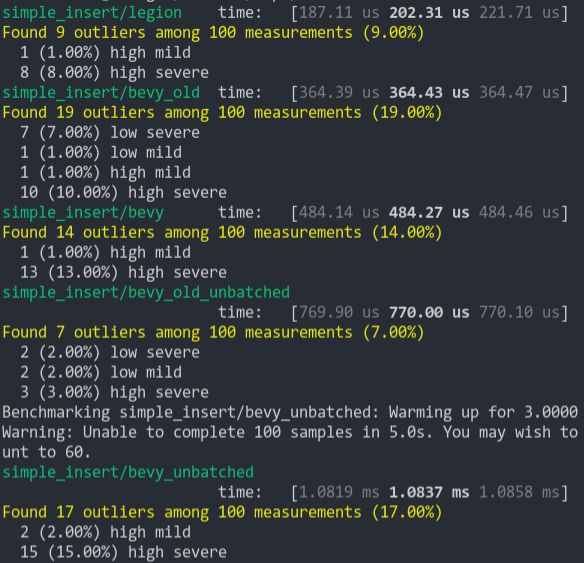
### Simpler Iter (from ecs_bench_suite)
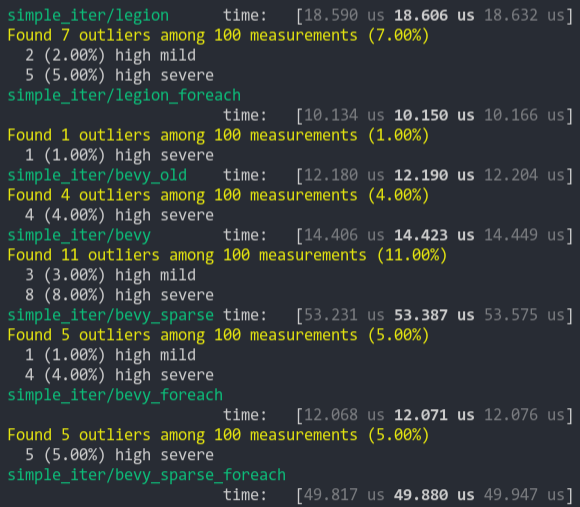
### Fragment Iter (from ecs_bench_suite)
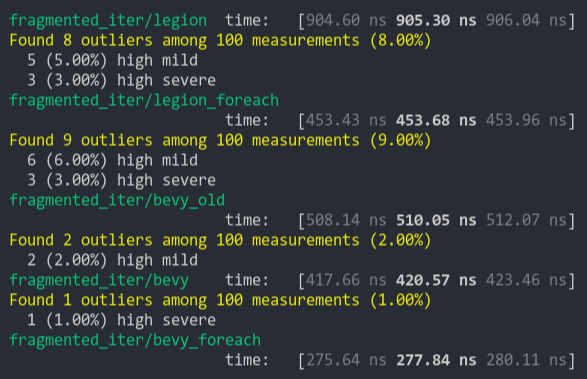
### Sparse Fragmented Iter
Iterate a query that matches 5 entities from a single matching archetype, but there are 100 unmatching archetypes
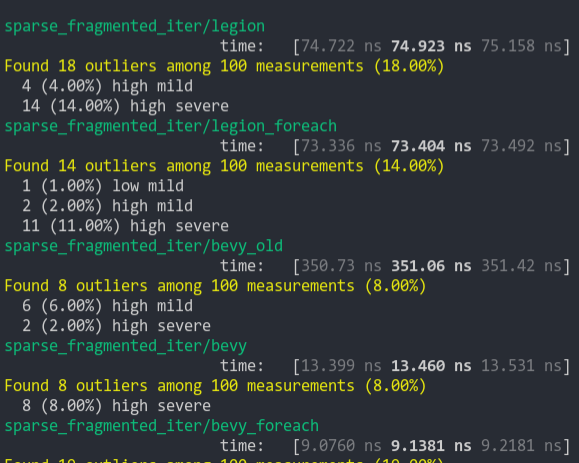
### Schedule (from ecs_bench_suite)
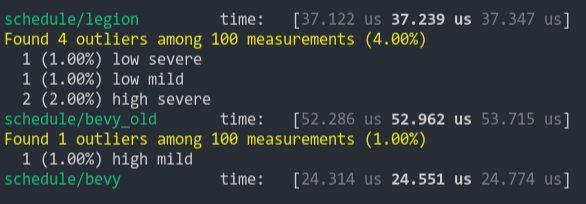
### Add Remove Component (from ecs_bench_suite)
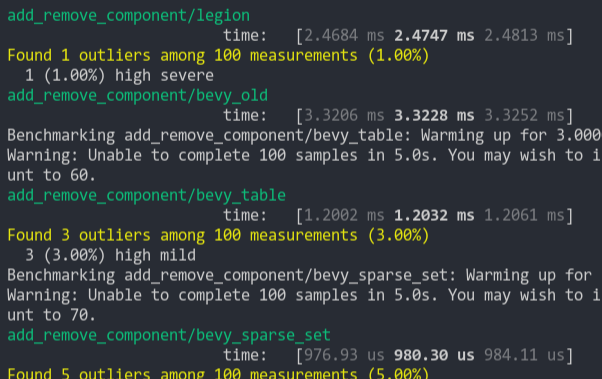
### Add Remove Component Big
Same as the test above, but each entity has 5 "large" matrix components and 1 "large" matrix component is added and removed
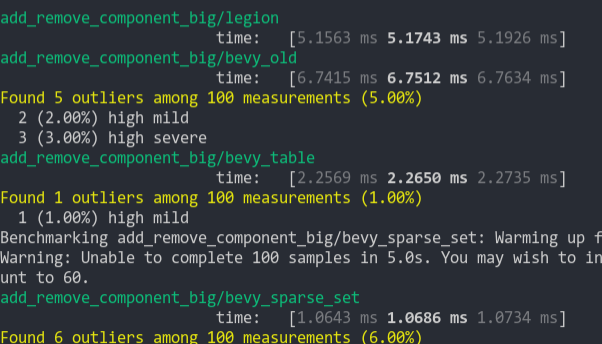
### Get Component
Looks up a single component value a large number of times
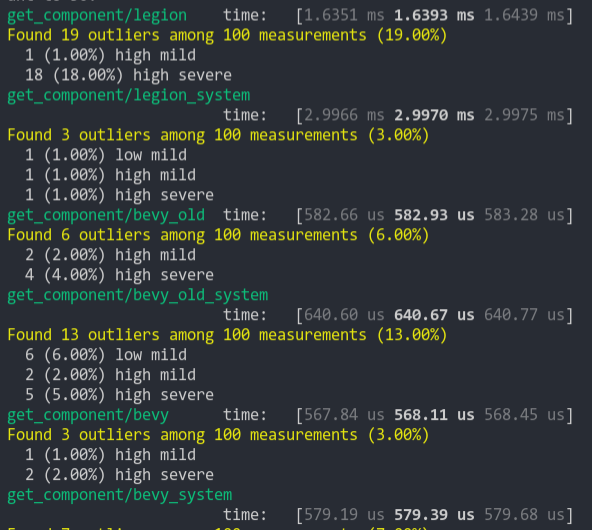
2021-03-05 07:54:35 +00:00
#[ inline ]
2022-12-11 23:04:04 +00:00
#[ allow(unused_variables) ]
Remove the `SystemParamState` trait and remove types like `ResState` (#6919)
Spiritual successor to #5205.
Actual successor to #6865.
# Objective
Currently, system params are defined using three traits: `SystemParam`, `ReadOnlySystemParam`, `SystemParamState`. The behavior for each param is specified by the `SystemParamState` trait, while `SystemParam` simply defers to the state.
Splitting the traits in this way makes it easier to implement within macros, but it increases the cognitive load. Worst of all, this approach requires each `MySystemParam` to have a public `MySystemParamState` type associated with it.
## Solution
* Merge the trait `SystemParamState` into `SystemParam`.
* Remove all trivial `SystemParam` state types.
* `OptionNonSendMutState<T>`: you will not be missed.
---
- [x] Fix/resolve the remaining test failure.
## Changelog
* Removed the trait `SystemParamState`, merging its functionality into `SystemParam`.
## Migration Guide
**Note**: this should replace the migration guide for #6865.
This is relative to Bevy 0.9, not main.
The traits `SystemParamState` and `SystemParamFetch` have been removed, and their functionality has been transferred to `SystemParam`.
```rust
// Before (0.9)
impl SystemParam for MyParam<'_, '_> {
type State = MyParamState;
}
unsafe impl SystemParamState for MyParamState {
fn init(world: &mut World, system_meta: &mut SystemMeta) -> Self { ... }
}
unsafe impl<'w, 's> SystemParamFetch<'w, 's> for MyParamState {
type Item = MyParam<'w, 's>;
fn get_param(&mut self, ...) -> Self::Item;
}
unsafe impl ReadOnlySystemParamFetch for MyParamState { }
// After (0.10)
unsafe impl SystemParam for MyParam<'_, '_> {
type State = MyParamState;
type Item<'w, 's> = MyParam<'w, 's>;
fn init_state(world: &mut World, system_meta: &mut SystemMeta) -> Self::State { ... }
fn get_param<'w, 's>(state: &mut Self::State, ...) -> Self::Item<'w, 's>;
}
unsafe impl ReadOnlySystemParam for MyParam<'_, '_> { }
```
The trait `ReadOnlySystemParamFetch` has been replaced with `ReadOnlySystemParam`.
```rust
// Before
unsafe impl ReadOnlySystemParamFetch for MyParamState {}
// After
unsafe impl ReadOnlySystemParam for MyParam<'_, '_> {}
```
2023-01-07 23:20:32 +00:00
fn apply ( state : & mut Self ::State , system_meta : & SystemMeta , world : & mut World ) { }
Add new SystemState and rename old SystemState to SystemMeta (#2283)
This enables `SystemParams` to be used outside of function systems. Anything can create and store `SystemState`, which enables efficient "param state cached" access to `SystemParams`.
It adds a `ReadOnlySystemParamFetch` trait, which enables safe `SystemState::get` calls without unique world access.
I renamed the old `SystemState` to `SystemMeta` to enable us to mirror the `QueryState` naming convention (but I'm happy to discuss alternative names if people have other ideas). I initially pitched this as `ParamState`, but given that it needs to include full system metadata, that doesn't feel like a particularly accurate name.
```rust
#[derive(Eq, PartialEq, Debug)]
struct A(usize);
#[derive(Eq, PartialEq, Debug)]
struct B(usize);
let mut world = World::default();
world.insert_resource(A(42));
world.spawn().insert(B(7));
// we get nice lifetime elision when declaring the type on the left hand side
let mut system_state: SystemState<(Res<A>, Query<&B>)> = SystemState::new(&mut world);
let (a, query) = system_state.get(&world);
assert_eq!(*a, A(42), "returned resource matches initial value");
assert_eq!(
*query.single().unwrap(),
B(7),
"returned component matches initial value"
);
// mutable system params require unique world access
let mut system_state: SystemState<(ResMut<A>, Query<&mut B>)> = SystemState::new(&mut world);
let (a, query) = system_state.get_mut(&mut world);
// static lifetimes are required when declaring inside of structs
struct SomeContainer {
state: SystemState<(Res<'static, A>, Res<'static, B>)>
}
// this can be shortened using type aliases, which will be useful for complex param tuples
type MyParams<'a> = (Res<'a, A>, Res<'a, B>);
struct SomeContainer {
state: SystemState<MyParams<'static>>
}
// It is the user's responsibility to call SystemState::apply(world) for parameters that queue up work
let mut system_state: SystemState<(Commands, Query<&B>)> = SystemState::new(&mut world);
{
let (mut commands, query) = system_state.get(&world);
commands.insert_resource(3.14);
}
system_state.apply(&mut world);
```
## Future Work
* Actually use SystemState inside FunctionSystem. This would be trivial, but it requires FunctionSystem to wrap SystemState in Option in its current form (which complicates system metadata lookup). I'd prefer to hold off until we adopt something like the later designs linked in #1364, which enable us to contruct Systems using a World reference (and also remove the need for `.system`).
* Consider a "scoped" approach to automatically call SystemState::apply when systems params are no longer being used (either a container type with a Drop impl, or a function that takes a closure for user logic operating on params).
2021-06-02 19:57:38 +00:00
2020-11-08 20:34:05 +00:00
/// # Safety
2021-04-15 20:36:16 +00:00
///
Remove the `SystemParamState` trait and remove types like `ResState` (#6919)
Spiritual successor to #5205.
Actual successor to #6865.
# Objective
Currently, system params are defined using three traits: `SystemParam`, `ReadOnlySystemParam`, `SystemParamState`. The behavior for each param is specified by the `SystemParamState` trait, while `SystemParam` simply defers to the state.
Splitting the traits in this way makes it easier to implement within macros, but it increases the cognitive load. Worst of all, this approach requires each `MySystemParam` to have a public `MySystemParamState` type associated with it.
## Solution
* Merge the trait `SystemParamState` into `SystemParam`.
* Remove all trivial `SystemParam` state types.
* `OptionNonSendMutState<T>`: you will not be missed.
---
- [x] Fix/resolve the remaining test failure.
## Changelog
* Removed the trait `SystemParamState`, merging its functionality into `SystemParam`.
## Migration Guide
**Note**: this should replace the migration guide for #6865.
This is relative to Bevy 0.9, not main.
The traits `SystemParamState` and `SystemParamFetch` have been removed, and their functionality has been transferred to `SystemParam`.
```rust
// Before (0.9)
impl SystemParam for MyParam<'_, '_> {
type State = MyParamState;
}
unsafe impl SystemParamState for MyParamState {
fn init(world: &mut World, system_meta: &mut SystemMeta) -> Self { ... }
}
unsafe impl<'w, 's> SystemParamFetch<'w, 's> for MyParamState {
type Item = MyParam<'w, 's>;
fn get_param(&mut self, ...) -> Self::Item;
}
unsafe impl ReadOnlySystemParamFetch for MyParamState { }
// After (0.10)
unsafe impl SystemParam for MyParam<'_, '_> {
type State = MyParamState;
type Item<'w, 's> = MyParam<'w, 's>;
fn init_state(world: &mut World, system_meta: &mut SystemMeta) -> Self::State { ... }
fn get_param<'w, 's>(state: &mut Self::State, ...) -> Self::Item<'w, 's>;
}
unsafe impl ReadOnlySystemParam for MyParam<'_, '_> { }
```
The trait `ReadOnlySystemParamFetch` has been replaced with `ReadOnlySystemParam`.
```rust
// Before
unsafe impl ReadOnlySystemParamFetch for MyParamState {}
// After
unsafe impl ReadOnlySystemParam for MyParam<'_, '_> {}
```
2023-01-07 23:20:32 +00:00
/// This call might use any of the [`World`] accesses that were registered in [`Self::init_state`].
2023-01-20 13:39:23 +00:00
/// - None of those accesses may conflict with any other [`SystemParam`]s
/// that exist at the same time, including those on other threads.
/// - `world` must be the same `World` that was used to initialize [`state`](SystemParam::init_state).
Simplify trait hierarchy for `SystemParam` (#6865)
# Objective
* Implementing a custom `SystemParam` by hand requires implementing three traits -- four if it is read-only.
* The trait `SystemParamFetch<'w, 's>` is a workaround from before we had generic associated types, and is no longer necessary.
## Solution
* Combine the trait `SystemParamFetch` with `SystemParamState`.
* I decided to remove the `Fetch` name and keep the `State` name, since the former was consistently conflated with the latter.
* Replace the trait `ReadOnlySystemParamFetch` with `ReadOnlySystemParam`, which simplifies trait bounds in generic code.
---
## Changelog
- Removed the trait `SystemParamFetch`, moving its functionality to `SystemParamState`.
- Replaced the trait `ReadOnlySystemParamFetch` with `ReadOnlySystemParam`.
## Migration Guide
The trait `SystemParamFetch` has been removed, and its functionality has been transferred to `SystemParamState`.
```rust
// Before
impl SystemParamState for MyParamState {
fn init(world: &mut World, system_meta: &mut SystemMeta) -> Self { ... }
}
impl<'w, 's> SystemParamFetch<'w, 's> for MyParamState {
type Item = MyParam<'w, 's>;
fn get_param(...) -> Self::Item;
}
// After
impl SystemParamState for MyParamState {
type Item<'w, 's> = MyParam<'w, 's>; // Generic associated types!
fn init(world: &mut World, system_meta: &mut SystemMeta) -> Self { ... }
fn get_param<'w, 's>(...) -> Self::Item<'w, 's>;
}
```
The trait `ReadOnlySystemParamFetch` has been replaced with `ReadOnlySystemParam`.
```rust
// Before
unsafe impl ReadOnlySystemParamFetch for MyParamState {}
// After
unsafe impl<'w, 's> ReadOnlySystemParam for MyParam<'w, 's> {}
```
2022-12-11 18:34:14 +00:00
unsafe fn get_param < ' world , ' state > (
Remove the `SystemParamState` trait and remove types like `ResState` (#6919)
Spiritual successor to #5205.
Actual successor to #6865.
# Objective
Currently, system params are defined using three traits: `SystemParam`, `ReadOnlySystemParam`, `SystemParamState`. The behavior for each param is specified by the `SystemParamState` trait, while `SystemParam` simply defers to the state.
Splitting the traits in this way makes it easier to implement within macros, but it increases the cognitive load. Worst of all, this approach requires each `MySystemParam` to have a public `MySystemParamState` type associated with it.
## Solution
* Merge the trait `SystemParamState` into `SystemParam`.
* Remove all trivial `SystemParam` state types.
* `OptionNonSendMutState<T>`: you will not be missed.
---
- [x] Fix/resolve the remaining test failure.
## Changelog
* Removed the trait `SystemParamState`, merging its functionality into `SystemParam`.
## Migration Guide
**Note**: this should replace the migration guide for #6865.
This is relative to Bevy 0.9, not main.
The traits `SystemParamState` and `SystemParamFetch` have been removed, and their functionality has been transferred to `SystemParam`.
```rust
// Before (0.9)
impl SystemParam for MyParam<'_, '_> {
type State = MyParamState;
}
unsafe impl SystemParamState for MyParamState {
fn init(world: &mut World, system_meta: &mut SystemMeta) -> Self { ... }
}
unsafe impl<'w, 's> SystemParamFetch<'w, 's> for MyParamState {
type Item = MyParam<'w, 's>;
fn get_param(&mut self, ...) -> Self::Item;
}
unsafe impl ReadOnlySystemParamFetch for MyParamState { }
// After (0.10)
unsafe impl SystemParam for MyParam<'_, '_> {
type State = MyParamState;
type Item<'w, 's> = MyParam<'w, 's>;
fn init_state(world: &mut World, system_meta: &mut SystemMeta) -> Self::State { ... }
fn get_param<'w, 's>(state: &mut Self::State, ...) -> Self::Item<'w, 's>;
}
unsafe impl ReadOnlySystemParam for MyParam<'_, '_> { }
```
The trait `ReadOnlySystemParamFetch` has been replaced with `ReadOnlySystemParam`.
```rust
// Before
unsafe impl ReadOnlySystemParamFetch for MyParamState {}
// After
unsafe impl ReadOnlySystemParam for MyParam<'_, '_> {}
```
2023-01-07 23:20:32 +00:00
state : & ' state mut Self ::State ,
Add new SystemState and rename old SystemState to SystemMeta (#2283)
This enables `SystemParams` to be used outside of function systems. Anything can create and store `SystemState`, which enables efficient "param state cached" access to `SystemParams`.
It adds a `ReadOnlySystemParamFetch` trait, which enables safe `SystemState::get` calls without unique world access.
I renamed the old `SystemState` to `SystemMeta` to enable us to mirror the `QueryState` naming convention (but I'm happy to discuss alternative names if people have other ideas). I initially pitched this as `ParamState`, but given that it needs to include full system metadata, that doesn't feel like a particularly accurate name.
```rust
#[derive(Eq, PartialEq, Debug)]
struct A(usize);
#[derive(Eq, PartialEq, Debug)]
struct B(usize);
let mut world = World::default();
world.insert_resource(A(42));
world.spawn().insert(B(7));
// we get nice lifetime elision when declaring the type on the left hand side
let mut system_state: SystemState<(Res<A>, Query<&B>)> = SystemState::new(&mut world);
let (a, query) = system_state.get(&world);
assert_eq!(*a, A(42), "returned resource matches initial value");
assert_eq!(
*query.single().unwrap(),
B(7),
"returned component matches initial value"
);
// mutable system params require unique world access
let mut system_state: SystemState<(ResMut<A>, Query<&mut B>)> = SystemState::new(&mut world);
let (a, query) = system_state.get_mut(&mut world);
// static lifetimes are required when declaring inside of structs
struct SomeContainer {
state: SystemState<(Res<'static, A>, Res<'static, B>)>
}
// this can be shortened using type aliases, which will be useful for complex param tuples
type MyParams<'a> = (Res<'a, A>, Res<'a, B>);
struct SomeContainer {
state: SystemState<MyParams<'static>>
}
// It is the user's responsibility to call SystemState::apply(world) for parameters that queue up work
let mut system_state: SystemState<(Commands, Query<&B>)> = SystemState::new(&mut world);
{
let (mut commands, query) = system_state.get(&world);
commands.insert_resource(3.14);
}
system_state.apply(&mut world);
```
## Future Work
* Actually use SystemState inside FunctionSystem. This would be trivial, but it requires FunctionSystem to wrap SystemState in Option in its current form (which complicates system metadata lookup). I'd prefer to hold off until we adopt something like the later designs linked in #1364, which enable us to contruct Systems using a World reference (and also remove the need for `.system`).
* Consider a "scoped" approach to automatically call SystemState::apply when systems params are no longer being used (either a container type with a Drop impl, or a function that takes a closure for user logic operating on params).
2021-06-02 19:57:38 +00:00
system_meta : & SystemMeta ,
System Param Lifetime Split (#2605)
# Objective
Enable using exact World lifetimes during read-only access . This is motivated by the new renderer's need to allow read-only world-only queries to outlive the query itself (but still be constrained by the world lifetime).
For example:
https://github.com/bevyengine/bevy/blob/115b170d1f11a91146bb6d6e9684dceb8b21f786/pipelined/bevy_pbr2/src/render/mod.rs#L774
## Solution
Split out SystemParam state and world lifetimes and pipe those lifetimes up to read-only Query ops (and add into_inner for Res). According to every safety test I've run so far (except one), this is safe (see the temporary safety test commit). Note that changing the mutable variants to the new lifetimes would allow aliased mutable pointers (try doing that to see how it affects the temporary safety tests).
The new state lifetime on SystemParam does make `#[derive(SystemParam)]` more cumbersome (the current impl requires PhantomData if you don't use both lifetimes). We can make this better by detecting whether or not a lifetime is used in the derive and adjusting accordingly, but that should probably be done in its own pr.
## Why is this a draft?
The new lifetimes break QuerySet safety in one very specific case (see the query_set system in system_safety_test). We need to solve this before we can use the lifetimes given.
This is due to the fact that QuerySet is just a wrapper over Query, which now relies on world lifetimes instead of `&self` lifetimes to prevent aliasing (but in systems, each Query has its own implied lifetime, not a centralized world lifetime). I believe the fix is to rewrite QuerySet to have its own World lifetime (and own the internal reference). This will complicate the impl a bit, but I think it is doable. I'm curious if anyone else has better ideas.
Personally, I think these new lifetimes need to happen. We've gotta have a way to directly tie read-only World queries to the World lifetime. The new renderer is the first place this has come up, but I doubt it will be the last. Worst case scenario we can come up with a second `WorldLifetimeQuery<Q, F = ()>` parameter to enable these read-only scenarios, but I'd rather not add another type to the type zoo.
2021-08-15 20:51:53 +00:00
world : & ' world World ,
Reliable change detection (#1471)
# Problem Definition
The current change tracking (via flags for both components and resources) fails to detect changes made by systems that are scheduled to run earlier in the frame than they are.
This issue is discussed at length in [#68](https://github.com/bevyengine/bevy/issues/68) and [#54](https://github.com/bevyengine/bevy/issues/54).
This is very much a draft PR, and contributions are welcome and needed.
# Criteria
1. Each change is detected at least once, no matter the ordering.
2. Each change is detected at most once, no matter the ordering.
3. Changes should be detected the same frame that they are made.
4. Competitive ergonomics. Ideally does not require opting-in.
5. Low CPU overhead of computation.
6. Memory efficient. This must not increase over time, except where the number of entities / resources does.
7. Changes should not be lost for systems that don't run.
8. A frame needs to act as a pure function. Given the same set of entities / components it needs to produce the same end state without side-effects.
**Exact** change-tracking proposals satisfy criteria 1 and 2.
**Conservative** change-tracking proposals satisfy criteria 1 but not 2.
**Flaky** change tracking proposals satisfy criteria 2 but not 1.
# Code Base Navigation
There are three types of flags:
- `Added`: A piece of data was added to an entity / `Resources`.
- `Mutated`: A piece of data was able to be modified, because its `DerefMut` was accessed
- `Changed`: The bitwise OR of `Added` and `Changed`
The special behavior of `ChangedRes`, with respect to the scheduler is being removed in [#1313](https://github.com/bevyengine/bevy/pull/1313) and does not need to be reproduced.
`ChangedRes` and friends can be found in "bevy_ecs/core/resources/resource_query.rs".
The `Flags` trait for Components can be found in "bevy_ecs/core/query.rs".
`ComponentFlags` are stored in "bevy_ecs/core/archetypes.rs", defined on line 446.
# Proposals
**Proposal 5 was selected for implementation.**
## Proposal 0: No Change Detection
The baseline, where computations are performed on everything regardless of whether it changed.
**Type:** Conservative
**Pros:**
- already implemented
- will never miss events
- no overhead
**Cons:**
- tons of repeated work
- doesn't allow users to avoid repeating work (or monitoring for other changes)
## Proposal 1: Earlier-This-Tick Change Detection
The current approach as of Bevy 0.4. Flags are set, and then flushed at the end of each frame.
**Type:** Flaky
**Pros:**
- already implemented
- simple to understand
- low memory overhead (2 bits per component)
- low time overhead (clear every flag once per frame)
**Cons:**
- misses systems based on ordering
- systems that don't run every frame miss changes
- duplicates detection when looping
- can lead to unresolvable circular dependencies
## Proposal 2: Two-Tick Change Detection
Flags persist for two frames, using a double-buffer system identical to that used in events.
A change is observed if it is found in either the current frame's list of changes or the previous frame's.
**Type:** Conservative
**Pros:**
- easy to understand
- easy to implement
- low memory overhead (4 bits per component)
- low time overhead (bit mask and shift every flag once per frame)
**Cons:**
- can result in a great deal of duplicated work
- systems that don't run every frame miss changes
- duplicates detection when looping
## Proposal 3: Last-Tick Change Detection
Flags persist for two frames, using a double-buffer system identical to that used in events.
A change is observed if it is found in the previous frame's list of changes.
**Type:** Exact
**Pros:**
- exact
- easy to understand
- easy to implement
- low memory overhead (4 bits per component)
- low time overhead (bit mask and shift every flag once per frame)
**Cons:**
- change detection is always delayed, possibly causing painful chained delays
- systems that don't run every frame miss changes
- duplicates detection when looping
## Proposal 4: Flag-Doubling Change Detection
Combine Proposal 2 and Proposal 3. Differentiate between `JustChanged` (current behavior) and `Changed` (Proposal 3).
Pack this data into the flags according to [this implementation proposal](https://github.com/bevyengine/bevy/issues/68#issuecomment-769174804).
**Type:** Flaky + Exact
**Pros:**
- allows users to acc
- easy to implement
- low memory overhead (4 bits per component)
- low time overhead (bit mask and shift every flag once per frame)
**Cons:**
- users must specify the type of change detection required
- still quite fragile to system ordering effects when using the flaky `JustChanged` form
- cannot get immediate + exact results
- systems that don't run every frame miss changes
- duplicates detection when looping
## [SELECTED] Proposal 5: Generation-Counter Change Detection
A global counter is increased after each system is run. Each component saves the time of last mutation, and each system saves the time of last execution. Mutation is detected when the component's counter is greater than the system's counter. Discussed [here](https://github.com/bevyengine/bevy/issues/68#issuecomment-769174804). How to handle addition detection is unsolved; the current proposal is to use the highest bit of the counter as in proposal 1.
**Type:** Exact (for mutations), flaky (for additions)
**Pros:**
- low time overhead (set component counter on access, set system counter after execution)
- robust to systems that don't run every frame
- robust to systems that loop
**Cons:**
- moderately complex implementation
- must be modified as systems are inserted dynamically
- medium memory overhead (4 bytes per component + system)
- unsolved addition detection
## Proposal 6: System-Data Change Detection
For each system, track which system's changes it has seen. This approach is only worth fully designing and implementing if Proposal 5 fails in some way.
**Type:** Exact
**Pros:**
- exact
- conceptually simple
**Cons:**
- requires storing data on each system
- implementation is complex
- must be modified as systems are inserted dynamically
## Proposal 7: Total-Order Change Detection
Discussed [here](https://github.com/bevyengine/bevy/issues/68#issuecomment-754326523). This proposal is somewhat complicated by the new scheduler, but I believe it should still be conceptually feasible. This approach is only worth fully designing and implementing if Proposal 5 fails in some way.
**Type:** Exact
**Pros:**
- exact
- efficient data storage relative to other exact proposals
**Cons:**
- requires access to the scheduler
- complex implementation and difficulty grokking
- must be modified as systems are inserted dynamically
# Tests
- We will need to verify properties 1, 2, 3, 7 and 8. Priority: 1 > 2 = 3 > 8 > 7
- Ideally we can use identical user-facing syntax for all proposals, allowing us to re-use the same syntax for each.
- When writing tests, we need to carefully specify order using explicit dependencies.
- These tests will need to be duplicated for both components and resources.
- We need to be sure to handle cases where ambiguous system orders exist.
`changing_system` is always the system that makes the changes, and `detecting_system` always detects the changes.
The component / resource changed will be simple boolean wrapper structs.
## Basic Added / Mutated / Changed
2 x 3 design:
- Resources vs. Components
- Added vs. Changed vs. Mutated
- `changing_system` runs before `detecting_system`
- verify at the end of tick 2
## At Least Once
2 x 3 design:
- Resources vs. Components
- Added vs. Changed vs. Mutated
- `changing_system` runs after `detecting_system`
- verify at the end of tick 2
## At Most Once
2 x 3 design:
- Resources vs. Components
- Added vs. Changed vs. Mutated
- `changing_system` runs once before `detecting_system`
- increment a counter based on the number of changes detected
- verify at the end of tick 2
## Fast Detection
2 x 3 design:
- Resources vs. Components
- Added vs. Changed vs. Mutated
- `changing_system` runs before `detecting_system`
- verify at the end of tick 1
## Ambiguous System Ordering Robustness
2 x 3 x 2 design:
- Resources vs. Components
- Added vs. Changed vs. Mutated
- `changing_system` runs [before/after] `detecting_system` in tick 1
- `changing_system` runs [after/before] `detecting_system` in tick 2
## System Pausing
2 x 3 design:
- Resources vs. Components
- Added vs. Changed vs. Mutated
- `changing_system` runs in tick 1, then is disabled by run criteria
- `detecting_system` is disabled by run criteria until it is run once during tick 3
- verify at the end of tick 3
## Addition Causes Mutation
2 design:
- Resources vs. Components
- `adding_system_1` adds a component / resource
- `adding system_2` adds the same component / resource
- verify the `Mutated` flag at the end of the tick
- verify the `Added` flag at the end of the tick
First check tests for: https://github.com/bevyengine/bevy/issues/333
Second check tests for: https://github.com/bevyengine/bevy/issues/1443
## Changes Made By Commands
- `adding_system` runs in Update in tick 1, and sends a command to add a component
- `detecting_system` runs in Update in tick 1 and 2, after `adding_system`
- We can't detect the changes in tick 1, since they haven't been processed yet
- If we were to track these changes as being emitted by `adding_system`, we can't detect the changes in tick 2 either, since `detecting_system` has already run once after `adding_system` :(
# Benchmarks
See: [general advice](https://github.com/bevyengine/bevy/blob/master/docs/profiling.md), [Criterion crate](https://github.com/bheisler/criterion.rs)
There are several critical parameters to vary:
1. entity count (1 to 10^9)
2. fraction of entities that are changed (0% to 100%)
3. cost to perform work on changed entities, i.e. workload (1 ns to 1s)
1 and 2 should be varied between benchmark runs. 3 can be added on computationally.
We want to measure:
- memory cost
- run time
We should collect these measurements across several frames (100?) to reduce bootup effects and accurately measure the mean, variance and drift.
Entity-component change detection is much more important to benchmark than resource change detection, due to the orders of magnitude higher number of pieces of data.
No change detection at all should be included in benchmarks as a second control for cases where missing changes is unacceptable.
## Graphs
1. y: performance, x: log_10(entity count), color: proposal, facet: performance metric. Set cost to perform work to 0.
2. y: run time, x: cost to perform work, color: proposal, facet: fraction changed. Set number of entities to 10^6
3. y: memory, x: frames, color: proposal
# Conclusions
1. Is the theoretical categorization of the proposals correct according to our tests?
2. How does the performance of the proposals compare without any load?
3. How does the performance of the proposals compare with realistic loads?
4. At what workload does more exact change tracking become worth the (presumably) higher overhead?
5. When does adding change-detection to save on work become worthwhile?
6. Is there enough divergence in performance between the best solutions in each class to ship more than one change-tracking solution?
# Implementation Plan
1. Write a test suite.
2. Verify that tests fail for existing approach.
3. Write a benchmark suite.
4. Get performance numbers for existing approach.
5. Implement, test and benchmark various solutions using a Git branch per proposal.
6. Create a draft PR with all solutions and present results to team.
7. Select a solution and replace existing change detection.
Co-authored-by: Brice DAVIER <bricedavier@gmail.com>
Co-authored-by: Carter Anderson <mcanders1@gmail.com>
2021-03-19 17:53:26 +00:00
change_tick : u32 ,
Simplify trait hierarchy for `SystemParam` (#6865)
# Objective
* Implementing a custom `SystemParam` by hand requires implementing three traits -- four if it is read-only.
* The trait `SystemParamFetch<'w, 's>` is a workaround from before we had generic associated types, and is no longer necessary.
## Solution
* Combine the trait `SystemParamFetch` with `SystemParamState`.
* I decided to remove the `Fetch` name and keep the `State` name, since the former was consistently conflated with the latter.
* Replace the trait `ReadOnlySystemParamFetch` with `ReadOnlySystemParam`, which simplifies trait bounds in generic code.
---
## Changelog
- Removed the trait `SystemParamFetch`, moving its functionality to `SystemParamState`.
- Replaced the trait `ReadOnlySystemParamFetch` with `ReadOnlySystemParam`.
## Migration Guide
The trait `SystemParamFetch` has been removed, and its functionality has been transferred to `SystemParamState`.
```rust
// Before
impl SystemParamState for MyParamState {
fn init(world: &mut World, system_meta: &mut SystemMeta) -> Self { ... }
}
impl<'w, 's> SystemParamFetch<'w, 's> for MyParamState {
type Item = MyParam<'w, 's>;
fn get_param(...) -> Self::Item;
}
// After
impl SystemParamState for MyParamState {
type Item<'w, 's> = MyParam<'w, 's>; // Generic associated types!
fn init(world: &mut World, system_meta: &mut SystemMeta) -> Self { ... }
fn get_param<'w, 's>(...) -> Self::Item<'w, 's>;
}
```
The trait `ReadOnlySystemParamFetch` has been replaced with `ReadOnlySystemParam`.
```rust
// Before
unsafe impl ReadOnlySystemParamFetch for MyParamState {}
// After
unsafe impl<'w, 's> ReadOnlySystemParam for MyParam<'w, 's> {}
```
2022-12-11 18:34:14 +00:00
) -> Self ::Item < ' world , ' state > ;
2020-12-16 05:57:16 +00:00
}
Simplify trait hierarchy for `SystemParam` (#6865)
# Objective
* Implementing a custom `SystemParam` by hand requires implementing three traits -- four if it is read-only.
* The trait `SystemParamFetch<'w, 's>` is a workaround from before we had generic associated types, and is no longer necessary.
## Solution
* Combine the trait `SystemParamFetch` with `SystemParamState`.
* I decided to remove the `Fetch` name and keep the `State` name, since the former was consistently conflated with the latter.
* Replace the trait `ReadOnlySystemParamFetch` with `ReadOnlySystemParam`, which simplifies trait bounds in generic code.
---
## Changelog
- Removed the trait `SystemParamFetch`, moving its functionality to `SystemParamState`.
- Replaced the trait `ReadOnlySystemParamFetch` with `ReadOnlySystemParam`.
## Migration Guide
The trait `SystemParamFetch` has been removed, and its functionality has been transferred to `SystemParamState`.
```rust
// Before
impl SystemParamState for MyParamState {
fn init(world: &mut World, system_meta: &mut SystemMeta) -> Self { ... }
}
impl<'w, 's> SystemParamFetch<'w, 's> for MyParamState {
type Item = MyParam<'w, 's>;
fn get_param(...) -> Self::Item;
}
// After
impl SystemParamState for MyParamState {
type Item<'w, 's> = MyParam<'w, 's>; // Generic associated types!
fn init(world: &mut World, system_meta: &mut SystemMeta) -> Self { ... }
fn get_param<'w, 's>(...) -> Self::Item<'w, 's>;
}
```
The trait `ReadOnlySystemParamFetch` has been replaced with `ReadOnlySystemParam`.
```rust
// Before
unsafe impl ReadOnlySystemParamFetch for MyParamState {}
// After
unsafe impl<'w, 's> ReadOnlySystemParam for MyParam<'w, 's> {}
```
2022-12-11 18:34:14 +00:00
/// A [`SystemParam`] that only reads a given [`World`].
///
/// # Safety
Remove the `SystemParamState` trait and remove types like `ResState` (#6919)
Spiritual successor to #5205.
Actual successor to #6865.
# Objective
Currently, system params are defined using three traits: `SystemParam`, `ReadOnlySystemParam`, `SystemParamState`. The behavior for each param is specified by the `SystemParamState` trait, while `SystemParam` simply defers to the state.
Splitting the traits in this way makes it easier to implement within macros, but it increases the cognitive load. Worst of all, this approach requires each `MySystemParam` to have a public `MySystemParamState` type associated with it.
## Solution
* Merge the trait `SystemParamState` into `SystemParam`.
* Remove all trivial `SystemParam` state types.
* `OptionNonSendMutState<T>`: you will not be missed.
---
- [x] Fix/resolve the remaining test failure.
## Changelog
* Removed the trait `SystemParamState`, merging its functionality into `SystemParam`.
## Migration Guide
**Note**: this should replace the migration guide for #6865.
This is relative to Bevy 0.9, not main.
The traits `SystemParamState` and `SystemParamFetch` have been removed, and their functionality has been transferred to `SystemParam`.
```rust
// Before (0.9)
impl SystemParam for MyParam<'_, '_> {
type State = MyParamState;
}
unsafe impl SystemParamState for MyParamState {
fn init(world: &mut World, system_meta: &mut SystemMeta) -> Self { ... }
}
unsafe impl<'w, 's> SystemParamFetch<'w, 's> for MyParamState {
type Item = MyParam<'w, 's>;
fn get_param(&mut self, ...) -> Self::Item;
}
unsafe impl ReadOnlySystemParamFetch for MyParamState { }
// After (0.10)
unsafe impl SystemParam for MyParam<'_, '_> {
type State = MyParamState;
type Item<'w, 's> = MyParam<'w, 's>;
fn init_state(world: &mut World, system_meta: &mut SystemMeta) -> Self::State { ... }
fn get_param<'w, 's>(state: &mut Self::State, ...) -> Self::Item<'w, 's>;
}
unsafe impl ReadOnlySystemParam for MyParam<'_, '_> { }
```
The trait `ReadOnlySystemParamFetch` has been replaced with `ReadOnlySystemParam`.
```rust
// Before
unsafe impl ReadOnlySystemParamFetch for MyParamState {}
// After
unsafe impl ReadOnlySystemParam for MyParam<'_, '_> {}
```
2023-01-07 23:20:32 +00:00
/// This must only be implemented for [`SystemParam`] impls that exclusively read the World passed in to [`SystemParam::get_param`]
Simplify trait hierarchy for `SystemParam` (#6865)
# Objective
* Implementing a custom `SystemParam` by hand requires implementing three traits -- four if it is read-only.
* The trait `SystemParamFetch<'w, 's>` is a workaround from before we had generic associated types, and is no longer necessary.
## Solution
* Combine the trait `SystemParamFetch` with `SystemParamState`.
* I decided to remove the `Fetch` name and keep the `State` name, since the former was consistently conflated with the latter.
* Replace the trait `ReadOnlySystemParamFetch` with `ReadOnlySystemParam`, which simplifies trait bounds in generic code.
---
## Changelog
- Removed the trait `SystemParamFetch`, moving its functionality to `SystemParamState`.
- Replaced the trait `ReadOnlySystemParamFetch` with `ReadOnlySystemParam`.
## Migration Guide
The trait `SystemParamFetch` has been removed, and its functionality has been transferred to `SystemParamState`.
```rust
// Before
impl SystemParamState for MyParamState {
fn init(world: &mut World, system_meta: &mut SystemMeta) -> Self { ... }
}
impl<'w, 's> SystemParamFetch<'w, 's> for MyParamState {
type Item = MyParam<'w, 's>;
fn get_param(...) -> Self::Item;
}
// After
impl SystemParamState for MyParamState {
type Item<'w, 's> = MyParam<'w, 's>; // Generic associated types!
fn init(world: &mut World, system_meta: &mut SystemMeta) -> Self { ... }
fn get_param<'w, 's>(...) -> Self::Item<'w, 's>;
}
```
The trait `ReadOnlySystemParamFetch` has been replaced with `ReadOnlySystemParam`.
```rust
// Before
unsafe impl ReadOnlySystemParamFetch for MyParamState {}
// After
unsafe impl<'w, 's> ReadOnlySystemParam for MyParam<'w, 's> {}
```
2022-12-11 18:34:14 +00:00
pub unsafe trait ReadOnlySystemParam : SystemParam { }
Remove the `SystemParamState` trait and remove types like `ResState` (#6919)
Spiritual successor to #5205.
Actual successor to #6865.
# Objective
Currently, system params are defined using three traits: `SystemParam`, `ReadOnlySystemParam`, `SystemParamState`. The behavior for each param is specified by the `SystemParamState` trait, while `SystemParam` simply defers to the state.
Splitting the traits in this way makes it easier to implement within macros, but it increases the cognitive load. Worst of all, this approach requires each `MySystemParam` to have a public `MySystemParamState` type associated with it.
## Solution
* Merge the trait `SystemParamState` into `SystemParam`.
* Remove all trivial `SystemParam` state types.
* `OptionNonSendMutState<T>`: you will not be missed.
---
- [x] Fix/resolve the remaining test failure.
## Changelog
* Removed the trait `SystemParamState`, merging its functionality into `SystemParam`.
## Migration Guide
**Note**: this should replace the migration guide for #6865.
This is relative to Bevy 0.9, not main.
The traits `SystemParamState` and `SystemParamFetch` have been removed, and their functionality has been transferred to `SystemParam`.
```rust
// Before (0.9)
impl SystemParam for MyParam<'_, '_> {
type State = MyParamState;
}
unsafe impl SystemParamState for MyParamState {
fn init(world: &mut World, system_meta: &mut SystemMeta) -> Self { ... }
}
unsafe impl<'w, 's> SystemParamFetch<'w, 's> for MyParamState {
type Item = MyParam<'w, 's>;
fn get_param(&mut self, ...) -> Self::Item;
}
unsafe impl ReadOnlySystemParamFetch for MyParamState { }
// After (0.10)
unsafe impl SystemParam for MyParam<'_, '_> {
type State = MyParamState;
type Item<'w, 's> = MyParam<'w, 's>;
fn init_state(world: &mut World, system_meta: &mut SystemMeta) -> Self::State { ... }
fn get_param<'w, 's>(state: &mut Self::State, ...) -> Self::Item<'w, 's>;
}
unsafe impl ReadOnlySystemParam for MyParam<'_, '_> { }
```
The trait `ReadOnlySystemParamFetch` has been replaced with `ReadOnlySystemParam`.
```rust
// Before
unsafe impl ReadOnlySystemParamFetch for MyParamState {}
// After
unsafe impl ReadOnlySystemParam for MyParam<'_, '_> {}
```
2023-01-07 23:20:32 +00:00
/// Shorthand way of accessing the associated type [`SystemParam::Item`] for a given [`SystemParam`].
pub type SystemParamItem < ' w , ' s , P > = < P as SystemParam > ::Item < ' w , ' s > ;
2020-11-08 20:34:05 +00:00
2022-07-04 14:44:24 +00:00
// SAFETY: QueryState is constrained to read-only fetches, so it only reads World.
Simplify trait hierarchy for `SystemParam` (#6865)
# Objective
* Implementing a custom `SystemParam` by hand requires implementing three traits -- four if it is read-only.
* The trait `SystemParamFetch<'w, 's>` is a workaround from before we had generic associated types, and is no longer necessary.
## Solution
* Combine the trait `SystemParamFetch` with `SystemParamState`.
* I decided to remove the `Fetch` name and keep the `State` name, since the former was consistently conflated with the latter.
* Replace the trait `ReadOnlySystemParamFetch` with `ReadOnlySystemParam`, which simplifies trait bounds in generic code.
---
## Changelog
- Removed the trait `SystemParamFetch`, moving its functionality to `SystemParamState`.
- Replaced the trait `ReadOnlySystemParamFetch` with `ReadOnlySystemParam`.
## Migration Guide
The trait `SystemParamFetch` has been removed, and its functionality has been transferred to `SystemParamState`.
```rust
// Before
impl SystemParamState for MyParamState {
fn init(world: &mut World, system_meta: &mut SystemMeta) -> Self { ... }
}
impl<'w, 's> SystemParamFetch<'w, 's> for MyParamState {
type Item = MyParam<'w, 's>;
fn get_param(...) -> Self::Item;
}
// After
impl SystemParamState for MyParamState {
type Item<'w, 's> = MyParam<'w, 's>; // Generic associated types!
fn init(world: &mut World, system_meta: &mut SystemMeta) -> Self { ... }
fn get_param<'w, 's>(...) -> Self::Item<'w, 's>;
}
```
The trait `ReadOnlySystemParamFetch` has been replaced with `ReadOnlySystemParam`.
```rust
// Before
unsafe impl ReadOnlySystemParamFetch for MyParamState {}
// After
unsafe impl<'w, 's> ReadOnlySystemParam for MyParam<'w, 's> {}
```
2022-12-11 18:34:14 +00:00
unsafe impl < ' w , ' s , Q : ReadOnlyWorldQuery + 'static , F : ReadOnlyWorldQuery + 'static >
ReadOnlySystemParam for Query < ' w , ' s , Q , F >
2022-09-18 23:52:01 +00:00
{
}
Add new SystemState and rename old SystemState to SystemMeta (#2283)
This enables `SystemParams` to be used outside of function systems. Anything can create and store `SystemState`, which enables efficient "param state cached" access to `SystemParams`.
It adds a `ReadOnlySystemParamFetch` trait, which enables safe `SystemState::get` calls without unique world access.
I renamed the old `SystemState` to `SystemMeta` to enable us to mirror the `QueryState` naming convention (but I'm happy to discuss alternative names if people have other ideas). I initially pitched this as `ParamState`, but given that it needs to include full system metadata, that doesn't feel like a particularly accurate name.
```rust
#[derive(Eq, PartialEq, Debug)]
struct A(usize);
#[derive(Eq, PartialEq, Debug)]
struct B(usize);
let mut world = World::default();
world.insert_resource(A(42));
world.spawn().insert(B(7));
// we get nice lifetime elision when declaring the type on the left hand side
let mut system_state: SystemState<(Res<A>, Query<&B>)> = SystemState::new(&mut world);
let (a, query) = system_state.get(&world);
assert_eq!(*a, A(42), "returned resource matches initial value");
assert_eq!(
*query.single().unwrap(),
B(7),
"returned component matches initial value"
);
// mutable system params require unique world access
let mut system_state: SystemState<(ResMut<A>, Query<&mut B>)> = SystemState::new(&mut world);
let (a, query) = system_state.get_mut(&mut world);
// static lifetimes are required when declaring inside of structs
struct SomeContainer {
state: SystemState<(Res<'static, A>, Res<'static, B>)>
}
// this can be shortened using type aliases, which will be useful for complex param tuples
type MyParams<'a> = (Res<'a, A>, Res<'a, B>);
struct SomeContainer {
state: SystemState<MyParams<'static>>
}
// It is the user's responsibility to call SystemState::apply(world) for parameters that queue up work
let mut system_state: SystemState<(Commands, Query<&B>)> = SystemState::new(&mut world);
{
let (mut commands, query) = system_state.get(&world);
commands.insert_resource(3.14);
}
system_state.apply(&mut world);
```
## Future Work
* Actually use SystemState inside FunctionSystem. This would be trivial, but it requires FunctionSystem to wrap SystemState in Option in its current form (which complicates system metadata lookup). I'd prefer to hold off until we adopt something like the later designs linked in #1364, which enable us to contruct Systems using a World reference (and also remove the need for `.system`).
* Consider a "scoped" approach to automatically call SystemState::apply when systems params are no longer being used (either a container type with a Drop impl, or a function that takes a closure for user logic operating on params).
2021-06-02 19:57:38 +00:00
2022-07-04 14:44:24 +00:00
// SAFETY: Relevant query ComponentId and ArchetypeComponentId access is applied to SystemMeta. If
Remove the `SystemParamState` trait and remove types like `ResState` (#6919)
Spiritual successor to #5205.
Actual successor to #6865.
# Objective
Currently, system params are defined using three traits: `SystemParam`, `ReadOnlySystemParam`, `SystemParamState`. The behavior for each param is specified by the `SystemParamState` trait, while `SystemParam` simply defers to the state.
Splitting the traits in this way makes it easier to implement within macros, but it increases the cognitive load. Worst of all, this approach requires each `MySystemParam` to have a public `MySystemParamState` type associated with it.
## Solution
* Merge the trait `SystemParamState` into `SystemParam`.
* Remove all trivial `SystemParam` state types.
* `OptionNonSendMutState<T>`: you will not be missed.
---
- [x] Fix/resolve the remaining test failure.
## Changelog
* Removed the trait `SystemParamState`, merging its functionality into `SystemParam`.
## Migration Guide
**Note**: this should replace the migration guide for #6865.
This is relative to Bevy 0.9, not main.
The traits `SystemParamState` and `SystemParamFetch` have been removed, and their functionality has been transferred to `SystemParam`.
```rust
// Before (0.9)
impl SystemParam for MyParam<'_, '_> {
type State = MyParamState;
}
unsafe impl SystemParamState for MyParamState {
fn init(world: &mut World, system_meta: &mut SystemMeta) -> Self { ... }
}
unsafe impl<'w, 's> SystemParamFetch<'w, 's> for MyParamState {
type Item = MyParam<'w, 's>;
fn get_param(&mut self, ...) -> Self::Item;
}
unsafe impl ReadOnlySystemParamFetch for MyParamState { }
// After (0.10)
unsafe impl SystemParam for MyParam<'_, '_> {
type State = MyParamState;
type Item<'w, 's> = MyParam<'w, 's>;
fn init_state(world: &mut World, system_meta: &mut SystemMeta) -> Self::State { ... }
fn get_param<'w, 's>(state: &mut Self::State, ...) -> Self::Item<'w, 's>;
}
unsafe impl ReadOnlySystemParam for MyParam<'_, '_> { }
```
The trait `ReadOnlySystemParamFetch` has been replaced with `ReadOnlySystemParam`.
```rust
// Before
unsafe impl ReadOnlySystemParamFetch for MyParamState {}
// After
unsafe impl ReadOnlySystemParam for MyParam<'_, '_> {}
```
2023-01-07 23:20:32 +00:00
// this Query conflicts with any prior access, a panic will occur.
unsafe impl < Q : WorldQuery + 'static , F : ReadOnlyWorldQuery + 'static > SystemParam
for Query < '_ , '_ , Q , F >
Bevy ECS V2 (#1525)
# Bevy ECS V2
This is a rewrite of Bevy ECS (basically everything but the new executor/schedule, which are already awesome). The overall goal was to improve the performance and versatility of Bevy ECS. Here is a quick bulleted list of changes before we dive into the details:
* Complete World rewrite
* Multiple component storage types:
* Tables: fast cache friendly iteration, slower add/removes (previously called Archetypes)
* Sparse Sets: fast add/remove, slower iteration
* Stateful Queries (caches query results for faster iteration. fragmented iteration is _fast_ now)
* Stateful System Params (caches expensive operations. inspired by @DJMcNab's work in #1364)
* Configurable System Params (users can set configuration when they construct their systems. once again inspired by @DJMcNab's work)
* Archetypes are now "just metadata", component storage is separate
* Archetype Graph (for faster archetype changes)
* Component Metadata
* Configure component storage type
* Retrieve information about component size/type/name/layout/send-ness/etc
* Components are uniquely identified by a densely packed ComponentId
* TypeIds are now totally optional (which should make implementing scripting easier)
* Super fast "for_each" query iterators
* Merged Resources into World. Resources are now just a special type of component
* EntityRef/EntityMut builder apis (more efficient and more ergonomic)
* Fast bitset-backed `Access<T>` replaces old hashmap-based approach everywhere
* Query conflicts are determined by component access instead of archetype component access (to avoid random failures at runtime)
* With/Without are still taken into account for conflicts, so this should still be comfy to use
* Much simpler `IntoSystem` impl
* Significantly reduced the amount of hashing throughout the ecs in favor of Sparse Sets (indexed by densely packed ArchetypeId, ComponentId, BundleId, and TableId)
* Safety Improvements
* Entity reservation uses a normal world reference instead of unsafe transmute
* QuerySets no longer transmute lifetimes
* Made traits "unsafe" where relevant
* More thorough safety docs
* WorldCell
* Exposes safe mutable access to multiple resources at a time in a World
* Replaced "catch all" `System::update_archetypes(world: &World)` with `System::new_archetype(archetype: &Archetype)`
* Simpler Bundle implementation
* Replaced slow "remove_bundle_one_by_one" used as fallback for Commands::remove_bundle with fast "remove_bundle_intersection"
* Removed `Mut<T>` query impl. it is better to only support one way: `&mut T`
* Removed with() from `Flags<T>` in favor of `Option<Flags<T>>`, which allows querying for flags to be "filtered" by default
* Components now have is_send property (currently only resources support non-send)
* More granular module organization
* New `RemovedComponents<T>` SystemParam that replaces `query.removed::<T>()`
* `world.resource_scope()` for mutable access to resources and world at the same time
* WorldQuery and QueryFilter traits unified. FilterFetch trait added to enable "short circuit" filtering. Auto impled for cases that don't need it
* Significantly slimmed down SystemState in favor of individual SystemParam state
* System Commands changed from `commands: &mut Commands` back to `mut commands: Commands` (to allow Commands to have a World reference)
Fixes #1320
## `World` Rewrite
This is a from-scratch rewrite of `World` that fills the niche that `hecs` used to. Yes, this means Bevy ECS is no longer a "fork" of hecs. We're going out our own!
(the only shared code between the projects is the entity id allocator, which is already basically ideal)
A huge shout out to @SanderMertens (author of [flecs](https://github.com/SanderMertens/flecs)) for sharing some great ideas with me (specifically hybrid ecs storage and archetype graphs). He also helped advise on a number of implementation details.
## Component Storage (The Problem)
Two ECS storage paradigms have gained a lot of traction over the years:
* **Archetypal ECS**:
* Stores components in "tables" with static schemas. Each "column" stores components of a given type. Each "row" is an entity.
* Each "archetype" has its own table. Adding/removing an entity's component changes the archetype.
* Enables super-fast Query iteration due to its cache-friendly data layout
* Comes at the cost of more expensive add/remove operations for an Entity's components, because all components need to be copied to the new archetype's "table"
* **Sparse Set ECS**:
* Stores components of the same type in densely packed arrays, which are sparsely indexed by densely packed unsigned integers (Entity ids)
* Query iteration is slower than Archetypal ECS because each entity's component could be at any position in the sparse set. This "random access" pattern isn't cache friendly. Additionally, there is an extra layer of indirection because you must first map the entity id to an index in the component array.
* Adding/removing components is a cheap, constant time operation
Bevy ECS V1, hecs, legion, flec, and Unity DOTS are all "archetypal ecs-es". I personally think "archetypal" storage is a good default for game engines. An entity's archetype doesn't need to change frequently in general, and it creates "fast by default" query iteration (which is a much more common operation). It is also "self optimizing". Users don't need to think about optimizing component layouts for iteration performance. It "just works" without any extra boilerplate.
Shipyard and EnTT are "sparse set ecs-es". They employ "packing" as a way to work around the "suboptimal by default" iteration performance for specific sets of components. This helps, but I didn't think this was a good choice for a general purpose engine like Bevy because:
1. "packs" conflict with each other. If bevy decides to internally pack the Transform and GlobalTransform components, users are then blocked if they want to pack some custom component with Transform.
2. users need to take manual action to optimize
Developers selecting an ECS framework are stuck with a hard choice. Select an "archetypal" framework with "fast iteration everywhere" but without the ability to cheaply add/remove components, or select a "sparse set" framework to cheaply add/remove components but with slower iteration performance.
## Hybrid Component Storage (The Solution)
In Bevy ECS V2, we get to have our cake and eat it too. It now has _both_ of the component storage types above (and more can be added later if needed):
* **Tables** (aka "archetypal" storage)
* The default storage. If you don't configure anything, this is what you get
* Fast iteration by default
* Slower add/remove operations
* **Sparse Sets**
* Opt-in
* Slower iteration
* Faster add/remove operations
These storage types complement each other perfectly. By default Query iteration is fast. If developers know that they want to add/remove a component at high frequencies, they can set the storage to "sparse set":
```rust
world.register_component(
ComponentDescriptor::new::<MyComponent>(StorageType::SparseSet)
).unwrap();
```
## Archetypes
Archetypes are now "just metadata" ... they no longer store components directly. They do store:
* The `ComponentId`s of each of the Archetype's components (and that component's storage type)
* Archetypes are uniquely defined by their component layouts
* For example: entities with "table" components `[A, B, C]` _and_ "sparse set" components `[D, E]` will always be in the same archetype.
* The `TableId` associated with the archetype
* For now each archetype has exactly one table (which can have no components),
* There is a 1->Many relationship from Tables->Archetypes. A given table could have any number of archetype components stored in it:
* Ex: an entity with "table storage" components `[A, B, C]` and "sparse set" components `[D, E]` will share the same `[A, B, C]` table as an entity with `[A, B, C]` table component and `[F]` sparse set components.
* This 1->Many relationship is how we preserve fast "cache friendly" iteration performance when possible (more on this later)
* A list of entities that are in the archetype and the row id of the table they are in
* ArchetypeComponentIds
* unique densely packed identifiers for (ArchetypeId, ComponentId) pairs
* used by the schedule executor for cheap system access control
* "Archetype Graph Edges" (see the next section)
## The "Archetype Graph"
Archetype changes in Bevy (and a number of other archetypal ecs-es) have historically been expensive to compute. First, you need to allocate a new vector of the entity's current component ids, add or remove components based on the operation performed, sort it (to ensure it is order-independent), then hash it to find the archetype (if it exists). And thats all before we get to the _already_ expensive full copy of all components to the new table storage.
The solution is to build a "graph" of archetypes to cache these results. @SanderMertens first exposed me to the idea (and he got it from @gjroelofs, who came up with it). They propose adding directed edges between archetypes for add/remove component operations. If `ComponentId`s are densely packed, you can use sparse sets to cheaply jump between archetypes.
Bevy takes this one step further by using add/remove `Bundle` edges instead of `Component` edges. Bevy encourages the use of `Bundles` to group add/remove operations. This is largely for "clearer game logic" reasons, but it also helps cut down on the number of archetype changes required. `Bundles` now also have densely-packed `BundleId`s. This allows us to use a _single_ edge for each bundle operation (rather than needing to traverse N edges ... one for each component). Single component operations are also bundles, so this is strictly an improvement over a "component only" graph.
As a result, an operation that used to be _heavy_ (both for allocations and compute) is now two dirt-cheap array lookups and zero allocations.
## Stateful Queries
World queries are now stateful. This allows us to:
1. Cache archetype (and table) matches
* This resolves another issue with (naive) archetypal ECS: query performance getting worse as the number of archetypes goes up (and fragmentation occurs).
2. Cache Fetch and Filter state
* The expensive parts of fetch/filter operations (such as hashing the TypeId to find the ComponentId) now only happen once when the Query is first constructed
3. Incrementally build up state
* When new archetypes are added, we only process the new archetypes (no need to rebuild state for old archetypes)
As a result, the direct `World` query api now looks like this:
```rust
let mut query = world.query::<(&A, &mut B)>();
for (a, mut b) in query.iter_mut(&mut world) {
}
```
Requiring `World` to generate stateful queries (rather than letting the `QueryState` type be constructed separately) allows us to ensure that _all_ queries are properly initialized (and the relevant world state, such as ComponentIds). This enables QueryState to remove branches from its operations that check for initialization status (and also enables query.iter() to take an immutable world reference because it doesn't need to initialize anything in world).
However in systems, this is a non-breaking change. State management is done internally by the relevant SystemParam.
## Stateful SystemParams
Like Queries, `SystemParams` now also cache state. For example, `Query` system params store the "stateful query" state mentioned above. Commands store their internal `CommandQueue`. This means you can now safely use as many separate `Commands` parameters in your system as you want. `Local<T>` system params store their `T` value in their state (instead of in Resources).
SystemParam state also enabled a significant slim-down of SystemState. It is much nicer to look at now.
Per-SystemParam state naturally insulates us from an "aliased mut" class of errors we have hit in the past (ex: using multiple `Commands` system params).
(credit goes to @DJMcNab for the initial idea and draft pr here #1364)
## Configurable SystemParams
@DJMcNab also had the great idea to make SystemParams configurable. This allows users to provide some initial configuration / values for system parameters (when possible). Most SystemParams have no config (the config type is `()`), but the `Local<T>` param now supports user-provided parameters:
```rust
fn foo(value: Local<usize>) {
}
app.add_system(foo.system().config(|c| c.0 = Some(10)));
```
## Uber Fast "for_each" Query Iterators
Developers now have the choice to use a fast "for_each" iterator, which yields ~1.5-3x iteration speed improvements for "fragmented iteration", and minor ~1.2x iteration speed improvements for unfragmented iteration.
```rust
fn system(query: Query<(&A, &mut B)>) {
// you now have the option to do this for a speed boost
query.for_each_mut(|(a, mut b)| {
});
// however normal iterators are still available
for (a, mut b) in query.iter_mut() {
}
}
```
I think in most cases we should continue to encourage "normal" iterators as they are more flexible and more "rust idiomatic". But when that extra "oomf" is needed, it makes sense to use `for_each`.
We should also consider using `for_each` for internal bevy systems to give our users a nice speed boost (but that should be a separate pr).
## Component Metadata
`World` now has a `Components` collection, which is accessible via `world.components()`. This stores mappings from `ComponentId` to `ComponentInfo`, as well as `TypeId` to `ComponentId` mappings (where relevant). `ComponentInfo` stores information about the component, such as ComponentId, TypeId, memory layout, send-ness (currently limited to resources), and storage type.
## Significantly Cheaper `Access<T>`
We used to use `TypeAccess<TypeId>` to manage read/write component/archetype-component access. This was expensive because TypeIds must be hashed and compared individually. The parallel executor got around this by "condensing" type ids into bitset-backed access types. This worked, but it had to be re-generated from the `TypeAccess<TypeId>`sources every time archetypes changed.
This pr removes TypeAccess in favor of faster bitset access everywhere. We can do this thanks to the move to densely packed `ComponentId`s and `ArchetypeComponentId`s.
## Merged Resources into World
Resources had a lot of redundant functionality with Components. They stored typed data, they had access control, they had unique ids, they were queryable via SystemParams, etc. In fact the _only_ major difference between them was that they were unique (and didn't correlate to an entity).
Separate resources also had the downside of requiring a separate set of access controls, which meant the parallel executor needed to compare more bitsets per system and manage more state.
I initially got the "separate resources" idea from `legion`. I think that design was motivated by the fact that it made the direct world query/resource lifetime interactions more manageable. It certainly made our lives easier when using Resources alongside hecs/bevy_ecs. However we already have a construct for safely and ergonomically managing in-world lifetimes: systems (which use `Access<T>` internally).
This pr merges Resources into World:
```rust
world.insert_resource(1);
world.insert_resource(2.0);
let a = world.get_resource::<i32>().unwrap();
let mut b = world.get_resource_mut::<f64>().unwrap();
*b = 3.0;
```
Resources are now just a special kind of component. They have their own ComponentIds (and their own resource TypeId->ComponentId scope, so they don't conflict wit components of the same type). They are stored in a special "resource archetype", which stores components inside the archetype using a new `unique_components` sparse set (note that this sparse set could later be used to implement Tags). This allows us to keep the code size small by reusing existing datastructures (namely Column, Archetype, ComponentFlags, and ComponentInfo). This allows us the executor to use a single `Access<ArchetypeComponentId>` per system. It should also make scripting language integration easier.
_But_ this merge did create problems for people directly interacting with `World`. What if you need mutable access to multiple resources at the same time? `world.get_resource_mut()` borrows World mutably!
## WorldCell
WorldCell applies the `Access<ArchetypeComponentId>` concept to direct world access:
```rust
let world_cell = world.cell();
let a = world_cell.get_resource_mut::<i32>().unwrap();
let b = world_cell.get_resource_mut::<f64>().unwrap();
```
This adds cheap runtime checks (a sparse set lookup of `ArchetypeComponentId` and a counter) to ensure that world accesses do not conflict with each other. Each operation returns a `WorldBorrow<'w, T>` or `WorldBorrowMut<'w, T>` wrapper type, which will release the relevant ArchetypeComponentId resources when dropped.
World caches the access sparse set (and only one cell can exist at a time), so `world.cell()` is a cheap operation.
WorldCell does _not_ use atomic operations. It is non-send, does a mutable borrow of world to prevent other accesses, and uses a simple `Rc<RefCell<ArchetypeComponentAccess>>` wrapper in each WorldBorrow pointer.
The api is currently limited to resource access, but it can and should be extended to queries / entity component access.
## Resource Scopes
WorldCell does not yet support component queries, and even when it does there are sometimes legitimate reasons to want a mutable world ref _and_ a mutable resource ref (ex: bevy_render and bevy_scene both need this). In these cases we could always drop down to the unsafe `world.get_resource_unchecked_mut()`, but that is not ideal!
Instead developers can use a "resource scope"
```rust
world.resource_scope(|world: &mut World, a: &mut A| {
})
```
This temporarily removes the `A` resource from `World`, provides mutable pointers to both, and re-adds A to World when finished. Thanks to the move to ComponentIds/sparse sets, this is a cheap operation.
If multiple resources are required, scopes can be nested. We could also consider adding a "resource tuple" to the api if this pattern becomes common and the boilerplate gets nasty.
## Query Conflicts Use ComponentId Instead of ArchetypeComponentId
For safety reasons, systems cannot contain queries that conflict with each other without wrapping them in a QuerySet. On bevy `main`, we use ArchetypeComponentIds to determine conflicts. This is nice because it can take into account filters:
```rust
// these queries will never conflict due to their filters
fn filter_system(a: Query<&mut A, With<B>>, b: Query<&mut B, Without<B>>) {
}
```
But it also has a significant downside:
```rust
// these queries will not conflict _until_ an entity with A, B, and C is spawned
fn maybe_conflicts_system(a: Query<(&mut A, &C)>, b: Query<(&mut A, &B)>) {
}
```
The system above will panic at runtime if an entity with A, B, and C is spawned. This makes it hard to trust that your game logic will run without crashing.
In this pr, I switched to using `ComponentId` instead. This _is_ more constraining. `maybe_conflicts_system` will now always fail, but it will do it consistently at startup. Naively, it would also _disallow_ `filter_system`, which would be a significant downgrade in usability. Bevy has a number of internal systems that rely on disjoint queries and I expect it to be a common pattern in userspace.
To resolve this, I added a new `FilteredAccess<T>` type, which wraps `Access<T>` and adds with/without filters. If two `FilteredAccess` have with/without values that prove they are disjoint, they will no longer conflict.
## EntityRef / EntityMut
World entity operations on `main` require that the user passes in an `entity` id to each operation:
```rust
let entity = world.spawn((A, )); // create a new entity with A
world.get::<A>(entity);
world.insert(entity, (B, C));
world.insert_one(entity, D);
```
This means that each operation needs to look up the entity location / verify its validity. The initial spawn operation also requires a Bundle as input. This can be awkward when no components are required (or one component is required).
These operations have been replaced by `EntityRef` and `EntityMut`, which are "builder-style" wrappers around world that provide read and read/write operations on a single, pre-validated entity:
```rust
// spawn now takes no inputs and returns an EntityMut
let entity = world.spawn()
.insert(A) // insert a single component into the entity
.insert_bundle((B, C)) // insert a bundle of components into the entity
.id() // id returns the Entity id
// Returns EntityMut (or panics if the entity does not exist)
world.entity_mut(entity)
.insert(D)
.insert_bundle(SomeBundle::default());
{
// returns EntityRef (or panics if the entity does not exist)
let d = world.entity(entity)
.get::<D>() // gets the D component
.unwrap();
// world.get still exists for ergonomics
let d = world.get::<D>(entity).unwrap();
}
// These variants return Options if you want to check existence instead of panicing
world.get_entity_mut(entity)
.unwrap()
.insert(E);
if let Some(entity_ref) = world.get_entity(entity) {
let d = entity_ref.get::<D>().unwrap();
}
```
This _does not_ affect the current Commands api or terminology. I think that should be a separate conversation as that is a much larger breaking change.
## Safety Improvements
* Entity reservation in Commands uses a normal world borrow instead of an unsafe transmute
* QuerySets no longer transmutes lifetimes
* Made traits "unsafe" when implementing a trait incorrectly could cause unsafety
* More thorough safety docs
## RemovedComponents SystemParam
The old approach to querying removed components: `query.removed:<T>()` was confusing because it had no connection to the query itself. I replaced it with the following, which is both clearer and allows us to cache the ComponentId mapping in the SystemParamState:
```rust
fn system(removed: RemovedComponents<T>) {
for entity in removed.iter() {
}
}
```
## Simpler Bundle implementation
Bundles are no longer responsible for sorting (or deduping) TypeInfo. They are just a simple ordered list of component types / data. This makes the implementation smaller and opens the door to an easy "nested bundle" implementation in the future (which i might even add in this pr). Duplicate detection is now done once per bundle type by World the first time a bundle is used.
## Unified WorldQuery and QueryFilter types
(don't worry they are still separate type _parameters_ in Queries .. this is a non-breaking change)
WorldQuery and QueryFilter were already basically identical apis. With the addition of `FetchState` and more storage-specific fetch methods, the overlap was even clearer (and the redundancy more painful).
QueryFilters are now just `F: WorldQuery where F::Fetch: FilterFetch`. FilterFetch requires `Fetch<Item = bool>` and adds new "short circuit" variants of fetch methods. This enables a filter tuple like `(With<A>, Without<B>, Changed<C>)` to stop evaluating the filter after the first mismatch is encountered. FilterFetch is automatically implemented for `Fetch` implementations that return bool.
This forces fetch implementations that return things like `(bool, bool, bool)` (such as the filter above) to manually implement FilterFetch and decide whether or not to short-circuit.
## More Granular Modules
World no longer globs all of the internal modules together. It now exports `core`, `system`, and `schedule` separately. I'm also considering exporting `core` submodules directly as that is still pretty "glob-ey" and unorganized (feedback welcome here).
## Remaining Draft Work (to be done in this pr)
* ~~panic on conflicting WorldQuery fetches (&A, &mut A)~~
* ~~bevy `main` and hecs both currently allow this, but we should protect against it if possible~~
* ~~batch_iter / par_iter (currently stubbed out)~~
* ~~ChangedRes~~
* ~~I skipped this while we sort out #1313. This pr should be adapted to account for whatever we land on there~~.
* ~~The `Archetypes` and `Tables` collections use hashes of sorted lists of component ids to uniquely identify each archetype/table. This hash is then used as the key in a HashMap to look up the relevant ArchetypeId or TableId. (which doesn't handle hash collisions properly)~~
* ~~It is currently unsafe to generate a Query from "World A", then use it on "World B" (despite the api claiming it is safe). We should probably close this gap. This could be done by adding a randomly generated WorldId to each world, then storing that id in each Query. They could then be compared to each other on each `query.do_thing(&world)` operation. This _does_ add an extra branch to each query operation, so I'm open to other suggestions if people have them.~~
* ~~Nested Bundles (if i find time)~~
## Potential Future Work
* Expand WorldCell to support queries.
* Consider not allocating in the empty archetype on `world.spawn()`
* ex: return something like EntityMutUninit, which turns into EntityMut after an `insert` or `insert_bundle` op
* this actually regressed performance last time i tried it, but in theory it should be faster
* Optimize SparseSet::insert (see `PERF` comment on insert)
* Replace SparseArray `Option<T>` with T::MAX to cut down on branching
* would enable cheaper get_unchecked() operations
* upstream fixedbitset optimizations
* fixedbitset could be allocation free for small block counts (store blocks in a SmallVec)
* fixedbitset could have a const constructor
* Consider implementing Tags (archetype-specific by-value data that affects archetype identity)
* ex: ArchetypeA could have `[A, B, C]` table components and `[D(1)]` "tag" component. ArchetypeB could have `[A, B, C]` table components and a `[D(2)]` tag component. The archetypes are different, despite both having D tags because the value inside D is different.
* this could potentially build on top of the `archetype.unique_components` added in this pr for resource storage.
* Consider reverting `all_tuples` proc macro in favor of the old `macro_rules` implementation
* all_tuples is more flexible and produces cleaner documentation (the macro_rules version produces weird type parameter orders due to parser constraints)
* but unfortunately all_tuples also appears to make Rust Analyzer sad/slow when working inside of `bevy_ecs` (does not affect user code)
* Consider "resource queries" and/or "mixed resource and entity component queries" as an alternative to WorldCell
* this is basically just "systems" so maybe it's not worth it
* Add more world ops
* `world.clear()`
* `world.reserve<T: Bundle>(count: usize)`
* Try using the old archetype allocation strategy (allocate new memory on resize and copy everything over). I expect this to improve batch insertion performance at the cost of unbatched performance. But thats just a guess. I'm not an allocation perf pro :)
* Adapt Commands apis for consistency with new World apis
## Benchmarks
key:
* `bevy_old`: bevy `main` branch
* `bevy`: this branch
* `_foreach`: uses an optimized for_each iterator
* ` _sparse`: uses sparse set storage (if unspecified assume table storage)
* `_system`: runs inside a system (if unspecified assume test happens via direct world ops)
### Simple Insert (from ecs_bench_suite)
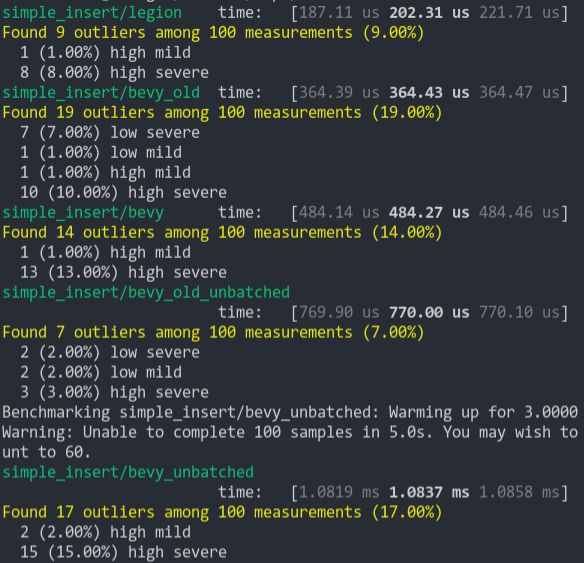
### Simpler Iter (from ecs_bench_suite)
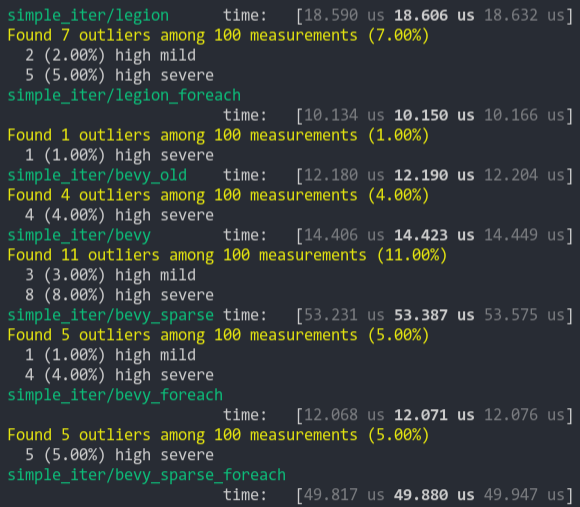
### Fragment Iter (from ecs_bench_suite)
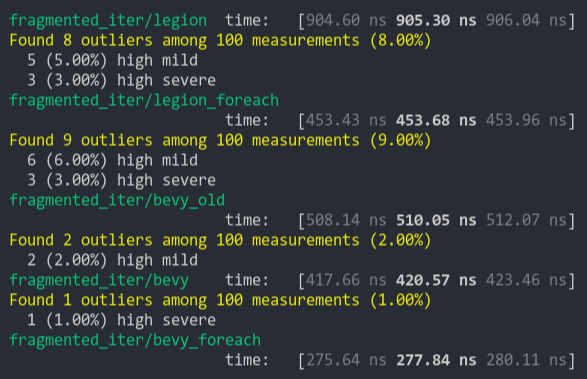
### Sparse Fragmented Iter
Iterate a query that matches 5 entities from a single matching archetype, but there are 100 unmatching archetypes
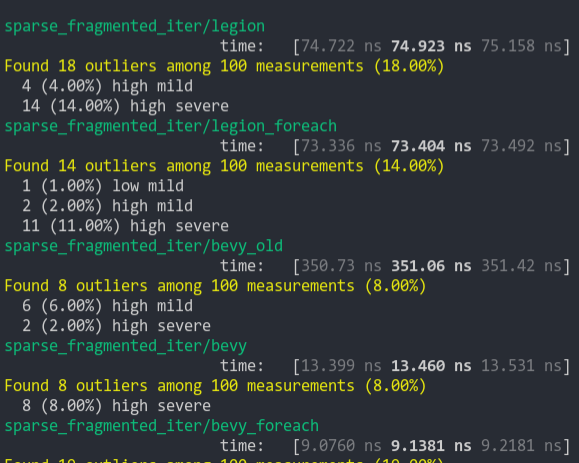
### Schedule (from ecs_bench_suite)
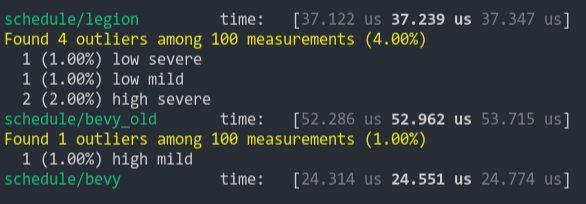
### Add Remove Component (from ecs_bench_suite)
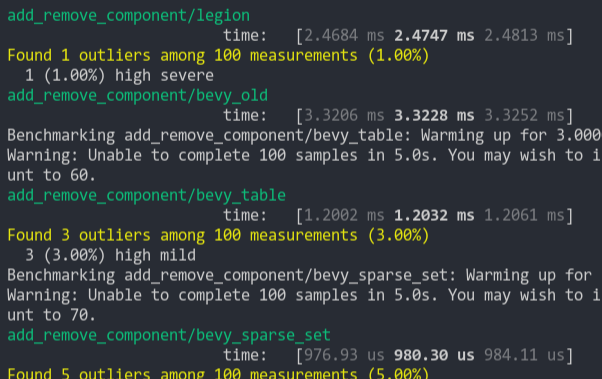
### Add Remove Component Big
Same as the test above, but each entity has 5 "large" matrix components and 1 "large" matrix component is added and removed
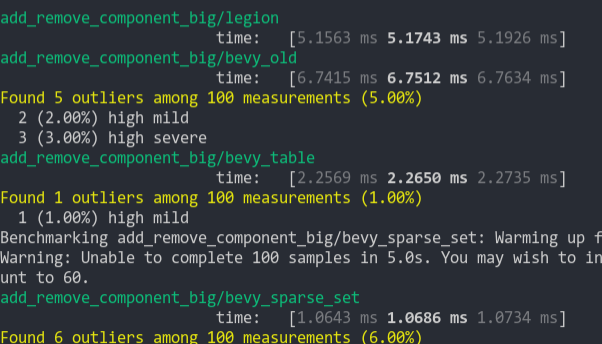
### Get Component
Looks up a single component value a large number of times
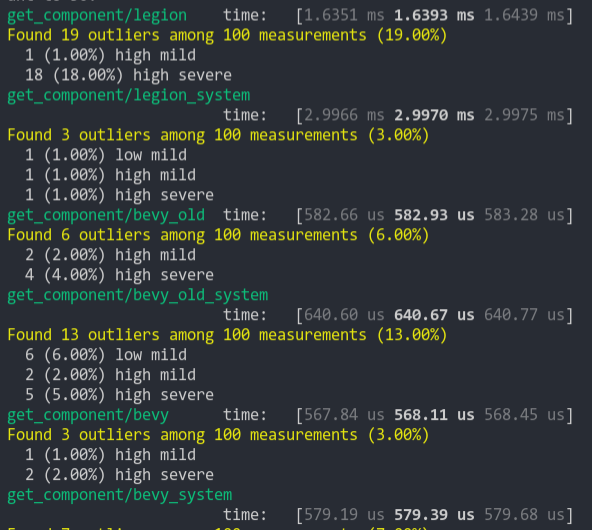
2021-03-05 07:54:35 +00:00
{
Remove the `SystemParamState` trait and remove types like `ResState` (#6919)
Spiritual successor to #5205.
Actual successor to #6865.
# Objective
Currently, system params are defined using three traits: `SystemParam`, `ReadOnlySystemParam`, `SystemParamState`. The behavior for each param is specified by the `SystemParamState` trait, while `SystemParam` simply defers to the state.
Splitting the traits in this way makes it easier to implement within macros, but it increases the cognitive load. Worst of all, this approach requires each `MySystemParam` to have a public `MySystemParamState` type associated with it.
## Solution
* Merge the trait `SystemParamState` into `SystemParam`.
* Remove all trivial `SystemParam` state types.
* `OptionNonSendMutState<T>`: you will not be missed.
---
- [x] Fix/resolve the remaining test failure.
## Changelog
* Removed the trait `SystemParamState`, merging its functionality into `SystemParam`.
## Migration Guide
**Note**: this should replace the migration guide for #6865.
This is relative to Bevy 0.9, not main.
The traits `SystemParamState` and `SystemParamFetch` have been removed, and their functionality has been transferred to `SystemParam`.
```rust
// Before (0.9)
impl SystemParam for MyParam<'_, '_> {
type State = MyParamState;
}
unsafe impl SystemParamState for MyParamState {
fn init(world: &mut World, system_meta: &mut SystemMeta) -> Self { ... }
}
unsafe impl<'w, 's> SystemParamFetch<'w, 's> for MyParamState {
type Item = MyParam<'w, 's>;
fn get_param(&mut self, ...) -> Self::Item;
}
unsafe impl ReadOnlySystemParamFetch for MyParamState { }
// After (0.10)
unsafe impl SystemParam for MyParam<'_, '_> {
type State = MyParamState;
type Item<'w, 's> = MyParam<'w, 's>;
fn init_state(world: &mut World, system_meta: &mut SystemMeta) -> Self::State { ... }
fn get_param<'w, 's>(state: &mut Self::State, ...) -> Self::Item<'w, 's>;
}
unsafe impl ReadOnlySystemParam for MyParam<'_, '_> { }
```
The trait `ReadOnlySystemParamFetch` has been replaced with `ReadOnlySystemParam`.
```rust
// Before
unsafe impl ReadOnlySystemParamFetch for MyParamState {}
// After
unsafe impl ReadOnlySystemParam for MyParam<'_, '_> {}
```
2023-01-07 23:20:32 +00:00
type State = QueryState < Q , F > ;
Simplify trait hierarchy for `SystemParam` (#6865)
# Objective
* Implementing a custom `SystemParam` by hand requires implementing three traits -- four if it is read-only.
* The trait `SystemParamFetch<'w, 's>` is a workaround from before we had generic associated types, and is no longer necessary.
## Solution
* Combine the trait `SystemParamFetch` with `SystemParamState`.
* I decided to remove the `Fetch` name and keep the `State` name, since the former was consistently conflated with the latter.
* Replace the trait `ReadOnlySystemParamFetch` with `ReadOnlySystemParam`, which simplifies trait bounds in generic code.
---
## Changelog
- Removed the trait `SystemParamFetch`, moving its functionality to `SystemParamState`.
- Replaced the trait `ReadOnlySystemParamFetch` with `ReadOnlySystemParam`.
## Migration Guide
The trait `SystemParamFetch` has been removed, and its functionality has been transferred to `SystemParamState`.
```rust
// Before
impl SystemParamState for MyParamState {
fn init(world: &mut World, system_meta: &mut SystemMeta) -> Self { ... }
}
impl<'w, 's> SystemParamFetch<'w, 's> for MyParamState {
type Item = MyParam<'w, 's>;
fn get_param(...) -> Self::Item;
}
// After
impl SystemParamState for MyParamState {
type Item<'w, 's> = MyParam<'w, 's>; // Generic associated types!
fn init(world: &mut World, system_meta: &mut SystemMeta) -> Self { ... }
fn get_param<'w, 's>(...) -> Self::Item<'w, 's>;
}
```
The trait `ReadOnlySystemParamFetch` has been replaced with `ReadOnlySystemParam`.
```rust
// Before
unsafe impl ReadOnlySystemParamFetch for MyParamState {}
// After
unsafe impl<'w, 's> ReadOnlySystemParam for MyParam<'w, 's> {}
```
2022-12-11 18:34:14 +00:00
type Item < ' w , ' s > = Query < ' w , ' s , Q , F > ;
Remove the `SystemParamState` trait and remove types like `ResState` (#6919)
Spiritual successor to #5205.
Actual successor to #6865.
# Objective
Currently, system params are defined using three traits: `SystemParam`, `ReadOnlySystemParam`, `SystemParamState`. The behavior for each param is specified by the `SystemParamState` trait, while `SystemParam` simply defers to the state.
Splitting the traits in this way makes it easier to implement within macros, but it increases the cognitive load. Worst of all, this approach requires each `MySystemParam` to have a public `MySystemParamState` type associated with it.
## Solution
* Merge the trait `SystemParamState` into `SystemParam`.
* Remove all trivial `SystemParam` state types.
* `OptionNonSendMutState<T>`: you will not be missed.
---
- [x] Fix/resolve the remaining test failure.
## Changelog
* Removed the trait `SystemParamState`, merging its functionality into `SystemParam`.
## Migration Guide
**Note**: this should replace the migration guide for #6865.
This is relative to Bevy 0.9, not main.
The traits `SystemParamState` and `SystemParamFetch` have been removed, and their functionality has been transferred to `SystemParam`.
```rust
// Before (0.9)
impl SystemParam for MyParam<'_, '_> {
type State = MyParamState;
}
unsafe impl SystemParamState for MyParamState {
fn init(world: &mut World, system_meta: &mut SystemMeta) -> Self { ... }
}
unsafe impl<'w, 's> SystemParamFetch<'w, 's> for MyParamState {
type Item = MyParam<'w, 's>;
fn get_param(&mut self, ...) -> Self::Item;
}
unsafe impl ReadOnlySystemParamFetch for MyParamState { }
// After (0.10)
unsafe impl SystemParam for MyParam<'_, '_> {
type State = MyParamState;
type Item<'w, 's> = MyParam<'w, 's>;
fn init_state(world: &mut World, system_meta: &mut SystemMeta) -> Self::State { ... }
fn get_param<'w, 's>(state: &mut Self::State, ...) -> Self::Item<'w, 's>;
}
unsafe impl ReadOnlySystemParam for MyParam<'_, '_> { }
```
The trait `ReadOnlySystemParamFetch` has been replaced with `ReadOnlySystemParam`.
```rust
// Before
unsafe impl ReadOnlySystemParamFetch for MyParamState {}
// After
unsafe impl ReadOnlySystemParam for MyParam<'_, '_> {}
```
2023-01-07 23:20:32 +00:00
fn init_state ( world : & mut World , system_meta : & mut SystemMeta ) -> Self ::State {
Bevy ECS V2 (#1525)
# Bevy ECS V2
This is a rewrite of Bevy ECS (basically everything but the new executor/schedule, which are already awesome). The overall goal was to improve the performance and versatility of Bevy ECS. Here is a quick bulleted list of changes before we dive into the details:
* Complete World rewrite
* Multiple component storage types:
* Tables: fast cache friendly iteration, slower add/removes (previously called Archetypes)
* Sparse Sets: fast add/remove, slower iteration
* Stateful Queries (caches query results for faster iteration. fragmented iteration is _fast_ now)
* Stateful System Params (caches expensive operations. inspired by @DJMcNab's work in #1364)
* Configurable System Params (users can set configuration when they construct their systems. once again inspired by @DJMcNab's work)
* Archetypes are now "just metadata", component storage is separate
* Archetype Graph (for faster archetype changes)
* Component Metadata
* Configure component storage type
* Retrieve information about component size/type/name/layout/send-ness/etc
* Components are uniquely identified by a densely packed ComponentId
* TypeIds are now totally optional (which should make implementing scripting easier)
* Super fast "for_each" query iterators
* Merged Resources into World. Resources are now just a special type of component
* EntityRef/EntityMut builder apis (more efficient and more ergonomic)
* Fast bitset-backed `Access<T>` replaces old hashmap-based approach everywhere
* Query conflicts are determined by component access instead of archetype component access (to avoid random failures at runtime)
* With/Without are still taken into account for conflicts, so this should still be comfy to use
* Much simpler `IntoSystem` impl
* Significantly reduced the amount of hashing throughout the ecs in favor of Sparse Sets (indexed by densely packed ArchetypeId, ComponentId, BundleId, and TableId)
* Safety Improvements
* Entity reservation uses a normal world reference instead of unsafe transmute
* QuerySets no longer transmute lifetimes
* Made traits "unsafe" where relevant
* More thorough safety docs
* WorldCell
* Exposes safe mutable access to multiple resources at a time in a World
* Replaced "catch all" `System::update_archetypes(world: &World)` with `System::new_archetype(archetype: &Archetype)`
* Simpler Bundle implementation
* Replaced slow "remove_bundle_one_by_one" used as fallback for Commands::remove_bundle with fast "remove_bundle_intersection"
* Removed `Mut<T>` query impl. it is better to only support one way: `&mut T`
* Removed with() from `Flags<T>` in favor of `Option<Flags<T>>`, which allows querying for flags to be "filtered" by default
* Components now have is_send property (currently only resources support non-send)
* More granular module organization
* New `RemovedComponents<T>` SystemParam that replaces `query.removed::<T>()`
* `world.resource_scope()` for mutable access to resources and world at the same time
* WorldQuery and QueryFilter traits unified. FilterFetch trait added to enable "short circuit" filtering. Auto impled for cases that don't need it
* Significantly slimmed down SystemState in favor of individual SystemParam state
* System Commands changed from `commands: &mut Commands` back to `mut commands: Commands` (to allow Commands to have a World reference)
Fixes #1320
## `World` Rewrite
This is a from-scratch rewrite of `World` that fills the niche that `hecs` used to. Yes, this means Bevy ECS is no longer a "fork" of hecs. We're going out our own!
(the only shared code between the projects is the entity id allocator, which is already basically ideal)
A huge shout out to @SanderMertens (author of [flecs](https://github.com/SanderMertens/flecs)) for sharing some great ideas with me (specifically hybrid ecs storage and archetype graphs). He also helped advise on a number of implementation details.
## Component Storage (The Problem)
Two ECS storage paradigms have gained a lot of traction over the years:
* **Archetypal ECS**:
* Stores components in "tables" with static schemas. Each "column" stores components of a given type. Each "row" is an entity.
* Each "archetype" has its own table. Adding/removing an entity's component changes the archetype.
* Enables super-fast Query iteration due to its cache-friendly data layout
* Comes at the cost of more expensive add/remove operations for an Entity's components, because all components need to be copied to the new archetype's "table"
* **Sparse Set ECS**:
* Stores components of the same type in densely packed arrays, which are sparsely indexed by densely packed unsigned integers (Entity ids)
* Query iteration is slower than Archetypal ECS because each entity's component could be at any position in the sparse set. This "random access" pattern isn't cache friendly. Additionally, there is an extra layer of indirection because you must first map the entity id to an index in the component array.
* Adding/removing components is a cheap, constant time operation
Bevy ECS V1, hecs, legion, flec, and Unity DOTS are all "archetypal ecs-es". I personally think "archetypal" storage is a good default for game engines. An entity's archetype doesn't need to change frequently in general, and it creates "fast by default" query iteration (which is a much more common operation). It is also "self optimizing". Users don't need to think about optimizing component layouts for iteration performance. It "just works" without any extra boilerplate.
Shipyard and EnTT are "sparse set ecs-es". They employ "packing" as a way to work around the "suboptimal by default" iteration performance for specific sets of components. This helps, but I didn't think this was a good choice for a general purpose engine like Bevy because:
1. "packs" conflict with each other. If bevy decides to internally pack the Transform and GlobalTransform components, users are then blocked if they want to pack some custom component with Transform.
2. users need to take manual action to optimize
Developers selecting an ECS framework are stuck with a hard choice. Select an "archetypal" framework with "fast iteration everywhere" but without the ability to cheaply add/remove components, or select a "sparse set" framework to cheaply add/remove components but with slower iteration performance.
## Hybrid Component Storage (The Solution)
In Bevy ECS V2, we get to have our cake and eat it too. It now has _both_ of the component storage types above (and more can be added later if needed):
* **Tables** (aka "archetypal" storage)
* The default storage. If you don't configure anything, this is what you get
* Fast iteration by default
* Slower add/remove operations
* **Sparse Sets**
* Opt-in
* Slower iteration
* Faster add/remove operations
These storage types complement each other perfectly. By default Query iteration is fast. If developers know that they want to add/remove a component at high frequencies, they can set the storage to "sparse set":
```rust
world.register_component(
ComponentDescriptor::new::<MyComponent>(StorageType::SparseSet)
).unwrap();
```
## Archetypes
Archetypes are now "just metadata" ... they no longer store components directly. They do store:
* The `ComponentId`s of each of the Archetype's components (and that component's storage type)
* Archetypes are uniquely defined by their component layouts
* For example: entities with "table" components `[A, B, C]` _and_ "sparse set" components `[D, E]` will always be in the same archetype.
* The `TableId` associated with the archetype
* For now each archetype has exactly one table (which can have no components),
* There is a 1->Many relationship from Tables->Archetypes. A given table could have any number of archetype components stored in it:
* Ex: an entity with "table storage" components `[A, B, C]` and "sparse set" components `[D, E]` will share the same `[A, B, C]` table as an entity with `[A, B, C]` table component and `[F]` sparse set components.
* This 1->Many relationship is how we preserve fast "cache friendly" iteration performance when possible (more on this later)
* A list of entities that are in the archetype and the row id of the table they are in
* ArchetypeComponentIds
* unique densely packed identifiers for (ArchetypeId, ComponentId) pairs
* used by the schedule executor for cheap system access control
* "Archetype Graph Edges" (see the next section)
## The "Archetype Graph"
Archetype changes in Bevy (and a number of other archetypal ecs-es) have historically been expensive to compute. First, you need to allocate a new vector of the entity's current component ids, add or remove components based on the operation performed, sort it (to ensure it is order-independent), then hash it to find the archetype (if it exists). And thats all before we get to the _already_ expensive full copy of all components to the new table storage.
The solution is to build a "graph" of archetypes to cache these results. @SanderMertens first exposed me to the idea (and he got it from @gjroelofs, who came up with it). They propose adding directed edges between archetypes for add/remove component operations. If `ComponentId`s are densely packed, you can use sparse sets to cheaply jump between archetypes.
Bevy takes this one step further by using add/remove `Bundle` edges instead of `Component` edges. Bevy encourages the use of `Bundles` to group add/remove operations. This is largely for "clearer game logic" reasons, but it also helps cut down on the number of archetype changes required. `Bundles` now also have densely-packed `BundleId`s. This allows us to use a _single_ edge for each bundle operation (rather than needing to traverse N edges ... one for each component). Single component operations are also bundles, so this is strictly an improvement over a "component only" graph.
As a result, an operation that used to be _heavy_ (both for allocations and compute) is now two dirt-cheap array lookups and zero allocations.
## Stateful Queries
World queries are now stateful. This allows us to:
1. Cache archetype (and table) matches
* This resolves another issue with (naive) archetypal ECS: query performance getting worse as the number of archetypes goes up (and fragmentation occurs).
2. Cache Fetch and Filter state
* The expensive parts of fetch/filter operations (such as hashing the TypeId to find the ComponentId) now only happen once when the Query is first constructed
3. Incrementally build up state
* When new archetypes are added, we only process the new archetypes (no need to rebuild state for old archetypes)
As a result, the direct `World` query api now looks like this:
```rust
let mut query = world.query::<(&A, &mut B)>();
for (a, mut b) in query.iter_mut(&mut world) {
}
```
Requiring `World` to generate stateful queries (rather than letting the `QueryState` type be constructed separately) allows us to ensure that _all_ queries are properly initialized (and the relevant world state, such as ComponentIds). This enables QueryState to remove branches from its operations that check for initialization status (and also enables query.iter() to take an immutable world reference because it doesn't need to initialize anything in world).
However in systems, this is a non-breaking change. State management is done internally by the relevant SystemParam.
## Stateful SystemParams
Like Queries, `SystemParams` now also cache state. For example, `Query` system params store the "stateful query" state mentioned above. Commands store their internal `CommandQueue`. This means you can now safely use as many separate `Commands` parameters in your system as you want. `Local<T>` system params store their `T` value in their state (instead of in Resources).
SystemParam state also enabled a significant slim-down of SystemState. It is much nicer to look at now.
Per-SystemParam state naturally insulates us from an "aliased mut" class of errors we have hit in the past (ex: using multiple `Commands` system params).
(credit goes to @DJMcNab for the initial idea and draft pr here #1364)
## Configurable SystemParams
@DJMcNab also had the great idea to make SystemParams configurable. This allows users to provide some initial configuration / values for system parameters (when possible). Most SystemParams have no config (the config type is `()`), but the `Local<T>` param now supports user-provided parameters:
```rust
fn foo(value: Local<usize>) {
}
app.add_system(foo.system().config(|c| c.0 = Some(10)));
```
## Uber Fast "for_each" Query Iterators
Developers now have the choice to use a fast "for_each" iterator, which yields ~1.5-3x iteration speed improvements for "fragmented iteration", and minor ~1.2x iteration speed improvements for unfragmented iteration.
```rust
fn system(query: Query<(&A, &mut B)>) {
// you now have the option to do this for a speed boost
query.for_each_mut(|(a, mut b)| {
});
// however normal iterators are still available
for (a, mut b) in query.iter_mut() {
}
}
```
I think in most cases we should continue to encourage "normal" iterators as they are more flexible and more "rust idiomatic". But when that extra "oomf" is needed, it makes sense to use `for_each`.
We should also consider using `for_each` for internal bevy systems to give our users a nice speed boost (but that should be a separate pr).
## Component Metadata
`World` now has a `Components` collection, which is accessible via `world.components()`. This stores mappings from `ComponentId` to `ComponentInfo`, as well as `TypeId` to `ComponentId` mappings (where relevant). `ComponentInfo` stores information about the component, such as ComponentId, TypeId, memory layout, send-ness (currently limited to resources), and storage type.
## Significantly Cheaper `Access<T>`
We used to use `TypeAccess<TypeId>` to manage read/write component/archetype-component access. This was expensive because TypeIds must be hashed and compared individually. The parallel executor got around this by "condensing" type ids into bitset-backed access types. This worked, but it had to be re-generated from the `TypeAccess<TypeId>`sources every time archetypes changed.
This pr removes TypeAccess in favor of faster bitset access everywhere. We can do this thanks to the move to densely packed `ComponentId`s and `ArchetypeComponentId`s.
## Merged Resources into World
Resources had a lot of redundant functionality with Components. They stored typed data, they had access control, they had unique ids, they were queryable via SystemParams, etc. In fact the _only_ major difference between them was that they were unique (and didn't correlate to an entity).
Separate resources also had the downside of requiring a separate set of access controls, which meant the parallel executor needed to compare more bitsets per system and manage more state.
I initially got the "separate resources" idea from `legion`. I think that design was motivated by the fact that it made the direct world query/resource lifetime interactions more manageable. It certainly made our lives easier when using Resources alongside hecs/bevy_ecs. However we already have a construct for safely and ergonomically managing in-world lifetimes: systems (which use `Access<T>` internally).
This pr merges Resources into World:
```rust
world.insert_resource(1);
world.insert_resource(2.0);
let a = world.get_resource::<i32>().unwrap();
let mut b = world.get_resource_mut::<f64>().unwrap();
*b = 3.0;
```
Resources are now just a special kind of component. They have their own ComponentIds (and their own resource TypeId->ComponentId scope, so they don't conflict wit components of the same type). They are stored in a special "resource archetype", which stores components inside the archetype using a new `unique_components` sparse set (note that this sparse set could later be used to implement Tags). This allows us to keep the code size small by reusing existing datastructures (namely Column, Archetype, ComponentFlags, and ComponentInfo). This allows us the executor to use a single `Access<ArchetypeComponentId>` per system. It should also make scripting language integration easier.
_But_ this merge did create problems for people directly interacting with `World`. What if you need mutable access to multiple resources at the same time? `world.get_resource_mut()` borrows World mutably!
## WorldCell
WorldCell applies the `Access<ArchetypeComponentId>` concept to direct world access:
```rust
let world_cell = world.cell();
let a = world_cell.get_resource_mut::<i32>().unwrap();
let b = world_cell.get_resource_mut::<f64>().unwrap();
```
This adds cheap runtime checks (a sparse set lookup of `ArchetypeComponentId` and a counter) to ensure that world accesses do not conflict with each other. Each operation returns a `WorldBorrow<'w, T>` or `WorldBorrowMut<'w, T>` wrapper type, which will release the relevant ArchetypeComponentId resources when dropped.
World caches the access sparse set (and only one cell can exist at a time), so `world.cell()` is a cheap operation.
WorldCell does _not_ use atomic operations. It is non-send, does a mutable borrow of world to prevent other accesses, and uses a simple `Rc<RefCell<ArchetypeComponentAccess>>` wrapper in each WorldBorrow pointer.
The api is currently limited to resource access, but it can and should be extended to queries / entity component access.
## Resource Scopes
WorldCell does not yet support component queries, and even when it does there are sometimes legitimate reasons to want a mutable world ref _and_ a mutable resource ref (ex: bevy_render and bevy_scene both need this). In these cases we could always drop down to the unsafe `world.get_resource_unchecked_mut()`, but that is not ideal!
Instead developers can use a "resource scope"
```rust
world.resource_scope(|world: &mut World, a: &mut A| {
})
```
This temporarily removes the `A` resource from `World`, provides mutable pointers to both, and re-adds A to World when finished. Thanks to the move to ComponentIds/sparse sets, this is a cheap operation.
If multiple resources are required, scopes can be nested. We could also consider adding a "resource tuple" to the api if this pattern becomes common and the boilerplate gets nasty.
## Query Conflicts Use ComponentId Instead of ArchetypeComponentId
For safety reasons, systems cannot contain queries that conflict with each other without wrapping them in a QuerySet. On bevy `main`, we use ArchetypeComponentIds to determine conflicts. This is nice because it can take into account filters:
```rust
// these queries will never conflict due to their filters
fn filter_system(a: Query<&mut A, With<B>>, b: Query<&mut B, Without<B>>) {
}
```
But it also has a significant downside:
```rust
// these queries will not conflict _until_ an entity with A, B, and C is spawned
fn maybe_conflicts_system(a: Query<(&mut A, &C)>, b: Query<(&mut A, &B)>) {
}
```
The system above will panic at runtime if an entity with A, B, and C is spawned. This makes it hard to trust that your game logic will run without crashing.
In this pr, I switched to using `ComponentId` instead. This _is_ more constraining. `maybe_conflicts_system` will now always fail, but it will do it consistently at startup. Naively, it would also _disallow_ `filter_system`, which would be a significant downgrade in usability. Bevy has a number of internal systems that rely on disjoint queries and I expect it to be a common pattern in userspace.
To resolve this, I added a new `FilteredAccess<T>` type, which wraps `Access<T>` and adds with/without filters. If two `FilteredAccess` have with/without values that prove they are disjoint, they will no longer conflict.
## EntityRef / EntityMut
World entity operations on `main` require that the user passes in an `entity` id to each operation:
```rust
let entity = world.spawn((A, )); // create a new entity with A
world.get::<A>(entity);
world.insert(entity, (B, C));
world.insert_one(entity, D);
```
This means that each operation needs to look up the entity location / verify its validity. The initial spawn operation also requires a Bundle as input. This can be awkward when no components are required (or one component is required).
These operations have been replaced by `EntityRef` and `EntityMut`, which are "builder-style" wrappers around world that provide read and read/write operations on a single, pre-validated entity:
```rust
// spawn now takes no inputs and returns an EntityMut
let entity = world.spawn()
.insert(A) // insert a single component into the entity
.insert_bundle((B, C)) // insert a bundle of components into the entity
.id() // id returns the Entity id
// Returns EntityMut (or panics if the entity does not exist)
world.entity_mut(entity)
.insert(D)
.insert_bundle(SomeBundle::default());
{
// returns EntityRef (or panics if the entity does not exist)
let d = world.entity(entity)
.get::<D>() // gets the D component
.unwrap();
// world.get still exists for ergonomics
let d = world.get::<D>(entity).unwrap();
}
// These variants return Options if you want to check existence instead of panicing
world.get_entity_mut(entity)
.unwrap()
.insert(E);
if let Some(entity_ref) = world.get_entity(entity) {
let d = entity_ref.get::<D>().unwrap();
}
```
This _does not_ affect the current Commands api or terminology. I think that should be a separate conversation as that is a much larger breaking change.
## Safety Improvements
* Entity reservation in Commands uses a normal world borrow instead of an unsafe transmute
* QuerySets no longer transmutes lifetimes
* Made traits "unsafe" when implementing a trait incorrectly could cause unsafety
* More thorough safety docs
## RemovedComponents SystemParam
The old approach to querying removed components: `query.removed:<T>()` was confusing because it had no connection to the query itself. I replaced it with the following, which is both clearer and allows us to cache the ComponentId mapping in the SystemParamState:
```rust
fn system(removed: RemovedComponents<T>) {
for entity in removed.iter() {
}
}
```
## Simpler Bundle implementation
Bundles are no longer responsible for sorting (or deduping) TypeInfo. They are just a simple ordered list of component types / data. This makes the implementation smaller and opens the door to an easy "nested bundle" implementation in the future (which i might even add in this pr). Duplicate detection is now done once per bundle type by World the first time a bundle is used.
## Unified WorldQuery and QueryFilter types
(don't worry they are still separate type _parameters_ in Queries .. this is a non-breaking change)
WorldQuery and QueryFilter were already basically identical apis. With the addition of `FetchState` and more storage-specific fetch methods, the overlap was even clearer (and the redundancy more painful).
QueryFilters are now just `F: WorldQuery where F::Fetch: FilterFetch`. FilterFetch requires `Fetch<Item = bool>` and adds new "short circuit" variants of fetch methods. This enables a filter tuple like `(With<A>, Without<B>, Changed<C>)` to stop evaluating the filter after the first mismatch is encountered. FilterFetch is automatically implemented for `Fetch` implementations that return bool.
This forces fetch implementations that return things like `(bool, bool, bool)` (such as the filter above) to manually implement FilterFetch and decide whether or not to short-circuit.
## More Granular Modules
World no longer globs all of the internal modules together. It now exports `core`, `system`, and `schedule` separately. I'm also considering exporting `core` submodules directly as that is still pretty "glob-ey" and unorganized (feedback welcome here).
## Remaining Draft Work (to be done in this pr)
* ~~panic on conflicting WorldQuery fetches (&A, &mut A)~~
* ~~bevy `main` and hecs both currently allow this, but we should protect against it if possible~~
* ~~batch_iter / par_iter (currently stubbed out)~~
* ~~ChangedRes~~
* ~~I skipped this while we sort out #1313. This pr should be adapted to account for whatever we land on there~~.
* ~~The `Archetypes` and `Tables` collections use hashes of sorted lists of component ids to uniquely identify each archetype/table. This hash is then used as the key in a HashMap to look up the relevant ArchetypeId or TableId. (which doesn't handle hash collisions properly)~~
* ~~It is currently unsafe to generate a Query from "World A", then use it on "World B" (despite the api claiming it is safe). We should probably close this gap. This could be done by adding a randomly generated WorldId to each world, then storing that id in each Query. They could then be compared to each other on each `query.do_thing(&world)` operation. This _does_ add an extra branch to each query operation, so I'm open to other suggestions if people have them.~~
* ~~Nested Bundles (if i find time)~~
## Potential Future Work
* Expand WorldCell to support queries.
* Consider not allocating in the empty archetype on `world.spawn()`
* ex: return something like EntityMutUninit, which turns into EntityMut after an `insert` or `insert_bundle` op
* this actually regressed performance last time i tried it, but in theory it should be faster
* Optimize SparseSet::insert (see `PERF` comment on insert)
* Replace SparseArray `Option<T>` with T::MAX to cut down on branching
* would enable cheaper get_unchecked() operations
* upstream fixedbitset optimizations
* fixedbitset could be allocation free for small block counts (store blocks in a SmallVec)
* fixedbitset could have a const constructor
* Consider implementing Tags (archetype-specific by-value data that affects archetype identity)
* ex: ArchetypeA could have `[A, B, C]` table components and `[D(1)]` "tag" component. ArchetypeB could have `[A, B, C]` table components and a `[D(2)]` tag component. The archetypes are different, despite both having D tags because the value inside D is different.
* this could potentially build on top of the `archetype.unique_components` added in this pr for resource storage.
* Consider reverting `all_tuples` proc macro in favor of the old `macro_rules` implementation
* all_tuples is more flexible and produces cleaner documentation (the macro_rules version produces weird type parameter orders due to parser constraints)
* but unfortunately all_tuples also appears to make Rust Analyzer sad/slow when working inside of `bevy_ecs` (does not affect user code)
* Consider "resource queries" and/or "mixed resource and entity component queries" as an alternative to WorldCell
* this is basically just "systems" so maybe it's not worth it
* Add more world ops
* `world.clear()`
* `world.reserve<T: Bundle>(count: usize)`
* Try using the old archetype allocation strategy (allocate new memory on resize and copy everything over). I expect this to improve batch insertion performance at the cost of unbatched performance. But thats just a guess. I'm not an allocation perf pro :)
* Adapt Commands apis for consistency with new World apis
## Benchmarks
key:
* `bevy_old`: bevy `main` branch
* `bevy`: this branch
* `_foreach`: uses an optimized for_each iterator
* ` _sparse`: uses sparse set storage (if unspecified assume table storage)
* `_system`: runs inside a system (if unspecified assume test happens via direct world ops)
### Simple Insert (from ecs_bench_suite)
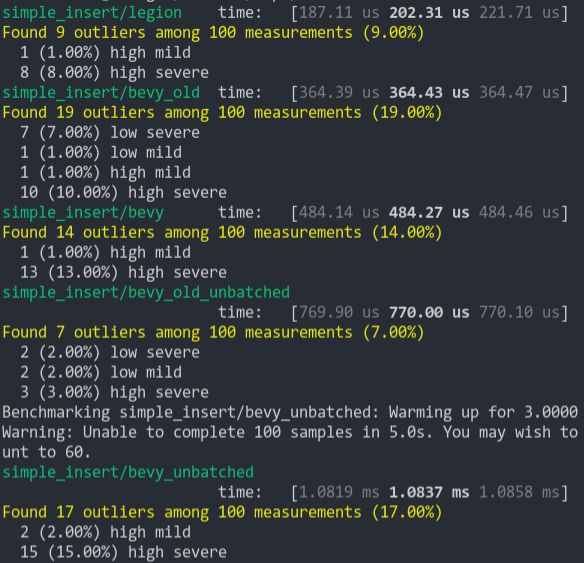
### Simpler Iter (from ecs_bench_suite)
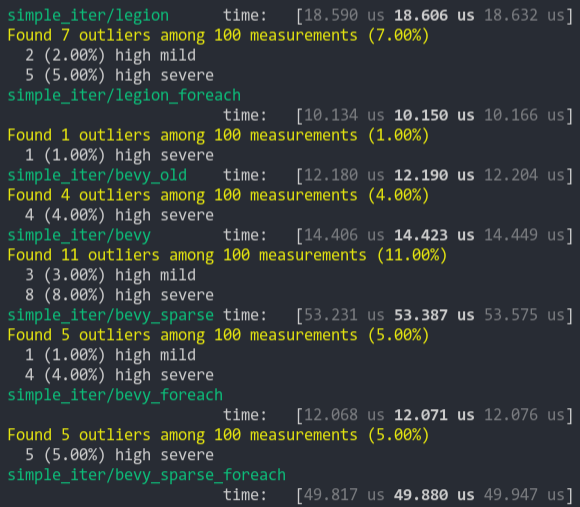
### Fragment Iter (from ecs_bench_suite)
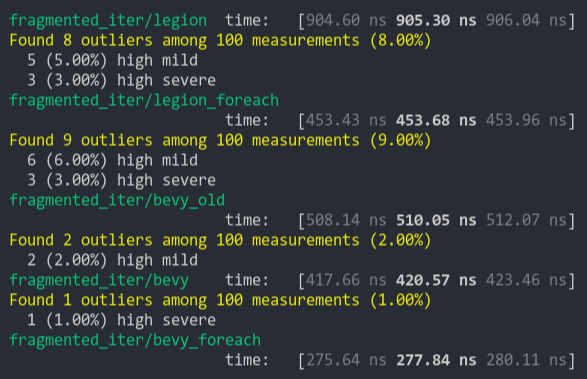
### Sparse Fragmented Iter
Iterate a query that matches 5 entities from a single matching archetype, but there are 100 unmatching archetypes
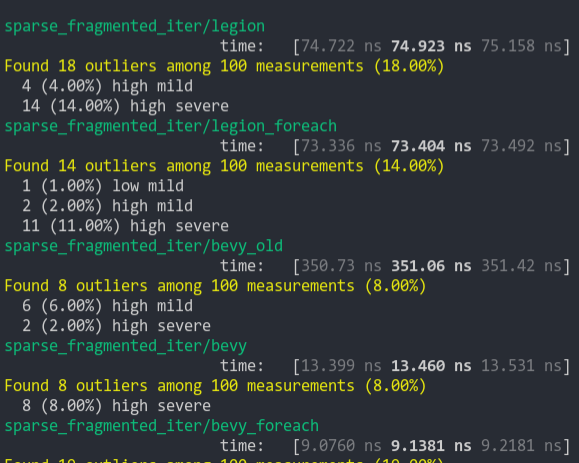
### Schedule (from ecs_bench_suite)
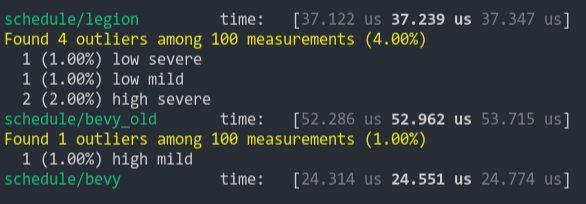
### Add Remove Component (from ecs_bench_suite)
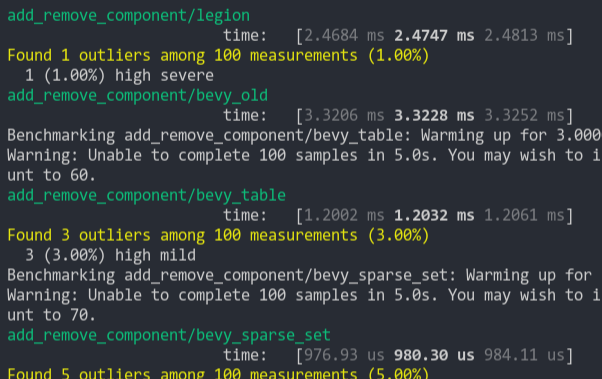
### Add Remove Component Big
Same as the test above, but each entity has 5 "large" matrix components and 1 "large" matrix component is added and removed
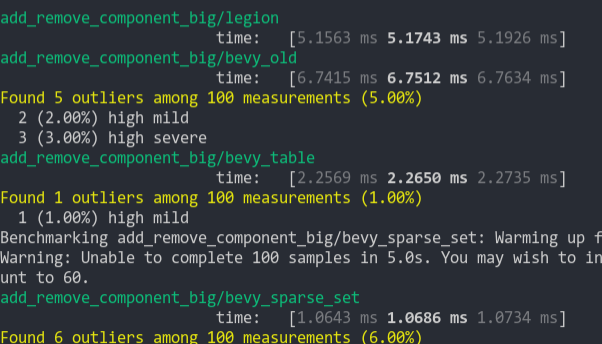
### Get Component
Looks up a single component value a large number of times
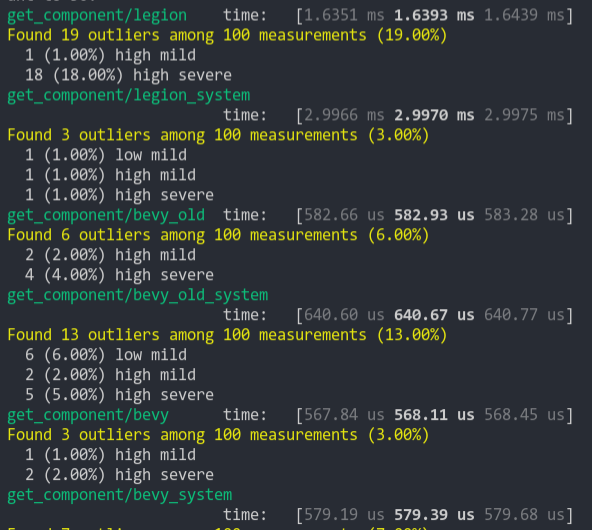
2021-03-05 07:54:35 +00:00
let state = QueryState ::new ( world ) ;
assert_component_access_compatibility (
Add new SystemState and rename old SystemState to SystemMeta (#2283)
This enables `SystemParams` to be used outside of function systems. Anything can create and store `SystemState`, which enables efficient "param state cached" access to `SystemParams`.
It adds a `ReadOnlySystemParamFetch` trait, which enables safe `SystemState::get` calls without unique world access.
I renamed the old `SystemState` to `SystemMeta` to enable us to mirror the `QueryState` naming convention (but I'm happy to discuss alternative names if people have other ideas). I initially pitched this as `ParamState`, but given that it needs to include full system metadata, that doesn't feel like a particularly accurate name.
```rust
#[derive(Eq, PartialEq, Debug)]
struct A(usize);
#[derive(Eq, PartialEq, Debug)]
struct B(usize);
let mut world = World::default();
world.insert_resource(A(42));
world.spawn().insert(B(7));
// we get nice lifetime elision when declaring the type on the left hand side
let mut system_state: SystemState<(Res<A>, Query<&B>)> = SystemState::new(&mut world);
let (a, query) = system_state.get(&world);
assert_eq!(*a, A(42), "returned resource matches initial value");
assert_eq!(
*query.single().unwrap(),
B(7),
"returned component matches initial value"
);
// mutable system params require unique world access
let mut system_state: SystemState<(ResMut<A>, Query<&mut B>)> = SystemState::new(&mut world);
let (a, query) = system_state.get_mut(&mut world);
// static lifetimes are required when declaring inside of structs
struct SomeContainer {
state: SystemState<(Res<'static, A>, Res<'static, B>)>
}
// this can be shortened using type aliases, which will be useful for complex param tuples
type MyParams<'a> = (Res<'a, A>, Res<'a, B>);
struct SomeContainer {
state: SystemState<MyParams<'static>>
}
// It is the user's responsibility to call SystemState::apply(world) for parameters that queue up work
let mut system_state: SystemState<(Commands, Query<&B>)> = SystemState::new(&mut world);
{
let (mut commands, query) = system_state.get(&world);
commands.insert_resource(3.14);
}
system_state.apply(&mut world);
```
## Future Work
* Actually use SystemState inside FunctionSystem. This would be trivial, but it requires FunctionSystem to wrap SystemState in Option in its current form (which complicates system metadata lookup). I'd prefer to hold off until we adopt something like the later designs linked in #1364, which enable us to contruct Systems using a World reference (and also remove the need for `.system`).
* Consider a "scoped" approach to automatically call SystemState::apply when systems params are no longer being used (either a container type with a Drop impl, or a function that takes a closure for user logic operating on params).
2021-06-02 19:57:38 +00:00
& system_meta . name ,
Bevy ECS V2 (#1525)
# Bevy ECS V2
This is a rewrite of Bevy ECS (basically everything but the new executor/schedule, which are already awesome). The overall goal was to improve the performance and versatility of Bevy ECS. Here is a quick bulleted list of changes before we dive into the details:
* Complete World rewrite
* Multiple component storage types:
* Tables: fast cache friendly iteration, slower add/removes (previously called Archetypes)
* Sparse Sets: fast add/remove, slower iteration
* Stateful Queries (caches query results for faster iteration. fragmented iteration is _fast_ now)
* Stateful System Params (caches expensive operations. inspired by @DJMcNab's work in #1364)
* Configurable System Params (users can set configuration when they construct their systems. once again inspired by @DJMcNab's work)
* Archetypes are now "just metadata", component storage is separate
* Archetype Graph (for faster archetype changes)
* Component Metadata
* Configure component storage type
* Retrieve information about component size/type/name/layout/send-ness/etc
* Components are uniquely identified by a densely packed ComponentId
* TypeIds are now totally optional (which should make implementing scripting easier)
* Super fast "for_each" query iterators
* Merged Resources into World. Resources are now just a special type of component
* EntityRef/EntityMut builder apis (more efficient and more ergonomic)
* Fast bitset-backed `Access<T>` replaces old hashmap-based approach everywhere
* Query conflicts are determined by component access instead of archetype component access (to avoid random failures at runtime)
* With/Without are still taken into account for conflicts, so this should still be comfy to use
* Much simpler `IntoSystem` impl
* Significantly reduced the amount of hashing throughout the ecs in favor of Sparse Sets (indexed by densely packed ArchetypeId, ComponentId, BundleId, and TableId)
* Safety Improvements
* Entity reservation uses a normal world reference instead of unsafe transmute
* QuerySets no longer transmute lifetimes
* Made traits "unsafe" where relevant
* More thorough safety docs
* WorldCell
* Exposes safe mutable access to multiple resources at a time in a World
* Replaced "catch all" `System::update_archetypes(world: &World)` with `System::new_archetype(archetype: &Archetype)`
* Simpler Bundle implementation
* Replaced slow "remove_bundle_one_by_one" used as fallback for Commands::remove_bundle with fast "remove_bundle_intersection"
* Removed `Mut<T>` query impl. it is better to only support one way: `&mut T`
* Removed with() from `Flags<T>` in favor of `Option<Flags<T>>`, which allows querying for flags to be "filtered" by default
* Components now have is_send property (currently only resources support non-send)
* More granular module organization
* New `RemovedComponents<T>` SystemParam that replaces `query.removed::<T>()`
* `world.resource_scope()` for mutable access to resources and world at the same time
* WorldQuery and QueryFilter traits unified. FilterFetch trait added to enable "short circuit" filtering. Auto impled for cases that don't need it
* Significantly slimmed down SystemState in favor of individual SystemParam state
* System Commands changed from `commands: &mut Commands` back to `mut commands: Commands` (to allow Commands to have a World reference)
Fixes #1320
## `World` Rewrite
This is a from-scratch rewrite of `World` that fills the niche that `hecs` used to. Yes, this means Bevy ECS is no longer a "fork" of hecs. We're going out our own!
(the only shared code between the projects is the entity id allocator, which is already basically ideal)
A huge shout out to @SanderMertens (author of [flecs](https://github.com/SanderMertens/flecs)) for sharing some great ideas with me (specifically hybrid ecs storage and archetype graphs). He also helped advise on a number of implementation details.
## Component Storage (The Problem)
Two ECS storage paradigms have gained a lot of traction over the years:
* **Archetypal ECS**:
* Stores components in "tables" with static schemas. Each "column" stores components of a given type. Each "row" is an entity.
* Each "archetype" has its own table. Adding/removing an entity's component changes the archetype.
* Enables super-fast Query iteration due to its cache-friendly data layout
* Comes at the cost of more expensive add/remove operations for an Entity's components, because all components need to be copied to the new archetype's "table"
* **Sparse Set ECS**:
* Stores components of the same type in densely packed arrays, which are sparsely indexed by densely packed unsigned integers (Entity ids)
* Query iteration is slower than Archetypal ECS because each entity's component could be at any position in the sparse set. This "random access" pattern isn't cache friendly. Additionally, there is an extra layer of indirection because you must first map the entity id to an index in the component array.
* Adding/removing components is a cheap, constant time operation
Bevy ECS V1, hecs, legion, flec, and Unity DOTS are all "archetypal ecs-es". I personally think "archetypal" storage is a good default for game engines. An entity's archetype doesn't need to change frequently in general, and it creates "fast by default" query iteration (which is a much more common operation). It is also "self optimizing". Users don't need to think about optimizing component layouts for iteration performance. It "just works" without any extra boilerplate.
Shipyard and EnTT are "sparse set ecs-es". They employ "packing" as a way to work around the "suboptimal by default" iteration performance for specific sets of components. This helps, but I didn't think this was a good choice for a general purpose engine like Bevy because:
1. "packs" conflict with each other. If bevy decides to internally pack the Transform and GlobalTransform components, users are then blocked if they want to pack some custom component with Transform.
2. users need to take manual action to optimize
Developers selecting an ECS framework are stuck with a hard choice. Select an "archetypal" framework with "fast iteration everywhere" but without the ability to cheaply add/remove components, or select a "sparse set" framework to cheaply add/remove components but with slower iteration performance.
## Hybrid Component Storage (The Solution)
In Bevy ECS V2, we get to have our cake and eat it too. It now has _both_ of the component storage types above (and more can be added later if needed):
* **Tables** (aka "archetypal" storage)
* The default storage. If you don't configure anything, this is what you get
* Fast iteration by default
* Slower add/remove operations
* **Sparse Sets**
* Opt-in
* Slower iteration
* Faster add/remove operations
These storage types complement each other perfectly. By default Query iteration is fast. If developers know that they want to add/remove a component at high frequencies, they can set the storage to "sparse set":
```rust
world.register_component(
ComponentDescriptor::new::<MyComponent>(StorageType::SparseSet)
).unwrap();
```
## Archetypes
Archetypes are now "just metadata" ... they no longer store components directly. They do store:
* The `ComponentId`s of each of the Archetype's components (and that component's storage type)
* Archetypes are uniquely defined by their component layouts
* For example: entities with "table" components `[A, B, C]` _and_ "sparse set" components `[D, E]` will always be in the same archetype.
* The `TableId` associated with the archetype
* For now each archetype has exactly one table (which can have no components),
* There is a 1->Many relationship from Tables->Archetypes. A given table could have any number of archetype components stored in it:
* Ex: an entity with "table storage" components `[A, B, C]` and "sparse set" components `[D, E]` will share the same `[A, B, C]` table as an entity with `[A, B, C]` table component and `[F]` sparse set components.
* This 1->Many relationship is how we preserve fast "cache friendly" iteration performance when possible (more on this later)
* A list of entities that are in the archetype and the row id of the table they are in
* ArchetypeComponentIds
* unique densely packed identifiers for (ArchetypeId, ComponentId) pairs
* used by the schedule executor for cheap system access control
* "Archetype Graph Edges" (see the next section)
## The "Archetype Graph"
Archetype changes in Bevy (and a number of other archetypal ecs-es) have historically been expensive to compute. First, you need to allocate a new vector of the entity's current component ids, add or remove components based on the operation performed, sort it (to ensure it is order-independent), then hash it to find the archetype (if it exists). And thats all before we get to the _already_ expensive full copy of all components to the new table storage.
The solution is to build a "graph" of archetypes to cache these results. @SanderMertens first exposed me to the idea (and he got it from @gjroelofs, who came up with it). They propose adding directed edges between archetypes for add/remove component operations. If `ComponentId`s are densely packed, you can use sparse sets to cheaply jump between archetypes.
Bevy takes this one step further by using add/remove `Bundle` edges instead of `Component` edges. Bevy encourages the use of `Bundles` to group add/remove operations. This is largely for "clearer game logic" reasons, but it also helps cut down on the number of archetype changes required. `Bundles` now also have densely-packed `BundleId`s. This allows us to use a _single_ edge for each bundle operation (rather than needing to traverse N edges ... one for each component). Single component operations are also bundles, so this is strictly an improvement over a "component only" graph.
As a result, an operation that used to be _heavy_ (both for allocations and compute) is now two dirt-cheap array lookups and zero allocations.
## Stateful Queries
World queries are now stateful. This allows us to:
1. Cache archetype (and table) matches
* This resolves another issue with (naive) archetypal ECS: query performance getting worse as the number of archetypes goes up (and fragmentation occurs).
2. Cache Fetch and Filter state
* The expensive parts of fetch/filter operations (such as hashing the TypeId to find the ComponentId) now only happen once when the Query is first constructed
3. Incrementally build up state
* When new archetypes are added, we only process the new archetypes (no need to rebuild state for old archetypes)
As a result, the direct `World` query api now looks like this:
```rust
let mut query = world.query::<(&A, &mut B)>();
for (a, mut b) in query.iter_mut(&mut world) {
}
```
Requiring `World` to generate stateful queries (rather than letting the `QueryState` type be constructed separately) allows us to ensure that _all_ queries are properly initialized (and the relevant world state, such as ComponentIds). This enables QueryState to remove branches from its operations that check for initialization status (and also enables query.iter() to take an immutable world reference because it doesn't need to initialize anything in world).
However in systems, this is a non-breaking change. State management is done internally by the relevant SystemParam.
## Stateful SystemParams
Like Queries, `SystemParams` now also cache state. For example, `Query` system params store the "stateful query" state mentioned above. Commands store their internal `CommandQueue`. This means you can now safely use as many separate `Commands` parameters in your system as you want. `Local<T>` system params store their `T` value in their state (instead of in Resources).
SystemParam state also enabled a significant slim-down of SystemState. It is much nicer to look at now.
Per-SystemParam state naturally insulates us from an "aliased mut" class of errors we have hit in the past (ex: using multiple `Commands` system params).
(credit goes to @DJMcNab for the initial idea and draft pr here #1364)
## Configurable SystemParams
@DJMcNab also had the great idea to make SystemParams configurable. This allows users to provide some initial configuration / values for system parameters (when possible). Most SystemParams have no config (the config type is `()`), but the `Local<T>` param now supports user-provided parameters:
```rust
fn foo(value: Local<usize>) {
}
app.add_system(foo.system().config(|c| c.0 = Some(10)));
```
## Uber Fast "for_each" Query Iterators
Developers now have the choice to use a fast "for_each" iterator, which yields ~1.5-3x iteration speed improvements for "fragmented iteration", and minor ~1.2x iteration speed improvements for unfragmented iteration.
```rust
fn system(query: Query<(&A, &mut B)>) {
// you now have the option to do this for a speed boost
query.for_each_mut(|(a, mut b)| {
});
// however normal iterators are still available
for (a, mut b) in query.iter_mut() {
}
}
```
I think in most cases we should continue to encourage "normal" iterators as they are more flexible and more "rust idiomatic". But when that extra "oomf" is needed, it makes sense to use `for_each`.
We should also consider using `for_each` for internal bevy systems to give our users a nice speed boost (but that should be a separate pr).
## Component Metadata
`World` now has a `Components` collection, which is accessible via `world.components()`. This stores mappings from `ComponentId` to `ComponentInfo`, as well as `TypeId` to `ComponentId` mappings (where relevant). `ComponentInfo` stores information about the component, such as ComponentId, TypeId, memory layout, send-ness (currently limited to resources), and storage type.
## Significantly Cheaper `Access<T>`
We used to use `TypeAccess<TypeId>` to manage read/write component/archetype-component access. This was expensive because TypeIds must be hashed and compared individually. The parallel executor got around this by "condensing" type ids into bitset-backed access types. This worked, but it had to be re-generated from the `TypeAccess<TypeId>`sources every time archetypes changed.
This pr removes TypeAccess in favor of faster bitset access everywhere. We can do this thanks to the move to densely packed `ComponentId`s and `ArchetypeComponentId`s.
## Merged Resources into World
Resources had a lot of redundant functionality with Components. They stored typed data, they had access control, they had unique ids, they were queryable via SystemParams, etc. In fact the _only_ major difference between them was that they were unique (and didn't correlate to an entity).
Separate resources also had the downside of requiring a separate set of access controls, which meant the parallel executor needed to compare more bitsets per system and manage more state.
I initially got the "separate resources" idea from `legion`. I think that design was motivated by the fact that it made the direct world query/resource lifetime interactions more manageable. It certainly made our lives easier when using Resources alongside hecs/bevy_ecs. However we already have a construct for safely and ergonomically managing in-world lifetimes: systems (which use `Access<T>` internally).
This pr merges Resources into World:
```rust
world.insert_resource(1);
world.insert_resource(2.0);
let a = world.get_resource::<i32>().unwrap();
let mut b = world.get_resource_mut::<f64>().unwrap();
*b = 3.0;
```
Resources are now just a special kind of component. They have their own ComponentIds (and their own resource TypeId->ComponentId scope, so they don't conflict wit components of the same type). They are stored in a special "resource archetype", which stores components inside the archetype using a new `unique_components` sparse set (note that this sparse set could later be used to implement Tags). This allows us to keep the code size small by reusing existing datastructures (namely Column, Archetype, ComponentFlags, and ComponentInfo). This allows us the executor to use a single `Access<ArchetypeComponentId>` per system. It should also make scripting language integration easier.
_But_ this merge did create problems for people directly interacting with `World`. What if you need mutable access to multiple resources at the same time? `world.get_resource_mut()` borrows World mutably!
## WorldCell
WorldCell applies the `Access<ArchetypeComponentId>` concept to direct world access:
```rust
let world_cell = world.cell();
let a = world_cell.get_resource_mut::<i32>().unwrap();
let b = world_cell.get_resource_mut::<f64>().unwrap();
```
This adds cheap runtime checks (a sparse set lookup of `ArchetypeComponentId` and a counter) to ensure that world accesses do not conflict with each other. Each operation returns a `WorldBorrow<'w, T>` or `WorldBorrowMut<'w, T>` wrapper type, which will release the relevant ArchetypeComponentId resources when dropped.
World caches the access sparse set (and only one cell can exist at a time), so `world.cell()` is a cheap operation.
WorldCell does _not_ use atomic operations. It is non-send, does a mutable borrow of world to prevent other accesses, and uses a simple `Rc<RefCell<ArchetypeComponentAccess>>` wrapper in each WorldBorrow pointer.
The api is currently limited to resource access, but it can and should be extended to queries / entity component access.
## Resource Scopes
WorldCell does not yet support component queries, and even when it does there are sometimes legitimate reasons to want a mutable world ref _and_ a mutable resource ref (ex: bevy_render and bevy_scene both need this). In these cases we could always drop down to the unsafe `world.get_resource_unchecked_mut()`, but that is not ideal!
Instead developers can use a "resource scope"
```rust
world.resource_scope(|world: &mut World, a: &mut A| {
})
```
This temporarily removes the `A` resource from `World`, provides mutable pointers to both, and re-adds A to World when finished. Thanks to the move to ComponentIds/sparse sets, this is a cheap operation.
If multiple resources are required, scopes can be nested. We could also consider adding a "resource tuple" to the api if this pattern becomes common and the boilerplate gets nasty.
## Query Conflicts Use ComponentId Instead of ArchetypeComponentId
For safety reasons, systems cannot contain queries that conflict with each other without wrapping them in a QuerySet. On bevy `main`, we use ArchetypeComponentIds to determine conflicts. This is nice because it can take into account filters:
```rust
// these queries will never conflict due to their filters
fn filter_system(a: Query<&mut A, With<B>>, b: Query<&mut B, Without<B>>) {
}
```
But it also has a significant downside:
```rust
// these queries will not conflict _until_ an entity with A, B, and C is spawned
fn maybe_conflicts_system(a: Query<(&mut A, &C)>, b: Query<(&mut A, &B)>) {
}
```
The system above will panic at runtime if an entity with A, B, and C is spawned. This makes it hard to trust that your game logic will run without crashing.
In this pr, I switched to using `ComponentId` instead. This _is_ more constraining. `maybe_conflicts_system` will now always fail, but it will do it consistently at startup. Naively, it would also _disallow_ `filter_system`, which would be a significant downgrade in usability. Bevy has a number of internal systems that rely on disjoint queries and I expect it to be a common pattern in userspace.
To resolve this, I added a new `FilteredAccess<T>` type, which wraps `Access<T>` and adds with/without filters. If two `FilteredAccess` have with/without values that prove they are disjoint, they will no longer conflict.
## EntityRef / EntityMut
World entity operations on `main` require that the user passes in an `entity` id to each operation:
```rust
let entity = world.spawn((A, )); // create a new entity with A
world.get::<A>(entity);
world.insert(entity, (B, C));
world.insert_one(entity, D);
```
This means that each operation needs to look up the entity location / verify its validity. The initial spawn operation also requires a Bundle as input. This can be awkward when no components are required (or one component is required).
These operations have been replaced by `EntityRef` and `EntityMut`, which are "builder-style" wrappers around world that provide read and read/write operations on a single, pre-validated entity:
```rust
// spawn now takes no inputs and returns an EntityMut
let entity = world.spawn()
.insert(A) // insert a single component into the entity
.insert_bundle((B, C)) // insert a bundle of components into the entity
.id() // id returns the Entity id
// Returns EntityMut (or panics if the entity does not exist)
world.entity_mut(entity)
.insert(D)
.insert_bundle(SomeBundle::default());
{
// returns EntityRef (or panics if the entity does not exist)
let d = world.entity(entity)
.get::<D>() // gets the D component
.unwrap();
// world.get still exists for ergonomics
let d = world.get::<D>(entity).unwrap();
}
// These variants return Options if you want to check existence instead of panicing
world.get_entity_mut(entity)
.unwrap()
.insert(E);
if let Some(entity_ref) = world.get_entity(entity) {
let d = entity_ref.get::<D>().unwrap();
}
```
This _does not_ affect the current Commands api or terminology. I think that should be a separate conversation as that is a much larger breaking change.
## Safety Improvements
* Entity reservation in Commands uses a normal world borrow instead of an unsafe transmute
* QuerySets no longer transmutes lifetimes
* Made traits "unsafe" when implementing a trait incorrectly could cause unsafety
* More thorough safety docs
## RemovedComponents SystemParam
The old approach to querying removed components: `query.removed:<T>()` was confusing because it had no connection to the query itself. I replaced it with the following, which is both clearer and allows us to cache the ComponentId mapping in the SystemParamState:
```rust
fn system(removed: RemovedComponents<T>) {
for entity in removed.iter() {
}
}
```
## Simpler Bundle implementation
Bundles are no longer responsible for sorting (or deduping) TypeInfo. They are just a simple ordered list of component types / data. This makes the implementation smaller and opens the door to an easy "nested bundle" implementation in the future (which i might even add in this pr). Duplicate detection is now done once per bundle type by World the first time a bundle is used.
## Unified WorldQuery and QueryFilter types
(don't worry they are still separate type _parameters_ in Queries .. this is a non-breaking change)
WorldQuery and QueryFilter were already basically identical apis. With the addition of `FetchState` and more storage-specific fetch methods, the overlap was even clearer (and the redundancy more painful).
QueryFilters are now just `F: WorldQuery where F::Fetch: FilterFetch`. FilterFetch requires `Fetch<Item = bool>` and adds new "short circuit" variants of fetch methods. This enables a filter tuple like `(With<A>, Without<B>, Changed<C>)` to stop evaluating the filter after the first mismatch is encountered. FilterFetch is automatically implemented for `Fetch` implementations that return bool.
This forces fetch implementations that return things like `(bool, bool, bool)` (such as the filter above) to manually implement FilterFetch and decide whether or not to short-circuit.
## More Granular Modules
World no longer globs all of the internal modules together. It now exports `core`, `system`, and `schedule` separately. I'm also considering exporting `core` submodules directly as that is still pretty "glob-ey" and unorganized (feedback welcome here).
## Remaining Draft Work (to be done in this pr)
* ~~panic on conflicting WorldQuery fetches (&A, &mut A)~~
* ~~bevy `main` and hecs both currently allow this, but we should protect against it if possible~~
* ~~batch_iter / par_iter (currently stubbed out)~~
* ~~ChangedRes~~
* ~~I skipped this while we sort out #1313. This pr should be adapted to account for whatever we land on there~~.
* ~~The `Archetypes` and `Tables` collections use hashes of sorted lists of component ids to uniquely identify each archetype/table. This hash is then used as the key in a HashMap to look up the relevant ArchetypeId or TableId. (which doesn't handle hash collisions properly)~~
* ~~It is currently unsafe to generate a Query from "World A", then use it on "World B" (despite the api claiming it is safe). We should probably close this gap. This could be done by adding a randomly generated WorldId to each world, then storing that id in each Query. They could then be compared to each other on each `query.do_thing(&world)` operation. This _does_ add an extra branch to each query operation, so I'm open to other suggestions if people have them.~~
* ~~Nested Bundles (if i find time)~~
## Potential Future Work
* Expand WorldCell to support queries.
* Consider not allocating in the empty archetype on `world.spawn()`
* ex: return something like EntityMutUninit, which turns into EntityMut after an `insert` or `insert_bundle` op
* this actually regressed performance last time i tried it, but in theory it should be faster
* Optimize SparseSet::insert (see `PERF` comment on insert)
* Replace SparseArray `Option<T>` with T::MAX to cut down on branching
* would enable cheaper get_unchecked() operations
* upstream fixedbitset optimizations
* fixedbitset could be allocation free for small block counts (store blocks in a SmallVec)
* fixedbitset could have a const constructor
* Consider implementing Tags (archetype-specific by-value data that affects archetype identity)
* ex: ArchetypeA could have `[A, B, C]` table components and `[D(1)]` "tag" component. ArchetypeB could have `[A, B, C]` table components and a `[D(2)]` tag component. The archetypes are different, despite both having D tags because the value inside D is different.
* this could potentially build on top of the `archetype.unique_components` added in this pr for resource storage.
* Consider reverting `all_tuples` proc macro in favor of the old `macro_rules` implementation
* all_tuples is more flexible and produces cleaner documentation (the macro_rules version produces weird type parameter orders due to parser constraints)
* but unfortunately all_tuples also appears to make Rust Analyzer sad/slow when working inside of `bevy_ecs` (does not affect user code)
* Consider "resource queries" and/or "mixed resource and entity component queries" as an alternative to WorldCell
* this is basically just "systems" so maybe it's not worth it
* Add more world ops
* `world.clear()`
* `world.reserve<T: Bundle>(count: usize)`
* Try using the old archetype allocation strategy (allocate new memory on resize and copy everything over). I expect this to improve batch insertion performance at the cost of unbatched performance. But thats just a guess. I'm not an allocation perf pro :)
* Adapt Commands apis for consistency with new World apis
## Benchmarks
key:
* `bevy_old`: bevy `main` branch
* `bevy`: this branch
* `_foreach`: uses an optimized for_each iterator
* ` _sparse`: uses sparse set storage (if unspecified assume table storage)
* `_system`: runs inside a system (if unspecified assume test happens via direct world ops)
### Simple Insert (from ecs_bench_suite)
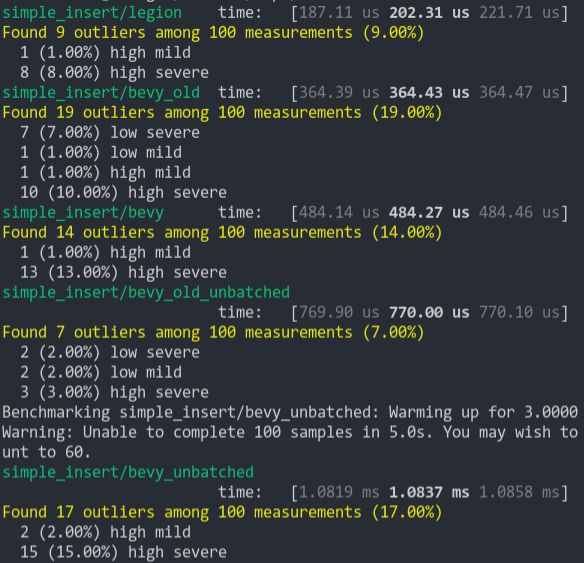
### Simpler Iter (from ecs_bench_suite)
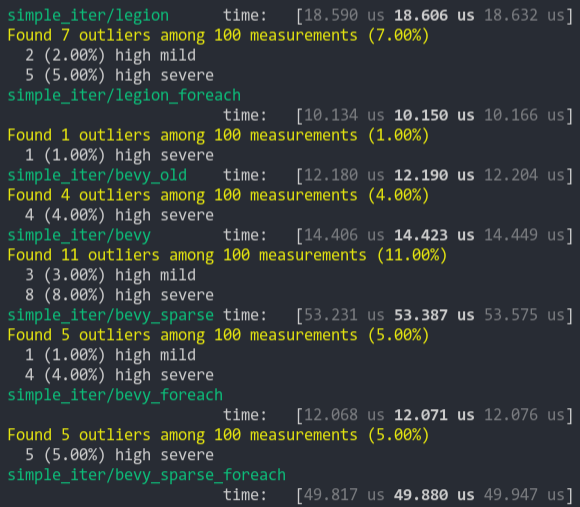
### Fragment Iter (from ecs_bench_suite)
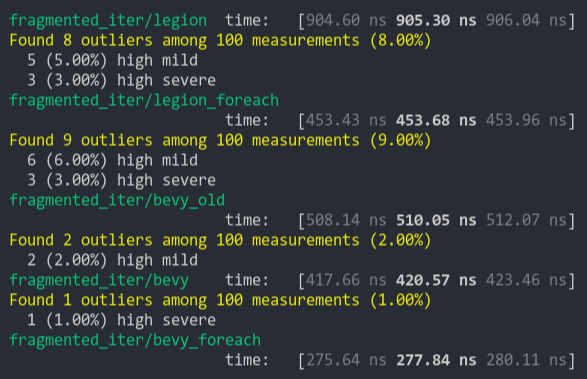
### Sparse Fragmented Iter
Iterate a query that matches 5 entities from a single matching archetype, but there are 100 unmatching archetypes
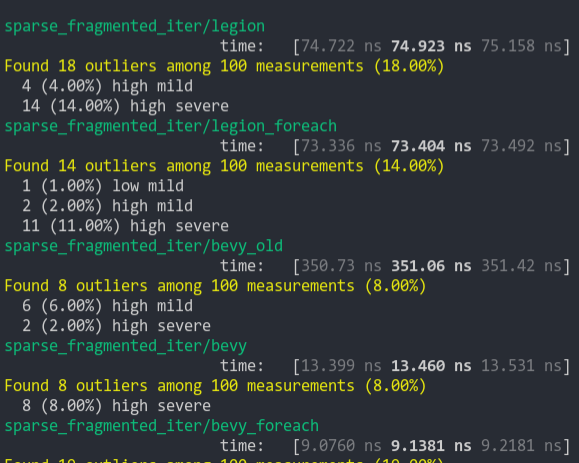
### Schedule (from ecs_bench_suite)
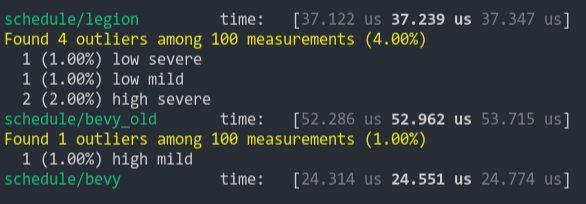
### Add Remove Component (from ecs_bench_suite)
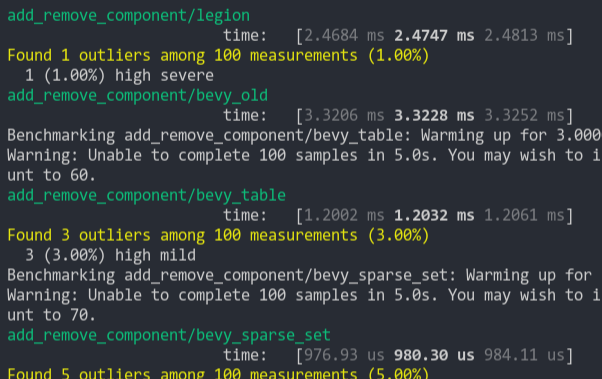
### Add Remove Component Big
Same as the test above, but each entity has 5 "large" matrix components and 1 "large" matrix component is added and removed
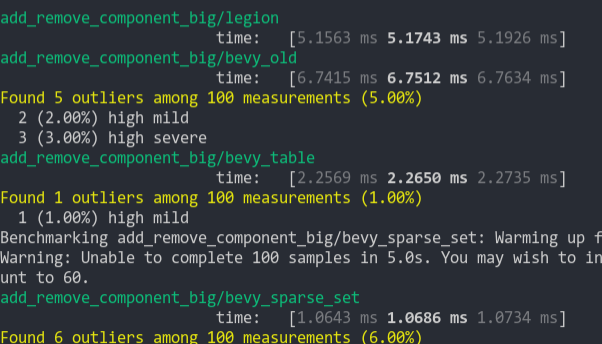
### Get Component
Looks up a single component value a large number of times
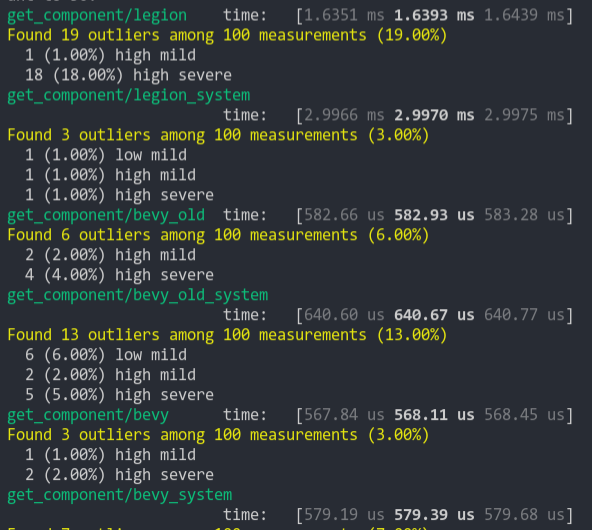
2021-03-05 07:54:35 +00:00
std ::any ::type_name ::< Q > ( ) ,
std ::any ::type_name ::< F > ( ) ,
Add new SystemState and rename old SystemState to SystemMeta (#2283)
This enables `SystemParams` to be used outside of function systems. Anything can create and store `SystemState`, which enables efficient "param state cached" access to `SystemParams`.
It adds a `ReadOnlySystemParamFetch` trait, which enables safe `SystemState::get` calls without unique world access.
I renamed the old `SystemState` to `SystemMeta` to enable us to mirror the `QueryState` naming convention (but I'm happy to discuss alternative names if people have other ideas). I initially pitched this as `ParamState`, but given that it needs to include full system metadata, that doesn't feel like a particularly accurate name.
```rust
#[derive(Eq, PartialEq, Debug)]
struct A(usize);
#[derive(Eq, PartialEq, Debug)]
struct B(usize);
let mut world = World::default();
world.insert_resource(A(42));
world.spawn().insert(B(7));
// we get nice lifetime elision when declaring the type on the left hand side
let mut system_state: SystemState<(Res<A>, Query<&B>)> = SystemState::new(&mut world);
let (a, query) = system_state.get(&world);
assert_eq!(*a, A(42), "returned resource matches initial value");
assert_eq!(
*query.single().unwrap(),
B(7),
"returned component matches initial value"
);
// mutable system params require unique world access
let mut system_state: SystemState<(ResMut<A>, Query<&mut B>)> = SystemState::new(&mut world);
let (a, query) = system_state.get_mut(&mut world);
// static lifetimes are required when declaring inside of structs
struct SomeContainer {
state: SystemState<(Res<'static, A>, Res<'static, B>)>
}
// this can be shortened using type aliases, which will be useful for complex param tuples
type MyParams<'a> = (Res<'a, A>, Res<'a, B>);
struct SomeContainer {
state: SystemState<MyParams<'static>>
}
// It is the user's responsibility to call SystemState::apply(world) for parameters that queue up work
let mut system_state: SystemState<(Commands, Query<&B>)> = SystemState::new(&mut world);
{
let (mut commands, query) = system_state.get(&world);
commands.insert_resource(3.14);
}
system_state.apply(&mut world);
```
## Future Work
* Actually use SystemState inside FunctionSystem. This would be trivial, but it requires FunctionSystem to wrap SystemState in Option in its current form (which complicates system metadata lookup). I'd prefer to hold off until we adopt something like the later designs linked in #1364, which enable us to contruct Systems using a World reference (and also remove the need for `.system`).
* Consider a "scoped" approach to automatically call SystemState::apply when systems params are no longer being used (either a container type with a Drop impl, or a function that takes a closure for user logic operating on params).
2021-06-02 19:57:38 +00:00
& system_meta . component_access_set ,
Bevy ECS V2 (#1525)
# Bevy ECS V2
This is a rewrite of Bevy ECS (basically everything but the new executor/schedule, which are already awesome). The overall goal was to improve the performance and versatility of Bevy ECS. Here is a quick bulleted list of changes before we dive into the details:
* Complete World rewrite
* Multiple component storage types:
* Tables: fast cache friendly iteration, slower add/removes (previously called Archetypes)
* Sparse Sets: fast add/remove, slower iteration
* Stateful Queries (caches query results for faster iteration. fragmented iteration is _fast_ now)
* Stateful System Params (caches expensive operations. inspired by @DJMcNab's work in #1364)
* Configurable System Params (users can set configuration when they construct their systems. once again inspired by @DJMcNab's work)
* Archetypes are now "just metadata", component storage is separate
* Archetype Graph (for faster archetype changes)
* Component Metadata
* Configure component storage type
* Retrieve information about component size/type/name/layout/send-ness/etc
* Components are uniquely identified by a densely packed ComponentId
* TypeIds are now totally optional (which should make implementing scripting easier)
* Super fast "for_each" query iterators
* Merged Resources into World. Resources are now just a special type of component
* EntityRef/EntityMut builder apis (more efficient and more ergonomic)
* Fast bitset-backed `Access<T>` replaces old hashmap-based approach everywhere
* Query conflicts are determined by component access instead of archetype component access (to avoid random failures at runtime)
* With/Without are still taken into account for conflicts, so this should still be comfy to use
* Much simpler `IntoSystem` impl
* Significantly reduced the amount of hashing throughout the ecs in favor of Sparse Sets (indexed by densely packed ArchetypeId, ComponentId, BundleId, and TableId)
* Safety Improvements
* Entity reservation uses a normal world reference instead of unsafe transmute
* QuerySets no longer transmute lifetimes
* Made traits "unsafe" where relevant
* More thorough safety docs
* WorldCell
* Exposes safe mutable access to multiple resources at a time in a World
* Replaced "catch all" `System::update_archetypes(world: &World)` with `System::new_archetype(archetype: &Archetype)`
* Simpler Bundle implementation
* Replaced slow "remove_bundle_one_by_one" used as fallback for Commands::remove_bundle with fast "remove_bundle_intersection"
* Removed `Mut<T>` query impl. it is better to only support one way: `&mut T`
* Removed with() from `Flags<T>` in favor of `Option<Flags<T>>`, which allows querying for flags to be "filtered" by default
* Components now have is_send property (currently only resources support non-send)
* More granular module organization
* New `RemovedComponents<T>` SystemParam that replaces `query.removed::<T>()`
* `world.resource_scope()` for mutable access to resources and world at the same time
* WorldQuery and QueryFilter traits unified. FilterFetch trait added to enable "short circuit" filtering. Auto impled for cases that don't need it
* Significantly slimmed down SystemState in favor of individual SystemParam state
* System Commands changed from `commands: &mut Commands` back to `mut commands: Commands` (to allow Commands to have a World reference)
Fixes #1320
## `World` Rewrite
This is a from-scratch rewrite of `World` that fills the niche that `hecs` used to. Yes, this means Bevy ECS is no longer a "fork" of hecs. We're going out our own!
(the only shared code between the projects is the entity id allocator, which is already basically ideal)
A huge shout out to @SanderMertens (author of [flecs](https://github.com/SanderMertens/flecs)) for sharing some great ideas with me (specifically hybrid ecs storage and archetype graphs). He also helped advise on a number of implementation details.
## Component Storage (The Problem)
Two ECS storage paradigms have gained a lot of traction over the years:
* **Archetypal ECS**:
* Stores components in "tables" with static schemas. Each "column" stores components of a given type. Each "row" is an entity.
* Each "archetype" has its own table. Adding/removing an entity's component changes the archetype.
* Enables super-fast Query iteration due to its cache-friendly data layout
* Comes at the cost of more expensive add/remove operations for an Entity's components, because all components need to be copied to the new archetype's "table"
* **Sparse Set ECS**:
* Stores components of the same type in densely packed arrays, which are sparsely indexed by densely packed unsigned integers (Entity ids)
* Query iteration is slower than Archetypal ECS because each entity's component could be at any position in the sparse set. This "random access" pattern isn't cache friendly. Additionally, there is an extra layer of indirection because you must first map the entity id to an index in the component array.
* Adding/removing components is a cheap, constant time operation
Bevy ECS V1, hecs, legion, flec, and Unity DOTS are all "archetypal ecs-es". I personally think "archetypal" storage is a good default for game engines. An entity's archetype doesn't need to change frequently in general, and it creates "fast by default" query iteration (which is a much more common operation). It is also "self optimizing". Users don't need to think about optimizing component layouts for iteration performance. It "just works" without any extra boilerplate.
Shipyard and EnTT are "sparse set ecs-es". They employ "packing" as a way to work around the "suboptimal by default" iteration performance for specific sets of components. This helps, but I didn't think this was a good choice for a general purpose engine like Bevy because:
1. "packs" conflict with each other. If bevy decides to internally pack the Transform and GlobalTransform components, users are then blocked if they want to pack some custom component with Transform.
2. users need to take manual action to optimize
Developers selecting an ECS framework are stuck with a hard choice. Select an "archetypal" framework with "fast iteration everywhere" but without the ability to cheaply add/remove components, or select a "sparse set" framework to cheaply add/remove components but with slower iteration performance.
## Hybrid Component Storage (The Solution)
In Bevy ECS V2, we get to have our cake and eat it too. It now has _both_ of the component storage types above (and more can be added later if needed):
* **Tables** (aka "archetypal" storage)
* The default storage. If you don't configure anything, this is what you get
* Fast iteration by default
* Slower add/remove operations
* **Sparse Sets**
* Opt-in
* Slower iteration
* Faster add/remove operations
These storage types complement each other perfectly. By default Query iteration is fast. If developers know that they want to add/remove a component at high frequencies, they can set the storage to "sparse set":
```rust
world.register_component(
ComponentDescriptor::new::<MyComponent>(StorageType::SparseSet)
).unwrap();
```
## Archetypes
Archetypes are now "just metadata" ... they no longer store components directly. They do store:
* The `ComponentId`s of each of the Archetype's components (and that component's storage type)
* Archetypes are uniquely defined by their component layouts
* For example: entities with "table" components `[A, B, C]` _and_ "sparse set" components `[D, E]` will always be in the same archetype.
* The `TableId` associated with the archetype
* For now each archetype has exactly one table (which can have no components),
* There is a 1->Many relationship from Tables->Archetypes. A given table could have any number of archetype components stored in it:
* Ex: an entity with "table storage" components `[A, B, C]` and "sparse set" components `[D, E]` will share the same `[A, B, C]` table as an entity with `[A, B, C]` table component and `[F]` sparse set components.
* This 1->Many relationship is how we preserve fast "cache friendly" iteration performance when possible (more on this later)
* A list of entities that are in the archetype and the row id of the table they are in
* ArchetypeComponentIds
* unique densely packed identifiers for (ArchetypeId, ComponentId) pairs
* used by the schedule executor for cheap system access control
* "Archetype Graph Edges" (see the next section)
## The "Archetype Graph"
Archetype changes in Bevy (and a number of other archetypal ecs-es) have historically been expensive to compute. First, you need to allocate a new vector of the entity's current component ids, add or remove components based on the operation performed, sort it (to ensure it is order-independent), then hash it to find the archetype (if it exists). And thats all before we get to the _already_ expensive full copy of all components to the new table storage.
The solution is to build a "graph" of archetypes to cache these results. @SanderMertens first exposed me to the idea (and he got it from @gjroelofs, who came up with it). They propose adding directed edges between archetypes for add/remove component operations. If `ComponentId`s are densely packed, you can use sparse sets to cheaply jump between archetypes.
Bevy takes this one step further by using add/remove `Bundle` edges instead of `Component` edges. Bevy encourages the use of `Bundles` to group add/remove operations. This is largely for "clearer game logic" reasons, but it also helps cut down on the number of archetype changes required. `Bundles` now also have densely-packed `BundleId`s. This allows us to use a _single_ edge for each bundle operation (rather than needing to traverse N edges ... one for each component). Single component operations are also bundles, so this is strictly an improvement over a "component only" graph.
As a result, an operation that used to be _heavy_ (both for allocations and compute) is now two dirt-cheap array lookups and zero allocations.
## Stateful Queries
World queries are now stateful. This allows us to:
1. Cache archetype (and table) matches
* This resolves another issue with (naive) archetypal ECS: query performance getting worse as the number of archetypes goes up (and fragmentation occurs).
2. Cache Fetch and Filter state
* The expensive parts of fetch/filter operations (such as hashing the TypeId to find the ComponentId) now only happen once when the Query is first constructed
3. Incrementally build up state
* When new archetypes are added, we only process the new archetypes (no need to rebuild state for old archetypes)
As a result, the direct `World` query api now looks like this:
```rust
let mut query = world.query::<(&A, &mut B)>();
for (a, mut b) in query.iter_mut(&mut world) {
}
```
Requiring `World` to generate stateful queries (rather than letting the `QueryState` type be constructed separately) allows us to ensure that _all_ queries are properly initialized (and the relevant world state, such as ComponentIds). This enables QueryState to remove branches from its operations that check for initialization status (and also enables query.iter() to take an immutable world reference because it doesn't need to initialize anything in world).
However in systems, this is a non-breaking change. State management is done internally by the relevant SystemParam.
## Stateful SystemParams
Like Queries, `SystemParams` now also cache state. For example, `Query` system params store the "stateful query" state mentioned above. Commands store their internal `CommandQueue`. This means you can now safely use as many separate `Commands` parameters in your system as you want. `Local<T>` system params store their `T` value in their state (instead of in Resources).
SystemParam state also enabled a significant slim-down of SystemState. It is much nicer to look at now.
Per-SystemParam state naturally insulates us from an "aliased mut" class of errors we have hit in the past (ex: using multiple `Commands` system params).
(credit goes to @DJMcNab for the initial idea and draft pr here #1364)
## Configurable SystemParams
@DJMcNab also had the great idea to make SystemParams configurable. This allows users to provide some initial configuration / values for system parameters (when possible). Most SystemParams have no config (the config type is `()`), but the `Local<T>` param now supports user-provided parameters:
```rust
fn foo(value: Local<usize>) {
}
app.add_system(foo.system().config(|c| c.0 = Some(10)));
```
## Uber Fast "for_each" Query Iterators
Developers now have the choice to use a fast "for_each" iterator, which yields ~1.5-3x iteration speed improvements for "fragmented iteration", and minor ~1.2x iteration speed improvements for unfragmented iteration.
```rust
fn system(query: Query<(&A, &mut B)>) {
// you now have the option to do this for a speed boost
query.for_each_mut(|(a, mut b)| {
});
// however normal iterators are still available
for (a, mut b) in query.iter_mut() {
}
}
```
I think in most cases we should continue to encourage "normal" iterators as they are more flexible and more "rust idiomatic". But when that extra "oomf" is needed, it makes sense to use `for_each`.
We should also consider using `for_each` for internal bevy systems to give our users a nice speed boost (but that should be a separate pr).
## Component Metadata
`World` now has a `Components` collection, which is accessible via `world.components()`. This stores mappings from `ComponentId` to `ComponentInfo`, as well as `TypeId` to `ComponentId` mappings (where relevant). `ComponentInfo` stores information about the component, such as ComponentId, TypeId, memory layout, send-ness (currently limited to resources), and storage type.
## Significantly Cheaper `Access<T>`
We used to use `TypeAccess<TypeId>` to manage read/write component/archetype-component access. This was expensive because TypeIds must be hashed and compared individually. The parallel executor got around this by "condensing" type ids into bitset-backed access types. This worked, but it had to be re-generated from the `TypeAccess<TypeId>`sources every time archetypes changed.
This pr removes TypeAccess in favor of faster bitset access everywhere. We can do this thanks to the move to densely packed `ComponentId`s and `ArchetypeComponentId`s.
## Merged Resources into World
Resources had a lot of redundant functionality with Components. They stored typed data, they had access control, they had unique ids, they were queryable via SystemParams, etc. In fact the _only_ major difference between them was that they were unique (and didn't correlate to an entity).
Separate resources also had the downside of requiring a separate set of access controls, which meant the parallel executor needed to compare more bitsets per system and manage more state.
I initially got the "separate resources" idea from `legion`. I think that design was motivated by the fact that it made the direct world query/resource lifetime interactions more manageable. It certainly made our lives easier when using Resources alongside hecs/bevy_ecs. However we already have a construct for safely and ergonomically managing in-world lifetimes: systems (which use `Access<T>` internally).
This pr merges Resources into World:
```rust
world.insert_resource(1);
world.insert_resource(2.0);
let a = world.get_resource::<i32>().unwrap();
let mut b = world.get_resource_mut::<f64>().unwrap();
*b = 3.0;
```
Resources are now just a special kind of component. They have their own ComponentIds (and their own resource TypeId->ComponentId scope, so they don't conflict wit components of the same type). They are stored in a special "resource archetype", which stores components inside the archetype using a new `unique_components` sparse set (note that this sparse set could later be used to implement Tags). This allows us to keep the code size small by reusing existing datastructures (namely Column, Archetype, ComponentFlags, and ComponentInfo). This allows us the executor to use a single `Access<ArchetypeComponentId>` per system. It should also make scripting language integration easier.
_But_ this merge did create problems for people directly interacting with `World`. What if you need mutable access to multiple resources at the same time? `world.get_resource_mut()` borrows World mutably!
## WorldCell
WorldCell applies the `Access<ArchetypeComponentId>` concept to direct world access:
```rust
let world_cell = world.cell();
let a = world_cell.get_resource_mut::<i32>().unwrap();
let b = world_cell.get_resource_mut::<f64>().unwrap();
```
This adds cheap runtime checks (a sparse set lookup of `ArchetypeComponentId` and a counter) to ensure that world accesses do not conflict with each other. Each operation returns a `WorldBorrow<'w, T>` or `WorldBorrowMut<'w, T>` wrapper type, which will release the relevant ArchetypeComponentId resources when dropped.
World caches the access sparse set (and only one cell can exist at a time), so `world.cell()` is a cheap operation.
WorldCell does _not_ use atomic operations. It is non-send, does a mutable borrow of world to prevent other accesses, and uses a simple `Rc<RefCell<ArchetypeComponentAccess>>` wrapper in each WorldBorrow pointer.
The api is currently limited to resource access, but it can and should be extended to queries / entity component access.
## Resource Scopes
WorldCell does not yet support component queries, and even when it does there are sometimes legitimate reasons to want a mutable world ref _and_ a mutable resource ref (ex: bevy_render and bevy_scene both need this). In these cases we could always drop down to the unsafe `world.get_resource_unchecked_mut()`, but that is not ideal!
Instead developers can use a "resource scope"
```rust
world.resource_scope(|world: &mut World, a: &mut A| {
})
```
This temporarily removes the `A` resource from `World`, provides mutable pointers to both, and re-adds A to World when finished. Thanks to the move to ComponentIds/sparse sets, this is a cheap operation.
If multiple resources are required, scopes can be nested. We could also consider adding a "resource tuple" to the api if this pattern becomes common and the boilerplate gets nasty.
## Query Conflicts Use ComponentId Instead of ArchetypeComponentId
For safety reasons, systems cannot contain queries that conflict with each other without wrapping them in a QuerySet. On bevy `main`, we use ArchetypeComponentIds to determine conflicts. This is nice because it can take into account filters:
```rust
// these queries will never conflict due to their filters
fn filter_system(a: Query<&mut A, With<B>>, b: Query<&mut B, Without<B>>) {
}
```
But it also has a significant downside:
```rust
// these queries will not conflict _until_ an entity with A, B, and C is spawned
fn maybe_conflicts_system(a: Query<(&mut A, &C)>, b: Query<(&mut A, &B)>) {
}
```
The system above will panic at runtime if an entity with A, B, and C is spawned. This makes it hard to trust that your game logic will run without crashing.
In this pr, I switched to using `ComponentId` instead. This _is_ more constraining. `maybe_conflicts_system` will now always fail, but it will do it consistently at startup. Naively, it would also _disallow_ `filter_system`, which would be a significant downgrade in usability. Bevy has a number of internal systems that rely on disjoint queries and I expect it to be a common pattern in userspace.
To resolve this, I added a new `FilteredAccess<T>` type, which wraps `Access<T>` and adds with/without filters. If two `FilteredAccess` have with/without values that prove they are disjoint, they will no longer conflict.
## EntityRef / EntityMut
World entity operations on `main` require that the user passes in an `entity` id to each operation:
```rust
let entity = world.spawn((A, )); // create a new entity with A
world.get::<A>(entity);
world.insert(entity, (B, C));
world.insert_one(entity, D);
```
This means that each operation needs to look up the entity location / verify its validity. The initial spawn operation also requires a Bundle as input. This can be awkward when no components are required (or one component is required).
These operations have been replaced by `EntityRef` and `EntityMut`, which are "builder-style" wrappers around world that provide read and read/write operations on a single, pre-validated entity:
```rust
// spawn now takes no inputs and returns an EntityMut
let entity = world.spawn()
.insert(A) // insert a single component into the entity
.insert_bundle((B, C)) // insert a bundle of components into the entity
.id() // id returns the Entity id
// Returns EntityMut (or panics if the entity does not exist)
world.entity_mut(entity)
.insert(D)
.insert_bundle(SomeBundle::default());
{
// returns EntityRef (or panics if the entity does not exist)
let d = world.entity(entity)
.get::<D>() // gets the D component
.unwrap();
// world.get still exists for ergonomics
let d = world.get::<D>(entity).unwrap();
}
// These variants return Options if you want to check existence instead of panicing
world.get_entity_mut(entity)
.unwrap()
.insert(E);
if let Some(entity_ref) = world.get_entity(entity) {
let d = entity_ref.get::<D>().unwrap();
}
```
This _does not_ affect the current Commands api or terminology. I think that should be a separate conversation as that is a much larger breaking change.
## Safety Improvements
* Entity reservation in Commands uses a normal world borrow instead of an unsafe transmute
* QuerySets no longer transmutes lifetimes
* Made traits "unsafe" when implementing a trait incorrectly could cause unsafety
* More thorough safety docs
## RemovedComponents SystemParam
The old approach to querying removed components: `query.removed:<T>()` was confusing because it had no connection to the query itself. I replaced it with the following, which is both clearer and allows us to cache the ComponentId mapping in the SystemParamState:
```rust
fn system(removed: RemovedComponents<T>) {
for entity in removed.iter() {
}
}
```
## Simpler Bundle implementation
Bundles are no longer responsible for sorting (or deduping) TypeInfo. They are just a simple ordered list of component types / data. This makes the implementation smaller and opens the door to an easy "nested bundle" implementation in the future (which i might even add in this pr). Duplicate detection is now done once per bundle type by World the first time a bundle is used.
## Unified WorldQuery and QueryFilter types
(don't worry they are still separate type _parameters_ in Queries .. this is a non-breaking change)
WorldQuery and QueryFilter were already basically identical apis. With the addition of `FetchState` and more storage-specific fetch methods, the overlap was even clearer (and the redundancy more painful).
QueryFilters are now just `F: WorldQuery where F::Fetch: FilterFetch`. FilterFetch requires `Fetch<Item = bool>` and adds new "short circuit" variants of fetch methods. This enables a filter tuple like `(With<A>, Without<B>, Changed<C>)` to stop evaluating the filter after the first mismatch is encountered. FilterFetch is automatically implemented for `Fetch` implementations that return bool.
This forces fetch implementations that return things like `(bool, bool, bool)` (such as the filter above) to manually implement FilterFetch and decide whether or not to short-circuit.
## More Granular Modules
World no longer globs all of the internal modules together. It now exports `core`, `system`, and `schedule` separately. I'm also considering exporting `core` submodules directly as that is still pretty "glob-ey" and unorganized (feedback welcome here).
## Remaining Draft Work (to be done in this pr)
* ~~panic on conflicting WorldQuery fetches (&A, &mut A)~~
* ~~bevy `main` and hecs both currently allow this, but we should protect against it if possible~~
* ~~batch_iter / par_iter (currently stubbed out)~~
* ~~ChangedRes~~
* ~~I skipped this while we sort out #1313. This pr should be adapted to account for whatever we land on there~~.
* ~~The `Archetypes` and `Tables` collections use hashes of sorted lists of component ids to uniquely identify each archetype/table. This hash is then used as the key in a HashMap to look up the relevant ArchetypeId or TableId. (which doesn't handle hash collisions properly)~~
* ~~It is currently unsafe to generate a Query from "World A", then use it on "World B" (despite the api claiming it is safe). We should probably close this gap. This could be done by adding a randomly generated WorldId to each world, then storing that id in each Query. They could then be compared to each other on each `query.do_thing(&world)` operation. This _does_ add an extra branch to each query operation, so I'm open to other suggestions if people have them.~~
* ~~Nested Bundles (if i find time)~~
## Potential Future Work
* Expand WorldCell to support queries.
* Consider not allocating in the empty archetype on `world.spawn()`
* ex: return something like EntityMutUninit, which turns into EntityMut after an `insert` or `insert_bundle` op
* this actually regressed performance last time i tried it, but in theory it should be faster
* Optimize SparseSet::insert (see `PERF` comment on insert)
* Replace SparseArray `Option<T>` with T::MAX to cut down on branching
* would enable cheaper get_unchecked() operations
* upstream fixedbitset optimizations
* fixedbitset could be allocation free for small block counts (store blocks in a SmallVec)
* fixedbitset could have a const constructor
* Consider implementing Tags (archetype-specific by-value data that affects archetype identity)
* ex: ArchetypeA could have `[A, B, C]` table components and `[D(1)]` "tag" component. ArchetypeB could have `[A, B, C]` table components and a `[D(2)]` tag component. The archetypes are different, despite both having D tags because the value inside D is different.
* this could potentially build on top of the `archetype.unique_components` added in this pr for resource storage.
* Consider reverting `all_tuples` proc macro in favor of the old `macro_rules` implementation
* all_tuples is more flexible and produces cleaner documentation (the macro_rules version produces weird type parameter orders due to parser constraints)
* but unfortunately all_tuples also appears to make Rust Analyzer sad/slow when working inside of `bevy_ecs` (does not affect user code)
* Consider "resource queries" and/or "mixed resource and entity component queries" as an alternative to WorldCell
* this is basically just "systems" so maybe it's not worth it
* Add more world ops
* `world.clear()`
* `world.reserve<T: Bundle>(count: usize)`
* Try using the old archetype allocation strategy (allocate new memory on resize and copy everything over). I expect this to improve batch insertion performance at the cost of unbatched performance. But thats just a guess. I'm not an allocation perf pro :)
* Adapt Commands apis for consistency with new World apis
## Benchmarks
key:
* `bevy_old`: bevy `main` branch
* `bevy`: this branch
* `_foreach`: uses an optimized for_each iterator
* ` _sparse`: uses sparse set storage (if unspecified assume table storage)
* `_system`: runs inside a system (if unspecified assume test happens via direct world ops)
### Simple Insert (from ecs_bench_suite)
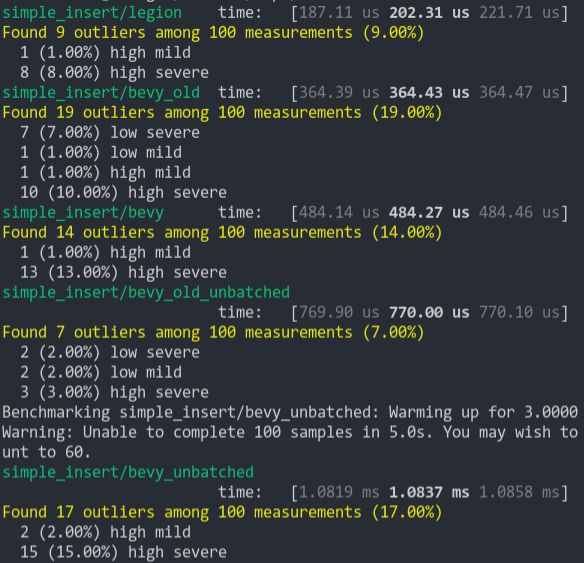
### Simpler Iter (from ecs_bench_suite)
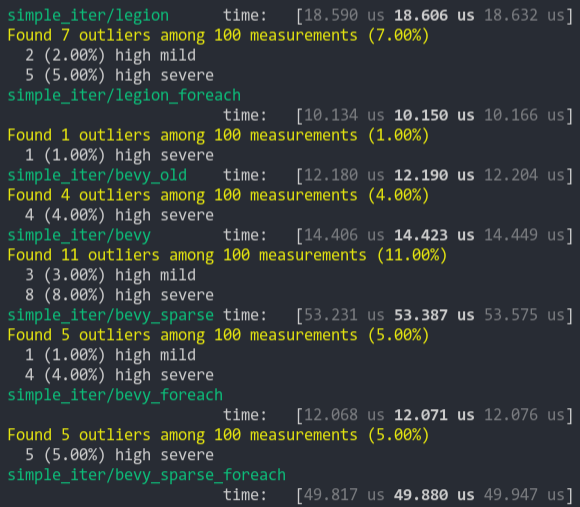
### Fragment Iter (from ecs_bench_suite)
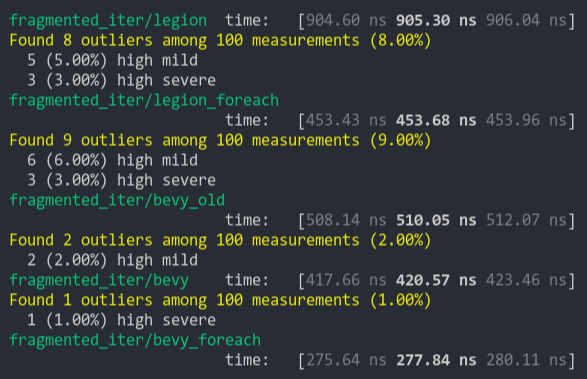
### Sparse Fragmented Iter
Iterate a query that matches 5 entities from a single matching archetype, but there are 100 unmatching archetypes
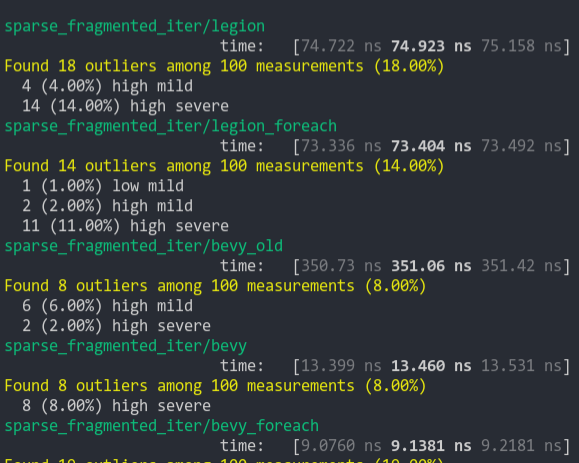
### Schedule (from ecs_bench_suite)
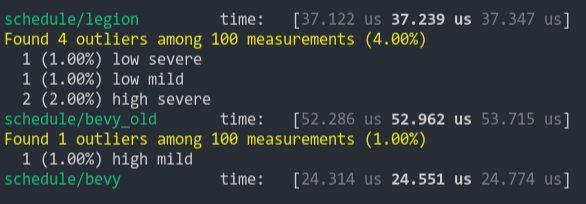
### Add Remove Component (from ecs_bench_suite)
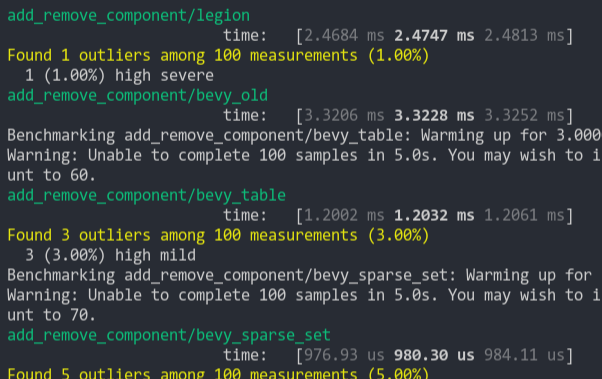
### Add Remove Component Big
Same as the test above, but each entity has 5 "large" matrix components and 1 "large" matrix component is added and removed
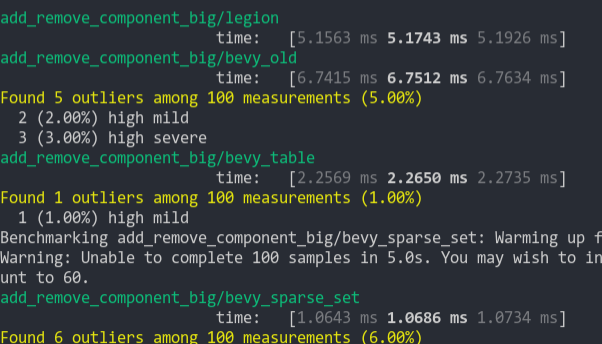
### Get Component
Looks up a single component value a large number of times
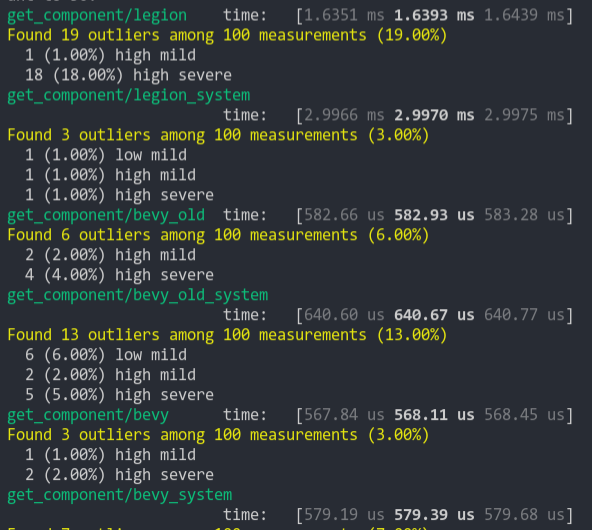
2021-03-05 07:54:35 +00:00
& state . component_access ,
world ,
) ;
Add new SystemState and rename old SystemState to SystemMeta (#2283)
This enables `SystemParams` to be used outside of function systems. Anything can create and store `SystemState`, which enables efficient "param state cached" access to `SystemParams`.
It adds a `ReadOnlySystemParamFetch` trait, which enables safe `SystemState::get` calls without unique world access.
I renamed the old `SystemState` to `SystemMeta` to enable us to mirror the `QueryState` naming convention (but I'm happy to discuss alternative names if people have other ideas). I initially pitched this as `ParamState`, but given that it needs to include full system metadata, that doesn't feel like a particularly accurate name.
```rust
#[derive(Eq, PartialEq, Debug)]
struct A(usize);
#[derive(Eq, PartialEq, Debug)]
struct B(usize);
let mut world = World::default();
world.insert_resource(A(42));
world.spawn().insert(B(7));
// we get nice lifetime elision when declaring the type on the left hand side
let mut system_state: SystemState<(Res<A>, Query<&B>)> = SystemState::new(&mut world);
let (a, query) = system_state.get(&world);
assert_eq!(*a, A(42), "returned resource matches initial value");
assert_eq!(
*query.single().unwrap(),
B(7),
"returned component matches initial value"
);
// mutable system params require unique world access
let mut system_state: SystemState<(ResMut<A>, Query<&mut B>)> = SystemState::new(&mut world);
let (a, query) = system_state.get_mut(&mut world);
// static lifetimes are required when declaring inside of structs
struct SomeContainer {
state: SystemState<(Res<'static, A>, Res<'static, B>)>
}
// this can be shortened using type aliases, which will be useful for complex param tuples
type MyParams<'a> = (Res<'a, A>, Res<'a, B>);
struct SomeContainer {
state: SystemState<MyParams<'static>>
}
// It is the user's responsibility to call SystemState::apply(world) for parameters that queue up work
let mut system_state: SystemState<(Commands, Query<&B>)> = SystemState::new(&mut world);
{
let (mut commands, query) = system_state.get(&world);
commands.insert_resource(3.14);
}
system_state.apply(&mut world);
```
## Future Work
* Actually use SystemState inside FunctionSystem. This would be trivial, but it requires FunctionSystem to wrap SystemState in Option in its current form (which complicates system metadata lookup). I'd prefer to hold off until we adopt something like the later designs linked in #1364, which enable us to contruct Systems using a World reference (and also remove the need for `.system`).
* Consider a "scoped" approach to automatically call SystemState::apply when systems params are no longer being used (either a container type with a Drop impl, or a function that takes a closure for user logic operating on params).
2021-06-02 19:57:38 +00:00
system_meta
Bevy ECS V2 (#1525)
# Bevy ECS V2
This is a rewrite of Bevy ECS (basically everything but the new executor/schedule, which are already awesome). The overall goal was to improve the performance and versatility of Bevy ECS. Here is a quick bulleted list of changes before we dive into the details:
* Complete World rewrite
* Multiple component storage types:
* Tables: fast cache friendly iteration, slower add/removes (previously called Archetypes)
* Sparse Sets: fast add/remove, slower iteration
* Stateful Queries (caches query results for faster iteration. fragmented iteration is _fast_ now)
* Stateful System Params (caches expensive operations. inspired by @DJMcNab's work in #1364)
* Configurable System Params (users can set configuration when they construct their systems. once again inspired by @DJMcNab's work)
* Archetypes are now "just metadata", component storage is separate
* Archetype Graph (for faster archetype changes)
* Component Metadata
* Configure component storage type
* Retrieve information about component size/type/name/layout/send-ness/etc
* Components are uniquely identified by a densely packed ComponentId
* TypeIds are now totally optional (which should make implementing scripting easier)
* Super fast "for_each" query iterators
* Merged Resources into World. Resources are now just a special type of component
* EntityRef/EntityMut builder apis (more efficient and more ergonomic)
* Fast bitset-backed `Access<T>` replaces old hashmap-based approach everywhere
* Query conflicts are determined by component access instead of archetype component access (to avoid random failures at runtime)
* With/Without are still taken into account for conflicts, so this should still be comfy to use
* Much simpler `IntoSystem` impl
* Significantly reduced the amount of hashing throughout the ecs in favor of Sparse Sets (indexed by densely packed ArchetypeId, ComponentId, BundleId, and TableId)
* Safety Improvements
* Entity reservation uses a normal world reference instead of unsafe transmute
* QuerySets no longer transmute lifetimes
* Made traits "unsafe" where relevant
* More thorough safety docs
* WorldCell
* Exposes safe mutable access to multiple resources at a time in a World
* Replaced "catch all" `System::update_archetypes(world: &World)` with `System::new_archetype(archetype: &Archetype)`
* Simpler Bundle implementation
* Replaced slow "remove_bundle_one_by_one" used as fallback for Commands::remove_bundle with fast "remove_bundle_intersection"
* Removed `Mut<T>` query impl. it is better to only support one way: `&mut T`
* Removed with() from `Flags<T>` in favor of `Option<Flags<T>>`, which allows querying for flags to be "filtered" by default
* Components now have is_send property (currently only resources support non-send)
* More granular module organization
* New `RemovedComponents<T>` SystemParam that replaces `query.removed::<T>()`
* `world.resource_scope()` for mutable access to resources and world at the same time
* WorldQuery and QueryFilter traits unified. FilterFetch trait added to enable "short circuit" filtering. Auto impled for cases that don't need it
* Significantly slimmed down SystemState in favor of individual SystemParam state
* System Commands changed from `commands: &mut Commands` back to `mut commands: Commands` (to allow Commands to have a World reference)
Fixes #1320
## `World` Rewrite
This is a from-scratch rewrite of `World` that fills the niche that `hecs` used to. Yes, this means Bevy ECS is no longer a "fork" of hecs. We're going out our own!
(the only shared code between the projects is the entity id allocator, which is already basically ideal)
A huge shout out to @SanderMertens (author of [flecs](https://github.com/SanderMertens/flecs)) for sharing some great ideas with me (specifically hybrid ecs storage and archetype graphs). He also helped advise on a number of implementation details.
## Component Storage (The Problem)
Two ECS storage paradigms have gained a lot of traction over the years:
* **Archetypal ECS**:
* Stores components in "tables" with static schemas. Each "column" stores components of a given type. Each "row" is an entity.
* Each "archetype" has its own table. Adding/removing an entity's component changes the archetype.
* Enables super-fast Query iteration due to its cache-friendly data layout
* Comes at the cost of more expensive add/remove operations for an Entity's components, because all components need to be copied to the new archetype's "table"
* **Sparse Set ECS**:
* Stores components of the same type in densely packed arrays, which are sparsely indexed by densely packed unsigned integers (Entity ids)
* Query iteration is slower than Archetypal ECS because each entity's component could be at any position in the sparse set. This "random access" pattern isn't cache friendly. Additionally, there is an extra layer of indirection because you must first map the entity id to an index in the component array.
* Adding/removing components is a cheap, constant time operation
Bevy ECS V1, hecs, legion, flec, and Unity DOTS are all "archetypal ecs-es". I personally think "archetypal" storage is a good default for game engines. An entity's archetype doesn't need to change frequently in general, and it creates "fast by default" query iteration (which is a much more common operation). It is also "self optimizing". Users don't need to think about optimizing component layouts for iteration performance. It "just works" without any extra boilerplate.
Shipyard and EnTT are "sparse set ecs-es". They employ "packing" as a way to work around the "suboptimal by default" iteration performance for specific sets of components. This helps, but I didn't think this was a good choice for a general purpose engine like Bevy because:
1. "packs" conflict with each other. If bevy decides to internally pack the Transform and GlobalTransform components, users are then blocked if they want to pack some custom component with Transform.
2. users need to take manual action to optimize
Developers selecting an ECS framework are stuck with a hard choice. Select an "archetypal" framework with "fast iteration everywhere" but without the ability to cheaply add/remove components, or select a "sparse set" framework to cheaply add/remove components but with slower iteration performance.
## Hybrid Component Storage (The Solution)
In Bevy ECS V2, we get to have our cake and eat it too. It now has _both_ of the component storage types above (and more can be added later if needed):
* **Tables** (aka "archetypal" storage)
* The default storage. If you don't configure anything, this is what you get
* Fast iteration by default
* Slower add/remove operations
* **Sparse Sets**
* Opt-in
* Slower iteration
* Faster add/remove operations
These storage types complement each other perfectly. By default Query iteration is fast. If developers know that they want to add/remove a component at high frequencies, they can set the storage to "sparse set":
```rust
world.register_component(
ComponentDescriptor::new::<MyComponent>(StorageType::SparseSet)
).unwrap();
```
## Archetypes
Archetypes are now "just metadata" ... they no longer store components directly. They do store:
* The `ComponentId`s of each of the Archetype's components (and that component's storage type)
* Archetypes are uniquely defined by their component layouts
* For example: entities with "table" components `[A, B, C]` _and_ "sparse set" components `[D, E]` will always be in the same archetype.
* The `TableId` associated with the archetype
* For now each archetype has exactly one table (which can have no components),
* There is a 1->Many relationship from Tables->Archetypes. A given table could have any number of archetype components stored in it:
* Ex: an entity with "table storage" components `[A, B, C]` and "sparse set" components `[D, E]` will share the same `[A, B, C]` table as an entity with `[A, B, C]` table component and `[F]` sparse set components.
* This 1->Many relationship is how we preserve fast "cache friendly" iteration performance when possible (more on this later)
* A list of entities that are in the archetype and the row id of the table they are in
* ArchetypeComponentIds
* unique densely packed identifiers for (ArchetypeId, ComponentId) pairs
* used by the schedule executor for cheap system access control
* "Archetype Graph Edges" (see the next section)
## The "Archetype Graph"
Archetype changes in Bevy (and a number of other archetypal ecs-es) have historically been expensive to compute. First, you need to allocate a new vector of the entity's current component ids, add or remove components based on the operation performed, sort it (to ensure it is order-independent), then hash it to find the archetype (if it exists). And thats all before we get to the _already_ expensive full copy of all components to the new table storage.
The solution is to build a "graph" of archetypes to cache these results. @SanderMertens first exposed me to the idea (and he got it from @gjroelofs, who came up with it). They propose adding directed edges between archetypes for add/remove component operations. If `ComponentId`s are densely packed, you can use sparse sets to cheaply jump between archetypes.
Bevy takes this one step further by using add/remove `Bundle` edges instead of `Component` edges. Bevy encourages the use of `Bundles` to group add/remove operations. This is largely for "clearer game logic" reasons, but it also helps cut down on the number of archetype changes required. `Bundles` now also have densely-packed `BundleId`s. This allows us to use a _single_ edge for each bundle operation (rather than needing to traverse N edges ... one for each component). Single component operations are also bundles, so this is strictly an improvement over a "component only" graph.
As a result, an operation that used to be _heavy_ (both for allocations and compute) is now two dirt-cheap array lookups and zero allocations.
## Stateful Queries
World queries are now stateful. This allows us to:
1. Cache archetype (and table) matches
* This resolves another issue with (naive) archetypal ECS: query performance getting worse as the number of archetypes goes up (and fragmentation occurs).
2. Cache Fetch and Filter state
* The expensive parts of fetch/filter operations (such as hashing the TypeId to find the ComponentId) now only happen once when the Query is first constructed
3. Incrementally build up state
* When new archetypes are added, we only process the new archetypes (no need to rebuild state for old archetypes)
As a result, the direct `World` query api now looks like this:
```rust
let mut query = world.query::<(&A, &mut B)>();
for (a, mut b) in query.iter_mut(&mut world) {
}
```
Requiring `World` to generate stateful queries (rather than letting the `QueryState` type be constructed separately) allows us to ensure that _all_ queries are properly initialized (and the relevant world state, such as ComponentIds). This enables QueryState to remove branches from its operations that check for initialization status (and also enables query.iter() to take an immutable world reference because it doesn't need to initialize anything in world).
However in systems, this is a non-breaking change. State management is done internally by the relevant SystemParam.
## Stateful SystemParams
Like Queries, `SystemParams` now also cache state. For example, `Query` system params store the "stateful query" state mentioned above. Commands store their internal `CommandQueue`. This means you can now safely use as many separate `Commands` parameters in your system as you want. `Local<T>` system params store their `T` value in their state (instead of in Resources).
SystemParam state also enabled a significant slim-down of SystemState. It is much nicer to look at now.
Per-SystemParam state naturally insulates us from an "aliased mut" class of errors we have hit in the past (ex: using multiple `Commands` system params).
(credit goes to @DJMcNab for the initial idea and draft pr here #1364)
## Configurable SystemParams
@DJMcNab also had the great idea to make SystemParams configurable. This allows users to provide some initial configuration / values for system parameters (when possible). Most SystemParams have no config (the config type is `()`), but the `Local<T>` param now supports user-provided parameters:
```rust
fn foo(value: Local<usize>) {
}
app.add_system(foo.system().config(|c| c.0 = Some(10)));
```
## Uber Fast "for_each" Query Iterators
Developers now have the choice to use a fast "for_each" iterator, which yields ~1.5-3x iteration speed improvements for "fragmented iteration", and minor ~1.2x iteration speed improvements for unfragmented iteration.
```rust
fn system(query: Query<(&A, &mut B)>) {
// you now have the option to do this for a speed boost
query.for_each_mut(|(a, mut b)| {
});
// however normal iterators are still available
for (a, mut b) in query.iter_mut() {
}
}
```
I think in most cases we should continue to encourage "normal" iterators as they are more flexible and more "rust idiomatic". But when that extra "oomf" is needed, it makes sense to use `for_each`.
We should also consider using `for_each` for internal bevy systems to give our users a nice speed boost (but that should be a separate pr).
## Component Metadata
`World` now has a `Components` collection, which is accessible via `world.components()`. This stores mappings from `ComponentId` to `ComponentInfo`, as well as `TypeId` to `ComponentId` mappings (where relevant). `ComponentInfo` stores information about the component, such as ComponentId, TypeId, memory layout, send-ness (currently limited to resources), and storage type.
## Significantly Cheaper `Access<T>`
We used to use `TypeAccess<TypeId>` to manage read/write component/archetype-component access. This was expensive because TypeIds must be hashed and compared individually. The parallel executor got around this by "condensing" type ids into bitset-backed access types. This worked, but it had to be re-generated from the `TypeAccess<TypeId>`sources every time archetypes changed.
This pr removes TypeAccess in favor of faster bitset access everywhere. We can do this thanks to the move to densely packed `ComponentId`s and `ArchetypeComponentId`s.
## Merged Resources into World
Resources had a lot of redundant functionality with Components. They stored typed data, they had access control, they had unique ids, they were queryable via SystemParams, etc. In fact the _only_ major difference between them was that they were unique (and didn't correlate to an entity).
Separate resources also had the downside of requiring a separate set of access controls, which meant the parallel executor needed to compare more bitsets per system and manage more state.
I initially got the "separate resources" idea from `legion`. I think that design was motivated by the fact that it made the direct world query/resource lifetime interactions more manageable. It certainly made our lives easier when using Resources alongside hecs/bevy_ecs. However we already have a construct for safely and ergonomically managing in-world lifetimes: systems (which use `Access<T>` internally).
This pr merges Resources into World:
```rust
world.insert_resource(1);
world.insert_resource(2.0);
let a = world.get_resource::<i32>().unwrap();
let mut b = world.get_resource_mut::<f64>().unwrap();
*b = 3.0;
```
Resources are now just a special kind of component. They have their own ComponentIds (and their own resource TypeId->ComponentId scope, so they don't conflict wit components of the same type). They are stored in a special "resource archetype", which stores components inside the archetype using a new `unique_components` sparse set (note that this sparse set could later be used to implement Tags). This allows us to keep the code size small by reusing existing datastructures (namely Column, Archetype, ComponentFlags, and ComponentInfo). This allows us the executor to use a single `Access<ArchetypeComponentId>` per system. It should also make scripting language integration easier.
_But_ this merge did create problems for people directly interacting with `World`. What if you need mutable access to multiple resources at the same time? `world.get_resource_mut()` borrows World mutably!
## WorldCell
WorldCell applies the `Access<ArchetypeComponentId>` concept to direct world access:
```rust
let world_cell = world.cell();
let a = world_cell.get_resource_mut::<i32>().unwrap();
let b = world_cell.get_resource_mut::<f64>().unwrap();
```
This adds cheap runtime checks (a sparse set lookup of `ArchetypeComponentId` and a counter) to ensure that world accesses do not conflict with each other. Each operation returns a `WorldBorrow<'w, T>` or `WorldBorrowMut<'w, T>` wrapper type, which will release the relevant ArchetypeComponentId resources when dropped.
World caches the access sparse set (and only one cell can exist at a time), so `world.cell()` is a cheap operation.
WorldCell does _not_ use atomic operations. It is non-send, does a mutable borrow of world to prevent other accesses, and uses a simple `Rc<RefCell<ArchetypeComponentAccess>>` wrapper in each WorldBorrow pointer.
The api is currently limited to resource access, but it can and should be extended to queries / entity component access.
## Resource Scopes
WorldCell does not yet support component queries, and even when it does there are sometimes legitimate reasons to want a mutable world ref _and_ a mutable resource ref (ex: bevy_render and bevy_scene both need this). In these cases we could always drop down to the unsafe `world.get_resource_unchecked_mut()`, but that is not ideal!
Instead developers can use a "resource scope"
```rust
world.resource_scope(|world: &mut World, a: &mut A| {
})
```
This temporarily removes the `A` resource from `World`, provides mutable pointers to both, and re-adds A to World when finished. Thanks to the move to ComponentIds/sparse sets, this is a cheap operation.
If multiple resources are required, scopes can be nested. We could also consider adding a "resource tuple" to the api if this pattern becomes common and the boilerplate gets nasty.
## Query Conflicts Use ComponentId Instead of ArchetypeComponentId
For safety reasons, systems cannot contain queries that conflict with each other without wrapping them in a QuerySet. On bevy `main`, we use ArchetypeComponentIds to determine conflicts. This is nice because it can take into account filters:
```rust
// these queries will never conflict due to their filters
fn filter_system(a: Query<&mut A, With<B>>, b: Query<&mut B, Without<B>>) {
}
```
But it also has a significant downside:
```rust
// these queries will not conflict _until_ an entity with A, B, and C is spawned
fn maybe_conflicts_system(a: Query<(&mut A, &C)>, b: Query<(&mut A, &B)>) {
}
```
The system above will panic at runtime if an entity with A, B, and C is spawned. This makes it hard to trust that your game logic will run without crashing.
In this pr, I switched to using `ComponentId` instead. This _is_ more constraining. `maybe_conflicts_system` will now always fail, but it will do it consistently at startup. Naively, it would also _disallow_ `filter_system`, which would be a significant downgrade in usability. Bevy has a number of internal systems that rely on disjoint queries and I expect it to be a common pattern in userspace.
To resolve this, I added a new `FilteredAccess<T>` type, which wraps `Access<T>` and adds with/without filters. If two `FilteredAccess` have with/without values that prove they are disjoint, they will no longer conflict.
## EntityRef / EntityMut
World entity operations on `main` require that the user passes in an `entity` id to each operation:
```rust
let entity = world.spawn((A, )); // create a new entity with A
world.get::<A>(entity);
world.insert(entity, (B, C));
world.insert_one(entity, D);
```
This means that each operation needs to look up the entity location / verify its validity. The initial spawn operation also requires a Bundle as input. This can be awkward when no components are required (or one component is required).
These operations have been replaced by `EntityRef` and `EntityMut`, which are "builder-style" wrappers around world that provide read and read/write operations on a single, pre-validated entity:
```rust
// spawn now takes no inputs and returns an EntityMut
let entity = world.spawn()
.insert(A) // insert a single component into the entity
.insert_bundle((B, C)) // insert a bundle of components into the entity
.id() // id returns the Entity id
// Returns EntityMut (or panics if the entity does not exist)
world.entity_mut(entity)
.insert(D)
.insert_bundle(SomeBundle::default());
{
// returns EntityRef (or panics if the entity does not exist)
let d = world.entity(entity)
.get::<D>() // gets the D component
.unwrap();
// world.get still exists for ergonomics
let d = world.get::<D>(entity).unwrap();
}
// These variants return Options if you want to check existence instead of panicing
world.get_entity_mut(entity)
.unwrap()
.insert(E);
if let Some(entity_ref) = world.get_entity(entity) {
let d = entity_ref.get::<D>().unwrap();
}
```
This _does not_ affect the current Commands api or terminology. I think that should be a separate conversation as that is a much larger breaking change.
## Safety Improvements
* Entity reservation in Commands uses a normal world borrow instead of an unsafe transmute
* QuerySets no longer transmutes lifetimes
* Made traits "unsafe" when implementing a trait incorrectly could cause unsafety
* More thorough safety docs
## RemovedComponents SystemParam
The old approach to querying removed components: `query.removed:<T>()` was confusing because it had no connection to the query itself. I replaced it with the following, which is both clearer and allows us to cache the ComponentId mapping in the SystemParamState:
```rust
fn system(removed: RemovedComponents<T>) {
for entity in removed.iter() {
}
}
```
## Simpler Bundle implementation
Bundles are no longer responsible for sorting (or deduping) TypeInfo. They are just a simple ordered list of component types / data. This makes the implementation smaller and opens the door to an easy "nested bundle" implementation in the future (which i might even add in this pr). Duplicate detection is now done once per bundle type by World the first time a bundle is used.
## Unified WorldQuery and QueryFilter types
(don't worry they are still separate type _parameters_ in Queries .. this is a non-breaking change)
WorldQuery and QueryFilter were already basically identical apis. With the addition of `FetchState` and more storage-specific fetch methods, the overlap was even clearer (and the redundancy more painful).
QueryFilters are now just `F: WorldQuery where F::Fetch: FilterFetch`. FilterFetch requires `Fetch<Item = bool>` and adds new "short circuit" variants of fetch methods. This enables a filter tuple like `(With<A>, Without<B>, Changed<C>)` to stop evaluating the filter after the first mismatch is encountered. FilterFetch is automatically implemented for `Fetch` implementations that return bool.
This forces fetch implementations that return things like `(bool, bool, bool)` (such as the filter above) to manually implement FilterFetch and decide whether or not to short-circuit.
## More Granular Modules
World no longer globs all of the internal modules together. It now exports `core`, `system`, and `schedule` separately. I'm also considering exporting `core` submodules directly as that is still pretty "glob-ey" and unorganized (feedback welcome here).
## Remaining Draft Work (to be done in this pr)
* ~~panic on conflicting WorldQuery fetches (&A, &mut A)~~
* ~~bevy `main` and hecs both currently allow this, but we should protect against it if possible~~
* ~~batch_iter / par_iter (currently stubbed out)~~
* ~~ChangedRes~~
* ~~I skipped this while we sort out #1313. This pr should be adapted to account for whatever we land on there~~.
* ~~The `Archetypes` and `Tables` collections use hashes of sorted lists of component ids to uniquely identify each archetype/table. This hash is then used as the key in a HashMap to look up the relevant ArchetypeId or TableId. (which doesn't handle hash collisions properly)~~
* ~~It is currently unsafe to generate a Query from "World A", then use it on "World B" (despite the api claiming it is safe). We should probably close this gap. This could be done by adding a randomly generated WorldId to each world, then storing that id in each Query. They could then be compared to each other on each `query.do_thing(&world)` operation. This _does_ add an extra branch to each query operation, so I'm open to other suggestions if people have them.~~
* ~~Nested Bundles (if i find time)~~
## Potential Future Work
* Expand WorldCell to support queries.
* Consider not allocating in the empty archetype on `world.spawn()`
* ex: return something like EntityMutUninit, which turns into EntityMut after an `insert` or `insert_bundle` op
* this actually regressed performance last time i tried it, but in theory it should be faster
* Optimize SparseSet::insert (see `PERF` comment on insert)
* Replace SparseArray `Option<T>` with T::MAX to cut down on branching
* would enable cheaper get_unchecked() operations
* upstream fixedbitset optimizations
* fixedbitset could be allocation free for small block counts (store blocks in a SmallVec)
* fixedbitset could have a const constructor
* Consider implementing Tags (archetype-specific by-value data that affects archetype identity)
* ex: ArchetypeA could have `[A, B, C]` table components and `[D(1)]` "tag" component. ArchetypeB could have `[A, B, C]` table components and a `[D(2)]` tag component. The archetypes are different, despite both having D tags because the value inside D is different.
* this could potentially build on top of the `archetype.unique_components` added in this pr for resource storage.
* Consider reverting `all_tuples` proc macro in favor of the old `macro_rules` implementation
* all_tuples is more flexible and produces cleaner documentation (the macro_rules version produces weird type parameter orders due to parser constraints)
* but unfortunately all_tuples also appears to make Rust Analyzer sad/slow when working inside of `bevy_ecs` (does not affect user code)
* Consider "resource queries" and/or "mixed resource and entity component queries" as an alternative to WorldCell
* this is basically just "systems" so maybe it's not worth it
* Add more world ops
* `world.clear()`
* `world.reserve<T: Bundle>(count: usize)`
* Try using the old archetype allocation strategy (allocate new memory on resize and copy everything over). I expect this to improve batch insertion performance at the cost of unbatched performance. But thats just a guess. I'm not an allocation perf pro :)
* Adapt Commands apis for consistency with new World apis
## Benchmarks
key:
* `bevy_old`: bevy `main` branch
* `bevy`: this branch
* `_foreach`: uses an optimized for_each iterator
* ` _sparse`: uses sparse set storage (if unspecified assume table storage)
* `_system`: runs inside a system (if unspecified assume test happens via direct world ops)
### Simple Insert (from ecs_bench_suite)
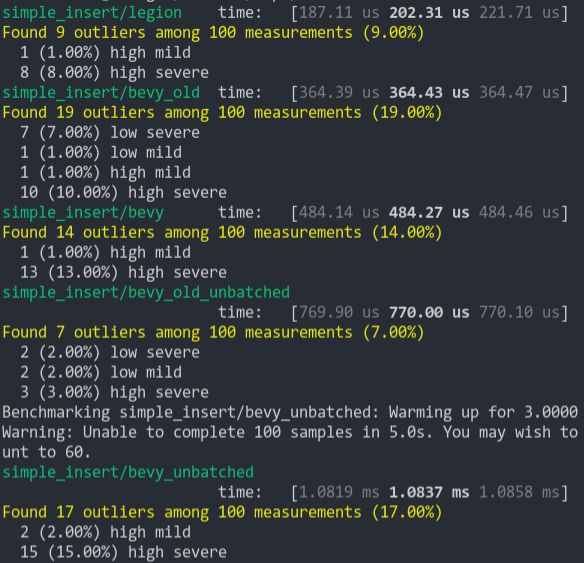
### Simpler Iter (from ecs_bench_suite)
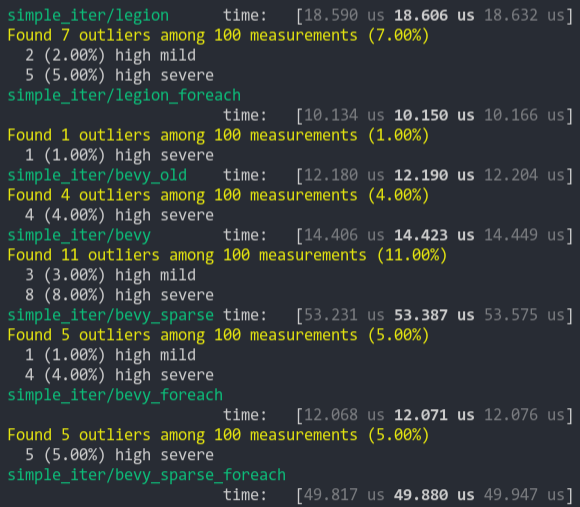
### Fragment Iter (from ecs_bench_suite)
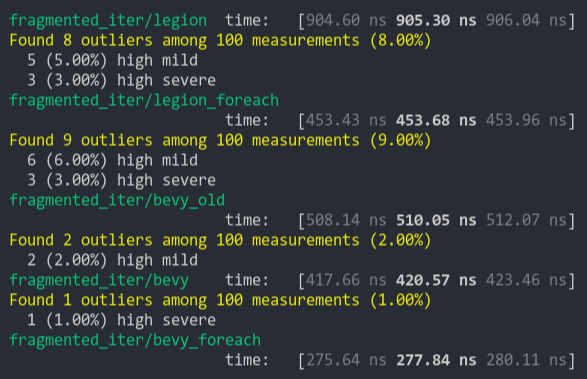
### Sparse Fragmented Iter
Iterate a query that matches 5 entities from a single matching archetype, but there are 100 unmatching archetypes
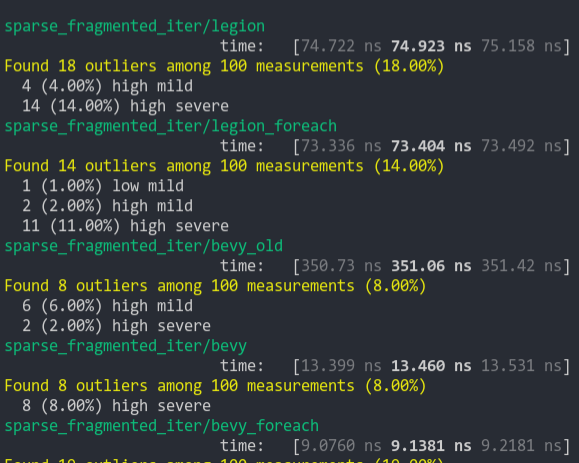
### Schedule (from ecs_bench_suite)
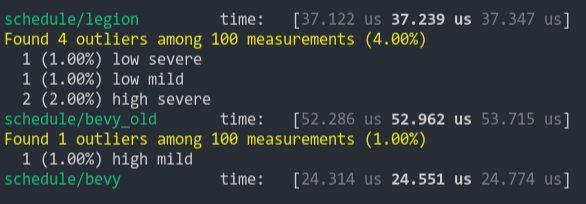
### Add Remove Component (from ecs_bench_suite)
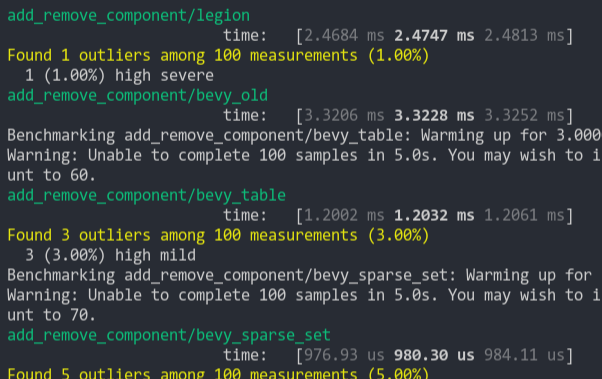
### Add Remove Component Big
Same as the test above, but each entity has 5 "large" matrix components and 1 "large" matrix component is added and removed
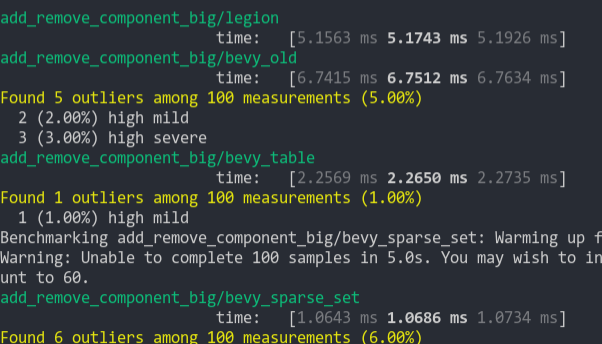
### Get Component
Looks up a single component value a large number of times
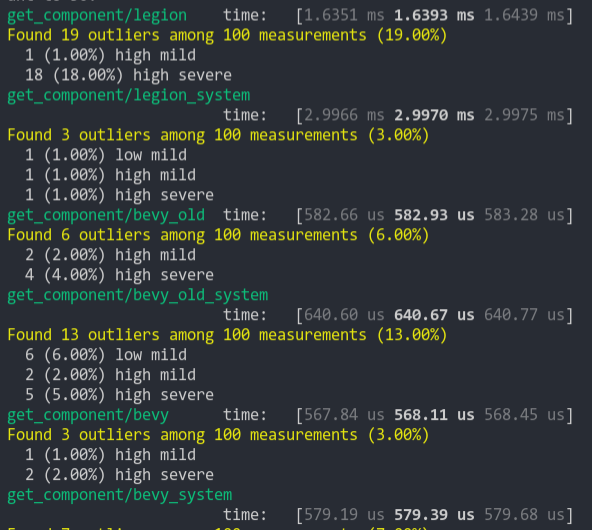
2021-03-05 07:54:35 +00:00
. component_access_set
. add ( state . component_access . clone ( ) ) ;
Add new SystemState and rename old SystemState to SystemMeta (#2283)
This enables `SystemParams` to be used outside of function systems. Anything can create and store `SystemState`, which enables efficient "param state cached" access to `SystemParams`.
It adds a `ReadOnlySystemParamFetch` trait, which enables safe `SystemState::get` calls without unique world access.
I renamed the old `SystemState` to `SystemMeta` to enable us to mirror the `QueryState` naming convention (but I'm happy to discuss alternative names if people have other ideas). I initially pitched this as `ParamState`, but given that it needs to include full system metadata, that doesn't feel like a particularly accurate name.
```rust
#[derive(Eq, PartialEq, Debug)]
struct A(usize);
#[derive(Eq, PartialEq, Debug)]
struct B(usize);
let mut world = World::default();
world.insert_resource(A(42));
world.spawn().insert(B(7));
// we get nice lifetime elision when declaring the type on the left hand side
let mut system_state: SystemState<(Res<A>, Query<&B>)> = SystemState::new(&mut world);
let (a, query) = system_state.get(&world);
assert_eq!(*a, A(42), "returned resource matches initial value");
assert_eq!(
*query.single().unwrap(),
B(7),
"returned component matches initial value"
);
// mutable system params require unique world access
let mut system_state: SystemState<(ResMut<A>, Query<&mut B>)> = SystemState::new(&mut world);
let (a, query) = system_state.get_mut(&mut world);
// static lifetimes are required when declaring inside of structs
struct SomeContainer {
state: SystemState<(Res<'static, A>, Res<'static, B>)>
}
// this can be shortened using type aliases, which will be useful for complex param tuples
type MyParams<'a> = (Res<'a, A>, Res<'a, B>);
struct SomeContainer {
state: SystemState<MyParams<'static>>
}
// It is the user's responsibility to call SystemState::apply(world) for parameters that queue up work
let mut system_state: SystemState<(Commands, Query<&B>)> = SystemState::new(&mut world);
{
let (mut commands, query) = system_state.get(&world);
commands.insert_resource(3.14);
}
system_state.apply(&mut world);
```
## Future Work
* Actually use SystemState inside FunctionSystem. This would be trivial, but it requires FunctionSystem to wrap SystemState in Option in its current form (which complicates system metadata lookup). I'd prefer to hold off until we adopt something like the later designs linked in #1364, which enable us to contruct Systems using a World reference (and also remove the need for `.system`).
* Consider a "scoped" approach to automatically call SystemState::apply when systems params are no longer being used (either a container type with a Drop impl, or a function that takes a closure for user logic operating on params).
2021-06-02 19:57:38 +00:00
system_meta
Bevy ECS V2 (#1525)
# Bevy ECS V2
This is a rewrite of Bevy ECS (basically everything but the new executor/schedule, which are already awesome). The overall goal was to improve the performance and versatility of Bevy ECS. Here is a quick bulleted list of changes before we dive into the details:
* Complete World rewrite
* Multiple component storage types:
* Tables: fast cache friendly iteration, slower add/removes (previously called Archetypes)
* Sparse Sets: fast add/remove, slower iteration
* Stateful Queries (caches query results for faster iteration. fragmented iteration is _fast_ now)
* Stateful System Params (caches expensive operations. inspired by @DJMcNab's work in #1364)
* Configurable System Params (users can set configuration when they construct their systems. once again inspired by @DJMcNab's work)
* Archetypes are now "just metadata", component storage is separate
* Archetype Graph (for faster archetype changes)
* Component Metadata
* Configure component storage type
* Retrieve information about component size/type/name/layout/send-ness/etc
* Components are uniquely identified by a densely packed ComponentId
* TypeIds are now totally optional (which should make implementing scripting easier)
* Super fast "for_each" query iterators
* Merged Resources into World. Resources are now just a special type of component
* EntityRef/EntityMut builder apis (more efficient and more ergonomic)
* Fast bitset-backed `Access<T>` replaces old hashmap-based approach everywhere
* Query conflicts are determined by component access instead of archetype component access (to avoid random failures at runtime)
* With/Without are still taken into account for conflicts, so this should still be comfy to use
* Much simpler `IntoSystem` impl
* Significantly reduced the amount of hashing throughout the ecs in favor of Sparse Sets (indexed by densely packed ArchetypeId, ComponentId, BundleId, and TableId)
* Safety Improvements
* Entity reservation uses a normal world reference instead of unsafe transmute
* QuerySets no longer transmute lifetimes
* Made traits "unsafe" where relevant
* More thorough safety docs
* WorldCell
* Exposes safe mutable access to multiple resources at a time in a World
* Replaced "catch all" `System::update_archetypes(world: &World)` with `System::new_archetype(archetype: &Archetype)`
* Simpler Bundle implementation
* Replaced slow "remove_bundle_one_by_one" used as fallback for Commands::remove_bundle with fast "remove_bundle_intersection"
* Removed `Mut<T>` query impl. it is better to only support one way: `&mut T`
* Removed with() from `Flags<T>` in favor of `Option<Flags<T>>`, which allows querying for flags to be "filtered" by default
* Components now have is_send property (currently only resources support non-send)
* More granular module organization
* New `RemovedComponents<T>` SystemParam that replaces `query.removed::<T>()`
* `world.resource_scope()` for mutable access to resources and world at the same time
* WorldQuery and QueryFilter traits unified. FilterFetch trait added to enable "short circuit" filtering. Auto impled for cases that don't need it
* Significantly slimmed down SystemState in favor of individual SystemParam state
* System Commands changed from `commands: &mut Commands` back to `mut commands: Commands` (to allow Commands to have a World reference)
Fixes #1320
## `World` Rewrite
This is a from-scratch rewrite of `World` that fills the niche that `hecs` used to. Yes, this means Bevy ECS is no longer a "fork" of hecs. We're going out our own!
(the only shared code between the projects is the entity id allocator, which is already basically ideal)
A huge shout out to @SanderMertens (author of [flecs](https://github.com/SanderMertens/flecs)) for sharing some great ideas with me (specifically hybrid ecs storage and archetype graphs). He also helped advise on a number of implementation details.
## Component Storage (The Problem)
Two ECS storage paradigms have gained a lot of traction over the years:
* **Archetypal ECS**:
* Stores components in "tables" with static schemas. Each "column" stores components of a given type. Each "row" is an entity.
* Each "archetype" has its own table. Adding/removing an entity's component changes the archetype.
* Enables super-fast Query iteration due to its cache-friendly data layout
* Comes at the cost of more expensive add/remove operations for an Entity's components, because all components need to be copied to the new archetype's "table"
* **Sparse Set ECS**:
* Stores components of the same type in densely packed arrays, which are sparsely indexed by densely packed unsigned integers (Entity ids)
* Query iteration is slower than Archetypal ECS because each entity's component could be at any position in the sparse set. This "random access" pattern isn't cache friendly. Additionally, there is an extra layer of indirection because you must first map the entity id to an index in the component array.
* Adding/removing components is a cheap, constant time operation
Bevy ECS V1, hecs, legion, flec, and Unity DOTS are all "archetypal ecs-es". I personally think "archetypal" storage is a good default for game engines. An entity's archetype doesn't need to change frequently in general, and it creates "fast by default" query iteration (which is a much more common operation). It is also "self optimizing". Users don't need to think about optimizing component layouts for iteration performance. It "just works" without any extra boilerplate.
Shipyard and EnTT are "sparse set ecs-es". They employ "packing" as a way to work around the "suboptimal by default" iteration performance for specific sets of components. This helps, but I didn't think this was a good choice for a general purpose engine like Bevy because:
1. "packs" conflict with each other. If bevy decides to internally pack the Transform and GlobalTransform components, users are then blocked if they want to pack some custom component with Transform.
2. users need to take manual action to optimize
Developers selecting an ECS framework are stuck with a hard choice. Select an "archetypal" framework with "fast iteration everywhere" but without the ability to cheaply add/remove components, or select a "sparse set" framework to cheaply add/remove components but with slower iteration performance.
## Hybrid Component Storage (The Solution)
In Bevy ECS V2, we get to have our cake and eat it too. It now has _both_ of the component storage types above (and more can be added later if needed):
* **Tables** (aka "archetypal" storage)
* The default storage. If you don't configure anything, this is what you get
* Fast iteration by default
* Slower add/remove operations
* **Sparse Sets**
* Opt-in
* Slower iteration
* Faster add/remove operations
These storage types complement each other perfectly. By default Query iteration is fast. If developers know that they want to add/remove a component at high frequencies, they can set the storage to "sparse set":
```rust
world.register_component(
ComponentDescriptor::new::<MyComponent>(StorageType::SparseSet)
).unwrap();
```
## Archetypes
Archetypes are now "just metadata" ... they no longer store components directly. They do store:
* The `ComponentId`s of each of the Archetype's components (and that component's storage type)
* Archetypes are uniquely defined by their component layouts
* For example: entities with "table" components `[A, B, C]` _and_ "sparse set" components `[D, E]` will always be in the same archetype.
* The `TableId` associated with the archetype
* For now each archetype has exactly one table (which can have no components),
* There is a 1->Many relationship from Tables->Archetypes. A given table could have any number of archetype components stored in it:
* Ex: an entity with "table storage" components `[A, B, C]` and "sparse set" components `[D, E]` will share the same `[A, B, C]` table as an entity with `[A, B, C]` table component and `[F]` sparse set components.
* This 1->Many relationship is how we preserve fast "cache friendly" iteration performance when possible (more on this later)
* A list of entities that are in the archetype and the row id of the table they are in
* ArchetypeComponentIds
* unique densely packed identifiers for (ArchetypeId, ComponentId) pairs
* used by the schedule executor for cheap system access control
* "Archetype Graph Edges" (see the next section)
## The "Archetype Graph"
Archetype changes in Bevy (and a number of other archetypal ecs-es) have historically been expensive to compute. First, you need to allocate a new vector of the entity's current component ids, add or remove components based on the operation performed, sort it (to ensure it is order-independent), then hash it to find the archetype (if it exists). And thats all before we get to the _already_ expensive full copy of all components to the new table storage.
The solution is to build a "graph" of archetypes to cache these results. @SanderMertens first exposed me to the idea (and he got it from @gjroelofs, who came up with it). They propose adding directed edges between archetypes for add/remove component operations. If `ComponentId`s are densely packed, you can use sparse sets to cheaply jump between archetypes.
Bevy takes this one step further by using add/remove `Bundle` edges instead of `Component` edges. Bevy encourages the use of `Bundles` to group add/remove operations. This is largely for "clearer game logic" reasons, but it also helps cut down on the number of archetype changes required. `Bundles` now also have densely-packed `BundleId`s. This allows us to use a _single_ edge for each bundle operation (rather than needing to traverse N edges ... one for each component). Single component operations are also bundles, so this is strictly an improvement over a "component only" graph.
As a result, an operation that used to be _heavy_ (both for allocations and compute) is now two dirt-cheap array lookups and zero allocations.
## Stateful Queries
World queries are now stateful. This allows us to:
1. Cache archetype (and table) matches
* This resolves another issue with (naive) archetypal ECS: query performance getting worse as the number of archetypes goes up (and fragmentation occurs).
2. Cache Fetch and Filter state
* The expensive parts of fetch/filter operations (such as hashing the TypeId to find the ComponentId) now only happen once when the Query is first constructed
3. Incrementally build up state
* When new archetypes are added, we only process the new archetypes (no need to rebuild state for old archetypes)
As a result, the direct `World` query api now looks like this:
```rust
let mut query = world.query::<(&A, &mut B)>();
for (a, mut b) in query.iter_mut(&mut world) {
}
```
Requiring `World` to generate stateful queries (rather than letting the `QueryState` type be constructed separately) allows us to ensure that _all_ queries are properly initialized (and the relevant world state, such as ComponentIds). This enables QueryState to remove branches from its operations that check for initialization status (and also enables query.iter() to take an immutable world reference because it doesn't need to initialize anything in world).
However in systems, this is a non-breaking change. State management is done internally by the relevant SystemParam.
## Stateful SystemParams
Like Queries, `SystemParams` now also cache state. For example, `Query` system params store the "stateful query" state mentioned above. Commands store their internal `CommandQueue`. This means you can now safely use as many separate `Commands` parameters in your system as you want. `Local<T>` system params store their `T` value in their state (instead of in Resources).
SystemParam state also enabled a significant slim-down of SystemState. It is much nicer to look at now.
Per-SystemParam state naturally insulates us from an "aliased mut" class of errors we have hit in the past (ex: using multiple `Commands` system params).
(credit goes to @DJMcNab for the initial idea and draft pr here #1364)
## Configurable SystemParams
@DJMcNab also had the great idea to make SystemParams configurable. This allows users to provide some initial configuration / values for system parameters (when possible). Most SystemParams have no config (the config type is `()`), but the `Local<T>` param now supports user-provided parameters:
```rust
fn foo(value: Local<usize>) {
}
app.add_system(foo.system().config(|c| c.0 = Some(10)));
```
## Uber Fast "for_each" Query Iterators
Developers now have the choice to use a fast "for_each" iterator, which yields ~1.5-3x iteration speed improvements for "fragmented iteration", and minor ~1.2x iteration speed improvements for unfragmented iteration.
```rust
fn system(query: Query<(&A, &mut B)>) {
// you now have the option to do this for a speed boost
query.for_each_mut(|(a, mut b)| {
});
// however normal iterators are still available
for (a, mut b) in query.iter_mut() {
}
}
```
I think in most cases we should continue to encourage "normal" iterators as they are more flexible and more "rust idiomatic". But when that extra "oomf" is needed, it makes sense to use `for_each`.
We should also consider using `for_each` for internal bevy systems to give our users a nice speed boost (but that should be a separate pr).
## Component Metadata
`World` now has a `Components` collection, which is accessible via `world.components()`. This stores mappings from `ComponentId` to `ComponentInfo`, as well as `TypeId` to `ComponentId` mappings (where relevant). `ComponentInfo` stores information about the component, such as ComponentId, TypeId, memory layout, send-ness (currently limited to resources), and storage type.
## Significantly Cheaper `Access<T>`
We used to use `TypeAccess<TypeId>` to manage read/write component/archetype-component access. This was expensive because TypeIds must be hashed and compared individually. The parallel executor got around this by "condensing" type ids into bitset-backed access types. This worked, but it had to be re-generated from the `TypeAccess<TypeId>`sources every time archetypes changed.
This pr removes TypeAccess in favor of faster bitset access everywhere. We can do this thanks to the move to densely packed `ComponentId`s and `ArchetypeComponentId`s.
## Merged Resources into World
Resources had a lot of redundant functionality with Components. They stored typed data, they had access control, they had unique ids, they were queryable via SystemParams, etc. In fact the _only_ major difference between them was that they were unique (and didn't correlate to an entity).
Separate resources also had the downside of requiring a separate set of access controls, which meant the parallel executor needed to compare more bitsets per system and manage more state.
I initially got the "separate resources" idea from `legion`. I think that design was motivated by the fact that it made the direct world query/resource lifetime interactions more manageable. It certainly made our lives easier when using Resources alongside hecs/bevy_ecs. However we already have a construct for safely and ergonomically managing in-world lifetimes: systems (which use `Access<T>` internally).
This pr merges Resources into World:
```rust
world.insert_resource(1);
world.insert_resource(2.0);
let a = world.get_resource::<i32>().unwrap();
let mut b = world.get_resource_mut::<f64>().unwrap();
*b = 3.0;
```
Resources are now just a special kind of component. They have their own ComponentIds (and their own resource TypeId->ComponentId scope, so they don't conflict wit components of the same type). They are stored in a special "resource archetype", which stores components inside the archetype using a new `unique_components` sparse set (note that this sparse set could later be used to implement Tags). This allows us to keep the code size small by reusing existing datastructures (namely Column, Archetype, ComponentFlags, and ComponentInfo). This allows us the executor to use a single `Access<ArchetypeComponentId>` per system. It should also make scripting language integration easier.
_But_ this merge did create problems for people directly interacting with `World`. What if you need mutable access to multiple resources at the same time? `world.get_resource_mut()` borrows World mutably!
## WorldCell
WorldCell applies the `Access<ArchetypeComponentId>` concept to direct world access:
```rust
let world_cell = world.cell();
let a = world_cell.get_resource_mut::<i32>().unwrap();
let b = world_cell.get_resource_mut::<f64>().unwrap();
```
This adds cheap runtime checks (a sparse set lookup of `ArchetypeComponentId` and a counter) to ensure that world accesses do not conflict with each other. Each operation returns a `WorldBorrow<'w, T>` or `WorldBorrowMut<'w, T>` wrapper type, which will release the relevant ArchetypeComponentId resources when dropped.
World caches the access sparse set (and only one cell can exist at a time), so `world.cell()` is a cheap operation.
WorldCell does _not_ use atomic operations. It is non-send, does a mutable borrow of world to prevent other accesses, and uses a simple `Rc<RefCell<ArchetypeComponentAccess>>` wrapper in each WorldBorrow pointer.
The api is currently limited to resource access, but it can and should be extended to queries / entity component access.
## Resource Scopes
WorldCell does not yet support component queries, and even when it does there are sometimes legitimate reasons to want a mutable world ref _and_ a mutable resource ref (ex: bevy_render and bevy_scene both need this). In these cases we could always drop down to the unsafe `world.get_resource_unchecked_mut()`, but that is not ideal!
Instead developers can use a "resource scope"
```rust
world.resource_scope(|world: &mut World, a: &mut A| {
})
```
This temporarily removes the `A` resource from `World`, provides mutable pointers to both, and re-adds A to World when finished. Thanks to the move to ComponentIds/sparse sets, this is a cheap operation.
If multiple resources are required, scopes can be nested. We could also consider adding a "resource tuple" to the api if this pattern becomes common and the boilerplate gets nasty.
## Query Conflicts Use ComponentId Instead of ArchetypeComponentId
For safety reasons, systems cannot contain queries that conflict with each other without wrapping them in a QuerySet. On bevy `main`, we use ArchetypeComponentIds to determine conflicts. This is nice because it can take into account filters:
```rust
// these queries will never conflict due to their filters
fn filter_system(a: Query<&mut A, With<B>>, b: Query<&mut B, Without<B>>) {
}
```
But it also has a significant downside:
```rust
// these queries will not conflict _until_ an entity with A, B, and C is spawned
fn maybe_conflicts_system(a: Query<(&mut A, &C)>, b: Query<(&mut A, &B)>) {
}
```
The system above will panic at runtime if an entity with A, B, and C is spawned. This makes it hard to trust that your game logic will run without crashing.
In this pr, I switched to using `ComponentId` instead. This _is_ more constraining. `maybe_conflicts_system` will now always fail, but it will do it consistently at startup. Naively, it would also _disallow_ `filter_system`, which would be a significant downgrade in usability. Bevy has a number of internal systems that rely on disjoint queries and I expect it to be a common pattern in userspace.
To resolve this, I added a new `FilteredAccess<T>` type, which wraps `Access<T>` and adds with/without filters. If two `FilteredAccess` have with/without values that prove they are disjoint, they will no longer conflict.
## EntityRef / EntityMut
World entity operations on `main` require that the user passes in an `entity` id to each operation:
```rust
let entity = world.spawn((A, )); // create a new entity with A
world.get::<A>(entity);
world.insert(entity, (B, C));
world.insert_one(entity, D);
```
This means that each operation needs to look up the entity location / verify its validity. The initial spawn operation also requires a Bundle as input. This can be awkward when no components are required (or one component is required).
These operations have been replaced by `EntityRef` and `EntityMut`, which are "builder-style" wrappers around world that provide read and read/write operations on a single, pre-validated entity:
```rust
// spawn now takes no inputs and returns an EntityMut
let entity = world.spawn()
.insert(A) // insert a single component into the entity
.insert_bundle((B, C)) // insert a bundle of components into the entity
.id() // id returns the Entity id
// Returns EntityMut (or panics if the entity does not exist)
world.entity_mut(entity)
.insert(D)
.insert_bundle(SomeBundle::default());
{
// returns EntityRef (or panics if the entity does not exist)
let d = world.entity(entity)
.get::<D>() // gets the D component
.unwrap();
// world.get still exists for ergonomics
let d = world.get::<D>(entity).unwrap();
}
// These variants return Options if you want to check existence instead of panicing
world.get_entity_mut(entity)
.unwrap()
.insert(E);
if let Some(entity_ref) = world.get_entity(entity) {
let d = entity_ref.get::<D>().unwrap();
}
```
This _does not_ affect the current Commands api or terminology. I think that should be a separate conversation as that is a much larger breaking change.
## Safety Improvements
* Entity reservation in Commands uses a normal world borrow instead of an unsafe transmute
* QuerySets no longer transmutes lifetimes
* Made traits "unsafe" when implementing a trait incorrectly could cause unsafety
* More thorough safety docs
## RemovedComponents SystemParam
The old approach to querying removed components: `query.removed:<T>()` was confusing because it had no connection to the query itself. I replaced it with the following, which is both clearer and allows us to cache the ComponentId mapping in the SystemParamState:
```rust
fn system(removed: RemovedComponents<T>) {
for entity in removed.iter() {
}
}
```
## Simpler Bundle implementation
Bundles are no longer responsible for sorting (or deduping) TypeInfo. They are just a simple ordered list of component types / data. This makes the implementation smaller and opens the door to an easy "nested bundle" implementation in the future (which i might even add in this pr). Duplicate detection is now done once per bundle type by World the first time a bundle is used.
## Unified WorldQuery and QueryFilter types
(don't worry they are still separate type _parameters_ in Queries .. this is a non-breaking change)
WorldQuery and QueryFilter were already basically identical apis. With the addition of `FetchState` and more storage-specific fetch methods, the overlap was even clearer (and the redundancy more painful).
QueryFilters are now just `F: WorldQuery where F::Fetch: FilterFetch`. FilterFetch requires `Fetch<Item = bool>` and adds new "short circuit" variants of fetch methods. This enables a filter tuple like `(With<A>, Without<B>, Changed<C>)` to stop evaluating the filter after the first mismatch is encountered. FilterFetch is automatically implemented for `Fetch` implementations that return bool.
This forces fetch implementations that return things like `(bool, bool, bool)` (such as the filter above) to manually implement FilterFetch and decide whether or not to short-circuit.
## More Granular Modules
World no longer globs all of the internal modules together. It now exports `core`, `system`, and `schedule` separately. I'm also considering exporting `core` submodules directly as that is still pretty "glob-ey" and unorganized (feedback welcome here).
## Remaining Draft Work (to be done in this pr)
* ~~panic on conflicting WorldQuery fetches (&A, &mut A)~~
* ~~bevy `main` and hecs both currently allow this, but we should protect against it if possible~~
* ~~batch_iter / par_iter (currently stubbed out)~~
* ~~ChangedRes~~
* ~~I skipped this while we sort out #1313. This pr should be adapted to account for whatever we land on there~~.
* ~~The `Archetypes` and `Tables` collections use hashes of sorted lists of component ids to uniquely identify each archetype/table. This hash is then used as the key in a HashMap to look up the relevant ArchetypeId or TableId. (which doesn't handle hash collisions properly)~~
* ~~It is currently unsafe to generate a Query from "World A", then use it on "World B" (despite the api claiming it is safe). We should probably close this gap. This could be done by adding a randomly generated WorldId to each world, then storing that id in each Query. They could then be compared to each other on each `query.do_thing(&world)` operation. This _does_ add an extra branch to each query operation, so I'm open to other suggestions if people have them.~~
* ~~Nested Bundles (if i find time)~~
## Potential Future Work
* Expand WorldCell to support queries.
* Consider not allocating in the empty archetype on `world.spawn()`
* ex: return something like EntityMutUninit, which turns into EntityMut after an `insert` or `insert_bundle` op
* this actually regressed performance last time i tried it, but in theory it should be faster
* Optimize SparseSet::insert (see `PERF` comment on insert)
* Replace SparseArray `Option<T>` with T::MAX to cut down on branching
* would enable cheaper get_unchecked() operations
* upstream fixedbitset optimizations
* fixedbitset could be allocation free for small block counts (store blocks in a SmallVec)
* fixedbitset could have a const constructor
* Consider implementing Tags (archetype-specific by-value data that affects archetype identity)
* ex: ArchetypeA could have `[A, B, C]` table components and `[D(1)]` "tag" component. ArchetypeB could have `[A, B, C]` table components and a `[D(2)]` tag component. The archetypes are different, despite both having D tags because the value inside D is different.
* this could potentially build on top of the `archetype.unique_components` added in this pr for resource storage.
* Consider reverting `all_tuples` proc macro in favor of the old `macro_rules` implementation
* all_tuples is more flexible and produces cleaner documentation (the macro_rules version produces weird type parameter orders due to parser constraints)
* but unfortunately all_tuples also appears to make Rust Analyzer sad/slow when working inside of `bevy_ecs` (does not affect user code)
* Consider "resource queries" and/or "mixed resource and entity component queries" as an alternative to WorldCell
* this is basically just "systems" so maybe it's not worth it
* Add more world ops
* `world.clear()`
* `world.reserve<T: Bundle>(count: usize)`
* Try using the old archetype allocation strategy (allocate new memory on resize and copy everything over). I expect this to improve batch insertion performance at the cost of unbatched performance. But thats just a guess. I'm not an allocation perf pro :)
* Adapt Commands apis for consistency with new World apis
## Benchmarks
key:
* `bevy_old`: bevy `main` branch
* `bevy`: this branch
* `_foreach`: uses an optimized for_each iterator
* ` _sparse`: uses sparse set storage (if unspecified assume table storage)
* `_system`: runs inside a system (if unspecified assume test happens via direct world ops)
### Simple Insert (from ecs_bench_suite)
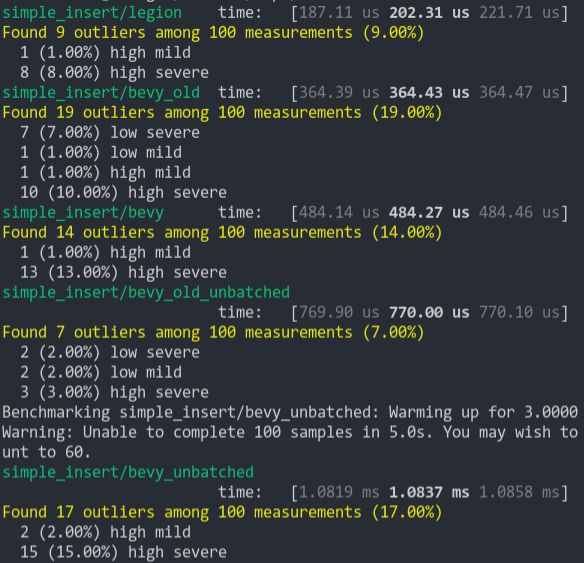
### Simpler Iter (from ecs_bench_suite)
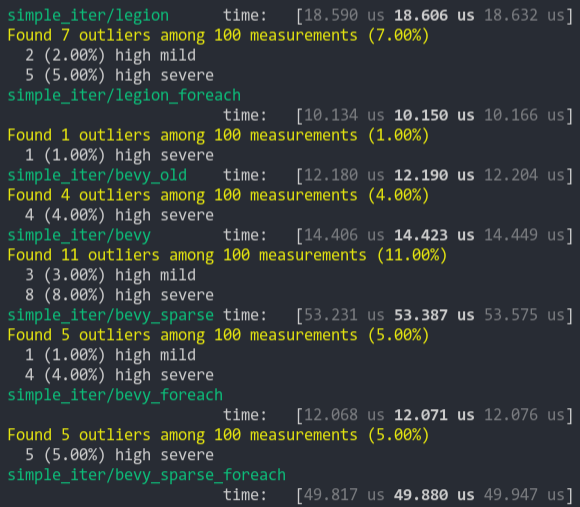
### Fragment Iter (from ecs_bench_suite)
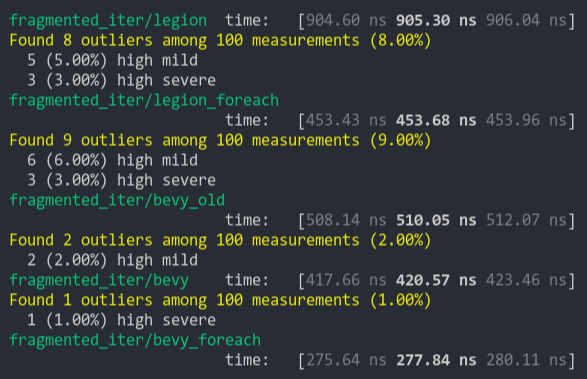
### Sparse Fragmented Iter
Iterate a query that matches 5 entities from a single matching archetype, but there are 100 unmatching archetypes
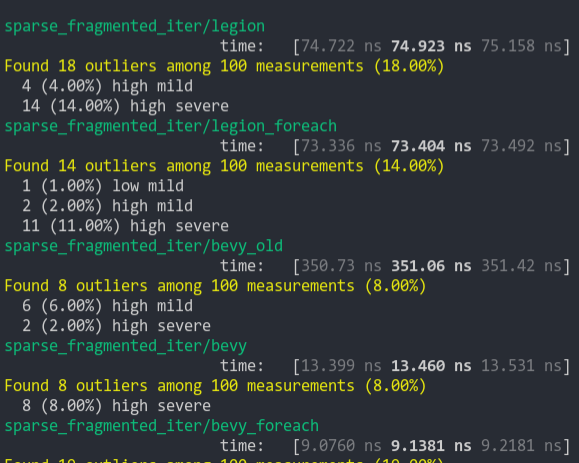
### Schedule (from ecs_bench_suite)
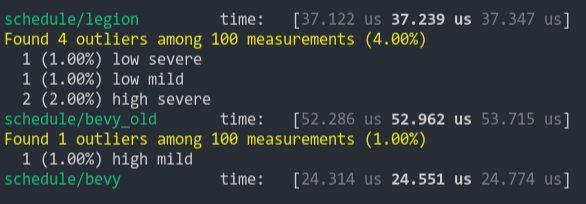
### Add Remove Component (from ecs_bench_suite)
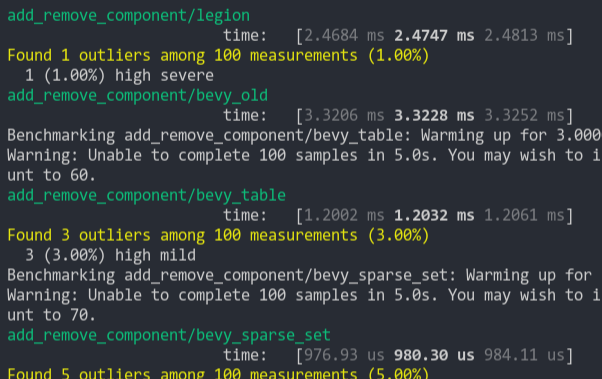
### Add Remove Component Big
Same as the test above, but each entity has 5 "large" matrix components and 1 "large" matrix component is added and removed
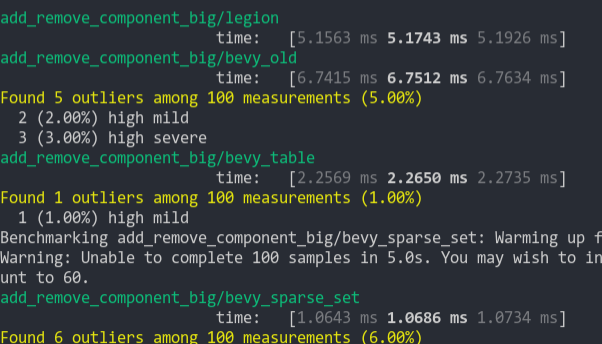
### Get Component
Looks up a single component value a large number of times
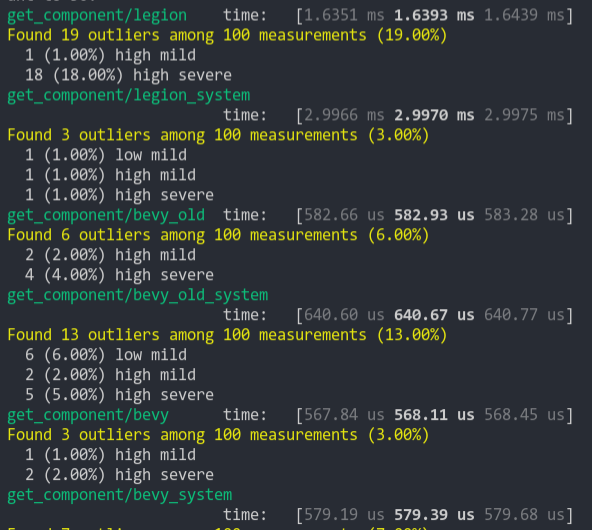
2021-03-05 07:54:35 +00:00
. archetype_component_access
. extend ( & state . archetype_component_access ) ;
state
}
Remove the `SystemParamState` trait and remove types like `ResState` (#6919)
Spiritual successor to #5205.
Actual successor to #6865.
# Objective
Currently, system params are defined using three traits: `SystemParam`, `ReadOnlySystemParam`, `SystemParamState`. The behavior for each param is specified by the `SystemParamState` trait, while `SystemParam` simply defers to the state.
Splitting the traits in this way makes it easier to implement within macros, but it increases the cognitive load. Worst of all, this approach requires each `MySystemParam` to have a public `MySystemParamState` type associated with it.
## Solution
* Merge the trait `SystemParamState` into `SystemParam`.
* Remove all trivial `SystemParam` state types.
* `OptionNonSendMutState<T>`: you will not be missed.
---
- [x] Fix/resolve the remaining test failure.
## Changelog
* Removed the trait `SystemParamState`, merging its functionality into `SystemParam`.
## Migration Guide
**Note**: this should replace the migration guide for #6865.
This is relative to Bevy 0.9, not main.
The traits `SystemParamState` and `SystemParamFetch` have been removed, and their functionality has been transferred to `SystemParam`.
```rust
// Before (0.9)
impl SystemParam for MyParam<'_, '_> {
type State = MyParamState;
}
unsafe impl SystemParamState for MyParamState {
fn init(world: &mut World, system_meta: &mut SystemMeta) -> Self { ... }
}
unsafe impl<'w, 's> SystemParamFetch<'w, 's> for MyParamState {
type Item = MyParam<'w, 's>;
fn get_param(&mut self, ...) -> Self::Item;
}
unsafe impl ReadOnlySystemParamFetch for MyParamState { }
// After (0.10)
unsafe impl SystemParam for MyParam<'_, '_> {
type State = MyParamState;
type Item<'w, 's> = MyParam<'w, 's>;
fn init_state(world: &mut World, system_meta: &mut SystemMeta) -> Self::State { ... }
fn get_param<'w, 's>(state: &mut Self::State, ...) -> Self::Item<'w, 's>;
}
unsafe impl ReadOnlySystemParam for MyParam<'_, '_> { }
```
The trait `ReadOnlySystemParamFetch` has been replaced with `ReadOnlySystemParam`.
```rust
// Before
unsafe impl ReadOnlySystemParamFetch for MyParamState {}
// After
unsafe impl ReadOnlySystemParam for MyParam<'_, '_> {}
```
2023-01-07 23:20:32 +00:00
fn new_archetype ( state : & mut Self ::State , archetype : & Archetype , system_meta : & mut SystemMeta ) {
state . new_archetype ( archetype ) ;
Add new SystemState and rename old SystemState to SystemMeta (#2283)
This enables `SystemParams` to be used outside of function systems. Anything can create and store `SystemState`, which enables efficient "param state cached" access to `SystemParams`.
It adds a `ReadOnlySystemParamFetch` trait, which enables safe `SystemState::get` calls without unique world access.
I renamed the old `SystemState` to `SystemMeta` to enable us to mirror the `QueryState` naming convention (but I'm happy to discuss alternative names if people have other ideas). I initially pitched this as `ParamState`, but given that it needs to include full system metadata, that doesn't feel like a particularly accurate name.
```rust
#[derive(Eq, PartialEq, Debug)]
struct A(usize);
#[derive(Eq, PartialEq, Debug)]
struct B(usize);
let mut world = World::default();
world.insert_resource(A(42));
world.spawn().insert(B(7));
// we get nice lifetime elision when declaring the type on the left hand side
let mut system_state: SystemState<(Res<A>, Query<&B>)> = SystemState::new(&mut world);
let (a, query) = system_state.get(&world);
assert_eq!(*a, A(42), "returned resource matches initial value");
assert_eq!(
*query.single().unwrap(),
B(7),
"returned component matches initial value"
);
// mutable system params require unique world access
let mut system_state: SystemState<(ResMut<A>, Query<&mut B>)> = SystemState::new(&mut world);
let (a, query) = system_state.get_mut(&mut world);
// static lifetimes are required when declaring inside of structs
struct SomeContainer {
state: SystemState<(Res<'static, A>, Res<'static, B>)>
}
// this can be shortened using type aliases, which will be useful for complex param tuples
type MyParams<'a> = (Res<'a, A>, Res<'a, B>);
struct SomeContainer {
state: SystemState<MyParams<'static>>
}
// It is the user's responsibility to call SystemState::apply(world) for parameters that queue up work
let mut system_state: SystemState<(Commands, Query<&B>)> = SystemState::new(&mut world);
{
let (mut commands, query) = system_state.get(&world);
commands.insert_resource(3.14);
}
system_state.apply(&mut world);
```
## Future Work
* Actually use SystemState inside FunctionSystem. This would be trivial, but it requires FunctionSystem to wrap SystemState in Option in its current form (which complicates system metadata lookup). I'd prefer to hold off until we adopt something like the later designs linked in #1364, which enable us to contruct Systems using a World reference (and also remove the need for `.system`).
* Consider a "scoped" approach to automatically call SystemState::apply when systems params are no longer being used (either a container type with a Drop impl, or a function that takes a closure for user logic operating on params).
2021-06-02 19:57:38 +00:00
system_meta
Bevy ECS V2 (#1525)
# Bevy ECS V2
This is a rewrite of Bevy ECS (basically everything but the new executor/schedule, which are already awesome). The overall goal was to improve the performance and versatility of Bevy ECS. Here is a quick bulleted list of changes before we dive into the details:
* Complete World rewrite
* Multiple component storage types:
* Tables: fast cache friendly iteration, slower add/removes (previously called Archetypes)
* Sparse Sets: fast add/remove, slower iteration
* Stateful Queries (caches query results for faster iteration. fragmented iteration is _fast_ now)
* Stateful System Params (caches expensive operations. inspired by @DJMcNab's work in #1364)
* Configurable System Params (users can set configuration when they construct their systems. once again inspired by @DJMcNab's work)
* Archetypes are now "just metadata", component storage is separate
* Archetype Graph (for faster archetype changes)
* Component Metadata
* Configure component storage type
* Retrieve information about component size/type/name/layout/send-ness/etc
* Components are uniquely identified by a densely packed ComponentId
* TypeIds are now totally optional (which should make implementing scripting easier)
* Super fast "for_each" query iterators
* Merged Resources into World. Resources are now just a special type of component
* EntityRef/EntityMut builder apis (more efficient and more ergonomic)
* Fast bitset-backed `Access<T>` replaces old hashmap-based approach everywhere
* Query conflicts are determined by component access instead of archetype component access (to avoid random failures at runtime)
* With/Without are still taken into account for conflicts, so this should still be comfy to use
* Much simpler `IntoSystem` impl
* Significantly reduced the amount of hashing throughout the ecs in favor of Sparse Sets (indexed by densely packed ArchetypeId, ComponentId, BundleId, and TableId)
* Safety Improvements
* Entity reservation uses a normal world reference instead of unsafe transmute
* QuerySets no longer transmute lifetimes
* Made traits "unsafe" where relevant
* More thorough safety docs
* WorldCell
* Exposes safe mutable access to multiple resources at a time in a World
* Replaced "catch all" `System::update_archetypes(world: &World)` with `System::new_archetype(archetype: &Archetype)`
* Simpler Bundle implementation
* Replaced slow "remove_bundle_one_by_one" used as fallback for Commands::remove_bundle with fast "remove_bundle_intersection"
* Removed `Mut<T>` query impl. it is better to only support one way: `&mut T`
* Removed with() from `Flags<T>` in favor of `Option<Flags<T>>`, which allows querying for flags to be "filtered" by default
* Components now have is_send property (currently only resources support non-send)
* More granular module organization
* New `RemovedComponents<T>` SystemParam that replaces `query.removed::<T>()`
* `world.resource_scope()` for mutable access to resources and world at the same time
* WorldQuery and QueryFilter traits unified. FilterFetch trait added to enable "short circuit" filtering. Auto impled for cases that don't need it
* Significantly slimmed down SystemState in favor of individual SystemParam state
* System Commands changed from `commands: &mut Commands` back to `mut commands: Commands` (to allow Commands to have a World reference)
Fixes #1320
## `World` Rewrite
This is a from-scratch rewrite of `World` that fills the niche that `hecs` used to. Yes, this means Bevy ECS is no longer a "fork" of hecs. We're going out our own!
(the only shared code between the projects is the entity id allocator, which is already basically ideal)
A huge shout out to @SanderMertens (author of [flecs](https://github.com/SanderMertens/flecs)) for sharing some great ideas with me (specifically hybrid ecs storage and archetype graphs). He also helped advise on a number of implementation details.
## Component Storage (The Problem)
Two ECS storage paradigms have gained a lot of traction over the years:
* **Archetypal ECS**:
* Stores components in "tables" with static schemas. Each "column" stores components of a given type. Each "row" is an entity.
* Each "archetype" has its own table. Adding/removing an entity's component changes the archetype.
* Enables super-fast Query iteration due to its cache-friendly data layout
* Comes at the cost of more expensive add/remove operations for an Entity's components, because all components need to be copied to the new archetype's "table"
* **Sparse Set ECS**:
* Stores components of the same type in densely packed arrays, which are sparsely indexed by densely packed unsigned integers (Entity ids)
* Query iteration is slower than Archetypal ECS because each entity's component could be at any position in the sparse set. This "random access" pattern isn't cache friendly. Additionally, there is an extra layer of indirection because you must first map the entity id to an index in the component array.
* Adding/removing components is a cheap, constant time operation
Bevy ECS V1, hecs, legion, flec, and Unity DOTS are all "archetypal ecs-es". I personally think "archetypal" storage is a good default for game engines. An entity's archetype doesn't need to change frequently in general, and it creates "fast by default" query iteration (which is a much more common operation). It is also "self optimizing". Users don't need to think about optimizing component layouts for iteration performance. It "just works" without any extra boilerplate.
Shipyard and EnTT are "sparse set ecs-es". They employ "packing" as a way to work around the "suboptimal by default" iteration performance for specific sets of components. This helps, but I didn't think this was a good choice for a general purpose engine like Bevy because:
1. "packs" conflict with each other. If bevy decides to internally pack the Transform and GlobalTransform components, users are then blocked if they want to pack some custom component with Transform.
2. users need to take manual action to optimize
Developers selecting an ECS framework are stuck with a hard choice. Select an "archetypal" framework with "fast iteration everywhere" but without the ability to cheaply add/remove components, or select a "sparse set" framework to cheaply add/remove components but with slower iteration performance.
## Hybrid Component Storage (The Solution)
In Bevy ECS V2, we get to have our cake and eat it too. It now has _both_ of the component storage types above (and more can be added later if needed):
* **Tables** (aka "archetypal" storage)
* The default storage. If you don't configure anything, this is what you get
* Fast iteration by default
* Slower add/remove operations
* **Sparse Sets**
* Opt-in
* Slower iteration
* Faster add/remove operations
These storage types complement each other perfectly. By default Query iteration is fast. If developers know that they want to add/remove a component at high frequencies, they can set the storage to "sparse set":
```rust
world.register_component(
ComponentDescriptor::new::<MyComponent>(StorageType::SparseSet)
).unwrap();
```
## Archetypes
Archetypes are now "just metadata" ... they no longer store components directly. They do store:
* The `ComponentId`s of each of the Archetype's components (and that component's storage type)
* Archetypes are uniquely defined by their component layouts
* For example: entities with "table" components `[A, B, C]` _and_ "sparse set" components `[D, E]` will always be in the same archetype.
* The `TableId` associated with the archetype
* For now each archetype has exactly one table (which can have no components),
* There is a 1->Many relationship from Tables->Archetypes. A given table could have any number of archetype components stored in it:
* Ex: an entity with "table storage" components `[A, B, C]` and "sparse set" components `[D, E]` will share the same `[A, B, C]` table as an entity with `[A, B, C]` table component and `[F]` sparse set components.
* This 1->Many relationship is how we preserve fast "cache friendly" iteration performance when possible (more on this later)
* A list of entities that are in the archetype and the row id of the table they are in
* ArchetypeComponentIds
* unique densely packed identifiers for (ArchetypeId, ComponentId) pairs
* used by the schedule executor for cheap system access control
* "Archetype Graph Edges" (see the next section)
## The "Archetype Graph"
Archetype changes in Bevy (and a number of other archetypal ecs-es) have historically been expensive to compute. First, you need to allocate a new vector of the entity's current component ids, add or remove components based on the operation performed, sort it (to ensure it is order-independent), then hash it to find the archetype (if it exists). And thats all before we get to the _already_ expensive full copy of all components to the new table storage.
The solution is to build a "graph" of archetypes to cache these results. @SanderMertens first exposed me to the idea (and he got it from @gjroelofs, who came up with it). They propose adding directed edges between archetypes for add/remove component operations. If `ComponentId`s are densely packed, you can use sparse sets to cheaply jump between archetypes.
Bevy takes this one step further by using add/remove `Bundle` edges instead of `Component` edges. Bevy encourages the use of `Bundles` to group add/remove operations. This is largely for "clearer game logic" reasons, but it also helps cut down on the number of archetype changes required. `Bundles` now also have densely-packed `BundleId`s. This allows us to use a _single_ edge for each bundle operation (rather than needing to traverse N edges ... one for each component). Single component operations are also bundles, so this is strictly an improvement over a "component only" graph.
As a result, an operation that used to be _heavy_ (both for allocations and compute) is now two dirt-cheap array lookups and zero allocations.
## Stateful Queries
World queries are now stateful. This allows us to:
1. Cache archetype (and table) matches
* This resolves another issue with (naive) archetypal ECS: query performance getting worse as the number of archetypes goes up (and fragmentation occurs).
2. Cache Fetch and Filter state
* The expensive parts of fetch/filter operations (such as hashing the TypeId to find the ComponentId) now only happen once when the Query is first constructed
3. Incrementally build up state
* When new archetypes are added, we only process the new archetypes (no need to rebuild state for old archetypes)
As a result, the direct `World` query api now looks like this:
```rust
let mut query = world.query::<(&A, &mut B)>();
for (a, mut b) in query.iter_mut(&mut world) {
}
```
Requiring `World` to generate stateful queries (rather than letting the `QueryState` type be constructed separately) allows us to ensure that _all_ queries are properly initialized (and the relevant world state, such as ComponentIds). This enables QueryState to remove branches from its operations that check for initialization status (and also enables query.iter() to take an immutable world reference because it doesn't need to initialize anything in world).
However in systems, this is a non-breaking change. State management is done internally by the relevant SystemParam.
## Stateful SystemParams
Like Queries, `SystemParams` now also cache state. For example, `Query` system params store the "stateful query" state mentioned above. Commands store their internal `CommandQueue`. This means you can now safely use as many separate `Commands` parameters in your system as you want. `Local<T>` system params store their `T` value in their state (instead of in Resources).
SystemParam state also enabled a significant slim-down of SystemState. It is much nicer to look at now.
Per-SystemParam state naturally insulates us from an "aliased mut" class of errors we have hit in the past (ex: using multiple `Commands` system params).
(credit goes to @DJMcNab for the initial idea and draft pr here #1364)
## Configurable SystemParams
@DJMcNab also had the great idea to make SystemParams configurable. This allows users to provide some initial configuration / values for system parameters (when possible). Most SystemParams have no config (the config type is `()`), but the `Local<T>` param now supports user-provided parameters:
```rust
fn foo(value: Local<usize>) {
}
app.add_system(foo.system().config(|c| c.0 = Some(10)));
```
## Uber Fast "for_each" Query Iterators
Developers now have the choice to use a fast "for_each" iterator, which yields ~1.5-3x iteration speed improvements for "fragmented iteration", and minor ~1.2x iteration speed improvements for unfragmented iteration.
```rust
fn system(query: Query<(&A, &mut B)>) {
// you now have the option to do this for a speed boost
query.for_each_mut(|(a, mut b)| {
});
// however normal iterators are still available
for (a, mut b) in query.iter_mut() {
}
}
```
I think in most cases we should continue to encourage "normal" iterators as they are more flexible and more "rust idiomatic". But when that extra "oomf" is needed, it makes sense to use `for_each`.
We should also consider using `for_each` for internal bevy systems to give our users a nice speed boost (but that should be a separate pr).
## Component Metadata
`World` now has a `Components` collection, which is accessible via `world.components()`. This stores mappings from `ComponentId` to `ComponentInfo`, as well as `TypeId` to `ComponentId` mappings (where relevant). `ComponentInfo` stores information about the component, such as ComponentId, TypeId, memory layout, send-ness (currently limited to resources), and storage type.
## Significantly Cheaper `Access<T>`
We used to use `TypeAccess<TypeId>` to manage read/write component/archetype-component access. This was expensive because TypeIds must be hashed and compared individually. The parallel executor got around this by "condensing" type ids into bitset-backed access types. This worked, but it had to be re-generated from the `TypeAccess<TypeId>`sources every time archetypes changed.
This pr removes TypeAccess in favor of faster bitset access everywhere. We can do this thanks to the move to densely packed `ComponentId`s and `ArchetypeComponentId`s.
## Merged Resources into World
Resources had a lot of redundant functionality with Components. They stored typed data, they had access control, they had unique ids, they were queryable via SystemParams, etc. In fact the _only_ major difference between them was that they were unique (and didn't correlate to an entity).
Separate resources also had the downside of requiring a separate set of access controls, which meant the parallel executor needed to compare more bitsets per system and manage more state.
I initially got the "separate resources" idea from `legion`. I think that design was motivated by the fact that it made the direct world query/resource lifetime interactions more manageable. It certainly made our lives easier when using Resources alongside hecs/bevy_ecs. However we already have a construct for safely and ergonomically managing in-world lifetimes: systems (which use `Access<T>` internally).
This pr merges Resources into World:
```rust
world.insert_resource(1);
world.insert_resource(2.0);
let a = world.get_resource::<i32>().unwrap();
let mut b = world.get_resource_mut::<f64>().unwrap();
*b = 3.0;
```
Resources are now just a special kind of component. They have their own ComponentIds (and their own resource TypeId->ComponentId scope, so they don't conflict wit components of the same type). They are stored in a special "resource archetype", which stores components inside the archetype using a new `unique_components` sparse set (note that this sparse set could later be used to implement Tags). This allows us to keep the code size small by reusing existing datastructures (namely Column, Archetype, ComponentFlags, and ComponentInfo). This allows us the executor to use a single `Access<ArchetypeComponentId>` per system. It should also make scripting language integration easier.
_But_ this merge did create problems for people directly interacting with `World`. What if you need mutable access to multiple resources at the same time? `world.get_resource_mut()` borrows World mutably!
## WorldCell
WorldCell applies the `Access<ArchetypeComponentId>` concept to direct world access:
```rust
let world_cell = world.cell();
let a = world_cell.get_resource_mut::<i32>().unwrap();
let b = world_cell.get_resource_mut::<f64>().unwrap();
```
This adds cheap runtime checks (a sparse set lookup of `ArchetypeComponentId` and a counter) to ensure that world accesses do not conflict with each other. Each operation returns a `WorldBorrow<'w, T>` or `WorldBorrowMut<'w, T>` wrapper type, which will release the relevant ArchetypeComponentId resources when dropped.
World caches the access sparse set (and only one cell can exist at a time), so `world.cell()` is a cheap operation.
WorldCell does _not_ use atomic operations. It is non-send, does a mutable borrow of world to prevent other accesses, and uses a simple `Rc<RefCell<ArchetypeComponentAccess>>` wrapper in each WorldBorrow pointer.
The api is currently limited to resource access, but it can and should be extended to queries / entity component access.
## Resource Scopes
WorldCell does not yet support component queries, and even when it does there are sometimes legitimate reasons to want a mutable world ref _and_ a mutable resource ref (ex: bevy_render and bevy_scene both need this). In these cases we could always drop down to the unsafe `world.get_resource_unchecked_mut()`, but that is not ideal!
Instead developers can use a "resource scope"
```rust
world.resource_scope(|world: &mut World, a: &mut A| {
})
```
This temporarily removes the `A` resource from `World`, provides mutable pointers to both, and re-adds A to World when finished. Thanks to the move to ComponentIds/sparse sets, this is a cheap operation.
If multiple resources are required, scopes can be nested. We could also consider adding a "resource tuple" to the api if this pattern becomes common and the boilerplate gets nasty.
## Query Conflicts Use ComponentId Instead of ArchetypeComponentId
For safety reasons, systems cannot contain queries that conflict with each other without wrapping them in a QuerySet. On bevy `main`, we use ArchetypeComponentIds to determine conflicts. This is nice because it can take into account filters:
```rust
// these queries will never conflict due to their filters
fn filter_system(a: Query<&mut A, With<B>>, b: Query<&mut B, Without<B>>) {
}
```
But it also has a significant downside:
```rust
// these queries will not conflict _until_ an entity with A, B, and C is spawned
fn maybe_conflicts_system(a: Query<(&mut A, &C)>, b: Query<(&mut A, &B)>) {
}
```
The system above will panic at runtime if an entity with A, B, and C is spawned. This makes it hard to trust that your game logic will run without crashing.
In this pr, I switched to using `ComponentId` instead. This _is_ more constraining. `maybe_conflicts_system` will now always fail, but it will do it consistently at startup. Naively, it would also _disallow_ `filter_system`, which would be a significant downgrade in usability. Bevy has a number of internal systems that rely on disjoint queries and I expect it to be a common pattern in userspace.
To resolve this, I added a new `FilteredAccess<T>` type, which wraps `Access<T>` and adds with/without filters. If two `FilteredAccess` have with/without values that prove they are disjoint, they will no longer conflict.
## EntityRef / EntityMut
World entity operations on `main` require that the user passes in an `entity` id to each operation:
```rust
let entity = world.spawn((A, )); // create a new entity with A
world.get::<A>(entity);
world.insert(entity, (B, C));
world.insert_one(entity, D);
```
This means that each operation needs to look up the entity location / verify its validity. The initial spawn operation also requires a Bundle as input. This can be awkward when no components are required (or one component is required).
These operations have been replaced by `EntityRef` and `EntityMut`, which are "builder-style" wrappers around world that provide read and read/write operations on a single, pre-validated entity:
```rust
// spawn now takes no inputs and returns an EntityMut
let entity = world.spawn()
.insert(A) // insert a single component into the entity
.insert_bundle((B, C)) // insert a bundle of components into the entity
.id() // id returns the Entity id
// Returns EntityMut (or panics if the entity does not exist)
world.entity_mut(entity)
.insert(D)
.insert_bundle(SomeBundle::default());
{
// returns EntityRef (or panics if the entity does not exist)
let d = world.entity(entity)
.get::<D>() // gets the D component
.unwrap();
// world.get still exists for ergonomics
let d = world.get::<D>(entity).unwrap();
}
// These variants return Options if you want to check existence instead of panicing
world.get_entity_mut(entity)
.unwrap()
.insert(E);
if let Some(entity_ref) = world.get_entity(entity) {
let d = entity_ref.get::<D>().unwrap();
}
```
This _does not_ affect the current Commands api or terminology. I think that should be a separate conversation as that is a much larger breaking change.
## Safety Improvements
* Entity reservation in Commands uses a normal world borrow instead of an unsafe transmute
* QuerySets no longer transmutes lifetimes
* Made traits "unsafe" when implementing a trait incorrectly could cause unsafety
* More thorough safety docs
## RemovedComponents SystemParam
The old approach to querying removed components: `query.removed:<T>()` was confusing because it had no connection to the query itself. I replaced it with the following, which is both clearer and allows us to cache the ComponentId mapping in the SystemParamState:
```rust
fn system(removed: RemovedComponents<T>) {
for entity in removed.iter() {
}
}
```
## Simpler Bundle implementation
Bundles are no longer responsible for sorting (or deduping) TypeInfo. They are just a simple ordered list of component types / data. This makes the implementation smaller and opens the door to an easy "nested bundle" implementation in the future (which i might even add in this pr). Duplicate detection is now done once per bundle type by World the first time a bundle is used.
## Unified WorldQuery and QueryFilter types
(don't worry they are still separate type _parameters_ in Queries .. this is a non-breaking change)
WorldQuery and QueryFilter were already basically identical apis. With the addition of `FetchState` and more storage-specific fetch methods, the overlap was even clearer (and the redundancy more painful).
QueryFilters are now just `F: WorldQuery where F::Fetch: FilterFetch`. FilterFetch requires `Fetch<Item = bool>` and adds new "short circuit" variants of fetch methods. This enables a filter tuple like `(With<A>, Without<B>, Changed<C>)` to stop evaluating the filter after the first mismatch is encountered. FilterFetch is automatically implemented for `Fetch` implementations that return bool.
This forces fetch implementations that return things like `(bool, bool, bool)` (such as the filter above) to manually implement FilterFetch and decide whether or not to short-circuit.
## More Granular Modules
World no longer globs all of the internal modules together. It now exports `core`, `system`, and `schedule` separately. I'm also considering exporting `core` submodules directly as that is still pretty "glob-ey" and unorganized (feedback welcome here).
## Remaining Draft Work (to be done in this pr)
* ~~panic on conflicting WorldQuery fetches (&A, &mut A)~~
* ~~bevy `main` and hecs both currently allow this, but we should protect against it if possible~~
* ~~batch_iter / par_iter (currently stubbed out)~~
* ~~ChangedRes~~
* ~~I skipped this while we sort out #1313. This pr should be adapted to account for whatever we land on there~~.
* ~~The `Archetypes` and `Tables` collections use hashes of sorted lists of component ids to uniquely identify each archetype/table. This hash is then used as the key in a HashMap to look up the relevant ArchetypeId or TableId. (which doesn't handle hash collisions properly)~~
* ~~It is currently unsafe to generate a Query from "World A", then use it on "World B" (despite the api claiming it is safe). We should probably close this gap. This could be done by adding a randomly generated WorldId to each world, then storing that id in each Query. They could then be compared to each other on each `query.do_thing(&world)` operation. This _does_ add an extra branch to each query operation, so I'm open to other suggestions if people have them.~~
* ~~Nested Bundles (if i find time)~~
## Potential Future Work
* Expand WorldCell to support queries.
* Consider not allocating in the empty archetype on `world.spawn()`
* ex: return something like EntityMutUninit, which turns into EntityMut after an `insert` or `insert_bundle` op
* this actually regressed performance last time i tried it, but in theory it should be faster
* Optimize SparseSet::insert (see `PERF` comment on insert)
* Replace SparseArray `Option<T>` with T::MAX to cut down on branching
* would enable cheaper get_unchecked() operations
* upstream fixedbitset optimizations
* fixedbitset could be allocation free for small block counts (store blocks in a SmallVec)
* fixedbitset could have a const constructor
* Consider implementing Tags (archetype-specific by-value data that affects archetype identity)
* ex: ArchetypeA could have `[A, B, C]` table components and `[D(1)]` "tag" component. ArchetypeB could have `[A, B, C]` table components and a `[D(2)]` tag component. The archetypes are different, despite both having D tags because the value inside D is different.
* this could potentially build on top of the `archetype.unique_components` added in this pr for resource storage.
* Consider reverting `all_tuples` proc macro in favor of the old `macro_rules` implementation
* all_tuples is more flexible and produces cleaner documentation (the macro_rules version produces weird type parameter orders due to parser constraints)
* but unfortunately all_tuples also appears to make Rust Analyzer sad/slow when working inside of `bevy_ecs` (does not affect user code)
* Consider "resource queries" and/or "mixed resource and entity component queries" as an alternative to WorldCell
* this is basically just "systems" so maybe it's not worth it
* Add more world ops
* `world.clear()`
* `world.reserve<T: Bundle>(count: usize)`
* Try using the old archetype allocation strategy (allocate new memory on resize and copy everything over). I expect this to improve batch insertion performance at the cost of unbatched performance. But thats just a guess. I'm not an allocation perf pro :)
* Adapt Commands apis for consistency with new World apis
## Benchmarks
key:
* `bevy_old`: bevy `main` branch
* `bevy`: this branch
* `_foreach`: uses an optimized for_each iterator
* ` _sparse`: uses sparse set storage (if unspecified assume table storage)
* `_system`: runs inside a system (if unspecified assume test happens via direct world ops)
### Simple Insert (from ecs_bench_suite)
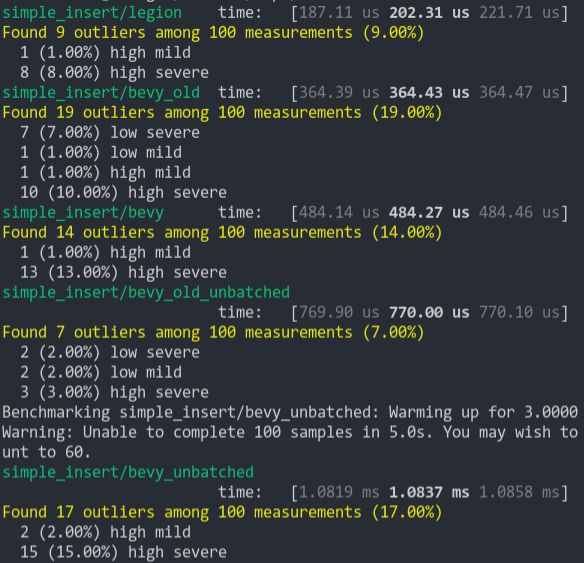
### Simpler Iter (from ecs_bench_suite)
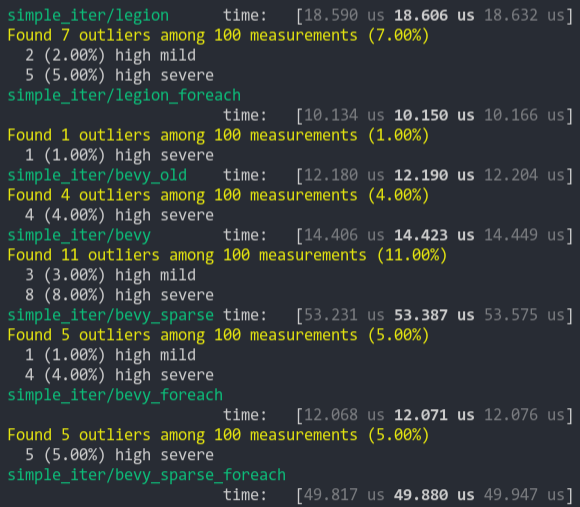
### Fragment Iter (from ecs_bench_suite)
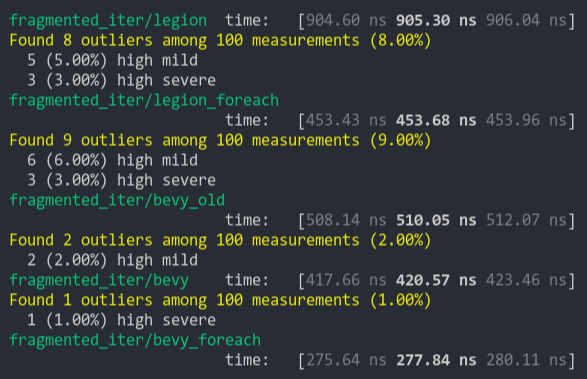
### Sparse Fragmented Iter
Iterate a query that matches 5 entities from a single matching archetype, but there are 100 unmatching archetypes
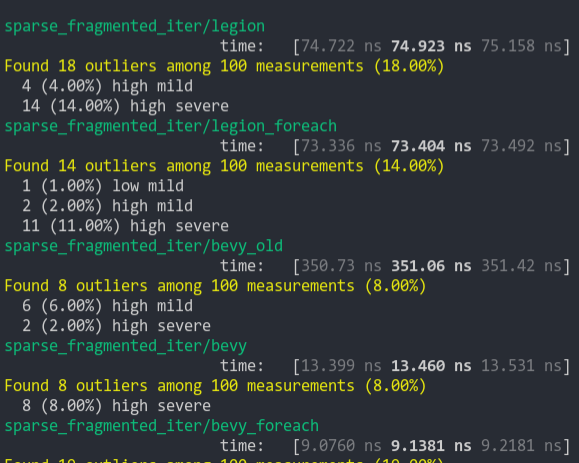
### Schedule (from ecs_bench_suite)
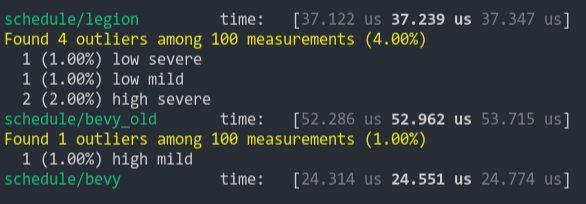
### Add Remove Component (from ecs_bench_suite)
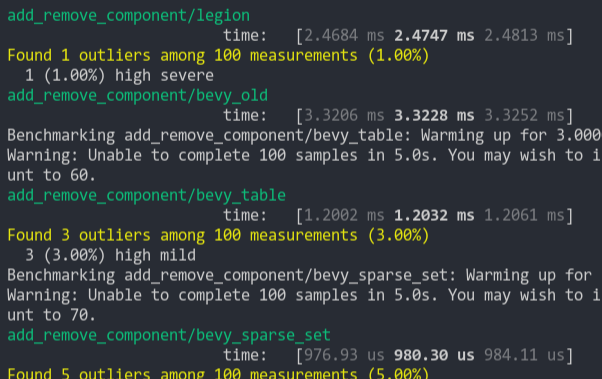
### Add Remove Component Big
Same as the test above, but each entity has 5 "large" matrix components and 1 "large" matrix component is added and removed
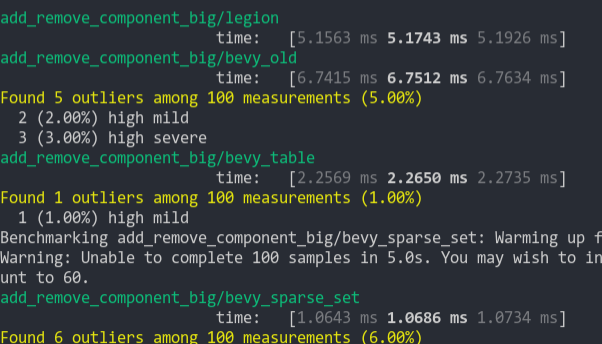
### Get Component
Looks up a single component value a large number of times
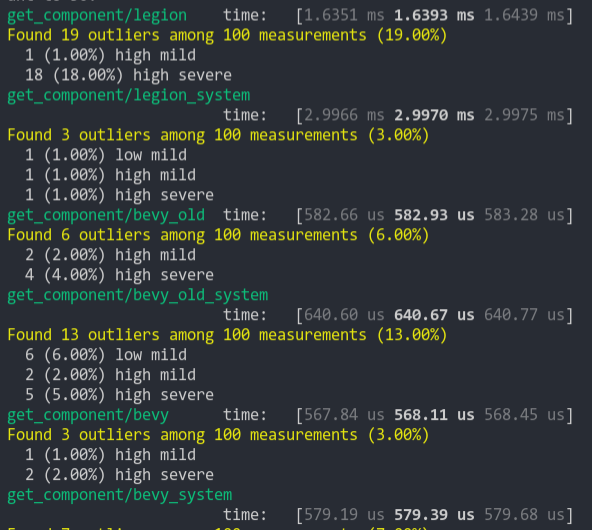
2021-03-05 07:54:35 +00:00
. archetype_component_access
Remove the `SystemParamState` trait and remove types like `ResState` (#6919)
Spiritual successor to #5205.
Actual successor to #6865.
# Objective
Currently, system params are defined using three traits: `SystemParam`, `ReadOnlySystemParam`, `SystemParamState`. The behavior for each param is specified by the `SystemParamState` trait, while `SystemParam` simply defers to the state.
Splitting the traits in this way makes it easier to implement within macros, but it increases the cognitive load. Worst of all, this approach requires each `MySystemParam` to have a public `MySystemParamState` type associated with it.
## Solution
* Merge the trait `SystemParamState` into `SystemParam`.
* Remove all trivial `SystemParam` state types.
* `OptionNonSendMutState<T>`: you will not be missed.
---
- [x] Fix/resolve the remaining test failure.
## Changelog
* Removed the trait `SystemParamState`, merging its functionality into `SystemParam`.
## Migration Guide
**Note**: this should replace the migration guide for #6865.
This is relative to Bevy 0.9, not main.
The traits `SystemParamState` and `SystemParamFetch` have been removed, and their functionality has been transferred to `SystemParam`.
```rust
// Before (0.9)
impl SystemParam for MyParam<'_, '_> {
type State = MyParamState;
}
unsafe impl SystemParamState for MyParamState {
fn init(world: &mut World, system_meta: &mut SystemMeta) -> Self { ... }
}
unsafe impl<'w, 's> SystemParamFetch<'w, 's> for MyParamState {
type Item = MyParam<'w, 's>;
fn get_param(&mut self, ...) -> Self::Item;
}
unsafe impl ReadOnlySystemParamFetch for MyParamState { }
// After (0.10)
unsafe impl SystemParam for MyParam<'_, '_> {
type State = MyParamState;
type Item<'w, 's> = MyParam<'w, 's>;
fn init_state(world: &mut World, system_meta: &mut SystemMeta) -> Self::State { ... }
fn get_param<'w, 's>(state: &mut Self::State, ...) -> Self::Item<'w, 's>;
}
unsafe impl ReadOnlySystemParam for MyParam<'_, '_> { }
```
The trait `ReadOnlySystemParamFetch` has been replaced with `ReadOnlySystemParam`.
```rust
// Before
unsafe impl ReadOnlySystemParamFetch for MyParamState {}
// After
unsafe impl ReadOnlySystemParam for MyParam<'_, '_> {}
```
2023-01-07 23:20:32 +00:00
. extend ( & state . archetype_component_access ) ;
Bevy ECS V2 (#1525)
# Bevy ECS V2
This is a rewrite of Bevy ECS (basically everything but the new executor/schedule, which are already awesome). The overall goal was to improve the performance and versatility of Bevy ECS. Here is a quick bulleted list of changes before we dive into the details:
* Complete World rewrite
* Multiple component storage types:
* Tables: fast cache friendly iteration, slower add/removes (previously called Archetypes)
* Sparse Sets: fast add/remove, slower iteration
* Stateful Queries (caches query results for faster iteration. fragmented iteration is _fast_ now)
* Stateful System Params (caches expensive operations. inspired by @DJMcNab's work in #1364)
* Configurable System Params (users can set configuration when they construct their systems. once again inspired by @DJMcNab's work)
* Archetypes are now "just metadata", component storage is separate
* Archetype Graph (for faster archetype changes)
* Component Metadata
* Configure component storage type
* Retrieve information about component size/type/name/layout/send-ness/etc
* Components are uniquely identified by a densely packed ComponentId
* TypeIds are now totally optional (which should make implementing scripting easier)
* Super fast "for_each" query iterators
* Merged Resources into World. Resources are now just a special type of component
* EntityRef/EntityMut builder apis (more efficient and more ergonomic)
* Fast bitset-backed `Access<T>` replaces old hashmap-based approach everywhere
* Query conflicts are determined by component access instead of archetype component access (to avoid random failures at runtime)
* With/Without are still taken into account for conflicts, so this should still be comfy to use
* Much simpler `IntoSystem` impl
* Significantly reduced the amount of hashing throughout the ecs in favor of Sparse Sets (indexed by densely packed ArchetypeId, ComponentId, BundleId, and TableId)
* Safety Improvements
* Entity reservation uses a normal world reference instead of unsafe transmute
* QuerySets no longer transmute lifetimes
* Made traits "unsafe" where relevant
* More thorough safety docs
* WorldCell
* Exposes safe mutable access to multiple resources at a time in a World
* Replaced "catch all" `System::update_archetypes(world: &World)` with `System::new_archetype(archetype: &Archetype)`
* Simpler Bundle implementation
* Replaced slow "remove_bundle_one_by_one" used as fallback for Commands::remove_bundle with fast "remove_bundle_intersection"
* Removed `Mut<T>` query impl. it is better to only support one way: `&mut T`
* Removed with() from `Flags<T>` in favor of `Option<Flags<T>>`, which allows querying for flags to be "filtered" by default
* Components now have is_send property (currently only resources support non-send)
* More granular module organization
* New `RemovedComponents<T>` SystemParam that replaces `query.removed::<T>()`
* `world.resource_scope()` for mutable access to resources and world at the same time
* WorldQuery and QueryFilter traits unified. FilterFetch trait added to enable "short circuit" filtering. Auto impled for cases that don't need it
* Significantly slimmed down SystemState in favor of individual SystemParam state
* System Commands changed from `commands: &mut Commands` back to `mut commands: Commands` (to allow Commands to have a World reference)
Fixes #1320
## `World` Rewrite
This is a from-scratch rewrite of `World` that fills the niche that `hecs` used to. Yes, this means Bevy ECS is no longer a "fork" of hecs. We're going out our own!
(the only shared code between the projects is the entity id allocator, which is already basically ideal)
A huge shout out to @SanderMertens (author of [flecs](https://github.com/SanderMertens/flecs)) for sharing some great ideas with me (specifically hybrid ecs storage and archetype graphs). He also helped advise on a number of implementation details.
## Component Storage (The Problem)
Two ECS storage paradigms have gained a lot of traction over the years:
* **Archetypal ECS**:
* Stores components in "tables" with static schemas. Each "column" stores components of a given type. Each "row" is an entity.
* Each "archetype" has its own table. Adding/removing an entity's component changes the archetype.
* Enables super-fast Query iteration due to its cache-friendly data layout
* Comes at the cost of more expensive add/remove operations for an Entity's components, because all components need to be copied to the new archetype's "table"
* **Sparse Set ECS**:
* Stores components of the same type in densely packed arrays, which are sparsely indexed by densely packed unsigned integers (Entity ids)
* Query iteration is slower than Archetypal ECS because each entity's component could be at any position in the sparse set. This "random access" pattern isn't cache friendly. Additionally, there is an extra layer of indirection because you must first map the entity id to an index in the component array.
* Adding/removing components is a cheap, constant time operation
Bevy ECS V1, hecs, legion, flec, and Unity DOTS are all "archetypal ecs-es". I personally think "archetypal" storage is a good default for game engines. An entity's archetype doesn't need to change frequently in general, and it creates "fast by default" query iteration (which is a much more common operation). It is also "self optimizing". Users don't need to think about optimizing component layouts for iteration performance. It "just works" without any extra boilerplate.
Shipyard and EnTT are "sparse set ecs-es". They employ "packing" as a way to work around the "suboptimal by default" iteration performance for specific sets of components. This helps, but I didn't think this was a good choice for a general purpose engine like Bevy because:
1. "packs" conflict with each other. If bevy decides to internally pack the Transform and GlobalTransform components, users are then blocked if they want to pack some custom component with Transform.
2. users need to take manual action to optimize
Developers selecting an ECS framework are stuck with a hard choice. Select an "archetypal" framework with "fast iteration everywhere" but without the ability to cheaply add/remove components, or select a "sparse set" framework to cheaply add/remove components but with slower iteration performance.
## Hybrid Component Storage (The Solution)
In Bevy ECS V2, we get to have our cake and eat it too. It now has _both_ of the component storage types above (and more can be added later if needed):
* **Tables** (aka "archetypal" storage)
* The default storage. If you don't configure anything, this is what you get
* Fast iteration by default
* Slower add/remove operations
* **Sparse Sets**
* Opt-in
* Slower iteration
* Faster add/remove operations
These storage types complement each other perfectly. By default Query iteration is fast. If developers know that they want to add/remove a component at high frequencies, they can set the storage to "sparse set":
```rust
world.register_component(
ComponentDescriptor::new::<MyComponent>(StorageType::SparseSet)
).unwrap();
```
## Archetypes
Archetypes are now "just metadata" ... they no longer store components directly. They do store:
* The `ComponentId`s of each of the Archetype's components (and that component's storage type)
* Archetypes are uniquely defined by their component layouts
* For example: entities with "table" components `[A, B, C]` _and_ "sparse set" components `[D, E]` will always be in the same archetype.
* The `TableId` associated with the archetype
* For now each archetype has exactly one table (which can have no components),
* There is a 1->Many relationship from Tables->Archetypes. A given table could have any number of archetype components stored in it:
* Ex: an entity with "table storage" components `[A, B, C]` and "sparse set" components `[D, E]` will share the same `[A, B, C]` table as an entity with `[A, B, C]` table component and `[F]` sparse set components.
* This 1->Many relationship is how we preserve fast "cache friendly" iteration performance when possible (more on this later)
* A list of entities that are in the archetype and the row id of the table they are in
* ArchetypeComponentIds
* unique densely packed identifiers for (ArchetypeId, ComponentId) pairs
* used by the schedule executor for cheap system access control
* "Archetype Graph Edges" (see the next section)
## The "Archetype Graph"
Archetype changes in Bevy (and a number of other archetypal ecs-es) have historically been expensive to compute. First, you need to allocate a new vector of the entity's current component ids, add or remove components based on the operation performed, sort it (to ensure it is order-independent), then hash it to find the archetype (if it exists). And thats all before we get to the _already_ expensive full copy of all components to the new table storage.
The solution is to build a "graph" of archetypes to cache these results. @SanderMertens first exposed me to the idea (and he got it from @gjroelofs, who came up with it). They propose adding directed edges between archetypes for add/remove component operations. If `ComponentId`s are densely packed, you can use sparse sets to cheaply jump between archetypes.
Bevy takes this one step further by using add/remove `Bundle` edges instead of `Component` edges. Bevy encourages the use of `Bundles` to group add/remove operations. This is largely for "clearer game logic" reasons, but it also helps cut down on the number of archetype changes required. `Bundles` now also have densely-packed `BundleId`s. This allows us to use a _single_ edge for each bundle operation (rather than needing to traverse N edges ... one for each component). Single component operations are also bundles, so this is strictly an improvement over a "component only" graph.
As a result, an operation that used to be _heavy_ (both for allocations and compute) is now two dirt-cheap array lookups and zero allocations.
## Stateful Queries
World queries are now stateful. This allows us to:
1. Cache archetype (and table) matches
* This resolves another issue with (naive) archetypal ECS: query performance getting worse as the number of archetypes goes up (and fragmentation occurs).
2. Cache Fetch and Filter state
* The expensive parts of fetch/filter operations (such as hashing the TypeId to find the ComponentId) now only happen once when the Query is first constructed
3. Incrementally build up state
* When new archetypes are added, we only process the new archetypes (no need to rebuild state for old archetypes)
As a result, the direct `World` query api now looks like this:
```rust
let mut query = world.query::<(&A, &mut B)>();
for (a, mut b) in query.iter_mut(&mut world) {
}
```
Requiring `World` to generate stateful queries (rather than letting the `QueryState` type be constructed separately) allows us to ensure that _all_ queries are properly initialized (and the relevant world state, such as ComponentIds). This enables QueryState to remove branches from its operations that check for initialization status (and also enables query.iter() to take an immutable world reference because it doesn't need to initialize anything in world).
However in systems, this is a non-breaking change. State management is done internally by the relevant SystemParam.
## Stateful SystemParams
Like Queries, `SystemParams` now also cache state. For example, `Query` system params store the "stateful query" state mentioned above. Commands store their internal `CommandQueue`. This means you can now safely use as many separate `Commands` parameters in your system as you want. `Local<T>` system params store their `T` value in their state (instead of in Resources).
SystemParam state also enabled a significant slim-down of SystemState. It is much nicer to look at now.
Per-SystemParam state naturally insulates us from an "aliased mut" class of errors we have hit in the past (ex: using multiple `Commands` system params).
(credit goes to @DJMcNab for the initial idea and draft pr here #1364)
## Configurable SystemParams
@DJMcNab also had the great idea to make SystemParams configurable. This allows users to provide some initial configuration / values for system parameters (when possible). Most SystemParams have no config (the config type is `()`), but the `Local<T>` param now supports user-provided parameters:
```rust
fn foo(value: Local<usize>) {
}
app.add_system(foo.system().config(|c| c.0 = Some(10)));
```
## Uber Fast "for_each" Query Iterators
Developers now have the choice to use a fast "for_each" iterator, which yields ~1.5-3x iteration speed improvements for "fragmented iteration", and minor ~1.2x iteration speed improvements for unfragmented iteration.
```rust
fn system(query: Query<(&A, &mut B)>) {
// you now have the option to do this for a speed boost
query.for_each_mut(|(a, mut b)| {
});
// however normal iterators are still available
for (a, mut b) in query.iter_mut() {
}
}
```
I think in most cases we should continue to encourage "normal" iterators as they are more flexible and more "rust idiomatic". But when that extra "oomf" is needed, it makes sense to use `for_each`.
We should also consider using `for_each` for internal bevy systems to give our users a nice speed boost (but that should be a separate pr).
## Component Metadata
`World` now has a `Components` collection, which is accessible via `world.components()`. This stores mappings from `ComponentId` to `ComponentInfo`, as well as `TypeId` to `ComponentId` mappings (where relevant). `ComponentInfo` stores information about the component, such as ComponentId, TypeId, memory layout, send-ness (currently limited to resources), and storage type.
## Significantly Cheaper `Access<T>`
We used to use `TypeAccess<TypeId>` to manage read/write component/archetype-component access. This was expensive because TypeIds must be hashed and compared individually. The parallel executor got around this by "condensing" type ids into bitset-backed access types. This worked, but it had to be re-generated from the `TypeAccess<TypeId>`sources every time archetypes changed.
This pr removes TypeAccess in favor of faster bitset access everywhere. We can do this thanks to the move to densely packed `ComponentId`s and `ArchetypeComponentId`s.
## Merged Resources into World
Resources had a lot of redundant functionality with Components. They stored typed data, they had access control, they had unique ids, they were queryable via SystemParams, etc. In fact the _only_ major difference between them was that they were unique (and didn't correlate to an entity).
Separate resources also had the downside of requiring a separate set of access controls, which meant the parallel executor needed to compare more bitsets per system and manage more state.
I initially got the "separate resources" idea from `legion`. I think that design was motivated by the fact that it made the direct world query/resource lifetime interactions more manageable. It certainly made our lives easier when using Resources alongside hecs/bevy_ecs. However we already have a construct for safely and ergonomically managing in-world lifetimes: systems (which use `Access<T>` internally).
This pr merges Resources into World:
```rust
world.insert_resource(1);
world.insert_resource(2.0);
let a = world.get_resource::<i32>().unwrap();
let mut b = world.get_resource_mut::<f64>().unwrap();
*b = 3.0;
```
Resources are now just a special kind of component. They have their own ComponentIds (and their own resource TypeId->ComponentId scope, so they don't conflict wit components of the same type). They are stored in a special "resource archetype", which stores components inside the archetype using a new `unique_components` sparse set (note that this sparse set could later be used to implement Tags). This allows us to keep the code size small by reusing existing datastructures (namely Column, Archetype, ComponentFlags, and ComponentInfo). This allows us the executor to use a single `Access<ArchetypeComponentId>` per system. It should also make scripting language integration easier.
_But_ this merge did create problems for people directly interacting with `World`. What if you need mutable access to multiple resources at the same time? `world.get_resource_mut()` borrows World mutably!
## WorldCell
WorldCell applies the `Access<ArchetypeComponentId>` concept to direct world access:
```rust
let world_cell = world.cell();
let a = world_cell.get_resource_mut::<i32>().unwrap();
let b = world_cell.get_resource_mut::<f64>().unwrap();
```
This adds cheap runtime checks (a sparse set lookup of `ArchetypeComponentId` and a counter) to ensure that world accesses do not conflict with each other. Each operation returns a `WorldBorrow<'w, T>` or `WorldBorrowMut<'w, T>` wrapper type, which will release the relevant ArchetypeComponentId resources when dropped.
World caches the access sparse set (and only one cell can exist at a time), so `world.cell()` is a cheap operation.
WorldCell does _not_ use atomic operations. It is non-send, does a mutable borrow of world to prevent other accesses, and uses a simple `Rc<RefCell<ArchetypeComponentAccess>>` wrapper in each WorldBorrow pointer.
The api is currently limited to resource access, but it can and should be extended to queries / entity component access.
## Resource Scopes
WorldCell does not yet support component queries, and even when it does there are sometimes legitimate reasons to want a mutable world ref _and_ a mutable resource ref (ex: bevy_render and bevy_scene both need this). In these cases we could always drop down to the unsafe `world.get_resource_unchecked_mut()`, but that is not ideal!
Instead developers can use a "resource scope"
```rust
world.resource_scope(|world: &mut World, a: &mut A| {
})
```
This temporarily removes the `A` resource from `World`, provides mutable pointers to both, and re-adds A to World when finished. Thanks to the move to ComponentIds/sparse sets, this is a cheap operation.
If multiple resources are required, scopes can be nested. We could also consider adding a "resource tuple" to the api if this pattern becomes common and the boilerplate gets nasty.
## Query Conflicts Use ComponentId Instead of ArchetypeComponentId
For safety reasons, systems cannot contain queries that conflict with each other without wrapping them in a QuerySet. On bevy `main`, we use ArchetypeComponentIds to determine conflicts. This is nice because it can take into account filters:
```rust
// these queries will never conflict due to their filters
fn filter_system(a: Query<&mut A, With<B>>, b: Query<&mut B, Without<B>>) {
}
```
But it also has a significant downside:
```rust
// these queries will not conflict _until_ an entity with A, B, and C is spawned
fn maybe_conflicts_system(a: Query<(&mut A, &C)>, b: Query<(&mut A, &B)>) {
}
```
The system above will panic at runtime if an entity with A, B, and C is spawned. This makes it hard to trust that your game logic will run without crashing.
In this pr, I switched to using `ComponentId` instead. This _is_ more constraining. `maybe_conflicts_system` will now always fail, but it will do it consistently at startup. Naively, it would also _disallow_ `filter_system`, which would be a significant downgrade in usability. Bevy has a number of internal systems that rely on disjoint queries and I expect it to be a common pattern in userspace.
To resolve this, I added a new `FilteredAccess<T>` type, which wraps `Access<T>` and adds with/without filters. If two `FilteredAccess` have with/without values that prove they are disjoint, they will no longer conflict.
## EntityRef / EntityMut
World entity operations on `main` require that the user passes in an `entity` id to each operation:
```rust
let entity = world.spawn((A, )); // create a new entity with A
world.get::<A>(entity);
world.insert(entity, (B, C));
world.insert_one(entity, D);
```
This means that each operation needs to look up the entity location / verify its validity. The initial spawn operation also requires a Bundle as input. This can be awkward when no components are required (or one component is required).
These operations have been replaced by `EntityRef` and `EntityMut`, which are "builder-style" wrappers around world that provide read and read/write operations on a single, pre-validated entity:
```rust
// spawn now takes no inputs and returns an EntityMut
let entity = world.spawn()
.insert(A) // insert a single component into the entity
.insert_bundle((B, C)) // insert a bundle of components into the entity
.id() // id returns the Entity id
// Returns EntityMut (or panics if the entity does not exist)
world.entity_mut(entity)
.insert(D)
.insert_bundle(SomeBundle::default());
{
// returns EntityRef (or panics if the entity does not exist)
let d = world.entity(entity)
.get::<D>() // gets the D component
.unwrap();
// world.get still exists for ergonomics
let d = world.get::<D>(entity).unwrap();
}
// These variants return Options if you want to check existence instead of panicing
world.get_entity_mut(entity)
.unwrap()
.insert(E);
if let Some(entity_ref) = world.get_entity(entity) {
let d = entity_ref.get::<D>().unwrap();
}
```
This _does not_ affect the current Commands api or terminology. I think that should be a separate conversation as that is a much larger breaking change.
## Safety Improvements
* Entity reservation in Commands uses a normal world borrow instead of an unsafe transmute
* QuerySets no longer transmutes lifetimes
* Made traits "unsafe" when implementing a trait incorrectly could cause unsafety
* More thorough safety docs
## RemovedComponents SystemParam
The old approach to querying removed components: `query.removed:<T>()` was confusing because it had no connection to the query itself. I replaced it with the following, which is both clearer and allows us to cache the ComponentId mapping in the SystemParamState:
```rust
fn system(removed: RemovedComponents<T>) {
for entity in removed.iter() {
}
}
```
## Simpler Bundle implementation
Bundles are no longer responsible for sorting (or deduping) TypeInfo. They are just a simple ordered list of component types / data. This makes the implementation smaller and opens the door to an easy "nested bundle" implementation in the future (which i might even add in this pr). Duplicate detection is now done once per bundle type by World the first time a bundle is used.
## Unified WorldQuery and QueryFilter types
(don't worry they are still separate type _parameters_ in Queries .. this is a non-breaking change)
WorldQuery and QueryFilter were already basically identical apis. With the addition of `FetchState` and more storage-specific fetch methods, the overlap was even clearer (and the redundancy more painful).
QueryFilters are now just `F: WorldQuery where F::Fetch: FilterFetch`. FilterFetch requires `Fetch<Item = bool>` and adds new "short circuit" variants of fetch methods. This enables a filter tuple like `(With<A>, Without<B>, Changed<C>)` to stop evaluating the filter after the first mismatch is encountered. FilterFetch is automatically implemented for `Fetch` implementations that return bool.
This forces fetch implementations that return things like `(bool, bool, bool)` (such as the filter above) to manually implement FilterFetch and decide whether or not to short-circuit.
## More Granular Modules
World no longer globs all of the internal modules together. It now exports `core`, `system`, and `schedule` separately. I'm also considering exporting `core` submodules directly as that is still pretty "glob-ey" and unorganized (feedback welcome here).
## Remaining Draft Work (to be done in this pr)
* ~~panic on conflicting WorldQuery fetches (&A, &mut A)~~
* ~~bevy `main` and hecs both currently allow this, but we should protect against it if possible~~
* ~~batch_iter / par_iter (currently stubbed out)~~
* ~~ChangedRes~~
* ~~I skipped this while we sort out #1313. This pr should be adapted to account for whatever we land on there~~.
* ~~The `Archetypes` and `Tables` collections use hashes of sorted lists of component ids to uniquely identify each archetype/table. This hash is then used as the key in a HashMap to look up the relevant ArchetypeId or TableId. (which doesn't handle hash collisions properly)~~
* ~~It is currently unsafe to generate a Query from "World A", then use it on "World B" (despite the api claiming it is safe). We should probably close this gap. This could be done by adding a randomly generated WorldId to each world, then storing that id in each Query. They could then be compared to each other on each `query.do_thing(&world)` operation. This _does_ add an extra branch to each query operation, so I'm open to other suggestions if people have them.~~
* ~~Nested Bundles (if i find time)~~
## Potential Future Work
* Expand WorldCell to support queries.
* Consider not allocating in the empty archetype on `world.spawn()`
* ex: return something like EntityMutUninit, which turns into EntityMut after an `insert` or `insert_bundle` op
* this actually regressed performance last time i tried it, but in theory it should be faster
* Optimize SparseSet::insert (see `PERF` comment on insert)
* Replace SparseArray `Option<T>` with T::MAX to cut down on branching
* would enable cheaper get_unchecked() operations
* upstream fixedbitset optimizations
* fixedbitset could be allocation free for small block counts (store blocks in a SmallVec)
* fixedbitset could have a const constructor
* Consider implementing Tags (archetype-specific by-value data that affects archetype identity)
* ex: ArchetypeA could have `[A, B, C]` table components and `[D(1)]` "tag" component. ArchetypeB could have `[A, B, C]` table components and a `[D(2)]` tag component. The archetypes are different, despite both having D tags because the value inside D is different.
* this could potentially build on top of the `archetype.unique_components` added in this pr for resource storage.
* Consider reverting `all_tuples` proc macro in favor of the old `macro_rules` implementation
* all_tuples is more flexible and produces cleaner documentation (the macro_rules version produces weird type parameter orders due to parser constraints)
* but unfortunately all_tuples also appears to make Rust Analyzer sad/slow when working inside of `bevy_ecs` (does not affect user code)
* Consider "resource queries" and/or "mixed resource and entity component queries" as an alternative to WorldCell
* this is basically just "systems" so maybe it's not worth it
* Add more world ops
* `world.clear()`
* `world.reserve<T: Bundle>(count: usize)`
* Try using the old archetype allocation strategy (allocate new memory on resize and copy everything over). I expect this to improve batch insertion performance at the cost of unbatched performance. But thats just a guess. I'm not an allocation perf pro :)
* Adapt Commands apis for consistency with new World apis
## Benchmarks
key:
* `bevy_old`: bevy `main` branch
* `bevy`: this branch
* `_foreach`: uses an optimized for_each iterator
* ` _sparse`: uses sparse set storage (if unspecified assume table storage)
* `_system`: runs inside a system (if unspecified assume test happens via direct world ops)
### Simple Insert (from ecs_bench_suite)
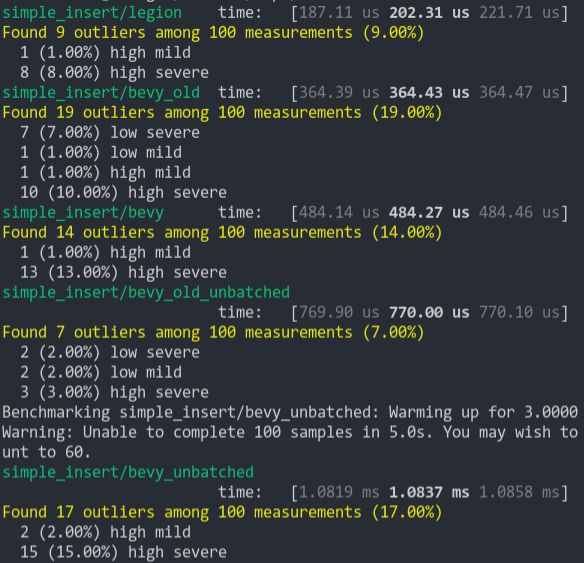
### Simpler Iter (from ecs_bench_suite)
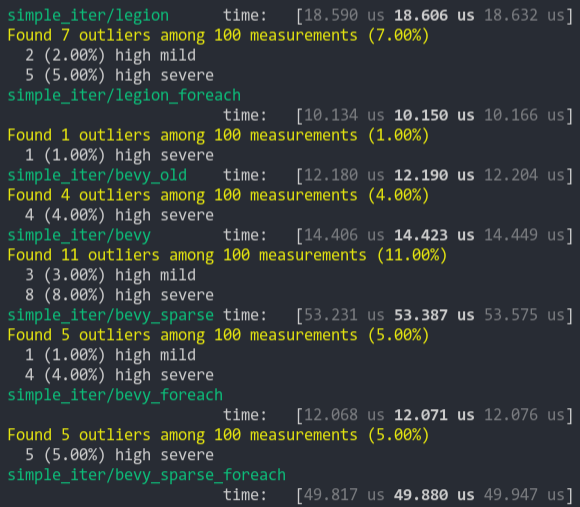
### Fragment Iter (from ecs_bench_suite)
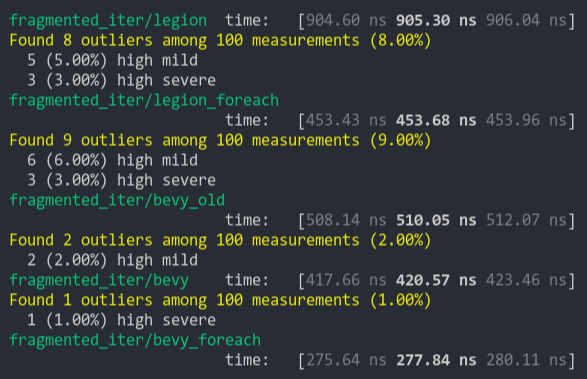
### Sparse Fragmented Iter
Iterate a query that matches 5 entities from a single matching archetype, but there are 100 unmatching archetypes
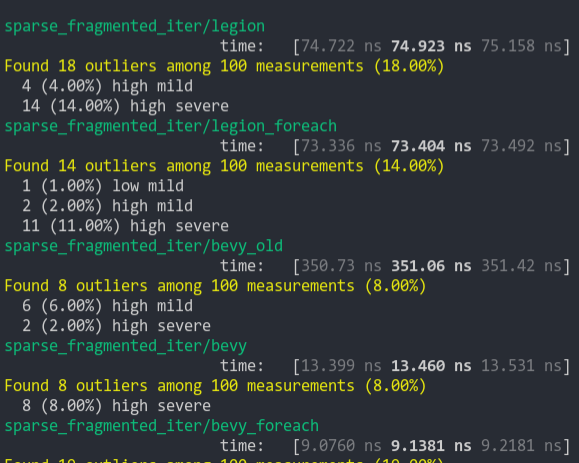
### Schedule (from ecs_bench_suite)
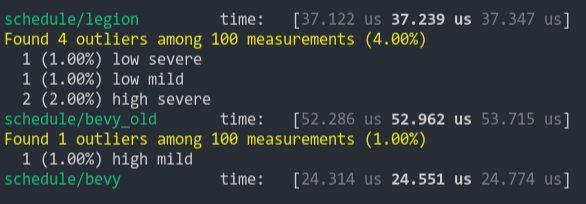
### Add Remove Component (from ecs_bench_suite)
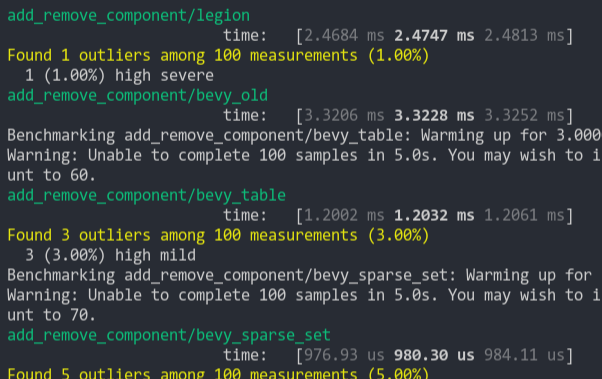
### Add Remove Component Big
Same as the test above, but each entity has 5 "large" matrix components and 1 "large" matrix component is added and removed
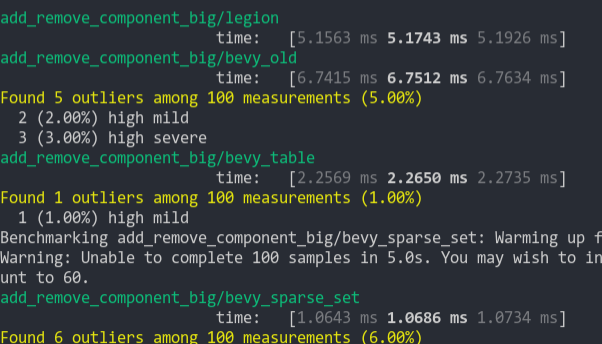
### Get Component
Looks up a single component value a large number of times
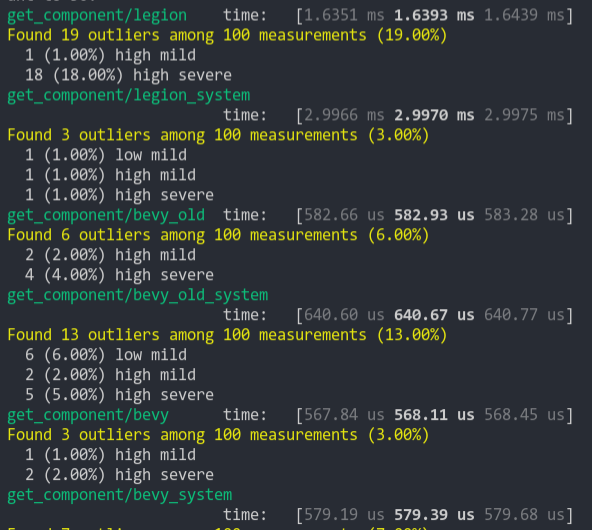
2021-03-05 07:54:35 +00:00
}
2020-12-16 05:57:16 +00:00
2020-11-08 20:34:05 +00:00
#[ inline ]
Simplify trait hierarchy for `SystemParam` (#6865)
# Objective
* Implementing a custom `SystemParam` by hand requires implementing three traits -- four if it is read-only.
* The trait `SystemParamFetch<'w, 's>` is a workaround from before we had generic associated types, and is no longer necessary.
## Solution
* Combine the trait `SystemParamFetch` with `SystemParamState`.
* I decided to remove the `Fetch` name and keep the `State` name, since the former was consistently conflated with the latter.
* Replace the trait `ReadOnlySystemParamFetch` with `ReadOnlySystemParam`, which simplifies trait bounds in generic code.
---
## Changelog
- Removed the trait `SystemParamFetch`, moving its functionality to `SystemParamState`.
- Replaced the trait `ReadOnlySystemParamFetch` with `ReadOnlySystemParam`.
## Migration Guide
The trait `SystemParamFetch` has been removed, and its functionality has been transferred to `SystemParamState`.
```rust
// Before
impl SystemParamState for MyParamState {
fn init(world: &mut World, system_meta: &mut SystemMeta) -> Self { ... }
}
impl<'w, 's> SystemParamFetch<'w, 's> for MyParamState {
type Item = MyParam<'w, 's>;
fn get_param(...) -> Self::Item;
}
// After
impl SystemParamState for MyParamState {
type Item<'w, 's> = MyParam<'w, 's>; // Generic associated types!
fn init(world: &mut World, system_meta: &mut SystemMeta) -> Self { ... }
fn get_param<'w, 's>(...) -> Self::Item<'w, 's>;
}
```
The trait `ReadOnlySystemParamFetch` has been replaced with `ReadOnlySystemParam`.
```rust
// Before
unsafe impl ReadOnlySystemParamFetch for MyParamState {}
// After
unsafe impl<'w, 's> ReadOnlySystemParam for MyParam<'w, 's> {}
```
2022-12-11 18:34:14 +00:00
unsafe fn get_param < ' w , ' s > (
Remove the `SystemParamState` trait and remove types like `ResState` (#6919)
Spiritual successor to #5205.
Actual successor to #6865.
# Objective
Currently, system params are defined using three traits: `SystemParam`, `ReadOnlySystemParam`, `SystemParamState`. The behavior for each param is specified by the `SystemParamState` trait, while `SystemParam` simply defers to the state.
Splitting the traits in this way makes it easier to implement within macros, but it increases the cognitive load. Worst of all, this approach requires each `MySystemParam` to have a public `MySystemParamState` type associated with it.
## Solution
* Merge the trait `SystemParamState` into `SystemParam`.
* Remove all trivial `SystemParam` state types.
* `OptionNonSendMutState<T>`: you will not be missed.
---
- [x] Fix/resolve the remaining test failure.
## Changelog
* Removed the trait `SystemParamState`, merging its functionality into `SystemParam`.
## Migration Guide
**Note**: this should replace the migration guide for #6865.
This is relative to Bevy 0.9, not main.
The traits `SystemParamState` and `SystemParamFetch` have been removed, and their functionality has been transferred to `SystemParam`.
```rust
// Before (0.9)
impl SystemParam for MyParam<'_, '_> {
type State = MyParamState;
}
unsafe impl SystemParamState for MyParamState {
fn init(world: &mut World, system_meta: &mut SystemMeta) -> Self { ... }
}
unsafe impl<'w, 's> SystemParamFetch<'w, 's> for MyParamState {
type Item = MyParam<'w, 's>;
fn get_param(&mut self, ...) -> Self::Item;
}
unsafe impl ReadOnlySystemParamFetch for MyParamState { }
// After (0.10)
unsafe impl SystemParam for MyParam<'_, '_> {
type State = MyParamState;
type Item<'w, 's> = MyParam<'w, 's>;
fn init_state(world: &mut World, system_meta: &mut SystemMeta) -> Self::State { ... }
fn get_param<'w, 's>(state: &mut Self::State, ...) -> Self::Item<'w, 's>;
}
unsafe impl ReadOnlySystemParam for MyParam<'_, '_> { }
```
The trait `ReadOnlySystemParamFetch` has been replaced with `ReadOnlySystemParam`.
```rust
// Before
unsafe impl ReadOnlySystemParamFetch for MyParamState {}
// After
unsafe impl ReadOnlySystemParam for MyParam<'_, '_> {}
```
2023-01-07 23:20:32 +00:00
state : & ' s mut Self ::State ,
Add new SystemState and rename old SystemState to SystemMeta (#2283)
This enables `SystemParams` to be used outside of function systems. Anything can create and store `SystemState`, which enables efficient "param state cached" access to `SystemParams`.
It adds a `ReadOnlySystemParamFetch` trait, which enables safe `SystemState::get` calls without unique world access.
I renamed the old `SystemState` to `SystemMeta` to enable us to mirror the `QueryState` naming convention (but I'm happy to discuss alternative names if people have other ideas). I initially pitched this as `ParamState`, but given that it needs to include full system metadata, that doesn't feel like a particularly accurate name.
```rust
#[derive(Eq, PartialEq, Debug)]
struct A(usize);
#[derive(Eq, PartialEq, Debug)]
struct B(usize);
let mut world = World::default();
world.insert_resource(A(42));
world.spawn().insert(B(7));
// we get nice lifetime elision when declaring the type on the left hand side
let mut system_state: SystemState<(Res<A>, Query<&B>)> = SystemState::new(&mut world);
let (a, query) = system_state.get(&world);
assert_eq!(*a, A(42), "returned resource matches initial value");
assert_eq!(
*query.single().unwrap(),
B(7),
"returned component matches initial value"
);
// mutable system params require unique world access
let mut system_state: SystemState<(ResMut<A>, Query<&mut B>)> = SystemState::new(&mut world);
let (a, query) = system_state.get_mut(&mut world);
// static lifetimes are required when declaring inside of structs
struct SomeContainer {
state: SystemState<(Res<'static, A>, Res<'static, B>)>
}
// this can be shortened using type aliases, which will be useful for complex param tuples
type MyParams<'a> = (Res<'a, A>, Res<'a, B>);
struct SomeContainer {
state: SystemState<MyParams<'static>>
}
// It is the user's responsibility to call SystemState::apply(world) for parameters that queue up work
let mut system_state: SystemState<(Commands, Query<&B>)> = SystemState::new(&mut world);
{
let (mut commands, query) = system_state.get(&world);
commands.insert_resource(3.14);
}
system_state.apply(&mut world);
```
## Future Work
* Actually use SystemState inside FunctionSystem. This would be trivial, but it requires FunctionSystem to wrap SystemState in Option in its current form (which complicates system metadata lookup). I'd prefer to hold off until we adopt something like the later designs linked in #1364, which enable us to contruct Systems using a World reference (and also remove the need for `.system`).
* Consider a "scoped" approach to automatically call SystemState::apply when systems params are no longer being used (either a container type with a Drop impl, or a function that takes a closure for user logic operating on params).
2021-06-02 19:57:38 +00:00
system_meta : & SystemMeta ,
System Param Lifetime Split (#2605)
# Objective
Enable using exact World lifetimes during read-only access . This is motivated by the new renderer's need to allow read-only world-only queries to outlive the query itself (but still be constrained by the world lifetime).
For example:
https://github.com/bevyengine/bevy/blob/115b170d1f11a91146bb6d6e9684dceb8b21f786/pipelined/bevy_pbr2/src/render/mod.rs#L774
## Solution
Split out SystemParam state and world lifetimes and pipe those lifetimes up to read-only Query ops (and add into_inner for Res). According to every safety test I've run so far (except one), this is safe (see the temporary safety test commit). Note that changing the mutable variants to the new lifetimes would allow aliased mutable pointers (try doing that to see how it affects the temporary safety tests).
The new state lifetime on SystemParam does make `#[derive(SystemParam)]` more cumbersome (the current impl requires PhantomData if you don't use both lifetimes). We can make this better by detecting whether or not a lifetime is used in the derive and adjusting accordingly, but that should probably be done in its own pr.
## Why is this a draft?
The new lifetimes break QuerySet safety in one very specific case (see the query_set system in system_safety_test). We need to solve this before we can use the lifetimes given.
This is due to the fact that QuerySet is just a wrapper over Query, which now relies on world lifetimes instead of `&self` lifetimes to prevent aliasing (but in systems, each Query has its own implied lifetime, not a centralized world lifetime). I believe the fix is to rewrite QuerySet to have its own World lifetime (and own the internal reference). This will complicate the impl a bit, but I think it is doable. I'm curious if anyone else has better ideas.
Personally, I think these new lifetimes need to happen. We've gotta have a way to directly tie read-only World queries to the World lifetime. The new renderer is the first place this has come up, but I doubt it will be the last. Worst case scenario we can come up with a second `WorldLifetimeQuery<Q, F = ()>` parameter to enable these read-only scenarios, but I'd rather not add another type to the type zoo.
2021-08-15 20:51:53 +00:00
world : & ' w World ,
Reliable change detection (#1471)
# Problem Definition
The current change tracking (via flags for both components and resources) fails to detect changes made by systems that are scheduled to run earlier in the frame than they are.
This issue is discussed at length in [#68](https://github.com/bevyengine/bevy/issues/68) and [#54](https://github.com/bevyengine/bevy/issues/54).
This is very much a draft PR, and contributions are welcome and needed.
# Criteria
1. Each change is detected at least once, no matter the ordering.
2. Each change is detected at most once, no matter the ordering.
3. Changes should be detected the same frame that they are made.
4. Competitive ergonomics. Ideally does not require opting-in.
5. Low CPU overhead of computation.
6. Memory efficient. This must not increase over time, except where the number of entities / resources does.
7. Changes should not be lost for systems that don't run.
8. A frame needs to act as a pure function. Given the same set of entities / components it needs to produce the same end state without side-effects.
**Exact** change-tracking proposals satisfy criteria 1 and 2.
**Conservative** change-tracking proposals satisfy criteria 1 but not 2.
**Flaky** change tracking proposals satisfy criteria 2 but not 1.
# Code Base Navigation
There are three types of flags:
- `Added`: A piece of data was added to an entity / `Resources`.
- `Mutated`: A piece of data was able to be modified, because its `DerefMut` was accessed
- `Changed`: The bitwise OR of `Added` and `Changed`
The special behavior of `ChangedRes`, with respect to the scheduler is being removed in [#1313](https://github.com/bevyengine/bevy/pull/1313) and does not need to be reproduced.
`ChangedRes` and friends can be found in "bevy_ecs/core/resources/resource_query.rs".
The `Flags` trait for Components can be found in "bevy_ecs/core/query.rs".
`ComponentFlags` are stored in "bevy_ecs/core/archetypes.rs", defined on line 446.
# Proposals
**Proposal 5 was selected for implementation.**
## Proposal 0: No Change Detection
The baseline, where computations are performed on everything regardless of whether it changed.
**Type:** Conservative
**Pros:**
- already implemented
- will never miss events
- no overhead
**Cons:**
- tons of repeated work
- doesn't allow users to avoid repeating work (or monitoring for other changes)
## Proposal 1: Earlier-This-Tick Change Detection
The current approach as of Bevy 0.4. Flags are set, and then flushed at the end of each frame.
**Type:** Flaky
**Pros:**
- already implemented
- simple to understand
- low memory overhead (2 bits per component)
- low time overhead (clear every flag once per frame)
**Cons:**
- misses systems based on ordering
- systems that don't run every frame miss changes
- duplicates detection when looping
- can lead to unresolvable circular dependencies
## Proposal 2: Two-Tick Change Detection
Flags persist for two frames, using a double-buffer system identical to that used in events.
A change is observed if it is found in either the current frame's list of changes or the previous frame's.
**Type:** Conservative
**Pros:**
- easy to understand
- easy to implement
- low memory overhead (4 bits per component)
- low time overhead (bit mask and shift every flag once per frame)
**Cons:**
- can result in a great deal of duplicated work
- systems that don't run every frame miss changes
- duplicates detection when looping
## Proposal 3: Last-Tick Change Detection
Flags persist for two frames, using a double-buffer system identical to that used in events.
A change is observed if it is found in the previous frame's list of changes.
**Type:** Exact
**Pros:**
- exact
- easy to understand
- easy to implement
- low memory overhead (4 bits per component)
- low time overhead (bit mask and shift every flag once per frame)
**Cons:**
- change detection is always delayed, possibly causing painful chained delays
- systems that don't run every frame miss changes
- duplicates detection when looping
## Proposal 4: Flag-Doubling Change Detection
Combine Proposal 2 and Proposal 3. Differentiate between `JustChanged` (current behavior) and `Changed` (Proposal 3).
Pack this data into the flags according to [this implementation proposal](https://github.com/bevyengine/bevy/issues/68#issuecomment-769174804).
**Type:** Flaky + Exact
**Pros:**
- allows users to acc
- easy to implement
- low memory overhead (4 bits per component)
- low time overhead (bit mask and shift every flag once per frame)
**Cons:**
- users must specify the type of change detection required
- still quite fragile to system ordering effects when using the flaky `JustChanged` form
- cannot get immediate + exact results
- systems that don't run every frame miss changes
- duplicates detection when looping
## [SELECTED] Proposal 5: Generation-Counter Change Detection
A global counter is increased after each system is run. Each component saves the time of last mutation, and each system saves the time of last execution. Mutation is detected when the component's counter is greater than the system's counter. Discussed [here](https://github.com/bevyengine/bevy/issues/68#issuecomment-769174804). How to handle addition detection is unsolved; the current proposal is to use the highest bit of the counter as in proposal 1.
**Type:** Exact (for mutations), flaky (for additions)
**Pros:**
- low time overhead (set component counter on access, set system counter after execution)
- robust to systems that don't run every frame
- robust to systems that loop
**Cons:**
- moderately complex implementation
- must be modified as systems are inserted dynamically
- medium memory overhead (4 bytes per component + system)
- unsolved addition detection
## Proposal 6: System-Data Change Detection
For each system, track which system's changes it has seen. This approach is only worth fully designing and implementing if Proposal 5 fails in some way.
**Type:** Exact
**Pros:**
- exact
- conceptually simple
**Cons:**
- requires storing data on each system
- implementation is complex
- must be modified as systems are inserted dynamically
## Proposal 7: Total-Order Change Detection
Discussed [here](https://github.com/bevyengine/bevy/issues/68#issuecomment-754326523). This proposal is somewhat complicated by the new scheduler, but I believe it should still be conceptually feasible. This approach is only worth fully designing and implementing if Proposal 5 fails in some way.
**Type:** Exact
**Pros:**
- exact
- efficient data storage relative to other exact proposals
**Cons:**
- requires access to the scheduler
- complex implementation and difficulty grokking
- must be modified as systems are inserted dynamically
# Tests
- We will need to verify properties 1, 2, 3, 7 and 8. Priority: 1 > 2 = 3 > 8 > 7
- Ideally we can use identical user-facing syntax for all proposals, allowing us to re-use the same syntax for each.
- When writing tests, we need to carefully specify order using explicit dependencies.
- These tests will need to be duplicated for both components and resources.
- We need to be sure to handle cases where ambiguous system orders exist.
`changing_system` is always the system that makes the changes, and `detecting_system` always detects the changes.
The component / resource changed will be simple boolean wrapper structs.
## Basic Added / Mutated / Changed
2 x 3 design:
- Resources vs. Components
- Added vs. Changed vs. Mutated
- `changing_system` runs before `detecting_system`
- verify at the end of tick 2
## At Least Once
2 x 3 design:
- Resources vs. Components
- Added vs. Changed vs. Mutated
- `changing_system` runs after `detecting_system`
- verify at the end of tick 2
## At Most Once
2 x 3 design:
- Resources vs. Components
- Added vs. Changed vs. Mutated
- `changing_system` runs once before `detecting_system`
- increment a counter based on the number of changes detected
- verify at the end of tick 2
## Fast Detection
2 x 3 design:
- Resources vs. Components
- Added vs. Changed vs. Mutated
- `changing_system` runs before `detecting_system`
- verify at the end of tick 1
## Ambiguous System Ordering Robustness
2 x 3 x 2 design:
- Resources vs. Components
- Added vs. Changed vs. Mutated
- `changing_system` runs [before/after] `detecting_system` in tick 1
- `changing_system` runs [after/before] `detecting_system` in tick 2
## System Pausing
2 x 3 design:
- Resources vs. Components
- Added vs. Changed vs. Mutated
- `changing_system` runs in tick 1, then is disabled by run criteria
- `detecting_system` is disabled by run criteria until it is run once during tick 3
- verify at the end of tick 3
## Addition Causes Mutation
2 design:
- Resources vs. Components
- `adding_system_1` adds a component / resource
- `adding system_2` adds the same component / resource
- verify the `Mutated` flag at the end of the tick
- verify the `Added` flag at the end of the tick
First check tests for: https://github.com/bevyengine/bevy/issues/333
Second check tests for: https://github.com/bevyengine/bevy/issues/1443
## Changes Made By Commands
- `adding_system` runs in Update in tick 1, and sends a command to add a component
- `detecting_system` runs in Update in tick 1 and 2, after `adding_system`
- We can't detect the changes in tick 1, since they haven't been processed yet
- If we were to track these changes as being emitted by `adding_system`, we can't detect the changes in tick 2 either, since `detecting_system` has already run once after `adding_system` :(
# Benchmarks
See: [general advice](https://github.com/bevyengine/bevy/blob/master/docs/profiling.md), [Criterion crate](https://github.com/bheisler/criterion.rs)
There are several critical parameters to vary:
1. entity count (1 to 10^9)
2. fraction of entities that are changed (0% to 100%)
3. cost to perform work on changed entities, i.e. workload (1 ns to 1s)
1 and 2 should be varied between benchmark runs. 3 can be added on computationally.
We want to measure:
- memory cost
- run time
We should collect these measurements across several frames (100?) to reduce bootup effects and accurately measure the mean, variance and drift.
Entity-component change detection is much more important to benchmark than resource change detection, due to the orders of magnitude higher number of pieces of data.
No change detection at all should be included in benchmarks as a second control for cases where missing changes is unacceptable.
## Graphs
1. y: performance, x: log_10(entity count), color: proposal, facet: performance metric. Set cost to perform work to 0.
2. y: run time, x: cost to perform work, color: proposal, facet: fraction changed. Set number of entities to 10^6
3. y: memory, x: frames, color: proposal
# Conclusions
1. Is the theoretical categorization of the proposals correct according to our tests?
2. How does the performance of the proposals compare without any load?
3. How does the performance of the proposals compare with realistic loads?
4. At what workload does more exact change tracking become worth the (presumably) higher overhead?
5. When does adding change-detection to save on work become worthwhile?
6. Is there enough divergence in performance between the best solutions in each class to ship more than one change-tracking solution?
# Implementation Plan
1. Write a test suite.
2. Verify that tests fail for existing approach.
3. Write a benchmark suite.
4. Get performance numbers for existing approach.
5. Implement, test and benchmark various solutions using a Git branch per proposal.
6. Create a draft PR with all solutions and present results to team.
7. Select a solution and replace existing change detection.
Co-authored-by: Brice DAVIER <bricedavier@gmail.com>
Co-authored-by: Carter Anderson <mcanders1@gmail.com>
2021-03-19 17:53:26 +00:00
change_tick : u32 ,
Simplify trait hierarchy for `SystemParam` (#6865)
# Objective
* Implementing a custom `SystemParam` by hand requires implementing three traits -- four if it is read-only.
* The trait `SystemParamFetch<'w, 's>` is a workaround from before we had generic associated types, and is no longer necessary.
## Solution
* Combine the trait `SystemParamFetch` with `SystemParamState`.
* I decided to remove the `Fetch` name and keep the `State` name, since the former was consistently conflated with the latter.
* Replace the trait `ReadOnlySystemParamFetch` with `ReadOnlySystemParam`, which simplifies trait bounds in generic code.
---
## Changelog
- Removed the trait `SystemParamFetch`, moving its functionality to `SystemParamState`.
- Replaced the trait `ReadOnlySystemParamFetch` with `ReadOnlySystemParam`.
## Migration Guide
The trait `SystemParamFetch` has been removed, and its functionality has been transferred to `SystemParamState`.
```rust
// Before
impl SystemParamState for MyParamState {
fn init(world: &mut World, system_meta: &mut SystemMeta) -> Self { ... }
}
impl<'w, 's> SystemParamFetch<'w, 's> for MyParamState {
type Item = MyParam<'w, 's>;
fn get_param(...) -> Self::Item;
}
// After
impl SystemParamState for MyParamState {
type Item<'w, 's> = MyParam<'w, 's>; // Generic associated types!
fn init(world: &mut World, system_meta: &mut SystemMeta) -> Self { ... }
fn get_param<'w, 's>(...) -> Self::Item<'w, 's>;
}
```
The trait `ReadOnlySystemParamFetch` has been replaced with `ReadOnlySystemParam`.
```rust
// Before
unsafe impl ReadOnlySystemParamFetch for MyParamState {}
// After
unsafe impl<'w, 's> ReadOnlySystemParam for MyParam<'w, 's> {}
```
2022-12-11 18:34:14 +00:00
) -> Self ::Item < ' w , ' s > {
2023-01-10 18:55:23 +00:00
Query ::new (
world ,
state ,
system_meta . last_change_tick ,
change_tick ,
false ,
)
2020-11-08 20:34:05 +00:00
}
Bevy ECS V2 (#1525)
# Bevy ECS V2
This is a rewrite of Bevy ECS (basically everything but the new executor/schedule, which are already awesome). The overall goal was to improve the performance and versatility of Bevy ECS. Here is a quick bulleted list of changes before we dive into the details:
* Complete World rewrite
* Multiple component storage types:
* Tables: fast cache friendly iteration, slower add/removes (previously called Archetypes)
* Sparse Sets: fast add/remove, slower iteration
* Stateful Queries (caches query results for faster iteration. fragmented iteration is _fast_ now)
* Stateful System Params (caches expensive operations. inspired by @DJMcNab's work in #1364)
* Configurable System Params (users can set configuration when they construct their systems. once again inspired by @DJMcNab's work)
* Archetypes are now "just metadata", component storage is separate
* Archetype Graph (for faster archetype changes)
* Component Metadata
* Configure component storage type
* Retrieve information about component size/type/name/layout/send-ness/etc
* Components are uniquely identified by a densely packed ComponentId
* TypeIds are now totally optional (which should make implementing scripting easier)
* Super fast "for_each" query iterators
* Merged Resources into World. Resources are now just a special type of component
* EntityRef/EntityMut builder apis (more efficient and more ergonomic)
* Fast bitset-backed `Access<T>` replaces old hashmap-based approach everywhere
* Query conflicts are determined by component access instead of archetype component access (to avoid random failures at runtime)
* With/Without are still taken into account for conflicts, so this should still be comfy to use
* Much simpler `IntoSystem` impl
* Significantly reduced the amount of hashing throughout the ecs in favor of Sparse Sets (indexed by densely packed ArchetypeId, ComponentId, BundleId, and TableId)
* Safety Improvements
* Entity reservation uses a normal world reference instead of unsafe transmute
* QuerySets no longer transmute lifetimes
* Made traits "unsafe" where relevant
* More thorough safety docs
* WorldCell
* Exposes safe mutable access to multiple resources at a time in a World
* Replaced "catch all" `System::update_archetypes(world: &World)` with `System::new_archetype(archetype: &Archetype)`
* Simpler Bundle implementation
* Replaced slow "remove_bundle_one_by_one" used as fallback for Commands::remove_bundle with fast "remove_bundle_intersection"
* Removed `Mut<T>` query impl. it is better to only support one way: `&mut T`
* Removed with() from `Flags<T>` in favor of `Option<Flags<T>>`, which allows querying for flags to be "filtered" by default
* Components now have is_send property (currently only resources support non-send)
* More granular module organization
* New `RemovedComponents<T>` SystemParam that replaces `query.removed::<T>()`
* `world.resource_scope()` for mutable access to resources and world at the same time
* WorldQuery and QueryFilter traits unified. FilterFetch trait added to enable "short circuit" filtering. Auto impled for cases that don't need it
* Significantly slimmed down SystemState in favor of individual SystemParam state
* System Commands changed from `commands: &mut Commands` back to `mut commands: Commands` (to allow Commands to have a World reference)
Fixes #1320
## `World` Rewrite
This is a from-scratch rewrite of `World` that fills the niche that `hecs` used to. Yes, this means Bevy ECS is no longer a "fork" of hecs. We're going out our own!
(the only shared code between the projects is the entity id allocator, which is already basically ideal)
A huge shout out to @SanderMertens (author of [flecs](https://github.com/SanderMertens/flecs)) for sharing some great ideas with me (specifically hybrid ecs storage and archetype graphs). He also helped advise on a number of implementation details.
## Component Storage (The Problem)
Two ECS storage paradigms have gained a lot of traction over the years:
* **Archetypal ECS**:
* Stores components in "tables" with static schemas. Each "column" stores components of a given type. Each "row" is an entity.
* Each "archetype" has its own table. Adding/removing an entity's component changes the archetype.
* Enables super-fast Query iteration due to its cache-friendly data layout
* Comes at the cost of more expensive add/remove operations for an Entity's components, because all components need to be copied to the new archetype's "table"
* **Sparse Set ECS**:
* Stores components of the same type in densely packed arrays, which are sparsely indexed by densely packed unsigned integers (Entity ids)
* Query iteration is slower than Archetypal ECS because each entity's component could be at any position in the sparse set. This "random access" pattern isn't cache friendly. Additionally, there is an extra layer of indirection because you must first map the entity id to an index in the component array.
* Adding/removing components is a cheap, constant time operation
Bevy ECS V1, hecs, legion, flec, and Unity DOTS are all "archetypal ecs-es". I personally think "archetypal" storage is a good default for game engines. An entity's archetype doesn't need to change frequently in general, and it creates "fast by default" query iteration (which is a much more common operation). It is also "self optimizing". Users don't need to think about optimizing component layouts for iteration performance. It "just works" without any extra boilerplate.
Shipyard and EnTT are "sparse set ecs-es". They employ "packing" as a way to work around the "suboptimal by default" iteration performance for specific sets of components. This helps, but I didn't think this was a good choice for a general purpose engine like Bevy because:
1. "packs" conflict with each other. If bevy decides to internally pack the Transform and GlobalTransform components, users are then blocked if they want to pack some custom component with Transform.
2. users need to take manual action to optimize
Developers selecting an ECS framework are stuck with a hard choice. Select an "archetypal" framework with "fast iteration everywhere" but without the ability to cheaply add/remove components, or select a "sparse set" framework to cheaply add/remove components but with slower iteration performance.
## Hybrid Component Storage (The Solution)
In Bevy ECS V2, we get to have our cake and eat it too. It now has _both_ of the component storage types above (and more can be added later if needed):
* **Tables** (aka "archetypal" storage)
* The default storage. If you don't configure anything, this is what you get
* Fast iteration by default
* Slower add/remove operations
* **Sparse Sets**
* Opt-in
* Slower iteration
* Faster add/remove operations
These storage types complement each other perfectly. By default Query iteration is fast. If developers know that they want to add/remove a component at high frequencies, they can set the storage to "sparse set":
```rust
world.register_component(
ComponentDescriptor::new::<MyComponent>(StorageType::SparseSet)
).unwrap();
```
## Archetypes
Archetypes are now "just metadata" ... they no longer store components directly. They do store:
* The `ComponentId`s of each of the Archetype's components (and that component's storage type)
* Archetypes are uniquely defined by their component layouts
* For example: entities with "table" components `[A, B, C]` _and_ "sparse set" components `[D, E]` will always be in the same archetype.
* The `TableId` associated with the archetype
* For now each archetype has exactly one table (which can have no components),
* There is a 1->Many relationship from Tables->Archetypes. A given table could have any number of archetype components stored in it:
* Ex: an entity with "table storage" components `[A, B, C]` and "sparse set" components `[D, E]` will share the same `[A, B, C]` table as an entity with `[A, B, C]` table component and `[F]` sparse set components.
* This 1->Many relationship is how we preserve fast "cache friendly" iteration performance when possible (more on this later)
* A list of entities that are in the archetype and the row id of the table they are in
* ArchetypeComponentIds
* unique densely packed identifiers for (ArchetypeId, ComponentId) pairs
* used by the schedule executor for cheap system access control
* "Archetype Graph Edges" (see the next section)
## The "Archetype Graph"
Archetype changes in Bevy (and a number of other archetypal ecs-es) have historically been expensive to compute. First, you need to allocate a new vector of the entity's current component ids, add or remove components based on the operation performed, sort it (to ensure it is order-independent), then hash it to find the archetype (if it exists). And thats all before we get to the _already_ expensive full copy of all components to the new table storage.
The solution is to build a "graph" of archetypes to cache these results. @SanderMertens first exposed me to the idea (and he got it from @gjroelofs, who came up with it). They propose adding directed edges between archetypes for add/remove component operations. If `ComponentId`s are densely packed, you can use sparse sets to cheaply jump between archetypes.
Bevy takes this one step further by using add/remove `Bundle` edges instead of `Component` edges. Bevy encourages the use of `Bundles` to group add/remove operations. This is largely for "clearer game logic" reasons, but it also helps cut down on the number of archetype changes required. `Bundles` now also have densely-packed `BundleId`s. This allows us to use a _single_ edge for each bundle operation (rather than needing to traverse N edges ... one for each component). Single component operations are also bundles, so this is strictly an improvement over a "component only" graph.
As a result, an operation that used to be _heavy_ (both for allocations and compute) is now two dirt-cheap array lookups and zero allocations.
## Stateful Queries
World queries are now stateful. This allows us to:
1. Cache archetype (and table) matches
* This resolves another issue with (naive) archetypal ECS: query performance getting worse as the number of archetypes goes up (and fragmentation occurs).
2. Cache Fetch and Filter state
* The expensive parts of fetch/filter operations (such as hashing the TypeId to find the ComponentId) now only happen once when the Query is first constructed
3. Incrementally build up state
* When new archetypes are added, we only process the new archetypes (no need to rebuild state for old archetypes)
As a result, the direct `World` query api now looks like this:
```rust
let mut query = world.query::<(&A, &mut B)>();
for (a, mut b) in query.iter_mut(&mut world) {
}
```
Requiring `World` to generate stateful queries (rather than letting the `QueryState` type be constructed separately) allows us to ensure that _all_ queries are properly initialized (and the relevant world state, such as ComponentIds). This enables QueryState to remove branches from its operations that check for initialization status (and also enables query.iter() to take an immutable world reference because it doesn't need to initialize anything in world).
However in systems, this is a non-breaking change. State management is done internally by the relevant SystemParam.
## Stateful SystemParams
Like Queries, `SystemParams` now also cache state. For example, `Query` system params store the "stateful query" state mentioned above. Commands store their internal `CommandQueue`. This means you can now safely use as many separate `Commands` parameters in your system as you want. `Local<T>` system params store their `T` value in their state (instead of in Resources).
SystemParam state also enabled a significant slim-down of SystemState. It is much nicer to look at now.
Per-SystemParam state naturally insulates us from an "aliased mut" class of errors we have hit in the past (ex: using multiple `Commands` system params).
(credit goes to @DJMcNab for the initial idea and draft pr here #1364)
## Configurable SystemParams
@DJMcNab also had the great idea to make SystemParams configurable. This allows users to provide some initial configuration / values for system parameters (when possible). Most SystemParams have no config (the config type is `()`), but the `Local<T>` param now supports user-provided parameters:
```rust
fn foo(value: Local<usize>) {
}
app.add_system(foo.system().config(|c| c.0 = Some(10)));
```
## Uber Fast "for_each" Query Iterators
Developers now have the choice to use a fast "for_each" iterator, which yields ~1.5-3x iteration speed improvements for "fragmented iteration", and minor ~1.2x iteration speed improvements for unfragmented iteration.
```rust
fn system(query: Query<(&A, &mut B)>) {
// you now have the option to do this for a speed boost
query.for_each_mut(|(a, mut b)| {
});
// however normal iterators are still available
for (a, mut b) in query.iter_mut() {
}
}
```
I think in most cases we should continue to encourage "normal" iterators as they are more flexible and more "rust idiomatic". But when that extra "oomf" is needed, it makes sense to use `for_each`.
We should also consider using `for_each` for internal bevy systems to give our users a nice speed boost (but that should be a separate pr).
## Component Metadata
`World` now has a `Components` collection, which is accessible via `world.components()`. This stores mappings from `ComponentId` to `ComponentInfo`, as well as `TypeId` to `ComponentId` mappings (where relevant). `ComponentInfo` stores information about the component, such as ComponentId, TypeId, memory layout, send-ness (currently limited to resources), and storage type.
## Significantly Cheaper `Access<T>`
We used to use `TypeAccess<TypeId>` to manage read/write component/archetype-component access. This was expensive because TypeIds must be hashed and compared individually. The parallel executor got around this by "condensing" type ids into bitset-backed access types. This worked, but it had to be re-generated from the `TypeAccess<TypeId>`sources every time archetypes changed.
This pr removes TypeAccess in favor of faster bitset access everywhere. We can do this thanks to the move to densely packed `ComponentId`s and `ArchetypeComponentId`s.
## Merged Resources into World
Resources had a lot of redundant functionality with Components. They stored typed data, they had access control, they had unique ids, they were queryable via SystemParams, etc. In fact the _only_ major difference between them was that they were unique (and didn't correlate to an entity).
Separate resources also had the downside of requiring a separate set of access controls, which meant the parallel executor needed to compare more bitsets per system and manage more state.
I initially got the "separate resources" idea from `legion`. I think that design was motivated by the fact that it made the direct world query/resource lifetime interactions more manageable. It certainly made our lives easier when using Resources alongside hecs/bevy_ecs. However we already have a construct for safely and ergonomically managing in-world lifetimes: systems (which use `Access<T>` internally).
This pr merges Resources into World:
```rust
world.insert_resource(1);
world.insert_resource(2.0);
let a = world.get_resource::<i32>().unwrap();
let mut b = world.get_resource_mut::<f64>().unwrap();
*b = 3.0;
```
Resources are now just a special kind of component. They have their own ComponentIds (and their own resource TypeId->ComponentId scope, so they don't conflict wit components of the same type). They are stored in a special "resource archetype", which stores components inside the archetype using a new `unique_components` sparse set (note that this sparse set could later be used to implement Tags). This allows us to keep the code size small by reusing existing datastructures (namely Column, Archetype, ComponentFlags, and ComponentInfo). This allows us the executor to use a single `Access<ArchetypeComponentId>` per system. It should also make scripting language integration easier.
_But_ this merge did create problems for people directly interacting with `World`. What if you need mutable access to multiple resources at the same time? `world.get_resource_mut()` borrows World mutably!
## WorldCell
WorldCell applies the `Access<ArchetypeComponentId>` concept to direct world access:
```rust
let world_cell = world.cell();
let a = world_cell.get_resource_mut::<i32>().unwrap();
let b = world_cell.get_resource_mut::<f64>().unwrap();
```
This adds cheap runtime checks (a sparse set lookup of `ArchetypeComponentId` and a counter) to ensure that world accesses do not conflict with each other. Each operation returns a `WorldBorrow<'w, T>` or `WorldBorrowMut<'w, T>` wrapper type, which will release the relevant ArchetypeComponentId resources when dropped.
World caches the access sparse set (and only one cell can exist at a time), so `world.cell()` is a cheap operation.
WorldCell does _not_ use atomic operations. It is non-send, does a mutable borrow of world to prevent other accesses, and uses a simple `Rc<RefCell<ArchetypeComponentAccess>>` wrapper in each WorldBorrow pointer.
The api is currently limited to resource access, but it can and should be extended to queries / entity component access.
## Resource Scopes
WorldCell does not yet support component queries, and even when it does there are sometimes legitimate reasons to want a mutable world ref _and_ a mutable resource ref (ex: bevy_render and bevy_scene both need this). In these cases we could always drop down to the unsafe `world.get_resource_unchecked_mut()`, but that is not ideal!
Instead developers can use a "resource scope"
```rust
world.resource_scope(|world: &mut World, a: &mut A| {
})
```
This temporarily removes the `A` resource from `World`, provides mutable pointers to both, and re-adds A to World when finished. Thanks to the move to ComponentIds/sparse sets, this is a cheap operation.
If multiple resources are required, scopes can be nested. We could also consider adding a "resource tuple" to the api if this pattern becomes common and the boilerplate gets nasty.
## Query Conflicts Use ComponentId Instead of ArchetypeComponentId
For safety reasons, systems cannot contain queries that conflict with each other without wrapping them in a QuerySet. On bevy `main`, we use ArchetypeComponentIds to determine conflicts. This is nice because it can take into account filters:
```rust
// these queries will never conflict due to their filters
fn filter_system(a: Query<&mut A, With<B>>, b: Query<&mut B, Without<B>>) {
}
```
But it also has a significant downside:
```rust
// these queries will not conflict _until_ an entity with A, B, and C is spawned
fn maybe_conflicts_system(a: Query<(&mut A, &C)>, b: Query<(&mut A, &B)>) {
}
```
The system above will panic at runtime if an entity with A, B, and C is spawned. This makes it hard to trust that your game logic will run without crashing.
In this pr, I switched to using `ComponentId` instead. This _is_ more constraining. `maybe_conflicts_system` will now always fail, but it will do it consistently at startup. Naively, it would also _disallow_ `filter_system`, which would be a significant downgrade in usability. Bevy has a number of internal systems that rely on disjoint queries and I expect it to be a common pattern in userspace.
To resolve this, I added a new `FilteredAccess<T>` type, which wraps `Access<T>` and adds with/without filters. If two `FilteredAccess` have with/without values that prove they are disjoint, they will no longer conflict.
## EntityRef / EntityMut
World entity operations on `main` require that the user passes in an `entity` id to each operation:
```rust
let entity = world.spawn((A, )); // create a new entity with A
world.get::<A>(entity);
world.insert(entity, (B, C));
world.insert_one(entity, D);
```
This means that each operation needs to look up the entity location / verify its validity. The initial spawn operation also requires a Bundle as input. This can be awkward when no components are required (or one component is required).
These operations have been replaced by `EntityRef` and `EntityMut`, which are "builder-style" wrappers around world that provide read and read/write operations on a single, pre-validated entity:
```rust
// spawn now takes no inputs and returns an EntityMut
let entity = world.spawn()
.insert(A) // insert a single component into the entity
.insert_bundle((B, C)) // insert a bundle of components into the entity
.id() // id returns the Entity id
// Returns EntityMut (or panics if the entity does not exist)
world.entity_mut(entity)
.insert(D)
.insert_bundle(SomeBundle::default());
{
// returns EntityRef (or panics if the entity does not exist)
let d = world.entity(entity)
.get::<D>() // gets the D component
.unwrap();
// world.get still exists for ergonomics
let d = world.get::<D>(entity).unwrap();
}
// These variants return Options if you want to check existence instead of panicing
world.get_entity_mut(entity)
.unwrap()
.insert(E);
if let Some(entity_ref) = world.get_entity(entity) {
let d = entity_ref.get::<D>().unwrap();
}
```
This _does not_ affect the current Commands api or terminology. I think that should be a separate conversation as that is a much larger breaking change.
## Safety Improvements
* Entity reservation in Commands uses a normal world borrow instead of an unsafe transmute
* QuerySets no longer transmutes lifetimes
* Made traits "unsafe" when implementing a trait incorrectly could cause unsafety
* More thorough safety docs
## RemovedComponents SystemParam
The old approach to querying removed components: `query.removed:<T>()` was confusing because it had no connection to the query itself. I replaced it with the following, which is both clearer and allows us to cache the ComponentId mapping in the SystemParamState:
```rust
fn system(removed: RemovedComponents<T>) {
for entity in removed.iter() {
}
}
```
## Simpler Bundle implementation
Bundles are no longer responsible for sorting (or deduping) TypeInfo. They are just a simple ordered list of component types / data. This makes the implementation smaller and opens the door to an easy "nested bundle" implementation in the future (which i might even add in this pr). Duplicate detection is now done once per bundle type by World the first time a bundle is used.
## Unified WorldQuery and QueryFilter types
(don't worry they are still separate type _parameters_ in Queries .. this is a non-breaking change)
WorldQuery and QueryFilter were already basically identical apis. With the addition of `FetchState` and more storage-specific fetch methods, the overlap was even clearer (and the redundancy more painful).
QueryFilters are now just `F: WorldQuery where F::Fetch: FilterFetch`. FilterFetch requires `Fetch<Item = bool>` and adds new "short circuit" variants of fetch methods. This enables a filter tuple like `(With<A>, Without<B>, Changed<C>)` to stop evaluating the filter after the first mismatch is encountered. FilterFetch is automatically implemented for `Fetch` implementations that return bool.
This forces fetch implementations that return things like `(bool, bool, bool)` (such as the filter above) to manually implement FilterFetch and decide whether or not to short-circuit.
## More Granular Modules
World no longer globs all of the internal modules together. It now exports `core`, `system`, and `schedule` separately. I'm also considering exporting `core` submodules directly as that is still pretty "glob-ey" and unorganized (feedback welcome here).
## Remaining Draft Work (to be done in this pr)
* ~~panic on conflicting WorldQuery fetches (&A, &mut A)~~
* ~~bevy `main` and hecs both currently allow this, but we should protect against it if possible~~
* ~~batch_iter / par_iter (currently stubbed out)~~
* ~~ChangedRes~~
* ~~I skipped this while we sort out #1313. This pr should be adapted to account for whatever we land on there~~.
* ~~The `Archetypes` and `Tables` collections use hashes of sorted lists of component ids to uniquely identify each archetype/table. This hash is then used as the key in a HashMap to look up the relevant ArchetypeId or TableId. (which doesn't handle hash collisions properly)~~
* ~~It is currently unsafe to generate a Query from "World A", then use it on "World B" (despite the api claiming it is safe). We should probably close this gap. This could be done by adding a randomly generated WorldId to each world, then storing that id in each Query. They could then be compared to each other on each `query.do_thing(&world)` operation. This _does_ add an extra branch to each query operation, so I'm open to other suggestions if people have them.~~
* ~~Nested Bundles (if i find time)~~
## Potential Future Work
* Expand WorldCell to support queries.
* Consider not allocating in the empty archetype on `world.spawn()`
* ex: return something like EntityMutUninit, which turns into EntityMut after an `insert` or `insert_bundle` op
* this actually regressed performance last time i tried it, but in theory it should be faster
* Optimize SparseSet::insert (see `PERF` comment on insert)
* Replace SparseArray `Option<T>` with T::MAX to cut down on branching
* would enable cheaper get_unchecked() operations
* upstream fixedbitset optimizations
* fixedbitset could be allocation free for small block counts (store blocks in a SmallVec)
* fixedbitset could have a const constructor
* Consider implementing Tags (archetype-specific by-value data that affects archetype identity)
* ex: ArchetypeA could have `[A, B, C]` table components and `[D(1)]` "tag" component. ArchetypeB could have `[A, B, C]` table components and a `[D(2)]` tag component. The archetypes are different, despite both having D tags because the value inside D is different.
* this could potentially build on top of the `archetype.unique_components` added in this pr for resource storage.
* Consider reverting `all_tuples` proc macro in favor of the old `macro_rules` implementation
* all_tuples is more flexible and produces cleaner documentation (the macro_rules version produces weird type parameter orders due to parser constraints)
* but unfortunately all_tuples also appears to make Rust Analyzer sad/slow when working inside of `bevy_ecs` (does not affect user code)
* Consider "resource queries" and/or "mixed resource and entity component queries" as an alternative to WorldCell
* this is basically just "systems" so maybe it's not worth it
* Add more world ops
* `world.clear()`
* `world.reserve<T: Bundle>(count: usize)`
* Try using the old archetype allocation strategy (allocate new memory on resize and copy everything over). I expect this to improve batch insertion performance at the cost of unbatched performance. But thats just a guess. I'm not an allocation perf pro :)
* Adapt Commands apis for consistency with new World apis
## Benchmarks
key:
* `bevy_old`: bevy `main` branch
* `bevy`: this branch
* `_foreach`: uses an optimized for_each iterator
* ` _sparse`: uses sparse set storage (if unspecified assume table storage)
* `_system`: runs inside a system (if unspecified assume test happens via direct world ops)
### Simple Insert (from ecs_bench_suite)
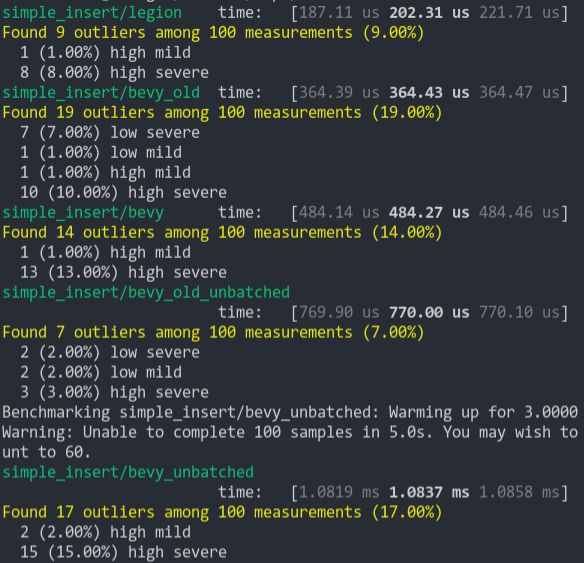
### Simpler Iter (from ecs_bench_suite)
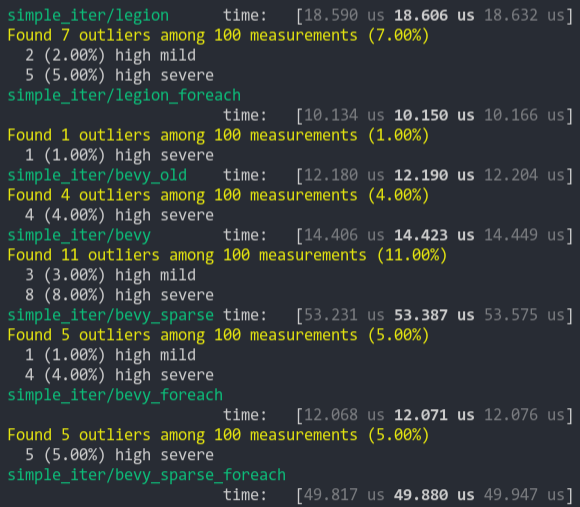
### Fragment Iter (from ecs_bench_suite)
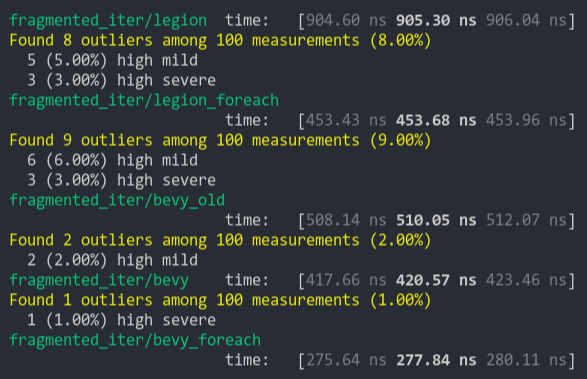
### Sparse Fragmented Iter
Iterate a query that matches 5 entities from a single matching archetype, but there are 100 unmatching archetypes
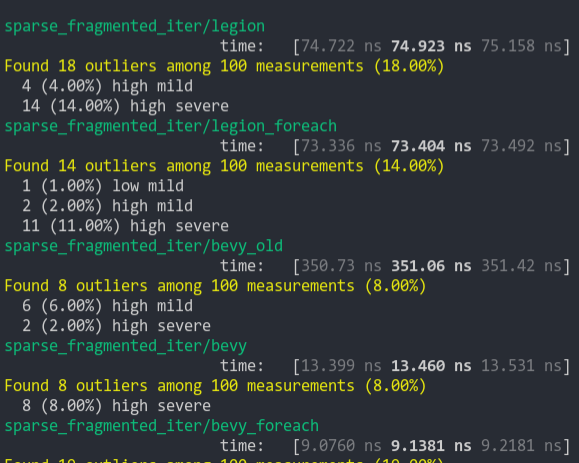
### Schedule (from ecs_bench_suite)
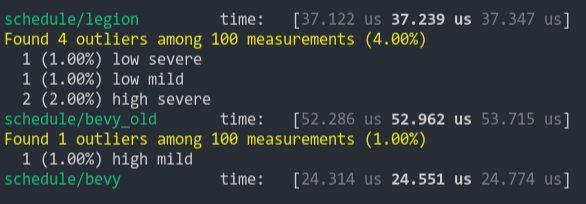
### Add Remove Component (from ecs_bench_suite)
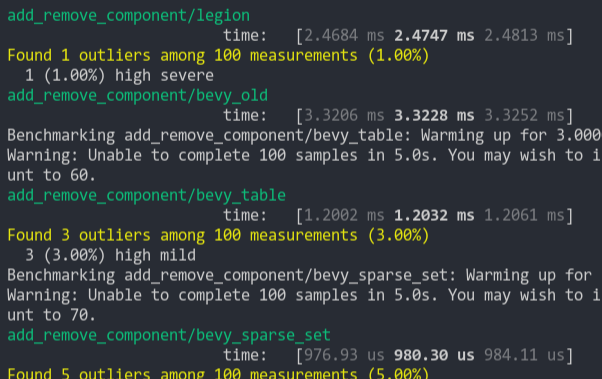
### Add Remove Component Big
Same as the test above, but each entity has 5 "large" matrix components and 1 "large" matrix component is added and removed
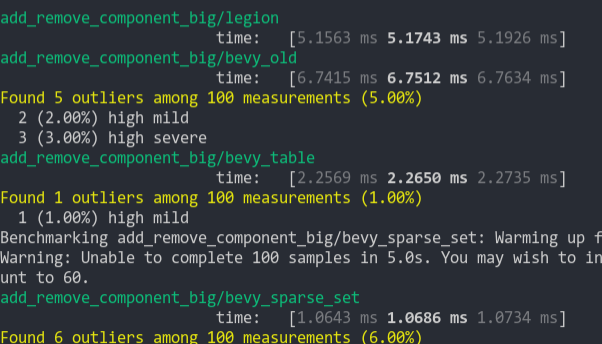
### Get Component
Looks up a single component value a large number of times
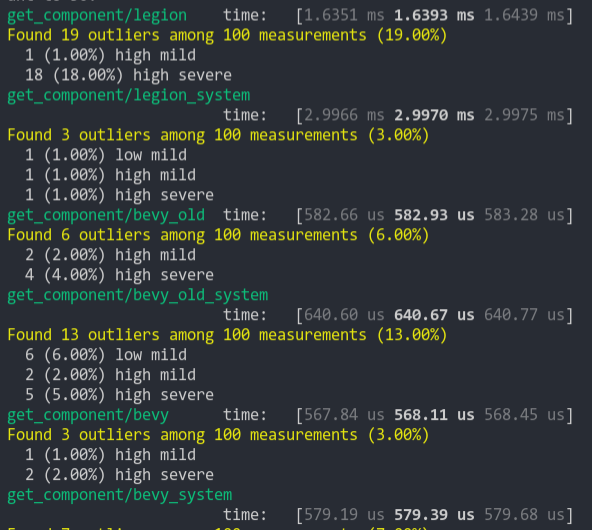
2021-03-05 07:54:35 +00:00
}
2020-11-08 20:34:05 +00:00
Bevy ECS V2 (#1525)
# Bevy ECS V2
This is a rewrite of Bevy ECS (basically everything but the new executor/schedule, which are already awesome). The overall goal was to improve the performance and versatility of Bevy ECS. Here is a quick bulleted list of changes before we dive into the details:
* Complete World rewrite
* Multiple component storage types:
* Tables: fast cache friendly iteration, slower add/removes (previously called Archetypes)
* Sparse Sets: fast add/remove, slower iteration
* Stateful Queries (caches query results for faster iteration. fragmented iteration is _fast_ now)
* Stateful System Params (caches expensive operations. inspired by @DJMcNab's work in #1364)
* Configurable System Params (users can set configuration when they construct their systems. once again inspired by @DJMcNab's work)
* Archetypes are now "just metadata", component storage is separate
* Archetype Graph (for faster archetype changes)
* Component Metadata
* Configure component storage type
* Retrieve information about component size/type/name/layout/send-ness/etc
* Components are uniquely identified by a densely packed ComponentId
* TypeIds are now totally optional (which should make implementing scripting easier)
* Super fast "for_each" query iterators
* Merged Resources into World. Resources are now just a special type of component
* EntityRef/EntityMut builder apis (more efficient and more ergonomic)
* Fast bitset-backed `Access<T>` replaces old hashmap-based approach everywhere
* Query conflicts are determined by component access instead of archetype component access (to avoid random failures at runtime)
* With/Without are still taken into account for conflicts, so this should still be comfy to use
* Much simpler `IntoSystem` impl
* Significantly reduced the amount of hashing throughout the ecs in favor of Sparse Sets (indexed by densely packed ArchetypeId, ComponentId, BundleId, and TableId)
* Safety Improvements
* Entity reservation uses a normal world reference instead of unsafe transmute
* QuerySets no longer transmute lifetimes
* Made traits "unsafe" where relevant
* More thorough safety docs
* WorldCell
* Exposes safe mutable access to multiple resources at a time in a World
* Replaced "catch all" `System::update_archetypes(world: &World)` with `System::new_archetype(archetype: &Archetype)`
* Simpler Bundle implementation
* Replaced slow "remove_bundle_one_by_one" used as fallback for Commands::remove_bundle with fast "remove_bundle_intersection"
* Removed `Mut<T>` query impl. it is better to only support one way: `&mut T`
* Removed with() from `Flags<T>` in favor of `Option<Flags<T>>`, which allows querying for flags to be "filtered" by default
* Components now have is_send property (currently only resources support non-send)
* More granular module organization
* New `RemovedComponents<T>` SystemParam that replaces `query.removed::<T>()`
* `world.resource_scope()` for mutable access to resources and world at the same time
* WorldQuery and QueryFilter traits unified. FilterFetch trait added to enable "short circuit" filtering. Auto impled for cases that don't need it
* Significantly slimmed down SystemState in favor of individual SystemParam state
* System Commands changed from `commands: &mut Commands` back to `mut commands: Commands` (to allow Commands to have a World reference)
Fixes #1320
## `World` Rewrite
This is a from-scratch rewrite of `World` that fills the niche that `hecs` used to. Yes, this means Bevy ECS is no longer a "fork" of hecs. We're going out our own!
(the only shared code between the projects is the entity id allocator, which is already basically ideal)
A huge shout out to @SanderMertens (author of [flecs](https://github.com/SanderMertens/flecs)) for sharing some great ideas with me (specifically hybrid ecs storage and archetype graphs). He also helped advise on a number of implementation details.
## Component Storage (The Problem)
Two ECS storage paradigms have gained a lot of traction over the years:
* **Archetypal ECS**:
* Stores components in "tables" with static schemas. Each "column" stores components of a given type. Each "row" is an entity.
* Each "archetype" has its own table. Adding/removing an entity's component changes the archetype.
* Enables super-fast Query iteration due to its cache-friendly data layout
* Comes at the cost of more expensive add/remove operations for an Entity's components, because all components need to be copied to the new archetype's "table"
* **Sparse Set ECS**:
* Stores components of the same type in densely packed arrays, which are sparsely indexed by densely packed unsigned integers (Entity ids)
* Query iteration is slower than Archetypal ECS because each entity's component could be at any position in the sparse set. This "random access" pattern isn't cache friendly. Additionally, there is an extra layer of indirection because you must first map the entity id to an index in the component array.
* Adding/removing components is a cheap, constant time operation
Bevy ECS V1, hecs, legion, flec, and Unity DOTS are all "archetypal ecs-es". I personally think "archetypal" storage is a good default for game engines. An entity's archetype doesn't need to change frequently in general, and it creates "fast by default" query iteration (which is a much more common operation). It is also "self optimizing". Users don't need to think about optimizing component layouts for iteration performance. It "just works" without any extra boilerplate.
Shipyard and EnTT are "sparse set ecs-es". They employ "packing" as a way to work around the "suboptimal by default" iteration performance for specific sets of components. This helps, but I didn't think this was a good choice for a general purpose engine like Bevy because:
1. "packs" conflict with each other. If bevy decides to internally pack the Transform and GlobalTransform components, users are then blocked if they want to pack some custom component with Transform.
2. users need to take manual action to optimize
Developers selecting an ECS framework are stuck with a hard choice. Select an "archetypal" framework with "fast iteration everywhere" but without the ability to cheaply add/remove components, or select a "sparse set" framework to cheaply add/remove components but with slower iteration performance.
## Hybrid Component Storage (The Solution)
In Bevy ECS V2, we get to have our cake and eat it too. It now has _both_ of the component storage types above (and more can be added later if needed):
* **Tables** (aka "archetypal" storage)
* The default storage. If you don't configure anything, this is what you get
* Fast iteration by default
* Slower add/remove operations
* **Sparse Sets**
* Opt-in
* Slower iteration
* Faster add/remove operations
These storage types complement each other perfectly. By default Query iteration is fast. If developers know that they want to add/remove a component at high frequencies, they can set the storage to "sparse set":
```rust
world.register_component(
ComponentDescriptor::new::<MyComponent>(StorageType::SparseSet)
).unwrap();
```
## Archetypes
Archetypes are now "just metadata" ... they no longer store components directly. They do store:
* The `ComponentId`s of each of the Archetype's components (and that component's storage type)
* Archetypes are uniquely defined by their component layouts
* For example: entities with "table" components `[A, B, C]` _and_ "sparse set" components `[D, E]` will always be in the same archetype.
* The `TableId` associated with the archetype
* For now each archetype has exactly one table (which can have no components),
* There is a 1->Many relationship from Tables->Archetypes. A given table could have any number of archetype components stored in it:
* Ex: an entity with "table storage" components `[A, B, C]` and "sparse set" components `[D, E]` will share the same `[A, B, C]` table as an entity with `[A, B, C]` table component and `[F]` sparse set components.
* This 1->Many relationship is how we preserve fast "cache friendly" iteration performance when possible (more on this later)
* A list of entities that are in the archetype and the row id of the table they are in
* ArchetypeComponentIds
* unique densely packed identifiers for (ArchetypeId, ComponentId) pairs
* used by the schedule executor for cheap system access control
* "Archetype Graph Edges" (see the next section)
## The "Archetype Graph"
Archetype changes in Bevy (and a number of other archetypal ecs-es) have historically been expensive to compute. First, you need to allocate a new vector of the entity's current component ids, add or remove components based on the operation performed, sort it (to ensure it is order-independent), then hash it to find the archetype (if it exists). And thats all before we get to the _already_ expensive full copy of all components to the new table storage.
The solution is to build a "graph" of archetypes to cache these results. @SanderMertens first exposed me to the idea (and he got it from @gjroelofs, who came up with it). They propose adding directed edges between archetypes for add/remove component operations. If `ComponentId`s are densely packed, you can use sparse sets to cheaply jump between archetypes.
Bevy takes this one step further by using add/remove `Bundle` edges instead of `Component` edges. Bevy encourages the use of `Bundles` to group add/remove operations. This is largely for "clearer game logic" reasons, but it also helps cut down on the number of archetype changes required. `Bundles` now also have densely-packed `BundleId`s. This allows us to use a _single_ edge for each bundle operation (rather than needing to traverse N edges ... one for each component). Single component operations are also bundles, so this is strictly an improvement over a "component only" graph.
As a result, an operation that used to be _heavy_ (both for allocations and compute) is now two dirt-cheap array lookups and zero allocations.
## Stateful Queries
World queries are now stateful. This allows us to:
1. Cache archetype (and table) matches
* This resolves another issue with (naive) archetypal ECS: query performance getting worse as the number of archetypes goes up (and fragmentation occurs).
2. Cache Fetch and Filter state
* The expensive parts of fetch/filter operations (such as hashing the TypeId to find the ComponentId) now only happen once when the Query is first constructed
3. Incrementally build up state
* When new archetypes are added, we only process the new archetypes (no need to rebuild state for old archetypes)
As a result, the direct `World` query api now looks like this:
```rust
let mut query = world.query::<(&A, &mut B)>();
for (a, mut b) in query.iter_mut(&mut world) {
}
```
Requiring `World` to generate stateful queries (rather than letting the `QueryState` type be constructed separately) allows us to ensure that _all_ queries are properly initialized (and the relevant world state, such as ComponentIds). This enables QueryState to remove branches from its operations that check for initialization status (and also enables query.iter() to take an immutable world reference because it doesn't need to initialize anything in world).
However in systems, this is a non-breaking change. State management is done internally by the relevant SystemParam.
## Stateful SystemParams
Like Queries, `SystemParams` now also cache state. For example, `Query` system params store the "stateful query" state mentioned above. Commands store their internal `CommandQueue`. This means you can now safely use as many separate `Commands` parameters in your system as you want. `Local<T>` system params store their `T` value in their state (instead of in Resources).
SystemParam state also enabled a significant slim-down of SystemState. It is much nicer to look at now.
Per-SystemParam state naturally insulates us from an "aliased mut" class of errors we have hit in the past (ex: using multiple `Commands` system params).
(credit goes to @DJMcNab for the initial idea and draft pr here #1364)
## Configurable SystemParams
@DJMcNab also had the great idea to make SystemParams configurable. This allows users to provide some initial configuration / values for system parameters (when possible). Most SystemParams have no config (the config type is `()`), but the `Local<T>` param now supports user-provided parameters:
```rust
fn foo(value: Local<usize>) {
}
app.add_system(foo.system().config(|c| c.0 = Some(10)));
```
## Uber Fast "for_each" Query Iterators
Developers now have the choice to use a fast "for_each" iterator, which yields ~1.5-3x iteration speed improvements for "fragmented iteration", and minor ~1.2x iteration speed improvements for unfragmented iteration.
```rust
fn system(query: Query<(&A, &mut B)>) {
// you now have the option to do this for a speed boost
query.for_each_mut(|(a, mut b)| {
});
// however normal iterators are still available
for (a, mut b) in query.iter_mut() {
}
}
```
I think in most cases we should continue to encourage "normal" iterators as they are more flexible and more "rust idiomatic". But when that extra "oomf" is needed, it makes sense to use `for_each`.
We should also consider using `for_each` for internal bevy systems to give our users a nice speed boost (but that should be a separate pr).
## Component Metadata
`World` now has a `Components` collection, which is accessible via `world.components()`. This stores mappings from `ComponentId` to `ComponentInfo`, as well as `TypeId` to `ComponentId` mappings (where relevant). `ComponentInfo` stores information about the component, such as ComponentId, TypeId, memory layout, send-ness (currently limited to resources), and storage type.
## Significantly Cheaper `Access<T>`
We used to use `TypeAccess<TypeId>` to manage read/write component/archetype-component access. This was expensive because TypeIds must be hashed and compared individually. The parallel executor got around this by "condensing" type ids into bitset-backed access types. This worked, but it had to be re-generated from the `TypeAccess<TypeId>`sources every time archetypes changed.
This pr removes TypeAccess in favor of faster bitset access everywhere. We can do this thanks to the move to densely packed `ComponentId`s and `ArchetypeComponentId`s.
## Merged Resources into World
Resources had a lot of redundant functionality with Components. They stored typed data, they had access control, they had unique ids, they were queryable via SystemParams, etc. In fact the _only_ major difference between them was that they were unique (and didn't correlate to an entity).
Separate resources also had the downside of requiring a separate set of access controls, which meant the parallel executor needed to compare more bitsets per system and manage more state.
I initially got the "separate resources" idea from `legion`. I think that design was motivated by the fact that it made the direct world query/resource lifetime interactions more manageable. It certainly made our lives easier when using Resources alongside hecs/bevy_ecs. However we already have a construct for safely and ergonomically managing in-world lifetimes: systems (which use `Access<T>` internally).
This pr merges Resources into World:
```rust
world.insert_resource(1);
world.insert_resource(2.0);
let a = world.get_resource::<i32>().unwrap();
let mut b = world.get_resource_mut::<f64>().unwrap();
*b = 3.0;
```
Resources are now just a special kind of component. They have their own ComponentIds (and their own resource TypeId->ComponentId scope, so they don't conflict wit components of the same type). They are stored in a special "resource archetype", which stores components inside the archetype using a new `unique_components` sparse set (note that this sparse set could later be used to implement Tags). This allows us to keep the code size small by reusing existing datastructures (namely Column, Archetype, ComponentFlags, and ComponentInfo). This allows us the executor to use a single `Access<ArchetypeComponentId>` per system. It should also make scripting language integration easier.
_But_ this merge did create problems for people directly interacting with `World`. What if you need mutable access to multiple resources at the same time? `world.get_resource_mut()` borrows World mutably!
## WorldCell
WorldCell applies the `Access<ArchetypeComponentId>` concept to direct world access:
```rust
let world_cell = world.cell();
let a = world_cell.get_resource_mut::<i32>().unwrap();
let b = world_cell.get_resource_mut::<f64>().unwrap();
```
This adds cheap runtime checks (a sparse set lookup of `ArchetypeComponentId` and a counter) to ensure that world accesses do not conflict with each other. Each operation returns a `WorldBorrow<'w, T>` or `WorldBorrowMut<'w, T>` wrapper type, which will release the relevant ArchetypeComponentId resources when dropped.
World caches the access sparse set (and only one cell can exist at a time), so `world.cell()` is a cheap operation.
WorldCell does _not_ use atomic operations. It is non-send, does a mutable borrow of world to prevent other accesses, and uses a simple `Rc<RefCell<ArchetypeComponentAccess>>` wrapper in each WorldBorrow pointer.
The api is currently limited to resource access, but it can and should be extended to queries / entity component access.
## Resource Scopes
WorldCell does not yet support component queries, and even when it does there are sometimes legitimate reasons to want a mutable world ref _and_ a mutable resource ref (ex: bevy_render and bevy_scene both need this). In these cases we could always drop down to the unsafe `world.get_resource_unchecked_mut()`, but that is not ideal!
Instead developers can use a "resource scope"
```rust
world.resource_scope(|world: &mut World, a: &mut A| {
})
```
This temporarily removes the `A` resource from `World`, provides mutable pointers to both, and re-adds A to World when finished. Thanks to the move to ComponentIds/sparse sets, this is a cheap operation.
If multiple resources are required, scopes can be nested. We could also consider adding a "resource tuple" to the api if this pattern becomes common and the boilerplate gets nasty.
## Query Conflicts Use ComponentId Instead of ArchetypeComponentId
For safety reasons, systems cannot contain queries that conflict with each other without wrapping them in a QuerySet. On bevy `main`, we use ArchetypeComponentIds to determine conflicts. This is nice because it can take into account filters:
```rust
// these queries will never conflict due to their filters
fn filter_system(a: Query<&mut A, With<B>>, b: Query<&mut B, Without<B>>) {
}
```
But it also has a significant downside:
```rust
// these queries will not conflict _until_ an entity with A, B, and C is spawned
fn maybe_conflicts_system(a: Query<(&mut A, &C)>, b: Query<(&mut A, &B)>) {
}
```
The system above will panic at runtime if an entity with A, B, and C is spawned. This makes it hard to trust that your game logic will run without crashing.
In this pr, I switched to using `ComponentId` instead. This _is_ more constraining. `maybe_conflicts_system` will now always fail, but it will do it consistently at startup. Naively, it would also _disallow_ `filter_system`, which would be a significant downgrade in usability. Bevy has a number of internal systems that rely on disjoint queries and I expect it to be a common pattern in userspace.
To resolve this, I added a new `FilteredAccess<T>` type, which wraps `Access<T>` and adds with/without filters. If two `FilteredAccess` have with/without values that prove they are disjoint, they will no longer conflict.
## EntityRef / EntityMut
World entity operations on `main` require that the user passes in an `entity` id to each operation:
```rust
let entity = world.spawn((A, )); // create a new entity with A
world.get::<A>(entity);
world.insert(entity, (B, C));
world.insert_one(entity, D);
```
This means that each operation needs to look up the entity location / verify its validity. The initial spawn operation also requires a Bundle as input. This can be awkward when no components are required (or one component is required).
These operations have been replaced by `EntityRef` and `EntityMut`, which are "builder-style" wrappers around world that provide read and read/write operations on a single, pre-validated entity:
```rust
// spawn now takes no inputs and returns an EntityMut
let entity = world.spawn()
.insert(A) // insert a single component into the entity
.insert_bundle((B, C)) // insert a bundle of components into the entity
.id() // id returns the Entity id
// Returns EntityMut (or panics if the entity does not exist)
world.entity_mut(entity)
.insert(D)
.insert_bundle(SomeBundle::default());
{
// returns EntityRef (or panics if the entity does not exist)
let d = world.entity(entity)
.get::<D>() // gets the D component
.unwrap();
// world.get still exists for ergonomics
let d = world.get::<D>(entity).unwrap();
}
// These variants return Options if you want to check existence instead of panicing
world.get_entity_mut(entity)
.unwrap()
.insert(E);
if let Some(entity_ref) = world.get_entity(entity) {
let d = entity_ref.get::<D>().unwrap();
}
```
This _does not_ affect the current Commands api or terminology. I think that should be a separate conversation as that is a much larger breaking change.
## Safety Improvements
* Entity reservation in Commands uses a normal world borrow instead of an unsafe transmute
* QuerySets no longer transmutes lifetimes
* Made traits "unsafe" when implementing a trait incorrectly could cause unsafety
* More thorough safety docs
## RemovedComponents SystemParam
The old approach to querying removed components: `query.removed:<T>()` was confusing because it had no connection to the query itself. I replaced it with the following, which is both clearer and allows us to cache the ComponentId mapping in the SystemParamState:
```rust
fn system(removed: RemovedComponents<T>) {
for entity in removed.iter() {
}
}
```
## Simpler Bundle implementation
Bundles are no longer responsible for sorting (or deduping) TypeInfo. They are just a simple ordered list of component types / data. This makes the implementation smaller and opens the door to an easy "nested bundle" implementation in the future (which i might even add in this pr). Duplicate detection is now done once per bundle type by World the first time a bundle is used.
## Unified WorldQuery and QueryFilter types
(don't worry they are still separate type _parameters_ in Queries .. this is a non-breaking change)
WorldQuery and QueryFilter were already basically identical apis. With the addition of `FetchState` and more storage-specific fetch methods, the overlap was even clearer (and the redundancy more painful).
QueryFilters are now just `F: WorldQuery where F::Fetch: FilterFetch`. FilterFetch requires `Fetch<Item = bool>` and adds new "short circuit" variants of fetch methods. This enables a filter tuple like `(With<A>, Without<B>, Changed<C>)` to stop evaluating the filter after the first mismatch is encountered. FilterFetch is automatically implemented for `Fetch` implementations that return bool.
This forces fetch implementations that return things like `(bool, bool, bool)` (such as the filter above) to manually implement FilterFetch and decide whether or not to short-circuit.
## More Granular Modules
World no longer globs all of the internal modules together. It now exports `core`, `system`, and `schedule` separately. I'm also considering exporting `core` submodules directly as that is still pretty "glob-ey" and unorganized (feedback welcome here).
## Remaining Draft Work (to be done in this pr)
* ~~panic on conflicting WorldQuery fetches (&A, &mut A)~~
* ~~bevy `main` and hecs both currently allow this, but we should protect against it if possible~~
* ~~batch_iter / par_iter (currently stubbed out)~~
* ~~ChangedRes~~
* ~~I skipped this while we sort out #1313. This pr should be adapted to account for whatever we land on there~~.
* ~~The `Archetypes` and `Tables` collections use hashes of sorted lists of component ids to uniquely identify each archetype/table. This hash is then used as the key in a HashMap to look up the relevant ArchetypeId or TableId. (which doesn't handle hash collisions properly)~~
* ~~It is currently unsafe to generate a Query from "World A", then use it on "World B" (despite the api claiming it is safe). We should probably close this gap. This could be done by adding a randomly generated WorldId to each world, then storing that id in each Query. They could then be compared to each other on each `query.do_thing(&world)` operation. This _does_ add an extra branch to each query operation, so I'm open to other suggestions if people have them.~~
* ~~Nested Bundles (if i find time)~~
## Potential Future Work
* Expand WorldCell to support queries.
* Consider not allocating in the empty archetype on `world.spawn()`
* ex: return something like EntityMutUninit, which turns into EntityMut after an `insert` or `insert_bundle` op
* this actually regressed performance last time i tried it, but in theory it should be faster
* Optimize SparseSet::insert (see `PERF` comment on insert)
* Replace SparseArray `Option<T>` with T::MAX to cut down on branching
* would enable cheaper get_unchecked() operations
* upstream fixedbitset optimizations
* fixedbitset could be allocation free for small block counts (store blocks in a SmallVec)
* fixedbitset could have a const constructor
* Consider implementing Tags (archetype-specific by-value data that affects archetype identity)
* ex: ArchetypeA could have `[A, B, C]` table components and `[D(1)]` "tag" component. ArchetypeB could have `[A, B, C]` table components and a `[D(2)]` tag component. The archetypes are different, despite both having D tags because the value inside D is different.
* this could potentially build on top of the `archetype.unique_components` added in this pr for resource storage.
* Consider reverting `all_tuples` proc macro in favor of the old `macro_rules` implementation
* all_tuples is more flexible and produces cleaner documentation (the macro_rules version produces weird type parameter orders due to parser constraints)
* but unfortunately all_tuples also appears to make Rust Analyzer sad/slow when working inside of `bevy_ecs` (does not affect user code)
* Consider "resource queries" and/or "mixed resource and entity component queries" as an alternative to WorldCell
* this is basically just "systems" so maybe it's not worth it
* Add more world ops
* `world.clear()`
* `world.reserve<T: Bundle>(count: usize)`
* Try using the old archetype allocation strategy (allocate new memory on resize and copy everything over). I expect this to improve batch insertion performance at the cost of unbatched performance. But thats just a guess. I'm not an allocation perf pro :)
* Adapt Commands apis for consistency with new World apis
## Benchmarks
key:
* `bevy_old`: bevy `main` branch
* `bevy`: this branch
* `_foreach`: uses an optimized for_each iterator
* ` _sparse`: uses sparse set storage (if unspecified assume table storage)
* `_system`: runs inside a system (if unspecified assume test happens via direct world ops)
### Simple Insert (from ecs_bench_suite)
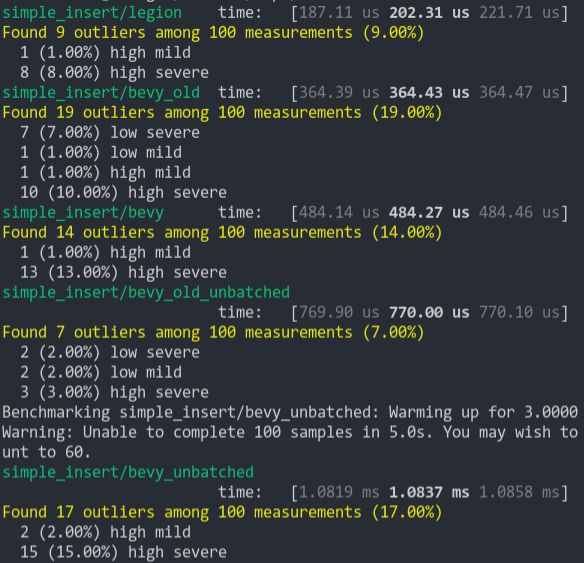
### Simpler Iter (from ecs_bench_suite)
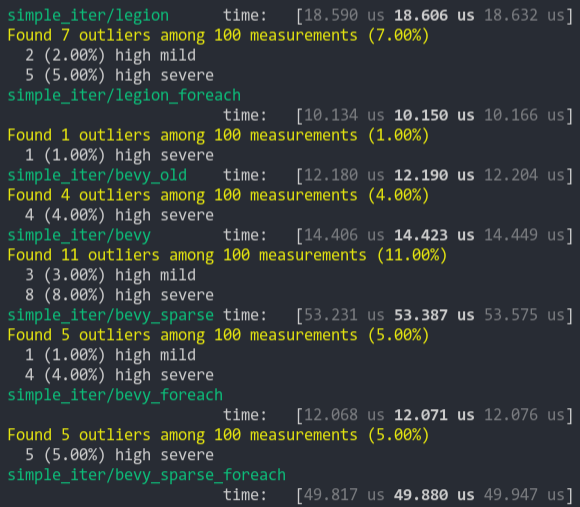
### Fragment Iter (from ecs_bench_suite)
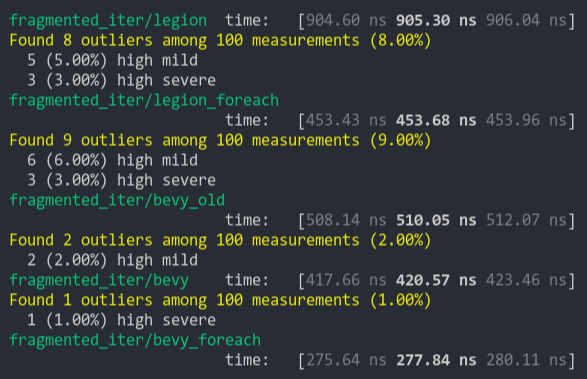
### Sparse Fragmented Iter
Iterate a query that matches 5 entities from a single matching archetype, but there are 100 unmatching archetypes
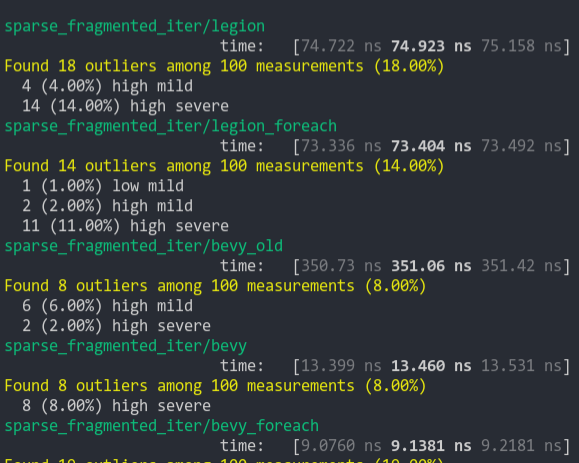
### Schedule (from ecs_bench_suite)
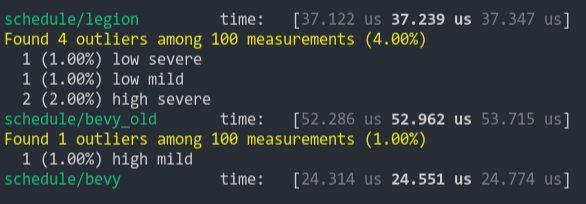
### Add Remove Component (from ecs_bench_suite)
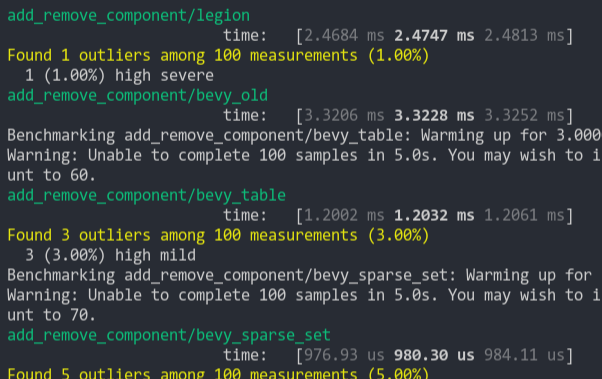
### Add Remove Component Big
Same as the test above, but each entity has 5 "large" matrix components and 1 "large" matrix component is added and removed
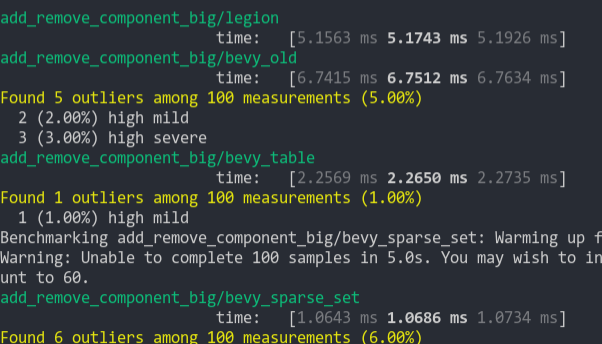
### Get Component
Looks up a single component value a large number of times
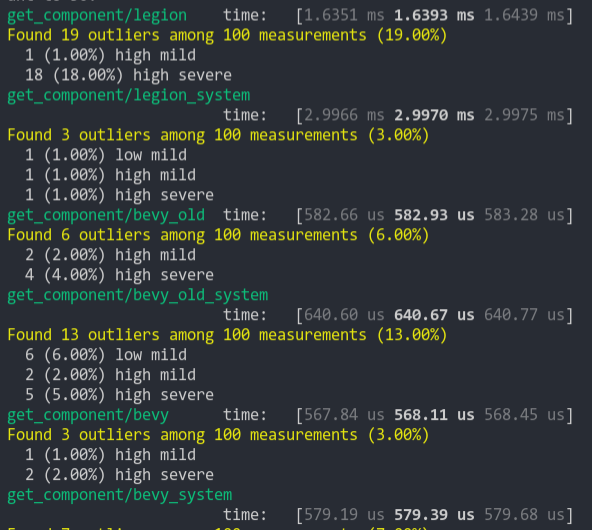
2021-03-05 07:54:35 +00:00
fn assert_component_access_compatibility (
system_name : & str ,
query_type : & 'static str ,
filter_type : & 'static str ,
system_access : & FilteredAccessSet < ComponentId > ,
current : & FilteredAccess < ComponentId > ,
world : & World ,
) {
2022-11-06 01:42:15 +00:00
let conflicts = system_access . get_conflicts_single ( current ) ;
Bevy ECS V2 (#1525)
# Bevy ECS V2
This is a rewrite of Bevy ECS (basically everything but the new executor/schedule, which are already awesome). The overall goal was to improve the performance and versatility of Bevy ECS. Here is a quick bulleted list of changes before we dive into the details:
* Complete World rewrite
* Multiple component storage types:
* Tables: fast cache friendly iteration, slower add/removes (previously called Archetypes)
* Sparse Sets: fast add/remove, slower iteration
* Stateful Queries (caches query results for faster iteration. fragmented iteration is _fast_ now)
* Stateful System Params (caches expensive operations. inspired by @DJMcNab's work in #1364)
* Configurable System Params (users can set configuration when they construct their systems. once again inspired by @DJMcNab's work)
* Archetypes are now "just metadata", component storage is separate
* Archetype Graph (for faster archetype changes)
* Component Metadata
* Configure component storage type
* Retrieve information about component size/type/name/layout/send-ness/etc
* Components are uniquely identified by a densely packed ComponentId
* TypeIds are now totally optional (which should make implementing scripting easier)
* Super fast "for_each" query iterators
* Merged Resources into World. Resources are now just a special type of component
* EntityRef/EntityMut builder apis (more efficient and more ergonomic)
* Fast bitset-backed `Access<T>` replaces old hashmap-based approach everywhere
* Query conflicts are determined by component access instead of archetype component access (to avoid random failures at runtime)
* With/Without are still taken into account for conflicts, so this should still be comfy to use
* Much simpler `IntoSystem` impl
* Significantly reduced the amount of hashing throughout the ecs in favor of Sparse Sets (indexed by densely packed ArchetypeId, ComponentId, BundleId, and TableId)
* Safety Improvements
* Entity reservation uses a normal world reference instead of unsafe transmute
* QuerySets no longer transmute lifetimes
* Made traits "unsafe" where relevant
* More thorough safety docs
* WorldCell
* Exposes safe mutable access to multiple resources at a time in a World
* Replaced "catch all" `System::update_archetypes(world: &World)` with `System::new_archetype(archetype: &Archetype)`
* Simpler Bundle implementation
* Replaced slow "remove_bundle_one_by_one" used as fallback for Commands::remove_bundle with fast "remove_bundle_intersection"
* Removed `Mut<T>` query impl. it is better to only support one way: `&mut T`
* Removed with() from `Flags<T>` in favor of `Option<Flags<T>>`, which allows querying for flags to be "filtered" by default
* Components now have is_send property (currently only resources support non-send)
* More granular module organization
* New `RemovedComponents<T>` SystemParam that replaces `query.removed::<T>()`
* `world.resource_scope()` for mutable access to resources and world at the same time
* WorldQuery and QueryFilter traits unified. FilterFetch trait added to enable "short circuit" filtering. Auto impled for cases that don't need it
* Significantly slimmed down SystemState in favor of individual SystemParam state
* System Commands changed from `commands: &mut Commands` back to `mut commands: Commands` (to allow Commands to have a World reference)
Fixes #1320
## `World` Rewrite
This is a from-scratch rewrite of `World` that fills the niche that `hecs` used to. Yes, this means Bevy ECS is no longer a "fork" of hecs. We're going out our own!
(the only shared code between the projects is the entity id allocator, which is already basically ideal)
A huge shout out to @SanderMertens (author of [flecs](https://github.com/SanderMertens/flecs)) for sharing some great ideas with me (specifically hybrid ecs storage and archetype graphs). He also helped advise on a number of implementation details.
## Component Storage (The Problem)
Two ECS storage paradigms have gained a lot of traction over the years:
* **Archetypal ECS**:
* Stores components in "tables" with static schemas. Each "column" stores components of a given type. Each "row" is an entity.
* Each "archetype" has its own table. Adding/removing an entity's component changes the archetype.
* Enables super-fast Query iteration due to its cache-friendly data layout
* Comes at the cost of more expensive add/remove operations for an Entity's components, because all components need to be copied to the new archetype's "table"
* **Sparse Set ECS**:
* Stores components of the same type in densely packed arrays, which are sparsely indexed by densely packed unsigned integers (Entity ids)
* Query iteration is slower than Archetypal ECS because each entity's component could be at any position in the sparse set. This "random access" pattern isn't cache friendly. Additionally, there is an extra layer of indirection because you must first map the entity id to an index in the component array.
* Adding/removing components is a cheap, constant time operation
Bevy ECS V1, hecs, legion, flec, and Unity DOTS are all "archetypal ecs-es". I personally think "archetypal" storage is a good default for game engines. An entity's archetype doesn't need to change frequently in general, and it creates "fast by default" query iteration (which is a much more common operation). It is also "self optimizing". Users don't need to think about optimizing component layouts for iteration performance. It "just works" without any extra boilerplate.
Shipyard and EnTT are "sparse set ecs-es". They employ "packing" as a way to work around the "suboptimal by default" iteration performance for specific sets of components. This helps, but I didn't think this was a good choice for a general purpose engine like Bevy because:
1. "packs" conflict with each other. If bevy decides to internally pack the Transform and GlobalTransform components, users are then blocked if they want to pack some custom component with Transform.
2. users need to take manual action to optimize
Developers selecting an ECS framework are stuck with a hard choice. Select an "archetypal" framework with "fast iteration everywhere" but without the ability to cheaply add/remove components, or select a "sparse set" framework to cheaply add/remove components but with slower iteration performance.
## Hybrid Component Storage (The Solution)
In Bevy ECS V2, we get to have our cake and eat it too. It now has _both_ of the component storage types above (and more can be added later if needed):
* **Tables** (aka "archetypal" storage)
* The default storage. If you don't configure anything, this is what you get
* Fast iteration by default
* Slower add/remove operations
* **Sparse Sets**
* Opt-in
* Slower iteration
* Faster add/remove operations
These storage types complement each other perfectly. By default Query iteration is fast. If developers know that they want to add/remove a component at high frequencies, they can set the storage to "sparse set":
```rust
world.register_component(
ComponentDescriptor::new::<MyComponent>(StorageType::SparseSet)
).unwrap();
```
## Archetypes
Archetypes are now "just metadata" ... they no longer store components directly. They do store:
* The `ComponentId`s of each of the Archetype's components (and that component's storage type)
* Archetypes are uniquely defined by their component layouts
* For example: entities with "table" components `[A, B, C]` _and_ "sparse set" components `[D, E]` will always be in the same archetype.
* The `TableId` associated with the archetype
* For now each archetype has exactly one table (which can have no components),
* There is a 1->Many relationship from Tables->Archetypes. A given table could have any number of archetype components stored in it:
* Ex: an entity with "table storage" components `[A, B, C]` and "sparse set" components `[D, E]` will share the same `[A, B, C]` table as an entity with `[A, B, C]` table component and `[F]` sparse set components.
* This 1->Many relationship is how we preserve fast "cache friendly" iteration performance when possible (more on this later)
* A list of entities that are in the archetype and the row id of the table they are in
* ArchetypeComponentIds
* unique densely packed identifiers for (ArchetypeId, ComponentId) pairs
* used by the schedule executor for cheap system access control
* "Archetype Graph Edges" (see the next section)
## The "Archetype Graph"
Archetype changes in Bevy (and a number of other archetypal ecs-es) have historically been expensive to compute. First, you need to allocate a new vector of the entity's current component ids, add or remove components based on the operation performed, sort it (to ensure it is order-independent), then hash it to find the archetype (if it exists). And thats all before we get to the _already_ expensive full copy of all components to the new table storage.
The solution is to build a "graph" of archetypes to cache these results. @SanderMertens first exposed me to the idea (and he got it from @gjroelofs, who came up with it). They propose adding directed edges between archetypes for add/remove component operations. If `ComponentId`s are densely packed, you can use sparse sets to cheaply jump between archetypes.
Bevy takes this one step further by using add/remove `Bundle` edges instead of `Component` edges. Bevy encourages the use of `Bundles` to group add/remove operations. This is largely for "clearer game logic" reasons, but it also helps cut down on the number of archetype changes required. `Bundles` now also have densely-packed `BundleId`s. This allows us to use a _single_ edge for each bundle operation (rather than needing to traverse N edges ... one for each component). Single component operations are also bundles, so this is strictly an improvement over a "component only" graph.
As a result, an operation that used to be _heavy_ (both for allocations and compute) is now two dirt-cheap array lookups and zero allocations.
## Stateful Queries
World queries are now stateful. This allows us to:
1. Cache archetype (and table) matches
* This resolves another issue with (naive) archetypal ECS: query performance getting worse as the number of archetypes goes up (and fragmentation occurs).
2. Cache Fetch and Filter state
* The expensive parts of fetch/filter operations (such as hashing the TypeId to find the ComponentId) now only happen once when the Query is first constructed
3. Incrementally build up state
* When new archetypes are added, we only process the new archetypes (no need to rebuild state for old archetypes)
As a result, the direct `World` query api now looks like this:
```rust
let mut query = world.query::<(&A, &mut B)>();
for (a, mut b) in query.iter_mut(&mut world) {
}
```
Requiring `World` to generate stateful queries (rather than letting the `QueryState` type be constructed separately) allows us to ensure that _all_ queries are properly initialized (and the relevant world state, such as ComponentIds). This enables QueryState to remove branches from its operations that check for initialization status (and also enables query.iter() to take an immutable world reference because it doesn't need to initialize anything in world).
However in systems, this is a non-breaking change. State management is done internally by the relevant SystemParam.
## Stateful SystemParams
Like Queries, `SystemParams` now also cache state. For example, `Query` system params store the "stateful query" state mentioned above. Commands store their internal `CommandQueue`. This means you can now safely use as many separate `Commands` parameters in your system as you want. `Local<T>` system params store their `T` value in their state (instead of in Resources).
SystemParam state also enabled a significant slim-down of SystemState. It is much nicer to look at now.
Per-SystemParam state naturally insulates us from an "aliased mut" class of errors we have hit in the past (ex: using multiple `Commands` system params).
(credit goes to @DJMcNab for the initial idea and draft pr here #1364)
## Configurable SystemParams
@DJMcNab also had the great idea to make SystemParams configurable. This allows users to provide some initial configuration / values for system parameters (when possible). Most SystemParams have no config (the config type is `()`), but the `Local<T>` param now supports user-provided parameters:
```rust
fn foo(value: Local<usize>) {
}
app.add_system(foo.system().config(|c| c.0 = Some(10)));
```
## Uber Fast "for_each" Query Iterators
Developers now have the choice to use a fast "for_each" iterator, which yields ~1.5-3x iteration speed improvements for "fragmented iteration", and minor ~1.2x iteration speed improvements for unfragmented iteration.
```rust
fn system(query: Query<(&A, &mut B)>) {
// you now have the option to do this for a speed boost
query.for_each_mut(|(a, mut b)| {
});
// however normal iterators are still available
for (a, mut b) in query.iter_mut() {
}
}
```
I think in most cases we should continue to encourage "normal" iterators as they are more flexible and more "rust idiomatic". But when that extra "oomf" is needed, it makes sense to use `for_each`.
We should also consider using `for_each` for internal bevy systems to give our users a nice speed boost (but that should be a separate pr).
## Component Metadata
`World` now has a `Components` collection, which is accessible via `world.components()`. This stores mappings from `ComponentId` to `ComponentInfo`, as well as `TypeId` to `ComponentId` mappings (where relevant). `ComponentInfo` stores information about the component, such as ComponentId, TypeId, memory layout, send-ness (currently limited to resources), and storage type.
## Significantly Cheaper `Access<T>`
We used to use `TypeAccess<TypeId>` to manage read/write component/archetype-component access. This was expensive because TypeIds must be hashed and compared individually. The parallel executor got around this by "condensing" type ids into bitset-backed access types. This worked, but it had to be re-generated from the `TypeAccess<TypeId>`sources every time archetypes changed.
This pr removes TypeAccess in favor of faster bitset access everywhere. We can do this thanks to the move to densely packed `ComponentId`s and `ArchetypeComponentId`s.
## Merged Resources into World
Resources had a lot of redundant functionality with Components. They stored typed data, they had access control, they had unique ids, they were queryable via SystemParams, etc. In fact the _only_ major difference between them was that they were unique (and didn't correlate to an entity).
Separate resources also had the downside of requiring a separate set of access controls, which meant the parallel executor needed to compare more bitsets per system and manage more state.
I initially got the "separate resources" idea from `legion`. I think that design was motivated by the fact that it made the direct world query/resource lifetime interactions more manageable. It certainly made our lives easier when using Resources alongside hecs/bevy_ecs. However we already have a construct for safely and ergonomically managing in-world lifetimes: systems (which use `Access<T>` internally).
This pr merges Resources into World:
```rust
world.insert_resource(1);
world.insert_resource(2.0);
let a = world.get_resource::<i32>().unwrap();
let mut b = world.get_resource_mut::<f64>().unwrap();
*b = 3.0;
```
Resources are now just a special kind of component. They have their own ComponentIds (and their own resource TypeId->ComponentId scope, so they don't conflict wit components of the same type). They are stored in a special "resource archetype", which stores components inside the archetype using a new `unique_components` sparse set (note that this sparse set could later be used to implement Tags). This allows us to keep the code size small by reusing existing datastructures (namely Column, Archetype, ComponentFlags, and ComponentInfo). This allows us the executor to use a single `Access<ArchetypeComponentId>` per system. It should also make scripting language integration easier.
_But_ this merge did create problems for people directly interacting with `World`. What if you need mutable access to multiple resources at the same time? `world.get_resource_mut()` borrows World mutably!
## WorldCell
WorldCell applies the `Access<ArchetypeComponentId>` concept to direct world access:
```rust
let world_cell = world.cell();
let a = world_cell.get_resource_mut::<i32>().unwrap();
let b = world_cell.get_resource_mut::<f64>().unwrap();
```
This adds cheap runtime checks (a sparse set lookup of `ArchetypeComponentId` and a counter) to ensure that world accesses do not conflict with each other. Each operation returns a `WorldBorrow<'w, T>` or `WorldBorrowMut<'w, T>` wrapper type, which will release the relevant ArchetypeComponentId resources when dropped.
World caches the access sparse set (and only one cell can exist at a time), so `world.cell()` is a cheap operation.
WorldCell does _not_ use atomic operations. It is non-send, does a mutable borrow of world to prevent other accesses, and uses a simple `Rc<RefCell<ArchetypeComponentAccess>>` wrapper in each WorldBorrow pointer.
The api is currently limited to resource access, but it can and should be extended to queries / entity component access.
## Resource Scopes
WorldCell does not yet support component queries, and even when it does there are sometimes legitimate reasons to want a mutable world ref _and_ a mutable resource ref (ex: bevy_render and bevy_scene both need this). In these cases we could always drop down to the unsafe `world.get_resource_unchecked_mut()`, but that is not ideal!
Instead developers can use a "resource scope"
```rust
world.resource_scope(|world: &mut World, a: &mut A| {
})
```
This temporarily removes the `A` resource from `World`, provides mutable pointers to both, and re-adds A to World when finished. Thanks to the move to ComponentIds/sparse sets, this is a cheap operation.
If multiple resources are required, scopes can be nested. We could also consider adding a "resource tuple" to the api if this pattern becomes common and the boilerplate gets nasty.
## Query Conflicts Use ComponentId Instead of ArchetypeComponentId
For safety reasons, systems cannot contain queries that conflict with each other without wrapping them in a QuerySet. On bevy `main`, we use ArchetypeComponentIds to determine conflicts. This is nice because it can take into account filters:
```rust
// these queries will never conflict due to their filters
fn filter_system(a: Query<&mut A, With<B>>, b: Query<&mut B, Without<B>>) {
}
```
But it also has a significant downside:
```rust
// these queries will not conflict _until_ an entity with A, B, and C is spawned
fn maybe_conflicts_system(a: Query<(&mut A, &C)>, b: Query<(&mut A, &B)>) {
}
```
The system above will panic at runtime if an entity with A, B, and C is spawned. This makes it hard to trust that your game logic will run without crashing.
In this pr, I switched to using `ComponentId` instead. This _is_ more constraining. `maybe_conflicts_system` will now always fail, but it will do it consistently at startup. Naively, it would also _disallow_ `filter_system`, which would be a significant downgrade in usability. Bevy has a number of internal systems that rely on disjoint queries and I expect it to be a common pattern in userspace.
To resolve this, I added a new `FilteredAccess<T>` type, which wraps `Access<T>` and adds with/without filters. If two `FilteredAccess` have with/without values that prove they are disjoint, they will no longer conflict.
## EntityRef / EntityMut
World entity operations on `main` require that the user passes in an `entity` id to each operation:
```rust
let entity = world.spawn((A, )); // create a new entity with A
world.get::<A>(entity);
world.insert(entity, (B, C));
world.insert_one(entity, D);
```
This means that each operation needs to look up the entity location / verify its validity. The initial spawn operation also requires a Bundle as input. This can be awkward when no components are required (or one component is required).
These operations have been replaced by `EntityRef` and `EntityMut`, which are "builder-style" wrappers around world that provide read and read/write operations on a single, pre-validated entity:
```rust
// spawn now takes no inputs and returns an EntityMut
let entity = world.spawn()
.insert(A) // insert a single component into the entity
.insert_bundle((B, C)) // insert a bundle of components into the entity
.id() // id returns the Entity id
// Returns EntityMut (or panics if the entity does not exist)
world.entity_mut(entity)
.insert(D)
.insert_bundle(SomeBundle::default());
{
// returns EntityRef (or panics if the entity does not exist)
let d = world.entity(entity)
.get::<D>() // gets the D component
.unwrap();
// world.get still exists for ergonomics
let d = world.get::<D>(entity).unwrap();
}
// These variants return Options if you want to check existence instead of panicing
world.get_entity_mut(entity)
.unwrap()
.insert(E);
if let Some(entity_ref) = world.get_entity(entity) {
let d = entity_ref.get::<D>().unwrap();
}
```
This _does not_ affect the current Commands api or terminology. I think that should be a separate conversation as that is a much larger breaking change.
## Safety Improvements
* Entity reservation in Commands uses a normal world borrow instead of an unsafe transmute
* QuerySets no longer transmutes lifetimes
* Made traits "unsafe" when implementing a trait incorrectly could cause unsafety
* More thorough safety docs
## RemovedComponents SystemParam
The old approach to querying removed components: `query.removed:<T>()` was confusing because it had no connection to the query itself. I replaced it with the following, which is both clearer and allows us to cache the ComponentId mapping in the SystemParamState:
```rust
fn system(removed: RemovedComponents<T>) {
for entity in removed.iter() {
}
}
```
## Simpler Bundle implementation
Bundles are no longer responsible for sorting (or deduping) TypeInfo. They are just a simple ordered list of component types / data. This makes the implementation smaller and opens the door to an easy "nested bundle" implementation in the future (which i might even add in this pr). Duplicate detection is now done once per bundle type by World the first time a bundle is used.
## Unified WorldQuery and QueryFilter types
(don't worry they are still separate type _parameters_ in Queries .. this is a non-breaking change)
WorldQuery and QueryFilter were already basically identical apis. With the addition of `FetchState` and more storage-specific fetch methods, the overlap was even clearer (and the redundancy more painful).
QueryFilters are now just `F: WorldQuery where F::Fetch: FilterFetch`. FilterFetch requires `Fetch<Item = bool>` and adds new "short circuit" variants of fetch methods. This enables a filter tuple like `(With<A>, Without<B>, Changed<C>)` to stop evaluating the filter after the first mismatch is encountered. FilterFetch is automatically implemented for `Fetch` implementations that return bool.
This forces fetch implementations that return things like `(bool, bool, bool)` (such as the filter above) to manually implement FilterFetch and decide whether or not to short-circuit.
## More Granular Modules
World no longer globs all of the internal modules together. It now exports `core`, `system`, and `schedule` separately. I'm also considering exporting `core` submodules directly as that is still pretty "glob-ey" and unorganized (feedback welcome here).
## Remaining Draft Work (to be done in this pr)
* ~~panic on conflicting WorldQuery fetches (&A, &mut A)~~
* ~~bevy `main` and hecs both currently allow this, but we should protect against it if possible~~
* ~~batch_iter / par_iter (currently stubbed out)~~
* ~~ChangedRes~~
* ~~I skipped this while we sort out #1313. This pr should be adapted to account for whatever we land on there~~.
* ~~The `Archetypes` and `Tables` collections use hashes of sorted lists of component ids to uniquely identify each archetype/table. This hash is then used as the key in a HashMap to look up the relevant ArchetypeId or TableId. (which doesn't handle hash collisions properly)~~
* ~~It is currently unsafe to generate a Query from "World A", then use it on "World B" (despite the api claiming it is safe). We should probably close this gap. This could be done by adding a randomly generated WorldId to each world, then storing that id in each Query. They could then be compared to each other on each `query.do_thing(&world)` operation. This _does_ add an extra branch to each query operation, so I'm open to other suggestions if people have them.~~
* ~~Nested Bundles (if i find time)~~
## Potential Future Work
* Expand WorldCell to support queries.
* Consider not allocating in the empty archetype on `world.spawn()`
* ex: return something like EntityMutUninit, which turns into EntityMut after an `insert` or `insert_bundle` op
* this actually regressed performance last time i tried it, but in theory it should be faster
* Optimize SparseSet::insert (see `PERF` comment on insert)
* Replace SparseArray `Option<T>` with T::MAX to cut down on branching
* would enable cheaper get_unchecked() operations
* upstream fixedbitset optimizations
* fixedbitset could be allocation free for small block counts (store blocks in a SmallVec)
* fixedbitset could have a const constructor
* Consider implementing Tags (archetype-specific by-value data that affects archetype identity)
* ex: ArchetypeA could have `[A, B, C]` table components and `[D(1)]` "tag" component. ArchetypeB could have `[A, B, C]` table components and a `[D(2)]` tag component. The archetypes are different, despite both having D tags because the value inside D is different.
* this could potentially build on top of the `archetype.unique_components` added in this pr for resource storage.
* Consider reverting `all_tuples` proc macro in favor of the old `macro_rules` implementation
* all_tuples is more flexible and produces cleaner documentation (the macro_rules version produces weird type parameter orders due to parser constraints)
* but unfortunately all_tuples also appears to make Rust Analyzer sad/slow when working inside of `bevy_ecs` (does not affect user code)
* Consider "resource queries" and/or "mixed resource and entity component queries" as an alternative to WorldCell
* this is basically just "systems" so maybe it's not worth it
* Add more world ops
* `world.clear()`
* `world.reserve<T: Bundle>(count: usize)`
* Try using the old archetype allocation strategy (allocate new memory on resize and copy everything over). I expect this to improve batch insertion performance at the cost of unbatched performance. But thats just a guess. I'm not an allocation perf pro :)
* Adapt Commands apis for consistency with new World apis
## Benchmarks
key:
* `bevy_old`: bevy `main` branch
* `bevy`: this branch
* `_foreach`: uses an optimized for_each iterator
* ` _sparse`: uses sparse set storage (if unspecified assume table storage)
* `_system`: runs inside a system (if unspecified assume test happens via direct world ops)
### Simple Insert (from ecs_bench_suite)
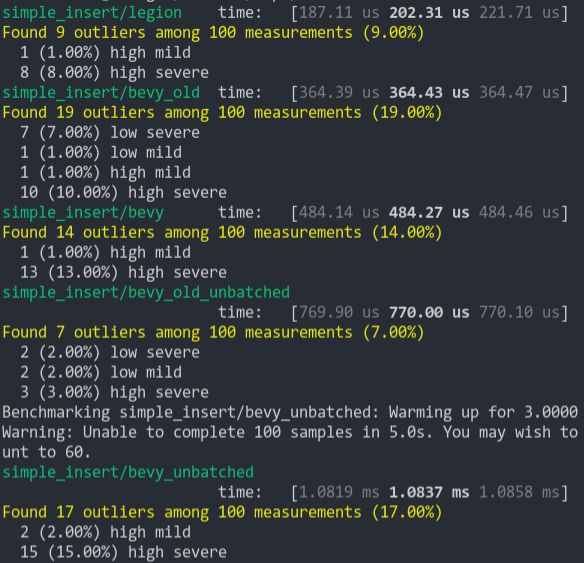
### Simpler Iter (from ecs_bench_suite)
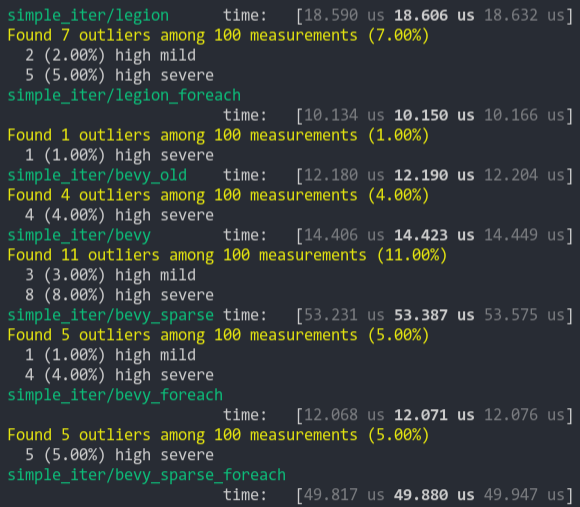
### Fragment Iter (from ecs_bench_suite)
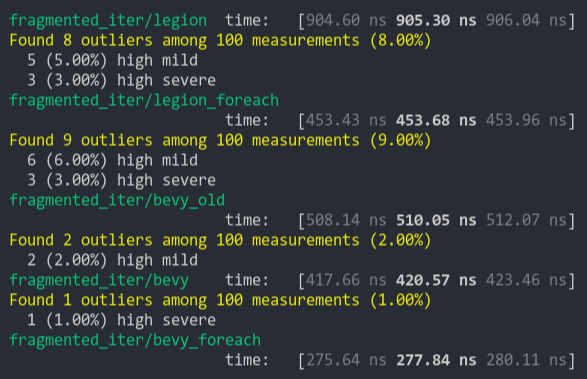
### Sparse Fragmented Iter
Iterate a query that matches 5 entities from a single matching archetype, but there are 100 unmatching archetypes
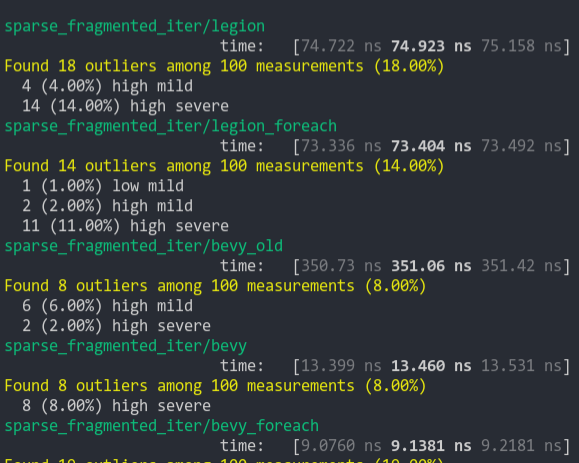
### Schedule (from ecs_bench_suite)
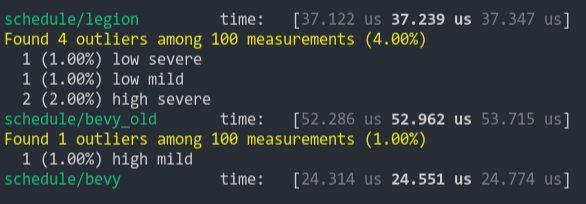
### Add Remove Component (from ecs_bench_suite)
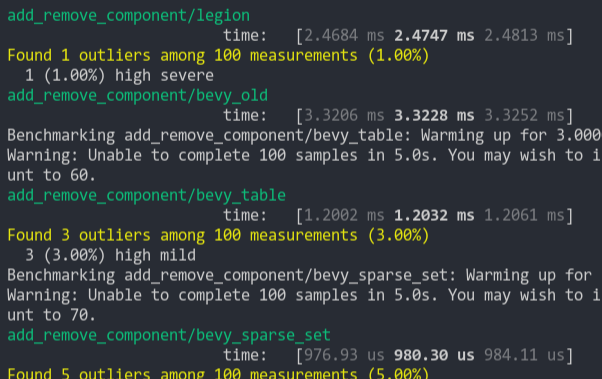
### Add Remove Component Big
Same as the test above, but each entity has 5 "large" matrix components and 1 "large" matrix component is added and removed
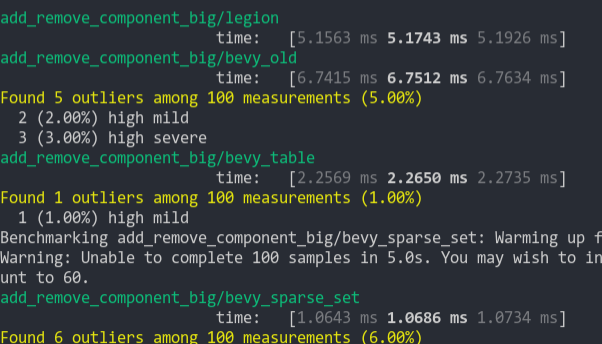
### Get Component
Looks up a single component value a large number of times
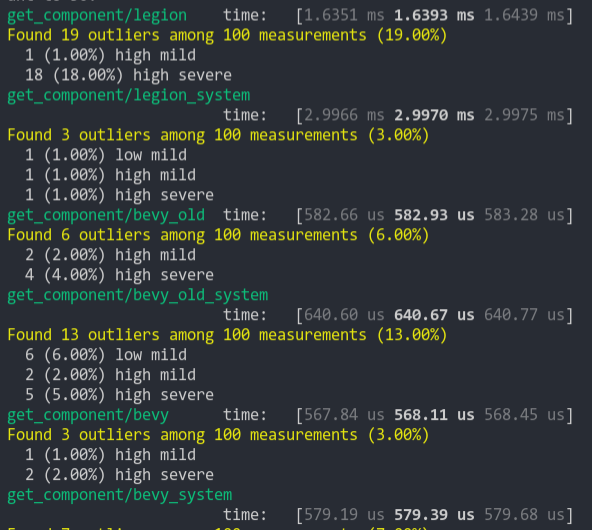
2021-03-05 07:54:35 +00:00
if conflicts . is_empty ( ) {
return ;
2020-11-08 20:34:05 +00:00
}
Bevy ECS V2 (#1525)
# Bevy ECS V2
This is a rewrite of Bevy ECS (basically everything but the new executor/schedule, which are already awesome). The overall goal was to improve the performance and versatility of Bevy ECS. Here is a quick bulleted list of changes before we dive into the details:
* Complete World rewrite
* Multiple component storage types:
* Tables: fast cache friendly iteration, slower add/removes (previously called Archetypes)
* Sparse Sets: fast add/remove, slower iteration
* Stateful Queries (caches query results for faster iteration. fragmented iteration is _fast_ now)
* Stateful System Params (caches expensive operations. inspired by @DJMcNab's work in #1364)
* Configurable System Params (users can set configuration when they construct their systems. once again inspired by @DJMcNab's work)
* Archetypes are now "just metadata", component storage is separate
* Archetype Graph (for faster archetype changes)
* Component Metadata
* Configure component storage type
* Retrieve information about component size/type/name/layout/send-ness/etc
* Components are uniquely identified by a densely packed ComponentId
* TypeIds are now totally optional (which should make implementing scripting easier)
* Super fast "for_each" query iterators
* Merged Resources into World. Resources are now just a special type of component
* EntityRef/EntityMut builder apis (more efficient and more ergonomic)
* Fast bitset-backed `Access<T>` replaces old hashmap-based approach everywhere
* Query conflicts are determined by component access instead of archetype component access (to avoid random failures at runtime)
* With/Without are still taken into account for conflicts, so this should still be comfy to use
* Much simpler `IntoSystem` impl
* Significantly reduced the amount of hashing throughout the ecs in favor of Sparse Sets (indexed by densely packed ArchetypeId, ComponentId, BundleId, and TableId)
* Safety Improvements
* Entity reservation uses a normal world reference instead of unsafe transmute
* QuerySets no longer transmute lifetimes
* Made traits "unsafe" where relevant
* More thorough safety docs
* WorldCell
* Exposes safe mutable access to multiple resources at a time in a World
* Replaced "catch all" `System::update_archetypes(world: &World)` with `System::new_archetype(archetype: &Archetype)`
* Simpler Bundle implementation
* Replaced slow "remove_bundle_one_by_one" used as fallback for Commands::remove_bundle with fast "remove_bundle_intersection"
* Removed `Mut<T>` query impl. it is better to only support one way: `&mut T`
* Removed with() from `Flags<T>` in favor of `Option<Flags<T>>`, which allows querying for flags to be "filtered" by default
* Components now have is_send property (currently only resources support non-send)
* More granular module organization
* New `RemovedComponents<T>` SystemParam that replaces `query.removed::<T>()`
* `world.resource_scope()` for mutable access to resources and world at the same time
* WorldQuery and QueryFilter traits unified. FilterFetch trait added to enable "short circuit" filtering. Auto impled for cases that don't need it
* Significantly slimmed down SystemState in favor of individual SystemParam state
* System Commands changed from `commands: &mut Commands` back to `mut commands: Commands` (to allow Commands to have a World reference)
Fixes #1320
## `World` Rewrite
This is a from-scratch rewrite of `World` that fills the niche that `hecs` used to. Yes, this means Bevy ECS is no longer a "fork" of hecs. We're going out our own!
(the only shared code between the projects is the entity id allocator, which is already basically ideal)
A huge shout out to @SanderMertens (author of [flecs](https://github.com/SanderMertens/flecs)) for sharing some great ideas with me (specifically hybrid ecs storage and archetype graphs). He also helped advise on a number of implementation details.
## Component Storage (The Problem)
Two ECS storage paradigms have gained a lot of traction over the years:
* **Archetypal ECS**:
* Stores components in "tables" with static schemas. Each "column" stores components of a given type. Each "row" is an entity.
* Each "archetype" has its own table. Adding/removing an entity's component changes the archetype.
* Enables super-fast Query iteration due to its cache-friendly data layout
* Comes at the cost of more expensive add/remove operations for an Entity's components, because all components need to be copied to the new archetype's "table"
* **Sparse Set ECS**:
* Stores components of the same type in densely packed arrays, which are sparsely indexed by densely packed unsigned integers (Entity ids)
* Query iteration is slower than Archetypal ECS because each entity's component could be at any position in the sparse set. This "random access" pattern isn't cache friendly. Additionally, there is an extra layer of indirection because you must first map the entity id to an index in the component array.
* Adding/removing components is a cheap, constant time operation
Bevy ECS V1, hecs, legion, flec, and Unity DOTS are all "archetypal ecs-es". I personally think "archetypal" storage is a good default for game engines. An entity's archetype doesn't need to change frequently in general, and it creates "fast by default" query iteration (which is a much more common operation). It is also "self optimizing". Users don't need to think about optimizing component layouts for iteration performance. It "just works" without any extra boilerplate.
Shipyard and EnTT are "sparse set ecs-es". They employ "packing" as a way to work around the "suboptimal by default" iteration performance for specific sets of components. This helps, but I didn't think this was a good choice for a general purpose engine like Bevy because:
1. "packs" conflict with each other. If bevy decides to internally pack the Transform and GlobalTransform components, users are then blocked if they want to pack some custom component with Transform.
2. users need to take manual action to optimize
Developers selecting an ECS framework are stuck with a hard choice. Select an "archetypal" framework with "fast iteration everywhere" but without the ability to cheaply add/remove components, or select a "sparse set" framework to cheaply add/remove components but with slower iteration performance.
## Hybrid Component Storage (The Solution)
In Bevy ECS V2, we get to have our cake and eat it too. It now has _both_ of the component storage types above (and more can be added later if needed):
* **Tables** (aka "archetypal" storage)
* The default storage. If you don't configure anything, this is what you get
* Fast iteration by default
* Slower add/remove operations
* **Sparse Sets**
* Opt-in
* Slower iteration
* Faster add/remove operations
These storage types complement each other perfectly. By default Query iteration is fast. If developers know that they want to add/remove a component at high frequencies, they can set the storage to "sparse set":
```rust
world.register_component(
ComponentDescriptor::new::<MyComponent>(StorageType::SparseSet)
).unwrap();
```
## Archetypes
Archetypes are now "just metadata" ... they no longer store components directly. They do store:
* The `ComponentId`s of each of the Archetype's components (and that component's storage type)
* Archetypes are uniquely defined by their component layouts
* For example: entities with "table" components `[A, B, C]` _and_ "sparse set" components `[D, E]` will always be in the same archetype.
* The `TableId` associated with the archetype
* For now each archetype has exactly one table (which can have no components),
* There is a 1->Many relationship from Tables->Archetypes. A given table could have any number of archetype components stored in it:
* Ex: an entity with "table storage" components `[A, B, C]` and "sparse set" components `[D, E]` will share the same `[A, B, C]` table as an entity with `[A, B, C]` table component and `[F]` sparse set components.
* This 1->Many relationship is how we preserve fast "cache friendly" iteration performance when possible (more on this later)
* A list of entities that are in the archetype and the row id of the table they are in
* ArchetypeComponentIds
* unique densely packed identifiers for (ArchetypeId, ComponentId) pairs
* used by the schedule executor for cheap system access control
* "Archetype Graph Edges" (see the next section)
## The "Archetype Graph"
Archetype changes in Bevy (and a number of other archetypal ecs-es) have historically been expensive to compute. First, you need to allocate a new vector of the entity's current component ids, add or remove components based on the operation performed, sort it (to ensure it is order-independent), then hash it to find the archetype (if it exists). And thats all before we get to the _already_ expensive full copy of all components to the new table storage.
The solution is to build a "graph" of archetypes to cache these results. @SanderMertens first exposed me to the idea (and he got it from @gjroelofs, who came up with it). They propose adding directed edges between archetypes for add/remove component operations. If `ComponentId`s are densely packed, you can use sparse sets to cheaply jump between archetypes.
Bevy takes this one step further by using add/remove `Bundle` edges instead of `Component` edges. Bevy encourages the use of `Bundles` to group add/remove operations. This is largely for "clearer game logic" reasons, but it also helps cut down on the number of archetype changes required. `Bundles` now also have densely-packed `BundleId`s. This allows us to use a _single_ edge for each bundle operation (rather than needing to traverse N edges ... one for each component). Single component operations are also bundles, so this is strictly an improvement over a "component only" graph.
As a result, an operation that used to be _heavy_ (both for allocations and compute) is now two dirt-cheap array lookups and zero allocations.
## Stateful Queries
World queries are now stateful. This allows us to:
1. Cache archetype (and table) matches
* This resolves another issue with (naive) archetypal ECS: query performance getting worse as the number of archetypes goes up (and fragmentation occurs).
2. Cache Fetch and Filter state
* The expensive parts of fetch/filter operations (such as hashing the TypeId to find the ComponentId) now only happen once when the Query is first constructed
3. Incrementally build up state
* When new archetypes are added, we only process the new archetypes (no need to rebuild state for old archetypes)
As a result, the direct `World` query api now looks like this:
```rust
let mut query = world.query::<(&A, &mut B)>();
for (a, mut b) in query.iter_mut(&mut world) {
}
```
Requiring `World` to generate stateful queries (rather than letting the `QueryState` type be constructed separately) allows us to ensure that _all_ queries are properly initialized (and the relevant world state, such as ComponentIds). This enables QueryState to remove branches from its operations that check for initialization status (and also enables query.iter() to take an immutable world reference because it doesn't need to initialize anything in world).
However in systems, this is a non-breaking change. State management is done internally by the relevant SystemParam.
## Stateful SystemParams
Like Queries, `SystemParams` now also cache state. For example, `Query` system params store the "stateful query" state mentioned above. Commands store their internal `CommandQueue`. This means you can now safely use as many separate `Commands` parameters in your system as you want. `Local<T>` system params store their `T` value in their state (instead of in Resources).
SystemParam state also enabled a significant slim-down of SystemState. It is much nicer to look at now.
Per-SystemParam state naturally insulates us from an "aliased mut" class of errors we have hit in the past (ex: using multiple `Commands` system params).
(credit goes to @DJMcNab for the initial idea and draft pr here #1364)
## Configurable SystemParams
@DJMcNab also had the great idea to make SystemParams configurable. This allows users to provide some initial configuration / values for system parameters (when possible). Most SystemParams have no config (the config type is `()`), but the `Local<T>` param now supports user-provided parameters:
```rust
fn foo(value: Local<usize>) {
}
app.add_system(foo.system().config(|c| c.0 = Some(10)));
```
## Uber Fast "for_each" Query Iterators
Developers now have the choice to use a fast "for_each" iterator, which yields ~1.5-3x iteration speed improvements for "fragmented iteration", and minor ~1.2x iteration speed improvements for unfragmented iteration.
```rust
fn system(query: Query<(&A, &mut B)>) {
// you now have the option to do this for a speed boost
query.for_each_mut(|(a, mut b)| {
});
// however normal iterators are still available
for (a, mut b) in query.iter_mut() {
}
}
```
I think in most cases we should continue to encourage "normal" iterators as they are more flexible and more "rust idiomatic". But when that extra "oomf" is needed, it makes sense to use `for_each`.
We should also consider using `for_each` for internal bevy systems to give our users a nice speed boost (but that should be a separate pr).
## Component Metadata
`World` now has a `Components` collection, which is accessible via `world.components()`. This stores mappings from `ComponentId` to `ComponentInfo`, as well as `TypeId` to `ComponentId` mappings (where relevant). `ComponentInfo` stores information about the component, such as ComponentId, TypeId, memory layout, send-ness (currently limited to resources), and storage type.
## Significantly Cheaper `Access<T>`
We used to use `TypeAccess<TypeId>` to manage read/write component/archetype-component access. This was expensive because TypeIds must be hashed and compared individually. The parallel executor got around this by "condensing" type ids into bitset-backed access types. This worked, but it had to be re-generated from the `TypeAccess<TypeId>`sources every time archetypes changed.
This pr removes TypeAccess in favor of faster bitset access everywhere. We can do this thanks to the move to densely packed `ComponentId`s and `ArchetypeComponentId`s.
## Merged Resources into World
Resources had a lot of redundant functionality with Components. They stored typed data, they had access control, they had unique ids, they were queryable via SystemParams, etc. In fact the _only_ major difference between them was that they were unique (and didn't correlate to an entity).
Separate resources also had the downside of requiring a separate set of access controls, which meant the parallel executor needed to compare more bitsets per system and manage more state.
I initially got the "separate resources" idea from `legion`. I think that design was motivated by the fact that it made the direct world query/resource lifetime interactions more manageable. It certainly made our lives easier when using Resources alongside hecs/bevy_ecs. However we already have a construct for safely and ergonomically managing in-world lifetimes: systems (which use `Access<T>` internally).
This pr merges Resources into World:
```rust
world.insert_resource(1);
world.insert_resource(2.0);
let a = world.get_resource::<i32>().unwrap();
let mut b = world.get_resource_mut::<f64>().unwrap();
*b = 3.0;
```
Resources are now just a special kind of component. They have their own ComponentIds (and their own resource TypeId->ComponentId scope, so they don't conflict wit components of the same type). They are stored in a special "resource archetype", which stores components inside the archetype using a new `unique_components` sparse set (note that this sparse set could later be used to implement Tags). This allows us to keep the code size small by reusing existing datastructures (namely Column, Archetype, ComponentFlags, and ComponentInfo). This allows us the executor to use a single `Access<ArchetypeComponentId>` per system. It should also make scripting language integration easier.
_But_ this merge did create problems for people directly interacting with `World`. What if you need mutable access to multiple resources at the same time? `world.get_resource_mut()` borrows World mutably!
## WorldCell
WorldCell applies the `Access<ArchetypeComponentId>` concept to direct world access:
```rust
let world_cell = world.cell();
let a = world_cell.get_resource_mut::<i32>().unwrap();
let b = world_cell.get_resource_mut::<f64>().unwrap();
```
This adds cheap runtime checks (a sparse set lookup of `ArchetypeComponentId` and a counter) to ensure that world accesses do not conflict with each other. Each operation returns a `WorldBorrow<'w, T>` or `WorldBorrowMut<'w, T>` wrapper type, which will release the relevant ArchetypeComponentId resources when dropped.
World caches the access sparse set (and only one cell can exist at a time), so `world.cell()` is a cheap operation.
WorldCell does _not_ use atomic operations. It is non-send, does a mutable borrow of world to prevent other accesses, and uses a simple `Rc<RefCell<ArchetypeComponentAccess>>` wrapper in each WorldBorrow pointer.
The api is currently limited to resource access, but it can and should be extended to queries / entity component access.
## Resource Scopes
WorldCell does not yet support component queries, and even when it does there are sometimes legitimate reasons to want a mutable world ref _and_ a mutable resource ref (ex: bevy_render and bevy_scene both need this). In these cases we could always drop down to the unsafe `world.get_resource_unchecked_mut()`, but that is not ideal!
Instead developers can use a "resource scope"
```rust
world.resource_scope(|world: &mut World, a: &mut A| {
})
```
This temporarily removes the `A` resource from `World`, provides mutable pointers to both, and re-adds A to World when finished. Thanks to the move to ComponentIds/sparse sets, this is a cheap operation.
If multiple resources are required, scopes can be nested. We could also consider adding a "resource tuple" to the api if this pattern becomes common and the boilerplate gets nasty.
## Query Conflicts Use ComponentId Instead of ArchetypeComponentId
For safety reasons, systems cannot contain queries that conflict with each other without wrapping them in a QuerySet. On bevy `main`, we use ArchetypeComponentIds to determine conflicts. This is nice because it can take into account filters:
```rust
// these queries will never conflict due to their filters
fn filter_system(a: Query<&mut A, With<B>>, b: Query<&mut B, Without<B>>) {
}
```
But it also has a significant downside:
```rust
// these queries will not conflict _until_ an entity with A, B, and C is spawned
fn maybe_conflicts_system(a: Query<(&mut A, &C)>, b: Query<(&mut A, &B)>) {
}
```
The system above will panic at runtime if an entity with A, B, and C is spawned. This makes it hard to trust that your game logic will run without crashing.
In this pr, I switched to using `ComponentId` instead. This _is_ more constraining. `maybe_conflicts_system` will now always fail, but it will do it consistently at startup. Naively, it would also _disallow_ `filter_system`, which would be a significant downgrade in usability. Bevy has a number of internal systems that rely on disjoint queries and I expect it to be a common pattern in userspace.
To resolve this, I added a new `FilteredAccess<T>` type, which wraps `Access<T>` and adds with/without filters. If two `FilteredAccess` have with/without values that prove they are disjoint, they will no longer conflict.
## EntityRef / EntityMut
World entity operations on `main` require that the user passes in an `entity` id to each operation:
```rust
let entity = world.spawn((A, )); // create a new entity with A
world.get::<A>(entity);
world.insert(entity, (B, C));
world.insert_one(entity, D);
```
This means that each operation needs to look up the entity location / verify its validity. The initial spawn operation also requires a Bundle as input. This can be awkward when no components are required (or one component is required).
These operations have been replaced by `EntityRef` and `EntityMut`, which are "builder-style" wrappers around world that provide read and read/write operations on a single, pre-validated entity:
```rust
// spawn now takes no inputs and returns an EntityMut
let entity = world.spawn()
.insert(A) // insert a single component into the entity
.insert_bundle((B, C)) // insert a bundle of components into the entity
.id() // id returns the Entity id
// Returns EntityMut (or panics if the entity does not exist)
world.entity_mut(entity)
.insert(D)
.insert_bundle(SomeBundle::default());
{
// returns EntityRef (or panics if the entity does not exist)
let d = world.entity(entity)
.get::<D>() // gets the D component
.unwrap();
// world.get still exists for ergonomics
let d = world.get::<D>(entity).unwrap();
}
// These variants return Options if you want to check existence instead of panicing
world.get_entity_mut(entity)
.unwrap()
.insert(E);
if let Some(entity_ref) = world.get_entity(entity) {
let d = entity_ref.get::<D>().unwrap();
}
```
This _does not_ affect the current Commands api or terminology. I think that should be a separate conversation as that is a much larger breaking change.
## Safety Improvements
* Entity reservation in Commands uses a normal world borrow instead of an unsafe transmute
* QuerySets no longer transmutes lifetimes
* Made traits "unsafe" when implementing a trait incorrectly could cause unsafety
* More thorough safety docs
## RemovedComponents SystemParam
The old approach to querying removed components: `query.removed:<T>()` was confusing because it had no connection to the query itself. I replaced it with the following, which is both clearer and allows us to cache the ComponentId mapping in the SystemParamState:
```rust
fn system(removed: RemovedComponents<T>) {
for entity in removed.iter() {
}
}
```
## Simpler Bundle implementation
Bundles are no longer responsible for sorting (or deduping) TypeInfo. They are just a simple ordered list of component types / data. This makes the implementation smaller and opens the door to an easy "nested bundle" implementation in the future (which i might even add in this pr). Duplicate detection is now done once per bundle type by World the first time a bundle is used.
## Unified WorldQuery and QueryFilter types
(don't worry they are still separate type _parameters_ in Queries .. this is a non-breaking change)
WorldQuery and QueryFilter were already basically identical apis. With the addition of `FetchState` and more storage-specific fetch methods, the overlap was even clearer (and the redundancy more painful).
QueryFilters are now just `F: WorldQuery where F::Fetch: FilterFetch`. FilterFetch requires `Fetch<Item = bool>` and adds new "short circuit" variants of fetch methods. This enables a filter tuple like `(With<A>, Without<B>, Changed<C>)` to stop evaluating the filter after the first mismatch is encountered. FilterFetch is automatically implemented for `Fetch` implementations that return bool.
This forces fetch implementations that return things like `(bool, bool, bool)` (such as the filter above) to manually implement FilterFetch and decide whether or not to short-circuit.
## More Granular Modules
World no longer globs all of the internal modules together. It now exports `core`, `system`, and `schedule` separately. I'm also considering exporting `core` submodules directly as that is still pretty "glob-ey" and unorganized (feedback welcome here).
## Remaining Draft Work (to be done in this pr)
* ~~panic on conflicting WorldQuery fetches (&A, &mut A)~~
* ~~bevy `main` and hecs both currently allow this, but we should protect against it if possible~~
* ~~batch_iter / par_iter (currently stubbed out)~~
* ~~ChangedRes~~
* ~~I skipped this while we sort out #1313. This pr should be adapted to account for whatever we land on there~~.
* ~~The `Archetypes` and `Tables` collections use hashes of sorted lists of component ids to uniquely identify each archetype/table. This hash is then used as the key in a HashMap to look up the relevant ArchetypeId or TableId. (which doesn't handle hash collisions properly)~~
* ~~It is currently unsafe to generate a Query from "World A", then use it on "World B" (despite the api claiming it is safe). We should probably close this gap. This could be done by adding a randomly generated WorldId to each world, then storing that id in each Query. They could then be compared to each other on each `query.do_thing(&world)` operation. This _does_ add an extra branch to each query operation, so I'm open to other suggestions if people have them.~~
* ~~Nested Bundles (if i find time)~~
## Potential Future Work
* Expand WorldCell to support queries.
* Consider not allocating in the empty archetype on `world.spawn()`
* ex: return something like EntityMutUninit, which turns into EntityMut after an `insert` or `insert_bundle` op
* this actually regressed performance last time i tried it, but in theory it should be faster
* Optimize SparseSet::insert (see `PERF` comment on insert)
* Replace SparseArray `Option<T>` with T::MAX to cut down on branching
* would enable cheaper get_unchecked() operations
* upstream fixedbitset optimizations
* fixedbitset could be allocation free for small block counts (store blocks in a SmallVec)
* fixedbitset could have a const constructor
* Consider implementing Tags (archetype-specific by-value data that affects archetype identity)
* ex: ArchetypeA could have `[A, B, C]` table components and `[D(1)]` "tag" component. ArchetypeB could have `[A, B, C]` table components and a `[D(2)]` tag component. The archetypes are different, despite both having D tags because the value inside D is different.
* this could potentially build on top of the `archetype.unique_components` added in this pr for resource storage.
* Consider reverting `all_tuples` proc macro in favor of the old `macro_rules` implementation
* all_tuples is more flexible and produces cleaner documentation (the macro_rules version produces weird type parameter orders due to parser constraints)
* but unfortunately all_tuples also appears to make Rust Analyzer sad/slow when working inside of `bevy_ecs` (does not affect user code)
* Consider "resource queries" and/or "mixed resource and entity component queries" as an alternative to WorldCell
* this is basically just "systems" so maybe it's not worth it
* Add more world ops
* `world.clear()`
* `world.reserve<T: Bundle>(count: usize)`
* Try using the old archetype allocation strategy (allocate new memory on resize and copy everything over). I expect this to improve batch insertion performance at the cost of unbatched performance. But thats just a guess. I'm not an allocation perf pro :)
* Adapt Commands apis for consistency with new World apis
## Benchmarks
key:
* `bevy_old`: bevy `main` branch
* `bevy`: this branch
* `_foreach`: uses an optimized for_each iterator
* ` _sparse`: uses sparse set storage (if unspecified assume table storage)
* `_system`: runs inside a system (if unspecified assume test happens via direct world ops)
### Simple Insert (from ecs_bench_suite)
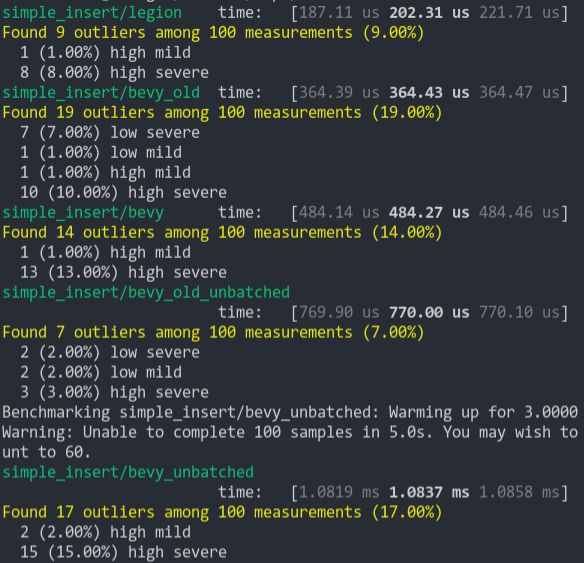
### Simpler Iter (from ecs_bench_suite)
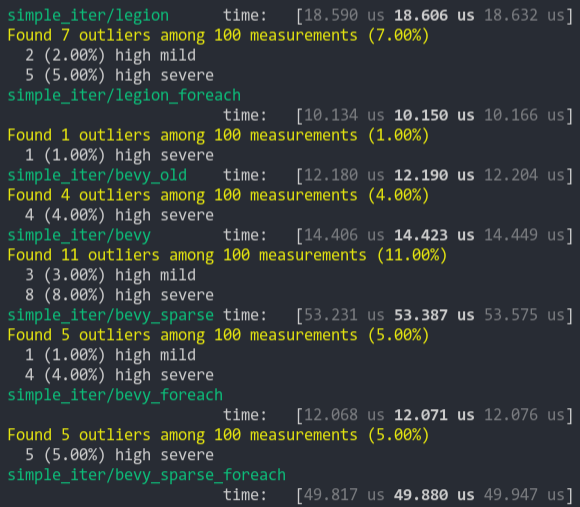
### Fragment Iter (from ecs_bench_suite)
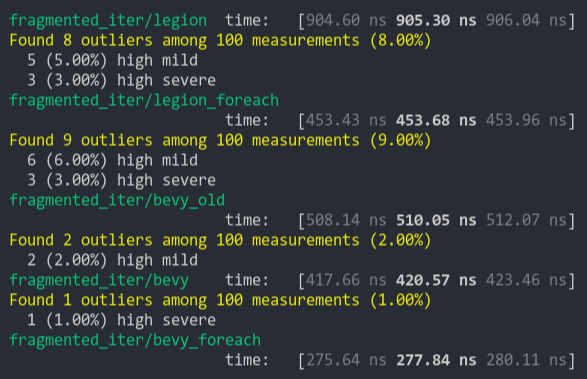
### Sparse Fragmented Iter
Iterate a query that matches 5 entities from a single matching archetype, but there are 100 unmatching archetypes
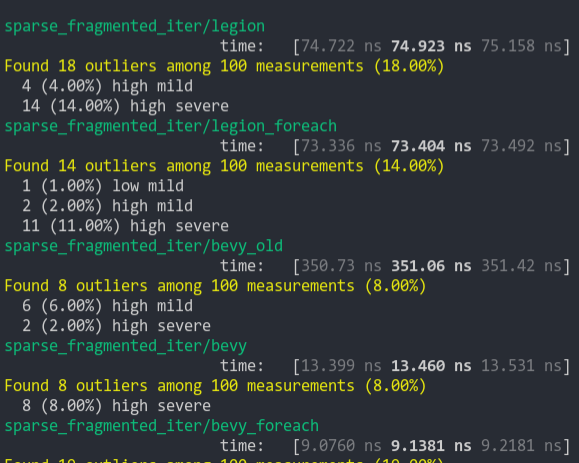
### Schedule (from ecs_bench_suite)
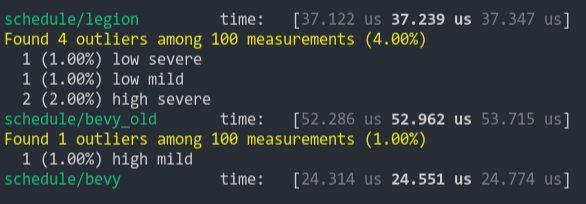
### Add Remove Component (from ecs_bench_suite)
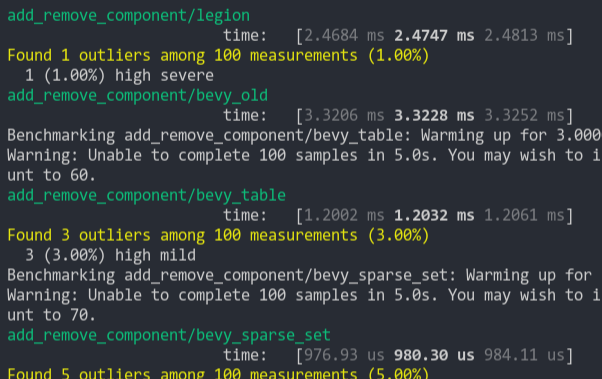
### Add Remove Component Big
Same as the test above, but each entity has 5 "large" matrix components and 1 "large" matrix component is added and removed
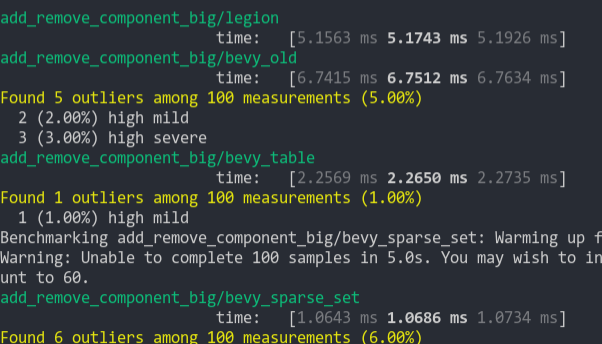
### Get Component
Looks up a single component value a large number of times
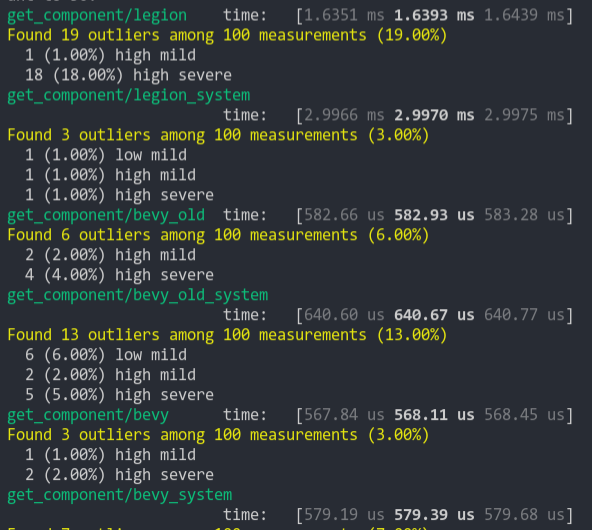
2021-03-05 07:54:35 +00:00
let conflicting_components = conflicts
2022-11-06 01:42:15 +00:00
. into_iter ( )
Bevy ECS V2 (#1525)
# Bevy ECS V2
This is a rewrite of Bevy ECS (basically everything but the new executor/schedule, which are already awesome). The overall goal was to improve the performance and versatility of Bevy ECS. Here is a quick bulleted list of changes before we dive into the details:
* Complete World rewrite
* Multiple component storage types:
* Tables: fast cache friendly iteration, slower add/removes (previously called Archetypes)
* Sparse Sets: fast add/remove, slower iteration
* Stateful Queries (caches query results for faster iteration. fragmented iteration is _fast_ now)
* Stateful System Params (caches expensive operations. inspired by @DJMcNab's work in #1364)
* Configurable System Params (users can set configuration when they construct their systems. once again inspired by @DJMcNab's work)
* Archetypes are now "just metadata", component storage is separate
* Archetype Graph (for faster archetype changes)
* Component Metadata
* Configure component storage type
* Retrieve information about component size/type/name/layout/send-ness/etc
* Components are uniquely identified by a densely packed ComponentId
* TypeIds are now totally optional (which should make implementing scripting easier)
* Super fast "for_each" query iterators
* Merged Resources into World. Resources are now just a special type of component
* EntityRef/EntityMut builder apis (more efficient and more ergonomic)
* Fast bitset-backed `Access<T>` replaces old hashmap-based approach everywhere
* Query conflicts are determined by component access instead of archetype component access (to avoid random failures at runtime)
* With/Without are still taken into account for conflicts, so this should still be comfy to use
* Much simpler `IntoSystem` impl
* Significantly reduced the amount of hashing throughout the ecs in favor of Sparse Sets (indexed by densely packed ArchetypeId, ComponentId, BundleId, and TableId)
* Safety Improvements
* Entity reservation uses a normal world reference instead of unsafe transmute
* QuerySets no longer transmute lifetimes
* Made traits "unsafe" where relevant
* More thorough safety docs
* WorldCell
* Exposes safe mutable access to multiple resources at a time in a World
* Replaced "catch all" `System::update_archetypes(world: &World)` with `System::new_archetype(archetype: &Archetype)`
* Simpler Bundle implementation
* Replaced slow "remove_bundle_one_by_one" used as fallback for Commands::remove_bundle with fast "remove_bundle_intersection"
* Removed `Mut<T>` query impl. it is better to only support one way: `&mut T`
* Removed with() from `Flags<T>` in favor of `Option<Flags<T>>`, which allows querying for flags to be "filtered" by default
* Components now have is_send property (currently only resources support non-send)
* More granular module organization
* New `RemovedComponents<T>` SystemParam that replaces `query.removed::<T>()`
* `world.resource_scope()` for mutable access to resources and world at the same time
* WorldQuery and QueryFilter traits unified. FilterFetch trait added to enable "short circuit" filtering. Auto impled for cases that don't need it
* Significantly slimmed down SystemState in favor of individual SystemParam state
* System Commands changed from `commands: &mut Commands` back to `mut commands: Commands` (to allow Commands to have a World reference)
Fixes #1320
## `World` Rewrite
This is a from-scratch rewrite of `World` that fills the niche that `hecs` used to. Yes, this means Bevy ECS is no longer a "fork" of hecs. We're going out our own!
(the only shared code between the projects is the entity id allocator, which is already basically ideal)
A huge shout out to @SanderMertens (author of [flecs](https://github.com/SanderMertens/flecs)) for sharing some great ideas with me (specifically hybrid ecs storage and archetype graphs). He also helped advise on a number of implementation details.
## Component Storage (The Problem)
Two ECS storage paradigms have gained a lot of traction over the years:
* **Archetypal ECS**:
* Stores components in "tables" with static schemas. Each "column" stores components of a given type. Each "row" is an entity.
* Each "archetype" has its own table. Adding/removing an entity's component changes the archetype.
* Enables super-fast Query iteration due to its cache-friendly data layout
* Comes at the cost of more expensive add/remove operations for an Entity's components, because all components need to be copied to the new archetype's "table"
* **Sparse Set ECS**:
* Stores components of the same type in densely packed arrays, which are sparsely indexed by densely packed unsigned integers (Entity ids)
* Query iteration is slower than Archetypal ECS because each entity's component could be at any position in the sparse set. This "random access" pattern isn't cache friendly. Additionally, there is an extra layer of indirection because you must first map the entity id to an index in the component array.
* Adding/removing components is a cheap, constant time operation
Bevy ECS V1, hecs, legion, flec, and Unity DOTS are all "archetypal ecs-es". I personally think "archetypal" storage is a good default for game engines. An entity's archetype doesn't need to change frequently in general, and it creates "fast by default" query iteration (which is a much more common operation). It is also "self optimizing". Users don't need to think about optimizing component layouts for iteration performance. It "just works" without any extra boilerplate.
Shipyard and EnTT are "sparse set ecs-es". They employ "packing" as a way to work around the "suboptimal by default" iteration performance for specific sets of components. This helps, but I didn't think this was a good choice for a general purpose engine like Bevy because:
1. "packs" conflict with each other. If bevy decides to internally pack the Transform and GlobalTransform components, users are then blocked if they want to pack some custom component with Transform.
2. users need to take manual action to optimize
Developers selecting an ECS framework are stuck with a hard choice. Select an "archetypal" framework with "fast iteration everywhere" but without the ability to cheaply add/remove components, or select a "sparse set" framework to cheaply add/remove components but with slower iteration performance.
## Hybrid Component Storage (The Solution)
In Bevy ECS V2, we get to have our cake and eat it too. It now has _both_ of the component storage types above (and more can be added later if needed):
* **Tables** (aka "archetypal" storage)
* The default storage. If you don't configure anything, this is what you get
* Fast iteration by default
* Slower add/remove operations
* **Sparse Sets**
* Opt-in
* Slower iteration
* Faster add/remove operations
These storage types complement each other perfectly. By default Query iteration is fast. If developers know that they want to add/remove a component at high frequencies, they can set the storage to "sparse set":
```rust
world.register_component(
ComponentDescriptor::new::<MyComponent>(StorageType::SparseSet)
).unwrap();
```
## Archetypes
Archetypes are now "just metadata" ... they no longer store components directly. They do store:
* The `ComponentId`s of each of the Archetype's components (and that component's storage type)
* Archetypes are uniquely defined by their component layouts
* For example: entities with "table" components `[A, B, C]` _and_ "sparse set" components `[D, E]` will always be in the same archetype.
* The `TableId` associated with the archetype
* For now each archetype has exactly one table (which can have no components),
* There is a 1->Many relationship from Tables->Archetypes. A given table could have any number of archetype components stored in it:
* Ex: an entity with "table storage" components `[A, B, C]` and "sparse set" components `[D, E]` will share the same `[A, B, C]` table as an entity with `[A, B, C]` table component and `[F]` sparse set components.
* This 1->Many relationship is how we preserve fast "cache friendly" iteration performance when possible (more on this later)
* A list of entities that are in the archetype and the row id of the table they are in
* ArchetypeComponentIds
* unique densely packed identifiers for (ArchetypeId, ComponentId) pairs
* used by the schedule executor for cheap system access control
* "Archetype Graph Edges" (see the next section)
## The "Archetype Graph"
Archetype changes in Bevy (and a number of other archetypal ecs-es) have historically been expensive to compute. First, you need to allocate a new vector of the entity's current component ids, add or remove components based on the operation performed, sort it (to ensure it is order-independent), then hash it to find the archetype (if it exists). And thats all before we get to the _already_ expensive full copy of all components to the new table storage.
The solution is to build a "graph" of archetypes to cache these results. @SanderMertens first exposed me to the idea (and he got it from @gjroelofs, who came up with it). They propose adding directed edges between archetypes for add/remove component operations. If `ComponentId`s are densely packed, you can use sparse sets to cheaply jump between archetypes.
Bevy takes this one step further by using add/remove `Bundle` edges instead of `Component` edges. Bevy encourages the use of `Bundles` to group add/remove operations. This is largely for "clearer game logic" reasons, but it also helps cut down on the number of archetype changes required. `Bundles` now also have densely-packed `BundleId`s. This allows us to use a _single_ edge for each bundle operation (rather than needing to traverse N edges ... one for each component). Single component operations are also bundles, so this is strictly an improvement over a "component only" graph.
As a result, an operation that used to be _heavy_ (both for allocations and compute) is now two dirt-cheap array lookups and zero allocations.
## Stateful Queries
World queries are now stateful. This allows us to:
1. Cache archetype (and table) matches
* This resolves another issue with (naive) archetypal ECS: query performance getting worse as the number of archetypes goes up (and fragmentation occurs).
2. Cache Fetch and Filter state
* The expensive parts of fetch/filter operations (such as hashing the TypeId to find the ComponentId) now only happen once when the Query is first constructed
3. Incrementally build up state
* When new archetypes are added, we only process the new archetypes (no need to rebuild state for old archetypes)
As a result, the direct `World` query api now looks like this:
```rust
let mut query = world.query::<(&A, &mut B)>();
for (a, mut b) in query.iter_mut(&mut world) {
}
```
Requiring `World` to generate stateful queries (rather than letting the `QueryState` type be constructed separately) allows us to ensure that _all_ queries are properly initialized (and the relevant world state, such as ComponentIds). This enables QueryState to remove branches from its operations that check for initialization status (and also enables query.iter() to take an immutable world reference because it doesn't need to initialize anything in world).
However in systems, this is a non-breaking change. State management is done internally by the relevant SystemParam.
## Stateful SystemParams
Like Queries, `SystemParams` now also cache state. For example, `Query` system params store the "stateful query" state mentioned above. Commands store their internal `CommandQueue`. This means you can now safely use as many separate `Commands` parameters in your system as you want. `Local<T>` system params store their `T` value in their state (instead of in Resources).
SystemParam state also enabled a significant slim-down of SystemState. It is much nicer to look at now.
Per-SystemParam state naturally insulates us from an "aliased mut" class of errors we have hit in the past (ex: using multiple `Commands` system params).
(credit goes to @DJMcNab for the initial idea and draft pr here #1364)
## Configurable SystemParams
@DJMcNab also had the great idea to make SystemParams configurable. This allows users to provide some initial configuration / values for system parameters (when possible). Most SystemParams have no config (the config type is `()`), but the `Local<T>` param now supports user-provided parameters:
```rust
fn foo(value: Local<usize>) {
}
app.add_system(foo.system().config(|c| c.0 = Some(10)));
```
## Uber Fast "for_each" Query Iterators
Developers now have the choice to use a fast "for_each" iterator, which yields ~1.5-3x iteration speed improvements for "fragmented iteration", and minor ~1.2x iteration speed improvements for unfragmented iteration.
```rust
fn system(query: Query<(&A, &mut B)>) {
// you now have the option to do this for a speed boost
query.for_each_mut(|(a, mut b)| {
});
// however normal iterators are still available
for (a, mut b) in query.iter_mut() {
}
}
```
I think in most cases we should continue to encourage "normal" iterators as they are more flexible and more "rust idiomatic". But when that extra "oomf" is needed, it makes sense to use `for_each`.
We should also consider using `for_each` for internal bevy systems to give our users a nice speed boost (but that should be a separate pr).
## Component Metadata
`World` now has a `Components` collection, which is accessible via `world.components()`. This stores mappings from `ComponentId` to `ComponentInfo`, as well as `TypeId` to `ComponentId` mappings (where relevant). `ComponentInfo` stores information about the component, such as ComponentId, TypeId, memory layout, send-ness (currently limited to resources), and storage type.
## Significantly Cheaper `Access<T>`
We used to use `TypeAccess<TypeId>` to manage read/write component/archetype-component access. This was expensive because TypeIds must be hashed and compared individually. The parallel executor got around this by "condensing" type ids into bitset-backed access types. This worked, but it had to be re-generated from the `TypeAccess<TypeId>`sources every time archetypes changed.
This pr removes TypeAccess in favor of faster bitset access everywhere. We can do this thanks to the move to densely packed `ComponentId`s and `ArchetypeComponentId`s.
## Merged Resources into World
Resources had a lot of redundant functionality with Components. They stored typed data, they had access control, they had unique ids, they were queryable via SystemParams, etc. In fact the _only_ major difference between them was that they were unique (and didn't correlate to an entity).
Separate resources also had the downside of requiring a separate set of access controls, which meant the parallel executor needed to compare more bitsets per system and manage more state.
I initially got the "separate resources" idea from `legion`. I think that design was motivated by the fact that it made the direct world query/resource lifetime interactions more manageable. It certainly made our lives easier when using Resources alongside hecs/bevy_ecs. However we already have a construct for safely and ergonomically managing in-world lifetimes: systems (which use `Access<T>` internally).
This pr merges Resources into World:
```rust
world.insert_resource(1);
world.insert_resource(2.0);
let a = world.get_resource::<i32>().unwrap();
let mut b = world.get_resource_mut::<f64>().unwrap();
*b = 3.0;
```
Resources are now just a special kind of component. They have their own ComponentIds (and their own resource TypeId->ComponentId scope, so they don't conflict wit components of the same type). They are stored in a special "resource archetype", which stores components inside the archetype using a new `unique_components` sparse set (note that this sparse set could later be used to implement Tags). This allows us to keep the code size small by reusing existing datastructures (namely Column, Archetype, ComponentFlags, and ComponentInfo). This allows us the executor to use a single `Access<ArchetypeComponentId>` per system. It should also make scripting language integration easier.
_But_ this merge did create problems for people directly interacting with `World`. What if you need mutable access to multiple resources at the same time? `world.get_resource_mut()` borrows World mutably!
## WorldCell
WorldCell applies the `Access<ArchetypeComponentId>` concept to direct world access:
```rust
let world_cell = world.cell();
let a = world_cell.get_resource_mut::<i32>().unwrap();
let b = world_cell.get_resource_mut::<f64>().unwrap();
```
This adds cheap runtime checks (a sparse set lookup of `ArchetypeComponentId` and a counter) to ensure that world accesses do not conflict with each other. Each operation returns a `WorldBorrow<'w, T>` or `WorldBorrowMut<'w, T>` wrapper type, which will release the relevant ArchetypeComponentId resources when dropped.
World caches the access sparse set (and only one cell can exist at a time), so `world.cell()` is a cheap operation.
WorldCell does _not_ use atomic operations. It is non-send, does a mutable borrow of world to prevent other accesses, and uses a simple `Rc<RefCell<ArchetypeComponentAccess>>` wrapper in each WorldBorrow pointer.
The api is currently limited to resource access, but it can and should be extended to queries / entity component access.
## Resource Scopes
WorldCell does not yet support component queries, and even when it does there are sometimes legitimate reasons to want a mutable world ref _and_ a mutable resource ref (ex: bevy_render and bevy_scene both need this). In these cases we could always drop down to the unsafe `world.get_resource_unchecked_mut()`, but that is not ideal!
Instead developers can use a "resource scope"
```rust
world.resource_scope(|world: &mut World, a: &mut A| {
})
```
This temporarily removes the `A` resource from `World`, provides mutable pointers to both, and re-adds A to World when finished. Thanks to the move to ComponentIds/sparse sets, this is a cheap operation.
If multiple resources are required, scopes can be nested. We could also consider adding a "resource tuple" to the api if this pattern becomes common and the boilerplate gets nasty.
## Query Conflicts Use ComponentId Instead of ArchetypeComponentId
For safety reasons, systems cannot contain queries that conflict with each other without wrapping them in a QuerySet. On bevy `main`, we use ArchetypeComponentIds to determine conflicts. This is nice because it can take into account filters:
```rust
// these queries will never conflict due to their filters
fn filter_system(a: Query<&mut A, With<B>>, b: Query<&mut B, Without<B>>) {
}
```
But it also has a significant downside:
```rust
// these queries will not conflict _until_ an entity with A, B, and C is spawned
fn maybe_conflicts_system(a: Query<(&mut A, &C)>, b: Query<(&mut A, &B)>) {
}
```
The system above will panic at runtime if an entity with A, B, and C is spawned. This makes it hard to trust that your game logic will run without crashing.
In this pr, I switched to using `ComponentId` instead. This _is_ more constraining. `maybe_conflicts_system` will now always fail, but it will do it consistently at startup. Naively, it would also _disallow_ `filter_system`, which would be a significant downgrade in usability. Bevy has a number of internal systems that rely on disjoint queries and I expect it to be a common pattern in userspace.
To resolve this, I added a new `FilteredAccess<T>` type, which wraps `Access<T>` and adds with/without filters. If two `FilteredAccess` have with/without values that prove they are disjoint, they will no longer conflict.
## EntityRef / EntityMut
World entity operations on `main` require that the user passes in an `entity` id to each operation:
```rust
let entity = world.spawn((A, )); // create a new entity with A
world.get::<A>(entity);
world.insert(entity, (B, C));
world.insert_one(entity, D);
```
This means that each operation needs to look up the entity location / verify its validity. The initial spawn operation also requires a Bundle as input. This can be awkward when no components are required (or one component is required).
These operations have been replaced by `EntityRef` and `EntityMut`, which are "builder-style" wrappers around world that provide read and read/write operations on a single, pre-validated entity:
```rust
// spawn now takes no inputs and returns an EntityMut
let entity = world.spawn()
.insert(A) // insert a single component into the entity
.insert_bundle((B, C)) // insert a bundle of components into the entity
.id() // id returns the Entity id
// Returns EntityMut (or panics if the entity does not exist)
world.entity_mut(entity)
.insert(D)
.insert_bundle(SomeBundle::default());
{
// returns EntityRef (or panics if the entity does not exist)
let d = world.entity(entity)
.get::<D>() // gets the D component
.unwrap();
// world.get still exists for ergonomics
let d = world.get::<D>(entity).unwrap();
}
// These variants return Options if you want to check existence instead of panicing
world.get_entity_mut(entity)
.unwrap()
.insert(E);
if let Some(entity_ref) = world.get_entity(entity) {
let d = entity_ref.get::<D>().unwrap();
}
```
This _does not_ affect the current Commands api or terminology. I think that should be a separate conversation as that is a much larger breaking change.
## Safety Improvements
* Entity reservation in Commands uses a normal world borrow instead of an unsafe transmute
* QuerySets no longer transmutes lifetimes
* Made traits "unsafe" when implementing a trait incorrectly could cause unsafety
* More thorough safety docs
## RemovedComponents SystemParam
The old approach to querying removed components: `query.removed:<T>()` was confusing because it had no connection to the query itself. I replaced it with the following, which is both clearer and allows us to cache the ComponentId mapping in the SystemParamState:
```rust
fn system(removed: RemovedComponents<T>) {
for entity in removed.iter() {
}
}
```
## Simpler Bundle implementation
Bundles are no longer responsible for sorting (or deduping) TypeInfo. They are just a simple ordered list of component types / data. This makes the implementation smaller and opens the door to an easy "nested bundle" implementation in the future (which i might even add in this pr). Duplicate detection is now done once per bundle type by World the first time a bundle is used.
## Unified WorldQuery and QueryFilter types
(don't worry they are still separate type _parameters_ in Queries .. this is a non-breaking change)
WorldQuery and QueryFilter were already basically identical apis. With the addition of `FetchState` and more storage-specific fetch methods, the overlap was even clearer (and the redundancy more painful).
QueryFilters are now just `F: WorldQuery where F::Fetch: FilterFetch`. FilterFetch requires `Fetch<Item = bool>` and adds new "short circuit" variants of fetch methods. This enables a filter tuple like `(With<A>, Without<B>, Changed<C>)` to stop evaluating the filter after the first mismatch is encountered. FilterFetch is automatically implemented for `Fetch` implementations that return bool.
This forces fetch implementations that return things like `(bool, bool, bool)` (such as the filter above) to manually implement FilterFetch and decide whether or not to short-circuit.
## More Granular Modules
World no longer globs all of the internal modules together. It now exports `core`, `system`, and `schedule` separately. I'm also considering exporting `core` submodules directly as that is still pretty "glob-ey" and unorganized (feedback welcome here).
## Remaining Draft Work (to be done in this pr)
* ~~panic on conflicting WorldQuery fetches (&A, &mut A)~~
* ~~bevy `main` and hecs both currently allow this, but we should protect against it if possible~~
* ~~batch_iter / par_iter (currently stubbed out)~~
* ~~ChangedRes~~
* ~~I skipped this while we sort out #1313. This pr should be adapted to account for whatever we land on there~~.
* ~~The `Archetypes` and `Tables` collections use hashes of sorted lists of component ids to uniquely identify each archetype/table. This hash is then used as the key in a HashMap to look up the relevant ArchetypeId or TableId. (which doesn't handle hash collisions properly)~~
* ~~It is currently unsafe to generate a Query from "World A", then use it on "World B" (despite the api claiming it is safe). We should probably close this gap. This could be done by adding a randomly generated WorldId to each world, then storing that id in each Query. They could then be compared to each other on each `query.do_thing(&world)` operation. This _does_ add an extra branch to each query operation, so I'm open to other suggestions if people have them.~~
* ~~Nested Bundles (if i find time)~~
## Potential Future Work
* Expand WorldCell to support queries.
* Consider not allocating in the empty archetype on `world.spawn()`
* ex: return something like EntityMutUninit, which turns into EntityMut after an `insert` or `insert_bundle` op
* this actually regressed performance last time i tried it, but in theory it should be faster
* Optimize SparseSet::insert (see `PERF` comment on insert)
* Replace SparseArray `Option<T>` with T::MAX to cut down on branching
* would enable cheaper get_unchecked() operations
* upstream fixedbitset optimizations
* fixedbitset could be allocation free for small block counts (store blocks in a SmallVec)
* fixedbitset could have a const constructor
* Consider implementing Tags (archetype-specific by-value data that affects archetype identity)
* ex: ArchetypeA could have `[A, B, C]` table components and `[D(1)]` "tag" component. ArchetypeB could have `[A, B, C]` table components and a `[D(2)]` tag component. The archetypes are different, despite both having D tags because the value inside D is different.
* this could potentially build on top of the `archetype.unique_components` added in this pr for resource storage.
* Consider reverting `all_tuples` proc macro in favor of the old `macro_rules` implementation
* all_tuples is more flexible and produces cleaner documentation (the macro_rules version produces weird type parameter orders due to parser constraints)
* but unfortunately all_tuples also appears to make Rust Analyzer sad/slow when working inside of `bevy_ecs` (does not affect user code)
* Consider "resource queries" and/or "mixed resource and entity component queries" as an alternative to WorldCell
* this is basically just "systems" so maybe it's not worth it
* Add more world ops
* `world.clear()`
* `world.reserve<T: Bundle>(count: usize)`
* Try using the old archetype allocation strategy (allocate new memory on resize and copy everything over). I expect this to improve batch insertion performance at the cost of unbatched performance. But thats just a guess. I'm not an allocation perf pro :)
* Adapt Commands apis for consistency with new World apis
## Benchmarks
key:
* `bevy_old`: bevy `main` branch
* `bevy`: this branch
* `_foreach`: uses an optimized for_each iterator
* ` _sparse`: uses sparse set storage (if unspecified assume table storage)
* `_system`: runs inside a system (if unspecified assume test happens via direct world ops)
### Simple Insert (from ecs_bench_suite)
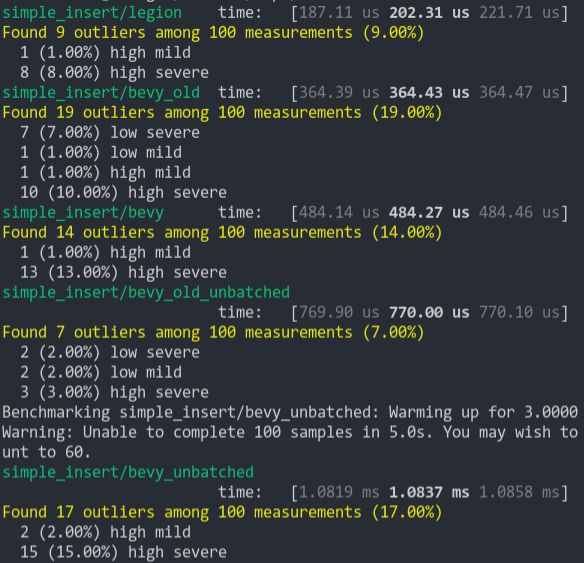
### Simpler Iter (from ecs_bench_suite)
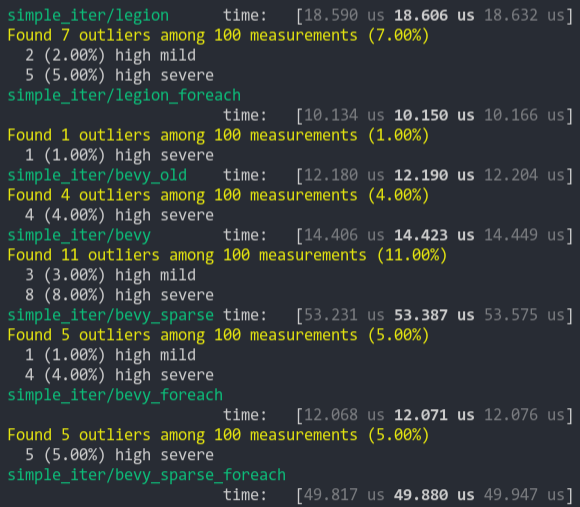
### Fragment Iter (from ecs_bench_suite)
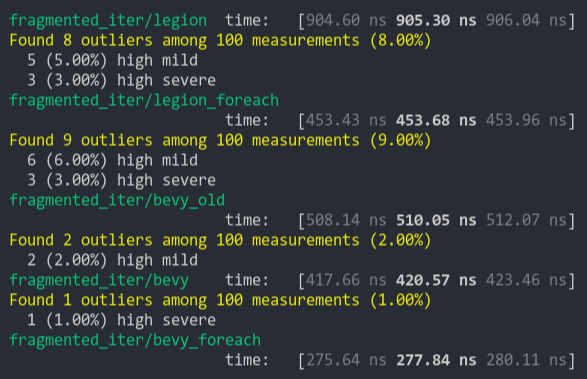
### Sparse Fragmented Iter
Iterate a query that matches 5 entities from a single matching archetype, but there are 100 unmatching archetypes
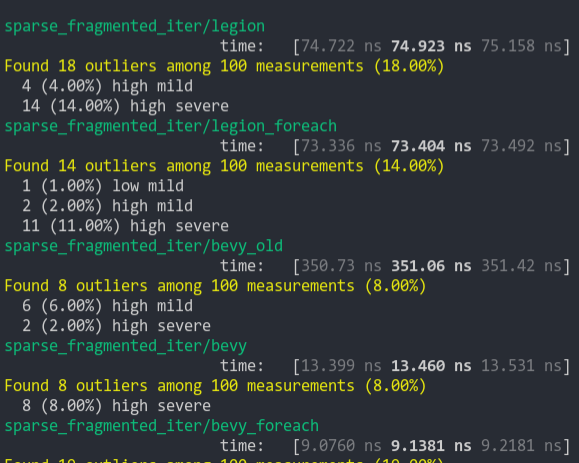
### Schedule (from ecs_bench_suite)
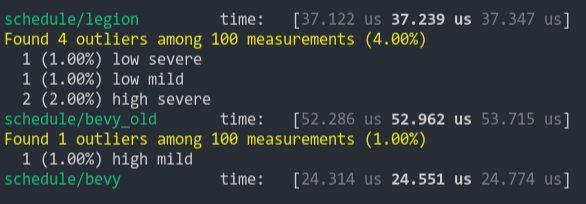
### Add Remove Component (from ecs_bench_suite)
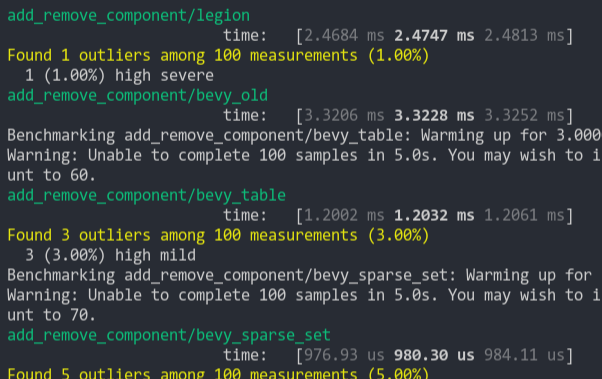
### Add Remove Component Big
Same as the test above, but each entity has 5 "large" matrix components and 1 "large" matrix component is added and removed
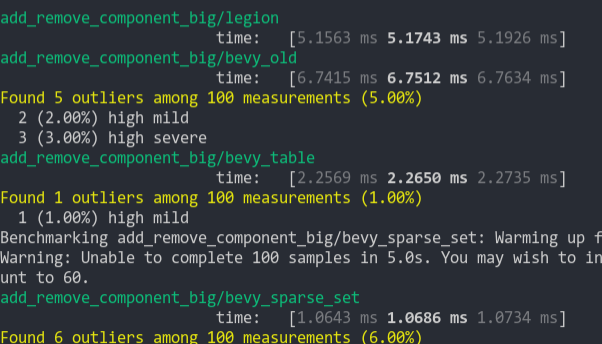
### Get Component
Looks up a single component value a large number of times
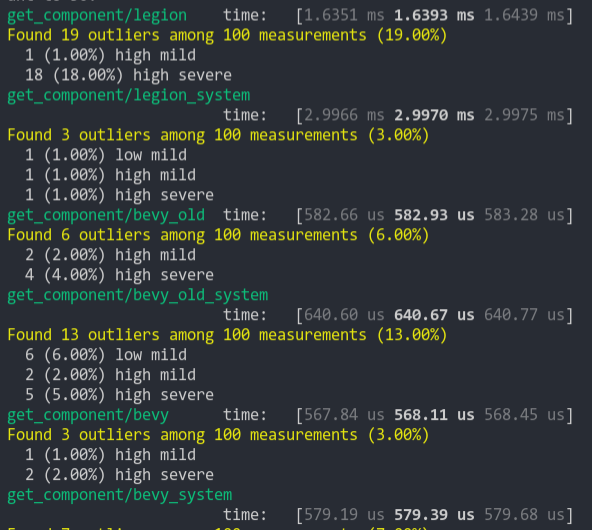
2021-03-05 07:54:35 +00:00
. map ( | component_id | world . components . get_info ( component_id ) . unwrap ( ) . name ( ) )
. collect ::< Vec < & str > > ( ) ;
let accesses = conflicting_components . join ( " , " ) ;
2023-01-11 09:51:22 +00:00
panic! ( " error[B0001]: Query< {query_type} , {filter_type} > in system {system_name} accesses component(s) {accesses} in a way that conflicts with a previous system parameter. Consider using `Without<T>` to create disjoint Queries or merging conflicting Queries into a `ParamSet`. " ) ;
2020-11-08 20:34:05 +00:00
}
2022-12-25 00:06:22 +00:00
/// A collection of potentially conflicting [`SystemParam`]s allowed by disjoint access.
///
/// Allows systems to safely access and interact with up to 8 mutually exclusive [`SystemParam`]s, such as
/// two queries that reference the same mutable data or an event reader and writer of the same type.
///
/// Each individual [`SystemParam`] can be accessed by using the functions `p0()`, `p1()`, ..., `p7()`,
/// according to the order they are defined in the `ParamSet`. This ensures that there's either
/// only one mutable reference to a parameter at a time or any number of immutable references.
///
/// # Examples
///
/// The following system mutably accesses the same component two times,
/// which is not allowed due to rust's mutability rules.
///
/// ```should_panic
/// # use bevy_ecs::prelude::*;
/// #
/// # #[derive(Component)]
/// # struct Health;
/// #
/// # #[derive(Component)]
/// # struct Enemy;
/// #
/// # #[derive(Component)]
/// # struct Ally;
/// #
/// // This will panic at runtime when the system gets initialized.
/// fn bad_system(
/// mut enemies: Query<&mut Health, With<Enemy>>,
/// mut allies: Query<&mut Health, With<Ally>>,
/// ) {
/// // ...
/// }
/// #
/// # let mut bad_system_system = bevy_ecs::system::IntoSystem::into_system(bad_system);
/// # let mut world = World::new();
/// # bad_system_system.initialize(&mut world);
/// # bad_system_system.run((), &mut world);
/// ```
///
2023-01-06 00:43:30 +00:00
/// Conflicting `SystemParam`s like these can be placed in a `ParamSet`,
2022-12-25 00:06:22 +00:00
/// which leverages the borrow checker to ensure that only one of the contained parameters are accessed at a given time.
///
/// ```
/// # use bevy_ecs::prelude::*;
/// #
/// # #[derive(Component)]
/// # struct Health;
/// #
/// # #[derive(Component)]
/// # struct Enemy;
/// #
/// # #[derive(Component)]
/// # struct Ally;
/// #
/// // Given the following system
/// fn fancy_system(
/// mut set: ParamSet<(
/// Query<&mut Health, With<Enemy>>,
/// Query<&mut Health, With<Ally>>,
/// )>
/// ) {
/// // This will access the first `SystemParam`.
/// for mut health in set.p0().iter_mut() {
/// // Do your fancy stuff here...
/// }
///
/// // The second `SystemParam`.
/// // This would fail to compile if the previous parameter was still borrowed.
/// for mut health in set.p1().iter_mut() {
/// // Do even fancier stuff here...
/// }
/// }
/// # bevy_ecs::system::assert_is_system(fancy_system);
/// ```
///
/// Of course, `ParamSet`s can be used with any kind of `SystemParam`, not just [queries](Query).
///
/// ```
/// # use bevy_ecs::prelude::*;
/// #
/// # struct MyEvent;
/// # impl MyEvent {
/// # pub fn new() -> Self { Self }
/// # }
/// fn event_system(
/// mut set: ParamSet<(
/// // `EventReader`s and `EventWriter`s conflict with each other,
/// // since they both access the event queue resource for `MyEvent`.
/// EventReader<MyEvent>,
/// EventWriter<MyEvent>,
/// // `&World` reads the entire world, so a `ParamSet` is the only way
/// // that it can be used in the same system as any mutable accesses.
/// &World,
/// )>,
/// ) {
/// for event in set.p0().iter() {
/// // ...
/// # let _event = event;
/// }
/// set.p1().send(MyEvent::new());
///
/// let entities = set.p2().entities();
/// // ...
/// # let _entities = entities;
/// }
/// # bevy_ecs::system::assert_is_system(event_system);
/// ```
2022-03-29 23:39:38 +00:00
pub struct ParamSet < ' w , ' s , T : SystemParam > {
Simplify trait hierarchy for `SystemParam` (#6865)
# Objective
* Implementing a custom `SystemParam` by hand requires implementing three traits -- four if it is read-only.
* The trait `SystemParamFetch<'w, 's>` is a workaround from before we had generic associated types, and is no longer necessary.
## Solution
* Combine the trait `SystemParamFetch` with `SystemParamState`.
* I decided to remove the `Fetch` name and keep the `State` name, since the former was consistently conflated with the latter.
* Replace the trait `ReadOnlySystemParamFetch` with `ReadOnlySystemParam`, which simplifies trait bounds in generic code.
---
## Changelog
- Removed the trait `SystemParamFetch`, moving its functionality to `SystemParamState`.
- Replaced the trait `ReadOnlySystemParamFetch` with `ReadOnlySystemParam`.
## Migration Guide
The trait `SystemParamFetch` has been removed, and its functionality has been transferred to `SystemParamState`.
```rust
// Before
impl SystemParamState for MyParamState {
fn init(world: &mut World, system_meta: &mut SystemMeta) -> Self { ... }
}
impl<'w, 's> SystemParamFetch<'w, 's> for MyParamState {
type Item = MyParam<'w, 's>;
fn get_param(...) -> Self::Item;
}
// After
impl SystemParamState for MyParamState {
type Item<'w, 's> = MyParam<'w, 's>; // Generic associated types!
fn init(world: &mut World, system_meta: &mut SystemMeta) -> Self { ... }
fn get_param<'w, 's>(...) -> Self::Item<'w, 's>;
}
```
The trait `ReadOnlySystemParamFetch` has been replaced with `ReadOnlySystemParam`.
```rust
// Before
unsafe impl ReadOnlySystemParamFetch for MyParamState {}
// After
unsafe impl<'w, 's> ReadOnlySystemParam for MyParam<'w, 's> {}
```
2022-12-11 18:34:14 +00:00
param_states : & ' s mut T ::State ,
System Param Lifetime Split (#2605)
# Objective
Enable using exact World lifetimes during read-only access . This is motivated by the new renderer's need to allow read-only world-only queries to outlive the query itself (but still be constrained by the world lifetime).
For example:
https://github.com/bevyengine/bevy/blob/115b170d1f11a91146bb6d6e9684dceb8b21f786/pipelined/bevy_pbr2/src/render/mod.rs#L774
## Solution
Split out SystemParam state and world lifetimes and pipe those lifetimes up to read-only Query ops (and add into_inner for Res). According to every safety test I've run so far (except one), this is safe (see the temporary safety test commit). Note that changing the mutable variants to the new lifetimes would allow aliased mutable pointers (try doing that to see how it affects the temporary safety tests).
The new state lifetime on SystemParam does make `#[derive(SystemParam)]` more cumbersome (the current impl requires PhantomData if you don't use both lifetimes). We can make this better by detecting whether or not a lifetime is used in the derive and adjusting accordingly, but that should probably be done in its own pr.
## Why is this a draft?
The new lifetimes break QuerySet safety in one very specific case (see the query_set system in system_safety_test). We need to solve this before we can use the lifetimes given.
This is due to the fact that QuerySet is just a wrapper over Query, which now relies on world lifetimes instead of `&self` lifetimes to prevent aliasing (but in systems, each Query has its own implied lifetime, not a centralized world lifetime). I believe the fix is to rewrite QuerySet to have its own World lifetime (and own the internal reference). This will complicate the impl a bit, but I think it is doable. I'm curious if anyone else has better ideas.
Personally, I think these new lifetimes need to happen. We've gotta have a way to directly tie read-only World queries to the World lifetime. The new renderer is the first place this has come up, but I doubt it will be the last. Worst case scenario we can come up with a second `WorldLifetimeQuery<Q, F = ()>` parameter to enable these read-only scenarios, but I'd rather not add another type to the type zoo.
2021-08-15 20:51:53 +00:00
world : & ' w World ,
2022-03-29 23:39:38 +00:00
system_meta : SystemMeta ,
System Param Lifetime Split (#2605)
# Objective
Enable using exact World lifetimes during read-only access . This is motivated by the new renderer's need to allow read-only world-only queries to outlive the query itself (but still be constrained by the world lifetime).
For example:
https://github.com/bevyengine/bevy/blob/115b170d1f11a91146bb6d6e9684dceb8b21f786/pipelined/bevy_pbr2/src/render/mod.rs#L774
## Solution
Split out SystemParam state and world lifetimes and pipe those lifetimes up to read-only Query ops (and add into_inner for Res). According to every safety test I've run so far (except one), this is safe (see the temporary safety test commit). Note that changing the mutable variants to the new lifetimes would allow aliased mutable pointers (try doing that to see how it affects the temporary safety tests).
The new state lifetime on SystemParam does make `#[derive(SystemParam)]` more cumbersome (the current impl requires PhantomData if you don't use both lifetimes). We can make this better by detecting whether or not a lifetime is used in the derive and adjusting accordingly, but that should probably be done in its own pr.
## Why is this a draft?
The new lifetimes break QuerySet safety in one very specific case (see the query_set system in system_safety_test). We need to solve this before we can use the lifetimes given.
This is due to the fact that QuerySet is just a wrapper over Query, which now relies on world lifetimes instead of `&self` lifetimes to prevent aliasing (but in systems, each Query has its own implied lifetime, not a centralized world lifetime). I believe the fix is to rewrite QuerySet to have its own World lifetime (and own the internal reference). This will complicate the impl a bit, but I think it is doable. I'm curious if anyone else has better ideas.
Personally, I think these new lifetimes need to happen. We've gotta have a way to directly tie read-only World queries to the World lifetime. The new renderer is the first place this has come up, but I doubt it will be the last. Worst case scenario we can come up with a second `WorldLifetimeQuery<Q, F = ()>` parameter to enable these read-only scenarios, but I'd rather not add another type to the type zoo.
2021-08-15 20:51:53 +00:00
change_tick : u32 ,
}
2022-03-29 23:39:38 +00:00
impl_param_set! ( ) ;
Bevy ECS V2 (#1525)
# Bevy ECS V2
This is a rewrite of Bevy ECS (basically everything but the new executor/schedule, which are already awesome). The overall goal was to improve the performance and versatility of Bevy ECS. Here is a quick bulleted list of changes before we dive into the details:
* Complete World rewrite
* Multiple component storage types:
* Tables: fast cache friendly iteration, slower add/removes (previously called Archetypes)
* Sparse Sets: fast add/remove, slower iteration
* Stateful Queries (caches query results for faster iteration. fragmented iteration is _fast_ now)
* Stateful System Params (caches expensive operations. inspired by @DJMcNab's work in #1364)
* Configurable System Params (users can set configuration when they construct their systems. once again inspired by @DJMcNab's work)
* Archetypes are now "just metadata", component storage is separate
* Archetype Graph (for faster archetype changes)
* Component Metadata
* Configure component storage type
* Retrieve information about component size/type/name/layout/send-ness/etc
* Components are uniquely identified by a densely packed ComponentId
* TypeIds are now totally optional (which should make implementing scripting easier)
* Super fast "for_each" query iterators
* Merged Resources into World. Resources are now just a special type of component
* EntityRef/EntityMut builder apis (more efficient and more ergonomic)
* Fast bitset-backed `Access<T>` replaces old hashmap-based approach everywhere
* Query conflicts are determined by component access instead of archetype component access (to avoid random failures at runtime)
* With/Without are still taken into account for conflicts, so this should still be comfy to use
* Much simpler `IntoSystem` impl
* Significantly reduced the amount of hashing throughout the ecs in favor of Sparse Sets (indexed by densely packed ArchetypeId, ComponentId, BundleId, and TableId)
* Safety Improvements
* Entity reservation uses a normal world reference instead of unsafe transmute
* QuerySets no longer transmute lifetimes
* Made traits "unsafe" where relevant
* More thorough safety docs
* WorldCell
* Exposes safe mutable access to multiple resources at a time in a World
* Replaced "catch all" `System::update_archetypes(world: &World)` with `System::new_archetype(archetype: &Archetype)`
* Simpler Bundle implementation
* Replaced slow "remove_bundle_one_by_one" used as fallback for Commands::remove_bundle with fast "remove_bundle_intersection"
* Removed `Mut<T>` query impl. it is better to only support one way: `&mut T`
* Removed with() from `Flags<T>` in favor of `Option<Flags<T>>`, which allows querying for flags to be "filtered" by default
* Components now have is_send property (currently only resources support non-send)
* More granular module organization
* New `RemovedComponents<T>` SystemParam that replaces `query.removed::<T>()`
* `world.resource_scope()` for mutable access to resources and world at the same time
* WorldQuery and QueryFilter traits unified. FilterFetch trait added to enable "short circuit" filtering. Auto impled for cases that don't need it
* Significantly slimmed down SystemState in favor of individual SystemParam state
* System Commands changed from `commands: &mut Commands` back to `mut commands: Commands` (to allow Commands to have a World reference)
Fixes #1320
## `World` Rewrite
This is a from-scratch rewrite of `World` that fills the niche that `hecs` used to. Yes, this means Bevy ECS is no longer a "fork" of hecs. We're going out our own!
(the only shared code between the projects is the entity id allocator, which is already basically ideal)
A huge shout out to @SanderMertens (author of [flecs](https://github.com/SanderMertens/flecs)) for sharing some great ideas with me (specifically hybrid ecs storage and archetype graphs). He also helped advise on a number of implementation details.
## Component Storage (The Problem)
Two ECS storage paradigms have gained a lot of traction over the years:
* **Archetypal ECS**:
* Stores components in "tables" with static schemas. Each "column" stores components of a given type. Each "row" is an entity.
* Each "archetype" has its own table. Adding/removing an entity's component changes the archetype.
* Enables super-fast Query iteration due to its cache-friendly data layout
* Comes at the cost of more expensive add/remove operations for an Entity's components, because all components need to be copied to the new archetype's "table"
* **Sparse Set ECS**:
* Stores components of the same type in densely packed arrays, which are sparsely indexed by densely packed unsigned integers (Entity ids)
* Query iteration is slower than Archetypal ECS because each entity's component could be at any position in the sparse set. This "random access" pattern isn't cache friendly. Additionally, there is an extra layer of indirection because you must first map the entity id to an index in the component array.
* Adding/removing components is a cheap, constant time operation
Bevy ECS V1, hecs, legion, flec, and Unity DOTS are all "archetypal ecs-es". I personally think "archetypal" storage is a good default for game engines. An entity's archetype doesn't need to change frequently in general, and it creates "fast by default" query iteration (which is a much more common operation). It is also "self optimizing". Users don't need to think about optimizing component layouts for iteration performance. It "just works" without any extra boilerplate.
Shipyard and EnTT are "sparse set ecs-es". They employ "packing" as a way to work around the "suboptimal by default" iteration performance for specific sets of components. This helps, but I didn't think this was a good choice for a general purpose engine like Bevy because:
1. "packs" conflict with each other. If bevy decides to internally pack the Transform and GlobalTransform components, users are then blocked if they want to pack some custom component with Transform.
2. users need to take manual action to optimize
Developers selecting an ECS framework are stuck with a hard choice. Select an "archetypal" framework with "fast iteration everywhere" but without the ability to cheaply add/remove components, or select a "sparse set" framework to cheaply add/remove components but with slower iteration performance.
## Hybrid Component Storage (The Solution)
In Bevy ECS V2, we get to have our cake and eat it too. It now has _both_ of the component storage types above (and more can be added later if needed):
* **Tables** (aka "archetypal" storage)
* The default storage. If you don't configure anything, this is what you get
* Fast iteration by default
* Slower add/remove operations
* **Sparse Sets**
* Opt-in
* Slower iteration
* Faster add/remove operations
These storage types complement each other perfectly. By default Query iteration is fast. If developers know that they want to add/remove a component at high frequencies, they can set the storage to "sparse set":
```rust
world.register_component(
ComponentDescriptor::new::<MyComponent>(StorageType::SparseSet)
).unwrap();
```
## Archetypes
Archetypes are now "just metadata" ... they no longer store components directly. They do store:
* The `ComponentId`s of each of the Archetype's components (and that component's storage type)
* Archetypes are uniquely defined by their component layouts
* For example: entities with "table" components `[A, B, C]` _and_ "sparse set" components `[D, E]` will always be in the same archetype.
* The `TableId` associated with the archetype
* For now each archetype has exactly one table (which can have no components),
* There is a 1->Many relationship from Tables->Archetypes. A given table could have any number of archetype components stored in it:
* Ex: an entity with "table storage" components `[A, B, C]` and "sparse set" components `[D, E]` will share the same `[A, B, C]` table as an entity with `[A, B, C]` table component and `[F]` sparse set components.
* This 1->Many relationship is how we preserve fast "cache friendly" iteration performance when possible (more on this later)
* A list of entities that are in the archetype and the row id of the table they are in
* ArchetypeComponentIds
* unique densely packed identifiers for (ArchetypeId, ComponentId) pairs
* used by the schedule executor for cheap system access control
* "Archetype Graph Edges" (see the next section)
## The "Archetype Graph"
Archetype changes in Bevy (and a number of other archetypal ecs-es) have historically been expensive to compute. First, you need to allocate a new vector of the entity's current component ids, add or remove components based on the operation performed, sort it (to ensure it is order-independent), then hash it to find the archetype (if it exists). And thats all before we get to the _already_ expensive full copy of all components to the new table storage.
The solution is to build a "graph" of archetypes to cache these results. @SanderMertens first exposed me to the idea (and he got it from @gjroelofs, who came up with it). They propose adding directed edges between archetypes for add/remove component operations. If `ComponentId`s are densely packed, you can use sparse sets to cheaply jump between archetypes.
Bevy takes this one step further by using add/remove `Bundle` edges instead of `Component` edges. Bevy encourages the use of `Bundles` to group add/remove operations. This is largely for "clearer game logic" reasons, but it also helps cut down on the number of archetype changes required. `Bundles` now also have densely-packed `BundleId`s. This allows us to use a _single_ edge for each bundle operation (rather than needing to traverse N edges ... one for each component). Single component operations are also bundles, so this is strictly an improvement over a "component only" graph.
As a result, an operation that used to be _heavy_ (both for allocations and compute) is now two dirt-cheap array lookups and zero allocations.
## Stateful Queries
World queries are now stateful. This allows us to:
1. Cache archetype (and table) matches
* This resolves another issue with (naive) archetypal ECS: query performance getting worse as the number of archetypes goes up (and fragmentation occurs).
2. Cache Fetch and Filter state
* The expensive parts of fetch/filter operations (such as hashing the TypeId to find the ComponentId) now only happen once when the Query is first constructed
3. Incrementally build up state
* When new archetypes are added, we only process the new archetypes (no need to rebuild state for old archetypes)
As a result, the direct `World` query api now looks like this:
```rust
let mut query = world.query::<(&A, &mut B)>();
for (a, mut b) in query.iter_mut(&mut world) {
}
```
Requiring `World` to generate stateful queries (rather than letting the `QueryState` type be constructed separately) allows us to ensure that _all_ queries are properly initialized (and the relevant world state, such as ComponentIds). This enables QueryState to remove branches from its operations that check for initialization status (and also enables query.iter() to take an immutable world reference because it doesn't need to initialize anything in world).
However in systems, this is a non-breaking change. State management is done internally by the relevant SystemParam.
## Stateful SystemParams
Like Queries, `SystemParams` now also cache state. For example, `Query` system params store the "stateful query" state mentioned above. Commands store their internal `CommandQueue`. This means you can now safely use as many separate `Commands` parameters in your system as you want. `Local<T>` system params store their `T` value in their state (instead of in Resources).
SystemParam state also enabled a significant slim-down of SystemState. It is much nicer to look at now.
Per-SystemParam state naturally insulates us from an "aliased mut" class of errors we have hit in the past (ex: using multiple `Commands` system params).
(credit goes to @DJMcNab for the initial idea and draft pr here #1364)
## Configurable SystemParams
@DJMcNab also had the great idea to make SystemParams configurable. This allows users to provide some initial configuration / values for system parameters (when possible). Most SystemParams have no config (the config type is `()`), but the `Local<T>` param now supports user-provided parameters:
```rust
fn foo(value: Local<usize>) {
}
app.add_system(foo.system().config(|c| c.0 = Some(10)));
```
## Uber Fast "for_each" Query Iterators
Developers now have the choice to use a fast "for_each" iterator, which yields ~1.5-3x iteration speed improvements for "fragmented iteration", and minor ~1.2x iteration speed improvements for unfragmented iteration.
```rust
fn system(query: Query<(&A, &mut B)>) {
// you now have the option to do this for a speed boost
query.for_each_mut(|(a, mut b)| {
});
// however normal iterators are still available
for (a, mut b) in query.iter_mut() {
}
}
```
I think in most cases we should continue to encourage "normal" iterators as they are more flexible and more "rust idiomatic". But when that extra "oomf" is needed, it makes sense to use `for_each`.
We should also consider using `for_each` for internal bevy systems to give our users a nice speed boost (but that should be a separate pr).
## Component Metadata
`World` now has a `Components` collection, which is accessible via `world.components()`. This stores mappings from `ComponentId` to `ComponentInfo`, as well as `TypeId` to `ComponentId` mappings (where relevant). `ComponentInfo` stores information about the component, such as ComponentId, TypeId, memory layout, send-ness (currently limited to resources), and storage type.
## Significantly Cheaper `Access<T>`
We used to use `TypeAccess<TypeId>` to manage read/write component/archetype-component access. This was expensive because TypeIds must be hashed and compared individually. The parallel executor got around this by "condensing" type ids into bitset-backed access types. This worked, but it had to be re-generated from the `TypeAccess<TypeId>`sources every time archetypes changed.
This pr removes TypeAccess in favor of faster bitset access everywhere. We can do this thanks to the move to densely packed `ComponentId`s and `ArchetypeComponentId`s.
## Merged Resources into World
Resources had a lot of redundant functionality with Components. They stored typed data, they had access control, they had unique ids, they were queryable via SystemParams, etc. In fact the _only_ major difference between them was that they were unique (and didn't correlate to an entity).
Separate resources also had the downside of requiring a separate set of access controls, which meant the parallel executor needed to compare more bitsets per system and manage more state.
I initially got the "separate resources" idea from `legion`. I think that design was motivated by the fact that it made the direct world query/resource lifetime interactions more manageable. It certainly made our lives easier when using Resources alongside hecs/bevy_ecs. However we already have a construct for safely and ergonomically managing in-world lifetimes: systems (which use `Access<T>` internally).
This pr merges Resources into World:
```rust
world.insert_resource(1);
world.insert_resource(2.0);
let a = world.get_resource::<i32>().unwrap();
let mut b = world.get_resource_mut::<f64>().unwrap();
*b = 3.0;
```
Resources are now just a special kind of component. They have their own ComponentIds (and their own resource TypeId->ComponentId scope, so they don't conflict wit components of the same type). They are stored in a special "resource archetype", which stores components inside the archetype using a new `unique_components` sparse set (note that this sparse set could later be used to implement Tags). This allows us to keep the code size small by reusing existing datastructures (namely Column, Archetype, ComponentFlags, and ComponentInfo). This allows us the executor to use a single `Access<ArchetypeComponentId>` per system. It should also make scripting language integration easier.
_But_ this merge did create problems for people directly interacting with `World`. What if you need mutable access to multiple resources at the same time? `world.get_resource_mut()` borrows World mutably!
## WorldCell
WorldCell applies the `Access<ArchetypeComponentId>` concept to direct world access:
```rust
let world_cell = world.cell();
let a = world_cell.get_resource_mut::<i32>().unwrap();
let b = world_cell.get_resource_mut::<f64>().unwrap();
```
This adds cheap runtime checks (a sparse set lookup of `ArchetypeComponentId` and a counter) to ensure that world accesses do not conflict with each other. Each operation returns a `WorldBorrow<'w, T>` or `WorldBorrowMut<'w, T>` wrapper type, which will release the relevant ArchetypeComponentId resources when dropped.
World caches the access sparse set (and only one cell can exist at a time), so `world.cell()` is a cheap operation.
WorldCell does _not_ use atomic operations. It is non-send, does a mutable borrow of world to prevent other accesses, and uses a simple `Rc<RefCell<ArchetypeComponentAccess>>` wrapper in each WorldBorrow pointer.
The api is currently limited to resource access, but it can and should be extended to queries / entity component access.
## Resource Scopes
WorldCell does not yet support component queries, and even when it does there are sometimes legitimate reasons to want a mutable world ref _and_ a mutable resource ref (ex: bevy_render and bevy_scene both need this). In these cases we could always drop down to the unsafe `world.get_resource_unchecked_mut()`, but that is not ideal!
Instead developers can use a "resource scope"
```rust
world.resource_scope(|world: &mut World, a: &mut A| {
})
```
This temporarily removes the `A` resource from `World`, provides mutable pointers to both, and re-adds A to World when finished. Thanks to the move to ComponentIds/sparse sets, this is a cheap operation.
If multiple resources are required, scopes can be nested. We could also consider adding a "resource tuple" to the api if this pattern becomes common and the boilerplate gets nasty.
## Query Conflicts Use ComponentId Instead of ArchetypeComponentId
For safety reasons, systems cannot contain queries that conflict with each other without wrapping them in a QuerySet. On bevy `main`, we use ArchetypeComponentIds to determine conflicts. This is nice because it can take into account filters:
```rust
// these queries will never conflict due to their filters
fn filter_system(a: Query<&mut A, With<B>>, b: Query<&mut B, Without<B>>) {
}
```
But it also has a significant downside:
```rust
// these queries will not conflict _until_ an entity with A, B, and C is spawned
fn maybe_conflicts_system(a: Query<(&mut A, &C)>, b: Query<(&mut A, &B)>) {
}
```
The system above will panic at runtime if an entity with A, B, and C is spawned. This makes it hard to trust that your game logic will run without crashing.
In this pr, I switched to using `ComponentId` instead. This _is_ more constraining. `maybe_conflicts_system` will now always fail, but it will do it consistently at startup. Naively, it would also _disallow_ `filter_system`, which would be a significant downgrade in usability. Bevy has a number of internal systems that rely on disjoint queries and I expect it to be a common pattern in userspace.
To resolve this, I added a new `FilteredAccess<T>` type, which wraps `Access<T>` and adds with/without filters. If two `FilteredAccess` have with/without values that prove they are disjoint, they will no longer conflict.
## EntityRef / EntityMut
World entity operations on `main` require that the user passes in an `entity` id to each operation:
```rust
let entity = world.spawn((A, )); // create a new entity with A
world.get::<A>(entity);
world.insert(entity, (B, C));
world.insert_one(entity, D);
```
This means that each operation needs to look up the entity location / verify its validity. The initial spawn operation also requires a Bundle as input. This can be awkward when no components are required (or one component is required).
These operations have been replaced by `EntityRef` and `EntityMut`, which are "builder-style" wrappers around world that provide read and read/write operations on a single, pre-validated entity:
```rust
// spawn now takes no inputs and returns an EntityMut
let entity = world.spawn()
.insert(A) // insert a single component into the entity
.insert_bundle((B, C)) // insert a bundle of components into the entity
.id() // id returns the Entity id
// Returns EntityMut (or panics if the entity does not exist)
world.entity_mut(entity)
.insert(D)
.insert_bundle(SomeBundle::default());
{
// returns EntityRef (or panics if the entity does not exist)
let d = world.entity(entity)
.get::<D>() // gets the D component
.unwrap();
// world.get still exists for ergonomics
let d = world.get::<D>(entity).unwrap();
}
// These variants return Options if you want to check existence instead of panicing
world.get_entity_mut(entity)
.unwrap()
.insert(E);
if let Some(entity_ref) = world.get_entity(entity) {
let d = entity_ref.get::<D>().unwrap();
}
```
This _does not_ affect the current Commands api or terminology. I think that should be a separate conversation as that is a much larger breaking change.
## Safety Improvements
* Entity reservation in Commands uses a normal world borrow instead of an unsafe transmute
* QuerySets no longer transmutes lifetimes
* Made traits "unsafe" when implementing a trait incorrectly could cause unsafety
* More thorough safety docs
## RemovedComponents SystemParam
The old approach to querying removed components: `query.removed:<T>()` was confusing because it had no connection to the query itself. I replaced it with the following, which is both clearer and allows us to cache the ComponentId mapping in the SystemParamState:
```rust
fn system(removed: RemovedComponents<T>) {
for entity in removed.iter() {
}
}
```
## Simpler Bundle implementation
Bundles are no longer responsible for sorting (or deduping) TypeInfo. They are just a simple ordered list of component types / data. This makes the implementation smaller and opens the door to an easy "nested bundle" implementation in the future (which i might even add in this pr). Duplicate detection is now done once per bundle type by World the first time a bundle is used.
## Unified WorldQuery and QueryFilter types
(don't worry they are still separate type _parameters_ in Queries .. this is a non-breaking change)
WorldQuery and QueryFilter were already basically identical apis. With the addition of `FetchState` and more storage-specific fetch methods, the overlap was even clearer (and the redundancy more painful).
QueryFilters are now just `F: WorldQuery where F::Fetch: FilterFetch`. FilterFetch requires `Fetch<Item = bool>` and adds new "short circuit" variants of fetch methods. This enables a filter tuple like `(With<A>, Without<B>, Changed<C>)` to stop evaluating the filter after the first mismatch is encountered. FilterFetch is automatically implemented for `Fetch` implementations that return bool.
This forces fetch implementations that return things like `(bool, bool, bool)` (such as the filter above) to manually implement FilterFetch and decide whether or not to short-circuit.
## More Granular Modules
World no longer globs all of the internal modules together. It now exports `core`, `system`, and `schedule` separately. I'm also considering exporting `core` submodules directly as that is still pretty "glob-ey" and unorganized (feedback welcome here).
## Remaining Draft Work (to be done in this pr)
* ~~panic on conflicting WorldQuery fetches (&A, &mut A)~~
* ~~bevy `main` and hecs both currently allow this, but we should protect against it if possible~~
* ~~batch_iter / par_iter (currently stubbed out)~~
* ~~ChangedRes~~
* ~~I skipped this while we sort out #1313. This pr should be adapted to account for whatever we land on there~~.
* ~~The `Archetypes` and `Tables` collections use hashes of sorted lists of component ids to uniquely identify each archetype/table. This hash is then used as the key in a HashMap to look up the relevant ArchetypeId or TableId. (which doesn't handle hash collisions properly)~~
* ~~It is currently unsafe to generate a Query from "World A", then use it on "World B" (despite the api claiming it is safe). We should probably close this gap. This could be done by adding a randomly generated WorldId to each world, then storing that id in each Query. They could then be compared to each other on each `query.do_thing(&world)` operation. This _does_ add an extra branch to each query operation, so I'm open to other suggestions if people have them.~~
* ~~Nested Bundles (if i find time)~~
## Potential Future Work
* Expand WorldCell to support queries.
* Consider not allocating in the empty archetype on `world.spawn()`
* ex: return something like EntityMutUninit, which turns into EntityMut after an `insert` or `insert_bundle` op
* this actually regressed performance last time i tried it, but in theory it should be faster
* Optimize SparseSet::insert (see `PERF` comment on insert)
* Replace SparseArray `Option<T>` with T::MAX to cut down on branching
* would enable cheaper get_unchecked() operations
* upstream fixedbitset optimizations
* fixedbitset could be allocation free for small block counts (store blocks in a SmallVec)
* fixedbitset could have a const constructor
* Consider implementing Tags (archetype-specific by-value data that affects archetype identity)
* ex: ArchetypeA could have `[A, B, C]` table components and `[D(1)]` "tag" component. ArchetypeB could have `[A, B, C]` table components and a `[D(2)]` tag component. The archetypes are different, despite both having D tags because the value inside D is different.
* this could potentially build on top of the `archetype.unique_components` added in this pr for resource storage.
* Consider reverting `all_tuples` proc macro in favor of the old `macro_rules` implementation
* all_tuples is more flexible and produces cleaner documentation (the macro_rules version produces weird type parameter orders due to parser constraints)
* but unfortunately all_tuples also appears to make Rust Analyzer sad/slow when working inside of `bevy_ecs` (does not affect user code)
* Consider "resource queries" and/or "mixed resource and entity component queries" as an alternative to WorldCell
* this is basically just "systems" so maybe it's not worth it
* Add more world ops
* `world.clear()`
* `world.reserve<T: Bundle>(count: usize)`
* Try using the old archetype allocation strategy (allocate new memory on resize and copy everything over). I expect this to improve batch insertion performance at the cost of unbatched performance. But thats just a guess. I'm not an allocation perf pro :)
* Adapt Commands apis for consistency with new World apis
## Benchmarks
key:
* `bevy_old`: bevy `main` branch
* `bevy`: this branch
* `_foreach`: uses an optimized for_each iterator
* ` _sparse`: uses sparse set storage (if unspecified assume table storage)
* `_system`: runs inside a system (if unspecified assume test happens via direct world ops)
### Simple Insert (from ecs_bench_suite)
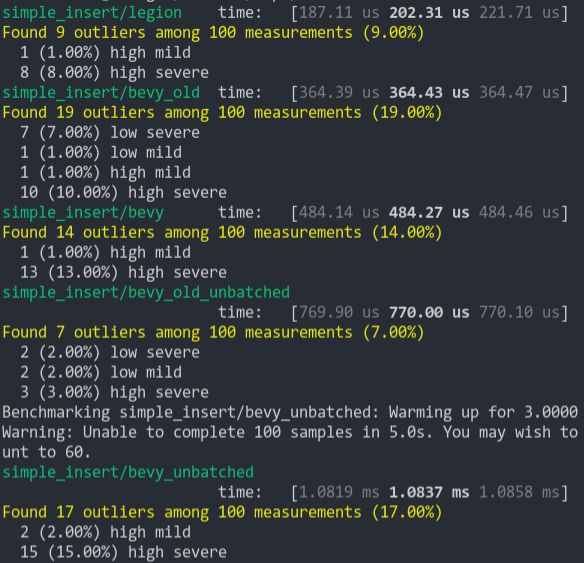
### Simpler Iter (from ecs_bench_suite)
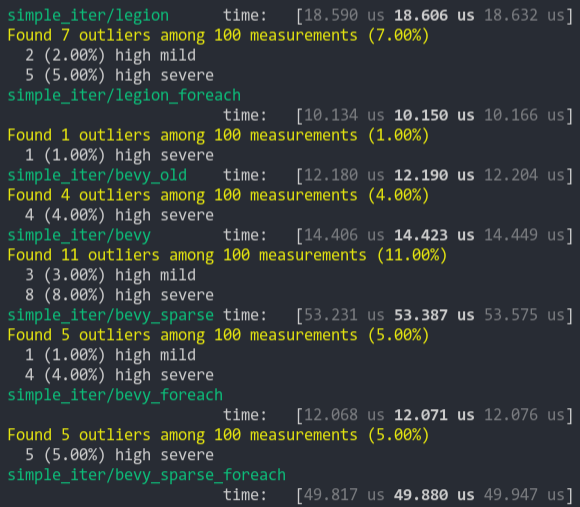
### Fragment Iter (from ecs_bench_suite)
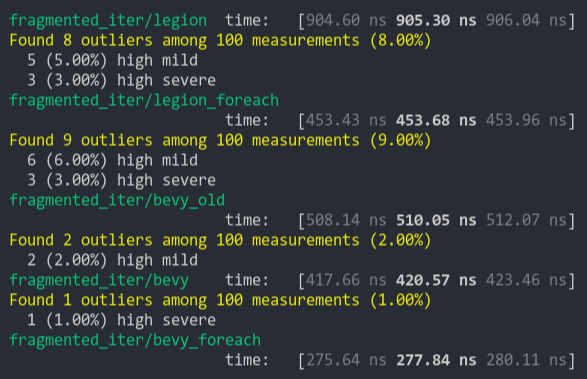
### Sparse Fragmented Iter
Iterate a query that matches 5 entities from a single matching archetype, but there are 100 unmatching archetypes
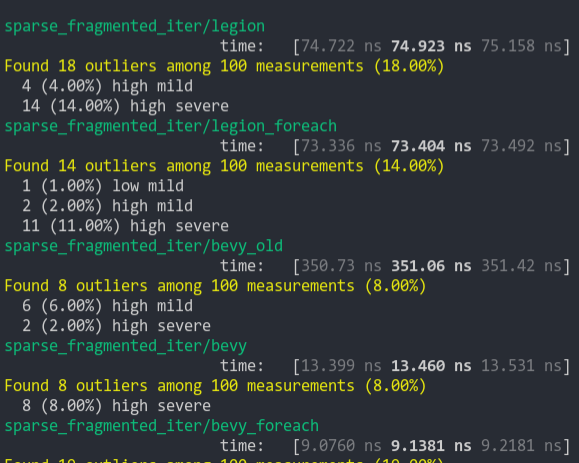
### Schedule (from ecs_bench_suite)
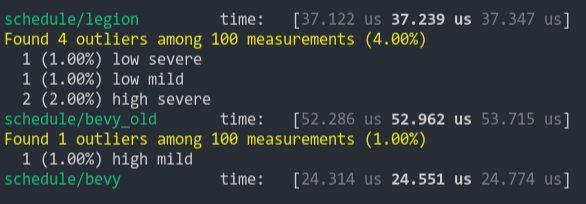
### Add Remove Component (from ecs_bench_suite)
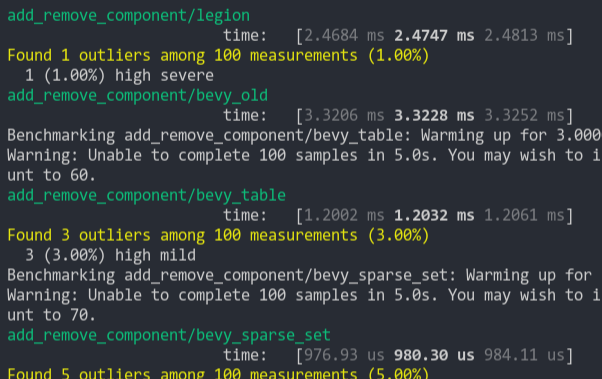
### Add Remove Component Big
Same as the test above, but each entity has 5 "large" matrix components and 1 "large" matrix component is added and removed
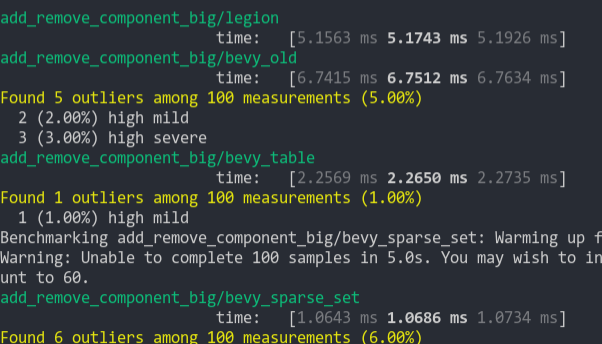
### Get Component
Looks up a single component value a large number of times
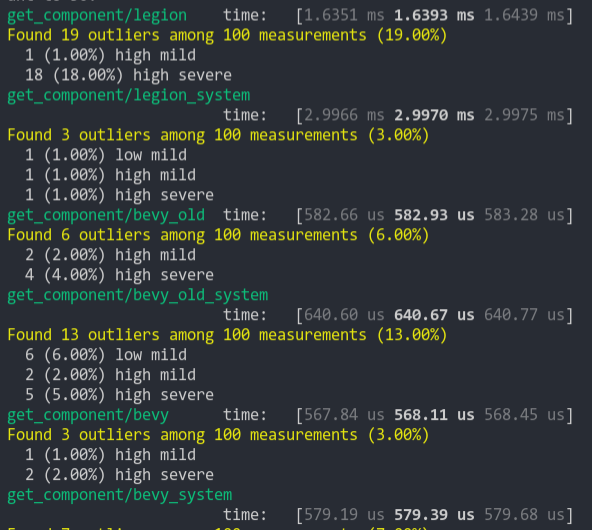
2021-03-05 07:54:35 +00:00
Make `Resource` trait opt-in, requiring `#[derive(Resource)]` V2 (#5577)
*This PR description is an edited copy of #5007, written by @alice-i-cecile.*
# Objective
Follow-up to https://github.com/bevyengine/bevy/pull/2254. The `Resource` trait currently has a blanket implementation for all types that meet its bounds.
While ergonomic, this results in several drawbacks:
* it is possible to make confusing, silent mistakes such as inserting a function pointer (Foo) rather than a value (Foo::Bar) as a resource
* it is challenging to discover if a type is intended to be used as a resource
* we cannot later add customization options (see the [RFC](https://github.com/bevyengine/rfcs/blob/main/rfcs/27-derive-component.md) for the equivalent choice for Component).
* dependencies can use the same Rust type as a resource in invisibly conflicting ways
* raw Rust types used as resources cannot preserve privacy appropriately, as anyone able to access that type can read and write to internal values
* we cannot capture a definitive list of possible resources to display to users in an editor
## Notes to reviewers
* Review this commit-by-commit; there's effectively no back-tracking and there's a lot of churn in some of these commits.
*ira: My commits are not as well organized :')*
* I've relaxed the bound on Local to Send + Sync + 'static: I don't think these concerns apply there, so this can keep things simple. Storing e.g. a u32 in a Local is fine, because there's a variable name attached explaining what it does.
* I think this is a bad place for the Resource trait to live, but I've left it in place to make reviewing easier. IMO that's best tackled with https://github.com/bevyengine/bevy/issues/4981.
## Changelog
`Resource` is no longer automatically implemented for all matching types. Instead, use the new `#[derive(Resource)]` macro.
## Migration Guide
Add `#[derive(Resource)]` to all types you are using as a resource.
If you are using a third party type as a resource, wrap it in a tuple struct to bypass orphan rules. Consider deriving `Deref` and `DerefMut` to improve ergonomics.
`ClearColor` no longer implements `Component`. Using `ClearColor` as a component in 0.8 did nothing.
Use the `ClearColorConfig` in the `Camera3d` and `Camera2d` components instead.
Co-authored-by: Alice <alice.i.cecile@gmail.com>
Co-authored-by: Alice Cecile <alice.i.cecile@gmail.com>
Co-authored-by: devil-ira <justthecooldude@gmail.com>
Co-authored-by: Carter Anderson <mcanders1@gmail.com>
2022-08-08 21:36:35 +00:00
/// A type that can be inserted into a [`World`] as a singleton.
///
/// You can access resource data in systems using the [`Res`] and [`ResMut`] system parameters
///
/// Only one resource of each type can be stored in a [`World`] at any given time.
///
/// # Examples
///
/// ```
/// # let mut world = World::default();
Migrate engine to Schedule v3 (#7267)
Huge thanks to @maniwani, @devil-ira, @hymm, @cart, @superdump and @jakobhellermann for the help with this PR.
# Objective
- Followup #6587.
- Minimal integration for the Stageless Scheduling RFC: https://github.com/bevyengine/rfcs/pull/45
## Solution
- [x] Remove old scheduling module
- [x] Migrate new methods to no longer use extension methods
- [x] Fix compiler errors
- [x] Fix benchmarks
- [x] Fix examples
- [x] Fix docs
- [x] Fix tests
## Changelog
### Added
- a large number of methods on `App` to work with schedules ergonomically
- the `CoreSchedule` enum
- `App::add_extract_system` via the `RenderingAppExtension` trait extension method
- the private `prepare_view_uniforms` system now has a public system set for scheduling purposes, called `ViewSet::PrepareUniforms`
### Removed
- stages, and all code that mentions stages
- states have been dramatically simplified, and no longer use a stack
- `RunCriteriaLabel`
- `AsSystemLabel` trait
- `on_hierarchy_reports_enabled` run criteria (now just uses an ad hoc resource checking run condition)
- systems in `RenderSet/Stage::Extract` no longer warn when they do not read data from the main world
- `RunCriteriaLabel`
- `transform_propagate_system_set`: this was a nonstandard pattern that didn't actually provide enough control. The systems are already `pub`: the docs have been updated to ensure that the third-party usage is clear.
### Changed
- `System::default_labels` is now `System::default_system_sets`.
- `App::add_default_labels` is now `App::add_default_sets`
- `CoreStage` and `StartupStage` enums are now `CoreSet` and `StartupSet`
- `App::add_system_set` was renamed to `App::add_systems`
- The `StartupSchedule` label is now defined as part of the `CoreSchedules` enum
- `.label(SystemLabel)` is now referred to as `.in_set(SystemSet)`
- `SystemLabel` trait was replaced by `SystemSet`
- `SystemTypeIdLabel<T>` was replaced by `SystemSetType<T>`
- The `ReportHierarchyIssue` resource now has a public constructor (`new`), and implements `PartialEq`
- Fixed time steps now use a schedule (`CoreSchedule::FixedTimeStep`) rather than a run criteria.
- Adding rendering extraction systems now panics rather than silently failing if no subapp with the `RenderApp` label is found.
- the `calculate_bounds` system, with the `CalculateBounds` label, is now in `CoreSet::Update`, rather than in `CoreSet::PostUpdate` before commands are applied.
- `SceneSpawnerSystem` now runs under `CoreSet::Update`, rather than `CoreStage::PreUpdate.at_end()`.
- `bevy_pbr::add_clusters` is no longer an exclusive system
- the top level `bevy_ecs::schedule` module was replaced with `bevy_ecs::scheduling`
- `tick_global_task_pools_on_main_thread` is no longer run as an exclusive system. Instead, it has been replaced by `tick_global_task_pools`, which uses a `NonSend` resource to force running on the main thread.
## Migration Guide
- Calls to `.label(MyLabel)` should be replaced with `.in_set(MySet)`
- Stages have been removed. Replace these with system sets, and then add command flushes using the `apply_system_buffers` exclusive system where needed.
- The `CoreStage`, `StartupStage, `RenderStage` and `AssetStage` enums have been replaced with `CoreSet`, `StartupSet, `RenderSet` and `AssetSet`. The same scheduling guarantees have been preserved.
- Systems are no longer added to `CoreSet::Update` by default. Add systems manually if this behavior is needed, although you should consider adding your game logic systems to `CoreSchedule::FixedTimestep` instead for more reliable framerate-independent behavior.
- Similarly, startup systems are no longer part of `StartupSet::Startup` by default. In most cases, this won't matter to you.
- For example, `add_system_to_stage(CoreStage::PostUpdate, my_system)` should be replaced with
- `add_system(my_system.in_set(CoreSet::PostUpdate)`
- When testing systems or otherwise running them in a headless fashion, simply construct and run a schedule using `Schedule::new()` and `World::run_schedule` rather than constructing stages
- Run criteria have been renamed to run conditions. These can now be combined with each other and with states.
- Looping run criteria and state stacks have been removed. Use an exclusive system that runs a schedule if you need this level of control over system control flow.
- For app-level control flow over which schedules get run when (such as for rollback networking), create your own schedule and insert it under the `CoreSchedule::Outer` label.
- Fixed timesteps are now evaluated in a schedule, rather than controlled via run criteria. The `run_fixed_timestep` system runs this schedule between `CoreSet::First` and `CoreSet::PreUpdate` by default.
- Command flush points introduced by `AssetStage` have been removed. If you were relying on these, add them back manually.
- Adding extract systems is now typically done directly on the main app. Make sure the `RenderingAppExtension` trait is in scope, then call `app.add_extract_system(my_system)`.
- the `calculate_bounds` system, with the `CalculateBounds` label, is now in `CoreSet::Update`, rather than in `CoreSet::PostUpdate` before commands are applied. You may need to order your movement systems to occur before this system in order to avoid system order ambiguities in culling behavior.
- the `RenderLabel` `AppLabel` was renamed to `RenderApp` for clarity
- `App::add_state` now takes 0 arguments: the starting state is set based on the `Default` impl.
- Instead of creating `SystemSet` containers for systems that run in stages, simply use `.on_enter::<State::Variant>()` or its `on_exit` or `on_update` siblings.
- `SystemLabel` derives should be replaced with `SystemSet`. You will also need to add the `Debug`, `PartialEq`, `Eq`, and `Hash` traits to satisfy the new trait bounds.
- `with_run_criteria` has been renamed to `run_if`. Run criteria have been renamed to run conditions for clarity, and should now simply return a bool.
- States have been dramatically simplified: there is no longer a "state stack". To queue a transition to the next state, call `NextState::set`
## TODO
- [x] remove dead methods on App and World
- [x] add `App::add_system_to_schedule` and `App::add_systems_to_schedule`
- [x] avoid adding the default system set at inappropriate times
- [x] remove any accidental cycles in the default plugins schedule
- [x] migrate benchmarks
- [x] expose explicit labels for the built-in command flush points
- [x] migrate engine code
- [x] remove all mentions of stages from the docs
- [x] verify docs for States
- [x] fix uses of exclusive systems that use .end / .at_start / .before_commands
- [x] migrate RenderStage and AssetStage
- [x] migrate examples
- [x] ensure that transform propagation is exported in a sufficiently public way (the systems are already pub)
- [x] ensure that on_enter schedules are run at least once before the main app
- [x] re-enable opt-in to execution order ambiguities
- [x] revert change to `update_bounds` to ensure it runs in `PostUpdate`
- [x] test all examples
- [x] unbreak directional lights
- [x] unbreak shadows (see 3d_scene, 3d_shape, lighting, transparaency_3d examples)
- [x] game menu example shows loading screen and menu simultaneously
- [x] display settings menu is a blank screen
- [x] `without_winit` example panics
- [x] ensure all tests pass
- [x] SubApp doc test fails
- [x] runs_spawn_local tasks fails
- [x] [Fix panic_when_hierachy_cycle test hanging](https://github.com/alice-i-cecile/bevy/pull/120)
## Points of Difficulty and Controversy
**Reviewers, please give feedback on these and look closely**
1. Default sets, from the RFC, have been removed. These added a tremendous amount of implicit complexity and result in hard to debug scheduling errors. They're going to be tackled in the form of "base sets" by @cart in a followup.
2. The outer schedule controls which schedule is run when `App::update` is called.
3. I implemented `Label for `Box<dyn Label>` for our label types. This enables us to store schedule labels in concrete form, and then later run them. I ran into the same set of problems when working with one-shot systems. We've previously investigated this pattern in depth, and it does not appear to lead to extra indirection with nested boxes.
4. `SubApp::update` simply runs the default schedule once. This sucks, but this whole API is incomplete and this was the minimal changeset.
5. `time_system` and `tick_global_task_pools_on_main_thread` no longer use exclusive systems to attempt to force scheduling order
6. Implemetnation strategy for fixed timesteps
7. `AssetStage` was migrated to `AssetSet` without reintroducing command flush points. These did not appear to be used, and it's nice to remove these bottlenecks.
8. Migration of `bevy_render/lib.rs` and pipelined rendering. The logic here is unusually tricky, as we have complex scheduling requirements.
## Future Work (ideally before 0.10)
- Rename schedule_v3 module to schedule or scheduling
- Add a derive macro to states, and likely a `EnumIter` trait of some form
- Figure out what exactly to do with the "systems added should basically work by default" problem
- Improve ergonomics for working with fixed timesteps and states
- Polish FixedTime API to match Time
- Rebase and merge #7415
- Resolve all internal ambiguities (blocked on better tools, especially #7442)
- Add "base sets" to replace the removed default sets.
2023-02-06 02:04:50 +00:00
/// # let mut schedule = Schedule::new();
Make `Resource` trait opt-in, requiring `#[derive(Resource)]` V2 (#5577)
*This PR description is an edited copy of #5007, written by @alice-i-cecile.*
# Objective
Follow-up to https://github.com/bevyengine/bevy/pull/2254. The `Resource` trait currently has a blanket implementation for all types that meet its bounds.
While ergonomic, this results in several drawbacks:
* it is possible to make confusing, silent mistakes such as inserting a function pointer (Foo) rather than a value (Foo::Bar) as a resource
* it is challenging to discover if a type is intended to be used as a resource
* we cannot later add customization options (see the [RFC](https://github.com/bevyengine/rfcs/blob/main/rfcs/27-derive-component.md) for the equivalent choice for Component).
* dependencies can use the same Rust type as a resource in invisibly conflicting ways
* raw Rust types used as resources cannot preserve privacy appropriately, as anyone able to access that type can read and write to internal values
* we cannot capture a definitive list of possible resources to display to users in an editor
## Notes to reviewers
* Review this commit-by-commit; there's effectively no back-tracking and there's a lot of churn in some of these commits.
*ira: My commits are not as well organized :')*
* I've relaxed the bound on Local to Send + Sync + 'static: I don't think these concerns apply there, so this can keep things simple. Storing e.g. a u32 in a Local is fine, because there's a variable name attached explaining what it does.
* I think this is a bad place for the Resource trait to live, but I've left it in place to make reviewing easier. IMO that's best tackled with https://github.com/bevyengine/bevy/issues/4981.
## Changelog
`Resource` is no longer automatically implemented for all matching types. Instead, use the new `#[derive(Resource)]` macro.
## Migration Guide
Add `#[derive(Resource)]` to all types you are using as a resource.
If you are using a third party type as a resource, wrap it in a tuple struct to bypass orphan rules. Consider deriving `Deref` and `DerefMut` to improve ergonomics.
`ClearColor` no longer implements `Component`. Using `ClearColor` as a component in 0.8 did nothing.
Use the `ClearColorConfig` in the `Camera3d` and `Camera2d` components instead.
Co-authored-by: Alice <alice.i.cecile@gmail.com>
Co-authored-by: Alice Cecile <alice.i.cecile@gmail.com>
Co-authored-by: devil-ira <justthecooldude@gmail.com>
Co-authored-by: Carter Anderson <mcanders1@gmail.com>
2022-08-08 21:36:35 +00:00
/// # use bevy_ecs::prelude::*;
/// #[derive(Resource)]
/// struct MyResource { value: u32 }
///
/// world.insert_resource(MyResource { value: 42 });
///
/// fn read_resource_system(resource: Res<MyResource>) {
/// assert_eq!(resource.value, 42);
/// }
///
/// fn write_resource_system(mut resource: ResMut<MyResource>) {
/// assert_eq!(resource.value, 42);
/// resource.value = 0;
/// assert_eq!(resource.value, 0);
/// }
Migrate engine to Schedule v3 (#7267)
Huge thanks to @maniwani, @devil-ira, @hymm, @cart, @superdump and @jakobhellermann for the help with this PR.
# Objective
- Followup #6587.
- Minimal integration for the Stageless Scheduling RFC: https://github.com/bevyengine/rfcs/pull/45
## Solution
- [x] Remove old scheduling module
- [x] Migrate new methods to no longer use extension methods
- [x] Fix compiler errors
- [x] Fix benchmarks
- [x] Fix examples
- [x] Fix docs
- [x] Fix tests
## Changelog
### Added
- a large number of methods on `App` to work with schedules ergonomically
- the `CoreSchedule` enum
- `App::add_extract_system` via the `RenderingAppExtension` trait extension method
- the private `prepare_view_uniforms` system now has a public system set for scheduling purposes, called `ViewSet::PrepareUniforms`
### Removed
- stages, and all code that mentions stages
- states have been dramatically simplified, and no longer use a stack
- `RunCriteriaLabel`
- `AsSystemLabel` trait
- `on_hierarchy_reports_enabled` run criteria (now just uses an ad hoc resource checking run condition)
- systems in `RenderSet/Stage::Extract` no longer warn when they do not read data from the main world
- `RunCriteriaLabel`
- `transform_propagate_system_set`: this was a nonstandard pattern that didn't actually provide enough control. The systems are already `pub`: the docs have been updated to ensure that the third-party usage is clear.
### Changed
- `System::default_labels` is now `System::default_system_sets`.
- `App::add_default_labels` is now `App::add_default_sets`
- `CoreStage` and `StartupStage` enums are now `CoreSet` and `StartupSet`
- `App::add_system_set` was renamed to `App::add_systems`
- The `StartupSchedule` label is now defined as part of the `CoreSchedules` enum
- `.label(SystemLabel)` is now referred to as `.in_set(SystemSet)`
- `SystemLabel` trait was replaced by `SystemSet`
- `SystemTypeIdLabel<T>` was replaced by `SystemSetType<T>`
- The `ReportHierarchyIssue` resource now has a public constructor (`new`), and implements `PartialEq`
- Fixed time steps now use a schedule (`CoreSchedule::FixedTimeStep`) rather than a run criteria.
- Adding rendering extraction systems now panics rather than silently failing if no subapp with the `RenderApp` label is found.
- the `calculate_bounds` system, with the `CalculateBounds` label, is now in `CoreSet::Update`, rather than in `CoreSet::PostUpdate` before commands are applied.
- `SceneSpawnerSystem` now runs under `CoreSet::Update`, rather than `CoreStage::PreUpdate.at_end()`.
- `bevy_pbr::add_clusters` is no longer an exclusive system
- the top level `bevy_ecs::schedule` module was replaced with `bevy_ecs::scheduling`
- `tick_global_task_pools_on_main_thread` is no longer run as an exclusive system. Instead, it has been replaced by `tick_global_task_pools`, which uses a `NonSend` resource to force running on the main thread.
## Migration Guide
- Calls to `.label(MyLabel)` should be replaced with `.in_set(MySet)`
- Stages have been removed. Replace these with system sets, and then add command flushes using the `apply_system_buffers` exclusive system where needed.
- The `CoreStage`, `StartupStage, `RenderStage` and `AssetStage` enums have been replaced with `CoreSet`, `StartupSet, `RenderSet` and `AssetSet`. The same scheduling guarantees have been preserved.
- Systems are no longer added to `CoreSet::Update` by default. Add systems manually if this behavior is needed, although you should consider adding your game logic systems to `CoreSchedule::FixedTimestep` instead for more reliable framerate-independent behavior.
- Similarly, startup systems are no longer part of `StartupSet::Startup` by default. In most cases, this won't matter to you.
- For example, `add_system_to_stage(CoreStage::PostUpdate, my_system)` should be replaced with
- `add_system(my_system.in_set(CoreSet::PostUpdate)`
- When testing systems or otherwise running them in a headless fashion, simply construct and run a schedule using `Schedule::new()` and `World::run_schedule` rather than constructing stages
- Run criteria have been renamed to run conditions. These can now be combined with each other and with states.
- Looping run criteria and state stacks have been removed. Use an exclusive system that runs a schedule if you need this level of control over system control flow.
- For app-level control flow over which schedules get run when (such as for rollback networking), create your own schedule and insert it under the `CoreSchedule::Outer` label.
- Fixed timesteps are now evaluated in a schedule, rather than controlled via run criteria. The `run_fixed_timestep` system runs this schedule between `CoreSet::First` and `CoreSet::PreUpdate` by default.
- Command flush points introduced by `AssetStage` have been removed. If you were relying on these, add them back manually.
- Adding extract systems is now typically done directly on the main app. Make sure the `RenderingAppExtension` trait is in scope, then call `app.add_extract_system(my_system)`.
- the `calculate_bounds` system, with the `CalculateBounds` label, is now in `CoreSet::Update`, rather than in `CoreSet::PostUpdate` before commands are applied. You may need to order your movement systems to occur before this system in order to avoid system order ambiguities in culling behavior.
- the `RenderLabel` `AppLabel` was renamed to `RenderApp` for clarity
- `App::add_state` now takes 0 arguments: the starting state is set based on the `Default` impl.
- Instead of creating `SystemSet` containers for systems that run in stages, simply use `.on_enter::<State::Variant>()` or its `on_exit` or `on_update` siblings.
- `SystemLabel` derives should be replaced with `SystemSet`. You will also need to add the `Debug`, `PartialEq`, `Eq`, and `Hash` traits to satisfy the new trait bounds.
- `with_run_criteria` has been renamed to `run_if`. Run criteria have been renamed to run conditions for clarity, and should now simply return a bool.
- States have been dramatically simplified: there is no longer a "state stack". To queue a transition to the next state, call `NextState::set`
## TODO
- [x] remove dead methods on App and World
- [x] add `App::add_system_to_schedule` and `App::add_systems_to_schedule`
- [x] avoid adding the default system set at inappropriate times
- [x] remove any accidental cycles in the default plugins schedule
- [x] migrate benchmarks
- [x] expose explicit labels for the built-in command flush points
- [x] migrate engine code
- [x] remove all mentions of stages from the docs
- [x] verify docs for States
- [x] fix uses of exclusive systems that use .end / .at_start / .before_commands
- [x] migrate RenderStage and AssetStage
- [x] migrate examples
- [x] ensure that transform propagation is exported in a sufficiently public way (the systems are already pub)
- [x] ensure that on_enter schedules are run at least once before the main app
- [x] re-enable opt-in to execution order ambiguities
- [x] revert change to `update_bounds` to ensure it runs in `PostUpdate`
- [x] test all examples
- [x] unbreak directional lights
- [x] unbreak shadows (see 3d_scene, 3d_shape, lighting, transparaency_3d examples)
- [x] game menu example shows loading screen and menu simultaneously
- [x] display settings menu is a blank screen
- [x] `without_winit` example panics
- [x] ensure all tests pass
- [x] SubApp doc test fails
- [x] runs_spawn_local tasks fails
- [x] [Fix panic_when_hierachy_cycle test hanging](https://github.com/alice-i-cecile/bevy/pull/120)
## Points of Difficulty and Controversy
**Reviewers, please give feedback on these and look closely**
1. Default sets, from the RFC, have been removed. These added a tremendous amount of implicit complexity and result in hard to debug scheduling errors. They're going to be tackled in the form of "base sets" by @cart in a followup.
2. The outer schedule controls which schedule is run when `App::update` is called.
3. I implemented `Label for `Box<dyn Label>` for our label types. This enables us to store schedule labels in concrete form, and then later run them. I ran into the same set of problems when working with one-shot systems. We've previously investigated this pattern in depth, and it does not appear to lead to extra indirection with nested boxes.
4. `SubApp::update` simply runs the default schedule once. This sucks, but this whole API is incomplete and this was the minimal changeset.
5. `time_system` and `tick_global_task_pools_on_main_thread` no longer use exclusive systems to attempt to force scheduling order
6. Implemetnation strategy for fixed timesteps
7. `AssetStage` was migrated to `AssetSet` without reintroducing command flush points. These did not appear to be used, and it's nice to remove these bottlenecks.
8. Migration of `bevy_render/lib.rs` and pipelined rendering. The logic here is unusually tricky, as we have complex scheduling requirements.
## Future Work (ideally before 0.10)
- Rename schedule_v3 module to schedule or scheduling
- Add a derive macro to states, and likely a `EnumIter` trait of some form
- Figure out what exactly to do with the "systems added should basically work by default" problem
- Improve ergonomics for working with fixed timesteps and states
- Polish FixedTime API to match Time
- Rebase and merge #7415
- Resolve all internal ambiguities (blocked on better tools, especially #7442)
- Add "base sets" to replace the removed default sets.
2023-02-06 02:04:50 +00:00
/// # schedule.add_system(read_resource_system);
/// # schedule.add_system(write_resource_system.after(read_resource_system));
/// # schedule.run(&mut world);
Make `Resource` trait opt-in, requiring `#[derive(Resource)]` V2 (#5577)
*This PR description is an edited copy of #5007, written by @alice-i-cecile.*
# Objective
Follow-up to https://github.com/bevyengine/bevy/pull/2254. The `Resource` trait currently has a blanket implementation for all types that meet its bounds.
While ergonomic, this results in several drawbacks:
* it is possible to make confusing, silent mistakes such as inserting a function pointer (Foo) rather than a value (Foo::Bar) as a resource
* it is challenging to discover if a type is intended to be used as a resource
* we cannot later add customization options (see the [RFC](https://github.com/bevyengine/rfcs/blob/main/rfcs/27-derive-component.md) for the equivalent choice for Component).
* dependencies can use the same Rust type as a resource in invisibly conflicting ways
* raw Rust types used as resources cannot preserve privacy appropriately, as anyone able to access that type can read and write to internal values
* we cannot capture a definitive list of possible resources to display to users in an editor
## Notes to reviewers
* Review this commit-by-commit; there's effectively no back-tracking and there's a lot of churn in some of these commits.
*ira: My commits are not as well organized :')*
* I've relaxed the bound on Local to Send + Sync + 'static: I don't think these concerns apply there, so this can keep things simple. Storing e.g. a u32 in a Local is fine, because there's a variable name attached explaining what it does.
* I think this is a bad place for the Resource trait to live, but I've left it in place to make reviewing easier. IMO that's best tackled with https://github.com/bevyengine/bevy/issues/4981.
## Changelog
`Resource` is no longer automatically implemented for all matching types. Instead, use the new `#[derive(Resource)]` macro.
## Migration Guide
Add `#[derive(Resource)]` to all types you are using as a resource.
If you are using a third party type as a resource, wrap it in a tuple struct to bypass orphan rules. Consider deriving `Deref` and `DerefMut` to improve ergonomics.
`ClearColor` no longer implements `Component`. Using `ClearColor` as a component in 0.8 did nothing.
Use the `ClearColorConfig` in the `Camera3d` and `Camera2d` components instead.
Co-authored-by: Alice <alice.i.cecile@gmail.com>
Co-authored-by: Alice Cecile <alice.i.cecile@gmail.com>
Co-authored-by: devil-ira <justthecooldude@gmail.com>
Co-authored-by: Carter Anderson <mcanders1@gmail.com>
2022-08-08 21:36:35 +00:00
/// ```
2021-10-03 19:23:44 +00:00
pub trait Resource : Send + Sync + 'static { }
Implement `WorldQuery` derive macro (#2713)
# Objective
- Closes #786
- Closes #2252
- Closes #2588
This PR implements a derive macro that allows users to define their queries as structs with named fields.
## Example
```rust
#[derive(WorldQuery)]
#[world_query(derive(Debug))]
struct NumQuery<'w, T: Component, P: Component> {
entity: Entity,
u: UNumQuery<'w>,
generic: GenericQuery<'w, T, P>,
}
#[derive(WorldQuery)]
#[world_query(derive(Debug))]
struct UNumQuery<'w> {
u_16: &'w u16,
u_32_opt: Option<&'w u32>,
}
#[derive(WorldQuery)]
#[world_query(derive(Debug))]
struct GenericQuery<'w, T: Component, P: Component> {
generic: (&'w T, &'w P),
}
#[derive(WorldQuery)]
#[world_query(filter)]
struct NumQueryFilter<T: Component, P: Component> {
_u_16: With<u16>,
_u_32: With<u32>,
_or: Or<(With<i16>, Changed<u16>, Added<u32>)>,
_generic_tuple: (With<T>, With<P>),
_without: Without<Option<u16>>,
_tp: PhantomData<(T, P)>,
}
fn print_nums_readonly(query: Query<NumQuery<u64, i64>, NumQueryFilter<u64, i64>>) {
for num in query.iter() {
println!("{:#?}", num);
}
}
#[derive(WorldQuery)]
#[world_query(mutable, derive(Debug))]
struct MutNumQuery<'w, T: Component, P: Component> {
i_16: &'w mut i16,
i_32_opt: Option<&'w mut i32>,
}
fn print_nums(mut query: Query<MutNumQuery, NumQueryFilter<u64, i64>>) {
for num in query.iter_mut() {
println!("{:#?}", num);
}
}
```
## TODOs:
- [x] Add support for `&T` and `&mut T`
- [x] Test
- [x] Add support for optional types
- [x] Test
- [x] Add support for `Entity`
- [x] Test
- [x] Add support for nested `WorldQuery`
- [x] Test
- [x] Add support for tuples
- [x] Test
- [x] Add support for generics
- [x] Test
- [x] Add support for query filters
- [x] Test
- [x] Add support for `PhantomData`
- [x] Test
- [x] Refactor `read_world_query_field_type_info`
- [x] Properly document `readonly` attribute for nested queries and the static assertions that guarantee safety
- [x] Test that we never implement `ReadOnlyFetch` for types that need mutable access
- [x] Test that we insert static assertions for nested `WorldQuery` that a user marked as readonly
2022-02-24 00:19:49 +00:00
Remove the `SystemParamState` trait and remove types like `ResState` (#6919)
Spiritual successor to #5205.
Actual successor to #6865.
# Objective
Currently, system params are defined using three traits: `SystemParam`, `ReadOnlySystemParam`, `SystemParamState`. The behavior for each param is specified by the `SystemParamState` trait, while `SystemParam` simply defers to the state.
Splitting the traits in this way makes it easier to implement within macros, but it increases the cognitive load. Worst of all, this approach requires each `MySystemParam` to have a public `MySystemParamState` type associated with it.
## Solution
* Merge the trait `SystemParamState` into `SystemParam`.
* Remove all trivial `SystemParam` state types.
* `OptionNonSendMutState<T>`: you will not be missed.
---
- [x] Fix/resolve the remaining test failure.
## Changelog
* Removed the trait `SystemParamState`, merging its functionality into `SystemParam`.
## Migration Guide
**Note**: this should replace the migration guide for #6865.
This is relative to Bevy 0.9, not main.
The traits `SystemParamState` and `SystemParamFetch` have been removed, and their functionality has been transferred to `SystemParam`.
```rust
// Before (0.9)
impl SystemParam for MyParam<'_, '_> {
type State = MyParamState;
}
unsafe impl SystemParamState for MyParamState {
fn init(world: &mut World, system_meta: &mut SystemMeta) -> Self { ... }
}
unsafe impl<'w, 's> SystemParamFetch<'w, 's> for MyParamState {
type Item = MyParam<'w, 's>;
fn get_param(&mut self, ...) -> Self::Item;
}
unsafe impl ReadOnlySystemParamFetch for MyParamState { }
// After (0.10)
unsafe impl SystemParam for MyParam<'_, '_> {
type State = MyParamState;
type Item<'w, 's> = MyParam<'w, 's>;
fn init_state(world: &mut World, system_meta: &mut SystemMeta) -> Self::State { ... }
fn get_param<'w, 's>(state: &mut Self::State, ...) -> Self::Item<'w, 's>;
}
unsafe impl ReadOnlySystemParam for MyParam<'_, '_> { }
```
The trait `ReadOnlySystemParamFetch` has been replaced with `ReadOnlySystemParam`.
```rust
// Before
unsafe impl ReadOnlySystemParamFetch for MyParamState {}
// After
unsafe impl ReadOnlySystemParam for MyParam<'_, '_> {}
```
2023-01-07 23:20:32 +00:00
// SAFETY: Res only reads a single World resource
unsafe impl < ' a , T : Resource > ReadOnlySystemParam for Res < ' a , T > { }
Bevy ECS V2 (#1525)
# Bevy ECS V2
This is a rewrite of Bevy ECS (basically everything but the new executor/schedule, which are already awesome). The overall goal was to improve the performance and versatility of Bevy ECS. Here is a quick bulleted list of changes before we dive into the details:
* Complete World rewrite
* Multiple component storage types:
* Tables: fast cache friendly iteration, slower add/removes (previously called Archetypes)
* Sparse Sets: fast add/remove, slower iteration
* Stateful Queries (caches query results for faster iteration. fragmented iteration is _fast_ now)
* Stateful System Params (caches expensive operations. inspired by @DJMcNab's work in #1364)
* Configurable System Params (users can set configuration when they construct their systems. once again inspired by @DJMcNab's work)
* Archetypes are now "just metadata", component storage is separate
* Archetype Graph (for faster archetype changes)
* Component Metadata
* Configure component storage type
* Retrieve information about component size/type/name/layout/send-ness/etc
* Components are uniquely identified by a densely packed ComponentId
* TypeIds are now totally optional (which should make implementing scripting easier)
* Super fast "for_each" query iterators
* Merged Resources into World. Resources are now just a special type of component
* EntityRef/EntityMut builder apis (more efficient and more ergonomic)
* Fast bitset-backed `Access<T>` replaces old hashmap-based approach everywhere
* Query conflicts are determined by component access instead of archetype component access (to avoid random failures at runtime)
* With/Without are still taken into account for conflicts, so this should still be comfy to use
* Much simpler `IntoSystem` impl
* Significantly reduced the amount of hashing throughout the ecs in favor of Sparse Sets (indexed by densely packed ArchetypeId, ComponentId, BundleId, and TableId)
* Safety Improvements
* Entity reservation uses a normal world reference instead of unsafe transmute
* QuerySets no longer transmute lifetimes
* Made traits "unsafe" where relevant
* More thorough safety docs
* WorldCell
* Exposes safe mutable access to multiple resources at a time in a World
* Replaced "catch all" `System::update_archetypes(world: &World)` with `System::new_archetype(archetype: &Archetype)`
* Simpler Bundle implementation
* Replaced slow "remove_bundle_one_by_one" used as fallback for Commands::remove_bundle with fast "remove_bundle_intersection"
* Removed `Mut<T>` query impl. it is better to only support one way: `&mut T`
* Removed with() from `Flags<T>` in favor of `Option<Flags<T>>`, which allows querying for flags to be "filtered" by default
* Components now have is_send property (currently only resources support non-send)
* More granular module organization
* New `RemovedComponents<T>` SystemParam that replaces `query.removed::<T>()`
* `world.resource_scope()` for mutable access to resources and world at the same time
* WorldQuery and QueryFilter traits unified. FilterFetch trait added to enable "short circuit" filtering. Auto impled for cases that don't need it
* Significantly slimmed down SystemState in favor of individual SystemParam state
* System Commands changed from `commands: &mut Commands` back to `mut commands: Commands` (to allow Commands to have a World reference)
Fixes #1320
## `World` Rewrite
This is a from-scratch rewrite of `World` that fills the niche that `hecs` used to. Yes, this means Bevy ECS is no longer a "fork" of hecs. We're going out our own!
(the only shared code between the projects is the entity id allocator, which is already basically ideal)
A huge shout out to @SanderMertens (author of [flecs](https://github.com/SanderMertens/flecs)) for sharing some great ideas with me (specifically hybrid ecs storage and archetype graphs). He also helped advise on a number of implementation details.
## Component Storage (The Problem)
Two ECS storage paradigms have gained a lot of traction over the years:
* **Archetypal ECS**:
* Stores components in "tables" with static schemas. Each "column" stores components of a given type. Each "row" is an entity.
* Each "archetype" has its own table. Adding/removing an entity's component changes the archetype.
* Enables super-fast Query iteration due to its cache-friendly data layout
* Comes at the cost of more expensive add/remove operations for an Entity's components, because all components need to be copied to the new archetype's "table"
* **Sparse Set ECS**:
* Stores components of the same type in densely packed arrays, which are sparsely indexed by densely packed unsigned integers (Entity ids)
* Query iteration is slower than Archetypal ECS because each entity's component could be at any position in the sparse set. This "random access" pattern isn't cache friendly. Additionally, there is an extra layer of indirection because you must first map the entity id to an index in the component array.
* Adding/removing components is a cheap, constant time operation
Bevy ECS V1, hecs, legion, flec, and Unity DOTS are all "archetypal ecs-es". I personally think "archetypal" storage is a good default for game engines. An entity's archetype doesn't need to change frequently in general, and it creates "fast by default" query iteration (which is a much more common operation). It is also "self optimizing". Users don't need to think about optimizing component layouts for iteration performance. It "just works" without any extra boilerplate.
Shipyard and EnTT are "sparse set ecs-es". They employ "packing" as a way to work around the "suboptimal by default" iteration performance for specific sets of components. This helps, but I didn't think this was a good choice for a general purpose engine like Bevy because:
1. "packs" conflict with each other. If bevy decides to internally pack the Transform and GlobalTransform components, users are then blocked if they want to pack some custom component with Transform.
2. users need to take manual action to optimize
Developers selecting an ECS framework are stuck with a hard choice. Select an "archetypal" framework with "fast iteration everywhere" but without the ability to cheaply add/remove components, or select a "sparse set" framework to cheaply add/remove components but with slower iteration performance.
## Hybrid Component Storage (The Solution)
In Bevy ECS V2, we get to have our cake and eat it too. It now has _both_ of the component storage types above (and more can be added later if needed):
* **Tables** (aka "archetypal" storage)
* The default storage. If you don't configure anything, this is what you get
* Fast iteration by default
* Slower add/remove operations
* **Sparse Sets**
* Opt-in
* Slower iteration
* Faster add/remove operations
These storage types complement each other perfectly. By default Query iteration is fast. If developers know that they want to add/remove a component at high frequencies, they can set the storage to "sparse set":
```rust
world.register_component(
ComponentDescriptor::new::<MyComponent>(StorageType::SparseSet)
).unwrap();
```
## Archetypes
Archetypes are now "just metadata" ... they no longer store components directly. They do store:
* The `ComponentId`s of each of the Archetype's components (and that component's storage type)
* Archetypes are uniquely defined by their component layouts
* For example: entities with "table" components `[A, B, C]` _and_ "sparse set" components `[D, E]` will always be in the same archetype.
* The `TableId` associated with the archetype
* For now each archetype has exactly one table (which can have no components),
* There is a 1->Many relationship from Tables->Archetypes. A given table could have any number of archetype components stored in it:
* Ex: an entity with "table storage" components `[A, B, C]` and "sparse set" components `[D, E]` will share the same `[A, B, C]` table as an entity with `[A, B, C]` table component and `[F]` sparse set components.
* This 1->Many relationship is how we preserve fast "cache friendly" iteration performance when possible (more on this later)
* A list of entities that are in the archetype and the row id of the table they are in
* ArchetypeComponentIds
* unique densely packed identifiers for (ArchetypeId, ComponentId) pairs
* used by the schedule executor for cheap system access control
* "Archetype Graph Edges" (see the next section)
## The "Archetype Graph"
Archetype changes in Bevy (and a number of other archetypal ecs-es) have historically been expensive to compute. First, you need to allocate a new vector of the entity's current component ids, add or remove components based on the operation performed, sort it (to ensure it is order-independent), then hash it to find the archetype (if it exists). And thats all before we get to the _already_ expensive full copy of all components to the new table storage.
The solution is to build a "graph" of archetypes to cache these results. @SanderMertens first exposed me to the idea (and he got it from @gjroelofs, who came up with it). They propose adding directed edges between archetypes for add/remove component operations. If `ComponentId`s are densely packed, you can use sparse sets to cheaply jump between archetypes.
Bevy takes this one step further by using add/remove `Bundle` edges instead of `Component` edges. Bevy encourages the use of `Bundles` to group add/remove operations. This is largely for "clearer game logic" reasons, but it also helps cut down on the number of archetype changes required. `Bundles` now also have densely-packed `BundleId`s. This allows us to use a _single_ edge for each bundle operation (rather than needing to traverse N edges ... one for each component). Single component operations are also bundles, so this is strictly an improvement over a "component only" graph.
As a result, an operation that used to be _heavy_ (both for allocations and compute) is now two dirt-cheap array lookups and zero allocations.
## Stateful Queries
World queries are now stateful. This allows us to:
1. Cache archetype (and table) matches
* This resolves another issue with (naive) archetypal ECS: query performance getting worse as the number of archetypes goes up (and fragmentation occurs).
2. Cache Fetch and Filter state
* The expensive parts of fetch/filter operations (such as hashing the TypeId to find the ComponentId) now only happen once when the Query is first constructed
3. Incrementally build up state
* When new archetypes are added, we only process the new archetypes (no need to rebuild state for old archetypes)
As a result, the direct `World` query api now looks like this:
```rust
let mut query = world.query::<(&A, &mut B)>();
for (a, mut b) in query.iter_mut(&mut world) {
}
```
Requiring `World` to generate stateful queries (rather than letting the `QueryState` type be constructed separately) allows us to ensure that _all_ queries are properly initialized (and the relevant world state, such as ComponentIds). This enables QueryState to remove branches from its operations that check for initialization status (and also enables query.iter() to take an immutable world reference because it doesn't need to initialize anything in world).
However in systems, this is a non-breaking change. State management is done internally by the relevant SystemParam.
## Stateful SystemParams
Like Queries, `SystemParams` now also cache state. For example, `Query` system params store the "stateful query" state mentioned above. Commands store their internal `CommandQueue`. This means you can now safely use as many separate `Commands` parameters in your system as you want. `Local<T>` system params store their `T` value in their state (instead of in Resources).
SystemParam state also enabled a significant slim-down of SystemState. It is much nicer to look at now.
Per-SystemParam state naturally insulates us from an "aliased mut" class of errors we have hit in the past (ex: using multiple `Commands` system params).
(credit goes to @DJMcNab for the initial idea and draft pr here #1364)
## Configurable SystemParams
@DJMcNab also had the great idea to make SystemParams configurable. This allows users to provide some initial configuration / values for system parameters (when possible). Most SystemParams have no config (the config type is `()`), but the `Local<T>` param now supports user-provided parameters:
```rust
fn foo(value: Local<usize>) {
}
app.add_system(foo.system().config(|c| c.0 = Some(10)));
```
## Uber Fast "for_each" Query Iterators
Developers now have the choice to use a fast "for_each" iterator, which yields ~1.5-3x iteration speed improvements for "fragmented iteration", and minor ~1.2x iteration speed improvements for unfragmented iteration.
```rust
fn system(query: Query<(&A, &mut B)>) {
// you now have the option to do this for a speed boost
query.for_each_mut(|(a, mut b)| {
});
// however normal iterators are still available
for (a, mut b) in query.iter_mut() {
}
}
```
I think in most cases we should continue to encourage "normal" iterators as they are more flexible and more "rust idiomatic". But when that extra "oomf" is needed, it makes sense to use `for_each`.
We should also consider using `for_each` for internal bevy systems to give our users a nice speed boost (but that should be a separate pr).
## Component Metadata
`World` now has a `Components` collection, which is accessible via `world.components()`. This stores mappings from `ComponentId` to `ComponentInfo`, as well as `TypeId` to `ComponentId` mappings (where relevant). `ComponentInfo` stores information about the component, such as ComponentId, TypeId, memory layout, send-ness (currently limited to resources), and storage type.
## Significantly Cheaper `Access<T>`
We used to use `TypeAccess<TypeId>` to manage read/write component/archetype-component access. This was expensive because TypeIds must be hashed and compared individually. The parallel executor got around this by "condensing" type ids into bitset-backed access types. This worked, but it had to be re-generated from the `TypeAccess<TypeId>`sources every time archetypes changed.
This pr removes TypeAccess in favor of faster bitset access everywhere. We can do this thanks to the move to densely packed `ComponentId`s and `ArchetypeComponentId`s.
## Merged Resources into World
Resources had a lot of redundant functionality with Components. They stored typed data, they had access control, they had unique ids, they were queryable via SystemParams, etc. In fact the _only_ major difference between them was that they were unique (and didn't correlate to an entity).
Separate resources also had the downside of requiring a separate set of access controls, which meant the parallel executor needed to compare more bitsets per system and manage more state.
I initially got the "separate resources" idea from `legion`. I think that design was motivated by the fact that it made the direct world query/resource lifetime interactions more manageable. It certainly made our lives easier when using Resources alongside hecs/bevy_ecs. However we already have a construct for safely and ergonomically managing in-world lifetimes: systems (which use `Access<T>` internally).
This pr merges Resources into World:
```rust
world.insert_resource(1);
world.insert_resource(2.0);
let a = world.get_resource::<i32>().unwrap();
let mut b = world.get_resource_mut::<f64>().unwrap();
*b = 3.0;
```
Resources are now just a special kind of component. They have their own ComponentIds (and their own resource TypeId->ComponentId scope, so they don't conflict wit components of the same type). They are stored in a special "resource archetype", which stores components inside the archetype using a new `unique_components` sparse set (note that this sparse set could later be used to implement Tags). This allows us to keep the code size small by reusing existing datastructures (namely Column, Archetype, ComponentFlags, and ComponentInfo). This allows us the executor to use a single `Access<ArchetypeComponentId>` per system. It should also make scripting language integration easier.
_But_ this merge did create problems for people directly interacting with `World`. What if you need mutable access to multiple resources at the same time? `world.get_resource_mut()` borrows World mutably!
## WorldCell
WorldCell applies the `Access<ArchetypeComponentId>` concept to direct world access:
```rust
let world_cell = world.cell();
let a = world_cell.get_resource_mut::<i32>().unwrap();
let b = world_cell.get_resource_mut::<f64>().unwrap();
```
This adds cheap runtime checks (a sparse set lookup of `ArchetypeComponentId` and a counter) to ensure that world accesses do not conflict with each other. Each operation returns a `WorldBorrow<'w, T>` or `WorldBorrowMut<'w, T>` wrapper type, which will release the relevant ArchetypeComponentId resources when dropped.
World caches the access sparse set (and only one cell can exist at a time), so `world.cell()` is a cheap operation.
WorldCell does _not_ use atomic operations. It is non-send, does a mutable borrow of world to prevent other accesses, and uses a simple `Rc<RefCell<ArchetypeComponentAccess>>` wrapper in each WorldBorrow pointer.
The api is currently limited to resource access, but it can and should be extended to queries / entity component access.
## Resource Scopes
WorldCell does not yet support component queries, and even when it does there are sometimes legitimate reasons to want a mutable world ref _and_ a mutable resource ref (ex: bevy_render and bevy_scene both need this). In these cases we could always drop down to the unsafe `world.get_resource_unchecked_mut()`, but that is not ideal!
Instead developers can use a "resource scope"
```rust
world.resource_scope(|world: &mut World, a: &mut A| {
})
```
This temporarily removes the `A` resource from `World`, provides mutable pointers to both, and re-adds A to World when finished. Thanks to the move to ComponentIds/sparse sets, this is a cheap operation.
If multiple resources are required, scopes can be nested. We could also consider adding a "resource tuple" to the api if this pattern becomes common and the boilerplate gets nasty.
## Query Conflicts Use ComponentId Instead of ArchetypeComponentId
For safety reasons, systems cannot contain queries that conflict with each other without wrapping them in a QuerySet. On bevy `main`, we use ArchetypeComponentIds to determine conflicts. This is nice because it can take into account filters:
```rust
// these queries will never conflict due to their filters
fn filter_system(a: Query<&mut A, With<B>>, b: Query<&mut B, Without<B>>) {
}
```
But it also has a significant downside:
```rust
// these queries will not conflict _until_ an entity with A, B, and C is spawned
fn maybe_conflicts_system(a: Query<(&mut A, &C)>, b: Query<(&mut A, &B)>) {
}
```
The system above will panic at runtime if an entity with A, B, and C is spawned. This makes it hard to trust that your game logic will run without crashing.
In this pr, I switched to using `ComponentId` instead. This _is_ more constraining. `maybe_conflicts_system` will now always fail, but it will do it consistently at startup. Naively, it would also _disallow_ `filter_system`, which would be a significant downgrade in usability. Bevy has a number of internal systems that rely on disjoint queries and I expect it to be a common pattern in userspace.
To resolve this, I added a new `FilteredAccess<T>` type, which wraps `Access<T>` and adds with/without filters. If two `FilteredAccess` have with/without values that prove they are disjoint, they will no longer conflict.
## EntityRef / EntityMut
World entity operations on `main` require that the user passes in an `entity` id to each operation:
```rust
let entity = world.spawn((A, )); // create a new entity with A
world.get::<A>(entity);
world.insert(entity, (B, C));
world.insert_one(entity, D);
```
This means that each operation needs to look up the entity location / verify its validity. The initial spawn operation also requires a Bundle as input. This can be awkward when no components are required (or one component is required).
These operations have been replaced by `EntityRef` and `EntityMut`, which are "builder-style" wrappers around world that provide read and read/write operations on a single, pre-validated entity:
```rust
// spawn now takes no inputs and returns an EntityMut
let entity = world.spawn()
.insert(A) // insert a single component into the entity
.insert_bundle((B, C)) // insert a bundle of components into the entity
.id() // id returns the Entity id
// Returns EntityMut (or panics if the entity does not exist)
world.entity_mut(entity)
.insert(D)
.insert_bundle(SomeBundle::default());
{
// returns EntityRef (or panics if the entity does not exist)
let d = world.entity(entity)
.get::<D>() // gets the D component
.unwrap();
// world.get still exists for ergonomics
let d = world.get::<D>(entity).unwrap();
}
// These variants return Options if you want to check existence instead of panicing
world.get_entity_mut(entity)
.unwrap()
.insert(E);
if let Some(entity_ref) = world.get_entity(entity) {
let d = entity_ref.get::<D>().unwrap();
}
```
This _does not_ affect the current Commands api or terminology. I think that should be a separate conversation as that is a much larger breaking change.
## Safety Improvements
* Entity reservation in Commands uses a normal world borrow instead of an unsafe transmute
* QuerySets no longer transmutes lifetimes
* Made traits "unsafe" when implementing a trait incorrectly could cause unsafety
* More thorough safety docs
## RemovedComponents SystemParam
The old approach to querying removed components: `query.removed:<T>()` was confusing because it had no connection to the query itself. I replaced it with the following, which is both clearer and allows us to cache the ComponentId mapping in the SystemParamState:
```rust
fn system(removed: RemovedComponents<T>) {
for entity in removed.iter() {
}
}
```
## Simpler Bundle implementation
Bundles are no longer responsible for sorting (or deduping) TypeInfo. They are just a simple ordered list of component types / data. This makes the implementation smaller and opens the door to an easy "nested bundle" implementation in the future (which i might even add in this pr). Duplicate detection is now done once per bundle type by World the first time a bundle is used.
## Unified WorldQuery and QueryFilter types
(don't worry they are still separate type _parameters_ in Queries .. this is a non-breaking change)
WorldQuery and QueryFilter were already basically identical apis. With the addition of `FetchState` and more storage-specific fetch methods, the overlap was even clearer (and the redundancy more painful).
QueryFilters are now just `F: WorldQuery where F::Fetch: FilterFetch`. FilterFetch requires `Fetch<Item = bool>` and adds new "short circuit" variants of fetch methods. This enables a filter tuple like `(With<A>, Without<B>, Changed<C>)` to stop evaluating the filter after the first mismatch is encountered. FilterFetch is automatically implemented for `Fetch` implementations that return bool.
This forces fetch implementations that return things like `(bool, bool, bool)` (such as the filter above) to manually implement FilterFetch and decide whether or not to short-circuit.
## More Granular Modules
World no longer globs all of the internal modules together. It now exports `core`, `system`, and `schedule` separately. I'm also considering exporting `core` submodules directly as that is still pretty "glob-ey" and unorganized (feedback welcome here).
## Remaining Draft Work (to be done in this pr)
* ~~panic on conflicting WorldQuery fetches (&A, &mut A)~~
* ~~bevy `main` and hecs both currently allow this, but we should protect against it if possible~~
* ~~batch_iter / par_iter (currently stubbed out)~~
* ~~ChangedRes~~
* ~~I skipped this while we sort out #1313. This pr should be adapted to account for whatever we land on there~~.
* ~~The `Archetypes` and `Tables` collections use hashes of sorted lists of component ids to uniquely identify each archetype/table. This hash is then used as the key in a HashMap to look up the relevant ArchetypeId or TableId. (which doesn't handle hash collisions properly)~~
* ~~It is currently unsafe to generate a Query from "World A", then use it on "World B" (despite the api claiming it is safe). We should probably close this gap. This could be done by adding a randomly generated WorldId to each world, then storing that id in each Query. They could then be compared to each other on each `query.do_thing(&world)` operation. This _does_ add an extra branch to each query operation, so I'm open to other suggestions if people have them.~~
* ~~Nested Bundles (if i find time)~~
## Potential Future Work
* Expand WorldCell to support queries.
* Consider not allocating in the empty archetype on `world.spawn()`
* ex: return something like EntityMutUninit, which turns into EntityMut after an `insert` or `insert_bundle` op
* this actually regressed performance last time i tried it, but in theory it should be faster
* Optimize SparseSet::insert (see `PERF` comment on insert)
* Replace SparseArray `Option<T>` with T::MAX to cut down on branching
* would enable cheaper get_unchecked() operations
* upstream fixedbitset optimizations
* fixedbitset could be allocation free for small block counts (store blocks in a SmallVec)
* fixedbitset could have a const constructor
* Consider implementing Tags (archetype-specific by-value data that affects archetype identity)
* ex: ArchetypeA could have `[A, B, C]` table components and `[D(1)]` "tag" component. ArchetypeB could have `[A, B, C]` table components and a `[D(2)]` tag component. The archetypes are different, despite both having D tags because the value inside D is different.
* this could potentially build on top of the `archetype.unique_components` added in this pr for resource storage.
* Consider reverting `all_tuples` proc macro in favor of the old `macro_rules` implementation
* all_tuples is more flexible and produces cleaner documentation (the macro_rules version produces weird type parameter orders due to parser constraints)
* but unfortunately all_tuples also appears to make Rust Analyzer sad/slow when working inside of `bevy_ecs` (does not affect user code)
* Consider "resource queries" and/or "mixed resource and entity component queries" as an alternative to WorldCell
* this is basically just "systems" so maybe it's not worth it
* Add more world ops
* `world.clear()`
* `world.reserve<T: Bundle>(count: usize)`
* Try using the old archetype allocation strategy (allocate new memory on resize and copy everything over). I expect this to improve batch insertion performance at the cost of unbatched performance. But thats just a guess. I'm not an allocation perf pro :)
* Adapt Commands apis for consistency with new World apis
## Benchmarks
key:
* `bevy_old`: bevy `main` branch
* `bevy`: this branch
* `_foreach`: uses an optimized for_each iterator
* ` _sparse`: uses sparse set storage (if unspecified assume table storage)
* `_system`: runs inside a system (if unspecified assume test happens via direct world ops)
### Simple Insert (from ecs_bench_suite)
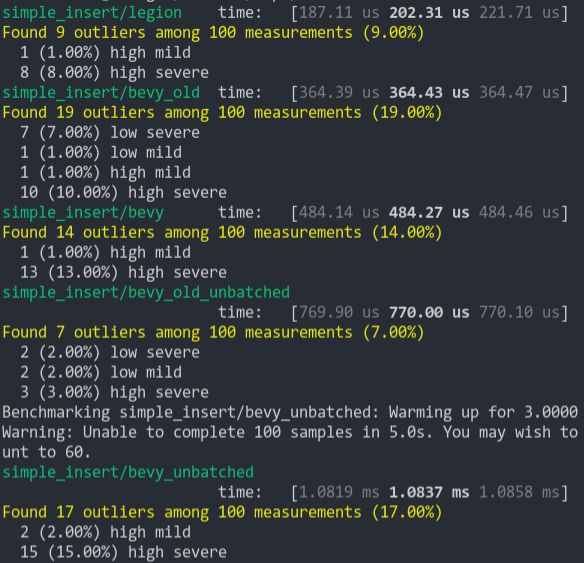
### Simpler Iter (from ecs_bench_suite)
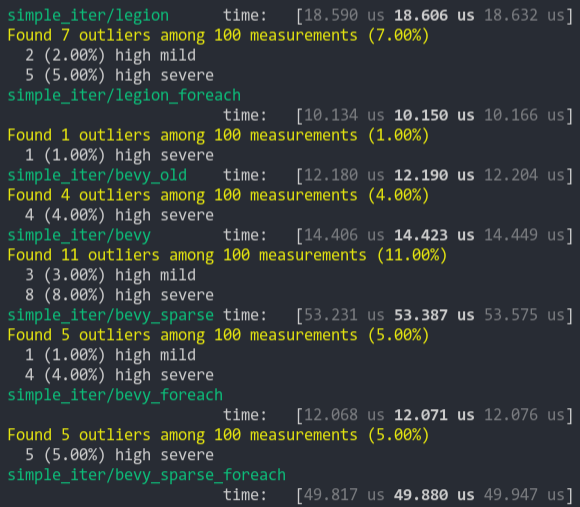
### Fragment Iter (from ecs_bench_suite)
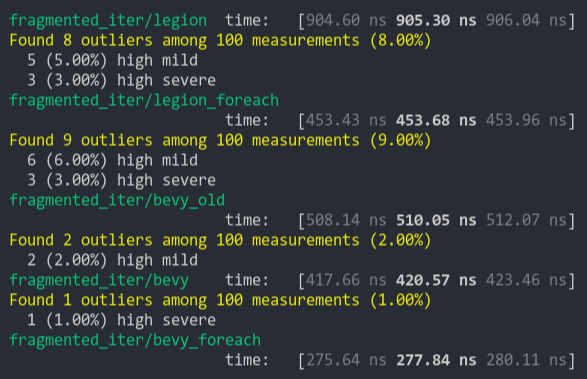
### Sparse Fragmented Iter
Iterate a query that matches 5 entities from a single matching archetype, but there are 100 unmatching archetypes
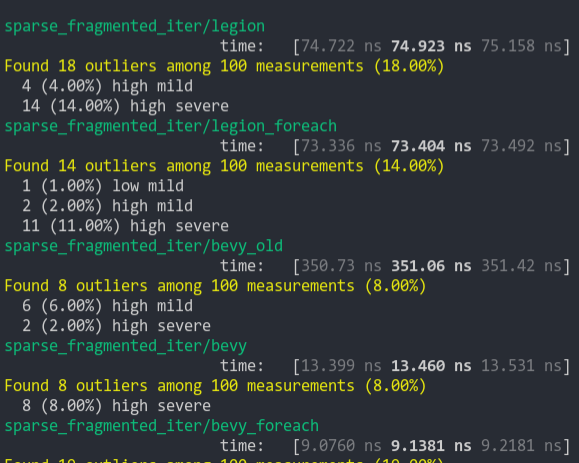
### Schedule (from ecs_bench_suite)
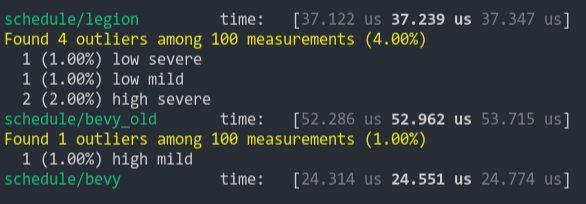
### Add Remove Component (from ecs_bench_suite)
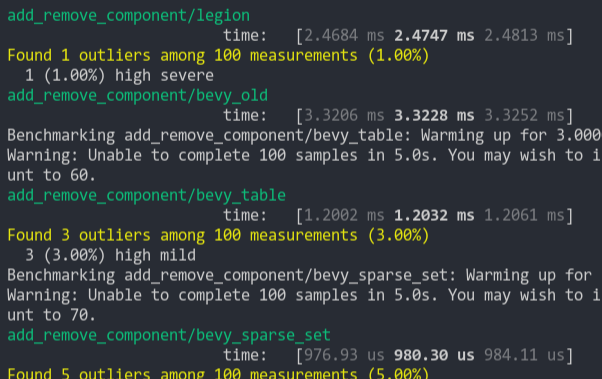
### Add Remove Component Big
Same as the test above, but each entity has 5 "large" matrix components and 1 "large" matrix component is added and removed
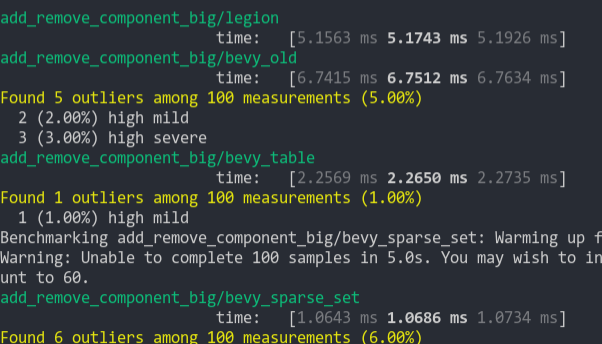
### Get Component
Looks up a single component value a large number of times
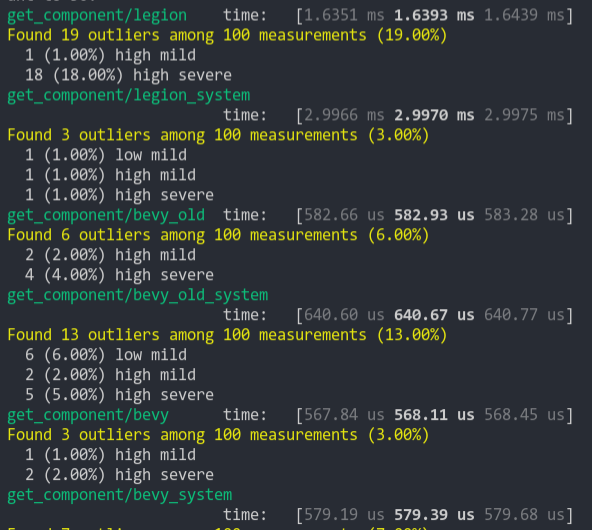
2021-03-05 07:54:35 +00:00
2022-07-04 14:44:24 +00:00
// SAFETY: Res ComponentId and ArchetypeComponentId access is applied to SystemMeta. If this Res
2021-03-11 00:27:30 +00:00
// conflicts with any prior access, a panic will occur.
Remove the `SystemParamState` trait and remove types like `ResState` (#6919)
Spiritual successor to #5205.
Actual successor to #6865.
# Objective
Currently, system params are defined using three traits: `SystemParam`, `ReadOnlySystemParam`, `SystemParamState`. The behavior for each param is specified by the `SystemParamState` trait, while `SystemParam` simply defers to the state.
Splitting the traits in this way makes it easier to implement within macros, but it increases the cognitive load. Worst of all, this approach requires each `MySystemParam` to have a public `MySystemParamState` type associated with it.
## Solution
* Merge the trait `SystemParamState` into `SystemParam`.
* Remove all trivial `SystemParam` state types.
* `OptionNonSendMutState<T>`: you will not be missed.
---
- [x] Fix/resolve the remaining test failure.
## Changelog
* Removed the trait `SystemParamState`, merging its functionality into `SystemParam`.
## Migration Guide
**Note**: this should replace the migration guide for #6865.
This is relative to Bevy 0.9, not main.
The traits `SystemParamState` and `SystemParamFetch` have been removed, and their functionality has been transferred to `SystemParam`.
```rust
// Before (0.9)
impl SystemParam for MyParam<'_, '_> {
type State = MyParamState;
}
unsafe impl SystemParamState for MyParamState {
fn init(world: &mut World, system_meta: &mut SystemMeta) -> Self { ... }
}
unsafe impl<'w, 's> SystemParamFetch<'w, 's> for MyParamState {
type Item = MyParam<'w, 's>;
fn get_param(&mut self, ...) -> Self::Item;
}
unsafe impl ReadOnlySystemParamFetch for MyParamState { }
// After (0.10)
unsafe impl SystemParam for MyParam<'_, '_> {
type State = MyParamState;
type Item<'w, 's> = MyParam<'w, 's>;
fn init_state(world: &mut World, system_meta: &mut SystemMeta) -> Self::State { ... }
fn get_param<'w, 's>(state: &mut Self::State, ...) -> Self::Item<'w, 's>;
}
unsafe impl ReadOnlySystemParam for MyParam<'_, '_> { }
```
The trait `ReadOnlySystemParamFetch` has been replaced with `ReadOnlySystemParam`.
```rust
// Before
unsafe impl ReadOnlySystemParamFetch for MyParamState {}
// After
unsafe impl ReadOnlySystemParam for MyParam<'_, '_> {}
```
2023-01-07 23:20:32 +00:00
unsafe impl < ' a , T : Resource > SystemParam for Res < ' a , T > {
type State = ComponentId ;
Simplify trait hierarchy for `SystemParam` (#6865)
# Objective
* Implementing a custom `SystemParam` by hand requires implementing three traits -- four if it is read-only.
* The trait `SystemParamFetch<'w, 's>` is a workaround from before we had generic associated types, and is no longer necessary.
## Solution
* Combine the trait `SystemParamFetch` with `SystemParamState`.
* I decided to remove the `Fetch` name and keep the `State` name, since the former was consistently conflated with the latter.
* Replace the trait `ReadOnlySystemParamFetch` with `ReadOnlySystemParam`, which simplifies trait bounds in generic code.
---
## Changelog
- Removed the trait `SystemParamFetch`, moving its functionality to `SystemParamState`.
- Replaced the trait `ReadOnlySystemParamFetch` with `ReadOnlySystemParam`.
## Migration Guide
The trait `SystemParamFetch` has been removed, and its functionality has been transferred to `SystemParamState`.
```rust
// Before
impl SystemParamState for MyParamState {
fn init(world: &mut World, system_meta: &mut SystemMeta) -> Self { ... }
}
impl<'w, 's> SystemParamFetch<'w, 's> for MyParamState {
type Item = MyParam<'w, 's>;
fn get_param(...) -> Self::Item;
}
// After
impl SystemParamState for MyParamState {
type Item<'w, 's> = MyParam<'w, 's>; // Generic associated types!
fn init(world: &mut World, system_meta: &mut SystemMeta) -> Self { ... }
fn get_param<'w, 's>(...) -> Self::Item<'w, 's>;
}
```
The trait `ReadOnlySystemParamFetch` has been replaced with `ReadOnlySystemParam`.
```rust
// Before
unsafe impl ReadOnlySystemParamFetch for MyParamState {}
// After
unsafe impl<'w, 's> ReadOnlySystemParam for MyParam<'w, 's> {}
```
2022-12-11 18:34:14 +00:00
type Item < ' w , ' s > = Res < ' w , T > ;
Remove the `SystemParamState` trait and remove types like `ResState` (#6919)
Spiritual successor to #5205.
Actual successor to #6865.
# Objective
Currently, system params are defined using three traits: `SystemParam`, `ReadOnlySystemParam`, `SystemParamState`. The behavior for each param is specified by the `SystemParamState` trait, while `SystemParam` simply defers to the state.
Splitting the traits in this way makes it easier to implement within macros, but it increases the cognitive load. Worst of all, this approach requires each `MySystemParam` to have a public `MySystemParamState` type associated with it.
## Solution
* Merge the trait `SystemParamState` into `SystemParam`.
* Remove all trivial `SystemParam` state types.
* `OptionNonSendMutState<T>`: you will not be missed.
---
- [x] Fix/resolve the remaining test failure.
## Changelog
* Removed the trait `SystemParamState`, merging its functionality into `SystemParam`.
## Migration Guide
**Note**: this should replace the migration guide for #6865.
This is relative to Bevy 0.9, not main.
The traits `SystemParamState` and `SystemParamFetch` have been removed, and their functionality has been transferred to `SystemParam`.
```rust
// Before (0.9)
impl SystemParam for MyParam<'_, '_> {
type State = MyParamState;
}
unsafe impl SystemParamState for MyParamState {
fn init(world: &mut World, system_meta: &mut SystemMeta) -> Self { ... }
}
unsafe impl<'w, 's> SystemParamFetch<'w, 's> for MyParamState {
type Item = MyParam<'w, 's>;
fn get_param(&mut self, ...) -> Self::Item;
}
unsafe impl ReadOnlySystemParamFetch for MyParamState { }
// After (0.10)
unsafe impl SystemParam for MyParam<'_, '_> {
type State = MyParamState;
type Item<'w, 's> = MyParam<'w, 's>;
fn init_state(world: &mut World, system_meta: &mut SystemMeta) -> Self::State { ... }
fn get_param<'w, 's>(state: &mut Self::State, ...) -> Self::Item<'w, 's>;
}
unsafe impl ReadOnlySystemParam for MyParam<'_, '_> { }
```
The trait `ReadOnlySystemParamFetch` has been replaced with `ReadOnlySystemParam`.
```rust
// Before
unsafe impl ReadOnlySystemParamFetch for MyParamState {}
// After
unsafe impl ReadOnlySystemParam for MyParam<'_, '_> {}
```
2023-01-07 23:20:32 +00:00
fn init_state ( world : & mut World , system_meta : & mut SystemMeta ) -> Self ::State {
Bevy ECS V2 (#1525)
# Bevy ECS V2
This is a rewrite of Bevy ECS (basically everything but the new executor/schedule, which are already awesome). The overall goal was to improve the performance and versatility of Bevy ECS. Here is a quick bulleted list of changes before we dive into the details:
* Complete World rewrite
* Multiple component storage types:
* Tables: fast cache friendly iteration, slower add/removes (previously called Archetypes)
* Sparse Sets: fast add/remove, slower iteration
* Stateful Queries (caches query results for faster iteration. fragmented iteration is _fast_ now)
* Stateful System Params (caches expensive operations. inspired by @DJMcNab's work in #1364)
* Configurable System Params (users can set configuration when they construct their systems. once again inspired by @DJMcNab's work)
* Archetypes are now "just metadata", component storage is separate
* Archetype Graph (for faster archetype changes)
* Component Metadata
* Configure component storage type
* Retrieve information about component size/type/name/layout/send-ness/etc
* Components are uniquely identified by a densely packed ComponentId
* TypeIds are now totally optional (which should make implementing scripting easier)
* Super fast "for_each" query iterators
* Merged Resources into World. Resources are now just a special type of component
* EntityRef/EntityMut builder apis (more efficient and more ergonomic)
* Fast bitset-backed `Access<T>` replaces old hashmap-based approach everywhere
* Query conflicts are determined by component access instead of archetype component access (to avoid random failures at runtime)
* With/Without are still taken into account for conflicts, so this should still be comfy to use
* Much simpler `IntoSystem` impl
* Significantly reduced the amount of hashing throughout the ecs in favor of Sparse Sets (indexed by densely packed ArchetypeId, ComponentId, BundleId, and TableId)
* Safety Improvements
* Entity reservation uses a normal world reference instead of unsafe transmute
* QuerySets no longer transmute lifetimes
* Made traits "unsafe" where relevant
* More thorough safety docs
* WorldCell
* Exposes safe mutable access to multiple resources at a time in a World
* Replaced "catch all" `System::update_archetypes(world: &World)` with `System::new_archetype(archetype: &Archetype)`
* Simpler Bundle implementation
* Replaced slow "remove_bundle_one_by_one" used as fallback for Commands::remove_bundle with fast "remove_bundle_intersection"
* Removed `Mut<T>` query impl. it is better to only support one way: `&mut T`
* Removed with() from `Flags<T>` in favor of `Option<Flags<T>>`, which allows querying for flags to be "filtered" by default
* Components now have is_send property (currently only resources support non-send)
* More granular module organization
* New `RemovedComponents<T>` SystemParam that replaces `query.removed::<T>()`
* `world.resource_scope()` for mutable access to resources and world at the same time
* WorldQuery and QueryFilter traits unified. FilterFetch trait added to enable "short circuit" filtering. Auto impled for cases that don't need it
* Significantly slimmed down SystemState in favor of individual SystemParam state
* System Commands changed from `commands: &mut Commands` back to `mut commands: Commands` (to allow Commands to have a World reference)
Fixes #1320
## `World` Rewrite
This is a from-scratch rewrite of `World` that fills the niche that `hecs` used to. Yes, this means Bevy ECS is no longer a "fork" of hecs. We're going out our own!
(the only shared code between the projects is the entity id allocator, which is already basically ideal)
A huge shout out to @SanderMertens (author of [flecs](https://github.com/SanderMertens/flecs)) for sharing some great ideas with me (specifically hybrid ecs storage and archetype graphs). He also helped advise on a number of implementation details.
## Component Storage (The Problem)
Two ECS storage paradigms have gained a lot of traction over the years:
* **Archetypal ECS**:
* Stores components in "tables" with static schemas. Each "column" stores components of a given type. Each "row" is an entity.
* Each "archetype" has its own table. Adding/removing an entity's component changes the archetype.
* Enables super-fast Query iteration due to its cache-friendly data layout
* Comes at the cost of more expensive add/remove operations for an Entity's components, because all components need to be copied to the new archetype's "table"
* **Sparse Set ECS**:
* Stores components of the same type in densely packed arrays, which are sparsely indexed by densely packed unsigned integers (Entity ids)
* Query iteration is slower than Archetypal ECS because each entity's component could be at any position in the sparse set. This "random access" pattern isn't cache friendly. Additionally, there is an extra layer of indirection because you must first map the entity id to an index in the component array.
* Adding/removing components is a cheap, constant time operation
Bevy ECS V1, hecs, legion, flec, and Unity DOTS are all "archetypal ecs-es". I personally think "archetypal" storage is a good default for game engines. An entity's archetype doesn't need to change frequently in general, and it creates "fast by default" query iteration (which is a much more common operation). It is also "self optimizing". Users don't need to think about optimizing component layouts for iteration performance. It "just works" without any extra boilerplate.
Shipyard and EnTT are "sparse set ecs-es". They employ "packing" as a way to work around the "suboptimal by default" iteration performance for specific sets of components. This helps, but I didn't think this was a good choice for a general purpose engine like Bevy because:
1. "packs" conflict with each other. If bevy decides to internally pack the Transform and GlobalTransform components, users are then blocked if they want to pack some custom component with Transform.
2. users need to take manual action to optimize
Developers selecting an ECS framework are stuck with a hard choice. Select an "archetypal" framework with "fast iteration everywhere" but without the ability to cheaply add/remove components, or select a "sparse set" framework to cheaply add/remove components but with slower iteration performance.
## Hybrid Component Storage (The Solution)
In Bevy ECS V2, we get to have our cake and eat it too. It now has _both_ of the component storage types above (and more can be added later if needed):
* **Tables** (aka "archetypal" storage)
* The default storage. If you don't configure anything, this is what you get
* Fast iteration by default
* Slower add/remove operations
* **Sparse Sets**
* Opt-in
* Slower iteration
* Faster add/remove operations
These storage types complement each other perfectly. By default Query iteration is fast. If developers know that they want to add/remove a component at high frequencies, they can set the storage to "sparse set":
```rust
world.register_component(
ComponentDescriptor::new::<MyComponent>(StorageType::SparseSet)
).unwrap();
```
## Archetypes
Archetypes are now "just metadata" ... they no longer store components directly. They do store:
* The `ComponentId`s of each of the Archetype's components (and that component's storage type)
* Archetypes are uniquely defined by their component layouts
* For example: entities with "table" components `[A, B, C]` _and_ "sparse set" components `[D, E]` will always be in the same archetype.
* The `TableId` associated with the archetype
* For now each archetype has exactly one table (which can have no components),
* There is a 1->Many relationship from Tables->Archetypes. A given table could have any number of archetype components stored in it:
* Ex: an entity with "table storage" components `[A, B, C]` and "sparse set" components `[D, E]` will share the same `[A, B, C]` table as an entity with `[A, B, C]` table component and `[F]` sparse set components.
* This 1->Many relationship is how we preserve fast "cache friendly" iteration performance when possible (more on this later)
* A list of entities that are in the archetype and the row id of the table they are in
* ArchetypeComponentIds
* unique densely packed identifiers for (ArchetypeId, ComponentId) pairs
* used by the schedule executor for cheap system access control
* "Archetype Graph Edges" (see the next section)
## The "Archetype Graph"
Archetype changes in Bevy (and a number of other archetypal ecs-es) have historically been expensive to compute. First, you need to allocate a new vector of the entity's current component ids, add or remove components based on the operation performed, sort it (to ensure it is order-independent), then hash it to find the archetype (if it exists). And thats all before we get to the _already_ expensive full copy of all components to the new table storage.
The solution is to build a "graph" of archetypes to cache these results. @SanderMertens first exposed me to the idea (and he got it from @gjroelofs, who came up with it). They propose adding directed edges between archetypes for add/remove component operations. If `ComponentId`s are densely packed, you can use sparse sets to cheaply jump between archetypes.
Bevy takes this one step further by using add/remove `Bundle` edges instead of `Component` edges. Bevy encourages the use of `Bundles` to group add/remove operations. This is largely for "clearer game logic" reasons, but it also helps cut down on the number of archetype changes required. `Bundles` now also have densely-packed `BundleId`s. This allows us to use a _single_ edge for each bundle operation (rather than needing to traverse N edges ... one for each component). Single component operations are also bundles, so this is strictly an improvement over a "component only" graph.
As a result, an operation that used to be _heavy_ (both for allocations and compute) is now two dirt-cheap array lookups and zero allocations.
## Stateful Queries
World queries are now stateful. This allows us to:
1. Cache archetype (and table) matches
* This resolves another issue with (naive) archetypal ECS: query performance getting worse as the number of archetypes goes up (and fragmentation occurs).
2. Cache Fetch and Filter state
* The expensive parts of fetch/filter operations (such as hashing the TypeId to find the ComponentId) now only happen once when the Query is first constructed
3. Incrementally build up state
* When new archetypes are added, we only process the new archetypes (no need to rebuild state for old archetypes)
As a result, the direct `World` query api now looks like this:
```rust
let mut query = world.query::<(&A, &mut B)>();
for (a, mut b) in query.iter_mut(&mut world) {
}
```
Requiring `World` to generate stateful queries (rather than letting the `QueryState` type be constructed separately) allows us to ensure that _all_ queries are properly initialized (and the relevant world state, such as ComponentIds). This enables QueryState to remove branches from its operations that check for initialization status (and also enables query.iter() to take an immutable world reference because it doesn't need to initialize anything in world).
However in systems, this is a non-breaking change. State management is done internally by the relevant SystemParam.
## Stateful SystemParams
Like Queries, `SystemParams` now also cache state. For example, `Query` system params store the "stateful query" state mentioned above. Commands store their internal `CommandQueue`. This means you can now safely use as many separate `Commands` parameters in your system as you want. `Local<T>` system params store their `T` value in their state (instead of in Resources).
SystemParam state also enabled a significant slim-down of SystemState. It is much nicer to look at now.
Per-SystemParam state naturally insulates us from an "aliased mut" class of errors we have hit in the past (ex: using multiple `Commands` system params).
(credit goes to @DJMcNab for the initial idea and draft pr here #1364)
## Configurable SystemParams
@DJMcNab also had the great idea to make SystemParams configurable. This allows users to provide some initial configuration / values for system parameters (when possible). Most SystemParams have no config (the config type is `()`), but the `Local<T>` param now supports user-provided parameters:
```rust
fn foo(value: Local<usize>) {
}
app.add_system(foo.system().config(|c| c.0 = Some(10)));
```
## Uber Fast "for_each" Query Iterators
Developers now have the choice to use a fast "for_each" iterator, which yields ~1.5-3x iteration speed improvements for "fragmented iteration", and minor ~1.2x iteration speed improvements for unfragmented iteration.
```rust
fn system(query: Query<(&A, &mut B)>) {
// you now have the option to do this for a speed boost
query.for_each_mut(|(a, mut b)| {
});
// however normal iterators are still available
for (a, mut b) in query.iter_mut() {
}
}
```
I think in most cases we should continue to encourage "normal" iterators as they are more flexible and more "rust idiomatic". But when that extra "oomf" is needed, it makes sense to use `for_each`.
We should also consider using `for_each` for internal bevy systems to give our users a nice speed boost (but that should be a separate pr).
## Component Metadata
`World` now has a `Components` collection, which is accessible via `world.components()`. This stores mappings from `ComponentId` to `ComponentInfo`, as well as `TypeId` to `ComponentId` mappings (where relevant). `ComponentInfo` stores information about the component, such as ComponentId, TypeId, memory layout, send-ness (currently limited to resources), and storage type.
## Significantly Cheaper `Access<T>`
We used to use `TypeAccess<TypeId>` to manage read/write component/archetype-component access. This was expensive because TypeIds must be hashed and compared individually. The parallel executor got around this by "condensing" type ids into bitset-backed access types. This worked, but it had to be re-generated from the `TypeAccess<TypeId>`sources every time archetypes changed.
This pr removes TypeAccess in favor of faster bitset access everywhere. We can do this thanks to the move to densely packed `ComponentId`s and `ArchetypeComponentId`s.
## Merged Resources into World
Resources had a lot of redundant functionality with Components. They stored typed data, they had access control, they had unique ids, they were queryable via SystemParams, etc. In fact the _only_ major difference between them was that they were unique (and didn't correlate to an entity).
Separate resources also had the downside of requiring a separate set of access controls, which meant the parallel executor needed to compare more bitsets per system and manage more state.
I initially got the "separate resources" idea from `legion`. I think that design was motivated by the fact that it made the direct world query/resource lifetime interactions more manageable. It certainly made our lives easier when using Resources alongside hecs/bevy_ecs. However we already have a construct for safely and ergonomically managing in-world lifetimes: systems (which use `Access<T>` internally).
This pr merges Resources into World:
```rust
world.insert_resource(1);
world.insert_resource(2.0);
let a = world.get_resource::<i32>().unwrap();
let mut b = world.get_resource_mut::<f64>().unwrap();
*b = 3.0;
```
Resources are now just a special kind of component. They have their own ComponentIds (and their own resource TypeId->ComponentId scope, so they don't conflict wit components of the same type). They are stored in a special "resource archetype", which stores components inside the archetype using a new `unique_components` sparse set (note that this sparse set could later be used to implement Tags). This allows us to keep the code size small by reusing existing datastructures (namely Column, Archetype, ComponentFlags, and ComponentInfo). This allows us the executor to use a single `Access<ArchetypeComponentId>` per system. It should also make scripting language integration easier.
_But_ this merge did create problems for people directly interacting with `World`. What if you need mutable access to multiple resources at the same time? `world.get_resource_mut()` borrows World mutably!
## WorldCell
WorldCell applies the `Access<ArchetypeComponentId>` concept to direct world access:
```rust
let world_cell = world.cell();
let a = world_cell.get_resource_mut::<i32>().unwrap();
let b = world_cell.get_resource_mut::<f64>().unwrap();
```
This adds cheap runtime checks (a sparse set lookup of `ArchetypeComponentId` and a counter) to ensure that world accesses do not conflict with each other. Each operation returns a `WorldBorrow<'w, T>` or `WorldBorrowMut<'w, T>` wrapper type, which will release the relevant ArchetypeComponentId resources when dropped.
World caches the access sparse set (and only one cell can exist at a time), so `world.cell()` is a cheap operation.
WorldCell does _not_ use atomic operations. It is non-send, does a mutable borrow of world to prevent other accesses, and uses a simple `Rc<RefCell<ArchetypeComponentAccess>>` wrapper in each WorldBorrow pointer.
The api is currently limited to resource access, but it can and should be extended to queries / entity component access.
## Resource Scopes
WorldCell does not yet support component queries, and even when it does there are sometimes legitimate reasons to want a mutable world ref _and_ a mutable resource ref (ex: bevy_render and bevy_scene both need this). In these cases we could always drop down to the unsafe `world.get_resource_unchecked_mut()`, but that is not ideal!
Instead developers can use a "resource scope"
```rust
world.resource_scope(|world: &mut World, a: &mut A| {
})
```
This temporarily removes the `A` resource from `World`, provides mutable pointers to both, and re-adds A to World when finished. Thanks to the move to ComponentIds/sparse sets, this is a cheap operation.
If multiple resources are required, scopes can be nested. We could also consider adding a "resource tuple" to the api if this pattern becomes common and the boilerplate gets nasty.
## Query Conflicts Use ComponentId Instead of ArchetypeComponentId
For safety reasons, systems cannot contain queries that conflict with each other without wrapping them in a QuerySet. On bevy `main`, we use ArchetypeComponentIds to determine conflicts. This is nice because it can take into account filters:
```rust
// these queries will never conflict due to their filters
fn filter_system(a: Query<&mut A, With<B>>, b: Query<&mut B, Without<B>>) {
}
```
But it also has a significant downside:
```rust
// these queries will not conflict _until_ an entity with A, B, and C is spawned
fn maybe_conflicts_system(a: Query<(&mut A, &C)>, b: Query<(&mut A, &B)>) {
}
```
The system above will panic at runtime if an entity with A, B, and C is spawned. This makes it hard to trust that your game logic will run without crashing.
In this pr, I switched to using `ComponentId` instead. This _is_ more constraining. `maybe_conflicts_system` will now always fail, but it will do it consistently at startup. Naively, it would also _disallow_ `filter_system`, which would be a significant downgrade in usability. Bevy has a number of internal systems that rely on disjoint queries and I expect it to be a common pattern in userspace.
To resolve this, I added a new `FilteredAccess<T>` type, which wraps `Access<T>` and adds with/without filters. If two `FilteredAccess` have with/without values that prove they are disjoint, they will no longer conflict.
## EntityRef / EntityMut
World entity operations on `main` require that the user passes in an `entity` id to each operation:
```rust
let entity = world.spawn((A, )); // create a new entity with A
world.get::<A>(entity);
world.insert(entity, (B, C));
world.insert_one(entity, D);
```
This means that each operation needs to look up the entity location / verify its validity. The initial spawn operation also requires a Bundle as input. This can be awkward when no components are required (or one component is required).
These operations have been replaced by `EntityRef` and `EntityMut`, which are "builder-style" wrappers around world that provide read and read/write operations on a single, pre-validated entity:
```rust
// spawn now takes no inputs and returns an EntityMut
let entity = world.spawn()
.insert(A) // insert a single component into the entity
.insert_bundle((B, C)) // insert a bundle of components into the entity
.id() // id returns the Entity id
// Returns EntityMut (or panics if the entity does not exist)
world.entity_mut(entity)
.insert(D)
.insert_bundle(SomeBundle::default());
{
// returns EntityRef (or panics if the entity does not exist)
let d = world.entity(entity)
.get::<D>() // gets the D component
.unwrap();
// world.get still exists for ergonomics
let d = world.get::<D>(entity).unwrap();
}
// These variants return Options if you want to check existence instead of panicing
world.get_entity_mut(entity)
.unwrap()
.insert(E);
if let Some(entity_ref) = world.get_entity(entity) {
let d = entity_ref.get::<D>().unwrap();
}
```
This _does not_ affect the current Commands api or terminology. I think that should be a separate conversation as that is a much larger breaking change.
## Safety Improvements
* Entity reservation in Commands uses a normal world borrow instead of an unsafe transmute
* QuerySets no longer transmutes lifetimes
* Made traits "unsafe" when implementing a trait incorrectly could cause unsafety
* More thorough safety docs
## RemovedComponents SystemParam
The old approach to querying removed components: `query.removed:<T>()` was confusing because it had no connection to the query itself. I replaced it with the following, which is both clearer and allows us to cache the ComponentId mapping in the SystemParamState:
```rust
fn system(removed: RemovedComponents<T>) {
for entity in removed.iter() {
}
}
```
## Simpler Bundle implementation
Bundles are no longer responsible for sorting (or deduping) TypeInfo. They are just a simple ordered list of component types / data. This makes the implementation smaller and opens the door to an easy "nested bundle" implementation in the future (which i might even add in this pr). Duplicate detection is now done once per bundle type by World the first time a bundle is used.
## Unified WorldQuery and QueryFilter types
(don't worry they are still separate type _parameters_ in Queries .. this is a non-breaking change)
WorldQuery and QueryFilter were already basically identical apis. With the addition of `FetchState` and more storage-specific fetch methods, the overlap was even clearer (and the redundancy more painful).
QueryFilters are now just `F: WorldQuery where F::Fetch: FilterFetch`. FilterFetch requires `Fetch<Item = bool>` and adds new "short circuit" variants of fetch methods. This enables a filter tuple like `(With<A>, Without<B>, Changed<C>)` to stop evaluating the filter after the first mismatch is encountered. FilterFetch is automatically implemented for `Fetch` implementations that return bool.
This forces fetch implementations that return things like `(bool, bool, bool)` (such as the filter above) to manually implement FilterFetch and decide whether or not to short-circuit.
## More Granular Modules
World no longer globs all of the internal modules together. It now exports `core`, `system`, and `schedule` separately. I'm also considering exporting `core` submodules directly as that is still pretty "glob-ey" and unorganized (feedback welcome here).
## Remaining Draft Work (to be done in this pr)
* ~~panic on conflicting WorldQuery fetches (&A, &mut A)~~
* ~~bevy `main` and hecs both currently allow this, but we should protect against it if possible~~
* ~~batch_iter / par_iter (currently stubbed out)~~
* ~~ChangedRes~~
* ~~I skipped this while we sort out #1313. This pr should be adapted to account for whatever we land on there~~.
* ~~The `Archetypes` and `Tables` collections use hashes of sorted lists of component ids to uniquely identify each archetype/table. This hash is then used as the key in a HashMap to look up the relevant ArchetypeId or TableId. (which doesn't handle hash collisions properly)~~
* ~~It is currently unsafe to generate a Query from "World A", then use it on "World B" (despite the api claiming it is safe). We should probably close this gap. This could be done by adding a randomly generated WorldId to each world, then storing that id in each Query. They could then be compared to each other on each `query.do_thing(&world)` operation. This _does_ add an extra branch to each query operation, so I'm open to other suggestions if people have them.~~
* ~~Nested Bundles (if i find time)~~
## Potential Future Work
* Expand WorldCell to support queries.
* Consider not allocating in the empty archetype on `world.spawn()`
* ex: return something like EntityMutUninit, which turns into EntityMut after an `insert` or `insert_bundle` op
* this actually regressed performance last time i tried it, but in theory it should be faster
* Optimize SparseSet::insert (see `PERF` comment on insert)
* Replace SparseArray `Option<T>` with T::MAX to cut down on branching
* would enable cheaper get_unchecked() operations
* upstream fixedbitset optimizations
* fixedbitset could be allocation free for small block counts (store blocks in a SmallVec)
* fixedbitset could have a const constructor
* Consider implementing Tags (archetype-specific by-value data that affects archetype identity)
* ex: ArchetypeA could have `[A, B, C]` table components and `[D(1)]` "tag" component. ArchetypeB could have `[A, B, C]` table components and a `[D(2)]` tag component. The archetypes are different, despite both having D tags because the value inside D is different.
* this could potentially build on top of the `archetype.unique_components` added in this pr for resource storage.
* Consider reverting `all_tuples` proc macro in favor of the old `macro_rules` implementation
* all_tuples is more flexible and produces cleaner documentation (the macro_rules version produces weird type parameter orders due to parser constraints)
* but unfortunately all_tuples also appears to make Rust Analyzer sad/slow when working inside of `bevy_ecs` (does not affect user code)
* Consider "resource queries" and/or "mixed resource and entity component queries" as an alternative to WorldCell
* this is basically just "systems" so maybe it's not worth it
* Add more world ops
* `world.clear()`
* `world.reserve<T: Bundle>(count: usize)`
* Try using the old archetype allocation strategy (allocate new memory on resize and copy everything over). I expect this to improve batch insertion performance at the cost of unbatched performance. But thats just a guess. I'm not an allocation perf pro :)
* Adapt Commands apis for consistency with new World apis
## Benchmarks
key:
* `bevy_old`: bevy `main` branch
* `bevy`: this branch
* `_foreach`: uses an optimized for_each iterator
* ` _sparse`: uses sparse set storage (if unspecified assume table storage)
* `_system`: runs inside a system (if unspecified assume test happens via direct world ops)
### Simple Insert (from ecs_bench_suite)
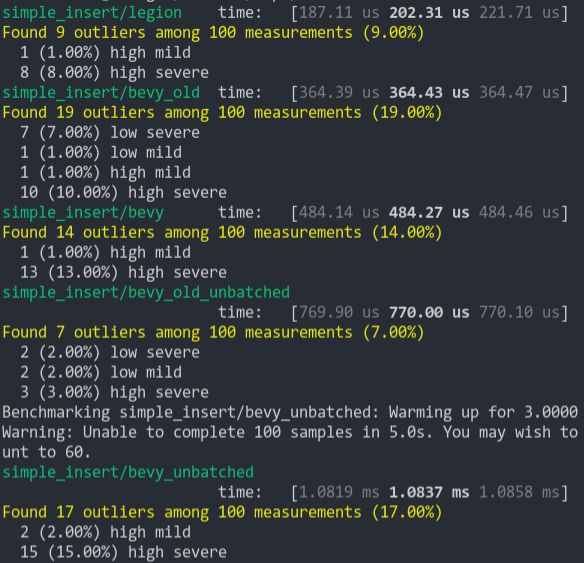
### Simpler Iter (from ecs_bench_suite)
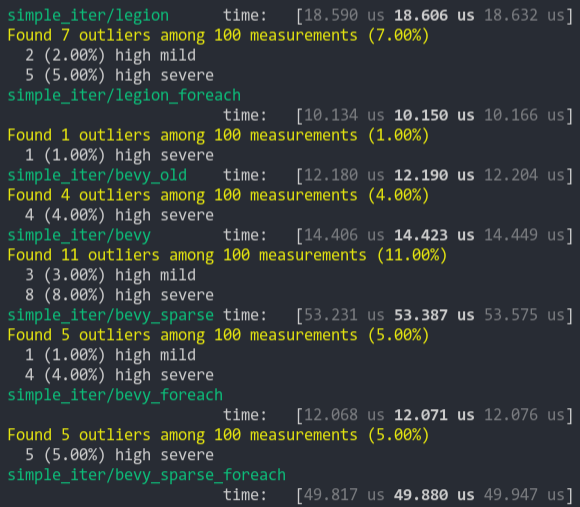
### Fragment Iter (from ecs_bench_suite)
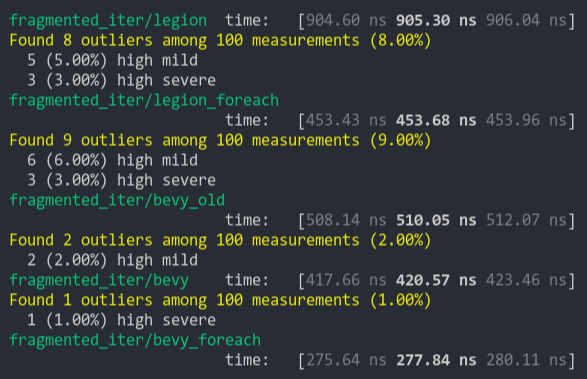
### Sparse Fragmented Iter
Iterate a query that matches 5 entities from a single matching archetype, but there are 100 unmatching archetypes
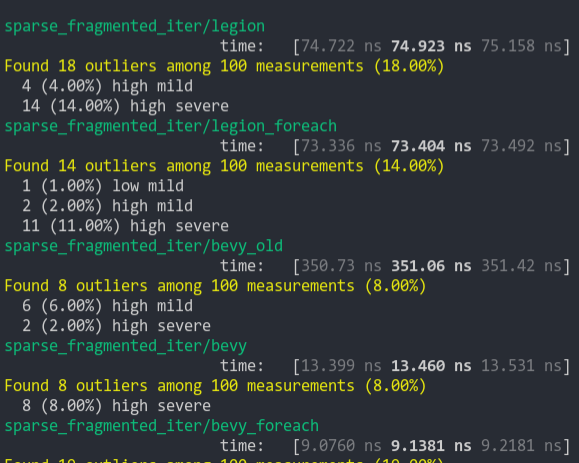
### Schedule (from ecs_bench_suite)
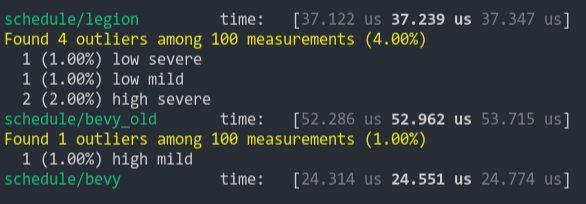
### Add Remove Component (from ecs_bench_suite)
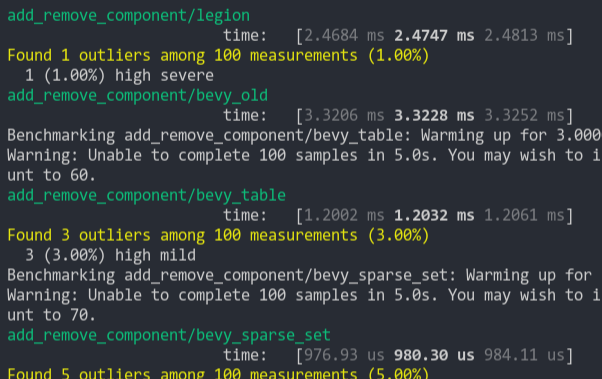
### Add Remove Component Big
Same as the test above, but each entity has 5 "large" matrix components and 1 "large" matrix component is added and removed
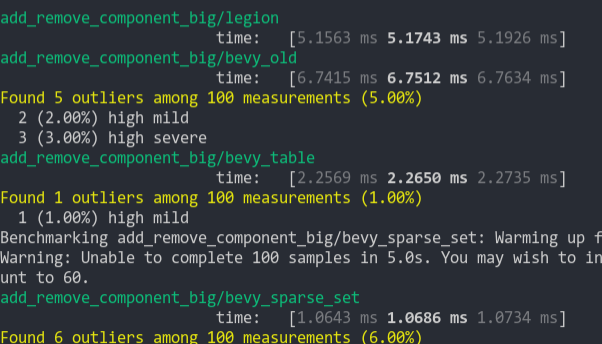
### Get Component
Looks up a single component value a large number of times
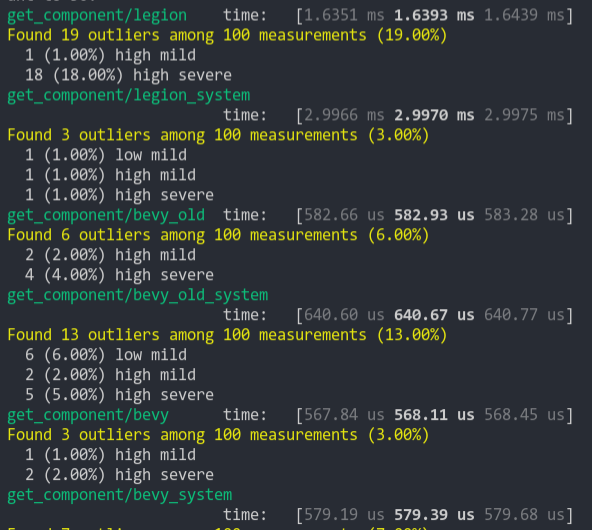
2021-03-05 07:54:35 +00:00
let component_id = world . initialize_resource ::< T > ( ) ;
Fix FilteredAccessSet get_conflicts inconsistency (#5105)
# Objective
* Enable `Res` and `Query` parameter mutual exclusion
* Required for https://github.com/bevyengine/bevy/pull/5080
The `FilteredAccessSet::get_conflicts` methods didn't work properly with
`Res` and `ResMut` parameters. Because those added their access by using
the `combined_access_mut` method and directly modifying the global
access state of the FilteredAccessSet. This caused an inconsistency,
because get_conflicts assumes that ALL added access have a corresponding
`FilteredAccess` added to the `filtered_accesses` field.
In practice, that means that SystemParam that adds their access through
the `Access` returned by `combined_access_mut` and the ones that add
their access using the `add` method lived in two different universes. As
a result, they could never be mutually exclusive.
## Solution
This commit fixes it by removing the `combined_access_mut` method. This
ensures that the `combined_access` field of FilteredAccessSet is always
updated consistently with the addition of a filter. When checking for
filtered access, it is now possible to account for `Res` and `ResMut`
invalid access. This is currently not needed, but might be in the
future.
We add the `add_unfiltered_{read,write}` methods to replace previous
usages of `combined_access_mut`.
We also add improved Debug implementations on FixedBitSet so that their
meaning is much clearer in debug output.
---
## Changelog
* Fix `Res` and `Query` parameter never being mutually exclusive.
## Migration Guide
Note: this mostly changes ECS internals, but since the API is public, it is technically breaking:
* Removed `FilteredAccessSet::combined_access_mut`
* Replace _immutable_ usage of those by `combined_access`
* For _mutable_ usages, use the new `add_unfiltered_{read,write}` methods instead of `combined_access_mut` followed by `add_{read,write}`
2022-11-16 11:05:48 +00:00
let combined_access = system_meta . component_access_set . combined_access ( ) ;
2022-02-13 22:33:55 +00:00
assert! (
! combined_access . has_write ( component_id ) ,
" error[B0002]: Res<{}> in system {} conflicts with a previous ResMut<{0}> access. Consider removing the duplicate access. " ,
std ::any ::type_name ::< T > ( ) ,
system_meta . name ,
) ;
Fix FilteredAccessSet get_conflicts inconsistency (#5105)
# Objective
* Enable `Res` and `Query` parameter mutual exclusion
* Required for https://github.com/bevyengine/bevy/pull/5080
The `FilteredAccessSet::get_conflicts` methods didn't work properly with
`Res` and `ResMut` parameters. Because those added their access by using
the `combined_access_mut` method and directly modifying the global
access state of the FilteredAccessSet. This caused an inconsistency,
because get_conflicts assumes that ALL added access have a corresponding
`FilteredAccess` added to the `filtered_accesses` field.
In practice, that means that SystemParam that adds their access through
the `Access` returned by `combined_access_mut` and the ones that add
their access using the `add` method lived in two different universes. As
a result, they could never be mutually exclusive.
## Solution
This commit fixes it by removing the `combined_access_mut` method. This
ensures that the `combined_access` field of FilteredAccessSet is always
updated consistently with the addition of a filter. When checking for
filtered access, it is now possible to account for `Res` and `ResMut`
invalid access. This is currently not needed, but might be in the
future.
We add the `add_unfiltered_{read,write}` methods to replace previous
usages of `combined_access_mut`.
We also add improved Debug implementations on FixedBitSet so that their
meaning is much clearer in debug output.
---
## Changelog
* Fix `Res` and `Query` parameter never being mutually exclusive.
## Migration Guide
Note: this mostly changes ECS internals, but since the API is public, it is technically breaking:
* Removed `FilteredAccessSet::combined_access_mut`
* Replace _immutable_ usage of those by `combined_access`
* For _mutable_ usages, use the new `add_unfiltered_{read,write}` methods instead of `combined_access_mut` followed by `add_{read,write}`
2022-11-16 11:05:48 +00:00
system_meta
. component_access_set
. add_unfiltered_read ( component_id ) ;
Bevy ECS V2 (#1525)
# Bevy ECS V2
This is a rewrite of Bevy ECS (basically everything but the new executor/schedule, which are already awesome). The overall goal was to improve the performance and versatility of Bevy ECS. Here is a quick bulleted list of changes before we dive into the details:
* Complete World rewrite
* Multiple component storage types:
* Tables: fast cache friendly iteration, slower add/removes (previously called Archetypes)
* Sparse Sets: fast add/remove, slower iteration
* Stateful Queries (caches query results for faster iteration. fragmented iteration is _fast_ now)
* Stateful System Params (caches expensive operations. inspired by @DJMcNab's work in #1364)
* Configurable System Params (users can set configuration when they construct their systems. once again inspired by @DJMcNab's work)
* Archetypes are now "just metadata", component storage is separate
* Archetype Graph (for faster archetype changes)
* Component Metadata
* Configure component storage type
* Retrieve information about component size/type/name/layout/send-ness/etc
* Components are uniquely identified by a densely packed ComponentId
* TypeIds are now totally optional (which should make implementing scripting easier)
* Super fast "for_each" query iterators
* Merged Resources into World. Resources are now just a special type of component
* EntityRef/EntityMut builder apis (more efficient and more ergonomic)
* Fast bitset-backed `Access<T>` replaces old hashmap-based approach everywhere
* Query conflicts are determined by component access instead of archetype component access (to avoid random failures at runtime)
* With/Without are still taken into account for conflicts, so this should still be comfy to use
* Much simpler `IntoSystem` impl
* Significantly reduced the amount of hashing throughout the ecs in favor of Sparse Sets (indexed by densely packed ArchetypeId, ComponentId, BundleId, and TableId)
* Safety Improvements
* Entity reservation uses a normal world reference instead of unsafe transmute
* QuerySets no longer transmute lifetimes
* Made traits "unsafe" where relevant
* More thorough safety docs
* WorldCell
* Exposes safe mutable access to multiple resources at a time in a World
* Replaced "catch all" `System::update_archetypes(world: &World)` with `System::new_archetype(archetype: &Archetype)`
* Simpler Bundle implementation
* Replaced slow "remove_bundle_one_by_one" used as fallback for Commands::remove_bundle with fast "remove_bundle_intersection"
* Removed `Mut<T>` query impl. it is better to only support one way: `&mut T`
* Removed with() from `Flags<T>` in favor of `Option<Flags<T>>`, which allows querying for flags to be "filtered" by default
* Components now have is_send property (currently only resources support non-send)
* More granular module organization
* New `RemovedComponents<T>` SystemParam that replaces `query.removed::<T>()`
* `world.resource_scope()` for mutable access to resources and world at the same time
* WorldQuery and QueryFilter traits unified. FilterFetch trait added to enable "short circuit" filtering. Auto impled for cases that don't need it
* Significantly slimmed down SystemState in favor of individual SystemParam state
* System Commands changed from `commands: &mut Commands` back to `mut commands: Commands` (to allow Commands to have a World reference)
Fixes #1320
## `World` Rewrite
This is a from-scratch rewrite of `World` that fills the niche that `hecs` used to. Yes, this means Bevy ECS is no longer a "fork" of hecs. We're going out our own!
(the only shared code between the projects is the entity id allocator, which is already basically ideal)
A huge shout out to @SanderMertens (author of [flecs](https://github.com/SanderMertens/flecs)) for sharing some great ideas with me (specifically hybrid ecs storage and archetype graphs). He also helped advise on a number of implementation details.
## Component Storage (The Problem)
Two ECS storage paradigms have gained a lot of traction over the years:
* **Archetypal ECS**:
* Stores components in "tables" with static schemas. Each "column" stores components of a given type. Each "row" is an entity.
* Each "archetype" has its own table. Adding/removing an entity's component changes the archetype.
* Enables super-fast Query iteration due to its cache-friendly data layout
* Comes at the cost of more expensive add/remove operations for an Entity's components, because all components need to be copied to the new archetype's "table"
* **Sparse Set ECS**:
* Stores components of the same type in densely packed arrays, which are sparsely indexed by densely packed unsigned integers (Entity ids)
* Query iteration is slower than Archetypal ECS because each entity's component could be at any position in the sparse set. This "random access" pattern isn't cache friendly. Additionally, there is an extra layer of indirection because you must first map the entity id to an index in the component array.
* Adding/removing components is a cheap, constant time operation
Bevy ECS V1, hecs, legion, flec, and Unity DOTS are all "archetypal ecs-es". I personally think "archetypal" storage is a good default for game engines. An entity's archetype doesn't need to change frequently in general, and it creates "fast by default" query iteration (which is a much more common operation). It is also "self optimizing". Users don't need to think about optimizing component layouts for iteration performance. It "just works" without any extra boilerplate.
Shipyard and EnTT are "sparse set ecs-es". They employ "packing" as a way to work around the "suboptimal by default" iteration performance for specific sets of components. This helps, but I didn't think this was a good choice for a general purpose engine like Bevy because:
1. "packs" conflict with each other. If bevy decides to internally pack the Transform and GlobalTransform components, users are then blocked if they want to pack some custom component with Transform.
2. users need to take manual action to optimize
Developers selecting an ECS framework are stuck with a hard choice. Select an "archetypal" framework with "fast iteration everywhere" but without the ability to cheaply add/remove components, or select a "sparse set" framework to cheaply add/remove components but with slower iteration performance.
## Hybrid Component Storage (The Solution)
In Bevy ECS V2, we get to have our cake and eat it too. It now has _both_ of the component storage types above (and more can be added later if needed):
* **Tables** (aka "archetypal" storage)
* The default storage. If you don't configure anything, this is what you get
* Fast iteration by default
* Slower add/remove operations
* **Sparse Sets**
* Opt-in
* Slower iteration
* Faster add/remove operations
These storage types complement each other perfectly. By default Query iteration is fast. If developers know that they want to add/remove a component at high frequencies, they can set the storage to "sparse set":
```rust
world.register_component(
ComponentDescriptor::new::<MyComponent>(StorageType::SparseSet)
).unwrap();
```
## Archetypes
Archetypes are now "just metadata" ... they no longer store components directly. They do store:
* The `ComponentId`s of each of the Archetype's components (and that component's storage type)
* Archetypes are uniquely defined by their component layouts
* For example: entities with "table" components `[A, B, C]` _and_ "sparse set" components `[D, E]` will always be in the same archetype.
* The `TableId` associated with the archetype
* For now each archetype has exactly one table (which can have no components),
* There is a 1->Many relationship from Tables->Archetypes. A given table could have any number of archetype components stored in it:
* Ex: an entity with "table storage" components `[A, B, C]` and "sparse set" components `[D, E]` will share the same `[A, B, C]` table as an entity with `[A, B, C]` table component and `[F]` sparse set components.
* This 1->Many relationship is how we preserve fast "cache friendly" iteration performance when possible (more on this later)
* A list of entities that are in the archetype and the row id of the table they are in
* ArchetypeComponentIds
* unique densely packed identifiers for (ArchetypeId, ComponentId) pairs
* used by the schedule executor for cheap system access control
* "Archetype Graph Edges" (see the next section)
## The "Archetype Graph"
Archetype changes in Bevy (and a number of other archetypal ecs-es) have historically been expensive to compute. First, you need to allocate a new vector of the entity's current component ids, add or remove components based on the operation performed, sort it (to ensure it is order-independent), then hash it to find the archetype (if it exists). And thats all before we get to the _already_ expensive full copy of all components to the new table storage.
The solution is to build a "graph" of archetypes to cache these results. @SanderMertens first exposed me to the idea (and he got it from @gjroelofs, who came up with it). They propose adding directed edges between archetypes for add/remove component operations. If `ComponentId`s are densely packed, you can use sparse sets to cheaply jump between archetypes.
Bevy takes this one step further by using add/remove `Bundle` edges instead of `Component` edges. Bevy encourages the use of `Bundles` to group add/remove operations. This is largely for "clearer game logic" reasons, but it also helps cut down on the number of archetype changes required. `Bundles` now also have densely-packed `BundleId`s. This allows us to use a _single_ edge for each bundle operation (rather than needing to traverse N edges ... one for each component). Single component operations are also bundles, so this is strictly an improvement over a "component only" graph.
As a result, an operation that used to be _heavy_ (both for allocations and compute) is now two dirt-cheap array lookups and zero allocations.
## Stateful Queries
World queries are now stateful. This allows us to:
1. Cache archetype (and table) matches
* This resolves another issue with (naive) archetypal ECS: query performance getting worse as the number of archetypes goes up (and fragmentation occurs).
2. Cache Fetch and Filter state
* The expensive parts of fetch/filter operations (such as hashing the TypeId to find the ComponentId) now only happen once when the Query is first constructed
3. Incrementally build up state
* When new archetypes are added, we only process the new archetypes (no need to rebuild state for old archetypes)
As a result, the direct `World` query api now looks like this:
```rust
let mut query = world.query::<(&A, &mut B)>();
for (a, mut b) in query.iter_mut(&mut world) {
}
```
Requiring `World` to generate stateful queries (rather than letting the `QueryState` type be constructed separately) allows us to ensure that _all_ queries are properly initialized (and the relevant world state, such as ComponentIds). This enables QueryState to remove branches from its operations that check for initialization status (and also enables query.iter() to take an immutable world reference because it doesn't need to initialize anything in world).
However in systems, this is a non-breaking change. State management is done internally by the relevant SystemParam.
## Stateful SystemParams
Like Queries, `SystemParams` now also cache state. For example, `Query` system params store the "stateful query" state mentioned above. Commands store their internal `CommandQueue`. This means you can now safely use as many separate `Commands` parameters in your system as you want. `Local<T>` system params store their `T` value in their state (instead of in Resources).
SystemParam state also enabled a significant slim-down of SystemState. It is much nicer to look at now.
Per-SystemParam state naturally insulates us from an "aliased mut" class of errors we have hit in the past (ex: using multiple `Commands` system params).
(credit goes to @DJMcNab for the initial idea and draft pr here #1364)
## Configurable SystemParams
@DJMcNab also had the great idea to make SystemParams configurable. This allows users to provide some initial configuration / values for system parameters (when possible). Most SystemParams have no config (the config type is `()`), but the `Local<T>` param now supports user-provided parameters:
```rust
fn foo(value: Local<usize>) {
}
app.add_system(foo.system().config(|c| c.0 = Some(10)));
```
## Uber Fast "for_each" Query Iterators
Developers now have the choice to use a fast "for_each" iterator, which yields ~1.5-3x iteration speed improvements for "fragmented iteration", and minor ~1.2x iteration speed improvements for unfragmented iteration.
```rust
fn system(query: Query<(&A, &mut B)>) {
// you now have the option to do this for a speed boost
query.for_each_mut(|(a, mut b)| {
});
// however normal iterators are still available
for (a, mut b) in query.iter_mut() {
}
}
```
I think in most cases we should continue to encourage "normal" iterators as they are more flexible and more "rust idiomatic". But when that extra "oomf" is needed, it makes sense to use `for_each`.
We should also consider using `for_each` for internal bevy systems to give our users a nice speed boost (but that should be a separate pr).
## Component Metadata
`World` now has a `Components` collection, which is accessible via `world.components()`. This stores mappings from `ComponentId` to `ComponentInfo`, as well as `TypeId` to `ComponentId` mappings (where relevant). `ComponentInfo` stores information about the component, such as ComponentId, TypeId, memory layout, send-ness (currently limited to resources), and storage type.
## Significantly Cheaper `Access<T>`
We used to use `TypeAccess<TypeId>` to manage read/write component/archetype-component access. This was expensive because TypeIds must be hashed and compared individually. The parallel executor got around this by "condensing" type ids into bitset-backed access types. This worked, but it had to be re-generated from the `TypeAccess<TypeId>`sources every time archetypes changed.
This pr removes TypeAccess in favor of faster bitset access everywhere. We can do this thanks to the move to densely packed `ComponentId`s and `ArchetypeComponentId`s.
## Merged Resources into World
Resources had a lot of redundant functionality with Components. They stored typed data, they had access control, they had unique ids, they were queryable via SystemParams, etc. In fact the _only_ major difference between them was that they were unique (and didn't correlate to an entity).
Separate resources also had the downside of requiring a separate set of access controls, which meant the parallel executor needed to compare more bitsets per system and manage more state.
I initially got the "separate resources" idea from `legion`. I think that design was motivated by the fact that it made the direct world query/resource lifetime interactions more manageable. It certainly made our lives easier when using Resources alongside hecs/bevy_ecs. However we already have a construct for safely and ergonomically managing in-world lifetimes: systems (which use `Access<T>` internally).
This pr merges Resources into World:
```rust
world.insert_resource(1);
world.insert_resource(2.0);
let a = world.get_resource::<i32>().unwrap();
let mut b = world.get_resource_mut::<f64>().unwrap();
*b = 3.0;
```
Resources are now just a special kind of component. They have their own ComponentIds (and their own resource TypeId->ComponentId scope, so they don't conflict wit components of the same type). They are stored in a special "resource archetype", which stores components inside the archetype using a new `unique_components` sparse set (note that this sparse set could later be used to implement Tags). This allows us to keep the code size small by reusing existing datastructures (namely Column, Archetype, ComponentFlags, and ComponentInfo). This allows us the executor to use a single `Access<ArchetypeComponentId>` per system. It should also make scripting language integration easier.
_But_ this merge did create problems for people directly interacting with `World`. What if you need mutable access to multiple resources at the same time? `world.get_resource_mut()` borrows World mutably!
## WorldCell
WorldCell applies the `Access<ArchetypeComponentId>` concept to direct world access:
```rust
let world_cell = world.cell();
let a = world_cell.get_resource_mut::<i32>().unwrap();
let b = world_cell.get_resource_mut::<f64>().unwrap();
```
This adds cheap runtime checks (a sparse set lookup of `ArchetypeComponentId` and a counter) to ensure that world accesses do not conflict with each other. Each operation returns a `WorldBorrow<'w, T>` or `WorldBorrowMut<'w, T>` wrapper type, which will release the relevant ArchetypeComponentId resources when dropped.
World caches the access sparse set (and only one cell can exist at a time), so `world.cell()` is a cheap operation.
WorldCell does _not_ use atomic operations. It is non-send, does a mutable borrow of world to prevent other accesses, and uses a simple `Rc<RefCell<ArchetypeComponentAccess>>` wrapper in each WorldBorrow pointer.
The api is currently limited to resource access, but it can and should be extended to queries / entity component access.
## Resource Scopes
WorldCell does not yet support component queries, and even when it does there are sometimes legitimate reasons to want a mutable world ref _and_ a mutable resource ref (ex: bevy_render and bevy_scene both need this). In these cases we could always drop down to the unsafe `world.get_resource_unchecked_mut()`, but that is not ideal!
Instead developers can use a "resource scope"
```rust
world.resource_scope(|world: &mut World, a: &mut A| {
})
```
This temporarily removes the `A` resource from `World`, provides mutable pointers to both, and re-adds A to World when finished. Thanks to the move to ComponentIds/sparse sets, this is a cheap operation.
If multiple resources are required, scopes can be nested. We could also consider adding a "resource tuple" to the api if this pattern becomes common and the boilerplate gets nasty.
## Query Conflicts Use ComponentId Instead of ArchetypeComponentId
For safety reasons, systems cannot contain queries that conflict with each other without wrapping them in a QuerySet. On bevy `main`, we use ArchetypeComponentIds to determine conflicts. This is nice because it can take into account filters:
```rust
// these queries will never conflict due to their filters
fn filter_system(a: Query<&mut A, With<B>>, b: Query<&mut B, Without<B>>) {
}
```
But it also has a significant downside:
```rust
// these queries will not conflict _until_ an entity with A, B, and C is spawned
fn maybe_conflicts_system(a: Query<(&mut A, &C)>, b: Query<(&mut A, &B)>) {
}
```
The system above will panic at runtime if an entity with A, B, and C is spawned. This makes it hard to trust that your game logic will run without crashing.
In this pr, I switched to using `ComponentId` instead. This _is_ more constraining. `maybe_conflicts_system` will now always fail, but it will do it consistently at startup. Naively, it would also _disallow_ `filter_system`, which would be a significant downgrade in usability. Bevy has a number of internal systems that rely on disjoint queries and I expect it to be a common pattern in userspace.
To resolve this, I added a new `FilteredAccess<T>` type, which wraps `Access<T>` and adds with/without filters. If two `FilteredAccess` have with/without values that prove they are disjoint, they will no longer conflict.
## EntityRef / EntityMut
World entity operations on `main` require that the user passes in an `entity` id to each operation:
```rust
let entity = world.spawn((A, )); // create a new entity with A
world.get::<A>(entity);
world.insert(entity, (B, C));
world.insert_one(entity, D);
```
This means that each operation needs to look up the entity location / verify its validity. The initial spawn operation also requires a Bundle as input. This can be awkward when no components are required (or one component is required).
These operations have been replaced by `EntityRef` and `EntityMut`, which are "builder-style" wrappers around world that provide read and read/write operations on a single, pre-validated entity:
```rust
// spawn now takes no inputs and returns an EntityMut
let entity = world.spawn()
.insert(A) // insert a single component into the entity
.insert_bundle((B, C)) // insert a bundle of components into the entity
.id() // id returns the Entity id
// Returns EntityMut (or panics if the entity does not exist)
world.entity_mut(entity)
.insert(D)
.insert_bundle(SomeBundle::default());
{
// returns EntityRef (or panics if the entity does not exist)
let d = world.entity(entity)
.get::<D>() // gets the D component
.unwrap();
// world.get still exists for ergonomics
let d = world.get::<D>(entity).unwrap();
}
// These variants return Options if you want to check existence instead of panicing
world.get_entity_mut(entity)
.unwrap()
.insert(E);
if let Some(entity_ref) = world.get_entity(entity) {
let d = entity_ref.get::<D>().unwrap();
}
```
This _does not_ affect the current Commands api or terminology. I think that should be a separate conversation as that is a much larger breaking change.
## Safety Improvements
* Entity reservation in Commands uses a normal world borrow instead of an unsafe transmute
* QuerySets no longer transmutes lifetimes
* Made traits "unsafe" when implementing a trait incorrectly could cause unsafety
* More thorough safety docs
## RemovedComponents SystemParam
The old approach to querying removed components: `query.removed:<T>()` was confusing because it had no connection to the query itself. I replaced it with the following, which is both clearer and allows us to cache the ComponentId mapping in the SystemParamState:
```rust
fn system(removed: RemovedComponents<T>) {
for entity in removed.iter() {
}
}
```
## Simpler Bundle implementation
Bundles are no longer responsible for sorting (or deduping) TypeInfo. They are just a simple ordered list of component types / data. This makes the implementation smaller and opens the door to an easy "nested bundle" implementation in the future (which i might even add in this pr). Duplicate detection is now done once per bundle type by World the first time a bundle is used.
## Unified WorldQuery and QueryFilter types
(don't worry they are still separate type _parameters_ in Queries .. this is a non-breaking change)
WorldQuery and QueryFilter were already basically identical apis. With the addition of `FetchState` and more storage-specific fetch methods, the overlap was even clearer (and the redundancy more painful).
QueryFilters are now just `F: WorldQuery where F::Fetch: FilterFetch`. FilterFetch requires `Fetch<Item = bool>` and adds new "short circuit" variants of fetch methods. This enables a filter tuple like `(With<A>, Without<B>, Changed<C>)` to stop evaluating the filter after the first mismatch is encountered. FilterFetch is automatically implemented for `Fetch` implementations that return bool.
This forces fetch implementations that return things like `(bool, bool, bool)` (such as the filter above) to manually implement FilterFetch and decide whether or not to short-circuit.
## More Granular Modules
World no longer globs all of the internal modules together. It now exports `core`, `system`, and `schedule` separately. I'm also considering exporting `core` submodules directly as that is still pretty "glob-ey" and unorganized (feedback welcome here).
## Remaining Draft Work (to be done in this pr)
* ~~panic on conflicting WorldQuery fetches (&A, &mut A)~~
* ~~bevy `main` and hecs both currently allow this, but we should protect against it if possible~~
* ~~batch_iter / par_iter (currently stubbed out)~~
* ~~ChangedRes~~
* ~~I skipped this while we sort out #1313. This pr should be adapted to account for whatever we land on there~~.
* ~~The `Archetypes` and `Tables` collections use hashes of sorted lists of component ids to uniquely identify each archetype/table. This hash is then used as the key in a HashMap to look up the relevant ArchetypeId or TableId. (which doesn't handle hash collisions properly)~~
* ~~It is currently unsafe to generate a Query from "World A", then use it on "World B" (despite the api claiming it is safe). We should probably close this gap. This could be done by adding a randomly generated WorldId to each world, then storing that id in each Query. They could then be compared to each other on each `query.do_thing(&world)` operation. This _does_ add an extra branch to each query operation, so I'm open to other suggestions if people have them.~~
* ~~Nested Bundles (if i find time)~~
## Potential Future Work
* Expand WorldCell to support queries.
* Consider not allocating in the empty archetype on `world.spawn()`
* ex: return something like EntityMutUninit, which turns into EntityMut after an `insert` or `insert_bundle` op
* this actually regressed performance last time i tried it, but in theory it should be faster
* Optimize SparseSet::insert (see `PERF` comment on insert)
* Replace SparseArray `Option<T>` with T::MAX to cut down on branching
* would enable cheaper get_unchecked() operations
* upstream fixedbitset optimizations
* fixedbitset could be allocation free for small block counts (store blocks in a SmallVec)
* fixedbitset could have a const constructor
* Consider implementing Tags (archetype-specific by-value data that affects archetype identity)
* ex: ArchetypeA could have `[A, B, C]` table components and `[D(1)]` "tag" component. ArchetypeB could have `[A, B, C]` table components and a `[D(2)]` tag component. The archetypes are different, despite both having D tags because the value inside D is different.
* this could potentially build on top of the `archetype.unique_components` added in this pr for resource storage.
* Consider reverting `all_tuples` proc macro in favor of the old `macro_rules` implementation
* all_tuples is more flexible and produces cleaner documentation (the macro_rules version produces weird type parameter orders due to parser constraints)
* but unfortunately all_tuples also appears to make Rust Analyzer sad/slow when working inside of `bevy_ecs` (does not affect user code)
* Consider "resource queries" and/or "mixed resource and entity component queries" as an alternative to WorldCell
* this is basically just "systems" so maybe it's not worth it
* Add more world ops
* `world.clear()`
* `world.reserve<T: Bundle>(count: usize)`
* Try using the old archetype allocation strategy (allocate new memory on resize and copy everything over). I expect this to improve batch insertion performance at the cost of unbatched performance. But thats just a guess. I'm not an allocation perf pro :)
* Adapt Commands apis for consistency with new World apis
## Benchmarks
key:
* `bevy_old`: bevy `main` branch
* `bevy`: this branch
* `_foreach`: uses an optimized for_each iterator
* ` _sparse`: uses sparse set storage (if unspecified assume table storage)
* `_system`: runs inside a system (if unspecified assume test happens via direct world ops)
### Simple Insert (from ecs_bench_suite)
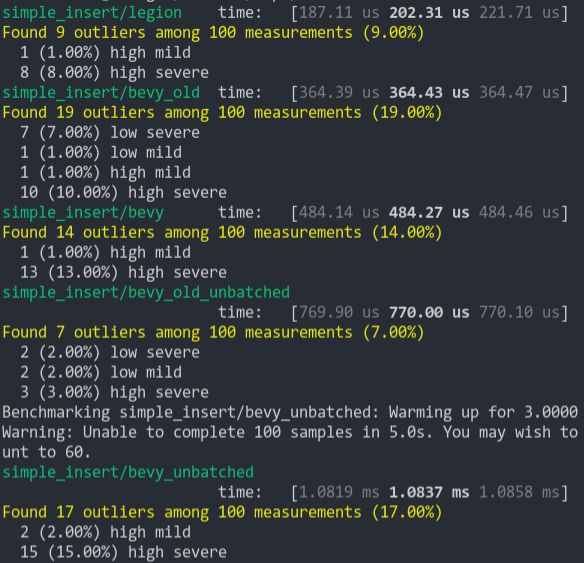
### Simpler Iter (from ecs_bench_suite)
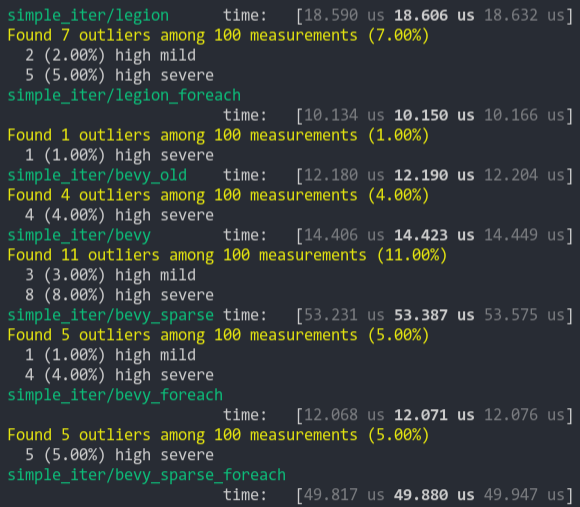
### Fragment Iter (from ecs_bench_suite)
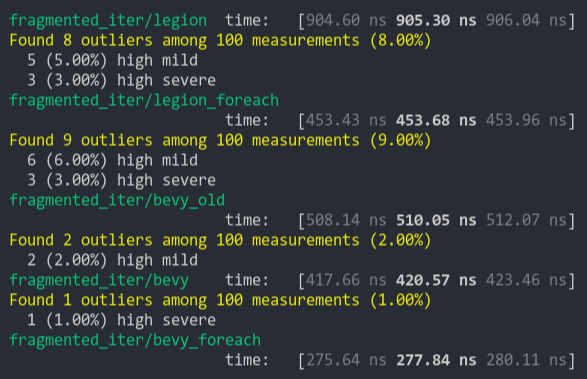
### Sparse Fragmented Iter
Iterate a query that matches 5 entities from a single matching archetype, but there are 100 unmatching archetypes
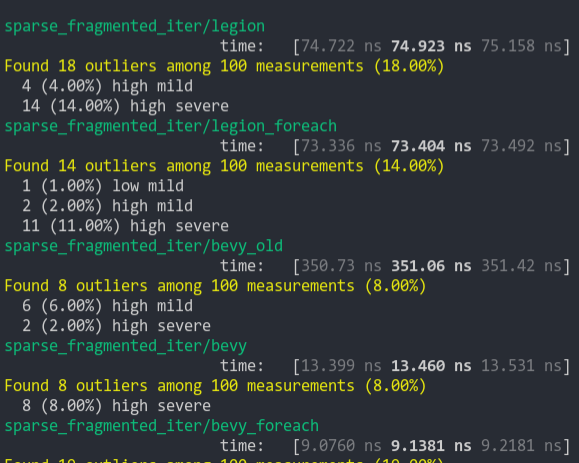
### Schedule (from ecs_bench_suite)
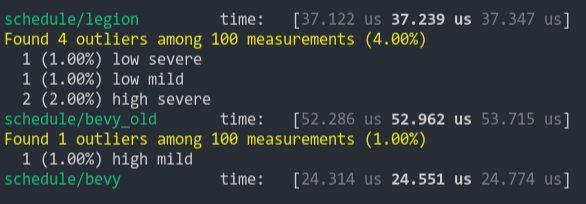
### Add Remove Component (from ecs_bench_suite)
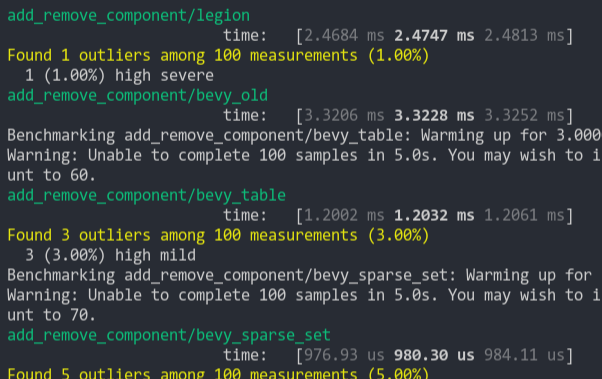
### Add Remove Component Big
Same as the test above, but each entity has 5 "large" matrix components and 1 "large" matrix component is added and removed
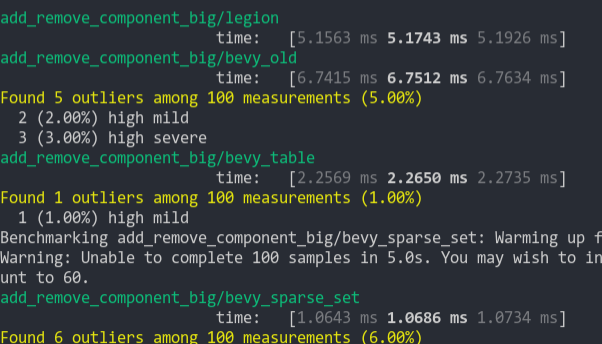
### Get Component
Looks up a single component value a large number of times
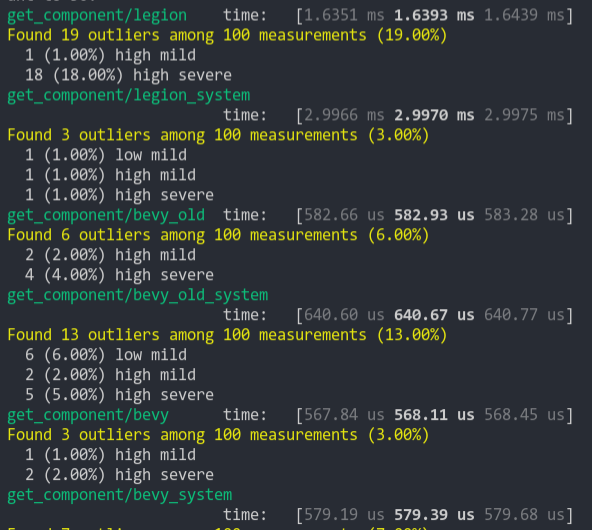
2021-03-05 07:54:35 +00:00
2022-10-24 13:46:36 +00:00
let archetype_component_id = world
. get_resource_archetype_component_id ( component_id )
Bevy ECS V2 (#1525)
# Bevy ECS V2
This is a rewrite of Bevy ECS (basically everything but the new executor/schedule, which are already awesome). The overall goal was to improve the performance and versatility of Bevy ECS. Here is a quick bulleted list of changes before we dive into the details:
* Complete World rewrite
* Multiple component storage types:
* Tables: fast cache friendly iteration, slower add/removes (previously called Archetypes)
* Sparse Sets: fast add/remove, slower iteration
* Stateful Queries (caches query results for faster iteration. fragmented iteration is _fast_ now)
* Stateful System Params (caches expensive operations. inspired by @DJMcNab's work in #1364)
* Configurable System Params (users can set configuration when they construct their systems. once again inspired by @DJMcNab's work)
* Archetypes are now "just metadata", component storage is separate
* Archetype Graph (for faster archetype changes)
* Component Metadata
* Configure component storage type
* Retrieve information about component size/type/name/layout/send-ness/etc
* Components are uniquely identified by a densely packed ComponentId
* TypeIds are now totally optional (which should make implementing scripting easier)
* Super fast "for_each" query iterators
* Merged Resources into World. Resources are now just a special type of component
* EntityRef/EntityMut builder apis (more efficient and more ergonomic)
* Fast bitset-backed `Access<T>` replaces old hashmap-based approach everywhere
* Query conflicts are determined by component access instead of archetype component access (to avoid random failures at runtime)
* With/Without are still taken into account for conflicts, so this should still be comfy to use
* Much simpler `IntoSystem` impl
* Significantly reduced the amount of hashing throughout the ecs in favor of Sparse Sets (indexed by densely packed ArchetypeId, ComponentId, BundleId, and TableId)
* Safety Improvements
* Entity reservation uses a normal world reference instead of unsafe transmute
* QuerySets no longer transmute lifetimes
* Made traits "unsafe" where relevant
* More thorough safety docs
* WorldCell
* Exposes safe mutable access to multiple resources at a time in a World
* Replaced "catch all" `System::update_archetypes(world: &World)` with `System::new_archetype(archetype: &Archetype)`
* Simpler Bundle implementation
* Replaced slow "remove_bundle_one_by_one" used as fallback for Commands::remove_bundle with fast "remove_bundle_intersection"
* Removed `Mut<T>` query impl. it is better to only support one way: `&mut T`
* Removed with() from `Flags<T>` in favor of `Option<Flags<T>>`, which allows querying for flags to be "filtered" by default
* Components now have is_send property (currently only resources support non-send)
* More granular module organization
* New `RemovedComponents<T>` SystemParam that replaces `query.removed::<T>()`
* `world.resource_scope()` for mutable access to resources and world at the same time
* WorldQuery and QueryFilter traits unified. FilterFetch trait added to enable "short circuit" filtering. Auto impled for cases that don't need it
* Significantly slimmed down SystemState in favor of individual SystemParam state
* System Commands changed from `commands: &mut Commands` back to `mut commands: Commands` (to allow Commands to have a World reference)
Fixes #1320
## `World` Rewrite
This is a from-scratch rewrite of `World` that fills the niche that `hecs` used to. Yes, this means Bevy ECS is no longer a "fork" of hecs. We're going out our own!
(the only shared code between the projects is the entity id allocator, which is already basically ideal)
A huge shout out to @SanderMertens (author of [flecs](https://github.com/SanderMertens/flecs)) for sharing some great ideas with me (specifically hybrid ecs storage and archetype graphs). He also helped advise on a number of implementation details.
## Component Storage (The Problem)
Two ECS storage paradigms have gained a lot of traction over the years:
* **Archetypal ECS**:
* Stores components in "tables" with static schemas. Each "column" stores components of a given type. Each "row" is an entity.
* Each "archetype" has its own table. Adding/removing an entity's component changes the archetype.
* Enables super-fast Query iteration due to its cache-friendly data layout
* Comes at the cost of more expensive add/remove operations for an Entity's components, because all components need to be copied to the new archetype's "table"
* **Sparse Set ECS**:
* Stores components of the same type in densely packed arrays, which are sparsely indexed by densely packed unsigned integers (Entity ids)
* Query iteration is slower than Archetypal ECS because each entity's component could be at any position in the sparse set. This "random access" pattern isn't cache friendly. Additionally, there is an extra layer of indirection because you must first map the entity id to an index in the component array.
* Adding/removing components is a cheap, constant time operation
Bevy ECS V1, hecs, legion, flec, and Unity DOTS are all "archetypal ecs-es". I personally think "archetypal" storage is a good default for game engines. An entity's archetype doesn't need to change frequently in general, and it creates "fast by default" query iteration (which is a much more common operation). It is also "self optimizing". Users don't need to think about optimizing component layouts for iteration performance. It "just works" without any extra boilerplate.
Shipyard and EnTT are "sparse set ecs-es". They employ "packing" as a way to work around the "suboptimal by default" iteration performance for specific sets of components. This helps, but I didn't think this was a good choice for a general purpose engine like Bevy because:
1. "packs" conflict with each other. If bevy decides to internally pack the Transform and GlobalTransform components, users are then blocked if they want to pack some custom component with Transform.
2. users need to take manual action to optimize
Developers selecting an ECS framework are stuck with a hard choice. Select an "archetypal" framework with "fast iteration everywhere" but without the ability to cheaply add/remove components, or select a "sparse set" framework to cheaply add/remove components but with slower iteration performance.
## Hybrid Component Storage (The Solution)
In Bevy ECS V2, we get to have our cake and eat it too. It now has _both_ of the component storage types above (and more can be added later if needed):
* **Tables** (aka "archetypal" storage)
* The default storage. If you don't configure anything, this is what you get
* Fast iteration by default
* Slower add/remove operations
* **Sparse Sets**
* Opt-in
* Slower iteration
* Faster add/remove operations
These storage types complement each other perfectly. By default Query iteration is fast. If developers know that they want to add/remove a component at high frequencies, they can set the storage to "sparse set":
```rust
world.register_component(
ComponentDescriptor::new::<MyComponent>(StorageType::SparseSet)
).unwrap();
```
## Archetypes
Archetypes are now "just metadata" ... they no longer store components directly. They do store:
* The `ComponentId`s of each of the Archetype's components (and that component's storage type)
* Archetypes are uniquely defined by their component layouts
* For example: entities with "table" components `[A, B, C]` _and_ "sparse set" components `[D, E]` will always be in the same archetype.
* The `TableId` associated with the archetype
* For now each archetype has exactly one table (which can have no components),
* There is a 1->Many relationship from Tables->Archetypes. A given table could have any number of archetype components stored in it:
* Ex: an entity with "table storage" components `[A, B, C]` and "sparse set" components `[D, E]` will share the same `[A, B, C]` table as an entity with `[A, B, C]` table component and `[F]` sparse set components.
* This 1->Many relationship is how we preserve fast "cache friendly" iteration performance when possible (more on this later)
* A list of entities that are in the archetype and the row id of the table they are in
* ArchetypeComponentIds
* unique densely packed identifiers for (ArchetypeId, ComponentId) pairs
* used by the schedule executor for cheap system access control
* "Archetype Graph Edges" (see the next section)
## The "Archetype Graph"
Archetype changes in Bevy (and a number of other archetypal ecs-es) have historically been expensive to compute. First, you need to allocate a new vector of the entity's current component ids, add or remove components based on the operation performed, sort it (to ensure it is order-independent), then hash it to find the archetype (if it exists). And thats all before we get to the _already_ expensive full copy of all components to the new table storage.
The solution is to build a "graph" of archetypes to cache these results. @SanderMertens first exposed me to the idea (and he got it from @gjroelofs, who came up with it). They propose adding directed edges between archetypes for add/remove component operations. If `ComponentId`s are densely packed, you can use sparse sets to cheaply jump between archetypes.
Bevy takes this one step further by using add/remove `Bundle` edges instead of `Component` edges. Bevy encourages the use of `Bundles` to group add/remove operations. This is largely for "clearer game logic" reasons, but it also helps cut down on the number of archetype changes required. `Bundles` now also have densely-packed `BundleId`s. This allows us to use a _single_ edge for each bundle operation (rather than needing to traverse N edges ... one for each component). Single component operations are also bundles, so this is strictly an improvement over a "component only" graph.
As a result, an operation that used to be _heavy_ (both for allocations and compute) is now two dirt-cheap array lookups and zero allocations.
## Stateful Queries
World queries are now stateful. This allows us to:
1. Cache archetype (and table) matches
* This resolves another issue with (naive) archetypal ECS: query performance getting worse as the number of archetypes goes up (and fragmentation occurs).
2. Cache Fetch and Filter state
* The expensive parts of fetch/filter operations (such as hashing the TypeId to find the ComponentId) now only happen once when the Query is first constructed
3. Incrementally build up state
* When new archetypes are added, we only process the new archetypes (no need to rebuild state for old archetypes)
As a result, the direct `World` query api now looks like this:
```rust
let mut query = world.query::<(&A, &mut B)>();
for (a, mut b) in query.iter_mut(&mut world) {
}
```
Requiring `World` to generate stateful queries (rather than letting the `QueryState` type be constructed separately) allows us to ensure that _all_ queries are properly initialized (and the relevant world state, such as ComponentIds). This enables QueryState to remove branches from its operations that check for initialization status (and also enables query.iter() to take an immutable world reference because it doesn't need to initialize anything in world).
However in systems, this is a non-breaking change. State management is done internally by the relevant SystemParam.
## Stateful SystemParams
Like Queries, `SystemParams` now also cache state. For example, `Query` system params store the "stateful query" state mentioned above. Commands store their internal `CommandQueue`. This means you can now safely use as many separate `Commands` parameters in your system as you want. `Local<T>` system params store their `T` value in their state (instead of in Resources).
SystemParam state also enabled a significant slim-down of SystemState. It is much nicer to look at now.
Per-SystemParam state naturally insulates us from an "aliased mut" class of errors we have hit in the past (ex: using multiple `Commands` system params).
(credit goes to @DJMcNab for the initial idea and draft pr here #1364)
## Configurable SystemParams
@DJMcNab also had the great idea to make SystemParams configurable. This allows users to provide some initial configuration / values for system parameters (when possible). Most SystemParams have no config (the config type is `()`), but the `Local<T>` param now supports user-provided parameters:
```rust
fn foo(value: Local<usize>) {
}
app.add_system(foo.system().config(|c| c.0 = Some(10)));
```
## Uber Fast "for_each" Query Iterators
Developers now have the choice to use a fast "for_each" iterator, which yields ~1.5-3x iteration speed improvements for "fragmented iteration", and minor ~1.2x iteration speed improvements for unfragmented iteration.
```rust
fn system(query: Query<(&A, &mut B)>) {
// you now have the option to do this for a speed boost
query.for_each_mut(|(a, mut b)| {
});
// however normal iterators are still available
for (a, mut b) in query.iter_mut() {
}
}
```
I think in most cases we should continue to encourage "normal" iterators as they are more flexible and more "rust idiomatic". But when that extra "oomf" is needed, it makes sense to use `for_each`.
We should also consider using `for_each` for internal bevy systems to give our users a nice speed boost (but that should be a separate pr).
## Component Metadata
`World` now has a `Components` collection, which is accessible via `world.components()`. This stores mappings from `ComponentId` to `ComponentInfo`, as well as `TypeId` to `ComponentId` mappings (where relevant). `ComponentInfo` stores information about the component, such as ComponentId, TypeId, memory layout, send-ness (currently limited to resources), and storage type.
## Significantly Cheaper `Access<T>`
We used to use `TypeAccess<TypeId>` to manage read/write component/archetype-component access. This was expensive because TypeIds must be hashed and compared individually. The parallel executor got around this by "condensing" type ids into bitset-backed access types. This worked, but it had to be re-generated from the `TypeAccess<TypeId>`sources every time archetypes changed.
This pr removes TypeAccess in favor of faster bitset access everywhere. We can do this thanks to the move to densely packed `ComponentId`s and `ArchetypeComponentId`s.
## Merged Resources into World
Resources had a lot of redundant functionality with Components. They stored typed data, they had access control, they had unique ids, they were queryable via SystemParams, etc. In fact the _only_ major difference between them was that they were unique (and didn't correlate to an entity).
Separate resources also had the downside of requiring a separate set of access controls, which meant the parallel executor needed to compare more bitsets per system and manage more state.
I initially got the "separate resources" idea from `legion`. I think that design was motivated by the fact that it made the direct world query/resource lifetime interactions more manageable. It certainly made our lives easier when using Resources alongside hecs/bevy_ecs. However we already have a construct for safely and ergonomically managing in-world lifetimes: systems (which use `Access<T>` internally).
This pr merges Resources into World:
```rust
world.insert_resource(1);
world.insert_resource(2.0);
let a = world.get_resource::<i32>().unwrap();
let mut b = world.get_resource_mut::<f64>().unwrap();
*b = 3.0;
```
Resources are now just a special kind of component. They have their own ComponentIds (and their own resource TypeId->ComponentId scope, so they don't conflict wit components of the same type). They are stored in a special "resource archetype", which stores components inside the archetype using a new `unique_components` sparse set (note that this sparse set could later be used to implement Tags). This allows us to keep the code size small by reusing existing datastructures (namely Column, Archetype, ComponentFlags, and ComponentInfo). This allows us the executor to use a single `Access<ArchetypeComponentId>` per system. It should also make scripting language integration easier.
_But_ this merge did create problems for people directly interacting with `World`. What if you need mutable access to multiple resources at the same time? `world.get_resource_mut()` borrows World mutably!
## WorldCell
WorldCell applies the `Access<ArchetypeComponentId>` concept to direct world access:
```rust
let world_cell = world.cell();
let a = world_cell.get_resource_mut::<i32>().unwrap();
let b = world_cell.get_resource_mut::<f64>().unwrap();
```
This adds cheap runtime checks (a sparse set lookup of `ArchetypeComponentId` and a counter) to ensure that world accesses do not conflict with each other. Each operation returns a `WorldBorrow<'w, T>` or `WorldBorrowMut<'w, T>` wrapper type, which will release the relevant ArchetypeComponentId resources when dropped.
World caches the access sparse set (and only one cell can exist at a time), so `world.cell()` is a cheap operation.
WorldCell does _not_ use atomic operations. It is non-send, does a mutable borrow of world to prevent other accesses, and uses a simple `Rc<RefCell<ArchetypeComponentAccess>>` wrapper in each WorldBorrow pointer.
The api is currently limited to resource access, but it can and should be extended to queries / entity component access.
## Resource Scopes
WorldCell does not yet support component queries, and even when it does there are sometimes legitimate reasons to want a mutable world ref _and_ a mutable resource ref (ex: bevy_render and bevy_scene both need this). In these cases we could always drop down to the unsafe `world.get_resource_unchecked_mut()`, but that is not ideal!
Instead developers can use a "resource scope"
```rust
world.resource_scope(|world: &mut World, a: &mut A| {
})
```
This temporarily removes the `A` resource from `World`, provides mutable pointers to both, and re-adds A to World when finished. Thanks to the move to ComponentIds/sparse sets, this is a cheap operation.
If multiple resources are required, scopes can be nested. We could also consider adding a "resource tuple" to the api if this pattern becomes common and the boilerplate gets nasty.
## Query Conflicts Use ComponentId Instead of ArchetypeComponentId
For safety reasons, systems cannot contain queries that conflict with each other without wrapping them in a QuerySet. On bevy `main`, we use ArchetypeComponentIds to determine conflicts. This is nice because it can take into account filters:
```rust
// these queries will never conflict due to their filters
fn filter_system(a: Query<&mut A, With<B>>, b: Query<&mut B, Without<B>>) {
}
```
But it also has a significant downside:
```rust
// these queries will not conflict _until_ an entity with A, B, and C is spawned
fn maybe_conflicts_system(a: Query<(&mut A, &C)>, b: Query<(&mut A, &B)>) {
}
```
The system above will panic at runtime if an entity with A, B, and C is spawned. This makes it hard to trust that your game logic will run without crashing.
In this pr, I switched to using `ComponentId` instead. This _is_ more constraining. `maybe_conflicts_system` will now always fail, but it will do it consistently at startup. Naively, it would also _disallow_ `filter_system`, which would be a significant downgrade in usability. Bevy has a number of internal systems that rely on disjoint queries and I expect it to be a common pattern in userspace.
To resolve this, I added a new `FilteredAccess<T>` type, which wraps `Access<T>` and adds with/without filters. If two `FilteredAccess` have with/without values that prove they are disjoint, they will no longer conflict.
## EntityRef / EntityMut
World entity operations on `main` require that the user passes in an `entity` id to each operation:
```rust
let entity = world.spawn((A, )); // create a new entity with A
world.get::<A>(entity);
world.insert(entity, (B, C));
world.insert_one(entity, D);
```
This means that each operation needs to look up the entity location / verify its validity. The initial spawn operation also requires a Bundle as input. This can be awkward when no components are required (or one component is required).
These operations have been replaced by `EntityRef` and `EntityMut`, which are "builder-style" wrappers around world that provide read and read/write operations on a single, pre-validated entity:
```rust
// spawn now takes no inputs and returns an EntityMut
let entity = world.spawn()
.insert(A) // insert a single component into the entity
.insert_bundle((B, C)) // insert a bundle of components into the entity
.id() // id returns the Entity id
// Returns EntityMut (or panics if the entity does not exist)
world.entity_mut(entity)
.insert(D)
.insert_bundle(SomeBundle::default());
{
// returns EntityRef (or panics if the entity does not exist)
let d = world.entity(entity)
.get::<D>() // gets the D component
.unwrap();
// world.get still exists for ergonomics
let d = world.get::<D>(entity).unwrap();
}
// These variants return Options if you want to check existence instead of panicing
world.get_entity_mut(entity)
.unwrap()
.insert(E);
if let Some(entity_ref) = world.get_entity(entity) {
let d = entity_ref.get::<D>().unwrap();
}
```
This _does not_ affect the current Commands api or terminology. I think that should be a separate conversation as that is a much larger breaking change.
## Safety Improvements
* Entity reservation in Commands uses a normal world borrow instead of an unsafe transmute
* QuerySets no longer transmutes lifetimes
* Made traits "unsafe" when implementing a trait incorrectly could cause unsafety
* More thorough safety docs
## RemovedComponents SystemParam
The old approach to querying removed components: `query.removed:<T>()` was confusing because it had no connection to the query itself. I replaced it with the following, which is both clearer and allows us to cache the ComponentId mapping in the SystemParamState:
```rust
fn system(removed: RemovedComponents<T>) {
for entity in removed.iter() {
}
}
```
## Simpler Bundle implementation
Bundles are no longer responsible for sorting (or deduping) TypeInfo. They are just a simple ordered list of component types / data. This makes the implementation smaller and opens the door to an easy "nested bundle" implementation in the future (which i might even add in this pr). Duplicate detection is now done once per bundle type by World the first time a bundle is used.
## Unified WorldQuery and QueryFilter types
(don't worry they are still separate type _parameters_ in Queries .. this is a non-breaking change)
WorldQuery and QueryFilter were already basically identical apis. With the addition of `FetchState` and more storage-specific fetch methods, the overlap was even clearer (and the redundancy more painful).
QueryFilters are now just `F: WorldQuery where F::Fetch: FilterFetch`. FilterFetch requires `Fetch<Item = bool>` and adds new "short circuit" variants of fetch methods. This enables a filter tuple like `(With<A>, Without<B>, Changed<C>)` to stop evaluating the filter after the first mismatch is encountered. FilterFetch is automatically implemented for `Fetch` implementations that return bool.
This forces fetch implementations that return things like `(bool, bool, bool)` (such as the filter above) to manually implement FilterFetch and decide whether or not to short-circuit.
## More Granular Modules
World no longer globs all of the internal modules together. It now exports `core`, `system`, and `schedule` separately. I'm also considering exporting `core` submodules directly as that is still pretty "glob-ey" and unorganized (feedback welcome here).
## Remaining Draft Work (to be done in this pr)
* ~~panic on conflicting WorldQuery fetches (&A, &mut A)~~
* ~~bevy `main` and hecs both currently allow this, but we should protect against it if possible~~
* ~~batch_iter / par_iter (currently stubbed out)~~
* ~~ChangedRes~~
* ~~I skipped this while we sort out #1313. This pr should be adapted to account for whatever we land on there~~.
* ~~The `Archetypes` and `Tables` collections use hashes of sorted lists of component ids to uniquely identify each archetype/table. This hash is then used as the key in a HashMap to look up the relevant ArchetypeId or TableId. (which doesn't handle hash collisions properly)~~
* ~~It is currently unsafe to generate a Query from "World A", then use it on "World B" (despite the api claiming it is safe). We should probably close this gap. This could be done by adding a randomly generated WorldId to each world, then storing that id in each Query. They could then be compared to each other on each `query.do_thing(&world)` operation. This _does_ add an extra branch to each query operation, so I'm open to other suggestions if people have them.~~
* ~~Nested Bundles (if i find time)~~
## Potential Future Work
* Expand WorldCell to support queries.
* Consider not allocating in the empty archetype on `world.spawn()`
* ex: return something like EntityMutUninit, which turns into EntityMut after an `insert` or `insert_bundle` op
* this actually regressed performance last time i tried it, but in theory it should be faster
* Optimize SparseSet::insert (see `PERF` comment on insert)
* Replace SparseArray `Option<T>` with T::MAX to cut down on branching
* would enable cheaper get_unchecked() operations
* upstream fixedbitset optimizations
* fixedbitset could be allocation free for small block counts (store blocks in a SmallVec)
* fixedbitset could have a const constructor
* Consider implementing Tags (archetype-specific by-value data that affects archetype identity)
* ex: ArchetypeA could have `[A, B, C]` table components and `[D(1)]` "tag" component. ArchetypeB could have `[A, B, C]` table components and a `[D(2)]` tag component. The archetypes are different, despite both having D tags because the value inside D is different.
* this could potentially build on top of the `archetype.unique_components` added in this pr for resource storage.
* Consider reverting `all_tuples` proc macro in favor of the old `macro_rules` implementation
* all_tuples is more flexible and produces cleaner documentation (the macro_rules version produces weird type parameter orders due to parser constraints)
* but unfortunately all_tuples also appears to make Rust Analyzer sad/slow when working inside of `bevy_ecs` (does not affect user code)
* Consider "resource queries" and/or "mixed resource and entity component queries" as an alternative to WorldCell
* this is basically just "systems" so maybe it's not worth it
* Add more world ops
* `world.clear()`
* `world.reserve<T: Bundle>(count: usize)`
* Try using the old archetype allocation strategy (allocate new memory on resize and copy everything over). I expect this to improve batch insertion performance at the cost of unbatched performance. But thats just a guess. I'm not an allocation perf pro :)
* Adapt Commands apis for consistency with new World apis
## Benchmarks
key:
* `bevy_old`: bevy `main` branch
* `bevy`: this branch
* `_foreach`: uses an optimized for_each iterator
* ` _sparse`: uses sparse set storage (if unspecified assume table storage)
* `_system`: runs inside a system (if unspecified assume test happens via direct world ops)
### Simple Insert (from ecs_bench_suite)
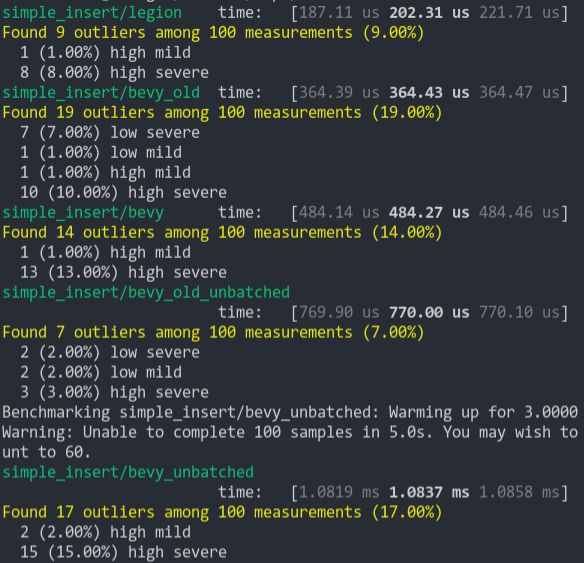
### Simpler Iter (from ecs_bench_suite)
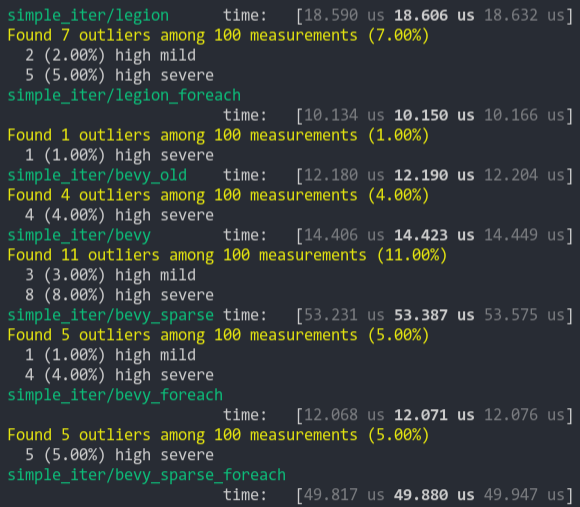
### Fragment Iter (from ecs_bench_suite)
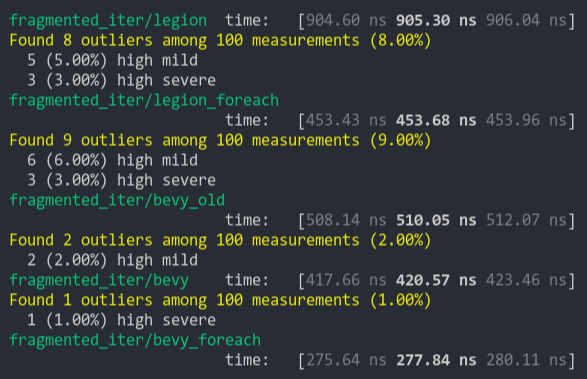
### Sparse Fragmented Iter
Iterate a query that matches 5 entities from a single matching archetype, but there are 100 unmatching archetypes
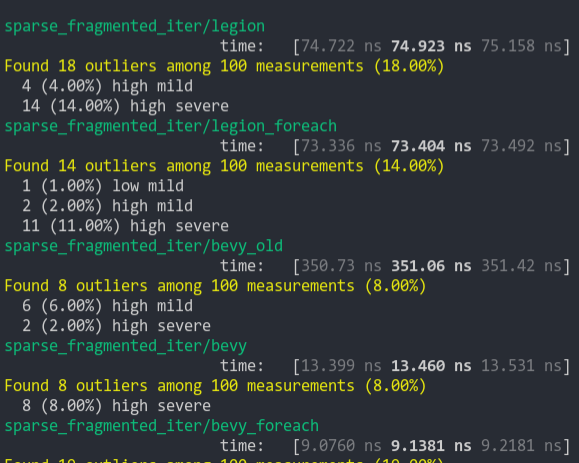
### Schedule (from ecs_bench_suite)
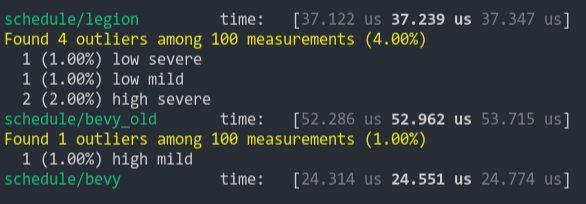
### Add Remove Component (from ecs_bench_suite)
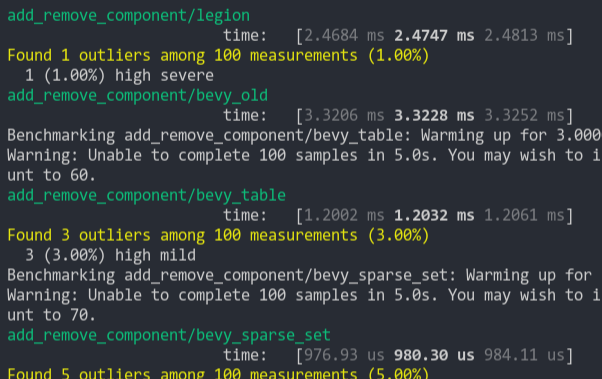
### Add Remove Component Big
Same as the test above, but each entity has 5 "large" matrix components and 1 "large" matrix component is added and removed
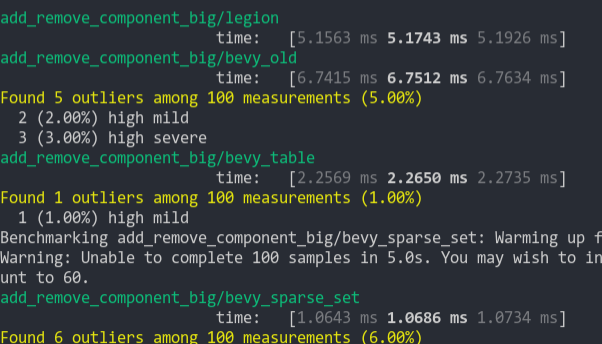
### Get Component
Looks up a single component value a large number of times
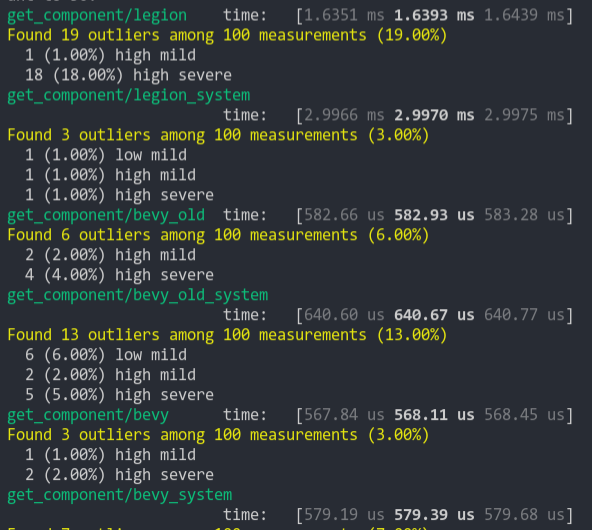
2021-03-05 07:54:35 +00:00
. unwrap ( ) ;
Add new SystemState and rename old SystemState to SystemMeta (#2283)
This enables `SystemParams` to be used outside of function systems. Anything can create and store `SystemState`, which enables efficient "param state cached" access to `SystemParams`.
It adds a `ReadOnlySystemParamFetch` trait, which enables safe `SystemState::get` calls without unique world access.
I renamed the old `SystemState` to `SystemMeta` to enable us to mirror the `QueryState` naming convention (but I'm happy to discuss alternative names if people have other ideas). I initially pitched this as `ParamState`, but given that it needs to include full system metadata, that doesn't feel like a particularly accurate name.
```rust
#[derive(Eq, PartialEq, Debug)]
struct A(usize);
#[derive(Eq, PartialEq, Debug)]
struct B(usize);
let mut world = World::default();
world.insert_resource(A(42));
world.spawn().insert(B(7));
// we get nice lifetime elision when declaring the type on the left hand side
let mut system_state: SystemState<(Res<A>, Query<&B>)> = SystemState::new(&mut world);
let (a, query) = system_state.get(&world);
assert_eq!(*a, A(42), "returned resource matches initial value");
assert_eq!(
*query.single().unwrap(),
B(7),
"returned component matches initial value"
);
// mutable system params require unique world access
let mut system_state: SystemState<(ResMut<A>, Query<&mut B>)> = SystemState::new(&mut world);
let (a, query) = system_state.get_mut(&mut world);
// static lifetimes are required when declaring inside of structs
struct SomeContainer {
state: SystemState<(Res<'static, A>, Res<'static, B>)>
}
// this can be shortened using type aliases, which will be useful for complex param tuples
type MyParams<'a> = (Res<'a, A>, Res<'a, B>);
struct SomeContainer {
state: SystemState<MyParams<'static>>
}
// It is the user's responsibility to call SystemState::apply(world) for parameters that queue up work
let mut system_state: SystemState<(Commands, Query<&B>)> = SystemState::new(&mut world);
{
let (mut commands, query) = system_state.get(&world);
commands.insert_resource(3.14);
}
system_state.apply(&mut world);
```
## Future Work
* Actually use SystemState inside FunctionSystem. This would be trivial, but it requires FunctionSystem to wrap SystemState in Option in its current form (which complicates system metadata lookup). I'd prefer to hold off until we adopt something like the later designs linked in #1364, which enable us to contruct Systems using a World reference (and also remove the need for `.system`).
* Consider a "scoped" approach to automatically call SystemState::apply when systems params are no longer being used (either a container type with a Drop impl, or a function that takes a closure for user logic operating on params).
2021-06-02 19:57:38 +00:00
system_meta
Bevy ECS V2 (#1525)
# Bevy ECS V2
This is a rewrite of Bevy ECS (basically everything but the new executor/schedule, which are already awesome). The overall goal was to improve the performance and versatility of Bevy ECS. Here is a quick bulleted list of changes before we dive into the details:
* Complete World rewrite
* Multiple component storage types:
* Tables: fast cache friendly iteration, slower add/removes (previously called Archetypes)
* Sparse Sets: fast add/remove, slower iteration
* Stateful Queries (caches query results for faster iteration. fragmented iteration is _fast_ now)
* Stateful System Params (caches expensive operations. inspired by @DJMcNab's work in #1364)
* Configurable System Params (users can set configuration when they construct their systems. once again inspired by @DJMcNab's work)
* Archetypes are now "just metadata", component storage is separate
* Archetype Graph (for faster archetype changes)
* Component Metadata
* Configure component storage type
* Retrieve information about component size/type/name/layout/send-ness/etc
* Components are uniquely identified by a densely packed ComponentId
* TypeIds are now totally optional (which should make implementing scripting easier)
* Super fast "for_each" query iterators
* Merged Resources into World. Resources are now just a special type of component
* EntityRef/EntityMut builder apis (more efficient and more ergonomic)
* Fast bitset-backed `Access<T>` replaces old hashmap-based approach everywhere
* Query conflicts are determined by component access instead of archetype component access (to avoid random failures at runtime)
* With/Without are still taken into account for conflicts, so this should still be comfy to use
* Much simpler `IntoSystem` impl
* Significantly reduced the amount of hashing throughout the ecs in favor of Sparse Sets (indexed by densely packed ArchetypeId, ComponentId, BundleId, and TableId)
* Safety Improvements
* Entity reservation uses a normal world reference instead of unsafe transmute
* QuerySets no longer transmute lifetimes
* Made traits "unsafe" where relevant
* More thorough safety docs
* WorldCell
* Exposes safe mutable access to multiple resources at a time in a World
* Replaced "catch all" `System::update_archetypes(world: &World)` with `System::new_archetype(archetype: &Archetype)`
* Simpler Bundle implementation
* Replaced slow "remove_bundle_one_by_one" used as fallback for Commands::remove_bundle with fast "remove_bundle_intersection"
* Removed `Mut<T>` query impl. it is better to only support one way: `&mut T`
* Removed with() from `Flags<T>` in favor of `Option<Flags<T>>`, which allows querying for flags to be "filtered" by default
* Components now have is_send property (currently only resources support non-send)
* More granular module organization
* New `RemovedComponents<T>` SystemParam that replaces `query.removed::<T>()`
* `world.resource_scope()` for mutable access to resources and world at the same time
* WorldQuery and QueryFilter traits unified. FilterFetch trait added to enable "short circuit" filtering. Auto impled for cases that don't need it
* Significantly slimmed down SystemState in favor of individual SystemParam state
* System Commands changed from `commands: &mut Commands` back to `mut commands: Commands` (to allow Commands to have a World reference)
Fixes #1320
## `World` Rewrite
This is a from-scratch rewrite of `World` that fills the niche that `hecs` used to. Yes, this means Bevy ECS is no longer a "fork" of hecs. We're going out our own!
(the only shared code between the projects is the entity id allocator, which is already basically ideal)
A huge shout out to @SanderMertens (author of [flecs](https://github.com/SanderMertens/flecs)) for sharing some great ideas with me (specifically hybrid ecs storage and archetype graphs). He also helped advise on a number of implementation details.
## Component Storage (The Problem)
Two ECS storage paradigms have gained a lot of traction over the years:
* **Archetypal ECS**:
* Stores components in "tables" with static schemas. Each "column" stores components of a given type. Each "row" is an entity.
* Each "archetype" has its own table. Adding/removing an entity's component changes the archetype.
* Enables super-fast Query iteration due to its cache-friendly data layout
* Comes at the cost of more expensive add/remove operations for an Entity's components, because all components need to be copied to the new archetype's "table"
* **Sparse Set ECS**:
* Stores components of the same type in densely packed arrays, which are sparsely indexed by densely packed unsigned integers (Entity ids)
* Query iteration is slower than Archetypal ECS because each entity's component could be at any position in the sparse set. This "random access" pattern isn't cache friendly. Additionally, there is an extra layer of indirection because you must first map the entity id to an index in the component array.
* Adding/removing components is a cheap, constant time operation
Bevy ECS V1, hecs, legion, flec, and Unity DOTS are all "archetypal ecs-es". I personally think "archetypal" storage is a good default for game engines. An entity's archetype doesn't need to change frequently in general, and it creates "fast by default" query iteration (which is a much more common operation). It is also "self optimizing". Users don't need to think about optimizing component layouts for iteration performance. It "just works" without any extra boilerplate.
Shipyard and EnTT are "sparse set ecs-es". They employ "packing" as a way to work around the "suboptimal by default" iteration performance for specific sets of components. This helps, but I didn't think this was a good choice for a general purpose engine like Bevy because:
1. "packs" conflict with each other. If bevy decides to internally pack the Transform and GlobalTransform components, users are then blocked if they want to pack some custom component with Transform.
2. users need to take manual action to optimize
Developers selecting an ECS framework are stuck with a hard choice. Select an "archetypal" framework with "fast iteration everywhere" but without the ability to cheaply add/remove components, or select a "sparse set" framework to cheaply add/remove components but with slower iteration performance.
## Hybrid Component Storage (The Solution)
In Bevy ECS V2, we get to have our cake and eat it too. It now has _both_ of the component storage types above (and more can be added later if needed):
* **Tables** (aka "archetypal" storage)
* The default storage. If you don't configure anything, this is what you get
* Fast iteration by default
* Slower add/remove operations
* **Sparse Sets**
* Opt-in
* Slower iteration
* Faster add/remove operations
These storage types complement each other perfectly. By default Query iteration is fast. If developers know that they want to add/remove a component at high frequencies, they can set the storage to "sparse set":
```rust
world.register_component(
ComponentDescriptor::new::<MyComponent>(StorageType::SparseSet)
).unwrap();
```
## Archetypes
Archetypes are now "just metadata" ... they no longer store components directly. They do store:
* The `ComponentId`s of each of the Archetype's components (and that component's storage type)
* Archetypes are uniquely defined by their component layouts
* For example: entities with "table" components `[A, B, C]` _and_ "sparse set" components `[D, E]` will always be in the same archetype.
* The `TableId` associated with the archetype
* For now each archetype has exactly one table (which can have no components),
* There is a 1->Many relationship from Tables->Archetypes. A given table could have any number of archetype components stored in it:
* Ex: an entity with "table storage" components `[A, B, C]` and "sparse set" components `[D, E]` will share the same `[A, B, C]` table as an entity with `[A, B, C]` table component and `[F]` sparse set components.
* This 1->Many relationship is how we preserve fast "cache friendly" iteration performance when possible (more on this later)
* A list of entities that are in the archetype and the row id of the table they are in
* ArchetypeComponentIds
* unique densely packed identifiers for (ArchetypeId, ComponentId) pairs
* used by the schedule executor for cheap system access control
* "Archetype Graph Edges" (see the next section)
## The "Archetype Graph"
Archetype changes in Bevy (and a number of other archetypal ecs-es) have historically been expensive to compute. First, you need to allocate a new vector of the entity's current component ids, add or remove components based on the operation performed, sort it (to ensure it is order-independent), then hash it to find the archetype (if it exists). And thats all before we get to the _already_ expensive full copy of all components to the new table storage.
The solution is to build a "graph" of archetypes to cache these results. @SanderMertens first exposed me to the idea (and he got it from @gjroelofs, who came up with it). They propose adding directed edges between archetypes for add/remove component operations. If `ComponentId`s are densely packed, you can use sparse sets to cheaply jump between archetypes.
Bevy takes this one step further by using add/remove `Bundle` edges instead of `Component` edges. Bevy encourages the use of `Bundles` to group add/remove operations. This is largely for "clearer game logic" reasons, but it also helps cut down on the number of archetype changes required. `Bundles` now also have densely-packed `BundleId`s. This allows us to use a _single_ edge for each bundle operation (rather than needing to traverse N edges ... one for each component). Single component operations are also bundles, so this is strictly an improvement over a "component only" graph.
As a result, an operation that used to be _heavy_ (both for allocations and compute) is now two dirt-cheap array lookups and zero allocations.
## Stateful Queries
World queries are now stateful. This allows us to:
1. Cache archetype (and table) matches
* This resolves another issue with (naive) archetypal ECS: query performance getting worse as the number of archetypes goes up (and fragmentation occurs).
2. Cache Fetch and Filter state
* The expensive parts of fetch/filter operations (such as hashing the TypeId to find the ComponentId) now only happen once when the Query is first constructed
3. Incrementally build up state
* When new archetypes are added, we only process the new archetypes (no need to rebuild state for old archetypes)
As a result, the direct `World` query api now looks like this:
```rust
let mut query = world.query::<(&A, &mut B)>();
for (a, mut b) in query.iter_mut(&mut world) {
}
```
Requiring `World` to generate stateful queries (rather than letting the `QueryState` type be constructed separately) allows us to ensure that _all_ queries are properly initialized (and the relevant world state, such as ComponentIds). This enables QueryState to remove branches from its operations that check for initialization status (and also enables query.iter() to take an immutable world reference because it doesn't need to initialize anything in world).
However in systems, this is a non-breaking change. State management is done internally by the relevant SystemParam.
## Stateful SystemParams
Like Queries, `SystemParams` now also cache state. For example, `Query` system params store the "stateful query" state mentioned above. Commands store their internal `CommandQueue`. This means you can now safely use as many separate `Commands` parameters in your system as you want. `Local<T>` system params store their `T` value in their state (instead of in Resources).
SystemParam state also enabled a significant slim-down of SystemState. It is much nicer to look at now.
Per-SystemParam state naturally insulates us from an "aliased mut" class of errors we have hit in the past (ex: using multiple `Commands` system params).
(credit goes to @DJMcNab for the initial idea and draft pr here #1364)
## Configurable SystemParams
@DJMcNab also had the great idea to make SystemParams configurable. This allows users to provide some initial configuration / values for system parameters (when possible). Most SystemParams have no config (the config type is `()`), but the `Local<T>` param now supports user-provided parameters:
```rust
fn foo(value: Local<usize>) {
}
app.add_system(foo.system().config(|c| c.0 = Some(10)));
```
## Uber Fast "for_each" Query Iterators
Developers now have the choice to use a fast "for_each" iterator, which yields ~1.5-3x iteration speed improvements for "fragmented iteration", and minor ~1.2x iteration speed improvements for unfragmented iteration.
```rust
fn system(query: Query<(&A, &mut B)>) {
// you now have the option to do this for a speed boost
query.for_each_mut(|(a, mut b)| {
});
// however normal iterators are still available
for (a, mut b) in query.iter_mut() {
}
}
```
I think in most cases we should continue to encourage "normal" iterators as they are more flexible and more "rust idiomatic". But when that extra "oomf" is needed, it makes sense to use `for_each`.
We should also consider using `for_each` for internal bevy systems to give our users a nice speed boost (but that should be a separate pr).
## Component Metadata
`World` now has a `Components` collection, which is accessible via `world.components()`. This stores mappings from `ComponentId` to `ComponentInfo`, as well as `TypeId` to `ComponentId` mappings (where relevant). `ComponentInfo` stores information about the component, such as ComponentId, TypeId, memory layout, send-ness (currently limited to resources), and storage type.
## Significantly Cheaper `Access<T>`
We used to use `TypeAccess<TypeId>` to manage read/write component/archetype-component access. This was expensive because TypeIds must be hashed and compared individually. The parallel executor got around this by "condensing" type ids into bitset-backed access types. This worked, but it had to be re-generated from the `TypeAccess<TypeId>`sources every time archetypes changed.
This pr removes TypeAccess in favor of faster bitset access everywhere. We can do this thanks to the move to densely packed `ComponentId`s and `ArchetypeComponentId`s.
## Merged Resources into World
Resources had a lot of redundant functionality with Components. They stored typed data, they had access control, they had unique ids, they were queryable via SystemParams, etc. In fact the _only_ major difference between them was that they were unique (and didn't correlate to an entity).
Separate resources also had the downside of requiring a separate set of access controls, which meant the parallel executor needed to compare more bitsets per system and manage more state.
I initially got the "separate resources" idea from `legion`. I think that design was motivated by the fact that it made the direct world query/resource lifetime interactions more manageable. It certainly made our lives easier when using Resources alongside hecs/bevy_ecs. However we already have a construct for safely and ergonomically managing in-world lifetimes: systems (which use `Access<T>` internally).
This pr merges Resources into World:
```rust
world.insert_resource(1);
world.insert_resource(2.0);
let a = world.get_resource::<i32>().unwrap();
let mut b = world.get_resource_mut::<f64>().unwrap();
*b = 3.0;
```
Resources are now just a special kind of component. They have their own ComponentIds (and their own resource TypeId->ComponentId scope, so they don't conflict wit components of the same type). They are stored in a special "resource archetype", which stores components inside the archetype using a new `unique_components` sparse set (note that this sparse set could later be used to implement Tags). This allows us to keep the code size small by reusing existing datastructures (namely Column, Archetype, ComponentFlags, and ComponentInfo). This allows us the executor to use a single `Access<ArchetypeComponentId>` per system. It should also make scripting language integration easier.
_But_ this merge did create problems for people directly interacting with `World`. What if you need mutable access to multiple resources at the same time? `world.get_resource_mut()` borrows World mutably!
## WorldCell
WorldCell applies the `Access<ArchetypeComponentId>` concept to direct world access:
```rust
let world_cell = world.cell();
let a = world_cell.get_resource_mut::<i32>().unwrap();
let b = world_cell.get_resource_mut::<f64>().unwrap();
```
This adds cheap runtime checks (a sparse set lookup of `ArchetypeComponentId` and a counter) to ensure that world accesses do not conflict with each other. Each operation returns a `WorldBorrow<'w, T>` or `WorldBorrowMut<'w, T>` wrapper type, which will release the relevant ArchetypeComponentId resources when dropped.
World caches the access sparse set (and only one cell can exist at a time), so `world.cell()` is a cheap operation.
WorldCell does _not_ use atomic operations. It is non-send, does a mutable borrow of world to prevent other accesses, and uses a simple `Rc<RefCell<ArchetypeComponentAccess>>` wrapper in each WorldBorrow pointer.
The api is currently limited to resource access, but it can and should be extended to queries / entity component access.
## Resource Scopes
WorldCell does not yet support component queries, and even when it does there are sometimes legitimate reasons to want a mutable world ref _and_ a mutable resource ref (ex: bevy_render and bevy_scene both need this). In these cases we could always drop down to the unsafe `world.get_resource_unchecked_mut()`, but that is not ideal!
Instead developers can use a "resource scope"
```rust
world.resource_scope(|world: &mut World, a: &mut A| {
})
```
This temporarily removes the `A` resource from `World`, provides mutable pointers to both, and re-adds A to World when finished. Thanks to the move to ComponentIds/sparse sets, this is a cheap operation.
If multiple resources are required, scopes can be nested. We could also consider adding a "resource tuple" to the api if this pattern becomes common and the boilerplate gets nasty.
## Query Conflicts Use ComponentId Instead of ArchetypeComponentId
For safety reasons, systems cannot contain queries that conflict with each other without wrapping them in a QuerySet. On bevy `main`, we use ArchetypeComponentIds to determine conflicts. This is nice because it can take into account filters:
```rust
// these queries will never conflict due to their filters
fn filter_system(a: Query<&mut A, With<B>>, b: Query<&mut B, Without<B>>) {
}
```
But it also has a significant downside:
```rust
// these queries will not conflict _until_ an entity with A, B, and C is spawned
fn maybe_conflicts_system(a: Query<(&mut A, &C)>, b: Query<(&mut A, &B)>) {
}
```
The system above will panic at runtime if an entity with A, B, and C is spawned. This makes it hard to trust that your game logic will run without crashing.
In this pr, I switched to using `ComponentId` instead. This _is_ more constraining. `maybe_conflicts_system` will now always fail, but it will do it consistently at startup. Naively, it would also _disallow_ `filter_system`, which would be a significant downgrade in usability. Bevy has a number of internal systems that rely on disjoint queries and I expect it to be a common pattern in userspace.
To resolve this, I added a new `FilteredAccess<T>` type, which wraps `Access<T>` and adds with/without filters. If two `FilteredAccess` have with/without values that prove they are disjoint, they will no longer conflict.
## EntityRef / EntityMut
World entity operations on `main` require that the user passes in an `entity` id to each operation:
```rust
let entity = world.spawn((A, )); // create a new entity with A
world.get::<A>(entity);
world.insert(entity, (B, C));
world.insert_one(entity, D);
```
This means that each operation needs to look up the entity location / verify its validity. The initial spawn operation also requires a Bundle as input. This can be awkward when no components are required (or one component is required).
These operations have been replaced by `EntityRef` and `EntityMut`, which are "builder-style" wrappers around world that provide read and read/write operations on a single, pre-validated entity:
```rust
// spawn now takes no inputs and returns an EntityMut
let entity = world.spawn()
.insert(A) // insert a single component into the entity
.insert_bundle((B, C)) // insert a bundle of components into the entity
.id() // id returns the Entity id
// Returns EntityMut (or panics if the entity does not exist)
world.entity_mut(entity)
.insert(D)
.insert_bundle(SomeBundle::default());
{
// returns EntityRef (or panics if the entity does not exist)
let d = world.entity(entity)
.get::<D>() // gets the D component
.unwrap();
// world.get still exists for ergonomics
let d = world.get::<D>(entity).unwrap();
}
// These variants return Options if you want to check existence instead of panicing
world.get_entity_mut(entity)
.unwrap()
.insert(E);
if let Some(entity_ref) = world.get_entity(entity) {
let d = entity_ref.get::<D>().unwrap();
}
```
This _does not_ affect the current Commands api or terminology. I think that should be a separate conversation as that is a much larger breaking change.
## Safety Improvements
* Entity reservation in Commands uses a normal world borrow instead of an unsafe transmute
* QuerySets no longer transmutes lifetimes
* Made traits "unsafe" when implementing a trait incorrectly could cause unsafety
* More thorough safety docs
## RemovedComponents SystemParam
The old approach to querying removed components: `query.removed:<T>()` was confusing because it had no connection to the query itself. I replaced it with the following, which is both clearer and allows us to cache the ComponentId mapping in the SystemParamState:
```rust
fn system(removed: RemovedComponents<T>) {
for entity in removed.iter() {
}
}
```
## Simpler Bundle implementation
Bundles are no longer responsible for sorting (or deduping) TypeInfo. They are just a simple ordered list of component types / data. This makes the implementation smaller and opens the door to an easy "nested bundle" implementation in the future (which i might even add in this pr). Duplicate detection is now done once per bundle type by World the first time a bundle is used.
## Unified WorldQuery and QueryFilter types
(don't worry they are still separate type _parameters_ in Queries .. this is a non-breaking change)
WorldQuery and QueryFilter were already basically identical apis. With the addition of `FetchState` and more storage-specific fetch methods, the overlap was even clearer (and the redundancy more painful).
QueryFilters are now just `F: WorldQuery where F::Fetch: FilterFetch`. FilterFetch requires `Fetch<Item = bool>` and adds new "short circuit" variants of fetch methods. This enables a filter tuple like `(With<A>, Without<B>, Changed<C>)` to stop evaluating the filter after the first mismatch is encountered. FilterFetch is automatically implemented for `Fetch` implementations that return bool.
This forces fetch implementations that return things like `(bool, bool, bool)` (such as the filter above) to manually implement FilterFetch and decide whether or not to short-circuit.
## More Granular Modules
World no longer globs all of the internal modules together. It now exports `core`, `system`, and `schedule` separately. I'm also considering exporting `core` submodules directly as that is still pretty "glob-ey" and unorganized (feedback welcome here).
## Remaining Draft Work (to be done in this pr)
* ~~panic on conflicting WorldQuery fetches (&A, &mut A)~~
* ~~bevy `main` and hecs both currently allow this, but we should protect against it if possible~~
* ~~batch_iter / par_iter (currently stubbed out)~~
* ~~ChangedRes~~
* ~~I skipped this while we sort out #1313. This pr should be adapted to account for whatever we land on there~~.
* ~~The `Archetypes` and `Tables` collections use hashes of sorted lists of component ids to uniquely identify each archetype/table. This hash is then used as the key in a HashMap to look up the relevant ArchetypeId or TableId. (which doesn't handle hash collisions properly)~~
* ~~It is currently unsafe to generate a Query from "World A", then use it on "World B" (despite the api claiming it is safe). We should probably close this gap. This could be done by adding a randomly generated WorldId to each world, then storing that id in each Query. They could then be compared to each other on each `query.do_thing(&world)` operation. This _does_ add an extra branch to each query operation, so I'm open to other suggestions if people have them.~~
* ~~Nested Bundles (if i find time)~~
## Potential Future Work
* Expand WorldCell to support queries.
* Consider not allocating in the empty archetype on `world.spawn()`
* ex: return something like EntityMutUninit, which turns into EntityMut after an `insert` or `insert_bundle` op
* this actually regressed performance last time i tried it, but in theory it should be faster
* Optimize SparseSet::insert (see `PERF` comment on insert)
* Replace SparseArray `Option<T>` with T::MAX to cut down on branching
* would enable cheaper get_unchecked() operations
* upstream fixedbitset optimizations
* fixedbitset could be allocation free for small block counts (store blocks in a SmallVec)
* fixedbitset could have a const constructor
* Consider implementing Tags (archetype-specific by-value data that affects archetype identity)
* ex: ArchetypeA could have `[A, B, C]` table components and `[D(1)]` "tag" component. ArchetypeB could have `[A, B, C]` table components and a `[D(2)]` tag component. The archetypes are different, despite both having D tags because the value inside D is different.
* this could potentially build on top of the `archetype.unique_components` added in this pr for resource storage.
* Consider reverting `all_tuples` proc macro in favor of the old `macro_rules` implementation
* all_tuples is more flexible and produces cleaner documentation (the macro_rules version produces weird type parameter orders due to parser constraints)
* but unfortunately all_tuples also appears to make Rust Analyzer sad/slow when working inside of `bevy_ecs` (does not affect user code)
* Consider "resource queries" and/or "mixed resource and entity component queries" as an alternative to WorldCell
* this is basically just "systems" so maybe it's not worth it
* Add more world ops
* `world.clear()`
* `world.reserve<T: Bundle>(count: usize)`
* Try using the old archetype allocation strategy (allocate new memory on resize and copy everything over). I expect this to improve batch insertion performance at the cost of unbatched performance. But thats just a guess. I'm not an allocation perf pro :)
* Adapt Commands apis for consistency with new World apis
## Benchmarks
key:
* `bevy_old`: bevy `main` branch
* `bevy`: this branch
* `_foreach`: uses an optimized for_each iterator
* ` _sparse`: uses sparse set storage (if unspecified assume table storage)
* `_system`: runs inside a system (if unspecified assume test happens via direct world ops)
### Simple Insert (from ecs_bench_suite)
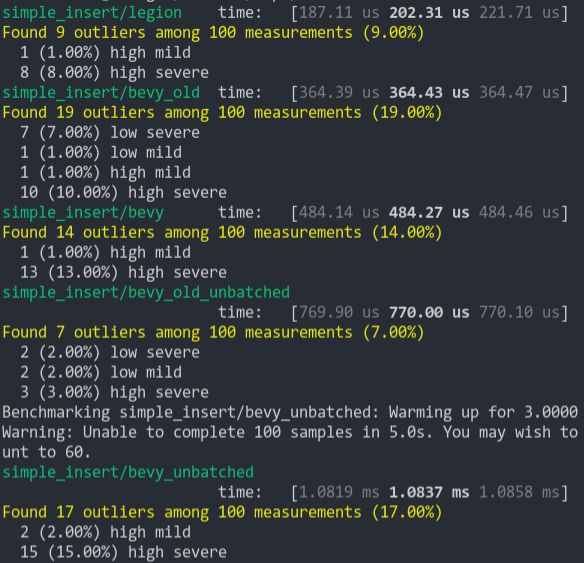
### Simpler Iter (from ecs_bench_suite)
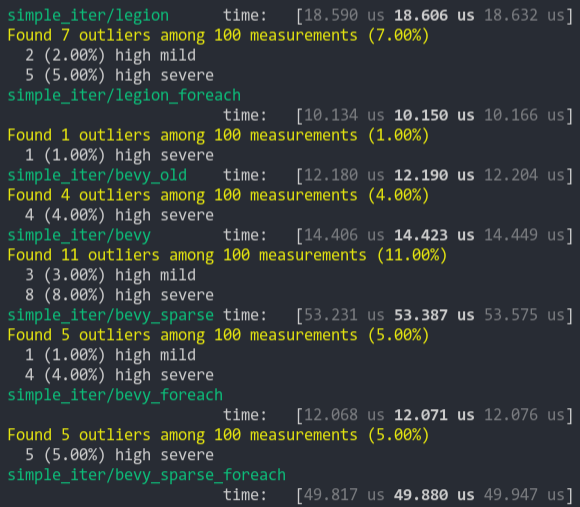
### Fragment Iter (from ecs_bench_suite)
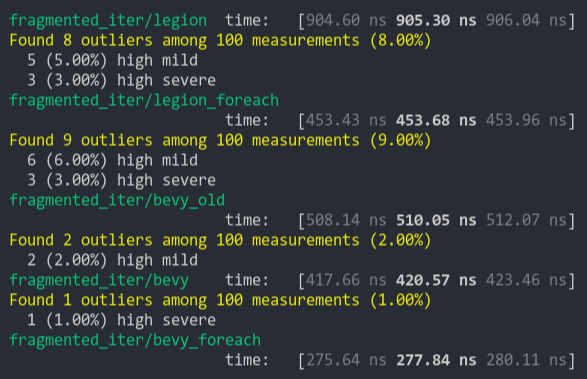
### Sparse Fragmented Iter
Iterate a query that matches 5 entities from a single matching archetype, but there are 100 unmatching archetypes
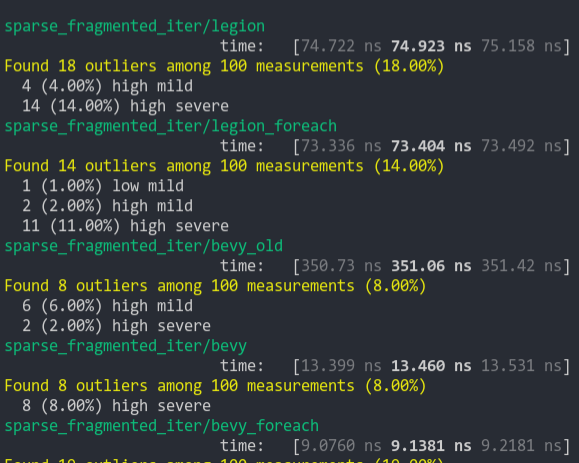
### Schedule (from ecs_bench_suite)
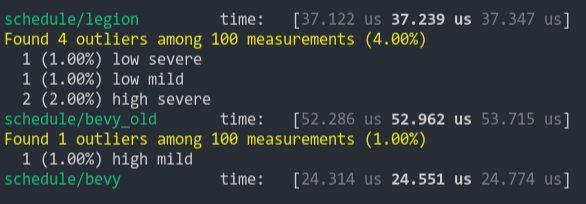
### Add Remove Component (from ecs_bench_suite)
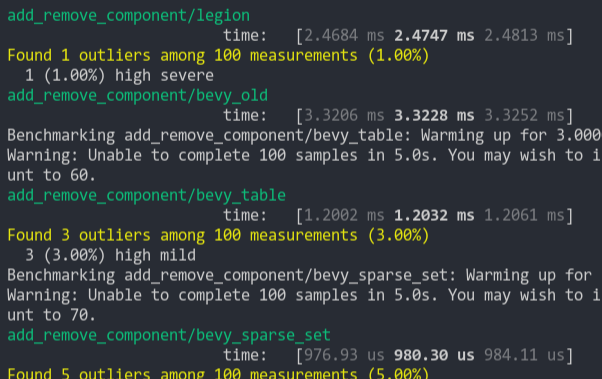
### Add Remove Component Big
Same as the test above, but each entity has 5 "large" matrix components and 1 "large" matrix component is added and removed
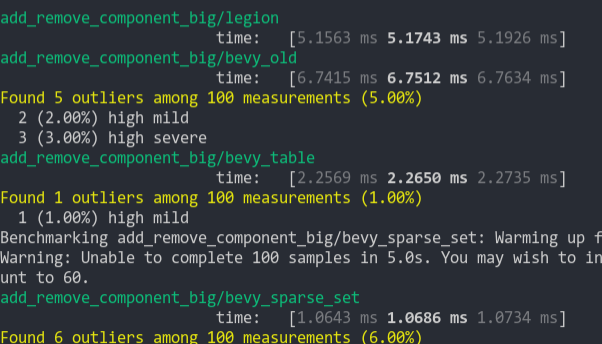
### Get Component
Looks up a single component value a large number of times
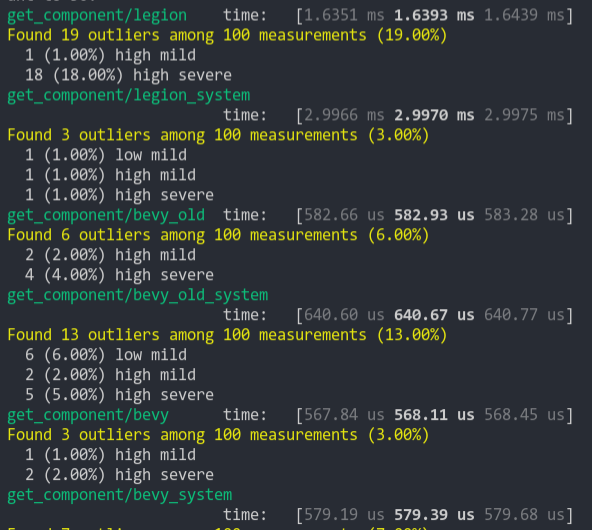
2021-03-05 07:54:35 +00:00
. archetype_component_access
. add_read ( archetype_component_id ) ;
Remove the `SystemParamState` trait and remove types like `ResState` (#6919)
Spiritual successor to #5205.
Actual successor to #6865.
# Objective
Currently, system params are defined using three traits: `SystemParam`, `ReadOnlySystemParam`, `SystemParamState`. The behavior for each param is specified by the `SystemParamState` trait, while `SystemParam` simply defers to the state.
Splitting the traits in this way makes it easier to implement within macros, but it increases the cognitive load. Worst of all, this approach requires each `MySystemParam` to have a public `MySystemParamState` type associated with it.
## Solution
* Merge the trait `SystemParamState` into `SystemParam`.
* Remove all trivial `SystemParam` state types.
* `OptionNonSendMutState<T>`: you will not be missed.
---
- [x] Fix/resolve the remaining test failure.
## Changelog
* Removed the trait `SystemParamState`, merging its functionality into `SystemParam`.
## Migration Guide
**Note**: this should replace the migration guide for #6865.
This is relative to Bevy 0.9, not main.
The traits `SystemParamState` and `SystemParamFetch` have been removed, and their functionality has been transferred to `SystemParam`.
```rust
// Before (0.9)
impl SystemParam for MyParam<'_, '_> {
type State = MyParamState;
}
unsafe impl SystemParamState for MyParamState {
fn init(world: &mut World, system_meta: &mut SystemMeta) -> Self { ... }
}
unsafe impl<'w, 's> SystemParamFetch<'w, 's> for MyParamState {
type Item = MyParam<'w, 's>;
fn get_param(&mut self, ...) -> Self::Item;
}
unsafe impl ReadOnlySystemParamFetch for MyParamState { }
// After (0.10)
unsafe impl SystemParam for MyParam<'_, '_> {
type State = MyParamState;
type Item<'w, 's> = MyParam<'w, 's>;
fn init_state(world: &mut World, system_meta: &mut SystemMeta) -> Self::State { ... }
fn get_param<'w, 's>(state: &mut Self::State, ...) -> Self::Item<'w, 's>;
}
unsafe impl ReadOnlySystemParam for MyParam<'_, '_> { }
```
The trait `ReadOnlySystemParamFetch` has been replaced with `ReadOnlySystemParam`.
```rust
// Before
unsafe impl ReadOnlySystemParamFetch for MyParamState {}
// After
unsafe impl ReadOnlySystemParam for MyParam<'_, '_> {}
```
2023-01-07 23:20:32 +00:00
component_id
Bevy ECS V2 (#1525)
# Bevy ECS V2
This is a rewrite of Bevy ECS (basically everything but the new executor/schedule, which are already awesome). The overall goal was to improve the performance and versatility of Bevy ECS. Here is a quick bulleted list of changes before we dive into the details:
* Complete World rewrite
* Multiple component storage types:
* Tables: fast cache friendly iteration, slower add/removes (previously called Archetypes)
* Sparse Sets: fast add/remove, slower iteration
* Stateful Queries (caches query results for faster iteration. fragmented iteration is _fast_ now)
* Stateful System Params (caches expensive operations. inspired by @DJMcNab's work in #1364)
* Configurable System Params (users can set configuration when they construct their systems. once again inspired by @DJMcNab's work)
* Archetypes are now "just metadata", component storage is separate
* Archetype Graph (for faster archetype changes)
* Component Metadata
* Configure component storage type
* Retrieve information about component size/type/name/layout/send-ness/etc
* Components are uniquely identified by a densely packed ComponentId
* TypeIds are now totally optional (which should make implementing scripting easier)
* Super fast "for_each" query iterators
* Merged Resources into World. Resources are now just a special type of component
* EntityRef/EntityMut builder apis (more efficient and more ergonomic)
* Fast bitset-backed `Access<T>` replaces old hashmap-based approach everywhere
* Query conflicts are determined by component access instead of archetype component access (to avoid random failures at runtime)
* With/Without are still taken into account for conflicts, so this should still be comfy to use
* Much simpler `IntoSystem` impl
* Significantly reduced the amount of hashing throughout the ecs in favor of Sparse Sets (indexed by densely packed ArchetypeId, ComponentId, BundleId, and TableId)
* Safety Improvements
* Entity reservation uses a normal world reference instead of unsafe transmute
* QuerySets no longer transmute lifetimes
* Made traits "unsafe" where relevant
* More thorough safety docs
* WorldCell
* Exposes safe mutable access to multiple resources at a time in a World
* Replaced "catch all" `System::update_archetypes(world: &World)` with `System::new_archetype(archetype: &Archetype)`
* Simpler Bundle implementation
* Replaced slow "remove_bundle_one_by_one" used as fallback for Commands::remove_bundle with fast "remove_bundle_intersection"
* Removed `Mut<T>` query impl. it is better to only support one way: `&mut T`
* Removed with() from `Flags<T>` in favor of `Option<Flags<T>>`, which allows querying for flags to be "filtered" by default
* Components now have is_send property (currently only resources support non-send)
* More granular module organization
* New `RemovedComponents<T>` SystemParam that replaces `query.removed::<T>()`
* `world.resource_scope()` for mutable access to resources and world at the same time
* WorldQuery and QueryFilter traits unified. FilterFetch trait added to enable "short circuit" filtering. Auto impled for cases that don't need it
* Significantly slimmed down SystemState in favor of individual SystemParam state
* System Commands changed from `commands: &mut Commands` back to `mut commands: Commands` (to allow Commands to have a World reference)
Fixes #1320
## `World` Rewrite
This is a from-scratch rewrite of `World` that fills the niche that `hecs` used to. Yes, this means Bevy ECS is no longer a "fork" of hecs. We're going out our own!
(the only shared code between the projects is the entity id allocator, which is already basically ideal)
A huge shout out to @SanderMertens (author of [flecs](https://github.com/SanderMertens/flecs)) for sharing some great ideas with me (specifically hybrid ecs storage and archetype graphs). He also helped advise on a number of implementation details.
## Component Storage (The Problem)
Two ECS storage paradigms have gained a lot of traction over the years:
* **Archetypal ECS**:
* Stores components in "tables" with static schemas. Each "column" stores components of a given type. Each "row" is an entity.
* Each "archetype" has its own table. Adding/removing an entity's component changes the archetype.
* Enables super-fast Query iteration due to its cache-friendly data layout
* Comes at the cost of more expensive add/remove operations for an Entity's components, because all components need to be copied to the new archetype's "table"
* **Sparse Set ECS**:
* Stores components of the same type in densely packed arrays, which are sparsely indexed by densely packed unsigned integers (Entity ids)
* Query iteration is slower than Archetypal ECS because each entity's component could be at any position in the sparse set. This "random access" pattern isn't cache friendly. Additionally, there is an extra layer of indirection because you must first map the entity id to an index in the component array.
* Adding/removing components is a cheap, constant time operation
Bevy ECS V1, hecs, legion, flec, and Unity DOTS are all "archetypal ecs-es". I personally think "archetypal" storage is a good default for game engines. An entity's archetype doesn't need to change frequently in general, and it creates "fast by default" query iteration (which is a much more common operation). It is also "self optimizing". Users don't need to think about optimizing component layouts for iteration performance. It "just works" without any extra boilerplate.
Shipyard and EnTT are "sparse set ecs-es". They employ "packing" as a way to work around the "suboptimal by default" iteration performance for specific sets of components. This helps, but I didn't think this was a good choice for a general purpose engine like Bevy because:
1. "packs" conflict with each other. If bevy decides to internally pack the Transform and GlobalTransform components, users are then blocked if they want to pack some custom component with Transform.
2. users need to take manual action to optimize
Developers selecting an ECS framework are stuck with a hard choice. Select an "archetypal" framework with "fast iteration everywhere" but without the ability to cheaply add/remove components, or select a "sparse set" framework to cheaply add/remove components but with slower iteration performance.
## Hybrid Component Storage (The Solution)
In Bevy ECS V2, we get to have our cake and eat it too. It now has _both_ of the component storage types above (and more can be added later if needed):
* **Tables** (aka "archetypal" storage)
* The default storage. If you don't configure anything, this is what you get
* Fast iteration by default
* Slower add/remove operations
* **Sparse Sets**
* Opt-in
* Slower iteration
* Faster add/remove operations
These storage types complement each other perfectly. By default Query iteration is fast. If developers know that they want to add/remove a component at high frequencies, they can set the storage to "sparse set":
```rust
world.register_component(
ComponentDescriptor::new::<MyComponent>(StorageType::SparseSet)
).unwrap();
```
## Archetypes
Archetypes are now "just metadata" ... they no longer store components directly. They do store:
* The `ComponentId`s of each of the Archetype's components (and that component's storage type)
* Archetypes are uniquely defined by their component layouts
* For example: entities with "table" components `[A, B, C]` _and_ "sparse set" components `[D, E]` will always be in the same archetype.
* The `TableId` associated with the archetype
* For now each archetype has exactly one table (which can have no components),
* There is a 1->Many relationship from Tables->Archetypes. A given table could have any number of archetype components stored in it:
* Ex: an entity with "table storage" components `[A, B, C]` and "sparse set" components `[D, E]` will share the same `[A, B, C]` table as an entity with `[A, B, C]` table component and `[F]` sparse set components.
* This 1->Many relationship is how we preserve fast "cache friendly" iteration performance when possible (more on this later)
* A list of entities that are in the archetype and the row id of the table they are in
* ArchetypeComponentIds
* unique densely packed identifiers for (ArchetypeId, ComponentId) pairs
* used by the schedule executor for cheap system access control
* "Archetype Graph Edges" (see the next section)
## The "Archetype Graph"
Archetype changes in Bevy (and a number of other archetypal ecs-es) have historically been expensive to compute. First, you need to allocate a new vector of the entity's current component ids, add or remove components based on the operation performed, sort it (to ensure it is order-independent), then hash it to find the archetype (if it exists). And thats all before we get to the _already_ expensive full copy of all components to the new table storage.
The solution is to build a "graph" of archetypes to cache these results. @SanderMertens first exposed me to the idea (and he got it from @gjroelofs, who came up with it). They propose adding directed edges between archetypes for add/remove component operations. If `ComponentId`s are densely packed, you can use sparse sets to cheaply jump between archetypes.
Bevy takes this one step further by using add/remove `Bundle` edges instead of `Component` edges. Bevy encourages the use of `Bundles` to group add/remove operations. This is largely for "clearer game logic" reasons, but it also helps cut down on the number of archetype changes required. `Bundles` now also have densely-packed `BundleId`s. This allows us to use a _single_ edge for each bundle operation (rather than needing to traverse N edges ... one for each component). Single component operations are also bundles, so this is strictly an improvement over a "component only" graph.
As a result, an operation that used to be _heavy_ (both for allocations and compute) is now two dirt-cheap array lookups and zero allocations.
## Stateful Queries
World queries are now stateful. This allows us to:
1. Cache archetype (and table) matches
* This resolves another issue with (naive) archetypal ECS: query performance getting worse as the number of archetypes goes up (and fragmentation occurs).
2. Cache Fetch and Filter state
* The expensive parts of fetch/filter operations (such as hashing the TypeId to find the ComponentId) now only happen once when the Query is first constructed
3. Incrementally build up state
* When new archetypes are added, we only process the new archetypes (no need to rebuild state for old archetypes)
As a result, the direct `World` query api now looks like this:
```rust
let mut query = world.query::<(&A, &mut B)>();
for (a, mut b) in query.iter_mut(&mut world) {
}
```
Requiring `World` to generate stateful queries (rather than letting the `QueryState` type be constructed separately) allows us to ensure that _all_ queries are properly initialized (and the relevant world state, such as ComponentIds). This enables QueryState to remove branches from its operations that check for initialization status (and also enables query.iter() to take an immutable world reference because it doesn't need to initialize anything in world).
However in systems, this is a non-breaking change. State management is done internally by the relevant SystemParam.
## Stateful SystemParams
Like Queries, `SystemParams` now also cache state. For example, `Query` system params store the "stateful query" state mentioned above. Commands store their internal `CommandQueue`. This means you can now safely use as many separate `Commands` parameters in your system as you want. `Local<T>` system params store their `T` value in their state (instead of in Resources).
SystemParam state also enabled a significant slim-down of SystemState. It is much nicer to look at now.
Per-SystemParam state naturally insulates us from an "aliased mut" class of errors we have hit in the past (ex: using multiple `Commands` system params).
(credit goes to @DJMcNab for the initial idea and draft pr here #1364)
## Configurable SystemParams
@DJMcNab also had the great idea to make SystemParams configurable. This allows users to provide some initial configuration / values for system parameters (when possible). Most SystemParams have no config (the config type is `()`), but the `Local<T>` param now supports user-provided parameters:
```rust
fn foo(value: Local<usize>) {
}
app.add_system(foo.system().config(|c| c.0 = Some(10)));
```
## Uber Fast "for_each" Query Iterators
Developers now have the choice to use a fast "for_each" iterator, which yields ~1.5-3x iteration speed improvements for "fragmented iteration", and minor ~1.2x iteration speed improvements for unfragmented iteration.
```rust
fn system(query: Query<(&A, &mut B)>) {
// you now have the option to do this for a speed boost
query.for_each_mut(|(a, mut b)| {
});
// however normal iterators are still available
for (a, mut b) in query.iter_mut() {
}
}
```
I think in most cases we should continue to encourage "normal" iterators as they are more flexible and more "rust idiomatic". But when that extra "oomf" is needed, it makes sense to use `for_each`.
We should also consider using `for_each` for internal bevy systems to give our users a nice speed boost (but that should be a separate pr).
## Component Metadata
`World` now has a `Components` collection, which is accessible via `world.components()`. This stores mappings from `ComponentId` to `ComponentInfo`, as well as `TypeId` to `ComponentId` mappings (where relevant). `ComponentInfo` stores information about the component, such as ComponentId, TypeId, memory layout, send-ness (currently limited to resources), and storage type.
## Significantly Cheaper `Access<T>`
We used to use `TypeAccess<TypeId>` to manage read/write component/archetype-component access. This was expensive because TypeIds must be hashed and compared individually. The parallel executor got around this by "condensing" type ids into bitset-backed access types. This worked, but it had to be re-generated from the `TypeAccess<TypeId>`sources every time archetypes changed.
This pr removes TypeAccess in favor of faster bitset access everywhere. We can do this thanks to the move to densely packed `ComponentId`s and `ArchetypeComponentId`s.
## Merged Resources into World
Resources had a lot of redundant functionality with Components. They stored typed data, they had access control, they had unique ids, they were queryable via SystemParams, etc. In fact the _only_ major difference between them was that they were unique (and didn't correlate to an entity).
Separate resources also had the downside of requiring a separate set of access controls, which meant the parallel executor needed to compare more bitsets per system and manage more state.
I initially got the "separate resources" idea from `legion`. I think that design was motivated by the fact that it made the direct world query/resource lifetime interactions more manageable. It certainly made our lives easier when using Resources alongside hecs/bevy_ecs. However we already have a construct for safely and ergonomically managing in-world lifetimes: systems (which use `Access<T>` internally).
This pr merges Resources into World:
```rust
world.insert_resource(1);
world.insert_resource(2.0);
let a = world.get_resource::<i32>().unwrap();
let mut b = world.get_resource_mut::<f64>().unwrap();
*b = 3.0;
```
Resources are now just a special kind of component. They have their own ComponentIds (and their own resource TypeId->ComponentId scope, so they don't conflict wit components of the same type). They are stored in a special "resource archetype", which stores components inside the archetype using a new `unique_components` sparse set (note that this sparse set could later be used to implement Tags). This allows us to keep the code size small by reusing existing datastructures (namely Column, Archetype, ComponentFlags, and ComponentInfo). This allows us the executor to use a single `Access<ArchetypeComponentId>` per system. It should also make scripting language integration easier.
_But_ this merge did create problems for people directly interacting with `World`. What if you need mutable access to multiple resources at the same time? `world.get_resource_mut()` borrows World mutably!
## WorldCell
WorldCell applies the `Access<ArchetypeComponentId>` concept to direct world access:
```rust
let world_cell = world.cell();
let a = world_cell.get_resource_mut::<i32>().unwrap();
let b = world_cell.get_resource_mut::<f64>().unwrap();
```
This adds cheap runtime checks (a sparse set lookup of `ArchetypeComponentId` and a counter) to ensure that world accesses do not conflict with each other. Each operation returns a `WorldBorrow<'w, T>` or `WorldBorrowMut<'w, T>` wrapper type, which will release the relevant ArchetypeComponentId resources when dropped.
World caches the access sparse set (and only one cell can exist at a time), so `world.cell()` is a cheap operation.
WorldCell does _not_ use atomic operations. It is non-send, does a mutable borrow of world to prevent other accesses, and uses a simple `Rc<RefCell<ArchetypeComponentAccess>>` wrapper in each WorldBorrow pointer.
The api is currently limited to resource access, but it can and should be extended to queries / entity component access.
## Resource Scopes
WorldCell does not yet support component queries, and even when it does there are sometimes legitimate reasons to want a mutable world ref _and_ a mutable resource ref (ex: bevy_render and bevy_scene both need this). In these cases we could always drop down to the unsafe `world.get_resource_unchecked_mut()`, but that is not ideal!
Instead developers can use a "resource scope"
```rust
world.resource_scope(|world: &mut World, a: &mut A| {
})
```
This temporarily removes the `A` resource from `World`, provides mutable pointers to both, and re-adds A to World when finished. Thanks to the move to ComponentIds/sparse sets, this is a cheap operation.
If multiple resources are required, scopes can be nested. We could also consider adding a "resource tuple" to the api if this pattern becomes common and the boilerplate gets nasty.
## Query Conflicts Use ComponentId Instead of ArchetypeComponentId
For safety reasons, systems cannot contain queries that conflict with each other without wrapping them in a QuerySet. On bevy `main`, we use ArchetypeComponentIds to determine conflicts. This is nice because it can take into account filters:
```rust
// these queries will never conflict due to their filters
fn filter_system(a: Query<&mut A, With<B>>, b: Query<&mut B, Without<B>>) {
}
```
But it also has a significant downside:
```rust
// these queries will not conflict _until_ an entity with A, B, and C is spawned
fn maybe_conflicts_system(a: Query<(&mut A, &C)>, b: Query<(&mut A, &B)>) {
}
```
The system above will panic at runtime if an entity with A, B, and C is spawned. This makes it hard to trust that your game logic will run without crashing.
In this pr, I switched to using `ComponentId` instead. This _is_ more constraining. `maybe_conflicts_system` will now always fail, but it will do it consistently at startup. Naively, it would also _disallow_ `filter_system`, which would be a significant downgrade in usability. Bevy has a number of internal systems that rely on disjoint queries and I expect it to be a common pattern in userspace.
To resolve this, I added a new `FilteredAccess<T>` type, which wraps `Access<T>` and adds with/without filters. If two `FilteredAccess` have with/without values that prove they are disjoint, they will no longer conflict.
## EntityRef / EntityMut
World entity operations on `main` require that the user passes in an `entity` id to each operation:
```rust
let entity = world.spawn((A, )); // create a new entity with A
world.get::<A>(entity);
world.insert(entity, (B, C));
world.insert_one(entity, D);
```
This means that each operation needs to look up the entity location / verify its validity. The initial spawn operation also requires a Bundle as input. This can be awkward when no components are required (or one component is required).
These operations have been replaced by `EntityRef` and `EntityMut`, which are "builder-style" wrappers around world that provide read and read/write operations on a single, pre-validated entity:
```rust
// spawn now takes no inputs and returns an EntityMut
let entity = world.spawn()
.insert(A) // insert a single component into the entity
.insert_bundle((B, C)) // insert a bundle of components into the entity
.id() // id returns the Entity id
// Returns EntityMut (or panics if the entity does not exist)
world.entity_mut(entity)
.insert(D)
.insert_bundle(SomeBundle::default());
{
// returns EntityRef (or panics if the entity does not exist)
let d = world.entity(entity)
.get::<D>() // gets the D component
.unwrap();
// world.get still exists for ergonomics
let d = world.get::<D>(entity).unwrap();
}
// These variants return Options if you want to check existence instead of panicing
world.get_entity_mut(entity)
.unwrap()
.insert(E);
if let Some(entity_ref) = world.get_entity(entity) {
let d = entity_ref.get::<D>().unwrap();
}
```
This _does not_ affect the current Commands api or terminology. I think that should be a separate conversation as that is a much larger breaking change.
## Safety Improvements
* Entity reservation in Commands uses a normal world borrow instead of an unsafe transmute
* QuerySets no longer transmutes lifetimes
* Made traits "unsafe" when implementing a trait incorrectly could cause unsafety
* More thorough safety docs
## RemovedComponents SystemParam
The old approach to querying removed components: `query.removed:<T>()` was confusing because it had no connection to the query itself. I replaced it with the following, which is both clearer and allows us to cache the ComponentId mapping in the SystemParamState:
```rust
fn system(removed: RemovedComponents<T>) {
for entity in removed.iter() {
}
}
```
## Simpler Bundle implementation
Bundles are no longer responsible for sorting (or deduping) TypeInfo. They are just a simple ordered list of component types / data. This makes the implementation smaller and opens the door to an easy "nested bundle" implementation in the future (which i might even add in this pr). Duplicate detection is now done once per bundle type by World the first time a bundle is used.
## Unified WorldQuery and QueryFilter types
(don't worry they are still separate type _parameters_ in Queries .. this is a non-breaking change)
WorldQuery and QueryFilter were already basically identical apis. With the addition of `FetchState` and more storage-specific fetch methods, the overlap was even clearer (and the redundancy more painful).
QueryFilters are now just `F: WorldQuery where F::Fetch: FilterFetch`. FilterFetch requires `Fetch<Item = bool>` and adds new "short circuit" variants of fetch methods. This enables a filter tuple like `(With<A>, Without<B>, Changed<C>)` to stop evaluating the filter after the first mismatch is encountered. FilterFetch is automatically implemented for `Fetch` implementations that return bool.
This forces fetch implementations that return things like `(bool, bool, bool)` (such as the filter above) to manually implement FilterFetch and decide whether or not to short-circuit.
## More Granular Modules
World no longer globs all of the internal modules together. It now exports `core`, `system`, and `schedule` separately. I'm also considering exporting `core` submodules directly as that is still pretty "glob-ey" and unorganized (feedback welcome here).
## Remaining Draft Work (to be done in this pr)
* ~~panic on conflicting WorldQuery fetches (&A, &mut A)~~
* ~~bevy `main` and hecs both currently allow this, but we should protect against it if possible~~
* ~~batch_iter / par_iter (currently stubbed out)~~
* ~~ChangedRes~~
* ~~I skipped this while we sort out #1313. This pr should be adapted to account for whatever we land on there~~.
* ~~The `Archetypes` and `Tables` collections use hashes of sorted lists of component ids to uniquely identify each archetype/table. This hash is then used as the key in a HashMap to look up the relevant ArchetypeId or TableId. (which doesn't handle hash collisions properly)~~
* ~~It is currently unsafe to generate a Query from "World A", then use it on "World B" (despite the api claiming it is safe). We should probably close this gap. This could be done by adding a randomly generated WorldId to each world, then storing that id in each Query. They could then be compared to each other on each `query.do_thing(&world)` operation. This _does_ add an extra branch to each query operation, so I'm open to other suggestions if people have them.~~
* ~~Nested Bundles (if i find time)~~
## Potential Future Work
* Expand WorldCell to support queries.
* Consider not allocating in the empty archetype on `world.spawn()`
* ex: return something like EntityMutUninit, which turns into EntityMut after an `insert` or `insert_bundle` op
* this actually regressed performance last time i tried it, but in theory it should be faster
* Optimize SparseSet::insert (see `PERF` comment on insert)
* Replace SparseArray `Option<T>` with T::MAX to cut down on branching
* would enable cheaper get_unchecked() operations
* upstream fixedbitset optimizations
* fixedbitset could be allocation free for small block counts (store blocks in a SmallVec)
* fixedbitset could have a const constructor
* Consider implementing Tags (archetype-specific by-value data that affects archetype identity)
* ex: ArchetypeA could have `[A, B, C]` table components and `[D(1)]` "tag" component. ArchetypeB could have `[A, B, C]` table components and a `[D(2)]` tag component. The archetypes are different, despite both having D tags because the value inside D is different.
* this could potentially build on top of the `archetype.unique_components` added in this pr for resource storage.
* Consider reverting `all_tuples` proc macro in favor of the old `macro_rules` implementation
* all_tuples is more flexible and produces cleaner documentation (the macro_rules version produces weird type parameter orders due to parser constraints)
* but unfortunately all_tuples also appears to make Rust Analyzer sad/slow when working inside of `bevy_ecs` (does not affect user code)
* Consider "resource queries" and/or "mixed resource and entity component queries" as an alternative to WorldCell
* this is basically just "systems" so maybe it's not worth it
* Add more world ops
* `world.clear()`
* `world.reserve<T: Bundle>(count: usize)`
* Try using the old archetype allocation strategy (allocate new memory on resize and copy everything over). I expect this to improve batch insertion performance at the cost of unbatched performance. But thats just a guess. I'm not an allocation perf pro :)
* Adapt Commands apis for consistency with new World apis
## Benchmarks
key:
* `bevy_old`: bevy `main` branch
* `bevy`: this branch
* `_foreach`: uses an optimized for_each iterator
* ` _sparse`: uses sparse set storage (if unspecified assume table storage)
* `_system`: runs inside a system (if unspecified assume test happens via direct world ops)
### Simple Insert (from ecs_bench_suite)
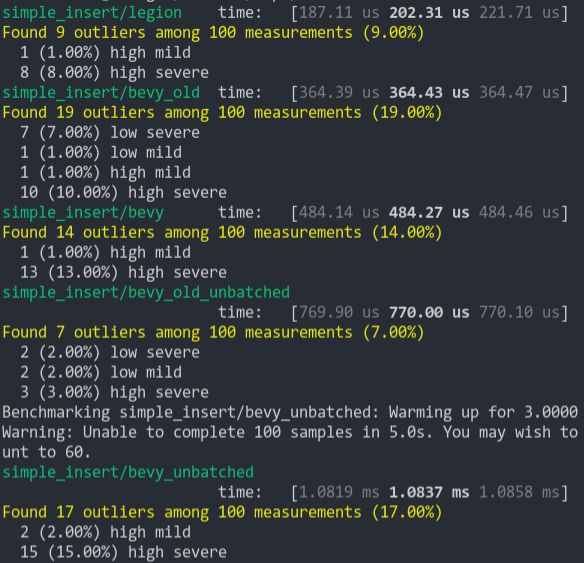
### Simpler Iter (from ecs_bench_suite)
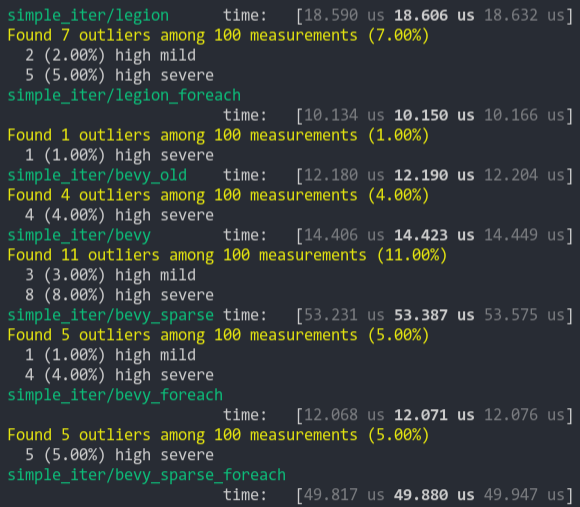
### Fragment Iter (from ecs_bench_suite)
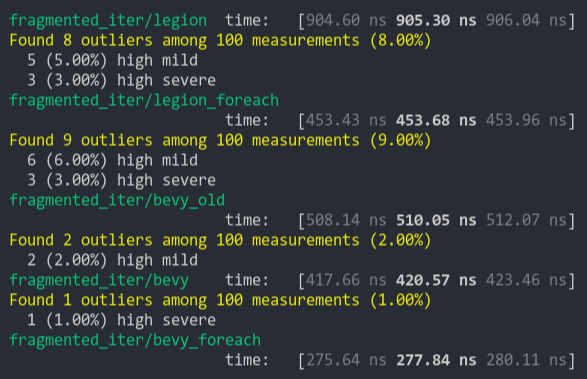
### Sparse Fragmented Iter
Iterate a query that matches 5 entities from a single matching archetype, but there are 100 unmatching archetypes
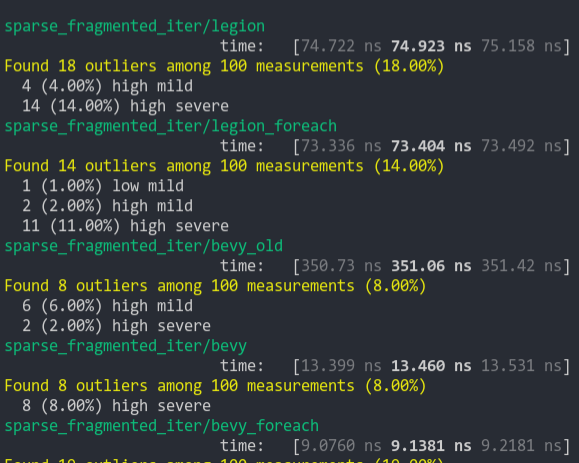
### Schedule (from ecs_bench_suite)
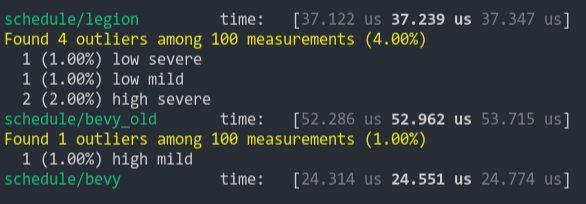
### Add Remove Component (from ecs_bench_suite)
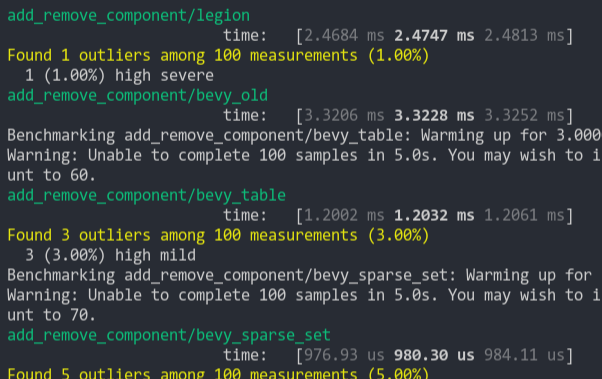
### Add Remove Component Big
Same as the test above, but each entity has 5 "large" matrix components and 1 "large" matrix component is added and removed
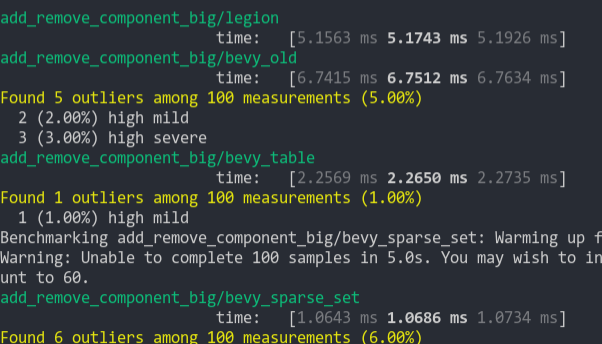
### Get Component
Looks up a single component value a large number of times
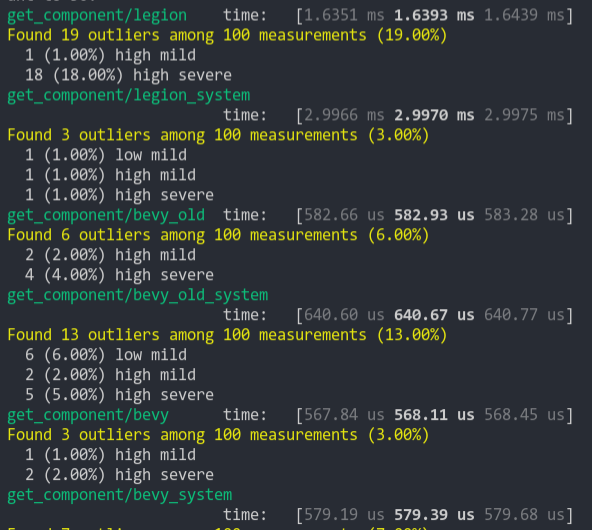
2021-03-05 07:54:35 +00:00
}
2020-12-16 05:57:16 +00:00
2020-11-08 20:34:05 +00:00
#[ inline ]
Simplify trait hierarchy for `SystemParam` (#6865)
# Objective
* Implementing a custom `SystemParam` by hand requires implementing three traits -- four if it is read-only.
* The trait `SystemParamFetch<'w, 's>` is a workaround from before we had generic associated types, and is no longer necessary.
## Solution
* Combine the trait `SystemParamFetch` with `SystemParamState`.
* I decided to remove the `Fetch` name and keep the `State` name, since the former was consistently conflated with the latter.
* Replace the trait `ReadOnlySystemParamFetch` with `ReadOnlySystemParam`, which simplifies trait bounds in generic code.
---
## Changelog
- Removed the trait `SystemParamFetch`, moving its functionality to `SystemParamState`.
- Replaced the trait `ReadOnlySystemParamFetch` with `ReadOnlySystemParam`.
## Migration Guide
The trait `SystemParamFetch` has been removed, and its functionality has been transferred to `SystemParamState`.
```rust
// Before
impl SystemParamState for MyParamState {
fn init(world: &mut World, system_meta: &mut SystemMeta) -> Self { ... }
}
impl<'w, 's> SystemParamFetch<'w, 's> for MyParamState {
type Item = MyParam<'w, 's>;
fn get_param(...) -> Self::Item;
}
// After
impl SystemParamState for MyParamState {
type Item<'w, 's> = MyParam<'w, 's>; // Generic associated types!
fn init(world: &mut World, system_meta: &mut SystemMeta) -> Self { ... }
fn get_param<'w, 's>(...) -> Self::Item<'w, 's>;
}
```
The trait `ReadOnlySystemParamFetch` has been replaced with `ReadOnlySystemParam`.
```rust
// Before
unsafe impl ReadOnlySystemParamFetch for MyParamState {}
// After
unsafe impl<'w, 's> ReadOnlySystemParam for MyParam<'w, 's> {}
```
2022-12-11 18:34:14 +00:00
unsafe fn get_param < ' w , ' s > (
Remove the `SystemParamState` trait and remove types like `ResState` (#6919)
Spiritual successor to #5205.
Actual successor to #6865.
# Objective
Currently, system params are defined using three traits: `SystemParam`, `ReadOnlySystemParam`, `SystemParamState`. The behavior for each param is specified by the `SystemParamState` trait, while `SystemParam` simply defers to the state.
Splitting the traits in this way makes it easier to implement within macros, but it increases the cognitive load. Worst of all, this approach requires each `MySystemParam` to have a public `MySystemParamState` type associated with it.
## Solution
* Merge the trait `SystemParamState` into `SystemParam`.
* Remove all trivial `SystemParam` state types.
* `OptionNonSendMutState<T>`: you will not be missed.
---
- [x] Fix/resolve the remaining test failure.
## Changelog
* Removed the trait `SystemParamState`, merging its functionality into `SystemParam`.
## Migration Guide
**Note**: this should replace the migration guide for #6865.
This is relative to Bevy 0.9, not main.
The traits `SystemParamState` and `SystemParamFetch` have been removed, and their functionality has been transferred to `SystemParam`.
```rust
// Before (0.9)
impl SystemParam for MyParam<'_, '_> {
type State = MyParamState;
}
unsafe impl SystemParamState for MyParamState {
fn init(world: &mut World, system_meta: &mut SystemMeta) -> Self { ... }
}
unsafe impl<'w, 's> SystemParamFetch<'w, 's> for MyParamState {
type Item = MyParam<'w, 's>;
fn get_param(&mut self, ...) -> Self::Item;
}
unsafe impl ReadOnlySystemParamFetch for MyParamState { }
// After (0.10)
unsafe impl SystemParam for MyParam<'_, '_> {
type State = MyParamState;
type Item<'w, 's> = MyParam<'w, 's>;
fn init_state(world: &mut World, system_meta: &mut SystemMeta) -> Self::State { ... }
fn get_param<'w, 's>(state: &mut Self::State, ...) -> Self::Item<'w, 's>;
}
unsafe impl ReadOnlySystemParam for MyParam<'_, '_> { }
```
The trait `ReadOnlySystemParamFetch` has been replaced with `ReadOnlySystemParam`.
```rust
// Before
unsafe impl ReadOnlySystemParamFetch for MyParamState {}
// After
unsafe impl ReadOnlySystemParam for MyParam<'_, '_> {}
```
2023-01-07 23:20:32 +00:00
& mut component_id : & ' s mut Self ::State ,
Add new SystemState and rename old SystemState to SystemMeta (#2283)
This enables `SystemParams` to be used outside of function systems. Anything can create and store `SystemState`, which enables efficient "param state cached" access to `SystemParams`.
It adds a `ReadOnlySystemParamFetch` trait, which enables safe `SystemState::get` calls without unique world access.
I renamed the old `SystemState` to `SystemMeta` to enable us to mirror the `QueryState` naming convention (but I'm happy to discuss alternative names if people have other ideas). I initially pitched this as `ParamState`, but given that it needs to include full system metadata, that doesn't feel like a particularly accurate name.
```rust
#[derive(Eq, PartialEq, Debug)]
struct A(usize);
#[derive(Eq, PartialEq, Debug)]
struct B(usize);
let mut world = World::default();
world.insert_resource(A(42));
world.spawn().insert(B(7));
// we get nice lifetime elision when declaring the type on the left hand side
let mut system_state: SystemState<(Res<A>, Query<&B>)> = SystemState::new(&mut world);
let (a, query) = system_state.get(&world);
assert_eq!(*a, A(42), "returned resource matches initial value");
assert_eq!(
*query.single().unwrap(),
B(7),
"returned component matches initial value"
);
// mutable system params require unique world access
let mut system_state: SystemState<(ResMut<A>, Query<&mut B>)> = SystemState::new(&mut world);
let (a, query) = system_state.get_mut(&mut world);
// static lifetimes are required when declaring inside of structs
struct SomeContainer {
state: SystemState<(Res<'static, A>, Res<'static, B>)>
}
// this can be shortened using type aliases, which will be useful for complex param tuples
type MyParams<'a> = (Res<'a, A>, Res<'a, B>);
struct SomeContainer {
state: SystemState<MyParams<'static>>
}
// It is the user's responsibility to call SystemState::apply(world) for parameters that queue up work
let mut system_state: SystemState<(Commands, Query<&B>)> = SystemState::new(&mut world);
{
let (mut commands, query) = system_state.get(&world);
commands.insert_resource(3.14);
}
system_state.apply(&mut world);
```
## Future Work
* Actually use SystemState inside FunctionSystem. This would be trivial, but it requires FunctionSystem to wrap SystemState in Option in its current form (which complicates system metadata lookup). I'd prefer to hold off until we adopt something like the later designs linked in #1364, which enable us to contruct Systems using a World reference (and also remove the need for `.system`).
* Consider a "scoped" approach to automatically call SystemState::apply when systems params are no longer being used (either a container type with a Drop impl, or a function that takes a closure for user logic operating on params).
2021-06-02 19:57:38 +00:00
system_meta : & SystemMeta ,
System Param Lifetime Split (#2605)
# Objective
Enable using exact World lifetimes during read-only access . This is motivated by the new renderer's need to allow read-only world-only queries to outlive the query itself (but still be constrained by the world lifetime).
For example:
https://github.com/bevyengine/bevy/blob/115b170d1f11a91146bb6d6e9684dceb8b21f786/pipelined/bevy_pbr2/src/render/mod.rs#L774
## Solution
Split out SystemParam state and world lifetimes and pipe those lifetimes up to read-only Query ops (and add into_inner for Res). According to every safety test I've run so far (except one), this is safe (see the temporary safety test commit). Note that changing the mutable variants to the new lifetimes would allow aliased mutable pointers (try doing that to see how it affects the temporary safety tests).
The new state lifetime on SystemParam does make `#[derive(SystemParam)]` more cumbersome (the current impl requires PhantomData if you don't use both lifetimes). We can make this better by detecting whether or not a lifetime is used in the derive and adjusting accordingly, but that should probably be done in its own pr.
## Why is this a draft?
The new lifetimes break QuerySet safety in one very specific case (see the query_set system in system_safety_test). We need to solve this before we can use the lifetimes given.
This is due to the fact that QuerySet is just a wrapper over Query, which now relies on world lifetimes instead of `&self` lifetimes to prevent aliasing (but in systems, each Query has its own implied lifetime, not a centralized world lifetime). I believe the fix is to rewrite QuerySet to have its own World lifetime (and own the internal reference). This will complicate the impl a bit, but I think it is doable. I'm curious if anyone else has better ideas.
Personally, I think these new lifetimes need to happen. We've gotta have a way to directly tie read-only World queries to the World lifetime. The new renderer is the first place this has come up, but I doubt it will be the last. Worst case scenario we can come up with a second `WorldLifetimeQuery<Q, F = ()>` parameter to enable these read-only scenarios, but I'd rather not add another type to the type zoo.
2021-08-15 20:51:53 +00:00
world : & ' w World ,
Reliable change detection (#1471)
# Problem Definition
The current change tracking (via flags for both components and resources) fails to detect changes made by systems that are scheduled to run earlier in the frame than they are.
This issue is discussed at length in [#68](https://github.com/bevyengine/bevy/issues/68) and [#54](https://github.com/bevyengine/bevy/issues/54).
This is very much a draft PR, and contributions are welcome and needed.
# Criteria
1. Each change is detected at least once, no matter the ordering.
2. Each change is detected at most once, no matter the ordering.
3. Changes should be detected the same frame that they are made.
4. Competitive ergonomics. Ideally does not require opting-in.
5. Low CPU overhead of computation.
6. Memory efficient. This must not increase over time, except where the number of entities / resources does.
7. Changes should not be lost for systems that don't run.
8. A frame needs to act as a pure function. Given the same set of entities / components it needs to produce the same end state without side-effects.
**Exact** change-tracking proposals satisfy criteria 1 and 2.
**Conservative** change-tracking proposals satisfy criteria 1 but not 2.
**Flaky** change tracking proposals satisfy criteria 2 but not 1.
# Code Base Navigation
There are three types of flags:
- `Added`: A piece of data was added to an entity / `Resources`.
- `Mutated`: A piece of data was able to be modified, because its `DerefMut` was accessed
- `Changed`: The bitwise OR of `Added` and `Changed`
The special behavior of `ChangedRes`, with respect to the scheduler is being removed in [#1313](https://github.com/bevyengine/bevy/pull/1313) and does not need to be reproduced.
`ChangedRes` and friends can be found in "bevy_ecs/core/resources/resource_query.rs".
The `Flags` trait for Components can be found in "bevy_ecs/core/query.rs".
`ComponentFlags` are stored in "bevy_ecs/core/archetypes.rs", defined on line 446.
# Proposals
**Proposal 5 was selected for implementation.**
## Proposal 0: No Change Detection
The baseline, where computations are performed on everything regardless of whether it changed.
**Type:** Conservative
**Pros:**
- already implemented
- will never miss events
- no overhead
**Cons:**
- tons of repeated work
- doesn't allow users to avoid repeating work (or monitoring for other changes)
## Proposal 1: Earlier-This-Tick Change Detection
The current approach as of Bevy 0.4. Flags are set, and then flushed at the end of each frame.
**Type:** Flaky
**Pros:**
- already implemented
- simple to understand
- low memory overhead (2 bits per component)
- low time overhead (clear every flag once per frame)
**Cons:**
- misses systems based on ordering
- systems that don't run every frame miss changes
- duplicates detection when looping
- can lead to unresolvable circular dependencies
## Proposal 2: Two-Tick Change Detection
Flags persist for two frames, using a double-buffer system identical to that used in events.
A change is observed if it is found in either the current frame's list of changes or the previous frame's.
**Type:** Conservative
**Pros:**
- easy to understand
- easy to implement
- low memory overhead (4 bits per component)
- low time overhead (bit mask and shift every flag once per frame)
**Cons:**
- can result in a great deal of duplicated work
- systems that don't run every frame miss changes
- duplicates detection when looping
## Proposal 3: Last-Tick Change Detection
Flags persist for two frames, using a double-buffer system identical to that used in events.
A change is observed if it is found in the previous frame's list of changes.
**Type:** Exact
**Pros:**
- exact
- easy to understand
- easy to implement
- low memory overhead (4 bits per component)
- low time overhead (bit mask and shift every flag once per frame)
**Cons:**
- change detection is always delayed, possibly causing painful chained delays
- systems that don't run every frame miss changes
- duplicates detection when looping
## Proposal 4: Flag-Doubling Change Detection
Combine Proposal 2 and Proposal 3. Differentiate between `JustChanged` (current behavior) and `Changed` (Proposal 3).
Pack this data into the flags according to [this implementation proposal](https://github.com/bevyengine/bevy/issues/68#issuecomment-769174804).
**Type:** Flaky + Exact
**Pros:**
- allows users to acc
- easy to implement
- low memory overhead (4 bits per component)
- low time overhead (bit mask and shift every flag once per frame)
**Cons:**
- users must specify the type of change detection required
- still quite fragile to system ordering effects when using the flaky `JustChanged` form
- cannot get immediate + exact results
- systems that don't run every frame miss changes
- duplicates detection when looping
## [SELECTED] Proposal 5: Generation-Counter Change Detection
A global counter is increased after each system is run. Each component saves the time of last mutation, and each system saves the time of last execution. Mutation is detected when the component's counter is greater than the system's counter. Discussed [here](https://github.com/bevyengine/bevy/issues/68#issuecomment-769174804). How to handle addition detection is unsolved; the current proposal is to use the highest bit of the counter as in proposal 1.
**Type:** Exact (for mutations), flaky (for additions)
**Pros:**
- low time overhead (set component counter on access, set system counter after execution)
- robust to systems that don't run every frame
- robust to systems that loop
**Cons:**
- moderately complex implementation
- must be modified as systems are inserted dynamically
- medium memory overhead (4 bytes per component + system)
- unsolved addition detection
## Proposal 6: System-Data Change Detection
For each system, track which system's changes it has seen. This approach is only worth fully designing and implementing if Proposal 5 fails in some way.
**Type:** Exact
**Pros:**
- exact
- conceptually simple
**Cons:**
- requires storing data on each system
- implementation is complex
- must be modified as systems are inserted dynamically
## Proposal 7: Total-Order Change Detection
Discussed [here](https://github.com/bevyengine/bevy/issues/68#issuecomment-754326523). This proposal is somewhat complicated by the new scheduler, but I believe it should still be conceptually feasible. This approach is only worth fully designing and implementing if Proposal 5 fails in some way.
**Type:** Exact
**Pros:**
- exact
- efficient data storage relative to other exact proposals
**Cons:**
- requires access to the scheduler
- complex implementation and difficulty grokking
- must be modified as systems are inserted dynamically
# Tests
- We will need to verify properties 1, 2, 3, 7 and 8. Priority: 1 > 2 = 3 > 8 > 7
- Ideally we can use identical user-facing syntax for all proposals, allowing us to re-use the same syntax for each.
- When writing tests, we need to carefully specify order using explicit dependencies.
- These tests will need to be duplicated for both components and resources.
- We need to be sure to handle cases where ambiguous system orders exist.
`changing_system` is always the system that makes the changes, and `detecting_system` always detects the changes.
The component / resource changed will be simple boolean wrapper structs.
## Basic Added / Mutated / Changed
2 x 3 design:
- Resources vs. Components
- Added vs. Changed vs. Mutated
- `changing_system` runs before `detecting_system`
- verify at the end of tick 2
## At Least Once
2 x 3 design:
- Resources vs. Components
- Added vs. Changed vs. Mutated
- `changing_system` runs after `detecting_system`
- verify at the end of tick 2
## At Most Once
2 x 3 design:
- Resources vs. Components
- Added vs. Changed vs. Mutated
- `changing_system` runs once before `detecting_system`
- increment a counter based on the number of changes detected
- verify at the end of tick 2
## Fast Detection
2 x 3 design:
- Resources vs. Components
- Added vs. Changed vs. Mutated
- `changing_system` runs before `detecting_system`
- verify at the end of tick 1
## Ambiguous System Ordering Robustness
2 x 3 x 2 design:
- Resources vs. Components
- Added vs. Changed vs. Mutated
- `changing_system` runs [before/after] `detecting_system` in tick 1
- `changing_system` runs [after/before] `detecting_system` in tick 2
## System Pausing
2 x 3 design:
- Resources vs. Components
- Added vs. Changed vs. Mutated
- `changing_system` runs in tick 1, then is disabled by run criteria
- `detecting_system` is disabled by run criteria until it is run once during tick 3
- verify at the end of tick 3
## Addition Causes Mutation
2 design:
- Resources vs. Components
- `adding_system_1` adds a component / resource
- `adding system_2` adds the same component / resource
- verify the `Mutated` flag at the end of the tick
- verify the `Added` flag at the end of the tick
First check tests for: https://github.com/bevyengine/bevy/issues/333
Second check tests for: https://github.com/bevyengine/bevy/issues/1443
## Changes Made By Commands
- `adding_system` runs in Update in tick 1, and sends a command to add a component
- `detecting_system` runs in Update in tick 1 and 2, after `adding_system`
- We can't detect the changes in tick 1, since they haven't been processed yet
- If we were to track these changes as being emitted by `adding_system`, we can't detect the changes in tick 2 either, since `detecting_system` has already run once after `adding_system` :(
# Benchmarks
See: [general advice](https://github.com/bevyengine/bevy/blob/master/docs/profiling.md), [Criterion crate](https://github.com/bheisler/criterion.rs)
There are several critical parameters to vary:
1. entity count (1 to 10^9)
2. fraction of entities that are changed (0% to 100%)
3. cost to perform work on changed entities, i.e. workload (1 ns to 1s)
1 and 2 should be varied between benchmark runs. 3 can be added on computationally.
We want to measure:
- memory cost
- run time
We should collect these measurements across several frames (100?) to reduce bootup effects and accurately measure the mean, variance and drift.
Entity-component change detection is much more important to benchmark than resource change detection, due to the orders of magnitude higher number of pieces of data.
No change detection at all should be included in benchmarks as a second control for cases where missing changes is unacceptable.
## Graphs
1. y: performance, x: log_10(entity count), color: proposal, facet: performance metric. Set cost to perform work to 0.
2. y: run time, x: cost to perform work, color: proposal, facet: fraction changed. Set number of entities to 10^6
3. y: memory, x: frames, color: proposal
# Conclusions
1. Is the theoretical categorization of the proposals correct according to our tests?
2. How does the performance of the proposals compare without any load?
3. How does the performance of the proposals compare with realistic loads?
4. At what workload does more exact change tracking become worth the (presumably) higher overhead?
5. When does adding change-detection to save on work become worthwhile?
6. Is there enough divergence in performance between the best solutions in each class to ship more than one change-tracking solution?
# Implementation Plan
1. Write a test suite.
2. Verify that tests fail for existing approach.
3. Write a benchmark suite.
4. Get performance numbers for existing approach.
5. Implement, test and benchmark various solutions using a Git branch per proposal.
6. Create a draft PR with all solutions and present results to team.
7. Select a solution and replace existing change detection.
Co-authored-by: Brice DAVIER <bricedavier@gmail.com>
Co-authored-by: Carter Anderson <mcanders1@gmail.com>
2021-03-19 17:53:26 +00:00
change_tick : u32 ,
Simplify trait hierarchy for `SystemParam` (#6865)
# Objective
* Implementing a custom `SystemParam` by hand requires implementing three traits -- four if it is read-only.
* The trait `SystemParamFetch<'w, 's>` is a workaround from before we had generic associated types, and is no longer necessary.
## Solution
* Combine the trait `SystemParamFetch` with `SystemParamState`.
* I decided to remove the `Fetch` name and keep the `State` name, since the former was consistently conflated with the latter.
* Replace the trait `ReadOnlySystemParamFetch` with `ReadOnlySystemParam`, which simplifies trait bounds in generic code.
---
## Changelog
- Removed the trait `SystemParamFetch`, moving its functionality to `SystemParamState`.
- Replaced the trait `ReadOnlySystemParamFetch` with `ReadOnlySystemParam`.
## Migration Guide
The trait `SystemParamFetch` has been removed, and its functionality has been transferred to `SystemParamState`.
```rust
// Before
impl SystemParamState for MyParamState {
fn init(world: &mut World, system_meta: &mut SystemMeta) -> Self { ... }
}
impl<'w, 's> SystemParamFetch<'w, 's> for MyParamState {
type Item = MyParam<'w, 's>;
fn get_param(...) -> Self::Item;
}
// After
impl SystemParamState for MyParamState {
type Item<'w, 's> = MyParam<'w, 's>; // Generic associated types!
fn init(world: &mut World, system_meta: &mut SystemMeta) -> Self { ... }
fn get_param<'w, 's>(...) -> Self::Item<'w, 's>;
}
```
The trait `ReadOnlySystemParamFetch` has been replaced with `ReadOnlySystemParam`.
```rust
// Before
unsafe impl ReadOnlySystemParamFetch for MyParamState {}
// After
unsafe impl<'w, 's> ReadOnlySystemParam for MyParam<'w, 's> {}
```
2022-12-11 18:34:14 +00:00
) -> Self ::Item < ' w , ' s > {
2022-10-24 13:46:36 +00:00
let ( ptr , ticks ) = world
2023-02-06 19:02:52 +00:00
. as_unsafe_world_cell_migration_internal ( )
Remove the `SystemParamState` trait and remove types like `ResState` (#6919)
Spiritual successor to #5205.
Actual successor to #6865.
# Objective
Currently, system params are defined using three traits: `SystemParam`, `ReadOnlySystemParam`, `SystemParamState`. The behavior for each param is specified by the `SystemParamState` trait, while `SystemParam` simply defers to the state.
Splitting the traits in this way makes it easier to implement within macros, but it increases the cognitive load. Worst of all, this approach requires each `MySystemParam` to have a public `MySystemParamState` type associated with it.
## Solution
* Merge the trait `SystemParamState` into `SystemParam`.
* Remove all trivial `SystemParam` state types.
* `OptionNonSendMutState<T>`: you will not be missed.
---
- [x] Fix/resolve the remaining test failure.
## Changelog
* Removed the trait `SystemParamState`, merging its functionality into `SystemParam`.
## Migration Guide
**Note**: this should replace the migration guide for #6865.
This is relative to Bevy 0.9, not main.
The traits `SystemParamState` and `SystemParamFetch` have been removed, and their functionality has been transferred to `SystemParam`.
```rust
// Before (0.9)
impl SystemParam for MyParam<'_, '_> {
type State = MyParamState;
}
unsafe impl SystemParamState for MyParamState {
fn init(world: &mut World, system_meta: &mut SystemMeta) -> Self { ... }
}
unsafe impl<'w, 's> SystemParamFetch<'w, 's> for MyParamState {
type Item = MyParam<'w, 's>;
fn get_param(&mut self, ...) -> Self::Item;
}
unsafe impl ReadOnlySystemParamFetch for MyParamState { }
// After (0.10)
unsafe impl SystemParam for MyParam<'_, '_> {
type State = MyParamState;
type Item<'w, 's> = MyParam<'w, 's>;
fn init_state(world: &mut World, system_meta: &mut SystemMeta) -> Self::State { ... }
fn get_param<'w, 's>(state: &mut Self::State, ...) -> Self::Item<'w, 's>;
}
unsafe impl ReadOnlySystemParam for MyParam<'_, '_> { }
```
The trait `ReadOnlySystemParamFetch` has been replaced with `ReadOnlySystemParam`.
```rust
// Before
unsafe impl ReadOnlySystemParamFetch for MyParamState {}
// After
unsafe impl ReadOnlySystemParam for MyParam<'_, '_> {}
```
2023-01-07 23:20:32 +00:00
. get_resource_with_ticks ( component_id )
2021-03-14 00:36:16 +00:00
. unwrap_or_else ( | | {
panic! (
2021-04-14 22:52:43 +00:00
" Resource requested by {} does not exist: {} " ,
Add new SystemState and rename old SystemState to SystemMeta (#2283)
This enables `SystemParams` to be used outside of function systems. Anything can create and store `SystemState`, which enables efficient "param state cached" access to `SystemParams`.
It adds a `ReadOnlySystemParamFetch` trait, which enables safe `SystemState::get` calls without unique world access.
I renamed the old `SystemState` to `SystemMeta` to enable us to mirror the `QueryState` naming convention (but I'm happy to discuss alternative names if people have other ideas). I initially pitched this as `ParamState`, but given that it needs to include full system metadata, that doesn't feel like a particularly accurate name.
```rust
#[derive(Eq, PartialEq, Debug)]
struct A(usize);
#[derive(Eq, PartialEq, Debug)]
struct B(usize);
let mut world = World::default();
world.insert_resource(A(42));
world.spawn().insert(B(7));
// we get nice lifetime elision when declaring the type on the left hand side
let mut system_state: SystemState<(Res<A>, Query<&B>)> = SystemState::new(&mut world);
let (a, query) = system_state.get(&world);
assert_eq!(*a, A(42), "returned resource matches initial value");
assert_eq!(
*query.single().unwrap(),
B(7),
"returned component matches initial value"
);
// mutable system params require unique world access
let mut system_state: SystemState<(ResMut<A>, Query<&mut B>)> = SystemState::new(&mut world);
let (a, query) = system_state.get_mut(&mut world);
// static lifetimes are required when declaring inside of structs
struct SomeContainer {
state: SystemState<(Res<'static, A>, Res<'static, B>)>
}
// this can be shortened using type aliases, which will be useful for complex param tuples
type MyParams<'a> = (Res<'a, A>, Res<'a, B>);
struct SomeContainer {
state: SystemState<MyParams<'static>>
}
// It is the user's responsibility to call SystemState::apply(world) for parameters that queue up work
let mut system_state: SystemState<(Commands, Query<&B>)> = SystemState::new(&mut world);
{
let (mut commands, query) = system_state.get(&world);
commands.insert_resource(3.14);
}
system_state.apply(&mut world);
```
## Future Work
* Actually use SystemState inside FunctionSystem. This would be trivial, but it requires FunctionSystem to wrap SystemState in Option in its current form (which complicates system metadata lookup). I'd prefer to hold off until we adopt something like the later designs linked in #1364, which enable us to contruct Systems using a World reference (and also remove the need for `.system`).
* Consider a "scoped" approach to automatically call SystemState::apply when systems params are no longer being used (either a container type with a Drop impl, or a function that takes a closure for user logic operating on params).
2021-06-02 19:57:38 +00:00
system_meta . name ,
2021-03-14 00:36:16 +00:00
std ::any ::type_name ::< T > ( )
)
} ) ;
Bevy ECS V2 (#1525)
# Bevy ECS V2
This is a rewrite of Bevy ECS (basically everything but the new executor/schedule, which are already awesome). The overall goal was to improve the performance and versatility of Bevy ECS. Here is a quick bulleted list of changes before we dive into the details:
* Complete World rewrite
* Multiple component storage types:
* Tables: fast cache friendly iteration, slower add/removes (previously called Archetypes)
* Sparse Sets: fast add/remove, slower iteration
* Stateful Queries (caches query results for faster iteration. fragmented iteration is _fast_ now)
* Stateful System Params (caches expensive operations. inspired by @DJMcNab's work in #1364)
* Configurable System Params (users can set configuration when they construct their systems. once again inspired by @DJMcNab's work)
* Archetypes are now "just metadata", component storage is separate
* Archetype Graph (for faster archetype changes)
* Component Metadata
* Configure component storage type
* Retrieve information about component size/type/name/layout/send-ness/etc
* Components are uniquely identified by a densely packed ComponentId
* TypeIds are now totally optional (which should make implementing scripting easier)
* Super fast "for_each" query iterators
* Merged Resources into World. Resources are now just a special type of component
* EntityRef/EntityMut builder apis (more efficient and more ergonomic)
* Fast bitset-backed `Access<T>` replaces old hashmap-based approach everywhere
* Query conflicts are determined by component access instead of archetype component access (to avoid random failures at runtime)
* With/Without are still taken into account for conflicts, so this should still be comfy to use
* Much simpler `IntoSystem` impl
* Significantly reduced the amount of hashing throughout the ecs in favor of Sparse Sets (indexed by densely packed ArchetypeId, ComponentId, BundleId, and TableId)
* Safety Improvements
* Entity reservation uses a normal world reference instead of unsafe transmute
* QuerySets no longer transmute lifetimes
* Made traits "unsafe" where relevant
* More thorough safety docs
* WorldCell
* Exposes safe mutable access to multiple resources at a time in a World
* Replaced "catch all" `System::update_archetypes(world: &World)` with `System::new_archetype(archetype: &Archetype)`
* Simpler Bundle implementation
* Replaced slow "remove_bundle_one_by_one" used as fallback for Commands::remove_bundle with fast "remove_bundle_intersection"
* Removed `Mut<T>` query impl. it is better to only support one way: `&mut T`
* Removed with() from `Flags<T>` in favor of `Option<Flags<T>>`, which allows querying for flags to be "filtered" by default
* Components now have is_send property (currently only resources support non-send)
* More granular module organization
* New `RemovedComponents<T>` SystemParam that replaces `query.removed::<T>()`
* `world.resource_scope()` for mutable access to resources and world at the same time
* WorldQuery and QueryFilter traits unified. FilterFetch trait added to enable "short circuit" filtering. Auto impled for cases that don't need it
* Significantly slimmed down SystemState in favor of individual SystemParam state
* System Commands changed from `commands: &mut Commands` back to `mut commands: Commands` (to allow Commands to have a World reference)
Fixes #1320
## `World` Rewrite
This is a from-scratch rewrite of `World` that fills the niche that `hecs` used to. Yes, this means Bevy ECS is no longer a "fork" of hecs. We're going out our own!
(the only shared code between the projects is the entity id allocator, which is already basically ideal)
A huge shout out to @SanderMertens (author of [flecs](https://github.com/SanderMertens/flecs)) for sharing some great ideas with me (specifically hybrid ecs storage and archetype graphs). He also helped advise on a number of implementation details.
## Component Storage (The Problem)
Two ECS storage paradigms have gained a lot of traction over the years:
* **Archetypal ECS**:
* Stores components in "tables" with static schemas. Each "column" stores components of a given type. Each "row" is an entity.
* Each "archetype" has its own table. Adding/removing an entity's component changes the archetype.
* Enables super-fast Query iteration due to its cache-friendly data layout
* Comes at the cost of more expensive add/remove operations for an Entity's components, because all components need to be copied to the new archetype's "table"
* **Sparse Set ECS**:
* Stores components of the same type in densely packed arrays, which are sparsely indexed by densely packed unsigned integers (Entity ids)
* Query iteration is slower than Archetypal ECS because each entity's component could be at any position in the sparse set. This "random access" pattern isn't cache friendly. Additionally, there is an extra layer of indirection because you must first map the entity id to an index in the component array.
* Adding/removing components is a cheap, constant time operation
Bevy ECS V1, hecs, legion, flec, and Unity DOTS are all "archetypal ecs-es". I personally think "archetypal" storage is a good default for game engines. An entity's archetype doesn't need to change frequently in general, and it creates "fast by default" query iteration (which is a much more common operation). It is also "self optimizing". Users don't need to think about optimizing component layouts for iteration performance. It "just works" without any extra boilerplate.
Shipyard and EnTT are "sparse set ecs-es". They employ "packing" as a way to work around the "suboptimal by default" iteration performance for specific sets of components. This helps, but I didn't think this was a good choice for a general purpose engine like Bevy because:
1. "packs" conflict with each other. If bevy decides to internally pack the Transform and GlobalTransform components, users are then blocked if they want to pack some custom component with Transform.
2. users need to take manual action to optimize
Developers selecting an ECS framework are stuck with a hard choice. Select an "archetypal" framework with "fast iteration everywhere" but without the ability to cheaply add/remove components, or select a "sparse set" framework to cheaply add/remove components but with slower iteration performance.
## Hybrid Component Storage (The Solution)
In Bevy ECS V2, we get to have our cake and eat it too. It now has _both_ of the component storage types above (and more can be added later if needed):
* **Tables** (aka "archetypal" storage)
* The default storage. If you don't configure anything, this is what you get
* Fast iteration by default
* Slower add/remove operations
* **Sparse Sets**
* Opt-in
* Slower iteration
* Faster add/remove operations
These storage types complement each other perfectly. By default Query iteration is fast. If developers know that they want to add/remove a component at high frequencies, they can set the storage to "sparse set":
```rust
world.register_component(
ComponentDescriptor::new::<MyComponent>(StorageType::SparseSet)
).unwrap();
```
## Archetypes
Archetypes are now "just metadata" ... they no longer store components directly. They do store:
* The `ComponentId`s of each of the Archetype's components (and that component's storage type)
* Archetypes are uniquely defined by their component layouts
* For example: entities with "table" components `[A, B, C]` _and_ "sparse set" components `[D, E]` will always be in the same archetype.
* The `TableId` associated with the archetype
* For now each archetype has exactly one table (which can have no components),
* There is a 1->Many relationship from Tables->Archetypes. A given table could have any number of archetype components stored in it:
* Ex: an entity with "table storage" components `[A, B, C]` and "sparse set" components `[D, E]` will share the same `[A, B, C]` table as an entity with `[A, B, C]` table component and `[F]` sparse set components.
* This 1->Many relationship is how we preserve fast "cache friendly" iteration performance when possible (more on this later)
* A list of entities that are in the archetype and the row id of the table they are in
* ArchetypeComponentIds
* unique densely packed identifiers for (ArchetypeId, ComponentId) pairs
* used by the schedule executor for cheap system access control
* "Archetype Graph Edges" (see the next section)
## The "Archetype Graph"
Archetype changes in Bevy (and a number of other archetypal ecs-es) have historically been expensive to compute. First, you need to allocate a new vector of the entity's current component ids, add or remove components based on the operation performed, sort it (to ensure it is order-independent), then hash it to find the archetype (if it exists). And thats all before we get to the _already_ expensive full copy of all components to the new table storage.
The solution is to build a "graph" of archetypes to cache these results. @SanderMertens first exposed me to the idea (and he got it from @gjroelofs, who came up with it). They propose adding directed edges between archetypes for add/remove component operations. If `ComponentId`s are densely packed, you can use sparse sets to cheaply jump between archetypes.
Bevy takes this one step further by using add/remove `Bundle` edges instead of `Component` edges. Bevy encourages the use of `Bundles` to group add/remove operations. This is largely for "clearer game logic" reasons, but it also helps cut down on the number of archetype changes required. `Bundles` now also have densely-packed `BundleId`s. This allows us to use a _single_ edge for each bundle operation (rather than needing to traverse N edges ... one for each component). Single component operations are also bundles, so this is strictly an improvement over a "component only" graph.
As a result, an operation that used to be _heavy_ (both for allocations and compute) is now two dirt-cheap array lookups and zero allocations.
## Stateful Queries
World queries are now stateful. This allows us to:
1. Cache archetype (and table) matches
* This resolves another issue with (naive) archetypal ECS: query performance getting worse as the number of archetypes goes up (and fragmentation occurs).
2. Cache Fetch and Filter state
* The expensive parts of fetch/filter operations (such as hashing the TypeId to find the ComponentId) now only happen once when the Query is first constructed
3. Incrementally build up state
* When new archetypes are added, we only process the new archetypes (no need to rebuild state for old archetypes)
As a result, the direct `World` query api now looks like this:
```rust
let mut query = world.query::<(&A, &mut B)>();
for (a, mut b) in query.iter_mut(&mut world) {
}
```
Requiring `World` to generate stateful queries (rather than letting the `QueryState` type be constructed separately) allows us to ensure that _all_ queries are properly initialized (and the relevant world state, such as ComponentIds). This enables QueryState to remove branches from its operations that check for initialization status (and also enables query.iter() to take an immutable world reference because it doesn't need to initialize anything in world).
However in systems, this is a non-breaking change. State management is done internally by the relevant SystemParam.
## Stateful SystemParams
Like Queries, `SystemParams` now also cache state. For example, `Query` system params store the "stateful query" state mentioned above. Commands store their internal `CommandQueue`. This means you can now safely use as many separate `Commands` parameters in your system as you want. `Local<T>` system params store their `T` value in their state (instead of in Resources).
SystemParam state also enabled a significant slim-down of SystemState. It is much nicer to look at now.
Per-SystemParam state naturally insulates us from an "aliased mut" class of errors we have hit in the past (ex: using multiple `Commands` system params).
(credit goes to @DJMcNab for the initial idea and draft pr here #1364)
## Configurable SystemParams
@DJMcNab also had the great idea to make SystemParams configurable. This allows users to provide some initial configuration / values for system parameters (when possible). Most SystemParams have no config (the config type is `()`), but the `Local<T>` param now supports user-provided parameters:
```rust
fn foo(value: Local<usize>) {
}
app.add_system(foo.system().config(|c| c.0 = Some(10)));
```
## Uber Fast "for_each" Query Iterators
Developers now have the choice to use a fast "for_each" iterator, which yields ~1.5-3x iteration speed improvements for "fragmented iteration", and minor ~1.2x iteration speed improvements for unfragmented iteration.
```rust
fn system(query: Query<(&A, &mut B)>) {
// you now have the option to do this for a speed boost
query.for_each_mut(|(a, mut b)| {
});
// however normal iterators are still available
for (a, mut b) in query.iter_mut() {
}
}
```
I think in most cases we should continue to encourage "normal" iterators as they are more flexible and more "rust idiomatic". But when that extra "oomf" is needed, it makes sense to use `for_each`.
We should also consider using `for_each` for internal bevy systems to give our users a nice speed boost (but that should be a separate pr).
## Component Metadata
`World` now has a `Components` collection, which is accessible via `world.components()`. This stores mappings from `ComponentId` to `ComponentInfo`, as well as `TypeId` to `ComponentId` mappings (where relevant). `ComponentInfo` stores information about the component, such as ComponentId, TypeId, memory layout, send-ness (currently limited to resources), and storage type.
## Significantly Cheaper `Access<T>`
We used to use `TypeAccess<TypeId>` to manage read/write component/archetype-component access. This was expensive because TypeIds must be hashed and compared individually. The parallel executor got around this by "condensing" type ids into bitset-backed access types. This worked, but it had to be re-generated from the `TypeAccess<TypeId>`sources every time archetypes changed.
This pr removes TypeAccess in favor of faster bitset access everywhere. We can do this thanks to the move to densely packed `ComponentId`s and `ArchetypeComponentId`s.
## Merged Resources into World
Resources had a lot of redundant functionality with Components. They stored typed data, they had access control, they had unique ids, they were queryable via SystemParams, etc. In fact the _only_ major difference between them was that they were unique (and didn't correlate to an entity).
Separate resources also had the downside of requiring a separate set of access controls, which meant the parallel executor needed to compare more bitsets per system and manage more state.
I initially got the "separate resources" idea from `legion`. I think that design was motivated by the fact that it made the direct world query/resource lifetime interactions more manageable. It certainly made our lives easier when using Resources alongside hecs/bevy_ecs. However we already have a construct for safely and ergonomically managing in-world lifetimes: systems (which use `Access<T>` internally).
This pr merges Resources into World:
```rust
world.insert_resource(1);
world.insert_resource(2.0);
let a = world.get_resource::<i32>().unwrap();
let mut b = world.get_resource_mut::<f64>().unwrap();
*b = 3.0;
```
Resources are now just a special kind of component. They have their own ComponentIds (and their own resource TypeId->ComponentId scope, so they don't conflict wit components of the same type). They are stored in a special "resource archetype", which stores components inside the archetype using a new `unique_components` sparse set (note that this sparse set could later be used to implement Tags). This allows us to keep the code size small by reusing existing datastructures (namely Column, Archetype, ComponentFlags, and ComponentInfo). This allows us the executor to use a single `Access<ArchetypeComponentId>` per system. It should also make scripting language integration easier.
_But_ this merge did create problems for people directly interacting with `World`. What if you need mutable access to multiple resources at the same time? `world.get_resource_mut()` borrows World mutably!
## WorldCell
WorldCell applies the `Access<ArchetypeComponentId>` concept to direct world access:
```rust
let world_cell = world.cell();
let a = world_cell.get_resource_mut::<i32>().unwrap();
let b = world_cell.get_resource_mut::<f64>().unwrap();
```
This adds cheap runtime checks (a sparse set lookup of `ArchetypeComponentId` and a counter) to ensure that world accesses do not conflict with each other. Each operation returns a `WorldBorrow<'w, T>` or `WorldBorrowMut<'w, T>` wrapper type, which will release the relevant ArchetypeComponentId resources when dropped.
World caches the access sparse set (and only one cell can exist at a time), so `world.cell()` is a cheap operation.
WorldCell does _not_ use atomic operations. It is non-send, does a mutable borrow of world to prevent other accesses, and uses a simple `Rc<RefCell<ArchetypeComponentAccess>>` wrapper in each WorldBorrow pointer.
The api is currently limited to resource access, but it can and should be extended to queries / entity component access.
## Resource Scopes
WorldCell does not yet support component queries, and even when it does there are sometimes legitimate reasons to want a mutable world ref _and_ a mutable resource ref (ex: bevy_render and bevy_scene both need this). In these cases we could always drop down to the unsafe `world.get_resource_unchecked_mut()`, but that is not ideal!
Instead developers can use a "resource scope"
```rust
world.resource_scope(|world: &mut World, a: &mut A| {
})
```
This temporarily removes the `A` resource from `World`, provides mutable pointers to both, and re-adds A to World when finished. Thanks to the move to ComponentIds/sparse sets, this is a cheap operation.
If multiple resources are required, scopes can be nested. We could also consider adding a "resource tuple" to the api if this pattern becomes common and the boilerplate gets nasty.
## Query Conflicts Use ComponentId Instead of ArchetypeComponentId
For safety reasons, systems cannot contain queries that conflict with each other without wrapping them in a QuerySet. On bevy `main`, we use ArchetypeComponentIds to determine conflicts. This is nice because it can take into account filters:
```rust
// these queries will never conflict due to their filters
fn filter_system(a: Query<&mut A, With<B>>, b: Query<&mut B, Without<B>>) {
}
```
But it also has a significant downside:
```rust
// these queries will not conflict _until_ an entity with A, B, and C is spawned
fn maybe_conflicts_system(a: Query<(&mut A, &C)>, b: Query<(&mut A, &B)>) {
}
```
The system above will panic at runtime if an entity with A, B, and C is spawned. This makes it hard to trust that your game logic will run without crashing.
In this pr, I switched to using `ComponentId` instead. This _is_ more constraining. `maybe_conflicts_system` will now always fail, but it will do it consistently at startup. Naively, it would also _disallow_ `filter_system`, which would be a significant downgrade in usability. Bevy has a number of internal systems that rely on disjoint queries and I expect it to be a common pattern in userspace.
To resolve this, I added a new `FilteredAccess<T>` type, which wraps `Access<T>` and adds with/without filters. If two `FilteredAccess` have with/without values that prove they are disjoint, they will no longer conflict.
## EntityRef / EntityMut
World entity operations on `main` require that the user passes in an `entity` id to each operation:
```rust
let entity = world.spawn((A, )); // create a new entity with A
world.get::<A>(entity);
world.insert(entity, (B, C));
world.insert_one(entity, D);
```
This means that each operation needs to look up the entity location / verify its validity. The initial spawn operation also requires a Bundle as input. This can be awkward when no components are required (or one component is required).
These operations have been replaced by `EntityRef` and `EntityMut`, which are "builder-style" wrappers around world that provide read and read/write operations on a single, pre-validated entity:
```rust
// spawn now takes no inputs and returns an EntityMut
let entity = world.spawn()
.insert(A) // insert a single component into the entity
.insert_bundle((B, C)) // insert a bundle of components into the entity
.id() // id returns the Entity id
// Returns EntityMut (or panics if the entity does not exist)
world.entity_mut(entity)
.insert(D)
.insert_bundle(SomeBundle::default());
{
// returns EntityRef (or panics if the entity does not exist)
let d = world.entity(entity)
.get::<D>() // gets the D component
.unwrap();
// world.get still exists for ergonomics
let d = world.get::<D>(entity).unwrap();
}
// These variants return Options if you want to check existence instead of panicing
world.get_entity_mut(entity)
.unwrap()
.insert(E);
if let Some(entity_ref) = world.get_entity(entity) {
let d = entity_ref.get::<D>().unwrap();
}
```
This _does not_ affect the current Commands api or terminology. I think that should be a separate conversation as that is a much larger breaking change.
## Safety Improvements
* Entity reservation in Commands uses a normal world borrow instead of an unsafe transmute
* QuerySets no longer transmutes lifetimes
* Made traits "unsafe" when implementing a trait incorrectly could cause unsafety
* More thorough safety docs
## RemovedComponents SystemParam
The old approach to querying removed components: `query.removed:<T>()` was confusing because it had no connection to the query itself. I replaced it with the following, which is both clearer and allows us to cache the ComponentId mapping in the SystemParamState:
```rust
fn system(removed: RemovedComponents<T>) {
for entity in removed.iter() {
}
}
```
## Simpler Bundle implementation
Bundles are no longer responsible for sorting (or deduping) TypeInfo. They are just a simple ordered list of component types / data. This makes the implementation smaller and opens the door to an easy "nested bundle" implementation in the future (which i might even add in this pr). Duplicate detection is now done once per bundle type by World the first time a bundle is used.
## Unified WorldQuery and QueryFilter types
(don't worry they are still separate type _parameters_ in Queries .. this is a non-breaking change)
WorldQuery and QueryFilter were already basically identical apis. With the addition of `FetchState` and more storage-specific fetch methods, the overlap was even clearer (and the redundancy more painful).
QueryFilters are now just `F: WorldQuery where F::Fetch: FilterFetch`. FilterFetch requires `Fetch<Item = bool>` and adds new "short circuit" variants of fetch methods. This enables a filter tuple like `(With<A>, Without<B>, Changed<C>)` to stop evaluating the filter after the first mismatch is encountered. FilterFetch is automatically implemented for `Fetch` implementations that return bool.
This forces fetch implementations that return things like `(bool, bool, bool)` (such as the filter above) to manually implement FilterFetch and decide whether or not to short-circuit.
## More Granular Modules
World no longer globs all of the internal modules together. It now exports `core`, `system`, and `schedule` separately. I'm also considering exporting `core` submodules directly as that is still pretty "glob-ey" and unorganized (feedback welcome here).
## Remaining Draft Work (to be done in this pr)
* ~~panic on conflicting WorldQuery fetches (&A, &mut A)~~
* ~~bevy `main` and hecs both currently allow this, but we should protect against it if possible~~
* ~~batch_iter / par_iter (currently stubbed out)~~
* ~~ChangedRes~~
* ~~I skipped this while we sort out #1313. This pr should be adapted to account for whatever we land on there~~.
* ~~The `Archetypes` and `Tables` collections use hashes of sorted lists of component ids to uniquely identify each archetype/table. This hash is then used as the key in a HashMap to look up the relevant ArchetypeId or TableId. (which doesn't handle hash collisions properly)~~
* ~~It is currently unsafe to generate a Query from "World A", then use it on "World B" (despite the api claiming it is safe). We should probably close this gap. This could be done by adding a randomly generated WorldId to each world, then storing that id in each Query. They could then be compared to each other on each `query.do_thing(&world)` operation. This _does_ add an extra branch to each query operation, so I'm open to other suggestions if people have them.~~
* ~~Nested Bundles (if i find time)~~
## Potential Future Work
* Expand WorldCell to support queries.
* Consider not allocating in the empty archetype on `world.spawn()`
* ex: return something like EntityMutUninit, which turns into EntityMut after an `insert` or `insert_bundle` op
* this actually regressed performance last time i tried it, but in theory it should be faster
* Optimize SparseSet::insert (see `PERF` comment on insert)
* Replace SparseArray `Option<T>` with T::MAX to cut down on branching
* would enable cheaper get_unchecked() operations
* upstream fixedbitset optimizations
* fixedbitset could be allocation free for small block counts (store blocks in a SmallVec)
* fixedbitset could have a const constructor
* Consider implementing Tags (archetype-specific by-value data that affects archetype identity)
* ex: ArchetypeA could have `[A, B, C]` table components and `[D(1)]` "tag" component. ArchetypeB could have `[A, B, C]` table components and a `[D(2)]` tag component. The archetypes are different, despite both having D tags because the value inside D is different.
* this could potentially build on top of the `archetype.unique_components` added in this pr for resource storage.
* Consider reverting `all_tuples` proc macro in favor of the old `macro_rules` implementation
* all_tuples is more flexible and produces cleaner documentation (the macro_rules version produces weird type parameter orders due to parser constraints)
* but unfortunately all_tuples also appears to make Rust Analyzer sad/slow when working inside of `bevy_ecs` (does not affect user code)
* Consider "resource queries" and/or "mixed resource and entity component queries" as an alternative to WorldCell
* this is basically just "systems" so maybe it's not worth it
* Add more world ops
* `world.clear()`
* `world.reserve<T: Bundle>(count: usize)`
* Try using the old archetype allocation strategy (allocate new memory on resize and copy everything over). I expect this to improve batch insertion performance at the cost of unbatched performance. But thats just a guess. I'm not an allocation perf pro :)
* Adapt Commands apis for consistency with new World apis
## Benchmarks
key:
* `bevy_old`: bevy `main` branch
* `bevy`: this branch
* `_foreach`: uses an optimized for_each iterator
* ` _sparse`: uses sparse set storage (if unspecified assume table storage)
* `_system`: runs inside a system (if unspecified assume test happens via direct world ops)
### Simple Insert (from ecs_bench_suite)
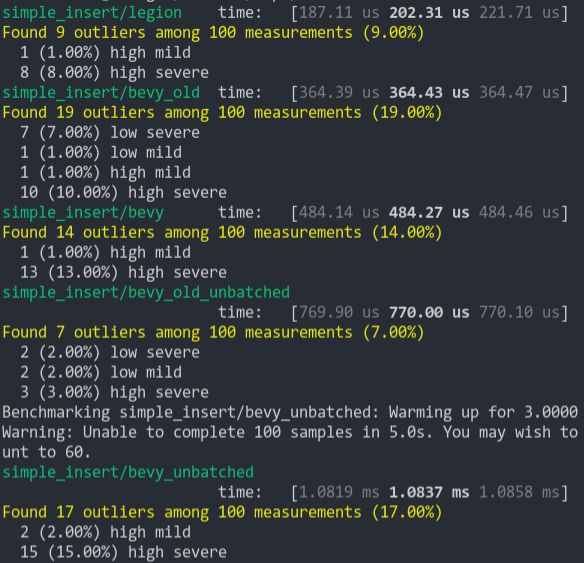
### Simpler Iter (from ecs_bench_suite)
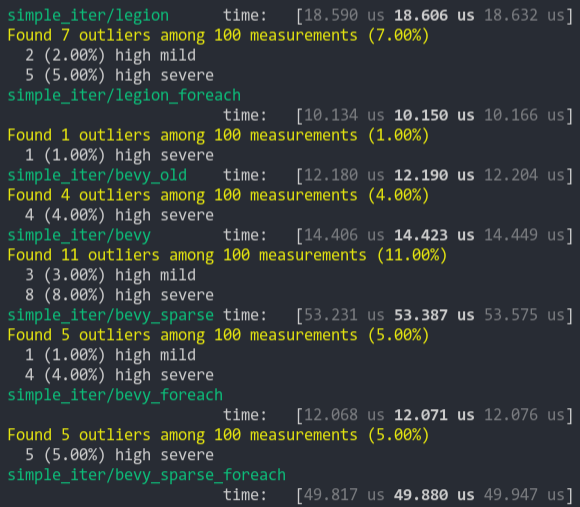
### Fragment Iter (from ecs_bench_suite)
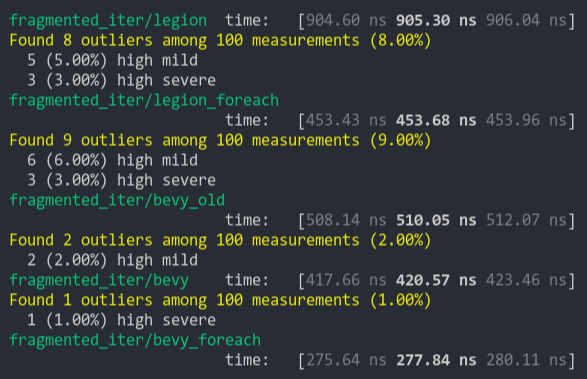
### Sparse Fragmented Iter
Iterate a query that matches 5 entities from a single matching archetype, but there are 100 unmatching archetypes
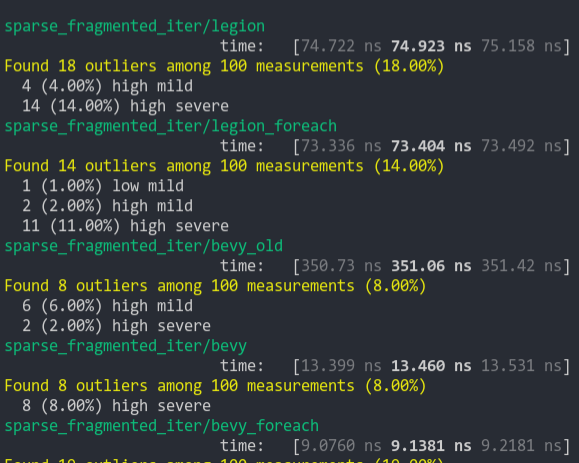
### Schedule (from ecs_bench_suite)
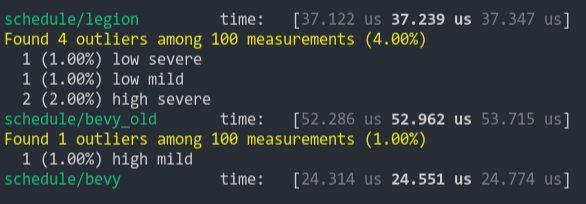
### Add Remove Component (from ecs_bench_suite)
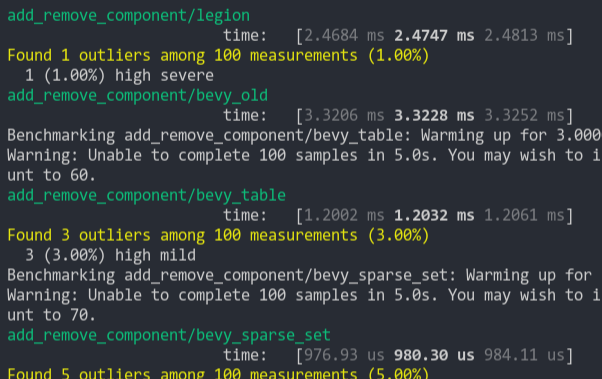
### Add Remove Component Big
Same as the test above, but each entity has 5 "large" matrix components and 1 "large" matrix component is added and removed
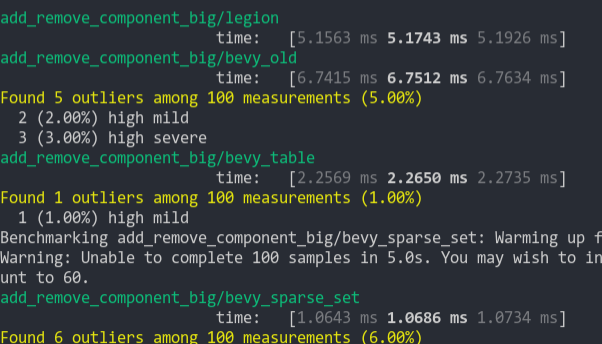
### Get Component
Looks up a single component value a large number of times
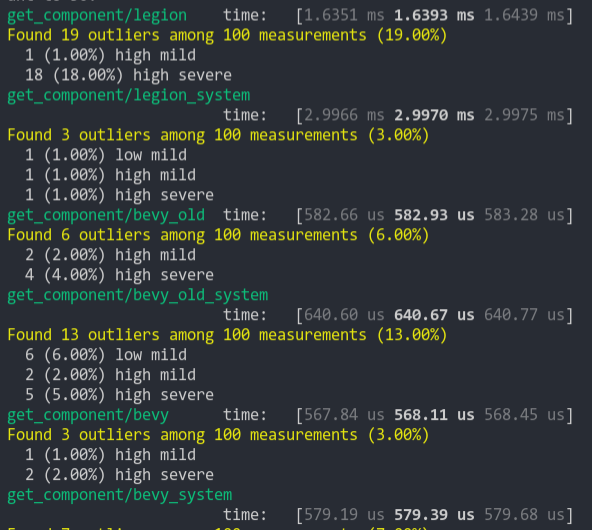
2021-03-05 07:54:35 +00:00
Res {
2022-10-24 13:46:36 +00:00
value : ptr . deref ( ) ,
2023-01-11 15:41:54 +00:00
ticks : Ticks {
added : ticks . added . deref ( ) ,
changed : ticks . changed . deref ( ) ,
last_change_tick : system_meta . last_change_tick ,
change_tick ,
} ,
Bevy ECS V2 (#1525)
# Bevy ECS V2
This is a rewrite of Bevy ECS (basically everything but the new executor/schedule, which are already awesome). The overall goal was to improve the performance and versatility of Bevy ECS. Here is a quick bulleted list of changes before we dive into the details:
* Complete World rewrite
* Multiple component storage types:
* Tables: fast cache friendly iteration, slower add/removes (previously called Archetypes)
* Sparse Sets: fast add/remove, slower iteration
* Stateful Queries (caches query results for faster iteration. fragmented iteration is _fast_ now)
* Stateful System Params (caches expensive operations. inspired by @DJMcNab's work in #1364)
* Configurable System Params (users can set configuration when they construct their systems. once again inspired by @DJMcNab's work)
* Archetypes are now "just metadata", component storage is separate
* Archetype Graph (for faster archetype changes)
* Component Metadata
* Configure component storage type
* Retrieve information about component size/type/name/layout/send-ness/etc
* Components are uniquely identified by a densely packed ComponentId
* TypeIds are now totally optional (which should make implementing scripting easier)
* Super fast "for_each" query iterators
* Merged Resources into World. Resources are now just a special type of component
* EntityRef/EntityMut builder apis (more efficient and more ergonomic)
* Fast bitset-backed `Access<T>` replaces old hashmap-based approach everywhere
* Query conflicts are determined by component access instead of archetype component access (to avoid random failures at runtime)
* With/Without are still taken into account for conflicts, so this should still be comfy to use
* Much simpler `IntoSystem` impl
* Significantly reduced the amount of hashing throughout the ecs in favor of Sparse Sets (indexed by densely packed ArchetypeId, ComponentId, BundleId, and TableId)
* Safety Improvements
* Entity reservation uses a normal world reference instead of unsafe transmute
* QuerySets no longer transmute lifetimes
* Made traits "unsafe" where relevant
* More thorough safety docs
* WorldCell
* Exposes safe mutable access to multiple resources at a time in a World
* Replaced "catch all" `System::update_archetypes(world: &World)` with `System::new_archetype(archetype: &Archetype)`
* Simpler Bundle implementation
* Replaced slow "remove_bundle_one_by_one" used as fallback for Commands::remove_bundle with fast "remove_bundle_intersection"
* Removed `Mut<T>` query impl. it is better to only support one way: `&mut T`
* Removed with() from `Flags<T>` in favor of `Option<Flags<T>>`, which allows querying for flags to be "filtered" by default
* Components now have is_send property (currently only resources support non-send)
* More granular module organization
* New `RemovedComponents<T>` SystemParam that replaces `query.removed::<T>()`
* `world.resource_scope()` for mutable access to resources and world at the same time
* WorldQuery and QueryFilter traits unified. FilterFetch trait added to enable "short circuit" filtering. Auto impled for cases that don't need it
* Significantly slimmed down SystemState in favor of individual SystemParam state
* System Commands changed from `commands: &mut Commands` back to `mut commands: Commands` (to allow Commands to have a World reference)
Fixes #1320
## `World` Rewrite
This is a from-scratch rewrite of `World` that fills the niche that `hecs` used to. Yes, this means Bevy ECS is no longer a "fork" of hecs. We're going out our own!
(the only shared code between the projects is the entity id allocator, which is already basically ideal)
A huge shout out to @SanderMertens (author of [flecs](https://github.com/SanderMertens/flecs)) for sharing some great ideas with me (specifically hybrid ecs storage and archetype graphs). He also helped advise on a number of implementation details.
## Component Storage (The Problem)
Two ECS storage paradigms have gained a lot of traction over the years:
* **Archetypal ECS**:
* Stores components in "tables" with static schemas. Each "column" stores components of a given type. Each "row" is an entity.
* Each "archetype" has its own table. Adding/removing an entity's component changes the archetype.
* Enables super-fast Query iteration due to its cache-friendly data layout
* Comes at the cost of more expensive add/remove operations for an Entity's components, because all components need to be copied to the new archetype's "table"
* **Sparse Set ECS**:
* Stores components of the same type in densely packed arrays, which are sparsely indexed by densely packed unsigned integers (Entity ids)
* Query iteration is slower than Archetypal ECS because each entity's component could be at any position in the sparse set. This "random access" pattern isn't cache friendly. Additionally, there is an extra layer of indirection because you must first map the entity id to an index in the component array.
* Adding/removing components is a cheap, constant time operation
Bevy ECS V1, hecs, legion, flec, and Unity DOTS are all "archetypal ecs-es". I personally think "archetypal" storage is a good default for game engines. An entity's archetype doesn't need to change frequently in general, and it creates "fast by default" query iteration (which is a much more common operation). It is also "self optimizing". Users don't need to think about optimizing component layouts for iteration performance. It "just works" without any extra boilerplate.
Shipyard and EnTT are "sparse set ecs-es". They employ "packing" as a way to work around the "suboptimal by default" iteration performance for specific sets of components. This helps, but I didn't think this was a good choice for a general purpose engine like Bevy because:
1. "packs" conflict with each other. If bevy decides to internally pack the Transform and GlobalTransform components, users are then blocked if they want to pack some custom component with Transform.
2. users need to take manual action to optimize
Developers selecting an ECS framework are stuck with a hard choice. Select an "archetypal" framework with "fast iteration everywhere" but without the ability to cheaply add/remove components, or select a "sparse set" framework to cheaply add/remove components but with slower iteration performance.
## Hybrid Component Storage (The Solution)
In Bevy ECS V2, we get to have our cake and eat it too. It now has _both_ of the component storage types above (and more can be added later if needed):
* **Tables** (aka "archetypal" storage)
* The default storage. If you don't configure anything, this is what you get
* Fast iteration by default
* Slower add/remove operations
* **Sparse Sets**
* Opt-in
* Slower iteration
* Faster add/remove operations
These storage types complement each other perfectly. By default Query iteration is fast. If developers know that they want to add/remove a component at high frequencies, they can set the storage to "sparse set":
```rust
world.register_component(
ComponentDescriptor::new::<MyComponent>(StorageType::SparseSet)
).unwrap();
```
## Archetypes
Archetypes are now "just metadata" ... they no longer store components directly. They do store:
* The `ComponentId`s of each of the Archetype's components (and that component's storage type)
* Archetypes are uniquely defined by their component layouts
* For example: entities with "table" components `[A, B, C]` _and_ "sparse set" components `[D, E]` will always be in the same archetype.
* The `TableId` associated with the archetype
* For now each archetype has exactly one table (which can have no components),
* There is a 1->Many relationship from Tables->Archetypes. A given table could have any number of archetype components stored in it:
* Ex: an entity with "table storage" components `[A, B, C]` and "sparse set" components `[D, E]` will share the same `[A, B, C]` table as an entity with `[A, B, C]` table component and `[F]` sparse set components.
* This 1->Many relationship is how we preserve fast "cache friendly" iteration performance when possible (more on this later)
* A list of entities that are in the archetype and the row id of the table they are in
* ArchetypeComponentIds
* unique densely packed identifiers for (ArchetypeId, ComponentId) pairs
* used by the schedule executor for cheap system access control
* "Archetype Graph Edges" (see the next section)
## The "Archetype Graph"
Archetype changes in Bevy (and a number of other archetypal ecs-es) have historically been expensive to compute. First, you need to allocate a new vector of the entity's current component ids, add or remove components based on the operation performed, sort it (to ensure it is order-independent), then hash it to find the archetype (if it exists). And thats all before we get to the _already_ expensive full copy of all components to the new table storage.
The solution is to build a "graph" of archetypes to cache these results. @SanderMertens first exposed me to the idea (and he got it from @gjroelofs, who came up with it). They propose adding directed edges between archetypes for add/remove component operations. If `ComponentId`s are densely packed, you can use sparse sets to cheaply jump between archetypes.
Bevy takes this one step further by using add/remove `Bundle` edges instead of `Component` edges. Bevy encourages the use of `Bundles` to group add/remove operations. This is largely for "clearer game logic" reasons, but it also helps cut down on the number of archetype changes required. `Bundles` now also have densely-packed `BundleId`s. This allows us to use a _single_ edge for each bundle operation (rather than needing to traverse N edges ... one for each component). Single component operations are also bundles, so this is strictly an improvement over a "component only" graph.
As a result, an operation that used to be _heavy_ (both for allocations and compute) is now two dirt-cheap array lookups and zero allocations.
## Stateful Queries
World queries are now stateful. This allows us to:
1. Cache archetype (and table) matches
* This resolves another issue with (naive) archetypal ECS: query performance getting worse as the number of archetypes goes up (and fragmentation occurs).
2. Cache Fetch and Filter state
* The expensive parts of fetch/filter operations (such as hashing the TypeId to find the ComponentId) now only happen once when the Query is first constructed
3. Incrementally build up state
* When new archetypes are added, we only process the new archetypes (no need to rebuild state for old archetypes)
As a result, the direct `World` query api now looks like this:
```rust
let mut query = world.query::<(&A, &mut B)>();
for (a, mut b) in query.iter_mut(&mut world) {
}
```
Requiring `World` to generate stateful queries (rather than letting the `QueryState` type be constructed separately) allows us to ensure that _all_ queries are properly initialized (and the relevant world state, such as ComponentIds). This enables QueryState to remove branches from its operations that check for initialization status (and also enables query.iter() to take an immutable world reference because it doesn't need to initialize anything in world).
However in systems, this is a non-breaking change. State management is done internally by the relevant SystemParam.
## Stateful SystemParams
Like Queries, `SystemParams` now also cache state. For example, `Query` system params store the "stateful query" state mentioned above. Commands store their internal `CommandQueue`. This means you can now safely use as many separate `Commands` parameters in your system as you want. `Local<T>` system params store their `T` value in their state (instead of in Resources).
SystemParam state also enabled a significant slim-down of SystemState. It is much nicer to look at now.
Per-SystemParam state naturally insulates us from an "aliased mut" class of errors we have hit in the past (ex: using multiple `Commands` system params).
(credit goes to @DJMcNab for the initial idea and draft pr here #1364)
## Configurable SystemParams
@DJMcNab also had the great idea to make SystemParams configurable. This allows users to provide some initial configuration / values for system parameters (when possible). Most SystemParams have no config (the config type is `()`), but the `Local<T>` param now supports user-provided parameters:
```rust
fn foo(value: Local<usize>) {
}
app.add_system(foo.system().config(|c| c.0 = Some(10)));
```
## Uber Fast "for_each" Query Iterators
Developers now have the choice to use a fast "for_each" iterator, which yields ~1.5-3x iteration speed improvements for "fragmented iteration", and minor ~1.2x iteration speed improvements for unfragmented iteration.
```rust
fn system(query: Query<(&A, &mut B)>) {
// you now have the option to do this for a speed boost
query.for_each_mut(|(a, mut b)| {
});
// however normal iterators are still available
for (a, mut b) in query.iter_mut() {
}
}
```
I think in most cases we should continue to encourage "normal" iterators as they are more flexible and more "rust idiomatic". But when that extra "oomf" is needed, it makes sense to use `for_each`.
We should also consider using `for_each` for internal bevy systems to give our users a nice speed boost (but that should be a separate pr).
## Component Metadata
`World` now has a `Components` collection, which is accessible via `world.components()`. This stores mappings from `ComponentId` to `ComponentInfo`, as well as `TypeId` to `ComponentId` mappings (where relevant). `ComponentInfo` stores information about the component, such as ComponentId, TypeId, memory layout, send-ness (currently limited to resources), and storage type.
## Significantly Cheaper `Access<T>`
We used to use `TypeAccess<TypeId>` to manage read/write component/archetype-component access. This was expensive because TypeIds must be hashed and compared individually. The parallel executor got around this by "condensing" type ids into bitset-backed access types. This worked, but it had to be re-generated from the `TypeAccess<TypeId>`sources every time archetypes changed.
This pr removes TypeAccess in favor of faster bitset access everywhere. We can do this thanks to the move to densely packed `ComponentId`s and `ArchetypeComponentId`s.
## Merged Resources into World
Resources had a lot of redundant functionality with Components. They stored typed data, they had access control, they had unique ids, they were queryable via SystemParams, etc. In fact the _only_ major difference between them was that they were unique (and didn't correlate to an entity).
Separate resources also had the downside of requiring a separate set of access controls, which meant the parallel executor needed to compare more bitsets per system and manage more state.
I initially got the "separate resources" idea from `legion`. I think that design was motivated by the fact that it made the direct world query/resource lifetime interactions more manageable. It certainly made our lives easier when using Resources alongside hecs/bevy_ecs. However we already have a construct for safely and ergonomically managing in-world lifetimes: systems (which use `Access<T>` internally).
This pr merges Resources into World:
```rust
world.insert_resource(1);
world.insert_resource(2.0);
let a = world.get_resource::<i32>().unwrap();
let mut b = world.get_resource_mut::<f64>().unwrap();
*b = 3.0;
```
Resources are now just a special kind of component. They have their own ComponentIds (and their own resource TypeId->ComponentId scope, so they don't conflict wit components of the same type). They are stored in a special "resource archetype", which stores components inside the archetype using a new `unique_components` sparse set (note that this sparse set could later be used to implement Tags). This allows us to keep the code size small by reusing existing datastructures (namely Column, Archetype, ComponentFlags, and ComponentInfo). This allows us the executor to use a single `Access<ArchetypeComponentId>` per system. It should also make scripting language integration easier.
_But_ this merge did create problems for people directly interacting with `World`. What if you need mutable access to multiple resources at the same time? `world.get_resource_mut()` borrows World mutably!
## WorldCell
WorldCell applies the `Access<ArchetypeComponentId>` concept to direct world access:
```rust
let world_cell = world.cell();
let a = world_cell.get_resource_mut::<i32>().unwrap();
let b = world_cell.get_resource_mut::<f64>().unwrap();
```
This adds cheap runtime checks (a sparse set lookup of `ArchetypeComponentId` and a counter) to ensure that world accesses do not conflict with each other. Each operation returns a `WorldBorrow<'w, T>` or `WorldBorrowMut<'w, T>` wrapper type, which will release the relevant ArchetypeComponentId resources when dropped.
World caches the access sparse set (and only one cell can exist at a time), so `world.cell()` is a cheap operation.
WorldCell does _not_ use atomic operations. It is non-send, does a mutable borrow of world to prevent other accesses, and uses a simple `Rc<RefCell<ArchetypeComponentAccess>>` wrapper in each WorldBorrow pointer.
The api is currently limited to resource access, but it can and should be extended to queries / entity component access.
## Resource Scopes
WorldCell does not yet support component queries, and even when it does there are sometimes legitimate reasons to want a mutable world ref _and_ a mutable resource ref (ex: bevy_render and bevy_scene both need this). In these cases we could always drop down to the unsafe `world.get_resource_unchecked_mut()`, but that is not ideal!
Instead developers can use a "resource scope"
```rust
world.resource_scope(|world: &mut World, a: &mut A| {
})
```
This temporarily removes the `A` resource from `World`, provides mutable pointers to both, and re-adds A to World when finished. Thanks to the move to ComponentIds/sparse sets, this is a cheap operation.
If multiple resources are required, scopes can be nested. We could also consider adding a "resource tuple" to the api if this pattern becomes common and the boilerplate gets nasty.
## Query Conflicts Use ComponentId Instead of ArchetypeComponentId
For safety reasons, systems cannot contain queries that conflict with each other without wrapping them in a QuerySet. On bevy `main`, we use ArchetypeComponentIds to determine conflicts. This is nice because it can take into account filters:
```rust
// these queries will never conflict due to their filters
fn filter_system(a: Query<&mut A, With<B>>, b: Query<&mut B, Without<B>>) {
}
```
But it also has a significant downside:
```rust
// these queries will not conflict _until_ an entity with A, B, and C is spawned
fn maybe_conflicts_system(a: Query<(&mut A, &C)>, b: Query<(&mut A, &B)>) {
}
```
The system above will panic at runtime if an entity with A, B, and C is spawned. This makes it hard to trust that your game logic will run without crashing.
In this pr, I switched to using `ComponentId` instead. This _is_ more constraining. `maybe_conflicts_system` will now always fail, but it will do it consistently at startup. Naively, it would also _disallow_ `filter_system`, which would be a significant downgrade in usability. Bevy has a number of internal systems that rely on disjoint queries and I expect it to be a common pattern in userspace.
To resolve this, I added a new `FilteredAccess<T>` type, which wraps `Access<T>` and adds with/without filters. If two `FilteredAccess` have with/without values that prove they are disjoint, they will no longer conflict.
## EntityRef / EntityMut
World entity operations on `main` require that the user passes in an `entity` id to each operation:
```rust
let entity = world.spawn((A, )); // create a new entity with A
world.get::<A>(entity);
world.insert(entity, (B, C));
world.insert_one(entity, D);
```
This means that each operation needs to look up the entity location / verify its validity. The initial spawn operation also requires a Bundle as input. This can be awkward when no components are required (or one component is required).
These operations have been replaced by `EntityRef` and `EntityMut`, which are "builder-style" wrappers around world that provide read and read/write operations on a single, pre-validated entity:
```rust
// spawn now takes no inputs and returns an EntityMut
let entity = world.spawn()
.insert(A) // insert a single component into the entity
.insert_bundle((B, C)) // insert a bundle of components into the entity
.id() // id returns the Entity id
// Returns EntityMut (or panics if the entity does not exist)
world.entity_mut(entity)
.insert(D)
.insert_bundle(SomeBundle::default());
{
// returns EntityRef (or panics if the entity does not exist)
let d = world.entity(entity)
.get::<D>() // gets the D component
.unwrap();
// world.get still exists for ergonomics
let d = world.get::<D>(entity).unwrap();
}
// These variants return Options if you want to check existence instead of panicing
world.get_entity_mut(entity)
.unwrap()
.insert(E);
if let Some(entity_ref) = world.get_entity(entity) {
let d = entity_ref.get::<D>().unwrap();
}
```
This _does not_ affect the current Commands api or terminology. I think that should be a separate conversation as that is a much larger breaking change.
## Safety Improvements
* Entity reservation in Commands uses a normal world borrow instead of an unsafe transmute
* QuerySets no longer transmutes lifetimes
* Made traits "unsafe" when implementing a trait incorrectly could cause unsafety
* More thorough safety docs
## RemovedComponents SystemParam
The old approach to querying removed components: `query.removed:<T>()` was confusing because it had no connection to the query itself. I replaced it with the following, which is both clearer and allows us to cache the ComponentId mapping in the SystemParamState:
```rust
fn system(removed: RemovedComponents<T>) {
for entity in removed.iter() {
}
}
```
## Simpler Bundle implementation
Bundles are no longer responsible for sorting (or deduping) TypeInfo. They are just a simple ordered list of component types / data. This makes the implementation smaller and opens the door to an easy "nested bundle" implementation in the future (which i might even add in this pr). Duplicate detection is now done once per bundle type by World the first time a bundle is used.
## Unified WorldQuery and QueryFilter types
(don't worry they are still separate type _parameters_ in Queries .. this is a non-breaking change)
WorldQuery and QueryFilter were already basically identical apis. With the addition of `FetchState` and more storage-specific fetch methods, the overlap was even clearer (and the redundancy more painful).
QueryFilters are now just `F: WorldQuery where F::Fetch: FilterFetch`. FilterFetch requires `Fetch<Item = bool>` and adds new "short circuit" variants of fetch methods. This enables a filter tuple like `(With<A>, Without<B>, Changed<C>)` to stop evaluating the filter after the first mismatch is encountered. FilterFetch is automatically implemented for `Fetch` implementations that return bool.
This forces fetch implementations that return things like `(bool, bool, bool)` (such as the filter above) to manually implement FilterFetch and decide whether or not to short-circuit.
## More Granular Modules
World no longer globs all of the internal modules together. It now exports `core`, `system`, and `schedule` separately. I'm also considering exporting `core` submodules directly as that is still pretty "glob-ey" and unorganized (feedback welcome here).
## Remaining Draft Work (to be done in this pr)
* ~~panic on conflicting WorldQuery fetches (&A, &mut A)~~
* ~~bevy `main` and hecs both currently allow this, but we should protect against it if possible~~
* ~~batch_iter / par_iter (currently stubbed out)~~
* ~~ChangedRes~~
* ~~I skipped this while we sort out #1313. This pr should be adapted to account for whatever we land on there~~.
* ~~The `Archetypes` and `Tables` collections use hashes of sorted lists of component ids to uniquely identify each archetype/table. This hash is then used as the key in a HashMap to look up the relevant ArchetypeId or TableId. (which doesn't handle hash collisions properly)~~
* ~~It is currently unsafe to generate a Query from "World A", then use it on "World B" (despite the api claiming it is safe). We should probably close this gap. This could be done by adding a randomly generated WorldId to each world, then storing that id in each Query. They could then be compared to each other on each `query.do_thing(&world)` operation. This _does_ add an extra branch to each query operation, so I'm open to other suggestions if people have them.~~
* ~~Nested Bundles (if i find time)~~
## Potential Future Work
* Expand WorldCell to support queries.
* Consider not allocating in the empty archetype on `world.spawn()`
* ex: return something like EntityMutUninit, which turns into EntityMut after an `insert` or `insert_bundle` op
* this actually regressed performance last time i tried it, but in theory it should be faster
* Optimize SparseSet::insert (see `PERF` comment on insert)
* Replace SparseArray `Option<T>` with T::MAX to cut down on branching
* would enable cheaper get_unchecked() operations
* upstream fixedbitset optimizations
* fixedbitset could be allocation free for small block counts (store blocks in a SmallVec)
* fixedbitset could have a const constructor
* Consider implementing Tags (archetype-specific by-value data that affects archetype identity)
* ex: ArchetypeA could have `[A, B, C]` table components and `[D(1)]` "tag" component. ArchetypeB could have `[A, B, C]` table components and a `[D(2)]` tag component. The archetypes are different, despite both having D tags because the value inside D is different.
* this could potentially build on top of the `archetype.unique_components` added in this pr for resource storage.
* Consider reverting `all_tuples` proc macro in favor of the old `macro_rules` implementation
* all_tuples is more flexible and produces cleaner documentation (the macro_rules version produces weird type parameter orders due to parser constraints)
* but unfortunately all_tuples also appears to make Rust Analyzer sad/slow when working inside of `bevy_ecs` (does not affect user code)
* Consider "resource queries" and/or "mixed resource and entity component queries" as an alternative to WorldCell
* this is basically just "systems" so maybe it's not worth it
* Add more world ops
* `world.clear()`
* `world.reserve<T: Bundle>(count: usize)`
* Try using the old archetype allocation strategy (allocate new memory on resize and copy everything over). I expect this to improve batch insertion performance at the cost of unbatched performance. But thats just a guess. I'm not an allocation perf pro :)
* Adapt Commands apis for consistency with new World apis
## Benchmarks
key:
* `bevy_old`: bevy `main` branch
* `bevy`: this branch
* `_foreach`: uses an optimized for_each iterator
* ` _sparse`: uses sparse set storage (if unspecified assume table storage)
* `_system`: runs inside a system (if unspecified assume test happens via direct world ops)
### Simple Insert (from ecs_bench_suite)
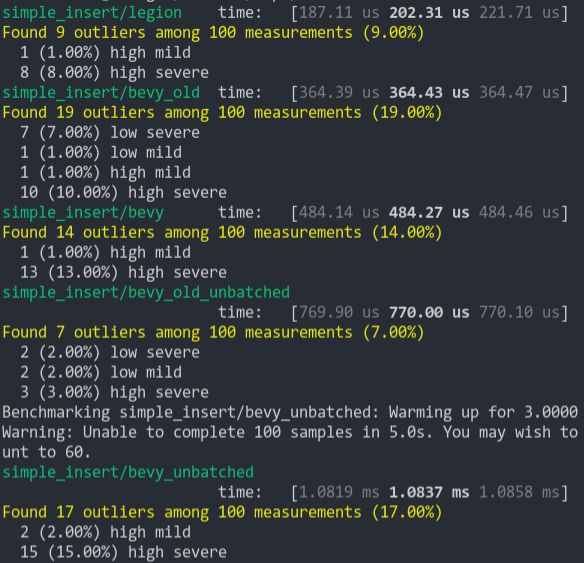
### Simpler Iter (from ecs_bench_suite)
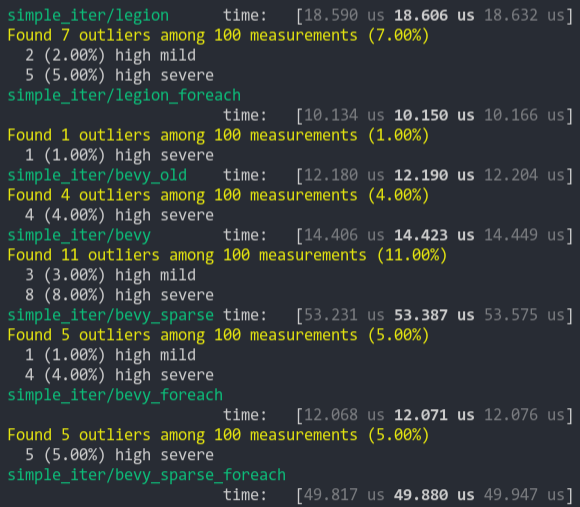
### Fragment Iter (from ecs_bench_suite)
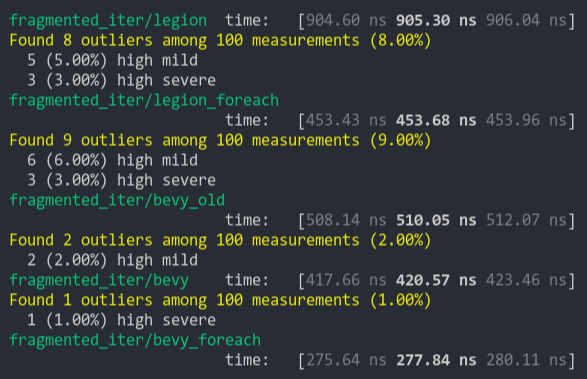
### Sparse Fragmented Iter
Iterate a query that matches 5 entities from a single matching archetype, but there are 100 unmatching archetypes
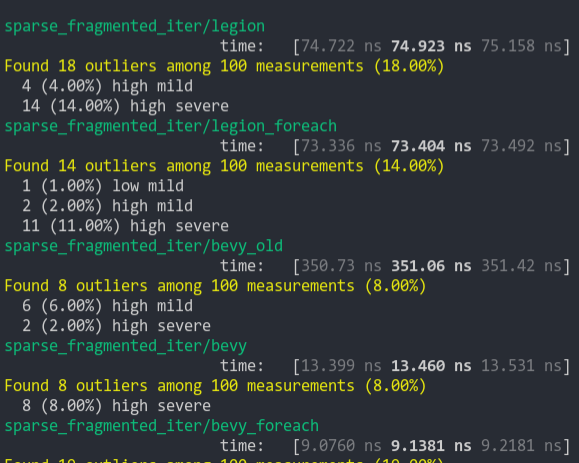
### Schedule (from ecs_bench_suite)
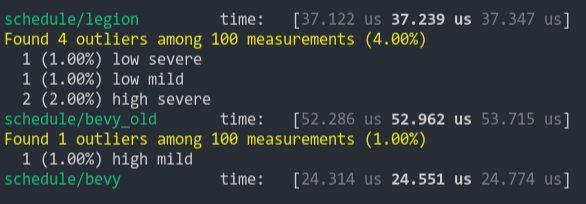
### Add Remove Component (from ecs_bench_suite)
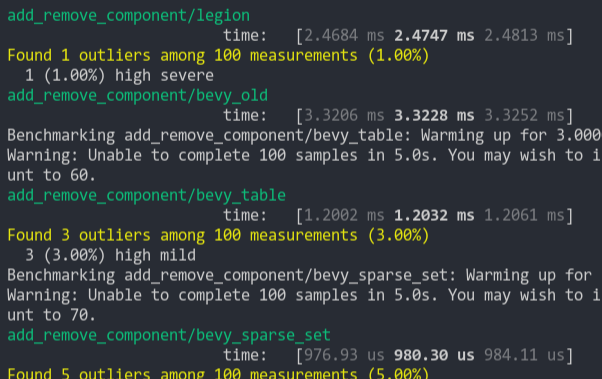
### Add Remove Component Big
Same as the test above, but each entity has 5 "large" matrix components and 1 "large" matrix component is added and removed
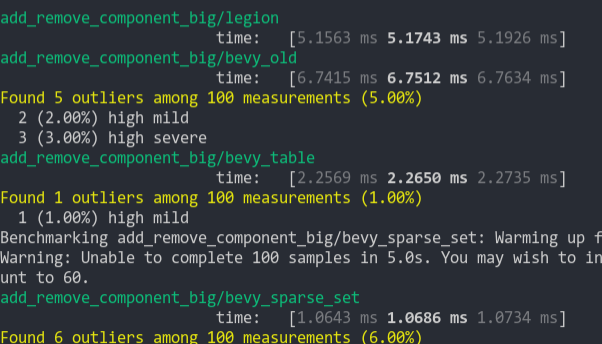
### Get Component
Looks up a single component value a large number of times
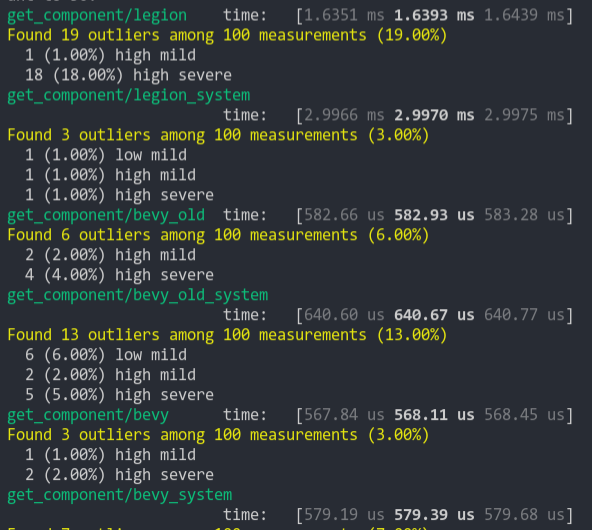
2021-03-05 07:54:35 +00:00
}
2020-11-08 20:34:05 +00:00
}
Bevy ECS V2 (#1525)
# Bevy ECS V2
This is a rewrite of Bevy ECS (basically everything but the new executor/schedule, which are already awesome). The overall goal was to improve the performance and versatility of Bevy ECS. Here is a quick bulleted list of changes before we dive into the details:
* Complete World rewrite
* Multiple component storage types:
* Tables: fast cache friendly iteration, slower add/removes (previously called Archetypes)
* Sparse Sets: fast add/remove, slower iteration
* Stateful Queries (caches query results for faster iteration. fragmented iteration is _fast_ now)
* Stateful System Params (caches expensive operations. inspired by @DJMcNab's work in #1364)
* Configurable System Params (users can set configuration when they construct their systems. once again inspired by @DJMcNab's work)
* Archetypes are now "just metadata", component storage is separate
* Archetype Graph (for faster archetype changes)
* Component Metadata
* Configure component storage type
* Retrieve information about component size/type/name/layout/send-ness/etc
* Components are uniquely identified by a densely packed ComponentId
* TypeIds are now totally optional (which should make implementing scripting easier)
* Super fast "for_each" query iterators
* Merged Resources into World. Resources are now just a special type of component
* EntityRef/EntityMut builder apis (more efficient and more ergonomic)
* Fast bitset-backed `Access<T>` replaces old hashmap-based approach everywhere
* Query conflicts are determined by component access instead of archetype component access (to avoid random failures at runtime)
* With/Without are still taken into account for conflicts, so this should still be comfy to use
* Much simpler `IntoSystem` impl
* Significantly reduced the amount of hashing throughout the ecs in favor of Sparse Sets (indexed by densely packed ArchetypeId, ComponentId, BundleId, and TableId)
* Safety Improvements
* Entity reservation uses a normal world reference instead of unsafe transmute
* QuerySets no longer transmute lifetimes
* Made traits "unsafe" where relevant
* More thorough safety docs
* WorldCell
* Exposes safe mutable access to multiple resources at a time in a World
* Replaced "catch all" `System::update_archetypes(world: &World)` with `System::new_archetype(archetype: &Archetype)`
* Simpler Bundle implementation
* Replaced slow "remove_bundle_one_by_one" used as fallback for Commands::remove_bundle with fast "remove_bundle_intersection"
* Removed `Mut<T>` query impl. it is better to only support one way: `&mut T`
* Removed with() from `Flags<T>` in favor of `Option<Flags<T>>`, which allows querying for flags to be "filtered" by default
* Components now have is_send property (currently only resources support non-send)
* More granular module organization
* New `RemovedComponents<T>` SystemParam that replaces `query.removed::<T>()`
* `world.resource_scope()` for mutable access to resources and world at the same time
* WorldQuery and QueryFilter traits unified. FilterFetch trait added to enable "short circuit" filtering. Auto impled for cases that don't need it
* Significantly slimmed down SystemState in favor of individual SystemParam state
* System Commands changed from `commands: &mut Commands` back to `mut commands: Commands` (to allow Commands to have a World reference)
Fixes #1320
## `World` Rewrite
This is a from-scratch rewrite of `World` that fills the niche that `hecs` used to. Yes, this means Bevy ECS is no longer a "fork" of hecs. We're going out our own!
(the only shared code between the projects is the entity id allocator, which is already basically ideal)
A huge shout out to @SanderMertens (author of [flecs](https://github.com/SanderMertens/flecs)) for sharing some great ideas with me (specifically hybrid ecs storage and archetype graphs). He also helped advise on a number of implementation details.
## Component Storage (The Problem)
Two ECS storage paradigms have gained a lot of traction over the years:
* **Archetypal ECS**:
* Stores components in "tables" with static schemas. Each "column" stores components of a given type. Each "row" is an entity.
* Each "archetype" has its own table. Adding/removing an entity's component changes the archetype.
* Enables super-fast Query iteration due to its cache-friendly data layout
* Comes at the cost of more expensive add/remove operations for an Entity's components, because all components need to be copied to the new archetype's "table"
* **Sparse Set ECS**:
* Stores components of the same type in densely packed arrays, which are sparsely indexed by densely packed unsigned integers (Entity ids)
* Query iteration is slower than Archetypal ECS because each entity's component could be at any position in the sparse set. This "random access" pattern isn't cache friendly. Additionally, there is an extra layer of indirection because you must first map the entity id to an index in the component array.
* Adding/removing components is a cheap, constant time operation
Bevy ECS V1, hecs, legion, flec, and Unity DOTS are all "archetypal ecs-es". I personally think "archetypal" storage is a good default for game engines. An entity's archetype doesn't need to change frequently in general, and it creates "fast by default" query iteration (which is a much more common operation). It is also "self optimizing". Users don't need to think about optimizing component layouts for iteration performance. It "just works" without any extra boilerplate.
Shipyard and EnTT are "sparse set ecs-es". They employ "packing" as a way to work around the "suboptimal by default" iteration performance for specific sets of components. This helps, but I didn't think this was a good choice for a general purpose engine like Bevy because:
1. "packs" conflict with each other. If bevy decides to internally pack the Transform and GlobalTransform components, users are then blocked if they want to pack some custom component with Transform.
2. users need to take manual action to optimize
Developers selecting an ECS framework are stuck with a hard choice. Select an "archetypal" framework with "fast iteration everywhere" but without the ability to cheaply add/remove components, or select a "sparse set" framework to cheaply add/remove components but with slower iteration performance.
## Hybrid Component Storage (The Solution)
In Bevy ECS V2, we get to have our cake and eat it too. It now has _both_ of the component storage types above (and more can be added later if needed):
* **Tables** (aka "archetypal" storage)
* The default storage. If you don't configure anything, this is what you get
* Fast iteration by default
* Slower add/remove operations
* **Sparse Sets**
* Opt-in
* Slower iteration
* Faster add/remove operations
These storage types complement each other perfectly. By default Query iteration is fast. If developers know that they want to add/remove a component at high frequencies, they can set the storage to "sparse set":
```rust
world.register_component(
ComponentDescriptor::new::<MyComponent>(StorageType::SparseSet)
).unwrap();
```
## Archetypes
Archetypes are now "just metadata" ... they no longer store components directly. They do store:
* The `ComponentId`s of each of the Archetype's components (and that component's storage type)
* Archetypes are uniquely defined by their component layouts
* For example: entities with "table" components `[A, B, C]` _and_ "sparse set" components `[D, E]` will always be in the same archetype.
* The `TableId` associated with the archetype
* For now each archetype has exactly one table (which can have no components),
* There is a 1->Many relationship from Tables->Archetypes. A given table could have any number of archetype components stored in it:
* Ex: an entity with "table storage" components `[A, B, C]` and "sparse set" components `[D, E]` will share the same `[A, B, C]` table as an entity with `[A, B, C]` table component and `[F]` sparse set components.
* This 1->Many relationship is how we preserve fast "cache friendly" iteration performance when possible (more on this later)
* A list of entities that are in the archetype and the row id of the table they are in
* ArchetypeComponentIds
* unique densely packed identifiers for (ArchetypeId, ComponentId) pairs
* used by the schedule executor for cheap system access control
* "Archetype Graph Edges" (see the next section)
## The "Archetype Graph"
Archetype changes in Bevy (and a number of other archetypal ecs-es) have historically been expensive to compute. First, you need to allocate a new vector of the entity's current component ids, add or remove components based on the operation performed, sort it (to ensure it is order-independent), then hash it to find the archetype (if it exists). And thats all before we get to the _already_ expensive full copy of all components to the new table storage.
The solution is to build a "graph" of archetypes to cache these results. @SanderMertens first exposed me to the idea (and he got it from @gjroelofs, who came up with it). They propose adding directed edges between archetypes for add/remove component operations. If `ComponentId`s are densely packed, you can use sparse sets to cheaply jump between archetypes.
Bevy takes this one step further by using add/remove `Bundle` edges instead of `Component` edges. Bevy encourages the use of `Bundles` to group add/remove operations. This is largely for "clearer game logic" reasons, but it also helps cut down on the number of archetype changes required. `Bundles` now also have densely-packed `BundleId`s. This allows us to use a _single_ edge for each bundle operation (rather than needing to traverse N edges ... one for each component). Single component operations are also bundles, so this is strictly an improvement over a "component only" graph.
As a result, an operation that used to be _heavy_ (both for allocations and compute) is now two dirt-cheap array lookups and zero allocations.
## Stateful Queries
World queries are now stateful. This allows us to:
1. Cache archetype (and table) matches
* This resolves another issue with (naive) archetypal ECS: query performance getting worse as the number of archetypes goes up (and fragmentation occurs).
2. Cache Fetch and Filter state
* The expensive parts of fetch/filter operations (such as hashing the TypeId to find the ComponentId) now only happen once when the Query is first constructed
3. Incrementally build up state
* When new archetypes are added, we only process the new archetypes (no need to rebuild state for old archetypes)
As a result, the direct `World` query api now looks like this:
```rust
let mut query = world.query::<(&A, &mut B)>();
for (a, mut b) in query.iter_mut(&mut world) {
}
```
Requiring `World` to generate stateful queries (rather than letting the `QueryState` type be constructed separately) allows us to ensure that _all_ queries are properly initialized (and the relevant world state, such as ComponentIds). This enables QueryState to remove branches from its operations that check for initialization status (and also enables query.iter() to take an immutable world reference because it doesn't need to initialize anything in world).
However in systems, this is a non-breaking change. State management is done internally by the relevant SystemParam.
## Stateful SystemParams
Like Queries, `SystemParams` now also cache state. For example, `Query` system params store the "stateful query" state mentioned above. Commands store their internal `CommandQueue`. This means you can now safely use as many separate `Commands` parameters in your system as you want. `Local<T>` system params store their `T` value in their state (instead of in Resources).
SystemParam state also enabled a significant slim-down of SystemState. It is much nicer to look at now.
Per-SystemParam state naturally insulates us from an "aliased mut" class of errors we have hit in the past (ex: using multiple `Commands` system params).
(credit goes to @DJMcNab for the initial idea and draft pr here #1364)
## Configurable SystemParams
@DJMcNab also had the great idea to make SystemParams configurable. This allows users to provide some initial configuration / values for system parameters (when possible). Most SystemParams have no config (the config type is `()`), but the `Local<T>` param now supports user-provided parameters:
```rust
fn foo(value: Local<usize>) {
}
app.add_system(foo.system().config(|c| c.0 = Some(10)));
```
## Uber Fast "for_each" Query Iterators
Developers now have the choice to use a fast "for_each" iterator, which yields ~1.5-3x iteration speed improvements for "fragmented iteration", and minor ~1.2x iteration speed improvements for unfragmented iteration.
```rust
fn system(query: Query<(&A, &mut B)>) {
// you now have the option to do this for a speed boost
query.for_each_mut(|(a, mut b)| {
});
// however normal iterators are still available
for (a, mut b) in query.iter_mut() {
}
}
```
I think in most cases we should continue to encourage "normal" iterators as they are more flexible and more "rust idiomatic". But when that extra "oomf" is needed, it makes sense to use `for_each`.
We should also consider using `for_each` for internal bevy systems to give our users a nice speed boost (but that should be a separate pr).
## Component Metadata
`World` now has a `Components` collection, which is accessible via `world.components()`. This stores mappings from `ComponentId` to `ComponentInfo`, as well as `TypeId` to `ComponentId` mappings (where relevant). `ComponentInfo` stores information about the component, such as ComponentId, TypeId, memory layout, send-ness (currently limited to resources), and storage type.
## Significantly Cheaper `Access<T>`
We used to use `TypeAccess<TypeId>` to manage read/write component/archetype-component access. This was expensive because TypeIds must be hashed and compared individually. The parallel executor got around this by "condensing" type ids into bitset-backed access types. This worked, but it had to be re-generated from the `TypeAccess<TypeId>`sources every time archetypes changed.
This pr removes TypeAccess in favor of faster bitset access everywhere. We can do this thanks to the move to densely packed `ComponentId`s and `ArchetypeComponentId`s.
## Merged Resources into World
Resources had a lot of redundant functionality with Components. They stored typed data, they had access control, they had unique ids, they were queryable via SystemParams, etc. In fact the _only_ major difference between them was that they were unique (and didn't correlate to an entity).
Separate resources also had the downside of requiring a separate set of access controls, which meant the parallel executor needed to compare more bitsets per system and manage more state.
I initially got the "separate resources" idea from `legion`. I think that design was motivated by the fact that it made the direct world query/resource lifetime interactions more manageable. It certainly made our lives easier when using Resources alongside hecs/bevy_ecs. However we already have a construct for safely and ergonomically managing in-world lifetimes: systems (which use `Access<T>` internally).
This pr merges Resources into World:
```rust
world.insert_resource(1);
world.insert_resource(2.0);
let a = world.get_resource::<i32>().unwrap();
let mut b = world.get_resource_mut::<f64>().unwrap();
*b = 3.0;
```
Resources are now just a special kind of component. They have their own ComponentIds (and their own resource TypeId->ComponentId scope, so they don't conflict wit components of the same type). They are stored in a special "resource archetype", which stores components inside the archetype using a new `unique_components` sparse set (note that this sparse set could later be used to implement Tags). This allows us to keep the code size small by reusing existing datastructures (namely Column, Archetype, ComponentFlags, and ComponentInfo). This allows us the executor to use a single `Access<ArchetypeComponentId>` per system. It should also make scripting language integration easier.
_But_ this merge did create problems for people directly interacting with `World`. What if you need mutable access to multiple resources at the same time? `world.get_resource_mut()` borrows World mutably!
## WorldCell
WorldCell applies the `Access<ArchetypeComponentId>` concept to direct world access:
```rust
let world_cell = world.cell();
let a = world_cell.get_resource_mut::<i32>().unwrap();
let b = world_cell.get_resource_mut::<f64>().unwrap();
```
This adds cheap runtime checks (a sparse set lookup of `ArchetypeComponentId` and a counter) to ensure that world accesses do not conflict with each other. Each operation returns a `WorldBorrow<'w, T>` or `WorldBorrowMut<'w, T>` wrapper type, which will release the relevant ArchetypeComponentId resources when dropped.
World caches the access sparse set (and only one cell can exist at a time), so `world.cell()` is a cheap operation.
WorldCell does _not_ use atomic operations. It is non-send, does a mutable borrow of world to prevent other accesses, and uses a simple `Rc<RefCell<ArchetypeComponentAccess>>` wrapper in each WorldBorrow pointer.
The api is currently limited to resource access, but it can and should be extended to queries / entity component access.
## Resource Scopes
WorldCell does not yet support component queries, and even when it does there are sometimes legitimate reasons to want a mutable world ref _and_ a mutable resource ref (ex: bevy_render and bevy_scene both need this). In these cases we could always drop down to the unsafe `world.get_resource_unchecked_mut()`, but that is not ideal!
Instead developers can use a "resource scope"
```rust
world.resource_scope(|world: &mut World, a: &mut A| {
})
```
This temporarily removes the `A` resource from `World`, provides mutable pointers to both, and re-adds A to World when finished. Thanks to the move to ComponentIds/sparse sets, this is a cheap operation.
If multiple resources are required, scopes can be nested. We could also consider adding a "resource tuple" to the api if this pattern becomes common and the boilerplate gets nasty.
## Query Conflicts Use ComponentId Instead of ArchetypeComponentId
For safety reasons, systems cannot contain queries that conflict with each other without wrapping them in a QuerySet. On bevy `main`, we use ArchetypeComponentIds to determine conflicts. This is nice because it can take into account filters:
```rust
// these queries will never conflict due to their filters
fn filter_system(a: Query<&mut A, With<B>>, b: Query<&mut B, Without<B>>) {
}
```
But it also has a significant downside:
```rust
// these queries will not conflict _until_ an entity with A, B, and C is spawned
fn maybe_conflicts_system(a: Query<(&mut A, &C)>, b: Query<(&mut A, &B)>) {
}
```
The system above will panic at runtime if an entity with A, B, and C is spawned. This makes it hard to trust that your game logic will run without crashing.
In this pr, I switched to using `ComponentId` instead. This _is_ more constraining. `maybe_conflicts_system` will now always fail, but it will do it consistently at startup. Naively, it would also _disallow_ `filter_system`, which would be a significant downgrade in usability. Bevy has a number of internal systems that rely on disjoint queries and I expect it to be a common pattern in userspace.
To resolve this, I added a new `FilteredAccess<T>` type, which wraps `Access<T>` and adds with/without filters. If two `FilteredAccess` have with/without values that prove they are disjoint, they will no longer conflict.
## EntityRef / EntityMut
World entity operations on `main` require that the user passes in an `entity` id to each operation:
```rust
let entity = world.spawn((A, )); // create a new entity with A
world.get::<A>(entity);
world.insert(entity, (B, C));
world.insert_one(entity, D);
```
This means that each operation needs to look up the entity location / verify its validity. The initial spawn operation also requires a Bundle as input. This can be awkward when no components are required (or one component is required).
These operations have been replaced by `EntityRef` and `EntityMut`, which are "builder-style" wrappers around world that provide read and read/write operations on a single, pre-validated entity:
```rust
// spawn now takes no inputs and returns an EntityMut
let entity = world.spawn()
.insert(A) // insert a single component into the entity
.insert_bundle((B, C)) // insert a bundle of components into the entity
.id() // id returns the Entity id
// Returns EntityMut (or panics if the entity does not exist)
world.entity_mut(entity)
.insert(D)
.insert_bundle(SomeBundle::default());
{
// returns EntityRef (or panics if the entity does not exist)
let d = world.entity(entity)
.get::<D>() // gets the D component
.unwrap();
// world.get still exists for ergonomics
let d = world.get::<D>(entity).unwrap();
}
// These variants return Options if you want to check existence instead of panicing
world.get_entity_mut(entity)
.unwrap()
.insert(E);
if let Some(entity_ref) = world.get_entity(entity) {
let d = entity_ref.get::<D>().unwrap();
}
```
This _does not_ affect the current Commands api or terminology. I think that should be a separate conversation as that is a much larger breaking change.
## Safety Improvements
* Entity reservation in Commands uses a normal world borrow instead of an unsafe transmute
* QuerySets no longer transmutes lifetimes
* Made traits "unsafe" when implementing a trait incorrectly could cause unsafety
* More thorough safety docs
## RemovedComponents SystemParam
The old approach to querying removed components: `query.removed:<T>()` was confusing because it had no connection to the query itself. I replaced it with the following, which is both clearer and allows us to cache the ComponentId mapping in the SystemParamState:
```rust
fn system(removed: RemovedComponents<T>) {
for entity in removed.iter() {
}
}
```
## Simpler Bundle implementation
Bundles are no longer responsible for sorting (or deduping) TypeInfo. They are just a simple ordered list of component types / data. This makes the implementation smaller and opens the door to an easy "nested bundle" implementation in the future (which i might even add in this pr). Duplicate detection is now done once per bundle type by World the first time a bundle is used.
## Unified WorldQuery and QueryFilter types
(don't worry they are still separate type _parameters_ in Queries .. this is a non-breaking change)
WorldQuery and QueryFilter were already basically identical apis. With the addition of `FetchState` and more storage-specific fetch methods, the overlap was even clearer (and the redundancy more painful).
QueryFilters are now just `F: WorldQuery where F::Fetch: FilterFetch`. FilterFetch requires `Fetch<Item = bool>` and adds new "short circuit" variants of fetch methods. This enables a filter tuple like `(With<A>, Without<B>, Changed<C>)` to stop evaluating the filter after the first mismatch is encountered. FilterFetch is automatically implemented for `Fetch` implementations that return bool.
This forces fetch implementations that return things like `(bool, bool, bool)` (such as the filter above) to manually implement FilterFetch and decide whether or not to short-circuit.
## More Granular Modules
World no longer globs all of the internal modules together. It now exports `core`, `system`, and `schedule` separately. I'm also considering exporting `core` submodules directly as that is still pretty "glob-ey" and unorganized (feedback welcome here).
## Remaining Draft Work (to be done in this pr)
* ~~panic on conflicting WorldQuery fetches (&A, &mut A)~~
* ~~bevy `main` and hecs both currently allow this, but we should protect against it if possible~~
* ~~batch_iter / par_iter (currently stubbed out)~~
* ~~ChangedRes~~
* ~~I skipped this while we sort out #1313. This pr should be adapted to account for whatever we land on there~~.
* ~~The `Archetypes` and `Tables` collections use hashes of sorted lists of component ids to uniquely identify each archetype/table. This hash is then used as the key in a HashMap to look up the relevant ArchetypeId or TableId. (which doesn't handle hash collisions properly)~~
* ~~It is currently unsafe to generate a Query from "World A", then use it on "World B" (despite the api claiming it is safe). We should probably close this gap. This could be done by adding a randomly generated WorldId to each world, then storing that id in each Query. They could then be compared to each other on each `query.do_thing(&world)` operation. This _does_ add an extra branch to each query operation, so I'm open to other suggestions if people have them.~~
* ~~Nested Bundles (if i find time)~~
## Potential Future Work
* Expand WorldCell to support queries.
* Consider not allocating in the empty archetype on `world.spawn()`
* ex: return something like EntityMutUninit, which turns into EntityMut after an `insert` or `insert_bundle` op
* this actually regressed performance last time i tried it, but in theory it should be faster
* Optimize SparseSet::insert (see `PERF` comment on insert)
* Replace SparseArray `Option<T>` with T::MAX to cut down on branching
* would enable cheaper get_unchecked() operations
* upstream fixedbitset optimizations
* fixedbitset could be allocation free for small block counts (store blocks in a SmallVec)
* fixedbitset could have a const constructor
* Consider implementing Tags (archetype-specific by-value data that affects archetype identity)
* ex: ArchetypeA could have `[A, B, C]` table components and `[D(1)]` "tag" component. ArchetypeB could have `[A, B, C]` table components and a `[D(2)]` tag component. The archetypes are different, despite both having D tags because the value inside D is different.
* this could potentially build on top of the `archetype.unique_components` added in this pr for resource storage.
* Consider reverting `all_tuples` proc macro in favor of the old `macro_rules` implementation
* all_tuples is more flexible and produces cleaner documentation (the macro_rules version produces weird type parameter orders due to parser constraints)
* but unfortunately all_tuples also appears to make Rust Analyzer sad/slow when working inside of `bevy_ecs` (does not affect user code)
* Consider "resource queries" and/or "mixed resource and entity component queries" as an alternative to WorldCell
* this is basically just "systems" so maybe it's not worth it
* Add more world ops
* `world.clear()`
* `world.reserve<T: Bundle>(count: usize)`
* Try using the old archetype allocation strategy (allocate new memory on resize and copy everything over). I expect this to improve batch insertion performance at the cost of unbatched performance. But thats just a guess. I'm not an allocation perf pro :)
* Adapt Commands apis for consistency with new World apis
## Benchmarks
key:
* `bevy_old`: bevy `main` branch
* `bevy`: this branch
* `_foreach`: uses an optimized for_each iterator
* ` _sparse`: uses sparse set storage (if unspecified assume table storage)
* `_system`: runs inside a system (if unspecified assume test happens via direct world ops)
### Simple Insert (from ecs_bench_suite)
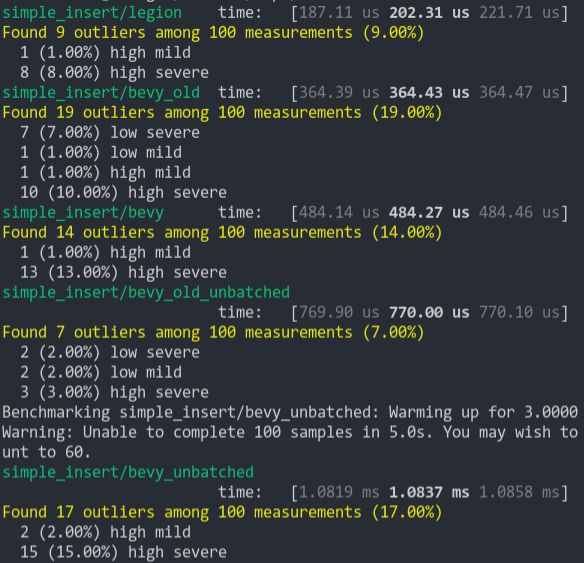
### Simpler Iter (from ecs_bench_suite)
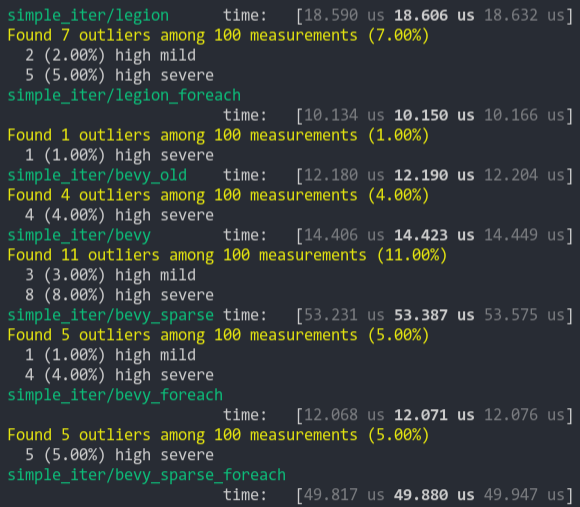
### Fragment Iter (from ecs_bench_suite)
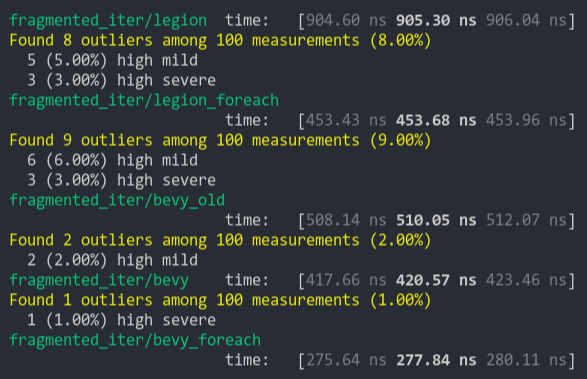
### Sparse Fragmented Iter
Iterate a query that matches 5 entities from a single matching archetype, but there are 100 unmatching archetypes
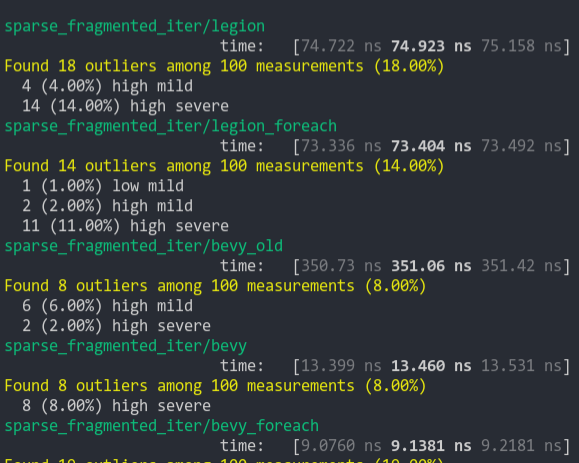
### Schedule (from ecs_bench_suite)
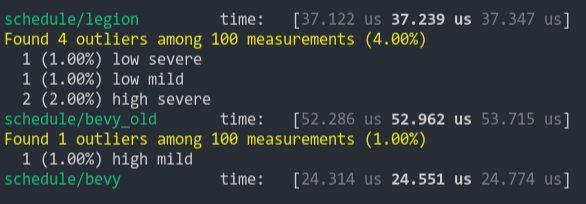
### Add Remove Component (from ecs_bench_suite)
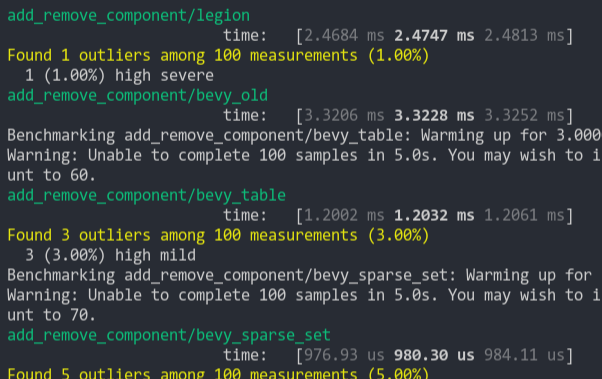
### Add Remove Component Big
Same as the test above, but each entity has 5 "large" matrix components and 1 "large" matrix component is added and removed
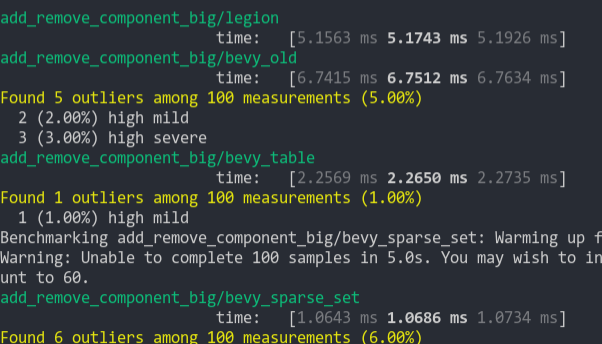
### Get Component
Looks up a single component value a large number of times
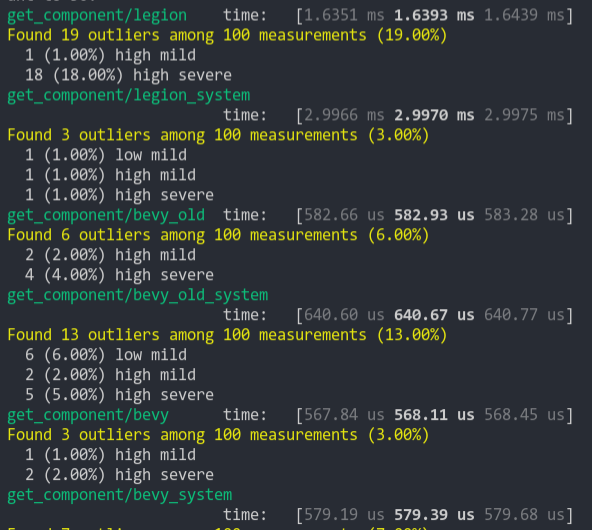
2021-03-05 07:54:35 +00:00
}
2020-11-08 20:34:05 +00:00
2022-07-04 14:44:24 +00:00
// SAFETY: Only reads a single World resource
Simplify trait hierarchy for `SystemParam` (#6865)
# Objective
* Implementing a custom `SystemParam` by hand requires implementing three traits -- four if it is read-only.
* The trait `SystemParamFetch<'w, 's>` is a workaround from before we had generic associated types, and is no longer necessary.
## Solution
* Combine the trait `SystemParamFetch` with `SystemParamState`.
* I decided to remove the `Fetch` name and keep the `State` name, since the former was consistently conflated with the latter.
* Replace the trait `ReadOnlySystemParamFetch` with `ReadOnlySystemParam`, which simplifies trait bounds in generic code.
---
## Changelog
- Removed the trait `SystemParamFetch`, moving its functionality to `SystemParamState`.
- Replaced the trait `ReadOnlySystemParamFetch` with `ReadOnlySystemParam`.
## Migration Guide
The trait `SystemParamFetch` has been removed, and its functionality has been transferred to `SystemParamState`.
```rust
// Before
impl SystemParamState for MyParamState {
fn init(world: &mut World, system_meta: &mut SystemMeta) -> Self { ... }
}
impl<'w, 's> SystemParamFetch<'w, 's> for MyParamState {
type Item = MyParam<'w, 's>;
fn get_param(...) -> Self::Item;
}
// After
impl SystemParamState for MyParamState {
type Item<'w, 's> = MyParam<'w, 's>; // Generic associated types!
fn init(world: &mut World, system_meta: &mut SystemMeta) -> Self { ... }
fn get_param<'w, 's>(...) -> Self::Item<'w, 's>;
}
```
The trait `ReadOnlySystemParamFetch` has been replaced with `ReadOnlySystemParam`.
```rust
// Before
unsafe impl ReadOnlySystemParamFetch for MyParamState {}
// After
unsafe impl<'w, 's> ReadOnlySystemParam for MyParam<'w, 's> {}
```
2022-12-11 18:34:14 +00:00
unsafe impl < ' a , T : Resource > ReadOnlySystemParam for Option < Res < ' a , T > > { }
Add new SystemState and rename old SystemState to SystemMeta (#2283)
This enables `SystemParams` to be used outside of function systems. Anything can create and store `SystemState`, which enables efficient "param state cached" access to `SystemParams`.
It adds a `ReadOnlySystemParamFetch` trait, which enables safe `SystemState::get` calls without unique world access.
I renamed the old `SystemState` to `SystemMeta` to enable us to mirror the `QueryState` naming convention (but I'm happy to discuss alternative names if people have other ideas). I initially pitched this as `ParamState`, but given that it needs to include full system metadata, that doesn't feel like a particularly accurate name.
```rust
#[derive(Eq, PartialEq, Debug)]
struct A(usize);
#[derive(Eq, PartialEq, Debug)]
struct B(usize);
let mut world = World::default();
world.insert_resource(A(42));
world.spawn().insert(B(7));
// we get nice lifetime elision when declaring the type on the left hand side
let mut system_state: SystemState<(Res<A>, Query<&B>)> = SystemState::new(&mut world);
let (a, query) = system_state.get(&world);
assert_eq!(*a, A(42), "returned resource matches initial value");
assert_eq!(
*query.single().unwrap(),
B(7),
"returned component matches initial value"
);
// mutable system params require unique world access
let mut system_state: SystemState<(ResMut<A>, Query<&mut B>)> = SystemState::new(&mut world);
let (a, query) = system_state.get_mut(&mut world);
// static lifetimes are required when declaring inside of structs
struct SomeContainer {
state: SystemState<(Res<'static, A>, Res<'static, B>)>
}
// this can be shortened using type aliases, which will be useful for complex param tuples
type MyParams<'a> = (Res<'a, A>, Res<'a, B>);
struct SomeContainer {
state: SystemState<MyParams<'static>>
}
// It is the user's responsibility to call SystemState::apply(world) for parameters that queue up work
let mut system_state: SystemState<(Commands, Query<&B>)> = SystemState::new(&mut world);
{
let (mut commands, query) = system_state.get(&world);
commands.insert_resource(3.14);
}
system_state.apply(&mut world);
```
## Future Work
* Actually use SystemState inside FunctionSystem. This would be trivial, but it requires FunctionSystem to wrap SystemState in Option in its current form (which complicates system metadata lookup). I'd prefer to hold off until we adopt something like the later designs linked in #1364, which enable us to contruct Systems using a World reference (and also remove the need for `.system`).
* Consider a "scoped" approach to automatically call SystemState::apply when systems params are no longer being used (either a container type with a Drop impl, or a function that takes a closure for user logic operating on params).
2021-06-02 19:57:38 +00:00
Remove the `SystemParamState` trait and remove types like `ResState` (#6919)
Spiritual successor to #5205.
Actual successor to #6865.
# Objective
Currently, system params are defined using three traits: `SystemParam`, `ReadOnlySystemParam`, `SystemParamState`. The behavior for each param is specified by the `SystemParamState` trait, while `SystemParam` simply defers to the state.
Splitting the traits in this way makes it easier to implement within macros, but it increases the cognitive load. Worst of all, this approach requires each `MySystemParam` to have a public `MySystemParamState` type associated with it.
## Solution
* Merge the trait `SystemParamState` into `SystemParam`.
* Remove all trivial `SystemParam` state types.
* `OptionNonSendMutState<T>`: you will not be missed.
---
- [x] Fix/resolve the remaining test failure.
## Changelog
* Removed the trait `SystemParamState`, merging its functionality into `SystemParam`.
## Migration Guide
**Note**: this should replace the migration guide for #6865.
This is relative to Bevy 0.9, not main.
The traits `SystemParamState` and `SystemParamFetch` have been removed, and their functionality has been transferred to `SystemParam`.
```rust
// Before (0.9)
impl SystemParam for MyParam<'_, '_> {
type State = MyParamState;
}
unsafe impl SystemParamState for MyParamState {
fn init(world: &mut World, system_meta: &mut SystemMeta) -> Self { ... }
}
unsafe impl<'w, 's> SystemParamFetch<'w, 's> for MyParamState {
type Item = MyParam<'w, 's>;
fn get_param(&mut self, ...) -> Self::Item;
}
unsafe impl ReadOnlySystemParamFetch for MyParamState { }
// After (0.10)
unsafe impl SystemParam for MyParam<'_, '_> {
type State = MyParamState;
type Item<'w, 's> = MyParam<'w, 's>;
fn init_state(world: &mut World, system_meta: &mut SystemMeta) -> Self::State { ... }
fn get_param<'w, 's>(state: &mut Self::State, ...) -> Self::Item<'w, 's>;
}
unsafe impl ReadOnlySystemParam for MyParam<'_, '_> { }
```
The trait `ReadOnlySystemParamFetch` has been replaced with `ReadOnlySystemParam`.
```rust
// Before
unsafe impl ReadOnlySystemParamFetch for MyParamState {}
// After
unsafe impl ReadOnlySystemParam for MyParam<'_, '_> {}
```
2023-01-07 23:20:32 +00:00
// SAFETY: this impl defers to `Res`, which initializes and validates the correct world access.
unsafe impl < ' a , T : Resource > SystemParam for Option < Res < ' a , T > > {
type State = ComponentId ;
Simplify trait hierarchy for `SystemParam` (#6865)
# Objective
* Implementing a custom `SystemParam` by hand requires implementing three traits -- four if it is read-only.
* The trait `SystemParamFetch<'w, 's>` is a workaround from before we had generic associated types, and is no longer necessary.
## Solution
* Combine the trait `SystemParamFetch` with `SystemParamState`.
* I decided to remove the `Fetch` name and keep the `State` name, since the former was consistently conflated with the latter.
* Replace the trait `ReadOnlySystemParamFetch` with `ReadOnlySystemParam`, which simplifies trait bounds in generic code.
---
## Changelog
- Removed the trait `SystemParamFetch`, moving its functionality to `SystemParamState`.
- Replaced the trait `ReadOnlySystemParamFetch` with `ReadOnlySystemParam`.
## Migration Guide
The trait `SystemParamFetch` has been removed, and its functionality has been transferred to `SystemParamState`.
```rust
// Before
impl SystemParamState for MyParamState {
fn init(world: &mut World, system_meta: &mut SystemMeta) -> Self { ... }
}
impl<'w, 's> SystemParamFetch<'w, 's> for MyParamState {
type Item = MyParam<'w, 's>;
fn get_param(...) -> Self::Item;
}
// After
impl SystemParamState for MyParamState {
type Item<'w, 's> = MyParam<'w, 's>; // Generic associated types!
fn init(world: &mut World, system_meta: &mut SystemMeta) -> Self { ... }
fn get_param<'w, 's>(...) -> Self::Item<'w, 's>;
}
```
The trait `ReadOnlySystemParamFetch` has been replaced with `ReadOnlySystemParam`.
```rust
// Before
unsafe impl ReadOnlySystemParamFetch for MyParamState {}
// After
unsafe impl<'w, 's> ReadOnlySystemParam for MyParam<'w, 's> {}
```
2022-12-11 18:34:14 +00:00
type Item < ' w , ' s > = Option < Res < ' w , T > > ;
Remove the `SystemParamState` trait and remove types like `ResState` (#6919)
Spiritual successor to #5205.
Actual successor to #6865.
# Objective
Currently, system params are defined using three traits: `SystemParam`, `ReadOnlySystemParam`, `SystemParamState`. The behavior for each param is specified by the `SystemParamState` trait, while `SystemParam` simply defers to the state.
Splitting the traits in this way makes it easier to implement within macros, but it increases the cognitive load. Worst of all, this approach requires each `MySystemParam` to have a public `MySystemParamState` type associated with it.
## Solution
* Merge the trait `SystemParamState` into `SystemParam`.
* Remove all trivial `SystemParam` state types.
* `OptionNonSendMutState<T>`: you will not be missed.
---
- [x] Fix/resolve the remaining test failure.
## Changelog
* Removed the trait `SystemParamState`, merging its functionality into `SystemParam`.
## Migration Guide
**Note**: this should replace the migration guide for #6865.
This is relative to Bevy 0.9, not main.
The traits `SystemParamState` and `SystemParamFetch` have been removed, and their functionality has been transferred to `SystemParam`.
```rust
// Before (0.9)
impl SystemParam for MyParam<'_, '_> {
type State = MyParamState;
}
unsafe impl SystemParamState for MyParamState {
fn init(world: &mut World, system_meta: &mut SystemMeta) -> Self { ... }
}
unsafe impl<'w, 's> SystemParamFetch<'w, 's> for MyParamState {
type Item = MyParam<'w, 's>;
fn get_param(&mut self, ...) -> Self::Item;
}
unsafe impl ReadOnlySystemParamFetch for MyParamState { }
// After (0.10)
unsafe impl SystemParam for MyParam<'_, '_> {
type State = MyParamState;
type Item<'w, 's> = MyParam<'w, 's>;
fn init_state(world: &mut World, system_meta: &mut SystemMeta) -> Self::State { ... }
fn get_param<'w, 's>(state: &mut Self::State, ...) -> Self::Item<'w, 's>;
}
unsafe impl ReadOnlySystemParam for MyParam<'_, '_> { }
```
The trait `ReadOnlySystemParamFetch` has been replaced with `ReadOnlySystemParam`.
```rust
// Before
unsafe impl ReadOnlySystemParamFetch for MyParamState {}
// After
unsafe impl ReadOnlySystemParam for MyParam<'_, '_> {}
```
2023-01-07 23:20:32 +00:00
fn init_state ( world : & mut World , system_meta : & mut SystemMeta ) -> Self ::State {
Res ::< T > ::init_state ( world , system_meta )
2021-03-08 20:12:22 +00:00
}
#[ inline ]
Simplify trait hierarchy for `SystemParam` (#6865)
# Objective
* Implementing a custom `SystemParam` by hand requires implementing three traits -- four if it is read-only.
* The trait `SystemParamFetch<'w, 's>` is a workaround from before we had generic associated types, and is no longer necessary.
## Solution
* Combine the trait `SystemParamFetch` with `SystemParamState`.
* I decided to remove the `Fetch` name and keep the `State` name, since the former was consistently conflated with the latter.
* Replace the trait `ReadOnlySystemParamFetch` with `ReadOnlySystemParam`, which simplifies trait bounds in generic code.
---
## Changelog
- Removed the trait `SystemParamFetch`, moving its functionality to `SystemParamState`.
- Replaced the trait `ReadOnlySystemParamFetch` with `ReadOnlySystemParam`.
## Migration Guide
The trait `SystemParamFetch` has been removed, and its functionality has been transferred to `SystemParamState`.
```rust
// Before
impl SystemParamState for MyParamState {
fn init(world: &mut World, system_meta: &mut SystemMeta) -> Self { ... }
}
impl<'w, 's> SystemParamFetch<'w, 's> for MyParamState {
type Item = MyParam<'w, 's>;
fn get_param(...) -> Self::Item;
}
// After
impl SystemParamState for MyParamState {
type Item<'w, 's> = MyParam<'w, 's>; // Generic associated types!
fn init(world: &mut World, system_meta: &mut SystemMeta) -> Self { ... }
fn get_param<'w, 's>(...) -> Self::Item<'w, 's>;
}
```
The trait `ReadOnlySystemParamFetch` has been replaced with `ReadOnlySystemParam`.
```rust
// Before
unsafe impl ReadOnlySystemParamFetch for MyParamState {}
// After
unsafe impl<'w, 's> ReadOnlySystemParam for MyParam<'w, 's> {}
```
2022-12-11 18:34:14 +00:00
unsafe fn get_param < ' w , ' s > (
Remove the `SystemParamState` trait and remove types like `ResState` (#6919)
Spiritual successor to #5205.
Actual successor to #6865.
# Objective
Currently, system params are defined using three traits: `SystemParam`, `ReadOnlySystemParam`, `SystemParamState`. The behavior for each param is specified by the `SystemParamState` trait, while `SystemParam` simply defers to the state.
Splitting the traits in this way makes it easier to implement within macros, but it increases the cognitive load. Worst of all, this approach requires each `MySystemParam` to have a public `MySystemParamState` type associated with it.
## Solution
* Merge the trait `SystemParamState` into `SystemParam`.
* Remove all trivial `SystemParam` state types.
* `OptionNonSendMutState<T>`: you will not be missed.
---
- [x] Fix/resolve the remaining test failure.
## Changelog
* Removed the trait `SystemParamState`, merging its functionality into `SystemParam`.
## Migration Guide
**Note**: this should replace the migration guide for #6865.
This is relative to Bevy 0.9, not main.
The traits `SystemParamState` and `SystemParamFetch` have been removed, and their functionality has been transferred to `SystemParam`.
```rust
// Before (0.9)
impl SystemParam for MyParam<'_, '_> {
type State = MyParamState;
}
unsafe impl SystemParamState for MyParamState {
fn init(world: &mut World, system_meta: &mut SystemMeta) -> Self { ... }
}
unsafe impl<'w, 's> SystemParamFetch<'w, 's> for MyParamState {
type Item = MyParam<'w, 's>;
fn get_param(&mut self, ...) -> Self::Item;
}
unsafe impl ReadOnlySystemParamFetch for MyParamState { }
// After (0.10)
unsafe impl SystemParam for MyParam<'_, '_> {
type State = MyParamState;
type Item<'w, 's> = MyParam<'w, 's>;
fn init_state(world: &mut World, system_meta: &mut SystemMeta) -> Self::State { ... }
fn get_param<'w, 's>(state: &mut Self::State, ...) -> Self::Item<'w, 's>;
}
unsafe impl ReadOnlySystemParam for MyParam<'_, '_> { }
```
The trait `ReadOnlySystemParamFetch` has been replaced with `ReadOnlySystemParam`.
```rust
// Before
unsafe impl ReadOnlySystemParamFetch for MyParamState {}
// After
unsafe impl ReadOnlySystemParam for MyParam<'_, '_> {}
```
2023-01-07 23:20:32 +00:00
& mut component_id : & ' s mut Self ::State ,
Add new SystemState and rename old SystemState to SystemMeta (#2283)
This enables `SystemParams` to be used outside of function systems. Anything can create and store `SystemState`, which enables efficient "param state cached" access to `SystemParams`.
It adds a `ReadOnlySystemParamFetch` trait, which enables safe `SystemState::get` calls without unique world access.
I renamed the old `SystemState` to `SystemMeta` to enable us to mirror the `QueryState` naming convention (but I'm happy to discuss alternative names if people have other ideas). I initially pitched this as `ParamState`, but given that it needs to include full system metadata, that doesn't feel like a particularly accurate name.
```rust
#[derive(Eq, PartialEq, Debug)]
struct A(usize);
#[derive(Eq, PartialEq, Debug)]
struct B(usize);
let mut world = World::default();
world.insert_resource(A(42));
world.spawn().insert(B(7));
// we get nice lifetime elision when declaring the type on the left hand side
let mut system_state: SystemState<(Res<A>, Query<&B>)> = SystemState::new(&mut world);
let (a, query) = system_state.get(&world);
assert_eq!(*a, A(42), "returned resource matches initial value");
assert_eq!(
*query.single().unwrap(),
B(7),
"returned component matches initial value"
);
// mutable system params require unique world access
let mut system_state: SystemState<(ResMut<A>, Query<&mut B>)> = SystemState::new(&mut world);
let (a, query) = system_state.get_mut(&mut world);
// static lifetimes are required when declaring inside of structs
struct SomeContainer {
state: SystemState<(Res<'static, A>, Res<'static, B>)>
}
// this can be shortened using type aliases, which will be useful for complex param tuples
type MyParams<'a> = (Res<'a, A>, Res<'a, B>);
struct SomeContainer {
state: SystemState<MyParams<'static>>
}
// It is the user's responsibility to call SystemState::apply(world) for parameters that queue up work
let mut system_state: SystemState<(Commands, Query<&B>)> = SystemState::new(&mut world);
{
let (mut commands, query) = system_state.get(&world);
commands.insert_resource(3.14);
}
system_state.apply(&mut world);
```
## Future Work
* Actually use SystemState inside FunctionSystem. This would be trivial, but it requires FunctionSystem to wrap SystemState in Option in its current form (which complicates system metadata lookup). I'd prefer to hold off until we adopt something like the later designs linked in #1364, which enable us to contruct Systems using a World reference (and also remove the need for `.system`).
* Consider a "scoped" approach to automatically call SystemState::apply when systems params are no longer being used (either a container type with a Drop impl, or a function that takes a closure for user logic operating on params).
2021-06-02 19:57:38 +00:00
system_meta : & SystemMeta ,
System Param Lifetime Split (#2605)
# Objective
Enable using exact World lifetimes during read-only access . This is motivated by the new renderer's need to allow read-only world-only queries to outlive the query itself (but still be constrained by the world lifetime).
For example:
https://github.com/bevyengine/bevy/blob/115b170d1f11a91146bb6d6e9684dceb8b21f786/pipelined/bevy_pbr2/src/render/mod.rs#L774
## Solution
Split out SystemParam state and world lifetimes and pipe those lifetimes up to read-only Query ops (and add into_inner for Res). According to every safety test I've run so far (except one), this is safe (see the temporary safety test commit). Note that changing the mutable variants to the new lifetimes would allow aliased mutable pointers (try doing that to see how it affects the temporary safety tests).
The new state lifetime on SystemParam does make `#[derive(SystemParam)]` more cumbersome (the current impl requires PhantomData if you don't use both lifetimes). We can make this better by detecting whether or not a lifetime is used in the derive and adjusting accordingly, but that should probably be done in its own pr.
## Why is this a draft?
The new lifetimes break QuerySet safety in one very specific case (see the query_set system in system_safety_test). We need to solve this before we can use the lifetimes given.
This is due to the fact that QuerySet is just a wrapper over Query, which now relies on world lifetimes instead of `&self` lifetimes to prevent aliasing (but in systems, each Query has its own implied lifetime, not a centralized world lifetime). I believe the fix is to rewrite QuerySet to have its own World lifetime (and own the internal reference). This will complicate the impl a bit, but I think it is doable. I'm curious if anyone else has better ideas.
Personally, I think these new lifetimes need to happen. We've gotta have a way to directly tie read-only World queries to the World lifetime. The new renderer is the first place this has come up, but I doubt it will be the last. Worst case scenario we can come up with a second `WorldLifetimeQuery<Q, F = ()>` parameter to enable these read-only scenarios, but I'd rather not add another type to the type zoo.
2021-08-15 20:51:53 +00:00
world : & ' w World ,
Reliable change detection (#1471)
# Problem Definition
The current change tracking (via flags for both components and resources) fails to detect changes made by systems that are scheduled to run earlier in the frame than they are.
This issue is discussed at length in [#68](https://github.com/bevyengine/bevy/issues/68) and [#54](https://github.com/bevyengine/bevy/issues/54).
This is very much a draft PR, and contributions are welcome and needed.
# Criteria
1. Each change is detected at least once, no matter the ordering.
2. Each change is detected at most once, no matter the ordering.
3. Changes should be detected the same frame that they are made.
4. Competitive ergonomics. Ideally does not require opting-in.
5. Low CPU overhead of computation.
6. Memory efficient. This must not increase over time, except where the number of entities / resources does.
7. Changes should not be lost for systems that don't run.
8. A frame needs to act as a pure function. Given the same set of entities / components it needs to produce the same end state without side-effects.
**Exact** change-tracking proposals satisfy criteria 1 and 2.
**Conservative** change-tracking proposals satisfy criteria 1 but not 2.
**Flaky** change tracking proposals satisfy criteria 2 but not 1.
# Code Base Navigation
There are three types of flags:
- `Added`: A piece of data was added to an entity / `Resources`.
- `Mutated`: A piece of data was able to be modified, because its `DerefMut` was accessed
- `Changed`: The bitwise OR of `Added` and `Changed`
The special behavior of `ChangedRes`, with respect to the scheduler is being removed in [#1313](https://github.com/bevyengine/bevy/pull/1313) and does not need to be reproduced.
`ChangedRes` and friends can be found in "bevy_ecs/core/resources/resource_query.rs".
The `Flags` trait for Components can be found in "bevy_ecs/core/query.rs".
`ComponentFlags` are stored in "bevy_ecs/core/archetypes.rs", defined on line 446.
# Proposals
**Proposal 5 was selected for implementation.**
## Proposal 0: No Change Detection
The baseline, where computations are performed on everything regardless of whether it changed.
**Type:** Conservative
**Pros:**
- already implemented
- will never miss events
- no overhead
**Cons:**
- tons of repeated work
- doesn't allow users to avoid repeating work (or monitoring for other changes)
## Proposal 1: Earlier-This-Tick Change Detection
The current approach as of Bevy 0.4. Flags are set, and then flushed at the end of each frame.
**Type:** Flaky
**Pros:**
- already implemented
- simple to understand
- low memory overhead (2 bits per component)
- low time overhead (clear every flag once per frame)
**Cons:**
- misses systems based on ordering
- systems that don't run every frame miss changes
- duplicates detection when looping
- can lead to unresolvable circular dependencies
## Proposal 2: Two-Tick Change Detection
Flags persist for two frames, using a double-buffer system identical to that used in events.
A change is observed if it is found in either the current frame's list of changes or the previous frame's.
**Type:** Conservative
**Pros:**
- easy to understand
- easy to implement
- low memory overhead (4 bits per component)
- low time overhead (bit mask and shift every flag once per frame)
**Cons:**
- can result in a great deal of duplicated work
- systems that don't run every frame miss changes
- duplicates detection when looping
## Proposal 3: Last-Tick Change Detection
Flags persist for two frames, using a double-buffer system identical to that used in events.
A change is observed if it is found in the previous frame's list of changes.
**Type:** Exact
**Pros:**
- exact
- easy to understand
- easy to implement
- low memory overhead (4 bits per component)
- low time overhead (bit mask and shift every flag once per frame)
**Cons:**
- change detection is always delayed, possibly causing painful chained delays
- systems that don't run every frame miss changes
- duplicates detection when looping
## Proposal 4: Flag-Doubling Change Detection
Combine Proposal 2 and Proposal 3. Differentiate between `JustChanged` (current behavior) and `Changed` (Proposal 3).
Pack this data into the flags according to [this implementation proposal](https://github.com/bevyengine/bevy/issues/68#issuecomment-769174804).
**Type:** Flaky + Exact
**Pros:**
- allows users to acc
- easy to implement
- low memory overhead (4 bits per component)
- low time overhead (bit mask and shift every flag once per frame)
**Cons:**
- users must specify the type of change detection required
- still quite fragile to system ordering effects when using the flaky `JustChanged` form
- cannot get immediate + exact results
- systems that don't run every frame miss changes
- duplicates detection when looping
## [SELECTED] Proposal 5: Generation-Counter Change Detection
A global counter is increased after each system is run. Each component saves the time of last mutation, and each system saves the time of last execution. Mutation is detected when the component's counter is greater than the system's counter. Discussed [here](https://github.com/bevyengine/bevy/issues/68#issuecomment-769174804). How to handle addition detection is unsolved; the current proposal is to use the highest bit of the counter as in proposal 1.
**Type:** Exact (for mutations), flaky (for additions)
**Pros:**
- low time overhead (set component counter on access, set system counter after execution)
- robust to systems that don't run every frame
- robust to systems that loop
**Cons:**
- moderately complex implementation
- must be modified as systems are inserted dynamically
- medium memory overhead (4 bytes per component + system)
- unsolved addition detection
## Proposal 6: System-Data Change Detection
For each system, track which system's changes it has seen. This approach is only worth fully designing and implementing if Proposal 5 fails in some way.
**Type:** Exact
**Pros:**
- exact
- conceptually simple
**Cons:**
- requires storing data on each system
- implementation is complex
- must be modified as systems are inserted dynamically
## Proposal 7: Total-Order Change Detection
Discussed [here](https://github.com/bevyengine/bevy/issues/68#issuecomment-754326523). This proposal is somewhat complicated by the new scheduler, but I believe it should still be conceptually feasible. This approach is only worth fully designing and implementing if Proposal 5 fails in some way.
**Type:** Exact
**Pros:**
- exact
- efficient data storage relative to other exact proposals
**Cons:**
- requires access to the scheduler
- complex implementation and difficulty grokking
- must be modified as systems are inserted dynamically
# Tests
- We will need to verify properties 1, 2, 3, 7 and 8. Priority: 1 > 2 = 3 > 8 > 7
- Ideally we can use identical user-facing syntax for all proposals, allowing us to re-use the same syntax for each.
- When writing tests, we need to carefully specify order using explicit dependencies.
- These tests will need to be duplicated for both components and resources.
- We need to be sure to handle cases where ambiguous system orders exist.
`changing_system` is always the system that makes the changes, and `detecting_system` always detects the changes.
The component / resource changed will be simple boolean wrapper structs.
## Basic Added / Mutated / Changed
2 x 3 design:
- Resources vs. Components
- Added vs. Changed vs. Mutated
- `changing_system` runs before `detecting_system`
- verify at the end of tick 2
## At Least Once
2 x 3 design:
- Resources vs. Components
- Added vs. Changed vs. Mutated
- `changing_system` runs after `detecting_system`
- verify at the end of tick 2
## At Most Once
2 x 3 design:
- Resources vs. Components
- Added vs. Changed vs. Mutated
- `changing_system` runs once before `detecting_system`
- increment a counter based on the number of changes detected
- verify at the end of tick 2
## Fast Detection
2 x 3 design:
- Resources vs. Components
- Added vs. Changed vs. Mutated
- `changing_system` runs before `detecting_system`
- verify at the end of tick 1
## Ambiguous System Ordering Robustness
2 x 3 x 2 design:
- Resources vs. Components
- Added vs. Changed vs. Mutated
- `changing_system` runs [before/after] `detecting_system` in tick 1
- `changing_system` runs [after/before] `detecting_system` in tick 2
## System Pausing
2 x 3 design:
- Resources vs. Components
- Added vs. Changed vs. Mutated
- `changing_system` runs in tick 1, then is disabled by run criteria
- `detecting_system` is disabled by run criteria until it is run once during tick 3
- verify at the end of tick 3
## Addition Causes Mutation
2 design:
- Resources vs. Components
- `adding_system_1` adds a component / resource
- `adding system_2` adds the same component / resource
- verify the `Mutated` flag at the end of the tick
- verify the `Added` flag at the end of the tick
First check tests for: https://github.com/bevyengine/bevy/issues/333
Second check tests for: https://github.com/bevyengine/bevy/issues/1443
## Changes Made By Commands
- `adding_system` runs in Update in tick 1, and sends a command to add a component
- `detecting_system` runs in Update in tick 1 and 2, after `adding_system`
- We can't detect the changes in tick 1, since they haven't been processed yet
- If we were to track these changes as being emitted by `adding_system`, we can't detect the changes in tick 2 either, since `detecting_system` has already run once after `adding_system` :(
# Benchmarks
See: [general advice](https://github.com/bevyengine/bevy/blob/master/docs/profiling.md), [Criterion crate](https://github.com/bheisler/criterion.rs)
There are several critical parameters to vary:
1. entity count (1 to 10^9)
2. fraction of entities that are changed (0% to 100%)
3. cost to perform work on changed entities, i.e. workload (1 ns to 1s)
1 and 2 should be varied between benchmark runs. 3 can be added on computationally.
We want to measure:
- memory cost
- run time
We should collect these measurements across several frames (100?) to reduce bootup effects and accurately measure the mean, variance and drift.
Entity-component change detection is much more important to benchmark than resource change detection, due to the orders of magnitude higher number of pieces of data.
No change detection at all should be included in benchmarks as a second control for cases where missing changes is unacceptable.
## Graphs
1. y: performance, x: log_10(entity count), color: proposal, facet: performance metric. Set cost to perform work to 0.
2. y: run time, x: cost to perform work, color: proposal, facet: fraction changed. Set number of entities to 10^6
3. y: memory, x: frames, color: proposal
# Conclusions
1. Is the theoretical categorization of the proposals correct according to our tests?
2. How does the performance of the proposals compare without any load?
3. How does the performance of the proposals compare with realistic loads?
4. At what workload does more exact change tracking become worth the (presumably) higher overhead?
5. When does adding change-detection to save on work become worthwhile?
6. Is there enough divergence in performance between the best solutions in each class to ship more than one change-tracking solution?
# Implementation Plan
1. Write a test suite.
2. Verify that tests fail for existing approach.
3. Write a benchmark suite.
4. Get performance numbers for existing approach.
5. Implement, test and benchmark various solutions using a Git branch per proposal.
6. Create a draft PR with all solutions and present results to team.
7. Select a solution and replace existing change detection.
Co-authored-by: Brice DAVIER <bricedavier@gmail.com>
Co-authored-by: Carter Anderson <mcanders1@gmail.com>
2021-03-19 17:53:26 +00:00
change_tick : u32 ,
Simplify trait hierarchy for `SystemParam` (#6865)
# Objective
* Implementing a custom `SystemParam` by hand requires implementing three traits -- four if it is read-only.
* The trait `SystemParamFetch<'w, 's>` is a workaround from before we had generic associated types, and is no longer necessary.
## Solution
* Combine the trait `SystemParamFetch` with `SystemParamState`.
* I decided to remove the `Fetch` name and keep the `State` name, since the former was consistently conflated with the latter.
* Replace the trait `ReadOnlySystemParamFetch` with `ReadOnlySystemParam`, which simplifies trait bounds in generic code.
---
## Changelog
- Removed the trait `SystemParamFetch`, moving its functionality to `SystemParamState`.
- Replaced the trait `ReadOnlySystemParamFetch` with `ReadOnlySystemParam`.
## Migration Guide
The trait `SystemParamFetch` has been removed, and its functionality has been transferred to `SystemParamState`.
```rust
// Before
impl SystemParamState for MyParamState {
fn init(world: &mut World, system_meta: &mut SystemMeta) -> Self { ... }
}
impl<'w, 's> SystemParamFetch<'w, 's> for MyParamState {
type Item = MyParam<'w, 's>;
fn get_param(...) -> Self::Item;
}
// After
impl SystemParamState for MyParamState {
type Item<'w, 's> = MyParam<'w, 's>; // Generic associated types!
fn init(world: &mut World, system_meta: &mut SystemMeta) -> Self { ... }
fn get_param<'w, 's>(...) -> Self::Item<'w, 's>;
}
```
The trait `ReadOnlySystemParamFetch` has been replaced with `ReadOnlySystemParam`.
```rust
// Before
unsafe impl ReadOnlySystemParamFetch for MyParamState {}
// After
unsafe impl<'w, 's> ReadOnlySystemParam for MyParam<'w, 's> {}
```
2022-12-11 18:34:14 +00:00
) -> Self ::Item < ' w , ' s > {
2021-03-08 20:12:22 +00:00
world
2023-02-06 19:02:52 +00:00
. as_unsafe_world_cell_migration_internal ( )
Remove the `SystemParamState` trait and remove types like `ResState` (#6919)
Spiritual successor to #5205.
Actual successor to #6865.
# Objective
Currently, system params are defined using three traits: `SystemParam`, `ReadOnlySystemParam`, `SystemParamState`. The behavior for each param is specified by the `SystemParamState` trait, while `SystemParam` simply defers to the state.
Splitting the traits in this way makes it easier to implement within macros, but it increases the cognitive load. Worst of all, this approach requires each `MySystemParam` to have a public `MySystemParamState` type associated with it.
## Solution
* Merge the trait `SystemParamState` into `SystemParam`.
* Remove all trivial `SystemParam` state types.
* `OptionNonSendMutState<T>`: you will not be missed.
---
- [x] Fix/resolve the remaining test failure.
## Changelog
* Removed the trait `SystemParamState`, merging its functionality into `SystemParam`.
## Migration Guide
**Note**: this should replace the migration guide for #6865.
This is relative to Bevy 0.9, not main.
The traits `SystemParamState` and `SystemParamFetch` have been removed, and their functionality has been transferred to `SystemParam`.
```rust
// Before (0.9)
impl SystemParam for MyParam<'_, '_> {
type State = MyParamState;
}
unsafe impl SystemParamState for MyParamState {
fn init(world: &mut World, system_meta: &mut SystemMeta) -> Self { ... }
}
unsafe impl<'w, 's> SystemParamFetch<'w, 's> for MyParamState {
type Item = MyParam<'w, 's>;
fn get_param(&mut self, ...) -> Self::Item;
}
unsafe impl ReadOnlySystemParamFetch for MyParamState { }
// After (0.10)
unsafe impl SystemParam for MyParam<'_, '_> {
type State = MyParamState;
type Item<'w, 's> = MyParam<'w, 's>;
fn init_state(world: &mut World, system_meta: &mut SystemMeta) -> Self::State { ... }
fn get_param<'w, 's>(state: &mut Self::State, ...) -> Self::Item<'w, 's>;
}
unsafe impl ReadOnlySystemParam for MyParam<'_, '_> { }
```
The trait `ReadOnlySystemParamFetch` has been replaced with `ReadOnlySystemParam`.
```rust
// Before
unsafe impl ReadOnlySystemParamFetch for MyParamState {}
// After
unsafe impl ReadOnlySystemParam for MyParam<'_, '_> {}
```
2023-01-07 23:20:32 +00:00
. get_resource_with_ticks ( component_id )
2022-10-24 13:46:36 +00:00
. map ( | ( ptr , ticks ) | Res {
value : ptr . deref ( ) ,
2023-01-11 15:41:54 +00:00
ticks : Ticks {
added : ticks . added . deref ( ) ,
changed : ticks . changed . deref ( ) ,
last_change_tick : system_meta . last_change_tick ,
change_tick ,
} ,
2021-03-08 20:12:22 +00:00
} )
}
}
2022-07-04 14:44:24 +00:00
// SAFETY: Res ComponentId and ArchetypeComponentId access is applied to SystemMeta. If this Res
2021-03-11 00:27:30 +00:00
// conflicts with any prior access, a panic will occur.
Remove the `SystemParamState` trait and remove types like `ResState` (#6919)
Spiritual successor to #5205.
Actual successor to #6865.
# Objective
Currently, system params are defined using three traits: `SystemParam`, `ReadOnlySystemParam`, `SystemParamState`. The behavior for each param is specified by the `SystemParamState` trait, while `SystemParam` simply defers to the state.
Splitting the traits in this way makes it easier to implement within macros, but it increases the cognitive load. Worst of all, this approach requires each `MySystemParam` to have a public `MySystemParamState` type associated with it.
## Solution
* Merge the trait `SystemParamState` into `SystemParam`.
* Remove all trivial `SystemParam` state types.
* `OptionNonSendMutState<T>`: you will not be missed.
---
- [x] Fix/resolve the remaining test failure.
## Changelog
* Removed the trait `SystemParamState`, merging its functionality into `SystemParam`.
## Migration Guide
**Note**: this should replace the migration guide for #6865.
This is relative to Bevy 0.9, not main.
The traits `SystemParamState` and `SystemParamFetch` have been removed, and their functionality has been transferred to `SystemParam`.
```rust
// Before (0.9)
impl SystemParam for MyParam<'_, '_> {
type State = MyParamState;
}
unsafe impl SystemParamState for MyParamState {
fn init(world: &mut World, system_meta: &mut SystemMeta) -> Self { ... }
}
unsafe impl<'w, 's> SystemParamFetch<'w, 's> for MyParamState {
type Item = MyParam<'w, 's>;
fn get_param(&mut self, ...) -> Self::Item;
}
unsafe impl ReadOnlySystemParamFetch for MyParamState { }
// After (0.10)
unsafe impl SystemParam for MyParam<'_, '_> {
type State = MyParamState;
type Item<'w, 's> = MyParam<'w, 's>;
fn init_state(world: &mut World, system_meta: &mut SystemMeta) -> Self::State { ... }
fn get_param<'w, 's>(state: &mut Self::State, ...) -> Self::Item<'w, 's>;
}
unsafe impl ReadOnlySystemParam for MyParam<'_, '_> { }
```
The trait `ReadOnlySystemParamFetch` has been replaced with `ReadOnlySystemParam`.
```rust
// Before
unsafe impl ReadOnlySystemParamFetch for MyParamState {}
// After
unsafe impl ReadOnlySystemParam for MyParam<'_, '_> {}
```
2023-01-07 23:20:32 +00:00
unsafe impl < ' a , T : Resource > SystemParam for ResMut < ' a , T > {
type State = ComponentId ;
Simplify trait hierarchy for `SystemParam` (#6865)
# Objective
* Implementing a custom `SystemParam` by hand requires implementing three traits -- four if it is read-only.
* The trait `SystemParamFetch<'w, 's>` is a workaround from before we had generic associated types, and is no longer necessary.
## Solution
* Combine the trait `SystemParamFetch` with `SystemParamState`.
* I decided to remove the `Fetch` name and keep the `State` name, since the former was consistently conflated with the latter.
* Replace the trait `ReadOnlySystemParamFetch` with `ReadOnlySystemParam`, which simplifies trait bounds in generic code.
---
## Changelog
- Removed the trait `SystemParamFetch`, moving its functionality to `SystemParamState`.
- Replaced the trait `ReadOnlySystemParamFetch` with `ReadOnlySystemParam`.
## Migration Guide
The trait `SystemParamFetch` has been removed, and its functionality has been transferred to `SystemParamState`.
```rust
// Before
impl SystemParamState for MyParamState {
fn init(world: &mut World, system_meta: &mut SystemMeta) -> Self { ... }
}
impl<'w, 's> SystemParamFetch<'w, 's> for MyParamState {
type Item = MyParam<'w, 's>;
fn get_param(...) -> Self::Item;
}
// After
impl SystemParamState for MyParamState {
type Item<'w, 's> = MyParam<'w, 's>; // Generic associated types!
fn init(world: &mut World, system_meta: &mut SystemMeta) -> Self { ... }
fn get_param<'w, 's>(...) -> Self::Item<'w, 's>;
}
```
The trait `ReadOnlySystemParamFetch` has been replaced with `ReadOnlySystemParam`.
```rust
// Before
unsafe impl ReadOnlySystemParamFetch for MyParamState {}
// After
unsafe impl<'w, 's> ReadOnlySystemParam for MyParam<'w, 's> {}
```
2022-12-11 18:34:14 +00:00
type Item < ' w , ' s > = ResMut < ' w , T > ;
Remove the `SystemParamState` trait and remove types like `ResState` (#6919)
Spiritual successor to #5205.
Actual successor to #6865.
# Objective
Currently, system params are defined using three traits: `SystemParam`, `ReadOnlySystemParam`, `SystemParamState`. The behavior for each param is specified by the `SystemParamState` trait, while `SystemParam` simply defers to the state.
Splitting the traits in this way makes it easier to implement within macros, but it increases the cognitive load. Worst of all, this approach requires each `MySystemParam` to have a public `MySystemParamState` type associated with it.
## Solution
* Merge the trait `SystemParamState` into `SystemParam`.
* Remove all trivial `SystemParam` state types.
* `OptionNonSendMutState<T>`: you will not be missed.
---
- [x] Fix/resolve the remaining test failure.
## Changelog
* Removed the trait `SystemParamState`, merging its functionality into `SystemParam`.
## Migration Guide
**Note**: this should replace the migration guide for #6865.
This is relative to Bevy 0.9, not main.
The traits `SystemParamState` and `SystemParamFetch` have been removed, and their functionality has been transferred to `SystemParam`.
```rust
// Before (0.9)
impl SystemParam for MyParam<'_, '_> {
type State = MyParamState;
}
unsafe impl SystemParamState for MyParamState {
fn init(world: &mut World, system_meta: &mut SystemMeta) -> Self { ... }
}
unsafe impl<'w, 's> SystemParamFetch<'w, 's> for MyParamState {
type Item = MyParam<'w, 's>;
fn get_param(&mut self, ...) -> Self::Item;
}
unsafe impl ReadOnlySystemParamFetch for MyParamState { }
// After (0.10)
unsafe impl SystemParam for MyParam<'_, '_> {
type State = MyParamState;
type Item<'w, 's> = MyParam<'w, 's>;
fn init_state(world: &mut World, system_meta: &mut SystemMeta) -> Self::State { ... }
fn get_param<'w, 's>(state: &mut Self::State, ...) -> Self::Item<'w, 's>;
}
unsafe impl ReadOnlySystemParam for MyParam<'_, '_> { }
```
The trait `ReadOnlySystemParamFetch` has been replaced with `ReadOnlySystemParam`.
```rust
// Before
unsafe impl ReadOnlySystemParamFetch for MyParamState {}
// After
unsafe impl ReadOnlySystemParam for MyParam<'_, '_> {}
```
2023-01-07 23:20:32 +00:00
fn init_state ( world : & mut World , system_meta : & mut SystemMeta ) -> Self ::State {
Bevy ECS V2 (#1525)
# Bevy ECS V2
This is a rewrite of Bevy ECS (basically everything but the new executor/schedule, which are already awesome). The overall goal was to improve the performance and versatility of Bevy ECS. Here is a quick bulleted list of changes before we dive into the details:
* Complete World rewrite
* Multiple component storage types:
* Tables: fast cache friendly iteration, slower add/removes (previously called Archetypes)
* Sparse Sets: fast add/remove, slower iteration
* Stateful Queries (caches query results for faster iteration. fragmented iteration is _fast_ now)
* Stateful System Params (caches expensive operations. inspired by @DJMcNab's work in #1364)
* Configurable System Params (users can set configuration when they construct their systems. once again inspired by @DJMcNab's work)
* Archetypes are now "just metadata", component storage is separate
* Archetype Graph (for faster archetype changes)
* Component Metadata
* Configure component storage type
* Retrieve information about component size/type/name/layout/send-ness/etc
* Components are uniquely identified by a densely packed ComponentId
* TypeIds are now totally optional (which should make implementing scripting easier)
* Super fast "for_each" query iterators
* Merged Resources into World. Resources are now just a special type of component
* EntityRef/EntityMut builder apis (more efficient and more ergonomic)
* Fast bitset-backed `Access<T>` replaces old hashmap-based approach everywhere
* Query conflicts are determined by component access instead of archetype component access (to avoid random failures at runtime)
* With/Without are still taken into account for conflicts, so this should still be comfy to use
* Much simpler `IntoSystem` impl
* Significantly reduced the amount of hashing throughout the ecs in favor of Sparse Sets (indexed by densely packed ArchetypeId, ComponentId, BundleId, and TableId)
* Safety Improvements
* Entity reservation uses a normal world reference instead of unsafe transmute
* QuerySets no longer transmute lifetimes
* Made traits "unsafe" where relevant
* More thorough safety docs
* WorldCell
* Exposes safe mutable access to multiple resources at a time in a World
* Replaced "catch all" `System::update_archetypes(world: &World)` with `System::new_archetype(archetype: &Archetype)`
* Simpler Bundle implementation
* Replaced slow "remove_bundle_one_by_one" used as fallback for Commands::remove_bundle with fast "remove_bundle_intersection"
* Removed `Mut<T>` query impl. it is better to only support one way: `&mut T`
* Removed with() from `Flags<T>` in favor of `Option<Flags<T>>`, which allows querying for flags to be "filtered" by default
* Components now have is_send property (currently only resources support non-send)
* More granular module organization
* New `RemovedComponents<T>` SystemParam that replaces `query.removed::<T>()`
* `world.resource_scope()` for mutable access to resources and world at the same time
* WorldQuery and QueryFilter traits unified. FilterFetch trait added to enable "short circuit" filtering. Auto impled for cases that don't need it
* Significantly slimmed down SystemState in favor of individual SystemParam state
* System Commands changed from `commands: &mut Commands` back to `mut commands: Commands` (to allow Commands to have a World reference)
Fixes #1320
## `World` Rewrite
This is a from-scratch rewrite of `World` that fills the niche that `hecs` used to. Yes, this means Bevy ECS is no longer a "fork" of hecs. We're going out our own!
(the only shared code between the projects is the entity id allocator, which is already basically ideal)
A huge shout out to @SanderMertens (author of [flecs](https://github.com/SanderMertens/flecs)) for sharing some great ideas with me (specifically hybrid ecs storage and archetype graphs). He also helped advise on a number of implementation details.
## Component Storage (The Problem)
Two ECS storage paradigms have gained a lot of traction over the years:
* **Archetypal ECS**:
* Stores components in "tables" with static schemas. Each "column" stores components of a given type. Each "row" is an entity.
* Each "archetype" has its own table. Adding/removing an entity's component changes the archetype.
* Enables super-fast Query iteration due to its cache-friendly data layout
* Comes at the cost of more expensive add/remove operations for an Entity's components, because all components need to be copied to the new archetype's "table"
* **Sparse Set ECS**:
* Stores components of the same type in densely packed arrays, which are sparsely indexed by densely packed unsigned integers (Entity ids)
* Query iteration is slower than Archetypal ECS because each entity's component could be at any position in the sparse set. This "random access" pattern isn't cache friendly. Additionally, there is an extra layer of indirection because you must first map the entity id to an index in the component array.
* Adding/removing components is a cheap, constant time operation
Bevy ECS V1, hecs, legion, flec, and Unity DOTS are all "archetypal ecs-es". I personally think "archetypal" storage is a good default for game engines. An entity's archetype doesn't need to change frequently in general, and it creates "fast by default" query iteration (which is a much more common operation). It is also "self optimizing". Users don't need to think about optimizing component layouts for iteration performance. It "just works" without any extra boilerplate.
Shipyard and EnTT are "sparse set ecs-es". They employ "packing" as a way to work around the "suboptimal by default" iteration performance for specific sets of components. This helps, but I didn't think this was a good choice for a general purpose engine like Bevy because:
1. "packs" conflict with each other. If bevy decides to internally pack the Transform and GlobalTransform components, users are then blocked if they want to pack some custom component with Transform.
2. users need to take manual action to optimize
Developers selecting an ECS framework are stuck with a hard choice. Select an "archetypal" framework with "fast iteration everywhere" but without the ability to cheaply add/remove components, or select a "sparse set" framework to cheaply add/remove components but with slower iteration performance.
## Hybrid Component Storage (The Solution)
In Bevy ECS V2, we get to have our cake and eat it too. It now has _both_ of the component storage types above (and more can be added later if needed):
* **Tables** (aka "archetypal" storage)
* The default storage. If you don't configure anything, this is what you get
* Fast iteration by default
* Slower add/remove operations
* **Sparse Sets**
* Opt-in
* Slower iteration
* Faster add/remove operations
These storage types complement each other perfectly. By default Query iteration is fast. If developers know that they want to add/remove a component at high frequencies, they can set the storage to "sparse set":
```rust
world.register_component(
ComponentDescriptor::new::<MyComponent>(StorageType::SparseSet)
).unwrap();
```
## Archetypes
Archetypes are now "just metadata" ... they no longer store components directly. They do store:
* The `ComponentId`s of each of the Archetype's components (and that component's storage type)
* Archetypes are uniquely defined by their component layouts
* For example: entities with "table" components `[A, B, C]` _and_ "sparse set" components `[D, E]` will always be in the same archetype.
* The `TableId` associated with the archetype
* For now each archetype has exactly one table (which can have no components),
* There is a 1->Many relationship from Tables->Archetypes. A given table could have any number of archetype components stored in it:
* Ex: an entity with "table storage" components `[A, B, C]` and "sparse set" components `[D, E]` will share the same `[A, B, C]` table as an entity with `[A, B, C]` table component and `[F]` sparse set components.
* This 1->Many relationship is how we preserve fast "cache friendly" iteration performance when possible (more on this later)
* A list of entities that are in the archetype and the row id of the table they are in
* ArchetypeComponentIds
* unique densely packed identifiers for (ArchetypeId, ComponentId) pairs
* used by the schedule executor for cheap system access control
* "Archetype Graph Edges" (see the next section)
## The "Archetype Graph"
Archetype changes in Bevy (and a number of other archetypal ecs-es) have historically been expensive to compute. First, you need to allocate a new vector of the entity's current component ids, add or remove components based on the operation performed, sort it (to ensure it is order-independent), then hash it to find the archetype (if it exists). And thats all before we get to the _already_ expensive full copy of all components to the new table storage.
The solution is to build a "graph" of archetypes to cache these results. @SanderMertens first exposed me to the idea (and he got it from @gjroelofs, who came up with it). They propose adding directed edges between archetypes for add/remove component operations. If `ComponentId`s are densely packed, you can use sparse sets to cheaply jump between archetypes.
Bevy takes this one step further by using add/remove `Bundle` edges instead of `Component` edges. Bevy encourages the use of `Bundles` to group add/remove operations. This is largely for "clearer game logic" reasons, but it also helps cut down on the number of archetype changes required. `Bundles` now also have densely-packed `BundleId`s. This allows us to use a _single_ edge for each bundle operation (rather than needing to traverse N edges ... one for each component). Single component operations are also bundles, so this is strictly an improvement over a "component only" graph.
As a result, an operation that used to be _heavy_ (both for allocations and compute) is now two dirt-cheap array lookups and zero allocations.
## Stateful Queries
World queries are now stateful. This allows us to:
1. Cache archetype (and table) matches
* This resolves another issue with (naive) archetypal ECS: query performance getting worse as the number of archetypes goes up (and fragmentation occurs).
2. Cache Fetch and Filter state
* The expensive parts of fetch/filter operations (such as hashing the TypeId to find the ComponentId) now only happen once when the Query is first constructed
3. Incrementally build up state
* When new archetypes are added, we only process the new archetypes (no need to rebuild state for old archetypes)
As a result, the direct `World` query api now looks like this:
```rust
let mut query = world.query::<(&A, &mut B)>();
for (a, mut b) in query.iter_mut(&mut world) {
}
```
Requiring `World` to generate stateful queries (rather than letting the `QueryState` type be constructed separately) allows us to ensure that _all_ queries are properly initialized (and the relevant world state, such as ComponentIds). This enables QueryState to remove branches from its operations that check for initialization status (and also enables query.iter() to take an immutable world reference because it doesn't need to initialize anything in world).
However in systems, this is a non-breaking change. State management is done internally by the relevant SystemParam.
## Stateful SystemParams
Like Queries, `SystemParams` now also cache state. For example, `Query` system params store the "stateful query" state mentioned above. Commands store their internal `CommandQueue`. This means you can now safely use as many separate `Commands` parameters in your system as you want. `Local<T>` system params store their `T` value in their state (instead of in Resources).
SystemParam state also enabled a significant slim-down of SystemState. It is much nicer to look at now.
Per-SystemParam state naturally insulates us from an "aliased mut" class of errors we have hit in the past (ex: using multiple `Commands` system params).
(credit goes to @DJMcNab for the initial idea and draft pr here #1364)
## Configurable SystemParams
@DJMcNab also had the great idea to make SystemParams configurable. This allows users to provide some initial configuration / values for system parameters (when possible). Most SystemParams have no config (the config type is `()`), but the `Local<T>` param now supports user-provided parameters:
```rust
fn foo(value: Local<usize>) {
}
app.add_system(foo.system().config(|c| c.0 = Some(10)));
```
## Uber Fast "for_each" Query Iterators
Developers now have the choice to use a fast "for_each" iterator, which yields ~1.5-3x iteration speed improvements for "fragmented iteration", and minor ~1.2x iteration speed improvements for unfragmented iteration.
```rust
fn system(query: Query<(&A, &mut B)>) {
// you now have the option to do this for a speed boost
query.for_each_mut(|(a, mut b)| {
});
// however normal iterators are still available
for (a, mut b) in query.iter_mut() {
}
}
```
I think in most cases we should continue to encourage "normal" iterators as they are more flexible and more "rust idiomatic". But when that extra "oomf" is needed, it makes sense to use `for_each`.
We should also consider using `for_each` for internal bevy systems to give our users a nice speed boost (but that should be a separate pr).
## Component Metadata
`World` now has a `Components` collection, which is accessible via `world.components()`. This stores mappings from `ComponentId` to `ComponentInfo`, as well as `TypeId` to `ComponentId` mappings (where relevant). `ComponentInfo` stores information about the component, such as ComponentId, TypeId, memory layout, send-ness (currently limited to resources), and storage type.
## Significantly Cheaper `Access<T>`
We used to use `TypeAccess<TypeId>` to manage read/write component/archetype-component access. This was expensive because TypeIds must be hashed and compared individually. The parallel executor got around this by "condensing" type ids into bitset-backed access types. This worked, but it had to be re-generated from the `TypeAccess<TypeId>`sources every time archetypes changed.
This pr removes TypeAccess in favor of faster bitset access everywhere. We can do this thanks to the move to densely packed `ComponentId`s and `ArchetypeComponentId`s.
## Merged Resources into World
Resources had a lot of redundant functionality with Components. They stored typed data, they had access control, they had unique ids, they were queryable via SystemParams, etc. In fact the _only_ major difference between them was that they were unique (and didn't correlate to an entity).
Separate resources also had the downside of requiring a separate set of access controls, which meant the parallel executor needed to compare more bitsets per system and manage more state.
I initially got the "separate resources" idea from `legion`. I think that design was motivated by the fact that it made the direct world query/resource lifetime interactions more manageable. It certainly made our lives easier when using Resources alongside hecs/bevy_ecs. However we already have a construct for safely and ergonomically managing in-world lifetimes: systems (which use `Access<T>` internally).
This pr merges Resources into World:
```rust
world.insert_resource(1);
world.insert_resource(2.0);
let a = world.get_resource::<i32>().unwrap();
let mut b = world.get_resource_mut::<f64>().unwrap();
*b = 3.0;
```
Resources are now just a special kind of component. They have their own ComponentIds (and their own resource TypeId->ComponentId scope, so they don't conflict wit components of the same type). They are stored in a special "resource archetype", which stores components inside the archetype using a new `unique_components` sparse set (note that this sparse set could later be used to implement Tags). This allows us to keep the code size small by reusing existing datastructures (namely Column, Archetype, ComponentFlags, and ComponentInfo). This allows us the executor to use a single `Access<ArchetypeComponentId>` per system. It should also make scripting language integration easier.
_But_ this merge did create problems for people directly interacting with `World`. What if you need mutable access to multiple resources at the same time? `world.get_resource_mut()` borrows World mutably!
## WorldCell
WorldCell applies the `Access<ArchetypeComponentId>` concept to direct world access:
```rust
let world_cell = world.cell();
let a = world_cell.get_resource_mut::<i32>().unwrap();
let b = world_cell.get_resource_mut::<f64>().unwrap();
```
This adds cheap runtime checks (a sparse set lookup of `ArchetypeComponentId` and a counter) to ensure that world accesses do not conflict with each other. Each operation returns a `WorldBorrow<'w, T>` or `WorldBorrowMut<'w, T>` wrapper type, which will release the relevant ArchetypeComponentId resources when dropped.
World caches the access sparse set (and only one cell can exist at a time), so `world.cell()` is a cheap operation.
WorldCell does _not_ use atomic operations. It is non-send, does a mutable borrow of world to prevent other accesses, and uses a simple `Rc<RefCell<ArchetypeComponentAccess>>` wrapper in each WorldBorrow pointer.
The api is currently limited to resource access, but it can and should be extended to queries / entity component access.
## Resource Scopes
WorldCell does not yet support component queries, and even when it does there are sometimes legitimate reasons to want a mutable world ref _and_ a mutable resource ref (ex: bevy_render and bevy_scene both need this). In these cases we could always drop down to the unsafe `world.get_resource_unchecked_mut()`, but that is not ideal!
Instead developers can use a "resource scope"
```rust
world.resource_scope(|world: &mut World, a: &mut A| {
})
```
This temporarily removes the `A` resource from `World`, provides mutable pointers to both, and re-adds A to World when finished. Thanks to the move to ComponentIds/sparse sets, this is a cheap operation.
If multiple resources are required, scopes can be nested. We could also consider adding a "resource tuple" to the api if this pattern becomes common and the boilerplate gets nasty.
## Query Conflicts Use ComponentId Instead of ArchetypeComponentId
For safety reasons, systems cannot contain queries that conflict with each other without wrapping them in a QuerySet. On bevy `main`, we use ArchetypeComponentIds to determine conflicts. This is nice because it can take into account filters:
```rust
// these queries will never conflict due to their filters
fn filter_system(a: Query<&mut A, With<B>>, b: Query<&mut B, Without<B>>) {
}
```
But it also has a significant downside:
```rust
// these queries will not conflict _until_ an entity with A, B, and C is spawned
fn maybe_conflicts_system(a: Query<(&mut A, &C)>, b: Query<(&mut A, &B)>) {
}
```
The system above will panic at runtime if an entity with A, B, and C is spawned. This makes it hard to trust that your game logic will run without crashing.
In this pr, I switched to using `ComponentId` instead. This _is_ more constraining. `maybe_conflicts_system` will now always fail, but it will do it consistently at startup. Naively, it would also _disallow_ `filter_system`, which would be a significant downgrade in usability. Bevy has a number of internal systems that rely on disjoint queries and I expect it to be a common pattern in userspace.
To resolve this, I added a new `FilteredAccess<T>` type, which wraps `Access<T>` and adds with/without filters. If two `FilteredAccess` have with/without values that prove they are disjoint, they will no longer conflict.
## EntityRef / EntityMut
World entity operations on `main` require that the user passes in an `entity` id to each operation:
```rust
let entity = world.spawn((A, )); // create a new entity with A
world.get::<A>(entity);
world.insert(entity, (B, C));
world.insert_one(entity, D);
```
This means that each operation needs to look up the entity location / verify its validity. The initial spawn operation also requires a Bundle as input. This can be awkward when no components are required (or one component is required).
These operations have been replaced by `EntityRef` and `EntityMut`, which are "builder-style" wrappers around world that provide read and read/write operations on a single, pre-validated entity:
```rust
// spawn now takes no inputs and returns an EntityMut
let entity = world.spawn()
.insert(A) // insert a single component into the entity
.insert_bundle((B, C)) // insert a bundle of components into the entity
.id() // id returns the Entity id
// Returns EntityMut (or panics if the entity does not exist)
world.entity_mut(entity)
.insert(D)
.insert_bundle(SomeBundle::default());
{
// returns EntityRef (or panics if the entity does not exist)
let d = world.entity(entity)
.get::<D>() // gets the D component
.unwrap();
// world.get still exists for ergonomics
let d = world.get::<D>(entity).unwrap();
}
// These variants return Options if you want to check existence instead of panicing
world.get_entity_mut(entity)
.unwrap()
.insert(E);
if let Some(entity_ref) = world.get_entity(entity) {
let d = entity_ref.get::<D>().unwrap();
}
```
This _does not_ affect the current Commands api or terminology. I think that should be a separate conversation as that is a much larger breaking change.
## Safety Improvements
* Entity reservation in Commands uses a normal world borrow instead of an unsafe transmute
* QuerySets no longer transmutes lifetimes
* Made traits "unsafe" when implementing a trait incorrectly could cause unsafety
* More thorough safety docs
## RemovedComponents SystemParam
The old approach to querying removed components: `query.removed:<T>()` was confusing because it had no connection to the query itself. I replaced it with the following, which is both clearer and allows us to cache the ComponentId mapping in the SystemParamState:
```rust
fn system(removed: RemovedComponents<T>) {
for entity in removed.iter() {
}
}
```
## Simpler Bundle implementation
Bundles are no longer responsible for sorting (or deduping) TypeInfo. They are just a simple ordered list of component types / data. This makes the implementation smaller and opens the door to an easy "nested bundle" implementation in the future (which i might even add in this pr). Duplicate detection is now done once per bundle type by World the first time a bundle is used.
## Unified WorldQuery and QueryFilter types
(don't worry they are still separate type _parameters_ in Queries .. this is a non-breaking change)
WorldQuery and QueryFilter were already basically identical apis. With the addition of `FetchState` and more storage-specific fetch methods, the overlap was even clearer (and the redundancy more painful).
QueryFilters are now just `F: WorldQuery where F::Fetch: FilterFetch`. FilterFetch requires `Fetch<Item = bool>` and adds new "short circuit" variants of fetch methods. This enables a filter tuple like `(With<A>, Without<B>, Changed<C>)` to stop evaluating the filter after the first mismatch is encountered. FilterFetch is automatically implemented for `Fetch` implementations that return bool.
This forces fetch implementations that return things like `(bool, bool, bool)` (such as the filter above) to manually implement FilterFetch and decide whether or not to short-circuit.
## More Granular Modules
World no longer globs all of the internal modules together. It now exports `core`, `system`, and `schedule` separately. I'm also considering exporting `core` submodules directly as that is still pretty "glob-ey" and unorganized (feedback welcome here).
## Remaining Draft Work (to be done in this pr)
* ~~panic on conflicting WorldQuery fetches (&A, &mut A)~~
* ~~bevy `main` and hecs both currently allow this, but we should protect against it if possible~~
* ~~batch_iter / par_iter (currently stubbed out)~~
* ~~ChangedRes~~
* ~~I skipped this while we sort out #1313. This pr should be adapted to account for whatever we land on there~~.
* ~~The `Archetypes` and `Tables` collections use hashes of sorted lists of component ids to uniquely identify each archetype/table. This hash is then used as the key in a HashMap to look up the relevant ArchetypeId or TableId. (which doesn't handle hash collisions properly)~~
* ~~It is currently unsafe to generate a Query from "World A", then use it on "World B" (despite the api claiming it is safe). We should probably close this gap. This could be done by adding a randomly generated WorldId to each world, then storing that id in each Query. They could then be compared to each other on each `query.do_thing(&world)` operation. This _does_ add an extra branch to each query operation, so I'm open to other suggestions if people have them.~~
* ~~Nested Bundles (if i find time)~~
## Potential Future Work
* Expand WorldCell to support queries.
* Consider not allocating in the empty archetype on `world.spawn()`
* ex: return something like EntityMutUninit, which turns into EntityMut after an `insert` or `insert_bundle` op
* this actually regressed performance last time i tried it, but in theory it should be faster
* Optimize SparseSet::insert (see `PERF` comment on insert)
* Replace SparseArray `Option<T>` with T::MAX to cut down on branching
* would enable cheaper get_unchecked() operations
* upstream fixedbitset optimizations
* fixedbitset could be allocation free for small block counts (store blocks in a SmallVec)
* fixedbitset could have a const constructor
* Consider implementing Tags (archetype-specific by-value data that affects archetype identity)
* ex: ArchetypeA could have `[A, B, C]` table components and `[D(1)]` "tag" component. ArchetypeB could have `[A, B, C]` table components and a `[D(2)]` tag component. The archetypes are different, despite both having D tags because the value inside D is different.
* this could potentially build on top of the `archetype.unique_components` added in this pr for resource storage.
* Consider reverting `all_tuples` proc macro in favor of the old `macro_rules` implementation
* all_tuples is more flexible and produces cleaner documentation (the macro_rules version produces weird type parameter orders due to parser constraints)
* but unfortunately all_tuples also appears to make Rust Analyzer sad/slow when working inside of `bevy_ecs` (does not affect user code)
* Consider "resource queries" and/or "mixed resource and entity component queries" as an alternative to WorldCell
* this is basically just "systems" so maybe it's not worth it
* Add more world ops
* `world.clear()`
* `world.reserve<T: Bundle>(count: usize)`
* Try using the old archetype allocation strategy (allocate new memory on resize and copy everything over). I expect this to improve batch insertion performance at the cost of unbatched performance. But thats just a guess. I'm not an allocation perf pro :)
* Adapt Commands apis for consistency with new World apis
## Benchmarks
key:
* `bevy_old`: bevy `main` branch
* `bevy`: this branch
* `_foreach`: uses an optimized for_each iterator
* ` _sparse`: uses sparse set storage (if unspecified assume table storage)
* `_system`: runs inside a system (if unspecified assume test happens via direct world ops)
### Simple Insert (from ecs_bench_suite)
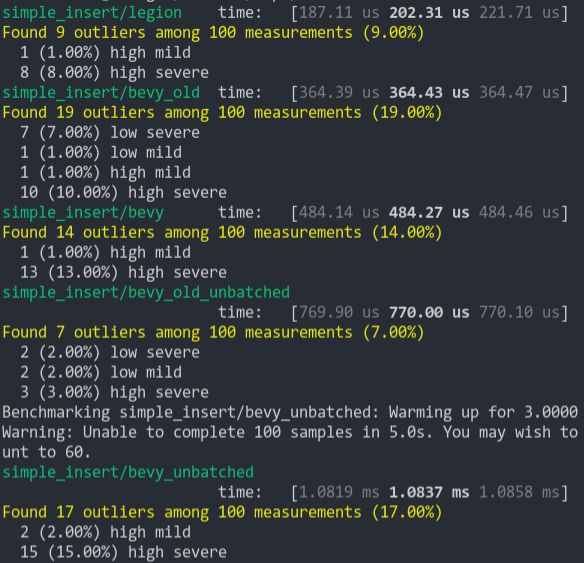
### Simpler Iter (from ecs_bench_suite)
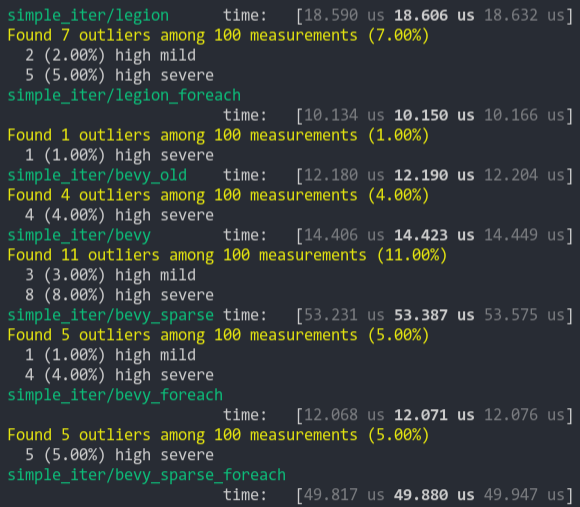
### Fragment Iter (from ecs_bench_suite)
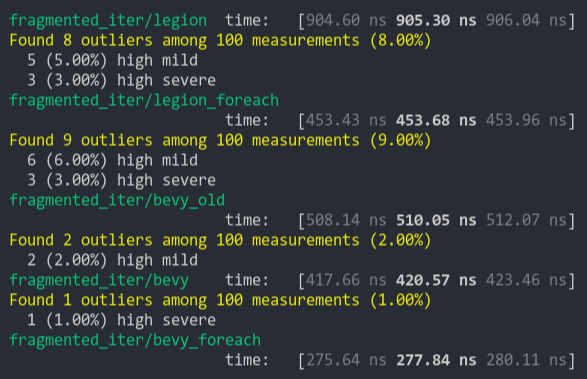
### Sparse Fragmented Iter
Iterate a query that matches 5 entities from a single matching archetype, but there are 100 unmatching archetypes
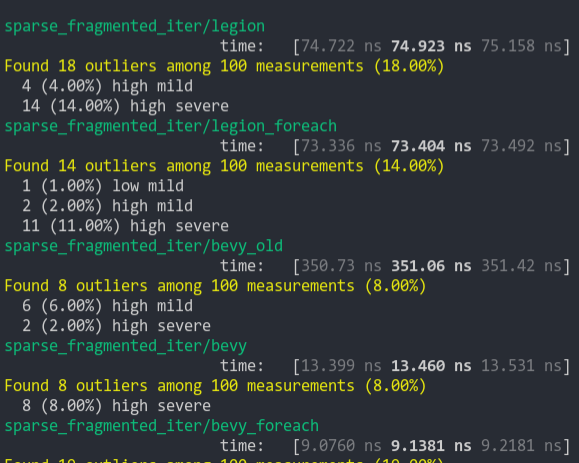
### Schedule (from ecs_bench_suite)
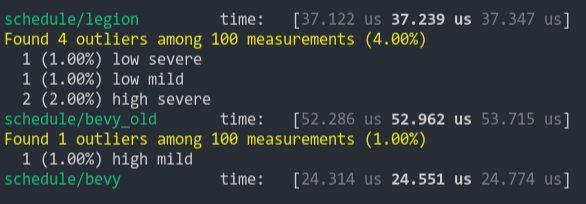
### Add Remove Component (from ecs_bench_suite)
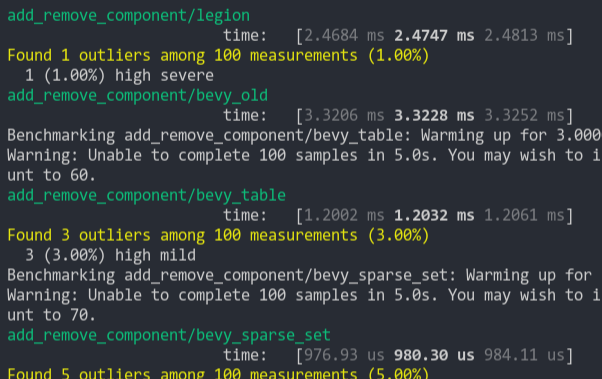
### Add Remove Component Big
Same as the test above, but each entity has 5 "large" matrix components and 1 "large" matrix component is added and removed
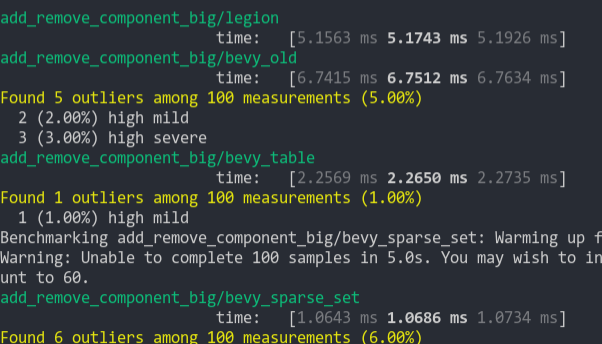
### Get Component
Looks up a single component value a large number of times
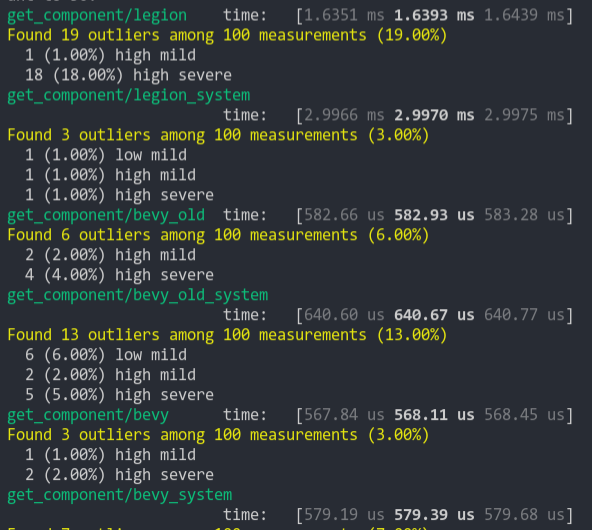
2021-03-05 07:54:35 +00:00
let component_id = world . initialize_resource ::< T > ( ) ;
Fix FilteredAccessSet get_conflicts inconsistency (#5105)
# Objective
* Enable `Res` and `Query` parameter mutual exclusion
* Required for https://github.com/bevyengine/bevy/pull/5080
The `FilteredAccessSet::get_conflicts` methods didn't work properly with
`Res` and `ResMut` parameters. Because those added their access by using
the `combined_access_mut` method and directly modifying the global
access state of the FilteredAccessSet. This caused an inconsistency,
because get_conflicts assumes that ALL added access have a corresponding
`FilteredAccess` added to the `filtered_accesses` field.
In practice, that means that SystemParam that adds their access through
the `Access` returned by `combined_access_mut` and the ones that add
their access using the `add` method lived in two different universes. As
a result, they could never be mutually exclusive.
## Solution
This commit fixes it by removing the `combined_access_mut` method. This
ensures that the `combined_access` field of FilteredAccessSet is always
updated consistently with the addition of a filter. When checking for
filtered access, it is now possible to account for `Res` and `ResMut`
invalid access. This is currently not needed, but might be in the
future.
We add the `add_unfiltered_{read,write}` methods to replace previous
usages of `combined_access_mut`.
We also add improved Debug implementations on FixedBitSet so that their
meaning is much clearer in debug output.
---
## Changelog
* Fix `Res` and `Query` parameter never being mutually exclusive.
## Migration Guide
Note: this mostly changes ECS internals, but since the API is public, it is technically breaking:
* Removed `FilteredAccessSet::combined_access_mut`
* Replace _immutable_ usage of those by `combined_access`
* For _mutable_ usages, use the new `add_unfiltered_{read,write}` methods instead of `combined_access_mut` followed by `add_{read,write}`
2022-11-16 11:05:48 +00:00
let combined_access = system_meta . component_access_set . combined_access ( ) ;
Bevy ECS V2 (#1525)
# Bevy ECS V2
This is a rewrite of Bevy ECS (basically everything but the new executor/schedule, which are already awesome). The overall goal was to improve the performance and versatility of Bevy ECS. Here is a quick bulleted list of changes before we dive into the details:
* Complete World rewrite
* Multiple component storage types:
* Tables: fast cache friendly iteration, slower add/removes (previously called Archetypes)
* Sparse Sets: fast add/remove, slower iteration
* Stateful Queries (caches query results for faster iteration. fragmented iteration is _fast_ now)
* Stateful System Params (caches expensive operations. inspired by @DJMcNab's work in #1364)
* Configurable System Params (users can set configuration when they construct their systems. once again inspired by @DJMcNab's work)
* Archetypes are now "just metadata", component storage is separate
* Archetype Graph (for faster archetype changes)
* Component Metadata
* Configure component storage type
* Retrieve information about component size/type/name/layout/send-ness/etc
* Components are uniquely identified by a densely packed ComponentId
* TypeIds are now totally optional (which should make implementing scripting easier)
* Super fast "for_each" query iterators
* Merged Resources into World. Resources are now just a special type of component
* EntityRef/EntityMut builder apis (more efficient and more ergonomic)
* Fast bitset-backed `Access<T>` replaces old hashmap-based approach everywhere
* Query conflicts are determined by component access instead of archetype component access (to avoid random failures at runtime)
* With/Without are still taken into account for conflicts, so this should still be comfy to use
* Much simpler `IntoSystem` impl
* Significantly reduced the amount of hashing throughout the ecs in favor of Sparse Sets (indexed by densely packed ArchetypeId, ComponentId, BundleId, and TableId)
* Safety Improvements
* Entity reservation uses a normal world reference instead of unsafe transmute
* QuerySets no longer transmute lifetimes
* Made traits "unsafe" where relevant
* More thorough safety docs
* WorldCell
* Exposes safe mutable access to multiple resources at a time in a World
* Replaced "catch all" `System::update_archetypes(world: &World)` with `System::new_archetype(archetype: &Archetype)`
* Simpler Bundle implementation
* Replaced slow "remove_bundle_one_by_one" used as fallback for Commands::remove_bundle with fast "remove_bundle_intersection"
* Removed `Mut<T>` query impl. it is better to only support one way: `&mut T`
* Removed with() from `Flags<T>` in favor of `Option<Flags<T>>`, which allows querying for flags to be "filtered" by default
* Components now have is_send property (currently only resources support non-send)
* More granular module organization
* New `RemovedComponents<T>` SystemParam that replaces `query.removed::<T>()`
* `world.resource_scope()` for mutable access to resources and world at the same time
* WorldQuery and QueryFilter traits unified. FilterFetch trait added to enable "short circuit" filtering. Auto impled for cases that don't need it
* Significantly slimmed down SystemState in favor of individual SystemParam state
* System Commands changed from `commands: &mut Commands` back to `mut commands: Commands` (to allow Commands to have a World reference)
Fixes #1320
## `World` Rewrite
This is a from-scratch rewrite of `World` that fills the niche that `hecs` used to. Yes, this means Bevy ECS is no longer a "fork" of hecs. We're going out our own!
(the only shared code between the projects is the entity id allocator, which is already basically ideal)
A huge shout out to @SanderMertens (author of [flecs](https://github.com/SanderMertens/flecs)) for sharing some great ideas with me (specifically hybrid ecs storage and archetype graphs). He also helped advise on a number of implementation details.
## Component Storage (The Problem)
Two ECS storage paradigms have gained a lot of traction over the years:
* **Archetypal ECS**:
* Stores components in "tables" with static schemas. Each "column" stores components of a given type. Each "row" is an entity.
* Each "archetype" has its own table. Adding/removing an entity's component changes the archetype.
* Enables super-fast Query iteration due to its cache-friendly data layout
* Comes at the cost of more expensive add/remove operations for an Entity's components, because all components need to be copied to the new archetype's "table"
* **Sparse Set ECS**:
* Stores components of the same type in densely packed arrays, which are sparsely indexed by densely packed unsigned integers (Entity ids)
* Query iteration is slower than Archetypal ECS because each entity's component could be at any position in the sparse set. This "random access" pattern isn't cache friendly. Additionally, there is an extra layer of indirection because you must first map the entity id to an index in the component array.
* Adding/removing components is a cheap, constant time operation
Bevy ECS V1, hecs, legion, flec, and Unity DOTS are all "archetypal ecs-es". I personally think "archetypal" storage is a good default for game engines. An entity's archetype doesn't need to change frequently in general, and it creates "fast by default" query iteration (which is a much more common operation). It is also "self optimizing". Users don't need to think about optimizing component layouts for iteration performance. It "just works" without any extra boilerplate.
Shipyard and EnTT are "sparse set ecs-es". They employ "packing" as a way to work around the "suboptimal by default" iteration performance for specific sets of components. This helps, but I didn't think this was a good choice for a general purpose engine like Bevy because:
1. "packs" conflict with each other. If bevy decides to internally pack the Transform and GlobalTransform components, users are then blocked if they want to pack some custom component with Transform.
2. users need to take manual action to optimize
Developers selecting an ECS framework are stuck with a hard choice. Select an "archetypal" framework with "fast iteration everywhere" but without the ability to cheaply add/remove components, or select a "sparse set" framework to cheaply add/remove components but with slower iteration performance.
## Hybrid Component Storage (The Solution)
In Bevy ECS V2, we get to have our cake and eat it too. It now has _both_ of the component storage types above (and more can be added later if needed):
* **Tables** (aka "archetypal" storage)
* The default storage. If you don't configure anything, this is what you get
* Fast iteration by default
* Slower add/remove operations
* **Sparse Sets**
* Opt-in
* Slower iteration
* Faster add/remove operations
These storage types complement each other perfectly. By default Query iteration is fast. If developers know that they want to add/remove a component at high frequencies, they can set the storage to "sparse set":
```rust
world.register_component(
ComponentDescriptor::new::<MyComponent>(StorageType::SparseSet)
).unwrap();
```
## Archetypes
Archetypes are now "just metadata" ... they no longer store components directly. They do store:
* The `ComponentId`s of each of the Archetype's components (and that component's storage type)
* Archetypes are uniquely defined by their component layouts
* For example: entities with "table" components `[A, B, C]` _and_ "sparse set" components `[D, E]` will always be in the same archetype.
* The `TableId` associated with the archetype
* For now each archetype has exactly one table (which can have no components),
* There is a 1->Many relationship from Tables->Archetypes. A given table could have any number of archetype components stored in it:
* Ex: an entity with "table storage" components `[A, B, C]` and "sparse set" components `[D, E]` will share the same `[A, B, C]` table as an entity with `[A, B, C]` table component and `[F]` sparse set components.
* This 1->Many relationship is how we preserve fast "cache friendly" iteration performance when possible (more on this later)
* A list of entities that are in the archetype and the row id of the table they are in
* ArchetypeComponentIds
* unique densely packed identifiers for (ArchetypeId, ComponentId) pairs
* used by the schedule executor for cheap system access control
* "Archetype Graph Edges" (see the next section)
## The "Archetype Graph"
Archetype changes in Bevy (and a number of other archetypal ecs-es) have historically been expensive to compute. First, you need to allocate a new vector of the entity's current component ids, add or remove components based on the operation performed, sort it (to ensure it is order-independent), then hash it to find the archetype (if it exists). And thats all before we get to the _already_ expensive full copy of all components to the new table storage.
The solution is to build a "graph" of archetypes to cache these results. @SanderMertens first exposed me to the idea (and he got it from @gjroelofs, who came up with it). They propose adding directed edges between archetypes for add/remove component operations. If `ComponentId`s are densely packed, you can use sparse sets to cheaply jump between archetypes.
Bevy takes this one step further by using add/remove `Bundle` edges instead of `Component` edges. Bevy encourages the use of `Bundles` to group add/remove operations. This is largely for "clearer game logic" reasons, but it also helps cut down on the number of archetype changes required. `Bundles` now also have densely-packed `BundleId`s. This allows us to use a _single_ edge for each bundle operation (rather than needing to traverse N edges ... one for each component). Single component operations are also bundles, so this is strictly an improvement over a "component only" graph.
As a result, an operation that used to be _heavy_ (both for allocations and compute) is now two dirt-cheap array lookups and zero allocations.
## Stateful Queries
World queries are now stateful. This allows us to:
1. Cache archetype (and table) matches
* This resolves another issue with (naive) archetypal ECS: query performance getting worse as the number of archetypes goes up (and fragmentation occurs).
2. Cache Fetch and Filter state
* The expensive parts of fetch/filter operations (such as hashing the TypeId to find the ComponentId) now only happen once when the Query is first constructed
3. Incrementally build up state
* When new archetypes are added, we only process the new archetypes (no need to rebuild state for old archetypes)
As a result, the direct `World` query api now looks like this:
```rust
let mut query = world.query::<(&A, &mut B)>();
for (a, mut b) in query.iter_mut(&mut world) {
}
```
Requiring `World` to generate stateful queries (rather than letting the `QueryState` type be constructed separately) allows us to ensure that _all_ queries are properly initialized (and the relevant world state, such as ComponentIds). This enables QueryState to remove branches from its operations that check for initialization status (and also enables query.iter() to take an immutable world reference because it doesn't need to initialize anything in world).
However in systems, this is a non-breaking change. State management is done internally by the relevant SystemParam.
## Stateful SystemParams
Like Queries, `SystemParams` now also cache state. For example, `Query` system params store the "stateful query" state mentioned above. Commands store their internal `CommandQueue`. This means you can now safely use as many separate `Commands` parameters in your system as you want. `Local<T>` system params store their `T` value in their state (instead of in Resources).
SystemParam state also enabled a significant slim-down of SystemState. It is much nicer to look at now.
Per-SystemParam state naturally insulates us from an "aliased mut" class of errors we have hit in the past (ex: using multiple `Commands` system params).
(credit goes to @DJMcNab for the initial idea and draft pr here #1364)
## Configurable SystemParams
@DJMcNab also had the great idea to make SystemParams configurable. This allows users to provide some initial configuration / values for system parameters (when possible). Most SystemParams have no config (the config type is `()`), but the `Local<T>` param now supports user-provided parameters:
```rust
fn foo(value: Local<usize>) {
}
app.add_system(foo.system().config(|c| c.0 = Some(10)));
```
## Uber Fast "for_each" Query Iterators
Developers now have the choice to use a fast "for_each" iterator, which yields ~1.5-3x iteration speed improvements for "fragmented iteration", and minor ~1.2x iteration speed improvements for unfragmented iteration.
```rust
fn system(query: Query<(&A, &mut B)>) {
// you now have the option to do this for a speed boost
query.for_each_mut(|(a, mut b)| {
});
// however normal iterators are still available
for (a, mut b) in query.iter_mut() {
}
}
```
I think in most cases we should continue to encourage "normal" iterators as they are more flexible and more "rust idiomatic". But when that extra "oomf" is needed, it makes sense to use `for_each`.
We should also consider using `for_each` for internal bevy systems to give our users a nice speed boost (but that should be a separate pr).
## Component Metadata
`World` now has a `Components` collection, which is accessible via `world.components()`. This stores mappings from `ComponentId` to `ComponentInfo`, as well as `TypeId` to `ComponentId` mappings (where relevant). `ComponentInfo` stores information about the component, such as ComponentId, TypeId, memory layout, send-ness (currently limited to resources), and storage type.
## Significantly Cheaper `Access<T>`
We used to use `TypeAccess<TypeId>` to manage read/write component/archetype-component access. This was expensive because TypeIds must be hashed and compared individually. The parallel executor got around this by "condensing" type ids into bitset-backed access types. This worked, but it had to be re-generated from the `TypeAccess<TypeId>`sources every time archetypes changed.
This pr removes TypeAccess in favor of faster bitset access everywhere. We can do this thanks to the move to densely packed `ComponentId`s and `ArchetypeComponentId`s.
## Merged Resources into World
Resources had a lot of redundant functionality with Components. They stored typed data, they had access control, they had unique ids, they were queryable via SystemParams, etc. In fact the _only_ major difference between them was that they were unique (and didn't correlate to an entity).
Separate resources also had the downside of requiring a separate set of access controls, which meant the parallel executor needed to compare more bitsets per system and manage more state.
I initially got the "separate resources" idea from `legion`. I think that design was motivated by the fact that it made the direct world query/resource lifetime interactions more manageable. It certainly made our lives easier when using Resources alongside hecs/bevy_ecs. However we already have a construct for safely and ergonomically managing in-world lifetimes: systems (which use `Access<T>` internally).
This pr merges Resources into World:
```rust
world.insert_resource(1);
world.insert_resource(2.0);
let a = world.get_resource::<i32>().unwrap();
let mut b = world.get_resource_mut::<f64>().unwrap();
*b = 3.0;
```
Resources are now just a special kind of component. They have their own ComponentIds (and their own resource TypeId->ComponentId scope, so they don't conflict wit components of the same type). They are stored in a special "resource archetype", which stores components inside the archetype using a new `unique_components` sparse set (note that this sparse set could later be used to implement Tags). This allows us to keep the code size small by reusing existing datastructures (namely Column, Archetype, ComponentFlags, and ComponentInfo). This allows us the executor to use a single `Access<ArchetypeComponentId>` per system. It should also make scripting language integration easier.
_But_ this merge did create problems for people directly interacting with `World`. What if you need mutable access to multiple resources at the same time? `world.get_resource_mut()` borrows World mutably!
## WorldCell
WorldCell applies the `Access<ArchetypeComponentId>` concept to direct world access:
```rust
let world_cell = world.cell();
let a = world_cell.get_resource_mut::<i32>().unwrap();
let b = world_cell.get_resource_mut::<f64>().unwrap();
```
This adds cheap runtime checks (a sparse set lookup of `ArchetypeComponentId` and a counter) to ensure that world accesses do not conflict with each other. Each operation returns a `WorldBorrow<'w, T>` or `WorldBorrowMut<'w, T>` wrapper type, which will release the relevant ArchetypeComponentId resources when dropped.
World caches the access sparse set (and only one cell can exist at a time), so `world.cell()` is a cheap operation.
WorldCell does _not_ use atomic operations. It is non-send, does a mutable borrow of world to prevent other accesses, and uses a simple `Rc<RefCell<ArchetypeComponentAccess>>` wrapper in each WorldBorrow pointer.
The api is currently limited to resource access, but it can and should be extended to queries / entity component access.
## Resource Scopes
WorldCell does not yet support component queries, and even when it does there are sometimes legitimate reasons to want a mutable world ref _and_ a mutable resource ref (ex: bevy_render and bevy_scene both need this). In these cases we could always drop down to the unsafe `world.get_resource_unchecked_mut()`, but that is not ideal!
Instead developers can use a "resource scope"
```rust
world.resource_scope(|world: &mut World, a: &mut A| {
})
```
This temporarily removes the `A` resource from `World`, provides mutable pointers to both, and re-adds A to World when finished. Thanks to the move to ComponentIds/sparse sets, this is a cheap operation.
If multiple resources are required, scopes can be nested. We could also consider adding a "resource tuple" to the api if this pattern becomes common and the boilerplate gets nasty.
## Query Conflicts Use ComponentId Instead of ArchetypeComponentId
For safety reasons, systems cannot contain queries that conflict with each other without wrapping them in a QuerySet. On bevy `main`, we use ArchetypeComponentIds to determine conflicts. This is nice because it can take into account filters:
```rust
// these queries will never conflict due to their filters
fn filter_system(a: Query<&mut A, With<B>>, b: Query<&mut B, Without<B>>) {
}
```
But it also has a significant downside:
```rust
// these queries will not conflict _until_ an entity with A, B, and C is spawned
fn maybe_conflicts_system(a: Query<(&mut A, &C)>, b: Query<(&mut A, &B)>) {
}
```
The system above will panic at runtime if an entity with A, B, and C is spawned. This makes it hard to trust that your game logic will run without crashing.
In this pr, I switched to using `ComponentId` instead. This _is_ more constraining. `maybe_conflicts_system` will now always fail, but it will do it consistently at startup. Naively, it would also _disallow_ `filter_system`, which would be a significant downgrade in usability. Bevy has a number of internal systems that rely on disjoint queries and I expect it to be a common pattern in userspace.
To resolve this, I added a new `FilteredAccess<T>` type, which wraps `Access<T>` and adds with/without filters. If two `FilteredAccess` have with/without values that prove they are disjoint, they will no longer conflict.
## EntityRef / EntityMut
World entity operations on `main` require that the user passes in an `entity` id to each operation:
```rust
let entity = world.spawn((A, )); // create a new entity with A
world.get::<A>(entity);
world.insert(entity, (B, C));
world.insert_one(entity, D);
```
This means that each operation needs to look up the entity location / verify its validity. The initial spawn operation also requires a Bundle as input. This can be awkward when no components are required (or one component is required).
These operations have been replaced by `EntityRef` and `EntityMut`, which are "builder-style" wrappers around world that provide read and read/write operations on a single, pre-validated entity:
```rust
// spawn now takes no inputs and returns an EntityMut
let entity = world.spawn()
.insert(A) // insert a single component into the entity
.insert_bundle((B, C)) // insert a bundle of components into the entity
.id() // id returns the Entity id
// Returns EntityMut (or panics if the entity does not exist)
world.entity_mut(entity)
.insert(D)
.insert_bundle(SomeBundle::default());
{
// returns EntityRef (or panics if the entity does not exist)
let d = world.entity(entity)
.get::<D>() // gets the D component
.unwrap();
// world.get still exists for ergonomics
let d = world.get::<D>(entity).unwrap();
}
// These variants return Options if you want to check existence instead of panicing
world.get_entity_mut(entity)
.unwrap()
.insert(E);
if let Some(entity_ref) = world.get_entity(entity) {
let d = entity_ref.get::<D>().unwrap();
}
```
This _does not_ affect the current Commands api or terminology. I think that should be a separate conversation as that is a much larger breaking change.
## Safety Improvements
* Entity reservation in Commands uses a normal world borrow instead of an unsafe transmute
* QuerySets no longer transmutes lifetimes
* Made traits "unsafe" when implementing a trait incorrectly could cause unsafety
* More thorough safety docs
## RemovedComponents SystemParam
The old approach to querying removed components: `query.removed:<T>()` was confusing because it had no connection to the query itself. I replaced it with the following, which is both clearer and allows us to cache the ComponentId mapping in the SystemParamState:
```rust
fn system(removed: RemovedComponents<T>) {
for entity in removed.iter() {
}
}
```
## Simpler Bundle implementation
Bundles are no longer responsible for sorting (or deduping) TypeInfo. They are just a simple ordered list of component types / data. This makes the implementation smaller and opens the door to an easy "nested bundle" implementation in the future (which i might even add in this pr). Duplicate detection is now done once per bundle type by World the first time a bundle is used.
## Unified WorldQuery and QueryFilter types
(don't worry they are still separate type _parameters_ in Queries .. this is a non-breaking change)
WorldQuery and QueryFilter were already basically identical apis. With the addition of `FetchState` and more storage-specific fetch methods, the overlap was even clearer (and the redundancy more painful).
QueryFilters are now just `F: WorldQuery where F::Fetch: FilterFetch`. FilterFetch requires `Fetch<Item = bool>` and adds new "short circuit" variants of fetch methods. This enables a filter tuple like `(With<A>, Without<B>, Changed<C>)` to stop evaluating the filter after the first mismatch is encountered. FilterFetch is automatically implemented for `Fetch` implementations that return bool.
This forces fetch implementations that return things like `(bool, bool, bool)` (such as the filter above) to manually implement FilterFetch and decide whether or not to short-circuit.
## More Granular Modules
World no longer globs all of the internal modules together. It now exports `core`, `system`, and `schedule` separately. I'm also considering exporting `core` submodules directly as that is still pretty "glob-ey" and unorganized (feedback welcome here).
## Remaining Draft Work (to be done in this pr)
* ~~panic on conflicting WorldQuery fetches (&A, &mut A)~~
* ~~bevy `main` and hecs both currently allow this, but we should protect against it if possible~~
* ~~batch_iter / par_iter (currently stubbed out)~~
* ~~ChangedRes~~
* ~~I skipped this while we sort out #1313. This pr should be adapted to account for whatever we land on there~~.
* ~~The `Archetypes` and `Tables` collections use hashes of sorted lists of component ids to uniquely identify each archetype/table. This hash is then used as the key in a HashMap to look up the relevant ArchetypeId or TableId. (which doesn't handle hash collisions properly)~~
* ~~It is currently unsafe to generate a Query from "World A", then use it on "World B" (despite the api claiming it is safe). We should probably close this gap. This could be done by adding a randomly generated WorldId to each world, then storing that id in each Query. They could then be compared to each other on each `query.do_thing(&world)` operation. This _does_ add an extra branch to each query operation, so I'm open to other suggestions if people have them.~~
* ~~Nested Bundles (if i find time)~~
## Potential Future Work
* Expand WorldCell to support queries.
* Consider not allocating in the empty archetype on `world.spawn()`
* ex: return something like EntityMutUninit, which turns into EntityMut after an `insert` or `insert_bundle` op
* this actually regressed performance last time i tried it, but in theory it should be faster
* Optimize SparseSet::insert (see `PERF` comment on insert)
* Replace SparseArray `Option<T>` with T::MAX to cut down on branching
* would enable cheaper get_unchecked() operations
* upstream fixedbitset optimizations
* fixedbitset could be allocation free for small block counts (store blocks in a SmallVec)
* fixedbitset could have a const constructor
* Consider implementing Tags (archetype-specific by-value data that affects archetype identity)
* ex: ArchetypeA could have `[A, B, C]` table components and `[D(1)]` "tag" component. ArchetypeB could have `[A, B, C]` table components and a `[D(2)]` tag component. The archetypes are different, despite both having D tags because the value inside D is different.
* this could potentially build on top of the `archetype.unique_components` added in this pr for resource storage.
* Consider reverting `all_tuples` proc macro in favor of the old `macro_rules` implementation
* all_tuples is more flexible and produces cleaner documentation (the macro_rules version produces weird type parameter orders due to parser constraints)
* but unfortunately all_tuples also appears to make Rust Analyzer sad/slow when working inside of `bevy_ecs` (does not affect user code)
* Consider "resource queries" and/or "mixed resource and entity component queries" as an alternative to WorldCell
* this is basically just "systems" so maybe it's not worth it
* Add more world ops
* `world.clear()`
* `world.reserve<T: Bundle>(count: usize)`
* Try using the old archetype allocation strategy (allocate new memory on resize and copy everything over). I expect this to improve batch insertion performance at the cost of unbatched performance. But thats just a guess. I'm not an allocation perf pro :)
* Adapt Commands apis for consistency with new World apis
## Benchmarks
key:
* `bevy_old`: bevy `main` branch
* `bevy`: this branch
* `_foreach`: uses an optimized for_each iterator
* ` _sparse`: uses sparse set storage (if unspecified assume table storage)
* `_system`: runs inside a system (if unspecified assume test happens via direct world ops)
### Simple Insert (from ecs_bench_suite)
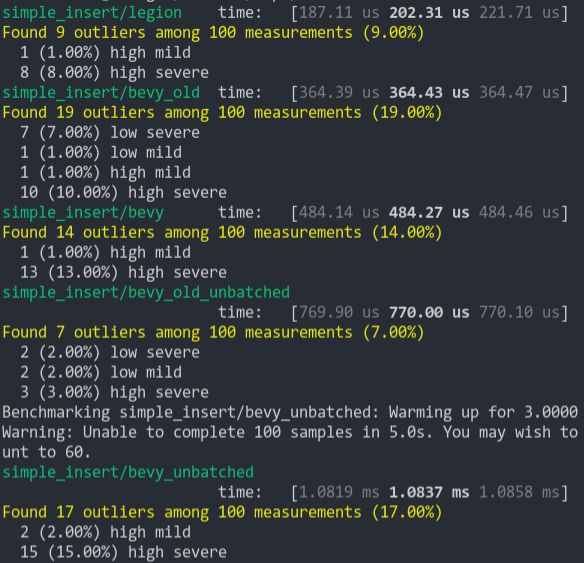
### Simpler Iter (from ecs_bench_suite)
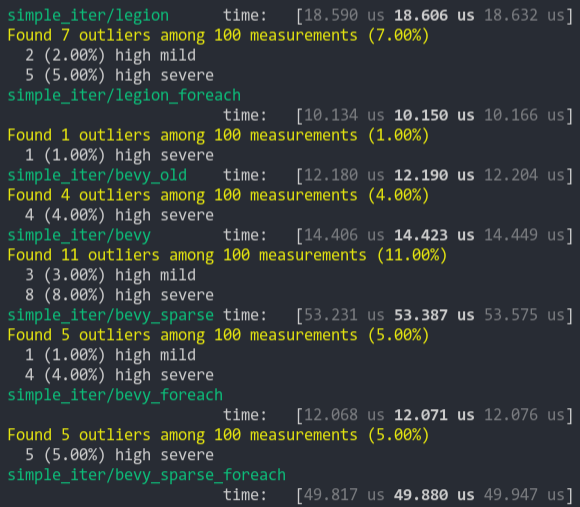
### Fragment Iter (from ecs_bench_suite)
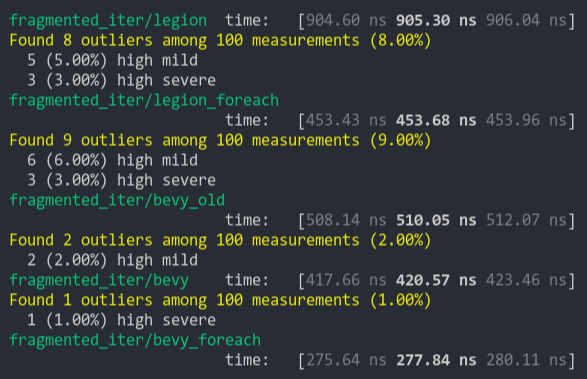
### Sparse Fragmented Iter
Iterate a query that matches 5 entities from a single matching archetype, but there are 100 unmatching archetypes
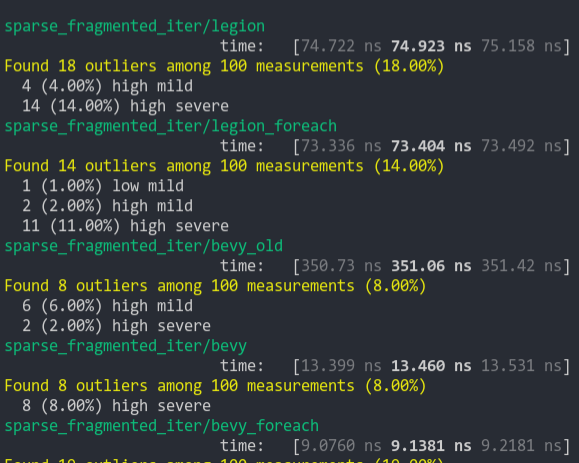
### Schedule (from ecs_bench_suite)
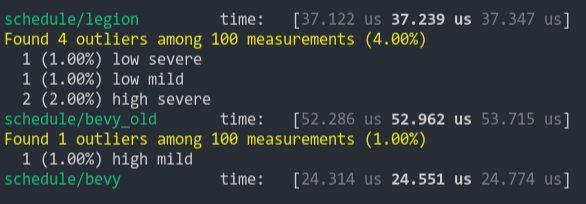
### Add Remove Component (from ecs_bench_suite)
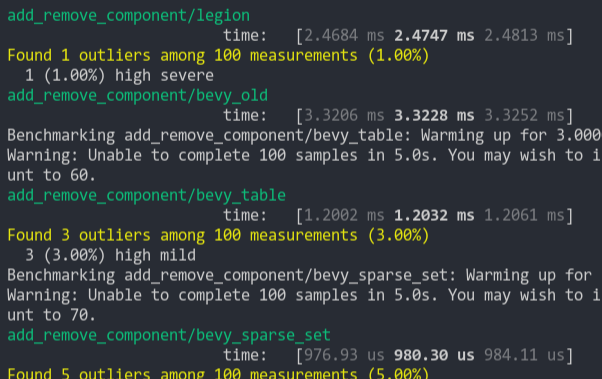
### Add Remove Component Big
Same as the test above, but each entity has 5 "large" matrix components and 1 "large" matrix component is added and removed
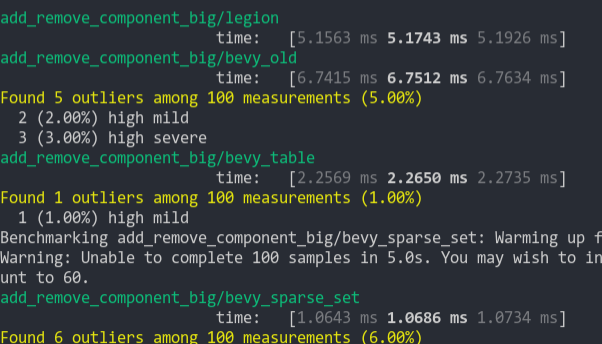
### Get Component
Looks up a single component value a large number of times
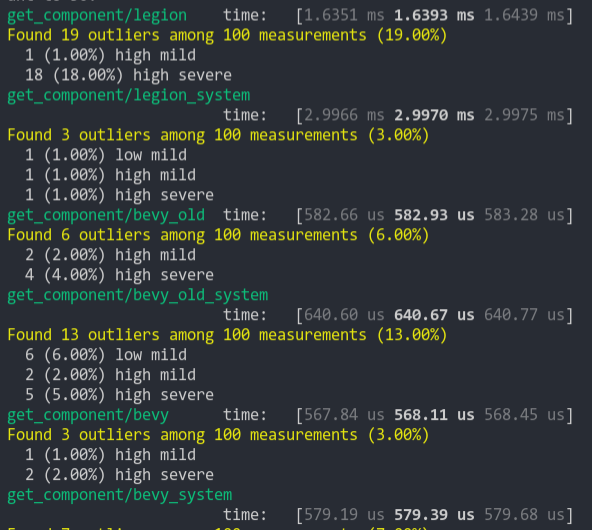
2021-03-05 07:54:35 +00:00
if combined_access . has_write ( component_id ) {
panic! (
2021-11-06 20:53:11 +00:00
" error[B0002]: ResMut<{}> in system {} conflicts with a previous ResMut<{0}> access. Consider removing the duplicate access. " ,
Add new SystemState and rename old SystemState to SystemMeta (#2283)
This enables `SystemParams` to be used outside of function systems. Anything can create and store `SystemState`, which enables efficient "param state cached" access to `SystemParams`.
It adds a `ReadOnlySystemParamFetch` trait, which enables safe `SystemState::get` calls without unique world access.
I renamed the old `SystemState` to `SystemMeta` to enable us to mirror the `QueryState` naming convention (but I'm happy to discuss alternative names if people have other ideas). I initially pitched this as `ParamState`, but given that it needs to include full system metadata, that doesn't feel like a particularly accurate name.
```rust
#[derive(Eq, PartialEq, Debug)]
struct A(usize);
#[derive(Eq, PartialEq, Debug)]
struct B(usize);
let mut world = World::default();
world.insert_resource(A(42));
world.spawn().insert(B(7));
// we get nice lifetime elision when declaring the type on the left hand side
let mut system_state: SystemState<(Res<A>, Query<&B>)> = SystemState::new(&mut world);
let (a, query) = system_state.get(&world);
assert_eq!(*a, A(42), "returned resource matches initial value");
assert_eq!(
*query.single().unwrap(),
B(7),
"returned component matches initial value"
);
// mutable system params require unique world access
let mut system_state: SystemState<(ResMut<A>, Query<&mut B>)> = SystemState::new(&mut world);
let (a, query) = system_state.get_mut(&mut world);
// static lifetimes are required when declaring inside of structs
struct SomeContainer {
state: SystemState<(Res<'static, A>, Res<'static, B>)>
}
// this can be shortened using type aliases, which will be useful for complex param tuples
type MyParams<'a> = (Res<'a, A>, Res<'a, B>);
struct SomeContainer {
state: SystemState<MyParams<'static>>
}
// It is the user's responsibility to call SystemState::apply(world) for parameters that queue up work
let mut system_state: SystemState<(Commands, Query<&B>)> = SystemState::new(&mut world);
{
let (mut commands, query) = system_state.get(&world);
commands.insert_resource(3.14);
}
system_state.apply(&mut world);
```
## Future Work
* Actually use SystemState inside FunctionSystem. This would be trivial, but it requires FunctionSystem to wrap SystemState in Option in its current form (which complicates system metadata lookup). I'd prefer to hold off until we adopt something like the later designs linked in #1364, which enable us to contruct Systems using a World reference (and also remove the need for `.system`).
* Consider a "scoped" approach to automatically call SystemState::apply when systems params are no longer being used (either a container type with a Drop impl, or a function that takes a closure for user logic operating on params).
2021-06-02 19:57:38 +00:00
std ::any ::type_name ::< T > ( ) , system_meta . name ) ;
Bevy ECS V2 (#1525)
# Bevy ECS V2
This is a rewrite of Bevy ECS (basically everything but the new executor/schedule, which are already awesome). The overall goal was to improve the performance and versatility of Bevy ECS. Here is a quick bulleted list of changes before we dive into the details:
* Complete World rewrite
* Multiple component storage types:
* Tables: fast cache friendly iteration, slower add/removes (previously called Archetypes)
* Sparse Sets: fast add/remove, slower iteration
* Stateful Queries (caches query results for faster iteration. fragmented iteration is _fast_ now)
* Stateful System Params (caches expensive operations. inspired by @DJMcNab's work in #1364)
* Configurable System Params (users can set configuration when they construct their systems. once again inspired by @DJMcNab's work)
* Archetypes are now "just metadata", component storage is separate
* Archetype Graph (for faster archetype changes)
* Component Metadata
* Configure component storage type
* Retrieve information about component size/type/name/layout/send-ness/etc
* Components are uniquely identified by a densely packed ComponentId
* TypeIds are now totally optional (which should make implementing scripting easier)
* Super fast "for_each" query iterators
* Merged Resources into World. Resources are now just a special type of component
* EntityRef/EntityMut builder apis (more efficient and more ergonomic)
* Fast bitset-backed `Access<T>` replaces old hashmap-based approach everywhere
* Query conflicts are determined by component access instead of archetype component access (to avoid random failures at runtime)
* With/Without are still taken into account for conflicts, so this should still be comfy to use
* Much simpler `IntoSystem` impl
* Significantly reduced the amount of hashing throughout the ecs in favor of Sparse Sets (indexed by densely packed ArchetypeId, ComponentId, BundleId, and TableId)
* Safety Improvements
* Entity reservation uses a normal world reference instead of unsafe transmute
* QuerySets no longer transmute lifetimes
* Made traits "unsafe" where relevant
* More thorough safety docs
* WorldCell
* Exposes safe mutable access to multiple resources at a time in a World
* Replaced "catch all" `System::update_archetypes(world: &World)` with `System::new_archetype(archetype: &Archetype)`
* Simpler Bundle implementation
* Replaced slow "remove_bundle_one_by_one" used as fallback for Commands::remove_bundle with fast "remove_bundle_intersection"
* Removed `Mut<T>` query impl. it is better to only support one way: `&mut T`
* Removed with() from `Flags<T>` in favor of `Option<Flags<T>>`, which allows querying for flags to be "filtered" by default
* Components now have is_send property (currently only resources support non-send)
* More granular module organization
* New `RemovedComponents<T>` SystemParam that replaces `query.removed::<T>()`
* `world.resource_scope()` for mutable access to resources and world at the same time
* WorldQuery and QueryFilter traits unified. FilterFetch trait added to enable "short circuit" filtering. Auto impled for cases that don't need it
* Significantly slimmed down SystemState in favor of individual SystemParam state
* System Commands changed from `commands: &mut Commands` back to `mut commands: Commands` (to allow Commands to have a World reference)
Fixes #1320
## `World` Rewrite
This is a from-scratch rewrite of `World` that fills the niche that `hecs` used to. Yes, this means Bevy ECS is no longer a "fork" of hecs. We're going out our own!
(the only shared code between the projects is the entity id allocator, which is already basically ideal)
A huge shout out to @SanderMertens (author of [flecs](https://github.com/SanderMertens/flecs)) for sharing some great ideas with me (specifically hybrid ecs storage and archetype graphs). He also helped advise on a number of implementation details.
## Component Storage (The Problem)
Two ECS storage paradigms have gained a lot of traction over the years:
* **Archetypal ECS**:
* Stores components in "tables" with static schemas. Each "column" stores components of a given type. Each "row" is an entity.
* Each "archetype" has its own table. Adding/removing an entity's component changes the archetype.
* Enables super-fast Query iteration due to its cache-friendly data layout
* Comes at the cost of more expensive add/remove operations for an Entity's components, because all components need to be copied to the new archetype's "table"
* **Sparse Set ECS**:
* Stores components of the same type in densely packed arrays, which are sparsely indexed by densely packed unsigned integers (Entity ids)
* Query iteration is slower than Archetypal ECS because each entity's component could be at any position in the sparse set. This "random access" pattern isn't cache friendly. Additionally, there is an extra layer of indirection because you must first map the entity id to an index in the component array.
* Adding/removing components is a cheap, constant time operation
Bevy ECS V1, hecs, legion, flec, and Unity DOTS are all "archetypal ecs-es". I personally think "archetypal" storage is a good default for game engines. An entity's archetype doesn't need to change frequently in general, and it creates "fast by default" query iteration (which is a much more common operation). It is also "self optimizing". Users don't need to think about optimizing component layouts for iteration performance. It "just works" without any extra boilerplate.
Shipyard and EnTT are "sparse set ecs-es". They employ "packing" as a way to work around the "suboptimal by default" iteration performance for specific sets of components. This helps, but I didn't think this was a good choice for a general purpose engine like Bevy because:
1. "packs" conflict with each other. If bevy decides to internally pack the Transform and GlobalTransform components, users are then blocked if they want to pack some custom component with Transform.
2. users need to take manual action to optimize
Developers selecting an ECS framework are stuck with a hard choice. Select an "archetypal" framework with "fast iteration everywhere" but without the ability to cheaply add/remove components, or select a "sparse set" framework to cheaply add/remove components but with slower iteration performance.
## Hybrid Component Storage (The Solution)
In Bevy ECS V2, we get to have our cake and eat it too. It now has _both_ of the component storage types above (and more can be added later if needed):
* **Tables** (aka "archetypal" storage)
* The default storage. If you don't configure anything, this is what you get
* Fast iteration by default
* Slower add/remove operations
* **Sparse Sets**
* Opt-in
* Slower iteration
* Faster add/remove operations
These storage types complement each other perfectly. By default Query iteration is fast. If developers know that they want to add/remove a component at high frequencies, they can set the storage to "sparse set":
```rust
world.register_component(
ComponentDescriptor::new::<MyComponent>(StorageType::SparseSet)
).unwrap();
```
## Archetypes
Archetypes are now "just metadata" ... they no longer store components directly. They do store:
* The `ComponentId`s of each of the Archetype's components (and that component's storage type)
* Archetypes are uniquely defined by their component layouts
* For example: entities with "table" components `[A, B, C]` _and_ "sparse set" components `[D, E]` will always be in the same archetype.
* The `TableId` associated with the archetype
* For now each archetype has exactly one table (which can have no components),
* There is a 1->Many relationship from Tables->Archetypes. A given table could have any number of archetype components stored in it:
* Ex: an entity with "table storage" components `[A, B, C]` and "sparse set" components `[D, E]` will share the same `[A, B, C]` table as an entity with `[A, B, C]` table component and `[F]` sparse set components.
* This 1->Many relationship is how we preserve fast "cache friendly" iteration performance when possible (more on this later)
* A list of entities that are in the archetype and the row id of the table they are in
* ArchetypeComponentIds
* unique densely packed identifiers for (ArchetypeId, ComponentId) pairs
* used by the schedule executor for cheap system access control
* "Archetype Graph Edges" (see the next section)
## The "Archetype Graph"
Archetype changes in Bevy (and a number of other archetypal ecs-es) have historically been expensive to compute. First, you need to allocate a new vector of the entity's current component ids, add or remove components based on the operation performed, sort it (to ensure it is order-independent), then hash it to find the archetype (if it exists). And thats all before we get to the _already_ expensive full copy of all components to the new table storage.
The solution is to build a "graph" of archetypes to cache these results. @SanderMertens first exposed me to the idea (and he got it from @gjroelofs, who came up with it). They propose adding directed edges between archetypes for add/remove component operations. If `ComponentId`s are densely packed, you can use sparse sets to cheaply jump between archetypes.
Bevy takes this one step further by using add/remove `Bundle` edges instead of `Component` edges. Bevy encourages the use of `Bundles` to group add/remove operations. This is largely for "clearer game logic" reasons, but it also helps cut down on the number of archetype changes required. `Bundles` now also have densely-packed `BundleId`s. This allows us to use a _single_ edge for each bundle operation (rather than needing to traverse N edges ... one for each component). Single component operations are also bundles, so this is strictly an improvement over a "component only" graph.
As a result, an operation that used to be _heavy_ (both for allocations and compute) is now two dirt-cheap array lookups and zero allocations.
## Stateful Queries
World queries are now stateful. This allows us to:
1. Cache archetype (and table) matches
* This resolves another issue with (naive) archetypal ECS: query performance getting worse as the number of archetypes goes up (and fragmentation occurs).
2. Cache Fetch and Filter state
* The expensive parts of fetch/filter operations (such as hashing the TypeId to find the ComponentId) now only happen once when the Query is first constructed
3. Incrementally build up state
* When new archetypes are added, we only process the new archetypes (no need to rebuild state for old archetypes)
As a result, the direct `World` query api now looks like this:
```rust
let mut query = world.query::<(&A, &mut B)>();
for (a, mut b) in query.iter_mut(&mut world) {
}
```
Requiring `World` to generate stateful queries (rather than letting the `QueryState` type be constructed separately) allows us to ensure that _all_ queries are properly initialized (and the relevant world state, such as ComponentIds). This enables QueryState to remove branches from its operations that check for initialization status (and also enables query.iter() to take an immutable world reference because it doesn't need to initialize anything in world).
However in systems, this is a non-breaking change. State management is done internally by the relevant SystemParam.
## Stateful SystemParams
Like Queries, `SystemParams` now also cache state. For example, `Query` system params store the "stateful query" state mentioned above. Commands store their internal `CommandQueue`. This means you can now safely use as many separate `Commands` parameters in your system as you want. `Local<T>` system params store their `T` value in their state (instead of in Resources).
SystemParam state also enabled a significant slim-down of SystemState. It is much nicer to look at now.
Per-SystemParam state naturally insulates us from an "aliased mut" class of errors we have hit in the past (ex: using multiple `Commands` system params).
(credit goes to @DJMcNab for the initial idea and draft pr here #1364)
## Configurable SystemParams
@DJMcNab also had the great idea to make SystemParams configurable. This allows users to provide some initial configuration / values for system parameters (when possible). Most SystemParams have no config (the config type is `()`), but the `Local<T>` param now supports user-provided parameters:
```rust
fn foo(value: Local<usize>) {
}
app.add_system(foo.system().config(|c| c.0 = Some(10)));
```
## Uber Fast "for_each" Query Iterators
Developers now have the choice to use a fast "for_each" iterator, which yields ~1.5-3x iteration speed improvements for "fragmented iteration", and minor ~1.2x iteration speed improvements for unfragmented iteration.
```rust
fn system(query: Query<(&A, &mut B)>) {
// you now have the option to do this for a speed boost
query.for_each_mut(|(a, mut b)| {
});
// however normal iterators are still available
for (a, mut b) in query.iter_mut() {
}
}
```
I think in most cases we should continue to encourage "normal" iterators as they are more flexible and more "rust idiomatic". But when that extra "oomf" is needed, it makes sense to use `for_each`.
We should also consider using `for_each` for internal bevy systems to give our users a nice speed boost (but that should be a separate pr).
## Component Metadata
`World` now has a `Components` collection, which is accessible via `world.components()`. This stores mappings from `ComponentId` to `ComponentInfo`, as well as `TypeId` to `ComponentId` mappings (where relevant). `ComponentInfo` stores information about the component, such as ComponentId, TypeId, memory layout, send-ness (currently limited to resources), and storage type.
## Significantly Cheaper `Access<T>`
We used to use `TypeAccess<TypeId>` to manage read/write component/archetype-component access. This was expensive because TypeIds must be hashed and compared individually. The parallel executor got around this by "condensing" type ids into bitset-backed access types. This worked, but it had to be re-generated from the `TypeAccess<TypeId>`sources every time archetypes changed.
This pr removes TypeAccess in favor of faster bitset access everywhere. We can do this thanks to the move to densely packed `ComponentId`s and `ArchetypeComponentId`s.
## Merged Resources into World
Resources had a lot of redundant functionality with Components. They stored typed data, they had access control, they had unique ids, they were queryable via SystemParams, etc. In fact the _only_ major difference between them was that they were unique (and didn't correlate to an entity).
Separate resources also had the downside of requiring a separate set of access controls, which meant the parallel executor needed to compare more bitsets per system and manage more state.
I initially got the "separate resources" idea from `legion`. I think that design was motivated by the fact that it made the direct world query/resource lifetime interactions more manageable. It certainly made our lives easier when using Resources alongside hecs/bevy_ecs. However we already have a construct for safely and ergonomically managing in-world lifetimes: systems (which use `Access<T>` internally).
This pr merges Resources into World:
```rust
world.insert_resource(1);
world.insert_resource(2.0);
let a = world.get_resource::<i32>().unwrap();
let mut b = world.get_resource_mut::<f64>().unwrap();
*b = 3.0;
```
Resources are now just a special kind of component. They have their own ComponentIds (and their own resource TypeId->ComponentId scope, so they don't conflict wit components of the same type). They are stored in a special "resource archetype", which stores components inside the archetype using a new `unique_components` sparse set (note that this sparse set could later be used to implement Tags). This allows us to keep the code size small by reusing existing datastructures (namely Column, Archetype, ComponentFlags, and ComponentInfo). This allows us the executor to use a single `Access<ArchetypeComponentId>` per system. It should also make scripting language integration easier.
_But_ this merge did create problems for people directly interacting with `World`. What if you need mutable access to multiple resources at the same time? `world.get_resource_mut()` borrows World mutably!
## WorldCell
WorldCell applies the `Access<ArchetypeComponentId>` concept to direct world access:
```rust
let world_cell = world.cell();
let a = world_cell.get_resource_mut::<i32>().unwrap();
let b = world_cell.get_resource_mut::<f64>().unwrap();
```
This adds cheap runtime checks (a sparse set lookup of `ArchetypeComponentId` and a counter) to ensure that world accesses do not conflict with each other. Each operation returns a `WorldBorrow<'w, T>` or `WorldBorrowMut<'w, T>` wrapper type, which will release the relevant ArchetypeComponentId resources when dropped.
World caches the access sparse set (and only one cell can exist at a time), so `world.cell()` is a cheap operation.
WorldCell does _not_ use atomic operations. It is non-send, does a mutable borrow of world to prevent other accesses, and uses a simple `Rc<RefCell<ArchetypeComponentAccess>>` wrapper in each WorldBorrow pointer.
The api is currently limited to resource access, but it can and should be extended to queries / entity component access.
## Resource Scopes
WorldCell does not yet support component queries, and even when it does there are sometimes legitimate reasons to want a mutable world ref _and_ a mutable resource ref (ex: bevy_render and bevy_scene both need this). In these cases we could always drop down to the unsafe `world.get_resource_unchecked_mut()`, but that is not ideal!
Instead developers can use a "resource scope"
```rust
world.resource_scope(|world: &mut World, a: &mut A| {
})
```
This temporarily removes the `A` resource from `World`, provides mutable pointers to both, and re-adds A to World when finished. Thanks to the move to ComponentIds/sparse sets, this is a cheap operation.
If multiple resources are required, scopes can be nested. We could also consider adding a "resource tuple" to the api if this pattern becomes common and the boilerplate gets nasty.
## Query Conflicts Use ComponentId Instead of ArchetypeComponentId
For safety reasons, systems cannot contain queries that conflict with each other without wrapping them in a QuerySet. On bevy `main`, we use ArchetypeComponentIds to determine conflicts. This is nice because it can take into account filters:
```rust
// these queries will never conflict due to their filters
fn filter_system(a: Query<&mut A, With<B>>, b: Query<&mut B, Without<B>>) {
}
```
But it also has a significant downside:
```rust
// these queries will not conflict _until_ an entity with A, B, and C is spawned
fn maybe_conflicts_system(a: Query<(&mut A, &C)>, b: Query<(&mut A, &B)>) {
}
```
The system above will panic at runtime if an entity with A, B, and C is spawned. This makes it hard to trust that your game logic will run without crashing.
In this pr, I switched to using `ComponentId` instead. This _is_ more constraining. `maybe_conflicts_system` will now always fail, but it will do it consistently at startup. Naively, it would also _disallow_ `filter_system`, which would be a significant downgrade in usability. Bevy has a number of internal systems that rely on disjoint queries and I expect it to be a common pattern in userspace.
To resolve this, I added a new `FilteredAccess<T>` type, which wraps `Access<T>` and adds with/without filters. If two `FilteredAccess` have with/without values that prove they are disjoint, they will no longer conflict.
## EntityRef / EntityMut
World entity operations on `main` require that the user passes in an `entity` id to each operation:
```rust
let entity = world.spawn((A, )); // create a new entity with A
world.get::<A>(entity);
world.insert(entity, (B, C));
world.insert_one(entity, D);
```
This means that each operation needs to look up the entity location / verify its validity. The initial spawn operation also requires a Bundle as input. This can be awkward when no components are required (or one component is required).
These operations have been replaced by `EntityRef` and `EntityMut`, which are "builder-style" wrappers around world that provide read and read/write operations on a single, pre-validated entity:
```rust
// spawn now takes no inputs and returns an EntityMut
let entity = world.spawn()
.insert(A) // insert a single component into the entity
.insert_bundle((B, C)) // insert a bundle of components into the entity
.id() // id returns the Entity id
// Returns EntityMut (or panics if the entity does not exist)
world.entity_mut(entity)
.insert(D)
.insert_bundle(SomeBundle::default());
{
// returns EntityRef (or panics if the entity does not exist)
let d = world.entity(entity)
.get::<D>() // gets the D component
.unwrap();
// world.get still exists for ergonomics
let d = world.get::<D>(entity).unwrap();
}
// These variants return Options if you want to check existence instead of panicing
world.get_entity_mut(entity)
.unwrap()
.insert(E);
if let Some(entity_ref) = world.get_entity(entity) {
let d = entity_ref.get::<D>().unwrap();
}
```
This _does not_ affect the current Commands api or terminology. I think that should be a separate conversation as that is a much larger breaking change.
## Safety Improvements
* Entity reservation in Commands uses a normal world borrow instead of an unsafe transmute
* QuerySets no longer transmutes lifetimes
* Made traits "unsafe" when implementing a trait incorrectly could cause unsafety
* More thorough safety docs
## RemovedComponents SystemParam
The old approach to querying removed components: `query.removed:<T>()` was confusing because it had no connection to the query itself. I replaced it with the following, which is both clearer and allows us to cache the ComponentId mapping in the SystemParamState:
```rust
fn system(removed: RemovedComponents<T>) {
for entity in removed.iter() {
}
}
```
## Simpler Bundle implementation
Bundles are no longer responsible for sorting (or deduping) TypeInfo. They are just a simple ordered list of component types / data. This makes the implementation smaller and opens the door to an easy "nested bundle" implementation in the future (which i might even add in this pr). Duplicate detection is now done once per bundle type by World the first time a bundle is used.
## Unified WorldQuery and QueryFilter types
(don't worry they are still separate type _parameters_ in Queries .. this is a non-breaking change)
WorldQuery and QueryFilter were already basically identical apis. With the addition of `FetchState` and more storage-specific fetch methods, the overlap was even clearer (and the redundancy more painful).
QueryFilters are now just `F: WorldQuery where F::Fetch: FilterFetch`. FilterFetch requires `Fetch<Item = bool>` and adds new "short circuit" variants of fetch methods. This enables a filter tuple like `(With<A>, Without<B>, Changed<C>)` to stop evaluating the filter after the first mismatch is encountered. FilterFetch is automatically implemented for `Fetch` implementations that return bool.
This forces fetch implementations that return things like `(bool, bool, bool)` (such as the filter above) to manually implement FilterFetch and decide whether or not to short-circuit.
## More Granular Modules
World no longer globs all of the internal modules together. It now exports `core`, `system`, and `schedule` separately. I'm also considering exporting `core` submodules directly as that is still pretty "glob-ey" and unorganized (feedback welcome here).
## Remaining Draft Work (to be done in this pr)
* ~~panic on conflicting WorldQuery fetches (&A, &mut A)~~
* ~~bevy `main` and hecs both currently allow this, but we should protect against it if possible~~
* ~~batch_iter / par_iter (currently stubbed out)~~
* ~~ChangedRes~~
* ~~I skipped this while we sort out #1313. This pr should be adapted to account for whatever we land on there~~.
* ~~The `Archetypes` and `Tables` collections use hashes of sorted lists of component ids to uniquely identify each archetype/table. This hash is then used as the key in a HashMap to look up the relevant ArchetypeId or TableId. (which doesn't handle hash collisions properly)~~
* ~~It is currently unsafe to generate a Query from "World A", then use it on "World B" (despite the api claiming it is safe). We should probably close this gap. This could be done by adding a randomly generated WorldId to each world, then storing that id in each Query. They could then be compared to each other on each `query.do_thing(&world)` operation. This _does_ add an extra branch to each query operation, so I'm open to other suggestions if people have them.~~
* ~~Nested Bundles (if i find time)~~
## Potential Future Work
* Expand WorldCell to support queries.
* Consider not allocating in the empty archetype on `world.spawn()`
* ex: return something like EntityMutUninit, which turns into EntityMut after an `insert` or `insert_bundle` op
* this actually regressed performance last time i tried it, but in theory it should be faster
* Optimize SparseSet::insert (see `PERF` comment on insert)
* Replace SparseArray `Option<T>` with T::MAX to cut down on branching
* would enable cheaper get_unchecked() operations
* upstream fixedbitset optimizations
* fixedbitset could be allocation free for small block counts (store blocks in a SmallVec)
* fixedbitset could have a const constructor
* Consider implementing Tags (archetype-specific by-value data that affects archetype identity)
* ex: ArchetypeA could have `[A, B, C]` table components and `[D(1)]` "tag" component. ArchetypeB could have `[A, B, C]` table components and a `[D(2)]` tag component. The archetypes are different, despite both having D tags because the value inside D is different.
* this could potentially build on top of the `archetype.unique_components` added in this pr for resource storage.
* Consider reverting `all_tuples` proc macro in favor of the old `macro_rules` implementation
* all_tuples is more flexible and produces cleaner documentation (the macro_rules version produces weird type parameter orders due to parser constraints)
* but unfortunately all_tuples also appears to make Rust Analyzer sad/slow when working inside of `bevy_ecs` (does not affect user code)
* Consider "resource queries" and/or "mixed resource and entity component queries" as an alternative to WorldCell
* this is basically just "systems" so maybe it's not worth it
* Add more world ops
* `world.clear()`
* `world.reserve<T: Bundle>(count: usize)`
* Try using the old archetype allocation strategy (allocate new memory on resize and copy everything over). I expect this to improve batch insertion performance at the cost of unbatched performance. But thats just a guess. I'm not an allocation perf pro :)
* Adapt Commands apis for consistency with new World apis
## Benchmarks
key:
* `bevy_old`: bevy `main` branch
* `bevy`: this branch
* `_foreach`: uses an optimized for_each iterator
* ` _sparse`: uses sparse set storage (if unspecified assume table storage)
* `_system`: runs inside a system (if unspecified assume test happens via direct world ops)
### Simple Insert (from ecs_bench_suite)
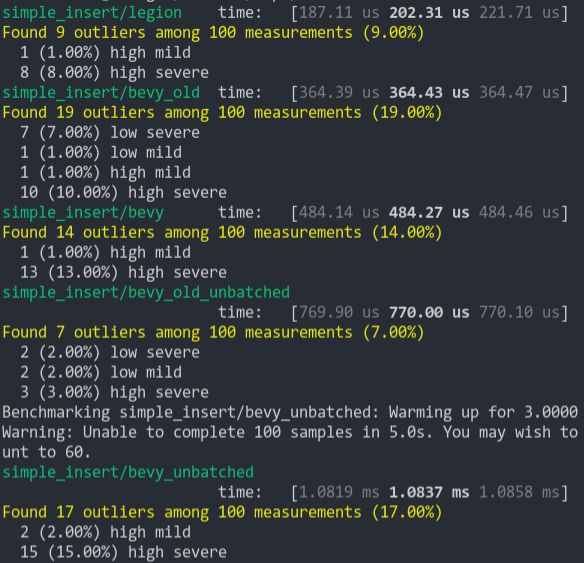
### Simpler Iter (from ecs_bench_suite)
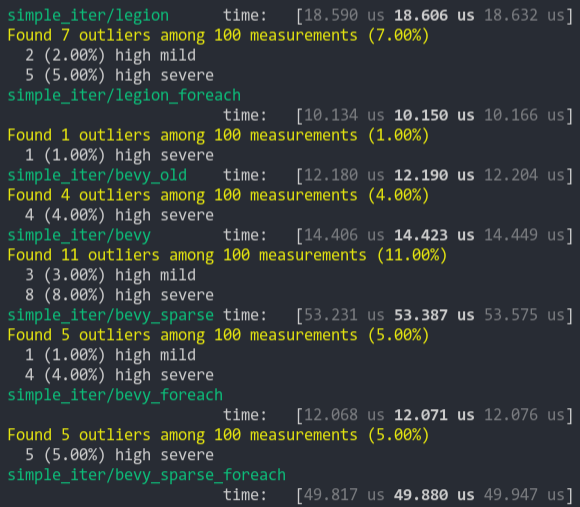
### Fragment Iter (from ecs_bench_suite)
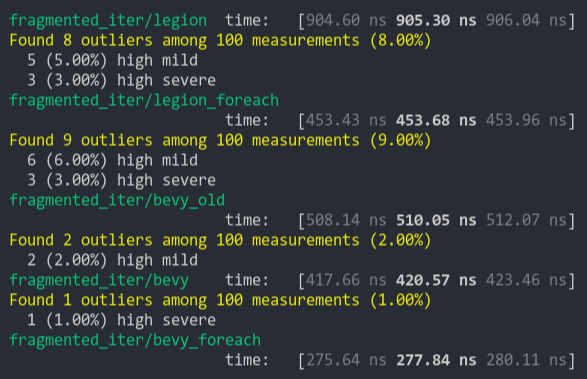
### Sparse Fragmented Iter
Iterate a query that matches 5 entities from a single matching archetype, but there are 100 unmatching archetypes
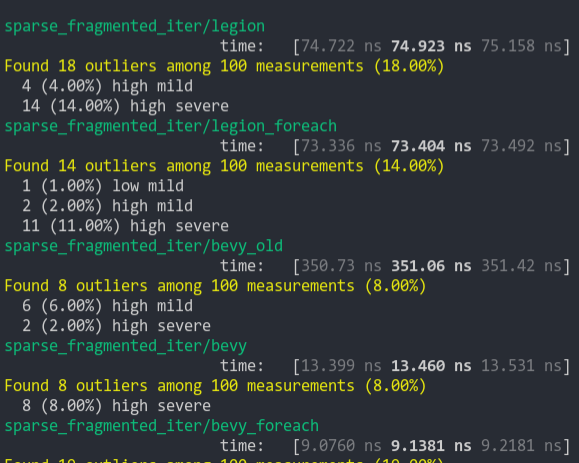
### Schedule (from ecs_bench_suite)
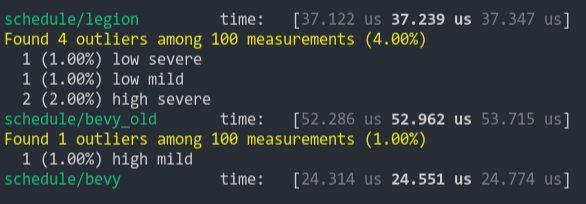
### Add Remove Component (from ecs_bench_suite)
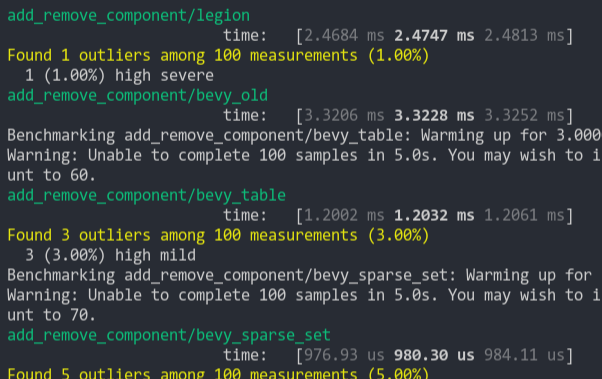
### Add Remove Component Big
Same as the test above, but each entity has 5 "large" matrix components and 1 "large" matrix component is added and removed
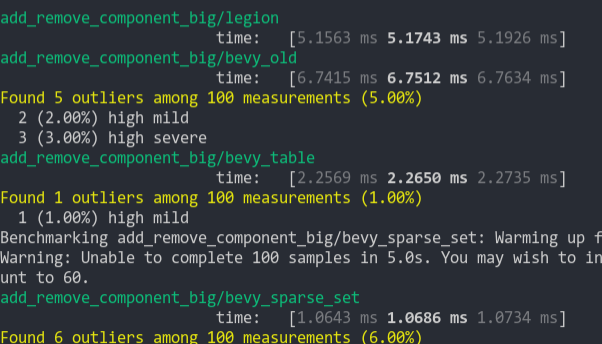
### Get Component
Looks up a single component value a large number of times
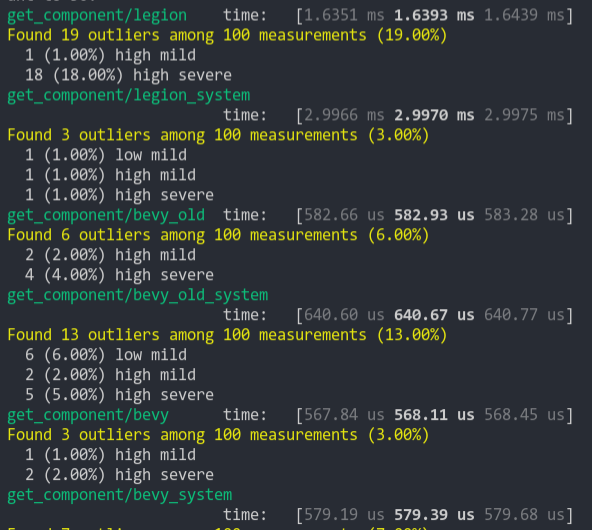
2021-03-05 07:54:35 +00:00
} else if combined_access . has_read ( component_id ) {
panic! (
2021-11-06 20:53:11 +00:00
" error[B0002]: ResMut<{}> in system {} conflicts with a previous Res<{0}> access. Consider removing the duplicate access. " ,
Add new SystemState and rename old SystemState to SystemMeta (#2283)
This enables `SystemParams` to be used outside of function systems. Anything can create and store `SystemState`, which enables efficient "param state cached" access to `SystemParams`.
It adds a `ReadOnlySystemParamFetch` trait, which enables safe `SystemState::get` calls without unique world access.
I renamed the old `SystemState` to `SystemMeta` to enable us to mirror the `QueryState` naming convention (but I'm happy to discuss alternative names if people have other ideas). I initially pitched this as `ParamState`, but given that it needs to include full system metadata, that doesn't feel like a particularly accurate name.
```rust
#[derive(Eq, PartialEq, Debug)]
struct A(usize);
#[derive(Eq, PartialEq, Debug)]
struct B(usize);
let mut world = World::default();
world.insert_resource(A(42));
world.spawn().insert(B(7));
// we get nice lifetime elision when declaring the type on the left hand side
let mut system_state: SystemState<(Res<A>, Query<&B>)> = SystemState::new(&mut world);
let (a, query) = system_state.get(&world);
assert_eq!(*a, A(42), "returned resource matches initial value");
assert_eq!(
*query.single().unwrap(),
B(7),
"returned component matches initial value"
);
// mutable system params require unique world access
let mut system_state: SystemState<(ResMut<A>, Query<&mut B>)> = SystemState::new(&mut world);
let (a, query) = system_state.get_mut(&mut world);
// static lifetimes are required when declaring inside of structs
struct SomeContainer {
state: SystemState<(Res<'static, A>, Res<'static, B>)>
}
// this can be shortened using type aliases, which will be useful for complex param tuples
type MyParams<'a> = (Res<'a, A>, Res<'a, B>);
struct SomeContainer {
state: SystemState<MyParams<'static>>
}
// It is the user's responsibility to call SystemState::apply(world) for parameters that queue up work
let mut system_state: SystemState<(Commands, Query<&B>)> = SystemState::new(&mut world);
{
let (mut commands, query) = system_state.get(&world);
commands.insert_resource(3.14);
}
system_state.apply(&mut world);
```
## Future Work
* Actually use SystemState inside FunctionSystem. This would be trivial, but it requires FunctionSystem to wrap SystemState in Option in its current form (which complicates system metadata lookup). I'd prefer to hold off until we adopt something like the later designs linked in #1364, which enable us to contruct Systems using a World reference (and also remove the need for `.system`).
* Consider a "scoped" approach to automatically call SystemState::apply when systems params are no longer being used (either a container type with a Drop impl, or a function that takes a closure for user logic operating on params).
2021-06-02 19:57:38 +00:00
std ::any ::type_name ::< T > ( ) , system_meta . name ) ;
2021-02-09 20:14:10 +00:00
}
Fix FilteredAccessSet get_conflicts inconsistency (#5105)
# Objective
* Enable `Res` and `Query` parameter mutual exclusion
* Required for https://github.com/bevyengine/bevy/pull/5080
The `FilteredAccessSet::get_conflicts` methods didn't work properly with
`Res` and `ResMut` parameters. Because those added their access by using
the `combined_access_mut` method and directly modifying the global
access state of the FilteredAccessSet. This caused an inconsistency,
because get_conflicts assumes that ALL added access have a corresponding
`FilteredAccess` added to the `filtered_accesses` field.
In practice, that means that SystemParam that adds their access through
the `Access` returned by `combined_access_mut` and the ones that add
their access using the `add` method lived in two different universes. As
a result, they could never be mutually exclusive.
## Solution
This commit fixes it by removing the `combined_access_mut` method. This
ensures that the `combined_access` field of FilteredAccessSet is always
updated consistently with the addition of a filter. When checking for
filtered access, it is now possible to account for `Res` and `ResMut`
invalid access. This is currently not needed, but might be in the
future.
We add the `add_unfiltered_{read,write}` methods to replace previous
usages of `combined_access_mut`.
We also add improved Debug implementations on FixedBitSet so that their
meaning is much clearer in debug output.
---
## Changelog
* Fix `Res` and `Query` parameter never being mutually exclusive.
## Migration Guide
Note: this mostly changes ECS internals, but since the API is public, it is technically breaking:
* Removed `FilteredAccessSet::combined_access_mut`
* Replace _immutable_ usage of those by `combined_access`
* For _mutable_ usages, use the new `add_unfiltered_{read,write}` methods instead of `combined_access_mut` followed by `add_{read,write}`
2022-11-16 11:05:48 +00:00
system_meta
. component_access_set
. add_unfiltered_write ( component_id ) ;
Bevy ECS V2 (#1525)
# Bevy ECS V2
This is a rewrite of Bevy ECS (basically everything but the new executor/schedule, which are already awesome). The overall goal was to improve the performance and versatility of Bevy ECS. Here is a quick bulleted list of changes before we dive into the details:
* Complete World rewrite
* Multiple component storage types:
* Tables: fast cache friendly iteration, slower add/removes (previously called Archetypes)
* Sparse Sets: fast add/remove, slower iteration
* Stateful Queries (caches query results for faster iteration. fragmented iteration is _fast_ now)
* Stateful System Params (caches expensive operations. inspired by @DJMcNab's work in #1364)
* Configurable System Params (users can set configuration when they construct their systems. once again inspired by @DJMcNab's work)
* Archetypes are now "just metadata", component storage is separate
* Archetype Graph (for faster archetype changes)
* Component Metadata
* Configure component storage type
* Retrieve information about component size/type/name/layout/send-ness/etc
* Components are uniquely identified by a densely packed ComponentId
* TypeIds are now totally optional (which should make implementing scripting easier)
* Super fast "for_each" query iterators
* Merged Resources into World. Resources are now just a special type of component
* EntityRef/EntityMut builder apis (more efficient and more ergonomic)
* Fast bitset-backed `Access<T>` replaces old hashmap-based approach everywhere
* Query conflicts are determined by component access instead of archetype component access (to avoid random failures at runtime)
* With/Without are still taken into account for conflicts, so this should still be comfy to use
* Much simpler `IntoSystem` impl
* Significantly reduced the amount of hashing throughout the ecs in favor of Sparse Sets (indexed by densely packed ArchetypeId, ComponentId, BundleId, and TableId)
* Safety Improvements
* Entity reservation uses a normal world reference instead of unsafe transmute
* QuerySets no longer transmute lifetimes
* Made traits "unsafe" where relevant
* More thorough safety docs
* WorldCell
* Exposes safe mutable access to multiple resources at a time in a World
* Replaced "catch all" `System::update_archetypes(world: &World)` with `System::new_archetype(archetype: &Archetype)`
* Simpler Bundle implementation
* Replaced slow "remove_bundle_one_by_one" used as fallback for Commands::remove_bundle with fast "remove_bundle_intersection"
* Removed `Mut<T>` query impl. it is better to only support one way: `&mut T`
* Removed with() from `Flags<T>` in favor of `Option<Flags<T>>`, which allows querying for flags to be "filtered" by default
* Components now have is_send property (currently only resources support non-send)
* More granular module organization
* New `RemovedComponents<T>` SystemParam that replaces `query.removed::<T>()`
* `world.resource_scope()` for mutable access to resources and world at the same time
* WorldQuery and QueryFilter traits unified. FilterFetch trait added to enable "short circuit" filtering. Auto impled for cases that don't need it
* Significantly slimmed down SystemState in favor of individual SystemParam state
* System Commands changed from `commands: &mut Commands` back to `mut commands: Commands` (to allow Commands to have a World reference)
Fixes #1320
## `World` Rewrite
This is a from-scratch rewrite of `World` that fills the niche that `hecs` used to. Yes, this means Bevy ECS is no longer a "fork" of hecs. We're going out our own!
(the only shared code between the projects is the entity id allocator, which is already basically ideal)
A huge shout out to @SanderMertens (author of [flecs](https://github.com/SanderMertens/flecs)) for sharing some great ideas with me (specifically hybrid ecs storage and archetype graphs). He also helped advise on a number of implementation details.
## Component Storage (The Problem)
Two ECS storage paradigms have gained a lot of traction over the years:
* **Archetypal ECS**:
* Stores components in "tables" with static schemas. Each "column" stores components of a given type. Each "row" is an entity.
* Each "archetype" has its own table. Adding/removing an entity's component changes the archetype.
* Enables super-fast Query iteration due to its cache-friendly data layout
* Comes at the cost of more expensive add/remove operations for an Entity's components, because all components need to be copied to the new archetype's "table"
* **Sparse Set ECS**:
* Stores components of the same type in densely packed arrays, which are sparsely indexed by densely packed unsigned integers (Entity ids)
* Query iteration is slower than Archetypal ECS because each entity's component could be at any position in the sparse set. This "random access" pattern isn't cache friendly. Additionally, there is an extra layer of indirection because you must first map the entity id to an index in the component array.
* Adding/removing components is a cheap, constant time operation
Bevy ECS V1, hecs, legion, flec, and Unity DOTS are all "archetypal ecs-es". I personally think "archetypal" storage is a good default for game engines. An entity's archetype doesn't need to change frequently in general, and it creates "fast by default" query iteration (which is a much more common operation). It is also "self optimizing". Users don't need to think about optimizing component layouts for iteration performance. It "just works" without any extra boilerplate.
Shipyard and EnTT are "sparse set ecs-es". They employ "packing" as a way to work around the "suboptimal by default" iteration performance for specific sets of components. This helps, but I didn't think this was a good choice for a general purpose engine like Bevy because:
1. "packs" conflict with each other. If bevy decides to internally pack the Transform and GlobalTransform components, users are then blocked if they want to pack some custom component with Transform.
2. users need to take manual action to optimize
Developers selecting an ECS framework are stuck with a hard choice. Select an "archetypal" framework with "fast iteration everywhere" but without the ability to cheaply add/remove components, or select a "sparse set" framework to cheaply add/remove components but with slower iteration performance.
## Hybrid Component Storage (The Solution)
In Bevy ECS V2, we get to have our cake and eat it too. It now has _both_ of the component storage types above (and more can be added later if needed):
* **Tables** (aka "archetypal" storage)
* The default storage. If you don't configure anything, this is what you get
* Fast iteration by default
* Slower add/remove operations
* **Sparse Sets**
* Opt-in
* Slower iteration
* Faster add/remove operations
These storage types complement each other perfectly. By default Query iteration is fast. If developers know that they want to add/remove a component at high frequencies, they can set the storage to "sparse set":
```rust
world.register_component(
ComponentDescriptor::new::<MyComponent>(StorageType::SparseSet)
).unwrap();
```
## Archetypes
Archetypes are now "just metadata" ... they no longer store components directly. They do store:
* The `ComponentId`s of each of the Archetype's components (and that component's storage type)
* Archetypes are uniquely defined by their component layouts
* For example: entities with "table" components `[A, B, C]` _and_ "sparse set" components `[D, E]` will always be in the same archetype.
* The `TableId` associated with the archetype
* For now each archetype has exactly one table (which can have no components),
* There is a 1->Many relationship from Tables->Archetypes. A given table could have any number of archetype components stored in it:
* Ex: an entity with "table storage" components `[A, B, C]` and "sparse set" components `[D, E]` will share the same `[A, B, C]` table as an entity with `[A, B, C]` table component and `[F]` sparse set components.
* This 1->Many relationship is how we preserve fast "cache friendly" iteration performance when possible (more on this later)
* A list of entities that are in the archetype and the row id of the table they are in
* ArchetypeComponentIds
* unique densely packed identifiers for (ArchetypeId, ComponentId) pairs
* used by the schedule executor for cheap system access control
* "Archetype Graph Edges" (see the next section)
## The "Archetype Graph"
Archetype changes in Bevy (and a number of other archetypal ecs-es) have historically been expensive to compute. First, you need to allocate a new vector of the entity's current component ids, add or remove components based on the operation performed, sort it (to ensure it is order-independent), then hash it to find the archetype (if it exists). And thats all before we get to the _already_ expensive full copy of all components to the new table storage.
The solution is to build a "graph" of archetypes to cache these results. @SanderMertens first exposed me to the idea (and he got it from @gjroelofs, who came up with it). They propose adding directed edges between archetypes for add/remove component operations. If `ComponentId`s are densely packed, you can use sparse sets to cheaply jump between archetypes.
Bevy takes this one step further by using add/remove `Bundle` edges instead of `Component` edges. Bevy encourages the use of `Bundles` to group add/remove operations. This is largely for "clearer game logic" reasons, but it also helps cut down on the number of archetype changes required. `Bundles` now also have densely-packed `BundleId`s. This allows us to use a _single_ edge for each bundle operation (rather than needing to traverse N edges ... one for each component). Single component operations are also bundles, so this is strictly an improvement over a "component only" graph.
As a result, an operation that used to be _heavy_ (both for allocations and compute) is now two dirt-cheap array lookups and zero allocations.
## Stateful Queries
World queries are now stateful. This allows us to:
1. Cache archetype (and table) matches
* This resolves another issue with (naive) archetypal ECS: query performance getting worse as the number of archetypes goes up (and fragmentation occurs).
2. Cache Fetch and Filter state
* The expensive parts of fetch/filter operations (such as hashing the TypeId to find the ComponentId) now only happen once when the Query is first constructed
3. Incrementally build up state
* When new archetypes are added, we only process the new archetypes (no need to rebuild state for old archetypes)
As a result, the direct `World` query api now looks like this:
```rust
let mut query = world.query::<(&A, &mut B)>();
for (a, mut b) in query.iter_mut(&mut world) {
}
```
Requiring `World` to generate stateful queries (rather than letting the `QueryState` type be constructed separately) allows us to ensure that _all_ queries are properly initialized (and the relevant world state, such as ComponentIds). This enables QueryState to remove branches from its operations that check for initialization status (and also enables query.iter() to take an immutable world reference because it doesn't need to initialize anything in world).
However in systems, this is a non-breaking change. State management is done internally by the relevant SystemParam.
## Stateful SystemParams
Like Queries, `SystemParams` now also cache state. For example, `Query` system params store the "stateful query" state mentioned above. Commands store their internal `CommandQueue`. This means you can now safely use as many separate `Commands` parameters in your system as you want. `Local<T>` system params store their `T` value in their state (instead of in Resources).
SystemParam state also enabled a significant slim-down of SystemState. It is much nicer to look at now.
Per-SystemParam state naturally insulates us from an "aliased mut" class of errors we have hit in the past (ex: using multiple `Commands` system params).
(credit goes to @DJMcNab for the initial idea and draft pr here #1364)
## Configurable SystemParams
@DJMcNab also had the great idea to make SystemParams configurable. This allows users to provide some initial configuration / values for system parameters (when possible). Most SystemParams have no config (the config type is `()`), but the `Local<T>` param now supports user-provided parameters:
```rust
fn foo(value: Local<usize>) {
}
app.add_system(foo.system().config(|c| c.0 = Some(10)));
```
## Uber Fast "for_each" Query Iterators
Developers now have the choice to use a fast "for_each" iterator, which yields ~1.5-3x iteration speed improvements for "fragmented iteration", and minor ~1.2x iteration speed improvements for unfragmented iteration.
```rust
fn system(query: Query<(&A, &mut B)>) {
// you now have the option to do this for a speed boost
query.for_each_mut(|(a, mut b)| {
});
// however normal iterators are still available
for (a, mut b) in query.iter_mut() {
}
}
```
I think in most cases we should continue to encourage "normal" iterators as they are more flexible and more "rust idiomatic". But when that extra "oomf" is needed, it makes sense to use `for_each`.
We should also consider using `for_each` for internal bevy systems to give our users a nice speed boost (but that should be a separate pr).
## Component Metadata
`World` now has a `Components` collection, which is accessible via `world.components()`. This stores mappings from `ComponentId` to `ComponentInfo`, as well as `TypeId` to `ComponentId` mappings (where relevant). `ComponentInfo` stores information about the component, such as ComponentId, TypeId, memory layout, send-ness (currently limited to resources), and storage type.
## Significantly Cheaper `Access<T>`
We used to use `TypeAccess<TypeId>` to manage read/write component/archetype-component access. This was expensive because TypeIds must be hashed and compared individually. The parallel executor got around this by "condensing" type ids into bitset-backed access types. This worked, but it had to be re-generated from the `TypeAccess<TypeId>`sources every time archetypes changed.
This pr removes TypeAccess in favor of faster bitset access everywhere. We can do this thanks to the move to densely packed `ComponentId`s and `ArchetypeComponentId`s.
## Merged Resources into World
Resources had a lot of redundant functionality with Components. They stored typed data, they had access control, they had unique ids, they were queryable via SystemParams, etc. In fact the _only_ major difference between them was that they were unique (and didn't correlate to an entity).
Separate resources also had the downside of requiring a separate set of access controls, which meant the parallel executor needed to compare more bitsets per system and manage more state.
I initially got the "separate resources" idea from `legion`. I think that design was motivated by the fact that it made the direct world query/resource lifetime interactions more manageable. It certainly made our lives easier when using Resources alongside hecs/bevy_ecs. However we already have a construct for safely and ergonomically managing in-world lifetimes: systems (which use `Access<T>` internally).
This pr merges Resources into World:
```rust
world.insert_resource(1);
world.insert_resource(2.0);
let a = world.get_resource::<i32>().unwrap();
let mut b = world.get_resource_mut::<f64>().unwrap();
*b = 3.0;
```
Resources are now just a special kind of component. They have their own ComponentIds (and their own resource TypeId->ComponentId scope, so they don't conflict wit components of the same type). They are stored in a special "resource archetype", which stores components inside the archetype using a new `unique_components` sparse set (note that this sparse set could later be used to implement Tags). This allows us to keep the code size small by reusing existing datastructures (namely Column, Archetype, ComponentFlags, and ComponentInfo). This allows us the executor to use a single `Access<ArchetypeComponentId>` per system. It should also make scripting language integration easier.
_But_ this merge did create problems for people directly interacting with `World`. What if you need mutable access to multiple resources at the same time? `world.get_resource_mut()` borrows World mutably!
## WorldCell
WorldCell applies the `Access<ArchetypeComponentId>` concept to direct world access:
```rust
let world_cell = world.cell();
let a = world_cell.get_resource_mut::<i32>().unwrap();
let b = world_cell.get_resource_mut::<f64>().unwrap();
```
This adds cheap runtime checks (a sparse set lookup of `ArchetypeComponentId` and a counter) to ensure that world accesses do not conflict with each other. Each operation returns a `WorldBorrow<'w, T>` or `WorldBorrowMut<'w, T>` wrapper type, which will release the relevant ArchetypeComponentId resources when dropped.
World caches the access sparse set (and only one cell can exist at a time), so `world.cell()` is a cheap operation.
WorldCell does _not_ use atomic operations. It is non-send, does a mutable borrow of world to prevent other accesses, and uses a simple `Rc<RefCell<ArchetypeComponentAccess>>` wrapper in each WorldBorrow pointer.
The api is currently limited to resource access, but it can and should be extended to queries / entity component access.
## Resource Scopes
WorldCell does not yet support component queries, and even when it does there are sometimes legitimate reasons to want a mutable world ref _and_ a mutable resource ref (ex: bevy_render and bevy_scene both need this). In these cases we could always drop down to the unsafe `world.get_resource_unchecked_mut()`, but that is not ideal!
Instead developers can use a "resource scope"
```rust
world.resource_scope(|world: &mut World, a: &mut A| {
})
```
This temporarily removes the `A` resource from `World`, provides mutable pointers to both, and re-adds A to World when finished. Thanks to the move to ComponentIds/sparse sets, this is a cheap operation.
If multiple resources are required, scopes can be nested. We could also consider adding a "resource tuple" to the api if this pattern becomes common and the boilerplate gets nasty.
## Query Conflicts Use ComponentId Instead of ArchetypeComponentId
For safety reasons, systems cannot contain queries that conflict with each other without wrapping them in a QuerySet. On bevy `main`, we use ArchetypeComponentIds to determine conflicts. This is nice because it can take into account filters:
```rust
// these queries will never conflict due to their filters
fn filter_system(a: Query<&mut A, With<B>>, b: Query<&mut B, Without<B>>) {
}
```
But it also has a significant downside:
```rust
// these queries will not conflict _until_ an entity with A, B, and C is spawned
fn maybe_conflicts_system(a: Query<(&mut A, &C)>, b: Query<(&mut A, &B)>) {
}
```
The system above will panic at runtime if an entity with A, B, and C is spawned. This makes it hard to trust that your game logic will run without crashing.
In this pr, I switched to using `ComponentId` instead. This _is_ more constraining. `maybe_conflicts_system` will now always fail, but it will do it consistently at startup. Naively, it would also _disallow_ `filter_system`, which would be a significant downgrade in usability. Bevy has a number of internal systems that rely on disjoint queries and I expect it to be a common pattern in userspace.
To resolve this, I added a new `FilteredAccess<T>` type, which wraps `Access<T>` and adds with/without filters. If two `FilteredAccess` have with/without values that prove they are disjoint, they will no longer conflict.
## EntityRef / EntityMut
World entity operations on `main` require that the user passes in an `entity` id to each operation:
```rust
let entity = world.spawn((A, )); // create a new entity with A
world.get::<A>(entity);
world.insert(entity, (B, C));
world.insert_one(entity, D);
```
This means that each operation needs to look up the entity location / verify its validity. The initial spawn operation also requires a Bundle as input. This can be awkward when no components are required (or one component is required).
These operations have been replaced by `EntityRef` and `EntityMut`, which are "builder-style" wrappers around world that provide read and read/write operations on a single, pre-validated entity:
```rust
// spawn now takes no inputs and returns an EntityMut
let entity = world.spawn()
.insert(A) // insert a single component into the entity
.insert_bundle((B, C)) // insert a bundle of components into the entity
.id() // id returns the Entity id
// Returns EntityMut (or panics if the entity does not exist)
world.entity_mut(entity)
.insert(D)
.insert_bundle(SomeBundle::default());
{
// returns EntityRef (or panics if the entity does not exist)
let d = world.entity(entity)
.get::<D>() // gets the D component
.unwrap();
// world.get still exists for ergonomics
let d = world.get::<D>(entity).unwrap();
}
// These variants return Options if you want to check existence instead of panicing
world.get_entity_mut(entity)
.unwrap()
.insert(E);
if let Some(entity_ref) = world.get_entity(entity) {
let d = entity_ref.get::<D>().unwrap();
}
```
This _does not_ affect the current Commands api or terminology. I think that should be a separate conversation as that is a much larger breaking change.
## Safety Improvements
* Entity reservation in Commands uses a normal world borrow instead of an unsafe transmute
* QuerySets no longer transmutes lifetimes
* Made traits "unsafe" when implementing a trait incorrectly could cause unsafety
* More thorough safety docs
## RemovedComponents SystemParam
The old approach to querying removed components: `query.removed:<T>()` was confusing because it had no connection to the query itself. I replaced it with the following, which is both clearer and allows us to cache the ComponentId mapping in the SystemParamState:
```rust
fn system(removed: RemovedComponents<T>) {
for entity in removed.iter() {
}
}
```
## Simpler Bundle implementation
Bundles are no longer responsible for sorting (or deduping) TypeInfo. They are just a simple ordered list of component types / data. This makes the implementation smaller and opens the door to an easy "nested bundle" implementation in the future (which i might even add in this pr). Duplicate detection is now done once per bundle type by World the first time a bundle is used.
## Unified WorldQuery and QueryFilter types
(don't worry they are still separate type _parameters_ in Queries .. this is a non-breaking change)
WorldQuery and QueryFilter were already basically identical apis. With the addition of `FetchState` and more storage-specific fetch methods, the overlap was even clearer (and the redundancy more painful).
QueryFilters are now just `F: WorldQuery where F::Fetch: FilterFetch`. FilterFetch requires `Fetch<Item = bool>` and adds new "short circuit" variants of fetch methods. This enables a filter tuple like `(With<A>, Without<B>, Changed<C>)` to stop evaluating the filter after the first mismatch is encountered. FilterFetch is automatically implemented for `Fetch` implementations that return bool.
This forces fetch implementations that return things like `(bool, bool, bool)` (such as the filter above) to manually implement FilterFetch and decide whether or not to short-circuit.
## More Granular Modules
World no longer globs all of the internal modules together. It now exports `core`, `system`, and `schedule` separately. I'm also considering exporting `core` submodules directly as that is still pretty "glob-ey" and unorganized (feedback welcome here).
## Remaining Draft Work (to be done in this pr)
* ~~panic on conflicting WorldQuery fetches (&A, &mut A)~~
* ~~bevy `main` and hecs both currently allow this, but we should protect against it if possible~~
* ~~batch_iter / par_iter (currently stubbed out)~~
* ~~ChangedRes~~
* ~~I skipped this while we sort out #1313. This pr should be adapted to account for whatever we land on there~~.
* ~~The `Archetypes` and `Tables` collections use hashes of sorted lists of component ids to uniquely identify each archetype/table. This hash is then used as the key in a HashMap to look up the relevant ArchetypeId or TableId. (which doesn't handle hash collisions properly)~~
* ~~It is currently unsafe to generate a Query from "World A", then use it on "World B" (despite the api claiming it is safe). We should probably close this gap. This could be done by adding a randomly generated WorldId to each world, then storing that id in each Query. They could then be compared to each other on each `query.do_thing(&world)` operation. This _does_ add an extra branch to each query operation, so I'm open to other suggestions if people have them.~~
* ~~Nested Bundles (if i find time)~~
## Potential Future Work
* Expand WorldCell to support queries.
* Consider not allocating in the empty archetype on `world.spawn()`
* ex: return something like EntityMutUninit, which turns into EntityMut after an `insert` or `insert_bundle` op
* this actually regressed performance last time i tried it, but in theory it should be faster
* Optimize SparseSet::insert (see `PERF` comment on insert)
* Replace SparseArray `Option<T>` with T::MAX to cut down on branching
* would enable cheaper get_unchecked() operations
* upstream fixedbitset optimizations
* fixedbitset could be allocation free for small block counts (store blocks in a SmallVec)
* fixedbitset could have a const constructor
* Consider implementing Tags (archetype-specific by-value data that affects archetype identity)
* ex: ArchetypeA could have `[A, B, C]` table components and `[D(1)]` "tag" component. ArchetypeB could have `[A, B, C]` table components and a `[D(2)]` tag component. The archetypes are different, despite both having D tags because the value inside D is different.
* this could potentially build on top of the `archetype.unique_components` added in this pr for resource storage.
* Consider reverting `all_tuples` proc macro in favor of the old `macro_rules` implementation
* all_tuples is more flexible and produces cleaner documentation (the macro_rules version produces weird type parameter orders due to parser constraints)
* but unfortunately all_tuples also appears to make Rust Analyzer sad/slow when working inside of `bevy_ecs` (does not affect user code)
* Consider "resource queries" and/or "mixed resource and entity component queries" as an alternative to WorldCell
* this is basically just "systems" so maybe it's not worth it
* Add more world ops
* `world.clear()`
* `world.reserve<T: Bundle>(count: usize)`
* Try using the old archetype allocation strategy (allocate new memory on resize and copy everything over). I expect this to improve batch insertion performance at the cost of unbatched performance. But thats just a guess. I'm not an allocation perf pro :)
* Adapt Commands apis for consistency with new World apis
## Benchmarks
key:
* `bevy_old`: bevy `main` branch
* `bevy`: this branch
* `_foreach`: uses an optimized for_each iterator
* ` _sparse`: uses sparse set storage (if unspecified assume table storage)
* `_system`: runs inside a system (if unspecified assume test happens via direct world ops)
### Simple Insert (from ecs_bench_suite)
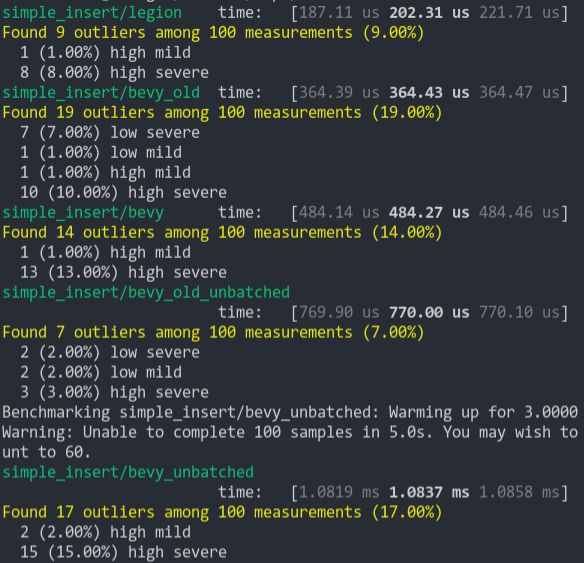
### Simpler Iter (from ecs_bench_suite)
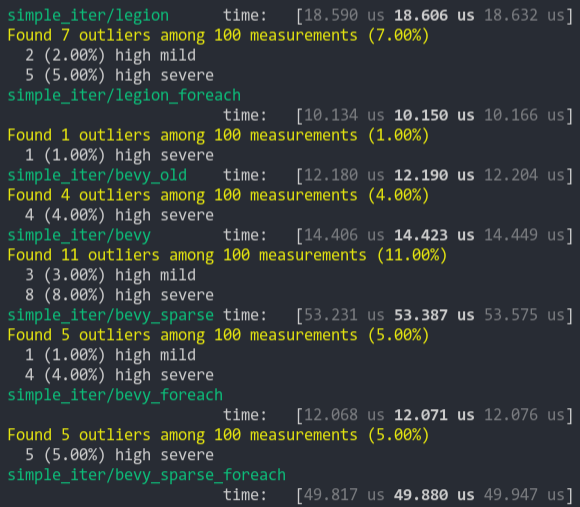
### Fragment Iter (from ecs_bench_suite)
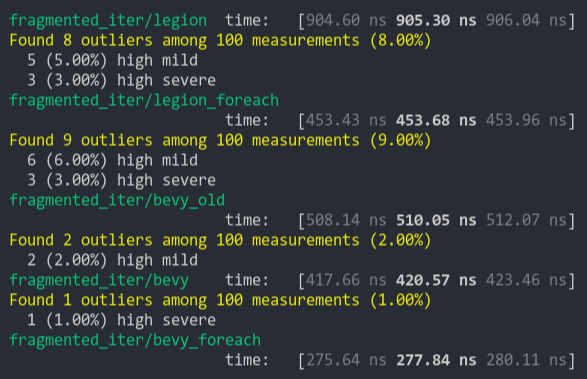
### Sparse Fragmented Iter
Iterate a query that matches 5 entities from a single matching archetype, but there are 100 unmatching archetypes
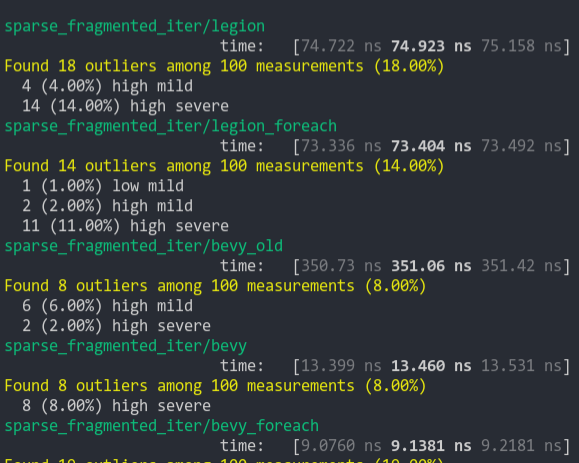
### Schedule (from ecs_bench_suite)
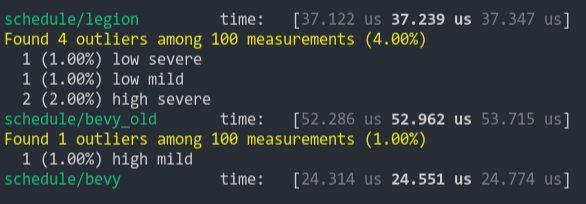
### Add Remove Component (from ecs_bench_suite)
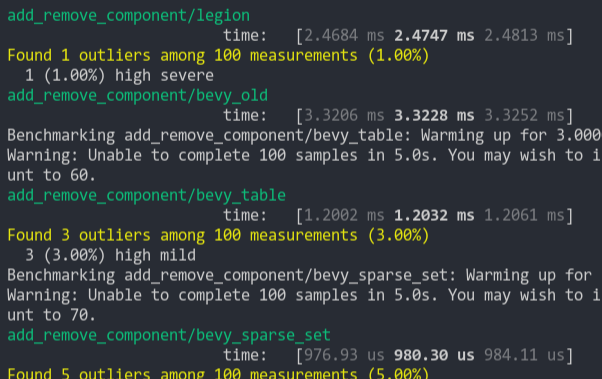
### Add Remove Component Big
Same as the test above, but each entity has 5 "large" matrix components and 1 "large" matrix component is added and removed
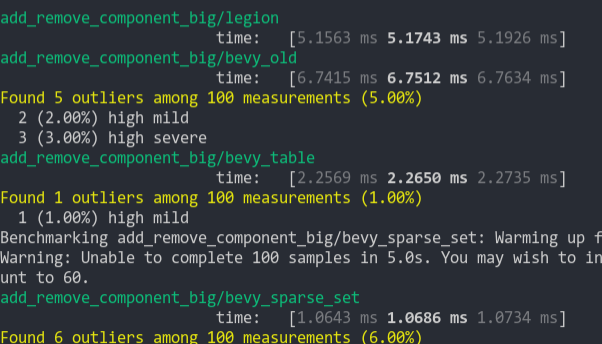
### Get Component
Looks up a single component value a large number of times
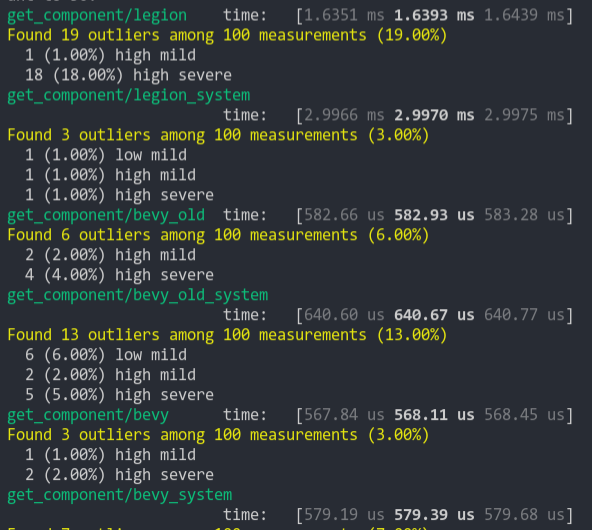
2021-03-05 07:54:35 +00:00
2022-10-24 13:46:36 +00:00
let archetype_component_id = world
. get_resource_archetype_component_id ( component_id )
Bevy ECS V2 (#1525)
# Bevy ECS V2
This is a rewrite of Bevy ECS (basically everything but the new executor/schedule, which are already awesome). The overall goal was to improve the performance and versatility of Bevy ECS. Here is a quick bulleted list of changes before we dive into the details:
* Complete World rewrite
* Multiple component storage types:
* Tables: fast cache friendly iteration, slower add/removes (previously called Archetypes)
* Sparse Sets: fast add/remove, slower iteration
* Stateful Queries (caches query results for faster iteration. fragmented iteration is _fast_ now)
* Stateful System Params (caches expensive operations. inspired by @DJMcNab's work in #1364)
* Configurable System Params (users can set configuration when they construct their systems. once again inspired by @DJMcNab's work)
* Archetypes are now "just metadata", component storage is separate
* Archetype Graph (for faster archetype changes)
* Component Metadata
* Configure component storage type
* Retrieve information about component size/type/name/layout/send-ness/etc
* Components are uniquely identified by a densely packed ComponentId
* TypeIds are now totally optional (which should make implementing scripting easier)
* Super fast "for_each" query iterators
* Merged Resources into World. Resources are now just a special type of component
* EntityRef/EntityMut builder apis (more efficient and more ergonomic)
* Fast bitset-backed `Access<T>` replaces old hashmap-based approach everywhere
* Query conflicts are determined by component access instead of archetype component access (to avoid random failures at runtime)
* With/Without are still taken into account for conflicts, so this should still be comfy to use
* Much simpler `IntoSystem` impl
* Significantly reduced the amount of hashing throughout the ecs in favor of Sparse Sets (indexed by densely packed ArchetypeId, ComponentId, BundleId, and TableId)
* Safety Improvements
* Entity reservation uses a normal world reference instead of unsafe transmute
* QuerySets no longer transmute lifetimes
* Made traits "unsafe" where relevant
* More thorough safety docs
* WorldCell
* Exposes safe mutable access to multiple resources at a time in a World
* Replaced "catch all" `System::update_archetypes(world: &World)` with `System::new_archetype(archetype: &Archetype)`
* Simpler Bundle implementation
* Replaced slow "remove_bundle_one_by_one" used as fallback for Commands::remove_bundle with fast "remove_bundle_intersection"
* Removed `Mut<T>` query impl. it is better to only support one way: `&mut T`
* Removed with() from `Flags<T>` in favor of `Option<Flags<T>>`, which allows querying for flags to be "filtered" by default
* Components now have is_send property (currently only resources support non-send)
* More granular module organization
* New `RemovedComponents<T>` SystemParam that replaces `query.removed::<T>()`
* `world.resource_scope()` for mutable access to resources and world at the same time
* WorldQuery and QueryFilter traits unified. FilterFetch trait added to enable "short circuit" filtering. Auto impled for cases that don't need it
* Significantly slimmed down SystemState in favor of individual SystemParam state
* System Commands changed from `commands: &mut Commands` back to `mut commands: Commands` (to allow Commands to have a World reference)
Fixes #1320
## `World` Rewrite
This is a from-scratch rewrite of `World` that fills the niche that `hecs` used to. Yes, this means Bevy ECS is no longer a "fork" of hecs. We're going out our own!
(the only shared code between the projects is the entity id allocator, which is already basically ideal)
A huge shout out to @SanderMertens (author of [flecs](https://github.com/SanderMertens/flecs)) for sharing some great ideas with me (specifically hybrid ecs storage and archetype graphs). He also helped advise on a number of implementation details.
## Component Storage (The Problem)
Two ECS storage paradigms have gained a lot of traction over the years:
* **Archetypal ECS**:
* Stores components in "tables" with static schemas. Each "column" stores components of a given type. Each "row" is an entity.
* Each "archetype" has its own table. Adding/removing an entity's component changes the archetype.
* Enables super-fast Query iteration due to its cache-friendly data layout
* Comes at the cost of more expensive add/remove operations for an Entity's components, because all components need to be copied to the new archetype's "table"
* **Sparse Set ECS**:
* Stores components of the same type in densely packed arrays, which are sparsely indexed by densely packed unsigned integers (Entity ids)
* Query iteration is slower than Archetypal ECS because each entity's component could be at any position in the sparse set. This "random access" pattern isn't cache friendly. Additionally, there is an extra layer of indirection because you must first map the entity id to an index in the component array.
* Adding/removing components is a cheap, constant time operation
Bevy ECS V1, hecs, legion, flec, and Unity DOTS are all "archetypal ecs-es". I personally think "archetypal" storage is a good default for game engines. An entity's archetype doesn't need to change frequently in general, and it creates "fast by default" query iteration (which is a much more common operation). It is also "self optimizing". Users don't need to think about optimizing component layouts for iteration performance. It "just works" without any extra boilerplate.
Shipyard and EnTT are "sparse set ecs-es". They employ "packing" as a way to work around the "suboptimal by default" iteration performance for specific sets of components. This helps, but I didn't think this was a good choice for a general purpose engine like Bevy because:
1. "packs" conflict with each other. If bevy decides to internally pack the Transform and GlobalTransform components, users are then blocked if they want to pack some custom component with Transform.
2. users need to take manual action to optimize
Developers selecting an ECS framework are stuck with a hard choice. Select an "archetypal" framework with "fast iteration everywhere" but without the ability to cheaply add/remove components, or select a "sparse set" framework to cheaply add/remove components but with slower iteration performance.
## Hybrid Component Storage (The Solution)
In Bevy ECS V2, we get to have our cake and eat it too. It now has _both_ of the component storage types above (and more can be added later if needed):
* **Tables** (aka "archetypal" storage)
* The default storage. If you don't configure anything, this is what you get
* Fast iteration by default
* Slower add/remove operations
* **Sparse Sets**
* Opt-in
* Slower iteration
* Faster add/remove operations
These storage types complement each other perfectly. By default Query iteration is fast. If developers know that they want to add/remove a component at high frequencies, they can set the storage to "sparse set":
```rust
world.register_component(
ComponentDescriptor::new::<MyComponent>(StorageType::SparseSet)
).unwrap();
```
## Archetypes
Archetypes are now "just metadata" ... they no longer store components directly. They do store:
* The `ComponentId`s of each of the Archetype's components (and that component's storage type)
* Archetypes are uniquely defined by their component layouts
* For example: entities with "table" components `[A, B, C]` _and_ "sparse set" components `[D, E]` will always be in the same archetype.
* The `TableId` associated with the archetype
* For now each archetype has exactly one table (which can have no components),
* There is a 1->Many relationship from Tables->Archetypes. A given table could have any number of archetype components stored in it:
* Ex: an entity with "table storage" components `[A, B, C]` and "sparse set" components `[D, E]` will share the same `[A, B, C]` table as an entity with `[A, B, C]` table component and `[F]` sparse set components.
* This 1->Many relationship is how we preserve fast "cache friendly" iteration performance when possible (more on this later)
* A list of entities that are in the archetype and the row id of the table they are in
* ArchetypeComponentIds
* unique densely packed identifiers for (ArchetypeId, ComponentId) pairs
* used by the schedule executor for cheap system access control
* "Archetype Graph Edges" (see the next section)
## The "Archetype Graph"
Archetype changes in Bevy (and a number of other archetypal ecs-es) have historically been expensive to compute. First, you need to allocate a new vector of the entity's current component ids, add or remove components based on the operation performed, sort it (to ensure it is order-independent), then hash it to find the archetype (if it exists). And thats all before we get to the _already_ expensive full copy of all components to the new table storage.
The solution is to build a "graph" of archetypes to cache these results. @SanderMertens first exposed me to the idea (and he got it from @gjroelofs, who came up with it). They propose adding directed edges between archetypes for add/remove component operations. If `ComponentId`s are densely packed, you can use sparse sets to cheaply jump between archetypes.
Bevy takes this one step further by using add/remove `Bundle` edges instead of `Component` edges. Bevy encourages the use of `Bundles` to group add/remove operations. This is largely for "clearer game logic" reasons, but it also helps cut down on the number of archetype changes required. `Bundles` now also have densely-packed `BundleId`s. This allows us to use a _single_ edge for each bundle operation (rather than needing to traverse N edges ... one for each component). Single component operations are also bundles, so this is strictly an improvement over a "component only" graph.
As a result, an operation that used to be _heavy_ (both for allocations and compute) is now two dirt-cheap array lookups and zero allocations.
## Stateful Queries
World queries are now stateful. This allows us to:
1. Cache archetype (and table) matches
* This resolves another issue with (naive) archetypal ECS: query performance getting worse as the number of archetypes goes up (and fragmentation occurs).
2. Cache Fetch and Filter state
* The expensive parts of fetch/filter operations (such as hashing the TypeId to find the ComponentId) now only happen once when the Query is first constructed
3. Incrementally build up state
* When new archetypes are added, we only process the new archetypes (no need to rebuild state for old archetypes)
As a result, the direct `World` query api now looks like this:
```rust
let mut query = world.query::<(&A, &mut B)>();
for (a, mut b) in query.iter_mut(&mut world) {
}
```
Requiring `World` to generate stateful queries (rather than letting the `QueryState` type be constructed separately) allows us to ensure that _all_ queries are properly initialized (and the relevant world state, such as ComponentIds). This enables QueryState to remove branches from its operations that check for initialization status (and also enables query.iter() to take an immutable world reference because it doesn't need to initialize anything in world).
However in systems, this is a non-breaking change. State management is done internally by the relevant SystemParam.
## Stateful SystemParams
Like Queries, `SystemParams` now also cache state. For example, `Query` system params store the "stateful query" state mentioned above. Commands store their internal `CommandQueue`. This means you can now safely use as many separate `Commands` parameters in your system as you want. `Local<T>` system params store their `T` value in their state (instead of in Resources).
SystemParam state also enabled a significant slim-down of SystemState. It is much nicer to look at now.
Per-SystemParam state naturally insulates us from an "aliased mut" class of errors we have hit in the past (ex: using multiple `Commands` system params).
(credit goes to @DJMcNab for the initial idea and draft pr here #1364)
## Configurable SystemParams
@DJMcNab also had the great idea to make SystemParams configurable. This allows users to provide some initial configuration / values for system parameters (when possible). Most SystemParams have no config (the config type is `()`), but the `Local<T>` param now supports user-provided parameters:
```rust
fn foo(value: Local<usize>) {
}
app.add_system(foo.system().config(|c| c.0 = Some(10)));
```
## Uber Fast "for_each" Query Iterators
Developers now have the choice to use a fast "for_each" iterator, which yields ~1.5-3x iteration speed improvements for "fragmented iteration", and minor ~1.2x iteration speed improvements for unfragmented iteration.
```rust
fn system(query: Query<(&A, &mut B)>) {
// you now have the option to do this for a speed boost
query.for_each_mut(|(a, mut b)| {
});
// however normal iterators are still available
for (a, mut b) in query.iter_mut() {
}
}
```
I think in most cases we should continue to encourage "normal" iterators as they are more flexible and more "rust idiomatic". But when that extra "oomf" is needed, it makes sense to use `for_each`.
We should also consider using `for_each` for internal bevy systems to give our users a nice speed boost (but that should be a separate pr).
## Component Metadata
`World` now has a `Components` collection, which is accessible via `world.components()`. This stores mappings from `ComponentId` to `ComponentInfo`, as well as `TypeId` to `ComponentId` mappings (where relevant). `ComponentInfo` stores information about the component, such as ComponentId, TypeId, memory layout, send-ness (currently limited to resources), and storage type.
## Significantly Cheaper `Access<T>`
We used to use `TypeAccess<TypeId>` to manage read/write component/archetype-component access. This was expensive because TypeIds must be hashed and compared individually. The parallel executor got around this by "condensing" type ids into bitset-backed access types. This worked, but it had to be re-generated from the `TypeAccess<TypeId>`sources every time archetypes changed.
This pr removes TypeAccess in favor of faster bitset access everywhere. We can do this thanks to the move to densely packed `ComponentId`s and `ArchetypeComponentId`s.
## Merged Resources into World
Resources had a lot of redundant functionality with Components. They stored typed data, they had access control, they had unique ids, they were queryable via SystemParams, etc. In fact the _only_ major difference between them was that they were unique (and didn't correlate to an entity).
Separate resources also had the downside of requiring a separate set of access controls, which meant the parallel executor needed to compare more bitsets per system and manage more state.
I initially got the "separate resources" idea from `legion`. I think that design was motivated by the fact that it made the direct world query/resource lifetime interactions more manageable. It certainly made our lives easier when using Resources alongside hecs/bevy_ecs. However we already have a construct for safely and ergonomically managing in-world lifetimes: systems (which use `Access<T>` internally).
This pr merges Resources into World:
```rust
world.insert_resource(1);
world.insert_resource(2.0);
let a = world.get_resource::<i32>().unwrap();
let mut b = world.get_resource_mut::<f64>().unwrap();
*b = 3.0;
```
Resources are now just a special kind of component. They have their own ComponentIds (and their own resource TypeId->ComponentId scope, so they don't conflict wit components of the same type). They are stored in a special "resource archetype", which stores components inside the archetype using a new `unique_components` sparse set (note that this sparse set could later be used to implement Tags). This allows us to keep the code size small by reusing existing datastructures (namely Column, Archetype, ComponentFlags, and ComponentInfo). This allows us the executor to use a single `Access<ArchetypeComponentId>` per system. It should also make scripting language integration easier.
_But_ this merge did create problems for people directly interacting with `World`. What if you need mutable access to multiple resources at the same time? `world.get_resource_mut()` borrows World mutably!
## WorldCell
WorldCell applies the `Access<ArchetypeComponentId>` concept to direct world access:
```rust
let world_cell = world.cell();
let a = world_cell.get_resource_mut::<i32>().unwrap();
let b = world_cell.get_resource_mut::<f64>().unwrap();
```
This adds cheap runtime checks (a sparse set lookup of `ArchetypeComponentId` and a counter) to ensure that world accesses do not conflict with each other. Each operation returns a `WorldBorrow<'w, T>` or `WorldBorrowMut<'w, T>` wrapper type, which will release the relevant ArchetypeComponentId resources when dropped.
World caches the access sparse set (and only one cell can exist at a time), so `world.cell()` is a cheap operation.
WorldCell does _not_ use atomic operations. It is non-send, does a mutable borrow of world to prevent other accesses, and uses a simple `Rc<RefCell<ArchetypeComponentAccess>>` wrapper in each WorldBorrow pointer.
The api is currently limited to resource access, but it can and should be extended to queries / entity component access.
## Resource Scopes
WorldCell does not yet support component queries, and even when it does there are sometimes legitimate reasons to want a mutable world ref _and_ a mutable resource ref (ex: bevy_render and bevy_scene both need this). In these cases we could always drop down to the unsafe `world.get_resource_unchecked_mut()`, but that is not ideal!
Instead developers can use a "resource scope"
```rust
world.resource_scope(|world: &mut World, a: &mut A| {
})
```
This temporarily removes the `A` resource from `World`, provides mutable pointers to both, and re-adds A to World when finished. Thanks to the move to ComponentIds/sparse sets, this is a cheap operation.
If multiple resources are required, scopes can be nested. We could also consider adding a "resource tuple" to the api if this pattern becomes common and the boilerplate gets nasty.
## Query Conflicts Use ComponentId Instead of ArchetypeComponentId
For safety reasons, systems cannot contain queries that conflict with each other without wrapping them in a QuerySet. On bevy `main`, we use ArchetypeComponentIds to determine conflicts. This is nice because it can take into account filters:
```rust
// these queries will never conflict due to their filters
fn filter_system(a: Query<&mut A, With<B>>, b: Query<&mut B, Without<B>>) {
}
```
But it also has a significant downside:
```rust
// these queries will not conflict _until_ an entity with A, B, and C is spawned
fn maybe_conflicts_system(a: Query<(&mut A, &C)>, b: Query<(&mut A, &B)>) {
}
```
The system above will panic at runtime if an entity with A, B, and C is spawned. This makes it hard to trust that your game logic will run without crashing.
In this pr, I switched to using `ComponentId` instead. This _is_ more constraining. `maybe_conflicts_system` will now always fail, but it will do it consistently at startup. Naively, it would also _disallow_ `filter_system`, which would be a significant downgrade in usability. Bevy has a number of internal systems that rely on disjoint queries and I expect it to be a common pattern in userspace.
To resolve this, I added a new `FilteredAccess<T>` type, which wraps `Access<T>` and adds with/without filters. If two `FilteredAccess` have with/without values that prove they are disjoint, they will no longer conflict.
## EntityRef / EntityMut
World entity operations on `main` require that the user passes in an `entity` id to each operation:
```rust
let entity = world.spawn((A, )); // create a new entity with A
world.get::<A>(entity);
world.insert(entity, (B, C));
world.insert_one(entity, D);
```
This means that each operation needs to look up the entity location / verify its validity. The initial spawn operation also requires a Bundle as input. This can be awkward when no components are required (or one component is required).
These operations have been replaced by `EntityRef` and `EntityMut`, which are "builder-style" wrappers around world that provide read and read/write operations on a single, pre-validated entity:
```rust
// spawn now takes no inputs and returns an EntityMut
let entity = world.spawn()
.insert(A) // insert a single component into the entity
.insert_bundle((B, C)) // insert a bundle of components into the entity
.id() // id returns the Entity id
// Returns EntityMut (or panics if the entity does not exist)
world.entity_mut(entity)
.insert(D)
.insert_bundle(SomeBundle::default());
{
// returns EntityRef (or panics if the entity does not exist)
let d = world.entity(entity)
.get::<D>() // gets the D component
.unwrap();
// world.get still exists for ergonomics
let d = world.get::<D>(entity).unwrap();
}
// These variants return Options if you want to check existence instead of panicing
world.get_entity_mut(entity)
.unwrap()
.insert(E);
if let Some(entity_ref) = world.get_entity(entity) {
let d = entity_ref.get::<D>().unwrap();
}
```
This _does not_ affect the current Commands api or terminology. I think that should be a separate conversation as that is a much larger breaking change.
## Safety Improvements
* Entity reservation in Commands uses a normal world borrow instead of an unsafe transmute
* QuerySets no longer transmutes lifetimes
* Made traits "unsafe" when implementing a trait incorrectly could cause unsafety
* More thorough safety docs
## RemovedComponents SystemParam
The old approach to querying removed components: `query.removed:<T>()` was confusing because it had no connection to the query itself. I replaced it with the following, which is both clearer and allows us to cache the ComponentId mapping in the SystemParamState:
```rust
fn system(removed: RemovedComponents<T>) {
for entity in removed.iter() {
}
}
```
## Simpler Bundle implementation
Bundles are no longer responsible for sorting (or deduping) TypeInfo. They are just a simple ordered list of component types / data. This makes the implementation smaller and opens the door to an easy "nested bundle" implementation in the future (which i might even add in this pr). Duplicate detection is now done once per bundle type by World the first time a bundle is used.
## Unified WorldQuery and QueryFilter types
(don't worry they are still separate type _parameters_ in Queries .. this is a non-breaking change)
WorldQuery and QueryFilter were already basically identical apis. With the addition of `FetchState` and more storage-specific fetch methods, the overlap was even clearer (and the redundancy more painful).
QueryFilters are now just `F: WorldQuery where F::Fetch: FilterFetch`. FilterFetch requires `Fetch<Item = bool>` and adds new "short circuit" variants of fetch methods. This enables a filter tuple like `(With<A>, Without<B>, Changed<C>)` to stop evaluating the filter after the first mismatch is encountered. FilterFetch is automatically implemented for `Fetch` implementations that return bool.
This forces fetch implementations that return things like `(bool, bool, bool)` (such as the filter above) to manually implement FilterFetch and decide whether or not to short-circuit.
## More Granular Modules
World no longer globs all of the internal modules together. It now exports `core`, `system`, and `schedule` separately. I'm also considering exporting `core` submodules directly as that is still pretty "glob-ey" and unorganized (feedback welcome here).
## Remaining Draft Work (to be done in this pr)
* ~~panic on conflicting WorldQuery fetches (&A, &mut A)~~
* ~~bevy `main` and hecs both currently allow this, but we should protect against it if possible~~
* ~~batch_iter / par_iter (currently stubbed out)~~
* ~~ChangedRes~~
* ~~I skipped this while we sort out #1313. This pr should be adapted to account for whatever we land on there~~.
* ~~The `Archetypes` and `Tables` collections use hashes of sorted lists of component ids to uniquely identify each archetype/table. This hash is then used as the key in a HashMap to look up the relevant ArchetypeId or TableId. (which doesn't handle hash collisions properly)~~
* ~~It is currently unsafe to generate a Query from "World A", then use it on "World B" (despite the api claiming it is safe). We should probably close this gap. This could be done by adding a randomly generated WorldId to each world, then storing that id in each Query. They could then be compared to each other on each `query.do_thing(&world)` operation. This _does_ add an extra branch to each query operation, so I'm open to other suggestions if people have them.~~
* ~~Nested Bundles (if i find time)~~
## Potential Future Work
* Expand WorldCell to support queries.
* Consider not allocating in the empty archetype on `world.spawn()`
* ex: return something like EntityMutUninit, which turns into EntityMut after an `insert` or `insert_bundle` op
* this actually regressed performance last time i tried it, but in theory it should be faster
* Optimize SparseSet::insert (see `PERF` comment on insert)
* Replace SparseArray `Option<T>` with T::MAX to cut down on branching
* would enable cheaper get_unchecked() operations
* upstream fixedbitset optimizations
* fixedbitset could be allocation free for small block counts (store blocks in a SmallVec)
* fixedbitset could have a const constructor
* Consider implementing Tags (archetype-specific by-value data that affects archetype identity)
* ex: ArchetypeA could have `[A, B, C]` table components and `[D(1)]` "tag" component. ArchetypeB could have `[A, B, C]` table components and a `[D(2)]` tag component. The archetypes are different, despite both having D tags because the value inside D is different.
* this could potentially build on top of the `archetype.unique_components` added in this pr for resource storage.
* Consider reverting `all_tuples` proc macro in favor of the old `macro_rules` implementation
* all_tuples is more flexible and produces cleaner documentation (the macro_rules version produces weird type parameter orders due to parser constraints)
* but unfortunately all_tuples also appears to make Rust Analyzer sad/slow when working inside of `bevy_ecs` (does not affect user code)
* Consider "resource queries" and/or "mixed resource and entity component queries" as an alternative to WorldCell
* this is basically just "systems" so maybe it's not worth it
* Add more world ops
* `world.clear()`
* `world.reserve<T: Bundle>(count: usize)`
* Try using the old archetype allocation strategy (allocate new memory on resize and copy everything over). I expect this to improve batch insertion performance at the cost of unbatched performance. But thats just a guess. I'm not an allocation perf pro :)
* Adapt Commands apis for consistency with new World apis
## Benchmarks
key:
* `bevy_old`: bevy `main` branch
* `bevy`: this branch
* `_foreach`: uses an optimized for_each iterator
* ` _sparse`: uses sparse set storage (if unspecified assume table storage)
* `_system`: runs inside a system (if unspecified assume test happens via direct world ops)
### Simple Insert (from ecs_bench_suite)
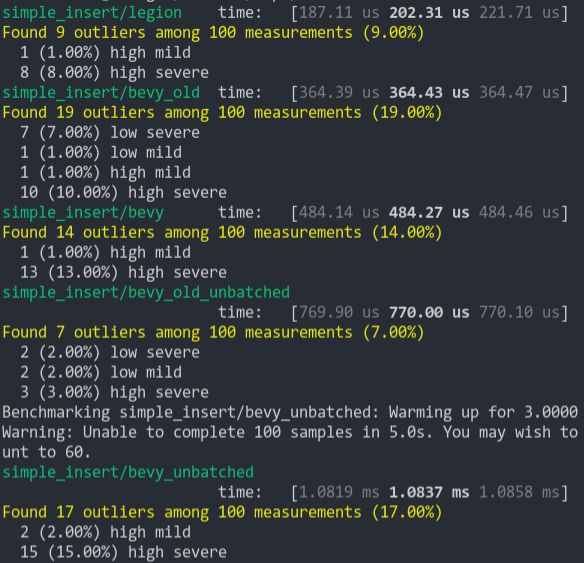
### Simpler Iter (from ecs_bench_suite)
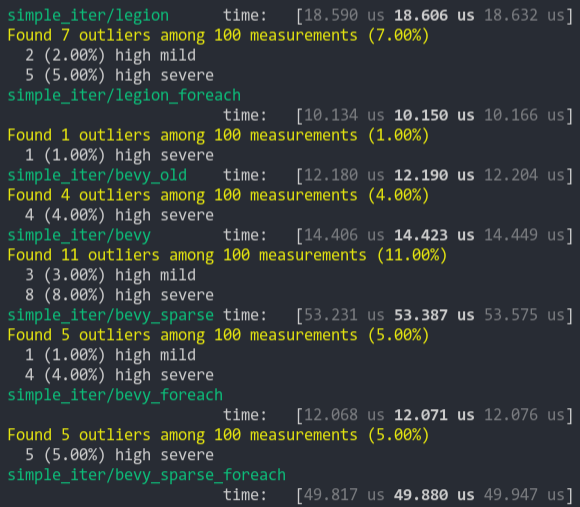
### Fragment Iter (from ecs_bench_suite)
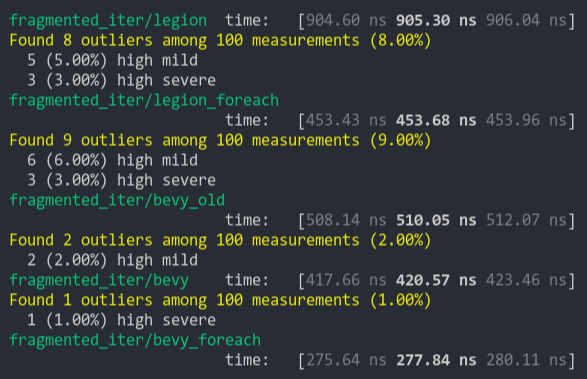
### Sparse Fragmented Iter
Iterate a query that matches 5 entities from a single matching archetype, but there are 100 unmatching archetypes
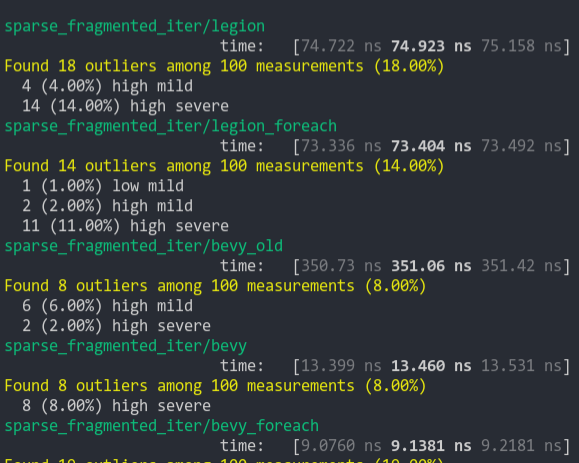
### Schedule (from ecs_bench_suite)
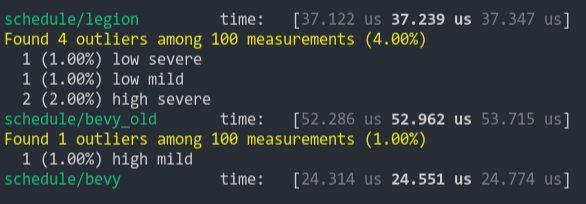
### Add Remove Component (from ecs_bench_suite)
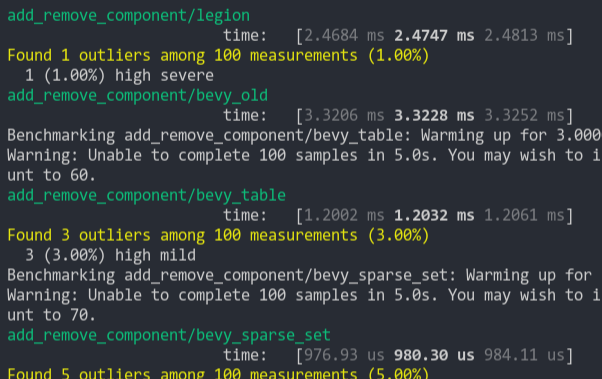
### Add Remove Component Big
Same as the test above, but each entity has 5 "large" matrix components and 1 "large" matrix component is added and removed
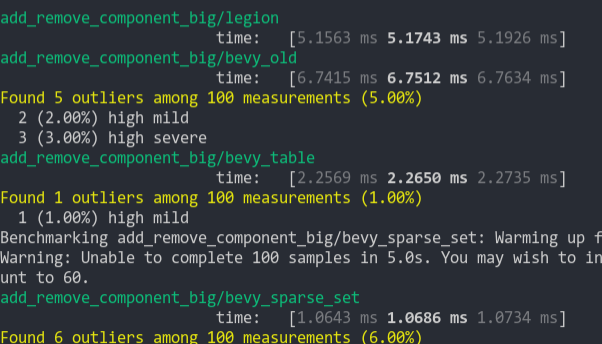
### Get Component
Looks up a single component value a large number of times
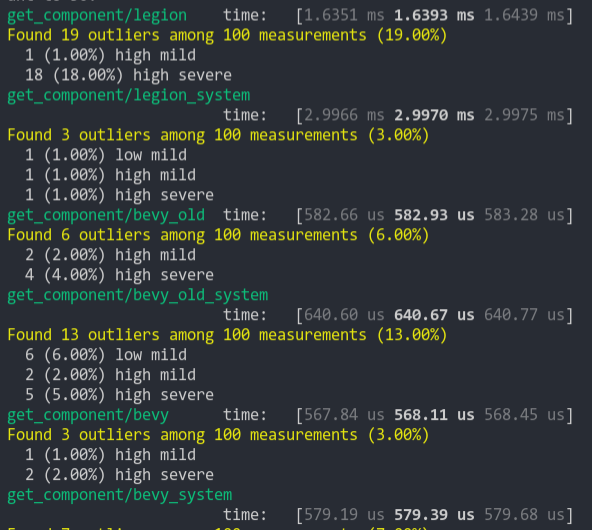
2021-03-05 07:54:35 +00:00
. unwrap ( ) ;
Add new SystemState and rename old SystemState to SystemMeta (#2283)
This enables `SystemParams` to be used outside of function systems. Anything can create and store `SystemState`, which enables efficient "param state cached" access to `SystemParams`.
It adds a `ReadOnlySystemParamFetch` trait, which enables safe `SystemState::get` calls without unique world access.
I renamed the old `SystemState` to `SystemMeta` to enable us to mirror the `QueryState` naming convention (but I'm happy to discuss alternative names if people have other ideas). I initially pitched this as `ParamState`, but given that it needs to include full system metadata, that doesn't feel like a particularly accurate name.
```rust
#[derive(Eq, PartialEq, Debug)]
struct A(usize);
#[derive(Eq, PartialEq, Debug)]
struct B(usize);
let mut world = World::default();
world.insert_resource(A(42));
world.spawn().insert(B(7));
// we get nice lifetime elision when declaring the type on the left hand side
let mut system_state: SystemState<(Res<A>, Query<&B>)> = SystemState::new(&mut world);
let (a, query) = system_state.get(&world);
assert_eq!(*a, A(42), "returned resource matches initial value");
assert_eq!(
*query.single().unwrap(),
B(7),
"returned component matches initial value"
);
// mutable system params require unique world access
let mut system_state: SystemState<(ResMut<A>, Query<&mut B>)> = SystemState::new(&mut world);
let (a, query) = system_state.get_mut(&mut world);
// static lifetimes are required when declaring inside of structs
struct SomeContainer {
state: SystemState<(Res<'static, A>, Res<'static, B>)>
}
// this can be shortened using type aliases, which will be useful for complex param tuples
type MyParams<'a> = (Res<'a, A>, Res<'a, B>);
struct SomeContainer {
state: SystemState<MyParams<'static>>
}
// It is the user's responsibility to call SystemState::apply(world) for parameters that queue up work
let mut system_state: SystemState<(Commands, Query<&B>)> = SystemState::new(&mut world);
{
let (mut commands, query) = system_state.get(&world);
commands.insert_resource(3.14);
}
system_state.apply(&mut world);
```
## Future Work
* Actually use SystemState inside FunctionSystem. This would be trivial, but it requires FunctionSystem to wrap SystemState in Option in its current form (which complicates system metadata lookup). I'd prefer to hold off until we adopt something like the later designs linked in #1364, which enable us to contruct Systems using a World reference (and also remove the need for `.system`).
* Consider a "scoped" approach to automatically call SystemState::apply when systems params are no longer being used (either a container type with a Drop impl, or a function that takes a closure for user logic operating on params).
2021-06-02 19:57:38 +00:00
system_meta
Bevy ECS V2 (#1525)
# Bevy ECS V2
This is a rewrite of Bevy ECS (basically everything but the new executor/schedule, which are already awesome). The overall goal was to improve the performance and versatility of Bevy ECS. Here is a quick bulleted list of changes before we dive into the details:
* Complete World rewrite
* Multiple component storage types:
* Tables: fast cache friendly iteration, slower add/removes (previously called Archetypes)
* Sparse Sets: fast add/remove, slower iteration
* Stateful Queries (caches query results for faster iteration. fragmented iteration is _fast_ now)
* Stateful System Params (caches expensive operations. inspired by @DJMcNab's work in #1364)
* Configurable System Params (users can set configuration when they construct their systems. once again inspired by @DJMcNab's work)
* Archetypes are now "just metadata", component storage is separate
* Archetype Graph (for faster archetype changes)
* Component Metadata
* Configure component storage type
* Retrieve information about component size/type/name/layout/send-ness/etc
* Components are uniquely identified by a densely packed ComponentId
* TypeIds are now totally optional (which should make implementing scripting easier)
* Super fast "for_each" query iterators
* Merged Resources into World. Resources are now just a special type of component
* EntityRef/EntityMut builder apis (more efficient and more ergonomic)
* Fast bitset-backed `Access<T>` replaces old hashmap-based approach everywhere
* Query conflicts are determined by component access instead of archetype component access (to avoid random failures at runtime)
* With/Without are still taken into account for conflicts, so this should still be comfy to use
* Much simpler `IntoSystem` impl
* Significantly reduced the amount of hashing throughout the ecs in favor of Sparse Sets (indexed by densely packed ArchetypeId, ComponentId, BundleId, and TableId)
* Safety Improvements
* Entity reservation uses a normal world reference instead of unsafe transmute
* QuerySets no longer transmute lifetimes
* Made traits "unsafe" where relevant
* More thorough safety docs
* WorldCell
* Exposes safe mutable access to multiple resources at a time in a World
* Replaced "catch all" `System::update_archetypes(world: &World)` with `System::new_archetype(archetype: &Archetype)`
* Simpler Bundle implementation
* Replaced slow "remove_bundle_one_by_one" used as fallback for Commands::remove_bundle with fast "remove_bundle_intersection"
* Removed `Mut<T>` query impl. it is better to only support one way: `&mut T`
* Removed with() from `Flags<T>` in favor of `Option<Flags<T>>`, which allows querying for flags to be "filtered" by default
* Components now have is_send property (currently only resources support non-send)
* More granular module organization
* New `RemovedComponents<T>` SystemParam that replaces `query.removed::<T>()`
* `world.resource_scope()` for mutable access to resources and world at the same time
* WorldQuery and QueryFilter traits unified. FilterFetch trait added to enable "short circuit" filtering. Auto impled for cases that don't need it
* Significantly slimmed down SystemState in favor of individual SystemParam state
* System Commands changed from `commands: &mut Commands` back to `mut commands: Commands` (to allow Commands to have a World reference)
Fixes #1320
## `World` Rewrite
This is a from-scratch rewrite of `World` that fills the niche that `hecs` used to. Yes, this means Bevy ECS is no longer a "fork" of hecs. We're going out our own!
(the only shared code between the projects is the entity id allocator, which is already basically ideal)
A huge shout out to @SanderMertens (author of [flecs](https://github.com/SanderMertens/flecs)) for sharing some great ideas with me (specifically hybrid ecs storage and archetype graphs). He also helped advise on a number of implementation details.
## Component Storage (The Problem)
Two ECS storage paradigms have gained a lot of traction over the years:
* **Archetypal ECS**:
* Stores components in "tables" with static schemas. Each "column" stores components of a given type. Each "row" is an entity.
* Each "archetype" has its own table. Adding/removing an entity's component changes the archetype.
* Enables super-fast Query iteration due to its cache-friendly data layout
* Comes at the cost of more expensive add/remove operations for an Entity's components, because all components need to be copied to the new archetype's "table"
* **Sparse Set ECS**:
* Stores components of the same type in densely packed arrays, which are sparsely indexed by densely packed unsigned integers (Entity ids)
* Query iteration is slower than Archetypal ECS because each entity's component could be at any position in the sparse set. This "random access" pattern isn't cache friendly. Additionally, there is an extra layer of indirection because you must first map the entity id to an index in the component array.
* Adding/removing components is a cheap, constant time operation
Bevy ECS V1, hecs, legion, flec, and Unity DOTS are all "archetypal ecs-es". I personally think "archetypal" storage is a good default for game engines. An entity's archetype doesn't need to change frequently in general, and it creates "fast by default" query iteration (which is a much more common operation). It is also "self optimizing". Users don't need to think about optimizing component layouts for iteration performance. It "just works" without any extra boilerplate.
Shipyard and EnTT are "sparse set ecs-es". They employ "packing" as a way to work around the "suboptimal by default" iteration performance for specific sets of components. This helps, but I didn't think this was a good choice for a general purpose engine like Bevy because:
1. "packs" conflict with each other. If bevy decides to internally pack the Transform and GlobalTransform components, users are then blocked if they want to pack some custom component with Transform.
2. users need to take manual action to optimize
Developers selecting an ECS framework are stuck with a hard choice. Select an "archetypal" framework with "fast iteration everywhere" but without the ability to cheaply add/remove components, or select a "sparse set" framework to cheaply add/remove components but with slower iteration performance.
## Hybrid Component Storage (The Solution)
In Bevy ECS V2, we get to have our cake and eat it too. It now has _both_ of the component storage types above (and more can be added later if needed):
* **Tables** (aka "archetypal" storage)
* The default storage. If you don't configure anything, this is what you get
* Fast iteration by default
* Slower add/remove operations
* **Sparse Sets**
* Opt-in
* Slower iteration
* Faster add/remove operations
These storage types complement each other perfectly. By default Query iteration is fast. If developers know that they want to add/remove a component at high frequencies, they can set the storage to "sparse set":
```rust
world.register_component(
ComponentDescriptor::new::<MyComponent>(StorageType::SparseSet)
).unwrap();
```
## Archetypes
Archetypes are now "just metadata" ... they no longer store components directly. They do store:
* The `ComponentId`s of each of the Archetype's components (and that component's storage type)
* Archetypes are uniquely defined by their component layouts
* For example: entities with "table" components `[A, B, C]` _and_ "sparse set" components `[D, E]` will always be in the same archetype.
* The `TableId` associated with the archetype
* For now each archetype has exactly one table (which can have no components),
* There is a 1->Many relationship from Tables->Archetypes. A given table could have any number of archetype components stored in it:
* Ex: an entity with "table storage" components `[A, B, C]` and "sparse set" components `[D, E]` will share the same `[A, B, C]` table as an entity with `[A, B, C]` table component and `[F]` sparse set components.
* This 1->Many relationship is how we preserve fast "cache friendly" iteration performance when possible (more on this later)
* A list of entities that are in the archetype and the row id of the table they are in
* ArchetypeComponentIds
* unique densely packed identifiers for (ArchetypeId, ComponentId) pairs
* used by the schedule executor for cheap system access control
* "Archetype Graph Edges" (see the next section)
## The "Archetype Graph"
Archetype changes in Bevy (and a number of other archetypal ecs-es) have historically been expensive to compute. First, you need to allocate a new vector of the entity's current component ids, add or remove components based on the operation performed, sort it (to ensure it is order-independent), then hash it to find the archetype (if it exists). And thats all before we get to the _already_ expensive full copy of all components to the new table storage.
The solution is to build a "graph" of archetypes to cache these results. @SanderMertens first exposed me to the idea (and he got it from @gjroelofs, who came up with it). They propose adding directed edges between archetypes for add/remove component operations. If `ComponentId`s are densely packed, you can use sparse sets to cheaply jump between archetypes.
Bevy takes this one step further by using add/remove `Bundle` edges instead of `Component` edges. Bevy encourages the use of `Bundles` to group add/remove operations. This is largely for "clearer game logic" reasons, but it also helps cut down on the number of archetype changes required. `Bundles` now also have densely-packed `BundleId`s. This allows us to use a _single_ edge for each bundle operation (rather than needing to traverse N edges ... one for each component). Single component operations are also bundles, so this is strictly an improvement over a "component only" graph.
As a result, an operation that used to be _heavy_ (both for allocations and compute) is now two dirt-cheap array lookups and zero allocations.
## Stateful Queries
World queries are now stateful. This allows us to:
1. Cache archetype (and table) matches
* This resolves another issue with (naive) archetypal ECS: query performance getting worse as the number of archetypes goes up (and fragmentation occurs).
2. Cache Fetch and Filter state
* The expensive parts of fetch/filter operations (such as hashing the TypeId to find the ComponentId) now only happen once when the Query is first constructed
3. Incrementally build up state
* When new archetypes are added, we only process the new archetypes (no need to rebuild state for old archetypes)
As a result, the direct `World` query api now looks like this:
```rust
let mut query = world.query::<(&A, &mut B)>();
for (a, mut b) in query.iter_mut(&mut world) {
}
```
Requiring `World` to generate stateful queries (rather than letting the `QueryState` type be constructed separately) allows us to ensure that _all_ queries are properly initialized (and the relevant world state, such as ComponentIds). This enables QueryState to remove branches from its operations that check for initialization status (and also enables query.iter() to take an immutable world reference because it doesn't need to initialize anything in world).
However in systems, this is a non-breaking change. State management is done internally by the relevant SystemParam.
## Stateful SystemParams
Like Queries, `SystemParams` now also cache state. For example, `Query` system params store the "stateful query" state mentioned above. Commands store their internal `CommandQueue`. This means you can now safely use as many separate `Commands` parameters in your system as you want. `Local<T>` system params store their `T` value in their state (instead of in Resources).
SystemParam state also enabled a significant slim-down of SystemState. It is much nicer to look at now.
Per-SystemParam state naturally insulates us from an "aliased mut" class of errors we have hit in the past (ex: using multiple `Commands` system params).
(credit goes to @DJMcNab for the initial idea and draft pr here #1364)
## Configurable SystemParams
@DJMcNab also had the great idea to make SystemParams configurable. This allows users to provide some initial configuration / values for system parameters (when possible). Most SystemParams have no config (the config type is `()`), but the `Local<T>` param now supports user-provided parameters:
```rust
fn foo(value: Local<usize>) {
}
app.add_system(foo.system().config(|c| c.0 = Some(10)));
```
## Uber Fast "for_each" Query Iterators
Developers now have the choice to use a fast "for_each" iterator, which yields ~1.5-3x iteration speed improvements for "fragmented iteration", and minor ~1.2x iteration speed improvements for unfragmented iteration.
```rust
fn system(query: Query<(&A, &mut B)>) {
// you now have the option to do this for a speed boost
query.for_each_mut(|(a, mut b)| {
});
// however normal iterators are still available
for (a, mut b) in query.iter_mut() {
}
}
```
I think in most cases we should continue to encourage "normal" iterators as they are more flexible and more "rust idiomatic". But when that extra "oomf" is needed, it makes sense to use `for_each`.
We should also consider using `for_each` for internal bevy systems to give our users a nice speed boost (but that should be a separate pr).
## Component Metadata
`World` now has a `Components` collection, which is accessible via `world.components()`. This stores mappings from `ComponentId` to `ComponentInfo`, as well as `TypeId` to `ComponentId` mappings (where relevant). `ComponentInfo` stores information about the component, such as ComponentId, TypeId, memory layout, send-ness (currently limited to resources), and storage type.
## Significantly Cheaper `Access<T>`
We used to use `TypeAccess<TypeId>` to manage read/write component/archetype-component access. This was expensive because TypeIds must be hashed and compared individually. The parallel executor got around this by "condensing" type ids into bitset-backed access types. This worked, but it had to be re-generated from the `TypeAccess<TypeId>`sources every time archetypes changed.
This pr removes TypeAccess in favor of faster bitset access everywhere. We can do this thanks to the move to densely packed `ComponentId`s and `ArchetypeComponentId`s.
## Merged Resources into World
Resources had a lot of redundant functionality with Components. They stored typed data, they had access control, they had unique ids, they were queryable via SystemParams, etc. In fact the _only_ major difference between them was that they were unique (and didn't correlate to an entity).
Separate resources also had the downside of requiring a separate set of access controls, which meant the parallel executor needed to compare more bitsets per system and manage more state.
I initially got the "separate resources" idea from `legion`. I think that design was motivated by the fact that it made the direct world query/resource lifetime interactions more manageable. It certainly made our lives easier when using Resources alongside hecs/bevy_ecs. However we already have a construct for safely and ergonomically managing in-world lifetimes: systems (which use `Access<T>` internally).
This pr merges Resources into World:
```rust
world.insert_resource(1);
world.insert_resource(2.0);
let a = world.get_resource::<i32>().unwrap();
let mut b = world.get_resource_mut::<f64>().unwrap();
*b = 3.0;
```
Resources are now just a special kind of component. They have their own ComponentIds (and their own resource TypeId->ComponentId scope, so they don't conflict wit components of the same type). They are stored in a special "resource archetype", which stores components inside the archetype using a new `unique_components` sparse set (note that this sparse set could later be used to implement Tags). This allows us to keep the code size small by reusing existing datastructures (namely Column, Archetype, ComponentFlags, and ComponentInfo). This allows us the executor to use a single `Access<ArchetypeComponentId>` per system. It should also make scripting language integration easier.
_But_ this merge did create problems for people directly interacting with `World`. What if you need mutable access to multiple resources at the same time? `world.get_resource_mut()` borrows World mutably!
## WorldCell
WorldCell applies the `Access<ArchetypeComponentId>` concept to direct world access:
```rust
let world_cell = world.cell();
let a = world_cell.get_resource_mut::<i32>().unwrap();
let b = world_cell.get_resource_mut::<f64>().unwrap();
```
This adds cheap runtime checks (a sparse set lookup of `ArchetypeComponentId` and a counter) to ensure that world accesses do not conflict with each other. Each operation returns a `WorldBorrow<'w, T>` or `WorldBorrowMut<'w, T>` wrapper type, which will release the relevant ArchetypeComponentId resources when dropped.
World caches the access sparse set (and only one cell can exist at a time), so `world.cell()` is a cheap operation.
WorldCell does _not_ use atomic operations. It is non-send, does a mutable borrow of world to prevent other accesses, and uses a simple `Rc<RefCell<ArchetypeComponentAccess>>` wrapper in each WorldBorrow pointer.
The api is currently limited to resource access, but it can and should be extended to queries / entity component access.
## Resource Scopes
WorldCell does not yet support component queries, and even when it does there are sometimes legitimate reasons to want a mutable world ref _and_ a mutable resource ref (ex: bevy_render and bevy_scene both need this). In these cases we could always drop down to the unsafe `world.get_resource_unchecked_mut()`, but that is not ideal!
Instead developers can use a "resource scope"
```rust
world.resource_scope(|world: &mut World, a: &mut A| {
})
```
This temporarily removes the `A` resource from `World`, provides mutable pointers to both, and re-adds A to World when finished. Thanks to the move to ComponentIds/sparse sets, this is a cheap operation.
If multiple resources are required, scopes can be nested. We could also consider adding a "resource tuple" to the api if this pattern becomes common and the boilerplate gets nasty.
## Query Conflicts Use ComponentId Instead of ArchetypeComponentId
For safety reasons, systems cannot contain queries that conflict with each other without wrapping them in a QuerySet. On bevy `main`, we use ArchetypeComponentIds to determine conflicts. This is nice because it can take into account filters:
```rust
// these queries will never conflict due to their filters
fn filter_system(a: Query<&mut A, With<B>>, b: Query<&mut B, Without<B>>) {
}
```
But it also has a significant downside:
```rust
// these queries will not conflict _until_ an entity with A, B, and C is spawned
fn maybe_conflicts_system(a: Query<(&mut A, &C)>, b: Query<(&mut A, &B)>) {
}
```
The system above will panic at runtime if an entity with A, B, and C is spawned. This makes it hard to trust that your game logic will run without crashing.
In this pr, I switched to using `ComponentId` instead. This _is_ more constraining. `maybe_conflicts_system` will now always fail, but it will do it consistently at startup. Naively, it would also _disallow_ `filter_system`, which would be a significant downgrade in usability. Bevy has a number of internal systems that rely on disjoint queries and I expect it to be a common pattern in userspace.
To resolve this, I added a new `FilteredAccess<T>` type, which wraps `Access<T>` and adds with/without filters. If two `FilteredAccess` have with/without values that prove they are disjoint, they will no longer conflict.
## EntityRef / EntityMut
World entity operations on `main` require that the user passes in an `entity` id to each operation:
```rust
let entity = world.spawn((A, )); // create a new entity with A
world.get::<A>(entity);
world.insert(entity, (B, C));
world.insert_one(entity, D);
```
This means that each operation needs to look up the entity location / verify its validity. The initial spawn operation also requires a Bundle as input. This can be awkward when no components are required (or one component is required).
These operations have been replaced by `EntityRef` and `EntityMut`, which are "builder-style" wrappers around world that provide read and read/write operations on a single, pre-validated entity:
```rust
// spawn now takes no inputs and returns an EntityMut
let entity = world.spawn()
.insert(A) // insert a single component into the entity
.insert_bundle((B, C)) // insert a bundle of components into the entity
.id() // id returns the Entity id
// Returns EntityMut (or panics if the entity does not exist)
world.entity_mut(entity)
.insert(D)
.insert_bundle(SomeBundle::default());
{
// returns EntityRef (or panics if the entity does not exist)
let d = world.entity(entity)
.get::<D>() // gets the D component
.unwrap();
// world.get still exists for ergonomics
let d = world.get::<D>(entity).unwrap();
}
// These variants return Options if you want to check existence instead of panicing
world.get_entity_mut(entity)
.unwrap()
.insert(E);
if let Some(entity_ref) = world.get_entity(entity) {
let d = entity_ref.get::<D>().unwrap();
}
```
This _does not_ affect the current Commands api or terminology. I think that should be a separate conversation as that is a much larger breaking change.
## Safety Improvements
* Entity reservation in Commands uses a normal world borrow instead of an unsafe transmute
* QuerySets no longer transmutes lifetimes
* Made traits "unsafe" when implementing a trait incorrectly could cause unsafety
* More thorough safety docs
## RemovedComponents SystemParam
The old approach to querying removed components: `query.removed:<T>()` was confusing because it had no connection to the query itself. I replaced it with the following, which is both clearer and allows us to cache the ComponentId mapping in the SystemParamState:
```rust
fn system(removed: RemovedComponents<T>) {
for entity in removed.iter() {
}
}
```
## Simpler Bundle implementation
Bundles are no longer responsible for sorting (or deduping) TypeInfo. They are just a simple ordered list of component types / data. This makes the implementation smaller and opens the door to an easy "nested bundle" implementation in the future (which i might even add in this pr). Duplicate detection is now done once per bundle type by World the first time a bundle is used.
## Unified WorldQuery and QueryFilter types
(don't worry they are still separate type _parameters_ in Queries .. this is a non-breaking change)
WorldQuery and QueryFilter were already basically identical apis. With the addition of `FetchState` and more storage-specific fetch methods, the overlap was even clearer (and the redundancy more painful).
QueryFilters are now just `F: WorldQuery where F::Fetch: FilterFetch`. FilterFetch requires `Fetch<Item = bool>` and adds new "short circuit" variants of fetch methods. This enables a filter tuple like `(With<A>, Without<B>, Changed<C>)` to stop evaluating the filter after the first mismatch is encountered. FilterFetch is automatically implemented for `Fetch` implementations that return bool.
This forces fetch implementations that return things like `(bool, bool, bool)` (such as the filter above) to manually implement FilterFetch and decide whether or not to short-circuit.
## More Granular Modules
World no longer globs all of the internal modules together. It now exports `core`, `system`, and `schedule` separately. I'm also considering exporting `core` submodules directly as that is still pretty "glob-ey" and unorganized (feedback welcome here).
## Remaining Draft Work (to be done in this pr)
* ~~panic on conflicting WorldQuery fetches (&A, &mut A)~~
* ~~bevy `main` and hecs both currently allow this, but we should protect against it if possible~~
* ~~batch_iter / par_iter (currently stubbed out)~~
* ~~ChangedRes~~
* ~~I skipped this while we sort out #1313. This pr should be adapted to account for whatever we land on there~~.
* ~~The `Archetypes` and `Tables` collections use hashes of sorted lists of component ids to uniquely identify each archetype/table. This hash is then used as the key in a HashMap to look up the relevant ArchetypeId or TableId. (which doesn't handle hash collisions properly)~~
* ~~It is currently unsafe to generate a Query from "World A", then use it on "World B" (despite the api claiming it is safe). We should probably close this gap. This could be done by adding a randomly generated WorldId to each world, then storing that id in each Query. They could then be compared to each other on each `query.do_thing(&world)` operation. This _does_ add an extra branch to each query operation, so I'm open to other suggestions if people have them.~~
* ~~Nested Bundles (if i find time)~~
## Potential Future Work
* Expand WorldCell to support queries.
* Consider not allocating in the empty archetype on `world.spawn()`
* ex: return something like EntityMutUninit, which turns into EntityMut after an `insert` or `insert_bundle` op
* this actually regressed performance last time i tried it, but in theory it should be faster
* Optimize SparseSet::insert (see `PERF` comment on insert)
* Replace SparseArray `Option<T>` with T::MAX to cut down on branching
* would enable cheaper get_unchecked() operations
* upstream fixedbitset optimizations
* fixedbitset could be allocation free for small block counts (store blocks in a SmallVec)
* fixedbitset could have a const constructor
* Consider implementing Tags (archetype-specific by-value data that affects archetype identity)
* ex: ArchetypeA could have `[A, B, C]` table components and `[D(1)]` "tag" component. ArchetypeB could have `[A, B, C]` table components and a `[D(2)]` tag component. The archetypes are different, despite both having D tags because the value inside D is different.
* this could potentially build on top of the `archetype.unique_components` added in this pr for resource storage.
* Consider reverting `all_tuples` proc macro in favor of the old `macro_rules` implementation
* all_tuples is more flexible and produces cleaner documentation (the macro_rules version produces weird type parameter orders due to parser constraints)
* but unfortunately all_tuples also appears to make Rust Analyzer sad/slow when working inside of `bevy_ecs` (does not affect user code)
* Consider "resource queries" and/or "mixed resource and entity component queries" as an alternative to WorldCell
* this is basically just "systems" so maybe it's not worth it
* Add more world ops
* `world.clear()`
* `world.reserve<T: Bundle>(count: usize)`
* Try using the old archetype allocation strategy (allocate new memory on resize and copy everything over). I expect this to improve batch insertion performance at the cost of unbatched performance. But thats just a guess. I'm not an allocation perf pro :)
* Adapt Commands apis for consistency with new World apis
## Benchmarks
key:
* `bevy_old`: bevy `main` branch
* `bevy`: this branch
* `_foreach`: uses an optimized for_each iterator
* ` _sparse`: uses sparse set storage (if unspecified assume table storage)
* `_system`: runs inside a system (if unspecified assume test happens via direct world ops)
### Simple Insert (from ecs_bench_suite)
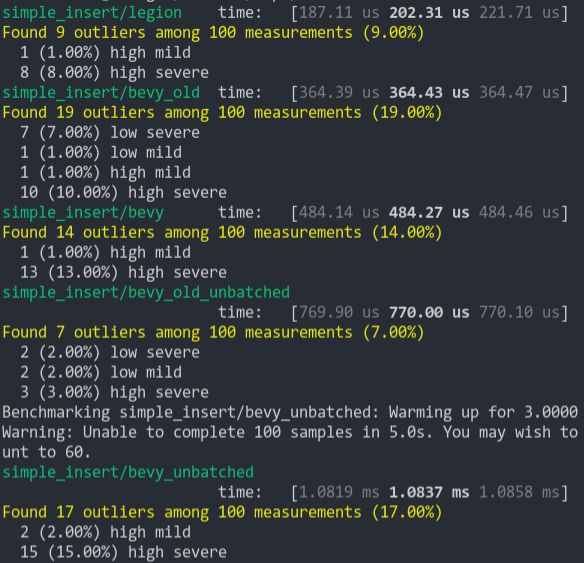
### Simpler Iter (from ecs_bench_suite)
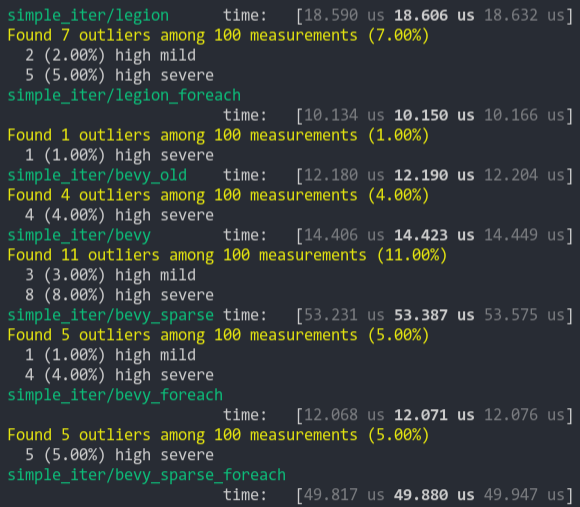
### Fragment Iter (from ecs_bench_suite)
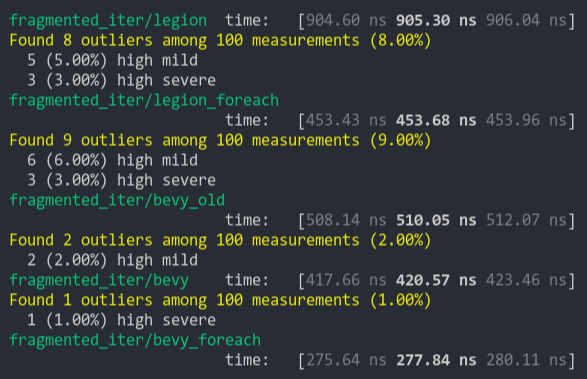
### Sparse Fragmented Iter
Iterate a query that matches 5 entities from a single matching archetype, but there are 100 unmatching archetypes
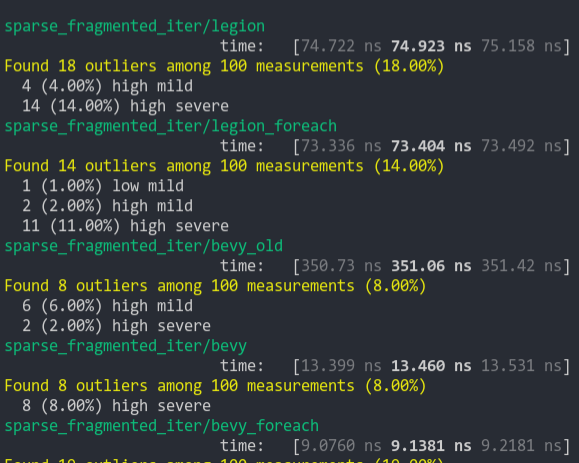
### Schedule (from ecs_bench_suite)
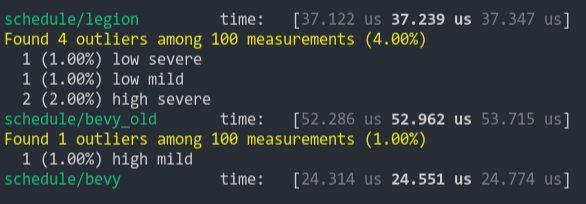
### Add Remove Component (from ecs_bench_suite)
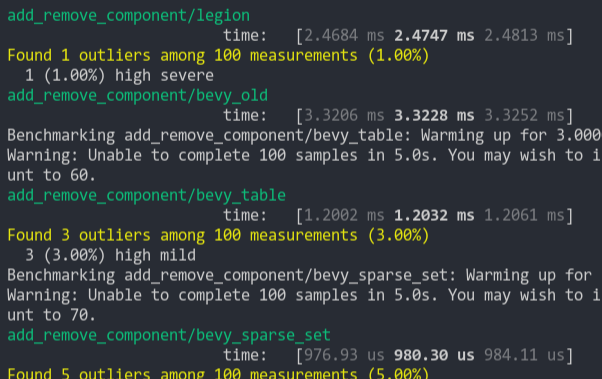
### Add Remove Component Big
Same as the test above, but each entity has 5 "large" matrix components and 1 "large" matrix component is added and removed
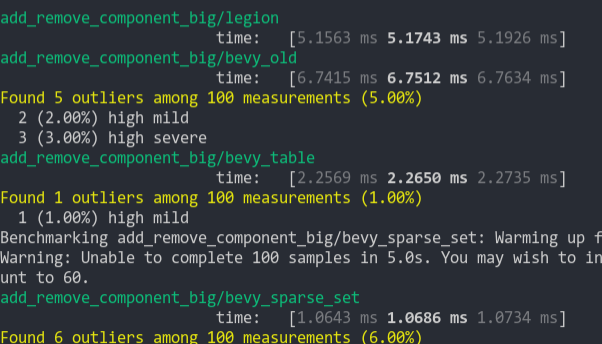
### Get Component
Looks up a single component value a large number of times
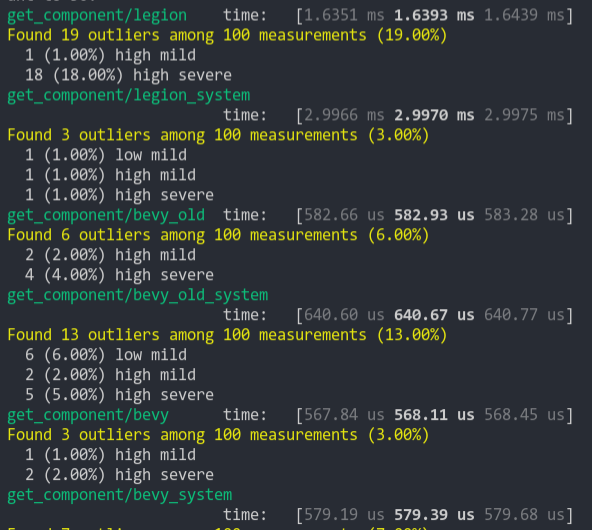
2021-03-05 07:54:35 +00:00
. archetype_component_access
. add_write ( archetype_component_id ) ;
Remove the `SystemParamState` trait and remove types like `ResState` (#6919)
Spiritual successor to #5205.
Actual successor to #6865.
# Objective
Currently, system params are defined using three traits: `SystemParam`, `ReadOnlySystemParam`, `SystemParamState`. The behavior for each param is specified by the `SystemParamState` trait, while `SystemParam` simply defers to the state.
Splitting the traits in this way makes it easier to implement within macros, but it increases the cognitive load. Worst of all, this approach requires each `MySystemParam` to have a public `MySystemParamState` type associated with it.
## Solution
* Merge the trait `SystemParamState` into `SystemParam`.
* Remove all trivial `SystemParam` state types.
* `OptionNonSendMutState<T>`: you will not be missed.
---
- [x] Fix/resolve the remaining test failure.
## Changelog
* Removed the trait `SystemParamState`, merging its functionality into `SystemParam`.
## Migration Guide
**Note**: this should replace the migration guide for #6865.
This is relative to Bevy 0.9, not main.
The traits `SystemParamState` and `SystemParamFetch` have been removed, and their functionality has been transferred to `SystemParam`.
```rust
// Before (0.9)
impl SystemParam for MyParam<'_, '_> {
type State = MyParamState;
}
unsafe impl SystemParamState for MyParamState {
fn init(world: &mut World, system_meta: &mut SystemMeta) -> Self { ... }
}
unsafe impl<'w, 's> SystemParamFetch<'w, 's> for MyParamState {
type Item = MyParam<'w, 's>;
fn get_param(&mut self, ...) -> Self::Item;
}
unsafe impl ReadOnlySystemParamFetch for MyParamState { }
// After (0.10)
unsafe impl SystemParam for MyParam<'_, '_> {
type State = MyParamState;
type Item<'w, 's> = MyParam<'w, 's>;
fn init_state(world: &mut World, system_meta: &mut SystemMeta) -> Self::State { ... }
fn get_param<'w, 's>(state: &mut Self::State, ...) -> Self::Item<'w, 's>;
}
unsafe impl ReadOnlySystemParam for MyParam<'_, '_> { }
```
The trait `ReadOnlySystemParamFetch` has been replaced with `ReadOnlySystemParam`.
```rust
// Before
unsafe impl ReadOnlySystemParamFetch for MyParamState {}
// After
unsafe impl ReadOnlySystemParam for MyParam<'_, '_> {}
```
2023-01-07 23:20:32 +00:00
component_id
2020-11-08 20:34:05 +00:00
}
Bevy ECS V2 (#1525)
# Bevy ECS V2
This is a rewrite of Bevy ECS (basically everything but the new executor/schedule, which are already awesome). The overall goal was to improve the performance and versatility of Bevy ECS. Here is a quick bulleted list of changes before we dive into the details:
* Complete World rewrite
* Multiple component storage types:
* Tables: fast cache friendly iteration, slower add/removes (previously called Archetypes)
* Sparse Sets: fast add/remove, slower iteration
* Stateful Queries (caches query results for faster iteration. fragmented iteration is _fast_ now)
* Stateful System Params (caches expensive operations. inspired by @DJMcNab's work in #1364)
* Configurable System Params (users can set configuration when they construct their systems. once again inspired by @DJMcNab's work)
* Archetypes are now "just metadata", component storage is separate
* Archetype Graph (for faster archetype changes)
* Component Metadata
* Configure component storage type
* Retrieve information about component size/type/name/layout/send-ness/etc
* Components are uniquely identified by a densely packed ComponentId
* TypeIds are now totally optional (which should make implementing scripting easier)
* Super fast "for_each" query iterators
* Merged Resources into World. Resources are now just a special type of component
* EntityRef/EntityMut builder apis (more efficient and more ergonomic)
* Fast bitset-backed `Access<T>` replaces old hashmap-based approach everywhere
* Query conflicts are determined by component access instead of archetype component access (to avoid random failures at runtime)
* With/Without are still taken into account for conflicts, so this should still be comfy to use
* Much simpler `IntoSystem` impl
* Significantly reduced the amount of hashing throughout the ecs in favor of Sparse Sets (indexed by densely packed ArchetypeId, ComponentId, BundleId, and TableId)
* Safety Improvements
* Entity reservation uses a normal world reference instead of unsafe transmute
* QuerySets no longer transmute lifetimes
* Made traits "unsafe" where relevant
* More thorough safety docs
* WorldCell
* Exposes safe mutable access to multiple resources at a time in a World
* Replaced "catch all" `System::update_archetypes(world: &World)` with `System::new_archetype(archetype: &Archetype)`
* Simpler Bundle implementation
* Replaced slow "remove_bundle_one_by_one" used as fallback for Commands::remove_bundle with fast "remove_bundle_intersection"
* Removed `Mut<T>` query impl. it is better to only support one way: `&mut T`
* Removed with() from `Flags<T>` in favor of `Option<Flags<T>>`, which allows querying for flags to be "filtered" by default
* Components now have is_send property (currently only resources support non-send)
* More granular module organization
* New `RemovedComponents<T>` SystemParam that replaces `query.removed::<T>()`
* `world.resource_scope()` for mutable access to resources and world at the same time
* WorldQuery and QueryFilter traits unified. FilterFetch trait added to enable "short circuit" filtering. Auto impled for cases that don't need it
* Significantly slimmed down SystemState in favor of individual SystemParam state
* System Commands changed from `commands: &mut Commands` back to `mut commands: Commands` (to allow Commands to have a World reference)
Fixes #1320
## `World` Rewrite
This is a from-scratch rewrite of `World` that fills the niche that `hecs` used to. Yes, this means Bevy ECS is no longer a "fork" of hecs. We're going out our own!
(the only shared code between the projects is the entity id allocator, which is already basically ideal)
A huge shout out to @SanderMertens (author of [flecs](https://github.com/SanderMertens/flecs)) for sharing some great ideas with me (specifically hybrid ecs storage and archetype graphs). He also helped advise on a number of implementation details.
## Component Storage (The Problem)
Two ECS storage paradigms have gained a lot of traction over the years:
* **Archetypal ECS**:
* Stores components in "tables" with static schemas. Each "column" stores components of a given type. Each "row" is an entity.
* Each "archetype" has its own table. Adding/removing an entity's component changes the archetype.
* Enables super-fast Query iteration due to its cache-friendly data layout
* Comes at the cost of more expensive add/remove operations for an Entity's components, because all components need to be copied to the new archetype's "table"
* **Sparse Set ECS**:
* Stores components of the same type in densely packed arrays, which are sparsely indexed by densely packed unsigned integers (Entity ids)
* Query iteration is slower than Archetypal ECS because each entity's component could be at any position in the sparse set. This "random access" pattern isn't cache friendly. Additionally, there is an extra layer of indirection because you must first map the entity id to an index in the component array.
* Adding/removing components is a cheap, constant time operation
Bevy ECS V1, hecs, legion, flec, and Unity DOTS are all "archetypal ecs-es". I personally think "archetypal" storage is a good default for game engines. An entity's archetype doesn't need to change frequently in general, and it creates "fast by default" query iteration (which is a much more common operation). It is also "self optimizing". Users don't need to think about optimizing component layouts for iteration performance. It "just works" without any extra boilerplate.
Shipyard and EnTT are "sparse set ecs-es". They employ "packing" as a way to work around the "suboptimal by default" iteration performance for specific sets of components. This helps, but I didn't think this was a good choice for a general purpose engine like Bevy because:
1. "packs" conflict with each other. If bevy decides to internally pack the Transform and GlobalTransform components, users are then blocked if they want to pack some custom component with Transform.
2. users need to take manual action to optimize
Developers selecting an ECS framework are stuck with a hard choice. Select an "archetypal" framework with "fast iteration everywhere" but without the ability to cheaply add/remove components, or select a "sparse set" framework to cheaply add/remove components but with slower iteration performance.
## Hybrid Component Storage (The Solution)
In Bevy ECS V2, we get to have our cake and eat it too. It now has _both_ of the component storage types above (and more can be added later if needed):
* **Tables** (aka "archetypal" storage)
* The default storage. If you don't configure anything, this is what you get
* Fast iteration by default
* Slower add/remove operations
* **Sparse Sets**
* Opt-in
* Slower iteration
* Faster add/remove operations
These storage types complement each other perfectly. By default Query iteration is fast. If developers know that they want to add/remove a component at high frequencies, they can set the storage to "sparse set":
```rust
world.register_component(
ComponentDescriptor::new::<MyComponent>(StorageType::SparseSet)
).unwrap();
```
## Archetypes
Archetypes are now "just metadata" ... they no longer store components directly. They do store:
* The `ComponentId`s of each of the Archetype's components (and that component's storage type)
* Archetypes are uniquely defined by their component layouts
* For example: entities with "table" components `[A, B, C]` _and_ "sparse set" components `[D, E]` will always be in the same archetype.
* The `TableId` associated with the archetype
* For now each archetype has exactly one table (which can have no components),
* There is a 1->Many relationship from Tables->Archetypes. A given table could have any number of archetype components stored in it:
* Ex: an entity with "table storage" components `[A, B, C]` and "sparse set" components `[D, E]` will share the same `[A, B, C]` table as an entity with `[A, B, C]` table component and `[F]` sparse set components.
* This 1->Many relationship is how we preserve fast "cache friendly" iteration performance when possible (more on this later)
* A list of entities that are in the archetype and the row id of the table they are in
* ArchetypeComponentIds
* unique densely packed identifiers for (ArchetypeId, ComponentId) pairs
* used by the schedule executor for cheap system access control
* "Archetype Graph Edges" (see the next section)
## The "Archetype Graph"
Archetype changes in Bevy (and a number of other archetypal ecs-es) have historically been expensive to compute. First, you need to allocate a new vector of the entity's current component ids, add or remove components based on the operation performed, sort it (to ensure it is order-independent), then hash it to find the archetype (if it exists). And thats all before we get to the _already_ expensive full copy of all components to the new table storage.
The solution is to build a "graph" of archetypes to cache these results. @SanderMertens first exposed me to the idea (and he got it from @gjroelofs, who came up with it). They propose adding directed edges between archetypes for add/remove component operations. If `ComponentId`s are densely packed, you can use sparse sets to cheaply jump between archetypes.
Bevy takes this one step further by using add/remove `Bundle` edges instead of `Component` edges. Bevy encourages the use of `Bundles` to group add/remove operations. This is largely for "clearer game logic" reasons, but it also helps cut down on the number of archetype changes required. `Bundles` now also have densely-packed `BundleId`s. This allows us to use a _single_ edge for each bundle operation (rather than needing to traverse N edges ... one for each component). Single component operations are also bundles, so this is strictly an improvement over a "component only" graph.
As a result, an operation that used to be _heavy_ (both for allocations and compute) is now two dirt-cheap array lookups and zero allocations.
## Stateful Queries
World queries are now stateful. This allows us to:
1. Cache archetype (and table) matches
* This resolves another issue with (naive) archetypal ECS: query performance getting worse as the number of archetypes goes up (and fragmentation occurs).
2. Cache Fetch and Filter state
* The expensive parts of fetch/filter operations (such as hashing the TypeId to find the ComponentId) now only happen once when the Query is first constructed
3. Incrementally build up state
* When new archetypes are added, we only process the new archetypes (no need to rebuild state for old archetypes)
As a result, the direct `World` query api now looks like this:
```rust
let mut query = world.query::<(&A, &mut B)>();
for (a, mut b) in query.iter_mut(&mut world) {
}
```
Requiring `World` to generate stateful queries (rather than letting the `QueryState` type be constructed separately) allows us to ensure that _all_ queries are properly initialized (and the relevant world state, such as ComponentIds). This enables QueryState to remove branches from its operations that check for initialization status (and also enables query.iter() to take an immutable world reference because it doesn't need to initialize anything in world).
However in systems, this is a non-breaking change. State management is done internally by the relevant SystemParam.
## Stateful SystemParams
Like Queries, `SystemParams` now also cache state. For example, `Query` system params store the "stateful query" state mentioned above. Commands store their internal `CommandQueue`. This means you can now safely use as many separate `Commands` parameters in your system as you want. `Local<T>` system params store their `T` value in their state (instead of in Resources).
SystemParam state also enabled a significant slim-down of SystemState. It is much nicer to look at now.
Per-SystemParam state naturally insulates us from an "aliased mut" class of errors we have hit in the past (ex: using multiple `Commands` system params).
(credit goes to @DJMcNab for the initial idea and draft pr here #1364)
## Configurable SystemParams
@DJMcNab also had the great idea to make SystemParams configurable. This allows users to provide some initial configuration / values for system parameters (when possible). Most SystemParams have no config (the config type is `()`), but the `Local<T>` param now supports user-provided parameters:
```rust
fn foo(value: Local<usize>) {
}
app.add_system(foo.system().config(|c| c.0 = Some(10)));
```
## Uber Fast "for_each" Query Iterators
Developers now have the choice to use a fast "for_each" iterator, which yields ~1.5-3x iteration speed improvements for "fragmented iteration", and minor ~1.2x iteration speed improvements for unfragmented iteration.
```rust
fn system(query: Query<(&A, &mut B)>) {
// you now have the option to do this for a speed boost
query.for_each_mut(|(a, mut b)| {
});
// however normal iterators are still available
for (a, mut b) in query.iter_mut() {
}
}
```
I think in most cases we should continue to encourage "normal" iterators as they are more flexible and more "rust idiomatic". But when that extra "oomf" is needed, it makes sense to use `for_each`.
We should also consider using `for_each` for internal bevy systems to give our users a nice speed boost (but that should be a separate pr).
## Component Metadata
`World` now has a `Components` collection, which is accessible via `world.components()`. This stores mappings from `ComponentId` to `ComponentInfo`, as well as `TypeId` to `ComponentId` mappings (where relevant). `ComponentInfo` stores information about the component, such as ComponentId, TypeId, memory layout, send-ness (currently limited to resources), and storage type.
## Significantly Cheaper `Access<T>`
We used to use `TypeAccess<TypeId>` to manage read/write component/archetype-component access. This was expensive because TypeIds must be hashed and compared individually. The parallel executor got around this by "condensing" type ids into bitset-backed access types. This worked, but it had to be re-generated from the `TypeAccess<TypeId>`sources every time archetypes changed.
This pr removes TypeAccess in favor of faster bitset access everywhere. We can do this thanks to the move to densely packed `ComponentId`s and `ArchetypeComponentId`s.
## Merged Resources into World
Resources had a lot of redundant functionality with Components. They stored typed data, they had access control, they had unique ids, they were queryable via SystemParams, etc. In fact the _only_ major difference between them was that they were unique (and didn't correlate to an entity).
Separate resources also had the downside of requiring a separate set of access controls, which meant the parallel executor needed to compare more bitsets per system and manage more state.
I initially got the "separate resources" idea from `legion`. I think that design was motivated by the fact that it made the direct world query/resource lifetime interactions more manageable. It certainly made our lives easier when using Resources alongside hecs/bevy_ecs. However we already have a construct for safely and ergonomically managing in-world lifetimes: systems (which use `Access<T>` internally).
This pr merges Resources into World:
```rust
world.insert_resource(1);
world.insert_resource(2.0);
let a = world.get_resource::<i32>().unwrap();
let mut b = world.get_resource_mut::<f64>().unwrap();
*b = 3.0;
```
Resources are now just a special kind of component. They have their own ComponentIds (and their own resource TypeId->ComponentId scope, so they don't conflict wit components of the same type). They are stored in a special "resource archetype", which stores components inside the archetype using a new `unique_components` sparse set (note that this sparse set could later be used to implement Tags). This allows us to keep the code size small by reusing existing datastructures (namely Column, Archetype, ComponentFlags, and ComponentInfo). This allows us the executor to use a single `Access<ArchetypeComponentId>` per system. It should also make scripting language integration easier.
_But_ this merge did create problems for people directly interacting with `World`. What if you need mutable access to multiple resources at the same time? `world.get_resource_mut()` borrows World mutably!
## WorldCell
WorldCell applies the `Access<ArchetypeComponentId>` concept to direct world access:
```rust
let world_cell = world.cell();
let a = world_cell.get_resource_mut::<i32>().unwrap();
let b = world_cell.get_resource_mut::<f64>().unwrap();
```
This adds cheap runtime checks (a sparse set lookup of `ArchetypeComponentId` and a counter) to ensure that world accesses do not conflict with each other. Each operation returns a `WorldBorrow<'w, T>` or `WorldBorrowMut<'w, T>` wrapper type, which will release the relevant ArchetypeComponentId resources when dropped.
World caches the access sparse set (and only one cell can exist at a time), so `world.cell()` is a cheap operation.
WorldCell does _not_ use atomic operations. It is non-send, does a mutable borrow of world to prevent other accesses, and uses a simple `Rc<RefCell<ArchetypeComponentAccess>>` wrapper in each WorldBorrow pointer.
The api is currently limited to resource access, but it can and should be extended to queries / entity component access.
## Resource Scopes
WorldCell does not yet support component queries, and even when it does there are sometimes legitimate reasons to want a mutable world ref _and_ a mutable resource ref (ex: bevy_render and bevy_scene both need this). In these cases we could always drop down to the unsafe `world.get_resource_unchecked_mut()`, but that is not ideal!
Instead developers can use a "resource scope"
```rust
world.resource_scope(|world: &mut World, a: &mut A| {
})
```
This temporarily removes the `A` resource from `World`, provides mutable pointers to both, and re-adds A to World when finished. Thanks to the move to ComponentIds/sparse sets, this is a cheap operation.
If multiple resources are required, scopes can be nested. We could also consider adding a "resource tuple" to the api if this pattern becomes common and the boilerplate gets nasty.
## Query Conflicts Use ComponentId Instead of ArchetypeComponentId
For safety reasons, systems cannot contain queries that conflict with each other without wrapping them in a QuerySet. On bevy `main`, we use ArchetypeComponentIds to determine conflicts. This is nice because it can take into account filters:
```rust
// these queries will never conflict due to their filters
fn filter_system(a: Query<&mut A, With<B>>, b: Query<&mut B, Without<B>>) {
}
```
But it also has a significant downside:
```rust
// these queries will not conflict _until_ an entity with A, B, and C is spawned
fn maybe_conflicts_system(a: Query<(&mut A, &C)>, b: Query<(&mut A, &B)>) {
}
```
The system above will panic at runtime if an entity with A, B, and C is spawned. This makes it hard to trust that your game logic will run without crashing.
In this pr, I switched to using `ComponentId` instead. This _is_ more constraining. `maybe_conflicts_system` will now always fail, but it will do it consistently at startup. Naively, it would also _disallow_ `filter_system`, which would be a significant downgrade in usability. Bevy has a number of internal systems that rely on disjoint queries and I expect it to be a common pattern in userspace.
To resolve this, I added a new `FilteredAccess<T>` type, which wraps `Access<T>` and adds with/without filters. If two `FilteredAccess` have with/without values that prove they are disjoint, they will no longer conflict.
## EntityRef / EntityMut
World entity operations on `main` require that the user passes in an `entity` id to each operation:
```rust
let entity = world.spawn((A, )); // create a new entity with A
world.get::<A>(entity);
world.insert(entity, (B, C));
world.insert_one(entity, D);
```
This means that each operation needs to look up the entity location / verify its validity. The initial spawn operation also requires a Bundle as input. This can be awkward when no components are required (or one component is required).
These operations have been replaced by `EntityRef` and `EntityMut`, which are "builder-style" wrappers around world that provide read and read/write operations on a single, pre-validated entity:
```rust
// spawn now takes no inputs and returns an EntityMut
let entity = world.spawn()
.insert(A) // insert a single component into the entity
.insert_bundle((B, C)) // insert a bundle of components into the entity
.id() // id returns the Entity id
// Returns EntityMut (or panics if the entity does not exist)
world.entity_mut(entity)
.insert(D)
.insert_bundle(SomeBundle::default());
{
// returns EntityRef (or panics if the entity does not exist)
let d = world.entity(entity)
.get::<D>() // gets the D component
.unwrap();
// world.get still exists for ergonomics
let d = world.get::<D>(entity).unwrap();
}
// These variants return Options if you want to check existence instead of panicing
world.get_entity_mut(entity)
.unwrap()
.insert(E);
if let Some(entity_ref) = world.get_entity(entity) {
let d = entity_ref.get::<D>().unwrap();
}
```
This _does not_ affect the current Commands api or terminology. I think that should be a separate conversation as that is a much larger breaking change.
## Safety Improvements
* Entity reservation in Commands uses a normal world borrow instead of an unsafe transmute
* QuerySets no longer transmutes lifetimes
* Made traits "unsafe" when implementing a trait incorrectly could cause unsafety
* More thorough safety docs
## RemovedComponents SystemParam
The old approach to querying removed components: `query.removed:<T>()` was confusing because it had no connection to the query itself. I replaced it with the following, which is both clearer and allows us to cache the ComponentId mapping in the SystemParamState:
```rust
fn system(removed: RemovedComponents<T>) {
for entity in removed.iter() {
}
}
```
## Simpler Bundle implementation
Bundles are no longer responsible for sorting (or deduping) TypeInfo. They are just a simple ordered list of component types / data. This makes the implementation smaller and opens the door to an easy "nested bundle" implementation in the future (which i might even add in this pr). Duplicate detection is now done once per bundle type by World the first time a bundle is used.
## Unified WorldQuery and QueryFilter types
(don't worry they are still separate type _parameters_ in Queries .. this is a non-breaking change)
WorldQuery and QueryFilter were already basically identical apis. With the addition of `FetchState` and more storage-specific fetch methods, the overlap was even clearer (and the redundancy more painful).
QueryFilters are now just `F: WorldQuery where F::Fetch: FilterFetch`. FilterFetch requires `Fetch<Item = bool>` and adds new "short circuit" variants of fetch methods. This enables a filter tuple like `(With<A>, Without<B>, Changed<C>)` to stop evaluating the filter after the first mismatch is encountered. FilterFetch is automatically implemented for `Fetch` implementations that return bool.
This forces fetch implementations that return things like `(bool, bool, bool)` (such as the filter above) to manually implement FilterFetch and decide whether or not to short-circuit.
## More Granular Modules
World no longer globs all of the internal modules together. It now exports `core`, `system`, and `schedule` separately. I'm also considering exporting `core` submodules directly as that is still pretty "glob-ey" and unorganized (feedback welcome here).
## Remaining Draft Work (to be done in this pr)
* ~~panic on conflicting WorldQuery fetches (&A, &mut A)~~
* ~~bevy `main` and hecs both currently allow this, but we should protect against it if possible~~
* ~~batch_iter / par_iter (currently stubbed out)~~
* ~~ChangedRes~~
* ~~I skipped this while we sort out #1313. This pr should be adapted to account for whatever we land on there~~.
* ~~The `Archetypes` and `Tables` collections use hashes of sorted lists of component ids to uniquely identify each archetype/table. This hash is then used as the key in a HashMap to look up the relevant ArchetypeId or TableId. (which doesn't handle hash collisions properly)~~
* ~~It is currently unsafe to generate a Query from "World A", then use it on "World B" (despite the api claiming it is safe). We should probably close this gap. This could be done by adding a randomly generated WorldId to each world, then storing that id in each Query. They could then be compared to each other on each `query.do_thing(&world)` operation. This _does_ add an extra branch to each query operation, so I'm open to other suggestions if people have them.~~
* ~~Nested Bundles (if i find time)~~
## Potential Future Work
* Expand WorldCell to support queries.
* Consider not allocating in the empty archetype on `world.spawn()`
* ex: return something like EntityMutUninit, which turns into EntityMut after an `insert` or `insert_bundle` op
* this actually regressed performance last time i tried it, but in theory it should be faster
* Optimize SparseSet::insert (see `PERF` comment on insert)
* Replace SparseArray `Option<T>` with T::MAX to cut down on branching
* would enable cheaper get_unchecked() operations
* upstream fixedbitset optimizations
* fixedbitset could be allocation free for small block counts (store blocks in a SmallVec)
* fixedbitset could have a const constructor
* Consider implementing Tags (archetype-specific by-value data that affects archetype identity)
* ex: ArchetypeA could have `[A, B, C]` table components and `[D(1)]` "tag" component. ArchetypeB could have `[A, B, C]` table components and a `[D(2)]` tag component. The archetypes are different, despite both having D tags because the value inside D is different.
* this could potentially build on top of the `archetype.unique_components` added in this pr for resource storage.
* Consider reverting `all_tuples` proc macro in favor of the old `macro_rules` implementation
* all_tuples is more flexible and produces cleaner documentation (the macro_rules version produces weird type parameter orders due to parser constraints)
* but unfortunately all_tuples also appears to make Rust Analyzer sad/slow when working inside of `bevy_ecs` (does not affect user code)
* Consider "resource queries" and/or "mixed resource and entity component queries" as an alternative to WorldCell
* this is basically just "systems" so maybe it's not worth it
* Add more world ops
* `world.clear()`
* `world.reserve<T: Bundle>(count: usize)`
* Try using the old archetype allocation strategy (allocate new memory on resize and copy everything over). I expect this to improve batch insertion performance at the cost of unbatched performance. But thats just a guess. I'm not an allocation perf pro :)
* Adapt Commands apis for consistency with new World apis
## Benchmarks
key:
* `bevy_old`: bevy `main` branch
* `bevy`: this branch
* `_foreach`: uses an optimized for_each iterator
* ` _sparse`: uses sparse set storage (if unspecified assume table storage)
* `_system`: runs inside a system (if unspecified assume test happens via direct world ops)
### Simple Insert (from ecs_bench_suite)
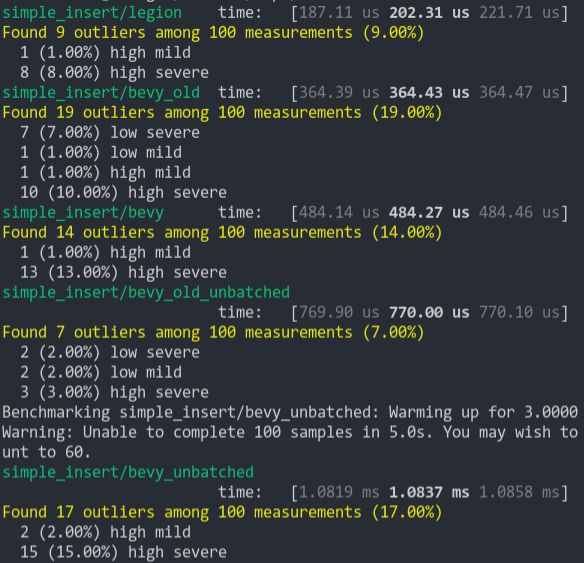
### Simpler Iter (from ecs_bench_suite)
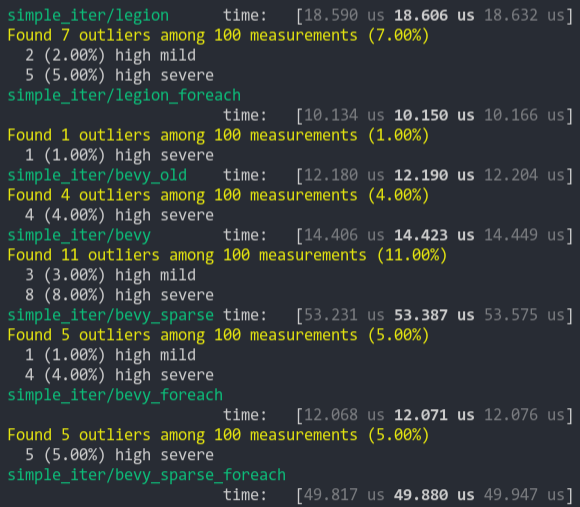
### Fragment Iter (from ecs_bench_suite)
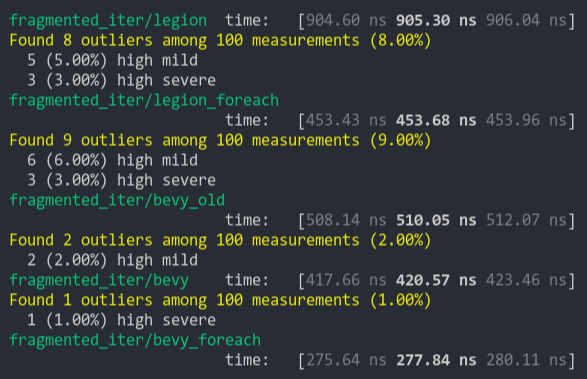
### Sparse Fragmented Iter
Iterate a query that matches 5 entities from a single matching archetype, but there are 100 unmatching archetypes
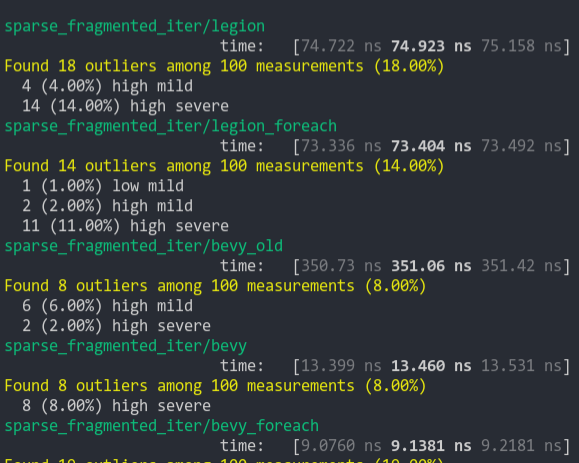
### Schedule (from ecs_bench_suite)
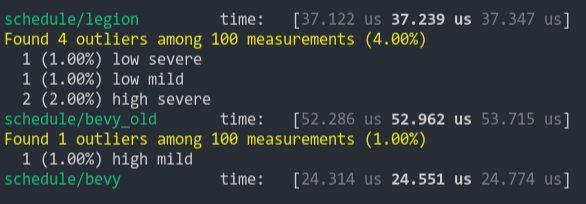
### Add Remove Component (from ecs_bench_suite)
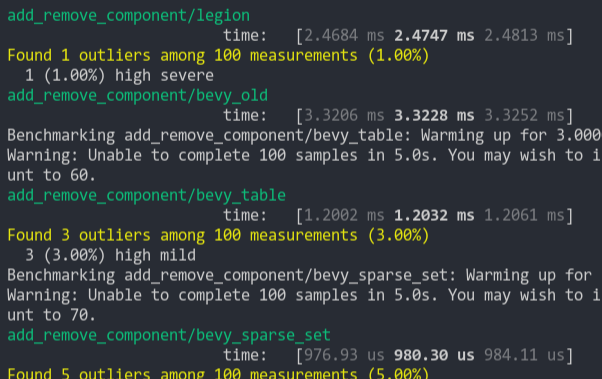
### Add Remove Component Big
Same as the test above, but each entity has 5 "large" matrix components and 1 "large" matrix component is added and removed
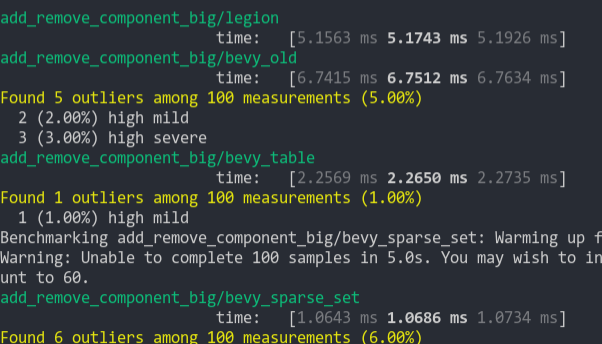
### Get Component
Looks up a single component value a large number of times
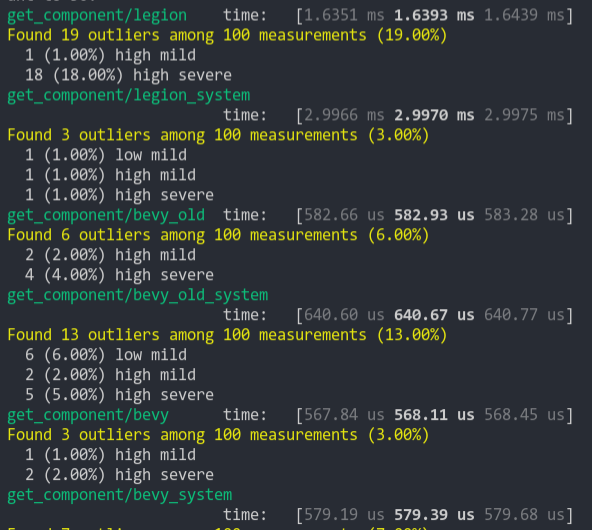
2021-03-05 07:54:35 +00:00
#[ inline ]
Simplify trait hierarchy for `SystemParam` (#6865)
# Objective
* Implementing a custom `SystemParam` by hand requires implementing three traits -- four if it is read-only.
* The trait `SystemParamFetch<'w, 's>` is a workaround from before we had generic associated types, and is no longer necessary.
## Solution
* Combine the trait `SystemParamFetch` with `SystemParamState`.
* I decided to remove the `Fetch` name and keep the `State` name, since the former was consistently conflated with the latter.
* Replace the trait `ReadOnlySystemParamFetch` with `ReadOnlySystemParam`, which simplifies trait bounds in generic code.
---
## Changelog
- Removed the trait `SystemParamFetch`, moving its functionality to `SystemParamState`.
- Replaced the trait `ReadOnlySystemParamFetch` with `ReadOnlySystemParam`.
## Migration Guide
The trait `SystemParamFetch` has been removed, and its functionality has been transferred to `SystemParamState`.
```rust
// Before
impl SystemParamState for MyParamState {
fn init(world: &mut World, system_meta: &mut SystemMeta) -> Self { ... }
}
impl<'w, 's> SystemParamFetch<'w, 's> for MyParamState {
type Item = MyParam<'w, 's>;
fn get_param(...) -> Self::Item;
}
// After
impl SystemParamState for MyParamState {
type Item<'w, 's> = MyParam<'w, 's>; // Generic associated types!
fn init(world: &mut World, system_meta: &mut SystemMeta) -> Self { ... }
fn get_param<'w, 's>(...) -> Self::Item<'w, 's>;
}
```
The trait `ReadOnlySystemParamFetch` has been replaced with `ReadOnlySystemParam`.
```rust
// Before
unsafe impl ReadOnlySystemParamFetch for MyParamState {}
// After
unsafe impl<'w, 's> ReadOnlySystemParam for MyParam<'w, 's> {}
```
2022-12-11 18:34:14 +00:00
unsafe fn get_param < ' w , ' s > (
Remove the `SystemParamState` trait and remove types like `ResState` (#6919)
Spiritual successor to #5205.
Actual successor to #6865.
# Objective
Currently, system params are defined using three traits: `SystemParam`, `ReadOnlySystemParam`, `SystemParamState`. The behavior for each param is specified by the `SystemParamState` trait, while `SystemParam` simply defers to the state.
Splitting the traits in this way makes it easier to implement within macros, but it increases the cognitive load. Worst of all, this approach requires each `MySystemParam` to have a public `MySystemParamState` type associated with it.
## Solution
* Merge the trait `SystemParamState` into `SystemParam`.
* Remove all trivial `SystemParam` state types.
* `OptionNonSendMutState<T>`: you will not be missed.
---
- [x] Fix/resolve the remaining test failure.
## Changelog
* Removed the trait `SystemParamState`, merging its functionality into `SystemParam`.
## Migration Guide
**Note**: this should replace the migration guide for #6865.
This is relative to Bevy 0.9, not main.
The traits `SystemParamState` and `SystemParamFetch` have been removed, and their functionality has been transferred to `SystemParam`.
```rust
// Before (0.9)
impl SystemParam for MyParam<'_, '_> {
type State = MyParamState;
}
unsafe impl SystemParamState for MyParamState {
fn init(world: &mut World, system_meta: &mut SystemMeta) -> Self { ... }
}
unsafe impl<'w, 's> SystemParamFetch<'w, 's> for MyParamState {
type Item = MyParam<'w, 's>;
fn get_param(&mut self, ...) -> Self::Item;
}
unsafe impl ReadOnlySystemParamFetch for MyParamState { }
// After (0.10)
unsafe impl SystemParam for MyParam<'_, '_> {
type State = MyParamState;
type Item<'w, 's> = MyParam<'w, 's>;
fn init_state(world: &mut World, system_meta: &mut SystemMeta) -> Self::State { ... }
fn get_param<'w, 's>(state: &mut Self::State, ...) -> Self::Item<'w, 's>;
}
unsafe impl ReadOnlySystemParam for MyParam<'_, '_> { }
```
The trait `ReadOnlySystemParamFetch` has been replaced with `ReadOnlySystemParam`.
```rust
// Before
unsafe impl ReadOnlySystemParamFetch for MyParamState {}
// After
unsafe impl ReadOnlySystemParam for MyParam<'_, '_> {}
```
2023-01-07 23:20:32 +00:00
& mut component_id : & ' s mut Self ::State ,
Add new SystemState and rename old SystemState to SystemMeta (#2283)
This enables `SystemParams` to be used outside of function systems. Anything can create and store `SystemState`, which enables efficient "param state cached" access to `SystemParams`.
It adds a `ReadOnlySystemParamFetch` trait, which enables safe `SystemState::get` calls without unique world access.
I renamed the old `SystemState` to `SystemMeta` to enable us to mirror the `QueryState` naming convention (but I'm happy to discuss alternative names if people have other ideas). I initially pitched this as `ParamState`, but given that it needs to include full system metadata, that doesn't feel like a particularly accurate name.
```rust
#[derive(Eq, PartialEq, Debug)]
struct A(usize);
#[derive(Eq, PartialEq, Debug)]
struct B(usize);
let mut world = World::default();
world.insert_resource(A(42));
world.spawn().insert(B(7));
// we get nice lifetime elision when declaring the type on the left hand side
let mut system_state: SystemState<(Res<A>, Query<&B>)> = SystemState::new(&mut world);
let (a, query) = system_state.get(&world);
assert_eq!(*a, A(42), "returned resource matches initial value");
assert_eq!(
*query.single().unwrap(),
B(7),
"returned component matches initial value"
);
// mutable system params require unique world access
let mut system_state: SystemState<(ResMut<A>, Query<&mut B>)> = SystemState::new(&mut world);
let (a, query) = system_state.get_mut(&mut world);
// static lifetimes are required when declaring inside of structs
struct SomeContainer {
state: SystemState<(Res<'static, A>, Res<'static, B>)>
}
// this can be shortened using type aliases, which will be useful for complex param tuples
type MyParams<'a> = (Res<'a, A>, Res<'a, B>);
struct SomeContainer {
state: SystemState<MyParams<'static>>
}
// It is the user's responsibility to call SystemState::apply(world) for parameters that queue up work
let mut system_state: SystemState<(Commands, Query<&B>)> = SystemState::new(&mut world);
{
let (mut commands, query) = system_state.get(&world);
commands.insert_resource(3.14);
}
system_state.apply(&mut world);
```
## Future Work
* Actually use SystemState inside FunctionSystem. This would be trivial, but it requires FunctionSystem to wrap SystemState in Option in its current form (which complicates system metadata lookup). I'd prefer to hold off until we adopt something like the later designs linked in #1364, which enable us to contruct Systems using a World reference (and also remove the need for `.system`).
* Consider a "scoped" approach to automatically call SystemState::apply when systems params are no longer being used (either a container type with a Drop impl, or a function that takes a closure for user logic operating on params).
2021-06-02 19:57:38 +00:00
system_meta : & SystemMeta ,
System Param Lifetime Split (#2605)
# Objective
Enable using exact World lifetimes during read-only access . This is motivated by the new renderer's need to allow read-only world-only queries to outlive the query itself (but still be constrained by the world lifetime).
For example:
https://github.com/bevyengine/bevy/blob/115b170d1f11a91146bb6d6e9684dceb8b21f786/pipelined/bevy_pbr2/src/render/mod.rs#L774
## Solution
Split out SystemParam state and world lifetimes and pipe those lifetimes up to read-only Query ops (and add into_inner for Res). According to every safety test I've run so far (except one), this is safe (see the temporary safety test commit). Note that changing the mutable variants to the new lifetimes would allow aliased mutable pointers (try doing that to see how it affects the temporary safety tests).
The new state lifetime on SystemParam does make `#[derive(SystemParam)]` more cumbersome (the current impl requires PhantomData if you don't use both lifetimes). We can make this better by detecting whether or not a lifetime is used in the derive and adjusting accordingly, but that should probably be done in its own pr.
## Why is this a draft?
The new lifetimes break QuerySet safety in one very specific case (see the query_set system in system_safety_test). We need to solve this before we can use the lifetimes given.
This is due to the fact that QuerySet is just a wrapper over Query, which now relies on world lifetimes instead of `&self` lifetimes to prevent aliasing (but in systems, each Query has its own implied lifetime, not a centralized world lifetime). I believe the fix is to rewrite QuerySet to have its own World lifetime (and own the internal reference). This will complicate the impl a bit, but I think it is doable. I'm curious if anyone else has better ideas.
Personally, I think these new lifetimes need to happen. We've gotta have a way to directly tie read-only World queries to the World lifetime. The new renderer is the first place this has come up, but I doubt it will be the last. Worst case scenario we can come up with a second `WorldLifetimeQuery<Q, F = ()>` parameter to enable these read-only scenarios, but I'd rather not add another type to the type zoo.
2021-08-15 20:51:53 +00:00
world : & ' w World ,
Reliable change detection (#1471)
# Problem Definition
The current change tracking (via flags for both components and resources) fails to detect changes made by systems that are scheduled to run earlier in the frame than they are.
This issue is discussed at length in [#68](https://github.com/bevyengine/bevy/issues/68) and [#54](https://github.com/bevyengine/bevy/issues/54).
This is very much a draft PR, and contributions are welcome and needed.
# Criteria
1. Each change is detected at least once, no matter the ordering.
2. Each change is detected at most once, no matter the ordering.
3. Changes should be detected the same frame that they are made.
4. Competitive ergonomics. Ideally does not require opting-in.
5. Low CPU overhead of computation.
6. Memory efficient. This must not increase over time, except where the number of entities / resources does.
7. Changes should not be lost for systems that don't run.
8. A frame needs to act as a pure function. Given the same set of entities / components it needs to produce the same end state without side-effects.
**Exact** change-tracking proposals satisfy criteria 1 and 2.
**Conservative** change-tracking proposals satisfy criteria 1 but not 2.
**Flaky** change tracking proposals satisfy criteria 2 but not 1.
# Code Base Navigation
There are three types of flags:
- `Added`: A piece of data was added to an entity / `Resources`.
- `Mutated`: A piece of data was able to be modified, because its `DerefMut` was accessed
- `Changed`: The bitwise OR of `Added` and `Changed`
The special behavior of `ChangedRes`, with respect to the scheduler is being removed in [#1313](https://github.com/bevyengine/bevy/pull/1313) and does not need to be reproduced.
`ChangedRes` and friends can be found in "bevy_ecs/core/resources/resource_query.rs".
The `Flags` trait for Components can be found in "bevy_ecs/core/query.rs".
`ComponentFlags` are stored in "bevy_ecs/core/archetypes.rs", defined on line 446.
# Proposals
**Proposal 5 was selected for implementation.**
## Proposal 0: No Change Detection
The baseline, where computations are performed on everything regardless of whether it changed.
**Type:** Conservative
**Pros:**
- already implemented
- will never miss events
- no overhead
**Cons:**
- tons of repeated work
- doesn't allow users to avoid repeating work (or monitoring for other changes)
## Proposal 1: Earlier-This-Tick Change Detection
The current approach as of Bevy 0.4. Flags are set, and then flushed at the end of each frame.
**Type:** Flaky
**Pros:**
- already implemented
- simple to understand
- low memory overhead (2 bits per component)
- low time overhead (clear every flag once per frame)
**Cons:**
- misses systems based on ordering
- systems that don't run every frame miss changes
- duplicates detection when looping
- can lead to unresolvable circular dependencies
## Proposal 2: Two-Tick Change Detection
Flags persist for two frames, using a double-buffer system identical to that used in events.
A change is observed if it is found in either the current frame's list of changes or the previous frame's.
**Type:** Conservative
**Pros:**
- easy to understand
- easy to implement
- low memory overhead (4 bits per component)
- low time overhead (bit mask and shift every flag once per frame)
**Cons:**
- can result in a great deal of duplicated work
- systems that don't run every frame miss changes
- duplicates detection when looping
## Proposal 3: Last-Tick Change Detection
Flags persist for two frames, using a double-buffer system identical to that used in events.
A change is observed if it is found in the previous frame's list of changes.
**Type:** Exact
**Pros:**
- exact
- easy to understand
- easy to implement
- low memory overhead (4 bits per component)
- low time overhead (bit mask and shift every flag once per frame)
**Cons:**
- change detection is always delayed, possibly causing painful chained delays
- systems that don't run every frame miss changes
- duplicates detection when looping
## Proposal 4: Flag-Doubling Change Detection
Combine Proposal 2 and Proposal 3. Differentiate between `JustChanged` (current behavior) and `Changed` (Proposal 3).
Pack this data into the flags according to [this implementation proposal](https://github.com/bevyengine/bevy/issues/68#issuecomment-769174804).
**Type:** Flaky + Exact
**Pros:**
- allows users to acc
- easy to implement
- low memory overhead (4 bits per component)
- low time overhead (bit mask and shift every flag once per frame)
**Cons:**
- users must specify the type of change detection required
- still quite fragile to system ordering effects when using the flaky `JustChanged` form
- cannot get immediate + exact results
- systems that don't run every frame miss changes
- duplicates detection when looping
## [SELECTED] Proposal 5: Generation-Counter Change Detection
A global counter is increased after each system is run. Each component saves the time of last mutation, and each system saves the time of last execution. Mutation is detected when the component's counter is greater than the system's counter. Discussed [here](https://github.com/bevyengine/bevy/issues/68#issuecomment-769174804). How to handle addition detection is unsolved; the current proposal is to use the highest bit of the counter as in proposal 1.
**Type:** Exact (for mutations), flaky (for additions)
**Pros:**
- low time overhead (set component counter on access, set system counter after execution)
- robust to systems that don't run every frame
- robust to systems that loop
**Cons:**
- moderately complex implementation
- must be modified as systems are inserted dynamically
- medium memory overhead (4 bytes per component + system)
- unsolved addition detection
## Proposal 6: System-Data Change Detection
For each system, track which system's changes it has seen. This approach is only worth fully designing and implementing if Proposal 5 fails in some way.
**Type:** Exact
**Pros:**
- exact
- conceptually simple
**Cons:**
- requires storing data on each system
- implementation is complex
- must be modified as systems are inserted dynamically
## Proposal 7: Total-Order Change Detection
Discussed [here](https://github.com/bevyengine/bevy/issues/68#issuecomment-754326523). This proposal is somewhat complicated by the new scheduler, but I believe it should still be conceptually feasible. This approach is only worth fully designing and implementing if Proposal 5 fails in some way.
**Type:** Exact
**Pros:**
- exact
- efficient data storage relative to other exact proposals
**Cons:**
- requires access to the scheduler
- complex implementation and difficulty grokking
- must be modified as systems are inserted dynamically
# Tests
- We will need to verify properties 1, 2, 3, 7 and 8. Priority: 1 > 2 = 3 > 8 > 7
- Ideally we can use identical user-facing syntax for all proposals, allowing us to re-use the same syntax for each.
- When writing tests, we need to carefully specify order using explicit dependencies.
- These tests will need to be duplicated for both components and resources.
- We need to be sure to handle cases where ambiguous system orders exist.
`changing_system` is always the system that makes the changes, and `detecting_system` always detects the changes.
The component / resource changed will be simple boolean wrapper structs.
## Basic Added / Mutated / Changed
2 x 3 design:
- Resources vs. Components
- Added vs. Changed vs. Mutated
- `changing_system` runs before `detecting_system`
- verify at the end of tick 2
## At Least Once
2 x 3 design:
- Resources vs. Components
- Added vs. Changed vs. Mutated
- `changing_system` runs after `detecting_system`
- verify at the end of tick 2
## At Most Once
2 x 3 design:
- Resources vs. Components
- Added vs. Changed vs. Mutated
- `changing_system` runs once before `detecting_system`
- increment a counter based on the number of changes detected
- verify at the end of tick 2
## Fast Detection
2 x 3 design:
- Resources vs. Components
- Added vs. Changed vs. Mutated
- `changing_system` runs before `detecting_system`
- verify at the end of tick 1
## Ambiguous System Ordering Robustness
2 x 3 x 2 design:
- Resources vs. Components
- Added vs. Changed vs. Mutated
- `changing_system` runs [before/after] `detecting_system` in tick 1
- `changing_system` runs [after/before] `detecting_system` in tick 2
## System Pausing
2 x 3 design:
- Resources vs. Components
- Added vs. Changed vs. Mutated
- `changing_system` runs in tick 1, then is disabled by run criteria
- `detecting_system` is disabled by run criteria until it is run once during tick 3
- verify at the end of tick 3
## Addition Causes Mutation
2 design:
- Resources vs. Components
- `adding_system_1` adds a component / resource
- `adding system_2` adds the same component / resource
- verify the `Mutated` flag at the end of the tick
- verify the `Added` flag at the end of the tick
First check tests for: https://github.com/bevyengine/bevy/issues/333
Second check tests for: https://github.com/bevyengine/bevy/issues/1443
## Changes Made By Commands
- `adding_system` runs in Update in tick 1, and sends a command to add a component
- `detecting_system` runs in Update in tick 1 and 2, after `adding_system`
- We can't detect the changes in tick 1, since they haven't been processed yet
- If we were to track these changes as being emitted by `adding_system`, we can't detect the changes in tick 2 either, since `detecting_system` has already run once after `adding_system` :(
# Benchmarks
See: [general advice](https://github.com/bevyengine/bevy/blob/master/docs/profiling.md), [Criterion crate](https://github.com/bheisler/criterion.rs)
There are several critical parameters to vary:
1. entity count (1 to 10^9)
2. fraction of entities that are changed (0% to 100%)
3. cost to perform work on changed entities, i.e. workload (1 ns to 1s)
1 and 2 should be varied between benchmark runs. 3 can be added on computationally.
We want to measure:
- memory cost
- run time
We should collect these measurements across several frames (100?) to reduce bootup effects and accurately measure the mean, variance and drift.
Entity-component change detection is much more important to benchmark than resource change detection, due to the orders of magnitude higher number of pieces of data.
No change detection at all should be included in benchmarks as a second control for cases where missing changes is unacceptable.
## Graphs
1. y: performance, x: log_10(entity count), color: proposal, facet: performance metric. Set cost to perform work to 0.
2. y: run time, x: cost to perform work, color: proposal, facet: fraction changed. Set number of entities to 10^6
3. y: memory, x: frames, color: proposal
# Conclusions
1. Is the theoretical categorization of the proposals correct according to our tests?
2. How does the performance of the proposals compare without any load?
3. How does the performance of the proposals compare with realistic loads?
4. At what workload does more exact change tracking become worth the (presumably) higher overhead?
5. When does adding change-detection to save on work become worthwhile?
6. Is there enough divergence in performance between the best solutions in each class to ship more than one change-tracking solution?
# Implementation Plan
1. Write a test suite.
2. Verify that tests fail for existing approach.
3. Write a benchmark suite.
4. Get performance numbers for existing approach.
5. Implement, test and benchmark various solutions using a Git branch per proposal.
6. Create a draft PR with all solutions and present results to team.
7. Select a solution and replace existing change detection.
Co-authored-by: Brice DAVIER <bricedavier@gmail.com>
Co-authored-by: Carter Anderson <mcanders1@gmail.com>
2021-03-19 17:53:26 +00:00
change_tick : u32 ,
Simplify trait hierarchy for `SystemParam` (#6865)
# Objective
* Implementing a custom `SystemParam` by hand requires implementing three traits -- four if it is read-only.
* The trait `SystemParamFetch<'w, 's>` is a workaround from before we had generic associated types, and is no longer necessary.
## Solution
* Combine the trait `SystemParamFetch` with `SystemParamState`.
* I decided to remove the `Fetch` name and keep the `State` name, since the former was consistently conflated with the latter.
* Replace the trait `ReadOnlySystemParamFetch` with `ReadOnlySystemParam`, which simplifies trait bounds in generic code.
---
## Changelog
- Removed the trait `SystemParamFetch`, moving its functionality to `SystemParamState`.
- Replaced the trait `ReadOnlySystemParamFetch` with `ReadOnlySystemParam`.
## Migration Guide
The trait `SystemParamFetch` has been removed, and its functionality has been transferred to `SystemParamState`.
```rust
// Before
impl SystemParamState for MyParamState {
fn init(world: &mut World, system_meta: &mut SystemMeta) -> Self { ... }
}
impl<'w, 's> SystemParamFetch<'w, 's> for MyParamState {
type Item = MyParam<'w, 's>;
fn get_param(...) -> Self::Item;
}
// After
impl SystemParamState for MyParamState {
type Item<'w, 's> = MyParam<'w, 's>; // Generic associated types!
fn init(world: &mut World, system_meta: &mut SystemMeta) -> Self { ... }
fn get_param<'w, 's>(...) -> Self::Item<'w, 's>;
}
```
The trait `ReadOnlySystemParamFetch` has been replaced with `ReadOnlySystemParam`.
```rust
// Before
unsafe impl ReadOnlySystemParamFetch for MyParamState {}
// After
unsafe impl<'w, 's> ReadOnlySystemParam for MyParam<'w, 's> {}
```
2022-12-11 18:34:14 +00:00
) -> Self ::Item < ' w , ' s > {
Bevy ECS V2 (#1525)
# Bevy ECS V2
This is a rewrite of Bevy ECS (basically everything but the new executor/schedule, which are already awesome). The overall goal was to improve the performance and versatility of Bevy ECS. Here is a quick bulleted list of changes before we dive into the details:
* Complete World rewrite
* Multiple component storage types:
* Tables: fast cache friendly iteration, slower add/removes (previously called Archetypes)
* Sparse Sets: fast add/remove, slower iteration
* Stateful Queries (caches query results for faster iteration. fragmented iteration is _fast_ now)
* Stateful System Params (caches expensive operations. inspired by @DJMcNab's work in #1364)
* Configurable System Params (users can set configuration when they construct their systems. once again inspired by @DJMcNab's work)
* Archetypes are now "just metadata", component storage is separate
* Archetype Graph (for faster archetype changes)
* Component Metadata
* Configure component storage type
* Retrieve information about component size/type/name/layout/send-ness/etc
* Components are uniquely identified by a densely packed ComponentId
* TypeIds are now totally optional (which should make implementing scripting easier)
* Super fast "for_each" query iterators
* Merged Resources into World. Resources are now just a special type of component
* EntityRef/EntityMut builder apis (more efficient and more ergonomic)
* Fast bitset-backed `Access<T>` replaces old hashmap-based approach everywhere
* Query conflicts are determined by component access instead of archetype component access (to avoid random failures at runtime)
* With/Without are still taken into account for conflicts, so this should still be comfy to use
* Much simpler `IntoSystem` impl
* Significantly reduced the amount of hashing throughout the ecs in favor of Sparse Sets (indexed by densely packed ArchetypeId, ComponentId, BundleId, and TableId)
* Safety Improvements
* Entity reservation uses a normal world reference instead of unsafe transmute
* QuerySets no longer transmute lifetimes
* Made traits "unsafe" where relevant
* More thorough safety docs
* WorldCell
* Exposes safe mutable access to multiple resources at a time in a World
* Replaced "catch all" `System::update_archetypes(world: &World)` with `System::new_archetype(archetype: &Archetype)`
* Simpler Bundle implementation
* Replaced slow "remove_bundle_one_by_one" used as fallback for Commands::remove_bundle with fast "remove_bundle_intersection"
* Removed `Mut<T>` query impl. it is better to only support one way: `&mut T`
* Removed with() from `Flags<T>` in favor of `Option<Flags<T>>`, which allows querying for flags to be "filtered" by default
* Components now have is_send property (currently only resources support non-send)
* More granular module organization
* New `RemovedComponents<T>` SystemParam that replaces `query.removed::<T>()`
* `world.resource_scope()` for mutable access to resources and world at the same time
* WorldQuery and QueryFilter traits unified. FilterFetch trait added to enable "short circuit" filtering. Auto impled for cases that don't need it
* Significantly slimmed down SystemState in favor of individual SystemParam state
* System Commands changed from `commands: &mut Commands` back to `mut commands: Commands` (to allow Commands to have a World reference)
Fixes #1320
## `World` Rewrite
This is a from-scratch rewrite of `World` that fills the niche that `hecs` used to. Yes, this means Bevy ECS is no longer a "fork" of hecs. We're going out our own!
(the only shared code between the projects is the entity id allocator, which is already basically ideal)
A huge shout out to @SanderMertens (author of [flecs](https://github.com/SanderMertens/flecs)) for sharing some great ideas with me (specifically hybrid ecs storage and archetype graphs). He also helped advise on a number of implementation details.
## Component Storage (The Problem)
Two ECS storage paradigms have gained a lot of traction over the years:
* **Archetypal ECS**:
* Stores components in "tables" with static schemas. Each "column" stores components of a given type. Each "row" is an entity.
* Each "archetype" has its own table. Adding/removing an entity's component changes the archetype.
* Enables super-fast Query iteration due to its cache-friendly data layout
* Comes at the cost of more expensive add/remove operations for an Entity's components, because all components need to be copied to the new archetype's "table"
* **Sparse Set ECS**:
* Stores components of the same type in densely packed arrays, which are sparsely indexed by densely packed unsigned integers (Entity ids)
* Query iteration is slower than Archetypal ECS because each entity's component could be at any position in the sparse set. This "random access" pattern isn't cache friendly. Additionally, there is an extra layer of indirection because you must first map the entity id to an index in the component array.
* Adding/removing components is a cheap, constant time operation
Bevy ECS V1, hecs, legion, flec, and Unity DOTS are all "archetypal ecs-es". I personally think "archetypal" storage is a good default for game engines. An entity's archetype doesn't need to change frequently in general, and it creates "fast by default" query iteration (which is a much more common operation). It is also "self optimizing". Users don't need to think about optimizing component layouts for iteration performance. It "just works" without any extra boilerplate.
Shipyard and EnTT are "sparse set ecs-es". They employ "packing" as a way to work around the "suboptimal by default" iteration performance for specific sets of components. This helps, but I didn't think this was a good choice for a general purpose engine like Bevy because:
1. "packs" conflict with each other. If bevy decides to internally pack the Transform and GlobalTransform components, users are then blocked if they want to pack some custom component with Transform.
2. users need to take manual action to optimize
Developers selecting an ECS framework are stuck with a hard choice. Select an "archetypal" framework with "fast iteration everywhere" but without the ability to cheaply add/remove components, or select a "sparse set" framework to cheaply add/remove components but with slower iteration performance.
## Hybrid Component Storage (The Solution)
In Bevy ECS V2, we get to have our cake and eat it too. It now has _both_ of the component storage types above (and more can be added later if needed):
* **Tables** (aka "archetypal" storage)
* The default storage. If you don't configure anything, this is what you get
* Fast iteration by default
* Slower add/remove operations
* **Sparse Sets**
* Opt-in
* Slower iteration
* Faster add/remove operations
These storage types complement each other perfectly. By default Query iteration is fast. If developers know that they want to add/remove a component at high frequencies, they can set the storage to "sparse set":
```rust
world.register_component(
ComponentDescriptor::new::<MyComponent>(StorageType::SparseSet)
).unwrap();
```
## Archetypes
Archetypes are now "just metadata" ... they no longer store components directly. They do store:
* The `ComponentId`s of each of the Archetype's components (and that component's storage type)
* Archetypes are uniquely defined by their component layouts
* For example: entities with "table" components `[A, B, C]` _and_ "sparse set" components `[D, E]` will always be in the same archetype.
* The `TableId` associated with the archetype
* For now each archetype has exactly one table (which can have no components),
* There is a 1->Many relationship from Tables->Archetypes. A given table could have any number of archetype components stored in it:
* Ex: an entity with "table storage" components `[A, B, C]` and "sparse set" components `[D, E]` will share the same `[A, B, C]` table as an entity with `[A, B, C]` table component and `[F]` sparse set components.
* This 1->Many relationship is how we preserve fast "cache friendly" iteration performance when possible (more on this later)
* A list of entities that are in the archetype and the row id of the table they are in
* ArchetypeComponentIds
* unique densely packed identifiers for (ArchetypeId, ComponentId) pairs
* used by the schedule executor for cheap system access control
* "Archetype Graph Edges" (see the next section)
## The "Archetype Graph"
Archetype changes in Bevy (and a number of other archetypal ecs-es) have historically been expensive to compute. First, you need to allocate a new vector of the entity's current component ids, add or remove components based on the operation performed, sort it (to ensure it is order-independent), then hash it to find the archetype (if it exists). And thats all before we get to the _already_ expensive full copy of all components to the new table storage.
The solution is to build a "graph" of archetypes to cache these results. @SanderMertens first exposed me to the idea (and he got it from @gjroelofs, who came up with it). They propose adding directed edges between archetypes for add/remove component operations. If `ComponentId`s are densely packed, you can use sparse sets to cheaply jump between archetypes.
Bevy takes this one step further by using add/remove `Bundle` edges instead of `Component` edges. Bevy encourages the use of `Bundles` to group add/remove operations. This is largely for "clearer game logic" reasons, but it also helps cut down on the number of archetype changes required. `Bundles` now also have densely-packed `BundleId`s. This allows us to use a _single_ edge for each bundle operation (rather than needing to traverse N edges ... one for each component). Single component operations are also bundles, so this is strictly an improvement over a "component only" graph.
As a result, an operation that used to be _heavy_ (both for allocations and compute) is now two dirt-cheap array lookups and zero allocations.
## Stateful Queries
World queries are now stateful. This allows us to:
1. Cache archetype (and table) matches
* This resolves another issue with (naive) archetypal ECS: query performance getting worse as the number of archetypes goes up (and fragmentation occurs).
2. Cache Fetch and Filter state
* The expensive parts of fetch/filter operations (such as hashing the TypeId to find the ComponentId) now only happen once when the Query is first constructed
3. Incrementally build up state
* When new archetypes are added, we only process the new archetypes (no need to rebuild state for old archetypes)
As a result, the direct `World` query api now looks like this:
```rust
let mut query = world.query::<(&A, &mut B)>();
for (a, mut b) in query.iter_mut(&mut world) {
}
```
Requiring `World` to generate stateful queries (rather than letting the `QueryState` type be constructed separately) allows us to ensure that _all_ queries are properly initialized (and the relevant world state, such as ComponentIds). This enables QueryState to remove branches from its operations that check for initialization status (and also enables query.iter() to take an immutable world reference because it doesn't need to initialize anything in world).
However in systems, this is a non-breaking change. State management is done internally by the relevant SystemParam.
## Stateful SystemParams
Like Queries, `SystemParams` now also cache state. For example, `Query` system params store the "stateful query" state mentioned above. Commands store their internal `CommandQueue`. This means you can now safely use as many separate `Commands` parameters in your system as you want. `Local<T>` system params store their `T` value in their state (instead of in Resources).
SystemParam state also enabled a significant slim-down of SystemState. It is much nicer to look at now.
Per-SystemParam state naturally insulates us from an "aliased mut" class of errors we have hit in the past (ex: using multiple `Commands` system params).
(credit goes to @DJMcNab for the initial idea and draft pr here #1364)
## Configurable SystemParams
@DJMcNab also had the great idea to make SystemParams configurable. This allows users to provide some initial configuration / values for system parameters (when possible). Most SystemParams have no config (the config type is `()`), but the `Local<T>` param now supports user-provided parameters:
```rust
fn foo(value: Local<usize>) {
}
app.add_system(foo.system().config(|c| c.0 = Some(10)));
```
## Uber Fast "for_each" Query Iterators
Developers now have the choice to use a fast "for_each" iterator, which yields ~1.5-3x iteration speed improvements for "fragmented iteration", and minor ~1.2x iteration speed improvements for unfragmented iteration.
```rust
fn system(query: Query<(&A, &mut B)>) {
// you now have the option to do this for a speed boost
query.for_each_mut(|(a, mut b)| {
});
// however normal iterators are still available
for (a, mut b) in query.iter_mut() {
}
}
```
I think in most cases we should continue to encourage "normal" iterators as they are more flexible and more "rust idiomatic". But when that extra "oomf" is needed, it makes sense to use `for_each`.
We should also consider using `for_each` for internal bevy systems to give our users a nice speed boost (but that should be a separate pr).
## Component Metadata
`World` now has a `Components` collection, which is accessible via `world.components()`. This stores mappings from `ComponentId` to `ComponentInfo`, as well as `TypeId` to `ComponentId` mappings (where relevant). `ComponentInfo` stores information about the component, such as ComponentId, TypeId, memory layout, send-ness (currently limited to resources), and storage type.
## Significantly Cheaper `Access<T>`
We used to use `TypeAccess<TypeId>` to manage read/write component/archetype-component access. This was expensive because TypeIds must be hashed and compared individually. The parallel executor got around this by "condensing" type ids into bitset-backed access types. This worked, but it had to be re-generated from the `TypeAccess<TypeId>`sources every time archetypes changed.
This pr removes TypeAccess in favor of faster bitset access everywhere. We can do this thanks to the move to densely packed `ComponentId`s and `ArchetypeComponentId`s.
## Merged Resources into World
Resources had a lot of redundant functionality with Components. They stored typed data, they had access control, they had unique ids, they were queryable via SystemParams, etc. In fact the _only_ major difference between them was that they were unique (and didn't correlate to an entity).
Separate resources also had the downside of requiring a separate set of access controls, which meant the parallel executor needed to compare more bitsets per system and manage more state.
I initially got the "separate resources" idea from `legion`. I think that design was motivated by the fact that it made the direct world query/resource lifetime interactions more manageable. It certainly made our lives easier when using Resources alongside hecs/bevy_ecs. However we already have a construct for safely and ergonomically managing in-world lifetimes: systems (which use `Access<T>` internally).
This pr merges Resources into World:
```rust
world.insert_resource(1);
world.insert_resource(2.0);
let a = world.get_resource::<i32>().unwrap();
let mut b = world.get_resource_mut::<f64>().unwrap();
*b = 3.0;
```
Resources are now just a special kind of component. They have their own ComponentIds (and their own resource TypeId->ComponentId scope, so they don't conflict wit components of the same type). They are stored in a special "resource archetype", which stores components inside the archetype using a new `unique_components` sparse set (note that this sparse set could later be used to implement Tags). This allows us to keep the code size small by reusing existing datastructures (namely Column, Archetype, ComponentFlags, and ComponentInfo). This allows us the executor to use a single `Access<ArchetypeComponentId>` per system. It should also make scripting language integration easier.
_But_ this merge did create problems for people directly interacting with `World`. What if you need mutable access to multiple resources at the same time? `world.get_resource_mut()` borrows World mutably!
## WorldCell
WorldCell applies the `Access<ArchetypeComponentId>` concept to direct world access:
```rust
let world_cell = world.cell();
let a = world_cell.get_resource_mut::<i32>().unwrap();
let b = world_cell.get_resource_mut::<f64>().unwrap();
```
This adds cheap runtime checks (a sparse set lookup of `ArchetypeComponentId` and a counter) to ensure that world accesses do not conflict with each other. Each operation returns a `WorldBorrow<'w, T>` or `WorldBorrowMut<'w, T>` wrapper type, which will release the relevant ArchetypeComponentId resources when dropped.
World caches the access sparse set (and only one cell can exist at a time), so `world.cell()` is a cheap operation.
WorldCell does _not_ use atomic operations. It is non-send, does a mutable borrow of world to prevent other accesses, and uses a simple `Rc<RefCell<ArchetypeComponentAccess>>` wrapper in each WorldBorrow pointer.
The api is currently limited to resource access, but it can and should be extended to queries / entity component access.
## Resource Scopes
WorldCell does not yet support component queries, and even when it does there are sometimes legitimate reasons to want a mutable world ref _and_ a mutable resource ref (ex: bevy_render and bevy_scene both need this). In these cases we could always drop down to the unsafe `world.get_resource_unchecked_mut()`, but that is not ideal!
Instead developers can use a "resource scope"
```rust
world.resource_scope(|world: &mut World, a: &mut A| {
})
```
This temporarily removes the `A` resource from `World`, provides mutable pointers to both, and re-adds A to World when finished. Thanks to the move to ComponentIds/sparse sets, this is a cheap operation.
If multiple resources are required, scopes can be nested. We could also consider adding a "resource tuple" to the api if this pattern becomes common and the boilerplate gets nasty.
## Query Conflicts Use ComponentId Instead of ArchetypeComponentId
For safety reasons, systems cannot contain queries that conflict with each other without wrapping them in a QuerySet. On bevy `main`, we use ArchetypeComponentIds to determine conflicts. This is nice because it can take into account filters:
```rust
// these queries will never conflict due to their filters
fn filter_system(a: Query<&mut A, With<B>>, b: Query<&mut B, Without<B>>) {
}
```
But it also has a significant downside:
```rust
// these queries will not conflict _until_ an entity with A, B, and C is spawned
fn maybe_conflicts_system(a: Query<(&mut A, &C)>, b: Query<(&mut A, &B)>) {
}
```
The system above will panic at runtime if an entity with A, B, and C is spawned. This makes it hard to trust that your game logic will run without crashing.
In this pr, I switched to using `ComponentId` instead. This _is_ more constraining. `maybe_conflicts_system` will now always fail, but it will do it consistently at startup. Naively, it would also _disallow_ `filter_system`, which would be a significant downgrade in usability. Bevy has a number of internal systems that rely on disjoint queries and I expect it to be a common pattern in userspace.
To resolve this, I added a new `FilteredAccess<T>` type, which wraps `Access<T>` and adds with/without filters. If two `FilteredAccess` have with/without values that prove they are disjoint, they will no longer conflict.
## EntityRef / EntityMut
World entity operations on `main` require that the user passes in an `entity` id to each operation:
```rust
let entity = world.spawn((A, )); // create a new entity with A
world.get::<A>(entity);
world.insert(entity, (B, C));
world.insert_one(entity, D);
```
This means that each operation needs to look up the entity location / verify its validity. The initial spawn operation also requires a Bundle as input. This can be awkward when no components are required (or one component is required).
These operations have been replaced by `EntityRef` and `EntityMut`, which are "builder-style" wrappers around world that provide read and read/write operations on a single, pre-validated entity:
```rust
// spawn now takes no inputs and returns an EntityMut
let entity = world.spawn()
.insert(A) // insert a single component into the entity
.insert_bundle((B, C)) // insert a bundle of components into the entity
.id() // id returns the Entity id
// Returns EntityMut (or panics if the entity does not exist)
world.entity_mut(entity)
.insert(D)
.insert_bundle(SomeBundle::default());
{
// returns EntityRef (or panics if the entity does not exist)
let d = world.entity(entity)
.get::<D>() // gets the D component
.unwrap();
// world.get still exists for ergonomics
let d = world.get::<D>(entity).unwrap();
}
// These variants return Options if you want to check existence instead of panicing
world.get_entity_mut(entity)
.unwrap()
.insert(E);
if let Some(entity_ref) = world.get_entity(entity) {
let d = entity_ref.get::<D>().unwrap();
}
```
This _does not_ affect the current Commands api or terminology. I think that should be a separate conversation as that is a much larger breaking change.
## Safety Improvements
* Entity reservation in Commands uses a normal world borrow instead of an unsafe transmute
* QuerySets no longer transmutes lifetimes
* Made traits "unsafe" when implementing a trait incorrectly could cause unsafety
* More thorough safety docs
## RemovedComponents SystemParam
The old approach to querying removed components: `query.removed:<T>()` was confusing because it had no connection to the query itself. I replaced it with the following, which is both clearer and allows us to cache the ComponentId mapping in the SystemParamState:
```rust
fn system(removed: RemovedComponents<T>) {
for entity in removed.iter() {
}
}
```
## Simpler Bundle implementation
Bundles are no longer responsible for sorting (or deduping) TypeInfo. They are just a simple ordered list of component types / data. This makes the implementation smaller and opens the door to an easy "nested bundle" implementation in the future (which i might even add in this pr). Duplicate detection is now done once per bundle type by World the first time a bundle is used.
## Unified WorldQuery and QueryFilter types
(don't worry they are still separate type _parameters_ in Queries .. this is a non-breaking change)
WorldQuery and QueryFilter were already basically identical apis. With the addition of `FetchState` and more storage-specific fetch methods, the overlap was even clearer (and the redundancy more painful).
QueryFilters are now just `F: WorldQuery where F::Fetch: FilterFetch`. FilterFetch requires `Fetch<Item = bool>` and adds new "short circuit" variants of fetch methods. This enables a filter tuple like `(With<A>, Without<B>, Changed<C>)` to stop evaluating the filter after the first mismatch is encountered. FilterFetch is automatically implemented for `Fetch` implementations that return bool.
This forces fetch implementations that return things like `(bool, bool, bool)` (such as the filter above) to manually implement FilterFetch and decide whether or not to short-circuit.
## More Granular Modules
World no longer globs all of the internal modules together. It now exports `core`, `system`, and `schedule` separately. I'm also considering exporting `core` submodules directly as that is still pretty "glob-ey" and unorganized (feedback welcome here).
## Remaining Draft Work (to be done in this pr)
* ~~panic on conflicting WorldQuery fetches (&A, &mut A)~~
* ~~bevy `main` and hecs both currently allow this, but we should protect against it if possible~~
* ~~batch_iter / par_iter (currently stubbed out)~~
* ~~ChangedRes~~
* ~~I skipped this while we sort out #1313. This pr should be adapted to account for whatever we land on there~~.
* ~~The `Archetypes` and `Tables` collections use hashes of sorted lists of component ids to uniquely identify each archetype/table. This hash is then used as the key in a HashMap to look up the relevant ArchetypeId or TableId. (which doesn't handle hash collisions properly)~~
* ~~It is currently unsafe to generate a Query from "World A", then use it on "World B" (despite the api claiming it is safe). We should probably close this gap. This could be done by adding a randomly generated WorldId to each world, then storing that id in each Query. They could then be compared to each other on each `query.do_thing(&world)` operation. This _does_ add an extra branch to each query operation, so I'm open to other suggestions if people have them.~~
* ~~Nested Bundles (if i find time)~~
## Potential Future Work
* Expand WorldCell to support queries.
* Consider not allocating in the empty archetype on `world.spawn()`
* ex: return something like EntityMutUninit, which turns into EntityMut after an `insert` or `insert_bundle` op
* this actually regressed performance last time i tried it, but in theory it should be faster
* Optimize SparseSet::insert (see `PERF` comment on insert)
* Replace SparseArray `Option<T>` with T::MAX to cut down on branching
* would enable cheaper get_unchecked() operations
* upstream fixedbitset optimizations
* fixedbitset could be allocation free for small block counts (store blocks in a SmallVec)
* fixedbitset could have a const constructor
* Consider implementing Tags (archetype-specific by-value data that affects archetype identity)
* ex: ArchetypeA could have `[A, B, C]` table components and `[D(1)]` "tag" component. ArchetypeB could have `[A, B, C]` table components and a `[D(2)]` tag component. The archetypes are different, despite both having D tags because the value inside D is different.
* this could potentially build on top of the `archetype.unique_components` added in this pr for resource storage.
* Consider reverting `all_tuples` proc macro in favor of the old `macro_rules` implementation
* all_tuples is more flexible and produces cleaner documentation (the macro_rules version produces weird type parameter orders due to parser constraints)
* but unfortunately all_tuples also appears to make Rust Analyzer sad/slow when working inside of `bevy_ecs` (does not affect user code)
* Consider "resource queries" and/or "mixed resource and entity component queries" as an alternative to WorldCell
* this is basically just "systems" so maybe it's not worth it
* Add more world ops
* `world.clear()`
* `world.reserve<T: Bundle>(count: usize)`
* Try using the old archetype allocation strategy (allocate new memory on resize and copy everything over). I expect this to improve batch insertion performance at the cost of unbatched performance. But thats just a guess. I'm not an allocation perf pro :)
* Adapt Commands apis for consistency with new World apis
## Benchmarks
key:
* `bevy_old`: bevy `main` branch
* `bevy`: this branch
* `_foreach`: uses an optimized for_each iterator
* ` _sparse`: uses sparse set storage (if unspecified assume table storage)
* `_system`: runs inside a system (if unspecified assume test happens via direct world ops)
### Simple Insert (from ecs_bench_suite)
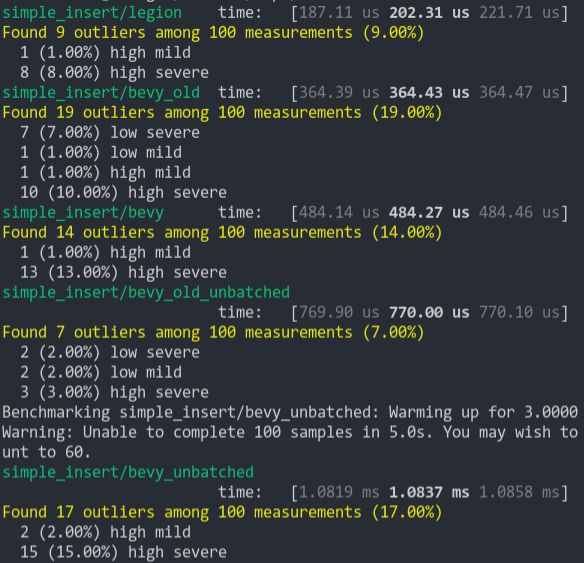
### Simpler Iter (from ecs_bench_suite)
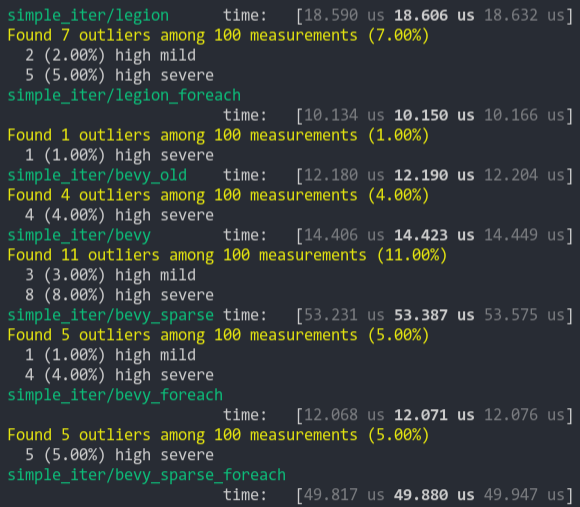
### Fragment Iter (from ecs_bench_suite)
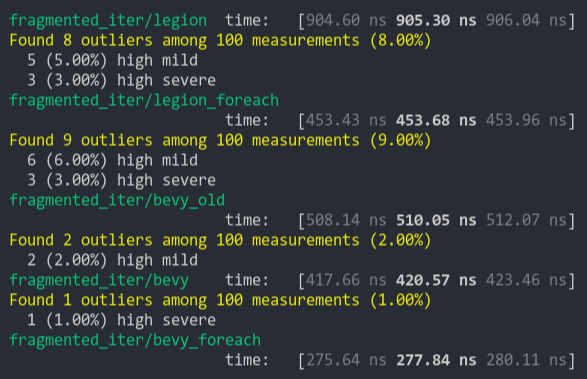
### Sparse Fragmented Iter
Iterate a query that matches 5 entities from a single matching archetype, but there are 100 unmatching archetypes
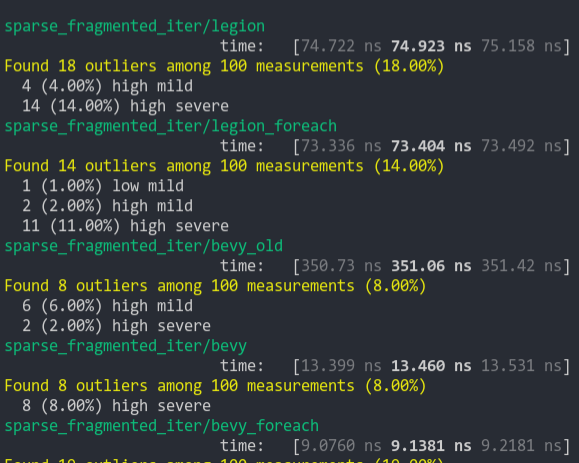
### Schedule (from ecs_bench_suite)
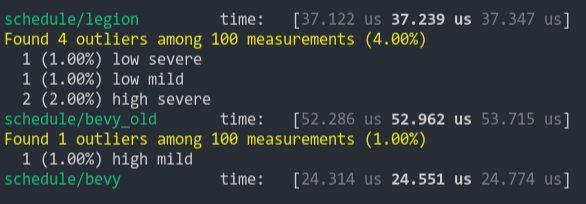
### Add Remove Component (from ecs_bench_suite)
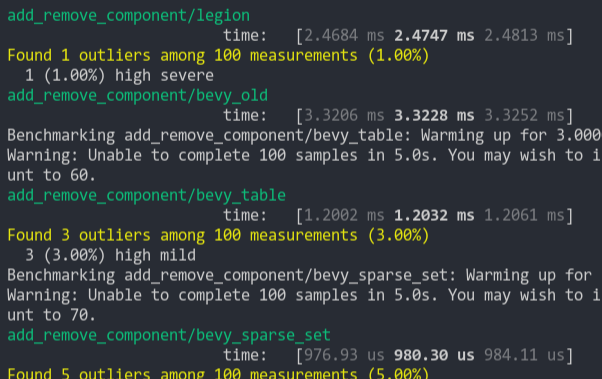
### Add Remove Component Big
Same as the test above, but each entity has 5 "large" matrix components and 1 "large" matrix component is added and removed
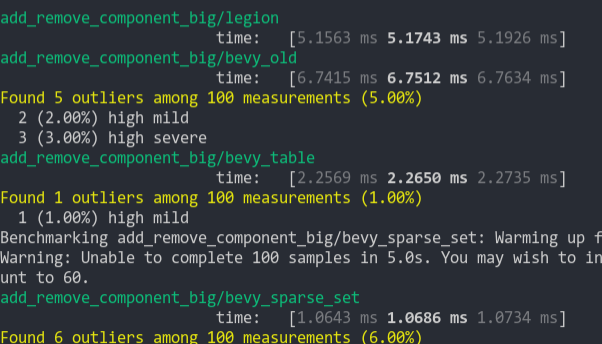
### Get Component
Looks up a single component value a large number of times
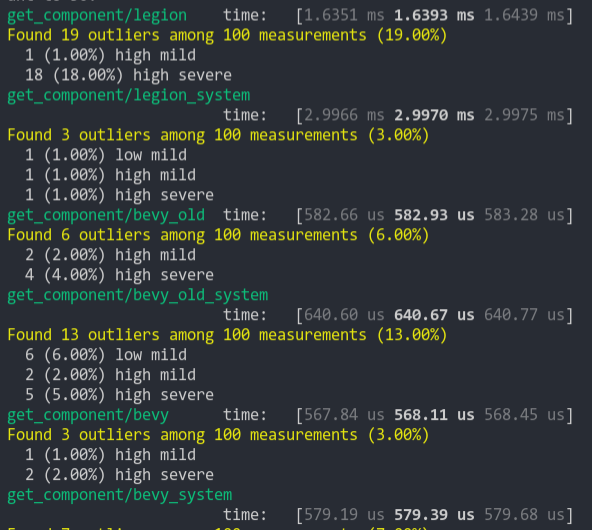
2021-03-05 07:54:35 +00:00
let value = world
add `UnsafeWorldCell` abstraction (#6404)
alternative to #5922, implements #5956
builds on top of https://github.com/bevyengine/bevy/pull/6402
# Objective
https://github.com/bevyengine/bevy/issues/5956 goes into more detail, but the TLDR is:
- bevy systems ensure disjoint accesses to resources and components, and for that to work there are methods `World::get_resource_unchecked_mut(&self)`, ..., `EntityRef::get_mut_unchecked(&self)` etc.
- we don't have these unchecked methods for `by_id` variants, so third-party crate authors cannot build their own safe disjoint-access abstractions with these
- having `_unchecked_mut` methods is not great, because in their presence safe code can accidentally violate subtle invariants. Having to go through `world.as_unsafe_world_cell().unsafe_method()` forces you to stop and think about what you want to write in your `// SAFETY` comment.
The alternative is to keep exposing `_unchecked_mut` variants for every operation that we want third-party crates to build upon, but we'd prefer to avoid using these methods alltogether: https://github.com/bevyengine/bevy/pull/5922#issuecomment-1241954543
Also, this is something that **cannot be implemented outside of bevy**, so having either this PR or #5922 as an escape hatch with lots of discouraging comments would be great.
## Solution
- add `UnsafeWorldCell` with `unsafe fn get_resource(&self)`, `unsafe fn get_resource_mut(&self)`
- add `fn World::as_unsafe_world_cell(&mut self) -> UnsafeWorldCell<'_>` (and `as_unsafe_world_cell_readonly(&self)`)
- add `UnsafeWorldCellEntityRef` with `unsafe fn get`, `unsafe fn get_mut` and the other utilities on `EntityRef` (no methods for spawning, despawning, insertion)
- use the `UnsafeWorldCell` abstraction in `ReflectComponent`, `ReflectResource` and `ReflectAsset`, so these APIs are easier to reason about
- remove `World::get_resource_mut_unchecked`, `EntityRef::get_mut_unchecked` and use `unsafe { world.as_unsafe_world_cell().get_mut() }` and `unsafe { world.as_unsafe_world_cell().get_entity(entity)?.get_mut() }` instead
This PR does **not** make use of `UnsafeWorldCell` for anywhere else in `bevy_ecs` such as `SystemParam` or `Query`. That is a much larger change, and I am convinced that having `UnsafeWorldCell` is already useful for third-party crates.
Implemented API:
```rust
struct World { .. }
impl World {
fn as_unsafe_world_cell(&self) -> UnsafeWorldCell<'_>;
}
struct UnsafeWorldCell<'w>(&'w World);
impl<'w> UnsafeWorldCell {
unsafe fn world(&self) -> &World;
fn get_entity(&self) -> UnsafeWorldCellEntityRef<'w>; // returns 'w which is `'self` of the `World::as_unsafe_world_cell(&'w self)`
unsafe fn get_resource<T>(&self) -> Option<&'w T>;
unsafe fn get_resource_by_id(&self, ComponentId) -> Option<&'w T>;
unsafe fn get_resource_mut<T>(&self) -> Option<Mut<'w, T>>;
unsafe fn get_resource_mut_by_id(&self) -> Option<MutUntyped<'w>>;
unsafe fn get_non_send_resource<T>(&self) -> Option<&'w T>;
unsafe fn get_non_send_resource_mut<T>(&self) -> Option<Mut<'w, T>>>;
// not included: remove, remove_resource, despawn, anything that might change archetypes
}
struct UnsafeWorldCellEntityRef<'w> { .. }
impl UnsafeWorldCellEntityRef<'w> {
unsafe fn get<T>(&self, Entity) -> Option<&'w T>;
unsafe fn get_by_id(&self, Entity, ComponentId) -> Option<Ptr<'w>>;
unsafe fn get_mut<T>(&self, Entity) -> Option<Mut<'w, T>>;
unsafe fn get_mut_by_id(&self, Entity, ComponentId) -> Option<MutUntyped<'w>>;
unsafe fn get_change_ticks<T>(&self, Entity) -> Option<Mut<'w, T>>;
// fn id, archetype, contains, contains_id, containts_type_id
}
```
<details>
<summary>UnsafeWorldCell docs</summary>
Variant of the [`World`] where resource and component accesses takes a `&World`, and the responsibility to avoid
aliasing violations are given to the caller instead of being checked at compile-time by rust's unique XOR shared rule.
### Rationale
In rust, having a `&mut World` means that there are absolutely no other references to the safe world alive at the same time,
without exceptions. Not even unsafe code can change this.
But there are situations where careful shared mutable access through a type is possible and safe. For this, rust provides the [`UnsafeCell`](std::cell::UnsafeCell)
escape hatch, which allows you to get a `*mut T` from a `&UnsafeCell<T>` and around which safe abstractions can be built.
Access to resources and components can be done uniquely using [`World::resource_mut`] and [`World::entity_mut`], and shared using [`World::resource`] and [`World::entity`].
These methods use lifetimes to check at compile time that no aliasing rules are being broken.
This alone is not enough to implement bevy systems where multiple systems can access *disjoint* parts of the world concurrently. For this, bevy stores all values of
resources and components (and [`ComponentTicks`](crate::component::ComponentTicks)) in [`UnsafeCell`](std::cell::UnsafeCell)s, and carefully validates disjoint access patterns using
APIs like [`System::component_access`](crate::system::System::component_access).
A system then can be executed using [`System::run_unsafe`](crate::system::System::run_unsafe) with a `&World` and use methods with interior mutability to access resource values.
access resource values.
### Example Usage
[`UnsafeWorldCell`] can be used as a building block for writing APIs that safely allow disjoint access into the world.
In the following example, the world is split into a resource access half and a component access half, where each one can
safely hand out mutable references.
```rust
use bevy_ecs::world::World;
use bevy_ecs::change_detection::Mut;
use bevy_ecs::system::Resource;
use bevy_ecs::world::unsafe_world_cell_world::UnsafeWorldCell;
// INVARIANT: existance of this struct means that users of it are the only ones being able to access resources in the world
struct OnlyResourceAccessWorld<'w>(UnsafeWorldCell<'w>);
// INVARIANT: existance of this struct means that users of it are the only ones being able to access components in the world
struct OnlyComponentAccessWorld<'w>(UnsafeWorldCell<'w>);
impl<'w> OnlyResourceAccessWorld<'w> {
fn get_resource_mut<T: Resource>(&mut self) -> Option<Mut<'w, T>> {
// SAFETY: resource access is allowed through this UnsafeWorldCell
unsafe { self.0.get_resource_mut::<T>() }
}
}
// impl<'w> OnlyComponentAccessWorld<'w> {
// ...
// }
// the two interior mutable worlds borrow from the `&mut World`, so it cannot be accessed while they are live
fn split_world_access(world: &mut World) -> (OnlyResourceAccessWorld<'_>, OnlyComponentAccessWorld<'_>) {
let resource_access = OnlyResourceAccessWorld(unsafe { world.as_unsafe_world_cell() });
let component_access = OnlyComponentAccessWorld(unsafe { world.as_unsafe_world_cell() });
(resource_access, component_access)
}
```
</details>
2023-01-27 00:12:13 +00:00
. as_unsafe_world_cell_migration_internal ( )
2023-02-06 19:02:52 +00:00
. get_resource_mut_by_id ( component_id )
2021-03-14 00:36:16 +00:00
. unwrap_or_else ( | | {
panic! (
2021-04-23 17:54:04 +00:00
" Resource requested by {} does not exist: {} " ,
Add new SystemState and rename old SystemState to SystemMeta (#2283)
This enables `SystemParams` to be used outside of function systems. Anything can create and store `SystemState`, which enables efficient "param state cached" access to `SystemParams`.
It adds a `ReadOnlySystemParamFetch` trait, which enables safe `SystemState::get` calls without unique world access.
I renamed the old `SystemState` to `SystemMeta` to enable us to mirror the `QueryState` naming convention (but I'm happy to discuss alternative names if people have other ideas). I initially pitched this as `ParamState`, but given that it needs to include full system metadata, that doesn't feel like a particularly accurate name.
```rust
#[derive(Eq, PartialEq, Debug)]
struct A(usize);
#[derive(Eq, PartialEq, Debug)]
struct B(usize);
let mut world = World::default();
world.insert_resource(A(42));
world.spawn().insert(B(7));
// we get nice lifetime elision when declaring the type on the left hand side
let mut system_state: SystemState<(Res<A>, Query<&B>)> = SystemState::new(&mut world);
let (a, query) = system_state.get(&world);
assert_eq!(*a, A(42), "returned resource matches initial value");
assert_eq!(
*query.single().unwrap(),
B(7),
"returned component matches initial value"
);
// mutable system params require unique world access
let mut system_state: SystemState<(ResMut<A>, Query<&mut B>)> = SystemState::new(&mut world);
let (a, query) = system_state.get_mut(&mut world);
// static lifetimes are required when declaring inside of structs
struct SomeContainer {
state: SystemState<(Res<'static, A>, Res<'static, B>)>
}
// this can be shortened using type aliases, which will be useful for complex param tuples
type MyParams<'a> = (Res<'a, A>, Res<'a, B>);
struct SomeContainer {
state: SystemState<MyParams<'static>>
}
// It is the user's responsibility to call SystemState::apply(world) for parameters that queue up work
let mut system_state: SystemState<(Commands, Query<&B>)> = SystemState::new(&mut world);
{
let (mut commands, query) = system_state.get(&world);
commands.insert_resource(3.14);
}
system_state.apply(&mut world);
```
## Future Work
* Actually use SystemState inside FunctionSystem. This would be trivial, but it requires FunctionSystem to wrap SystemState in Option in its current form (which complicates system metadata lookup). I'd prefer to hold off until we adopt something like the later designs linked in #1364, which enable us to contruct Systems using a World reference (and also remove the need for `.system`).
* Consider a "scoped" approach to automatically call SystemState::apply when systems params are no longer being used (either a container type with a Drop impl, or a function that takes a closure for user logic operating on params).
2021-06-02 19:57:38 +00:00
system_meta . name ,
2021-03-14 00:36:16 +00:00
std ::any ::type_name ::< T > ( )
)
} ) ;
Bevy ECS V2 (#1525)
# Bevy ECS V2
This is a rewrite of Bevy ECS (basically everything but the new executor/schedule, which are already awesome). The overall goal was to improve the performance and versatility of Bevy ECS. Here is a quick bulleted list of changes before we dive into the details:
* Complete World rewrite
* Multiple component storage types:
* Tables: fast cache friendly iteration, slower add/removes (previously called Archetypes)
* Sparse Sets: fast add/remove, slower iteration
* Stateful Queries (caches query results for faster iteration. fragmented iteration is _fast_ now)
* Stateful System Params (caches expensive operations. inspired by @DJMcNab's work in #1364)
* Configurable System Params (users can set configuration when they construct their systems. once again inspired by @DJMcNab's work)
* Archetypes are now "just metadata", component storage is separate
* Archetype Graph (for faster archetype changes)
* Component Metadata
* Configure component storage type
* Retrieve information about component size/type/name/layout/send-ness/etc
* Components are uniquely identified by a densely packed ComponentId
* TypeIds are now totally optional (which should make implementing scripting easier)
* Super fast "for_each" query iterators
* Merged Resources into World. Resources are now just a special type of component
* EntityRef/EntityMut builder apis (more efficient and more ergonomic)
* Fast bitset-backed `Access<T>` replaces old hashmap-based approach everywhere
* Query conflicts are determined by component access instead of archetype component access (to avoid random failures at runtime)
* With/Without are still taken into account for conflicts, so this should still be comfy to use
* Much simpler `IntoSystem` impl
* Significantly reduced the amount of hashing throughout the ecs in favor of Sparse Sets (indexed by densely packed ArchetypeId, ComponentId, BundleId, and TableId)
* Safety Improvements
* Entity reservation uses a normal world reference instead of unsafe transmute
* QuerySets no longer transmute lifetimes
* Made traits "unsafe" where relevant
* More thorough safety docs
* WorldCell
* Exposes safe mutable access to multiple resources at a time in a World
* Replaced "catch all" `System::update_archetypes(world: &World)` with `System::new_archetype(archetype: &Archetype)`
* Simpler Bundle implementation
* Replaced slow "remove_bundle_one_by_one" used as fallback for Commands::remove_bundle with fast "remove_bundle_intersection"
* Removed `Mut<T>` query impl. it is better to only support one way: `&mut T`
* Removed with() from `Flags<T>` in favor of `Option<Flags<T>>`, which allows querying for flags to be "filtered" by default
* Components now have is_send property (currently only resources support non-send)
* More granular module organization
* New `RemovedComponents<T>` SystemParam that replaces `query.removed::<T>()`
* `world.resource_scope()` for mutable access to resources and world at the same time
* WorldQuery and QueryFilter traits unified. FilterFetch trait added to enable "short circuit" filtering. Auto impled for cases that don't need it
* Significantly slimmed down SystemState in favor of individual SystemParam state
* System Commands changed from `commands: &mut Commands` back to `mut commands: Commands` (to allow Commands to have a World reference)
Fixes #1320
## `World` Rewrite
This is a from-scratch rewrite of `World` that fills the niche that `hecs` used to. Yes, this means Bevy ECS is no longer a "fork" of hecs. We're going out our own!
(the only shared code between the projects is the entity id allocator, which is already basically ideal)
A huge shout out to @SanderMertens (author of [flecs](https://github.com/SanderMertens/flecs)) for sharing some great ideas with me (specifically hybrid ecs storage and archetype graphs). He also helped advise on a number of implementation details.
## Component Storage (The Problem)
Two ECS storage paradigms have gained a lot of traction over the years:
* **Archetypal ECS**:
* Stores components in "tables" with static schemas. Each "column" stores components of a given type. Each "row" is an entity.
* Each "archetype" has its own table. Adding/removing an entity's component changes the archetype.
* Enables super-fast Query iteration due to its cache-friendly data layout
* Comes at the cost of more expensive add/remove operations for an Entity's components, because all components need to be copied to the new archetype's "table"
* **Sparse Set ECS**:
* Stores components of the same type in densely packed arrays, which are sparsely indexed by densely packed unsigned integers (Entity ids)
* Query iteration is slower than Archetypal ECS because each entity's component could be at any position in the sparse set. This "random access" pattern isn't cache friendly. Additionally, there is an extra layer of indirection because you must first map the entity id to an index in the component array.
* Adding/removing components is a cheap, constant time operation
Bevy ECS V1, hecs, legion, flec, and Unity DOTS are all "archetypal ecs-es". I personally think "archetypal" storage is a good default for game engines. An entity's archetype doesn't need to change frequently in general, and it creates "fast by default" query iteration (which is a much more common operation). It is also "self optimizing". Users don't need to think about optimizing component layouts for iteration performance. It "just works" without any extra boilerplate.
Shipyard and EnTT are "sparse set ecs-es". They employ "packing" as a way to work around the "suboptimal by default" iteration performance for specific sets of components. This helps, but I didn't think this was a good choice for a general purpose engine like Bevy because:
1. "packs" conflict with each other. If bevy decides to internally pack the Transform and GlobalTransform components, users are then blocked if they want to pack some custom component with Transform.
2. users need to take manual action to optimize
Developers selecting an ECS framework are stuck with a hard choice. Select an "archetypal" framework with "fast iteration everywhere" but without the ability to cheaply add/remove components, or select a "sparse set" framework to cheaply add/remove components but with slower iteration performance.
## Hybrid Component Storage (The Solution)
In Bevy ECS V2, we get to have our cake and eat it too. It now has _both_ of the component storage types above (and more can be added later if needed):
* **Tables** (aka "archetypal" storage)
* The default storage. If you don't configure anything, this is what you get
* Fast iteration by default
* Slower add/remove operations
* **Sparse Sets**
* Opt-in
* Slower iteration
* Faster add/remove operations
These storage types complement each other perfectly. By default Query iteration is fast. If developers know that they want to add/remove a component at high frequencies, they can set the storage to "sparse set":
```rust
world.register_component(
ComponentDescriptor::new::<MyComponent>(StorageType::SparseSet)
).unwrap();
```
## Archetypes
Archetypes are now "just metadata" ... they no longer store components directly. They do store:
* The `ComponentId`s of each of the Archetype's components (and that component's storage type)
* Archetypes are uniquely defined by their component layouts
* For example: entities with "table" components `[A, B, C]` _and_ "sparse set" components `[D, E]` will always be in the same archetype.
* The `TableId` associated with the archetype
* For now each archetype has exactly one table (which can have no components),
* There is a 1->Many relationship from Tables->Archetypes. A given table could have any number of archetype components stored in it:
* Ex: an entity with "table storage" components `[A, B, C]` and "sparse set" components `[D, E]` will share the same `[A, B, C]` table as an entity with `[A, B, C]` table component and `[F]` sparse set components.
* This 1->Many relationship is how we preserve fast "cache friendly" iteration performance when possible (more on this later)
* A list of entities that are in the archetype and the row id of the table they are in
* ArchetypeComponentIds
* unique densely packed identifiers for (ArchetypeId, ComponentId) pairs
* used by the schedule executor for cheap system access control
* "Archetype Graph Edges" (see the next section)
## The "Archetype Graph"
Archetype changes in Bevy (and a number of other archetypal ecs-es) have historically been expensive to compute. First, you need to allocate a new vector of the entity's current component ids, add or remove components based on the operation performed, sort it (to ensure it is order-independent), then hash it to find the archetype (if it exists). And thats all before we get to the _already_ expensive full copy of all components to the new table storage.
The solution is to build a "graph" of archetypes to cache these results. @SanderMertens first exposed me to the idea (and he got it from @gjroelofs, who came up with it). They propose adding directed edges between archetypes for add/remove component operations. If `ComponentId`s are densely packed, you can use sparse sets to cheaply jump between archetypes.
Bevy takes this one step further by using add/remove `Bundle` edges instead of `Component` edges. Bevy encourages the use of `Bundles` to group add/remove operations. This is largely for "clearer game logic" reasons, but it also helps cut down on the number of archetype changes required. `Bundles` now also have densely-packed `BundleId`s. This allows us to use a _single_ edge for each bundle operation (rather than needing to traverse N edges ... one for each component). Single component operations are also bundles, so this is strictly an improvement over a "component only" graph.
As a result, an operation that used to be _heavy_ (both for allocations and compute) is now two dirt-cheap array lookups and zero allocations.
## Stateful Queries
World queries are now stateful. This allows us to:
1. Cache archetype (and table) matches
* This resolves another issue with (naive) archetypal ECS: query performance getting worse as the number of archetypes goes up (and fragmentation occurs).
2. Cache Fetch and Filter state
* The expensive parts of fetch/filter operations (such as hashing the TypeId to find the ComponentId) now only happen once when the Query is first constructed
3. Incrementally build up state
* When new archetypes are added, we only process the new archetypes (no need to rebuild state for old archetypes)
As a result, the direct `World` query api now looks like this:
```rust
let mut query = world.query::<(&A, &mut B)>();
for (a, mut b) in query.iter_mut(&mut world) {
}
```
Requiring `World` to generate stateful queries (rather than letting the `QueryState` type be constructed separately) allows us to ensure that _all_ queries are properly initialized (and the relevant world state, such as ComponentIds). This enables QueryState to remove branches from its operations that check for initialization status (and also enables query.iter() to take an immutable world reference because it doesn't need to initialize anything in world).
However in systems, this is a non-breaking change. State management is done internally by the relevant SystemParam.
## Stateful SystemParams
Like Queries, `SystemParams` now also cache state. For example, `Query` system params store the "stateful query" state mentioned above. Commands store their internal `CommandQueue`. This means you can now safely use as many separate `Commands` parameters in your system as you want. `Local<T>` system params store their `T` value in their state (instead of in Resources).
SystemParam state also enabled a significant slim-down of SystemState. It is much nicer to look at now.
Per-SystemParam state naturally insulates us from an "aliased mut" class of errors we have hit in the past (ex: using multiple `Commands` system params).
(credit goes to @DJMcNab for the initial idea and draft pr here #1364)
## Configurable SystemParams
@DJMcNab also had the great idea to make SystemParams configurable. This allows users to provide some initial configuration / values for system parameters (when possible). Most SystemParams have no config (the config type is `()`), but the `Local<T>` param now supports user-provided parameters:
```rust
fn foo(value: Local<usize>) {
}
app.add_system(foo.system().config(|c| c.0 = Some(10)));
```
## Uber Fast "for_each" Query Iterators
Developers now have the choice to use a fast "for_each" iterator, which yields ~1.5-3x iteration speed improvements for "fragmented iteration", and minor ~1.2x iteration speed improvements for unfragmented iteration.
```rust
fn system(query: Query<(&A, &mut B)>) {
// you now have the option to do this for a speed boost
query.for_each_mut(|(a, mut b)| {
});
// however normal iterators are still available
for (a, mut b) in query.iter_mut() {
}
}
```
I think in most cases we should continue to encourage "normal" iterators as they are more flexible and more "rust idiomatic". But when that extra "oomf" is needed, it makes sense to use `for_each`.
We should also consider using `for_each` for internal bevy systems to give our users a nice speed boost (but that should be a separate pr).
## Component Metadata
`World` now has a `Components` collection, which is accessible via `world.components()`. This stores mappings from `ComponentId` to `ComponentInfo`, as well as `TypeId` to `ComponentId` mappings (where relevant). `ComponentInfo` stores information about the component, such as ComponentId, TypeId, memory layout, send-ness (currently limited to resources), and storage type.
## Significantly Cheaper `Access<T>`
We used to use `TypeAccess<TypeId>` to manage read/write component/archetype-component access. This was expensive because TypeIds must be hashed and compared individually. The parallel executor got around this by "condensing" type ids into bitset-backed access types. This worked, but it had to be re-generated from the `TypeAccess<TypeId>`sources every time archetypes changed.
This pr removes TypeAccess in favor of faster bitset access everywhere. We can do this thanks to the move to densely packed `ComponentId`s and `ArchetypeComponentId`s.
## Merged Resources into World
Resources had a lot of redundant functionality with Components. They stored typed data, they had access control, they had unique ids, they were queryable via SystemParams, etc. In fact the _only_ major difference between them was that they were unique (and didn't correlate to an entity).
Separate resources also had the downside of requiring a separate set of access controls, which meant the parallel executor needed to compare more bitsets per system and manage more state.
I initially got the "separate resources" idea from `legion`. I think that design was motivated by the fact that it made the direct world query/resource lifetime interactions more manageable. It certainly made our lives easier when using Resources alongside hecs/bevy_ecs. However we already have a construct for safely and ergonomically managing in-world lifetimes: systems (which use `Access<T>` internally).
This pr merges Resources into World:
```rust
world.insert_resource(1);
world.insert_resource(2.0);
let a = world.get_resource::<i32>().unwrap();
let mut b = world.get_resource_mut::<f64>().unwrap();
*b = 3.0;
```
Resources are now just a special kind of component. They have their own ComponentIds (and their own resource TypeId->ComponentId scope, so they don't conflict wit components of the same type). They are stored in a special "resource archetype", which stores components inside the archetype using a new `unique_components` sparse set (note that this sparse set could later be used to implement Tags). This allows us to keep the code size small by reusing existing datastructures (namely Column, Archetype, ComponentFlags, and ComponentInfo). This allows us the executor to use a single `Access<ArchetypeComponentId>` per system. It should also make scripting language integration easier.
_But_ this merge did create problems for people directly interacting with `World`. What if you need mutable access to multiple resources at the same time? `world.get_resource_mut()` borrows World mutably!
## WorldCell
WorldCell applies the `Access<ArchetypeComponentId>` concept to direct world access:
```rust
let world_cell = world.cell();
let a = world_cell.get_resource_mut::<i32>().unwrap();
let b = world_cell.get_resource_mut::<f64>().unwrap();
```
This adds cheap runtime checks (a sparse set lookup of `ArchetypeComponentId` and a counter) to ensure that world accesses do not conflict with each other. Each operation returns a `WorldBorrow<'w, T>` or `WorldBorrowMut<'w, T>` wrapper type, which will release the relevant ArchetypeComponentId resources when dropped.
World caches the access sparse set (and only one cell can exist at a time), so `world.cell()` is a cheap operation.
WorldCell does _not_ use atomic operations. It is non-send, does a mutable borrow of world to prevent other accesses, and uses a simple `Rc<RefCell<ArchetypeComponentAccess>>` wrapper in each WorldBorrow pointer.
The api is currently limited to resource access, but it can and should be extended to queries / entity component access.
## Resource Scopes
WorldCell does not yet support component queries, and even when it does there are sometimes legitimate reasons to want a mutable world ref _and_ a mutable resource ref (ex: bevy_render and bevy_scene both need this). In these cases we could always drop down to the unsafe `world.get_resource_unchecked_mut()`, but that is not ideal!
Instead developers can use a "resource scope"
```rust
world.resource_scope(|world: &mut World, a: &mut A| {
})
```
This temporarily removes the `A` resource from `World`, provides mutable pointers to both, and re-adds A to World when finished. Thanks to the move to ComponentIds/sparse sets, this is a cheap operation.
If multiple resources are required, scopes can be nested. We could also consider adding a "resource tuple" to the api if this pattern becomes common and the boilerplate gets nasty.
## Query Conflicts Use ComponentId Instead of ArchetypeComponentId
For safety reasons, systems cannot contain queries that conflict with each other without wrapping them in a QuerySet. On bevy `main`, we use ArchetypeComponentIds to determine conflicts. This is nice because it can take into account filters:
```rust
// these queries will never conflict due to their filters
fn filter_system(a: Query<&mut A, With<B>>, b: Query<&mut B, Without<B>>) {
}
```
But it also has a significant downside:
```rust
// these queries will not conflict _until_ an entity with A, B, and C is spawned
fn maybe_conflicts_system(a: Query<(&mut A, &C)>, b: Query<(&mut A, &B)>) {
}
```
The system above will panic at runtime if an entity with A, B, and C is spawned. This makes it hard to trust that your game logic will run without crashing.
In this pr, I switched to using `ComponentId` instead. This _is_ more constraining. `maybe_conflicts_system` will now always fail, but it will do it consistently at startup. Naively, it would also _disallow_ `filter_system`, which would be a significant downgrade in usability. Bevy has a number of internal systems that rely on disjoint queries and I expect it to be a common pattern in userspace.
To resolve this, I added a new `FilteredAccess<T>` type, which wraps `Access<T>` and adds with/without filters. If two `FilteredAccess` have with/without values that prove they are disjoint, they will no longer conflict.
## EntityRef / EntityMut
World entity operations on `main` require that the user passes in an `entity` id to each operation:
```rust
let entity = world.spawn((A, )); // create a new entity with A
world.get::<A>(entity);
world.insert(entity, (B, C));
world.insert_one(entity, D);
```
This means that each operation needs to look up the entity location / verify its validity. The initial spawn operation also requires a Bundle as input. This can be awkward when no components are required (or one component is required).
These operations have been replaced by `EntityRef` and `EntityMut`, which are "builder-style" wrappers around world that provide read and read/write operations on a single, pre-validated entity:
```rust
// spawn now takes no inputs and returns an EntityMut
let entity = world.spawn()
.insert(A) // insert a single component into the entity
.insert_bundle((B, C)) // insert a bundle of components into the entity
.id() // id returns the Entity id
// Returns EntityMut (or panics if the entity does not exist)
world.entity_mut(entity)
.insert(D)
.insert_bundle(SomeBundle::default());
{
// returns EntityRef (or panics if the entity does not exist)
let d = world.entity(entity)
.get::<D>() // gets the D component
.unwrap();
// world.get still exists for ergonomics
let d = world.get::<D>(entity).unwrap();
}
// These variants return Options if you want to check existence instead of panicing
world.get_entity_mut(entity)
.unwrap()
.insert(E);
if let Some(entity_ref) = world.get_entity(entity) {
let d = entity_ref.get::<D>().unwrap();
}
```
This _does not_ affect the current Commands api or terminology. I think that should be a separate conversation as that is a much larger breaking change.
## Safety Improvements
* Entity reservation in Commands uses a normal world borrow instead of an unsafe transmute
* QuerySets no longer transmutes lifetimes
* Made traits "unsafe" when implementing a trait incorrectly could cause unsafety
* More thorough safety docs
## RemovedComponents SystemParam
The old approach to querying removed components: `query.removed:<T>()` was confusing because it had no connection to the query itself. I replaced it with the following, which is both clearer and allows us to cache the ComponentId mapping in the SystemParamState:
```rust
fn system(removed: RemovedComponents<T>) {
for entity in removed.iter() {
}
}
```
## Simpler Bundle implementation
Bundles are no longer responsible for sorting (or deduping) TypeInfo. They are just a simple ordered list of component types / data. This makes the implementation smaller and opens the door to an easy "nested bundle" implementation in the future (which i might even add in this pr). Duplicate detection is now done once per bundle type by World the first time a bundle is used.
## Unified WorldQuery and QueryFilter types
(don't worry they are still separate type _parameters_ in Queries .. this is a non-breaking change)
WorldQuery and QueryFilter were already basically identical apis. With the addition of `FetchState` and more storage-specific fetch methods, the overlap was even clearer (and the redundancy more painful).
QueryFilters are now just `F: WorldQuery where F::Fetch: FilterFetch`. FilterFetch requires `Fetch<Item = bool>` and adds new "short circuit" variants of fetch methods. This enables a filter tuple like `(With<A>, Without<B>, Changed<C>)` to stop evaluating the filter after the first mismatch is encountered. FilterFetch is automatically implemented for `Fetch` implementations that return bool.
This forces fetch implementations that return things like `(bool, bool, bool)` (such as the filter above) to manually implement FilterFetch and decide whether or not to short-circuit.
## More Granular Modules
World no longer globs all of the internal modules together. It now exports `core`, `system`, and `schedule` separately. I'm also considering exporting `core` submodules directly as that is still pretty "glob-ey" and unorganized (feedback welcome here).
## Remaining Draft Work (to be done in this pr)
* ~~panic on conflicting WorldQuery fetches (&A, &mut A)~~
* ~~bevy `main` and hecs both currently allow this, but we should protect against it if possible~~
* ~~batch_iter / par_iter (currently stubbed out)~~
* ~~ChangedRes~~
* ~~I skipped this while we sort out #1313. This pr should be adapted to account for whatever we land on there~~.
* ~~The `Archetypes` and `Tables` collections use hashes of sorted lists of component ids to uniquely identify each archetype/table. This hash is then used as the key in a HashMap to look up the relevant ArchetypeId or TableId. (which doesn't handle hash collisions properly)~~
* ~~It is currently unsafe to generate a Query from "World A", then use it on "World B" (despite the api claiming it is safe). We should probably close this gap. This could be done by adding a randomly generated WorldId to each world, then storing that id in each Query. They could then be compared to each other on each `query.do_thing(&world)` operation. This _does_ add an extra branch to each query operation, so I'm open to other suggestions if people have them.~~
* ~~Nested Bundles (if i find time)~~
## Potential Future Work
* Expand WorldCell to support queries.
* Consider not allocating in the empty archetype on `world.spawn()`
* ex: return something like EntityMutUninit, which turns into EntityMut after an `insert` or `insert_bundle` op
* this actually regressed performance last time i tried it, but in theory it should be faster
* Optimize SparseSet::insert (see `PERF` comment on insert)
* Replace SparseArray `Option<T>` with T::MAX to cut down on branching
* would enable cheaper get_unchecked() operations
* upstream fixedbitset optimizations
* fixedbitset could be allocation free for small block counts (store blocks in a SmallVec)
* fixedbitset could have a const constructor
* Consider implementing Tags (archetype-specific by-value data that affects archetype identity)
* ex: ArchetypeA could have `[A, B, C]` table components and `[D(1)]` "tag" component. ArchetypeB could have `[A, B, C]` table components and a `[D(2)]` tag component. The archetypes are different, despite both having D tags because the value inside D is different.
* this could potentially build on top of the `archetype.unique_components` added in this pr for resource storage.
* Consider reverting `all_tuples` proc macro in favor of the old `macro_rules` implementation
* all_tuples is more flexible and produces cleaner documentation (the macro_rules version produces weird type parameter orders due to parser constraints)
* but unfortunately all_tuples also appears to make Rust Analyzer sad/slow when working inside of `bevy_ecs` (does not affect user code)
* Consider "resource queries" and/or "mixed resource and entity component queries" as an alternative to WorldCell
* this is basically just "systems" so maybe it's not worth it
* Add more world ops
* `world.clear()`
* `world.reserve<T: Bundle>(count: usize)`
* Try using the old archetype allocation strategy (allocate new memory on resize and copy everything over). I expect this to improve batch insertion performance at the cost of unbatched performance. But thats just a guess. I'm not an allocation perf pro :)
* Adapt Commands apis for consistency with new World apis
## Benchmarks
key:
* `bevy_old`: bevy `main` branch
* `bevy`: this branch
* `_foreach`: uses an optimized for_each iterator
* ` _sparse`: uses sparse set storage (if unspecified assume table storage)
* `_system`: runs inside a system (if unspecified assume test happens via direct world ops)
### Simple Insert (from ecs_bench_suite)
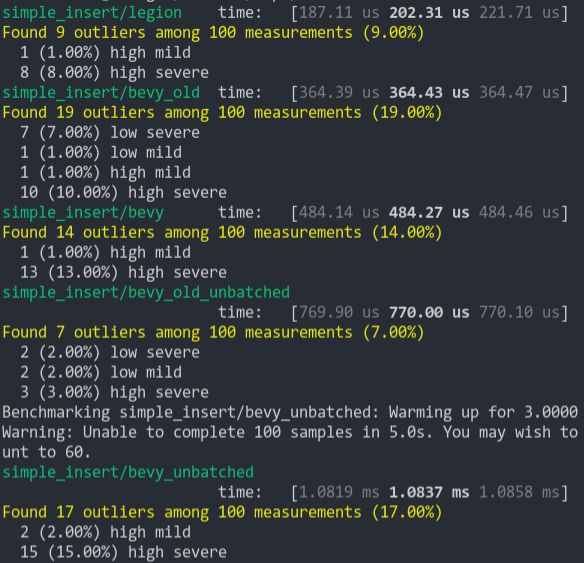
### Simpler Iter (from ecs_bench_suite)
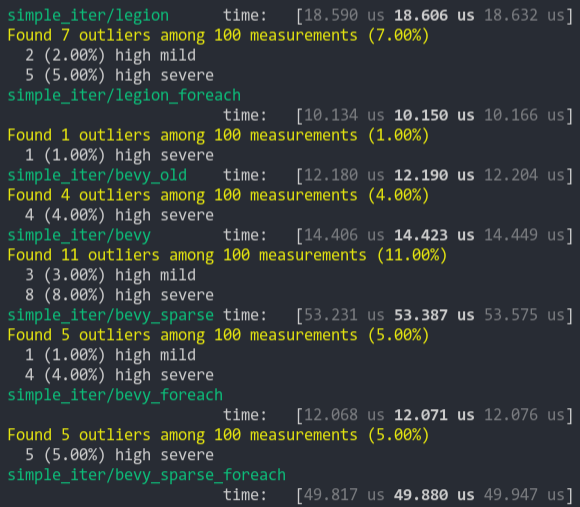
### Fragment Iter (from ecs_bench_suite)
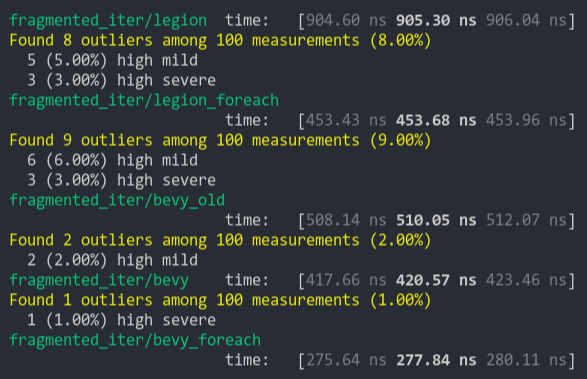
### Sparse Fragmented Iter
Iterate a query that matches 5 entities from a single matching archetype, but there are 100 unmatching archetypes
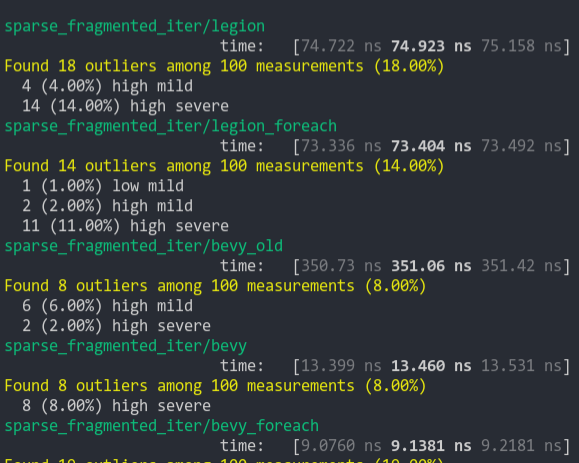
### Schedule (from ecs_bench_suite)
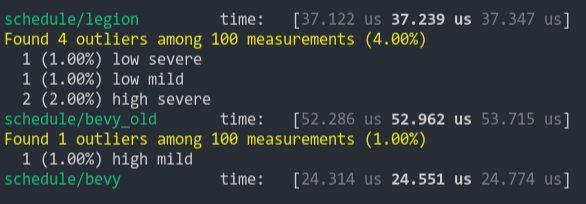
### Add Remove Component (from ecs_bench_suite)
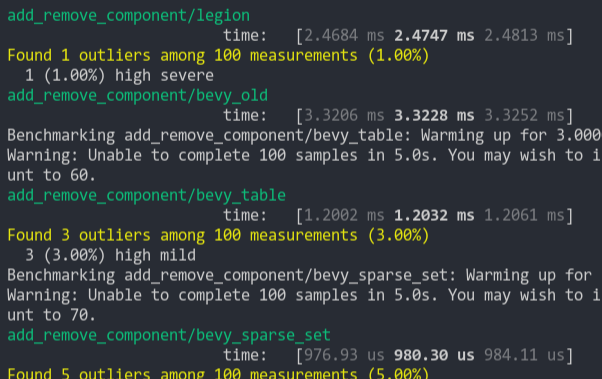
### Add Remove Component Big
Same as the test above, but each entity has 5 "large" matrix components and 1 "large" matrix component is added and removed
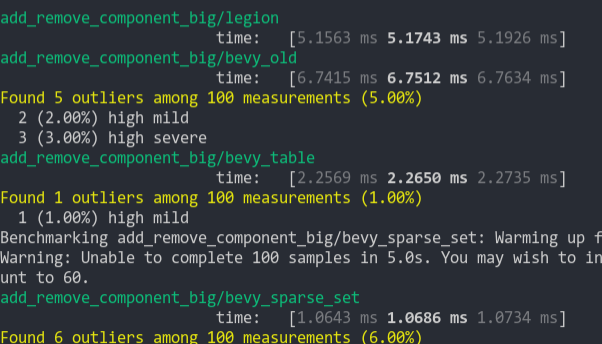
### Get Component
Looks up a single component value a large number of times
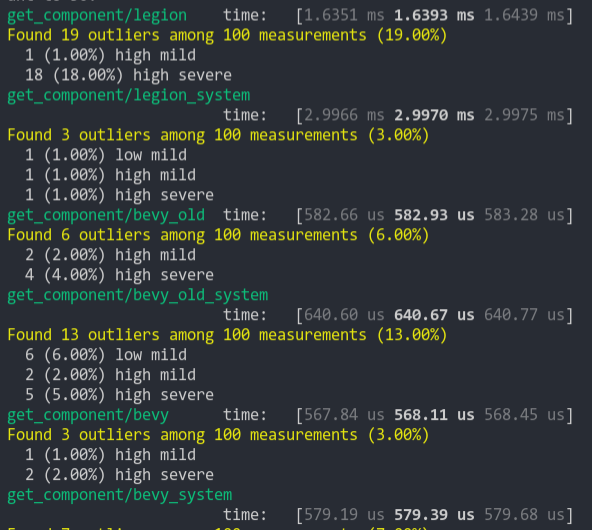
2021-03-05 07:54:35 +00:00
ResMut {
2023-02-06 19:02:52 +00:00
value : value . value . deref_mut ::< T > ( ) ,
2023-01-11 15:41:54 +00:00
ticks : TicksMut {
2022-11-21 12:59:09 +00:00
added : value . ticks . added ,
changed : value . ticks . changed ,
Add new SystemState and rename old SystemState to SystemMeta (#2283)
This enables `SystemParams` to be used outside of function systems. Anything can create and store `SystemState`, which enables efficient "param state cached" access to `SystemParams`.
It adds a `ReadOnlySystemParamFetch` trait, which enables safe `SystemState::get` calls without unique world access.
I renamed the old `SystemState` to `SystemMeta` to enable us to mirror the `QueryState` naming convention (but I'm happy to discuss alternative names if people have other ideas). I initially pitched this as `ParamState`, but given that it needs to include full system metadata, that doesn't feel like a particularly accurate name.
```rust
#[derive(Eq, PartialEq, Debug)]
struct A(usize);
#[derive(Eq, PartialEq, Debug)]
struct B(usize);
let mut world = World::default();
world.insert_resource(A(42));
world.spawn().insert(B(7));
// we get nice lifetime elision when declaring the type on the left hand side
let mut system_state: SystemState<(Res<A>, Query<&B>)> = SystemState::new(&mut world);
let (a, query) = system_state.get(&world);
assert_eq!(*a, A(42), "returned resource matches initial value");
assert_eq!(
*query.single().unwrap(),
B(7),
"returned component matches initial value"
);
// mutable system params require unique world access
let mut system_state: SystemState<(ResMut<A>, Query<&mut B>)> = SystemState::new(&mut world);
let (a, query) = system_state.get_mut(&mut world);
// static lifetimes are required when declaring inside of structs
struct SomeContainer {
state: SystemState<(Res<'static, A>, Res<'static, B>)>
}
// this can be shortened using type aliases, which will be useful for complex param tuples
type MyParams<'a> = (Res<'a, A>, Res<'a, B>);
struct SomeContainer {
state: SystemState<MyParams<'static>>
}
// It is the user's responsibility to call SystemState::apply(world) for parameters that queue up work
let mut system_state: SystemState<(Commands, Query<&B>)> = SystemState::new(&mut world);
{
let (mut commands, query) = system_state.get(&world);
commands.insert_resource(3.14);
}
system_state.apply(&mut world);
```
## Future Work
* Actually use SystemState inside FunctionSystem. This would be trivial, but it requires FunctionSystem to wrap SystemState in Option in its current form (which complicates system metadata lookup). I'd prefer to hold off until we adopt something like the later designs linked in #1364, which enable us to contruct Systems using a World reference (and also remove the need for `.system`).
* Consider a "scoped" approach to automatically call SystemState::apply when systems params are no longer being used (either a container type with a Drop impl, or a function that takes a closure for user logic operating on params).
2021-06-02 19:57:38 +00:00
last_change_tick : system_meta . last_change_tick ,
2021-05-30 19:29:31 +00:00
change_tick ,
} ,
Bevy ECS V2 (#1525)
# Bevy ECS V2
This is a rewrite of Bevy ECS (basically everything but the new executor/schedule, which are already awesome). The overall goal was to improve the performance and versatility of Bevy ECS. Here is a quick bulleted list of changes before we dive into the details:
* Complete World rewrite
* Multiple component storage types:
* Tables: fast cache friendly iteration, slower add/removes (previously called Archetypes)
* Sparse Sets: fast add/remove, slower iteration
* Stateful Queries (caches query results for faster iteration. fragmented iteration is _fast_ now)
* Stateful System Params (caches expensive operations. inspired by @DJMcNab's work in #1364)
* Configurable System Params (users can set configuration when they construct their systems. once again inspired by @DJMcNab's work)
* Archetypes are now "just metadata", component storage is separate
* Archetype Graph (for faster archetype changes)
* Component Metadata
* Configure component storage type
* Retrieve information about component size/type/name/layout/send-ness/etc
* Components are uniquely identified by a densely packed ComponentId
* TypeIds are now totally optional (which should make implementing scripting easier)
* Super fast "for_each" query iterators
* Merged Resources into World. Resources are now just a special type of component
* EntityRef/EntityMut builder apis (more efficient and more ergonomic)
* Fast bitset-backed `Access<T>` replaces old hashmap-based approach everywhere
* Query conflicts are determined by component access instead of archetype component access (to avoid random failures at runtime)
* With/Without are still taken into account for conflicts, so this should still be comfy to use
* Much simpler `IntoSystem` impl
* Significantly reduced the amount of hashing throughout the ecs in favor of Sparse Sets (indexed by densely packed ArchetypeId, ComponentId, BundleId, and TableId)
* Safety Improvements
* Entity reservation uses a normal world reference instead of unsafe transmute
* QuerySets no longer transmute lifetimes
* Made traits "unsafe" where relevant
* More thorough safety docs
* WorldCell
* Exposes safe mutable access to multiple resources at a time in a World
* Replaced "catch all" `System::update_archetypes(world: &World)` with `System::new_archetype(archetype: &Archetype)`
* Simpler Bundle implementation
* Replaced slow "remove_bundle_one_by_one" used as fallback for Commands::remove_bundle with fast "remove_bundle_intersection"
* Removed `Mut<T>` query impl. it is better to only support one way: `&mut T`
* Removed with() from `Flags<T>` in favor of `Option<Flags<T>>`, which allows querying for flags to be "filtered" by default
* Components now have is_send property (currently only resources support non-send)
* More granular module organization
* New `RemovedComponents<T>` SystemParam that replaces `query.removed::<T>()`
* `world.resource_scope()` for mutable access to resources and world at the same time
* WorldQuery and QueryFilter traits unified. FilterFetch trait added to enable "short circuit" filtering. Auto impled for cases that don't need it
* Significantly slimmed down SystemState in favor of individual SystemParam state
* System Commands changed from `commands: &mut Commands` back to `mut commands: Commands` (to allow Commands to have a World reference)
Fixes #1320
## `World` Rewrite
This is a from-scratch rewrite of `World` that fills the niche that `hecs` used to. Yes, this means Bevy ECS is no longer a "fork" of hecs. We're going out our own!
(the only shared code between the projects is the entity id allocator, which is already basically ideal)
A huge shout out to @SanderMertens (author of [flecs](https://github.com/SanderMertens/flecs)) for sharing some great ideas with me (specifically hybrid ecs storage and archetype graphs). He also helped advise on a number of implementation details.
## Component Storage (The Problem)
Two ECS storage paradigms have gained a lot of traction over the years:
* **Archetypal ECS**:
* Stores components in "tables" with static schemas. Each "column" stores components of a given type. Each "row" is an entity.
* Each "archetype" has its own table. Adding/removing an entity's component changes the archetype.
* Enables super-fast Query iteration due to its cache-friendly data layout
* Comes at the cost of more expensive add/remove operations for an Entity's components, because all components need to be copied to the new archetype's "table"
* **Sparse Set ECS**:
* Stores components of the same type in densely packed arrays, which are sparsely indexed by densely packed unsigned integers (Entity ids)
* Query iteration is slower than Archetypal ECS because each entity's component could be at any position in the sparse set. This "random access" pattern isn't cache friendly. Additionally, there is an extra layer of indirection because you must first map the entity id to an index in the component array.
* Adding/removing components is a cheap, constant time operation
Bevy ECS V1, hecs, legion, flec, and Unity DOTS are all "archetypal ecs-es". I personally think "archetypal" storage is a good default for game engines. An entity's archetype doesn't need to change frequently in general, and it creates "fast by default" query iteration (which is a much more common operation). It is also "self optimizing". Users don't need to think about optimizing component layouts for iteration performance. It "just works" without any extra boilerplate.
Shipyard and EnTT are "sparse set ecs-es". They employ "packing" as a way to work around the "suboptimal by default" iteration performance for specific sets of components. This helps, but I didn't think this was a good choice for a general purpose engine like Bevy because:
1. "packs" conflict with each other. If bevy decides to internally pack the Transform and GlobalTransform components, users are then blocked if they want to pack some custom component with Transform.
2. users need to take manual action to optimize
Developers selecting an ECS framework are stuck with a hard choice. Select an "archetypal" framework with "fast iteration everywhere" but without the ability to cheaply add/remove components, or select a "sparse set" framework to cheaply add/remove components but with slower iteration performance.
## Hybrid Component Storage (The Solution)
In Bevy ECS V2, we get to have our cake and eat it too. It now has _both_ of the component storage types above (and more can be added later if needed):
* **Tables** (aka "archetypal" storage)
* The default storage. If you don't configure anything, this is what you get
* Fast iteration by default
* Slower add/remove operations
* **Sparse Sets**
* Opt-in
* Slower iteration
* Faster add/remove operations
These storage types complement each other perfectly. By default Query iteration is fast. If developers know that they want to add/remove a component at high frequencies, they can set the storage to "sparse set":
```rust
world.register_component(
ComponentDescriptor::new::<MyComponent>(StorageType::SparseSet)
).unwrap();
```
## Archetypes
Archetypes are now "just metadata" ... they no longer store components directly. They do store:
* The `ComponentId`s of each of the Archetype's components (and that component's storage type)
* Archetypes are uniquely defined by their component layouts
* For example: entities with "table" components `[A, B, C]` _and_ "sparse set" components `[D, E]` will always be in the same archetype.
* The `TableId` associated with the archetype
* For now each archetype has exactly one table (which can have no components),
* There is a 1->Many relationship from Tables->Archetypes. A given table could have any number of archetype components stored in it:
* Ex: an entity with "table storage" components `[A, B, C]` and "sparse set" components `[D, E]` will share the same `[A, B, C]` table as an entity with `[A, B, C]` table component and `[F]` sparse set components.
* This 1->Many relationship is how we preserve fast "cache friendly" iteration performance when possible (more on this later)
* A list of entities that are in the archetype and the row id of the table they are in
* ArchetypeComponentIds
* unique densely packed identifiers for (ArchetypeId, ComponentId) pairs
* used by the schedule executor for cheap system access control
* "Archetype Graph Edges" (see the next section)
## The "Archetype Graph"
Archetype changes in Bevy (and a number of other archetypal ecs-es) have historically been expensive to compute. First, you need to allocate a new vector of the entity's current component ids, add or remove components based on the operation performed, sort it (to ensure it is order-independent), then hash it to find the archetype (if it exists). And thats all before we get to the _already_ expensive full copy of all components to the new table storage.
The solution is to build a "graph" of archetypes to cache these results. @SanderMertens first exposed me to the idea (and he got it from @gjroelofs, who came up with it). They propose adding directed edges between archetypes for add/remove component operations. If `ComponentId`s are densely packed, you can use sparse sets to cheaply jump between archetypes.
Bevy takes this one step further by using add/remove `Bundle` edges instead of `Component` edges. Bevy encourages the use of `Bundles` to group add/remove operations. This is largely for "clearer game logic" reasons, but it also helps cut down on the number of archetype changes required. `Bundles` now also have densely-packed `BundleId`s. This allows us to use a _single_ edge for each bundle operation (rather than needing to traverse N edges ... one for each component). Single component operations are also bundles, so this is strictly an improvement over a "component only" graph.
As a result, an operation that used to be _heavy_ (both for allocations and compute) is now two dirt-cheap array lookups and zero allocations.
## Stateful Queries
World queries are now stateful. This allows us to:
1. Cache archetype (and table) matches
* This resolves another issue with (naive) archetypal ECS: query performance getting worse as the number of archetypes goes up (and fragmentation occurs).
2. Cache Fetch and Filter state
* The expensive parts of fetch/filter operations (such as hashing the TypeId to find the ComponentId) now only happen once when the Query is first constructed
3. Incrementally build up state
* When new archetypes are added, we only process the new archetypes (no need to rebuild state for old archetypes)
As a result, the direct `World` query api now looks like this:
```rust
let mut query = world.query::<(&A, &mut B)>();
for (a, mut b) in query.iter_mut(&mut world) {
}
```
Requiring `World` to generate stateful queries (rather than letting the `QueryState` type be constructed separately) allows us to ensure that _all_ queries are properly initialized (and the relevant world state, such as ComponentIds). This enables QueryState to remove branches from its operations that check for initialization status (and also enables query.iter() to take an immutable world reference because it doesn't need to initialize anything in world).
However in systems, this is a non-breaking change. State management is done internally by the relevant SystemParam.
## Stateful SystemParams
Like Queries, `SystemParams` now also cache state. For example, `Query` system params store the "stateful query" state mentioned above. Commands store their internal `CommandQueue`. This means you can now safely use as many separate `Commands` parameters in your system as you want. `Local<T>` system params store their `T` value in their state (instead of in Resources).
SystemParam state also enabled a significant slim-down of SystemState. It is much nicer to look at now.
Per-SystemParam state naturally insulates us from an "aliased mut" class of errors we have hit in the past (ex: using multiple `Commands` system params).
(credit goes to @DJMcNab for the initial idea and draft pr here #1364)
## Configurable SystemParams
@DJMcNab also had the great idea to make SystemParams configurable. This allows users to provide some initial configuration / values for system parameters (when possible). Most SystemParams have no config (the config type is `()`), but the `Local<T>` param now supports user-provided parameters:
```rust
fn foo(value: Local<usize>) {
}
app.add_system(foo.system().config(|c| c.0 = Some(10)));
```
## Uber Fast "for_each" Query Iterators
Developers now have the choice to use a fast "for_each" iterator, which yields ~1.5-3x iteration speed improvements for "fragmented iteration", and minor ~1.2x iteration speed improvements for unfragmented iteration.
```rust
fn system(query: Query<(&A, &mut B)>) {
// you now have the option to do this for a speed boost
query.for_each_mut(|(a, mut b)| {
});
// however normal iterators are still available
for (a, mut b) in query.iter_mut() {
}
}
```
I think in most cases we should continue to encourage "normal" iterators as they are more flexible and more "rust idiomatic". But when that extra "oomf" is needed, it makes sense to use `for_each`.
We should also consider using `for_each` for internal bevy systems to give our users a nice speed boost (but that should be a separate pr).
## Component Metadata
`World` now has a `Components` collection, which is accessible via `world.components()`. This stores mappings from `ComponentId` to `ComponentInfo`, as well as `TypeId` to `ComponentId` mappings (where relevant). `ComponentInfo` stores information about the component, such as ComponentId, TypeId, memory layout, send-ness (currently limited to resources), and storage type.
## Significantly Cheaper `Access<T>`
We used to use `TypeAccess<TypeId>` to manage read/write component/archetype-component access. This was expensive because TypeIds must be hashed and compared individually. The parallel executor got around this by "condensing" type ids into bitset-backed access types. This worked, but it had to be re-generated from the `TypeAccess<TypeId>`sources every time archetypes changed.
This pr removes TypeAccess in favor of faster bitset access everywhere. We can do this thanks to the move to densely packed `ComponentId`s and `ArchetypeComponentId`s.
## Merged Resources into World
Resources had a lot of redundant functionality with Components. They stored typed data, they had access control, they had unique ids, they were queryable via SystemParams, etc. In fact the _only_ major difference between them was that they were unique (and didn't correlate to an entity).
Separate resources also had the downside of requiring a separate set of access controls, which meant the parallel executor needed to compare more bitsets per system and manage more state.
I initially got the "separate resources" idea from `legion`. I think that design was motivated by the fact that it made the direct world query/resource lifetime interactions more manageable. It certainly made our lives easier when using Resources alongside hecs/bevy_ecs. However we already have a construct for safely and ergonomically managing in-world lifetimes: systems (which use `Access<T>` internally).
This pr merges Resources into World:
```rust
world.insert_resource(1);
world.insert_resource(2.0);
let a = world.get_resource::<i32>().unwrap();
let mut b = world.get_resource_mut::<f64>().unwrap();
*b = 3.0;
```
Resources are now just a special kind of component. They have their own ComponentIds (and their own resource TypeId->ComponentId scope, so they don't conflict wit components of the same type). They are stored in a special "resource archetype", which stores components inside the archetype using a new `unique_components` sparse set (note that this sparse set could later be used to implement Tags). This allows us to keep the code size small by reusing existing datastructures (namely Column, Archetype, ComponentFlags, and ComponentInfo). This allows us the executor to use a single `Access<ArchetypeComponentId>` per system. It should also make scripting language integration easier.
_But_ this merge did create problems for people directly interacting with `World`. What if you need mutable access to multiple resources at the same time? `world.get_resource_mut()` borrows World mutably!
## WorldCell
WorldCell applies the `Access<ArchetypeComponentId>` concept to direct world access:
```rust
let world_cell = world.cell();
let a = world_cell.get_resource_mut::<i32>().unwrap();
let b = world_cell.get_resource_mut::<f64>().unwrap();
```
This adds cheap runtime checks (a sparse set lookup of `ArchetypeComponentId` and a counter) to ensure that world accesses do not conflict with each other. Each operation returns a `WorldBorrow<'w, T>` or `WorldBorrowMut<'w, T>` wrapper type, which will release the relevant ArchetypeComponentId resources when dropped.
World caches the access sparse set (and only one cell can exist at a time), so `world.cell()` is a cheap operation.
WorldCell does _not_ use atomic operations. It is non-send, does a mutable borrow of world to prevent other accesses, and uses a simple `Rc<RefCell<ArchetypeComponentAccess>>` wrapper in each WorldBorrow pointer.
The api is currently limited to resource access, but it can and should be extended to queries / entity component access.
## Resource Scopes
WorldCell does not yet support component queries, and even when it does there are sometimes legitimate reasons to want a mutable world ref _and_ a mutable resource ref (ex: bevy_render and bevy_scene both need this). In these cases we could always drop down to the unsafe `world.get_resource_unchecked_mut()`, but that is not ideal!
Instead developers can use a "resource scope"
```rust
world.resource_scope(|world: &mut World, a: &mut A| {
})
```
This temporarily removes the `A` resource from `World`, provides mutable pointers to both, and re-adds A to World when finished. Thanks to the move to ComponentIds/sparse sets, this is a cheap operation.
If multiple resources are required, scopes can be nested. We could also consider adding a "resource tuple" to the api if this pattern becomes common and the boilerplate gets nasty.
## Query Conflicts Use ComponentId Instead of ArchetypeComponentId
For safety reasons, systems cannot contain queries that conflict with each other without wrapping them in a QuerySet. On bevy `main`, we use ArchetypeComponentIds to determine conflicts. This is nice because it can take into account filters:
```rust
// these queries will never conflict due to their filters
fn filter_system(a: Query<&mut A, With<B>>, b: Query<&mut B, Without<B>>) {
}
```
But it also has a significant downside:
```rust
// these queries will not conflict _until_ an entity with A, B, and C is spawned
fn maybe_conflicts_system(a: Query<(&mut A, &C)>, b: Query<(&mut A, &B)>) {
}
```
The system above will panic at runtime if an entity with A, B, and C is spawned. This makes it hard to trust that your game logic will run without crashing.
In this pr, I switched to using `ComponentId` instead. This _is_ more constraining. `maybe_conflicts_system` will now always fail, but it will do it consistently at startup. Naively, it would also _disallow_ `filter_system`, which would be a significant downgrade in usability. Bevy has a number of internal systems that rely on disjoint queries and I expect it to be a common pattern in userspace.
To resolve this, I added a new `FilteredAccess<T>` type, which wraps `Access<T>` and adds with/without filters. If two `FilteredAccess` have with/without values that prove they are disjoint, they will no longer conflict.
## EntityRef / EntityMut
World entity operations on `main` require that the user passes in an `entity` id to each operation:
```rust
let entity = world.spawn((A, )); // create a new entity with A
world.get::<A>(entity);
world.insert(entity, (B, C));
world.insert_one(entity, D);
```
This means that each operation needs to look up the entity location / verify its validity. The initial spawn operation also requires a Bundle as input. This can be awkward when no components are required (or one component is required).
These operations have been replaced by `EntityRef` and `EntityMut`, which are "builder-style" wrappers around world that provide read and read/write operations on a single, pre-validated entity:
```rust
// spawn now takes no inputs and returns an EntityMut
let entity = world.spawn()
.insert(A) // insert a single component into the entity
.insert_bundle((B, C)) // insert a bundle of components into the entity
.id() // id returns the Entity id
// Returns EntityMut (or panics if the entity does not exist)
world.entity_mut(entity)
.insert(D)
.insert_bundle(SomeBundle::default());
{
// returns EntityRef (or panics if the entity does not exist)
let d = world.entity(entity)
.get::<D>() // gets the D component
.unwrap();
// world.get still exists for ergonomics
let d = world.get::<D>(entity).unwrap();
}
// These variants return Options if you want to check existence instead of panicing
world.get_entity_mut(entity)
.unwrap()
.insert(E);
if let Some(entity_ref) = world.get_entity(entity) {
let d = entity_ref.get::<D>().unwrap();
}
```
This _does not_ affect the current Commands api or terminology. I think that should be a separate conversation as that is a much larger breaking change.
## Safety Improvements
* Entity reservation in Commands uses a normal world borrow instead of an unsafe transmute
* QuerySets no longer transmutes lifetimes
* Made traits "unsafe" when implementing a trait incorrectly could cause unsafety
* More thorough safety docs
## RemovedComponents SystemParam
The old approach to querying removed components: `query.removed:<T>()` was confusing because it had no connection to the query itself. I replaced it with the following, which is both clearer and allows us to cache the ComponentId mapping in the SystemParamState:
```rust
fn system(removed: RemovedComponents<T>) {
for entity in removed.iter() {
}
}
```
## Simpler Bundle implementation
Bundles are no longer responsible for sorting (or deduping) TypeInfo. They are just a simple ordered list of component types / data. This makes the implementation smaller and opens the door to an easy "nested bundle" implementation in the future (which i might even add in this pr). Duplicate detection is now done once per bundle type by World the first time a bundle is used.
## Unified WorldQuery and QueryFilter types
(don't worry they are still separate type _parameters_ in Queries .. this is a non-breaking change)
WorldQuery and QueryFilter were already basically identical apis. With the addition of `FetchState` and more storage-specific fetch methods, the overlap was even clearer (and the redundancy more painful).
QueryFilters are now just `F: WorldQuery where F::Fetch: FilterFetch`. FilterFetch requires `Fetch<Item = bool>` and adds new "short circuit" variants of fetch methods. This enables a filter tuple like `(With<A>, Without<B>, Changed<C>)` to stop evaluating the filter after the first mismatch is encountered. FilterFetch is automatically implemented for `Fetch` implementations that return bool.
This forces fetch implementations that return things like `(bool, bool, bool)` (such as the filter above) to manually implement FilterFetch and decide whether or not to short-circuit.
## More Granular Modules
World no longer globs all of the internal modules together. It now exports `core`, `system`, and `schedule` separately. I'm also considering exporting `core` submodules directly as that is still pretty "glob-ey" and unorganized (feedback welcome here).
## Remaining Draft Work (to be done in this pr)
* ~~panic on conflicting WorldQuery fetches (&A, &mut A)~~
* ~~bevy `main` and hecs both currently allow this, but we should protect against it if possible~~
* ~~batch_iter / par_iter (currently stubbed out)~~
* ~~ChangedRes~~
* ~~I skipped this while we sort out #1313. This pr should be adapted to account for whatever we land on there~~.
* ~~The `Archetypes` and `Tables` collections use hashes of sorted lists of component ids to uniquely identify each archetype/table. This hash is then used as the key in a HashMap to look up the relevant ArchetypeId or TableId. (which doesn't handle hash collisions properly)~~
* ~~It is currently unsafe to generate a Query from "World A", then use it on "World B" (despite the api claiming it is safe). We should probably close this gap. This could be done by adding a randomly generated WorldId to each world, then storing that id in each Query. They could then be compared to each other on each `query.do_thing(&world)` operation. This _does_ add an extra branch to each query operation, so I'm open to other suggestions if people have them.~~
* ~~Nested Bundles (if i find time)~~
## Potential Future Work
* Expand WorldCell to support queries.
* Consider not allocating in the empty archetype on `world.spawn()`
* ex: return something like EntityMutUninit, which turns into EntityMut after an `insert` or `insert_bundle` op
* this actually regressed performance last time i tried it, but in theory it should be faster
* Optimize SparseSet::insert (see `PERF` comment on insert)
* Replace SparseArray `Option<T>` with T::MAX to cut down on branching
* would enable cheaper get_unchecked() operations
* upstream fixedbitset optimizations
* fixedbitset could be allocation free for small block counts (store blocks in a SmallVec)
* fixedbitset could have a const constructor
* Consider implementing Tags (archetype-specific by-value data that affects archetype identity)
* ex: ArchetypeA could have `[A, B, C]` table components and `[D(1)]` "tag" component. ArchetypeB could have `[A, B, C]` table components and a `[D(2)]` tag component. The archetypes are different, despite both having D tags because the value inside D is different.
* this could potentially build on top of the `archetype.unique_components` added in this pr for resource storage.
* Consider reverting `all_tuples` proc macro in favor of the old `macro_rules` implementation
* all_tuples is more flexible and produces cleaner documentation (the macro_rules version produces weird type parameter orders due to parser constraints)
* but unfortunately all_tuples also appears to make Rust Analyzer sad/slow when working inside of `bevy_ecs` (does not affect user code)
* Consider "resource queries" and/or "mixed resource and entity component queries" as an alternative to WorldCell
* this is basically just "systems" so maybe it's not worth it
* Add more world ops
* `world.clear()`
* `world.reserve<T: Bundle>(count: usize)`
* Try using the old archetype allocation strategy (allocate new memory on resize and copy everything over). I expect this to improve batch insertion performance at the cost of unbatched performance. But thats just a guess. I'm not an allocation perf pro :)
* Adapt Commands apis for consistency with new World apis
## Benchmarks
key:
* `bevy_old`: bevy `main` branch
* `bevy`: this branch
* `_foreach`: uses an optimized for_each iterator
* ` _sparse`: uses sparse set storage (if unspecified assume table storage)
* `_system`: runs inside a system (if unspecified assume test happens via direct world ops)
### Simple Insert (from ecs_bench_suite)
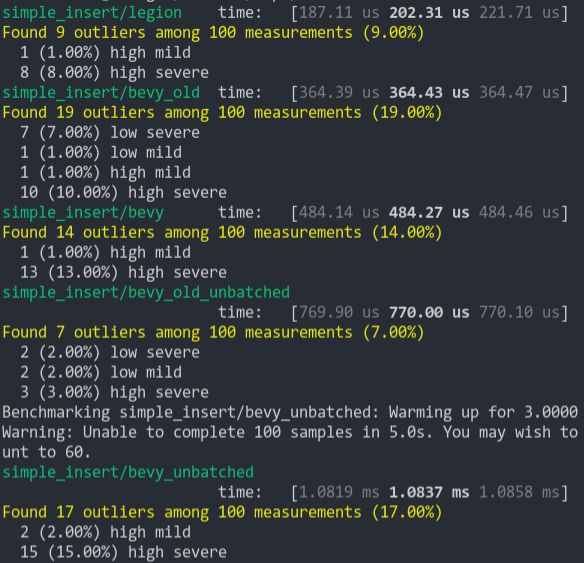
### Simpler Iter (from ecs_bench_suite)
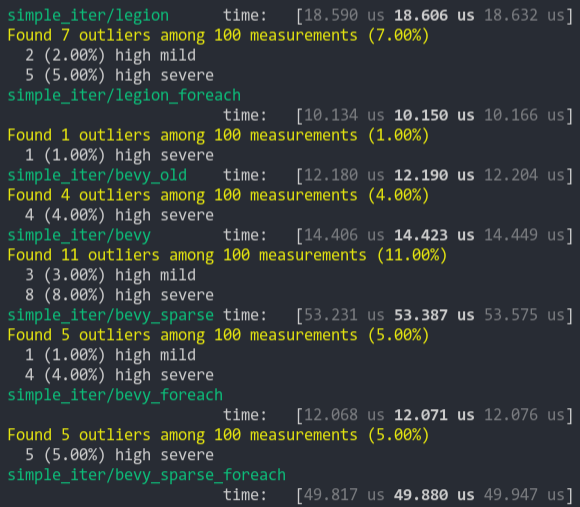
### Fragment Iter (from ecs_bench_suite)
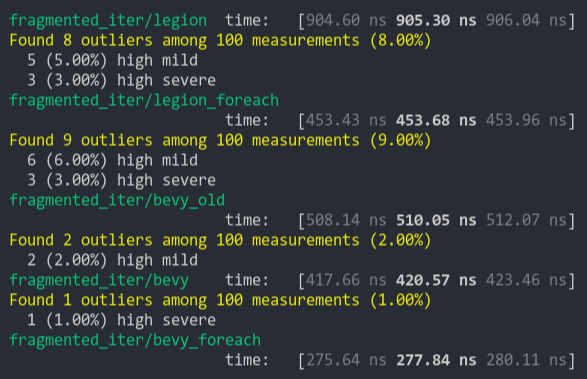
### Sparse Fragmented Iter
Iterate a query that matches 5 entities from a single matching archetype, but there are 100 unmatching archetypes
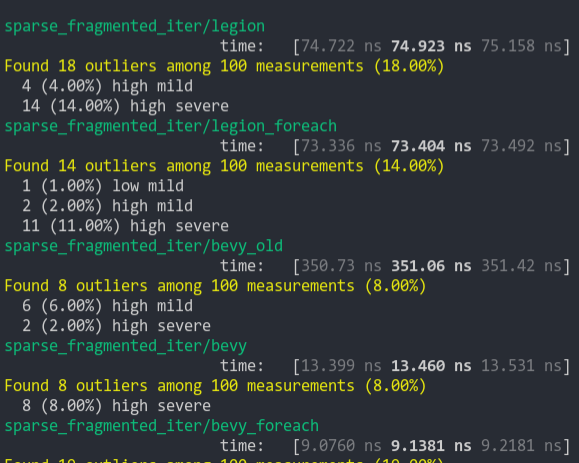
### Schedule (from ecs_bench_suite)
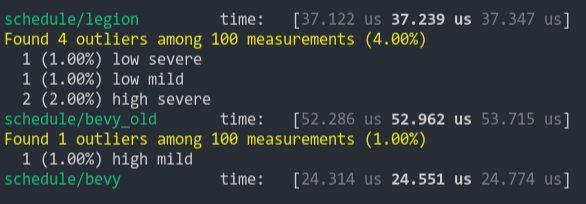
### Add Remove Component (from ecs_bench_suite)
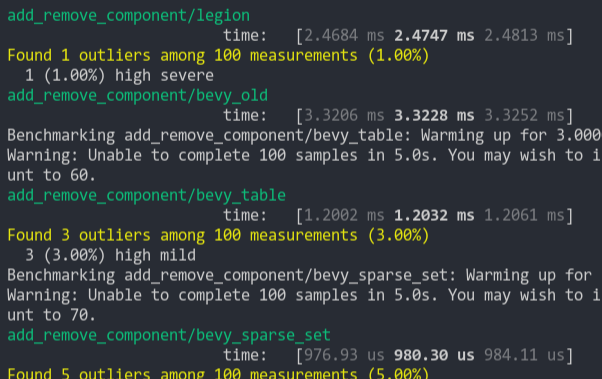
### Add Remove Component Big
Same as the test above, but each entity has 5 "large" matrix components and 1 "large" matrix component is added and removed
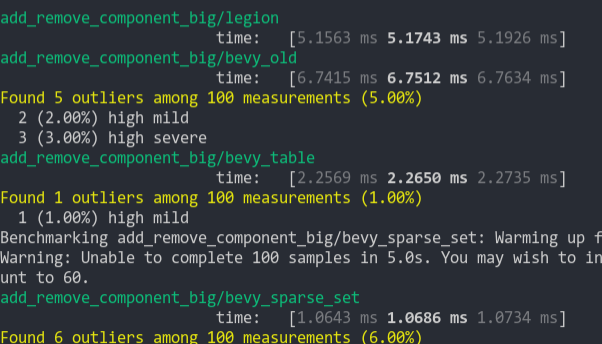
### Get Component
Looks up a single component value a large number of times
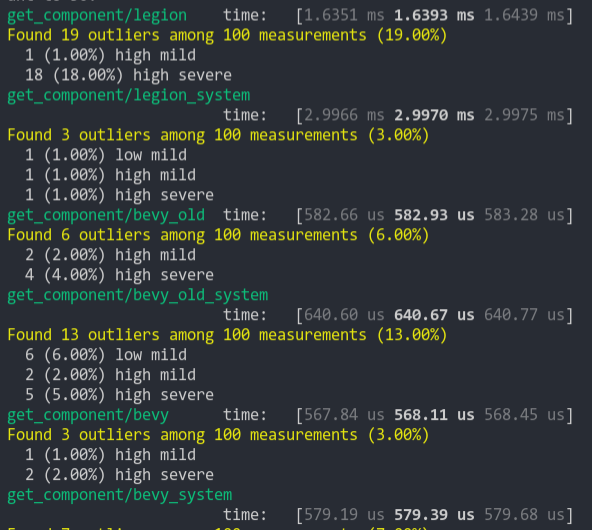
2021-03-05 07:54:35 +00:00
}
}
}
2020-12-16 05:57:16 +00:00
Remove the `SystemParamState` trait and remove types like `ResState` (#6919)
Spiritual successor to #5205.
Actual successor to #6865.
# Objective
Currently, system params are defined using three traits: `SystemParam`, `ReadOnlySystemParam`, `SystemParamState`. The behavior for each param is specified by the `SystemParamState` trait, while `SystemParam` simply defers to the state.
Splitting the traits in this way makes it easier to implement within macros, but it increases the cognitive load. Worst of all, this approach requires each `MySystemParam` to have a public `MySystemParamState` type associated with it.
## Solution
* Merge the trait `SystemParamState` into `SystemParam`.
* Remove all trivial `SystemParam` state types.
* `OptionNonSendMutState<T>`: you will not be missed.
---
- [x] Fix/resolve the remaining test failure.
## Changelog
* Removed the trait `SystemParamState`, merging its functionality into `SystemParam`.
## Migration Guide
**Note**: this should replace the migration guide for #6865.
This is relative to Bevy 0.9, not main.
The traits `SystemParamState` and `SystemParamFetch` have been removed, and their functionality has been transferred to `SystemParam`.
```rust
// Before (0.9)
impl SystemParam for MyParam<'_, '_> {
type State = MyParamState;
}
unsafe impl SystemParamState for MyParamState {
fn init(world: &mut World, system_meta: &mut SystemMeta) -> Self { ... }
}
unsafe impl<'w, 's> SystemParamFetch<'w, 's> for MyParamState {
type Item = MyParam<'w, 's>;
fn get_param(&mut self, ...) -> Self::Item;
}
unsafe impl ReadOnlySystemParamFetch for MyParamState { }
// After (0.10)
unsafe impl SystemParam for MyParam<'_, '_> {
type State = MyParamState;
type Item<'w, 's> = MyParam<'w, 's>;
fn init_state(world: &mut World, system_meta: &mut SystemMeta) -> Self::State { ... }
fn get_param<'w, 's>(state: &mut Self::State, ...) -> Self::Item<'w, 's>;
}
unsafe impl ReadOnlySystemParam for MyParam<'_, '_> { }
```
The trait `ReadOnlySystemParamFetch` has been replaced with `ReadOnlySystemParam`.
```rust
// Before
unsafe impl ReadOnlySystemParamFetch for MyParamState {}
// After
unsafe impl ReadOnlySystemParam for MyParam<'_, '_> {}
```
2023-01-07 23:20:32 +00:00
// SAFETY: this impl defers to `ResMut`, which initializes and validates the correct world access.
unsafe impl < ' a , T : Resource > SystemParam for Option < ResMut < ' a , T > > {
type State = ComponentId ;
Simplify trait hierarchy for `SystemParam` (#6865)
# Objective
* Implementing a custom `SystemParam` by hand requires implementing three traits -- four if it is read-only.
* The trait `SystemParamFetch<'w, 's>` is a workaround from before we had generic associated types, and is no longer necessary.
## Solution
* Combine the trait `SystemParamFetch` with `SystemParamState`.
* I decided to remove the `Fetch` name and keep the `State` name, since the former was consistently conflated with the latter.
* Replace the trait `ReadOnlySystemParamFetch` with `ReadOnlySystemParam`, which simplifies trait bounds in generic code.
---
## Changelog
- Removed the trait `SystemParamFetch`, moving its functionality to `SystemParamState`.
- Replaced the trait `ReadOnlySystemParamFetch` with `ReadOnlySystemParam`.
## Migration Guide
The trait `SystemParamFetch` has been removed, and its functionality has been transferred to `SystemParamState`.
```rust
// Before
impl SystemParamState for MyParamState {
fn init(world: &mut World, system_meta: &mut SystemMeta) -> Self { ... }
}
impl<'w, 's> SystemParamFetch<'w, 's> for MyParamState {
type Item = MyParam<'w, 's>;
fn get_param(...) -> Self::Item;
}
// After
impl SystemParamState for MyParamState {
type Item<'w, 's> = MyParam<'w, 's>; // Generic associated types!
fn init(world: &mut World, system_meta: &mut SystemMeta) -> Self { ... }
fn get_param<'w, 's>(...) -> Self::Item<'w, 's>;
}
```
The trait `ReadOnlySystemParamFetch` has been replaced with `ReadOnlySystemParam`.
```rust
// Before
unsafe impl ReadOnlySystemParamFetch for MyParamState {}
// After
unsafe impl<'w, 's> ReadOnlySystemParam for MyParam<'w, 's> {}
```
2022-12-11 18:34:14 +00:00
type Item < ' w , ' s > = Option < ResMut < ' w , T > > ;
Remove the `SystemParamState` trait and remove types like `ResState` (#6919)
Spiritual successor to #5205.
Actual successor to #6865.
# Objective
Currently, system params are defined using three traits: `SystemParam`, `ReadOnlySystemParam`, `SystemParamState`. The behavior for each param is specified by the `SystemParamState` trait, while `SystemParam` simply defers to the state.
Splitting the traits in this way makes it easier to implement within macros, but it increases the cognitive load. Worst of all, this approach requires each `MySystemParam` to have a public `MySystemParamState` type associated with it.
## Solution
* Merge the trait `SystemParamState` into `SystemParam`.
* Remove all trivial `SystemParam` state types.
* `OptionNonSendMutState<T>`: you will not be missed.
---
- [x] Fix/resolve the remaining test failure.
## Changelog
* Removed the trait `SystemParamState`, merging its functionality into `SystemParam`.
## Migration Guide
**Note**: this should replace the migration guide for #6865.
This is relative to Bevy 0.9, not main.
The traits `SystemParamState` and `SystemParamFetch` have been removed, and their functionality has been transferred to `SystemParam`.
```rust
// Before (0.9)
impl SystemParam for MyParam<'_, '_> {
type State = MyParamState;
}
unsafe impl SystemParamState for MyParamState {
fn init(world: &mut World, system_meta: &mut SystemMeta) -> Self { ... }
}
unsafe impl<'w, 's> SystemParamFetch<'w, 's> for MyParamState {
type Item = MyParam<'w, 's>;
fn get_param(&mut self, ...) -> Self::Item;
}
unsafe impl ReadOnlySystemParamFetch for MyParamState { }
// After (0.10)
unsafe impl SystemParam for MyParam<'_, '_> {
type State = MyParamState;
type Item<'w, 's> = MyParam<'w, 's>;
fn init_state(world: &mut World, system_meta: &mut SystemMeta) -> Self::State { ... }
fn get_param<'w, 's>(state: &mut Self::State, ...) -> Self::Item<'w, 's>;
}
unsafe impl ReadOnlySystemParam for MyParam<'_, '_> { }
```
The trait `ReadOnlySystemParamFetch` has been replaced with `ReadOnlySystemParam`.
```rust
// Before
unsafe impl ReadOnlySystemParamFetch for MyParamState {}
// After
unsafe impl ReadOnlySystemParam for MyParam<'_, '_> {}
```
2023-01-07 23:20:32 +00:00
fn init_state ( world : & mut World , system_meta : & mut SystemMeta ) -> Self ::State {
ResMut ::< T > ::init_state ( world , system_meta )
2021-03-08 20:12:22 +00:00
}
#[ inline ]
Simplify trait hierarchy for `SystemParam` (#6865)
# Objective
* Implementing a custom `SystemParam` by hand requires implementing three traits -- four if it is read-only.
* The trait `SystemParamFetch<'w, 's>` is a workaround from before we had generic associated types, and is no longer necessary.
## Solution
* Combine the trait `SystemParamFetch` with `SystemParamState`.
* I decided to remove the `Fetch` name and keep the `State` name, since the former was consistently conflated with the latter.
* Replace the trait `ReadOnlySystemParamFetch` with `ReadOnlySystemParam`, which simplifies trait bounds in generic code.
---
## Changelog
- Removed the trait `SystemParamFetch`, moving its functionality to `SystemParamState`.
- Replaced the trait `ReadOnlySystemParamFetch` with `ReadOnlySystemParam`.
## Migration Guide
The trait `SystemParamFetch` has been removed, and its functionality has been transferred to `SystemParamState`.
```rust
// Before
impl SystemParamState for MyParamState {
fn init(world: &mut World, system_meta: &mut SystemMeta) -> Self { ... }
}
impl<'w, 's> SystemParamFetch<'w, 's> for MyParamState {
type Item = MyParam<'w, 's>;
fn get_param(...) -> Self::Item;
}
// After
impl SystemParamState for MyParamState {
type Item<'w, 's> = MyParam<'w, 's>; // Generic associated types!
fn init(world: &mut World, system_meta: &mut SystemMeta) -> Self { ... }
fn get_param<'w, 's>(...) -> Self::Item<'w, 's>;
}
```
The trait `ReadOnlySystemParamFetch` has been replaced with `ReadOnlySystemParam`.
```rust
// Before
unsafe impl ReadOnlySystemParamFetch for MyParamState {}
// After
unsafe impl<'w, 's> ReadOnlySystemParam for MyParam<'w, 's> {}
```
2022-12-11 18:34:14 +00:00
unsafe fn get_param < ' w , ' s > (
Remove the `SystemParamState` trait and remove types like `ResState` (#6919)
Spiritual successor to #5205.
Actual successor to #6865.
# Objective
Currently, system params are defined using three traits: `SystemParam`, `ReadOnlySystemParam`, `SystemParamState`. The behavior for each param is specified by the `SystemParamState` trait, while `SystemParam` simply defers to the state.
Splitting the traits in this way makes it easier to implement within macros, but it increases the cognitive load. Worst of all, this approach requires each `MySystemParam` to have a public `MySystemParamState` type associated with it.
## Solution
* Merge the trait `SystemParamState` into `SystemParam`.
* Remove all trivial `SystemParam` state types.
* `OptionNonSendMutState<T>`: you will not be missed.
---
- [x] Fix/resolve the remaining test failure.
## Changelog
* Removed the trait `SystemParamState`, merging its functionality into `SystemParam`.
## Migration Guide
**Note**: this should replace the migration guide for #6865.
This is relative to Bevy 0.9, not main.
The traits `SystemParamState` and `SystemParamFetch` have been removed, and their functionality has been transferred to `SystemParam`.
```rust
// Before (0.9)
impl SystemParam for MyParam<'_, '_> {
type State = MyParamState;
}
unsafe impl SystemParamState for MyParamState {
fn init(world: &mut World, system_meta: &mut SystemMeta) -> Self { ... }
}
unsafe impl<'w, 's> SystemParamFetch<'w, 's> for MyParamState {
type Item = MyParam<'w, 's>;
fn get_param(&mut self, ...) -> Self::Item;
}
unsafe impl ReadOnlySystemParamFetch for MyParamState { }
// After (0.10)
unsafe impl SystemParam for MyParam<'_, '_> {
type State = MyParamState;
type Item<'w, 's> = MyParam<'w, 's>;
fn init_state(world: &mut World, system_meta: &mut SystemMeta) -> Self::State { ... }
fn get_param<'w, 's>(state: &mut Self::State, ...) -> Self::Item<'w, 's>;
}
unsafe impl ReadOnlySystemParam for MyParam<'_, '_> { }
```
The trait `ReadOnlySystemParamFetch` has been replaced with `ReadOnlySystemParam`.
```rust
// Before
unsafe impl ReadOnlySystemParamFetch for MyParamState {}
// After
unsafe impl ReadOnlySystemParam for MyParam<'_, '_> {}
```
2023-01-07 23:20:32 +00:00
& mut component_id : & ' s mut Self ::State ,
Add new SystemState and rename old SystemState to SystemMeta (#2283)
This enables `SystemParams` to be used outside of function systems. Anything can create and store `SystemState`, which enables efficient "param state cached" access to `SystemParams`.
It adds a `ReadOnlySystemParamFetch` trait, which enables safe `SystemState::get` calls without unique world access.
I renamed the old `SystemState` to `SystemMeta` to enable us to mirror the `QueryState` naming convention (but I'm happy to discuss alternative names if people have other ideas). I initially pitched this as `ParamState`, but given that it needs to include full system metadata, that doesn't feel like a particularly accurate name.
```rust
#[derive(Eq, PartialEq, Debug)]
struct A(usize);
#[derive(Eq, PartialEq, Debug)]
struct B(usize);
let mut world = World::default();
world.insert_resource(A(42));
world.spawn().insert(B(7));
// we get nice lifetime elision when declaring the type on the left hand side
let mut system_state: SystemState<(Res<A>, Query<&B>)> = SystemState::new(&mut world);
let (a, query) = system_state.get(&world);
assert_eq!(*a, A(42), "returned resource matches initial value");
assert_eq!(
*query.single().unwrap(),
B(7),
"returned component matches initial value"
);
// mutable system params require unique world access
let mut system_state: SystemState<(ResMut<A>, Query<&mut B>)> = SystemState::new(&mut world);
let (a, query) = system_state.get_mut(&mut world);
// static lifetimes are required when declaring inside of structs
struct SomeContainer {
state: SystemState<(Res<'static, A>, Res<'static, B>)>
}
// this can be shortened using type aliases, which will be useful for complex param tuples
type MyParams<'a> = (Res<'a, A>, Res<'a, B>);
struct SomeContainer {
state: SystemState<MyParams<'static>>
}
// It is the user's responsibility to call SystemState::apply(world) for parameters that queue up work
let mut system_state: SystemState<(Commands, Query<&B>)> = SystemState::new(&mut world);
{
let (mut commands, query) = system_state.get(&world);
commands.insert_resource(3.14);
}
system_state.apply(&mut world);
```
## Future Work
* Actually use SystemState inside FunctionSystem. This would be trivial, but it requires FunctionSystem to wrap SystemState in Option in its current form (which complicates system metadata lookup). I'd prefer to hold off until we adopt something like the later designs linked in #1364, which enable us to contruct Systems using a World reference (and also remove the need for `.system`).
* Consider a "scoped" approach to automatically call SystemState::apply when systems params are no longer being used (either a container type with a Drop impl, or a function that takes a closure for user logic operating on params).
2021-06-02 19:57:38 +00:00
system_meta : & SystemMeta ,
System Param Lifetime Split (#2605)
# Objective
Enable using exact World lifetimes during read-only access . This is motivated by the new renderer's need to allow read-only world-only queries to outlive the query itself (but still be constrained by the world lifetime).
For example:
https://github.com/bevyengine/bevy/blob/115b170d1f11a91146bb6d6e9684dceb8b21f786/pipelined/bevy_pbr2/src/render/mod.rs#L774
## Solution
Split out SystemParam state and world lifetimes and pipe those lifetimes up to read-only Query ops (and add into_inner for Res). According to every safety test I've run so far (except one), this is safe (see the temporary safety test commit). Note that changing the mutable variants to the new lifetimes would allow aliased mutable pointers (try doing that to see how it affects the temporary safety tests).
The new state lifetime on SystemParam does make `#[derive(SystemParam)]` more cumbersome (the current impl requires PhantomData if you don't use both lifetimes). We can make this better by detecting whether or not a lifetime is used in the derive and adjusting accordingly, but that should probably be done in its own pr.
## Why is this a draft?
The new lifetimes break QuerySet safety in one very specific case (see the query_set system in system_safety_test). We need to solve this before we can use the lifetimes given.
This is due to the fact that QuerySet is just a wrapper over Query, which now relies on world lifetimes instead of `&self` lifetimes to prevent aliasing (but in systems, each Query has its own implied lifetime, not a centralized world lifetime). I believe the fix is to rewrite QuerySet to have its own World lifetime (and own the internal reference). This will complicate the impl a bit, but I think it is doable. I'm curious if anyone else has better ideas.
Personally, I think these new lifetimes need to happen. We've gotta have a way to directly tie read-only World queries to the World lifetime. The new renderer is the first place this has come up, but I doubt it will be the last. Worst case scenario we can come up with a second `WorldLifetimeQuery<Q, F = ()>` parameter to enable these read-only scenarios, but I'd rather not add another type to the type zoo.
2021-08-15 20:51:53 +00:00
world : & ' w World ,
Reliable change detection (#1471)
# Problem Definition
The current change tracking (via flags for both components and resources) fails to detect changes made by systems that are scheduled to run earlier in the frame than they are.
This issue is discussed at length in [#68](https://github.com/bevyengine/bevy/issues/68) and [#54](https://github.com/bevyengine/bevy/issues/54).
This is very much a draft PR, and contributions are welcome and needed.
# Criteria
1. Each change is detected at least once, no matter the ordering.
2. Each change is detected at most once, no matter the ordering.
3. Changes should be detected the same frame that they are made.
4. Competitive ergonomics. Ideally does not require opting-in.
5. Low CPU overhead of computation.
6. Memory efficient. This must not increase over time, except where the number of entities / resources does.
7. Changes should not be lost for systems that don't run.
8. A frame needs to act as a pure function. Given the same set of entities / components it needs to produce the same end state without side-effects.
**Exact** change-tracking proposals satisfy criteria 1 and 2.
**Conservative** change-tracking proposals satisfy criteria 1 but not 2.
**Flaky** change tracking proposals satisfy criteria 2 but not 1.
# Code Base Navigation
There are three types of flags:
- `Added`: A piece of data was added to an entity / `Resources`.
- `Mutated`: A piece of data was able to be modified, because its `DerefMut` was accessed
- `Changed`: The bitwise OR of `Added` and `Changed`
The special behavior of `ChangedRes`, with respect to the scheduler is being removed in [#1313](https://github.com/bevyengine/bevy/pull/1313) and does not need to be reproduced.
`ChangedRes` and friends can be found in "bevy_ecs/core/resources/resource_query.rs".
The `Flags` trait for Components can be found in "bevy_ecs/core/query.rs".
`ComponentFlags` are stored in "bevy_ecs/core/archetypes.rs", defined on line 446.
# Proposals
**Proposal 5 was selected for implementation.**
## Proposal 0: No Change Detection
The baseline, where computations are performed on everything regardless of whether it changed.
**Type:** Conservative
**Pros:**
- already implemented
- will never miss events
- no overhead
**Cons:**
- tons of repeated work
- doesn't allow users to avoid repeating work (or monitoring for other changes)
## Proposal 1: Earlier-This-Tick Change Detection
The current approach as of Bevy 0.4. Flags are set, and then flushed at the end of each frame.
**Type:** Flaky
**Pros:**
- already implemented
- simple to understand
- low memory overhead (2 bits per component)
- low time overhead (clear every flag once per frame)
**Cons:**
- misses systems based on ordering
- systems that don't run every frame miss changes
- duplicates detection when looping
- can lead to unresolvable circular dependencies
## Proposal 2: Two-Tick Change Detection
Flags persist for two frames, using a double-buffer system identical to that used in events.
A change is observed if it is found in either the current frame's list of changes or the previous frame's.
**Type:** Conservative
**Pros:**
- easy to understand
- easy to implement
- low memory overhead (4 bits per component)
- low time overhead (bit mask and shift every flag once per frame)
**Cons:**
- can result in a great deal of duplicated work
- systems that don't run every frame miss changes
- duplicates detection when looping
## Proposal 3: Last-Tick Change Detection
Flags persist for two frames, using a double-buffer system identical to that used in events.
A change is observed if it is found in the previous frame's list of changes.
**Type:** Exact
**Pros:**
- exact
- easy to understand
- easy to implement
- low memory overhead (4 bits per component)
- low time overhead (bit mask and shift every flag once per frame)
**Cons:**
- change detection is always delayed, possibly causing painful chained delays
- systems that don't run every frame miss changes
- duplicates detection when looping
## Proposal 4: Flag-Doubling Change Detection
Combine Proposal 2 and Proposal 3. Differentiate between `JustChanged` (current behavior) and `Changed` (Proposal 3).
Pack this data into the flags according to [this implementation proposal](https://github.com/bevyengine/bevy/issues/68#issuecomment-769174804).
**Type:** Flaky + Exact
**Pros:**
- allows users to acc
- easy to implement
- low memory overhead (4 bits per component)
- low time overhead (bit mask and shift every flag once per frame)
**Cons:**
- users must specify the type of change detection required
- still quite fragile to system ordering effects when using the flaky `JustChanged` form
- cannot get immediate + exact results
- systems that don't run every frame miss changes
- duplicates detection when looping
## [SELECTED] Proposal 5: Generation-Counter Change Detection
A global counter is increased after each system is run. Each component saves the time of last mutation, and each system saves the time of last execution. Mutation is detected when the component's counter is greater than the system's counter. Discussed [here](https://github.com/bevyengine/bevy/issues/68#issuecomment-769174804). How to handle addition detection is unsolved; the current proposal is to use the highest bit of the counter as in proposal 1.
**Type:** Exact (for mutations), flaky (for additions)
**Pros:**
- low time overhead (set component counter on access, set system counter after execution)
- robust to systems that don't run every frame
- robust to systems that loop
**Cons:**
- moderately complex implementation
- must be modified as systems are inserted dynamically
- medium memory overhead (4 bytes per component + system)
- unsolved addition detection
## Proposal 6: System-Data Change Detection
For each system, track which system's changes it has seen. This approach is only worth fully designing and implementing if Proposal 5 fails in some way.
**Type:** Exact
**Pros:**
- exact
- conceptually simple
**Cons:**
- requires storing data on each system
- implementation is complex
- must be modified as systems are inserted dynamically
## Proposal 7: Total-Order Change Detection
Discussed [here](https://github.com/bevyengine/bevy/issues/68#issuecomment-754326523). This proposal is somewhat complicated by the new scheduler, but I believe it should still be conceptually feasible. This approach is only worth fully designing and implementing if Proposal 5 fails in some way.
**Type:** Exact
**Pros:**
- exact
- efficient data storage relative to other exact proposals
**Cons:**
- requires access to the scheduler
- complex implementation and difficulty grokking
- must be modified as systems are inserted dynamically
# Tests
- We will need to verify properties 1, 2, 3, 7 and 8. Priority: 1 > 2 = 3 > 8 > 7
- Ideally we can use identical user-facing syntax for all proposals, allowing us to re-use the same syntax for each.
- When writing tests, we need to carefully specify order using explicit dependencies.
- These tests will need to be duplicated for both components and resources.
- We need to be sure to handle cases where ambiguous system orders exist.
`changing_system` is always the system that makes the changes, and `detecting_system` always detects the changes.
The component / resource changed will be simple boolean wrapper structs.
## Basic Added / Mutated / Changed
2 x 3 design:
- Resources vs. Components
- Added vs. Changed vs. Mutated
- `changing_system` runs before `detecting_system`
- verify at the end of tick 2
## At Least Once
2 x 3 design:
- Resources vs. Components
- Added vs. Changed vs. Mutated
- `changing_system` runs after `detecting_system`
- verify at the end of tick 2
## At Most Once
2 x 3 design:
- Resources vs. Components
- Added vs. Changed vs. Mutated
- `changing_system` runs once before `detecting_system`
- increment a counter based on the number of changes detected
- verify at the end of tick 2
## Fast Detection
2 x 3 design:
- Resources vs. Components
- Added vs. Changed vs. Mutated
- `changing_system` runs before `detecting_system`
- verify at the end of tick 1
## Ambiguous System Ordering Robustness
2 x 3 x 2 design:
- Resources vs. Components
- Added vs. Changed vs. Mutated
- `changing_system` runs [before/after] `detecting_system` in tick 1
- `changing_system` runs [after/before] `detecting_system` in tick 2
## System Pausing
2 x 3 design:
- Resources vs. Components
- Added vs. Changed vs. Mutated
- `changing_system` runs in tick 1, then is disabled by run criteria
- `detecting_system` is disabled by run criteria until it is run once during tick 3
- verify at the end of tick 3
## Addition Causes Mutation
2 design:
- Resources vs. Components
- `adding_system_1` adds a component / resource
- `adding system_2` adds the same component / resource
- verify the `Mutated` flag at the end of the tick
- verify the `Added` flag at the end of the tick
First check tests for: https://github.com/bevyengine/bevy/issues/333
Second check tests for: https://github.com/bevyengine/bevy/issues/1443
## Changes Made By Commands
- `adding_system` runs in Update in tick 1, and sends a command to add a component
- `detecting_system` runs in Update in tick 1 and 2, after `adding_system`
- We can't detect the changes in tick 1, since they haven't been processed yet
- If we were to track these changes as being emitted by `adding_system`, we can't detect the changes in tick 2 either, since `detecting_system` has already run once after `adding_system` :(
# Benchmarks
See: [general advice](https://github.com/bevyengine/bevy/blob/master/docs/profiling.md), [Criterion crate](https://github.com/bheisler/criterion.rs)
There are several critical parameters to vary:
1. entity count (1 to 10^9)
2. fraction of entities that are changed (0% to 100%)
3. cost to perform work on changed entities, i.e. workload (1 ns to 1s)
1 and 2 should be varied between benchmark runs. 3 can be added on computationally.
We want to measure:
- memory cost
- run time
We should collect these measurements across several frames (100?) to reduce bootup effects and accurately measure the mean, variance and drift.
Entity-component change detection is much more important to benchmark than resource change detection, due to the orders of magnitude higher number of pieces of data.
No change detection at all should be included in benchmarks as a second control for cases where missing changes is unacceptable.
## Graphs
1. y: performance, x: log_10(entity count), color: proposal, facet: performance metric. Set cost to perform work to 0.
2. y: run time, x: cost to perform work, color: proposal, facet: fraction changed. Set number of entities to 10^6
3. y: memory, x: frames, color: proposal
# Conclusions
1. Is the theoretical categorization of the proposals correct according to our tests?
2. How does the performance of the proposals compare without any load?
3. How does the performance of the proposals compare with realistic loads?
4. At what workload does more exact change tracking become worth the (presumably) higher overhead?
5. When does adding change-detection to save on work become worthwhile?
6. Is there enough divergence in performance between the best solutions in each class to ship more than one change-tracking solution?
# Implementation Plan
1. Write a test suite.
2. Verify that tests fail for existing approach.
3. Write a benchmark suite.
4. Get performance numbers for existing approach.
5. Implement, test and benchmark various solutions using a Git branch per proposal.
6. Create a draft PR with all solutions and present results to team.
7. Select a solution and replace existing change detection.
Co-authored-by: Brice DAVIER <bricedavier@gmail.com>
Co-authored-by: Carter Anderson <mcanders1@gmail.com>
2021-03-19 17:53:26 +00:00
change_tick : u32 ,
Simplify trait hierarchy for `SystemParam` (#6865)
# Objective
* Implementing a custom `SystemParam` by hand requires implementing three traits -- four if it is read-only.
* The trait `SystemParamFetch<'w, 's>` is a workaround from before we had generic associated types, and is no longer necessary.
## Solution
* Combine the trait `SystemParamFetch` with `SystemParamState`.
* I decided to remove the `Fetch` name and keep the `State` name, since the former was consistently conflated with the latter.
* Replace the trait `ReadOnlySystemParamFetch` with `ReadOnlySystemParam`, which simplifies trait bounds in generic code.
---
## Changelog
- Removed the trait `SystemParamFetch`, moving its functionality to `SystemParamState`.
- Replaced the trait `ReadOnlySystemParamFetch` with `ReadOnlySystemParam`.
## Migration Guide
The trait `SystemParamFetch` has been removed, and its functionality has been transferred to `SystemParamState`.
```rust
// Before
impl SystemParamState for MyParamState {
fn init(world: &mut World, system_meta: &mut SystemMeta) -> Self { ... }
}
impl<'w, 's> SystemParamFetch<'w, 's> for MyParamState {
type Item = MyParam<'w, 's>;
fn get_param(...) -> Self::Item;
}
// After
impl SystemParamState for MyParamState {
type Item<'w, 's> = MyParam<'w, 's>; // Generic associated types!
fn init(world: &mut World, system_meta: &mut SystemMeta) -> Self { ... }
fn get_param<'w, 's>(...) -> Self::Item<'w, 's>;
}
```
The trait `ReadOnlySystemParamFetch` has been replaced with `ReadOnlySystemParam`.
```rust
// Before
unsafe impl ReadOnlySystemParamFetch for MyParamState {}
// After
unsafe impl<'w, 's> ReadOnlySystemParam for MyParam<'w, 's> {}
```
2022-12-11 18:34:14 +00:00
) -> Self ::Item < ' w , ' s > {
2021-03-08 20:12:22 +00:00
world
add `UnsafeWorldCell` abstraction (#6404)
alternative to #5922, implements #5956
builds on top of https://github.com/bevyengine/bevy/pull/6402
# Objective
https://github.com/bevyengine/bevy/issues/5956 goes into more detail, but the TLDR is:
- bevy systems ensure disjoint accesses to resources and components, and for that to work there are methods `World::get_resource_unchecked_mut(&self)`, ..., `EntityRef::get_mut_unchecked(&self)` etc.
- we don't have these unchecked methods for `by_id` variants, so third-party crate authors cannot build their own safe disjoint-access abstractions with these
- having `_unchecked_mut` methods is not great, because in their presence safe code can accidentally violate subtle invariants. Having to go through `world.as_unsafe_world_cell().unsafe_method()` forces you to stop and think about what you want to write in your `// SAFETY` comment.
The alternative is to keep exposing `_unchecked_mut` variants for every operation that we want third-party crates to build upon, but we'd prefer to avoid using these methods alltogether: https://github.com/bevyengine/bevy/pull/5922#issuecomment-1241954543
Also, this is something that **cannot be implemented outside of bevy**, so having either this PR or #5922 as an escape hatch with lots of discouraging comments would be great.
## Solution
- add `UnsafeWorldCell` with `unsafe fn get_resource(&self)`, `unsafe fn get_resource_mut(&self)`
- add `fn World::as_unsafe_world_cell(&mut self) -> UnsafeWorldCell<'_>` (and `as_unsafe_world_cell_readonly(&self)`)
- add `UnsafeWorldCellEntityRef` with `unsafe fn get`, `unsafe fn get_mut` and the other utilities on `EntityRef` (no methods for spawning, despawning, insertion)
- use the `UnsafeWorldCell` abstraction in `ReflectComponent`, `ReflectResource` and `ReflectAsset`, so these APIs are easier to reason about
- remove `World::get_resource_mut_unchecked`, `EntityRef::get_mut_unchecked` and use `unsafe { world.as_unsafe_world_cell().get_mut() }` and `unsafe { world.as_unsafe_world_cell().get_entity(entity)?.get_mut() }` instead
This PR does **not** make use of `UnsafeWorldCell` for anywhere else in `bevy_ecs` such as `SystemParam` or `Query`. That is a much larger change, and I am convinced that having `UnsafeWorldCell` is already useful for third-party crates.
Implemented API:
```rust
struct World { .. }
impl World {
fn as_unsafe_world_cell(&self) -> UnsafeWorldCell<'_>;
}
struct UnsafeWorldCell<'w>(&'w World);
impl<'w> UnsafeWorldCell {
unsafe fn world(&self) -> &World;
fn get_entity(&self) -> UnsafeWorldCellEntityRef<'w>; // returns 'w which is `'self` of the `World::as_unsafe_world_cell(&'w self)`
unsafe fn get_resource<T>(&self) -> Option<&'w T>;
unsafe fn get_resource_by_id(&self, ComponentId) -> Option<&'w T>;
unsafe fn get_resource_mut<T>(&self) -> Option<Mut<'w, T>>;
unsafe fn get_resource_mut_by_id(&self) -> Option<MutUntyped<'w>>;
unsafe fn get_non_send_resource<T>(&self) -> Option<&'w T>;
unsafe fn get_non_send_resource_mut<T>(&self) -> Option<Mut<'w, T>>>;
// not included: remove, remove_resource, despawn, anything that might change archetypes
}
struct UnsafeWorldCellEntityRef<'w> { .. }
impl UnsafeWorldCellEntityRef<'w> {
unsafe fn get<T>(&self, Entity) -> Option<&'w T>;
unsafe fn get_by_id(&self, Entity, ComponentId) -> Option<Ptr<'w>>;
unsafe fn get_mut<T>(&self, Entity) -> Option<Mut<'w, T>>;
unsafe fn get_mut_by_id(&self, Entity, ComponentId) -> Option<MutUntyped<'w>>;
unsafe fn get_change_ticks<T>(&self, Entity) -> Option<Mut<'w, T>>;
// fn id, archetype, contains, contains_id, containts_type_id
}
```
<details>
<summary>UnsafeWorldCell docs</summary>
Variant of the [`World`] where resource and component accesses takes a `&World`, and the responsibility to avoid
aliasing violations are given to the caller instead of being checked at compile-time by rust's unique XOR shared rule.
### Rationale
In rust, having a `&mut World` means that there are absolutely no other references to the safe world alive at the same time,
without exceptions. Not even unsafe code can change this.
But there are situations where careful shared mutable access through a type is possible and safe. For this, rust provides the [`UnsafeCell`](std::cell::UnsafeCell)
escape hatch, which allows you to get a `*mut T` from a `&UnsafeCell<T>` and around which safe abstractions can be built.
Access to resources and components can be done uniquely using [`World::resource_mut`] and [`World::entity_mut`], and shared using [`World::resource`] and [`World::entity`].
These methods use lifetimes to check at compile time that no aliasing rules are being broken.
This alone is not enough to implement bevy systems where multiple systems can access *disjoint* parts of the world concurrently. For this, bevy stores all values of
resources and components (and [`ComponentTicks`](crate::component::ComponentTicks)) in [`UnsafeCell`](std::cell::UnsafeCell)s, and carefully validates disjoint access patterns using
APIs like [`System::component_access`](crate::system::System::component_access).
A system then can be executed using [`System::run_unsafe`](crate::system::System::run_unsafe) with a `&World` and use methods with interior mutability to access resource values.
access resource values.
### Example Usage
[`UnsafeWorldCell`] can be used as a building block for writing APIs that safely allow disjoint access into the world.
In the following example, the world is split into a resource access half and a component access half, where each one can
safely hand out mutable references.
```rust
use bevy_ecs::world::World;
use bevy_ecs::change_detection::Mut;
use bevy_ecs::system::Resource;
use bevy_ecs::world::unsafe_world_cell_world::UnsafeWorldCell;
// INVARIANT: existance of this struct means that users of it are the only ones being able to access resources in the world
struct OnlyResourceAccessWorld<'w>(UnsafeWorldCell<'w>);
// INVARIANT: existance of this struct means that users of it are the only ones being able to access components in the world
struct OnlyComponentAccessWorld<'w>(UnsafeWorldCell<'w>);
impl<'w> OnlyResourceAccessWorld<'w> {
fn get_resource_mut<T: Resource>(&mut self) -> Option<Mut<'w, T>> {
// SAFETY: resource access is allowed through this UnsafeWorldCell
unsafe { self.0.get_resource_mut::<T>() }
}
}
// impl<'w> OnlyComponentAccessWorld<'w> {
// ...
// }
// the two interior mutable worlds borrow from the `&mut World`, so it cannot be accessed while they are live
fn split_world_access(world: &mut World) -> (OnlyResourceAccessWorld<'_>, OnlyComponentAccessWorld<'_>) {
let resource_access = OnlyResourceAccessWorld(unsafe { world.as_unsafe_world_cell() });
let component_access = OnlyComponentAccessWorld(unsafe { world.as_unsafe_world_cell() });
(resource_access, component_access)
}
```
</details>
2023-01-27 00:12:13 +00:00
. as_unsafe_world_cell_migration_internal ( )
2023-02-06 19:02:52 +00:00
. get_resource_mut_by_id ( component_id )
2021-03-08 20:12:22 +00:00
. map ( | value | ResMut {
2023-02-06 19:02:52 +00:00
value : value . value . deref_mut ::< T > ( ) ,
2023-01-11 15:41:54 +00:00
ticks : TicksMut {
2022-11-21 12:59:09 +00:00
added : value . ticks . added ,
changed : value . ticks . changed ,
Add new SystemState and rename old SystemState to SystemMeta (#2283)
This enables `SystemParams` to be used outside of function systems. Anything can create and store `SystemState`, which enables efficient "param state cached" access to `SystemParams`.
It adds a `ReadOnlySystemParamFetch` trait, which enables safe `SystemState::get` calls without unique world access.
I renamed the old `SystemState` to `SystemMeta` to enable us to mirror the `QueryState` naming convention (but I'm happy to discuss alternative names if people have other ideas). I initially pitched this as `ParamState`, but given that it needs to include full system metadata, that doesn't feel like a particularly accurate name.
```rust
#[derive(Eq, PartialEq, Debug)]
struct A(usize);
#[derive(Eq, PartialEq, Debug)]
struct B(usize);
let mut world = World::default();
world.insert_resource(A(42));
world.spawn().insert(B(7));
// we get nice lifetime elision when declaring the type on the left hand side
let mut system_state: SystemState<(Res<A>, Query<&B>)> = SystemState::new(&mut world);
let (a, query) = system_state.get(&world);
assert_eq!(*a, A(42), "returned resource matches initial value");
assert_eq!(
*query.single().unwrap(),
B(7),
"returned component matches initial value"
);
// mutable system params require unique world access
let mut system_state: SystemState<(ResMut<A>, Query<&mut B>)> = SystemState::new(&mut world);
let (a, query) = system_state.get_mut(&mut world);
// static lifetimes are required when declaring inside of structs
struct SomeContainer {
state: SystemState<(Res<'static, A>, Res<'static, B>)>
}
// this can be shortened using type aliases, which will be useful for complex param tuples
type MyParams<'a> = (Res<'a, A>, Res<'a, B>);
struct SomeContainer {
state: SystemState<MyParams<'static>>
}
// It is the user's responsibility to call SystemState::apply(world) for parameters that queue up work
let mut system_state: SystemState<(Commands, Query<&B>)> = SystemState::new(&mut world);
{
let (mut commands, query) = system_state.get(&world);
commands.insert_resource(3.14);
}
system_state.apply(&mut world);
```
## Future Work
* Actually use SystemState inside FunctionSystem. This would be trivial, but it requires FunctionSystem to wrap SystemState in Option in its current form (which complicates system metadata lookup). I'd prefer to hold off until we adopt something like the later designs linked in #1364, which enable us to contruct Systems using a World reference (and also remove the need for `.system`).
* Consider a "scoped" approach to automatically call SystemState::apply when systems params are no longer being used (either a container type with a Drop impl, or a function that takes a closure for user logic operating on params).
2021-06-02 19:57:38 +00:00
last_change_tick : system_meta . last_change_tick ,
2021-05-30 19:29:31 +00:00
change_tick ,
} ,
2021-03-08 20:12:22 +00:00
} )
}
}
2022-07-04 14:44:24 +00:00
/// SAFETY: only reads world
Simplify trait hierarchy for `SystemParam` (#6865)
# Objective
* Implementing a custom `SystemParam` by hand requires implementing three traits -- four if it is read-only.
* The trait `SystemParamFetch<'w, 's>` is a workaround from before we had generic associated types, and is no longer necessary.
## Solution
* Combine the trait `SystemParamFetch` with `SystemParamState`.
* I decided to remove the `Fetch` name and keep the `State` name, since the former was consistently conflated with the latter.
* Replace the trait `ReadOnlySystemParamFetch` with `ReadOnlySystemParam`, which simplifies trait bounds in generic code.
---
## Changelog
- Removed the trait `SystemParamFetch`, moving its functionality to `SystemParamState`.
- Replaced the trait `ReadOnlySystemParamFetch` with `ReadOnlySystemParam`.
## Migration Guide
The trait `SystemParamFetch` has been removed, and its functionality has been transferred to `SystemParamState`.
```rust
// Before
impl SystemParamState for MyParamState {
fn init(world: &mut World, system_meta: &mut SystemMeta) -> Self { ... }
}
impl<'w, 's> SystemParamFetch<'w, 's> for MyParamState {
type Item = MyParam<'w, 's>;
fn get_param(...) -> Self::Item;
}
// After
impl SystemParamState for MyParamState {
type Item<'w, 's> = MyParam<'w, 's>; // Generic associated types!
fn init(world: &mut World, system_meta: &mut SystemMeta) -> Self { ... }
fn get_param<'w, 's>(...) -> Self::Item<'w, 's>;
}
```
The trait `ReadOnlySystemParamFetch` has been replaced with `ReadOnlySystemParam`.
```rust
// Before
unsafe impl ReadOnlySystemParamFetch for MyParamState {}
// After
unsafe impl<'w, 's> ReadOnlySystemParam for MyParam<'w, 's> {}
```
2022-12-11 18:34:14 +00:00
unsafe impl < ' w > ReadOnlySystemParam for & ' w World { }
2022-02-03 23:43:25 +00:00
2022-07-04 14:44:24 +00:00
// SAFETY: `read_all` access is set and conflicts result in a panic
Remove the `SystemParamState` trait and remove types like `ResState` (#6919)
Spiritual successor to #5205.
Actual successor to #6865.
# Objective
Currently, system params are defined using three traits: `SystemParam`, `ReadOnlySystemParam`, `SystemParamState`. The behavior for each param is specified by the `SystemParamState` trait, while `SystemParam` simply defers to the state.
Splitting the traits in this way makes it easier to implement within macros, but it increases the cognitive load. Worst of all, this approach requires each `MySystemParam` to have a public `MySystemParamState` type associated with it.
## Solution
* Merge the trait `SystemParamState` into `SystemParam`.
* Remove all trivial `SystemParam` state types.
* `OptionNonSendMutState<T>`: you will not be missed.
---
- [x] Fix/resolve the remaining test failure.
## Changelog
* Removed the trait `SystemParamState`, merging its functionality into `SystemParam`.
## Migration Guide
**Note**: this should replace the migration guide for #6865.
This is relative to Bevy 0.9, not main.
The traits `SystemParamState` and `SystemParamFetch` have been removed, and their functionality has been transferred to `SystemParam`.
```rust
// Before (0.9)
impl SystemParam for MyParam<'_, '_> {
type State = MyParamState;
}
unsafe impl SystemParamState for MyParamState {
fn init(world: &mut World, system_meta: &mut SystemMeta) -> Self { ... }
}
unsafe impl<'w, 's> SystemParamFetch<'w, 's> for MyParamState {
type Item = MyParam<'w, 's>;
fn get_param(&mut self, ...) -> Self::Item;
}
unsafe impl ReadOnlySystemParamFetch for MyParamState { }
// After (0.10)
unsafe impl SystemParam for MyParam<'_, '_> {
type State = MyParamState;
type Item<'w, 's> = MyParam<'w, 's>;
fn init_state(world: &mut World, system_meta: &mut SystemMeta) -> Self::State { ... }
fn get_param<'w, 's>(state: &mut Self::State, ...) -> Self::Item<'w, 's>;
}
unsafe impl ReadOnlySystemParam for MyParam<'_, '_> { }
```
The trait `ReadOnlySystemParamFetch` has been replaced with `ReadOnlySystemParam`.
```rust
// Before
unsafe impl ReadOnlySystemParamFetch for MyParamState {}
// After
unsafe impl ReadOnlySystemParam for MyParam<'_, '_> {}
```
2023-01-07 23:20:32 +00:00
unsafe impl SystemParam for & '_ World {
type State = ( ) ;
Simplify trait hierarchy for `SystemParam` (#6865)
# Objective
* Implementing a custom `SystemParam` by hand requires implementing three traits -- four if it is read-only.
* The trait `SystemParamFetch<'w, 's>` is a workaround from before we had generic associated types, and is no longer necessary.
## Solution
* Combine the trait `SystemParamFetch` with `SystemParamState`.
* I decided to remove the `Fetch` name and keep the `State` name, since the former was consistently conflated with the latter.
* Replace the trait `ReadOnlySystemParamFetch` with `ReadOnlySystemParam`, which simplifies trait bounds in generic code.
---
## Changelog
- Removed the trait `SystemParamFetch`, moving its functionality to `SystemParamState`.
- Replaced the trait `ReadOnlySystemParamFetch` with `ReadOnlySystemParam`.
## Migration Guide
The trait `SystemParamFetch` has been removed, and its functionality has been transferred to `SystemParamState`.
```rust
// Before
impl SystemParamState for MyParamState {
fn init(world: &mut World, system_meta: &mut SystemMeta) -> Self { ... }
}
impl<'w, 's> SystemParamFetch<'w, 's> for MyParamState {
type Item = MyParam<'w, 's>;
fn get_param(...) -> Self::Item;
}
// After
impl SystemParamState for MyParamState {
type Item<'w, 's> = MyParam<'w, 's>; // Generic associated types!
fn init(world: &mut World, system_meta: &mut SystemMeta) -> Self { ... }
fn get_param<'w, 's>(...) -> Self::Item<'w, 's>;
}
```
The trait `ReadOnlySystemParamFetch` has been replaced with `ReadOnlySystemParam`.
```rust
// Before
unsafe impl ReadOnlySystemParamFetch for MyParamState {}
// After
unsafe impl<'w, 's> ReadOnlySystemParam for MyParam<'w, 's> {}
```
2022-12-11 18:34:14 +00:00
type Item < ' w , ' s > = & ' w World ;
Remove the `SystemParamState` trait and remove types like `ResState` (#6919)
Spiritual successor to #5205.
Actual successor to #6865.
# Objective
Currently, system params are defined using three traits: `SystemParam`, `ReadOnlySystemParam`, `SystemParamState`. The behavior for each param is specified by the `SystemParamState` trait, while `SystemParam` simply defers to the state.
Splitting the traits in this way makes it easier to implement within macros, but it increases the cognitive load. Worst of all, this approach requires each `MySystemParam` to have a public `MySystemParamState` type associated with it.
## Solution
* Merge the trait `SystemParamState` into `SystemParam`.
* Remove all trivial `SystemParam` state types.
* `OptionNonSendMutState<T>`: you will not be missed.
---
- [x] Fix/resolve the remaining test failure.
## Changelog
* Removed the trait `SystemParamState`, merging its functionality into `SystemParam`.
## Migration Guide
**Note**: this should replace the migration guide for #6865.
This is relative to Bevy 0.9, not main.
The traits `SystemParamState` and `SystemParamFetch` have been removed, and their functionality has been transferred to `SystemParam`.
```rust
// Before (0.9)
impl SystemParam for MyParam<'_, '_> {
type State = MyParamState;
}
unsafe impl SystemParamState for MyParamState {
fn init(world: &mut World, system_meta: &mut SystemMeta) -> Self { ... }
}
unsafe impl<'w, 's> SystemParamFetch<'w, 's> for MyParamState {
type Item = MyParam<'w, 's>;
fn get_param(&mut self, ...) -> Self::Item;
}
unsafe impl ReadOnlySystemParamFetch for MyParamState { }
// After (0.10)
unsafe impl SystemParam for MyParam<'_, '_> {
type State = MyParamState;
type Item<'w, 's> = MyParam<'w, 's>;
fn init_state(world: &mut World, system_meta: &mut SystemMeta) -> Self::State { ... }
fn get_param<'w, 's>(state: &mut Self::State, ...) -> Self::Item<'w, 's>;
}
unsafe impl ReadOnlySystemParam for MyParam<'_, '_> { }
```
The trait `ReadOnlySystemParamFetch` has been replaced with `ReadOnlySystemParam`.
```rust
// Before
unsafe impl ReadOnlySystemParamFetch for MyParamState {}
// After
unsafe impl ReadOnlySystemParam for MyParam<'_, '_> {}
```
2023-01-07 23:20:32 +00:00
fn init_state ( _world : & mut World , system_meta : & mut SystemMeta ) -> Self ::State {
2022-02-03 23:43:25 +00:00
let mut access = Access ::default ( ) ;
access . read_all ( ) ;
if ! system_meta
. archetype_component_access
. is_compatible ( & access )
{
panic! ( " &World conflicts with a previous mutable system parameter. Allowing this would break Rust's mutability rules " ) ;
}
system_meta . archetype_component_access . extend ( & access ) ;
let mut filtered_access = FilteredAccess ::default ( ) ;
filtered_access . read_all ( ) ;
if ! system_meta
. component_access_set
2022-05-09 14:39:22 +00:00
. get_conflicts_single ( & filtered_access )
2022-02-03 23:43:25 +00:00
. is_empty ( )
{
panic! ( " &World conflicts with a previous mutable system parameter. Allowing this would break Rust's mutability rules " ) ;
}
system_meta . component_access_set . add ( filtered_access ) ;
}
Simplify trait hierarchy for `SystemParam` (#6865)
# Objective
* Implementing a custom `SystemParam` by hand requires implementing three traits -- four if it is read-only.
* The trait `SystemParamFetch<'w, 's>` is a workaround from before we had generic associated types, and is no longer necessary.
## Solution
* Combine the trait `SystemParamFetch` with `SystemParamState`.
* I decided to remove the `Fetch` name and keep the `State` name, since the former was consistently conflated with the latter.
* Replace the trait `ReadOnlySystemParamFetch` with `ReadOnlySystemParam`, which simplifies trait bounds in generic code.
---
## Changelog
- Removed the trait `SystemParamFetch`, moving its functionality to `SystemParamState`.
- Replaced the trait `ReadOnlySystemParamFetch` with `ReadOnlySystemParam`.
## Migration Guide
The trait `SystemParamFetch` has been removed, and its functionality has been transferred to `SystemParamState`.
```rust
// Before
impl SystemParamState for MyParamState {
fn init(world: &mut World, system_meta: &mut SystemMeta) -> Self { ... }
}
impl<'w, 's> SystemParamFetch<'w, 's> for MyParamState {
type Item = MyParam<'w, 's>;
fn get_param(...) -> Self::Item;
}
// After
impl SystemParamState for MyParamState {
type Item<'w, 's> = MyParam<'w, 's>; // Generic associated types!
fn init(world: &mut World, system_meta: &mut SystemMeta) -> Self { ... }
fn get_param<'w, 's>(...) -> Self::Item<'w, 's>;
}
```
The trait `ReadOnlySystemParamFetch` has been replaced with `ReadOnlySystemParam`.
```rust
// Before
unsafe impl ReadOnlySystemParamFetch for MyParamState {}
// After
unsafe impl<'w, 's> ReadOnlySystemParam for MyParam<'w, 's> {}
```
2022-12-11 18:34:14 +00:00
unsafe fn get_param < ' w , ' s > (
Remove the `SystemParamState` trait and remove types like `ResState` (#6919)
Spiritual successor to #5205.
Actual successor to #6865.
# Objective
Currently, system params are defined using three traits: `SystemParam`, `ReadOnlySystemParam`, `SystemParamState`. The behavior for each param is specified by the `SystemParamState` trait, while `SystemParam` simply defers to the state.
Splitting the traits in this way makes it easier to implement within macros, but it increases the cognitive load. Worst of all, this approach requires each `MySystemParam` to have a public `MySystemParamState` type associated with it.
## Solution
* Merge the trait `SystemParamState` into `SystemParam`.
* Remove all trivial `SystemParam` state types.
* `OptionNonSendMutState<T>`: you will not be missed.
---
- [x] Fix/resolve the remaining test failure.
## Changelog
* Removed the trait `SystemParamState`, merging its functionality into `SystemParam`.
## Migration Guide
**Note**: this should replace the migration guide for #6865.
This is relative to Bevy 0.9, not main.
The traits `SystemParamState` and `SystemParamFetch` have been removed, and their functionality has been transferred to `SystemParam`.
```rust
// Before (0.9)
impl SystemParam for MyParam<'_, '_> {
type State = MyParamState;
}
unsafe impl SystemParamState for MyParamState {
fn init(world: &mut World, system_meta: &mut SystemMeta) -> Self { ... }
}
unsafe impl<'w, 's> SystemParamFetch<'w, 's> for MyParamState {
type Item = MyParam<'w, 's>;
fn get_param(&mut self, ...) -> Self::Item;
}
unsafe impl ReadOnlySystemParamFetch for MyParamState { }
// After (0.10)
unsafe impl SystemParam for MyParam<'_, '_> {
type State = MyParamState;
type Item<'w, 's> = MyParam<'w, 's>;
fn init_state(world: &mut World, system_meta: &mut SystemMeta) -> Self::State { ... }
fn get_param<'w, 's>(state: &mut Self::State, ...) -> Self::Item<'w, 's>;
}
unsafe impl ReadOnlySystemParam for MyParam<'_, '_> { }
```
The trait `ReadOnlySystemParamFetch` has been replaced with `ReadOnlySystemParam`.
```rust
// Before
unsafe impl ReadOnlySystemParamFetch for MyParamState {}
// After
unsafe impl ReadOnlySystemParam for MyParam<'_, '_> {}
```
2023-01-07 23:20:32 +00:00
_state : & ' s mut Self ::State ,
2022-02-03 23:43:25 +00:00
_system_meta : & SystemMeta ,
world : & ' w World ,
_change_tick : u32 ,
Simplify trait hierarchy for `SystemParam` (#6865)
# Objective
* Implementing a custom `SystemParam` by hand requires implementing three traits -- four if it is read-only.
* The trait `SystemParamFetch<'w, 's>` is a workaround from before we had generic associated types, and is no longer necessary.
## Solution
* Combine the trait `SystemParamFetch` with `SystemParamState`.
* I decided to remove the `Fetch` name and keep the `State` name, since the former was consistently conflated with the latter.
* Replace the trait `ReadOnlySystemParamFetch` with `ReadOnlySystemParam`, which simplifies trait bounds in generic code.
---
## Changelog
- Removed the trait `SystemParamFetch`, moving its functionality to `SystemParamState`.
- Replaced the trait `ReadOnlySystemParamFetch` with `ReadOnlySystemParam`.
## Migration Guide
The trait `SystemParamFetch` has been removed, and its functionality has been transferred to `SystemParamState`.
```rust
// Before
impl SystemParamState for MyParamState {
fn init(world: &mut World, system_meta: &mut SystemMeta) -> Self { ... }
}
impl<'w, 's> SystemParamFetch<'w, 's> for MyParamState {
type Item = MyParam<'w, 's>;
fn get_param(...) -> Self::Item;
}
// After
impl SystemParamState for MyParamState {
type Item<'w, 's> = MyParam<'w, 's>; // Generic associated types!
fn init(world: &mut World, system_meta: &mut SystemMeta) -> Self { ... }
fn get_param<'w, 's>(...) -> Self::Item<'w, 's>;
}
```
The trait `ReadOnlySystemParamFetch` has been replaced with `ReadOnlySystemParam`.
```rust
// Before
unsafe impl ReadOnlySystemParamFetch for MyParamState {}
// After
unsafe impl<'w, 's> ReadOnlySystemParam for MyParam<'w, 's> {}
```
2022-12-11 18:34:14 +00:00
) -> Self ::Item < ' w , ' s > {
2022-02-03 23:43:25 +00:00
world
}
}
2021-04-15 20:36:16 +00:00
/// A system local [`SystemParam`].
///
/// A local may only be accessed by the system itself and is therefore not visible to other systems.
/// If two or more systems specify the same local type each will have their own unique local.
2023-01-06 15:40:10 +00:00
/// If multiple [`SystemParam`]s within the same system each specify the same local type
/// each will get their own distinct data storage.
///
/// The supplied lifetime parameter is the [`SystemParam`]s `'s` lifetime.
2021-04-15 20:36:16 +00:00
///
/// # Examples
///
/// ```
/// # use bevy_ecs::prelude::*;
/// # let world = &mut World::default();
/// fn write_to_local(mut local: Local<usize>) {
/// *local = 42;
/// }
/// fn read_from_local(local: Local<usize>) -> usize {
/// *local
/// }
2022-02-08 04:00:58 +00:00
/// let mut write_system = IntoSystem::into_system(write_to_local);
/// let mut read_system = IntoSystem::into_system(read_from_local);
2021-04-15 20:36:16 +00:00
/// write_system.initialize(world);
/// read_system.initialize(world);
///
/// assert_eq!(read_system.run((), world), 0);
/// write_system.run((), world);
/// // Note how the read local is still 0 due to the locals not being shared.
/// assert_eq!(read_system.run((), world), 0);
/// ```
2022-02-25 03:10:59 +00:00
///
/// N.B. A [`Local`]s value cannot be read or written to outside of the containing system.
/// To add configuration to a system, convert a capturing closure into the system instead:
///
/// ```
/// # use bevy_ecs::prelude::*;
/// # use bevy_ecs::system::assert_is_system;
/// struct Config(u32);
Make `Resource` trait opt-in, requiring `#[derive(Resource)]` V2 (#5577)
*This PR description is an edited copy of #5007, written by @alice-i-cecile.*
# Objective
Follow-up to https://github.com/bevyengine/bevy/pull/2254. The `Resource` trait currently has a blanket implementation for all types that meet its bounds.
While ergonomic, this results in several drawbacks:
* it is possible to make confusing, silent mistakes such as inserting a function pointer (Foo) rather than a value (Foo::Bar) as a resource
* it is challenging to discover if a type is intended to be used as a resource
* we cannot later add customization options (see the [RFC](https://github.com/bevyengine/rfcs/blob/main/rfcs/27-derive-component.md) for the equivalent choice for Component).
* dependencies can use the same Rust type as a resource in invisibly conflicting ways
* raw Rust types used as resources cannot preserve privacy appropriately, as anyone able to access that type can read and write to internal values
* we cannot capture a definitive list of possible resources to display to users in an editor
## Notes to reviewers
* Review this commit-by-commit; there's effectively no back-tracking and there's a lot of churn in some of these commits.
*ira: My commits are not as well organized :')*
* I've relaxed the bound on Local to Send + Sync + 'static: I don't think these concerns apply there, so this can keep things simple. Storing e.g. a u32 in a Local is fine, because there's a variable name attached explaining what it does.
* I think this is a bad place for the Resource trait to live, but I've left it in place to make reviewing easier. IMO that's best tackled with https://github.com/bevyengine/bevy/issues/4981.
## Changelog
`Resource` is no longer automatically implemented for all matching types. Instead, use the new `#[derive(Resource)]` macro.
## Migration Guide
Add `#[derive(Resource)]` to all types you are using as a resource.
If you are using a third party type as a resource, wrap it in a tuple struct to bypass orphan rules. Consider deriving `Deref` and `DerefMut` to improve ergonomics.
`ClearColor` no longer implements `Component`. Using `ClearColor` as a component in 0.8 did nothing.
Use the `ClearColorConfig` in the `Camera3d` and `Camera2d` components instead.
Co-authored-by: Alice <alice.i.cecile@gmail.com>
Co-authored-by: Alice Cecile <alice.i.cecile@gmail.com>
Co-authored-by: devil-ira <justthecooldude@gmail.com>
Co-authored-by: Carter Anderson <mcanders1@gmail.com>
2022-08-08 21:36:35 +00:00
/// #[derive(Resource)]
2022-02-25 03:10:59 +00:00
/// struct Myu32Wrapper(u32);
/// fn reset_to_system(value: Config) -> impl FnMut(ResMut<Myu32Wrapper>) {
/// move |mut val| val.0 = value.0
/// }
///
/// // .add_system(reset_to_system(my_config))
/// # assert_is_system(reset_to_system(Config(10)));
/// ```
2023-01-06 15:40:10 +00:00
pub struct Local < ' s , T : FromWorld + Send + 'static > ( pub ( crate ) & ' s mut T ) ;
Bevy ECS V2 (#1525)
# Bevy ECS V2
This is a rewrite of Bevy ECS (basically everything but the new executor/schedule, which are already awesome). The overall goal was to improve the performance and versatility of Bevy ECS. Here is a quick bulleted list of changes before we dive into the details:
* Complete World rewrite
* Multiple component storage types:
* Tables: fast cache friendly iteration, slower add/removes (previously called Archetypes)
* Sparse Sets: fast add/remove, slower iteration
* Stateful Queries (caches query results for faster iteration. fragmented iteration is _fast_ now)
* Stateful System Params (caches expensive operations. inspired by @DJMcNab's work in #1364)
* Configurable System Params (users can set configuration when they construct their systems. once again inspired by @DJMcNab's work)
* Archetypes are now "just metadata", component storage is separate
* Archetype Graph (for faster archetype changes)
* Component Metadata
* Configure component storage type
* Retrieve information about component size/type/name/layout/send-ness/etc
* Components are uniquely identified by a densely packed ComponentId
* TypeIds are now totally optional (which should make implementing scripting easier)
* Super fast "for_each" query iterators
* Merged Resources into World. Resources are now just a special type of component
* EntityRef/EntityMut builder apis (more efficient and more ergonomic)
* Fast bitset-backed `Access<T>` replaces old hashmap-based approach everywhere
* Query conflicts are determined by component access instead of archetype component access (to avoid random failures at runtime)
* With/Without are still taken into account for conflicts, so this should still be comfy to use
* Much simpler `IntoSystem` impl
* Significantly reduced the amount of hashing throughout the ecs in favor of Sparse Sets (indexed by densely packed ArchetypeId, ComponentId, BundleId, and TableId)
* Safety Improvements
* Entity reservation uses a normal world reference instead of unsafe transmute
* QuerySets no longer transmute lifetimes
* Made traits "unsafe" where relevant
* More thorough safety docs
* WorldCell
* Exposes safe mutable access to multiple resources at a time in a World
* Replaced "catch all" `System::update_archetypes(world: &World)` with `System::new_archetype(archetype: &Archetype)`
* Simpler Bundle implementation
* Replaced slow "remove_bundle_one_by_one" used as fallback for Commands::remove_bundle with fast "remove_bundle_intersection"
* Removed `Mut<T>` query impl. it is better to only support one way: `&mut T`
* Removed with() from `Flags<T>` in favor of `Option<Flags<T>>`, which allows querying for flags to be "filtered" by default
* Components now have is_send property (currently only resources support non-send)
* More granular module organization
* New `RemovedComponents<T>` SystemParam that replaces `query.removed::<T>()`
* `world.resource_scope()` for mutable access to resources and world at the same time
* WorldQuery and QueryFilter traits unified. FilterFetch trait added to enable "short circuit" filtering. Auto impled for cases that don't need it
* Significantly slimmed down SystemState in favor of individual SystemParam state
* System Commands changed from `commands: &mut Commands` back to `mut commands: Commands` (to allow Commands to have a World reference)
Fixes #1320
## `World` Rewrite
This is a from-scratch rewrite of `World` that fills the niche that `hecs` used to. Yes, this means Bevy ECS is no longer a "fork" of hecs. We're going out our own!
(the only shared code between the projects is the entity id allocator, which is already basically ideal)
A huge shout out to @SanderMertens (author of [flecs](https://github.com/SanderMertens/flecs)) for sharing some great ideas with me (specifically hybrid ecs storage and archetype graphs). He also helped advise on a number of implementation details.
## Component Storage (The Problem)
Two ECS storage paradigms have gained a lot of traction over the years:
* **Archetypal ECS**:
* Stores components in "tables" with static schemas. Each "column" stores components of a given type. Each "row" is an entity.
* Each "archetype" has its own table. Adding/removing an entity's component changes the archetype.
* Enables super-fast Query iteration due to its cache-friendly data layout
* Comes at the cost of more expensive add/remove operations for an Entity's components, because all components need to be copied to the new archetype's "table"
* **Sparse Set ECS**:
* Stores components of the same type in densely packed arrays, which are sparsely indexed by densely packed unsigned integers (Entity ids)
* Query iteration is slower than Archetypal ECS because each entity's component could be at any position in the sparse set. This "random access" pattern isn't cache friendly. Additionally, there is an extra layer of indirection because you must first map the entity id to an index in the component array.
* Adding/removing components is a cheap, constant time operation
Bevy ECS V1, hecs, legion, flec, and Unity DOTS are all "archetypal ecs-es". I personally think "archetypal" storage is a good default for game engines. An entity's archetype doesn't need to change frequently in general, and it creates "fast by default" query iteration (which is a much more common operation). It is also "self optimizing". Users don't need to think about optimizing component layouts for iteration performance. It "just works" without any extra boilerplate.
Shipyard and EnTT are "sparse set ecs-es". They employ "packing" as a way to work around the "suboptimal by default" iteration performance for specific sets of components. This helps, but I didn't think this was a good choice for a general purpose engine like Bevy because:
1. "packs" conflict with each other. If bevy decides to internally pack the Transform and GlobalTransform components, users are then blocked if they want to pack some custom component with Transform.
2. users need to take manual action to optimize
Developers selecting an ECS framework are stuck with a hard choice. Select an "archetypal" framework with "fast iteration everywhere" but without the ability to cheaply add/remove components, or select a "sparse set" framework to cheaply add/remove components but with slower iteration performance.
## Hybrid Component Storage (The Solution)
In Bevy ECS V2, we get to have our cake and eat it too. It now has _both_ of the component storage types above (and more can be added later if needed):
* **Tables** (aka "archetypal" storage)
* The default storage. If you don't configure anything, this is what you get
* Fast iteration by default
* Slower add/remove operations
* **Sparse Sets**
* Opt-in
* Slower iteration
* Faster add/remove operations
These storage types complement each other perfectly. By default Query iteration is fast. If developers know that they want to add/remove a component at high frequencies, they can set the storage to "sparse set":
```rust
world.register_component(
ComponentDescriptor::new::<MyComponent>(StorageType::SparseSet)
).unwrap();
```
## Archetypes
Archetypes are now "just metadata" ... they no longer store components directly. They do store:
* The `ComponentId`s of each of the Archetype's components (and that component's storage type)
* Archetypes are uniquely defined by their component layouts
* For example: entities with "table" components `[A, B, C]` _and_ "sparse set" components `[D, E]` will always be in the same archetype.
* The `TableId` associated with the archetype
* For now each archetype has exactly one table (which can have no components),
* There is a 1->Many relationship from Tables->Archetypes. A given table could have any number of archetype components stored in it:
* Ex: an entity with "table storage" components `[A, B, C]` and "sparse set" components `[D, E]` will share the same `[A, B, C]` table as an entity with `[A, B, C]` table component and `[F]` sparse set components.
* This 1->Many relationship is how we preserve fast "cache friendly" iteration performance when possible (more on this later)
* A list of entities that are in the archetype and the row id of the table they are in
* ArchetypeComponentIds
* unique densely packed identifiers for (ArchetypeId, ComponentId) pairs
* used by the schedule executor for cheap system access control
* "Archetype Graph Edges" (see the next section)
## The "Archetype Graph"
Archetype changes in Bevy (and a number of other archetypal ecs-es) have historically been expensive to compute. First, you need to allocate a new vector of the entity's current component ids, add or remove components based on the operation performed, sort it (to ensure it is order-independent), then hash it to find the archetype (if it exists). And thats all before we get to the _already_ expensive full copy of all components to the new table storage.
The solution is to build a "graph" of archetypes to cache these results. @SanderMertens first exposed me to the idea (and he got it from @gjroelofs, who came up with it). They propose adding directed edges between archetypes for add/remove component operations. If `ComponentId`s are densely packed, you can use sparse sets to cheaply jump between archetypes.
Bevy takes this one step further by using add/remove `Bundle` edges instead of `Component` edges. Bevy encourages the use of `Bundles` to group add/remove operations. This is largely for "clearer game logic" reasons, but it also helps cut down on the number of archetype changes required. `Bundles` now also have densely-packed `BundleId`s. This allows us to use a _single_ edge for each bundle operation (rather than needing to traverse N edges ... one for each component). Single component operations are also bundles, so this is strictly an improvement over a "component only" graph.
As a result, an operation that used to be _heavy_ (both for allocations and compute) is now two dirt-cheap array lookups and zero allocations.
## Stateful Queries
World queries are now stateful. This allows us to:
1. Cache archetype (and table) matches
* This resolves another issue with (naive) archetypal ECS: query performance getting worse as the number of archetypes goes up (and fragmentation occurs).
2. Cache Fetch and Filter state
* The expensive parts of fetch/filter operations (such as hashing the TypeId to find the ComponentId) now only happen once when the Query is first constructed
3. Incrementally build up state
* When new archetypes are added, we only process the new archetypes (no need to rebuild state for old archetypes)
As a result, the direct `World` query api now looks like this:
```rust
let mut query = world.query::<(&A, &mut B)>();
for (a, mut b) in query.iter_mut(&mut world) {
}
```
Requiring `World` to generate stateful queries (rather than letting the `QueryState` type be constructed separately) allows us to ensure that _all_ queries are properly initialized (and the relevant world state, such as ComponentIds). This enables QueryState to remove branches from its operations that check for initialization status (and also enables query.iter() to take an immutable world reference because it doesn't need to initialize anything in world).
However in systems, this is a non-breaking change. State management is done internally by the relevant SystemParam.
## Stateful SystemParams
Like Queries, `SystemParams` now also cache state. For example, `Query` system params store the "stateful query" state mentioned above. Commands store their internal `CommandQueue`. This means you can now safely use as many separate `Commands` parameters in your system as you want. `Local<T>` system params store their `T` value in their state (instead of in Resources).
SystemParam state also enabled a significant slim-down of SystemState. It is much nicer to look at now.
Per-SystemParam state naturally insulates us from an "aliased mut" class of errors we have hit in the past (ex: using multiple `Commands` system params).
(credit goes to @DJMcNab for the initial idea and draft pr here #1364)
## Configurable SystemParams
@DJMcNab also had the great idea to make SystemParams configurable. This allows users to provide some initial configuration / values for system parameters (when possible). Most SystemParams have no config (the config type is `()`), but the `Local<T>` param now supports user-provided parameters:
```rust
fn foo(value: Local<usize>) {
}
app.add_system(foo.system().config(|c| c.0 = Some(10)));
```
## Uber Fast "for_each" Query Iterators
Developers now have the choice to use a fast "for_each" iterator, which yields ~1.5-3x iteration speed improvements for "fragmented iteration", and minor ~1.2x iteration speed improvements for unfragmented iteration.
```rust
fn system(query: Query<(&A, &mut B)>) {
// you now have the option to do this for a speed boost
query.for_each_mut(|(a, mut b)| {
});
// however normal iterators are still available
for (a, mut b) in query.iter_mut() {
}
}
```
I think in most cases we should continue to encourage "normal" iterators as they are more flexible and more "rust idiomatic". But when that extra "oomf" is needed, it makes sense to use `for_each`.
We should also consider using `for_each` for internal bevy systems to give our users a nice speed boost (but that should be a separate pr).
## Component Metadata
`World` now has a `Components` collection, which is accessible via `world.components()`. This stores mappings from `ComponentId` to `ComponentInfo`, as well as `TypeId` to `ComponentId` mappings (where relevant). `ComponentInfo` stores information about the component, such as ComponentId, TypeId, memory layout, send-ness (currently limited to resources), and storage type.
## Significantly Cheaper `Access<T>`
We used to use `TypeAccess<TypeId>` to manage read/write component/archetype-component access. This was expensive because TypeIds must be hashed and compared individually. The parallel executor got around this by "condensing" type ids into bitset-backed access types. This worked, but it had to be re-generated from the `TypeAccess<TypeId>`sources every time archetypes changed.
This pr removes TypeAccess in favor of faster bitset access everywhere. We can do this thanks to the move to densely packed `ComponentId`s and `ArchetypeComponentId`s.
## Merged Resources into World
Resources had a lot of redundant functionality with Components. They stored typed data, they had access control, they had unique ids, they were queryable via SystemParams, etc. In fact the _only_ major difference between them was that they were unique (and didn't correlate to an entity).
Separate resources also had the downside of requiring a separate set of access controls, which meant the parallel executor needed to compare more bitsets per system and manage more state.
I initially got the "separate resources" idea from `legion`. I think that design was motivated by the fact that it made the direct world query/resource lifetime interactions more manageable. It certainly made our lives easier when using Resources alongside hecs/bevy_ecs. However we already have a construct for safely and ergonomically managing in-world lifetimes: systems (which use `Access<T>` internally).
This pr merges Resources into World:
```rust
world.insert_resource(1);
world.insert_resource(2.0);
let a = world.get_resource::<i32>().unwrap();
let mut b = world.get_resource_mut::<f64>().unwrap();
*b = 3.0;
```
Resources are now just a special kind of component. They have their own ComponentIds (and their own resource TypeId->ComponentId scope, so they don't conflict wit components of the same type). They are stored in a special "resource archetype", which stores components inside the archetype using a new `unique_components` sparse set (note that this sparse set could later be used to implement Tags). This allows us to keep the code size small by reusing existing datastructures (namely Column, Archetype, ComponentFlags, and ComponentInfo). This allows us the executor to use a single `Access<ArchetypeComponentId>` per system. It should also make scripting language integration easier.
_But_ this merge did create problems for people directly interacting with `World`. What if you need mutable access to multiple resources at the same time? `world.get_resource_mut()` borrows World mutably!
## WorldCell
WorldCell applies the `Access<ArchetypeComponentId>` concept to direct world access:
```rust
let world_cell = world.cell();
let a = world_cell.get_resource_mut::<i32>().unwrap();
let b = world_cell.get_resource_mut::<f64>().unwrap();
```
This adds cheap runtime checks (a sparse set lookup of `ArchetypeComponentId` and a counter) to ensure that world accesses do not conflict with each other. Each operation returns a `WorldBorrow<'w, T>` or `WorldBorrowMut<'w, T>` wrapper type, which will release the relevant ArchetypeComponentId resources when dropped.
World caches the access sparse set (and only one cell can exist at a time), so `world.cell()` is a cheap operation.
WorldCell does _not_ use atomic operations. It is non-send, does a mutable borrow of world to prevent other accesses, and uses a simple `Rc<RefCell<ArchetypeComponentAccess>>` wrapper in each WorldBorrow pointer.
The api is currently limited to resource access, but it can and should be extended to queries / entity component access.
## Resource Scopes
WorldCell does not yet support component queries, and even when it does there are sometimes legitimate reasons to want a mutable world ref _and_ a mutable resource ref (ex: bevy_render and bevy_scene both need this). In these cases we could always drop down to the unsafe `world.get_resource_unchecked_mut()`, but that is not ideal!
Instead developers can use a "resource scope"
```rust
world.resource_scope(|world: &mut World, a: &mut A| {
})
```
This temporarily removes the `A` resource from `World`, provides mutable pointers to both, and re-adds A to World when finished. Thanks to the move to ComponentIds/sparse sets, this is a cheap operation.
If multiple resources are required, scopes can be nested. We could also consider adding a "resource tuple" to the api if this pattern becomes common and the boilerplate gets nasty.
## Query Conflicts Use ComponentId Instead of ArchetypeComponentId
For safety reasons, systems cannot contain queries that conflict with each other without wrapping them in a QuerySet. On bevy `main`, we use ArchetypeComponentIds to determine conflicts. This is nice because it can take into account filters:
```rust
// these queries will never conflict due to their filters
fn filter_system(a: Query<&mut A, With<B>>, b: Query<&mut B, Without<B>>) {
}
```
But it also has a significant downside:
```rust
// these queries will not conflict _until_ an entity with A, B, and C is spawned
fn maybe_conflicts_system(a: Query<(&mut A, &C)>, b: Query<(&mut A, &B)>) {
}
```
The system above will panic at runtime if an entity with A, B, and C is spawned. This makes it hard to trust that your game logic will run without crashing.
In this pr, I switched to using `ComponentId` instead. This _is_ more constraining. `maybe_conflicts_system` will now always fail, but it will do it consistently at startup. Naively, it would also _disallow_ `filter_system`, which would be a significant downgrade in usability. Bevy has a number of internal systems that rely on disjoint queries and I expect it to be a common pattern in userspace.
To resolve this, I added a new `FilteredAccess<T>` type, which wraps `Access<T>` and adds with/without filters. If two `FilteredAccess` have with/without values that prove they are disjoint, they will no longer conflict.
## EntityRef / EntityMut
World entity operations on `main` require that the user passes in an `entity` id to each operation:
```rust
let entity = world.spawn((A, )); // create a new entity with A
world.get::<A>(entity);
world.insert(entity, (B, C));
world.insert_one(entity, D);
```
This means that each operation needs to look up the entity location / verify its validity. The initial spawn operation also requires a Bundle as input. This can be awkward when no components are required (or one component is required).
These operations have been replaced by `EntityRef` and `EntityMut`, which are "builder-style" wrappers around world that provide read and read/write operations on a single, pre-validated entity:
```rust
// spawn now takes no inputs and returns an EntityMut
let entity = world.spawn()
.insert(A) // insert a single component into the entity
.insert_bundle((B, C)) // insert a bundle of components into the entity
.id() // id returns the Entity id
// Returns EntityMut (or panics if the entity does not exist)
world.entity_mut(entity)
.insert(D)
.insert_bundle(SomeBundle::default());
{
// returns EntityRef (or panics if the entity does not exist)
let d = world.entity(entity)
.get::<D>() // gets the D component
.unwrap();
// world.get still exists for ergonomics
let d = world.get::<D>(entity).unwrap();
}
// These variants return Options if you want to check existence instead of panicing
world.get_entity_mut(entity)
.unwrap()
.insert(E);
if let Some(entity_ref) = world.get_entity(entity) {
let d = entity_ref.get::<D>().unwrap();
}
```
This _does not_ affect the current Commands api or terminology. I think that should be a separate conversation as that is a much larger breaking change.
## Safety Improvements
* Entity reservation in Commands uses a normal world borrow instead of an unsafe transmute
* QuerySets no longer transmutes lifetimes
* Made traits "unsafe" when implementing a trait incorrectly could cause unsafety
* More thorough safety docs
## RemovedComponents SystemParam
The old approach to querying removed components: `query.removed:<T>()` was confusing because it had no connection to the query itself. I replaced it with the following, which is both clearer and allows us to cache the ComponentId mapping in the SystemParamState:
```rust
fn system(removed: RemovedComponents<T>) {
for entity in removed.iter() {
}
}
```
## Simpler Bundle implementation
Bundles are no longer responsible for sorting (or deduping) TypeInfo. They are just a simple ordered list of component types / data. This makes the implementation smaller and opens the door to an easy "nested bundle" implementation in the future (which i might even add in this pr). Duplicate detection is now done once per bundle type by World the first time a bundle is used.
## Unified WorldQuery and QueryFilter types
(don't worry they are still separate type _parameters_ in Queries .. this is a non-breaking change)
WorldQuery and QueryFilter were already basically identical apis. With the addition of `FetchState` and more storage-specific fetch methods, the overlap was even clearer (and the redundancy more painful).
QueryFilters are now just `F: WorldQuery where F::Fetch: FilterFetch`. FilterFetch requires `Fetch<Item = bool>` and adds new "short circuit" variants of fetch methods. This enables a filter tuple like `(With<A>, Without<B>, Changed<C>)` to stop evaluating the filter after the first mismatch is encountered. FilterFetch is automatically implemented for `Fetch` implementations that return bool.
This forces fetch implementations that return things like `(bool, bool, bool)` (such as the filter above) to manually implement FilterFetch and decide whether or not to short-circuit.
## More Granular Modules
World no longer globs all of the internal modules together. It now exports `core`, `system`, and `schedule` separately. I'm also considering exporting `core` submodules directly as that is still pretty "glob-ey" and unorganized (feedback welcome here).
## Remaining Draft Work (to be done in this pr)
* ~~panic on conflicting WorldQuery fetches (&A, &mut A)~~
* ~~bevy `main` and hecs both currently allow this, but we should protect against it if possible~~
* ~~batch_iter / par_iter (currently stubbed out)~~
* ~~ChangedRes~~
* ~~I skipped this while we sort out #1313. This pr should be adapted to account for whatever we land on there~~.
* ~~The `Archetypes` and `Tables` collections use hashes of sorted lists of component ids to uniquely identify each archetype/table. This hash is then used as the key in a HashMap to look up the relevant ArchetypeId or TableId. (which doesn't handle hash collisions properly)~~
* ~~It is currently unsafe to generate a Query from "World A", then use it on "World B" (despite the api claiming it is safe). We should probably close this gap. This could be done by adding a randomly generated WorldId to each world, then storing that id in each Query. They could then be compared to each other on each `query.do_thing(&world)` operation. This _does_ add an extra branch to each query operation, so I'm open to other suggestions if people have them.~~
* ~~Nested Bundles (if i find time)~~
## Potential Future Work
* Expand WorldCell to support queries.
* Consider not allocating in the empty archetype on `world.spawn()`
* ex: return something like EntityMutUninit, which turns into EntityMut after an `insert` or `insert_bundle` op
* this actually regressed performance last time i tried it, but in theory it should be faster
* Optimize SparseSet::insert (see `PERF` comment on insert)
* Replace SparseArray `Option<T>` with T::MAX to cut down on branching
* would enable cheaper get_unchecked() operations
* upstream fixedbitset optimizations
* fixedbitset could be allocation free for small block counts (store blocks in a SmallVec)
* fixedbitset could have a const constructor
* Consider implementing Tags (archetype-specific by-value data that affects archetype identity)
* ex: ArchetypeA could have `[A, B, C]` table components and `[D(1)]` "tag" component. ArchetypeB could have `[A, B, C]` table components and a `[D(2)]` tag component. The archetypes are different, despite both having D tags because the value inside D is different.
* this could potentially build on top of the `archetype.unique_components` added in this pr for resource storage.
* Consider reverting `all_tuples` proc macro in favor of the old `macro_rules` implementation
* all_tuples is more flexible and produces cleaner documentation (the macro_rules version produces weird type parameter orders due to parser constraints)
* but unfortunately all_tuples also appears to make Rust Analyzer sad/slow when working inside of `bevy_ecs` (does not affect user code)
* Consider "resource queries" and/or "mixed resource and entity component queries" as an alternative to WorldCell
* this is basically just "systems" so maybe it's not worth it
* Add more world ops
* `world.clear()`
* `world.reserve<T: Bundle>(count: usize)`
* Try using the old archetype allocation strategy (allocate new memory on resize and copy everything over). I expect this to improve batch insertion performance at the cost of unbatched performance. But thats just a guess. I'm not an allocation perf pro :)
* Adapt Commands apis for consistency with new World apis
## Benchmarks
key:
* `bevy_old`: bevy `main` branch
* `bevy`: this branch
* `_foreach`: uses an optimized for_each iterator
* ` _sparse`: uses sparse set storage (if unspecified assume table storage)
* `_system`: runs inside a system (if unspecified assume test happens via direct world ops)
### Simple Insert (from ecs_bench_suite)
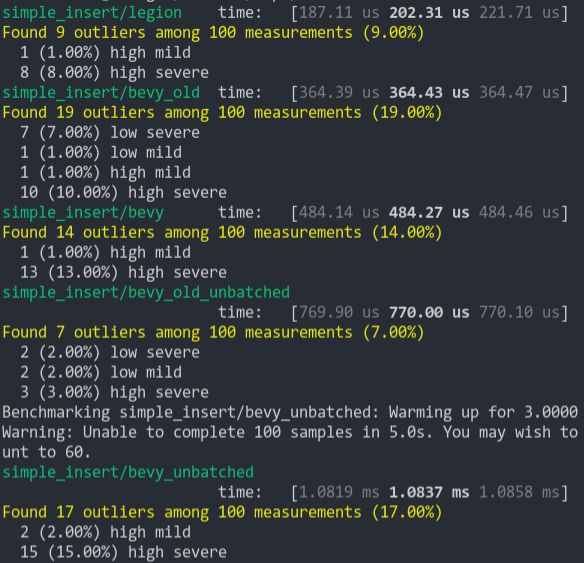
### Simpler Iter (from ecs_bench_suite)
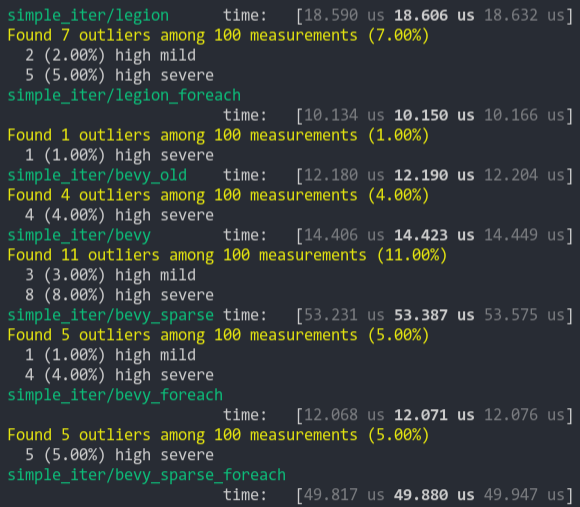
### Fragment Iter (from ecs_bench_suite)
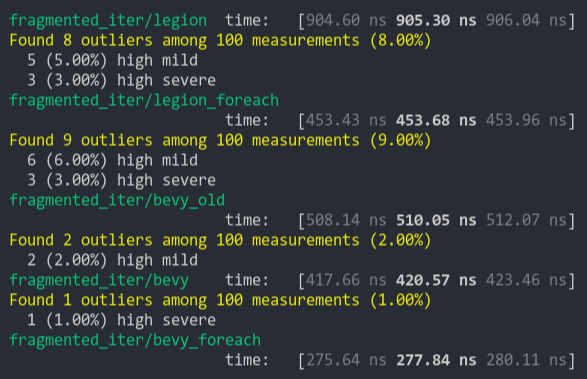
### Sparse Fragmented Iter
Iterate a query that matches 5 entities from a single matching archetype, but there are 100 unmatching archetypes
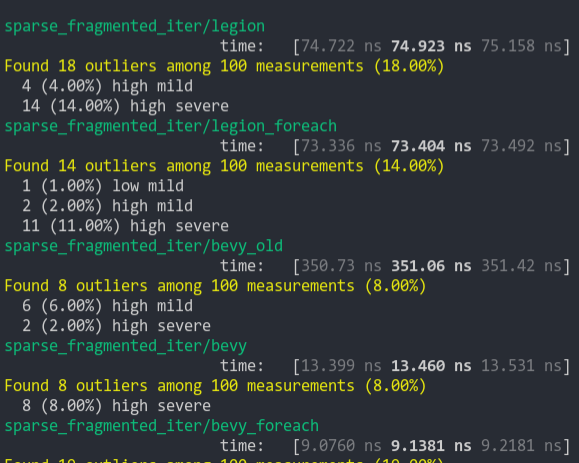
### Schedule (from ecs_bench_suite)
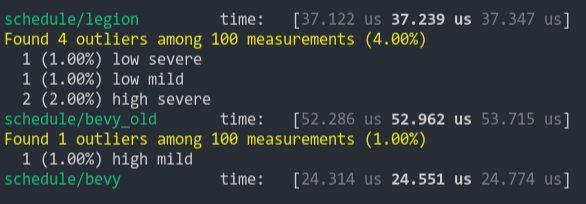
### Add Remove Component (from ecs_bench_suite)
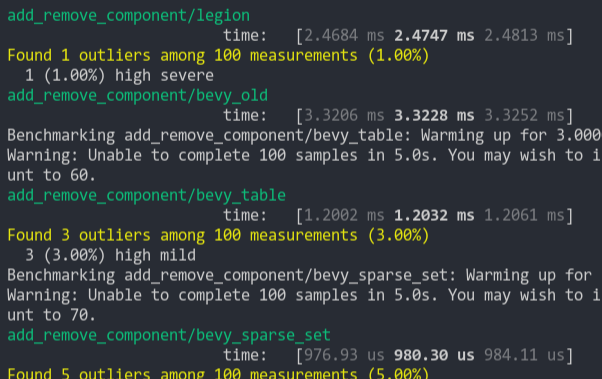
### Add Remove Component Big
Same as the test above, but each entity has 5 "large" matrix components and 1 "large" matrix component is added and removed
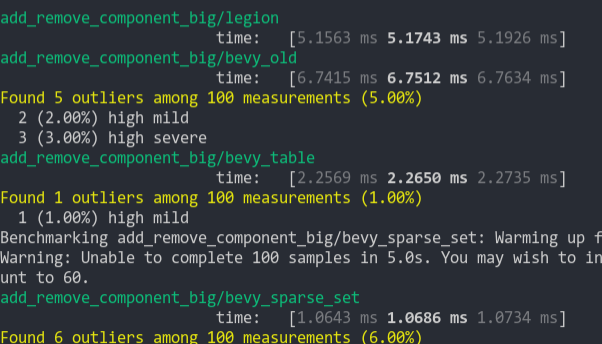
### Get Component
Looks up a single component value a large number of times
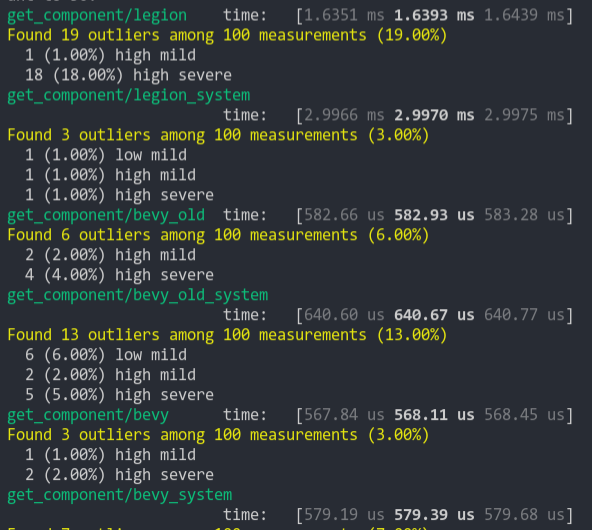
2021-03-05 07:54:35 +00:00
2022-08-13 10:51:19 +00:00
// SAFETY: Local only accesses internal state
2023-01-06 15:40:10 +00:00
unsafe impl < ' s , T : FromWorld + Send + 'static > ReadOnlySystemParam for Local < ' s , T > { }
Add new SystemState and rename old SystemState to SystemMeta (#2283)
This enables `SystemParams` to be used outside of function systems. Anything can create and store `SystemState`, which enables efficient "param state cached" access to `SystemParams`.
It adds a `ReadOnlySystemParamFetch` trait, which enables safe `SystemState::get` calls without unique world access.
I renamed the old `SystemState` to `SystemMeta` to enable us to mirror the `QueryState` naming convention (but I'm happy to discuss alternative names if people have other ideas). I initially pitched this as `ParamState`, but given that it needs to include full system metadata, that doesn't feel like a particularly accurate name.
```rust
#[derive(Eq, PartialEq, Debug)]
struct A(usize);
#[derive(Eq, PartialEq, Debug)]
struct B(usize);
let mut world = World::default();
world.insert_resource(A(42));
world.spawn().insert(B(7));
// we get nice lifetime elision when declaring the type on the left hand side
let mut system_state: SystemState<(Res<A>, Query<&B>)> = SystemState::new(&mut world);
let (a, query) = system_state.get(&world);
assert_eq!(*a, A(42), "returned resource matches initial value");
assert_eq!(
*query.single().unwrap(),
B(7),
"returned component matches initial value"
);
// mutable system params require unique world access
let mut system_state: SystemState<(ResMut<A>, Query<&mut B>)> = SystemState::new(&mut world);
let (a, query) = system_state.get_mut(&mut world);
// static lifetimes are required when declaring inside of structs
struct SomeContainer {
state: SystemState<(Res<'static, A>, Res<'static, B>)>
}
// this can be shortened using type aliases, which will be useful for complex param tuples
type MyParams<'a> = (Res<'a, A>, Res<'a, B>);
struct SomeContainer {
state: SystemState<MyParams<'static>>
}
// It is the user's responsibility to call SystemState::apply(world) for parameters that queue up work
let mut system_state: SystemState<(Commands, Query<&B>)> = SystemState::new(&mut world);
{
let (mut commands, query) = system_state.get(&world);
commands.insert_resource(3.14);
}
system_state.apply(&mut world);
```
## Future Work
* Actually use SystemState inside FunctionSystem. This would be trivial, but it requires FunctionSystem to wrap SystemState in Option in its current form (which complicates system metadata lookup). I'd prefer to hold off until we adopt something like the later designs linked in #1364, which enable us to contruct Systems using a World reference (and also remove the need for `.system`).
* Consider a "scoped" approach to automatically call SystemState::apply when systems params are no longer being used (either a container type with a Drop impl, or a function that takes a closure for user logic operating on params).
2021-06-02 19:57:38 +00:00
2023-01-06 15:40:10 +00:00
impl < ' s , T : FromWorld + Send + Sync + 'static > Debug for Local < ' s , T >
2021-05-01 02:32:32 +00:00
where
T : Debug ,
{
fn fmt ( & self , f : & mut std ::fmt ::Formatter < '_ > ) -> std ::fmt ::Result {
f . debug_tuple ( " Local " ) . field ( & self . 0 ) . finish ( )
}
}
2023-01-06 15:40:10 +00:00
impl < ' s , T : FromWorld + Send + Sync + 'static > Deref for Local < ' s , T > {
Bevy ECS V2 (#1525)
# Bevy ECS V2
This is a rewrite of Bevy ECS (basically everything but the new executor/schedule, which are already awesome). The overall goal was to improve the performance and versatility of Bevy ECS. Here is a quick bulleted list of changes before we dive into the details:
* Complete World rewrite
* Multiple component storage types:
* Tables: fast cache friendly iteration, slower add/removes (previously called Archetypes)
* Sparse Sets: fast add/remove, slower iteration
* Stateful Queries (caches query results for faster iteration. fragmented iteration is _fast_ now)
* Stateful System Params (caches expensive operations. inspired by @DJMcNab's work in #1364)
* Configurable System Params (users can set configuration when they construct their systems. once again inspired by @DJMcNab's work)
* Archetypes are now "just metadata", component storage is separate
* Archetype Graph (for faster archetype changes)
* Component Metadata
* Configure component storage type
* Retrieve information about component size/type/name/layout/send-ness/etc
* Components are uniquely identified by a densely packed ComponentId
* TypeIds are now totally optional (which should make implementing scripting easier)
* Super fast "for_each" query iterators
* Merged Resources into World. Resources are now just a special type of component
* EntityRef/EntityMut builder apis (more efficient and more ergonomic)
* Fast bitset-backed `Access<T>` replaces old hashmap-based approach everywhere
* Query conflicts are determined by component access instead of archetype component access (to avoid random failures at runtime)
* With/Without are still taken into account for conflicts, so this should still be comfy to use
* Much simpler `IntoSystem` impl
* Significantly reduced the amount of hashing throughout the ecs in favor of Sparse Sets (indexed by densely packed ArchetypeId, ComponentId, BundleId, and TableId)
* Safety Improvements
* Entity reservation uses a normal world reference instead of unsafe transmute
* QuerySets no longer transmute lifetimes
* Made traits "unsafe" where relevant
* More thorough safety docs
* WorldCell
* Exposes safe mutable access to multiple resources at a time in a World
* Replaced "catch all" `System::update_archetypes(world: &World)` with `System::new_archetype(archetype: &Archetype)`
* Simpler Bundle implementation
* Replaced slow "remove_bundle_one_by_one" used as fallback for Commands::remove_bundle with fast "remove_bundle_intersection"
* Removed `Mut<T>` query impl. it is better to only support one way: `&mut T`
* Removed with() from `Flags<T>` in favor of `Option<Flags<T>>`, which allows querying for flags to be "filtered" by default
* Components now have is_send property (currently only resources support non-send)
* More granular module organization
* New `RemovedComponents<T>` SystemParam that replaces `query.removed::<T>()`
* `world.resource_scope()` for mutable access to resources and world at the same time
* WorldQuery and QueryFilter traits unified. FilterFetch trait added to enable "short circuit" filtering. Auto impled for cases that don't need it
* Significantly slimmed down SystemState in favor of individual SystemParam state
* System Commands changed from `commands: &mut Commands` back to `mut commands: Commands` (to allow Commands to have a World reference)
Fixes #1320
## `World` Rewrite
This is a from-scratch rewrite of `World` that fills the niche that `hecs` used to. Yes, this means Bevy ECS is no longer a "fork" of hecs. We're going out our own!
(the only shared code between the projects is the entity id allocator, which is already basically ideal)
A huge shout out to @SanderMertens (author of [flecs](https://github.com/SanderMertens/flecs)) for sharing some great ideas with me (specifically hybrid ecs storage and archetype graphs). He also helped advise on a number of implementation details.
## Component Storage (The Problem)
Two ECS storage paradigms have gained a lot of traction over the years:
* **Archetypal ECS**:
* Stores components in "tables" with static schemas. Each "column" stores components of a given type. Each "row" is an entity.
* Each "archetype" has its own table. Adding/removing an entity's component changes the archetype.
* Enables super-fast Query iteration due to its cache-friendly data layout
* Comes at the cost of more expensive add/remove operations for an Entity's components, because all components need to be copied to the new archetype's "table"
* **Sparse Set ECS**:
* Stores components of the same type in densely packed arrays, which are sparsely indexed by densely packed unsigned integers (Entity ids)
* Query iteration is slower than Archetypal ECS because each entity's component could be at any position in the sparse set. This "random access" pattern isn't cache friendly. Additionally, there is an extra layer of indirection because you must first map the entity id to an index in the component array.
* Adding/removing components is a cheap, constant time operation
Bevy ECS V1, hecs, legion, flec, and Unity DOTS are all "archetypal ecs-es". I personally think "archetypal" storage is a good default for game engines. An entity's archetype doesn't need to change frequently in general, and it creates "fast by default" query iteration (which is a much more common operation). It is also "self optimizing". Users don't need to think about optimizing component layouts for iteration performance. It "just works" without any extra boilerplate.
Shipyard and EnTT are "sparse set ecs-es". They employ "packing" as a way to work around the "suboptimal by default" iteration performance for specific sets of components. This helps, but I didn't think this was a good choice for a general purpose engine like Bevy because:
1. "packs" conflict with each other. If bevy decides to internally pack the Transform and GlobalTransform components, users are then blocked if they want to pack some custom component with Transform.
2. users need to take manual action to optimize
Developers selecting an ECS framework are stuck with a hard choice. Select an "archetypal" framework with "fast iteration everywhere" but without the ability to cheaply add/remove components, or select a "sparse set" framework to cheaply add/remove components but with slower iteration performance.
## Hybrid Component Storage (The Solution)
In Bevy ECS V2, we get to have our cake and eat it too. It now has _both_ of the component storage types above (and more can be added later if needed):
* **Tables** (aka "archetypal" storage)
* The default storage. If you don't configure anything, this is what you get
* Fast iteration by default
* Slower add/remove operations
* **Sparse Sets**
* Opt-in
* Slower iteration
* Faster add/remove operations
These storage types complement each other perfectly. By default Query iteration is fast. If developers know that they want to add/remove a component at high frequencies, they can set the storage to "sparse set":
```rust
world.register_component(
ComponentDescriptor::new::<MyComponent>(StorageType::SparseSet)
).unwrap();
```
## Archetypes
Archetypes are now "just metadata" ... they no longer store components directly. They do store:
* The `ComponentId`s of each of the Archetype's components (and that component's storage type)
* Archetypes are uniquely defined by their component layouts
* For example: entities with "table" components `[A, B, C]` _and_ "sparse set" components `[D, E]` will always be in the same archetype.
* The `TableId` associated with the archetype
* For now each archetype has exactly one table (which can have no components),
* There is a 1->Many relationship from Tables->Archetypes. A given table could have any number of archetype components stored in it:
* Ex: an entity with "table storage" components `[A, B, C]` and "sparse set" components `[D, E]` will share the same `[A, B, C]` table as an entity with `[A, B, C]` table component and `[F]` sparse set components.
* This 1->Many relationship is how we preserve fast "cache friendly" iteration performance when possible (more on this later)
* A list of entities that are in the archetype and the row id of the table they are in
* ArchetypeComponentIds
* unique densely packed identifiers for (ArchetypeId, ComponentId) pairs
* used by the schedule executor for cheap system access control
* "Archetype Graph Edges" (see the next section)
## The "Archetype Graph"
Archetype changes in Bevy (and a number of other archetypal ecs-es) have historically been expensive to compute. First, you need to allocate a new vector of the entity's current component ids, add or remove components based on the operation performed, sort it (to ensure it is order-independent), then hash it to find the archetype (if it exists). And thats all before we get to the _already_ expensive full copy of all components to the new table storage.
The solution is to build a "graph" of archetypes to cache these results. @SanderMertens first exposed me to the idea (and he got it from @gjroelofs, who came up with it). They propose adding directed edges between archetypes for add/remove component operations. If `ComponentId`s are densely packed, you can use sparse sets to cheaply jump between archetypes.
Bevy takes this one step further by using add/remove `Bundle` edges instead of `Component` edges. Bevy encourages the use of `Bundles` to group add/remove operations. This is largely for "clearer game logic" reasons, but it also helps cut down on the number of archetype changes required. `Bundles` now also have densely-packed `BundleId`s. This allows us to use a _single_ edge for each bundle operation (rather than needing to traverse N edges ... one for each component). Single component operations are also bundles, so this is strictly an improvement over a "component only" graph.
As a result, an operation that used to be _heavy_ (both for allocations and compute) is now two dirt-cheap array lookups and zero allocations.
## Stateful Queries
World queries are now stateful. This allows us to:
1. Cache archetype (and table) matches
* This resolves another issue with (naive) archetypal ECS: query performance getting worse as the number of archetypes goes up (and fragmentation occurs).
2. Cache Fetch and Filter state
* The expensive parts of fetch/filter operations (such as hashing the TypeId to find the ComponentId) now only happen once when the Query is first constructed
3. Incrementally build up state
* When new archetypes are added, we only process the new archetypes (no need to rebuild state for old archetypes)
As a result, the direct `World` query api now looks like this:
```rust
let mut query = world.query::<(&A, &mut B)>();
for (a, mut b) in query.iter_mut(&mut world) {
}
```
Requiring `World` to generate stateful queries (rather than letting the `QueryState` type be constructed separately) allows us to ensure that _all_ queries are properly initialized (and the relevant world state, such as ComponentIds). This enables QueryState to remove branches from its operations that check for initialization status (and also enables query.iter() to take an immutable world reference because it doesn't need to initialize anything in world).
However in systems, this is a non-breaking change. State management is done internally by the relevant SystemParam.
## Stateful SystemParams
Like Queries, `SystemParams` now also cache state. For example, `Query` system params store the "stateful query" state mentioned above. Commands store their internal `CommandQueue`. This means you can now safely use as many separate `Commands` parameters in your system as you want. `Local<T>` system params store their `T` value in their state (instead of in Resources).
SystemParam state also enabled a significant slim-down of SystemState. It is much nicer to look at now.
Per-SystemParam state naturally insulates us from an "aliased mut" class of errors we have hit in the past (ex: using multiple `Commands` system params).
(credit goes to @DJMcNab for the initial idea and draft pr here #1364)
## Configurable SystemParams
@DJMcNab also had the great idea to make SystemParams configurable. This allows users to provide some initial configuration / values for system parameters (when possible). Most SystemParams have no config (the config type is `()`), but the `Local<T>` param now supports user-provided parameters:
```rust
fn foo(value: Local<usize>) {
}
app.add_system(foo.system().config(|c| c.0 = Some(10)));
```
## Uber Fast "for_each" Query Iterators
Developers now have the choice to use a fast "for_each" iterator, which yields ~1.5-3x iteration speed improvements for "fragmented iteration", and minor ~1.2x iteration speed improvements for unfragmented iteration.
```rust
fn system(query: Query<(&A, &mut B)>) {
// you now have the option to do this for a speed boost
query.for_each_mut(|(a, mut b)| {
});
// however normal iterators are still available
for (a, mut b) in query.iter_mut() {
}
}
```
I think in most cases we should continue to encourage "normal" iterators as they are more flexible and more "rust idiomatic". But when that extra "oomf" is needed, it makes sense to use `for_each`.
We should also consider using `for_each` for internal bevy systems to give our users a nice speed boost (but that should be a separate pr).
## Component Metadata
`World` now has a `Components` collection, which is accessible via `world.components()`. This stores mappings from `ComponentId` to `ComponentInfo`, as well as `TypeId` to `ComponentId` mappings (where relevant). `ComponentInfo` stores information about the component, such as ComponentId, TypeId, memory layout, send-ness (currently limited to resources), and storage type.
## Significantly Cheaper `Access<T>`
We used to use `TypeAccess<TypeId>` to manage read/write component/archetype-component access. This was expensive because TypeIds must be hashed and compared individually. The parallel executor got around this by "condensing" type ids into bitset-backed access types. This worked, but it had to be re-generated from the `TypeAccess<TypeId>`sources every time archetypes changed.
This pr removes TypeAccess in favor of faster bitset access everywhere. We can do this thanks to the move to densely packed `ComponentId`s and `ArchetypeComponentId`s.
## Merged Resources into World
Resources had a lot of redundant functionality with Components. They stored typed data, they had access control, they had unique ids, they were queryable via SystemParams, etc. In fact the _only_ major difference between them was that they were unique (and didn't correlate to an entity).
Separate resources also had the downside of requiring a separate set of access controls, which meant the parallel executor needed to compare more bitsets per system and manage more state.
I initially got the "separate resources" idea from `legion`. I think that design was motivated by the fact that it made the direct world query/resource lifetime interactions more manageable. It certainly made our lives easier when using Resources alongside hecs/bevy_ecs. However we already have a construct for safely and ergonomically managing in-world lifetimes: systems (which use `Access<T>` internally).
This pr merges Resources into World:
```rust
world.insert_resource(1);
world.insert_resource(2.0);
let a = world.get_resource::<i32>().unwrap();
let mut b = world.get_resource_mut::<f64>().unwrap();
*b = 3.0;
```
Resources are now just a special kind of component. They have their own ComponentIds (and their own resource TypeId->ComponentId scope, so they don't conflict wit components of the same type). They are stored in a special "resource archetype", which stores components inside the archetype using a new `unique_components` sparse set (note that this sparse set could later be used to implement Tags). This allows us to keep the code size small by reusing existing datastructures (namely Column, Archetype, ComponentFlags, and ComponentInfo). This allows us the executor to use a single `Access<ArchetypeComponentId>` per system. It should also make scripting language integration easier.
_But_ this merge did create problems for people directly interacting with `World`. What if you need mutable access to multiple resources at the same time? `world.get_resource_mut()` borrows World mutably!
## WorldCell
WorldCell applies the `Access<ArchetypeComponentId>` concept to direct world access:
```rust
let world_cell = world.cell();
let a = world_cell.get_resource_mut::<i32>().unwrap();
let b = world_cell.get_resource_mut::<f64>().unwrap();
```
This adds cheap runtime checks (a sparse set lookup of `ArchetypeComponentId` and a counter) to ensure that world accesses do not conflict with each other. Each operation returns a `WorldBorrow<'w, T>` or `WorldBorrowMut<'w, T>` wrapper type, which will release the relevant ArchetypeComponentId resources when dropped.
World caches the access sparse set (and only one cell can exist at a time), so `world.cell()` is a cheap operation.
WorldCell does _not_ use atomic operations. It is non-send, does a mutable borrow of world to prevent other accesses, and uses a simple `Rc<RefCell<ArchetypeComponentAccess>>` wrapper in each WorldBorrow pointer.
The api is currently limited to resource access, but it can and should be extended to queries / entity component access.
## Resource Scopes
WorldCell does not yet support component queries, and even when it does there are sometimes legitimate reasons to want a mutable world ref _and_ a mutable resource ref (ex: bevy_render and bevy_scene both need this). In these cases we could always drop down to the unsafe `world.get_resource_unchecked_mut()`, but that is not ideal!
Instead developers can use a "resource scope"
```rust
world.resource_scope(|world: &mut World, a: &mut A| {
})
```
This temporarily removes the `A` resource from `World`, provides mutable pointers to both, and re-adds A to World when finished. Thanks to the move to ComponentIds/sparse sets, this is a cheap operation.
If multiple resources are required, scopes can be nested. We could also consider adding a "resource tuple" to the api if this pattern becomes common and the boilerplate gets nasty.
## Query Conflicts Use ComponentId Instead of ArchetypeComponentId
For safety reasons, systems cannot contain queries that conflict with each other without wrapping them in a QuerySet. On bevy `main`, we use ArchetypeComponentIds to determine conflicts. This is nice because it can take into account filters:
```rust
// these queries will never conflict due to their filters
fn filter_system(a: Query<&mut A, With<B>>, b: Query<&mut B, Without<B>>) {
}
```
But it also has a significant downside:
```rust
// these queries will not conflict _until_ an entity with A, B, and C is spawned
fn maybe_conflicts_system(a: Query<(&mut A, &C)>, b: Query<(&mut A, &B)>) {
}
```
The system above will panic at runtime if an entity with A, B, and C is spawned. This makes it hard to trust that your game logic will run without crashing.
In this pr, I switched to using `ComponentId` instead. This _is_ more constraining. `maybe_conflicts_system` will now always fail, but it will do it consistently at startup. Naively, it would also _disallow_ `filter_system`, which would be a significant downgrade in usability. Bevy has a number of internal systems that rely on disjoint queries and I expect it to be a common pattern in userspace.
To resolve this, I added a new `FilteredAccess<T>` type, which wraps `Access<T>` and adds with/without filters. If two `FilteredAccess` have with/without values that prove they are disjoint, they will no longer conflict.
## EntityRef / EntityMut
World entity operations on `main` require that the user passes in an `entity` id to each operation:
```rust
let entity = world.spawn((A, )); // create a new entity with A
world.get::<A>(entity);
world.insert(entity, (B, C));
world.insert_one(entity, D);
```
This means that each operation needs to look up the entity location / verify its validity. The initial spawn operation also requires a Bundle as input. This can be awkward when no components are required (or one component is required).
These operations have been replaced by `EntityRef` and `EntityMut`, which are "builder-style" wrappers around world that provide read and read/write operations on a single, pre-validated entity:
```rust
// spawn now takes no inputs and returns an EntityMut
let entity = world.spawn()
.insert(A) // insert a single component into the entity
.insert_bundle((B, C)) // insert a bundle of components into the entity
.id() // id returns the Entity id
// Returns EntityMut (or panics if the entity does not exist)
world.entity_mut(entity)
.insert(D)
.insert_bundle(SomeBundle::default());
{
// returns EntityRef (or panics if the entity does not exist)
let d = world.entity(entity)
.get::<D>() // gets the D component
.unwrap();
// world.get still exists for ergonomics
let d = world.get::<D>(entity).unwrap();
}
// These variants return Options if you want to check existence instead of panicing
world.get_entity_mut(entity)
.unwrap()
.insert(E);
if let Some(entity_ref) = world.get_entity(entity) {
let d = entity_ref.get::<D>().unwrap();
}
```
This _does not_ affect the current Commands api or terminology. I think that should be a separate conversation as that is a much larger breaking change.
## Safety Improvements
* Entity reservation in Commands uses a normal world borrow instead of an unsafe transmute
* QuerySets no longer transmutes lifetimes
* Made traits "unsafe" when implementing a trait incorrectly could cause unsafety
* More thorough safety docs
## RemovedComponents SystemParam
The old approach to querying removed components: `query.removed:<T>()` was confusing because it had no connection to the query itself. I replaced it with the following, which is both clearer and allows us to cache the ComponentId mapping in the SystemParamState:
```rust
fn system(removed: RemovedComponents<T>) {
for entity in removed.iter() {
}
}
```
## Simpler Bundle implementation
Bundles are no longer responsible for sorting (or deduping) TypeInfo. They are just a simple ordered list of component types / data. This makes the implementation smaller and opens the door to an easy "nested bundle" implementation in the future (which i might even add in this pr). Duplicate detection is now done once per bundle type by World the first time a bundle is used.
## Unified WorldQuery and QueryFilter types
(don't worry they are still separate type _parameters_ in Queries .. this is a non-breaking change)
WorldQuery and QueryFilter were already basically identical apis. With the addition of `FetchState` and more storage-specific fetch methods, the overlap was even clearer (and the redundancy more painful).
QueryFilters are now just `F: WorldQuery where F::Fetch: FilterFetch`. FilterFetch requires `Fetch<Item = bool>` and adds new "short circuit" variants of fetch methods. This enables a filter tuple like `(With<A>, Without<B>, Changed<C>)` to stop evaluating the filter after the first mismatch is encountered. FilterFetch is automatically implemented for `Fetch` implementations that return bool.
This forces fetch implementations that return things like `(bool, bool, bool)` (such as the filter above) to manually implement FilterFetch and decide whether or not to short-circuit.
## More Granular Modules
World no longer globs all of the internal modules together. It now exports `core`, `system`, and `schedule` separately. I'm also considering exporting `core` submodules directly as that is still pretty "glob-ey" and unorganized (feedback welcome here).
## Remaining Draft Work (to be done in this pr)
* ~~panic on conflicting WorldQuery fetches (&A, &mut A)~~
* ~~bevy `main` and hecs both currently allow this, but we should protect against it if possible~~
* ~~batch_iter / par_iter (currently stubbed out)~~
* ~~ChangedRes~~
* ~~I skipped this while we sort out #1313. This pr should be adapted to account for whatever we land on there~~.
* ~~The `Archetypes` and `Tables` collections use hashes of sorted lists of component ids to uniquely identify each archetype/table. This hash is then used as the key in a HashMap to look up the relevant ArchetypeId or TableId. (which doesn't handle hash collisions properly)~~
* ~~It is currently unsafe to generate a Query from "World A", then use it on "World B" (despite the api claiming it is safe). We should probably close this gap. This could be done by adding a randomly generated WorldId to each world, then storing that id in each Query. They could then be compared to each other on each `query.do_thing(&world)` operation. This _does_ add an extra branch to each query operation, so I'm open to other suggestions if people have them.~~
* ~~Nested Bundles (if i find time)~~
## Potential Future Work
* Expand WorldCell to support queries.
* Consider not allocating in the empty archetype on `world.spawn()`
* ex: return something like EntityMutUninit, which turns into EntityMut after an `insert` or `insert_bundle` op
* this actually regressed performance last time i tried it, but in theory it should be faster
* Optimize SparseSet::insert (see `PERF` comment on insert)
* Replace SparseArray `Option<T>` with T::MAX to cut down on branching
* would enable cheaper get_unchecked() operations
* upstream fixedbitset optimizations
* fixedbitset could be allocation free for small block counts (store blocks in a SmallVec)
* fixedbitset could have a const constructor
* Consider implementing Tags (archetype-specific by-value data that affects archetype identity)
* ex: ArchetypeA could have `[A, B, C]` table components and `[D(1)]` "tag" component. ArchetypeB could have `[A, B, C]` table components and a `[D(2)]` tag component. The archetypes are different, despite both having D tags because the value inside D is different.
* this could potentially build on top of the `archetype.unique_components` added in this pr for resource storage.
* Consider reverting `all_tuples` proc macro in favor of the old `macro_rules` implementation
* all_tuples is more flexible and produces cleaner documentation (the macro_rules version produces weird type parameter orders due to parser constraints)
* but unfortunately all_tuples also appears to make Rust Analyzer sad/slow when working inside of `bevy_ecs` (does not affect user code)
* Consider "resource queries" and/or "mixed resource and entity component queries" as an alternative to WorldCell
* this is basically just "systems" so maybe it's not worth it
* Add more world ops
* `world.clear()`
* `world.reserve<T: Bundle>(count: usize)`
* Try using the old archetype allocation strategy (allocate new memory on resize and copy everything over). I expect this to improve batch insertion performance at the cost of unbatched performance. But thats just a guess. I'm not an allocation perf pro :)
* Adapt Commands apis for consistency with new World apis
## Benchmarks
key:
* `bevy_old`: bevy `main` branch
* `bevy`: this branch
* `_foreach`: uses an optimized for_each iterator
* ` _sparse`: uses sparse set storage (if unspecified assume table storage)
* `_system`: runs inside a system (if unspecified assume test happens via direct world ops)
### Simple Insert (from ecs_bench_suite)
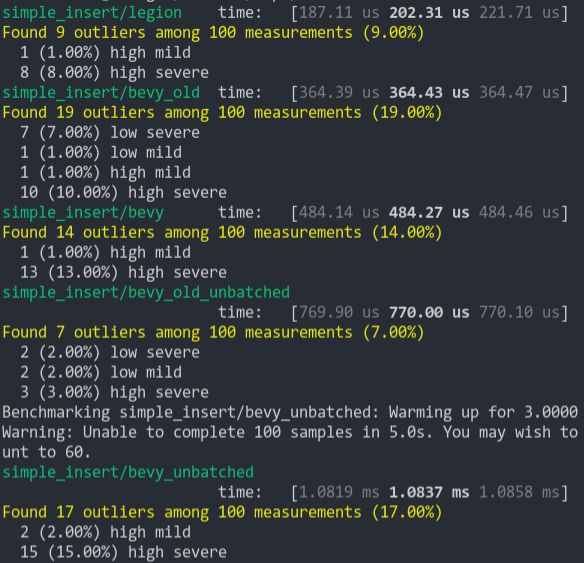
### Simpler Iter (from ecs_bench_suite)
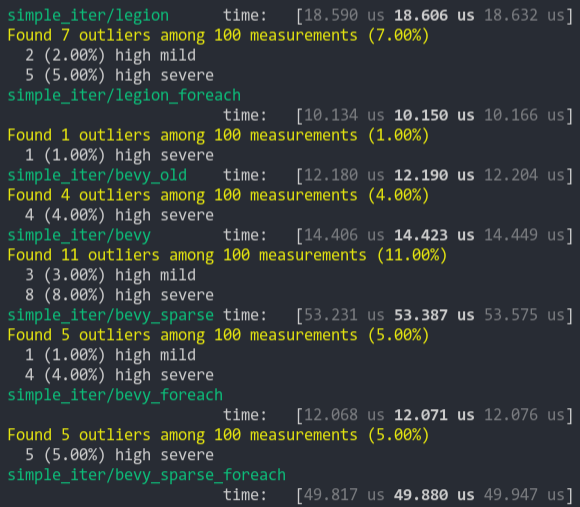
### Fragment Iter (from ecs_bench_suite)
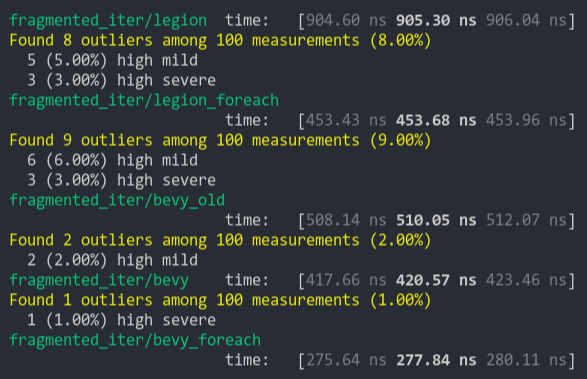
### Sparse Fragmented Iter
Iterate a query that matches 5 entities from a single matching archetype, but there are 100 unmatching archetypes
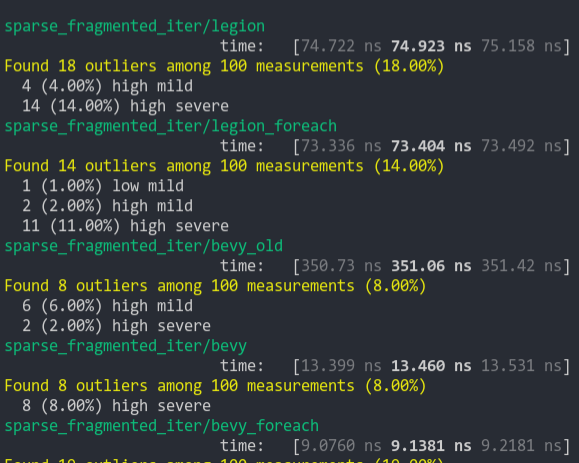
### Schedule (from ecs_bench_suite)
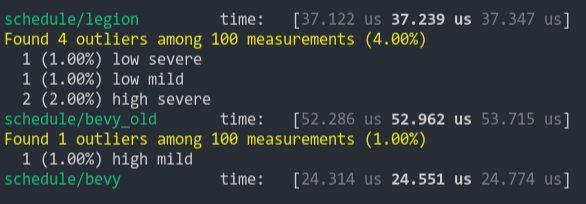
### Add Remove Component (from ecs_bench_suite)
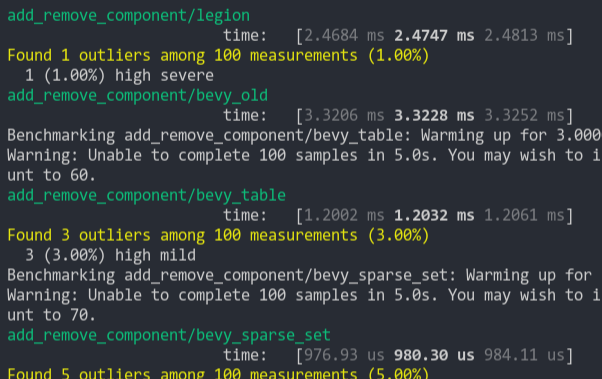
### Add Remove Component Big
Same as the test above, but each entity has 5 "large" matrix components and 1 "large" matrix component is added and removed
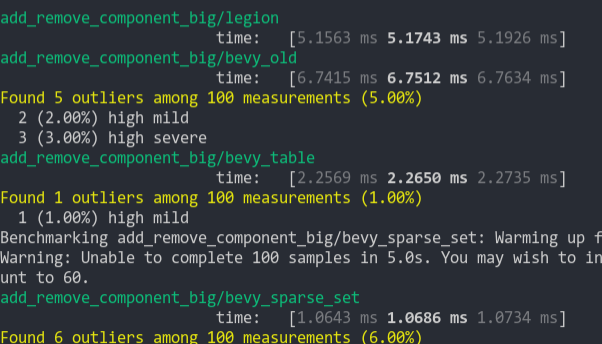
### Get Component
Looks up a single component value a large number of times
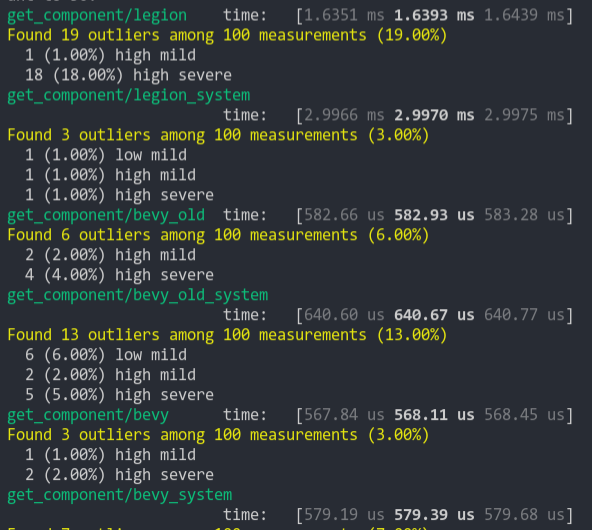
2021-03-05 07:54:35 +00:00
type Target = T ;
#[ inline ]
fn deref ( & self ) -> & Self ::Target {
self . 0
}
}
2023-01-06 15:40:10 +00:00
impl < ' s , T : FromWorld + Send + Sync + 'static > DerefMut for Local < ' s , T > {
Bevy ECS V2 (#1525)
# Bevy ECS V2
This is a rewrite of Bevy ECS (basically everything but the new executor/schedule, which are already awesome). The overall goal was to improve the performance and versatility of Bevy ECS. Here is a quick bulleted list of changes before we dive into the details:
* Complete World rewrite
* Multiple component storage types:
* Tables: fast cache friendly iteration, slower add/removes (previously called Archetypes)
* Sparse Sets: fast add/remove, slower iteration
* Stateful Queries (caches query results for faster iteration. fragmented iteration is _fast_ now)
* Stateful System Params (caches expensive operations. inspired by @DJMcNab's work in #1364)
* Configurable System Params (users can set configuration when they construct their systems. once again inspired by @DJMcNab's work)
* Archetypes are now "just metadata", component storage is separate
* Archetype Graph (for faster archetype changes)
* Component Metadata
* Configure component storage type
* Retrieve information about component size/type/name/layout/send-ness/etc
* Components are uniquely identified by a densely packed ComponentId
* TypeIds are now totally optional (which should make implementing scripting easier)
* Super fast "for_each" query iterators
* Merged Resources into World. Resources are now just a special type of component
* EntityRef/EntityMut builder apis (more efficient and more ergonomic)
* Fast bitset-backed `Access<T>` replaces old hashmap-based approach everywhere
* Query conflicts are determined by component access instead of archetype component access (to avoid random failures at runtime)
* With/Without are still taken into account for conflicts, so this should still be comfy to use
* Much simpler `IntoSystem` impl
* Significantly reduced the amount of hashing throughout the ecs in favor of Sparse Sets (indexed by densely packed ArchetypeId, ComponentId, BundleId, and TableId)
* Safety Improvements
* Entity reservation uses a normal world reference instead of unsafe transmute
* QuerySets no longer transmute lifetimes
* Made traits "unsafe" where relevant
* More thorough safety docs
* WorldCell
* Exposes safe mutable access to multiple resources at a time in a World
* Replaced "catch all" `System::update_archetypes(world: &World)` with `System::new_archetype(archetype: &Archetype)`
* Simpler Bundle implementation
* Replaced slow "remove_bundle_one_by_one" used as fallback for Commands::remove_bundle with fast "remove_bundle_intersection"
* Removed `Mut<T>` query impl. it is better to only support one way: `&mut T`
* Removed with() from `Flags<T>` in favor of `Option<Flags<T>>`, which allows querying for flags to be "filtered" by default
* Components now have is_send property (currently only resources support non-send)
* More granular module organization
* New `RemovedComponents<T>` SystemParam that replaces `query.removed::<T>()`
* `world.resource_scope()` for mutable access to resources and world at the same time
* WorldQuery and QueryFilter traits unified. FilterFetch trait added to enable "short circuit" filtering. Auto impled for cases that don't need it
* Significantly slimmed down SystemState in favor of individual SystemParam state
* System Commands changed from `commands: &mut Commands` back to `mut commands: Commands` (to allow Commands to have a World reference)
Fixes #1320
## `World` Rewrite
This is a from-scratch rewrite of `World` that fills the niche that `hecs` used to. Yes, this means Bevy ECS is no longer a "fork" of hecs. We're going out our own!
(the only shared code between the projects is the entity id allocator, which is already basically ideal)
A huge shout out to @SanderMertens (author of [flecs](https://github.com/SanderMertens/flecs)) for sharing some great ideas with me (specifically hybrid ecs storage and archetype graphs). He also helped advise on a number of implementation details.
## Component Storage (The Problem)
Two ECS storage paradigms have gained a lot of traction over the years:
* **Archetypal ECS**:
* Stores components in "tables" with static schemas. Each "column" stores components of a given type. Each "row" is an entity.
* Each "archetype" has its own table. Adding/removing an entity's component changes the archetype.
* Enables super-fast Query iteration due to its cache-friendly data layout
* Comes at the cost of more expensive add/remove operations for an Entity's components, because all components need to be copied to the new archetype's "table"
* **Sparse Set ECS**:
* Stores components of the same type in densely packed arrays, which are sparsely indexed by densely packed unsigned integers (Entity ids)
* Query iteration is slower than Archetypal ECS because each entity's component could be at any position in the sparse set. This "random access" pattern isn't cache friendly. Additionally, there is an extra layer of indirection because you must first map the entity id to an index in the component array.
* Adding/removing components is a cheap, constant time operation
Bevy ECS V1, hecs, legion, flec, and Unity DOTS are all "archetypal ecs-es". I personally think "archetypal" storage is a good default for game engines. An entity's archetype doesn't need to change frequently in general, and it creates "fast by default" query iteration (which is a much more common operation). It is also "self optimizing". Users don't need to think about optimizing component layouts for iteration performance. It "just works" without any extra boilerplate.
Shipyard and EnTT are "sparse set ecs-es". They employ "packing" as a way to work around the "suboptimal by default" iteration performance for specific sets of components. This helps, but I didn't think this was a good choice for a general purpose engine like Bevy because:
1. "packs" conflict with each other. If bevy decides to internally pack the Transform and GlobalTransform components, users are then blocked if they want to pack some custom component with Transform.
2. users need to take manual action to optimize
Developers selecting an ECS framework are stuck with a hard choice. Select an "archetypal" framework with "fast iteration everywhere" but without the ability to cheaply add/remove components, or select a "sparse set" framework to cheaply add/remove components but with slower iteration performance.
## Hybrid Component Storage (The Solution)
In Bevy ECS V2, we get to have our cake and eat it too. It now has _both_ of the component storage types above (and more can be added later if needed):
* **Tables** (aka "archetypal" storage)
* The default storage. If you don't configure anything, this is what you get
* Fast iteration by default
* Slower add/remove operations
* **Sparse Sets**
* Opt-in
* Slower iteration
* Faster add/remove operations
These storage types complement each other perfectly. By default Query iteration is fast. If developers know that they want to add/remove a component at high frequencies, they can set the storage to "sparse set":
```rust
world.register_component(
ComponentDescriptor::new::<MyComponent>(StorageType::SparseSet)
).unwrap();
```
## Archetypes
Archetypes are now "just metadata" ... they no longer store components directly. They do store:
* The `ComponentId`s of each of the Archetype's components (and that component's storage type)
* Archetypes are uniquely defined by their component layouts
* For example: entities with "table" components `[A, B, C]` _and_ "sparse set" components `[D, E]` will always be in the same archetype.
* The `TableId` associated with the archetype
* For now each archetype has exactly one table (which can have no components),
* There is a 1->Many relationship from Tables->Archetypes. A given table could have any number of archetype components stored in it:
* Ex: an entity with "table storage" components `[A, B, C]` and "sparse set" components `[D, E]` will share the same `[A, B, C]` table as an entity with `[A, B, C]` table component and `[F]` sparse set components.
* This 1->Many relationship is how we preserve fast "cache friendly" iteration performance when possible (more on this later)
* A list of entities that are in the archetype and the row id of the table they are in
* ArchetypeComponentIds
* unique densely packed identifiers for (ArchetypeId, ComponentId) pairs
* used by the schedule executor for cheap system access control
* "Archetype Graph Edges" (see the next section)
## The "Archetype Graph"
Archetype changes in Bevy (and a number of other archetypal ecs-es) have historically been expensive to compute. First, you need to allocate a new vector of the entity's current component ids, add or remove components based on the operation performed, sort it (to ensure it is order-independent), then hash it to find the archetype (if it exists). And thats all before we get to the _already_ expensive full copy of all components to the new table storage.
The solution is to build a "graph" of archetypes to cache these results. @SanderMertens first exposed me to the idea (and he got it from @gjroelofs, who came up with it). They propose adding directed edges between archetypes for add/remove component operations. If `ComponentId`s are densely packed, you can use sparse sets to cheaply jump between archetypes.
Bevy takes this one step further by using add/remove `Bundle` edges instead of `Component` edges. Bevy encourages the use of `Bundles` to group add/remove operations. This is largely for "clearer game logic" reasons, but it also helps cut down on the number of archetype changes required. `Bundles` now also have densely-packed `BundleId`s. This allows us to use a _single_ edge for each bundle operation (rather than needing to traverse N edges ... one for each component). Single component operations are also bundles, so this is strictly an improvement over a "component only" graph.
As a result, an operation that used to be _heavy_ (both for allocations and compute) is now two dirt-cheap array lookups and zero allocations.
## Stateful Queries
World queries are now stateful. This allows us to:
1. Cache archetype (and table) matches
* This resolves another issue with (naive) archetypal ECS: query performance getting worse as the number of archetypes goes up (and fragmentation occurs).
2. Cache Fetch and Filter state
* The expensive parts of fetch/filter operations (such as hashing the TypeId to find the ComponentId) now only happen once when the Query is first constructed
3. Incrementally build up state
* When new archetypes are added, we only process the new archetypes (no need to rebuild state for old archetypes)
As a result, the direct `World` query api now looks like this:
```rust
let mut query = world.query::<(&A, &mut B)>();
for (a, mut b) in query.iter_mut(&mut world) {
}
```
Requiring `World` to generate stateful queries (rather than letting the `QueryState` type be constructed separately) allows us to ensure that _all_ queries are properly initialized (and the relevant world state, such as ComponentIds). This enables QueryState to remove branches from its operations that check for initialization status (and also enables query.iter() to take an immutable world reference because it doesn't need to initialize anything in world).
However in systems, this is a non-breaking change. State management is done internally by the relevant SystemParam.
## Stateful SystemParams
Like Queries, `SystemParams` now also cache state. For example, `Query` system params store the "stateful query" state mentioned above. Commands store their internal `CommandQueue`. This means you can now safely use as many separate `Commands` parameters in your system as you want. `Local<T>` system params store their `T` value in their state (instead of in Resources).
SystemParam state also enabled a significant slim-down of SystemState. It is much nicer to look at now.
Per-SystemParam state naturally insulates us from an "aliased mut" class of errors we have hit in the past (ex: using multiple `Commands` system params).
(credit goes to @DJMcNab for the initial idea and draft pr here #1364)
## Configurable SystemParams
@DJMcNab also had the great idea to make SystemParams configurable. This allows users to provide some initial configuration / values for system parameters (when possible). Most SystemParams have no config (the config type is `()`), but the `Local<T>` param now supports user-provided parameters:
```rust
fn foo(value: Local<usize>) {
}
app.add_system(foo.system().config(|c| c.0 = Some(10)));
```
## Uber Fast "for_each" Query Iterators
Developers now have the choice to use a fast "for_each" iterator, which yields ~1.5-3x iteration speed improvements for "fragmented iteration", and minor ~1.2x iteration speed improvements for unfragmented iteration.
```rust
fn system(query: Query<(&A, &mut B)>) {
// you now have the option to do this for a speed boost
query.for_each_mut(|(a, mut b)| {
});
// however normal iterators are still available
for (a, mut b) in query.iter_mut() {
}
}
```
I think in most cases we should continue to encourage "normal" iterators as they are more flexible and more "rust idiomatic". But when that extra "oomf" is needed, it makes sense to use `for_each`.
We should also consider using `for_each` for internal bevy systems to give our users a nice speed boost (but that should be a separate pr).
## Component Metadata
`World` now has a `Components` collection, which is accessible via `world.components()`. This stores mappings from `ComponentId` to `ComponentInfo`, as well as `TypeId` to `ComponentId` mappings (where relevant). `ComponentInfo` stores information about the component, such as ComponentId, TypeId, memory layout, send-ness (currently limited to resources), and storage type.
## Significantly Cheaper `Access<T>`
We used to use `TypeAccess<TypeId>` to manage read/write component/archetype-component access. This was expensive because TypeIds must be hashed and compared individually. The parallel executor got around this by "condensing" type ids into bitset-backed access types. This worked, but it had to be re-generated from the `TypeAccess<TypeId>`sources every time archetypes changed.
This pr removes TypeAccess in favor of faster bitset access everywhere. We can do this thanks to the move to densely packed `ComponentId`s and `ArchetypeComponentId`s.
## Merged Resources into World
Resources had a lot of redundant functionality with Components. They stored typed data, they had access control, they had unique ids, they were queryable via SystemParams, etc. In fact the _only_ major difference between them was that they were unique (and didn't correlate to an entity).
Separate resources also had the downside of requiring a separate set of access controls, which meant the parallel executor needed to compare more bitsets per system and manage more state.
I initially got the "separate resources" idea from `legion`. I think that design was motivated by the fact that it made the direct world query/resource lifetime interactions more manageable. It certainly made our lives easier when using Resources alongside hecs/bevy_ecs. However we already have a construct for safely and ergonomically managing in-world lifetimes: systems (which use `Access<T>` internally).
This pr merges Resources into World:
```rust
world.insert_resource(1);
world.insert_resource(2.0);
let a = world.get_resource::<i32>().unwrap();
let mut b = world.get_resource_mut::<f64>().unwrap();
*b = 3.0;
```
Resources are now just a special kind of component. They have their own ComponentIds (and their own resource TypeId->ComponentId scope, so they don't conflict wit components of the same type). They are stored in a special "resource archetype", which stores components inside the archetype using a new `unique_components` sparse set (note that this sparse set could later be used to implement Tags). This allows us to keep the code size small by reusing existing datastructures (namely Column, Archetype, ComponentFlags, and ComponentInfo). This allows us the executor to use a single `Access<ArchetypeComponentId>` per system. It should also make scripting language integration easier.
_But_ this merge did create problems for people directly interacting with `World`. What if you need mutable access to multiple resources at the same time? `world.get_resource_mut()` borrows World mutably!
## WorldCell
WorldCell applies the `Access<ArchetypeComponentId>` concept to direct world access:
```rust
let world_cell = world.cell();
let a = world_cell.get_resource_mut::<i32>().unwrap();
let b = world_cell.get_resource_mut::<f64>().unwrap();
```
This adds cheap runtime checks (a sparse set lookup of `ArchetypeComponentId` and a counter) to ensure that world accesses do not conflict with each other. Each operation returns a `WorldBorrow<'w, T>` or `WorldBorrowMut<'w, T>` wrapper type, which will release the relevant ArchetypeComponentId resources when dropped.
World caches the access sparse set (and only one cell can exist at a time), so `world.cell()` is a cheap operation.
WorldCell does _not_ use atomic operations. It is non-send, does a mutable borrow of world to prevent other accesses, and uses a simple `Rc<RefCell<ArchetypeComponentAccess>>` wrapper in each WorldBorrow pointer.
The api is currently limited to resource access, but it can and should be extended to queries / entity component access.
## Resource Scopes
WorldCell does not yet support component queries, and even when it does there are sometimes legitimate reasons to want a mutable world ref _and_ a mutable resource ref (ex: bevy_render and bevy_scene both need this). In these cases we could always drop down to the unsafe `world.get_resource_unchecked_mut()`, but that is not ideal!
Instead developers can use a "resource scope"
```rust
world.resource_scope(|world: &mut World, a: &mut A| {
})
```
This temporarily removes the `A` resource from `World`, provides mutable pointers to both, and re-adds A to World when finished. Thanks to the move to ComponentIds/sparse sets, this is a cheap operation.
If multiple resources are required, scopes can be nested. We could also consider adding a "resource tuple" to the api if this pattern becomes common and the boilerplate gets nasty.
## Query Conflicts Use ComponentId Instead of ArchetypeComponentId
For safety reasons, systems cannot contain queries that conflict with each other without wrapping them in a QuerySet. On bevy `main`, we use ArchetypeComponentIds to determine conflicts. This is nice because it can take into account filters:
```rust
// these queries will never conflict due to their filters
fn filter_system(a: Query<&mut A, With<B>>, b: Query<&mut B, Without<B>>) {
}
```
But it also has a significant downside:
```rust
// these queries will not conflict _until_ an entity with A, B, and C is spawned
fn maybe_conflicts_system(a: Query<(&mut A, &C)>, b: Query<(&mut A, &B)>) {
}
```
The system above will panic at runtime if an entity with A, B, and C is spawned. This makes it hard to trust that your game logic will run without crashing.
In this pr, I switched to using `ComponentId` instead. This _is_ more constraining. `maybe_conflicts_system` will now always fail, but it will do it consistently at startup. Naively, it would also _disallow_ `filter_system`, which would be a significant downgrade in usability. Bevy has a number of internal systems that rely on disjoint queries and I expect it to be a common pattern in userspace.
To resolve this, I added a new `FilteredAccess<T>` type, which wraps `Access<T>` and adds with/without filters. If two `FilteredAccess` have with/without values that prove they are disjoint, they will no longer conflict.
## EntityRef / EntityMut
World entity operations on `main` require that the user passes in an `entity` id to each operation:
```rust
let entity = world.spawn((A, )); // create a new entity with A
world.get::<A>(entity);
world.insert(entity, (B, C));
world.insert_one(entity, D);
```
This means that each operation needs to look up the entity location / verify its validity. The initial spawn operation also requires a Bundle as input. This can be awkward when no components are required (or one component is required).
These operations have been replaced by `EntityRef` and `EntityMut`, which are "builder-style" wrappers around world that provide read and read/write operations on a single, pre-validated entity:
```rust
// spawn now takes no inputs and returns an EntityMut
let entity = world.spawn()
.insert(A) // insert a single component into the entity
.insert_bundle((B, C)) // insert a bundle of components into the entity
.id() // id returns the Entity id
// Returns EntityMut (or panics if the entity does not exist)
world.entity_mut(entity)
.insert(D)
.insert_bundle(SomeBundle::default());
{
// returns EntityRef (or panics if the entity does not exist)
let d = world.entity(entity)
.get::<D>() // gets the D component
.unwrap();
// world.get still exists for ergonomics
let d = world.get::<D>(entity).unwrap();
}
// These variants return Options if you want to check existence instead of panicing
world.get_entity_mut(entity)
.unwrap()
.insert(E);
if let Some(entity_ref) = world.get_entity(entity) {
let d = entity_ref.get::<D>().unwrap();
}
```
This _does not_ affect the current Commands api or terminology. I think that should be a separate conversation as that is a much larger breaking change.
## Safety Improvements
* Entity reservation in Commands uses a normal world borrow instead of an unsafe transmute
* QuerySets no longer transmutes lifetimes
* Made traits "unsafe" when implementing a trait incorrectly could cause unsafety
* More thorough safety docs
## RemovedComponents SystemParam
The old approach to querying removed components: `query.removed:<T>()` was confusing because it had no connection to the query itself. I replaced it with the following, which is both clearer and allows us to cache the ComponentId mapping in the SystemParamState:
```rust
fn system(removed: RemovedComponents<T>) {
for entity in removed.iter() {
}
}
```
## Simpler Bundle implementation
Bundles are no longer responsible for sorting (or deduping) TypeInfo. They are just a simple ordered list of component types / data. This makes the implementation smaller and opens the door to an easy "nested bundle" implementation in the future (which i might even add in this pr). Duplicate detection is now done once per bundle type by World the first time a bundle is used.
## Unified WorldQuery and QueryFilter types
(don't worry they are still separate type _parameters_ in Queries .. this is a non-breaking change)
WorldQuery and QueryFilter were already basically identical apis. With the addition of `FetchState` and more storage-specific fetch methods, the overlap was even clearer (and the redundancy more painful).
QueryFilters are now just `F: WorldQuery where F::Fetch: FilterFetch`. FilterFetch requires `Fetch<Item = bool>` and adds new "short circuit" variants of fetch methods. This enables a filter tuple like `(With<A>, Without<B>, Changed<C>)` to stop evaluating the filter after the first mismatch is encountered. FilterFetch is automatically implemented for `Fetch` implementations that return bool.
This forces fetch implementations that return things like `(bool, bool, bool)` (such as the filter above) to manually implement FilterFetch and decide whether or not to short-circuit.
## More Granular Modules
World no longer globs all of the internal modules together. It now exports `core`, `system`, and `schedule` separately. I'm also considering exporting `core` submodules directly as that is still pretty "glob-ey" and unorganized (feedback welcome here).
## Remaining Draft Work (to be done in this pr)
* ~~panic on conflicting WorldQuery fetches (&A, &mut A)~~
* ~~bevy `main` and hecs both currently allow this, but we should protect against it if possible~~
* ~~batch_iter / par_iter (currently stubbed out)~~
* ~~ChangedRes~~
* ~~I skipped this while we sort out #1313. This pr should be adapted to account for whatever we land on there~~.
* ~~The `Archetypes` and `Tables` collections use hashes of sorted lists of component ids to uniquely identify each archetype/table. This hash is then used as the key in a HashMap to look up the relevant ArchetypeId or TableId. (which doesn't handle hash collisions properly)~~
* ~~It is currently unsafe to generate a Query from "World A", then use it on "World B" (despite the api claiming it is safe). We should probably close this gap. This could be done by adding a randomly generated WorldId to each world, then storing that id in each Query. They could then be compared to each other on each `query.do_thing(&world)` operation. This _does_ add an extra branch to each query operation, so I'm open to other suggestions if people have them.~~
* ~~Nested Bundles (if i find time)~~
## Potential Future Work
* Expand WorldCell to support queries.
* Consider not allocating in the empty archetype on `world.spawn()`
* ex: return something like EntityMutUninit, which turns into EntityMut after an `insert` or `insert_bundle` op
* this actually regressed performance last time i tried it, but in theory it should be faster
* Optimize SparseSet::insert (see `PERF` comment on insert)
* Replace SparseArray `Option<T>` with T::MAX to cut down on branching
* would enable cheaper get_unchecked() operations
* upstream fixedbitset optimizations
* fixedbitset could be allocation free for small block counts (store blocks in a SmallVec)
* fixedbitset could have a const constructor
* Consider implementing Tags (archetype-specific by-value data that affects archetype identity)
* ex: ArchetypeA could have `[A, B, C]` table components and `[D(1)]` "tag" component. ArchetypeB could have `[A, B, C]` table components and a `[D(2)]` tag component. The archetypes are different, despite both having D tags because the value inside D is different.
* this could potentially build on top of the `archetype.unique_components` added in this pr for resource storage.
* Consider reverting `all_tuples` proc macro in favor of the old `macro_rules` implementation
* all_tuples is more flexible and produces cleaner documentation (the macro_rules version produces weird type parameter orders due to parser constraints)
* but unfortunately all_tuples also appears to make Rust Analyzer sad/slow when working inside of `bevy_ecs` (does not affect user code)
* Consider "resource queries" and/or "mixed resource and entity component queries" as an alternative to WorldCell
* this is basically just "systems" so maybe it's not worth it
* Add more world ops
* `world.clear()`
* `world.reserve<T: Bundle>(count: usize)`
* Try using the old archetype allocation strategy (allocate new memory on resize and copy everything over). I expect this to improve batch insertion performance at the cost of unbatched performance. But thats just a guess. I'm not an allocation perf pro :)
* Adapt Commands apis for consistency with new World apis
## Benchmarks
key:
* `bevy_old`: bevy `main` branch
* `bevy`: this branch
* `_foreach`: uses an optimized for_each iterator
* ` _sparse`: uses sparse set storage (if unspecified assume table storage)
* `_system`: runs inside a system (if unspecified assume test happens via direct world ops)
### Simple Insert (from ecs_bench_suite)
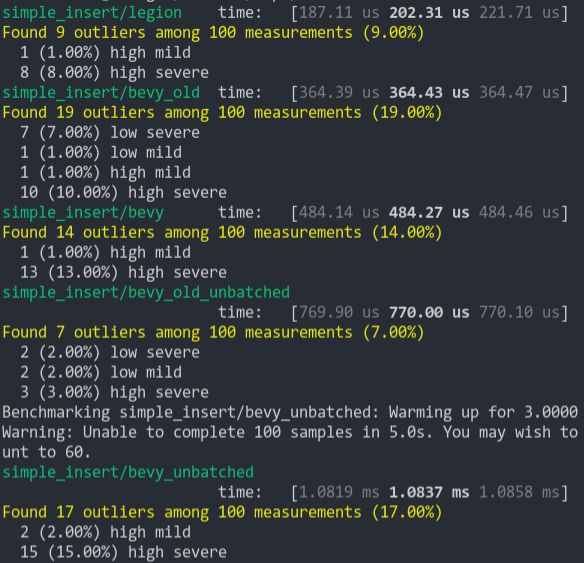
### Simpler Iter (from ecs_bench_suite)
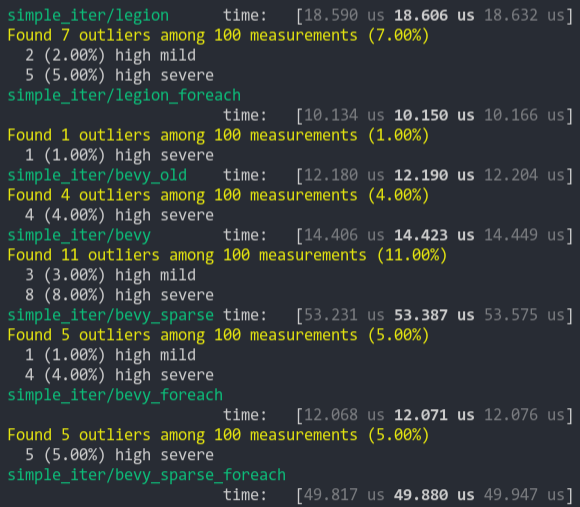
### Fragment Iter (from ecs_bench_suite)
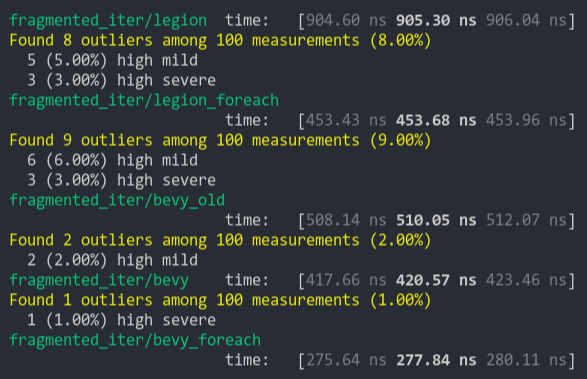
### Sparse Fragmented Iter
Iterate a query that matches 5 entities from a single matching archetype, but there are 100 unmatching archetypes
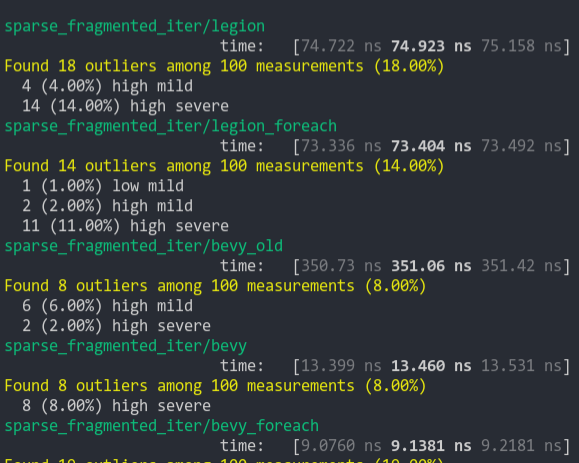
### Schedule (from ecs_bench_suite)
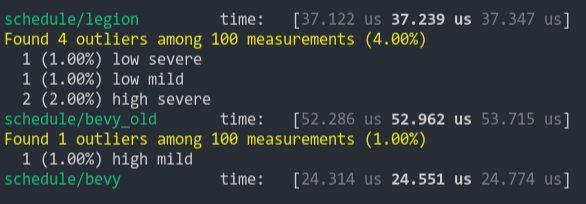
### Add Remove Component (from ecs_bench_suite)
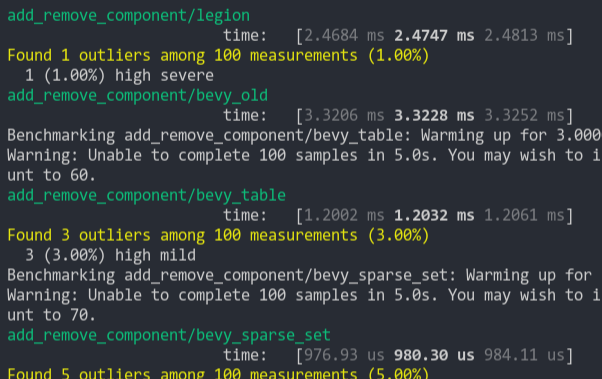
### Add Remove Component Big
Same as the test above, but each entity has 5 "large" matrix components and 1 "large" matrix component is added and removed
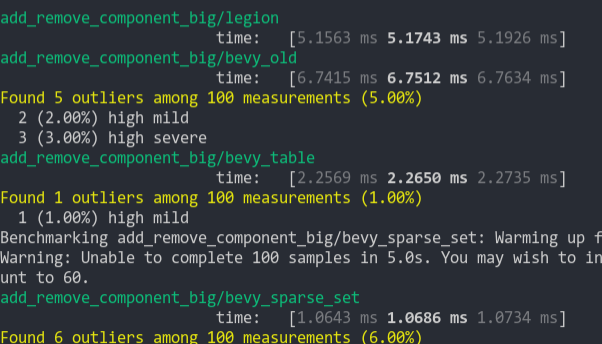
### Get Component
Looks up a single component value a large number of times
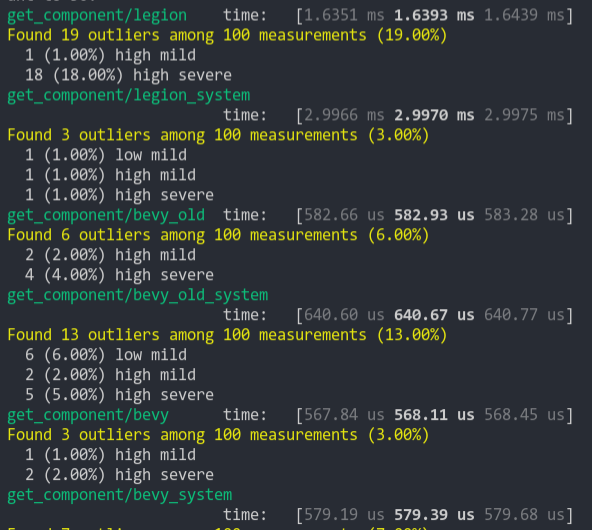
2021-03-05 07:54:35 +00:00
#[ inline ]
fn deref_mut ( & mut self ) -> & mut Self ::Target {
self . 0
}
2020-12-16 05:57:16 +00:00
}
2023-01-06 15:40:10 +00:00
impl < ' s , ' a , T : FromWorld + Send + 'static > IntoIterator for & ' a Local < ' s , T >
2022-10-24 21:01:08 +00:00
where
& ' a T : IntoIterator ,
{
type Item = < & ' a T as IntoIterator > ::Item ;
type IntoIter = < & ' a T as IntoIterator > ::IntoIter ;
fn into_iter ( self ) -> Self ::IntoIter {
self . 0. into_iter ( )
}
}
2023-01-06 15:40:10 +00:00
impl < ' s , ' a , T : FromWorld + Send + 'static > IntoIterator for & ' a mut Local < ' s , T >
2022-10-24 21:01:08 +00:00
where
& ' a mut T : IntoIterator ,
{
type Item = < & ' a mut T as IntoIterator > ::Item ;
type IntoIter = < & ' a mut T as IntoIterator > ::IntoIter ;
fn into_iter ( self ) -> Self ::IntoIter {
self . 0. into_iter ( )
}
}
2022-08-13 10:51:19 +00:00
// SAFETY: only local state is accessed
Remove the `SystemParamState` trait and remove types like `ResState` (#6919)
Spiritual successor to #5205.
Actual successor to #6865.
# Objective
Currently, system params are defined using three traits: `SystemParam`, `ReadOnlySystemParam`, `SystemParamState`. The behavior for each param is specified by the `SystemParamState` trait, while `SystemParam` simply defers to the state.
Splitting the traits in this way makes it easier to implement within macros, but it increases the cognitive load. Worst of all, this approach requires each `MySystemParam` to have a public `MySystemParamState` type associated with it.
## Solution
* Merge the trait `SystemParamState` into `SystemParam`.
* Remove all trivial `SystemParam` state types.
* `OptionNonSendMutState<T>`: you will not be missed.
---
- [x] Fix/resolve the remaining test failure.
## Changelog
* Removed the trait `SystemParamState`, merging its functionality into `SystemParam`.
## Migration Guide
**Note**: this should replace the migration guide for #6865.
This is relative to Bevy 0.9, not main.
The traits `SystemParamState` and `SystemParamFetch` have been removed, and their functionality has been transferred to `SystemParam`.
```rust
// Before (0.9)
impl SystemParam for MyParam<'_, '_> {
type State = MyParamState;
}
unsafe impl SystemParamState for MyParamState {
fn init(world: &mut World, system_meta: &mut SystemMeta) -> Self { ... }
}
unsafe impl<'w, 's> SystemParamFetch<'w, 's> for MyParamState {
type Item = MyParam<'w, 's>;
fn get_param(&mut self, ...) -> Self::Item;
}
unsafe impl ReadOnlySystemParamFetch for MyParamState { }
// After (0.10)
unsafe impl SystemParam for MyParam<'_, '_> {
type State = MyParamState;
type Item<'w, 's> = MyParam<'w, 's>;
fn init_state(world: &mut World, system_meta: &mut SystemMeta) -> Self::State { ... }
fn get_param<'w, 's>(state: &mut Self::State, ...) -> Self::Item<'w, 's>;
}
unsafe impl ReadOnlySystemParam for MyParam<'_, '_> { }
```
The trait `ReadOnlySystemParamFetch` has been replaced with `ReadOnlySystemParam`.
```rust
// Before
unsafe impl ReadOnlySystemParamFetch for MyParamState {}
// After
unsafe impl ReadOnlySystemParam for MyParam<'_, '_> {}
```
2023-01-07 23:20:32 +00:00
unsafe impl < ' a , T : FromWorld + Send + 'static > SystemParam for Local < ' a , T > {
type State = SyncCell < T > ;
Simplify trait hierarchy for `SystemParam` (#6865)
# Objective
* Implementing a custom `SystemParam` by hand requires implementing three traits -- four if it is read-only.
* The trait `SystemParamFetch<'w, 's>` is a workaround from before we had generic associated types, and is no longer necessary.
## Solution
* Combine the trait `SystemParamFetch` with `SystemParamState`.
* I decided to remove the `Fetch` name and keep the `State` name, since the former was consistently conflated with the latter.
* Replace the trait `ReadOnlySystemParamFetch` with `ReadOnlySystemParam`, which simplifies trait bounds in generic code.
---
## Changelog
- Removed the trait `SystemParamFetch`, moving its functionality to `SystemParamState`.
- Replaced the trait `ReadOnlySystemParamFetch` with `ReadOnlySystemParam`.
## Migration Guide
The trait `SystemParamFetch` has been removed, and its functionality has been transferred to `SystemParamState`.
```rust
// Before
impl SystemParamState for MyParamState {
fn init(world: &mut World, system_meta: &mut SystemMeta) -> Self { ... }
}
impl<'w, 's> SystemParamFetch<'w, 's> for MyParamState {
type Item = MyParam<'w, 's>;
fn get_param(...) -> Self::Item;
}
// After
impl SystemParamState for MyParamState {
type Item<'w, 's> = MyParam<'w, 's>; // Generic associated types!
fn init(world: &mut World, system_meta: &mut SystemMeta) -> Self { ... }
fn get_param<'w, 's>(...) -> Self::Item<'w, 's>;
}
```
The trait `ReadOnlySystemParamFetch` has been replaced with `ReadOnlySystemParam`.
```rust
// Before
unsafe impl ReadOnlySystemParamFetch for MyParamState {}
// After
unsafe impl<'w, 's> ReadOnlySystemParam for MyParam<'w, 's> {}
```
2022-12-11 18:34:14 +00:00
type Item < ' w , ' s > = Local < ' s , T > ;
Remove the `SystemParamState` trait and remove types like `ResState` (#6919)
Spiritual successor to #5205.
Actual successor to #6865.
# Objective
Currently, system params are defined using three traits: `SystemParam`, `ReadOnlySystemParam`, `SystemParamState`. The behavior for each param is specified by the `SystemParamState` trait, while `SystemParam` simply defers to the state.
Splitting the traits in this way makes it easier to implement within macros, but it increases the cognitive load. Worst of all, this approach requires each `MySystemParam` to have a public `MySystemParamState` type associated with it.
## Solution
* Merge the trait `SystemParamState` into `SystemParam`.
* Remove all trivial `SystemParam` state types.
* `OptionNonSendMutState<T>`: you will not be missed.
---
- [x] Fix/resolve the remaining test failure.
## Changelog
* Removed the trait `SystemParamState`, merging its functionality into `SystemParam`.
## Migration Guide
**Note**: this should replace the migration guide for #6865.
This is relative to Bevy 0.9, not main.
The traits `SystemParamState` and `SystemParamFetch` have been removed, and their functionality has been transferred to `SystemParam`.
```rust
// Before (0.9)
impl SystemParam for MyParam<'_, '_> {
type State = MyParamState;
}
unsafe impl SystemParamState for MyParamState {
fn init(world: &mut World, system_meta: &mut SystemMeta) -> Self { ... }
}
unsafe impl<'w, 's> SystemParamFetch<'w, 's> for MyParamState {
type Item = MyParam<'w, 's>;
fn get_param(&mut self, ...) -> Self::Item;
}
unsafe impl ReadOnlySystemParamFetch for MyParamState { }
// After (0.10)
unsafe impl SystemParam for MyParam<'_, '_> {
type State = MyParamState;
type Item<'w, 's> = MyParam<'w, 's>;
fn init_state(world: &mut World, system_meta: &mut SystemMeta) -> Self::State { ... }
fn get_param<'w, 's>(state: &mut Self::State, ...) -> Self::Item<'w, 's>;
}
unsafe impl ReadOnlySystemParam for MyParam<'_, '_> { }
```
The trait `ReadOnlySystemParamFetch` has been replaced with `ReadOnlySystemParam`.
```rust
// Before
unsafe impl ReadOnlySystemParamFetch for MyParamState {}
// After
unsafe impl ReadOnlySystemParam for MyParam<'_, '_> {}
```
2023-01-07 23:20:32 +00:00
fn init_state ( world : & mut World , _system_meta : & mut SystemMeta ) -> Self ::State {
SyncCell ::new ( T ::from_world ( world ) )
2021-04-03 23:13:54 +00:00
}
2020-11-08 20:34:05 +00:00
#[ inline ]
Simplify trait hierarchy for `SystemParam` (#6865)
# Objective
* Implementing a custom `SystemParam` by hand requires implementing three traits -- four if it is read-only.
* The trait `SystemParamFetch<'w, 's>` is a workaround from before we had generic associated types, and is no longer necessary.
## Solution
* Combine the trait `SystemParamFetch` with `SystemParamState`.
* I decided to remove the `Fetch` name and keep the `State` name, since the former was consistently conflated with the latter.
* Replace the trait `ReadOnlySystemParamFetch` with `ReadOnlySystemParam`, which simplifies trait bounds in generic code.
---
## Changelog
- Removed the trait `SystemParamFetch`, moving its functionality to `SystemParamState`.
- Replaced the trait `ReadOnlySystemParamFetch` with `ReadOnlySystemParam`.
## Migration Guide
The trait `SystemParamFetch` has been removed, and its functionality has been transferred to `SystemParamState`.
```rust
// Before
impl SystemParamState for MyParamState {
fn init(world: &mut World, system_meta: &mut SystemMeta) -> Self { ... }
}
impl<'w, 's> SystemParamFetch<'w, 's> for MyParamState {
type Item = MyParam<'w, 's>;
fn get_param(...) -> Self::Item;
}
// After
impl SystemParamState for MyParamState {
type Item<'w, 's> = MyParam<'w, 's>; // Generic associated types!
fn init(world: &mut World, system_meta: &mut SystemMeta) -> Self { ... }
fn get_param<'w, 's>(...) -> Self::Item<'w, 's>;
}
```
The trait `ReadOnlySystemParamFetch` has been replaced with `ReadOnlySystemParam`.
```rust
// Before
unsafe impl ReadOnlySystemParamFetch for MyParamState {}
// After
unsafe impl<'w, 's> ReadOnlySystemParam for MyParam<'w, 's> {}
```
2022-12-11 18:34:14 +00:00
unsafe fn get_param < ' w , ' s > (
Remove the `SystemParamState` trait and remove types like `ResState` (#6919)
Spiritual successor to #5205.
Actual successor to #6865.
# Objective
Currently, system params are defined using three traits: `SystemParam`, `ReadOnlySystemParam`, `SystemParamState`. The behavior for each param is specified by the `SystemParamState` trait, while `SystemParam` simply defers to the state.
Splitting the traits in this way makes it easier to implement within macros, but it increases the cognitive load. Worst of all, this approach requires each `MySystemParam` to have a public `MySystemParamState` type associated with it.
## Solution
* Merge the trait `SystemParamState` into `SystemParam`.
* Remove all trivial `SystemParam` state types.
* `OptionNonSendMutState<T>`: you will not be missed.
---
- [x] Fix/resolve the remaining test failure.
## Changelog
* Removed the trait `SystemParamState`, merging its functionality into `SystemParam`.
## Migration Guide
**Note**: this should replace the migration guide for #6865.
This is relative to Bevy 0.9, not main.
The traits `SystemParamState` and `SystemParamFetch` have been removed, and their functionality has been transferred to `SystemParam`.
```rust
// Before (0.9)
impl SystemParam for MyParam<'_, '_> {
type State = MyParamState;
}
unsafe impl SystemParamState for MyParamState {
fn init(world: &mut World, system_meta: &mut SystemMeta) -> Self { ... }
}
unsafe impl<'w, 's> SystemParamFetch<'w, 's> for MyParamState {
type Item = MyParam<'w, 's>;
fn get_param(&mut self, ...) -> Self::Item;
}
unsafe impl ReadOnlySystemParamFetch for MyParamState { }
// After (0.10)
unsafe impl SystemParam for MyParam<'_, '_> {
type State = MyParamState;
type Item<'w, 's> = MyParam<'w, 's>;
fn init_state(world: &mut World, system_meta: &mut SystemMeta) -> Self::State { ... }
fn get_param<'w, 's>(state: &mut Self::State, ...) -> Self::Item<'w, 's>;
}
unsafe impl ReadOnlySystemParam for MyParam<'_, '_> { }
```
The trait `ReadOnlySystemParamFetch` has been replaced with `ReadOnlySystemParam`.
```rust
// Before
unsafe impl ReadOnlySystemParamFetch for MyParamState {}
// After
unsafe impl ReadOnlySystemParam for MyParam<'_, '_> {}
```
2023-01-07 23:20:32 +00:00
state : & ' s mut Self ::State ,
Add new SystemState and rename old SystemState to SystemMeta (#2283)
This enables `SystemParams` to be used outside of function systems. Anything can create and store `SystemState`, which enables efficient "param state cached" access to `SystemParams`.
It adds a `ReadOnlySystemParamFetch` trait, which enables safe `SystemState::get` calls without unique world access.
I renamed the old `SystemState` to `SystemMeta` to enable us to mirror the `QueryState` naming convention (but I'm happy to discuss alternative names if people have other ideas). I initially pitched this as `ParamState`, but given that it needs to include full system metadata, that doesn't feel like a particularly accurate name.
```rust
#[derive(Eq, PartialEq, Debug)]
struct A(usize);
#[derive(Eq, PartialEq, Debug)]
struct B(usize);
let mut world = World::default();
world.insert_resource(A(42));
world.spawn().insert(B(7));
// we get nice lifetime elision when declaring the type on the left hand side
let mut system_state: SystemState<(Res<A>, Query<&B>)> = SystemState::new(&mut world);
let (a, query) = system_state.get(&world);
assert_eq!(*a, A(42), "returned resource matches initial value");
assert_eq!(
*query.single().unwrap(),
B(7),
"returned component matches initial value"
);
// mutable system params require unique world access
let mut system_state: SystemState<(ResMut<A>, Query<&mut B>)> = SystemState::new(&mut world);
let (a, query) = system_state.get_mut(&mut world);
// static lifetimes are required when declaring inside of structs
struct SomeContainer {
state: SystemState<(Res<'static, A>, Res<'static, B>)>
}
// this can be shortened using type aliases, which will be useful for complex param tuples
type MyParams<'a> = (Res<'a, A>, Res<'a, B>);
struct SomeContainer {
state: SystemState<MyParams<'static>>
}
// It is the user's responsibility to call SystemState::apply(world) for parameters that queue up work
let mut system_state: SystemState<(Commands, Query<&B>)> = SystemState::new(&mut world);
{
let (mut commands, query) = system_state.get(&world);
commands.insert_resource(3.14);
}
system_state.apply(&mut world);
```
## Future Work
* Actually use SystemState inside FunctionSystem. This would be trivial, but it requires FunctionSystem to wrap SystemState in Option in its current form (which complicates system metadata lookup). I'd prefer to hold off until we adopt something like the later designs linked in #1364, which enable us to contruct Systems using a World reference (and also remove the need for `.system`).
* Consider a "scoped" approach to automatically call SystemState::apply when systems params are no longer being used (either a container type with a Drop impl, or a function that takes a closure for user logic operating on params).
2021-06-02 19:57:38 +00:00
_system_meta : & SystemMeta ,
System Param Lifetime Split (#2605)
# Objective
Enable using exact World lifetimes during read-only access . This is motivated by the new renderer's need to allow read-only world-only queries to outlive the query itself (but still be constrained by the world lifetime).
For example:
https://github.com/bevyengine/bevy/blob/115b170d1f11a91146bb6d6e9684dceb8b21f786/pipelined/bevy_pbr2/src/render/mod.rs#L774
## Solution
Split out SystemParam state and world lifetimes and pipe those lifetimes up to read-only Query ops (and add into_inner for Res). According to every safety test I've run so far (except one), this is safe (see the temporary safety test commit). Note that changing the mutable variants to the new lifetimes would allow aliased mutable pointers (try doing that to see how it affects the temporary safety tests).
The new state lifetime on SystemParam does make `#[derive(SystemParam)]` more cumbersome (the current impl requires PhantomData if you don't use both lifetimes). We can make this better by detecting whether or not a lifetime is used in the derive and adjusting accordingly, but that should probably be done in its own pr.
## Why is this a draft?
The new lifetimes break QuerySet safety in one very specific case (see the query_set system in system_safety_test). We need to solve this before we can use the lifetimes given.
This is due to the fact that QuerySet is just a wrapper over Query, which now relies on world lifetimes instead of `&self` lifetimes to prevent aliasing (but in systems, each Query has its own implied lifetime, not a centralized world lifetime). I believe the fix is to rewrite QuerySet to have its own World lifetime (and own the internal reference). This will complicate the impl a bit, but I think it is doable. I'm curious if anyone else has better ideas.
Personally, I think these new lifetimes need to happen. We've gotta have a way to directly tie read-only World queries to the World lifetime. The new renderer is the first place this has come up, but I doubt it will be the last. Worst case scenario we can come up with a second `WorldLifetimeQuery<Q, F = ()>` parameter to enable these read-only scenarios, but I'd rather not add another type to the type zoo.
2021-08-15 20:51:53 +00:00
_world : & ' w World ,
Reliable change detection (#1471)
# Problem Definition
The current change tracking (via flags for both components and resources) fails to detect changes made by systems that are scheduled to run earlier in the frame than they are.
This issue is discussed at length in [#68](https://github.com/bevyengine/bevy/issues/68) and [#54](https://github.com/bevyengine/bevy/issues/54).
This is very much a draft PR, and contributions are welcome and needed.
# Criteria
1. Each change is detected at least once, no matter the ordering.
2. Each change is detected at most once, no matter the ordering.
3. Changes should be detected the same frame that they are made.
4. Competitive ergonomics. Ideally does not require opting-in.
5. Low CPU overhead of computation.
6. Memory efficient. This must not increase over time, except where the number of entities / resources does.
7. Changes should not be lost for systems that don't run.
8. A frame needs to act as a pure function. Given the same set of entities / components it needs to produce the same end state without side-effects.
**Exact** change-tracking proposals satisfy criteria 1 and 2.
**Conservative** change-tracking proposals satisfy criteria 1 but not 2.
**Flaky** change tracking proposals satisfy criteria 2 but not 1.
# Code Base Navigation
There are three types of flags:
- `Added`: A piece of data was added to an entity / `Resources`.
- `Mutated`: A piece of data was able to be modified, because its `DerefMut` was accessed
- `Changed`: The bitwise OR of `Added` and `Changed`
The special behavior of `ChangedRes`, with respect to the scheduler is being removed in [#1313](https://github.com/bevyengine/bevy/pull/1313) and does not need to be reproduced.
`ChangedRes` and friends can be found in "bevy_ecs/core/resources/resource_query.rs".
The `Flags` trait for Components can be found in "bevy_ecs/core/query.rs".
`ComponentFlags` are stored in "bevy_ecs/core/archetypes.rs", defined on line 446.
# Proposals
**Proposal 5 was selected for implementation.**
## Proposal 0: No Change Detection
The baseline, where computations are performed on everything regardless of whether it changed.
**Type:** Conservative
**Pros:**
- already implemented
- will never miss events
- no overhead
**Cons:**
- tons of repeated work
- doesn't allow users to avoid repeating work (or monitoring for other changes)
## Proposal 1: Earlier-This-Tick Change Detection
The current approach as of Bevy 0.4. Flags are set, and then flushed at the end of each frame.
**Type:** Flaky
**Pros:**
- already implemented
- simple to understand
- low memory overhead (2 bits per component)
- low time overhead (clear every flag once per frame)
**Cons:**
- misses systems based on ordering
- systems that don't run every frame miss changes
- duplicates detection when looping
- can lead to unresolvable circular dependencies
## Proposal 2: Two-Tick Change Detection
Flags persist for two frames, using a double-buffer system identical to that used in events.
A change is observed if it is found in either the current frame's list of changes or the previous frame's.
**Type:** Conservative
**Pros:**
- easy to understand
- easy to implement
- low memory overhead (4 bits per component)
- low time overhead (bit mask and shift every flag once per frame)
**Cons:**
- can result in a great deal of duplicated work
- systems that don't run every frame miss changes
- duplicates detection when looping
## Proposal 3: Last-Tick Change Detection
Flags persist for two frames, using a double-buffer system identical to that used in events.
A change is observed if it is found in the previous frame's list of changes.
**Type:** Exact
**Pros:**
- exact
- easy to understand
- easy to implement
- low memory overhead (4 bits per component)
- low time overhead (bit mask and shift every flag once per frame)
**Cons:**
- change detection is always delayed, possibly causing painful chained delays
- systems that don't run every frame miss changes
- duplicates detection when looping
## Proposal 4: Flag-Doubling Change Detection
Combine Proposal 2 and Proposal 3. Differentiate between `JustChanged` (current behavior) and `Changed` (Proposal 3).
Pack this data into the flags according to [this implementation proposal](https://github.com/bevyengine/bevy/issues/68#issuecomment-769174804).
**Type:** Flaky + Exact
**Pros:**
- allows users to acc
- easy to implement
- low memory overhead (4 bits per component)
- low time overhead (bit mask and shift every flag once per frame)
**Cons:**
- users must specify the type of change detection required
- still quite fragile to system ordering effects when using the flaky `JustChanged` form
- cannot get immediate + exact results
- systems that don't run every frame miss changes
- duplicates detection when looping
## [SELECTED] Proposal 5: Generation-Counter Change Detection
A global counter is increased after each system is run. Each component saves the time of last mutation, and each system saves the time of last execution. Mutation is detected when the component's counter is greater than the system's counter. Discussed [here](https://github.com/bevyengine/bevy/issues/68#issuecomment-769174804). How to handle addition detection is unsolved; the current proposal is to use the highest bit of the counter as in proposal 1.
**Type:** Exact (for mutations), flaky (for additions)
**Pros:**
- low time overhead (set component counter on access, set system counter after execution)
- robust to systems that don't run every frame
- robust to systems that loop
**Cons:**
- moderately complex implementation
- must be modified as systems are inserted dynamically
- medium memory overhead (4 bytes per component + system)
- unsolved addition detection
## Proposal 6: System-Data Change Detection
For each system, track which system's changes it has seen. This approach is only worth fully designing and implementing if Proposal 5 fails in some way.
**Type:** Exact
**Pros:**
- exact
- conceptually simple
**Cons:**
- requires storing data on each system
- implementation is complex
- must be modified as systems are inserted dynamically
## Proposal 7: Total-Order Change Detection
Discussed [here](https://github.com/bevyengine/bevy/issues/68#issuecomment-754326523). This proposal is somewhat complicated by the new scheduler, but I believe it should still be conceptually feasible. This approach is only worth fully designing and implementing if Proposal 5 fails in some way.
**Type:** Exact
**Pros:**
- exact
- efficient data storage relative to other exact proposals
**Cons:**
- requires access to the scheduler
- complex implementation and difficulty grokking
- must be modified as systems are inserted dynamically
# Tests
- We will need to verify properties 1, 2, 3, 7 and 8. Priority: 1 > 2 = 3 > 8 > 7
- Ideally we can use identical user-facing syntax for all proposals, allowing us to re-use the same syntax for each.
- When writing tests, we need to carefully specify order using explicit dependencies.
- These tests will need to be duplicated for both components and resources.
- We need to be sure to handle cases where ambiguous system orders exist.
`changing_system` is always the system that makes the changes, and `detecting_system` always detects the changes.
The component / resource changed will be simple boolean wrapper structs.
## Basic Added / Mutated / Changed
2 x 3 design:
- Resources vs. Components
- Added vs. Changed vs. Mutated
- `changing_system` runs before `detecting_system`
- verify at the end of tick 2
## At Least Once
2 x 3 design:
- Resources vs. Components
- Added vs. Changed vs. Mutated
- `changing_system` runs after `detecting_system`
- verify at the end of tick 2
## At Most Once
2 x 3 design:
- Resources vs. Components
- Added vs. Changed vs. Mutated
- `changing_system` runs once before `detecting_system`
- increment a counter based on the number of changes detected
- verify at the end of tick 2
## Fast Detection
2 x 3 design:
- Resources vs. Components
- Added vs. Changed vs. Mutated
- `changing_system` runs before `detecting_system`
- verify at the end of tick 1
## Ambiguous System Ordering Robustness
2 x 3 x 2 design:
- Resources vs. Components
- Added vs. Changed vs. Mutated
- `changing_system` runs [before/after] `detecting_system` in tick 1
- `changing_system` runs [after/before] `detecting_system` in tick 2
## System Pausing
2 x 3 design:
- Resources vs. Components
- Added vs. Changed vs. Mutated
- `changing_system` runs in tick 1, then is disabled by run criteria
- `detecting_system` is disabled by run criteria until it is run once during tick 3
- verify at the end of tick 3
## Addition Causes Mutation
2 design:
- Resources vs. Components
- `adding_system_1` adds a component / resource
- `adding system_2` adds the same component / resource
- verify the `Mutated` flag at the end of the tick
- verify the `Added` flag at the end of the tick
First check tests for: https://github.com/bevyengine/bevy/issues/333
Second check tests for: https://github.com/bevyengine/bevy/issues/1443
## Changes Made By Commands
- `adding_system` runs in Update in tick 1, and sends a command to add a component
- `detecting_system` runs in Update in tick 1 and 2, after `adding_system`
- We can't detect the changes in tick 1, since they haven't been processed yet
- If we were to track these changes as being emitted by `adding_system`, we can't detect the changes in tick 2 either, since `detecting_system` has already run once after `adding_system` :(
# Benchmarks
See: [general advice](https://github.com/bevyengine/bevy/blob/master/docs/profiling.md), [Criterion crate](https://github.com/bheisler/criterion.rs)
There are several critical parameters to vary:
1. entity count (1 to 10^9)
2. fraction of entities that are changed (0% to 100%)
3. cost to perform work on changed entities, i.e. workload (1 ns to 1s)
1 and 2 should be varied between benchmark runs. 3 can be added on computationally.
We want to measure:
- memory cost
- run time
We should collect these measurements across several frames (100?) to reduce bootup effects and accurately measure the mean, variance and drift.
Entity-component change detection is much more important to benchmark than resource change detection, due to the orders of magnitude higher number of pieces of data.
No change detection at all should be included in benchmarks as a second control for cases where missing changes is unacceptable.
## Graphs
1. y: performance, x: log_10(entity count), color: proposal, facet: performance metric. Set cost to perform work to 0.
2. y: run time, x: cost to perform work, color: proposal, facet: fraction changed. Set number of entities to 10^6
3. y: memory, x: frames, color: proposal
# Conclusions
1. Is the theoretical categorization of the proposals correct according to our tests?
2. How does the performance of the proposals compare without any load?
3. How does the performance of the proposals compare with realistic loads?
4. At what workload does more exact change tracking become worth the (presumably) higher overhead?
5. When does adding change-detection to save on work become worthwhile?
6. Is there enough divergence in performance between the best solutions in each class to ship more than one change-tracking solution?
# Implementation Plan
1. Write a test suite.
2. Verify that tests fail for existing approach.
3. Write a benchmark suite.
4. Get performance numbers for existing approach.
5. Implement, test and benchmark various solutions using a Git branch per proposal.
6. Create a draft PR with all solutions and present results to team.
7. Select a solution and replace existing change detection.
Co-authored-by: Brice DAVIER <bricedavier@gmail.com>
Co-authored-by: Carter Anderson <mcanders1@gmail.com>
2021-03-19 17:53:26 +00:00
_change_tick : u32 ,
Simplify trait hierarchy for `SystemParam` (#6865)
# Objective
* Implementing a custom `SystemParam` by hand requires implementing three traits -- four if it is read-only.
* The trait `SystemParamFetch<'w, 's>` is a workaround from before we had generic associated types, and is no longer necessary.
## Solution
* Combine the trait `SystemParamFetch` with `SystemParamState`.
* I decided to remove the `Fetch` name and keep the `State` name, since the former was consistently conflated with the latter.
* Replace the trait `ReadOnlySystemParamFetch` with `ReadOnlySystemParam`, which simplifies trait bounds in generic code.
---
## Changelog
- Removed the trait `SystemParamFetch`, moving its functionality to `SystemParamState`.
- Replaced the trait `ReadOnlySystemParamFetch` with `ReadOnlySystemParam`.
## Migration Guide
The trait `SystemParamFetch` has been removed, and its functionality has been transferred to `SystemParamState`.
```rust
// Before
impl SystemParamState for MyParamState {
fn init(world: &mut World, system_meta: &mut SystemMeta) -> Self { ... }
}
impl<'w, 's> SystemParamFetch<'w, 's> for MyParamState {
type Item = MyParam<'w, 's>;
fn get_param(...) -> Self::Item;
}
// After
impl SystemParamState for MyParamState {
type Item<'w, 's> = MyParam<'w, 's>; // Generic associated types!
fn init(world: &mut World, system_meta: &mut SystemMeta) -> Self { ... }
fn get_param<'w, 's>(...) -> Self::Item<'w, 's>;
}
```
The trait `ReadOnlySystemParamFetch` has been replaced with `ReadOnlySystemParam`.
```rust
// Before
unsafe impl ReadOnlySystemParamFetch for MyParamState {}
// After
unsafe impl<'w, 's> ReadOnlySystemParam for MyParam<'w, 's> {}
```
2022-12-11 18:34:14 +00:00
) -> Self ::Item < ' w , ' s > {
Remove the `SystemParamState` trait and remove types like `ResState` (#6919)
Spiritual successor to #5205.
Actual successor to #6865.
# Objective
Currently, system params are defined using three traits: `SystemParam`, `ReadOnlySystemParam`, `SystemParamState`. The behavior for each param is specified by the `SystemParamState` trait, while `SystemParam` simply defers to the state.
Splitting the traits in this way makes it easier to implement within macros, but it increases the cognitive load. Worst of all, this approach requires each `MySystemParam` to have a public `MySystemParamState` type associated with it.
## Solution
* Merge the trait `SystemParamState` into `SystemParam`.
* Remove all trivial `SystemParam` state types.
* `OptionNonSendMutState<T>`: you will not be missed.
---
- [x] Fix/resolve the remaining test failure.
## Changelog
* Removed the trait `SystemParamState`, merging its functionality into `SystemParam`.
## Migration Guide
**Note**: this should replace the migration guide for #6865.
This is relative to Bevy 0.9, not main.
The traits `SystemParamState` and `SystemParamFetch` have been removed, and their functionality has been transferred to `SystemParam`.
```rust
// Before (0.9)
impl SystemParam for MyParam<'_, '_> {
type State = MyParamState;
}
unsafe impl SystemParamState for MyParamState {
fn init(world: &mut World, system_meta: &mut SystemMeta) -> Self { ... }
}
unsafe impl<'w, 's> SystemParamFetch<'w, 's> for MyParamState {
type Item = MyParam<'w, 's>;
fn get_param(&mut self, ...) -> Self::Item;
}
unsafe impl ReadOnlySystemParamFetch for MyParamState { }
// After (0.10)
unsafe impl SystemParam for MyParam<'_, '_> {
type State = MyParamState;
type Item<'w, 's> = MyParam<'w, 's>;
fn init_state(world: &mut World, system_meta: &mut SystemMeta) -> Self::State { ... }
fn get_param<'w, 's>(state: &mut Self::State, ...) -> Self::Item<'w, 's>;
}
unsafe impl ReadOnlySystemParam for MyParam<'_, '_> { }
```
The trait `ReadOnlySystemParamFetch` has been replaced with `ReadOnlySystemParam`.
```rust
// Before
unsafe impl ReadOnlySystemParamFetch for MyParamState {}
// After
unsafe impl ReadOnlySystemParam for MyParam<'_, '_> {}
```
2023-01-07 23:20:32 +00:00
Local ( state . get ( ) )
2020-11-08 20:34:05 +00:00
}
}
Add a `SystemParam` primitive for deferred mutations; allow `#[derive]`ing more types of SystemParam (#6817)
# Objective
One pattern to increase parallelism is deferred mutation: instead of directly mutating the world (and preventing other systems from running at the same time), you queue up operations to be applied to the world at the end of the stage. The most common example of this pattern uses the `Commands` SystemParam.
In order to avoid the overhead associated with commands, some power users may want to add their own deferred mutation behavior. To do this, you must implement the unsafe trait `SystemParam`, which interfaces with engine internals in a way that we'd like users to be able to avoid.
## Solution
Add the `Deferred<T>` primitive `SystemParam`, which encapsulates the deferred mutation pattern.
This can be combined with other types of `SystemParam` to safely and ergonomically create powerful custom types.
Essentially, this is just a variant of `Local<T>` which can run code at the end of the stage.
This type is used in the engine to derive `Commands` and `ParallelCommands`, which removes a bunch of unsafe boilerplate.
### Example
```rust
use bevy_ecs::system::{Deferred, SystemBuffer};
/// Sends events with a delay, but may run in parallel with other event writers.
#[derive(SystemParam)]
pub struct BufferedEventWriter<'s, E: Event> {
queue: Deferred<'s, EventQueue<E>>,
}
struct EventQueue<E>(Vec<E>);
impl<'s, E: Event> BufferedEventWriter<'s, E> {
/// Queues up an event to be sent at the end of this stage.
pub fn send(&mut self, event: E) {
self.queue.0.push(event);
}
}
// The `SystemBuffer` trait controls how [`Deferred`] gets applied at the end of the stage.
impl<E: Event> SystemBuffer for EventQueue<E> {
fn apply(&mut self, world: &mut World) {
let mut events = world.resource_mut::<Events<E>>();
for e in self.0.drain(..) {
events.send(e);
}
}
}
```
---
## Changelog
+ Added the `SystemParam` type `Deferred<T>`, which can be used to defer `World` mutations. Powered by the new trait `SystemBuffer`.
2023-02-06 21:57:57 +00:00
/// Types that can be used with [`Deferred<T>`] in systems.
/// This allows storing system-local data which is used to defer [`World`] mutations.
///
/// Types that implement `SystemBuffer` should take care to perform as many
/// computations up-front as possible. Buffers cannot be applied in parallel,
/// so you should try to minimize the time spent in [`SystemBuffer::apply`].
pub trait SystemBuffer : FromWorld + Send + 'static {
/// Applies any deferred mutations to the [`World`].
fn apply ( & mut self , system_meta : & SystemMeta , world : & mut World ) ;
}
/// A [`SystemParam`] that stores a buffer which gets applied to the [`World`] at the end of a stage.
/// This is used internally by [`Commands`] to defer `World` mutations.
///
/// [`Commands`]: crate::system::Commands
///
/// # Examples
///
/// By using this type to defer mutations, you can avoid mutable `World` access within
/// a system, which allows it to run in parallel with more systems.
///
/// Note that deferring mutations is *not* free, and should only be used if
/// the gains in parallelization outweigh the time it takes to apply deferred mutations.
/// In general, [`Deferred`] should only be used for mutations that are infrequent,
/// or which otherwise take up a small portion of a system's run-time.
///
/// ```
/// # use bevy_ecs::prelude::*;
/// // Tracks whether or not there is a threat the player should be aware of.
/// #[derive(Resource, Default)]
/// pub struct Alarm(bool);
///
/// #[derive(Component)]
/// pub struct Settlement {
/// // ...
/// }
///
/// // A threat from inside the settlement.
/// #[derive(Component)]
/// pub struct Criminal;
///
/// // A threat from outside the settlement.
/// #[derive(Component)]
/// pub struct Monster;
///
/// # impl Criminal { pub fn is_threat(&self, _: &Settlement) -> bool { true } }
///
/// use bevy_ecs::system::{Deferred, SystemBuffer, SystemMeta};
///
/// // Uses deferred mutations to allow signalling the alarm from multiple systems in parallel.
/// #[derive(Resource, Default)]
/// struct AlarmFlag(bool);
///
/// impl AlarmFlag {
/// /// Sounds the alarm at the end of the current stage.
/// pub fn flag(&mut self) {
/// self.0 = true;
/// }
/// }
///
/// impl SystemBuffer for AlarmFlag {
/// // When `AlarmFlag` is used in a system, this function will get
/// // called at the end of the system's stage.
/// fn apply(&mut self, system_meta: &SystemMeta, world: &mut World) {
/// if self.0 {
/// world.resource_mut::<Alarm>().0 = true;
/// self.0 = false;
/// }
/// }
/// }
///
/// // Sound the alarm if there are any criminals who pose a threat.
/// fn alert_criminal(
/// settlements: Query<&Settlement>,
/// criminals: Query<&Criminal>,
/// mut alarm: Deferred<AlarmFlag>
/// ) {
/// let settlement = settlements.single();
/// for criminal in &criminals {
/// // Only sound the alarm if the criminal is a threat.
/// // For this example, assume that this check is expensive to run.
/// // Since the majority of this system's run-time is dominated
/// // by calling `is_threat()`, we defer sounding the alarm to
/// // allow this system to run in parallel with other alarm systems.
/// if criminal.is_threat(settlement) {
/// alarm.flag();
/// }
/// }
/// }
///
/// // Sound the alarm if there is a monster.
/// fn alert_monster(
/// monsters: Query<&Monster>,
/// mut alarm: ResMut<Alarm>
/// ) {
/// if monsters.iter().next().is_some() {
/// // Since this system does nothing except for sounding the alarm,
/// // it would be pointless to defer it, so we sound the alarm directly.
/// alarm.0 = true;
/// }
/// }
///
/// let mut world = World::new();
/// world.init_resource::<Alarm>();
/// world.spawn(Settlement {
/// // ...
/// });
///
/// let mut schedule = Schedule::new();
/// schedule
/// // These two systems have no conflicts and will run in parallel.
/// .add_system(alert_criminal)
/// .add_system(alert_monster);
///
/// // There are no criminals or monsters, so the alarm is not sounded.
/// schedule.run(&mut world);
/// assert_eq!(world.resource::<Alarm>().0, false);
///
/// // Spawn a monster, which will cause the alarm to be sounded.
/// let m_id = world.spawn(Monster).id();
/// schedule.run(&mut world);
/// assert_eq!(world.resource::<Alarm>().0, true);
///
/// // Remove the monster and reset the alarm.
/// world.entity_mut(m_id).despawn();
/// world.resource_mut::<Alarm>().0 = false;
///
/// // Spawn a criminal, which will cause the alarm to be sounded.
/// world.spawn(Criminal);
/// schedule.run(&mut world);
/// assert_eq!(world.resource::<Alarm>().0, true);
/// ```
pub struct Deferred < ' a , T : SystemBuffer > ( pub ( crate ) & ' a mut T ) ;
impl < ' a , T : SystemBuffer > Deref for Deferred < ' a , T > {
type Target = T ;
#[ inline ]
fn deref ( & self ) -> & Self ::Target {
self . 0
}
}
impl < ' a , T : SystemBuffer > DerefMut for Deferred < ' a , T > {
#[ inline ]
fn deref_mut ( & mut self ) -> & mut Self ::Target {
self . 0
}
}
// SAFETY: Only local state is accessed.
unsafe impl < T : SystemBuffer > ReadOnlySystemParam for Deferred < '_ , T > { }
// SAFETY: Only local state is accessed.
unsafe impl < T : SystemBuffer > SystemParam for Deferred < '_ , T > {
type State = SyncCell < T > ;
type Item < ' w , ' s > = Deferred < ' s , T > ;
fn init_state ( world : & mut World , _system_meta : & mut SystemMeta ) -> Self ::State {
SyncCell ::new ( T ::from_world ( world ) )
}
fn apply ( state : & mut Self ::State , system_meta : & SystemMeta , world : & mut World ) {
state . get ( ) . apply ( system_meta , world ) ;
}
unsafe fn get_param < ' w , ' s > (
state : & ' s mut Self ::State ,
_system_meta : & SystemMeta ,
_world : & ' w World ,
_change_tick : u32 ,
) -> Self ::Item < ' w , ' s > {
Deferred ( state . get ( ) )
}
}
2021-04-15 20:36:16 +00:00
/// Shared borrow of a non-[`Send`] resource.
///
/// Only `Send` resources may be accessed with the [`Res`] [`SystemParam`]. In case that the
/// resource does not implement `Send`, this `SystemParam` wrapper can be used. This will instruct
/// the scheduler to instead run the system on the main thread so that it doesn't send the resource
/// over to another thread.
///
/// # Panics
///
/// Panics when used as a `SystemParameter` if the resource does not exist.
2021-06-26 19:29:38 +00:00
///
/// Use `Option<NonSend<T>>` instead if the resource might not always exist.
2021-06-09 19:01:59 +00:00
pub struct NonSend < ' w , T : 'static > {
Bevy ECS V2 (#1525)
# Bevy ECS V2
This is a rewrite of Bevy ECS (basically everything but the new executor/schedule, which are already awesome). The overall goal was to improve the performance and versatility of Bevy ECS. Here is a quick bulleted list of changes before we dive into the details:
* Complete World rewrite
* Multiple component storage types:
* Tables: fast cache friendly iteration, slower add/removes (previously called Archetypes)
* Sparse Sets: fast add/remove, slower iteration
* Stateful Queries (caches query results for faster iteration. fragmented iteration is _fast_ now)
* Stateful System Params (caches expensive operations. inspired by @DJMcNab's work in #1364)
* Configurable System Params (users can set configuration when they construct their systems. once again inspired by @DJMcNab's work)
* Archetypes are now "just metadata", component storage is separate
* Archetype Graph (for faster archetype changes)
* Component Metadata
* Configure component storage type
* Retrieve information about component size/type/name/layout/send-ness/etc
* Components are uniquely identified by a densely packed ComponentId
* TypeIds are now totally optional (which should make implementing scripting easier)
* Super fast "for_each" query iterators
* Merged Resources into World. Resources are now just a special type of component
* EntityRef/EntityMut builder apis (more efficient and more ergonomic)
* Fast bitset-backed `Access<T>` replaces old hashmap-based approach everywhere
* Query conflicts are determined by component access instead of archetype component access (to avoid random failures at runtime)
* With/Without are still taken into account for conflicts, so this should still be comfy to use
* Much simpler `IntoSystem` impl
* Significantly reduced the amount of hashing throughout the ecs in favor of Sparse Sets (indexed by densely packed ArchetypeId, ComponentId, BundleId, and TableId)
* Safety Improvements
* Entity reservation uses a normal world reference instead of unsafe transmute
* QuerySets no longer transmute lifetimes
* Made traits "unsafe" where relevant
* More thorough safety docs
* WorldCell
* Exposes safe mutable access to multiple resources at a time in a World
* Replaced "catch all" `System::update_archetypes(world: &World)` with `System::new_archetype(archetype: &Archetype)`
* Simpler Bundle implementation
* Replaced slow "remove_bundle_one_by_one" used as fallback for Commands::remove_bundle with fast "remove_bundle_intersection"
* Removed `Mut<T>` query impl. it is better to only support one way: `&mut T`
* Removed with() from `Flags<T>` in favor of `Option<Flags<T>>`, which allows querying for flags to be "filtered" by default
* Components now have is_send property (currently only resources support non-send)
* More granular module organization
* New `RemovedComponents<T>` SystemParam that replaces `query.removed::<T>()`
* `world.resource_scope()` for mutable access to resources and world at the same time
* WorldQuery and QueryFilter traits unified. FilterFetch trait added to enable "short circuit" filtering. Auto impled for cases that don't need it
* Significantly slimmed down SystemState in favor of individual SystemParam state
* System Commands changed from `commands: &mut Commands` back to `mut commands: Commands` (to allow Commands to have a World reference)
Fixes #1320
## `World` Rewrite
This is a from-scratch rewrite of `World` that fills the niche that `hecs` used to. Yes, this means Bevy ECS is no longer a "fork" of hecs. We're going out our own!
(the only shared code between the projects is the entity id allocator, which is already basically ideal)
A huge shout out to @SanderMertens (author of [flecs](https://github.com/SanderMertens/flecs)) for sharing some great ideas with me (specifically hybrid ecs storage and archetype graphs). He also helped advise on a number of implementation details.
## Component Storage (The Problem)
Two ECS storage paradigms have gained a lot of traction over the years:
* **Archetypal ECS**:
* Stores components in "tables" with static schemas. Each "column" stores components of a given type. Each "row" is an entity.
* Each "archetype" has its own table. Adding/removing an entity's component changes the archetype.
* Enables super-fast Query iteration due to its cache-friendly data layout
* Comes at the cost of more expensive add/remove operations for an Entity's components, because all components need to be copied to the new archetype's "table"
* **Sparse Set ECS**:
* Stores components of the same type in densely packed arrays, which are sparsely indexed by densely packed unsigned integers (Entity ids)
* Query iteration is slower than Archetypal ECS because each entity's component could be at any position in the sparse set. This "random access" pattern isn't cache friendly. Additionally, there is an extra layer of indirection because you must first map the entity id to an index in the component array.
* Adding/removing components is a cheap, constant time operation
Bevy ECS V1, hecs, legion, flec, and Unity DOTS are all "archetypal ecs-es". I personally think "archetypal" storage is a good default for game engines. An entity's archetype doesn't need to change frequently in general, and it creates "fast by default" query iteration (which is a much more common operation). It is also "self optimizing". Users don't need to think about optimizing component layouts for iteration performance. It "just works" without any extra boilerplate.
Shipyard and EnTT are "sparse set ecs-es". They employ "packing" as a way to work around the "suboptimal by default" iteration performance for specific sets of components. This helps, but I didn't think this was a good choice for a general purpose engine like Bevy because:
1. "packs" conflict with each other. If bevy decides to internally pack the Transform and GlobalTransform components, users are then blocked if they want to pack some custom component with Transform.
2. users need to take manual action to optimize
Developers selecting an ECS framework are stuck with a hard choice. Select an "archetypal" framework with "fast iteration everywhere" but without the ability to cheaply add/remove components, or select a "sparse set" framework to cheaply add/remove components but with slower iteration performance.
## Hybrid Component Storage (The Solution)
In Bevy ECS V2, we get to have our cake and eat it too. It now has _both_ of the component storage types above (and more can be added later if needed):
* **Tables** (aka "archetypal" storage)
* The default storage. If you don't configure anything, this is what you get
* Fast iteration by default
* Slower add/remove operations
* **Sparse Sets**
* Opt-in
* Slower iteration
* Faster add/remove operations
These storage types complement each other perfectly. By default Query iteration is fast. If developers know that they want to add/remove a component at high frequencies, they can set the storage to "sparse set":
```rust
world.register_component(
ComponentDescriptor::new::<MyComponent>(StorageType::SparseSet)
).unwrap();
```
## Archetypes
Archetypes are now "just metadata" ... they no longer store components directly. They do store:
* The `ComponentId`s of each of the Archetype's components (and that component's storage type)
* Archetypes are uniquely defined by their component layouts
* For example: entities with "table" components `[A, B, C]` _and_ "sparse set" components `[D, E]` will always be in the same archetype.
* The `TableId` associated with the archetype
* For now each archetype has exactly one table (which can have no components),
* There is a 1->Many relationship from Tables->Archetypes. A given table could have any number of archetype components stored in it:
* Ex: an entity with "table storage" components `[A, B, C]` and "sparse set" components `[D, E]` will share the same `[A, B, C]` table as an entity with `[A, B, C]` table component and `[F]` sparse set components.
* This 1->Many relationship is how we preserve fast "cache friendly" iteration performance when possible (more on this later)
* A list of entities that are in the archetype and the row id of the table they are in
* ArchetypeComponentIds
* unique densely packed identifiers for (ArchetypeId, ComponentId) pairs
* used by the schedule executor for cheap system access control
* "Archetype Graph Edges" (see the next section)
## The "Archetype Graph"
Archetype changes in Bevy (and a number of other archetypal ecs-es) have historically been expensive to compute. First, you need to allocate a new vector of the entity's current component ids, add or remove components based on the operation performed, sort it (to ensure it is order-independent), then hash it to find the archetype (if it exists). And thats all before we get to the _already_ expensive full copy of all components to the new table storage.
The solution is to build a "graph" of archetypes to cache these results. @SanderMertens first exposed me to the idea (and he got it from @gjroelofs, who came up with it). They propose adding directed edges between archetypes for add/remove component operations. If `ComponentId`s are densely packed, you can use sparse sets to cheaply jump between archetypes.
Bevy takes this one step further by using add/remove `Bundle` edges instead of `Component` edges. Bevy encourages the use of `Bundles` to group add/remove operations. This is largely for "clearer game logic" reasons, but it also helps cut down on the number of archetype changes required. `Bundles` now also have densely-packed `BundleId`s. This allows us to use a _single_ edge for each bundle operation (rather than needing to traverse N edges ... one for each component). Single component operations are also bundles, so this is strictly an improvement over a "component only" graph.
As a result, an operation that used to be _heavy_ (both for allocations and compute) is now two dirt-cheap array lookups and zero allocations.
## Stateful Queries
World queries are now stateful. This allows us to:
1. Cache archetype (and table) matches
* This resolves another issue with (naive) archetypal ECS: query performance getting worse as the number of archetypes goes up (and fragmentation occurs).
2. Cache Fetch and Filter state
* The expensive parts of fetch/filter operations (such as hashing the TypeId to find the ComponentId) now only happen once when the Query is first constructed
3. Incrementally build up state
* When new archetypes are added, we only process the new archetypes (no need to rebuild state for old archetypes)
As a result, the direct `World` query api now looks like this:
```rust
let mut query = world.query::<(&A, &mut B)>();
for (a, mut b) in query.iter_mut(&mut world) {
}
```
Requiring `World` to generate stateful queries (rather than letting the `QueryState` type be constructed separately) allows us to ensure that _all_ queries are properly initialized (and the relevant world state, such as ComponentIds). This enables QueryState to remove branches from its operations that check for initialization status (and also enables query.iter() to take an immutable world reference because it doesn't need to initialize anything in world).
However in systems, this is a non-breaking change. State management is done internally by the relevant SystemParam.
## Stateful SystemParams
Like Queries, `SystemParams` now also cache state. For example, `Query` system params store the "stateful query" state mentioned above. Commands store their internal `CommandQueue`. This means you can now safely use as many separate `Commands` parameters in your system as you want. `Local<T>` system params store their `T` value in their state (instead of in Resources).
SystemParam state also enabled a significant slim-down of SystemState. It is much nicer to look at now.
Per-SystemParam state naturally insulates us from an "aliased mut" class of errors we have hit in the past (ex: using multiple `Commands` system params).
(credit goes to @DJMcNab for the initial idea and draft pr here #1364)
## Configurable SystemParams
@DJMcNab also had the great idea to make SystemParams configurable. This allows users to provide some initial configuration / values for system parameters (when possible). Most SystemParams have no config (the config type is `()`), but the `Local<T>` param now supports user-provided parameters:
```rust
fn foo(value: Local<usize>) {
}
app.add_system(foo.system().config(|c| c.0 = Some(10)));
```
## Uber Fast "for_each" Query Iterators
Developers now have the choice to use a fast "for_each" iterator, which yields ~1.5-3x iteration speed improvements for "fragmented iteration", and minor ~1.2x iteration speed improvements for unfragmented iteration.
```rust
fn system(query: Query<(&A, &mut B)>) {
// you now have the option to do this for a speed boost
query.for_each_mut(|(a, mut b)| {
});
// however normal iterators are still available
for (a, mut b) in query.iter_mut() {
}
}
```
I think in most cases we should continue to encourage "normal" iterators as they are more flexible and more "rust idiomatic". But when that extra "oomf" is needed, it makes sense to use `for_each`.
We should also consider using `for_each` for internal bevy systems to give our users a nice speed boost (but that should be a separate pr).
## Component Metadata
`World` now has a `Components` collection, which is accessible via `world.components()`. This stores mappings from `ComponentId` to `ComponentInfo`, as well as `TypeId` to `ComponentId` mappings (where relevant). `ComponentInfo` stores information about the component, such as ComponentId, TypeId, memory layout, send-ness (currently limited to resources), and storage type.
## Significantly Cheaper `Access<T>`
We used to use `TypeAccess<TypeId>` to manage read/write component/archetype-component access. This was expensive because TypeIds must be hashed and compared individually. The parallel executor got around this by "condensing" type ids into bitset-backed access types. This worked, but it had to be re-generated from the `TypeAccess<TypeId>`sources every time archetypes changed.
This pr removes TypeAccess in favor of faster bitset access everywhere. We can do this thanks to the move to densely packed `ComponentId`s and `ArchetypeComponentId`s.
## Merged Resources into World
Resources had a lot of redundant functionality with Components. They stored typed data, they had access control, they had unique ids, they were queryable via SystemParams, etc. In fact the _only_ major difference between them was that they were unique (and didn't correlate to an entity).
Separate resources also had the downside of requiring a separate set of access controls, which meant the parallel executor needed to compare more bitsets per system and manage more state.
I initially got the "separate resources" idea from `legion`. I think that design was motivated by the fact that it made the direct world query/resource lifetime interactions more manageable. It certainly made our lives easier when using Resources alongside hecs/bevy_ecs. However we already have a construct for safely and ergonomically managing in-world lifetimes: systems (which use `Access<T>` internally).
This pr merges Resources into World:
```rust
world.insert_resource(1);
world.insert_resource(2.0);
let a = world.get_resource::<i32>().unwrap();
let mut b = world.get_resource_mut::<f64>().unwrap();
*b = 3.0;
```
Resources are now just a special kind of component. They have their own ComponentIds (and their own resource TypeId->ComponentId scope, so they don't conflict wit components of the same type). They are stored in a special "resource archetype", which stores components inside the archetype using a new `unique_components` sparse set (note that this sparse set could later be used to implement Tags). This allows us to keep the code size small by reusing existing datastructures (namely Column, Archetype, ComponentFlags, and ComponentInfo). This allows us the executor to use a single `Access<ArchetypeComponentId>` per system. It should also make scripting language integration easier.
_But_ this merge did create problems for people directly interacting with `World`. What if you need mutable access to multiple resources at the same time? `world.get_resource_mut()` borrows World mutably!
## WorldCell
WorldCell applies the `Access<ArchetypeComponentId>` concept to direct world access:
```rust
let world_cell = world.cell();
let a = world_cell.get_resource_mut::<i32>().unwrap();
let b = world_cell.get_resource_mut::<f64>().unwrap();
```
This adds cheap runtime checks (a sparse set lookup of `ArchetypeComponentId` and a counter) to ensure that world accesses do not conflict with each other. Each operation returns a `WorldBorrow<'w, T>` or `WorldBorrowMut<'w, T>` wrapper type, which will release the relevant ArchetypeComponentId resources when dropped.
World caches the access sparse set (and only one cell can exist at a time), so `world.cell()` is a cheap operation.
WorldCell does _not_ use atomic operations. It is non-send, does a mutable borrow of world to prevent other accesses, and uses a simple `Rc<RefCell<ArchetypeComponentAccess>>` wrapper in each WorldBorrow pointer.
The api is currently limited to resource access, but it can and should be extended to queries / entity component access.
## Resource Scopes
WorldCell does not yet support component queries, and even when it does there are sometimes legitimate reasons to want a mutable world ref _and_ a mutable resource ref (ex: bevy_render and bevy_scene both need this). In these cases we could always drop down to the unsafe `world.get_resource_unchecked_mut()`, but that is not ideal!
Instead developers can use a "resource scope"
```rust
world.resource_scope(|world: &mut World, a: &mut A| {
})
```
This temporarily removes the `A` resource from `World`, provides mutable pointers to both, and re-adds A to World when finished. Thanks to the move to ComponentIds/sparse sets, this is a cheap operation.
If multiple resources are required, scopes can be nested. We could also consider adding a "resource tuple" to the api if this pattern becomes common and the boilerplate gets nasty.
## Query Conflicts Use ComponentId Instead of ArchetypeComponentId
For safety reasons, systems cannot contain queries that conflict with each other without wrapping them in a QuerySet. On bevy `main`, we use ArchetypeComponentIds to determine conflicts. This is nice because it can take into account filters:
```rust
// these queries will never conflict due to their filters
fn filter_system(a: Query<&mut A, With<B>>, b: Query<&mut B, Without<B>>) {
}
```
But it also has a significant downside:
```rust
// these queries will not conflict _until_ an entity with A, B, and C is spawned
fn maybe_conflicts_system(a: Query<(&mut A, &C)>, b: Query<(&mut A, &B)>) {
}
```
The system above will panic at runtime if an entity with A, B, and C is spawned. This makes it hard to trust that your game logic will run without crashing.
In this pr, I switched to using `ComponentId` instead. This _is_ more constraining. `maybe_conflicts_system` will now always fail, but it will do it consistently at startup. Naively, it would also _disallow_ `filter_system`, which would be a significant downgrade in usability. Bevy has a number of internal systems that rely on disjoint queries and I expect it to be a common pattern in userspace.
To resolve this, I added a new `FilteredAccess<T>` type, which wraps `Access<T>` and adds with/without filters. If two `FilteredAccess` have with/without values that prove they are disjoint, they will no longer conflict.
## EntityRef / EntityMut
World entity operations on `main` require that the user passes in an `entity` id to each operation:
```rust
let entity = world.spawn((A, )); // create a new entity with A
world.get::<A>(entity);
world.insert(entity, (B, C));
world.insert_one(entity, D);
```
This means that each operation needs to look up the entity location / verify its validity. The initial spawn operation also requires a Bundle as input. This can be awkward when no components are required (or one component is required).
These operations have been replaced by `EntityRef` and `EntityMut`, which are "builder-style" wrappers around world that provide read and read/write operations on a single, pre-validated entity:
```rust
// spawn now takes no inputs and returns an EntityMut
let entity = world.spawn()
.insert(A) // insert a single component into the entity
.insert_bundle((B, C)) // insert a bundle of components into the entity
.id() // id returns the Entity id
// Returns EntityMut (or panics if the entity does not exist)
world.entity_mut(entity)
.insert(D)
.insert_bundle(SomeBundle::default());
{
// returns EntityRef (or panics if the entity does not exist)
let d = world.entity(entity)
.get::<D>() // gets the D component
.unwrap();
// world.get still exists for ergonomics
let d = world.get::<D>(entity).unwrap();
}
// These variants return Options if you want to check existence instead of panicing
world.get_entity_mut(entity)
.unwrap()
.insert(E);
if let Some(entity_ref) = world.get_entity(entity) {
let d = entity_ref.get::<D>().unwrap();
}
```
This _does not_ affect the current Commands api or terminology. I think that should be a separate conversation as that is a much larger breaking change.
## Safety Improvements
* Entity reservation in Commands uses a normal world borrow instead of an unsafe transmute
* QuerySets no longer transmutes lifetimes
* Made traits "unsafe" when implementing a trait incorrectly could cause unsafety
* More thorough safety docs
## RemovedComponents SystemParam
The old approach to querying removed components: `query.removed:<T>()` was confusing because it had no connection to the query itself. I replaced it with the following, which is both clearer and allows us to cache the ComponentId mapping in the SystemParamState:
```rust
fn system(removed: RemovedComponents<T>) {
for entity in removed.iter() {
}
}
```
## Simpler Bundle implementation
Bundles are no longer responsible for sorting (or deduping) TypeInfo. They are just a simple ordered list of component types / data. This makes the implementation smaller and opens the door to an easy "nested bundle" implementation in the future (which i might even add in this pr). Duplicate detection is now done once per bundle type by World the first time a bundle is used.
## Unified WorldQuery and QueryFilter types
(don't worry they are still separate type _parameters_ in Queries .. this is a non-breaking change)
WorldQuery and QueryFilter were already basically identical apis. With the addition of `FetchState` and more storage-specific fetch methods, the overlap was even clearer (and the redundancy more painful).
QueryFilters are now just `F: WorldQuery where F::Fetch: FilterFetch`. FilterFetch requires `Fetch<Item = bool>` and adds new "short circuit" variants of fetch methods. This enables a filter tuple like `(With<A>, Without<B>, Changed<C>)` to stop evaluating the filter after the first mismatch is encountered. FilterFetch is automatically implemented for `Fetch` implementations that return bool.
This forces fetch implementations that return things like `(bool, bool, bool)` (such as the filter above) to manually implement FilterFetch and decide whether or not to short-circuit.
## More Granular Modules
World no longer globs all of the internal modules together. It now exports `core`, `system`, and `schedule` separately. I'm also considering exporting `core` submodules directly as that is still pretty "glob-ey" and unorganized (feedback welcome here).
## Remaining Draft Work (to be done in this pr)
* ~~panic on conflicting WorldQuery fetches (&A, &mut A)~~
* ~~bevy `main` and hecs both currently allow this, but we should protect against it if possible~~
* ~~batch_iter / par_iter (currently stubbed out)~~
* ~~ChangedRes~~
* ~~I skipped this while we sort out #1313. This pr should be adapted to account for whatever we land on there~~.
* ~~The `Archetypes` and `Tables` collections use hashes of sorted lists of component ids to uniquely identify each archetype/table. This hash is then used as the key in a HashMap to look up the relevant ArchetypeId or TableId. (which doesn't handle hash collisions properly)~~
* ~~It is currently unsafe to generate a Query from "World A", then use it on "World B" (despite the api claiming it is safe). We should probably close this gap. This could be done by adding a randomly generated WorldId to each world, then storing that id in each Query. They could then be compared to each other on each `query.do_thing(&world)` operation. This _does_ add an extra branch to each query operation, so I'm open to other suggestions if people have them.~~
* ~~Nested Bundles (if i find time)~~
## Potential Future Work
* Expand WorldCell to support queries.
* Consider not allocating in the empty archetype on `world.spawn()`
* ex: return something like EntityMutUninit, which turns into EntityMut after an `insert` or `insert_bundle` op
* this actually regressed performance last time i tried it, but in theory it should be faster
* Optimize SparseSet::insert (see `PERF` comment on insert)
* Replace SparseArray `Option<T>` with T::MAX to cut down on branching
* would enable cheaper get_unchecked() operations
* upstream fixedbitset optimizations
* fixedbitset could be allocation free for small block counts (store blocks in a SmallVec)
* fixedbitset could have a const constructor
* Consider implementing Tags (archetype-specific by-value data that affects archetype identity)
* ex: ArchetypeA could have `[A, B, C]` table components and `[D(1)]` "tag" component. ArchetypeB could have `[A, B, C]` table components and a `[D(2)]` tag component. The archetypes are different, despite both having D tags because the value inside D is different.
* this could potentially build on top of the `archetype.unique_components` added in this pr for resource storage.
* Consider reverting `all_tuples` proc macro in favor of the old `macro_rules` implementation
* all_tuples is more flexible and produces cleaner documentation (the macro_rules version produces weird type parameter orders due to parser constraints)
* but unfortunately all_tuples also appears to make Rust Analyzer sad/slow when working inside of `bevy_ecs` (does not affect user code)
* Consider "resource queries" and/or "mixed resource and entity component queries" as an alternative to WorldCell
* this is basically just "systems" so maybe it's not worth it
* Add more world ops
* `world.clear()`
* `world.reserve<T: Bundle>(count: usize)`
* Try using the old archetype allocation strategy (allocate new memory on resize and copy everything over). I expect this to improve batch insertion performance at the cost of unbatched performance. But thats just a guess. I'm not an allocation perf pro :)
* Adapt Commands apis for consistency with new World apis
## Benchmarks
key:
* `bevy_old`: bevy `main` branch
* `bevy`: this branch
* `_foreach`: uses an optimized for_each iterator
* ` _sparse`: uses sparse set storage (if unspecified assume table storage)
* `_system`: runs inside a system (if unspecified assume test happens via direct world ops)
### Simple Insert (from ecs_bench_suite)
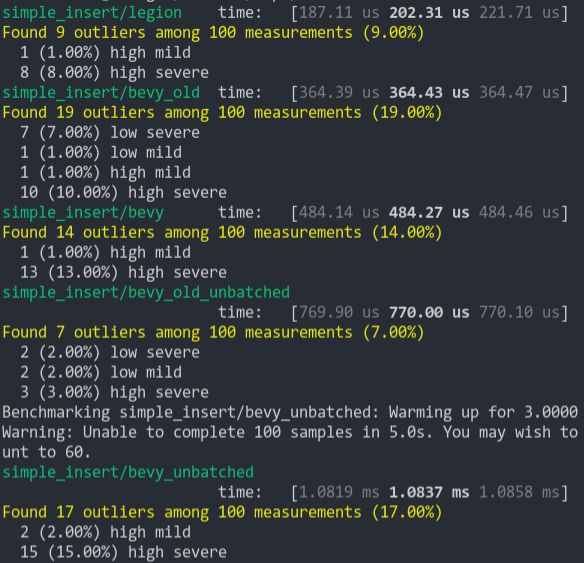
### Simpler Iter (from ecs_bench_suite)
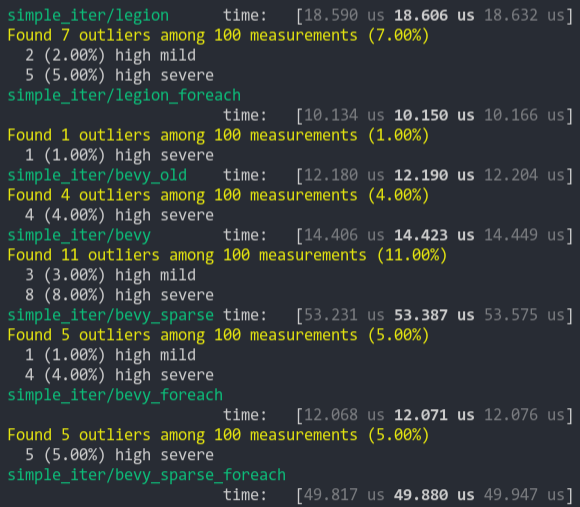
### Fragment Iter (from ecs_bench_suite)
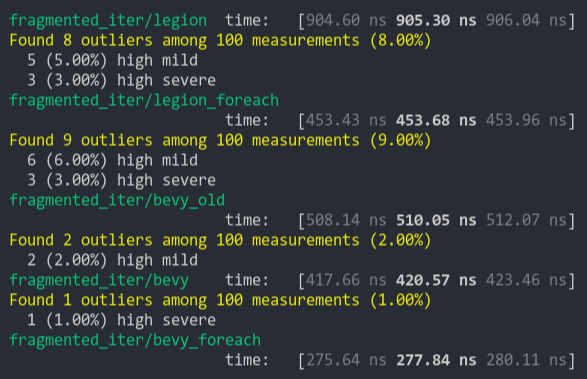
### Sparse Fragmented Iter
Iterate a query that matches 5 entities from a single matching archetype, but there are 100 unmatching archetypes
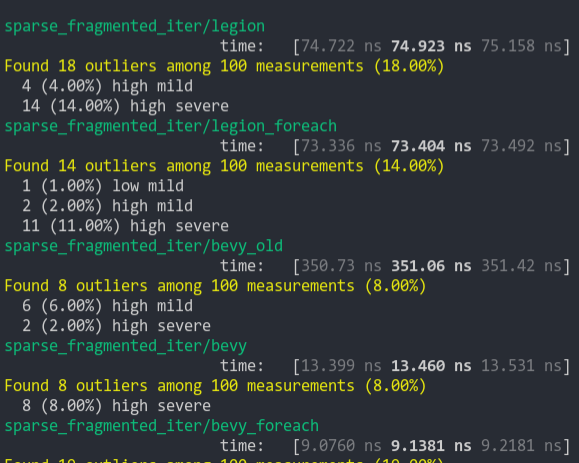
### Schedule (from ecs_bench_suite)
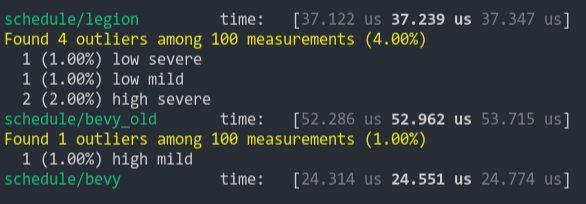
### Add Remove Component (from ecs_bench_suite)
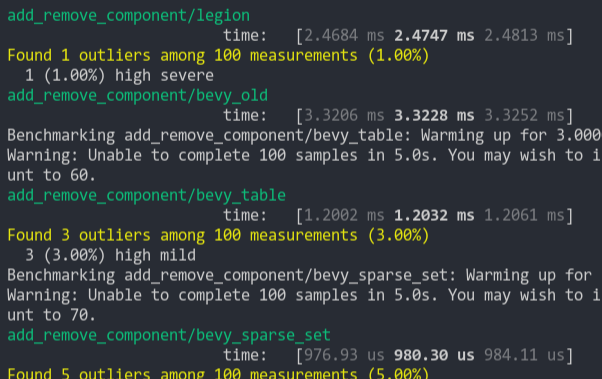
### Add Remove Component Big
Same as the test above, but each entity has 5 "large" matrix components and 1 "large" matrix component is added and removed
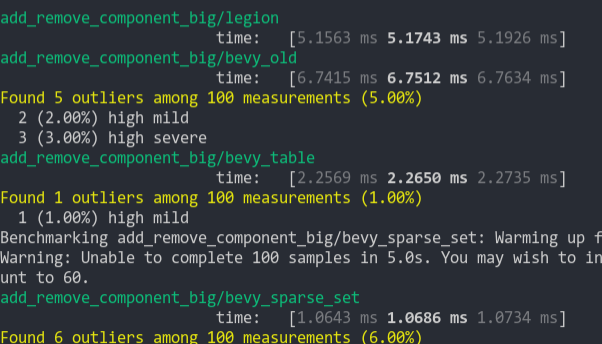
### Get Component
Looks up a single component value a large number of times
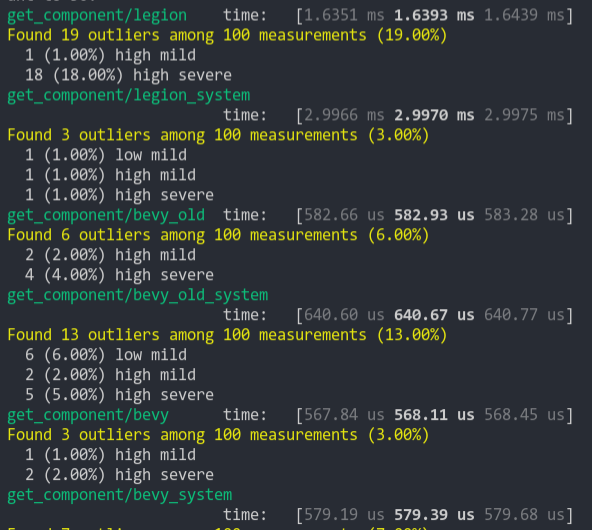
2021-03-05 07:54:35 +00:00
pub ( crate ) value : & ' w T ,
Reliable change detection (#1471)
# Problem Definition
The current change tracking (via flags for both components and resources) fails to detect changes made by systems that are scheduled to run earlier in the frame than they are.
This issue is discussed at length in [#68](https://github.com/bevyengine/bevy/issues/68) and [#54](https://github.com/bevyengine/bevy/issues/54).
This is very much a draft PR, and contributions are welcome and needed.
# Criteria
1. Each change is detected at least once, no matter the ordering.
2. Each change is detected at most once, no matter the ordering.
3. Changes should be detected the same frame that they are made.
4. Competitive ergonomics. Ideally does not require opting-in.
5. Low CPU overhead of computation.
6. Memory efficient. This must not increase over time, except where the number of entities / resources does.
7. Changes should not be lost for systems that don't run.
8. A frame needs to act as a pure function. Given the same set of entities / components it needs to produce the same end state without side-effects.
**Exact** change-tracking proposals satisfy criteria 1 and 2.
**Conservative** change-tracking proposals satisfy criteria 1 but not 2.
**Flaky** change tracking proposals satisfy criteria 2 but not 1.
# Code Base Navigation
There are three types of flags:
- `Added`: A piece of data was added to an entity / `Resources`.
- `Mutated`: A piece of data was able to be modified, because its `DerefMut` was accessed
- `Changed`: The bitwise OR of `Added` and `Changed`
The special behavior of `ChangedRes`, with respect to the scheduler is being removed in [#1313](https://github.com/bevyengine/bevy/pull/1313) and does not need to be reproduced.
`ChangedRes` and friends can be found in "bevy_ecs/core/resources/resource_query.rs".
The `Flags` trait for Components can be found in "bevy_ecs/core/query.rs".
`ComponentFlags` are stored in "bevy_ecs/core/archetypes.rs", defined on line 446.
# Proposals
**Proposal 5 was selected for implementation.**
## Proposal 0: No Change Detection
The baseline, where computations are performed on everything regardless of whether it changed.
**Type:** Conservative
**Pros:**
- already implemented
- will never miss events
- no overhead
**Cons:**
- tons of repeated work
- doesn't allow users to avoid repeating work (or monitoring for other changes)
## Proposal 1: Earlier-This-Tick Change Detection
The current approach as of Bevy 0.4. Flags are set, and then flushed at the end of each frame.
**Type:** Flaky
**Pros:**
- already implemented
- simple to understand
- low memory overhead (2 bits per component)
- low time overhead (clear every flag once per frame)
**Cons:**
- misses systems based on ordering
- systems that don't run every frame miss changes
- duplicates detection when looping
- can lead to unresolvable circular dependencies
## Proposal 2: Two-Tick Change Detection
Flags persist for two frames, using a double-buffer system identical to that used in events.
A change is observed if it is found in either the current frame's list of changes or the previous frame's.
**Type:** Conservative
**Pros:**
- easy to understand
- easy to implement
- low memory overhead (4 bits per component)
- low time overhead (bit mask and shift every flag once per frame)
**Cons:**
- can result in a great deal of duplicated work
- systems that don't run every frame miss changes
- duplicates detection when looping
## Proposal 3: Last-Tick Change Detection
Flags persist for two frames, using a double-buffer system identical to that used in events.
A change is observed if it is found in the previous frame's list of changes.
**Type:** Exact
**Pros:**
- exact
- easy to understand
- easy to implement
- low memory overhead (4 bits per component)
- low time overhead (bit mask and shift every flag once per frame)
**Cons:**
- change detection is always delayed, possibly causing painful chained delays
- systems that don't run every frame miss changes
- duplicates detection when looping
## Proposal 4: Flag-Doubling Change Detection
Combine Proposal 2 and Proposal 3. Differentiate between `JustChanged` (current behavior) and `Changed` (Proposal 3).
Pack this data into the flags according to [this implementation proposal](https://github.com/bevyengine/bevy/issues/68#issuecomment-769174804).
**Type:** Flaky + Exact
**Pros:**
- allows users to acc
- easy to implement
- low memory overhead (4 bits per component)
- low time overhead (bit mask and shift every flag once per frame)
**Cons:**
- users must specify the type of change detection required
- still quite fragile to system ordering effects when using the flaky `JustChanged` form
- cannot get immediate + exact results
- systems that don't run every frame miss changes
- duplicates detection when looping
## [SELECTED] Proposal 5: Generation-Counter Change Detection
A global counter is increased after each system is run. Each component saves the time of last mutation, and each system saves the time of last execution. Mutation is detected when the component's counter is greater than the system's counter. Discussed [here](https://github.com/bevyengine/bevy/issues/68#issuecomment-769174804). How to handle addition detection is unsolved; the current proposal is to use the highest bit of the counter as in proposal 1.
**Type:** Exact (for mutations), flaky (for additions)
**Pros:**
- low time overhead (set component counter on access, set system counter after execution)
- robust to systems that don't run every frame
- robust to systems that loop
**Cons:**
- moderately complex implementation
- must be modified as systems are inserted dynamically
- medium memory overhead (4 bytes per component + system)
- unsolved addition detection
## Proposal 6: System-Data Change Detection
For each system, track which system's changes it has seen. This approach is only worth fully designing and implementing if Proposal 5 fails in some way.
**Type:** Exact
**Pros:**
- exact
- conceptually simple
**Cons:**
- requires storing data on each system
- implementation is complex
- must be modified as systems are inserted dynamically
## Proposal 7: Total-Order Change Detection
Discussed [here](https://github.com/bevyengine/bevy/issues/68#issuecomment-754326523). This proposal is somewhat complicated by the new scheduler, but I believe it should still be conceptually feasible. This approach is only worth fully designing and implementing if Proposal 5 fails in some way.
**Type:** Exact
**Pros:**
- exact
- efficient data storage relative to other exact proposals
**Cons:**
- requires access to the scheduler
- complex implementation and difficulty grokking
- must be modified as systems are inserted dynamically
# Tests
- We will need to verify properties 1, 2, 3, 7 and 8. Priority: 1 > 2 = 3 > 8 > 7
- Ideally we can use identical user-facing syntax for all proposals, allowing us to re-use the same syntax for each.
- When writing tests, we need to carefully specify order using explicit dependencies.
- These tests will need to be duplicated for both components and resources.
- We need to be sure to handle cases where ambiguous system orders exist.
`changing_system` is always the system that makes the changes, and `detecting_system` always detects the changes.
The component / resource changed will be simple boolean wrapper structs.
## Basic Added / Mutated / Changed
2 x 3 design:
- Resources vs. Components
- Added vs. Changed vs. Mutated
- `changing_system` runs before `detecting_system`
- verify at the end of tick 2
## At Least Once
2 x 3 design:
- Resources vs. Components
- Added vs. Changed vs. Mutated
- `changing_system` runs after `detecting_system`
- verify at the end of tick 2
## At Most Once
2 x 3 design:
- Resources vs. Components
- Added vs. Changed vs. Mutated
- `changing_system` runs once before `detecting_system`
- increment a counter based on the number of changes detected
- verify at the end of tick 2
## Fast Detection
2 x 3 design:
- Resources vs. Components
- Added vs. Changed vs. Mutated
- `changing_system` runs before `detecting_system`
- verify at the end of tick 1
## Ambiguous System Ordering Robustness
2 x 3 x 2 design:
- Resources vs. Components
- Added vs. Changed vs. Mutated
- `changing_system` runs [before/after] `detecting_system` in tick 1
- `changing_system` runs [after/before] `detecting_system` in tick 2
## System Pausing
2 x 3 design:
- Resources vs. Components
- Added vs. Changed vs. Mutated
- `changing_system` runs in tick 1, then is disabled by run criteria
- `detecting_system` is disabled by run criteria until it is run once during tick 3
- verify at the end of tick 3
## Addition Causes Mutation
2 design:
- Resources vs. Components
- `adding_system_1` adds a component / resource
- `adding system_2` adds the same component / resource
- verify the `Mutated` flag at the end of the tick
- verify the `Added` flag at the end of the tick
First check tests for: https://github.com/bevyengine/bevy/issues/333
Second check tests for: https://github.com/bevyengine/bevy/issues/1443
## Changes Made By Commands
- `adding_system` runs in Update in tick 1, and sends a command to add a component
- `detecting_system` runs in Update in tick 1 and 2, after `adding_system`
- We can't detect the changes in tick 1, since they haven't been processed yet
- If we were to track these changes as being emitted by `adding_system`, we can't detect the changes in tick 2 either, since `detecting_system` has already run once after `adding_system` :(
# Benchmarks
See: [general advice](https://github.com/bevyengine/bevy/blob/master/docs/profiling.md), [Criterion crate](https://github.com/bheisler/criterion.rs)
There are several critical parameters to vary:
1. entity count (1 to 10^9)
2. fraction of entities that are changed (0% to 100%)
3. cost to perform work on changed entities, i.e. workload (1 ns to 1s)
1 and 2 should be varied between benchmark runs. 3 can be added on computationally.
We want to measure:
- memory cost
- run time
We should collect these measurements across several frames (100?) to reduce bootup effects and accurately measure the mean, variance and drift.
Entity-component change detection is much more important to benchmark than resource change detection, due to the orders of magnitude higher number of pieces of data.
No change detection at all should be included in benchmarks as a second control for cases where missing changes is unacceptable.
## Graphs
1. y: performance, x: log_10(entity count), color: proposal, facet: performance metric. Set cost to perform work to 0.
2. y: run time, x: cost to perform work, color: proposal, facet: fraction changed. Set number of entities to 10^6
3. y: memory, x: frames, color: proposal
# Conclusions
1. Is the theoretical categorization of the proposals correct according to our tests?
2. How does the performance of the proposals compare without any load?
3. How does the performance of the proposals compare with realistic loads?
4. At what workload does more exact change tracking become worth the (presumably) higher overhead?
5. When does adding change-detection to save on work become worthwhile?
6. Is there enough divergence in performance between the best solutions in each class to ship more than one change-tracking solution?
# Implementation Plan
1. Write a test suite.
2. Verify that tests fail for existing approach.
3. Write a benchmark suite.
4. Get performance numbers for existing approach.
5. Implement, test and benchmark various solutions using a Git branch per proposal.
6. Create a draft PR with all solutions and present results to team.
7. Select a solution and replace existing change detection.
Co-authored-by: Brice DAVIER <bricedavier@gmail.com>
Co-authored-by: Carter Anderson <mcanders1@gmail.com>
2021-03-19 17:53:26 +00:00
ticks : ComponentTicks ,
last_change_tick : u32 ,
change_tick : u32 ,
}
2022-07-04 14:44:24 +00:00
// SAFETY: Only reads a single World non-send resource
Simplify trait hierarchy for `SystemParam` (#6865)
# Objective
* Implementing a custom `SystemParam` by hand requires implementing three traits -- four if it is read-only.
* The trait `SystemParamFetch<'w, 's>` is a workaround from before we had generic associated types, and is no longer necessary.
## Solution
* Combine the trait `SystemParamFetch` with `SystemParamState`.
* I decided to remove the `Fetch` name and keep the `State` name, since the former was consistently conflated with the latter.
* Replace the trait `ReadOnlySystemParamFetch` with `ReadOnlySystemParam`, which simplifies trait bounds in generic code.
---
## Changelog
- Removed the trait `SystemParamFetch`, moving its functionality to `SystemParamState`.
- Replaced the trait `ReadOnlySystemParamFetch` with `ReadOnlySystemParam`.
## Migration Guide
The trait `SystemParamFetch` has been removed, and its functionality has been transferred to `SystemParamState`.
```rust
// Before
impl SystemParamState for MyParamState {
fn init(world: &mut World, system_meta: &mut SystemMeta) -> Self { ... }
}
impl<'w, 's> SystemParamFetch<'w, 's> for MyParamState {
type Item = MyParam<'w, 's>;
fn get_param(...) -> Self::Item;
}
// After
impl SystemParamState for MyParamState {
type Item<'w, 's> = MyParam<'w, 's>; // Generic associated types!
fn init(world: &mut World, system_meta: &mut SystemMeta) -> Self { ... }
fn get_param<'w, 's>(...) -> Self::Item<'w, 's>;
}
```
The trait `ReadOnlySystemParamFetch` has been replaced with `ReadOnlySystemParam`.
```rust
// Before
unsafe impl ReadOnlySystemParamFetch for MyParamState {}
// After
unsafe impl<'w, 's> ReadOnlySystemParam for MyParam<'w, 's> {}
```
2022-12-11 18:34:14 +00:00
unsafe impl < ' w , T > ReadOnlySystemParam for NonSend < ' w , T > { }
Add new SystemState and rename old SystemState to SystemMeta (#2283)
This enables `SystemParams` to be used outside of function systems. Anything can create and store `SystemState`, which enables efficient "param state cached" access to `SystemParams`.
It adds a `ReadOnlySystemParamFetch` trait, which enables safe `SystemState::get` calls without unique world access.
I renamed the old `SystemState` to `SystemMeta` to enable us to mirror the `QueryState` naming convention (but I'm happy to discuss alternative names if people have other ideas). I initially pitched this as `ParamState`, but given that it needs to include full system metadata, that doesn't feel like a particularly accurate name.
```rust
#[derive(Eq, PartialEq, Debug)]
struct A(usize);
#[derive(Eq, PartialEq, Debug)]
struct B(usize);
let mut world = World::default();
world.insert_resource(A(42));
world.spawn().insert(B(7));
// we get nice lifetime elision when declaring the type on the left hand side
let mut system_state: SystemState<(Res<A>, Query<&B>)> = SystemState::new(&mut world);
let (a, query) = system_state.get(&world);
assert_eq!(*a, A(42), "returned resource matches initial value");
assert_eq!(
*query.single().unwrap(),
B(7),
"returned component matches initial value"
);
// mutable system params require unique world access
let mut system_state: SystemState<(ResMut<A>, Query<&mut B>)> = SystemState::new(&mut world);
let (a, query) = system_state.get_mut(&mut world);
// static lifetimes are required when declaring inside of structs
struct SomeContainer {
state: SystemState<(Res<'static, A>, Res<'static, B>)>
}
// this can be shortened using type aliases, which will be useful for complex param tuples
type MyParams<'a> = (Res<'a, A>, Res<'a, B>);
struct SomeContainer {
state: SystemState<MyParams<'static>>
}
// It is the user's responsibility to call SystemState::apply(world) for parameters that queue up work
let mut system_state: SystemState<(Commands, Query<&B>)> = SystemState::new(&mut world);
{
let (mut commands, query) = system_state.get(&world);
commands.insert_resource(3.14);
}
system_state.apply(&mut world);
```
## Future Work
* Actually use SystemState inside FunctionSystem. This would be trivial, but it requires FunctionSystem to wrap SystemState in Option in its current form (which complicates system metadata lookup). I'd prefer to hold off until we adopt something like the later designs linked in #1364, which enable us to contruct Systems using a World reference (and also remove the need for `.system`).
* Consider a "scoped" approach to automatically call SystemState::apply when systems params are no longer being used (either a container type with a Drop impl, or a function that takes a closure for user logic operating on params).
2021-06-02 19:57:38 +00:00
2021-05-01 02:32:32 +00:00
impl < ' w , T > Debug for NonSend < ' w , T >
where
T : Debug ,
{
fn fmt ( & self , f : & mut std ::fmt ::Formatter < '_ > ) -> std ::fmt ::Result {
f . debug_tuple ( " NonSend " ) . field ( & self . value ) . finish ( )
}
}
2021-06-09 19:01:59 +00:00
impl < ' w , T : 'static > NonSend < ' w , T > {
Make change lifespan deterministic and update docs (#3956)
## Objective
- ~~Make absurdly long-lived changes stay detectable for even longer (without leveling up to `u64`).~~
- Give all changes a consistent maximum lifespan.
- Improve code clarity.
## Solution
- ~~Increase the frequency of `check_tick` scans to increase the oldest reliably-detectable change.~~
(Deferred until we can benchmark the cost of a scan.)
- Ignore changes older than the maximum reliably-detectable age.
- General refactoring—name the constants, use them everywhere, and update the docs.
- Update test cases to check for the specified behavior.
## Related
This PR addresses (at least partially) the concerns raised in:
- #3071
- #3082 (and associated PR #3084)
## Background
- #1471
Given the minimum interval between `check_ticks` scans, `N`, the oldest reliably-detectable change is `u32::MAX - (2 * N - 1)` (or `MAX_CHANGE_AGE`). Reducing `N` from ~530 million (current value) to something like ~2 million would extend the lifetime of changes by a billion.
| minimum `check_ticks` interval | oldest reliably-detectable change | usable % of `u32::MAX` |
| --- | --- | --- |
| `u32::MAX / 8` (536,870,911) | `(u32::MAX / 4) * 3` | 75.0% |
| `2_000_000` | `u32::MAX - 3_999_999` | 99.9% |
Similarly, changes are still allowed to be between `MAX_CHANGE_AGE`-old and `u32::MAX`-old in the interim between `check_tick` scans. While we prevent their age from overflowing, the test to detect changes still compares raw values. This makes failure ultimately unreliable, since when ancient changes stop being detected varies depending on when the next scan occurs.
## Open Question
Currently, systems and system states are incorrectly initialized with their `last_change_tick` set to `0`, which doesn't handle wraparound correctly.
For consistent behavior, they should either be initialized to the world's `last_change_tick` (and detect no changes) or to `MAX_CHANGE_AGE` behind the world's current `change_tick` (and detect everything as a change). I've currently gone with the latter since that was closer to the existing behavior.
## Follow-up Work
(Edited: entire section)
We haven't actually profiled how long a `check_ticks` scan takes on a "large" `World` , so we don't know if it's safe to increase their frequency. However, we are currently relying on play sessions not lasting long enough to trigger a scan and apps not having enough entities/archetypes for it to be "expensive" (our assumption). That isn't a real solution. (Either scanning never costs enough to impact frame times or we provide an option to use `u64` change ticks. Nobody will accept random hiccups.)
To further extend the lifetime of changes, we actually only need to increment the world tick if a system has `Fetch: !ReadOnlySystemParamFetch`. The behavior will be identical because all writes are sequenced, but I'm not sure how to implement that in a way that the compiler can optimize the branch out.
Also, since having no false positives depends on a `check_ticks` scan running at least every `2 * N - 1` ticks, a `last_check_tick` should also be stored in the `World` so that any lull in system execution (like a command flush) could trigger a scan if needed. To be completely robust, all the systems initialized on the world should be scanned, not just those in the current stage.
2022-05-09 14:00:16 +00:00
/// Returns `true` if the resource was added after the system last ran.
Reliable change detection (#1471)
# Problem Definition
The current change tracking (via flags for both components and resources) fails to detect changes made by systems that are scheduled to run earlier in the frame than they are.
This issue is discussed at length in [#68](https://github.com/bevyengine/bevy/issues/68) and [#54](https://github.com/bevyengine/bevy/issues/54).
This is very much a draft PR, and contributions are welcome and needed.
# Criteria
1. Each change is detected at least once, no matter the ordering.
2. Each change is detected at most once, no matter the ordering.
3. Changes should be detected the same frame that they are made.
4. Competitive ergonomics. Ideally does not require opting-in.
5. Low CPU overhead of computation.
6. Memory efficient. This must not increase over time, except where the number of entities / resources does.
7. Changes should not be lost for systems that don't run.
8. A frame needs to act as a pure function. Given the same set of entities / components it needs to produce the same end state without side-effects.
**Exact** change-tracking proposals satisfy criteria 1 and 2.
**Conservative** change-tracking proposals satisfy criteria 1 but not 2.
**Flaky** change tracking proposals satisfy criteria 2 but not 1.
# Code Base Navigation
There are three types of flags:
- `Added`: A piece of data was added to an entity / `Resources`.
- `Mutated`: A piece of data was able to be modified, because its `DerefMut` was accessed
- `Changed`: The bitwise OR of `Added` and `Changed`
The special behavior of `ChangedRes`, with respect to the scheduler is being removed in [#1313](https://github.com/bevyengine/bevy/pull/1313) and does not need to be reproduced.
`ChangedRes` and friends can be found in "bevy_ecs/core/resources/resource_query.rs".
The `Flags` trait for Components can be found in "bevy_ecs/core/query.rs".
`ComponentFlags` are stored in "bevy_ecs/core/archetypes.rs", defined on line 446.
# Proposals
**Proposal 5 was selected for implementation.**
## Proposal 0: No Change Detection
The baseline, where computations are performed on everything regardless of whether it changed.
**Type:** Conservative
**Pros:**
- already implemented
- will never miss events
- no overhead
**Cons:**
- tons of repeated work
- doesn't allow users to avoid repeating work (or monitoring for other changes)
## Proposal 1: Earlier-This-Tick Change Detection
The current approach as of Bevy 0.4. Flags are set, and then flushed at the end of each frame.
**Type:** Flaky
**Pros:**
- already implemented
- simple to understand
- low memory overhead (2 bits per component)
- low time overhead (clear every flag once per frame)
**Cons:**
- misses systems based on ordering
- systems that don't run every frame miss changes
- duplicates detection when looping
- can lead to unresolvable circular dependencies
## Proposal 2: Two-Tick Change Detection
Flags persist for two frames, using a double-buffer system identical to that used in events.
A change is observed if it is found in either the current frame's list of changes or the previous frame's.
**Type:** Conservative
**Pros:**
- easy to understand
- easy to implement
- low memory overhead (4 bits per component)
- low time overhead (bit mask and shift every flag once per frame)
**Cons:**
- can result in a great deal of duplicated work
- systems that don't run every frame miss changes
- duplicates detection when looping
## Proposal 3: Last-Tick Change Detection
Flags persist for two frames, using a double-buffer system identical to that used in events.
A change is observed if it is found in the previous frame's list of changes.
**Type:** Exact
**Pros:**
- exact
- easy to understand
- easy to implement
- low memory overhead (4 bits per component)
- low time overhead (bit mask and shift every flag once per frame)
**Cons:**
- change detection is always delayed, possibly causing painful chained delays
- systems that don't run every frame miss changes
- duplicates detection when looping
## Proposal 4: Flag-Doubling Change Detection
Combine Proposal 2 and Proposal 3. Differentiate between `JustChanged` (current behavior) and `Changed` (Proposal 3).
Pack this data into the flags according to [this implementation proposal](https://github.com/bevyengine/bevy/issues/68#issuecomment-769174804).
**Type:** Flaky + Exact
**Pros:**
- allows users to acc
- easy to implement
- low memory overhead (4 bits per component)
- low time overhead (bit mask and shift every flag once per frame)
**Cons:**
- users must specify the type of change detection required
- still quite fragile to system ordering effects when using the flaky `JustChanged` form
- cannot get immediate + exact results
- systems that don't run every frame miss changes
- duplicates detection when looping
## [SELECTED] Proposal 5: Generation-Counter Change Detection
A global counter is increased after each system is run. Each component saves the time of last mutation, and each system saves the time of last execution. Mutation is detected when the component's counter is greater than the system's counter. Discussed [here](https://github.com/bevyengine/bevy/issues/68#issuecomment-769174804). How to handle addition detection is unsolved; the current proposal is to use the highest bit of the counter as in proposal 1.
**Type:** Exact (for mutations), flaky (for additions)
**Pros:**
- low time overhead (set component counter on access, set system counter after execution)
- robust to systems that don't run every frame
- robust to systems that loop
**Cons:**
- moderately complex implementation
- must be modified as systems are inserted dynamically
- medium memory overhead (4 bytes per component + system)
- unsolved addition detection
## Proposal 6: System-Data Change Detection
For each system, track which system's changes it has seen. This approach is only worth fully designing and implementing if Proposal 5 fails in some way.
**Type:** Exact
**Pros:**
- exact
- conceptually simple
**Cons:**
- requires storing data on each system
- implementation is complex
- must be modified as systems are inserted dynamically
## Proposal 7: Total-Order Change Detection
Discussed [here](https://github.com/bevyengine/bevy/issues/68#issuecomment-754326523). This proposal is somewhat complicated by the new scheduler, but I believe it should still be conceptually feasible. This approach is only worth fully designing and implementing if Proposal 5 fails in some way.
**Type:** Exact
**Pros:**
- exact
- efficient data storage relative to other exact proposals
**Cons:**
- requires access to the scheduler
- complex implementation and difficulty grokking
- must be modified as systems are inserted dynamically
# Tests
- We will need to verify properties 1, 2, 3, 7 and 8. Priority: 1 > 2 = 3 > 8 > 7
- Ideally we can use identical user-facing syntax for all proposals, allowing us to re-use the same syntax for each.
- When writing tests, we need to carefully specify order using explicit dependencies.
- These tests will need to be duplicated for both components and resources.
- We need to be sure to handle cases where ambiguous system orders exist.
`changing_system` is always the system that makes the changes, and `detecting_system` always detects the changes.
The component / resource changed will be simple boolean wrapper structs.
## Basic Added / Mutated / Changed
2 x 3 design:
- Resources vs. Components
- Added vs. Changed vs. Mutated
- `changing_system` runs before `detecting_system`
- verify at the end of tick 2
## At Least Once
2 x 3 design:
- Resources vs. Components
- Added vs. Changed vs. Mutated
- `changing_system` runs after `detecting_system`
- verify at the end of tick 2
## At Most Once
2 x 3 design:
- Resources vs. Components
- Added vs. Changed vs. Mutated
- `changing_system` runs once before `detecting_system`
- increment a counter based on the number of changes detected
- verify at the end of tick 2
## Fast Detection
2 x 3 design:
- Resources vs. Components
- Added vs. Changed vs. Mutated
- `changing_system` runs before `detecting_system`
- verify at the end of tick 1
## Ambiguous System Ordering Robustness
2 x 3 x 2 design:
- Resources vs. Components
- Added vs. Changed vs. Mutated
- `changing_system` runs [before/after] `detecting_system` in tick 1
- `changing_system` runs [after/before] `detecting_system` in tick 2
## System Pausing
2 x 3 design:
- Resources vs. Components
- Added vs. Changed vs. Mutated
- `changing_system` runs in tick 1, then is disabled by run criteria
- `detecting_system` is disabled by run criteria until it is run once during tick 3
- verify at the end of tick 3
## Addition Causes Mutation
2 design:
- Resources vs. Components
- `adding_system_1` adds a component / resource
- `adding system_2` adds the same component / resource
- verify the `Mutated` flag at the end of the tick
- verify the `Added` flag at the end of the tick
First check tests for: https://github.com/bevyengine/bevy/issues/333
Second check tests for: https://github.com/bevyengine/bevy/issues/1443
## Changes Made By Commands
- `adding_system` runs in Update in tick 1, and sends a command to add a component
- `detecting_system` runs in Update in tick 1 and 2, after `adding_system`
- We can't detect the changes in tick 1, since they haven't been processed yet
- If we were to track these changes as being emitted by `adding_system`, we can't detect the changes in tick 2 either, since `detecting_system` has already run once after `adding_system` :(
# Benchmarks
See: [general advice](https://github.com/bevyengine/bevy/blob/master/docs/profiling.md), [Criterion crate](https://github.com/bheisler/criterion.rs)
There are several critical parameters to vary:
1. entity count (1 to 10^9)
2. fraction of entities that are changed (0% to 100%)
3. cost to perform work on changed entities, i.e. workload (1 ns to 1s)
1 and 2 should be varied between benchmark runs. 3 can be added on computationally.
We want to measure:
- memory cost
- run time
We should collect these measurements across several frames (100?) to reduce bootup effects and accurately measure the mean, variance and drift.
Entity-component change detection is much more important to benchmark than resource change detection, due to the orders of magnitude higher number of pieces of data.
No change detection at all should be included in benchmarks as a second control for cases where missing changes is unacceptable.
## Graphs
1. y: performance, x: log_10(entity count), color: proposal, facet: performance metric. Set cost to perform work to 0.
2. y: run time, x: cost to perform work, color: proposal, facet: fraction changed. Set number of entities to 10^6
3. y: memory, x: frames, color: proposal
# Conclusions
1. Is the theoretical categorization of the proposals correct according to our tests?
2. How does the performance of the proposals compare without any load?
3. How does the performance of the proposals compare with realistic loads?
4. At what workload does more exact change tracking become worth the (presumably) higher overhead?
5. When does adding change-detection to save on work become worthwhile?
6. Is there enough divergence in performance between the best solutions in each class to ship more than one change-tracking solution?
# Implementation Plan
1. Write a test suite.
2. Verify that tests fail for existing approach.
3. Write a benchmark suite.
4. Get performance numbers for existing approach.
5. Implement, test and benchmark various solutions using a Git branch per proposal.
6. Create a draft PR with all solutions and present results to team.
7. Select a solution and replace existing change detection.
Co-authored-by: Brice DAVIER <bricedavier@gmail.com>
Co-authored-by: Carter Anderson <mcanders1@gmail.com>
2021-03-19 17:53:26 +00:00
pub fn is_added ( & self ) -> bool {
self . ticks . is_added ( self . last_change_tick , self . change_tick )
}
Make change lifespan deterministic and update docs (#3956)
## Objective
- ~~Make absurdly long-lived changes stay detectable for even longer (without leveling up to `u64`).~~
- Give all changes a consistent maximum lifespan.
- Improve code clarity.
## Solution
- ~~Increase the frequency of `check_tick` scans to increase the oldest reliably-detectable change.~~
(Deferred until we can benchmark the cost of a scan.)
- Ignore changes older than the maximum reliably-detectable age.
- General refactoring—name the constants, use them everywhere, and update the docs.
- Update test cases to check for the specified behavior.
## Related
This PR addresses (at least partially) the concerns raised in:
- #3071
- #3082 (and associated PR #3084)
## Background
- #1471
Given the minimum interval between `check_ticks` scans, `N`, the oldest reliably-detectable change is `u32::MAX - (2 * N - 1)` (or `MAX_CHANGE_AGE`). Reducing `N` from ~530 million (current value) to something like ~2 million would extend the lifetime of changes by a billion.
| minimum `check_ticks` interval | oldest reliably-detectable change | usable % of `u32::MAX` |
| --- | --- | --- |
| `u32::MAX / 8` (536,870,911) | `(u32::MAX / 4) * 3` | 75.0% |
| `2_000_000` | `u32::MAX - 3_999_999` | 99.9% |
Similarly, changes are still allowed to be between `MAX_CHANGE_AGE`-old and `u32::MAX`-old in the interim between `check_tick` scans. While we prevent their age from overflowing, the test to detect changes still compares raw values. This makes failure ultimately unreliable, since when ancient changes stop being detected varies depending on when the next scan occurs.
## Open Question
Currently, systems and system states are incorrectly initialized with their `last_change_tick` set to `0`, which doesn't handle wraparound correctly.
For consistent behavior, they should either be initialized to the world's `last_change_tick` (and detect no changes) or to `MAX_CHANGE_AGE` behind the world's current `change_tick` (and detect everything as a change). I've currently gone with the latter since that was closer to the existing behavior.
## Follow-up Work
(Edited: entire section)
We haven't actually profiled how long a `check_ticks` scan takes on a "large" `World` , so we don't know if it's safe to increase their frequency. However, we are currently relying on play sessions not lasting long enough to trigger a scan and apps not having enough entities/archetypes for it to be "expensive" (our assumption). That isn't a real solution. (Either scanning never costs enough to impact frame times or we provide an option to use `u64` change ticks. Nobody will accept random hiccups.)
To further extend the lifetime of changes, we actually only need to increment the world tick if a system has `Fetch: !ReadOnlySystemParamFetch`. The behavior will be identical because all writes are sequenced, but I'm not sure how to implement that in a way that the compiler can optimize the branch out.
Also, since having no false positives depends on a `check_ticks` scan running at least every `2 * N - 1` ticks, a `last_check_tick` should also be stored in the `World` so that any lull in system execution (like a command flush) could trigger a scan if needed. To be completely robust, all the systems initialized on the world should be scanned, not just those in the current stage.
2022-05-09 14:00:16 +00:00
/// Returns `true` if the resource was added or mutably dereferenced after the system last ran.
Reliable change detection (#1471)
# Problem Definition
The current change tracking (via flags for both components and resources) fails to detect changes made by systems that are scheduled to run earlier in the frame than they are.
This issue is discussed at length in [#68](https://github.com/bevyengine/bevy/issues/68) and [#54](https://github.com/bevyengine/bevy/issues/54).
This is very much a draft PR, and contributions are welcome and needed.
# Criteria
1. Each change is detected at least once, no matter the ordering.
2. Each change is detected at most once, no matter the ordering.
3. Changes should be detected the same frame that they are made.
4. Competitive ergonomics. Ideally does not require opting-in.
5. Low CPU overhead of computation.
6. Memory efficient. This must not increase over time, except where the number of entities / resources does.
7. Changes should not be lost for systems that don't run.
8. A frame needs to act as a pure function. Given the same set of entities / components it needs to produce the same end state without side-effects.
**Exact** change-tracking proposals satisfy criteria 1 and 2.
**Conservative** change-tracking proposals satisfy criteria 1 but not 2.
**Flaky** change tracking proposals satisfy criteria 2 but not 1.
# Code Base Navigation
There are three types of flags:
- `Added`: A piece of data was added to an entity / `Resources`.
- `Mutated`: A piece of data was able to be modified, because its `DerefMut` was accessed
- `Changed`: The bitwise OR of `Added` and `Changed`
The special behavior of `ChangedRes`, with respect to the scheduler is being removed in [#1313](https://github.com/bevyengine/bevy/pull/1313) and does not need to be reproduced.
`ChangedRes` and friends can be found in "bevy_ecs/core/resources/resource_query.rs".
The `Flags` trait for Components can be found in "bevy_ecs/core/query.rs".
`ComponentFlags` are stored in "bevy_ecs/core/archetypes.rs", defined on line 446.
# Proposals
**Proposal 5 was selected for implementation.**
## Proposal 0: No Change Detection
The baseline, where computations are performed on everything regardless of whether it changed.
**Type:** Conservative
**Pros:**
- already implemented
- will never miss events
- no overhead
**Cons:**
- tons of repeated work
- doesn't allow users to avoid repeating work (or monitoring for other changes)
## Proposal 1: Earlier-This-Tick Change Detection
The current approach as of Bevy 0.4. Flags are set, and then flushed at the end of each frame.
**Type:** Flaky
**Pros:**
- already implemented
- simple to understand
- low memory overhead (2 bits per component)
- low time overhead (clear every flag once per frame)
**Cons:**
- misses systems based on ordering
- systems that don't run every frame miss changes
- duplicates detection when looping
- can lead to unresolvable circular dependencies
## Proposal 2: Two-Tick Change Detection
Flags persist for two frames, using a double-buffer system identical to that used in events.
A change is observed if it is found in either the current frame's list of changes or the previous frame's.
**Type:** Conservative
**Pros:**
- easy to understand
- easy to implement
- low memory overhead (4 bits per component)
- low time overhead (bit mask and shift every flag once per frame)
**Cons:**
- can result in a great deal of duplicated work
- systems that don't run every frame miss changes
- duplicates detection when looping
## Proposal 3: Last-Tick Change Detection
Flags persist for two frames, using a double-buffer system identical to that used in events.
A change is observed if it is found in the previous frame's list of changes.
**Type:** Exact
**Pros:**
- exact
- easy to understand
- easy to implement
- low memory overhead (4 bits per component)
- low time overhead (bit mask and shift every flag once per frame)
**Cons:**
- change detection is always delayed, possibly causing painful chained delays
- systems that don't run every frame miss changes
- duplicates detection when looping
## Proposal 4: Flag-Doubling Change Detection
Combine Proposal 2 and Proposal 3. Differentiate between `JustChanged` (current behavior) and `Changed` (Proposal 3).
Pack this data into the flags according to [this implementation proposal](https://github.com/bevyengine/bevy/issues/68#issuecomment-769174804).
**Type:** Flaky + Exact
**Pros:**
- allows users to acc
- easy to implement
- low memory overhead (4 bits per component)
- low time overhead (bit mask and shift every flag once per frame)
**Cons:**
- users must specify the type of change detection required
- still quite fragile to system ordering effects when using the flaky `JustChanged` form
- cannot get immediate + exact results
- systems that don't run every frame miss changes
- duplicates detection when looping
## [SELECTED] Proposal 5: Generation-Counter Change Detection
A global counter is increased after each system is run. Each component saves the time of last mutation, and each system saves the time of last execution. Mutation is detected when the component's counter is greater than the system's counter. Discussed [here](https://github.com/bevyengine/bevy/issues/68#issuecomment-769174804). How to handle addition detection is unsolved; the current proposal is to use the highest bit of the counter as in proposal 1.
**Type:** Exact (for mutations), flaky (for additions)
**Pros:**
- low time overhead (set component counter on access, set system counter after execution)
- robust to systems that don't run every frame
- robust to systems that loop
**Cons:**
- moderately complex implementation
- must be modified as systems are inserted dynamically
- medium memory overhead (4 bytes per component + system)
- unsolved addition detection
## Proposal 6: System-Data Change Detection
For each system, track which system's changes it has seen. This approach is only worth fully designing and implementing if Proposal 5 fails in some way.
**Type:** Exact
**Pros:**
- exact
- conceptually simple
**Cons:**
- requires storing data on each system
- implementation is complex
- must be modified as systems are inserted dynamically
## Proposal 7: Total-Order Change Detection
Discussed [here](https://github.com/bevyengine/bevy/issues/68#issuecomment-754326523). This proposal is somewhat complicated by the new scheduler, but I believe it should still be conceptually feasible. This approach is only worth fully designing and implementing if Proposal 5 fails in some way.
**Type:** Exact
**Pros:**
- exact
- efficient data storage relative to other exact proposals
**Cons:**
- requires access to the scheduler
- complex implementation and difficulty grokking
- must be modified as systems are inserted dynamically
# Tests
- We will need to verify properties 1, 2, 3, 7 and 8. Priority: 1 > 2 = 3 > 8 > 7
- Ideally we can use identical user-facing syntax for all proposals, allowing us to re-use the same syntax for each.
- When writing tests, we need to carefully specify order using explicit dependencies.
- These tests will need to be duplicated for both components and resources.
- We need to be sure to handle cases where ambiguous system orders exist.
`changing_system` is always the system that makes the changes, and `detecting_system` always detects the changes.
The component / resource changed will be simple boolean wrapper structs.
## Basic Added / Mutated / Changed
2 x 3 design:
- Resources vs. Components
- Added vs. Changed vs. Mutated
- `changing_system` runs before `detecting_system`
- verify at the end of tick 2
## At Least Once
2 x 3 design:
- Resources vs. Components
- Added vs. Changed vs. Mutated
- `changing_system` runs after `detecting_system`
- verify at the end of tick 2
## At Most Once
2 x 3 design:
- Resources vs. Components
- Added vs. Changed vs. Mutated
- `changing_system` runs once before `detecting_system`
- increment a counter based on the number of changes detected
- verify at the end of tick 2
## Fast Detection
2 x 3 design:
- Resources vs. Components
- Added vs. Changed vs. Mutated
- `changing_system` runs before `detecting_system`
- verify at the end of tick 1
## Ambiguous System Ordering Robustness
2 x 3 x 2 design:
- Resources vs. Components
- Added vs. Changed vs. Mutated
- `changing_system` runs [before/after] `detecting_system` in tick 1
- `changing_system` runs [after/before] `detecting_system` in tick 2
## System Pausing
2 x 3 design:
- Resources vs. Components
- Added vs. Changed vs. Mutated
- `changing_system` runs in tick 1, then is disabled by run criteria
- `detecting_system` is disabled by run criteria until it is run once during tick 3
- verify at the end of tick 3
## Addition Causes Mutation
2 design:
- Resources vs. Components
- `adding_system_1` adds a component / resource
- `adding system_2` adds the same component / resource
- verify the `Mutated` flag at the end of the tick
- verify the `Added` flag at the end of the tick
First check tests for: https://github.com/bevyengine/bevy/issues/333
Second check tests for: https://github.com/bevyengine/bevy/issues/1443
## Changes Made By Commands
- `adding_system` runs in Update in tick 1, and sends a command to add a component
- `detecting_system` runs in Update in tick 1 and 2, after `adding_system`
- We can't detect the changes in tick 1, since they haven't been processed yet
- If we were to track these changes as being emitted by `adding_system`, we can't detect the changes in tick 2 either, since `detecting_system` has already run once after `adding_system` :(
# Benchmarks
See: [general advice](https://github.com/bevyengine/bevy/blob/master/docs/profiling.md), [Criterion crate](https://github.com/bheisler/criterion.rs)
There are several critical parameters to vary:
1. entity count (1 to 10^9)
2. fraction of entities that are changed (0% to 100%)
3. cost to perform work on changed entities, i.e. workload (1 ns to 1s)
1 and 2 should be varied between benchmark runs. 3 can be added on computationally.
We want to measure:
- memory cost
- run time
We should collect these measurements across several frames (100?) to reduce bootup effects and accurately measure the mean, variance and drift.
Entity-component change detection is much more important to benchmark than resource change detection, due to the orders of magnitude higher number of pieces of data.
No change detection at all should be included in benchmarks as a second control for cases where missing changes is unacceptable.
## Graphs
1. y: performance, x: log_10(entity count), color: proposal, facet: performance metric. Set cost to perform work to 0.
2. y: run time, x: cost to perform work, color: proposal, facet: fraction changed. Set number of entities to 10^6
3. y: memory, x: frames, color: proposal
# Conclusions
1. Is the theoretical categorization of the proposals correct according to our tests?
2. How does the performance of the proposals compare without any load?
3. How does the performance of the proposals compare with realistic loads?
4. At what workload does more exact change tracking become worth the (presumably) higher overhead?
5. When does adding change-detection to save on work become worthwhile?
6. Is there enough divergence in performance between the best solutions in each class to ship more than one change-tracking solution?
# Implementation Plan
1. Write a test suite.
2. Verify that tests fail for existing approach.
3. Write a benchmark suite.
4. Get performance numbers for existing approach.
5. Implement, test and benchmark various solutions using a Git branch per proposal.
6. Create a draft PR with all solutions and present results to team.
7. Select a solution and replace existing change detection.
Co-authored-by: Brice DAVIER <bricedavier@gmail.com>
Co-authored-by: Carter Anderson <mcanders1@gmail.com>
2021-03-19 17:53:26 +00:00
pub fn is_changed ( & self ) -> bool {
self . ticks
. is_changed ( self . last_change_tick , self . change_tick )
}
Bevy ECS V2 (#1525)
# Bevy ECS V2
This is a rewrite of Bevy ECS (basically everything but the new executor/schedule, which are already awesome). The overall goal was to improve the performance and versatility of Bevy ECS. Here is a quick bulleted list of changes before we dive into the details:
* Complete World rewrite
* Multiple component storage types:
* Tables: fast cache friendly iteration, slower add/removes (previously called Archetypes)
* Sparse Sets: fast add/remove, slower iteration
* Stateful Queries (caches query results for faster iteration. fragmented iteration is _fast_ now)
* Stateful System Params (caches expensive operations. inspired by @DJMcNab's work in #1364)
* Configurable System Params (users can set configuration when they construct their systems. once again inspired by @DJMcNab's work)
* Archetypes are now "just metadata", component storage is separate
* Archetype Graph (for faster archetype changes)
* Component Metadata
* Configure component storage type
* Retrieve information about component size/type/name/layout/send-ness/etc
* Components are uniquely identified by a densely packed ComponentId
* TypeIds are now totally optional (which should make implementing scripting easier)
* Super fast "for_each" query iterators
* Merged Resources into World. Resources are now just a special type of component
* EntityRef/EntityMut builder apis (more efficient and more ergonomic)
* Fast bitset-backed `Access<T>` replaces old hashmap-based approach everywhere
* Query conflicts are determined by component access instead of archetype component access (to avoid random failures at runtime)
* With/Without are still taken into account for conflicts, so this should still be comfy to use
* Much simpler `IntoSystem` impl
* Significantly reduced the amount of hashing throughout the ecs in favor of Sparse Sets (indexed by densely packed ArchetypeId, ComponentId, BundleId, and TableId)
* Safety Improvements
* Entity reservation uses a normal world reference instead of unsafe transmute
* QuerySets no longer transmute lifetimes
* Made traits "unsafe" where relevant
* More thorough safety docs
* WorldCell
* Exposes safe mutable access to multiple resources at a time in a World
* Replaced "catch all" `System::update_archetypes(world: &World)` with `System::new_archetype(archetype: &Archetype)`
* Simpler Bundle implementation
* Replaced slow "remove_bundle_one_by_one" used as fallback for Commands::remove_bundle with fast "remove_bundle_intersection"
* Removed `Mut<T>` query impl. it is better to only support one way: `&mut T`
* Removed with() from `Flags<T>` in favor of `Option<Flags<T>>`, which allows querying for flags to be "filtered" by default
* Components now have is_send property (currently only resources support non-send)
* More granular module organization
* New `RemovedComponents<T>` SystemParam that replaces `query.removed::<T>()`
* `world.resource_scope()` for mutable access to resources and world at the same time
* WorldQuery and QueryFilter traits unified. FilterFetch trait added to enable "short circuit" filtering. Auto impled for cases that don't need it
* Significantly slimmed down SystemState in favor of individual SystemParam state
* System Commands changed from `commands: &mut Commands` back to `mut commands: Commands` (to allow Commands to have a World reference)
Fixes #1320
## `World` Rewrite
This is a from-scratch rewrite of `World` that fills the niche that `hecs` used to. Yes, this means Bevy ECS is no longer a "fork" of hecs. We're going out our own!
(the only shared code between the projects is the entity id allocator, which is already basically ideal)
A huge shout out to @SanderMertens (author of [flecs](https://github.com/SanderMertens/flecs)) for sharing some great ideas with me (specifically hybrid ecs storage and archetype graphs). He also helped advise on a number of implementation details.
## Component Storage (The Problem)
Two ECS storage paradigms have gained a lot of traction over the years:
* **Archetypal ECS**:
* Stores components in "tables" with static schemas. Each "column" stores components of a given type. Each "row" is an entity.
* Each "archetype" has its own table. Adding/removing an entity's component changes the archetype.
* Enables super-fast Query iteration due to its cache-friendly data layout
* Comes at the cost of more expensive add/remove operations for an Entity's components, because all components need to be copied to the new archetype's "table"
* **Sparse Set ECS**:
* Stores components of the same type in densely packed arrays, which are sparsely indexed by densely packed unsigned integers (Entity ids)
* Query iteration is slower than Archetypal ECS because each entity's component could be at any position in the sparse set. This "random access" pattern isn't cache friendly. Additionally, there is an extra layer of indirection because you must first map the entity id to an index in the component array.
* Adding/removing components is a cheap, constant time operation
Bevy ECS V1, hecs, legion, flec, and Unity DOTS are all "archetypal ecs-es". I personally think "archetypal" storage is a good default for game engines. An entity's archetype doesn't need to change frequently in general, and it creates "fast by default" query iteration (which is a much more common operation). It is also "self optimizing". Users don't need to think about optimizing component layouts for iteration performance. It "just works" without any extra boilerplate.
Shipyard and EnTT are "sparse set ecs-es". They employ "packing" as a way to work around the "suboptimal by default" iteration performance for specific sets of components. This helps, but I didn't think this was a good choice for a general purpose engine like Bevy because:
1. "packs" conflict with each other. If bevy decides to internally pack the Transform and GlobalTransform components, users are then blocked if they want to pack some custom component with Transform.
2. users need to take manual action to optimize
Developers selecting an ECS framework are stuck with a hard choice. Select an "archetypal" framework with "fast iteration everywhere" but without the ability to cheaply add/remove components, or select a "sparse set" framework to cheaply add/remove components but with slower iteration performance.
## Hybrid Component Storage (The Solution)
In Bevy ECS V2, we get to have our cake and eat it too. It now has _both_ of the component storage types above (and more can be added later if needed):
* **Tables** (aka "archetypal" storage)
* The default storage. If you don't configure anything, this is what you get
* Fast iteration by default
* Slower add/remove operations
* **Sparse Sets**
* Opt-in
* Slower iteration
* Faster add/remove operations
These storage types complement each other perfectly. By default Query iteration is fast. If developers know that they want to add/remove a component at high frequencies, they can set the storage to "sparse set":
```rust
world.register_component(
ComponentDescriptor::new::<MyComponent>(StorageType::SparseSet)
).unwrap();
```
## Archetypes
Archetypes are now "just metadata" ... they no longer store components directly. They do store:
* The `ComponentId`s of each of the Archetype's components (and that component's storage type)
* Archetypes are uniquely defined by their component layouts
* For example: entities with "table" components `[A, B, C]` _and_ "sparse set" components `[D, E]` will always be in the same archetype.
* The `TableId` associated with the archetype
* For now each archetype has exactly one table (which can have no components),
* There is a 1->Many relationship from Tables->Archetypes. A given table could have any number of archetype components stored in it:
* Ex: an entity with "table storage" components `[A, B, C]` and "sparse set" components `[D, E]` will share the same `[A, B, C]` table as an entity with `[A, B, C]` table component and `[F]` sparse set components.
* This 1->Many relationship is how we preserve fast "cache friendly" iteration performance when possible (more on this later)
* A list of entities that are in the archetype and the row id of the table they are in
* ArchetypeComponentIds
* unique densely packed identifiers for (ArchetypeId, ComponentId) pairs
* used by the schedule executor for cheap system access control
* "Archetype Graph Edges" (see the next section)
## The "Archetype Graph"
Archetype changes in Bevy (and a number of other archetypal ecs-es) have historically been expensive to compute. First, you need to allocate a new vector of the entity's current component ids, add or remove components based on the operation performed, sort it (to ensure it is order-independent), then hash it to find the archetype (if it exists). And thats all before we get to the _already_ expensive full copy of all components to the new table storage.
The solution is to build a "graph" of archetypes to cache these results. @SanderMertens first exposed me to the idea (and he got it from @gjroelofs, who came up with it). They propose adding directed edges between archetypes for add/remove component operations. If `ComponentId`s are densely packed, you can use sparse sets to cheaply jump between archetypes.
Bevy takes this one step further by using add/remove `Bundle` edges instead of `Component` edges. Bevy encourages the use of `Bundles` to group add/remove operations. This is largely for "clearer game logic" reasons, but it also helps cut down on the number of archetype changes required. `Bundles` now also have densely-packed `BundleId`s. This allows us to use a _single_ edge for each bundle operation (rather than needing to traverse N edges ... one for each component). Single component operations are also bundles, so this is strictly an improvement over a "component only" graph.
As a result, an operation that used to be _heavy_ (both for allocations and compute) is now two dirt-cheap array lookups and zero allocations.
## Stateful Queries
World queries are now stateful. This allows us to:
1. Cache archetype (and table) matches
* This resolves another issue with (naive) archetypal ECS: query performance getting worse as the number of archetypes goes up (and fragmentation occurs).
2. Cache Fetch and Filter state
* The expensive parts of fetch/filter operations (such as hashing the TypeId to find the ComponentId) now only happen once when the Query is first constructed
3. Incrementally build up state
* When new archetypes are added, we only process the new archetypes (no need to rebuild state for old archetypes)
As a result, the direct `World` query api now looks like this:
```rust
let mut query = world.query::<(&A, &mut B)>();
for (a, mut b) in query.iter_mut(&mut world) {
}
```
Requiring `World` to generate stateful queries (rather than letting the `QueryState` type be constructed separately) allows us to ensure that _all_ queries are properly initialized (and the relevant world state, such as ComponentIds). This enables QueryState to remove branches from its operations that check for initialization status (and also enables query.iter() to take an immutable world reference because it doesn't need to initialize anything in world).
However in systems, this is a non-breaking change. State management is done internally by the relevant SystemParam.
## Stateful SystemParams
Like Queries, `SystemParams` now also cache state. For example, `Query` system params store the "stateful query" state mentioned above. Commands store their internal `CommandQueue`. This means you can now safely use as many separate `Commands` parameters in your system as you want. `Local<T>` system params store their `T` value in their state (instead of in Resources).
SystemParam state also enabled a significant slim-down of SystemState. It is much nicer to look at now.
Per-SystemParam state naturally insulates us from an "aliased mut" class of errors we have hit in the past (ex: using multiple `Commands` system params).
(credit goes to @DJMcNab for the initial idea and draft pr here #1364)
## Configurable SystemParams
@DJMcNab also had the great idea to make SystemParams configurable. This allows users to provide some initial configuration / values for system parameters (when possible). Most SystemParams have no config (the config type is `()`), but the `Local<T>` param now supports user-provided parameters:
```rust
fn foo(value: Local<usize>) {
}
app.add_system(foo.system().config(|c| c.0 = Some(10)));
```
## Uber Fast "for_each" Query Iterators
Developers now have the choice to use a fast "for_each" iterator, which yields ~1.5-3x iteration speed improvements for "fragmented iteration", and minor ~1.2x iteration speed improvements for unfragmented iteration.
```rust
fn system(query: Query<(&A, &mut B)>) {
// you now have the option to do this for a speed boost
query.for_each_mut(|(a, mut b)| {
});
// however normal iterators are still available
for (a, mut b) in query.iter_mut() {
}
}
```
I think in most cases we should continue to encourage "normal" iterators as they are more flexible and more "rust idiomatic". But when that extra "oomf" is needed, it makes sense to use `for_each`.
We should also consider using `for_each` for internal bevy systems to give our users a nice speed boost (but that should be a separate pr).
## Component Metadata
`World` now has a `Components` collection, which is accessible via `world.components()`. This stores mappings from `ComponentId` to `ComponentInfo`, as well as `TypeId` to `ComponentId` mappings (where relevant). `ComponentInfo` stores information about the component, such as ComponentId, TypeId, memory layout, send-ness (currently limited to resources), and storage type.
## Significantly Cheaper `Access<T>`
We used to use `TypeAccess<TypeId>` to manage read/write component/archetype-component access. This was expensive because TypeIds must be hashed and compared individually. The parallel executor got around this by "condensing" type ids into bitset-backed access types. This worked, but it had to be re-generated from the `TypeAccess<TypeId>`sources every time archetypes changed.
This pr removes TypeAccess in favor of faster bitset access everywhere. We can do this thanks to the move to densely packed `ComponentId`s and `ArchetypeComponentId`s.
## Merged Resources into World
Resources had a lot of redundant functionality with Components. They stored typed data, they had access control, they had unique ids, they were queryable via SystemParams, etc. In fact the _only_ major difference between them was that they were unique (and didn't correlate to an entity).
Separate resources also had the downside of requiring a separate set of access controls, which meant the parallel executor needed to compare more bitsets per system and manage more state.
I initially got the "separate resources" idea from `legion`. I think that design was motivated by the fact that it made the direct world query/resource lifetime interactions more manageable. It certainly made our lives easier when using Resources alongside hecs/bevy_ecs. However we already have a construct for safely and ergonomically managing in-world lifetimes: systems (which use `Access<T>` internally).
This pr merges Resources into World:
```rust
world.insert_resource(1);
world.insert_resource(2.0);
let a = world.get_resource::<i32>().unwrap();
let mut b = world.get_resource_mut::<f64>().unwrap();
*b = 3.0;
```
Resources are now just a special kind of component. They have their own ComponentIds (and their own resource TypeId->ComponentId scope, so they don't conflict wit components of the same type). They are stored in a special "resource archetype", which stores components inside the archetype using a new `unique_components` sparse set (note that this sparse set could later be used to implement Tags). This allows us to keep the code size small by reusing existing datastructures (namely Column, Archetype, ComponentFlags, and ComponentInfo). This allows us the executor to use a single `Access<ArchetypeComponentId>` per system. It should also make scripting language integration easier.
_But_ this merge did create problems for people directly interacting with `World`. What if you need mutable access to multiple resources at the same time? `world.get_resource_mut()` borrows World mutably!
## WorldCell
WorldCell applies the `Access<ArchetypeComponentId>` concept to direct world access:
```rust
let world_cell = world.cell();
let a = world_cell.get_resource_mut::<i32>().unwrap();
let b = world_cell.get_resource_mut::<f64>().unwrap();
```
This adds cheap runtime checks (a sparse set lookup of `ArchetypeComponentId` and a counter) to ensure that world accesses do not conflict with each other. Each operation returns a `WorldBorrow<'w, T>` or `WorldBorrowMut<'w, T>` wrapper type, which will release the relevant ArchetypeComponentId resources when dropped.
World caches the access sparse set (and only one cell can exist at a time), so `world.cell()` is a cheap operation.
WorldCell does _not_ use atomic operations. It is non-send, does a mutable borrow of world to prevent other accesses, and uses a simple `Rc<RefCell<ArchetypeComponentAccess>>` wrapper in each WorldBorrow pointer.
The api is currently limited to resource access, but it can and should be extended to queries / entity component access.
## Resource Scopes
WorldCell does not yet support component queries, and even when it does there are sometimes legitimate reasons to want a mutable world ref _and_ a mutable resource ref (ex: bevy_render and bevy_scene both need this). In these cases we could always drop down to the unsafe `world.get_resource_unchecked_mut()`, but that is not ideal!
Instead developers can use a "resource scope"
```rust
world.resource_scope(|world: &mut World, a: &mut A| {
})
```
This temporarily removes the `A` resource from `World`, provides mutable pointers to both, and re-adds A to World when finished. Thanks to the move to ComponentIds/sparse sets, this is a cheap operation.
If multiple resources are required, scopes can be nested. We could also consider adding a "resource tuple" to the api if this pattern becomes common and the boilerplate gets nasty.
## Query Conflicts Use ComponentId Instead of ArchetypeComponentId
For safety reasons, systems cannot contain queries that conflict with each other without wrapping them in a QuerySet. On bevy `main`, we use ArchetypeComponentIds to determine conflicts. This is nice because it can take into account filters:
```rust
// these queries will never conflict due to their filters
fn filter_system(a: Query<&mut A, With<B>>, b: Query<&mut B, Without<B>>) {
}
```
But it also has a significant downside:
```rust
// these queries will not conflict _until_ an entity with A, B, and C is spawned
fn maybe_conflicts_system(a: Query<(&mut A, &C)>, b: Query<(&mut A, &B)>) {
}
```
The system above will panic at runtime if an entity with A, B, and C is spawned. This makes it hard to trust that your game logic will run without crashing.
In this pr, I switched to using `ComponentId` instead. This _is_ more constraining. `maybe_conflicts_system` will now always fail, but it will do it consistently at startup. Naively, it would also _disallow_ `filter_system`, which would be a significant downgrade in usability. Bevy has a number of internal systems that rely on disjoint queries and I expect it to be a common pattern in userspace.
To resolve this, I added a new `FilteredAccess<T>` type, which wraps `Access<T>` and adds with/without filters. If two `FilteredAccess` have with/without values that prove they are disjoint, they will no longer conflict.
## EntityRef / EntityMut
World entity operations on `main` require that the user passes in an `entity` id to each operation:
```rust
let entity = world.spawn((A, )); // create a new entity with A
world.get::<A>(entity);
world.insert(entity, (B, C));
world.insert_one(entity, D);
```
This means that each operation needs to look up the entity location / verify its validity. The initial spawn operation also requires a Bundle as input. This can be awkward when no components are required (or one component is required).
These operations have been replaced by `EntityRef` and `EntityMut`, which are "builder-style" wrappers around world that provide read and read/write operations on a single, pre-validated entity:
```rust
// spawn now takes no inputs and returns an EntityMut
let entity = world.spawn()
.insert(A) // insert a single component into the entity
.insert_bundle((B, C)) // insert a bundle of components into the entity
.id() // id returns the Entity id
// Returns EntityMut (or panics if the entity does not exist)
world.entity_mut(entity)
.insert(D)
.insert_bundle(SomeBundle::default());
{
// returns EntityRef (or panics if the entity does not exist)
let d = world.entity(entity)
.get::<D>() // gets the D component
.unwrap();
// world.get still exists for ergonomics
let d = world.get::<D>(entity).unwrap();
}
// These variants return Options if you want to check existence instead of panicing
world.get_entity_mut(entity)
.unwrap()
.insert(E);
if let Some(entity_ref) = world.get_entity(entity) {
let d = entity_ref.get::<D>().unwrap();
}
```
This _does not_ affect the current Commands api or terminology. I think that should be a separate conversation as that is a much larger breaking change.
## Safety Improvements
* Entity reservation in Commands uses a normal world borrow instead of an unsafe transmute
* QuerySets no longer transmutes lifetimes
* Made traits "unsafe" when implementing a trait incorrectly could cause unsafety
* More thorough safety docs
## RemovedComponents SystemParam
The old approach to querying removed components: `query.removed:<T>()` was confusing because it had no connection to the query itself. I replaced it with the following, which is both clearer and allows us to cache the ComponentId mapping in the SystemParamState:
```rust
fn system(removed: RemovedComponents<T>) {
for entity in removed.iter() {
}
}
```
## Simpler Bundle implementation
Bundles are no longer responsible for sorting (or deduping) TypeInfo. They are just a simple ordered list of component types / data. This makes the implementation smaller and opens the door to an easy "nested bundle" implementation in the future (which i might even add in this pr). Duplicate detection is now done once per bundle type by World the first time a bundle is used.
## Unified WorldQuery and QueryFilter types
(don't worry they are still separate type _parameters_ in Queries .. this is a non-breaking change)
WorldQuery and QueryFilter were already basically identical apis. With the addition of `FetchState` and more storage-specific fetch methods, the overlap was even clearer (and the redundancy more painful).
QueryFilters are now just `F: WorldQuery where F::Fetch: FilterFetch`. FilterFetch requires `Fetch<Item = bool>` and adds new "short circuit" variants of fetch methods. This enables a filter tuple like `(With<A>, Without<B>, Changed<C>)` to stop evaluating the filter after the first mismatch is encountered. FilterFetch is automatically implemented for `Fetch` implementations that return bool.
This forces fetch implementations that return things like `(bool, bool, bool)` (such as the filter above) to manually implement FilterFetch and decide whether or not to short-circuit.
## More Granular Modules
World no longer globs all of the internal modules together. It now exports `core`, `system`, and `schedule` separately. I'm also considering exporting `core` submodules directly as that is still pretty "glob-ey" and unorganized (feedback welcome here).
## Remaining Draft Work (to be done in this pr)
* ~~panic on conflicting WorldQuery fetches (&A, &mut A)~~
* ~~bevy `main` and hecs both currently allow this, but we should protect against it if possible~~
* ~~batch_iter / par_iter (currently stubbed out)~~
* ~~ChangedRes~~
* ~~I skipped this while we sort out #1313. This pr should be adapted to account for whatever we land on there~~.
* ~~The `Archetypes` and `Tables` collections use hashes of sorted lists of component ids to uniquely identify each archetype/table. This hash is then used as the key in a HashMap to look up the relevant ArchetypeId or TableId. (which doesn't handle hash collisions properly)~~
* ~~It is currently unsafe to generate a Query from "World A", then use it on "World B" (despite the api claiming it is safe). We should probably close this gap. This could be done by adding a randomly generated WorldId to each world, then storing that id in each Query. They could then be compared to each other on each `query.do_thing(&world)` operation. This _does_ add an extra branch to each query operation, so I'm open to other suggestions if people have them.~~
* ~~Nested Bundles (if i find time)~~
## Potential Future Work
* Expand WorldCell to support queries.
* Consider not allocating in the empty archetype on `world.spawn()`
* ex: return something like EntityMutUninit, which turns into EntityMut after an `insert` or `insert_bundle` op
* this actually regressed performance last time i tried it, but in theory it should be faster
* Optimize SparseSet::insert (see `PERF` comment on insert)
* Replace SparseArray `Option<T>` with T::MAX to cut down on branching
* would enable cheaper get_unchecked() operations
* upstream fixedbitset optimizations
* fixedbitset could be allocation free for small block counts (store blocks in a SmallVec)
* fixedbitset could have a const constructor
* Consider implementing Tags (archetype-specific by-value data that affects archetype identity)
* ex: ArchetypeA could have `[A, B, C]` table components and `[D(1)]` "tag" component. ArchetypeB could have `[A, B, C]` table components and a `[D(2)]` tag component. The archetypes are different, despite both having D tags because the value inside D is different.
* this could potentially build on top of the `archetype.unique_components` added in this pr for resource storage.
* Consider reverting `all_tuples` proc macro in favor of the old `macro_rules` implementation
* all_tuples is more flexible and produces cleaner documentation (the macro_rules version produces weird type parameter orders due to parser constraints)
* but unfortunately all_tuples also appears to make Rust Analyzer sad/slow when working inside of `bevy_ecs` (does not affect user code)
* Consider "resource queries" and/or "mixed resource and entity component queries" as an alternative to WorldCell
* this is basically just "systems" so maybe it's not worth it
* Add more world ops
* `world.clear()`
* `world.reserve<T: Bundle>(count: usize)`
* Try using the old archetype allocation strategy (allocate new memory on resize and copy everything over). I expect this to improve batch insertion performance at the cost of unbatched performance. But thats just a guess. I'm not an allocation perf pro :)
* Adapt Commands apis for consistency with new World apis
## Benchmarks
key:
* `bevy_old`: bevy `main` branch
* `bevy`: this branch
* `_foreach`: uses an optimized for_each iterator
* ` _sparse`: uses sparse set storage (if unspecified assume table storage)
* `_system`: runs inside a system (if unspecified assume test happens via direct world ops)
### Simple Insert (from ecs_bench_suite)
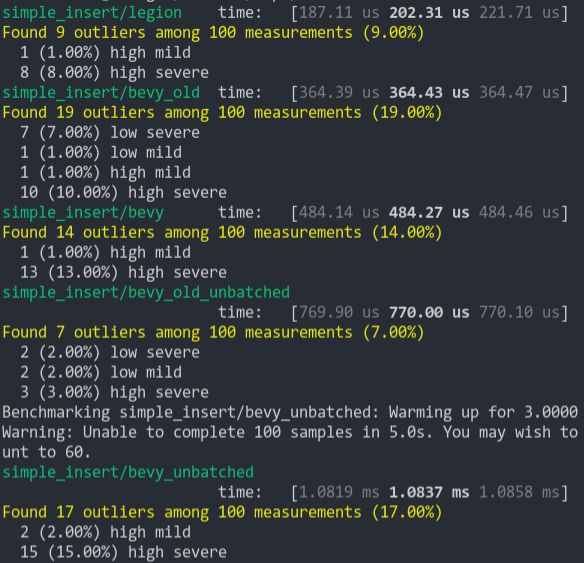
### Simpler Iter (from ecs_bench_suite)
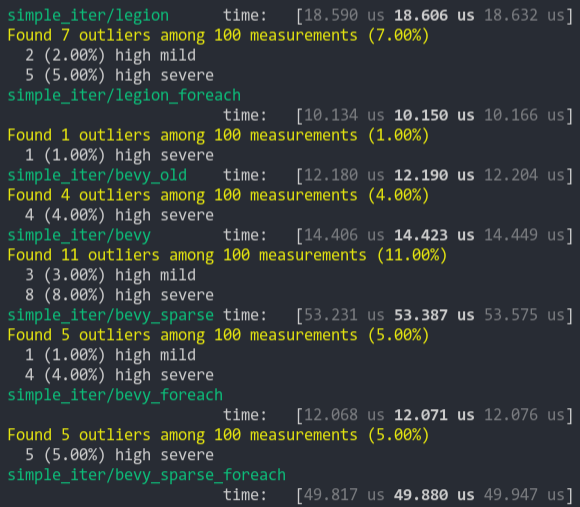
### Fragment Iter (from ecs_bench_suite)
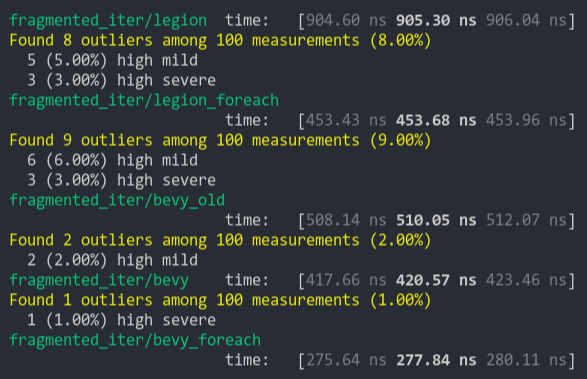
### Sparse Fragmented Iter
Iterate a query that matches 5 entities from a single matching archetype, but there are 100 unmatching archetypes
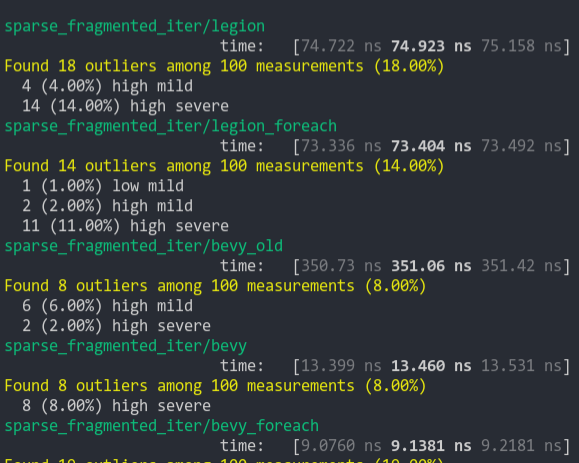
### Schedule (from ecs_bench_suite)
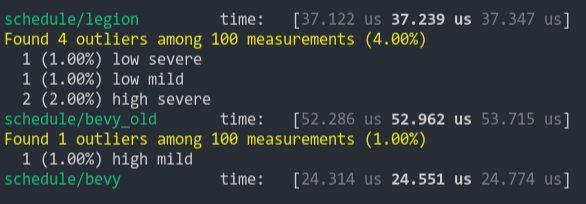
### Add Remove Component (from ecs_bench_suite)
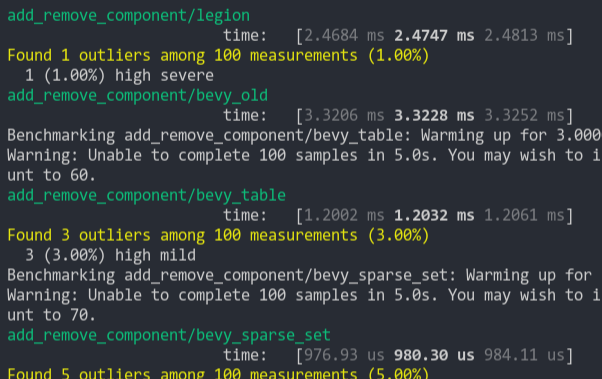
### Add Remove Component Big
Same as the test above, but each entity has 5 "large" matrix components and 1 "large" matrix component is added and removed
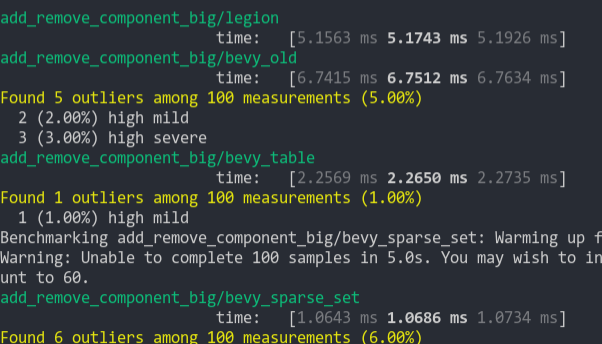
### Get Component
Looks up a single component value a large number of times
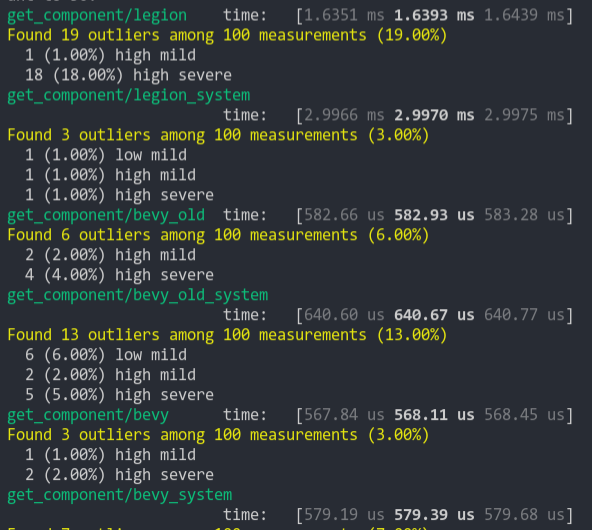
2021-03-05 07:54:35 +00:00
}
2021-10-03 19:23:44 +00:00
impl < ' w , T > Deref for NonSend < ' w , T > {
Bevy ECS V2 (#1525)
# Bevy ECS V2
This is a rewrite of Bevy ECS (basically everything but the new executor/schedule, which are already awesome). The overall goal was to improve the performance and versatility of Bevy ECS. Here is a quick bulleted list of changes before we dive into the details:
* Complete World rewrite
* Multiple component storage types:
* Tables: fast cache friendly iteration, slower add/removes (previously called Archetypes)
* Sparse Sets: fast add/remove, slower iteration
* Stateful Queries (caches query results for faster iteration. fragmented iteration is _fast_ now)
* Stateful System Params (caches expensive operations. inspired by @DJMcNab's work in #1364)
* Configurable System Params (users can set configuration when they construct their systems. once again inspired by @DJMcNab's work)
* Archetypes are now "just metadata", component storage is separate
* Archetype Graph (for faster archetype changes)
* Component Metadata
* Configure component storage type
* Retrieve information about component size/type/name/layout/send-ness/etc
* Components are uniquely identified by a densely packed ComponentId
* TypeIds are now totally optional (which should make implementing scripting easier)
* Super fast "for_each" query iterators
* Merged Resources into World. Resources are now just a special type of component
* EntityRef/EntityMut builder apis (more efficient and more ergonomic)
* Fast bitset-backed `Access<T>` replaces old hashmap-based approach everywhere
* Query conflicts are determined by component access instead of archetype component access (to avoid random failures at runtime)
* With/Without are still taken into account for conflicts, so this should still be comfy to use
* Much simpler `IntoSystem` impl
* Significantly reduced the amount of hashing throughout the ecs in favor of Sparse Sets (indexed by densely packed ArchetypeId, ComponentId, BundleId, and TableId)
* Safety Improvements
* Entity reservation uses a normal world reference instead of unsafe transmute
* QuerySets no longer transmute lifetimes
* Made traits "unsafe" where relevant
* More thorough safety docs
* WorldCell
* Exposes safe mutable access to multiple resources at a time in a World
* Replaced "catch all" `System::update_archetypes(world: &World)` with `System::new_archetype(archetype: &Archetype)`
* Simpler Bundle implementation
* Replaced slow "remove_bundle_one_by_one" used as fallback for Commands::remove_bundle with fast "remove_bundle_intersection"
* Removed `Mut<T>` query impl. it is better to only support one way: `&mut T`
* Removed with() from `Flags<T>` in favor of `Option<Flags<T>>`, which allows querying for flags to be "filtered" by default
* Components now have is_send property (currently only resources support non-send)
* More granular module organization
* New `RemovedComponents<T>` SystemParam that replaces `query.removed::<T>()`
* `world.resource_scope()` for mutable access to resources and world at the same time
* WorldQuery and QueryFilter traits unified. FilterFetch trait added to enable "short circuit" filtering. Auto impled for cases that don't need it
* Significantly slimmed down SystemState in favor of individual SystemParam state
* System Commands changed from `commands: &mut Commands` back to `mut commands: Commands` (to allow Commands to have a World reference)
Fixes #1320
## `World` Rewrite
This is a from-scratch rewrite of `World` that fills the niche that `hecs` used to. Yes, this means Bevy ECS is no longer a "fork" of hecs. We're going out our own!
(the only shared code between the projects is the entity id allocator, which is already basically ideal)
A huge shout out to @SanderMertens (author of [flecs](https://github.com/SanderMertens/flecs)) for sharing some great ideas with me (specifically hybrid ecs storage and archetype graphs). He also helped advise on a number of implementation details.
## Component Storage (The Problem)
Two ECS storage paradigms have gained a lot of traction over the years:
* **Archetypal ECS**:
* Stores components in "tables" with static schemas. Each "column" stores components of a given type. Each "row" is an entity.
* Each "archetype" has its own table. Adding/removing an entity's component changes the archetype.
* Enables super-fast Query iteration due to its cache-friendly data layout
* Comes at the cost of more expensive add/remove operations for an Entity's components, because all components need to be copied to the new archetype's "table"
* **Sparse Set ECS**:
* Stores components of the same type in densely packed arrays, which are sparsely indexed by densely packed unsigned integers (Entity ids)
* Query iteration is slower than Archetypal ECS because each entity's component could be at any position in the sparse set. This "random access" pattern isn't cache friendly. Additionally, there is an extra layer of indirection because you must first map the entity id to an index in the component array.
* Adding/removing components is a cheap, constant time operation
Bevy ECS V1, hecs, legion, flec, and Unity DOTS are all "archetypal ecs-es". I personally think "archetypal" storage is a good default for game engines. An entity's archetype doesn't need to change frequently in general, and it creates "fast by default" query iteration (which is a much more common operation). It is also "self optimizing". Users don't need to think about optimizing component layouts for iteration performance. It "just works" without any extra boilerplate.
Shipyard and EnTT are "sparse set ecs-es". They employ "packing" as a way to work around the "suboptimal by default" iteration performance for specific sets of components. This helps, but I didn't think this was a good choice for a general purpose engine like Bevy because:
1. "packs" conflict with each other. If bevy decides to internally pack the Transform and GlobalTransform components, users are then blocked if they want to pack some custom component with Transform.
2. users need to take manual action to optimize
Developers selecting an ECS framework are stuck with a hard choice. Select an "archetypal" framework with "fast iteration everywhere" but without the ability to cheaply add/remove components, or select a "sparse set" framework to cheaply add/remove components but with slower iteration performance.
## Hybrid Component Storage (The Solution)
In Bevy ECS V2, we get to have our cake and eat it too. It now has _both_ of the component storage types above (and more can be added later if needed):
* **Tables** (aka "archetypal" storage)
* The default storage. If you don't configure anything, this is what you get
* Fast iteration by default
* Slower add/remove operations
* **Sparse Sets**
* Opt-in
* Slower iteration
* Faster add/remove operations
These storage types complement each other perfectly. By default Query iteration is fast. If developers know that they want to add/remove a component at high frequencies, they can set the storage to "sparse set":
```rust
world.register_component(
ComponentDescriptor::new::<MyComponent>(StorageType::SparseSet)
).unwrap();
```
## Archetypes
Archetypes are now "just metadata" ... they no longer store components directly. They do store:
* The `ComponentId`s of each of the Archetype's components (and that component's storage type)
* Archetypes are uniquely defined by their component layouts
* For example: entities with "table" components `[A, B, C]` _and_ "sparse set" components `[D, E]` will always be in the same archetype.
* The `TableId` associated with the archetype
* For now each archetype has exactly one table (which can have no components),
* There is a 1->Many relationship from Tables->Archetypes. A given table could have any number of archetype components stored in it:
* Ex: an entity with "table storage" components `[A, B, C]` and "sparse set" components `[D, E]` will share the same `[A, B, C]` table as an entity with `[A, B, C]` table component and `[F]` sparse set components.
* This 1->Many relationship is how we preserve fast "cache friendly" iteration performance when possible (more on this later)
* A list of entities that are in the archetype and the row id of the table they are in
* ArchetypeComponentIds
* unique densely packed identifiers for (ArchetypeId, ComponentId) pairs
* used by the schedule executor for cheap system access control
* "Archetype Graph Edges" (see the next section)
## The "Archetype Graph"
Archetype changes in Bevy (and a number of other archetypal ecs-es) have historically been expensive to compute. First, you need to allocate a new vector of the entity's current component ids, add or remove components based on the operation performed, sort it (to ensure it is order-independent), then hash it to find the archetype (if it exists). And thats all before we get to the _already_ expensive full copy of all components to the new table storage.
The solution is to build a "graph" of archetypes to cache these results. @SanderMertens first exposed me to the idea (and he got it from @gjroelofs, who came up with it). They propose adding directed edges between archetypes for add/remove component operations. If `ComponentId`s are densely packed, you can use sparse sets to cheaply jump between archetypes.
Bevy takes this one step further by using add/remove `Bundle` edges instead of `Component` edges. Bevy encourages the use of `Bundles` to group add/remove operations. This is largely for "clearer game logic" reasons, but it also helps cut down on the number of archetype changes required. `Bundles` now also have densely-packed `BundleId`s. This allows us to use a _single_ edge for each bundle operation (rather than needing to traverse N edges ... one for each component). Single component operations are also bundles, so this is strictly an improvement over a "component only" graph.
As a result, an operation that used to be _heavy_ (both for allocations and compute) is now two dirt-cheap array lookups and zero allocations.
## Stateful Queries
World queries are now stateful. This allows us to:
1. Cache archetype (and table) matches
* This resolves another issue with (naive) archetypal ECS: query performance getting worse as the number of archetypes goes up (and fragmentation occurs).
2. Cache Fetch and Filter state
* The expensive parts of fetch/filter operations (such as hashing the TypeId to find the ComponentId) now only happen once when the Query is first constructed
3. Incrementally build up state
* When new archetypes are added, we only process the new archetypes (no need to rebuild state for old archetypes)
As a result, the direct `World` query api now looks like this:
```rust
let mut query = world.query::<(&A, &mut B)>();
for (a, mut b) in query.iter_mut(&mut world) {
}
```
Requiring `World` to generate stateful queries (rather than letting the `QueryState` type be constructed separately) allows us to ensure that _all_ queries are properly initialized (and the relevant world state, such as ComponentIds). This enables QueryState to remove branches from its operations that check for initialization status (and also enables query.iter() to take an immutable world reference because it doesn't need to initialize anything in world).
However in systems, this is a non-breaking change. State management is done internally by the relevant SystemParam.
## Stateful SystemParams
Like Queries, `SystemParams` now also cache state. For example, `Query` system params store the "stateful query" state mentioned above. Commands store their internal `CommandQueue`. This means you can now safely use as many separate `Commands` parameters in your system as you want. `Local<T>` system params store their `T` value in their state (instead of in Resources).
SystemParam state also enabled a significant slim-down of SystemState. It is much nicer to look at now.
Per-SystemParam state naturally insulates us from an "aliased mut" class of errors we have hit in the past (ex: using multiple `Commands` system params).
(credit goes to @DJMcNab for the initial idea and draft pr here #1364)
## Configurable SystemParams
@DJMcNab also had the great idea to make SystemParams configurable. This allows users to provide some initial configuration / values for system parameters (when possible). Most SystemParams have no config (the config type is `()`), but the `Local<T>` param now supports user-provided parameters:
```rust
fn foo(value: Local<usize>) {
}
app.add_system(foo.system().config(|c| c.0 = Some(10)));
```
## Uber Fast "for_each" Query Iterators
Developers now have the choice to use a fast "for_each" iterator, which yields ~1.5-3x iteration speed improvements for "fragmented iteration", and minor ~1.2x iteration speed improvements for unfragmented iteration.
```rust
fn system(query: Query<(&A, &mut B)>) {
// you now have the option to do this for a speed boost
query.for_each_mut(|(a, mut b)| {
});
// however normal iterators are still available
for (a, mut b) in query.iter_mut() {
}
}
```
I think in most cases we should continue to encourage "normal" iterators as they are more flexible and more "rust idiomatic". But when that extra "oomf" is needed, it makes sense to use `for_each`.
We should also consider using `for_each` for internal bevy systems to give our users a nice speed boost (but that should be a separate pr).
## Component Metadata
`World` now has a `Components` collection, which is accessible via `world.components()`. This stores mappings from `ComponentId` to `ComponentInfo`, as well as `TypeId` to `ComponentId` mappings (where relevant). `ComponentInfo` stores information about the component, such as ComponentId, TypeId, memory layout, send-ness (currently limited to resources), and storage type.
## Significantly Cheaper `Access<T>`
We used to use `TypeAccess<TypeId>` to manage read/write component/archetype-component access. This was expensive because TypeIds must be hashed and compared individually. The parallel executor got around this by "condensing" type ids into bitset-backed access types. This worked, but it had to be re-generated from the `TypeAccess<TypeId>`sources every time archetypes changed.
This pr removes TypeAccess in favor of faster bitset access everywhere. We can do this thanks to the move to densely packed `ComponentId`s and `ArchetypeComponentId`s.
## Merged Resources into World
Resources had a lot of redundant functionality with Components. They stored typed data, they had access control, they had unique ids, they were queryable via SystemParams, etc. In fact the _only_ major difference between them was that they were unique (and didn't correlate to an entity).
Separate resources also had the downside of requiring a separate set of access controls, which meant the parallel executor needed to compare more bitsets per system and manage more state.
I initially got the "separate resources" idea from `legion`. I think that design was motivated by the fact that it made the direct world query/resource lifetime interactions more manageable. It certainly made our lives easier when using Resources alongside hecs/bevy_ecs. However we already have a construct for safely and ergonomically managing in-world lifetimes: systems (which use `Access<T>` internally).
This pr merges Resources into World:
```rust
world.insert_resource(1);
world.insert_resource(2.0);
let a = world.get_resource::<i32>().unwrap();
let mut b = world.get_resource_mut::<f64>().unwrap();
*b = 3.0;
```
Resources are now just a special kind of component. They have their own ComponentIds (and their own resource TypeId->ComponentId scope, so they don't conflict wit components of the same type). They are stored in a special "resource archetype", which stores components inside the archetype using a new `unique_components` sparse set (note that this sparse set could later be used to implement Tags). This allows us to keep the code size small by reusing existing datastructures (namely Column, Archetype, ComponentFlags, and ComponentInfo). This allows us the executor to use a single `Access<ArchetypeComponentId>` per system. It should also make scripting language integration easier.
_But_ this merge did create problems for people directly interacting with `World`. What if you need mutable access to multiple resources at the same time? `world.get_resource_mut()` borrows World mutably!
## WorldCell
WorldCell applies the `Access<ArchetypeComponentId>` concept to direct world access:
```rust
let world_cell = world.cell();
let a = world_cell.get_resource_mut::<i32>().unwrap();
let b = world_cell.get_resource_mut::<f64>().unwrap();
```
This adds cheap runtime checks (a sparse set lookup of `ArchetypeComponentId` and a counter) to ensure that world accesses do not conflict with each other. Each operation returns a `WorldBorrow<'w, T>` or `WorldBorrowMut<'w, T>` wrapper type, which will release the relevant ArchetypeComponentId resources when dropped.
World caches the access sparse set (and only one cell can exist at a time), so `world.cell()` is a cheap operation.
WorldCell does _not_ use atomic operations. It is non-send, does a mutable borrow of world to prevent other accesses, and uses a simple `Rc<RefCell<ArchetypeComponentAccess>>` wrapper in each WorldBorrow pointer.
The api is currently limited to resource access, but it can and should be extended to queries / entity component access.
## Resource Scopes
WorldCell does not yet support component queries, and even when it does there are sometimes legitimate reasons to want a mutable world ref _and_ a mutable resource ref (ex: bevy_render and bevy_scene both need this). In these cases we could always drop down to the unsafe `world.get_resource_unchecked_mut()`, but that is not ideal!
Instead developers can use a "resource scope"
```rust
world.resource_scope(|world: &mut World, a: &mut A| {
})
```
This temporarily removes the `A` resource from `World`, provides mutable pointers to both, and re-adds A to World when finished. Thanks to the move to ComponentIds/sparse sets, this is a cheap operation.
If multiple resources are required, scopes can be nested. We could also consider adding a "resource tuple" to the api if this pattern becomes common and the boilerplate gets nasty.
## Query Conflicts Use ComponentId Instead of ArchetypeComponentId
For safety reasons, systems cannot contain queries that conflict with each other without wrapping them in a QuerySet. On bevy `main`, we use ArchetypeComponentIds to determine conflicts. This is nice because it can take into account filters:
```rust
// these queries will never conflict due to their filters
fn filter_system(a: Query<&mut A, With<B>>, b: Query<&mut B, Without<B>>) {
}
```
But it also has a significant downside:
```rust
// these queries will not conflict _until_ an entity with A, B, and C is spawned
fn maybe_conflicts_system(a: Query<(&mut A, &C)>, b: Query<(&mut A, &B)>) {
}
```
The system above will panic at runtime if an entity with A, B, and C is spawned. This makes it hard to trust that your game logic will run without crashing.
In this pr, I switched to using `ComponentId` instead. This _is_ more constraining. `maybe_conflicts_system` will now always fail, but it will do it consistently at startup. Naively, it would also _disallow_ `filter_system`, which would be a significant downgrade in usability. Bevy has a number of internal systems that rely on disjoint queries and I expect it to be a common pattern in userspace.
To resolve this, I added a new `FilteredAccess<T>` type, which wraps `Access<T>` and adds with/without filters. If two `FilteredAccess` have with/without values that prove they are disjoint, they will no longer conflict.
## EntityRef / EntityMut
World entity operations on `main` require that the user passes in an `entity` id to each operation:
```rust
let entity = world.spawn((A, )); // create a new entity with A
world.get::<A>(entity);
world.insert(entity, (B, C));
world.insert_one(entity, D);
```
This means that each operation needs to look up the entity location / verify its validity. The initial spawn operation also requires a Bundle as input. This can be awkward when no components are required (or one component is required).
These operations have been replaced by `EntityRef` and `EntityMut`, which are "builder-style" wrappers around world that provide read and read/write operations on a single, pre-validated entity:
```rust
// spawn now takes no inputs and returns an EntityMut
let entity = world.spawn()
.insert(A) // insert a single component into the entity
.insert_bundle((B, C)) // insert a bundle of components into the entity
.id() // id returns the Entity id
// Returns EntityMut (or panics if the entity does not exist)
world.entity_mut(entity)
.insert(D)
.insert_bundle(SomeBundle::default());
{
// returns EntityRef (or panics if the entity does not exist)
let d = world.entity(entity)
.get::<D>() // gets the D component
.unwrap();
// world.get still exists for ergonomics
let d = world.get::<D>(entity).unwrap();
}
// These variants return Options if you want to check existence instead of panicing
world.get_entity_mut(entity)
.unwrap()
.insert(E);
if let Some(entity_ref) = world.get_entity(entity) {
let d = entity_ref.get::<D>().unwrap();
}
```
This _does not_ affect the current Commands api or terminology. I think that should be a separate conversation as that is a much larger breaking change.
## Safety Improvements
* Entity reservation in Commands uses a normal world borrow instead of an unsafe transmute
* QuerySets no longer transmutes lifetimes
* Made traits "unsafe" when implementing a trait incorrectly could cause unsafety
* More thorough safety docs
## RemovedComponents SystemParam
The old approach to querying removed components: `query.removed:<T>()` was confusing because it had no connection to the query itself. I replaced it with the following, which is both clearer and allows us to cache the ComponentId mapping in the SystemParamState:
```rust
fn system(removed: RemovedComponents<T>) {
for entity in removed.iter() {
}
}
```
## Simpler Bundle implementation
Bundles are no longer responsible for sorting (or deduping) TypeInfo. They are just a simple ordered list of component types / data. This makes the implementation smaller and opens the door to an easy "nested bundle" implementation in the future (which i might even add in this pr). Duplicate detection is now done once per bundle type by World the first time a bundle is used.
## Unified WorldQuery and QueryFilter types
(don't worry they are still separate type _parameters_ in Queries .. this is a non-breaking change)
WorldQuery and QueryFilter were already basically identical apis. With the addition of `FetchState` and more storage-specific fetch methods, the overlap was even clearer (and the redundancy more painful).
QueryFilters are now just `F: WorldQuery where F::Fetch: FilterFetch`. FilterFetch requires `Fetch<Item = bool>` and adds new "short circuit" variants of fetch methods. This enables a filter tuple like `(With<A>, Without<B>, Changed<C>)` to stop evaluating the filter after the first mismatch is encountered. FilterFetch is automatically implemented for `Fetch` implementations that return bool.
This forces fetch implementations that return things like `(bool, bool, bool)` (such as the filter above) to manually implement FilterFetch and decide whether or not to short-circuit.
## More Granular Modules
World no longer globs all of the internal modules together. It now exports `core`, `system`, and `schedule` separately. I'm also considering exporting `core` submodules directly as that is still pretty "glob-ey" and unorganized (feedback welcome here).
## Remaining Draft Work (to be done in this pr)
* ~~panic on conflicting WorldQuery fetches (&A, &mut A)~~
* ~~bevy `main` and hecs both currently allow this, but we should protect against it if possible~~
* ~~batch_iter / par_iter (currently stubbed out)~~
* ~~ChangedRes~~
* ~~I skipped this while we sort out #1313. This pr should be adapted to account for whatever we land on there~~.
* ~~The `Archetypes` and `Tables` collections use hashes of sorted lists of component ids to uniquely identify each archetype/table. This hash is then used as the key in a HashMap to look up the relevant ArchetypeId or TableId. (which doesn't handle hash collisions properly)~~
* ~~It is currently unsafe to generate a Query from "World A", then use it on "World B" (despite the api claiming it is safe). We should probably close this gap. This could be done by adding a randomly generated WorldId to each world, then storing that id in each Query. They could then be compared to each other on each `query.do_thing(&world)` operation. This _does_ add an extra branch to each query operation, so I'm open to other suggestions if people have them.~~
* ~~Nested Bundles (if i find time)~~
## Potential Future Work
* Expand WorldCell to support queries.
* Consider not allocating in the empty archetype on `world.spawn()`
* ex: return something like EntityMutUninit, which turns into EntityMut after an `insert` or `insert_bundle` op
* this actually regressed performance last time i tried it, but in theory it should be faster
* Optimize SparseSet::insert (see `PERF` comment on insert)
* Replace SparseArray `Option<T>` with T::MAX to cut down on branching
* would enable cheaper get_unchecked() operations
* upstream fixedbitset optimizations
* fixedbitset could be allocation free for small block counts (store blocks in a SmallVec)
* fixedbitset could have a const constructor
* Consider implementing Tags (archetype-specific by-value data that affects archetype identity)
* ex: ArchetypeA could have `[A, B, C]` table components and `[D(1)]` "tag" component. ArchetypeB could have `[A, B, C]` table components and a `[D(2)]` tag component. The archetypes are different, despite both having D tags because the value inside D is different.
* this could potentially build on top of the `archetype.unique_components` added in this pr for resource storage.
* Consider reverting `all_tuples` proc macro in favor of the old `macro_rules` implementation
* all_tuples is more flexible and produces cleaner documentation (the macro_rules version produces weird type parameter orders due to parser constraints)
* but unfortunately all_tuples also appears to make Rust Analyzer sad/slow when working inside of `bevy_ecs` (does not affect user code)
* Consider "resource queries" and/or "mixed resource and entity component queries" as an alternative to WorldCell
* this is basically just "systems" so maybe it's not worth it
* Add more world ops
* `world.clear()`
* `world.reserve<T: Bundle>(count: usize)`
* Try using the old archetype allocation strategy (allocate new memory on resize and copy everything over). I expect this to improve batch insertion performance at the cost of unbatched performance. But thats just a guess. I'm not an allocation perf pro :)
* Adapt Commands apis for consistency with new World apis
## Benchmarks
key:
* `bevy_old`: bevy `main` branch
* `bevy`: this branch
* `_foreach`: uses an optimized for_each iterator
* ` _sparse`: uses sparse set storage (if unspecified assume table storage)
* `_system`: runs inside a system (if unspecified assume test happens via direct world ops)
### Simple Insert (from ecs_bench_suite)
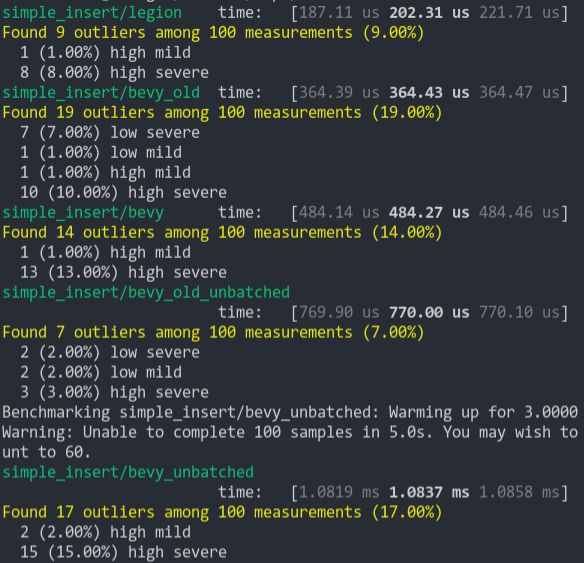
### Simpler Iter (from ecs_bench_suite)
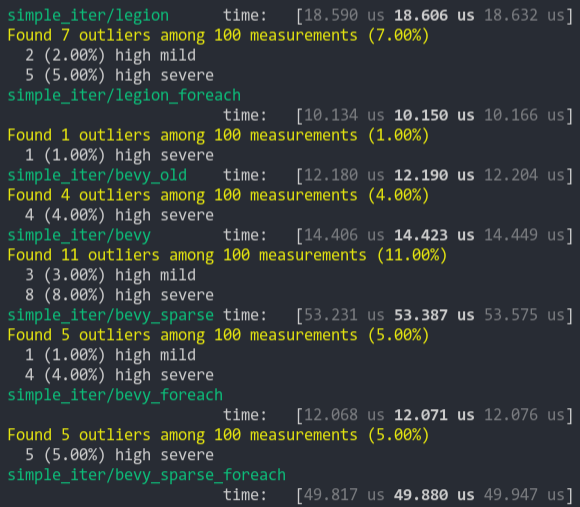
### Fragment Iter (from ecs_bench_suite)
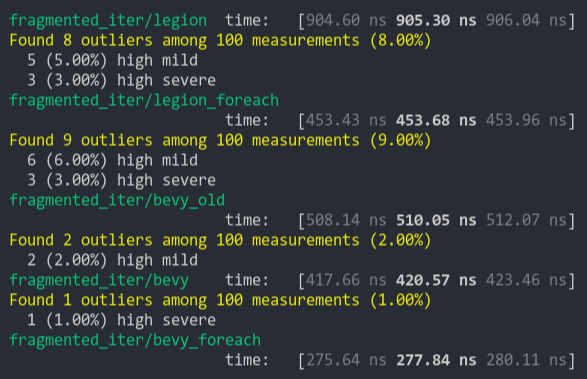
### Sparse Fragmented Iter
Iterate a query that matches 5 entities from a single matching archetype, but there are 100 unmatching archetypes
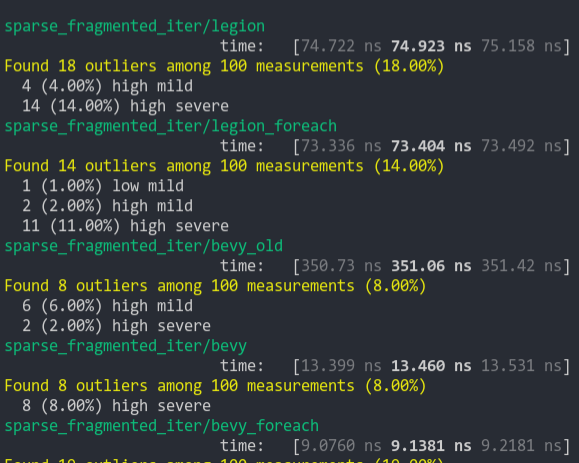
### Schedule (from ecs_bench_suite)
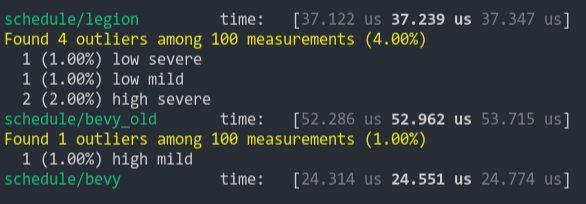
### Add Remove Component (from ecs_bench_suite)
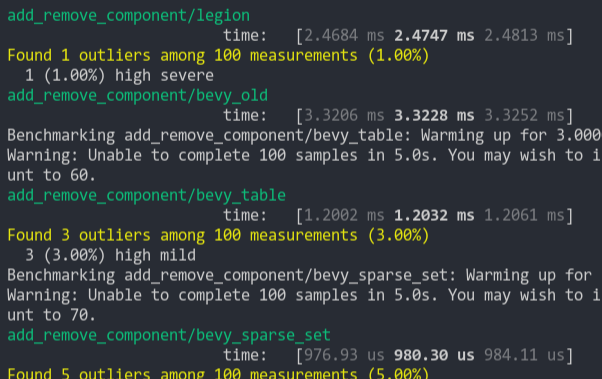
### Add Remove Component Big
Same as the test above, but each entity has 5 "large" matrix components and 1 "large" matrix component is added and removed
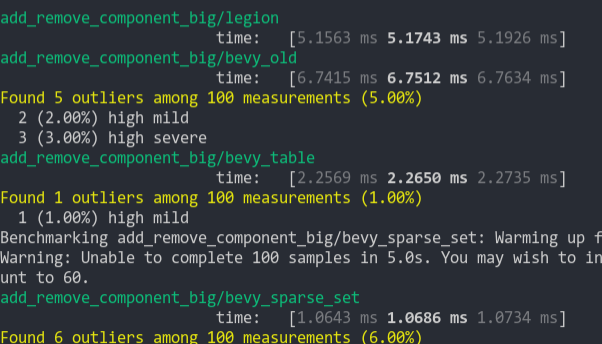
### Get Component
Looks up a single component value a large number of times
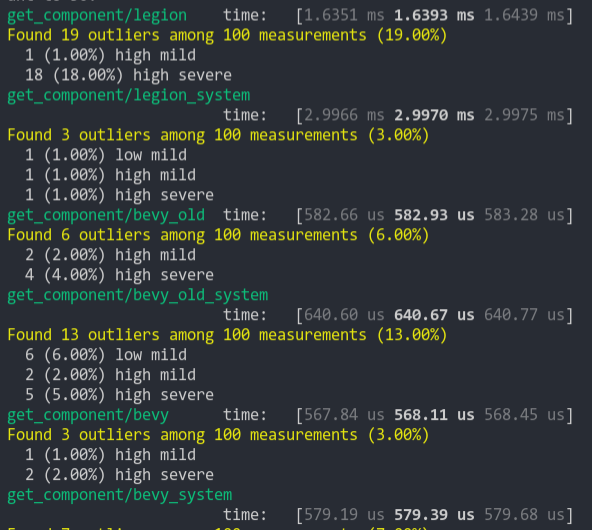
2021-03-05 07:54:35 +00:00
type Target = T ;
2020-12-16 05:57:16 +00:00
Bevy ECS V2 (#1525)
# Bevy ECS V2
This is a rewrite of Bevy ECS (basically everything but the new executor/schedule, which are already awesome). The overall goal was to improve the performance and versatility of Bevy ECS. Here is a quick bulleted list of changes before we dive into the details:
* Complete World rewrite
* Multiple component storage types:
* Tables: fast cache friendly iteration, slower add/removes (previously called Archetypes)
* Sparse Sets: fast add/remove, slower iteration
* Stateful Queries (caches query results for faster iteration. fragmented iteration is _fast_ now)
* Stateful System Params (caches expensive operations. inspired by @DJMcNab's work in #1364)
* Configurable System Params (users can set configuration when they construct their systems. once again inspired by @DJMcNab's work)
* Archetypes are now "just metadata", component storage is separate
* Archetype Graph (for faster archetype changes)
* Component Metadata
* Configure component storage type
* Retrieve information about component size/type/name/layout/send-ness/etc
* Components are uniquely identified by a densely packed ComponentId
* TypeIds are now totally optional (which should make implementing scripting easier)
* Super fast "for_each" query iterators
* Merged Resources into World. Resources are now just a special type of component
* EntityRef/EntityMut builder apis (more efficient and more ergonomic)
* Fast bitset-backed `Access<T>` replaces old hashmap-based approach everywhere
* Query conflicts are determined by component access instead of archetype component access (to avoid random failures at runtime)
* With/Without are still taken into account for conflicts, so this should still be comfy to use
* Much simpler `IntoSystem` impl
* Significantly reduced the amount of hashing throughout the ecs in favor of Sparse Sets (indexed by densely packed ArchetypeId, ComponentId, BundleId, and TableId)
* Safety Improvements
* Entity reservation uses a normal world reference instead of unsafe transmute
* QuerySets no longer transmute lifetimes
* Made traits "unsafe" where relevant
* More thorough safety docs
* WorldCell
* Exposes safe mutable access to multiple resources at a time in a World
* Replaced "catch all" `System::update_archetypes(world: &World)` with `System::new_archetype(archetype: &Archetype)`
* Simpler Bundle implementation
* Replaced slow "remove_bundle_one_by_one" used as fallback for Commands::remove_bundle with fast "remove_bundle_intersection"
* Removed `Mut<T>` query impl. it is better to only support one way: `&mut T`
* Removed with() from `Flags<T>` in favor of `Option<Flags<T>>`, which allows querying for flags to be "filtered" by default
* Components now have is_send property (currently only resources support non-send)
* More granular module organization
* New `RemovedComponents<T>` SystemParam that replaces `query.removed::<T>()`
* `world.resource_scope()` for mutable access to resources and world at the same time
* WorldQuery and QueryFilter traits unified. FilterFetch trait added to enable "short circuit" filtering. Auto impled for cases that don't need it
* Significantly slimmed down SystemState in favor of individual SystemParam state
* System Commands changed from `commands: &mut Commands` back to `mut commands: Commands` (to allow Commands to have a World reference)
Fixes #1320
## `World` Rewrite
This is a from-scratch rewrite of `World` that fills the niche that `hecs` used to. Yes, this means Bevy ECS is no longer a "fork" of hecs. We're going out our own!
(the only shared code between the projects is the entity id allocator, which is already basically ideal)
A huge shout out to @SanderMertens (author of [flecs](https://github.com/SanderMertens/flecs)) for sharing some great ideas with me (specifically hybrid ecs storage and archetype graphs). He also helped advise on a number of implementation details.
## Component Storage (The Problem)
Two ECS storage paradigms have gained a lot of traction over the years:
* **Archetypal ECS**:
* Stores components in "tables" with static schemas. Each "column" stores components of a given type. Each "row" is an entity.
* Each "archetype" has its own table. Adding/removing an entity's component changes the archetype.
* Enables super-fast Query iteration due to its cache-friendly data layout
* Comes at the cost of more expensive add/remove operations for an Entity's components, because all components need to be copied to the new archetype's "table"
* **Sparse Set ECS**:
* Stores components of the same type in densely packed arrays, which are sparsely indexed by densely packed unsigned integers (Entity ids)
* Query iteration is slower than Archetypal ECS because each entity's component could be at any position in the sparse set. This "random access" pattern isn't cache friendly. Additionally, there is an extra layer of indirection because you must first map the entity id to an index in the component array.
* Adding/removing components is a cheap, constant time operation
Bevy ECS V1, hecs, legion, flec, and Unity DOTS are all "archetypal ecs-es". I personally think "archetypal" storage is a good default for game engines. An entity's archetype doesn't need to change frequently in general, and it creates "fast by default" query iteration (which is a much more common operation). It is also "self optimizing". Users don't need to think about optimizing component layouts for iteration performance. It "just works" without any extra boilerplate.
Shipyard and EnTT are "sparse set ecs-es". They employ "packing" as a way to work around the "suboptimal by default" iteration performance for specific sets of components. This helps, but I didn't think this was a good choice for a general purpose engine like Bevy because:
1. "packs" conflict with each other. If bevy decides to internally pack the Transform and GlobalTransform components, users are then blocked if they want to pack some custom component with Transform.
2. users need to take manual action to optimize
Developers selecting an ECS framework are stuck with a hard choice. Select an "archetypal" framework with "fast iteration everywhere" but without the ability to cheaply add/remove components, or select a "sparse set" framework to cheaply add/remove components but with slower iteration performance.
## Hybrid Component Storage (The Solution)
In Bevy ECS V2, we get to have our cake and eat it too. It now has _both_ of the component storage types above (and more can be added later if needed):
* **Tables** (aka "archetypal" storage)
* The default storage. If you don't configure anything, this is what you get
* Fast iteration by default
* Slower add/remove operations
* **Sparse Sets**
* Opt-in
* Slower iteration
* Faster add/remove operations
These storage types complement each other perfectly. By default Query iteration is fast. If developers know that they want to add/remove a component at high frequencies, they can set the storage to "sparse set":
```rust
world.register_component(
ComponentDescriptor::new::<MyComponent>(StorageType::SparseSet)
).unwrap();
```
## Archetypes
Archetypes are now "just metadata" ... they no longer store components directly. They do store:
* The `ComponentId`s of each of the Archetype's components (and that component's storage type)
* Archetypes are uniquely defined by their component layouts
* For example: entities with "table" components `[A, B, C]` _and_ "sparse set" components `[D, E]` will always be in the same archetype.
* The `TableId` associated with the archetype
* For now each archetype has exactly one table (which can have no components),
* There is a 1->Many relationship from Tables->Archetypes. A given table could have any number of archetype components stored in it:
* Ex: an entity with "table storage" components `[A, B, C]` and "sparse set" components `[D, E]` will share the same `[A, B, C]` table as an entity with `[A, B, C]` table component and `[F]` sparse set components.
* This 1->Many relationship is how we preserve fast "cache friendly" iteration performance when possible (more on this later)
* A list of entities that are in the archetype and the row id of the table they are in
* ArchetypeComponentIds
* unique densely packed identifiers for (ArchetypeId, ComponentId) pairs
* used by the schedule executor for cheap system access control
* "Archetype Graph Edges" (see the next section)
## The "Archetype Graph"
Archetype changes in Bevy (and a number of other archetypal ecs-es) have historically been expensive to compute. First, you need to allocate a new vector of the entity's current component ids, add or remove components based on the operation performed, sort it (to ensure it is order-independent), then hash it to find the archetype (if it exists). And thats all before we get to the _already_ expensive full copy of all components to the new table storage.
The solution is to build a "graph" of archetypes to cache these results. @SanderMertens first exposed me to the idea (and he got it from @gjroelofs, who came up with it). They propose adding directed edges between archetypes for add/remove component operations. If `ComponentId`s are densely packed, you can use sparse sets to cheaply jump between archetypes.
Bevy takes this one step further by using add/remove `Bundle` edges instead of `Component` edges. Bevy encourages the use of `Bundles` to group add/remove operations. This is largely for "clearer game logic" reasons, but it also helps cut down on the number of archetype changes required. `Bundles` now also have densely-packed `BundleId`s. This allows us to use a _single_ edge for each bundle operation (rather than needing to traverse N edges ... one for each component). Single component operations are also bundles, so this is strictly an improvement over a "component only" graph.
As a result, an operation that used to be _heavy_ (both for allocations and compute) is now two dirt-cheap array lookups and zero allocations.
## Stateful Queries
World queries are now stateful. This allows us to:
1. Cache archetype (and table) matches
* This resolves another issue with (naive) archetypal ECS: query performance getting worse as the number of archetypes goes up (and fragmentation occurs).
2. Cache Fetch and Filter state
* The expensive parts of fetch/filter operations (such as hashing the TypeId to find the ComponentId) now only happen once when the Query is first constructed
3. Incrementally build up state
* When new archetypes are added, we only process the new archetypes (no need to rebuild state for old archetypes)
As a result, the direct `World` query api now looks like this:
```rust
let mut query = world.query::<(&A, &mut B)>();
for (a, mut b) in query.iter_mut(&mut world) {
}
```
Requiring `World` to generate stateful queries (rather than letting the `QueryState` type be constructed separately) allows us to ensure that _all_ queries are properly initialized (and the relevant world state, such as ComponentIds). This enables QueryState to remove branches from its operations that check for initialization status (and also enables query.iter() to take an immutable world reference because it doesn't need to initialize anything in world).
However in systems, this is a non-breaking change. State management is done internally by the relevant SystemParam.
## Stateful SystemParams
Like Queries, `SystemParams` now also cache state. For example, `Query` system params store the "stateful query" state mentioned above. Commands store their internal `CommandQueue`. This means you can now safely use as many separate `Commands` parameters in your system as you want. `Local<T>` system params store their `T` value in their state (instead of in Resources).
SystemParam state also enabled a significant slim-down of SystemState. It is much nicer to look at now.
Per-SystemParam state naturally insulates us from an "aliased mut" class of errors we have hit in the past (ex: using multiple `Commands` system params).
(credit goes to @DJMcNab for the initial idea and draft pr here #1364)
## Configurable SystemParams
@DJMcNab also had the great idea to make SystemParams configurable. This allows users to provide some initial configuration / values for system parameters (when possible). Most SystemParams have no config (the config type is `()`), but the `Local<T>` param now supports user-provided parameters:
```rust
fn foo(value: Local<usize>) {
}
app.add_system(foo.system().config(|c| c.0 = Some(10)));
```
## Uber Fast "for_each" Query Iterators
Developers now have the choice to use a fast "for_each" iterator, which yields ~1.5-3x iteration speed improvements for "fragmented iteration", and minor ~1.2x iteration speed improvements for unfragmented iteration.
```rust
fn system(query: Query<(&A, &mut B)>) {
// you now have the option to do this for a speed boost
query.for_each_mut(|(a, mut b)| {
});
// however normal iterators are still available
for (a, mut b) in query.iter_mut() {
}
}
```
I think in most cases we should continue to encourage "normal" iterators as they are more flexible and more "rust idiomatic". But when that extra "oomf" is needed, it makes sense to use `for_each`.
We should also consider using `for_each` for internal bevy systems to give our users a nice speed boost (but that should be a separate pr).
## Component Metadata
`World` now has a `Components` collection, which is accessible via `world.components()`. This stores mappings from `ComponentId` to `ComponentInfo`, as well as `TypeId` to `ComponentId` mappings (where relevant). `ComponentInfo` stores information about the component, such as ComponentId, TypeId, memory layout, send-ness (currently limited to resources), and storage type.
## Significantly Cheaper `Access<T>`
We used to use `TypeAccess<TypeId>` to manage read/write component/archetype-component access. This was expensive because TypeIds must be hashed and compared individually. The parallel executor got around this by "condensing" type ids into bitset-backed access types. This worked, but it had to be re-generated from the `TypeAccess<TypeId>`sources every time archetypes changed.
This pr removes TypeAccess in favor of faster bitset access everywhere. We can do this thanks to the move to densely packed `ComponentId`s and `ArchetypeComponentId`s.
## Merged Resources into World
Resources had a lot of redundant functionality with Components. They stored typed data, they had access control, they had unique ids, they were queryable via SystemParams, etc. In fact the _only_ major difference between them was that they were unique (and didn't correlate to an entity).
Separate resources also had the downside of requiring a separate set of access controls, which meant the parallel executor needed to compare more bitsets per system and manage more state.
I initially got the "separate resources" idea from `legion`. I think that design was motivated by the fact that it made the direct world query/resource lifetime interactions more manageable. It certainly made our lives easier when using Resources alongside hecs/bevy_ecs. However we already have a construct for safely and ergonomically managing in-world lifetimes: systems (which use `Access<T>` internally).
This pr merges Resources into World:
```rust
world.insert_resource(1);
world.insert_resource(2.0);
let a = world.get_resource::<i32>().unwrap();
let mut b = world.get_resource_mut::<f64>().unwrap();
*b = 3.0;
```
Resources are now just a special kind of component. They have their own ComponentIds (and their own resource TypeId->ComponentId scope, so they don't conflict wit components of the same type). They are stored in a special "resource archetype", which stores components inside the archetype using a new `unique_components` sparse set (note that this sparse set could later be used to implement Tags). This allows us to keep the code size small by reusing existing datastructures (namely Column, Archetype, ComponentFlags, and ComponentInfo). This allows us the executor to use a single `Access<ArchetypeComponentId>` per system. It should also make scripting language integration easier.
_But_ this merge did create problems for people directly interacting with `World`. What if you need mutable access to multiple resources at the same time? `world.get_resource_mut()` borrows World mutably!
## WorldCell
WorldCell applies the `Access<ArchetypeComponentId>` concept to direct world access:
```rust
let world_cell = world.cell();
let a = world_cell.get_resource_mut::<i32>().unwrap();
let b = world_cell.get_resource_mut::<f64>().unwrap();
```
This adds cheap runtime checks (a sparse set lookup of `ArchetypeComponentId` and a counter) to ensure that world accesses do not conflict with each other. Each operation returns a `WorldBorrow<'w, T>` or `WorldBorrowMut<'w, T>` wrapper type, which will release the relevant ArchetypeComponentId resources when dropped.
World caches the access sparse set (and only one cell can exist at a time), so `world.cell()` is a cheap operation.
WorldCell does _not_ use atomic operations. It is non-send, does a mutable borrow of world to prevent other accesses, and uses a simple `Rc<RefCell<ArchetypeComponentAccess>>` wrapper in each WorldBorrow pointer.
The api is currently limited to resource access, but it can and should be extended to queries / entity component access.
## Resource Scopes
WorldCell does not yet support component queries, and even when it does there are sometimes legitimate reasons to want a mutable world ref _and_ a mutable resource ref (ex: bevy_render and bevy_scene both need this). In these cases we could always drop down to the unsafe `world.get_resource_unchecked_mut()`, but that is not ideal!
Instead developers can use a "resource scope"
```rust
world.resource_scope(|world: &mut World, a: &mut A| {
})
```
This temporarily removes the `A` resource from `World`, provides mutable pointers to both, and re-adds A to World when finished. Thanks to the move to ComponentIds/sparse sets, this is a cheap operation.
If multiple resources are required, scopes can be nested. We could also consider adding a "resource tuple" to the api if this pattern becomes common and the boilerplate gets nasty.
## Query Conflicts Use ComponentId Instead of ArchetypeComponentId
For safety reasons, systems cannot contain queries that conflict with each other without wrapping them in a QuerySet. On bevy `main`, we use ArchetypeComponentIds to determine conflicts. This is nice because it can take into account filters:
```rust
// these queries will never conflict due to their filters
fn filter_system(a: Query<&mut A, With<B>>, b: Query<&mut B, Without<B>>) {
}
```
But it also has a significant downside:
```rust
// these queries will not conflict _until_ an entity with A, B, and C is spawned
fn maybe_conflicts_system(a: Query<(&mut A, &C)>, b: Query<(&mut A, &B)>) {
}
```
The system above will panic at runtime if an entity with A, B, and C is spawned. This makes it hard to trust that your game logic will run without crashing.
In this pr, I switched to using `ComponentId` instead. This _is_ more constraining. `maybe_conflicts_system` will now always fail, but it will do it consistently at startup. Naively, it would also _disallow_ `filter_system`, which would be a significant downgrade in usability. Bevy has a number of internal systems that rely on disjoint queries and I expect it to be a common pattern in userspace.
To resolve this, I added a new `FilteredAccess<T>` type, which wraps `Access<T>` and adds with/without filters. If two `FilteredAccess` have with/without values that prove they are disjoint, they will no longer conflict.
## EntityRef / EntityMut
World entity operations on `main` require that the user passes in an `entity` id to each operation:
```rust
let entity = world.spawn((A, )); // create a new entity with A
world.get::<A>(entity);
world.insert(entity, (B, C));
world.insert_one(entity, D);
```
This means that each operation needs to look up the entity location / verify its validity. The initial spawn operation also requires a Bundle as input. This can be awkward when no components are required (or one component is required).
These operations have been replaced by `EntityRef` and `EntityMut`, which are "builder-style" wrappers around world that provide read and read/write operations on a single, pre-validated entity:
```rust
// spawn now takes no inputs and returns an EntityMut
let entity = world.spawn()
.insert(A) // insert a single component into the entity
.insert_bundle((B, C)) // insert a bundle of components into the entity
.id() // id returns the Entity id
// Returns EntityMut (or panics if the entity does not exist)
world.entity_mut(entity)
.insert(D)
.insert_bundle(SomeBundle::default());
{
// returns EntityRef (or panics if the entity does not exist)
let d = world.entity(entity)
.get::<D>() // gets the D component
.unwrap();
// world.get still exists for ergonomics
let d = world.get::<D>(entity).unwrap();
}
// These variants return Options if you want to check existence instead of panicing
world.get_entity_mut(entity)
.unwrap()
.insert(E);
if let Some(entity_ref) = world.get_entity(entity) {
let d = entity_ref.get::<D>().unwrap();
}
```
This _does not_ affect the current Commands api or terminology. I think that should be a separate conversation as that is a much larger breaking change.
## Safety Improvements
* Entity reservation in Commands uses a normal world borrow instead of an unsafe transmute
* QuerySets no longer transmutes lifetimes
* Made traits "unsafe" when implementing a trait incorrectly could cause unsafety
* More thorough safety docs
## RemovedComponents SystemParam
The old approach to querying removed components: `query.removed:<T>()` was confusing because it had no connection to the query itself. I replaced it with the following, which is both clearer and allows us to cache the ComponentId mapping in the SystemParamState:
```rust
fn system(removed: RemovedComponents<T>) {
for entity in removed.iter() {
}
}
```
## Simpler Bundle implementation
Bundles are no longer responsible for sorting (or deduping) TypeInfo. They are just a simple ordered list of component types / data. This makes the implementation smaller and opens the door to an easy "nested bundle" implementation in the future (which i might even add in this pr). Duplicate detection is now done once per bundle type by World the first time a bundle is used.
## Unified WorldQuery and QueryFilter types
(don't worry they are still separate type _parameters_ in Queries .. this is a non-breaking change)
WorldQuery and QueryFilter were already basically identical apis. With the addition of `FetchState` and more storage-specific fetch methods, the overlap was even clearer (and the redundancy more painful).
QueryFilters are now just `F: WorldQuery where F::Fetch: FilterFetch`. FilterFetch requires `Fetch<Item = bool>` and adds new "short circuit" variants of fetch methods. This enables a filter tuple like `(With<A>, Without<B>, Changed<C>)` to stop evaluating the filter after the first mismatch is encountered. FilterFetch is automatically implemented for `Fetch` implementations that return bool.
This forces fetch implementations that return things like `(bool, bool, bool)` (such as the filter above) to manually implement FilterFetch and decide whether or not to short-circuit.
## More Granular Modules
World no longer globs all of the internal modules together. It now exports `core`, `system`, and `schedule` separately. I'm also considering exporting `core` submodules directly as that is still pretty "glob-ey" and unorganized (feedback welcome here).
## Remaining Draft Work (to be done in this pr)
* ~~panic on conflicting WorldQuery fetches (&A, &mut A)~~
* ~~bevy `main` and hecs both currently allow this, but we should protect against it if possible~~
* ~~batch_iter / par_iter (currently stubbed out)~~
* ~~ChangedRes~~
* ~~I skipped this while we sort out #1313. This pr should be adapted to account for whatever we land on there~~.
* ~~The `Archetypes` and `Tables` collections use hashes of sorted lists of component ids to uniquely identify each archetype/table. This hash is then used as the key in a HashMap to look up the relevant ArchetypeId or TableId. (which doesn't handle hash collisions properly)~~
* ~~It is currently unsafe to generate a Query from "World A", then use it on "World B" (despite the api claiming it is safe). We should probably close this gap. This could be done by adding a randomly generated WorldId to each world, then storing that id in each Query. They could then be compared to each other on each `query.do_thing(&world)` operation. This _does_ add an extra branch to each query operation, so I'm open to other suggestions if people have them.~~
* ~~Nested Bundles (if i find time)~~
## Potential Future Work
* Expand WorldCell to support queries.
* Consider not allocating in the empty archetype on `world.spawn()`
* ex: return something like EntityMutUninit, which turns into EntityMut after an `insert` or `insert_bundle` op
* this actually regressed performance last time i tried it, but in theory it should be faster
* Optimize SparseSet::insert (see `PERF` comment on insert)
* Replace SparseArray `Option<T>` with T::MAX to cut down on branching
* would enable cheaper get_unchecked() operations
* upstream fixedbitset optimizations
* fixedbitset could be allocation free for small block counts (store blocks in a SmallVec)
* fixedbitset could have a const constructor
* Consider implementing Tags (archetype-specific by-value data that affects archetype identity)
* ex: ArchetypeA could have `[A, B, C]` table components and `[D(1)]` "tag" component. ArchetypeB could have `[A, B, C]` table components and a `[D(2)]` tag component. The archetypes are different, despite both having D tags because the value inside D is different.
* this could potentially build on top of the `archetype.unique_components` added in this pr for resource storage.
* Consider reverting `all_tuples` proc macro in favor of the old `macro_rules` implementation
* all_tuples is more flexible and produces cleaner documentation (the macro_rules version produces weird type parameter orders due to parser constraints)
* but unfortunately all_tuples also appears to make Rust Analyzer sad/slow when working inside of `bevy_ecs` (does not affect user code)
* Consider "resource queries" and/or "mixed resource and entity component queries" as an alternative to WorldCell
* this is basically just "systems" so maybe it's not worth it
* Add more world ops
* `world.clear()`
* `world.reserve<T: Bundle>(count: usize)`
* Try using the old archetype allocation strategy (allocate new memory on resize and copy everything over). I expect this to improve batch insertion performance at the cost of unbatched performance. But thats just a guess. I'm not an allocation perf pro :)
* Adapt Commands apis for consistency with new World apis
## Benchmarks
key:
* `bevy_old`: bevy `main` branch
* `bevy`: this branch
* `_foreach`: uses an optimized for_each iterator
* ` _sparse`: uses sparse set storage (if unspecified assume table storage)
* `_system`: runs inside a system (if unspecified assume test happens via direct world ops)
### Simple Insert (from ecs_bench_suite)
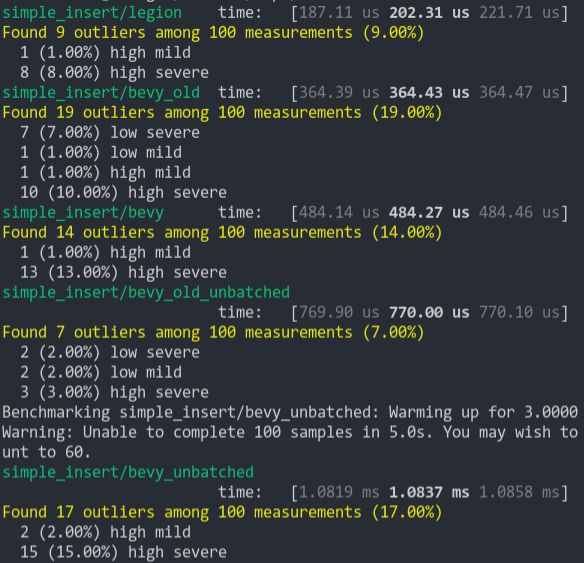
### Simpler Iter (from ecs_bench_suite)
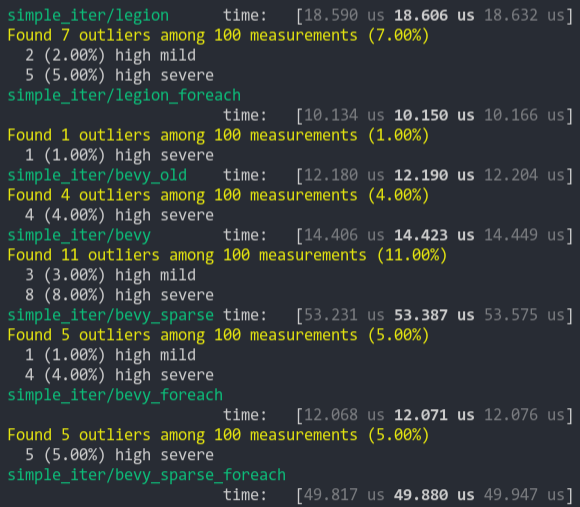
### Fragment Iter (from ecs_bench_suite)
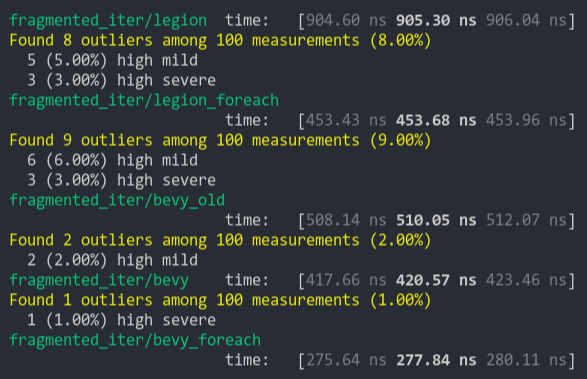
### Sparse Fragmented Iter
Iterate a query that matches 5 entities from a single matching archetype, but there are 100 unmatching archetypes
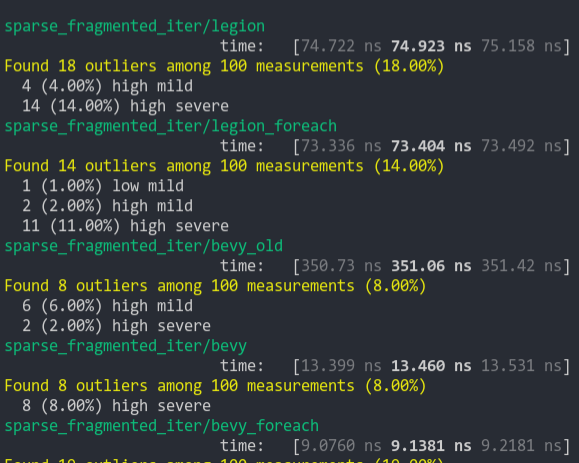
### Schedule (from ecs_bench_suite)
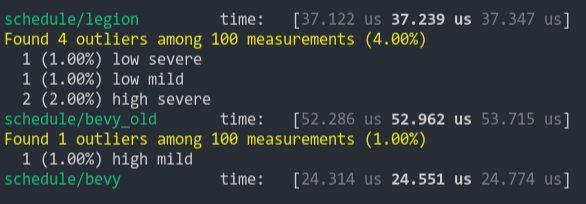
### Add Remove Component (from ecs_bench_suite)
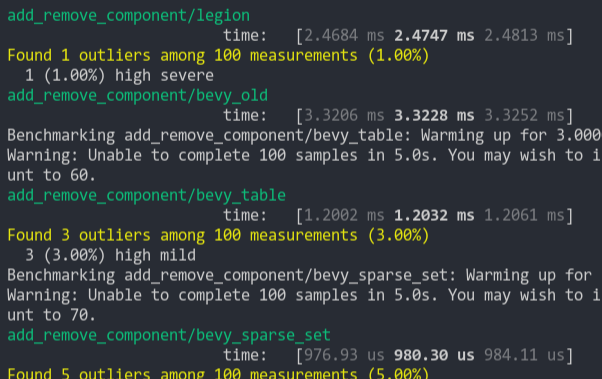
### Add Remove Component Big
Same as the test above, but each entity has 5 "large" matrix components and 1 "large" matrix component is added and removed
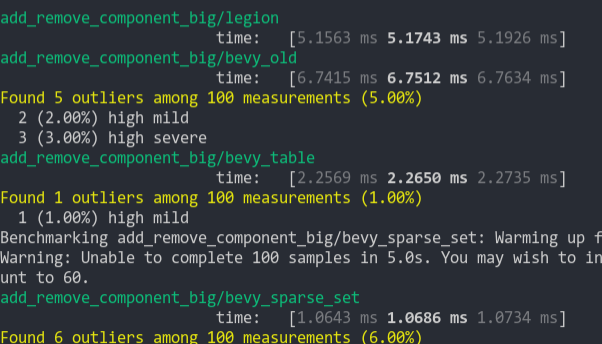
### Get Component
Looks up a single component value a large number of times
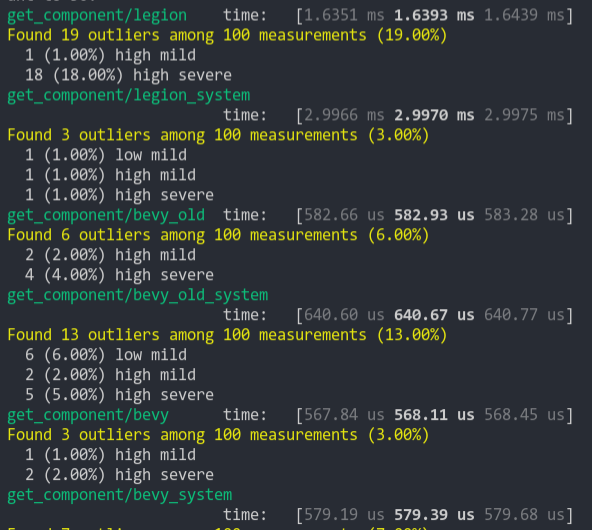
2021-03-05 07:54:35 +00:00
fn deref ( & self ) -> & Self ::Target {
self . value
}
2020-12-16 05:57:16 +00:00
}
2022-02-04 03:07:21 +00:00
impl < ' a , T > From < NonSendMut < ' a , T > > for NonSend < ' a , T > {
fn from ( nsm : NonSendMut < ' a , T > ) -> Self {
Self {
value : nsm . value ,
2022-11-21 12:59:09 +00:00
ticks : ComponentTicks {
added : nsm . ticks . added . to_owned ( ) ,
changed : nsm . ticks . changed . to_owned ( ) ,
} ,
2022-02-04 03:07:21 +00:00
change_tick : nsm . ticks . change_tick ,
last_change_tick : nsm . ticks . last_change_tick ,
}
}
}
2020-12-16 05:57:16 +00:00
2022-07-04 14:44:24 +00:00
// SAFETY: NonSendComponentId and ArchetypeComponentId access is applied to SystemMeta. If this
2021-03-11 00:27:30 +00:00
// NonSend conflicts with any prior access, a panic will occur.
Remove the `SystemParamState` trait and remove types like `ResState` (#6919)
Spiritual successor to #5205.
Actual successor to #6865.
# Objective
Currently, system params are defined using three traits: `SystemParam`, `ReadOnlySystemParam`, `SystemParamState`. The behavior for each param is specified by the `SystemParamState` trait, while `SystemParam` simply defers to the state.
Splitting the traits in this way makes it easier to implement within macros, but it increases the cognitive load. Worst of all, this approach requires each `MySystemParam` to have a public `MySystemParamState` type associated with it.
## Solution
* Merge the trait `SystemParamState` into `SystemParam`.
* Remove all trivial `SystemParam` state types.
* `OptionNonSendMutState<T>`: you will not be missed.
---
- [x] Fix/resolve the remaining test failure.
## Changelog
* Removed the trait `SystemParamState`, merging its functionality into `SystemParam`.
## Migration Guide
**Note**: this should replace the migration guide for #6865.
This is relative to Bevy 0.9, not main.
The traits `SystemParamState` and `SystemParamFetch` have been removed, and their functionality has been transferred to `SystemParam`.
```rust
// Before (0.9)
impl SystemParam for MyParam<'_, '_> {
type State = MyParamState;
}
unsafe impl SystemParamState for MyParamState {
fn init(world: &mut World, system_meta: &mut SystemMeta) -> Self { ... }
}
unsafe impl<'w, 's> SystemParamFetch<'w, 's> for MyParamState {
type Item = MyParam<'w, 's>;
fn get_param(&mut self, ...) -> Self::Item;
}
unsafe impl ReadOnlySystemParamFetch for MyParamState { }
// After (0.10)
unsafe impl SystemParam for MyParam<'_, '_> {
type State = MyParamState;
type Item<'w, 's> = MyParam<'w, 's>;
fn init_state(world: &mut World, system_meta: &mut SystemMeta) -> Self::State { ... }
fn get_param<'w, 's>(state: &mut Self::State, ...) -> Self::Item<'w, 's>;
}
unsafe impl ReadOnlySystemParam for MyParam<'_, '_> { }
```
The trait `ReadOnlySystemParamFetch` has been replaced with `ReadOnlySystemParam`.
```rust
// Before
unsafe impl ReadOnlySystemParamFetch for MyParamState {}
// After
unsafe impl ReadOnlySystemParam for MyParam<'_, '_> {}
```
2023-01-07 23:20:32 +00:00
unsafe impl < ' a , T : 'static > SystemParam for NonSend < ' a , T > {
type State = ComponentId ;
Simplify trait hierarchy for `SystemParam` (#6865)
# Objective
* Implementing a custom `SystemParam` by hand requires implementing three traits -- four if it is read-only.
* The trait `SystemParamFetch<'w, 's>` is a workaround from before we had generic associated types, and is no longer necessary.
## Solution
* Combine the trait `SystemParamFetch` with `SystemParamState`.
* I decided to remove the `Fetch` name and keep the `State` name, since the former was consistently conflated with the latter.
* Replace the trait `ReadOnlySystemParamFetch` with `ReadOnlySystemParam`, which simplifies trait bounds in generic code.
---
## Changelog
- Removed the trait `SystemParamFetch`, moving its functionality to `SystemParamState`.
- Replaced the trait `ReadOnlySystemParamFetch` with `ReadOnlySystemParam`.
## Migration Guide
The trait `SystemParamFetch` has been removed, and its functionality has been transferred to `SystemParamState`.
```rust
// Before
impl SystemParamState for MyParamState {
fn init(world: &mut World, system_meta: &mut SystemMeta) -> Self { ... }
}
impl<'w, 's> SystemParamFetch<'w, 's> for MyParamState {
type Item = MyParam<'w, 's>;
fn get_param(...) -> Self::Item;
}
// After
impl SystemParamState for MyParamState {
type Item<'w, 's> = MyParam<'w, 's>; // Generic associated types!
fn init(world: &mut World, system_meta: &mut SystemMeta) -> Self { ... }
fn get_param<'w, 's>(...) -> Self::Item<'w, 's>;
}
```
The trait `ReadOnlySystemParamFetch` has been replaced with `ReadOnlySystemParam`.
```rust
// Before
unsafe impl ReadOnlySystemParamFetch for MyParamState {}
// After
unsafe impl<'w, 's> ReadOnlySystemParam for MyParam<'w, 's> {}
```
2022-12-11 18:34:14 +00:00
type Item < ' w , ' s > = NonSend < ' w , T > ;
Remove the `SystemParamState` trait and remove types like `ResState` (#6919)
Spiritual successor to #5205.
Actual successor to #6865.
# Objective
Currently, system params are defined using three traits: `SystemParam`, `ReadOnlySystemParam`, `SystemParamState`. The behavior for each param is specified by the `SystemParamState` trait, while `SystemParam` simply defers to the state.
Splitting the traits in this way makes it easier to implement within macros, but it increases the cognitive load. Worst of all, this approach requires each `MySystemParam` to have a public `MySystemParamState` type associated with it.
## Solution
* Merge the trait `SystemParamState` into `SystemParam`.
* Remove all trivial `SystemParam` state types.
* `OptionNonSendMutState<T>`: you will not be missed.
---
- [x] Fix/resolve the remaining test failure.
## Changelog
* Removed the trait `SystemParamState`, merging its functionality into `SystemParam`.
## Migration Guide
**Note**: this should replace the migration guide for #6865.
This is relative to Bevy 0.9, not main.
The traits `SystemParamState` and `SystemParamFetch` have been removed, and their functionality has been transferred to `SystemParam`.
```rust
// Before (0.9)
impl SystemParam for MyParam<'_, '_> {
type State = MyParamState;
}
unsafe impl SystemParamState for MyParamState {
fn init(world: &mut World, system_meta: &mut SystemMeta) -> Self { ... }
}
unsafe impl<'w, 's> SystemParamFetch<'w, 's> for MyParamState {
type Item = MyParam<'w, 's>;
fn get_param(&mut self, ...) -> Self::Item;
}
unsafe impl ReadOnlySystemParamFetch for MyParamState { }
// After (0.10)
unsafe impl SystemParam for MyParam<'_, '_> {
type State = MyParamState;
type Item<'w, 's> = MyParam<'w, 's>;
fn init_state(world: &mut World, system_meta: &mut SystemMeta) -> Self::State { ... }
fn get_param<'w, 's>(state: &mut Self::State, ...) -> Self::Item<'w, 's>;
}
unsafe impl ReadOnlySystemParam for MyParam<'_, '_> { }
```
The trait `ReadOnlySystemParamFetch` has been replaced with `ReadOnlySystemParam`.
```rust
// Before
unsafe impl ReadOnlySystemParamFetch for MyParamState {}
// After
unsafe impl ReadOnlySystemParam for MyParam<'_, '_> {}
```
2023-01-07 23:20:32 +00:00
fn init_state ( world : & mut World , system_meta : & mut SystemMeta ) -> Self ::State {
Add new SystemState and rename old SystemState to SystemMeta (#2283)
This enables `SystemParams` to be used outside of function systems. Anything can create and store `SystemState`, which enables efficient "param state cached" access to `SystemParams`.
It adds a `ReadOnlySystemParamFetch` trait, which enables safe `SystemState::get` calls without unique world access.
I renamed the old `SystemState` to `SystemMeta` to enable us to mirror the `QueryState` naming convention (but I'm happy to discuss alternative names if people have other ideas). I initially pitched this as `ParamState`, but given that it needs to include full system metadata, that doesn't feel like a particularly accurate name.
```rust
#[derive(Eq, PartialEq, Debug)]
struct A(usize);
#[derive(Eq, PartialEq, Debug)]
struct B(usize);
let mut world = World::default();
world.insert_resource(A(42));
world.spawn().insert(B(7));
// we get nice lifetime elision when declaring the type on the left hand side
let mut system_state: SystemState<(Res<A>, Query<&B>)> = SystemState::new(&mut world);
let (a, query) = system_state.get(&world);
assert_eq!(*a, A(42), "returned resource matches initial value");
assert_eq!(
*query.single().unwrap(),
B(7),
"returned component matches initial value"
);
// mutable system params require unique world access
let mut system_state: SystemState<(ResMut<A>, Query<&mut B>)> = SystemState::new(&mut world);
let (a, query) = system_state.get_mut(&mut world);
// static lifetimes are required when declaring inside of structs
struct SomeContainer {
state: SystemState<(Res<'static, A>, Res<'static, B>)>
}
// this can be shortened using type aliases, which will be useful for complex param tuples
type MyParams<'a> = (Res<'a, A>, Res<'a, B>);
struct SomeContainer {
state: SystemState<MyParams<'static>>
}
// It is the user's responsibility to call SystemState::apply(world) for parameters that queue up work
let mut system_state: SystemState<(Commands, Query<&B>)> = SystemState::new(&mut world);
{
let (mut commands, query) = system_state.get(&world);
commands.insert_resource(3.14);
}
system_state.apply(&mut world);
```
## Future Work
* Actually use SystemState inside FunctionSystem. This would be trivial, but it requires FunctionSystem to wrap SystemState in Option in its current form (which complicates system metadata lookup). I'd prefer to hold off until we adopt something like the later designs linked in #1364, which enable us to contruct Systems using a World reference (and also remove the need for `.system`).
* Consider a "scoped" approach to automatically call SystemState::apply when systems params are no longer being used (either a container type with a Drop impl, or a function that takes a closure for user logic operating on params).
2021-06-02 19:57:38 +00:00
system_meta . set_non_send ( ) ;
Bevy ECS V2 (#1525)
# Bevy ECS V2
This is a rewrite of Bevy ECS (basically everything but the new executor/schedule, which are already awesome). The overall goal was to improve the performance and versatility of Bevy ECS. Here is a quick bulleted list of changes before we dive into the details:
* Complete World rewrite
* Multiple component storage types:
* Tables: fast cache friendly iteration, slower add/removes (previously called Archetypes)
* Sparse Sets: fast add/remove, slower iteration
* Stateful Queries (caches query results for faster iteration. fragmented iteration is _fast_ now)
* Stateful System Params (caches expensive operations. inspired by @DJMcNab's work in #1364)
* Configurable System Params (users can set configuration when they construct their systems. once again inspired by @DJMcNab's work)
* Archetypes are now "just metadata", component storage is separate
* Archetype Graph (for faster archetype changes)
* Component Metadata
* Configure component storage type
* Retrieve information about component size/type/name/layout/send-ness/etc
* Components are uniquely identified by a densely packed ComponentId
* TypeIds are now totally optional (which should make implementing scripting easier)
* Super fast "for_each" query iterators
* Merged Resources into World. Resources are now just a special type of component
* EntityRef/EntityMut builder apis (more efficient and more ergonomic)
* Fast bitset-backed `Access<T>` replaces old hashmap-based approach everywhere
* Query conflicts are determined by component access instead of archetype component access (to avoid random failures at runtime)
* With/Without are still taken into account for conflicts, so this should still be comfy to use
* Much simpler `IntoSystem` impl
* Significantly reduced the amount of hashing throughout the ecs in favor of Sparse Sets (indexed by densely packed ArchetypeId, ComponentId, BundleId, and TableId)
* Safety Improvements
* Entity reservation uses a normal world reference instead of unsafe transmute
* QuerySets no longer transmute lifetimes
* Made traits "unsafe" where relevant
* More thorough safety docs
* WorldCell
* Exposes safe mutable access to multiple resources at a time in a World
* Replaced "catch all" `System::update_archetypes(world: &World)` with `System::new_archetype(archetype: &Archetype)`
* Simpler Bundle implementation
* Replaced slow "remove_bundle_one_by_one" used as fallback for Commands::remove_bundle with fast "remove_bundle_intersection"
* Removed `Mut<T>` query impl. it is better to only support one way: `&mut T`
* Removed with() from `Flags<T>` in favor of `Option<Flags<T>>`, which allows querying for flags to be "filtered" by default
* Components now have is_send property (currently only resources support non-send)
* More granular module organization
* New `RemovedComponents<T>` SystemParam that replaces `query.removed::<T>()`
* `world.resource_scope()` for mutable access to resources and world at the same time
* WorldQuery and QueryFilter traits unified. FilterFetch trait added to enable "short circuit" filtering. Auto impled for cases that don't need it
* Significantly slimmed down SystemState in favor of individual SystemParam state
* System Commands changed from `commands: &mut Commands` back to `mut commands: Commands` (to allow Commands to have a World reference)
Fixes #1320
## `World` Rewrite
This is a from-scratch rewrite of `World` that fills the niche that `hecs` used to. Yes, this means Bevy ECS is no longer a "fork" of hecs. We're going out our own!
(the only shared code between the projects is the entity id allocator, which is already basically ideal)
A huge shout out to @SanderMertens (author of [flecs](https://github.com/SanderMertens/flecs)) for sharing some great ideas with me (specifically hybrid ecs storage and archetype graphs). He also helped advise on a number of implementation details.
## Component Storage (The Problem)
Two ECS storage paradigms have gained a lot of traction over the years:
* **Archetypal ECS**:
* Stores components in "tables" with static schemas. Each "column" stores components of a given type. Each "row" is an entity.
* Each "archetype" has its own table. Adding/removing an entity's component changes the archetype.
* Enables super-fast Query iteration due to its cache-friendly data layout
* Comes at the cost of more expensive add/remove operations for an Entity's components, because all components need to be copied to the new archetype's "table"
* **Sparse Set ECS**:
* Stores components of the same type in densely packed arrays, which are sparsely indexed by densely packed unsigned integers (Entity ids)
* Query iteration is slower than Archetypal ECS because each entity's component could be at any position in the sparse set. This "random access" pattern isn't cache friendly. Additionally, there is an extra layer of indirection because you must first map the entity id to an index in the component array.
* Adding/removing components is a cheap, constant time operation
Bevy ECS V1, hecs, legion, flec, and Unity DOTS are all "archetypal ecs-es". I personally think "archetypal" storage is a good default for game engines. An entity's archetype doesn't need to change frequently in general, and it creates "fast by default" query iteration (which is a much more common operation). It is also "self optimizing". Users don't need to think about optimizing component layouts for iteration performance. It "just works" without any extra boilerplate.
Shipyard and EnTT are "sparse set ecs-es". They employ "packing" as a way to work around the "suboptimal by default" iteration performance for specific sets of components. This helps, but I didn't think this was a good choice for a general purpose engine like Bevy because:
1. "packs" conflict with each other. If bevy decides to internally pack the Transform and GlobalTransform components, users are then blocked if they want to pack some custom component with Transform.
2. users need to take manual action to optimize
Developers selecting an ECS framework are stuck with a hard choice. Select an "archetypal" framework with "fast iteration everywhere" but without the ability to cheaply add/remove components, or select a "sparse set" framework to cheaply add/remove components but with slower iteration performance.
## Hybrid Component Storage (The Solution)
In Bevy ECS V2, we get to have our cake and eat it too. It now has _both_ of the component storage types above (and more can be added later if needed):
* **Tables** (aka "archetypal" storage)
* The default storage. If you don't configure anything, this is what you get
* Fast iteration by default
* Slower add/remove operations
* **Sparse Sets**
* Opt-in
* Slower iteration
* Faster add/remove operations
These storage types complement each other perfectly. By default Query iteration is fast. If developers know that they want to add/remove a component at high frequencies, they can set the storage to "sparse set":
```rust
world.register_component(
ComponentDescriptor::new::<MyComponent>(StorageType::SparseSet)
).unwrap();
```
## Archetypes
Archetypes are now "just metadata" ... they no longer store components directly. They do store:
* The `ComponentId`s of each of the Archetype's components (and that component's storage type)
* Archetypes are uniquely defined by their component layouts
* For example: entities with "table" components `[A, B, C]` _and_ "sparse set" components `[D, E]` will always be in the same archetype.
* The `TableId` associated with the archetype
* For now each archetype has exactly one table (which can have no components),
* There is a 1->Many relationship from Tables->Archetypes. A given table could have any number of archetype components stored in it:
* Ex: an entity with "table storage" components `[A, B, C]` and "sparse set" components `[D, E]` will share the same `[A, B, C]` table as an entity with `[A, B, C]` table component and `[F]` sparse set components.
* This 1->Many relationship is how we preserve fast "cache friendly" iteration performance when possible (more on this later)
* A list of entities that are in the archetype and the row id of the table they are in
* ArchetypeComponentIds
* unique densely packed identifiers for (ArchetypeId, ComponentId) pairs
* used by the schedule executor for cheap system access control
* "Archetype Graph Edges" (see the next section)
## The "Archetype Graph"
Archetype changes in Bevy (and a number of other archetypal ecs-es) have historically been expensive to compute. First, you need to allocate a new vector of the entity's current component ids, add or remove components based on the operation performed, sort it (to ensure it is order-independent), then hash it to find the archetype (if it exists). And thats all before we get to the _already_ expensive full copy of all components to the new table storage.
The solution is to build a "graph" of archetypes to cache these results. @SanderMertens first exposed me to the idea (and he got it from @gjroelofs, who came up with it). They propose adding directed edges between archetypes for add/remove component operations. If `ComponentId`s are densely packed, you can use sparse sets to cheaply jump between archetypes.
Bevy takes this one step further by using add/remove `Bundle` edges instead of `Component` edges. Bevy encourages the use of `Bundles` to group add/remove operations. This is largely for "clearer game logic" reasons, but it also helps cut down on the number of archetype changes required. `Bundles` now also have densely-packed `BundleId`s. This allows us to use a _single_ edge for each bundle operation (rather than needing to traverse N edges ... one for each component). Single component operations are also bundles, so this is strictly an improvement over a "component only" graph.
As a result, an operation that used to be _heavy_ (both for allocations and compute) is now two dirt-cheap array lookups and zero allocations.
## Stateful Queries
World queries are now stateful. This allows us to:
1. Cache archetype (and table) matches
* This resolves another issue with (naive) archetypal ECS: query performance getting worse as the number of archetypes goes up (and fragmentation occurs).
2. Cache Fetch and Filter state
* The expensive parts of fetch/filter operations (such as hashing the TypeId to find the ComponentId) now only happen once when the Query is first constructed
3. Incrementally build up state
* When new archetypes are added, we only process the new archetypes (no need to rebuild state for old archetypes)
As a result, the direct `World` query api now looks like this:
```rust
let mut query = world.query::<(&A, &mut B)>();
for (a, mut b) in query.iter_mut(&mut world) {
}
```
Requiring `World` to generate stateful queries (rather than letting the `QueryState` type be constructed separately) allows us to ensure that _all_ queries are properly initialized (and the relevant world state, such as ComponentIds). This enables QueryState to remove branches from its operations that check for initialization status (and also enables query.iter() to take an immutable world reference because it doesn't need to initialize anything in world).
However in systems, this is a non-breaking change. State management is done internally by the relevant SystemParam.
## Stateful SystemParams
Like Queries, `SystemParams` now also cache state. For example, `Query` system params store the "stateful query" state mentioned above. Commands store their internal `CommandQueue`. This means you can now safely use as many separate `Commands` parameters in your system as you want. `Local<T>` system params store their `T` value in their state (instead of in Resources).
SystemParam state also enabled a significant slim-down of SystemState. It is much nicer to look at now.
Per-SystemParam state naturally insulates us from an "aliased mut" class of errors we have hit in the past (ex: using multiple `Commands` system params).
(credit goes to @DJMcNab for the initial idea and draft pr here #1364)
## Configurable SystemParams
@DJMcNab also had the great idea to make SystemParams configurable. This allows users to provide some initial configuration / values for system parameters (when possible). Most SystemParams have no config (the config type is `()`), but the `Local<T>` param now supports user-provided parameters:
```rust
fn foo(value: Local<usize>) {
}
app.add_system(foo.system().config(|c| c.0 = Some(10)));
```
## Uber Fast "for_each" Query Iterators
Developers now have the choice to use a fast "for_each" iterator, which yields ~1.5-3x iteration speed improvements for "fragmented iteration", and minor ~1.2x iteration speed improvements for unfragmented iteration.
```rust
fn system(query: Query<(&A, &mut B)>) {
// you now have the option to do this for a speed boost
query.for_each_mut(|(a, mut b)| {
});
// however normal iterators are still available
for (a, mut b) in query.iter_mut() {
}
}
```
I think in most cases we should continue to encourage "normal" iterators as they are more flexible and more "rust idiomatic". But when that extra "oomf" is needed, it makes sense to use `for_each`.
We should also consider using `for_each` for internal bevy systems to give our users a nice speed boost (but that should be a separate pr).
## Component Metadata
`World` now has a `Components` collection, which is accessible via `world.components()`. This stores mappings from `ComponentId` to `ComponentInfo`, as well as `TypeId` to `ComponentId` mappings (where relevant). `ComponentInfo` stores information about the component, such as ComponentId, TypeId, memory layout, send-ness (currently limited to resources), and storage type.
## Significantly Cheaper `Access<T>`
We used to use `TypeAccess<TypeId>` to manage read/write component/archetype-component access. This was expensive because TypeIds must be hashed and compared individually. The parallel executor got around this by "condensing" type ids into bitset-backed access types. This worked, but it had to be re-generated from the `TypeAccess<TypeId>`sources every time archetypes changed.
This pr removes TypeAccess in favor of faster bitset access everywhere. We can do this thanks to the move to densely packed `ComponentId`s and `ArchetypeComponentId`s.
## Merged Resources into World
Resources had a lot of redundant functionality with Components. They stored typed data, they had access control, they had unique ids, they were queryable via SystemParams, etc. In fact the _only_ major difference between them was that they were unique (and didn't correlate to an entity).
Separate resources also had the downside of requiring a separate set of access controls, which meant the parallel executor needed to compare more bitsets per system and manage more state.
I initially got the "separate resources" idea from `legion`. I think that design was motivated by the fact that it made the direct world query/resource lifetime interactions more manageable. It certainly made our lives easier when using Resources alongside hecs/bevy_ecs. However we already have a construct for safely and ergonomically managing in-world lifetimes: systems (which use `Access<T>` internally).
This pr merges Resources into World:
```rust
world.insert_resource(1);
world.insert_resource(2.0);
let a = world.get_resource::<i32>().unwrap();
let mut b = world.get_resource_mut::<f64>().unwrap();
*b = 3.0;
```
Resources are now just a special kind of component. They have their own ComponentIds (and their own resource TypeId->ComponentId scope, so they don't conflict wit components of the same type). They are stored in a special "resource archetype", which stores components inside the archetype using a new `unique_components` sparse set (note that this sparse set could later be used to implement Tags). This allows us to keep the code size small by reusing existing datastructures (namely Column, Archetype, ComponentFlags, and ComponentInfo). This allows us the executor to use a single `Access<ArchetypeComponentId>` per system. It should also make scripting language integration easier.
_But_ this merge did create problems for people directly interacting with `World`. What if you need mutable access to multiple resources at the same time? `world.get_resource_mut()` borrows World mutably!
## WorldCell
WorldCell applies the `Access<ArchetypeComponentId>` concept to direct world access:
```rust
let world_cell = world.cell();
let a = world_cell.get_resource_mut::<i32>().unwrap();
let b = world_cell.get_resource_mut::<f64>().unwrap();
```
This adds cheap runtime checks (a sparse set lookup of `ArchetypeComponentId` and a counter) to ensure that world accesses do not conflict with each other. Each operation returns a `WorldBorrow<'w, T>` or `WorldBorrowMut<'w, T>` wrapper type, which will release the relevant ArchetypeComponentId resources when dropped.
World caches the access sparse set (and only one cell can exist at a time), so `world.cell()` is a cheap operation.
WorldCell does _not_ use atomic operations. It is non-send, does a mutable borrow of world to prevent other accesses, and uses a simple `Rc<RefCell<ArchetypeComponentAccess>>` wrapper in each WorldBorrow pointer.
The api is currently limited to resource access, but it can and should be extended to queries / entity component access.
## Resource Scopes
WorldCell does not yet support component queries, and even when it does there are sometimes legitimate reasons to want a mutable world ref _and_ a mutable resource ref (ex: bevy_render and bevy_scene both need this). In these cases we could always drop down to the unsafe `world.get_resource_unchecked_mut()`, but that is not ideal!
Instead developers can use a "resource scope"
```rust
world.resource_scope(|world: &mut World, a: &mut A| {
})
```
This temporarily removes the `A` resource from `World`, provides mutable pointers to both, and re-adds A to World when finished. Thanks to the move to ComponentIds/sparse sets, this is a cheap operation.
If multiple resources are required, scopes can be nested. We could also consider adding a "resource tuple" to the api if this pattern becomes common and the boilerplate gets nasty.
## Query Conflicts Use ComponentId Instead of ArchetypeComponentId
For safety reasons, systems cannot contain queries that conflict with each other without wrapping them in a QuerySet. On bevy `main`, we use ArchetypeComponentIds to determine conflicts. This is nice because it can take into account filters:
```rust
// these queries will never conflict due to their filters
fn filter_system(a: Query<&mut A, With<B>>, b: Query<&mut B, Without<B>>) {
}
```
But it also has a significant downside:
```rust
// these queries will not conflict _until_ an entity with A, B, and C is spawned
fn maybe_conflicts_system(a: Query<(&mut A, &C)>, b: Query<(&mut A, &B)>) {
}
```
The system above will panic at runtime if an entity with A, B, and C is spawned. This makes it hard to trust that your game logic will run without crashing.
In this pr, I switched to using `ComponentId` instead. This _is_ more constraining. `maybe_conflicts_system` will now always fail, but it will do it consistently at startup. Naively, it would also _disallow_ `filter_system`, which would be a significant downgrade in usability. Bevy has a number of internal systems that rely on disjoint queries and I expect it to be a common pattern in userspace.
To resolve this, I added a new `FilteredAccess<T>` type, which wraps `Access<T>` and adds with/without filters. If two `FilteredAccess` have with/without values that prove they are disjoint, they will no longer conflict.
## EntityRef / EntityMut
World entity operations on `main` require that the user passes in an `entity` id to each operation:
```rust
let entity = world.spawn((A, )); // create a new entity with A
world.get::<A>(entity);
world.insert(entity, (B, C));
world.insert_one(entity, D);
```
This means that each operation needs to look up the entity location / verify its validity. The initial spawn operation also requires a Bundle as input. This can be awkward when no components are required (or one component is required).
These operations have been replaced by `EntityRef` and `EntityMut`, which are "builder-style" wrappers around world that provide read and read/write operations on a single, pre-validated entity:
```rust
// spawn now takes no inputs and returns an EntityMut
let entity = world.spawn()
.insert(A) // insert a single component into the entity
.insert_bundle((B, C)) // insert a bundle of components into the entity
.id() // id returns the Entity id
// Returns EntityMut (or panics if the entity does not exist)
world.entity_mut(entity)
.insert(D)
.insert_bundle(SomeBundle::default());
{
// returns EntityRef (or panics if the entity does not exist)
let d = world.entity(entity)
.get::<D>() // gets the D component
.unwrap();
// world.get still exists for ergonomics
let d = world.get::<D>(entity).unwrap();
}
// These variants return Options if you want to check existence instead of panicing
world.get_entity_mut(entity)
.unwrap()
.insert(E);
if let Some(entity_ref) = world.get_entity(entity) {
let d = entity_ref.get::<D>().unwrap();
}
```
This _does not_ affect the current Commands api or terminology. I think that should be a separate conversation as that is a much larger breaking change.
## Safety Improvements
* Entity reservation in Commands uses a normal world borrow instead of an unsafe transmute
* QuerySets no longer transmutes lifetimes
* Made traits "unsafe" when implementing a trait incorrectly could cause unsafety
* More thorough safety docs
## RemovedComponents SystemParam
The old approach to querying removed components: `query.removed:<T>()` was confusing because it had no connection to the query itself. I replaced it with the following, which is both clearer and allows us to cache the ComponentId mapping in the SystemParamState:
```rust
fn system(removed: RemovedComponents<T>) {
for entity in removed.iter() {
}
}
```
## Simpler Bundle implementation
Bundles are no longer responsible for sorting (or deduping) TypeInfo. They are just a simple ordered list of component types / data. This makes the implementation smaller and opens the door to an easy "nested bundle" implementation in the future (which i might even add in this pr). Duplicate detection is now done once per bundle type by World the first time a bundle is used.
## Unified WorldQuery and QueryFilter types
(don't worry they are still separate type _parameters_ in Queries .. this is a non-breaking change)
WorldQuery and QueryFilter were already basically identical apis. With the addition of `FetchState` and more storage-specific fetch methods, the overlap was even clearer (and the redundancy more painful).
QueryFilters are now just `F: WorldQuery where F::Fetch: FilterFetch`. FilterFetch requires `Fetch<Item = bool>` and adds new "short circuit" variants of fetch methods. This enables a filter tuple like `(With<A>, Without<B>, Changed<C>)` to stop evaluating the filter after the first mismatch is encountered. FilterFetch is automatically implemented for `Fetch` implementations that return bool.
This forces fetch implementations that return things like `(bool, bool, bool)` (such as the filter above) to manually implement FilterFetch and decide whether or not to short-circuit.
## More Granular Modules
World no longer globs all of the internal modules together. It now exports `core`, `system`, and `schedule` separately. I'm also considering exporting `core` submodules directly as that is still pretty "glob-ey" and unorganized (feedback welcome here).
## Remaining Draft Work (to be done in this pr)
* ~~panic on conflicting WorldQuery fetches (&A, &mut A)~~
* ~~bevy `main` and hecs both currently allow this, but we should protect against it if possible~~
* ~~batch_iter / par_iter (currently stubbed out)~~
* ~~ChangedRes~~
* ~~I skipped this while we sort out #1313. This pr should be adapted to account for whatever we land on there~~.
* ~~The `Archetypes` and `Tables` collections use hashes of sorted lists of component ids to uniquely identify each archetype/table. This hash is then used as the key in a HashMap to look up the relevant ArchetypeId or TableId. (which doesn't handle hash collisions properly)~~
* ~~It is currently unsafe to generate a Query from "World A", then use it on "World B" (despite the api claiming it is safe). We should probably close this gap. This could be done by adding a randomly generated WorldId to each world, then storing that id in each Query. They could then be compared to each other on each `query.do_thing(&world)` operation. This _does_ add an extra branch to each query operation, so I'm open to other suggestions if people have them.~~
* ~~Nested Bundles (if i find time)~~
## Potential Future Work
* Expand WorldCell to support queries.
* Consider not allocating in the empty archetype on `world.spawn()`
* ex: return something like EntityMutUninit, which turns into EntityMut after an `insert` or `insert_bundle` op
* this actually regressed performance last time i tried it, but in theory it should be faster
* Optimize SparseSet::insert (see `PERF` comment on insert)
* Replace SparseArray `Option<T>` with T::MAX to cut down on branching
* would enable cheaper get_unchecked() operations
* upstream fixedbitset optimizations
* fixedbitset could be allocation free for small block counts (store blocks in a SmallVec)
* fixedbitset could have a const constructor
* Consider implementing Tags (archetype-specific by-value data that affects archetype identity)
* ex: ArchetypeA could have `[A, B, C]` table components and `[D(1)]` "tag" component. ArchetypeB could have `[A, B, C]` table components and a `[D(2)]` tag component. The archetypes are different, despite both having D tags because the value inside D is different.
* this could potentially build on top of the `archetype.unique_components` added in this pr for resource storage.
* Consider reverting `all_tuples` proc macro in favor of the old `macro_rules` implementation
* all_tuples is more flexible and produces cleaner documentation (the macro_rules version produces weird type parameter orders due to parser constraints)
* but unfortunately all_tuples also appears to make Rust Analyzer sad/slow when working inside of `bevy_ecs` (does not affect user code)
* Consider "resource queries" and/or "mixed resource and entity component queries" as an alternative to WorldCell
* this is basically just "systems" so maybe it's not worth it
* Add more world ops
* `world.clear()`
* `world.reserve<T: Bundle>(count: usize)`
* Try using the old archetype allocation strategy (allocate new memory on resize and copy everything over). I expect this to improve batch insertion performance at the cost of unbatched performance. But thats just a guess. I'm not an allocation perf pro :)
* Adapt Commands apis for consistency with new World apis
## Benchmarks
key:
* `bevy_old`: bevy `main` branch
* `bevy`: this branch
* `_foreach`: uses an optimized for_each iterator
* ` _sparse`: uses sparse set storage (if unspecified assume table storage)
* `_system`: runs inside a system (if unspecified assume test happens via direct world ops)
### Simple Insert (from ecs_bench_suite)
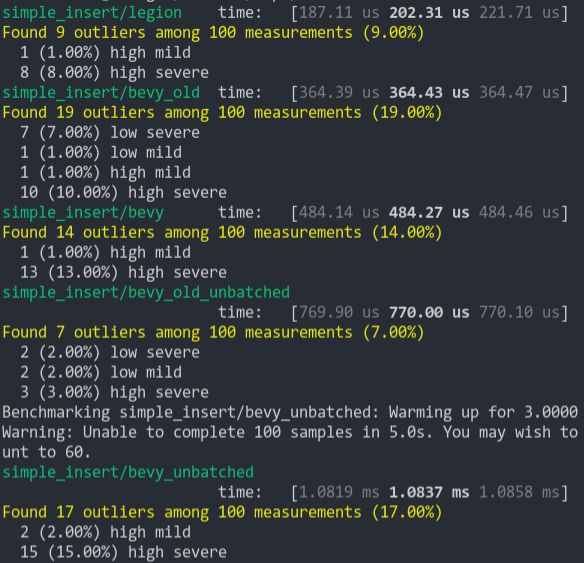
### Simpler Iter (from ecs_bench_suite)
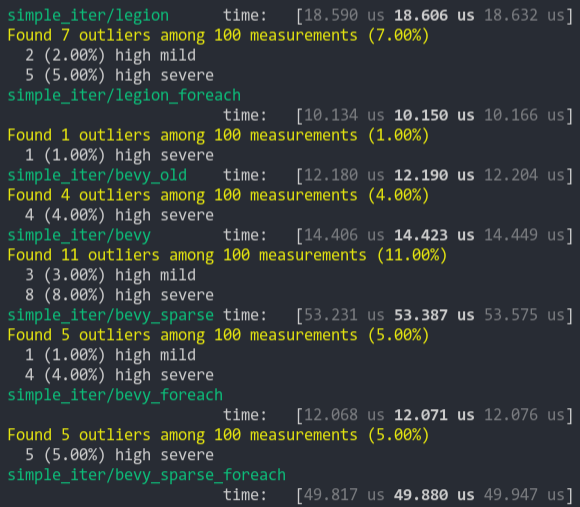
### Fragment Iter (from ecs_bench_suite)
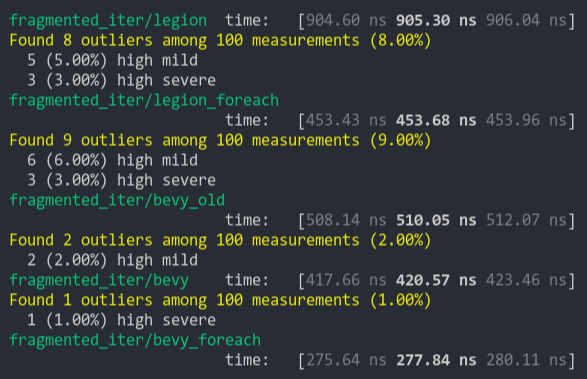
### Sparse Fragmented Iter
Iterate a query that matches 5 entities from a single matching archetype, but there are 100 unmatching archetypes
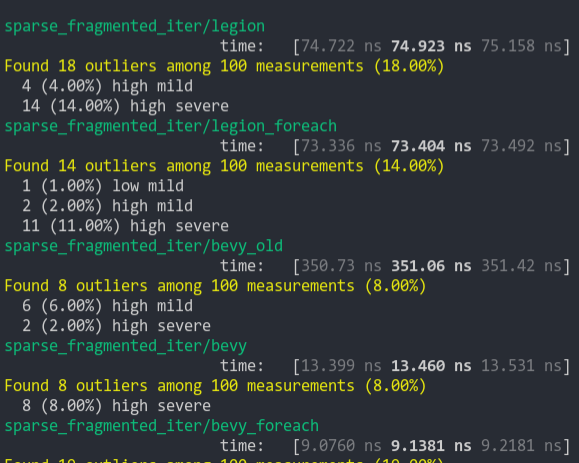
### Schedule (from ecs_bench_suite)
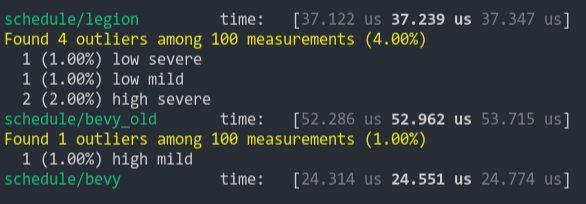
### Add Remove Component (from ecs_bench_suite)
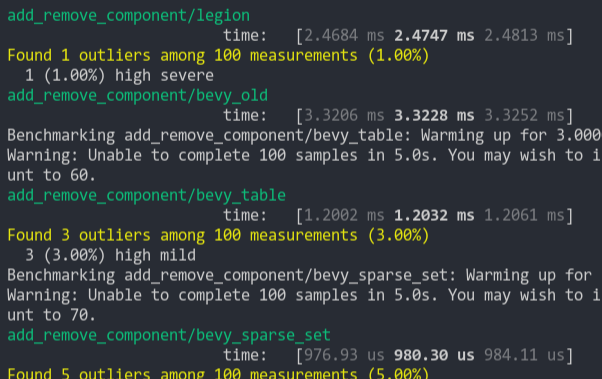
### Add Remove Component Big
Same as the test above, but each entity has 5 "large" matrix components and 1 "large" matrix component is added and removed
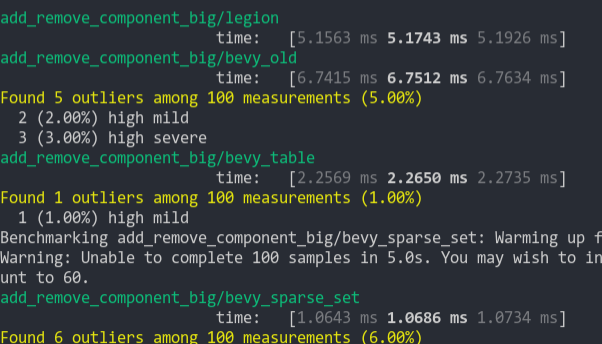
### Get Component
Looks up a single component value a large number of times
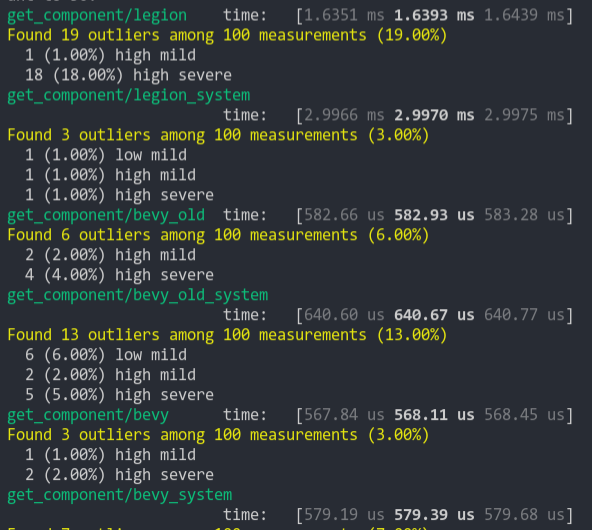
2021-03-05 07:54:35 +00:00
let component_id = world . initialize_non_send_resource ::< T > ( ) ;
Fix FilteredAccessSet get_conflicts inconsistency (#5105)
# Objective
* Enable `Res` and `Query` parameter mutual exclusion
* Required for https://github.com/bevyengine/bevy/pull/5080
The `FilteredAccessSet::get_conflicts` methods didn't work properly with
`Res` and `ResMut` parameters. Because those added their access by using
the `combined_access_mut` method and directly modifying the global
access state of the FilteredAccessSet. This caused an inconsistency,
because get_conflicts assumes that ALL added access have a corresponding
`FilteredAccess` added to the `filtered_accesses` field.
In practice, that means that SystemParam that adds their access through
the `Access` returned by `combined_access_mut` and the ones that add
their access using the `add` method lived in two different universes. As
a result, they could never be mutually exclusive.
## Solution
This commit fixes it by removing the `combined_access_mut` method. This
ensures that the `combined_access` field of FilteredAccessSet is always
updated consistently with the addition of a filter. When checking for
filtered access, it is now possible to account for `Res` and `ResMut`
invalid access. This is currently not needed, but might be in the
future.
We add the `add_unfiltered_{read,write}` methods to replace previous
usages of `combined_access_mut`.
We also add improved Debug implementations on FixedBitSet so that their
meaning is much clearer in debug output.
---
## Changelog
* Fix `Res` and `Query` parameter never being mutually exclusive.
## Migration Guide
Note: this mostly changes ECS internals, but since the API is public, it is technically breaking:
* Removed `FilteredAccessSet::combined_access_mut`
* Replace _immutable_ usage of those by `combined_access`
* For _mutable_ usages, use the new `add_unfiltered_{read,write}` methods instead of `combined_access_mut` followed by `add_{read,write}`
2022-11-16 11:05:48 +00:00
let combined_access = system_meta . component_access_set . combined_access ( ) ;
2022-02-13 22:33:55 +00:00
assert! (
! combined_access . has_write ( component_id ) ,
" error[B0002]: NonSend<{}> in system {} conflicts with a previous mutable resource access ({0}). Consider removing the duplicate access. " ,
std ::any ::type_name ::< T > ( ) ,
system_meta . name ,
) ;
Fix FilteredAccessSet get_conflicts inconsistency (#5105)
# Objective
* Enable `Res` and `Query` parameter mutual exclusion
* Required for https://github.com/bevyengine/bevy/pull/5080
The `FilteredAccessSet::get_conflicts` methods didn't work properly with
`Res` and `ResMut` parameters. Because those added their access by using
the `combined_access_mut` method and directly modifying the global
access state of the FilteredAccessSet. This caused an inconsistency,
because get_conflicts assumes that ALL added access have a corresponding
`FilteredAccess` added to the `filtered_accesses` field.
In practice, that means that SystemParam that adds their access through
the `Access` returned by `combined_access_mut` and the ones that add
their access using the `add` method lived in two different universes. As
a result, they could never be mutually exclusive.
## Solution
This commit fixes it by removing the `combined_access_mut` method. This
ensures that the `combined_access` field of FilteredAccessSet is always
updated consistently with the addition of a filter. When checking for
filtered access, it is now possible to account for `Res` and `ResMut`
invalid access. This is currently not needed, but might be in the
future.
We add the `add_unfiltered_{read,write}` methods to replace previous
usages of `combined_access_mut`.
We also add improved Debug implementations on FixedBitSet so that their
meaning is much clearer in debug output.
---
## Changelog
* Fix `Res` and `Query` parameter never being mutually exclusive.
## Migration Guide
Note: this mostly changes ECS internals, but since the API is public, it is technically breaking:
* Removed `FilteredAccessSet::combined_access_mut`
* Replace _immutable_ usage of those by `combined_access`
* For _mutable_ usages, use the new `add_unfiltered_{read,write}` methods instead of `combined_access_mut` followed by `add_{read,write}`
2022-11-16 11:05:48 +00:00
system_meta
. component_access_set
. add_unfiltered_read ( component_id ) ;
Bevy ECS V2 (#1525)
# Bevy ECS V2
This is a rewrite of Bevy ECS (basically everything but the new executor/schedule, which are already awesome). The overall goal was to improve the performance and versatility of Bevy ECS. Here is a quick bulleted list of changes before we dive into the details:
* Complete World rewrite
* Multiple component storage types:
* Tables: fast cache friendly iteration, slower add/removes (previously called Archetypes)
* Sparse Sets: fast add/remove, slower iteration
* Stateful Queries (caches query results for faster iteration. fragmented iteration is _fast_ now)
* Stateful System Params (caches expensive operations. inspired by @DJMcNab's work in #1364)
* Configurable System Params (users can set configuration when they construct their systems. once again inspired by @DJMcNab's work)
* Archetypes are now "just metadata", component storage is separate
* Archetype Graph (for faster archetype changes)
* Component Metadata
* Configure component storage type
* Retrieve information about component size/type/name/layout/send-ness/etc
* Components are uniquely identified by a densely packed ComponentId
* TypeIds are now totally optional (which should make implementing scripting easier)
* Super fast "for_each" query iterators
* Merged Resources into World. Resources are now just a special type of component
* EntityRef/EntityMut builder apis (more efficient and more ergonomic)
* Fast bitset-backed `Access<T>` replaces old hashmap-based approach everywhere
* Query conflicts are determined by component access instead of archetype component access (to avoid random failures at runtime)
* With/Without are still taken into account for conflicts, so this should still be comfy to use
* Much simpler `IntoSystem` impl
* Significantly reduced the amount of hashing throughout the ecs in favor of Sparse Sets (indexed by densely packed ArchetypeId, ComponentId, BundleId, and TableId)
* Safety Improvements
* Entity reservation uses a normal world reference instead of unsafe transmute
* QuerySets no longer transmute lifetimes
* Made traits "unsafe" where relevant
* More thorough safety docs
* WorldCell
* Exposes safe mutable access to multiple resources at a time in a World
* Replaced "catch all" `System::update_archetypes(world: &World)` with `System::new_archetype(archetype: &Archetype)`
* Simpler Bundle implementation
* Replaced slow "remove_bundle_one_by_one" used as fallback for Commands::remove_bundle with fast "remove_bundle_intersection"
* Removed `Mut<T>` query impl. it is better to only support one way: `&mut T`
* Removed with() from `Flags<T>` in favor of `Option<Flags<T>>`, which allows querying for flags to be "filtered" by default
* Components now have is_send property (currently only resources support non-send)
* More granular module organization
* New `RemovedComponents<T>` SystemParam that replaces `query.removed::<T>()`
* `world.resource_scope()` for mutable access to resources and world at the same time
* WorldQuery and QueryFilter traits unified. FilterFetch trait added to enable "short circuit" filtering. Auto impled for cases that don't need it
* Significantly slimmed down SystemState in favor of individual SystemParam state
* System Commands changed from `commands: &mut Commands` back to `mut commands: Commands` (to allow Commands to have a World reference)
Fixes #1320
## `World` Rewrite
This is a from-scratch rewrite of `World` that fills the niche that `hecs` used to. Yes, this means Bevy ECS is no longer a "fork" of hecs. We're going out our own!
(the only shared code between the projects is the entity id allocator, which is already basically ideal)
A huge shout out to @SanderMertens (author of [flecs](https://github.com/SanderMertens/flecs)) for sharing some great ideas with me (specifically hybrid ecs storage and archetype graphs). He also helped advise on a number of implementation details.
## Component Storage (The Problem)
Two ECS storage paradigms have gained a lot of traction over the years:
* **Archetypal ECS**:
* Stores components in "tables" with static schemas. Each "column" stores components of a given type. Each "row" is an entity.
* Each "archetype" has its own table. Adding/removing an entity's component changes the archetype.
* Enables super-fast Query iteration due to its cache-friendly data layout
* Comes at the cost of more expensive add/remove operations for an Entity's components, because all components need to be copied to the new archetype's "table"
* **Sparse Set ECS**:
* Stores components of the same type in densely packed arrays, which are sparsely indexed by densely packed unsigned integers (Entity ids)
* Query iteration is slower than Archetypal ECS because each entity's component could be at any position in the sparse set. This "random access" pattern isn't cache friendly. Additionally, there is an extra layer of indirection because you must first map the entity id to an index in the component array.
* Adding/removing components is a cheap, constant time operation
Bevy ECS V1, hecs, legion, flec, and Unity DOTS are all "archetypal ecs-es". I personally think "archetypal" storage is a good default for game engines. An entity's archetype doesn't need to change frequently in general, and it creates "fast by default" query iteration (which is a much more common operation). It is also "self optimizing". Users don't need to think about optimizing component layouts for iteration performance. It "just works" without any extra boilerplate.
Shipyard and EnTT are "sparse set ecs-es". They employ "packing" as a way to work around the "suboptimal by default" iteration performance for specific sets of components. This helps, but I didn't think this was a good choice for a general purpose engine like Bevy because:
1. "packs" conflict with each other. If bevy decides to internally pack the Transform and GlobalTransform components, users are then blocked if they want to pack some custom component with Transform.
2. users need to take manual action to optimize
Developers selecting an ECS framework are stuck with a hard choice. Select an "archetypal" framework with "fast iteration everywhere" but without the ability to cheaply add/remove components, or select a "sparse set" framework to cheaply add/remove components but with slower iteration performance.
## Hybrid Component Storage (The Solution)
In Bevy ECS V2, we get to have our cake and eat it too. It now has _both_ of the component storage types above (and more can be added later if needed):
* **Tables** (aka "archetypal" storage)
* The default storage. If you don't configure anything, this is what you get
* Fast iteration by default
* Slower add/remove operations
* **Sparse Sets**
* Opt-in
* Slower iteration
* Faster add/remove operations
These storage types complement each other perfectly. By default Query iteration is fast. If developers know that they want to add/remove a component at high frequencies, they can set the storage to "sparse set":
```rust
world.register_component(
ComponentDescriptor::new::<MyComponent>(StorageType::SparseSet)
).unwrap();
```
## Archetypes
Archetypes are now "just metadata" ... they no longer store components directly. They do store:
* The `ComponentId`s of each of the Archetype's components (and that component's storage type)
* Archetypes are uniquely defined by their component layouts
* For example: entities with "table" components `[A, B, C]` _and_ "sparse set" components `[D, E]` will always be in the same archetype.
* The `TableId` associated with the archetype
* For now each archetype has exactly one table (which can have no components),
* There is a 1->Many relationship from Tables->Archetypes. A given table could have any number of archetype components stored in it:
* Ex: an entity with "table storage" components `[A, B, C]` and "sparse set" components `[D, E]` will share the same `[A, B, C]` table as an entity with `[A, B, C]` table component and `[F]` sparse set components.
* This 1->Many relationship is how we preserve fast "cache friendly" iteration performance when possible (more on this later)
* A list of entities that are in the archetype and the row id of the table they are in
* ArchetypeComponentIds
* unique densely packed identifiers for (ArchetypeId, ComponentId) pairs
* used by the schedule executor for cheap system access control
* "Archetype Graph Edges" (see the next section)
## The "Archetype Graph"
Archetype changes in Bevy (and a number of other archetypal ecs-es) have historically been expensive to compute. First, you need to allocate a new vector of the entity's current component ids, add or remove components based on the operation performed, sort it (to ensure it is order-independent), then hash it to find the archetype (if it exists). And thats all before we get to the _already_ expensive full copy of all components to the new table storage.
The solution is to build a "graph" of archetypes to cache these results. @SanderMertens first exposed me to the idea (and he got it from @gjroelofs, who came up with it). They propose adding directed edges between archetypes for add/remove component operations. If `ComponentId`s are densely packed, you can use sparse sets to cheaply jump between archetypes.
Bevy takes this one step further by using add/remove `Bundle` edges instead of `Component` edges. Bevy encourages the use of `Bundles` to group add/remove operations. This is largely for "clearer game logic" reasons, but it also helps cut down on the number of archetype changes required. `Bundles` now also have densely-packed `BundleId`s. This allows us to use a _single_ edge for each bundle operation (rather than needing to traverse N edges ... one for each component). Single component operations are also bundles, so this is strictly an improvement over a "component only" graph.
As a result, an operation that used to be _heavy_ (both for allocations and compute) is now two dirt-cheap array lookups and zero allocations.
## Stateful Queries
World queries are now stateful. This allows us to:
1. Cache archetype (and table) matches
* This resolves another issue with (naive) archetypal ECS: query performance getting worse as the number of archetypes goes up (and fragmentation occurs).
2. Cache Fetch and Filter state
* The expensive parts of fetch/filter operations (such as hashing the TypeId to find the ComponentId) now only happen once when the Query is first constructed
3. Incrementally build up state
* When new archetypes are added, we only process the new archetypes (no need to rebuild state for old archetypes)
As a result, the direct `World` query api now looks like this:
```rust
let mut query = world.query::<(&A, &mut B)>();
for (a, mut b) in query.iter_mut(&mut world) {
}
```
Requiring `World` to generate stateful queries (rather than letting the `QueryState` type be constructed separately) allows us to ensure that _all_ queries are properly initialized (and the relevant world state, such as ComponentIds). This enables QueryState to remove branches from its operations that check for initialization status (and also enables query.iter() to take an immutable world reference because it doesn't need to initialize anything in world).
However in systems, this is a non-breaking change. State management is done internally by the relevant SystemParam.
## Stateful SystemParams
Like Queries, `SystemParams` now also cache state. For example, `Query` system params store the "stateful query" state mentioned above. Commands store their internal `CommandQueue`. This means you can now safely use as many separate `Commands` parameters in your system as you want. `Local<T>` system params store their `T` value in their state (instead of in Resources).
SystemParam state also enabled a significant slim-down of SystemState. It is much nicer to look at now.
Per-SystemParam state naturally insulates us from an "aliased mut" class of errors we have hit in the past (ex: using multiple `Commands` system params).
(credit goes to @DJMcNab for the initial idea and draft pr here #1364)
## Configurable SystemParams
@DJMcNab also had the great idea to make SystemParams configurable. This allows users to provide some initial configuration / values for system parameters (when possible). Most SystemParams have no config (the config type is `()`), but the `Local<T>` param now supports user-provided parameters:
```rust
fn foo(value: Local<usize>) {
}
app.add_system(foo.system().config(|c| c.0 = Some(10)));
```
## Uber Fast "for_each" Query Iterators
Developers now have the choice to use a fast "for_each" iterator, which yields ~1.5-3x iteration speed improvements for "fragmented iteration", and minor ~1.2x iteration speed improvements for unfragmented iteration.
```rust
fn system(query: Query<(&A, &mut B)>) {
// you now have the option to do this for a speed boost
query.for_each_mut(|(a, mut b)| {
});
// however normal iterators are still available
for (a, mut b) in query.iter_mut() {
}
}
```
I think in most cases we should continue to encourage "normal" iterators as they are more flexible and more "rust idiomatic". But when that extra "oomf" is needed, it makes sense to use `for_each`.
We should also consider using `for_each` for internal bevy systems to give our users a nice speed boost (but that should be a separate pr).
## Component Metadata
`World` now has a `Components` collection, which is accessible via `world.components()`. This stores mappings from `ComponentId` to `ComponentInfo`, as well as `TypeId` to `ComponentId` mappings (where relevant). `ComponentInfo` stores information about the component, such as ComponentId, TypeId, memory layout, send-ness (currently limited to resources), and storage type.
## Significantly Cheaper `Access<T>`
We used to use `TypeAccess<TypeId>` to manage read/write component/archetype-component access. This was expensive because TypeIds must be hashed and compared individually. The parallel executor got around this by "condensing" type ids into bitset-backed access types. This worked, but it had to be re-generated from the `TypeAccess<TypeId>`sources every time archetypes changed.
This pr removes TypeAccess in favor of faster bitset access everywhere. We can do this thanks to the move to densely packed `ComponentId`s and `ArchetypeComponentId`s.
## Merged Resources into World
Resources had a lot of redundant functionality with Components. They stored typed data, they had access control, they had unique ids, they were queryable via SystemParams, etc. In fact the _only_ major difference between them was that they were unique (and didn't correlate to an entity).
Separate resources also had the downside of requiring a separate set of access controls, which meant the parallel executor needed to compare more bitsets per system and manage more state.
I initially got the "separate resources" idea from `legion`. I think that design was motivated by the fact that it made the direct world query/resource lifetime interactions more manageable. It certainly made our lives easier when using Resources alongside hecs/bevy_ecs. However we already have a construct for safely and ergonomically managing in-world lifetimes: systems (which use `Access<T>` internally).
This pr merges Resources into World:
```rust
world.insert_resource(1);
world.insert_resource(2.0);
let a = world.get_resource::<i32>().unwrap();
let mut b = world.get_resource_mut::<f64>().unwrap();
*b = 3.0;
```
Resources are now just a special kind of component. They have their own ComponentIds (and their own resource TypeId->ComponentId scope, so they don't conflict wit components of the same type). They are stored in a special "resource archetype", which stores components inside the archetype using a new `unique_components` sparse set (note that this sparse set could later be used to implement Tags). This allows us to keep the code size small by reusing existing datastructures (namely Column, Archetype, ComponentFlags, and ComponentInfo). This allows us the executor to use a single `Access<ArchetypeComponentId>` per system. It should also make scripting language integration easier.
_But_ this merge did create problems for people directly interacting with `World`. What if you need mutable access to multiple resources at the same time? `world.get_resource_mut()` borrows World mutably!
## WorldCell
WorldCell applies the `Access<ArchetypeComponentId>` concept to direct world access:
```rust
let world_cell = world.cell();
let a = world_cell.get_resource_mut::<i32>().unwrap();
let b = world_cell.get_resource_mut::<f64>().unwrap();
```
This adds cheap runtime checks (a sparse set lookup of `ArchetypeComponentId` and a counter) to ensure that world accesses do not conflict with each other. Each operation returns a `WorldBorrow<'w, T>` or `WorldBorrowMut<'w, T>` wrapper type, which will release the relevant ArchetypeComponentId resources when dropped.
World caches the access sparse set (and only one cell can exist at a time), so `world.cell()` is a cheap operation.
WorldCell does _not_ use atomic operations. It is non-send, does a mutable borrow of world to prevent other accesses, and uses a simple `Rc<RefCell<ArchetypeComponentAccess>>` wrapper in each WorldBorrow pointer.
The api is currently limited to resource access, but it can and should be extended to queries / entity component access.
## Resource Scopes
WorldCell does not yet support component queries, and even when it does there are sometimes legitimate reasons to want a mutable world ref _and_ a mutable resource ref (ex: bevy_render and bevy_scene both need this). In these cases we could always drop down to the unsafe `world.get_resource_unchecked_mut()`, but that is not ideal!
Instead developers can use a "resource scope"
```rust
world.resource_scope(|world: &mut World, a: &mut A| {
})
```
This temporarily removes the `A` resource from `World`, provides mutable pointers to both, and re-adds A to World when finished. Thanks to the move to ComponentIds/sparse sets, this is a cheap operation.
If multiple resources are required, scopes can be nested. We could also consider adding a "resource tuple" to the api if this pattern becomes common and the boilerplate gets nasty.
## Query Conflicts Use ComponentId Instead of ArchetypeComponentId
For safety reasons, systems cannot contain queries that conflict with each other without wrapping them in a QuerySet. On bevy `main`, we use ArchetypeComponentIds to determine conflicts. This is nice because it can take into account filters:
```rust
// these queries will never conflict due to their filters
fn filter_system(a: Query<&mut A, With<B>>, b: Query<&mut B, Without<B>>) {
}
```
But it also has a significant downside:
```rust
// these queries will not conflict _until_ an entity with A, B, and C is spawned
fn maybe_conflicts_system(a: Query<(&mut A, &C)>, b: Query<(&mut A, &B)>) {
}
```
The system above will panic at runtime if an entity with A, B, and C is spawned. This makes it hard to trust that your game logic will run without crashing.
In this pr, I switched to using `ComponentId` instead. This _is_ more constraining. `maybe_conflicts_system` will now always fail, but it will do it consistently at startup. Naively, it would also _disallow_ `filter_system`, which would be a significant downgrade in usability. Bevy has a number of internal systems that rely on disjoint queries and I expect it to be a common pattern in userspace.
To resolve this, I added a new `FilteredAccess<T>` type, which wraps `Access<T>` and adds with/without filters. If two `FilteredAccess` have with/without values that prove they are disjoint, they will no longer conflict.
## EntityRef / EntityMut
World entity operations on `main` require that the user passes in an `entity` id to each operation:
```rust
let entity = world.spawn((A, )); // create a new entity with A
world.get::<A>(entity);
world.insert(entity, (B, C));
world.insert_one(entity, D);
```
This means that each operation needs to look up the entity location / verify its validity. The initial spawn operation also requires a Bundle as input. This can be awkward when no components are required (or one component is required).
These operations have been replaced by `EntityRef` and `EntityMut`, which are "builder-style" wrappers around world that provide read and read/write operations on a single, pre-validated entity:
```rust
// spawn now takes no inputs and returns an EntityMut
let entity = world.spawn()
.insert(A) // insert a single component into the entity
.insert_bundle((B, C)) // insert a bundle of components into the entity
.id() // id returns the Entity id
// Returns EntityMut (or panics if the entity does not exist)
world.entity_mut(entity)
.insert(D)
.insert_bundle(SomeBundle::default());
{
// returns EntityRef (or panics if the entity does not exist)
let d = world.entity(entity)
.get::<D>() // gets the D component
.unwrap();
// world.get still exists for ergonomics
let d = world.get::<D>(entity).unwrap();
}
// These variants return Options if you want to check existence instead of panicing
world.get_entity_mut(entity)
.unwrap()
.insert(E);
if let Some(entity_ref) = world.get_entity(entity) {
let d = entity_ref.get::<D>().unwrap();
}
```
This _does not_ affect the current Commands api or terminology. I think that should be a separate conversation as that is a much larger breaking change.
## Safety Improvements
* Entity reservation in Commands uses a normal world borrow instead of an unsafe transmute
* QuerySets no longer transmutes lifetimes
* Made traits "unsafe" when implementing a trait incorrectly could cause unsafety
* More thorough safety docs
## RemovedComponents SystemParam
The old approach to querying removed components: `query.removed:<T>()` was confusing because it had no connection to the query itself. I replaced it with the following, which is both clearer and allows us to cache the ComponentId mapping in the SystemParamState:
```rust
fn system(removed: RemovedComponents<T>) {
for entity in removed.iter() {
}
}
```
## Simpler Bundle implementation
Bundles are no longer responsible for sorting (or deduping) TypeInfo. They are just a simple ordered list of component types / data. This makes the implementation smaller and opens the door to an easy "nested bundle" implementation in the future (which i might even add in this pr). Duplicate detection is now done once per bundle type by World the first time a bundle is used.
## Unified WorldQuery and QueryFilter types
(don't worry they are still separate type _parameters_ in Queries .. this is a non-breaking change)
WorldQuery and QueryFilter were already basically identical apis. With the addition of `FetchState` and more storage-specific fetch methods, the overlap was even clearer (and the redundancy more painful).
QueryFilters are now just `F: WorldQuery where F::Fetch: FilterFetch`. FilterFetch requires `Fetch<Item = bool>` and adds new "short circuit" variants of fetch methods. This enables a filter tuple like `(With<A>, Without<B>, Changed<C>)` to stop evaluating the filter after the first mismatch is encountered. FilterFetch is automatically implemented for `Fetch` implementations that return bool.
This forces fetch implementations that return things like `(bool, bool, bool)` (such as the filter above) to manually implement FilterFetch and decide whether or not to short-circuit.
## More Granular Modules
World no longer globs all of the internal modules together. It now exports `core`, `system`, and `schedule` separately. I'm also considering exporting `core` submodules directly as that is still pretty "glob-ey" and unorganized (feedback welcome here).
## Remaining Draft Work (to be done in this pr)
* ~~panic on conflicting WorldQuery fetches (&A, &mut A)~~
* ~~bevy `main` and hecs both currently allow this, but we should protect against it if possible~~
* ~~batch_iter / par_iter (currently stubbed out)~~
* ~~ChangedRes~~
* ~~I skipped this while we sort out #1313. This pr should be adapted to account for whatever we land on there~~.
* ~~The `Archetypes` and `Tables` collections use hashes of sorted lists of component ids to uniquely identify each archetype/table. This hash is then used as the key in a HashMap to look up the relevant ArchetypeId or TableId. (which doesn't handle hash collisions properly)~~
* ~~It is currently unsafe to generate a Query from "World A", then use it on "World B" (despite the api claiming it is safe). We should probably close this gap. This could be done by adding a randomly generated WorldId to each world, then storing that id in each Query. They could then be compared to each other on each `query.do_thing(&world)` operation. This _does_ add an extra branch to each query operation, so I'm open to other suggestions if people have them.~~
* ~~Nested Bundles (if i find time)~~
## Potential Future Work
* Expand WorldCell to support queries.
* Consider not allocating in the empty archetype on `world.spawn()`
* ex: return something like EntityMutUninit, which turns into EntityMut after an `insert` or `insert_bundle` op
* this actually regressed performance last time i tried it, but in theory it should be faster
* Optimize SparseSet::insert (see `PERF` comment on insert)
* Replace SparseArray `Option<T>` with T::MAX to cut down on branching
* would enable cheaper get_unchecked() operations
* upstream fixedbitset optimizations
* fixedbitset could be allocation free for small block counts (store blocks in a SmallVec)
* fixedbitset could have a const constructor
* Consider implementing Tags (archetype-specific by-value data that affects archetype identity)
* ex: ArchetypeA could have `[A, B, C]` table components and `[D(1)]` "tag" component. ArchetypeB could have `[A, B, C]` table components and a `[D(2)]` tag component. The archetypes are different, despite both having D tags because the value inside D is different.
* this could potentially build on top of the `archetype.unique_components` added in this pr for resource storage.
* Consider reverting `all_tuples` proc macro in favor of the old `macro_rules` implementation
* all_tuples is more flexible and produces cleaner documentation (the macro_rules version produces weird type parameter orders due to parser constraints)
* but unfortunately all_tuples also appears to make Rust Analyzer sad/slow when working inside of `bevy_ecs` (does not affect user code)
* Consider "resource queries" and/or "mixed resource and entity component queries" as an alternative to WorldCell
* this is basically just "systems" so maybe it's not worth it
* Add more world ops
* `world.clear()`
* `world.reserve<T: Bundle>(count: usize)`
* Try using the old archetype allocation strategy (allocate new memory on resize and copy everything over). I expect this to improve batch insertion performance at the cost of unbatched performance. But thats just a guess. I'm not an allocation perf pro :)
* Adapt Commands apis for consistency with new World apis
## Benchmarks
key:
* `bevy_old`: bevy `main` branch
* `bevy`: this branch
* `_foreach`: uses an optimized for_each iterator
* ` _sparse`: uses sparse set storage (if unspecified assume table storage)
* `_system`: runs inside a system (if unspecified assume test happens via direct world ops)
### Simple Insert (from ecs_bench_suite)
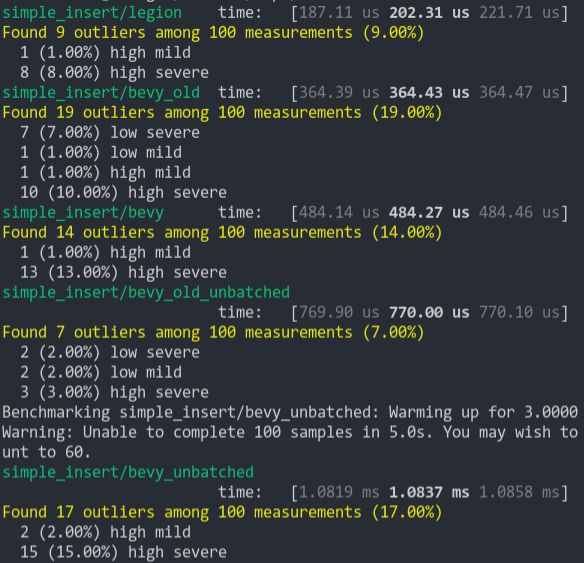
### Simpler Iter (from ecs_bench_suite)
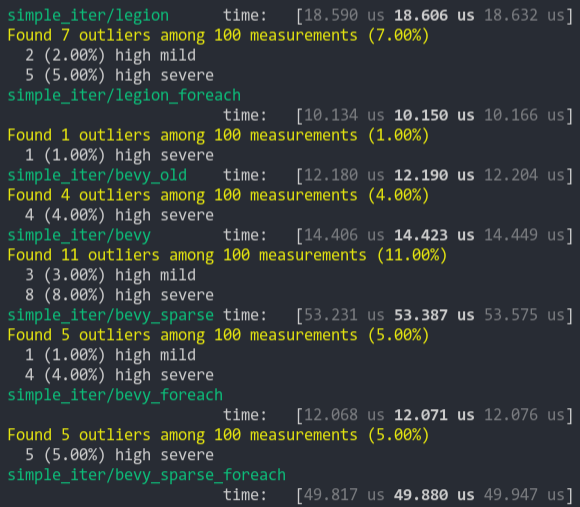
### Fragment Iter (from ecs_bench_suite)
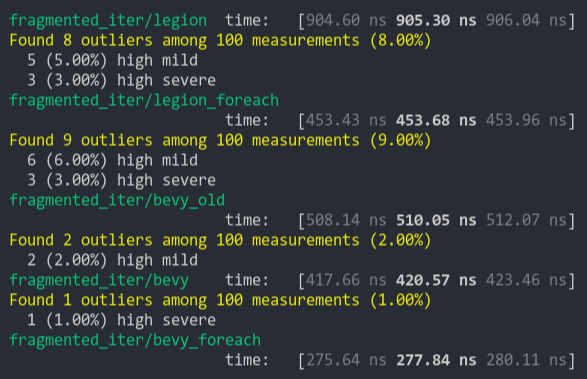
### Sparse Fragmented Iter
Iterate a query that matches 5 entities from a single matching archetype, but there are 100 unmatching archetypes
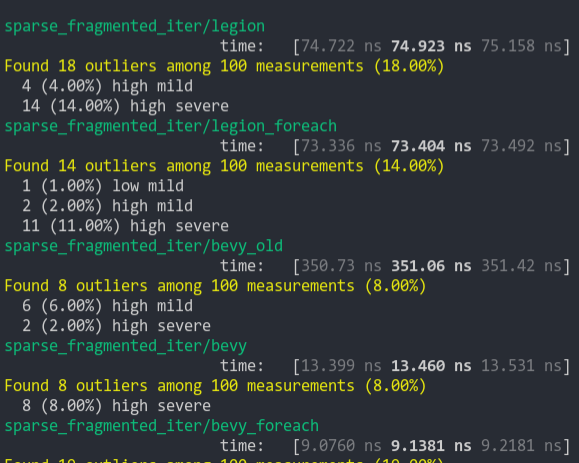
### Schedule (from ecs_bench_suite)
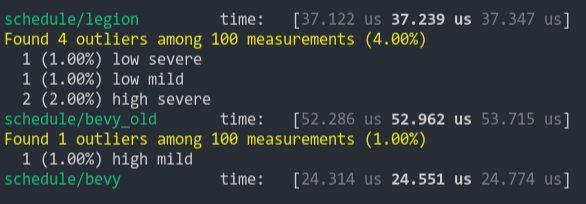
### Add Remove Component (from ecs_bench_suite)
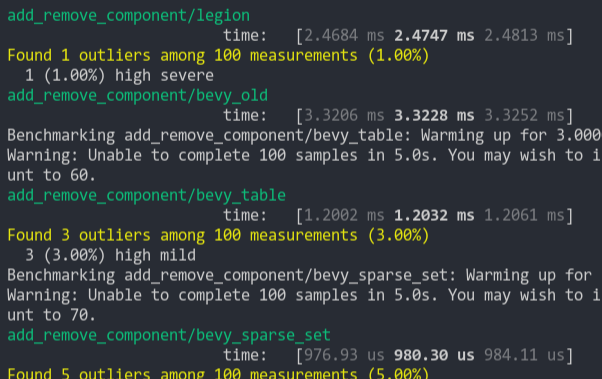
### Add Remove Component Big
Same as the test above, but each entity has 5 "large" matrix components and 1 "large" matrix component is added and removed
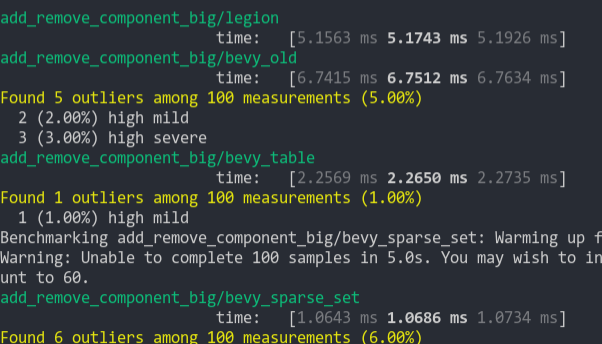
### Get Component
Looks up a single component value a large number of times
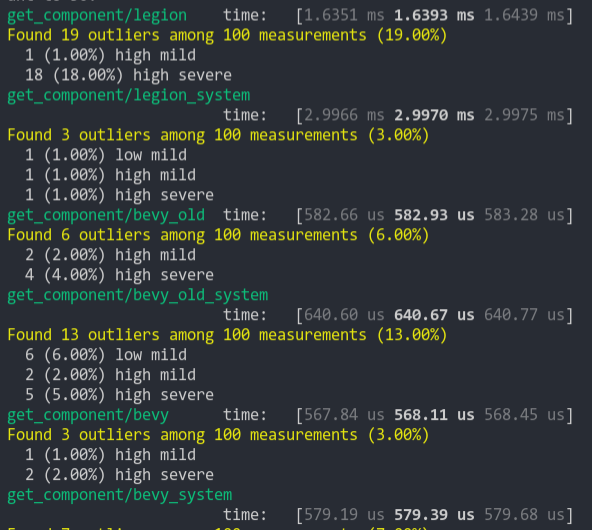
2021-03-05 07:54:35 +00:00
2022-10-24 13:46:36 +00:00
let archetype_component_id = world
Panic on dropping NonSend in non-origin thread. (#6534)
# Objective
Fixes #3310. Fixes #6282. Fixes #6278. Fixes #3666.
## Solution
Split out `!Send` resources into `NonSendResources`. Add a `origin_thread_id` to all `!Send` Resources, check it on dropping `NonSendResourceData`, if there's a mismatch, panic. Moved all of the checks that `MainThreadValidator` would do into `NonSendResources` instead.
All `!Send` resources now individually track which thread they were inserted from. This is validated against for every access, mutation, and drop that could be done against the value.
A regression test using an altered version of the example from #3310 has been added.
This is a stopgap solution for the current status quo. A full solution may involve fully removing `!Send` resources/components from `World`, which will likely require a much more thorough design on how to handle the existing in-engine and ecosystem use cases.
This PR also introduces another breaking change:
```rust
use bevy_ecs::prelude::*;
#[derive(Resource)]
struct Resource(u32);
fn main() {
let mut world = World::new();
world.insert_resource(Resource(1));
world.insert_non_send_resource(Resource(2));
let res = world.get_resource_mut::<Resource>().unwrap();
assert_eq!(res.0, 2);
}
```
This code will run correctly on 0.9.1 but not with this PR, since NonSend resources and normal resources have become actual distinct concepts storage wise.
## Changelog
Changed: Fix soundness bug with `World: Send`. Dropping a `World` that contains a `!Send` resource on the wrong thread will now panic.
## Migration Guide
Normal resources and `NonSend` resources no longer share the same backing storage. If `R: Resource`, then `NonSend<R>` and `Res<R>` will return different instances from each other. If you are using both `Res<T>` and `NonSend<T>` (or their mutable variants), to fetch the same resources, it's strongly advised to use `Res<T>`.
2023-01-09 20:40:34 +00:00
. get_non_send_archetype_component_id ( component_id )
Bevy ECS V2 (#1525)
# Bevy ECS V2
This is a rewrite of Bevy ECS (basically everything but the new executor/schedule, which are already awesome). The overall goal was to improve the performance and versatility of Bevy ECS. Here is a quick bulleted list of changes before we dive into the details:
* Complete World rewrite
* Multiple component storage types:
* Tables: fast cache friendly iteration, slower add/removes (previously called Archetypes)
* Sparse Sets: fast add/remove, slower iteration
* Stateful Queries (caches query results for faster iteration. fragmented iteration is _fast_ now)
* Stateful System Params (caches expensive operations. inspired by @DJMcNab's work in #1364)
* Configurable System Params (users can set configuration when they construct their systems. once again inspired by @DJMcNab's work)
* Archetypes are now "just metadata", component storage is separate
* Archetype Graph (for faster archetype changes)
* Component Metadata
* Configure component storage type
* Retrieve information about component size/type/name/layout/send-ness/etc
* Components are uniquely identified by a densely packed ComponentId
* TypeIds are now totally optional (which should make implementing scripting easier)
* Super fast "for_each" query iterators
* Merged Resources into World. Resources are now just a special type of component
* EntityRef/EntityMut builder apis (more efficient and more ergonomic)
* Fast bitset-backed `Access<T>` replaces old hashmap-based approach everywhere
* Query conflicts are determined by component access instead of archetype component access (to avoid random failures at runtime)
* With/Without are still taken into account for conflicts, so this should still be comfy to use
* Much simpler `IntoSystem` impl
* Significantly reduced the amount of hashing throughout the ecs in favor of Sparse Sets (indexed by densely packed ArchetypeId, ComponentId, BundleId, and TableId)
* Safety Improvements
* Entity reservation uses a normal world reference instead of unsafe transmute
* QuerySets no longer transmute lifetimes
* Made traits "unsafe" where relevant
* More thorough safety docs
* WorldCell
* Exposes safe mutable access to multiple resources at a time in a World
* Replaced "catch all" `System::update_archetypes(world: &World)` with `System::new_archetype(archetype: &Archetype)`
* Simpler Bundle implementation
* Replaced slow "remove_bundle_one_by_one" used as fallback for Commands::remove_bundle with fast "remove_bundle_intersection"
* Removed `Mut<T>` query impl. it is better to only support one way: `&mut T`
* Removed with() from `Flags<T>` in favor of `Option<Flags<T>>`, which allows querying for flags to be "filtered" by default
* Components now have is_send property (currently only resources support non-send)
* More granular module organization
* New `RemovedComponents<T>` SystemParam that replaces `query.removed::<T>()`
* `world.resource_scope()` for mutable access to resources and world at the same time
* WorldQuery and QueryFilter traits unified. FilterFetch trait added to enable "short circuit" filtering. Auto impled for cases that don't need it
* Significantly slimmed down SystemState in favor of individual SystemParam state
* System Commands changed from `commands: &mut Commands` back to `mut commands: Commands` (to allow Commands to have a World reference)
Fixes #1320
## `World` Rewrite
This is a from-scratch rewrite of `World` that fills the niche that `hecs` used to. Yes, this means Bevy ECS is no longer a "fork" of hecs. We're going out our own!
(the only shared code between the projects is the entity id allocator, which is already basically ideal)
A huge shout out to @SanderMertens (author of [flecs](https://github.com/SanderMertens/flecs)) for sharing some great ideas with me (specifically hybrid ecs storage and archetype graphs). He also helped advise on a number of implementation details.
## Component Storage (The Problem)
Two ECS storage paradigms have gained a lot of traction over the years:
* **Archetypal ECS**:
* Stores components in "tables" with static schemas. Each "column" stores components of a given type. Each "row" is an entity.
* Each "archetype" has its own table. Adding/removing an entity's component changes the archetype.
* Enables super-fast Query iteration due to its cache-friendly data layout
* Comes at the cost of more expensive add/remove operations for an Entity's components, because all components need to be copied to the new archetype's "table"
* **Sparse Set ECS**:
* Stores components of the same type in densely packed arrays, which are sparsely indexed by densely packed unsigned integers (Entity ids)
* Query iteration is slower than Archetypal ECS because each entity's component could be at any position in the sparse set. This "random access" pattern isn't cache friendly. Additionally, there is an extra layer of indirection because you must first map the entity id to an index in the component array.
* Adding/removing components is a cheap, constant time operation
Bevy ECS V1, hecs, legion, flec, and Unity DOTS are all "archetypal ecs-es". I personally think "archetypal" storage is a good default for game engines. An entity's archetype doesn't need to change frequently in general, and it creates "fast by default" query iteration (which is a much more common operation). It is also "self optimizing". Users don't need to think about optimizing component layouts for iteration performance. It "just works" without any extra boilerplate.
Shipyard and EnTT are "sparse set ecs-es". They employ "packing" as a way to work around the "suboptimal by default" iteration performance for specific sets of components. This helps, but I didn't think this was a good choice for a general purpose engine like Bevy because:
1. "packs" conflict with each other. If bevy decides to internally pack the Transform and GlobalTransform components, users are then blocked if they want to pack some custom component with Transform.
2. users need to take manual action to optimize
Developers selecting an ECS framework are stuck with a hard choice. Select an "archetypal" framework with "fast iteration everywhere" but without the ability to cheaply add/remove components, or select a "sparse set" framework to cheaply add/remove components but with slower iteration performance.
## Hybrid Component Storage (The Solution)
In Bevy ECS V2, we get to have our cake and eat it too. It now has _both_ of the component storage types above (and more can be added later if needed):
* **Tables** (aka "archetypal" storage)
* The default storage. If you don't configure anything, this is what you get
* Fast iteration by default
* Slower add/remove operations
* **Sparse Sets**
* Opt-in
* Slower iteration
* Faster add/remove operations
These storage types complement each other perfectly. By default Query iteration is fast. If developers know that they want to add/remove a component at high frequencies, they can set the storage to "sparse set":
```rust
world.register_component(
ComponentDescriptor::new::<MyComponent>(StorageType::SparseSet)
).unwrap();
```
## Archetypes
Archetypes are now "just metadata" ... they no longer store components directly. They do store:
* The `ComponentId`s of each of the Archetype's components (and that component's storage type)
* Archetypes are uniquely defined by their component layouts
* For example: entities with "table" components `[A, B, C]` _and_ "sparse set" components `[D, E]` will always be in the same archetype.
* The `TableId` associated with the archetype
* For now each archetype has exactly one table (which can have no components),
* There is a 1->Many relationship from Tables->Archetypes. A given table could have any number of archetype components stored in it:
* Ex: an entity with "table storage" components `[A, B, C]` and "sparse set" components `[D, E]` will share the same `[A, B, C]` table as an entity with `[A, B, C]` table component and `[F]` sparse set components.
* This 1->Many relationship is how we preserve fast "cache friendly" iteration performance when possible (more on this later)
* A list of entities that are in the archetype and the row id of the table they are in
* ArchetypeComponentIds
* unique densely packed identifiers for (ArchetypeId, ComponentId) pairs
* used by the schedule executor for cheap system access control
* "Archetype Graph Edges" (see the next section)
## The "Archetype Graph"
Archetype changes in Bevy (and a number of other archetypal ecs-es) have historically been expensive to compute. First, you need to allocate a new vector of the entity's current component ids, add or remove components based on the operation performed, sort it (to ensure it is order-independent), then hash it to find the archetype (if it exists). And thats all before we get to the _already_ expensive full copy of all components to the new table storage.
The solution is to build a "graph" of archetypes to cache these results. @SanderMertens first exposed me to the idea (and he got it from @gjroelofs, who came up with it). They propose adding directed edges between archetypes for add/remove component operations. If `ComponentId`s are densely packed, you can use sparse sets to cheaply jump between archetypes.
Bevy takes this one step further by using add/remove `Bundle` edges instead of `Component` edges. Bevy encourages the use of `Bundles` to group add/remove operations. This is largely for "clearer game logic" reasons, but it also helps cut down on the number of archetype changes required. `Bundles` now also have densely-packed `BundleId`s. This allows us to use a _single_ edge for each bundle operation (rather than needing to traverse N edges ... one for each component). Single component operations are also bundles, so this is strictly an improvement over a "component only" graph.
As a result, an operation that used to be _heavy_ (both for allocations and compute) is now two dirt-cheap array lookups and zero allocations.
## Stateful Queries
World queries are now stateful. This allows us to:
1. Cache archetype (and table) matches
* This resolves another issue with (naive) archetypal ECS: query performance getting worse as the number of archetypes goes up (and fragmentation occurs).
2. Cache Fetch and Filter state
* The expensive parts of fetch/filter operations (such as hashing the TypeId to find the ComponentId) now only happen once when the Query is first constructed
3. Incrementally build up state
* When new archetypes are added, we only process the new archetypes (no need to rebuild state for old archetypes)
As a result, the direct `World` query api now looks like this:
```rust
let mut query = world.query::<(&A, &mut B)>();
for (a, mut b) in query.iter_mut(&mut world) {
}
```
Requiring `World` to generate stateful queries (rather than letting the `QueryState` type be constructed separately) allows us to ensure that _all_ queries are properly initialized (and the relevant world state, such as ComponentIds). This enables QueryState to remove branches from its operations that check for initialization status (and also enables query.iter() to take an immutable world reference because it doesn't need to initialize anything in world).
However in systems, this is a non-breaking change. State management is done internally by the relevant SystemParam.
## Stateful SystemParams
Like Queries, `SystemParams` now also cache state. For example, `Query` system params store the "stateful query" state mentioned above. Commands store their internal `CommandQueue`. This means you can now safely use as many separate `Commands` parameters in your system as you want. `Local<T>` system params store their `T` value in their state (instead of in Resources).
SystemParam state also enabled a significant slim-down of SystemState. It is much nicer to look at now.
Per-SystemParam state naturally insulates us from an "aliased mut" class of errors we have hit in the past (ex: using multiple `Commands` system params).
(credit goes to @DJMcNab for the initial idea and draft pr here #1364)
## Configurable SystemParams
@DJMcNab also had the great idea to make SystemParams configurable. This allows users to provide some initial configuration / values for system parameters (when possible). Most SystemParams have no config (the config type is `()`), but the `Local<T>` param now supports user-provided parameters:
```rust
fn foo(value: Local<usize>) {
}
app.add_system(foo.system().config(|c| c.0 = Some(10)));
```
## Uber Fast "for_each" Query Iterators
Developers now have the choice to use a fast "for_each" iterator, which yields ~1.5-3x iteration speed improvements for "fragmented iteration", and minor ~1.2x iteration speed improvements for unfragmented iteration.
```rust
fn system(query: Query<(&A, &mut B)>) {
// you now have the option to do this for a speed boost
query.for_each_mut(|(a, mut b)| {
});
// however normal iterators are still available
for (a, mut b) in query.iter_mut() {
}
}
```
I think in most cases we should continue to encourage "normal" iterators as they are more flexible and more "rust idiomatic". But when that extra "oomf" is needed, it makes sense to use `for_each`.
We should also consider using `for_each` for internal bevy systems to give our users a nice speed boost (but that should be a separate pr).
## Component Metadata
`World` now has a `Components` collection, which is accessible via `world.components()`. This stores mappings from `ComponentId` to `ComponentInfo`, as well as `TypeId` to `ComponentId` mappings (where relevant). `ComponentInfo` stores information about the component, such as ComponentId, TypeId, memory layout, send-ness (currently limited to resources), and storage type.
## Significantly Cheaper `Access<T>`
We used to use `TypeAccess<TypeId>` to manage read/write component/archetype-component access. This was expensive because TypeIds must be hashed and compared individually. The parallel executor got around this by "condensing" type ids into bitset-backed access types. This worked, but it had to be re-generated from the `TypeAccess<TypeId>`sources every time archetypes changed.
This pr removes TypeAccess in favor of faster bitset access everywhere. We can do this thanks to the move to densely packed `ComponentId`s and `ArchetypeComponentId`s.
## Merged Resources into World
Resources had a lot of redundant functionality with Components. They stored typed data, they had access control, they had unique ids, they were queryable via SystemParams, etc. In fact the _only_ major difference between them was that they were unique (and didn't correlate to an entity).
Separate resources also had the downside of requiring a separate set of access controls, which meant the parallel executor needed to compare more bitsets per system and manage more state.
I initially got the "separate resources" idea from `legion`. I think that design was motivated by the fact that it made the direct world query/resource lifetime interactions more manageable. It certainly made our lives easier when using Resources alongside hecs/bevy_ecs. However we already have a construct for safely and ergonomically managing in-world lifetimes: systems (which use `Access<T>` internally).
This pr merges Resources into World:
```rust
world.insert_resource(1);
world.insert_resource(2.0);
let a = world.get_resource::<i32>().unwrap();
let mut b = world.get_resource_mut::<f64>().unwrap();
*b = 3.0;
```
Resources are now just a special kind of component. They have their own ComponentIds (and their own resource TypeId->ComponentId scope, so they don't conflict wit components of the same type). They are stored in a special "resource archetype", which stores components inside the archetype using a new `unique_components` sparse set (note that this sparse set could later be used to implement Tags). This allows us to keep the code size small by reusing existing datastructures (namely Column, Archetype, ComponentFlags, and ComponentInfo). This allows us the executor to use a single `Access<ArchetypeComponentId>` per system. It should also make scripting language integration easier.
_But_ this merge did create problems for people directly interacting with `World`. What if you need mutable access to multiple resources at the same time? `world.get_resource_mut()` borrows World mutably!
## WorldCell
WorldCell applies the `Access<ArchetypeComponentId>` concept to direct world access:
```rust
let world_cell = world.cell();
let a = world_cell.get_resource_mut::<i32>().unwrap();
let b = world_cell.get_resource_mut::<f64>().unwrap();
```
This adds cheap runtime checks (a sparse set lookup of `ArchetypeComponentId` and a counter) to ensure that world accesses do not conflict with each other. Each operation returns a `WorldBorrow<'w, T>` or `WorldBorrowMut<'w, T>` wrapper type, which will release the relevant ArchetypeComponentId resources when dropped.
World caches the access sparse set (and only one cell can exist at a time), so `world.cell()` is a cheap operation.
WorldCell does _not_ use atomic operations. It is non-send, does a mutable borrow of world to prevent other accesses, and uses a simple `Rc<RefCell<ArchetypeComponentAccess>>` wrapper in each WorldBorrow pointer.
The api is currently limited to resource access, but it can and should be extended to queries / entity component access.
## Resource Scopes
WorldCell does not yet support component queries, and even when it does there are sometimes legitimate reasons to want a mutable world ref _and_ a mutable resource ref (ex: bevy_render and bevy_scene both need this). In these cases we could always drop down to the unsafe `world.get_resource_unchecked_mut()`, but that is not ideal!
Instead developers can use a "resource scope"
```rust
world.resource_scope(|world: &mut World, a: &mut A| {
})
```
This temporarily removes the `A` resource from `World`, provides mutable pointers to both, and re-adds A to World when finished. Thanks to the move to ComponentIds/sparse sets, this is a cheap operation.
If multiple resources are required, scopes can be nested. We could also consider adding a "resource tuple" to the api if this pattern becomes common and the boilerplate gets nasty.
## Query Conflicts Use ComponentId Instead of ArchetypeComponentId
For safety reasons, systems cannot contain queries that conflict with each other without wrapping them in a QuerySet. On bevy `main`, we use ArchetypeComponentIds to determine conflicts. This is nice because it can take into account filters:
```rust
// these queries will never conflict due to their filters
fn filter_system(a: Query<&mut A, With<B>>, b: Query<&mut B, Without<B>>) {
}
```
But it also has a significant downside:
```rust
// these queries will not conflict _until_ an entity with A, B, and C is spawned
fn maybe_conflicts_system(a: Query<(&mut A, &C)>, b: Query<(&mut A, &B)>) {
}
```
The system above will panic at runtime if an entity with A, B, and C is spawned. This makes it hard to trust that your game logic will run without crashing.
In this pr, I switched to using `ComponentId` instead. This _is_ more constraining. `maybe_conflicts_system` will now always fail, but it will do it consistently at startup. Naively, it would also _disallow_ `filter_system`, which would be a significant downgrade in usability. Bevy has a number of internal systems that rely on disjoint queries and I expect it to be a common pattern in userspace.
To resolve this, I added a new `FilteredAccess<T>` type, which wraps `Access<T>` and adds with/without filters. If two `FilteredAccess` have with/without values that prove they are disjoint, they will no longer conflict.
## EntityRef / EntityMut
World entity operations on `main` require that the user passes in an `entity` id to each operation:
```rust
let entity = world.spawn((A, )); // create a new entity with A
world.get::<A>(entity);
world.insert(entity, (B, C));
world.insert_one(entity, D);
```
This means that each operation needs to look up the entity location / verify its validity. The initial spawn operation also requires a Bundle as input. This can be awkward when no components are required (or one component is required).
These operations have been replaced by `EntityRef` and `EntityMut`, which are "builder-style" wrappers around world that provide read and read/write operations on a single, pre-validated entity:
```rust
// spawn now takes no inputs and returns an EntityMut
let entity = world.spawn()
.insert(A) // insert a single component into the entity
.insert_bundle((B, C)) // insert a bundle of components into the entity
.id() // id returns the Entity id
// Returns EntityMut (or panics if the entity does not exist)
world.entity_mut(entity)
.insert(D)
.insert_bundle(SomeBundle::default());
{
// returns EntityRef (or panics if the entity does not exist)
let d = world.entity(entity)
.get::<D>() // gets the D component
.unwrap();
// world.get still exists for ergonomics
let d = world.get::<D>(entity).unwrap();
}
// These variants return Options if you want to check existence instead of panicing
world.get_entity_mut(entity)
.unwrap()
.insert(E);
if let Some(entity_ref) = world.get_entity(entity) {
let d = entity_ref.get::<D>().unwrap();
}
```
This _does not_ affect the current Commands api or terminology. I think that should be a separate conversation as that is a much larger breaking change.
## Safety Improvements
* Entity reservation in Commands uses a normal world borrow instead of an unsafe transmute
* QuerySets no longer transmutes lifetimes
* Made traits "unsafe" when implementing a trait incorrectly could cause unsafety
* More thorough safety docs
## RemovedComponents SystemParam
The old approach to querying removed components: `query.removed:<T>()` was confusing because it had no connection to the query itself. I replaced it with the following, which is both clearer and allows us to cache the ComponentId mapping in the SystemParamState:
```rust
fn system(removed: RemovedComponents<T>) {
for entity in removed.iter() {
}
}
```
## Simpler Bundle implementation
Bundles are no longer responsible for sorting (or deduping) TypeInfo. They are just a simple ordered list of component types / data. This makes the implementation smaller and opens the door to an easy "nested bundle" implementation in the future (which i might even add in this pr). Duplicate detection is now done once per bundle type by World the first time a bundle is used.
## Unified WorldQuery and QueryFilter types
(don't worry they are still separate type _parameters_ in Queries .. this is a non-breaking change)
WorldQuery and QueryFilter were already basically identical apis. With the addition of `FetchState` and more storage-specific fetch methods, the overlap was even clearer (and the redundancy more painful).
QueryFilters are now just `F: WorldQuery where F::Fetch: FilterFetch`. FilterFetch requires `Fetch<Item = bool>` and adds new "short circuit" variants of fetch methods. This enables a filter tuple like `(With<A>, Without<B>, Changed<C>)` to stop evaluating the filter after the first mismatch is encountered. FilterFetch is automatically implemented for `Fetch` implementations that return bool.
This forces fetch implementations that return things like `(bool, bool, bool)` (such as the filter above) to manually implement FilterFetch and decide whether or not to short-circuit.
## More Granular Modules
World no longer globs all of the internal modules together. It now exports `core`, `system`, and `schedule` separately. I'm also considering exporting `core` submodules directly as that is still pretty "glob-ey" and unorganized (feedback welcome here).
## Remaining Draft Work (to be done in this pr)
* ~~panic on conflicting WorldQuery fetches (&A, &mut A)~~
* ~~bevy `main` and hecs both currently allow this, but we should protect against it if possible~~
* ~~batch_iter / par_iter (currently stubbed out)~~
* ~~ChangedRes~~
* ~~I skipped this while we sort out #1313. This pr should be adapted to account for whatever we land on there~~.
* ~~The `Archetypes` and `Tables` collections use hashes of sorted lists of component ids to uniquely identify each archetype/table. This hash is then used as the key in a HashMap to look up the relevant ArchetypeId or TableId. (which doesn't handle hash collisions properly)~~
* ~~It is currently unsafe to generate a Query from "World A", then use it on "World B" (despite the api claiming it is safe). We should probably close this gap. This could be done by adding a randomly generated WorldId to each world, then storing that id in each Query. They could then be compared to each other on each `query.do_thing(&world)` operation. This _does_ add an extra branch to each query operation, so I'm open to other suggestions if people have them.~~
* ~~Nested Bundles (if i find time)~~
## Potential Future Work
* Expand WorldCell to support queries.
* Consider not allocating in the empty archetype on `world.spawn()`
* ex: return something like EntityMutUninit, which turns into EntityMut after an `insert` or `insert_bundle` op
* this actually regressed performance last time i tried it, but in theory it should be faster
* Optimize SparseSet::insert (see `PERF` comment on insert)
* Replace SparseArray `Option<T>` with T::MAX to cut down on branching
* would enable cheaper get_unchecked() operations
* upstream fixedbitset optimizations
* fixedbitset could be allocation free for small block counts (store blocks in a SmallVec)
* fixedbitset could have a const constructor
* Consider implementing Tags (archetype-specific by-value data that affects archetype identity)
* ex: ArchetypeA could have `[A, B, C]` table components and `[D(1)]` "tag" component. ArchetypeB could have `[A, B, C]` table components and a `[D(2)]` tag component. The archetypes are different, despite both having D tags because the value inside D is different.
* this could potentially build on top of the `archetype.unique_components` added in this pr for resource storage.
* Consider reverting `all_tuples` proc macro in favor of the old `macro_rules` implementation
* all_tuples is more flexible and produces cleaner documentation (the macro_rules version produces weird type parameter orders due to parser constraints)
* but unfortunately all_tuples also appears to make Rust Analyzer sad/slow when working inside of `bevy_ecs` (does not affect user code)
* Consider "resource queries" and/or "mixed resource and entity component queries" as an alternative to WorldCell
* this is basically just "systems" so maybe it's not worth it
* Add more world ops
* `world.clear()`
* `world.reserve<T: Bundle>(count: usize)`
* Try using the old archetype allocation strategy (allocate new memory on resize and copy everything over). I expect this to improve batch insertion performance at the cost of unbatched performance. But thats just a guess. I'm not an allocation perf pro :)
* Adapt Commands apis for consistency with new World apis
## Benchmarks
key:
* `bevy_old`: bevy `main` branch
* `bevy`: this branch
* `_foreach`: uses an optimized for_each iterator
* ` _sparse`: uses sparse set storage (if unspecified assume table storage)
* `_system`: runs inside a system (if unspecified assume test happens via direct world ops)
### Simple Insert (from ecs_bench_suite)
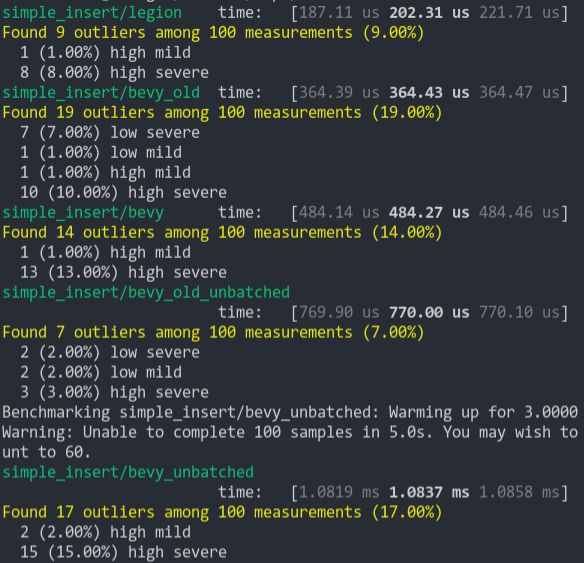
### Simpler Iter (from ecs_bench_suite)
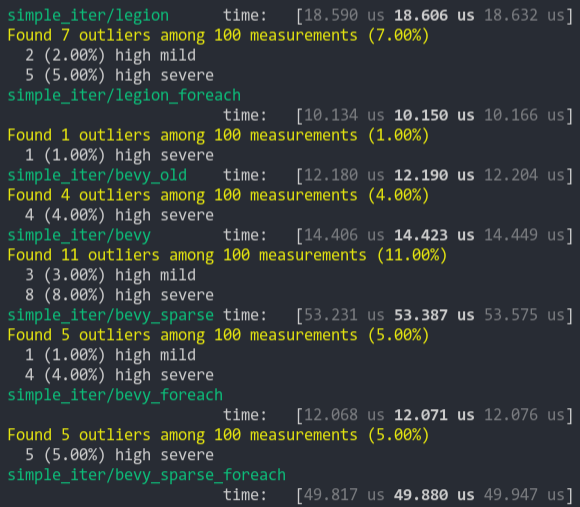
### Fragment Iter (from ecs_bench_suite)
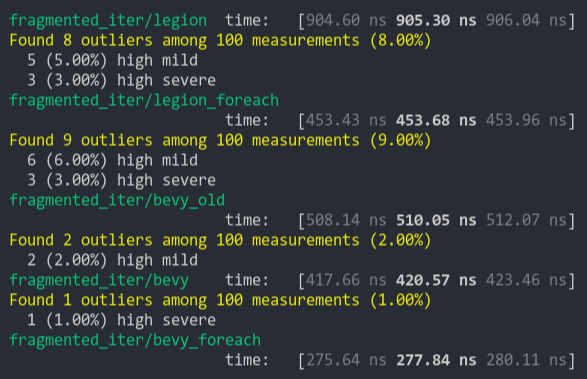
### Sparse Fragmented Iter
Iterate a query that matches 5 entities from a single matching archetype, but there are 100 unmatching archetypes
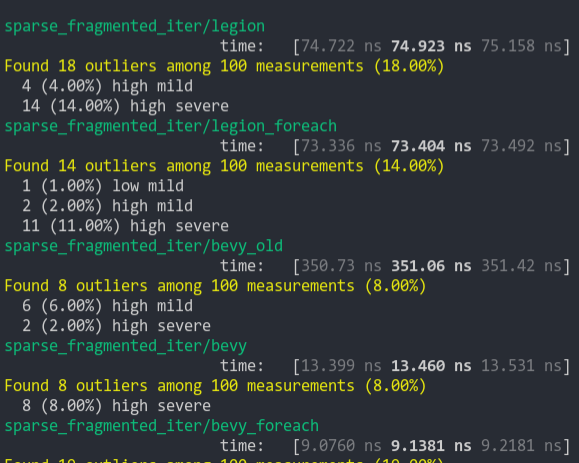
### Schedule (from ecs_bench_suite)
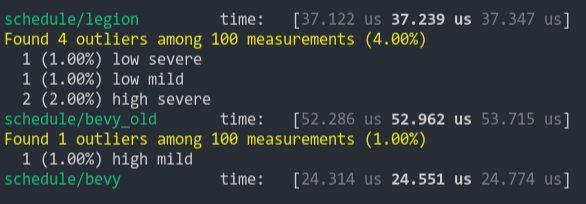
### Add Remove Component (from ecs_bench_suite)
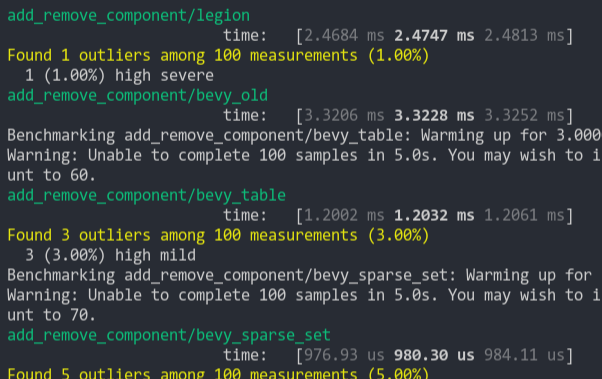
### Add Remove Component Big
Same as the test above, but each entity has 5 "large" matrix components and 1 "large" matrix component is added and removed
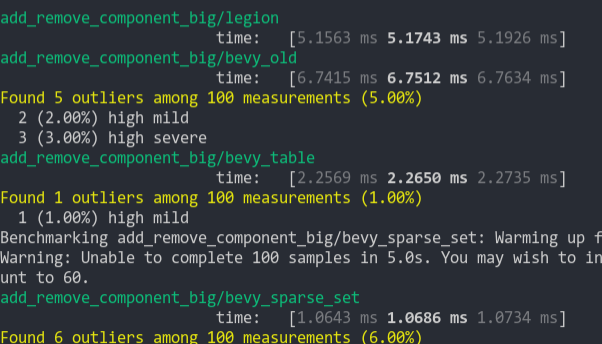
### Get Component
Looks up a single component value a large number of times
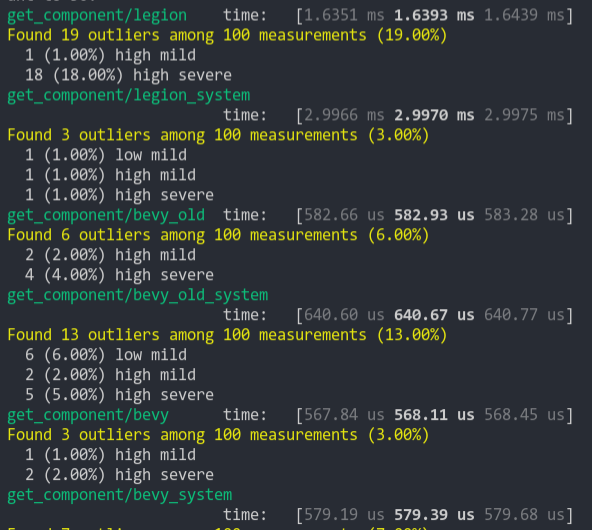
2021-03-05 07:54:35 +00:00
. unwrap ( ) ;
Add new SystemState and rename old SystemState to SystemMeta (#2283)
This enables `SystemParams` to be used outside of function systems. Anything can create and store `SystemState`, which enables efficient "param state cached" access to `SystemParams`.
It adds a `ReadOnlySystemParamFetch` trait, which enables safe `SystemState::get` calls without unique world access.
I renamed the old `SystemState` to `SystemMeta` to enable us to mirror the `QueryState` naming convention (but I'm happy to discuss alternative names if people have other ideas). I initially pitched this as `ParamState`, but given that it needs to include full system metadata, that doesn't feel like a particularly accurate name.
```rust
#[derive(Eq, PartialEq, Debug)]
struct A(usize);
#[derive(Eq, PartialEq, Debug)]
struct B(usize);
let mut world = World::default();
world.insert_resource(A(42));
world.spawn().insert(B(7));
// we get nice lifetime elision when declaring the type on the left hand side
let mut system_state: SystemState<(Res<A>, Query<&B>)> = SystemState::new(&mut world);
let (a, query) = system_state.get(&world);
assert_eq!(*a, A(42), "returned resource matches initial value");
assert_eq!(
*query.single().unwrap(),
B(7),
"returned component matches initial value"
);
// mutable system params require unique world access
let mut system_state: SystemState<(ResMut<A>, Query<&mut B>)> = SystemState::new(&mut world);
let (a, query) = system_state.get_mut(&mut world);
// static lifetimes are required when declaring inside of structs
struct SomeContainer {
state: SystemState<(Res<'static, A>, Res<'static, B>)>
}
// this can be shortened using type aliases, which will be useful for complex param tuples
type MyParams<'a> = (Res<'a, A>, Res<'a, B>);
struct SomeContainer {
state: SystemState<MyParams<'static>>
}
// It is the user's responsibility to call SystemState::apply(world) for parameters that queue up work
let mut system_state: SystemState<(Commands, Query<&B>)> = SystemState::new(&mut world);
{
let (mut commands, query) = system_state.get(&world);
commands.insert_resource(3.14);
}
system_state.apply(&mut world);
```
## Future Work
* Actually use SystemState inside FunctionSystem. This would be trivial, but it requires FunctionSystem to wrap SystemState in Option in its current form (which complicates system metadata lookup). I'd prefer to hold off until we adopt something like the later designs linked in #1364, which enable us to contruct Systems using a World reference (and also remove the need for `.system`).
* Consider a "scoped" approach to automatically call SystemState::apply when systems params are no longer being used (either a container type with a Drop impl, or a function that takes a closure for user logic operating on params).
2021-06-02 19:57:38 +00:00
system_meta
Bevy ECS V2 (#1525)
# Bevy ECS V2
This is a rewrite of Bevy ECS (basically everything but the new executor/schedule, which are already awesome). The overall goal was to improve the performance and versatility of Bevy ECS. Here is a quick bulleted list of changes before we dive into the details:
* Complete World rewrite
* Multiple component storage types:
* Tables: fast cache friendly iteration, slower add/removes (previously called Archetypes)
* Sparse Sets: fast add/remove, slower iteration
* Stateful Queries (caches query results for faster iteration. fragmented iteration is _fast_ now)
* Stateful System Params (caches expensive operations. inspired by @DJMcNab's work in #1364)
* Configurable System Params (users can set configuration when they construct their systems. once again inspired by @DJMcNab's work)
* Archetypes are now "just metadata", component storage is separate
* Archetype Graph (for faster archetype changes)
* Component Metadata
* Configure component storage type
* Retrieve information about component size/type/name/layout/send-ness/etc
* Components are uniquely identified by a densely packed ComponentId
* TypeIds are now totally optional (which should make implementing scripting easier)
* Super fast "for_each" query iterators
* Merged Resources into World. Resources are now just a special type of component
* EntityRef/EntityMut builder apis (more efficient and more ergonomic)
* Fast bitset-backed `Access<T>` replaces old hashmap-based approach everywhere
* Query conflicts are determined by component access instead of archetype component access (to avoid random failures at runtime)
* With/Without are still taken into account for conflicts, so this should still be comfy to use
* Much simpler `IntoSystem` impl
* Significantly reduced the amount of hashing throughout the ecs in favor of Sparse Sets (indexed by densely packed ArchetypeId, ComponentId, BundleId, and TableId)
* Safety Improvements
* Entity reservation uses a normal world reference instead of unsafe transmute
* QuerySets no longer transmute lifetimes
* Made traits "unsafe" where relevant
* More thorough safety docs
* WorldCell
* Exposes safe mutable access to multiple resources at a time in a World
* Replaced "catch all" `System::update_archetypes(world: &World)` with `System::new_archetype(archetype: &Archetype)`
* Simpler Bundle implementation
* Replaced slow "remove_bundle_one_by_one" used as fallback for Commands::remove_bundle with fast "remove_bundle_intersection"
* Removed `Mut<T>` query impl. it is better to only support one way: `&mut T`
* Removed with() from `Flags<T>` in favor of `Option<Flags<T>>`, which allows querying for flags to be "filtered" by default
* Components now have is_send property (currently only resources support non-send)
* More granular module organization
* New `RemovedComponents<T>` SystemParam that replaces `query.removed::<T>()`
* `world.resource_scope()` for mutable access to resources and world at the same time
* WorldQuery and QueryFilter traits unified. FilterFetch trait added to enable "short circuit" filtering. Auto impled for cases that don't need it
* Significantly slimmed down SystemState in favor of individual SystemParam state
* System Commands changed from `commands: &mut Commands` back to `mut commands: Commands` (to allow Commands to have a World reference)
Fixes #1320
## `World` Rewrite
This is a from-scratch rewrite of `World` that fills the niche that `hecs` used to. Yes, this means Bevy ECS is no longer a "fork" of hecs. We're going out our own!
(the only shared code between the projects is the entity id allocator, which is already basically ideal)
A huge shout out to @SanderMertens (author of [flecs](https://github.com/SanderMertens/flecs)) for sharing some great ideas with me (specifically hybrid ecs storage and archetype graphs). He also helped advise on a number of implementation details.
## Component Storage (The Problem)
Two ECS storage paradigms have gained a lot of traction over the years:
* **Archetypal ECS**:
* Stores components in "tables" with static schemas. Each "column" stores components of a given type. Each "row" is an entity.
* Each "archetype" has its own table. Adding/removing an entity's component changes the archetype.
* Enables super-fast Query iteration due to its cache-friendly data layout
* Comes at the cost of more expensive add/remove operations for an Entity's components, because all components need to be copied to the new archetype's "table"
* **Sparse Set ECS**:
* Stores components of the same type in densely packed arrays, which are sparsely indexed by densely packed unsigned integers (Entity ids)
* Query iteration is slower than Archetypal ECS because each entity's component could be at any position in the sparse set. This "random access" pattern isn't cache friendly. Additionally, there is an extra layer of indirection because you must first map the entity id to an index in the component array.
* Adding/removing components is a cheap, constant time operation
Bevy ECS V1, hecs, legion, flec, and Unity DOTS are all "archetypal ecs-es". I personally think "archetypal" storage is a good default for game engines. An entity's archetype doesn't need to change frequently in general, and it creates "fast by default" query iteration (which is a much more common operation). It is also "self optimizing". Users don't need to think about optimizing component layouts for iteration performance. It "just works" without any extra boilerplate.
Shipyard and EnTT are "sparse set ecs-es". They employ "packing" as a way to work around the "suboptimal by default" iteration performance for specific sets of components. This helps, but I didn't think this was a good choice for a general purpose engine like Bevy because:
1. "packs" conflict with each other. If bevy decides to internally pack the Transform and GlobalTransform components, users are then blocked if they want to pack some custom component with Transform.
2. users need to take manual action to optimize
Developers selecting an ECS framework are stuck with a hard choice. Select an "archetypal" framework with "fast iteration everywhere" but without the ability to cheaply add/remove components, or select a "sparse set" framework to cheaply add/remove components but with slower iteration performance.
## Hybrid Component Storage (The Solution)
In Bevy ECS V2, we get to have our cake and eat it too. It now has _both_ of the component storage types above (and more can be added later if needed):
* **Tables** (aka "archetypal" storage)
* The default storage. If you don't configure anything, this is what you get
* Fast iteration by default
* Slower add/remove operations
* **Sparse Sets**
* Opt-in
* Slower iteration
* Faster add/remove operations
These storage types complement each other perfectly. By default Query iteration is fast. If developers know that they want to add/remove a component at high frequencies, they can set the storage to "sparse set":
```rust
world.register_component(
ComponentDescriptor::new::<MyComponent>(StorageType::SparseSet)
).unwrap();
```
## Archetypes
Archetypes are now "just metadata" ... they no longer store components directly. They do store:
* The `ComponentId`s of each of the Archetype's components (and that component's storage type)
* Archetypes are uniquely defined by their component layouts
* For example: entities with "table" components `[A, B, C]` _and_ "sparse set" components `[D, E]` will always be in the same archetype.
* The `TableId` associated with the archetype
* For now each archetype has exactly one table (which can have no components),
* There is a 1->Many relationship from Tables->Archetypes. A given table could have any number of archetype components stored in it:
* Ex: an entity with "table storage" components `[A, B, C]` and "sparse set" components `[D, E]` will share the same `[A, B, C]` table as an entity with `[A, B, C]` table component and `[F]` sparse set components.
* This 1->Many relationship is how we preserve fast "cache friendly" iteration performance when possible (more on this later)
* A list of entities that are in the archetype and the row id of the table they are in
* ArchetypeComponentIds
* unique densely packed identifiers for (ArchetypeId, ComponentId) pairs
* used by the schedule executor for cheap system access control
* "Archetype Graph Edges" (see the next section)
## The "Archetype Graph"
Archetype changes in Bevy (and a number of other archetypal ecs-es) have historically been expensive to compute. First, you need to allocate a new vector of the entity's current component ids, add or remove components based on the operation performed, sort it (to ensure it is order-independent), then hash it to find the archetype (if it exists). And thats all before we get to the _already_ expensive full copy of all components to the new table storage.
The solution is to build a "graph" of archetypes to cache these results. @SanderMertens first exposed me to the idea (and he got it from @gjroelofs, who came up with it). They propose adding directed edges between archetypes for add/remove component operations. If `ComponentId`s are densely packed, you can use sparse sets to cheaply jump between archetypes.
Bevy takes this one step further by using add/remove `Bundle` edges instead of `Component` edges. Bevy encourages the use of `Bundles` to group add/remove operations. This is largely for "clearer game logic" reasons, but it also helps cut down on the number of archetype changes required. `Bundles` now also have densely-packed `BundleId`s. This allows us to use a _single_ edge for each bundle operation (rather than needing to traverse N edges ... one for each component). Single component operations are also bundles, so this is strictly an improvement over a "component only" graph.
As a result, an operation that used to be _heavy_ (both for allocations and compute) is now two dirt-cheap array lookups and zero allocations.
## Stateful Queries
World queries are now stateful. This allows us to:
1. Cache archetype (and table) matches
* This resolves another issue with (naive) archetypal ECS: query performance getting worse as the number of archetypes goes up (and fragmentation occurs).
2. Cache Fetch and Filter state
* The expensive parts of fetch/filter operations (such as hashing the TypeId to find the ComponentId) now only happen once when the Query is first constructed
3. Incrementally build up state
* When new archetypes are added, we only process the new archetypes (no need to rebuild state for old archetypes)
As a result, the direct `World` query api now looks like this:
```rust
let mut query = world.query::<(&A, &mut B)>();
for (a, mut b) in query.iter_mut(&mut world) {
}
```
Requiring `World` to generate stateful queries (rather than letting the `QueryState` type be constructed separately) allows us to ensure that _all_ queries are properly initialized (and the relevant world state, such as ComponentIds). This enables QueryState to remove branches from its operations that check for initialization status (and also enables query.iter() to take an immutable world reference because it doesn't need to initialize anything in world).
However in systems, this is a non-breaking change. State management is done internally by the relevant SystemParam.
## Stateful SystemParams
Like Queries, `SystemParams` now also cache state. For example, `Query` system params store the "stateful query" state mentioned above. Commands store their internal `CommandQueue`. This means you can now safely use as many separate `Commands` parameters in your system as you want. `Local<T>` system params store their `T` value in their state (instead of in Resources).
SystemParam state also enabled a significant slim-down of SystemState. It is much nicer to look at now.
Per-SystemParam state naturally insulates us from an "aliased mut" class of errors we have hit in the past (ex: using multiple `Commands` system params).
(credit goes to @DJMcNab for the initial idea and draft pr here #1364)
## Configurable SystemParams
@DJMcNab also had the great idea to make SystemParams configurable. This allows users to provide some initial configuration / values for system parameters (when possible). Most SystemParams have no config (the config type is `()`), but the `Local<T>` param now supports user-provided parameters:
```rust
fn foo(value: Local<usize>) {
}
app.add_system(foo.system().config(|c| c.0 = Some(10)));
```
## Uber Fast "for_each" Query Iterators
Developers now have the choice to use a fast "for_each" iterator, which yields ~1.5-3x iteration speed improvements for "fragmented iteration", and minor ~1.2x iteration speed improvements for unfragmented iteration.
```rust
fn system(query: Query<(&A, &mut B)>) {
// you now have the option to do this for a speed boost
query.for_each_mut(|(a, mut b)| {
});
// however normal iterators are still available
for (a, mut b) in query.iter_mut() {
}
}
```
I think in most cases we should continue to encourage "normal" iterators as they are more flexible and more "rust idiomatic". But when that extra "oomf" is needed, it makes sense to use `for_each`.
We should also consider using `for_each` for internal bevy systems to give our users a nice speed boost (but that should be a separate pr).
## Component Metadata
`World` now has a `Components` collection, which is accessible via `world.components()`. This stores mappings from `ComponentId` to `ComponentInfo`, as well as `TypeId` to `ComponentId` mappings (where relevant). `ComponentInfo` stores information about the component, such as ComponentId, TypeId, memory layout, send-ness (currently limited to resources), and storage type.
## Significantly Cheaper `Access<T>`
We used to use `TypeAccess<TypeId>` to manage read/write component/archetype-component access. This was expensive because TypeIds must be hashed and compared individually. The parallel executor got around this by "condensing" type ids into bitset-backed access types. This worked, but it had to be re-generated from the `TypeAccess<TypeId>`sources every time archetypes changed.
This pr removes TypeAccess in favor of faster bitset access everywhere. We can do this thanks to the move to densely packed `ComponentId`s and `ArchetypeComponentId`s.
## Merged Resources into World
Resources had a lot of redundant functionality with Components. They stored typed data, they had access control, they had unique ids, they were queryable via SystemParams, etc. In fact the _only_ major difference between them was that they were unique (and didn't correlate to an entity).
Separate resources also had the downside of requiring a separate set of access controls, which meant the parallel executor needed to compare more bitsets per system and manage more state.
I initially got the "separate resources" idea from `legion`. I think that design was motivated by the fact that it made the direct world query/resource lifetime interactions more manageable. It certainly made our lives easier when using Resources alongside hecs/bevy_ecs. However we already have a construct for safely and ergonomically managing in-world lifetimes: systems (which use `Access<T>` internally).
This pr merges Resources into World:
```rust
world.insert_resource(1);
world.insert_resource(2.0);
let a = world.get_resource::<i32>().unwrap();
let mut b = world.get_resource_mut::<f64>().unwrap();
*b = 3.0;
```
Resources are now just a special kind of component. They have their own ComponentIds (and their own resource TypeId->ComponentId scope, so they don't conflict wit components of the same type). They are stored in a special "resource archetype", which stores components inside the archetype using a new `unique_components` sparse set (note that this sparse set could later be used to implement Tags). This allows us to keep the code size small by reusing existing datastructures (namely Column, Archetype, ComponentFlags, and ComponentInfo). This allows us the executor to use a single `Access<ArchetypeComponentId>` per system. It should also make scripting language integration easier.
_But_ this merge did create problems for people directly interacting with `World`. What if you need mutable access to multiple resources at the same time? `world.get_resource_mut()` borrows World mutably!
## WorldCell
WorldCell applies the `Access<ArchetypeComponentId>` concept to direct world access:
```rust
let world_cell = world.cell();
let a = world_cell.get_resource_mut::<i32>().unwrap();
let b = world_cell.get_resource_mut::<f64>().unwrap();
```
This adds cheap runtime checks (a sparse set lookup of `ArchetypeComponentId` and a counter) to ensure that world accesses do not conflict with each other. Each operation returns a `WorldBorrow<'w, T>` or `WorldBorrowMut<'w, T>` wrapper type, which will release the relevant ArchetypeComponentId resources when dropped.
World caches the access sparse set (and only one cell can exist at a time), so `world.cell()` is a cheap operation.
WorldCell does _not_ use atomic operations. It is non-send, does a mutable borrow of world to prevent other accesses, and uses a simple `Rc<RefCell<ArchetypeComponentAccess>>` wrapper in each WorldBorrow pointer.
The api is currently limited to resource access, but it can and should be extended to queries / entity component access.
## Resource Scopes
WorldCell does not yet support component queries, and even when it does there are sometimes legitimate reasons to want a mutable world ref _and_ a mutable resource ref (ex: bevy_render and bevy_scene both need this). In these cases we could always drop down to the unsafe `world.get_resource_unchecked_mut()`, but that is not ideal!
Instead developers can use a "resource scope"
```rust
world.resource_scope(|world: &mut World, a: &mut A| {
})
```
This temporarily removes the `A` resource from `World`, provides mutable pointers to both, and re-adds A to World when finished. Thanks to the move to ComponentIds/sparse sets, this is a cheap operation.
If multiple resources are required, scopes can be nested. We could also consider adding a "resource tuple" to the api if this pattern becomes common and the boilerplate gets nasty.
## Query Conflicts Use ComponentId Instead of ArchetypeComponentId
For safety reasons, systems cannot contain queries that conflict with each other without wrapping them in a QuerySet. On bevy `main`, we use ArchetypeComponentIds to determine conflicts. This is nice because it can take into account filters:
```rust
// these queries will never conflict due to their filters
fn filter_system(a: Query<&mut A, With<B>>, b: Query<&mut B, Without<B>>) {
}
```
But it also has a significant downside:
```rust
// these queries will not conflict _until_ an entity with A, B, and C is spawned
fn maybe_conflicts_system(a: Query<(&mut A, &C)>, b: Query<(&mut A, &B)>) {
}
```
The system above will panic at runtime if an entity with A, B, and C is spawned. This makes it hard to trust that your game logic will run without crashing.
In this pr, I switched to using `ComponentId` instead. This _is_ more constraining. `maybe_conflicts_system` will now always fail, but it will do it consistently at startup. Naively, it would also _disallow_ `filter_system`, which would be a significant downgrade in usability. Bevy has a number of internal systems that rely on disjoint queries and I expect it to be a common pattern in userspace.
To resolve this, I added a new `FilteredAccess<T>` type, which wraps `Access<T>` and adds with/without filters. If two `FilteredAccess` have with/without values that prove they are disjoint, they will no longer conflict.
## EntityRef / EntityMut
World entity operations on `main` require that the user passes in an `entity` id to each operation:
```rust
let entity = world.spawn((A, )); // create a new entity with A
world.get::<A>(entity);
world.insert(entity, (B, C));
world.insert_one(entity, D);
```
This means that each operation needs to look up the entity location / verify its validity. The initial spawn operation also requires a Bundle as input. This can be awkward when no components are required (or one component is required).
These operations have been replaced by `EntityRef` and `EntityMut`, which are "builder-style" wrappers around world that provide read and read/write operations on a single, pre-validated entity:
```rust
// spawn now takes no inputs and returns an EntityMut
let entity = world.spawn()
.insert(A) // insert a single component into the entity
.insert_bundle((B, C)) // insert a bundle of components into the entity
.id() // id returns the Entity id
// Returns EntityMut (or panics if the entity does not exist)
world.entity_mut(entity)
.insert(D)
.insert_bundle(SomeBundle::default());
{
// returns EntityRef (or panics if the entity does not exist)
let d = world.entity(entity)
.get::<D>() // gets the D component
.unwrap();
// world.get still exists for ergonomics
let d = world.get::<D>(entity).unwrap();
}
// These variants return Options if you want to check existence instead of panicing
world.get_entity_mut(entity)
.unwrap()
.insert(E);
if let Some(entity_ref) = world.get_entity(entity) {
let d = entity_ref.get::<D>().unwrap();
}
```
This _does not_ affect the current Commands api or terminology. I think that should be a separate conversation as that is a much larger breaking change.
## Safety Improvements
* Entity reservation in Commands uses a normal world borrow instead of an unsafe transmute
* QuerySets no longer transmutes lifetimes
* Made traits "unsafe" when implementing a trait incorrectly could cause unsafety
* More thorough safety docs
## RemovedComponents SystemParam
The old approach to querying removed components: `query.removed:<T>()` was confusing because it had no connection to the query itself. I replaced it with the following, which is both clearer and allows us to cache the ComponentId mapping in the SystemParamState:
```rust
fn system(removed: RemovedComponents<T>) {
for entity in removed.iter() {
}
}
```
## Simpler Bundle implementation
Bundles are no longer responsible for sorting (or deduping) TypeInfo. They are just a simple ordered list of component types / data. This makes the implementation smaller and opens the door to an easy "nested bundle" implementation in the future (which i might even add in this pr). Duplicate detection is now done once per bundle type by World the first time a bundle is used.
## Unified WorldQuery and QueryFilter types
(don't worry they are still separate type _parameters_ in Queries .. this is a non-breaking change)
WorldQuery and QueryFilter were already basically identical apis. With the addition of `FetchState` and more storage-specific fetch methods, the overlap was even clearer (and the redundancy more painful).
QueryFilters are now just `F: WorldQuery where F::Fetch: FilterFetch`. FilterFetch requires `Fetch<Item = bool>` and adds new "short circuit" variants of fetch methods. This enables a filter tuple like `(With<A>, Without<B>, Changed<C>)` to stop evaluating the filter after the first mismatch is encountered. FilterFetch is automatically implemented for `Fetch` implementations that return bool.
This forces fetch implementations that return things like `(bool, bool, bool)` (such as the filter above) to manually implement FilterFetch and decide whether or not to short-circuit.
## More Granular Modules
World no longer globs all of the internal modules together. It now exports `core`, `system`, and `schedule` separately. I'm also considering exporting `core` submodules directly as that is still pretty "glob-ey" and unorganized (feedback welcome here).
## Remaining Draft Work (to be done in this pr)
* ~~panic on conflicting WorldQuery fetches (&A, &mut A)~~
* ~~bevy `main` and hecs both currently allow this, but we should protect against it if possible~~
* ~~batch_iter / par_iter (currently stubbed out)~~
* ~~ChangedRes~~
* ~~I skipped this while we sort out #1313. This pr should be adapted to account for whatever we land on there~~.
* ~~The `Archetypes` and `Tables` collections use hashes of sorted lists of component ids to uniquely identify each archetype/table. This hash is then used as the key in a HashMap to look up the relevant ArchetypeId or TableId. (which doesn't handle hash collisions properly)~~
* ~~It is currently unsafe to generate a Query from "World A", then use it on "World B" (despite the api claiming it is safe). We should probably close this gap. This could be done by adding a randomly generated WorldId to each world, then storing that id in each Query. They could then be compared to each other on each `query.do_thing(&world)` operation. This _does_ add an extra branch to each query operation, so I'm open to other suggestions if people have them.~~
* ~~Nested Bundles (if i find time)~~
## Potential Future Work
* Expand WorldCell to support queries.
* Consider not allocating in the empty archetype on `world.spawn()`
* ex: return something like EntityMutUninit, which turns into EntityMut after an `insert` or `insert_bundle` op
* this actually regressed performance last time i tried it, but in theory it should be faster
* Optimize SparseSet::insert (see `PERF` comment on insert)
* Replace SparseArray `Option<T>` with T::MAX to cut down on branching
* would enable cheaper get_unchecked() operations
* upstream fixedbitset optimizations
* fixedbitset could be allocation free for small block counts (store blocks in a SmallVec)
* fixedbitset could have a const constructor
* Consider implementing Tags (archetype-specific by-value data that affects archetype identity)
* ex: ArchetypeA could have `[A, B, C]` table components and `[D(1)]` "tag" component. ArchetypeB could have `[A, B, C]` table components and a `[D(2)]` tag component. The archetypes are different, despite both having D tags because the value inside D is different.
* this could potentially build on top of the `archetype.unique_components` added in this pr for resource storage.
* Consider reverting `all_tuples` proc macro in favor of the old `macro_rules` implementation
* all_tuples is more flexible and produces cleaner documentation (the macro_rules version produces weird type parameter orders due to parser constraints)
* but unfortunately all_tuples also appears to make Rust Analyzer sad/slow when working inside of `bevy_ecs` (does not affect user code)
* Consider "resource queries" and/or "mixed resource and entity component queries" as an alternative to WorldCell
* this is basically just "systems" so maybe it's not worth it
* Add more world ops
* `world.clear()`
* `world.reserve<T: Bundle>(count: usize)`
* Try using the old archetype allocation strategy (allocate new memory on resize and copy everything over). I expect this to improve batch insertion performance at the cost of unbatched performance. But thats just a guess. I'm not an allocation perf pro :)
* Adapt Commands apis for consistency with new World apis
## Benchmarks
key:
* `bevy_old`: bevy `main` branch
* `bevy`: this branch
* `_foreach`: uses an optimized for_each iterator
* ` _sparse`: uses sparse set storage (if unspecified assume table storage)
* `_system`: runs inside a system (if unspecified assume test happens via direct world ops)
### Simple Insert (from ecs_bench_suite)
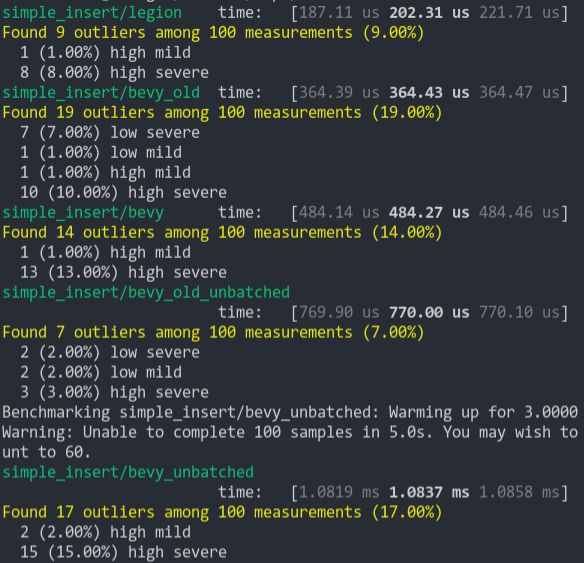
### Simpler Iter (from ecs_bench_suite)
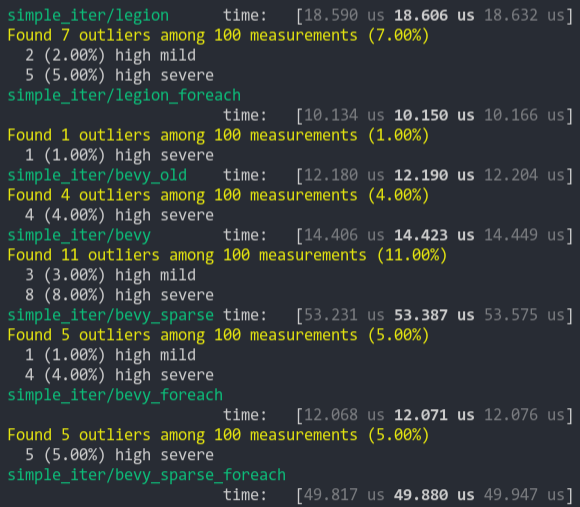
### Fragment Iter (from ecs_bench_suite)
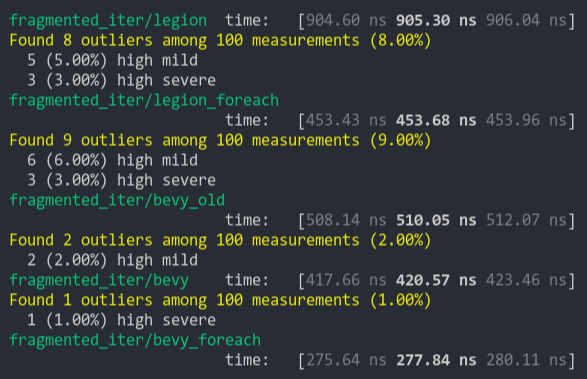
### Sparse Fragmented Iter
Iterate a query that matches 5 entities from a single matching archetype, but there are 100 unmatching archetypes
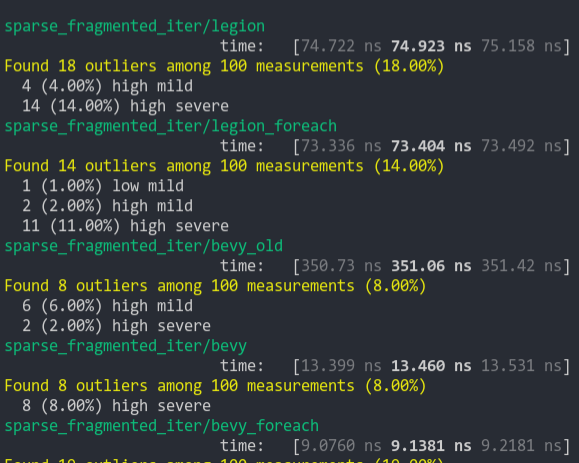
### Schedule (from ecs_bench_suite)
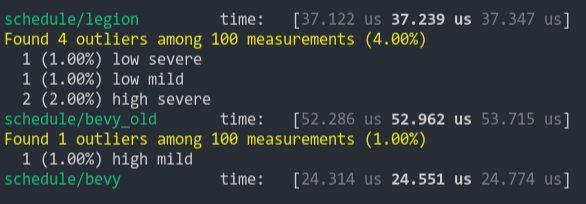
### Add Remove Component (from ecs_bench_suite)
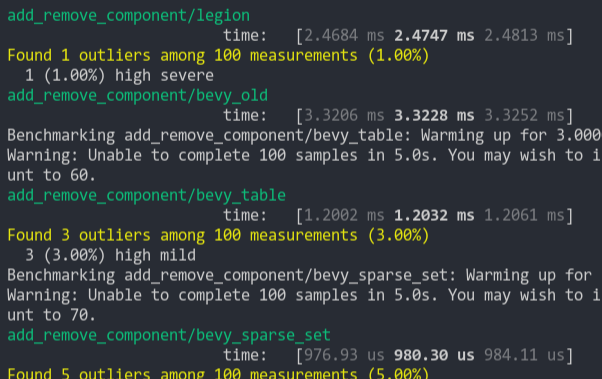
### Add Remove Component Big
Same as the test above, but each entity has 5 "large" matrix components and 1 "large" matrix component is added and removed
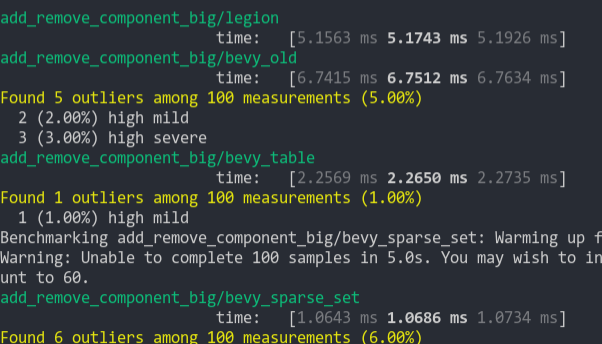
### Get Component
Looks up a single component value a large number of times
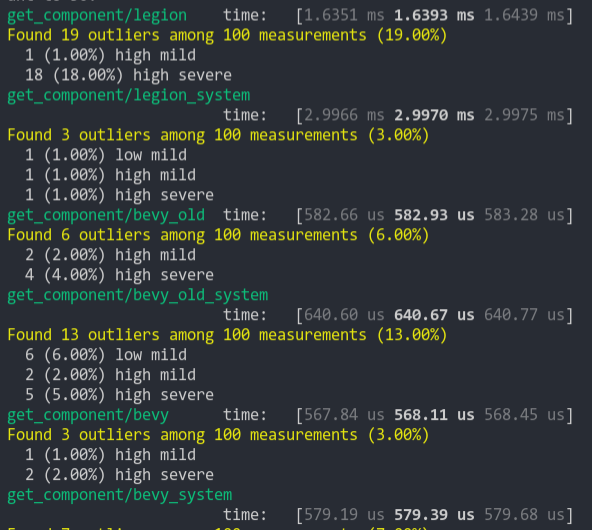
2021-03-05 07:54:35 +00:00
. archetype_component_access
. add_read ( archetype_component_id ) ;
Remove the `SystemParamState` trait and remove types like `ResState` (#6919)
Spiritual successor to #5205.
Actual successor to #6865.
# Objective
Currently, system params are defined using three traits: `SystemParam`, `ReadOnlySystemParam`, `SystemParamState`. The behavior for each param is specified by the `SystemParamState` trait, while `SystemParam` simply defers to the state.
Splitting the traits in this way makes it easier to implement within macros, but it increases the cognitive load. Worst of all, this approach requires each `MySystemParam` to have a public `MySystemParamState` type associated with it.
## Solution
* Merge the trait `SystemParamState` into `SystemParam`.
* Remove all trivial `SystemParam` state types.
* `OptionNonSendMutState<T>`: you will not be missed.
---
- [x] Fix/resolve the remaining test failure.
## Changelog
* Removed the trait `SystemParamState`, merging its functionality into `SystemParam`.
## Migration Guide
**Note**: this should replace the migration guide for #6865.
This is relative to Bevy 0.9, not main.
The traits `SystemParamState` and `SystemParamFetch` have been removed, and their functionality has been transferred to `SystemParam`.
```rust
// Before (0.9)
impl SystemParam for MyParam<'_, '_> {
type State = MyParamState;
}
unsafe impl SystemParamState for MyParamState {
fn init(world: &mut World, system_meta: &mut SystemMeta) -> Self { ... }
}
unsafe impl<'w, 's> SystemParamFetch<'w, 's> for MyParamState {
type Item = MyParam<'w, 's>;
fn get_param(&mut self, ...) -> Self::Item;
}
unsafe impl ReadOnlySystemParamFetch for MyParamState { }
// After (0.10)
unsafe impl SystemParam for MyParam<'_, '_> {
type State = MyParamState;
type Item<'w, 's> = MyParam<'w, 's>;
fn init_state(world: &mut World, system_meta: &mut SystemMeta) -> Self::State { ... }
fn get_param<'w, 's>(state: &mut Self::State, ...) -> Self::Item<'w, 's>;
}
unsafe impl ReadOnlySystemParam for MyParam<'_, '_> { }
```
The trait `ReadOnlySystemParamFetch` has been replaced with `ReadOnlySystemParam`.
```rust
// Before
unsafe impl ReadOnlySystemParamFetch for MyParamState {}
// After
unsafe impl ReadOnlySystemParam for MyParam<'_, '_> {}
```
2023-01-07 23:20:32 +00:00
component_id
2020-11-08 20:34:05 +00:00
}
#[ inline ]
Simplify trait hierarchy for `SystemParam` (#6865)
# Objective
* Implementing a custom `SystemParam` by hand requires implementing three traits -- four if it is read-only.
* The trait `SystemParamFetch<'w, 's>` is a workaround from before we had generic associated types, and is no longer necessary.
## Solution
* Combine the trait `SystemParamFetch` with `SystemParamState`.
* I decided to remove the `Fetch` name and keep the `State` name, since the former was consistently conflated with the latter.
* Replace the trait `ReadOnlySystemParamFetch` with `ReadOnlySystemParam`, which simplifies trait bounds in generic code.
---
## Changelog
- Removed the trait `SystemParamFetch`, moving its functionality to `SystemParamState`.
- Replaced the trait `ReadOnlySystemParamFetch` with `ReadOnlySystemParam`.
## Migration Guide
The trait `SystemParamFetch` has been removed, and its functionality has been transferred to `SystemParamState`.
```rust
// Before
impl SystemParamState for MyParamState {
fn init(world: &mut World, system_meta: &mut SystemMeta) -> Self { ... }
}
impl<'w, 's> SystemParamFetch<'w, 's> for MyParamState {
type Item = MyParam<'w, 's>;
fn get_param(...) -> Self::Item;
}
// After
impl SystemParamState for MyParamState {
type Item<'w, 's> = MyParam<'w, 's>; // Generic associated types!
fn init(world: &mut World, system_meta: &mut SystemMeta) -> Self { ... }
fn get_param<'w, 's>(...) -> Self::Item<'w, 's>;
}
```
The trait `ReadOnlySystemParamFetch` has been replaced with `ReadOnlySystemParam`.
```rust
// Before
unsafe impl ReadOnlySystemParamFetch for MyParamState {}
// After
unsafe impl<'w, 's> ReadOnlySystemParam for MyParam<'w, 's> {}
```
2022-12-11 18:34:14 +00:00
unsafe fn get_param < ' w , ' s > (
Remove the `SystemParamState` trait and remove types like `ResState` (#6919)
Spiritual successor to #5205.
Actual successor to #6865.
# Objective
Currently, system params are defined using three traits: `SystemParam`, `ReadOnlySystemParam`, `SystemParamState`. The behavior for each param is specified by the `SystemParamState` trait, while `SystemParam` simply defers to the state.
Splitting the traits in this way makes it easier to implement within macros, but it increases the cognitive load. Worst of all, this approach requires each `MySystemParam` to have a public `MySystemParamState` type associated with it.
## Solution
* Merge the trait `SystemParamState` into `SystemParam`.
* Remove all trivial `SystemParam` state types.
* `OptionNonSendMutState<T>`: you will not be missed.
---
- [x] Fix/resolve the remaining test failure.
## Changelog
* Removed the trait `SystemParamState`, merging its functionality into `SystemParam`.
## Migration Guide
**Note**: this should replace the migration guide for #6865.
This is relative to Bevy 0.9, not main.
The traits `SystemParamState` and `SystemParamFetch` have been removed, and their functionality has been transferred to `SystemParam`.
```rust
// Before (0.9)
impl SystemParam for MyParam<'_, '_> {
type State = MyParamState;
}
unsafe impl SystemParamState for MyParamState {
fn init(world: &mut World, system_meta: &mut SystemMeta) -> Self { ... }
}
unsafe impl<'w, 's> SystemParamFetch<'w, 's> for MyParamState {
type Item = MyParam<'w, 's>;
fn get_param(&mut self, ...) -> Self::Item;
}
unsafe impl ReadOnlySystemParamFetch for MyParamState { }
// After (0.10)
unsafe impl SystemParam for MyParam<'_, '_> {
type State = MyParamState;
type Item<'w, 's> = MyParam<'w, 's>;
fn init_state(world: &mut World, system_meta: &mut SystemMeta) -> Self::State { ... }
fn get_param<'w, 's>(state: &mut Self::State, ...) -> Self::Item<'w, 's>;
}
unsafe impl ReadOnlySystemParam for MyParam<'_, '_> { }
```
The trait `ReadOnlySystemParamFetch` has been replaced with `ReadOnlySystemParam`.
```rust
// Before
unsafe impl ReadOnlySystemParamFetch for MyParamState {}
// After
unsafe impl ReadOnlySystemParam for MyParam<'_, '_> {}
```
2023-01-07 23:20:32 +00:00
& mut component_id : & ' s mut Self ::State ,
Add new SystemState and rename old SystemState to SystemMeta (#2283)
This enables `SystemParams` to be used outside of function systems. Anything can create and store `SystemState`, which enables efficient "param state cached" access to `SystemParams`.
It adds a `ReadOnlySystemParamFetch` trait, which enables safe `SystemState::get` calls without unique world access.
I renamed the old `SystemState` to `SystemMeta` to enable us to mirror the `QueryState` naming convention (but I'm happy to discuss alternative names if people have other ideas). I initially pitched this as `ParamState`, but given that it needs to include full system metadata, that doesn't feel like a particularly accurate name.
```rust
#[derive(Eq, PartialEq, Debug)]
struct A(usize);
#[derive(Eq, PartialEq, Debug)]
struct B(usize);
let mut world = World::default();
world.insert_resource(A(42));
world.spawn().insert(B(7));
// we get nice lifetime elision when declaring the type on the left hand side
let mut system_state: SystemState<(Res<A>, Query<&B>)> = SystemState::new(&mut world);
let (a, query) = system_state.get(&world);
assert_eq!(*a, A(42), "returned resource matches initial value");
assert_eq!(
*query.single().unwrap(),
B(7),
"returned component matches initial value"
);
// mutable system params require unique world access
let mut system_state: SystemState<(ResMut<A>, Query<&mut B>)> = SystemState::new(&mut world);
let (a, query) = system_state.get_mut(&mut world);
// static lifetimes are required when declaring inside of structs
struct SomeContainer {
state: SystemState<(Res<'static, A>, Res<'static, B>)>
}
// this can be shortened using type aliases, which will be useful for complex param tuples
type MyParams<'a> = (Res<'a, A>, Res<'a, B>);
struct SomeContainer {
state: SystemState<MyParams<'static>>
}
// It is the user's responsibility to call SystemState::apply(world) for parameters that queue up work
let mut system_state: SystemState<(Commands, Query<&B>)> = SystemState::new(&mut world);
{
let (mut commands, query) = system_state.get(&world);
commands.insert_resource(3.14);
}
system_state.apply(&mut world);
```
## Future Work
* Actually use SystemState inside FunctionSystem. This would be trivial, but it requires FunctionSystem to wrap SystemState in Option in its current form (which complicates system metadata lookup). I'd prefer to hold off until we adopt something like the later designs linked in #1364, which enable us to contruct Systems using a World reference (and also remove the need for `.system`).
* Consider a "scoped" approach to automatically call SystemState::apply when systems params are no longer being used (either a container type with a Drop impl, or a function that takes a closure for user logic operating on params).
2021-06-02 19:57:38 +00:00
system_meta : & SystemMeta ,
System Param Lifetime Split (#2605)
# Objective
Enable using exact World lifetimes during read-only access . This is motivated by the new renderer's need to allow read-only world-only queries to outlive the query itself (but still be constrained by the world lifetime).
For example:
https://github.com/bevyengine/bevy/blob/115b170d1f11a91146bb6d6e9684dceb8b21f786/pipelined/bevy_pbr2/src/render/mod.rs#L774
## Solution
Split out SystemParam state and world lifetimes and pipe those lifetimes up to read-only Query ops (and add into_inner for Res). According to every safety test I've run so far (except one), this is safe (see the temporary safety test commit). Note that changing the mutable variants to the new lifetimes would allow aliased mutable pointers (try doing that to see how it affects the temporary safety tests).
The new state lifetime on SystemParam does make `#[derive(SystemParam)]` more cumbersome (the current impl requires PhantomData if you don't use both lifetimes). We can make this better by detecting whether or not a lifetime is used in the derive and adjusting accordingly, but that should probably be done in its own pr.
## Why is this a draft?
The new lifetimes break QuerySet safety in one very specific case (see the query_set system in system_safety_test). We need to solve this before we can use the lifetimes given.
This is due to the fact that QuerySet is just a wrapper over Query, which now relies on world lifetimes instead of `&self` lifetimes to prevent aliasing (but in systems, each Query has its own implied lifetime, not a centralized world lifetime). I believe the fix is to rewrite QuerySet to have its own World lifetime (and own the internal reference). This will complicate the impl a bit, but I think it is doable. I'm curious if anyone else has better ideas.
Personally, I think these new lifetimes need to happen. We've gotta have a way to directly tie read-only World queries to the World lifetime. The new renderer is the first place this has come up, but I doubt it will be the last. Worst case scenario we can come up with a second `WorldLifetimeQuery<Q, F = ()>` parameter to enable these read-only scenarios, but I'd rather not add another type to the type zoo.
2021-08-15 20:51:53 +00:00
world : & ' w World ,
Reliable change detection (#1471)
# Problem Definition
The current change tracking (via flags for both components and resources) fails to detect changes made by systems that are scheduled to run earlier in the frame than they are.
This issue is discussed at length in [#68](https://github.com/bevyengine/bevy/issues/68) and [#54](https://github.com/bevyengine/bevy/issues/54).
This is very much a draft PR, and contributions are welcome and needed.
# Criteria
1. Each change is detected at least once, no matter the ordering.
2. Each change is detected at most once, no matter the ordering.
3. Changes should be detected the same frame that they are made.
4. Competitive ergonomics. Ideally does not require opting-in.
5. Low CPU overhead of computation.
6. Memory efficient. This must not increase over time, except where the number of entities / resources does.
7. Changes should not be lost for systems that don't run.
8. A frame needs to act as a pure function. Given the same set of entities / components it needs to produce the same end state without side-effects.
**Exact** change-tracking proposals satisfy criteria 1 and 2.
**Conservative** change-tracking proposals satisfy criteria 1 but not 2.
**Flaky** change tracking proposals satisfy criteria 2 but not 1.
# Code Base Navigation
There are three types of flags:
- `Added`: A piece of data was added to an entity / `Resources`.
- `Mutated`: A piece of data was able to be modified, because its `DerefMut` was accessed
- `Changed`: The bitwise OR of `Added` and `Changed`
The special behavior of `ChangedRes`, with respect to the scheduler is being removed in [#1313](https://github.com/bevyengine/bevy/pull/1313) and does not need to be reproduced.
`ChangedRes` and friends can be found in "bevy_ecs/core/resources/resource_query.rs".
The `Flags` trait for Components can be found in "bevy_ecs/core/query.rs".
`ComponentFlags` are stored in "bevy_ecs/core/archetypes.rs", defined on line 446.
# Proposals
**Proposal 5 was selected for implementation.**
## Proposal 0: No Change Detection
The baseline, where computations are performed on everything regardless of whether it changed.
**Type:** Conservative
**Pros:**
- already implemented
- will never miss events
- no overhead
**Cons:**
- tons of repeated work
- doesn't allow users to avoid repeating work (or monitoring for other changes)
## Proposal 1: Earlier-This-Tick Change Detection
The current approach as of Bevy 0.4. Flags are set, and then flushed at the end of each frame.
**Type:** Flaky
**Pros:**
- already implemented
- simple to understand
- low memory overhead (2 bits per component)
- low time overhead (clear every flag once per frame)
**Cons:**
- misses systems based on ordering
- systems that don't run every frame miss changes
- duplicates detection when looping
- can lead to unresolvable circular dependencies
## Proposal 2: Two-Tick Change Detection
Flags persist for two frames, using a double-buffer system identical to that used in events.
A change is observed if it is found in either the current frame's list of changes or the previous frame's.
**Type:** Conservative
**Pros:**
- easy to understand
- easy to implement
- low memory overhead (4 bits per component)
- low time overhead (bit mask and shift every flag once per frame)
**Cons:**
- can result in a great deal of duplicated work
- systems that don't run every frame miss changes
- duplicates detection when looping
## Proposal 3: Last-Tick Change Detection
Flags persist for two frames, using a double-buffer system identical to that used in events.
A change is observed if it is found in the previous frame's list of changes.
**Type:** Exact
**Pros:**
- exact
- easy to understand
- easy to implement
- low memory overhead (4 bits per component)
- low time overhead (bit mask and shift every flag once per frame)
**Cons:**
- change detection is always delayed, possibly causing painful chained delays
- systems that don't run every frame miss changes
- duplicates detection when looping
## Proposal 4: Flag-Doubling Change Detection
Combine Proposal 2 and Proposal 3. Differentiate between `JustChanged` (current behavior) and `Changed` (Proposal 3).
Pack this data into the flags according to [this implementation proposal](https://github.com/bevyengine/bevy/issues/68#issuecomment-769174804).
**Type:** Flaky + Exact
**Pros:**
- allows users to acc
- easy to implement
- low memory overhead (4 bits per component)
- low time overhead (bit mask and shift every flag once per frame)
**Cons:**
- users must specify the type of change detection required
- still quite fragile to system ordering effects when using the flaky `JustChanged` form
- cannot get immediate + exact results
- systems that don't run every frame miss changes
- duplicates detection when looping
## [SELECTED] Proposal 5: Generation-Counter Change Detection
A global counter is increased after each system is run. Each component saves the time of last mutation, and each system saves the time of last execution. Mutation is detected when the component's counter is greater than the system's counter. Discussed [here](https://github.com/bevyengine/bevy/issues/68#issuecomment-769174804). How to handle addition detection is unsolved; the current proposal is to use the highest bit of the counter as in proposal 1.
**Type:** Exact (for mutations), flaky (for additions)
**Pros:**
- low time overhead (set component counter on access, set system counter after execution)
- robust to systems that don't run every frame
- robust to systems that loop
**Cons:**
- moderately complex implementation
- must be modified as systems are inserted dynamically
- medium memory overhead (4 bytes per component + system)
- unsolved addition detection
## Proposal 6: System-Data Change Detection
For each system, track which system's changes it has seen. This approach is only worth fully designing and implementing if Proposal 5 fails in some way.
**Type:** Exact
**Pros:**
- exact
- conceptually simple
**Cons:**
- requires storing data on each system
- implementation is complex
- must be modified as systems are inserted dynamically
## Proposal 7: Total-Order Change Detection
Discussed [here](https://github.com/bevyengine/bevy/issues/68#issuecomment-754326523). This proposal is somewhat complicated by the new scheduler, but I believe it should still be conceptually feasible. This approach is only worth fully designing and implementing if Proposal 5 fails in some way.
**Type:** Exact
**Pros:**
- exact
- efficient data storage relative to other exact proposals
**Cons:**
- requires access to the scheduler
- complex implementation and difficulty grokking
- must be modified as systems are inserted dynamically
# Tests
- We will need to verify properties 1, 2, 3, 7 and 8. Priority: 1 > 2 = 3 > 8 > 7
- Ideally we can use identical user-facing syntax for all proposals, allowing us to re-use the same syntax for each.
- When writing tests, we need to carefully specify order using explicit dependencies.
- These tests will need to be duplicated for both components and resources.
- We need to be sure to handle cases where ambiguous system orders exist.
`changing_system` is always the system that makes the changes, and `detecting_system` always detects the changes.
The component / resource changed will be simple boolean wrapper structs.
## Basic Added / Mutated / Changed
2 x 3 design:
- Resources vs. Components
- Added vs. Changed vs. Mutated
- `changing_system` runs before `detecting_system`
- verify at the end of tick 2
## At Least Once
2 x 3 design:
- Resources vs. Components
- Added vs. Changed vs. Mutated
- `changing_system` runs after `detecting_system`
- verify at the end of tick 2
## At Most Once
2 x 3 design:
- Resources vs. Components
- Added vs. Changed vs. Mutated
- `changing_system` runs once before `detecting_system`
- increment a counter based on the number of changes detected
- verify at the end of tick 2
## Fast Detection
2 x 3 design:
- Resources vs. Components
- Added vs. Changed vs. Mutated
- `changing_system` runs before `detecting_system`
- verify at the end of tick 1
## Ambiguous System Ordering Robustness
2 x 3 x 2 design:
- Resources vs. Components
- Added vs. Changed vs. Mutated
- `changing_system` runs [before/after] `detecting_system` in tick 1
- `changing_system` runs [after/before] `detecting_system` in tick 2
## System Pausing
2 x 3 design:
- Resources vs. Components
- Added vs. Changed vs. Mutated
- `changing_system` runs in tick 1, then is disabled by run criteria
- `detecting_system` is disabled by run criteria until it is run once during tick 3
- verify at the end of tick 3
## Addition Causes Mutation
2 design:
- Resources vs. Components
- `adding_system_1` adds a component / resource
- `adding system_2` adds the same component / resource
- verify the `Mutated` flag at the end of the tick
- verify the `Added` flag at the end of the tick
First check tests for: https://github.com/bevyengine/bevy/issues/333
Second check tests for: https://github.com/bevyengine/bevy/issues/1443
## Changes Made By Commands
- `adding_system` runs in Update in tick 1, and sends a command to add a component
- `detecting_system` runs in Update in tick 1 and 2, after `adding_system`
- We can't detect the changes in tick 1, since they haven't been processed yet
- If we were to track these changes as being emitted by `adding_system`, we can't detect the changes in tick 2 either, since `detecting_system` has already run once after `adding_system` :(
# Benchmarks
See: [general advice](https://github.com/bevyengine/bevy/blob/master/docs/profiling.md), [Criterion crate](https://github.com/bheisler/criterion.rs)
There are several critical parameters to vary:
1. entity count (1 to 10^9)
2. fraction of entities that are changed (0% to 100%)
3. cost to perform work on changed entities, i.e. workload (1 ns to 1s)
1 and 2 should be varied between benchmark runs. 3 can be added on computationally.
We want to measure:
- memory cost
- run time
We should collect these measurements across several frames (100?) to reduce bootup effects and accurately measure the mean, variance and drift.
Entity-component change detection is much more important to benchmark than resource change detection, due to the orders of magnitude higher number of pieces of data.
No change detection at all should be included in benchmarks as a second control for cases where missing changes is unacceptable.
## Graphs
1. y: performance, x: log_10(entity count), color: proposal, facet: performance metric. Set cost to perform work to 0.
2. y: run time, x: cost to perform work, color: proposal, facet: fraction changed. Set number of entities to 10^6
3. y: memory, x: frames, color: proposal
# Conclusions
1. Is the theoretical categorization of the proposals correct according to our tests?
2. How does the performance of the proposals compare without any load?
3. How does the performance of the proposals compare with realistic loads?
4. At what workload does more exact change tracking become worth the (presumably) higher overhead?
5. When does adding change-detection to save on work become worthwhile?
6. Is there enough divergence in performance between the best solutions in each class to ship more than one change-tracking solution?
# Implementation Plan
1. Write a test suite.
2. Verify that tests fail for existing approach.
3. Write a benchmark suite.
4. Get performance numbers for existing approach.
5. Implement, test and benchmark various solutions using a Git branch per proposal.
6. Create a draft PR with all solutions and present results to team.
7. Select a solution and replace existing change detection.
Co-authored-by: Brice DAVIER <bricedavier@gmail.com>
Co-authored-by: Carter Anderson <mcanders1@gmail.com>
2021-03-19 17:53:26 +00:00
change_tick : u32 ,
Simplify trait hierarchy for `SystemParam` (#6865)
# Objective
* Implementing a custom `SystemParam` by hand requires implementing three traits -- four if it is read-only.
* The trait `SystemParamFetch<'w, 's>` is a workaround from before we had generic associated types, and is no longer necessary.
## Solution
* Combine the trait `SystemParamFetch` with `SystemParamState`.
* I decided to remove the `Fetch` name and keep the `State` name, since the former was consistently conflated with the latter.
* Replace the trait `ReadOnlySystemParamFetch` with `ReadOnlySystemParam`, which simplifies trait bounds in generic code.
---
## Changelog
- Removed the trait `SystemParamFetch`, moving its functionality to `SystemParamState`.
- Replaced the trait `ReadOnlySystemParamFetch` with `ReadOnlySystemParam`.
## Migration Guide
The trait `SystemParamFetch` has been removed, and its functionality has been transferred to `SystemParamState`.
```rust
// Before
impl SystemParamState for MyParamState {
fn init(world: &mut World, system_meta: &mut SystemMeta) -> Self { ... }
}
impl<'w, 's> SystemParamFetch<'w, 's> for MyParamState {
type Item = MyParam<'w, 's>;
fn get_param(...) -> Self::Item;
}
// After
impl SystemParamState for MyParamState {
type Item<'w, 's> = MyParam<'w, 's>; // Generic associated types!
fn init(world: &mut World, system_meta: &mut SystemMeta) -> Self { ... }
fn get_param<'w, 's>(...) -> Self::Item<'w, 's>;
}
```
The trait `ReadOnlySystemParamFetch` has been replaced with `ReadOnlySystemParam`.
```rust
// Before
unsafe impl ReadOnlySystemParamFetch for MyParamState {}
// After
unsafe impl<'w, 's> ReadOnlySystemParam for MyParam<'w, 's> {}
```
2022-12-11 18:34:14 +00:00
) -> Self ::Item < ' w , ' s > {
2022-10-24 13:46:36 +00:00
let ( ptr , ticks ) = world
2023-02-06 19:02:52 +00:00
. as_unsafe_world_cell_migration_internal ( )
Panic on dropping NonSend in non-origin thread. (#6534)
# Objective
Fixes #3310. Fixes #6282. Fixes #6278. Fixes #3666.
## Solution
Split out `!Send` resources into `NonSendResources`. Add a `origin_thread_id` to all `!Send` Resources, check it on dropping `NonSendResourceData`, if there's a mismatch, panic. Moved all of the checks that `MainThreadValidator` would do into `NonSendResources` instead.
All `!Send` resources now individually track which thread they were inserted from. This is validated against for every access, mutation, and drop that could be done against the value.
A regression test using an altered version of the example from #3310 has been added.
This is a stopgap solution for the current status quo. A full solution may involve fully removing `!Send` resources/components from `World`, which will likely require a much more thorough design on how to handle the existing in-engine and ecosystem use cases.
This PR also introduces another breaking change:
```rust
use bevy_ecs::prelude::*;
#[derive(Resource)]
struct Resource(u32);
fn main() {
let mut world = World::new();
world.insert_resource(Resource(1));
world.insert_non_send_resource(Resource(2));
let res = world.get_resource_mut::<Resource>().unwrap();
assert_eq!(res.0, 2);
}
```
This code will run correctly on 0.9.1 but not with this PR, since NonSend resources and normal resources have become actual distinct concepts storage wise.
## Changelog
Changed: Fix soundness bug with `World: Send`. Dropping a `World` that contains a `!Send` resource on the wrong thread will now panic.
## Migration Guide
Normal resources and `NonSend` resources no longer share the same backing storage. If `R: Resource`, then `NonSend<R>` and `Res<R>` will return different instances from each other. If you are using both `Res<T>` and `NonSend<T>` (or their mutable variants), to fetch the same resources, it's strongly advised to use `Res<T>`.
2023-01-09 20:40:34 +00:00
. get_non_send_with_ticks ( component_id )
Reliable change detection (#1471)
# Problem Definition
The current change tracking (via flags for both components and resources) fails to detect changes made by systems that are scheduled to run earlier in the frame than they are.
This issue is discussed at length in [#68](https://github.com/bevyengine/bevy/issues/68) and [#54](https://github.com/bevyengine/bevy/issues/54).
This is very much a draft PR, and contributions are welcome and needed.
# Criteria
1. Each change is detected at least once, no matter the ordering.
2. Each change is detected at most once, no matter the ordering.
3. Changes should be detected the same frame that they are made.
4. Competitive ergonomics. Ideally does not require opting-in.
5. Low CPU overhead of computation.
6. Memory efficient. This must not increase over time, except where the number of entities / resources does.
7. Changes should not be lost for systems that don't run.
8. A frame needs to act as a pure function. Given the same set of entities / components it needs to produce the same end state without side-effects.
**Exact** change-tracking proposals satisfy criteria 1 and 2.
**Conservative** change-tracking proposals satisfy criteria 1 but not 2.
**Flaky** change tracking proposals satisfy criteria 2 but not 1.
# Code Base Navigation
There are three types of flags:
- `Added`: A piece of data was added to an entity / `Resources`.
- `Mutated`: A piece of data was able to be modified, because its `DerefMut` was accessed
- `Changed`: The bitwise OR of `Added` and `Changed`
The special behavior of `ChangedRes`, with respect to the scheduler is being removed in [#1313](https://github.com/bevyengine/bevy/pull/1313) and does not need to be reproduced.
`ChangedRes` and friends can be found in "bevy_ecs/core/resources/resource_query.rs".
The `Flags` trait for Components can be found in "bevy_ecs/core/query.rs".
`ComponentFlags` are stored in "bevy_ecs/core/archetypes.rs", defined on line 446.
# Proposals
**Proposal 5 was selected for implementation.**
## Proposal 0: No Change Detection
The baseline, where computations are performed on everything regardless of whether it changed.
**Type:** Conservative
**Pros:**
- already implemented
- will never miss events
- no overhead
**Cons:**
- tons of repeated work
- doesn't allow users to avoid repeating work (or monitoring for other changes)
## Proposal 1: Earlier-This-Tick Change Detection
The current approach as of Bevy 0.4. Flags are set, and then flushed at the end of each frame.
**Type:** Flaky
**Pros:**
- already implemented
- simple to understand
- low memory overhead (2 bits per component)
- low time overhead (clear every flag once per frame)
**Cons:**
- misses systems based on ordering
- systems that don't run every frame miss changes
- duplicates detection when looping
- can lead to unresolvable circular dependencies
## Proposal 2: Two-Tick Change Detection
Flags persist for two frames, using a double-buffer system identical to that used in events.
A change is observed if it is found in either the current frame's list of changes or the previous frame's.
**Type:** Conservative
**Pros:**
- easy to understand
- easy to implement
- low memory overhead (4 bits per component)
- low time overhead (bit mask and shift every flag once per frame)
**Cons:**
- can result in a great deal of duplicated work
- systems that don't run every frame miss changes
- duplicates detection when looping
## Proposal 3: Last-Tick Change Detection
Flags persist for two frames, using a double-buffer system identical to that used in events.
A change is observed if it is found in the previous frame's list of changes.
**Type:** Exact
**Pros:**
- exact
- easy to understand
- easy to implement
- low memory overhead (4 bits per component)
- low time overhead (bit mask and shift every flag once per frame)
**Cons:**
- change detection is always delayed, possibly causing painful chained delays
- systems that don't run every frame miss changes
- duplicates detection when looping
## Proposal 4: Flag-Doubling Change Detection
Combine Proposal 2 and Proposal 3. Differentiate between `JustChanged` (current behavior) and `Changed` (Proposal 3).
Pack this data into the flags according to [this implementation proposal](https://github.com/bevyengine/bevy/issues/68#issuecomment-769174804).
**Type:** Flaky + Exact
**Pros:**
- allows users to acc
- easy to implement
- low memory overhead (4 bits per component)
- low time overhead (bit mask and shift every flag once per frame)
**Cons:**
- users must specify the type of change detection required
- still quite fragile to system ordering effects when using the flaky `JustChanged` form
- cannot get immediate + exact results
- systems that don't run every frame miss changes
- duplicates detection when looping
## [SELECTED] Proposal 5: Generation-Counter Change Detection
A global counter is increased after each system is run. Each component saves the time of last mutation, and each system saves the time of last execution. Mutation is detected when the component's counter is greater than the system's counter. Discussed [here](https://github.com/bevyengine/bevy/issues/68#issuecomment-769174804). How to handle addition detection is unsolved; the current proposal is to use the highest bit of the counter as in proposal 1.
**Type:** Exact (for mutations), flaky (for additions)
**Pros:**
- low time overhead (set component counter on access, set system counter after execution)
- robust to systems that don't run every frame
- robust to systems that loop
**Cons:**
- moderately complex implementation
- must be modified as systems are inserted dynamically
- medium memory overhead (4 bytes per component + system)
- unsolved addition detection
## Proposal 6: System-Data Change Detection
For each system, track which system's changes it has seen. This approach is only worth fully designing and implementing if Proposal 5 fails in some way.
**Type:** Exact
**Pros:**
- exact
- conceptually simple
**Cons:**
- requires storing data on each system
- implementation is complex
- must be modified as systems are inserted dynamically
## Proposal 7: Total-Order Change Detection
Discussed [here](https://github.com/bevyengine/bevy/issues/68#issuecomment-754326523). This proposal is somewhat complicated by the new scheduler, but I believe it should still be conceptually feasible. This approach is only worth fully designing and implementing if Proposal 5 fails in some way.
**Type:** Exact
**Pros:**
- exact
- efficient data storage relative to other exact proposals
**Cons:**
- requires access to the scheduler
- complex implementation and difficulty grokking
- must be modified as systems are inserted dynamically
# Tests
- We will need to verify properties 1, 2, 3, 7 and 8. Priority: 1 > 2 = 3 > 8 > 7
- Ideally we can use identical user-facing syntax for all proposals, allowing us to re-use the same syntax for each.
- When writing tests, we need to carefully specify order using explicit dependencies.
- These tests will need to be duplicated for both components and resources.
- We need to be sure to handle cases where ambiguous system orders exist.
`changing_system` is always the system that makes the changes, and `detecting_system` always detects the changes.
The component / resource changed will be simple boolean wrapper structs.
## Basic Added / Mutated / Changed
2 x 3 design:
- Resources vs. Components
- Added vs. Changed vs. Mutated
- `changing_system` runs before `detecting_system`
- verify at the end of tick 2
## At Least Once
2 x 3 design:
- Resources vs. Components
- Added vs. Changed vs. Mutated
- `changing_system` runs after `detecting_system`
- verify at the end of tick 2
## At Most Once
2 x 3 design:
- Resources vs. Components
- Added vs. Changed vs. Mutated
- `changing_system` runs once before `detecting_system`
- increment a counter based on the number of changes detected
- verify at the end of tick 2
## Fast Detection
2 x 3 design:
- Resources vs. Components
- Added vs. Changed vs. Mutated
- `changing_system` runs before `detecting_system`
- verify at the end of tick 1
## Ambiguous System Ordering Robustness
2 x 3 x 2 design:
- Resources vs. Components
- Added vs. Changed vs. Mutated
- `changing_system` runs [before/after] `detecting_system` in tick 1
- `changing_system` runs [after/before] `detecting_system` in tick 2
## System Pausing
2 x 3 design:
- Resources vs. Components
- Added vs. Changed vs. Mutated
- `changing_system` runs in tick 1, then is disabled by run criteria
- `detecting_system` is disabled by run criteria until it is run once during tick 3
- verify at the end of tick 3
## Addition Causes Mutation
2 design:
- Resources vs. Components
- `adding_system_1` adds a component / resource
- `adding system_2` adds the same component / resource
- verify the `Mutated` flag at the end of the tick
- verify the `Added` flag at the end of the tick
First check tests for: https://github.com/bevyengine/bevy/issues/333
Second check tests for: https://github.com/bevyengine/bevy/issues/1443
## Changes Made By Commands
- `adding_system` runs in Update in tick 1, and sends a command to add a component
- `detecting_system` runs in Update in tick 1 and 2, after `adding_system`
- We can't detect the changes in tick 1, since they haven't been processed yet
- If we were to track these changes as being emitted by `adding_system`, we can't detect the changes in tick 2 either, since `detecting_system` has already run once after `adding_system` :(
# Benchmarks
See: [general advice](https://github.com/bevyengine/bevy/blob/master/docs/profiling.md), [Criterion crate](https://github.com/bheisler/criterion.rs)
There are several critical parameters to vary:
1. entity count (1 to 10^9)
2. fraction of entities that are changed (0% to 100%)
3. cost to perform work on changed entities, i.e. workload (1 ns to 1s)
1 and 2 should be varied between benchmark runs. 3 can be added on computationally.
We want to measure:
- memory cost
- run time
We should collect these measurements across several frames (100?) to reduce bootup effects and accurately measure the mean, variance and drift.
Entity-component change detection is much more important to benchmark than resource change detection, due to the orders of magnitude higher number of pieces of data.
No change detection at all should be included in benchmarks as a second control for cases where missing changes is unacceptable.
## Graphs
1. y: performance, x: log_10(entity count), color: proposal, facet: performance metric. Set cost to perform work to 0.
2. y: run time, x: cost to perform work, color: proposal, facet: fraction changed. Set number of entities to 10^6
3. y: memory, x: frames, color: proposal
# Conclusions
1. Is the theoretical categorization of the proposals correct according to our tests?
2. How does the performance of the proposals compare without any load?
3. How does the performance of the proposals compare with realistic loads?
4. At what workload does more exact change tracking become worth the (presumably) higher overhead?
5. When does adding change-detection to save on work become worthwhile?
6. Is there enough divergence in performance between the best solutions in each class to ship more than one change-tracking solution?
# Implementation Plan
1. Write a test suite.
2. Verify that tests fail for existing approach.
3. Write a benchmark suite.
4. Get performance numbers for existing approach.
5. Implement, test and benchmark various solutions using a Git branch per proposal.
6. Create a draft PR with all solutions and present results to team.
7. Select a solution and replace existing change detection.
Co-authored-by: Brice DAVIER <bricedavier@gmail.com>
Co-authored-by: Carter Anderson <mcanders1@gmail.com>
2021-03-19 17:53:26 +00:00
. unwrap_or_else ( | | {
panic! (
2021-04-23 17:54:04 +00:00
" Non-send resource requested by {} does not exist: {} " ,
Add new SystemState and rename old SystemState to SystemMeta (#2283)
This enables `SystemParams` to be used outside of function systems. Anything can create and store `SystemState`, which enables efficient "param state cached" access to `SystemParams`.
It adds a `ReadOnlySystemParamFetch` trait, which enables safe `SystemState::get` calls without unique world access.
I renamed the old `SystemState` to `SystemMeta` to enable us to mirror the `QueryState` naming convention (but I'm happy to discuss alternative names if people have other ideas). I initially pitched this as `ParamState`, but given that it needs to include full system metadata, that doesn't feel like a particularly accurate name.
```rust
#[derive(Eq, PartialEq, Debug)]
struct A(usize);
#[derive(Eq, PartialEq, Debug)]
struct B(usize);
let mut world = World::default();
world.insert_resource(A(42));
world.spawn().insert(B(7));
// we get nice lifetime elision when declaring the type on the left hand side
let mut system_state: SystemState<(Res<A>, Query<&B>)> = SystemState::new(&mut world);
let (a, query) = system_state.get(&world);
assert_eq!(*a, A(42), "returned resource matches initial value");
assert_eq!(
*query.single().unwrap(),
B(7),
"returned component matches initial value"
);
// mutable system params require unique world access
let mut system_state: SystemState<(ResMut<A>, Query<&mut B>)> = SystemState::new(&mut world);
let (a, query) = system_state.get_mut(&mut world);
// static lifetimes are required when declaring inside of structs
struct SomeContainer {
state: SystemState<(Res<'static, A>, Res<'static, B>)>
}
// this can be shortened using type aliases, which will be useful for complex param tuples
type MyParams<'a> = (Res<'a, A>, Res<'a, B>);
struct SomeContainer {
state: SystemState<MyParams<'static>>
}
// It is the user's responsibility to call SystemState::apply(world) for parameters that queue up work
let mut system_state: SystemState<(Commands, Query<&B>)> = SystemState::new(&mut world);
{
let (mut commands, query) = system_state.get(&world);
commands.insert_resource(3.14);
}
system_state.apply(&mut world);
```
## Future Work
* Actually use SystemState inside FunctionSystem. This would be trivial, but it requires FunctionSystem to wrap SystemState in Option in its current form (which complicates system metadata lookup). I'd prefer to hold off until we adopt something like the later designs linked in #1364, which enable us to contruct Systems using a World reference (and also remove the need for `.system`).
* Consider a "scoped" approach to automatically call SystemState::apply when systems params are no longer being used (either a container type with a Drop impl, or a function that takes a closure for user logic operating on params).
2021-06-02 19:57:38 +00:00
system_meta . name ,
Reliable change detection (#1471)
# Problem Definition
The current change tracking (via flags for both components and resources) fails to detect changes made by systems that are scheduled to run earlier in the frame than they are.
This issue is discussed at length in [#68](https://github.com/bevyengine/bevy/issues/68) and [#54](https://github.com/bevyengine/bevy/issues/54).
This is very much a draft PR, and contributions are welcome and needed.
# Criteria
1. Each change is detected at least once, no matter the ordering.
2. Each change is detected at most once, no matter the ordering.
3. Changes should be detected the same frame that they are made.
4. Competitive ergonomics. Ideally does not require opting-in.
5. Low CPU overhead of computation.
6. Memory efficient. This must not increase over time, except where the number of entities / resources does.
7. Changes should not be lost for systems that don't run.
8. A frame needs to act as a pure function. Given the same set of entities / components it needs to produce the same end state without side-effects.
**Exact** change-tracking proposals satisfy criteria 1 and 2.
**Conservative** change-tracking proposals satisfy criteria 1 but not 2.
**Flaky** change tracking proposals satisfy criteria 2 but not 1.
# Code Base Navigation
There are three types of flags:
- `Added`: A piece of data was added to an entity / `Resources`.
- `Mutated`: A piece of data was able to be modified, because its `DerefMut` was accessed
- `Changed`: The bitwise OR of `Added` and `Changed`
The special behavior of `ChangedRes`, with respect to the scheduler is being removed in [#1313](https://github.com/bevyengine/bevy/pull/1313) and does not need to be reproduced.
`ChangedRes` and friends can be found in "bevy_ecs/core/resources/resource_query.rs".
The `Flags` trait for Components can be found in "bevy_ecs/core/query.rs".
`ComponentFlags` are stored in "bevy_ecs/core/archetypes.rs", defined on line 446.
# Proposals
**Proposal 5 was selected for implementation.**
## Proposal 0: No Change Detection
The baseline, where computations are performed on everything regardless of whether it changed.
**Type:** Conservative
**Pros:**
- already implemented
- will never miss events
- no overhead
**Cons:**
- tons of repeated work
- doesn't allow users to avoid repeating work (or monitoring for other changes)
## Proposal 1: Earlier-This-Tick Change Detection
The current approach as of Bevy 0.4. Flags are set, and then flushed at the end of each frame.
**Type:** Flaky
**Pros:**
- already implemented
- simple to understand
- low memory overhead (2 bits per component)
- low time overhead (clear every flag once per frame)
**Cons:**
- misses systems based on ordering
- systems that don't run every frame miss changes
- duplicates detection when looping
- can lead to unresolvable circular dependencies
## Proposal 2: Two-Tick Change Detection
Flags persist for two frames, using a double-buffer system identical to that used in events.
A change is observed if it is found in either the current frame's list of changes or the previous frame's.
**Type:** Conservative
**Pros:**
- easy to understand
- easy to implement
- low memory overhead (4 bits per component)
- low time overhead (bit mask and shift every flag once per frame)
**Cons:**
- can result in a great deal of duplicated work
- systems that don't run every frame miss changes
- duplicates detection when looping
## Proposal 3: Last-Tick Change Detection
Flags persist for two frames, using a double-buffer system identical to that used in events.
A change is observed if it is found in the previous frame's list of changes.
**Type:** Exact
**Pros:**
- exact
- easy to understand
- easy to implement
- low memory overhead (4 bits per component)
- low time overhead (bit mask and shift every flag once per frame)
**Cons:**
- change detection is always delayed, possibly causing painful chained delays
- systems that don't run every frame miss changes
- duplicates detection when looping
## Proposal 4: Flag-Doubling Change Detection
Combine Proposal 2 and Proposal 3. Differentiate between `JustChanged` (current behavior) and `Changed` (Proposal 3).
Pack this data into the flags according to [this implementation proposal](https://github.com/bevyengine/bevy/issues/68#issuecomment-769174804).
**Type:** Flaky + Exact
**Pros:**
- allows users to acc
- easy to implement
- low memory overhead (4 bits per component)
- low time overhead (bit mask and shift every flag once per frame)
**Cons:**
- users must specify the type of change detection required
- still quite fragile to system ordering effects when using the flaky `JustChanged` form
- cannot get immediate + exact results
- systems that don't run every frame miss changes
- duplicates detection when looping
## [SELECTED] Proposal 5: Generation-Counter Change Detection
A global counter is increased after each system is run. Each component saves the time of last mutation, and each system saves the time of last execution. Mutation is detected when the component's counter is greater than the system's counter. Discussed [here](https://github.com/bevyengine/bevy/issues/68#issuecomment-769174804). How to handle addition detection is unsolved; the current proposal is to use the highest bit of the counter as in proposal 1.
**Type:** Exact (for mutations), flaky (for additions)
**Pros:**
- low time overhead (set component counter on access, set system counter after execution)
- robust to systems that don't run every frame
- robust to systems that loop
**Cons:**
- moderately complex implementation
- must be modified as systems are inserted dynamically
- medium memory overhead (4 bytes per component + system)
- unsolved addition detection
## Proposal 6: System-Data Change Detection
For each system, track which system's changes it has seen. This approach is only worth fully designing and implementing if Proposal 5 fails in some way.
**Type:** Exact
**Pros:**
- exact
- conceptually simple
**Cons:**
- requires storing data on each system
- implementation is complex
- must be modified as systems are inserted dynamically
## Proposal 7: Total-Order Change Detection
Discussed [here](https://github.com/bevyengine/bevy/issues/68#issuecomment-754326523). This proposal is somewhat complicated by the new scheduler, but I believe it should still be conceptually feasible. This approach is only worth fully designing and implementing if Proposal 5 fails in some way.
**Type:** Exact
**Pros:**
- exact
- efficient data storage relative to other exact proposals
**Cons:**
- requires access to the scheduler
- complex implementation and difficulty grokking
- must be modified as systems are inserted dynamically
# Tests
- We will need to verify properties 1, 2, 3, 7 and 8. Priority: 1 > 2 = 3 > 8 > 7
- Ideally we can use identical user-facing syntax for all proposals, allowing us to re-use the same syntax for each.
- When writing tests, we need to carefully specify order using explicit dependencies.
- These tests will need to be duplicated for both components and resources.
- We need to be sure to handle cases where ambiguous system orders exist.
`changing_system` is always the system that makes the changes, and `detecting_system` always detects the changes.
The component / resource changed will be simple boolean wrapper structs.
## Basic Added / Mutated / Changed
2 x 3 design:
- Resources vs. Components
- Added vs. Changed vs. Mutated
- `changing_system` runs before `detecting_system`
- verify at the end of tick 2
## At Least Once
2 x 3 design:
- Resources vs. Components
- Added vs. Changed vs. Mutated
- `changing_system` runs after `detecting_system`
- verify at the end of tick 2
## At Most Once
2 x 3 design:
- Resources vs. Components
- Added vs. Changed vs. Mutated
- `changing_system` runs once before `detecting_system`
- increment a counter based on the number of changes detected
- verify at the end of tick 2
## Fast Detection
2 x 3 design:
- Resources vs. Components
- Added vs. Changed vs. Mutated
- `changing_system` runs before `detecting_system`
- verify at the end of tick 1
## Ambiguous System Ordering Robustness
2 x 3 x 2 design:
- Resources vs. Components
- Added vs. Changed vs. Mutated
- `changing_system` runs [before/after] `detecting_system` in tick 1
- `changing_system` runs [after/before] `detecting_system` in tick 2
## System Pausing
2 x 3 design:
- Resources vs. Components
- Added vs. Changed vs. Mutated
- `changing_system` runs in tick 1, then is disabled by run criteria
- `detecting_system` is disabled by run criteria until it is run once during tick 3
- verify at the end of tick 3
## Addition Causes Mutation
2 design:
- Resources vs. Components
- `adding_system_1` adds a component / resource
- `adding system_2` adds the same component / resource
- verify the `Mutated` flag at the end of the tick
- verify the `Added` flag at the end of the tick
First check tests for: https://github.com/bevyengine/bevy/issues/333
Second check tests for: https://github.com/bevyengine/bevy/issues/1443
## Changes Made By Commands
- `adding_system` runs in Update in tick 1, and sends a command to add a component
- `detecting_system` runs in Update in tick 1 and 2, after `adding_system`
- We can't detect the changes in tick 1, since they haven't been processed yet
- If we were to track these changes as being emitted by `adding_system`, we can't detect the changes in tick 2 either, since `detecting_system` has already run once after `adding_system` :(
# Benchmarks
See: [general advice](https://github.com/bevyengine/bevy/blob/master/docs/profiling.md), [Criterion crate](https://github.com/bheisler/criterion.rs)
There are several critical parameters to vary:
1. entity count (1 to 10^9)
2. fraction of entities that are changed (0% to 100%)
3. cost to perform work on changed entities, i.e. workload (1 ns to 1s)
1 and 2 should be varied between benchmark runs. 3 can be added on computationally.
We want to measure:
- memory cost
- run time
We should collect these measurements across several frames (100?) to reduce bootup effects and accurately measure the mean, variance and drift.
Entity-component change detection is much more important to benchmark than resource change detection, due to the orders of magnitude higher number of pieces of data.
No change detection at all should be included in benchmarks as a second control for cases where missing changes is unacceptable.
## Graphs
1. y: performance, x: log_10(entity count), color: proposal, facet: performance metric. Set cost to perform work to 0.
2. y: run time, x: cost to perform work, color: proposal, facet: fraction changed. Set number of entities to 10^6
3. y: memory, x: frames, color: proposal
# Conclusions
1. Is the theoretical categorization of the proposals correct according to our tests?
2. How does the performance of the proposals compare without any load?
3. How does the performance of the proposals compare with realistic loads?
4. At what workload does more exact change tracking become worth the (presumably) higher overhead?
5. When does adding change-detection to save on work become worthwhile?
6. Is there enough divergence in performance between the best solutions in each class to ship more than one change-tracking solution?
# Implementation Plan
1. Write a test suite.
2. Verify that tests fail for existing approach.
3. Write a benchmark suite.
4. Get performance numbers for existing approach.
5. Implement, test and benchmark various solutions using a Git branch per proposal.
6. Create a draft PR with all solutions and present results to team.
7. Select a solution and replace existing change detection.
Co-authored-by: Brice DAVIER <bricedavier@gmail.com>
Co-authored-by: Carter Anderson <mcanders1@gmail.com>
2021-03-19 17:53:26 +00:00
std ::any ::type_name ::< T > ( )
)
} ) ;
2021-05-30 20:15:40 +00:00
Bevy ECS V2 (#1525)
# Bevy ECS V2
This is a rewrite of Bevy ECS (basically everything but the new executor/schedule, which are already awesome). The overall goal was to improve the performance and versatility of Bevy ECS. Here is a quick bulleted list of changes before we dive into the details:
* Complete World rewrite
* Multiple component storage types:
* Tables: fast cache friendly iteration, slower add/removes (previously called Archetypes)
* Sparse Sets: fast add/remove, slower iteration
* Stateful Queries (caches query results for faster iteration. fragmented iteration is _fast_ now)
* Stateful System Params (caches expensive operations. inspired by @DJMcNab's work in #1364)
* Configurable System Params (users can set configuration when they construct their systems. once again inspired by @DJMcNab's work)
* Archetypes are now "just metadata", component storage is separate
* Archetype Graph (for faster archetype changes)
* Component Metadata
* Configure component storage type
* Retrieve information about component size/type/name/layout/send-ness/etc
* Components are uniquely identified by a densely packed ComponentId
* TypeIds are now totally optional (which should make implementing scripting easier)
* Super fast "for_each" query iterators
* Merged Resources into World. Resources are now just a special type of component
* EntityRef/EntityMut builder apis (more efficient and more ergonomic)
* Fast bitset-backed `Access<T>` replaces old hashmap-based approach everywhere
* Query conflicts are determined by component access instead of archetype component access (to avoid random failures at runtime)
* With/Without are still taken into account for conflicts, so this should still be comfy to use
* Much simpler `IntoSystem` impl
* Significantly reduced the amount of hashing throughout the ecs in favor of Sparse Sets (indexed by densely packed ArchetypeId, ComponentId, BundleId, and TableId)
* Safety Improvements
* Entity reservation uses a normal world reference instead of unsafe transmute
* QuerySets no longer transmute lifetimes
* Made traits "unsafe" where relevant
* More thorough safety docs
* WorldCell
* Exposes safe mutable access to multiple resources at a time in a World
* Replaced "catch all" `System::update_archetypes(world: &World)` with `System::new_archetype(archetype: &Archetype)`
* Simpler Bundle implementation
* Replaced slow "remove_bundle_one_by_one" used as fallback for Commands::remove_bundle with fast "remove_bundle_intersection"
* Removed `Mut<T>` query impl. it is better to only support one way: `&mut T`
* Removed with() from `Flags<T>` in favor of `Option<Flags<T>>`, which allows querying for flags to be "filtered" by default
* Components now have is_send property (currently only resources support non-send)
* More granular module organization
* New `RemovedComponents<T>` SystemParam that replaces `query.removed::<T>()`
* `world.resource_scope()` for mutable access to resources and world at the same time
* WorldQuery and QueryFilter traits unified. FilterFetch trait added to enable "short circuit" filtering. Auto impled for cases that don't need it
* Significantly slimmed down SystemState in favor of individual SystemParam state
* System Commands changed from `commands: &mut Commands` back to `mut commands: Commands` (to allow Commands to have a World reference)
Fixes #1320
## `World` Rewrite
This is a from-scratch rewrite of `World` that fills the niche that `hecs` used to. Yes, this means Bevy ECS is no longer a "fork" of hecs. We're going out our own!
(the only shared code between the projects is the entity id allocator, which is already basically ideal)
A huge shout out to @SanderMertens (author of [flecs](https://github.com/SanderMertens/flecs)) for sharing some great ideas with me (specifically hybrid ecs storage and archetype graphs). He also helped advise on a number of implementation details.
## Component Storage (The Problem)
Two ECS storage paradigms have gained a lot of traction over the years:
* **Archetypal ECS**:
* Stores components in "tables" with static schemas. Each "column" stores components of a given type. Each "row" is an entity.
* Each "archetype" has its own table. Adding/removing an entity's component changes the archetype.
* Enables super-fast Query iteration due to its cache-friendly data layout
* Comes at the cost of more expensive add/remove operations for an Entity's components, because all components need to be copied to the new archetype's "table"
* **Sparse Set ECS**:
* Stores components of the same type in densely packed arrays, which are sparsely indexed by densely packed unsigned integers (Entity ids)
* Query iteration is slower than Archetypal ECS because each entity's component could be at any position in the sparse set. This "random access" pattern isn't cache friendly. Additionally, there is an extra layer of indirection because you must first map the entity id to an index in the component array.
* Adding/removing components is a cheap, constant time operation
Bevy ECS V1, hecs, legion, flec, and Unity DOTS are all "archetypal ecs-es". I personally think "archetypal" storage is a good default for game engines. An entity's archetype doesn't need to change frequently in general, and it creates "fast by default" query iteration (which is a much more common operation). It is also "self optimizing". Users don't need to think about optimizing component layouts for iteration performance. It "just works" without any extra boilerplate.
Shipyard and EnTT are "sparse set ecs-es". They employ "packing" as a way to work around the "suboptimal by default" iteration performance for specific sets of components. This helps, but I didn't think this was a good choice for a general purpose engine like Bevy because:
1. "packs" conflict with each other. If bevy decides to internally pack the Transform and GlobalTransform components, users are then blocked if they want to pack some custom component with Transform.
2. users need to take manual action to optimize
Developers selecting an ECS framework are stuck with a hard choice. Select an "archetypal" framework with "fast iteration everywhere" but without the ability to cheaply add/remove components, or select a "sparse set" framework to cheaply add/remove components but with slower iteration performance.
## Hybrid Component Storage (The Solution)
In Bevy ECS V2, we get to have our cake and eat it too. It now has _both_ of the component storage types above (and more can be added later if needed):
* **Tables** (aka "archetypal" storage)
* The default storage. If you don't configure anything, this is what you get
* Fast iteration by default
* Slower add/remove operations
* **Sparse Sets**
* Opt-in
* Slower iteration
* Faster add/remove operations
These storage types complement each other perfectly. By default Query iteration is fast. If developers know that they want to add/remove a component at high frequencies, they can set the storage to "sparse set":
```rust
world.register_component(
ComponentDescriptor::new::<MyComponent>(StorageType::SparseSet)
).unwrap();
```
## Archetypes
Archetypes are now "just metadata" ... they no longer store components directly. They do store:
* The `ComponentId`s of each of the Archetype's components (and that component's storage type)
* Archetypes are uniquely defined by their component layouts
* For example: entities with "table" components `[A, B, C]` _and_ "sparse set" components `[D, E]` will always be in the same archetype.
* The `TableId` associated with the archetype
* For now each archetype has exactly one table (which can have no components),
* There is a 1->Many relationship from Tables->Archetypes. A given table could have any number of archetype components stored in it:
* Ex: an entity with "table storage" components `[A, B, C]` and "sparse set" components `[D, E]` will share the same `[A, B, C]` table as an entity with `[A, B, C]` table component and `[F]` sparse set components.
* This 1->Many relationship is how we preserve fast "cache friendly" iteration performance when possible (more on this later)
* A list of entities that are in the archetype and the row id of the table they are in
* ArchetypeComponentIds
* unique densely packed identifiers for (ArchetypeId, ComponentId) pairs
* used by the schedule executor for cheap system access control
* "Archetype Graph Edges" (see the next section)
## The "Archetype Graph"
Archetype changes in Bevy (and a number of other archetypal ecs-es) have historically been expensive to compute. First, you need to allocate a new vector of the entity's current component ids, add or remove components based on the operation performed, sort it (to ensure it is order-independent), then hash it to find the archetype (if it exists). And thats all before we get to the _already_ expensive full copy of all components to the new table storage.
The solution is to build a "graph" of archetypes to cache these results. @SanderMertens first exposed me to the idea (and he got it from @gjroelofs, who came up with it). They propose adding directed edges between archetypes for add/remove component operations. If `ComponentId`s are densely packed, you can use sparse sets to cheaply jump between archetypes.
Bevy takes this one step further by using add/remove `Bundle` edges instead of `Component` edges. Bevy encourages the use of `Bundles` to group add/remove operations. This is largely for "clearer game logic" reasons, but it also helps cut down on the number of archetype changes required. `Bundles` now also have densely-packed `BundleId`s. This allows us to use a _single_ edge for each bundle operation (rather than needing to traverse N edges ... one for each component). Single component operations are also bundles, so this is strictly an improvement over a "component only" graph.
As a result, an operation that used to be _heavy_ (both for allocations and compute) is now two dirt-cheap array lookups and zero allocations.
## Stateful Queries
World queries are now stateful. This allows us to:
1. Cache archetype (and table) matches
* This resolves another issue with (naive) archetypal ECS: query performance getting worse as the number of archetypes goes up (and fragmentation occurs).
2. Cache Fetch and Filter state
* The expensive parts of fetch/filter operations (such as hashing the TypeId to find the ComponentId) now only happen once when the Query is first constructed
3. Incrementally build up state
* When new archetypes are added, we only process the new archetypes (no need to rebuild state for old archetypes)
As a result, the direct `World` query api now looks like this:
```rust
let mut query = world.query::<(&A, &mut B)>();
for (a, mut b) in query.iter_mut(&mut world) {
}
```
Requiring `World` to generate stateful queries (rather than letting the `QueryState` type be constructed separately) allows us to ensure that _all_ queries are properly initialized (and the relevant world state, such as ComponentIds). This enables QueryState to remove branches from its operations that check for initialization status (and also enables query.iter() to take an immutable world reference because it doesn't need to initialize anything in world).
However in systems, this is a non-breaking change. State management is done internally by the relevant SystemParam.
## Stateful SystemParams
Like Queries, `SystemParams` now also cache state. For example, `Query` system params store the "stateful query" state mentioned above. Commands store their internal `CommandQueue`. This means you can now safely use as many separate `Commands` parameters in your system as you want. `Local<T>` system params store their `T` value in their state (instead of in Resources).
SystemParam state also enabled a significant slim-down of SystemState. It is much nicer to look at now.
Per-SystemParam state naturally insulates us from an "aliased mut" class of errors we have hit in the past (ex: using multiple `Commands` system params).
(credit goes to @DJMcNab for the initial idea and draft pr here #1364)
## Configurable SystemParams
@DJMcNab also had the great idea to make SystemParams configurable. This allows users to provide some initial configuration / values for system parameters (when possible). Most SystemParams have no config (the config type is `()`), but the `Local<T>` param now supports user-provided parameters:
```rust
fn foo(value: Local<usize>) {
}
app.add_system(foo.system().config(|c| c.0 = Some(10)));
```
## Uber Fast "for_each" Query Iterators
Developers now have the choice to use a fast "for_each" iterator, which yields ~1.5-3x iteration speed improvements for "fragmented iteration", and minor ~1.2x iteration speed improvements for unfragmented iteration.
```rust
fn system(query: Query<(&A, &mut B)>) {
// you now have the option to do this for a speed boost
query.for_each_mut(|(a, mut b)| {
});
// however normal iterators are still available
for (a, mut b) in query.iter_mut() {
}
}
```
I think in most cases we should continue to encourage "normal" iterators as they are more flexible and more "rust idiomatic". But when that extra "oomf" is needed, it makes sense to use `for_each`.
We should also consider using `for_each` for internal bevy systems to give our users a nice speed boost (but that should be a separate pr).
## Component Metadata
`World` now has a `Components` collection, which is accessible via `world.components()`. This stores mappings from `ComponentId` to `ComponentInfo`, as well as `TypeId` to `ComponentId` mappings (where relevant). `ComponentInfo` stores information about the component, such as ComponentId, TypeId, memory layout, send-ness (currently limited to resources), and storage type.
## Significantly Cheaper `Access<T>`
We used to use `TypeAccess<TypeId>` to manage read/write component/archetype-component access. This was expensive because TypeIds must be hashed and compared individually. The parallel executor got around this by "condensing" type ids into bitset-backed access types. This worked, but it had to be re-generated from the `TypeAccess<TypeId>`sources every time archetypes changed.
This pr removes TypeAccess in favor of faster bitset access everywhere. We can do this thanks to the move to densely packed `ComponentId`s and `ArchetypeComponentId`s.
## Merged Resources into World
Resources had a lot of redundant functionality with Components. They stored typed data, they had access control, they had unique ids, they were queryable via SystemParams, etc. In fact the _only_ major difference between them was that they were unique (and didn't correlate to an entity).
Separate resources also had the downside of requiring a separate set of access controls, which meant the parallel executor needed to compare more bitsets per system and manage more state.
I initially got the "separate resources" idea from `legion`. I think that design was motivated by the fact that it made the direct world query/resource lifetime interactions more manageable. It certainly made our lives easier when using Resources alongside hecs/bevy_ecs. However we already have a construct for safely and ergonomically managing in-world lifetimes: systems (which use `Access<T>` internally).
This pr merges Resources into World:
```rust
world.insert_resource(1);
world.insert_resource(2.0);
let a = world.get_resource::<i32>().unwrap();
let mut b = world.get_resource_mut::<f64>().unwrap();
*b = 3.0;
```
Resources are now just a special kind of component. They have their own ComponentIds (and their own resource TypeId->ComponentId scope, so they don't conflict wit components of the same type). They are stored in a special "resource archetype", which stores components inside the archetype using a new `unique_components` sparse set (note that this sparse set could later be used to implement Tags). This allows us to keep the code size small by reusing existing datastructures (namely Column, Archetype, ComponentFlags, and ComponentInfo). This allows us the executor to use a single `Access<ArchetypeComponentId>` per system. It should also make scripting language integration easier.
_But_ this merge did create problems for people directly interacting with `World`. What if you need mutable access to multiple resources at the same time? `world.get_resource_mut()` borrows World mutably!
## WorldCell
WorldCell applies the `Access<ArchetypeComponentId>` concept to direct world access:
```rust
let world_cell = world.cell();
let a = world_cell.get_resource_mut::<i32>().unwrap();
let b = world_cell.get_resource_mut::<f64>().unwrap();
```
This adds cheap runtime checks (a sparse set lookup of `ArchetypeComponentId` and a counter) to ensure that world accesses do not conflict with each other. Each operation returns a `WorldBorrow<'w, T>` or `WorldBorrowMut<'w, T>` wrapper type, which will release the relevant ArchetypeComponentId resources when dropped.
World caches the access sparse set (and only one cell can exist at a time), so `world.cell()` is a cheap operation.
WorldCell does _not_ use atomic operations. It is non-send, does a mutable borrow of world to prevent other accesses, and uses a simple `Rc<RefCell<ArchetypeComponentAccess>>` wrapper in each WorldBorrow pointer.
The api is currently limited to resource access, but it can and should be extended to queries / entity component access.
## Resource Scopes
WorldCell does not yet support component queries, and even when it does there are sometimes legitimate reasons to want a mutable world ref _and_ a mutable resource ref (ex: bevy_render and bevy_scene both need this). In these cases we could always drop down to the unsafe `world.get_resource_unchecked_mut()`, but that is not ideal!
Instead developers can use a "resource scope"
```rust
world.resource_scope(|world: &mut World, a: &mut A| {
})
```
This temporarily removes the `A` resource from `World`, provides mutable pointers to both, and re-adds A to World when finished. Thanks to the move to ComponentIds/sparse sets, this is a cheap operation.
If multiple resources are required, scopes can be nested. We could also consider adding a "resource tuple" to the api if this pattern becomes common and the boilerplate gets nasty.
## Query Conflicts Use ComponentId Instead of ArchetypeComponentId
For safety reasons, systems cannot contain queries that conflict with each other without wrapping them in a QuerySet. On bevy `main`, we use ArchetypeComponentIds to determine conflicts. This is nice because it can take into account filters:
```rust
// these queries will never conflict due to their filters
fn filter_system(a: Query<&mut A, With<B>>, b: Query<&mut B, Without<B>>) {
}
```
But it also has a significant downside:
```rust
// these queries will not conflict _until_ an entity with A, B, and C is spawned
fn maybe_conflicts_system(a: Query<(&mut A, &C)>, b: Query<(&mut A, &B)>) {
}
```
The system above will panic at runtime if an entity with A, B, and C is spawned. This makes it hard to trust that your game logic will run without crashing.
In this pr, I switched to using `ComponentId` instead. This _is_ more constraining. `maybe_conflicts_system` will now always fail, but it will do it consistently at startup. Naively, it would also _disallow_ `filter_system`, which would be a significant downgrade in usability. Bevy has a number of internal systems that rely on disjoint queries and I expect it to be a common pattern in userspace.
To resolve this, I added a new `FilteredAccess<T>` type, which wraps `Access<T>` and adds with/without filters. If two `FilteredAccess` have with/without values that prove they are disjoint, they will no longer conflict.
## EntityRef / EntityMut
World entity operations on `main` require that the user passes in an `entity` id to each operation:
```rust
let entity = world.spawn((A, )); // create a new entity with A
world.get::<A>(entity);
world.insert(entity, (B, C));
world.insert_one(entity, D);
```
This means that each operation needs to look up the entity location / verify its validity. The initial spawn operation also requires a Bundle as input. This can be awkward when no components are required (or one component is required).
These operations have been replaced by `EntityRef` and `EntityMut`, which are "builder-style" wrappers around world that provide read and read/write operations on a single, pre-validated entity:
```rust
// spawn now takes no inputs and returns an EntityMut
let entity = world.spawn()
.insert(A) // insert a single component into the entity
.insert_bundle((B, C)) // insert a bundle of components into the entity
.id() // id returns the Entity id
// Returns EntityMut (or panics if the entity does not exist)
world.entity_mut(entity)
.insert(D)
.insert_bundle(SomeBundle::default());
{
// returns EntityRef (or panics if the entity does not exist)
let d = world.entity(entity)
.get::<D>() // gets the D component
.unwrap();
// world.get still exists for ergonomics
let d = world.get::<D>(entity).unwrap();
}
// These variants return Options if you want to check existence instead of panicing
world.get_entity_mut(entity)
.unwrap()
.insert(E);
if let Some(entity_ref) = world.get_entity(entity) {
let d = entity_ref.get::<D>().unwrap();
}
```
This _does not_ affect the current Commands api or terminology. I think that should be a separate conversation as that is a much larger breaking change.
## Safety Improvements
* Entity reservation in Commands uses a normal world borrow instead of an unsafe transmute
* QuerySets no longer transmutes lifetimes
* Made traits "unsafe" when implementing a trait incorrectly could cause unsafety
* More thorough safety docs
## RemovedComponents SystemParam
The old approach to querying removed components: `query.removed:<T>()` was confusing because it had no connection to the query itself. I replaced it with the following, which is both clearer and allows us to cache the ComponentId mapping in the SystemParamState:
```rust
fn system(removed: RemovedComponents<T>) {
for entity in removed.iter() {
}
}
```
## Simpler Bundle implementation
Bundles are no longer responsible for sorting (or deduping) TypeInfo. They are just a simple ordered list of component types / data. This makes the implementation smaller and opens the door to an easy "nested bundle" implementation in the future (which i might even add in this pr). Duplicate detection is now done once per bundle type by World the first time a bundle is used.
## Unified WorldQuery and QueryFilter types
(don't worry they are still separate type _parameters_ in Queries .. this is a non-breaking change)
WorldQuery and QueryFilter were already basically identical apis. With the addition of `FetchState` and more storage-specific fetch methods, the overlap was even clearer (and the redundancy more painful).
QueryFilters are now just `F: WorldQuery where F::Fetch: FilterFetch`. FilterFetch requires `Fetch<Item = bool>` and adds new "short circuit" variants of fetch methods. This enables a filter tuple like `(With<A>, Without<B>, Changed<C>)` to stop evaluating the filter after the first mismatch is encountered. FilterFetch is automatically implemented for `Fetch` implementations that return bool.
This forces fetch implementations that return things like `(bool, bool, bool)` (such as the filter above) to manually implement FilterFetch and decide whether or not to short-circuit.
## More Granular Modules
World no longer globs all of the internal modules together. It now exports `core`, `system`, and `schedule` separately. I'm also considering exporting `core` submodules directly as that is still pretty "glob-ey" and unorganized (feedback welcome here).
## Remaining Draft Work (to be done in this pr)
* ~~panic on conflicting WorldQuery fetches (&A, &mut A)~~
* ~~bevy `main` and hecs both currently allow this, but we should protect against it if possible~~
* ~~batch_iter / par_iter (currently stubbed out)~~
* ~~ChangedRes~~
* ~~I skipped this while we sort out #1313. This pr should be adapted to account for whatever we land on there~~.
* ~~The `Archetypes` and `Tables` collections use hashes of sorted lists of component ids to uniquely identify each archetype/table. This hash is then used as the key in a HashMap to look up the relevant ArchetypeId or TableId. (which doesn't handle hash collisions properly)~~
* ~~It is currently unsafe to generate a Query from "World A", then use it on "World B" (despite the api claiming it is safe). We should probably close this gap. This could be done by adding a randomly generated WorldId to each world, then storing that id in each Query. They could then be compared to each other on each `query.do_thing(&world)` operation. This _does_ add an extra branch to each query operation, so I'm open to other suggestions if people have them.~~
* ~~Nested Bundles (if i find time)~~
## Potential Future Work
* Expand WorldCell to support queries.
* Consider not allocating in the empty archetype on `world.spawn()`
* ex: return something like EntityMutUninit, which turns into EntityMut after an `insert` or `insert_bundle` op
* this actually regressed performance last time i tried it, but in theory it should be faster
* Optimize SparseSet::insert (see `PERF` comment on insert)
* Replace SparseArray `Option<T>` with T::MAX to cut down on branching
* would enable cheaper get_unchecked() operations
* upstream fixedbitset optimizations
* fixedbitset could be allocation free for small block counts (store blocks in a SmallVec)
* fixedbitset could have a const constructor
* Consider implementing Tags (archetype-specific by-value data that affects archetype identity)
* ex: ArchetypeA could have `[A, B, C]` table components and `[D(1)]` "tag" component. ArchetypeB could have `[A, B, C]` table components and a `[D(2)]` tag component. The archetypes are different, despite both having D tags because the value inside D is different.
* this could potentially build on top of the `archetype.unique_components` added in this pr for resource storage.
* Consider reverting `all_tuples` proc macro in favor of the old `macro_rules` implementation
* all_tuples is more flexible and produces cleaner documentation (the macro_rules version produces weird type parameter orders due to parser constraints)
* but unfortunately all_tuples also appears to make Rust Analyzer sad/slow when working inside of `bevy_ecs` (does not affect user code)
* Consider "resource queries" and/or "mixed resource and entity component queries" as an alternative to WorldCell
* this is basically just "systems" so maybe it's not worth it
* Add more world ops
* `world.clear()`
* `world.reserve<T: Bundle>(count: usize)`
* Try using the old archetype allocation strategy (allocate new memory on resize and copy everything over). I expect this to improve batch insertion performance at the cost of unbatched performance. But thats just a guess. I'm not an allocation perf pro :)
* Adapt Commands apis for consistency with new World apis
## Benchmarks
key:
* `bevy_old`: bevy `main` branch
* `bevy`: this branch
* `_foreach`: uses an optimized for_each iterator
* ` _sparse`: uses sparse set storage (if unspecified assume table storage)
* `_system`: runs inside a system (if unspecified assume test happens via direct world ops)
### Simple Insert (from ecs_bench_suite)
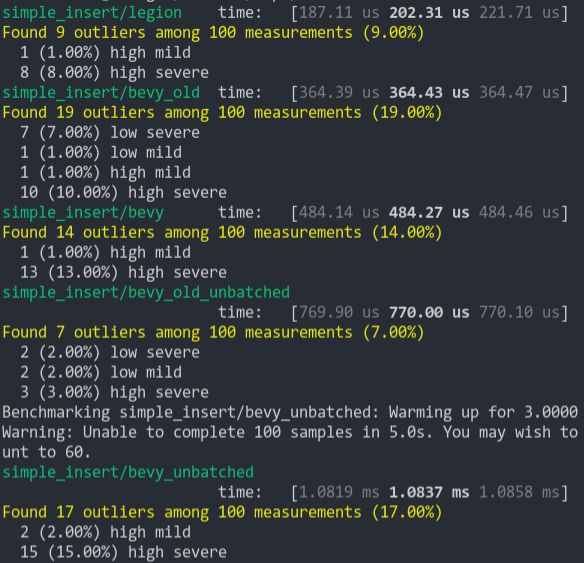
### Simpler Iter (from ecs_bench_suite)
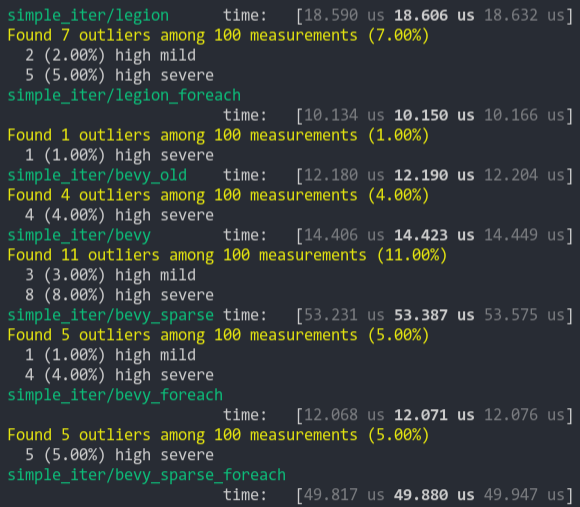
### Fragment Iter (from ecs_bench_suite)
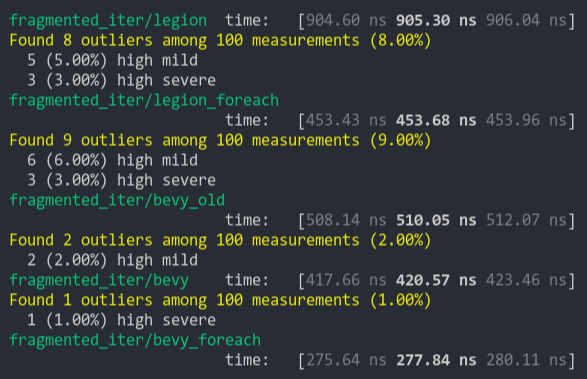
### Sparse Fragmented Iter
Iterate a query that matches 5 entities from a single matching archetype, but there are 100 unmatching archetypes
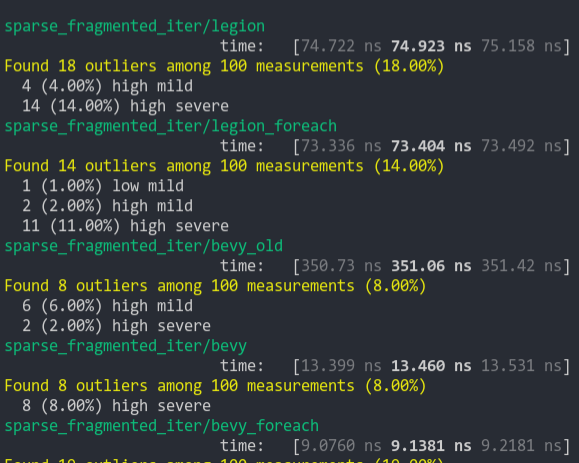
### Schedule (from ecs_bench_suite)
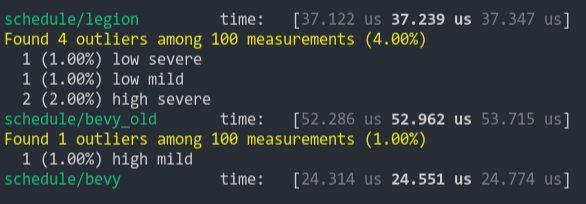
### Add Remove Component (from ecs_bench_suite)
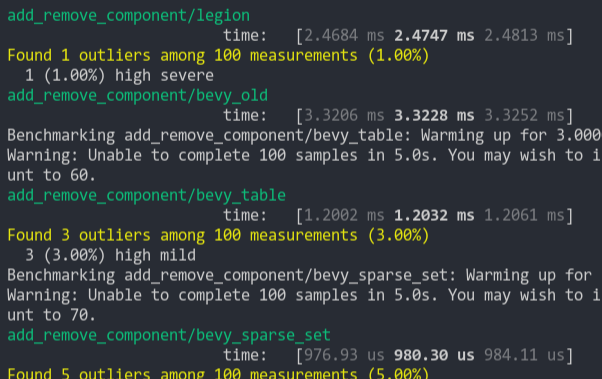
### Add Remove Component Big
Same as the test above, but each entity has 5 "large" matrix components and 1 "large" matrix component is added and removed
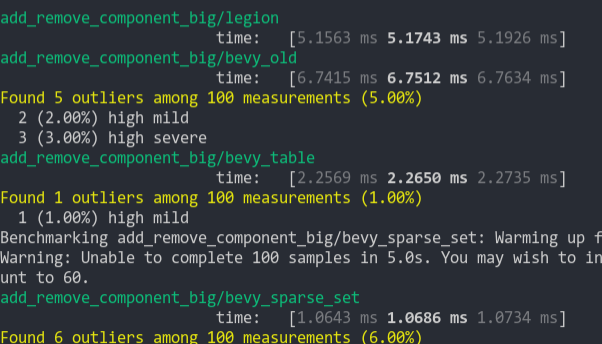
### Get Component
Looks up a single component value a large number of times
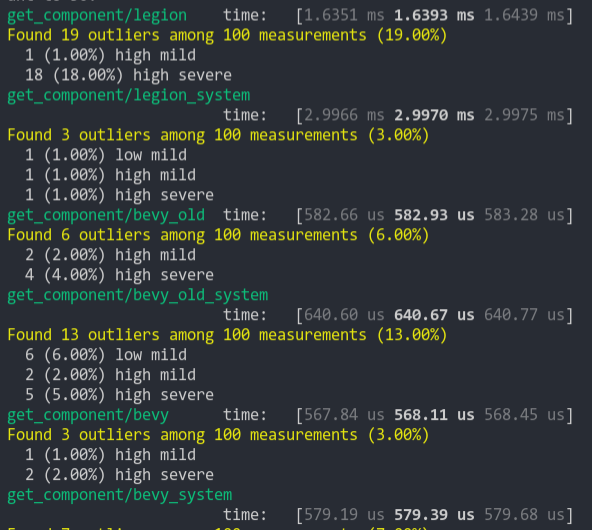
2021-03-05 07:54:35 +00:00
NonSend {
2022-10-24 13:46:36 +00:00
value : ptr . deref ( ) ,
ticks : ticks . read ( ) ,
Add new SystemState and rename old SystemState to SystemMeta (#2283)
This enables `SystemParams` to be used outside of function systems. Anything can create and store `SystemState`, which enables efficient "param state cached" access to `SystemParams`.
It adds a `ReadOnlySystemParamFetch` trait, which enables safe `SystemState::get` calls without unique world access.
I renamed the old `SystemState` to `SystemMeta` to enable us to mirror the `QueryState` naming convention (but I'm happy to discuss alternative names if people have other ideas). I initially pitched this as `ParamState`, but given that it needs to include full system metadata, that doesn't feel like a particularly accurate name.
```rust
#[derive(Eq, PartialEq, Debug)]
struct A(usize);
#[derive(Eq, PartialEq, Debug)]
struct B(usize);
let mut world = World::default();
world.insert_resource(A(42));
world.spawn().insert(B(7));
// we get nice lifetime elision when declaring the type on the left hand side
let mut system_state: SystemState<(Res<A>, Query<&B>)> = SystemState::new(&mut world);
let (a, query) = system_state.get(&world);
assert_eq!(*a, A(42), "returned resource matches initial value");
assert_eq!(
*query.single().unwrap(),
B(7),
"returned component matches initial value"
);
// mutable system params require unique world access
let mut system_state: SystemState<(ResMut<A>, Query<&mut B>)> = SystemState::new(&mut world);
let (a, query) = system_state.get_mut(&mut world);
// static lifetimes are required when declaring inside of structs
struct SomeContainer {
state: SystemState<(Res<'static, A>, Res<'static, B>)>
}
// this can be shortened using type aliases, which will be useful for complex param tuples
type MyParams<'a> = (Res<'a, A>, Res<'a, B>);
struct SomeContainer {
state: SystemState<MyParams<'static>>
}
// It is the user's responsibility to call SystemState::apply(world) for parameters that queue up work
let mut system_state: SystemState<(Commands, Query<&B>)> = SystemState::new(&mut world);
{
let (mut commands, query) = system_state.get(&world);
commands.insert_resource(3.14);
}
system_state.apply(&mut world);
```
## Future Work
* Actually use SystemState inside FunctionSystem. This would be trivial, but it requires FunctionSystem to wrap SystemState in Option in its current form (which complicates system metadata lookup). I'd prefer to hold off until we adopt something like the later designs linked in #1364, which enable us to contruct Systems using a World reference (and also remove the need for `.system`).
* Consider a "scoped" approach to automatically call SystemState::apply when systems params are no longer being used (either a container type with a Drop impl, or a function that takes a closure for user logic operating on params).
2021-06-02 19:57:38 +00:00
last_change_tick : system_meta . last_change_tick ,
Reliable change detection (#1471)
# Problem Definition
The current change tracking (via flags for both components and resources) fails to detect changes made by systems that are scheduled to run earlier in the frame than they are.
This issue is discussed at length in [#68](https://github.com/bevyengine/bevy/issues/68) and [#54](https://github.com/bevyengine/bevy/issues/54).
This is very much a draft PR, and contributions are welcome and needed.
# Criteria
1. Each change is detected at least once, no matter the ordering.
2. Each change is detected at most once, no matter the ordering.
3. Changes should be detected the same frame that they are made.
4. Competitive ergonomics. Ideally does not require opting-in.
5. Low CPU overhead of computation.
6. Memory efficient. This must not increase over time, except where the number of entities / resources does.
7. Changes should not be lost for systems that don't run.
8. A frame needs to act as a pure function. Given the same set of entities / components it needs to produce the same end state without side-effects.
**Exact** change-tracking proposals satisfy criteria 1 and 2.
**Conservative** change-tracking proposals satisfy criteria 1 but not 2.
**Flaky** change tracking proposals satisfy criteria 2 but not 1.
# Code Base Navigation
There are three types of flags:
- `Added`: A piece of data was added to an entity / `Resources`.
- `Mutated`: A piece of data was able to be modified, because its `DerefMut` was accessed
- `Changed`: The bitwise OR of `Added` and `Changed`
The special behavior of `ChangedRes`, with respect to the scheduler is being removed in [#1313](https://github.com/bevyengine/bevy/pull/1313) and does not need to be reproduced.
`ChangedRes` and friends can be found in "bevy_ecs/core/resources/resource_query.rs".
The `Flags` trait for Components can be found in "bevy_ecs/core/query.rs".
`ComponentFlags` are stored in "bevy_ecs/core/archetypes.rs", defined on line 446.
# Proposals
**Proposal 5 was selected for implementation.**
## Proposal 0: No Change Detection
The baseline, where computations are performed on everything regardless of whether it changed.
**Type:** Conservative
**Pros:**
- already implemented
- will never miss events
- no overhead
**Cons:**
- tons of repeated work
- doesn't allow users to avoid repeating work (or monitoring for other changes)
## Proposal 1: Earlier-This-Tick Change Detection
The current approach as of Bevy 0.4. Flags are set, and then flushed at the end of each frame.
**Type:** Flaky
**Pros:**
- already implemented
- simple to understand
- low memory overhead (2 bits per component)
- low time overhead (clear every flag once per frame)
**Cons:**
- misses systems based on ordering
- systems that don't run every frame miss changes
- duplicates detection when looping
- can lead to unresolvable circular dependencies
## Proposal 2: Two-Tick Change Detection
Flags persist for two frames, using a double-buffer system identical to that used in events.
A change is observed if it is found in either the current frame's list of changes or the previous frame's.
**Type:** Conservative
**Pros:**
- easy to understand
- easy to implement
- low memory overhead (4 bits per component)
- low time overhead (bit mask and shift every flag once per frame)
**Cons:**
- can result in a great deal of duplicated work
- systems that don't run every frame miss changes
- duplicates detection when looping
## Proposal 3: Last-Tick Change Detection
Flags persist for two frames, using a double-buffer system identical to that used in events.
A change is observed if it is found in the previous frame's list of changes.
**Type:** Exact
**Pros:**
- exact
- easy to understand
- easy to implement
- low memory overhead (4 bits per component)
- low time overhead (bit mask and shift every flag once per frame)
**Cons:**
- change detection is always delayed, possibly causing painful chained delays
- systems that don't run every frame miss changes
- duplicates detection when looping
## Proposal 4: Flag-Doubling Change Detection
Combine Proposal 2 and Proposal 3. Differentiate between `JustChanged` (current behavior) and `Changed` (Proposal 3).
Pack this data into the flags according to [this implementation proposal](https://github.com/bevyengine/bevy/issues/68#issuecomment-769174804).
**Type:** Flaky + Exact
**Pros:**
- allows users to acc
- easy to implement
- low memory overhead (4 bits per component)
- low time overhead (bit mask and shift every flag once per frame)
**Cons:**
- users must specify the type of change detection required
- still quite fragile to system ordering effects when using the flaky `JustChanged` form
- cannot get immediate + exact results
- systems that don't run every frame miss changes
- duplicates detection when looping
## [SELECTED] Proposal 5: Generation-Counter Change Detection
A global counter is increased after each system is run. Each component saves the time of last mutation, and each system saves the time of last execution. Mutation is detected when the component's counter is greater than the system's counter. Discussed [here](https://github.com/bevyengine/bevy/issues/68#issuecomment-769174804). How to handle addition detection is unsolved; the current proposal is to use the highest bit of the counter as in proposal 1.
**Type:** Exact (for mutations), flaky (for additions)
**Pros:**
- low time overhead (set component counter on access, set system counter after execution)
- robust to systems that don't run every frame
- robust to systems that loop
**Cons:**
- moderately complex implementation
- must be modified as systems are inserted dynamically
- medium memory overhead (4 bytes per component + system)
- unsolved addition detection
## Proposal 6: System-Data Change Detection
For each system, track which system's changes it has seen. This approach is only worth fully designing and implementing if Proposal 5 fails in some way.
**Type:** Exact
**Pros:**
- exact
- conceptually simple
**Cons:**
- requires storing data on each system
- implementation is complex
- must be modified as systems are inserted dynamically
## Proposal 7: Total-Order Change Detection
Discussed [here](https://github.com/bevyengine/bevy/issues/68#issuecomment-754326523). This proposal is somewhat complicated by the new scheduler, but I believe it should still be conceptually feasible. This approach is only worth fully designing and implementing if Proposal 5 fails in some way.
**Type:** Exact
**Pros:**
- exact
- efficient data storage relative to other exact proposals
**Cons:**
- requires access to the scheduler
- complex implementation and difficulty grokking
- must be modified as systems are inserted dynamically
# Tests
- We will need to verify properties 1, 2, 3, 7 and 8. Priority: 1 > 2 = 3 > 8 > 7
- Ideally we can use identical user-facing syntax for all proposals, allowing us to re-use the same syntax for each.
- When writing tests, we need to carefully specify order using explicit dependencies.
- These tests will need to be duplicated for both components and resources.
- We need to be sure to handle cases where ambiguous system orders exist.
`changing_system` is always the system that makes the changes, and `detecting_system` always detects the changes.
The component / resource changed will be simple boolean wrapper structs.
## Basic Added / Mutated / Changed
2 x 3 design:
- Resources vs. Components
- Added vs. Changed vs. Mutated
- `changing_system` runs before `detecting_system`
- verify at the end of tick 2
## At Least Once
2 x 3 design:
- Resources vs. Components
- Added vs. Changed vs. Mutated
- `changing_system` runs after `detecting_system`
- verify at the end of tick 2
## At Most Once
2 x 3 design:
- Resources vs. Components
- Added vs. Changed vs. Mutated
- `changing_system` runs once before `detecting_system`
- increment a counter based on the number of changes detected
- verify at the end of tick 2
## Fast Detection
2 x 3 design:
- Resources vs. Components
- Added vs. Changed vs. Mutated
- `changing_system` runs before `detecting_system`
- verify at the end of tick 1
## Ambiguous System Ordering Robustness
2 x 3 x 2 design:
- Resources vs. Components
- Added vs. Changed vs. Mutated
- `changing_system` runs [before/after] `detecting_system` in tick 1
- `changing_system` runs [after/before] `detecting_system` in tick 2
## System Pausing
2 x 3 design:
- Resources vs. Components
- Added vs. Changed vs. Mutated
- `changing_system` runs in tick 1, then is disabled by run criteria
- `detecting_system` is disabled by run criteria until it is run once during tick 3
- verify at the end of tick 3
## Addition Causes Mutation
2 design:
- Resources vs. Components
- `adding_system_1` adds a component / resource
- `adding system_2` adds the same component / resource
- verify the `Mutated` flag at the end of the tick
- verify the `Added` flag at the end of the tick
First check tests for: https://github.com/bevyengine/bevy/issues/333
Second check tests for: https://github.com/bevyengine/bevy/issues/1443
## Changes Made By Commands
- `adding_system` runs in Update in tick 1, and sends a command to add a component
- `detecting_system` runs in Update in tick 1 and 2, after `adding_system`
- We can't detect the changes in tick 1, since they haven't been processed yet
- If we were to track these changes as being emitted by `adding_system`, we can't detect the changes in tick 2 either, since `detecting_system` has already run once after `adding_system` :(
# Benchmarks
See: [general advice](https://github.com/bevyengine/bevy/blob/master/docs/profiling.md), [Criterion crate](https://github.com/bheisler/criterion.rs)
There are several critical parameters to vary:
1. entity count (1 to 10^9)
2. fraction of entities that are changed (0% to 100%)
3. cost to perform work on changed entities, i.e. workload (1 ns to 1s)
1 and 2 should be varied between benchmark runs. 3 can be added on computationally.
We want to measure:
- memory cost
- run time
We should collect these measurements across several frames (100?) to reduce bootup effects and accurately measure the mean, variance and drift.
Entity-component change detection is much more important to benchmark than resource change detection, due to the orders of magnitude higher number of pieces of data.
No change detection at all should be included in benchmarks as a second control for cases where missing changes is unacceptable.
## Graphs
1. y: performance, x: log_10(entity count), color: proposal, facet: performance metric. Set cost to perform work to 0.
2. y: run time, x: cost to perform work, color: proposal, facet: fraction changed. Set number of entities to 10^6
3. y: memory, x: frames, color: proposal
# Conclusions
1. Is the theoretical categorization of the proposals correct according to our tests?
2. How does the performance of the proposals compare without any load?
3. How does the performance of the proposals compare with realistic loads?
4. At what workload does more exact change tracking become worth the (presumably) higher overhead?
5. When does adding change-detection to save on work become worthwhile?
6. Is there enough divergence in performance between the best solutions in each class to ship more than one change-tracking solution?
# Implementation Plan
1. Write a test suite.
2. Verify that tests fail for existing approach.
3. Write a benchmark suite.
4. Get performance numbers for existing approach.
5. Implement, test and benchmark various solutions using a Git branch per proposal.
6. Create a draft PR with all solutions and present results to team.
7. Select a solution and replace existing change detection.
Co-authored-by: Brice DAVIER <bricedavier@gmail.com>
Co-authored-by: Carter Anderson <mcanders1@gmail.com>
2021-03-19 17:53:26 +00:00
change_tick ,
Bevy ECS V2 (#1525)
# Bevy ECS V2
This is a rewrite of Bevy ECS (basically everything but the new executor/schedule, which are already awesome). The overall goal was to improve the performance and versatility of Bevy ECS. Here is a quick bulleted list of changes before we dive into the details:
* Complete World rewrite
* Multiple component storage types:
* Tables: fast cache friendly iteration, slower add/removes (previously called Archetypes)
* Sparse Sets: fast add/remove, slower iteration
* Stateful Queries (caches query results for faster iteration. fragmented iteration is _fast_ now)
* Stateful System Params (caches expensive operations. inspired by @DJMcNab's work in #1364)
* Configurable System Params (users can set configuration when they construct their systems. once again inspired by @DJMcNab's work)
* Archetypes are now "just metadata", component storage is separate
* Archetype Graph (for faster archetype changes)
* Component Metadata
* Configure component storage type
* Retrieve information about component size/type/name/layout/send-ness/etc
* Components are uniquely identified by a densely packed ComponentId
* TypeIds are now totally optional (which should make implementing scripting easier)
* Super fast "for_each" query iterators
* Merged Resources into World. Resources are now just a special type of component
* EntityRef/EntityMut builder apis (more efficient and more ergonomic)
* Fast bitset-backed `Access<T>` replaces old hashmap-based approach everywhere
* Query conflicts are determined by component access instead of archetype component access (to avoid random failures at runtime)
* With/Without are still taken into account for conflicts, so this should still be comfy to use
* Much simpler `IntoSystem` impl
* Significantly reduced the amount of hashing throughout the ecs in favor of Sparse Sets (indexed by densely packed ArchetypeId, ComponentId, BundleId, and TableId)
* Safety Improvements
* Entity reservation uses a normal world reference instead of unsafe transmute
* QuerySets no longer transmute lifetimes
* Made traits "unsafe" where relevant
* More thorough safety docs
* WorldCell
* Exposes safe mutable access to multiple resources at a time in a World
* Replaced "catch all" `System::update_archetypes(world: &World)` with `System::new_archetype(archetype: &Archetype)`
* Simpler Bundle implementation
* Replaced slow "remove_bundle_one_by_one" used as fallback for Commands::remove_bundle with fast "remove_bundle_intersection"
* Removed `Mut<T>` query impl. it is better to only support one way: `&mut T`
* Removed with() from `Flags<T>` in favor of `Option<Flags<T>>`, which allows querying for flags to be "filtered" by default
* Components now have is_send property (currently only resources support non-send)
* More granular module organization
* New `RemovedComponents<T>` SystemParam that replaces `query.removed::<T>()`
* `world.resource_scope()` for mutable access to resources and world at the same time
* WorldQuery and QueryFilter traits unified. FilterFetch trait added to enable "short circuit" filtering. Auto impled for cases that don't need it
* Significantly slimmed down SystemState in favor of individual SystemParam state
* System Commands changed from `commands: &mut Commands` back to `mut commands: Commands` (to allow Commands to have a World reference)
Fixes #1320
## `World` Rewrite
This is a from-scratch rewrite of `World` that fills the niche that `hecs` used to. Yes, this means Bevy ECS is no longer a "fork" of hecs. We're going out our own!
(the only shared code between the projects is the entity id allocator, which is already basically ideal)
A huge shout out to @SanderMertens (author of [flecs](https://github.com/SanderMertens/flecs)) for sharing some great ideas with me (specifically hybrid ecs storage and archetype graphs). He also helped advise on a number of implementation details.
## Component Storage (The Problem)
Two ECS storage paradigms have gained a lot of traction over the years:
* **Archetypal ECS**:
* Stores components in "tables" with static schemas. Each "column" stores components of a given type. Each "row" is an entity.
* Each "archetype" has its own table. Adding/removing an entity's component changes the archetype.
* Enables super-fast Query iteration due to its cache-friendly data layout
* Comes at the cost of more expensive add/remove operations for an Entity's components, because all components need to be copied to the new archetype's "table"
* **Sparse Set ECS**:
* Stores components of the same type in densely packed arrays, which are sparsely indexed by densely packed unsigned integers (Entity ids)
* Query iteration is slower than Archetypal ECS because each entity's component could be at any position in the sparse set. This "random access" pattern isn't cache friendly. Additionally, there is an extra layer of indirection because you must first map the entity id to an index in the component array.
* Adding/removing components is a cheap, constant time operation
Bevy ECS V1, hecs, legion, flec, and Unity DOTS are all "archetypal ecs-es". I personally think "archetypal" storage is a good default for game engines. An entity's archetype doesn't need to change frequently in general, and it creates "fast by default" query iteration (which is a much more common operation). It is also "self optimizing". Users don't need to think about optimizing component layouts for iteration performance. It "just works" without any extra boilerplate.
Shipyard and EnTT are "sparse set ecs-es". They employ "packing" as a way to work around the "suboptimal by default" iteration performance for specific sets of components. This helps, but I didn't think this was a good choice for a general purpose engine like Bevy because:
1. "packs" conflict with each other. If bevy decides to internally pack the Transform and GlobalTransform components, users are then blocked if they want to pack some custom component with Transform.
2. users need to take manual action to optimize
Developers selecting an ECS framework are stuck with a hard choice. Select an "archetypal" framework with "fast iteration everywhere" but without the ability to cheaply add/remove components, or select a "sparse set" framework to cheaply add/remove components but with slower iteration performance.
## Hybrid Component Storage (The Solution)
In Bevy ECS V2, we get to have our cake and eat it too. It now has _both_ of the component storage types above (and more can be added later if needed):
* **Tables** (aka "archetypal" storage)
* The default storage. If you don't configure anything, this is what you get
* Fast iteration by default
* Slower add/remove operations
* **Sparse Sets**
* Opt-in
* Slower iteration
* Faster add/remove operations
These storage types complement each other perfectly. By default Query iteration is fast. If developers know that they want to add/remove a component at high frequencies, they can set the storage to "sparse set":
```rust
world.register_component(
ComponentDescriptor::new::<MyComponent>(StorageType::SparseSet)
).unwrap();
```
## Archetypes
Archetypes are now "just metadata" ... they no longer store components directly. They do store:
* The `ComponentId`s of each of the Archetype's components (and that component's storage type)
* Archetypes are uniquely defined by their component layouts
* For example: entities with "table" components `[A, B, C]` _and_ "sparse set" components `[D, E]` will always be in the same archetype.
* The `TableId` associated with the archetype
* For now each archetype has exactly one table (which can have no components),
* There is a 1->Many relationship from Tables->Archetypes. A given table could have any number of archetype components stored in it:
* Ex: an entity with "table storage" components `[A, B, C]` and "sparse set" components `[D, E]` will share the same `[A, B, C]` table as an entity with `[A, B, C]` table component and `[F]` sparse set components.
* This 1->Many relationship is how we preserve fast "cache friendly" iteration performance when possible (more on this later)
* A list of entities that are in the archetype and the row id of the table they are in
* ArchetypeComponentIds
* unique densely packed identifiers for (ArchetypeId, ComponentId) pairs
* used by the schedule executor for cheap system access control
* "Archetype Graph Edges" (see the next section)
## The "Archetype Graph"
Archetype changes in Bevy (and a number of other archetypal ecs-es) have historically been expensive to compute. First, you need to allocate a new vector of the entity's current component ids, add or remove components based on the operation performed, sort it (to ensure it is order-independent), then hash it to find the archetype (if it exists). And thats all before we get to the _already_ expensive full copy of all components to the new table storage.
The solution is to build a "graph" of archetypes to cache these results. @SanderMertens first exposed me to the idea (and he got it from @gjroelofs, who came up with it). They propose adding directed edges between archetypes for add/remove component operations. If `ComponentId`s are densely packed, you can use sparse sets to cheaply jump between archetypes.
Bevy takes this one step further by using add/remove `Bundle` edges instead of `Component` edges. Bevy encourages the use of `Bundles` to group add/remove operations. This is largely for "clearer game logic" reasons, but it also helps cut down on the number of archetype changes required. `Bundles` now also have densely-packed `BundleId`s. This allows us to use a _single_ edge for each bundle operation (rather than needing to traverse N edges ... one for each component). Single component operations are also bundles, so this is strictly an improvement over a "component only" graph.
As a result, an operation that used to be _heavy_ (both for allocations and compute) is now two dirt-cheap array lookups and zero allocations.
## Stateful Queries
World queries are now stateful. This allows us to:
1. Cache archetype (and table) matches
* This resolves another issue with (naive) archetypal ECS: query performance getting worse as the number of archetypes goes up (and fragmentation occurs).
2. Cache Fetch and Filter state
* The expensive parts of fetch/filter operations (such as hashing the TypeId to find the ComponentId) now only happen once when the Query is first constructed
3. Incrementally build up state
* When new archetypes are added, we only process the new archetypes (no need to rebuild state for old archetypes)
As a result, the direct `World` query api now looks like this:
```rust
let mut query = world.query::<(&A, &mut B)>();
for (a, mut b) in query.iter_mut(&mut world) {
}
```
Requiring `World` to generate stateful queries (rather than letting the `QueryState` type be constructed separately) allows us to ensure that _all_ queries are properly initialized (and the relevant world state, such as ComponentIds). This enables QueryState to remove branches from its operations that check for initialization status (and also enables query.iter() to take an immutable world reference because it doesn't need to initialize anything in world).
However in systems, this is a non-breaking change. State management is done internally by the relevant SystemParam.
## Stateful SystemParams
Like Queries, `SystemParams` now also cache state. For example, `Query` system params store the "stateful query" state mentioned above. Commands store their internal `CommandQueue`. This means you can now safely use as many separate `Commands` parameters in your system as you want. `Local<T>` system params store their `T` value in their state (instead of in Resources).
SystemParam state also enabled a significant slim-down of SystemState. It is much nicer to look at now.
Per-SystemParam state naturally insulates us from an "aliased mut" class of errors we have hit in the past (ex: using multiple `Commands` system params).
(credit goes to @DJMcNab for the initial idea and draft pr here #1364)
## Configurable SystemParams
@DJMcNab also had the great idea to make SystemParams configurable. This allows users to provide some initial configuration / values for system parameters (when possible). Most SystemParams have no config (the config type is `()`), but the `Local<T>` param now supports user-provided parameters:
```rust
fn foo(value: Local<usize>) {
}
app.add_system(foo.system().config(|c| c.0 = Some(10)));
```
## Uber Fast "for_each" Query Iterators
Developers now have the choice to use a fast "for_each" iterator, which yields ~1.5-3x iteration speed improvements for "fragmented iteration", and minor ~1.2x iteration speed improvements for unfragmented iteration.
```rust
fn system(query: Query<(&A, &mut B)>) {
// you now have the option to do this for a speed boost
query.for_each_mut(|(a, mut b)| {
});
// however normal iterators are still available
for (a, mut b) in query.iter_mut() {
}
}
```
I think in most cases we should continue to encourage "normal" iterators as they are more flexible and more "rust idiomatic". But when that extra "oomf" is needed, it makes sense to use `for_each`.
We should also consider using `for_each` for internal bevy systems to give our users a nice speed boost (but that should be a separate pr).
## Component Metadata
`World` now has a `Components` collection, which is accessible via `world.components()`. This stores mappings from `ComponentId` to `ComponentInfo`, as well as `TypeId` to `ComponentId` mappings (where relevant). `ComponentInfo` stores information about the component, such as ComponentId, TypeId, memory layout, send-ness (currently limited to resources), and storage type.
## Significantly Cheaper `Access<T>`
We used to use `TypeAccess<TypeId>` to manage read/write component/archetype-component access. This was expensive because TypeIds must be hashed and compared individually. The parallel executor got around this by "condensing" type ids into bitset-backed access types. This worked, but it had to be re-generated from the `TypeAccess<TypeId>`sources every time archetypes changed.
This pr removes TypeAccess in favor of faster bitset access everywhere. We can do this thanks to the move to densely packed `ComponentId`s and `ArchetypeComponentId`s.
## Merged Resources into World
Resources had a lot of redundant functionality with Components. They stored typed data, they had access control, they had unique ids, they were queryable via SystemParams, etc. In fact the _only_ major difference between them was that they were unique (and didn't correlate to an entity).
Separate resources also had the downside of requiring a separate set of access controls, which meant the parallel executor needed to compare more bitsets per system and manage more state.
I initially got the "separate resources" idea from `legion`. I think that design was motivated by the fact that it made the direct world query/resource lifetime interactions more manageable. It certainly made our lives easier when using Resources alongside hecs/bevy_ecs. However we already have a construct for safely and ergonomically managing in-world lifetimes: systems (which use `Access<T>` internally).
This pr merges Resources into World:
```rust
world.insert_resource(1);
world.insert_resource(2.0);
let a = world.get_resource::<i32>().unwrap();
let mut b = world.get_resource_mut::<f64>().unwrap();
*b = 3.0;
```
Resources are now just a special kind of component. They have their own ComponentIds (and their own resource TypeId->ComponentId scope, so they don't conflict wit components of the same type). They are stored in a special "resource archetype", which stores components inside the archetype using a new `unique_components` sparse set (note that this sparse set could later be used to implement Tags). This allows us to keep the code size small by reusing existing datastructures (namely Column, Archetype, ComponentFlags, and ComponentInfo). This allows us the executor to use a single `Access<ArchetypeComponentId>` per system. It should also make scripting language integration easier.
_But_ this merge did create problems for people directly interacting with `World`. What if you need mutable access to multiple resources at the same time? `world.get_resource_mut()` borrows World mutably!
## WorldCell
WorldCell applies the `Access<ArchetypeComponentId>` concept to direct world access:
```rust
let world_cell = world.cell();
let a = world_cell.get_resource_mut::<i32>().unwrap();
let b = world_cell.get_resource_mut::<f64>().unwrap();
```
This adds cheap runtime checks (a sparse set lookup of `ArchetypeComponentId` and a counter) to ensure that world accesses do not conflict with each other. Each operation returns a `WorldBorrow<'w, T>` or `WorldBorrowMut<'w, T>` wrapper type, which will release the relevant ArchetypeComponentId resources when dropped.
World caches the access sparse set (and only one cell can exist at a time), so `world.cell()` is a cheap operation.
WorldCell does _not_ use atomic operations. It is non-send, does a mutable borrow of world to prevent other accesses, and uses a simple `Rc<RefCell<ArchetypeComponentAccess>>` wrapper in each WorldBorrow pointer.
The api is currently limited to resource access, but it can and should be extended to queries / entity component access.
## Resource Scopes
WorldCell does not yet support component queries, and even when it does there are sometimes legitimate reasons to want a mutable world ref _and_ a mutable resource ref (ex: bevy_render and bevy_scene both need this). In these cases we could always drop down to the unsafe `world.get_resource_unchecked_mut()`, but that is not ideal!
Instead developers can use a "resource scope"
```rust
world.resource_scope(|world: &mut World, a: &mut A| {
})
```
This temporarily removes the `A` resource from `World`, provides mutable pointers to both, and re-adds A to World when finished. Thanks to the move to ComponentIds/sparse sets, this is a cheap operation.
If multiple resources are required, scopes can be nested. We could also consider adding a "resource tuple" to the api if this pattern becomes common and the boilerplate gets nasty.
## Query Conflicts Use ComponentId Instead of ArchetypeComponentId
For safety reasons, systems cannot contain queries that conflict with each other without wrapping them in a QuerySet. On bevy `main`, we use ArchetypeComponentIds to determine conflicts. This is nice because it can take into account filters:
```rust
// these queries will never conflict due to their filters
fn filter_system(a: Query<&mut A, With<B>>, b: Query<&mut B, Without<B>>) {
}
```
But it also has a significant downside:
```rust
// these queries will not conflict _until_ an entity with A, B, and C is spawned
fn maybe_conflicts_system(a: Query<(&mut A, &C)>, b: Query<(&mut A, &B)>) {
}
```
The system above will panic at runtime if an entity with A, B, and C is spawned. This makes it hard to trust that your game logic will run without crashing.
In this pr, I switched to using `ComponentId` instead. This _is_ more constraining. `maybe_conflicts_system` will now always fail, but it will do it consistently at startup. Naively, it would also _disallow_ `filter_system`, which would be a significant downgrade in usability. Bevy has a number of internal systems that rely on disjoint queries and I expect it to be a common pattern in userspace.
To resolve this, I added a new `FilteredAccess<T>` type, which wraps `Access<T>` and adds with/without filters. If two `FilteredAccess` have with/without values that prove they are disjoint, they will no longer conflict.
## EntityRef / EntityMut
World entity operations on `main` require that the user passes in an `entity` id to each operation:
```rust
let entity = world.spawn((A, )); // create a new entity with A
world.get::<A>(entity);
world.insert(entity, (B, C));
world.insert_one(entity, D);
```
This means that each operation needs to look up the entity location / verify its validity. The initial spawn operation also requires a Bundle as input. This can be awkward when no components are required (or one component is required).
These operations have been replaced by `EntityRef` and `EntityMut`, which are "builder-style" wrappers around world that provide read and read/write operations on a single, pre-validated entity:
```rust
// spawn now takes no inputs and returns an EntityMut
let entity = world.spawn()
.insert(A) // insert a single component into the entity
.insert_bundle((B, C)) // insert a bundle of components into the entity
.id() // id returns the Entity id
// Returns EntityMut (or panics if the entity does not exist)
world.entity_mut(entity)
.insert(D)
.insert_bundle(SomeBundle::default());
{
// returns EntityRef (or panics if the entity does not exist)
let d = world.entity(entity)
.get::<D>() // gets the D component
.unwrap();
// world.get still exists for ergonomics
let d = world.get::<D>(entity).unwrap();
}
// These variants return Options if you want to check existence instead of panicing
world.get_entity_mut(entity)
.unwrap()
.insert(E);
if let Some(entity_ref) = world.get_entity(entity) {
let d = entity_ref.get::<D>().unwrap();
}
```
This _does not_ affect the current Commands api or terminology. I think that should be a separate conversation as that is a much larger breaking change.
## Safety Improvements
* Entity reservation in Commands uses a normal world borrow instead of an unsafe transmute
* QuerySets no longer transmutes lifetimes
* Made traits "unsafe" when implementing a trait incorrectly could cause unsafety
* More thorough safety docs
## RemovedComponents SystemParam
The old approach to querying removed components: `query.removed:<T>()` was confusing because it had no connection to the query itself. I replaced it with the following, which is both clearer and allows us to cache the ComponentId mapping in the SystemParamState:
```rust
fn system(removed: RemovedComponents<T>) {
for entity in removed.iter() {
}
}
```
## Simpler Bundle implementation
Bundles are no longer responsible for sorting (or deduping) TypeInfo. They are just a simple ordered list of component types / data. This makes the implementation smaller and opens the door to an easy "nested bundle" implementation in the future (which i might even add in this pr). Duplicate detection is now done once per bundle type by World the first time a bundle is used.
## Unified WorldQuery and QueryFilter types
(don't worry they are still separate type _parameters_ in Queries .. this is a non-breaking change)
WorldQuery and QueryFilter were already basically identical apis. With the addition of `FetchState` and more storage-specific fetch methods, the overlap was even clearer (and the redundancy more painful).
QueryFilters are now just `F: WorldQuery where F::Fetch: FilterFetch`. FilterFetch requires `Fetch<Item = bool>` and adds new "short circuit" variants of fetch methods. This enables a filter tuple like `(With<A>, Without<B>, Changed<C>)` to stop evaluating the filter after the first mismatch is encountered. FilterFetch is automatically implemented for `Fetch` implementations that return bool.
This forces fetch implementations that return things like `(bool, bool, bool)` (such as the filter above) to manually implement FilterFetch and decide whether or not to short-circuit.
## More Granular Modules
World no longer globs all of the internal modules together. It now exports `core`, `system`, and `schedule` separately. I'm also considering exporting `core` submodules directly as that is still pretty "glob-ey" and unorganized (feedback welcome here).
## Remaining Draft Work (to be done in this pr)
* ~~panic on conflicting WorldQuery fetches (&A, &mut A)~~
* ~~bevy `main` and hecs both currently allow this, but we should protect against it if possible~~
* ~~batch_iter / par_iter (currently stubbed out)~~
* ~~ChangedRes~~
* ~~I skipped this while we sort out #1313. This pr should be adapted to account for whatever we land on there~~.
* ~~The `Archetypes` and `Tables` collections use hashes of sorted lists of component ids to uniquely identify each archetype/table. This hash is then used as the key in a HashMap to look up the relevant ArchetypeId or TableId. (which doesn't handle hash collisions properly)~~
* ~~It is currently unsafe to generate a Query from "World A", then use it on "World B" (despite the api claiming it is safe). We should probably close this gap. This could be done by adding a randomly generated WorldId to each world, then storing that id in each Query. They could then be compared to each other on each `query.do_thing(&world)` operation. This _does_ add an extra branch to each query operation, so I'm open to other suggestions if people have them.~~
* ~~Nested Bundles (if i find time)~~
## Potential Future Work
* Expand WorldCell to support queries.
* Consider not allocating in the empty archetype on `world.spawn()`
* ex: return something like EntityMutUninit, which turns into EntityMut after an `insert` or `insert_bundle` op
* this actually regressed performance last time i tried it, but in theory it should be faster
* Optimize SparseSet::insert (see `PERF` comment on insert)
* Replace SparseArray `Option<T>` with T::MAX to cut down on branching
* would enable cheaper get_unchecked() operations
* upstream fixedbitset optimizations
* fixedbitset could be allocation free for small block counts (store blocks in a SmallVec)
* fixedbitset could have a const constructor
* Consider implementing Tags (archetype-specific by-value data that affects archetype identity)
* ex: ArchetypeA could have `[A, B, C]` table components and `[D(1)]` "tag" component. ArchetypeB could have `[A, B, C]` table components and a `[D(2)]` tag component. The archetypes are different, despite both having D tags because the value inside D is different.
* this could potentially build on top of the `archetype.unique_components` added in this pr for resource storage.
* Consider reverting `all_tuples` proc macro in favor of the old `macro_rules` implementation
* all_tuples is more flexible and produces cleaner documentation (the macro_rules version produces weird type parameter orders due to parser constraints)
* but unfortunately all_tuples also appears to make Rust Analyzer sad/slow when working inside of `bevy_ecs` (does not affect user code)
* Consider "resource queries" and/or "mixed resource and entity component queries" as an alternative to WorldCell
* this is basically just "systems" so maybe it's not worth it
* Add more world ops
* `world.clear()`
* `world.reserve<T: Bundle>(count: usize)`
* Try using the old archetype allocation strategy (allocate new memory on resize and copy everything over). I expect this to improve batch insertion performance at the cost of unbatched performance. But thats just a guess. I'm not an allocation perf pro :)
* Adapt Commands apis for consistency with new World apis
## Benchmarks
key:
* `bevy_old`: bevy `main` branch
* `bevy`: this branch
* `_foreach`: uses an optimized for_each iterator
* ` _sparse`: uses sparse set storage (if unspecified assume table storage)
* `_system`: runs inside a system (if unspecified assume test happens via direct world ops)
### Simple Insert (from ecs_bench_suite)
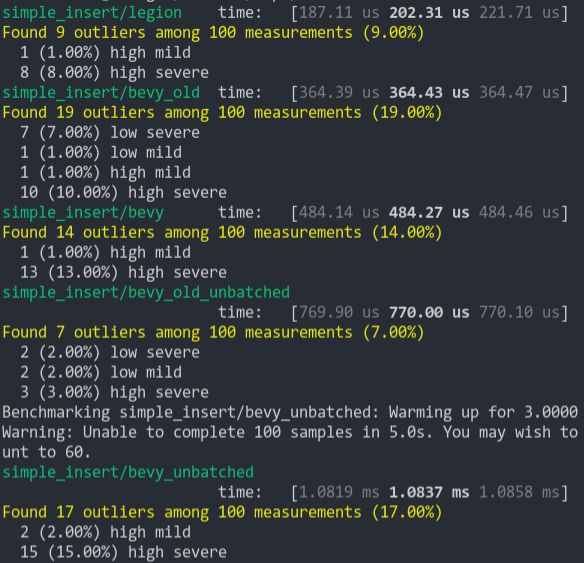
### Simpler Iter (from ecs_bench_suite)
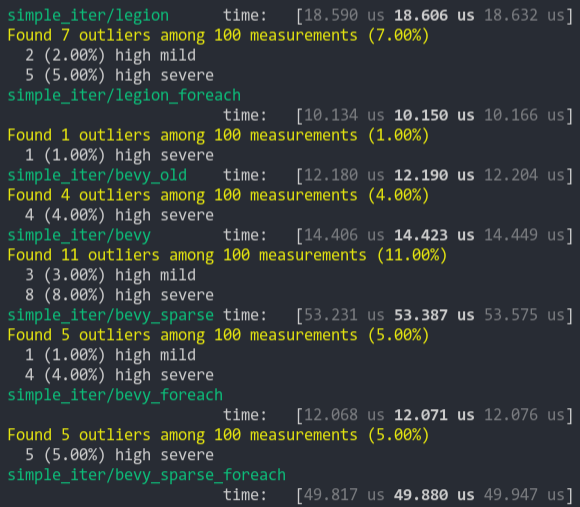
### Fragment Iter (from ecs_bench_suite)
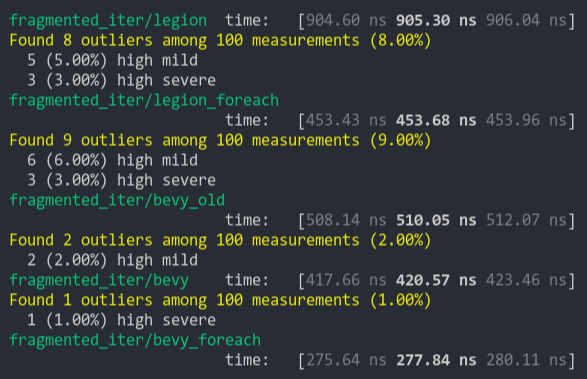
### Sparse Fragmented Iter
Iterate a query that matches 5 entities from a single matching archetype, but there are 100 unmatching archetypes
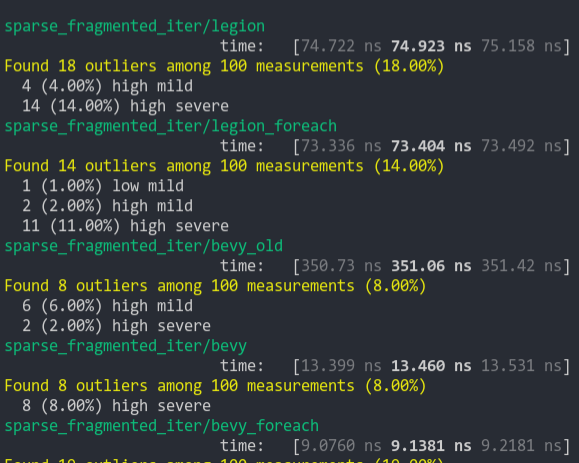
### Schedule (from ecs_bench_suite)
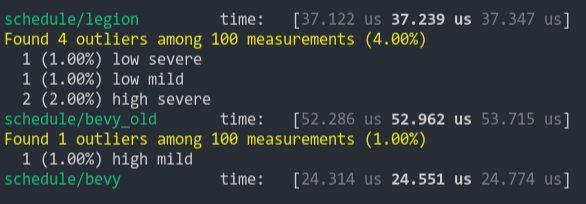
### Add Remove Component (from ecs_bench_suite)
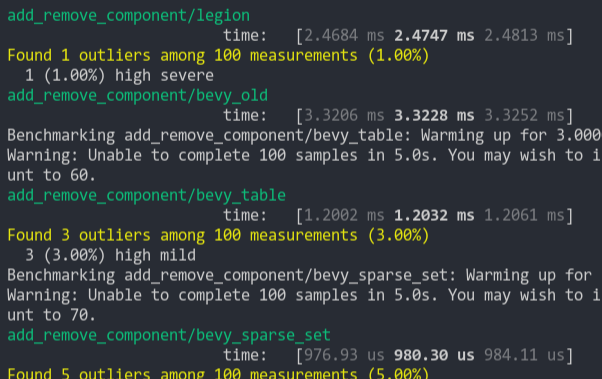
### Add Remove Component Big
Same as the test above, but each entity has 5 "large" matrix components and 1 "large" matrix component is added and removed
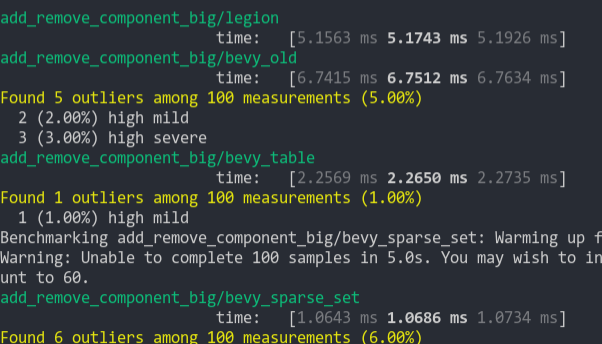
### Get Component
Looks up a single component value a large number of times
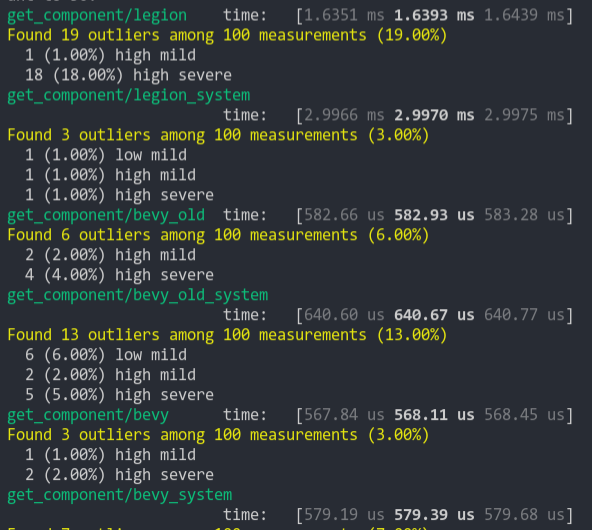
2021-03-05 07:54:35 +00:00
}
2020-11-08 20:34:05 +00:00
}
}
2023-01-17 03:29:08 +00:00
// SAFETY: Only reads a single World non-send resource
unsafe impl < T : 'static > ReadOnlySystemParam for Option < NonSend < '_ , T > > { }
Remove the `SystemParamState` trait and remove types like `ResState` (#6919)
Spiritual successor to #5205.
Actual successor to #6865.
# Objective
Currently, system params are defined using three traits: `SystemParam`, `ReadOnlySystemParam`, `SystemParamState`. The behavior for each param is specified by the `SystemParamState` trait, while `SystemParam` simply defers to the state.
Splitting the traits in this way makes it easier to implement within macros, but it increases the cognitive load. Worst of all, this approach requires each `MySystemParam` to have a public `MySystemParamState` type associated with it.
## Solution
* Merge the trait `SystemParamState` into `SystemParam`.
* Remove all trivial `SystemParam` state types.
* `OptionNonSendMutState<T>`: you will not be missed.
---
- [x] Fix/resolve the remaining test failure.
## Changelog
* Removed the trait `SystemParamState`, merging its functionality into `SystemParam`.
## Migration Guide
**Note**: this should replace the migration guide for #6865.
This is relative to Bevy 0.9, not main.
The traits `SystemParamState` and `SystemParamFetch` have been removed, and their functionality has been transferred to `SystemParam`.
```rust
// Before (0.9)
impl SystemParam for MyParam<'_, '_> {
type State = MyParamState;
}
unsafe impl SystemParamState for MyParamState {
fn init(world: &mut World, system_meta: &mut SystemMeta) -> Self { ... }
}
unsafe impl<'w, 's> SystemParamFetch<'w, 's> for MyParamState {
type Item = MyParam<'w, 's>;
fn get_param(&mut self, ...) -> Self::Item;
}
unsafe impl ReadOnlySystemParamFetch for MyParamState { }
// After (0.10)
unsafe impl SystemParam for MyParam<'_, '_> {
type State = MyParamState;
type Item<'w, 's> = MyParam<'w, 's>;
fn init_state(world: &mut World, system_meta: &mut SystemMeta) -> Self::State { ... }
fn get_param<'w, 's>(state: &mut Self::State, ...) -> Self::Item<'w, 's>;
}
unsafe impl ReadOnlySystemParam for MyParam<'_, '_> { }
```
The trait `ReadOnlySystemParamFetch` has been replaced with `ReadOnlySystemParam`.
```rust
// Before
unsafe impl ReadOnlySystemParamFetch for MyParamState {}
// After
unsafe impl ReadOnlySystemParam for MyParam<'_, '_> {}
```
2023-01-07 23:20:32 +00:00
// SAFETY: this impl defers to `NonSend`, which initializes and validates the correct world access.
unsafe impl < T : 'static > SystemParam for Option < NonSend < '_ , T > > {
type State = ComponentId ;
Simplify trait hierarchy for `SystemParam` (#6865)
# Objective
* Implementing a custom `SystemParam` by hand requires implementing three traits -- four if it is read-only.
* The trait `SystemParamFetch<'w, 's>` is a workaround from before we had generic associated types, and is no longer necessary.
## Solution
* Combine the trait `SystemParamFetch` with `SystemParamState`.
* I decided to remove the `Fetch` name and keep the `State` name, since the former was consistently conflated with the latter.
* Replace the trait `ReadOnlySystemParamFetch` with `ReadOnlySystemParam`, which simplifies trait bounds in generic code.
---
## Changelog
- Removed the trait `SystemParamFetch`, moving its functionality to `SystemParamState`.
- Replaced the trait `ReadOnlySystemParamFetch` with `ReadOnlySystemParam`.
## Migration Guide
The trait `SystemParamFetch` has been removed, and its functionality has been transferred to `SystemParamState`.
```rust
// Before
impl SystemParamState for MyParamState {
fn init(world: &mut World, system_meta: &mut SystemMeta) -> Self { ... }
}
impl<'w, 's> SystemParamFetch<'w, 's> for MyParamState {
type Item = MyParam<'w, 's>;
fn get_param(...) -> Self::Item;
}
// After
impl SystemParamState for MyParamState {
type Item<'w, 's> = MyParam<'w, 's>; // Generic associated types!
fn init(world: &mut World, system_meta: &mut SystemMeta) -> Self { ... }
fn get_param<'w, 's>(...) -> Self::Item<'w, 's>;
}
```
The trait `ReadOnlySystemParamFetch` has been replaced with `ReadOnlySystemParam`.
```rust
// Before
unsafe impl ReadOnlySystemParamFetch for MyParamState {}
// After
unsafe impl<'w, 's> ReadOnlySystemParam for MyParam<'w, 's> {}
```
2022-12-11 18:34:14 +00:00
type Item < ' w , ' s > = Option < NonSend < ' w , T > > ;
Remove the `SystemParamState` trait and remove types like `ResState` (#6919)
Spiritual successor to #5205.
Actual successor to #6865.
# Objective
Currently, system params are defined using three traits: `SystemParam`, `ReadOnlySystemParam`, `SystemParamState`. The behavior for each param is specified by the `SystemParamState` trait, while `SystemParam` simply defers to the state.
Splitting the traits in this way makes it easier to implement within macros, but it increases the cognitive load. Worst of all, this approach requires each `MySystemParam` to have a public `MySystemParamState` type associated with it.
## Solution
* Merge the trait `SystemParamState` into `SystemParam`.
* Remove all trivial `SystemParam` state types.
* `OptionNonSendMutState<T>`: you will not be missed.
---
- [x] Fix/resolve the remaining test failure.
## Changelog
* Removed the trait `SystemParamState`, merging its functionality into `SystemParam`.
## Migration Guide
**Note**: this should replace the migration guide for #6865.
This is relative to Bevy 0.9, not main.
The traits `SystemParamState` and `SystemParamFetch` have been removed, and their functionality has been transferred to `SystemParam`.
```rust
// Before (0.9)
impl SystemParam for MyParam<'_, '_> {
type State = MyParamState;
}
unsafe impl SystemParamState for MyParamState {
fn init(world: &mut World, system_meta: &mut SystemMeta) -> Self { ... }
}
unsafe impl<'w, 's> SystemParamFetch<'w, 's> for MyParamState {
type Item = MyParam<'w, 's>;
fn get_param(&mut self, ...) -> Self::Item;
}
unsafe impl ReadOnlySystemParamFetch for MyParamState { }
// After (0.10)
unsafe impl SystemParam for MyParam<'_, '_> {
type State = MyParamState;
type Item<'w, 's> = MyParam<'w, 's>;
fn init_state(world: &mut World, system_meta: &mut SystemMeta) -> Self::State { ... }
fn get_param<'w, 's>(state: &mut Self::State, ...) -> Self::Item<'w, 's>;
}
unsafe impl ReadOnlySystemParam for MyParam<'_, '_> { }
```
The trait `ReadOnlySystemParamFetch` has been replaced with `ReadOnlySystemParam`.
```rust
// Before
unsafe impl ReadOnlySystemParamFetch for MyParamState {}
// After
unsafe impl ReadOnlySystemParam for MyParam<'_, '_> {}
```
2023-01-07 23:20:32 +00:00
fn init_state ( world : & mut World , system_meta : & mut SystemMeta ) -> Self ::State {
NonSend ::< T > ::init_state ( world , system_meta )
2021-06-26 19:29:38 +00:00
}
#[ inline ]
Simplify trait hierarchy for `SystemParam` (#6865)
# Objective
* Implementing a custom `SystemParam` by hand requires implementing three traits -- four if it is read-only.
* The trait `SystemParamFetch<'w, 's>` is a workaround from before we had generic associated types, and is no longer necessary.
## Solution
* Combine the trait `SystemParamFetch` with `SystemParamState`.
* I decided to remove the `Fetch` name and keep the `State` name, since the former was consistently conflated with the latter.
* Replace the trait `ReadOnlySystemParamFetch` with `ReadOnlySystemParam`, which simplifies trait bounds in generic code.
---
## Changelog
- Removed the trait `SystemParamFetch`, moving its functionality to `SystemParamState`.
- Replaced the trait `ReadOnlySystemParamFetch` with `ReadOnlySystemParam`.
## Migration Guide
The trait `SystemParamFetch` has been removed, and its functionality has been transferred to `SystemParamState`.
```rust
// Before
impl SystemParamState for MyParamState {
fn init(world: &mut World, system_meta: &mut SystemMeta) -> Self { ... }
}
impl<'w, 's> SystemParamFetch<'w, 's> for MyParamState {
type Item = MyParam<'w, 's>;
fn get_param(...) -> Self::Item;
}
// After
impl SystemParamState for MyParamState {
type Item<'w, 's> = MyParam<'w, 's>; // Generic associated types!
fn init(world: &mut World, system_meta: &mut SystemMeta) -> Self { ... }
fn get_param<'w, 's>(...) -> Self::Item<'w, 's>;
}
```
The trait `ReadOnlySystemParamFetch` has been replaced with `ReadOnlySystemParam`.
```rust
// Before
unsafe impl ReadOnlySystemParamFetch for MyParamState {}
// After
unsafe impl<'w, 's> ReadOnlySystemParam for MyParam<'w, 's> {}
```
2022-12-11 18:34:14 +00:00
unsafe fn get_param < ' w , ' s > (
Remove the `SystemParamState` trait and remove types like `ResState` (#6919)
Spiritual successor to #5205.
Actual successor to #6865.
# Objective
Currently, system params are defined using three traits: `SystemParam`, `ReadOnlySystemParam`, `SystemParamState`. The behavior for each param is specified by the `SystemParamState` trait, while `SystemParam` simply defers to the state.
Splitting the traits in this way makes it easier to implement within macros, but it increases the cognitive load. Worst of all, this approach requires each `MySystemParam` to have a public `MySystemParamState` type associated with it.
## Solution
* Merge the trait `SystemParamState` into `SystemParam`.
* Remove all trivial `SystemParam` state types.
* `OptionNonSendMutState<T>`: you will not be missed.
---
- [x] Fix/resolve the remaining test failure.
## Changelog
* Removed the trait `SystemParamState`, merging its functionality into `SystemParam`.
## Migration Guide
**Note**: this should replace the migration guide for #6865.
This is relative to Bevy 0.9, not main.
The traits `SystemParamState` and `SystemParamFetch` have been removed, and their functionality has been transferred to `SystemParam`.
```rust
// Before (0.9)
impl SystemParam for MyParam<'_, '_> {
type State = MyParamState;
}
unsafe impl SystemParamState for MyParamState {
fn init(world: &mut World, system_meta: &mut SystemMeta) -> Self { ... }
}
unsafe impl<'w, 's> SystemParamFetch<'w, 's> for MyParamState {
type Item = MyParam<'w, 's>;
fn get_param(&mut self, ...) -> Self::Item;
}
unsafe impl ReadOnlySystemParamFetch for MyParamState { }
// After (0.10)
unsafe impl SystemParam for MyParam<'_, '_> {
type State = MyParamState;
type Item<'w, 's> = MyParam<'w, 's>;
fn init_state(world: &mut World, system_meta: &mut SystemMeta) -> Self::State { ... }
fn get_param<'w, 's>(state: &mut Self::State, ...) -> Self::Item<'w, 's>;
}
unsafe impl ReadOnlySystemParam for MyParam<'_, '_> { }
```
The trait `ReadOnlySystemParamFetch` has been replaced with `ReadOnlySystemParam`.
```rust
// Before
unsafe impl ReadOnlySystemParamFetch for MyParamState {}
// After
unsafe impl ReadOnlySystemParam for MyParam<'_, '_> {}
```
2023-01-07 23:20:32 +00:00
& mut component_id : & ' s mut Self ::State ,
2021-06-26 19:29:38 +00:00
system_meta : & SystemMeta ,
System Param Lifetime Split (#2605)
# Objective
Enable using exact World lifetimes during read-only access . This is motivated by the new renderer's need to allow read-only world-only queries to outlive the query itself (but still be constrained by the world lifetime).
For example:
https://github.com/bevyengine/bevy/blob/115b170d1f11a91146bb6d6e9684dceb8b21f786/pipelined/bevy_pbr2/src/render/mod.rs#L774
## Solution
Split out SystemParam state and world lifetimes and pipe those lifetimes up to read-only Query ops (and add into_inner for Res). According to every safety test I've run so far (except one), this is safe (see the temporary safety test commit). Note that changing the mutable variants to the new lifetimes would allow aliased mutable pointers (try doing that to see how it affects the temporary safety tests).
The new state lifetime on SystemParam does make `#[derive(SystemParam)]` more cumbersome (the current impl requires PhantomData if you don't use both lifetimes). We can make this better by detecting whether or not a lifetime is used in the derive and adjusting accordingly, but that should probably be done in its own pr.
## Why is this a draft?
The new lifetimes break QuerySet safety in one very specific case (see the query_set system in system_safety_test). We need to solve this before we can use the lifetimes given.
This is due to the fact that QuerySet is just a wrapper over Query, which now relies on world lifetimes instead of `&self` lifetimes to prevent aliasing (but in systems, each Query has its own implied lifetime, not a centralized world lifetime). I believe the fix is to rewrite QuerySet to have its own World lifetime (and own the internal reference). This will complicate the impl a bit, but I think it is doable. I'm curious if anyone else has better ideas.
Personally, I think these new lifetimes need to happen. We've gotta have a way to directly tie read-only World queries to the World lifetime. The new renderer is the first place this has come up, but I doubt it will be the last. Worst case scenario we can come up with a second `WorldLifetimeQuery<Q, F = ()>` parameter to enable these read-only scenarios, but I'd rather not add another type to the type zoo.
2021-08-15 20:51:53 +00:00
world : & ' w World ,
2021-06-26 19:29:38 +00:00
change_tick : u32 ,
Simplify trait hierarchy for `SystemParam` (#6865)
# Objective
* Implementing a custom `SystemParam` by hand requires implementing three traits -- four if it is read-only.
* The trait `SystemParamFetch<'w, 's>` is a workaround from before we had generic associated types, and is no longer necessary.
## Solution
* Combine the trait `SystemParamFetch` with `SystemParamState`.
* I decided to remove the `Fetch` name and keep the `State` name, since the former was consistently conflated with the latter.
* Replace the trait `ReadOnlySystemParamFetch` with `ReadOnlySystemParam`, which simplifies trait bounds in generic code.
---
## Changelog
- Removed the trait `SystemParamFetch`, moving its functionality to `SystemParamState`.
- Replaced the trait `ReadOnlySystemParamFetch` with `ReadOnlySystemParam`.
## Migration Guide
The trait `SystemParamFetch` has been removed, and its functionality has been transferred to `SystemParamState`.
```rust
// Before
impl SystemParamState for MyParamState {
fn init(world: &mut World, system_meta: &mut SystemMeta) -> Self { ... }
}
impl<'w, 's> SystemParamFetch<'w, 's> for MyParamState {
type Item = MyParam<'w, 's>;
fn get_param(...) -> Self::Item;
}
// After
impl SystemParamState for MyParamState {
type Item<'w, 's> = MyParam<'w, 's>; // Generic associated types!
fn init(world: &mut World, system_meta: &mut SystemMeta) -> Self { ... }
fn get_param<'w, 's>(...) -> Self::Item<'w, 's>;
}
```
The trait `ReadOnlySystemParamFetch` has been replaced with `ReadOnlySystemParam`.
```rust
// Before
unsafe impl ReadOnlySystemParamFetch for MyParamState {}
// After
unsafe impl<'w, 's> ReadOnlySystemParam for MyParam<'w, 's> {}
```
2022-12-11 18:34:14 +00:00
) -> Self ::Item < ' w , ' s > {
2021-06-26 19:29:38 +00:00
world
2023-02-06 19:02:52 +00:00
. as_unsafe_world_cell_migration_internal ( )
Panic on dropping NonSend in non-origin thread. (#6534)
# Objective
Fixes #3310. Fixes #6282. Fixes #6278. Fixes #3666.
## Solution
Split out `!Send` resources into `NonSendResources`. Add a `origin_thread_id` to all `!Send` Resources, check it on dropping `NonSendResourceData`, if there's a mismatch, panic. Moved all of the checks that `MainThreadValidator` would do into `NonSendResources` instead.
All `!Send` resources now individually track which thread they were inserted from. This is validated against for every access, mutation, and drop that could be done against the value.
A regression test using an altered version of the example from #3310 has been added.
This is a stopgap solution for the current status quo. A full solution may involve fully removing `!Send` resources/components from `World`, which will likely require a much more thorough design on how to handle the existing in-engine and ecosystem use cases.
This PR also introduces another breaking change:
```rust
use bevy_ecs::prelude::*;
#[derive(Resource)]
struct Resource(u32);
fn main() {
let mut world = World::new();
world.insert_resource(Resource(1));
world.insert_non_send_resource(Resource(2));
let res = world.get_resource_mut::<Resource>().unwrap();
assert_eq!(res.0, 2);
}
```
This code will run correctly on 0.9.1 but not with this PR, since NonSend resources and normal resources have become actual distinct concepts storage wise.
## Changelog
Changed: Fix soundness bug with `World: Send`. Dropping a `World` that contains a `!Send` resource on the wrong thread will now panic.
## Migration Guide
Normal resources and `NonSend` resources no longer share the same backing storage. If `R: Resource`, then `NonSend<R>` and `Res<R>` will return different instances from each other. If you are using both `Res<T>` and `NonSend<T>` (or their mutable variants), to fetch the same resources, it's strongly advised to use `Res<T>`.
2023-01-09 20:40:34 +00:00
. get_non_send_with_ticks ( component_id )
2022-10-24 13:46:36 +00:00
. map ( | ( ptr , ticks ) | NonSend {
value : ptr . deref ( ) ,
ticks : ticks . read ( ) ,
2021-06-26 19:29:38 +00:00
last_change_tick : system_meta . last_change_tick ,
change_tick ,
} )
}
}
2022-07-04 14:44:24 +00:00
// SAFETY: NonSendMut ComponentId and ArchetypeComponentId access is applied to SystemMeta. If this
2021-03-11 00:27:30 +00:00
// NonSendMut conflicts with any prior access, a panic will occur.
Remove the `SystemParamState` trait and remove types like `ResState` (#6919)
Spiritual successor to #5205.
Actual successor to #6865.
# Objective
Currently, system params are defined using three traits: `SystemParam`, `ReadOnlySystemParam`, `SystemParamState`. The behavior for each param is specified by the `SystemParamState` trait, while `SystemParam` simply defers to the state.
Splitting the traits in this way makes it easier to implement within macros, but it increases the cognitive load. Worst of all, this approach requires each `MySystemParam` to have a public `MySystemParamState` type associated with it.
## Solution
* Merge the trait `SystemParamState` into `SystemParam`.
* Remove all trivial `SystemParam` state types.
* `OptionNonSendMutState<T>`: you will not be missed.
---
- [x] Fix/resolve the remaining test failure.
## Changelog
* Removed the trait `SystemParamState`, merging its functionality into `SystemParam`.
## Migration Guide
**Note**: this should replace the migration guide for #6865.
This is relative to Bevy 0.9, not main.
The traits `SystemParamState` and `SystemParamFetch` have been removed, and their functionality has been transferred to `SystemParam`.
```rust
// Before (0.9)
impl SystemParam for MyParam<'_, '_> {
type State = MyParamState;
}
unsafe impl SystemParamState for MyParamState {
fn init(world: &mut World, system_meta: &mut SystemMeta) -> Self { ... }
}
unsafe impl<'w, 's> SystemParamFetch<'w, 's> for MyParamState {
type Item = MyParam<'w, 's>;
fn get_param(&mut self, ...) -> Self::Item;
}
unsafe impl ReadOnlySystemParamFetch for MyParamState { }
// After (0.10)
unsafe impl SystemParam for MyParam<'_, '_> {
type State = MyParamState;
type Item<'w, 's> = MyParam<'w, 's>;
fn init_state(world: &mut World, system_meta: &mut SystemMeta) -> Self::State { ... }
fn get_param<'w, 's>(state: &mut Self::State, ...) -> Self::Item<'w, 's>;
}
unsafe impl ReadOnlySystemParam for MyParam<'_, '_> { }
```
The trait `ReadOnlySystemParamFetch` has been replaced with `ReadOnlySystemParam`.
```rust
// Before
unsafe impl ReadOnlySystemParamFetch for MyParamState {}
// After
unsafe impl ReadOnlySystemParam for MyParam<'_, '_> {}
```
2023-01-07 23:20:32 +00:00
unsafe impl < ' a , T : 'static > SystemParam for NonSendMut < ' a , T > {
type State = ComponentId ;
Simplify trait hierarchy for `SystemParam` (#6865)
# Objective
* Implementing a custom `SystemParam` by hand requires implementing three traits -- four if it is read-only.
* The trait `SystemParamFetch<'w, 's>` is a workaround from before we had generic associated types, and is no longer necessary.
## Solution
* Combine the trait `SystemParamFetch` with `SystemParamState`.
* I decided to remove the `Fetch` name and keep the `State` name, since the former was consistently conflated with the latter.
* Replace the trait `ReadOnlySystemParamFetch` with `ReadOnlySystemParam`, which simplifies trait bounds in generic code.
---
## Changelog
- Removed the trait `SystemParamFetch`, moving its functionality to `SystemParamState`.
- Replaced the trait `ReadOnlySystemParamFetch` with `ReadOnlySystemParam`.
## Migration Guide
The trait `SystemParamFetch` has been removed, and its functionality has been transferred to `SystemParamState`.
```rust
// Before
impl SystemParamState for MyParamState {
fn init(world: &mut World, system_meta: &mut SystemMeta) -> Self { ... }
}
impl<'w, 's> SystemParamFetch<'w, 's> for MyParamState {
type Item = MyParam<'w, 's>;
fn get_param(...) -> Self::Item;
}
// After
impl SystemParamState for MyParamState {
type Item<'w, 's> = MyParam<'w, 's>; // Generic associated types!
fn init(world: &mut World, system_meta: &mut SystemMeta) -> Self { ... }
fn get_param<'w, 's>(...) -> Self::Item<'w, 's>;
}
```
The trait `ReadOnlySystemParamFetch` has been replaced with `ReadOnlySystemParam`.
```rust
// Before
unsafe impl ReadOnlySystemParamFetch for MyParamState {}
// After
unsafe impl<'w, 's> ReadOnlySystemParam for MyParam<'w, 's> {}
```
2022-12-11 18:34:14 +00:00
type Item < ' w , ' s > = NonSendMut < ' w , T > ;
Remove the `SystemParamState` trait and remove types like `ResState` (#6919)
Spiritual successor to #5205.
Actual successor to #6865.
# Objective
Currently, system params are defined using three traits: `SystemParam`, `ReadOnlySystemParam`, `SystemParamState`. The behavior for each param is specified by the `SystemParamState` trait, while `SystemParam` simply defers to the state.
Splitting the traits in this way makes it easier to implement within macros, but it increases the cognitive load. Worst of all, this approach requires each `MySystemParam` to have a public `MySystemParamState` type associated with it.
## Solution
* Merge the trait `SystemParamState` into `SystemParam`.
* Remove all trivial `SystemParam` state types.
* `OptionNonSendMutState<T>`: you will not be missed.
---
- [x] Fix/resolve the remaining test failure.
## Changelog
* Removed the trait `SystemParamState`, merging its functionality into `SystemParam`.
## Migration Guide
**Note**: this should replace the migration guide for #6865.
This is relative to Bevy 0.9, not main.
The traits `SystemParamState` and `SystemParamFetch` have been removed, and their functionality has been transferred to `SystemParam`.
```rust
// Before (0.9)
impl SystemParam for MyParam<'_, '_> {
type State = MyParamState;
}
unsafe impl SystemParamState for MyParamState {
fn init(world: &mut World, system_meta: &mut SystemMeta) -> Self { ... }
}
unsafe impl<'w, 's> SystemParamFetch<'w, 's> for MyParamState {
type Item = MyParam<'w, 's>;
fn get_param(&mut self, ...) -> Self::Item;
}
unsafe impl ReadOnlySystemParamFetch for MyParamState { }
// After (0.10)
unsafe impl SystemParam for MyParam<'_, '_> {
type State = MyParamState;
type Item<'w, 's> = MyParam<'w, 's>;
fn init_state(world: &mut World, system_meta: &mut SystemMeta) -> Self::State { ... }
fn get_param<'w, 's>(state: &mut Self::State, ...) -> Self::Item<'w, 's>;
}
unsafe impl ReadOnlySystemParam for MyParam<'_, '_> { }
```
The trait `ReadOnlySystemParamFetch` has been replaced with `ReadOnlySystemParam`.
```rust
// Before
unsafe impl ReadOnlySystemParamFetch for MyParamState {}
// After
unsafe impl ReadOnlySystemParam for MyParam<'_, '_> {}
```
2023-01-07 23:20:32 +00:00
fn init_state ( world : & mut World , system_meta : & mut SystemMeta ) -> Self ::State {
Add new SystemState and rename old SystemState to SystemMeta (#2283)
This enables `SystemParams` to be used outside of function systems. Anything can create and store `SystemState`, which enables efficient "param state cached" access to `SystemParams`.
It adds a `ReadOnlySystemParamFetch` trait, which enables safe `SystemState::get` calls without unique world access.
I renamed the old `SystemState` to `SystemMeta` to enable us to mirror the `QueryState` naming convention (but I'm happy to discuss alternative names if people have other ideas). I initially pitched this as `ParamState`, but given that it needs to include full system metadata, that doesn't feel like a particularly accurate name.
```rust
#[derive(Eq, PartialEq, Debug)]
struct A(usize);
#[derive(Eq, PartialEq, Debug)]
struct B(usize);
let mut world = World::default();
world.insert_resource(A(42));
world.spawn().insert(B(7));
// we get nice lifetime elision when declaring the type on the left hand side
let mut system_state: SystemState<(Res<A>, Query<&B>)> = SystemState::new(&mut world);
let (a, query) = system_state.get(&world);
assert_eq!(*a, A(42), "returned resource matches initial value");
assert_eq!(
*query.single().unwrap(),
B(7),
"returned component matches initial value"
);
// mutable system params require unique world access
let mut system_state: SystemState<(ResMut<A>, Query<&mut B>)> = SystemState::new(&mut world);
let (a, query) = system_state.get_mut(&mut world);
// static lifetimes are required when declaring inside of structs
struct SomeContainer {
state: SystemState<(Res<'static, A>, Res<'static, B>)>
}
// this can be shortened using type aliases, which will be useful for complex param tuples
type MyParams<'a> = (Res<'a, A>, Res<'a, B>);
struct SomeContainer {
state: SystemState<MyParams<'static>>
}
// It is the user's responsibility to call SystemState::apply(world) for parameters that queue up work
let mut system_state: SystemState<(Commands, Query<&B>)> = SystemState::new(&mut world);
{
let (mut commands, query) = system_state.get(&world);
commands.insert_resource(3.14);
}
system_state.apply(&mut world);
```
## Future Work
* Actually use SystemState inside FunctionSystem. This would be trivial, but it requires FunctionSystem to wrap SystemState in Option in its current form (which complicates system metadata lookup). I'd prefer to hold off until we adopt something like the later designs linked in #1364, which enable us to contruct Systems using a World reference (and also remove the need for `.system`).
* Consider a "scoped" approach to automatically call SystemState::apply when systems params are no longer being used (either a container type with a Drop impl, or a function that takes a closure for user logic operating on params).
2021-06-02 19:57:38 +00:00
system_meta . set_non_send ( ) ;
Bevy ECS V2 (#1525)
# Bevy ECS V2
This is a rewrite of Bevy ECS (basically everything but the new executor/schedule, which are already awesome). The overall goal was to improve the performance and versatility of Bevy ECS. Here is a quick bulleted list of changes before we dive into the details:
* Complete World rewrite
* Multiple component storage types:
* Tables: fast cache friendly iteration, slower add/removes (previously called Archetypes)
* Sparse Sets: fast add/remove, slower iteration
* Stateful Queries (caches query results for faster iteration. fragmented iteration is _fast_ now)
* Stateful System Params (caches expensive operations. inspired by @DJMcNab's work in #1364)
* Configurable System Params (users can set configuration when they construct their systems. once again inspired by @DJMcNab's work)
* Archetypes are now "just metadata", component storage is separate
* Archetype Graph (for faster archetype changes)
* Component Metadata
* Configure component storage type
* Retrieve information about component size/type/name/layout/send-ness/etc
* Components are uniquely identified by a densely packed ComponentId
* TypeIds are now totally optional (which should make implementing scripting easier)
* Super fast "for_each" query iterators
* Merged Resources into World. Resources are now just a special type of component
* EntityRef/EntityMut builder apis (more efficient and more ergonomic)
* Fast bitset-backed `Access<T>` replaces old hashmap-based approach everywhere
* Query conflicts are determined by component access instead of archetype component access (to avoid random failures at runtime)
* With/Without are still taken into account for conflicts, so this should still be comfy to use
* Much simpler `IntoSystem` impl
* Significantly reduced the amount of hashing throughout the ecs in favor of Sparse Sets (indexed by densely packed ArchetypeId, ComponentId, BundleId, and TableId)
* Safety Improvements
* Entity reservation uses a normal world reference instead of unsafe transmute
* QuerySets no longer transmute lifetimes
* Made traits "unsafe" where relevant
* More thorough safety docs
* WorldCell
* Exposes safe mutable access to multiple resources at a time in a World
* Replaced "catch all" `System::update_archetypes(world: &World)` with `System::new_archetype(archetype: &Archetype)`
* Simpler Bundle implementation
* Replaced slow "remove_bundle_one_by_one" used as fallback for Commands::remove_bundle with fast "remove_bundle_intersection"
* Removed `Mut<T>` query impl. it is better to only support one way: `&mut T`
* Removed with() from `Flags<T>` in favor of `Option<Flags<T>>`, which allows querying for flags to be "filtered" by default
* Components now have is_send property (currently only resources support non-send)
* More granular module organization
* New `RemovedComponents<T>` SystemParam that replaces `query.removed::<T>()`
* `world.resource_scope()` for mutable access to resources and world at the same time
* WorldQuery and QueryFilter traits unified. FilterFetch trait added to enable "short circuit" filtering. Auto impled for cases that don't need it
* Significantly slimmed down SystemState in favor of individual SystemParam state
* System Commands changed from `commands: &mut Commands` back to `mut commands: Commands` (to allow Commands to have a World reference)
Fixes #1320
## `World` Rewrite
This is a from-scratch rewrite of `World` that fills the niche that `hecs` used to. Yes, this means Bevy ECS is no longer a "fork" of hecs. We're going out our own!
(the only shared code between the projects is the entity id allocator, which is already basically ideal)
A huge shout out to @SanderMertens (author of [flecs](https://github.com/SanderMertens/flecs)) for sharing some great ideas with me (specifically hybrid ecs storage and archetype graphs). He also helped advise on a number of implementation details.
## Component Storage (The Problem)
Two ECS storage paradigms have gained a lot of traction over the years:
* **Archetypal ECS**:
* Stores components in "tables" with static schemas. Each "column" stores components of a given type. Each "row" is an entity.
* Each "archetype" has its own table. Adding/removing an entity's component changes the archetype.
* Enables super-fast Query iteration due to its cache-friendly data layout
* Comes at the cost of more expensive add/remove operations for an Entity's components, because all components need to be copied to the new archetype's "table"
* **Sparse Set ECS**:
* Stores components of the same type in densely packed arrays, which are sparsely indexed by densely packed unsigned integers (Entity ids)
* Query iteration is slower than Archetypal ECS because each entity's component could be at any position in the sparse set. This "random access" pattern isn't cache friendly. Additionally, there is an extra layer of indirection because you must first map the entity id to an index in the component array.
* Adding/removing components is a cheap, constant time operation
Bevy ECS V1, hecs, legion, flec, and Unity DOTS are all "archetypal ecs-es". I personally think "archetypal" storage is a good default for game engines. An entity's archetype doesn't need to change frequently in general, and it creates "fast by default" query iteration (which is a much more common operation). It is also "self optimizing". Users don't need to think about optimizing component layouts for iteration performance. It "just works" without any extra boilerplate.
Shipyard and EnTT are "sparse set ecs-es". They employ "packing" as a way to work around the "suboptimal by default" iteration performance for specific sets of components. This helps, but I didn't think this was a good choice for a general purpose engine like Bevy because:
1. "packs" conflict with each other. If bevy decides to internally pack the Transform and GlobalTransform components, users are then blocked if they want to pack some custom component with Transform.
2. users need to take manual action to optimize
Developers selecting an ECS framework are stuck with a hard choice. Select an "archetypal" framework with "fast iteration everywhere" but without the ability to cheaply add/remove components, or select a "sparse set" framework to cheaply add/remove components but with slower iteration performance.
## Hybrid Component Storage (The Solution)
In Bevy ECS V2, we get to have our cake and eat it too. It now has _both_ of the component storage types above (and more can be added later if needed):
* **Tables** (aka "archetypal" storage)
* The default storage. If you don't configure anything, this is what you get
* Fast iteration by default
* Slower add/remove operations
* **Sparse Sets**
* Opt-in
* Slower iteration
* Faster add/remove operations
These storage types complement each other perfectly. By default Query iteration is fast. If developers know that they want to add/remove a component at high frequencies, they can set the storage to "sparse set":
```rust
world.register_component(
ComponentDescriptor::new::<MyComponent>(StorageType::SparseSet)
).unwrap();
```
## Archetypes
Archetypes are now "just metadata" ... they no longer store components directly. They do store:
* The `ComponentId`s of each of the Archetype's components (and that component's storage type)
* Archetypes are uniquely defined by their component layouts
* For example: entities with "table" components `[A, B, C]` _and_ "sparse set" components `[D, E]` will always be in the same archetype.
* The `TableId` associated with the archetype
* For now each archetype has exactly one table (which can have no components),
* There is a 1->Many relationship from Tables->Archetypes. A given table could have any number of archetype components stored in it:
* Ex: an entity with "table storage" components `[A, B, C]` and "sparse set" components `[D, E]` will share the same `[A, B, C]` table as an entity with `[A, B, C]` table component and `[F]` sparse set components.
* This 1->Many relationship is how we preserve fast "cache friendly" iteration performance when possible (more on this later)
* A list of entities that are in the archetype and the row id of the table they are in
* ArchetypeComponentIds
* unique densely packed identifiers for (ArchetypeId, ComponentId) pairs
* used by the schedule executor for cheap system access control
* "Archetype Graph Edges" (see the next section)
## The "Archetype Graph"
Archetype changes in Bevy (and a number of other archetypal ecs-es) have historically been expensive to compute. First, you need to allocate a new vector of the entity's current component ids, add or remove components based on the operation performed, sort it (to ensure it is order-independent), then hash it to find the archetype (if it exists). And thats all before we get to the _already_ expensive full copy of all components to the new table storage.
The solution is to build a "graph" of archetypes to cache these results. @SanderMertens first exposed me to the idea (and he got it from @gjroelofs, who came up with it). They propose adding directed edges between archetypes for add/remove component operations. If `ComponentId`s are densely packed, you can use sparse sets to cheaply jump between archetypes.
Bevy takes this one step further by using add/remove `Bundle` edges instead of `Component` edges. Bevy encourages the use of `Bundles` to group add/remove operations. This is largely for "clearer game logic" reasons, but it also helps cut down on the number of archetype changes required. `Bundles` now also have densely-packed `BundleId`s. This allows us to use a _single_ edge for each bundle operation (rather than needing to traverse N edges ... one for each component). Single component operations are also bundles, so this is strictly an improvement over a "component only" graph.
As a result, an operation that used to be _heavy_ (both for allocations and compute) is now two dirt-cheap array lookups and zero allocations.
## Stateful Queries
World queries are now stateful. This allows us to:
1. Cache archetype (and table) matches
* This resolves another issue with (naive) archetypal ECS: query performance getting worse as the number of archetypes goes up (and fragmentation occurs).
2. Cache Fetch and Filter state
* The expensive parts of fetch/filter operations (such as hashing the TypeId to find the ComponentId) now only happen once when the Query is first constructed
3. Incrementally build up state
* When new archetypes are added, we only process the new archetypes (no need to rebuild state for old archetypes)
As a result, the direct `World` query api now looks like this:
```rust
let mut query = world.query::<(&A, &mut B)>();
for (a, mut b) in query.iter_mut(&mut world) {
}
```
Requiring `World` to generate stateful queries (rather than letting the `QueryState` type be constructed separately) allows us to ensure that _all_ queries are properly initialized (and the relevant world state, such as ComponentIds). This enables QueryState to remove branches from its operations that check for initialization status (and also enables query.iter() to take an immutable world reference because it doesn't need to initialize anything in world).
However in systems, this is a non-breaking change. State management is done internally by the relevant SystemParam.
## Stateful SystemParams
Like Queries, `SystemParams` now also cache state. For example, `Query` system params store the "stateful query" state mentioned above. Commands store their internal `CommandQueue`. This means you can now safely use as many separate `Commands` parameters in your system as you want. `Local<T>` system params store their `T` value in their state (instead of in Resources).
SystemParam state also enabled a significant slim-down of SystemState. It is much nicer to look at now.
Per-SystemParam state naturally insulates us from an "aliased mut" class of errors we have hit in the past (ex: using multiple `Commands` system params).
(credit goes to @DJMcNab for the initial idea and draft pr here #1364)
## Configurable SystemParams
@DJMcNab also had the great idea to make SystemParams configurable. This allows users to provide some initial configuration / values for system parameters (when possible). Most SystemParams have no config (the config type is `()`), but the `Local<T>` param now supports user-provided parameters:
```rust
fn foo(value: Local<usize>) {
}
app.add_system(foo.system().config(|c| c.0 = Some(10)));
```
## Uber Fast "for_each" Query Iterators
Developers now have the choice to use a fast "for_each" iterator, which yields ~1.5-3x iteration speed improvements for "fragmented iteration", and minor ~1.2x iteration speed improvements for unfragmented iteration.
```rust
fn system(query: Query<(&A, &mut B)>) {
// you now have the option to do this for a speed boost
query.for_each_mut(|(a, mut b)| {
});
// however normal iterators are still available
for (a, mut b) in query.iter_mut() {
}
}
```
I think in most cases we should continue to encourage "normal" iterators as they are more flexible and more "rust idiomatic". But when that extra "oomf" is needed, it makes sense to use `for_each`.
We should also consider using `for_each` for internal bevy systems to give our users a nice speed boost (but that should be a separate pr).
## Component Metadata
`World` now has a `Components` collection, which is accessible via `world.components()`. This stores mappings from `ComponentId` to `ComponentInfo`, as well as `TypeId` to `ComponentId` mappings (where relevant). `ComponentInfo` stores information about the component, such as ComponentId, TypeId, memory layout, send-ness (currently limited to resources), and storage type.
## Significantly Cheaper `Access<T>`
We used to use `TypeAccess<TypeId>` to manage read/write component/archetype-component access. This was expensive because TypeIds must be hashed and compared individually. The parallel executor got around this by "condensing" type ids into bitset-backed access types. This worked, but it had to be re-generated from the `TypeAccess<TypeId>`sources every time archetypes changed.
This pr removes TypeAccess in favor of faster bitset access everywhere. We can do this thanks to the move to densely packed `ComponentId`s and `ArchetypeComponentId`s.
## Merged Resources into World
Resources had a lot of redundant functionality with Components. They stored typed data, they had access control, they had unique ids, they were queryable via SystemParams, etc. In fact the _only_ major difference between them was that they were unique (and didn't correlate to an entity).
Separate resources also had the downside of requiring a separate set of access controls, which meant the parallel executor needed to compare more bitsets per system and manage more state.
I initially got the "separate resources" idea from `legion`. I think that design was motivated by the fact that it made the direct world query/resource lifetime interactions more manageable. It certainly made our lives easier when using Resources alongside hecs/bevy_ecs. However we already have a construct for safely and ergonomically managing in-world lifetimes: systems (which use `Access<T>` internally).
This pr merges Resources into World:
```rust
world.insert_resource(1);
world.insert_resource(2.0);
let a = world.get_resource::<i32>().unwrap();
let mut b = world.get_resource_mut::<f64>().unwrap();
*b = 3.0;
```
Resources are now just a special kind of component. They have their own ComponentIds (and their own resource TypeId->ComponentId scope, so they don't conflict wit components of the same type). They are stored in a special "resource archetype", which stores components inside the archetype using a new `unique_components` sparse set (note that this sparse set could later be used to implement Tags). This allows us to keep the code size small by reusing existing datastructures (namely Column, Archetype, ComponentFlags, and ComponentInfo). This allows us the executor to use a single `Access<ArchetypeComponentId>` per system. It should also make scripting language integration easier.
_But_ this merge did create problems for people directly interacting with `World`. What if you need mutable access to multiple resources at the same time? `world.get_resource_mut()` borrows World mutably!
## WorldCell
WorldCell applies the `Access<ArchetypeComponentId>` concept to direct world access:
```rust
let world_cell = world.cell();
let a = world_cell.get_resource_mut::<i32>().unwrap();
let b = world_cell.get_resource_mut::<f64>().unwrap();
```
This adds cheap runtime checks (a sparse set lookup of `ArchetypeComponentId` and a counter) to ensure that world accesses do not conflict with each other. Each operation returns a `WorldBorrow<'w, T>` or `WorldBorrowMut<'w, T>` wrapper type, which will release the relevant ArchetypeComponentId resources when dropped.
World caches the access sparse set (and only one cell can exist at a time), so `world.cell()` is a cheap operation.
WorldCell does _not_ use atomic operations. It is non-send, does a mutable borrow of world to prevent other accesses, and uses a simple `Rc<RefCell<ArchetypeComponentAccess>>` wrapper in each WorldBorrow pointer.
The api is currently limited to resource access, but it can and should be extended to queries / entity component access.
## Resource Scopes
WorldCell does not yet support component queries, and even when it does there are sometimes legitimate reasons to want a mutable world ref _and_ a mutable resource ref (ex: bevy_render and bevy_scene both need this). In these cases we could always drop down to the unsafe `world.get_resource_unchecked_mut()`, but that is not ideal!
Instead developers can use a "resource scope"
```rust
world.resource_scope(|world: &mut World, a: &mut A| {
})
```
This temporarily removes the `A` resource from `World`, provides mutable pointers to both, and re-adds A to World when finished. Thanks to the move to ComponentIds/sparse sets, this is a cheap operation.
If multiple resources are required, scopes can be nested. We could also consider adding a "resource tuple" to the api if this pattern becomes common and the boilerplate gets nasty.
## Query Conflicts Use ComponentId Instead of ArchetypeComponentId
For safety reasons, systems cannot contain queries that conflict with each other without wrapping them in a QuerySet. On bevy `main`, we use ArchetypeComponentIds to determine conflicts. This is nice because it can take into account filters:
```rust
// these queries will never conflict due to their filters
fn filter_system(a: Query<&mut A, With<B>>, b: Query<&mut B, Without<B>>) {
}
```
But it also has a significant downside:
```rust
// these queries will not conflict _until_ an entity with A, B, and C is spawned
fn maybe_conflicts_system(a: Query<(&mut A, &C)>, b: Query<(&mut A, &B)>) {
}
```
The system above will panic at runtime if an entity with A, B, and C is spawned. This makes it hard to trust that your game logic will run without crashing.
In this pr, I switched to using `ComponentId` instead. This _is_ more constraining. `maybe_conflicts_system` will now always fail, but it will do it consistently at startup. Naively, it would also _disallow_ `filter_system`, which would be a significant downgrade in usability. Bevy has a number of internal systems that rely on disjoint queries and I expect it to be a common pattern in userspace.
To resolve this, I added a new `FilteredAccess<T>` type, which wraps `Access<T>` and adds with/without filters. If two `FilteredAccess` have with/without values that prove they are disjoint, they will no longer conflict.
## EntityRef / EntityMut
World entity operations on `main` require that the user passes in an `entity` id to each operation:
```rust
let entity = world.spawn((A, )); // create a new entity with A
world.get::<A>(entity);
world.insert(entity, (B, C));
world.insert_one(entity, D);
```
This means that each operation needs to look up the entity location / verify its validity. The initial spawn operation also requires a Bundle as input. This can be awkward when no components are required (or one component is required).
These operations have been replaced by `EntityRef` and `EntityMut`, which are "builder-style" wrappers around world that provide read and read/write operations on a single, pre-validated entity:
```rust
// spawn now takes no inputs and returns an EntityMut
let entity = world.spawn()
.insert(A) // insert a single component into the entity
.insert_bundle((B, C)) // insert a bundle of components into the entity
.id() // id returns the Entity id
// Returns EntityMut (or panics if the entity does not exist)
world.entity_mut(entity)
.insert(D)
.insert_bundle(SomeBundle::default());
{
// returns EntityRef (or panics if the entity does not exist)
let d = world.entity(entity)
.get::<D>() // gets the D component
.unwrap();
// world.get still exists for ergonomics
let d = world.get::<D>(entity).unwrap();
}
// These variants return Options if you want to check existence instead of panicing
world.get_entity_mut(entity)
.unwrap()
.insert(E);
if let Some(entity_ref) = world.get_entity(entity) {
let d = entity_ref.get::<D>().unwrap();
}
```
This _does not_ affect the current Commands api or terminology. I think that should be a separate conversation as that is a much larger breaking change.
## Safety Improvements
* Entity reservation in Commands uses a normal world borrow instead of an unsafe transmute
* QuerySets no longer transmutes lifetimes
* Made traits "unsafe" when implementing a trait incorrectly could cause unsafety
* More thorough safety docs
## RemovedComponents SystemParam
The old approach to querying removed components: `query.removed:<T>()` was confusing because it had no connection to the query itself. I replaced it with the following, which is both clearer and allows us to cache the ComponentId mapping in the SystemParamState:
```rust
fn system(removed: RemovedComponents<T>) {
for entity in removed.iter() {
}
}
```
## Simpler Bundle implementation
Bundles are no longer responsible for sorting (or deduping) TypeInfo. They are just a simple ordered list of component types / data. This makes the implementation smaller and opens the door to an easy "nested bundle" implementation in the future (which i might even add in this pr). Duplicate detection is now done once per bundle type by World the first time a bundle is used.
## Unified WorldQuery and QueryFilter types
(don't worry they are still separate type _parameters_ in Queries .. this is a non-breaking change)
WorldQuery and QueryFilter were already basically identical apis. With the addition of `FetchState` and more storage-specific fetch methods, the overlap was even clearer (and the redundancy more painful).
QueryFilters are now just `F: WorldQuery where F::Fetch: FilterFetch`. FilterFetch requires `Fetch<Item = bool>` and adds new "short circuit" variants of fetch methods. This enables a filter tuple like `(With<A>, Without<B>, Changed<C>)` to stop evaluating the filter after the first mismatch is encountered. FilterFetch is automatically implemented for `Fetch` implementations that return bool.
This forces fetch implementations that return things like `(bool, bool, bool)` (such as the filter above) to manually implement FilterFetch and decide whether or not to short-circuit.
## More Granular Modules
World no longer globs all of the internal modules together. It now exports `core`, `system`, and `schedule` separately. I'm also considering exporting `core` submodules directly as that is still pretty "glob-ey" and unorganized (feedback welcome here).
## Remaining Draft Work (to be done in this pr)
* ~~panic on conflicting WorldQuery fetches (&A, &mut A)~~
* ~~bevy `main` and hecs both currently allow this, but we should protect against it if possible~~
* ~~batch_iter / par_iter (currently stubbed out)~~
* ~~ChangedRes~~
* ~~I skipped this while we sort out #1313. This pr should be adapted to account for whatever we land on there~~.
* ~~The `Archetypes` and `Tables` collections use hashes of sorted lists of component ids to uniquely identify each archetype/table. This hash is then used as the key in a HashMap to look up the relevant ArchetypeId or TableId. (which doesn't handle hash collisions properly)~~
* ~~It is currently unsafe to generate a Query from "World A", then use it on "World B" (despite the api claiming it is safe). We should probably close this gap. This could be done by adding a randomly generated WorldId to each world, then storing that id in each Query. They could then be compared to each other on each `query.do_thing(&world)` operation. This _does_ add an extra branch to each query operation, so I'm open to other suggestions if people have them.~~
* ~~Nested Bundles (if i find time)~~
## Potential Future Work
* Expand WorldCell to support queries.
* Consider not allocating in the empty archetype on `world.spawn()`
* ex: return something like EntityMutUninit, which turns into EntityMut after an `insert` or `insert_bundle` op
* this actually regressed performance last time i tried it, but in theory it should be faster
* Optimize SparseSet::insert (see `PERF` comment on insert)
* Replace SparseArray `Option<T>` with T::MAX to cut down on branching
* would enable cheaper get_unchecked() operations
* upstream fixedbitset optimizations
* fixedbitset could be allocation free for small block counts (store blocks in a SmallVec)
* fixedbitset could have a const constructor
* Consider implementing Tags (archetype-specific by-value data that affects archetype identity)
* ex: ArchetypeA could have `[A, B, C]` table components and `[D(1)]` "tag" component. ArchetypeB could have `[A, B, C]` table components and a `[D(2)]` tag component. The archetypes are different, despite both having D tags because the value inside D is different.
* this could potentially build on top of the `archetype.unique_components` added in this pr for resource storage.
* Consider reverting `all_tuples` proc macro in favor of the old `macro_rules` implementation
* all_tuples is more flexible and produces cleaner documentation (the macro_rules version produces weird type parameter orders due to parser constraints)
* but unfortunately all_tuples also appears to make Rust Analyzer sad/slow when working inside of `bevy_ecs` (does not affect user code)
* Consider "resource queries" and/or "mixed resource and entity component queries" as an alternative to WorldCell
* this is basically just "systems" so maybe it's not worth it
* Add more world ops
* `world.clear()`
* `world.reserve<T: Bundle>(count: usize)`
* Try using the old archetype allocation strategy (allocate new memory on resize and copy everything over). I expect this to improve batch insertion performance at the cost of unbatched performance. But thats just a guess. I'm not an allocation perf pro :)
* Adapt Commands apis for consistency with new World apis
## Benchmarks
key:
* `bevy_old`: bevy `main` branch
* `bevy`: this branch
* `_foreach`: uses an optimized for_each iterator
* ` _sparse`: uses sparse set storage (if unspecified assume table storage)
* `_system`: runs inside a system (if unspecified assume test happens via direct world ops)
### Simple Insert (from ecs_bench_suite)
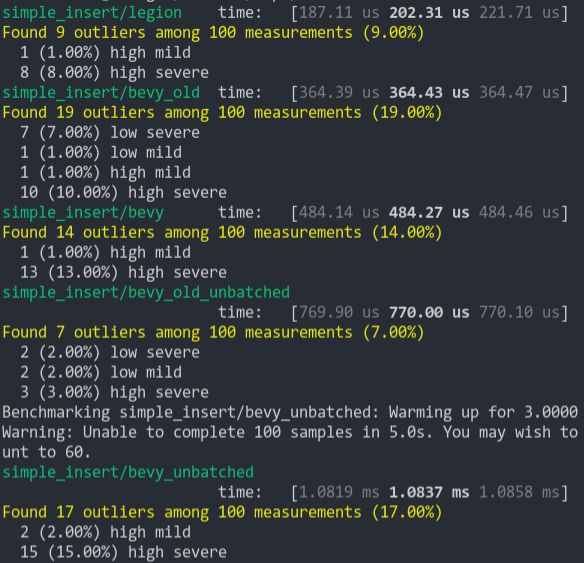
### Simpler Iter (from ecs_bench_suite)
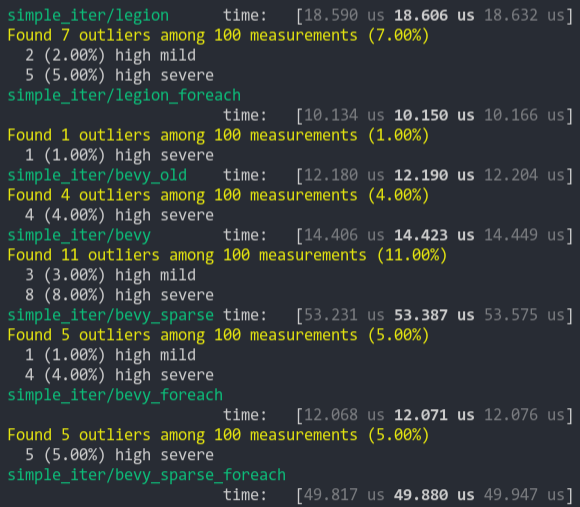
### Fragment Iter (from ecs_bench_suite)
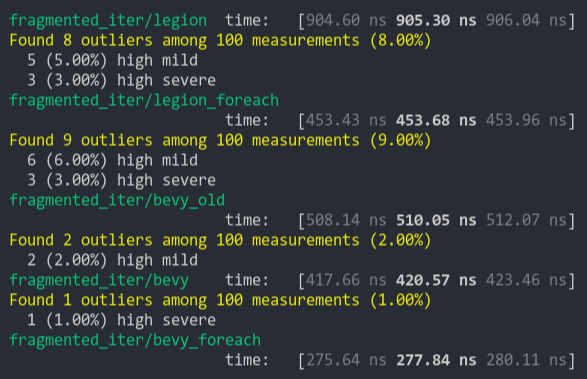
### Sparse Fragmented Iter
Iterate a query that matches 5 entities from a single matching archetype, but there are 100 unmatching archetypes
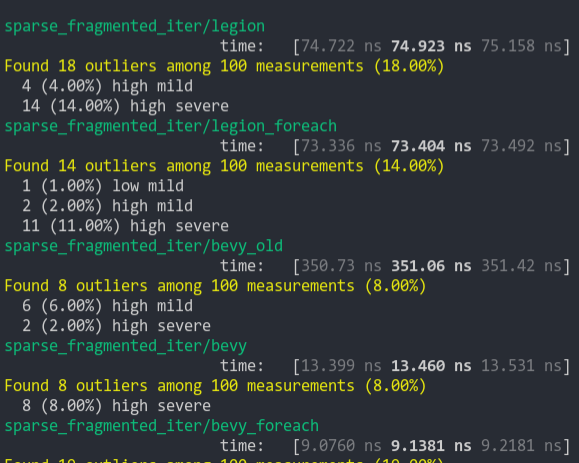
### Schedule (from ecs_bench_suite)
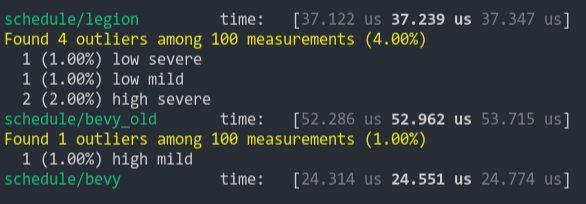
### Add Remove Component (from ecs_bench_suite)
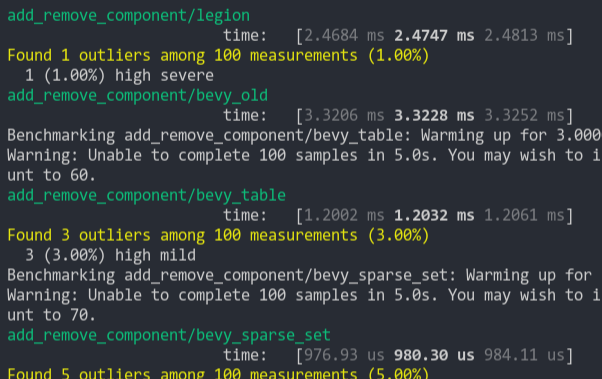
### Add Remove Component Big
Same as the test above, but each entity has 5 "large" matrix components and 1 "large" matrix component is added and removed
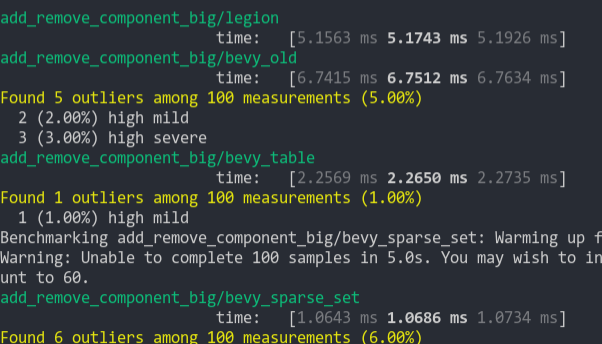
### Get Component
Looks up a single component value a large number of times
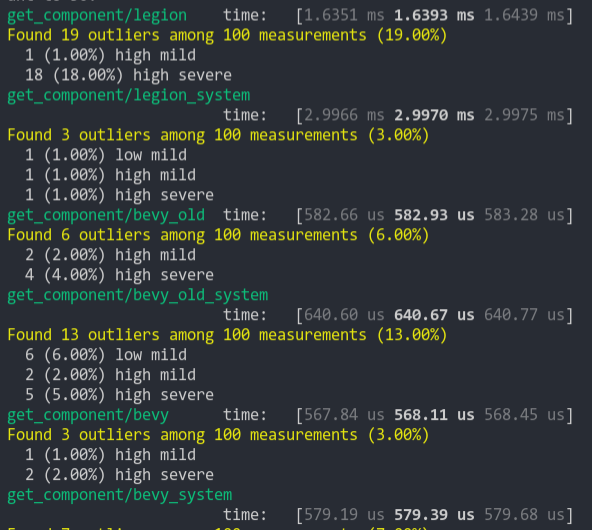
2021-03-05 07:54:35 +00:00
2021-08-31 20:52:21 +00:00
let component_id = world . initialize_non_send_resource ::< T > ( ) ;
Fix FilteredAccessSet get_conflicts inconsistency (#5105)
# Objective
* Enable `Res` and `Query` parameter mutual exclusion
* Required for https://github.com/bevyengine/bevy/pull/5080
The `FilteredAccessSet::get_conflicts` methods didn't work properly with
`Res` and `ResMut` parameters. Because those added their access by using
the `combined_access_mut` method and directly modifying the global
access state of the FilteredAccessSet. This caused an inconsistency,
because get_conflicts assumes that ALL added access have a corresponding
`FilteredAccess` added to the `filtered_accesses` field.
In practice, that means that SystemParam that adds their access through
the `Access` returned by `combined_access_mut` and the ones that add
their access using the `add` method lived in two different universes. As
a result, they could never be mutually exclusive.
## Solution
This commit fixes it by removing the `combined_access_mut` method. This
ensures that the `combined_access` field of FilteredAccessSet is always
updated consistently with the addition of a filter. When checking for
filtered access, it is now possible to account for `Res` and `ResMut`
invalid access. This is currently not needed, but might be in the
future.
We add the `add_unfiltered_{read,write}` methods to replace previous
usages of `combined_access_mut`.
We also add improved Debug implementations on FixedBitSet so that their
meaning is much clearer in debug output.
---
## Changelog
* Fix `Res` and `Query` parameter never being mutually exclusive.
## Migration Guide
Note: this mostly changes ECS internals, but since the API is public, it is technically breaking:
* Removed `FilteredAccessSet::combined_access_mut`
* Replace _immutable_ usage of those by `combined_access`
* For _mutable_ usages, use the new `add_unfiltered_{read,write}` methods instead of `combined_access_mut` followed by `add_{read,write}`
2022-11-16 11:05:48 +00:00
let combined_access = system_meta . component_access_set . combined_access ( ) ;
Bevy ECS V2 (#1525)
# Bevy ECS V2
This is a rewrite of Bevy ECS (basically everything but the new executor/schedule, which are already awesome). The overall goal was to improve the performance and versatility of Bevy ECS. Here is a quick bulleted list of changes before we dive into the details:
* Complete World rewrite
* Multiple component storage types:
* Tables: fast cache friendly iteration, slower add/removes (previously called Archetypes)
* Sparse Sets: fast add/remove, slower iteration
* Stateful Queries (caches query results for faster iteration. fragmented iteration is _fast_ now)
* Stateful System Params (caches expensive operations. inspired by @DJMcNab's work in #1364)
* Configurable System Params (users can set configuration when they construct their systems. once again inspired by @DJMcNab's work)
* Archetypes are now "just metadata", component storage is separate
* Archetype Graph (for faster archetype changes)
* Component Metadata
* Configure component storage type
* Retrieve information about component size/type/name/layout/send-ness/etc
* Components are uniquely identified by a densely packed ComponentId
* TypeIds are now totally optional (which should make implementing scripting easier)
* Super fast "for_each" query iterators
* Merged Resources into World. Resources are now just a special type of component
* EntityRef/EntityMut builder apis (more efficient and more ergonomic)
* Fast bitset-backed `Access<T>` replaces old hashmap-based approach everywhere
* Query conflicts are determined by component access instead of archetype component access (to avoid random failures at runtime)
* With/Without are still taken into account for conflicts, so this should still be comfy to use
* Much simpler `IntoSystem` impl
* Significantly reduced the amount of hashing throughout the ecs in favor of Sparse Sets (indexed by densely packed ArchetypeId, ComponentId, BundleId, and TableId)
* Safety Improvements
* Entity reservation uses a normal world reference instead of unsafe transmute
* QuerySets no longer transmute lifetimes
* Made traits "unsafe" where relevant
* More thorough safety docs
* WorldCell
* Exposes safe mutable access to multiple resources at a time in a World
* Replaced "catch all" `System::update_archetypes(world: &World)` with `System::new_archetype(archetype: &Archetype)`
* Simpler Bundle implementation
* Replaced slow "remove_bundle_one_by_one" used as fallback for Commands::remove_bundle with fast "remove_bundle_intersection"
* Removed `Mut<T>` query impl. it is better to only support one way: `&mut T`
* Removed with() from `Flags<T>` in favor of `Option<Flags<T>>`, which allows querying for flags to be "filtered" by default
* Components now have is_send property (currently only resources support non-send)
* More granular module organization
* New `RemovedComponents<T>` SystemParam that replaces `query.removed::<T>()`
* `world.resource_scope()` for mutable access to resources and world at the same time
* WorldQuery and QueryFilter traits unified. FilterFetch trait added to enable "short circuit" filtering. Auto impled for cases that don't need it
* Significantly slimmed down SystemState in favor of individual SystemParam state
* System Commands changed from `commands: &mut Commands` back to `mut commands: Commands` (to allow Commands to have a World reference)
Fixes #1320
## `World` Rewrite
This is a from-scratch rewrite of `World` that fills the niche that `hecs` used to. Yes, this means Bevy ECS is no longer a "fork" of hecs. We're going out our own!
(the only shared code between the projects is the entity id allocator, which is already basically ideal)
A huge shout out to @SanderMertens (author of [flecs](https://github.com/SanderMertens/flecs)) for sharing some great ideas with me (specifically hybrid ecs storage and archetype graphs). He also helped advise on a number of implementation details.
## Component Storage (The Problem)
Two ECS storage paradigms have gained a lot of traction over the years:
* **Archetypal ECS**:
* Stores components in "tables" with static schemas. Each "column" stores components of a given type. Each "row" is an entity.
* Each "archetype" has its own table. Adding/removing an entity's component changes the archetype.
* Enables super-fast Query iteration due to its cache-friendly data layout
* Comes at the cost of more expensive add/remove operations for an Entity's components, because all components need to be copied to the new archetype's "table"
* **Sparse Set ECS**:
* Stores components of the same type in densely packed arrays, which are sparsely indexed by densely packed unsigned integers (Entity ids)
* Query iteration is slower than Archetypal ECS because each entity's component could be at any position in the sparse set. This "random access" pattern isn't cache friendly. Additionally, there is an extra layer of indirection because you must first map the entity id to an index in the component array.
* Adding/removing components is a cheap, constant time operation
Bevy ECS V1, hecs, legion, flec, and Unity DOTS are all "archetypal ecs-es". I personally think "archetypal" storage is a good default for game engines. An entity's archetype doesn't need to change frequently in general, and it creates "fast by default" query iteration (which is a much more common operation). It is also "self optimizing". Users don't need to think about optimizing component layouts for iteration performance. It "just works" without any extra boilerplate.
Shipyard and EnTT are "sparse set ecs-es". They employ "packing" as a way to work around the "suboptimal by default" iteration performance for specific sets of components. This helps, but I didn't think this was a good choice for a general purpose engine like Bevy because:
1. "packs" conflict with each other. If bevy decides to internally pack the Transform and GlobalTransform components, users are then blocked if they want to pack some custom component with Transform.
2. users need to take manual action to optimize
Developers selecting an ECS framework are stuck with a hard choice. Select an "archetypal" framework with "fast iteration everywhere" but without the ability to cheaply add/remove components, or select a "sparse set" framework to cheaply add/remove components but with slower iteration performance.
## Hybrid Component Storage (The Solution)
In Bevy ECS V2, we get to have our cake and eat it too. It now has _both_ of the component storage types above (and more can be added later if needed):
* **Tables** (aka "archetypal" storage)
* The default storage. If you don't configure anything, this is what you get
* Fast iteration by default
* Slower add/remove operations
* **Sparse Sets**
* Opt-in
* Slower iteration
* Faster add/remove operations
These storage types complement each other perfectly. By default Query iteration is fast. If developers know that they want to add/remove a component at high frequencies, they can set the storage to "sparse set":
```rust
world.register_component(
ComponentDescriptor::new::<MyComponent>(StorageType::SparseSet)
).unwrap();
```
## Archetypes
Archetypes are now "just metadata" ... they no longer store components directly. They do store:
* The `ComponentId`s of each of the Archetype's components (and that component's storage type)
* Archetypes are uniquely defined by their component layouts
* For example: entities with "table" components `[A, B, C]` _and_ "sparse set" components `[D, E]` will always be in the same archetype.
* The `TableId` associated with the archetype
* For now each archetype has exactly one table (which can have no components),
* There is a 1->Many relationship from Tables->Archetypes. A given table could have any number of archetype components stored in it:
* Ex: an entity with "table storage" components `[A, B, C]` and "sparse set" components `[D, E]` will share the same `[A, B, C]` table as an entity with `[A, B, C]` table component and `[F]` sparse set components.
* This 1->Many relationship is how we preserve fast "cache friendly" iteration performance when possible (more on this later)
* A list of entities that are in the archetype and the row id of the table they are in
* ArchetypeComponentIds
* unique densely packed identifiers for (ArchetypeId, ComponentId) pairs
* used by the schedule executor for cheap system access control
* "Archetype Graph Edges" (see the next section)
## The "Archetype Graph"
Archetype changes in Bevy (and a number of other archetypal ecs-es) have historically been expensive to compute. First, you need to allocate a new vector of the entity's current component ids, add or remove components based on the operation performed, sort it (to ensure it is order-independent), then hash it to find the archetype (if it exists). And thats all before we get to the _already_ expensive full copy of all components to the new table storage.
The solution is to build a "graph" of archetypes to cache these results. @SanderMertens first exposed me to the idea (and he got it from @gjroelofs, who came up with it). They propose adding directed edges between archetypes for add/remove component operations. If `ComponentId`s are densely packed, you can use sparse sets to cheaply jump between archetypes.
Bevy takes this one step further by using add/remove `Bundle` edges instead of `Component` edges. Bevy encourages the use of `Bundles` to group add/remove operations. This is largely for "clearer game logic" reasons, but it also helps cut down on the number of archetype changes required. `Bundles` now also have densely-packed `BundleId`s. This allows us to use a _single_ edge for each bundle operation (rather than needing to traverse N edges ... one for each component). Single component operations are also bundles, so this is strictly an improvement over a "component only" graph.
As a result, an operation that used to be _heavy_ (both for allocations and compute) is now two dirt-cheap array lookups and zero allocations.
## Stateful Queries
World queries are now stateful. This allows us to:
1. Cache archetype (and table) matches
* This resolves another issue with (naive) archetypal ECS: query performance getting worse as the number of archetypes goes up (and fragmentation occurs).
2. Cache Fetch and Filter state
* The expensive parts of fetch/filter operations (such as hashing the TypeId to find the ComponentId) now only happen once when the Query is first constructed
3. Incrementally build up state
* When new archetypes are added, we only process the new archetypes (no need to rebuild state for old archetypes)
As a result, the direct `World` query api now looks like this:
```rust
let mut query = world.query::<(&A, &mut B)>();
for (a, mut b) in query.iter_mut(&mut world) {
}
```
Requiring `World` to generate stateful queries (rather than letting the `QueryState` type be constructed separately) allows us to ensure that _all_ queries are properly initialized (and the relevant world state, such as ComponentIds). This enables QueryState to remove branches from its operations that check for initialization status (and also enables query.iter() to take an immutable world reference because it doesn't need to initialize anything in world).
However in systems, this is a non-breaking change. State management is done internally by the relevant SystemParam.
## Stateful SystemParams
Like Queries, `SystemParams` now also cache state. For example, `Query` system params store the "stateful query" state mentioned above. Commands store their internal `CommandQueue`. This means you can now safely use as many separate `Commands` parameters in your system as you want. `Local<T>` system params store their `T` value in their state (instead of in Resources).
SystemParam state also enabled a significant slim-down of SystemState. It is much nicer to look at now.
Per-SystemParam state naturally insulates us from an "aliased mut" class of errors we have hit in the past (ex: using multiple `Commands` system params).
(credit goes to @DJMcNab for the initial idea and draft pr here #1364)
## Configurable SystemParams
@DJMcNab also had the great idea to make SystemParams configurable. This allows users to provide some initial configuration / values for system parameters (when possible). Most SystemParams have no config (the config type is `()`), but the `Local<T>` param now supports user-provided parameters:
```rust
fn foo(value: Local<usize>) {
}
app.add_system(foo.system().config(|c| c.0 = Some(10)));
```
## Uber Fast "for_each" Query Iterators
Developers now have the choice to use a fast "for_each" iterator, which yields ~1.5-3x iteration speed improvements for "fragmented iteration", and minor ~1.2x iteration speed improvements for unfragmented iteration.
```rust
fn system(query: Query<(&A, &mut B)>) {
// you now have the option to do this for a speed boost
query.for_each_mut(|(a, mut b)| {
});
// however normal iterators are still available
for (a, mut b) in query.iter_mut() {
}
}
```
I think in most cases we should continue to encourage "normal" iterators as they are more flexible and more "rust idiomatic". But when that extra "oomf" is needed, it makes sense to use `for_each`.
We should also consider using `for_each` for internal bevy systems to give our users a nice speed boost (but that should be a separate pr).
## Component Metadata
`World` now has a `Components` collection, which is accessible via `world.components()`. This stores mappings from `ComponentId` to `ComponentInfo`, as well as `TypeId` to `ComponentId` mappings (where relevant). `ComponentInfo` stores information about the component, such as ComponentId, TypeId, memory layout, send-ness (currently limited to resources), and storage type.
## Significantly Cheaper `Access<T>`
We used to use `TypeAccess<TypeId>` to manage read/write component/archetype-component access. This was expensive because TypeIds must be hashed and compared individually. The parallel executor got around this by "condensing" type ids into bitset-backed access types. This worked, but it had to be re-generated from the `TypeAccess<TypeId>`sources every time archetypes changed.
This pr removes TypeAccess in favor of faster bitset access everywhere. We can do this thanks to the move to densely packed `ComponentId`s and `ArchetypeComponentId`s.
## Merged Resources into World
Resources had a lot of redundant functionality with Components. They stored typed data, they had access control, they had unique ids, they were queryable via SystemParams, etc. In fact the _only_ major difference between them was that they were unique (and didn't correlate to an entity).
Separate resources also had the downside of requiring a separate set of access controls, which meant the parallel executor needed to compare more bitsets per system and manage more state.
I initially got the "separate resources" idea from `legion`. I think that design was motivated by the fact that it made the direct world query/resource lifetime interactions more manageable. It certainly made our lives easier when using Resources alongside hecs/bevy_ecs. However we already have a construct for safely and ergonomically managing in-world lifetimes: systems (which use `Access<T>` internally).
This pr merges Resources into World:
```rust
world.insert_resource(1);
world.insert_resource(2.0);
let a = world.get_resource::<i32>().unwrap();
let mut b = world.get_resource_mut::<f64>().unwrap();
*b = 3.0;
```
Resources are now just a special kind of component. They have their own ComponentIds (and their own resource TypeId->ComponentId scope, so they don't conflict wit components of the same type). They are stored in a special "resource archetype", which stores components inside the archetype using a new `unique_components` sparse set (note that this sparse set could later be used to implement Tags). This allows us to keep the code size small by reusing existing datastructures (namely Column, Archetype, ComponentFlags, and ComponentInfo). This allows us the executor to use a single `Access<ArchetypeComponentId>` per system. It should also make scripting language integration easier.
_But_ this merge did create problems for people directly interacting with `World`. What if you need mutable access to multiple resources at the same time? `world.get_resource_mut()` borrows World mutably!
## WorldCell
WorldCell applies the `Access<ArchetypeComponentId>` concept to direct world access:
```rust
let world_cell = world.cell();
let a = world_cell.get_resource_mut::<i32>().unwrap();
let b = world_cell.get_resource_mut::<f64>().unwrap();
```
This adds cheap runtime checks (a sparse set lookup of `ArchetypeComponentId` and a counter) to ensure that world accesses do not conflict with each other. Each operation returns a `WorldBorrow<'w, T>` or `WorldBorrowMut<'w, T>` wrapper type, which will release the relevant ArchetypeComponentId resources when dropped.
World caches the access sparse set (and only one cell can exist at a time), so `world.cell()` is a cheap operation.
WorldCell does _not_ use atomic operations. It is non-send, does a mutable borrow of world to prevent other accesses, and uses a simple `Rc<RefCell<ArchetypeComponentAccess>>` wrapper in each WorldBorrow pointer.
The api is currently limited to resource access, but it can and should be extended to queries / entity component access.
## Resource Scopes
WorldCell does not yet support component queries, and even when it does there are sometimes legitimate reasons to want a mutable world ref _and_ a mutable resource ref (ex: bevy_render and bevy_scene both need this). In these cases we could always drop down to the unsafe `world.get_resource_unchecked_mut()`, but that is not ideal!
Instead developers can use a "resource scope"
```rust
world.resource_scope(|world: &mut World, a: &mut A| {
})
```
This temporarily removes the `A` resource from `World`, provides mutable pointers to both, and re-adds A to World when finished. Thanks to the move to ComponentIds/sparse sets, this is a cheap operation.
If multiple resources are required, scopes can be nested. We could also consider adding a "resource tuple" to the api if this pattern becomes common and the boilerplate gets nasty.
## Query Conflicts Use ComponentId Instead of ArchetypeComponentId
For safety reasons, systems cannot contain queries that conflict with each other without wrapping them in a QuerySet. On bevy `main`, we use ArchetypeComponentIds to determine conflicts. This is nice because it can take into account filters:
```rust
// these queries will never conflict due to their filters
fn filter_system(a: Query<&mut A, With<B>>, b: Query<&mut B, Without<B>>) {
}
```
But it also has a significant downside:
```rust
// these queries will not conflict _until_ an entity with A, B, and C is spawned
fn maybe_conflicts_system(a: Query<(&mut A, &C)>, b: Query<(&mut A, &B)>) {
}
```
The system above will panic at runtime if an entity with A, B, and C is spawned. This makes it hard to trust that your game logic will run without crashing.
In this pr, I switched to using `ComponentId` instead. This _is_ more constraining. `maybe_conflicts_system` will now always fail, but it will do it consistently at startup. Naively, it would also _disallow_ `filter_system`, which would be a significant downgrade in usability. Bevy has a number of internal systems that rely on disjoint queries and I expect it to be a common pattern in userspace.
To resolve this, I added a new `FilteredAccess<T>` type, which wraps `Access<T>` and adds with/without filters. If two `FilteredAccess` have with/without values that prove they are disjoint, they will no longer conflict.
## EntityRef / EntityMut
World entity operations on `main` require that the user passes in an `entity` id to each operation:
```rust
let entity = world.spawn((A, )); // create a new entity with A
world.get::<A>(entity);
world.insert(entity, (B, C));
world.insert_one(entity, D);
```
This means that each operation needs to look up the entity location / verify its validity. The initial spawn operation also requires a Bundle as input. This can be awkward when no components are required (or one component is required).
These operations have been replaced by `EntityRef` and `EntityMut`, which are "builder-style" wrappers around world that provide read and read/write operations on a single, pre-validated entity:
```rust
// spawn now takes no inputs and returns an EntityMut
let entity = world.spawn()
.insert(A) // insert a single component into the entity
.insert_bundle((B, C)) // insert a bundle of components into the entity
.id() // id returns the Entity id
// Returns EntityMut (or panics if the entity does not exist)
world.entity_mut(entity)
.insert(D)
.insert_bundle(SomeBundle::default());
{
// returns EntityRef (or panics if the entity does not exist)
let d = world.entity(entity)
.get::<D>() // gets the D component
.unwrap();
// world.get still exists for ergonomics
let d = world.get::<D>(entity).unwrap();
}
// These variants return Options if you want to check existence instead of panicing
world.get_entity_mut(entity)
.unwrap()
.insert(E);
if let Some(entity_ref) = world.get_entity(entity) {
let d = entity_ref.get::<D>().unwrap();
}
```
This _does not_ affect the current Commands api or terminology. I think that should be a separate conversation as that is a much larger breaking change.
## Safety Improvements
* Entity reservation in Commands uses a normal world borrow instead of an unsafe transmute
* QuerySets no longer transmutes lifetimes
* Made traits "unsafe" when implementing a trait incorrectly could cause unsafety
* More thorough safety docs
## RemovedComponents SystemParam
The old approach to querying removed components: `query.removed:<T>()` was confusing because it had no connection to the query itself. I replaced it with the following, which is both clearer and allows us to cache the ComponentId mapping in the SystemParamState:
```rust
fn system(removed: RemovedComponents<T>) {
for entity in removed.iter() {
}
}
```
## Simpler Bundle implementation
Bundles are no longer responsible for sorting (or deduping) TypeInfo. They are just a simple ordered list of component types / data. This makes the implementation smaller and opens the door to an easy "nested bundle" implementation in the future (which i might even add in this pr). Duplicate detection is now done once per bundle type by World the first time a bundle is used.
## Unified WorldQuery and QueryFilter types
(don't worry they are still separate type _parameters_ in Queries .. this is a non-breaking change)
WorldQuery and QueryFilter were already basically identical apis. With the addition of `FetchState` and more storage-specific fetch methods, the overlap was even clearer (and the redundancy more painful).
QueryFilters are now just `F: WorldQuery where F::Fetch: FilterFetch`. FilterFetch requires `Fetch<Item = bool>` and adds new "short circuit" variants of fetch methods. This enables a filter tuple like `(With<A>, Without<B>, Changed<C>)` to stop evaluating the filter after the first mismatch is encountered. FilterFetch is automatically implemented for `Fetch` implementations that return bool.
This forces fetch implementations that return things like `(bool, bool, bool)` (such as the filter above) to manually implement FilterFetch and decide whether or not to short-circuit.
## More Granular Modules
World no longer globs all of the internal modules together. It now exports `core`, `system`, and `schedule` separately. I'm also considering exporting `core` submodules directly as that is still pretty "glob-ey" and unorganized (feedback welcome here).
## Remaining Draft Work (to be done in this pr)
* ~~panic on conflicting WorldQuery fetches (&A, &mut A)~~
* ~~bevy `main` and hecs both currently allow this, but we should protect against it if possible~~
* ~~batch_iter / par_iter (currently stubbed out)~~
* ~~ChangedRes~~
* ~~I skipped this while we sort out #1313. This pr should be adapted to account for whatever we land on there~~.
* ~~The `Archetypes` and `Tables` collections use hashes of sorted lists of component ids to uniquely identify each archetype/table. This hash is then used as the key in a HashMap to look up the relevant ArchetypeId or TableId. (which doesn't handle hash collisions properly)~~
* ~~It is currently unsafe to generate a Query from "World A", then use it on "World B" (despite the api claiming it is safe). We should probably close this gap. This could be done by adding a randomly generated WorldId to each world, then storing that id in each Query. They could then be compared to each other on each `query.do_thing(&world)` operation. This _does_ add an extra branch to each query operation, so I'm open to other suggestions if people have them.~~
* ~~Nested Bundles (if i find time)~~
## Potential Future Work
* Expand WorldCell to support queries.
* Consider not allocating in the empty archetype on `world.spawn()`
* ex: return something like EntityMutUninit, which turns into EntityMut after an `insert` or `insert_bundle` op
* this actually regressed performance last time i tried it, but in theory it should be faster
* Optimize SparseSet::insert (see `PERF` comment on insert)
* Replace SparseArray `Option<T>` with T::MAX to cut down on branching
* would enable cheaper get_unchecked() operations
* upstream fixedbitset optimizations
* fixedbitset could be allocation free for small block counts (store blocks in a SmallVec)
* fixedbitset could have a const constructor
* Consider implementing Tags (archetype-specific by-value data that affects archetype identity)
* ex: ArchetypeA could have `[A, B, C]` table components and `[D(1)]` "tag" component. ArchetypeB could have `[A, B, C]` table components and a `[D(2)]` tag component. The archetypes are different, despite both having D tags because the value inside D is different.
* this could potentially build on top of the `archetype.unique_components` added in this pr for resource storage.
* Consider reverting `all_tuples` proc macro in favor of the old `macro_rules` implementation
* all_tuples is more flexible and produces cleaner documentation (the macro_rules version produces weird type parameter orders due to parser constraints)
* but unfortunately all_tuples also appears to make Rust Analyzer sad/slow when working inside of `bevy_ecs` (does not affect user code)
* Consider "resource queries" and/or "mixed resource and entity component queries" as an alternative to WorldCell
* this is basically just "systems" so maybe it's not worth it
* Add more world ops
* `world.clear()`
* `world.reserve<T: Bundle>(count: usize)`
* Try using the old archetype allocation strategy (allocate new memory on resize and copy everything over). I expect this to improve batch insertion performance at the cost of unbatched performance. But thats just a guess. I'm not an allocation perf pro :)
* Adapt Commands apis for consistency with new World apis
## Benchmarks
key:
* `bevy_old`: bevy `main` branch
* `bevy`: this branch
* `_foreach`: uses an optimized for_each iterator
* ` _sparse`: uses sparse set storage (if unspecified assume table storage)
* `_system`: runs inside a system (if unspecified assume test happens via direct world ops)
### Simple Insert (from ecs_bench_suite)
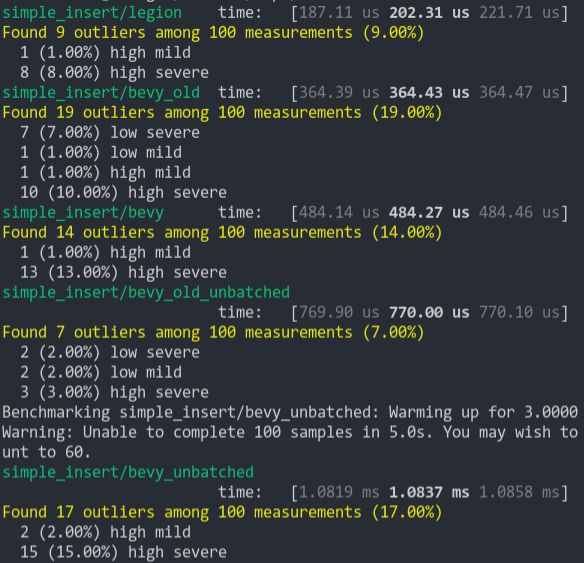
### Simpler Iter (from ecs_bench_suite)
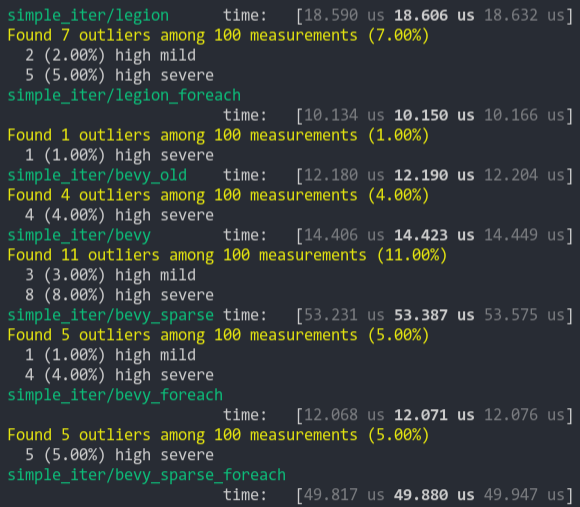
### Fragment Iter (from ecs_bench_suite)
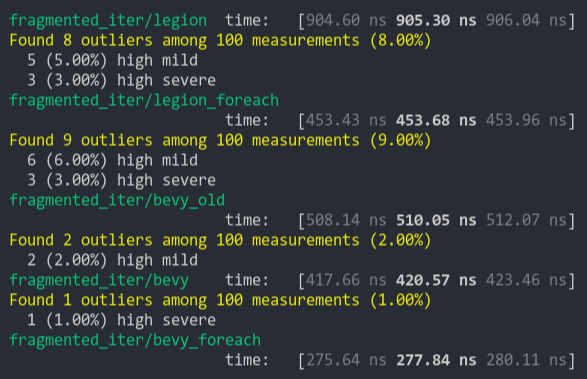
### Sparse Fragmented Iter
Iterate a query that matches 5 entities from a single matching archetype, but there are 100 unmatching archetypes
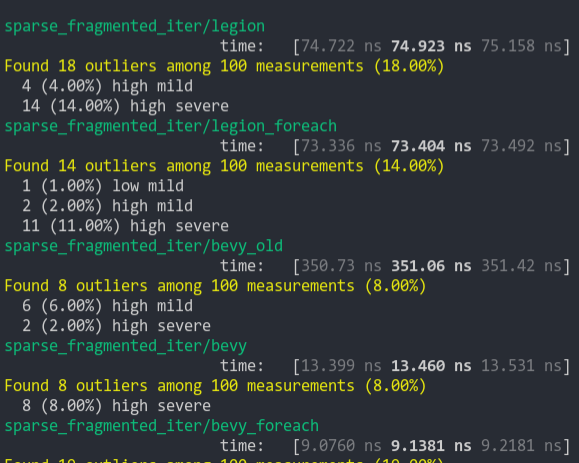
### Schedule (from ecs_bench_suite)
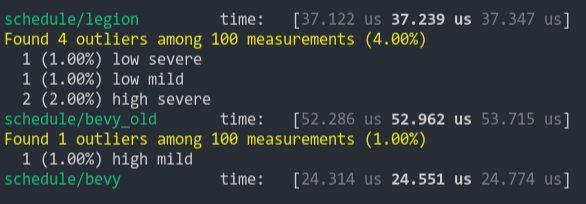
### Add Remove Component (from ecs_bench_suite)
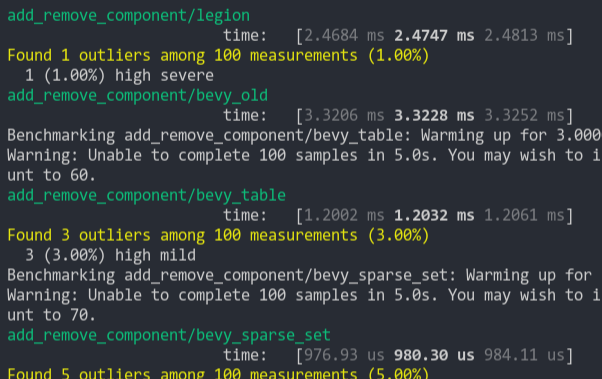
### Add Remove Component Big
Same as the test above, but each entity has 5 "large" matrix components and 1 "large" matrix component is added and removed
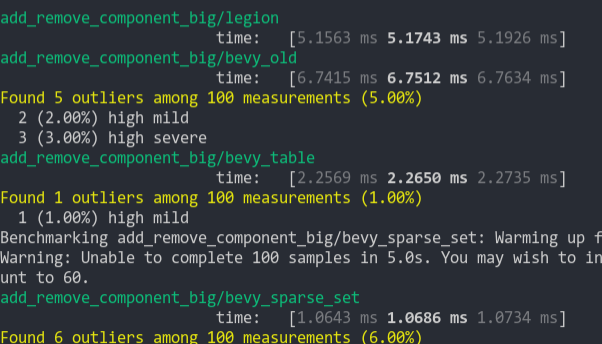
### Get Component
Looks up a single component value a large number of times
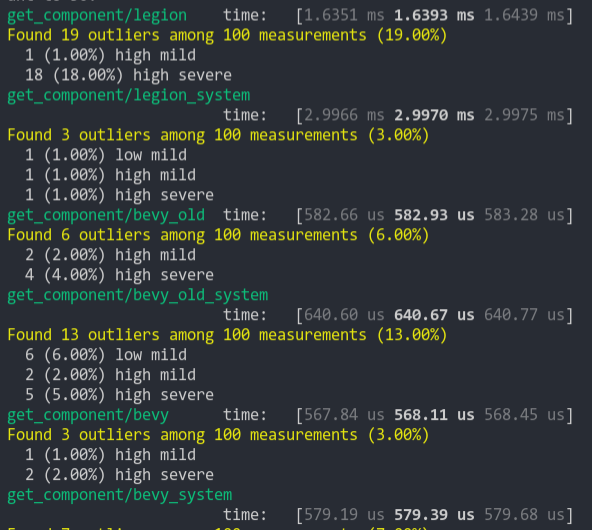
2021-03-05 07:54:35 +00:00
if combined_access . has_write ( component_id ) {
2020-11-15 20:42:43 +00:00
panic! (
2021-11-06 20:53:11 +00:00
" error[B0002]: NonSendMut<{}> in system {} conflicts with a previous mutable resource access ({0}). Consider removing the duplicate access. " ,
Add new SystemState and rename old SystemState to SystemMeta (#2283)
This enables `SystemParams` to be used outside of function systems. Anything can create and store `SystemState`, which enables efficient "param state cached" access to `SystemParams`.
It adds a `ReadOnlySystemParamFetch` trait, which enables safe `SystemState::get` calls without unique world access.
I renamed the old `SystemState` to `SystemMeta` to enable us to mirror the `QueryState` naming convention (but I'm happy to discuss alternative names if people have other ideas). I initially pitched this as `ParamState`, but given that it needs to include full system metadata, that doesn't feel like a particularly accurate name.
```rust
#[derive(Eq, PartialEq, Debug)]
struct A(usize);
#[derive(Eq, PartialEq, Debug)]
struct B(usize);
let mut world = World::default();
world.insert_resource(A(42));
world.spawn().insert(B(7));
// we get nice lifetime elision when declaring the type on the left hand side
let mut system_state: SystemState<(Res<A>, Query<&B>)> = SystemState::new(&mut world);
let (a, query) = system_state.get(&world);
assert_eq!(*a, A(42), "returned resource matches initial value");
assert_eq!(
*query.single().unwrap(),
B(7),
"returned component matches initial value"
);
// mutable system params require unique world access
let mut system_state: SystemState<(ResMut<A>, Query<&mut B>)> = SystemState::new(&mut world);
let (a, query) = system_state.get_mut(&mut world);
// static lifetimes are required when declaring inside of structs
struct SomeContainer {
state: SystemState<(Res<'static, A>, Res<'static, B>)>
}
// this can be shortened using type aliases, which will be useful for complex param tuples
type MyParams<'a> = (Res<'a, A>, Res<'a, B>);
struct SomeContainer {
state: SystemState<MyParams<'static>>
}
// It is the user's responsibility to call SystemState::apply(world) for parameters that queue up work
let mut system_state: SystemState<(Commands, Query<&B>)> = SystemState::new(&mut world);
{
let (mut commands, query) = system_state.get(&world);
commands.insert_resource(3.14);
}
system_state.apply(&mut world);
```
## Future Work
* Actually use SystemState inside FunctionSystem. This would be trivial, but it requires FunctionSystem to wrap SystemState in Option in its current form (which complicates system metadata lookup). I'd prefer to hold off until we adopt something like the later designs linked in #1364, which enable us to contruct Systems using a World reference (and also remove the need for `.system`).
* Consider a "scoped" approach to automatically call SystemState::apply when systems params are no longer being used (either a container type with a Drop impl, or a function that takes a closure for user logic operating on params).
2021-06-02 19:57:38 +00:00
std ::any ::type_name ::< T > ( ) , system_meta . name ) ;
Bevy ECS V2 (#1525)
# Bevy ECS V2
This is a rewrite of Bevy ECS (basically everything but the new executor/schedule, which are already awesome). The overall goal was to improve the performance and versatility of Bevy ECS. Here is a quick bulleted list of changes before we dive into the details:
* Complete World rewrite
* Multiple component storage types:
* Tables: fast cache friendly iteration, slower add/removes (previously called Archetypes)
* Sparse Sets: fast add/remove, slower iteration
* Stateful Queries (caches query results for faster iteration. fragmented iteration is _fast_ now)
* Stateful System Params (caches expensive operations. inspired by @DJMcNab's work in #1364)
* Configurable System Params (users can set configuration when they construct their systems. once again inspired by @DJMcNab's work)
* Archetypes are now "just metadata", component storage is separate
* Archetype Graph (for faster archetype changes)
* Component Metadata
* Configure component storage type
* Retrieve information about component size/type/name/layout/send-ness/etc
* Components are uniquely identified by a densely packed ComponentId
* TypeIds are now totally optional (which should make implementing scripting easier)
* Super fast "for_each" query iterators
* Merged Resources into World. Resources are now just a special type of component
* EntityRef/EntityMut builder apis (more efficient and more ergonomic)
* Fast bitset-backed `Access<T>` replaces old hashmap-based approach everywhere
* Query conflicts are determined by component access instead of archetype component access (to avoid random failures at runtime)
* With/Without are still taken into account for conflicts, so this should still be comfy to use
* Much simpler `IntoSystem` impl
* Significantly reduced the amount of hashing throughout the ecs in favor of Sparse Sets (indexed by densely packed ArchetypeId, ComponentId, BundleId, and TableId)
* Safety Improvements
* Entity reservation uses a normal world reference instead of unsafe transmute
* QuerySets no longer transmute lifetimes
* Made traits "unsafe" where relevant
* More thorough safety docs
* WorldCell
* Exposes safe mutable access to multiple resources at a time in a World
* Replaced "catch all" `System::update_archetypes(world: &World)` with `System::new_archetype(archetype: &Archetype)`
* Simpler Bundle implementation
* Replaced slow "remove_bundle_one_by_one" used as fallback for Commands::remove_bundle with fast "remove_bundle_intersection"
* Removed `Mut<T>` query impl. it is better to only support one way: `&mut T`
* Removed with() from `Flags<T>` in favor of `Option<Flags<T>>`, which allows querying for flags to be "filtered" by default
* Components now have is_send property (currently only resources support non-send)
* More granular module organization
* New `RemovedComponents<T>` SystemParam that replaces `query.removed::<T>()`
* `world.resource_scope()` for mutable access to resources and world at the same time
* WorldQuery and QueryFilter traits unified. FilterFetch trait added to enable "short circuit" filtering. Auto impled for cases that don't need it
* Significantly slimmed down SystemState in favor of individual SystemParam state
* System Commands changed from `commands: &mut Commands` back to `mut commands: Commands` (to allow Commands to have a World reference)
Fixes #1320
## `World` Rewrite
This is a from-scratch rewrite of `World` that fills the niche that `hecs` used to. Yes, this means Bevy ECS is no longer a "fork" of hecs. We're going out our own!
(the only shared code between the projects is the entity id allocator, which is already basically ideal)
A huge shout out to @SanderMertens (author of [flecs](https://github.com/SanderMertens/flecs)) for sharing some great ideas with me (specifically hybrid ecs storage and archetype graphs). He also helped advise on a number of implementation details.
## Component Storage (The Problem)
Two ECS storage paradigms have gained a lot of traction over the years:
* **Archetypal ECS**:
* Stores components in "tables" with static schemas. Each "column" stores components of a given type. Each "row" is an entity.
* Each "archetype" has its own table. Adding/removing an entity's component changes the archetype.
* Enables super-fast Query iteration due to its cache-friendly data layout
* Comes at the cost of more expensive add/remove operations for an Entity's components, because all components need to be copied to the new archetype's "table"
* **Sparse Set ECS**:
* Stores components of the same type in densely packed arrays, which are sparsely indexed by densely packed unsigned integers (Entity ids)
* Query iteration is slower than Archetypal ECS because each entity's component could be at any position in the sparse set. This "random access" pattern isn't cache friendly. Additionally, there is an extra layer of indirection because you must first map the entity id to an index in the component array.
* Adding/removing components is a cheap, constant time operation
Bevy ECS V1, hecs, legion, flec, and Unity DOTS are all "archetypal ecs-es". I personally think "archetypal" storage is a good default for game engines. An entity's archetype doesn't need to change frequently in general, and it creates "fast by default" query iteration (which is a much more common operation). It is also "self optimizing". Users don't need to think about optimizing component layouts for iteration performance. It "just works" without any extra boilerplate.
Shipyard and EnTT are "sparse set ecs-es". They employ "packing" as a way to work around the "suboptimal by default" iteration performance for specific sets of components. This helps, but I didn't think this was a good choice for a general purpose engine like Bevy because:
1. "packs" conflict with each other. If bevy decides to internally pack the Transform and GlobalTransform components, users are then blocked if they want to pack some custom component with Transform.
2. users need to take manual action to optimize
Developers selecting an ECS framework are stuck with a hard choice. Select an "archetypal" framework with "fast iteration everywhere" but without the ability to cheaply add/remove components, or select a "sparse set" framework to cheaply add/remove components but with slower iteration performance.
## Hybrid Component Storage (The Solution)
In Bevy ECS V2, we get to have our cake and eat it too. It now has _both_ of the component storage types above (and more can be added later if needed):
* **Tables** (aka "archetypal" storage)
* The default storage. If you don't configure anything, this is what you get
* Fast iteration by default
* Slower add/remove operations
* **Sparse Sets**
* Opt-in
* Slower iteration
* Faster add/remove operations
These storage types complement each other perfectly. By default Query iteration is fast. If developers know that they want to add/remove a component at high frequencies, they can set the storage to "sparse set":
```rust
world.register_component(
ComponentDescriptor::new::<MyComponent>(StorageType::SparseSet)
).unwrap();
```
## Archetypes
Archetypes are now "just metadata" ... they no longer store components directly. They do store:
* The `ComponentId`s of each of the Archetype's components (and that component's storage type)
* Archetypes are uniquely defined by their component layouts
* For example: entities with "table" components `[A, B, C]` _and_ "sparse set" components `[D, E]` will always be in the same archetype.
* The `TableId` associated with the archetype
* For now each archetype has exactly one table (which can have no components),
* There is a 1->Many relationship from Tables->Archetypes. A given table could have any number of archetype components stored in it:
* Ex: an entity with "table storage" components `[A, B, C]` and "sparse set" components `[D, E]` will share the same `[A, B, C]` table as an entity with `[A, B, C]` table component and `[F]` sparse set components.
* This 1->Many relationship is how we preserve fast "cache friendly" iteration performance when possible (more on this later)
* A list of entities that are in the archetype and the row id of the table they are in
* ArchetypeComponentIds
* unique densely packed identifiers for (ArchetypeId, ComponentId) pairs
* used by the schedule executor for cheap system access control
* "Archetype Graph Edges" (see the next section)
## The "Archetype Graph"
Archetype changes in Bevy (and a number of other archetypal ecs-es) have historically been expensive to compute. First, you need to allocate a new vector of the entity's current component ids, add or remove components based on the operation performed, sort it (to ensure it is order-independent), then hash it to find the archetype (if it exists). And thats all before we get to the _already_ expensive full copy of all components to the new table storage.
The solution is to build a "graph" of archetypes to cache these results. @SanderMertens first exposed me to the idea (and he got it from @gjroelofs, who came up with it). They propose adding directed edges between archetypes for add/remove component operations. If `ComponentId`s are densely packed, you can use sparse sets to cheaply jump between archetypes.
Bevy takes this one step further by using add/remove `Bundle` edges instead of `Component` edges. Bevy encourages the use of `Bundles` to group add/remove operations. This is largely for "clearer game logic" reasons, but it also helps cut down on the number of archetype changes required. `Bundles` now also have densely-packed `BundleId`s. This allows us to use a _single_ edge for each bundle operation (rather than needing to traverse N edges ... one for each component). Single component operations are also bundles, so this is strictly an improvement over a "component only" graph.
As a result, an operation that used to be _heavy_ (both for allocations and compute) is now two dirt-cheap array lookups and zero allocations.
## Stateful Queries
World queries are now stateful. This allows us to:
1. Cache archetype (and table) matches
* This resolves another issue with (naive) archetypal ECS: query performance getting worse as the number of archetypes goes up (and fragmentation occurs).
2. Cache Fetch and Filter state
* The expensive parts of fetch/filter operations (such as hashing the TypeId to find the ComponentId) now only happen once when the Query is first constructed
3. Incrementally build up state
* When new archetypes are added, we only process the new archetypes (no need to rebuild state for old archetypes)
As a result, the direct `World` query api now looks like this:
```rust
let mut query = world.query::<(&A, &mut B)>();
for (a, mut b) in query.iter_mut(&mut world) {
}
```
Requiring `World` to generate stateful queries (rather than letting the `QueryState` type be constructed separately) allows us to ensure that _all_ queries are properly initialized (and the relevant world state, such as ComponentIds). This enables QueryState to remove branches from its operations that check for initialization status (and also enables query.iter() to take an immutable world reference because it doesn't need to initialize anything in world).
However in systems, this is a non-breaking change. State management is done internally by the relevant SystemParam.
## Stateful SystemParams
Like Queries, `SystemParams` now also cache state. For example, `Query` system params store the "stateful query" state mentioned above. Commands store their internal `CommandQueue`. This means you can now safely use as many separate `Commands` parameters in your system as you want. `Local<T>` system params store their `T` value in their state (instead of in Resources).
SystemParam state also enabled a significant slim-down of SystemState. It is much nicer to look at now.
Per-SystemParam state naturally insulates us from an "aliased mut" class of errors we have hit in the past (ex: using multiple `Commands` system params).
(credit goes to @DJMcNab for the initial idea and draft pr here #1364)
## Configurable SystemParams
@DJMcNab also had the great idea to make SystemParams configurable. This allows users to provide some initial configuration / values for system parameters (when possible). Most SystemParams have no config (the config type is `()`), but the `Local<T>` param now supports user-provided parameters:
```rust
fn foo(value: Local<usize>) {
}
app.add_system(foo.system().config(|c| c.0 = Some(10)));
```
## Uber Fast "for_each" Query Iterators
Developers now have the choice to use a fast "for_each" iterator, which yields ~1.5-3x iteration speed improvements for "fragmented iteration", and minor ~1.2x iteration speed improvements for unfragmented iteration.
```rust
fn system(query: Query<(&A, &mut B)>) {
// you now have the option to do this for a speed boost
query.for_each_mut(|(a, mut b)| {
});
// however normal iterators are still available
for (a, mut b) in query.iter_mut() {
}
}
```
I think in most cases we should continue to encourage "normal" iterators as they are more flexible and more "rust idiomatic". But when that extra "oomf" is needed, it makes sense to use `for_each`.
We should also consider using `for_each` for internal bevy systems to give our users a nice speed boost (but that should be a separate pr).
## Component Metadata
`World` now has a `Components` collection, which is accessible via `world.components()`. This stores mappings from `ComponentId` to `ComponentInfo`, as well as `TypeId` to `ComponentId` mappings (where relevant). `ComponentInfo` stores information about the component, such as ComponentId, TypeId, memory layout, send-ness (currently limited to resources), and storage type.
## Significantly Cheaper `Access<T>`
We used to use `TypeAccess<TypeId>` to manage read/write component/archetype-component access. This was expensive because TypeIds must be hashed and compared individually. The parallel executor got around this by "condensing" type ids into bitset-backed access types. This worked, but it had to be re-generated from the `TypeAccess<TypeId>`sources every time archetypes changed.
This pr removes TypeAccess in favor of faster bitset access everywhere. We can do this thanks to the move to densely packed `ComponentId`s and `ArchetypeComponentId`s.
## Merged Resources into World
Resources had a lot of redundant functionality with Components. They stored typed data, they had access control, they had unique ids, they were queryable via SystemParams, etc. In fact the _only_ major difference between them was that they were unique (and didn't correlate to an entity).
Separate resources also had the downside of requiring a separate set of access controls, which meant the parallel executor needed to compare more bitsets per system and manage more state.
I initially got the "separate resources" idea from `legion`. I think that design was motivated by the fact that it made the direct world query/resource lifetime interactions more manageable. It certainly made our lives easier when using Resources alongside hecs/bevy_ecs. However we already have a construct for safely and ergonomically managing in-world lifetimes: systems (which use `Access<T>` internally).
This pr merges Resources into World:
```rust
world.insert_resource(1);
world.insert_resource(2.0);
let a = world.get_resource::<i32>().unwrap();
let mut b = world.get_resource_mut::<f64>().unwrap();
*b = 3.0;
```
Resources are now just a special kind of component. They have their own ComponentIds (and their own resource TypeId->ComponentId scope, so they don't conflict wit components of the same type). They are stored in a special "resource archetype", which stores components inside the archetype using a new `unique_components` sparse set (note that this sparse set could later be used to implement Tags). This allows us to keep the code size small by reusing existing datastructures (namely Column, Archetype, ComponentFlags, and ComponentInfo). This allows us the executor to use a single `Access<ArchetypeComponentId>` per system. It should also make scripting language integration easier.
_But_ this merge did create problems for people directly interacting with `World`. What if you need mutable access to multiple resources at the same time? `world.get_resource_mut()` borrows World mutably!
## WorldCell
WorldCell applies the `Access<ArchetypeComponentId>` concept to direct world access:
```rust
let world_cell = world.cell();
let a = world_cell.get_resource_mut::<i32>().unwrap();
let b = world_cell.get_resource_mut::<f64>().unwrap();
```
This adds cheap runtime checks (a sparse set lookup of `ArchetypeComponentId` and a counter) to ensure that world accesses do not conflict with each other. Each operation returns a `WorldBorrow<'w, T>` or `WorldBorrowMut<'w, T>` wrapper type, which will release the relevant ArchetypeComponentId resources when dropped.
World caches the access sparse set (and only one cell can exist at a time), so `world.cell()` is a cheap operation.
WorldCell does _not_ use atomic operations. It is non-send, does a mutable borrow of world to prevent other accesses, and uses a simple `Rc<RefCell<ArchetypeComponentAccess>>` wrapper in each WorldBorrow pointer.
The api is currently limited to resource access, but it can and should be extended to queries / entity component access.
## Resource Scopes
WorldCell does not yet support component queries, and even when it does there are sometimes legitimate reasons to want a mutable world ref _and_ a mutable resource ref (ex: bevy_render and bevy_scene both need this). In these cases we could always drop down to the unsafe `world.get_resource_unchecked_mut()`, but that is not ideal!
Instead developers can use a "resource scope"
```rust
world.resource_scope(|world: &mut World, a: &mut A| {
})
```
This temporarily removes the `A` resource from `World`, provides mutable pointers to both, and re-adds A to World when finished. Thanks to the move to ComponentIds/sparse sets, this is a cheap operation.
If multiple resources are required, scopes can be nested. We could also consider adding a "resource tuple" to the api if this pattern becomes common and the boilerplate gets nasty.
## Query Conflicts Use ComponentId Instead of ArchetypeComponentId
For safety reasons, systems cannot contain queries that conflict with each other without wrapping them in a QuerySet. On bevy `main`, we use ArchetypeComponentIds to determine conflicts. This is nice because it can take into account filters:
```rust
// these queries will never conflict due to their filters
fn filter_system(a: Query<&mut A, With<B>>, b: Query<&mut B, Without<B>>) {
}
```
But it also has a significant downside:
```rust
// these queries will not conflict _until_ an entity with A, B, and C is spawned
fn maybe_conflicts_system(a: Query<(&mut A, &C)>, b: Query<(&mut A, &B)>) {
}
```
The system above will panic at runtime if an entity with A, B, and C is spawned. This makes it hard to trust that your game logic will run without crashing.
In this pr, I switched to using `ComponentId` instead. This _is_ more constraining. `maybe_conflicts_system` will now always fail, but it will do it consistently at startup. Naively, it would also _disallow_ `filter_system`, which would be a significant downgrade in usability. Bevy has a number of internal systems that rely on disjoint queries and I expect it to be a common pattern in userspace.
To resolve this, I added a new `FilteredAccess<T>` type, which wraps `Access<T>` and adds with/without filters. If two `FilteredAccess` have with/without values that prove they are disjoint, they will no longer conflict.
## EntityRef / EntityMut
World entity operations on `main` require that the user passes in an `entity` id to each operation:
```rust
let entity = world.spawn((A, )); // create a new entity with A
world.get::<A>(entity);
world.insert(entity, (B, C));
world.insert_one(entity, D);
```
This means that each operation needs to look up the entity location / verify its validity. The initial spawn operation also requires a Bundle as input. This can be awkward when no components are required (or one component is required).
These operations have been replaced by `EntityRef` and `EntityMut`, which are "builder-style" wrappers around world that provide read and read/write operations on a single, pre-validated entity:
```rust
// spawn now takes no inputs and returns an EntityMut
let entity = world.spawn()
.insert(A) // insert a single component into the entity
.insert_bundle((B, C)) // insert a bundle of components into the entity
.id() // id returns the Entity id
// Returns EntityMut (or panics if the entity does not exist)
world.entity_mut(entity)
.insert(D)
.insert_bundle(SomeBundle::default());
{
// returns EntityRef (or panics if the entity does not exist)
let d = world.entity(entity)
.get::<D>() // gets the D component
.unwrap();
// world.get still exists for ergonomics
let d = world.get::<D>(entity).unwrap();
}
// These variants return Options if you want to check existence instead of panicing
world.get_entity_mut(entity)
.unwrap()
.insert(E);
if let Some(entity_ref) = world.get_entity(entity) {
let d = entity_ref.get::<D>().unwrap();
}
```
This _does not_ affect the current Commands api or terminology. I think that should be a separate conversation as that is a much larger breaking change.
## Safety Improvements
* Entity reservation in Commands uses a normal world borrow instead of an unsafe transmute
* QuerySets no longer transmutes lifetimes
* Made traits "unsafe" when implementing a trait incorrectly could cause unsafety
* More thorough safety docs
## RemovedComponents SystemParam
The old approach to querying removed components: `query.removed:<T>()` was confusing because it had no connection to the query itself. I replaced it with the following, which is both clearer and allows us to cache the ComponentId mapping in the SystemParamState:
```rust
fn system(removed: RemovedComponents<T>) {
for entity in removed.iter() {
}
}
```
## Simpler Bundle implementation
Bundles are no longer responsible for sorting (or deduping) TypeInfo. They are just a simple ordered list of component types / data. This makes the implementation smaller and opens the door to an easy "nested bundle" implementation in the future (which i might even add in this pr). Duplicate detection is now done once per bundle type by World the first time a bundle is used.
## Unified WorldQuery and QueryFilter types
(don't worry they are still separate type _parameters_ in Queries .. this is a non-breaking change)
WorldQuery and QueryFilter were already basically identical apis. With the addition of `FetchState` and more storage-specific fetch methods, the overlap was even clearer (and the redundancy more painful).
QueryFilters are now just `F: WorldQuery where F::Fetch: FilterFetch`. FilterFetch requires `Fetch<Item = bool>` and adds new "short circuit" variants of fetch methods. This enables a filter tuple like `(With<A>, Without<B>, Changed<C>)` to stop evaluating the filter after the first mismatch is encountered. FilterFetch is automatically implemented for `Fetch` implementations that return bool.
This forces fetch implementations that return things like `(bool, bool, bool)` (such as the filter above) to manually implement FilterFetch and decide whether or not to short-circuit.
## More Granular Modules
World no longer globs all of the internal modules together. It now exports `core`, `system`, and `schedule` separately. I'm also considering exporting `core` submodules directly as that is still pretty "glob-ey" and unorganized (feedback welcome here).
## Remaining Draft Work (to be done in this pr)
* ~~panic on conflicting WorldQuery fetches (&A, &mut A)~~
* ~~bevy `main` and hecs both currently allow this, but we should protect against it if possible~~
* ~~batch_iter / par_iter (currently stubbed out)~~
* ~~ChangedRes~~
* ~~I skipped this while we sort out #1313. This pr should be adapted to account for whatever we land on there~~.
* ~~The `Archetypes` and `Tables` collections use hashes of sorted lists of component ids to uniquely identify each archetype/table. This hash is then used as the key in a HashMap to look up the relevant ArchetypeId or TableId. (which doesn't handle hash collisions properly)~~
* ~~It is currently unsafe to generate a Query from "World A", then use it on "World B" (despite the api claiming it is safe). We should probably close this gap. This could be done by adding a randomly generated WorldId to each world, then storing that id in each Query. They could then be compared to each other on each `query.do_thing(&world)` operation. This _does_ add an extra branch to each query operation, so I'm open to other suggestions if people have them.~~
* ~~Nested Bundles (if i find time)~~
## Potential Future Work
* Expand WorldCell to support queries.
* Consider not allocating in the empty archetype on `world.spawn()`
* ex: return something like EntityMutUninit, which turns into EntityMut after an `insert` or `insert_bundle` op
* this actually regressed performance last time i tried it, but in theory it should be faster
* Optimize SparseSet::insert (see `PERF` comment on insert)
* Replace SparseArray `Option<T>` with T::MAX to cut down on branching
* would enable cheaper get_unchecked() operations
* upstream fixedbitset optimizations
* fixedbitset could be allocation free for small block counts (store blocks in a SmallVec)
* fixedbitset could have a const constructor
* Consider implementing Tags (archetype-specific by-value data that affects archetype identity)
* ex: ArchetypeA could have `[A, B, C]` table components and `[D(1)]` "tag" component. ArchetypeB could have `[A, B, C]` table components and a `[D(2)]` tag component. The archetypes are different, despite both having D tags because the value inside D is different.
* this could potentially build on top of the `archetype.unique_components` added in this pr for resource storage.
* Consider reverting `all_tuples` proc macro in favor of the old `macro_rules` implementation
* all_tuples is more flexible and produces cleaner documentation (the macro_rules version produces weird type parameter orders due to parser constraints)
* but unfortunately all_tuples also appears to make Rust Analyzer sad/slow when working inside of `bevy_ecs` (does not affect user code)
* Consider "resource queries" and/or "mixed resource and entity component queries" as an alternative to WorldCell
* this is basically just "systems" so maybe it's not worth it
* Add more world ops
* `world.clear()`
* `world.reserve<T: Bundle>(count: usize)`
* Try using the old archetype allocation strategy (allocate new memory on resize and copy everything over). I expect this to improve batch insertion performance at the cost of unbatched performance. But thats just a guess. I'm not an allocation perf pro :)
* Adapt Commands apis for consistency with new World apis
## Benchmarks
key:
* `bevy_old`: bevy `main` branch
* `bevy`: this branch
* `_foreach`: uses an optimized for_each iterator
* ` _sparse`: uses sparse set storage (if unspecified assume table storage)
* `_system`: runs inside a system (if unspecified assume test happens via direct world ops)
### Simple Insert (from ecs_bench_suite)
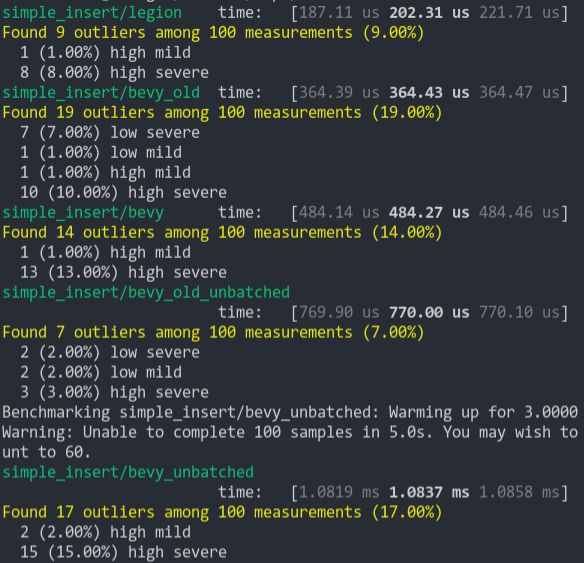
### Simpler Iter (from ecs_bench_suite)
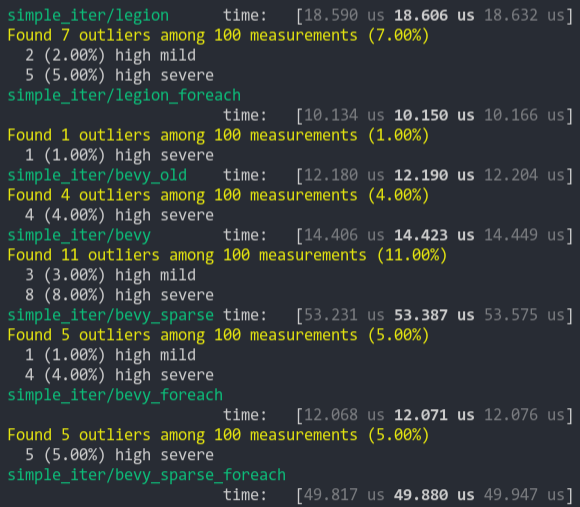
### Fragment Iter (from ecs_bench_suite)
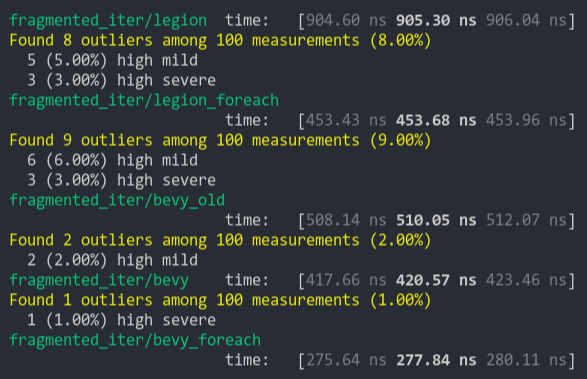
### Sparse Fragmented Iter
Iterate a query that matches 5 entities from a single matching archetype, but there are 100 unmatching archetypes
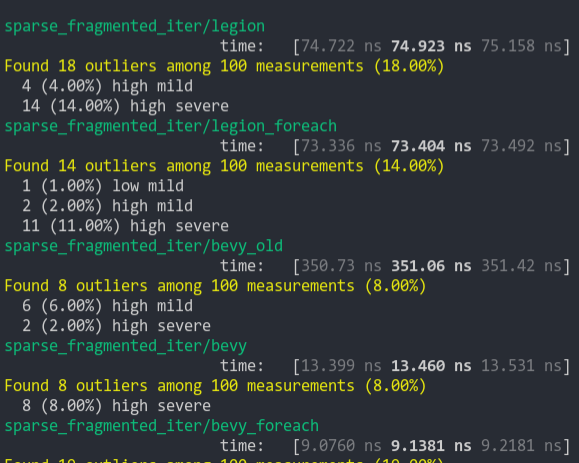
### Schedule (from ecs_bench_suite)
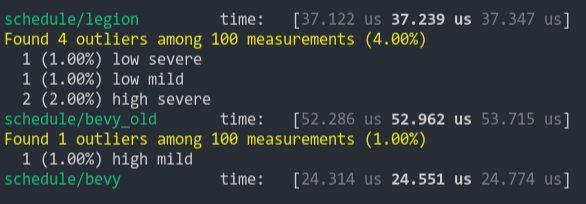
### Add Remove Component (from ecs_bench_suite)
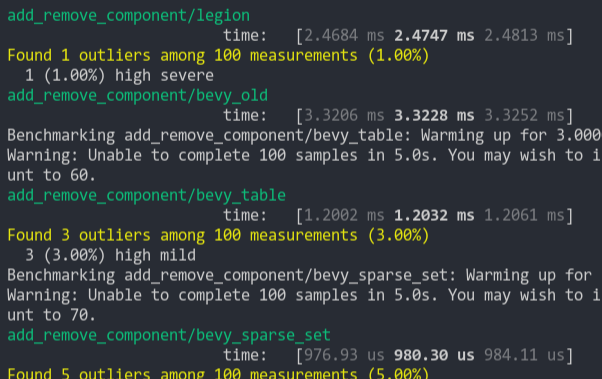
### Add Remove Component Big
Same as the test above, but each entity has 5 "large" matrix components and 1 "large" matrix component is added and removed
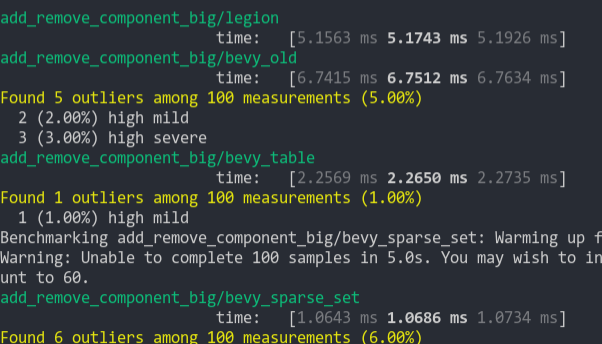
### Get Component
Looks up a single component value a large number of times
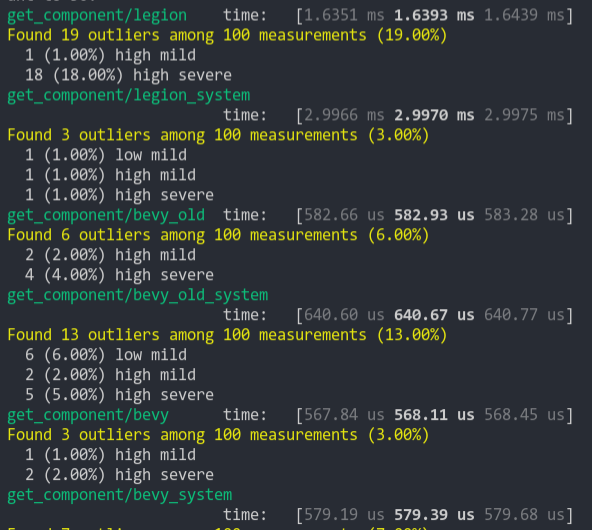
2021-03-05 07:54:35 +00:00
} else if combined_access . has_read ( component_id ) {
panic! (
2021-11-06 20:53:11 +00:00
" error[B0002]: NonSendMut<{}> in system {} conflicts with a previous immutable resource access ({0}). Consider removing the duplicate access. " ,
Add new SystemState and rename old SystemState to SystemMeta (#2283)
This enables `SystemParams` to be used outside of function systems. Anything can create and store `SystemState`, which enables efficient "param state cached" access to `SystemParams`.
It adds a `ReadOnlySystemParamFetch` trait, which enables safe `SystemState::get` calls without unique world access.
I renamed the old `SystemState` to `SystemMeta` to enable us to mirror the `QueryState` naming convention (but I'm happy to discuss alternative names if people have other ideas). I initially pitched this as `ParamState`, but given that it needs to include full system metadata, that doesn't feel like a particularly accurate name.
```rust
#[derive(Eq, PartialEq, Debug)]
struct A(usize);
#[derive(Eq, PartialEq, Debug)]
struct B(usize);
let mut world = World::default();
world.insert_resource(A(42));
world.spawn().insert(B(7));
// we get nice lifetime elision when declaring the type on the left hand side
let mut system_state: SystemState<(Res<A>, Query<&B>)> = SystemState::new(&mut world);
let (a, query) = system_state.get(&world);
assert_eq!(*a, A(42), "returned resource matches initial value");
assert_eq!(
*query.single().unwrap(),
B(7),
"returned component matches initial value"
);
// mutable system params require unique world access
let mut system_state: SystemState<(ResMut<A>, Query<&mut B>)> = SystemState::new(&mut world);
let (a, query) = system_state.get_mut(&mut world);
// static lifetimes are required when declaring inside of structs
struct SomeContainer {
state: SystemState<(Res<'static, A>, Res<'static, B>)>
}
// this can be shortened using type aliases, which will be useful for complex param tuples
type MyParams<'a> = (Res<'a, A>, Res<'a, B>);
struct SomeContainer {
state: SystemState<MyParams<'static>>
}
// It is the user's responsibility to call SystemState::apply(world) for parameters that queue up work
let mut system_state: SystemState<(Commands, Query<&B>)> = SystemState::new(&mut world);
{
let (mut commands, query) = system_state.get(&world);
commands.insert_resource(3.14);
}
system_state.apply(&mut world);
```
## Future Work
* Actually use SystemState inside FunctionSystem. This would be trivial, but it requires FunctionSystem to wrap SystemState in Option in its current form (which complicates system metadata lookup). I'd prefer to hold off until we adopt something like the later designs linked in #1364, which enable us to contruct Systems using a World reference (and also remove the need for `.system`).
* Consider a "scoped" approach to automatically call SystemState::apply when systems params are no longer being used (either a container type with a Drop impl, or a function that takes a closure for user logic operating on params).
2021-06-02 19:57:38 +00:00
std ::any ::type_name ::< T > ( ) , system_meta . name ) ;
2020-11-15 20:42:43 +00:00
}
Fix FilteredAccessSet get_conflicts inconsistency (#5105)
# Objective
* Enable `Res` and `Query` parameter mutual exclusion
* Required for https://github.com/bevyengine/bevy/pull/5080
The `FilteredAccessSet::get_conflicts` methods didn't work properly with
`Res` and `ResMut` parameters. Because those added their access by using
the `combined_access_mut` method and directly modifying the global
access state of the FilteredAccessSet. This caused an inconsistency,
because get_conflicts assumes that ALL added access have a corresponding
`FilteredAccess` added to the `filtered_accesses` field.
In practice, that means that SystemParam that adds their access through
the `Access` returned by `combined_access_mut` and the ones that add
their access using the `add` method lived in two different universes. As
a result, they could never be mutually exclusive.
## Solution
This commit fixes it by removing the `combined_access_mut` method. This
ensures that the `combined_access` field of FilteredAccessSet is always
updated consistently with the addition of a filter. When checking for
filtered access, it is now possible to account for `Res` and `ResMut`
invalid access. This is currently not needed, but might be in the
future.
We add the `add_unfiltered_{read,write}` methods to replace previous
usages of `combined_access_mut`.
We also add improved Debug implementations on FixedBitSet so that their
meaning is much clearer in debug output.
---
## Changelog
* Fix `Res` and `Query` parameter never being mutually exclusive.
## Migration Guide
Note: this mostly changes ECS internals, but since the API is public, it is technically breaking:
* Removed `FilteredAccessSet::combined_access_mut`
* Replace _immutable_ usage of those by `combined_access`
* For _mutable_ usages, use the new `add_unfiltered_{read,write}` methods instead of `combined_access_mut` followed by `add_{read,write}`
2022-11-16 11:05:48 +00:00
system_meta
. component_access_set
. add_unfiltered_write ( component_id ) ;
2020-11-15 20:42:43 +00:00
2022-10-24 13:46:36 +00:00
let archetype_component_id = world
Panic on dropping NonSend in non-origin thread. (#6534)
# Objective
Fixes #3310. Fixes #6282. Fixes #6278. Fixes #3666.
## Solution
Split out `!Send` resources into `NonSendResources`. Add a `origin_thread_id` to all `!Send` Resources, check it on dropping `NonSendResourceData`, if there's a mismatch, panic. Moved all of the checks that `MainThreadValidator` would do into `NonSendResources` instead.
All `!Send` resources now individually track which thread they were inserted from. This is validated against for every access, mutation, and drop that could be done against the value.
A regression test using an altered version of the example from #3310 has been added.
This is a stopgap solution for the current status quo. A full solution may involve fully removing `!Send` resources/components from `World`, which will likely require a much more thorough design on how to handle the existing in-engine and ecosystem use cases.
This PR also introduces another breaking change:
```rust
use bevy_ecs::prelude::*;
#[derive(Resource)]
struct Resource(u32);
fn main() {
let mut world = World::new();
world.insert_resource(Resource(1));
world.insert_non_send_resource(Resource(2));
let res = world.get_resource_mut::<Resource>().unwrap();
assert_eq!(res.0, 2);
}
```
This code will run correctly on 0.9.1 but not with this PR, since NonSend resources and normal resources have become actual distinct concepts storage wise.
## Changelog
Changed: Fix soundness bug with `World: Send`. Dropping a `World` that contains a `!Send` resource on the wrong thread will now panic.
## Migration Guide
Normal resources and `NonSend` resources no longer share the same backing storage. If `R: Resource`, then `NonSend<R>` and `Res<R>` will return different instances from each other. If you are using both `Res<T>` and `NonSend<T>` (or their mutable variants), to fetch the same resources, it's strongly advised to use `Res<T>`.
2023-01-09 20:40:34 +00:00
. get_non_send_archetype_component_id ( component_id )
Bevy ECS V2 (#1525)
# Bevy ECS V2
This is a rewrite of Bevy ECS (basically everything but the new executor/schedule, which are already awesome). The overall goal was to improve the performance and versatility of Bevy ECS. Here is a quick bulleted list of changes before we dive into the details:
* Complete World rewrite
* Multiple component storage types:
* Tables: fast cache friendly iteration, slower add/removes (previously called Archetypes)
* Sparse Sets: fast add/remove, slower iteration
* Stateful Queries (caches query results for faster iteration. fragmented iteration is _fast_ now)
* Stateful System Params (caches expensive operations. inspired by @DJMcNab's work in #1364)
* Configurable System Params (users can set configuration when they construct their systems. once again inspired by @DJMcNab's work)
* Archetypes are now "just metadata", component storage is separate
* Archetype Graph (for faster archetype changes)
* Component Metadata
* Configure component storage type
* Retrieve information about component size/type/name/layout/send-ness/etc
* Components are uniquely identified by a densely packed ComponentId
* TypeIds are now totally optional (which should make implementing scripting easier)
* Super fast "for_each" query iterators
* Merged Resources into World. Resources are now just a special type of component
* EntityRef/EntityMut builder apis (more efficient and more ergonomic)
* Fast bitset-backed `Access<T>` replaces old hashmap-based approach everywhere
* Query conflicts are determined by component access instead of archetype component access (to avoid random failures at runtime)
* With/Without are still taken into account for conflicts, so this should still be comfy to use
* Much simpler `IntoSystem` impl
* Significantly reduced the amount of hashing throughout the ecs in favor of Sparse Sets (indexed by densely packed ArchetypeId, ComponentId, BundleId, and TableId)
* Safety Improvements
* Entity reservation uses a normal world reference instead of unsafe transmute
* QuerySets no longer transmute lifetimes
* Made traits "unsafe" where relevant
* More thorough safety docs
* WorldCell
* Exposes safe mutable access to multiple resources at a time in a World
* Replaced "catch all" `System::update_archetypes(world: &World)` with `System::new_archetype(archetype: &Archetype)`
* Simpler Bundle implementation
* Replaced slow "remove_bundle_one_by_one" used as fallback for Commands::remove_bundle with fast "remove_bundle_intersection"
* Removed `Mut<T>` query impl. it is better to only support one way: `&mut T`
* Removed with() from `Flags<T>` in favor of `Option<Flags<T>>`, which allows querying for flags to be "filtered" by default
* Components now have is_send property (currently only resources support non-send)
* More granular module organization
* New `RemovedComponents<T>` SystemParam that replaces `query.removed::<T>()`
* `world.resource_scope()` for mutable access to resources and world at the same time
* WorldQuery and QueryFilter traits unified. FilterFetch trait added to enable "short circuit" filtering. Auto impled for cases that don't need it
* Significantly slimmed down SystemState in favor of individual SystemParam state
* System Commands changed from `commands: &mut Commands` back to `mut commands: Commands` (to allow Commands to have a World reference)
Fixes #1320
## `World` Rewrite
This is a from-scratch rewrite of `World` that fills the niche that `hecs` used to. Yes, this means Bevy ECS is no longer a "fork" of hecs. We're going out our own!
(the only shared code between the projects is the entity id allocator, which is already basically ideal)
A huge shout out to @SanderMertens (author of [flecs](https://github.com/SanderMertens/flecs)) for sharing some great ideas with me (specifically hybrid ecs storage and archetype graphs). He also helped advise on a number of implementation details.
## Component Storage (The Problem)
Two ECS storage paradigms have gained a lot of traction over the years:
* **Archetypal ECS**:
* Stores components in "tables" with static schemas. Each "column" stores components of a given type. Each "row" is an entity.
* Each "archetype" has its own table. Adding/removing an entity's component changes the archetype.
* Enables super-fast Query iteration due to its cache-friendly data layout
* Comes at the cost of more expensive add/remove operations for an Entity's components, because all components need to be copied to the new archetype's "table"
* **Sparse Set ECS**:
* Stores components of the same type in densely packed arrays, which are sparsely indexed by densely packed unsigned integers (Entity ids)
* Query iteration is slower than Archetypal ECS because each entity's component could be at any position in the sparse set. This "random access" pattern isn't cache friendly. Additionally, there is an extra layer of indirection because you must first map the entity id to an index in the component array.
* Adding/removing components is a cheap, constant time operation
Bevy ECS V1, hecs, legion, flec, and Unity DOTS are all "archetypal ecs-es". I personally think "archetypal" storage is a good default for game engines. An entity's archetype doesn't need to change frequently in general, and it creates "fast by default" query iteration (which is a much more common operation). It is also "self optimizing". Users don't need to think about optimizing component layouts for iteration performance. It "just works" without any extra boilerplate.
Shipyard and EnTT are "sparse set ecs-es". They employ "packing" as a way to work around the "suboptimal by default" iteration performance for specific sets of components. This helps, but I didn't think this was a good choice for a general purpose engine like Bevy because:
1. "packs" conflict with each other. If bevy decides to internally pack the Transform and GlobalTransform components, users are then blocked if they want to pack some custom component with Transform.
2. users need to take manual action to optimize
Developers selecting an ECS framework are stuck with a hard choice. Select an "archetypal" framework with "fast iteration everywhere" but without the ability to cheaply add/remove components, or select a "sparse set" framework to cheaply add/remove components but with slower iteration performance.
## Hybrid Component Storage (The Solution)
In Bevy ECS V2, we get to have our cake and eat it too. It now has _both_ of the component storage types above (and more can be added later if needed):
* **Tables** (aka "archetypal" storage)
* The default storage. If you don't configure anything, this is what you get
* Fast iteration by default
* Slower add/remove operations
* **Sparse Sets**
* Opt-in
* Slower iteration
* Faster add/remove operations
These storage types complement each other perfectly. By default Query iteration is fast. If developers know that they want to add/remove a component at high frequencies, they can set the storage to "sparse set":
```rust
world.register_component(
ComponentDescriptor::new::<MyComponent>(StorageType::SparseSet)
).unwrap();
```
## Archetypes
Archetypes are now "just metadata" ... they no longer store components directly. They do store:
* The `ComponentId`s of each of the Archetype's components (and that component's storage type)
* Archetypes are uniquely defined by their component layouts
* For example: entities with "table" components `[A, B, C]` _and_ "sparse set" components `[D, E]` will always be in the same archetype.
* The `TableId` associated with the archetype
* For now each archetype has exactly one table (which can have no components),
* There is a 1->Many relationship from Tables->Archetypes. A given table could have any number of archetype components stored in it:
* Ex: an entity with "table storage" components `[A, B, C]` and "sparse set" components `[D, E]` will share the same `[A, B, C]` table as an entity with `[A, B, C]` table component and `[F]` sparse set components.
* This 1->Many relationship is how we preserve fast "cache friendly" iteration performance when possible (more on this later)
* A list of entities that are in the archetype and the row id of the table they are in
* ArchetypeComponentIds
* unique densely packed identifiers for (ArchetypeId, ComponentId) pairs
* used by the schedule executor for cheap system access control
* "Archetype Graph Edges" (see the next section)
## The "Archetype Graph"
Archetype changes in Bevy (and a number of other archetypal ecs-es) have historically been expensive to compute. First, you need to allocate a new vector of the entity's current component ids, add or remove components based on the operation performed, sort it (to ensure it is order-independent), then hash it to find the archetype (if it exists). And thats all before we get to the _already_ expensive full copy of all components to the new table storage.
The solution is to build a "graph" of archetypes to cache these results. @SanderMertens first exposed me to the idea (and he got it from @gjroelofs, who came up with it). They propose adding directed edges between archetypes for add/remove component operations. If `ComponentId`s are densely packed, you can use sparse sets to cheaply jump between archetypes.
Bevy takes this one step further by using add/remove `Bundle` edges instead of `Component` edges. Bevy encourages the use of `Bundles` to group add/remove operations. This is largely for "clearer game logic" reasons, but it also helps cut down on the number of archetype changes required. `Bundles` now also have densely-packed `BundleId`s. This allows us to use a _single_ edge for each bundle operation (rather than needing to traverse N edges ... one for each component). Single component operations are also bundles, so this is strictly an improvement over a "component only" graph.
As a result, an operation that used to be _heavy_ (both for allocations and compute) is now two dirt-cheap array lookups and zero allocations.
## Stateful Queries
World queries are now stateful. This allows us to:
1. Cache archetype (and table) matches
* This resolves another issue with (naive) archetypal ECS: query performance getting worse as the number of archetypes goes up (and fragmentation occurs).
2. Cache Fetch and Filter state
* The expensive parts of fetch/filter operations (such as hashing the TypeId to find the ComponentId) now only happen once when the Query is first constructed
3. Incrementally build up state
* When new archetypes are added, we only process the new archetypes (no need to rebuild state for old archetypes)
As a result, the direct `World` query api now looks like this:
```rust
let mut query = world.query::<(&A, &mut B)>();
for (a, mut b) in query.iter_mut(&mut world) {
}
```
Requiring `World` to generate stateful queries (rather than letting the `QueryState` type be constructed separately) allows us to ensure that _all_ queries are properly initialized (and the relevant world state, such as ComponentIds). This enables QueryState to remove branches from its operations that check for initialization status (and also enables query.iter() to take an immutable world reference because it doesn't need to initialize anything in world).
However in systems, this is a non-breaking change. State management is done internally by the relevant SystemParam.
## Stateful SystemParams
Like Queries, `SystemParams` now also cache state. For example, `Query` system params store the "stateful query" state mentioned above. Commands store their internal `CommandQueue`. This means you can now safely use as many separate `Commands` parameters in your system as you want. `Local<T>` system params store their `T` value in their state (instead of in Resources).
SystemParam state also enabled a significant slim-down of SystemState. It is much nicer to look at now.
Per-SystemParam state naturally insulates us from an "aliased mut" class of errors we have hit in the past (ex: using multiple `Commands` system params).
(credit goes to @DJMcNab for the initial idea and draft pr here #1364)
## Configurable SystemParams
@DJMcNab also had the great idea to make SystemParams configurable. This allows users to provide some initial configuration / values for system parameters (when possible). Most SystemParams have no config (the config type is `()`), but the `Local<T>` param now supports user-provided parameters:
```rust
fn foo(value: Local<usize>) {
}
app.add_system(foo.system().config(|c| c.0 = Some(10)));
```
## Uber Fast "for_each" Query Iterators
Developers now have the choice to use a fast "for_each" iterator, which yields ~1.5-3x iteration speed improvements for "fragmented iteration", and minor ~1.2x iteration speed improvements for unfragmented iteration.
```rust
fn system(query: Query<(&A, &mut B)>) {
// you now have the option to do this for a speed boost
query.for_each_mut(|(a, mut b)| {
});
// however normal iterators are still available
for (a, mut b) in query.iter_mut() {
}
}
```
I think in most cases we should continue to encourage "normal" iterators as they are more flexible and more "rust idiomatic". But when that extra "oomf" is needed, it makes sense to use `for_each`.
We should also consider using `for_each` for internal bevy systems to give our users a nice speed boost (but that should be a separate pr).
## Component Metadata
`World` now has a `Components` collection, which is accessible via `world.components()`. This stores mappings from `ComponentId` to `ComponentInfo`, as well as `TypeId` to `ComponentId` mappings (where relevant). `ComponentInfo` stores information about the component, such as ComponentId, TypeId, memory layout, send-ness (currently limited to resources), and storage type.
## Significantly Cheaper `Access<T>`
We used to use `TypeAccess<TypeId>` to manage read/write component/archetype-component access. This was expensive because TypeIds must be hashed and compared individually. The parallel executor got around this by "condensing" type ids into bitset-backed access types. This worked, but it had to be re-generated from the `TypeAccess<TypeId>`sources every time archetypes changed.
This pr removes TypeAccess in favor of faster bitset access everywhere. We can do this thanks to the move to densely packed `ComponentId`s and `ArchetypeComponentId`s.
## Merged Resources into World
Resources had a lot of redundant functionality with Components. They stored typed data, they had access control, they had unique ids, they were queryable via SystemParams, etc. In fact the _only_ major difference between them was that they were unique (and didn't correlate to an entity).
Separate resources also had the downside of requiring a separate set of access controls, which meant the parallel executor needed to compare more bitsets per system and manage more state.
I initially got the "separate resources" idea from `legion`. I think that design was motivated by the fact that it made the direct world query/resource lifetime interactions more manageable. It certainly made our lives easier when using Resources alongside hecs/bevy_ecs. However we already have a construct for safely and ergonomically managing in-world lifetimes: systems (which use `Access<T>` internally).
This pr merges Resources into World:
```rust
world.insert_resource(1);
world.insert_resource(2.0);
let a = world.get_resource::<i32>().unwrap();
let mut b = world.get_resource_mut::<f64>().unwrap();
*b = 3.0;
```
Resources are now just a special kind of component. They have their own ComponentIds (and their own resource TypeId->ComponentId scope, so they don't conflict wit components of the same type). They are stored in a special "resource archetype", which stores components inside the archetype using a new `unique_components` sparse set (note that this sparse set could later be used to implement Tags). This allows us to keep the code size small by reusing existing datastructures (namely Column, Archetype, ComponentFlags, and ComponentInfo). This allows us the executor to use a single `Access<ArchetypeComponentId>` per system. It should also make scripting language integration easier.
_But_ this merge did create problems for people directly interacting with `World`. What if you need mutable access to multiple resources at the same time? `world.get_resource_mut()` borrows World mutably!
## WorldCell
WorldCell applies the `Access<ArchetypeComponentId>` concept to direct world access:
```rust
let world_cell = world.cell();
let a = world_cell.get_resource_mut::<i32>().unwrap();
let b = world_cell.get_resource_mut::<f64>().unwrap();
```
This adds cheap runtime checks (a sparse set lookup of `ArchetypeComponentId` and a counter) to ensure that world accesses do not conflict with each other. Each operation returns a `WorldBorrow<'w, T>` or `WorldBorrowMut<'w, T>` wrapper type, which will release the relevant ArchetypeComponentId resources when dropped.
World caches the access sparse set (and only one cell can exist at a time), so `world.cell()` is a cheap operation.
WorldCell does _not_ use atomic operations. It is non-send, does a mutable borrow of world to prevent other accesses, and uses a simple `Rc<RefCell<ArchetypeComponentAccess>>` wrapper in each WorldBorrow pointer.
The api is currently limited to resource access, but it can and should be extended to queries / entity component access.
## Resource Scopes
WorldCell does not yet support component queries, and even when it does there are sometimes legitimate reasons to want a mutable world ref _and_ a mutable resource ref (ex: bevy_render and bevy_scene both need this). In these cases we could always drop down to the unsafe `world.get_resource_unchecked_mut()`, but that is not ideal!
Instead developers can use a "resource scope"
```rust
world.resource_scope(|world: &mut World, a: &mut A| {
})
```
This temporarily removes the `A` resource from `World`, provides mutable pointers to both, and re-adds A to World when finished. Thanks to the move to ComponentIds/sparse sets, this is a cheap operation.
If multiple resources are required, scopes can be nested. We could also consider adding a "resource tuple" to the api if this pattern becomes common and the boilerplate gets nasty.
## Query Conflicts Use ComponentId Instead of ArchetypeComponentId
For safety reasons, systems cannot contain queries that conflict with each other without wrapping them in a QuerySet. On bevy `main`, we use ArchetypeComponentIds to determine conflicts. This is nice because it can take into account filters:
```rust
// these queries will never conflict due to their filters
fn filter_system(a: Query<&mut A, With<B>>, b: Query<&mut B, Without<B>>) {
}
```
But it also has a significant downside:
```rust
// these queries will not conflict _until_ an entity with A, B, and C is spawned
fn maybe_conflicts_system(a: Query<(&mut A, &C)>, b: Query<(&mut A, &B)>) {
}
```
The system above will panic at runtime if an entity with A, B, and C is spawned. This makes it hard to trust that your game logic will run without crashing.
In this pr, I switched to using `ComponentId` instead. This _is_ more constraining. `maybe_conflicts_system` will now always fail, but it will do it consistently at startup. Naively, it would also _disallow_ `filter_system`, which would be a significant downgrade in usability. Bevy has a number of internal systems that rely on disjoint queries and I expect it to be a common pattern in userspace.
To resolve this, I added a new `FilteredAccess<T>` type, which wraps `Access<T>` and adds with/without filters. If two `FilteredAccess` have with/without values that prove they are disjoint, they will no longer conflict.
## EntityRef / EntityMut
World entity operations on `main` require that the user passes in an `entity` id to each operation:
```rust
let entity = world.spawn((A, )); // create a new entity with A
world.get::<A>(entity);
world.insert(entity, (B, C));
world.insert_one(entity, D);
```
This means that each operation needs to look up the entity location / verify its validity. The initial spawn operation also requires a Bundle as input. This can be awkward when no components are required (or one component is required).
These operations have been replaced by `EntityRef` and `EntityMut`, which are "builder-style" wrappers around world that provide read and read/write operations on a single, pre-validated entity:
```rust
// spawn now takes no inputs and returns an EntityMut
let entity = world.spawn()
.insert(A) // insert a single component into the entity
.insert_bundle((B, C)) // insert a bundle of components into the entity
.id() // id returns the Entity id
// Returns EntityMut (or panics if the entity does not exist)
world.entity_mut(entity)
.insert(D)
.insert_bundle(SomeBundle::default());
{
// returns EntityRef (or panics if the entity does not exist)
let d = world.entity(entity)
.get::<D>() // gets the D component
.unwrap();
// world.get still exists for ergonomics
let d = world.get::<D>(entity).unwrap();
}
// These variants return Options if you want to check existence instead of panicing
world.get_entity_mut(entity)
.unwrap()
.insert(E);
if let Some(entity_ref) = world.get_entity(entity) {
let d = entity_ref.get::<D>().unwrap();
}
```
This _does not_ affect the current Commands api or terminology. I think that should be a separate conversation as that is a much larger breaking change.
## Safety Improvements
* Entity reservation in Commands uses a normal world borrow instead of an unsafe transmute
* QuerySets no longer transmutes lifetimes
* Made traits "unsafe" when implementing a trait incorrectly could cause unsafety
* More thorough safety docs
## RemovedComponents SystemParam
The old approach to querying removed components: `query.removed:<T>()` was confusing because it had no connection to the query itself. I replaced it with the following, which is both clearer and allows us to cache the ComponentId mapping in the SystemParamState:
```rust
fn system(removed: RemovedComponents<T>) {
for entity in removed.iter() {
}
}
```
## Simpler Bundle implementation
Bundles are no longer responsible for sorting (or deduping) TypeInfo. They are just a simple ordered list of component types / data. This makes the implementation smaller and opens the door to an easy "nested bundle" implementation in the future (which i might even add in this pr). Duplicate detection is now done once per bundle type by World the first time a bundle is used.
## Unified WorldQuery and QueryFilter types
(don't worry they are still separate type _parameters_ in Queries .. this is a non-breaking change)
WorldQuery and QueryFilter were already basically identical apis. With the addition of `FetchState` and more storage-specific fetch methods, the overlap was even clearer (and the redundancy more painful).
QueryFilters are now just `F: WorldQuery where F::Fetch: FilterFetch`. FilterFetch requires `Fetch<Item = bool>` and adds new "short circuit" variants of fetch methods. This enables a filter tuple like `(With<A>, Without<B>, Changed<C>)` to stop evaluating the filter after the first mismatch is encountered. FilterFetch is automatically implemented for `Fetch` implementations that return bool.
This forces fetch implementations that return things like `(bool, bool, bool)` (such as the filter above) to manually implement FilterFetch and decide whether or not to short-circuit.
## More Granular Modules
World no longer globs all of the internal modules together. It now exports `core`, `system`, and `schedule` separately. I'm also considering exporting `core` submodules directly as that is still pretty "glob-ey" and unorganized (feedback welcome here).
## Remaining Draft Work (to be done in this pr)
* ~~panic on conflicting WorldQuery fetches (&A, &mut A)~~
* ~~bevy `main` and hecs both currently allow this, but we should protect against it if possible~~
* ~~batch_iter / par_iter (currently stubbed out)~~
* ~~ChangedRes~~
* ~~I skipped this while we sort out #1313. This pr should be adapted to account for whatever we land on there~~.
* ~~The `Archetypes` and `Tables` collections use hashes of sorted lists of component ids to uniquely identify each archetype/table. This hash is then used as the key in a HashMap to look up the relevant ArchetypeId or TableId. (which doesn't handle hash collisions properly)~~
* ~~It is currently unsafe to generate a Query from "World A", then use it on "World B" (despite the api claiming it is safe). We should probably close this gap. This could be done by adding a randomly generated WorldId to each world, then storing that id in each Query. They could then be compared to each other on each `query.do_thing(&world)` operation. This _does_ add an extra branch to each query operation, so I'm open to other suggestions if people have them.~~
* ~~Nested Bundles (if i find time)~~
## Potential Future Work
* Expand WorldCell to support queries.
* Consider not allocating in the empty archetype on `world.spawn()`
* ex: return something like EntityMutUninit, which turns into EntityMut after an `insert` or `insert_bundle` op
* this actually regressed performance last time i tried it, but in theory it should be faster
* Optimize SparseSet::insert (see `PERF` comment on insert)
* Replace SparseArray `Option<T>` with T::MAX to cut down on branching
* would enable cheaper get_unchecked() operations
* upstream fixedbitset optimizations
* fixedbitset could be allocation free for small block counts (store blocks in a SmallVec)
* fixedbitset could have a const constructor
* Consider implementing Tags (archetype-specific by-value data that affects archetype identity)
* ex: ArchetypeA could have `[A, B, C]` table components and `[D(1)]` "tag" component. ArchetypeB could have `[A, B, C]` table components and a `[D(2)]` tag component. The archetypes are different, despite both having D tags because the value inside D is different.
* this could potentially build on top of the `archetype.unique_components` added in this pr for resource storage.
* Consider reverting `all_tuples` proc macro in favor of the old `macro_rules` implementation
* all_tuples is more flexible and produces cleaner documentation (the macro_rules version produces weird type parameter orders due to parser constraints)
* but unfortunately all_tuples also appears to make Rust Analyzer sad/slow when working inside of `bevy_ecs` (does not affect user code)
* Consider "resource queries" and/or "mixed resource and entity component queries" as an alternative to WorldCell
* this is basically just "systems" so maybe it's not worth it
* Add more world ops
* `world.clear()`
* `world.reserve<T: Bundle>(count: usize)`
* Try using the old archetype allocation strategy (allocate new memory on resize and copy everything over). I expect this to improve batch insertion performance at the cost of unbatched performance. But thats just a guess. I'm not an allocation perf pro :)
* Adapt Commands apis for consistency with new World apis
## Benchmarks
key:
* `bevy_old`: bevy `main` branch
* `bevy`: this branch
* `_foreach`: uses an optimized for_each iterator
* ` _sparse`: uses sparse set storage (if unspecified assume table storage)
* `_system`: runs inside a system (if unspecified assume test happens via direct world ops)
### Simple Insert (from ecs_bench_suite)
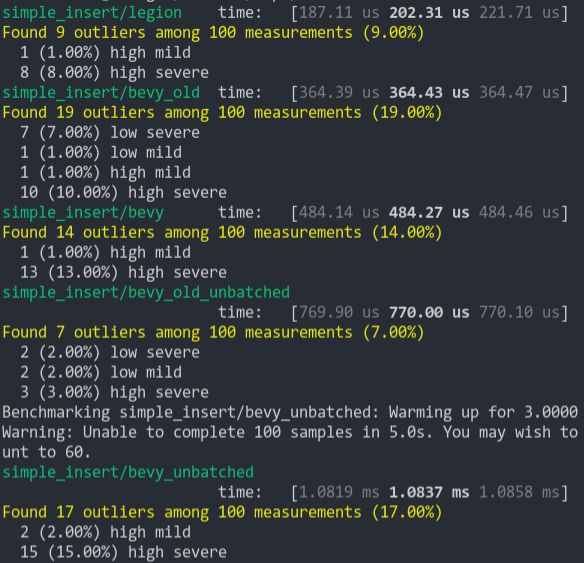
### Simpler Iter (from ecs_bench_suite)
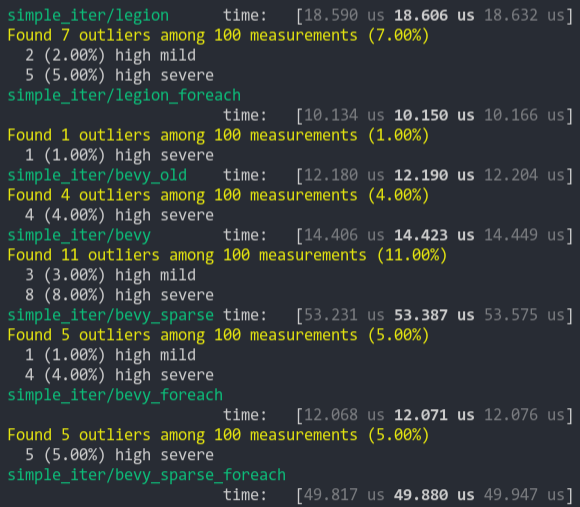
### Fragment Iter (from ecs_bench_suite)
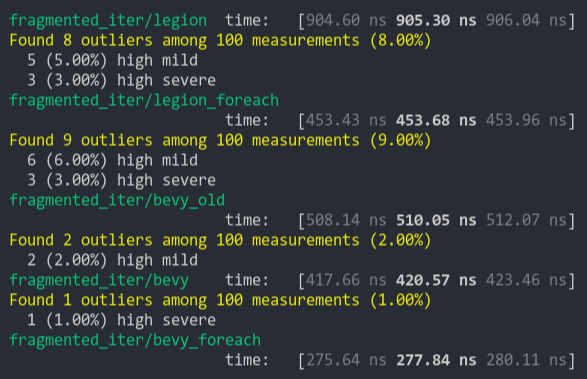
### Sparse Fragmented Iter
Iterate a query that matches 5 entities from a single matching archetype, but there are 100 unmatching archetypes
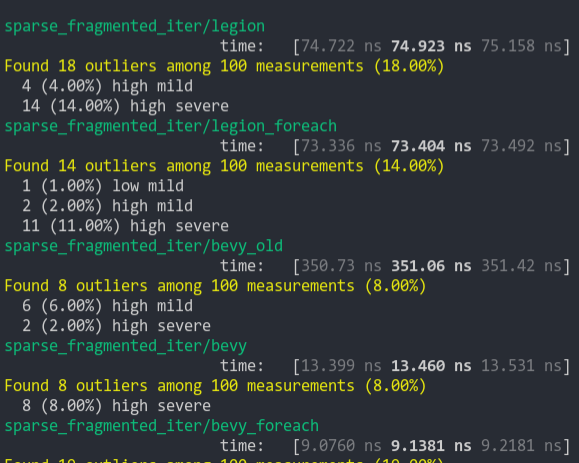
### Schedule (from ecs_bench_suite)
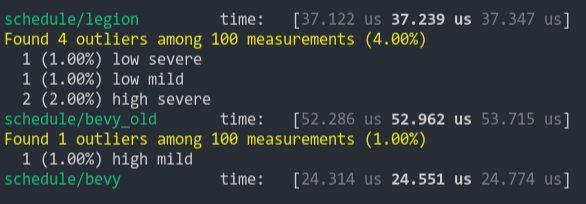
### Add Remove Component (from ecs_bench_suite)
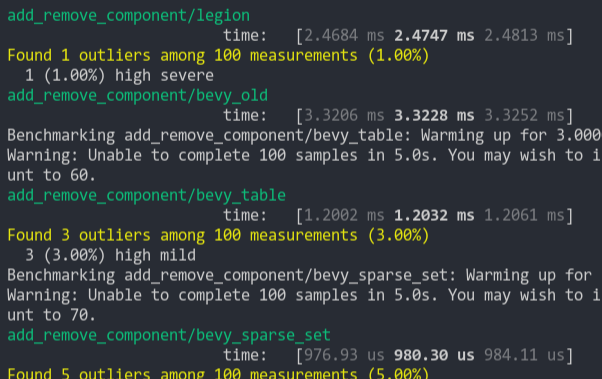
### Add Remove Component Big
Same as the test above, but each entity has 5 "large" matrix components and 1 "large" matrix component is added and removed
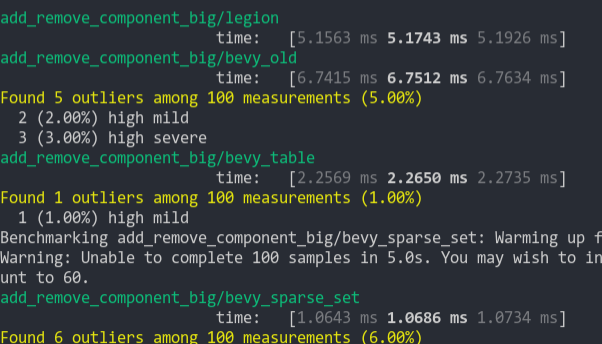
### Get Component
Looks up a single component value a large number of times
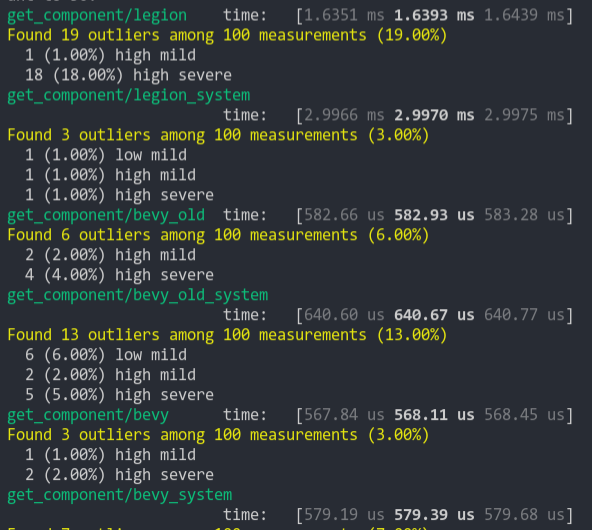
2021-03-05 07:54:35 +00:00
. unwrap ( ) ;
Add new SystemState and rename old SystemState to SystemMeta (#2283)
This enables `SystemParams` to be used outside of function systems. Anything can create and store `SystemState`, which enables efficient "param state cached" access to `SystemParams`.
It adds a `ReadOnlySystemParamFetch` trait, which enables safe `SystemState::get` calls without unique world access.
I renamed the old `SystemState` to `SystemMeta` to enable us to mirror the `QueryState` naming convention (but I'm happy to discuss alternative names if people have other ideas). I initially pitched this as `ParamState`, but given that it needs to include full system metadata, that doesn't feel like a particularly accurate name.
```rust
#[derive(Eq, PartialEq, Debug)]
struct A(usize);
#[derive(Eq, PartialEq, Debug)]
struct B(usize);
let mut world = World::default();
world.insert_resource(A(42));
world.spawn().insert(B(7));
// we get nice lifetime elision when declaring the type on the left hand side
let mut system_state: SystemState<(Res<A>, Query<&B>)> = SystemState::new(&mut world);
let (a, query) = system_state.get(&world);
assert_eq!(*a, A(42), "returned resource matches initial value");
assert_eq!(
*query.single().unwrap(),
B(7),
"returned component matches initial value"
);
// mutable system params require unique world access
let mut system_state: SystemState<(ResMut<A>, Query<&mut B>)> = SystemState::new(&mut world);
let (a, query) = system_state.get_mut(&mut world);
// static lifetimes are required when declaring inside of structs
struct SomeContainer {
state: SystemState<(Res<'static, A>, Res<'static, B>)>
}
// this can be shortened using type aliases, which will be useful for complex param tuples
type MyParams<'a> = (Res<'a, A>, Res<'a, B>);
struct SomeContainer {
state: SystemState<MyParams<'static>>
}
// It is the user's responsibility to call SystemState::apply(world) for parameters that queue up work
let mut system_state: SystemState<(Commands, Query<&B>)> = SystemState::new(&mut world);
{
let (mut commands, query) = system_state.get(&world);
commands.insert_resource(3.14);
}
system_state.apply(&mut world);
```
## Future Work
* Actually use SystemState inside FunctionSystem. This would be trivial, but it requires FunctionSystem to wrap SystemState in Option in its current form (which complicates system metadata lookup). I'd prefer to hold off until we adopt something like the later designs linked in #1364, which enable us to contruct Systems using a World reference (and also remove the need for `.system`).
* Consider a "scoped" approach to automatically call SystemState::apply when systems params are no longer being used (either a container type with a Drop impl, or a function that takes a closure for user logic operating on params).
2021-06-02 19:57:38 +00:00
system_meta
Bevy ECS V2 (#1525)
# Bevy ECS V2
This is a rewrite of Bevy ECS (basically everything but the new executor/schedule, which are already awesome). The overall goal was to improve the performance and versatility of Bevy ECS. Here is a quick bulleted list of changes before we dive into the details:
* Complete World rewrite
* Multiple component storage types:
* Tables: fast cache friendly iteration, slower add/removes (previously called Archetypes)
* Sparse Sets: fast add/remove, slower iteration
* Stateful Queries (caches query results for faster iteration. fragmented iteration is _fast_ now)
* Stateful System Params (caches expensive operations. inspired by @DJMcNab's work in #1364)
* Configurable System Params (users can set configuration when they construct their systems. once again inspired by @DJMcNab's work)
* Archetypes are now "just metadata", component storage is separate
* Archetype Graph (for faster archetype changes)
* Component Metadata
* Configure component storage type
* Retrieve information about component size/type/name/layout/send-ness/etc
* Components are uniquely identified by a densely packed ComponentId
* TypeIds are now totally optional (which should make implementing scripting easier)
* Super fast "for_each" query iterators
* Merged Resources into World. Resources are now just a special type of component
* EntityRef/EntityMut builder apis (more efficient and more ergonomic)
* Fast bitset-backed `Access<T>` replaces old hashmap-based approach everywhere
* Query conflicts are determined by component access instead of archetype component access (to avoid random failures at runtime)
* With/Without are still taken into account for conflicts, so this should still be comfy to use
* Much simpler `IntoSystem` impl
* Significantly reduced the amount of hashing throughout the ecs in favor of Sparse Sets (indexed by densely packed ArchetypeId, ComponentId, BundleId, and TableId)
* Safety Improvements
* Entity reservation uses a normal world reference instead of unsafe transmute
* QuerySets no longer transmute lifetimes
* Made traits "unsafe" where relevant
* More thorough safety docs
* WorldCell
* Exposes safe mutable access to multiple resources at a time in a World
* Replaced "catch all" `System::update_archetypes(world: &World)` with `System::new_archetype(archetype: &Archetype)`
* Simpler Bundle implementation
* Replaced slow "remove_bundle_one_by_one" used as fallback for Commands::remove_bundle with fast "remove_bundle_intersection"
* Removed `Mut<T>` query impl. it is better to only support one way: `&mut T`
* Removed with() from `Flags<T>` in favor of `Option<Flags<T>>`, which allows querying for flags to be "filtered" by default
* Components now have is_send property (currently only resources support non-send)
* More granular module organization
* New `RemovedComponents<T>` SystemParam that replaces `query.removed::<T>()`
* `world.resource_scope()` for mutable access to resources and world at the same time
* WorldQuery and QueryFilter traits unified. FilterFetch trait added to enable "short circuit" filtering. Auto impled for cases that don't need it
* Significantly slimmed down SystemState in favor of individual SystemParam state
* System Commands changed from `commands: &mut Commands` back to `mut commands: Commands` (to allow Commands to have a World reference)
Fixes #1320
## `World` Rewrite
This is a from-scratch rewrite of `World` that fills the niche that `hecs` used to. Yes, this means Bevy ECS is no longer a "fork" of hecs. We're going out our own!
(the only shared code between the projects is the entity id allocator, which is already basically ideal)
A huge shout out to @SanderMertens (author of [flecs](https://github.com/SanderMertens/flecs)) for sharing some great ideas with me (specifically hybrid ecs storage and archetype graphs). He also helped advise on a number of implementation details.
## Component Storage (The Problem)
Two ECS storage paradigms have gained a lot of traction over the years:
* **Archetypal ECS**:
* Stores components in "tables" with static schemas. Each "column" stores components of a given type. Each "row" is an entity.
* Each "archetype" has its own table. Adding/removing an entity's component changes the archetype.
* Enables super-fast Query iteration due to its cache-friendly data layout
* Comes at the cost of more expensive add/remove operations for an Entity's components, because all components need to be copied to the new archetype's "table"
* **Sparse Set ECS**:
* Stores components of the same type in densely packed arrays, which are sparsely indexed by densely packed unsigned integers (Entity ids)
* Query iteration is slower than Archetypal ECS because each entity's component could be at any position in the sparse set. This "random access" pattern isn't cache friendly. Additionally, there is an extra layer of indirection because you must first map the entity id to an index in the component array.
* Adding/removing components is a cheap, constant time operation
Bevy ECS V1, hecs, legion, flec, and Unity DOTS are all "archetypal ecs-es". I personally think "archetypal" storage is a good default for game engines. An entity's archetype doesn't need to change frequently in general, and it creates "fast by default" query iteration (which is a much more common operation). It is also "self optimizing". Users don't need to think about optimizing component layouts for iteration performance. It "just works" without any extra boilerplate.
Shipyard and EnTT are "sparse set ecs-es". They employ "packing" as a way to work around the "suboptimal by default" iteration performance for specific sets of components. This helps, but I didn't think this was a good choice for a general purpose engine like Bevy because:
1. "packs" conflict with each other. If bevy decides to internally pack the Transform and GlobalTransform components, users are then blocked if they want to pack some custom component with Transform.
2. users need to take manual action to optimize
Developers selecting an ECS framework are stuck with a hard choice. Select an "archetypal" framework with "fast iteration everywhere" but without the ability to cheaply add/remove components, or select a "sparse set" framework to cheaply add/remove components but with slower iteration performance.
## Hybrid Component Storage (The Solution)
In Bevy ECS V2, we get to have our cake and eat it too. It now has _both_ of the component storage types above (and more can be added later if needed):
* **Tables** (aka "archetypal" storage)
* The default storage. If you don't configure anything, this is what you get
* Fast iteration by default
* Slower add/remove operations
* **Sparse Sets**
* Opt-in
* Slower iteration
* Faster add/remove operations
These storage types complement each other perfectly. By default Query iteration is fast. If developers know that they want to add/remove a component at high frequencies, they can set the storage to "sparse set":
```rust
world.register_component(
ComponentDescriptor::new::<MyComponent>(StorageType::SparseSet)
).unwrap();
```
## Archetypes
Archetypes are now "just metadata" ... they no longer store components directly. They do store:
* The `ComponentId`s of each of the Archetype's components (and that component's storage type)
* Archetypes are uniquely defined by their component layouts
* For example: entities with "table" components `[A, B, C]` _and_ "sparse set" components `[D, E]` will always be in the same archetype.
* The `TableId` associated with the archetype
* For now each archetype has exactly one table (which can have no components),
* There is a 1->Many relationship from Tables->Archetypes. A given table could have any number of archetype components stored in it:
* Ex: an entity with "table storage" components `[A, B, C]` and "sparse set" components `[D, E]` will share the same `[A, B, C]` table as an entity with `[A, B, C]` table component and `[F]` sparse set components.
* This 1->Many relationship is how we preserve fast "cache friendly" iteration performance when possible (more on this later)
* A list of entities that are in the archetype and the row id of the table they are in
* ArchetypeComponentIds
* unique densely packed identifiers for (ArchetypeId, ComponentId) pairs
* used by the schedule executor for cheap system access control
* "Archetype Graph Edges" (see the next section)
## The "Archetype Graph"
Archetype changes in Bevy (and a number of other archetypal ecs-es) have historically been expensive to compute. First, you need to allocate a new vector of the entity's current component ids, add or remove components based on the operation performed, sort it (to ensure it is order-independent), then hash it to find the archetype (if it exists). And thats all before we get to the _already_ expensive full copy of all components to the new table storage.
The solution is to build a "graph" of archetypes to cache these results. @SanderMertens first exposed me to the idea (and he got it from @gjroelofs, who came up with it). They propose adding directed edges between archetypes for add/remove component operations. If `ComponentId`s are densely packed, you can use sparse sets to cheaply jump between archetypes.
Bevy takes this one step further by using add/remove `Bundle` edges instead of `Component` edges. Bevy encourages the use of `Bundles` to group add/remove operations. This is largely for "clearer game logic" reasons, but it also helps cut down on the number of archetype changes required. `Bundles` now also have densely-packed `BundleId`s. This allows us to use a _single_ edge for each bundle operation (rather than needing to traverse N edges ... one for each component). Single component operations are also bundles, so this is strictly an improvement over a "component only" graph.
As a result, an operation that used to be _heavy_ (both for allocations and compute) is now two dirt-cheap array lookups and zero allocations.
## Stateful Queries
World queries are now stateful. This allows us to:
1. Cache archetype (and table) matches
* This resolves another issue with (naive) archetypal ECS: query performance getting worse as the number of archetypes goes up (and fragmentation occurs).
2. Cache Fetch and Filter state
* The expensive parts of fetch/filter operations (such as hashing the TypeId to find the ComponentId) now only happen once when the Query is first constructed
3. Incrementally build up state
* When new archetypes are added, we only process the new archetypes (no need to rebuild state for old archetypes)
As a result, the direct `World` query api now looks like this:
```rust
let mut query = world.query::<(&A, &mut B)>();
for (a, mut b) in query.iter_mut(&mut world) {
}
```
Requiring `World` to generate stateful queries (rather than letting the `QueryState` type be constructed separately) allows us to ensure that _all_ queries are properly initialized (and the relevant world state, such as ComponentIds). This enables QueryState to remove branches from its operations that check for initialization status (and also enables query.iter() to take an immutable world reference because it doesn't need to initialize anything in world).
However in systems, this is a non-breaking change. State management is done internally by the relevant SystemParam.
## Stateful SystemParams
Like Queries, `SystemParams` now also cache state. For example, `Query` system params store the "stateful query" state mentioned above. Commands store their internal `CommandQueue`. This means you can now safely use as many separate `Commands` parameters in your system as you want. `Local<T>` system params store their `T` value in their state (instead of in Resources).
SystemParam state also enabled a significant slim-down of SystemState. It is much nicer to look at now.
Per-SystemParam state naturally insulates us from an "aliased mut" class of errors we have hit in the past (ex: using multiple `Commands` system params).
(credit goes to @DJMcNab for the initial idea and draft pr here #1364)
## Configurable SystemParams
@DJMcNab also had the great idea to make SystemParams configurable. This allows users to provide some initial configuration / values for system parameters (when possible). Most SystemParams have no config (the config type is `()`), but the `Local<T>` param now supports user-provided parameters:
```rust
fn foo(value: Local<usize>) {
}
app.add_system(foo.system().config(|c| c.0 = Some(10)));
```
## Uber Fast "for_each" Query Iterators
Developers now have the choice to use a fast "for_each" iterator, which yields ~1.5-3x iteration speed improvements for "fragmented iteration", and minor ~1.2x iteration speed improvements for unfragmented iteration.
```rust
fn system(query: Query<(&A, &mut B)>) {
// you now have the option to do this for a speed boost
query.for_each_mut(|(a, mut b)| {
});
// however normal iterators are still available
for (a, mut b) in query.iter_mut() {
}
}
```
I think in most cases we should continue to encourage "normal" iterators as they are more flexible and more "rust idiomatic". But when that extra "oomf" is needed, it makes sense to use `for_each`.
We should also consider using `for_each` for internal bevy systems to give our users a nice speed boost (but that should be a separate pr).
## Component Metadata
`World` now has a `Components` collection, which is accessible via `world.components()`. This stores mappings from `ComponentId` to `ComponentInfo`, as well as `TypeId` to `ComponentId` mappings (where relevant). `ComponentInfo` stores information about the component, such as ComponentId, TypeId, memory layout, send-ness (currently limited to resources), and storage type.
## Significantly Cheaper `Access<T>`
We used to use `TypeAccess<TypeId>` to manage read/write component/archetype-component access. This was expensive because TypeIds must be hashed and compared individually. The parallel executor got around this by "condensing" type ids into bitset-backed access types. This worked, but it had to be re-generated from the `TypeAccess<TypeId>`sources every time archetypes changed.
This pr removes TypeAccess in favor of faster bitset access everywhere. We can do this thanks to the move to densely packed `ComponentId`s and `ArchetypeComponentId`s.
## Merged Resources into World
Resources had a lot of redundant functionality with Components. They stored typed data, they had access control, they had unique ids, they were queryable via SystemParams, etc. In fact the _only_ major difference between them was that they were unique (and didn't correlate to an entity).
Separate resources also had the downside of requiring a separate set of access controls, which meant the parallel executor needed to compare more bitsets per system and manage more state.
I initially got the "separate resources" idea from `legion`. I think that design was motivated by the fact that it made the direct world query/resource lifetime interactions more manageable. It certainly made our lives easier when using Resources alongside hecs/bevy_ecs. However we already have a construct for safely and ergonomically managing in-world lifetimes: systems (which use `Access<T>` internally).
This pr merges Resources into World:
```rust
world.insert_resource(1);
world.insert_resource(2.0);
let a = world.get_resource::<i32>().unwrap();
let mut b = world.get_resource_mut::<f64>().unwrap();
*b = 3.0;
```
Resources are now just a special kind of component. They have their own ComponentIds (and their own resource TypeId->ComponentId scope, so they don't conflict wit components of the same type). They are stored in a special "resource archetype", which stores components inside the archetype using a new `unique_components` sparse set (note that this sparse set could later be used to implement Tags). This allows us to keep the code size small by reusing existing datastructures (namely Column, Archetype, ComponentFlags, and ComponentInfo). This allows us the executor to use a single `Access<ArchetypeComponentId>` per system. It should also make scripting language integration easier.
_But_ this merge did create problems for people directly interacting with `World`. What if you need mutable access to multiple resources at the same time? `world.get_resource_mut()` borrows World mutably!
## WorldCell
WorldCell applies the `Access<ArchetypeComponentId>` concept to direct world access:
```rust
let world_cell = world.cell();
let a = world_cell.get_resource_mut::<i32>().unwrap();
let b = world_cell.get_resource_mut::<f64>().unwrap();
```
This adds cheap runtime checks (a sparse set lookup of `ArchetypeComponentId` and a counter) to ensure that world accesses do not conflict with each other. Each operation returns a `WorldBorrow<'w, T>` or `WorldBorrowMut<'w, T>` wrapper type, which will release the relevant ArchetypeComponentId resources when dropped.
World caches the access sparse set (and only one cell can exist at a time), so `world.cell()` is a cheap operation.
WorldCell does _not_ use atomic operations. It is non-send, does a mutable borrow of world to prevent other accesses, and uses a simple `Rc<RefCell<ArchetypeComponentAccess>>` wrapper in each WorldBorrow pointer.
The api is currently limited to resource access, but it can and should be extended to queries / entity component access.
## Resource Scopes
WorldCell does not yet support component queries, and even when it does there are sometimes legitimate reasons to want a mutable world ref _and_ a mutable resource ref (ex: bevy_render and bevy_scene both need this). In these cases we could always drop down to the unsafe `world.get_resource_unchecked_mut()`, but that is not ideal!
Instead developers can use a "resource scope"
```rust
world.resource_scope(|world: &mut World, a: &mut A| {
})
```
This temporarily removes the `A` resource from `World`, provides mutable pointers to both, and re-adds A to World when finished. Thanks to the move to ComponentIds/sparse sets, this is a cheap operation.
If multiple resources are required, scopes can be nested. We could also consider adding a "resource tuple" to the api if this pattern becomes common and the boilerplate gets nasty.
## Query Conflicts Use ComponentId Instead of ArchetypeComponentId
For safety reasons, systems cannot contain queries that conflict with each other without wrapping them in a QuerySet. On bevy `main`, we use ArchetypeComponentIds to determine conflicts. This is nice because it can take into account filters:
```rust
// these queries will never conflict due to their filters
fn filter_system(a: Query<&mut A, With<B>>, b: Query<&mut B, Without<B>>) {
}
```
But it also has a significant downside:
```rust
// these queries will not conflict _until_ an entity with A, B, and C is spawned
fn maybe_conflicts_system(a: Query<(&mut A, &C)>, b: Query<(&mut A, &B)>) {
}
```
The system above will panic at runtime if an entity with A, B, and C is spawned. This makes it hard to trust that your game logic will run without crashing.
In this pr, I switched to using `ComponentId` instead. This _is_ more constraining. `maybe_conflicts_system` will now always fail, but it will do it consistently at startup. Naively, it would also _disallow_ `filter_system`, which would be a significant downgrade in usability. Bevy has a number of internal systems that rely on disjoint queries and I expect it to be a common pattern in userspace.
To resolve this, I added a new `FilteredAccess<T>` type, which wraps `Access<T>` and adds with/without filters. If two `FilteredAccess` have with/without values that prove they are disjoint, they will no longer conflict.
## EntityRef / EntityMut
World entity operations on `main` require that the user passes in an `entity` id to each operation:
```rust
let entity = world.spawn((A, )); // create a new entity with A
world.get::<A>(entity);
world.insert(entity, (B, C));
world.insert_one(entity, D);
```
This means that each operation needs to look up the entity location / verify its validity. The initial spawn operation also requires a Bundle as input. This can be awkward when no components are required (or one component is required).
These operations have been replaced by `EntityRef` and `EntityMut`, which are "builder-style" wrappers around world that provide read and read/write operations on a single, pre-validated entity:
```rust
// spawn now takes no inputs and returns an EntityMut
let entity = world.spawn()
.insert(A) // insert a single component into the entity
.insert_bundle((B, C)) // insert a bundle of components into the entity
.id() // id returns the Entity id
// Returns EntityMut (or panics if the entity does not exist)
world.entity_mut(entity)
.insert(D)
.insert_bundle(SomeBundle::default());
{
// returns EntityRef (or panics if the entity does not exist)
let d = world.entity(entity)
.get::<D>() // gets the D component
.unwrap();
// world.get still exists for ergonomics
let d = world.get::<D>(entity).unwrap();
}
// These variants return Options if you want to check existence instead of panicing
world.get_entity_mut(entity)
.unwrap()
.insert(E);
if let Some(entity_ref) = world.get_entity(entity) {
let d = entity_ref.get::<D>().unwrap();
}
```
This _does not_ affect the current Commands api or terminology. I think that should be a separate conversation as that is a much larger breaking change.
## Safety Improvements
* Entity reservation in Commands uses a normal world borrow instead of an unsafe transmute
* QuerySets no longer transmutes lifetimes
* Made traits "unsafe" when implementing a trait incorrectly could cause unsafety
* More thorough safety docs
## RemovedComponents SystemParam
The old approach to querying removed components: `query.removed:<T>()` was confusing because it had no connection to the query itself. I replaced it with the following, which is both clearer and allows us to cache the ComponentId mapping in the SystemParamState:
```rust
fn system(removed: RemovedComponents<T>) {
for entity in removed.iter() {
}
}
```
## Simpler Bundle implementation
Bundles are no longer responsible for sorting (or deduping) TypeInfo. They are just a simple ordered list of component types / data. This makes the implementation smaller and opens the door to an easy "nested bundle" implementation in the future (which i might even add in this pr). Duplicate detection is now done once per bundle type by World the first time a bundle is used.
## Unified WorldQuery and QueryFilter types
(don't worry they are still separate type _parameters_ in Queries .. this is a non-breaking change)
WorldQuery and QueryFilter were already basically identical apis. With the addition of `FetchState` and more storage-specific fetch methods, the overlap was even clearer (and the redundancy more painful).
QueryFilters are now just `F: WorldQuery where F::Fetch: FilterFetch`. FilterFetch requires `Fetch<Item = bool>` and adds new "short circuit" variants of fetch methods. This enables a filter tuple like `(With<A>, Without<B>, Changed<C>)` to stop evaluating the filter after the first mismatch is encountered. FilterFetch is automatically implemented for `Fetch` implementations that return bool.
This forces fetch implementations that return things like `(bool, bool, bool)` (such as the filter above) to manually implement FilterFetch and decide whether or not to short-circuit.
## More Granular Modules
World no longer globs all of the internal modules together. It now exports `core`, `system`, and `schedule` separately. I'm also considering exporting `core` submodules directly as that is still pretty "glob-ey" and unorganized (feedback welcome here).
## Remaining Draft Work (to be done in this pr)
* ~~panic on conflicting WorldQuery fetches (&A, &mut A)~~
* ~~bevy `main` and hecs both currently allow this, but we should protect against it if possible~~
* ~~batch_iter / par_iter (currently stubbed out)~~
* ~~ChangedRes~~
* ~~I skipped this while we sort out #1313. This pr should be adapted to account for whatever we land on there~~.
* ~~The `Archetypes` and `Tables` collections use hashes of sorted lists of component ids to uniquely identify each archetype/table. This hash is then used as the key in a HashMap to look up the relevant ArchetypeId or TableId. (which doesn't handle hash collisions properly)~~
* ~~It is currently unsafe to generate a Query from "World A", then use it on "World B" (despite the api claiming it is safe). We should probably close this gap. This could be done by adding a randomly generated WorldId to each world, then storing that id in each Query. They could then be compared to each other on each `query.do_thing(&world)` operation. This _does_ add an extra branch to each query operation, so I'm open to other suggestions if people have them.~~
* ~~Nested Bundles (if i find time)~~
## Potential Future Work
* Expand WorldCell to support queries.
* Consider not allocating in the empty archetype on `world.spawn()`
* ex: return something like EntityMutUninit, which turns into EntityMut after an `insert` or `insert_bundle` op
* this actually regressed performance last time i tried it, but in theory it should be faster
* Optimize SparseSet::insert (see `PERF` comment on insert)
* Replace SparseArray `Option<T>` with T::MAX to cut down on branching
* would enable cheaper get_unchecked() operations
* upstream fixedbitset optimizations
* fixedbitset could be allocation free for small block counts (store blocks in a SmallVec)
* fixedbitset could have a const constructor
* Consider implementing Tags (archetype-specific by-value data that affects archetype identity)
* ex: ArchetypeA could have `[A, B, C]` table components and `[D(1)]` "tag" component. ArchetypeB could have `[A, B, C]` table components and a `[D(2)]` tag component. The archetypes are different, despite both having D tags because the value inside D is different.
* this could potentially build on top of the `archetype.unique_components` added in this pr for resource storage.
* Consider reverting `all_tuples` proc macro in favor of the old `macro_rules` implementation
* all_tuples is more flexible and produces cleaner documentation (the macro_rules version produces weird type parameter orders due to parser constraints)
* but unfortunately all_tuples also appears to make Rust Analyzer sad/slow when working inside of `bevy_ecs` (does not affect user code)
* Consider "resource queries" and/or "mixed resource and entity component queries" as an alternative to WorldCell
* this is basically just "systems" so maybe it's not worth it
* Add more world ops
* `world.clear()`
* `world.reserve<T: Bundle>(count: usize)`
* Try using the old archetype allocation strategy (allocate new memory on resize and copy everything over). I expect this to improve batch insertion performance at the cost of unbatched performance. But thats just a guess. I'm not an allocation perf pro :)
* Adapt Commands apis for consistency with new World apis
## Benchmarks
key:
* `bevy_old`: bevy `main` branch
* `bevy`: this branch
* `_foreach`: uses an optimized for_each iterator
* ` _sparse`: uses sparse set storage (if unspecified assume table storage)
* `_system`: runs inside a system (if unspecified assume test happens via direct world ops)
### Simple Insert (from ecs_bench_suite)
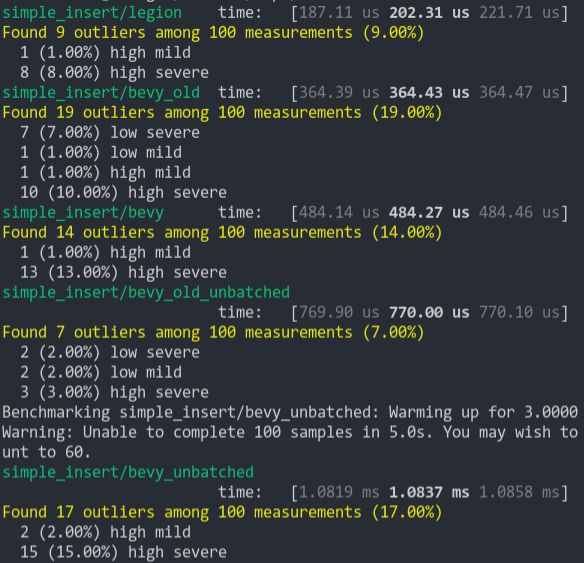
### Simpler Iter (from ecs_bench_suite)
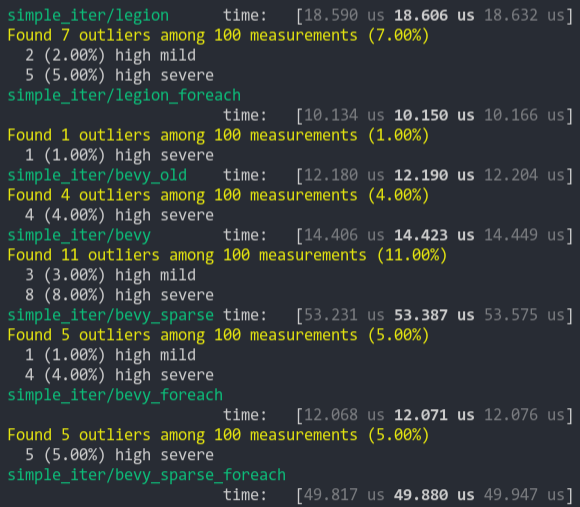
### Fragment Iter (from ecs_bench_suite)
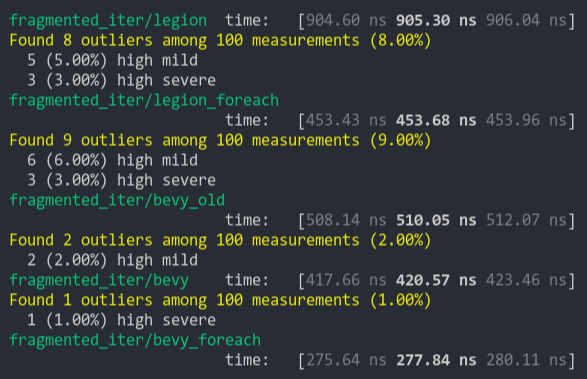
### Sparse Fragmented Iter
Iterate a query that matches 5 entities from a single matching archetype, but there are 100 unmatching archetypes
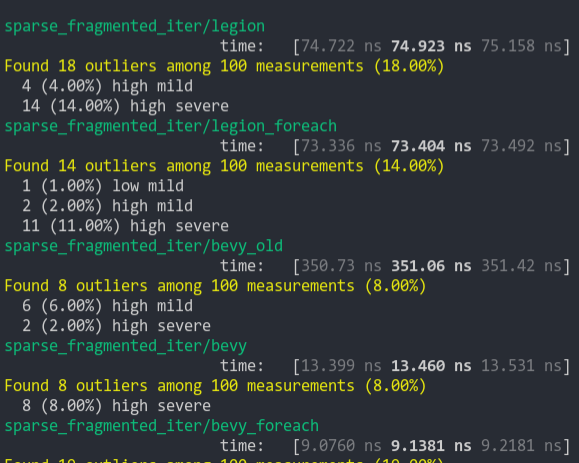
### Schedule (from ecs_bench_suite)
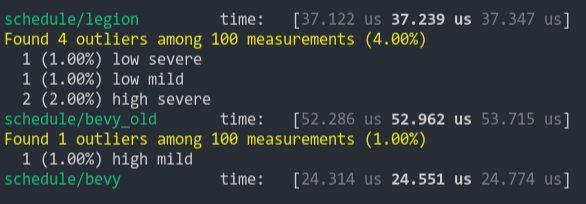
### Add Remove Component (from ecs_bench_suite)
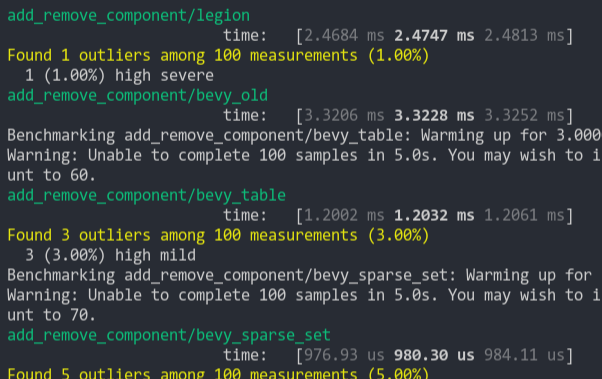
### Add Remove Component Big
Same as the test above, but each entity has 5 "large" matrix components and 1 "large" matrix component is added and removed
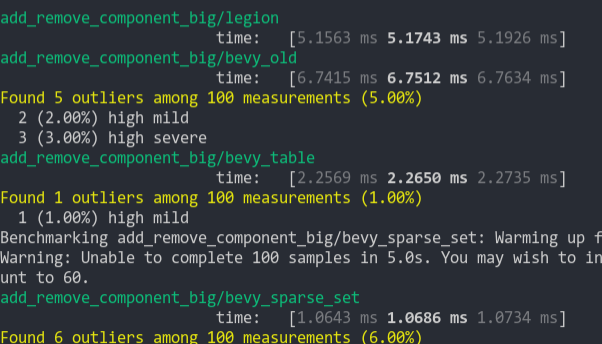
### Get Component
Looks up a single component value a large number of times
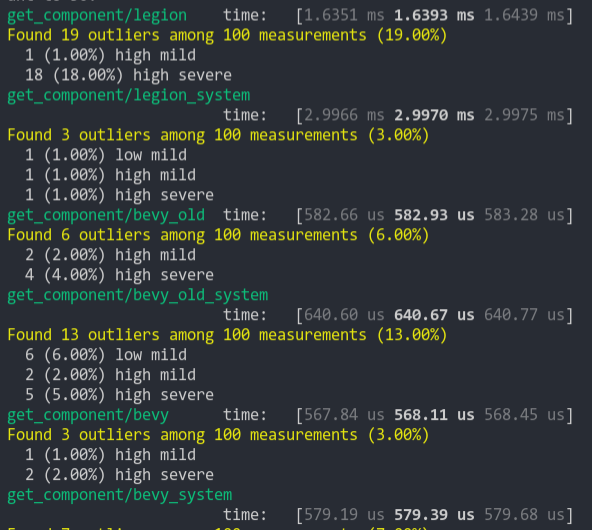
2021-03-05 07:54:35 +00:00
. archetype_component_access
. add_write ( archetype_component_id ) ;
Remove the `SystemParamState` trait and remove types like `ResState` (#6919)
Spiritual successor to #5205.
Actual successor to #6865.
# Objective
Currently, system params are defined using three traits: `SystemParam`, `ReadOnlySystemParam`, `SystemParamState`. The behavior for each param is specified by the `SystemParamState` trait, while `SystemParam` simply defers to the state.
Splitting the traits in this way makes it easier to implement within macros, but it increases the cognitive load. Worst of all, this approach requires each `MySystemParam` to have a public `MySystemParamState` type associated with it.
## Solution
* Merge the trait `SystemParamState` into `SystemParam`.
* Remove all trivial `SystemParam` state types.
* `OptionNonSendMutState<T>`: you will not be missed.
---
- [x] Fix/resolve the remaining test failure.
## Changelog
* Removed the trait `SystemParamState`, merging its functionality into `SystemParam`.
## Migration Guide
**Note**: this should replace the migration guide for #6865.
This is relative to Bevy 0.9, not main.
The traits `SystemParamState` and `SystemParamFetch` have been removed, and their functionality has been transferred to `SystemParam`.
```rust
// Before (0.9)
impl SystemParam for MyParam<'_, '_> {
type State = MyParamState;
}
unsafe impl SystemParamState for MyParamState {
fn init(world: &mut World, system_meta: &mut SystemMeta) -> Self { ... }
}
unsafe impl<'w, 's> SystemParamFetch<'w, 's> for MyParamState {
type Item = MyParam<'w, 's>;
fn get_param(&mut self, ...) -> Self::Item;
}
unsafe impl ReadOnlySystemParamFetch for MyParamState { }
// After (0.10)
unsafe impl SystemParam for MyParam<'_, '_> {
type State = MyParamState;
type Item<'w, 's> = MyParam<'w, 's>;
fn init_state(world: &mut World, system_meta: &mut SystemMeta) -> Self::State { ... }
fn get_param<'w, 's>(state: &mut Self::State, ...) -> Self::Item<'w, 's>;
}
unsafe impl ReadOnlySystemParam for MyParam<'_, '_> { }
```
The trait `ReadOnlySystemParamFetch` has been replaced with `ReadOnlySystemParam`.
```rust
// Before
unsafe impl ReadOnlySystemParamFetch for MyParamState {}
// After
unsafe impl ReadOnlySystemParam for MyParam<'_, '_> {}
```
2023-01-07 23:20:32 +00:00
component_id
Bevy ECS V2 (#1525)
# Bevy ECS V2
This is a rewrite of Bevy ECS (basically everything but the new executor/schedule, which are already awesome). The overall goal was to improve the performance and versatility of Bevy ECS. Here is a quick bulleted list of changes before we dive into the details:
* Complete World rewrite
* Multiple component storage types:
* Tables: fast cache friendly iteration, slower add/removes (previously called Archetypes)
* Sparse Sets: fast add/remove, slower iteration
* Stateful Queries (caches query results for faster iteration. fragmented iteration is _fast_ now)
* Stateful System Params (caches expensive operations. inspired by @DJMcNab's work in #1364)
* Configurable System Params (users can set configuration when they construct their systems. once again inspired by @DJMcNab's work)
* Archetypes are now "just metadata", component storage is separate
* Archetype Graph (for faster archetype changes)
* Component Metadata
* Configure component storage type
* Retrieve information about component size/type/name/layout/send-ness/etc
* Components are uniquely identified by a densely packed ComponentId
* TypeIds are now totally optional (which should make implementing scripting easier)
* Super fast "for_each" query iterators
* Merged Resources into World. Resources are now just a special type of component
* EntityRef/EntityMut builder apis (more efficient and more ergonomic)
* Fast bitset-backed `Access<T>` replaces old hashmap-based approach everywhere
* Query conflicts are determined by component access instead of archetype component access (to avoid random failures at runtime)
* With/Without are still taken into account for conflicts, so this should still be comfy to use
* Much simpler `IntoSystem` impl
* Significantly reduced the amount of hashing throughout the ecs in favor of Sparse Sets (indexed by densely packed ArchetypeId, ComponentId, BundleId, and TableId)
* Safety Improvements
* Entity reservation uses a normal world reference instead of unsafe transmute
* QuerySets no longer transmute lifetimes
* Made traits "unsafe" where relevant
* More thorough safety docs
* WorldCell
* Exposes safe mutable access to multiple resources at a time in a World
* Replaced "catch all" `System::update_archetypes(world: &World)` with `System::new_archetype(archetype: &Archetype)`
* Simpler Bundle implementation
* Replaced slow "remove_bundle_one_by_one" used as fallback for Commands::remove_bundle with fast "remove_bundle_intersection"
* Removed `Mut<T>` query impl. it is better to only support one way: `&mut T`
* Removed with() from `Flags<T>` in favor of `Option<Flags<T>>`, which allows querying for flags to be "filtered" by default
* Components now have is_send property (currently only resources support non-send)
* More granular module organization
* New `RemovedComponents<T>` SystemParam that replaces `query.removed::<T>()`
* `world.resource_scope()` for mutable access to resources and world at the same time
* WorldQuery and QueryFilter traits unified. FilterFetch trait added to enable "short circuit" filtering. Auto impled for cases that don't need it
* Significantly slimmed down SystemState in favor of individual SystemParam state
* System Commands changed from `commands: &mut Commands` back to `mut commands: Commands` (to allow Commands to have a World reference)
Fixes #1320
## `World` Rewrite
This is a from-scratch rewrite of `World` that fills the niche that `hecs` used to. Yes, this means Bevy ECS is no longer a "fork" of hecs. We're going out our own!
(the only shared code between the projects is the entity id allocator, which is already basically ideal)
A huge shout out to @SanderMertens (author of [flecs](https://github.com/SanderMertens/flecs)) for sharing some great ideas with me (specifically hybrid ecs storage and archetype graphs). He also helped advise on a number of implementation details.
## Component Storage (The Problem)
Two ECS storage paradigms have gained a lot of traction over the years:
* **Archetypal ECS**:
* Stores components in "tables" with static schemas. Each "column" stores components of a given type. Each "row" is an entity.
* Each "archetype" has its own table. Adding/removing an entity's component changes the archetype.
* Enables super-fast Query iteration due to its cache-friendly data layout
* Comes at the cost of more expensive add/remove operations for an Entity's components, because all components need to be copied to the new archetype's "table"
* **Sparse Set ECS**:
* Stores components of the same type in densely packed arrays, which are sparsely indexed by densely packed unsigned integers (Entity ids)
* Query iteration is slower than Archetypal ECS because each entity's component could be at any position in the sparse set. This "random access" pattern isn't cache friendly. Additionally, there is an extra layer of indirection because you must first map the entity id to an index in the component array.
* Adding/removing components is a cheap, constant time operation
Bevy ECS V1, hecs, legion, flec, and Unity DOTS are all "archetypal ecs-es". I personally think "archetypal" storage is a good default for game engines. An entity's archetype doesn't need to change frequently in general, and it creates "fast by default" query iteration (which is a much more common operation). It is also "self optimizing". Users don't need to think about optimizing component layouts for iteration performance. It "just works" without any extra boilerplate.
Shipyard and EnTT are "sparse set ecs-es". They employ "packing" as a way to work around the "suboptimal by default" iteration performance for specific sets of components. This helps, but I didn't think this was a good choice for a general purpose engine like Bevy because:
1. "packs" conflict with each other. If bevy decides to internally pack the Transform and GlobalTransform components, users are then blocked if they want to pack some custom component with Transform.
2. users need to take manual action to optimize
Developers selecting an ECS framework are stuck with a hard choice. Select an "archetypal" framework with "fast iteration everywhere" but without the ability to cheaply add/remove components, or select a "sparse set" framework to cheaply add/remove components but with slower iteration performance.
## Hybrid Component Storage (The Solution)
In Bevy ECS V2, we get to have our cake and eat it too. It now has _both_ of the component storage types above (and more can be added later if needed):
* **Tables** (aka "archetypal" storage)
* The default storage. If you don't configure anything, this is what you get
* Fast iteration by default
* Slower add/remove operations
* **Sparse Sets**
* Opt-in
* Slower iteration
* Faster add/remove operations
These storage types complement each other perfectly. By default Query iteration is fast. If developers know that they want to add/remove a component at high frequencies, they can set the storage to "sparse set":
```rust
world.register_component(
ComponentDescriptor::new::<MyComponent>(StorageType::SparseSet)
).unwrap();
```
## Archetypes
Archetypes are now "just metadata" ... they no longer store components directly. They do store:
* The `ComponentId`s of each of the Archetype's components (and that component's storage type)
* Archetypes are uniquely defined by their component layouts
* For example: entities with "table" components `[A, B, C]` _and_ "sparse set" components `[D, E]` will always be in the same archetype.
* The `TableId` associated with the archetype
* For now each archetype has exactly one table (which can have no components),
* There is a 1->Many relationship from Tables->Archetypes. A given table could have any number of archetype components stored in it:
* Ex: an entity with "table storage" components `[A, B, C]` and "sparse set" components `[D, E]` will share the same `[A, B, C]` table as an entity with `[A, B, C]` table component and `[F]` sparse set components.
* This 1->Many relationship is how we preserve fast "cache friendly" iteration performance when possible (more on this later)
* A list of entities that are in the archetype and the row id of the table they are in
* ArchetypeComponentIds
* unique densely packed identifiers for (ArchetypeId, ComponentId) pairs
* used by the schedule executor for cheap system access control
* "Archetype Graph Edges" (see the next section)
## The "Archetype Graph"
Archetype changes in Bevy (and a number of other archetypal ecs-es) have historically been expensive to compute. First, you need to allocate a new vector of the entity's current component ids, add or remove components based on the operation performed, sort it (to ensure it is order-independent), then hash it to find the archetype (if it exists). And thats all before we get to the _already_ expensive full copy of all components to the new table storage.
The solution is to build a "graph" of archetypes to cache these results. @SanderMertens first exposed me to the idea (and he got it from @gjroelofs, who came up with it). They propose adding directed edges between archetypes for add/remove component operations. If `ComponentId`s are densely packed, you can use sparse sets to cheaply jump between archetypes.
Bevy takes this one step further by using add/remove `Bundle` edges instead of `Component` edges. Bevy encourages the use of `Bundles` to group add/remove operations. This is largely for "clearer game logic" reasons, but it also helps cut down on the number of archetype changes required. `Bundles` now also have densely-packed `BundleId`s. This allows us to use a _single_ edge for each bundle operation (rather than needing to traverse N edges ... one for each component). Single component operations are also bundles, so this is strictly an improvement over a "component only" graph.
As a result, an operation that used to be _heavy_ (both for allocations and compute) is now two dirt-cheap array lookups and zero allocations.
## Stateful Queries
World queries are now stateful. This allows us to:
1. Cache archetype (and table) matches
* This resolves another issue with (naive) archetypal ECS: query performance getting worse as the number of archetypes goes up (and fragmentation occurs).
2. Cache Fetch and Filter state
* The expensive parts of fetch/filter operations (such as hashing the TypeId to find the ComponentId) now only happen once when the Query is first constructed
3. Incrementally build up state
* When new archetypes are added, we only process the new archetypes (no need to rebuild state for old archetypes)
As a result, the direct `World` query api now looks like this:
```rust
let mut query = world.query::<(&A, &mut B)>();
for (a, mut b) in query.iter_mut(&mut world) {
}
```
Requiring `World` to generate stateful queries (rather than letting the `QueryState` type be constructed separately) allows us to ensure that _all_ queries are properly initialized (and the relevant world state, such as ComponentIds). This enables QueryState to remove branches from its operations that check for initialization status (and also enables query.iter() to take an immutable world reference because it doesn't need to initialize anything in world).
However in systems, this is a non-breaking change. State management is done internally by the relevant SystemParam.
## Stateful SystemParams
Like Queries, `SystemParams` now also cache state. For example, `Query` system params store the "stateful query" state mentioned above. Commands store their internal `CommandQueue`. This means you can now safely use as many separate `Commands` parameters in your system as you want. `Local<T>` system params store their `T` value in their state (instead of in Resources).
SystemParam state also enabled a significant slim-down of SystemState. It is much nicer to look at now.
Per-SystemParam state naturally insulates us from an "aliased mut" class of errors we have hit in the past (ex: using multiple `Commands` system params).
(credit goes to @DJMcNab for the initial idea and draft pr here #1364)
## Configurable SystemParams
@DJMcNab also had the great idea to make SystemParams configurable. This allows users to provide some initial configuration / values for system parameters (when possible). Most SystemParams have no config (the config type is `()`), but the `Local<T>` param now supports user-provided parameters:
```rust
fn foo(value: Local<usize>) {
}
app.add_system(foo.system().config(|c| c.0 = Some(10)));
```
## Uber Fast "for_each" Query Iterators
Developers now have the choice to use a fast "for_each" iterator, which yields ~1.5-3x iteration speed improvements for "fragmented iteration", and minor ~1.2x iteration speed improvements for unfragmented iteration.
```rust
fn system(query: Query<(&A, &mut B)>) {
// you now have the option to do this for a speed boost
query.for_each_mut(|(a, mut b)| {
});
// however normal iterators are still available
for (a, mut b) in query.iter_mut() {
}
}
```
I think in most cases we should continue to encourage "normal" iterators as they are more flexible and more "rust idiomatic". But when that extra "oomf" is needed, it makes sense to use `for_each`.
We should also consider using `for_each` for internal bevy systems to give our users a nice speed boost (but that should be a separate pr).
## Component Metadata
`World` now has a `Components` collection, which is accessible via `world.components()`. This stores mappings from `ComponentId` to `ComponentInfo`, as well as `TypeId` to `ComponentId` mappings (where relevant). `ComponentInfo` stores information about the component, such as ComponentId, TypeId, memory layout, send-ness (currently limited to resources), and storage type.
## Significantly Cheaper `Access<T>`
We used to use `TypeAccess<TypeId>` to manage read/write component/archetype-component access. This was expensive because TypeIds must be hashed and compared individually. The parallel executor got around this by "condensing" type ids into bitset-backed access types. This worked, but it had to be re-generated from the `TypeAccess<TypeId>`sources every time archetypes changed.
This pr removes TypeAccess in favor of faster bitset access everywhere. We can do this thanks to the move to densely packed `ComponentId`s and `ArchetypeComponentId`s.
## Merged Resources into World
Resources had a lot of redundant functionality with Components. They stored typed data, they had access control, they had unique ids, they were queryable via SystemParams, etc. In fact the _only_ major difference between them was that they were unique (and didn't correlate to an entity).
Separate resources also had the downside of requiring a separate set of access controls, which meant the parallel executor needed to compare more bitsets per system and manage more state.
I initially got the "separate resources" idea from `legion`. I think that design was motivated by the fact that it made the direct world query/resource lifetime interactions more manageable. It certainly made our lives easier when using Resources alongside hecs/bevy_ecs. However we already have a construct for safely and ergonomically managing in-world lifetimes: systems (which use `Access<T>` internally).
This pr merges Resources into World:
```rust
world.insert_resource(1);
world.insert_resource(2.0);
let a = world.get_resource::<i32>().unwrap();
let mut b = world.get_resource_mut::<f64>().unwrap();
*b = 3.0;
```
Resources are now just a special kind of component. They have their own ComponentIds (and their own resource TypeId->ComponentId scope, so they don't conflict wit components of the same type). They are stored in a special "resource archetype", which stores components inside the archetype using a new `unique_components` sparse set (note that this sparse set could later be used to implement Tags). This allows us to keep the code size small by reusing existing datastructures (namely Column, Archetype, ComponentFlags, and ComponentInfo). This allows us the executor to use a single `Access<ArchetypeComponentId>` per system. It should also make scripting language integration easier.
_But_ this merge did create problems for people directly interacting with `World`. What if you need mutable access to multiple resources at the same time? `world.get_resource_mut()` borrows World mutably!
## WorldCell
WorldCell applies the `Access<ArchetypeComponentId>` concept to direct world access:
```rust
let world_cell = world.cell();
let a = world_cell.get_resource_mut::<i32>().unwrap();
let b = world_cell.get_resource_mut::<f64>().unwrap();
```
This adds cheap runtime checks (a sparse set lookup of `ArchetypeComponentId` and a counter) to ensure that world accesses do not conflict with each other. Each operation returns a `WorldBorrow<'w, T>` or `WorldBorrowMut<'w, T>` wrapper type, which will release the relevant ArchetypeComponentId resources when dropped.
World caches the access sparse set (and only one cell can exist at a time), so `world.cell()` is a cheap operation.
WorldCell does _not_ use atomic operations. It is non-send, does a mutable borrow of world to prevent other accesses, and uses a simple `Rc<RefCell<ArchetypeComponentAccess>>` wrapper in each WorldBorrow pointer.
The api is currently limited to resource access, but it can and should be extended to queries / entity component access.
## Resource Scopes
WorldCell does not yet support component queries, and even when it does there are sometimes legitimate reasons to want a mutable world ref _and_ a mutable resource ref (ex: bevy_render and bevy_scene both need this). In these cases we could always drop down to the unsafe `world.get_resource_unchecked_mut()`, but that is not ideal!
Instead developers can use a "resource scope"
```rust
world.resource_scope(|world: &mut World, a: &mut A| {
})
```
This temporarily removes the `A` resource from `World`, provides mutable pointers to both, and re-adds A to World when finished. Thanks to the move to ComponentIds/sparse sets, this is a cheap operation.
If multiple resources are required, scopes can be nested. We could also consider adding a "resource tuple" to the api if this pattern becomes common and the boilerplate gets nasty.
## Query Conflicts Use ComponentId Instead of ArchetypeComponentId
For safety reasons, systems cannot contain queries that conflict with each other without wrapping them in a QuerySet. On bevy `main`, we use ArchetypeComponentIds to determine conflicts. This is nice because it can take into account filters:
```rust
// these queries will never conflict due to their filters
fn filter_system(a: Query<&mut A, With<B>>, b: Query<&mut B, Without<B>>) {
}
```
But it also has a significant downside:
```rust
// these queries will not conflict _until_ an entity with A, B, and C is spawned
fn maybe_conflicts_system(a: Query<(&mut A, &C)>, b: Query<(&mut A, &B)>) {
}
```
The system above will panic at runtime if an entity with A, B, and C is spawned. This makes it hard to trust that your game logic will run without crashing.
In this pr, I switched to using `ComponentId` instead. This _is_ more constraining. `maybe_conflicts_system` will now always fail, but it will do it consistently at startup. Naively, it would also _disallow_ `filter_system`, which would be a significant downgrade in usability. Bevy has a number of internal systems that rely on disjoint queries and I expect it to be a common pattern in userspace.
To resolve this, I added a new `FilteredAccess<T>` type, which wraps `Access<T>` and adds with/without filters. If two `FilteredAccess` have with/without values that prove they are disjoint, they will no longer conflict.
## EntityRef / EntityMut
World entity operations on `main` require that the user passes in an `entity` id to each operation:
```rust
let entity = world.spawn((A, )); // create a new entity with A
world.get::<A>(entity);
world.insert(entity, (B, C));
world.insert_one(entity, D);
```
This means that each operation needs to look up the entity location / verify its validity. The initial spawn operation also requires a Bundle as input. This can be awkward when no components are required (or one component is required).
These operations have been replaced by `EntityRef` and `EntityMut`, which are "builder-style" wrappers around world that provide read and read/write operations on a single, pre-validated entity:
```rust
// spawn now takes no inputs and returns an EntityMut
let entity = world.spawn()
.insert(A) // insert a single component into the entity
.insert_bundle((B, C)) // insert a bundle of components into the entity
.id() // id returns the Entity id
// Returns EntityMut (or panics if the entity does not exist)
world.entity_mut(entity)
.insert(D)
.insert_bundle(SomeBundle::default());
{
// returns EntityRef (or panics if the entity does not exist)
let d = world.entity(entity)
.get::<D>() // gets the D component
.unwrap();
// world.get still exists for ergonomics
let d = world.get::<D>(entity).unwrap();
}
// These variants return Options if you want to check existence instead of panicing
world.get_entity_mut(entity)
.unwrap()
.insert(E);
if let Some(entity_ref) = world.get_entity(entity) {
let d = entity_ref.get::<D>().unwrap();
}
```
This _does not_ affect the current Commands api or terminology. I think that should be a separate conversation as that is a much larger breaking change.
## Safety Improvements
* Entity reservation in Commands uses a normal world borrow instead of an unsafe transmute
* QuerySets no longer transmutes lifetimes
* Made traits "unsafe" when implementing a trait incorrectly could cause unsafety
* More thorough safety docs
## RemovedComponents SystemParam
The old approach to querying removed components: `query.removed:<T>()` was confusing because it had no connection to the query itself. I replaced it with the following, which is both clearer and allows us to cache the ComponentId mapping in the SystemParamState:
```rust
fn system(removed: RemovedComponents<T>) {
for entity in removed.iter() {
}
}
```
## Simpler Bundle implementation
Bundles are no longer responsible for sorting (or deduping) TypeInfo. They are just a simple ordered list of component types / data. This makes the implementation smaller and opens the door to an easy "nested bundle" implementation in the future (which i might even add in this pr). Duplicate detection is now done once per bundle type by World the first time a bundle is used.
## Unified WorldQuery and QueryFilter types
(don't worry they are still separate type _parameters_ in Queries .. this is a non-breaking change)
WorldQuery and QueryFilter were already basically identical apis. With the addition of `FetchState` and more storage-specific fetch methods, the overlap was even clearer (and the redundancy more painful).
QueryFilters are now just `F: WorldQuery where F::Fetch: FilterFetch`. FilterFetch requires `Fetch<Item = bool>` and adds new "short circuit" variants of fetch methods. This enables a filter tuple like `(With<A>, Without<B>, Changed<C>)` to stop evaluating the filter after the first mismatch is encountered. FilterFetch is automatically implemented for `Fetch` implementations that return bool.
This forces fetch implementations that return things like `(bool, bool, bool)` (such as the filter above) to manually implement FilterFetch and decide whether or not to short-circuit.
## More Granular Modules
World no longer globs all of the internal modules together. It now exports `core`, `system`, and `schedule` separately. I'm also considering exporting `core` submodules directly as that is still pretty "glob-ey" and unorganized (feedback welcome here).
## Remaining Draft Work (to be done in this pr)
* ~~panic on conflicting WorldQuery fetches (&A, &mut A)~~
* ~~bevy `main` and hecs both currently allow this, but we should protect against it if possible~~
* ~~batch_iter / par_iter (currently stubbed out)~~
* ~~ChangedRes~~
* ~~I skipped this while we sort out #1313. This pr should be adapted to account for whatever we land on there~~.
* ~~The `Archetypes` and `Tables` collections use hashes of sorted lists of component ids to uniquely identify each archetype/table. This hash is then used as the key in a HashMap to look up the relevant ArchetypeId or TableId. (which doesn't handle hash collisions properly)~~
* ~~It is currently unsafe to generate a Query from "World A", then use it on "World B" (despite the api claiming it is safe). We should probably close this gap. This could be done by adding a randomly generated WorldId to each world, then storing that id in each Query. They could then be compared to each other on each `query.do_thing(&world)` operation. This _does_ add an extra branch to each query operation, so I'm open to other suggestions if people have them.~~
* ~~Nested Bundles (if i find time)~~
## Potential Future Work
* Expand WorldCell to support queries.
* Consider not allocating in the empty archetype on `world.spawn()`
* ex: return something like EntityMutUninit, which turns into EntityMut after an `insert` or `insert_bundle` op
* this actually regressed performance last time i tried it, but in theory it should be faster
* Optimize SparseSet::insert (see `PERF` comment on insert)
* Replace SparseArray `Option<T>` with T::MAX to cut down on branching
* would enable cheaper get_unchecked() operations
* upstream fixedbitset optimizations
* fixedbitset could be allocation free for small block counts (store blocks in a SmallVec)
* fixedbitset could have a const constructor
* Consider implementing Tags (archetype-specific by-value data that affects archetype identity)
* ex: ArchetypeA could have `[A, B, C]` table components and `[D(1)]` "tag" component. ArchetypeB could have `[A, B, C]` table components and a `[D(2)]` tag component. The archetypes are different, despite both having D tags because the value inside D is different.
* this could potentially build on top of the `archetype.unique_components` added in this pr for resource storage.
* Consider reverting `all_tuples` proc macro in favor of the old `macro_rules` implementation
* all_tuples is more flexible and produces cleaner documentation (the macro_rules version produces weird type parameter orders due to parser constraints)
* but unfortunately all_tuples also appears to make Rust Analyzer sad/slow when working inside of `bevy_ecs` (does not affect user code)
* Consider "resource queries" and/or "mixed resource and entity component queries" as an alternative to WorldCell
* this is basically just "systems" so maybe it's not worth it
* Add more world ops
* `world.clear()`
* `world.reserve<T: Bundle>(count: usize)`
* Try using the old archetype allocation strategy (allocate new memory on resize and copy everything over). I expect this to improve batch insertion performance at the cost of unbatched performance. But thats just a guess. I'm not an allocation perf pro :)
* Adapt Commands apis for consistency with new World apis
## Benchmarks
key:
* `bevy_old`: bevy `main` branch
* `bevy`: this branch
* `_foreach`: uses an optimized for_each iterator
* ` _sparse`: uses sparse set storage (if unspecified assume table storage)
* `_system`: runs inside a system (if unspecified assume test happens via direct world ops)
### Simple Insert (from ecs_bench_suite)
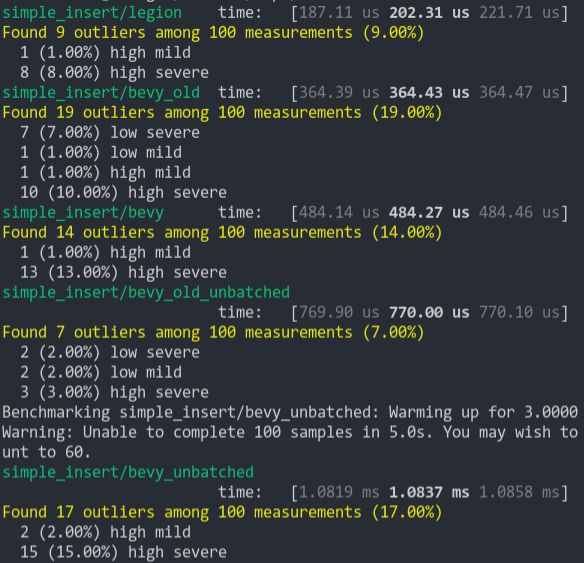
### Simpler Iter (from ecs_bench_suite)
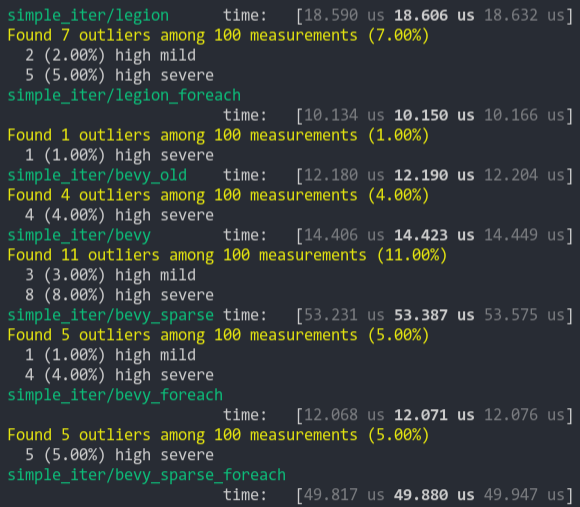
### Fragment Iter (from ecs_bench_suite)
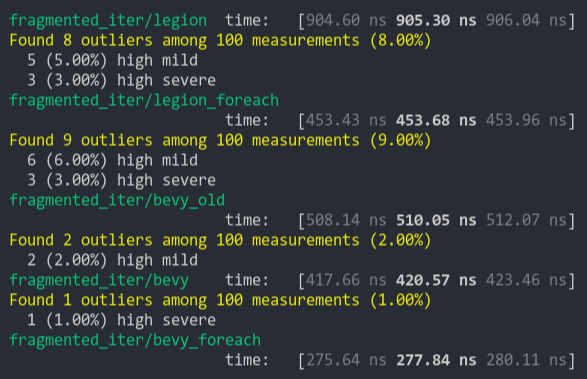
### Sparse Fragmented Iter
Iterate a query that matches 5 entities from a single matching archetype, but there are 100 unmatching archetypes
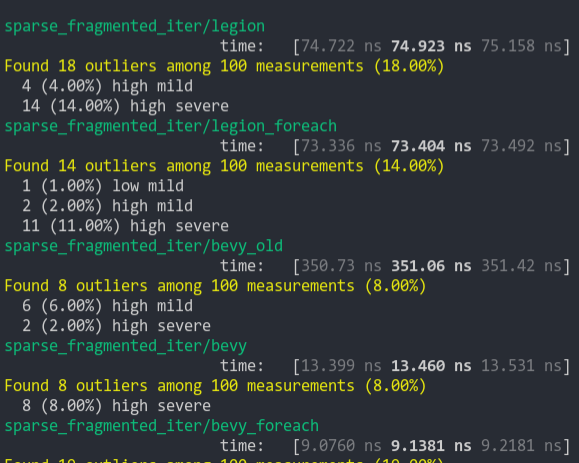
### Schedule (from ecs_bench_suite)
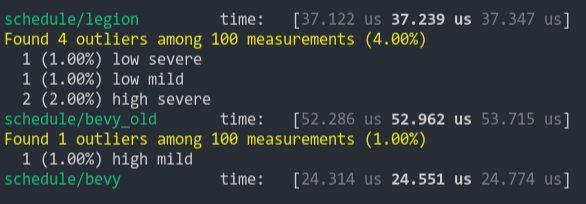
### Add Remove Component (from ecs_bench_suite)
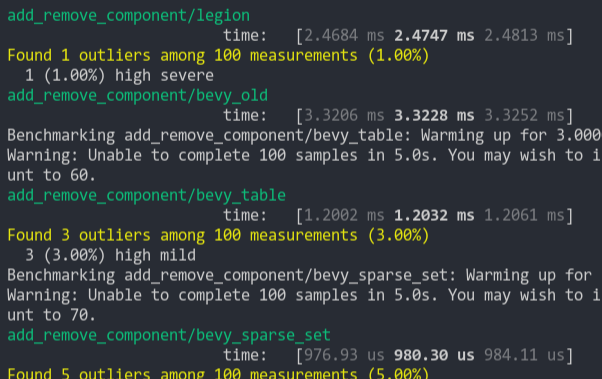
### Add Remove Component Big
Same as the test above, but each entity has 5 "large" matrix components and 1 "large" matrix component is added and removed
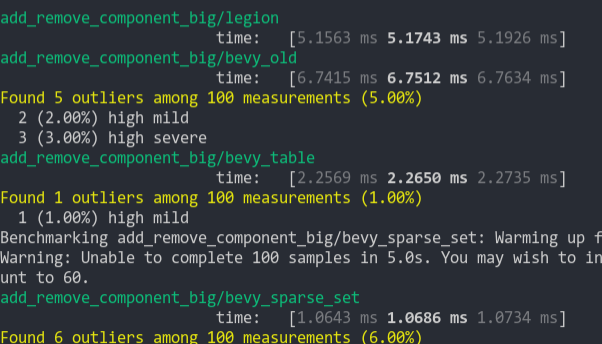
### Get Component
Looks up a single component value a large number of times
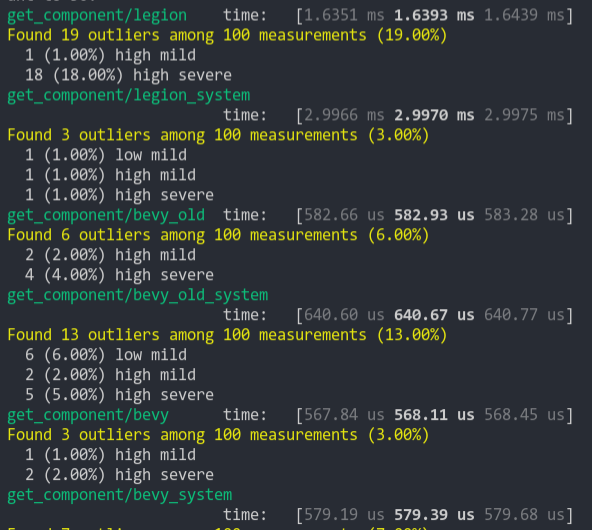
2021-03-05 07:54:35 +00:00
}
#[ inline ]
Simplify trait hierarchy for `SystemParam` (#6865)
# Objective
* Implementing a custom `SystemParam` by hand requires implementing three traits -- four if it is read-only.
* The trait `SystemParamFetch<'w, 's>` is a workaround from before we had generic associated types, and is no longer necessary.
## Solution
* Combine the trait `SystemParamFetch` with `SystemParamState`.
* I decided to remove the `Fetch` name and keep the `State` name, since the former was consistently conflated with the latter.
* Replace the trait `ReadOnlySystemParamFetch` with `ReadOnlySystemParam`, which simplifies trait bounds in generic code.
---
## Changelog
- Removed the trait `SystemParamFetch`, moving its functionality to `SystemParamState`.
- Replaced the trait `ReadOnlySystemParamFetch` with `ReadOnlySystemParam`.
## Migration Guide
The trait `SystemParamFetch` has been removed, and its functionality has been transferred to `SystemParamState`.
```rust
// Before
impl SystemParamState for MyParamState {
fn init(world: &mut World, system_meta: &mut SystemMeta) -> Self { ... }
}
impl<'w, 's> SystemParamFetch<'w, 's> for MyParamState {
type Item = MyParam<'w, 's>;
fn get_param(...) -> Self::Item;
}
// After
impl SystemParamState for MyParamState {
type Item<'w, 's> = MyParam<'w, 's>; // Generic associated types!
fn init(world: &mut World, system_meta: &mut SystemMeta) -> Self { ... }
fn get_param<'w, 's>(...) -> Self::Item<'w, 's>;
}
```
The trait `ReadOnlySystemParamFetch` has been replaced with `ReadOnlySystemParam`.
```rust
// Before
unsafe impl ReadOnlySystemParamFetch for MyParamState {}
// After
unsafe impl<'w, 's> ReadOnlySystemParam for MyParam<'w, 's> {}
```
2022-12-11 18:34:14 +00:00
unsafe fn get_param < ' w , ' s > (
Remove the `SystemParamState` trait and remove types like `ResState` (#6919)
Spiritual successor to #5205.
Actual successor to #6865.
# Objective
Currently, system params are defined using three traits: `SystemParam`, `ReadOnlySystemParam`, `SystemParamState`. The behavior for each param is specified by the `SystemParamState` trait, while `SystemParam` simply defers to the state.
Splitting the traits in this way makes it easier to implement within macros, but it increases the cognitive load. Worst of all, this approach requires each `MySystemParam` to have a public `MySystemParamState` type associated with it.
## Solution
* Merge the trait `SystemParamState` into `SystemParam`.
* Remove all trivial `SystemParam` state types.
* `OptionNonSendMutState<T>`: you will not be missed.
---
- [x] Fix/resolve the remaining test failure.
## Changelog
* Removed the trait `SystemParamState`, merging its functionality into `SystemParam`.
## Migration Guide
**Note**: this should replace the migration guide for #6865.
This is relative to Bevy 0.9, not main.
The traits `SystemParamState` and `SystemParamFetch` have been removed, and their functionality has been transferred to `SystemParam`.
```rust
// Before (0.9)
impl SystemParam for MyParam<'_, '_> {
type State = MyParamState;
}
unsafe impl SystemParamState for MyParamState {
fn init(world: &mut World, system_meta: &mut SystemMeta) -> Self { ... }
}
unsafe impl<'w, 's> SystemParamFetch<'w, 's> for MyParamState {
type Item = MyParam<'w, 's>;
fn get_param(&mut self, ...) -> Self::Item;
}
unsafe impl ReadOnlySystemParamFetch for MyParamState { }
// After (0.10)
unsafe impl SystemParam for MyParam<'_, '_> {
type State = MyParamState;
type Item<'w, 's> = MyParam<'w, 's>;
fn init_state(world: &mut World, system_meta: &mut SystemMeta) -> Self::State { ... }
fn get_param<'w, 's>(state: &mut Self::State, ...) -> Self::Item<'w, 's>;
}
unsafe impl ReadOnlySystemParam for MyParam<'_, '_> { }
```
The trait `ReadOnlySystemParamFetch` has been replaced with `ReadOnlySystemParam`.
```rust
// Before
unsafe impl ReadOnlySystemParamFetch for MyParamState {}
// After
unsafe impl ReadOnlySystemParam for MyParam<'_, '_> {}
```
2023-01-07 23:20:32 +00:00
& mut component_id : & ' s mut Self ::State ,
Add new SystemState and rename old SystemState to SystemMeta (#2283)
This enables `SystemParams` to be used outside of function systems. Anything can create and store `SystemState`, which enables efficient "param state cached" access to `SystemParams`.
It adds a `ReadOnlySystemParamFetch` trait, which enables safe `SystemState::get` calls without unique world access.
I renamed the old `SystemState` to `SystemMeta` to enable us to mirror the `QueryState` naming convention (but I'm happy to discuss alternative names if people have other ideas). I initially pitched this as `ParamState`, but given that it needs to include full system metadata, that doesn't feel like a particularly accurate name.
```rust
#[derive(Eq, PartialEq, Debug)]
struct A(usize);
#[derive(Eq, PartialEq, Debug)]
struct B(usize);
let mut world = World::default();
world.insert_resource(A(42));
world.spawn().insert(B(7));
// we get nice lifetime elision when declaring the type on the left hand side
let mut system_state: SystemState<(Res<A>, Query<&B>)> = SystemState::new(&mut world);
let (a, query) = system_state.get(&world);
assert_eq!(*a, A(42), "returned resource matches initial value");
assert_eq!(
*query.single().unwrap(),
B(7),
"returned component matches initial value"
);
// mutable system params require unique world access
let mut system_state: SystemState<(ResMut<A>, Query<&mut B>)> = SystemState::new(&mut world);
let (a, query) = system_state.get_mut(&mut world);
// static lifetimes are required when declaring inside of structs
struct SomeContainer {
state: SystemState<(Res<'static, A>, Res<'static, B>)>
}
// this can be shortened using type aliases, which will be useful for complex param tuples
type MyParams<'a> = (Res<'a, A>, Res<'a, B>);
struct SomeContainer {
state: SystemState<MyParams<'static>>
}
// It is the user's responsibility to call SystemState::apply(world) for parameters that queue up work
let mut system_state: SystemState<(Commands, Query<&B>)> = SystemState::new(&mut world);
{
let (mut commands, query) = system_state.get(&world);
commands.insert_resource(3.14);
}
system_state.apply(&mut world);
```
## Future Work
* Actually use SystemState inside FunctionSystem. This would be trivial, but it requires FunctionSystem to wrap SystemState in Option in its current form (which complicates system metadata lookup). I'd prefer to hold off until we adopt something like the later designs linked in #1364, which enable us to contruct Systems using a World reference (and also remove the need for `.system`).
* Consider a "scoped" approach to automatically call SystemState::apply when systems params are no longer being used (either a container type with a Drop impl, or a function that takes a closure for user logic operating on params).
2021-06-02 19:57:38 +00:00
system_meta : & SystemMeta ,
System Param Lifetime Split (#2605)
# Objective
Enable using exact World lifetimes during read-only access . This is motivated by the new renderer's need to allow read-only world-only queries to outlive the query itself (but still be constrained by the world lifetime).
For example:
https://github.com/bevyengine/bevy/blob/115b170d1f11a91146bb6d6e9684dceb8b21f786/pipelined/bevy_pbr2/src/render/mod.rs#L774
## Solution
Split out SystemParam state and world lifetimes and pipe those lifetimes up to read-only Query ops (and add into_inner for Res). According to every safety test I've run so far (except one), this is safe (see the temporary safety test commit). Note that changing the mutable variants to the new lifetimes would allow aliased mutable pointers (try doing that to see how it affects the temporary safety tests).
The new state lifetime on SystemParam does make `#[derive(SystemParam)]` more cumbersome (the current impl requires PhantomData if you don't use both lifetimes). We can make this better by detecting whether or not a lifetime is used in the derive and adjusting accordingly, but that should probably be done in its own pr.
## Why is this a draft?
The new lifetimes break QuerySet safety in one very specific case (see the query_set system in system_safety_test). We need to solve this before we can use the lifetimes given.
This is due to the fact that QuerySet is just a wrapper over Query, which now relies on world lifetimes instead of `&self` lifetimes to prevent aliasing (but in systems, each Query has its own implied lifetime, not a centralized world lifetime). I believe the fix is to rewrite QuerySet to have its own World lifetime (and own the internal reference). This will complicate the impl a bit, but I think it is doable. I'm curious if anyone else has better ideas.
Personally, I think these new lifetimes need to happen. We've gotta have a way to directly tie read-only World queries to the World lifetime. The new renderer is the first place this has come up, but I doubt it will be the last. Worst case scenario we can come up with a second `WorldLifetimeQuery<Q, F = ()>` parameter to enable these read-only scenarios, but I'd rather not add another type to the type zoo.
2021-08-15 20:51:53 +00:00
world : & ' w World ,
Reliable change detection (#1471)
# Problem Definition
The current change tracking (via flags for both components and resources) fails to detect changes made by systems that are scheduled to run earlier in the frame than they are.
This issue is discussed at length in [#68](https://github.com/bevyengine/bevy/issues/68) and [#54](https://github.com/bevyengine/bevy/issues/54).
This is very much a draft PR, and contributions are welcome and needed.
# Criteria
1. Each change is detected at least once, no matter the ordering.
2. Each change is detected at most once, no matter the ordering.
3. Changes should be detected the same frame that they are made.
4. Competitive ergonomics. Ideally does not require opting-in.
5. Low CPU overhead of computation.
6. Memory efficient. This must not increase over time, except where the number of entities / resources does.
7. Changes should not be lost for systems that don't run.
8. A frame needs to act as a pure function. Given the same set of entities / components it needs to produce the same end state without side-effects.
**Exact** change-tracking proposals satisfy criteria 1 and 2.
**Conservative** change-tracking proposals satisfy criteria 1 but not 2.
**Flaky** change tracking proposals satisfy criteria 2 but not 1.
# Code Base Navigation
There are three types of flags:
- `Added`: A piece of data was added to an entity / `Resources`.
- `Mutated`: A piece of data was able to be modified, because its `DerefMut` was accessed
- `Changed`: The bitwise OR of `Added` and `Changed`
The special behavior of `ChangedRes`, with respect to the scheduler is being removed in [#1313](https://github.com/bevyengine/bevy/pull/1313) and does not need to be reproduced.
`ChangedRes` and friends can be found in "bevy_ecs/core/resources/resource_query.rs".
The `Flags` trait for Components can be found in "bevy_ecs/core/query.rs".
`ComponentFlags` are stored in "bevy_ecs/core/archetypes.rs", defined on line 446.
# Proposals
**Proposal 5 was selected for implementation.**
## Proposal 0: No Change Detection
The baseline, where computations are performed on everything regardless of whether it changed.
**Type:** Conservative
**Pros:**
- already implemented
- will never miss events
- no overhead
**Cons:**
- tons of repeated work
- doesn't allow users to avoid repeating work (or monitoring for other changes)
## Proposal 1: Earlier-This-Tick Change Detection
The current approach as of Bevy 0.4. Flags are set, and then flushed at the end of each frame.
**Type:** Flaky
**Pros:**
- already implemented
- simple to understand
- low memory overhead (2 bits per component)
- low time overhead (clear every flag once per frame)
**Cons:**
- misses systems based on ordering
- systems that don't run every frame miss changes
- duplicates detection when looping
- can lead to unresolvable circular dependencies
## Proposal 2: Two-Tick Change Detection
Flags persist for two frames, using a double-buffer system identical to that used in events.
A change is observed if it is found in either the current frame's list of changes or the previous frame's.
**Type:** Conservative
**Pros:**
- easy to understand
- easy to implement
- low memory overhead (4 bits per component)
- low time overhead (bit mask and shift every flag once per frame)
**Cons:**
- can result in a great deal of duplicated work
- systems that don't run every frame miss changes
- duplicates detection when looping
## Proposal 3: Last-Tick Change Detection
Flags persist for two frames, using a double-buffer system identical to that used in events.
A change is observed if it is found in the previous frame's list of changes.
**Type:** Exact
**Pros:**
- exact
- easy to understand
- easy to implement
- low memory overhead (4 bits per component)
- low time overhead (bit mask and shift every flag once per frame)
**Cons:**
- change detection is always delayed, possibly causing painful chained delays
- systems that don't run every frame miss changes
- duplicates detection when looping
## Proposal 4: Flag-Doubling Change Detection
Combine Proposal 2 and Proposal 3. Differentiate between `JustChanged` (current behavior) and `Changed` (Proposal 3).
Pack this data into the flags according to [this implementation proposal](https://github.com/bevyengine/bevy/issues/68#issuecomment-769174804).
**Type:** Flaky + Exact
**Pros:**
- allows users to acc
- easy to implement
- low memory overhead (4 bits per component)
- low time overhead (bit mask and shift every flag once per frame)
**Cons:**
- users must specify the type of change detection required
- still quite fragile to system ordering effects when using the flaky `JustChanged` form
- cannot get immediate + exact results
- systems that don't run every frame miss changes
- duplicates detection when looping
## [SELECTED] Proposal 5: Generation-Counter Change Detection
A global counter is increased after each system is run. Each component saves the time of last mutation, and each system saves the time of last execution. Mutation is detected when the component's counter is greater than the system's counter. Discussed [here](https://github.com/bevyengine/bevy/issues/68#issuecomment-769174804). How to handle addition detection is unsolved; the current proposal is to use the highest bit of the counter as in proposal 1.
**Type:** Exact (for mutations), flaky (for additions)
**Pros:**
- low time overhead (set component counter on access, set system counter after execution)
- robust to systems that don't run every frame
- robust to systems that loop
**Cons:**
- moderately complex implementation
- must be modified as systems are inserted dynamically
- medium memory overhead (4 bytes per component + system)
- unsolved addition detection
## Proposal 6: System-Data Change Detection
For each system, track which system's changes it has seen. This approach is only worth fully designing and implementing if Proposal 5 fails in some way.
**Type:** Exact
**Pros:**
- exact
- conceptually simple
**Cons:**
- requires storing data on each system
- implementation is complex
- must be modified as systems are inserted dynamically
## Proposal 7: Total-Order Change Detection
Discussed [here](https://github.com/bevyengine/bevy/issues/68#issuecomment-754326523). This proposal is somewhat complicated by the new scheduler, but I believe it should still be conceptually feasible. This approach is only worth fully designing and implementing if Proposal 5 fails in some way.
**Type:** Exact
**Pros:**
- exact
- efficient data storage relative to other exact proposals
**Cons:**
- requires access to the scheduler
- complex implementation and difficulty grokking
- must be modified as systems are inserted dynamically
# Tests
- We will need to verify properties 1, 2, 3, 7 and 8. Priority: 1 > 2 = 3 > 8 > 7
- Ideally we can use identical user-facing syntax for all proposals, allowing us to re-use the same syntax for each.
- When writing tests, we need to carefully specify order using explicit dependencies.
- These tests will need to be duplicated for both components and resources.
- We need to be sure to handle cases where ambiguous system orders exist.
`changing_system` is always the system that makes the changes, and `detecting_system` always detects the changes.
The component / resource changed will be simple boolean wrapper structs.
## Basic Added / Mutated / Changed
2 x 3 design:
- Resources vs. Components
- Added vs. Changed vs. Mutated
- `changing_system` runs before `detecting_system`
- verify at the end of tick 2
## At Least Once
2 x 3 design:
- Resources vs. Components
- Added vs. Changed vs. Mutated
- `changing_system` runs after `detecting_system`
- verify at the end of tick 2
## At Most Once
2 x 3 design:
- Resources vs. Components
- Added vs. Changed vs. Mutated
- `changing_system` runs once before `detecting_system`
- increment a counter based on the number of changes detected
- verify at the end of tick 2
## Fast Detection
2 x 3 design:
- Resources vs. Components
- Added vs. Changed vs. Mutated
- `changing_system` runs before `detecting_system`
- verify at the end of tick 1
## Ambiguous System Ordering Robustness
2 x 3 x 2 design:
- Resources vs. Components
- Added vs. Changed vs. Mutated
- `changing_system` runs [before/after] `detecting_system` in tick 1
- `changing_system` runs [after/before] `detecting_system` in tick 2
## System Pausing
2 x 3 design:
- Resources vs. Components
- Added vs. Changed vs. Mutated
- `changing_system` runs in tick 1, then is disabled by run criteria
- `detecting_system` is disabled by run criteria until it is run once during tick 3
- verify at the end of tick 3
## Addition Causes Mutation
2 design:
- Resources vs. Components
- `adding_system_1` adds a component / resource
- `adding system_2` adds the same component / resource
- verify the `Mutated` flag at the end of the tick
- verify the `Added` flag at the end of the tick
First check tests for: https://github.com/bevyengine/bevy/issues/333
Second check tests for: https://github.com/bevyengine/bevy/issues/1443
## Changes Made By Commands
- `adding_system` runs in Update in tick 1, and sends a command to add a component
- `detecting_system` runs in Update in tick 1 and 2, after `adding_system`
- We can't detect the changes in tick 1, since they haven't been processed yet
- If we were to track these changes as being emitted by `adding_system`, we can't detect the changes in tick 2 either, since `detecting_system` has already run once after `adding_system` :(
# Benchmarks
See: [general advice](https://github.com/bevyengine/bevy/blob/master/docs/profiling.md), [Criterion crate](https://github.com/bheisler/criterion.rs)
There are several critical parameters to vary:
1. entity count (1 to 10^9)
2. fraction of entities that are changed (0% to 100%)
3. cost to perform work on changed entities, i.e. workload (1 ns to 1s)
1 and 2 should be varied between benchmark runs. 3 can be added on computationally.
We want to measure:
- memory cost
- run time
We should collect these measurements across several frames (100?) to reduce bootup effects and accurately measure the mean, variance and drift.
Entity-component change detection is much more important to benchmark than resource change detection, due to the orders of magnitude higher number of pieces of data.
No change detection at all should be included in benchmarks as a second control for cases where missing changes is unacceptable.
## Graphs
1. y: performance, x: log_10(entity count), color: proposal, facet: performance metric. Set cost to perform work to 0.
2. y: run time, x: cost to perform work, color: proposal, facet: fraction changed. Set number of entities to 10^6
3. y: memory, x: frames, color: proposal
# Conclusions
1. Is the theoretical categorization of the proposals correct according to our tests?
2. How does the performance of the proposals compare without any load?
3. How does the performance of the proposals compare with realistic loads?
4. At what workload does more exact change tracking become worth the (presumably) higher overhead?
5. When does adding change-detection to save on work become worthwhile?
6. Is there enough divergence in performance between the best solutions in each class to ship more than one change-tracking solution?
# Implementation Plan
1. Write a test suite.
2. Verify that tests fail for existing approach.
3. Write a benchmark suite.
4. Get performance numbers for existing approach.
5. Implement, test and benchmark various solutions using a Git branch per proposal.
6. Create a draft PR with all solutions and present results to team.
7. Select a solution and replace existing change detection.
Co-authored-by: Brice DAVIER <bricedavier@gmail.com>
Co-authored-by: Carter Anderson <mcanders1@gmail.com>
2021-03-19 17:53:26 +00:00
change_tick : u32 ,
Simplify trait hierarchy for `SystemParam` (#6865)
# Objective
* Implementing a custom `SystemParam` by hand requires implementing three traits -- four if it is read-only.
* The trait `SystemParamFetch<'w, 's>` is a workaround from before we had generic associated types, and is no longer necessary.
## Solution
* Combine the trait `SystemParamFetch` with `SystemParamState`.
* I decided to remove the `Fetch` name and keep the `State` name, since the former was consistently conflated with the latter.
* Replace the trait `ReadOnlySystemParamFetch` with `ReadOnlySystemParam`, which simplifies trait bounds in generic code.
---
## Changelog
- Removed the trait `SystemParamFetch`, moving its functionality to `SystemParamState`.
- Replaced the trait `ReadOnlySystemParamFetch` with `ReadOnlySystemParam`.
## Migration Guide
The trait `SystemParamFetch` has been removed, and its functionality has been transferred to `SystemParamState`.
```rust
// Before
impl SystemParamState for MyParamState {
fn init(world: &mut World, system_meta: &mut SystemMeta) -> Self { ... }
}
impl<'w, 's> SystemParamFetch<'w, 's> for MyParamState {
type Item = MyParam<'w, 's>;
fn get_param(...) -> Self::Item;
}
// After
impl SystemParamState for MyParamState {
type Item<'w, 's> = MyParam<'w, 's>; // Generic associated types!
fn init(world: &mut World, system_meta: &mut SystemMeta) -> Self { ... }
fn get_param<'w, 's>(...) -> Self::Item<'w, 's>;
}
```
The trait `ReadOnlySystemParamFetch` has been replaced with `ReadOnlySystemParam`.
```rust
// Before
unsafe impl ReadOnlySystemParamFetch for MyParamState {}
// After
unsafe impl<'w, 's> ReadOnlySystemParam for MyParam<'w, 's> {}
```
2022-12-11 18:34:14 +00:00
) -> Self ::Item < ' w , ' s > {
2022-10-24 13:46:36 +00:00
let ( ptr , ticks ) = world
2023-02-06 19:02:52 +00:00
. as_unsafe_world_cell_migration_internal ( )
Panic on dropping NonSend in non-origin thread. (#6534)
# Objective
Fixes #3310. Fixes #6282. Fixes #6278. Fixes #3666.
## Solution
Split out `!Send` resources into `NonSendResources`. Add a `origin_thread_id` to all `!Send` Resources, check it on dropping `NonSendResourceData`, if there's a mismatch, panic. Moved all of the checks that `MainThreadValidator` would do into `NonSendResources` instead.
All `!Send` resources now individually track which thread they were inserted from. This is validated against for every access, mutation, and drop that could be done against the value.
A regression test using an altered version of the example from #3310 has been added.
This is a stopgap solution for the current status quo. A full solution may involve fully removing `!Send` resources/components from `World`, which will likely require a much more thorough design on how to handle the existing in-engine and ecosystem use cases.
This PR also introduces another breaking change:
```rust
use bevy_ecs::prelude::*;
#[derive(Resource)]
struct Resource(u32);
fn main() {
let mut world = World::new();
world.insert_resource(Resource(1));
world.insert_non_send_resource(Resource(2));
let res = world.get_resource_mut::<Resource>().unwrap();
assert_eq!(res.0, 2);
}
```
This code will run correctly on 0.9.1 but not with this PR, since NonSend resources and normal resources have become actual distinct concepts storage wise.
## Changelog
Changed: Fix soundness bug with `World: Send`. Dropping a `World` that contains a `!Send` resource on the wrong thread will now panic.
## Migration Guide
Normal resources and `NonSend` resources no longer share the same backing storage. If `R: Resource`, then `NonSend<R>` and `Res<R>` will return different instances from each other. If you are using both `Res<T>` and `NonSend<T>` (or their mutable variants), to fetch the same resources, it's strongly advised to use `Res<T>`.
2023-01-09 20:40:34 +00:00
. get_non_send_with_ticks ( component_id )
2021-03-14 00:36:16 +00:00
. unwrap_or_else ( | | {
panic! (
2021-04-23 17:54:04 +00:00
" Non-send resource requested by {} does not exist: {} " ,
Add new SystemState and rename old SystemState to SystemMeta (#2283)
This enables `SystemParams` to be used outside of function systems. Anything can create and store `SystemState`, which enables efficient "param state cached" access to `SystemParams`.
It adds a `ReadOnlySystemParamFetch` trait, which enables safe `SystemState::get` calls without unique world access.
I renamed the old `SystemState` to `SystemMeta` to enable us to mirror the `QueryState` naming convention (but I'm happy to discuss alternative names if people have other ideas). I initially pitched this as `ParamState`, but given that it needs to include full system metadata, that doesn't feel like a particularly accurate name.
```rust
#[derive(Eq, PartialEq, Debug)]
struct A(usize);
#[derive(Eq, PartialEq, Debug)]
struct B(usize);
let mut world = World::default();
world.insert_resource(A(42));
world.spawn().insert(B(7));
// we get nice lifetime elision when declaring the type on the left hand side
let mut system_state: SystemState<(Res<A>, Query<&B>)> = SystemState::new(&mut world);
let (a, query) = system_state.get(&world);
assert_eq!(*a, A(42), "returned resource matches initial value");
assert_eq!(
*query.single().unwrap(),
B(7),
"returned component matches initial value"
);
// mutable system params require unique world access
let mut system_state: SystemState<(ResMut<A>, Query<&mut B>)> = SystemState::new(&mut world);
let (a, query) = system_state.get_mut(&mut world);
// static lifetimes are required when declaring inside of structs
struct SomeContainer {
state: SystemState<(Res<'static, A>, Res<'static, B>)>
}
// this can be shortened using type aliases, which will be useful for complex param tuples
type MyParams<'a> = (Res<'a, A>, Res<'a, B>);
struct SomeContainer {
state: SystemState<MyParams<'static>>
}
// It is the user's responsibility to call SystemState::apply(world) for parameters that queue up work
let mut system_state: SystemState<(Commands, Query<&B>)> = SystemState::new(&mut world);
{
let (mut commands, query) = system_state.get(&world);
commands.insert_resource(3.14);
}
system_state.apply(&mut world);
```
## Future Work
* Actually use SystemState inside FunctionSystem. This would be trivial, but it requires FunctionSystem to wrap SystemState in Option in its current form (which complicates system metadata lookup). I'd prefer to hold off until we adopt something like the later designs linked in #1364, which enable us to contruct Systems using a World reference (and also remove the need for `.system`).
* Consider a "scoped" approach to automatically call SystemState::apply when systems params are no longer being used (either a container type with a Drop impl, or a function that takes a closure for user logic operating on params).
2021-06-02 19:57:38 +00:00
system_meta . name ,
2021-03-14 00:36:16 +00:00
std ::any ::type_name ::< T > ( )
)
} ) ;
Bevy ECS V2 (#1525)
# Bevy ECS V2
This is a rewrite of Bevy ECS (basically everything but the new executor/schedule, which are already awesome). The overall goal was to improve the performance and versatility of Bevy ECS. Here is a quick bulleted list of changes before we dive into the details:
* Complete World rewrite
* Multiple component storage types:
* Tables: fast cache friendly iteration, slower add/removes (previously called Archetypes)
* Sparse Sets: fast add/remove, slower iteration
* Stateful Queries (caches query results for faster iteration. fragmented iteration is _fast_ now)
* Stateful System Params (caches expensive operations. inspired by @DJMcNab's work in #1364)
* Configurable System Params (users can set configuration when they construct their systems. once again inspired by @DJMcNab's work)
* Archetypes are now "just metadata", component storage is separate
* Archetype Graph (for faster archetype changes)
* Component Metadata
* Configure component storage type
* Retrieve information about component size/type/name/layout/send-ness/etc
* Components are uniquely identified by a densely packed ComponentId
* TypeIds are now totally optional (which should make implementing scripting easier)
* Super fast "for_each" query iterators
* Merged Resources into World. Resources are now just a special type of component
* EntityRef/EntityMut builder apis (more efficient and more ergonomic)
* Fast bitset-backed `Access<T>` replaces old hashmap-based approach everywhere
* Query conflicts are determined by component access instead of archetype component access (to avoid random failures at runtime)
* With/Without are still taken into account for conflicts, so this should still be comfy to use
* Much simpler `IntoSystem` impl
* Significantly reduced the amount of hashing throughout the ecs in favor of Sparse Sets (indexed by densely packed ArchetypeId, ComponentId, BundleId, and TableId)
* Safety Improvements
* Entity reservation uses a normal world reference instead of unsafe transmute
* QuerySets no longer transmute lifetimes
* Made traits "unsafe" where relevant
* More thorough safety docs
* WorldCell
* Exposes safe mutable access to multiple resources at a time in a World
* Replaced "catch all" `System::update_archetypes(world: &World)` with `System::new_archetype(archetype: &Archetype)`
* Simpler Bundle implementation
* Replaced slow "remove_bundle_one_by_one" used as fallback for Commands::remove_bundle with fast "remove_bundle_intersection"
* Removed `Mut<T>` query impl. it is better to only support one way: `&mut T`
* Removed with() from `Flags<T>` in favor of `Option<Flags<T>>`, which allows querying for flags to be "filtered" by default
* Components now have is_send property (currently only resources support non-send)
* More granular module organization
* New `RemovedComponents<T>` SystemParam that replaces `query.removed::<T>()`
* `world.resource_scope()` for mutable access to resources and world at the same time
* WorldQuery and QueryFilter traits unified. FilterFetch trait added to enable "short circuit" filtering. Auto impled for cases that don't need it
* Significantly slimmed down SystemState in favor of individual SystemParam state
* System Commands changed from `commands: &mut Commands` back to `mut commands: Commands` (to allow Commands to have a World reference)
Fixes #1320
## `World` Rewrite
This is a from-scratch rewrite of `World` that fills the niche that `hecs` used to. Yes, this means Bevy ECS is no longer a "fork" of hecs. We're going out our own!
(the only shared code between the projects is the entity id allocator, which is already basically ideal)
A huge shout out to @SanderMertens (author of [flecs](https://github.com/SanderMertens/flecs)) for sharing some great ideas with me (specifically hybrid ecs storage and archetype graphs). He also helped advise on a number of implementation details.
## Component Storage (The Problem)
Two ECS storage paradigms have gained a lot of traction over the years:
* **Archetypal ECS**:
* Stores components in "tables" with static schemas. Each "column" stores components of a given type. Each "row" is an entity.
* Each "archetype" has its own table. Adding/removing an entity's component changes the archetype.
* Enables super-fast Query iteration due to its cache-friendly data layout
* Comes at the cost of more expensive add/remove operations for an Entity's components, because all components need to be copied to the new archetype's "table"
* **Sparse Set ECS**:
* Stores components of the same type in densely packed arrays, which are sparsely indexed by densely packed unsigned integers (Entity ids)
* Query iteration is slower than Archetypal ECS because each entity's component could be at any position in the sparse set. This "random access" pattern isn't cache friendly. Additionally, there is an extra layer of indirection because you must first map the entity id to an index in the component array.
* Adding/removing components is a cheap, constant time operation
Bevy ECS V1, hecs, legion, flec, and Unity DOTS are all "archetypal ecs-es". I personally think "archetypal" storage is a good default for game engines. An entity's archetype doesn't need to change frequently in general, and it creates "fast by default" query iteration (which is a much more common operation). It is also "self optimizing". Users don't need to think about optimizing component layouts for iteration performance. It "just works" without any extra boilerplate.
Shipyard and EnTT are "sparse set ecs-es". They employ "packing" as a way to work around the "suboptimal by default" iteration performance for specific sets of components. This helps, but I didn't think this was a good choice for a general purpose engine like Bevy because:
1. "packs" conflict with each other. If bevy decides to internally pack the Transform and GlobalTransform components, users are then blocked if they want to pack some custom component with Transform.
2. users need to take manual action to optimize
Developers selecting an ECS framework are stuck with a hard choice. Select an "archetypal" framework with "fast iteration everywhere" but without the ability to cheaply add/remove components, or select a "sparse set" framework to cheaply add/remove components but with slower iteration performance.
## Hybrid Component Storage (The Solution)
In Bevy ECS V2, we get to have our cake and eat it too. It now has _both_ of the component storage types above (and more can be added later if needed):
* **Tables** (aka "archetypal" storage)
* The default storage. If you don't configure anything, this is what you get
* Fast iteration by default
* Slower add/remove operations
* **Sparse Sets**
* Opt-in
* Slower iteration
* Faster add/remove operations
These storage types complement each other perfectly. By default Query iteration is fast. If developers know that they want to add/remove a component at high frequencies, they can set the storage to "sparse set":
```rust
world.register_component(
ComponentDescriptor::new::<MyComponent>(StorageType::SparseSet)
).unwrap();
```
## Archetypes
Archetypes are now "just metadata" ... they no longer store components directly. They do store:
* The `ComponentId`s of each of the Archetype's components (and that component's storage type)
* Archetypes are uniquely defined by their component layouts
* For example: entities with "table" components `[A, B, C]` _and_ "sparse set" components `[D, E]` will always be in the same archetype.
* The `TableId` associated with the archetype
* For now each archetype has exactly one table (which can have no components),
* There is a 1->Many relationship from Tables->Archetypes. A given table could have any number of archetype components stored in it:
* Ex: an entity with "table storage" components `[A, B, C]` and "sparse set" components `[D, E]` will share the same `[A, B, C]` table as an entity with `[A, B, C]` table component and `[F]` sparse set components.
* This 1->Many relationship is how we preserve fast "cache friendly" iteration performance when possible (more on this later)
* A list of entities that are in the archetype and the row id of the table they are in
* ArchetypeComponentIds
* unique densely packed identifiers for (ArchetypeId, ComponentId) pairs
* used by the schedule executor for cheap system access control
* "Archetype Graph Edges" (see the next section)
## The "Archetype Graph"
Archetype changes in Bevy (and a number of other archetypal ecs-es) have historically been expensive to compute. First, you need to allocate a new vector of the entity's current component ids, add or remove components based on the operation performed, sort it (to ensure it is order-independent), then hash it to find the archetype (if it exists). And thats all before we get to the _already_ expensive full copy of all components to the new table storage.
The solution is to build a "graph" of archetypes to cache these results. @SanderMertens first exposed me to the idea (and he got it from @gjroelofs, who came up with it). They propose adding directed edges between archetypes for add/remove component operations. If `ComponentId`s are densely packed, you can use sparse sets to cheaply jump between archetypes.
Bevy takes this one step further by using add/remove `Bundle` edges instead of `Component` edges. Bevy encourages the use of `Bundles` to group add/remove operations. This is largely for "clearer game logic" reasons, but it also helps cut down on the number of archetype changes required. `Bundles` now also have densely-packed `BundleId`s. This allows us to use a _single_ edge for each bundle operation (rather than needing to traverse N edges ... one for each component). Single component operations are also bundles, so this is strictly an improvement over a "component only" graph.
As a result, an operation that used to be _heavy_ (both for allocations and compute) is now two dirt-cheap array lookups and zero allocations.
## Stateful Queries
World queries are now stateful. This allows us to:
1. Cache archetype (and table) matches
* This resolves another issue with (naive) archetypal ECS: query performance getting worse as the number of archetypes goes up (and fragmentation occurs).
2. Cache Fetch and Filter state
* The expensive parts of fetch/filter operations (such as hashing the TypeId to find the ComponentId) now only happen once when the Query is first constructed
3. Incrementally build up state
* When new archetypes are added, we only process the new archetypes (no need to rebuild state for old archetypes)
As a result, the direct `World` query api now looks like this:
```rust
let mut query = world.query::<(&A, &mut B)>();
for (a, mut b) in query.iter_mut(&mut world) {
}
```
Requiring `World` to generate stateful queries (rather than letting the `QueryState` type be constructed separately) allows us to ensure that _all_ queries are properly initialized (and the relevant world state, such as ComponentIds). This enables QueryState to remove branches from its operations that check for initialization status (and also enables query.iter() to take an immutable world reference because it doesn't need to initialize anything in world).
However in systems, this is a non-breaking change. State management is done internally by the relevant SystemParam.
## Stateful SystemParams
Like Queries, `SystemParams` now also cache state. For example, `Query` system params store the "stateful query" state mentioned above. Commands store their internal `CommandQueue`. This means you can now safely use as many separate `Commands` parameters in your system as you want. `Local<T>` system params store their `T` value in their state (instead of in Resources).
SystemParam state also enabled a significant slim-down of SystemState. It is much nicer to look at now.
Per-SystemParam state naturally insulates us from an "aliased mut" class of errors we have hit in the past (ex: using multiple `Commands` system params).
(credit goes to @DJMcNab for the initial idea and draft pr here #1364)
## Configurable SystemParams
@DJMcNab also had the great idea to make SystemParams configurable. This allows users to provide some initial configuration / values for system parameters (when possible). Most SystemParams have no config (the config type is `()`), but the `Local<T>` param now supports user-provided parameters:
```rust
fn foo(value: Local<usize>) {
}
app.add_system(foo.system().config(|c| c.0 = Some(10)));
```
## Uber Fast "for_each" Query Iterators
Developers now have the choice to use a fast "for_each" iterator, which yields ~1.5-3x iteration speed improvements for "fragmented iteration", and minor ~1.2x iteration speed improvements for unfragmented iteration.
```rust
fn system(query: Query<(&A, &mut B)>) {
// you now have the option to do this for a speed boost
query.for_each_mut(|(a, mut b)| {
});
// however normal iterators are still available
for (a, mut b) in query.iter_mut() {
}
}
```
I think in most cases we should continue to encourage "normal" iterators as they are more flexible and more "rust idiomatic". But when that extra "oomf" is needed, it makes sense to use `for_each`.
We should also consider using `for_each` for internal bevy systems to give our users a nice speed boost (but that should be a separate pr).
## Component Metadata
`World` now has a `Components` collection, which is accessible via `world.components()`. This stores mappings from `ComponentId` to `ComponentInfo`, as well as `TypeId` to `ComponentId` mappings (where relevant). `ComponentInfo` stores information about the component, such as ComponentId, TypeId, memory layout, send-ness (currently limited to resources), and storage type.
## Significantly Cheaper `Access<T>`
We used to use `TypeAccess<TypeId>` to manage read/write component/archetype-component access. This was expensive because TypeIds must be hashed and compared individually. The parallel executor got around this by "condensing" type ids into bitset-backed access types. This worked, but it had to be re-generated from the `TypeAccess<TypeId>`sources every time archetypes changed.
This pr removes TypeAccess in favor of faster bitset access everywhere. We can do this thanks to the move to densely packed `ComponentId`s and `ArchetypeComponentId`s.
## Merged Resources into World
Resources had a lot of redundant functionality with Components. They stored typed data, they had access control, they had unique ids, they were queryable via SystemParams, etc. In fact the _only_ major difference between them was that they were unique (and didn't correlate to an entity).
Separate resources also had the downside of requiring a separate set of access controls, which meant the parallel executor needed to compare more bitsets per system and manage more state.
I initially got the "separate resources" idea from `legion`. I think that design was motivated by the fact that it made the direct world query/resource lifetime interactions more manageable. It certainly made our lives easier when using Resources alongside hecs/bevy_ecs. However we already have a construct for safely and ergonomically managing in-world lifetimes: systems (which use `Access<T>` internally).
This pr merges Resources into World:
```rust
world.insert_resource(1);
world.insert_resource(2.0);
let a = world.get_resource::<i32>().unwrap();
let mut b = world.get_resource_mut::<f64>().unwrap();
*b = 3.0;
```
Resources are now just a special kind of component. They have their own ComponentIds (and their own resource TypeId->ComponentId scope, so they don't conflict wit components of the same type). They are stored in a special "resource archetype", which stores components inside the archetype using a new `unique_components` sparse set (note that this sparse set could later be used to implement Tags). This allows us to keep the code size small by reusing existing datastructures (namely Column, Archetype, ComponentFlags, and ComponentInfo). This allows us the executor to use a single `Access<ArchetypeComponentId>` per system. It should also make scripting language integration easier.
_But_ this merge did create problems for people directly interacting with `World`. What if you need mutable access to multiple resources at the same time? `world.get_resource_mut()` borrows World mutably!
## WorldCell
WorldCell applies the `Access<ArchetypeComponentId>` concept to direct world access:
```rust
let world_cell = world.cell();
let a = world_cell.get_resource_mut::<i32>().unwrap();
let b = world_cell.get_resource_mut::<f64>().unwrap();
```
This adds cheap runtime checks (a sparse set lookup of `ArchetypeComponentId` and a counter) to ensure that world accesses do not conflict with each other. Each operation returns a `WorldBorrow<'w, T>` or `WorldBorrowMut<'w, T>` wrapper type, which will release the relevant ArchetypeComponentId resources when dropped.
World caches the access sparse set (and only one cell can exist at a time), so `world.cell()` is a cheap operation.
WorldCell does _not_ use atomic operations. It is non-send, does a mutable borrow of world to prevent other accesses, and uses a simple `Rc<RefCell<ArchetypeComponentAccess>>` wrapper in each WorldBorrow pointer.
The api is currently limited to resource access, but it can and should be extended to queries / entity component access.
## Resource Scopes
WorldCell does not yet support component queries, and even when it does there are sometimes legitimate reasons to want a mutable world ref _and_ a mutable resource ref (ex: bevy_render and bevy_scene both need this). In these cases we could always drop down to the unsafe `world.get_resource_unchecked_mut()`, but that is not ideal!
Instead developers can use a "resource scope"
```rust
world.resource_scope(|world: &mut World, a: &mut A| {
})
```
This temporarily removes the `A` resource from `World`, provides mutable pointers to both, and re-adds A to World when finished. Thanks to the move to ComponentIds/sparse sets, this is a cheap operation.
If multiple resources are required, scopes can be nested. We could also consider adding a "resource tuple" to the api if this pattern becomes common and the boilerplate gets nasty.
## Query Conflicts Use ComponentId Instead of ArchetypeComponentId
For safety reasons, systems cannot contain queries that conflict with each other without wrapping them in a QuerySet. On bevy `main`, we use ArchetypeComponentIds to determine conflicts. This is nice because it can take into account filters:
```rust
// these queries will never conflict due to their filters
fn filter_system(a: Query<&mut A, With<B>>, b: Query<&mut B, Without<B>>) {
}
```
But it also has a significant downside:
```rust
// these queries will not conflict _until_ an entity with A, B, and C is spawned
fn maybe_conflicts_system(a: Query<(&mut A, &C)>, b: Query<(&mut A, &B)>) {
}
```
The system above will panic at runtime if an entity with A, B, and C is spawned. This makes it hard to trust that your game logic will run without crashing.
In this pr, I switched to using `ComponentId` instead. This _is_ more constraining. `maybe_conflicts_system` will now always fail, but it will do it consistently at startup. Naively, it would also _disallow_ `filter_system`, which would be a significant downgrade in usability. Bevy has a number of internal systems that rely on disjoint queries and I expect it to be a common pattern in userspace.
To resolve this, I added a new `FilteredAccess<T>` type, which wraps `Access<T>` and adds with/without filters. If two `FilteredAccess` have with/without values that prove they are disjoint, they will no longer conflict.
## EntityRef / EntityMut
World entity operations on `main` require that the user passes in an `entity` id to each operation:
```rust
let entity = world.spawn((A, )); // create a new entity with A
world.get::<A>(entity);
world.insert(entity, (B, C));
world.insert_one(entity, D);
```
This means that each operation needs to look up the entity location / verify its validity. The initial spawn operation also requires a Bundle as input. This can be awkward when no components are required (or one component is required).
These operations have been replaced by `EntityRef` and `EntityMut`, which are "builder-style" wrappers around world that provide read and read/write operations on a single, pre-validated entity:
```rust
// spawn now takes no inputs and returns an EntityMut
let entity = world.spawn()
.insert(A) // insert a single component into the entity
.insert_bundle((B, C)) // insert a bundle of components into the entity
.id() // id returns the Entity id
// Returns EntityMut (or panics if the entity does not exist)
world.entity_mut(entity)
.insert(D)
.insert_bundle(SomeBundle::default());
{
// returns EntityRef (or panics if the entity does not exist)
let d = world.entity(entity)
.get::<D>() // gets the D component
.unwrap();
// world.get still exists for ergonomics
let d = world.get::<D>(entity).unwrap();
}
// These variants return Options if you want to check existence instead of panicing
world.get_entity_mut(entity)
.unwrap()
.insert(E);
if let Some(entity_ref) = world.get_entity(entity) {
let d = entity_ref.get::<D>().unwrap();
}
```
This _does not_ affect the current Commands api or terminology. I think that should be a separate conversation as that is a much larger breaking change.
## Safety Improvements
* Entity reservation in Commands uses a normal world borrow instead of an unsafe transmute
* QuerySets no longer transmutes lifetimes
* Made traits "unsafe" when implementing a trait incorrectly could cause unsafety
* More thorough safety docs
## RemovedComponents SystemParam
The old approach to querying removed components: `query.removed:<T>()` was confusing because it had no connection to the query itself. I replaced it with the following, which is both clearer and allows us to cache the ComponentId mapping in the SystemParamState:
```rust
fn system(removed: RemovedComponents<T>) {
for entity in removed.iter() {
}
}
```
## Simpler Bundle implementation
Bundles are no longer responsible for sorting (or deduping) TypeInfo. They are just a simple ordered list of component types / data. This makes the implementation smaller and opens the door to an easy "nested bundle" implementation in the future (which i might even add in this pr). Duplicate detection is now done once per bundle type by World the first time a bundle is used.
## Unified WorldQuery and QueryFilter types
(don't worry they are still separate type _parameters_ in Queries .. this is a non-breaking change)
WorldQuery and QueryFilter were already basically identical apis. With the addition of `FetchState` and more storage-specific fetch methods, the overlap was even clearer (and the redundancy more painful).
QueryFilters are now just `F: WorldQuery where F::Fetch: FilterFetch`. FilterFetch requires `Fetch<Item = bool>` and adds new "short circuit" variants of fetch methods. This enables a filter tuple like `(With<A>, Without<B>, Changed<C>)` to stop evaluating the filter after the first mismatch is encountered. FilterFetch is automatically implemented for `Fetch` implementations that return bool.
This forces fetch implementations that return things like `(bool, bool, bool)` (such as the filter above) to manually implement FilterFetch and decide whether or not to short-circuit.
## More Granular Modules
World no longer globs all of the internal modules together. It now exports `core`, `system`, and `schedule` separately. I'm also considering exporting `core` submodules directly as that is still pretty "glob-ey" and unorganized (feedback welcome here).
## Remaining Draft Work (to be done in this pr)
* ~~panic on conflicting WorldQuery fetches (&A, &mut A)~~
* ~~bevy `main` and hecs both currently allow this, but we should protect against it if possible~~
* ~~batch_iter / par_iter (currently stubbed out)~~
* ~~ChangedRes~~
* ~~I skipped this while we sort out #1313. This pr should be adapted to account for whatever we land on there~~.
* ~~The `Archetypes` and `Tables` collections use hashes of sorted lists of component ids to uniquely identify each archetype/table. This hash is then used as the key in a HashMap to look up the relevant ArchetypeId or TableId. (which doesn't handle hash collisions properly)~~
* ~~It is currently unsafe to generate a Query from "World A", then use it on "World B" (despite the api claiming it is safe). We should probably close this gap. This could be done by adding a randomly generated WorldId to each world, then storing that id in each Query. They could then be compared to each other on each `query.do_thing(&world)` operation. This _does_ add an extra branch to each query operation, so I'm open to other suggestions if people have them.~~
* ~~Nested Bundles (if i find time)~~
## Potential Future Work
* Expand WorldCell to support queries.
* Consider not allocating in the empty archetype on `world.spawn()`
* ex: return something like EntityMutUninit, which turns into EntityMut after an `insert` or `insert_bundle` op
* this actually regressed performance last time i tried it, but in theory it should be faster
* Optimize SparseSet::insert (see `PERF` comment on insert)
* Replace SparseArray `Option<T>` with T::MAX to cut down on branching
* would enable cheaper get_unchecked() operations
* upstream fixedbitset optimizations
* fixedbitset could be allocation free for small block counts (store blocks in a SmallVec)
* fixedbitset could have a const constructor
* Consider implementing Tags (archetype-specific by-value data that affects archetype identity)
* ex: ArchetypeA could have `[A, B, C]` table components and `[D(1)]` "tag" component. ArchetypeB could have `[A, B, C]` table components and a `[D(2)]` tag component. The archetypes are different, despite both having D tags because the value inside D is different.
* this could potentially build on top of the `archetype.unique_components` added in this pr for resource storage.
* Consider reverting `all_tuples` proc macro in favor of the old `macro_rules` implementation
* all_tuples is more flexible and produces cleaner documentation (the macro_rules version produces weird type parameter orders due to parser constraints)
* but unfortunately all_tuples also appears to make Rust Analyzer sad/slow when working inside of `bevy_ecs` (does not affect user code)
* Consider "resource queries" and/or "mixed resource and entity component queries" as an alternative to WorldCell
* this is basically just "systems" so maybe it's not worth it
* Add more world ops
* `world.clear()`
* `world.reserve<T: Bundle>(count: usize)`
* Try using the old archetype allocation strategy (allocate new memory on resize and copy everything over). I expect this to improve batch insertion performance at the cost of unbatched performance. But thats just a guess. I'm not an allocation perf pro :)
* Adapt Commands apis for consistency with new World apis
## Benchmarks
key:
* `bevy_old`: bevy `main` branch
* `bevy`: this branch
* `_foreach`: uses an optimized for_each iterator
* ` _sparse`: uses sparse set storage (if unspecified assume table storage)
* `_system`: runs inside a system (if unspecified assume test happens via direct world ops)
### Simple Insert (from ecs_bench_suite)
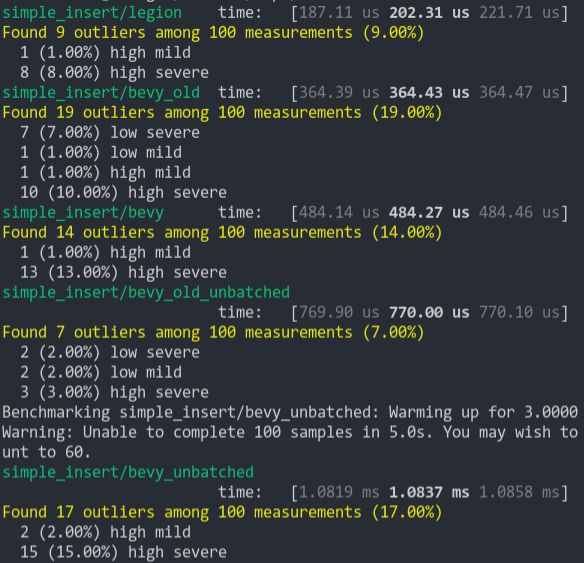
### Simpler Iter (from ecs_bench_suite)
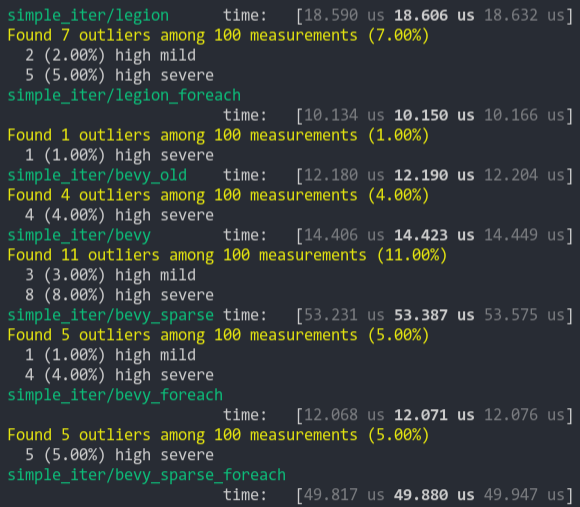
### Fragment Iter (from ecs_bench_suite)
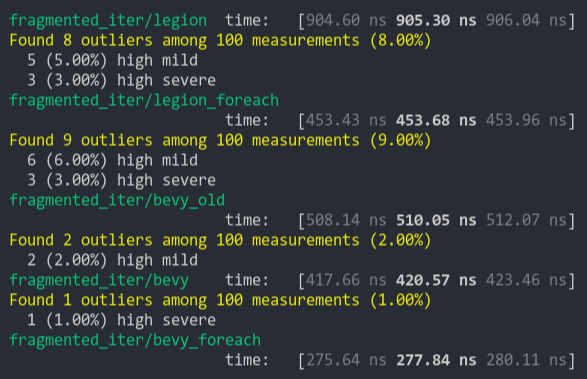
### Sparse Fragmented Iter
Iterate a query that matches 5 entities from a single matching archetype, but there are 100 unmatching archetypes
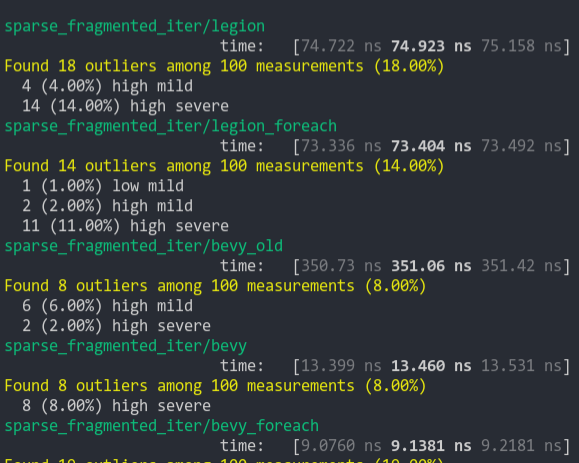
### Schedule (from ecs_bench_suite)
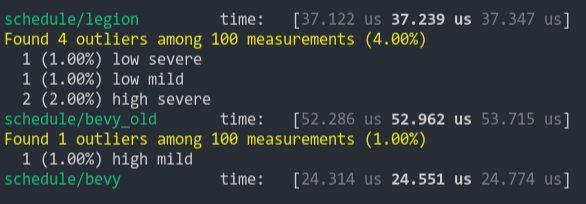
### Add Remove Component (from ecs_bench_suite)
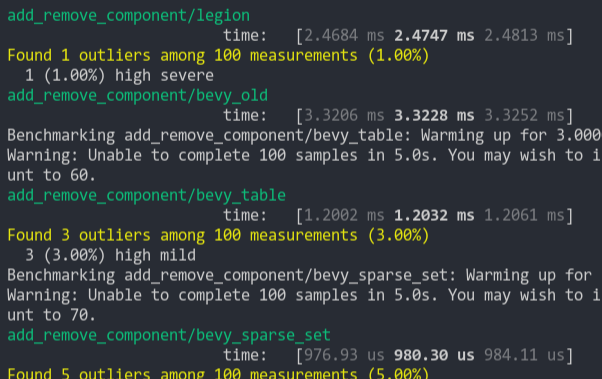
### Add Remove Component Big
Same as the test above, but each entity has 5 "large" matrix components and 1 "large" matrix component is added and removed
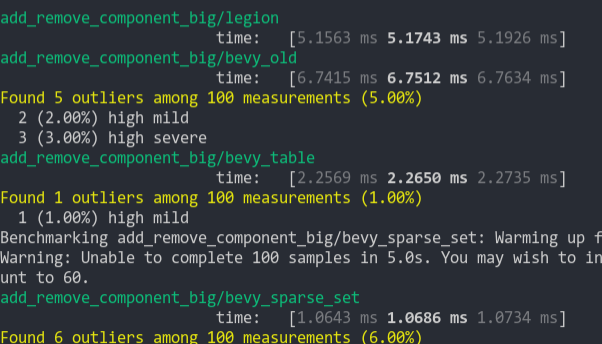
### Get Component
Looks up a single component value a large number of times
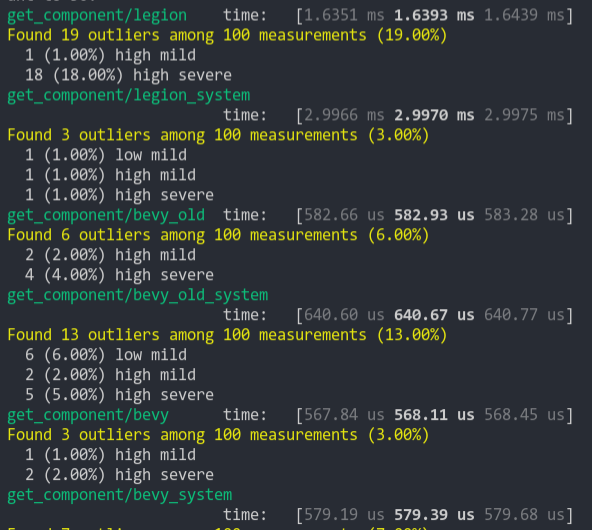
2021-03-05 07:54:35 +00:00
NonSendMut {
2022-10-24 13:46:36 +00:00
value : ptr . assert_unique ( ) . deref_mut ( ) ,
2023-01-11 15:41:54 +00:00
ticks : TicksMut ::from_tick_cells ( ticks , system_meta . last_change_tick , change_tick ) ,
Bevy ECS V2 (#1525)
# Bevy ECS V2
This is a rewrite of Bevy ECS (basically everything but the new executor/schedule, which are already awesome). The overall goal was to improve the performance and versatility of Bevy ECS. Here is a quick bulleted list of changes before we dive into the details:
* Complete World rewrite
* Multiple component storage types:
* Tables: fast cache friendly iteration, slower add/removes (previously called Archetypes)
* Sparse Sets: fast add/remove, slower iteration
* Stateful Queries (caches query results for faster iteration. fragmented iteration is _fast_ now)
* Stateful System Params (caches expensive operations. inspired by @DJMcNab's work in #1364)
* Configurable System Params (users can set configuration when they construct their systems. once again inspired by @DJMcNab's work)
* Archetypes are now "just metadata", component storage is separate
* Archetype Graph (for faster archetype changes)
* Component Metadata
* Configure component storage type
* Retrieve information about component size/type/name/layout/send-ness/etc
* Components are uniquely identified by a densely packed ComponentId
* TypeIds are now totally optional (which should make implementing scripting easier)
* Super fast "for_each" query iterators
* Merged Resources into World. Resources are now just a special type of component
* EntityRef/EntityMut builder apis (more efficient and more ergonomic)
* Fast bitset-backed `Access<T>` replaces old hashmap-based approach everywhere
* Query conflicts are determined by component access instead of archetype component access (to avoid random failures at runtime)
* With/Without are still taken into account for conflicts, so this should still be comfy to use
* Much simpler `IntoSystem` impl
* Significantly reduced the amount of hashing throughout the ecs in favor of Sparse Sets (indexed by densely packed ArchetypeId, ComponentId, BundleId, and TableId)
* Safety Improvements
* Entity reservation uses a normal world reference instead of unsafe transmute
* QuerySets no longer transmute lifetimes
* Made traits "unsafe" where relevant
* More thorough safety docs
* WorldCell
* Exposes safe mutable access to multiple resources at a time in a World
* Replaced "catch all" `System::update_archetypes(world: &World)` with `System::new_archetype(archetype: &Archetype)`
* Simpler Bundle implementation
* Replaced slow "remove_bundle_one_by_one" used as fallback for Commands::remove_bundle with fast "remove_bundle_intersection"
* Removed `Mut<T>` query impl. it is better to only support one way: `&mut T`
* Removed with() from `Flags<T>` in favor of `Option<Flags<T>>`, which allows querying for flags to be "filtered" by default
* Components now have is_send property (currently only resources support non-send)
* More granular module organization
* New `RemovedComponents<T>` SystemParam that replaces `query.removed::<T>()`
* `world.resource_scope()` for mutable access to resources and world at the same time
* WorldQuery and QueryFilter traits unified. FilterFetch trait added to enable "short circuit" filtering. Auto impled for cases that don't need it
* Significantly slimmed down SystemState in favor of individual SystemParam state
* System Commands changed from `commands: &mut Commands` back to `mut commands: Commands` (to allow Commands to have a World reference)
Fixes #1320
## `World` Rewrite
This is a from-scratch rewrite of `World` that fills the niche that `hecs` used to. Yes, this means Bevy ECS is no longer a "fork" of hecs. We're going out our own!
(the only shared code between the projects is the entity id allocator, which is already basically ideal)
A huge shout out to @SanderMertens (author of [flecs](https://github.com/SanderMertens/flecs)) for sharing some great ideas with me (specifically hybrid ecs storage and archetype graphs). He also helped advise on a number of implementation details.
## Component Storage (The Problem)
Two ECS storage paradigms have gained a lot of traction over the years:
* **Archetypal ECS**:
* Stores components in "tables" with static schemas. Each "column" stores components of a given type. Each "row" is an entity.
* Each "archetype" has its own table. Adding/removing an entity's component changes the archetype.
* Enables super-fast Query iteration due to its cache-friendly data layout
* Comes at the cost of more expensive add/remove operations for an Entity's components, because all components need to be copied to the new archetype's "table"
* **Sparse Set ECS**:
* Stores components of the same type in densely packed arrays, which are sparsely indexed by densely packed unsigned integers (Entity ids)
* Query iteration is slower than Archetypal ECS because each entity's component could be at any position in the sparse set. This "random access" pattern isn't cache friendly. Additionally, there is an extra layer of indirection because you must first map the entity id to an index in the component array.
* Adding/removing components is a cheap, constant time operation
Bevy ECS V1, hecs, legion, flec, and Unity DOTS are all "archetypal ecs-es". I personally think "archetypal" storage is a good default for game engines. An entity's archetype doesn't need to change frequently in general, and it creates "fast by default" query iteration (which is a much more common operation). It is also "self optimizing". Users don't need to think about optimizing component layouts for iteration performance. It "just works" without any extra boilerplate.
Shipyard and EnTT are "sparse set ecs-es". They employ "packing" as a way to work around the "suboptimal by default" iteration performance for specific sets of components. This helps, but I didn't think this was a good choice for a general purpose engine like Bevy because:
1. "packs" conflict with each other. If bevy decides to internally pack the Transform and GlobalTransform components, users are then blocked if they want to pack some custom component with Transform.
2. users need to take manual action to optimize
Developers selecting an ECS framework are stuck with a hard choice. Select an "archetypal" framework with "fast iteration everywhere" but without the ability to cheaply add/remove components, or select a "sparse set" framework to cheaply add/remove components but with slower iteration performance.
## Hybrid Component Storage (The Solution)
In Bevy ECS V2, we get to have our cake and eat it too. It now has _both_ of the component storage types above (and more can be added later if needed):
* **Tables** (aka "archetypal" storage)
* The default storage. If you don't configure anything, this is what you get
* Fast iteration by default
* Slower add/remove operations
* **Sparse Sets**
* Opt-in
* Slower iteration
* Faster add/remove operations
These storage types complement each other perfectly. By default Query iteration is fast. If developers know that they want to add/remove a component at high frequencies, they can set the storage to "sparse set":
```rust
world.register_component(
ComponentDescriptor::new::<MyComponent>(StorageType::SparseSet)
).unwrap();
```
## Archetypes
Archetypes are now "just metadata" ... they no longer store components directly. They do store:
* The `ComponentId`s of each of the Archetype's components (and that component's storage type)
* Archetypes are uniquely defined by their component layouts
* For example: entities with "table" components `[A, B, C]` _and_ "sparse set" components `[D, E]` will always be in the same archetype.
* The `TableId` associated with the archetype
* For now each archetype has exactly one table (which can have no components),
* There is a 1->Many relationship from Tables->Archetypes. A given table could have any number of archetype components stored in it:
* Ex: an entity with "table storage" components `[A, B, C]` and "sparse set" components `[D, E]` will share the same `[A, B, C]` table as an entity with `[A, B, C]` table component and `[F]` sparse set components.
* This 1->Many relationship is how we preserve fast "cache friendly" iteration performance when possible (more on this later)
* A list of entities that are in the archetype and the row id of the table they are in
* ArchetypeComponentIds
* unique densely packed identifiers for (ArchetypeId, ComponentId) pairs
* used by the schedule executor for cheap system access control
* "Archetype Graph Edges" (see the next section)
## The "Archetype Graph"
Archetype changes in Bevy (and a number of other archetypal ecs-es) have historically been expensive to compute. First, you need to allocate a new vector of the entity's current component ids, add or remove components based on the operation performed, sort it (to ensure it is order-independent), then hash it to find the archetype (if it exists). And thats all before we get to the _already_ expensive full copy of all components to the new table storage.
The solution is to build a "graph" of archetypes to cache these results. @SanderMertens first exposed me to the idea (and he got it from @gjroelofs, who came up with it). They propose adding directed edges between archetypes for add/remove component operations. If `ComponentId`s are densely packed, you can use sparse sets to cheaply jump between archetypes.
Bevy takes this one step further by using add/remove `Bundle` edges instead of `Component` edges. Bevy encourages the use of `Bundles` to group add/remove operations. This is largely for "clearer game logic" reasons, but it also helps cut down on the number of archetype changes required. `Bundles` now also have densely-packed `BundleId`s. This allows us to use a _single_ edge for each bundle operation (rather than needing to traverse N edges ... one for each component). Single component operations are also bundles, so this is strictly an improvement over a "component only" graph.
As a result, an operation that used to be _heavy_ (both for allocations and compute) is now two dirt-cheap array lookups and zero allocations.
## Stateful Queries
World queries are now stateful. This allows us to:
1. Cache archetype (and table) matches
* This resolves another issue with (naive) archetypal ECS: query performance getting worse as the number of archetypes goes up (and fragmentation occurs).
2. Cache Fetch and Filter state
* The expensive parts of fetch/filter operations (such as hashing the TypeId to find the ComponentId) now only happen once when the Query is first constructed
3. Incrementally build up state
* When new archetypes are added, we only process the new archetypes (no need to rebuild state for old archetypes)
As a result, the direct `World` query api now looks like this:
```rust
let mut query = world.query::<(&A, &mut B)>();
for (a, mut b) in query.iter_mut(&mut world) {
}
```
Requiring `World` to generate stateful queries (rather than letting the `QueryState` type be constructed separately) allows us to ensure that _all_ queries are properly initialized (and the relevant world state, such as ComponentIds). This enables QueryState to remove branches from its operations that check for initialization status (and also enables query.iter() to take an immutable world reference because it doesn't need to initialize anything in world).
However in systems, this is a non-breaking change. State management is done internally by the relevant SystemParam.
## Stateful SystemParams
Like Queries, `SystemParams` now also cache state. For example, `Query` system params store the "stateful query" state mentioned above. Commands store their internal `CommandQueue`. This means you can now safely use as many separate `Commands` parameters in your system as you want. `Local<T>` system params store their `T` value in their state (instead of in Resources).
SystemParam state also enabled a significant slim-down of SystemState. It is much nicer to look at now.
Per-SystemParam state naturally insulates us from an "aliased mut" class of errors we have hit in the past (ex: using multiple `Commands` system params).
(credit goes to @DJMcNab for the initial idea and draft pr here #1364)
## Configurable SystemParams
@DJMcNab also had the great idea to make SystemParams configurable. This allows users to provide some initial configuration / values for system parameters (when possible). Most SystemParams have no config (the config type is `()`), but the `Local<T>` param now supports user-provided parameters:
```rust
fn foo(value: Local<usize>) {
}
app.add_system(foo.system().config(|c| c.0 = Some(10)));
```
## Uber Fast "for_each" Query Iterators
Developers now have the choice to use a fast "for_each" iterator, which yields ~1.5-3x iteration speed improvements for "fragmented iteration", and minor ~1.2x iteration speed improvements for unfragmented iteration.
```rust
fn system(query: Query<(&A, &mut B)>) {
// you now have the option to do this for a speed boost
query.for_each_mut(|(a, mut b)| {
});
// however normal iterators are still available
for (a, mut b) in query.iter_mut() {
}
}
```
I think in most cases we should continue to encourage "normal" iterators as they are more flexible and more "rust idiomatic". But when that extra "oomf" is needed, it makes sense to use `for_each`.
We should also consider using `for_each` for internal bevy systems to give our users a nice speed boost (but that should be a separate pr).
## Component Metadata
`World` now has a `Components` collection, which is accessible via `world.components()`. This stores mappings from `ComponentId` to `ComponentInfo`, as well as `TypeId` to `ComponentId` mappings (where relevant). `ComponentInfo` stores information about the component, such as ComponentId, TypeId, memory layout, send-ness (currently limited to resources), and storage type.
## Significantly Cheaper `Access<T>`
We used to use `TypeAccess<TypeId>` to manage read/write component/archetype-component access. This was expensive because TypeIds must be hashed and compared individually. The parallel executor got around this by "condensing" type ids into bitset-backed access types. This worked, but it had to be re-generated from the `TypeAccess<TypeId>`sources every time archetypes changed.
This pr removes TypeAccess in favor of faster bitset access everywhere. We can do this thanks to the move to densely packed `ComponentId`s and `ArchetypeComponentId`s.
## Merged Resources into World
Resources had a lot of redundant functionality with Components. They stored typed data, they had access control, they had unique ids, they were queryable via SystemParams, etc. In fact the _only_ major difference between them was that they were unique (and didn't correlate to an entity).
Separate resources also had the downside of requiring a separate set of access controls, which meant the parallel executor needed to compare more bitsets per system and manage more state.
I initially got the "separate resources" idea from `legion`. I think that design was motivated by the fact that it made the direct world query/resource lifetime interactions more manageable. It certainly made our lives easier when using Resources alongside hecs/bevy_ecs. However we already have a construct for safely and ergonomically managing in-world lifetimes: systems (which use `Access<T>` internally).
This pr merges Resources into World:
```rust
world.insert_resource(1);
world.insert_resource(2.0);
let a = world.get_resource::<i32>().unwrap();
let mut b = world.get_resource_mut::<f64>().unwrap();
*b = 3.0;
```
Resources are now just a special kind of component. They have their own ComponentIds (and their own resource TypeId->ComponentId scope, so they don't conflict wit components of the same type). They are stored in a special "resource archetype", which stores components inside the archetype using a new `unique_components` sparse set (note that this sparse set could later be used to implement Tags). This allows us to keep the code size small by reusing existing datastructures (namely Column, Archetype, ComponentFlags, and ComponentInfo). This allows us the executor to use a single `Access<ArchetypeComponentId>` per system. It should also make scripting language integration easier.
_But_ this merge did create problems for people directly interacting with `World`. What if you need mutable access to multiple resources at the same time? `world.get_resource_mut()` borrows World mutably!
## WorldCell
WorldCell applies the `Access<ArchetypeComponentId>` concept to direct world access:
```rust
let world_cell = world.cell();
let a = world_cell.get_resource_mut::<i32>().unwrap();
let b = world_cell.get_resource_mut::<f64>().unwrap();
```
This adds cheap runtime checks (a sparse set lookup of `ArchetypeComponentId` and a counter) to ensure that world accesses do not conflict with each other. Each operation returns a `WorldBorrow<'w, T>` or `WorldBorrowMut<'w, T>` wrapper type, which will release the relevant ArchetypeComponentId resources when dropped.
World caches the access sparse set (and only one cell can exist at a time), so `world.cell()` is a cheap operation.
WorldCell does _not_ use atomic operations. It is non-send, does a mutable borrow of world to prevent other accesses, and uses a simple `Rc<RefCell<ArchetypeComponentAccess>>` wrapper in each WorldBorrow pointer.
The api is currently limited to resource access, but it can and should be extended to queries / entity component access.
## Resource Scopes
WorldCell does not yet support component queries, and even when it does there are sometimes legitimate reasons to want a mutable world ref _and_ a mutable resource ref (ex: bevy_render and bevy_scene both need this). In these cases we could always drop down to the unsafe `world.get_resource_unchecked_mut()`, but that is not ideal!
Instead developers can use a "resource scope"
```rust
world.resource_scope(|world: &mut World, a: &mut A| {
})
```
This temporarily removes the `A` resource from `World`, provides mutable pointers to both, and re-adds A to World when finished. Thanks to the move to ComponentIds/sparse sets, this is a cheap operation.
If multiple resources are required, scopes can be nested. We could also consider adding a "resource tuple" to the api if this pattern becomes common and the boilerplate gets nasty.
## Query Conflicts Use ComponentId Instead of ArchetypeComponentId
For safety reasons, systems cannot contain queries that conflict with each other without wrapping them in a QuerySet. On bevy `main`, we use ArchetypeComponentIds to determine conflicts. This is nice because it can take into account filters:
```rust
// these queries will never conflict due to their filters
fn filter_system(a: Query<&mut A, With<B>>, b: Query<&mut B, Without<B>>) {
}
```
But it also has a significant downside:
```rust
// these queries will not conflict _until_ an entity with A, B, and C is spawned
fn maybe_conflicts_system(a: Query<(&mut A, &C)>, b: Query<(&mut A, &B)>) {
}
```
The system above will panic at runtime if an entity with A, B, and C is spawned. This makes it hard to trust that your game logic will run without crashing.
In this pr, I switched to using `ComponentId` instead. This _is_ more constraining. `maybe_conflicts_system` will now always fail, but it will do it consistently at startup. Naively, it would also _disallow_ `filter_system`, which would be a significant downgrade in usability. Bevy has a number of internal systems that rely on disjoint queries and I expect it to be a common pattern in userspace.
To resolve this, I added a new `FilteredAccess<T>` type, which wraps `Access<T>` and adds with/without filters. If two `FilteredAccess` have with/without values that prove they are disjoint, they will no longer conflict.
## EntityRef / EntityMut
World entity operations on `main` require that the user passes in an `entity` id to each operation:
```rust
let entity = world.spawn((A, )); // create a new entity with A
world.get::<A>(entity);
world.insert(entity, (B, C));
world.insert_one(entity, D);
```
This means that each operation needs to look up the entity location / verify its validity. The initial spawn operation also requires a Bundle as input. This can be awkward when no components are required (or one component is required).
These operations have been replaced by `EntityRef` and `EntityMut`, which are "builder-style" wrappers around world that provide read and read/write operations on a single, pre-validated entity:
```rust
// spawn now takes no inputs and returns an EntityMut
let entity = world.spawn()
.insert(A) // insert a single component into the entity
.insert_bundle((B, C)) // insert a bundle of components into the entity
.id() // id returns the Entity id
// Returns EntityMut (or panics if the entity does not exist)
world.entity_mut(entity)
.insert(D)
.insert_bundle(SomeBundle::default());
{
// returns EntityRef (or panics if the entity does not exist)
let d = world.entity(entity)
.get::<D>() // gets the D component
.unwrap();
// world.get still exists for ergonomics
let d = world.get::<D>(entity).unwrap();
}
// These variants return Options if you want to check existence instead of panicing
world.get_entity_mut(entity)
.unwrap()
.insert(E);
if let Some(entity_ref) = world.get_entity(entity) {
let d = entity_ref.get::<D>().unwrap();
}
```
This _does not_ affect the current Commands api or terminology. I think that should be a separate conversation as that is a much larger breaking change.
## Safety Improvements
* Entity reservation in Commands uses a normal world borrow instead of an unsafe transmute
* QuerySets no longer transmutes lifetimes
* Made traits "unsafe" when implementing a trait incorrectly could cause unsafety
* More thorough safety docs
## RemovedComponents SystemParam
The old approach to querying removed components: `query.removed:<T>()` was confusing because it had no connection to the query itself. I replaced it with the following, which is both clearer and allows us to cache the ComponentId mapping in the SystemParamState:
```rust
fn system(removed: RemovedComponents<T>) {
for entity in removed.iter() {
}
}
```
## Simpler Bundle implementation
Bundles are no longer responsible for sorting (or deduping) TypeInfo. They are just a simple ordered list of component types / data. This makes the implementation smaller and opens the door to an easy "nested bundle" implementation in the future (which i might even add in this pr). Duplicate detection is now done once per bundle type by World the first time a bundle is used.
## Unified WorldQuery and QueryFilter types
(don't worry they are still separate type _parameters_ in Queries .. this is a non-breaking change)
WorldQuery and QueryFilter were already basically identical apis. With the addition of `FetchState` and more storage-specific fetch methods, the overlap was even clearer (and the redundancy more painful).
QueryFilters are now just `F: WorldQuery where F::Fetch: FilterFetch`. FilterFetch requires `Fetch<Item = bool>` and adds new "short circuit" variants of fetch methods. This enables a filter tuple like `(With<A>, Without<B>, Changed<C>)` to stop evaluating the filter after the first mismatch is encountered. FilterFetch is automatically implemented for `Fetch` implementations that return bool.
This forces fetch implementations that return things like `(bool, bool, bool)` (such as the filter above) to manually implement FilterFetch and decide whether or not to short-circuit.
## More Granular Modules
World no longer globs all of the internal modules together. It now exports `core`, `system`, and `schedule` separately. I'm also considering exporting `core` submodules directly as that is still pretty "glob-ey" and unorganized (feedback welcome here).
## Remaining Draft Work (to be done in this pr)
* ~~panic on conflicting WorldQuery fetches (&A, &mut A)~~
* ~~bevy `main` and hecs both currently allow this, but we should protect against it if possible~~
* ~~batch_iter / par_iter (currently stubbed out)~~
* ~~ChangedRes~~
* ~~I skipped this while we sort out #1313. This pr should be adapted to account for whatever we land on there~~.
* ~~The `Archetypes` and `Tables` collections use hashes of sorted lists of component ids to uniquely identify each archetype/table. This hash is then used as the key in a HashMap to look up the relevant ArchetypeId or TableId. (which doesn't handle hash collisions properly)~~
* ~~It is currently unsafe to generate a Query from "World A", then use it on "World B" (despite the api claiming it is safe). We should probably close this gap. This could be done by adding a randomly generated WorldId to each world, then storing that id in each Query. They could then be compared to each other on each `query.do_thing(&world)` operation. This _does_ add an extra branch to each query operation, so I'm open to other suggestions if people have them.~~
* ~~Nested Bundles (if i find time)~~
## Potential Future Work
* Expand WorldCell to support queries.
* Consider not allocating in the empty archetype on `world.spawn()`
* ex: return something like EntityMutUninit, which turns into EntityMut after an `insert` or `insert_bundle` op
* this actually regressed performance last time i tried it, but in theory it should be faster
* Optimize SparseSet::insert (see `PERF` comment on insert)
* Replace SparseArray `Option<T>` with T::MAX to cut down on branching
* would enable cheaper get_unchecked() operations
* upstream fixedbitset optimizations
* fixedbitset could be allocation free for small block counts (store blocks in a SmallVec)
* fixedbitset could have a const constructor
* Consider implementing Tags (archetype-specific by-value data that affects archetype identity)
* ex: ArchetypeA could have `[A, B, C]` table components and `[D(1)]` "tag" component. ArchetypeB could have `[A, B, C]` table components and a `[D(2)]` tag component. The archetypes are different, despite both having D tags because the value inside D is different.
* this could potentially build on top of the `archetype.unique_components` added in this pr for resource storage.
* Consider reverting `all_tuples` proc macro in favor of the old `macro_rules` implementation
* all_tuples is more flexible and produces cleaner documentation (the macro_rules version produces weird type parameter orders due to parser constraints)
* but unfortunately all_tuples also appears to make Rust Analyzer sad/slow when working inside of `bevy_ecs` (does not affect user code)
* Consider "resource queries" and/or "mixed resource and entity component queries" as an alternative to WorldCell
* this is basically just "systems" so maybe it's not worth it
* Add more world ops
* `world.clear()`
* `world.reserve<T: Bundle>(count: usize)`
* Try using the old archetype allocation strategy (allocate new memory on resize and copy everything over). I expect this to improve batch insertion performance at the cost of unbatched performance. But thats just a guess. I'm not an allocation perf pro :)
* Adapt Commands apis for consistency with new World apis
## Benchmarks
key:
* `bevy_old`: bevy `main` branch
* `bevy`: this branch
* `_foreach`: uses an optimized for_each iterator
* ` _sparse`: uses sparse set storage (if unspecified assume table storage)
* `_system`: runs inside a system (if unspecified assume test happens via direct world ops)
### Simple Insert (from ecs_bench_suite)
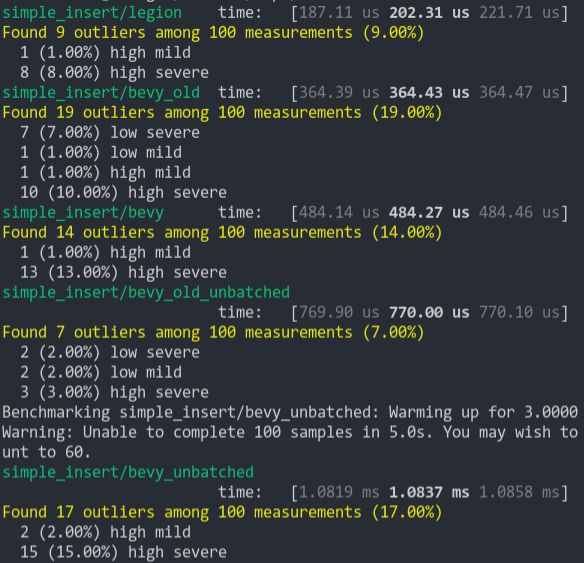
### Simpler Iter (from ecs_bench_suite)
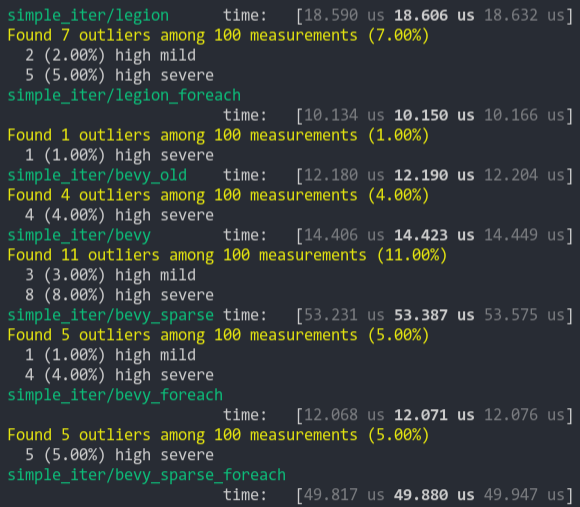
### Fragment Iter (from ecs_bench_suite)
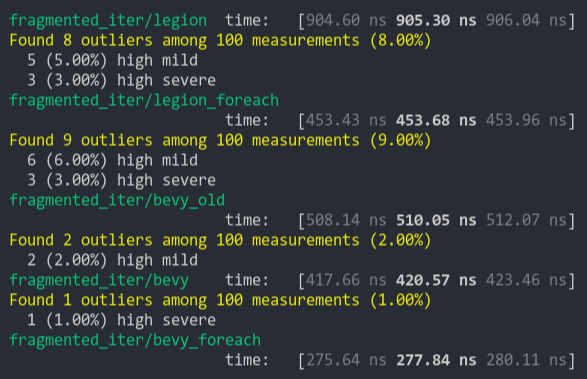
### Sparse Fragmented Iter
Iterate a query that matches 5 entities from a single matching archetype, but there are 100 unmatching archetypes
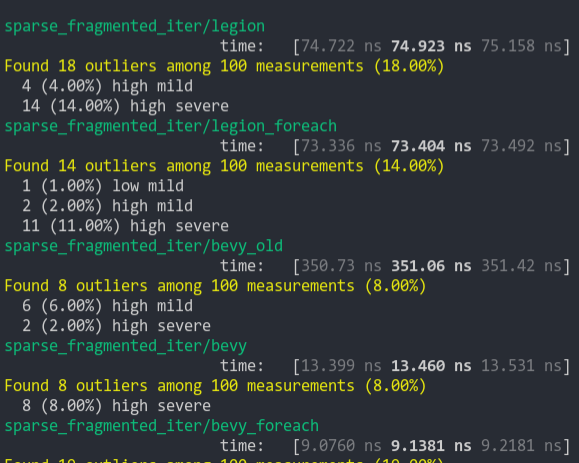
### Schedule (from ecs_bench_suite)
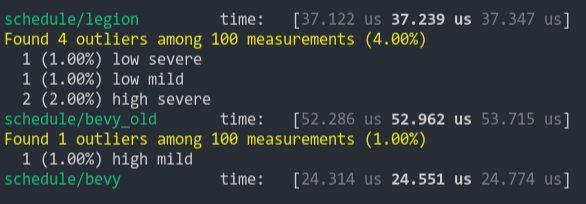
### Add Remove Component (from ecs_bench_suite)
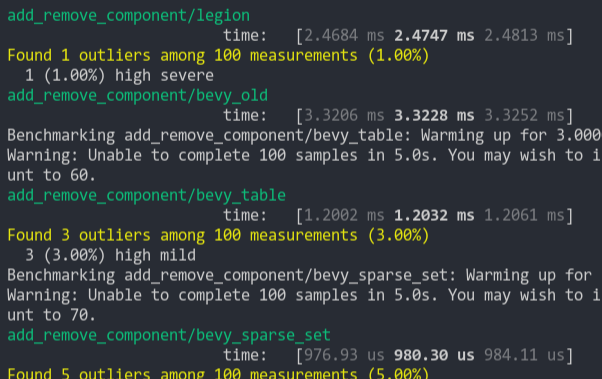
### Add Remove Component Big
Same as the test above, but each entity has 5 "large" matrix components and 1 "large" matrix component is added and removed
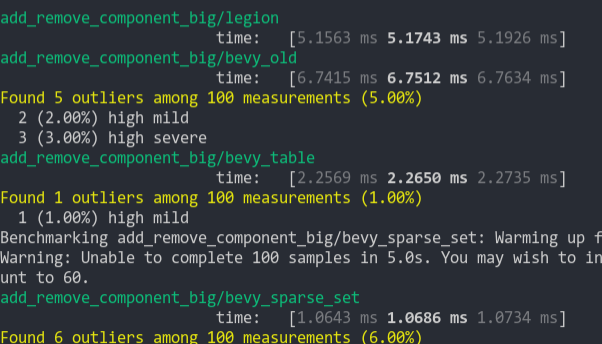
### Get Component
Looks up a single component value a large number of times
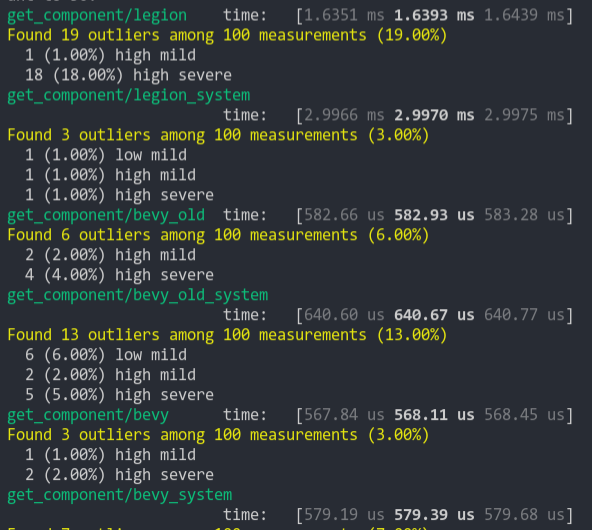
2021-03-05 07:54:35 +00:00
}
2020-11-08 20:34:05 +00:00
}
Bevy ECS V2 (#1525)
# Bevy ECS V2
This is a rewrite of Bevy ECS (basically everything but the new executor/schedule, which are already awesome). The overall goal was to improve the performance and versatility of Bevy ECS. Here is a quick bulleted list of changes before we dive into the details:
* Complete World rewrite
* Multiple component storage types:
* Tables: fast cache friendly iteration, slower add/removes (previously called Archetypes)
* Sparse Sets: fast add/remove, slower iteration
* Stateful Queries (caches query results for faster iteration. fragmented iteration is _fast_ now)
* Stateful System Params (caches expensive operations. inspired by @DJMcNab's work in #1364)
* Configurable System Params (users can set configuration when they construct their systems. once again inspired by @DJMcNab's work)
* Archetypes are now "just metadata", component storage is separate
* Archetype Graph (for faster archetype changes)
* Component Metadata
* Configure component storage type
* Retrieve information about component size/type/name/layout/send-ness/etc
* Components are uniquely identified by a densely packed ComponentId
* TypeIds are now totally optional (which should make implementing scripting easier)
* Super fast "for_each" query iterators
* Merged Resources into World. Resources are now just a special type of component
* EntityRef/EntityMut builder apis (more efficient and more ergonomic)
* Fast bitset-backed `Access<T>` replaces old hashmap-based approach everywhere
* Query conflicts are determined by component access instead of archetype component access (to avoid random failures at runtime)
* With/Without are still taken into account for conflicts, so this should still be comfy to use
* Much simpler `IntoSystem` impl
* Significantly reduced the amount of hashing throughout the ecs in favor of Sparse Sets (indexed by densely packed ArchetypeId, ComponentId, BundleId, and TableId)
* Safety Improvements
* Entity reservation uses a normal world reference instead of unsafe transmute
* QuerySets no longer transmute lifetimes
* Made traits "unsafe" where relevant
* More thorough safety docs
* WorldCell
* Exposes safe mutable access to multiple resources at a time in a World
* Replaced "catch all" `System::update_archetypes(world: &World)` with `System::new_archetype(archetype: &Archetype)`
* Simpler Bundle implementation
* Replaced slow "remove_bundle_one_by_one" used as fallback for Commands::remove_bundle with fast "remove_bundle_intersection"
* Removed `Mut<T>` query impl. it is better to only support one way: `&mut T`
* Removed with() from `Flags<T>` in favor of `Option<Flags<T>>`, which allows querying for flags to be "filtered" by default
* Components now have is_send property (currently only resources support non-send)
* More granular module organization
* New `RemovedComponents<T>` SystemParam that replaces `query.removed::<T>()`
* `world.resource_scope()` for mutable access to resources and world at the same time
* WorldQuery and QueryFilter traits unified. FilterFetch trait added to enable "short circuit" filtering. Auto impled for cases that don't need it
* Significantly slimmed down SystemState in favor of individual SystemParam state
* System Commands changed from `commands: &mut Commands` back to `mut commands: Commands` (to allow Commands to have a World reference)
Fixes #1320
## `World` Rewrite
This is a from-scratch rewrite of `World` that fills the niche that `hecs` used to. Yes, this means Bevy ECS is no longer a "fork" of hecs. We're going out our own!
(the only shared code between the projects is the entity id allocator, which is already basically ideal)
A huge shout out to @SanderMertens (author of [flecs](https://github.com/SanderMertens/flecs)) for sharing some great ideas with me (specifically hybrid ecs storage and archetype graphs). He also helped advise on a number of implementation details.
## Component Storage (The Problem)
Two ECS storage paradigms have gained a lot of traction over the years:
* **Archetypal ECS**:
* Stores components in "tables" with static schemas. Each "column" stores components of a given type. Each "row" is an entity.
* Each "archetype" has its own table. Adding/removing an entity's component changes the archetype.
* Enables super-fast Query iteration due to its cache-friendly data layout
* Comes at the cost of more expensive add/remove operations for an Entity's components, because all components need to be copied to the new archetype's "table"
* **Sparse Set ECS**:
* Stores components of the same type in densely packed arrays, which are sparsely indexed by densely packed unsigned integers (Entity ids)
* Query iteration is slower than Archetypal ECS because each entity's component could be at any position in the sparse set. This "random access" pattern isn't cache friendly. Additionally, there is an extra layer of indirection because you must first map the entity id to an index in the component array.
* Adding/removing components is a cheap, constant time operation
Bevy ECS V1, hecs, legion, flec, and Unity DOTS are all "archetypal ecs-es". I personally think "archetypal" storage is a good default for game engines. An entity's archetype doesn't need to change frequently in general, and it creates "fast by default" query iteration (which is a much more common operation). It is also "self optimizing". Users don't need to think about optimizing component layouts for iteration performance. It "just works" without any extra boilerplate.
Shipyard and EnTT are "sparse set ecs-es". They employ "packing" as a way to work around the "suboptimal by default" iteration performance for specific sets of components. This helps, but I didn't think this was a good choice for a general purpose engine like Bevy because:
1. "packs" conflict with each other. If bevy decides to internally pack the Transform and GlobalTransform components, users are then blocked if they want to pack some custom component with Transform.
2. users need to take manual action to optimize
Developers selecting an ECS framework are stuck with a hard choice. Select an "archetypal" framework with "fast iteration everywhere" but without the ability to cheaply add/remove components, or select a "sparse set" framework to cheaply add/remove components but with slower iteration performance.
## Hybrid Component Storage (The Solution)
In Bevy ECS V2, we get to have our cake and eat it too. It now has _both_ of the component storage types above (and more can be added later if needed):
* **Tables** (aka "archetypal" storage)
* The default storage. If you don't configure anything, this is what you get
* Fast iteration by default
* Slower add/remove operations
* **Sparse Sets**
* Opt-in
* Slower iteration
* Faster add/remove operations
These storage types complement each other perfectly. By default Query iteration is fast. If developers know that they want to add/remove a component at high frequencies, they can set the storage to "sparse set":
```rust
world.register_component(
ComponentDescriptor::new::<MyComponent>(StorageType::SparseSet)
).unwrap();
```
## Archetypes
Archetypes are now "just metadata" ... they no longer store components directly. They do store:
* The `ComponentId`s of each of the Archetype's components (and that component's storage type)
* Archetypes are uniquely defined by their component layouts
* For example: entities with "table" components `[A, B, C]` _and_ "sparse set" components `[D, E]` will always be in the same archetype.
* The `TableId` associated with the archetype
* For now each archetype has exactly one table (which can have no components),
* There is a 1->Many relationship from Tables->Archetypes. A given table could have any number of archetype components stored in it:
* Ex: an entity with "table storage" components `[A, B, C]` and "sparse set" components `[D, E]` will share the same `[A, B, C]` table as an entity with `[A, B, C]` table component and `[F]` sparse set components.
* This 1->Many relationship is how we preserve fast "cache friendly" iteration performance when possible (more on this later)
* A list of entities that are in the archetype and the row id of the table they are in
* ArchetypeComponentIds
* unique densely packed identifiers for (ArchetypeId, ComponentId) pairs
* used by the schedule executor for cheap system access control
* "Archetype Graph Edges" (see the next section)
## The "Archetype Graph"
Archetype changes in Bevy (and a number of other archetypal ecs-es) have historically been expensive to compute. First, you need to allocate a new vector of the entity's current component ids, add or remove components based on the operation performed, sort it (to ensure it is order-independent), then hash it to find the archetype (if it exists). And thats all before we get to the _already_ expensive full copy of all components to the new table storage.
The solution is to build a "graph" of archetypes to cache these results. @SanderMertens first exposed me to the idea (and he got it from @gjroelofs, who came up with it). They propose adding directed edges between archetypes for add/remove component operations. If `ComponentId`s are densely packed, you can use sparse sets to cheaply jump between archetypes.
Bevy takes this one step further by using add/remove `Bundle` edges instead of `Component` edges. Bevy encourages the use of `Bundles` to group add/remove operations. This is largely for "clearer game logic" reasons, but it also helps cut down on the number of archetype changes required. `Bundles` now also have densely-packed `BundleId`s. This allows us to use a _single_ edge for each bundle operation (rather than needing to traverse N edges ... one for each component). Single component operations are also bundles, so this is strictly an improvement over a "component only" graph.
As a result, an operation that used to be _heavy_ (both for allocations and compute) is now two dirt-cheap array lookups and zero allocations.
## Stateful Queries
World queries are now stateful. This allows us to:
1. Cache archetype (and table) matches
* This resolves another issue with (naive) archetypal ECS: query performance getting worse as the number of archetypes goes up (and fragmentation occurs).
2. Cache Fetch and Filter state
* The expensive parts of fetch/filter operations (such as hashing the TypeId to find the ComponentId) now only happen once when the Query is first constructed
3. Incrementally build up state
* When new archetypes are added, we only process the new archetypes (no need to rebuild state for old archetypes)
As a result, the direct `World` query api now looks like this:
```rust
let mut query = world.query::<(&A, &mut B)>();
for (a, mut b) in query.iter_mut(&mut world) {
}
```
Requiring `World` to generate stateful queries (rather than letting the `QueryState` type be constructed separately) allows us to ensure that _all_ queries are properly initialized (and the relevant world state, such as ComponentIds). This enables QueryState to remove branches from its operations that check for initialization status (and also enables query.iter() to take an immutable world reference because it doesn't need to initialize anything in world).
However in systems, this is a non-breaking change. State management is done internally by the relevant SystemParam.
## Stateful SystemParams
Like Queries, `SystemParams` now also cache state. For example, `Query` system params store the "stateful query" state mentioned above. Commands store their internal `CommandQueue`. This means you can now safely use as many separate `Commands` parameters in your system as you want. `Local<T>` system params store their `T` value in their state (instead of in Resources).
SystemParam state also enabled a significant slim-down of SystemState. It is much nicer to look at now.
Per-SystemParam state naturally insulates us from an "aliased mut" class of errors we have hit in the past (ex: using multiple `Commands` system params).
(credit goes to @DJMcNab for the initial idea and draft pr here #1364)
## Configurable SystemParams
@DJMcNab also had the great idea to make SystemParams configurable. This allows users to provide some initial configuration / values for system parameters (when possible). Most SystemParams have no config (the config type is `()`), but the `Local<T>` param now supports user-provided parameters:
```rust
fn foo(value: Local<usize>) {
}
app.add_system(foo.system().config(|c| c.0 = Some(10)));
```
## Uber Fast "for_each" Query Iterators
Developers now have the choice to use a fast "for_each" iterator, which yields ~1.5-3x iteration speed improvements for "fragmented iteration", and minor ~1.2x iteration speed improvements for unfragmented iteration.
```rust
fn system(query: Query<(&A, &mut B)>) {
// you now have the option to do this for a speed boost
query.for_each_mut(|(a, mut b)| {
});
// however normal iterators are still available
for (a, mut b) in query.iter_mut() {
}
}
```
I think in most cases we should continue to encourage "normal" iterators as they are more flexible and more "rust idiomatic". But when that extra "oomf" is needed, it makes sense to use `for_each`.
We should also consider using `for_each` for internal bevy systems to give our users a nice speed boost (but that should be a separate pr).
## Component Metadata
`World` now has a `Components` collection, which is accessible via `world.components()`. This stores mappings from `ComponentId` to `ComponentInfo`, as well as `TypeId` to `ComponentId` mappings (where relevant). `ComponentInfo` stores information about the component, such as ComponentId, TypeId, memory layout, send-ness (currently limited to resources), and storage type.
## Significantly Cheaper `Access<T>`
We used to use `TypeAccess<TypeId>` to manage read/write component/archetype-component access. This was expensive because TypeIds must be hashed and compared individually. The parallel executor got around this by "condensing" type ids into bitset-backed access types. This worked, but it had to be re-generated from the `TypeAccess<TypeId>`sources every time archetypes changed.
This pr removes TypeAccess in favor of faster bitset access everywhere. We can do this thanks to the move to densely packed `ComponentId`s and `ArchetypeComponentId`s.
## Merged Resources into World
Resources had a lot of redundant functionality with Components. They stored typed data, they had access control, they had unique ids, they were queryable via SystemParams, etc. In fact the _only_ major difference between them was that they were unique (and didn't correlate to an entity).
Separate resources also had the downside of requiring a separate set of access controls, which meant the parallel executor needed to compare more bitsets per system and manage more state.
I initially got the "separate resources" idea from `legion`. I think that design was motivated by the fact that it made the direct world query/resource lifetime interactions more manageable. It certainly made our lives easier when using Resources alongside hecs/bevy_ecs. However we already have a construct for safely and ergonomically managing in-world lifetimes: systems (which use `Access<T>` internally).
This pr merges Resources into World:
```rust
world.insert_resource(1);
world.insert_resource(2.0);
let a = world.get_resource::<i32>().unwrap();
let mut b = world.get_resource_mut::<f64>().unwrap();
*b = 3.0;
```
Resources are now just a special kind of component. They have their own ComponentIds (and their own resource TypeId->ComponentId scope, so they don't conflict wit components of the same type). They are stored in a special "resource archetype", which stores components inside the archetype using a new `unique_components` sparse set (note that this sparse set could later be used to implement Tags). This allows us to keep the code size small by reusing existing datastructures (namely Column, Archetype, ComponentFlags, and ComponentInfo). This allows us the executor to use a single `Access<ArchetypeComponentId>` per system. It should also make scripting language integration easier.
_But_ this merge did create problems for people directly interacting with `World`. What if you need mutable access to multiple resources at the same time? `world.get_resource_mut()` borrows World mutably!
## WorldCell
WorldCell applies the `Access<ArchetypeComponentId>` concept to direct world access:
```rust
let world_cell = world.cell();
let a = world_cell.get_resource_mut::<i32>().unwrap();
let b = world_cell.get_resource_mut::<f64>().unwrap();
```
This adds cheap runtime checks (a sparse set lookup of `ArchetypeComponentId` and a counter) to ensure that world accesses do not conflict with each other. Each operation returns a `WorldBorrow<'w, T>` or `WorldBorrowMut<'w, T>` wrapper type, which will release the relevant ArchetypeComponentId resources when dropped.
World caches the access sparse set (and only one cell can exist at a time), so `world.cell()` is a cheap operation.
WorldCell does _not_ use atomic operations. It is non-send, does a mutable borrow of world to prevent other accesses, and uses a simple `Rc<RefCell<ArchetypeComponentAccess>>` wrapper in each WorldBorrow pointer.
The api is currently limited to resource access, but it can and should be extended to queries / entity component access.
## Resource Scopes
WorldCell does not yet support component queries, and even when it does there are sometimes legitimate reasons to want a mutable world ref _and_ a mutable resource ref (ex: bevy_render and bevy_scene both need this). In these cases we could always drop down to the unsafe `world.get_resource_unchecked_mut()`, but that is not ideal!
Instead developers can use a "resource scope"
```rust
world.resource_scope(|world: &mut World, a: &mut A| {
})
```
This temporarily removes the `A` resource from `World`, provides mutable pointers to both, and re-adds A to World when finished. Thanks to the move to ComponentIds/sparse sets, this is a cheap operation.
If multiple resources are required, scopes can be nested. We could also consider adding a "resource tuple" to the api if this pattern becomes common and the boilerplate gets nasty.
## Query Conflicts Use ComponentId Instead of ArchetypeComponentId
For safety reasons, systems cannot contain queries that conflict with each other without wrapping them in a QuerySet. On bevy `main`, we use ArchetypeComponentIds to determine conflicts. This is nice because it can take into account filters:
```rust
// these queries will never conflict due to their filters
fn filter_system(a: Query<&mut A, With<B>>, b: Query<&mut B, Without<B>>) {
}
```
But it also has a significant downside:
```rust
// these queries will not conflict _until_ an entity with A, B, and C is spawned
fn maybe_conflicts_system(a: Query<(&mut A, &C)>, b: Query<(&mut A, &B)>) {
}
```
The system above will panic at runtime if an entity with A, B, and C is spawned. This makes it hard to trust that your game logic will run without crashing.
In this pr, I switched to using `ComponentId` instead. This _is_ more constraining. `maybe_conflicts_system` will now always fail, but it will do it consistently at startup. Naively, it would also _disallow_ `filter_system`, which would be a significant downgrade in usability. Bevy has a number of internal systems that rely on disjoint queries and I expect it to be a common pattern in userspace.
To resolve this, I added a new `FilteredAccess<T>` type, which wraps `Access<T>` and adds with/without filters. If two `FilteredAccess` have with/without values that prove they are disjoint, they will no longer conflict.
## EntityRef / EntityMut
World entity operations on `main` require that the user passes in an `entity` id to each operation:
```rust
let entity = world.spawn((A, )); // create a new entity with A
world.get::<A>(entity);
world.insert(entity, (B, C));
world.insert_one(entity, D);
```
This means that each operation needs to look up the entity location / verify its validity. The initial spawn operation also requires a Bundle as input. This can be awkward when no components are required (or one component is required).
These operations have been replaced by `EntityRef` and `EntityMut`, which are "builder-style" wrappers around world that provide read and read/write operations on a single, pre-validated entity:
```rust
// spawn now takes no inputs and returns an EntityMut
let entity = world.spawn()
.insert(A) // insert a single component into the entity
.insert_bundle((B, C)) // insert a bundle of components into the entity
.id() // id returns the Entity id
// Returns EntityMut (or panics if the entity does not exist)
world.entity_mut(entity)
.insert(D)
.insert_bundle(SomeBundle::default());
{
// returns EntityRef (or panics if the entity does not exist)
let d = world.entity(entity)
.get::<D>() // gets the D component
.unwrap();
// world.get still exists for ergonomics
let d = world.get::<D>(entity).unwrap();
}
// These variants return Options if you want to check existence instead of panicing
world.get_entity_mut(entity)
.unwrap()
.insert(E);
if let Some(entity_ref) = world.get_entity(entity) {
let d = entity_ref.get::<D>().unwrap();
}
```
This _does not_ affect the current Commands api or terminology. I think that should be a separate conversation as that is a much larger breaking change.
## Safety Improvements
* Entity reservation in Commands uses a normal world borrow instead of an unsafe transmute
* QuerySets no longer transmutes lifetimes
* Made traits "unsafe" when implementing a trait incorrectly could cause unsafety
* More thorough safety docs
## RemovedComponents SystemParam
The old approach to querying removed components: `query.removed:<T>()` was confusing because it had no connection to the query itself. I replaced it with the following, which is both clearer and allows us to cache the ComponentId mapping in the SystemParamState:
```rust
fn system(removed: RemovedComponents<T>) {
for entity in removed.iter() {
}
}
```
## Simpler Bundle implementation
Bundles are no longer responsible for sorting (or deduping) TypeInfo. They are just a simple ordered list of component types / data. This makes the implementation smaller and opens the door to an easy "nested bundle" implementation in the future (which i might even add in this pr). Duplicate detection is now done once per bundle type by World the first time a bundle is used.
## Unified WorldQuery and QueryFilter types
(don't worry they are still separate type _parameters_ in Queries .. this is a non-breaking change)
WorldQuery and QueryFilter were already basically identical apis. With the addition of `FetchState` and more storage-specific fetch methods, the overlap was even clearer (and the redundancy more painful).
QueryFilters are now just `F: WorldQuery where F::Fetch: FilterFetch`. FilterFetch requires `Fetch<Item = bool>` and adds new "short circuit" variants of fetch methods. This enables a filter tuple like `(With<A>, Without<B>, Changed<C>)` to stop evaluating the filter after the first mismatch is encountered. FilterFetch is automatically implemented for `Fetch` implementations that return bool.
This forces fetch implementations that return things like `(bool, bool, bool)` (such as the filter above) to manually implement FilterFetch and decide whether or not to short-circuit.
## More Granular Modules
World no longer globs all of the internal modules together. It now exports `core`, `system`, and `schedule` separately. I'm also considering exporting `core` submodules directly as that is still pretty "glob-ey" and unorganized (feedback welcome here).
## Remaining Draft Work (to be done in this pr)
* ~~panic on conflicting WorldQuery fetches (&A, &mut A)~~
* ~~bevy `main` and hecs both currently allow this, but we should protect against it if possible~~
* ~~batch_iter / par_iter (currently stubbed out)~~
* ~~ChangedRes~~
* ~~I skipped this while we sort out #1313. This pr should be adapted to account for whatever we land on there~~.
* ~~The `Archetypes` and `Tables` collections use hashes of sorted lists of component ids to uniquely identify each archetype/table. This hash is then used as the key in a HashMap to look up the relevant ArchetypeId or TableId. (which doesn't handle hash collisions properly)~~
* ~~It is currently unsafe to generate a Query from "World A", then use it on "World B" (despite the api claiming it is safe). We should probably close this gap. This could be done by adding a randomly generated WorldId to each world, then storing that id in each Query. They could then be compared to each other on each `query.do_thing(&world)` operation. This _does_ add an extra branch to each query operation, so I'm open to other suggestions if people have them.~~
* ~~Nested Bundles (if i find time)~~
## Potential Future Work
* Expand WorldCell to support queries.
* Consider not allocating in the empty archetype on `world.spawn()`
* ex: return something like EntityMutUninit, which turns into EntityMut after an `insert` or `insert_bundle` op
* this actually regressed performance last time i tried it, but in theory it should be faster
* Optimize SparseSet::insert (see `PERF` comment on insert)
* Replace SparseArray `Option<T>` with T::MAX to cut down on branching
* would enable cheaper get_unchecked() operations
* upstream fixedbitset optimizations
* fixedbitset could be allocation free for small block counts (store blocks in a SmallVec)
* fixedbitset could have a const constructor
* Consider implementing Tags (archetype-specific by-value data that affects archetype identity)
* ex: ArchetypeA could have `[A, B, C]` table components and `[D(1)]` "tag" component. ArchetypeB could have `[A, B, C]` table components and a `[D(2)]` tag component. The archetypes are different, despite both having D tags because the value inside D is different.
* this could potentially build on top of the `archetype.unique_components` added in this pr for resource storage.
* Consider reverting `all_tuples` proc macro in favor of the old `macro_rules` implementation
* all_tuples is more flexible and produces cleaner documentation (the macro_rules version produces weird type parameter orders due to parser constraints)
* but unfortunately all_tuples also appears to make Rust Analyzer sad/slow when working inside of `bevy_ecs` (does not affect user code)
* Consider "resource queries" and/or "mixed resource and entity component queries" as an alternative to WorldCell
* this is basically just "systems" so maybe it's not worth it
* Add more world ops
* `world.clear()`
* `world.reserve<T: Bundle>(count: usize)`
* Try using the old archetype allocation strategy (allocate new memory on resize and copy everything over). I expect this to improve batch insertion performance at the cost of unbatched performance. But thats just a guess. I'm not an allocation perf pro :)
* Adapt Commands apis for consistency with new World apis
## Benchmarks
key:
* `bevy_old`: bevy `main` branch
* `bevy`: this branch
* `_foreach`: uses an optimized for_each iterator
* ` _sparse`: uses sparse set storage (if unspecified assume table storage)
* `_system`: runs inside a system (if unspecified assume test happens via direct world ops)
### Simple Insert (from ecs_bench_suite)
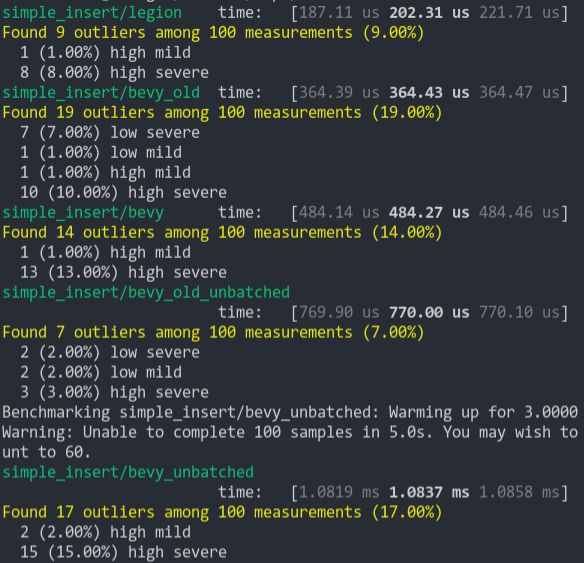
### Simpler Iter (from ecs_bench_suite)
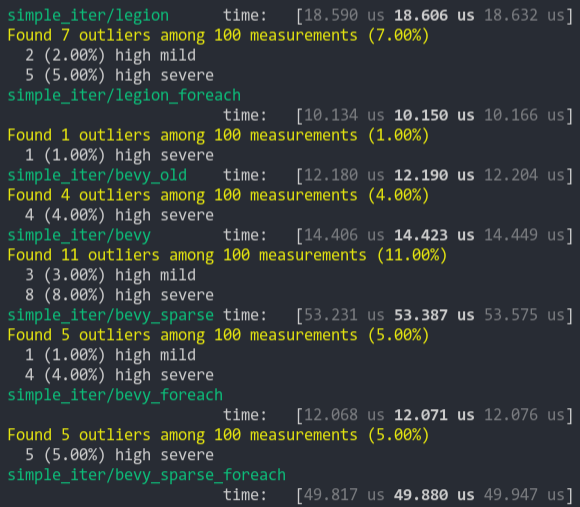
### Fragment Iter (from ecs_bench_suite)
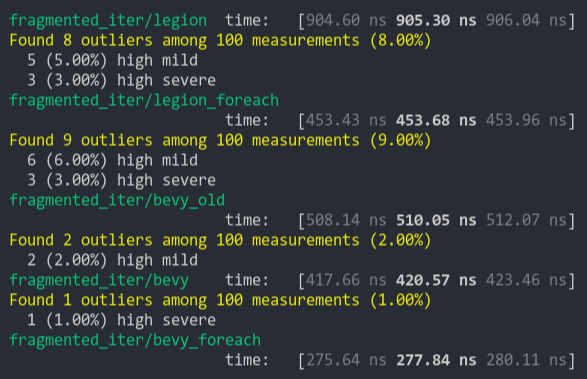
### Sparse Fragmented Iter
Iterate a query that matches 5 entities from a single matching archetype, but there are 100 unmatching archetypes
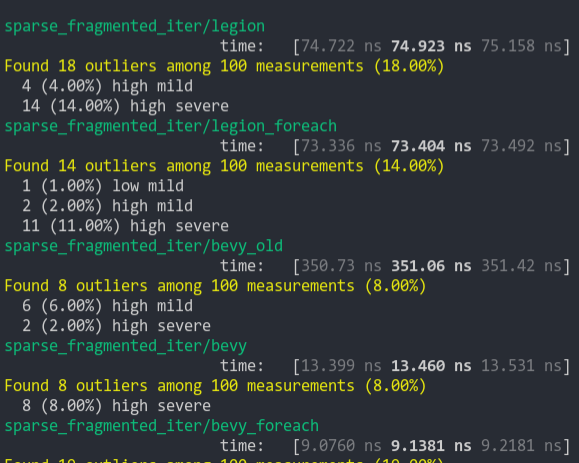
### Schedule (from ecs_bench_suite)
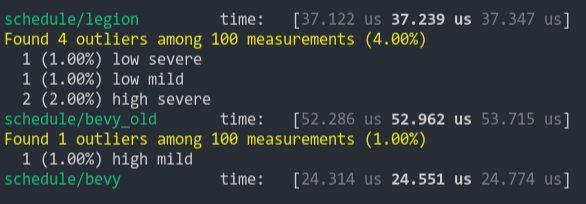
### Add Remove Component (from ecs_bench_suite)
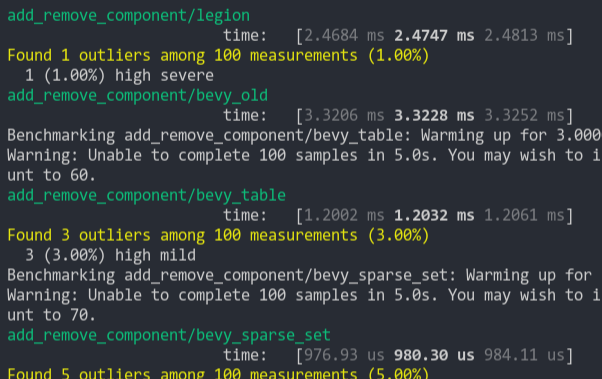
### Add Remove Component Big
Same as the test above, but each entity has 5 "large" matrix components and 1 "large" matrix component is added and removed
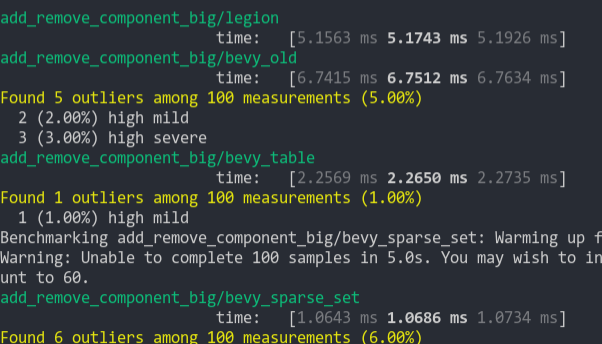
### Get Component
Looks up a single component value a large number of times
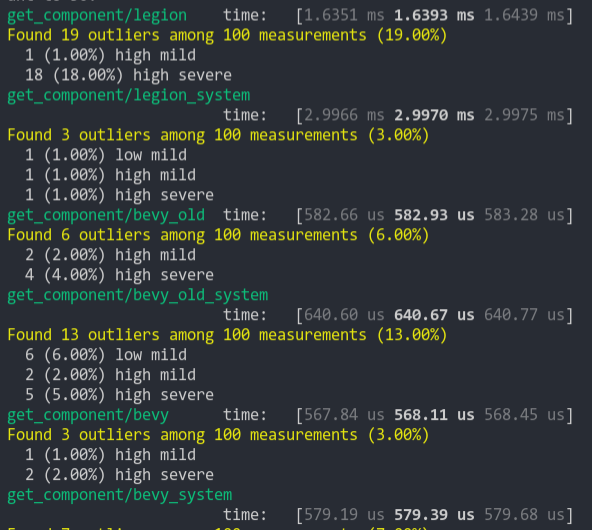
2021-03-05 07:54:35 +00:00
}
Remove the `SystemParamState` trait and remove types like `ResState` (#6919)
Spiritual successor to #5205.
Actual successor to #6865.
# Objective
Currently, system params are defined using three traits: `SystemParam`, `ReadOnlySystemParam`, `SystemParamState`. The behavior for each param is specified by the `SystemParamState` trait, while `SystemParam` simply defers to the state.
Splitting the traits in this way makes it easier to implement within macros, but it increases the cognitive load. Worst of all, this approach requires each `MySystemParam` to have a public `MySystemParamState` type associated with it.
## Solution
* Merge the trait `SystemParamState` into `SystemParam`.
* Remove all trivial `SystemParam` state types.
* `OptionNonSendMutState<T>`: you will not be missed.
---
- [x] Fix/resolve the remaining test failure.
## Changelog
* Removed the trait `SystemParamState`, merging its functionality into `SystemParam`.
## Migration Guide
**Note**: this should replace the migration guide for #6865.
This is relative to Bevy 0.9, not main.
The traits `SystemParamState` and `SystemParamFetch` have been removed, and their functionality has been transferred to `SystemParam`.
```rust
// Before (0.9)
impl SystemParam for MyParam<'_, '_> {
type State = MyParamState;
}
unsafe impl SystemParamState for MyParamState {
fn init(world: &mut World, system_meta: &mut SystemMeta) -> Self { ... }
}
unsafe impl<'w, 's> SystemParamFetch<'w, 's> for MyParamState {
type Item = MyParam<'w, 's>;
fn get_param(&mut self, ...) -> Self::Item;
}
unsafe impl ReadOnlySystemParamFetch for MyParamState { }
// After (0.10)
unsafe impl SystemParam for MyParam<'_, '_> {
type State = MyParamState;
type Item<'w, 's> = MyParam<'w, 's>;
fn init_state(world: &mut World, system_meta: &mut SystemMeta) -> Self::State { ... }
fn get_param<'w, 's>(state: &mut Self::State, ...) -> Self::Item<'w, 's>;
}
unsafe impl ReadOnlySystemParam for MyParam<'_, '_> { }
```
The trait `ReadOnlySystemParamFetch` has been replaced with `ReadOnlySystemParam`.
```rust
// Before
unsafe impl ReadOnlySystemParamFetch for MyParamState {}
// After
unsafe impl ReadOnlySystemParam for MyParam<'_, '_> {}
```
2023-01-07 23:20:32 +00:00
// SAFETY: this impl defers to `NonSendMut`, which initializes and validates the correct world access.
unsafe impl < ' a , T : 'static > SystemParam for Option < NonSendMut < ' a , T > > {
type State = ComponentId ;
Simplify trait hierarchy for `SystemParam` (#6865)
# Objective
* Implementing a custom `SystemParam` by hand requires implementing three traits -- four if it is read-only.
* The trait `SystemParamFetch<'w, 's>` is a workaround from before we had generic associated types, and is no longer necessary.
## Solution
* Combine the trait `SystemParamFetch` with `SystemParamState`.
* I decided to remove the `Fetch` name and keep the `State` name, since the former was consistently conflated with the latter.
* Replace the trait `ReadOnlySystemParamFetch` with `ReadOnlySystemParam`, which simplifies trait bounds in generic code.
---
## Changelog
- Removed the trait `SystemParamFetch`, moving its functionality to `SystemParamState`.
- Replaced the trait `ReadOnlySystemParamFetch` with `ReadOnlySystemParam`.
## Migration Guide
The trait `SystemParamFetch` has been removed, and its functionality has been transferred to `SystemParamState`.
```rust
// Before
impl SystemParamState for MyParamState {
fn init(world: &mut World, system_meta: &mut SystemMeta) -> Self { ... }
}
impl<'w, 's> SystemParamFetch<'w, 's> for MyParamState {
type Item = MyParam<'w, 's>;
fn get_param(...) -> Self::Item;
}
// After
impl SystemParamState for MyParamState {
type Item<'w, 's> = MyParam<'w, 's>; // Generic associated types!
fn init(world: &mut World, system_meta: &mut SystemMeta) -> Self { ... }
fn get_param<'w, 's>(...) -> Self::Item<'w, 's>;
}
```
The trait `ReadOnlySystemParamFetch` has been replaced with `ReadOnlySystemParam`.
```rust
// Before
unsafe impl ReadOnlySystemParamFetch for MyParamState {}
// After
unsafe impl<'w, 's> ReadOnlySystemParam for MyParam<'w, 's> {}
```
2022-12-11 18:34:14 +00:00
type Item < ' w , ' s > = Option < NonSendMut < ' w , T > > ;
Remove the `SystemParamState` trait and remove types like `ResState` (#6919)
Spiritual successor to #5205.
Actual successor to #6865.
# Objective
Currently, system params are defined using three traits: `SystemParam`, `ReadOnlySystemParam`, `SystemParamState`. The behavior for each param is specified by the `SystemParamState` trait, while `SystemParam` simply defers to the state.
Splitting the traits in this way makes it easier to implement within macros, but it increases the cognitive load. Worst of all, this approach requires each `MySystemParam` to have a public `MySystemParamState` type associated with it.
## Solution
* Merge the trait `SystemParamState` into `SystemParam`.
* Remove all trivial `SystemParam` state types.
* `OptionNonSendMutState<T>`: you will not be missed.
---
- [x] Fix/resolve the remaining test failure.
## Changelog
* Removed the trait `SystemParamState`, merging its functionality into `SystemParam`.
## Migration Guide
**Note**: this should replace the migration guide for #6865.
This is relative to Bevy 0.9, not main.
The traits `SystemParamState` and `SystemParamFetch` have been removed, and their functionality has been transferred to `SystemParam`.
```rust
// Before (0.9)
impl SystemParam for MyParam<'_, '_> {
type State = MyParamState;
}
unsafe impl SystemParamState for MyParamState {
fn init(world: &mut World, system_meta: &mut SystemMeta) -> Self { ... }
}
unsafe impl<'w, 's> SystemParamFetch<'w, 's> for MyParamState {
type Item = MyParam<'w, 's>;
fn get_param(&mut self, ...) -> Self::Item;
}
unsafe impl ReadOnlySystemParamFetch for MyParamState { }
// After (0.10)
unsafe impl SystemParam for MyParam<'_, '_> {
type State = MyParamState;
type Item<'w, 's> = MyParam<'w, 's>;
fn init_state(world: &mut World, system_meta: &mut SystemMeta) -> Self::State { ... }
fn get_param<'w, 's>(state: &mut Self::State, ...) -> Self::Item<'w, 's>;
}
unsafe impl ReadOnlySystemParam for MyParam<'_, '_> { }
```
The trait `ReadOnlySystemParamFetch` has been replaced with `ReadOnlySystemParam`.
```rust
// Before
unsafe impl ReadOnlySystemParamFetch for MyParamState {}
// After
unsafe impl ReadOnlySystemParam for MyParam<'_, '_> {}
```
2023-01-07 23:20:32 +00:00
fn init_state ( world : & mut World , system_meta : & mut SystemMeta ) -> Self ::State {
NonSendMut ::< T > ::init_state ( world , system_meta )
2021-06-26 19:29:38 +00:00
}
#[ inline ]
Simplify trait hierarchy for `SystemParam` (#6865)
# Objective
* Implementing a custom `SystemParam` by hand requires implementing three traits -- four if it is read-only.
* The trait `SystemParamFetch<'w, 's>` is a workaround from before we had generic associated types, and is no longer necessary.
## Solution
* Combine the trait `SystemParamFetch` with `SystemParamState`.
* I decided to remove the `Fetch` name and keep the `State` name, since the former was consistently conflated with the latter.
* Replace the trait `ReadOnlySystemParamFetch` with `ReadOnlySystemParam`, which simplifies trait bounds in generic code.
---
## Changelog
- Removed the trait `SystemParamFetch`, moving its functionality to `SystemParamState`.
- Replaced the trait `ReadOnlySystemParamFetch` with `ReadOnlySystemParam`.
## Migration Guide
The trait `SystemParamFetch` has been removed, and its functionality has been transferred to `SystemParamState`.
```rust
// Before
impl SystemParamState for MyParamState {
fn init(world: &mut World, system_meta: &mut SystemMeta) -> Self { ... }
}
impl<'w, 's> SystemParamFetch<'w, 's> for MyParamState {
type Item = MyParam<'w, 's>;
fn get_param(...) -> Self::Item;
}
// After
impl SystemParamState for MyParamState {
type Item<'w, 's> = MyParam<'w, 's>; // Generic associated types!
fn init(world: &mut World, system_meta: &mut SystemMeta) -> Self { ... }
fn get_param<'w, 's>(...) -> Self::Item<'w, 's>;
}
```
The trait `ReadOnlySystemParamFetch` has been replaced with `ReadOnlySystemParam`.
```rust
// Before
unsafe impl ReadOnlySystemParamFetch for MyParamState {}
// After
unsafe impl<'w, 's> ReadOnlySystemParam for MyParam<'w, 's> {}
```
2022-12-11 18:34:14 +00:00
unsafe fn get_param < ' w , ' s > (
Remove the `SystemParamState` trait and remove types like `ResState` (#6919)
Spiritual successor to #5205.
Actual successor to #6865.
# Objective
Currently, system params are defined using three traits: `SystemParam`, `ReadOnlySystemParam`, `SystemParamState`. The behavior for each param is specified by the `SystemParamState` trait, while `SystemParam` simply defers to the state.
Splitting the traits in this way makes it easier to implement within macros, but it increases the cognitive load. Worst of all, this approach requires each `MySystemParam` to have a public `MySystemParamState` type associated with it.
## Solution
* Merge the trait `SystemParamState` into `SystemParam`.
* Remove all trivial `SystemParam` state types.
* `OptionNonSendMutState<T>`: you will not be missed.
---
- [x] Fix/resolve the remaining test failure.
## Changelog
* Removed the trait `SystemParamState`, merging its functionality into `SystemParam`.
## Migration Guide
**Note**: this should replace the migration guide for #6865.
This is relative to Bevy 0.9, not main.
The traits `SystemParamState` and `SystemParamFetch` have been removed, and their functionality has been transferred to `SystemParam`.
```rust
// Before (0.9)
impl SystemParam for MyParam<'_, '_> {
type State = MyParamState;
}
unsafe impl SystemParamState for MyParamState {
fn init(world: &mut World, system_meta: &mut SystemMeta) -> Self { ... }
}
unsafe impl<'w, 's> SystemParamFetch<'w, 's> for MyParamState {
type Item = MyParam<'w, 's>;
fn get_param(&mut self, ...) -> Self::Item;
}
unsafe impl ReadOnlySystemParamFetch for MyParamState { }
// After (0.10)
unsafe impl SystemParam for MyParam<'_, '_> {
type State = MyParamState;
type Item<'w, 's> = MyParam<'w, 's>;
fn init_state(world: &mut World, system_meta: &mut SystemMeta) -> Self::State { ... }
fn get_param<'w, 's>(state: &mut Self::State, ...) -> Self::Item<'w, 's>;
}
unsafe impl ReadOnlySystemParam for MyParam<'_, '_> { }
```
The trait `ReadOnlySystemParamFetch` has been replaced with `ReadOnlySystemParam`.
```rust
// Before
unsafe impl ReadOnlySystemParamFetch for MyParamState {}
// After
unsafe impl ReadOnlySystemParam for MyParam<'_, '_> {}
```
2023-01-07 23:20:32 +00:00
& mut component_id : & ' s mut Self ::State ,
2021-06-26 19:29:38 +00:00
system_meta : & SystemMeta ,
System Param Lifetime Split (#2605)
# Objective
Enable using exact World lifetimes during read-only access . This is motivated by the new renderer's need to allow read-only world-only queries to outlive the query itself (but still be constrained by the world lifetime).
For example:
https://github.com/bevyengine/bevy/blob/115b170d1f11a91146bb6d6e9684dceb8b21f786/pipelined/bevy_pbr2/src/render/mod.rs#L774
## Solution
Split out SystemParam state and world lifetimes and pipe those lifetimes up to read-only Query ops (and add into_inner for Res). According to every safety test I've run so far (except one), this is safe (see the temporary safety test commit). Note that changing the mutable variants to the new lifetimes would allow aliased mutable pointers (try doing that to see how it affects the temporary safety tests).
The new state lifetime on SystemParam does make `#[derive(SystemParam)]` more cumbersome (the current impl requires PhantomData if you don't use both lifetimes). We can make this better by detecting whether or not a lifetime is used in the derive and adjusting accordingly, but that should probably be done in its own pr.
## Why is this a draft?
The new lifetimes break QuerySet safety in one very specific case (see the query_set system in system_safety_test). We need to solve this before we can use the lifetimes given.
This is due to the fact that QuerySet is just a wrapper over Query, which now relies on world lifetimes instead of `&self` lifetimes to prevent aliasing (but in systems, each Query has its own implied lifetime, not a centralized world lifetime). I believe the fix is to rewrite QuerySet to have its own World lifetime (and own the internal reference). This will complicate the impl a bit, but I think it is doable. I'm curious if anyone else has better ideas.
Personally, I think these new lifetimes need to happen. We've gotta have a way to directly tie read-only World queries to the World lifetime. The new renderer is the first place this has come up, but I doubt it will be the last. Worst case scenario we can come up with a second `WorldLifetimeQuery<Q, F = ()>` parameter to enable these read-only scenarios, but I'd rather not add another type to the type zoo.
2021-08-15 20:51:53 +00:00
world : & ' w World ,
2021-06-26 19:29:38 +00:00
change_tick : u32 ,
Simplify trait hierarchy for `SystemParam` (#6865)
# Objective
* Implementing a custom `SystemParam` by hand requires implementing three traits -- four if it is read-only.
* The trait `SystemParamFetch<'w, 's>` is a workaround from before we had generic associated types, and is no longer necessary.
## Solution
* Combine the trait `SystemParamFetch` with `SystemParamState`.
* I decided to remove the `Fetch` name and keep the `State` name, since the former was consistently conflated with the latter.
* Replace the trait `ReadOnlySystemParamFetch` with `ReadOnlySystemParam`, which simplifies trait bounds in generic code.
---
## Changelog
- Removed the trait `SystemParamFetch`, moving its functionality to `SystemParamState`.
- Replaced the trait `ReadOnlySystemParamFetch` with `ReadOnlySystemParam`.
## Migration Guide
The trait `SystemParamFetch` has been removed, and its functionality has been transferred to `SystemParamState`.
```rust
// Before
impl SystemParamState for MyParamState {
fn init(world: &mut World, system_meta: &mut SystemMeta) -> Self { ... }
}
impl<'w, 's> SystemParamFetch<'w, 's> for MyParamState {
type Item = MyParam<'w, 's>;
fn get_param(...) -> Self::Item;
}
// After
impl SystemParamState for MyParamState {
type Item<'w, 's> = MyParam<'w, 's>; // Generic associated types!
fn init(world: &mut World, system_meta: &mut SystemMeta) -> Self { ... }
fn get_param<'w, 's>(...) -> Self::Item<'w, 's>;
}
```
The trait `ReadOnlySystemParamFetch` has been replaced with `ReadOnlySystemParam`.
```rust
// Before
unsafe impl ReadOnlySystemParamFetch for MyParamState {}
// After
unsafe impl<'w, 's> ReadOnlySystemParam for MyParam<'w, 's> {}
```
2022-12-11 18:34:14 +00:00
) -> Self ::Item < ' w , ' s > {
2021-06-26 19:29:38 +00:00
world
2023-02-06 19:02:52 +00:00
. as_unsafe_world_cell_migration_internal ( )
Panic on dropping NonSend in non-origin thread. (#6534)
# Objective
Fixes #3310. Fixes #6282. Fixes #6278. Fixes #3666.
## Solution
Split out `!Send` resources into `NonSendResources`. Add a `origin_thread_id` to all `!Send` Resources, check it on dropping `NonSendResourceData`, if there's a mismatch, panic. Moved all of the checks that `MainThreadValidator` would do into `NonSendResources` instead.
All `!Send` resources now individually track which thread they were inserted from. This is validated against for every access, mutation, and drop that could be done against the value.
A regression test using an altered version of the example from #3310 has been added.
This is a stopgap solution for the current status quo. A full solution may involve fully removing `!Send` resources/components from `World`, which will likely require a much more thorough design on how to handle the existing in-engine and ecosystem use cases.
This PR also introduces another breaking change:
```rust
use bevy_ecs::prelude::*;
#[derive(Resource)]
struct Resource(u32);
fn main() {
let mut world = World::new();
world.insert_resource(Resource(1));
world.insert_non_send_resource(Resource(2));
let res = world.get_resource_mut::<Resource>().unwrap();
assert_eq!(res.0, 2);
}
```
This code will run correctly on 0.9.1 but not with this PR, since NonSend resources and normal resources have become actual distinct concepts storage wise.
## Changelog
Changed: Fix soundness bug with `World: Send`. Dropping a `World` that contains a `!Send` resource on the wrong thread will now panic.
## Migration Guide
Normal resources and `NonSend` resources no longer share the same backing storage. If `R: Resource`, then `NonSend<R>` and `Res<R>` will return different instances from each other. If you are using both `Res<T>` and `NonSend<T>` (or their mutable variants), to fetch the same resources, it's strongly advised to use `Res<T>`.
2023-01-09 20:40:34 +00:00
. get_non_send_with_ticks ( component_id )
2022-10-24 13:46:36 +00:00
. map ( | ( ptr , ticks ) | NonSendMut {
value : ptr . assert_unique ( ) . deref_mut ( ) ,
2023-01-11 15:41:54 +00:00
ticks : TicksMut ::from_tick_cells ( ticks , system_meta . last_change_tick , change_tick ) ,
2021-06-26 19:29:38 +00:00
} )
}
}
2022-07-04 14:44:24 +00:00
// SAFETY: Only reads World archetypes
Simplify trait hierarchy for `SystemParam` (#6865)
# Objective
* Implementing a custom `SystemParam` by hand requires implementing three traits -- four if it is read-only.
* The trait `SystemParamFetch<'w, 's>` is a workaround from before we had generic associated types, and is no longer necessary.
## Solution
* Combine the trait `SystemParamFetch` with `SystemParamState`.
* I decided to remove the `Fetch` name and keep the `State` name, since the former was consistently conflated with the latter.
* Replace the trait `ReadOnlySystemParamFetch` with `ReadOnlySystemParam`, which simplifies trait bounds in generic code.
---
## Changelog
- Removed the trait `SystemParamFetch`, moving its functionality to `SystemParamState`.
- Replaced the trait `ReadOnlySystemParamFetch` with `ReadOnlySystemParam`.
## Migration Guide
The trait `SystemParamFetch` has been removed, and its functionality has been transferred to `SystemParamState`.
```rust
// Before
impl SystemParamState for MyParamState {
fn init(world: &mut World, system_meta: &mut SystemMeta) -> Self { ... }
}
impl<'w, 's> SystemParamFetch<'w, 's> for MyParamState {
type Item = MyParam<'w, 's>;
fn get_param(...) -> Self::Item;
}
// After
impl SystemParamState for MyParamState {
type Item<'w, 's> = MyParam<'w, 's>; // Generic associated types!
fn init(world: &mut World, system_meta: &mut SystemMeta) -> Self { ... }
fn get_param<'w, 's>(...) -> Self::Item<'w, 's>;
}
```
The trait `ReadOnlySystemParamFetch` has been replaced with `ReadOnlySystemParam`.
```rust
// Before
unsafe impl ReadOnlySystemParamFetch for MyParamState {}
// After
unsafe impl<'w, 's> ReadOnlySystemParam for MyParam<'w, 's> {}
```
2022-12-11 18:34:14 +00:00
unsafe impl < ' a > ReadOnlySystemParam for & ' a Archetypes { }
Add new SystemState and rename old SystemState to SystemMeta (#2283)
This enables `SystemParams` to be used outside of function systems. Anything can create and store `SystemState`, which enables efficient "param state cached" access to `SystemParams`.
It adds a `ReadOnlySystemParamFetch` trait, which enables safe `SystemState::get` calls without unique world access.
I renamed the old `SystemState` to `SystemMeta` to enable us to mirror the `QueryState` naming convention (but I'm happy to discuss alternative names if people have other ideas). I initially pitched this as `ParamState`, but given that it needs to include full system metadata, that doesn't feel like a particularly accurate name.
```rust
#[derive(Eq, PartialEq, Debug)]
struct A(usize);
#[derive(Eq, PartialEq, Debug)]
struct B(usize);
let mut world = World::default();
world.insert_resource(A(42));
world.spawn().insert(B(7));
// we get nice lifetime elision when declaring the type on the left hand side
let mut system_state: SystemState<(Res<A>, Query<&B>)> = SystemState::new(&mut world);
let (a, query) = system_state.get(&world);
assert_eq!(*a, A(42), "returned resource matches initial value");
assert_eq!(
*query.single().unwrap(),
B(7),
"returned component matches initial value"
);
// mutable system params require unique world access
let mut system_state: SystemState<(ResMut<A>, Query<&mut B>)> = SystemState::new(&mut world);
let (a, query) = system_state.get_mut(&mut world);
// static lifetimes are required when declaring inside of structs
struct SomeContainer {
state: SystemState<(Res<'static, A>, Res<'static, B>)>
}
// this can be shortened using type aliases, which will be useful for complex param tuples
type MyParams<'a> = (Res<'a, A>, Res<'a, B>);
struct SomeContainer {
state: SystemState<MyParams<'static>>
}
// It is the user's responsibility to call SystemState::apply(world) for parameters that queue up work
let mut system_state: SystemState<(Commands, Query<&B>)> = SystemState::new(&mut world);
{
let (mut commands, query) = system_state.get(&world);
commands.insert_resource(3.14);
}
system_state.apply(&mut world);
```
## Future Work
* Actually use SystemState inside FunctionSystem. This would be trivial, but it requires FunctionSystem to wrap SystemState in Option in its current form (which complicates system metadata lookup). I'd prefer to hold off until we adopt something like the later designs linked in #1364, which enable us to contruct Systems using a World reference (and also remove the need for `.system`).
* Consider a "scoped" approach to automatically call SystemState::apply when systems params are no longer being used (either a container type with a Drop impl, or a function that takes a closure for user logic operating on params).
2021-06-02 19:57:38 +00:00
2022-07-04 14:44:24 +00:00
// SAFETY: no component value access
Remove the `SystemParamState` trait and remove types like `ResState` (#6919)
Spiritual successor to #5205.
Actual successor to #6865.
# Objective
Currently, system params are defined using three traits: `SystemParam`, `ReadOnlySystemParam`, `SystemParamState`. The behavior for each param is specified by the `SystemParamState` trait, while `SystemParam` simply defers to the state.
Splitting the traits in this way makes it easier to implement within macros, but it increases the cognitive load. Worst of all, this approach requires each `MySystemParam` to have a public `MySystemParamState` type associated with it.
## Solution
* Merge the trait `SystemParamState` into `SystemParam`.
* Remove all trivial `SystemParam` state types.
* `OptionNonSendMutState<T>`: you will not be missed.
---
- [x] Fix/resolve the remaining test failure.
## Changelog
* Removed the trait `SystemParamState`, merging its functionality into `SystemParam`.
## Migration Guide
**Note**: this should replace the migration guide for #6865.
This is relative to Bevy 0.9, not main.
The traits `SystemParamState` and `SystemParamFetch` have been removed, and their functionality has been transferred to `SystemParam`.
```rust
// Before (0.9)
impl SystemParam for MyParam<'_, '_> {
type State = MyParamState;
}
unsafe impl SystemParamState for MyParamState {
fn init(world: &mut World, system_meta: &mut SystemMeta) -> Self { ... }
}
unsafe impl<'w, 's> SystemParamFetch<'w, 's> for MyParamState {
type Item = MyParam<'w, 's>;
fn get_param(&mut self, ...) -> Self::Item;
}
unsafe impl ReadOnlySystemParamFetch for MyParamState { }
// After (0.10)
unsafe impl SystemParam for MyParam<'_, '_> {
type State = MyParamState;
type Item<'w, 's> = MyParam<'w, 's>;
fn init_state(world: &mut World, system_meta: &mut SystemMeta) -> Self::State { ... }
fn get_param<'w, 's>(state: &mut Self::State, ...) -> Self::Item<'w, 's>;
}
unsafe impl ReadOnlySystemParam for MyParam<'_, '_> { }
```
The trait `ReadOnlySystemParamFetch` has been replaced with `ReadOnlySystemParam`.
```rust
// Before
unsafe impl ReadOnlySystemParamFetch for MyParamState {}
// After
unsafe impl ReadOnlySystemParam for MyParam<'_, '_> {}
```
2023-01-07 23:20:32 +00:00
unsafe impl < ' a > SystemParam for & ' a Archetypes {
type State = ( ) ;
Simplify trait hierarchy for `SystemParam` (#6865)
# Objective
* Implementing a custom `SystemParam` by hand requires implementing three traits -- four if it is read-only.
* The trait `SystemParamFetch<'w, 's>` is a workaround from before we had generic associated types, and is no longer necessary.
## Solution
* Combine the trait `SystemParamFetch` with `SystemParamState`.
* I decided to remove the `Fetch` name and keep the `State` name, since the former was consistently conflated with the latter.
* Replace the trait `ReadOnlySystemParamFetch` with `ReadOnlySystemParam`, which simplifies trait bounds in generic code.
---
## Changelog
- Removed the trait `SystemParamFetch`, moving its functionality to `SystemParamState`.
- Replaced the trait `ReadOnlySystemParamFetch` with `ReadOnlySystemParam`.
## Migration Guide
The trait `SystemParamFetch` has been removed, and its functionality has been transferred to `SystemParamState`.
```rust
// Before
impl SystemParamState for MyParamState {
fn init(world: &mut World, system_meta: &mut SystemMeta) -> Self { ... }
}
impl<'w, 's> SystemParamFetch<'w, 's> for MyParamState {
type Item = MyParam<'w, 's>;
fn get_param(...) -> Self::Item;
}
// After
impl SystemParamState for MyParamState {
type Item<'w, 's> = MyParam<'w, 's>; // Generic associated types!
fn init(world: &mut World, system_meta: &mut SystemMeta) -> Self { ... }
fn get_param<'w, 's>(...) -> Self::Item<'w, 's>;
}
```
The trait `ReadOnlySystemParamFetch` has been replaced with `ReadOnlySystemParam`.
```rust
// Before
unsafe impl ReadOnlySystemParamFetch for MyParamState {}
// After
unsafe impl<'w, 's> ReadOnlySystemParam for MyParam<'w, 's> {}
```
2022-12-11 18:34:14 +00:00
type Item < ' w , ' s > = & ' w Archetypes ;
Remove the `SystemParamState` trait and remove types like `ResState` (#6919)
Spiritual successor to #5205.
Actual successor to #6865.
# Objective
Currently, system params are defined using three traits: `SystemParam`, `ReadOnlySystemParam`, `SystemParamState`. The behavior for each param is specified by the `SystemParamState` trait, while `SystemParam` simply defers to the state.
Splitting the traits in this way makes it easier to implement within macros, but it increases the cognitive load. Worst of all, this approach requires each `MySystemParam` to have a public `MySystemParamState` type associated with it.
## Solution
* Merge the trait `SystemParamState` into `SystemParam`.
* Remove all trivial `SystemParam` state types.
* `OptionNonSendMutState<T>`: you will not be missed.
---
- [x] Fix/resolve the remaining test failure.
## Changelog
* Removed the trait `SystemParamState`, merging its functionality into `SystemParam`.
## Migration Guide
**Note**: this should replace the migration guide for #6865.
This is relative to Bevy 0.9, not main.
The traits `SystemParamState` and `SystemParamFetch` have been removed, and their functionality has been transferred to `SystemParam`.
```rust
// Before (0.9)
impl SystemParam for MyParam<'_, '_> {
type State = MyParamState;
}
unsafe impl SystemParamState for MyParamState {
fn init(world: &mut World, system_meta: &mut SystemMeta) -> Self { ... }
}
unsafe impl<'w, 's> SystemParamFetch<'w, 's> for MyParamState {
type Item = MyParam<'w, 's>;
fn get_param(&mut self, ...) -> Self::Item;
}
unsafe impl ReadOnlySystemParamFetch for MyParamState { }
// After (0.10)
unsafe impl SystemParam for MyParam<'_, '_> {
type State = MyParamState;
type Item<'w, 's> = MyParam<'w, 's>;
fn init_state(world: &mut World, system_meta: &mut SystemMeta) -> Self::State { ... }
fn get_param<'w, 's>(state: &mut Self::State, ...) -> Self::Item<'w, 's>;
}
unsafe impl ReadOnlySystemParam for MyParam<'_, '_> { }
```
The trait `ReadOnlySystemParamFetch` has been replaced with `ReadOnlySystemParam`.
```rust
// Before
unsafe impl ReadOnlySystemParamFetch for MyParamState {}
// After
unsafe impl ReadOnlySystemParam for MyParam<'_, '_> {}
```
2023-01-07 23:20:32 +00:00
fn init_state ( _world : & mut World , _system_meta : & mut SystemMeta ) -> Self ::State { }
2020-11-08 20:34:05 +00:00
#[ inline ]
Simplify trait hierarchy for `SystemParam` (#6865)
# Objective
* Implementing a custom `SystemParam` by hand requires implementing three traits -- four if it is read-only.
* The trait `SystemParamFetch<'w, 's>` is a workaround from before we had generic associated types, and is no longer necessary.
## Solution
* Combine the trait `SystemParamFetch` with `SystemParamState`.
* I decided to remove the `Fetch` name and keep the `State` name, since the former was consistently conflated with the latter.
* Replace the trait `ReadOnlySystemParamFetch` with `ReadOnlySystemParam`, which simplifies trait bounds in generic code.
---
## Changelog
- Removed the trait `SystemParamFetch`, moving its functionality to `SystemParamState`.
- Replaced the trait `ReadOnlySystemParamFetch` with `ReadOnlySystemParam`.
## Migration Guide
The trait `SystemParamFetch` has been removed, and its functionality has been transferred to `SystemParamState`.
```rust
// Before
impl SystemParamState for MyParamState {
fn init(world: &mut World, system_meta: &mut SystemMeta) -> Self { ... }
}
impl<'w, 's> SystemParamFetch<'w, 's> for MyParamState {
type Item = MyParam<'w, 's>;
fn get_param(...) -> Self::Item;
}
// After
impl SystemParamState for MyParamState {
type Item<'w, 's> = MyParam<'w, 's>; // Generic associated types!
fn init(world: &mut World, system_meta: &mut SystemMeta) -> Self { ... }
fn get_param<'w, 's>(...) -> Self::Item<'w, 's>;
}
```
The trait `ReadOnlySystemParamFetch` has been replaced with `ReadOnlySystemParam`.
```rust
// Before
unsafe impl ReadOnlySystemParamFetch for MyParamState {}
// After
unsafe impl<'w, 's> ReadOnlySystemParam for MyParam<'w, 's> {}
```
2022-12-11 18:34:14 +00:00
unsafe fn get_param < ' w , ' s > (
Remove the `SystemParamState` trait and remove types like `ResState` (#6919)
Spiritual successor to #5205.
Actual successor to #6865.
# Objective
Currently, system params are defined using three traits: `SystemParam`, `ReadOnlySystemParam`, `SystemParamState`. The behavior for each param is specified by the `SystemParamState` trait, while `SystemParam` simply defers to the state.
Splitting the traits in this way makes it easier to implement within macros, but it increases the cognitive load. Worst of all, this approach requires each `MySystemParam` to have a public `MySystemParamState` type associated with it.
## Solution
* Merge the trait `SystemParamState` into `SystemParam`.
* Remove all trivial `SystemParam` state types.
* `OptionNonSendMutState<T>`: you will not be missed.
---
- [x] Fix/resolve the remaining test failure.
## Changelog
* Removed the trait `SystemParamState`, merging its functionality into `SystemParam`.
## Migration Guide
**Note**: this should replace the migration guide for #6865.
This is relative to Bevy 0.9, not main.
The traits `SystemParamState` and `SystemParamFetch` have been removed, and their functionality has been transferred to `SystemParam`.
```rust
// Before (0.9)
impl SystemParam for MyParam<'_, '_> {
type State = MyParamState;
}
unsafe impl SystemParamState for MyParamState {
fn init(world: &mut World, system_meta: &mut SystemMeta) -> Self { ... }
}
unsafe impl<'w, 's> SystemParamFetch<'w, 's> for MyParamState {
type Item = MyParam<'w, 's>;
fn get_param(&mut self, ...) -> Self::Item;
}
unsafe impl ReadOnlySystemParamFetch for MyParamState { }
// After (0.10)
unsafe impl SystemParam for MyParam<'_, '_> {
type State = MyParamState;
type Item<'w, 's> = MyParam<'w, 's>;
fn init_state(world: &mut World, system_meta: &mut SystemMeta) -> Self::State { ... }
fn get_param<'w, 's>(state: &mut Self::State, ...) -> Self::Item<'w, 's>;
}
unsafe impl ReadOnlySystemParam for MyParam<'_, '_> { }
```
The trait `ReadOnlySystemParamFetch` has been replaced with `ReadOnlySystemParam`.
```rust
// Before
unsafe impl ReadOnlySystemParamFetch for MyParamState {}
// After
unsafe impl ReadOnlySystemParam for MyParam<'_, '_> {}
```
2023-01-07 23:20:32 +00:00
_state : & ' s mut Self ::State ,
Add new SystemState and rename old SystemState to SystemMeta (#2283)
This enables `SystemParams` to be used outside of function systems. Anything can create and store `SystemState`, which enables efficient "param state cached" access to `SystemParams`.
It adds a `ReadOnlySystemParamFetch` trait, which enables safe `SystemState::get` calls without unique world access.
I renamed the old `SystemState` to `SystemMeta` to enable us to mirror the `QueryState` naming convention (but I'm happy to discuss alternative names if people have other ideas). I initially pitched this as `ParamState`, but given that it needs to include full system metadata, that doesn't feel like a particularly accurate name.
```rust
#[derive(Eq, PartialEq, Debug)]
struct A(usize);
#[derive(Eq, PartialEq, Debug)]
struct B(usize);
let mut world = World::default();
world.insert_resource(A(42));
world.spawn().insert(B(7));
// we get nice lifetime elision when declaring the type on the left hand side
let mut system_state: SystemState<(Res<A>, Query<&B>)> = SystemState::new(&mut world);
let (a, query) = system_state.get(&world);
assert_eq!(*a, A(42), "returned resource matches initial value");
assert_eq!(
*query.single().unwrap(),
B(7),
"returned component matches initial value"
);
// mutable system params require unique world access
let mut system_state: SystemState<(ResMut<A>, Query<&mut B>)> = SystemState::new(&mut world);
let (a, query) = system_state.get_mut(&mut world);
// static lifetimes are required when declaring inside of structs
struct SomeContainer {
state: SystemState<(Res<'static, A>, Res<'static, B>)>
}
// this can be shortened using type aliases, which will be useful for complex param tuples
type MyParams<'a> = (Res<'a, A>, Res<'a, B>);
struct SomeContainer {
state: SystemState<MyParams<'static>>
}
// It is the user's responsibility to call SystemState::apply(world) for parameters that queue up work
let mut system_state: SystemState<(Commands, Query<&B>)> = SystemState::new(&mut world);
{
let (mut commands, query) = system_state.get(&world);
commands.insert_resource(3.14);
}
system_state.apply(&mut world);
```
## Future Work
* Actually use SystemState inside FunctionSystem. This would be trivial, but it requires FunctionSystem to wrap SystemState in Option in its current form (which complicates system metadata lookup). I'd prefer to hold off until we adopt something like the later designs linked in #1364, which enable us to contruct Systems using a World reference (and also remove the need for `.system`).
* Consider a "scoped" approach to automatically call SystemState::apply when systems params are no longer being used (either a container type with a Drop impl, or a function that takes a closure for user logic operating on params).
2021-06-02 19:57:38 +00:00
_system_meta : & SystemMeta ,
System Param Lifetime Split (#2605)
# Objective
Enable using exact World lifetimes during read-only access . This is motivated by the new renderer's need to allow read-only world-only queries to outlive the query itself (but still be constrained by the world lifetime).
For example:
https://github.com/bevyengine/bevy/blob/115b170d1f11a91146bb6d6e9684dceb8b21f786/pipelined/bevy_pbr2/src/render/mod.rs#L774
## Solution
Split out SystemParam state and world lifetimes and pipe those lifetimes up to read-only Query ops (and add into_inner for Res). According to every safety test I've run so far (except one), this is safe (see the temporary safety test commit). Note that changing the mutable variants to the new lifetimes would allow aliased mutable pointers (try doing that to see how it affects the temporary safety tests).
The new state lifetime on SystemParam does make `#[derive(SystemParam)]` more cumbersome (the current impl requires PhantomData if you don't use both lifetimes). We can make this better by detecting whether or not a lifetime is used in the derive and adjusting accordingly, but that should probably be done in its own pr.
## Why is this a draft?
The new lifetimes break QuerySet safety in one very specific case (see the query_set system in system_safety_test). We need to solve this before we can use the lifetimes given.
This is due to the fact that QuerySet is just a wrapper over Query, which now relies on world lifetimes instead of `&self` lifetimes to prevent aliasing (but in systems, each Query has its own implied lifetime, not a centralized world lifetime). I believe the fix is to rewrite QuerySet to have its own World lifetime (and own the internal reference). This will complicate the impl a bit, but I think it is doable. I'm curious if anyone else has better ideas.
Personally, I think these new lifetimes need to happen. We've gotta have a way to directly tie read-only World queries to the World lifetime. The new renderer is the first place this has come up, but I doubt it will be the last. Worst case scenario we can come up with a second `WorldLifetimeQuery<Q, F = ()>` parameter to enable these read-only scenarios, but I'd rather not add another type to the type zoo.
2021-08-15 20:51:53 +00:00
world : & ' w World ,
Reliable change detection (#1471)
# Problem Definition
The current change tracking (via flags for both components and resources) fails to detect changes made by systems that are scheduled to run earlier in the frame than they are.
This issue is discussed at length in [#68](https://github.com/bevyengine/bevy/issues/68) and [#54](https://github.com/bevyengine/bevy/issues/54).
This is very much a draft PR, and contributions are welcome and needed.
# Criteria
1. Each change is detected at least once, no matter the ordering.
2. Each change is detected at most once, no matter the ordering.
3. Changes should be detected the same frame that they are made.
4. Competitive ergonomics. Ideally does not require opting-in.
5. Low CPU overhead of computation.
6. Memory efficient. This must not increase over time, except where the number of entities / resources does.
7. Changes should not be lost for systems that don't run.
8. A frame needs to act as a pure function. Given the same set of entities / components it needs to produce the same end state without side-effects.
**Exact** change-tracking proposals satisfy criteria 1 and 2.
**Conservative** change-tracking proposals satisfy criteria 1 but not 2.
**Flaky** change tracking proposals satisfy criteria 2 but not 1.
# Code Base Navigation
There are three types of flags:
- `Added`: A piece of data was added to an entity / `Resources`.
- `Mutated`: A piece of data was able to be modified, because its `DerefMut` was accessed
- `Changed`: The bitwise OR of `Added` and `Changed`
The special behavior of `ChangedRes`, with respect to the scheduler is being removed in [#1313](https://github.com/bevyengine/bevy/pull/1313) and does not need to be reproduced.
`ChangedRes` and friends can be found in "bevy_ecs/core/resources/resource_query.rs".
The `Flags` trait for Components can be found in "bevy_ecs/core/query.rs".
`ComponentFlags` are stored in "bevy_ecs/core/archetypes.rs", defined on line 446.
# Proposals
**Proposal 5 was selected for implementation.**
## Proposal 0: No Change Detection
The baseline, where computations are performed on everything regardless of whether it changed.
**Type:** Conservative
**Pros:**
- already implemented
- will never miss events
- no overhead
**Cons:**
- tons of repeated work
- doesn't allow users to avoid repeating work (or monitoring for other changes)
## Proposal 1: Earlier-This-Tick Change Detection
The current approach as of Bevy 0.4. Flags are set, and then flushed at the end of each frame.
**Type:** Flaky
**Pros:**
- already implemented
- simple to understand
- low memory overhead (2 bits per component)
- low time overhead (clear every flag once per frame)
**Cons:**
- misses systems based on ordering
- systems that don't run every frame miss changes
- duplicates detection when looping
- can lead to unresolvable circular dependencies
## Proposal 2: Two-Tick Change Detection
Flags persist for two frames, using a double-buffer system identical to that used in events.
A change is observed if it is found in either the current frame's list of changes or the previous frame's.
**Type:** Conservative
**Pros:**
- easy to understand
- easy to implement
- low memory overhead (4 bits per component)
- low time overhead (bit mask and shift every flag once per frame)
**Cons:**
- can result in a great deal of duplicated work
- systems that don't run every frame miss changes
- duplicates detection when looping
## Proposal 3: Last-Tick Change Detection
Flags persist for two frames, using a double-buffer system identical to that used in events.
A change is observed if it is found in the previous frame's list of changes.
**Type:** Exact
**Pros:**
- exact
- easy to understand
- easy to implement
- low memory overhead (4 bits per component)
- low time overhead (bit mask and shift every flag once per frame)
**Cons:**
- change detection is always delayed, possibly causing painful chained delays
- systems that don't run every frame miss changes
- duplicates detection when looping
## Proposal 4: Flag-Doubling Change Detection
Combine Proposal 2 and Proposal 3. Differentiate between `JustChanged` (current behavior) and `Changed` (Proposal 3).
Pack this data into the flags according to [this implementation proposal](https://github.com/bevyengine/bevy/issues/68#issuecomment-769174804).
**Type:** Flaky + Exact
**Pros:**
- allows users to acc
- easy to implement
- low memory overhead (4 bits per component)
- low time overhead (bit mask and shift every flag once per frame)
**Cons:**
- users must specify the type of change detection required
- still quite fragile to system ordering effects when using the flaky `JustChanged` form
- cannot get immediate + exact results
- systems that don't run every frame miss changes
- duplicates detection when looping
## [SELECTED] Proposal 5: Generation-Counter Change Detection
A global counter is increased after each system is run. Each component saves the time of last mutation, and each system saves the time of last execution. Mutation is detected when the component's counter is greater than the system's counter. Discussed [here](https://github.com/bevyengine/bevy/issues/68#issuecomment-769174804). How to handle addition detection is unsolved; the current proposal is to use the highest bit of the counter as in proposal 1.
**Type:** Exact (for mutations), flaky (for additions)
**Pros:**
- low time overhead (set component counter on access, set system counter after execution)
- robust to systems that don't run every frame
- robust to systems that loop
**Cons:**
- moderately complex implementation
- must be modified as systems are inserted dynamically
- medium memory overhead (4 bytes per component + system)
- unsolved addition detection
## Proposal 6: System-Data Change Detection
For each system, track which system's changes it has seen. This approach is only worth fully designing and implementing if Proposal 5 fails in some way.
**Type:** Exact
**Pros:**
- exact
- conceptually simple
**Cons:**
- requires storing data on each system
- implementation is complex
- must be modified as systems are inserted dynamically
## Proposal 7: Total-Order Change Detection
Discussed [here](https://github.com/bevyengine/bevy/issues/68#issuecomment-754326523). This proposal is somewhat complicated by the new scheduler, but I believe it should still be conceptually feasible. This approach is only worth fully designing and implementing if Proposal 5 fails in some way.
**Type:** Exact
**Pros:**
- exact
- efficient data storage relative to other exact proposals
**Cons:**
- requires access to the scheduler
- complex implementation and difficulty grokking
- must be modified as systems are inserted dynamically
# Tests
- We will need to verify properties 1, 2, 3, 7 and 8. Priority: 1 > 2 = 3 > 8 > 7
- Ideally we can use identical user-facing syntax for all proposals, allowing us to re-use the same syntax for each.
- When writing tests, we need to carefully specify order using explicit dependencies.
- These tests will need to be duplicated for both components and resources.
- We need to be sure to handle cases where ambiguous system orders exist.
`changing_system` is always the system that makes the changes, and `detecting_system` always detects the changes.
The component / resource changed will be simple boolean wrapper structs.
## Basic Added / Mutated / Changed
2 x 3 design:
- Resources vs. Components
- Added vs. Changed vs. Mutated
- `changing_system` runs before `detecting_system`
- verify at the end of tick 2
## At Least Once
2 x 3 design:
- Resources vs. Components
- Added vs. Changed vs. Mutated
- `changing_system` runs after `detecting_system`
- verify at the end of tick 2
## At Most Once
2 x 3 design:
- Resources vs. Components
- Added vs. Changed vs. Mutated
- `changing_system` runs once before `detecting_system`
- increment a counter based on the number of changes detected
- verify at the end of tick 2
## Fast Detection
2 x 3 design:
- Resources vs. Components
- Added vs. Changed vs. Mutated
- `changing_system` runs before `detecting_system`
- verify at the end of tick 1
## Ambiguous System Ordering Robustness
2 x 3 x 2 design:
- Resources vs. Components
- Added vs. Changed vs. Mutated
- `changing_system` runs [before/after] `detecting_system` in tick 1
- `changing_system` runs [after/before] `detecting_system` in tick 2
## System Pausing
2 x 3 design:
- Resources vs. Components
- Added vs. Changed vs. Mutated
- `changing_system` runs in tick 1, then is disabled by run criteria
- `detecting_system` is disabled by run criteria until it is run once during tick 3
- verify at the end of tick 3
## Addition Causes Mutation
2 design:
- Resources vs. Components
- `adding_system_1` adds a component / resource
- `adding system_2` adds the same component / resource
- verify the `Mutated` flag at the end of the tick
- verify the `Added` flag at the end of the tick
First check tests for: https://github.com/bevyengine/bevy/issues/333
Second check tests for: https://github.com/bevyengine/bevy/issues/1443
## Changes Made By Commands
- `adding_system` runs in Update in tick 1, and sends a command to add a component
- `detecting_system` runs in Update in tick 1 and 2, after `adding_system`
- We can't detect the changes in tick 1, since they haven't been processed yet
- If we were to track these changes as being emitted by `adding_system`, we can't detect the changes in tick 2 either, since `detecting_system` has already run once after `adding_system` :(
# Benchmarks
See: [general advice](https://github.com/bevyengine/bevy/blob/master/docs/profiling.md), [Criterion crate](https://github.com/bheisler/criterion.rs)
There are several critical parameters to vary:
1. entity count (1 to 10^9)
2. fraction of entities that are changed (0% to 100%)
3. cost to perform work on changed entities, i.e. workload (1 ns to 1s)
1 and 2 should be varied between benchmark runs. 3 can be added on computationally.
We want to measure:
- memory cost
- run time
We should collect these measurements across several frames (100?) to reduce bootup effects and accurately measure the mean, variance and drift.
Entity-component change detection is much more important to benchmark than resource change detection, due to the orders of magnitude higher number of pieces of data.
No change detection at all should be included in benchmarks as a second control for cases where missing changes is unacceptable.
## Graphs
1. y: performance, x: log_10(entity count), color: proposal, facet: performance metric. Set cost to perform work to 0.
2. y: run time, x: cost to perform work, color: proposal, facet: fraction changed. Set number of entities to 10^6
3. y: memory, x: frames, color: proposal
# Conclusions
1. Is the theoretical categorization of the proposals correct according to our tests?
2. How does the performance of the proposals compare without any load?
3. How does the performance of the proposals compare with realistic loads?
4. At what workload does more exact change tracking become worth the (presumably) higher overhead?
5. When does adding change-detection to save on work become worthwhile?
6. Is there enough divergence in performance between the best solutions in each class to ship more than one change-tracking solution?
# Implementation Plan
1. Write a test suite.
2. Verify that tests fail for existing approach.
3. Write a benchmark suite.
4. Get performance numbers for existing approach.
5. Implement, test and benchmark various solutions using a Git branch per proposal.
6. Create a draft PR with all solutions and present results to team.
7. Select a solution and replace existing change detection.
Co-authored-by: Brice DAVIER <bricedavier@gmail.com>
Co-authored-by: Carter Anderson <mcanders1@gmail.com>
2021-03-19 17:53:26 +00:00
_change_tick : u32 ,
Simplify trait hierarchy for `SystemParam` (#6865)
# Objective
* Implementing a custom `SystemParam` by hand requires implementing three traits -- four if it is read-only.
* The trait `SystemParamFetch<'w, 's>` is a workaround from before we had generic associated types, and is no longer necessary.
## Solution
* Combine the trait `SystemParamFetch` with `SystemParamState`.
* I decided to remove the `Fetch` name and keep the `State` name, since the former was consistently conflated with the latter.
* Replace the trait `ReadOnlySystemParamFetch` with `ReadOnlySystemParam`, which simplifies trait bounds in generic code.
---
## Changelog
- Removed the trait `SystemParamFetch`, moving its functionality to `SystemParamState`.
- Replaced the trait `ReadOnlySystemParamFetch` with `ReadOnlySystemParam`.
## Migration Guide
The trait `SystemParamFetch` has been removed, and its functionality has been transferred to `SystemParamState`.
```rust
// Before
impl SystemParamState for MyParamState {
fn init(world: &mut World, system_meta: &mut SystemMeta) -> Self { ... }
}
impl<'w, 's> SystemParamFetch<'w, 's> for MyParamState {
type Item = MyParam<'w, 's>;
fn get_param(...) -> Self::Item;
}
// After
impl SystemParamState for MyParamState {
type Item<'w, 's> = MyParam<'w, 's>; // Generic associated types!
fn init(world: &mut World, system_meta: &mut SystemMeta) -> Self { ... }
fn get_param<'w, 's>(...) -> Self::Item<'w, 's>;
}
```
The trait `ReadOnlySystemParamFetch` has been replaced with `ReadOnlySystemParam`.
```rust
// Before
unsafe impl ReadOnlySystemParamFetch for MyParamState {}
// After
unsafe impl<'w, 's> ReadOnlySystemParam for MyParam<'w, 's> {}
```
2022-12-11 18:34:14 +00:00
) -> Self ::Item < ' w , ' s > {
Bevy ECS V2 (#1525)
# Bevy ECS V2
This is a rewrite of Bevy ECS (basically everything but the new executor/schedule, which are already awesome). The overall goal was to improve the performance and versatility of Bevy ECS. Here is a quick bulleted list of changes before we dive into the details:
* Complete World rewrite
* Multiple component storage types:
* Tables: fast cache friendly iteration, slower add/removes (previously called Archetypes)
* Sparse Sets: fast add/remove, slower iteration
* Stateful Queries (caches query results for faster iteration. fragmented iteration is _fast_ now)
* Stateful System Params (caches expensive operations. inspired by @DJMcNab's work in #1364)
* Configurable System Params (users can set configuration when they construct their systems. once again inspired by @DJMcNab's work)
* Archetypes are now "just metadata", component storage is separate
* Archetype Graph (for faster archetype changes)
* Component Metadata
* Configure component storage type
* Retrieve information about component size/type/name/layout/send-ness/etc
* Components are uniquely identified by a densely packed ComponentId
* TypeIds are now totally optional (which should make implementing scripting easier)
* Super fast "for_each" query iterators
* Merged Resources into World. Resources are now just a special type of component
* EntityRef/EntityMut builder apis (more efficient and more ergonomic)
* Fast bitset-backed `Access<T>` replaces old hashmap-based approach everywhere
* Query conflicts are determined by component access instead of archetype component access (to avoid random failures at runtime)
* With/Without are still taken into account for conflicts, so this should still be comfy to use
* Much simpler `IntoSystem` impl
* Significantly reduced the amount of hashing throughout the ecs in favor of Sparse Sets (indexed by densely packed ArchetypeId, ComponentId, BundleId, and TableId)
* Safety Improvements
* Entity reservation uses a normal world reference instead of unsafe transmute
* QuerySets no longer transmute lifetimes
* Made traits "unsafe" where relevant
* More thorough safety docs
* WorldCell
* Exposes safe mutable access to multiple resources at a time in a World
* Replaced "catch all" `System::update_archetypes(world: &World)` with `System::new_archetype(archetype: &Archetype)`
* Simpler Bundle implementation
* Replaced slow "remove_bundle_one_by_one" used as fallback for Commands::remove_bundle with fast "remove_bundle_intersection"
* Removed `Mut<T>` query impl. it is better to only support one way: `&mut T`
* Removed with() from `Flags<T>` in favor of `Option<Flags<T>>`, which allows querying for flags to be "filtered" by default
* Components now have is_send property (currently only resources support non-send)
* More granular module organization
* New `RemovedComponents<T>` SystemParam that replaces `query.removed::<T>()`
* `world.resource_scope()` for mutable access to resources and world at the same time
* WorldQuery and QueryFilter traits unified. FilterFetch trait added to enable "short circuit" filtering. Auto impled for cases that don't need it
* Significantly slimmed down SystemState in favor of individual SystemParam state
* System Commands changed from `commands: &mut Commands` back to `mut commands: Commands` (to allow Commands to have a World reference)
Fixes #1320
## `World` Rewrite
This is a from-scratch rewrite of `World` that fills the niche that `hecs` used to. Yes, this means Bevy ECS is no longer a "fork" of hecs. We're going out our own!
(the only shared code between the projects is the entity id allocator, which is already basically ideal)
A huge shout out to @SanderMertens (author of [flecs](https://github.com/SanderMertens/flecs)) for sharing some great ideas with me (specifically hybrid ecs storage and archetype graphs). He also helped advise on a number of implementation details.
## Component Storage (The Problem)
Two ECS storage paradigms have gained a lot of traction over the years:
* **Archetypal ECS**:
* Stores components in "tables" with static schemas. Each "column" stores components of a given type. Each "row" is an entity.
* Each "archetype" has its own table. Adding/removing an entity's component changes the archetype.
* Enables super-fast Query iteration due to its cache-friendly data layout
* Comes at the cost of more expensive add/remove operations for an Entity's components, because all components need to be copied to the new archetype's "table"
* **Sparse Set ECS**:
* Stores components of the same type in densely packed arrays, which are sparsely indexed by densely packed unsigned integers (Entity ids)
* Query iteration is slower than Archetypal ECS because each entity's component could be at any position in the sparse set. This "random access" pattern isn't cache friendly. Additionally, there is an extra layer of indirection because you must first map the entity id to an index in the component array.
* Adding/removing components is a cheap, constant time operation
Bevy ECS V1, hecs, legion, flec, and Unity DOTS are all "archetypal ecs-es". I personally think "archetypal" storage is a good default for game engines. An entity's archetype doesn't need to change frequently in general, and it creates "fast by default" query iteration (which is a much more common operation). It is also "self optimizing". Users don't need to think about optimizing component layouts for iteration performance. It "just works" without any extra boilerplate.
Shipyard and EnTT are "sparse set ecs-es". They employ "packing" as a way to work around the "suboptimal by default" iteration performance for specific sets of components. This helps, but I didn't think this was a good choice for a general purpose engine like Bevy because:
1. "packs" conflict with each other. If bevy decides to internally pack the Transform and GlobalTransform components, users are then blocked if they want to pack some custom component with Transform.
2. users need to take manual action to optimize
Developers selecting an ECS framework are stuck with a hard choice. Select an "archetypal" framework with "fast iteration everywhere" but without the ability to cheaply add/remove components, or select a "sparse set" framework to cheaply add/remove components but with slower iteration performance.
## Hybrid Component Storage (The Solution)
In Bevy ECS V2, we get to have our cake and eat it too. It now has _both_ of the component storage types above (and more can be added later if needed):
* **Tables** (aka "archetypal" storage)
* The default storage. If you don't configure anything, this is what you get
* Fast iteration by default
* Slower add/remove operations
* **Sparse Sets**
* Opt-in
* Slower iteration
* Faster add/remove operations
These storage types complement each other perfectly. By default Query iteration is fast. If developers know that they want to add/remove a component at high frequencies, they can set the storage to "sparse set":
```rust
world.register_component(
ComponentDescriptor::new::<MyComponent>(StorageType::SparseSet)
).unwrap();
```
## Archetypes
Archetypes are now "just metadata" ... they no longer store components directly. They do store:
* The `ComponentId`s of each of the Archetype's components (and that component's storage type)
* Archetypes are uniquely defined by their component layouts
* For example: entities with "table" components `[A, B, C]` _and_ "sparse set" components `[D, E]` will always be in the same archetype.
* The `TableId` associated with the archetype
* For now each archetype has exactly one table (which can have no components),
* There is a 1->Many relationship from Tables->Archetypes. A given table could have any number of archetype components stored in it:
* Ex: an entity with "table storage" components `[A, B, C]` and "sparse set" components `[D, E]` will share the same `[A, B, C]` table as an entity with `[A, B, C]` table component and `[F]` sparse set components.
* This 1->Many relationship is how we preserve fast "cache friendly" iteration performance when possible (more on this later)
* A list of entities that are in the archetype and the row id of the table they are in
* ArchetypeComponentIds
* unique densely packed identifiers for (ArchetypeId, ComponentId) pairs
* used by the schedule executor for cheap system access control
* "Archetype Graph Edges" (see the next section)
## The "Archetype Graph"
Archetype changes in Bevy (and a number of other archetypal ecs-es) have historically been expensive to compute. First, you need to allocate a new vector of the entity's current component ids, add or remove components based on the operation performed, sort it (to ensure it is order-independent), then hash it to find the archetype (if it exists). And thats all before we get to the _already_ expensive full copy of all components to the new table storage.
The solution is to build a "graph" of archetypes to cache these results. @SanderMertens first exposed me to the idea (and he got it from @gjroelofs, who came up with it). They propose adding directed edges between archetypes for add/remove component operations. If `ComponentId`s are densely packed, you can use sparse sets to cheaply jump between archetypes.
Bevy takes this one step further by using add/remove `Bundle` edges instead of `Component` edges. Bevy encourages the use of `Bundles` to group add/remove operations. This is largely for "clearer game logic" reasons, but it also helps cut down on the number of archetype changes required. `Bundles` now also have densely-packed `BundleId`s. This allows us to use a _single_ edge for each bundle operation (rather than needing to traverse N edges ... one for each component). Single component operations are also bundles, so this is strictly an improvement over a "component only" graph.
As a result, an operation that used to be _heavy_ (both for allocations and compute) is now two dirt-cheap array lookups and zero allocations.
## Stateful Queries
World queries are now stateful. This allows us to:
1. Cache archetype (and table) matches
* This resolves another issue with (naive) archetypal ECS: query performance getting worse as the number of archetypes goes up (and fragmentation occurs).
2. Cache Fetch and Filter state
* The expensive parts of fetch/filter operations (such as hashing the TypeId to find the ComponentId) now only happen once when the Query is first constructed
3. Incrementally build up state
* When new archetypes are added, we only process the new archetypes (no need to rebuild state for old archetypes)
As a result, the direct `World` query api now looks like this:
```rust
let mut query = world.query::<(&A, &mut B)>();
for (a, mut b) in query.iter_mut(&mut world) {
}
```
Requiring `World` to generate stateful queries (rather than letting the `QueryState` type be constructed separately) allows us to ensure that _all_ queries are properly initialized (and the relevant world state, such as ComponentIds). This enables QueryState to remove branches from its operations that check for initialization status (and also enables query.iter() to take an immutable world reference because it doesn't need to initialize anything in world).
However in systems, this is a non-breaking change. State management is done internally by the relevant SystemParam.
## Stateful SystemParams
Like Queries, `SystemParams` now also cache state. For example, `Query` system params store the "stateful query" state mentioned above. Commands store their internal `CommandQueue`. This means you can now safely use as many separate `Commands` parameters in your system as you want. `Local<T>` system params store their `T` value in their state (instead of in Resources).
SystemParam state also enabled a significant slim-down of SystemState. It is much nicer to look at now.
Per-SystemParam state naturally insulates us from an "aliased mut" class of errors we have hit in the past (ex: using multiple `Commands` system params).
(credit goes to @DJMcNab for the initial idea and draft pr here #1364)
## Configurable SystemParams
@DJMcNab also had the great idea to make SystemParams configurable. This allows users to provide some initial configuration / values for system parameters (when possible). Most SystemParams have no config (the config type is `()`), but the `Local<T>` param now supports user-provided parameters:
```rust
fn foo(value: Local<usize>) {
}
app.add_system(foo.system().config(|c| c.0 = Some(10)));
```
## Uber Fast "for_each" Query Iterators
Developers now have the choice to use a fast "for_each" iterator, which yields ~1.5-3x iteration speed improvements for "fragmented iteration", and minor ~1.2x iteration speed improvements for unfragmented iteration.
```rust
fn system(query: Query<(&A, &mut B)>) {
// you now have the option to do this for a speed boost
query.for_each_mut(|(a, mut b)| {
});
// however normal iterators are still available
for (a, mut b) in query.iter_mut() {
}
}
```
I think in most cases we should continue to encourage "normal" iterators as they are more flexible and more "rust idiomatic". But when that extra "oomf" is needed, it makes sense to use `for_each`.
We should also consider using `for_each` for internal bevy systems to give our users a nice speed boost (but that should be a separate pr).
## Component Metadata
`World` now has a `Components` collection, which is accessible via `world.components()`. This stores mappings from `ComponentId` to `ComponentInfo`, as well as `TypeId` to `ComponentId` mappings (where relevant). `ComponentInfo` stores information about the component, such as ComponentId, TypeId, memory layout, send-ness (currently limited to resources), and storage type.
## Significantly Cheaper `Access<T>`
We used to use `TypeAccess<TypeId>` to manage read/write component/archetype-component access. This was expensive because TypeIds must be hashed and compared individually. The parallel executor got around this by "condensing" type ids into bitset-backed access types. This worked, but it had to be re-generated from the `TypeAccess<TypeId>`sources every time archetypes changed.
This pr removes TypeAccess in favor of faster bitset access everywhere. We can do this thanks to the move to densely packed `ComponentId`s and `ArchetypeComponentId`s.
## Merged Resources into World
Resources had a lot of redundant functionality with Components. They stored typed data, they had access control, they had unique ids, they were queryable via SystemParams, etc. In fact the _only_ major difference between them was that they were unique (and didn't correlate to an entity).
Separate resources also had the downside of requiring a separate set of access controls, which meant the parallel executor needed to compare more bitsets per system and manage more state.
I initially got the "separate resources" idea from `legion`. I think that design was motivated by the fact that it made the direct world query/resource lifetime interactions more manageable. It certainly made our lives easier when using Resources alongside hecs/bevy_ecs. However we already have a construct for safely and ergonomically managing in-world lifetimes: systems (which use `Access<T>` internally).
This pr merges Resources into World:
```rust
world.insert_resource(1);
world.insert_resource(2.0);
let a = world.get_resource::<i32>().unwrap();
let mut b = world.get_resource_mut::<f64>().unwrap();
*b = 3.0;
```
Resources are now just a special kind of component. They have their own ComponentIds (and their own resource TypeId->ComponentId scope, so they don't conflict wit components of the same type). They are stored in a special "resource archetype", which stores components inside the archetype using a new `unique_components` sparse set (note that this sparse set could later be used to implement Tags). This allows us to keep the code size small by reusing existing datastructures (namely Column, Archetype, ComponentFlags, and ComponentInfo). This allows us the executor to use a single `Access<ArchetypeComponentId>` per system. It should also make scripting language integration easier.
_But_ this merge did create problems for people directly interacting with `World`. What if you need mutable access to multiple resources at the same time? `world.get_resource_mut()` borrows World mutably!
## WorldCell
WorldCell applies the `Access<ArchetypeComponentId>` concept to direct world access:
```rust
let world_cell = world.cell();
let a = world_cell.get_resource_mut::<i32>().unwrap();
let b = world_cell.get_resource_mut::<f64>().unwrap();
```
This adds cheap runtime checks (a sparse set lookup of `ArchetypeComponentId` and a counter) to ensure that world accesses do not conflict with each other. Each operation returns a `WorldBorrow<'w, T>` or `WorldBorrowMut<'w, T>` wrapper type, which will release the relevant ArchetypeComponentId resources when dropped.
World caches the access sparse set (and only one cell can exist at a time), so `world.cell()` is a cheap operation.
WorldCell does _not_ use atomic operations. It is non-send, does a mutable borrow of world to prevent other accesses, and uses a simple `Rc<RefCell<ArchetypeComponentAccess>>` wrapper in each WorldBorrow pointer.
The api is currently limited to resource access, but it can and should be extended to queries / entity component access.
## Resource Scopes
WorldCell does not yet support component queries, and even when it does there are sometimes legitimate reasons to want a mutable world ref _and_ a mutable resource ref (ex: bevy_render and bevy_scene both need this). In these cases we could always drop down to the unsafe `world.get_resource_unchecked_mut()`, but that is not ideal!
Instead developers can use a "resource scope"
```rust
world.resource_scope(|world: &mut World, a: &mut A| {
})
```
This temporarily removes the `A` resource from `World`, provides mutable pointers to both, and re-adds A to World when finished. Thanks to the move to ComponentIds/sparse sets, this is a cheap operation.
If multiple resources are required, scopes can be nested. We could also consider adding a "resource tuple" to the api if this pattern becomes common and the boilerplate gets nasty.
## Query Conflicts Use ComponentId Instead of ArchetypeComponentId
For safety reasons, systems cannot contain queries that conflict with each other without wrapping them in a QuerySet. On bevy `main`, we use ArchetypeComponentIds to determine conflicts. This is nice because it can take into account filters:
```rust
// these queries will never conflict due to their filters
fn filter_system(a: Query<&mut A, With<B>>, b: Query<&mut B, Without<B>>) {
}
```
But it also has a significant downside:
```rust
// these queries will not conflict _until_ an entity with A, B, and C is spawned
fn maybe_conflicts_system(a: Query<(&mut A, &C)>, b: Query<(&mut A, &B)>) {
}
```
The system above will panic at runtime if an entity with A, B, and C is spawned. This makes it hard to trust that your game logic will run without crashing.
In this pr, I switched to using `ComponentId` instead. This _is_ more constraining. `maybe_conflicts_system` will now always fail, but it will do it consistently at startup. Naively, it would also _disallow_ `filter_system`, which would be a significant downgrade in usability. Bevy has a number of internal systems that rely on disjoint queries and I expect it to be a common pattern in userspace.
To resolve this, I added a new `FilteredAccess<T>` type, which wraps `Access<T>` and adds with/without filters. If two `FilteredAccess` have with/without values that prove they are disjoint, they will no longer conflict.
## EntityRef / EntityMut
World entity operations on `main` require that the user passes in an `entity` id to each operation:
```rust
let entity = world.spawn((A, )); // create a new entity with A
world.get::<A>(entity);
world.insert(entity, (B, C));
world.insert_one(entity, D);
```
This means that each operation needs to look up the entity location / verify its validity. The initial spawn operation also requires a Bundle as input. This can be awkward when no components are required (or one component is required).
These operations have been replaced by `EntityRef` and `EntityMut`, which are "builder-style" wrappers around world that provide read and read/write operations on a single, pre-validated entity:
```rust
// spawn now takes no inputs and returns an EntityMut
let entity = world.spawn()
.insert(A) // insert a single component into the entity
.insert_bundle((B, C)) // insert a bundle of components into the entity
.id() // id returns the Entity id
// Returns EntityMut (or panics if the entity does not exist)
world.entity_mut(entity)
.insert(D)
.insert_bundle(SomeBundle::default());
{
// returns EntityRef (or panics if the entity does not exist)
let d = world.entity(entity)
.get::<D>() // gets the D component
.unwrap();
// world.get still exists for ergonomics
let d = world.get::<D>(entity).unwrap();
}
// These variants return Options if you want to check existence instead of panicing
world.get_entity_mut(entity)
.unwrap()
.insert(E);
if let Some(entity_ref) = world.get_entity(entity) {
let d = entity_ref.get::<D>().unwrap();
}
```
This _does not_ affect the current Commands api or terminology. I think that should be a separate conversation as that is a much larger breaking change.
## Safety Improvements
* Entity reservation in Commands uses a normal world borrow instead of an unsafe transmute
* QuerySets no longer transmutes lifetimes
* Made traits "unsafe" when implementing a trait incorrectly could cause unsafety
* More thorough safety docs
## RemovedComponents SystemParam
The old approach to querying removed components: `query.removed:<T>()` was confusing because it had no connection to the query itself. I replaced it with the following, which is both clearer and allows us to cache the ComponentId mapping in the SystemParamState:
```rust
fn system(removed: RemovedComponents<T>) {
for entity in removed.iter() {
}
}
```
## Simpler Bundle implementation
Bundles are no longer responsible for sorting (or deduping) TypeInfo. They are just a simple ordered list of component types / data. This makes the implementation smaller and opens the door to an easy "nested bundle" implementation in the future (which i might even add in this pr). Duplicate detection is now done once per bundle type by World the first time a bundle is used.
## Unified WorldQuery and QueryFilter types
(don't worry they are still separate type _parameters_ in Queries .. this is a non-breaking change)
WorldQuery and QueryFilter were already basically identical apis. With the addition of `FetchState` and more storage-specific fetch methods, the overlap was even clearer (and the redundancy more painful).
QueryFilters are now just `F: WorldQuery where F::Fetch: FilterFetch`. FilterFetch requires `Fetch<Item = bool>` and adds new "short circuit" variants of fetch methods. This enables a filter tuple like `(With<A>, Without<B>, Changed<C>)` to stop evaluating the filter after the first mismatch is encountered. FilterFetch is automatically implemented for `Fetch` implementations that return bool.
This forces fetch implementations that return things like `(bool, bool, bool)` (such as the filter above) to manually implement FilterFetch and decide whether or not to short-circuit.
## More Granular Modules
World no longer globs all of the internal modules together. It now exports `core`, `system`, and `schedule` separately. I'm also considering exporting `core` submodules directly as that is still pretty "glob-ey" and unorganized (feedback welcome here).
## Remaining Draft Work (to be done in this pr)
* ~~panic on conflicting WorldQuery fetches (&A, &mut A)~~
* ~~bevy `main` and hecs both currently allow this, but we should protect against it if possible~~
* ~~batch_iter / par_iter (currently stubbed out)~~
* ~~ChangedRes~~
* ~~I skipped this while we sort out #1313. This pr should be adapted to account for whatever we land on there~~.
* ~~The `Archetypes` and `Tables` collections use hashes of sorted lists of component ids to uniquely identify each archetype/table. This hash is then used as the key in a HashMap to look up the relevant ArchetypeId or TableId. (which doesn't handle hash collisions properly)~~
* ~~It is currently unsafe to generate a Query from "World A", then use it on "World B" (despite the api claiming it is safe). We should probably close this gap. This could be done by adding a randomly generated WorldId to each world, then storing that id in each Query. They could then be compared to each other on each `query.do_thing(&world)` operation. This _does_ add an extra branch to each query operation, so I'm open to other suggestions if people have them.~~
* ~~Nested Bundles (if i find time)~~
## Potential Future Work
* Expand WorldCell to support queries.
* Consider not allocating in the empty archetype on `world.spawn()`
* ex: return something like EntityMutUninit, which turns into EntityMut after an `insert` or `insert_bundle` op
* this actually regressed performance last time i tried it, but in theory it should be faster
* Optimize SparseSet::insert (see `PERF` comment on insert)
* Replace SparseArray `Option<T>` with T::MAX to cut down on branching
* would enable cheaper get_unchecked() operations
* upstream fixedbitset optimizations
* fixedbitset could be allocation free for small block counts (store blocks in a SmallVec)
* fixedbitset could have a const constructor
* Consider implementing Tags (archetype-specific by-value data that affects archetype identity)
* ex: ArchetypeA could have `[A, B, C]` table components and `[D(1)]` "tag" component. ArchetypeB could have `[A, B, C]` table components and a `[D(2)]` tag component. The archetypes are different, despite both having D tags because the value inside D is different.
* this could potentially build on top of the `archetype.unique_components` added in this pr for resource storage.
* Consider reverting `all_tuples` proc macro in favor of the old `macro_rules` implementation
* all_tuples is more flexible and produces cleaner documentation (the macro_rules version produces weird type parameter orders due to parser constraints)
* but unfortunately all_tuples also appears to make Rust Analyzer sad/slow when working inside of `bevy_ecs` (does not affect user code)
* Consider "resource queries" and/or "mixed resource and entity component queries" as an alternative to WorldCell
* this is basically just "systems" so maybe it's not worth it
* Add more world ops
* `world.clear()`
* `world.reserve<T: Bundle>(count: usize)`
* Try using the old archetype allocation strategy (allocate new memory on resize and copy everything over). I expect this to improve batch insertion performance at the cost of unbatched performance. But thats just a guess. I'm not an allocation perf pro :)
* Adapt Commands apis for consistency with new World apis
## Benchmarks
key:
* `bevy_old`: bevy `main` branch
* `bevy`: this branch
* `_foreach`: uses an optimized for_each iterator
* ` _sparse`: uses sparse set storage (if unspecified assume table storage)
* `_system`: runs inside a system (if unspecified assume test happens via direct world ops)
### Simple Insert (from ecs_bench_suite)
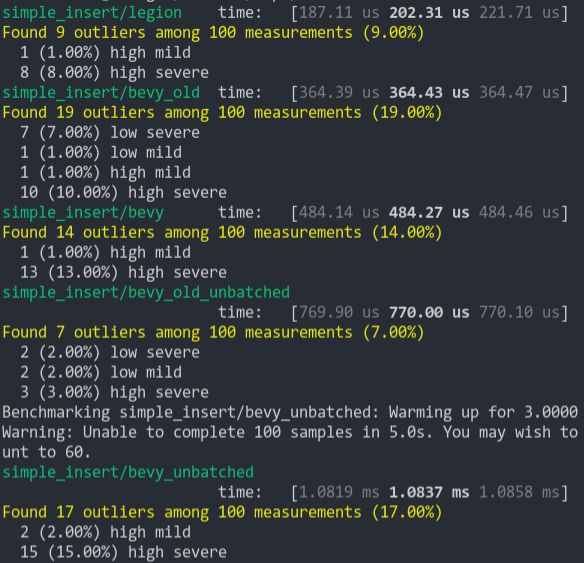
### Simpler Iter (from ecs_bench_suite)
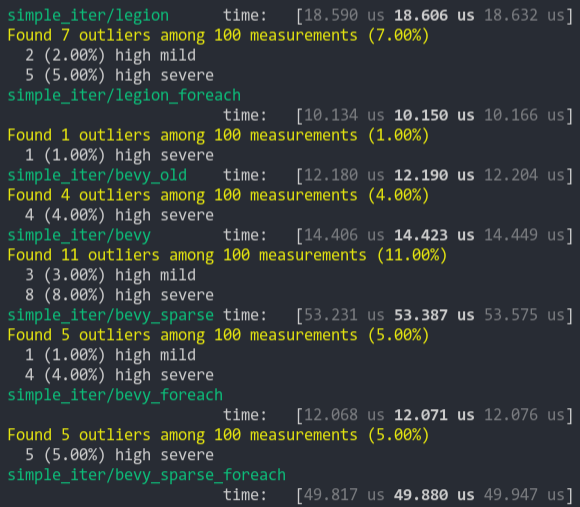
### Fragment Iter (from ecs_bench_suite)
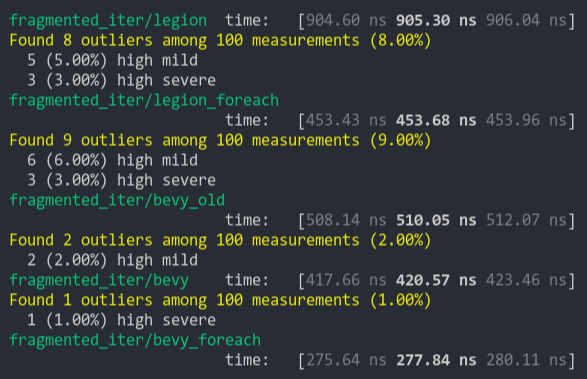
### Sparse Fragmented Iter
Iterate a query that matches 5 entities from a single matching archetype, but there are 100 unmatching archetypes
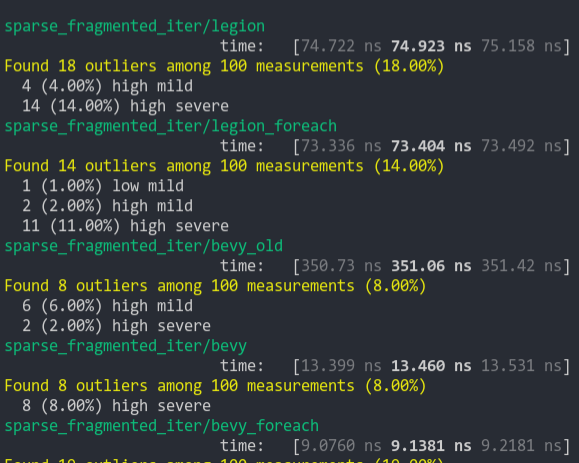
### Schedule (from ecs_bench_suite)
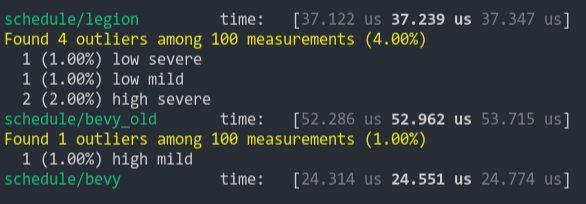
### Add Remove Component (from ecs_bench_suite)
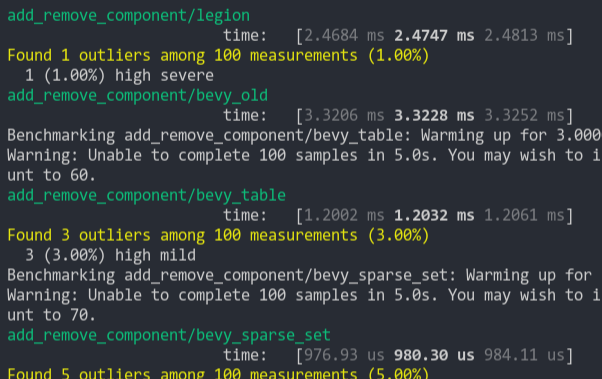
### Add Remove Component Big
Same as the test above, but each entity has 5 "large" matrix components and 1 "large" matrix component is added and removed
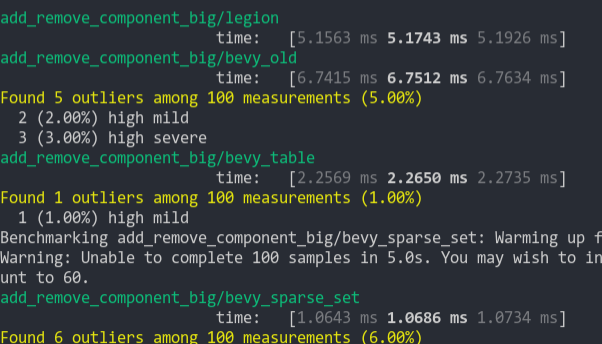
### Get Component
Looks up a single component value a large number of times
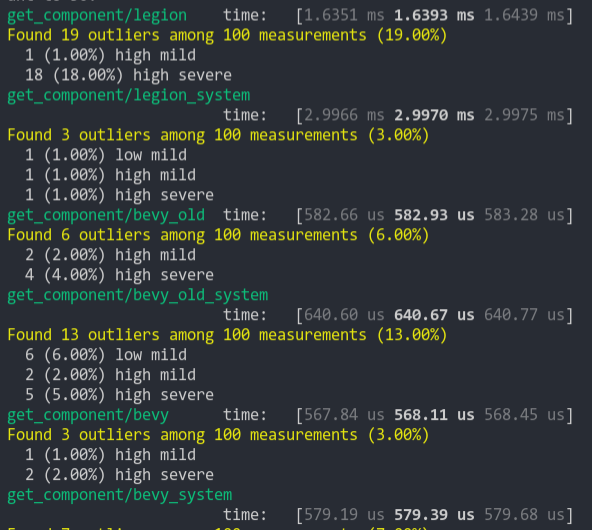
2021-03-05 07:54:35 +00:00
world . archetypes ( )
2020-11-08 20:34:05 +00:00
}
}
2022-07-04 14:44:24 +00:00
// SAFETY: Only reads World components
Simplify trait hierarchy for `SystemParam` (#6865)
# Objective
* Implementing a custom `SystemParam` by hand requires implementing three traits -- four if it is read-only.
* The trait `SystemParamFetch<'w, 's>` is a workaround from before we had generic associated types, and is no longer necessary.
## Solution
* Combine the trait `SystemParamFetch` with `SystemParamState`.
* I decided to remove the `Fetch` name and keep the `State` name, since the former was consistently conflated with the latter.
* Replace the trait `ReadOnlySystemParamFetch` with `ReadOnlySystemParam`, which simplifies trait bounds in generic code.
---
## Changelog
- Removed the trait `SystemParamFetch`, moving its functionality to `SystemParamState`.
- Replaced the trait `ReadOnlySystemParamFetch` with `ReadOnlySystemParam`.
## Migration Guide
The trait `SystemParamFetch` has been removed, and its functionality has been transferred to `SystemParamState`.
```rust
// Before
impl SystemParamState for MyParamState {
fn init(world: &mut World, system_meta: &mut SystemMeta) -> Self { ... }
}
impl<'w, 's> SystemParamFetch<'w, 's> for MyParamState {
type Item = MyParam<'w, 's>;
fn get_param(...) -> Self::Item;
}
// After
impl SystemParamState for MyParamState {
type Item<'w, 's> = MyParam<'w, 's>; // Generic associated types!
fn init(world: &mut World, system_meta: &mut SystemMeta) -> Self { ... }
fn get_param<'w, 's>(...) -> Self::Item<'w, 's>;
}
```
The trait `ReadOnlySystemParamFetch` has been replaced with `ReadOnlySystemParam`.
```rust
// Before
unsafe impl ReadOnlySystemParamFetch for MyParamState {}
// After
unsafe impl<'w, 's> ReadOnlySystemParam for MyParam<'w, 's> {}
```
2022-12-11 18:34:14 +00:00
unsafe impl < ' a > ReadOnlySystemParam for & ' a Components { }
Add new SystemState and rename old SystemState to SystemMeta (#2283)
This enables `SystemParams` to be used outside of function systems. Anything can create and store `SystemState`, which enables efficient "param state cached" access to `SystemParams`.
It adds a `ReadOnlySystemParamFetch` trait, which enables safe `SystemState::get` calls without unique world access.
I renamed the old `SystemState` to `SystemMeta` to enable us to mirror the `QueryState` naming convention (but I'm happy to discuss alternative names if people have other ideas). I initially pitched this as `ParamState`, but given that it needs to include full system metadata, that doesn't feel like a particularly accurate name.
```rust
#[derive(Eq, PartialEq, Debug)]
struct A(usize);
#[derive(Eq, PartialEq, Debug)]
struct B(usize);
let mut world = World::default();
world.insert_resource(A(42));
world.spawn().insert(B(7));
// we get nice lifetime elision when declaring the type on the left hand side
let mut system_state: SystemState<(Res<A>, Query<&B>)> = SystemState::new(&mut world);
let (a, query) = system_state.get(&world);
assert_eq!(*a, A(42), "returned resource matches initial value");
assert_eq!(
*query.single().unwrap(),
B(7),
"returned component matches initial value"
);
// mutable system params require unique world access
let mut system_state: SystemState<(ResMut<A>, Query<&mut B>)> = SystemState::new(&mut world);
let (a, query) = system_state.get_mut(&mut world);
// static lifetimes are required when declaring inside of structs
struct SomeContainer {
state: SystemState<(Res<'static, A>, Res<'static, B>)>
}
// this can be shortened using type aliases, which will be useful for complex param tuples
type MyParams<'a> = (Res<'a, A>, Res<'a, B>);
struct SomeContainer {
state: SystemState<MyParams<'static>>
}
// It is the user's responsibility to call SystemState::apply(world) for parameters that queue up work
let mut system_state: SystemState<(Commands, Query<&B>)> = SystemState::new(&mut world);
{
let (mut commands, query) = system_state.get(&world);
commands.insert_resource(3.14);
}
system_state.apply(&mut world);
```
## Future Work
* Actually use SystemState inside FunctionSystem. This would be trivial, but it requires FunctionSystem to wrap SystemState in Option in its current form (which complicates system metadata lookup). I'd prefer to hold off until we adopt something like the later designs linked in #1364, which enable us to contruct Systems using a World reference (and also remove the need for `.system`).
* Consider a "scoped" approach to automatically call SystemState::apply when systems params are no longer being used (either a container type with a Drop impl, or a function that takes a closure for user logic operating on params).
2021-06-02 19:57:38 +00:00
2022-07-04 14:44:24 +00:00
// SAFETY: no component value access
Remove the `SystemParamState` trait and remove types like `ResState` (#6919)
Spiritual successor to #5205.
Actual successor to #6865.
# Objective
Currently, system params are defined using three traits: `SystemParam`, `ReadOnlySystemParam`, `SystemParamState`. The behavior for each param is specified by the `SystemParamState` trait, while `SystemParam` simply defers to the state.
Splitting the traits in this way makes it easier to implement within macros, but it increases the cognitive load. Worst of all, this approach requires each `MySystemParam` to have a public `MySystemParamState` type associated with it.
## Solution
* Merge the trait `SystemParamState` into `SystemParam`.
* Remove all trivial `SystemParam` state types.
* `OptionNonSendMutState<T>`: you will not be missed.
---
- [x] Fix/resolve the remaining test failure.
## Changelog
* Removed the trait `SystemParamState`, merging its functionality into `SystemParam`.
## Migration Guide
**Note**: this should replace the migration guide for #6865.
This is relative to Bevy 0.9, not main.
The traits `SystemParamState` and `SystemParamFetch` have been removed, and their functionality has been transferred to `SystemParam`.
```rust
// Before (0.9)
impl SystemParam for MyParam<'_, '_> {
type State = MyParamState;
}
unsafe impl SystemParamState for MyParamState {
fn init(world: &mut World, system_meta: &mut SystemMeta) -> Self { ... }
}
unsafe impl<'w, 's> SystemParamFetch<'w, 's> for MyParamState {
type Item = MyParam<'w, 's>;
fn get_param(&mut self, ...) -> Self::Item;
}
unsafe impl ReadOnlySystemParamFetch for MyParamState { }
// After (0.10)
unsafe impl SystemParam for MyParam<'_, '_> {
type State = MyParamState;
type Item<'w, 's> = MyParam<'w, 's>;
fn init_state(world: &mut World, system_meta: &mut SystemMeta) -> Self::State { ... }
fn get_param<'w, 's>(state: &mut Self::State, ...) -> Self::Item<'w, 's>;
}
unsafe impl ReadOnlySystemParam for MyParam<'_, '_> { }
```
The trait `ReadOnlySystemParamFetch` has been replaced with `ReadOnlySystemParam`.
```rust
// Before
unsafe impl ReadOnlySystemParamFetch for MyParamState {}
// After
unsafe impl ReadOnlySystemParam for MyParam<'_, '_> {}
```
2023-01-07 23:20:32 +00:00
unsafe impl < ' a > SystemParam for & ' a Components {
type State = ( ) ;
Simplify trait hierarchy for `SystemParam` (#6865)
# Objective
* Implementing a custom `SystemParam` by hand requires implementing three traits -- four if it is read-only.
* The trait `SystemParamFetch<'w, 's>` is a workaround from before we had generic associated types, and is no longer necessary.
## Solution
* Combine the trait `SystemParamFetch` with `SystemParamState`.
* I decided to remove the `Fetch` name and keep the `State` name, since the former was consistently conflated with the latter.
* Replace the trait `ReadOnlySystemParamFetch` with `ReadOnlySystemParam`, which simplifies trait bounds in generic code.
---
## Changelog
- Removed the trait `SystemParamFetch`, moving its functionality to `SystemParamState`.
- Replaced the trait `ReadOnlySystemParamFetch` with `ReadOnlySystemParam`.
## Migration Guide
The trait `SystemParamFetch` has been removed, and its functionality has been transferred to `SystemParamState`.
```rust
// Before
impl SystemParamState for MyParamState {
fn init(world: &mut World, system_meta: &mut SystemMeta) -> Self { ... }
}
impl<'w, 's> SystemParamFetch<'w, 's> for MyParamState {
type Item = MyParam<'w, 's>;
fn get_param(...) -> Self::Item;
}
// After
impl SystemParamState for MyParamState {
type Item<'w, 's> = MyParam<'w, 's>; // Generic associated types!
fn init(world: &mut World, system_meta: &mut SystemMeta) -> Self { ... }
fn get_param<'w, 's>(...) -> Self::Item<'w, 's>;
}
```
The trait `ReadOnlySystemParamFetch` has been replaced with `ReadOnlySystemParam`.
```rust
// Before
unsafe impl ReadOnlySystemParamFetch for MyParamState {}
// After
unsafe impl<'w, 's> ReadOnlySystemParam for MyParam<'w, 's> {}
```
2022-12-11 18:34:14 +00:00
type Item < ' w , ' s > = & ' w Components ;
Remove the `SystemParamState` trait and remove types like `ResState` (#6919)
Spiritual successor to #5205.
Actual successor to #6865.
# Objective
Currently, system params are defined using three traits: `SystemParam`, `ReadOnlySystemParam`, `SystemParamState`. The behavior for each param is specified by the `SystemParamState` trait, while `SystemParam` simply defers to the state.
Splitting the traits in this way makes it easier to implement within macros, but it increases the cognitive load. Worst of all, this approach requires each `MySystemParam` to have a public `MySystemParamState` type associated with it.
## Solution
* Merge the trait `SystemParamState` into `SystemParam`.
* Remove all trivial `SystemParam` state types.
* `OptionNonSendMutState<T>`: you will not be missed.
---
- [x] Fix/resolve the remaining test failure.
## Changelog
* Removed the trait `SystemParamState`, merging its functionality into `SystemParam`.
## Migration Guide
**Note**: this should replace the migration guide for #6865.
This is relative to Bevy 0.9, not main.
The traits `SystemParamState` and `SystemParamFetch` have been removed, and their functionality has been transferred to `SystemParam`.
```rust
// Before (0.9)
impl SystemParam for MyParam<'_, '_> {
type State = MyParamState;
}
unsafe impl SystemParamState for MyParamState {
fn init(world: &mut World, system_meta: &mut SystemMeta) -> Self { ... }
}
unsafe impl<'w, 's> SystemParamFetch<'w, 's> for MyParamState {
type Item = MyParam<'w, 's>;
fn get_param(&mut self, ...) -> Self::Item;
}
unsafe impl ReadOnlySystemParamFetch for MyParamState { }
// After (0.10)
unsafe impl SystemParam for MyParam<'_, '_> {
type State = MyParamState;
type Item<'w, 's> = MyParam<'w, 's>;
fn init_state(world: &mut World, system_meta: &mut SystemMeta) -> Self::State { ... }
fn get_param<'w, 's>(state: &mut Self::State, ...) -> Self::Item<'w, 's>;
}
unsafe impl ReadOnlySystemParam for MyParam<'_, '_> { }
```
The trait `ReadOnlySystemParamFetch` has been replaced with `ReadOnlySystemParam`.
```rust
// Before
unsafe impl ReadOnlySystemParamFetch for MyParamState {}
// After
unsafe impl ReadOnlySystemParam for MyParam<'_, '_> {}
```
2023-01-07 23:20:32 +00:00
fn init_state ( _world : & mut World , _system_meta : & mut SystemMeta ) -> Self ::State { }
Bevy ECS V2 (#1525)
# Bevy ECS V2
This is a rewrite of Bevy ECS (basically everything but the new executor/schedule, which are already awesome). The overall goal was to improve the performance and versatility of Bevy ECS. Here is a quick bulleted list of changes before we dive into the details:
* Complete World rewrite
* Multiple component storage types:
* Tables: fast cache friendly iteration, slower add/removes (previously called Archetypes)
* Sparse Sets: fast add/remove, slower iteration
* Stateful Queries (caches query results for faster iteration. fragmented iteration is _fast_ now)
* Stateful System Params (caches expensive operations. inspired by @DJMcNab's work in #1364)
* Configurable System Params (users can set configuration when they construct their systems. once again inspired by @DJMcNab's work)
* Archetypes are now "just metadata", component storage is separate
* Archetype Graph (for faster archetype changes)
* Component Metadata
* Configure component storage type
* Retrieve information about component size/type/name/layout/send-ness/etc
* Components are uniquely identified by a densely packed ComponentId
* TypeIds are now totally optional (which should make implementing scripting easier)
* Super fast "for_each" query iterators
* Merged Resources into World. Resources are now just a special type of component
* EntityRef/EntityMut builder apis (more efficient and more ergonomic)
* Fast bitset-backed `Access<T>` replaces old hashmap-based approach everywhere
* Query conflicts are determined by component access instead of archetype component access (to avoid random failures at runtime)
* With/Without are still taken into account for conflicts, so this should still be comfy to use
* Much simpler `IntoSystem` impl
* Significantly reduced the amount of hashing throughout the ecs in favor of Sparse Sets (indexed by densely packed ArchetypeId, ComponentId, BundleId, and TableId)
* Safety Improvements
* Entity reservation uses a normal world reference instead of unsafe transmute
* QuerySets no longer transmute lifetimes
* Made traits "unsafe" where relevant
* More thorough safety docs
* WorldCell
* Exposes safe mutable access to multiple resources at a time in a World
* Replaced "catch all" `System::update_archetypes(world: &World)` with `System::new_archetype(archetype: &Archetype)`
* Simpler Bundle implementation
* Replaced slow "remove_bundle_one_by_one" used as fallback for Commands::remove_bundle with fast "remove_bundle_intersection"
* Removed `Mut<T>` query impl. it is better to only support one way: `&mut T`
* Removed with() from `Flags<T>` in favor of `Option<Flags<T>>`, which allows querying for flags to be "filtered" by default
* Components now have is_send property (currently only resources support non-send)
* More granular module organization
* New `RemovedComponents<T>` SystemParam that replaces `query.removed::<T>()`
* `world.resource_scope()` for mutable access to resources and world at the same time
* WorldQuery and QueryFilter traits unified. FilterFetch trait added to enable "short circuit" filtering. Auto impled for cases that don't need it
* Significantly slimmed down SystemState in favor of individual SystemParam state
* System Commands changed from `commands: &mut Commands` back to `mut commands: Commands` (to allow Commands to have a World reference)
Fixes #1320
## `World` Rewrite
This is a from-scratch rewrite of `World` that fills the niche that `hecs` used to. Yes, this means Bevy ECS is no longer a "fork" of hecs. We're going out our own!
(the only shared code between the projects is the entity id allocator, which is already basically ideal)
A huge shout out to @SanderMertens (author of [flecs](https://github.com/SanderMertens/flecs)) for sharing some great ideas with me (specifically hybrid ecs storage and archetype graphs). He also helped advise on a number of implementation details.
## Component Storage (The Problem)
Two ECS storage paradigms have gained a lot of traction over the years:
* **Archetypal ECS**:
* Stores components in "tables" with static schemas. Each "column" stores components of a given type. Each "row" is an entity.
* Each "archetype" has its own table. Adding/removing an entity's component changes the archetype.
* Enables super-fast Query iteration due to its cache-friendly data layout
* Comes at the cost of more expensive add/remove operations for an Entity's components, because all components need to be copied to the new archetype's "table"
* **Sparse Set ECS**:
* Stores components of the same type in densely packed arrays, which are sparsely indexed by densely packed unsigned integers (Entity ids)
* Query iteration is slower than Archetypal ECS because each entity's component could be at any position in the sparse set. This "random access" pattern isn't cache friendly. Additionally, there is an extra layer of indirection because you must first map the entity id to an index in the component array.
* Adding/removing components is a cheap, constant time operation
Bevy ECS V1, hecs, legion, flec, and Unity DOTS are all "archetypal ecs-es". I personally think "archetypal" storage is a good default for game engines. An entity's archetype doesn't need to change frequently in general, and it creates "fast by default" query iteration (which is a much more common operation). It is also "self optimizing". Users don't need to think about optimizing component layouts for iteration performance. It "just works" without any extra boilerplate.
Shipyard and EnTT are "sparse set ecs-es". They employ "packing" as a way to work around the "suboptimal by default" iteration performance for specific sets of components. This helps, but I didn't think this was a good choice for a general purpose engine like Bevy because:
1. "packs" conflict with each other. If bevy decides to internally pack the Transform and GlobalTransform components, users are then blocked if they want to pack some custom component with Transform.
2. users need to take manual action to optimize
Developers selecting an ECS framework are stuck with a hard choice. Select an "archetypal" framework with "fast iteration everywhere" but without the ability to cheaply add/remove components, or select a "sparse set" framework to cheaply add/remove components but with slower iteration performance.
## Hybrid Component Storage (The Solution)
In Bevy ECS V2, we get to have our cake and eat it too. It now has _both_ of the component storage types above (and more can be added later if needed):
* **Tables** (aka "archetypal" storage)
* The default storage. If you don't configure anything, this is what you get
* Fast iteration by default
* Slower add/remove operations
* **Sparse Sets**
* Opt-in
* Slower iteration
* Faster add/remove operations
These storage types complement each other perfectly. By default Query iteration is fast. If developers know that they want to add/remove a component at high frequencies, they can set the storage to "sparse set":
```rust
world.register_component(
ComponentDescriptor::new::<MyComponent>(StorageType::SparseSet)
).unwrap();
```
## Archetypes
Archetypes are now "just metadata" ... they no longer store components directly. They do store:
* The `ComponentId`s of each of the Archetype's components (and that component's storage type)
* Archetypes are uniquely defined by their component layouts
* For example: entities with "table" components `[A, B, C]` _and_ "sparse set" components `[D, E]` will always be in the same archetype.
* The `TableId` associated with the archetype
* For now each archetype has exactly one table (which can have no components),
* There is a 1->Many relationship from Tables->Archetypes. A given table could have any number of archetype components stored in it:
* Ex: an entity with "table storage" components `[A, B, C]` and "sparse set" components `[D, E]` will share the same `[A, B, C]` table as an entity with `[A, B, C]` table component and `[F]` sparse set components.
* This 1->Many relationship is how we preserve fast "cache friendly" iteration performance when possible (more on this later)
* A list of entities that are in the archetype and the row id of the table they are in
* ArchetypeComponentIds
* unique densely packed identifiers for (ArchetypeId, ComponentId) pairs
* used by the schedule executor for cheap system access control
* "Archetype Graph Edges" (see the next section)
## The "Archetype Graph"
Archetype changes in Bevy (and a number of other archetypal ecs-es) have historically been expensive to compute. First, you need to allocate a new vector of the entity's current component ids, add or remove components based on the operation performed, sort it (to ensure it is order-independent), then hash it to find the archetype (if it exists). And thats all before we get to the _already_ expensive full copy of all components to the new table storage.
The solution is to build a "graph" of archetypes to cache these results. @SanderMertens first exposed me to the idea (and he got it from @gjroelofs, who came up with it). They propose adding directed edges between archetypes for add/remove component operations. If `ComponentId`s are densely packed, you can use sparse sets to cheaply jump between archetypes.
Bevy takes this one step further by using add/remove `Bundle` edges instead of `Component` edges. Bevy encourages the use of `Bundles` to group add/remove operations. This is largely for "clearer game logic" reasons, but it also helps cut down on the number of archetype changes required. `Bundles` now also have densely-packed `BundleId`s. This allows us to use a _single_ edge for each bundle operation (rather than needing to traverse N edges ... one for each component). Single component operations are also bundles, so this is strictly an improvement over a "component only" graph.
As a result, an operation that used to be _heavy_ (both for allocations and compute) is now two dirt-cheap array lookups and zero allocations.
## Stateful Queries
World queries are now stateful. This allows us to:
1. Cache archetype (and table) matches
* This resolves another issue with (naive) archetypal ECS: query performance getting worse as the number of archetypes goes up (and fragmentation occurs).
2. Cache Fetch and Filter state
* The expensive parts of fetch/filter operations (such as hashing the TypeId to find the ComponentId) now only happen once when the Query is first constructed
3. Incrementally build up state
* When new archetypes are added, we only process the new archetypes (no need to rebuild state for old archetypes)
As a result, the direct `World` query api now looks like this:
```rust
let mut query = world.query::<(&A, &mut B)>();
for (a, mut b) in query.iter_mut(&mut world) {
}
```
Requiring `World` to generate stateful queries (rather than letting the `QueryState` type be constructed separately) allows us to ensure that _all_ queries are properly initialized (and the relevant world state, such as ComponentIds). This enables QueryState to remove branches from its operations that check for initialization status (and also enables query.iter() to take an immutable world reference because it doesn't need to initialize anything in world).
However in systems, this is a non-breaking change. State management is done internally by the relevant SystemParam.
## Stateful SystemParams
Like Queries, `SystemParams` now also cache state. For example, `Query` system params store the "stateful query" state mentioned above. Commands store their internal `CommandQueue`. This means you can now safely use as many separate `Commands` parameters in your system as you want. `Local<T>` system params store their `T` value in their state (instead of in Resources).
SystemParam state also enabled a significant slim-down of SystemState. It is much nicer to look at now.
Per-SystemParam state naturally insulates us from an "aliased mut" class of errors we have hit in the past (ex: using multiple `Commands` system params).
(credit goes to @DJMcNab for the initial idea and draft pr here #1364)
## Configurable SystemParams
@DJMcNab also had the great idea to make SystemParams configurable. This allows users to provide some initial configuration / values for system parameters (when possible). Most SystemParams have no config (the config type is `()`), but the `Local<T>` param now supports user-provided parameters:
```rust
fn foo(value: Local<usize>) {
}
app.add_system(foo.system().config(|c| c.0 = Some(10)));
```
## Uber Fast "for_each" Query Iterators
Developers now have the choice to use a fast "for_each" iterator, which yields ~1.5-3x iteration speed improvements for "fragmented iteration", and minor ~1.2x iteration speed improvements for unfragmented iteration.
```rust
fn system(query: Query<(&A, &mut B)>) {
// you now have the option to do this for a speed boost
query.for_each_mut(|(a, mut b)| {
});
// however normal iterators are still available
for (a, mut b) in query.iter_mut() {
}
}
```
I think in most cases we should continue to encourage "normal" iterators as they are more flexible and more "rust idiomatic". But when that extra "oomf" is needed, it makes sense to use `for_each`.
We should also consider using `for_each` for internal bevy systems to give our users a nice speed boost (but that should be a separate pr).
## Component Metadata
`World` now has a `Components` collection, which is accessible via `world.components()`. This stores mappings from `ComponentId` to `ComponentInfo`, as well as `TypeId` to `ComponentId` mappings (where relevant). `ComponentInfo` stores information about the component, such as ComponentId, TypeId, memory layout, send-ness (currently limited to resources), and storage type.
## Significantly Cheaper `Access<T>`
We used to use `TypeAccess<TypeId>` to manage read/write component/archetype-component access. This was expensive because TypeIds must be hashed and compared individually. The parallel executor got around this by "condensing" type ids into bitset-backed access types. This worked, but it had to be re-generated from the `TypeAccess<TypeId>`sources every time archetypes changed.
This pr removes TypeAccess in favor of faster bitset access everywhere. We can do this thanks to the move to densely packed `ComponentId`s and `ArchetypeComponentId`s.
## Merged Resources into World
Resources had a lot of redundant functionality with Components. They stored typed data, they had access control, they had unique ids, they were queryable via SystemParams, etc. In fact the _only_ major difference between them was that they were unique (and didn't correlate to an entity).
Separate resources also had the downside of requiring a separate set of access controls, which meant the parallel executor needed to compare more bitsets per system and manage more state.
I initially got the "separate resources" idea from `legion`. I think that design was motivated by the fact that it made the direct world query/resource lifetime interactions more manageable. It certainly made our lives easier when using Resources alongside hecs/bevy_ecs. However we already have a construct for safely and ergonomically managing in-world lifetimes: systems (which use `Access<T>` internally).
This pr merges Resources into World:
```rust
world.insert_resource(1);
world.insert_resource(2.0);
let a = world.get_resource::<i32>().unwrap();
let mut b = world.get_resource_mut::<f64>().unwrap();
*b = 3.0;
```
Resources are now just a special kind of component. They have their own ComponentIds (and their own resource TypeId->ComponentId scope, so they don't conflict wit components of the same type). They are stored in a special "resource archetype", which stores components inside the archetype using a new `unique_components` sparse set (note that this sparse set could later be used to implement Tags). This allows us to keep the code size small by reusing existing datastructures (namely Column, Archetype, ComponentFlags, and ComponentInfo). This allows us the executor to use a single `Access<ArchetypeComponentId>` per system. It should also make scripting language integration easier.
_But_ this merge did create problems for people directly interacting with `World`. What if you need mutable access to multiple resources at the same time? `world.get_resource_mut()` borrows World mutably!
## WorldCell
WorldCell applies the `Access<ArchetypeComponentId>` concept to direct world access:
```rust
let world_cell = world.cell();
let a = world_cell.get_resource_mut::<i32>().unwrap();
let b = world_cell.get_resource_mut::<f64>().unwrap();
```
This adds cheap runtime checks (a sparse set lookup of `ArchetypeComponentId` and a counter) to ensure that world accesses do not conflict with each other. Each operation returns a `WorldBorrow<'w, T>` or `WorldBorrowMut<'w, T>` wrapper type, which will release the relevant ArchetypeComponentId resources when dropped.
World caches the access sparse set (and only one cell can exist at a time), so `world.cell()` is a cheap operation.
WorldCell does _not_ use atomic operations. It is non-send, does a mutable borrow of world to prevent other accesses, and uses a simple `Rc<RefCell<ArchetypeComponentAccess>>` wrapper in each WorldBorrow pointer.
The api is currently limited to resource access, but it can and should be extended to queries / entity component access.
## Resource Scopes
WorldCell does not yet support component queries, and even when it does there are sometimes legitimate reasons to want a mutable world ref _and_ a mutable resource ref (ex: bevy_render and bevy_scene both need this). In these cases we could always drop down to the unsafe `world.get_resource_unchecked_mut()`, but that is not ideal!
Instead developers can use a "resource scope"
```rust
world.resource_scope(|world: &mut World, a: &mut A| {
})
```
This temporarily removes the `A` resource from `World`, provides mutable pointers to both, and re-adds A to World when finished. Thanks to the move to ComponentIds/sparse sets, this is a cheap operation.
If multiple resources are required, scopes can be nested. We could also consider adding a "resource tuple" to the api if this pattern becomes common and the boilerplate gets nasty.
## Query Conflicts Use ComponentId Instead of ArchetypeComponentId
For safety reasons, systems cannot contain queries that conflict with each other without wrapping them in a QuerySet. On bevy `main`, we use ArchetypeComponentIds to determine conflicts. This is nice because it can take into account filters:
```rust
// these queries will never conflict due to their filters
fn filter_system(a: Query<&mut A, With<B>>, b: Query<&mut B, Without<B>>) {
}
```
But it also has a significant downside:
```rust
// these queries will not conflict _until_ an entity with A, B, and C is spawned
fn maybe_conflicts_system(a: Query<(&mut A, &C)>, b: Query<(&mut A, &B)>) {
}
```
The system above will panic at runtime if an entity with A, B, and C is spawned. This makes it hard to trust that your game logic will run without crashing.
In this pr, I switched to using `ComponentId` instead. This _is_ more constraining. `maybe_conflicts_system` will now always fail, but it will do it consistently at startup. Naively, it would also _disallow_ `filter_system`, which would be a significant downgrade in usability. Bevy has a number of internal systems that rely on disjoint queries and I expect it to be a common pattern in userspace.
To resolve this, I added a new `FilteredAccess<T>` type, which wraps `Access<T>` and adds with/without filters. If two `FilteredAccess` have with/without values that prove they are disjoint, they will no longer conflict.
## EntityRef / EntityMut
World entity operations on `main` require that the user passes in an `entity` id to each operation:
```rust
let entity = world.spawn((A, )); // create a new entity with A
world.get::<A>(entity);
world.insert(entity, (B, C));
world.insert_one(entity, D);
```
This means that each operation needs to look up the entity location / verify its validity. The initial spawn operation also requires a Bundle as input. This can be awkward when no components are required (or one component is required).
These operations have been replaced by `EntityRef` and `EntityMut`, which are "builder-style" wrappers around world that provide read and read/write operations on a single, pre-validated entity:
```rust
// spawn now takes no inputs and returns an EntityMut
let entity = world.spawn()
.insert(A) // insert a single component into the entity
.insert_bundle((B, C)) // insert a bundle of components into the entity
.id() // id returns the Entity id
// Returns EntityMut (or panics if the entity does not exist)
world.entity_mut(entity)
.insert(D)
.insert_bundle(SomeBundle::default());
{
// returns EntityRef (or panics if the entity does not exist)
let d = world.entity(entity)
.get::<D>() // gets the D component
.unwrap();
// world.get still exists for ergonomics
let d = world.get::<D>(entity).unwrap();
}
// These variants return Options if you want to check existence instead of panicing
world.get_entity_mut(entity)
.unwrap()
.insert(E);
if let Some(entity_ref) = world.get_entity(entity) {
let d = entity_ref.get::<D>().unwrap();
}
```
This _does not_ affect the current Commands api or terminology. I think that should be a separate conversation as that is a much larger breaking change.
## Safety Improvements
* Entity reservation in Commands uses a normal world borrow instead of an unsafe transmute
* QuerySets no longer transmutes lifetimes
* Made traits "unsafe" when implementing a trait incorrectly could cause unsafety
* More thorough safety docs
## RemovedComponents SystemParam
The old approach to querying removed components: `query.removed:<T>()` was confusing because it had no connection to the query itself. I replaced it with the following, which is both clearer and allows us to cache the ComponentId mapping in the SystemParamState:
```rust
fn system(removed: RemovedComponents<T>) {
for entity in removed.iter() {
}
}
```
## Simpler Bundle implementation
Bundles are no longer responsible for sorting (or deduping) TypeInfo. They are just a simple ordered list of component types / data. This makes the implementation smaller and opens the door to an easy "nested bundle" implementation in the future (which i might even add in this pr). Duplicate detection is now done once per bundle type by World the first time a bundle is used.
## Unified WorldQuery and QueryFilter types
(don't worry they are still separate type _parameters_ in Queries .. this is a non-breaking change)
WorldQuery and QueryFilter were already basically identical apis. With the addition of `FetchState` and more storage-specific fetch methods, the overlap was even clearer (and the redundancy more painful).
QueryFilters are now just `F: WorldQuery where F::Fetch: FilterFetch`. FilterFetch requires `Fetch<Item = bool>` and adds new "short circuit" variants of fetch methods. This enables a filter tuple like `(With<A>, Without<B>, Changed<C>)` to stop evaluating the filter after the first mismatch is encountered. FilterFetch is automatically implemented for `Fetch` implementations that return bool.
This forces fetch implementations that return things like `(bool, bool, bool)` (such as the filter above) to manually implement FilterFetch and decide whether or not to short-circuit.
## More Granular Modules
World no longer globs all of the internal modules together. It now exports `core`, `system`, and `schedule` separately. I'm also considering exporting `core` submodules directly as that is still pretty "glob-ey" and unorganized (feedback welcome here).
## Remaining Draft Work (to be done in this pr)
* ~~panic on conflicting WorldQuery fetches (&A, &mut A)~~
* ~~bevy `main` and hecs both currently allow this, but we should protect against it if possible~~
* ~~batch_iter / par_iter (currently stubbed out)~~
* ~~ChangedRes~~
* ~~I skipped this while we sort out #1313. This pr should be adapted to account for whatever we land on there~~.
* ~~The `Archetypes` and `Tables` collections use hashes of sorted lists of component ids to uniquely identify each archetype/table. This hash is then used as the key in a HashMap to look up the relevant ArchetypeId or TableId. (which doesn't handle hash collisions properly)~~
* ~~It is currently unsafe to generate a Query from "World A", then use it on "World B" (despite the api claiming it is safe). We should probably close this gap. This could be done by adding a randomly generated WorldId to each world, then storing that id in each Query. They could then be compared to each other on each `query.do_thing(&world)` operation. This _does_ add an extra branch to each query operation, so I'm open to other suggestions if people have them.~~
* ~~Nested Bundles (if i find time)~~
## Potential Future Work
* Expand WorldCell to support queries.
* Consider not allocating in the empty archetype on `world.spawn()`
* ex: return something like EntityMutUninit, which turns into EntityMut after an `insert` or `insert_bundle` op
* this actually regressed performance last time i tried it, but in theory it should be faster
* Optimize SparseSet::insert (see `PERF` comment on insert)
* Replace SparseArray `Option<T>` with T::MAX to cut down on branching
* would enable cheaper get_unchecked() operations
* upstream fixedbitset optimizations
* fixedbitset could be allocation free for small block counts (store blocks in a SmallVec)
* fixedbitset could have a const constructor
* Consider implementing Tags (archetype-specific by-value data that affects archetype identity)
* ex: ArchetypeA could have `[A, B, C]` table components and `[D(1)]` "tag" component. ArchetypeB could have `[A, B, C]` table components and a `[D(2)]` tag component. The archetypes are different, despite both having D tags because the value inside D is different.
* this could potentially build on top of the `archetype.unique_components` added in this pr for resource storage.
* Consider reverting `all_tuples` proc macro in favor of the old `macro_rules` implementation
* all_tuples is more flexible and produces cleaner documentation (the macro_rules version produces weird type parameter orders due to parser constraints)
* but unfortunately all_tuples also appears to make Rust Analyzer sad/slow when working inside of `bevy_ecs` (does not affect user code)
* Consider "resource queries" and/or "mixed resource and entity component queries" as an alternative to WorldCell
* this is basically just "systems" so maybe it's not worth it
* Add more world ops
* `world.clear()`
* `world.reserve<T: Bundle>(count: usize)`
* Try using the old archetype allocation strategy (allocate new memory on resize and copy everything over). I expect this to improve batch insertion performance at the cost of unbatched performance. But thats just a guess. I'm not an allocation perf pro :)
* Adapt Commands apis for consistency with new World apis
## Benchmarks
key:
* `bevy_old`: bevy `main` branch
* `bevy`: this branch
* `_foreach`: uses an optimized for_each iterator
* ` _sparse`: uses sparse set storage (if unspecified assume table storage)
* `_system`: runs inside a system (if unspecified assume test happens via direct world ops)
### Simple Insert (from ecs_bench_suite)
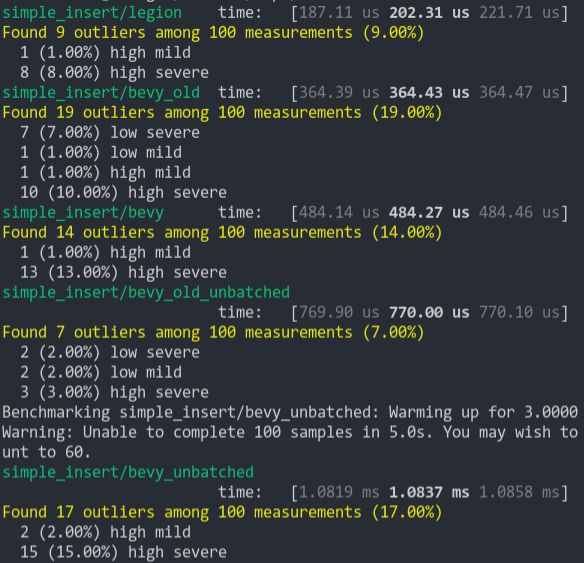
### Simpler Iter (from ecs_bench_suite)
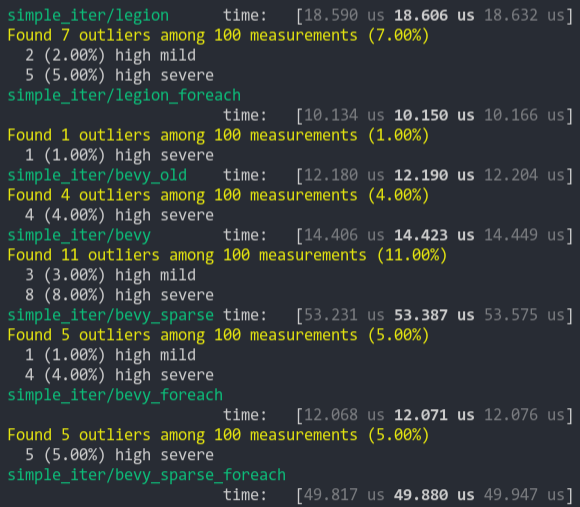
### Fragment Iter (from ecs_bench_suite)
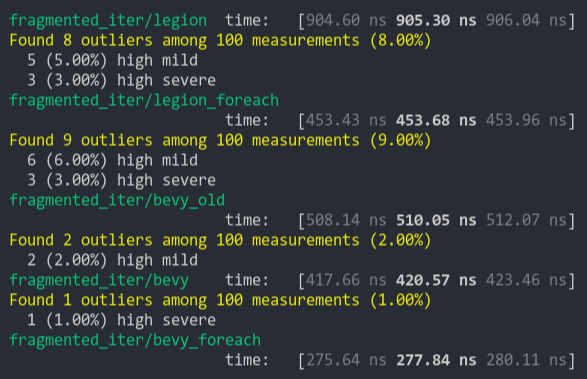
### Sparse Fragmented Iter
Iterate a query that matches 5 entities from a single matching archetype, but there are 100 unmatching archetypes
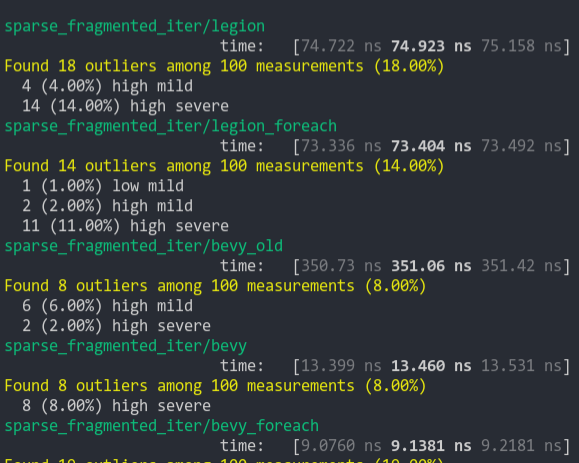
### Schedule (from ecs_bench_suite)
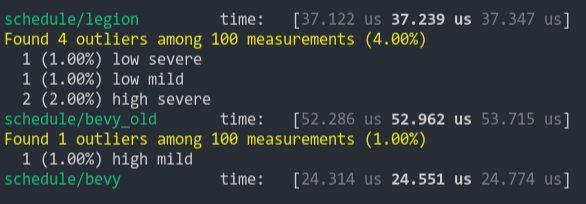
### Add Remove Component (from ecs_bench_suite)
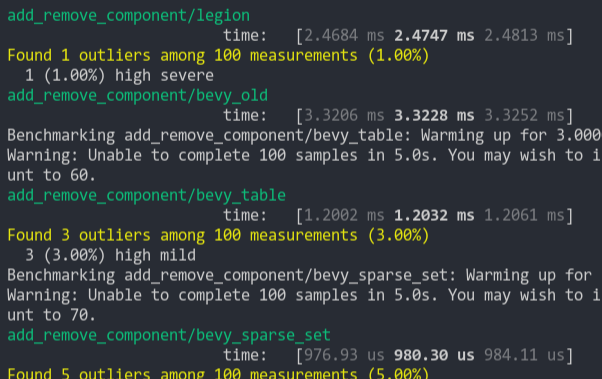
### Add Remove Component Big
Same as the test above, but each entity has 5 "large" matrix components and 1 "large" matrix component is added and removed
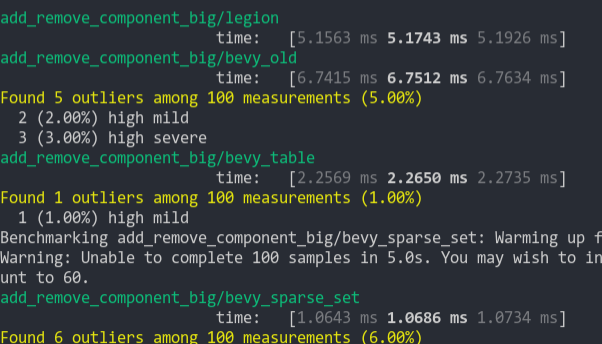
### Get Component
Looks up a single component value a large number of times
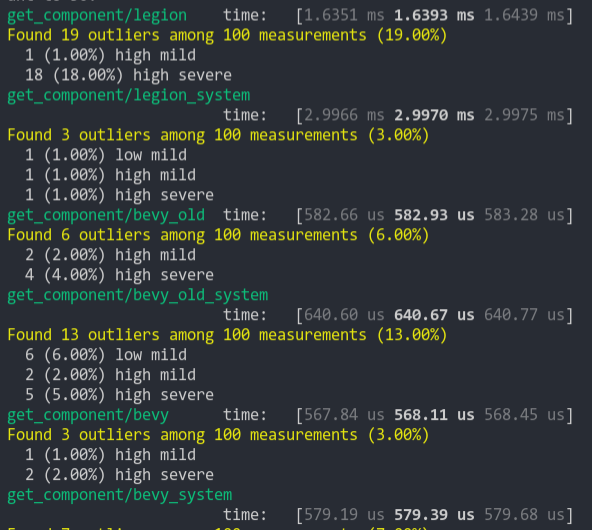
2021-03-05 07:54:35 +00:00
#[ inline ]
Simplify trait hierarchy for `SystemParam` (#6865)
# Objective
* Implementing a custom `SystemParam` by hand requires implementing three traits -- four if it is read-only.
* The trait `SystemParamFetch<'w, 's>` is a workaround from before we had generic associated types, and is no longer necessary.
## Solution
* Combine the trait `SystemParamFetch` with `SystemParamState`.
* I decided to remove the `Fetch` name and keep the `State` name, since the former was consistently conflated with the latter.
* Replace the trait `ReadOnlySystemParamFetch` with `ReadOnlySystemParam`, which simplifies trait bounds in generic code.
---
## Changelog
- Removed the trait `SystemParamFetch`, moving its functionality to `SystemParamState`.
- Replaced the trait `ReadOnlySystemParamFetch` with `ReadOnlySystemParam`.
## Migration Guide
The trait `SystemParamFetch` has been removed, and its functionality has been transferred to `SystemParamState`.
```rust
// Before
impl SystemParamState for MyParamState {
fn init(world: &mut World, system_meta: &mut SystemMeta) -> Self { ... }
}
impl<'w, 's> SystemParamFetch<'w, 's> for MyParamState {
type Item = MyParam<'w, 's>;
fn get_param(...) -> Self::Item;
}
// After
impl SystemParamState for MyParamState {
type Item<'w, 's> = MyParam<'w, 's>; // Generic associated types!
fn init(world: &mut World, system_meta: &mut SystemMeta) -> Self { ... }
fn get_param<'w, 's>(...) -> Self::Item<'w, 's>;
}
```
The trait `ReadOnlySystemParamFetch` has been replaced with `ReadOnlySystemParam`.
```rust
// Before
unsafe impl ReadOnlySystemParamFetch for MyParamState {}
// After
unsafe impl<'w, 's> ReadOnlySystemParam for MyParam<'w, 's> {}
```
2022-12-11 18:34:14 +00:00
unsafe fn get_param < ' w , ' s > (
Remove the `SystemParamState` trait and remove types like `ResState` (#6919)
Spiritual successor to #5205.
Actual successor to #6865.
# Objective
Currently, system params are defined using three traits: `SystemParam`, `ReadOnlySystemParam`, `SystemParamState`. The behavior for each param is specified by the `SystemParamState` trait, while `SystemParam` simply defers to the state.
Splitting the traits in this way makes it easier to implement within macros, but it increases the cognitive load. Worst of all, this approach requires each `MySystemParam` to have a public `MySystemParamState` type associated with it.
## Solution
* Merge the trait `SystemParamState` into `SystemParam`.
* Remove all trivial `SystemParam` state types.
* `OptionNonSendMutState<T>`: you will not be missed.
---
- [x] Fix/resolve the remaining test failure.
## Changelog
* Removed the trait `SystemParamState`, merging its functionality into `SystemParam`.
## Migration Guide
**Note**: this should replace the migration guide for #6865.
This is relative to Bevy 0.9, not main.
The traits `SystemParamState` and `SystemParamFetch` have been removed, and their functionality has been transferred to `SystemParam`.
```rust
// Before (0.9)
impl SystemParam for MyParam<'_, '_> {
type State = MyParamState;
}
unsafe impl SystemParamState for MyParamState {
fn init(world: &mut World, system_meta: &mut SystemMeta) -> Self { ... }
}
unsafe impl<'w, 's> SystemParamFetch<'w, 's> for MyParamState {
type Item = MyParam<'w, 's>;
fn get_param(&mut self, ...) -> Self::Item;
}
unsafe impl ReadOnlySystemParamFetch for MyParamState { }
// After (0.10)
unsafe impl SystemParam for MyParam<'_, '_> {
type State = MyParamState;
type Item<'w, 's> = MyParam<'w, 's>;
fn init_state(world: &mut World, system_meta: &mut SystemMeta) -> Self::State { ... }
fn get_param<'w, 's>(state: &mut Self::State, ...) -> Self::Item<'w, 's>;
}
unsafe impl ReadOnlySystemParam for MyParam<'_, '_> { }
```
The trait `ReadOnlySystemParamFetch` has been replaced with `ReadOnlySystemParam`.
```rust
// Before
unsafe impl ReadOnlySystemParamFetch for MyParamState {}
// After
unsafe impl ReadOnlySystemParam for MyParam<'_, '_> {}
```
2023-01-07 23:20:32 +00:00
_state : & ' s mut Self ::State ,
Add new SystemState and rename old SystemState to SystemMeta (#2283)
This enables `SystemParams` to be used outside of function systems. Anything can create and store `SystemState`, which enables efficient "param state cached" access to `SystemParams`.
It adds a `ReadOnlySystemParamFetch` trait, which enables safe `SystemState::get` calls without unique world access.
I renamed the old `SystemState` to `SystemMeta` to enable us to mirror the `QueryState` naming convention (but I'm happy to discuss alternative names if people have other ideas). I initially pitched this as `ParamState`, but given that it needs to include full system metadata, that doesn't feel like a particularly accurate name.
```rust
#[derive(Eq, PartialEq, Debug)]
struct A(usize);
#[derive(Eq, PartialEq, Debug)]
struct B(usize);
let mut world = World::default();
world.insert_resource(A(42));
world.spawn().insert(B(7));
// we get nice lifetime elision when declaring the type on the left hand side
let mut system_state: SystemState<(Res<A>, Query<&B>)> = SystemState::new(&mut world);
let (a, query) = system_state.get(&world);
assert_eq!(*a, A(42), "returned resource matches initial value");
assert_eq!(
*query.single().unwrap(),
B(7),
"returned component matches initial value"
);
// mutable system params require unique world access
let mut system_state: SystemState<(ResMut<A>, Query<&mut B>)> = SystemState::new(&mut world);
let (a, query) = system_state.get_mut(&mut world);
// static lifetimes are required when declaring inside of structs
struct SomeContainer {
state: SystemState<(Res<'static, A>, Res<'static, B>)>
}
// this can be shortened using type aliases, which will be useful for complex param tuples
type MyParams<'a> = (Res<'a, A>, Res<'a, B>);
struct SomeContainer {
state: SystemState<MyParams<'static>>
}
// It is the user's responsibility to call SystemState::apply(world) for parameters that queue up work
let mut system_state: SystemState<(Commands, Query<&B>)> = SystemState::new(&mut world);
{
let (mut commands, query) = system_state.get(&world);
commands.insert_resource(3.14);
}
system_state.apply(&mut world);
```
## Future Work
* Actually use SystemState inside FunctionSystem. This would be trivial, but it requires FunctionSystem to wrap SystemState in Option in its current form (which complicates system metadata lookup). I'd prefer to hold off until we adopt something like the later designs linked in #1364, which enable us to contruct Systems using a World reference (and also remove the need for `.system`).
* Consider a "scoped" approach to automatically call SystemState::apply when systems params are no longer being used (either a container type with a Drop impl, or a function that takes a closure for user logic operating on params).
2021-06-02 19:57:38 +00:00
_system_meta : & SystemMeta ,
System Param Lifetime Split (#2605)
# Objective
Enable using exact World lifetimes during read-only access . This is motivated by the new renderer's need to allow read-only world-only queries to outlive the query itself (but still be constrained by the world lifetime).
For example:
https://github.com/bevyengine/bevy/blob/115b170d1f11a91146bb6d6e9684dceb8b21f786/pipelined/bevy_pbr2/src/render/mod.rs#L774
## Solution
Split out SystemParam state and world lifetimes and pipe those lifetimes up to read-only Query ops (and add into_inner for Res). According to every safety test I've run so far (except one), this is safe (see the temporary safety test commit). Note that changing the mutable variants to the new lifetimes would allow aliased mutable pointers (try doing that to see how it affects the temporary safety tests).
The new state lifetime on SystemParam does make `#[derive(SystemParam)]` more cumbersome (the current impl requires PhantomData if you don't use both lifetimes). We can make this better by detecting whether or not a lifetime is used in the derive and adjusting accordingly, but that should probably be done in its own pr.
## Why is this a draft?
The new lifetimes break QuerySet safety in one very specific case (see the query_set system in system_safety_test). We need to solve this before we can use the lifetimes given.
This is due to the fact that QuerySet is just a wrapper over Query, which now relies on world lifetimes instead of `&self` lifetimes to prevent aliasing (but in systems, each Query has its own implied lifetime, not a centralized world lifetime). I believe the fix is to rewrite QuerySet to have its own World lifetime (and own the internal reference). This will complicate the impl a bit, but I think it is doable. I'm curious if anyone else has better ideas.
Personally, I think these new lifetimes need to happen. We've gotta have a way to directly tie read-only World queries to the World lifetime. The new renderer is the first place this has come up, but I doubt it will be the last. Worst case scenario we can come up with a second `WorldLifetimeQuery<Q, F = ()>` parameter to enable these read-only scenarios, but I'd rather not add another type to the type zoo.
2021-08-15 20:51:53 +00:00
world : & ' w World ,
Reliable change detection (#1471)
# Problem Definition
The current change tracking (via flags for both components and resources) fails to detect changes made by systems that are scheduled to run earlier in the frame than they are.
This issue is discussed at length in [#68](https://github.com/bevyengine/bevy/issues/68) and [#54](https://github.com/bevyengine/bevy/issues/54).
This is very much a draft PR, and contributions are welcome and needed.
# Criteria
1. Each change is detected at least once, no matter the ordering.
2. Each change is detected at most once, no matter the ordering.
3. Changes should be detected the same frame that they are made.
4. Competitive ergonomics. Ideally does not require opting-in.
5. Low CPU overhead of computation.
6. Memory efficient. This must not increase over time, except where the number of entities / resources does.
7. Changes should not be lost for systems that don't run.
8. A frame needs to act as a pure function. Given the same set of entities / components it needs to produce the same end state without side-effects.
**Exact** change-tracking proposals satisfy criteria 1 and 2.
**Conservative** change-tracking proposals satisfy criteria 1 but not 2.
**Flaky** change tracking proposals satisfy criteria 2 but not 1.
# Code Base Navigation
There are three types of flags:
- `Added`: A piece of data was added to an entity / `Resources`.
- `Mutated`: A piece of data was able to be modified, because its `DerefMut` was accessed
- `Changed`: The bitwise OR of `Added` and `Changed`
The special behavior of `ChangedRes`, with respect to the scheduler is being removed in [#1313](https://github.com/bevyengine/bevy/pull/1313) and does not need to be reproduced.
`ChangedRes` and friends can be found in "bevy_ecs/core/resources/resource_query.rs".
The `Flags` trait for Components can be found in "bevy_ecs/core/query.rs".
`ComponentFlags` are stored in "bevy_ecs/core/archetypes.rs", defined on line 446.
# Proposals
**Proposal 5 was selected for implementation.**
## Proposal 0: No Change Detection
The baseline, where computations are performed on everything regardless of whether it changed.
**Type:** Conservative
**Pros:**
- already implemented
- will never miss events
- no overhead
**Cons:**
- tons of repeated work
- doesn't allow users to avoid repeating work (or monitoring for other changes)
## Proposal 1: Earlier-This-Tick Change Detection
The current approach as of Bevy 0.4. Flags are set, and then flushed at the end of each frame.
**Type:** Flaky
**Pros:**
- already implemented
- simple to understand
- low memory overhead (2 bits per component)
- low time overhead (clear every flag once per frame)
**Cons:**
- misses systems based on ordering
- systems that don't run every frame miss changes
- duplicates detection when looping
- can lead to unresolvable circular dependencies
## Proposal 2: Two-Tick Change Detection
Flags persist for two frames, using a double-buffer system identical to that used in events.
A change is observed if it is found in either the current frame's list of changes or the previous frame's.
**Type:** Conservative
**Pros:**
- easy to understand
- easy to implement
- low memory overhead (4 bits per component)
- low time overhead (bit mask and shift every flag once per frame)
**Cons:**
- can result in a great deal of duplicated work
- systems that don't run every frame miss changes
- duplicates detection when looping
## Proposal 3: Last-Tick Change Detection
Flags persist for two frames, using a double-buffer system identical to that used in events.
A change is observed if it is found in the previous frame's list of changes.
**Type:** Exact
**Pros:**
- exact
- easy to understand
- easy to implement
- low memory overhead (4 bits per component)
- low time overhead (bit mask and shift every flag once per frame)
**Cons:**
- change detection is always delayed, possibly causing painful chained delays
- systems that don't run every frame miss changes
- duplicates detection when looping
## Proposal 4: Flag-Doubling Change Detection
Combine Proposal 2 and Proposal 3. Differentiate between `JustChanged` (current behavior) and `Changed` (Proposal 3).
Pack this data into the flags according to [this implementation proposal](https://github.com/bevyengine/bevy/issues/68#issuecomment-769174804).
**Type:** Flaky + Exact
**Pros:**
- allows users to acc
- easy to implement
- low memory overhead (4 bits per component)
- low time overhead (bit mask and shift every flag once per frame)
**Cons:**
- users must specify the type of change detection required
- still quite fragile to system ordering effects when using the flaky `JustChanged` form
- cannot get immediate + exact results
- systems that don't run every frame miss changes
- duplicates detection when looping
## [SELECTED] Proposal 5: Generation-Counter Change Detection
A global counter is increased after each system is run. Each component saves the time of last mutation, and each system saves the time of last execution. Mutation is detected when the component's counter is greater than the system's counter. Discussed [here](https://github.com/bevyengine/bevy/issues/68#issuecomment-769174804). How to handle addition detection is unsolved; the current proposal is to use the highest bit of the counter as in proposal 1.
**Type:** Exact (for mutations), flaky (for additions)
**Pros:**
- low time overhead (set component counter on access, set system counter after execution)
- robust to systems that don't run every frame
- robust to systems that loop
**Cons:**
- moderately complex implementation
- must be modified as systems are inserted dynamically
- medium memory overhead (4 bytes per component + system)
- unsolved addition detection
## Proposal 6: System-Data Change Detection
For each system, track which system's changes it has seen. This approach is only worth fully designing and implementing if Proposal 5 fails in some way.
**Type:** Exact
**Pros:**
- exact
- conceptually simple
**Cons:**
- requires storing data on each system
- implementation is complex
- must be modified as systems are inserted dynamically
## Proposal 7: Total-Order Change Detection
Discussed [here](https://github.com/bevyengine/bevy/issues/68#issuecomment-754326523). This proposal is somewhat complicated by the new scheduler, but I believe it should still be conceptually feasible. This approach is only worth fully designing and implementing if Proposal 5 fails in some way.
**Type:** Exact
**Pros:**
- exact
- efficient data storage relative to other exact proposals
**Cons:**
- requires access to the scheduler
- complex implementation and difficulty grokking
- must be modified as systems are inserted dynamically
# Tests
- We will need to verify properties 1, 2, 3, 7 and 8. Priority: 1 > 2 = 3 > 8 > 7
- Ideally we can use identical user-facing syntax for all proposals, allowing us to re-use the same syntax for each.
- When writing tests, we need to carefully specify order using explicit dependencies.
- These tests will need to be duplicated for both components and resources.
- We need to be sure to handle cases where ambiguous system orders exist.
`changing_system` is always the system that makes the changes, and `detecting_system` always detects the changes.
The component / resource changed will be simple boolean wrapper structs.
## Basic Added / Mutated / Changed
2 x 3 design:
- Resources vs. Components
- Added vs. Changed vs. Mutated
- `changing_system` runs before `detecting_system`
- verify at the end of tick 2
## At Least Once
2 x 3 design:
- Resources vs. Components
- Added vs. Changed vs. Mutated
- `changing_system` runs after `detecting_system`
- verify at the end of tick 2
## At Most Once
2 x 3 design:
- Resources vs. Components
- Added vs. Changed vs. Mutated
- `changing_system` runs once before `detecting_system`
- increment a counter based on the number of changes detected
- verify at the end of tick 2
## Fast Detection
2 x 3 design:
- Resources vs. Components
- Added vs. Changed vs. Mutated
- `changing_system` runs before `detecting_system`
- verify at the end of tick 1
## Ambiguous System Ordering Robustness
2 x 3 x 2 design:
- Resources vs. Components
- Added vs. Changed vs. Mutated
- `changing_system` runs [before/after] `detecting_system` in tick 1
- `changing_system` runs [after/before] `detecting_system` in tick 2
## System Pausing
2 x 3 design:
- Resources vs. Components
- Added vs. Changed vs. Mutated
- `changing_system` runs in tick 1, then is disabled by run criteria
- `detecting_system` is disabled by run criteria until it is run once during tick 3
- verify at the end of tick 3
## Addition Causes Mutation
2 design:
- Resources vs. Components
- `adding_system_1` adds a component / resource
- `adding system_2` adds the same component / resource
- verify the `Mutated` flag at the end of the tick
- verify the `Added` flag at the end of the tick
First check tests for: https://github.com/bevyengine/bevy/issues/333
Second check tests for: https://github.com/bevyengine/bevy/issues/1443
## Changes Made By Commands
- `adding_system` runs in Update in tick 1, and sends a command to add a component
- `detecting_system` runs in Update in tick 1 and 2, after `adding_system`
- We can't detect the changes in tick 1, since they haven't been processed yet
- If we were to track these changes as being emitted by `adding_system`, we can't detect the changes in tick 2 either, since `detecting_system` has already run once after `adding_system` :(
# Benchmarks
See: [general advice](https://github.com/bevyengine/bevy/blob/master/docs/profiling.md), [Criterion crate](https://github.com/bheisler/criterion.rs)
There are several critical parameters to vary:
1. entity count (1 to 10^9)
2. fraction of entities that are changed (0% to 100%)
3. cost to perform work on changed entities, i.e. workload (1 ns to 1s)
1 and 2 should be varied between benchmark runs. 3 can be added on computationally.
We want to measure:
- memory cost
- run time
We should collect these measurements across several frames (100?) to reduce bootup effects and accurately measure the mean, variance and drift.
Entity-component change detection is much more important to benchmark than resource change detection, due to the orders of magnitude higher number of pieces of data.
No change detection at all should be included in benchmarks as a second control for cases where missing changes is unacceptable.
## Graphs
1. y: performance, x: log_10(entity count), color: proposal, facet: performance metric. Set cost to perform work to 0.
2. y: run time, x: cost to perform work, color: proposal, facet: fraction changed. Set number of entities to 10^6
3. y: memory, x: frames, color: proposal
# Conclusions
1. Is the theoretical categorization of the proposals correct according to our tests?
2. How does the performance of the proposals compare without any load?
3. How does the performance of the proposals compare with realistic loads?
4. At what workload does more exact change tracking become worth the (presumably) higher overhead?
5. When does adding change-detection to save on work become worthwhile?
6. Is there enough divergence in performance between the best solutions in each class to ship more than one change-tracking solution?
# Implementation Plan
1. Write a test suite.
2. Verify that tests fail for existing approach.
3. Write a benchmark suite.
4. Get performance numbers for existing approach.
5. Implement, test and benchmark various solutions using a Git branch per proposal.
6. Create a draft PR with all solutions and present results to team.
7. Select a solution and replace existing change detection.
Co-authored-by: Brice DAVIER <bricedavier@gmail.com>
Co-authored-by: Carter Anderson <mcanders1@gmail.com>
2021-03-19 17:53:26 +00:00
_change_tick : u32 ,
Simplify trait hierarchy for `SystemParam` (#6865)
# Objective
* Implementing a custom `SystemParam` by hand requires implementing three traits -- four if it is read-only.
* The trait `SystemParamFetch<'w, 's>` is a workaround from before we had generic associated types, and is no longer necessary.
## Solution
* Combine the trait `SystemParamFetch` with `SystemParamState`.
* I decided to remove the `Fetch` name and keep the `State` name, since the former was consistently conflated with the latter.
* Replace the trait `ReadOnlySystemParamFetch` with `ReadOnlySystemParam`, which simplifies trait bounds in generic code.
---
## Changelog
- Removed the trait `SystemParamFetch`, moving its functionality to `SystemParamState`.
- Replaced the trait `ReadOnlySystemParamFetch` with `ReadOnlySystemParam`.
## Migration Guide
The trait `SystemParamFetch` has been removed, and its functionality has been transferred to `SystemParamState`.
```rust
// Before
impl SystemParamState for MyParamState {
fn init(world: &mut World, system_meta: &mut SystemMeta) -> Self { ... }
}
impl<'w, 's> SystemParamFetch<'w, 's> for MyParamState {
type Item = MyParam<'w, 's>;
fn get_param(...) -> Self::Item;
}
// After
impl SystemParamState for MyParamState {
type Item<'w, 's> = MyParam<'w, 's>; // Generic associated types!
fn init(world: &mut World, system_meta: &mut SystemMeta) -> Self { ... }
fn get_param<'w, 's>(...) -> Self::Item<'w, 's>;
}
```
The trait `ReadOnlySystemParamFetch` has been replaced with `ReadOnlySystemParam`.
```rust
// Before
unsafe impl ReadOnlySystemParamFetch for MyParamState {}
// After
unsafe impl<'w, 's> ReadOnlySystemParam for MyParam<'w, 's> {}
```
2022-12-11 18:34:14 +00:00
) -> Self ::Item < ' w , ' s > {
Bevy ECS V2 (#1525)
# Bevy ECS V2
This is a rewrite of Bevy ECS (basically everything but the new executor/schedule, which are already awesome). The overall goal was to improve the performance and versatility of Bevy ECS. Here is a quick bulleted list of changes before we dive into the details:
* Complete World rewrite
* Multiple component storage types:
* Tables: fast cache friendly iteration, slower add/removes (previously called Archetypes)
* Sparse Sets: fast add/remove, slower iteration
* Stateful Queries (caches query results for faster iteration. fragmented iteration is _fast_ now)
* Stateful System Params (caches expensive operations. inspired by @DJMcNab's work in #1364)
* Configurable System Params (users can set configuration when they construct their systems. once again inspired by @DJMcNab's work)
* Archetypes are now "just metadata", component storage is separate
* Archetype Graph (for faster archetype changes)
* Component Metadata
* Configure component storage type
* Retrieve information about component size/type/name/layout/send-ness/etc
* Components are uniquely identified by a densely packed ComponentId
* TypeIds are now totally optional (which should make implementing scripting easier)
* Super fast "for_each" query iterators
* Merged Resources into World. Resources are now just a special type of component
* EntityRef/EntityMut builder apis (more efficient and more ergonomic)
* Fast bitset-backed `Access<T>` replaces old hashmap-based approach everywhere
* Query conflicts are determined by component access instead of archetype component access (to avoid random failures at runtime)
* With/Without are still taken into account for conflicts, so this should still be comfy to use
* Much simpler `IntoSystem` impl
* Significantly reduced the amount of hashing throughout the ecs in favor of Sparse Sets (indexed by densely packed ArchetypeId, ComponentId, BundleId, and TableId)
* Safety Improvements
* Entity reservation uses a normal world reference instead of unsafe transmute
* QuerySets no longer transmute lifetimes
* Made traits "unsafe" where relevant
* More thorough safety docs
* WorldCell
* Exposes safe mutable access to multiple resources at a time in a World
* Replaced "catch all" `System::update_archetypes(world: &World)` with `System::new_archetype(archetype: &Archetype)`
* Simpler Bundle implementation
* Replaced slow "remove_bundle_one_by_one" used as fallback for Commands::remove_bundle with fast "remove_bundle_intersection"
* Removed `Mut<T>` query impl. it is better to only support one way: `&mut T`
* Removed with() from `Flags<T>` in favor of `Option<Flags<T>>`, which allows querying for flags to be "filtered" by default
* Components now have is_send property (currently only resources support non-send)
* More granular module organization
* New `RemovedComponents<T>` SystemParam that replaces `query.removed::<T>()`
* `world.resource_scope()` for mutable access to resources and world at the same time
* WorldQuery and QueryFilter traits unified. FilterFetch trait added to enable "short circuit" filtering. Auto impled for cases that don't need it
* Significantly slimmed down SystemState in favor of individual SystemParam state
* System Commands changed from `commands: &mut Commands` back to `mut commands: Commands` (to allow Commands to have a World reference)
Fixes #1320
## `World` Rewrite
This is a from-scratch rewrite of `World` that fills the niche that `hecs` used to. Yes, this means Bevy ECS is no longer a "fork" of hecs. We're going out our own!
(the only shared code between the projects is the entity id allocator, which is already basically ideal)
A huge shout out to @SanderMertens (author of [flecs](https://github.com/SanderMertens/flecs)) for sharing some great ideas with me (specifically hybrid ecs storage and archetype graphs). He also helped advise on a number of implementation details.
## Component Storage (The Problem)
Two ECS storage paradigms have gained a lot of traction over the years:
* **Archetypal ECS**:
* Stores components in "tables" with static schemas. Each "column" stores components of a given type. Each "row" is an entity.
* Each "archetype" has its own table. Adding/removing an entity's component changes the archetype.
* Enables super-fast Query iteration due to its cache-friendly data layout
* Comes at the cost of more expensive add/remove operations for an Entity's components, because all components need to be copied to the new archetype's "table"
* **Sparse Set ECS**:
* Stores components of the same type in densely packed arrays, which are sparsely indexed by densely packed unsigned integers (Entity ids)
* Query iteration is slower than Archetypal ECS because each entity's component could be at any position in the sparse set. This "random access" pattern isn't cache friendly. Additionally, there is an extra layer of indirection because you must first map the entity id to an index in the component array.
* Adding/removing components is a cheap, constant time operation
Bevy ECS V1, hecs, legion, flec, and Unity DOTS are all "archetypal ecs-es". I personally think "archetypal" storage is a good default for game engines. An entity's archetype doesn't need to change frequently in general, and it creates "fast by default" query iteration (which is a much more common operation). It is also "self optimizing". Users don't need to think about optimizing component layouts for iteration performance. It "just works" without any extra boilerplate.
Shipyard and EnTT are "sparse set ecs-es". They employ "packing" as a way to work around the "suboptimal by default" iteration performance for specific sets of components. This helps, but I didn't think this was a good choice for a general purpose engine like Bevy because:
1. "packs" conflict with each other. If bevy decides to internally pack the Transform and GlobalTransform components, users are then blocked if they want to pack some custom component with Transform.
2. users need to take manual action to optimize
Developers selecting an ECS framework are stuck with a hard choice. Select an "archetypal" framework with "fast iteration everywhere" but without the ability to cheaply add/remove components, or select a "sparse set" framework to cheaply add/remove components but with slower iteration performance.
## Hybrid Component Storage (The Solution)
In Bevy ECS V2, we get to have our cake and eat it too. It now has _both_ of the component storage types above (and more can be added later if needed):
* **Tables** (aka "archetypal" storage)
* The default storage. If you don't configure anything, this is what you get
* Fast iteration by default
* Slower add/remove operations
* **Sparse Sets**
* Opt-in
* Slower iteration
* Faster add/remove operations
These storage types complement each other perfectly. By default Query iteration is fast. If developers know that they want to add/remove a component at high frequencies, they can set the storage to "sparse set":
```rust
world.register_component(
ComponentDescriptor::new::<MyComponent>(StorageType::SparseSet)
).unwrap();
```
## Archetypes
Archetypes are now "just metadata" ... they no longer store components directly. They do store:
* The `ComponentId`s of each of the Archetype's components (and that component's storage type)
* Archetypes are uniquely defined by their component layouts
* For example: entities with "table" components `[A, B, C]` _and_ "sparse set" components `[D, E]` will always be in the same archetype.
* The `TableId` associated with the archetype
* For now each archetype has exactly one table (which can have no components),
* There is a 1->Many relationship from Tables->Archetypes. A given table could have any number of archetype components stored in it:
* Ex: an entity with "table storage" components `[A, B, C]` and "sparse set" components `[D, E]` will share the same `[A, B, C]` table as an entity with `[A, B, C]` table component and `[F]` sparse set components.
* This 1->Many relationship is how we preserve fast "cache friendly" iteration performance when possible (more on this later)
* A list of entities that are in the archetype and the row id of the table they are in
* ArchetypeComponentIds
* unique densely packed identifiers for (ArchetypeId, ComponentId) pairs
* used by the schedule executor for cheap system access control
* "Archetype Graph Edges" (see the next section)
## The "Archetype Graph"
Archetype changes in Bevy (and a number of other archetypal ecs-es) have historically been expensive to compute. First, you need to allocate a new vector of the entity's current component ids, add or remove components based on the operation performed, sort it (to ensure it is order-independent), then hash it to find the archetype (if it exists). And thats all before we get to the _already_ expensive full copy of all components to the new table storage.
The solution is to build a "graph" of archetypes to cache these results. @SanderMertens first exposed me to the idea (and he got it from @gjroelofs, who came up with it). They propose adding directed edges between archetypes for add/remove component operations. If `ComponentId`s are densely packed, you can use sparse sets to cheaply jump between archetypes.
Bevy takes this one step further by using add/remove `Bundle` edges instead of `Component` edges. Bevy encourages the use of `Bundles` to group add/remove operations. This is largely for "clearer game logic" reasons, but it also helps cut down on the number of archetype changes required. `Bundles` now also have densely-packed `BundleId`s. This allows us to use a _single_ edge for each bundle operation (rather than needing to traverse N edges ... one for each component). Single component operations are also bundles, so this is strictly an improvement over a "component only" graph.
As a result, an operation that used to be _heavy_ (both for allocations and compute) is now two dirt-cheap array lookups and zero allocations.
## Stateful Queries
World queries are now stateful. This allows us to:
1. Cache archetype (and table) matches
* This resolves another issue with (naive) archetypal ECS: query performance getting worse as the number of archetypes goes up (and fragmentation occurs).
2. Cache Fetch and Filter state
* The expensive parts of fetch/filter operations (such as hashing the TypeId to find the ComponentId) now only happen once when the Query is first constructed
3. Incrementally build up state
* When new archetypes are added, we only process the new archetypes (no need to rebuild state for old archetypes)
As a result, the direct `World` query api now looks like this:
```rust
let mut query = world.query::<(&A, &mut B)>();
for (a, mut b) in query.iter_mut(&mut world) {
}
```
Requiring `World` to generate stateful queries (rather than letting the `QueryState` type be constructed separately) allows us to ensure that _all_ queries are properly initialized (and the relevant world state, such as ComponentIds). This enables QueryState to remove branches from its operations that check for initialization status (and also enables query.iter() to take an immutable world reference because it doesn't need to initialize anything in world).
However in systems, this is a non-breaking change. State management is done internally by the relevant SystemParam.
## Stateful SystemParams
Like Queries, `SystemParams` now also cache state. For example, `Query` system params store the "stateful query" state mentioned above. Commands store their internal `CommandQueue`. This means you can now safely use as many separate `Commands` parameters in your system as you want. `Local<T>` system params store their `T` value in their state (instead of in Resources).
SystemParam state also enabled a significant slim-down of SystemState. It is much nicer to look at now.
Per-SystemParam state naturally insulates us from an "aliased mut" class of errors we have hit in the past (ex: using multiple `Commands` system params).
(credit goes to @DJMcNab for the initial idea and draft pr here #1364)
## Configurable SystemParams
@DJMcNab also had the great idea to make SystemParams configurable. This allows users to provide some initial configuration / values for system parameters (when possible). Most SystemParams have no config (the config type is `()`), but the `Local<T>` param now supports user-provided parameters:
```rust
fn foo(value: Local<usize>) {
}
app.add_system(foo.system().config(|c| c.0 = Some(10)));
```
## Uber Fast "for_each" Query Iterators
Developers now have the choice to use a fast "for_each" iterator, which yields ~1.5-3x iteration speed improvements for "fragmented iteration", and minor ~1.2x iteration speed improvements for unfragmented iteration.
```rust
fn system(query: Query<(&A, &mut B)>) {
// you now have the option to do this for a speed boost
query.for_each_mut(|(a, mut b)| {
});
// however normal iterators are still available
for (a, mut b) in query.iter_mut() {
}
}
```
I think in most cases we should continue to encourage "normal" iterators as they are more flexible and more "rust idiomatic". But when that extra "oomf" is needed, it makes sense to use `for_each`.
We should also consider using `for_each` for internal bevy systems to give our users a nice speed boost (but that should be a separate pr).
## Component Metadata
`World` now has a `Components` collection, which is accessible via `world.components()`. This stores mappings from `ComponentId` to `ComponentInfo`, as well as `TypeId` to `ComponentId` mappings (where relevant). `ComponentInfo` stores information about the component, such as ComponentId, TypeId, memory layout, send-ness (currently limited to resources), and storage type.
## Significantly Cheaper `Access<T>`
We used to use `TypeAccess<TypeId>` to manage read/write component/archetype-component access. This was expensive because TypeIds must be hashed and compared individually. The parallel executor got around this by "condensing" type ids into bitset-backed access types. This worked, but it had to be re-generated from the `TypeAccess<TypeId>`sources every time archetypes changed.
This pr removes TypeAccess in favor of faster bitset access everywhere. We can do this thanks to the move to densely packed `ComponentId`s and `ArchetypeComponentId`s.
## Merged Resources into World
Resources had a lot of redundant functionality with Components. They stored typed data, they had access control, they had unique ids, they were queryable via SystemParams, etc. In fact the _only_ major difference between them was that they were unique (and didn't correlate to an entity).
Separate resources also had the downside of requiring a separate set of access controls, which meant the parallel executor needed to compare more bitsets per system and manage more state.
I initially got the "separate resources" idea from `legion`. I think that design was motivated by the fact that it made the direct world query/resource lifetime interactions more manageable. It certainly made our lives easier when using Resources alongside hecs/bevy_ecs. However we already have a construct for safely and ergonomically managing in-world lifetimes: systems (which use `Access<T>` internally).
This pr merges Resources into World:
```rust
world.insert_resource(1);
world.insert_resource(2.0);
let a = world.get_resource::<i32>().unwrap();
let mut b = world.get_resource_mut::<f64>().unwrap();
*b = 3.0;
```
Resources are now just a special kind of component. They have their own ComponentIds (and their own resource TypeId->ComponentId scope, so they don't conflict wit components of the same type). They are stored in a special "resource archetype", which stores components inside the archetype using a new `unique_components` sparse set (note that this sparse set could later be used to implement Tags). This allows us to keep the code size small by reusing existing datastructures (namely Column, Archetype, ComponentFlags, and ComponentInfo). This allows us the executor to use a single `Access<ArchetypeComponentId>` per system. It should also make scripting language integration easier.
_But_ this merge did create problems for people directly interacting with `World`. What if you need mutable access to multiple resources at the same time? `world.get_resource_mut()` borrows World mutably!
## WorldCell
WorldCell applies the `Access<ArchetypeComponentId>` concept to direct world access:
```rust
let world_cell = world.cell();
let a = world_cell.get_resource_mut::<i32>().unwrap();
let b = world_cell.get_resource_mut::<f64>().unwrap();
```
This adds cheap runtime checks (a sparse set lookup of `ArchetypeComponentId` and a counter) to ensure that world accesses do not conflict with each other. Each operation returns a `WorldBorrow<'w, T>` or `WorldBorrowMut<'w, T>` wrapper type, which will release the relevant ArchetypeComponentId resources when dropped.
World caches the access sparse set (and only one cell can exist at a time), so `world.cell()` is a cheap operation.
WorldCell does _not_ use atomic operations. It is non-send, does a mutable borrow of world to prevent other accesses, and uses a simple `Rc<RefCell<ArchetypeComponentAccess>>` wrapper in each WorldBorrow pointer.
The api is currently limited to resource access, but it can and should be extended to queries / entity component access.
## Resource Scopes
WorldCell does not yet support component queries, and even when it does there are sometimes legitimate reasons to want a mutable world ref _and_ a mutable resource ref (ex: bevy_render and bevy_scene both need this). In these cases we could always drop down to the unsafe `world.get_resource_unchecked_mut()`, but that is not ideal!
Instead developers can use a "resource scope"
```rust
world.resource_scope(|world: &mut World, a: &mut A| {
})
```
This temporarily removes the `A` resource from `World`, provides mutable pointers to both, and re-adds A to World when finished. Thanks to the move to ComponentIds/sparse sets, this is a cheap operation.
If multiple resources are required, scopes can be nested. We could also consider adding a "resource tuple" to the api if this pattern becomes common and the boilerplate gets nasty.
## Query Conflicts Use ComponentId Instead of ArchetypeComponentId
For safety reasons, systems cannot contain queries that conflict with each other without wrapping them in a QuerySet. On bevy `main`, we use ArchetypeComponentIds to determine conflicts. This is nice because it can take into account filters:
```rust
// these queries will never conflict due to their filters
fn filter_system(a: Query<&mut A, With<B>>, b: Query<&mut B, Without<B>>) {
}
```
But it also has a significant downside:
```rust
// these queries will not conflict _until_ an entity with A, B, and C is spawned
fn maybe_conflicts_system(a: Query<(&mut A, &C)>, b: Query<(&mut A, &B)>) {
}
```
The system above will panic at runtime if an entity with A, B, and C is spawned. This makes it hard to trust that your game logic will run without crashing.
In this pr, I switched to using `ComponentId` instead. This _is_ more constraining. `maybe_conflicts_system` will now always fail, but it will do it consistently at startup. Naively, it would also _disallow_ `filter_system`, which would be a significant downgrade in usability. Bevy has a number of internal systems that rely on disjoint queries and I expect it to be a common pattern in userspace.
To resolve this, I added a new `FilteredAccess<T>` type, which wraps `Access<T>` and adds with/without filters. If two `FilteredAccess` have with/without values that prove they are disjoint, they will no longer conflict.
## EntityRef / EntityMut
World entity operations on `main` require that the user passes in an `entity` id to each operation:
```rust
let entity = world.spawn((A, )); // create a new entity with A
world.get::<A>(entity);
world.insert(entity, (B, C));
world.insert_one(entity, D);
```
This means that each operation needs to look up the entity location / verify its validity. The initial spawn operation also requires a Bundle as input. This can be awkward when no components are required (or one component is required).
These operations have been replaced by `EntityRef` and `EntityMut`, which are "builder-style" wrappers around world that provide read and read/write operations on a single, pre-validated entity:
```rust
// spawn now takes no inputs and returns an EntityMut
let entity = world.spawn()
.insert(A) // insert a single component into the entity
.insert_bundle((B, C)) // insert a bundle of components into the entity
.id() // id returns the Entity id
// Returns EntityMut (or panics if the entity does not exist)
world.entity_mut(entity)
.insert(D)
.insert_bundle(SomeBundle::default());
{
// returns EntityRef (or panics if the entity does not exist)
let d = world.entity(entity)
.get::<D>() // gets the D component
.unwrap();
// world.get still exists for ergonomics
let d = world.get::<D>(entity).unwrap();
}
// These variants return Options if you want to check existence instead of panicing
world.get_entity_mut(entity)
.unwrap()
.insert(E);
if let Some(entity_ref) = world.get_entity(entity) {
let d = entity_ref.get::<D>().unwrap();
}
```
This _does not_ affect the current Commands api or terminology. I think that should be a separate conversation as that is a much larger breaking change.
## Safety Improvements
* Entity reservation in Commands uses a normal world borrow instead of an unsafe transmute
* QuerySets no longer transmutes lifetimes
* Made traits "unsafe" when implementing a trait incorrectly could cause unsafety
* More thorough safety docs
## RemovedComponents SystemParam
The old approach to querying removed components: `query.removed:<T>()` was confusing because it had no connection to the query itself. I replaced it with the following, which is both clearer and allows us to cache the ComponentId mapping in the SystemParamState:
```rust
fn system(removed: RemovedComponents<T>) {
for entity in removed.iter() {
}
}
```
## Simpler Bundle implementation
Bundles are no longer responsible for sorting (or deduping) TypeInfo. They are just a simple ordered list of component types / data. This makes the implementation smaller and opens the door to an easy "nested bundle" implementation in the future (which i might even add in this pr). Duplicate detection is now done once per bundle type by World the first time a bundle is used.
## Unified WorldQuery and QueryFilter types
(don't worry they are still separate type _parameters_ in Queries .. this is a non-breaking change)
WorldQuery and QueryFilter were already basically identical apis. With the addition of `FetchState` and more storage-specific fetch methods, the overlap was even clearer (and the redundancy more painful).
QueryFilters are now just `F: WorldQuery where F::Fetch: FilterFetch`. FilterFetch requires `Fetch<Item = bool>` and adds new "short circuit" variants of fetch methods. This enables a filter tuple like `(With<A>, Without<B>, Changed<C>)` to stop evaluating the filter after the first mismatch is encountered. FilterFetch is automatically implemented for `Fetch` implementations that return bool.
This forces fetch implementations that return things like `(bool, bool, bool)` (such as the filter above) to manually implement FilterFetch and decide whether or not to short-circuit.
## More Granular Modules
World no longer globs all of the internal modules together. It now exports `core`, `system`, and `schedule` separately. I'm also considering exporting `core` submodules directly as that is still pretty "glob-ey" and unorganized (feedback welcome here).
## Remaining Draft Work (to be done in this pr)
* ~~panic on conflicting WorldQuery fetches (&A, &mut A)~~
* ~~bevy `main` and hecs both currently allow this, but we should protect against it if possible~~
* ~~batch_iter / par_iter (currently stubbed out)~~
* ~~ChangedRes~~
* ~~I skipped this while we sort out #1313. This pr should be adapted to account for whatever we land on there~~.
* ~~The `Archetypes` and `Tables` collections use hashes of sorted lists of component ids to uniquely identify each archetype/table. This hash is then used as the key in a HashMap to look up the relevant ArchetypeId or TableId. (which doesn't handle hash collisions properly)~~
* ~~It is currently unsafe to generate a Query from "World A", then use it on "World B" (despite the api claiming it is safe). We should probably close this gap. This could be done by adding a randomly generated WorldId to each world, then storing that id in each Query. They could then be compared to each other on each `query.do_thing(&world)` operation. This _does_ add an extra branch to each query operation, so I'm open to other suggestions if people have them.~~
* ~~Nested Bundles (if i find time)~~
## Potential Future Work
* Expand WorldCell to support queries.
* Consider not allocating in the empty archetype on `world.spawn()`
* ex: return something like EntityMutUninit, which turns into EntityMut after an `insert` or `insert_bundle` op
* this actually regressed performance last time i tried it, but in theory it should be faster
* Optimize SparseSet::insert (see `PERF` comment on insert)
* Replace SparseArray `Option<T>` with T::MAX to cut down on branching
* would enable cheaper get_unchecked() operations
* upstream fixedbitset optimizations
* fixedbitset could be allocation free for small block counts (store blocks in a SmallVec)
* fixedbitset could have a const constructor
* Consider implementing Tags (archetype-specific by-value data that affects archetype identity)
* ex: ArchetypeA could have `[A, B, C]` table components and `[D(1)]` "tag" component. ArchetypeB could have `[A, B, C]` table components and a `[D(2)]` tag component. The archetypes are different, despite both having D tags because the value inside D is different.
* this could potentially build on top of the `archetype.unique_components` added in this pr for resource storage.
* Consider reverting `all_tuples` proc macro in favor of the old `macro_rules` implementation
* all_tuples is more flexible and produces cleaner documentation (the macro_rules version produces weird type parameter orders due to parser constraints)
* but unfortunately all_tuples also appears to make Rust Analyzer sad/slow when working inside of `bevy_ecs` (does not affect user code)
* Consider "resource queries" and/or "mixed resource and entity component queries" as an alternative to WorldCell
* this is basically just "systems" so maybe it's not worth it
* Add more world ops
* `world.clear()`
* `world.reserve<T: Bundle>(count: usize)`
* Try using the old archetype allocation strategy (allocate new memory on resize and copy everything over). I expect this to improve batch insertion performance at the cost of unbatched performance. But thats just a guess. I'm not an allocation perf pro :)
* Adapt Commands apis for consistency with new World apis
## Benchmarks
key:
* `bevy_old`: bevy `main` branch
* `bevy`: this branch
* `_foreach`: uses an optimized for_each iterator
* ` _sparse`: uses sparse set storage (if unspecified assume table storage)
* `_system`: runs inside a system (if unspecified assume test happens via direct world ops)
### Simple Insert (from ecs_bench_suite)
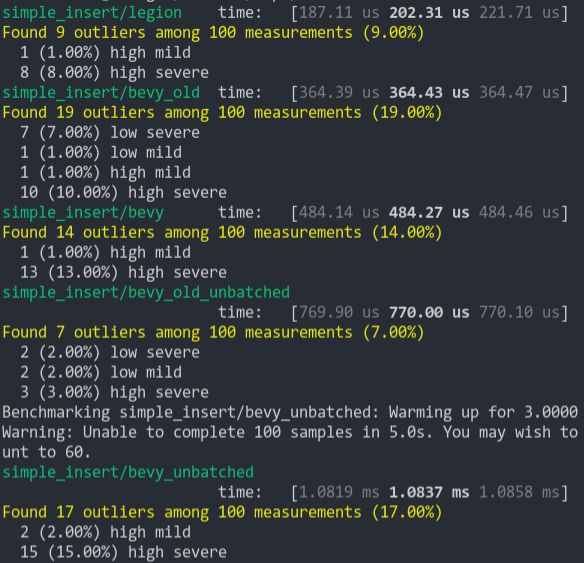
### Simpler Iter (from ecs_bench_suite)
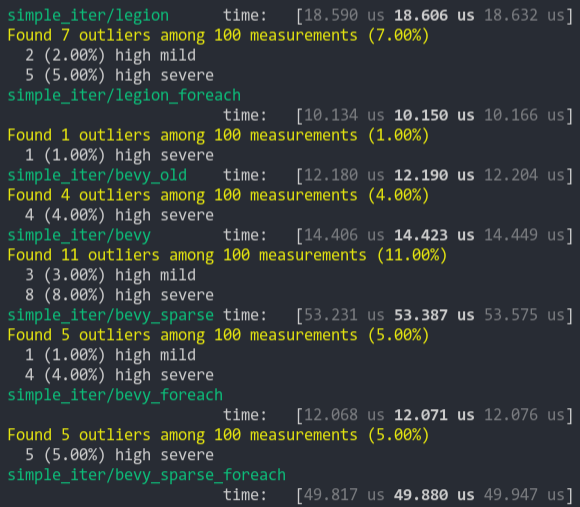
### Fragment Iter (from ecs_bench_suite)
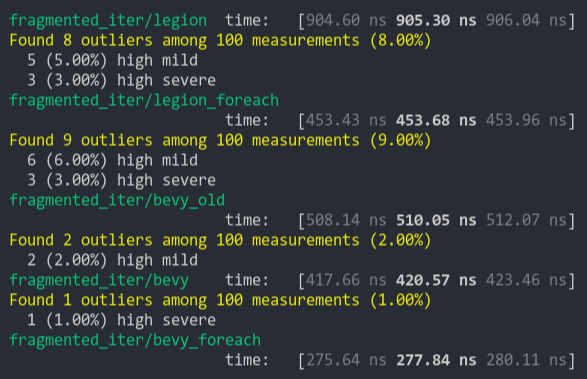
### Sparse Fragmented Iter
Iterate a query that matches 5 entities from a single matching archetype, but there are 100 unmatching archetypes
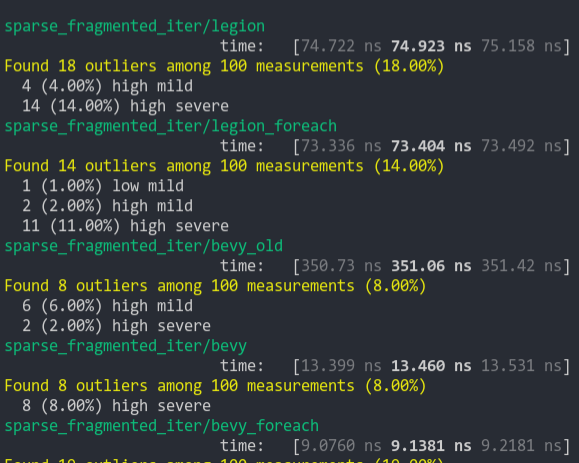
### Schedule (from ecs_bench_suite)
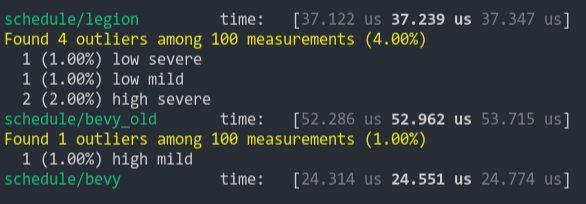
### Add Remove Component (from ecs_bench_suite)
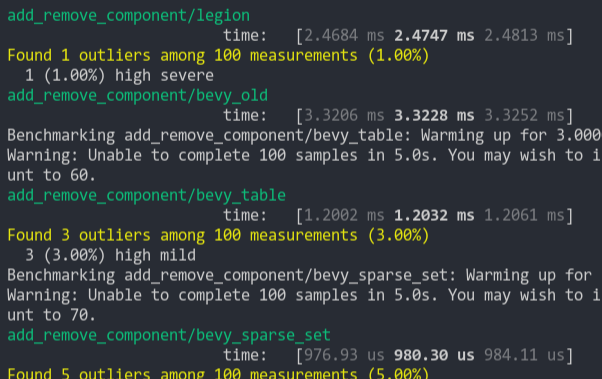
### Add Remove Component Big
Same as the test above, but each entity has 5 "large" matrix components and 1 "large" matrix component is added and removed
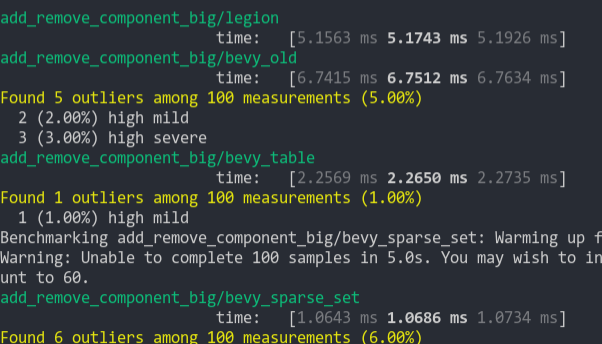
### Get Component
Looks up a single component value a large number of times
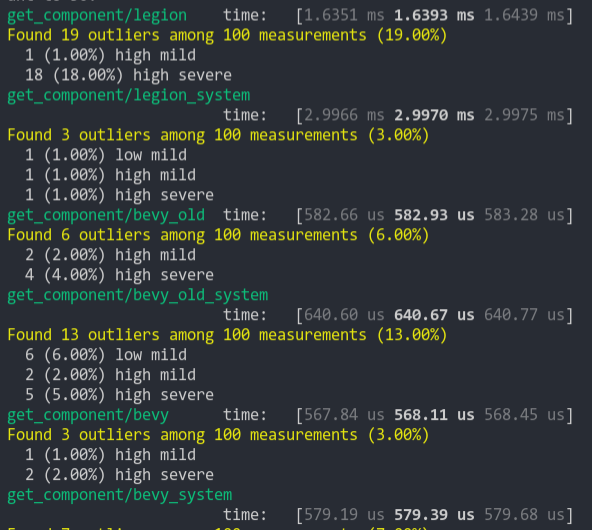
2021-03-05 07:54:35 +00:00
world . components ( )
}
}
2022-07-04 14:44:24 +00:00
// SAFETY: Only reads World entities
Simplify trait hierarchy for `SystemParam` (#6865)
# Objective
* Implementing a custom `SystemParam` by hand requires implementing three traits -- four if it is read-only.
* The trait `SystemParamFetch<'w, 's>` is a workaround from before we had generic associated types, and is no longer necessary.
## Solution
* Combine the trait `SystemParamFetch` with `SystemParamState`.
* I decided to remove the `Fetch` name and keep the `State` name, since the former was consistently conflated with the latter.
* Replace the trait `ReadOnlySystemParamFetch` with `ReadOnlySystemParam`, which simplifies trait bounds in generic code.
---
## Changelog
- Removed the trait `SystemParamFetch`, moving its functionality to `SystemParamState`.
- Replaced the trait `ReadOnlySystemParamFetch` with `ReadOnlySystemParam`.
## Migration Guide
The trait `SystemParamFetch` has been removed, and its functionality has been transferred to `SystemParamState`.
```rust
// Before
impl SystemParamState for MyParamState {
fn init(world: &mut World, system_meta: &mut SystemMeta) -> Self { ... }
}
impl<'w, 's> SystemParamFetch<'w, 's> for MyParamState {
type Item = MyParam<'w, 's>;
fn get_param(...) -> Self::Item;
}
// After
impl SystemParamState for MyParamState {
type Item<'w, 's> = MyParam<'w, 's>; // Generic associated types!
fn init(world: &mut World, system_meta: &mut SystemMeta) -> Self { ... }
fn get_param<'w, 's>(...) -> Self::Item<'w, 's>;
}
```
The trait `ReadOnlySystemParamFetch` has been replaced with `ReadOnlySystemParam`.
```rust
// Before
unsafe impl ReadOnlySystemParamFetch for MyParamState {}
// After
unsafe impl<'w, 's> ReadOnlySystemParam for MyParam<'w, 's> {}
```
2022-12-11 18:34:14 +00:00
unsafe impl < ' a > ReadOnlySystemParam for & ' a Entities { }
Add new SystemState and rename old SystemState to SystemMeta (#2283)
This enables `SystemParams` to be used outside of function systems. Anything can create and store `SystemState`, which enables efficient "param state cached" access to `SystemParams`.
It adds a `ReadOnlySystemParamFetch` trait, which enables safe `SystemState::get` calls without unique world access.
I renamed the old `SystemState` to `SystemMeta` to enable us to mirror the `QueryState` naming convention (but I'm happy to discuss alternative names if people have other ideas). I initially pitched this as `ParamState`, but given that it needs to include full system metadata, that doesn't feel like a particularly accurate name.
```rust
#[derive(Eq, PartialEq, Debug)]
struct A(usize);
#[derive(Eq, PartialEq, Debug)]
struct B(usize);
let mut world = World::default();
world.insert_resource(A(42));
world.spawn().insert(B(7));
// we get nice lifetime elision when declaring the type on the left hand side
let mut system_state: SystemState<(Res<A>, Query<&B>)> = SystemState::new(&mut world);
let (a, query) = system_state.get(&world);
assert_eq!(*a, A(42), "returned resource matches initial value");
assert_eq!(
*query.single().unwrap(),
B(7),
"returned component matches initial value"
);
// mutable system params require unique world access
let mut system_state: SystemState<(ResMut<A>, Query<&mut B>)> = SystemState::new(&mut world);
let (a, query) = system_state.get_mut(&mut world);
// static lifetimes are required when declaring inside of structs
struct SomeContainer {
state: SystemState<(Res<'static, A>, Res<'static, B>)>
}
// this can be shortened using type aliases, which will be useful for complex param tuples
type MyParams<'a> = (Res<'a, A>, Res<'a, B>);
struct SomeContainer {
state: SystemState<MyParams<'static>>
}
// It is the user's responsibility to call SystemState::apply(world) for parameters that queue up work
let mut system_state: SystemState<(Commands, Query<&B>)> = SystemState::new(&mut world);
{
let (mut commands, query) = system_state.get(&world);
commands.insert_resource(3.14);
}
system_state.apply(&mut world);
```
## Future Work
* Actually use SystemState inside FunctionSystem. This would be trivial, but it requires FunctionSystem to wrap SystemState in Option in its current form (which complicates system metadata lookup). I'd prefer to hold off until we adopt something like the later designs linked in #1364, which enable us to contruct Systems using a World reference (and also remove the need for `.system`).
* Consider a "scoped" approach to automatically call SystemState::apply when systems params are no longer being used (either a container type with a Drop impl, or a function that takes a closure for user logic operating on params).
2021-06-02 19:57:38 +00:00
2022-07-04 14:44:24 +00:00
// SAFETY: no component value access
Remove the `SystemParamState` trait and remove types like `ResState` (#6919)
Spiritual successor to #5205.
Actual successor to #6865.
# Objective
Currently, system params are defined using three traits: `SystemParam`, `ReadOnlySystemParam`, `SystemParamState`. The behavior for each param is specified by the `SystemParamState` trait, while `SystemParam` simply defers to the state.
Splitting the traits in this way makes it easier to implement within macros, but it increases the cognitive load. Worst of all, this approach requires each `MySystemParam` to have a public `MySystemParamState` type associated with it.
## Solution
* Merge the trait `SystemParamState` into `SystemParam`.
* Remove all trivial `SystemParam` state types.
* `OptionNonSendMutState<T>`: you will not be missed.
---
- [x] Fix/resolve the remaining test failure.
## Changelog
* Removed the trait `SystemParamState`, merging its functionality into `SystemParam`.
## Migration Guide
**Note**: this should replace the migration guide for #6865.
This is relative to Bevy 0.9, not main.
The traits `SystemParamState` and `SystemParamFetch` have been removed, and their functionality has been transferred to `SystemParam`.
```rust
// Before (0.9)
impl SystemParam for MyParam<'_, '_> {
type State = MyParamState;
}
unsafe impl SystemParamState for MyParamState {
fn init(world: &mut World, system_meta: &mut SystemMeta) -> Self { ... }
}
unsafe impl<'w, 's> SystemParamFetch<'w, 's> for MyParamState {
type Item = MyParam<'w, 's>;
fn get_param(&mut self, ...) -> Self::Item;
}
unsafe impl ReadOnlySystemParamFetch for MyParamState { }
// After (0.10)
unsafe impl SystemParam for MyParam<'_, '_> {
type State = MyParamState;
type Item<'w, 's> = MyParam<'w, 's>;
fn init_state(world: &mut World, system_meta: &mut SystemMeta) -> Self::State { ... }
fn get_param<'w, 's>(state: &mut Self::State, ...) -> Self::Item<'w, 's>;
}
unsafe impl ReadOnlySystemParam for MyParam<'_, '_> { }
```
The trait `ReadOnlySystemParamFetch` has been replaced with `ReadOnlySystemParam`.
```rust
// Before
unsafe impl ReadOnlySystemParamFetch for MyParamState {}
// After
unsafe impl ReadOnlySystemParam for MyParam<'_, '_> {}
```
2023-01-07 23:20:32 +00:00
unsafe impl < ' a > SystemParam for & ' a Entities {
type State = ( ) ;
Simplify trait hierarchy for `SystemParam` (#6865)
# Objective
* Implementing a custom `SystemParam` by hand requires implementing three traits -- four if it is read-only.
* The trait `SystemParamFetch<'w, 's>` is a workaround from before we had generic associated types, and is no longer necessary.
## Solution
* Combine the trait `SystemParamFetch` with `SystemParamState`.
* I decided to remove the `Fetch` name and keep the `State` name, since the former was consistently conflated with the latter.
* Replace the trait `ReadOnlySystemParamFetch` with `ReadOnlySystemParam`, which simplifies trait bounds in generic code.
---
## Changelog
- Removed the trait `SystemParamFetch`, moving its functionality to `SystemParamState`.
- Replaced the trait `ReadOnlySystemParamFetch` with `ReadOnlySystemParam`.
## Migration Guide
The trait `SystemParamFetch` has been removed, and its functionality has been transferred to `SystemParamState`.
```rust
// Before
impl SystemParamState for MyParamState {
fn init(world: &mut World, system_meta: &mut SystemMeta) -> Self { ... }
}
impl<'w, 's> SystemParamFetch<'w, 's> for MyParamState {
type Item = MyParam<'w, 's>;
fn get_param(...) -> Self::Item;
}
// After
impl SystemParamState for MyParamState {
type Item<'w, 's> = MyParam<'w, 's>; // Generic associated types!
fn init(world: &mut World, system_meta: &mut SystemMeta) -> Self { ... }
fn get_param<'w, 's>(...) -> Self::Item<'w, 's>;
}
```
The trait `ReadOnlySystemParamFetch` has been replaced with `ReadOnlySystemParam`.
```rust
// Before
unsafe impl ReadOnlySystemParamFetch for MyParamState {}
// After
unsafe impl<'w, 's> ReadOnlySystemParam for MyParam<'w, 's> {}
```
2022-12-11 18:34:14 +00:00
type Item < ' w , ' s > = & ' w Entities ;
Remove the `SystemParamState` trait and remove types like `ResState` (#6919)
Spiritual successor to #5205.
Actual successor to #6865.
# Objective
Currently, system params are defined using three traits: `SystemParam`, `ReadOnlySystemParam`, `SystemParamState`. The behavior for each param is specified by the `SystemParamState` trait, while `SystemParam` simply defers to the state.
Splitting the traits in this way makes it easier to implement within macros, but it increases the cognitive load. Worst of all, this approach requires each `MySystemParam` to have a public `MySystemParamState` type associated with it.
## Solution
* Merge the trait `SystemParamState` into `SystemParam`.
* Remove all trivial `SystemParam` state types.
* `OptionNonSendMutState<T>`: you will not be missed.
---
- [x] Fix/resolve the remaining test failure.
## Changelog
* Removed the trait `SystemParamState`, merging its functionality into `SystemParam`.
## Migration Guide
**Note**: this should replace the migration guide for #6865.
This is relative to Bevy 0.9, not main.
The traits `SystemParamState` and `SystemParamFetch` have been removed, and their functionality has been transferred to `SystemParam`.
```rust
// Before (0.9)
impl SystemParam for MyParam<'_, '_> {
type State = MyParamState;
}
unsafe impl SystemParamState for MyParamState {
fn init(world: &mut World, system_meta: &mut SystemMeta) -> Self { ... }
}
unsafe impl<'w, 's> SystemParamFetch<'w, 's> for MyParamState {
type Item = MyParam<'w, 's>;
fn get_param(&mut self, ...) -> Self::Item;
}
unsafe impl ReadOnlySystemParamFetch for MyParamState { }
// After (0.10)
unsafe impl SystemParam for MyParam<'_, '_> {
type State = MyParamState;
type Item<'w, 's> = MyParam<'w, 's>;
fn init_state(world: &mut World, system_meta: &mut SystemMeta) -> Self::State { ... }
fn get_param<'w, 's>(state: &mut Self::State, ...) -> Self::Item<'w, 's>;
}
unsafe impl ReadOnlySystemParam for MyParam<'_, '_> { }
```
The trait `ReadOnlySystemParamFetch` has been replaced with `ReadOnlySystemParam`.
```rust
// Before
unsafe impl ReadOnlySystemParamFetch for MyParamState {}
// After
unsafe impl ReadOnlySystemParam for MyParam<'_, '_> {}
```
2023-01-07 23:20:32 +00:00
fn init_state ( _world : & mut World , _system_meta : & mut SystemMeta ) -> Self ::State { }
2021-02-09 20:14:10 +00:00
#[ inline ]
Simplify trait hierarchy for `SystemParam` (#6865)
# Objective
* Implementing a custom `SystemParam` by hand requires implementing three traits -- four if it is read-only.
* The trait `SystemParamFetch<'w, 's>` is a workaround from before we had generic associated types, and is no longer necessary.
## Solution
* Combine the trait `SystemParamFetch` with `SystemParamState`.
* I decided to remove the `Fetch` name and keep the `State` name, since the former was consistently conflated with the latter.
* Replace the trait `ReadOnlySystemParamFetch` with `ReadOnlySystemParam`, which simplifies trait bounds in generic code.
---
## Changelog
- Removed the trait `SystemParamFetch`, moving its functionality to `SystemParamState`.
- Replaced the trait `ReadOnlySystemParamFetch` with `ReadOnlySystemParam`.
## Migration Guide
The trait `SystemParamFetch` has been removed, and its functionality has been transferred to `SystemParamState`.
```rust
// Before
impl SystemParamState for MyParamState {
fn init(world: &mut World, system_meta: &mut SystemMeta) -> Self { ... }
}
impl<'w, 's> SystemParamFetch<'w, 's> for MyParamState {
type Item = MyParam<'w, 's>;
fn get_param(...) -> Self::Item;
}
// After
impl SystemParamState for MyParamState {
type Item<'w, 's> = MyParam<'w, 's>; // Generic associated types!
fn init(world: &mut World, system_meta: &mut SystemMeta) -> Self { ... }
fn get_param<'w, 's>(...) -> Self::Item<'w, 's>;
}
```
The trait `ReadOnlySystemParamFetch` has been replaced with `ReadOnlySystemParam`.
```rust
// Before
unsafe impl ReadOnlySystemParamFetch for MyParamState {}
// After
unsafe impl<'w, 's> ReadOnlySystemParam for MyParam<'w, 's> {}
```
2022-12-11 18:34:14 +00:00
unsafe fn get_param < ' w , ' s > (
Remove the `SystemParamState` trait and remove types like `ResState` (#6919)
Spiritual successor to #5205.
Actual successor to #6865.
# Objective
Currently, system params are defined using three traits: `SystemParam`, `ReadOnlySystemParam`, `SystemParamState`. The behavior for each param is specified by the `SystemParamState` trait, while `SystemParam` simply defers to the state.
Splitting the traits in this way makes it easier to implement within macros, but it increases the cognitive load. Worst of all, this approach requires each `MySystemParam` to have a public `MySystemParamState` type associated with it.
## Solution
* Merge the trait `SystemParamState` into `SystemParam`.
* Remove all trivial `SystemParam` state types.
* `OptionNonSendMutState<T>`: you will not be missed.
---
- [x] Fix/resolve the remaining test failure.
## Changelog
* Removed the trait `SystemParamState`, merging its functionality into `SystemParam`.
## Migration Guide
**Note**: this should replace the migration guide for #6865.
This is relative to Bevy 0.9, not main.
The traits `SystemParamState` and `SystemParamFetch` have been removed, and their functionality has been transferred to `SystemParam`.
```rust
// Before (0.9)
impl SystemParam for MyParam<'_, '_> {
type State = MyParamState;
}
unsafe impl SystemParamState for MyParamState {
fn init(world: &mut World, system_meta: &mut SystemMeta) -> Self { ... }
}
unsafe impl<'w, 's> SystemParamFetch<'w, 's> for MyParamState {
type Item = MyParam<'w, 's>;
fn get_param(&mut self, ...) -> Self::Item;
}
unsafe impl ReadOnlySystemParamFetch for MyParamState { }
// After (0.10)
unsafe impl SystemParam for MyParam<'_, '_> {
type State = MyParamState;
type Item<'w, 's> = MyParam<'w, 's>;
fn init_state(world: &mut World, system_meta: &mut SystemMeta) -> Self::State { ... }
fn get_param<'w, 's>(state: &mut Self::State, ...) -> Self::Item<'w, 's>;
}
unsafe impl ReadOnlySystemParam for MyParam<'_, '_> { }
```
The trait `ReadOnlySystemParamFetch` has been replaced with `ReadOnlySystemParam`.
```rust
// Before
unsafe impl ReadOnlySystemParamFetch for MyParamState {}
// After
unsafe impl ReadOnlySystemParam for MyParam<'_, '_> {}
```
2023-01-07 23:20:32 +00:00
_state : & ' s mut Self ::State ,
Add new SystemState and rename old SystemState to SystemMeta (#2283)
This enables `SystemParams` to be used outside of function systems. Anything can create and store `SystemState`, which enables efficient "param state cached" access to `SystemParams`.
It adds a `ReadOnlySystemParamFetch` trait, which enables safe `SystemState::get` calls without unique world access.
I renamed the old `SystemState` to `SystemMeta` to enable us to mirror the `QueryState` naming convention (but I'm happy to discuss alternative names if people have other ideas). I initially pitched this as `ParamState`, but given that it needs to include full system metadata, that doesn't feel like a particularly accurate name.
```rust
#[derive(Eq, PartialEq, Debug)]
struct A(usize);
#[derive(Eq, PartialEq, Debug)]
struct B(usize);
let mut world = World::default();
world.insert_resource(A(42));
world.spawn().insert(B(7));
// we get nice lifetime elision when declaring the type on the left hand side
let mut system_state: SystemState<(Res<A>, Query<&B>)> = SystemState::new(&mut world);
let (a, query) = system_state.get(&world);
assert_eq!(*a, A(42), "returned resource matches initial value");
assert_eq!(
*query.single().unwrap(),
B(7),
"returned component matches initial value"
);
// mutable system params require unique world access
let mut system_state: SystemState<(ResMut<A>, Query<&mut B>)> = SystemState::new(&mut world);
let (a, query) = system_state.get_mut(&mut world);
// static lifetimes are required when declaring inside of structs
struct SomeContainer {
state: SystemState<(Res<'static, A>, Res<'static, B>)>
}
// this can be shortened using type aliases, which will be useful for complex param tuples
type MyParams<'a> = (Res<'a, A>, Res<'a, B>);
struct SomeContainer {
state: SystemState<MyParams<'static>>
}
// It is the user's responsibility to call SystemState::apply(world) for parameters that queue up work
let mut system_state: SystemState<(Commands, Query<&B>)> = SystemState::new(&mut world);
{
let (mut commands, query) = system_state.get(&world);
commands.insert_resource(3.14);
}
system_state.apply(&mut world);
```
## Future Work
* Actually use SystemState inside FunctionSystem. This would be trivial, but it requires FunctionSystem to wrap SystemState in Option in its current form (which complicates system metadata lookup). I'd prefer to hold off until we adopt something like the later designs linked in #1364, which enable us to contruct Systems using a World reference (and also remove the need for `.system`).
* Consider a "scoped" approach to automatically call SystemState::apply when systems params are no longer being used (either a container type with a Drop impl, or a function that takes a closure for user logic operating on params).
2021-06-02 19:57:38 +00:00
_system_meta : & SystemMeta ,
System Param Lifetime Split (#2605)
# Objective
Enable using exact World lifetimes during read-only access . This is motivated by the new renderer's need to allow read-only world-only queries to outlive the query itself (but still be constrained by the world lifetime).
For example:
https://github.com/bevyengine/bevy/blob/115b170d1f11a91146bb6d6e9684dceb8b21f786/pipelined/bevy_pbr2/src/render/mod.rs#L774
## Solution
Split out SystemParam state and world lifetimes and pipe those lifetimes up to read-only Query ops (and add into_inner for Res). According to every safety test I've run so far (except one), this is safe (see the temporary safety test commit). Note that changing the mutable variants to the new lifetimes would allow aliased mutable pointers (try doing that to see how it affects the temporary safety tests).
The new state lifetime on SystemParam does make `#[derive(SystemParam)]` more cumbersome (the current impl requires PhantomData if you don't use both lifetimes). We can make this better by detecting whether or not a lifetime is used in the derive and adjusting accordingly, but that should probably be done in its own pr.
## Why is this a draft?
The new lifetimes break QuerySet safety in one very specific case (see the query_set system in system_safety_test). We need to solve this before we can use the lifetimes given.
This is due to the fact that QuerySet is just a wrapper over Query, which now relies on world lifetimes instead of `&self` lifetimes to prevent aliasing (but in systems, each Query has its own implied lifetime, not a centralized world lifetime). I believe the fix is to rewrite QuerySet to have its own World lifetime (and own the internal reference). This will complicate the impl a bit, but I think it is doable. I'm curious if anyone else has better ideas.
Personally, I think these new lifetimes need to happen. We've gotta have a way to directly tie read-only World queries to the World lifetime. The new renderer is the first place this has come up, but I doubt it will be the last. Worst case scenario we can come up with a second `WorldLifetimeQuery<Q, F = ()>` parameter to enable these read-only scenarios, but I'd rather not add another type to the type zoo.
2021-08-15 20:51:53 +00:00
world : & ' w World ,
Reliable change detection (#1471)
# Problem Definition
The current change tracking (via flags for both components and resources) fails to detect changes made by systems that are scheduled to run earlier in the frame than they are.
This issue is discussed at length in [#68](https://github.com/bevyengine/bevy/issues/68) and [#54](https://github.com/bevyengine/bevy/issues/54).
This is very much a draft PR, and contributions are welcome and needed.
# Criteria
1. Each change is detected at least once, no matter the ordering.
2. Each change is detected at most once, no matter the ordering.
3. Changes should be detected the same frame that they are made.
4. Competitive ergonomics. Ideally does not require opting-in.
5. Low CPU overhead of computation.
6. Memory efficient. This must not increase over time, except where the number of entities / resources does.
7. Changes should not be lost for systems that don't run.
8. A frame needs to act as a pure function. Given the same set of entities / components it needs to produce the same end state without side-effects.
**Exact** change-tracking proposals satisfy criteria 1 and 2.
**Conservative** change-tracking proposals satisfy criteria 1 but not 2.
**Flaky** change tracking proposals satisfy criteria 2 but not 1.
# Code Base Navigation
There are three types of flags:
- `Added`: A piece of data was added to an entity / `Resources`.
- `Mutated`: A piece of data was able to be modified, because its `DerefMut` was accessed
- `Changed`: The bitwise OR of `Added` and `Changed`
The special behavior of `ChangedRes`, with respect to the scheduler is being removed in [#1313](https://github.com/bevyengine/bevy/pull/1313) and does not need to be reproduced.
`ChangedRes` and friends can be found in "bevy_ecs/core/resources/resource_query.rs".
The `Flags` trait for Components can be found in "bevy_ecs/core/query.rs".
`ComponentFlags` are stored in "bevy_ecs/core/archetypes.rs", defined on line 446.
# Proposals
**Proposal 5 was selected for implementation.**
## Proposal 0: No Change Detection
The baseline, where computations are performed on everything regardless of whether it changed.
**Type:** Conservative
**Pros:**
- already implemented
- will never miss events
- no overhead
**Cons:**
- tons of repeated work
- doesn't allow users to avoid repeating work (or monitoring for other changes)
## Proposal 1: Earlier-This-Tick Change Detection
The current approach as of Bevy 0.4. Flags are set, and then flushed at the end of each frame.
**Type:** Flaky
**Pros:**
- already implemented
- simple to understand
- low memory overhead (2 bits per component)
- low time overhead (clear every flag once per frame)
**Cons:**
- misses systems based on ordering
- systems that don't run every frame miss changes
- duplicates detection when looping
- can lead to unresolvable circular dependencies
## Proposal 2: Two-Tick Change Detection
Flags persist for two frames, using a double-buffer system identical to that used in events.
A change is observed if it is found in either the current frame's list of changes or the previous frame's.
**Type:** Conservative
**Pros:**
- easy to understand
- easy to implement
- low memory overhead (4 bits per component)
- low time overhead (bit mask and shift every flag once per frame)
**Cons:**
- can result in a great deal of duplicated work
- systems that don't run every frame miss changes
- duplicates detection when looping
## Proposal 3: Last-Tick Change Detection
Flags persist for two frames, using a double-buffer system identical to that used in events.
A change is observed if it is found in the previous frame's list of changes.
**Type:** Exact
**Pros:**
- exact
- easy to understand
- easy to implement
- low memory overhead (4 bits per component)
- low time overhead (bit mask and shift every flag once per frame)
**Cons:**
- change detection is always delayed, possibly causing painful chained delays
- systems that don't run every frame miss changes
- duplicates detection when looping
## Proposal 4: Flag-Doubling Change Detection
Combine Proposal 2 and Proposal 3. Differentiate between `JustChanged` (current behavior) and `Changed` (Proposal 3).
Pack this data into the flags according to [this implementation proposal](https://github.com/bevyengine/bevy/issues/68#issuecomment-769174804).
**Type:** Flaky + Exact
**Pros:**
- allows users to acc
- easy to implement
- low memory overhead (4 bits per component)
- low time overhead (bit mask and shift every flag once per frame)
**Cons:**
- users must specify the type of change detection required
- still quite fragile to system ordering effects when using the flaky `JustChanged` form
- cannot get immediate + exact results
- systems that don't run every frame miss changes
- duplicates detection when looping
## [SELECTED] Proposal 5: Generation-Counter Change Detection
A global counter is increased after each system is run. Each component saves the time of last mutation, and each system saves the time of last execution. Mutation is detected when the component's counter is greater than the system's counter. Discussed [here](https://github.com/bevyengine/bevy/issues/68#issuecomment-769174804). How to handle addition detection is unsolved; the current proposal is to use the highest bit of the counter as in proposal 1.
**Type:** Exact (for mutations), flaky (for additions)
**Pros:**
- low time overhead (set component counter on access, set system counter after execution)
- robust to systems that don't run every frame
- robust to systems that loop
**Cons:**
- moderately complex implementation
- must be modified as systems are inserted dynamically
- medium memory overhead (4 bytes per component + system)
- unsolved addition detection
## Proposal 6: System-Data Change Detection
For each system, track which system's changes it has seen. This approach is only worth fully designing and implementing if Proposal 5 fails in some way.
**Type:** Exact
**Pros:**
- exact
- conceptually simple
**Cons:**
- requires storing data on each system
- implementation is complex
- must be modified as systems are inserted dynamically
## Proposal 7: Total-Order Change Detection
Discussed [here](https://github.com/bevyengine/bevy/issues/68#issuecomment-754326523). This proposal is somewhat complicated by the new scheduler, but I believe it should still be conceptually feasible. This approach is only worth fully designing and implementing if Proposal 5 fails in some way.
**Type:** Exact
**Pros:**
- exact
- efficient data storage relative to other exact proposals
**Cons:**
- requires access to the scheduler
- complex implementation and difficulty grokking
- must be modified as systems are inserted dynamically
# Tests
- We will need to verify properties 1, 2, 3, 7 and 8. Priority: 1 > 2 = 3 > 8 > 7
- Ideally we can use identical user-facing syntax for all proposals, allowing us to re-use the same syntax for each.
- When writing tests, we need to carefully specify order using explicit dependencies.
- These tests will need to be duplicated for both components and resources.
- We need to be sure to handle cases where ambiguous system orders exist.
`changing_system` is always the system that makes the changes, and `detecting_system` always detects the changes.
The component / resource changed will be simple boolean wrapper structs.
## Basic Added / Mutated / Changed
2 x 3 design:
- Resources vs. Components
- Added vs. Changed vs. Mutated
- `changing_system` runs before `detecting_system`
- verify at the end of tick 2
## At Least Once
2 x 3 design:
- Resources vs. Components
- Added vs. Changed vs. Mutated
- `changing_system` runs after `detecting_system`
- verify at the end of tick 2
## At Most Once
2 x 3 design:
- Resources vs. Components
- Added vs. Changed vs. Mutated
- `changing_system` runs once before `detecting_system`
- increment a counter based on the number of changes detected
- verify at the end of tick 2
## Fast Detection
2 x 3 design:
- Resources vs. Components
- Added vs. Changed vs. Mutated
- `changing_system` runs before `detecting_system`
- verify at the end of tick 1
## Ambiguous System Ordering Robustness
2 x 3 x 2 design:
- Resources vs. Components
- Added vs. Changed vs. Mutated
- `changing_system` runs [before/after] `detecting_system` in tick 1
- `changing_system` runs [after/before] `detecting_system` in tick 2
## System Pausing
2 x 3 design:
- Resources vs. Components
- Added vs. Changed vs. Mutated
- `changing_system` runs in tick 1, then is disabled by run criteria
- `detecting_system` is disabled by run criteria until it is run once during tick 3
- verify at the end of tick 3
## Addition Causes Mutation
2 design:
- Resources vs. Components
- `adding_system_1` adds a component / resource
- `adding system_2` adds the same component / resource
- verify the `Mutated` flag at the end of the tick
- verify the `Added` flag at the end of the tick
First check tests for: https://github.com/bevyengine/bevy/issues/333
Second check tests for: https://github.com/bevyengine/bevy/issues/1443
## Changes Made By Commands
- `adding_system` runs in Update in tick 1, and sends a command to add a component
- `detecting_system` runs in Update in tick 1 and 2, after `adding_system`
- We can't detect the changes in tick 1, since they haven't been processed yet
- If we were to track these changes as being emitted by `adding_system`, we can't detect the changes in tick 2 either, since `detecting_system` has already run once after `adding_system` :(
# Benchmarks
See: [general advice](https://github.com/bevyengine/bevy/blob/master/docs/profiling.md), [Criterion crate](https://github.com/bheisler/criterion.rs)
There are several critical parameters to vary:
1. entity count (1 to 10^9)
2. fraction of entities that are changed (0% to 100%)
3. cost to perform work on changed entities, i.e. workload (1 ns to 1s)
1 and 2 should be varied between benchmark runs. 3 can be added on computationally.
We want to measure:
- memory cost
- run time
We should collect these measurements across several frames (100?) to reduce bootup effects and accurately measure the mean, variance and drift.
Entity-component change detection is much more important to benchmark than resource change detection, due to the orders of magnitude higher number of pieces of data.
No change detection at all should be included in benchmarks as a second control for cases where missing changes is unacceptable.
## Graphs
1. y: performance, x: log_10(entity count), color: proposal, facet: performance metric. Set cost to perform work to 0.
2. y: run time, x: cost to perform work, color: proposal, facet: fraction changed. Set number of entities to 10^6
3. y: memory, x: frames, color: proposal
# Conclusions
1. Is the theoretical categorization of the proposals correct according to our tests?
2. How does the performance of the proposals compare without any load?
3. How does the performance of the proposals compare with realistic loads?
4. At what workload does more exact change tracking become worth the (presumably) higher overhead?
5. When does adding change-detection to save on work become worthwhile?
6. Is there enough divergence in performance between the best solutions in each class to ship more than one change-tracking solution?
# Implementation Plan
1. Write a test suite.
2. Verify that tests fail for existing approach.
3. Write a benchmark suite.
4. Get performance numbers for existing approach.
5. Implement, test and benchmark various solutions using a Git branch per proposal.
6. Create a draft PR with all solutions and present results to team.
7. Select a solution and replace existing change detection.
Co-authored-by: Brice DAVIER <bricedavier@gmail.com>
Co-authored-by: Carter Anderson <mcanders1@gmail.com>
2021-03-19 17:53:26 +00:00
_change_tick : u32 ,
Simplify trait hierarchy for `SystemParam` (#6865)
# Objective
* Implementing a custom `SystemParam` by hand requires implementing three traits -- four if it is read-only.
* The trait `SystemParamFetch<'w, 's>` is a workaround from before we had generic associated types, and is no longer necessary.
## Solution
* Combine the trait `SystemParamFetch` with `SystemParamState`.
* I decided to remove the `Fetch` name and keep the `State` name, since the former was consistently conflated with the latter.
* Replace the trait `ReadOnlySystemParamFetch` with `ReadOnlySystemParam`, which simplifies trait bounds in generic code.
---
## Changelog
- Removed the trait `SystemParamFetch`, moving its functionality to `SystemParamState`.
- Replaced the trait `ReadOnlySystemParamFetch` with `ReadOnlySystemParam`.
## Migration Guide
The trait `SystemParamFetch` has been removed, and its functionality has been transferred to `SystemParamState`.
```rust
// Before
impl SystemParamState for MyParamState {
fn init(world: &mut World, system_meta: &mut SystemMeta) -> Self { ... }
}
impl<'w, 's> SystemParamFetch<'w, 's> for MyParamState {
type Item = MyParam<'w, 's>;
fn get_param(...) -> Self::Item;
}
// After
impl SystemParamState for MyParamState {
type Item<'w, 's> = MyParam<'w, 's>; // Generic associated types!
fn init(world: &mut World, system_meta: &mut SystemMeta) -> Self { ... }
fn get_param<'w, 's>(...) -> Self::Item<'w, 's>;
}
```
The trait `ReadOnlySystemParamFetch` has been replaced with `ReadOnlySystemParam`.
```rust
// Before
unsafe impl ReadOnlySystemParamFetch for MyParamState {}
// After
unsafe impl<'w, 's> ReadOnlySystemParam for MyParam<'w, 's> {}
```
2022-12-11 18:34:14 +00:00
) -> Self ::Item < ' w , ' s > {
Bevy ECS V2 (#1525)
# Bevy ECS V2
This is a rewrite of Bevy ECS (basically everything but the new executor/schedule, which are already awesome). The overall goal was to improve the performance and versatility of Bevy ECS. Here is a quick bulleted list of changes before we dive into the details:
* Complete World rewrite
* Multiple component storage types:
* Tables: fast cache friendly iteration, slower add/removes (previously called Archetypes)
* Sparse Sets: fast add/remove, slower iteration
* Stateful Queries (caches query results for faster iteration. fragmented iteration is _fast_ now)
* Stateful System Params (caches expensive operations. inspired by @DJMcNab's work in #1364)
* Configurable System Params (users can set configuration when they construct their systems. once again inspired by @DJMcNab's work)
* Archetypes are now "just metadata", component storage is separate
* Archetype Graph (for faster archetype changes)
* Component Metadata
* Configure component storage type
* Retrieve information about component size/type/name/layout/send-ness/etc
* Components are uniquely identified by a densely packed ComponentId
* TypeIds are now totally optional (which should make implementing scripting easier)
* Super fast "for_each" query iterators
* Merged Resources into World. Resources are now just a special type of component
* EntityRef/EntityMut builder apis (more efficient and more ergonomic)
* Fast bitset-backed `Access<T>` replaces old hashmap-based approach everywhere
* Query conflicts are determined by component access instead of archetype component access (to avoid random failures at runtime)
* With/Without are still taken into account for conflicts, so this should still be comfy to use
* Much simpler `IntoSystem` impl
* Significantly reduced the amount of hashing throughout the ecs in favor of Sparse Sets (indexed by densely packed ArchetypeId, ComponentId, BundleId, and TableId)
* Safety Improvements
* Entity reservation uses a normal world reference instead of unsafe transmute
* QuerySets no longer transmute lifetimes
* Made traits "unsafe" where relevant
* More thorough safety docs
* WorldCell
* Exposes safe mutable access to multiple resources at a time in a World
* Replaced "catch all" `System::update_archetypes(world: &World)` with `System::new_archetype(archetype: &Archetype)`
* Simpler Bundle implementation
* Replaced slow "remove_bundle_one_by_one" used as fallback for Commands::remove_bundle with fast "remove_bundle_intersection"
* Removed `Mut<T>` query impl. it is better to only support one way: `&mut T`
* Removed with() from `Flags<T>` in favor of `Option<Flags<T>>`, which allows querying for flags to be "filtered" by default
* Components now have is_send property (currently only resources support non-send)
* More granular module organization
* New `RemovedComponents<T>` SystemParam that replaces `query.removed::<T>()`
* `world.resource_scope()` for mutable access to resources and world at the same time
* WorldQuery and QueryFilter traits unified. FilterFetch trait added to enable "short circuit" filtering. Auto impled for cases that don't need it
* Significantly slimmed down SystemState in favor of individual SystemParam state
* System Commands changed from `commands: &mut Commands` back to `mut commands: Commands` (to allow Commands to have a World reference)
Fixes #1320
## `World` Rewrite
This is a from-scratch rewrite of `World` that fills the niche that `hecs` used to. Yes, this means Bevy ECS is no longer a "fork" of hecs. We're going out our own!
(the only shared code between the projects is the entity id allocator, which is already basically ideal)
A huge shout out to @SanderMertens (author of [flecs](https://github.com/SanderMertens/flecs)) for sharing some great ideas with me (specifically hybrid ecs storage and archetype graphs). He also helped advise on a number of implementation details.
## Component Storage (The Problem)
Two ECS storage paradigms have gained a lot of traction over the years:
* **Archetypal ECS**:
* Stores components in "tables" with static schemas. Each "column" stores components of a given type. Each "row" is an entity.
* Each "archetype" has its own table. Adding/removing an entity's component changes the archetype.
* Enables super-fast Query iteration due to its cache-friendly data layout
* Comes at the cost of more expensive add/remove operations for an Entity's components, because all components need to be copied to the new archetype's "table"
* **Sparse Set ECS**:
* Stores components of the same type in densely packed arrays, which are sparsely indexed by densely packed unsigned integers (Entity ids)
* Query iteration is slower than Archetypal ECS because each entity's component could be at any position in the sparse set. This "random access" pattern isn't cache friendly. Additionally, there is an extra layer of indirection because you must first map the entity id to an index in the component array.
* Adding/removing components is a cheap, constant time operation
Bevy ECS V1, hecs, legion, flec, and Unity DOTS are all "archetypal ecs-es". I personally think "archetypal" storage is a good default for game engines. An entity's archetype doesn't need to change frequently in general, and it creates "fast by default" query iteration (which is a much more common operation). It is also "self optimizing". Users don't need to think about optimizing component layouts for iteration performance. It "just works" without any extra boilerplate.
Shipyard and EnTT are "sparse set ecs-es". They employ "packing" as a way to work around the "suboptimal by default" iteration performance for specific sets of components. This helps, but I didn't think this was a good choice for a general purpose engine like Bevy because:
1. "packs" conflict with each other. If bevy decides to internally pack the Transform and GlobalTransform components, users are then blocked if they want to pack some custom component with Transform.
2. users need to take manual action to optimize
Developers selecting an ECS framework are stuck with a hard choice. Select an "archetypal" framework with "fast iteration everywhere" but without the ability to cheaply add/remove components, or select a "sparse set" framework to cheaply add/remove components but with slower iteration performance.
## Hybrid Component Storage (The Solution)
In Bevy ECS V2, we get to have our cake and eat it too. It now has _both_ of the component storage types above (and more can be added later if needed):
* **Tables** (aka "archetypal" storage)
* The default storage. If you don't configure anything, this is what you get
* Fast iteration by default
* Slower add/remove operations
* **Sparse Sets**
* Opt-in
* Slower iteration
* Faster add/remove operations
These storage types complement each other perfectly. By default Query iteration is fast. If developers know that they want to add/remove a component at high frequencies, they can set the storage to "sparse set":
```rust
world.register_component(
ComponentDescriptor::new::<MyComponent>(StorageType::SparseSet)
).unwrap();
```
## Archetypes
Archetypes are now "just metadata" ... they no longer store components directly. They do store:
* The `ComponentId`s of each of the Archetype's components (and that component's storage type)
* Archetypes are uniquely defined by their component layouts
* For example: entities with "table" components `[A, B, C]` _and_ "sparse set" components `[D, E]` will always be in the same archetype.
* The `TableId` associated with the archetype
* For now each archetype has exactly one table (which can have no components),
* There is a 1->Many relationship from Tables->Archetypes. A given table could have any number of archetype components stored in it:
* Ex: an entity with "table storage" components `[A, B, C]` and "sparse set" components `[D, E]` will share the same `[A, B, C]` table as an entity with `[A, B, C]` table component and `[F]` sparse set components.
* This 1->Many relationship is how we preserve fast "cache friendly" iteration performance when possible (more on this later)
* A list of entities that are in the archetype and the row id of the table they are in
* ArchetypeComponentIds
* unique densely packed identifiers for (ArchetypeId, ComponentId) pairs
* used by the schedule executor for cheap system access control
* "Archetype Graph Edges" (see the next section)
## The "Archetype Graph"
Archetype changes in Bevy (and a number of other archetypal ecs-es) have historically been expensive to compute. First, you need to allocate a new vector of the entity's current component ids, add or remove components based on the operation performed, sort it (to ensure it is order-independent), then hash it to find the archetype (if it exists). And thats all before we get to the _already_ expensive full copy of all components to the new table storage.
The solution is to build a "graph" of archetypes to cache these results. @SanderMertens first exposed me to the idea (and he got it from @gjroelofs, who came up with it). They propose adding directed edges between archetypes for add/remove component operations. If `ComponentId`s are densely packed, you can use sparse sets to cheaply jump between archetypes.
Bevy takes this one step further by using add/remove `Bundle` edges instead of `Component` edges. Bevy encourages the use of `Bundles` to group add/remove operations. This is largely for "clearer game logic" reasons, but it also helps cut down on the number of archetype changes required. `Bundles` now also have densely-packed `BundleId`s. This allows us to use a _single_ edge for each bundle operation (rather than needing to traverse N edges ... one for each component). Single component operations are also bundles, so this is strictly an improvement over a "component only" graph.
As a result, an operation that used to be _heavy_ (both for allocations and compute) is now two dirt-cheap array lookups and zero allocations.
## Stateful Queries
World queries are now stateful. This allows us to:
1. Cache archetype (and table) matches
* This resolves another issue with (naive) archetypal ECS: query performance getting worse as the number of archetypes goes up (and fragmentation occurs).
2. Cache Fetch and Filter state
* The expensive parts of fetch/filter operations (such as hashing the TypeId to find the ComponentId) now only happen once when the Query is first constructed
3. Incrementally build up state
* When new archetypes are added, we only process the new archetypes (no need to rebuild state for old archetypes)
As a result, the direct `World` query api now looks like this:
```rust
let mut query = world.query::<(&A, &mut B)>();
for (a, mut b) in query.iter_mut(&mut world) {
}
```
Requiring `World` to generate stateful queries (rather than letting the `QueryState` type be constructed separately) allows us to ensure that _all_ queries are properly initialized (and the relevant world state, such as ComponentIds). This enables QueryState to remove branches from its operations that check for initialization status (and also enables query.iter() to take an immutable world reference because it doesn't need to initialize anything in world).
However in systems, this is a non-breaking change. State management is done internally by the relevant SystemParam.
## Stateful SystemParams
Like Queries, `SystemParams` now also cache state. For example, `Query` system params store the "stateful query" state mentioned above. Commands store their internal `CommandQueue`. This means you can now safely use as many separate `Commands` parameters in your system as you want. `Local<T>` system params store their `T` value in their state (instead of in Resources).
SystemParam state also enabled a significant slim-down of SystemState. It is much nicer to look at now.
Per-SystemParam state naturally insulates us from an "aliased mut" class of errors we have hit in the past (ex: using multiple `Commands` system params).
(credit goes to @DJMcNab for the initial idea and draft pr here #1364)
## Configurable SystemParams
@DJMcNab also had the great idea to make SystemParams configurable. This allows users to provide some initial configuration / values for system parameters (when possible). Most SystemParams have no config (the config type is `()`), but the `Local<T>` param now supports user-provided parameters:
```rust
fn foo(value: Local<usize>) {
}
app.add_system(foo.system().config(|c| c.0 = Some(10)));
```
## Uber Fast "for_each" Query Iterators
Developers now have the choice to use a fast "for_each" iterator, which yields ~1.5-3x iteration speed improvements for "fragmented iteration", and minor ~1.2x iteration speed improvements for unfragmented iteration.
```rust
fn system(query: Query<(&A, &mut B)>) {
// you now have the option to do this for a speed boost
query.for_each_mut(|(a, mut b)| {
});
// however normal iterators are still available
for (a, mut b) in query.iter_mut() {
}
}
```
I think in most cases we should continue to encourage "normal" iterators as they are more flexible and more "rust idiomatic". But when that extra "oomf" is needed, it makes sense to use `for_each`.
We should also consider using `for_each` for internal bevy systems to give our users a nice speed boost (but that should be a separate pr).
## Component Metadata
`World` now has a `Components` collection, which is accessible via `world.components()`. This stores mappings from `ComponentId` to `ComponentInfo`, as well as `TypeId` to `ComponentId` mappings (where relevant). `ComponentInfo` stores information about the component, such as ComponentId, TypeId, memory layout, send-ness (currently limited to resources), and storage type.
## Significantly Cheaper `Access<T>`
We used to use `TypeAccess<TypeId>` to manage read/write component/archetype-component access. This was expensive because TypeIds must be hashed and compared individually. The parallel executor got around this by "condensing" type ids into bitset-backed access types. This worked, but it had to be re-generated from the `TypeAccess<TypeId>`sources every time archetypes changed.
This pr removes TypeAccess in favor of faster bitset access everywhere. We can do this thanks to the move to densely packed `ComponentId`s and `ArchetypeComponentId`s.
## Merged Resources into World
Resources had a lot of redundant functionality with Components. They stored typed data, they had access control, they had unique ids, they were queryable via SystemParams, etc. In fact the _only_ major difference between them was that they were unique (and didn't correlate to an entity).
Separate resources also had the downside of requiring a separate set of access controls, which meant the parallel executor needed to compare more bitsets per system and manage more state.
I initially got the "separate resources" idea from `legion`. I think that design was motivated by the fact that it made the direct world query/resource lifetime interactions more manageable. It certainly made our lives easier when using Resources alongside hecs/bevy_ecs. However we already have a construct for safely and ergonomically managing in-world lifetimes: systems (which use `Access<T>` internally).
This pr merges Resources into World:
```rust
world.insert_resource(1);
world.insert_resource(2.0);
let a = world.get_resource::<i32>().unwrap();
let mut b = world.get_resource_mut::<f64>().unwrap();
*b = 3.0;
```
Resources are now just a special kind of component. They have their own ComponentIds (and their own resource TypeId->ComponentId scope, so they don't conflict wit components of the same type). They are stored in a special "resource archetype", which stores components inside the archetype using a new `unique_components` sparse set (note that this sparse set could later be used to implement Tags). This allows us to keep the code size small by reusing existing datastructures (namely Column, Archetype, ComponentFlags, and ComponentInfo). This allows us the executor to use a single `Access<ArchetypeComponentId>` per system. It should also make scripting language integration easier.
_But_ this merge did create problems for people directly interacting with `World`. What if you need mutable access to multiple resources at the same time? `world.get_resource_mut()` borrows World mutably!
## WorldCell
WorldCell applies the `Access<ArchetypeComponentId>` concept to direct world access:
```rust
let world_cell = world.cell();
let a = world_cell.get_resource_mut::<i32>().unwrap();
let b = world_cell.get_resource_mut::<f64>().unwrap();
```
This adds cheap runtime checks (a sparse set lookup of `ArchetypeComponentId` and a counter) to ensure that world accesses do not conflict with each other. Each operation returns a `WorldBorrow<'w, T>` or `WorldBorrowMut<'w, T>` wrapper type, which will release the relevant ArchetypeComponentId resources when dropped.
World caches the access sparse set (and only one cell can exist at a time), so `world.cell()` is a cheap operation.
WorldCell does _not_ use atomic operations. It is non-send, does a mutable borrow of world to prevent other accesses, and uses a simple `Rc<RefCell<ArchetypeComponentAccess>>` wrapper in each WorldBorrow pointer.
The api is currently limited to resource access, but it can and should be extended to queries / entity component access.
## Resource Scopes
WorldCell does not yet support component queries, and even when it does there are sometimes legitimate reasons to want a mutable world ref _and_ a mutable resource ref (ex: bevy_render and bevy_scene both need this). In these cases we could always drop down to the unsafe `world.get_resource_unchecked_mut()`, but that is not ideal!
Instead developers can use a "resource scope"
```rust
world.resource_scope(|world: &mut World, a: &mut A| {
})
```
This temporarily removes the `A` resource from `World`, provides mutable pointers to both, and re-adds A to World when finished. Thanks to the move to ComponentIds/sparse sets, this is a cheap operation.
If multiple resources are required, scopes can be nested. We could also consider adding a "resource tuple" to the api if this pattern becomes common and the boilerplate gets nasty.
## Query Conflicts Use ComponentId Instead of ArchetypeComponentId
For safety reasons, systems cannot contain queries that conflict with each other without wrapping them in a QuerySet. On bevy `main`, we use ArchetypeComponentIds to determine conflicts. This is nice because it can take into account filters:
```rust
// these queries will never conflict due to their filters
fn filter_system(a: Query<&mut A, With<B>>, b: Query<&mut B, Without<B>>) {
}
```
But it also has a significant downside:
```rust
// these queries will not conflict _until_ an entity with A, B, and C is spawned
fn maybe_conflicts_system(a: Query<(&mut A, &C)>, b: Query<(&mut A, &B)>) {
}
```
The system above will panic at runtime if an entity with A, B, and C is spawned. This makes it hard to trust that your game logic will run without crashing.
In this pr, I switched to using `ComponentId` instead. This _is_ more constraining. `maybe_conflicts_system` will now always fail, but it will do it consistently at startup. Naively, it would also _disallow_ `filter_system`, which would be a significant downgrade in usability. Bevy has a number of internal systems that rely on disjoint queries and I expect it to be a common pattern in userspace.
To resolve this, I added a new `FilteredAccess<T>` type, which wraps `Access<T>` and adds with/without filters. If two `FilteredAccess` have with/without values that prove they are disjoint, they will no longer conflict.
## EntityRef / EntityMut
World entity operations on `main` require that the user passes in an `entity` id to each operation:
```rust
let entity = world.spawn((A, )); // create a new entity with A
world.get::<A>(entity);
world.insert(entity, (B, C));
world.insert_one(entity, D);
```
This means that each operation needs to look up the entity location / verify its validity. The initial spawn operation also requires a Bundle as input. This can be awkward when no components are required (or one component is required).
These operations have been replaced by `EntityRef` and `EntityMut`, which are "builder-style" wrappers around world that provide read and read/write operations on a single, pre-validated entity:
```rust
// spawn now takes no inputs and returns an EntityMut
let entity = world.spawn()
.insert(A) // insert a single component into the entity
.insert_bundle((B, C)) // insert a bundle of components into the entity
.id() // id returns the Entity id
// Returns EntityMut (or panics if the entity does not exist)
world.entity_mut(entity)
.insert(D)
.insert_bundle(SomeBundle::default());
{
// returns EntityRef (or panics if the entity does not exist)
let d = world.entity(entity)
.get::<D>() // gets the D component
.unwrap();
// world.get still exists for ergonomics
let d = world.get::<D>(entity).unwrap();
}
// These variants return Options if you want to check existence instead of panicing
world.get_entity_mut(entity)
.unwrap()
.insert(E);
if let Some(entity_ref) = world.get_entity(entity) {
let d = entity_ref.get::<D>().unwrap();
}
```
This _does not_ affect the current Commands api or terminology. I think that should be a separate conversation as that is a much larger breaking change.
## Safety Improvements
* Entity reservation in Commands uses a normal world borrow instead of an unsafe transmute
* QuerySets no longer transmutes lifetimes
* Made traits "unsafe" when implementing a trait incorrectly could cause unsafety
* More thorough safety docs
## RemovedComponents SystemParam
The old approach to querying removed components: `query.removed:<T>()` was confusing because it had no connection to the query itself. I replaced it with the following, which is both clearer and allows us to cache the ComponentId mapping in the SystemParamState:
```rust
fn system(removed: RemovedComponents<T>) {
for entity in removed.iter() {
}
}
```
## Simpler Bundle implementation
Bundles are no longer responsible for sorting (or deduping) TypeInfo. They are just a simple ordered list of component types / data. This makes the implementation smaller and opens the door to an easy "nested bundle" implementation in the future (which i might even add in this pr). Duplicate detection is now done once per bundle type by World the first time a bundle is used.
## Unified WorldQuery and QueryFilter types
(don't worry they are still separate type _parameters_ in Queries .. this is a non-breaking change)
WorldQuery and QueryFilter were already basically identical apis. With the addition of `FetchState` and more storage-specific fetch methods, the overlap was even clearer (and the redundancy more painful).
QueryFilters are now just `F: WorldQuery where F::Fetch: FilterFetch`. FilterFetch requires `Fetch<Item = bool>` and adds new "short circuit" variants of fetch methods. This enables a filter tuple like `(With<A>, Without<B>, Changed<C>)` to stop evaluating the filter after the first mismatch is encountered. FilterFetch is automatically implemented for `Fetch` implementations that return bool.
This forces fetch implementations that return things like `(bool, bool, bool)` (such as the filter above) to manually implement FilterFetch and decide whether or not to short-circuit.
## More Granular Modules
World no longer globs all of the internal modules together. It now exports `core`, `system`, and `schedule` separately. I'm also considering exporting `core` submodules directly as that is still pretty "glob-ey" and unorganized (feedback welcome here).
## Remaining Draft Work (to be done in this pr)
* ~~panic on conflicting WorldQuery fetches (&A, &mut A)~~
* ~~bevy `main` and hecs both currently allow this, but we should protect against it if possible~~
* ~~batch_iter / par_iter (currently stubbed out)~~
* ~~ChangedRes~~
* ~~I skipped this while we sort out #1313. This pr should be adapted to account for whatever we land on there~~.
* ~~The `Archetypes` and `Tables` collections use hashes of sorted lists of component ids to uniquely identify each archetype/table. This hash is then used as the key in a HashMap to look up the relevant ArchetypeId or TableId. (which doesn't handle hash collisions properly)~~
* ~~It is currently unsafe to generate a Query from "World A", then use it on "World B" (despite the api claiming it is safe). We should probably close this gap. This could be done by adding a randomly generated WorldId to each world, then storing that id in each Query. They could then be compared to each other on each `query.do_thing(&world)` operation. This _does_ add an extra branch to each query operation, so I'm open to other suggestions if people have them.~~
* ~~Nested Bundles (if i find time)~~
## Potential Future Work
* Expand WorldCell to support queries.
* Consider not allocating in the empty archetype on `world.spawn()`
* ex: return something like EntityMutUninit, which turns into EntityMut after an `insert` or `insert_bundle` op
* this actually regressed performance last time i tried it, but in theory it should be faster
* Optimize SparseSet::insert (see `PERF` comment on insert)
* Replace SparseArray `Option<T>` with T::MAX to cut down on branching
* would enable cheaper get_unchecked() operations
* upstream fixedbitset optimizations
* fixedbitset could be allocation free for small block counts (store blocks in a SmallVec)
* fixedbitset could have a const constructor
* Consider implementing Tags (archetype-specific by-value data that affects archetype identity)
* ex: ArchetypeA could have `[A, B, C]` table components and `[D(1)]` "tag" component. ArchetypeB could have `[A, B, C]` table components and a `[D(2)]` tag component. The archetypes are different, despite both having D tags because the value inside D is different.
* this could potentially build on top of the `archetype.unique_components` added in this pr for resource storage.
* Consider reverting `all_tuples` proc macro in favor of the old `macro_rules` implementation
* all_tuples is more flexible and produces cleaner documentation (the macro_rules version produces weird type parameter orders due to parser constraints)
* but unfortunately all_tuples also appears to make Rust Analyzer sad/slow when working inside of `bevy_ecs` (does not affect user code)
* Consider "resource queries" and/or "mixed resource and entity component queries" as an alternative to WorldCell
* this is basically just "systems" so maybe it's not worth it
* Add more world ops
* `world.clear()`
* `world.reserve<T: Bundle>(count: usize)`
* Try using the old archetype allocation strategy (allocate new memory on resize and copy everything over). I expect this to improve batch insertion performance at the cost of unbatched performance. But thats just a guess. I'm not an allocation perf pro :)
* Adapt Commands apis for consistency with new World apis
## Benchmarks
key:
* `bevy_old`: bevy `main` branch
* `bevy`: this branch
* `_foreach`: uses an optimized for_each iterator
* ` _sparse`: uses sparse set storage (if unspecified assume table storage)
* `_system`: runs inside a system (if unspecified assume test happens via direct world ops)
### Simple Insert (from ecs_bench_suite)
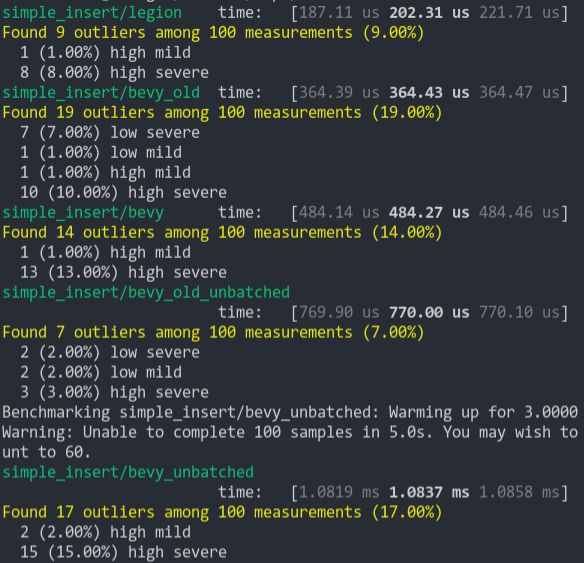
### Simpler Iter (from ecs_bench_suite)
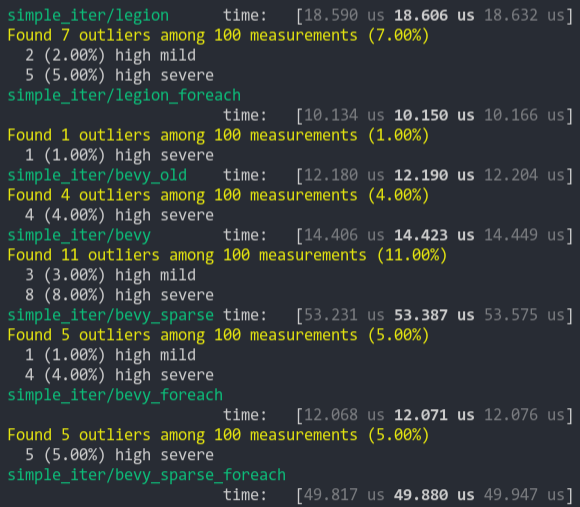
### Fragment Iter (from ecs_bench_suite)
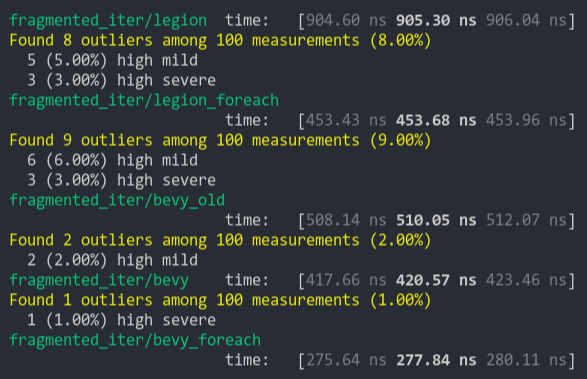
### Sparse Fragmented Iter
Iterate a query that matches 5 entities from a single matching archetype, but there are 100 unmatching archetypes
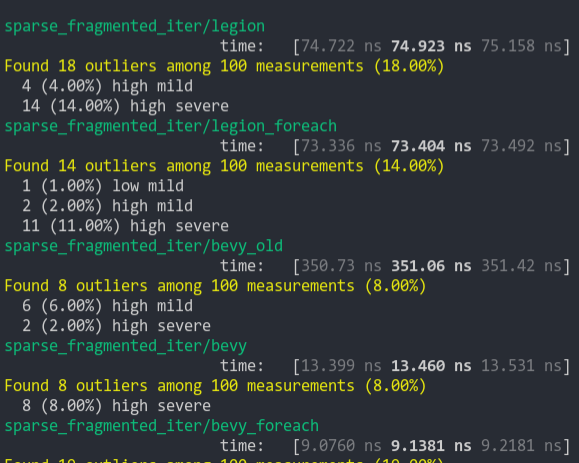
### Schedule (from ecs_bench_suite)
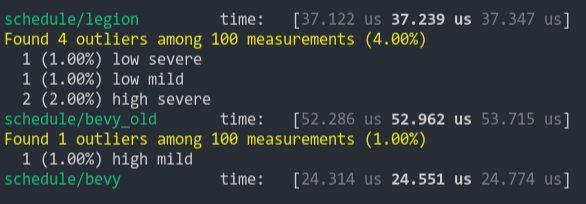
### Add Remove Component (from ecs_bench_suite)
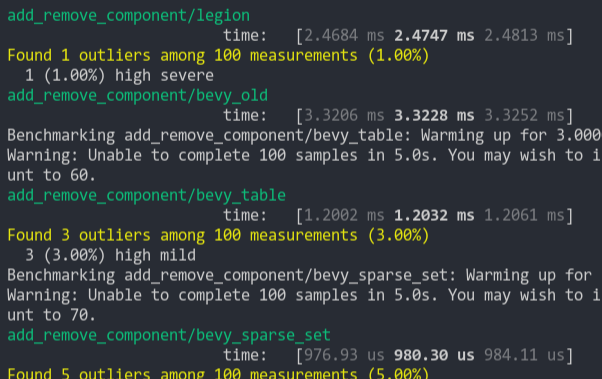
### Add Remove Component Big
Same as the test above, but each entity has 5 "large" matrix components and 1 "large" matrix component is added and removed
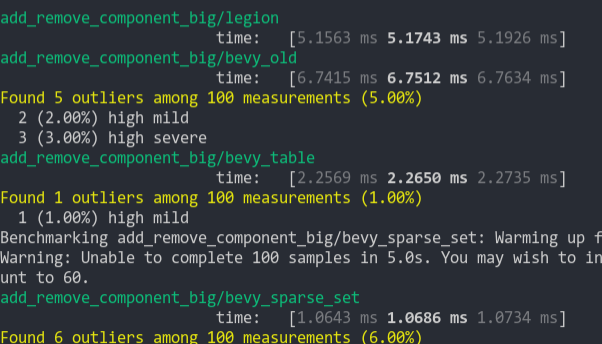
### Get Component
Looks up a single component value a large number of times
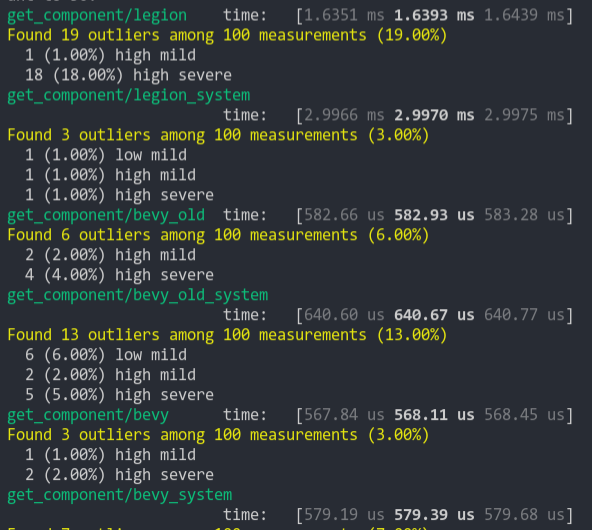
2021-03-05 07:54:35 +00:00
world . entities ( )
2021-02-09 20:14:10 +00:00
}
}
2022-07-04 14:44:24 +00:00
// SAFETY: Only reads World bundles
Simplify trait hierarchy for `SystemParam` (#6865)
# Objective
* Implementing a custom `SystemParam` by hand requires implementing three traits -- four if it is read-only.
* The trait `SystemParamFetch<'w, 's>` is a workaround from before we had generic associated types, and is no longer necessary.
## Solution
* Combine the trait `SystemParamFetch` with `SystemParamState`.
* I decided to remove the `Fetch` name and keep the `State` name, since the former was consistently conflated with the latter.
* Replace the trait `ReadOnlySystemParamFetch` with `ReadOnlySystemParam`, which simplifies trait bounds in generic code.
---
## Changelog
- Removed the trait `SystemParamFetch`, moving its functionality to `SystemParamState`.
- Replaced the trait `ReadOnlySystemParamFetch` with `ReadOnlySystemParam`.
## Migration Guide
The trait `SystemParamFetch` has been removed, and its functionality has been transferred to `SystemParamState`.
```rust
// Before
impl SystemParamState for MyParamState {
fn init(world: &mut World, system_meta: &mut SystemMeta) -> Self { ... }
}
impl<'w, 's> SystemParamFetch<'w, 's> for MyParamState {
type Item = MyParam<'w, 's>;
fn get_param(...) -> Self::Item;
}
// After
impl SystemParamState for MyParamState {
type Item<'w, 's> = MyParam<'w, 's>; // Generic associated types!
fn init(world: &mut World, system_meta: &mut SystemMeta) -> Self { ... }
fn get_param<'w, 's>(...) -> Self::Item<'w, 's>;
}
```
The trait `ReadOnlySystemParamFetch` has been replaced with `ReadOnlySystemParam`.
```rust
// Before
unsafe impl ReadOnlySystemParamFetch for MyParamState {}
// After
unsafe impl<'w, 's> ReadOnlySystemParam for MyParam<'w, 's> {}
```
2022-12-11 18:34:14 +00:00
unsafe impl < ' a > ReadOnlySystemParam for & ' a Bundles { }
Add new SystemState and rename old SystemState to SystemMeta (#2283)
This enables `SystemParams` to be used outside of function systems. Anything can create and store `SystemState`, which enables efficient "param state cached" access to `SystemParams`.
It adds a `ReadOnlySystemParamFetch` trait, which enables safe `SystemState::get` calls without unique world access.
I renamed the old `SystemState` to `SystemMeta` to enable us to mirror the `QueryState` naming convention (but I'm happy to discuss alternative names if people have other ideas). I initially pitched this as `ParamState`, but given that it needs to include full system metadata, that doesn't feel like a particularly accurate name.
```rust
#[derive(Eq, PartialEq, Debug)]
struct A(usize);
#[derive(Eq, PartialEq, Debug)]
struct B(usize);
let mut world = World::default();
world.insert_resource(A(42));
world.spawn().insert(B(7));
// we get nice lifetime elision when declaring the type on the left hand side
let mut system_state: SystemState<(Res<A>, Query<&B>)> = SystemState::new(&mut world);
let (a, query) = system_state.get(&world);
assert_eq!(*a, A(42), "returned resource matches initial value");
assert_eq!(
*query.single().unwrap(),
B(7),
"returned component matches initial value"
);
// mutable system params require unique world access
let mut system_state: SystemState<(ResMut<A>, Query<&mut B>)> = SystemState::new(&mut world);
let (a, query) = system_state.get_mut(&mut world);
// static lifetimes are required when declaring inside of structs
struct SomeContainer {
state: SystemState<(Res<'static, A>, Res<'static, B>)>
}
// this can be shortened using type aliases, which will be useful for complex param tuples
type MyParams<'a> = (Res<'a, A>, Res<'a, B>);
struct SomeContainer {
state: SystemState<MyParams<'static>>
}
// It is the user's responsibility to call SystemState::apply(world) for parameters that queue up work
let mut system_state: SystemState<(Commands, Query<&B>)> = SystemState::new(&mut world);
{
let (mut commands, query) = system_state.get(&world);
commands.insert_resource(3.14);
}
system_state.apply(&mut world);
```
## Future Work
* Actually use SystemState inside FunctionSystem. This would be trivial, but it requires FunctionSystem to wrap SystemState in Option in its current form (which complicates system metadata lookup). I'd prefer to hold off until we adopt something like the later designs linked in #1364, which enable us to contruct Systems using a World reference (and also remove the need for `.system`).
* Consider a "scoped" approach to automatically call SystemState::apply when systems params are no longer being used (either a container type with a Drop impl, or a function that takes a closure for user logic operating on params).
2021-06-02 19:57:38 +00:00
2022-07-04 14:44:24 +00:00
// SAFETY: no component value access
Remove the `SystemParamState` trait and remove types like `ResState` (#6919)
Spiritual successor to #5205.
Actual successor to #6865.
# Objective
Currently, system params are defined using three traits: `SystemParam`, `ReadOnlySystemParam`, `SystemParamState`. The behavior for each param is specified by the `SystemParamState` trait, while `SystemParam` simply defers to the state.
Splitting the traits in this way makes it easier to implement within macros, but it increases the cognitive load. Worst of all, this approach requires each `MySystemParam` to have a public `MySystemParamState` type associated with it.
## Solution
* Merge the trait `SystemParamState` into `SystemParam`.
* Remove all trivial `SystemParam` state types.
* `OptionNonSendMutState<T>`: you will not be missed.
---
- [x] Fix/resolve the remaining test failure.
## Changelog
* Removed the trait `SystemParamState`, merging its functionality into `SystemParam`.
## Migration Guide
**Note**: this should replace the migration guide for #6865.
This is relative to Bevy 0.9, not main.
The traits `SystemParamState` and `SystemParamFetch` have been removed, and their functionality has been transferred to `SystemParam`.
```rust
// Before (0.9)
impl SystemParam for MyParam<'_, '_> {
type State = MyParamState;
}
unsafe impl SystemParamState for MyParamState {
fn init(world: &mut World, system_meta: &mut SystemMeta) -> Self { ... }
}
unsafe impl<'w, 's> SystemParamFetch<'w, 's> for MyParamState {
type Item = MyParam<'w, 's>;
fn get_param(&mut self, ...) -> Self::Item;
}
unsafe impl ReadOnlySystemParamFetch for MyParamState { }
// After (0.10)
unsafe impl SystemParam for MyParam<'_, '_> {
type State = MyParamState;
type Item<'w, 's> = MyParam<'w, 's>;
fn init_state(world: &mut World, system_meta: &mut SystemMeta) -> Self::State { ... }
fn get_param<'w, 's>(state: &mut Self::State, ...) -> Self::Item<'w, 's>;
}
unsafe impl ReadOnlySystemParam for MyParam<'_, '_> { }
```
The trait `ReadOnlySystemParamFetch` has been replaced with `ReadOnlySystemParam`.
```rust
// Before
unsafe impl ReadOnlySystemParamFetch for MyParamState {}
// After
unsafe impl ReadOnlySystemParam for MyParam<'_, '_> {}
```
2023-01-07 23:20:32 +00:00
unsafe impl < ' a > SystemParam for & ' a Bundles {
type State = ( ) ;
Simplify trait hierarchy for `SystemParam` (#6865)
# Objective
* Implementing a custom `SystemParam` by hand requires implementing three traits -- four if it is read-only.
* The trait `SystemParamFetch<'w, 's>` is a workaround from before we had generic associated types, and is no longer necessary.
## Solution
* Combine the trait `SystemParamFetch` with `SystemParamState`.
* I decided to remove the `Fetch` name and keep the `State` name, since the former was consistently conflated with the latter.
* Replace the trait `ReadOnlySystemParamFetch` with `ReadOnlySystemParam`, which simplifies trait bounds in generic code.
---
## Changelog
- Removed the trait `SystemParamFetch`, moving its functionality to `SystemParamState`.
- Replaced the trait `ReadOnlySystemParamFetch` with `ReadOnlySystemParam`.
## Migration Guide
The trait `SystemParamFetch` has been removed, and its functionality has been transferred to `SystemParamState`.
```rust
// Before
impl SystemParamState for MyParamState {
fn init(world: &mut World, system_meta: &mut SystemMeta) -> Self { ... }
}
impl<'w, 's> SystemParamFetch<'w, 's> for MyParamState {
type Item = MyParam<'w, 's>;
fn get_param(...) -> Self::Item;
}
// After
impl SystemParamState for MyParamState {
type Item<'w, 's> = MyParam<'w, 's>; // Generic associated types!
fn init(world: &mut World, system_meta: &mut SystemMeta) -> Self { ... }
fn get_param<'w, 's>(...) -> Self::Item<'w, 's>;
}
```
The trait `ReadOnlySystemParamFetch` has been replaced with `ReadOnlySystemParam`.
```rust
// Before
unsafe impl ReadOnlySystemParamFetch for MyParamState {}
// After
unsafe impl<'w, 's> ReadOnlySystemParam for MyParam<'w, 's> {}
```
2022-12-11 18:34:14 +00:00
type Item < ' w , ' s > = & ' w Bundles ;
Remove the `SystemParamState` trait and remove types like `ResState` (#6919)
Spiritual successor to #5205.
Actual successor to #6865.
# Objective
Currently, system params are defined using three traits: `SystemParam`, `ReadOnlySystemParam`, `SystemParamState`. The behavior for each param is specified by the `SystemParamState` trait, while `SystemParam` simply defers to the state.
Splitting the traits in this way makes it easier to implement within macros, but it increases the cognitive load. Worst of all, this approach requires each `MySystemParam` to have a public `MySystemParamState` type associated with it.
## Solution
* Merge the trait `SystemParamState` into `SystemParam`.
* Remove all trivial `SystemParam` state types.
* `OptionNonSendMutState<T>`: you will not be missed.
---
- [x] Fix/resolve the remaining test failure.
## Changelog
* Removed the trait `SystemParamState`, merging its functionality into `SystemParam`.
## Migration Guide
**Note**: this should replace the migration guide for #6865.
This is relative to Bevy 0.9, not main.
The traits `SystemParamState` and `SystemParamFetch` have been removed, and their functionality has been transferred to `SystemParam`.
```rust
// Before (0.9)
impl SystemParam for MyParam<'_, '_> {
type State = MyParamState;
}
unsafe impl SystemParamState for MyParamState {
fn init(world: &mut World, system_meta: &mut SystemMeta) -> Self { ... }
}
unsafe impl<'w, 's> SystemParamFetch<'w, 's> for MyParamState {
type Item = MyParam<'w, 's>;
fn get_param(&mut self, ...) -> Self::Item;
}
unsafe impl ReadOnlySystemParamFetch for MyParamState { }
// After (0.10)
unsafe impl SystemParam for MyParam<'_, '_> {
type State = MyParamState;
type Item<'w, 's> = MyParam<'w, 's>;
fn init_state(world: &mut World, system_meta: &mut SystemMeta) -> Self::State { ... }
fn get_param<'w, 's>(state: &mut Self::State, ...) -> Self::Item<'w, 's>;
}
unsafe impl ReadOnlySystemParam for MyParam<'_, '_> { }
```
The trait `ReadOnlySystemParamFetch` has been replaced with `ReadOnlySystemParam`.
```rust
// Before
unsafe impl ReadOnlySystemParamFetch for MyParamState {}
// After
unsafe impl ReadOnlySystemParam for MyParam<'_, '_> {}
```
2023-01-07 23:20:32 +00:00
fn init_state ( _world : & mut World , _system_meta : & mut SystemMeta ) -> Self ::State { }
Bevy ECS V2 (#1525)
# Bevy ECS V2
This is a rewrite of Bevy ECS (basically everything but the new executor/schedule, which are already awesome). The overall goal was to improve the performance and versatility of Bevy ECS. Here is a quick bulleted list of changes before we dive into the details:
* Complete World rewrite
* Multiple component storage types:
* Tables: fast cache friendly iteration, slower add/removes (previously called Archetypes)
* Sparse Sets: fast add/remove, slower iteration
* Stateful Queries (caches query results for faster iteration. fragmented iteration is _fast_ now)
* Stateful System Params (caches expensive operations. inspired by @DJMcNab's work in #1364)
* Configurable System Params (users can set configuration when they construct their systems. once again inspired by @DJMcNab's work)
* Archetypes are now "just metadata", component storage is separate
* Archetype Graph (for faster archetype changes)
* Component Metadata
* Configure component storage type
* Retrieve information about component size/type/name/layout/send-ness/etc
* Components are uniquely identified by a densely packed ComponentId
* TypeIds are now totally optional (which should make implementing scripting easier)
* Super fast "for_each" query iterators
* Merged Resources into World. Resources are now just a special type of component
* EntityRef/EntityMut builder apis (more efficient and more ergonomic)
* Fast bitset-backed `Access<T>` replaces old hashmap-based approach everywhere
* Query conflicts are determined by component access instead of archetype component access (to avoid random failures at runtime)
* With/Without are still taken into account for conflicts, so this should still be comfy to use
* Much simpler `IntoSystem` impl
* Significantly reduced the amount of hashing throughout the ecs in favor of Sparse Sets (indexed by densely packed ArchetypeId, ComponentId, BundleId, and TableId)
* Safety Improvements
* Entity reservation uses a normal world reference instead of unsafe transmute
* QuerySets no longer transmute lifetimes
* Made traits "unsafe" where relevant
* More thorough safety docs
* WorldCell
* Exposes safe mutable access to multiple resources at a time in a World
* Replaced "catch all" `System::update_archetypes(world: &World)` with `System::new_archetype(archetype: &Archetype)`
* Simpler Bundle implementation
* Replaced slow "remove_bundle_one_by_one" used as fallback for Commands::remove_bundle with fast "remove_bundle_intersection"
* Removed `Mut<T>` query impl. it is better to only support one way: `&mut T`
* Removed with() from `Flags<T>` in favor of `Option<Flags<T>>`, which allows querying for flags to be "filtered" by default
* Components now have is_send property (currently only resources support non-send)
* More granular module organization
* New `RemovedComponents<T>` SystemParam that replaces `query.removed::<T>()`
* `world.resource_scope()` for mutable access to resources and world at the same time
* WorldQuery and QueryFilter traits unified. FilterFetch trait added to enable "short circuit" filtering. Auto impled for cases that don't need it
* Significantly slimmed down SystemState in favor of individual SystemParam state
* System Commands changed from `commands: &mut Commands` back to `mut commands: Commands` (to allow Commands to have a World reference)
Fixes #1320
## `World` Rewrite
This is a from-scratch rewrite of `World` that fills the niche that `hecs` used to. Yes, this means Bevy ECS is no longer a "fork" of hecs. We're going out our own!
(the only shared code between the projects is the entity id allocator, which is already basically ideal)
A huge shout out to @SanderMertens (author of [flecs](https://github.com/SanderMertens/flecs)) for sharing some great ideas with me (specifically hybrid ecs storage and archetype graphs). He also helped advise on a number of implementation details.
## Component Storage (The Problem)
Two ECS storage paradigms have gained a lot of traction over the years:
* **Archetypal ECS**:
* Stores components in "tables" with static schemas. Each "column" stores components of a given type. Each "row" is an entity.
* Each "archetype" has its own table. Adding/removing an entity's component changes the archetype.
* Enables super-fast Query iteration due to its cache-friendly data layout
* Comes at the cost of more expensive add/remove operations for an Entity's components, because all components need to be copied to the new archetype's "table"
* **Sparse Set ECS**:
* Stores components of the same type in densely packed arrays, which are sparsely indexed by densely packed unsigned integers (Entity ids)
* Query iteration is slower than Archetypal ECS because each entity's component could be at any position in the sparse set. This "random access" pattern isn't cache friendly. Additionally, there is an extra layer of indirection because you must first map the entity id to an index in the component array.
* Adding/removing components is a cheap, constant time operation
Bevy ECS V1, hecs, legion, flec, and Unity DOTS are all "archetypal ecs-es". I personally think "archetypal" storage is a good default for game engines. An entity's archetype doesn't need to change frequently in general, and it creates "fast by default" query iteration (which is a much more common operation). It is also "self optimizing". Users don't need to think about optimizing component layouts for iteration performance. It "just works" without any extra boilerplate.
Shipyard and EnTT are "sparse set ecs-es". They employ "packing" as a way to work around the "suboptimal by default" iteration performance for specific sets of components. This helps, but I didn't think this was a good choice for a general purpose engine like Bevy because:
1. "packs" conflict with each other. If bevy decides to internally pack the Transform and GlobalTransform components, users are then blocked if they want to pack some custom component with Transform.
2. users need to take manual action to optimize
Developers selecting an ECS framework are stuck with a hard choice. Select an "archetypal" framework with "fast iteration everywhere" but without the ability to cheaply add/remove components, or select a "sparse set" framework to cheaply add/remove components but with slower iteration performance.
## Hybrid Component Storage (The Solution)
In Bevy ECS V2, we get to have our cake and eat it too. It now has _both_ of the component storage types above (and more can be added later if needed):
* **Tables** (aka "archetypal" storage)
* The default storage. If you don't configure anything, this is what you get
* Fast iteration by default
* Slower add/remove operations
* **Sparse Sets**
* Opt-in
* Slower iteration
* Faster add/remove operations
These storage types complement each other perfectly. By default Query iteration is fast. If developers know that they want to add/remove a component at high frequencies, they can set the storage to "sparse set":
```rust
world.register_component(
ComponentDescriptor::new::<MyComponent>(StorageType::SparseSet)
).unwrap();
```
## Archetypes
Archetypes are now "just metadata" ... they no longer store components directly. They do store:
* The `ComponentId`s of each of the Archetype's components (and that component's storage type)
* Archetypes are uniquely defined by their component layouts
* For example: entities with "table" components `[A, B, C]` _and_ "sparse set" components `[D, E]` will always be in the same archetype.
* The `TableId` associated with the archetype
* For now each archetype has exactly one table (which can have no components),
* There is a 1->Many relationship from Tables->Archetypes. A given table could have any number of archetype components stored in it:
* Ex: an entity with "table storage" components `[A, B, C]` and "sparse set" components `[D, E]` will share the same `[A, B, C]` table as an entity with `[A, B, C]` table component and `[F]` sparse set components.
* This 1->Many relationship is how we preserve fast "cache friendly" iteration performance when possible (more on this later)
* A list of entities that are in the archetype and the row id of the table they are in
* ArchetypeComponentIds
* unique densely packed identifiers for (ArchetypeId, ComponentId) pairs
* used by the schedule executor for cheap system access control
* "Archetype Graph Edges" (see the next section)
## The "Archetype Graph"
Archetype changes in Bevy (and a number of other archetypal ecs-es) have historically been expensive to compute. First, you need to allocate a new vector of the entity's current component ids, add or remove components based on the operation performed, sort it (to ensure it is order-independent), then hash it to find the archetype (if it exists). And thats all before we get to the _already_ expensive full copy of all components to the new table storage.
The solution is to build a "graph" of archetypes to cache these results. @SanderMertens first exposed me to the idea (and he got it from @gjroelofs, who came up with it). They propose adding directed edges between archetypes for add/remove component operations. If `ComponentId`s are densely packed, you can use sparse sets to cheaply jump between archetypes.
Bevy takes this one step further by using add/remove `Bundle` edges instead of `Component` edges. Bevy encourages the use of `Bundles` to group add/remove operations. This is largely for "clearer game logic" reasons, but it also helps cut down on the number of archetype changes required. `Bundles` now also have densely-packed `BundleId`s. This allows us to use a _single_ edge for each bundle operation (rather than needing to traverse N edges ... one for each component). Single component operations are also bundles, so this is strictly an improvement over a "component only" graph.
As a result, an operation that used to be _heavy_ (both for allocations and compute) is now two dirt-cheap array lookups and zero allocations.
## Stateful Queries
World queries are now stateful. This allows us to:
1. Cache archetype (and table) matches
* This resolves another issue with (naive) archetypal ECS: query performance getting worse as the number of archetypes goes up (and fragmentation occurs).
2. Cache Fetch and Filter state
* The expensive parts of fetch/filter operations (such as hashing the TypeId to find the ComponentId) now only happen once when the Query is first constructed
3. Incrementally build up state
* When new archetypes are added, we only process the new archetypes (no need to rebuild state for old archetypes)
As a result, the direct `World` query api now looks like this:
```rust
let mut query = world.query::<(&A, &mut B)>();
for (a, mut b) in query.iter_mut(&mut world) {
}
```
Requiring `World` to generate stateful queries (rather than letting the `QueryState` type be constructed separately) allows us to ensure that _all_ queries are properly initialized (and the relevant world state, such as ComponentIds). This enables QueryState to remove branches from its operations that check for initialization status (and also enables query.iter() to take an immutable world reference because it doesn't need to initialize anything in world).
However in systems, this is a non-breaking change. State management is done internally by the relevant SystemParam.
## Stateful SystemParams
Like Queries, `SystemParams` now also cache state. For example, `Query` system params store the "stateful query" state mentioned above. Commands store their internal `CommandQueue`. This means you can now safely use as many separate `Commands` parameters in your system as you want. `Local<T>` system params store their `T` value in their state (instead of in Resources).
SystemParam state also enabled a significant slim-down of SystemState. It is much nicer to look at now.
Per-SystemParam state naturally insulates us from an "aliased mut" class of errors we have hit in the past (ex: using multiple `Commands` system params).
(credit goes to @DJMcNab for the initial idea and draft pr here #1364)
## Configurable SystemParams
@DJMcNab also had the great idea to make SystemParams configurable. This allows users to provide some initial configuration / values for system parameters (when possible). Most SystemParams have no config (the config type is `()`), but the `Local<T>` param now supports user-provided parameters:
```rust
fn foo(value: Local<usize>) {
}
app.add_system(foo.system().config(|c| c.0 = Some(10)));
```
## Uber Fast "for_each" Query Iterators
Developers now have the choice to use a fast "for_each" iterator, which yields ~1.5-3x iteration speed improvements for "fragmented iteration", and minor ~1.2x iteration speed improvements for unfragmented iteration.
```rust
fn system(query: Query<(&A, &mut B)>) {
// you now have the option to do this for a speed boost
query.for_each_mut(|(a, mut b)| {
});
// however normal iterators are still available
for (a, mut b) in query.iter_mut() {
}
}
```
I think in most cases we should continue to encourage "normal" iterators as they are more flexible and more "rust idiomatic". But when that extra "oomf" is needed, it makes sense to use `for_each`.
We should also consider using `for_each` for internal bevy systems to give our users a nice speed boost (but that should be a separate pr).
## Component Metadata
`World` now has a `Components` collection, which is accessible via `world.components()`. This stores mappings from `ComponentId` to `ComponentInfo`, as well as `TypeId` to `ComponentId` mappings (where relevant). `ComponentInfo` stores information about the component, such as ComponentId, TypeId, memory layout, send-ness (currently limited to resources), and storage type.
## Significantly Cheaper `Access<T>`
We used to use `TypeAccess<TypeId>` to manage read/write component/archetype-component access. This was expensive because TypeIds must be hashed and compared individually. The parallel executor got around this by "condensing" type ids into bitset-backed access types. This worked, but it had to be re-generated from the `TypeAccess<TypeId>`sources every time archetypes changed.
This pr removes TypeAccess in favor of faster bitset access everywhere. We can do this thanks to the move to densely packed `ComponentId`s and `ArchetypeComponentId`s.
## Merged Resources into World
Resources had a lot of redundant functionality with Components. They stored typed data, they had access control, they had unique ids, they were queryable via SystemParams, etc. In fact the _only_ major difference between them was that they were unique (and didn't correlate to an entity).
Separate resources also had the downside of requiring a separate set of access controls, which meant the parallel executor needed to compare more bitsets per system and manage more state.
I initially got the "separate resources" idea from `legion`. I think that design was motivated by the fact that it made the direct world query/resource lifetime interactions more manageable. It certainly made our lives easier when using Resources alongside hecs/bevy_ecs. However we already have a construct for safely and ergonomically managing in-world lifetimes: systems (which use `Access<T>` internally).
This pr merges Resources into World:
```rust
world.insert_resource(1);
world.insert_resource(2.0);
let a = world.get_resource::<i32>().unwrap();
let mut b = world.get_resource_mut::<f64>().unwrap();
*b = 3.0;
```
Resources are now just a special kind of component. They have their own ComponentIds (and their own resource TypeId->ComponentId scope, so they don't conflict wit components of the same type). They are stored in a special "resource archetype", which stores components inside the archetype using a new `unique_components` sparse set (note that this sparse set could later be used to implement Tags). This allows us to keep the code size small by reusing existing datastructures (namely Column, Archetype, ComponentFlags, and ComponentInfo). This allows us the executor to use a single `Access<ArchetypeComponentId>` per system. It should also make scripting language integration easier.
_But_ this merge did create problems for people directly interacting with `World`. What if you need mutable access to multiple resources at the same time? `world.get_resource_mut()` borrows World mutably!
## WorldCell
WorldCell applies the `Access<ArchetypeComponentId>` concept to direct world access:
```rust
let world_cell = world.cell();
let a = world_cell.get_resource_mut::<i32>().unwrap();
let b = world_cell.get_resource_mut::<f64>().unwrap();
```
This adds cheap runtime checks (a sparse set lookup of `ArchetypeComponentId` and a counter) to ensure that world accesses do not conflict with each other. Each operation returns a `WorldBorrow<'w, T>` or `WorldBorrowMut<'w, T>` wrapper type, which will release the relevant ArchetypeComponentId resources when dropped.
World caches the access sparse set (and only one cell can exist at a time), so `world.cell()` is a cheap operation.
WorldCell does _not_ use atomic operations. It is non-send, does a mutable borrow of world to prevent other accesses, and uses a simple `Rc<RefCell<ArchetypeComponentAccess>>` wrapper in each WorldBorrow pointer.
The api is currently limited to resource access, but it can and should be extended to queries / entity component access.
## Resource Scopes
WorldCell does not yet support component queries, and even when it does there are sometimes legitimate reasons to want a mutable world ref _and_ a mutable resource ref (ex: bevy_render and bevy_scene both need this). In these cases we could always drop down to the unsafe `world.get_resource_unchecked_mut()`, but that is not ideal!
Instead developers can use a "resource scope"
```rust
world.resource_scope(|world: &mut World, a: &mut A| {
})
```
This temporarily removes the `A` resource from `World`, provides mutable pointers to both, and re-adds A to World when finished. Thanks to the move to ComponentIds/sparse sets, this is a cheap operation.
If multiple resources are required, scopes can be nested. We could also consider adding a "resource tuple" to the api if this pattern becomes common and the boilerplate gets nasty.
## Query Conflicts Use ComponentId Instead of ArchetypeComponentId
For safety reasons, systems cannot contain queries that conflict with each other without wrapping them in a QuerySet. On bevy `main`, we use ArchetypeComponentIds to determine conflicts. This is nice because it can take into account filters:
```rust
// these queries will never conflict due to their filters
fn filter_system(a: Query<&mut A, With<B>>, b: Query<&mut B, Without<B>>) {
}
```
But it also has a significant downside:
```rust
// these queries will not conflict _until_ an entity with A, B, and C is spawned
fn maybe_conflicts_system(a: Query<(&mut A, &C)>, b: Query<(&mut A, &B)>) {
}
```
The system above will panic at runtime if an entity with A, B, and C is spawned. This makes it hard to trust that your game logic will run without crashing.
In this pr, I switched to using `ComponentId` instead. This _is_ more constraining. `maybe_conflicts_system` will now always fail, but it will do it consistently at startup. Naively, it would also _disallow_ `filter_system`, which would be a significant downgrade in usability. Bevy has a number of internal systems that rely on disjoint queries and I expect it to be a common pattern in userspace.
To resolve this, I added a new `FilteredAccess<T>` type, which wraps `Access<T>` and adds with/without filters. If two `FilteredAccess` have with/without values that prove they are disjoint, they will no longer conflict.
## EntityRef / EntityMut
World entity operations on `main` require that the user passes in an `entity` id to each operation:
```rust
let entity = world.spawn((A, )); // create a new entity with A
world.get::<A>(entity);
world.insert(entity, (B, C));
world.insert_one(entity, D);
```
This means that each operation needs to look up the entity location / verify its validity. The initial spawn operation also requires a Bundle as input. This can be awkward when no components are required (or one component is required).
These operations have been replaced by `EntityRef` and `EntityMut`, which are "builder-style" wrappers around world that provide read and read/write operations on a single, pre-validated entity:
```rust
// spawn now takes no inputs and returns an EntityMut
let entity = world.spawn()
.insert(A) // insert a single component into the entity
.insert_bundle((B, C)) // insert a bundle of components into the entity
.id() // id returns the Entity id
// Returns EntityMut (or panics if the entity does not exist)
world.entity_mut(entity)
.insert(D)
.insert_bundle(SomeBundle::default());
{
// returns EntityRef (or panics if the entity does not exist)
let d = world.entity(entity)
.get::<D>() // gets the D component
.unwrap();
// world.get still exists for ergonomics
let d = world.get::<D>(entity).unwrap();
}
// These variants return Options if you want to check existence instead of panicing
world.get_entity_mut(entity)
.unwrap()
.insert(E);
if let Some(entity_ref) = world.get_entity(entity) {
let d = entity_ref.get::<D>().unwrap();
}
```
This _does not_ affect the current Commands api or terminology. I think that should be a separate conversation as that is a much larger breaking change.
## Safety Improvements
* Entity reservation in Commands uses a normal world borrow instead of an unsafe transmute
* QuerySets no longer transmutes lifetimes
* Made traits "unsafe" when implementing a trait incorrectly could cause unsafety
* More thorough safety docs
## RemovedComponents SystemParam
The old approach to querying removed components: `query.removed:<T>()` was confusing because it had no connection to the query itself. I replaced it with the following, which is both clearer and allows us to cache the ComponentId mapping in the SystemParamState:
```rust
fn system(removed: RemovedComponents<T>) {
for entity in removed.iter() {
}
}
```
## Simpler Bundle implementation
Bundles are no longer responsible for sorting (or deduping) TypeInfo. They are just a simple ordered list of component types / data. This makes the implementation smaller and opens the door to an easy "nested bundle" implementation in the future (which i might even add in this pr). Duplicate detection is now done once per bundle type by World the first time a bundle is used.
## Unified WorldQuery and QueryFilter types
(don't worry they are still separate type _parameters_ in Queries .. this is a non-breaking change)
WorldQuery and QueryFilter were already basically identical apis. With the addition of `FetchState` and more storage-specific fetch methods, the overlap was even clearer (and the redundancy more painful).
QueryFilters are now just `F: WorldQuery where F::Fetch: FilterFetch`. FilterFetch requires `Fetch<Item = bool>` and adds new "short circuit" variants of fetch methods. This enables a filter tuple like `(With<A>, Without<B>, Changed<C>)` to stop evaluating the filter after the first mismatch is encountered. FilterFetch is automatically implemented for `Fetch` implementations that return bool.
This forces fetch implementations that return things like `(bool, bool, bool)` (such as the filter above) to manually implement FilterFetch and decide whether or not to short-circuit.
## More Granular Modules
World no longer globs all of the internal modules together. It now exports `core`, `system`, and `schedule` separately. I'm also considering exporting `core` submodules directly as that is still pretty "glob-ey" and unorganized (feedback welcome here).
## Remaining Draft Work (to be done in this pr)
* ~~panic on conflicting WorldQuery fetches (&A, &mut A)~~
* ~~bevy `main` and hecs both currently allow this, but we should protect against it if possible~~
* ~~batch_iter / par_iter (currently stubbed out)~~
* ~~ChangedRes~~
* ~~I skipped this while we sort out #1313. This pr should be adapted to account for whatever we land on there~~.
* ~~The `Archetypes` and `Tables` collections use hashes of sorted lists of component ids to uniquely identify each archetype/table. This hash is then used as the key in a HashMap to look up the relevant ArchetypeId or TableId. (which doesn't handle hash collisions properly)~~
* ~~It is currently unsafe to generate a Query from "World A", then use it on "World B" (despite the api claiming it is safe). We should probably close this gap. This could be done by adding a randomly generated WorldId to each world, then storing that id in each Query. They could then be compared to each other on each `query.do_thing(&world)` operation. This _does_ add an extra branch to each query operation, so I'm open to other suggestions if people have them.~~
* ~~Nested Bundles (if i find time)~~
## Potential Future Work
* Expand WorldCell to support queries.
* Consider not allocating in the empty archetype on `world.spawn()`
* ex: return something like EntityMutUninit, which turns into EntityMut after an `insert` or `insert_bundle` op
* this actually regressed performance last time i tried it, but in theory it should be faster
* Optimize SparseSet::insert (see `PERF` comment on insert)
* Replace SparseArray `Option<T>` with T::MAX to cut down on branching
* would enable cheaper get_unchecked() operations
* upstream fixedbitset optimizations
* fixedbitset could be allocation free for small block counts (store blocks in a SmallVec)
* fixedbitset could have a const constructor
* Consider implementing Tags (archetype-specific by-value data that affects archetype identity)
* ex: ArchetypeA could have `[A, B, C]` table components and `[D(1)]` "tag" component. ArchetypeB could have `[A, B, C]` table components and a `[D(2)]` tag component. The archetypes are different, despite both having D tags because the value inside D is different.
* this could potentially build on top of the `archetype.unique_components` added in this pr for resource storage.
* Consider reverting `all_tuples` proc macro in favor of the old `macro_rules` implementation
* all_tuples is more flexible and produces cleaner documentation (the macro_rules version produces weird type parameter orders due to parser constraints)
* but unfortunately all_tuples also appears to make Rust Analyzer sad/slow when working inside of `bevy_ecs` (does not affect user code)
* Consider "resource queries" and/or "mixed resource and entity component queries" as an alternative to WorldCell
* this is basically just "systems" so maybe it's not worth it
* Add more world ops
* `world.clear()`
* `world.reserve<T: Bundle>(count: usize)`
* Try using the old archetype allocation strategy (allocate new memory on resize and copy everything over). I expect this to improve batch insertion performance at the cost of unbatched performance. But thats just a guess. I'm not an allocation perf pro :)
* Adapt Commands apis for consistency with new World apis
## Benchmarks
key:
* `bevy_old`: bevy `main` branch
* `bevy`: this branch
* `_foreach`: uses an optimized for_each iterator
* ` _sparse`: uses sparse set storage (if unspecified assume table storage)
* `_system`: runs inside a system (if unspecified assume test happens via direct world ops)
### Simple Insert (from ecs_bench_suite)
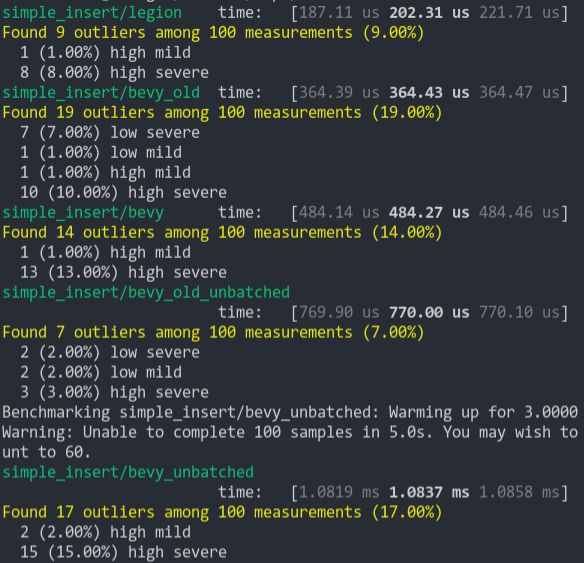
### Simpler Iter (from ecs_bench_suite)
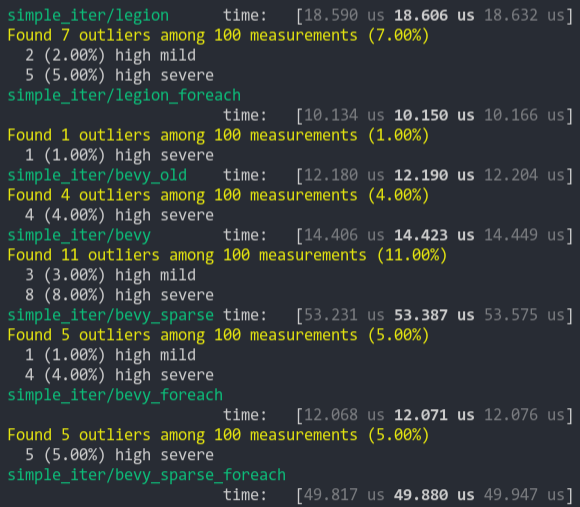
### Fragment Iter (from ecs_bench_suite)
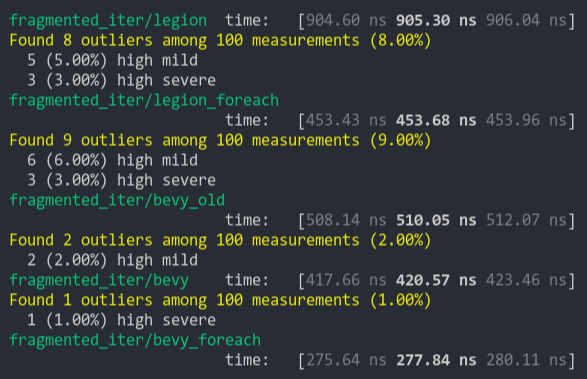
### Sparse Fragmented Iter
Iterate a query that matches 5 entities from a single matching archetype, but there are 100 unmatching archetypes
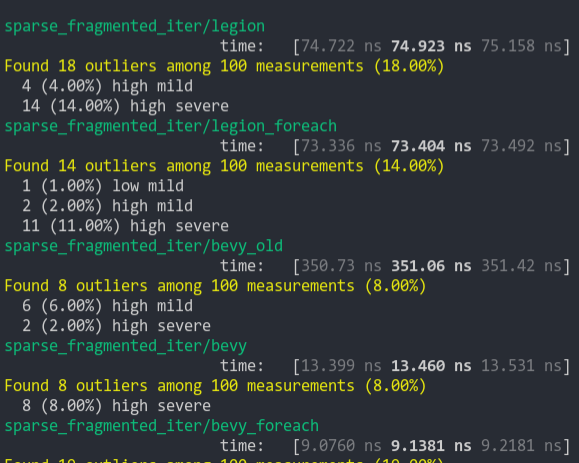
### Schedule (from ecs_bench_suite)
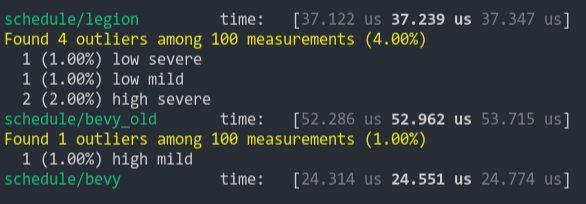
### Add Remove Component (from ecs_bench_suite)
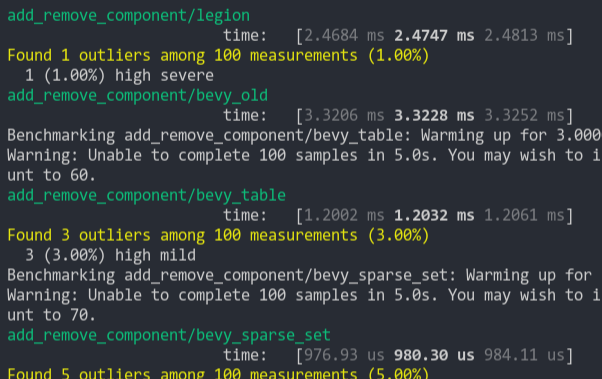
### Add Remove Component Big
Same as the test above, but each entity has 5 "large" matrix components and 1 "large" matrix component is added and removed
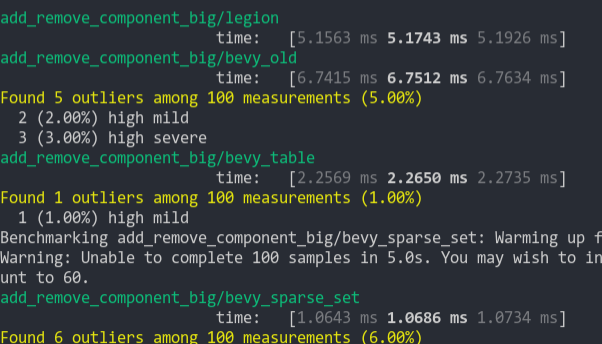
### Get Component
Looks up a single component value a large number of times
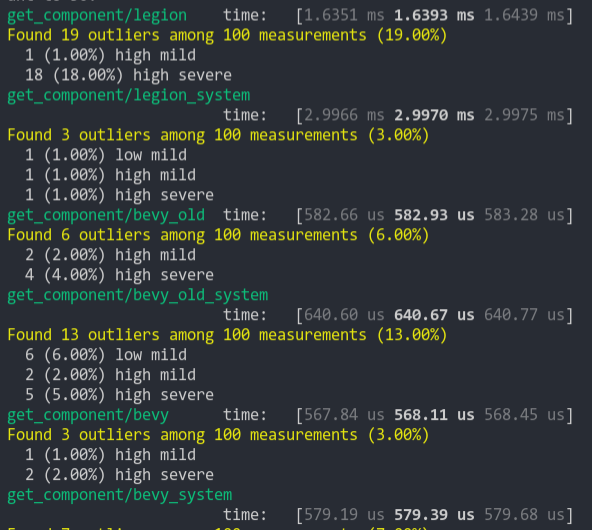
2021-03-05 07:54:35 +00:00
#[ inline ]
Simplify trait hierarchy for `SystemParam` (#6865)
# Objective
* Implementing a custom `SystemParam` by hand requires implementing three traits -- four if it is read-only.
* The trait `SystemParamFetch<'w, 's>` is a workaround from before we had generic associated types, and is no longer necessary.
## Solution
* Combine the trait `SystemParamFetch` with `SystemParamState`.
* I decided to remove the `Fetch` name and keep the `State` name, since the former was consistently conflated with the latter.
* Replace the trait `ReadOnlySystemParamFetch` with `ReadOnlySystemParam`, which simplifies trait bounds in generic code.
---
## Changelog
- Removed the trait `SystemParamFetch`, moving its functionality to `SystemParamState`.
- Replaced the trait `ReadOnlySystemParamFetch` with `ReadOnlySystemParam`.
## Migration Guide
The trait `SystemParamFetch` has been removed, and its functionality has been transferred to `SystemParamState`.
```rust
// Before
impl SystemParamState for MyParamState {
fn init(world: &mut World, system_meta: &mut SystemMeta) -> Self { ... }
}
impl<'w, 's> SystemParamFetch<'w, 's> for MyParamState {
type Item = MyParam<'w, 's>;
fn get_param(...) -> Self::Item;
}
// After
impl SystemParamState for MyParamState {
type Item<'w, 's> = MyParam<'w, 's>; // Generic associated types!
fn init(world: &mut World, system_meta: &mut SystemMeta) -> Self { ... }
fn get_param<'w, 's>(...) -> Self::Item<'w, 's>;
}
```
The trait `ReadOnlySystemParamFetch` has been replaced with `ReadOnlySystemParam`.
```rust
// Before
unsafe impl ReadOnlySystemParamFetch for MyParamState {}
// After
unsafe impl<'w, 's> ReadOnlySystemParam for MyParam<'w, 's> {}
```
2022-12-11 18:34:14 +00:00
unsafe fn get_param < ' w , ' s > (
Remove the `SystemParamState` trait and remove types like `ResState` (#6919)
Spiritual successor to #5205.
Actual successor to #6865.
# Objective
Currently, system params are defined using three traits: `SystemParam`, `ReadOnlySystemParam`, `SystemParamState`. The behavior for each param is specified by the `SystemParamState` trait, while `SystemParam` simply defers to the state.
Splitting the traits in this way makes it easier to implement within macros, but it increases the cognitive load. Worst of all, this approach requires each `MySystemParam` to have a public `MySystemParamState` type associated with it.
## Solution
* Merge the trait `SystemParamState` into `SystemParam`.
* Remove all trivial `SystemParam` state types.
* `OptionNonSendMutState<T>`: you will not be missed.
---
- [x] Fix/resolve the remaining test failure.
## Changelog
* Removed the trait `SystemParamState`, merging its functionality into `SystemParam`.
## Migration Guide
**Note**: this should replace the migration guide for #6865.
This is relative to Bevy 0.9, not main.
The traits `SystemParamState` and `SystemParamFetch` have been removed, and their functionality has been transferred to `SystemParam`.
```rust
// Before (0.9)
impl SystemParam for MyParam<'_, '_> {
type State = MyParamState;
}
unsafe impl SystemParamState for MyParamState {
fn init(world: &mut World, system_meta: &mut SystemMeta) -> Self { ... }
}
unsafe impl<'w, 's> SystemParamFetch<'w, 's> for MyParamState {
type Item = MyParam<'w, 's>;
fn get_param(&mut self, ...) -> Self::Item;
}
unsafe impl ReadOnlySystemParamFetch for MyParamState { }
// After (0.10)
unsafe impl SystemParam for MyParam<'_, '_> {
type State = MyParamState;
type Item<'w, 's> = MyParam<'w, 's>;
fn init_state(world: &mut World, system_meta: &mut SystemMeta) -> Self::State { ... }
fn get_param<'w, 's>(state: &mut Self::State, ...) -> Self::Item<'w, 's>;
}
unsafe impl ReadOnlySystemParam for MyParam<'_, '_> { }
```
The trait `ReadOnlySystemParamFetch` has been replaced with `ReadOnlySystemParam`.
```rust
// Before
unsafe impl ReadOnlySystemParamFetch for MyParamState {}
// After
unsafe impl ReadOnlySystemParam for MyParam<'_, '_> {}
```
2023-01-07 23:20:32 +00:00
_state : & ' s mut Self ::State ,
Add new SystemState and rename old SystemState to SystemMeta (#2283)
This enables `SystemParams` to be used outside of function systems. Anything can create and store `SystemState`, which enables efficient "param state cached" access to `SystemParams`.
It adds a `ReadOnlySystemParamFetch` trait, which enables safe `SystemState::get` calls without unique world access.
I renamed the old `SystemState` to `SystemMeta` to enable us to mirror the `QueryState` naming convention (but I'm happy to discuss alternative names if people have other ideas). I initially pitched this as `ParamState`, but given that it needs to include full system metadata, that doesn't feel like a particularly accurate name.
```rust
#[derive(Eq, PartialEq, Debug)]
struct A(usize);
#[derive(Eq, PartialEq, Debug)]
struct B(usize);
let mut world = World::default();
world.insert_resource(A(42));
world.spawn().insert(B(7));
// we get nice lifetime elision when declaring the type on the left hand side
let mut system_state: SystemState<(Res<A>, Query<&B>)> = SystemState::new(&mut world);
let (a, query) = system_state.get(&world);
assert_eq!(*a, A(42), "returned resource matches initial value");
assert_eq!(
*query.single().unwrap(),
B(7),
"returned component matches initial value"
);
// mutable system params require unique world access
let mut system_state: SystemState<(ResMut<A>, Query<&mut B>)> = SystemState::new(&mut world);
let (a, query) = system_state.get_mut(&mut world);
// static lifetimes are required when declaring inside of structs
struct SomeContainer {
state: SystemState<(Res<'static, A>, Res<'static, B>)>
}
// this can be shortened using type aliases, which will be useful for complex param tuples
type MyParams<'a> = (Res<'a, A>, Res<'a, B>);
struct SomeContainer {
state: SystemState<MyParams<'static>>
}
// It is the user's responsibility to call SystemState::apply(world) for parameters that queue up work
let mut system_state: SystemState<(Commands, Query<&B>)> = SystemState::new(&mut world);
{
let (mut commands, query) = system_state.get(&world);
commands.insert_resource(3.14);
}
system_state.apply(&mut world);
```
## Future Work
* Actually use SystemState inside FunctionSystem. This would be trivial, but it requires FunctionSystem to wrap SystemState in Option in its current form (which complicates system metadata lookup). I'd prefer to hold off until we adopt something like the later designs linked in #1364, which enable us to contruct Systems using a World reference (and also remove the need for `.system`).
* Consider a "scoped" approach to automatically call SystemState::apply when systems params are no longer being used (either a container type with a Drop impl, or a function that takes a closure for user logic operating on params).
2021-06-02 19:57:38 +00:00
_system_meta : & SystemMeta ,
System Param Lifetime Split (#2605)
# Objective
Enable using exact World lifetimes during read-only access . This is motivated by the new renderer's need to allow read-only world-only queries to outlive the query itself (but still be constrained by the world lifetime).
For example:
https://github.com/bevyengine/bevy/blob/115b170d1f11a91146bb6d6e9684dceb8b21f786/pipelined/bevy_pbr2/src/render/mod.rs#L774
## Solution
Split out SystemParam state and world lifetimes and pipe those lifetimes up to read-only Query ops (and add into_inner for Res). According to every safety test I've run so far (except one), this is safe (see the temporary safety test commit). Note that changing the mutable variants to the new lifetimes would allow aliased mutable pointers (try doing that to see how it affects the temporary safety tests).
The new state lifetime on SystemParam does make `#[derive(SystemParam)]` more cumbersome (the current impl requires PhantomData if you don't use both lifetimes). We can make this better by detecting whether or not a lifetime is used in the derive and adjusting accordingly, but that should probably be done in its own pr.
## Why is this a draft?
The new lifetimes break QuerySet safety in one very specific case (see the query_set system in system_safety_test). We need to solve this before we can use the lifetimes given.
This is due to the fact that QuerySet is just a wrapper over Query, which now relies on world lifetimes instead of `&self` lifetimes to prevent aliasing (but in systems, each Query has its own implied lifetime, not a centralized world lifetime). I believe the fix is to rewrite QuerySet to have its own World lifetime (and own the internal reference). This will complicate the impl a bit, but I think it is doable. I'm curious if anyone else has better ideas.
Personally, I think these new lifetimes need to happen. We've gotta have a way to directly tie read-only World queries to the World lifetime. The new renderer is the first place this has come up, but I doubt it will be the last. Worst case scenario we can come up with a second `WorldLifetimeQuery<Q, F = ()>` parameter to enable these read-only scenarios, but I'd rather not add another type to the type zoo.
2021-08-15 20:51:53 +00:00
world : & ' w World ,
Reliable change detection (#1471)
# Problem Definition
The current change tracking (via flags for both components and resources) fails to detect changes made by systems that are scheduled to run earlier in the frame than they are.
This issue is discussed at length in [#68](https://github.com/bevyengine/bevy/issues/68) and [#54](https://github.com/bevyengine/bevy/issues/54).
This is very much a draft PR, and contributions are welcome and needed.
# Criteria
1. Each change is detected at least once, no matter the ordering.
2. Each change is detected at most once, no matter the ordering.
3. Changes should be detected the same frame that they are made.
4. Competitive ergonomics. Ideally does not require opting-in.
5. Low CPU overhead of computation.
6. Memory efficient. This must not increase over time, except where the number of entities / resources does.
7. Changes should not be lost for systems that don't run.
8. A frame needs to act as a pure function. Given the same set of entities / components it needs to produce the same end state without side-effects.
**Exact** change-tracking proposals satisfy criteria 1 and 2.
**Conservative** change-tracking proposals satisfy criteria 1 but not 2.
**Flaky** change tracking proposals satisfy criteria 2 but not 1.
# Code Base Navigation
There are three types of flags:
- `Added`: A piece of data was added to an entity / `Resources`.
- `Mutated`: A piece of data was able to be modified, because its `DerefMut` was accessed
- `Changed`: The bitwise OR of `Added` and `Changed`
The special behavior of `ChangedRes`, with respect to the scheduler is being removed in [#1313](https://github.com/bevyengine/bevy/pull/1313) and does not need to be reproduced.
`ChangedRes` and friends can be found in "bevy_ecs/core/resources/resource_query.rs".
The `Flags` trait for Components can be found in "bevy_ecs/core/query.rs".
`ComponentFlags` are stored in "bevy_ecs/core/archetypes.rs", defined on line 446.
# Proposals
**Proposal 5 was selected for implementation.**
## Proposal 0: No Change Detection
The baseline, where computations are performed on everything regardless of whether it changed.
**Type:** Conservative
**Pros:**
- already implemented
- will never miss events
- no overhead
**Cons:**
- tons of repeated work
- doesn't allow users to avoid repeating work (or monitoring for other changes)
## Proposal 1: Earlier-This-Tick Change Detection
The current approach as of Bevy 0.4. Flags are set, and then flushed at the end of each frame.
**Type:** Flaky
**Pros:**
- already implemented
- simple to understand
- low memory overhead (2 bits per component)
- low time overhead (clear every flag once per frame)
**Cons:**
- misses systems based on ordering
- systems that don't run every frame miss changes
- duplicates detection when looping
- can lead to unresolvable circular dependencies
## Proposal 2: Two-Tick Change Detection
Flags persist for two frames, using a double-buffer system identical to that used in events.
A change is observed if it is found in either the current frame's list of changes or the previous frame's.
**Type:** Conservative
**Pros:**
- easy to understand
- easy to implement
- low memory overhead (4 bits per component)
- low time overhead (bit mask and shift every flag once per frame)
**Cons:**
- can result in a great deal of duplicated work
- systems that don't run every frame miss changes
- duplicates detection when looping
## Proposal 3: Last-Tick Change Detection
Flags persist for two frames, using a double-buffer system identical to that used in events.
A change is observed if it is found in the previous frame's list of changes.
**Type:** Exact
**Pros:**
- exact
- easy to understand
- easy to implement
- low memory overhead (4 bits per component)
- low time overhead (bit mask and shift every flag once per frame)
**Cons:**
- change detection is always delayed, possibly causing painful chained delays
- systems that don't run every frame miss changes
- duplicates detection when looping
## Proposal 4: Flag-Doubling Change Detection
Combine Proposal 2 and Proposal 3. Differentiate between `JustChanged` (current behavior) and `Changed` (Proposal 3).
Pack this data into the flags according to [this implementation proposal](https://github.com/bevyengine/bevy/issues/68#issuecomment-769174804).
**Type:** Flaky + Exact
**Pros:**
- allows users to acc
- easy to implement
- low memory overhead (4 bits per component)
- low time overhead (bit mask and shift every flag once per frame)
**Cons:**
- users must specify the type of change detection required
- still quite fragile to system ordering effects when using the flaky `JustChanged` form
- cannot get immediate + exact results
- systems that don't run every frame miss changes
- duplicates detection when looping
## [SELECTED] Proposal 5: Generation-Counter Change Detection
A global counter is increased after each system is run. Each component saves the time of last mutation, and each system saves the time of last execution. Mutation is detected when the component's counter is greater than the system's counter. Discussed [here](https://github.com/bevyengine/bevy/issues/68#issuecomment-769174804). How to handle addition detection is unsolved; the current proposal is to use the highest bit of the counter as in proposal 1.
**Type:** Exact (for mutations), flaky (for additions)
**Pros:**
- low time overhead (set component counter on access, set system counter after execution)
- robust to systems that don't run every frame
- robust to systems that loop
**Cons:**
- moderately complex implementation
- must be modified as systems are inserted dynamically
- medium memory overhead (4 bytes per component + system)
- unsolved addition detection
## Proposal 6: System-Data Change Detection
For each system, track which system's changes it has seen. This approach is only worth fully designing and implementing if Proposal 5 fails in some way.
**Type:** Exact
**Pros:**
- exact
- conceptually simple
**Cons:**
- requires storing data on each system
- implementation is complex
- must be modified as systems are inserted dynamically
## Proposal 7: Total-Order Change Detection
Discussed [here](https://github.com/bevyengine/bevy/issues/68#issuecomment-754326523). This proposal is somewhat complicated by the new scheduler, but I believe it should still be conceptually feasible. This approach is only worth fully designing and implementing if Proposal 5 fails in some way.
**Type:** Exact
**Pros:**
- exact
- efficient data storage relative to other exact proposals
**Cons:**
- requires access to the scheduler
- complex implementation and difficulty grokking
- must be modified as systems are inserted dynamically
# Tests
- We will need to verify properties 1, 2, 3, 7 and 8. Priority: 1 > 2 = 3 > 8 > 7
- Ideally we can use identical user-facing syntax for all proposals, allowing us to re-use the same syntax for each.
- When writing tests, we need to carefully specify order using explicit dependencies.
- These tests will need to be duplicated for both components and resources.
- We need to be sure to handle cases where ambiguous system orders exist.
`changing_system` is always the system that makes the changes, and `detecting_system` always detects the changes.
The component / resource changed will be simple boolean wrapper structs.
## Basic Added / Mutated / Changed
2 x 3 design:
- Resources vs. Components
- Added vs. Changed vs. Mutated
- `changing_system` runs before `detecting_system`
- verify at the end of tick 2
## At Least Once
2 x 3 design:
- Resources vs. Components
- Added vs. Changed vs. Mutated
- `changing_system` runs after `detecting_system`
- verify at the end of tick 2
## At Most Once
2 x 3 design:
- Resources vs. Components
- Added vs. Changed vs. Mutated
- `changing_system` runs once before `detecting_system`
- increment a counter based on the number of changes detected
- verify at the end of tick 2
## Fast Detection
2 x 3 design:
- Resources vs. Components
- Added vs. Changed vs. Mutated
- `changing_system` runs before `detecting_system`
- verify at the end of tick 1
## Ambiguous System Ordering Robustness
2 x 3 x 2 design:
- Resources vs. Components
- Added vs. Changed vs. Mutated
- `changing_system` runs [before/after] `detecting_system` in tick 1
- `changing_system` runs [after/before] `detecting_system` in tick 2
## System Pausing
2 x 3 design:
- Resources vs. Components
- Added vs. Changed vs. Mutated
- `changing_system` runs in tick 1, then is disabled by run criteria
- `detecting_system` is disabled by run criteria until it is run once during tick 3
- verify at the end of tick 3
## Addition Causes Mutation
2 design:
- Resources vs. Components
- `adding_system_1` adds a component / resource
- `adding system_2` adds the same component / resource
- verify the `Mutated` flag at the end of the tick
- verify the `Added` flag at the end of the tick
First check tests for: https://github.com/bevyengine/bevy/issues/333
Second check tests for: https://github.com/bevyengine/bevy/issues/1443
## Changes Made By Commands
- `adding_system` runs in Update in tick 1, and sends a command to add a component
- `detecting_system` runs in Update in tick 1 and 2, after `adding_system`
- We can't detect the changes in tick 1, since they haven't been processed yet
- If we were to track these changes as being emitted by `adding_system`, we can't detect the changes in tick 2 either, since `detecting_system` has already run once after `adding_system` :(
# Benchmarks
See: [general advice](https://github.com/bevyengine/bevy/blob/master/docs/profiling.md), [Criterion crate](https://github.com/bheisler/criterion.rs)
There are several critical parameters to vary:
1. entity count (1 to 10^9)
2. fraction of entities that are changed (0% to 100%)
3. cost to perform work on changed entities, i.e. workload (1 ns to 1s)
1 and 2 should be varied between benchmark runs. 3 can be added on computationally.
We want to measure:
- memory cost
- run time
We should collect these measurements across several frames (100?) to reduce bootup effects and accurately measure the mean, variance and drift.
Entity-component change detection is much more important to benchmark than resource change detection, due to the orders of magnitude higher number of pieces of data.
No change detection at all should be included in benchmarks as a second control for cases where missing changes is unacceptable.
## Graphs
1. y: performance, x: log_10(entity count), color: proposal, facet: performance metric. Set cost to perform work to 0.
2. y: run time, x: cost to perform work, color: proposal, facet: fraction changed. Set number of entities to 10^6
3. y: memory, x: frames, color: proposal
# Conclusions
1. Is the theoretical categorization of the proposals correct according to our tests?
2. How does the performance of the proposals compare without any load?
3. How does the performance of the proposals compare with realistic loads?
4. At what workload does more exact change tracking become worth the (presumably) higher overhead?
5. When does adding change-detection to save on work become worthwhile?
6. Is there enough divergence in performance between the best solutions in each class to ship more than one change-tracking solution?
# Implementation Plan
1. Write a test suite.
2. Verify that tests fail for existing approach.
3. Write a benchmark suite.
4. Get performance numbers for existing approach.
5. Implement, test and benchmark various solutions using a Git branch per proposal.
6. Create a draft PR with all solutions and present results to team.
7. Select a solution and replace existing change detection.
Co-authored-by: Brice DAVIER <bricedavier@gmail.com>
Co-authored-by: Carter Anderson <mcanders1@gmail.com>
2021-03-19 17:53:26 +00:00
_change_tick : u32 ,
Simplify trait hierarchy for `SystemParam` (#6865)
# Objective
* Implementing a custom `SystemParam` by hand requires implementing three traits -- four if it is read-only.
* The trait `SystemParamFetch<'w, 's>` is a workaround from before we had generic associated types, and is no longer necessary.
## Solution
* Combine the trait `SystemParamFetch` with `SystemParamState`.
* I decided to remove the `Fetch` name and keep the `State` name, since the former was consistently conflated with the latter.
* Replace the trait `ReadOnlySystemParamFetch` with `ReadOnlySystemParam`, which simplifies trait bounds in generic code.
---
## Changelog
- Removed the trait `SystemParamFetch`, moving its functionality to `SystemParamState`.
- Replaced the trait `ReadOnlySystemParamFetch` with `ReadOnlySystemParam`.
## Migration Guide
The trait `SystemParamFetch` has been removed, and its functionality has been transferred to `SystemParamState`.
```rust
// Before
impl SystemParamState for MyParamState {
fn init(world: &mut World, system_meta: &mut SystemMeta) -> Self { ... }
}
impl<'w, 's> SystemParamFetch<'w, 's> for MyParamState {
type Item = MyParam<'w, 's>;
fn get_param(...) -> Self::Item;
}
// After
impl SystemParamState for MyParamState {
type Item<'w, 's> = MyParam<'w, 's>; // Generic associated types!
fn init(world: &mut World, system_meta: &mut SystemMeta) -> Self { ... }
fn get_param<'w, 's>(...) -> Self::Item<'w, 's>;
}
```
The trait `ReadOnlySystemParamFetch` has been replaced with `ReadOnlySystemParam`.
```rust
// Before
unsafe impl ReadOnlySystemParamFetch for MyParamState {}
// After
unsafe impl<'w, 's> ReadOnlySystemParam for MyParam<'w, 's> {}
```
2022-12-11 18:34:14 +00:00
) -> Self ::Item < ' w , ' s > {
Bevy ECS V2 (#1525)
# Bevy ECS V2
This is a rewrite of Bevy ECS (basically everything but the new executor/schedule, which are already awesome). The overall goal was to improve the performance and versatility of Bevy ECS. Here is a quick bulleted list of changes before we dive into the details:
* Complete World rewrite
* Multiple component storage types:
* Tables: fast cache friendly iteration, slower add/removes (previously called Archetypes)
* Sparse Sets: fast add/remove, slower iteration
* Stateful Queries (caches query results for faster iteration. fragmented iteration is _fast_ now)
* Stateful System Params (caches expensive operations. inspired by @DJMcNab's work in #1364)
* Configurable System Params (users can set configuration when they construct their systems. once again inspired by @DJMcNab's work)
* Archetypes are now "just metadata", component storage is separate
* Archetype Graph (for faster archetype changes)
* Component Metadata
* Configure component storage type
* Retrieve information about component size/type/name/layout/send-ness/etc
* Components are uniquely identified by a densely packed ComponentId
* TypeIds are now totally optional (which should make implementing scripting easier)
* Super fast "for_each" query iterators
* Merged Resources into World. Resources are now just a special type of component
* EntityRef/EntityMut builder apis (more efficient and more ergonomic)
* Fast bitset-backed `Access<T>` replaces old hashmap-based approach everywhere
* Query conflicts are determined by component access instead of archetype component access (to avoid random failures at runtime)
* With/Without are still taken into account for conflicts, so this should still be comfy to use
* Much simpler `IntoSystem` impl
* Significantly reduced the amount of hashing throughout the ecs in favor of Sparse Sets (indexed by densely packed ArchetypeId, ComponentId, BundleId, and TableId)
* Safety Improvements
* Entity reservation uses a normal world reference instead of unsafe transmute
* QuerySets no longer transmute lifetimes
* Made traits "unsafe" where relevant
* More thorough safety docs
* WorldCell
* Exposes safe mutable access to multiple resources at a time in a World
* Replaced "catch all" `System::update_archetypes(world: &World)` with `System::new_archetype(archetype: &Archetype)`
* Simpler Bundle implementation
* Replaced slow "remove_bundle_one_by_one" used as fallback for Commands::remove_bundle with fast "remove_bundle_intersection"
* Removed `Mut<T>` query impl. it is better to only support one way: `&mut T`
* Removed with() from `Flags<T>` in favor of `Option<Flags<T>>`, which allows querying for flags to be "filtered" by default
* Components now have is_send property (currently only resources support non-send)
* More granular module organization
* New `RemovedComponents<T>` SystemParam that replaces `query.removed::<T>()`
* `world.resource_scope()` for mutable access to resources and world at the same time
* WorldQuery and QueryFilter traits unified. FilterFetch trait added to enable "short circuit" filtering. Auto impled for cases that don't need it
* Significantly slimmed down SystemState in favor of individual SystemParam state
* System Commands changed from `commands: &mut Commands` back to `mut commands: Commands` (to allow Commands to have a World reference)
Fixes #1320
## `World` Rewrite
This is a from-scratch rewrite of `World` that fills the niche that `hecs` used to. Yes, this means Bevy ECS is no longer a "fork" of hecs. We're going out our own!
(the only shared code between the projects is the entity id allocator, which is already basically ideal)
A huge shout out to @SanderMertens (author of [flecs](https://github.com/SanderMertens/flecs)) for sharing some great ideas with me (specifically hybrid ecs storage and archetype graphs). He also helped advise on a number of implementation details.
## Component Storage (The Problem)
Two ECS storage paradigms have gained a lot of traction over the years:
* **Archetypal ECS**:
* Stores components in "tables" with static schemas. Each "column" stores components of a given type. Each "row" is an entity.
* Each "archetype" has its own table. Adding/removing an entity's component changes the archetype.
* Enables super-fast Query iteration due to its cache-friendly data layout
* Comes at the cost of more expensive add/remove operations for an Entity's components, because all components need to be copied to the new archetype's "table"
* **Sparse Set ECS**:
* Stores components of the same type in densely packed arrays, which are sparsely indexed by densely packed unsigned integers (Entity ids)
* Query iteration is slower than Archetypal ECS because each entity's component could be at any position in the sparse set. This "random access" pattern isn't cache friendly. Additionally, there is an extra layer of indirection because you must first map the entity id to an index in the component array.
* Adding/removing components is a cheap, constant time operation
Bevy ECS V1, hecs, legion, flec, and Unity DOTS are all "archetypal ecs-es". I personally think "archetypal" storage is a good default for game engines. An entity's archetype doesn't need to change frequently in general, and it creates "fast by default" query iteration (which is a much more common operation). It is also "self optimizing". Users don't need to think about optimizing component layouts for iteration performance. It "just works" without any extra boilerplate.
Shipyard and EnTT are "sparse set ecs-es". They employ "packing" as a way to work around the "suboptimal by default" iteration performance for specific sets of components. This helps, but I didn't think this was a good choice for a general purpose engine like Bevy because:
1. "packs" conflict with each other. If bevy decides to internally pack the Transform and GlobalTransform components, users are then blocked if they want to pack some custom component with Transform.
2. users need to take manual action to optimize
Developers selecting an ECS framework are stuck with a hard choice. Select an "archetypal" framework with "fast iteration everywhere" but without the ability to cheaply add/remove components, or select a "sparse set" framework to cheaply add/remove components but with slower iteration performance.
## Hybrid Component Storage (The Solution)
In Bevy ECS V2, we get to have our cake and eat it too. It now has _both_ of the component storage types above (and more can be added later if needed):
* **Tables** (aka "archetypal" storage)
* The default storage. If you don't configure anything, this is what you get
* Fast iteration by default
* Slower add/remove operations
* **Sparse Sets**
* Opt-in
* Slower iteration
* Faster add/remove operations
These storage types complement each other perfectly. By default Query iteration is fast. If developers know that they want to add/remove a component at high frequencies, they can set the storage to "sparse set":
```rust
world.register_component(
ComponentDescriptor::new::<MyComponent>(StorageType::SparseSet)
).unwrap();
```
## Archetypes
Archetypes are now "just metadata" ... they no longer store components directly. They do store:
* The `ComponentId`s of each of the Archetype's components (and that component's storage type)
* Archetypes are uniquely defined by their component layouts
* For example: entities with "table" components `[A, B, C]` _and_ "sparse set" components `[D, E]` will always be in the same archetype.
* The `TableId` associated with the archetype
* For now each archetype has exactly one table (which can have no components),
* There is a 1->Many relationship from Tables->Archetypes. A given table could have any number of archetype components stored in it:
* Ex: an entity with "table storage" components `[A, B, C]` and "sparse set" components `[D, E]` will share the same `[A, B, C]` table as an entity with `[A, B, C]` table component and `[F]` sparse set components.
* This 1->Many relationship is how we preserve fast "cache friendly" iteration performance when possible (more on this later)
* A list of entities that are in the archetype and the row id of the table they are in
* ArchetypeComponentIds
* unique densely packed identifiers for (ArchetypeId, ComponentId) pairs
* used by the schedule executor for cheap system access control
* "Archetype Graph Edges" (see the next section)
## The "Archetype Graph"
Archetype changes in Bevy (and a number of other archetypal ecs-es) have historically been expensive to compute. First, you need to allocate a new vector of the entity's current component ids, add or remove components based on the operation performed, sort it (to ensure it is order-independent), then hash it to find the archetype (if it exists). And thats all before we get to the _already_ expensive full copy of all components to the new table storage.
The solution is to build a "graph" of archetypes to cache these results. @SanderMertens first exposed me to the idea (and he got it from @gjroelofs, who came up with it). They propose adding directed edges between archetypes for add/remove component operations. If `ComponentId`s are densely packed, you can use sparse sets to cheaply jump between archetypes.
Bevy takes this one step further by using add/remove `Bundle` edges instead of `Component` edges. Bevy encourages the use of `Bundles` to group add/remove operations. This is largely for "clearer game logic" reasons, but it also helps cut down on the number of archetype changes required. `Bundles` now also have densely-packed `BundleId`s. This allows us to use a _single_ edge for each bundle operation (rather than needing to traverse N edges ... one for each component). Single component operations are also bundles, so this is strictly an improvement over a "component only" graph.
As a result, an operation that used to be _heavy_ (both for allocations and compute) is now two dirt-cheap array lookups and zero allocations.
## Stateful Queries
World queries are now stateful. This allows us to:
1. Cache archetype (and table) matches
* This resolves another issue with (naive) archetypal ECS: query performance getting worse as the number of archetypes goes up (and fragmentation occurs).
2. Cache Fetch and Filter state
* The expensive parts of fetch/filter operations (such as hashing the TypeId to find the ComponentId) now only happen once when the Query is first constructed
3. Incrementally build up state
* When new archetypes are added, we only process the new archetypes (no need to rebuild state for old archetypes)
As a result, the direct `World` query api now looks like this:
```rust
let mut query = world.query::<(&A, &mut B)>();
for (a, mut b) in query.iter_mut(&mut world) {
}
```
Requiring `World` to generate stateful queries (rather than letting the `QueryState` type be constructed separately) allows us to ensure that _all_ queries are properly initialized (and the relevant world state, such as ComponentIds). This enables QueryState to remove branches from its operations that check for initialization status (and also enables query.iter() to take an immutable world reference because it doesn't need to initialize anything in world).
However in systems, this is a non-breaking change. State management is done internally by the relevant SystemParam.
## Stateful SystemParams
Like Queries, `SystemParams` now also cache state. For example, `Query` system params store the "stateful query" state mentioned above. Commands store their internal `CommandQueue`. This means you can now safely use as many separate `Commands` parameters in your system as you want. `Local<T>` system params store their `T` value in their state (instead of in Resources).
SystemParam state also enabled a significant slim-down of SystemState. It is much nicer to look at now.
Per-SystemParam state naturally insulates us from an "aliased mut" class of errors we have hit in the past (ex: using multiple `Commands` system params).
(credit goes to @DJMcNab for the initial idea and draft pr here #1364)
## Configurable SystemParams
@DJMcNab also had the great idea to make SystemParams configurable. This allows users to provide some initial configuration / values for system parameters (when possible). Most SystemParams have no config (the config type is `()`), but the `Local<T>` param now supports user-provided parameters:
```rust
fn foo(value: Local<usize>) {
}
app.add_system(foo.system().config(|c| c.0 = Some(10)));
```
## Uber Fast "for_each" Query Iterators
Developers now have the choice to use a fast "for_each" iterator, which yields ~1.5-3x iteration speed improvements for "fragmented iteration", and minor ~1.2x iteration speed improvements for unfragmented iteration.
```rust
fn system(query: Query<(&A, &mut B)>) {
// you now have the option to do this for a speed boost
query.for_each_mut(|(a, mut b)| {
});
// however normal iterators are still available
for (a, mut b) in query.iter_mut() {
}
}
```
I think in most cases we should continue to encourage "normal" iterators as they are more flexible and more "rust idiomatic". But when that extra "oomf" is needed, it makes sense to use `for_each`.
We should also consider using `for_each` for internal bevy systems to give our users a nice speed boost (but that should be a separate pr).
## Component Metadata
`World` now has a `Components` collection, which is accessible via `world.components()`. This stores mappings from `ComponentId` to `ComponentInfo`, as well as `TypeId` to `ComponentId` mappings (where relevant). `ComponentInfo` stores information about the component, such as ComponentId, TypeId, memory layout, send-ness (currently limited to resources), and storage type.
## Significantly Cheaper `Access<T>`
We used to use `TypeAccess<TypeId>` to manage read/write component/archetype-component access. This was expensive because TypeIds must be hashed and compared individually. The parallel executor got around this by "condensing" type ids into bitset-backed access types. This worked, but it had to be re-generated from the `TypeAccess<TypeId>`sources every time archetypes changed.
This pr removes TypeAccess in favor of faster bitset access everywhere. We can do this thanks to the move to densely packed `ComponentId`s and `ArchetypeComponentId`s.
## Merged Resources into World
Resources had a lot of redundant functionality with Components. They stored typed data, they had access control, they had unique ids, they were queryable via SystemParams, etc. In fact the _only_ major difference between them was that they were unique (and didn't correlate to an entity).
Separate resources also had the downside of requiring a separate set of access controls, which meant the parallel executor needed to compare more bitsets per system and manage more state.
I initially got the "separate resources" idea from `legion`. I think that design was motivated by the fact that it made the direct world query/resource lifetime interactions more manageable. It certainly made our lives easier when using Resources alongside hecs/bevy_ecs. However we already have a construct for safely and ergonomically managing in-world lifetimes: systems (which use `Access<T>` internally).
This pr merges Resources into World:
```rust
world.insert_resource(1);
world.insert_resource(2.0);
let a = world.get_resource::<i32>().unwrap();
let mut b = world.get_resource_mut::<f64>().unwrap();
*b = 3.0;
```
Resources are now just a special kind of component. They have their own ComponentIds (and their own resource TypeId->ComponentId scope, so they don't conflict wit components of the same type). They are stored in a special "resource archetype", which stores components inside the archetype using a new `unique_components` sparse set (note that this sparse set could later be used to implement Tags). This allows us to keep the code size small by reusing existing datastructures (namely Column, Archetype, ComponentFlags, and ComponentInfo). This allows us the executor to use a single `Access<ArchetypeComponentId>` per system. It should also make scripting language integration easier.
_But_ this merge did create problems for people directly interacting with `World`. What if you need mutable access to multiple resources at the same time? `world.get_resource_mut()` borrows World mutably!
## WorldCell
WorldCell applies the `Access<ArchetypeComponentId>` concept to direct world access:
```rust
let world_cell = world.cell();
let a = world_cell.get_resource_mut::<i32>().unwrap();
let b = world_cell.get_resource_mut::<f64>().unwrap();
```
This adds cheap runtime checks (a sparse set lookup of `ArchetypeComponentId` and a counter) to ensure that world accesses do not conflict with each other. Each operation returns a `WorldBorrow<'w, T>` or `WorldBorrowMut<'w, T>` wrapper type, which will release the relevant ArchetypeComponentId resources when dropped.
World caches the access sparse set (and only one cell can exist at a time), so `world.cell()` is a cheap operation.
WorldCell does _not_ use atomic operations. It is non-send, does a mutable borrow of world to prevent other accesses, and uses a simple `Rc<RefCell<ArchetypeComponentAccess>>` wrapper in each WorldBorrow pointer.
The api is currently limited to resource access, but it can and should be extended to queries / entity component access.
## Resource Scopes
WorldCell does not yet support component queries, and even when it does there are sometimes legitimate reasons to want a mutable world ref _and_ a mutable resource ref (ex: bevy_render and bevy_scene both need this). In these cases we could always drop down to the unsafe `world.get_resource_unchecked_mut()`, but that is not ideal!
Instead developers can use a "resource scope"
```rust
world.resource_scope(|world: &mut World, a: &mut A| {
})
```
This temporarily removes the `A` resource from `World`, provides mutable pointers to both, and re-adds A to World when finished. Thanks to the move to ComponentIds/sparse sets, this is a cheap operation.
If multiple resources are required, scopes can be nested. We could also consider adding a "resource tuple" to the api if this pattern becomes common and the boilerplate gets nasty.
## Query Conflicts Use ComponentId Instead of ArchetypeComponentId
For safety reasons, systems cannot contain queries that conflict with each other without wrapping them in a QuerySet. On bevy `main`, we use ArchetypeComponentIds to determine conflicts. This is nice because it can take into account filters:
```rust
// these queries will never conflict due to their filters
fn filter_system(a: Query<&mut A, With<B>>, b: Query<&mut B, Without<B>>) {
}
```
But it also has a significant downside:
```rust
// these queries will not conflict _until_ an entity with A, B, and C is spawned
fn maybe_conflicts_system(a: Query<(&mut A, &C)>, b: Query<(&mut A, &B)>) {
}
```
The system above will panic at runtime if an entity with A, B, and C is spawned. This makes it hard to trust that your game logic will run without crashing.
In this pr, I switched to using `ComponentId` instead. This _is_ more constraining. `maybe_conflicts_system` will now always fail, but it will do it consistently at startup. Naively, it would also _disallow_ `filter_system`, which would be a significant downgrade in usability. Bevy has a number of internal systems that rely on disjoint queries and I expect it to be a common pattern in userspace.
To resolve this, I added a new `FilteredAccess<T>` type, which wraps `Access<T>` and adds with/without filters. If two `FilteredAccess` have with/without values that prove they are disjoint, they will no longer conflict.
## EntityRef / EntityMut
World entity operations on `main` require that the user passes in an `entity` id to each operation:
```rust
let entity = world.spawn((A, )); // create a new entity with A
world.get::<A>(entity);
world.insert(entity, (B, C));
world.insert_one(entity, D);
```
This means that each operation needs to look up the entity location / verify its validity. The initial spawn operation also requires a Bundle as input. This can be awkward when no components are required (or one component is required).
These operations have been replaced by `EntityRef` and `EntityMut`, which are "builder-style" wrappers around world that provide read and read/write operations on a single, pre-validated entity:
```rust
// spawn now takes no inputs and returns an EntityMut
let entity = world.spawn()
.insert(A) // insert a single component into the entity
.insert_bundle((B, C)) // insert a bundle of components into the entity
.id() // id returns the Entity id
// Returns EntityMut (or panics if the entity does not exist)
world.entity_mut(entity)
.insert(D)
.insert_bundle(SomeBundle::default());
{
// returns EntityRef (or panics if the entity does not exist)
let d = world.entity(entity)
.get::<D>() // gets the D component
.unwrap();
// world.get still exists for ergonomics
let d = world.get::<D>(entity).unwrap();
}
// These variants return Options if you want to check existence instead of panicing
world.get_entity_mut(entity)
.unwrap()
.insert(E);
if let Some(entity_ref) = world.get_entity(entity) {
let d = entity_ref.get::<D>().unwrap();
}
```
This _does not_ affect the current Commands api or terminology. I think that should be a separate conversation as that is a much larger breaking change.
## Safety Improvements
* Entity reservation in Commands uses a normal world borrow instead of an unsafe transmute
* QuerySets no longer transmutes lifetimes
* Made traits "unsafe" when implementing a trait incorrectly could cause unsafety
* More thorough safety docs
## RemovedComponents SystemParam
The old approach to querying removed components: `query.removed:<T>()` was confusing because it had no connection to the query itself. I replaced it with the following, which is both clearer and allows us to cache the ComponentId mapping in the SystemParamState:
```rust
fn system(removed: RemovedComponents<T>) {
for entity in removed.iter() {
}
}
```
## Simpler Bundle implementation
Bundles are no longer responsible for sorting (or deduping) TypeInfo. They are just a simple ordered list of component types / data. This makes the implementation smaller and opens the door to an easy "nested bundle" implementation in the future (which i might even add in this pr). Duplicate detection is now done once per bundle type by World the first time a bundle is used.
## Unified WorldQuery and QueryFilter types
(don't worry they are still separate type _parameters_ in Queries .. this is a non-breaking change)
WorldQuery and QueryFilter were already basically identical apis. With the addition of `FetchState` and more storage-specific fetch methods, the overlap was even clearer (and the redundancy more painful).
QueryFilters are now just `F: WorldQuery where F::Fetch: FilterFetch`. FilterFetch requires `Fetch<Item = bool>` and adds new "short circuit" variants of fetch methods. This enables a filter tuple like `(With<A>, Without<B>, Changed<C>)` to stop evaluating the filter after the first mismatch is encountered. FilterFetch is automatically implemented for `Fetch` implementations that return bool.
This forces fetch implementations that return things like `(bool, bool, bool)` (such as the filter above) to manually implement FilterFetch and decide whether or not to short-circuit.
## More Granular Modules
World no longer globs all of the internal modules together. It now exports `core`, `system`, and `schedule` separately. I'm also considering exporting `core` submodules directly as that is still pretty "glob-ey" and unorganized (feedback welcome here).
## Remaining Draft Work (to be done in this pr)
* ~~panic on conflicting WorldQuery fetches (&A, &mut A)~~
* ~~bevy `main` and hecs both currently allow this, but we should protect against it if possible~~
* ~~batch_iter / par_iter (currently stubbed out)~~
* ~~ChangedRes~~
* ~~I skipped this while we sort out #1313. This pr should be adapted to account for whatever we land on there~~.
* ~~The `Archetypes` and `Tables` collections use hashes of sorted lists of component ids to uniquely identify each archetype/table. This hash is then used as the key in a HashMap to look up the relevant ArchetypeId or TableId. (which doesn't handle hash collisions properly)~~
* ~~It is currently unsafe to generate a Query from "World A", then use it on "World B" (despite the api claiming it is safe). We should probably close this gap. This could be done by adding a randomly generated WorldId to each world, then storing that id in each Query. They could then be compared to each other on each `query.do_thing(&world)` operation. This _does_ add an extra branch to each query operation, so I'm open to other suggestions if people have them.~~
* ~~Nested Bundles (if i find time)~~
## Potential Future Work
* Expand WorldCell to support queries.
* Consider not allocating in the empty archetype on `world.spawn()`
* ex: return something like EntityMutUninit, which turns into EntityMut after an `insert` or `insert_bundle` op
* this actually regressed performance last time i tried it, but in theory it should be faster
* Optimize SparseSet::insert (see `PERF` comment on insert)
* Replace SparseArray `Option<T>` with T::MAX to cut down on branching
* would enable cheaper get_unchecked() operations
* upstream fixedbitset optimizations
* fixedbitset could be allocation free for small block counts (store blocks in a SmallVec)
* fixedbitset could have a const constructor
* Consider implementing Tags (archetype-specific by-value data that affects archetype identity)
* ex: ArchetypeA could have `[A, B, C]` table components and `[D(1)]` "tag" component. ArchetypeB could have `[A, B, C]` table components and a `[D(2)]` tag component. The archetypes are different, despite both having D tags because the value inside D is different.
* this could potentially build on top of the `archetype.unique_components` added in this pr for resource storage.
* Consider reverting `all_tuples` proc macro in favor of the old `macro_rules` implementation
* all_tuples is more flexible and produces cleaner documentation (the macro_rules version produces weird type parameter orders due to parser constraints)
* but unfortunately all_tuples also appears to make Rust Analyzer sad/slow when working inside of `bevy_ecs` (does not affect user code)
* Consider "resource queries" and/or "mixed resource and entity component queries" as an alternative to WorldCell
* this is basically just "systems" so maybe it's not worth it
* Add more world ops
* `world.clear()`
* `world.reserve<T: Bundle>(count: usize)`
* Try using the old archetype allocation strategy (allocate new memory on resize and copy everything over). I expect this to improve batch insertion performance at the cost of unbatched performance. But thats just a guess. I'm not an allocation perf pro :)
* Adapt Commands apis for consistency with new World apis
## Benchmarks
key:
* `bevy_old`: bevy `main` branch
* `bevy`: this branch
* `_foreach`: uses an optimized for_each iterator
* ` _sparse`: uses sparse set storage (if unspecified assume table storage)
* `_system`: runs inside a system (if unspecified assume test happens via direct world ops)
### Simple Insert (from ecs_bench_suite)
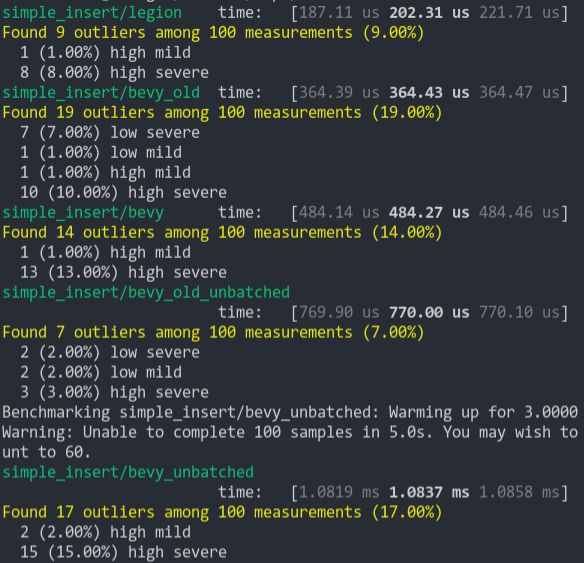
### Simpler Iter (from ecs_bench_suite)
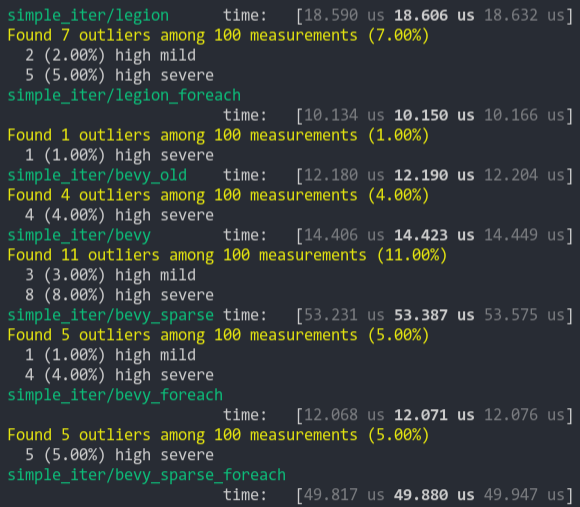
### Fragment Iter (from ecs_bench_suite)
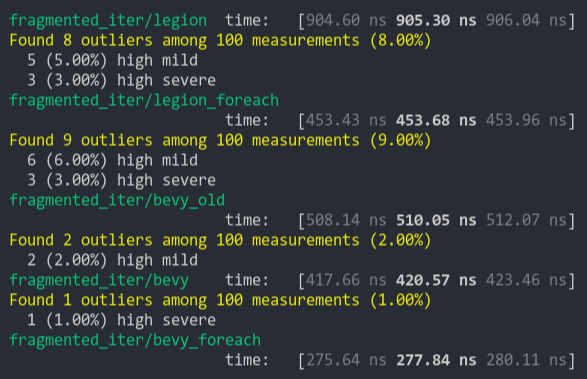
### Sparse Fragmented Iter
Iterate a query that matches 5 entities from a single matching archetype, but there are 100 unmatching archetypes
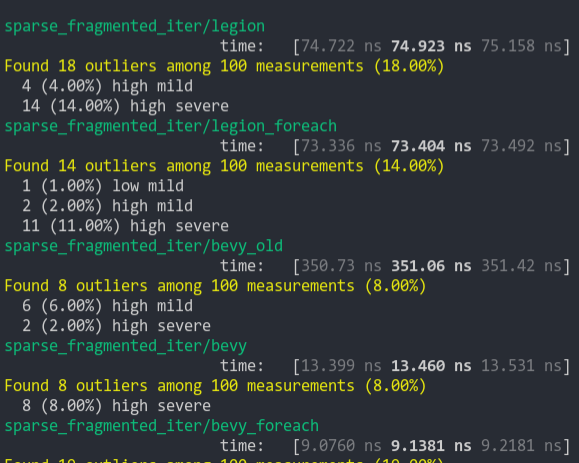
### Schedule (from ecs_bench_suite)
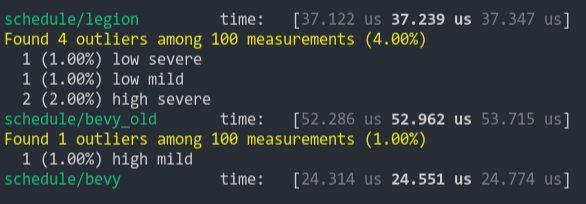
### Add Remove Component (from ecs_bench_suite)
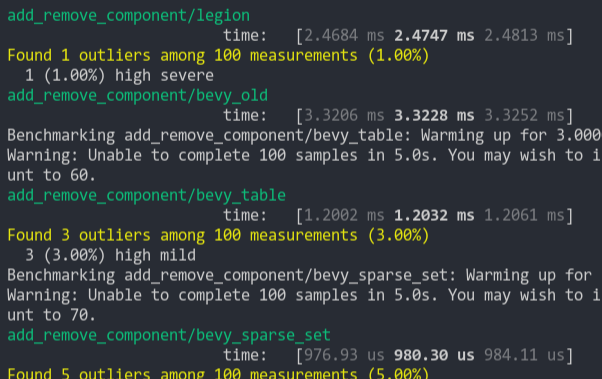
### Add Remove Component Big
Same as the test above, but each entity has 5 "large" matrix components and 1 "large" matrix component is added and removed
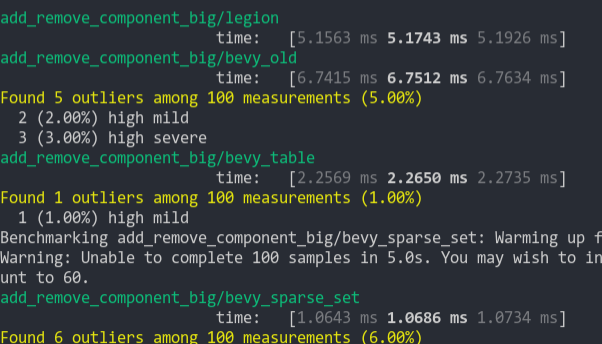
### Get Component
Looks up a single component value a large number of times
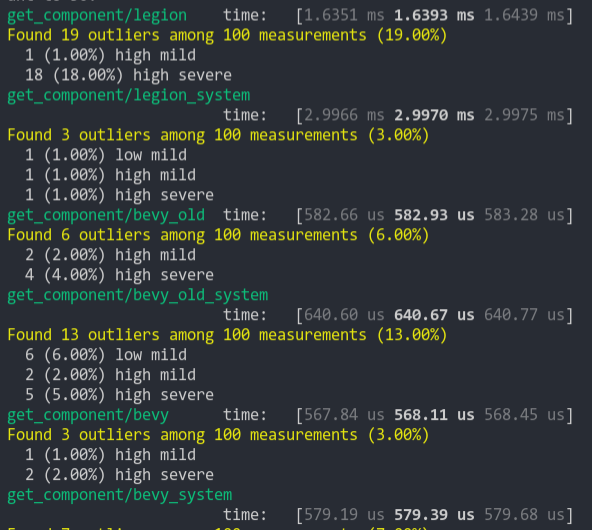
2021-03-05 07:54:35 +00:00
world . bundles ( )
}
}
2020-12-16 05:57:16 +00:00
2022-05-02 18:26:52 +00:00
/// A [`SystemParam`] that reads the previous and current change ticks of the system.
///
/// A system's change ticks are updated each time it runs:
/// - `last_change_tick` copies the previous value of `change_tick`
/// - `change_tick` copies the current value of [`World::read_change_tick`]
///
/// Component change ticks that are more recent than `last_change_tick` will be detected by the system.
/// Those can be read by calling [`last_changed`](crate::change_detection::DetectChanges::last_changed)
/// on a [`Mut<T>`](crate::change_detection::Mut) or [`ResMut<T>`](crate::change_detection::ResMut).
Reliable change detection (#1471)
# Problem Definition
The current change tracking (via flags for both components and resources) fails to detect changes made by systems that are scheduled to run earlier in the frame than they are.
This issue is discussed at length in [#68](https://github.com/bevyengine/bevy/issues/68) and [#54](https://github.com/bevyengine/bevy/issues/54).
This is very much a draft PR, and contributions are welcome and needed.
# Criteria
1. Each change is detected at least once, no matter the ordering.
2. Each change is detected at most once, no matter the ordering.
3. Changes should be detected the same frame that they are made.
4. Competitive ergonomics. Ideally does not require opting-in.
5. Low CPU overhead of computation.
6. Memory efficient. This must not increase over time, except where the number of entities / resources does.
7. Changes should not be lost for systems that don't run.
8. A frame needs to act as a pure function. Given the same set of entities / components it needs to produce the same end state without side-effects.
**Exact** change-tracking proposals satisfy criteria 1 and 2.
**Conservative** change-tracking proposals satisfy criteria 1 but not 2.
**Flaky** change tracking proposals satisfy criteria 2 but not 1.
# Code Base Navigation
There are three types of flags:
- `Added`: A piece of data was added to an entity / `Resources`.
- `Mutated`: A piece of data was able to be modified, because its `DerefMut` was accessed
- `Changed`: The bitwise OR of `Added` and `Changed`
The special behavior of `ChangedRes`, with respect to the scheduler is being removed in [#1313](https://github.com/bevyengine/bevy/pull/1313) and does not need to be reproduced.
`ChangedRes` and friends can be found in "bevy_ecs/core/resources/resource_query.rs".
The `Flags` trait for Components can be found in "bevy_ecs/core/query.rs".
`ComponentFlags` are stored in "bevy_ecs/core/archetypes.rs", defined on line 446.
# Proposals
**Proposal 5 was selected for implementation.**
## Proposal 0: No Change Detection
The baseline, where computations are performed on everything regardless of whether it changed.
**Type:** Conservative
**Pros:**
- already implemented
- will never miss events
- no overhead
**Cons:**
- tons of repeated work
- doesn't allow users to avoid repeating work (or monitoring for other changes)
## Proposal 1: Earlier-This-Tick Change Detection
The current approach as of Bevy 0.4. Flags are set, and then flushed at the end of each frame.
**Type:** Flaky
**Pros:**
- already implemented
- simple to understand
- low memory overhead (2 bits per component)
- low time overhead (clear every flag once per frame)
**Cons:**
- misses systems based on ordering
- systems that don't run every frame miss changes
- duplicates detection when looping
- can lead to unresolvable circular dependencies
## Proposal 2: Two-Tick Change Detection
Flags persist for two frames, using a double-buffer system identical to that used in events.
A change is observed if it is found in either the current frame's list of changes or the previous frame's.
**Type:** Conservative
**Pros:**
- easy to understand
- easy to implement
- low memory overhead (4 bits per component)
- low time overhead (bit mask and shift every flag once per frame)
**Cons:**
- can result in a great deal of duplicated work
- systems that don't run every frame miss changes
- duplicates detection when looping
## Proposal 3: Last-Tick Change Detection
Flags persist for two frames, using a double-buffer system identical to that used in events.
A change is observed if it is found in the previous frame's list of changes.
**Type:** Exact
**Pros:**
- exact
- easy to understand
- easy to implement
- low memory overhead (4 bits per component)
- low time overhead (bit mask and shift every flag once per frame)
**Cons:**
- change detection is always delayed, possibly causing painful chained delays
- systems that don't run every frame miss changes
- duplicates detection when looping
## Proposal 4: Flag-Doubling Change Detection
Combine Proposal 2 and Proposal 3. Differentiate between `JustChanged` (current behavior) and `Changed` (Proposal 3).
Pack this data into the flags according to [this implementation proposal](https://github.com/bevyengine/bevy/issues/68#issuecomment-769174804).
**Type:** Flaky + Exact
**Pros:**
- allows users to acc
- easy to implement
- low memory overhead (4 bits per component)
- low time overhead (bit mask and shift every flag once per frame)
**Cons:**
- users must specify the type of change detection required
- still quite fragile to system ordering effects when using the flaky `JustChanged` form
- cannot get immediate + exact results
- systems that don't run every frame miss changes
- duplicates detection when looping
## [SELECTED] Proposal 5: Generation-Counter Change Detection
A global counter is increased after each system is run. Each component saves the time of last mutation, and each system saves the time of last execution. Mutation is detected when the component's counter is greater than the system's counter. Discussed [here](https://github.com/bevyengine/bevy/issues/68#issuecomment-769174804). How to handle addition detection is unsolved; the current proposal is to use the highest bit of the counter as in proposal 1.
**Type:** Exact (for mutations), flaky (for additions)
**Pros:**
- low time overhead (set component counter on access, set system counter after execution)
- robust to systems that don't run every frame
- robust to systems that loop
**Cons:**
- moderately complex implementation
- must be modified as systems are inserted dynamically
- medium memory overhead (4 bytes per component + system)
- unsolved addition detection
## Proposal 6: System-Data Change Detection
For each system, track which system's changes it has seen. This approach is only worth fully designing and implementing if Proposal 5 fails in some way.
**Type:** Exact
**Pros:**
- exact
- conceptually simple
**Cons:**
- requires storing data on each system
- implementation is complex
- must be modified as systems are inserted dynamically
## Proposal 7: Total-Order Change Detection
Discussed [here](https://github.com/bevyengine/bevy/issues/68#issuecomment-754326523). This proposal is somewhat complicated by the new scheduler, but I believe it should still be conceptually feasible. This approach is only worth fully designing and implementing if Proposal 5 fails in some way.
**Type:** Exact
**Pros:**
- exact
- efficient data storage relative to other exact proposals
**Cons:**
- requires access to the scheduler
- complex implementation and difficulty grokking
- must be modified as systems are inserted dynamically
# Tests
- We will need to verify properties 1, 2, 3, 7 and 8. Priority: 1 > 2 = 3 > 8 > 7
- Ideally we can use identical user-facing syntax for all proposals, allowing us to re-use the same syntax for each.
- When writing tests, we need to carefully specify order using explicit dependencies.
- These tests will need to be duplicated for both components and resources.
- We need to be sure to handle cases where ambiguous system orders exist.
`changing_system` is always the system that makes the changes, and `detecting_system` always detects the changes.
The component / resource changed will be simple boolean wrapper structs.
## Basic Added / Mutated / Changed
2 x 3 design:
- Resources vs. Components
- Added vs. Changed vs. Mutated
- `changing_system` runs before `detecting_system`
- verify at the end of tick 2
## At Least Once
2 x 3 design:
- Resources vs. Components
- Added vs. Changed vs. Mutated
- `changing_system` runs after `detecting_system`
- verify at the end of tick 2
## At Most Once
2 x 3 design:
- Resources vs. Components
- Added vs. Changed vs. Mutated
- `changing_system` runs once before `detecting_system`
- increment a counter based on the number of changes detected
- verify at the end of tick 2
## Fast Detection
2 x 3 design:
- Resources vs. Components
- Added vs. Changed vs. Mutated
- `changing_system` runs before `detecting_system`
- verify at the end of tick 1
## Ambiguous System Ordering Robustness
2 x 3 x 2 design:
- Resources vs. Components
- Added vs. Changed vs. Mutated
- `changing_system` runs [before/after] `detecting_system` in tick 1
- `changing_system` runs [after/before] `detecting_system` in tick 2
## System Pausing
2 x 3 design:
- Resources vs. Components
- Added vs. Changed vs. Mutated
- `changing_system` runs in tick 1, then is disabled by run criteria
- `detecting_system` is disabled by run criteria until it is run once during tick 3
- verify at the end of tick 3
## Addition Causes Mutation
2 design:
- Resources vs. Components
- `adding_system_1` adds a component / resource
- `adding system_2` adds the same component / resource
- verify the `Mutated` flag at the end of the tick
- verify the `Added` flag at the end of the tick
First check tests for: https://github.com/bevyengine/bevy/issues/333
Second check tests for: https://github.com/bevyengine/bevy/issues/1443
## Changes Made By Commands
- `adding_system` runs in Update in tick 1, and sends a command to add a component
- `detecting_system` runs in Update in tick 1 and 2, after `adding_system`
- We can't detect the changes in tick 1, since they haven't been processed yet
- If we were to track these changes as being emitted by `adding_system`, we can't detect the changes in tick 2 either, since `detecting_system` has already run once after `adding_system` :(
# Benchmarks
See: [general advice](https://github.com/bevyengine/bevy/blob/master/docs/profiling.md), [Criterion crate](https://github.com/bheisler/criterion.rs)
There are several critical parameters to vary:
1. entity count (1 to 10^9)
2. fraction of entities that are changed (0% to 100%)
3. cost to perform work on changed entities, i.e. workload (1 ns to 1s)
1 and 2 should be varied between benchmark runs. 3 can be added on computationally.
We want to measure:
- memory cost
- run time
We should collect these measurements across several frames (100?) to reduce bootup effects and accurately measure the mean, variance and drift.
Entity-component change detection is much more important to benchmark than resource change detection, due to the orders of magnitude higher number of pieces of data.
No change detection at all should be included in benchmarks as a second control for cases where missing changes is unacceptable.
## Graphs
1. y: performance, x: log_10(entity count), color: proposal, facet: performance metric. Set cost to perform work to 0.
2. y: run time, x: cost to perform work, color: proposal, facet: fraction changed. Set number of entities to 10^6
3. y: memory, x: frames, color: proposal
# Conclusions
1. Is the theoretical categorization of the proposals correct according to our tests?
2. How does the performance of the proposals compare without any load?
3. How does the performance of the proposals compare with realistic loads?
4. At what workload does more exact change tracking become worth the (presumably) higher overhead?
5. When does adding change-detection to save on work become worthwhile?
6. Is there enough divergence in performance between the best solutions in each class to ship more than one change-tracking solution?
# Implementation Plan
1. Write a test suite.
2. Verify that tests fail for existing approach.
3. Write a benchmark suite.
4. Get performance numbers for existing approach.
5. Implement, test and benchmark various solutions using a Git branch per proposal.
6. Create a draft PR with all solutions and present results to team.
7. Select a solution and replace existing change detection.
Co-authored-by: Brice DAVIER <bricedavier@gmail.com>
Co-authored-by: Carter Anderson <mcanders1@gmail.com>
2021-03-19 17:53:26 +00:00
#[ derive(Debug) ]
pub struct SystemChangeTick {
2022-05-02 18:26:52 +00:00
last_change_tick : u32 ,
change_tick : u32 ,
}
impl SystemChangeTick {
/// Returns the current [`World`] change tick seen by the system.
#[ inline ]
pub fn change_tick ( & self ) -> u32 {
self . change_tick
}
/// Returns the [`World`] change tick seen by the system the previous time it ran.
#[ inline ]
pub fn last_change_tick ( & self ) -> u32 {
self . last_change_tick
}
Reliable change detection (#1471)
# Problem Definition
The current change tracking (via flags for both components and resources) fails to detect changes made by systems that are scheduled to run earlier in the frame than they are.
This issue is discussed at length in [#68](https://github.com/bevyengine/bevy/issues/68) and [#54](https://github.com/bevyengine/bevy/issues/54).
This is very much a draft PR, and contributions are welcome and needed.
# Criteria
1. Each change is detected at least once, no matter the ordering.
2. Each change is detected at most once, no matter the ordering.
3. Changes should be detected the same frame that they are made.
4. Competitive ergonomics. Ideally does not require opting-in.
5. Low CPU overhead of computation.
6. Memory efficient. This must not increase over time, except where the number of entities / resources does.
7. Changes should not be lost for systems that don't run.
8. A frame needs to act as a pure function. Given the same set of entities / components it needs to produce the same end state without side-effects.
**Exact** change-tracking proposals satisfy criteria 1 and 2.
**Conservative** change-tracking proposals satisfy criteria 1 but not 2.
**Flaky** change tracking proposals satisfy criteria 2 but not 1.
# Code Base Navigation
There are three types of flags:
- `Added`: A piece of data was added to an entity / `Resources`.
- `Mutated`: A piece of data was able to be modified, because its `DerefMut` was accessed
- `Changed`: The bitwise OR of `Added` and `Changed`
The special behavior of `ChangedRes`, with respect to the scheduler is being removed in [#1313](https://github.com/bevyengine/bevy/pull/1313) and does not need to be reproduced.
`ChangedRes` and friends can be found in "bevy_ecs/core/resources/resource_query.rs".
The `Flags` trait for Components can be found in "bevy_ecs/core/query.rs".
`ComponentFlags` are stored in "bevy_ecs/core/archetypes.rs", defined on line 446.
# Proposals
**Proposal 5 was selected for implementation.**
## Proposal 0: No Change Detection
The baseline, where computations are performed on everything regardless of whether it changed.
**Type:** Conservative
**Pros:**
- already implemented
- will never miss events
- no overhead
**Cons:**
- tons of repeated work
- doesn't allow users to avoid repeating work (or monitoring for other changes)
## Proposal 1: Earlier-This-Tick Change Detection
The current approach as of Bevy 0.4. Flags are set, and then flushed at the end of each frame.
**Type:** Flaky
**Pros:**
- already implemented
- simple to understand
- low memory overhead (2 bits per component)
- low time overhead (clear every flag once per frame)
**Cons:**
- misses systems based on ordering
- systems that don't run every frame miss changes
- duplicates detection when looping
- can lead to unresolvable circular dependencies
## Proposal 2: Two-Tick Change Detection
Flags persist for two frames, using a double-buffer system identical to that used in events.
A change is observed if it is found in either the current frame's list of changes or the previous frame's.
**Type:** Conservative
**Pros:**
- easy to understand
- easy to implement
- low memory overhead (4 bits per component)
- low time overhead (bit mask and shift every flag once per frame)
**Cons:**
- can result in a great deal of duplicated work
- systems that don't run every frame miss changes
- duplicates detection when looping
## Proposal 3: Last-Tick Change Detection
Flags persist for two frames, using a double-buffer system identical to that used in events.
A change is observed if it is found in the previous frame's list of changes.
**Type:** Exact
**Pros:**
- exact
- easy to understand
- easy to implement
- low memory overhead (4 bits per component)
- low time overhead (bit mask and shift every flag once per frame)
**Cons:**
- change detection is always delayed, possibly causing painful chained delays
- systems that don't run every frame miss changes
- duplicates detection when looping
## Proposal 4: Flag-Doubling Change Detection
Combine Proposal 2 and Proposal 3. Differentiate between `JustChanged` (current behavior) and `Changed` (Proposal 3).
Pack this data into the flags according to [this implementation proposal](https://github.com/bevyengine/bevy/issues/68#issuecomment-769174804).
**Type:** Flaky + Exact
**Pros:**
- allows users to acc
- easy to implement
- low memory overhead (4 bits per component)
- low time overhead (bit mask and shift every flag once per frame)
**Cons:**
- users must specify the type of change detection required
- still quite fragile to system ordering effects when using the flaky `JustChanged` form
- cannot get immediate + exact results
- systems that don't run every frame miss changes
- duplicates detection when looping
## [SELECTED] Proposal 5: Generation-Counter Change Detection
A global counter is increased after each system is run. Each component saves the time of last mutation, and each system saves the time of last execution. Mutation is detected when the component's counter is greater than the system's counter. Discussed [here](https://github.com/bevyengine/bevy/issues/68#issuecomment-769174804). How to handle addition detection is unsolved; the current proposal is to use the highest bit of the counter as in proposal 1.
**Type:** Exact (for mutations), flaky (for additions)
**Pros:**
- low time overhead (set component counter on access, set system counter after execution)
- robust to systems that don't run every frame
- robust to systems that loop
**Cons:**
- moderately complex implementation
- must be modified as systems are inserted dynamically
- medium memory overhead (4 bytes per component + system)
- unsolved addition detection
## Proposal 6: System-Data Change Detection
For each system, track which system's changes it has seen. This approach is only worth fully designing and implementing if Proposal 5 fails in some way.
**Type:** Exact
**Pros:**
- exact
- conceptually simple
**Cons:**
- requires storing data on each system
- implementation is complex
- must be modified as systems are inserted dynamically
## Proposal 7: Total-Order Change Detection
Discussed [here](https://github.com/bevyengine/bevy/issues/68#issuecomment-754326523). This proposal is somewhat complicated by the new scheduler, but I believe it should still be conceptually feasible. This approach is only worth fully designing and implementing if Proposal 5 fails in some way.
**Type:** Exact
**Pros:**
- exact
- efficient data storage relative to other exact proposals
**Cons:**
- requires access to the scheduler
- complex implementation and difficulty grokking
- must be modified as systems are inserted dynamically
# Tests
- We will need to verify properties 1, 2, 3, 7 and 8. Priority: 1 > 2 = 3 > 8 > 7
- Ideally we can use identical user-facing syntax for all proposals, allowing us to re-use the same syntax for each.
- When writing tests, we need to carefully specify order using explicit dependencies.
- These tests will need to be duplicated for both components and resources.
- We need to be sure to handle cases where ambiguous system orders exist.
`changing_system` is always the system that makes the changes, and `detecting_system` always detects the changes.
The component / resource changed will be simple boolean wrapper structs.
## Basic Added / Mutated / Changed
2 x 3 design:
- Resources vs. Components
- Added vs. Changed vs. Mutated
- `changing_system` runs before `detecting_system`
- verify at the end of tick 2
## At Least Once
2 x 3 design:
- Resources vs. Components
- Added vs. Changed vs. Mutated
- `changing_system` runs after `detecting_system`
- verify at the end of tick 2
## At Most Once
2 x 3 design:
- Resources vs. Components
- Added vs. Changed vs. Mutated
- `changing_system` runs once before `detecting_system`
- increment a counter based on the number of changes detected
- verify at the end of tick 2
## Fast Detection
2 x 3 design:
- Resources vs. Components
- Added vs. Changed vs. Mutated
- `changing_system` runs before `detecting_system`
- verify at the end of tick 1
## Ambiguous System Ordering Robustness
2 x 3 x 2 design:
- Resources vs. Components
- Added vs. Changed vs. Mutated
- `changing_system` runs [before/after] `detecting_system` in tick 1
- `changing_system` runs [after/before] `detecting_system` in tick 2
## System Pausing
2 x 3 design:
- Resources vs. Components
- Added vs. Changed vs. Mutated
- `changing_system` runs in tick 1, then is disabled by run criteria
- `detecting_system` is disabled by run criteria until it is run once during tick 3
- verify at the end of tick 3
## Addition Causes Mutation
2 design:
- Resources vs. Components
- `adding_system_1` adds a component / resource
- `adding system_2` adds the same component / resource
- verify the `Mutated` flag at the end of the tick
- verify the `Added` flag at the end of the tick
First check tests for: https://github.com/bevyengine/bevy/issues/333
Second check tests for: https://github.com/bevyengine/bevy/issues/1443
## Changes Made By Commands
- `adding_system` runs in Update in tick 1, and sends a command to add a component
- `detecting_system` runs in Update in tick 1 and 2, after `adding_system`
- We can't detect the changes in tick 1, since they haven't been processed yet
- If we were to track these changes as being emitted by `adding_system`, we can't detect the changes in tick 2 either, since `detecting_system` has already run once after `adding_system` :(
# Benchmarks
See: [general advice](https://github.com/bevyengine/bevy/blob/master/docs/profiling.md), [Criterion crate](https://github.com/bheisler/criterion.rs)
There are several critical parameters to vary:
1. entity count (1 to 10^9)
2. fraction of entities that are changed (0% to 100%)
3. cost to perform work on changed entities, i.e. workload (1 ns to 1s)
1 and 2 should be varied between benchmark runs. 3 can be added on computationally.
We want to measure:
- memory cost
- run time
We should collect these measurements across several frames (100?) to reduce bootup effects and accurately measure the mean, variance and drift.
Entity-component change detection is much more important to benchmark than resource change detection, due to the orders of magnitude higher number of pieces of data.
No change detection at all should be included in benchmarks as a second control for cases where missing changes is unacceptable.
## Graphs
1. y: performance, x: log_10(entity count), color: proposal, facet: performance metric. Set cost to perform work to 0.
2. y: run time, x: cost to perform work, color: proposal, facet: fraction changed. Set number of entities to 10^6
3. y: memory, x: frames, color: proposal
# Conclusions
1. Is the theoretical categorization of the proposals correct according to our tests?
2. How does the performance of the proposals compare without any load?
3. How does the performance of the proposals compare with realistic loads?
4. At what workload does more exact change tracking become worth the (presumably) higher overhead?
5. When does adding change-detection to save on work become worthwhile?
6. Is there enough divergence in performance between the best solutions in each class to ship more than one change-tracking solution?
# Implementation Plan
1. Write a test suite.
2. Verify that tests fail for existing approach.
3. Write a benchmark suite.
4. Get performance numbers for existing approach.
5. Implement, test and benchmark various solutions using a Git branch per proposal.
6. Create a draft PR with all solutions and present results to team.
7. Select a solution and replace existing change detection.
Co-authored-by: Brice DAVIER <bricedavier@gmail.com>
Co-authored-by: Carter Anderson <mcanders1@gmail.com>
2021-03-19 17:53:26 +00:00
}
2022-07-04 14:44:24 +00:00
// SAFETY: Only reads internal system state
Simplify trait hierarchy for `SystemParam` (#6865)
# Objective
* Implementing a custom `SystemParam` by hand requires implementing three traits -- four if it is read-only.
* The trait `SystemParamFetch<'w, 's>` is a workaround from before we had generic associated types, and is no longer necessary.
## Solution
* Combine the trait `SystemParamFetch` with `SystemParamState`.
* I decided to remove the `Fetch` name and keep the `State` name, since the former was consistently conflated with the latter.
* Replace the trait `ReadOnlySystemParamFetch` with `ReadOnlySystemParam`, which simplifies trait bounds in generic code.
---
## Changelog
- Removed the trait `SystemParamFetch`, moving its functionality to `SystemParamState`.
- Replaced the trait `ReadOnlySystemParamFetch` with `ReadOnlySystemParam`.
## Migration Guide
The trait `SystemParamFetch` has been removed, and its functionality has been transferred to `SystemParamState`.
```rust
// Before
impl SystemParamState for MyParamState {
fn init(world: &mut World, system_meta: &mut SystemMeta) -> Self { ... }
}
impl<'w, 's> SystemParamFetch<'w, 's> for MyParamState {
type Item = MyParam<'w, 's>;
fn get_param(...) -> Self::Item;
}
// After
impl SystemParamState for MyParamState {
type Item<'w, 's> = MyParam<'w, 's>; // Generic associated types!
fn init(world: &mut World, system_meta: &mut SystemMeta) -> Self { ... }
fn get_param<'w, 's>(...) -> Self::Item<'w, 's>;
}
```
The trait `ReadOnlySystemParamFetch` has been replaced with `ReadOnlySystemParam`.
```rust
// Before
unsafe impl ReadOnlySystemParamFetch for MyParamState {}
// After
unsafe impl<'w, 's> ReadOnlySystemParam for MyParam<'w, 's> {}
```
2022-12-11 18:34:14 +00:00
unsafe impl ReadOnlySystemParam for SystemChangeTick { }
Add new SystemState and rename old SystemState to SystemMeta (#2283)
This enables `SystemParams` to be used outside of function systems. Anything can create and store `SystemState`, which enables efficient "param state cached" access to `SystemParams`.
It adds a `ReadOnlySystemParamFetch` trait, which enables safe `SystemState::get` calls without unique world access.
I renamed the old `SystemState` to `SystemMeta` to enable us to mirror the `QueryState` naming convention (but I'm happy to discuss alternative names if people have other ideas). I initially pitched this as `ParamState`, but given that it needs to include full system metadata, that doesn't feel like a particularly accurate name.
```rust
#[derive(Eq, PartialEq, Debug)]
struct A(usize);
#[derive(Eq, PartialEq, Debug)]
struct B(usize);
let mut world = World::default();
world.insert_resource(A(42));
world.spawn().insert(B(7));
// we get nice lifetime elision when declaring the type on the left hand side
let mut system_state: SystemState<(Res<A>, Query<&B>)> = SystemState::new(&mut world);
let (a, query) = system_state.get(&world);
assert_eq!(*a, A(42), "returned resource matches initial value");
assert_eq!(
*query.single().unwrap(),
B(7),
"returned component matches initial value"
);
// mutable system params require unique world access
let mut system_state: SystemState<(ResMut<A>, Query<&mut B>)> = SystemState::new(&mut world);
let (a, query) = system_state.get_mut(&mut world);
// static lifetimes are required when declaring inside of structs
struct SomeContainer {
state: SystemState<(Res<'static, A>, Res<'static, B>)>
}
// this can be shortened using type aliases, which will be useful for complex param tuples
type MyParams<'a> = (Res<'a, A>, Res<'a, B>);
struct SomeContainer {
state: SystemState<MyParams<'static>>
}
// It is the user's responsibility to call SystemState::apply(world) for parameters that queue up work
let mut system_state: SystemState<(Commands, Query<&B>)> = SystemState::new(&mut world);
{
let (mut commands, query) = system_state.get(&world);
commands.insert_resource(3.14);
}
system_state.apply(&mut world);
```
## Future Work
* Actually use SystemState inside FunctionSystem. This would be trivial, but it requires FunctionSystem to wrap SystemState in Option in its current form (which complicates system metadata lookup). I'd prefer to hold off until we adopt something like the later designs linked in #1364, which enable us to contruct Systems using a World reference (and also remove the need for `.system`).
* Consider a "scoped" approach to automatically call SystemState::apply when systems params are no longer being used (either a container type with a Drop impl, or a function that takes a closure for user logic operating on params).
2021-06-02 19:57:38 +00:00
Remove the `SystemParamState` trait and remove types like `ResState` (#6919)
Spiritual successor to #5205.
Actual successor to #6865.
# Objective
Currently, system params are defined using three traits: `SystemParam`, `ReadOnlySystemParam`, `SystemParamState`. The behavior for each param is specified by the `SystemParamState` trait, while `SystemParam` simply defers to the state.
Splitting the traits in this way makes it easier to implement within macros, but it increases the cognitive load. Worst of all, this approach requires each `MySystemParam` to have a public `MySystemParamState` type associated with it.
## Solution
* Merge the trait `SystemParamState` into `SystemParam`.
* Remove all trivial `SystemParam` state types.
* `OptionNonSendMutState<T>`: you will not be missed.
---
- [x] Fix/resolve the remaining test failure.
## Changelog
* Removed the trait `SystemParamState`, merging its functionality into `SystemParam`.
## Migration Guide
**Note**: this should replace the migration guide for #6865.
This is relative to Bevy 0.9, not main.
The traits `SystemParamState` and `SystemParamFetch` have been removed, and their functionality has been transferred to `SystemParam`.
```rust
// Before (0.9)
impl SystemParam for MyParam<'_, '_> {
type State = MyParamState;
}
unsafe impl SystemParamState for MyParamState {
fn init(world: &mut World, system_meta: &mut SystemMeta) -> Self { ... }
}
unsafe impl<'w, 's> SystemParamFetch<'w, 's> for MyParamState {
type Item = MyParam<'w, 's>;
fn get_param(&mut self, ...) -> Self::Item;
}
unsafe impl ReadOnlySystemParamFetch for MyParamState { }
// After (0.10)
unsafe impl SystemParam for MyParam<'_, '_> {
type State = MyParamState;
type Item<'w, 's> = MyParam<'w, 's>;
fn init_state(world: &mut World, system_meta: &mut SystemMeta) -> Self::State { ... }
fn get_param<'w, 's>(state: &mut Self::State, ...) -> Self::Item<'w, 's>;
}
unsafe impl ReadOnlySystemParam for MyParam<'_, '_> { }
```
The trait `ReadOnlySystemParamFetch` has been replaced with `ReadOnlySystemParam`.
```rust
// Before
unsafe impl ReadOnlySystemParamFetch for MyParamState {}
// After
unsafe impl ReadOnlySystemParam for MyParam<'_, '_> {}
```
2023-01-07 23:20:32 +00:00
// SAFETY: `SystemChangeTick` doesn't require any world access
unsafe impl SystemParam for SystemChangeTick {
type State = ( ) ;
Simplify trait hierarchy for `SystemParam` (#6865)
# Objective
* Implementing a custom `SystemParam` by hand requires implementing three traits -- four if it is read-only.
* The trait `SystemParamFetch<'w, 's>` is a workaround from before we had generic associated types, and is no longer necessary.
## Solution
* Combine the trait `SystemParamFetch` with `SystemParamState`.
* I decided to remove the `Fetch` name and keep the `State` name, since the former was consistently conflated with the latter.
* Replace the trait `ReadOnlySystemParamFetch` with `ReadOnlySystemParam`, which simplifies trait bounds in generic code.
---
## Changelog
- Removed the trait `SystemParamFetch`, moving its functionality to `SystemParamState`.
- Replaced the trait `ReadOnlySystemParamFetch` with `ReadOnlySystemParam`.
## Migration Guide
The trait `SystemParamFetch` has been removed, and its functionality has been transferred to `SystemParamState`.
```rust
// Before
impl SystemParamState for MyParamState {
fn init(world: &mut World, system_meta: &mut SystemMeta) -> Self { ... }
}
impl<'w, 's> SystemParamFetch<'w, 's> for MyParamState {
type Item = MyParam<'w, 's>;
fn get_param(...) -> Self::Item;
}
// After
impl SystemParamState for MyParamState {
type Item<'w, 's> = MyParam<'w, 's>; // Generic associated types!
fn init(world: &mut World, system_meta: &mut SystemMeta) -> Self { ... }
fn get_param<'w, 's>(...) -> Self::Item<'w, 's>;
}
```
The trait `ReadOnlySystemParamFetch` has been replaced with `ReadOnlySystemParam`.
```rust
// Before
unsafe impl ReadOnlySystemParamFetch for MyParamState {}
// After
unsafe impl<'w, 's> ReadOnlySystemParam for MyParam<'w, 's> {}
```
2022-12-11 18:34:14 +00:00
type Item < ' w , ' s > = SystemChangeTick ;
Remove the `SystemParamState` trait and remove types like `ResState` (#6919)
Spiritual successor to #5205.
Actual successor to #6865.
# Objective
Currently, system params are defined using three traits: `SystemParam`, `ReadOnlySystemParam`, `SystemParamState`. The behavior for each param is specified by the `SystemParamState` trait, while `SystemParam` simply defers to the state.
Splitting the traits in this way makes it easier to implement within macros, but it increases the cognitive load. Worst of all, this approach requires each `MySystemParam` to have a public `MySystemParamState` type associated with it.
## Solution
* Merge the trait `SystemParamState` into `SystemParam`.
* Remove all trivial `SystemParam` state types.
* `OptionNonSendMutState<T>`: you will not be missed.
---
- [x] Fix/resolve the remaining test failure.
## Changelog
* Removed the trait `SystemParamState`, merging its functionality into `SystemParam`.
## Migration Guide
**Note**: this should replace the migration guide for #6865.
This is relative to Bevy 0.9, not main.
The traits `SystemParamState` and `SystemParamFetch` have been removed, and their functionality has been transferred to `SystemParam`.
```rust
// Before (0.9)
impl SystemParam for MyParam<'_, '_> {
type State = MyParamState;
}
unsafe impl SystemParamState for MyParamState {
fn init(world: &mut World, system_meta: &mut SystemMeta) -> Self { ... }
}
unsafe impl<'w, 's> SystemParamFetch<'w, 's> for MyParamState {
type Item = MyParam<'w, 's>;
fn get_param(&mut self, ...) -> Self::Item;
}
unsafe impl ReadOnlySystemParamFetch for MyParamState { }
// After (0.10)
unsafe impl SystemParam for MyParam<'_, '_> {
type State = MyParamState;
type Item<'w, 's> = MyParam<'w, 's>;
fn init_state(world: &mut World, system_meta: &mut SystemMeta) -> Self::State { ... }
fn get_param<'w, 's>(state: &mut Self::State, ...) -> Self::Item<'w, 's>;
}
unsafe impl ReadOnlySystemParam for MyParam<'_, '_> { }
```
The trait `ReadOnlySystemParamFetch` has been replaced with `ReadOnlySystemParam`.
```rust
// Before
unsafe impl ReadOnlySystemParamFetch for MyParamState {}
// After
unsafe impl ReadOnlySystemParam for MyParam<'_, '_> {}
```
2023-01-07 23:20:32 +00:00
fn init_state ( _world : & mut World , _system_meta : & mut SystemMeta ) -> Self ::State { }
Reliable change detection (#1471)
# Problem Definition
The current change tracking (via flags for both components and resources) fails to detect changes made by systems that are scheduled to run earlier in the frame than they are.
This issue is discussed at length in [#68](https://github.com/bevyengine/bevy/issues/68) and [#54](https://github.com/bevyengine/bevy/issues/54).
This is very much a draft PR, and contributions are welcome and needed.
# Criteria
1. Each change is detected at least once, no matter the ordering.
2. Each change is detected at most once, no matter the ordering.
3. Changes should be detected the same frame that they are made.
4. Competitive ergonomics. Ideally does not require opting-in.
5. Low CPU overhead of computation.
6. Memory efficient. This must not increase over time, except where the number of entities / resources does.
7. Changes should not be lost for systems that don't run.
8. A frame needs to act as a pure function. Given the same set of entities / components it needs to produce the same end state without side-effects.
**Exact** change-tracking proposals satisfy criteria 1 and 2.
**Conservative** change-tracking proposals satisfy criteria 1 but not 2.
**Flaky** change tracking proposals satisfy criteria 2 but not 1.
# Code Base Navigation
There are three types of flags:
- `Added`: A piece of data was added to an entity / `Resources`.
- `Mutated`: A piece of data was able to be modified, because its `DerefMut` was accessed
- `Changed`: The bitwise OR of `Added` and `Changed`
The special behavior of `ChangedRes`, with respect to the scheduler is being removed in [#1313](https://github.com/bevyengine/bevy/pull/1313) and does not need to be reproduced.
`ChangedRes` and friends can be found in "bevy_ecs/core/resources/resource_query.rs".
The `Flags` trait for Components can be found in "bevy_ecs/core/query.rs".
`ComponentFlags` are stored in "bevy_ecs/core/archetypes.rs", defined on line 446.
# Proposals
**Proposal 5 was selected for implementation.**
## Proposal 0: No Change Detection
The baseline, where computations are performed on everything regardless of whether it changed.
**Type:** Conservative
**Pros:**
- already implemented
- will never miss events
- no overhead
**Cons:**
- tons of repeated work
- doesn't allow users to avoid repeating work (or monitoring for other changes)
## Proposal 1: Earlier-This-Tick Change Detection
The current approach as of Bevy 0.4. Flags are set, and then flushed at the end of each frame.
**Type:** Flaky
**Pros:**
- already implemented
- simple to understand
- low memory overhead (2 bits per component)
- low time overhead (clear every flag once per frame)
**Cons:**
- misses systems based on ordering
- systems that don't run every frame miss changes
- duplicates detection when looping
- can lead to unresolvable circular dependencies
## Proposal 2: Two-Tick Change Detection
Flags persist for two frames, using a double-buffer system identical to that used in events.
A change is observed if it is found in either the current frame's list of changes or the previous frame's.
**Type:** Conservative
**Pros:**
- easy to understand
- easy to implement
- low memory overhead (4 bits per component)
- low time overhead (bit mask and shift every flag once per frame)
**Cons:**
- can result in a great deal of duplicated work
- systems that don't run every frame miss changes
- duplicates detection when looping
## Proposal 3: Last-Tick Change Detection
Flags persist for two frames, using a double-buffer system identical to that used in events.
A change is observed if it is found in the previous frame's list of changes.
**Type:** Exact
**Pros:**
- exact
- easy to understand
- easy to implement
- low memory overhead (4 bits per component)
- low time overhead (bit mask and shift every flag once per frame)
**Cons:**
- change detection is always delayed, possibly causing painful chained delays
- systems that don't run every frame miss changes
- duplicates detection when looping
## Proposal 4: Flag-Doubling Change Detection
Combine Proposal 2 and Proposal 3. Differentiate between `JustChanged` (current behavior) and `Changed` (Proposal 3).
Pack this data into the flags according to [this implementation proposal](https://github.com/bevyengine/bevy/issues/68#issuecomment-769174804).
**Type:** Flaky + Exact
**Pros:**
- allows users to acc
- easy to implement
- low memory overhead (4 bits per component)
- low time overhead (bit mask and shift every flag once per frame)
**Cons:**
- users must specify the type of change detection required
- still quite fragile to system ordering effects when using the flaky `JustChanged` form
- cannot get immediate + exact results
- systems that don't run every frame miss changes
- duplicates detection when looping
## [SELECTED] Proposal 5: Generation-Counter Change Detection
A global counter is increased after each system is run. Each component saves the time of last mutation, and each system saves the time of last execution. Mutation is detected when the component's counter is greater than the system's counter. Discussed [here](https://github.com/bevyengine/bevy/issues/68#issuecomment-769174804). How to handle addition detection is unsolved; the current proposal is to use the highest bit of the counter as in proposal 1.
**Type:** Exact (for mutations), flaky (for additions)
**Pros:**
- low time overhead (set component counter on access, set system counter after execution)
- robust to systems that don't run every frame
- robust to systems that loop
**Cons:**
- moderately complex implementation
- must be modified as systems are inserted dynamically
- medium memory overhead (4 bytes per component + system)
- unsolved addition detection
## Proposal 6: System-Data Change Detection
For each system, track which system's changes it has seen. This approach is only worth fully designing and implementing if Proposal 5 fails in some way.
**Type:** Exact
**Pros:**
- exact
- conceptually simple
**Cons:**
- requires storing data on each system
- implementation is complex
- must be modified as systems are inserted dynamically
## Proposal 7: Total-Order Change Detection
Discussed [here](https://github.com/bevyengine/bevy/issues/68#issuecomment-754326523). This proposal is somewhat complicated by the new scheduler, but I believe it should still be conceptually feasible. This approach is only worth fully designing and implementing if Proposal 5 fails in some way.
**Type:** Exact
**Pros:**
- exact
- efficient data storage relative to other exact proposals
**Cons:**
- requires access to the scheduler
- complex implementation and difficulty grokking
- must be modified as systems are inserted dynamically
# Tests
- We will need to verify properties 1, 2, 3, 7 and 8. Priority: 1 > 2 = 3 > 8 > 7
- Ideally we can use identical user-facing syntax for all proposals, allowing us to re-use the same syntax for each.
- When writing tests, we need to carefully specify order using explicit dependencies.
- These tests will need to be duplicated for both components and resources.
- We need to be sure to handle cases where ambiguous system orders exist.
`changing_system` is always the system that makes the changes, and `detecting_system` always detects the changes.
The component / resource changed will be simple boolean wrapper structs.
## Basic Added / Mutated / Changed
2 x 3 design:
- Resources vs. Components
- Added vs. Changed vs. Mutated
- `changing_system` runs before `detecting_system`
- verify at the end of tick 2
## At Least Once
2 x 3 design:
- Resources vs. Components
- Added vs. Changed vs. Mutated
- `changing_system` runs after `detecting_system`
- verify at the end of tick 2
## At Most Once
2 x 3 design:
- Resources vs. Components
- Added vs. Changed vs. Mutated
- `changing_system` runs once before `detecting_system`
- increment a counter based on the number of changes detected
- verify at the end of tick 2
## Fast Detection
2 x 3 design:
- Resources vs. Components
- Added vs. Changed vs. Mutated
- `changing_system` runs before `detecting_system`
- verify at the end of tick 1
## Ambiguous System Ordering Robustness
2 x 3 x 2 design:
- Resources vs. Components
- Added vs. Changed vs. Mutated
- `changing_system` runs [before/after] `detecting_system` in tick 1
- `changing_system` runs [after/before] `detecting_system` in tick 2
## System Pausing
2 x 3 design:
- Resources vs. Components
- Added vs. Changed vs. Mutated
- `changing_system` runs in tick 1, then is disabled by run criteria
- `detecting_system` is disabled by run criteria until it is run once during tick 3
- verify at the end of tick 3
## Addition Causes Mutation
2 design:
- Resources vs. Components
- `adding_system_1` adds a component / resource
- `adding system_2` adds the same component / resource
- verify the `Mutated` flag at the end of the tick
- verify the `Added` flag at the end of the tick
First check tests for: https://github.com/bevyengine/bevy/issues/333
Second check tests for: https://github.com/bevyengine/bevy/issues/1443
## Changes Made By Commands
- `adding_system` runs in Update in tick 1, and sends a command to add a component
- `detecting_system` runs in Update in tick 1 and 2, after `adding_system`
- We can't detect the changes in tick 1, since they haven't been processed yet
- If we were to track these changes as being emitted by `adding_system`, we can't detect the changes in tick 2 either, since `detecting_system` has already run once after `adding_system` :(
# Benchmarks
See: [general advice](https://github.com/bevyengine/bevy/blob/master/docs/profiling.md), [Criterion crate](https://github.com/bheisler/criterion.rs)
There are several critical parameters to vary:
1. entity count (1 to 10^9)
2. fraction of entities that are changed (0% to 100%)
3. cost to perform work on changed entities, i.e. workload (1 ns to 1s)
1 and 2 should be varied between benchmark runs. 3 can be added on computationally.
We want to measure:
- memory cost
- run time
We should collect these measurements across several frames (100?) to reduce bootup effects and accurately measure the mean, variance and drift.
Entity-component change detection is much more important to benchmark than resource change detection, due to the orders of magnitude higher number of pieces of data.
No change detection at all should be included in benchmarks as a second control for cases where missing changes is unacceptable.
## Graphs
1. y: performance, x: log_10(entity count), color: proposal, facet: performance metric. Set cost to perform work to 0.
2. y: run time, x: cost to perform work, color: proposal, facet: fraction changed. Set number of entities to 10^6
3. y: memory, x: frames, color: proposal
# Conclusions
1. Is the theoretical categorization of the proposals correct according to our tests?
2. How does the performance of the proposals compare without any load?
3. How does the performance of the proposals compare with realistic loads?
4. At what workload does more exact change tracking become worth the (presumably) higher overhead?
5. When does adding change-detection to save on work become worthwhile?
6. Is there enough divergence in performance between the best solutions in each class to ship more than one change-tracking solution?
# Implementation Plan
1. Write a test suite.
2. Verify that tests fail for existing approach.
3. Write a benchmark suite.
4. Get performance numbers for existing approach.
5. Implement, test and benchmark various solutions using a Git branch per proposal.
6. Create a draft PR with all solutions and present results to team.
7. Select a solution and replace existing change detection.
Co-authored-by: Brice DAVIER <bricedavier@gmail.com>
Co-authored-by: Carter Anderson <mcanders1@gmail.com>
2021-03-19 17:53:26 +00:00
Simplify trait hierarchy for `SystemParam` (#6865)
# Objective
* Implementing a custom `SystemParam` by hand requires implementing three traits -- four if it is read-only.
* The trait `SystemParamFetch<'w, 's>` is a workaround from before we had generic associated types, and is no longer necessary.
## Solution
* Combine the trait `SystemParamFetch` with `SystemParamState`.
* I decided to remove the `Fetch` name and keep the `State` name, since the former was consistently conflated with the latter.
* Replace the trait `ReadOnlySystemParamFetch` with `ReadOnlySystemParam`, which simplifies trait bounds in generic code.
---
## Changelog
- Removed the trait `SystemParamFetch`, moving its functionality to `SystemParamState`.
- Replaced the trait `ReadOnlySystemParamFetch` with `ReadOnlySystemParam`.
## Migration Guide
The trait `SystemParamFetch` has been removed, and its functionality has been transferred to `SystemParamState`.
```rust
// Before
impl SystemParamState for MyParamState {
fn init(world: &mut World, system_meta: &mut SystemMeta) -> Self { ... }
}
impl<'w, 's> SystemParamFetch<'w, 's> for MyParamState {
type Item = MyParam<'w, 's>;
fn get_param(...) -> Self::Item;
}
// After
impl SystemParamState for MyParamState {
type Item<'w, 's> = MyParam<'w, 's>; // Generic associated types!
fn init(world: &mut World, system_meta: &mut SystemMeta) -> Self { ... }
fn get_param<'w, 's>(...) -> Self::Item<'w, 's>;
}
```
The trait `ReadOnlySystemParamFetch` has been replaced with `ReadOnlySystemParam`.
```rust
// Before
unsafe impl ReadOnlySystemParamFetch for MyParamState {}
// After
unsafe impl<'w, 's> ReadOnlySystemParam for MyParam<'w, 's> {}
```
2022-12-11 18:34:14 +00:00
unsafe fn get_param < ' w , ' s > (
Remove the `SystemParamState` trait and remove types like `ResState` (#6919)
Spiritual successor to #5205.
Actual successor to #6865.
# Objective
Currently, system params are defined using three traits: `SystemParam`, `ReadOnlySystemParam`, `SystemParamState`. The behavior for each param is specified by the `SystemParamState` trait, while `SystemParam` simply defers to the state.
Splitting the traits in this way makes it easier to implement within macros, but it increases the cognitive load. Worst of all, this approach requires each `MySystemParam` to have a public `MySystemParamState` type associated with it.
## Solution
* Merge the trait `SystemParamState` into `SystemParam`.
* Remove all trivial `SystemParam` state types.
* `OptionNonSendMutState<T>`: you will not be missed.
---
- [x] Fix/resolve the remaining test failure.
## Changelog
* Removed the trait `SystemParamState`, merging its functionality into `SystemParam`.
## Migration Guide
**Note**: this should replace the migration guide for #6865.
This is relative to Bevy 0.9, not main.
The traits `SystemParamState` and `SystemParamFetch` have been removed, and their functionality has been transferred to `SystemParam`.
```rust
// Before (0.9)
impl SystemParam for MyParam<'_, '_> {
type State = MyParamState;
}
unsafe impl SystemParamState for MyParamState {
fn init(world: &mut World, system_meta: &mut SystemMeta) -> Self { ... }
}
unsafe impl<'w, 's> SystemParamFetch<'w, 's> for MyParamState {
type Item = MyParam<'w, 's>;
fn get_param(&mut self, ...) -> Self::Item;
}
unsafe impl ReadOnlySystemParamFetch for MyParamState { }
// After (0.10)
unsafe impl SystemParam for MyParam<'_, '_> {
type State = MyParamState;
type Item<'w, 's> = MyParam<'w, 's>;
fn init_state(world: &mut World, system_meta: &mut SystemMeta) -> Self::State { ... }
fn get_param<'w, 's>(state: &mut Self::State, ...) -> Self::Item<'w, 's>;
}
unsafe impl ReadOnlySystemParam for MyParam<'_, '_> { }
```
The trait `ReadOnlySystemParamFetch` has been replaced with `ReadOnlySystemParam`.
```rust
// Before
unsafe impl ReadOnlySystemParamFetch for MyParamState {}
// After
unsafe impl ReadOnlySystemParam for MyParam<'_, '_> {}
```
2023-01-07 23:20:32 +00:00
_state : & ' s mut Self ::State ,
Add new SystemState and rename old SystemState to SystemMeta (#2283)
This enables `SystemParams` to be used outside of function systems. Anything can create and store `SystemState`, which enables efficient "param state cached" access to `SystemParams`.
It adds a `ReadOnlySystemParamFetch` trait, which enables safe `SystemState::get` calls without unique world access.
I renamed the old `SystemState` to `SystemMeta` to enable us to mirror the `QueryState` naming convention (but I'm happy to discuss alternative names if people have other ideas). I initially pitched this as `ParamState`, but given that it needs to include full system metadata, that doesn't feel like a particularly accurate name.
```rust
#[derive(Eq, PartialEq, Debug)]
struct A(usize);
#[derive(Eq, PartialEq, Debug)]
struct B(usize);
let mut world = World::default();
world.insert_resource(A(42));
world.spawn().insert(B(7));
// we get nice lifetime elision when declaring the type on the left hand side
let mut system_state: SystemState<(Res<A>, Query<&B>)> = SystemState::new(&mut world);
let (a, query) = system_state.get(&world);
assert_eq!(*a, A(42), "returned resource matches initial value");
assert_eq!(
*query.single().unwrap(),
B(7),
"returned component matches initial value"
);
// mutable system params require unique world access
let mut system_state: SystemState<(ResMut<A>, Query<&mut B>)> = SystemState::new(&mut world);
let (a, query) = system_state.get_mut(&mut world);
// static lifetimes are required when declaring inside of structs
struct SomeContainer {
state: SystemState<(Res<'static, A>, Res<'static, B>)>
}
// this can be shortened using type aliases, which will be useful for complex param tuples
type MyParams<'a> = (Res<'a, A>, Res<'a, B>);
struct SomeContainer {
state: SystemState<MyParams<'static>>
}
// It is the user's responsibility to call SystemState::apply(world) for parameters that queue up work
let mut system_state: SystemState<(Commands, Query<&B>)> = SystemState::new(&mut world);
{
let (mut commands, query) = system_state.get(&world);
commands.insert_resource(3.14);
}
system_state.apply(&mut world);
```
## Future Work
* Actually use SystemState inside FunctionSystem. This would be trivial, but it requires FunctionSystem to wrap SystemState in Option in its current form (which complicates system metadata lookup). I'd prefer to hold off until we adopt something like the later designs linked in #1364, which enable us to contruct Systems using a World reference (and also remove the need for `.system`).
* Consider a "scoped" approach to automatically call SystemState::apply when systems params are no longer being used (either a container type with a Drop impl, or a function that takes a closure for user logic operating on params).
2021-06-02 19:57:38 +00:00
system_meta : & SystemMeta ,
System Param Lifetime Split (#2605)
# Objective
Enable using exact World lifetimes during read-only access . This is motivated by the new renderer's need to allow read-only world-only queries to outlive the query itself (but still be constrained by the world lifetime).
For example:
https://github.com/bevyengine/bevy/blob/115b170d1f11a91146bb6d6e9684dceb8b21f786/pipelined/bevy_pbr2/src/render/mod.rs#L774
## Solution
Split out SystemParam state and world lifetimes and pipe those lifetimes up to read-only Query ops (and add into_inner for Res). According to every safety test I've run so far (except one), this is safe (see the temporary safety test commit). Note that changing the mutable variants to the new lifetimes would allow aliased mutable pointers (try doing that to see how it affects the temporary safety tests).
The new state lifetime on SystemParam does make `#[derive(SystemParam)]` more cumbersome (the current impl requires PhantomData if you don't use both lifetimes). We can make this better by detecting whether or not a lifetime is used in the derive and adjusting accordingly, but that should probably be done in its own pr.
## Why is this a draft?
The new lifetimes break QuerySet safety in one very specific case (see the query_set system in system_safety_test). We need to solve this before we can use the lifetimes given.
This is due to the fact that QuerySet is just a wrapper over Query, which now relies on world lifetimes instead of `&self` lifetimes to prevent aliasing (but in systems, each Query has its own implied lifetime, not a centralized world lifetime). I believe the fix is to rewrite QuerySet to have its own World lifetime (and own the internal reference). This will complicate the impl a bit, but I think it is doable. I'm curious if anyone else has better ideas.
Personally, I think these new lifetimes need to happen. We've gotta have a way to directly tie read-only World queries to the World lifetime. The new renderer is the first place this has come up, but I doubt it will be the last. Worst case scenario we can come up with a second `WorldLifetimeQuery<Q, F = ()>` parameter to enable these read-only scenarios, but I'd rather not add another type to the type zoo.
2021-08-15 20:51:53 +00:00
_world : & ' w World ,
Reliable change detection (#1471)
# Problem Definition
The current change tracking (via flags for both components and resources) fails to detect changes made by systems that are scheduled to run earlier in the frame than they are.
This issue is discussed at length in [#68](https://github.com/bevyengine/bevy/issues/68) and [#54](https://github.com/bevyengine/bevy/issues/54).
This is very much a draft PR, and contributions are welcome and needed.
# Criteria
1. Each change is detected at least once, no matter the ordering.
2. Each change is detected at most once, no matter the ordering.
3. Changes should be detected the same frame that they are made.
4. Competitive ergonomics. Ideally does not require opting-in.
5. Low CPU overhead of computation.
6. Memory efficient. This must not increase over time, except where the number of entities / resources does.
7. Changes should not be lost for systems that don't run.
8. A frame needs to act as a pure function. Given the same set of entities / components it needs to produce the same end state without side-effects.
**Exact** change-tracking proposals satisfy criteria 1 and 2.
**Conservative** change-tracking proposals satisfy criteria 1 but not 2.
**Flaky** change tracking proposals satisfy criteria 2 but not 1.
# Code Base Navigation
There are three types of flags:
- `Added`: A piece of data was added to an entity / `Resources`.
- `Mutated`: A piece of data was able to be modified, because its `DerefMut` was accessed
- `Changed`: The bitwise OR of `Added` and `Changed`
The special behavior of `ChangedRes`, with respect to the scheduler is being removed in [#1313](https://github.com/bevyengine/bevy/pull/1313) and does not need to be reproduced.
`ChangedRes` and friends can be found in "bevy_ecs/core/resources/resource_query.rs".
The `Flags` trait for Components can be found in "bevy_ecs/core/query.rs".
`ComponentFlags` are stored in "bevy_ecs/core/archetypes.rs", defined on line 446.
# Proposals
**Proposal 5 was selected for implementation.**
## Proposal 0: No Change Detection
The baseline, where computations are performed on everything regardless of whether it changed.
**Type:** Conservative
**Pros:**
- already implemented
- will never miss events
- no overhead
**Cons:**
- tons of repeated work
- doesn't allow users to avoid repeating work (or monitoring for other changes)
## Proposal 1: Earlier-This-Tick Change Detection
The current approach as of Bevy 0.4. Flags are set, and then flushed at the end of each frame.
**Type:** Flaky
**Pros:**
- already implemented
- simple to understand
- low memory overhead (2 bits per component)
- low time overhead (clear every flag once per frame)
**Cons:**
- misses systems based on ordering
- systems that don't run every frame miss changes
- duplicates detection when looping
- can lead to unresolvable circular dependencies
## Proposal 2: Two-Tick Change Detection
Flags persist for two frames, using a double-buffer system identical to that used in events.
A change is observed if it is found in either the current frame's list of changes or the previous frame's.
**Type:** Conservative
**Pros:**
- easy to understand
- easy to implement
- low memory overhead (4 bits per component)
- low time overhead (bit mask and shift every flag once per frame)
**Cons:**
- can result in a great deal of duplicated work
- systems that don't run every frame miss changes
- duplicates detection when looping
## Proposal 3: Last-Tick Change Detection
Flags persist for two frames, using a double-buffer system identical to that used in events.
A change is observed if it is found in the previous frame's list of changes.
**Type:** Exact
**Pros:**
- exact
- easy to understand
- easy to implement
- low memory overhead (4 bits per component)
- low time overhead (bit mask and shift every flag once per frame)
**Cons:**
- change detection is always delayed, possibly causing painful chained delays
- systems that don't run every frame miss changes
- duplicates detection when looping
## Proposal 4: Flag-Doubling Change Detection
Combine Proposal 2 and Proposal 3. Differentiate between `JustChanged` (current behavior) and `Changed` (Proposal 3).
Pack this data into the flags according to [this implementation proposal](https://github.com/bevyengine/bevy/issues/68#issuecomment-769174804).
**Type:** Flaky + Exact
**Pros:**
- allows users to acc
- easy to implement
- low memory overhead (4 bits per component)
- low time overhead (bit mask and shift every flag once per frame)
**Cons:**
- users must specify the type of change detection required
- still quite fragile to system ordering effects when using the flaky `JustChanged` form
- cannot get immediate + exact results
- systems that don't run every frame miss changes
- duplicates detection when looping
## [SELECTED] Proposal 5: Generation-Counter Change Detection
A global counter is increased after each system is run. Each component saves the time of last mutation, and each system saves the time of last execution. Mutation is detected when the component's counter is greater than the system's counter. Discussed [here](https://github.com/bevyengine/bevy/issues/68#issuecomment-769174804). How to handle addition detection is unsolved; the current proposal is to use the highest bit of the counter as in proposal 1.
**Type:** Exact (for mutations), flaky (for additions)
**Pros:**
- low time overhead (set component counter on access, set system counter after execution)
- robust to systems that don't run every frame
- robust to systems that loop
**Cons:**
- moderately complex implementation
- must be modified as systems are inserted dynamically
- medium memory overhead (4 bytes per component + system)
- unsolved addition detection
## Proposal 6: System-Data Change Detection
For each system, track which system's changes it has seen. This approach is only worth fully designing and implementing if Proposal 5 fails in some way.
**Type:** Exact
**Pros:**
- exact
- conceptually simple
**Cons:**
- requires storing data on each system
- implementation is complex
- must be modified as systems are inserted dynamically
## Proposal 7: Total-Order Change Detection
Discussed [here](https://github.com/bevyengine/bevy/issues/68#issuecomment-754326523). This proposal is somewhat complicated by the new scheduler, but I believe it should still be conceptually feasible. This approach is only worth fully designing and implementing if Proposal 5 fails in some way.
**Type:** Exact
**Pros:**
- exact
- efficient data storage relative to other exact proposals
**Cons:**
- requires access to the scheduler
- complex implementation and difficulty grokking
- must be modified as systems are inserted dynamically
# Tests
- We will need to verify properties 1, 2, 3, 7 and 8. Priority: 1 > 2 = 3 > 8 > 7
- Ideally we can use identical user-facing syntax for all proposals, allowing us to re-use the same syntax for each.
- When writing tests, we need to carefully specify order using explicit dependencies.
- These tests will need to be duplicated for both components and resources.
- We need to be sure to handle cases where ambiguous system orders exist.
`changing_system` is always the system that makes the changes, and `detecting_system` always detects the changes.
The component / resource changed will be simple boolean wrapper structs.
## Basic Added / Mutated / Changed
2 x 3 design:
- Resources vs. Components
- Added vs. Changed vs. Mutated
- `changing_system` runs before `detecting_system`
- verify at the end of tick 2
## At Least Once
2 x 3 design:
- Resources vs. Components
- Added vs. Changed vs. Mutated
- `changing_system` runs after `detecting_system`
- verify at the end of tick 2
## At Most Once
2 x 3 design:
- Resources vs. Components
- Added vs. Changed vs. Mutated
- `changing_system` runs once before `detecting_system`
- increment a counter based on the number of changes detected
- verify at the end of tick 2
## Fast Detection
2 x 3 design:
- Resources vs. Components
- Added vs. Changed vs. Mutated
- `changing_system` runs before `detecting_system`
- verify at the end of tick 1
## Ambiguous System Ordering Robustness
2 x 3 x 2 design:
- Resources vs. Components
- Added vs. Changed vs. Mutated
- `changing_system` runs [before/after] `detecting_system` in tick 1
- `changing_system` runs [after/before] `detecting_system` in tick 2
## System Pausing
2 x 3 design:
- Resources vs. Components
- Added vs. Changed vs. Mutated
- `changing_system` runs in tick 1, then is disabled by run criteria
- `detecting_system` is disabled by run criteria until it is run once during tick 3
- verify at the end of tick 3
## Addition Causes Mutation
2 design:
- Resources vs. Components
- `adding_system_1` adds a component / resource
- `adding system_2` adds the same component / resource
- verify the `Mutated` flag at the end of the tick
- verify the `Added` flag at the end of the tick
First check tests for: https://github.com/bevyengine/bevy/issues/333
Second check tests for: https://github.com/bevyengine/bevy/issues/1443
## Changes Made By Commands
- `adding_system` runs in Update in tick 1, and sends a command to add a component
- `detecting_system` runs in Update in tick 1 and 2, after `adding_system`
- We can't detect the changes in tick 1, since they haven't been processed yet
- If we were to track these changes as being emitted by `adding_system`, we can't detect the changes in tick 2 either, since `detecting_system` has already run once after `adding_system` :(
# Benchmarks
See: [general advice](https://github.com/bevyengine/bevy/blob/master/docs/profiling.md), [Criterion crate](https://github.com/bheisler/criterion.rs)
There are several critical parameters to vary:
1. entity count (1 to 10^9)
2. fraction of entities that are changed (0% to 100%)
3. cost to perform work on changed entities, i.e. workload (1 ns to 1s)
1 and 2 should be varied between benchmark runs. 3 can be added on computationally.
We want to measure:
- memory cost
- run time
We should collect these measurements across several frames (100?) to reduce bootup effects and accurately measure the mean, variance and drift.
Entity-component change detection is much more important to benchmark than resource change detection, due to the orders of magnitude higher number of pieces of data.
No change detection at all should be included in benchmarks as a second control for cases where missing changes is unacceptable.
## Graphs
1. y: performance, x: log_10(entity count), color: proposal, facet: performance metric. Set cost to perform work to 0.
2. y: run time, x: cost to perform work, color: proposal, facet: fraction changed. Set number of entities to 10^6
3. y: memory, x: frames, color: proposal
# Conclusions
1. Is the theoretical categorization of the proposals correct according to our tests?
2. How does the performance of the proposals compare without any load?
3. How does the performance of the proposals compare with realistic loads?
4. At what workload does more exact change tracking become worth the (presumably) higher overhead?
5. When does adding change-detection to save on work become worthwhile?
6. Is there enough divergence in performance between the best solutions in each class to ship more than one change-tracking solution?
# Implementation Plan
1. Write a test suite.
2. Verify that tests fail for existing approach.
3. Write a benchmark suite.
4. Get performance numbers for existing approach.
5. Implement, test and benchmark various solutions using a Git branch per proposal.
6. Create a draft PR with all solutions and present results to team.
7. Select a solution and replace existing change detection.
Co-authored-by: Brice DAVIER <bricedavier@gmail.com>
Co-authored-by: Carter Anderson <mcanders1@gmail.com>
2021-03-19 17:53:26 +00:00
change_tick : u32 ,
Simplify trait hierarchy for `SystemParam` (#6865)
# Objective
* Implementing a custom `SystemParam` by hand requires implementing three traits -- four if it is read-only.
* The trait `SystemParamFetch<'w, 's>` is a workaround from before we had generic associated types, and is no longer necessary.
## Solution
* Combine the trait `SystemParamFetch` with `SystemParamState`.
* I decided to remove the `Fetch` name and keep the `State` name, since the former was consistently conflated with the latter.
* Replace the trait `ReadOnlySystemParamFetch` with `ReadOnlySystemParam`, which simplifies trait bounds in generic code.
---
## Changelog
- Removed the trait `SystemParamFetch`, moving its functionality to `SystemParamState`.
- Replaced the trait `ReadOnlySystemParamFetch` with `ReadOnlySystemParam`.
## Migration Guide
The trait `SystemParamFetch` has been removed, and its functionality has been transferred to `SystemParamState`.
```rust
// Before
impl SystemParamState for MyParamState {
fn init(world: &mut World, system_meta: &mut SystemMeta) -> Self { ... }
}
impl<'w, 's> SystemParamFetch<'w, 's> for MyParamState {
type Item = MyParam<'w, 's>;
fn get_param(...) -> Self::Item;
}
// After
impl SystemParamState for MyParamState {
type Item<'w, 's> = MyParam<'w, 's>; // Generic associated types!
fn init(world: &mut World, system_meta: &mut SystemMeta) -> Self { ... }
fn get_param<'w, 's>(...) -> Self::Item<'w, 's>;
}
```
The trait `ReadOnlySystemParamFetch` has been replaced with `ReadOnlySystemParam`.
```rust
// Before
unsafe impl ReadOnlySystemParamFetch for MyParamState {}
// After
unsafe impl<'w, 's> ReadOnlySystemParam for MyParam<'w, 's> {}
```
2022-12-11 18:34:14 +00:00
) -> Self ::Item < ' w , ' s > {
Reliable change detection (#1471)
# Problem Definition
The current change tracking (via flags for both components and resources) fails to detect changes made by systems that are scheduled to run earlier in the frame than they are.
This issue is discussed at length in [#68](https://github.com/bevyengine/bevy/issues/68) and [#54](https://github.com/bevyengine/bevy/issues/54).
This is very much a draft PR, and contributions are welcome and needed.
# Criteria
1. Each change is detected at least once, no matter the ordering.
2. Each change is detected at most once, no matter the ordering.
3. Changes should be detected the same frame that they are made.
4. Competitive ergonomics. Ideally does not require opting-in.
5. Low CPU overhead of computation.
6. Memory efficient. This must not increase over time, except where the number of entities / resources does.
7. Changes should not be lost for systems that don't run.
8. A frame needs to act as a pure function. Given the same set of entities / components it needs to produce the same end state without side-effects.
**Exact** change-tracking proposals satisfy criteria 1 and 2.
**Conservative** change-tracking proposals satisfy criteria 1 but not 2.
**Flaky** change tracking proposals satisfy criteria 2 but not 1.
# Code Base Navigation
There are three types of flags:
- `Added`: A piece of data was added to an entity / `Resources`.
- `Mutated`: A piece of data was able to be modified, because its `DerefMut` was accessed
- `Changed`: The bitwise OR of `Added` and `Changed`
The special behavior of `ChangedRes`, with respect to the scheduler is being removed in [#1313](https://github.com/bevyengine/bevy/pull/1313) and does not need to be reproduced.
`ChangedRes` and friends can be found in "bevy_ecs/core/resources/resource_query.rs".
The `Flags` trait for Components can be found in "bevy_ecs/core/query.rs".
`ComponentFlags` are stored in "bevy_ecs/core/archetypes.rs", defined on line 446.
# Proposals
**Proposal 5 was selected for implementation.**
## Proposal 0: No Change Detection
The baseline, where computations are performed on everything regardless of whether it changed.
**Type:** Conservative
**Pros:**
- already implemented
- will never miss events
- no overhead
**Cons:**
- tons of repeated work
- doesn't allow users to avoid repeating work (or monitoring for other changes)
## Proposal 1: Earlier-This-Tick Change Detection
The current approach as of Bevy 0.4. Flags are set, and then flushed at the end of each frame.
**Type:** Flaky
**Pros:**
- already implemented
- simple to understand
- low memory overhead (2 bits per component)
- low time overhead (clear every flag once per frame)
**Cons:**
- misses systems based on ordering
- systems that don't run every frame miss changes
- duplicates detection when looping
- can lead to unresolvable circular dependencies
## Proposal 2: Two-Tick Change Detection
Flags persist for two frames, using a double-buffer system identical to that used in events.
A change is observed if it is found in either the current frame's list of changes or the previous frame's.
**Type:** Conservative
**Pros:**
- easy to understand
- easy to implement
- low memory overhead (4 bits per component)
- low time overhead (bit mask and shift every flag once per frame)
**Cons:**
- can result in a great deal of duplicated work
- systems that don't run every frame miss changes
- duplicates detection when looping
## Proposal 3: Last-Tick Change Detection
Flags persist for two frames, using a double-buffer system identical to that used in events.
A change is observed if it is found in the previous frame's list of changes.
**Type:** Exact
**Pros:**
- exact
- easy to understand
- easy to implement
- low memory overhead (4 bits per component)
- low time overhead (bit mask and shift every flag once per frame)
**Cons:**
- change detection is always delayed, possibly causing painful chained delays
- systems that don't run every frame miss changes
- duplicates detection when looping
## Proposal 4: Flag-Doubling Change Detection
Combine Proposal 2 and Proposal 3. Differentiate between `JustChanged` (current behavior) and `Changed` (Proposal 3).
Pack this data into the flags according to [this implementation proposal](https://github.com/bevyengine/bevy/issues/68#issuecomment-769174804).
**Type:** Flaky + Exact
**Pros:**
- allows users to acc
- easy to implement
- low memory overhead (4 bits per component)
- low time overhead (bit mask and shift every flag once per frame)
**Cons:**
- users must specify the type of change detection required
- still quite fragile to system ordering effects when using the flaky `JustChanged` form
- cannot get immediate + exact results
- systems that don't run every frame miss changes
- duplicates detection when looping
## [SELECTED] Proposal 5: Generation-Counter Change Detection
A global counter is increased after each system is run. Each component saves the time of last mutation, and each system saves the time of last execution. Mutation is detected when the component's counter is greater than the system's counter. Discussed [here](https://github.com/bevyengine/bevy/issues/68#issuecomment-769174804). How to handle addition detection is unsolved; the current proposal is to use the highest bit of the counter as in proposal 1.
**Type:** Exact (for mutations), flaky (for additions)
**Pros:**
- low time overhead (set component counter on access, set system counter after execution)
- robust to systems that don't run every frame
- robust to systems that loop
**Cons:**
- moderately complex implementation
- must be modified as systems are inserted dynamically
- medium memory overhead (4 bytes per component + system)
- unsolved addition detection
## Proposal 6: System-Data Change Detection
For each system, track which system's changes it has seen. This approach is only worth fully designing and implementing if Proposal 5 fails in some way.
**Type:** Exact
**Pros:**
- exact
- conceptually simple
**Cons:**
- requires storing data on each system
- implementation is complex
- must be modified as systems are inserted dynamically
## Proposal 7: Total-Order Change Detection
Discussed [here](https://github.com/bevyengine/bevy/issues/68#issuecomment-754326523). This proposal is somewhat complicated by the new scheduler, but I believe it should still be conceptually feasible. This approach is only worth fully designing and implementing if Proposal 5 fails in some way.
**Type:** Exact
**Pros:**
- exact
- efficient data storage relative to other exact proposals
**Cons:**
- requires access to the scheduler
- complex implementation and difficulty grokking
- must be modified as systems are inserted dynamically
# Tests
- We will need to verify properties 1, 2, 3, 7 and 8. Priority: 1 > 2 = 3 > 8 > 7
- Ideally we can use identical user-facing syntax for all proposals, allowing us to re-use the same syntax for each.
- When writing tests, we need to carefully specify order using explicit dependencies.
- These tests will need to be duplicated for both components and resources.
- We need to be sure to handle cases where ambiguous system orders exist.
`changing_system` is always the system that makes the changes, and `detecting_system` always detects the changes.
The component / resource changed will be simple boolean wrapper structs.
## Basic Added / Mutated / Changed
2 x 3 design:
- Resources vs. Components
- Added vs. Changed vs. Mutated
- `changing_system` runs before `detecting_system`
- verify at the end of tick 2
## At Least Once
2 x 3 design:
- Resources vs. Components
- Added vs. Changed vs. Mutated
- `changing_system` runs after `detecting_system`
- verify at the end of tick 2
## At Most Once
2 x 3 design:
- Resources vs. Components
- Added vs. Changed vs. Mutated
- `changing_system` runs once before `detecting_system`
- increment a counter based on the number of changes detected
- verify at the end of tick 2
## Fast Detection
2 x 3 design:
- Resources vs. Components
- Added vs. Changed vs. Mutated
- `changing_system` runs before `detecting_system`
- verify at the end of tick 1
## Ambiguous System Ordering Robustness
2 x 3 x 2 design:
- Resources vs. Components
- Added vs. Changed vs. Mutated
- `changing_system` runs [before/after] `detecting_system` in tick 1
- `changing_system` runs [after/before] `detecting_system` in tick 2
## System Pausing
2 x 3 design:
- Resources vs. Components
- Added vs. Changed vs. Mutated
- `changing_system` runs in tick 1, then is disabled by run criteria
- `detecting_system` is disabled by run criteria until it is run once during tick 3
- verify at the end of tick 3
## Addition Causes Mutation
2 design:
- Resources vs. Components
- `adding_system_1` adds a component / resource
- `adding system_2` adds the same component / resource
- verify the `Mutated` flag at the end of the tick
- verify the `Added` flag at the end of the tick
First check tests for: https://github.com/bevyengine/bevy/issues/333
Second check tests for: https://github.com/bevyengine/bevy/issues/1443
## Changes Made By Commands
- `adding_system` runs in Update in tick 1, and sends a command to add a component
- `detecting_system` runs in Update in tick 1 and 2, after `adding_system`
- We can't detect the changes in tick 1, since they haven't been processed yet
- If we were to track these changes as being emitted by `adding_system`, we can't detect the changes in tick 2 either, since `detecting_system` has already run once after `adding_system` :(
# Benchmarks
See: [general advice](https://github.com/bevyengine/bevy/blob/master/docs/profiling.md), [Criterion crate](https://github.com/bheisler/criterion.rs)
There are several critical parameters to vary:
1. entity count (1 to 10^9)
2. fraction of entities that are changed (0% to 100%)
3. cost to perform work on changed entities, i.e. workload (1 ns to 1s)
1 and 2 should be varied between benchmark runs. 3 can be added on computationally.
We want to measure:
- memory cost
- run time
We should collect these measurements across several frames (100?) to reduce bootup effects and accurately measure the mean, variance and drift.
Entity-component change detection is much more important to benchmark than resource change detection, due to the orders of magnitude higher number of pieces of data.
No change detection at all should be included in benchmarks as a second control for cases where missing changes is unacceptable.
## Graphs
1. y: performance, x: log_10(entity count), color: proposal, facet: performance metric. Set cost to perform work to 0.
2. y: run time, x: cost to perform work, color: proposal, facet: fraction changed. Set number of entities to 10^6
3. y: memory, x: frames, color: proposal
# Conclusions
1. Is the theoretical categorization of the proposals correct according to our tests?
2. How does the performance of the proposals compare without any load?
3. How does the performance of the proposals compare with realistic loads?
4. At what workload does more exact change tracking become worth the (presumably) higher overhead?
5. When does adding change-detection to save on work become worthwhile?
6. Is there enough divergence in performance between the best solutions in each class to ship more than one change-tracking solution?
# Implementation Plan
1. Write a test suite.
2. Verify that tests fail for existing approach.
3. Write a benchmark suite.
4. Get performance numbers for existing approach.
5. Implement, test and benchmark various solutions using a Git branch per proposal.
6. Create a draft PR with all solutions and present results to team.
7. Select a solution and replace existing change detection.
Co-authored-by: Brice DAVIER <bricedavier@gmail.com>
Co-authored-by: Carter Anderson <mcanders1@gmail.com>
2021-03-19 17:53:26 +00:00
SystemChangeTick {
Add new SystemState and rename old SystemState to SystemMeta (#2283)
This enables `SystemParams` to be used outside of function systems. Anything can create and store `SystemState`, which enables efficient "param state cached" access to `SystemParams`.
It adds a `ReadOnlySystemParamFetch` trait, which enables safe `SystemState::get` calls without unique world access.
I renamed the old `SystemState` to `SystemMeta` to enable us to mirror the `QueryState` naming convention (but I'm happy to discuss alternative names if people have other ideas). I initially pitched this as `ParamState`, but given that it needs to include full system metadata, that doesn't feel like a particularly accurate name.
```rust
#[derive(Eq, PartialEq, Debug)]
struct A(usize);
#[derive(Eq, PartialEq, Debug)]
struct B(usize);
let mut world = World::default();
world.insert_resource(A(42));
world.spawn().insert(B(7));
// we get nice lifetime elision when declaring the type on the left hand side
let mut system_state: SystemState<(Res<A>, Query<&B>)> = SystemState::new(&mut world);
let (a, query) = system_state.get(&world);
assert_eq!(*a, A(42), "returned resource matches initial value");
assert_eq!(
*query.single().unwrap(),
B(7),
"returned component matches initial value"
);
// mutable system params require unique world access
let mut system_state: SystemState<(ResMut<A>, Query<&mut B>)> = SystemState::new(&mut world);
let (a, query) = system_state.get_mut(&mut world);
// static lifetimes are required when declaring inside of structs
struct SomeContainer {
state: SystemState<(Res<'static, A>, Res<'static, B>)>
}
// this can be shortened using type aliases, which will be useful for complex param tuples
type MyParams<'a> = (Res<'a, A>, Res<'a, B>);
struct SomeContainer {
state: SystemState<MyParams<'static>>
}
// It is the user's responsibility to call SystemState::apply(world) for parameters that queue up work
let mut system_state: SystemState<(Commands, Query<&B>)> = SystemState::new(&mut world);
{
let (mut commands, query) = system_state.get(&world);
commands.insert_resource(3.14);
}
system_state.apply(&mut world);
```
## Future Work
* Actually use SystemState inside FunctionSystem. This would be trivial, but it requires FunctionSystem to wrap SystemState in Option in its current form (which complicates system metadata lookup). I'd prefer to hold off until we adopt something like the later designs linked in #1364, which enable us to contruct Systems using a World reference (and also remove the need for `.system`).
* Consider a "scoped" approach to automatically call SystemState::apply when systems params are no longer being used (either a container type with a Drop impl, or a function that takes a closure for user logic operating on params).
2021-06-02 19:57:38 +00:00
last_change_tick : system_meta . last_change_tick ,
Reliable change detection (#1471)
# Problem Definition
The current change tracking (via flags for both components and resources) fails to detect changes made by systems that are scheduled to run earlier in the frame than they are.
This issue is discussed at length in [#68](https://github.com/bevyengine/bevy/issues/68) and [#54](https://github.com/bevyengine/bevy/issues/54).
This is very much a draft PR, and contributions are welcome and needed.
# Criteria
1. Each change is detected at least once, no matter the ordering.
2. Each change is detected at most once, no matter the ordering.
3. Changes should be detected the same frame that they are made.
4. Competitive ergonomics. Ideally does not require opting-in.
5. Low CPU overhead of computation.
6. Memory efficient. This must not increase over time, except where the number of entities / resources does.
7. Changes should not be lost for systems that don't run.
8. A frame needs to act as a pure function. Given the same set of entities / components it needs to produce the same end state without side-effects.
**Exact** change-tracking proposals satisfy criteria 1 and 2.
**Conservative** change-tracking proposals satisfy criteria 1 but not 2.
**Flaky** change tracking proposals satisfy criteria 2 but not 1.
# Code Base Navigation
There are three types of flags:
- `Added`: A piece of data was added to an entity / `Resources`.
- `Mutated`: A piece of data was able to be modified, because its `DerefMut` was accessed
- `Changed`: The bitwise OR of `Added` and `Changed`
The special behavior of `ChangedRes`, with respect to the scheduler is being removed in [#1313](https://github.com/bevyengine/bevy/pull/1313) and does not need to be reproduced.
`ChangedRes` and friends can be found in "bevy_ecs/core/resources/resource_query.rs".
The `Flags` trait for Components can be found in "bevy_ecs/core/query.rs".
`ComponentFlags` are stored in "bevy_ecs/core/archetypes.rs", defined on line 446.
# Proposals
**Proposal 5 was selected for implementation.**
## Proposal 0: No Change Detection
The baseline, where computations are performed on everything regardless of whether it changed.
**Type:** Conservative
**Pros:**
- already implemented
- will never miss events
- no overhead
**Cons:**
- tons of repeated work
- doesn't allow users to avoid repeating work (or monitoring for other changes)
## Proposal 1: Earlier-This-Tick Change Detection
The current approach as of Bevy 0.4. Flags are set, and then flushed at the end of each frame.
**Type:** Flaky
**Pros:**
- already implemented
- simple to understand
- low memory overhead (2 bits per component)
- low time overhead (clear every flag once per frame)
**Cons:**
- misses systems based on ordering
- systems that don't run every frame miss changes
- duplicates detection when looping
- can lead to unresolvable circular dependencies
## Proposal 2: Two-Tick Change Detection
Flags persist for two frames, using a double-buffer system identical to that used in events.
A change is observed if it is found in either the current frame's list of changes or the previous frame's.
**Type:** Conservative
**Pros:**
- easy to understand
- easy to implement
- low memory overhead (4 bits per component)
- low time overhead (bit mask and shift every flag once per frame)
**Cons:**
- can result in a great deal of duplicated work
- systems that don't run every frame miss changes
- duplicates detection when looping
## Proposal 3: Last-Tick Change Detection
Flags persist for two frames, using a double-buffer system identical to that used in events.
A change is observed if it is found in the previous frame's list of changes.
**Type:** Exact
**Pros:**
- exact
- easy to understand
- easy to implement
- low memory overhead (4 bits per component)
- low time overhead (bit mask and shift every flag once per frame)
**Cons:**
- change detection is always delayed, possibly causing painful chained delays
- systems that don't run every frame miss changes
- duplicates detection when looping
## Proposal 4: Flag-Doubling Change Detection
Combine Proposal 2 and Proposal 3. Differentiate between `JustChanged` (current behavior) and `Changed` (Proposal 3).
Pack this data into the flags according to [this implementation proposal](https://github.com/bevyengine/bevy/issues/68#issuecomment-769174804).
**Type:** Flaky + Exact
**Pros:**
- allows users to acc
- easy to implement
- low memory overhead (4 bits per component)
- low time overhead (bit mask and shift every flag once per frame)
**Cons:**
- users must specify the type of change detection required
- still quite fragile to system ordering effects when using the flaky `JustChanged` form
- cannot get immediate + exact results
- systems that don't run every frame miss changes
- duplicates detection when looping
## [SELECTED] Proposal 5: Generation-Counter Change Detection
A global counter is increased after each system is run. Each component saves the time of last mutation, and each system saves the time of last execution. Mutation is detected when the component's counter is greater than the system's counter. Discussed [here](https://github.com/bevyengine/bevy/issues/68#issuecomment-769174804). How to handle addition detection is unsolved; the current proposal is to use the highest bit of the counter as in proposal 1.
**Type:** Exact (for mutations), flaky (for additions)
**Pros:**
- low time overhead (set component counter on access, set system counter after execution)
- robust to systems that don't run every frame
- robust to systems that loop
**Cons:**
- moderately complex implementation
- must be modified as systems are inserted dynamically
- medium memory overhead (4 bytes per component + system)
- unsolved addition detection
## Proposal 6: System-Data Change Detection
For each system, track which system's changes it has seen. This approach is only worth fully designing and implementing if Proposal 5 fails in some way.
**Type:** Exact
**Pros:**
- exact
- conceptually simple
**Cons:**
- requires storing data on each system
- implementation is complex
- must be modified as systems are inserted dynamically
## Proposal 7: Total-Order Change Detection
Discussed [here](https://github.com/bevyengine/bevy/issues/68#issuecomment-754326523). This proposal is somewhat complicated by the new scheduler, but I believe it should still be conceptually feasible. This approach is only worth fully designing and implementing if Proposal 5 fails in some way.
**Type:** Exact
**Pros:**
- exact
- efficient data storage relative to other exact proposals
**Cons:**
- requires access to the scheduler
- complex implementation and difficulty grokking
- must be modified as systems are inserted dynamically
# Tests
- We will need to verify properties 1, 2, 3, 7 and 8. Priority: 1 > 2 = 3 > 8 > 7
- Ideally we can use identical user-facing syntax for all proposals, allowing us to re-use the same syntax for each.
- When writing tests, we need to carefully specify order using explicit dependencies.
- These tests will need to be duplicated for both components and resources.
- We need to be sure to handle cases where ambiguous system orders exist.
`changing_system` is always the system that makes the changes, and `detecting_system` always detects the changes.
The component / resource changed will be simple boolean wrapper structs.
## Basic Added / Mutated / Changed
2 x 3 design:
- Resources vs. Components
- Added vs. Changed vs. Mutated
- `changing_system` runs before `detecting_system`
- verify at the end of tick 2
## At Least Once
2 x 3 design:
- Resources vs. Components
- Added vs. Changed vs. Mutated
- `changing_system` runs after `detecting_system`
- verify at the end of tick 2
## At Most Once
2 x 3 design:
- Resources vs. Components
- Added vs. Changed vs. Mutated
- `changing_system` runs once before `detecting_system`
- increment a counter based on the number of changes detected
- verify at the end of tick 2
## Fast Detection
2 x 3 design:
- Resources vs. Components
- Added vs. Changed vs. Mutated
- `changing_system` runs before `detecting_system`
- verify at the end of tick 1
## Ambiguous System Ordering Robustness
2 x 3 x 2 design:
- Resources vs. Components
- Added vs. Changed vs. Mutated
- `changing_system` runs [before/after] `detecting_system` in tick 1
- `changing_system` runs [after/before] `detecting_system` in tick 2
## System Pausing
2 x 3 design:
- Resources vs. Components
- Added vs. Changed vs. Mutated
- `changing_system` runs in tick 1, then is disabled by run criteria
- `detecting_system` is disabled by run criteria until it is run once during tick 3
- verify at the end of tick 3
## Addition Causes Mutation
2 design:
- Resources vs. Components
- `adding_system_1` adds a component / resource
- `adding system_2` adds the same component / resource
- verify the `Mutated` flag at the end of the tick
- verify the `Added` flag at the end of the tick
First check tests for: https://github.com/bevyengine/bevy/issues/333
Second check tests for: https://github.com/bevyengine/bevy/issues/1443
## Changes Made By Commands
- `adding_system` runs in Update in tick 1, and sends a command to add a component
- `detecting_system` runs in Update in tick 1 and 2, after `adding_system`
- We can't detect the changes in tick 1, since they haven't been processed yet
- If we were to track these changes as being emitted by `adding_system`, we can't detect the changes in tick 2 either, since `detecting_system` has already run once after `adding_system` :(
# Benchmarks
See: [general advice](https://github.com/bevyengine/bevy/blob/master/docs/profiling.md), [Criterion crate](https://github.com/bheisler/criterion.rs)
There are several critical parameters to vary:
1. entity count (1 to 10^9)
2. fraction of entities that are changed (0% to 100%)
3. cost to perform work on changed entities, i.e. workload (1 ns to 1s)
1 and 2 should be varied between benchmark runs. 3 can be added on computationally.
We want to measure:
- memory cost
- run time
We should collect these measurements across several frames (100?) to reduce bootup effects and accurately measure the mean, variance and drift.
Entity-component change detection is much more important to benchmark than resource change detection, due to the orders of magnitude higher number of pieces of data.
No change detection at all should be included in benchmarks as a second control for cases where missing changes is unacceptable.
## Graphs
1. y: performance, x: log_10(entity count), color: proposal, facet: performance metric. Set cost to perform work to 0.
2. y: run time, x: cost to perform work, color: proposal, facet: fraction changed. Set number of entities to 10^6
3. y: memory, x: frames, color: proposal
# Conclusions
1. Is the theoretical categorization of the proposals correct according to our tests?
2. How does the performance of the proposals compare without any load?
3. How does the performance of the proposals compare with realistic loads?
4. At what workload does more exact change tracking become worth the (presumably) higher overhead?
5. When does adding change-detection to save on work become worthwhile?
6. Is there enough divergence in performance between the best solutions in each class to ship more than one change-tracking solution?
# Implementation Plan
1. Write a test suite.
2. Verify that tests fail for existing approach.
3. Write a benchmark suite.
4. Get performance numbers for existing approach.
5. Implement, test and benchmark various solutions using a Git branch per proposal.
6. Create a draft PR with all solutions and present results to team.
7. Select a solution and replace existing change detection.
Co-authored-by: Brice DAVIER <bricedavier@gmail.com>
Co-authored-by: Carter Anderson <mcanders1@gmail.com>
2021-03-19 17:53:26 +00:00
change_tick ,
}
}
}
2022-08-18 18:31:12 +00:00
/// Name of the system that corresponds to this [`crate::system::SystemState`].
///
/// This is not a reliable identifier, it is more so useful for debugging
/// purposes of finding where a system parameter is being used incorrectly.
pub struct SystemName < ' s > {
name : & ' s str ,
}
impl < ' s > SystemName < ' s > {
pub fn name ( & self ) -> & str {
self . name
}
}
impl < ' s > Deref for SystemName < ' s > {
type Target = str ;
fn deref ( & self ) -> & Self ::Target {
self . name ( )
}
}
impl < ' s > AsRef < str > for SystemName < ' s > {
fn as_ref ( & self ) -> & str {
self . name ( )
}
}
impl < ' s > From < SystemName < ' s > > for & ' s str {
fn from ( name : SystemName < ' s > ) -> & ' s str {
name . name
}
}
impl < ' s > std ::fmt ::Debug for SystemName < ' s > {
#[ inline(always) ]
fn fmt ( & self , f : & mut std ::fmt ::Formatter ) -> std ::fmt ::Result {
f . debug_tuple ( " SystemName " ) . field ( & self . name ( ) ) . finish ( )
}
}
impl < ' s > std ::fmt ::Display for SystemName < ' s > {
#[ inline(always) ]
fn fmt ( & self , f : & mut std ::fmt ::Formatter ) -> std ::fmt ::Result {
std ::fmt ::Display ::fmt ( & self . name ( ) , f )
}
}
// SAFETY: no component value access
Remove the `SystemParamState` trait and remove types like `ResState` (#6919)
Spiritual successor to #5205.
Actual successor to #6865.
# Objective
Currently, system params are defined using three traits: `SystemParam`, `ReadOnlySystemParam`, `SystemParamState`. The behavior for each param is specified by the `SystemParamState` trait, while `SystemParam` simply defers to the state.
Splitting the traits in this way makes it easier to implement within macros, but it increases the cognitive load. Worst of all, this approach requires each `MySystemParam` to have a public `MySystemParamState` type associated with it.
## Solution
* Merge the trait `SystemParamState` into `SystemParam`.
* Remove all trivial `SystemParam` state types.
* `OptionNonSendMutState<T>`: you will not be missed.
---
- [x] Fix/resolve the remaining test failure.
## Changelog
* Removed the trait `SystemParamState`, merging its functionality into `SystemParam`.
## Migration Guide
**Note**: this should replace the migration guide for #6865.
This is relative to Bevy 0.9, not main.
The traits `SystemParamState` and `SystemParamFetch` have been removed, and their functionality has been transferred to `SystemParam`.
```rust
// Before (0.9)
impl SystemParam for MyParam<'_, '_> {
type State = MyParamState;
}
unsafe impl SystemParamState for MyParamState {
fn init(world: &mut World, system_meta: &mut SystemMeta) -> Self { ... }
}
unsafe impl<'w, 's> SystemParamFetch<'w, 's> for MyParamState {
type Item = MyParam<'w, 's>;
fn get_param(&mut self, ...) -> Self::Item;
}
unsafe impl ReadOnlySystemParamFetch for MyParamState { }
// After (0.10)
unsafe impl SystemParam for MyParam<'_, '_> {
type State = MyParamState;
type Item<'w, 's> = MyParam<'w, 's>;
fn init_state(world: &mut World, system_meta: &mut SystemMeta) -> Self::State { ... }
fn get_param<'w, 's>(state: &mut Self::State, ...) -> Self::Item<'w, 's>;
}
unsafe impl ReadOnlySystemParam for MyParam<'_, '_> { }
```
The trait `ReadOnlySystemParamFetch` has been replaced with `ReadOnlySystemParam`.
```rust
// Before
unsafe impl ReadOnlySystemParamFetch for MyParamState {}
// After
unsafe impl ReadOnlySystemParam for MyParam<'_, '_> {}
```
2023-01-07 23:20:32 +00:00
unsafe impl SystemParam for SystemName < '_ > {
type State = Cow < 'static , str > ;
Simplify trait hierarchy for `SystemParam` (#6865)
# Objective
* Implementing a custom `SystemParam` by hand requires implementing three traits -- four if it is read-only.
* The trait `SystemParamFetch<'w, 's>` is a workaround from before we had generic associated types, and is no longer necessary.
## Solution
* Combine the trait `SystemParamFetch` with `SystemParamState`.
* I decided to remove the `Fetch` name and keep the `State` name, since the former was consistently conflated with the latter.
* Replace the trait `ReadOnlySystemParamFetch` with `ReadOnlySystemParam`, which simplifies trait bounds in generic code.
---
## Changelog
- Removed the trait `SystemParamFetch`, moving its functionality to `SystemParamState`.
- Replaced the trait `ReadOnlySystemParamFetch` with `ReadOnlySystemParam`.
## Migration Guide
The trait `SystemParamFetch` has been removed, and its functionality has been transferred to `SystemParamState`.
```rust
// Before
impl SystemParamState for MyParamState {
fn init(world: &mut World, system_meta: &mut SystemMeta) -> Self { ... }
}
impl<'w, 's> SystemParamFetch<'w, 's> for MyParamState {
type Item = MyParam<'w, 's>;
fn get_param(...) -> Self::Item;
}
// After
impl SystemParamState for MyParamState {
type Item<'w, 's> = MyParam<'w, 's>; // Generic associated types!
fn init(world: &mut World, system_meta: &mut SystemMeta) -> Self { ... }
fn get_param<'w, 's>(...) -> Self::Item<'w, 's>;
}
```
The trait `ReadOnlySystemParamFetch` has been replaced with `ReadOnlySystemParam`.
```rust
// Before
unsafe impl ReadOnlySystemParamFetch for MyParamState {}
// After
unsafe impl<'w, 's> ReadOnlySystemParam for MyParam<'w, 's> {}
```
2022-12-11 18:34:14 +00:00
type Item < ' w , ' s > = SystemName < ' s > ;
Remove the `SystemParamState` trait and remove types like `ResState` (#6919)
Spiritual successor to #5205.
Actual successor to #6865.
# Objective
Currently, system params are defined using three traits: `SystemParam`, `ReadOnlySystemParam`, `SystemParamState`. The behavior for each param is specified by the `SystemParamState` trait, while `SystemParam` simply defers to the state.
Splitting the traits in this way makes it easier to implement within macros, but it increases the cognitive load. Worst of all, this approach requires each `MySystemParam` to have a public `MySystemParamState` type associated with it.
## Solution
* Merge the trait `SystemParamState` into `SystemParam`.
* Remove all trivial `SystemParam` state types.
* `OptionNonSendMutState<T>`: you will not be missed.
---
- [x] Fix/resolve the remaining test failure.
## Changelog
* Removed the trait `SystemParamState`, merging its functionality into `SystemParam`.
## Migration Guide
**Note**: this should replace the migration guide for #6865.
This is relative to Bevy 0.9, not main.
The traits `SystemParamState` and `SystemParamFetch` have been removed, and their functionality has been transferred to `SystemParam`.
```rust
// Before (0.9)
impl SystemParam for MyParam<'_, '_> {
type State = MyParamState;
}
unsafe impl SystemParamState for MyParamState {
fn init(world: &mut World, system_meta: &mut SystemMeta) -> Self { ... }
}
unsafe impl<'w, 's> SystemParamFetch<'w, 's> for MyParamState {
type Item = MyParam<'w, 's>;
fn get_param(&mut self, ...) -> Self::Item;
}
unsafe impl ReadOnlySystemParamFetch for MyParamState { }
// After (0.10)
unsafe impl SystemParam for MyParam<'_, '_> {
type State = MyParamState;
type Item<'w, 's> = MyParam<'w, 's>;
fn init_state(world: &mut World, system_meta: &mut SystemMeta) -> Self::State { ... }
fn get_param<'w, 's>(state: &mut Self::State, ...) -> Self::Item<'w, 's>;
}
unsafe impl ReadOnlySystemParam for MyParam<'_, '_> { }
```
The trait `ReadOnlySystemParamFetch` has been replaced with `ReadOnlySystemParam`.
```rust
// Before
unsafe impl ReadOnlySystemParamFetch for MyParamState {}
// After
unsafe impl ReadOnlySystemParam for MyParam<'_, '_> {}
```
2023-01-07 23:20:32 +00:00
fn init_state ( _world : & mut World , system_meta : & mut SystemMeta ) -> Self ::State {
system_meta . name . clone ( )
2022-08-18 18:31:12 +00:00
}
#[ inline ]
Simplify trait hierarchy for `SystemParam` (#6865)
# Objective
* Implementing a custom `SystemParam` by hand requires implementing three traits -- four if it is read-only.
* The trait `SystemParamFetch<'w, 's>` is a workaround from before we had generic associated types, and is no longer necessary.
## Solution
* Combine the trait `SystemParamFetch` with `SystemParamState`.
* I decided to remove the `Fetch` name and keep the `State` name, since the former was consistently conflated with the latter.
* Replace the trait `ReadOnlySystemParamFetch` with `ReadOnlySystemParam`, which simplifies trait bounds in generic code.
---
## Changelog
- Removed the trait `SystemParamFetch`, moving its functionality to `SystemParamState`.
- Replaced the trait `ReadOnlySystemParamFetch` with `ReadOnlySystemParam`.
## Migration Guide
The trait `SystemParamFetch` has been removed, and its functionality has been transferred to `SystemParamState`.
```rust
// Before
impl SystemParamState for MyParamState {
fn init(world: &mut World, system_meta: &mut SystemMeta) -> Self { ... }
}
impl<'w, 's> SystemParamFetch<'w, 's> for MyParamState {
type Item = MyParam<'w, 's>;
fn get_param(...) -> Self::Item;
}
// After
impl SystemParamState for MyParamState {
type Item<'w, 's> = MyParam<'w, 's>; // Generic associated types!
fn init(world: &mut World, system_meta: &mut SystemMeta) -> Self { ... }
fn get_param<'w, 's>(...) -> Self::Item<'w, 's>;
}
```
The trait `ReadOnlySystemParamFetch` has been replaced with `ReadOnlySystemParam`.
```rust
// Before
unsafe impl ReadOnlySystemParamFetch for MyParamState {}
// After
unsafe impl<'w, 's> ReadOnlySystemParam for MyParam<'w, 's> {}
```
2022-12-11 18:34:14 +00:00
unsafe fn get_param < ' w , ' s > (
Remove the `SystemParamState` trait and remove types like `ResState` (#6919)
Spiritual successor to #5205.
Actual successor to #6865.
# Objective
Currently, system params are defined using three traits: `SystemParam`, `ReadOnlySystemParam`, `SystemParamState`. The behavior for each param is specified by the `SystemParamState` trait, while `SystemParam` simply defers to the state.
Splitting the traits in this way makes it easier to implement within macros, but it increases the cognitive load. Worst of all, this approach requires each `MySystemParam` to have a public `MySystemParamState` type associated with it.
## Solution
* Merge the trait `SystemParamState` into `SystemParam`.
* Remove all trivial `SystemParam` state types.
* `OptionNonSendMutState<T>`: you will not be missed.
---
- [x] Fix/resolve the remaining test failure.
## Changelog
* Removed the trait `SystemParamState`, merging its functionality into `SystemParam`.
## Migration Guide
**Note**: this should replace the migration guide for #6865.
This is relative to Bevy 0.9, not main.
The traits `SystemParamState` and `SystemParamFetch` have been removed, and their functionality has been transferred to `SystemParam`.
```rust
// Before (0.9)
impl SystemParam for MyParam<'_, '_> {
type State = MyParamState;
}
unsafe impl SystemParamState for MyParamState {
fn init(world: &mut World, system_meta: &mut SystemMeta) -> Self { ... }
}
unsafe impl<'w, 's> SystemParamFetch<'w, 's> for MyParamState {
type Item = MyParam<'w, 's>;
fn get_param(&mut self, ...) -> Self::Item;
}
unsafe impl ReadOnlySystemParamFetch for MyParamState { }
// After (0.10)
unsafe impl SystemParam for MyParam<'_, '_> {
type State = MyParamState;
type Item<'w, 's> = MyParam<'w, 's>;
fn init_state(world: &mut World, system_meta: &mut SystemMeta) -> Self::State { ... }
fn get_param<'w, 's>(state: &mut Self::State, ...) -> Self::Item<'w, 's>;
}
unsafe impl ReadOnlySystemParam for MyParam<'_, '_> { }
```
The trait `ReadOnlySystemParamFetch` has been replaced with `ReadOnlySystemParam`.
```rust
// Before
unsafe impl ReadOnlySystemParamFetch for MyParamState {}
// After
unsafe impl ReadOnlySystemParam for MyParam<'_, '_> {}
```
2023-01-07 23:20:32 +00:00
name : & ' s mut Self ::State ,
2022-08-18 18:31:12 +00:00
_system_meta : & SystemMeta ,
_world : & ' w World ,
_change_tick : u32 ,
Simplify trait hierarchy for `SystemParam` (#6865)
# Objective
* Implementing a custom `SystemParam` by hand requires implementing three traits -- four if it is read-only.
* The trait `SystemParamFetch<'w, 's>` is a workaround from before we had generic associated types, and is no longer necessary.
## Solution
* Combine the trait `SystemParamFetch` with `SystemParamState`.
* I decided to remove the `Fetch` name and keep the `State` name, since the former was consistently conflated with the latter.
* Replace the trait `ReadOnlySystemParamFetch` with `ReadOnlySystemParam`, which simplifies trait bounds in generic code.
---
## Changelog
- Removed the trait `SystemParamFetch`, moving its functionality to `SystemParamState`.
- Replaced the trait `ReadOnlySystemParamFetch` with `ReadOnlySystemParam`.
## Migration Guide
The trait `SystemParamFetch` has been removed, and its functionality has been transferred to `SystemParamState`.
```rust
// Before
impl SystemParamState for MyParamState {
fn init(world: &mut World, system_meta: &mut SystemMeta) -> Self { ... }
}
impl<'w, 's> SystemParamFetch<'w, 's> for MyParamState {
type Item = MyParam<'w, 's>;
fn get_param(...) -> Self::Item;
}
// After
impl SystemParamState for MyParamState {
type Item<'w, 's> = MyParam<'w, 's>; // Generic associated types!
fn init(world: &mut World, system_meta: &mut SystemMeta) -> Self { ... }
fn get_param<'w, 's>(...) -> Self::Item<'w, 's>;
}
```
The trait `ReadOnlySystemParamFetch` has been replaced with `ReadOnlySystemParam`.
```rust
// Before
unsafe impl ReadOnlySystemParamFetch for MyParamState {}
// After
unsafe impl<'w, 's> ReadOnlySystemParam for MyParam<'w, 's> {}
```
2022-12-11 18:34:14 +00:00
) -> Self ::Item < ' w , ' s > {
Remove the `SystemParamState` trait and remove types like `ResState` (#6919)
Spiritual successor to #5205.
Actual successor to #6865.
# Objective
Currently, system params are defined using three traits: `SystemParam`, `ReadOnlySystemParam`, `SystemParamState`. The behavior for each param is specified by the `SystemParamState` trait, while `SystemParam` simply defers to the state.
Splitting the traits in this way makes it easier to implement within macros, but it increases the cognitive load. Worst of all, this approach requires each `MySystemParam` to have a public `MySystemParamState` type associated with it.
## Solution
* Merge the trait `SystemParamState` into `SystemParam`.
* Remove all trivial `SystemParam` state types.
* `OptionNonSendMutState<T>`: you will not be missed.
---
- [x] Fix/resolve the remaining test failure.
## Changelog
* Removed the trait `SystemParamState`, merging its functionality into `SystemParam`.
## Migration Guide
**Note**: this should replace the migration guide for #6865.
This is relative to Bevy 0.9, not main.
The traits `SystemParamState` and `SystemParamFetch` have been removed, and their functionality has been transferred to `SystemParam`.
```rust
// Before (0.9)
impl SystemParam for MyParam<'_, '_> {
type State = MyParamState;
}
unsafe impl SystemParamState for MyParamState {
fn init(world: &mut World, system_meta: &mut SystemMeta) -> Self { ... }
}
unsafe impl<'w, 's> SystemParamFetch<'w, 's> for MyParamState {
type Item = MyParam<'w, 's>;
fn get_param(&mut self, ...) -> Self::Item;
}
unsafe impl ReadOnlySystemParamFetch for MyParamState { }
// After (0.10)
unsafe impl SystemParam for MyParam<'_, '_> {
type State = MyParamState;
type Item<'w, 's> = MyParam<'w, 's>;
fn init_state(world: &mut World, system_meta: &mut SystemMeta) -> Self::State { ... }
fn get_param<'w, 's>(state: &mut Self::State, ...) -> Self::Item<'w, 's>;
}
unsafe impl ReadOnlySystemParam for MyParam<'_, '_> { }
```
The trait `ReadOnlySystemParamFetch` has been replaced with `ReadOnlySystemParam`.
```rust
// Before
unsafe impl ReadOnlySystemParamFetch for MyParamState {}
// After
unsafe impl ReadOnlySystemParam for MyParam<'_, '_> {}
```
2023-01-07 23:20:32 +00:00
SystemName { name }
2022-08-18 18:31:12 +00:00
}
}
Remove the `SystemParamState` trait and remove types like `ResState` (#6919)
Spiritual successor to #5205.
Actual successor to #6865.
# Objective
Currently, system params are defined using three traits: `SystemParam`, `ReadOnlySystemParam`, `SystemParamState`. The behavior for each param is specified by the `SystemParamState` trait, while `SystemParam` simply defers to the state.
Splitting the traits in this way makes it easier to implement within macros, but it increases the cognitive load. Worst of all, this approach requires each `MySystemParam` to have a public `MySystemParamState` type associated with it.
## Solution
* Merge the trait `SystemParamState` into `SystemParam`.
* Remove all trivial `SystemParam` state types.
* `OptionNonSendMutState<T>`: you will not be missed.
---
- [x] Fix/resolve the remaining test failure.
## Changelog
* Removed the trait `SystemParamState`, merging its functionality into `SystemParam`.
## Migration Guide
**Note**: this should replace the migration guide for #6865.
This is relative to Bevy 0.9, not main.
The traits `SystemParamState` and `SystemParamFetch` have been removed, and their functionality has been transferred to `SystemParam`.
```rust
// Before (0.9)
impl SystemParam for MyParam<'_, '_> {
type State = MyParamState;
}
unsafe impl SystemParamState for MyParamState {
fn init(world: &mut World, system_meta: &mut SystemMeta) -> Self { ... }
}
unsafe impl<'w, 's> SystemParamFetch<'w, 's> for MyParamState {
type Item = MyParam<'w, 's>;
fn get_param(&mut self, ...) -> Self::Item;
}
unsafe impl ReadOnlySystemParamFetch for MyParamState { }
// After (0.10)
unsafe impl SystemParam for MyParam<'_, '_> {
type State = MyParamState;
type Item<'w, 's> = MyParam<'w, 's>;
fn init_state(world: &mut World, system_meta: &mut SystemMeta) -> Self::State { ... }
fn get_param<'w, 's>(state: &mut Self::State, ...) -> Self::Item<'w, 's>;
}
unsafe impl ReadOnlySystemParam for MyParam<'_, '_> { }
```
The trait `ReadOnlySystemParamFetch` has been replaced with `ReadOnlySystemParam`.
```rust
// Before
unsafe impl ReadOnlySystemParamFetch for MyParamState {}
// After
unsafe impl ReadOnlySystemParam for MyParam<'_, '_> {}
```
2023-01-07 23:20:32 +00:00
// SAFETY: Only reads internal system state
unsafe impl < ' s > ReadOnlySystemParam for SystemName < ' s > { }
2020-11-08 20:34:05 +00:00
macro_rules ! impl_system_param_tuple {
( $( $param : ident ) , * ) = > {
Simplify trait hierarchy for `SystemParam` (#6865)
# Objective
* Implementing a custom `SystemParam` by hand requires implementing three traits -- four if it is read-only.
* The trait `SystemParamFetch<'w, 's>` is a workaround from before we had generic associated types, and is no longer necessary.
## Solution
* Combine the trait `SystemParamFetch` with `SystemParamState`.
* I decided to remove the `Fetch` name and keep the `State` name, since the former was consistently conflated with the latter.
* Replace the trait `ReadOnlySystemParamFetch` with `ReadOnlySystemParam`, which simplifies trait bounds in generic code.
---
## Changelog
- Removed the trait `SystemParamFetch`, moving its functionality to `SystemParamState`.
- Replaced the trait `ReadOnlySystemParamFetch` with `ReadOnlySystemParam`.
## Migration Guide
The trait `SystemParamFetch` has been removed, and its functionality has been transferred to `SystemParamState`.
```rust
// Before
impl SystemParamState for MyParamState {
fn init(world: &mut World, system_meta: &mut SystemMeta) -> Self { ... }
}
impl<'w, 's> SystemParamFetch<'w, 's> for MyParamState {
type Item = MyParam<'w, 's>;
fn get_param(...) -> Self::Item;
}
// After
impl SystemParamState for MyParamState {
type Item<'w, 's> = MyParam<'w, 's>; // Generic associated types!
fn init(world: &mut World, system_meta: &mut SystemMeta) -> Self { ... }
fn get_param<'w, 's>(...) -> Self::Item<'w, 's>;
}
```
The trait `ReadOnlySystemParamFetch` has been replaced with `ReadOnlySystemParam`.
```rust
// Before
unsafe impl ReadOnlySystemParamFetch for MyParamState {}
// After
unsafe impl<'w, 's> ReadOnlySystemParam for MyParam<'w, 's> {}
```
2022-12-11 18:34:14 +00:00
// SAFETY: tuple consists only of ReadOnlySystemParams
unsafe impl < $( $param : ReadOnlySystemParam ) , * > ReadOnlySystemParam for ( $( $param , ) * ) { }
Add new SystemState and rename old SystemState to SystemMeta (#2283)
This enables `SystemParams` to be used outside of function systems. Anything can create and store `SystemState`, which enables efficient "param state cached" access to `SystemParams`.
It adds a `ReadOnlySystemParamFetch` trait, which enables safe `SystemState::get` calls without unique world access.
I renamed the old `SystemState` to `SystemMeta` to enable us to mirror the `QueryState` naming convention (but I'm happy to discuss alternative names if people have other ideas). I initially pitched this as `ParamState`, but given that it needs to include full system metadata, that doesn't feel like a particularly accurate name.
```rust
#[derive(Eq, PartialEq, Debug)]
struct A(usize);
#[derive(Eq, PartialEq, Debug)]
struct B(usize);
let mut world = World::default();
world.insert_resource(A(42));
world.spawn().insert(B(7));
// we get nice lifetime elision when declaring the type on the left hand side
let mut system_state: SystemState<(Res<A>, Query<&B>)> = SystemState::new(&mut world);
let (a, query) = system_state.get(&world);
assert_eq!(*a, A(42), "returned resource matches initial value");
assert_eq!(
*query.single().unwrap(),
B(7),
"returned component matches initial value"
);
// mutable system params require unique world access
let mut system_state: SystemState<(ResMut<A>, Query<&mut B>)> = SystemState::new(&mut world);
let (a, query) = system_state.get_mut(&mut world);
// static lifetimes are required when declaring inside of structs
struct SomeContainer {
state: SystemState<(Res<'static, A>, Res<'static, B>)>
}
// this can be shortened using type aliases, which will be useful for complex param tuples
type MyParams<'a> = (Res<'a, A>, Res<'a, B>);
struct SomeContainer {
state: SystemState<MyParams<'static>>
}
// It is the user's responsibility to call SystemState::apply(world) for parameters that queue up work
let mut system_state: SystemState<(Commands, Query<&B>)> = SystemState::new(&mut world);
{
let (mut commands, query) = system_state.get(&world);
commands.insert_resource(3.14);
}
system_state.apply(&mut world);
```
## Future Work
* Actually use SystemState inside FunctionSystem. This would be trivial, but it requires FunctionSystem to wrap SystemState in Option in its current form (which complicates system metadata lookup). I'd prefer to hold off until we adopt something like the later designs linked in #1364, which enable us to contruct Systems using a World reference (and also remove the need for `.system`).
* Consider a "scoped" approach to automatically call SystemState::apply when systems params are no longer being used (either a container type with a Drop impl, or a function that takes a closure for user logic operating on params).
2021-06-02 19:57:38 +00:00
Remove the `SystemParamState` trait and remove types like `ResState` (#6919)
Spiritual successor to #5205.
Actual successor to #6865.
# Objective
Currently, system params are defined using three traits: `SystemParam`, `ReadOnlySystemParam`, `SystemParamState`. The behavior for each param is specified by the `SystemParamState` trait, while `SystemParam` simply defers to the state.
Splitting the traits in this way makes it easier to implement within macros, but it increases the cognitive load. Worst of all, this approach requires each `MySystemParam` to have a public `MySystemParamState` type associated with it.
## Solution
* Merge the trait `SystemParamState` into `SystemParam`.
* Remove all trivial `SystemParam` state types.
* `OptionNonSendMutState<T>`: you will not be missed.
---
- [x] Fix/resolve the remaining test failure.
## Changelog
* Removed the trait `SystemParamState`, merging its functionality into `SystemParam`.
## Migration Guide
**Note**: this should replace the migration guide for #6865.
This is relative to Bevy 0.9, not main.
The traits `SystemParamState` and `SystemParamFetch` have been removed, and their functionality has been transferred to `SystemParam`.
```rust
// Before (0.9)
impl SystemParam for MyParam<'_, '_> {
type State = MyParamState;
}
unsafe impl SystemParamState for MyParamState {
fn init(world: &mut World, system_meta: &mut SystemMeta) -> Self { ... }
}
unsafe impl<'w, 's> SystemParamFetch<'w, 's> for MyParamState {
type Item = MyParam<'w, 's>;
fn get_param(&mut self, ...) -> Self::Item;
}
unsafe impl ReadOnlySystemParamFetch for MyParamState { }
// After (0.10)
unsafe impl SystemParam for MyParam<'_, '_> {
type State = MyParamState;
type Item<'w, 's> = MyParam<'w, 's>;
fn init_state(world: &mut World, system_meta: &mut SystemMeta) -> Self::State { ... }
fn get_param<'w, 's>(state: &mut Self::State, ...) -> Self::Item<'w, 's>;
}
unsafe impl ReadOnlySystemParam for MyParam<'_, '_> { }
```
The trait `ReadOnlySystemParamFetch` has been replaced with `ReadOnlySystemParam`.
```rust
// Before
unsafe impl ReadOnlySystemParamFetch for MyParamState {}
// After
unsafe impl ReadOnlySystemParam for MyParam<'_, '_> {}
```
2023-01-07 23:20:32 +00:00
// SAFETY: implementors of each `SystemParam` in the tuple have validated their impls
2022-07-04 14:44:24 +00:00
#[ allow(clippy::undocumented_unsafe_blocks) ] // false positive by clippy
2020-11-08 20:34:05 +00:00
#[ allow(non_snake_case) ]
Remove the `SystemParamState` trait and remove types like `ResState` (#6919)
Spiritual successor to #5205.
Actual successor to #6865.
# Objective
Currently, system params are defined using three traits: `SystemParam`, `ReadOnlySystemParam`, `SystemParamState`. The behavior for each param is specified by the `SystemParamState` trait, while `SystemParam` simply defers to the state.
Splitting the traits in this way makes it easier to implement within macros, but it increases the cognitive load. Worst of all, this approach requires each `MySystemParam` to have a public `MySystemParamState` type associated with it.
## Solution
* Merge the trait `SystemParamState` into `SystemParam`.
* Remove all trivial `SystemParam` state types.
* `OptionNonSendMutState<T>`: you will not be missed.
---
- [x] Fix/resolve the remaining test failure.
## Changelog
* Removed the trait `SystemParamState`, merging its functionality into `SystemParam`.
## Migration Guide
**Note**: this should replace the migration guide for #6865.
This is relative to Bevy 0.9, not main.
The traits `SystemParamState` and `SystemParamFetch` have been removed, and their functionality has been transferred to `SystemParam`.
```rust
// Before (0.9)
impl SystemParam for MyParam<'_, '_> {
type State = MyParamState;
}
unsafe impl SystemParamState for MyParamState {
fn init(world: &mut World, system_meta: &mut SystemMeta) -> Self { ... }
}
unsafe impl<'w, 's> SystemParamFetch<'w, 's> for MyParamState {
type Item = MyParam<'w, 's>;
fn get_param(&mut self, ...) -> Self::Item;
}
unsafe impl ReadOnlySystemParamFetch for MyParamState { }
// After (0.10)
unsafe impl SystemParam for MyParam<'_, '_> {
type State = MyParamState;
type Item<'w, 's> = MyParam<'w, 's>;
fn init_state(world: &mut World, system_meta: &mut SystemMeta) -> Self::State { ... }
fn get_param<'w, 's>(state: &mut Self::State, ...) -> Self::Item<'w, 's>;
}
unsafe impl ReadOnlySystemParam for MyParam<'_, '_> { }
```
The trait `ReadOnlySystemParamFetch` has been replaced with `ReadOnlySystemParam`.
```rust
// Before
unsafe impl ReadOnlySystemParamFetch for MyParamState {}
// After
unsafe impl ReadOnlySystemParam for MyParam<'_, '_> {}
```
2023-01-07 23:20:32 +00:00
unsafe impl < $( $param : SystemParam ) , * > SystemParam for ( $( $param , ) * ) {
type State = ( $( $param ::State , ) * ) ;
Simplify trait hierarchy for `SystemParam` (#6865)
# Objective
* Implementing a custom `SystemParam` by hand requires implementing three traits -- four if it is read-only.
* The trait `SystemParamFetch<'w, 's>` is a workaround from before we had generic associated types, and is no longer necessary.
## Solution
* Combine the trait `SystemParamFetch` with `SystemParamState`.
* I decided to remove the `Fetch` name and keep the `State` name, since the former was consistently conflated with the latter.
* Replace the trait `ReadOnlySystemParamFetch` with `ReadOnlySystemParam`, which simplifies trait bounds in generic code.
---
## Changelog
- Removed the trait `SystemParamFetch`, moving its functionality to `SystemParamState`.
- Replaced the trait `ReadOnlySystemParamFetch` with `ReadOnlySystemParam`.
## Migration Guide
The trait `SystemParamFetch` has been removed, and its functionality has been transferred to `SystemParamState`.
```rust
// Before
impl SystemParamState for MyParamState {
fn init(world: &mut World, system_meta: &mut SystemMeta) -> Self { ... }
}
impl<'w, 's> SystemParamFetch<'w, 's> for MyParamState {
type Item = MyParam<'w, 's>;
fn get_param(...) -> Self::Item;
}
// After
impl SystemParamState for MyParamState {
type Item<'w, 's> = MyParam<'w, 's>; // Generic associated types!
fn init(world: &mut World, system_meta: &mut SystemMeta) -> Self { ... }
fn get_param<'w, 's>(...) -> Self::Item<'w, 's>;
}
```
The trait `ReadOnlySystemParamFetch` has been replaced with `ReadOnlySystemParam`.
```rust
// Before
unsafe impl ReadOnlySystemParamFetch for MyParamState {}
// After
unsafe impl<'w, 's> ReadOnlySystemParam for MyParam<'w, 's> {}
```
2022-12-11 18:34:14 +00:00
type Item < ' w , ' s > = ( $( $param ::Item ::< ' w , ' s > , ) * ) ;
Bevy ECS V2 (#1525)
# Bevy ECS V2
This is a rewrite of Bevy ECS (basically everything but the new executor/schedule, which are already awesome). The overall goal was to improve the performance and versatility of Bevy ECS. Here is a quick bulleted list of changes before we dive into the details:
* Complete World rewrite
* Multiple component storage types:
* Tables: fast cache friendly iteration, slower add/removes (previously called Archetypes)
* Sparse Sets: fast add/remove, slower iteration
* Stateful Queries (caches query results for faster iteration. fragmented iteration is _fast_ now)
* Stateful System Params (caches expensive operations. inspired by @DJMcNab's work in #1364)
* Configurable System Params (users can set configuration when they construct their systems. once again inspired by @DJMcNab's work)
* Archetypes are now "just metadata", component storage is separate
* Archetype Graph (for faster archetype changes)
* Component Metadata
* Configure component storage type
* Retrieve information about component size/type/name/layout/send-ness/etc
* Components are uniquely identified by a densely packed ComponentId
* TypeIds are now totally optional (which should make implementing scripting easier)
* Super fast "for_each" query iterators
* Merged Resources into World. Resources are now just a special type of component
* EntityRef/EntityMut builder apis (more efficient and more ergonomic)
* Fast bitset-backed `Access<T>` replaces old hashmap-based approach everywhere
* Query conflicts are determined by component access instead of archetype component access (to avoid random failures at runtime)
* With/Without are still taken into account for conflicts, so this should still be comfy to use
* Much simpler `IntoSystem` impl
* Significantly reduced the amount of hashing throughout the ecs in favor of Sparse Sets (indexed by densely packed ArchetypeId, ComponentId, BundleId, and TableId)
* Safety Improvements
* Entity reservation uses a normal world reference instead of unsafe transmute
* QuerySets no longer transmute lifetimes
* Made traits "unsafe" where relevant
* More thorough safety docs
* WorldCell
* Exposes safe mutable access to multiple resources at a time in a World
* Replaced "catch all" `System::update_archetypes(world: &World)` with `System::new_archetype(archetype: &Archetype)`
* Simpler Bundle implementation
* Replaced slow "remove_bundle_one_by_one" used as fallback for Commands::remove_bundle with fast "remove_bundle_intersection"
* Removed `Mut<T>` query impl. it is better to only support one way: `&mut T`
* Removed with() from `Flags<T>` in favor of `Option<Flags<T>>`, which allows querying for flags to be "filtered" by default
* Components now have is_send property (currently only resources support non-send)
* More granular module organization
* New `RemovedComponents<T>` SystemParam that replaces `query.removed::<T>()`
* `world.resource_scope()` for mutable access to resources and world at the same time
* WorldQuery and QueryFilter traits unified. FilterFetch trait added to enable "short circuit" filtering. Auto impled for cases that don't need it
* Significantly slimmed down SystemState in favor of individual SystemParam state
* System Commands changed from `commands: &mut Commands` back to `mut commands: Commands` (to allow Commands to have a World reference)
Fixes #1320
## `World` Rewrite
This is a from-scratch rewrite of `World` that fills the niche that `hecs` used to. Yes, this means Bevy ECS is no longer a "fork" of hecs. We're going out our own!
(the only shared code between the projects is the entity id allocator, which is already basically ideal)
A huge shout out to @SanderMertens (author of [flecs](https://github.com/SanderMertens/flecs)) for sharing some great ideas with me (specifically hybrid ecs storage and archetype graphs). He also helped advise on a number of implementation details.
## Component Storage (The Problem)
Two ECS storage paradigms have gained a lot of traction over the years:
* **Archetypal ECS**:
* Stores components in "tables" with static schemas. Each "column" stores components of a given type. Each "row" is an entity.
* Each "archetype" has its own table. Adding/removing an entity's component changes the archetype.
* Enables super-fast Query iteration due to its cache-friendly data layout
* Comes at the cost of more expensive add/remove operations for an Entity's components, because all components need to be copied to the new archetype's "table"
* **Sparse Set ECS**:
* Stores components of the same type in densely packed arrays, which are sparsely indexed by densely packed unsigned integers (Entity ids)
* Query iteration is slower than Archetypal ECS because each entity's component could be at any position in the sparse set. This "random access" pattern isn't cache friendly. Additionally, there is an extra layer of indirection because you must first map the entity id to an index in the component array.
* Adding/removing components is a cheap, constant time operation
Bevy ECS V1, hecs, legion, flec, and Unity DOTS are all "archetypal ecs-es". I personally think "archetypal" storage is a good default for game engines. An entity's archetype doesn't need to change frequently in general, and it creates "fast by default" query iteration (which is a much more common operation). It is also "self optimizing". Users don't need to think about optimizing component layouts for iteration performance. It "just works" without any extra boilerplate.
Shipyard and EnTT are "sparse set ecs-es". They employ "packing" as a way to work around the "suboptimal by default" iteration performance for specific sets of components. This helps, but I didn't think this was a good choice for a general purpose engine like Bevy because:
1. "packs" conflict with each other. If bevy decides to internally pack the Transform and GlobalTransform components, users are then blocked if they want to pack some custom component with Transform.
2. users need to take manual action to optimize
Developers selecting an ECS framework are stuck with a hard choice. Select an "archetypal" framework with "fast iteration everywhere" but without the ability to cheaply add/remove components, or select a "sparse set" framework to cheaply add/remove components but with slower iteration performance.
## Hybrid Component Storage (The Solution)
In Bevy ECS V2, we get to have our cake and eat it too. It now has _both_ of the component storage types above (and more can be added later if needed):
* **Tables** (aka "archetypal" storage)
* The default storage. If you don't configure anything, this is what you get
* Fast iteration by default
* Slower add/remove operations
* **Sparse Sets**
* Opt-in
* Slower iteration
* Faster add/remove operations
These storage types complement each other perfectly. By default Query iteration is fast. If developers know that they want to add/remove a component at high frequencies, they can set the storage to "sparse set":
```rust
world.register_component(
ComponentDescriptor::new::<MyComponent>(StorageType::SparseSet)
).unwrap();
```
## Archetypes
Archetypes are now "just metadata" ... they no longer store components directly. They do store:
* The `ComponentId`s of each of the Archetype's components (and that component's storage type)
* Archetypes are uniquely defined by their component layouts
* For example: entities with "table" components `[A, B, C]` _and_ "sparse set" components `[D, E]` will always be in the same archetype.
* The `TableId` associated with the archetype
* For now each archetype has exactly one table (which can have no components),
* There is a 1->Many relationship from Tables->Archetypes. A given table could have any number of archetype components stored in it:
* Ex: an entity with "table storage" components `[A, B, C]` and "sparse set" components `[D, E]` will share the same `[A, B, C]` table as an entity with `[A, B, C]` table component and `[F]` sparse set components.
* This 1->Many relationship is how we preserve fast "cache friendly" iteration performance when possible (more on this later)
* A list of entities that are in the archetype and the row id of the table they are in
* ArchetypeComponentIds
* unique densely packed identifiers for (ArchetypeId, ComponentId) pairs
* used by the schedule executor for cheap system access control
* "Archetype Graph Edges" (see the next section)
## The "Archetype Graph"
Archetype changes in Bevy (and a number of other archetypal ecs-es) have historically been expensive to compute. First, you need to allocate a new vector of the entity's current component ids, add or remove components based on the operation performed, sort it (to ensure it is order-independent), then hash it to find the archetype (if it exists). And thats all before we get to the _already_ expensive full copy of all components to the new table storage.
The solution is to build a "graph" of archetypes to cache these results. @SanderMertens first exposed me to the idea (and he got it from @gjroelofs, who came up with it). They propose adding directed edges between archetypes for add/remove component operations. If `ComponentId`s are densely packed, you can use sparse sets to cheaply jump between archetypes.
Bevy takes this one step further by using add/remove `Bundle` edges instead of `Component` edges. Bevy encourages the use of `Bundles` to group add/remove operations. This is largely for "clearer game logic" reasons, but it also helps cut down on the number of archetype changes required. `Bundles` now also have densely-packed `BundleId`s. This allows us to use a _single_ edge for each bundle operation (rather than needing to traverse N edges ... one for each component). Single component operations are also bundles, so this is strictly an improvement over a "component only" graph.
As a result, an operation that used to be _heavy_ (both for allocations and compute) is now two dirt-cheap array lookups and zero allocations.
## Stateful Queries
World queries are now stateful. This allows us to:
1. Cache archetype (and table) matches
* This resolves another issue with (naive) archetypal ECS: query performance getting worse as the number of archetypes goes up (and fragmentation occurs).
2. Cache Fetch and Filter state
* The expensive parts of fetch/filter operations (such as hashing the TypeId to find the ComponentId) now only happen once when the Query is first constructed
3. Incrementally build up state
* When new archetypes are added, we only process the new archetypes (no need to rebuild state for old archetypes)
As a result, the direct `World` query api now looks like this:
```rust
let mut query = world.query::<(&A, &mut B)>();
for (a, mut b) in query.iter_mut(&mut world) {
}
```
Requiring `World` to generate stateful queries (rather than letting the `QueryState` type be constructed separately) allows us to ensure that _all_ queries are properly initialized (and the relevant world state, such as ComponentIds). This enables QueryState to remove branches from its operations that check for initialization status (and also enables query.iter() to take an immutable world reference because it doesn't need to initialize anything in world).
However in systems, this is a non-breaking change. State management is done internally by the relevant SystemParam.
## Stateful SystemParams
Like Queries, `SystemParams` now also cache state. For example, `Query` system params store the "stateful query" state mentioned above. Commands store their internal `CommandQueue`. This means you can now safely use as many separate `Commands` parameters in your system as you want. `Local<T>` system params store their `T` value in their state (instead of in Resources).
SystemParam state also enabled a significant slim-down of SystemState. It is much nicer to look at now.
Per-SystemParam state naturally insulates us from an "aliased mut" class of errors we have hit in the past (ex: using multiple `Commands` system params).
(credit goes to @DJMcNab for the initial idea and draft pr here #1364)
## Configurable SystemParams
@DJMcNab also had the great idea to make SystemParams configurable. This allows users to provide some initial configuration / values for system parameters (when possible). Most SystemParams have no config (the config type is `()`), but the `Local<T>` param now supports user-provided parameters:
```rust
fn foo(value: Local<usize>) {
}
app.add_system(foo.system().config(|c| c.0 = Some(10)));
```
## Uber Fast "for_each" Query Iterators
Developers now have the choice to use a fast "for_each" iterator, which yields ~1.5-3x iteration speed improvements for "fragmented iteration", and minor ~1.2x iteration speed improvements for unfragmented iteration.
```rust
fn system(query: Query<(&A, &mut B)>) {
// you now have the option to do this for a speed boost
query.for_each_mut(|(a, mut b)| {
});
// however normal iterators are still available
for (a, mut b) in query.iter_mut() {
}
}
```
I think in most cases we should continue to encourage "normal" iterators as they are more flexible and more "rust idiomatic". But when that extra "oomf" is needed, it makes sense to use `for_each`.
We should also consider using `for_each` for internal bevy systems to give our users a nice speed boost (but that should be a separate pr).
## Component Metadata
`World` now has a `Components` collection, which is accessible via `world.components()`. This stores mappings from `ComponentId` to `ComponentInfo`, as well as `TypeId` to `ComponentId` mappings (where relevant). `ComponentInfo` stores information about the component, such as ComponentId, TypeId, memory layout, send-ness (currently limited to resources), and storage type.
## Significantly Cheaper `Access<T>`
We used to use `TypeAccess<TypeId>` to manage read/write component/archetype-component access. This was expensive because TypeIds must be hashed and compared individually. The parallel executor got around this by "condensing" type ids into bitset-backed access types. This worked, but it had to be re-generated from the `TypeAccess<TypeId>`sources every time archetypes changed.
This pr removes TypeAccess in favor of faster bitset access everywhere. We can do this thanks to the move to densely packed `ComponentId`s and `ArchetypeComponentId`s.
## Merged Resources into World
Resources had a lot of redundant functionality with Components. They stored typed data, they had access control, they had unique ids, they were queryable via SystemParams, etc. In fact the _only_ major difference between them was that they were unique (and didn't correlate to an entity).
Separate resources also had the downside of requiring a separate set of access controls, which meant the parallel executor needed to compare more bitsets per system and manage more state.
I initially got the "separate resources" idea from `legion`. I think that design was motivated by the fact that it made the direct world query/resource lifetime interactions more manageable. It certainly made our lives easier when using Resources alongside hecs/bevy_ecs. However we already have a construct for safely and ergonomically managing in-world lifetimes: systems (which use `Access<T>` internally).
This pr merges Resources into World:
```rust
world.insert_resource(1);
world.insert_resource(2.0);
let a = world.get_resource::<i32>().unwrap();
let mut b = world.get_resource_mut::<f64>().unwrap();
*b = 3.0;
```
Resources are now just a special kind of component. They have their own ComponentIds (and their own resource TypeId->ComponentId scope, so they don't conflict wit components of the same type). They are stored in a special "resource archetype", which stores components inside the archetype using a new `unique_components` sparse set (note that this sparse set could later be used to implement Tags). This allows us to keep the code size small by reusing existing datastructures (namely Column, Archetype, ComponentFlags, and ComponentInfo). This allows us the executor to use a single `Access<ArchetypeComponentId>` per system. It should also make scripting language integration easier.
_But_ this merge did create problems for people directly interacting with `World`. What if you need mutable access to multiple resources at the same time? `world.get_resource_mut()` borrows World mutably!
## WorldCell
WorldCell applies the `Access<ArchetypeComponentId>` concept to direct world access:
```rust
let world_cell = world.cell();
let a = world_cell.get_resource_mut::<i32>().unwrap();
let b = world_cell.get_resource_mut::<f64>().unwrap();
```
This adds cheap runtime checks (a sparse set lookup of `ArchetypeComponentId` and a counter) to ensure that world accesses do not conflict with each other. Each operation returns a `WorldBorrow<'w, T>` or `WorldBorrowMut<'w, T>` wrapper type, which will release the relevant ArchetypeComponentId resources when dropped.
World caches the access sparse set (and only one cell can exist at a time), so `world.cell()` is a cheap operation.
WorldCell does _not_ use atomic operations. It is non-send, does a mutable borrow of world to prevent other accesses, and uses a simple `Rc<RefCell<ArchetypeComponentAccess>>` wrapper in each WorldBorrow pointer.
The api is currently limited to resource access, but it can and should be extended to queries / entity component access.
## Resource Scopes
WorldCell does not yet support component queries, and even when it does there are sometimes legitimate reasons to want a mutable world ref _and_ a mutable resource ref (ex: bevy_render and bevy_scene both need this). In these cases we could always drop down to the unsafe `world.get_resource_unchecked_mut()`, but that is not ideal!
Instead developers can use a "resource scope"
```rust
world.resource_scope(|world: &mut World, a: &mut A| {
})
```
This temporarily removes the `A` resource from `World`, provides mutable pointers to both, and re-adds A to World when finished. Thanks to the move to ComponentIds/sparse sets, this is a cheap operation.
If multiple resources are required, scopes can be nested. We could also consider adding a "resource tuple" to the api if this pattern becomes common and the boilerplate gets nasty.
## Query Conflicts Use ComponentId Instead of ArchetypeComponentId
For safety reasons, systems cannot contain queries that conflict with each other without wrapping them in a QuerySet. On bevy `main`, we use ArchetypeComponentIds to determine conflicts. This is nice because it can take into account filters:
```rust
// these queries will never conflict due to their filters
fn filter_system(a: Query<&mut A, With<B>>, b: Query<&mut B, Without<B>>) {
}
```
But it also has a significant downside:
```rust
// these queries will not conflict _until_ an entity with A, B, and C is spawned
fn maybe_conflicts_system(a: Query<(&mut A, &C)>, b: Query<(&mut A, &B)>) {
}
```
The system above will panic at runtime if an entity with A, B, and C is spawned. This makes it hard to trust that your game logic will run without crashing.
In this pr, I switched to using `ComponentId` instead. This _is_ more constraining. `maybe_conflicts_system` will now always fail, but it will do it consistently at startup. Naively, it would also _disallow_ `filter_system`, which would be a significant downgrade in usability. Bevy has a number of internal systems that rely on disjoint queries and I expect it to be a common pattern in userspace.
To resolve this, I added a new `FilteredAccess<T>` type, which wraps `Access<T>` and adds with/without filters. If two `FilteredAccess` have with/without values that prove they are disjoint, they will no longer conflict.
## EntityRef / EntityMut
World entity operations on `main` require that the user passes in an `entity` id to each operation:
```rust
let entity = world.spawn((A, )); // create a new entity with A
world.get::<A>(entity);
world.insert(entity, (B, C));
world.insert_one(entity, D);
```
This means that each operation needs to look up the entity location / verify its validity. The initial spawn operation also requires a Bundle as input. This can be awkward when no components are required (or one component is required).
These operations have been replaced by `EntityRef` and `EntityMut`, which are "builder-style" wrappers around world that provide read and read/write operations on a single, pre-validated entity:
```rust
// spawn now takes no inputs and returns an EntityMut
let entity = world.spawn()
.insert(A) // insert a single component into the entity
.insert_bundle((B, C)) // insert a bundle of components into the entity
.id() // id returns the Entity id
// Returns EntityMut (or panics if the entity does not exist)
world.entity_mut(entity)
.insert(D)
.insert_bundle(SomeBundle::default());
{
// returns EntityRef (or panics if the entity does not exist)
let d = world.entity(entity)
.get::<D>() // gets the D component
.unwrap();
// world.get still exists for ergonomics
let d = world.get::<D>(entity).unwrap();
}
// These variants return Options if you want to check existence instead of panicing
world.get_entity_mut(entity)
.unwrap()
.insert(E);
if let Some(entity_ref) = world.get_entity(entity) {
let d = entity_ref.get::<D>().unwrap();
}
```
This _does not_ affect the current Commands api or terminology. I think that should be a separate conversation as that is a much larger breaking change.
## Safety Improvements
* Entity reservation in Commands uses a normal world borrow instead of an unsafe transmute
* QuerySets no longer transmutes lifetimes
* Made traits "unsafe" when implementing a trait incorrectly could cause unsafety
* More thorough safety docs
## RemovedComponents SystemParam
The old approach to querying removed components: `query.removed:<T>()` was confusing because it had no connection to the query itself. I replaced it with the following, which is both clearer and allows us to cache the ComponentId mapping in the SystemParamState:
```rust
fn system(removed: RemovedComponents<T>) {
for entity in removed.iter() {
}
}
```
## Simpler Bundle implementation
Bundles are no longer responsible for sorting (or deduping) TypeInfo. They are just a simple ordered list of component types / data. This makes the implementation smaller and opens the door to an easy "nested bundle" implementation in the future (which i might even add in this pr). Duplicate detection is now done once per bundle type by World the first time a bundle is used.
## Unified WorldQuery and QueryFilter types
(don't worry they are still separate type _parameters_ in Queries .. this is a non-breaking change)
WorldQuery and QueryFilter were already basically identical apis. With the addition of `FetchState` and more storage-specific fetch methods, the overlap was even clearer (and the redundancy more painful).
QueryFilters are now just `F: WorldQuery where F::Fetch: FilterFetch`. FilterFetch requires `Fetch<Item = bool>` and adds new "short circuit" variants of fetch methods. This enables a filter tuple like `(With<A>, Without<B>, Changed<C>)` to stop evaluating the filter after the first mismatch is encountered. FilterFetch is automatically implemented for `Fetch` implementations that return bool.
This forces fetch implementations that return things like `(bool, bool, bool)` (such as the filter above) to manually implement FilterFetch and decide whether or not to short-circuit.
## More Granular Modules
World no longer globs all of the internal modules together. It now exports `core`, `system`, and `schedule` separately. I'm also considering exporting `core` submodules directly as that is still pretty "glob-ey" and unorganized (feedback welcome here).
## Remaining Draft Work (to be done in this pr)
* ~~panic on conflicting WorldQuery fetches (&A, &mut A)~~
* ~~bevy `main` and hecs both currently allow this, but we should protect against it if possible~~
* ~~batch_iter / par_iter (currently stubbed out)~~
* ~~ChangedRes~~
* ~~I skipped this while we sort out #1313. This pr should be adapted to account for whatever we land on there~~.
* ~~The `Archetypes` and `Tables` collections use hashes of sorted lists of component ids to uniquely identify each archetype/table. This hash is then used as the key in a HashMap to look up the relevant ArchetypeId or TableId. (which doesn't handle hash collisions properly)~~
* ~~It is currently unsafe to generate a Query from "World A", then use it on "World B" (despite the api claiming it is safe). We should probably close this gap. This could be done by adding a randomly generated WorldId to each world, then storing that id in each Query. They could then be compared to each other on each `query.do_thing(&world)` operation. This _does_ add an extra branch to each query operation, so I'm open to other suggestions if people have them.~~
* ~~Nested Bundles (if i find time)~~
## Potential Future Work
* Expand WorldCell to support queries.
* Consider not allocating in the empty archetype on `world.spawn()`
* ex: return something like EntityMutUninit, which turns into EntityMut after an `insert` or `insert_bundle` op
* this actually regressed performance last time i tried it, but in theory it should be faster
* Optimize SparseSet::insert (see `PERF` comment on insert)
* Replace SparseArray `Option<T>` with T::MAX to cut down on branching
* would enable cheaper get_unchecked() operations
* upstream fixedbitset optimizations
* fixedbitset could be allocation free for small block counts (store blocks in a SmallVec)
* fixedbitset could have a const constructor
* Consider implementing Tags (archetype-specific by-value data that affects archetype identity)
* ex: ArchetypeA could have `[A, B, C]` table components and `[D(1)]` "tag" component. ArchetypeB could have `[A, B, C]` table components and a `[D(2)]` tag component. The archetypes are different, despite both having D tags because the value inside D is different.
* this could potentially build on top of the `archetype.unique_components` added in this pr for resource storage.
* Consider reverting `all_tuples` proc macro in favor of the old `macro_rules` implementation
* all_tuples is more flexible and produces cleaner documentation (the macro_rules version produces weird type parameter orders due to parser constraints)
* but unfortunately all_tuples also appears to make Rust Analyzer sad/slow when working inside of `bevy_ecs` (does not affect user code)
* Consider "resource queries" and/or "mixed resource and entity component queries" as an alternative to WorldCell
* this is basically just "systems" so maybe it's not worth it
* Add more world ops
* `world.clear()`
* `world.reserve<T: Bundle>(count: usize)`
* Try using the old archetype allocation strategy (allocate new memory on resize and copy everything over). I expect this to improve batch insertion performance at the cost of unbatched performance. But thats just a guess. I'm not an allocation perf pro :)
* Adapt Commands apis for consistency with new World apis
## Benchmarks
key:
* `bevy_old`: bevy `main` branch
* `bevy`: this branch
* `_foreach`: uses an optimized for_each iterator
* ` _sparse`: uses sparse set storage (if unspecified assume table storage)
* `_system`: runs inside a system (if unspecified assume test happens via direct world ops)
### Simple Insert (from ecs_bench_suite)
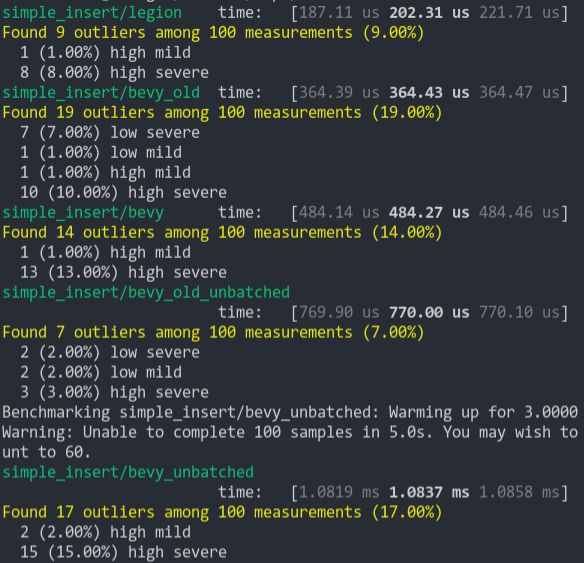
### Simpler Iter (from ecs_bench_suite)
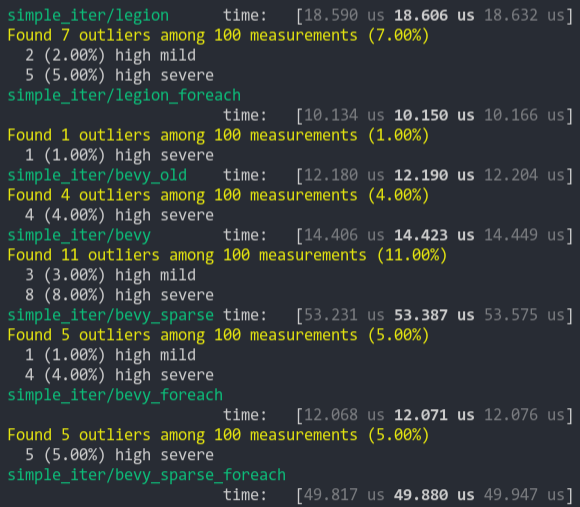
### Fragment Iter (from ecs_bench_suite)
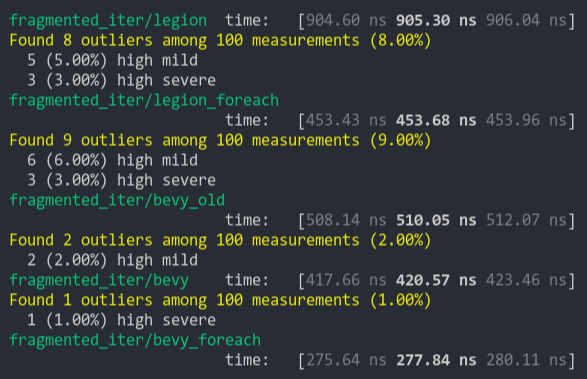
### Sparse Fragmented Iter
Iterate a query that matches 5 entities from a single matching archetype, but there are 100 unmatching archetypes
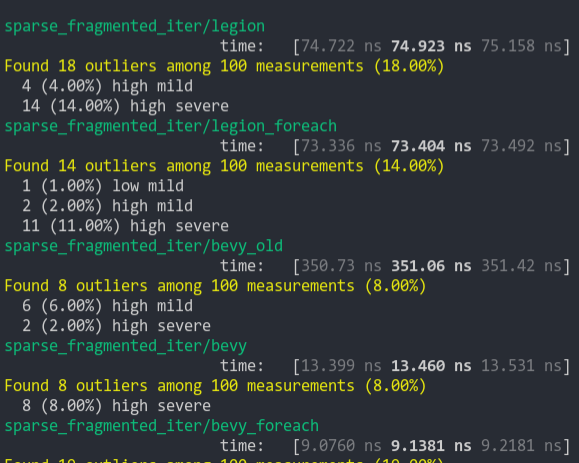
### Schedule (from ecs_bench_suite)
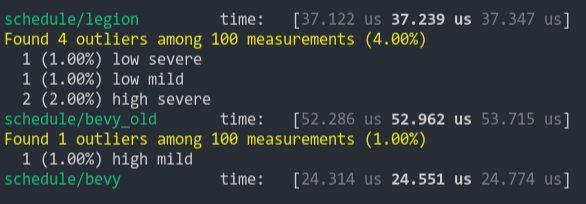
### Add Remove Component (from ecs_bench_suite)
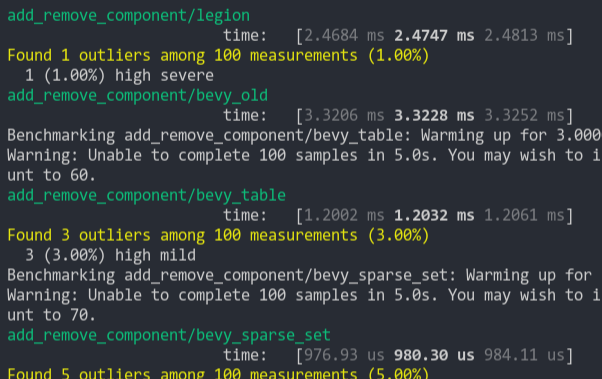
### Add Remove Component Big
Same as the test above, but each entity has 5 "large" matrix components and 1 "large" matrix component is added and removed
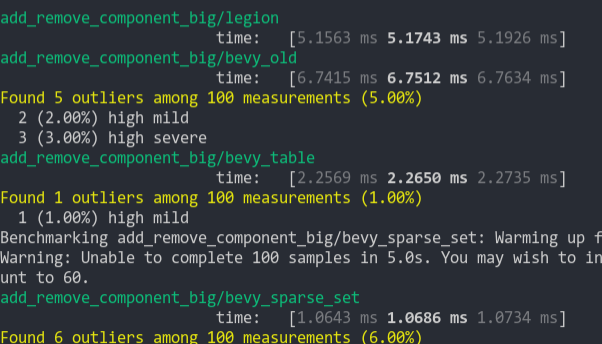
### Get Component
Looks up a single component value a large number of times
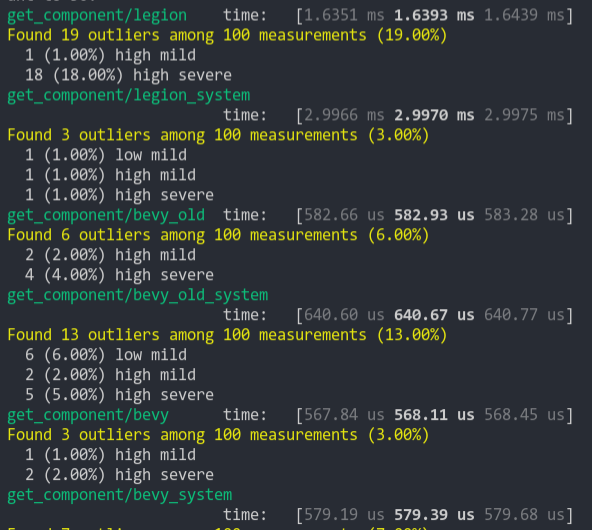
2021-03-05 07:54:35 +00:00
#[ inline ]
Remove the `SystemParamState` trait and remove types like `ResState` (#6919)
Spiritual successor to #5205.
Actual successor to #6865.
# Objective
Currently, system params are defined using three traits: `SystemParam`, `ReadOnlySystemParam`, `SystemParamState`. The behavior for each param is specified by the `SystemParamState` trait, while `SystemParam` simply defers to the state.
Splitting the traits in this way makes it easier to implement within macros, but it increases the cognitive load. Worst of all, this approach requires each `MySystemParam` to have a public `MySystemParamState` type associated with it.
## Solution
* Merge the trait `SystemParamState` into `SystemParam`.
* Remove all trivial `SystemParam` state types.
* `OptionNonSendMutState<T>`: you will not be missed.
---
- [x] Fix/resolve the remaining test failure.
## Changelog
* Removed the trait `SystemParamState`, merging its functionality into `SystemParam`.
## Migration Guide
**Note**: this should replace the migration guide for #6865.
This is relative to Bevy 0.9, not main.
The traits `SystemParamState` and `SystemParamFetch` have been removed, and their functionality has been transferred to `SystemParam`.
```rust
// Before (0.9)
impl SystemParam for MyParam<'_, '_> {
type State = MyParamState;
}
unsafe impl SystemParamState for MyParamState {
fn init(world: &mut World, system_meta: &mut SystemMeta) -> Self { ... }
}
unsafe impl<'w, 's> SystemParamFetch<'w, 's> for MyParamState {
type Item = MyParam<'w, 's>;
fn get_param(&mut self, ...) -> Self::Item;
}
unsafe impl ReadOnlySystemParamFetch for MyParamState { }
// After (0.10)
unsafe impl SystemParam for MyParam<'_, '_> {
type State = MyParamState;
type Item<'w, 's> = MyParam<'w, 's>;
fn init_state(world: &mut World, system_meta: &mut SystemMeta) -> Self::State { ... }
fn get_param<'w, 's>(state: &mut Self::State, ...) -> Self::Item<'w, 's>;
}
unsafe impl ReadOnlySystemParam for MyParam<'_, '_> { }
```
The trait `ReadOnlySystemParamFetch` has been replaced with `ReadOnlySystemParam`.
```rust
// Before
unsafe impl ReadOnlySystemParamFetch for MyParamState {}
// After
unsafe impl ReadOnlySystemParam for MyParam<'_, '_> {}
```
2023-01-07 23:20:32 +00:00
fn init_state ( _world : & mut World , _system_meta : & mut SystemMeta ) -> Self ::State {
( ( $( $param ::init_state ( _world , _system_meta ) , ) * ) )
2020-11-08 20:34:05 +00:00
}
#[ inline ]
Remove the `SystemParamState` trait and remove types like `ResState` (#6919)
Spiritual successor to #5205.
Actual successor to #6865.
# Objective
Currently, system params are defined using three traits: `SystemParam`, `ReadOnlySystemParam`, `SystemParamState`. The behavior for each param is specified by the `SystemParamState` trait, while `SystemParam` simply defers to the state.
Splitting the traits in this way makes it easier to implement within macros, but it increases the cognitive load. Worst of all, this approach requires each `MySystemParam` to have a public `MySystemParamState` type associated with it.
## Solution
* Merge the trait `SystemParamState` into `SystemParam`.
* Remove all trivial `SystemParam` state types.
* `OptionNonSendMutState<T>`: you will not be missed.
---
- [x] Fix/resolve the remaining test failure.
## Changelog
* Removed the trait `SystemParamState`, merging its functionality into `SystemParam`.
## Migration Guide
**Note**: this should replace the migration guide for #6865.
This is relative to Bevy 0.9, not main.
The traits `SystemParamState` and `SystemParamFetch` have been removed, and their functionality has been transferred to `SystemParam`.
```rust
// Before (0.9)
impl SystemParam for MyParam<'_, '_> {
type State = MyParamState;
}
unsafe impl SystemParamState for MyParamState {
fn init(world: &mut World, system_meta: &mut SystemMeta) -> Self { ... }
}
unsafe impl<'w, 's> SystemParamFetch<'w, 's> for MyParamState {
type Item = MyParam<'w, 's>;
fn get_param(&mut self, ...) -> Self::Item;
}
unsafe impl ReadOnlySystemParamFetch for MyParamState { }
// After (0.10)
unsafe impl SystemParam for MyParam<'_, '_> {
type State = MyParamState;
type Item<'w, 's> = MyParam<'w, 's>;
fn init_state(world: &mut World, system_meta: &mut SystemMeta) -> Self::State { ... }
fn get_param<'w, 's>(state: &mut Self::State, ...) -> Self::Item<'w, 's>;
}
unsafe impl ReadOnlySystemParam for MyParam<'_, '_> { }
```
The trait `ReadOnlySystemParamFetch` has been replaced with `ReadOnlySystemParam`.
```rust
// Before
unsafe impl ReadOnlySystemParamFetch for MyParamState {}
// After
unsafe impl ReadOnlySystemParam for MyParam<'_, '_> {}
```
2023-01-07 23:20:32 +00:00
fn new_archetype ( ( $( $param , ) * ) : & mut Self ::State , _archetype : & Archetype , _system_meta : & mut SystemMeta ) {
$( $param ::new_archetype ( $param , _archetype , _system_meta ) ; ) *
Bevy ECS V2 (#1525)
# Bevy ECS V2
This is a rewrite of Bevy ECS (basically everything but the new executor/schedule, which are already awesome). The overall goal was to improve the performance and versatility of Bevy ECS. Here is a quick bulleted list of changes before we dive into the details:
* Complete World rewrite
* Multiple component storage types:
* Tables: fast cache friendly iteration, slower add/removes (previously called Archetypes)
* Sparse Sets: fast add/remove, slower iteration
* Stateful Queries (caches query results for faster iteration. fragmented iteration is _fast_ now)
* Stateful System Params (caches expensive operations. inspired by @DJMcNab's work in #1364)
* Configurable System Params (users can set configuration when they construct their systems. once again inspired by @DJMcNab's work)
* Archetypes are now "just metadata", component storage is separate
* Archetype Graph (for faster archetype changes)
* Component Metadata
* Configure component storage type
* Retrieve information about component size/type/name/layout/send-ness/etc
* Components are uniquely identified by a densely packed ComponentId
* TypeIds are now totally optional (which should make implementing scripting easier)
* Super fast "for_each" query iterators
* Merged Resources into World. Resources are now just a special type of component
* EntityRef/EntityMut builder apis (more efficient and more ergonomic)
* Fast bitset-backed `Access<T>` replaces old hashmap-based approach everywhere
* Query conflicts are determined by component access instead of archetype component access (to avoid random failures at runtime)
* With/Without are still taken into account for conflicts, so this should still be comfy to use
* Much simpler `IntoSystem` impl
* Significantly reduced the amount of hashing throughout the ecs in favor of Sparse Sets (indexed by densely packed ArchetypeId, ComponentId, BundleId, and TableId)
* Safety Improvements
* Entity reservation uses a normal world reference instead of unsafe transmute
* QuerySets no longer transmute lifetimes
* Made traits "unsafe" where relevant
* More thorough safety docs
* WorldCell
* Exposes safe mutable access to multiple resources at a time in a World
* Replaced "catch all" `System::update_archetypes(world: &World)` with `System::new_archetype(archetype: &Archetype)`
* Simpler Bundle implementation
* Replaced slow "remove_bundle_one_by_one" used as fallback for Commands::remove_bundle with fast "remove_bundle_intersection"
* Removed `Mut<T>` query impl. it is better to only support one way: `&mut T`
* Removed with() from `Flags<T>` in favor of `Option<Flags<T>>`, which allows querying for flags to be "filtered" by default
* Components now have is_send property (currently only resources support non-send)
* More granular module organization
* New `RemovedComponents<T>` SystemParam that replaces `query.removed::<T>()`
* `world.resource_scope()` for mutable access to resources and world at the same time
* WorldQuery and QueryFilter traits unified. FilterFetch trait added to enable "short circuit" filtering. Auto impled for cases that don't need it
* Significantly slimmed down SystemState in favor of individual SystemParam state
* System Commands changed from `commands: &mut Commands` back to `mut commands: Commands` (to allow Commands to have a World reference)
Fixes #1320
## `World` Rewrite
This is a from-scratch rewrite of `World` that fills the niche that `hecs` used to. Yes, this means Bevy ECS is no longer a "fork" of hecs. We're going out our own!
(the only shared code between the projects is the entity id allocator, which is already basically ideal)
A huge shout out to @SanderMertens (author of [flecs](https://github.com/SanderMertens/flecs)) for sharing some great ideas with me (specifically hybrid ecs storage and archetype graphs). He also helped advise on a number of implementation details.
## Component Storage (The Problem)
Two ECS storage paradigms have gained a lot of traction over the years:
* **Archetypal ECS**:
* Stores components in "tables" with static schemas. Each "column" stores components of a given type. Each "row" is an entity.
* Each "archetype" has its own table. Adding/removing an entity's component changes the archetype.
* Enables super-fast Query iteration due to its cache-friendly data layout
* Comes at the cost of more expensive add/remove operations for an Entity's components, because all components need to be copied to the new archetype's "table"
* **Sparse Set ECS**:
* Stores components of the same type in densely packed arrays, which are sparsely indexed by densely packed unsigned integers (Entity ids)
* Query iteration is slower than Archetypal ECS because each entity's component could be at any position in the sparse set. This "random access" pattern isn't cache friendly. Additionally, there is an extra layer of indirection because you must first map the entity id to an index in the component array.
* Adding/removing components is a cheap, constant time operation
Bevy ECS V1, hecs, legion, flec, and Unity DOTS are all "archetypal ecs-es". I personally think "archetypal" storage is a good default for game engines. An entity's archetype doesn't need to change frequently in general, and it creates "fast by default" query iteration (which is a much more common operation). It is also "self optimizing". Users don't need to think about optimizing component layouts for iteration performance. It "just works" without any extra boilerplate.
Shipyard and EnTT are "sparse set ecs-es". They employ "packing" as a way to work around the "suboptimal by default" iteration performance for specific sets of components. This helps, but I didn't think this was a good choice for a general purpose engine like Bevy because:
1. "packs" conflict with each other. If bevy decides to internally pack the Transform and GlobalTransform components, users are then blocked if they want to pack some custom component with Transform.
2. users need to take manual action to optimize
Developers selecting an ECS framework are stuck with a hard choice. Select an "archetypal" framework with "fast iteration everywhere" but without the ability to cheaply add/remove components, or select a "sparse set" framework to cheaply add/remove components but with slower iteration performance.
## Hybrid Component Storage (The Solution)
In Bevy ECS V2, we get to have our cake and eat it too. It now has _both_ of the component storage types above (and more can be added later if needed):
* **Tables** (aka "archetypal" storage)
* The default storage. If you don't configure anything, this is what you get
* Fast iteration by default
* Slower add/remove operations
* **Sparse Sets**
* Opt-in
* Slower iteration
* Faster add/remove operations
These storage types complement each other perfectly. By default Query iteration is fast. If developers know that they want to add/remove a component at high frequencies, they can set the storage to "sparse set":
```rust
world.register_component(
ComponentDescriptor::new::<MyComponent>(StorageType::SparseSet)
).unwrap();
```
## Archetypes
Archetypes are now "just metadata" ... they no longer store components directly. They do store:
* The `ComponentId`s of each of the Archetype's components (and that component's storage type)
* Archetypes are uniquely defined by their component layouts
* For example: entities with "table" components `[A, B, C]` _and_ "sparse set" components `[D, E]` will always be in the same archetype.
* The `TableId` associated with the archetype
* For now each archetype has exactly one table (which can have no components),
* There is a 1->Many relationship from Tables->Archetypes. A given table could have any number of archetype components stored in it:
* Ex: an entity with "table storage" components `[A, B, C]` and "sparse set" components `[D, E]` will share the same `[A, B, C]` table as an entity with `[A, B, C]` table component and `[F]` sparse set components.
* This 1->Many relationship is how we preserve fast "cache friendly" iteration performance when possible (more on this later)
* A list of entities that are in the archetype and the row id of the table they are in
* ArchetypeComponentIds
* unique densely packed identifiers for (ArchetypeId, ComponentId) pairs
* used by the schedule executor for cheap system access control
* "Archetype Graph Edges" (see the next section)
## The "Archetype Graph"
Archetype changes in Bevy (and a number of other archetypal ecs-es) have historically been expensive to compute. First, you need to allocate a new vector of the entity's current component ids, add or remove components based on the operation performed, sort it (to ensure it is order-independent), then hash it to find the archetype (if it exists). And thats all before we get to the _already_ expensive full copy of all components to the new table storage.
The solution is to build a "graph" of archetypes to cache these results. @SanderMertens first exposed me to the idea (and he got it from @gjroelofs, who came up with it). They propose adding directed edges between archetypes for add/remove component operations. If `ComponentId`s are densely packed, you can use sparse sets to cheaply jump between archetypes.
Bevy takes this one step further by using add/remove `Bundle` edges instead of `Component` edges. Bevy encourages the use of `Bundles` to group add/remove operations. This is largely for "clearer game logic" reasons, but it also helps cut down on the number of archetype changes required. `Bundles` now also have densely-packed `BundleId`s. This allows us to use a _single_ edge for each bundle operation (rather than needing to traverse N edges ... one for each component). Single component operations are also bundles, so this is strictly an improvement over a "component only" graph.
As a result, an operation that used to be _heavy_ (both for allocations and compute) is now two dirt-cheap array lookups and zero allocations.
## Stateful Queries
World queries are now stateful. This allows us to:
1. Cache archetype (and table) matches
* This resolves another issue with (naive) archetypal ECS: query performance getting worse as the number of archetypes goes up (and fragmentation occurs).
2. Cache Fetch and Filter state
* The expensive parts of fetch/filter operations (such as hashing the TypeId to find the ComponentId) now only happen once when the Query is first constructed
3. Incrementally build up state
* When new archetypes are added, we only process the new archetypes (no need to rebuild state for old archetypes)
As a result, the direct `World` query api now looks like this:
```rust
let mut query = world.query::<(&A, &mut B)>();
for (a, mut b) in query.iter_mut(&mut world) {
}
```
Requiring `World` to generate stateful queries (rather than letting the `QueryState` type be constructed separately) allows us to ensure that _all_ queries are properly initialized (and the relevant world state, such as ComponentIds). This enables QueryState to remove branches from its operations that check for initialization status (and also enables query.iter() to take an immutable world reference because it doesn't need to initialize anything in world).
However in systems, this is a non-breaking change. State management is done internally by the relevant SystemParam.
## Stateful SystemParams
Like Queries, `SystemParams` now also cache state. For example, `Query` system params store the "stateful query" state mentioned above. Commands store their internal `CommandQueue`. This means you can now safely use as many separate `Commands` parameters in your system as you want. `Local<T>` system params store their `T` value in their state (instead of in Resources).
SystemParam state also enabled a significant slim-down of SystemState. It is much nicer to look at now.
Per-SystemParam state naturally insulates us from an "aliased mut" class of errors we have hit in the past (ex: using multiple `Commands` system params).
(credit goes to @DJMcNab for the initial idea and draft pr here #1364)
## Configurable SystemParams
@DJMcNab also had the great idea to make SystemParams configurable. This allows users to provide some initial configuration / values for system parameters (when possible). Most SystemParams have no config (the config type is `()`), but the `Local<T>` param now supports user-provided parameters:
```rust
fn foo(value: Local<usize>) {
}
app.add_system(foo.system().config(|c| c.0 = Some(10)));
```
## Uber Fast "for_each" Query Iterators
Developers now have the choice to use a fast "for_each" iterator, which yields ~1.5-3x iteration speed improvements for "fragmented iteration", and minor ~1.2x iteration speed improvements for unfragmented iteration.
```rust
fn system(query: Query<(&A, &mut B)>) {
// you now have the option to do this for a speed boost
query.for_each_mut(|(a, mut b)| {
});
// however normal iterators are still available
for (a, mut b) in query.iter_mut() {
}
}
```
I think in most cases we should continue to encourage "normal" iterators as they are more flexible and more "rust idiomatic". But when that extra "oomf" is needed, it makes sense to use `for_each`.
We should also consider using `for_each` for internal bevy systems to give our users a nice speed boost (but that should be a separate pr).
## Component Metadata
`World` now has a `Components` collection, which is accessible via `world.components()`. This stores mappings from `ComponentId` to `ComponentInfo`, as well as `TypeId` to `ComponentId` mappings (where relevant). `ComponentInfo` stores information about the component, such as ComponentId, TypeId, memory layout, send-ness (currently limited to resources), and storage type.
## Significantly Cheaper `Access<T>`
We used to use `TypeAccess<TypeId>` to manage read/write component/archetype-component access. This was expensive because TypeIds must be hashed and compared individually. The parallel executor got around this by "condensing" type ids into bitset-backed access types. This worked, but it had to be re-generated from the `TypeAccess<TypeId>`sources every time archetypes changed.
This pr removes TypeAccess in favor of faster bitset access everywhere. We can do this thanks to the move to densely packed `ComponentId`s and `ArchetypeComponentId`s.
## Merged Resources into World
Resources had a lot of redundant functionality with Components. They stored typed data, they had access control, they had unique ids, they were queryable via SystemParams, etc. In fact the _only_ major difference between them was that they were unique (and didn't correlate to an entity).
Separate resources also had the downside of requiring a separate set of access controls, which meant the parallel executor needed to compare more bitsets per system and manage more state.
I initially got the "separate resources" idea from `legion`. I think that design was motivated by the fact that it made the direct world query/resource lifetime interactions more manageable. It certainly made our lives easier when using Resources alongside hecs/bevy_ecs. However we already have a construct for safely and ergonomically managing in-world lifetimes: systems (which use `Access<T>` internally).
This pr merges Resources into World:
```rust
world.insert_resource(1);
world.insert_resource(2.0);
let a = world.get_resource::<i32>().unwrap();
let mut b = world.get_resource_mut::<f64>().unwrap();
*b = 3.0;
```
Resources are now just a special kind of component. They have their own ComponentIds (and their own resource TypeId->ComponentId scope, so they don't conflict wit components of the same type). They are stored in a special "resource archetype", which stores components inside the archetype using a new `unique_components` sparse set (note that this sparse set could later be used to implement Tags). This allows us to keep the code size small by reusing existing datastructures (namely Column, Archetype, ComponentFlags, and ComponentInfo). This allows us the executor to use a single `Access<ArchetypeComponentId>` per system. It should also make scripting language integration easier.
_But_ this merge did create problems for people directly interacting with `World`. What if you need mutable access to multiple resources at the same time? `world.get_resource_mut()` borrows World mutably!
## WorldCell
WorldCell applies the `Access<ArchetypeComponentId>` concept to direct world access:
```rust
let world_cell = world.cell();
let a = world_cell.get_resource_mut::<i32>().unwrap();
let b = world_cell.get_resource_mut::<f64>().unwrap();
```
This adds cheap runtime checks (a sparse set lookup of `ArchetypeComponentId` and a counter) to ensure that world accesses do not conflict with each other. Each operation returns a `WorldBorrow<'w, T>` or `WorldBorrowMut<'w, T>` wrapper type, which will release the relevant ArchetypeComponentId resources when dropped.
World caches the access sparse set (and only one cell can exist at a time), so `world.cell()` is a cheap operation.
WorldCell does _not_ use atomic operations. It is non-send, does a mutable borrow of world to prevent other accesses, and uses a simple `Rc<RefCell<ArchetypeComponentAccess>>` wrapper in each WorldBorrow pointer.
The api is currently limited to resource access, but it can and should be extended to queries / entity component access.
## Resource Scopes
WorldCell does not yet support component queries, and even when it does there are sometimes legitimate reasons to want a mutable world ref _and_ a mutable resource ref (ex: bevy_render and bevy_scene both need this). In these cases we could always drop down to the unsafe `world.get_resource_unchecked_mut()`, but that is not ideal!
Instead developers can use a "resource scope"
```rust
world.resource_scope(|world: &mut World, a: &mut A| {
})
```
This temporarily removes the `A` resource from `World`, provides mutable pointers to both, and re-adds A to World when finished. Thanks to the move to ComponentIds/sparse sets, this is a cheap operation.
If multiple resources are required, scopes can be nested. We could also consider adding a "resource tuple" to the api if this pattern becomes common and the boilerplate gets nasty.
## Query Conflicts Use ComponentId Instead of ArchetypeComponentId
For safety reasons, systems cannot contain queries that conflict with each other without wrapping them in a QuerySet. On bevy `main`, we use ArchetypeComponentIds to determine conflicts. This is nice because it can take into account filters:
```rust
// these queries will never conflict due to their filters
fn filter_system(a: Query<&mut A, With<B>>, b: Query<&mut B, Without<B>>) {
}
```
But it also has a significant downside:
```rust
// these queries will not conflict _until_ an entity with A, B, and C is spawned
fn maybe_conflicts_system(a: Query<(&mut A, &C)>, b: Query<(&mut A, &B)>) {
}
```
The system above will panic at runtime if an entity with A, B, and C is spawned. This makes it hard to trust that your game logic will run without crashing.
In this pr, I switched to using `ComponentId` instead. This _is_ more constraining. `maybe_conflicts_system` will now always fail, but it will do it consistently at startup. Naively, it would also _disallow_ `filter_system`, which would be a significant downgrade in usability. Bevy has a number of internal systems that rely on disjoint queries and I expect it to be a common pattern in userspace.
To resolve this, I added a new `FilteredAccess<T>` type, which wraps `Access<T>` and adds with/without filters. If two `FilteredAccess` have with/without values that prove they are disjoint, they will no longer conflict.
## EntityRef / EntityMut
World entity operations on `main` require that the user passes in an `entity` id to each operation:
```rust
let entity = world.spawn((A, )); // create a new entity with A
world.get::<A>(entity);
world.insert(entity, (B, C));
world.insert_one(entity, D);
```
This means that each operation needs to look up the entity location / verify its validity. The initial spawn operation also requires a Bundle as input. This can be awkward when no components are required (or one component is required).
These operations have been replaced by `EntityRef` and `EntityMut`, which are "builder-style" wrappers around world that provide read and read/write operations on a single, pre-validated entity:
```rust
// spawn now takes no inputs and returns an EntityMut
let entity = world.spawn()
.insert(A) // insert a single component into the entity
.insert_bundle((B, C)) // insert a bundle of components into the entity
.id() // id returns the Entity id
// Returns EntityMut (or panics if the entity does not exist)
world.entity_mut(entity)
.insert(D)
.insert_bundle(SomeBundle::default());
{
// returns EntityRef (or panics if the entity does not exist)
let d = world.entity(entity)
.get::<D>() // gets the D component
.unwrap();
// world.get still exists for ergonomics
let d = world.get::<D>(entity).unwrap();
}
// These variants return Options if you want to check existence instead of panicing
world.get_entity_mut(entity)
.unwrap()
.insert(E);
if let Some(entity_ref) = world.get_entity(entity) {
let d = entity_ref.get::<D>().unwrap();
}
```
This _does not_ affect the current Commands api or terminology. I think that should be a separate conversation as that is a much larger breaking change.
## Safety Improvements
* Entity reservation in Commands uses a normal world borrow instead of an unsafe transmute
* QuerySets no longer transmutes lifetimes
* Made traits "unsafe" when implementing a trait incorrectly could cause unsafety
* More thorough safety docs
## RemovedComponents SystemParam
The old approach to querying removed components: `query.removed:<T>()` was confusing because it had no connection to the query itself. I replaced it with the following, which is both clearer and allows us to cache the ComponentId mapping in the SystemParamState:
```rust
fn system(removed: RemovedComponents<T>) {
for entity in removed.iter() {
}
}
```
## Simpler Bundle implementation
Bundles are no longer responsible for sorting (or deduping) TypeInfo. They are just a simple ordered list of component types / data. This makes the implementation smaller and opens the door to an easy "nested bundle" implementation in the future (which i might even add in this pr). Duplicate detection is now done once per bundle type by World the first time a bundle is used.
## Unified WorldQuery and QueryFilter types
(don't worry they are still separate type _parameters_ in Queries .. this is a non-breaking change)
WorldQuery and QueryFilter were already basically identical apis. With the addition of `FetchState` and more storage-specific fetch methods, the overlap was even clearer (and the redundancy more painful).
QueryFilters are now just `F: WorldQuery where F::Fetch: FilterFetch`. FilterFetch requires `Fetch<Item = bool>` and adds new "short circuit" variants of fetch methods. This enables a filter tuple like `(With<A>, Without<B>, Changed<C>)` to stop evaluating the filter after the first mismatch is encountered. FilterFetch is automatically implemented for `Fetch` implementations that return bool.
This forces fetch implementations that return things like `(bool, bool, bool)` (such as the filter above) to manually implement FilterFetch and decide whether or not to short-circuit.
## More Granular Modules
World no longer globs all of the internal modules together. It now exports `core`, `system`, and `schedule` separately. I'm also considering exporting `core` submodules directly as that is still pretty "glob-ey" and unorganized (feedback welcome here).
## Remaining Draft Work (to be done in this pr)
* ~~panic on conflicting WorldQuery fetches (&A, &mut A)~~
* ~~bevy `main` and hecs both currently allow this, but we should protect against it if possible~~
* ~~batch_iter / par_iter (currently stubbed out)~~
* ~~ChangedRes~~
* ~~I skipped this while we sort out #1313. This pr should be adapted to account for whatever we land on there~~.
* ~~The `Archetypes` and `Tables` collections use hashes of sorted lists of component ids to uniquely identify each archetype/table. This hash is then used as the key in a HashMap to look up the relevant ArchetypeId or TableId. (which doesn't handle hash collisions properly)~~
* ~~It is currently unsafe to generate a Query from "World A", then use it on "World B" (despite the api claiming it is safe). We should probably close this gap. This could be done by adding a randomly generated WorldId to each world, then storing that id in each Query. They could then be compared to each other on each `query.do_thing(&world)` operation. This _does_ add an extra branch to each query operation, so I'm open to other suggestions if people have them.~~
* ~~Nested Bundles (if i find time)~~
## Potential Future Work
* Expand WorldCell to support queries.
* Consider not allocating in the empty archetype on `world.spawn()`
* ex: return something like EntityMutUninit, which turns into EntityMut after an `insert` or `insert_bundle` op
* this actually regressed performance last time i tried it, but in theory it should be faster
* Optimize SparseSet::insert (see `PERF` comment on insert)
* Replace SparseArray `Option<T>` with T::MAX to cut down on branching
* would enable cheaper get_unchecked() operations
* upstream fixedbitset optimizations
* fixedbitset could be allocation free for small block counts (store blocks in a SmallVec)
* fixedbitset could have a const constructor
* Consider implementing Tags (archetype-specific by-value data that affects archetype identity)
* ex: ArchetypeA could have `[A, B, C]` table components and `[D(1)]` "tag" component. ArchetypeB could have `[A, B, C]` table components and a `[D(2)]` tag component. The archetypes are different, despite both having D tags because the value inside D is different.
* this could potentially build on top of the `archetype.unique_components` added in this pr for resource storage.
* Consider reverting `all_tuples` proc macro in favor of the old `macro_rules` implementation
* all_tuples is more flexible and produces cleaner documentation (the macro_rules version produces weird type parameter orders due to parser constraints)
* but unfortunately all_tuples also appears to make Rust Analyzer sad/slow when working inside of `bevy_ecs` (does not affect user code)
* Consider "resource queries" and/or "mixed resource and entity component queries" as an alternative to WorldCell
* this is basically just "systems" so maybe it's not worth it
* Add more world ops
* `world.clear()`
* `world.reserve<T: Bundle>(count: usize)`
* Try using the old archetype allocation strategy (allocate new memory on resize and copy everything over). I expect this to improve batch insertion performance at the cost of unbatched performance. But thats just a guess. I'm not an allocation perf pro :)
* Adapt Commands apis for consistency with new World apis
## Benchmarks
key:
* `bevy_old`: bevy `main` branch
* `bevy`: this branch
* `_foreach`: uses an optimized for_each iterator
* ` _sparse`: uses sparse set storage (if unspecified assume table storage)
* `_system`: runs inside a system (if unspecified assume test happens via direct world ops)
### Simple Insert (from ecs_bench_suite)
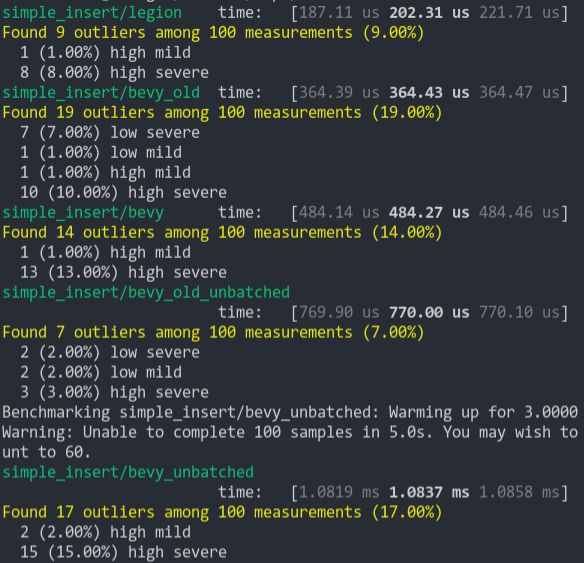
### Simpler Iter (from ecs_bench_suite)
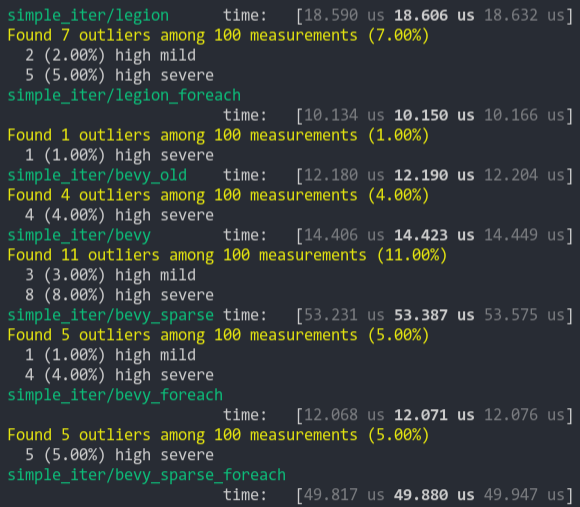
### Fragment Iter (from ecs_bench_suite)
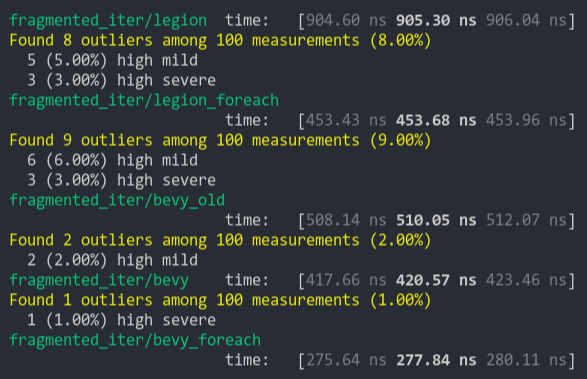
### Sparse Fragmented Iter
Iterate a query that matches 5 entities from a single matching archetype, but there are 100 unmatching archetypes
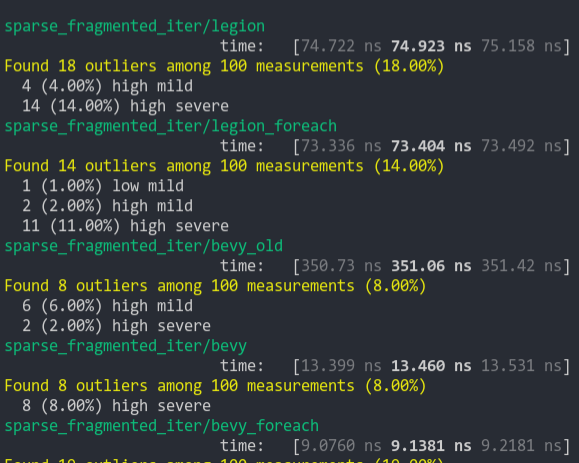
### Schedule (from ecs_bench_suite)
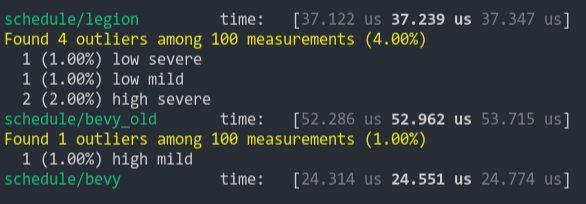
### Add Remove Component (from ecs_bench_suite)
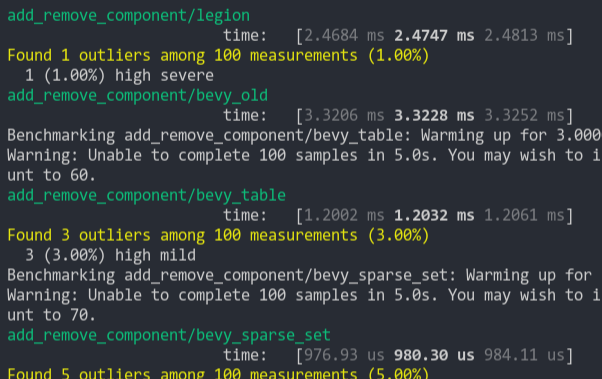
### Add Remove Component Big
Same as the test above, but each entity has 5 "large" matrix components and 1 "large" matrix component is added and removed
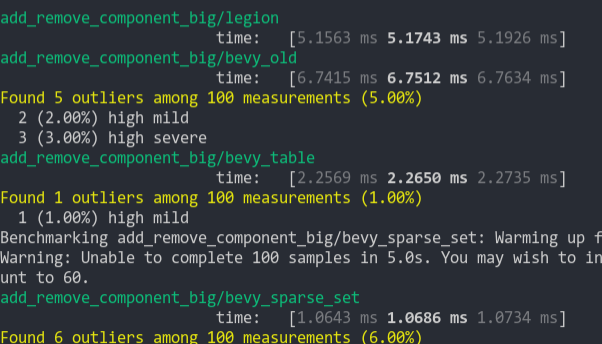
### Get Component
Looks up a single component value a large number of times
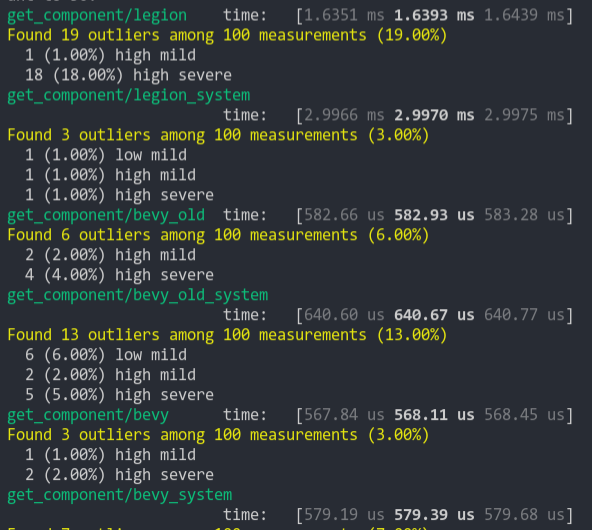
2021-03-05 07:54:35 +00:00
}
#[ inline ]
Remove the `SystemParamState` trait and remove types like `ResState` (#6919)
Spiritual successor to #5205.
Actual successor to #6865.
# Objective
Currently, system params are defined using three traits: `SystemParam`, `ReadOnlySystemParam`, `SystemParamState`. The behavior for each param is specified by the `SystemParamState` trait, while `SystemParam` simply defers to the state.
Splitting the traits in this way makes it easier to implement within macros, but it increases the cognitive load. Worst of all, this approach requires each `MySystemParam` to have a public `MySystemParamState` type associated with it.
## Solution
* Merge the trait `SystemParamState` into `SystemParam`.
* Remove all trivial `SystemParam` state types.
* `OptionNonSendMutState<T>`: you will not be missed.
---
- [x] Fix/resolve the remaining test failure.
## Changelog
* Removed the trait `SystemParamState`, merging its functionality into `SystemParam`.
## Migration Guide
**Note**: this should replace the migration guide for #6865.
This is relative to Bevy 0.9, not main.
The traits `SystemParamState` and `SystemParamFetch` have been removed, and their functionality has been transferred to `SystemParam`.
```rust
// Before (0.9)
impl SystemParam for MyParam<'_, '_> {
type State = MyParamState;
}
unsafe impl SystemParamState for MyParamState {
fn init(world: &mut World, system_meta: &mut SystemMeta) -> Self { ... }
}
unsafe impl<'w, 's> SystemParamFetch<'w, 's> for MyParamState {
type Item = MyParam<'w, 's>;
fn get_param(&mut self, ...) -> Self::Item;
}
unsafe impl ReadOnlySystemParamFetch for MyParamState { }
// After (0.10)
unsafe impl SystemParam for MyParam<'_, '_> {
type State = MyParamState;
type Item<'w, 's> = MyParam<'w, 's>;
fn init_state(world: &mut World, system_meta: &mut SystemMeta) -> Self::State { ... }
fn get_param<'w, 's>(state: &mut Self::State, ...) -> Self::Item<'w, 's>;
}
unsafe impl ReadOnlySystemParam for MyParam<'_, '_> { }
```
The trait `ReadOnlySystemParamFetch` has been replaced with `ReadOnlySystemParam`.
```rust
// Before
unsafe impl ReadOnlySystemParamFetch for MyParamState {}
// After
unsafe impl ReadOnlySystemParam for MyParam<'_, '_> {}
```
2023-01-07 23:20:32 +00:00
fn apply ( ( $( $param , ) * ) : & mut Self ::State , _system_meta : & SystemMeta , _world : & mut World ) {
$( $param ::apply ( $param , _system_meta , _world ) ; ) *
2020-11-08 20:34:05 +00:00
}
Simplify trait hierarchy for `SystemParam` (#6865)
# Objective
* Implementing a custom `SystemParam` by hand requires implementing three traits -- four if it is read-only.
* The trait `SystemParamFetch<'w, 's>` is a workaround from before we had generic associated types, and is no longer necessary.
## Solution
* Combine the trait `SystemParamFetch` with `SystemParamState`.
* I decided to remove the `Fetch` name and keep the `State` name, since the former was consistently conflated with the latter.
* Replace the trait `ReadOnlySystemParamFetch` with `ReadOnlySystemParam`, which simplifies trait bounds in generic code.
---
## Changelog
- Removed the trait `SystemParamFetch`, moving its functionality to `SystemParamState`.
- Replaced the trait `ReadOnlySystemParamFetch` with `ReadOnlySystemParam`.
## Migration Guide
The trait `SystemParamFetch` has been removed, and its functionality has been transferred to `SystemParamState`.
```rust
// Before
impl SystemParamState for MyParamState {
fn init(world: &mut World, system_meta: &mut SystemMeta) -> Self { ... }
}
impl<'w, 's> SystemParamFetch<'w, 's> for MyParamState {
type Item = MyParam<'w, 's>;
fn get_param(...) -> Self::Item;
}
// After
impl SystemParamState for MyParamState {
type Item<'w, 's> = MyParam<'w, 's>; // Generic associated types!
fn init(world: &mut World, system_meta: &mut SystemMeta) -> Self { ... }
fn get_param<'w, 's>(...) -> Self::Item<'w, 's>;
}
```
The trait `ReadOnlySystemParamFetch` has been replaced with `ReadOnlySystemParam`.
```rust
// Before
unsafe impl ReadOnlySystemParamFetch for MyParamState {}
// After
unsafe impl<'w, 's> ReadOnlySystemParam for MyParam<'w, 's> {}
```
2022-12-11 18:34:14 +00:00
#[ inline ]
#[ allow(clippy::unused_unit) ]
unsafe fn get_param < ' w , ' s > (
Remove the `SystemParamState` trait and remove types like `ResState` (#6919)
Spiritual successor to #5205.
Actual successor to #6865.
# Objective
Currently, system params are defined using three traits: `SystemParam`, `ReadOnlySystemParam`, `SystemParamState`. The behavior for each param is specified by the `SystemParamState` trait, while `SystemParam` simply defers to the state.
Splitting the traits in this way makes it easier to implement within macros, but it increases the cognitive load. Worst of all, this approach requires each `MySystemParam` to have a public `MySystemParamState` type associated with it.
## Solution
* Merge the trait `SystemParamState` into `SystemParam`.
* Remove all trivial `SystemParam` state types.
* `OptionNonSendMutState<T>`: you will not be missed.
---
- [x] Fix/resolve the remaining test failure.
## Changelog
* Removed the trait `SystemParamState`, merging its functionality into `SystemParam`.
## Migration Guide
**Note**: this should replace the migration guide for #6865.
This is relative to Bevy 0.9, not main.
The traits `SystemParamState` and `SystemParamFetch` have been removed, and their functionality has been transferred to `SystemParam`.
```rust
// Before (0.9)
impl SystemParam for MyParam<'_, '_> {
type State = MyParamState;
}
unsafe impl SystemParamState for MyParamState {
fn init(world: &mut World, system_meta: &mut SystemMeta) -> Self { ... }
}
unsafe impl<'w, 's> SystemParamFetch<'w, 's> for MyParamState {
type Item = MyParam<'w, 's>;
fn get_param(&mut self, ...) -> Self::Item;
}
unsafe impl ReadOnlySystemParamFetch for MyParamState { }
// After (0.10)
unsafe impl SystemParam for MyParam<'_, '_> {
type State = MyParamState;
type Item<'w, 's> = MyParam<'w, 's>;
fn init_state(world: &mut World, system_meta: &mut SystemMeta) -> Self::State { ... }
fn get_param<'w, 's>(state: &mut Self::State, ...) -> Self::Item<'w, 's>;
}
unsafe impl ReadOnlySystemParam for MyParam<'_, '_> { }
```
The trait `ReadOnlySystemParamFetch` has been replaced with `ReadOnlySystemParam`.
```rust
// Before
unsafe impl ReadOnlySystemParamFetch for MyParamState {}
// After
unsafe impl ReadOnlySystemParam for MyParam<'_, '_> {}
```
2023-01-07 23:20:32 +00:00
state : & ' s mut Self ::State ,
Simplify trait hierarchy for `SystemParam` (#6865)
# Objective
* Implementing a custom `SystemParam` by hand requires implementing three traits -- four if it is read-only.
* The trait `SystemParamFetch<'w, 's>` is a workaround from before we had generic associated types, and is no longer necessary.
## Solution
* Combine the trait `SystemParamFetch` with `SystemParamState`.
* I decided to remove the `Fetch` name and keep the `State` name, since the former was consistently conflated with the latter.
* Replace the trait `ReadOnlySystemParamFetch` with `ReadOnlySystemParam`, which simplifies trait bounds in generic code.
---
## Changelog
- Removed the trait `SystemParamFetch`, moving its functionality to `SystemParamState`.
- Replaced the trait `ReadOnlySystemParamFetch` with `ReadOnlySystemParam`.
## Migration Guide
The trait `SystemParamFetch` has been removed, and its functionality has been transferred to `SystemParamState`.
```rust
// Before
impl SystemParamState for MyParamState {
fn init(world: &mut World, system_meta: &mut SystemMeta) -> Self { ... }
}
impl<'w, 's> SystemParamFetch<'w, 's> for MyParamState {
type Item = MyParam<'w, 's>;
fn get_param(...) -> Self::Item;
}
// After
impl SystemParamState for MyParamState {
type Item<'w, 's> = MyParam<'w, 's>; // Generic associated types!
fn init(world: &mut World, system_meta: &mut SystemMeta) -> Self { ... }
fn get_param<'w, 's>(...) -> Self::Item<'w, 's>;
}
```
The trait `ReadOnlySystemParamFetch` has been replaced with `ReadOnlySystemParam`.
```rust
// Before
unsafe impl ReadOnlySystemParamFetch for MyParamState {}
// After
unsafe impl<'w, 's> ReadOnlySystemParam for MyParam<'w, 's> {}
```
2022-12-11 18:34:14 +00:00
_system_meta : & SystemMeta ,
_world : & ' w World ,
_change_tick : u32 ,
) -> Self ::Item < ' w , ' s > {
let ( $( $param , ) * ) = state ;
( $( $param ::get_param ( $param , _system_meta , _world , _change_tick ) , ) * )
}
2020-11-08 20:34:05 +00:00
}
} ;
}
2021-04-03 23:13:54 +00:00
all_tuples! ( impl_system_param_tuple , 0 , 16 , P ) ;
Modular Rendering (#2831)
This changes how render logic is composed to make it much more modular. Previously, all extraction logic was centralized for a given "type" of rendered thing. For example, we extracted meshes into a vector of ExtractedMesh, which contained the mesh and material asset handles, the transform, etc. We looked up bindings for "drawn things" using their index in the `Vec<ExtractedMesh>`. This worked fine for built in rendering, but made it hard to reuse logic for "custom" rendering. It also prevented us from reusing things like "extracted transforms" across contexts.
To make rendering more modular, I made a number of changes:
* Entities now drive rendering:
* We extract "render components" from "app components" and store them _on_ entities. No more centralized uber lists! We now have true "ECS-driven rendering"
* To make this perform well, I implemented #2673 in upstream Bevy for fast batch insertions into specific entities. This was merged into the `pipelined-rendering` branch here: #2815
* Reworked the `Draw` abstraction:
* Generic `PhaseItems`: each draw phase can define its own type of "rendered thing", which can define its own "sort key"
* Ported the 2d, 3d, and shadow phases to the new PhaseItem impl (currently Transparent2d, Transparent3d, and Shadow PhaseItems)
* `Draw` trait and and `DrawFunctions` are now generic on PhaseItem
* Modular / Ergonomic `DrawFunctions` via `RenderCommands`
* RenderCommand is a trait that runs an ECS query and produces one or more RenderPass calls. Types implementing this trait can be composed to create a final DrawFunction. For example the DrawPbr DrawFunction is created from the following DrawCommand tuple. Const generics are used to set specific bind group locations:
```rust
pub type DrawPbr = (
SetPbrPipeline,
SetMeshViewBindGroup<0>,
SetStandardMaterialBindGroup<1>,
SetTransformBindGroup<2>,
DrawMesh,
);
```
* The new `custom_shader_pipelined` example illustrates how the commands above can be reused to create a custom draw function:
```rust
type DrawCustom = (
SetCustomMaterialPipeline,
SetMeshViewBindGroup<0>,
SetTransformBindGroup<2>,
DrawMesh,
);
```
* ExtractComponentPlugin and UniformComponentPlugin:
* Simple, standardized ways to easily extract individual components and write them to GPU buffers
* Ported PBR and Sprite rendering to the new primitives above.
* Removed staging buffer from UniformVec in favor of direct Queue usage
* Makes UniformVec much easier to use and more ergonomic. Completely removes the need for custom render graph nodes in these contexts (see the PbrNode and view Node removals and the much simpler call patterns in the relevant Prepare systems).
* Added a many_cubes_pipelined example to benchmark baseline 3d rendering performance and ensure there were no major regressions during this port. Avoiding regressions was challenging given that the old approach of extracting into centralized vectors is basically the "optimal" approach. However thanks to a various ECS optimizations and render logic rephrasing, we pretty much break even on this benchmark!
* Lifetimeless SystemParams: this will be a bit divisive, but as we continue to embrace "trait driven systems" (ex: ExtractComponentPlugin, UniformComponentPlugin, DrawCommand), the ergonomics of `(Query<'static, 'static, (&'static A, &'static B, &'static)>, Res<'static, C>)` were getting very hard to bear. As a compromise, I added "static type aliases" for the relevant SystemParams. The previous example can now be expressed like this: `(SQuery<(Read<A>, Read<B>)>, SRes<C>)`. If anyone has better ideas / conflicting opinions, please let me know!
* RunSystem trait: a way to define Systems via a trait with a SystemParam associated type. This is used to implement the various plugins mentioned above. I also added SystemParamItem and QueryItem type aliases to make "trait stye" ecs interactions nicer on the eyes (and fingers).
* RenderAsset retrying: ensures that render assets are only created when they are "ready" and allows us to create bind groups directly inside render assets (which significantly simplified the StandardMaterial code). I think ultimately we should swap this out on "asset dependency" events to wait for dependencies to load, but this will require significant asset system changes.
* Updated some built in shaders to account for missing MeshUniform fields
2021-09-23 06:16:11 +00:00
pub mod lifetimeless {
pub type SQuery < Q , F = ( ) > = super ::Query < 'static , 'static , Q , F > ;
pub type Read < T > = & 'static T ;
pub type Write < T > = & 'static mut T ;
pub type SRes < T > = super ::Res < 'static , T > ;
pub type SResMut < T > = super ::ResMut < 'static , T > ;
pub type SCommands = crate ::system ::Commands < 'static , 'static > ;
}
2022-02-03 03:32:02 +00:00
2022-03-15 02:16:55 +00:00
/// A helper for using system parameters in generic contexts
///
/// This type is a [`SystemParam`] adapter which always has
Simplify trait hierarchy for `SystemParam` (#6865)
# Objective
* Implementing a custom `SystemParam` by hand requires implementing three traits -- four if it is read-only.
* The trait `SystemParamFetch<'w, 's>` is a workaround from before we had generic associated types, and is no longer necessary.
## Solution
* Combine the trait `SystemParamFetch` with `SystemParamState`.
* I decided to remove the `Fetch` name and keep the `State` name, since the former was consistently conflated with the latter.
* Replace the trait `ReadOnlySystemParamFetch` with `ReadOnlySystemParam`, which simplifies trait bounds in generic code.
---
## Changelog
- Removed the trait `SystemParamFetch`, moving its functionality to `SystemParamState`.
- Replaced the trait `ReadOnlySystemParamFetch` with `ReadOnlySystemParam`.
## Migration Guide
The trait `SystemParamFetch` has been removed, and its functionality has been transferred to `SystemParamState`.
```rust
// Before
impl SystemParamState for MyParamState {
fn init(world: &mut World, system_meta: &mut SystemMeta) -> Self { ... }
}
impl<'w, 's> SystemParamFetch<'w, 's> for MyParamState {
type Item = MyParam<'w, 's>;
fn get_param(...) -> Self::Item;
}
// After
impl SystemParamState for MyParamState {
type Item<'w, 's> = MyParam<'w, 's>; // Generic associated types!
fn init(world: &mut World, system_meta: &mut SystemMeta) -> Self { ... }
fn get_param<'w, 's>(...) -> Self::Item<'w, 's>;
}
```
The trait `ReadOnlySystemParamFetch` has been replaced with `ReadOnlySystemParam`.
```rust
// Before
unsafe impl ReadOnlySystemParamFetch for MyParamState {}
// After
unsafe impl<'w, 's> ReadOnlySystemParam for MyParam<'w, 's> {}
```
2022-12-11 18:34:14 +00:00
/// `Self::State::Item == Self` (ignoring lifetimes for brevity),
2022-03-15 02:16:55 +00:00
/// no matter the argument [`SystemParam`] (`P`) (other than
/// that `P` must be `'static`)
///
/// This makes it useful for having arbitrary [`SystemParam`] type arguments
/// to function systems, or for generic types using the [`derive@SystemParam`]
/// derive:
///
/// ```
/// # use bevy_ecs::prelude::*;
/// use bevy_ecs::system::{SystemParam, StaticSystemParam};
/// #[derive(SystemParam)]
/// struct GenericParam<'w,'s, T: SystemParam + 'static> {
/// field: StaticSystemParam<'w, 's, T>,
/// }
/// fn do_thing_generically<T: SystemParam + 'static>(t: StaticSystemParam<T>) {}
///
/// fn check_always_is_system<T: SystemParam + 'static>(){
/// bevy_ecs::system::assert_is_system(do_thing_generically::<T>);
/// }
/// ```
/// Note that in a real case you'd generally want
/// additional bounds on `P`, for your use of the parameter
/// to have a reason to be generic.
///
/// For example, using this would allow a type to be generic over
/// whether a resource is accessed mutably or not, with
/// impls being bounded on [`P: Deref<Target=MyType>`](Deref), and
/// [`P: DerefMut<Target=MyType>`](DerefMut) depending on whether the
/// method requires mutable access or not.
///
/// The method which doesn't use this type will not compile:
/// ```compile_fail
/// # use bevy_ecs::prelude::*;
/// # use bevy_ecs::system::{SystemParam, StaticSystemParam};
///
/// fn do_thing_generically<T: SystemParam + 'static>(t: T) {}
///
/// #[derive(SystemParam)]
Simplify trait hierarchy for `SystemParam` (#6865)
# Objective
* Implementing a custom `SystemParam` by hand requires implementing three traits -- four if it is read-only.
* The trait `SystemParamFetch<'w, 's>` is a workaround from before we had generic associated types, and is no longer necessary.
## Solution
* Combine the trait `SystemParamFetch` with `SystemParamState`.
* I decided to remove the `Fetch` name and keep the `State` name, since the former was consistently conflated with the latter.
* Replace the trait `ReadOnlySystemParamFetch` with `ReadOnlySystemParam`, which simplifies trait bounds in generic code.
---
## Changelog
- Removed the trait `SystemParamFetch`, moving its functionality to `SystemParamState`.
- Replaced the trait `ReadOnlySystemParamFetch` with `ReadOnlySystemParam`.
## Migration Guide
The trait `SystemParamFetch` has been removed, and its functionality has been transferred to `SystemParamState`.
```rust
// Before
impl SystemParamState for MyParamState {
fn init(world: &mut World, system_meta: &mut SystemMeta) -> Self { ... }
}
impl<'w, 's> SystemParamFetch<'w, 's> for MyParamState {
type Item = MyParam<'w, 's>;
fn get_param(...) -> Self::Item;
}
// After
impl SystemParamState for MyParamState {
type Item<'w, 's> = MyParam<'w, 's>; // Generic associated types!
fn init(world: &mut World, system_meta: &mut SystemMeta) -> Self { ... }
fn get_param<'w, 's>(...) -> Self::Item<'w, 's>;
}
```
The trait `ReadOnlySystemParamFetch` has been replaced with `ReadOnlySystemParam`.
```rust
// Before
unsafe impl ReadOnlySystemParamFetch for MyParamState {}
// After
unsafe impl<'w, 's> ReadOnlySystemParam for MyParam<'w, 's> {}
```
2022-12-11 18:34:14 +00:00
/// struct GenericParam<'w, 's, T: SystemParam> {
2022-03-15 02:16:55 +00:00
/// field: T,
/// #[system_param(ignore)]
Make the `SystemParam` derive macro more flexible (#6694)
# Objective
Currently, the `SystemParam` derive forces you to declare the lifetime parameters `<'w, 's>`, even if you don't use them.
If you don't follow this structure, the error message is quite nasty.
### Example (before):
```rust
#[derive(SystemParam)]
pub struct EventWriter<'w, 's, E: Event> {
events: ResMut<'w, Events<E>>,
// The derive forces us to declare the `'s` lifetime even though we don't use it,
// so we have to add this `PhantomData` to please rustc.
#[system_param(ignore)]
_marker: PhantomData<&'s ()>,
}
```
## Solution
* Allow the user to omit either lifetime.
* Emit a descriptive error if any lifetimes used are invalid.
### Example (after):
```rust
#[derive(SystemParam)]
pub struct EventWriter<'w, E: Event> {
events: ResMut<'w, Events<E>>,
}
```
---
## Changelog
* The `SystemParam` derive is now more flexible, allowing you to omit unused lifetime parameters.
2022-12-05 20:15:03 +00:00
/// // Use the lifetimes in this type, or they will be unbound.
2022-03-15 02:16:55 +00:00
/// phantom: core::marker::PhantomData<&'w &'s ()>
/// }
/// # fn check_always_is_system<T: SystemParam + 'static>(){
/// # bevy_ecs::system::assert_is_system(do_thing_generically::<T>);
/// # }
/// ```
///
pub struct StaticSystemParam < ' w , ' s , P : SystemParam > ( SystemParamItem < ' w , ' s , P > ) ;
impl < ' w , ' s , P : SystemParam > Deref for StaticSystemParam < ' w , ' s , P > {
type Target = SystemParamItem < ' w , ' s , P > ;
fn deref ( & self ) -> & Self ::Target {
& self . 0
}
}
impl < ' w , ' s , P : SystemParam > DerefMut for StaticSystemParam < ' w , ' s , P > {
fn deref_mut ( & mut self ) -> & mut Self ::Target {
& mut self . 0
}
}
impl < ' w , ' s , P : SystemParam > StaticSystemParam < ' w , ' s , P > {
/// Get the value of the parameter
pub fn into_inner ( self ) -> SystemParamItem < ' w , ' s , P > {
self . 0
}
}
2022-07-04 14:44:24 +00:00
// SAFETY: This doesn't add any more reads, and the delegated fetch confirms it
Simplify trait hierarchy for `SystemParam` (#6865)
# Objective
* Implementing a custom `SystemParam` by hand requires implementing three traits -- four if it is read-only.
* The trait `SystemParamFetch<'w, 's>` is a workaround from before we had generic associated types, and is no longer necessary.
## Solution
* Combine the trait `SystemParamFetch` with `SystemParamState`.
* I decided to remove the `Fetch` name and keep the `State` name, since the former was consistently conflated with the latter.
* Replace the trait `ReadOnlySystemParamFetch` with `ReadOnlySystemParam`, which simplifies trait bounds in generic code.
---
## Changelog
- Removed the trait `SystemParamFetch`, moving its functionality to `SystemParamState`.
- Replaced the trait `ReadOnlySystemParamFetch` with `ReadOnlySystemParam`.
## Migration Guide
The trait `SystemParamFetch` has been removed, and its functionality has been transferred to `SystemParamState`.
```rust
// Before
impl SystemParamState for MyParamState {
fn init(world: &mut World, system_meta: &mut SystemMeta) -> Self { ... }
}
impl<'w, 's> SystemParamFetch<'w, 's> for MyParamState {
type Item = MyParam<'w, 's>;
fn get_param(...) -> Self::Item;
}
// After
impl SystemParamState for MyParamState {
type Item<'w, 's> = MyParam<'w, 's>; // Generic associated types!
fn init(world: &mut World, system_meta: &mut SystemMeta) -> Self { ... }
fn get_param<'w, 's>(...) -> Self::Item<'w, 's>;
}
```
The trait `ReadOnlySystemParamFetch` has been replaced with `ReadOnlySystemParam`.
```rust
// Before
unsafe impl ReadOnlySystemParamFetch for MyParamState {}
// After
unsafe impl<'w, 's> ReadOnlySystemParam for MyParam<'w, 's> {}
```
2022-12-11 18:34:14 +00:00
unsafe impl < ' w , ' s , P : ReadOnlySystemParam + 'static > ReadOnlySystemParam
for StaticSystemParam < ' w , ' s , P >
2022-03-15 02:16:55 +00:00
{
}
Remove the `SystemParamState` trait and remove types like `ResState` (#6919)
Spiritual successor to #5205.
Actual successor to #6865.
# Objective
Currently, system params are defined using three traits: `SystemParam`, `ReadOnlySystemParam`, `SystemParamState`. The behavior for each param is specified by the `SystemParamState` trait, while `SystemParam` simply defers to the state.
Splitting the traits in this way makes it easier to implement within macros, but it increases the cognitive load. Worst of all, this approach requires each `MySystemParam` to have a public `MySystemParamState` type associated with it.
## Solution
* Merge the trait `SystemParamState` into `SystemParam`.
* Remove all trivial `SystemParam` state types.
* `OptionNonSendMutState<T>`: you will not be missed.
---
- [x] Fix/resolve the remaining test failure.
## Changelog
* Removed the trait `SystemParamState`, merging its functionality into `SystemParam`.
## Migration Guide
**Note**: this should replace the migration guide for #6865.
This is relative to Bevy 0.9, not main.
The traits `SystemParamState` and `SystemParamFetch` have been removed, and their functionality has been transferred to `SystemParam`.
```rust
// Before (0.9)
impl SystemParam for MyParam<'_, '_> {
type State = MyParamState;
}
unsafe impl SystemParamState for MyParamState {
fn init(world: &mut World, system_meta: &mut SystemMeta) -> Self { ... }
}
unsafe impl<'w, 's> SystemParamFetch<'w, 's> for MyParamState {
type Item = MyParam<'w, 's>;
fn get_param(&mut self, ...) -> Self::Item;
}
unsafe impl ReadOnlySystemParamFetch for MyParamState { }
// After (0.10)
unsafe impl SystemParam for MyParam<'_, '_> {
type State = MyParamState;
type Item<'w, 's> = MyParam<'w, 's>;
fn init_state(world: &mut World, system_meta: &mut SystemMeta) -> Self::State { ... }
fn get_param<'w, 's>(state: &mut Self::State, ...) -> Self::Item<'w, 's>;
}
unsafe impl ReadOnlySystemParam for MyParam<'_, '_> { }
```
The trait `ReadOnlySystemParamFetch` has been replaced with `ReadOnlySystemParam`.
```rust
// Before
unsafe impl ReadOnlySystemParamFetch for MyParamState {}
// After
unsafe impl ReadOnlySystemParam for MyParam<'_, '_> {}
```
2023-01-07 23:20:32 +00:00
// SAFETY: all methods are just delegated to `P`'s `SystemParam` implementation
unsafe impl < P : SystemParam + 'static > SystemParam for StaticSystemParam < '_ , '_ , P > {
type State = P ::State ;
Simplify trait hierarchy for `SystemParam` (#6865)
# Objective
* Implementing a custom `SystemParam` by hand requires implementing three traits -- four if it is read-only.
* The trait `SystemParamFetch<'w, 's>` is a workaround from before we had generic associated types, and is no longer necessary.
## Solution
* Combine the trait `SystemParamFetch` with `SystemParamState`.
* I decided to remove the `Fetch` name and keep the `State` name, since the former was consistently conflated with the latter.
* Replace the trait `ReadOnlySystemParamFetch` with `ReadOnlySystemParam`, which simplifies trait bounds in generic code.
---
## Changelog
- Removed the trait `SystemParamFetch`, moving its functionality to `SystemParamState`.
- Replaced the trait `ReadOnlySystemParamFetch` with `ReadOnlySystemParam`.
## Migration Guide
The trait `SystemParamFetch` has been removed, and its functionality has been transferred to `SystemParamState`.
```rust
// Before
impl SystemParamState for MyParamState {
fn init(world: &mut World, system_meta: &mut SystemMeta) -> Self { ... }
}
impl<'w, 's> SystemParamFetch<'w, 's> for MyParamState {
type Item = MyParam<'w, 's>;
fn get_param(...) -> Self::Item;
}
// After
impl SystemParamState for MyParamState {
type Item<'w, 's> = MyParam<'w, 's>; // Generic associated types!
fn init(world: &mut World, system_meta: &mut SystemMeta) -> Self { ... }
fn get_param<'w, 's>(...) -> Self::Item<'w, 's>;
}
```
The trait `ReadOnlySystemParamFetch` has been replaced with `ReadOnlySystemParam`.
```rust
// Before
unsafe impl ReadOnlySystemParamFetch for MyParamState {}
// After
unsafe impl<'w, 's> ReadOnlySystemParam for MyParam<'w, 's> {}
```
2022-12-11 18:34:14 +00:00
type Item < ' world , ' state > = StaticSystemParam < ' world , ' state , P > ;
Remove the `SystemParamState` trait and remove types like `ResState` (#6919)
Spiritual successor to #5205.
Actual successor to #6865.
# Objective
Currently, system params are defined using three traits: `SystemParam`, `ReadOnlySystemParam`, `SystemParamState`. The behavior for each param is specified by the `SystemParamState` trait, while `SystemParam` simply defers to the state.
Splitting the traits in this way makes it easier to implement within macros, but it increases the cognitive load. Worst of all, this approach requires each `MySystemParam` to have a public `MySystemParamState` type associated with it.
## Solution
* Merge the trait `SystemParamState` into `SystemParam`.
* Remove all trivial `SystemParam` state types.
* `OptionNonSendMutState<T>`: you will not be missed.
---
- [x] Fix/resolve the remaining test failure.
## Changelog
* Removed the trait `SystemParamState`, merging its functionality into `SystemParam`.
## Migration Guide
**Note**: this should replace the migration guide for #6865.
This is relative to Bevy 0.9, not main.
The traits `SystemParamState` and `SystemParamFetch` have been removed, and their functionality has been transferred to `SystemParam`.
```rust
// Before (0.9)
impl SystemParam for MyParam<'_, '_> {
type State = MyParamState;
}
unsafe impl SystemParamState for MyParamState {
fn init(world: &mut World, system_meta: &mut SystemMeta) -> Self { ... }
}
unsafe impl<'w, 's> SystemParamFetch<'w, 's> for MyParamState {
type Item = MyParam<'w, 's>;
fn get_param(&mut self, ...) -> Self::Item;
}
unsafe impl ReadOnlySystemParamFetch for MyParamState { }
// After (0.10)
unsafe impl SystemParam for MyParam<'_, '_> {
type State = MyParamState;
type Item<'w, 's> = MyParam<'w, 's>;
fn init_state(world: &mut World, system_meta: &mut SystemMeta) -> Self::State { ... }
fn get_param<'w, 's>(state: &mut Self::State, ...) -> Self::Item<'w, 's>;
}
unsafe impl ReadOnlySystemParam for MyParam<'_, '_> { }
```
The trait `ReadOnlySystemParamFetch` has been replaced with `ReadOnlySystemParam`.
```rust
// Before
unsafe impl ReadOnlySystemParamFetch for MyParamState {}
// After
unsafe impl ReadOnlySystemParam for MyParam<'_, '_> {}
```
2023-01-07 23:20:32 +00:00
fn init_state ( world : & mut World , system_meta : & mut SystemMeta ) -> Self ::State {
P ::init_state ( world , system_meta )
2022-03-15 02:16:55 +00:00
}
Remove the `SystemParamState` trait and remove types like `ResState` (#6919)
Spiritual successor to #5205.
Actual successor to #6865.
# Objective
Currently, system params are defined using three traits: `SystemParam`, `ReadOnlySystemParam`, `SystemParamState`. The behavior for each param is specified by the `SystemParamState` trait, while `SystemParam` simply defers to the state.
Splitting the traits in this way makes it easier to implement within macros, but it increases the cognitive load. Worst of all, this approach requires each `MySystemParam` to have a public `MySystemParamState` type associated with it.
## Solution
* Merge the trait `SystemParamState` into `SystemParam`.
* Remove all trivial `SystemParam` state types.
* `OptionNonSendMutState<T>`: you will not be missed.
---
- [x] Fix/resolve the remaining test failure.
## Changelog
* Removed the trait `SystemParamState`, merging its functionality into `SystemParam`.
## Migration Guide
**Note**: this should replace the migration guide for #6865.
This is relative to Bevy 0.9, not main.
The traits `SystemParamState` and `SystemParamFetch` have been removed, and their functionality has been transferred to `SystemParam`.
```rust
// Before (0.9)
impl SystemParam for MyParam<'_, '_> {
type State = MyParamState;
}
unsafe impl SystemParamState for MyParamState {
fn init(world: &mut World, system_meta: &mut SystemMeta) -> Self { ... }
}
unsafe impl<'w, 's> SystemParamFetch<'w, 's> for MyParamState {
type Item = MyParam<'w, 's>;
fn get_param(&mut self, ...) -> Self::Item;
}
unsafe impl ReadOnlySystemParamFetch for MyParamState { }
// After (0.10)
unsafe impl SystemParam for MyParam<'_, '_> {
type State = MyParamState;
type Item<'w, 's> = MyParam<'w, 's>;
fn init_state(world: &mut World, system_meta: &mut SystemMeta) -> Self::State { ... }
fn get_param<'w, 's>(state: &mut Self::State, ...) -> Self::Item<'w, 's>;
}
unsafe impl ReadOnlySystemParam for MyParam<'_, '_> { }
```
The trait `ReadOnlySystemParamFetch` has been replaced with `ReadOnlySystemParam`.
```rust
// Before
unsafe impl ReadOnlySystemParamFetch for MyParamState {}
// After
unsafe impl ReadOnlySystemParam for MyParam<'_, '_> {}
```
2023-01-07 23:20:32 +00:00
fn new_archetype ( state : & mut Self ::State , archetype : & Archetype , system_meta : & mut SystemMeta ) {
P ::new_archetype ( state , archetype , system_meta ) ;
2022-03-15 02:16:55 +00:00
}
Remove the `SystemParamState` trait and remove types like `ResState` (#6919)
Spiritual successor to #5205.
Actual successor to #6865.
# Objective
Currently, system params are defined using three traits: `SystemParam`, `ReadOnlySystemParam`, `SystemParamState`. The behavior for each param is specified by the `SystemParamState` trait, while `SystemParam` simply defers to the state.
Splitting the traits in this way makes it easier to implement within macros, but it increases the cognitive load. Worst of all, this approach requires each `MySystemParam` to have a public `MySystemParamState` type associated with it.
## Solution
* Merge the trait `SystemParamState` into `SystemParam`.
* Remove all trivial `SystemParam` state types.
* `OptionNonSendMutState<T>`: you will not be missed.
---
- [x] Fix/resolve the remaining test failure.
## Changelog
* Removed the trait `SystemParamState`, merging its functionality into `SystemParam`.
## Migration Guide
**Note**: this should replace the migration guide for #6865.
This is relative to Bevy 0.9, not main.
The traits `SystemParamState` and `SystemParamFetch` have been removed, and their functionality has been transferred to `SystemParam`.
```rust
// Before (0.9)
impl SystemParam for MyParam<'_, '_> {
type State = MyParamState;
}
unsafe impl SystemParamState for MyParamState {
fn init(world: &mut World, system_meta: &mut SystemMeta) -> Self { ... }
}
unsafe impl<'w, 's> SystemParamFetch<'w, 's> for MyParamState {
type Item = MyParam<'w, 's>;
fn get_param(&mut self, ...) -> Self::Item;
}
unsafe impl ReadOnlySystemParamFetch for MyParamState { }
// After (0.10)
unsafe impl SystemParam for MyParam<'_, '_> {
type State = MyParamState;
type Item<'w, 's> = MyParam<'w, 's>;
fn init_state(world: &mut World, system_meta: &mut SystemMeta) -> Self::State { ... }
fn get_param<'w, 's>(state: &mut Self::State, ...) -> Self::Item<'w, 's>;
}
unsafe impl ReadOnlySystemParam for MyParam<'_, '_> { }
```
The trait `ReadOnlySystemParamFetch` has been replaced with `ReadOnlySystemParam`.
```rust
// Before
unsafe impl ReadOnlySystemParamFetch for MyParamState {}
// After
unsafe impl ReadOnlySystemParam for MyParam<'_, '_> {}
```
2023-01-07 23:20:32 +00:00
fn apply ( state : & mut Self ::State , system_meta : & SystemMeta , world : & mut World ) {
P ::apply ( state , system_meta , world ) ;
2022-03-15 02:16:55 +00:00
}
Simplify trait hierarchy for `SystemParam` (#6865)
# Objective
* Implementing a custom `SystemParam` by hand requires implementing three traits -- four if it is read-only.
* The trait `SystemParamFetch<'w, 's>` is a workaround from before we had generic associated types, and is no longer necessary.
## Solution
* Combine the trait `SystemParamFetch` with `SystemParamState`.
* I decided to remove the `Fetch` name and keep the `State` name, since the former was consistently conflated with the latter.
* Replace the trait `ReadOnlySystemParamFetch` with `ReadOnlySystemParam`, which simplifies trait bounds in generic code.
---
## Changelog
- Removed the trait `SystemParamFetch`, moving its functionality to `SystemParamState`.
- Replaced the trait `ReadOnlySystemParamFetch` with `ReadOnlySystemParam`.
## Migration Guide
The trait `SystemParamFetch` has been removed, and its functionality has been transferred to `SystemParamState`.
```rust
// Before
impl SystemParamState for MyParamState {
fn init(world: &mut World, system_meta: &mut SystemMeta) -> Self { ... }
}
impl<'w, 's> SystemParamFetch<'w, 's> for MyParamState {
type Item = MyParam<'w, 's>;
fn get_param(...) -> Self::Item;
}
// After
impl SystemParamState for MyParamState {
type Item<'w, 's> = MyParam<'w, 's>; // Generic associated types!
fn init(world: &mut World, system_meta: &mut SystemMeta) -> Self { ... }
fn get_param<'w, 's>(...) -> Self::Item<'w, 's>;
}
```
The trait `ReadOnlySystemParamFetch` has been replaced with `ReadOnlySystemParam`.
```rust
// Before
unsafe impl ReadOnlySystemParamFetch for MyParamState {}
// After
unsafe impl<'w, 's> ReadOnlySystemParam for MyParam<'w, 's> {}
```
2022-12-11 18:34:14 +00:00
unsafe fn get_param < ' world , ' state > (
Remove the `SystemParamState` trait and remove types like `ResState` (#6919)
Spiritual successor to #5205.
Actual successor to #6865.
# Objective
Currently, system params are defined using three traits: `SystemParam`, `ReadOnlySystemParam`, `SystemParamState`. The behavior for each param is specified by the `SystemParamState` trait, while `SystemParam` simply defers to the state.
Splitting the traits in this way makes it easier to implement within macros, but it increases the cognitive load. Worst of all, this approach requires each `MySystemParam` to have a public `MySystemParamState` type associated with it.
## Solution
* Merge the trait `SystemParamState` into `SystemParam`.
* Remove all trivial `SystemParam` state types.
* `OptionNonSendMutState<T>`: you will not be missed.
---
- [x] Fix/resolve the remaining test failure.
## Changelog
* Removed the trait `SystemParamState`, merging its functionality into `SystemParam`.
## Migration Guide
**Note**: this should replace the migration guide for #6865.
This is relative to Bevy 0.9, not main.
The traits `SystemParamState` and `SystemParamFetch` have been removed, and their functionality has been transferred to `SystemParam`.
```rust
// Before (0.9)
impl SystemParam for MyParam<'_, '_> {
type State = MyParamState;
}
unsafe impl SystemParamState for MyParamState {
fn init(world: &mut World, system_meta: &mut SystemMeta) -> Self { ... }
}
unsafe impl<'w, 's> SystemParamFetch<'w, 's> for MyParamState {
type Item = MyParam<'w, 's>;
fn get_param(&mut self, ...) -> Self::Item;
}
unsafe impl ReadOnlySystemParamFetch for MyParamState { }
// After (0.10)
unsafe impl SystemParam for MyParam<'_, '_> {
type State = MyParamState;
type Item<'w, 's> = MyParam<'w, 's>;
fn init_state(world: &mut World, system_meta: &mut SystemMeta) -> Self::State { ... }
fn get_param<'w, 's>(state: &mut Self::State, ...) -> Self::Item<'w, 's>;
}
unsafe impl ReadOnlySystemParam for MyParam<'_, '_> { }
```
The trait `ReadOnlySystemParamFetch` has been replaced with `ReadOnlySystemParam`.
```rust
// Before
unsafe impl ReadOnlySystemParamFetch for MyParamState {}
// After
unsafe impl ReadOnlySystemParam for MyParam<'_, '_> {}
```
2023-01-07 23:20:32 +00:00
state : & ' state mut Self ::State ,
Simplify trait hierarchy for `SystemParam` (#6865)
# Objective
* Implementing a custom `SystemParam` by hand requires implementing three traits -- four if it is read-only.
* The trait `SystemParamFetch<'w, 's>` is a workaround from before we had generic associated types, and is no longer necessary.
## Solution
* Combine the trait `SystemParamFetch` with `SystemParamState`.
* I decided to remove the `Fetch` name and keep the `State` name, since the former was consistently conflated with the latter.
* Replace the trait `ReadOnlySystemParamFetch` with `ReadOnlySystemParam`, which simplifies trait bounds in generic code.
---
## Changelog
- Removed the trait `SystemParamFetch`, moving its functionality to `SystemParamState`.
- Replaced the trait `ReadOnlySystemParamFetch` with `ReadOnlySystemParam`.
## Migration Guide
The trait `SystemParamFetch` has been removed, and its functionality has been transferred to `SystemParamState`.
```rust
// Before
impl SystemParamState for MyParamState {
fn init(world: &mut World, system_meta: &mut SystemMeta) -> Self { ... }
}
impl<'w, 's> SystemParamFetch<'w, 's> for MyParamState {
type Item = MyParam<'w, 's>;
fn get_param(...) -> Self::Item;
}
// After
impl SystemParamState for MyParamState {
type Item<'w, 's> = MyParam<'w, 's>; // Generic associated types!
fn init(world: &mut World, system_meta: &mut SystemMeta) -> Self { ... }
fn get_param<'w, 's>(...) -> Self::Item<'w, 's>;
}
```
The trait `ReadOnlySystemParamFetch` has been replaced with `ReadOnlySystemParam`.
```rust
// Before
unsafe impl ReadOnlySystemParamFetch for MyParamState {}
// After
unsafe impl<'w, 's> ReadOnlySystemParam for MyParam<'w, 's> {}
```
2022-12-11 18:34:14 +00:00
system_meta : & SystemMeta ,
world : & ' world World ,
change_tick : u32 ,
) -> Self ::Item < ' world , ' state > {
Remove the `SystemParamState` trait and remove types like `ResState` (#6919)
Spiritual successor to #5205.
Actual successor to #6865.
# Objective
Currently, system params are defined using three traits: `SystemParam`, `ReadOnlySystemParam`, `SystemParamState`. The behavior for each param is specified by the `SystemParamState` trait, while `SystemParam` simply defers to the state.
Splitting the traits in this way makes it easier to implement within macros, but it increases the cognitive load. Worst of all, this approach requires each `MySystemParam` to have a public `MySystemParamState` type associated with it.
## Solution
* Merge the trait `SystemParamState` into `SystemParam`.
* Remove all trivial `SystemParam` state types.
* `OptionNonSendMutState<T>`: you will not be missed.
---
- [x] Fix/resolve the remaining test failure.
## Changelog
* Removed the trait `SystemParamState`, merging its functionality into `SystemParam`.
## Migration Guide
**Note**: this should replace the migration guide for #6865.
This is relative to Bevy 0.9, not main.
The traits `SystemParamState` and `SystemParamFetch` have been removed, and their functionality has been transferred to `SystemParam`.
```rust
// Before (0.9)
impl SystemParam for MyParam<'_, '_> {
type State = MyParamState;
}
unsafe impl SystemParamState for MyParamState {
fn init(world: &mut World, system_meta: &mut SystemMeta) -> Self { ... }
}
unsafe impl<'w, 's> SystemParamFetch<'w, 's> for MyParamState {
type Item = MyParam<'w, 's>;
fn get_param(&mut self, ...) -> Self::Item;
}
unsafe impl ReadOnlySystemParamFetch for MyParamState { }
// After (0.10)
unsafe impl SystemParam for MyParam<'_, '_> {
type State = MyParamState;
type Item<'w, 's> = MyParam<'w, 's>;
fn init_state(world: &mut World, system_meta: &mut SystemMeta) -> Self::State { ... }
fn get_param<'w, 's>(state: &mut Self::State, ...) -> Self::Item<'w, 's>;
}
unsafe impl ReadOnlySystemParam for MyParam<'_, '_> { }
```
The trait `ReadOnlySystemParamFetch` has been replaced with `ReadOnlySystemParam`.
```rust
// Before
unsafe impl ReadOnlySystemParamFetch for MyParamState {}
// After
unsafe impl ReadOnlySystemParam for MyParam<'_, '_> {}
```
2023-01-07 23:20:32 +00:00
// SAFETY: Defer to the safety of P::SystemParam
StaticSystemParam ( P ::get_param ( state , system_meta , world , change_tick ) )
Simplify trait hierarchy for `SystemParam` (#6865)
# Objective
* Implementing a custom `SystemParam` by hand requires implementing three traits -- four if it is read-only.
* The trait `SystemParamFetch<'w, 's>` is a workaround from before we had generic associated types, and is no longer necessary.
## Solution
* Combine the trait `SystemParamFetch` with `SystemParamState`.
* I decided to remove the `Fetch` name and keep the `State` name, since the former was consistently conflated with the latter.
* Replace the trait `ReadOnlySystemParamFetch` with `ReadOnlySystemParam`, which simplifies trait bounds in generic code.
---
## Changelog
- Removed the trait `SystemParamFetch`, moving its functionality to `SystemParamState`.
- Replaced the trait `ReadOnlySystemParamFetch` with `ReadOnlySystemParam`.
## Migration Guide
The trait `SystemParamFetch` has been removed, and its functionality has been transferred to `SystemParamState`.
```rust
// Before
impl SystemParamState for MyParamState {
fn init(world: &mut World, system_meta: &mut SystemMeta) -> Self { ... }
}
impl<'w, 's> SystemParamFetch<'w, 's> for MyParamState {
type Item = MyParam<'w, 's>;
fn get_param(...) -> Self::Item;
}
// After
impl SystemParamState for MyParamState {
type Item<'w, 's> = MyParam<'w, 's>; // Generic associated types!
fn init(world: &mut World, system_meta: &mut SystemMeta) -> Self { ... }
fn get_param<'w, 's>(...) -> Self::Item<'w, 's>;
}
```
The trait `ReadOnlySystemParamFetch` has been replaced with `ReadOnlySystemParam`.
```rust
// Before
unsafe impl ReadOnlySystemParamFetch for MyParamState {}
// After
unsafe impl<'w, 's> ReadOnlySystemParam for MyParam<'w, 's> {}
```
2022-12-11 18:34:14 +00:00
}
2022-03-15 02:16:55 +00:00
}
2022-02-03 03:32:02 +00:00
#[ cfg(test) ]
mod tests {
Make the `SystemParam` derive macro more flexible (#6694)
# Objective
Currently, the `SystemParam` derive forces you to declare the lifetime parameters `<'w, 's>`, even if you don't use them.
If you don't follow this structure, the error message is quite nasty.
### Example (before):
```rust
#[derive(SystemParam)]
pub struct EventWriter<'w, 's, E: Event> {
events: ResMut<'w, Events<E>>,
// The derive forces us to declare the `'s` lifetime even though we don't use it,
// so we have to add this `PhantomData` to please rustc.
#[system_param(ignore)]
_marker: PhantomData<&'s ()>,
}
```
## Solution
* Allow the user to omit either lifetime.
* Emit a descriptive error if any lifetimes used are invalid.
### Example (after):
```rust
#[derive(SystemParam)]
pub struct EventWriter<'w, E: Event> {
events: ResMut<'w, Events<E>>,
}
```
---
## Changelog
* The `SystemParam` derive is now more flexible, allowing you to omit unused lifetime parameters.
2022-12-05 20:15:03 +00:00
use super ::* ;
2022-02-03 03:32:02 +00:00
use crate ::{
self as bevy_ecs , // Necessary for the `SystemParam` Derive when used inside `bevy_ecs`.
2022-09-18 23:52:01 +00:00
query ::{ ReadOnlyWorldQuery , WorldQuery } ,
2022-02-03 03:32:02 +00:00
system ::Query ,
} ;
2023-02-04 20:53:37 +00:00
use std ::marker ::PhantomData ;
2022-02-03 03:32:02 +00:00
2023-01-23 22:34:32 +00:00
// Compile test for https://github.com/bevyengine/bevy/pull/2838.
2022-02-03 03:32:02 +00:00
#[ derive(SystemParam) ]
pub struct SpecialQuery <
' w ,
' s ,
Q : WorldQuery + Send + Sync + 'static ,
2022-09-18 23:52:01 +00:00
F : ReadOnlyWorldQuery + Send + Sync + 'static = ( ) ,
Use lifetimed, type erased pointers in bevy_ecs (#3001)
# Objective
`bevy_ecs` has large amounts of unsafe code which is hard to get right and makes it difficult to audit for soundness.
## Solution
Introduce lifetimed, type-erased pointers: `Ptr<'a>` `PtrMut<'a>` `OwningPtr<'a>'` and `ThinSlicePtr<'a, T>` which are newtypes around a raw pointer with a lifetime and conceptually representing strong invariants about the pointee and validity of the pointer.
The process of converting bevy_ecs to use these has already caught multiple cases of unsound behavior.
## Changelog
TL;DR for release notes: `bevy_ecs` now uses lifetimed, type-erased pointers internally, significantly improving safety and legibility without sacrificing performance. This should have approximately no end user impact, unless you were meddling with the (unfortunately public) internals of `bevy_ecs`.
- `Fetch`, `FilterFetch` and `ReadOnlyFetch` trait no longer have a `'state` lifetime
- this was unneeded
- `ReadOnly/Fetch` associated types on `WorldQuery` are now on a new `WorldQueryGats<'world>` trait
- was required to work around lack of Generic Associated Types (we wish to express `type Fetch<'a>: Fetch<'a>`)
- `derive(WorldQuery)` no longer requires `'w` lifetime on struct
- this was unneeded, and improves the end user experience
- `EntityMut::get_unchecked_mut` returns `&'_ mut T` not `&'w mut T`
- allows easier use of unsafe API with less footguns, and can be worked around via lifetime transmutery as a user
- `Bundle::from_components` now takes a `ctx` parameter to pass to the `FnMut` closure
- required because closure return types can't borrow from captures
- `Fetch::init` takes `&'world World`, `Fetch::set_archetype` takes `&'world Archetype` and `&'world Tables`, `Fetch::set_table` takes `&'world Table`
- allows types implementing `Fetch` to store borrows into world
- `WorldQuery` trait now has a `shrink` fn to shorten the lifetime in `Fetch::<'a>::Item`
- this works around lack of subtyping of assoc types, rust doesnt allow you to turn `<T as Fetch<'static>>::Item'` into `<T as Fetch<'a>>::Item'`
- `QueryCombinationsIter` requires this
- Most types implementing `Fetch` now have a lifetime `'w`
- allows the fetches to store borrows of world data instead of using raw pointers
## Migration guide
- `EntityMut::get_unchecked_mut` returns a more restricted lifetime, there is no general way to migrate this as it depends on your code
- `Bundle::from_components` implementations must pass the `ctx` arg to `func`
- `Bundle::from_components` callers have to use a fn arg instead of closure captures for borrowing from world
- Remove lifetime args on `derive(WorldQuery)` structs as it is nonsensical
- `<Q as WorldQuery>::ReadOnly/Fetch` should be changed to either `RO/QueryFetch<'world>` or `<Q as WorldQueryGats<'world>>::ReadOnly/Fetch`
- `<F as Fetch<'w, 's>>` should be changed to `<F as Fetch<'w>>`
- Change the fn sigs of `Fetch::init/set_archetype/set_table` to match respective trait fn sigs
- Implement the required `fn shrink` on any `WorldQuery` implementations
- Move assoc types `Fetch` and `ReadOnlyFetch` on `WorldQuery` impls to `WorldQueryGats` impls
- Pass an appropriate `'world` lifetime to whatever fetch struct you are for some reason using
### Type inference regression
in some cases rustc may give spurrious errors when attempting to infer the `F` parameter on a query/querystate this can be fixed by manually specifying the type, i.e. `QueryState::new::<_, ()>(world)`. The error is rather confusing:
```rust=
error[E0271]: type mismatch resolving `<() as Fetch<'_>>::Item == bool`
--> crates/bevy_pbr/src/render/light.rs:1413:30
|
1413 | main_view_query: QueryState::new(world),
| ^^^^^^^^^^^^^^^ expected `bool`, found `()`
|
= note: required because of the requirements on the impl of `for<'x> FilterFetch<'x>` for `<() as WorldQueryGats<'x>>::Fetch`
note: required by a bound in `bevy_ecs::query::QueryState::<Q, F>::new`
--> crates/bevy_ecs/src/query/state.rs:49:32
|
49 | for<'x> QueryFetch<'x, F>: FilterFetch<'x>,
| ^^^^^^^^^^^^^^^ required by this bound in `bevy_ecs::query::QueryState::<Q, F>::new`
```
---
Made with help from @BoxyUwU and @alice-i-cecile
Co-authored-by: Boxy <supbscripter@gmail.com>
2022-04-27 23:44:06 +00:00
> {
2022-02-03 03:32:02 +00:00
_query : Query < ' w , ' s , Q , F > ,
}
Make the `SystemParam` derive macro more flexible (#6694)
# Objective
Currently, the `SystemParam` derive forces you to declare the lifetime parameters `<'w, 's>`, even if you don't use them.
If you don't follow this structure, the error message is quite nasty.
### Example (before):
```rust
#[derive(SystemParam)]
pub struct EventWriter<'w, 's, E: Event> {
events: ResMut<'w, Events<E>>,
// The derive forces us to declare the `'s` lifetime even though we don't use it,
// so we have to add this `PhantomData` to please rustc.
#[system_param(ignore)]
_marker: PhantomData<&'s ()>,
}
```
## Solution
* Allow the user to omit either lifetime.
* Emit a descriptive error if any lifetimes used are invalid.
### Example (after):
```rust
#[derive(SystemParam)]
pub struct EventWriter<'w, E: Event> {
events: ResMut<'w, Events<E>>,
}
```
---
## Changelog
* The `SystemParam` derive is now more flexible, allowing you to omit unused lifetime parameters.
2022-12-05 20:15:03 +00:00
2023-01-23 22:34:32 +00:00
// Compile tests for https://github.com/bevyengine/bevy/pull/6694.
Make the `SystemParam` derive macro more flexible (#6694)
# Objective
Currently, the `SystemParam` derive forces you to declare the lifetime parameters `<'w, 's>`, even if you don't use them.
If you don't follow this structure, the error message is quite nasty.
### Example (before):
```rust
#[derive(SystemParam)]
pub struct EventWriter<'w, 's, E: Event> {
events: ResMut<'w, Events<E>>,
// The derive forces us to declare the `'s` lifetime even though we don't use it,
// so we have to add this `PhantomData` to please rustc.
#[system_param(ignore)]
_marker: PhantomData<&'s ()>,
}
```
## Solution
* Allow the user to omit either lifetime.
* Emit a descriptive error if any lifetimes used are invalid.
### Example (after):
```rust
#[derive(SystemParam)]
pub struct EventWriter<'w, E: Event> {
events: ResMut<'w, Events<E>>,
}
```
---
## Changelog
* The `SystemParam` derive is now more flexible, allowing you to omit unused lifetime parameters.
2022-12-05 20:15:03 +00:00
#[ derive(SystemParam) ]
pub struct SpecialRes < ' w , T : Resource > {
_res : Res < ' w , T > ,
}
#[ derive(SystemParam) ]
pub struct SpecialLocal < ' s , T : FromWorld + Send + 'static > {
_local : Local < ' s , T > ,
}
2022-12-21 01:54:10 +00:00
#[ derive(Resource) ]
pub struct R < const I : usize > ;
2023-01-23 22:34:32 +00:00
// Compile test for https://github.com/bevyengine/bevy/pull/7001.
2022-12-25 00:06:23 +00:00
#[ derive(SystemParam) ]
pub struct ConstGenericParam < ' w , const I : usize > ( Res < ' w , R < I > > ) ;
2023-01-23 22:34:32 +00:00
// Compile test for https://github.com/bevyengine/bevy/pull/6867.
2022-12-21 01:54:10 +00:00
#[ derive(SystemParam) ]
pub struct LongParam < ' w > {
_r0 : Res < ' w , R < 0 > > ,
_r1 : Res < ' w , R < 1 > > ,
_r2 : Res < ' w , R < 2 > > ,
_r3 : Res < ' w , R < 3 > > ,
_r4 : Res < ' w , R < 4 > > ,
_r5 : Res < ' w , R < 5 > > ,
_r6 : Res < ' w , R < 6 > > ,
_r7 : Res < ' w , R < 7 > > ,
_r8 : Res < ' w , R < 8 > > ,
_r9 : Res < ' w , R < 9 > > ,
_r10 : Res < ' w , R < 10 > > ,
_r11 : Res < ' w , R < 11 > > ,
_r12 : Res < ' w , R < 12 > > ,
_r13 : Res < ' w , R < 13 > > ,
_r14 : Res < ' w , R < 14 > > ,
_r15 : Res < ' w , R < 15 > > ,
_r16 : Res < ' w , R < 16 > > ,
}
#[ allow(dead_code) ]
fn long_system ( _param : LongParam ) {
crate ::system ::assert_is_system ( long_system ) ;
}
2023-01-23 22:34:32 +00:00
// Compile test for https://github.com/bevyengine/bevy/pull/6919.
2023-02-03 09:17:48 +00:00
// Regression test for https://github.com/bevyengine/bevy/issues/7447.
Remove the `SystemParamState` trait and remove types like `ResState` (#6919)
Spiritual successor to #5205.
Actual successor to #6865.
# Objective
Currently, system params are defined using three traits: `SystemParam`, `ReadOnlySystemParam`, `SystemParamState`. The behavior for each param is specified by the `SystemParamState` trait, while `SystemParam` simply defers to the state.
Splitting the traits in this way makes it easier to implement within macros, but it increases the cognitive load. Worst of all, this approach requires each `MySystemParam` to have a public `MySystemParamState` type associated with it.
## Solution
* Merge the trait `SystemParamState` into `SystemParam`.
* Remove all trivial `SystemParam` state types.
* `OptionNonSendMutState<T>`: you will not be missed.
---
- [x] Fix/resolve the remaining test failure.
## Changelog
* Removed the trait `SystemParamState`, merging its functionality into `SystemParam`.
## Migration Guide
**Note**: this should replace the migration guide for #6865.
This is relative to Bevy 0.9, not main.
The traits `SystemParamState` and `SystemParamFetch` have been removed, and their functionality has been transferred to `SystemParam`.
```rust
// Before (0.9)
impl SystemParam for MyParam<'_, '_> {
type State = MyParamState;
}
unsafe impl SystemParamState for MyParamState {
fn init(world: &mut World, system_meta: &mut SystemMeta) -> Self { ... }
}
unsafe impl<'w, 's> SystemParamFetch<'w, 's> for MyParamState {
type Item = MyParam<'w, 's>;
fn get_param(&mut self, ...) -> Self::Item;
}
unsafe impl ReadOnlySystemParamFetch for MyParamState { }
// After (0.10)
unsafe impl SystemParam for MyParam<'_, '_> {
type State = MyParamState;
type Item<'w, 's> = MyParam<'w, 's>;
fn init_state(world: &mut World, system_meta: &mut SystemMeta) -> Self::State { ... }
fn get_param<'w, 's>(state: &mut Self::State, ...) -> Self::Item<'w, 's>;
}
unsafe impl ReadOnlySystemParam for MyParam<'_, '_> { }
```
The trait `ReadOnlySystemParamFetch` has been replaced with `ReadOnlySystemParam`.
```rust
// Before
unsafe impl ReadOnlySystemParamFetch for MyParamState {}
// After
unsafe impl ReadOnlySystemParam for MyParam<'_, '_> {}
```
2023-01-07 23:20:32 +00:00
#[ derive(SystemParam) ]
2023-02-03 09:17:48 +00:00
struct IgnoredParam < ' w , T : Resource , Marker : 'static > {
Remove the `SystemParamState` trait and remove types like `ResState` (#6919)
Spiritual successor to #5205.
Actual successor to #6865.
# Objective
Currently, system params are defined using three traits: `SystemParam`, `ReadOnlySystemParam`, `SystemParamState`. The behavior for each param is specified by the `SystemParamState` trait, while `SystemParam` simply defers to the state.
Splitting the traits in this way makes it easier to implement within macros, but it increases the cognitive load. Worst of all, this approach requires each `MySystemParam` to have a public `MySystemParamState` type associated with it.
## Solution
* Merge the trait `SystemParamState` into `SystemParam`.
* Remove all trivial `SystemParam` state types.
* `OptionNonSendMutState<T>`: you will not be missed.
---
- [x] Fix/resolve the remaining test failure.
## Changelog
* Removed the trait `SystemParamState`, merging its functionality into `SystemParam`.
## Migration Guide
**Note**: this should replace the migration guide for #6865.
This is relative to Bevy 0.9, not main.
The traits `SystemParamState` and `SystemParamFetch` have been removed, and their functionality has been transferred to `SystemParam`.
```rust
// Before (0.9)
impl SystemParam for MyParam<'_, '_> {
type State = MyParamState;
}
unsafe impl SystemParamState for MyParamState {
fn init(world: &mut World, system_meta: &mut SystemMeta) -> Self { ... }
}
unsafe impl<'w, 's> SystemParamFetch<'w, 's> for MyParamState {
type Item = MyParam<'w, 's>;
fn get_param(&mut self, ...) -> Self::Item;
}
unsafe impl ReadOnlySystemParamFetch for MyParamState { }
// After (0.10)
unsafe impl SystemParam for MyParam<'_, '_> {
type State = MyParamState;
type Item<'w, 's> = MyParam<'w, 's>;
fn init_state(world: &mut World, system_meta: &mut SystemMeta) -> Self::State { ... }
fn get_param<'w, 's>(state: &mut Self::State, ...) -> Self::Item<'w, 's>;
}
unsafe impl ReadOnlySystemParam for MyParam<'_, '_> { }
```
The trait `ReadOnlySystemParamFetch` has been replaced with `ReadOnlySystemParam`.
```rust
// Before
unsafe impl ReadOnlySystemParamFetch for MyParamState {}
// After
unsafe impl ReadOnlySystemParam for MyParam<'_, '_> {}
```
2023-01-07 23:20:32 +00:00
_foo : Res < ' w , T > ,
#[ system_param(ignore) ]
2023-02-03 09:17:48 +00:00
marker : PhantomData < & ' w Marker > ,
Remove the `SystemParamState` trait and remove types like `ResState` (#6919)
Spiritual successor to #5205.
Actual successor to #6865.
# Objective
Currently, system params are defined using three traits: `SystemParam`, `ReadOnlySystemParam`, `SystemParamState`. The behavior for each param is specified by the `SystemParamState` trait, while `SystemParam` simply defers to the state.
Splitting the traits in this way makes it easier to implement within macros, but it increases the cognitive load. Worst of all, this approach requires each `MySystemParam` to have a public `MySystemParamState` type associated with it.
## Solution
* Merge the trait `SystemParamState` into `SystemParam`.
* Remove all trivial `SystemParam` state types.
* `OptionNonSendMutState<T>`: you will not be missed.
---
- [x] Fix/resolve the remaining test failure.
## Changelog
* Removed the trait `SystemParamState`, merging its functionality into `SystemParam`.
## Migration Guide
**Note**: this should replace the migration guide for #6865.
This is relative to Bevy 0.9, not main.
The traits `SystemParamState` and `SystemParamFetch` have been removed, and their functionality has been transferred to `SystemParam`.
```rust
// Before (0.9)
impl SystemParam for MyParam<'_, '_> {
type State = MyParamState;
}
unsafe impl SystemParamState for MyParamState {
fn init(world: &mut World, system_meta: &mut SystemMeta) -> Self { ... }
}
unsafe impl<'w, 's> SystemParamFetch<'w, 's> for MyParamState {
type Item = MyParam<'w, 's>;
fn get_param(&mut self, ...) -> Self::Item;
}
unsafe impl ReadOnlySystemParamFetch for MyParamState { }
// After (0.10)
unsafe impl SystemParam for MyParam<'_, '_> {
type State = MyParamState;
type Item<'w, 's> = MyParam<'w, 's>;
fn init_state(world: &mut World, system_meta: &mut SystemMeta) -> Self::State { ... }
fn get_param<'w, 's>(state: &mut Self::State, ...) -> Self::Item<'w, 's>;
}
unsafe impl ReadOnlySystemParam for MyParam<'_, '_> { }
```
The trait `ReadOnlySystemParamFetch` has been replaced with `ReadOnlySystemParam`.
```rust
// Before
unsafe impl ReadOnlySystemParamFetch for MyParamState {}
// After
unsafe impl ReadOnlySystemParam for MyParam<'_, '_> {}
```
2023-01-07 23:20:32 +00:00
}
2023-01-23 22:34:32 +00:00
// Compile tests for https://github.com/bevyengine/bevy/pull/6957.
Make the `SystemParam` derive macro more flexible (#6694)
# Objective
Currently, the `SystemParam` derive forces you to declare the lifetime parameters `<'w, 's>`, even if you don't use them.
If you don't follow this structure, the error message is quite nasty.
### Example (before):
```rust
#[derive(SystemParam)]
pub struct EventWriter<'w, 's, E: Event> {
events: ResMut<'w, Events<E>>,
// The derive forces us to declare the `'s` lifetime even though we don't use it,
// so we have to add this `PhantomData` to please rustc.
#[system_param(ignore)]
_marker: PhantomData<&'s ()>,
}
```
## Solution
* Allow the user to omit either lifetime.
* Emit a descriptive error if any lifetimes used are invalid.
### Example (after):
```rust
#[derive(SystemParam)]
pub struct EventWriter<'w, E: Event> {
events: ResMut<'w, Events<E>>,
}
```
---
## Changelog
* The `SystemParam` derive is now more flexible, allowing you to omit unused lifetime parameters.
2022-12-05 20:15:03 +00:00
#[ derive(SystemParam) ]
2022-12-20 23:45:44 +00:00
pub struct UnitParam ;
#[ derive(SystemParam) ]
pub struct TupleParam < ' w , ' s , R : Resource , L : FromWorld + Send + 'static > (
Res < ' w , R > ,
Local < ' s , L > ,
) ;
2023-01-04 23:25:36 +00:00
#[ derive(Resource) ]
struct PrivateResource ;
2023-01-23 22:34:32 +00:00
// Regression test for https://github.com/bevyengine/bevy/issues/4200.
2023-01-04 23:25:36 +00:00
#[ derive(SystemParam) ]
pub struct EncapsulatedParam < ' w > ( Res < ' w , PrivateResource > ) ;
2023-01-10 18:41:50 +00:00
// regression test for https://github.com/bevyengine/bevy/issues/7103.
#[ derive(SystemParam) ]
pub struct WhereParam < ' w , ' s , Q >
where
Q : 'static + WorldQuery ,
{
_q : Query < ' w , ' s , Q , ( ) > ,
}
2022-02-03 03:32:02 +00:00
}